- 1Emirates Nature - World Wide Fund for Nature, Abu Dhabi, United Arab Emirates
- 2National Oceanography Centre, Southampton, United Kingdom
- 3Oxford Seascape Ecology Lab, School of Geography and the Environment, University of Oxford, Oxford, United Kingdom
- 4Seascape Analytics Ltd, Plymouth, United Kingdom
- 5Department of Biology, Chemistry and Environmental Sciences, College of Arts and Sciences, American University of Sharjah, Sharjah, United Arab Emirates
- 6Environment Agency-Abu Dhabi, Abu Dhabi, United Arab Emirates
- 7Seascape Solutions, Mililani, HI, United States
Coastal ecosystems are integral to global carbon cycling and are increasingly recognised for their role in mitigating climate change. Within these ecosystems, the dynamics of carbon storage are diverse, varying significantly across different habitats. However, existing management strategies often focus predominantly on vegetated habitats neglecting the contributions of non-vegetated areas. We address this knowledge gap by providing a quantitative spatial assessment of carbon storage across coastal seascapes varying in plant biomass. Our comprehensive multi-habitat inventory of carbon stocks in the United Arab Emirates confirmed that mangroves are the largest carbon-storing habitat per hectare (94.3 t/ha), followed by saltmarshes (63.6 t/ha), microbial mats (51.6 t/ha), mudflats (46.8 t/ha), seagrass (32.5 t/ha), and coastal sabkha (31.0 t/ha).Mean carbon content in the top 50 cm of mangrove soils (53.9 t/ha) was similar to saltmarshes (52.7 t/ha), microbial mats (51.6 t/ha), and mudflats (46.8 t/ha). We highlight the importance of including non-vegetated habitats in carbon accounting and management strategies. Our findings suggest that a more context-specific whole-system approach is essential for guiding effective ecosystem management and designing ecologically meaningful Nature-based Solutions (NbS). Adopting this broader perspective in NbS can ensure more comprehensive conservation and restoration outcomes, which not only protect and enhance blue carbon ecosystems but also contribute to broader ecological and social benefits. This approach is pivotal for advancing our understanding of interconnected coastal ecosystems and their role in climate change mitigation.
Introduction
Coastal ecosystems are highly biodiverse and productive and have gained recognition for their role as rapid and long-term carbon sinks. Carbon sequestered in marine ecosystems, termed “blue carbon”, has been highlighted for its potential to mitigate climate change by removing carbon from the atmosphere (Howard et al., 2014). Coastal zones that support productive and diverse ecosystems are prime areas to support climate mitigation efforts through the implementation of nature-based solutions (NbS) (Buelow et al., 2022; O’Leary et al., 2022; Pittman et al., 2022) NbS are defined by the International Union for Conservation of Nature (IUCN), as “actions that protect, sustainably manage, and restore natural or modified ecosystems, that address societal challenges effectively and adaptively simultaneously providing human well-being and biodiversity benefits”(Cohen-Shacham et al., 2019).
Substantial recent attention has been given to the climate mitigation and adaptation function of coastal blue carbon ecosystems through nature-based climate solutions and policy (Macreadie et al., 2021; Howard et al., 2023). However, a spatially comprehensive understanding of the distribution of blue carbon both within individual habitat types and among geographically discrete seascapes and regions remains a significant challenge (Sánchez-Arcilla et al., 2022; Williamson and Gattuso, 2022). Research has increasingly recognised non-vegetated habitat types, including supratidal areas and mudflats (Brown et al., 2021; Chen and Lee, 2022), as important carbon sinks. Yet, studies have tended to focus on single habitat types resulting in very few sampling designs that extend across highly heterogeneous seascapes, especially in arid regions. This knowledge gap limits the effective design of ecologically meaningful NbS, despite being increasingly advocated for addressing the global crises of accelerated climate change and biodiversity loss (Seddon et al., 2020).
In the United Arab Emirates (UAE) and other arid countries, coastal ecosystems are the major carbon sinks, owing to the sparseness of vegetation in the terrestrial system. However, these coastal areas face multiple pressures from urbanisation and sea level rise that increase the vulnerability of blue carbon habitats (Mills et al., 2016; Lovelock and Reef, 2020; Mateos-Molina et al., 2024). The UAE also faces numerous environmental challenges associated with global warming, including increasing air and water temperatures (Hereher, 2020), diminishing freshwater resources (Savarani, 2023), and the loss of marine habitats and species (Wabnitz et al., 2018).
As a major regulatory ecosystem service, inventories of carbon stocks are essential for assessing the natural capital of coastal regions, together with the valuation of contributions to fisheries, tourism, and biodiversity (Hilmi et al., 2021). Such evidenced-based inventories are crucial to consider when prioritising locations to optimise and refine NbS to be impactful for climate mitigation while also delivering positive outcomes to biodiversity and co-benefits to people. This is especially relevant given the growing integration of NbS in key international agreements and frameworks such as the Nationally Determined Contributions of the Paris Agreement (Guimaraes et al., 2019; Seddon et al., 2019), Ramsar Convention targets (Metcalfe et al., 2018), and the United Nations Sustainable Development Goals (Keesstra et al., 2018; Sørup et al., 2019; Seifollahi-Aghmiuni et al., 2019). In 2022, the Kunming-Montreal Global Biodiversity Framework (COP15) recognised the role of NbS in achieving key targets, including Target 8, which focuses on minimising the impact of climate change on biodiversity, and Target 11, which aims to restore, maintain, and enhance nature’s contributions to people (CBD/COP/15/L.25).
In response, the UAE has recognised the importance of coastal zones and blue carbon habitats, a focus that is reflected in the UAE’s Climate Change Plan and Nationally Determined Contributions (NDC) (MOCCAE, 2023). The UAE has developed the National Climate Change Plan 2050 (MOCCAE, 2017) that aims to achieve net zero carbon emissions by 2050. The plan recognises the protection and restoration of coastal ecosystems as a key mechanism for implementation. Furthermore, the country’s NDCs, supported by the National Carbon Sequestration Project, outline targets for the significant expansion of mangrove forests as NbS to enhance natural ecosystem carbon sequestration in the country.
Previously, global NbS interventions for climate mitigation have focused on individual habitat types and carbon averages, which together with a strong knowledge base have led to a dominant focus on mangroves as targets for interventions (Howard et al., 2023). In the Arabian Gulf region, however, evidence is equivocal on whether mangroves are the largest carbon-storing habitat in the region. While some studies have found that mangroves have the largest carbon stores of blue carbon habitats in the region (Schile et al., 2017; Chatting et al., 2020), other studies present contrasting results (Campbell et al., 2015; Cusack et al., 2018).
Carbon accounting focused on individual habitat types overlooks the potential benefits derived from NbS that consider the collective contribution of interconnected habitat types in coastal lagoons (Macreadie et al., 2017; Mishra and Apte, 2020; Keith et al., 2021; Moritsch et al., 2021). Taking this consideration into account allows for a wider range of tailored NbS strategies and interventions that account for specific habitat characteristics, their synergistic interactions, and ecological processes at multiple scales. This aligns with the global conceptual shift in restoration ecology, moving from single habitat-focused restoration to whole-system approaches (Gilby et al., 2018; Lester et al., 2020; Pittman et al., 2021).
Furthermore, it is crucial to expand our understanding beyond only organic carbon, as biogeochemical and diagenetic processes convert organic carbon into inorganic forms (Bouillon et al., 2008). Therefore, studying the relative concentrations of organic to inorganic stocks can provide further insights into the overall carbon dynamics within habitat types. With the growing interest in mangroves as the only viable natural climate solution in the UAE, we designed our study to test whether mangroves do serve as the greatest coastal blue carbon function in the region. We only determined if any significant difference was evident between other habitat types and whether the results vary between two geographically discrete lagoons.
To evaluate our hypothesis, we quantified the carbon stocks of all the major mapped habitat types with two distinct coastal lagoons in the UAE. We explored how the variability of carbon storage within- and between seascapes can be used to inform NbS. We present a multi-habitat inventory and spatially comparative assessment of marine ecosystem carbon stocks from two large lagoonal seascapes in the UAE that have been prioritised for pioneering evidence-based NbS (Pittman et al., 2022). We present the first estimates of carbon stocks for intertidal mudflats and inorganic carbon stocks in arid environments. These results help to understand the variability of carbon storage within- and between seascapes.
Materials and methods
Study site
The study was conducted in two coastal lagoons in the UAE: Khor Faridah in Abu Dhabi and Umm Al Quwain (UAQ) lagoon in the emirate of UAQ. In the UAE, coastal lagoons (locally known as ‘Khors’) are shallow coastal water bodies that are partially separated from the sea and are known for their high productivity and biodiversity (Mateos-Molina et al., 2021a; Mateos-Molina et al., 2024). Khor Faridah is located on the eastern side of Abu Dhabi and covers an area of approximately 254 km2, while the UAQ seascape covers an area of about 138 km2 (Figure 1). The UAQ seascape is a Key Biodiversity Area (KBA Partnership, 2023), and both lagoons are recognised as Areas of Particular Importance for Marine Biodiversity (Ben Lamine et al., 2021). These lagoons have been prioritised for NbS based on their potential for climate change mitigation, the importance for biodiversity conservation, and socio-economic co-benefits to local communities (Pittman et al., 2022). The lagoons are also being examined for the potential for crop production using salt-tolerant plants native to the region (Elouafi et al., 2020).
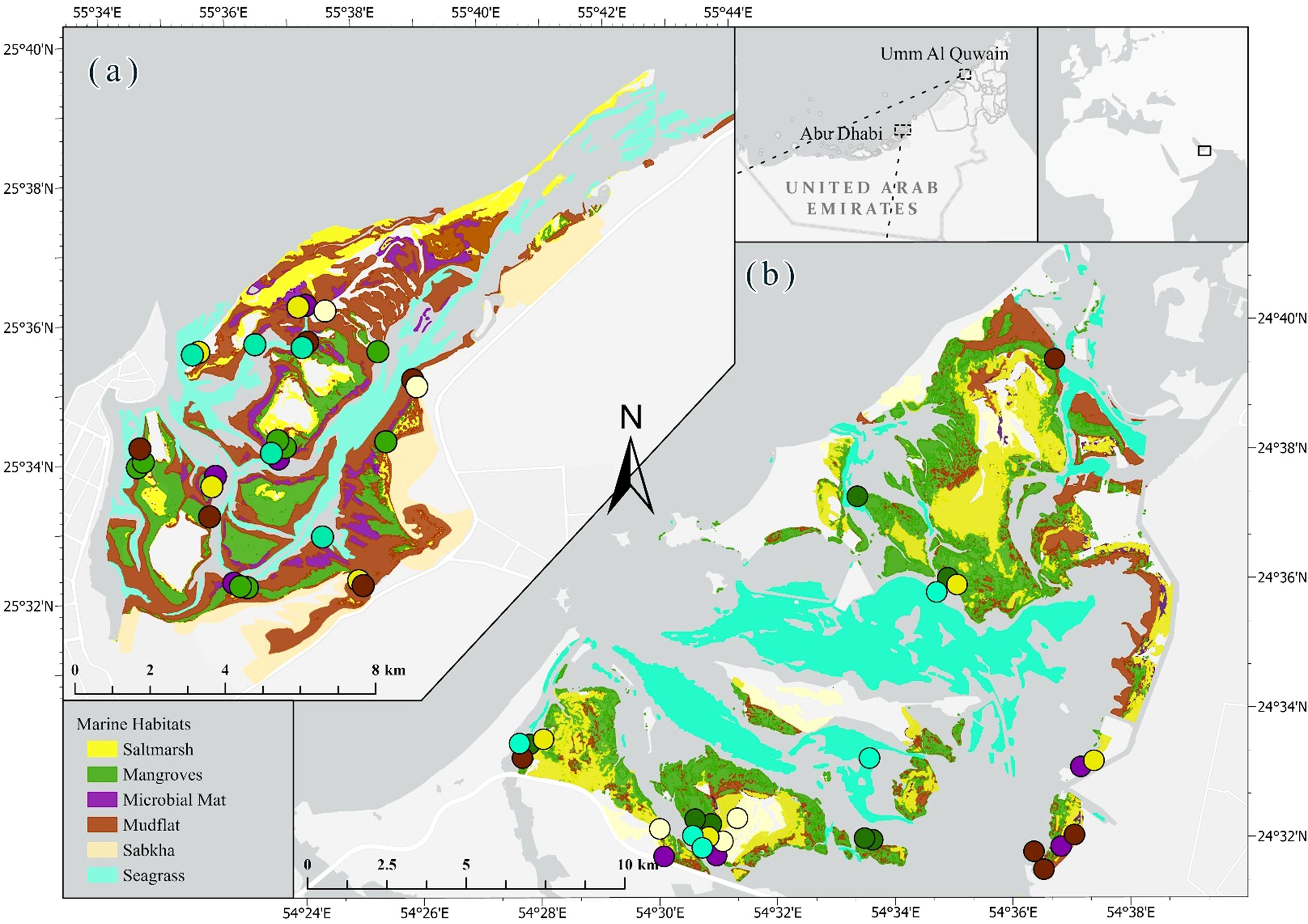
Figure 1 Site map and the sample locations of (A) Khor Faridah, Abu Dhabi emirate and (B) the UAQ seascape, Umm Al Quwain emirate. The samples are positioned to optimise visualisation and thus represent their approximate locations.
