- Red Sea Research Center, Division of Biological and Environmental Science and Engineering, King Abdullah University of Science and Technology, Thuwal, Saudi Arabia
Damselfishes (family Pomacentridae) are widely distributed reef-associated marine fishes. The family’s diversity, iconic nesting behaviors, and high environmental adaptability contribute to the popularity of damselfishes as aquarium species, tourist attractions, and model scientific study organisms. In the present study, we conducted a series of in situ underwater observations on two Red Sea damselfishes: the Red Sea anemonefish (Amphiprion bicinctus) and the Maldives damselfish (Amblyglyphidodon indicus). We followed the egg development processes and provided an in situ photo time series for both species. In our observations, some Amblyglyphidodon eggs began hatching as early as 120 hours post-spawning, whereas Amphiprion eggs never hatched until at least 144 hours post-spawning. Some of the key morphological and developmental features that could be indicative of the developmental stage included eye structure and pigmentation/appearance, the proportion of the embryo to the yolk sack, spinal structures, and jaw/mouth structures. By referencing the time scale provided herein, in situ observations could roughly indicate the developmental stage of the eggs in the nest and how much time is remaining before hatching. This would also help to decrease the underwater observation time of divers and minimize interference to nesting damselfishes but still enable the back-calculation of spawning times. The incorporation of this information with longer-term environmental data can provide important insight into factors driving reproductive cycles.
1 Introduction
Damselfishes (family Pomacentridae) are widely distributed reef-associated marine fish species with 423 extant species recognized in at least 29 genera (Parenti, 2021; Fricke et al., 2023). The family’s diversity, iconic nesting behaviors, and high environmental adaptability contribute to the popularity of damselfishes as aquarium species, tourist attractions, and model scientific study organisms (Fautin and Allen, 1992; Muñoz-Cordovez et al., 2019; Laudet and Ravasi, 2022). Early life-history stage descriptions (i.e., eggs, embryos, and larvae) have been conducted in only approximately 50 species of damselfish (Kavanagh and Alford, 2003; Muñoz-Cordovez et al., 2019). More ecological observations, descriptions, and studies, therefore, are needed to provide a better understanding of damselfish behavior, ecology, adaptation, and evolution.
The presence of scuba divers has been found to change the short-term nest-guarding behavior of damselfish, resulting in a 92% increase in the frequency of opportunistic predation on eggs in their nests (Giglio et al., 2022). Knowledge of the specific spawning or hatching date is very useful in the determination of environmental influences on reproductive behaviors (Pressley, 1980) and potential larval dispersal trajectories (Nanninga et al., 2015; Robitzch et al., 2020). To decrease the necessary underwater observation time by divers and therefore minimize interference to the nesting damselfishes, a reliable key to determine the stage of development from a single in situ photo could be very useful. Previous studies about damselfish egg development were mainly conducted in laboratory conditions (Gopakumar et al., 2002; Ghosh et al., 2012; Uthayasiva et al., 2014; Krishna et al., 2016; Anzeer et al., 2019; Muñoz-Cordovez et al., 2019), and very few of them have time-scaled in situ illustrations depicting stages of egg development (Beldade et al., 2022).
In the present study, we conducted a series of in situ underwater observations on two Red Sea damselfishes: the Red Sea anemonefish (Amphiprion bicinctus) and the Maldives damselfish (Amblyglyphidodon indicus). We traced both species’ egg development processes and provided a photo series with a time scale.
2 Method
The underwater observations were conducted from November 2021 to April 2023. The survey area is approximately 300 m along the coast from the entry location on a fringing reef on the north side of Jeddah, Saudi Arabia (21°45′26″N; 39°03′02″E). The Red Sea anemonefish (A. bicinctus) and the Maldives damselfish (A. indicus) were nesting within the same area from approximately 5 m to 30 m in depth. Once the nesting and spawning activities had been observed, observations were repeated approximately every 24 hours for the duration of the development and until hatching occurred. For each observation, macro and super-macro in situ photos were taken of the eggs showing the visual traits at each time step. The macro setting of the camera was similar to the fine limit of the bare human eye, while the super-macro photos at each development stage would show more details than typical human eyesight (approaching microscope-scale images).
