- 1Fisheries College, Jimei University, Xiamen, China
- 2Fujian Provincial Key Laboratory of Marine Fishery Resources and Eco-environment, Fisheries College of Jimei University, Xiamen, China
Laminarin has antioxidant and immunomodulatory properties and favorably impacts gut microbial composition, providing a potential solution for the treatment of intestinal diseases in fish. The aim of this study was to investigate the effects of laminarin on the growth and intestinal health of juvenile spotted seabass, Lateolabrax maculatus. A total of 450 juveniles (initial body weight: 7.14 ± 0.10 g) were randomly divided into 6 groups with 3 replicates per group and 25 fish per replicate. Six diets were prepared with laminarin supplementation at doses of 0% (Control), 0.4% (P0.4), 0.8% (P0.8), 1.2% (P1.2), 1.6% (P1.6), and 2% (P2). Each group was fed the corresponding diet for 8 weeks. The results indicated that dietary laminarin supplementation of 0.4-1.6% enhanced the specific growth rate (SGR), weight gain rate (WGR), and feed conversion ratio (FCR) of juvenile spotted seabass, and the difference was significant in the P0.8 group (P<0.05). Significantly higher intestinal amylase activity was measured in P0.8 compared with the control group. Trypsin activity was significantly increased in P0.4 and P0.8 groups in contrast to the control (P<0.05). Lipase activity was significantly increased in P0.4, P0.8, P1.6, and P2 groups in contrast to the control (P<0.05). Total antioxidant capacity was significantly increased in the P0.8, P1.2, and P1.6 groups compared to the control group (P<0.05). The P0.8 group exhibited significant increases in reduced glutathione, alkaline phosphatase, and lysozyme levels (P<0.05), whereas the concentrations of diamine oxidase and D-lactate were significantly decreased (P<0.05). Furthermore, intestinal villus height, villus width, and crypt depth were significantly increased in P0.8 and P2 groups (P<0.05), and muscular thickness was significantly increased in the P1.2 group (P<0.05). Intestinal microbial analysis revealed that the alpha diversity of the laminarin supplemented groups was significantly higher than that of the control group. Moreover, the abundance of intestinal beneficial bacteria Lactobacillus and Klebsiella in P0.4 and P0.8 groups was significantly increased (P<0.05), indicating that laminarin altered the composition of intestinal flora and the abundance of dominant bacteria, with a low dose being more conducive to the formation of beneficial bacteria. In conclusion, dietary laminarin supplementation can improve the growth performance and intestinal function of juvenile spotted seabass. Based on the regression analyses of weight gain rate and specific growth rate, the optimal supplemental level of laminarin was estimated to be 0.97% and 0.98%, respectively.
1 Introduction
Spotted seabass (Lateolabrax maculatus) is a carnivorous fish with fast growth and delicious meat (Cheng et al., 2021). Spotted seabass is an economically important fish in China, with a production exceeding 199,000 tons in 2021 (Cheng et al., 2021; Zhou et al., 2023). Nevertheless, with the increasing breeding scale and density, there are numerous challenges encountered during the breeding process of spotted seabass, such as pollution of aquaculture water, improper feed feeding, and the high proportion of plant protein instead of fish meal in the feed, which may cause intestinal diseases (Zhou et al., 2023). Intestinal diseases have high morbidity and mortality rates, which has become a key factor restricting the development of aquaculture industry (Hou and Ma, 2023). Before 2020, China primarily used antibiotics and chemicals to prevent and treat intestinal diseases (Hou and Ma, 2023). However, the problem of food safety caused by drug use is becoming increasingly prominent. In order to improve the safety of aquatic foods, the use of various antibiotics and chemicals in aquaculture is prohibited, which increases the difficulty of fish intestinal disease prevention and control (Yang et al., 2023). Therefore, it became urgent for aquaculture production to find alternatives to antibiotics in order to maintain gut health.
The intestine is an important immune organ for fish, with the crucial function of preventing intestinal endotoxin and bacterial invasion (Li et al., 2020). Additionally, the gut serves as the primary site for nutrient absorption in fish, and its digestive and absorptive capacities are closely linked to their growth (Liu et al., 2022a). Intestinal damage may lead to immune system disorders, weakened disease resistance, decreased appetite, and slow growth in fish (Tian et al., 2018). In general, intestinal health includes the efficiency of intestinal digestion and absorption, the balance of intestinal microbiota, and effective intestinal barrier and immune function (Bischoff, 2011).
Brown algae contain a variety of bioactive substances, such as proteins, minerals, vitamins, polysaccharides, polyunsaturated fatty acids, etc (Dobrinčić et al., 2020). Algae polysaccharides have attracted much attention due to their antibacterial, antiviral, anticoagulant, immunomodulating, antioxidant, and anticarcinogenic activities, especially laminarin (Dobrinčić et al., 2020). Laminarin is a carbohydrate containing β-1, 3-glucan, which can be formed by combining β-1, 3-glucoside and β-1, 6-glucoside (Morales-Lange et al., 2015). It exists in the intercellular and cytoplasmic spaces of laminaria, and its content varies with the season and habitat, up to 32% of dry weight (Yin et al., 2014). Laminarin not only exhibits various pharmacological activities such as anti-oxidation, anti-tumor, anti-coagulation, anti-cancer, immune regulation, anti-obesity, anti-diabetes, anti-inflammatory and regulation of intestinal flora, but also has the characteristics of biodegradation, good biocompatibility and natural low toxicity (Vidhya Hindu et al., 2019; Karuppusamy et al., 2022). Studies have demonstrated that dietary laminarin can significantly improve the growth performance and immune capacity of grouper (Epinephelus coioides) and rainbow trout (Oncorhynchus mykiss) (Morales-Lange et al., 2015; Abdel-Mawla et al., 2023). Likewise, the addition of 4 g kg-1 and 8 g kg-1 laminarin augmented antioxidant enzyme and digestive enzyme activities and promoted the growth of Ictalurus punctatu (Jiang et al., 2021). Additionally, it has been reported that laminarin exerts a beneficial impact on the intestinal health of animals (Devillé et al., 2007). McDonnell et al. and Walsh et al. found that dietary laminarin can improve intestinal morphology and increase the number of Lactobacillus in piglets (McDonnell et al., 2010; Walsh et al., 2013). In aquatic animals, Wu et al. found that dietary supplementation of 5 g Kg-1 laminarin significantly increased the beneficial intestinal bacteria Bacteroide, Comamonas and Mycoplasma abundance in juvenile largemouth bass (Wu et al., 2023). Abdel-Mawla et al. (2023) reported that the addition of laminarin could improve the intestinal morphology and increase the number of intestinal goblet cells of Thinlip Grey Mullet (Liza ramada). These studies suggest that laminaria polysaccharide holds promising potential for enhancing the growth of animals and promoting intestinal health maintenance. At present, there are few studies on the effect of laminarin on intestinal function of aquatic animals, and there is no report on whether laminarin has promoting effect on intestinal health of spotted seabass. The present study aimed to explore the effect of different levels of laminarin supplementation on the growth and intestinal health of juvenile spotted seabass by evaluating changes in intestinal morphology, growth indices, intestinal digestive enzyme indexes, antioxidant indexes, immune indexes, mucosal permeability indexes, and intestinal flora.
2 Materials and methods
2.1 Experimental diets
Laminarin was purchased from Xi ‘an Qingzhi Biotechnology Co., LTD., with a purity of at least 50%. Laminarin was extracted from laminaria by the warm-water extraction method. As shown in Table 1, a basal diet with a protein content of 46% and a fat content of 10% was prepared with reference to the nutritional requirements of spotted seabass (Ai et al., 2004). Six diets were prepared with the supplementation of laminarin: 0 (control group), 0.4% (P0.4 group), 0.8% (P0.8 group), 1.2% (P1.2 group), 1.6% (P1.6 group) and 2% (P2 group). Laminarin was used at the expense of wheat flour. All powder raw materials were mashed with a grinder (FJ-300, Ruizong Machinery Manufacturing Co., Ltd., Jiangyin, China) and passed through a 200-μm screen. Mix powdered raw materials according to the formula proportion, then add fish oil, soybean oil, and about 35% water to mix. Laminarin added to each group was completely dissolved in water and added to feed materials. Hard pellet feed with diameter 2.5 mm was produced by a double-screw extruder (F-76, Guangzhou Huagong Optical Mechanical and Electrical Technology Co., Ltd., China). Finally, the experimental feed was dried in an oven at 55°C (WGL–625B, Tianjin Test Instrument Co., Ltd.) to about 10% moisture and then stored at -20°C.
2.2 Fish and feeding management
Spotted seabass juveniles were purchased from a commercial farm (Zhangpu Jin Xing Farm, Zhangzhou, Fujian, China). The fish were fed with the basic diet for 3 weeks to accommodate fish to the experimental diets in a 1200 L tank. After 3 weeks of temporary breeding of spotted seabass, 450 healthy fish with an initial body weight of 7.14 ± 0.10 g were randomly allocated into six groups, each with three replicates and 25 fish per replicate. During the experiment, the fish were hand-fed to apparent satiety twice daily (7:00 and 17:00) for 8 weeks. Daily water changes were performed at a rate of approximately 30% per tank to maintain optimal water quality. Throughout the experiment, physicochemical parameters were closely monitored and maintained, with water temperature was kept at about 29 ± 1°C, salinity ranging between 0.5 and 2 ppt, pH levels between 7.6 and 8, dissolved oxygen levels at 7 mg/L, and ammonia nitrogen concentration consistently below 0.3 mg/L.
