- 1Department of Marine Science and Technology, University of Oviedo, Gijon, Spain
- 2Port Authority of Gijon, Gijon, Spain
- 3Department of Functional Biology, University of Oviedo, Oviedo, Spain
Introduction: The majority of studies on marine biosecurity focus on aquatic species, but invaders can also use dry ship compartments for dispersal; this pathway has been less explored than fouling or ballast water. Here we analysed the maritime traffic of the international Port of Gijon (south Bay of Biscay, Spain) as a case study.
Methods: Main donor countries were identified from traffic data. From the Global Invasive Species Database, we detected a similar proportion of expected global alien invasive species (AIS) from fouling and containers pathways to be actually settled in the recipient region.
Results: The AIS diversity transported via fouling was higher than that transported by containers. No significant difference between Australia, Brazil, Canada and South Africa in the proportion of global AIS actually transferred to Bay of Biscay supports the vision of port networks as a new ecosystem with multiple demes. Different AIS catalogues in the donor regions may explain a higher proportion of terrestrial AIS introduced via containers from Brazil and South Africa and marine AIS introduced via fouling.
Discussion: A more careful surveillance of biota in dry ship compartments, including it in a future revision in the International Convention for Safe Containers, is recommended to reduce the contribution of shipping to biological invasions.
1 Introduction
Biological invasions cause multiple pervasive effects on ecosystems, potentially disrupting the interactions of species, native biodiversity, and global ecological processes such as changes in the food web and drastic diversity reductions (e.g., Simberloff et al., 2013). Some invaders may even transform the habitat like ecosystem engineers (Darrigran and Damborenea, 2011). Invasions are producing a certain decline of species at the local and regional levels (Macdougall et al., 2009); biological invasions are the second driver of the extinction of species worldwide, after habitat destruction (Simberloff, 2001). Although the change of distribution and colonization of new habitats have always been fundamental for species survival, yet the rapid pace at which this change is occurring today, largely driven by human activities, is a cause for concern (e.g., Ojaveer et al., 2017). When the link between donor and recipient ecosystems is determined from human activities, indeed not all the species arriving in a new place will settle there and become invasive (e.g., Van Kleunen et al., 2010); only a few of them will be able to adapt and grow enough to cause an impact in the recipient region. The impact of invasive species on biodiversity and habitats cannot be generalized since it may vary in different areas and times (Ricciardi and Cohen, 2007). The problem is magnified in marine systems where complex life cycles facilitate connectivity between distant environments. Marine human-mediated invasions have been so pervasive that we may never fully know how the biota of the continental margins looked like before ships (Carlton, 1989). Once invasive species are established, they are virtually impossible to eradicate, and preventing their spread of dispersal can be very costly (Giangrande et al., 2020).
The intensity of maritime traffic is the main driver of alien species introduction since the most frequently used routes encompass the greatest risk of becoming highways for stowaway species (Keller et al., 2011). The problem has grown in the last decades due to the increase in the size and number of ships worldwide. International shipping currently moves approximately 11 billion tons per year and is expected to grow by at least 240% by 2050, which will encompass at least a three-fold increase in biological invasions (Sardain et al., 2019). A recognized example is the case of the Mediterranean Sea, where half of the almost 700 multicellular non-indigenous species (NIS) have been introduced through the Suez Canal since 1869 (Galil et al., 2014; Lucy et al., 2016). This canal is crossed by an average of more than 19,000 ships a year and accounts for 12% of international trade.
Among the different vectors of the introduction of alien species analyzed (Katsanevakis et al., 2013; Galil et al., 2014; Nunes et al., 2014), ships are one of the most important because the NIS can be transported in different compartments such as ballast tanks, hull biofouling, sea chests, and cargo pallets (e.g., Godwin, 2003; Niimi, 2004; Hulme et al., 2008; Hewitt et al., 2009). Commercial ports with intense maritime traffic are important gateways for new species and could be considered as “alien hotspots” (e.g., Pearce, 2013; Ibabe et al., 2021); secondary dispersal vectors like recreational boats (Kelly et al., 2012) or floating litter (Fernandez et al., 2022) may expand the NIS to the surrounding areas.
Hewitt et al. (2009) described ballast water and fouling as major pathways for exotic biota to enter ports. For this reason, the International Maritime Organization (IMO) has increased policies to reduce the effects of shipping worldwide through different regulations related to ballast water and fouling. Ballast water moves species on a global scale transporting them along the ships’ routes (ballasting and de-ballasting). It has been a main vector of invasive species in many regions (e.g., Verna et al., 2016; Carney et al., 2017). The International Convention for the Control and Management of Ships’ Ballast Water and Sediments, BWM Convention (entry into force: 8 September 2017, https://www.imo.org/en/About/Conventions/Pages/International-Convention-for-the-Control-and-Management-of-Ships%27-Ballast-Water-and-Sediments-(BWM).aspx, accessed in June 2023), is the instrument used globally to avoid biological pollution for ballast water. One of the main measures is the change of ballast water after 200 miles from the coast, avoiding shallow waters where the biota is much more abundant. Another measure is treating ballast water before arriving at the port of destination by removing all species that could be biologically contaminated after discharge. For the prevention of biological pollution by fouling, the International Convention on the Control of Harmful Anti-Fouling Systems on Ships, AFS Convention (entry into force: 17 September 2008; https://www.imo.org/en/About/Conventions/Pages/International-Convention-on-the-Control-of-Harmful-Anti-fouling-Systems-on-Ships-(AFS).aspx, accessed in June 2023), contains regulations on the antifouling paints to be applied to the hulls of ships. These paints must be changed after a maximum of 5 years when the ship is maintained in dry dock. Many shipping companies carry out intermediate hull cleanings between shipyard maintenances to reduce hull friction and increase speed.
The measures adopted by the IMO help prevent biopollution and reduce the problem since limiting the introduction of new species is an effective way of preventing invasions (Simberloff, 2013). The eradication of invaders is extremely difficult, sometimes impossible, and expensive (Pimentel et al., 2005; Olson, 2006; Senator and Rozenberg, 2017). Therefore, the knowledge of the introduction pathways, and the main donor areas of non-indigenous species that may eventually become invasive in a region, is a priority. Knowing the geographical origin of the species and their current distribution is very important and should be carefully considered for appropriate management and risk prediction (Kolar and Lodge, 2001; Olenin et al., 2011; Buckley and Catford, 2016). However, the biology and dynamics of invasions from shipping pathways are still poorly understood (Carney et al., 2017). The intensity of maritime traffic (the more the higher the risk), the distance from the source population (the shorter the higher the risk), and the ecosystem similarity (the more the higher the risk) are key predictors of the risks of invasion (e.g., Seebens et al., 2016). However, there are exceptions. As an example, on long journeys, predictions suggest a higher probability of introduction after arrival at the destination, when organisms can survive the transit (Saul et al., 2017). This may cause the paradoxical introduction via shipping of Antipodes’ organisms—the longest possible journey—not only for the resemblance of environmental conditions in donor and recipient habitats but also because survivors are likely more tolerant (Miralles et al., 2018).
Most models developed to predict the introduction of alien species via shipping focus on aquatic organisms that can travel in ballast water or fouling (e.g., Seebens et al., 2016; Sardain et al., 2019; Miralles et al., 2021), but the dry parts of the ships also shelter many terrestrial organisms (Hulme et al., 2008). Container vessels are also important as potential vectors of the transfer of species because they represented approximately 13% of the world fleet in 2020. Eight of the 10 ports handling the largest volumes of international cargo are cargo containers (Niimi, 2004). Hulme (2021) emphasizes the importance of freight containers for the long-distance transport of many species that find a good environment therein. Examples are invasive plants (McCullough et al., 2006) or mosquitoes that can be vectors of dangerous pathogens (Stanaway et al., 2001; Tatem et al., 2006). Despite these examples, terrestrial species that can be transported as stowaways in containers are less frequently considered. In contrast with the IMO conventions for ballast water and fouling control, the International Convention for Safe Containers does not even mention the problem of biological invaders nor establishes measures for preventing their inadvertent transport (entry into force: 6 September 1977; https://www.imo.org/en/About/Conventions/Pages/International-Convention-for-Safe-Containers-(CSC).aspx, accessed in June 2023). The relative weight of the different ship compartments (dry containers, hull fouling, ballast water, bilge water) in the introduction of species outside their native range is not well understood yet. In one of the few studies addressing this issue, Faulkner et al. (2017) identified a higher contribution of terrestrial than marine species to the alien invasive species (AIS) establishment debt in South Africa via shipping routes (40% more, i.e., 14.28 versus 10.04, respectively), principally from Asia. From the increasing importance of large ship containers in international trade (Sardain et al., 2019; Hulme, 2021), the actual introductions of alien species via cargo containers may be higher than expected, at least in some regions.
In this exploratory study in the south-central Bay of Biscay, the relative proportion of AIS introduced from ballast water, fouling, or containers that are actually established in the recipient region (realized introduction success, or establishment debt in Faulkner et al., 2017) has been estimated. Ship size, port of origin, pathway (ship compartments, like ballast tanks or containers), and days docked at the destination port were considered to estimate AIS introduction pressure from donor countries. Information available on the Internet in public, user-friendly databases (of maritime traffic and invasive species) was chosen to align with practical procedures demanded by stakeholders (Bayliss et al., 2013).