The underwater photos of Red Sea anemonefish eggs were taken using a DSLR camera Canon 5D Mark III with Canon EF 100mm F2.8L IS Macro Lens and Nauticam SMC-2 super-macro (X4) add-on lens. The whole camera set was enclosed in a Nexus underwater camera housing. Illumination and color restoration for each shot were provided by two INON 330 strobes with float arms.
The underwater photos of the Maldives damselfish eggs were taken using a full-frame mirrorless camera Sony A7RIV with Sony SEL90M28G FE 90mm F2.8 Macro Lens and Nauticam SMC-2 super-macro (X4) add-on lens. The camera set was enclosed in a Marelux underwater camera housing. Two Weefine Focus underwater strobes with float arms provided illumination and color restoration for each photo shot.
The focus length of both cameras with these macro lenses and super-macro add-on lenses is limited to approximately 7 cm from the subject. The relative sizes of each focal object are thus of comparable scale, and this can be calibrated as an underwater super-macro photo of a ruler.
3 Results
3.1 Red Sea anemonefish (A. bicinctus) observation
The spawning and egg-hatching process of the Red Sea anemonefish was observed from late November 2021 to early April 2022. The nesting depth ranged from 4 m to 29 m deep.
For the first 24 hours after the female Red Sea anemonefish finished egg laying (Figures 1A, B), each egg in the nest looked like a red-orange capsule with a white dot on the top (Figure 1B). These newly laid eggs had no obvious embryo formed; only a few divided cells formed an obvious white dot of the immature embryo tissues (Figure 1B). The red yolk sack filled up the majority space of each egg (Figure 1B).
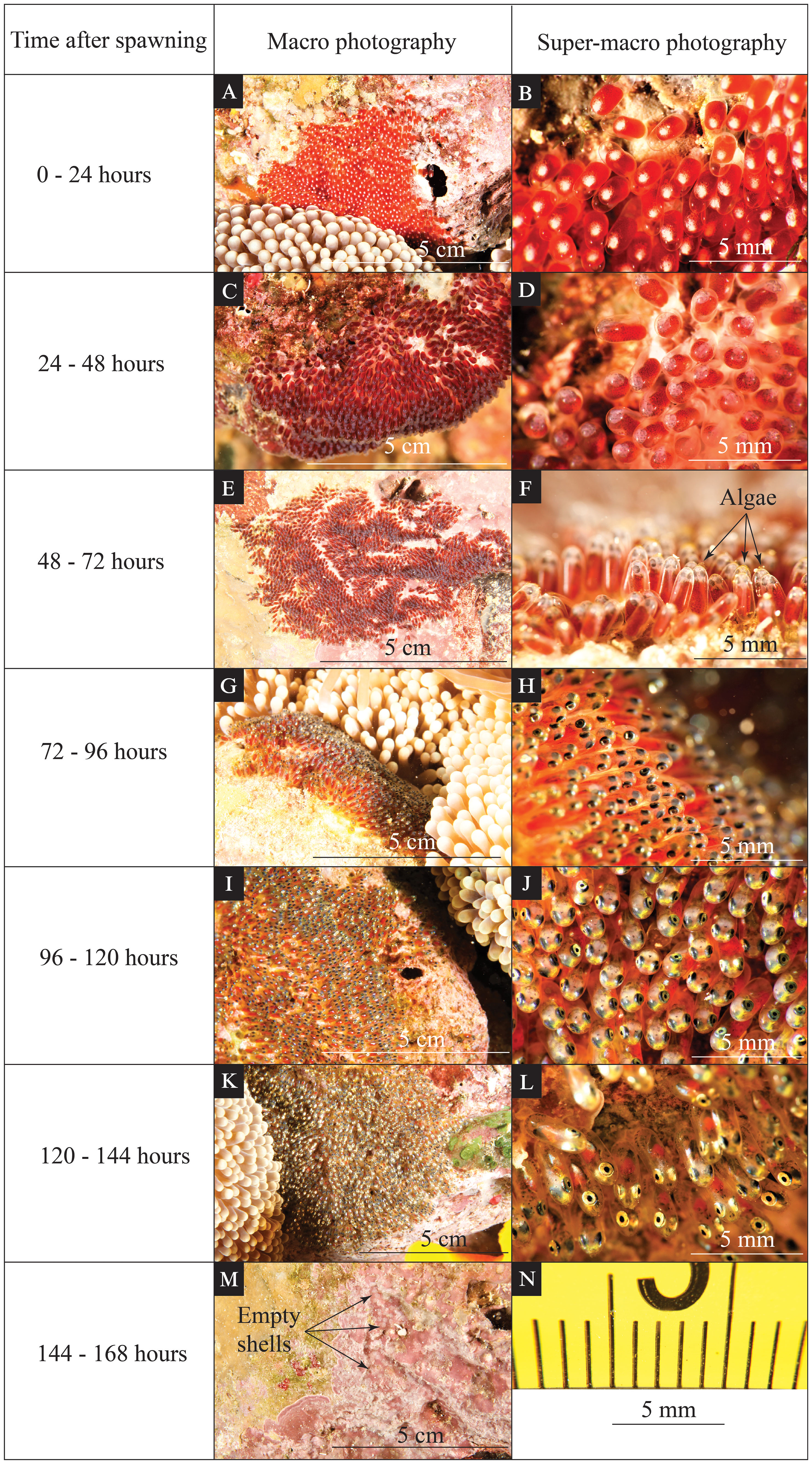
Figure 1 Time-series photographs of various visible changes during egg development for Red Sea anemonefish (Amphiprion bicinctus). The left column indicates the time after spawning. The middle column (A, C, E, G, I, K, M) shows an in situ macro photo highlighting the coloration and features of the eggs that can be seen by the naked eye. The right column (B, D, F, H, J, L) shows an in situ super-macro photo of the same clutch during the same dive. The scales for macro photos are indicated on each panel. Super-macro photos have minor image distortion at the periphery of the image (N); however, due to a fixed focal length, the central portion of the image has a reliable size scale.