2.3 Sample collection
After being fed for 8 weeks, the fish were fasted for 24 hours and then anesthetized with eugenol (1:10000). The total number of fish in each tank was recorded, and the body length and weight of each fish were measured for calculating weight gain (WG), specific growth rate (SGR), feed conversion ratio (FCR), and condition factor (CF). Eight randomly selected fish from each tank were used to collect blood samples from the caudal vein using a syringe pre-treated with heparin. The blood sample was transferred to a 1.0 mL sterile centrifuge tube and placed at 4°C for 20 hours. Serum was separated after centrifugation at 4°C and 836×g for 10 min and immediately stored at -80 °C until analysis. After collecting the blood sample, the surface of each fish was gently wiped with a 70% alcohol-soaked cotton ball. Subsequently, the fish (8 fish) were dissected, and the midgut samples were carefully collected. The midgut samples of 2 fish were fixed in a 4% paraformaldehyde solution for 24 hours to prepare tissue slices, and the midgut samples of the other 6 fish were washed with 0.9% normal saline and stored at -80°C to detect biochemical indices and microbial diversity (Three fish were utilized for the detection of biochemical indices in midgut samples, while the remaining three fish were used for the analysis of intestinal microbial composition).
2.4 Growth performance evaluation
The growth performance indexes in this experiment included weight gain (WG), specific growth rate (SGR), condition factor (CF), and feed conversion ratio (FCR), and the calculation formula for each index was as follows:
In the formula, W0 and WT respectively represent the initial body weight (g) and final body weight (g) of the experimental fish; d is the number of feeding days (d); WB and L were wet weight (g) and body length (cm) of experimental fish, respectively; F is the total food intake of experimental fish during feeding (g).
2.5 Biochemical indices analysis
Serum contents of diamine oxidase (DAO) and D-lactate (D-Lac) were measured spectrophotometrically using commercial test kits according to manufacturers (Nanjing, Nanjing Jiancheng Bioengineering Institute. Catalog No: DAO: A088-2-1, D-Lac: H263-1-2). Intestinal samples were homogenized with normal saline (0.86% concentration) at a ratio of 1:9, and the supernatant was used to determine the contents of total antioxidant capacity (T-AOC), superoxide dismutase (SOD), malondialdehyde (MDA), reduced glutathione (GSH), alkaline phosphatase (AKP), acid phosphatase (ACP), lysozyme (LZM), amylase (AMS), trypsin (TRS), and lipase (LPS) with commercial kits. All kits in this study were purchased from the Nanjing Jiancheng Bioengineering Institute (Catalog No: T-AOC: A015-2-1, SOD: A001-3, MDA: A003-1, GSH:A006-2-1, AKP:A059-2-2, ACP:A060-2-2, LZM:A050-1-1, AMS: C016-1-1, LPS: A054-2-1,TRS: A080-2).
2.6 Histological structure of the intestine
Two fish was randomly selected from each tank for dissection, and the midgut was taken out and fixed with 4% formaldehyde for 24h. Intestinal specimens were dehydrated with ethanol and then embedded in paraffin. After the paraffin was cured, the tissue sections were sliced into 5μm thickness using a microtome (LEiCA RM2016, Leica, China). Subsequently, the sections were flattened in water at 40°C and dried in an oven at 60°C. After drying, the paraffin sections were dyed with hematoxylin and eosin solutions, and the dye solution was rinsed with water. After drying, it was wrapped with neutral glue to make HE slices. The sections were placed under an optical microscope (NIKON ECLIPSE CI, Nikon Japan) to observe intestinal morphological parameters, and then Image pro plus 6.0 software was used to measure intestinal villus height (VH), villus width (VW), muscle thickness (MT), and crypt depth.
2.7 Intestinal microbiota DNA extraction and sequencing
Total intestinal bacterial DNA was extracted using a PowerSoil DNA Isolation Kit (MoBio Laboratories, Carlsbad, CA) according to the manufacturer’s instructions. DNA quality and concentration are tested using a microspectrophotometer (Nano800, Jiapeng, China). Primers 338F (5 ‘-ACTCCTACGGGAGGCAGCAG-3’) and 806R (5 ‘-GGACTACNNGGG TATCTAAT-3’) were used to amplify the V3-V4 hypervariant region of bacterial 16S rRNA gene. The amplification system was as follows: 3μL template DNA, 1μL forward primers and reverse primers, 3μL BSA, 12.5μL 2xTaq Plus Master Mix, and 4.5μL ddH2O. The procedure of amplification reaction was 95 °C for 5 min, followed by 95 °C for 45 s (32 cycles), 55 °C for 50 s (32 cycles), 72 °C for 45 s (32 cycles), and 72 °C for 10 min. The amplified products were purified using AMPure XP nucleic acid purification Kit (Agencourt, France). After that, the samples were sent to Beijing Allgwegene Technology Co., Ltd. for intestinal microbial sequencing and analysis.
2.8 Statistical analysis
Excel 2018 software (Microsoft Corp., USA) was used to collect the experimental data, and SPSS 22 (International Business Machines Corp., USA) software was used to perform one-way ANOVA to compare the differences among the groups. If there are significant differences in the analysis results, Duncan’s multiple is used for testing, P<0.05 is considered a significant difference. Results are shown using mean ± standard deviation.
3 Results
3.1 Growth performance
The effects of laminarin on the growth performance of juvenile spotted seabass are shown in Table 2. WG and SGR in P0.4, P0.8, P1.2 and P1.6 group were improved compared with the control group, and significantly in P0.8 group (P<0.05). FCR decreased to different degrees in laminarin supplemented groups, and P0.8 group was significantly lower than control group (P<0.05). In addition, the supplementation of laminarin had no significant effect on CF of juvenile spotted seabass (P>0.05). Based on the regression analyses of weight gain rate and specific growth rate, the optimal supplemental level of dietary laminarin was estimated to be 0.97% and 0.98% respectively (Figure 1).
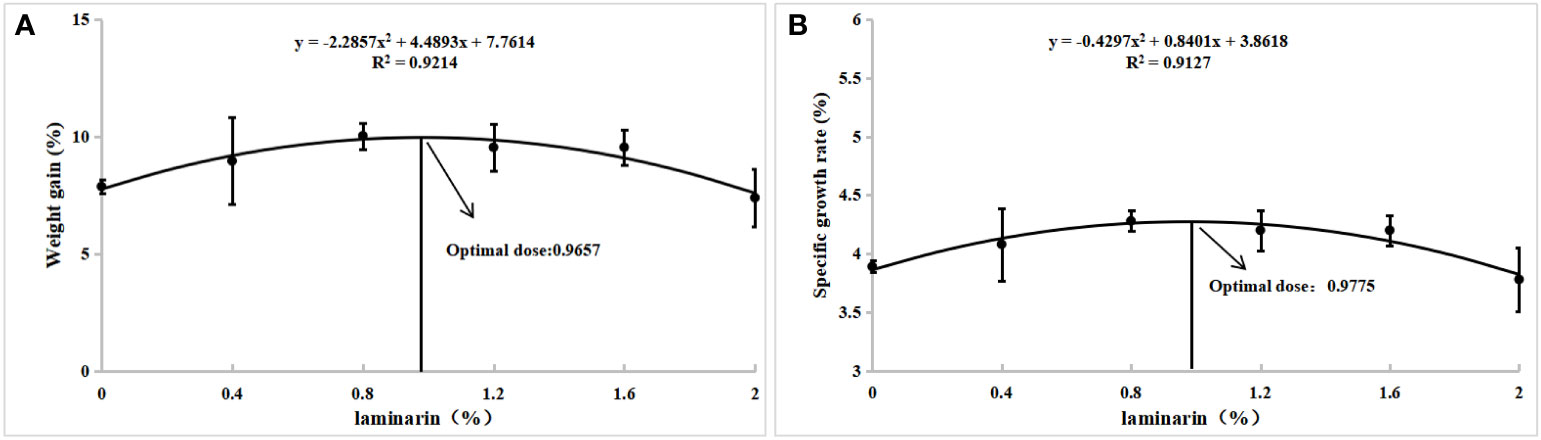
Figure 1 With weight gain rate (A) and specific growth rate (B) as evaluation indexes, quadratic polynomial regression analysis was used to determine the optimal additive amount of laminarin in the diet of L. maculatus.
3.2 Intestinal digestive enzyme activity and intestinal barrier function
The effects of laminarin on the intestinal digestive enzyme activities of spotted seabass are shown in Table 3. Significantly higher intestinal amylase activity was measured in P0.8 compared with the control group. Trypsin activity was significantly increased in P0.4 and P0.8 groups in contrast to the control. The LPS activity of P0.4, P0.8, P1.6, and P2 groups were significantly increased (P<0.05). Moreover, dietary supplementation with laminarin reduced the contents of DAO and D-Lac in serum, which were significantly decreased in the P0.8 group (P<0.05) (Table 3).
