- 1Department of Agro-Industrial Technology, Faculty of Applied Science, Food and Agro-Industrial Research Center, King Mongkut’s University of Technology North Bangkok, Bangkok, Thailand
- 2Research and Education Center “Industrial Biotechnologies”, Immanuel Kant Baltic Federal University, Kaliningrad, Russia
- 3Borneo Marine Research Institute, Universiti Malaysia Sabah, Kota Kinabalu, Malaysia
- 4Persian Gulf and Oman Sea Ecological Research Center, Iranian Fisheries Science Research Institute (IFSRI), Agricultural Research Education and Extension Organization (AREEO), Bandar Abbas, Iran
Collagens are abundant structural proteins found in connective tissues such as bones, swim bladder, skin, blood vessels, intestines, and cartilage. They make up around 30% of the total protein. The purpose of this paper is to provide a summary of the current knowledge about collagen isolated from marine organisms and its possible applications. Collagen is widely used in pharmaceuticals, food, biomedical and cosmetic industries due to its cell adhesion, biocompatibility, and safety properties. This review discusses various methods for extracting collagen from marine vertebrates and its physicochemical properties. Enzymatic extractions might be a more effective at extracting collagen than acidic extractions. Peptides derived from collagen hydrolysates have biological activity that promotes health and relieves symptoms caused by chronic diseases. Aquaculture can help with collagen availability but an integrated technology for processing raw materials is necessary to address the negative effects of production waste. Marine collagen has many benefits over terrestrial sources including its versatility in healing skin damage and slowing down the aging process. The advantages of marine collagen over terrestrial sources are discussed along with its potential biotherapeutic applications in bone and skin injuries. The development of effective cosmetic products can become a strategic direction for technological development.
Introduction
Collagens are proteins that provide structural support to connective tissues like bone, skin, and cartilage. There are 29 types of collagen present in the human body and they account for about 30% of the total protein in the body (Di Lullo et al., 2002; Rossert and de Crombrugghe, 2002; Müller, 2003). Type I is the most common and can be found in various tissues such as bone, heart, and skin. It is used extensively in pharmaceuticals, food, biomedical products, and cosmetics due to its cell adhesion properties, biocompatibility, safety, low antigenicity, and biodegradability (Aruta et al., 2009; Yamada et al., 2014; Pal et al., 2015; Wang, 2021). Type III is the second most common type present in connective tissues like organs, skin, and lungs. Collagen types V and XI are less abundant but can be found along with types I and II in cartilage, bone, and other tissues (Daboor et al., 2010). Breakdown of interstitial collagens is important for biological processes like wound healing and tissue remodeling (Brett, 2008). Collagen hydrolysates are used to produce liquid matrices while controlled rate freezing methods are used for tissue engineering purposes like skin regeneration or bone reconstruction (Dai et al., 2013). Collagen plays a role in various pathologies such as tumor cell spreading or periodontal disease (Khan and Khan, 2013). As we age, collagen synthesis in our bodies decreases. This puts more strain on our bones, hair tissue, and skin. However, collagen is considered a promising anti-aging material with rejuvenating properties (Kapuler et al., 2015; Xu et al., 2021).
The commercial collagen available now is typically derived from the skins and bones of calves and pigs. Unfortunately, there have been outbreaks of animal diseases such as spongiform encephalopathy and foot-and-mouth disease that have affected these sources of collagen and their derived products (Pal et al., 2015). Additionally, some countries prohibit the use of bovine/porcine collagen due to religious beliefs. Because purifying this type of protein is difficult and poses a risk for transmissible diseases, mammalian collagen has become less popular compared to marine collagen (Yemisken et al., 2023). Marine organisms like fish, jellyfish, sponges, and other invertebrates are a great source of bioavailable collagen that lack religious constraints and animal pathogens (Coppola et al., 2020b). Although marine collagen is both safe and easy to obtain, it does have a lower denaturation temperature than other sources which can limit its beneficial effects (Barzkar et al., 2018; Rajabimashhadi et al., 2023).
Even though, studies have shown that marine-origin collagenous materials are biocompatible and have good potential for tissue engineering applications compared to terrestrial organisms-derived collagen (Lim et al., 2019; Martins et al., 2022; Rajabimashhadi et al., 2023). This makes it an ideal ingredient for not only wound healing devices but also cosmeceuticals, dietary supplements and nutraceuticals (Martins et al., 2023; Rigogliuso et al., 2023). Further studies demonstrated that type I collagen matrix from tilapia scales has similar light scatter and transmission to the human cornea, as well as good biocompatibility in various animal models. BioCornea, a fish-scale-derived collagen matrix, is currently undergoing phase I clinical trials (van Essen et al., 2013; Rajabimashhadi et al., 2023). Additionally, type II collagen from the cartilage of the Peru jumbo flying squid Dosidicus gigas has shown promise in reducing pro-inflammatory mediators and relieving symptoms of osteoarthritis (Dai et al., 2018).
This review provides brief information about properties of marine collagen and its potential applications due to its importance. The review’s strengths are that it demonstrates that marine sources of collagen have numerous advantages over terrestrial and other sources. Marine collagen is widely available, does not have any religious restriction, and there have been only few reports on its toxicity (van Essen et al., 2013). In addition to what we have discussed so far, the use of marine collagen is environmentally friendly and safe. Furthermore, collagen has many applications in many fields, such as drug delivery, wound healing, skin aging, and tissue regeneration. Marine collagen has been reported to be more susceptible to hydrolysis than mammalian collagen, making it suitable for further processing into peptide derivatives (Dai et al., 2018). Collagen has been shown to have structural and functional properties that make it a natural substrate for cell adhesion, cell growth, and differentiation (Li et al., 2020). However, it should be noted that marine collagen has less residual proline and hydrochloroproline than bovine collagen and has not been shown to have less thermal stability than bovine collagen (Diogo et al., 2021). Moreover, most studies involve examining the effects of marine collagen in vitro or in animal models. However, further studies investigating the efficacy and possible side effects of marine collagen in humans should be mentioned.
In Russia, due to sanctions by the European Union, the U.S., and other allies, imports of marine raw materials and products have been significantly reduced. Therefore, it is relevant to search for replacement of foreign raw materials with domestic ones. According to the authors (Chen et al., 2022), collagen can be derived from byproducts and wastes generated during the deep processing of marine organisms. The use of such sources contributes to environmental protection and meets the principles of resource conservation and innovation in technological solutions. The maximum and rational use of marine organisms is supported by the Government of the Russian Federation, which indicates the relevance and urgency of such research (Liu et al., 2010).
There are three main methods of collagen extraction: neutral salt solubilized collagen, acid solubilized collagen, and pepsin solubilized collagen (Barzideh et al., 2014; Li et al., 2020). Loosely cross-linked collagen molecules are extracted with neutral salt solutions (Attaran Fariman et al., 2016). The extracted material is purified by dialysis, sedimentation, and centrifugation. Dilute acidic solvents such as citrate buffer, 0.5 M acetic acid, or hydrochloric acid (pH 2-3) are more effective than neutral salt solutions. Collagen from bone, cartilage, or material from aged organisms contains a higher percentage of keto-imine bonds and has lower solubility in dilute acidic solvents (Blanco et al., 2017).
Significantly higher yields compared to acid extraction can be achieved by taking advantage of the fact that the triple helix of collagen is relatively resistant to the action of proteases, i.e., pepsin or chymotrypsin, below approximately 20°C (Mizuta et al., 1994). Figure 1 shows a flow chart of collagen extraction from marine sources.
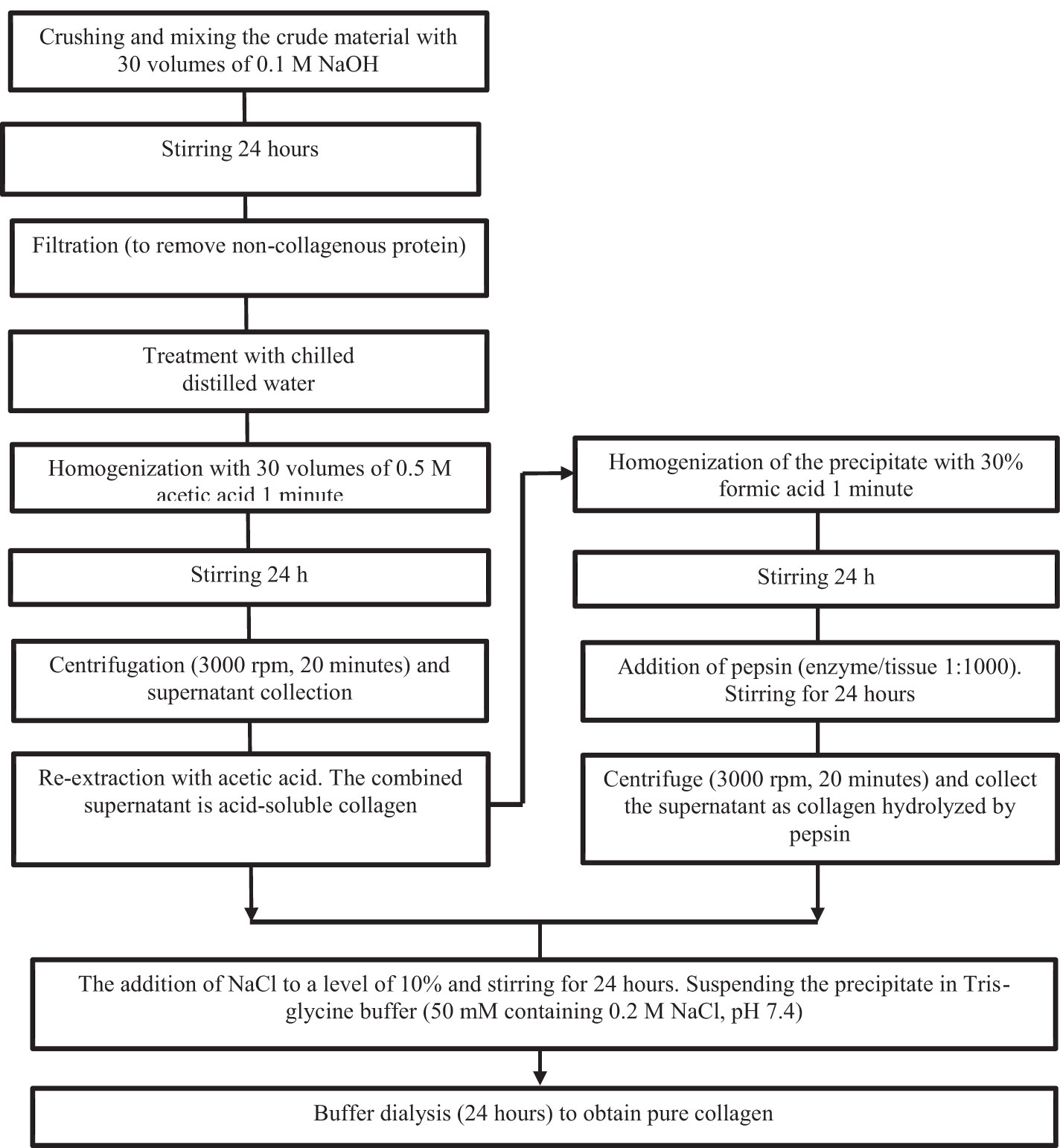
Figure 1 Flowchart of collagen extraction from marine sources [Venkatesan, J. & Anil, Sukumaran & Kim, Se-Kwon & Shim, Min. (2017). Marine Fish Proteins and Peptides for Cosmeceuticals: A Review. Marine Drugs. 15. 143. 10.3390/md15050143.].
Marine sources of collagen
To date, collagen has been found in the varied range of marine organisms. Marine sources of collagen are presented in Table 1. Figure 2 presents technical function flowsheet for producing soluble collagen. A significant number of commercial and aquaculture fish species, as well as non-fish species, are used as a source of marine collagen. Among them the following can be noted (Lin et al., 2019; Ahmed et al., 2020a; Coppola et al., 2020a; Han et al., 2021):
- Ray-finned fishes: Priacanthidae Günther, Sciaenidae Cuvier, Latidae Jordan, Lutjanidae Gill, Nemipteridae Regan, Sparidae Rafinesque, Acropomatiformes, Aulopiformes, Gonorynchiformes, Tetraodontiformes, Syngnathiformes, Pleuronectiformes, Cypriniformes, Osmeriformes, Salmoniformes, Perciformes, Acipenseriformes, Clupeiformes, Scombriformes, Siluriformes, Carangiformes, Gadiformes, Anguilliformes, Cichliformes, Esociformes;
- Chondrichthyes: Orectolobiformes, Carcharhiniformes, Heterodontiformes, Rajiformes;
- Mammals: representatives of the Cetartiodactyla order;
- Reptiles: representatives of the alligator family (Alligatoridae Gray);
- Cephalopods: Oegopsida, Octopoda, Sepiida;
- Bivalvia: representatives of the Pectinida order;
- Starfishes: representatives of the Comatulida order.
Physicochemical properties of marine collagen
Different methods for collagen extraction from marine organisms result in varying yields and physiochemical properties of the extracted collagens. Two common methods are acid soluble collagen (ASC) extraction and pepsin-solubilized (PSC) extraction (Mizuta et al., 1994; Liu et al., 2010; Barzideh et al., 2014; Attaran Fariman et al., 2016; Blanco et al., 2017; Li et al., 2020; Diogo et al., 2021; Chen et al., 2022). The acid-collagen reaction utilized in ASC extraction increases collagen extraction efficiency by breaking crosslinks in the collagen helix and increasing repulsion among tropocollagen molecules (Niu et al., 2016). PSC, also known as atelo-collagen, shows increased purity and reduced antigenicity compared to ASC due to pepsin treatment that removes telopeptide regions and related non-collagenous proteins (Kim et al., 2013). Enzymatic treatment using pepsin in combination with the acids has been shown by researchers to improve the yield of extracted collagen in multiple studies (Kim et al., 2013; Niu et al., 2016; Hadfi and Sarbon, 2019).
Marine collagen hydrolyzed with industrial potency
Peptides derived from collagen hydrolysates possess not only nutritional properties, but also have biological activities and regulatory roles that can alleviate symptoms related to chronic diseases and promote good health. Clinical studies and drug development have shown various peptides with hyperlipidemic, immuno-modulatory, chelating/absorbing metals, and anti-osteoporotic activity (Hadfi and Sarbon, 2019). Moreover, short peptides (<5 kDa) from hydrolyzed collagen of the squid Dosidicus gigas exhibit antioxidant and anti-inflammatory properties (Ogawa et al., 2003). Collagen also increases fibroblast proliferation and hyaluronic acid synthesis while detectable in human blood at molar concentrations after consuming collagen hydrolysate. These are some of the positive effects of collagen on human health (Wahyu and Widjanarko, 2018). Table 2 lists the applications for animal and marine collagen in Europe.
Hydrolyzed collagen has various biological activities that are useful in nutrition, food, industry, and medicine (Mizuta et al., 1994; Morishige et al., 2011; Ahmed et al., 2020a). Additionally, it is believed that hydrolysate can have a positive impact on treating osteoporosis, diabetes mellitus, gastric ulceration, skin hydration, hypertension and preservatives (Lima et al., 2011a; Santos et al., 2013; Barzkar and Sohail, 2020). Collagen is becoming an increasingly popular ingredient in drugs, food, drinks, cosmetics, tissue engineering and health care products due to its wide range of industrial applications (Liu et al., 2010; Bilek and Bayram, 2015; Hadfi and Sarbon, 2019; Carvalho et al., 2020a). The reason for the extensive use of collagen in medical and pharmacological industries is due to its exceptional biological properties like hemostatic activity, biodegradability and low antigenicity (Ogawa et al., 2003).