Lagoons in the study area comprise a diverse range of habitats, from the subtidal to the supratidal zone, each of which contributes to the overall blue carbon storage potential. These habitats include coastal sabkha, saltmarshes, microbial mats, mangroves, mudflats, and seagrass beds.
Coastal sabkha, occupying the supratidal zone, are hypersaline salt flat environments characterised by anoxic unvegetated soils. Saltmarshes thrive in the transitional zone between mangroves, mudflats, and coastal sabkha, often with sparsely distributed succulent shrubs, most commonly Salicornia persica, Arthrocnemum macrostachyum, Halocnemum strobilaceum, Halopeplis perfoliata (Gairola et al., 2019; Jamdade et al., 2022; Leandro et al., 2022).
Much of the upper intertidal areas are dominated by mangroves consisting of a single species, Avicennia marina (Grey mangrove) or qurm in Arabic. Microbial mats, also found on intertidal and supratidal mudflats, are complex, multilayered sessile communities of micro-organisms that are attached to a surface or buried firmly in an extracellular matrix (Roy et al., 2018). They encompass several types of mats that include dried polygonal mats in the upper intertidal zone (Prieto-Barajas et al., 2018), and blue-green cyanobacteria microbial mats in the lower intertidal zone (John and George, 2004).
Further seaward, the lower intertidal seabed is characterised by mudflats, often containing thick tidal sediments. In the shallow subtidal areas, seagrass (Halodule uninervis, Halophila ovalis, and Halophila stipulacea) is the dominant vegetation (Campbell et al., 2015).
Sampling design
Existing coastal habitat maps (Mateos-Molina et al., 2020; Mateos-Molina et al., 2021b) were updated using a new map of mangroves and saltmarshes, classified using spectral analysis (near-infrared for saltmarshes and Transformed Difference Vegetation Index for mangroves) applied to 10 m Sentinel-2 and 3 m Planet Lab satellite data acquired in 2021. A map accuracy assessment was carried out using a random-stratified allocation of 175 and 94 points across the mapped habitat type in Khor Faridah seascape and UAQ seascapes, respectively, with the allocation of samples being proportional to the area of habitat type. Each point was classified into a habitat type by a habitat mapping expert using the very high-resolution (30 to 60 cm pixels) imagery provided by the ESRI World Imagery layer (ESRI, 2022). An error matrix was calculated, resulting in an overall map accuracy of 72% in the Khor Faridah seascape and 78% for the UAQ seascape (Supplementary Tables S1 and S2, Supplementary Materials). The same habitat map was used to develop the blue carbon sampling strategy and illustrate the carbon distribution across the seascape.
Stratified random sampling was employed to select 10 points from each habitat type within the study sites using ArcGIS Pro 3.0. Of these 10 locations, one point per habitat was selected based on accessibility, land tenure and tidal regime, whilst minimising the distance travelled between points (Figure 1). To capture carbon variability caused by differences in tree density in mangrove habitats, two locations surrounding the sampled point were also sampled (one densely and one sparsely vegetated). Furthermore, samples were collected from both intertidal and supratidal areas in saltmarsh habitats and from polygonal, olive, and green mats in microbial mat habitats. In total, 54 cores were sampled across both lagoons.
Field and laboratory methods
The blue carbon assessment considered three main carbon pools: 1) aboveground living biomass (plants and pneumatophores), 2) belowground living biomass (roots) and 3) soil carbon. Little to no aboveground dead biomass (litter, downed wood, dead trees) was observed and thus was not included in the assessment. The survey methods (Figure 2) followed the guidelines of the Blue Carbon Initiative (Howard et al., 2014).
Living biomass estimates
Generally, saltmarshes consisted of the halophytic species A. macrostachyum, across the intertidal to supratidal zone distributed parallel to the shore. The height and density of halophytic plants were collected to estimate above and belowground living biomass using local allometric equations (Supplementary Table S3, Supplementary Material). Height and elliptical crown area were measured in a 25 m2 plot for more spatially homogeneous saltmarshes (unbroken vegetative cover) and four 100 m2 plots in more heterogeneous areas.
A. marina tree height and main stem diameter were recorded at breast height within 10 m2 mangrove sample plots. Two allometric equations were used for mangroves of different sizes (Table S4) and the carbon content was estimated using global tree carbon percentages of 48% and 39% for above- and belowground biomass, respectively (Kauffman and Donato, 2012). Pneumatophores were included in the aboveground biomass calculations by recording the density and height in each area. The density was quantified within a 10 cm wide band inside four randomly placed 1m quadrats. Four pneumatophores were cut at ground level at each corner of the quadrat to calculate an allometric equation from its oven-dried mass (dried for 72 hours at 60 °C). An equation was developed using the pneumatophore height to predict biomass and then multiplied by a carbon conversion factor of 39% (Kauffman and Donato, 2012).
The aboveground biomass of seagrass was collected using a spade to sample two 170 cm2 samples from the top layer of sediment. The samples were washed free of sediment and separated into living aboveground and belowground components at the point of greenery. Belowground carbon in the living component was disregarded due to the minimal mass of root and rhizome content compared to the aboveground component. The carbon content was determined by oven-drying the samples and multiplying the plant biomass by a carbon conversion factor of 34% (Duarte, 1990).
Soil carbon content and grain size estimates
Carbon stock estimates were derived from each sample location, resulting in 54 sediment cores and 244 samples (Table 1). Sediment cores were obtained using PVC pipes of 80 cm (8 cm diameter) or 150 cm in length (10 cm diameter), based on the depth of refusal achievable through manual force. Soil compression was minimised by gently hammering the core and repeating the core extraction where a high level of compaction was recorded. Any soil compaction still recorded was addressed by using a uniform correction factor for the entire core. This was determined by measuring the core, before and after extraction (Howard et al., 2014). Soil depth was defined as the maximum distance to which the core could be inserted.
Cores were photographed and the soil type, colour, and presence of shells, roots, and rhizomes were recorded. Samples were extracted using a syringe and their volume was recorded before being placed into test tubes for drying. Sampling occurred at depth intervals of approximately 0-2, 8-10, 15-17, 26-28, and 40-42 cm, with an additional sample taken at the distal end of the core, reflecting variations in carbon content in the upper 20-50 cm of soil (Howard et al., 2014).
Sub-samples were measured for dry bulk density (dry mass per unit volume) by dividing the mass of the dry soil by the original volume of the sample using a syringe. Sub-samples were analysed for organic (Corg) and inorganic (Cinorg) carbon concentrations. After accounting for compaction, the top 50 cm of soil was determined by multiplying carbon content by the thickness of the depth interval between two samples.
Since core depth varied, comparisons across habitat types in both lagoons required standardising measurements of carbon storage to the top 50 cm of sediment. For shallower cores, values were extrapolated to 50 cm depth, based on the assumption of negligible degradation of Corg with depth. The average length of extrapolation was 20 cm across 16 samples. This approach provided a reliable estimate of soil carbon while minimising the potential for inaccuracy associated with extrapolating to 1 m, where Corg concentration varies considerably.
Carbon content was determined using a Flash 2000 Elemental Analyzer (Thermo-Scientific). After measuring total carbon (Ctot), organic carbon was measured by removing inorganic carbon from a duplicate sub-sample using 3 M hydrochloric acid. Cinorg was determined by subtracting Corg from Ctot. Analytical precision for Corg and Ctot was 0.17 wt. %. For each sub-sample, reverse osmosis water was first used to remove any contaminants and floating organic materials. Following this purification, the sub-sample underwent grain size analysis using a Mastersizer 3000 laser microgranulometer (Malvern). All statistical analysis was conducted in R (build 353). Statistical significance between the total carbon across the habitat types was investigated using a Kruskal-Wallis and Dunn’s posthoc test, using a significant threshold of 0.05. A PERMANOVA (Permutational Multivariate Analysis of Variance) using Euclidean distance, 9999 permutations, and 5 degrees of freedom was used to reduce bias and yield the best power for a model with numerous independent variables and a low number of samples (Anderson and Braak, 2003). The PERMANOVA test was used to explore differences between soil carbon concentrations across individual soil samples for each of the habitat types.
A carbon distribution map was derived by multiplying the average carbon content values by the area of each habitat type in the habitat map. The total carbon stored in the lagoon was estimated by summing the carbon content across all blue carbon habitats. The associated error was determined by calculating the standard error between the highest and lowest estimates of total carbon using the User Accuracy (%) of the habitat map and the standard deviation of the carbon stock estimates.
Results
Carbon stocks in Khor Faridah
In Khor Faridah, saltmarshes stored the largest (90.4 ± 17.0 t/ha) amount of carbon per hectare of any individual habitat type (Figure 3). Mangroves were the second largest (74.7 ± 9.2 t/ha) carbon store per hectare, due to the presence of greater plant biomass. Approximately 88% of carbon in saltmarshes was stored in the soil, which stored almost 30 t/ha more than mangroves and seagrass soils. Microbial mats contained the second largest store of soil carbon (64.9 ± 33.0 t/ha). Mangroves, seagrass, and mudflats stored similar amounts of carbon in the soils (between 48.6 – 54.5 t/ha), with the carbon content in seagrass and mudflats hugely variable (with standard deviations of 20.8 t/ha and 33.8 t/ha, respectively). Coastal sabkha stored the least carbon of all habitat types sampled (31.1 ± 6.2 t/ha). There were significant differences in the total carbon stored across the habitat types (Kruskal-Wallis, p. Adjusted <0.001, eta-squared = 0.36). The effect size was large, indicating that approximately 36% of the variance in the total carbon stock could be explained by differences between the habitats. However, no significant differences were observed between the total carbon stock in each habitat type (Dunn’s, p. Adjusted >0.05), or the soil carbon (Kruskal-Wallis, 0.15; Dunn’s, p. Adjusted >0.05).