2 Methods and data
2.1 The case study: Port of Gijon
The Port of Gijon is one of the 46 international ports under the national Spanish Authority and holds roughly less than 5% of the total maritime traffic of those ports (4% in 2017; official traffic statistics of 2017 can be found at https://www.puertos.es/es-es/estadisticas/RestoEstad%C3%ADsticas/anuariosestadisticos/Paginas/2017.aspx). In the southcentral Bay of Biscay (region of Asturias), there is another much smaller international port, Avilés (0.88% of the total Spanish port traffic in 2017; Gijon accounted for 81.9% of the total maritime traffic in Asturias that year), that was not taken into account in this analysis. Approximately 1,000 ships arrive in the Port of Gijon annually, generating an average annual movement of more than 20 million tons of goods (Port Authority of Gijon, https://www.puertogijon.es/en/, accessed in June 2023). The port has 7,000 linear meters of berths distributed into four terminals. It mainly specializes in the discharge of solid bulks (iron ore, coal, others) and also in the discharge of liquid bulks (product tankers and LPG). Main loading operations involve cement carrier vessels, general cargo vessels (principally steel products), and containers (loading and unloading). From the dimensions of the docks described above, they can berth vessels of up to 300 m in length. In addition, some fishing vessels operate in the port. It has infrastructures adapted to the new requirements of Article 5 of the Ballast Water Management Convention about sediment reception facilities at terminals, to clean ballast tanks or do repair work. The BWM Convention, ratified by Spain in the Official State Gazette Number 282, 22 of November of 2016, requires that all ships carrying ballast water must change and treat ballast at sea in order to reduce biological pollution. For this reason, we limited this study to the time period 2004–2017, before the entry into force of the Convention.
The Port Authority of Gijon prepared a database with all maritime traffic data from 2004 to 2017. This database compiles all the information related to the operations carried out by the vessels: type of vessel, size, port of departure, type of cargo, next port, time of entrance in and departure from Gijon, etc. The following sections explain in detail the analysis carried out in this study to determine the AIS introduction pressure based on the traffic data collected in the database. The raw data can be consulted online at http://www.puertos.es/en-us (accessed in June 2023), following a link to the Traffic Statistics where the data of the ships that have visited all the ports in Spain since 1962 are available.
2.2 Introduction pressure estimations
To determine the pressure of AIS introduction by ballast water (BW hereafter), only vessels carrying ballast that arrive in Gijon should be taken into account. The data from ships that arrive loaded without BW are not used in this part. The ships carrying BW are those that arrive empty or partially empty of cargo, to perform operations of loading or transit and loading. Vessels working in the Bay of Gijon, such as tugboats or small fishing boats, sailboats, or recreational craft have not been taken into account because these types of vessels do not use BW; if they were to use it, since they do not sail many miles from the port, they cannot generate AIS introduction pressure anyway. In the absence of information on the real volume of BW transported by ships, an approximation was made according to their size. The public database available on the Internet gives the value of the gross tonnage (GT). The term GT is a dimensionless measurement, to indicate the volume of all the internal spaces of the ship and is normally used as a means for categorizing commercial vessels, especially those used for shipping. The higher the GT, the larger the vessel and therefore the more BW it carries and the more introduction pressure it generates. The AIS introduction pressure from each port was thus calculated from the GT of each vessel and maritime traffic statistics, summing the vessels’ GT by origin port.
For biofouling risk and other storage compartments, all vessels coming for any of the following operations were considered: loading, transit, and loading; unloading or transit; and unloading. As in the case of BW, ships operating in nearby areas and recreational vessels were not considered, assuming that these vessels cannot pollute biologically as they move within a few miles of the port. Hence, the study focused on cargo vessels that arrive from ports outside the recipient region.
To determine the AIS introduction pressure from biofouling or dry compartments, we used two variables: the GT (as an approximation to the ship size) and the time at the recipient port. The bigger the GT, the larger the vessel and the hull surface in contact with the water. Regarding the time at port, the more time, the bigger the chances that the species attached to its hull establish themselves in Gijon. The same rationale can be applied to the biota transported in other storage compartments (containers, holds, anchors, etc.). The unit “GT × t,” gross tonnage (GT) multiplied by time (t) in port, in days, was employed.
We set a minimum threshold at 30,000,000 GT × t for a port to be considered a main AIS donor, based on the maritime traffic statistics of Gijon port during the considered period that—as will be seen in the Results—included many vessels coming from routes scarcely frequented.
2.3 GIS analysis
The Geographical Information System (GIS) was employed in this study for the graphical representation of the maritime routes that generate the highest risk in the Port of Gijon. In order to determine these routes, an analysis of the ports from which the ships generating the biggest risk originated was carried out. The software used was “Global Mapper 18,” which offers access to a very extensive range of spatial data and provides the appropriate level of functionality for this project.
The exact routes or stops made by the vessels are not known, but the risk map shows, as realistically as possible, an assumed non-stop route from the port of origin to Gijon. For the representation of the routes, we used as reference the tracks followed by ships in the AMVER, which is a worldwide voluntary ship reporting system operated by the US Coast Guard (https://www.amver.com/, accessed in July 2023). In addition, as the GIS employed does not have nautical charts, Navionics online nautical charts were introduced to represent the routes following traffic separation devices, beaconing, channels, port entrances, etc. («Navionics», https://www.navionics.com/esp/, accessed June 2023).
2.4 Reference database for exotic and invasive organisms
The AIS that can theoretically arrive in the recipient region through Gijon port from the areas identified as main donors in the analysis of maritime traffic were taken from the Global Invasive Species Database (GISD) (Pagad et al., 2015). This database is managed by the Invasive Species Specialist Group (ISSG) of the International Union for Conservation of Nature (IUCN) Species Survival Commission. It is openly available at http://www.iucngisd.org/gisd/ accessed in June 2023 (ISSG, 2015). This database was chosen because it was employed by Faulkner et al. (2017), our reference for the departure hypothesis; it has a global geographical scope and is easy to use. The search can be done by taxonomic groups, geographical location, system (= habitat, like freshwater, marine, terrestrial), pathway, impact, and management, applying the corresponding filters. For this study, we used the tool “Advanced search” applying the filters indicated in Table 1.
The taxonomic nomenclature followed the World Register of Marine Species (WoRMS) database (Ahyong et al., 2023) at http://www.marinespecies.org/, accessed in June 2023. The names of the species contained in the ISSG database were updated accordingly in this study. The aim of this register is to provide an authoritative and comprehensive list of names of marine organisms. This register of marine species grew out of the European Register of Marine Species (ERMS), in combination with several other species registers maintained at the Flanders Marine Institute (VLIZ). WoRMS includes information from Aphia, a database developed by the VLIZ, with other authoritative lists of marine species such as AlgaeBase (Guiry and Guiry, 2023) or FishBase (Froese and Pauly, 2023).
2.5 Biota inventories of the recipient region
To identify marine and terrestrial AIS present in the southwest Bay of Biscay region, we checked current official national and regional inventories of alien invasive species, published reports, and research articles. The Spanish catalog can be found at https://www.miteco.gob.es/es/biodiversidad/temas/conservacion-de-especies/especies-exoticas-invasoras/ce-eei-catalogo.aspx, with additional information about particular species at http://invasiber2.org/fitxa_llista.php?taxonomic=2 and https://medioambiente.asturias.es/inicio. These three databases are compared with each other to categorize the species already present in the Bay of Biscay area. Access to these databases was in March 2020.
The expected species from potential donor regions and species already present in the recipient region were classified in relation to the south Bay of Biscay area as exotic (AIS), cryptogenic (no clear native distribution), cosmopolitan (native to many locations), or native (to the recipient region). It is worth mentioning that, after decades of interchange (via shipping and other transport ways), many species present in the donor regions, or in the recipient region, were not native in the past but are now treated as such (= naturalized species). The official species status as in 2020 was considered.
2.6 Data analysis
Potential and current AIS in the region were classified according to their donor region/s (some global AIS are present in many regions), its presence or absence in the recipient region, the most likely introduction pathway via shipping (i.e., dry containers, fouling, ballast water), and the large taxonomic group (phylum). To visualize the diversity of AIS found in the region and put this jointly with the potential introduction paths estimated from shipping information, we employed non-metric multidimensional scaling (nMDS) with 9,999 bootstraps. It is a classification method based on a distance or dissimilarity matrix of different parameters. In this study, we considered geographical origin (main donor or recipient region), habitat (aquatic = freshwater + marine; terrestrial), and introduction pathway, for which the value 1 or 0 was defined. Jaccard similarity was employed for the data that were binary (Chung et al., 2019).
The taxonomic diversity of the AIS introduced from each pathway was calculated using the index Shannon–Weiner, with the number of species per phylum as a unit. Comparison between pathways for AIS diversity was conducted using t-tests.
The expected AIS from a given pathway were estimated from the list of AIS in GISD (NL) and the list of AIS observed in the recipient region (NO), multiplying the total NO by the proportion of AIS that use that pathway. The same was done for the AIS habitat (aquatic versus terrestrial). Comparisons between the expected and observed AIS in the region (categorized by pathway or habitat) were made using contingency chi-square tests with Cramer’s V as a proxy of effect size. In addition, the exact p-value obtained from 9,999 Monte Carlo permutations was used in some tests.
To check if the AIS present in Spain arriving by different pathways differed between main donor regions, we did a multivariate ANOSIM test with 9,999 permutations and Bray–Curtis distance, giving each AIS 1/0 values for its presence/absence in each donor region. This test is a non-parametric analysis of similarities based on ranked dissimilarity matrices that in ecology can be used as an ANOVA-like test (Clarke, 1993).
The standard significance threshold of p <0.05 was adopted. Statistical analyses were done using the free software PAST (Hammer et al., 2001).