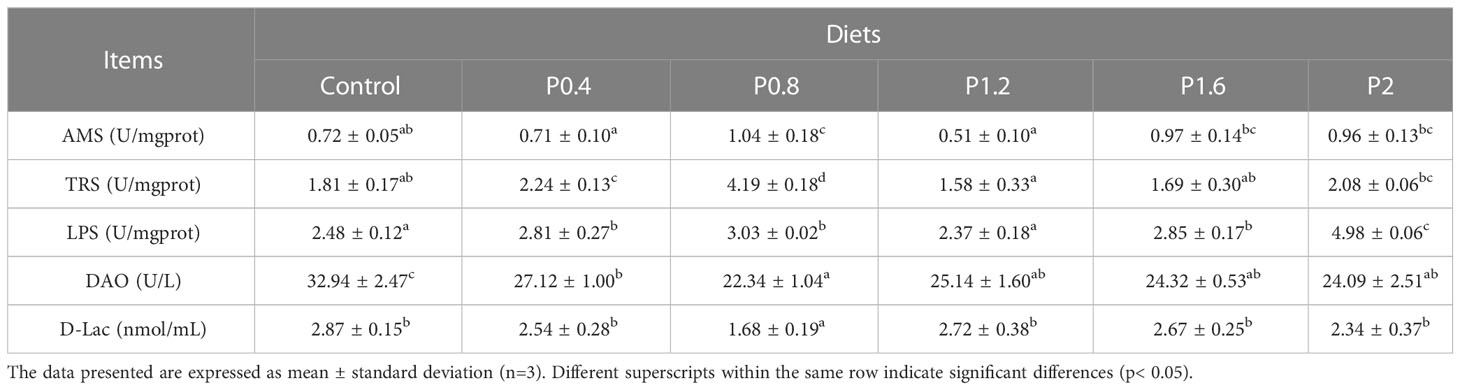
Table 3 Effects of laminarin on intestinal digestive enzyme indices and serum intestinal barrier function-related indices in L. maculatus.
3.3 Intestinal antioxidant capacity
The T-AOC of juvenile spotted seabass was significantly higher in the P0.4, P0.8, P1.2, and P1.6 groups in contrast to the control (P<0.05). Dietary laminarin increased intestinal GSH activity in spotted seabass, and it was significantly increased in the P0.8 group (P<0.05) (Table 4). No significant differences were observed in the activities of SOD and MDA among the dietary treatments (P > 0.05) (Table 4).
3.4 Intestinal immunity status
As shown in Table 5. Dietary laminarin can significantly affect intestinal AKP and LZM activities. Compared with the control group, intestinal AKP activity was significantly increased in the P0.4, P0.8, P1.6, and P2 groups (P<0.05). Intestinal LZM activity increased significantly among the laminarin supplemented groups (P<0.05). However, dietary laminarin had no impact on intestinal ACP activity (P > 0.05).
3.5 Morphological observation of intestine
Morphological observations of the intestine are shown in Figure 2. Compared with the control group, dietary laminarin increased intestinal villus height, villus width, and crypt depth, which were significantly increased in the P0.8 and P2 groups (P<0.05) (Table 6). Muscle thickness in the P1.2 group was significantly higher than that in the control group (P<0.05) (Table 6).
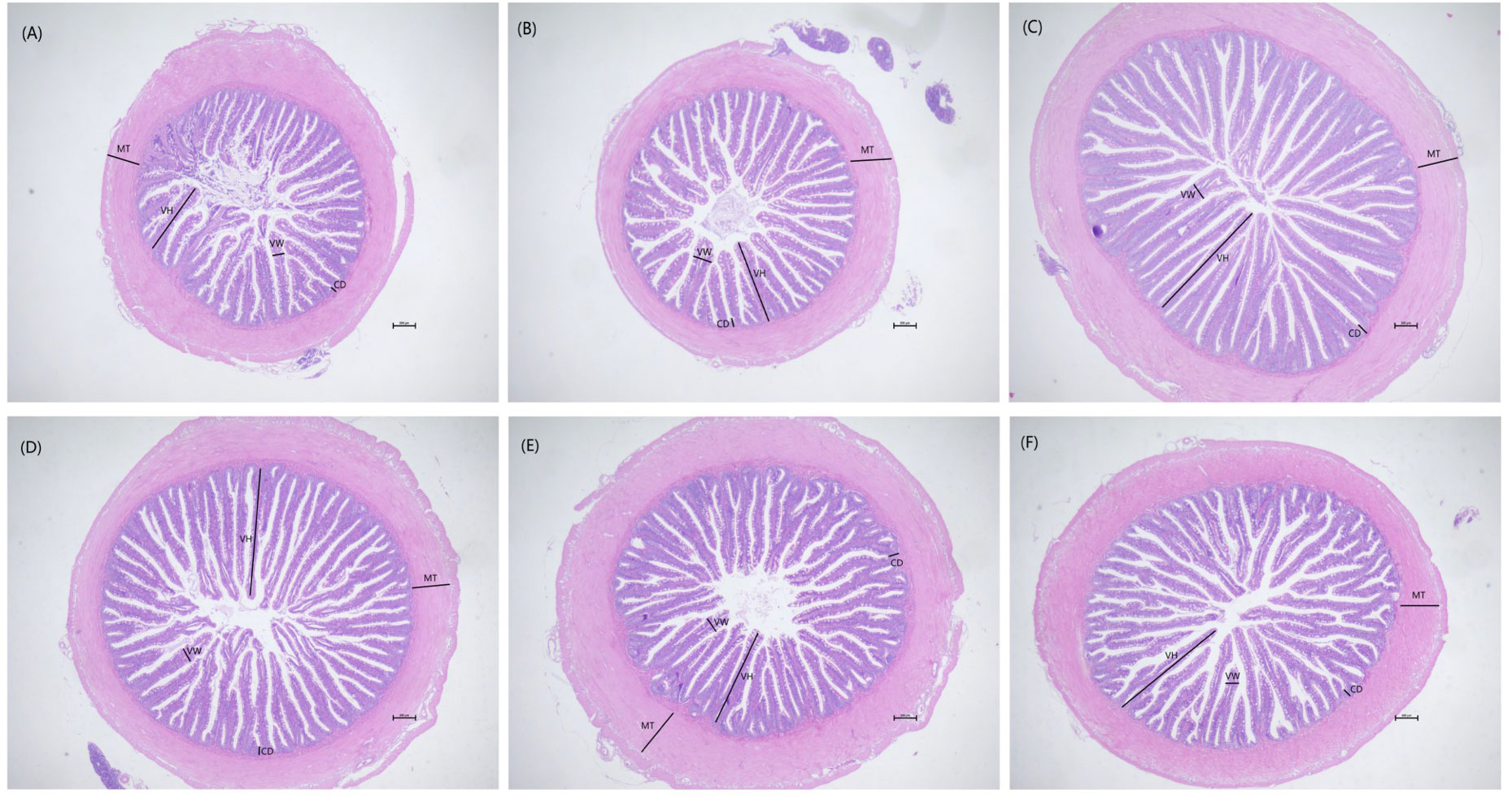
Figure 2 Effects of 0% (A), 0.4% (B), 0.8% (C), 1.2% (D), 1.6% (E), 2% (F) of laminarin on intestinal morphology of L. maculatus (HE slice, magnification × 40). VH: villus height, VW: villus width, CD: crypt depth, MT: muscle thickness.
3.6 Microbiota community characterization in the intestine
As shown in Figure 3, the amount of OTU in the Control, P0.4, P0.8, P1.2, P1.6, and P2 groups was 43, 41, 38, 45, 57, and 50, respectively. And there were 19 identical outs among all groups. The alpha diversity index was used to evaluate the microbial diversity of different feeding groups. Compared with the control group, the chao1 index in the P1.6 group was significantly increased, and the Shannon and Simpson indexes were significantly increased among the polysaccharide groups (P<0.05) (Table 7). PCA analysis showed that there was a significant separation between the polysaccharide groups and the control group, and the similarity from high to low was as follows: P0.4>P1.2>P2>P0.8>P1.6 (Figure 4).
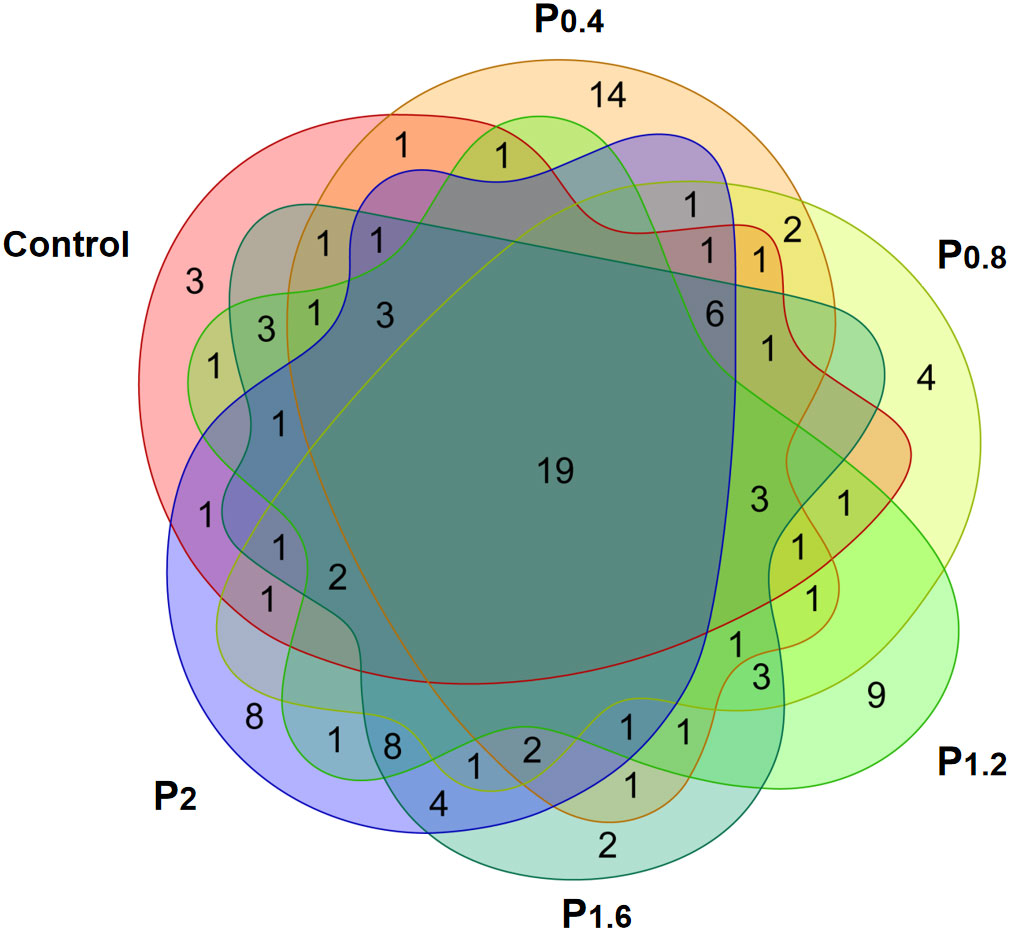
Figure 3 Effects of laminarin on the number of OTUs in the intestinal microbe of L. maculatus, the common covered part represented the number of OUTs common among the groups, and the uncovered part represented the number of OUTs unique to the group.