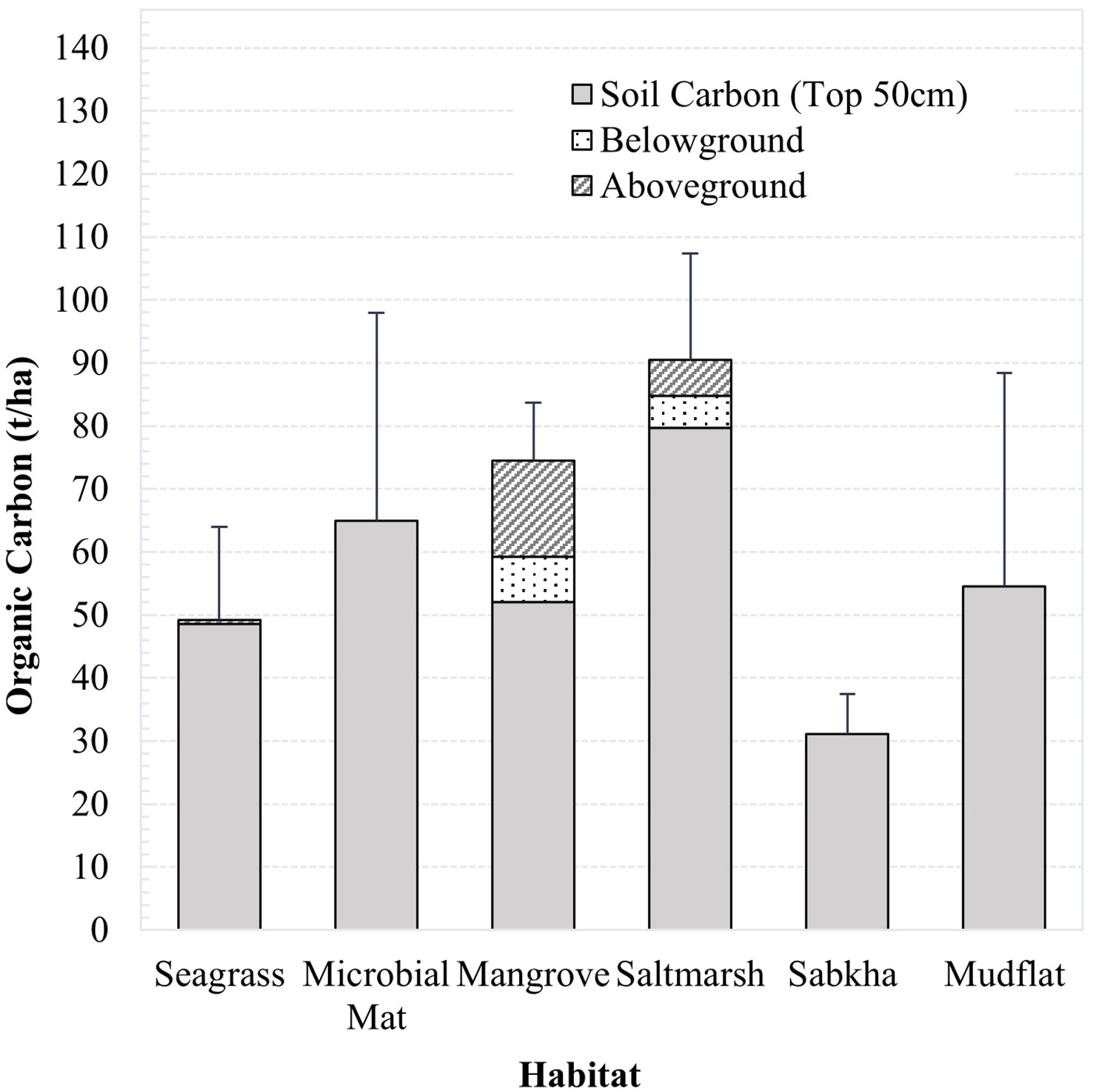
Figure 3 Graph of organic carbon content of each habitat in Khor Faridah for the living and soil components at 50 cm.
Assuming within-habitat homogeneity, most carbon was concentrated in three geographical regions: the eastern side of Saadiyat Island, Ramhan Island, and the eastern area of Ras Ghurab Island which were characterised by extensive mangrove and saltmarsh coverage (Figure 4; Supplementary Table S4, Supplementary Material). Seagrass covered the central areas of the lagoon and was found to be the second-largest contributor of carbon in the seascape, owing to its extensive spatial extent that was approximately twice that of the saltmarshes. In contrast, the less extensive microbial mats, and coastal sabkha exhibited a far smaller contribution to the total carbon stored in the seascape. Mudflats exhibited a high variability with a standard deviation of 52,792 tonnes compared to its carbon stock of 47,102 tonnes. Mangroves were the highest carbon contributors with 249,224 tonnes, but the standard deviation was lower in comparison to its total at 73,074 tonnes. Based on the average carbon store in each habitat type, it is estimated the Khor Faridah seascape stores 600, 878 ± 181,259 tonnes of organic carbon in all the carbon pools down to 50 cm of soil.
Carbon stocks in the UAQ seascape
Mangroves were the largest (113.8 ± 32.4 t/ha) carbon store per hectare of all habitat types sampled, of which 51% was attributed to the aboveground biomass (Figure 5). Microbial mats and mudflats stored similar amounts of carbon contents in their soil (38.3 ± 11.9 t/ha and 39.1 ± 21.7 t/ha, respectively). Approximately 30% of the carbon in saltmarshes was stored in the living biomass, with the soil carbon contribution similar to coastal sabkha (25.8 ± 9.7 t/ha and 30.9 ± 5.2 t/ha, respectively). Seagrass soil carbon storage was lower than all other habitat types (15.0 ± 9.5 t/ha), but statistically significant differences were only detected between mangroves and seagrass (Dunn’s, p=0.0001). There were significant differences in the total carbon stock across the habitat types (Kruskal-Wallis, p<0.001). The effect size was large (eta-squared = 0.77), indicating that approximately 77% of the variance in total carbon stock could be explained by differences between the habitat types. Differences between the soils of each habitat type were significantly different (Kruskal-Wallis, 0.01; eta-squared = 0.47), with only mangroves and seagrass observed as significantly different (Dunn’s, p=0.01).
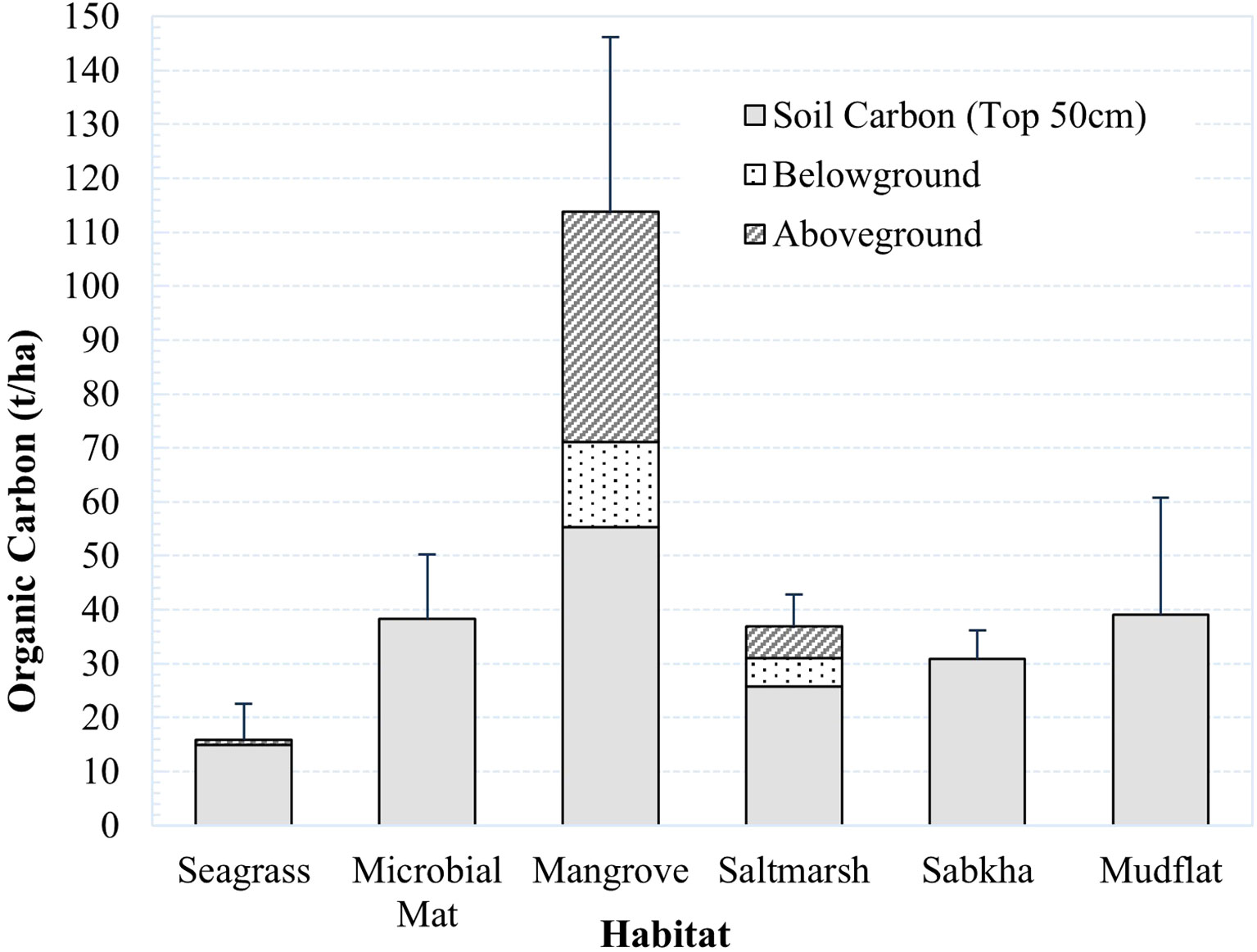
Figure 5 Graph of organic carbon content of each habitat in the UAQ seascape for the living and soil components at 50 cm.
Geographically, our findings indicate that most carbon was concentrated in the vicinity of the central islands, namely Jazirat al Ghallah, Akab, and Siniya which were characterised by extensive mangrove coverage (Figure 6; Supplementary Table S4, Supplementary Material). Mudflat extent was more than twice that of mangroves and contributed the largest proportion of carbon storage in the seascape. In contrast, saltmarshes and coastal sabkha covered a similar area to mangroves but with approximately half of the carbon content. Estimates of total carbon stocks for saltmarshes and coastal sabkha have a particularly low error when compared to seagrass and mangroves. Microbial mats were less extensive than seagrass, yet they stored over 2000 tonnes more carbon across the seascape. Based on the average carbon stored in each habitat type, the UAQ seascape is estimated to store 299, 885 ± 70,917 tonnes of organic carbon in all the carbon pools down to 50 cm of soil.
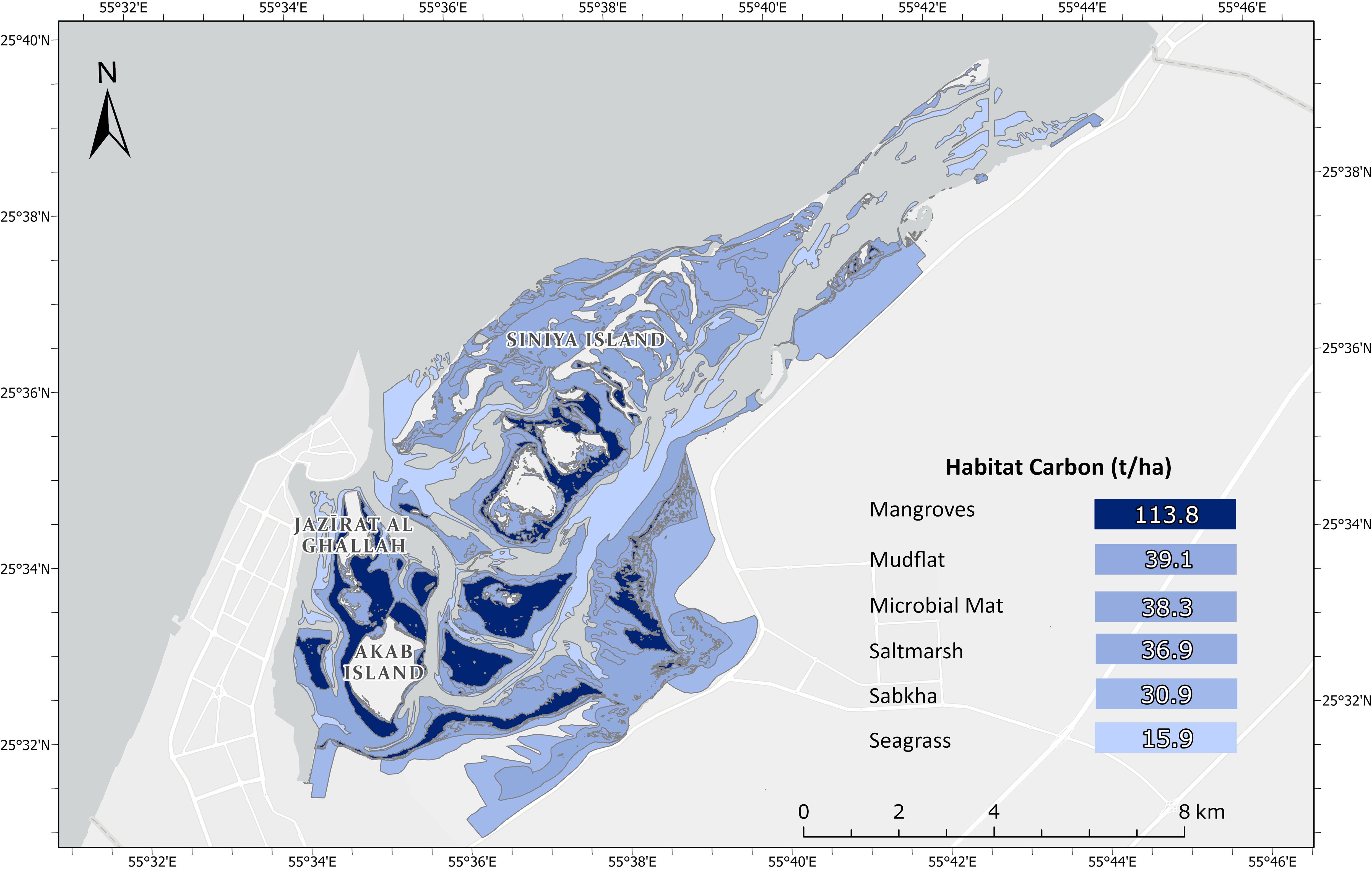
Figure 6 Map of carbon storage in the living biomass and soil carbon at 50 cm across the UAQ seascape.