3 Results
3.1 Maritime traffic analysis
The ports of Vigo, A Coruña, and Bilbao are those that generate the most ballast water imports in the Port of Gijon (Supplementary Figure 1 and the numerical values in Supplementary Table 1). These ports are all in northwest Spain (southwest Bay of Biscay), and the ballast water is uploaded from the same region. The reason is that in Asturias there is only one shipping company that has Gijon as a port of loading for its ships, and the company has fixed routes in the north of Spain only. The majority of ships arriving in the Port of Gijon come to unload goods; thus, they do not carry ballast water. Therefore, AIS carried by ballast water were not taken into account in this study.
Supplementary Figure 2 (numerical values in Supplementary Table 2) shows the ports of origin of the ships that generate a pressure of AIS import apart from ballast water. The five ports with the highest AIS introduction pressure (over the threshold set in this study of 30,000,000 (GT × t) × 103) corresponded to Ponta de Madeira (90,978.6 (GT × t) × 103) and Tubarao (76,528.9 (GT × t) × 103) in Brazil; then Richards Bay (4,0917.9 (GT × t) × 103) in KwaZulu Natal, South Africa; Ports of Hay Point (39,043.2 (GT × t) × 103) and Dalrymple Bay (35,145.3 (GT × t) × 103) in Australia; and Port Cartier (34,694.7 (GT × t) × 103) in Canada (Figure 1; Supplementary Table 2). All these places correspond to important mineral terminals. Gijon has a large traffic of bulk carriers coming to unload coal and iron ore for the steel industry of Asturias and for distribution in the surrounding regions. The nautical routes followed by the ships departing from those ports are mapped in Figure 1. The total sum of GT arriving from these ports represented 48.5% of the total maritime traffic arriving in Gijon port in the analyzed period (Supplementary Table 2).
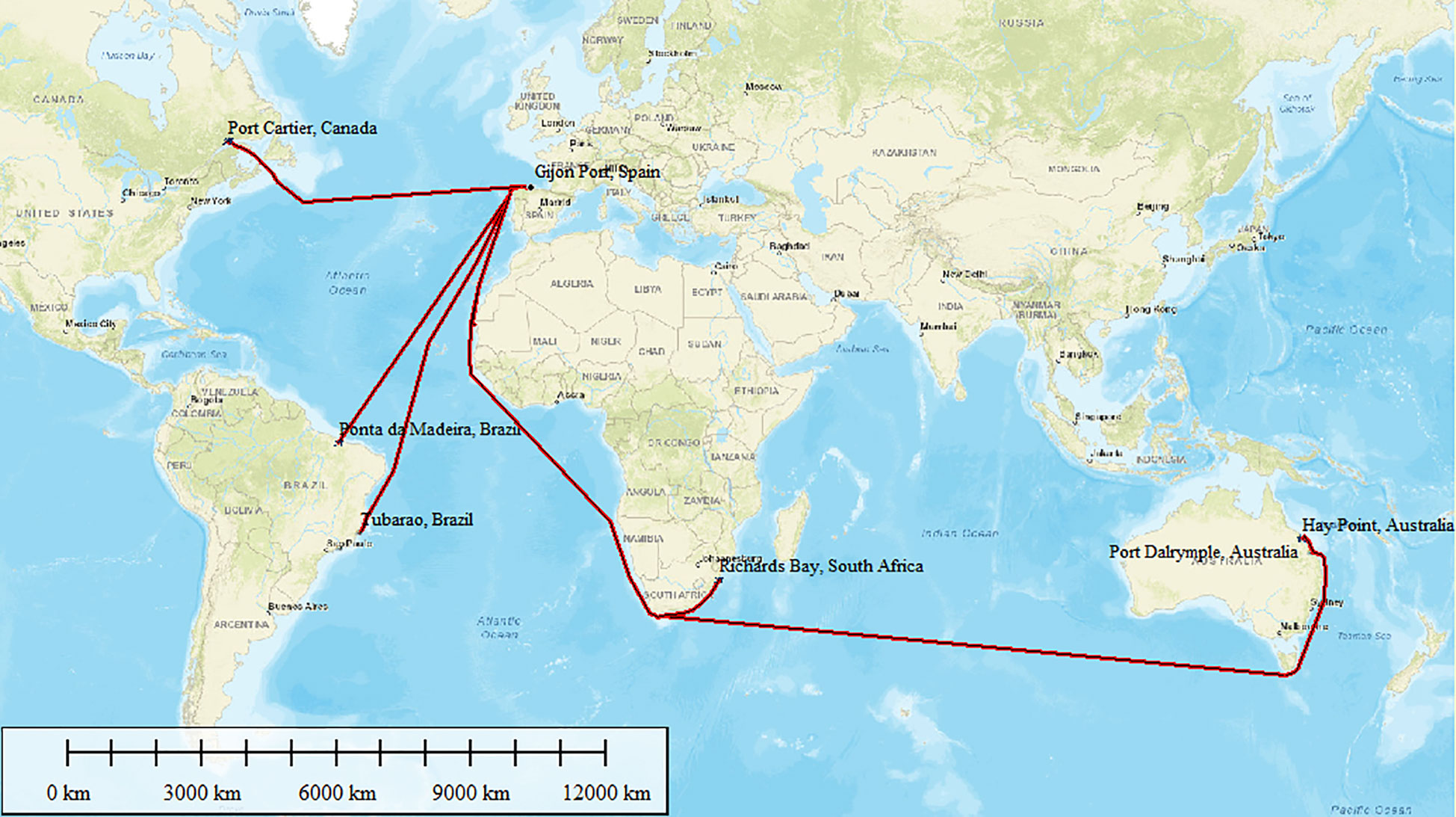
Figure 1 Routes from the ports that generate the highest AIS introduction pressure by biofouling and dry compartments.
As shown in Figure 1, the main donor ports are located at very different latitudes, have different climate, and are located in different marine ecoregions (Spalding et al., 2007), from the coldest and driest Port Cartier in the Temperate Northern Atlantic realm to the warmest and most humid Ponta Madeira in the Tropical Atlantic realm (Table 2). The number of global AIS that can use fouling and/or containers as introduction pathways documented in GISD was higher for Australia (45 AIS) than for the other three donor regions. On the other hand, the proportion of global AIS using the container pathway reported for South Africa (82%), and for Brazil to a minor extent (58%), was significantly higher than that reported for Australia (49%) and Canada (43%) donor regions (χ2 = 8.41, 3 d.f., p = 0.03 < 0.05, Cramer’s V = 0.26; Monte Carlo p = 0.041 with 9,999 permutations) (Table 2).
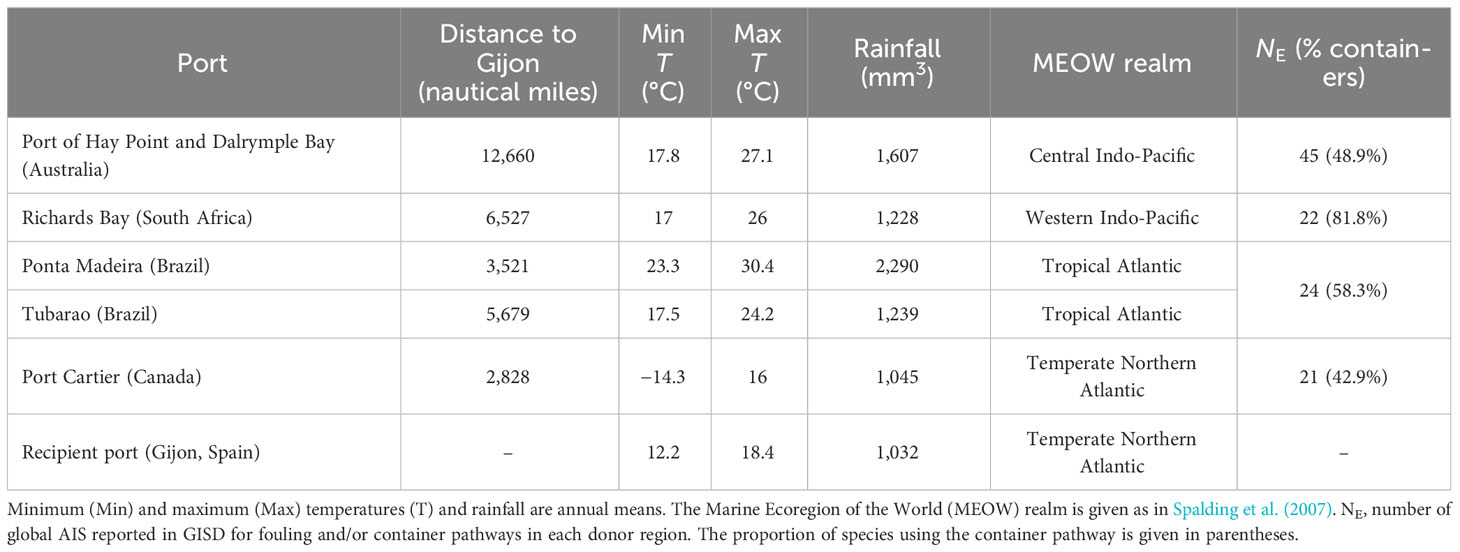
Table 2 Climate indicators, distance to the Port of Gijon, and expected AIS from the main donor ports as identified from maritime traffic data.