The purpose of the 196th study was to examine the significance of collagen-derived peptide secondary and tertiary structure after removal of fatty acids. The hydrolyzate efficiency of fish collagen reached 70%, with peptides (8.2-9.7 kDa) produced in the form of polyloline 2 (PP-II) at a concentration of at least 1 mg/mL and pH levels between 7-8. Moreover, the antioxidant activity of CF-CH increased as the ionic stability of aggregates increased, and protein isolation led to a decrease in antioxidant activity from 84.5% to 98.9%. After six months, soybean oil shelf life was extended by five times. Collagen’s unique charge distribution, protonation of amino acid residues, and affinity through the fiber optic network contribute to its high potency. Fish are high in fat energy-wise (Porfírio and Fanaro, 2016).
It was observed that copper had a higher chelating activity in intact collagen hydrolysis (Sinthusamran et al., 2013). The collagen hydrolyzate extracted from the gastric phase showed moderate ACE inhibitory activity with an IC50 value of 2.92 ± 0.22 mg/mL, which significantly increased to 0.49 ± 0.02 mg/mg after intestinal digestion (Lima et al., 2011b; Lima et al., 2015). Upon SGID, the inhibitory activity of collagen hydrolyzate against DPP IV was higher than that of collagen hydrolyzate trypsin (IC50 2.59 ± 0.04 mg/mL). The antioxidant activity of collagen and CTH after SGID was measured to be 0.87 ± 0.10 and 1.27 ± 0.03 μmol TE g–1, respectively (Lin et al., 2011; Pozzolini et al., 2018a; Zheng and Zheng, 2019). Out of the four skin conditions linked to inflammation or oxidative stress, dermatitis is the most common. Research has demonstrated that fish collagen peptide (FSCP) safeguards human keratinocyte-derived HaCaT cells against CoCl2-induced cytotoxicity and TNF-α-induced inflammatory responses. In HaCaT cells, key inflammatory cytokines, such as TNF-α, IL-1β, IL-8, and iNOS, lessen cellular oxidative damage. As a result of FSCP gene expression, caspase activity and cytochrome C release inhibit and reduce apoptosis. When exposed to CoCl2 or TNF-α, HaCaT cells increase Bcl-2 protein levels and ROS, MAPK (p38/MAPK, ERK and JNK). This study has revealed how marine collagen peptides (MCP) protect carotenoid endothelial cells (CAVEC) in type 2 diabetes mellitus (DM2), as well as the mechanisms behind this process. For an in vivo experiment involving diabetic patients, four groups were created randomly: a diabetic control group and three diabetic groups treated with MCP (2.25 g/kg bw/day, 4.5 g/kg bw/day or 9.0 g/kg bw/day). To serve as controls, 10 healthy mice were used. Human umbilical vein endothelial cells (HUVEC) were subjected to normal and high glucose levels as well as MCP (3.0, 15.0 and 3.0 mg/mL, respectively) for 24, 48, or 72 hours in vitro experiments. With the use of CAVEC, vascular/endocrine patterns, inflammation and related molecular biomarkers were detected and analyzed. The results of the study showed that MCP treatment was able to reduce blood glucose levels in rat coronary artery cells for four weeks and decreased endothelial fibrosis and inflammation (Langasco et al., 2017; Parisi et al., 2020). In vitro experiments showed that high glucose exposure caused a significant increase in cellular apoptosis in HUVEC while medium to high doses of MCP (4.5 and 9.0 g/kg bw/day respectively) inhibited this high glucose-mediated apoptosis (Ehrlich, 2010). Finally, moderate oral doses of MCP (0.5-4.5 g/kg bw/day) inhibited apoptosis, decreased binding factor and microbial expression, and reduced the early stages of type 2 diabetes which is a new treatment option to prevent cardiovascular complications (Ehrlich et al., 2006; Ehrlich et al., 2011).
A study was conducted to explore the therapeutic benefits of marine collagen peptides (MCP) from fish hydrolysates on Chinese patients diagnosed with type 2 diabetes mellitus (DM2). The study involved 100 diabetic patients and 50 healthy individuals. The diabetic patients were randomly assigned to either a treatment group or a control group. For three months, the treatment group received 13g of MCP every day. Blood samples were collected from all participants before treatment, at 1.5 months, and at 3 months after treatment to assess glucose and lipid metabolism (Heinemann et al., 2007). The researchers also measured serum levels of highly sensitive C-reactive protein (hs-CRP), nitric oxide (NO), bradykinin, prostacyclin (PGI2), and lipids. Results showed that fasting blood glucose, human glycated hemoglobin A1c (GHbA1c), fasting insulin, triglycerides, total cholesterol, low-density lipoprotein, and free fatty acids were all significantly lower in type 2 diabetes patients who received MCP treatment compared to those in the control group. Additionally, insulin sensitivity index and HDL levels improved. Interestingly, hs-CRP and NO levels decreased significantly while bradykinin, PGI2, and adiponectin levels were increased in MCP-treated T2DM patients compared with baseline or control levels (p < 0.1). MCP treatment improves glucose and lipid metabolism in diabetic patients (Lahoud, 2010).
In order to monitor the neurodegenerative effects of marine collagen peptides (MCPs) obtained from chum salmon skin through enzymatic hydrolysis, 20-month-old C57BL/6J mice were given 0.22%, 0.44%, or 1.32% (1.2% w/w) MCP for a period of three months (Pei et al., 2010). The researchers then used a step test and the Morris water maze to evaluate negative avoidance, spatial memory, and learning abilities in comparison to an older adult control group. Interestingly, there were no discernible differences between the MCP-treated group and the same-age control group in terms of learning and memory scores, even at doses as high as 0.44% and 1.32%. However, the MCP-treated group did exhibit alleviation of oxidative stress, reduced autophagy, and increased levels of brain-derived neurotrophic factor (BDNF) and post-operative density protein 95 (PSD95) when compared to the elderly control group (Xue et al., 2022). Despite these positive outcomes, there was still no significant difference between the MCP group and the same-age control group overall. Nonetheless, these findings suggest that MCP could be a potential functional food candidate for improving aging-related memory loss (Zhao et al., 2020).
In order to investigate how marine collagen peptides (MCP) derived from salmon skin (Oncorhynchus keta) impact lifespan and spontaneous carcinogenesis, a group of Sprague-Dawley rats were given varying concentrations of MCP mixed with their feed. There were 40 mice in each group, maintaining an equal male to female ratio. The study found that MCP had no significant effect on the body weight or food intake of male and female rats over the course of their lives (Burkel et al., 2016; Rajabimashhadi et al., 2023). However, it did inhibit the age-related decrease in antioxidant enzyme activity and lipid peroxidation in both sexes, resulting in an increase in maximum survival time. Interestingly, the incidence of spontaneous tumors decreased by 4.5% in males and 9% in females who were treated with MCP. Additionally, tumor mortality was significantly reduced compared to the control group for both males and females who received MCP treatment. As a result, it was concluded that MCP has a dose-dependent effect on increasing lifespan and reducing spontaneous tumorigenesis in Sprague-Dawley rats. The antioxidant properties of MCP may also play a role in prolonging life and preventing tumorigenesis (Burkel et al., 2016; Zhao et al., 2020; Xue et al., 2022).
A new approach to the use of marine collagen: collagen hydrogels
Collagen hydrogels are prepared from collagen solutions by polymerization at room temperature for 10-15 minutes. Gels should become opaque after polymerization. After they become opaque, the cup is moved to 37°C for another 45-60 minutes to complete polymerization. After 45-60 min, 2-3 mL of medium is added and gels are released from the walls of the cup by running the tip of a p200 pipette around the perimeter of the cup. The mixture is gently shaken to release the gel. The collagen gel should float in the medium (Govindharaj et al., 2019). Collagen hydrogels are a type of three-dimensional network that can absorb and hold large amounts of water (Di Lullo et al., 2002). This structure has many advantages, including biocompatibility, fluidity, and the ability to accommodate various biotherapeutic agents. These hydrogels are suitable for cell cultures, tissue engineering, drug delivery, and softgels. Marine polymers have emerged as an excellent natural alternative for creating new biomedical materials over the past decade (Jahromi and Barzkar 2018 a, b; Barzkar et al., 2019; Barzkar, 2020; Barzkar et al., 2021 a, b; Barzkar et al., 2022 a, b; Sankarapandian et al., 2022; Barzkar et al., 2023; Sankarapandian et al., 2023). Many collagen biopolymers can be obtained from marine by-products like fish skin or untapped resources like jellyfish, which can add value to biomaterials as part of circular economics strategies. Additionally, using marine resources like collagen can help reduce the risk of infection and boost immunity (Govindharaj et al., 2019; Venmathi Maran et al., 2023).
The physical characteristics of collagen hydrogels are directly influenced by certain factors such as porosity, molecular weight, density, and cross-linking between side chains. It is important to consider these factors when using collagen for therapeutic purposes (Im et al., 2017; Diogo et al., 2020). To gain a better understanding of the relationship between biopolymer structure and mechanical properties, research is needed on natural ionic ring polymer hydrogel formulations (Im et al., 2017).
When predicting the impact of collagen biopolymers on scaffold mechanical properties, it’s important to consider factors such as molecular weight and solution viscosity (Sousa et al., 2020). The collagen biopolymer, which has a high molecular weight of approximately 260 kDa, differs from other polymers like chitosan and fucoidan due to the extraction process. During this process, smaller molecules are removed while higher molecular weight ones are retained (Gao et al., 2023). Factors such as source, extraction method, life cycle, environment, and collection location can all affect the chemical composition and molecular weight of collagen. Additionally, there is growing interest in the potential health benefits of collagen due to its antioxidant, anti-inflammatory, antiviral, wound-healing properties and more (Liang et al., 2010).
In a study conducted in (Liang et al., 2011), the relationship between the texture/composition and rheological characteristics of hydrogels made from collagen, chitosan, and fucoidan was investigated. Hydrogels containing various mixtures of these three biopolymers generally exhibited better mechanical properties than others. When comparing hydrogels made from two marine polymers, those with a higher polymer concentration had better mechanical properties (Liang et al., 2011). The presence of chitosan did not significantly affect the mechanical properties of these hydrogels, which is consistent with previous studies on two-component hydrogels. The study confirmed that these hydrogels have a well-structured microenvironment that can support cell growth and stimulate cell migration because the structural pores are larger than the size of cells (Wang et al., 2015). These marine-based hydrogel systems have potential applications in tissue engineering and regenerative medicine, particularly in treating articular cartilage (Liang et al., 2014). Additionally, using marine collagen in these hydrogel structures is considered a “green” technology that can be scaled up without negative environmental impacts (Hema et al., 2017). The rheological properties and relative density of terrestrial and marine collagen-glycosaminoglycan scaffolds were determined in a study (Hema et al., 2017). Data from Harley et al. is presented in Table 3. Collagen’s original purpose was to provide stability and strength to body tissues by forming a support network for cellular structures. This function has led to many applications, including cosmetics, due to its unique properties such as biocompatibility, biodegradation, biomimicry, and hemostasis (Das et al., 2021). Collagen can also form cross-linked matrices when dissolved, similar to gelatin (Tran et al., 2020). The use of fibrillar collagen in cosmetics is based on its functional purpose, while types I-III and V are used for their largest functional component and market dominance (Tran et al., 2020). The main collagen functions are summarized in Table 4.
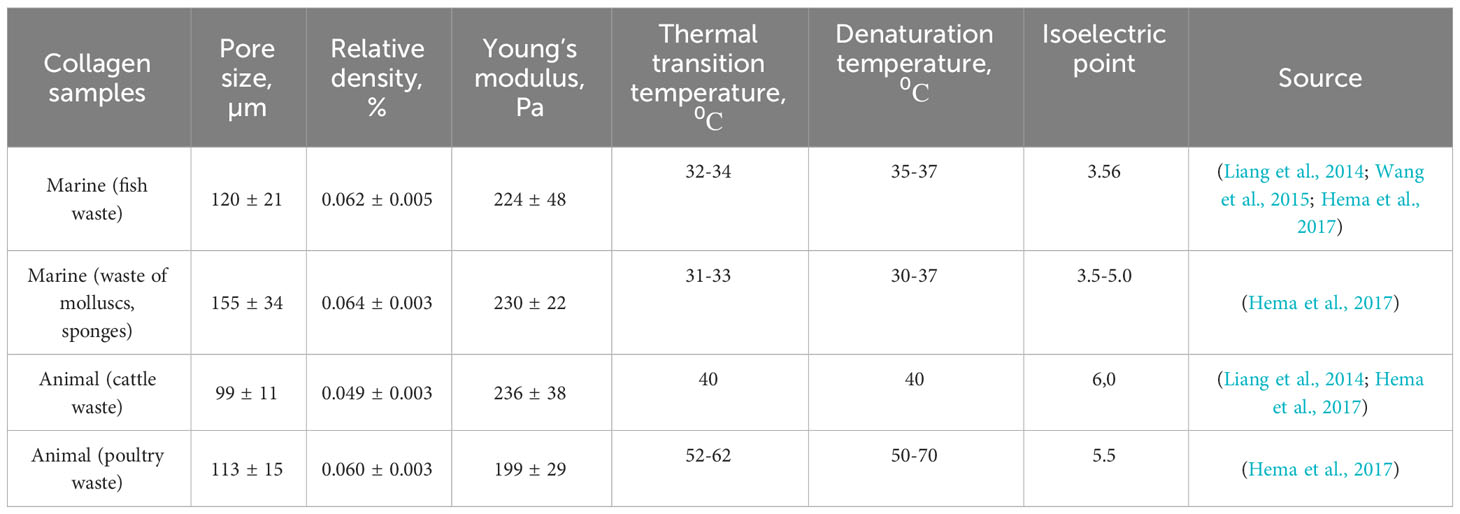
Table 3 Rheological properties (Young’s modulus) and relative density of collagen-glycosaminoglycan scaffolds.
The extracellular matrix (ECM) is crucial for maintaining cell integrity and aiding in cell functions like proliferation, differentiation, migration, and adhesion (Fischer et al., 2019; Carvalho et al., 2021). Marine organisms, such as fish, jellyfish, sponges, and other invertebrates, offer a valuable source of collagen that is free from religious restrictions and animal pathogens. This type of collagen is metabolically compatible and has clear advantages over other sources (Oryan et al., 2018). Fish skin is a popular choice for extracting type I collagen because it’s abundant and not suitable for industrial use. Overall, marine sources of collagen are a safe, convenient, and promising option. The combination of biomaterials and single gene delivery has shown promising potential for tissue engineering. Skin lesions can be slow to heal and may not heal completely, but studies have found that marine collagen from organisms like fish, jellyfish, and sponges can promote wound healing, enhance blood circulation, and prevent infection (Coppola et al., 2020b; Wang, 2021). In addition to these benefits, marine collagen has anti-aging properties that have been demonstrated in mice with osteoporosis (Wang, 2021). It can increase bone mineral density, protect against bone loss and osteoarthritis, induce plastic differentiation, and even improve skin elasticity while slowing the aging process (Wang, 2021). Finally, marine collagen is also used for immobilization and drug delivery within the human body (Mantha et al., 2019).