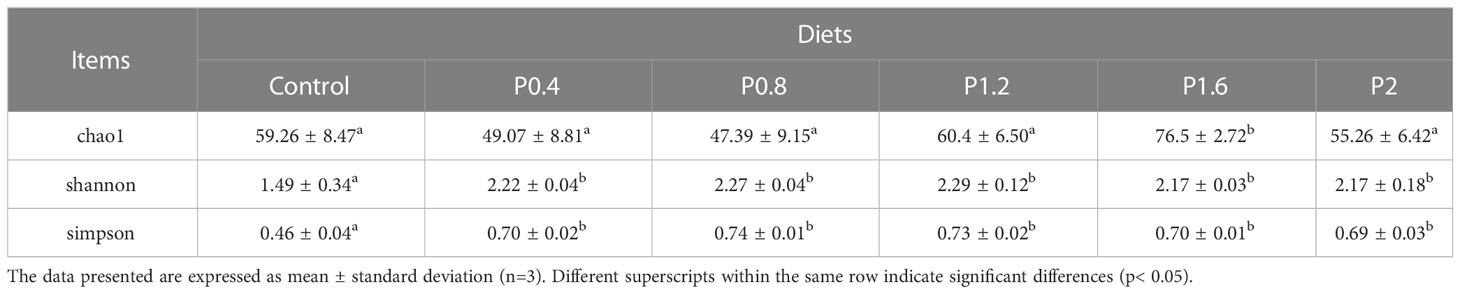
Table 7 Effects of laminarin on the alpha diversity indices (Chao1, Shannon and Simpson) of intestinal microbiome in L. maculatus.
In this study, Firmicutes, Proteobacteria, Bacteroidetes, Fusobacteria, Chloroflexi, Actinobacteria, and other unidentified bacteria were detected in the intestinal tract of the spotted seabass (Figure 5). Proteobacteria and Firmicutes were the dominant bacteria in the guts of spotted seabass at the phylum level, accounting for 15.9% and 83.9% of the total flora in the control group, respectively. The abundance of Proteobacteria increased significantly when the supplemental level reached 1.2% and above, while the abundance of Firmicutes decreased significantly when the supplemental level reached 1.2% and above (P<0.05) (Figure 6). At the genus level, compared with the control group, the abundance of beneficial bacteria Lactobacillus was significantly increased in the P0.4, P0.8, P1.6, and P2 groups, the abundance of Klebsiella was significantly increased in the P0.4 and P0.8 groups, and the abundance of Bacillus was significantly decreased among the polysaccharide groups(P<0.05) (Figures 7, 8).
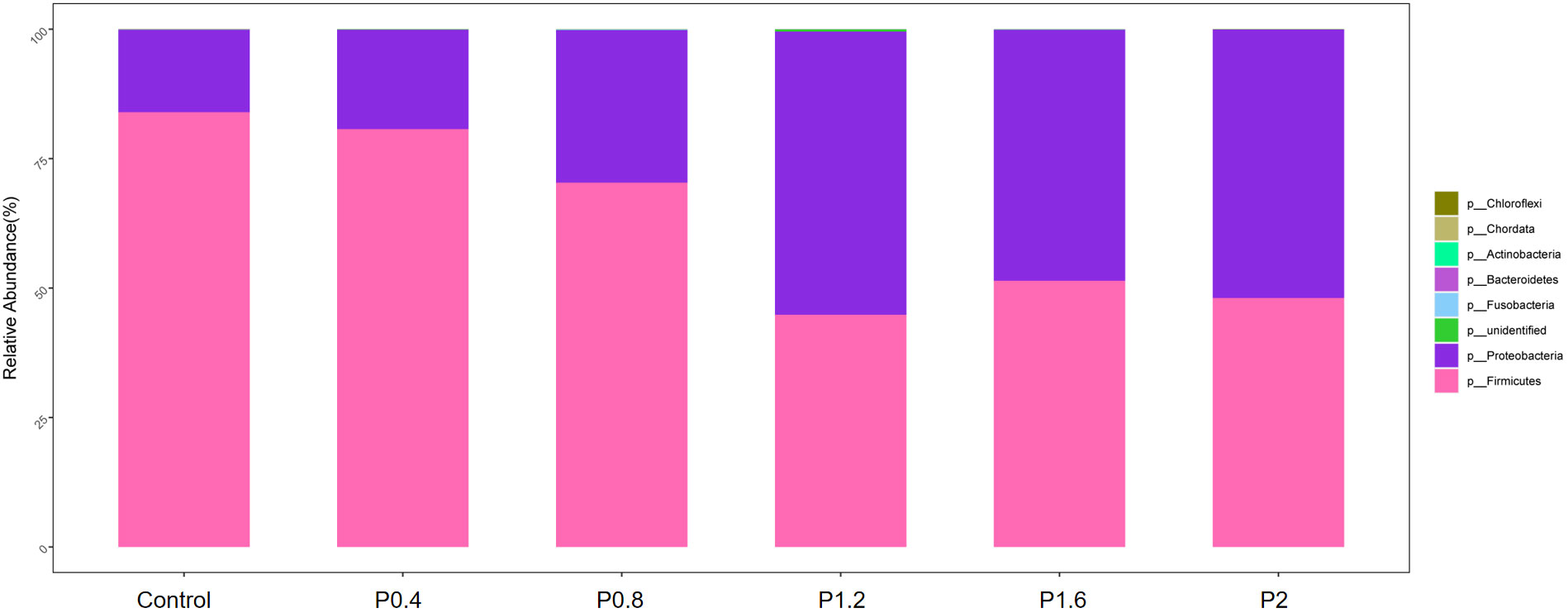
Figure 5 The effects of laminarin on species composition and abundance at the phylum levels of the gut microbiota of spotted sea bass.
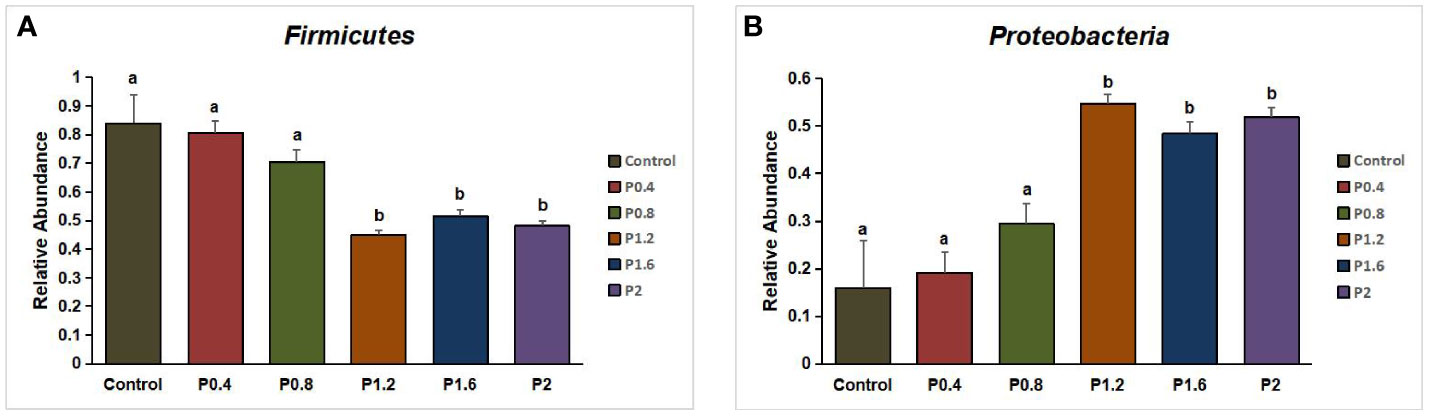
Figure 6 Effect of laminarin on the abundance of Firmicutes (A) and Proteobacteria (B) in L. maculatus. The data presented are expressed as mean ± standard deviation (n=3). Different superscripts within the same row indicate significant differences (p< 0.05).