3.2 AIS analysis from databases
After discarding the BW pathway for its scarce contribution to AIS in this specific case, ship fouling and dry containers were considered for AIS analysis. From the GISD search with the filters indicated in Table 1, a total of 77 globally invasive species able to use ship containers and/or fouling as introduction pathway occur in the donor regions (listed in Supplementary Table 3). Of these, 17 are considered native to Spain and may be present in the donor countries either as a consequence of introductions or because they are cosmopolitan. The other 60 AIS are exotic or cryptogenic for Spain (Figure 2). This figure, 60 AIS, represents 30.2% of the total 199 AIS (including fungi) cataloged in the country.
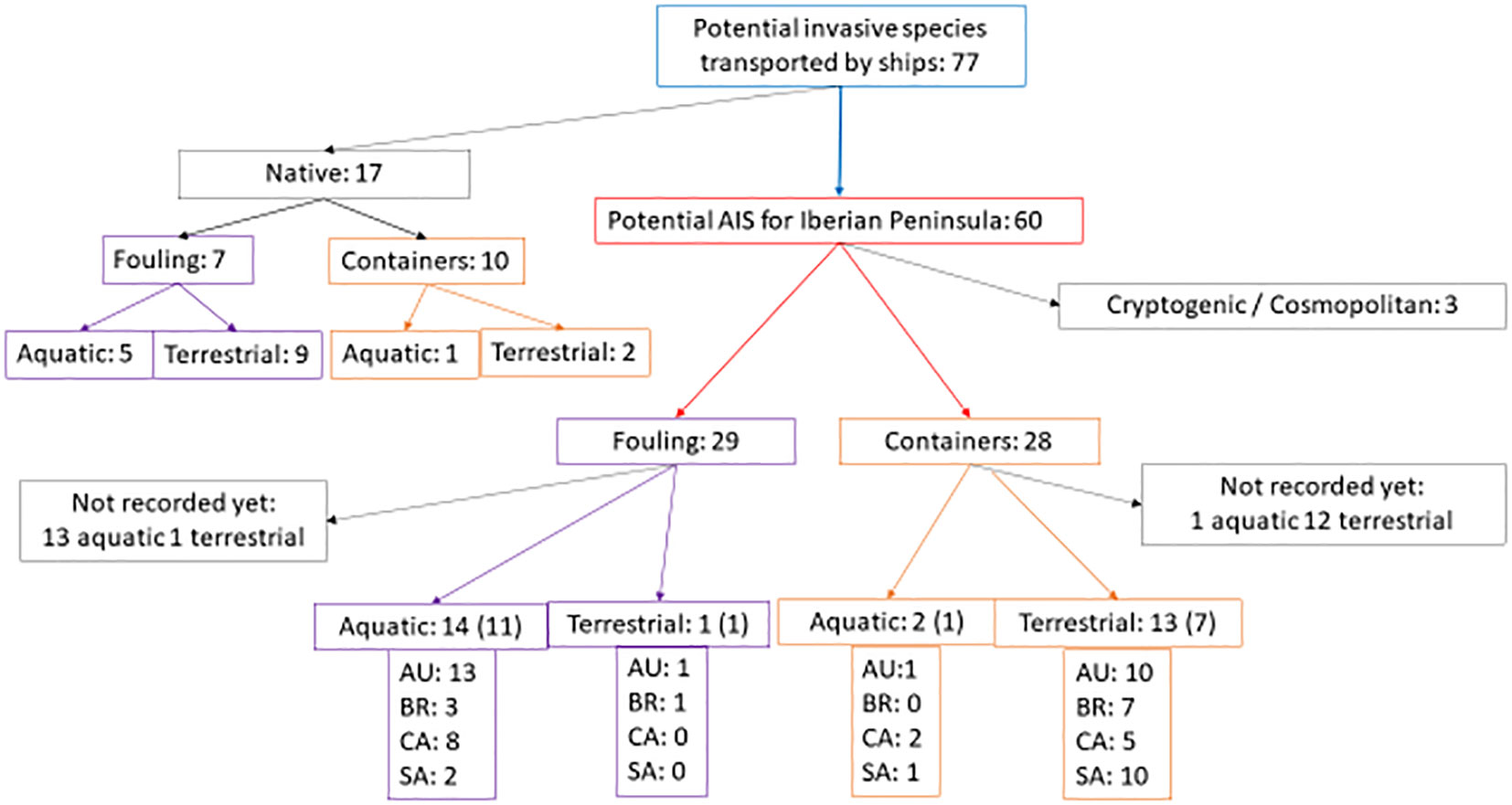
Figure 2 Flow diagram showing the number of invasive species found in the donor regions and in Spain that can be transported by ship fouling or dry containers. The number of species and type of habitat (aquatic or terrestrial) present at the same time in Spain and each donor port are indicated. AU, Australia; BR, Brazil; CA, Canada; SA, South Africa. The number of species reported from Asturias appears in parenthesis.
Cosmopolitan/cryptogenic species, like the domestic cat Felis catus and the rats Rattus norvegicus and Rattus rattus that are considered invasive in the Canary Islands but not in the study region, were excluded. Of the remaining AIS, 28 are reported to be transported in ship containers (Figure 2; Supplementary Table 3). Of those, 15 AIS (53.6%) have already been reported from the recipient region (8) or other areas within Spain (7). These are also considered here because a species introduced in a location may move and settle down in neighboring or even more distant regions where the habitat is suitable.
Of the other 29 AIS that can be transported by fouling (Figure 2; Supplementary Table 3), 15 (51.7%) are present in the Iberian Peninsula: 12 in the Asturias region and 3 in other zones in Spain. Thus, in the surroundings of Gijon port, a total of 20 AIS that could arrive in ships calling in Gijon port were actually present in 2020. The difference between the proportion of AIS present in the region (versus those not recorded yet) transported by fouling and those transported by containers was not significant (χ2 = 1.64, 1 d.f., p = 0.20 > 0.05, n.s.; Cramer’s V = 0.16), which indicates a similar importance of the two pathways. The 17 native AIS that could be exported to other countries had also a similar pathway profile, with 7 species transported by fouling and 10 by containers (Figure 2, upper left).
The habitat of the 30 AIS found in Spain was balanced between terrestrial (14) and aquatic species (16), from which 8 and 12, respectively, are already in Asturias (Figure 2, bottom). The habitat profile of the 17 native AIS was a little bit different with a dominance of terrestrial (11) over aquatic AIS (6) (Figure 2, upper left). However, the difference between native and imported AIS for the habitat type was not significant (χ2 = 1.42, 1 d.f., p = 0.23 > 0.05, n.s.; Cramer’s V = 0.17).
Regarding the donor regions, 25 of the 30 AIS present in Spain are also found in Australia (83.3%), 15 in Canada (50%), 13 in South Africa (43.3%), and 11 in Brazil (36.7%) (Figure 2, bottom). As expected for globally invasive species, many of the imported AIS occur in two or more donor countries; therefore, the sum of AIS by donor region appearing in Figure 2 is higher than the total number of AIS. Only three fouling animals from Australia and one plant associated to containers from Canada belong to one single donor region (Supplementary Table 3). The most frequent combination in this study was Australia–South Africa for hitchhikers in containers and Australia–Canada for fouling AIS (Supplementary Table 3).
The nMDS of the donor country (Australia, Brazil, Canada, South Africa), introduction pathway, and AIS habitat (aquatic, terrestrial) for the 30 AIS had a moderate stress of 0.11 with r2 of axis 1 = 0.74 and axis 2 = 0.02. In the scatter plot (Figure 3), Canadian and Australian AIS were connected by the fouling pathway and aquatic habitat, while Brazil and South Africa were connected by the container pathway and terrestrial habitat. This indicates that AIS imported from South Africa and Brazil are mainly terrestrial and transported by containers, while those imported from Canada and Australia are aquatic fouling species.
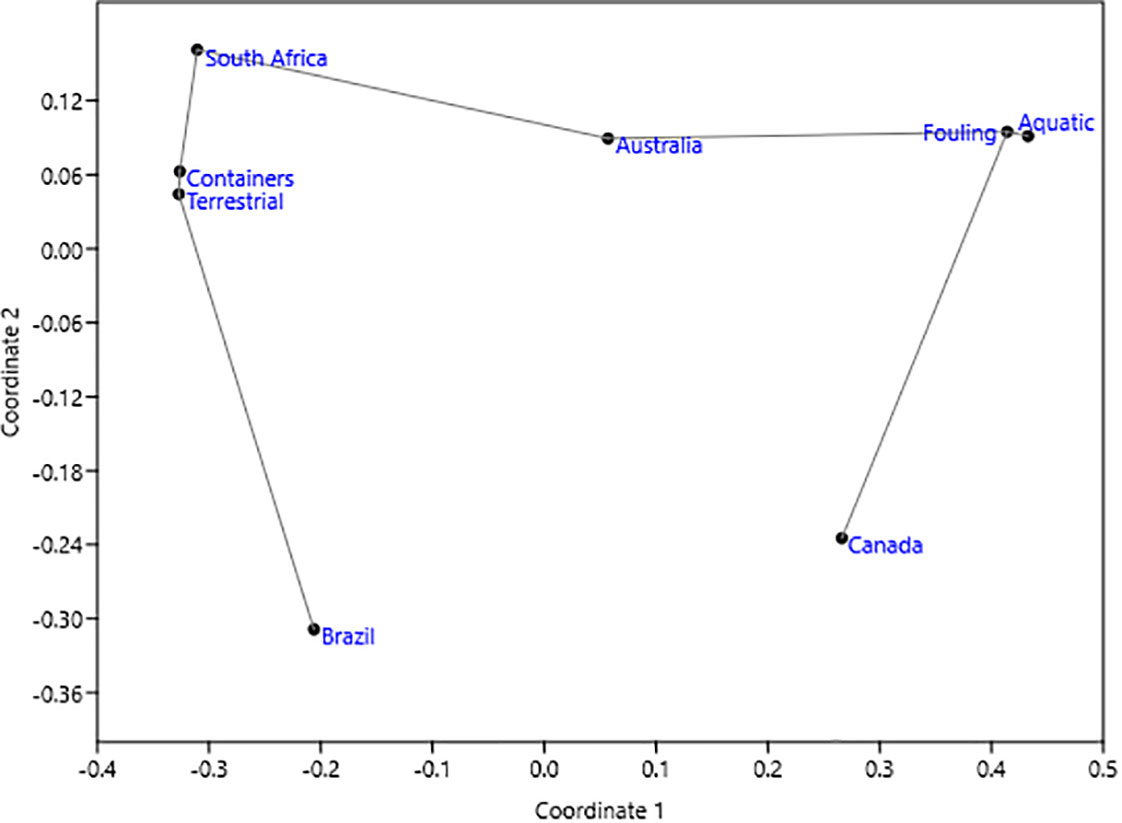
Figure 3 nMDS results. Scatter plot with minimum spanning tree constructed from 99,999 replicates and Jaccard similarity index.