Marine collagen hydroxylates, have been extracted and refined from the Chondrosia reniformis (Pozzolini et al., 2018b). In vitro tests were conducted using collagen peptide fragments at a concentration of 50 mg/mL, with cell examination at varying time intervals. The treated cells displayed signs of fibroblast and keratinocyte migration and proliferation, as well as increased wound adhesion between the skin and infected cells compared to the control group. These results demonstrate that marine collagen hydroxylates isolated from C. reniformis possess promising wound healing abilities. Similarly, hydrolyzed peptide collagen isolated from the jellyfish Rhopilema esculentum has also shown wound healing activity in both in vitro and in vivo experiments. In one study, collagen peptides were seen to increase cell migration and wound closure in a dose-dependent manner using the scratch-healing method. Additionally, a separate research on injured mice showed that collagen peptides partially promoted wound healing by stimulating chemokines such as β-FGF and TGF-β1, which protect wounds from infection by attracting inflammatory cells that also control the migration of fibroblasts and keratinocytes. Hence, promoting wound healing (Pozzolini et al., 2018b).
The anti-aging industry widely utilizes collagen for skin regeneration, as the aging process negatively impacts the aesthetic aspects of the skin structure. Collagen and elastane fibers are responsible for maintaining skin elasticity, mechanical strength, and general structure (García-Quintero and Palencia, 2021). Marine collagen is known for its antioxidant properties as it can protect skin cells from harmful free radicals and oxidants that damage cell membranes, DNA, and macromolecules, which contribute to skin aging (Carvalho et al., 2020b). To prevent oxidative stress, antioxidant enzymes such as superoxide dismutase and glutathione peroxidase play a crucial role by inhibiting free radicals and other dangerous oxygen species. Acid-soluble collagen isolated by Chi et al (Balitaan et al., 2020). demonstrated the antioxidant activity of three collagen peptides and also showed its protective effect against other radicals. Additionally, collagen peptides (ACH-P1, P2, P3) were studied for their effects on oxidation and the formation of oxidative particles. The results showed that lipid peroxidation was significantly reduced compared to controls due to decreased uptake, which is a measure of oxidation. Collagen peptides exhibit similar effects as effective antioxidants in preventing oxidative damage (Balitaan et al., 2020).
Marine collagen biopharmaceuticals have the potential to promote cartilage regeneration, in addition to improving skin and bone health. Osteoarthritis is a condition that lacks regenerative ability and is characterized by joint pain and stiffness due to cartilage degeneration. The exposure of subchondral bone further lowers the quality of life for those with OA (Harley et al., 2008; Sinthusamran et al., 2013). However, studies have shown that marine collagen can induce chondrogenic differentiation and potentially facilitate cartilage regeneration (Sionkowska et al., 2020). Researchers like Raabe et al. have found that hydrolyzed fish collagen and growth factor TGFB1 can stimulate protein and collagen fiber synthesis, while fish collagen has been shown to induce chondrogenic differentiation (Gómez-Ordóñez and Rupérez, 2011).
Bourdon et al. conducted a study on fish skin and cartilage chondrocytes to analyze the impact of three collagen hydrolysates (Wang, 2021). A particular experiment revealed that concentrations of 0.5, 50, and 100 µg/mL of collagen hydrolyzate increased collagen I and collagen II levels. Additionally, the use of collagen resulted in decreased expression of protein markers such as Htra1, Mmp103, Adamts5, and Cox2. These markers are known to be associated with OA development (Rahman, 2019). In another study by Ohnishi et al., rabbits injected with a combination of fish collagen peptides and glucosamine showed protection against cartilage damage while the control group developed OA (Townsend and Gannon, 2019). Although glucosamine and fish collagen peptides have some individual protective effects against OA, their combined effect provides the greatest protection (Chen et al., 2019; Townsend and Gannon, 2019; Geahchan et al., 2022). Collagen has also been found to have bifunctional properties (Allouche et al., 2020). The bifunctional properties of marine collagen are summarized in Table 5.
Collagen in functional foods
It is essential to improve the existing technology of collagen materials with different functions, the role of collagen in nutrition, create original products, engage in unconventional development, and maximize the conversion of collagen-rich resources into functional products. A significant share of collagen proteins is contained in subproducts of the I and II categories; the latter have long been considered of low value and were used in food production to a limited extent. Collagen is of particular interest and potential in strengthening the meat industry’s raw material base, providing animal protein, developing waste-free environmentally friendly technologies, increasing biological value, aesthetic appearance of products, reducing losses, and maximizing and rational use of meat raw materials (Bourdon et al., 2021).
At the moment, collagen-containing meat products with a variety of technical and physiological properties that stimulate the digestive process, absorb toxins and radioactive substances, and provide high performance, technical, and rheological properties of food products are being produced (Bourdon et al., 2021). The ability of collagen to bind many toxins is relevant in the field of deep processing of collagen raw materials with separation of target components, and theoretically justified and effective technical solutions are still insufficient. The most important challenge facing the food industry is to provide not only affordable food products to all segments, but also to maximize the functionality of these products. In this sense, functional foods should absorb and/or inhibit negative environmental factors affecting the human body by binding and releasing them. One of the most aggressive negative factors is heavy metal ions and radionuclides (Ferrario et al., 2020).
In this regard, an essential condition for increasing the functionality of food products is the inclusion of active components that are biologically neutral in relation to intra- and intercellular biochemical processes of the human body while also possessing a pronounced ability to at least sorb, and at most bind in low or non-dissociating complexes of polyvalent metal ions and radionuclides, demonstrating selectivity of action. To ensure the immune and physiological state of the organism in all spheres of life activity, including unfavorable and chronic conditions, it is necessary to create special, preventive, therapeutic, and generally strengthening nutrition, ingredients, biopreparations, and other products (Zhuang et al., 2009).
When assessing the use of food additives and ingredients based on modified collagen in meat product technology, dietary fibers and their connective tissue are also the most logical way to enrich food products, and the expansion of sources for their selective isolation in the form of isolated preparations of a given functionality for further use in the production of enriched products should be recognized (Ferrario et al., 2020).
Collagen for skin regeneration
Tissue engineering and regenerative medicine is a rapidly growing interdisciplinary field that integrates materials science, biotechnology, medicine, cell biology, pharmacology, and chemistry to repair damaged tissues and organs (Aziz et al., 2016; Geahchan et al., 2022). The widely accepted concept of tissue engineering and regenerative medicine includes three major components: the selection of suitable stem cells, the identification of signaling pathways for repair or regeneration of specific tissues or organs (Chi et al., 2015). Biomass is a key component for the production of scaffolds. The use of appropriate biopreparations improves adhesion, migration, proliferation, and differentiation of stem cells, increasing their ability to repair and regenerate damaged tissues and organs (Caruso et al., 2020).
Among all natural polymers, collagen is one of the most studied and widely used in clinical practice. Major biomedical applications involving collagen include biomaterials development, tissue engineering, absorbable surgical threads, hematopoiesis, and burn/wound treatment. To address societal ethical and nutritional concerns and the risk of spreading animal diseases such as bovine spongiform encephalopathy and foot-and-mouth disease, the scientific community is exploring the potential of using marine collagen as a substitute for mammalian collagen (Gauza-Włodarczyk et al., 2017). Another important argument in favor of using marine collagen is that collagen accounts for approximately 75% of the total weight of fish, indicating the abundance of this type of collagen (Raabe et al., 2010). During processing, approximately three-quarters of the fish, including skin, fins, bone system, and head, are discarded (Ito et al., 2018). Seafood is also an important source of valuable organic and inorganic materials used in various industries such as nutrition, cosmetics, regenerative medicine, and pharmaceuticals. Marine collagen applications include but are not limited to tissue engineering, wound dressing, cosmetics, and drug delivery (Ohnishi et al., 2013). Numerous studies have shown that hydrolyzed fish collagen exhibits biological activities such as regenerative, antioxidant, immunomodulatory, antibacterial, anti-inflammatory, and angiotensin-converting enzyme inhibitor.
Collagen in cosmetics
Collagen has a wide range of applications. It is widely used in cosmetic, pharmaceutical, medical, and food industries because of its high biocompatibility, non-toxicity, and biodegradability (Hu et al., 2021). Collagen is a key component of many cosmetic formulations because of its moisturizing properties. Because the cosmetic industry is always looking for new and effective products, the source of collagen is an important research question (Dhatchayani et al., 2020). The potential of marine collagen was discovered about 70 years ago during the study of marine sponges (Gómez-Guillén et al., 2011). The research contributed to the study of structural and physicochemical features of collagen of marine origin.
Skin is a tissue composed mainly of type I, III, and V collagens. The predominant type of collagen in the skin is type I (Tang et al., 2022). Studies have shown that this type of collagen is identical to marine collagen (Ito et al., 2018). Thus, marine collagen is the most popular source of collagen in the cosmetic industry. Studies on collagen from tilapia skin have shown that it has typical properties of type I collagen, along with acid-soluble collagen (ASC) and pepsin-soluble collagen (PSC). ASC has a dissociation temperature of 36.1°C and PSC has a dissociation temperature of 34.4°C (Tang et al., 2023). Acid-soluble collagen isolated from the skin of the fathead minnow (Hypohalftalmichthys molitrix) contained type I collagen as shown by SDS-PAGE (Mukherjee et al., 2023). Column chromatography confirmed three chains: α1, α2 and α3. The amount of collagen in Atlantic salmon (Salmo salar L.) corresponds to types I and V (Caddeo et al., 2017). Circulating dichroism (CD) raised the circulation temperature of salmon collagen to 27°C. A study of collagen content in cod also confirmed the presence of type I and type V collagens (Cossu et al., 2018). Experiments on adult bigeye snapper (Priacanthus tayenus) tissues (skin and bone) revealed two different types of α-chains, α1 and α2 in the form of type I collagen. The electrophoretic spectra of bigeye snapper skin and bone are very similar (La Noce et al., 2014). Both ASC and PSC were derived from hybridized fungi and confirmed by SDS-PAGE and FTIR. The dissociation temperatures of ASC measured by circular dichroism (CD) and differential scanning calorimetry (DSC) were 26.8°C and 26.5°C, respectively. Natural immature collagen is in great demand for cosmetic and biomedical purposes. However, since the temperature of fish collagen is low, there is a limitation to emulsification by heating water and oil. Hydrolyzed collagen is used in many cosmetic products because it can be used as an emulsion with a high emulsification temperature while maintaining the moisturizing properties of collagen (Das et al., 2021).
Conclusion
Collagen is a type of protein that makes up 30% of the body’s total protein and can be found in various tissues like bones, teeth, skin, blood vessels, intestines, and cartilage. A recent study reviewed the current knowledge about collagen isolated from marine organisms and discussed its potential applications. Due to its reliability, low antigenicity, and biodegradability, collagen is commonly used in various industries such as pharmaceuticals, food, biopharmaceuticals, and cosmetics. The article also provides an overview of the physico-chemical properties of marine collagen and the best methods for extracting it from marine organisms. These methods have been found to have positive effects on health and relieve symptoms caused by chronic diseases. If aquaculture can find a solution to the availability of collagen, waste disposal can be integrated into the processing of raw materials to solve production waste problems. One potential direction for advancing marine collagen technologies is manufacturing certified skincare products.
There are many biologically active substances found in marine organisms that can be utilized in the pharmaceutical and cosmetic industries. Research is currently advancing into the numerous applications of collagen derived from these organisms. The main source of marine collagen extractions are fish (skin, bones, scales, swim bladders, and cartilages), mollusks (mesogloea, muscle organ), marine invertebrates: jellyfish (dome, muscle tissue), sea cucumber (body walls), sea urchin (hard cover, connective tissue), polyps, octopus, and squid (muscle, tentacles, skin).
Marine collagen is a biomaterial that is water-soluble, metabolized, and easily obtainable. A literature review has shown that marine collagen is a versatile substance that can aid in treating skin lesions of varying severity and delay the aging process. Collagen has proven to stimulate the migration of keratinocytes and fibromuscular tissue, as well as cutaneous angiogenesis in both cases. Studies have also demonstrated that marine collagen and its derivatives are useful in preventing and treating osteoporosis and osteoarthritis, as well as other bone diseases. This is attributed to the fact that collagen promotes bone mineral density, mineral deposition, and inhibits the development and spread of osteoporosis. The advantages of marine collagen over terrestrial sources were discussed along with its potential biotherapeutic properties for skin and bone injuries. In keeping with the growing trend of replacing synthetic agents with more natural ones, collagen has found new applications as emulsifiers, foaming agents, colloidal stabilizers, hydrogels, clarifiers, biodegradable packaging materials, microencapsulating agents, and bioactive peptides. Furthermore, using fish processing waste to produce marine collagen for use in cosmetics has an environmental benefit by reducing their environmental impact as they are one of the food industry’s strategic environmental components.
Furthermore, the functional properties of marine collagen hydrolysates are focused on the production of bioactive peptides with a number of biological activities (antioxidant, antibacterial, and chondroprotective). On the other hand, the great development of modern analytical methods allows for a deeper characterization of marine collagen properties, and its application for species identification may be of particular interest.
Author contributions
Conceptualization, writing and original draft preparation: NB. Original draft preparation: SS, OB. Editing: BAVM, STJ. All authors have read and agreed to the published version of the manuscript.
Conflict of interest
The authors declare that the research was conducted in the absence of any commercial or financial relationships that could be construed as a potential conflict of interest.
Publisher’s note
All claims expressed in this article are solely those of the authors and do not necessarily represent those of their affiliated organizations, or those of the publisher, the editors and the reviewers. Any product that may be evaluated in this article, or claim that may be made by its manufacturer, is not guaranteed or endorsed by the publisher.
References
Abedin M. Z., Karim A. A., Latiff A. A., Gan C. Y., Ghazali F. C., Barzideh Z., et al. (2014). Biochemical and radical-scavenging properties of sea cucumber (Stichopus vastus) collagen hydrolysates. Natural Product Res. 28 (16), 1302–1305. doi: 10.1080/14786419.2014.900617
Addad S., Exposito J. Y., Faye C., Ricard-Blum S., Lethias C. (2011). Isolation, characterization and biological evaluation of jellyfish collagen for use in biomedical applications. Mar. Drugs 9, 967–983. doi: 10.3390/md9060967
Adibzadeh N., Aminzadeh S., Jamili S., Karkhane A. A., Farrokhi N. (2014). Purification and characterization of pepsin-solubilized collagen from skin of sea cucumber Holothuria parva. Appl. Biochem. Biotechnol. 173 (1), 143–154. doi: 10.1007/s12010-014-0823-4
Ahmed R., Getachew A. T., Cho Y., Chun B. (2018). Application of bacterial collagenolytic proteases for the extraction of type I collagen from the skin of bigeye tuna (Thunnus obesus). LWT 89, 44–51. doi: 10.1016/j.lwt.2017.10.024
Ahmed M., Verma A. K., Patel R. (2020a). Collagen extraction and recent activities of collagen peptides derived from seafood waste: a review. Sustain. Chem. Pharm. 18, 13. doi: 10.1016/j.scp.2020.100315
Ahmed Z., Powell L. C., Matin N., Mearns-Spragg A., Thornton C. A., Khan I. M., et al. (2021). Jellyfish collagen: A biocompatible collagen source for 3D scaffold fabrication and enhanced chondrogenicity. Mar. Drugs 19, 1–19. doi: 10.3390/md19080405
Ahmed R., Haq M., Chun B.-S. (2019). Characterization of marine derived collagen extracted from the by-products of bigeye tuna (Thunnus obesus). Int. J. Biol. Macromolecules 135, 668–676. doi: 10.1016/j.ijbiomac.2019.05.213
Ahmed M., Verma A. K., Patel R. (2020b). Collagen extraction and recent biological activities of collagen peptides derived from sea-food waste: A review. Sustain. Chem. Pharm. 18, 100315. doi: 10.1016/j.scp.2020.100315
Allouche M., et al. (2020). Laboratory bioassay exploring the effects of anti-aging skincare products on free-living marine nematodes: a case study of collagen. Environ. Sci. pollut. Res. 27, 11403–11412. doi: 10.1007/s11356-020-07655-1
Almuqoddas E., Hambyah I., Rizqiyanti R., Subagio A., Ketut Umiati N. A. (2019). “Characterisation of collagen from the skin of catfish (Pangasius sp) for innovative PVA-collagen nanofiber,” in E3S Web of Conferences, EDP Sciences.