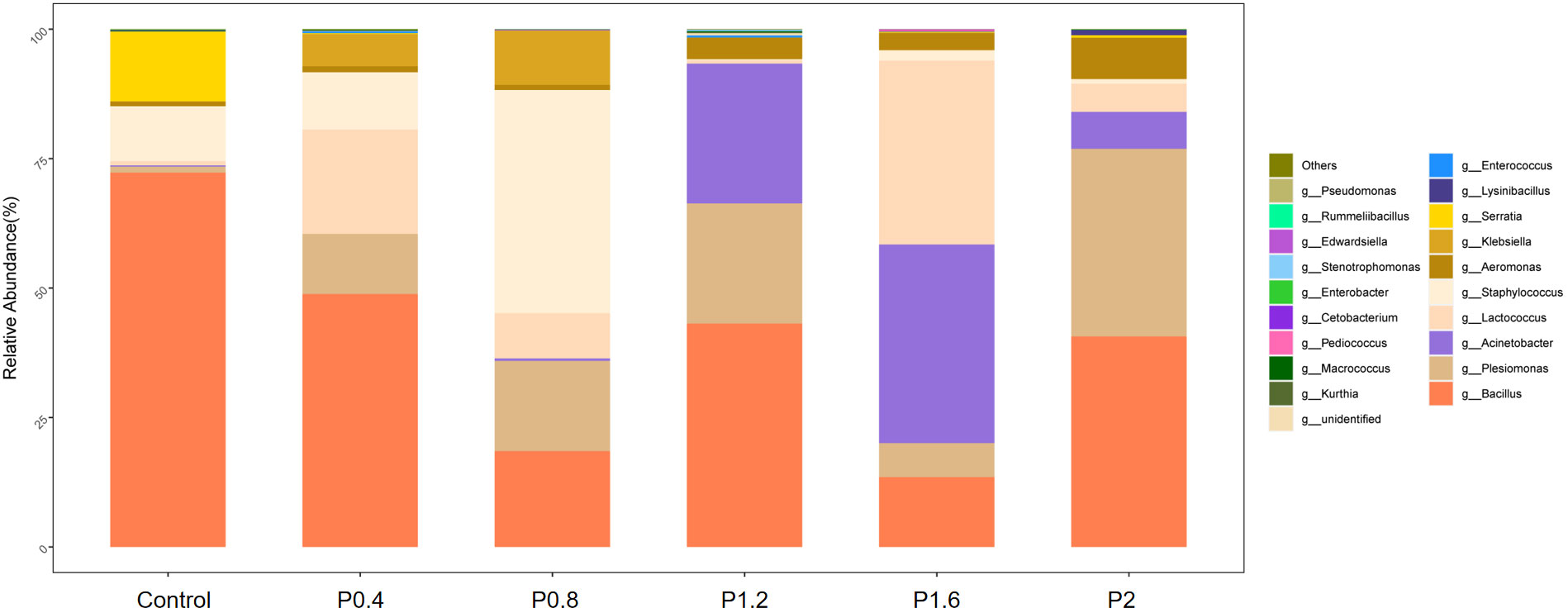
Figure 7 The effects of laminarin on species composition and abundance at the genus levels of the gut microbiota of L. maculatus.
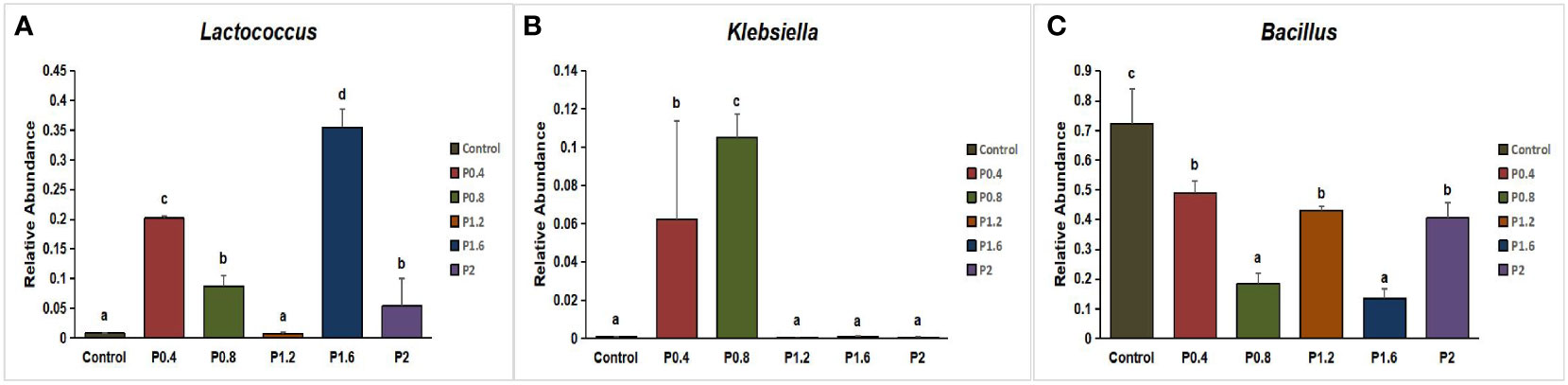
Figure 8 Effect of laminarin on the abundance of beneficial bacteria Lactobacillus (A), Klebsiella (B) and Bacillus (C) at the genus level in the gut of L. maculatus. The data presented are expressed as mean ± standard deviation (n=3). Different superscripts within the same row indicate significant differences (p< 0.05).
4 Discussion
Laminarin is a functional polysaccharide with a variety of biological activities, including antibacterial, antioxidant, and anti-inflammatory, which has been reported to improve growth performance in animals such as piglets and broilers (Heim et al., 2014; Rattigan et al., 2020; Venardou et al., 2021). In aquatic animals, studies have shown that dietary 0.5% laminarin can significantly increase the weight gain rate and condition factor of Epinephelus coioides (Li et al., 2015b). Jiang et al. (2021) found that dietary supplementation of 4g kg−1 and 8g kg−1 laminarin significantly increased the weight gain rate and specific growth rate of Ictalurus punctatus. Yin et al. (2014) found that supplementation of laminarin (0.5%-1.5%) in the basic diet regulated the immune response and stimulated the growth of the grouper, Epinephelus coioides. In this study, dietary supplementation of 0.4-1.6% laminarin increased the weight gain rate and specific growth rate of the spotted seabass, and reduce its feed coefficient, and the effect was significant in the P0.8 group (0.8%). The results are similar to those of previous studies. Additionally, in a recent study by Wu et al. (2023), the effects of dietary supplementation of laminarin at levels of 0.5%, 1%, and 1.5% were investigated in largemouth bass over a duration of 28 days. Surprisingly, the results indicated that laminarin had no significant impact on the growth of the fish. The contrasting outcome of their study compared to our findings may be attributed to variations in culture cycle and fish species. Furthermore, our study unveiled also that an elevated dietary concentration of laminarin hindered the growth of spotted seabass, indicating the potentially deleterious consequences of incorporating a higher dose of laminarin (2%) on the fish’s growth and metabolic processes. Similarly, the growth performance of the largemouth bass was decreased by a high dietary dose of laminarin (1.5%) (Wu et al., 2023). In conclusion, our study suggests that dietary supplementation with laminarin promotes the growth of spotted seabass. The regression analysis results, utilizing weight gain rate and specific growth rate as evaluation indicators, have revealed that the optimal level of laminarin supplementation to the basal diet for spotted seabass is determined to be 0.97%.
The activity of fish digestive enzymes is an important index to measure the nutrient composition, digestion, and absorption of feed (Deng et al., 2021). Previous studies have reported that algal polysaccharides can improve immune function and digestive enzyme activity in aquatic animals to promote growth, including Apostichopus japonicu, Litopenaeus vanname, Paralichthys olivaceus, and Penaeus monodon (Ragaza et al., 2015; Sivagnanavelmurugan et al., 2015; Díaz et al., 2017; Shahabuddin et al., 2017). In this study, the supplementation of 0.8% laminarin significantly increased the intestinal amylase and trypsin activities of spotted seabass. The results are comparable with those of Abdel-Mawla et al, who stated found that dietary supplementation of 338-761 mg kg-1 laminarin enhanced the digestive enzyme activity of Liza ramada (Abdel-Mawla et al., 2023). At the same time, the results of this study were consistent with the results of growth performance, indicating that an appropriate amount of laminarin can enhance digestive enzyme secretion and improve nutrient digestion and absorption in spotted seabass, thereby promoting their growth. Further, Karuppusamy et al. reported that laminarin can regulate fat metabolism and reduce fat deposition (Karuppusamy et al., 2022). The lipase activity in the P0.4, P0.8, P1.6, and P2 groups was significantly increased in this study, indicating that laminarin promoted fat conversion, which confirmed Karuppusamy’s view.