3.3 Inferred effect of donor country and introduction pathway in the study region
The proportion of species present in Asturias over the expected from each donor country (NE in Table 2) was 0.4, 0.29, 0.43, and 0.36 for Australia, Brazil, Canada, and South Africa, respectively (see the species details in Table 3). The difference between donor countries was not significant (χ2 = 1.1, 3 d.f., p = 0.78 > 0.05, n.s., Cramer’s V = 0.1).
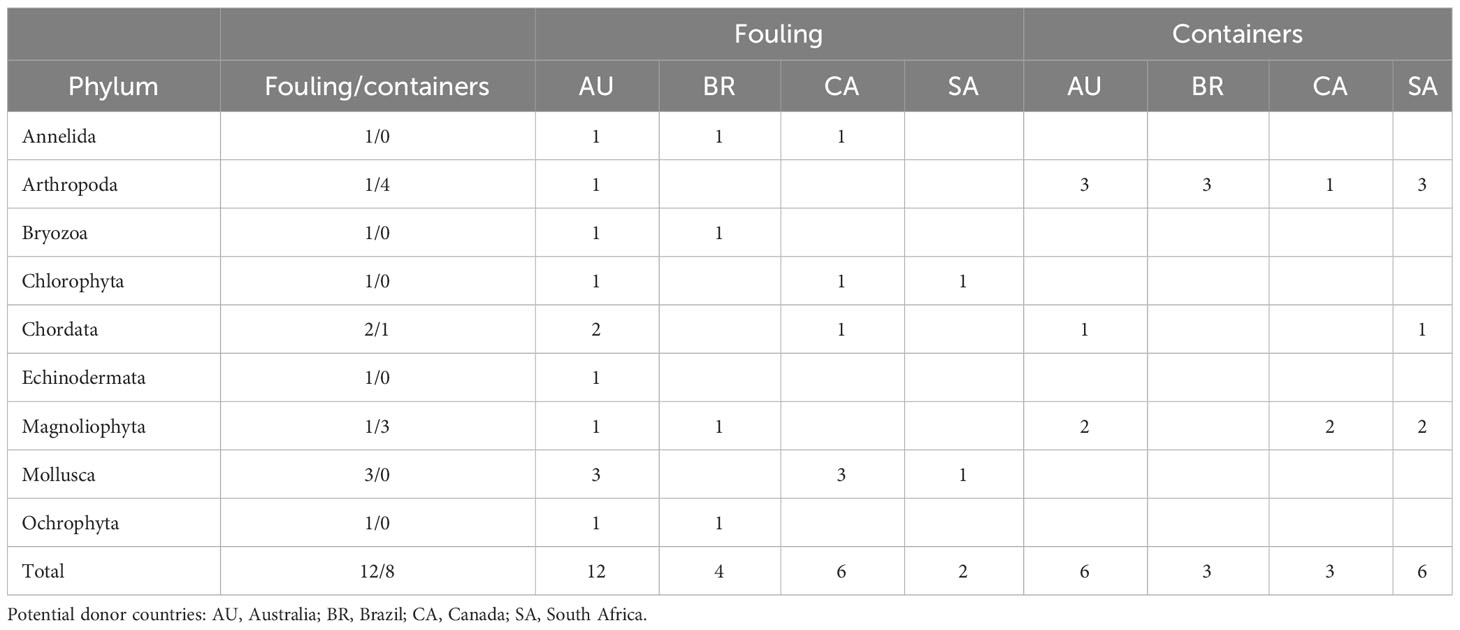
Table 3 Distribution of the imported AIS observed in Asturias by phylum, potential donor country, and pathway.
For the introduction pathway, the AIS imported from the four donor countries did not use fouling and container pathways with the same probability (Table 3; Supplementary Table 3), as revealed by the significant ANOSIM (R = 0.223, p = 0.014 < 0.05). The AIS from South Africa used containers more than fouling, while the AIS from Australia and Canada used fouling more than containers (Table 3). These results were indeed consistent with the results of the nMDS (Figure 3).
The AIS transported by fouling were more diverse, with nine phyla and Shannon–Weiner index H = 2.095 (variance 0.045), than those transported by containers that were only three phyla and had H = 0.974 (variance 0.04). The difference in diversity between the two pathways was highly significant: t = 3.81, 19.7 d.f., p = 0.001. The taxonomic catalog of the AIS was not identical, with more arthropods and macrophytes transported by containers, while mollusks and other taxa were transported only by fouling (Table 3).
In the analysis by country comparing the proportion of expected AIS (NE) with those actually present in Asturias (NO) for each pathway, no significant differences were found for any country (see contingency analysis and Monte Carlo values in Supplementary Table 4, all not significant). Including all the AIS present in the rest of Spain by country and pathway, the results were similar, with no significant differences between the two pathways by country (data not shown): the observed AIS did not differ from the expected ones. The same happened with habitat: no significant differences between expected and observed terrestrial and aquatic AIS were found for any donor country (Supplementary Table 4). In summary, a bias in AIS establishment success by pathway or habitat was not found in the present data. Thus, the significant ANOSIM showing differences between countries was likely due to differences in the country’s lists of organisms transported by each pathway, with more AIS that use containers listed in GISD for Brazil and South Africa and more ship fouling AIS listed for Australia and Canada (see NL in Supplementary Table 4).
3.4 Diversity of AIS native to Asturias
The AIS native to Asturias could be potentially exported to other countries connected by maritime traffic. The 17 native AIS listed in GDIS (Table 4) belonged to seven phyla. Those traveling by fouling were of six phyla with H = 1.75 (variance 0.07), and those traveling in containers were of four phyla with H = 1.5 (variance 0.04). The difference in diversity between the two pathways was not significant (t = 0.76, 14.2 d.f., p = 0.46). As in the case of imported AIS, Magnoliophyta (macrophytes) were preferentially transported by containers, and the listed mollusk (the Mediterranean mussel Mytilus galloprovincialis) was transported by fouling. Native and imported diversities of fouling AIS were not significantly different (t = 1.04, 15.91 d.f., p = 0.32). The diversity of native AIS transported by containers was higher than that of imported AIS that use this pathway (H = 1.5 versus 0.97), but the difference was only marginally significant (t = 1.899, 17.71 d.f., p = 0.07).
4 Discussion
The main novelty of this study was to confirm the important role of dry ship compartments—containers—in the introduction of AIS in the southwest Bay of Biscay. Most studies that investigate marine biosecurity focus on aquatic species (e.g., Seebens et al., 2016; Miralles et al., 2021). However, supporting Faulkner et al. (2017), terrestrial species that are transported in dry ship compartments represent an important part of the introduced species. The tiger mosquito Aedes albopictus, which is a vector of yellow fever and dengue viruses, and highly invasive plants like the South African ragwort Senecio inaequidens are examples of species transported in ship containers that are already established in the region studied in this work (Cano et al., 2007; Delacour et al., 2015).
This case study was focused on globally invasive AIS, unlike other investigations that consider all non-indigenous species regardless of their invasiveness in this (Miralles et al., 2016; Miralles et al., 2021) and other regions (e.g., Georgiades et al., 2020). This procedure indeed decreased the number of potential introductions in the model, but the results highlighted the different status of the considered donor countries regarding the number of biosecurity risk species reported in global databases. Australia was by far the country with more reports. This has been discussed by Nuñez et al. (2022): the whole issue of maritime biosecurity is especially important for island countries like Australia and New Zealand, that for their unique endemic biota are more vulnerable to the impact of introduced species (Lougheed, 2007). Consequently, they invest much effort in the research on biological invasions and report AIS carefully, something that is still needed in other regions, especially in Africa and South Asia but also in South America (Nuñez et al., 2022).
The results also suggest a bias in the pathways reported from each region. The results of our small study would indicate that investigations in South Africa are more focused on containers and terrestrial species than on fouling aquatic ones since there is no reason to assume that this country is more vulnerable to terrestrial than to aquatic invasions—at least in comparison to the Australian donor region that is located at a similar latitude (Figure 1). The watch list of species invasive to South Africa created from Faulkner et al. (2016) from published reports and databases contains a much higher number of terrestrial than aquatic species, supporting this vision in some way. Interestingly, the small list of AIS native to Spain that are also present in the four donor countries considered in this study (perhaps arrived there by ships traveling from Gijon port) also contains a higher number of species traveling in containers than by fouling and more terrestrial than aquatic species. This would suggest a taxonomic bias in the list of AIS toward terrestrial species that are easier to inventory in temperate regions.