Andersen C. M., Wold J. P. (2003). Fluorescence of muscle and connective tissue from cod and salmon. J. Agric. Food Chem. 51 (2), 470–476. doi: 10.1021/jf020524d
Arbex P. M., Sezgin Arslan T., Derkus B., Emregul E., Emregul K. C. (2018). Extruded sorghum flour (Sorghum bicolor L.) modulate adiposity and inflammation in high fat diet-induced obese rats. J. Funct. foods 42, 346–355. doi: 10.1016/j.jff.2018.01.010
Arslan Y. E., Sezgin Arslan T., Derkus B., Emregul E., Emregul K. C. (2017). Fabrication of human hair keratin/jellyfish collagen/eggshell-derived hydroxyapatite osteoinductive biocomposite scaffolds for bone tissue engineering: From waste to regenerative medicine products. Colloids Surfaces B: Biointerfaces 154, 160–170. doi: 10.1016/j.colsurfb.2017.03.034
Arumugam G. K. S., Sharma D., Balakrishnan R. M., Ettiyappan J. B. P. (2018). Extraction, optimization and characterization of collagen from sole fish skin. Sustain. Chem. Pharm. 9, 19–26. doi: 10.1016/j.scp.2018.04.003
Aruta C. G., Croce M. A., Quaglino D., Guerra D., Tiozzo R., et al. (2009). Biocompatibility of collagen membranes assessed by culturing human J111 macrophage cells. Materials 2 (3), 945–957. doi: 10.3390/ma2030945
Asaduzzaman A., Getachew A. T., Cho Y.-J., Park J.-S., Haq M., Chun B.-S., et al. (2020). Characterization of pepsin-solubilised collagen recovered from mackerel (Scomber japonicus) bone and skin using subcritical water hydrolysis. Int. J. Biol. macromolecules 148, 1290–1297. doi: 10.1016/j.ijbiomac.2019.10.104
Attaran Fariman G., Taheri A., Barzkar N. (2016). Sea cucumber (Stichopus horrens) body wall collagen of Chabahar bay and its gelatin properties. J. Food Sci. Technol. (2008-8787) 13 (52), 81–91. Available at: http://fsct.modares.ac.ir/article-7-7999-en.html
Aziz J., Shezali H., Radzi Z., Yahya N. A., Kassim N. H.A., Czernuszka J., et al. (2016). Molecular mechanisms of stress-responsive changes in collagen and elastin networks in skin. Skin Pharmacol. Physiol. 29 (4), 190–203. doi: 10.1159/000447017
Bairati A., De Biasi S., Cheli F., Oggioni A. (1987). The head cartilage of cephalopods. I. Architecture and ultrastructure of the extracellular matrix. Tissue Cell 19 (5), 673–685. doi: 10.1016/0040-8166(87)90074-7
Bal Z., Korkusuz F., Ishiguro H., Okada R., Kushioka J., Chijimatsu R., et al. (2021). A novel nano-hydroxyapatite/synthetic polymer/bone morphogenetic protein-2 composite for efficient bone regeneration. Spine J. 21 (5), 865–873. doi: 10.1016/j.spinee.2021.01.019
Balitaan J. N. I., Yeh J.-M., Santiago K. S. (2020). Marine waste to a functional biomaterial: Green facile synthesis of modified-β-chitin from Uroteuthis duvauceli pens (gladius). Int. J. Biol. macromolecules 154, 1565–1575. doi: 10.1016/j.ijbiomac.2019.11.041
Barzideh Z., Latiff A. A., Gan C. Y., Benjakul S., Karim A. A. (2014). Isolation and characterisation of collagen from the ribbon jellyfish (C hrysaora sp.). Int. J. Food Sci. Technol. 49 (6), 1490–1499. doi: 10.1111/ijfs.12464
Barzkar N. (2020). Marine microbial alkaline protease: an efficient and essential tool for various industrial applications. Int. J. Biol. Macromolecules. 161, 1216–1229. doi: 10.1016/j.ijbiomac.2020.06.072
Barzkar N., Homaei A., Hemmati R., Patel S. (2018). Thermostable marine microbial proteases for industrial applications: scopes and risks. Extremophiles 22, 335–346. doi: 10.1007/s00792-018-1009-8
Barzkar N., Jahromi S. T., Poorsaheli H. B., Vianello F. (2019). Metabolites from marine microorganisms, micro, and macroalgae: immense scope for pharmacology. Mar. Drugs 17, 464. doi: 10.3390/md17080464
Barzkar N., Jahromi S. T., Vianello F. (2022a). Marine microbial fibrinolytic enzymes: an overview of source, production, biochemical properties and thrombolytic activity. Mar. Drugs 20, 46. doi: 10.3390/md20010046
Barzkar N., Khan Z., Jahromi S. T., Pourmozaffar S., Gozari M., Nahavandi R. (2021a). A critical review on marine serine protease and its inhibitors: a new wave of drugs? Int. J. Biol. Macromolecules. 170, 674–687. doi: 10.1016/j.ijbiomac.2020.12.134
Barzkar N., Rungsardthong V., Tamadoni Jahromi S., Laraib Q., Das R., Babich O., et al. (2023). A recent update on fucoidonase: source, isolation methods and its enzymatic activity. Front. Mar. Sci. 10. doi: 10.3389/fmars.2023.1129982
Barzkar N., Sheng R., Sohail M., Jahromi S. T., Babich O., Sukhikh S., et al. (2022b). Alginate lyases from marine bacteria: an enzyme ocean for sustainable future. Molecules 27, 3375. doi: 10.3390/molecules27113375
Barzkar N., Sohail M. (2020). An overview on marine cellulolytic enzymes and their potential applications. Appl. Microbiol. Biotechnol. 104, 6873–6892. doi: 10.1007/s00253-020-10692-y
Barzkar N., Sohail M., Tamadoni Jahromi S., Gozari M., Poormozaffar S., Nahavandi R., et al. (2021b). Marine bacterial esterases: emerging biocatalysts for industrial applications. Appl. Biochem. Biotechnol. 193, 1187–1214. doi: 10.1007/s12010-020-03483-8
Benedetto C. D., et al. (2014). Production, characterization and biocompatibility of marine collagen matrices from an alternative and sustainable source: the sea urchin paracentrotus lividus. Mar. Drugs 12, 4912–4933. doi: 10.3390/md12094912
Benjakul S., et al. (2010). Extraction and characterisation of pepsin-solubilised collagens from the skin of bigeye snapper (Priacanthus tayenus and Priacanthus macracanthus). J. Sci. Food Agric. 90 (1), 132–138. doi: 10.1002/jsfa.3795
Bilek S. E., Bayram S. K. (2015). Fruit juice drink production containing hydrolyzed collagen. J. Funct. foods 14, 562–569. doi: 10.1016/j.jff.2015.02.024
Blanco M., Vázquez J. A., Pérez-Martín R. I., Sotelo C. G. (2017). Hydrolysates of fish skin collagen: An opportunity for valorizing fish industry byproducts. Mar. Drugs 15 (5), 131. doi: 10.3390/md15050131
Bourdon B., Contentin R., Cassé F., Maspimby C., Oddoux S., Noël A., et al. (2021). Marine collagen hydrolysates downregulate the synthesis of pro-catabolic and pro-inflammatory markers of osteoarthritis and favor collagen production and metabolic activity in equine articular chondrocyte organoids. Int. J. Mol. Sci. 22 (2), 580. doi: 10.3390/ijms22020580
Brunt E., Burgess J. (2018). The promise of marine molecules as cosmetic active ingredients. Int. J. cosmetic Sci. 40 (1), 1–15. doi: 10.1111/ics.12435
Burkel B., Morris B. A., Ponik S. M., Riching K. M., Eliceiri K. W., Keely P. J., et al. (2016). Preparation of 3D collagen gels and microchannels for the study of 3D interactions in vivo. J. visualized experiments JoVE 111, 53989. doi: 10.3791/53989
Caddeo S., Boffito M., Sartori S. (2017). Tissue engineering approaches in the design of healthy and pathological in vitro tissue models. Front. bioengineering Biotechnol. 5, 40. doi: 10.3389/fbioe.2017.00040
Caruso G., Floris R., Serangeli C., Di Paola L. (2020). Fishery wastes as a yet undiscovered treasure from the sea: Biomolecules sources, extraction methods and valorization. Mar. Drugs 18 (12), 622. doi: 10.3390/md18120622
Carvalho D. N., López-Cebral R., Sousa R. O., Alves A. L., Reys L. L., Silva S. S., et al. (2020a). Marine collagen-chitosan-fucoidan cryogels as cell-laden biocomposites envisaging tissue engineering. Biomed. Materials 15 (5), 055030. doi: 10.1088/1748-605X/ab9f04
Carvalho D. N., Inácio A. R., Sousa R. O., Reis R. L., Silva T. H. (2020b). “Seaweed polysaccharides as sustainable building blocks for biomaterials in tissue engineering,” in Sustainable seaweed technologies (Elsevier), 543–587. doi: 10.1016/B978-0-12-817943-7.00019-6
Carvalho D. N., Gonçalves C., Oliveira J. M., Williams D. S., Mearns-Spragg A., Reis R. L., et al. (2021). Innovative methodology for marine collagen–chitosan–fucoidan hydrogels production, tailoring rheological properties towards biomedical application. Green Chem. 23 (18), 7016–7029. doi: 10.1039/D1GC02223G
Chen J., Li L., Yi R., Xu N., Gao R., Hong B. (2016). Extraction and characterization of acid-soluble collagen from scales and skin of tilapia (Oreochromis niloticus). LWT - Food Sci. Technol. 66, 453–459. doi: 10.1016/j.lwt.2015.10.070
Chen S., Chen H., Xie Q., Hong B., Chen J., Hua F., et al. (2016). Rapid isolation of high purity pepsin-soluble type I collagen from scales of red drum fish (Sciaenops ocellatus). Food Hydrocolloids 52, 468–477. doi: 10.1016/j.foodhyd.2015.07.027
Chen Y.-P., Liang C.-H., Wu H.-T., Pang H.-Y., Chen C., Wang G.-H., et al. (2018a). Antioxidant and anti-inflammatory capacities of collagen peptides from milkfish (Chanos chanos) scales. J. Food Sci. Technol. 55 (6), 2310–2317. doi: 10.1007/s13197-018-3148-4
Chen Y.-P., Wu H.-T., Wang G.-H., Liang C.-H. (2018b). Improvement of skin condition on skin moisture and anti-melanogenesis by collagen peptides from milkfish (Chanos chanos) scales. IOP Conf. Series: Materials Sci. Eng. 382 (2), 022067. doi: 10.1088/1757-899X/382/2/022067
Chen J., Li L., Yi R., Gao R., He J. (2018). Release kinetics of Tilapia scale collagen I peptides during tryptic hydrolysis. Food Hydrocolloids 77, 931–936. doi: 10.1016/j.foodhyd.2017.11.040
Chen J., Gao K., Liu S., Wang S., Elango J., Bao B., et al. (2019). Fish collagen surgical compress repairing characteristics on wound healing process in vivo. Mar. Drugs 17 (1), 33. doi: 10.3390/md17010033
Chen Y., Jin H., Yang F., Jin S., Liu C., Zhang L., et al. (2019). Physicochemical, antioxidant properties of giant croaker (Nibea japonica) swim bladders collagen and wound healing evaluation. Int. J. Biol. macromolecules 138, 483–491. doi: 10.1016/j.ijbiomac.2019.07.111
Chen S., Hong Z., Wen H., Hong B., Lin R., Chen W., et al. (2022). Compositional and structural characteristics of pepsin-soluble type I collagen from the scales of red drum fish, Sciaenops ocellatus. Food Hydrocolloids 123, 107111. doi: 10.1016/j.foodhyd.2021.107111
Cheng X., Shao Z., Li C., Yu L., Raja MA., Liu C. (2017). Isolation, characterization and evaluation of collagen from jellyfish rhopilema esculentum kishinouye for use in hemostatic applications. PloS One 12 (1), e0169731. doi: 10.1371/journal.pone.0169731
Chi C.-F., Hu F.-Y., Wang B., Ren X.-J., Deng S.-G., Wu C.-W. (2015). Purification and characterization of three antioxidant peptides from protein hydrolyzate of croceine croaker (Pseudosciaena crocea) muscle. Food Chem. 168, 662–667. doi: 10.1016/j.foodchem.2014.07.117
Cicciù M. (2017). Real opportunity for the present and a forward step for the future of bone tissue engineering. J. Craniofacial Surg. 28 (3), 592–593. doi: 10.1097/SCS.0000000000003595
Coppola D., Oliviero M., Vitale G. A., Lauritano C., D Ambra I., Iannace S., et al. (2020a). Marine collagen from alternative and sustainable sources: extraction, processing and applications. Mar. Drugs 18 (214), 23. doi: 10.3390/md18040214
Coppola D., Oliviero M., Vitale G. A., Lauritano C., D Ambra I., Iannace S., et al. (2020b). Marine collagen from alternative and sustainable sources: extraction, processing and applications. Mar. Drugs 18, 214. doi: 10.3390/md18040214
Cossu G., et al. (2018). Lancet Commission: Stem cells and regenerative medicine. Lancet 391 (10123), 883–910. doi: 10.1016/S0140-6736(17)31366-1
Crini G., Lichtfouse E., Chanet G., Morin-Crini N. (2020). Applications of hemp in textiles, paper industry, insulation and building materials, horticulture, animal nutrition, food and beverages, nutraceuticals, cosmetics and hygiene, medicine, agrochemistry, energy production and environment: A review. Environmental Chemistry Letters 18 (5), 1451–1476. doi: 10.1007/s10311-020-01029-2
Cruz-López H., Rodríguez-Morales S., Enríquez-Paredes L. M., Villarreal-Gómez L. J., Olivera-Castillo L., Cortes-Santiago Y., et al. (2021). Comparison of collagen characteristic from the skin and swim bladder of Gulf corvina (Cynoscion othonopterus). Tissue Cell 72, 101593. doi: 10.1016/j.tice.2021.101593
Cui F.-x., Xue C.-H., Li Z.-J., Zhang Y.-Q., Dong P., Fu X.-Y., et al. (2007). Characterization and subunit composition of collagen from the body wall of sea cucumber Stichopus japonicus. Food Chem. 100 (3), 1120–1125. doi: 10.1016/j.foodchem.2005.11.019