The intestine is the main place for fish to utilize feed nutrients, and it is also an important immune organ for fish, whose function depends on the integrity of the intestinal physical barrier (Li et al., 2015a). The physical barrier is mainly composed of intestinal epithelial cells and tightly connected complexes between cells (Torrecillas et al., 2014). As an important part of the intestinal physical barrier, intestinal epithelial cells are vulnerable to oxidative damage, which may damage the structural integrity of the intestinal physical barrier and reduce intestinal immune function (Hoyle et al., 2007; Chen et al., 2009). Antioxidant defense is the basic cellular protection mechanism of fish tissue against oxidative stress (Oruc et al., 2003; Olsvik et al., 2005). Abdel-Mawla et al. (2023) illustrated that laminarin enhanced the antioxidative capacity (SOD, CAT, and GPx) while decreasing the level of MDA in Thinlip Grey Mulle. Wu et al. (2023) found that supplementation of 5.0 g kg-1 laminarin significantly enhanced the activities of T-AOC, SOD, and GSH, while supplementation of high dose (10 and 15 g kg-1) significantly decreased the activities of CAT and SOD. Jiang et al. (2021) found that SOD and CAT activities of Ictalurus punctatus increased with the dosage of laminarin increasing, and have a significant difference at the dosage of 4 g kg-1 and 8 g kg-1. Consistent with the results of these studies, in our study, dietary laminarin enhanced the intestinal T-AOC and GSH activities of spotted seabass, and the GSH activity was significantly increased in the P0.8 group, while the intestinal T-AOC was significantly increased in the P0.4, P0.8, P1.2, and P1.8 groups. The results indicated that Laminin could enhance the antioxidant capacity of the intestinal tract, thereby preventing intestinal damage caused by reactive oxygen species.
AKP, ACP and LZM are all related indexes to evaluate the nonspecific immunity of fish. Studies have reported that AKP is one of the important indicators to evaluate intestinal health when studying intestinal nutrition metabolism, and high AKP activity is conducive to the absorption of nutrients in a healthy state of the body (Yan et al., 2020). In addition, LZM has antibacterial and antiviral effects and can promote the phagocytic activity of phagocytic cells (Song et al., 2021). In this study, the intestinal AKP and LZM activities of spotted seabass in polysaccharide groups were higher than those in control group, which indicated that dietary laminarin could promote intestinal digestion and absorption of nutrients and improve intestinal immunity of spotted seabass. Previous research has found that laminarin reduced the AKP activity of Ictalurus punctatus, which was different from the results of this study (Jiang et al., 2021). It is speculated that different species may have different absorption and metabolism of laminarin, leading to different biological effects.
DAO and D-Lac contents are important indices to measure the functional integrity of intestinal mucosa. DAO is a sensitive intestinal epithelial cell enzyme with high activity in the upper villi of the intestinal mucosa (Ma et al., 2020). When intestinal mucosal cells are damaged and intestinal mucosal permeability increases, a large amount of DAO will be released into the blood, resulting in increased DAO content in the blood (Ma et al., 2020). D-Lac is the product of intestinal bacterial metabolism and does not participate in body metabolism (Wen et al., 2014). When intestinal mucosa is damaged, D-Lac will flow into the blood from the intestine, resulting in increased blood D-Lac content (Wen et al., 2014). Liu et al. found that high levels of non-starch polysaccharides (15%, 18%) significantly increased the activities of DAO and D-Lac in serum of largemouth bass, and damaged the intestinal barrier(Liu et al., 2022b). Yang et al. found that supplementation of 1.25% xylan in the diet improved the intestinal barrier function of juvenile turbot (Yang et al., 2019). In addition, Zhang et al. found that algin gum in laminarin reduced the contents of DAO and D-Lac in mouse plasma and repaired intestinal mucosal damage (Zhang et al., 2018). In our work, the supplementation of laminarin decreased the content of DAO and D-Lac in serum, and the effect was significant in the P0.8 group, indicating that laminarin had a certain repairing effect on the intestinal mucosal barrier of spotted seabass.
Intestines is an important place for the digestion and absorption of fish nutrients, and its morphological and structural integrity is directly proportional to the body’s digestion and absorption of nutrients (Li et al., 2021). The villus length, villous width, crypt depth, and muscular thickness all have important effects on the nutrient absorption capacity of the intestine (Fang et al., 2019). The increased length and width of intestinal villi can promote the contact area between intestine and nutrients and enhance the absorption capacity of nutrients (Caspary, 1992; Al-Fataftah and Abdelqader, 2014; Zhai et al., 2016). The increase in muscle thickness may encourage intestinal motility (Li et al., 2014). Crypt depth is mainly related to the renewal of intestinal epithelial cells, and the shallow crypt depth indicates that the renewal of epithelial cells is slower (Wang et al., 2003). At present, laminarin has more effects on the intestinal morphology of piglets. Walsh et al. (2013) found that dietary supplementation with 300 ppm laminarin significantly increased the duodenal villus height of weaned piglets. Rattigan et al. (2020) found that laminarin not only increased the villus height and width, but also increased V/C (villus height/crypt depth) value of weaned piglets. There are few studies on aquatic animals. Abdel-Mawla et al. (2023) found that dietary laminarin can improve intestinal villi morphology and increase the number of goblet cells in Liza ramada. This is similar to the results of previous studies, as the present experiment demonstrated that the crypt depth, villi length, and villi width of the intestine in spotted seabass were increased in all polysaccharide groups, and the muscular thickness was significantly increased in the P1.2 group. The findings suggest that laminarin supplementation can enhance intestinal morphology, stimulate the regeneration of intestinal epithelial cells, and facilitate nutrient digestion and absorption, which may account for the improved growth performance observed in spotted seabass fed with laminarin.
The intestinal microbiota is a symbiotic microbial community residing in the host body, which plays an important role in regulating metabolism, immunity, the endocrine system, and other physiological functions of the body (Zhou et al., 2022). It has been reported that laminarin can maintain the balance of intestinal flora by adjusting the content of short-chain fatty acids to change the proportion of specific bacteria (Xu et al., 2021). Previous studies have found that dietary laminarin can reduce the number of E. coli in the cecum and colon and increase the number of Lactobacillus in the colon in weaned piglets (Reilly et al., 2008; Walsh et al., 2013; Rattigan et al., 2020). In this study, alpha diversity results showed that dietary laminarin significantly increased the intestinal microbial community diversity of seabass (Simpson and Shannon index). Lin et al. analyzed the intestinal flora of the spotted seabass, and the results showed that Firmicutes, Proteobacteria, Bacterioidetes, and Fusobacteria were the main dominant bacteria in the intestinal tract of the fish (Lin et al., 2021). In the present work, Firmicutes and Proteobacteria were the dominant phyla in the control group and in each polysaccharide group, which was consistent with the results of previous studies, indicating that Firmicutes and Proteobacteria were the prime phyla in the intestinal tract of spotted seabass. In general, Proteobacteria are considered a sign of instability in the gut microbial structure, which can lead to metabolic confusion (Shin et al., 2015). Wu et al. (2023) reported that dietary supplementation with high levels of laminarin (1% and 1.5%) significantly increased proteobacteria abundance in the gut of largemouth bass. In this study, Proteobacteria abundance increased significantly in the P1.2, P1.6, and P2 groups, which was similar to the results of Wu et al., indicating that high doses of laminarin may induce intestinal metabolic disorder in fish. At the genus level, as a beneficial bacterium in the intestine, the abundance of Bacillus decreased to different degrees in all polysaccharide groups, indicating that laminarin had an inhibitory effect on the intestinal bacillus. However, we found that Lactobacillus abundance increased significantly in the P0.4, P0.8, P1.6, and P2 groups. Lactobacillus is thought to reflect the structural changes of beneficial flora, which can stimulate bile acid and short-chain fatty acid metabolism, improve nutrient availability, and enhance intestinal barrier function (Valeriano et al., 2017). Moreover, Klebsiella abundance was increased in the P0.4 and P0.8 groups. Klebsiella can promote the synthesis of short-chain fatty acids and produce beneficial metabolites (Hou and Ma, 2023). In conclusion, low doses of laminarin (0.4%, 0.8%) are more beneficial to the intestinal health of spotted seabass, regulating intestinal flora and promoting the formation of beneficial bacteria communities, while excessive additions of laminarin (1.2%, 1.6%, 2%) can increase the number of intestinal pathogenic bacteria in spotted seabass.
5 Conclusion
In conclusion, dietary laminarin not only enhanced the growth performance of juvenile sea bass but also augmented the abundance of beneficial bacteria Lactobacillus and Klebsiella in the intestine, while improving intestinal tissue morphology, barrier function, antioxidant capacity, and immune response. Using specific growth rate as the evaluation criterion, the optimal level of laminarin supplementation in spotted seabass diets was estimated to be 0.97 - 0.98%. These findings provide valuable insights for the practical application of laminarin in aquaculture.
Data availability statement
The datasets presented in this study has been stored in the NCBI gene database, its login link to https://www.ncbi.nlm.nih.gov/sra/PRJNA1001850, the accession number was PRJNA1001850.
Ethics statement
This study was approved by the Animal Ethics Committee of Jimei University (Fujian, China). The study was conducted in accordance with the local legislation and institutional requirements.
Author contributions
HQ, ZH, and ZBL conceived and designed the experiments. HQ, ZH, ZYL, JM, LK, YL, HL, and SZ performed the experiments. HQ analyzed the data, wrote the paper, and prepared figures and tables. HQ, ZH, and ZBL discuss the result together. ZH and ZBL reviewed drafts of the paper. All authors have read and approved this version of the article, and due care has been taken to ensure the integrity of the work.
Funding
This experiment was funded by Science and Technology Planning Project in Fujian, China (Grant No. 2015N0010) and Science and Technology Planning Project in Xiamen, China (Grant No. 3502Z20143017).
Conflict of interest
The authors declare that the research was conducted in the absence of any commercial or financial relationships that could be construed as a potential conflict of interest.
Publisher’s note
All claims expressed in this article are solely those of the authors and do not necessarily represent those of their affiliated organizations, or those of the publisher, the editors and the reviewers. Any product that may be evaluated in this article, or claim that may be made by its manufacturer, is not guaranteed or endorsed by the publisher.