The results obtained here were only partially consistent with those found for marine invertebrates (Miralles et al., 2018), with a high proportion of NIS introduced from Australia that could be unexpected considering the long travel distance and not the high intensity of maritime traffic (in traffic, it was the second country in this study, far behind Brazil). Miralles et al. (2018) explained it from a similar climate, in Asturias and its antipodes, and possibly a higher survivors’ resistance to such a long travel, according to the scenario predicted by Muirhead et al. (2015) of higher survival (at arrival) of propagules after long journeys. However, considering marine and terrestrial AIS, here, we did not find a significantly higher proportion of species from Australia than from Brazil if we take into account the expected NE listed in databases. This happened despite a more similar climate in Australia and the Bay of Biscay and a possible higher resistance of propagules surviving the longer travel. Much more intense maritime traffic between Brazil and Gijon would compensate for the loss of AIS due to adaptation failures to a much different ecosystem.
It is also possible that some global AIS are becoming truly cosmopolitan, for the globalization of maritime traffic that enables not only their dispersal but also multiple invasion hits (e.g., Ba et al., 2010; Banks et al., 2015; Muthukrishnan and Larkin, 2020). Gijon is a port of relatively modest size among 46 international ports under the Spanish National Authority (five in the Bay of Biscay) and many more marinas and fishing ports along 8,000 km of coast in Spain. Indeed, other international ports of Spain receive ships from the same donor countries and will contribute to the introduction of the same AIS. Supporting Touchard et al. (2023), the network of international ports would represent a partially isolated ecosystem where native and invasive species join in a melting pot, connected with fishing ports and marinas by local maritime traffic. The many ships transporting ballast water from neighboring ports would also transport fouling organisms that could stay for a time in those ports after being introduced from distant connections. In this sense, it is interesting to remark that in this case study, considering only one international port in Spain and four donor countries that represent roughly half of the total maritime traffic of the port, we found 30 AIS that could have entered Spain via that port, 20 of them established in Asturias region. This is 18.3% of a total number of 164 aquatic, terrestrial, and microbial AIS cataloged in Spain (excluding those specific to the islands) entering by any possible pathway (https://www.miteco.gob.es/es/biodiversidad/temas/conservacion-de-especies/especies-exoticas-invasoras/ce-eei-catalogo.aspx). Moreover, half of the recognized AIS are terrestrial. This would add interesting new connections between terrestrial communities via shipping, enabling the dispersal of organisms between otherwise clearly discontinuous demes. This connectivity way would deserve further exploration for its likely role in biota globalization.
Another interesting result of this study was the difference in taxonomic diversity between the groups of AIS using fouling and container pathways. Muthukrishnan and Larkin (2020) showed the importance of biological invasions for biotic homogenization, which is the reduction of distinctness between separate communities caused at least partially by the species moved across regions. In this case study, in Asturias, more taxa would have been imported via fouling (nine) than using freight containers (three); thus, fouling would contribute to increase diversity more than containers. This could be explained by the direct exposure of fouling organisms to the progressively changing conditions of seawater during the travel: they would have time for adaptation to warmer or colder conditions, as suggested by Miralles et al. (2018) for explaining a higher introduction of organisms surviving long travels. In contrast, organisms traveling in containers are enclosed there and go directly to the destination, without intermediate exposure to changing environments. When the container is open, they are suddenly exposed to the new conditions in the recipient region. One could expect a higher mortality, or at least a more difficult adaptation, in the second case. However, there was no significant difference between pathways in the proportion of AIS that were effectively introduced in the region (over the expected), which suggests that there is no clear difference in the ability of adaptation of AIS that use one or the other pathway; we should remember that we are working with globally invasive AIS with a high adaptation capacity. A taxonomic bias or incompleteness in the AIS catalogs of the countries that provided the majority of those terrestrial species transported in containers, Brazil and South Africa, could explain the observed difference in diversity. Nuñez et al. (2022) described far fewer reports of invasive species from these countries than from Australia and North America; thus, this type of taxonomic biases could be expected.
A relatively high diversity of imported AIS, similar to that of native species that are AIS elsewhere, indeed does not imply the beneficial effects of AIS. The introduction of AIS via shipping will homogenize marine biota (and terrestrial biota as well, as we see in this study) between donor and recipient regions. The net outcome of AIS introduction will be reduced diversity at large scales and perhaps reduced ecosystem resilience, even when the recipient community exhibits a high diversity (Cadotte, 2023).
4.1 Limitations of this study
This study does not intend to be an exhaustive analysis of all the potential risks of AIS introduction but a comparison between pathways followed from main donor countries (as for maritime traffic) to contribute to the AIS establishment debt in a small region. For this limitation, the study could be considered as a proof of concept. Only one reference database of globally invasive species such as GISD was employed, which is convenient for its standardized framework of pathway categories (Pagad et al., 2015). Other authoritative databases could be employed in addition to GISD, especially those that contain information centered in Europe like DAISIE (Roy et al., 2020) or NOBANIS (available from http://www.NOBANIS.org, accessed 20 June 2023) because the recipient region is European in this case.
Here, only global AIS were the focus, not non-indigenous species or locally invasive species whose potential as global invaders is not recognized yet. However, other studies consider all NIS as potentially invasive species since NIS may become invasive depending on the local conditions (e.g., Kenworthy et al., 2018): the composition of the local communities (e.g., Riera et al., 2018), if they find niches to occupy (e.g., Levine et al., 2004; González-Ortegón et al., 2010; Pack et al., 2022), or even if they have traits that facilitate invasions (e.g., Quell et al., 2021; Rato et al., 2021). This could be considered in further studies.
For the importance of ecological conditions in the establishment of AIS, another limitation was not introducing the ecological similarity between donor and recipient regions, as other studies do (e.g., Seebens et al., 2016; Sardain et al., 2019). The aim of this study was not to develop a model for the prediction of future invasions but to infer the possible pathway of AIS actually found in the recipient area, i.e., the establishment debt (Faulkner et al., 2017). Further studies focusing on protocols for the detection of ships coming from ports with a high risk of introducing AIS should consider the ecology of the donor and recipient region, at least salinity and temperature (e.g., Sardain et al., 2019).
4.2 Management recommendations
From the results obtained in this study, the dry parts of ships should be considered as AIS vectors in future marine policies addressing the issue of biological invasions and biosecurity. Such is the case of the International Convention on Container Security (CSC) https://www.imo.org/en/About/Conventions/Pages/International-Convention-for-Safe-Containers-(CSC).aspx (accessed June 2023), which refers to issues related to the safety of human life in the transport and handling of containers but does not make any reference to AIS transported in containers. Although global trade and shipping are vital to our society today, following the entry into force of international ballast water and fouling agreements, the need to adopt effective sustainable practices has become evident. As this study shows, containers are another vector to be taken into account in the introduction of AIS. It is important to introduce this issue in future revisions of the CSC. Shipping must seek to minimize as far as possible unwanted impacts and potential long-term consequences on our seas and oceans. Everyone wants healthy and productive oceans, and this requires the implementation of new measures to safeguard biological diversity.
GISD (ISSG, 2015) was employed here as a reference for the inference of expected AIS. Other international databases contain global information about biological invaders like CABI Compendium (CABI, 2023) employed by Seebens et al. (2016) and many other authors, DAISIE (Roy et al., 2020) employed for example by Quell et al. (2021), and more. The maintenance and improvement of this type of database is highly recommended because reliable references that consider the introduction pathways are essential for both coast and port managers.
Future research should improve the list of AIS present in different countries with special emphasis on underrepresented habitats and countries. Since the port networks could be considered a sort of ecosystems, international collaborations between the countries connected by maritime routes could be a way to undertake this task without putting an excessive toll on developing countries.
5 Conclusions
From the maritime traffic in the Port of Gijón (southwest Bay of Biscay) during the period 2004–2017, the vessels that most influenced the introduction of AIS in the region are bulk carriers from fouling and other compartments. The risk generated by ballast water is lower due to the type of trade in the port, with exports mainly to nearby ports in the Iberian Peninsula.
The freight containers would contribute to the introduction of terrestrial AIS, while biofouling would contribute more to the introduction of aquatic species. The contribution from each pathway to the established AIS was not significantly different.
Australian and Canadian ports were the main AIS donors by fouling, and Brazil and South Africa seem to be the main donors by containers.
AIS imported by fouling were taxonomically more diverse than those imported by the container pathway and similar in diversity to native species that are AIS elsewhere. The diversity would likely not influence their invasion success.
Data availability statement
The original contributions presented in the study are included in the article/Supplementary Material. Further inquiries can be directed to the corresponding author.
Author contributions
AA: analysis of biopollution and writing. DM-T: analysis of maritime traffic and writing. EG-V: conceptualization, analysis of biopollution, and writing. HM: conceptualization and analysis of maritime traffic. MB: analysis of maritime traffic and writing. MG: analysis of maritime traffic. VS-L: analysis of maritime traffic and writing. All authors contributed to the article and approved the submitted version.
Funding
This study has been funded by the Spanish Ministry of Science and Innovation, Grants PDC2021-120939-I00 and PID2022-138523OB-I00, and the Principality of Asturias (Spain) with the Grant IDI/AYUD2021/50967.
Acknowledgments
We are grateful to two reviewers for their help with the manuscript.