CunhaNeves A., Harnedy-Rothwell P. A., FitzGerald R. J. (2022). In vitro angiotensin-converting enzyme and dipeptidyl peptidase-IV inhibitory, and antioxidant activity of blue mussel (Mytilus edulis) byssus collagen hydrolysates. Eur. Food Res. Technol. 248 (7), 1721–1732. doi: 10.1007/s00217-022-04000-3
Daboor S. M., Budge S. M., Ghaly A. E., Brooks S., Dave D. (2010). Extraction and purification of collagenase enzymes: a critical review. Am. J. Biochem. Biotech. 6 (4), 239–263. doi: 10.3844/ajbbsp.2010.239.263
Dai W., Yao Z., Dong J., Kawazoe N., Zhang C., Chen G. (2013). Cartilage tissue engineering with controllable shape using a poly (lactic-co-glycolic acid)/collagen hybrid scaffold. J. bioactive compatible polymers 28 (3), 247–257. doi: 10.1177/0883911513484205
Dai M., Sui B., Xue Y., Liu X., Sun J. (2018). Cartilage repair in degenerative osteoarthritis mediated by squid type II collagen via immunomodulating activation of M2 macrophages, inhibiting apoptosis and hypertrophy of chondrocytes. Biomaterials 180, 91–103. doi: 10.1016/j.biomaterials.2018.07.011
Das S. K., Parandhaman T., Dey M. D. (2021). Biomolecule-assisted synthesis of biomimetic nanocomposite hydrogel for hemostatic and wound healing applications. Green Chem. 23 (2), 629–669. doi: 10.1039/D0GC03010D
De Luca C., Mikhal chik E. V., Suprun M. V., Papacharalambous M., Truhanov A. I., Korkina L. G. (2016). Skin antiageing and systemic redox effects of supplementation with marine collagen peptides and plant-derived antioxidants: A single-blind case-control clinical study. Oxid. Med. Cell. Longevity 2016, 1–14. doi: 10.1155/2016/4389410
Derkus B., Arslan Y. E., Bayrac A. T., Kantarcioglu I., Emregul K. C., Emregul E. (2016). Development of a novel aptasensor using jellyfish collagen as matrix and thrombin detection in blood samples obtained from patients with various neurodisease. Sensors Actuators B: Chem. 228, 725–736. doi: 10.1016/j.snb.2016.01.095
Devita L., Nurilmala M., Lioe H. N., Suhartono M. T. (2021). Chemical and antioxidant characteristics of skin-derived collagen obtained by acid-enzymatic hydrolysis of bigeye tuna (Thunnus obesus). Mar. Drugs 19, 1–19. doi: 10.3390/md19040222
Dhatchayani S., Vijayakumar S., Sarala N., Vaseeharan B., Sankaranarayanan K. (2020). Effect of curcumin sorbed selenite substituted hydroxyapatite on osteosarcoma cells: An in vitro study. J. Drug Delivery Sci. Technol. 60, 101963. doi: 10.1016/j.jddst.2020.101963
Di Lullo G. A., Sweeney S. M., Korkko J., Ala-Kokko L., San Antonio J. D. (2002). Mapping the ligand-binding sites and disease-associated mutations on the most abundant protein in the human, type I collagen. J. Biol. Chem. 277 (6), 4223–4231. doi: 10.1074/jbc.M110709200
Ding J.-F., Li Y.-Y., Xu J.-J., Su X.-R., Gao X., Yue F.-P. (2011). Study on effect of jellyfish collagen hydrolysate on anti-fatigue and anti-oxidation. Food Hydrocolloids 25 (5), 1350–1353. doi: 10.1016/j.foodhyd.2010.12.013
Ding D., Du B., Zhang C., Zaman F., Huang Y. (2019). Isolation and identification of an antioxidant collagen peptide from skipjack tuna (Katsuwonus pelamis) bone. RSC Adv. 9 (46), 27032–27041. doi: 10.1039/C9RA04665H
Diogo G. S., Marques C. F., Sotelo C. G., Pérez-Martín R. I., Pirraco R. P., Reis R. L., et al. (2020). Cell-laden biomimetically mineralized shark-skin-collagen-based 3D printed hydrogels for the engineering of hard tissues. ACS Biomaterials Sci. Eng. 6 (6), 3664–3672. doi: 10.1021/acsbiomaterials.0c00436
Diogo G. S., Carneiro F., Freitas-Ribeiro S., Sotelo C. G., Pérez-Martín R. I., Pirraco R. P., et al. (2021). Prionace glauca skin collagen bioengineered constructs as a promising approach to trigger cartilage regeneration. Materials Sci. Engineering: C 120, 111587. doi: 10.1016/j.msec.2020.111587
Dong X., Zhu B., Sun L., Zheng J., Jiang D., Zhou D., et al. (2011). Changes of collagen in sea cucumber (Stichopus japonicas) during cooking. Food Sci. Biotechnol. 20 (4), 1137. doi: 10.1007/s10068-011-0155-x
Ehrlich H. (2010). Chitin and collagen as universal and alternative templates in biomineralization. Int. Geology Rev. 52 (7-8), 661–699. doi: 10.1080/00206811003679521
Ehrlich H., Ereskovskii A., Drozdov A., Krylova D., Hanke T., Meissner H., et al. (2006). A modern approach to demineralization of spicules in glass sponges (Porifera: Hexactinellida) for the purpose of extraction and examination of the protein matrix. Russian J. Mar. Biol. 32, 186–193. doi: 10.1134/S1063074006030060
Ehrlich H., Brunner E., Simon P., Bazhenov V. V., Botting J. P., Tabachnick K. R., et al. (2011). Calcite reinforced silica–silica joints in the biocomposite skeleton of deep-sea glass sponges. Advanced Funct. Materials 21 (18), 3473–3481. doi: 10.1002/adfm.201100749
El-Rashidy A. A., Gad A., Abu-Hussein A. E.-H. G., Habib S. I., Badr N. A., Hashem A. A. (2015). Chemical and biological evaluation of Egyptian Nile Tilapia (Oreochromis niloticas) fish scale collagen. Int. J. Biol. Macromolecules 79, 618–626. doi: 10.1016/j.ijbiomac.2015.05.019
Ezquerra-Brauer J. M., Márquez-Ríos E, López-Corona BE, Ocaño-Higuera VM, Ramírez-Guerra HE, Cota-Arriola O, et al. (2018). Physicochemical changes of pepsin-solubilized and insoluble collagen in jumbo squid (Dosidicus gigas) muscle after cooking process. Int. J. Food Properties 21 (1), 821–834. doi: 10.1080/10942912.2018.1477159
Farage M. A., Miller K. W., Elsner P., Maibach H. I. (2013). Characteristics of the aging skin. Adv. Wound Care 2 (1), 5–10. doi: 10.1089/wound.2011.0356
Felician F. F., Yu R.-H., Li M.-Z., Li C.-J., Chen H.-Q., Jiang Y., et al. (2019). The wound healing potential of collagen peptides derived from the jellyfish Rhopilema esculentum. Chin. J. Traumatology 22 (01), 12–20. doi: 10.1016/j.cjtee.2018.10.004
Feng X., Zhang X., Li S., Zheng Y., Shi X., Li F., et al. (2020). Preparation of aminated fish scale collagen and oxidized sodium alginate hybrid hydrogel for enhanced full-thickness wound healing. Int. J. Biol. macromolecules 164, 626–637. doi: 10.1016/j.ijbiomac.2020.07.058
Feng Y., Shi Y., Tian Y., Yang Y., Wang J., Guo H., et al. (2023). The Collagen-Based Scaffolds for Bone Regeneration: A Journey through Electrospun Composites Integrated with Organic and Inorganic Additives. Processes 11 (7), 2105. doi: 10.3390/pr11072105
Ferrario C., Rusconi F., Pulaj A., Macchi R., Landini P., Paroni M., et al. (2020). From food waste to innovative biomaterial: Sea urchin-derived collagen for applications in skin regenerative medicine. Mar. Drugs 18 (8), 414. doi: 10.3390/md18080414
Fischer T., Hayn A., Mierke C. T. (2019). Fast and reliable advanced two-step pore-size analysis of biomimetic 3D extracellular matrix scaffolds. Sci. Rep. 9 (1), 1–10. doi: 10.1038/s41598-019-44764-5
Fu Y., Li C., Wang Q., Gao R., Cai X., Wang S., et al. (2022). The protective effect of collagen peptides from bigeye tuna (Thunnus obesus) skin and bone to attenuate UVB-induced photoaging via MAPK and TGF-β signaling pathways. J. Funct. Foods 93, 105101. doi: 10.1016/j.jff.2022.105101
Gao X., He J., Chen J., Zheng Y., Li Y., Ye T. (2023). Double-spotted pufferfish (Takifugu bimaculatus) skin collagen: Preparation, structure, cytocompatibility, rheological, and functional properties. Arabian J. Chem. 16 (1), 104402. doi: 10.1016/j.arabjc.2022.104402
García-Quintero A., Palencia M. (2021). A critical analysis of environmental sustainability metrics applied to green synthesis of nanomaterials and the assessment of environmental risks associated with the nanotechnology. Sci. Total Environ. 793, 148524. doi: 10.1016/j.scitotenv.2021.148524
Gauza-Włodarczyk M., Kubisz L., Mielcarek S., Włodarczyk D. (2017). Comparison of thermal properties of fish collagen and bovine collagen in the temperature range 298–670 K. Materials Sci. Engineering: C 80, 468–471. doi: 10.1016/j.msec.2017.06.012
Geahchan S., Baharlouei P., Rahman A. (2022). Marine collagen: a promising biomaterial for wound healing, skin anti-aging, and bone regeneration. Mar. Drugs 20 (1), 61. doi: 10.3390/md20010061
Goldring M. B., Otero M. (2011). Inflammation in osteoarthritis. Curr. Opin. Rheumatol. 23 (5), 471. doi: 10.1097/BOR.0b013e328349c2b1
Gómez-Guillén M., Giménez B., López-Caballero M. A., Montero M. (2011). Functional and bioactive properties of collagen and gelatin from alternative sources: A review. Food hydrocolloids 25 (8), 1813–1827. doi: 10.1016/j.foodhyd.2011.02.007
Gómez-Ordóñez E., Rupérez P. (2011). FTIR-ATR spectroscopy as a tool for polysaccharide identification in edible brown and red seaweeds. Food hydrocolloids 25 (6), 1514–1520. doi: 10.1016/j.foodhyd.2011.02.009
Govindharaj M., Roopavath U. K., Rath S. N. (2019). Valorization of discarded Marine Eel fish skin for collagen extraction as a 3D printable blue biomaterial for tissue engineering. J. Cleaner Production 230, 412–419. doi: 10.1016/j.jclepro.2019.05.082
Hadfi N., Sarbon N. (2019). Physicochemical properties of silver catfish (Pangasius sp.) skin collagen as influenced by acetic acid concentration. Food Res. 3 (6), 783–790. doi: 10.26656/fr.2017.3(6).130
Han Y., Ahn J.-R., Woo J.-W., Jung C.-K., Cho S.-M., Lee Y.-B., et al. (2010). Processing optimization and physicochemical characteristics of collagen from scales of yellowfin tuna (Thunnus albacares). Fisheries Aquat. Sci. 13 (2), 102–111. doi: 10.5657/fas.2010.13.2.102
Han S.-B., Won B., Yang S.-C., Kim D.-H. (2021). Asterias pectinifera derived collagen peptide-encapsulating elastic nanopolisomes for the cosmetic application. J. Ind. Eng. Chem. 98, 289–297. doi: 10.1016/j.jiec.2021.03.039
Haq M., et al. (2020). Biofunctional properties of bacterial collagenolytic protease-extracted collagen hydrolysates obtained using catalysts-assisted subcritical water hydrolysis. J. Ind. Eng. Chem. 81, 332–339. doi: 10.1016/j.jiec.2019.09.023
Harley B. A., Kim H.-D., Zaman M. H., Yannas I. V., Lauffenburger D. A., Gibson L. J. (2008). Microarchitecture of three-dimensional scaffolds influences cell migration behavior via junction interactions. Biophys. J. 95 (8), 4013–4024. doi: 10.1529/biophysj.107.122598
He X., Xie L., Zhang X., Lin F., Wen X., Teng B. (2021). The structural characteristics of collagen in swim bladders with 25-year sequence aging: the impact of age. Appl. Sci. 11 (10), 4578. doi: 10.3390/app11104578
Heinemann S., Ehrlich H., Douglas T., Heinemann C., Worch H., Schatton W., et al. (2007). Ultrastructural studies on the collagen of the marine sponge Chondrosia reniformis Nardo. Biomacromolecules 8 (11), 3452–3457. doi: 10.1021/bm700574y
Hema G., Joshy C., Shyni K., Chatterjee N. S., Ninan G., Mathew S. (2017). Optimization of process parameters for the production of collagen peptides from fish skin (Epinephelus malabaricus) using response surface methodology and its characterization. J. Food Sci. Technol. 54 (2), 488–496. doi: 10.1007/s13197-017-2490-2
Hoe N. K. M. (2014). PRODUCTION AND CHARACTERIZATION OF COLLAGEN AND GELATIN FROM EDIBLE JELLYFISH (Acromitus hardenbergi stiasny, 1934) FOR FABRICATION OF BIOFUNCTIONAL SCAFFOLD. PhD thesis, Universiti Putra Malaysia. Available at: http://psasir.upm.edu.my/id/eprint/56806.
Hoyer B., Bernhardt A, Lode A, Heinemann S, Sewing J, Klinger M, et al. (2014). Jellyfish collagen scaffolds for cartilage tissue engineering. Acta Biomaterialia 10 (2), 883–892. doi: 10.1016/j.actbio.2013.10.022
Hu Z., Tang Q., Yan D., Zheng G., Gu M., Luo Z., et al. (2021). A multi-functionalized calcitriol sustainable delivery system for promoting osteoporotic bone regeneration both in vitro and in vivo. Appl. Materials Today 22, 100906. doi: 10.1016/j.apmt.2020.100906
Hukmi N. M. M., Sarbon N. M. (2018). Isolation and characterization of acid soluble collagen (ASC) and pepsin soluble collagen (PSC) extracted from silver catfish (Pangasius sp.) skin. Int. Food Res. J. 5 (25), 1785–1791.