References
Abdel-Mawla M. S., Magouz F. I., Khalafalla M. M., Amer A. A., Soliman A. A., Zaineldin A. I., et al. (2023). Growth performance, intestinal morphology, blood biomarkers, and immune response of Thinlip Grey Mullet (Liza ramada) fed dietary laminarin supplement. J. Appl. Phycol. 35 (4), 1801–1811. doi: 10.1007/s10811-023-02973-6
Ai Q., Mai K., Li H., Zhang C., Zhang L., Duan Q., et al. (2004). Effects of dietary protein to energy ratios on growth and body composition of juvenile Japanese seabass, Lateolabrax japonicus. Aquaculture 230 (1), 507–516. doi: 10.1016/j.aquaculture.2003.09.040
Al-Fataftah A.-R., Abdelqader A. (2014). Effects of dietary Bacillus subtilis on heat-stressed broilers performance, intestinal morphology and microflora composition. Anim. Feed Sci. Technol. 198 (2014), 279–285. doi: 10.1016/j.anifeedsci.2014.10.012
Bischoff S. C. (2011). ‘Gut health’: a new objective in medicine? BMC Med. 9 (1), 24. doi: 10.1186/1741-7015-9-24
Caspary W. F. (1992). Physiology and pathophysiology of intestinal absorption. Am. J. Clin. Nutr. 55 (1), 299S–308S. doi: 10.1093/ajcn/55.1.299s
Chen J., Zhou X.-q., Feng L., Liu Y., Jiang J. (2009). Effects of glutamine on hydrogen peroxide-induced oxidative damage in intestinal epithelial cells of Jian carp (Cyprinus carpio var. Jian). Aquaculture 288 (3-4), 285–289. doi: 10.1016/j.aquaculture.2008.10.053
Cheng Y., Li X., Wang L., Lu K., Song K., Ai Q., et al. (2021). Effects of dietary arginine levels on growth, immune function of physical barriers and serum parameters of spotted seabass (Lateolabrax maculatus) reared at different water temperatures. Aquaculture 541, 736812. doi: 10.1016/j.aquaculture.2021.736812
Deng J., Zhang X., Sun Y., Mi H., Zhang L. (2021). Effects of different types of non-starch polysaccharides on growth, digestive enzyme activity, intestinal barrier function and antioxidant activity of rainbow trout (Oncorhynchus mykiss). Aquacult. Rep. 21, 100864. doi: 10.1016/j.aqrep.2021.100864
Devillé C., Gharbi M., Dandrifosse G., Peulen O. (2007). Study on the effects of laminarin, a polysaccharide from seaweed, on gut characteristics. J. Sci. Food Agric. 87 (9), 1717–1725. doi: 10.1002/jsfa.2901
Díaz A. C., Espino M. L., Arzoz N. S., Velurtas S. M., Ponce N. M. A., Stortz C. A., et al. (2017). Free radical scavenging activity of extracts from seaweeds Macrocystis pyrifera and Undaria pinnatifida: applications as functional food in the diet of prawn Artemesia longinaris. Latin Am. J. Aquat. Res. 45 (1), 104–112. doi: 10.3856/vol45-issue1-fulltext-10
Dobrinčić A., Balbino S., Zorić Z., Pedisić S., Bursać Kovačević D., Elez Garofulić I., et al. (2020). Advanced technologies for the extraction of marine brown algal polysaccharides. Mar. Drugs 18 (3), 168. doi: 10.3390/md18030168
Fang H., Xie J., Liao S., Guo T., Xie S., Liu Y., et al. (2019). Effects of Dietary Inclusion of Shrimp Paste on Growth Performance, Digestive Enzymes Activities, Antioxidant and Immunological Status and Intestinal Morphology of Hybrid Snakehead (Channa maculata ♀ × Channa argus ♂). Front. Physiol. 10. doi: 10.3389/fphys.2019.01027
Heim G., Walsh A. M., Sweeney T., Doyle D. N., O’Shea C. J., Ryan M. T., et al. (2014). Effect of seaweed-derived laminarin and fucoidan and zinc oxide on gut morphology, nutrient transporters, nutrient digestibility, growth performance and selected microbial populations in weaned pigs. Br. J. Nutr. 111 (9), 1577–1585. doi: 10.1017/S0007114513004224
Hou P., Ma J. (2023). Research progress on the effect of brown seaweed polysaccharide altering micro-ecology of animal gut. Chin. J. Anim. Sci. 59 (4), 69–74. doi: 10.19556/j.0258-7033.20220322-01
Hoyle I., Shaw B. J., Handy R. D. (2007). Dietary copper exposure in the African walking catfish, Clarias gariepinus: Transient osmoregulatory disturbances and oxidative stress. Aquat. Toxicol. 83 (1), 62–72. doi: 10.1016/j.aquatox.2007.03.014
Jiang H., Wang M., Zheng Y., Chen F., Fu L., Zhong L., et al. (2021). Dietary laminarin administration to enhance the immune responses, promote growing and strengthen physique in Ictalurus punctatus. Aquacult. Nutr. 27 (4), 1181–1191. doi: 10.1111/anu.13258
Karuppusamy S., Rajauria G., Fitzpatrick S., Lyons H., McMahon H., Curtin J., et al. (2022). Biological properties and health-promoting functions of laminarin: A comprehensive review of preclinical and clinical studies. Mar. Drugs 20 (12), 772. doi: 10.3390/md20120772
Li L., Feng L., Jiang W.-D., Jiang J., Wu P., Kuang S.-Y., et al. (2015a). Dietary pantothenic acid deficiency and excess depress the growth, intestinal mucosal immune and physical functions by regulating NF-κB, TOR, Nrf2 and MLCK signaling pathways in grass carp (Ctenopharyngodon idella). Fish Shellfish Immunol. 45 (2), 399–413. doi: 10.1016/j.fsi.2015.04.030
Li H., Lu L., Wu M., Xiong X., Luo L., Ma Y., et al. (2020). The effects of dietary extract of mulberry leaf on growth performance, hypoxia-reoxygenation stress and biochemical parameters in various organs of fish. Aquacult. Rep. 18, 100494. doi: 10.1016/j.aqrep.2020.100494
Li J., Wang C., Zhang Y., Wu D., Fan Z., Wang L. (2021). Effect of arginine supplementation in high starch diets on intestinal digestive enzyme activities and intestinal morphology of songpu mirror carp. J. Guangdong Ocean Univ. 41 (01), 39–46. doi: 10.3969/j.issn.1673-9159.2021.01.006
Li J., Xu Q., Wang C., Luo L., Zhao Z. (2014). Effects of glutamine and its precursors on intestinal digestive enzyme activity and intestinal morphology of songpu mirror carp (Cyprinus carpio specularis). Chin. J. Anim. Nutr. 26 (5), 1347–1352. doi: 10.3969/j.issn.1006-267x.2014.05.028
Li W., Yin G., Lin X., Huang Z., Lin J. (2015b). Effects of laminarin on growth performance and digestive enzyme activity in gastrointestinal tract of Epinephelus coioides. Feed Res. 4), 59–62. doi: 10.13557/j.cnki.issn1002-2813.2015.04.014
Lin N., Pan Y., Xu B., Gong H., Zeng H. (2021). Microbial diversity of intestinal contents and mucus in cultured seabass (Lateolabrax maculatus). J. Aquacult. 42 (6), 1–7. doi: 10.3969/j.issn.1004-2091.2021.06.001
Liu Y., Fan J., Huang H., Zhou H., Cao Y., Zhang Y., et al. (2022a). High dietary non-starch polysaccharides detrimental to nutrient digestibility, digestive enzyme activity, growth performance, and intestinal morphology in largemouth bass, Micropterus salmoides. Front. Nutr. 9. doi: 10.3389/fnut.2022.1015371
Liu Y., Huang H., Fan J., Zhou H., Zhang Y., Cao Y., et al. (2022b). Effects of dietary non-starch polysaccharides level on the growth, intestinal flora and intestinal health of juvenile largemouth bass Micropterus salmoides. Aquaculture 557 (2022), 738343. doi: 10.1016/J.AQUACULTURE.2022.738343
Ma J., Shah A. M., Wang Z. S., Hu R., Zou H. W., Wang X. Y., et al. (2020). Comparing the gastrointestinal barrier function between growth-retarded and normal yaks on the Qinghai-Tibetan Plateau. PEERJ 8, e9851. doi: 10.7717/peerj.9851
McDonnell P., Figat S., O’Doherty J. V. (2010). The effect of dietary laminarin and fucoidan in the diet of the weanling piglet on performance, selected faecal microbial populations and volatile fatty acid concentrations. animal 4 (4), 579–585. doi: 10.1017/S1751731109991376
Morales-Lange B., Bethke J., Schmitt P., Mercado L. (2015). Phenotypical parameters as a tool to evaluate the immunostimulatory effects of laminarin in Oncorhynchus mykiss. Aquacult. Res. 46 (11), 2707–2715. doi: 10.1111/are.12426
Olsvik P. A., Kristensen T., Waagbø R., Rosseland B. O., Tollefsen K.-E., Baeverfjord G., et al. (2005). mRNA expression of antioxidant enzymes (SOD, CAT and GSH-Px) and lipid peroxidative stress in liver of Atlantic salmon (Salmo salar) exposed to hyperoxic water during smoltification. Comp. Biochem. Physiol. Part A 141 (3), 314–323. doi: 10.1016/j.cbpa.2005.07.016