Conflict of interest
The authors declare that the research was conducted in the absence of any commercial or financial relationships that could be construed as a potential conflict of interest.
Publisher’s note
All claims expressed in this article are solely those of the authors and do not necessarily represent those of their affiliated organizations, or those of the publisher, the editors and the reviewers. Any product that may be evaluated in this article, or claim that may be made by its manufacturer, is not guaranteed or endorsed by the publisher.
Supplementary material
The Supplementary Material for this article can be found online at: https://www.frontiersin.org/articles/10.3389/fmars.2023.1245320/full#supplementary-material
References
Ahyong S., Boyko C. B., Bailly N., Bernot J., Bieler R., Brandão S. N., et al. (2023) World register of marine species (VLIZ). Available at: https://www.marinespecies.org (Accessed 2023-06-19).
Ba J., Hou Z., Platvoet D., Zhu L., Li S. (2010). Is Gammarus tigrinus (Crustacea, Amphipoda) becoming cosmopolitan through shipping? Predicting its potential invasive range using ecological niche modeling. Hydrobiologia 649, 183–194. doi: 10.1007/s10750-010-0244-5
Banks N. C., Paini D. R., Bayliss K. L., Hodda M. (2015). The role of global trade and transport network topology in the human-mediated dispersal of alien species. Ecol. Lett. 18 (2), 188–199. doi: 10.1111/ele.12397
Bayliss H., Stewart G., Wilcox A., Randall N. (2013). A perceived gap between invasive species research and stakeholder priorities. Neobiota 19, 68–72. doi: 10.3897/neobiota.19.4897
Buckley Y. M., Catford J. (2016). Does the biogeographic origin of species matter? Ecological effects of native and non-native species and the use of origin to guide management. J. Ecol. 104, 4–17. doi: 10.1111/1365-2745.12501
CABI. (2023). CABI compendium (Wallingford, UK: CAB International). Available at: https://www.cabidigitallibrary.org/journal/cabicompendium (Accessed June 2023).
Cadotte M. W. (2023). Quantifying and linking mechanism scenarios to invasive species impact. Ecol. Appl. 33 (2), e2777. doi: 10.1002/eap.2777
Cano L., Escarre J., Sans F. X. (2007). Factors affecting the invasion success of Senecio inaequidens and S pterophorus in Mediterranean plant communities. J. Vegetation Sci. 18 (2), 281–288. doi: 10.1111/j.1654-1103.2007.tb02539.x
Carlton J. T. (1989). Man's role in changing the face of the ocean: biological invasions and implications for conservation of near-shore environments. Conserv. Biol. 3 (3), 265–273. doi: 10.1111/J.1523-1739.1989.TB00086.X
Carney K. J., Minton M. S., Holzer K. K., Miller A. W., McCann L. D., Ruiz G. M. (2017). Evaluating the combined effects of ballast water management and trade dynamics on transfers of marine organisms by ships. PloS One 12, 3. doi: 10.1371/journal.pone.0172468
Chung N. C., Miasojedow B., Startek M., Gambin A. (2019). Jaccard/Tanimoto similarity test and estimation methods for biological presence-absence data. BMC Bioinf. 20 (15), 644. doi: 10.1186/s12859-019-3118-5
Clarke K. R. (1993). Non-parametric multivariate analyses of changes in community structure. Austral Ecol. 18 (1), 117–143. doi: 10.1111/j.1442-9993.1993.tb00438.x
Darrigran G., Damborenea C. (2011). Ecosystem engineering impact of limnoperna fortunei in south america. Zoolog. Sci. 28, 1–7. doi: 10.2108/zsj.28.1
Delacour S., Barandika J. F., García-Pérez A. L., Collantes F., Ruiz-Arrondo I., Alarcón-Elbal P. M., et al. (2015). Detección temprana de mosquito tigre, Aedes albopictus (Skuse 1894), en el País Vasco (España). Anales Biología 37, 25–30. doi: 10.6018/analesbio.37.3
Faulkner K. T., Robertson M. P., Rouget M., Wilson J. R. U. (2016). Understanding and managing the introduction pathways of alien taxa: South Africa as a case study. Biol. Invasions 18, 73–87. doi: 10.1007/s10530-015-0990-4
Faulkner K. T., Robertson M. P., Rouget M., Wilson J. R. U. (2017). Prioritising surveillance for alien organisms transported as stowaways on ships travelling to South Africa. PloS One 12, 20. doi: 10.1371/journal.pone.0173340
Fernandez F., Ibabe A., Rayon-Viña F., Ardura A., Bartolomé M., Borrell Y. J., et al. (2022). Flotsam, an overlooked vector of alien dispersal from ports. Estuarine Coast. Shelf Sci. 271, 107879. doi: 10.1016/j.ecss.2022.107879
Froese R., Pauly D. (Eds.) (2023). FishBase (Paris, France: World Wide Web electronic publication). Available at: www.fishbase.org.
Galil B. S., Marchini A., Occhipinti-Ambrogi A., Minchin D., Narščius A., Ojaveer H., et al. (2014). International arrivals: widespread bioinvasions in European Seas. Ethol. Ecol. Evol. 26, 152–171. doi: 10.1080/03949370.2014.897651
Georgiades E., Kluza D., Bates T., Lubarsky K., Brunton J., Growcott A., et al. (2020). Regulating vessel biofouling to support New Zealand’s marine biosecurity system – a blue print for evidence-based decision making. Front. Mar. Sci. 7. doi: 10.3389/fmars.2020.00390
Giangrande A., Pierri C., Pasqua M. D., Gravili C., Gambi M. C., Gravina M. F. (2020). The Mediterranean in check: Biological invasions in a changing sea. Mar. Ecol. 41, e12583. doi: 10.1111/maec.12583
Godwin L. S. (2003). Hull fouling of maritime vessels as a pathway for marine species invasions to the Hawaiian Islands. Biofouling 19, 123–131. doi: 10.1080/0892701031000061750
González-Ortegón E., Cuesta J. A., Pascual E., Drake P. (2010). Assessment of the interaction between the white shrimp, Palaemon longirostris, and the exotic oriental shrimp, Palaemon macrodactylus, in a European estuary (SW Spain). Biol. Invasions 12, 1731–1745. doi: 10.1007/s10530-009-9585-2
Guiry M. D., Guiry G. M. (2023). AlgaeBase (Galway: World-wide electronic publication, National University of Ireland). Available at: https://www.algaebase.org.
Hammer O., Harper D. A. T., Ryan P. D. (2001). PAST: paleontological statistics software package for education and data analysis. Palaeontol. Electron. 4 (1), 1–9.
Hewitt C. L., Minchin D., Gollasch S. (2009). The vessel as a vector – biofouling, ballast water and sediments. Biol. Invasions Mar. Ecosyst. 204, 117–131.
Hulme P. E. (2021). Unwelcome exchange: International trade as a direct and indirect driver of biological invasions worldwide. One Earth 4, 666–679. doi: 10.1016/j.oneear.2021.04.015
Hulme P. E., Bacher S., Kenis M., Klotz S., Kuhn I., Minchin D., et al. (2008). Grasping at the routes of biological invasions: a framework for integrating pathways into policy. J. Appl. Ecol. 45, 403–414. doi: 10.1111/j.1365-2664.2007.01442.x
Ibabe A., Miralles L., Carleos C. E., Soto-López V., Menéndez-Teleña D., Bartolomé M., et al. (2021). Building on gAMBI in ports for a challenging biological invasions scenario: Blue-gNIS as a proof of concept. Mar. Environ. Res. 169, 105340. doi: 10.1016/j.marenvres.2021.105340
ISSG. (2015). The global invasive species database. Available at: http://www.iucngisd.org/gisd/.