Collective carbon content from both seascapes
Across both seascapes, mangroves were found to be the highest carbon-storing habitat type with 94.3 ± 19.6 t/ha of carbon, of which approximately 40% was accounted for by the living biomass components (Figure 7). Saltmarshes ranked second with a carbon storage capacity of 63.6 ± 18.3 t/ha, of which 17% was attributed to living biomass. The carbon stored in mudflats and microbial mats was similar, just above and below 50 t/ha respectively. Seagrass and coastal sabkha stored lower carbon stocks in the sediment, just above 30 t/ha. The living components of seagrass contributed 2% of the total carbon stored in the habitat type. There were differences in the total carbon storage among the habitat types (Kruskal-Wallis test p. Adjusted < 0.001, eta-squared = 0.44). The effect size was large, indicating that approximately 44% of the variance in total carbon could be explained by differences between the discrete habitat types. The total carbon storage of mangroves differed from that of mudflats, coastal sabkha, and seagrass (Dunn’s, p. Adjusted = 0.03, 0.003, 0.0001, respectively).
There were differences observed within the total carbon stored in saltmarsh and microbial mats. Within saltmarshes, the mean total carbon was higher for intertidal (76.3 ± 36.5 t/ha) than supratidal (51.1 ± 17.4 t/ha) samples. This difference was more pronounced in the Khor Faridah seascape, where intertidal saltmarshes exceeded the supratidal areas by a difference of 43.8 t/ha. Despite this variance, the differences were not statistically different (Kruskal-Wallis, p. Adjusted = 0.25). Within microbial mats, polygonal mats stored more than double the carbon in the soil (62.8 ± 32.1 t/ha) than regularly inundated green and olive mats (25.4 ± 0 t/ha).
Soil carbon stocks in the top 50 cm did not show a statistical difference between habitats (Kruskal-Wallis, p. Adjusted = 0.13). Soil carbon content was similar across mangroves (53.8 ± 21.1 t/ha), saltmarshes (52.7 ± 31.6), and microbial mats (51.6 ± 24.5 t/ha). Soil carbon stocks were lower in mudflats (46.8 ± 25.3 t/ha) and the lowest in seagrass and coastal sabkha (31.8 ± 20.3 and 31.0 ± 3.9 t/ha, respectively).
Approximately 6% of the samples analysed for organic carbon fell below the analytical precision of the elemental analyser. Accordingly, caution should be exercised when interpreting any observed differences in the lowest carbon-storing samples.
Carbon content and variation across the depth profile
The soil depths sampled varied widely across the seascapes, with the highest range recorded for seagrass and the lowest for saltmarshes (Table 2). The soil depths were greatest for seagrass and lowest for coastal sabkha. When integrating the complete core, and assuming the soil depth captured the entire carbon profile, the soil organic carbon content was highest amongst mangroves and saltmarshes and lowest in coastal sabkha, whilst the content in the remaining habitats was similar. Mangroves exhibited the largest grain size range (178-879 µm) and the highest mean of 353 µm, followed by saltmarshes (322 µm), seagrass (273 µm), coastal sabkha (263 µm), mudflats (261 µm), and microbial mats (259 µm). The addition of carbon derived from the living components raised the total carbon in mangroves and saltmarshes to 98.8 ± 31.1 t/ha and 69.0 ± 23.1 t/ha, respectively. In seagrass, the deeper seagrass soils had a similar total carbon content (53.4 ± 38.5 t/ha) to the microbial mats and mudflats (53.4 ± 40.6 and 50.8 ± 34.9 t/ha, respectively). The complete core values for each seascape are given in the Supplementary Materials (Supplementary Table S5).
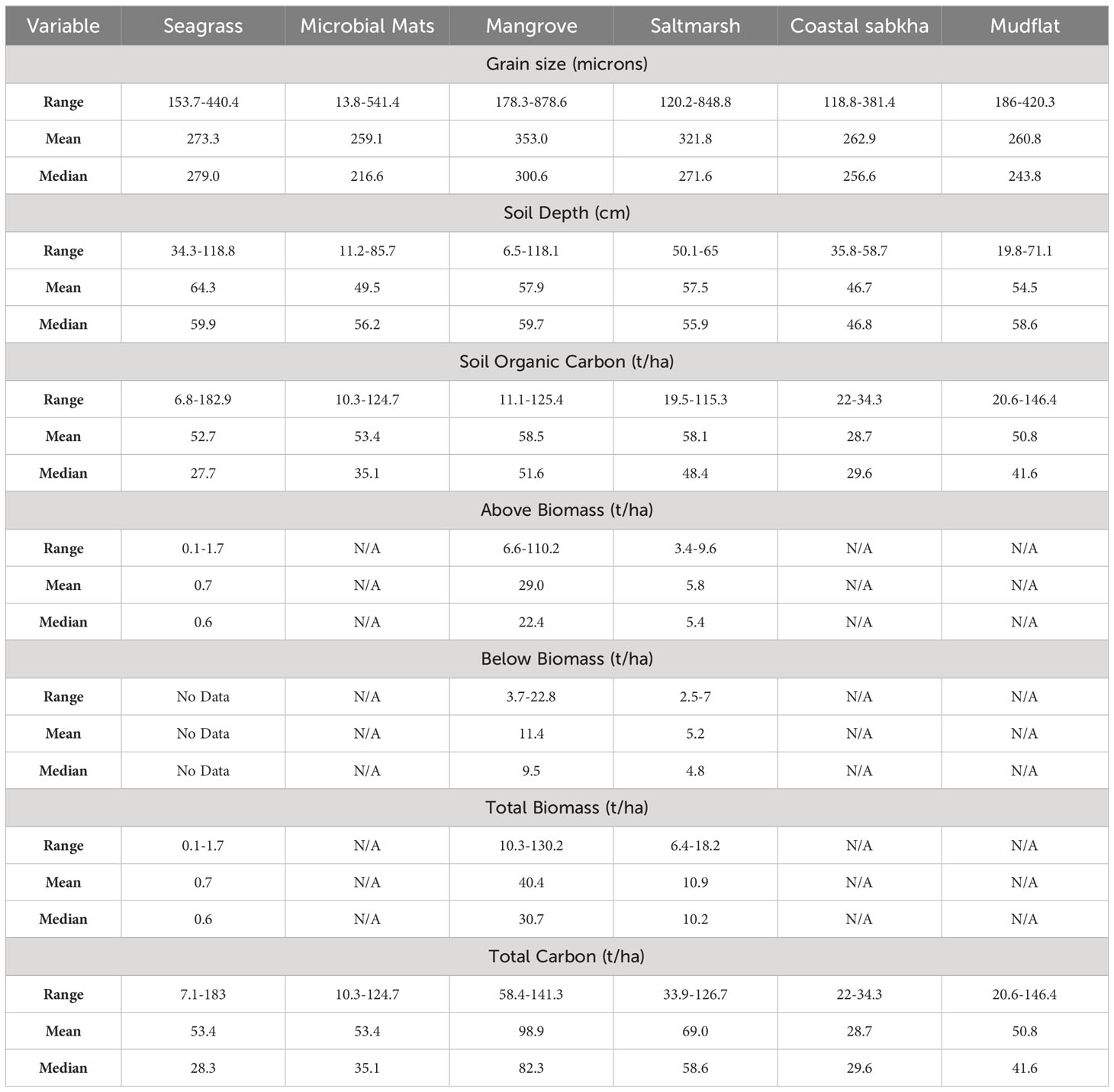
Table 2 Summary of soil depth, grain size and organic carbon stocks for the entire core collected with no extrapolation for cores less than 50 cm.
When all the soil samples across the depth profile were compared (n = 244), significant differences were detected between the habitat types (pseudo-F = 4.20, p-perm= 0.001). Coastal sabkha and seagrass were similar to each other (p perm = 0.61), but different from all other habitat types (p-perm < 0.05). The rest of the habitat types were statistically similar (p perm > 0.05).
Overall, soil carbon stocks tended to decline gradually with depth, except in seagrass (Figure 8). Carbon content varied considerably over depth for every habitat type apart from coastal sabkha, where carbon was consistently low. In general, mangrove soil carbon declined gradually with depth, with the top layers more variable than the deeper layers. In microbial mats, green and olive mats generally exhibited a decrease in carbon content with depth, whereas polygonal mats generally increased in deeper soils (Supplementary Figure S1, Supplementary Material).
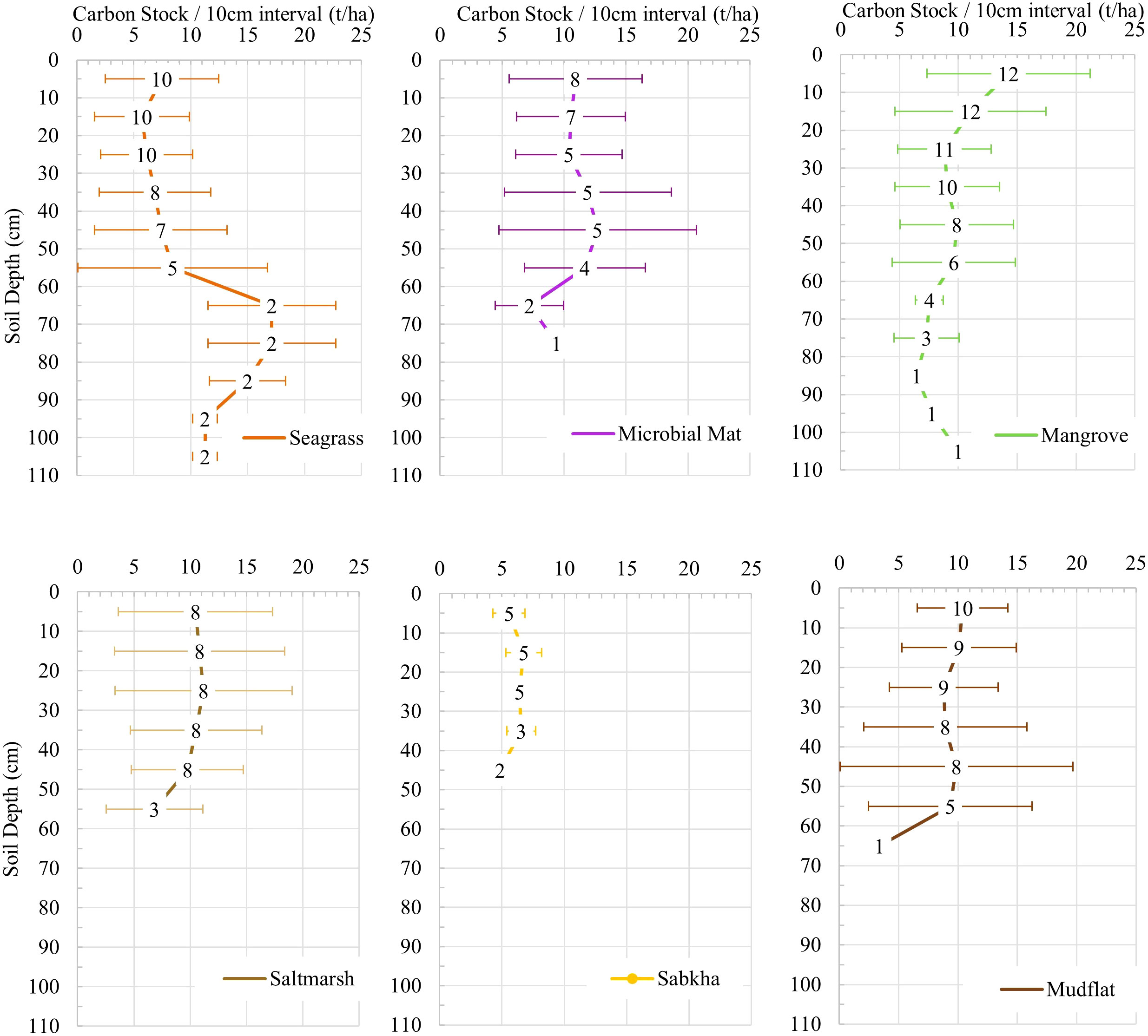
Figure 8 Average organic carbon storage in each habitat across the depth profile. Error bars are standard deviations and labels are the number of cores at each depth interval.