Oruc E. O., Sevgiler Y., Uner N. (2003). Tissue-specific oxidative stress responses in fish exposed to 2,4-D and azinphosmethyl. Comp. Biochem. Physiol. Part C 137 (1), 43–51. doi: 10.1016/j.cca.2003.11.006
Ragaza J. A., Koshio S., Mamauag R. E., Ishikawa M., Yokoyama S., Villamor S. S. (2015). Dietary supplemental effects of red seaweed Eucheuma denticulatum on growth performance, carcass composition and blood chemistry of juvenile Japanese flounder, Paralichthys olivaceus. Aquacult. Res. 46 (3), 647–657. doi: 10.1111/are.12211
Rattigan R., Sweeney T., Maher S., Thornton K., Rajauria G., O’Doherty J. V. (2020). Laminarin-rich extract improves growth performance, small intestinal morphology, gene expression of nutrient transporters and the large intestinal microbial composition of piglets during the critical post-weaning period. Br. J. Nutr. 123 (3), 255–263. doi: 10.1017/S0007114519002678
Reilly P., O’Doherty J. V., Pierce K. M., Callan J. J., O’Sullivan J. T., Sweeney T. (2008). The effects of seaweed extract inclusion on gut morphology, selected intestinal microbiota, nutrient digestibility, volatile fatty acid concentrations and the immune status of the weaned pig. Animal 2 (10), 1465–1473. doi: 10.1017/S1751731108002711
Shahabuddin A. M., Khan M. N. D., Mikami K., Araki T., Yoshimatsu T. (2017). Dietary supplementation of red alga Pyropia spheroplasts on growth, feed utilization and body composition of sea cucumber, Apostichopus japonicus (Selenka). Aquacult. Res. 48 (10), 5363–5372. doi: 10.1111/are.13350
Shin N.-R., Whon T. W., Bae J.-W. (2015). Proteobacteria: microbial signature of dysbiosis in gut microbiota. Trends Biotechnol. 33 (9), 496–503. doi: 10.1016/j.tibtech.2015.06.011
Sivagnanavelmurugan M., Karthik Ramnath G., Jude Thaddaeus B., Palavesam A., Immanuel G. (2015). Effect of Sargassum wightii fucoidan on growth and disease resistance to Vibrio parahaemolyticus in Penaeus monodon post-larvae. Aquacult. Nutr. 21 (6), 960–969. doi: 10.1111/anu.12217
Song Q., Xiao Y., Xiao Z., Liu T., Li J., Li P., et al. (2021). Lysozymes in fish. J. Agric. Food Chem. 69 (50), 15039–15051. doi: 10.1021/acs.jafc.1c06676
Tian J., Gao W., Wen H. (2018). Research advances: intestinal health and feed additives in aquatic animals. Chin. J. Anim. Nutr. 30 (1), 7–13. doi: 10.3969/j.issn.1006-267x.2018.01.002
Torrecillas S., Montero D., Izquierdo M. (2014). Improved health and growth of fish fed mannan oligosaccharides: Potential mode of action. Fish Shellfish Immunol. 36 (2), 525–544. doi: 10.1016/j.fsi.2013.12.029
Valeriano V. D. V., Balolong M. P., Kang D. K. (2017). Probiotic roles of Lactobacillus sp. in swine: insights from gut microbiota. J. Appl. Microbiol. 122 (3), 554–567. doi: 10.1111/jam.13364
Venardou B., O’Doherty J. V., Vigors S., O’Shea C. J., Burton E. J., Ryan M. T., et al. (2021). Effects of dietary supplementation with a laminarin-rich extract on the growth performance and gastrointestinal health in broilers. Poultry Sci. 100 (7), 101179. doi: 10.1016/j.psj.2021.101179
Vidhya Hindu S., Chandrasekaran N., Mukherjee A., Thomas J. (2019). A review on the impact of seaweed polysaccharide on the growth of probiotic bacteria and its application in aquaculture. Aquacult. Int. 27 (1), 227–238. doi: 10.1007/s10499-018-0318-3
Walsh A. M., Sweeney T., O’Shea C. J., Doyle D. N., O’Doherty J. V. (2013). Effect of dietary laminarin and fucoidan on selected microbiota, intestinal morphology and immune status of the newly weaned pig. Br. J. Nutr. 110 (9), 1630–1638. doi: 10.1017/S0007114513000834
Wang Z., She R., Chen Y., Yuan L., Xu J., Kang D. (2003). Effect of different levels of zinc and selenium in diet on the structure of mucosa epithelium in broiler small intestine. Chin. J. Vet. Sci. Technol. 07), 18–21. doi: 10.16656/j.issn.1673-4696.2003.07.005
Wen Z., Zhong-Yang S., Hong-Li S., Yang Y., Ben-Juan W., Nan-Nan F., et al. (2014). Protective effect of bone marrow mesenchymal stem cells in intestinal barrier permeability after heterotopic intestinal transplantation. World J. Gastroenterol. 20 (23), 7442–7451. doi: 10.3748/wjg.v20.i23.7442
Wu Y., Cheng Y., Qian S., Zhang W., Huang M., Yang S., et al. (2023). An evaluation of laminarin additive in the diets of Juvenile Largemouth Bass (Micropterus salmoides): Growth, Antioxidant Capacity, Immune Response and Intestinal Microbiota. Animals 13 (3), 459. doi: 10.3390/ani13030459
Xu Y., Zheng X., Wang Z. (2021). Effect of laminaria japonica polysaccharide on intestinal health and its application in pig production. FEED Res. 44 (17), 149–153. doi: 10.13557/j.cnki.issn1002-2813.2021.17.036
Yan X.-b., Dong X.-h., Tan B.-p., Zhang S., Chi S.-y., Qi-huiYang, et al. (2020). Influence of different oil sources on growth, disease resistance, immune response and immune-related gene expression on the hybrid grouper (♀ Epinephelus fuscoguttatus × ♂ E. lanceolatu), to Vibrio parahaemolyticus challenge. Fish Shellfish Immunol. 99 (C), 310–321. doi: 10.1016/j.fsi.2020.02.025
Yang P., Hu H., Li Y., Ai Q., Zhang W., Zhang Y., et al. (2019). Effect of dietary xylan on immune response, tight junction protein expression and bacterial community in the intestine of juvenile turbot (Scophthalmus maximus L.). Aquaculture 512 (2019), 734361. doi: 10.1016/j.aquaculture.2019.734361
Yang K., Xun P., Huang J., Jiang K., Huang X., Yu W., et al. (2023). Effects of dietary clostridium butyricum on the growth performance and intestinal health of hybrid groupers (Epinephelus lanceolatus♂ × E. fuscoguttatus♀). Aquacult. Rep. 30, 101588. doi: 10.1016/j.aqrep.2023.101588
Yin G., Li W., Lin Q., Lin X., Lin J., Zhu Q., et al. (2014). Dietary administration of laminarin improves the growth performance and immune responses in Epinephelus coioides. Fish Shellfish Immunol. 41 (2), 402–406. doi: 10.1016/j.fsi.2014.09.027
Zhai S., Shi Q., Chen X. (2016). Effect of dietary antimicrobial peptides-surfactin supplementation on parameters of intestinal health indices of genetically improved farmed tilapia (gift, oreochromis niloticus). Acta Hydrobiol. Sin. 40 (4), 823–829. doi: 10.7541/2016.106
Zhang T., Xue M., Liu J., Liu Y., Liang Z., Liang H. (2018). Protective effect of fucoidan on intestinal barrier in rats with breast cancer. Acta Nutrimenta Sin. 40 (1), 59–63. doi: 10.13325/j.cnki.acta.nutr.sin.2018.01.017
Zhou Q., Binghu F., Gao L., Zhong Y., Lin C. (2022). Interaction between gut microbiota and natural plant extracts. Chin. J. Vet. Sci. 42 (4), 836–840. doi: 10.16303/j.cnki.1005-4545.2022.04.35
Keywords: Lateolabrax maculatus, laminarin, digestive enzyme activity, intestinal barrier function, intestinal morphology, intestinal microbiome
Citation: Qin H, Long Z, Ma J, Kong L, Lin H, Zhou S, Lin Y, Huang Z, Liu L and Li Z (2023) Growth performance, digestive capacity and intestinal health of juvenile spotted seabass (Lateolabrax maculatus) fed dietary laminarin supplement. Front. Mar. Sci. 10:1242175. doi: 10.3389/fmars.2023.1242175
Received: 18 June 2023; Accepted: 31 July 2023;
Published: 17 August 2023.
Edited by:
Amalia Pérez-Jiménez, University of Granada, SpainReviewed by:
H-Michael Habte-Tsion, University of Maine, United StatesErick Perera, Spanish National Research Council (CSIC), Spain
Samad Rahimnejad, University of Murcia, Spain
Copyright © 2023 Qin, Long, Ma, Kong, Lin, Zhou, Lin, Huang, Liu and Li. This is an open-access article distributed under the terms of the Creative Commons Attribution License (CC BY). The use, distribution or reproduction in other forums is permitted, provided the original author(s) and the copyright owner(s) are credited and that the original publication in this journal is cited, in accordance with accepted academic practice. No use, distribution or reproduction is permitted which does not comply with these terms.
*Correspondence: Zhongbao Li, bGl6aG9uZ2Jhb0BqbXUuZWR1LmNu