Katsanevakis S., Zenetos A., Belchior C., Cardoso A. C. (2013). Invading European Seas: Assessing pathways of introduction of marine aliens. Ocean Coast. Manage. 76, 64–74. doi: 10.1016/j.ocecoaman.2013.02.024
Keller R. P., Drake J. M., Drew M. B., Lodge D. M. (2011). Linking environmental conditions and ship movements to estimate invasive species transport across the global shipping network. Divers. Distrib. 17, 93–102. doi: 10.1111/j.1472-4642.2010.00696.x
Kelly N., Wantola K., Weisz E., Yan N. (2012). Recreational boats as a vector of secondary spread for aquatic invasive species and native crustacean zooplankton. Biol. Invasions 15, 509–519. doi: 10.1007/s10530-012-0303-0
Kenworthy J., Rolland G., Samadi S., Lejeusne C. (2018). Local variation within marinas: effects of pollutants and implications for invasive species. Mar. pollut. Bull. 133, 96–106. doi: 10.1016/j.marpolbul.2018.05.001ff
Kolar C. S., Lodge D. M. (2001). Progress in invasion biology: predicting invaders. Trends Ecol. Evol. 16, 199–204. doi: 10.1016/s0169-5347(01)02101-2
Levine J. M., Adler P. B., Yelenik S. G. (2004). A meta-analysis of biotic resistance to exotic plant invasions. Ecol. Lett. 7, 975–989. doi: 10.1111/j.1461-0248.2004.00657.x
Lougheed T. (2007). Rooting out invasive species: lessons from down under. Environ. Health Perspect. 115 (7), A352–A357. doi: 10.1289/ehp.115-a352
Lucy F. E., Roy H., Simpson A., Carlton J. T., Hanson J. M., Magellan K., et al. (2016). INVASIVESNET towards an international association for open knowledge on invasive alien species. Manage. Biol. Invasions 7, 131–139. doi: 10.3391/mbi.2016.7.2.01
Macdougall A., Gilbert B., Levine J. (2009). Plant invasions and the niche. J. Ecol. 97, 609–615. doi: 10.1111/j.1365-2745.2009.01514.x
McCullough D. G., Work T. T., Cavey J. F., Liebhold A. M., Marshall D. (2006). Interceptions of nonindigenous plant pests at US ports of entry and border crossings over a 17-year period. Biol. Invasions 8 (4), 611–630. doi: 10.1007/s10530-005-1798-4
Miralles L., Ardura A., Arias A., Borrell Y. J., Clusa DL., Dopico E., et al (2016). Barcodes of marine invertebrates from north Iberian ports: Native diversity and resistance to biological invasions. Mar. Poll. Bull. 112, 183–188. doi: 10.1016/j.marpolbul.2016.08.022
Miralles L., Ardura A., Clusa L., García-Vazquez E. (2018). DNA barcodes of Antipode marine invertebrates in Bay of Biscay and Gulf of Lion ports suggest new biofouling challenges. Sci. Rep. 8, 11. doi: 10.1038/s41598-018-34447-y
Miralles L., Ibabe A., Gonzalez M., Garcia-Vazquez E., Borrell Y. J. (2021). “If you know the enemy and know yourself”: addressing the problem of biological invasions in ports through a new NIS Invasion Threat Score, routine monitoring and preventive action plans. Front. Mar. Sci. 8. doi: 10.3389/fmars.2021.633118
Muirhead J. R., Minton M. S., Miller W. A., Ruiz G. M. (2015). Projected effects of the Panama Canal expansion on shipping traffic and biological invasions. Divers. Distrib. 21, 75–87. doi: 10.1111/ddi.12260
Muthukrishnan R., Larkin D. J. (2020). Invasive species and biotic homogenization in temperate aquatic plant communities. Global Ecol. Biogeography 29, 656–667. doi: 10.1111/geb.13053
Niimi A. J. (2004). Role of container vessels in the introduction of exotic species. Mar. Pollut. Bull. 49, 778–782. doi: 10.1016/j.marpolbul.2004.06.006
Nunes A. L., Katsanevakis S., Zenetos A., Cardoso A. C. (2014). Gateways to alien invasions in the European seas. Aquat. Invasions 9, 133–144. doi: 10.3391/ai.2014.9.2.02
Nuñez M. A., Chiuffo M. C., Seebens H., Sara Kuebbing S., Matthew A. McCary M. A., Lieurance D., et al. (2022). Two decades of data reveal that Biological Invasions needs to increase participation beyond North America, Europe, and Australasia. Biol. Invasions 24, 333–340. doi: 10.1007/s10530-021-02666-6
Ojaveer H., Olenin S., Narščius A., Florin A.-B., Ezhova E., Gollasch S., et al. (2017). Dynamics of biological invasions and pathways over time: a case study of a temperate coastal sea. Biol. Invasions 19, 799–813. doi: 10.1007/s10530-016-1316-x
Olenin S., Elliott M., Bysveen I., Culverhouse P. F., Daunys D., Dubelaar G. B. J., et al. (2011). Recommendations on methods for the detection and control of biological pollution in marine coastal waters. Mar. Pollut. Bull. 62, 2598–2604. doi: 10.1016/j.marpolbul.2011.08.011
Olson L. J. (2006). The economics of terrestrial invasive species: a review of the literature. Agric. Resour. Econ. Rev. 35, 178–194. doi: 10.1017/S1068280500010145
Pack K. E., Mieszkowska N., Rius M. (2022). Rapid niche shifts as drivers for the spread of a non-indigenous species under novel environmental conditions. Diversity Distributions 28, 596–610. doi: 10.1111/ddi.13471
Pagad S., Genovesi P., Carnevali L., Scalera R., Clout M. (2015). IUCN SSC Invasive Species Specialist Group: invasive alien species information management supporting practitioners, policy makers and decision takers. Manage. Biol. Invasions 6, 127–135. doi: 10.3391/mbi.2015.6.2.03
Pearce F. (2013). 20 ports pose highest risk for alien invasions. New Scientist 218 (2916), 15. doi: 10.1016/S0262-4079(13)61166-5
Pimentel D., Zuniga R., Morrison D. (2005). Update on the environmental and economic costs associated with alien-invasive species in the United States. Ecol. Econ. 52, 273–288. doi: 10.1016/j.ecolecon.2004.10.002
Quell F., Schratzberger M., Beauchard O., Bruggeman J., Webb T. (2021). Biological trait profiles discriminate between native and non-indigenous marine invertebrates. Aquat. Invasions 16 (4), 571–600. doi: 10.3391/ai.2021.16.4.01
Rato L. D., Crespo D., Lemos M. F. L. (2021). Mechanisms of bioinvasions by coastal crabs using integrative approaches – A conceptual review. Ecol. Indic. 125, 107578. doi: 10.1016/j.ecolind.2021.107578
Ricciardi A., Cohen J. (2007). The invasiveness of an introduced species does not predict its impact. Biol. Invasions 9, 309–315. doi: 10.1007/s10530-006-9034-4
Riera L., Ramalhosa P., Canning-Clode J., Gestoso I. (2018). Variability in the settlement of non-indigenous species in benthic communities from an oceanic island. Helgoland Mar. Res. 72, 15. doi: 10.1186/s10152-018-0517-3
Roy D., Alderman D., Anastasiu P., Arianoutsou M., Augustin S., Bacher S., et al. (2020). DAISIE - Inventory of alien invasive species in Europe. Version 1.7 (Copenhagen, Denmark: Research Institute for Nature and Forest (INBO)). doi: 10.15468/ybwd3x
Sardain A., Sardain E., Leung B. (2019). Global forecasts of shipping traffic and biological invasions to 2050. Nat. Sustainability 2 (4), 274–282. doi: 10.1038/s41893-019-0245-y
Saul W.-C., Roy H. E., Booy O., Carnevali L., Chen H.-J., Genovesi P., et al. (2017). Assessing patterns in introduction pathways of alien species by linking major invasion data bases. J. Appl. Ecol. 54, 657–669. doi: 10.1111/1365-2664.12819
Seebens H., Schwartz N., Schupp P. J., Blasius B. (2016). Predicting the spread of marine species introduced by global shipping. Proc. Natl. Acad. Sci. U. S. A. 113, 5646–5651. doi: 10.1073/pnas.1524427113
Senator S. A., Rozenberg A. G. (2017). Assessment of economic and environmental impact of invasive plant species. Biol. Bull. Rev. 7 (4), 273–278. doi: 10.1134/S2079086417040089
Simberloff D. (2001). Biological invasions - How are they affecting us, and what can we do about them? West. North Am. Nat. 61, 308–315.
Simberloff D. (2013). Invasive species: what everyone needs to know (Oxford, United Kingdom: Oxford University Press).
Simberloff D., Martin J.-L., Genovesi P., Maris V., Wardle D. A., Aronson J., et al. (2013). Impacts of biological invasions: what's what and the way forward. Trends Ecol. Evol. 28 (1), 58–66. doi: 10.1016/j.tree.2012.07.013
Spalding M. D., Fox H. E., Allen G. R., Davidson N., Ferdaña Z. A., Finlayson M., et al. (2007). Marine ecoregions of the world: a bioregionalization of coastal and shelf areas. BioScience 57 (7), 573–583. doi: 10.1641/B570707
Stanaway M. A., Zalucki M. P., Gillespie P. S., Rodriguez C. M., Maynard G. V. (2001). Pest risk assessment of insects in sea cargo containers. Aust. J. Entomol. 40, 180–192.
Tatem A. J., Hay S. I., Rogers D. J. (2006). Global traffic and disease vector dispersal. Proc. Natl. Acad. Sci. U. S. A. 103 (16), 6242–6247. doi: 10.1073/pnas.0508391103
Touchard F., Simon A., Bierne N., Viard F. (2023). Urban rendezvous along the seashore: Ports as Darwinian field labs for studying marine evolution in the Anthropocene. Evolutionary Appl. 16, 560–579. doi: 10.1111/eva.13443
Van Kleunen M., Weber, Fischer (2010). A meta-analysis of trait diferences between invasive and non-invasive plant species. Ecol. Lett. 13, 235–245.
Keywords: shipping, biofouling, invasive species, ship containers, maritime traffic
Citation: Menéndez-Teleña D, Soto-López V, Bartolomé M, González Arenales M, Montes HJ, Ardura A and Garcia-Vazquez E (2023) Alien species established in the Bay of Biscay suggest significant contribution of both fouling and dry ship compartments. Front. Mar. Sci. 10:1245320. doi: 10.3389/fmars.2023.1245320
Received: 23 June 2023; Accepted: 23 August 2023;
Published: 19 September 2023.
Edited by:
Erik Ytreberg, Chalmers University of Technology, SwedenReviewed by:
Francesco Tiralongo, University of Catania, ItalyEmily Ralston, Florida Institute of Technology, United States
Copyright © 2023 Menéndez-Teleña, Soto-López, Bartolomé, González Arenales, Montes, Ardura and Garcia-Vazquez. This is an open-access article distributed under the terms of the Creative Commons Attribution License (CC BY). The use, distribution or reproduction in other forums is permitted, provided the original author(s) and the copyright owner(s) are credited and that the original publication in this journal is cited, in accordance with accepted academic practice. No use, distribution or reproduction is permitted which does not comply with these terms.
*Correspondence: Eva Garcia-Vazquez, egv@uniovi.es
†These authors have contributed equally to this work
‡Deceased