Inorganic carbon content across both seascapes
There was no correlation between organic and inorganic carbon when including all soil samples across the seascape. However, a negative correlation was observed within seagrass (Pearson’s coefficient = -0.54, R-squared = 0.51, p = 0.01) and mangroves (Pearson’s coefficient = -0.80, R-squared = 0.70, p = 0.01) when including all the soil samples across the depth profile (Supplementary Figure S2, Supplementary Material).
Discussion
This comprehensive, multi-habitat blue carbon stock assessment of two UAE seascapes addresses key knowledge gaps in the coastal management of lagoonal seascapes. This assessment provides a robust baseline for implementing the country’s National Climate Change Plan 2050 (MOCCAE, 2017) and Nationally Determined Contributions (MOCCAE, 2023). Carbon stock estimates are provided for six different coastal habitat types, including mudflats for the first time in an arid region. These estimates are essential for evaluating and shaping coastal NbS.
Our research findings support the hypothesis that, despite variations, each habitat type plays a distinct and important role in carbon storage. The soil carbon content across these habitats did not exhibit significant differences, except for sabkha, indicating a level of similarity in soil carbon storage among these varied ecosystems. The data confirm the importance of mangrove forests as the most carbon-rich habitat type encountered when including all carbon pools and underscores the important carbon storage function of the less-well recognised blue carbon habitat types of saltmarshes, seagrass, mudflats, and microbial mats that also support ecosystem integrity, connectivity, and biodiversity.
Our findings highlight the importance of including unvegetated habitat types as well as mangroves in conservation strategies such as NbS. We discovered that mudflats store almost as much carbon in the soil as vegetated habitats and microbial mats. The assessment revealed that saltmarshes can exceed carbon stored in mangroves at some sites, suggesting that often overlooked components of arid coastal ecosystems can contribute substantially to carbon sequestration. To effectively enhance carbon sequestration and biodiversity gains, future coastal climate change mitigation initiatives would benefit from a holistic approach that restores and safeguards the different components of coastal ecosystems.
Carbon stocks in the Khor Faridah seascape
NbS solutions often prioritise single habitat types based on mean carbon storage potential. However, in the Khor Faridah seascape, a range of discrete habitat types store comparable amounts of carbon in the top 50 cm of soil, despite significant differences in above and below ground biomass and soil composition. While vegetative habitats likely contribute significantly to carbon storage, it appears that storage is more dependent on local ecological characteristics that are often insufficiently measured in blue carbon assessments (Macreadie et al., 2019). These characteristics may encompass factors such as hydrodynamic energy, topography, and habitat structure, rather than inter-habitat variations (Samper-Villarreal et al., 2016). This influence is expected to be more pronounced in arid environments, which generally exhibit lower vegetation diversity and often lack riverine systems (Quoreshi et al., 2022) when compared to tropical areas (Brown et al., 2016; Radabaugh et al., 2018).
Carbon stocks in the UAQ seascape
In the UAQ seascape, mangroves are crucial in maintaining the largest organic carbon stores within the area. The high density of trees and tall pneumatophores found in these mangroves (Supplementary Table S6, Supplementary Material) contributes to the substantial carbon content derived from the living biomass. The high aboveground biomass in mangroves may be an adaptation to anoxic soil conditions (Al-Khayat and Alatalo, 2021) in shallow soils (mean core depth of 44.5 cm) across the seascape, which likely increases the oxygen demand.
Whilst mangroves are an important contributor to carbon storage in the lagoon, other biochemical processes are likely influencing the carbon content in the soils. Blue carbon sediments are prone to mineralisation, a process that converts organic carbon to inorganic stores, resulting in the deposition of calcium carbonate and releasing carbon dioxide (Howard et al., 2018; Filgueira et al., 2019). Notably, a substantial presence of bivalves, which sequester, calcify, and respire carbon, was observed, indicating they may contribute to soil carbon storage or result in carbon emission when dissolved.
In both seagrass and mangrove habitats, mineralisation appears to be an active process, supported by evidence of the inverse trend observed in the inorganic to organic depth profile (Supplementary Figure S2, Supplementary Material). This mineralisation process may contribute to variations in organic carbon content within and between habitats, particularly when inorganic carbon constitutes a substantially larger proportion of the total carbon in the soils (Supplementary Figure S4, Supplementary Material). This may explain lower organic carbon storage in the seagrass habitats of UAQ compared to mudflats, despite their ability to facilitate the deposition of suspended sediment (Fonseca and Fisher, 1986; Kennedy et al., 2010).
Carbon stocks across both seascapes
Carbon storage varies significantly across all habitats in both lagoons, except for coastal sabkha. Contrary to a previous study in the region (Schile et al., 2017), the average soil carbon content in saltmarshes was found to be as large as mangroves. This finding, also observed in Saudi Arabia (Cusack et al., 2018), could be attributable to the enhanced sediment trapping abilities of saltmarshes (Chen et al., 2018), leading to the increased deposition of finer sediments. In support, our data showed a reduction in mean grain size by 8.8% in saltmarshes compared to mangroves. When considering the carbon storage in intertidal areas, saltmarsh soils were found to store more carbon than mangroves. Intertidal areas, being more frequently inundated by tidal waters, create more anoxic conditions that impede decomposition, thereby promoting carbon retention (Kirwan et al., 2009). Further research is required to explain the role of saltmarsh vegetation in carbon storage dynamics between intertidal and supratidal saltmarshes. Nonetheless, these findings emphasise the significance of saltmarshes as blue carbon reservoirs in arid ecosystems, especially those that are intertidal.
In certain mangrove and saltmarsh areas, a reduction in carbon content was noted within the uppermost 0-30 cm of their sediment layers (Supplementary Figure S3, Supplementary Material). By examining the environmental conditions captured in sample photographs and comparing the samples with stable carbon content, an association was observed between the reduction in carbon in the topsoil and the high density of crab burrows. Previous research has documented that crab burrows facilitate soil aeration and promote the oxidation of organic carbon (Kristensen et al., 2008) and the release of methane and nitrous oxide, both potent but short-lived greenhouse gases (Rosentreter et al., 2018; Agusto et al., 2022; Grow et al., 2022; Alongi, 2023). Therefore, it is expected that these burrows yield more labile carbon, leading to a more rapid reduction in carbon in this horizon. Interestingly, the core that contained the highest carbon content throughout the entire seascape was in an intertidal saltmarsh with minimal crab burrowing.
In agreement with previous work, microbial mats are an important habitat for carbon storage (Schile et al., 2017). Nonetheless, it is imperative to consider the algal mat type in the development of management policies, as the carbon storage potential varies significantly among different algal mat types. Consistent with prior studies, olive/green mats present in the lower tidal zone contained less carbon than polygonal-cracked dry mats in the upper tidal zone (Abed et al., 2008). The drier conditions and reduced microbial activity in the upper intertidal zone create an environment conducive to the preservation of organic matter via the formation of a biofilm layer. This preservation process can both increase carbon storage and elevate organic carbon content in deeper soil horizons (Bhaskar and Bhosle, 2005). When designing NbS in coastal zones, conservation efforts should be prioritised towards polygonal microbial mats, given their crucial role in carbon storage.
Comparing carbon storage across literature in different habitats and lagoons poses challenges due to the spatial and depth-related variability of carbon content. This challenge is further complicated by using average carbon contents quantified from different soil depths. Previous studies have reported higher total carbon stocks in mangroves in the UAE compared to the findings of this study. On average, carbon stocks in mangroves across the UAE range from 229 t/ha (Arabian Gulf) to 389 t/ha (UAE East Coast), with an average soil depth of 71 cm (Schile et al., 2017). In contrast, along the west coast of Saudi Arabia, the reported carbon storage is 123 t/ha to a soil depth of 50 cm (Eid et al., 2020). In comparison, carbon stocks in mangroves in Qatar and the east coast of Saudi Arabia are lower, storing 46 t/ha and 43-76 t/ha to 1 m of sediments, respectively (Cusack et al., 2018; Chatting et al., 2020). Nevertheless, soil carbon stocks in mangroves were less than those reported in tropical and temperate regions (Thorhaug et al., 2020; Banda et al., 2021). The seagrass biomass in the studied area marginally exceeds the mean value of 0.4 t/ha estimated in Abu Dhabi (Campbell et al., 2015), but remains lower than global averages (Fourqurean et al., 2012).
This study also corroborates recent observations that mangrove trees are particularly important for carbon storage in arid environments (Schile et al., 2017). Our results showed that mangrove trees in the UAE stored more carbon in their biomass than sites sampled in Qatar (27.5 t/ha; Chatting et al., 2020) and a similar amount to mangroves in a tropical estuary in West Africa (47.5 t/ha; Kauffman and Bhomia, 2017). However, living biomass in the UAE remains lower than those in the majority of areas in the Indo-Pacific region (Donato et al., 2011).
Implementing NbS to optimise carbon storage
This study’s results have significant implications for NbS development in both lagoons. In Khor Faridah, where conversion of habitat is occurring through urbanisation, it is important to highlight the risk of lost carbon storage function for habitat types previously undervalued for their blue carbon function. In addition to carbon estimates per hectare, policies should consider the abundance of each habitat type when preserving carbon across the seascape. For example, seagrass in Khor Faridah is one of the lowest carbon stores per hectare, but the extensive coverage makes it the second-largest contributor of carbon in the lagoon. Subsequently, NbS should focus on solutions that protect or enhance seagrass across the whole lagoon, such as protected area designation, improving fishing practices, and managing dredging practices. Dredging is likely to impact blue carbon storage in seagrass by altering environmental conditions including turbidity, wave height, and water depth, especially in sheltered areas known for higher carbon storage (Samper-Villarreal et al., 2016).
Some studies have shown that restoring areas known to support mangroves provide greater carbon storage and seedling survival rates compared with the afforestation of non-mangrove mudflats (Lovelock et al., 2022; Song et al., 2023). When planning mangrove planting initiatives, it is crucial to consider the potential impacts on mudflats that are proven carbon sinks (Howard et al., 2023) and provide habitat for waterbirds (Javed et al., 2019). Further, there is a risk that the conservation of mangroves may lead to the de-prioritisation of saltmarshes that are considerable carbon stores, promoting leakage elsewhere in the lagoon (Wylie et al., 2016). Thus, NbS initiatives should focus on protecting and enhancing the carbon storage potential of existing habitats, including both vegetated and non-vegetated types, and local ecological conditions and management impacts. A nuanced, context-dependent, and adaptive approach is needed, highlighting the unique characteristics of ecosystems and habitats involved, that considers the potential for biodiversity-positive outcomes and co-benefits for people.