From 24 hours to 48 hours after egg laying (Figures 1C, D), each egg capsule became dark red in the nest. A half-transparent embryo was formed during this period, and some dark pigmentation started to develop on both the yolk sack and embryo (Figure 1D). The bright red-orange color of the yolk sack in the previous period was shaded by these newly developed features, resulting in overall coloration or the egg becoming dark red (Figure 1D).
From 48 hours to 72 hours after egg laying (Figures 1E, F), gray shades from eye pigmentation appeared on the tip of the dark red yolk sack in the macro photo records (Figure 1F). Eye pigmentation began during this period, and the yolk sack consisted of the most egg volume. Algae growth started to be obvious on the tip of eggs (see black arrows in Figure 1F).
From 72 hours to 96 hours after egg laying (Figures 1G, H), black shades from eye pigmentation were conspicuous on the tip of the dark red yolk sack (Figure 1H). The yolk sack still consisted of more than half of the egg volume. The eye pigmentation was more developed than in the previous period. Obvious pigmentation could be seen on the head. The spine structure started to be visible (Figure 1H).
From 96 hours to 120 hours after egg laying (Figures 1I, J), the glistening appearance of the eyes dominated the tip of the dark red egg capsule (Figure 1J). Obvious pigmentation could be seen all over the embryo. The yolk sack consisted of half or less half of the egg (Figure 1J).
From 120 hours to 144 hours after egg laying (Figures 1K, L), the eyes were glistening, and the developed embryos surrounded the yolk sack (Figure 1L). The yolk sack consisted of less than one-third of the egg, and the red-orange color of the yolk sack was almost totally covered by the dark-gray embryo (Figure 1L). Eye structure and pigmentation were well developed. The spine structures were visible. The jaw/mouth structure could be observed easily in the super-macro photo records (Figure 1L).
The actual hatching event was expected to happen within 24 hours of the previous period (i.e., 144–168 hours after egg laying). Empty shells could be observed shortly after the hatching event (see black arrows in Figure 1M).
From 48 hours of egg laying to hatching, the biofouling of algae on the tip of the eggs was obvious under super-macro photo records (Figure 2A). From the period of 120 to 144 hours after egg laying, a marine whelk (Pisania sp.) was observed to scrape and consume the fouling algae on the tip of the eggs (Figure 2B); however, this was only observed on very rare occasions (two out of 53 observation dives). The observed whelks appeared to be small individuals (~3 mm in length) compared to the typical adult sizes of Pisania spp. (longer than 1 cm in length at least) (Marais and Seccombe, 2010).