Idrus S., Hadinoto S., Kolanus J. (2018). Karakterisasi kolagen gelembung renang tuna sirip kuning (Thunnus albacares) dari perairan maluku menggunakan ekstraksi asam (Collagen characterization from swim bladder of yellowfin tuna (Thunnus albacares) from maluku using acid extraction). Biopropal Industri 9 (2), 87–94. doi: 10.36974/jbi.v9i2.4020
Ikoma T., Kobayashi H., Tanaka J., Walsh D., Mann S. (2003). Physical properties of type I collagen extracted from fish scales of Pagrus major and Oreochromis niloticas. Int. J. Biol. Macromolecules 32 (3), 199–204. doi: 10.1016/S0141-8130(03)00054-0
Im J., Choi C. H., Mun F., Lee J., Kim H., Jung W.-K., et al. (2017). A polycaprolactone/fish collagen/alginate biocomposite supplemented with phlorotannin for hard tissue regeneration. RSC Adv. 7 (4), 2009–2018. doi: 10.1039/C6RA25182J
Ito N., Seki S., Ueda F. (2018). Effects of composite supplement containing collagen peptide and ornithine on skin conditions and plasma IGF-1 levels—A randomized, double-blind, placebo-controlled trial. Mar. Drugs 16 (12), 482. doi: 10.3390/md16120482
Jahromi S. T., Barzkar N. (2018a). Future direction in marine bacterial agarases for industrial applications. Appl. Microbiol. Biotechnol. 102, 6847–6863. doi: 10.1007/s00253-018-9156-5
Jahromi S. T., Barzkar N. (2018b). Marine bacterial chitinase as sources of energy, eco-friendly agent, and industrial biocatalyst. Int. J. Biol. macromolecules. 120, 2147–2154. doi: 10.1016/j.ijbiomac.2018.09.083
Jaziri A. A., Shapawi R., Mokhtar R. A., Noordin W. N. M., Huda N. (2022). Physicochemical and microstructural analyses of pepsin-soluble collagens derived from lizardfish (Saurida tumbil bloch, 1795) skin, bone and scales. Gels 8, 1–18. doi: 10.3390/gels8080471
Jeong H.-S., Venkatesan J., Kim S.-K. (2013). Isolation and characterization of collagen from marine fish (Thunnus obesus). Biotechnol. Bioprocess Eng. 18 (6), 1185–1191. doi: 10.1007/s12257-013-0316-2
Jia J., Sun S., Zhou X., Miao N., Zhu Y., Wu Q. (2020). Effect of ultrasonic treatment on self-assembly behavior and physicochemical properties of collagen from Carassius auratus skin. Shipin Kexue/Food Sci. 41 (19), 98–104. doi: 10.7506/spkx1002-6630-20190826-275
Jin H.-X., Xu H.-P., Li Y., Zhang Q.-W., Xie H. (2019). Preparation and evaluation of peptides with potential antioxidant activity by microwave assisted enzymatic hydrolysis of collagen from sea cucumber Acaudina molpadioides obtained from Zhejiang province in China. Mar. Drugs 17, 1–14. doi: 10.3390/md17030169
Jongjareonrak A. (2006). Characterization and functional properties of collagen and Gelatin from bigeye snapper (Priacanthus macracanthus) and brownstripe red snapper (Lutjanus vitta) skins (Thailand: Prince of Songkla University). Available at: http://kb.psu.ac.th/psukb/handle/2553/1563
Jongjareonrak A., Senaratne L., Park P.-J., Kim S.-K. (2005). Isolation and characterization of collagen from bigeye snapper (Priacanthus macracanthus) skin. J. Sci. Food Agric. 85 (7), 1203–1210. doi: 10.1002/jsfa.2072
Kaewdang O., Benjakul S., Kaewmanee T., Kishimura H. (2014). Characteristics of collagens from the swim bladders of yellowfin tuna (Thunnus albacares). Food Chem. 155, 264–270. doi: 10.1016/j.foodchem.2014.01.076
Kapuler O., Selskaya B, Galeeva A, Kamllov F. (2015). Metabolism of collagen fibers in the presence of age-related changes. Vrach 26 (8), 64–69. Available at: https://journals.eco-vector.com/0236-3054/article/view/116433.
Katzman R. L., Halford M. H., Reinhold V. N., Jeanloz R. W. (1972). Invertebrate connective tissue. IX. Isolation and structure determination of glucosylgalactosylhydroxylysine from sponge and sea anemone collagen. Biochemistry 11 (7), 1161–1167. doi: 10.1021/bi00757a008
Khan R., Khan M. H. (2013). Use of collagen as a biomaterial: An update. J. Indian Soc. Periodontology 17 (4), 539–542. doi: 10.4103/0972-124X.118333
Khong N. M., Tabakaeva O. V., Tabakaev A. V., Piekoszewski W. (2016). Nutritional composition and total collagen content of three commercially important edible jellyfish. Food Chem. 196, 953–960. doi: 10.1016/j.foodchem.2015.09.094
Khong N. M., Yusoff F. M., Jamilah B., Basri M., Maznah I., Chan K. W., et al. (2018). Improved collagen extraction from jellyfish (Acromitus hardenbergi) with increased physical-induced solubilization processes. Food Chem. 251, 41–50. doi: 10.1016/j.foodchem.2017.12.083
Kim H. K., Kim Y. H., Kim Y. J., Park H. J., Lee N. H. (2012). Effects of ultrasonic treatment on collagen extraction from skins of the sea bass Lateolabrax japonicus. Fisheries Sci. 78 (2), 485–490. doi: 10.1007/s12562-012-0472-x
Kim H. K., Kim Y. H., Park H. J., Lee N. H. (2013). Application of ultrasonic treatment to extraction of collagen from the skins of sea bass Lateolabrax japonicus. Fisheries Sci. 79, 849–856. doi: 10.1007/s12562-013-0648-z
Kittiphattanabawon P., Benjakul S., Visessanguan W., Nagai T., Tanaka M. (2005). Characterisation of acid-soluble collagen from skin and bone of bigeye snapper (Priacanthus tayenus). Food Chem. 89 (3), 363–372. doi: 10.1016/j.foodchem.2004.02.042
Kozlowska J., Sionkowska A., Skopinska-Wisniewska J., Piechowicz K. (2015). Northern pike (Esox lucius) collagen: Extraction, characterization and potential application. Int. J. Biol. Macromolecules 81, 220–227. doi: 10.1016/j.ijbiomac.2015.08.002
Krishnamoorthi J., Ramasamy P., Shanmugam V., Shanmugam A. (2017). Isolation and partial characterization of collagen from outer skin of Sepia pharaonis (Ehrenberg, 1831) from Puducherry coast. Biochem. Biophys. Rep. 10, 39–45. doi: 10.1016/j.bbrep.2017.02.006
Kusumaningtyas E., Nurilmala M., Sibarani D. (2019). Antioxidant and antifungal activities of collagen hydrolysates from skin of milkfish (Chanos chanos) hydrolyzed using various bacillus proteases. IOP Conf. Series: Earth Environ. Sci. 278 (1), 012040. doi: 10.1088/1755-1315/278/1/012040
Langasco R., Cadeddu B, Formato M, Lepedda AJ, Cossu M, Giunchedi P, et al. (2017). Natural collagenic skeleton of marine sponges in pharmaceutics: Innovative biomaterial for topical drug delivery. Materials Sci. Engineering: C 70, 710–720. doi: 10.1016/j.msec.2016.09.041
La Noce M., Paino F., Spina A., Naddeo P., Montella R., Desiderio V., et al. (2014). Dental pulp stem cells: state of the art and suggestions for a true translation of research into therapy. J. dentistry 42 (7), 761–768. doi: 10.1016/j.jdent.2014.02.018
Lee L., Caldwell S., Gibbons J. (1997). Development of a cell line from skin of goldfish, Carassius auratus, and effects of ascorbic acid on collagen deposition. Histochemical J. 29 (1), 31–43. doi: 10.1023/A:1026412817431
Li P.-H., Lu W.-C., Chan Y.-J., Ko W.-C., Jung C.-C., Le Huynh D. T., et al. (2020). Extraction and characterization of collagen from sea cucumber (Holothuria cinerascens) and its potential application in moisturizing cosmetics. Aquaculture 515, 734590. doi: 10.1016/j.aquaculture.2019.734590
Liang J., Pei X.-R., Wang N., Zhang Z.-F., Wang J.-B., Li Y. (2010). Marine collagen peptides prepared from chum salmon (Oncorhynchus keta) skin extend the life span and inhibit spontaneous tumor incidence in sprague-dawley rats. J. medicinal Food 13 (4), 757–770. doi: 10.1089/jmf.2009.1279
Liang J., Pei X.-R., Zhang Z.-F., Wang N., Wang J.-B., Li Y. (2011). A chronic oral toxicity study of marine collagen peptides preparation from chum salmon (Oncorhynchus keta) skin using Sprague-Dawley rat. Mar. Drugs 10 (1), 20–34. doi: 10.3390/md10010020
Liang J., Li Q., Lin B., Yu Y., Ding Y., Dai X., et al. (2014). Comparative studies of oral administration of marine collagen peptides from Chum Salmon (Oncorhynchus keta) pre-and post-acute ethanol intoxication in female Sprague-Dawley rats. Food Funct. 5 (9), 2078–2085. doi: 10.1039/C4FO00161C
Lim Y.-S., Ok Y-J, Hwang S-Y, Kwak J-Y, Yoon S. (2019). Marine collagen as A promising biomaterial for biomedical applications. Mar. Drugs 17, 1–32. doi: 10.3390/md17080467
Lima C. A., Filho J. L. L., Neto B. B., Converti A., Carneiro da Cunha M. G., Porto A. L., et al. (2011a). Production and characterization of a collagenolytic serine proteinase by Penicillium aurantiogriseum URM 4622: A factorial study. Biotechnol. Bioprocess Eng. 16, 549–560. doi: 10.1007/s12257-010-0247-0
Lima C. A., Viana Marques D. A., Neto B. B., Lima Filho J. L., Carneiro-da-Cunha M. G., Porto ALLima C.A., et al. (2011b). Fermentation medium for collagenase production by Penicillium aurantiogriseum URM4622. Biotechnol. Prog. 27 (5), 1470–1477. doi: 10.1002/btpr.664
Lima C. A., Campos J. F., Filho J. L.L., Converti A., da Cunha M. G.C., Porto A. L. (2015). Antimicrobial and radical scavenging properties of bovine collagen hydrolysates produced by Penicillium aurantiogriseum URM 4622 collagenase. J. Food Sci. Technol. 52, 4459–4466. doi: 10.1007/s13197-014-1463-y
Lin Z., Solomon K. L., Zhang X., Pavlos N. J., Abel T., Willers C., et al. (2011). In vitro evaluation of natural marine sponge collagen as a scaffold for bone tissue engineering. Int. J. Biol. Sci. 7 (7), 968–977. doi: 10.7150/ijbs.7.968
Lin S., Xue Y.-P., San E., Keong T. C., Chen L., Zheng Y.-G. (2017). Extraction and characterization of pepsin soluble collagen from the body wall of sea cucumber Acaudina leucoprocta. J. Aquat. Food Product Technol. 26 (5), 502–515. doi: 10.1080/10498850.2016.1222560
Lin X., Chen Y., Jin H., Zhao Q., Liu C., Li R., et al. (2019). Collagen extracted from bigeye tuna (Thunnus obesus) skin by isoelectric precipitation: physicochemical properties, proliferation and migration activities. Mar. Drugs 17, 12. doi: 10.3390/md17050261
Lin F., Rong H., Lin J., Yuan Y., Yu J., Yu C., et al. (2020). Enhancement of collagen deposition in swim bladder of Chu's croaker (Nibea coibor) by proline: View from in-vitro and in-vivo study. Aquaculture 523, 735175. doi: 10.1016/j.aquaculture.2020.735175
Liu L., Ma M., Cai Z., Yang X., Wang W. (2010). Purification and properties of a collagenolytic protease produced by Bacillus cereus MBL13 strain. Food Technol. Biotechnol. 48 (2), 151. Available at: https://hrcak.srce.hr/53624
Liu Z.-q., Tuo F.-Y., Song L., Liu Y.-X., Dong X.-P., Li D.-M., et al. (2018). Action of trypsin on structural changes of collagen fibres from sea cucumber (Stichopus japonicus). Food Chem. 256, 113–118. doi: 10.1016/j.foodchem.2018.02.117
Liu Z., Oliveira A. C., Su Y.-C. (2010). Purification and characterization of pepsin-solubilized collagen from skin and connective tissue of giant red sea cucumber (Parastichopus californicus). J. Agric. Food Chem. 58 (2), 1270–1274. doi: 10.1021/jf9032415
Lu W.-C., Chiu C.-S., Chan Y.-J., Guo T.-P., Lin C.-C., Wang P.-C., et al. (2022). An in vivo study to evaluate the efficacy of blue shark (Prionace glauca) cartilage collagen as a cosmetic. Mar. Drugs 20 (10), 633. doi: 10.3390/md20100633
Luo J., Zhou Z., Yao X., Fu Y. (2020). Mineral-chelating peptides derived from fish collagen: Preparation, bioactivity and bioavailability. Lwt 134, 110209. doi: 10.1016/j.lwt.2020.110209
Mantha S., Pillai S., Khayambashi P., Upadhyay A., Zhang Y., Tao O., et al. (2019). Smart hydrogels in tissue engineering and regenerative medicine. Materials 12 (20), 3323. doi: 10.3390/ma12203323
Martins E., Diogo G. S., Pires R., Reis R. L., Silva T. H. (2022). 3D biocomposites comprising marine collagen and silica-based materials inspired on the composition of marine sponge skeletons envisaging bone tissue regeneration. Mar. Drugs 20, 1–24. doi: 10.3390/md20110718
Martins E., Reis R. L., Silva T. H. (2023). In vivo skin hydrating efficacy of fish collagen from Greenland halibut as a high-value active ingredient for cosmetic applications. Mar. Drugs 21, 1–19. doi: 10.3390/md21020057
Matmaroh K., Benjakul S., Prodpran T., Encarnacion A. B., Kishimura H. (2011). Characteristics of acid soluble collagen and pepsin soluble collagen from scale of spotted golden goatfish (Parupeneus heptacanthus). Food Chem. 129 (3), 1179–1186. doi: 10.1016/j.foodchem.2011.05.099
Melotti L., Martinello T., Perazzi A., Iacopetti I., Ferrario C., Sugni M., et al. (2021). A prototype skin substitute, made of recycled marine collagen, improves the skin regeneration of sheep. Animals 11 (5), 1219. doi: 10.3390/ani11051219