In the UAQ seascape, NbS that aims to maximise carbon will be directed towards planting mangroves due to their high carbon storage as indicated by the large proportion of carbon in the living biomass. When effectively designed, mangrove restoration also offers high feasibility in terms of cost and success rates (Friess et al., 2022b; Lovelock et al., 2022). However, before converting other coastal habitats to mangroves, decision makers should consider identifying what drives carbon storage in the highest storing areas and plan management and conservation areas accordingly. For example, management plans could prevent habitat fragmentation which has been shown to increase carbon stores whilst maintaining species diversity (Ricart et al., 2017; Gullström et al., 2018).
In addition to mangroves, incorporating other NbS strategies can enhance carbon storage and promote biodiversity including saltmarsh establishment, wetland restoration and macroalgae farming (Pessarrodona et al., 2023). These solutions not only enhance carbon sequestration but offer cobenefits to ecosystems and local communities, such as providing nursery habitats for commercially valuable fish species and improving water quality (Stewart-Sinclair et al., 2021; Jankowska et al., 2022). Nevertheless, any NbS intervention for coastal ecosystems must consider changing habitat suitability induced by climate change particularly changes to sea level, sedimentation, and salinity. In some parts of the world, mangroves and saltmarsh will struggle to keep pace with sea level rise and will be increasingly prone to more severe impacts from storms and thermal stress (Friess et al., 2022a; Saintilan et al., 2023).
Future research
To enhance our understanding of carbon storage patterns in habitats with similar ecological configurations, future research should aim to increase the number of samples collected. More samples help to discriminate the carbon content in each habitat type, where significant differences in this study were limited to those between mangroves and the lowest-storing habitats. In dynamic environments, increasing the number of samples over the core would provide a clearer understanding of how the stability of an individual habitat type influences carbon storage. For example, it would address questions regarding the time required for the formation of a microbial mat biofilm layer to enhance carbon sequestration in underlying soils. Regarding mangroves specifically, the observed carbon variability may have been influenced by methodological uncertainties when measuring Avicennia marina trees. This species often exhibits multiple branches that fork below the direct breast height, introducing measurement challenges. To address this issue, it is recommended to employ allometric equations based on canopy cover and/or height.
The average carbon content for each habitat, while informative, requires consideration of variability to avoid inaccuracies when extrapolating data to other arid lagoons. To enhance the precision of carbon stock mapping across the lagoon, when compared to area-based estimations, ensemble regression models could be used if data on soil composition, climate, vegetation, relief, or geology are available in the area. (Ladd et al., 2022). To fully understand this variability, further research is needed to explore how local ecological configuration, composition, and connectivity influence carbon stocks (Huxham et al., 2018; Asplund et al., 2021).
Accurate estimates of carbon sequestration rates and a greater exchange of knowledge and data among scientists across Gulf countries is essential (Fawzi et al., 2022). Across each habitat type are essential to design NbS aimed at maximising future carbon storage. In arid regions that are likely to have lower Corg content, it is recommended to employ dating alternatives to Corg such as 210Pb used in previous studies in the region to retrieve reliable dating estimates (Almahasheer et al., 2017). The use of gouge or Russian corers may also reduce the effect of compaction when compared to PVC cores (Huxham et al., 2018).
In addition, given the large proportion of inorganic carbon present in the soil, further research is needed to understand its role in carbon dynamics. Specifically, whether inorganic carbon contributes to elevating sequestration rates or offset them by dissolving being released back into the atmosphere. Furthermore, there is limited information on how burrowing fauna affect carbon dynamics in arid environments. These organisms can negatively impact carbon storage by releasing CO2 and more potent greenhouse gases such as methane and nitrous oxide, thereby reducing the net contribution of mangroves in mitigating climate change (Rosentreter et al., 2021).
Conclusion
Our study highlights the importance of adopting a multi-habitat perspective in assessing blue carbon stocks in arid seascapes. This has direct implications for the development of NbS strategies, which should account for differences in carbon dynamics at the seascape-level. Our findings in the Khor Faridah seascape show that carbon stocks across blue carbon habitats are similar and emphasise the need to value carbon storage in the seascape based on ecological characteristics and context rather than simply reporting mean values for single habitat types. Whilst in the UAQ seascape, mangrove trees contribute a substantial proportion of the carbon across the seascape and should be prioritised for conservation accordingly. In both seascapes, the non-vegetated habitats can store large carbon deposits, comparable to well-recognised sources of blue carbon. In particular, intertidal saltmarshes play a crucial role in carbon storage in arid lagoons. Further research is needed to identify the key ecological drivers of carbon storage in arid contexts and ecological consequences of climate change to refine NbS strategies.
This multi-habitat blue carbon assessment is essential for realigning NbS projects towards a more holistic whole-system approach that protects and enhances carbon storage across the seascape as part of ecosystem-based management. The study provides a scientific baseline to inform an evidence-based approach for designing and implementing NbS, supporting their expansion for climate action at the Emirate and national levels. Our study contributes to the broader scientific understanding of the role of coastal ecosystems in climate change mitigation, aligning with the global movement to restore coastal habitats. In doing so, this research addresses a crucial knowledge gap and lays the groundwork for informed decision-making in the pursuit of sustainable development and the associated ecological and societal benefits.
Data availability statement
The original contributions presented in the study are included in the article/Supplementary Material. Further inquiries can be directed to the corresponding author.
Author contributions
The research was led by SC, who wrote the initial draft of the paper. The project was conceived and led by DM-M and MA. CE, SP, and KS contributed to the design of the carbon accounting methods and sampling design and provided input to writing and revising the manuscript. Additionally, IB, HD, MM, KP, and FS provided valuable guidance throughout the project, supported fieldwork activities, shared data, and contributed edits to enhance subsequent versions of the manuscript. All authors contributed to the article and approved the submitted version.
Funding
This project was funded by HSBC through the global initiative Climate Solutions Partnership together with WWF and WRI. The funder had no role in study design, data collection and analysis, decision to publish, or preparation of the manuscript.
Acknowledgments
This study was carried out within the framework of the Nature Based Solutions project for climate, biodiversity, and people (2021-2025) conducted in the UAE by a consortium of partners and collaborators. HSBC provided the funding for this work which is a partnership between the Minister of Climate Change and Environment (MOCCAE), the Minister of Economy (MoE), the Environment Agency – Abu Dhabi (EAD), the Government of Umm Al Quwain, Emirates Nature-WWF and the International Center for Biosaline Agriculture (ICBA). The study is a component of the Nature-based Solution project led by Emirates Nature-WWF with local partners and part of the Climate Solution Partnership between HSBC, World Resources Institute (WRI) and WWF. A special thanks to His Highness Sheikh Majid Al Mualla, Chairman of Umm Al Quwain Tourism and Archaeology, Her Excellency Dr Shaikha Salem Al Dhaheri, Secretary General of EAD, and Ahmed Al Hashmi, Acting Executive Director of Terrestrial and Marine Biodiversity at EAD, for their advice and support throughout this study. Thanks to Ibrahim A. Bugla and Maitha Al Hameli for their expertise and support in this study. Furthermore, special thanks are due to Mr Mohammad from Kashta Boat Rentals and Mr Abdallah from Creek Adventures for their support during the fieldwork. We are grateful for the hard work and support from the field, lab assistants and statisticians and colleagues; Sherif Abdullah, Arabella Willing, Ahmed Abusamra, Waqas Ahmed, Norhan Ahmed, Dana Hilles and Meera Alrasai.
Conflict of interest
Author SP was employed by the company Seascape Analytics Ltd. Author KS was employed by the company Seascape Solutions LLC.
The remaining authors declare that the research was conducted in the absence of any commercial or financial relationships that could be construed as a potential conflict of interest.
Publisher’s note
All claims expressed in this article are solely those of the authors and do not necessarily represent those of their affiliated organizations, or those of the publisher, the editors and the reviewers. Any product that may be evaluated in this article, or claim that may be made by its manufacturer, is not guaranteed or endorsed by the publisher.
Supplementary material
The Supplementary Material for this article can be found online at: https://www.frontiersin.org/articles/10.3389/fmars.2023.1239904/full#supplementary-material
References
Abed R. M. M., Kohls K., Schoon R., Scherf A.-K., Schacht M., Palinska K. A., et al. (2008). Lipid biomarkers, pigments and cyanobacterial diversity of microbial mats across intertidal flats of the arid coast of the Arabian Gulf (Abu Dhabi, UAE). FEMS Microbiol. Ecol. 65, 449–462. doi: 10.1111/j.1574-6941.2008.00537.x
Agusto L. E., Qin G., Thibodeau B., Tang J., Zhang J., Zhou J., et al. (2022). Fiddling with the blue carbon: Fiddler crab burrows enhance CO2 and CH4 efflux in saltmarsh. Ecol. Indic 144, 109538. doi: 10.1016/j.ecolind.2022.109538
Al-Khayat J. A., Alatalo J. M. (2021). Relationship between tree size, sediment mud content, oxygen levels, and pneumatophore abundance in the mangrove tree species Avicennia marina (Forssk.) Vierh. J. Mar. Sci. Eng. 9, 100. doi: 10.3390/jmse9010100
Almahasheer H., Serrano O., Duarte C. M., Arias-Ortiz A., Masque P., Irigoien X. (2017). Low carbon sink capacity of Red Sea mangroves. Sci. Rep. 7, 9700. doi: 10.1038/s41598-017-10424-9
Alongi D. M. (2023). Current status and emerging perspectives of coastal blue carbon ecosystems. doi: 10.20517/cf.2023.04
Anderson M., Braak C.T. (2003). Permutation tests for multi-factorial analysis of variance. J. Stat. Comput. Simul. 73, 85–113. doi: 10.1080/00949650215733
Asplund M. E., Dahl M., Ismail R. O., Arias-Ortiz A., Deyanova D., Franco J. N., et al. (2021). Dynamics and fate of blue carbon in a mangrove–seagrass seascape: influence of landscape configuration and land-use change. Landsc. Ecol. 36, 1489–1509. doi: 10.1007/s10980-021-01216-8
Banda S. P., Adams J. B., Rajkaran A., Johnson J. L., Raw J. L. (2021). “Chapter 18 - Blue carbon storage comparing mangroves with saltmarsh and seagrass habitats at a warm temperate continental limit,” in Dynamic Sedimentary Environments of Mangrove Coasts, eds. Sidik F., Friess D. A. (Elsevier), 447–471. doi: 10.1016/B978-0-12-816437-2.00008-2
Ben Lamine E., Mateos-Molina D., Antonopoulou M., Burt J. A., Das H. S., Salim J., et al. (2021). Correction to: Identifying coastal and marine priority areas for conservation in the United Arab Emirates. Biodivers Conserv. 30, 2277. doi: 10.1007/s10531-021-02181-z
Bhaskar P. V., Bhosle N. B. (2005). Microbial extracellular polymeric substances in marine biogeochemical processes. Curr. Sci. 88, 45–53. Available at: http://www.jstor.org/stable/24110092.
Bouillon S., Borges A. V., Castañeda-Moya E., Diele K., Dittmar T., Duke N. C., et al. (2008). Mangrove production and carbon sinks: a revision of global budget estimates. Global Biogeochem. Cycles 22. doi: 10.1029/2007GB003052
Brown D. R., Conrad S., Akkerman K., Fairfax S., Fredericks J., Hanrio E., et al. (2016). Seagrass, mangrove and saltmarsh sedimentary carbon stocks in an urban estuary; Coffs Harbour, Australia. Reg. Stud. Mar. Sci. 8, 1–6. doi: 10.1016/j.rsma.2016.08.005
Brown D. R., Marotta H., Peixoto R. B., Enrich-Prast A., Barroso G. C., Soares M. L. G., et al. (2021). Hypersaline tidal flats as important “blue carbon” systems: a case study from three ecosystems. Biogeosciences 18, 2527–2538. doi: 10.5194/bg-18-2527-2021
Buelow C. A., Connolly R. M., Turschwell M. P., Adame M. F., Ahmadia G. N., Andradi-Brown D. A., et al. (2022). Ambitious global targets for mangrove and seagrass recovery. Curr. Biol. 32, 1641–1649. doi: 10.1016/j.cub.2022.02.013
Campbell J. E., Lacey E. A., Decker R. A., Crooks S., Fourqurean J. W. (2015). Carbon storage in seagrass beds of Abu Dhabi, United Arab emirates. Estuaries Coasts 38, 242–251. doi: 10.1007/s12237-014-9802-9
Chatting M., LeVay L., Walton M., Skov M. W., Kennedy H., Wilson S., et al. (2020). Mangrove carbon stocks and biomass partitioning in an extreme environment. Estuar. Coast. Shelf Sci. 244, 106940. doi: 10.1016/j.ecss.2020.106940
Chen Z. L., Lee S. Y. (2022). Tidal flats as a significant carbon reservoir in global coastal ecosystems. Front. Mar. Sci. 9, 900896. doi: 10.3389/fmars.2022.900896
Chen Y., Li Y., Thompson C., Wang X., Cai T., Chang Y. (2018). Differential sediment trapping abilities of mangrove and saltmarsh vegetation in a subtropical estuary. Geomorphology 318, 270–282. doi: 10.1016/j.geomorph.2018.06.018
Cohen-Shacham E., Andrade A., Dalton J., Dudley N., Jones M., Kumar C., et al. (2019). Core principles for successfully implementing and upscaling Nature-based Solutions. Environ. Sci. Policy 98, 20–29. doi: 10.1016/j.envsci.2019.04.014
Cusack M., Saderne V., Arias-Ortiz A., Masque P., Krishnakumar P. K., Rabaoui L., et al. (2018). Organic carbon sequestration and storage in vegetated coastal habitats along the western coast of the Arabian Gulf. Environ. Res. Lett. 13, 074007. doi: 10.1088/1748-9326/aac899
Donato D. C., Kauffman J. B., Murdiyarso D., Kurnianto S., Stidham M., Kanninen M. (2011). Mangroves among the most carbon-rich forests in the tropics. Nat. Geosci 4, 293–297. doi: 10.1038/ngeo1123
Duarte C. M. (1990). Seagrass nutrient content. Mar. Ecol. Prog. Ser. 67, 201–207. doi: 10.3354/meps067201
Eid E. M., Khedher K. M., Ayed H., Arshad M., Moatamed A., Mouldi A. (2020). Evaluation of carbon stock in the sediment of two mangrove species, Avicennia marina and Rhizophora mucronata, growing in the Farasan Islands, Saudi Arabia. Oceanologia 62, 200–213. doi: 10.1016/j.oceano.2019.12.001
Elouafi I., Shahid M. A., Begmuratov A., Hirich A. (2020). The contribution of alternative crops to food security in marginal environments, in Emerging Research in Alternative Crops, eds Hirich A., Choukr-Allah R., Ragab R. (Cham: Springer International Publishing), 1–23. doi: 10.1007/978-3-319-90472-6_1
ESRI (2022). “World imagery” [basemap]. 14.12.22. Scale not given. “World imagery map”. Environmental Systems Research Institute, Redlands, California, USA.
Fawzi N. A.-M., Fieseler C. M., Helmuth B., Leitão A., Al-Ainsi M., Al Mukaimi M., et al. (2022). Diplomacy for the world’s hottest sea. Science 376, 1389–1390. doi: 10.1126/science.add1555
Filgueira R., Strohmeier T., Strand Ø. (2019). “Regulating services of bivalve molluscs in the context of the carbon cycle and implications for ecosystem valuation,”. Smaal A., Ferreira J., Grant J., Petersen J., Strand Ø. (eds) Goods Serv. Mar. bivalves (Cham: Springer), 231–251. doi: 10.1007/978-3-319-96776-9_12
Fonseca M. S., Fisher J. S. (1986). A comparison of canopy friction and sediment movement between four species of seagrass with reference to their ecology and restoration. Mar. Ecol. Prog. Ser. 29, 5–22. doi: 10.3354/meps029015
Fourqurean J. W., Duarte C. M., Kennedy H., Marbà N., Holmer M., Mateo M. A., et al. (2012). Seagrass ecosystems as a globally significant carbon stock. Nat. Geosci 5, 505–509. doi: 10.1038/ngeo1477
Friess D. A., Adame M. F., Adams J. B., Lovelock C. E. (2022a). Mangrove forests under climate change in a 2 C world. Wiley Interdiscip Rev. Clim. Change 13, e792. doi: 10.1002/wcc.792
Friess D. A., Gatt Y. M., Ahmad R., Brown B. M., Sidik F., Wodehouse D. (2022b). Achieving ambitious mangrove restoration targets will need a transdisciplinary and evidence-informed approach. One Earth 5, 456–460. doi: 10.1016/j.oneear.2022.04.013
Gairola S., Mahmoud T., Shabana H. A. (2019). Diversity and distribution of salt-tolerant plants of the United Arab Emirates: perspectives for sustainable utilization and future research. Sabkha Ecosystems: Volume VI: Asia/Pacific, 349–362. doi: 10.1007/978-3-030-04417-6_21
Gilby B. L., Olds A. D., Connolly R. M., Henderson C. J., Schlacher T. A. (2018). Spatial restoration ecology: placing restoration in a landscape context. Bioscience 68, 1007–1019. doi: 10.1093/biosci/biy126
Grow A. K., Schutte C. A., Roberts B. J. (2022). Fiddler crab burrowing increases salt marsh greenhouse gas emissions. Biogeochemistry 158, 73–90. doi: 10.1007/s10533-021-00886-5
Guimaraes L., Hugel B., Jover N., Paz-Rivera C., Design K. T., Koserowski K. (2019) Pathway for Increasing Nature-based Solutions in NDCs: A Seven-Step Approach for Enhancing Nationally Determined Contributions through Nature-based Solutions. Available at: https://www.nytimes.com/2019/05/28/climate/united-nations-climate-pledges.html.
Gullström M., Lyimo L. D., Dahl M., Samuelsson G. S., Eggertsen M., Anderberg E., et al. (2018). Blue carbon storage in tropical seagrass meadows relates to carbonate stock dynamics, plant–sediment processes, and landscape context: insights from the western Indian Ocean. Ecosystems 21, 551–566. doi: 10.1007/s10021-017-0170-8
Hereher M. E. (2020). Assessment of climate change impacts on sea surface temperatures and sea level rise—The Arabian Gulf. Climate 8, 50. doi: 10.3390/cli8040050
Hilmi N., Chami R., Sutherland M. D., Hall-Spencer J. M., Lebleu L., Benitez M. B., et al. (2021). The role of blue carbon in climate change mitigation and carbon stock conservation. Front. Climate 102. doi: 10.3389/fclim.2021.710546
Howard J. L., Creed J. C., Aguiar M. V. P., Fourqurean J. W. (2018). CO2 released by carbonate sediment production in some coastal areas may offset the benefits of seagrass “Blue Carbon” storage. Limnol Oceanogr. 63, 160–172. doi: 10.1002/lno.10621
Howard J., Hoyt S., Isensee K., Telszewski M., Pidgeon E. (2014). Coastal blue carbon: Methods for assessing carbon stocks and emissions factors in mangroves, tidal salt marshes, and seagrasses (Arlington, Virginia, USA: Conservation International, Intergovernmental Oceanographic Commission of UNESCO, International Union for Conservation of Nature).
Howard J., Sutton-Grier A. E., Smart L. S., Lopes C. C., Hamilton J., Kleypas J., et al. (2023). Blue carbon pathways for climate mitigation: Known, emerging and unlikely. Mar. Policy 156, 105788. doi: 10.1016/j.marpol.2023.105788
Huxham M., Whitlock D., Githaiga M., Dencer-Brown A. (2018). Carbon in the coastal seascape: how interactions between mangrove forests, seagrass meadows and tidal marshes influence carbon storage. Curr. Forestry Rep. 4, 101–110. doi: 10.1007/s40725-018-0077-4
Jamdade R., Al-Shaer K., Al-Sallani M., Al-Harthi E., Mahmoud T., Gairola S., et al. (2022). Multilocus marker-based delimitation of Salicornia persica and its population discrimination assisted by supervised machine learning approach. PloS One 17, e0270463. doi: 10.1371/journal.pone.0270463
Jankowska E., Pelc R., Alvarez J., Mehra M., Frischmann C. J. (2022). Climate benefits from establishing marine protected areas targeted at blue carbon solutions. Proc. Natl. Acad. Sci. 119, e2121705119. doi: 10.1073/pnas.2121705119
Javed S., ElAlQamy H., Khan S. B., Ahmed S., Al Dhaheri S. S., Al Hammadi A., et al. (2019). Using greater flamingo tracking and count data in delineating marine protected areas in the coastal zone of Abu Dhabi, United Arab Emirates: Conservation planning in an economically important area. Glob Ecol. Conserv. 17, e00557. doi: 10.1016/j.gecco.2019.e00557
John D. M., George J. D. (2004). Intertidal and subtidal benthic communities in Abu Dhabi, United Arab Emirates (Abu Dhabi, UAE: Marine Atlas of Abu Dhabi. Emirates Heritage Club), 94–123.
Kauffman J. B., Bhomia R. K. (2017). Ecosystem carbon stocks of mangroves across broad environmental gradients in West-Central Africa: global and regional comparisons. PloS One 12, e0187749. doi: 10.1371/journal.pone.0187749
Kauffman J. B., Donato D. C. (2012). Protocols for the measurement, monitoring and reporting of structure, biomass and carbon stocks in mangrove forests (Indonesia: Cifor Bogor).
Keesstra S., Mol G., De Leeuw J., Okx J., De Cleen M., Visser S. (2018). Soil-related sustainable development goals: Four concepts to make land degradation neutrality and restoration work. Land (Basel) 7, 133. doi: 10.3390/land7040133
Keith H., Vardon M., Obst C., Young V., Houghton R. A., Mackey B. (2021). Evaluating nature-based solutions for climate mitigation and conservation requires comprehensive carbon accounting. Sci. Total Environ. 769, 144341. doi: 10.1016/j.scitotenv.2020.144341
Kennedy H., Beggins J., Duarte C. M., Fourqurean J. W., Holmer M., Marbà N., et al. (2010). Seagrass sediments as a global carbon sink: Isotopic constraints. Global Biogeochem. Cycles 24, GB4026. doi: 10.1029/2010GB003848
Kirwan M. L., Guntenspergen G. R., Morris J. T. (2009). Latitudinal trends in Spartina alterniflora productivity and the response of coastal marshes to global change. Glob Chang Biol. 15, 1982–1989. doi: 10.1111/j.1365-2486.2008.01834.x
Kristensen E., Bouillon S., Dittmar T., Marchand C. (2008). Organic carbon dynamics in mangrove ecosystems: a review. Aquat Bot. 89, 201–219. doi: 10.1016/j.aquabot.2007.12.005
Ladd C. J. T., Smeaton C., Skov M. W., Austin W. E. N. (2022). Best practice for upscaling soil organic carbon stocks in salt marshes. Geoderma 428, 116188. doi: 10.1016/j.geoderma.2022.116188
Leandro P., Knuteson S. L., Bartholomew A. (2022). The vegetation of the United Arab Emirates gulf coast: description and analysis. J. Coast. Res. 38, 951–967. doi: 10.2112/JCOASTRES-D-21-00162.1
Lester S. E., Dubel A. K., Hernán G., McHenry J., Rassweiler A. (2020). Spatial planning Principles for marine ecosystem restoration. Front. Mar. Sci. 7, 328. doi: 10.3389/fmars.2020.00328
Lovelock C. E., Barbier E., Duarte C. M. (2022). Tackling the mangrove restoration challenge. PloS Biol. 20, e3001836. doi: 10.1371/journal.pbio.3001836
Lovelock C. E., Reef R. (2020). Variable impacts of climate change on blue carbon. One Earth 3, 195–211. doi: 10.1016/j.oneear.2020.07.010
Macreadie P. I., Anton A., Raven J. A., Beaumont N., Connolly R. M., Friess D. A., et al. (2019). The future of Blue Carbon science. Nat. Commun. 10, 3998. doi: 10.1038/s41467-019-11693-w
Macreadie P. I., Costa M. D. P., Atwood T. B., Friess D. A., Kelleway J. J., Kennedy H., et al. (2021). Blue carbon as a natural climate solution. Nat. Rev. Earth Environ. 2, 826–839. doi: 10.1038/s43017-021-00224-1
Macreadie P. I., Serrano O., Maher D. T., Duarte C. M., Beardall J. (2017). Addressing calcium carbonate cycling in blue carbon accounting. Limnol Oceanogr Lett. 2, 195–201. doi: 10.1002/lol2.10052
Mateos-Molina D., Antonopoulou M., Baldwin R., Bejarano I., Burt J. A., García-Charton J. A., et al. (2020). Applying an integrated approach to coastal marine habitat mapping in the north-western United Arab Emirates. Mar. Environ. Res. 161, 105095. doi: 10.1016/j.marenvres.2020.105095
Mateos-Molina D., Lamine E. B., Antonopoulou M., Burt J. A., Das H. S., Javed S., et al. (2021a). Synthesis and evaluation of coastal and marine biodiversity spatial information in the United Arab Emirates for ecosystem-based management. Mar Pollut Bull 167, 112319.