Menezes M., Ribeiro H. L., Flávia de Oliveira M., de Andrade Feitosa J. P. (2020). Optimization of the collagen extraction from Nile tilapia skin (Oreochromis niloticus) and its hydrogel with hyaluronic acid. Colloids Surfaces B: Biointerfaces 189, 110852. doi: 10.1016/j.colsurfb.2020.110852
Meng K., Chen L., Xia G. (2021). Effects of zinc sulfate and zinc lactate on the properties of tilapia (Oreochromis Niloticus) skin collagen peptide chelate zinc. Food Chem. 347, 129043. doi: 10.1016/j.foodchem.2021.129043
Minh Thuy L. T., Okazaki E., Osako K. (2014). Isolation and characterization of acid-soluble collagen from the scales of marine fishes from Japan and Vietnam. Food Chem. 149, 264–270. doi: 10.1016/j.foodchem.2013.10.094
Mizuta S., Yoshinaka R., Sato M., Sakaguchi M. (1994). Characterization of collagen in the muscle of several crustacean species in association with raw meat texture. Fisheries Sci. 60 (3), 323–328. doi: 10.2331/fishsci.60.323
Mizuta S., Miyagi T., Nishimiya T., Yoshinaka R. (2004). Partial characterization of collagen in several bivalve molluscs. Food Chem. 87 (1), 83–88. doi: 10.1016/j.foodchem.2003.10.021
Mizuta S., Tanaka T., Yokoyama Y., Yoshinaka R. (2009). Hot-water solubility of mantle collagens in several cephalopod molluscs. Fisheries Sci. 75 (5), 1337–1344. doi: 10.1007/s12562-009-0150-9
Mohd Zaffarin A. S., Ng S.-F., Ng M. H., Hassan H., Alias E. (2021). Nano-hydroxyapatite as a delivery system for promoting bone regeneration in vivo: a systematic review. Nanomaterials 11 (10), 2569. doi: 10.3390/nano11102569
Moral A., Morales J., Ruíz-Capillas C., Montero P. (2002). Muscle protein solubility of some cephalopods (pota and octopus) during frozen storage. J. Sci. Food Agric. 82 (6), 663–668. doi: 10.1002/jsfa.1088
Morales J., Montero P., Moral A. (2000). Isolation and partial characterization of two types of muscle collagen in some cephalopods. J. Agric. Food Chem. 48 (6), 2142–2148. doi: 10.1021/jf990711k
Morishige H., Sugahara T., Nishimoto S., Muranaka A., Ohno F., Shiraishi R., et al. (2011). Immunostimulatory effects of collagen from jellyfish in vivo. Cytotechnology 63, 481–492. doi: 10.1007/s10616-011-9371-8
Mukherjee C., Varghese D., Krishna J., Boominathan T., Rakeshkumar R., Dineshkumar S., et al. (2023). Recent advances in biodegradable polymers–properties, applications and future prospects. Eur. Polymer J. p, 112068. doi: 10.1016/j.eurpolymj.2023.112068
Müller W. E. (2003). The origin of metazoan complexity: Porifera as integrated animals. Integr. Comp. Biol. 43 (1), 3–10. doi: 10.1093/icb/43.1.3
Muralidharan N., Jeya Shakila R., Sukumar D., Jeyasekaran G. (2013). Skin, bone and muscle collagen extraction from the trash fish, leather jacket (Odonus Niger) and their characterization. J. Food Sci. Technol. 50 (6), 1106–1113. doi: 10.1007/s13197-011-0440-y
Nagai T. (2004a). Characterization of collagen from Japanese sea bass caudal fin as waste material. Eur. Food Res. Technol. 218 (5), 424–427. doi: 10.1007/s00217-004-0892-7
Nagai T. (2004b). Collagen from Diamondback Squid (Thysanoteuthis rhombus) Outer Skin. Z Naturforsch C J Biosci. 59 (3-4), 271–5. doi: 1515/znc-2004-3-426
Nagai T., Worawattanamateekul W., Suzuki N., Nakamura T., Ito T., Fujiki K., et al. (2000). Isolation and characterization of collagen from rhizostomous jellyfish (Rhopilema asamushi). Food Chem. 70 (2), 205–208. doi: 10.1016/S0308-8146(00)00081-9
Nagai T., Suzuki N. (2000). Partial characterization of collagen from purple sea urchin (Anthocidaris crassispina) test. Int. J. Food Sci. Technol. 35 (5), 497–501. doi: 10.1046/j.1365-2621.2000.00406.x
Nalinanon S., Benjakul S., Visessanguan W., Kishimura H. (2007). Use of pepsin for collagen extraction from the skin of bigeye snapper (Priacanthus tayenus). Food Chem. 104 (2), 593–601. doi: 10.1016/j.foodchem.2006.12.035
Natsir H., Dali S., Arif A. (2019). “Activity and kinetics of α-glucosidase inhibition by collagen hydrolysate from Thunnus albacares bone,” in Journal of Physics: Conference Series, IOP Publishing. Volume 1341 (3). doi: 10.1088/1742-6596/1341/3/032015
Nguyen B. C., Kha T. C., Nguyen K. H. N., Nguyen H. M. X. (2021). Optimization of enzymatic hydrolysis of collagen from yellowfin tuna skin (Thunnus albacares) by response surface methodology and properties of hydrolyzed collagen. J. Food Process. Preservation 45 (4), e15319. doi: 10.1111/jfpp.15319
Niu H., Wang Z., Hou H., Zhang Z., Li B. (2016). Protective effect of cod (Gadus macrocephalus) skin collagen peptides on acetic acid-induced gastric ulcer in rats. J. Food Sci. 81 (7), H1807–H1815. doi: 10.1111/1750-3841.13332
Nomura Y., Oohashi K., Watanabe M., Kasugai S. (2005). Increase in bone mineral density through oral administration of shark gelatin to ovariectomized rats. Nutrition 21 (11-12), 1120–1126. doi: 10.1016/j.nut.2005.03.007
Nordwig A., Nowack H., Hieber-Rogall E. (1973). Sea anemone collagen: Further evidence for the existence of only oneα-chain type. J. Mol. Evol. 2 (2), 175–180. doi: 10.1007/BF01653997
Nurilmala M., Pertiwi R. M., Nurhayati T., Fauzi S., Batubara I., Ochiai Y. (2019a). Characterization of collagen and its hydrolysate from yellowfin tuna Thunnus albacares skin and their potencies as antioxidant and antiglycation agents. Fisheries Sci. 85 (3), 591–599. doi: 10.1007/s12562-019-01303-5
Nurilmala M., Fauzi S., Mayasari D., Batubara I.. (2019b). Collagen extraction from yellowfin tuna (Thunnus albacares) skin and its antioxidant activity. Jurnal Teknologi 81 (2), 140–149. doi: 10.11113/jt.v81.11614
Nurilmala M., Hizbullah H. H., Karnia E., Kusumaningtyas E., Ochiai Y.. (2020). Characterization and antioxidant activity of collagen, gelatin, and the derived peptides from yellowfin tuna (Thunnus albacares) skin. Mar. Drugs 18 (2), 98. doi: 10.3390/md18020098
Ogawa M., Moody M. W., Portier R. J., Bell J., Schexnayder M. A., Losso J. N. (2003). Biochemical properties of black drum and sheepshead seabream skin collagen. J. Agric. Food Chem. 51 (27), 8088–8092. doi: 10.1021/jf034350r
Ogawa M., Portier R. J., Moody M. W., Bell J., Schexnayder M. A., Losso J. N. (2004). Biochemical properties of bone and scale collagens isolated from the subtropical fish black drum (Pogonia cromis) and sheepshead seabream (Archosargus probatocephalus). Food Chem. 88 (4), 495–501. doi: 10.1016/j.foodchem.2004.02.006
Ohnishi A., Osaki T., Matahira Y., Tsuka T., Imagawa T., Okamoto Y., et al. (2013). Evaluation of the chondroprotective effects of glucosamine and fish collagen peptide on a rabbit ACLT model using serum biomarkers. J. Veterinary Med. Sci. 75 (4), 421–429. doi: 10.1292/jvms.12-0240
Oryan A., Kamali A., Moshiri A., Baharvand H., Daemi H. (2018). Chemical crosslinking of biopolymeric scaffolds: Current knowledge and future directions of crosslinked engineered bone scaffolds. Int. J. Biol. macromolecules 107, 678–688. doi: 10.1016/j.ijbiomac.2017.08.184
Oslan S. N. H., Shapawi R., Mokhtar R. A. M., Noordin W. N. M., Huda N. (2022). Characterization of acid-and pepsin-soluble collagen extracted from the skin of purple-spotted bigeye snapper. Gels 8 (10), 665. doi: 10.3390/gels8100665
Pal G. K., Nidheesh T., Suresh P. (2015). Comparative study on characteristics and in vitro fibril formation ability of acid and pepsin soluble collagen from the skin of catla (Catla catla) and rohu (Labeo rohita). Food Res. Int. 76, 804–812. doi: 10.1016/j.foodres.2015.07.018
Parisi J. R., Fernandes KR, Aparecida do Vale GC, de França Santana A, de Almeida Cruz M, Fortulan CA, et al. (2020). Marine spongin incorporation into Biosilicate® for tissue engineering applications: An in vivo study. J. Biomaterials Appl. 35 (2), 205–214. doi: 10.1177/0885328220922161
Park S.-Y., Lim H. K., Lee S., Hwang H. C., Cho S. K., Cho M. (2012). Pepsin-solubilised collagen (PSC) from Red Sea cucumber (Stichopus japonicus) regulates cell cycle and the fibronectin synthesis in HaCaT cell migration. Food Chem. 132 (1), 487–492. doi: 10.1016/j.foodchem.2011.11.032
Pei X., Yang R., Zhang Z., Gao L., Wang J., Xu Y., et al. (2010). Marine collagen peptide isolated from Chum Salmon (Oncorhynchus keta) skin facilitates learning and memory in aged C57BL/6J mice. Food Chem. 118 (2), 333–340. doi: 10.1016/j.foodchem.2009.04.120
Porfírio E., Fanaro G. B. (2016). Collagen supplementation as a complementary therapy for the prevention and treatment of osteoporosis and osteoarthritis: a systematic review. Rev. Bras. Geriatria e Gerontologia 19, 153–164. doi: 10.1590/1809-9823.2016.14145
Pozzolini M., Scarfì S, Gallus L, Castellano M, Vicini S, Cortese K, et al. (2018a). Production, characterization and biocompatibility evaluation of collagen membranes derived from marine sponge Chondrosia reniformis Nardo, 1847. Mar. Drugs 16 (4), 111. doi: 10.3390/md16040111
Pozzolini M., Millo E., Oliveri C., Mirata S., Salis A., Damonte G., et al. (2018b). Elicited ROS scavenging activity, photoprotective, and wound-healing properties of collagen-derived peptides from the marine sponge chondrosia reniformis. Mar. Drugs 16, 465. doi: 10.3390/md16120465
Pullar J. M., Carr A. C., Vissers M. C. (2017). The roles of vitamin C in skin health. Nutrients 9 (8), 866. doi: 10.3390/nu9080866
Putra A. B. N., Nishi K., Shiraishi R., Doi M., Sugahara T. (2014). Jellyfish collagen stimulates production of TNF-α and IL-6 by J774.1 cells through activation of NF-κB and JNK via TLR4 signaling pathway. Mol. Immunol. 58 (1), 32–37. doi: 10.1016/j.molimm.2013.11.003
Raabe O., Reich C., Wenisch S., Hild A., Burg-Roderfeld M., Siebert H.-C., et al. (2010). Hydrolyzed fish collagen induced chondrogenic differentiation of equine adipose tissue-derived stromal cells. Histochem. Cell Biol. 134, 545–554. doi: 10.1007/s00418-010-0760-4
Rachmawati R., Hidayat M., Permatasari N., Widyarti S. (2021). Potential effect of jellyfish aurelia aurita collagen scaffold induced alveolar bone regeneration in periodontal disease. Syst. Rev. Pharm. 12, 1397–1404.
Rahman M. A. (2019). Collagen of extracellular matrix from marine invertebrates and its medical applications. Mar. Drugs 17 (2), 118. doi: 10.3390/md17020118
Rajabimashhadi Z., Gallo N., Salvatore L., Lionetto F. (2023). Collagen derived from fish industry waste: progresses and challenges. Polymers 15, 1–28. doi: 10.3390/polym15030544
Rastian Z., Pütz S., Wang Y., Kumar S., Fleissner F., Weidner T., et al. (2018). Type I collagen from jellyfish catostylus mosaicus for biomaterial applications. ACS Biomaterials Sci. Eng. 4 (6), 2115–2125. doi: 10.1021/acsbiomaterials.7b00979
Rigogliuso S., Campora S., Notarbartolo M., Ghersi G. (2023). Recovery of bioactive compounds from marine organisms: focus on the future perspectives for pharmacological, biomedical and regenerative medicine applications of marine collagen. Molecules 28 (3), 1152. doi: 10.3390/molecules28031152
Rodríguez F. V., Morán L, González G, Troncoso E, Zúñiga RN. (2015). Physicochemical characterization of pepsin-soluble collagen extracted from the byssus of Chilean mussels (Mytilus Chilensis). (Núm: Revista de la Facultad de Ciencias Químicas) 10 (2014).
Rodríguez F., Morán L., González G., Troncoso E., Zúñiga R. N. (2017). Collagen extraction from mussel byssus: a new marine collagen source with physicochemical properties of industrial interest. J. Food Sci. Technol. 54 (5), 1228–1238. doi: 10.1007/s13197-017-2566-z
Rossert J., de Crombrugghe B. (2002). Type I collagen: structure, synthesis, and regulation, in Principles of bone biology (San Diego: Academic Press), 189–XVIII. doi: 10.1016/B978-012098652-1.50114-1
Saallah S., Roslan J., Julius F. S., Saallah S., Mohamad Razali U. H., Pindi W., et al. (2021). Comparative Study of The Yield and Physicochemical Properties of Collagen from Sea Cucumber (Holothuria scabra), Obtained through Dialysis and the Ultrafiltration Membrane. Molecules 26. doi: 10.3390/molecules26092564
Sampath Kumar N. S., Nazeer R. A. (2013). Characterization of Acid and Pepsin Soluble Collagen from the Skin of Horse Mackerels (Magalaspis cordyla) and Croaker (Otolithes ruber). Int. J. Food Properties 16 (3), 613–621. doi: 10.1080/10942912.2011.557796
Sankarapandian V., Jothirajan B., Arasu S. P., Subramaniam S., Venmathi Maran B. A. (2023). “Marine biotechnology and its applications in drug discovery,” in Marine biotechnology: Applications in food, drugs and energy (Springer), 189–208.
Sankarapandian V., Venmathi Maran B. A., Rajendran R. L., Jogalekar M. P., Gurunagarajan S., Krishnamoorthy R., et al (2022). An update on the effectiveness of probiotics in the prevention and treatment of cancer. Life 12, 59. doi: 10.3390/life12010059
Santos M. H., Silva R. M., Dumont V. C., Neves J. S., Mansur H. S., Heneine L. G. D. (2013). Extraction and characterization of highly purified collagen from bovine pericardium for potential bioengineering applications. Materials Sci. Engineering: C 33 (2), 790–800. doi: 10.1016/j.msec.2012.11.003
Sarabia-Sainz H. M., Torres-Arreola W., Ezquerra-Brauer J. M. (2018). Spectroscopic imaging: Nuclear magnetic resonance and Raman spectrometry for the detection of collagen cross-linking from giant squid mantle, fin, and tentacle tissues. Instrumentation Sci. Technol. 46 (5), 567–581. doi: 10.1080/10739149.2017.1421221
Seixas M. J., Martins E., Reis R. L., Silva T. H. (2020). Extraction and characterization of collagen from elasmobranch byproducts for potential biomaterial use. Mar. Drugs 18 (12), 617. doi: 10.3390/md18120617
Sewing J., Klinger M., Notbohm H. (2017). Jellyfish collagen matrices conserve the chondrogenic phenotype in two- and three-dimensional collagen matrices. J. Tissue Eng. Regenerative Med. 11 (3), 916–925. doi: 10.1002/term.1993
Shalaby M., Agwa M., Saeed H., Khedr S. M., Morsy O., El-Demellawy M. A. (2020). Fish scale collagen preparation, characterization and its application in wound healing. J. Polymers Environ. 28, 166–178. doi: 10.1007/s10924-019-01594-w
Shimizu K., Amemiya S., Yoshizato K. (1990). Biochemical and immunological characterization of collagen molecules from echinothurioid sea urchin Asthenosoma ijimai. Biochim. Biophys. Acta (BBA) - Protein Structure Mol. Enzymology 1038 (1), 39–46. doi: 10.1016/0167-4838(90)90007-3
Sinthusamran S., Benjakul S., Kishimura H. (2013). Comparative study on molecular characteristics of acid soluble collagens from skin and swim bladder of seabass (Lates calcarifer). Food Chem. 138 (4), 2435–2441. doi: 10.1016/j.foodchem.2012.11.136
Sionkowska A., Adamiak K., Musiał K., Gadomska M. (2020). Collagen based materials in cosmetic applications: A review. Materials 13 (19), 4217. doi: 10.3390/ma13194217
Sivakumar P., Suguna L., Chandrakasan G. (2000). Molecular species of collagen in the intramuscular connective tissues of the marine crab, Scylla serrata. Comp. Biochem. Physiol. Part B: Biochem. Mol. Biol. 125 (4), 555–562. doi: 10.1016/S0305-0491(00)00167-X
Sivakumar P., Suguna L., Chandrakasan G. (2003). Similarity between the major collagens of cuttlefish cranial cartilage and cornea. Comp. Biochem. Physiol. Part B: Biochem. Mol. Biol. 134 (1), 171–180. doi: 10.1016/S1096-4959(02)00224-5
Song X., Si L., Sun X., Zhu X., Li Z., Li Y., et al. (2022). Rheological properties, thermal stability and conformational changes of collagen from sea cucumber (Apostichopus japonicas). Food Chem. 389, 133033. doi: 10.1016/j.foodchem.2022.133033
Sousa R. O., et al. (2020). Collagen from Atlantic cod (Gadus morhua) skins extracted using CO2 acidified water with potential application in healthcare. J. Polymer Res. 27 (3), 73. doi: 10.1007/s10965-020-02048-x
Suarez-Jimenez B., Albajes-Eizagirre A., Lazarov A., Zhu X., Harrison B. J., Radua J., et al. (2020). Neural signatures of conditioning, extinction learning, and extinction recall in posttraumatic stress disorder: a meta-analysis of functional magnetic resonance imaging studies. psychol. Med. 50 (9), 1442–1451. doi: 10.1017/S0033291719001387
Sugahara T., Ueno M, Goto Y, Shiraishi R, Doi M, Akiyama K, et al. (2006). Immunostimulation effect of jellyfish collagen. Bioscience Biotechnology Biochem. 70 (9), 2131–2137. doi: 10.1271/bbb.60076
Sugiura H., Yunoki S., Kondo E., Ikoma T., Tanaka J., Yasuda K. (2009). In vivo biological responses and bioresorption of tilapia scale collagen as a potential biomaterial. J. Biomaterials Science Polymer Edition 20 (10), 1353–1368. doi: 10.1163/092050609X12457418396658
Susanti E., Lutfiana N., Suharti, Retnosari R. (2019). Screening of proteolytic bacteria from tauco Surabaya based on pathogenicity and selectivity of its protease on milky fish (Chanos chanos) scales for healthy and halal collagen production. IOP Conf. Series: Materials Sci. Eng. 509 (1), 012044. doi: 10.1088/1757-899X/509/1/012044
Tabakaeva O. V., Tabakaev A. V., Piekoszewski W. (2018). Nutritional composition and total collagen content of two commercially important edible bivalve molluscs from the Sea of Japan coast. J. Food Sci. Technol. 55 (12), 4877–4886. doi: 10.1007/s13197-018-3422-5
Tamilmozhi S., Veeruraj A., Arumugam M. (2013). Isolation and characterization of acid and pepsin-solubilized collagen from the skin of sailfish (Istiophorus platypterus). Food Res. Int. 54 (2), 1499–1505. doi: 10.1016/j.foodres.2013.10.002
Tan Y., Chang S. K. (2018). Isolation and characterization of collagen extracted from channel catfish (Ictalurus punctatus) skin. Food Chem. 242, 147–155. doi: 10.1016/j.foodchem.2017.09.013
Tang Y., Jin S., Li X., Li X., Hu X., Chen Y., et al. (2018). Physicochemical properties and biocompatibility evaluation of collagen from the skin of giant croaker (Nibea japonica). Mar. Drugs 16 (7), 222. doi: 10.3390/md16070222
Tang C., Zhou K., Zhu Y., Zhang W., Xie Y., Wang Z., et al. (2022). Collagen and its derivatives: From structure and properties to their applications in food industry. Food Hydrocolloids 131, 107748. doi: 10.1016/j.foodhyd.2022.107748
Tang C., Xu Y., Zhou K., Xie Y., Ma Y., Li C., et al. (2023). Mechanism behind the deterioration in gel properties of collagen gel induced by high-temperature treatments: A molecular perspective. Food Res. Int. 171, 112985. doi: 10.1016/j.foodres.2023.112985
Tian M., Xue C., Chang Y., Shen J., Zhang Y., Li Z., et al. (2020). Collagen fibrils of sea cucumber (Apostichopus japonicus) are heterotypic. Food Chem. 316, 126272. doi: 10.1016/j.foodchem.2020.126272
Townsend S. E., Gannon M. (2019). Extracellular matrix–associated factors play critical roles in regulating pancreatic β-cell proliferation and survival. Endocrinology 160 (8), 1885–1894. doi: 10.1210/en.2019-00206
Tran H. D., Park K. D., Ching Y. C., Huynh C., Nguyen D. H. (2020). A comprehensive review on polymeric hydrogel and its composite: Matrices of choice for bone and cartilage tissue engineering. J. Ind. Eng. Chem. 89, 58–82. doi: 10.1016/j.jiec.2020.06.017
Vallejos N., González G, Troncoso E, Zúñiga RN. (2014). Acid and enzyme-aided collagen extraction from the byssus of Chilean mussels (Mytilus Chilensis): effect of process parameters on extraction performance. Food Biophysics 9 (4), 322–331. doi: 10.1007/s11483-014-9339-2
van Essen T. H., et al. (2013). A fish scale–derived collagen matrix as artificial cornea in rats: properties and potential. Invest. Ophthalmol. Visual Sci. 54 (5), 3224–3233. doi: 10.1167/iovs.13-11799
Veeruraj A., Liu L., Zheng J., Wu J., Arumugam M. (2019). Evaluation of astaxanthin incorporated collagen film developed from the outer skin waste of squid Doryteuthis singhalensis for wound healing and tissue regenerative applications. Materials Sci. Engineering: C 95, 29–42. doi: 10.1016/j.msec.2018.10.055
Venmathi Maran B. A., Jackson A., Ananthan T., Kumar M. (2023). “Biotechnological applications of jellyfish-derived products,” In Marine biotechnology: Applications in food, drugs and energy (Springer) 245–270. doi: 10.1007/978-981-99-0624-6_12
Viji P., Phannendra T., Jesmi D., Madhusudana Rao B., Dhiju Das P., George N. (2019). Functional and antioxidant properties of gelatin hydrolysates prepared from skin and scale of sole fish. J. Aquat. Food Product Technol. 28 (10), 976–986. doi: 10.1080/10498850.2019.1672845
Wahyu Y. I., Widjanarko S. B. (2018). Extraction optimization and characterization of acid soluble collagen from milkfish scales (Chanos chanos Forskal). Carpathian J. Food Sci. Technol. 10 (1), 125–135.
Wang H. (2021). A review of the effects of collagen treatment in clinical studies. Polymers 13 (22), 3868. doi: 10.3390/polym13223868
Wang J., Xu M., Liang R., Zhao M., Zhang Z., Li Y. (2015). Oral administration of marine collagen peptides prepared from chum salmon (Oncorhynchus keta) improves wound healing following cesarean section in rats. Food Nutr. Res. 59 (1), 26411. doi: 10.3402/fnr.v59.26411
Wang J., Chang Y., Wu F., Xu X., Xue C. (2018). Fucosylated chondroitin sulfate is covalently associated with collagen fibrils in sea cucumber Apostichopus japonicus body wall. Carbohydr. Polymers 186, 439–444. doi: 10.1016/j.carbpol.2018.01.041
Wibawa S. F., Retnoningrum D. S., Suhartono M. T. (2015). acid soluble collagen from skin of common carp (Cyprinus carpio L), red snapper (Lutjanus sp.) and milkfish (Chanos chanos). World Appl. Sci. J. 33 (6), 990–995. doi: 10.5829/idosi.wasj.2015.33.06.209
Woo J.-W., Yu S-J, Cho S-M, Lee Y-B, Kim S-B. (2008). Extraction optimization and properties of collagen from yellowfin tuna (Thunnus albacares) dorsal skin. Food Hydrocolloids 22 (5), 879–887. doi: 10.1016/j.foodhyd.2007.04.015
Wu J., Guo X., Liu H., Chen L. (2019). Isolation and comparative study on the characterization of guanidine hydrochloride soluble collagen and pepsin soluble collagen from the body of surf clam shell (Coelomactra antiquata). Foods 8, 1–13. doi: 10.3390/foods8010011
Xu Q., Torres JE, Hakim M, Babiak PM, Pal P, Battistoni CM, et al. (2021). Collagen-and hyaluronic acid-based hydrogels and their biomedical applications. Materials Sci. Engineering: R: Rep. 146, 100641. doi: 10.1016/j.mser.2021.100641
Xue Z., Shui M., Lin X., Sun Y., Liu J., Wei C., et al. (2022). Role of BDNF/proBDNF imbalance in postoperative cognitive dysfunction by modulating synaptic plasticity in aged mice. Front. Aging Neurosci. 14. doi: 10.3389/fnagi.2022.780972
Yamada S., Yoshizawa Y., Kawakubo A., Ikeda T., Yanagiguchi K., Hayashi Y. (2013). Early gene and protein expression associated with osteoblast differentiation in response to fish collagen peptides powder. Dental Materials J. 32 (2), 233–240. doi: 10.4012/dmj.2012-188
Yamada S., Yamamoto K., Ikeda T., Yanagiguchi K., Hayashi Y. (2014). Potency of fish collagen as a scaffold for regenerative medicine. BioMed. Res. Int. 2014, 1–8. doi: 10.1155/2014/302932
Yan M., Li B, Zhao X, Ren G, Zhuang Y, Hou H, et al. (2008). Characterization of acid-soluble collagen from the skin of walleye pollock (Theragra chalcogramma). Food Chem. 107 (4), 1581–1586. doi: 10.1016/j.foodchem.2007.10.027
Yang R. Q., Chen YL, Sun LC, Ou W, Liu HY, Zhang LJ, et al. (2022). Involvement of MMP-9 in collagen degradation of sea bass (Lateolabrax japonicus): Cloning, expression, and characterization. J. Food Sci. 88 (2), 638–649. doi: 10.1111/1750-3841.16402
Yemisken E., Jiménez-Rosado M, Perez-Puyana V, Sancar S, Bektaş S, Yildiz T, et al. (2023). Alternative sources of marine bioactive compounds from the Black Sea: Isolation and characterization of fish skin collagen from Neogobius melanostomus (Pallas 1814)(Perciformes: Gobiidae). Regional Stud. Mar. Sci. 60, 102887. doi: 10.1016/j.rsma.2023.102887
Yoo S.-J., Woo CHo S.-M., Kim J.-W., Han S.-H., Ahn Y.-N., J. -R., et al. (2008). Processing and physicochemical properties of collagen from yellowfin tuna (Thunnus albacares) abdominal skin. Korean J. Fisheries Aquat. Sci. 41 (6), 427–434. doi: 10.5657/kfas.2008.41.6.427
Youn H. S., Shin T. J. (2009). Supramolecular assembly of collagen fibrils into collagen fiber in fish scales of red seabream, Pagrus major. J. Struct. Biol. 168 (2), 332–336. doi: 10.1016/j.jsb.2009.08.001
Zaelani B., Safithri M., Tarman K., Setyaningsih I. (2019). “Collagen isolation with acid soluble method from the skin of Red Snapper (lutjanus sp.),” in IOP Conference Series: Earth and Environmental Science. The 2nd International Conference on Integrated Coastal Management and Marine Biotechnology, (West Java, Indonesia: IOP Publishing) 241, 23–24. doi: 10.1088/1755-1315/241/1/012033
Zeng S.-k., Zhang C.-H., Lin H., Yang P., Hong P.-Z., Jiang Z. (2009). Isolation and characterisation of acid-solubilised collagen from the skin of Nile tilapia (Oreochromis niloticus). Food Chem. 116 (4), 879–883. doi: 10.1016/j.foodchem.2009.03.038
Zhang Z., Wang J., Ding Y., Dai X., Li Y. (2011). Oral administration of marine collagen peptides from Chum Salmon skin enhances cutaneous wound healing and angiogenesis in rats. J. Sci. Food Agric. 91 (12), 2173–2179. doi: 10.1002/jsfa.4435
Zhao Z., Yao M., Wei L., Ge S. (2020). Obesity caused by a high-fat diet regulates the Sirt1/PGC-1α/FNDC5/BDNF pathway to exacerbate isoflurane-induced postoperative cognitive dysfunction in older mice. Nutr. Neurosci. 23 (12), 971–982. doi: 10.1080/1028415X.2019.1581460
Zheng M.-H., Zheng J. (2019). Sponge (Porifera) collagen for bone tissue engineering. Marine-Derived Biomaterials Tissue Eng. Appl. p, 247–283. doi: 10.1007/978-981-13-8855-2_12
Zhong M., Chen T., Hu C., Ren C. (2015). Isolation and characterization of collagen from the body wall of sea cucumber stichopus monotuberculatus. J. Food Sci. 80 (4), C671–C679. doi: 10.1111/1750-3841
Zhu B.-W., Dong X-P, Zhou D-Y, Gao Y, Yang J-F, Li D-M, et al. (2012). Physicochemical properties and radical scavenging capacities of pepsin-solubilized collagen from sea cucumber Stichopus japonicus. Food Hydrocolloids 28 (1), 182–188. doi: 10.1016/j.foodhyd.2011.12.010
Zhuang Y., Hou H., Zhao X., Zhang Z., Li B. (2009). Effects of collagen and collagen hydrolysate from jellyfish (Rhopilema esculentum) on mice skin photoaging induced by UV irradiation. J. Food Sci. 74 (6), H183–H188. doi: 10.1111/j.1750-3841.2009.01236.x
Keywords: collagen, marine organisms, collagen production, collagen application, application prospects
Citation: Barzkar N, Sukhikh S, Babich O, Venmathi Maran BA and Tamadoni Jahromi S (2023) Marine collagen: purification, properties and application. Front. Mar. Sci. 10:1245077. doi: 10.3389/fmars.2023.1245077
Received: 23 June 2023; Accepted: 04 September 2023;
Published: 28 September 2023.
Edited by:
Saravana Periaswamy Sivagnanam, Teagasc Food Research Centre, IrelandReviewed by:
Selvakumari Ulagesan, Pukyong National University, Republic of KoreaMonjurul Haq, Jashore University of Science and Technology, Bangladesh
Eva Martins, Universidade Católica Portuguesa, Portugal
Copyright © 2023 Barzkar, Sukhikh, Babich, Venmathi Maran and Tamadoni Jahromi. This is an open-access article distributed under the terms of the Creative Commons Attribution License (CC BY). The use, distribution or reproduction in other forums is permitted, provided the original author(s) and the copyright owner(s) are credited and that the original publication in this journal is cited, in accordance with accepted academic practice. No use, distribution or reproduction is permitted which does not comply with these terms.
*Correspondence: Noora Barzkar, bm9vcmEuYmFyemthckBnbWFpbC5jb20=; YmFyemthci5waGRAaG9ybW96Z2FuLmFjLmly