Mateos-Molina D., Pittman S. J., Antonopoulou M., Baldwin R., Chakraborty A., García-Charton J. A., et al. (2021b). An integrative and participatory coastal habitat mapping framework for sustainable development actions in the United Arab Emirates. Appl. Geogr. 136, 102568. doi: 10.1016/j.apgeog.2021.102568
Mateos-Molina D., Pittman S. J., Antonopoulou M., Carpenter S., Möller M., Muzafar S. B., et al. (2024). Coastal Lagoons (Khors) of the Emirates. In: Burt J. A. (eds) A Natural History of the Emirates. Springer, Cham. doi: 10.1007/978-3-031-37397-8_8
Metcalfe C. D., Nagabhatla N., Fitzgerald S. K. (2018). Multifunctional wetlands: pollution abatement by natural and constructed wetlands, in: Nagabhatla N., Metcalfe C. D. (Eds.), Multifunctional Wetlands: Pollution Abatement and Other Ecological Services from Natural and Constructed Wetlands. Springer International Publishing, Cham, pp. 1–14. doi: 10.1007/978-3-319-674162_1
Mills M., Leon J. X., Saunders M. I., Bell J., Liu Y., O’Mara J., et al. (2016). Reconciling development and conservation under coastal squeeze from rising sea level. Conserv. Lett. 9, 361–368. doi: 10.1111/conl.12213
Mishra A. K., Apte D. (2020). Ecological connectivity with mangroves influences tropical seagrass population longevity and meadow traits within an island ecosystem. Mar. Ecol. Prog. Ser. 644, 47–63. doi: 10.3354/meps13349
MOCCAE (2023) Third update of second nationally determined contribution for the UAE. Available at: https://unfccc.int/sites/default/files/NDC/2023-07/Third%20Update%20of%20Second%20NDC%20for%20the%20UAE_v15.pdf (Accessed February 14, 2023).
Moritsch M. M., Young M., Carnell P., Macreadie P. I., Lovelock C., Nicholson E., et al. (2021). Estimating blue carbon sequestration under coastal management scenarios. Sci. Total Environ. 777, 145962. doi: 10.1016/j.scitotenv.2021.145962
O’Leary B. C., Fonseca C., Cornet C. C., de Vries M. B., Degia A. K., Failler P., et al. (2022). Embracing nature-based solutions to promote resilient marine and coastal ecosystems. Nature-Based Solutions 3, 100044. doi: 10.1016/j.nbsj.2022.100044
Pessarrodona A., Franco-Santos R. M., Wright L. S., Vanderklift M. A., Howard J., Pidgeon E., et al. (2023). Carbon sequestration and climate change mitigation using macroalgae: a state of knowledge review. Biol. Rev. 98, 1945–1971. doi: 10.1111/brv.12990
Pittman S. J., Stamoulis K. A., Antonopoulou M., Das H. S., Shahid M., Delevaux J. M. S., et al. (2022). Rapid site selection to prioritize coastal seascapes for nature-based solutions with multiple benefits. Front. Mar. Sci. 9. doi: 10.3389/FMARS.2022.832480/BIBTEX
Pittman S. J., Yates K. L., Bouchet P. J., Alvarez-Berastegui D., Andréfouët S., Bell S. S., et al. (2021). Seascape ecology: identifying research priorities for an emerging ocean sustainability science. Mar. Ecol. Prog. Ser. 663, 1–29. doi: 10.3354/meps13661
Prieto-Barajas C. M., Valencia-Cantero E., Santoyo G. (2018). Microbial mat ecosystems: Structure types, functional diversity, and biotechnological application. Electronic J. Biotechnol. 31, 48–56. doi: 10.1016/j.ejbt.2017.11.001
Quoreshi A. M., Kumar V., Adeleke R., Qu L., Atangana A. R. (2022). Soils and vegetation in desert and arid regions: Soil system processes, biodiversity and ecosystem functioning, and restoration. Front. Environ. Sci. 10. doi: 10.3389/fenvs.2022.962905
Radabaugh K. R., Moyer R. P., Chappel A. R., Powell C. E., Bociu I., Clark B. C., et al. (2018). Coastal blue carbon assessment of mangroves, salt marshes, and salt barrens in Tampa Bay, Florida, USA. Estuaries Coasts 41, 1496–1510. doi: 10.1007/s12237-017-0362-7
Ricart A. M., Pérez M., Romero J. (2017). Landscape configuration modulates carbon storage in seagrass sediments. Estuar. Coast. Shelf Sci. 185, 69–76. doi: 10.1016/j.ecss.2016.12.011
Rosentreter J. A., Al-Haj A. N., Fulweiler R. W., Williamson P. (2021). Methane and nitrous oxide emissions complicate coastal blue carbon assessments. Global Biogeochem. Cycles 35, e2020GB006858. doi: 10.1029/2020GB006858
Rosentreter J. A., Maher D. T., Erler D. V., Murray R. H., Eyre B. D. (2018). Methane emissions partially offset “blue carbon” burial in mangroves. Sci. Adv. 4, eaao4985. doi: 10.1126/sciadv.aao4985
Roy R., Tiwari M., Donelli G., Tiwari V. (2018). Strategies for combating bacterial biofilms: A focus on anti-biofilm agents and their mechanisms of action. Virulence 9, 522–554. doi: 10.1080/21505594.2017.1313372
Saintilan N., Horton B., Törnqvist T. E., Ashe E. L., Khan N. S., Schuerch M., et al. (2023). Widespread retreat of coastal habitat is likely at warming levels above 1.5° C. Nature 621, 112–119. doi: 10.1038/s41586-023-06448-z
Samper-Villarreal J., Lovelock C. E., Saunders M. I., Roelfsema C., Mumby P. J. (2016). Organic carbon in seagrass sediments is influenced by seagrass canopy complexity, turbidity, wave height, and water depth. Limnol Oceanogr. 61, 938–952. doi: 10.1002/lno.10262
Sánchez-Arcilla A., Cáceres I., Le Roux X., Hinkel J., Schuerch M., Nicholls R. J., et al. (2022). Barriers and enablers for upscaling coastal restoration. Nature-Based Solutions 2, 100032. doi: 10.1016/j.nbsj.2022.100032
Savarani S. (2023). “Restoring freshwater resources in Abu Dhabi: challenges and solutions,” in City Governments and Our Environmental Future, eds. Spiegel-Feld D., Wyman K. M., Coughlin J. J. (New York University Press), 86–102. doi: 10.18574/nyu/9781479805716.003.0007
Schile L. M., Kauffman J. B., Crooks S., Fourqurean J. W., Glavan J., Megonigal J. P. (2017). Limits on carbon sequestration in arid blue carbon ecosystems. Ecol. Appl. 27 (3), 859–874. doi: 10.1002/eap.1489
Seddon N., Chausson A., Berry P., Girardin C. A. J., Smith A., Turner B. (2020). Understanding the value and limits of nature-based solutions to climate change and other global challenges. Philos. Trans. R. Soc. B 375, 20190120. doi: 10.1098/rstb.2019.0120
Seddon N., Sengupta S., García-Espinosa M., Hauler I., Herr D., Rizvi A. R. (2019). Nature-based solutions in nationally determined contributions (Gland, Switzerland and Oxford: IUCN and University of Oxford).
Seifollahi-Aghmiuni S., Nockrach M., Kalantari Z. (2019). The potential of wetlands in achieving the sustainable development goals of the 2030 Agenda. Water (Basel) 11, 609. doi: 10.3390/w11030609
Song S., Ding Y., Li W., Meng Y., Zhou J., Gou R., et al. (2023). Mangrove reforestation provides greater blue carbon benefit than afforestation for mitigating global climate change. Nat. Commun. 14, 756. doi: 10.1038/s41467-023-36477-1
Sørup H. J. D., Fryd O., Liu L., Arnbjerg-Nielsen K., Jensen M. B. (2019). An SDG-based framework for assessing urban stormwater management systems. Blue-Green Syst. 1, 102–118. doi: 10.2166/bgs.2019.922
Stewart-Sinclair P. J., Klein C. J., Bateman I. J., Lovelock C. E. (2021). Spatial cost–benefit analysis of blue restoration and factors driving net benefits globally. Conserv. Biol. 35, 1850–1860. doi: 10.1111/cobi.13742
Thorhaug A., Gallagher J. B., Kiswara W., Prathep A., Huang X., Yap T.-K., et al. (2020). Coastal and estuarine blue carbon stocks in the greater Southeast Asia region: seagrasses and mangroves per nation and sum of total. Mar. pollut. Bull. 160, 111168. doi: 10.1016/j.marpolbul.2020.111168
Wabnitz C. C. C., Lam V. W. Y., Reygondeau G., Teh L. C. L., Al-Abdulrazzak D., Khalfallah M., et al. (2018). Climate change impacts on marine biodiversity, fisheries and society in the Arabian Gulf. PloS One 13, e0194537. doi: 10.1371/journal.pone.0194537
Williamson P., Gattuso J.-P. (2022). Carbon removal using coastal blue carbon ecosystems is uncertain and unreliable, with questionable climatic cost-effectiveness. Front. Climate 4, 130. doi: 10.3389/fclim.2022.853666
Keywords: blue carbon, carbon accounting, United Arab Emirates, climate change, coastal ecosystems, carbon dynamics, conservation, ecosystem services
Citation: Carpenter S, Evans C, Pittman SJ, Antonopoulou M, Bejarano I, Das HS, Möller M, Peel K, Samara F, Stamoulis KA and Mateos-Molina D (2023) Multi-habitat carbon stock assessments to inform nature-based solutions for coastal seascapes in arid regions. Front. Mar. Sci. 10:1239904. doi: 10.3389/fmars.2023.1239904
Received: 14 June 2023; Accepted: 16 November 2023;
Published: 01 December 2023.
Edited by:
J. Severino Pino Ibánhez, Spanish National Research Council (CSIC), SpainReviewed by:
Craig Smeaton, University of St Andrews, United KingdomPedro Beca-Carretero, Spanish National Research Council (CSIC), Spain
Copyright © 2023 Carpenter, Evans, Pittman, Antonopoulou, Bejarano, Das, Möller, Peel, Samara, Stamoulis and Mateos-Molina. This is an open-access article distributed under the terms of the Creative Commons Attribution License (CC BY). The use, distribution or reproduction in other forums is permitted, provided the original author(s) and the copyright owner(s) are credited and that the original publication in this journal is cited, in accordance with accepted academic practice. No use, distribution or reproduction is permitted which does not comply with these terms.
*Correspondence: Stephen Carpenter, scarpenter@enwwf.ae