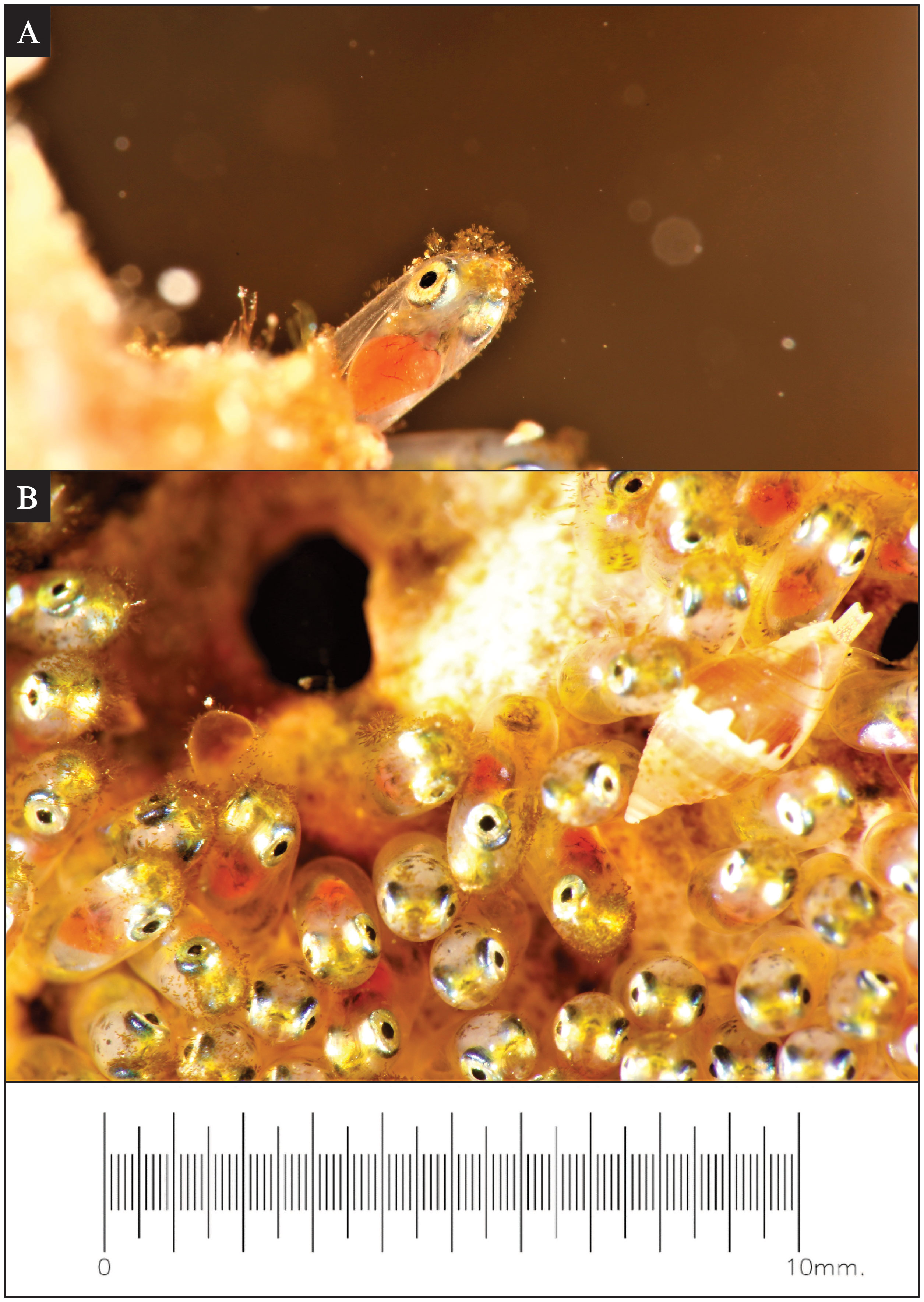
Figure 2 Environmental interactions observed during Red Sea anemonefish (Amphiprion bicinctus) egg development. (A) A super-macro photo of algae growth commonly observed on the tip of eggs after approximately 120 hours post-spawning. On some clutches at this stage, marine whelks (Pisania sp.) (B) were observed consuming the fouling algae off of the tip of the eggs. The scale is the same for both images.
3.2 Maldives damselfish (A. indicus) observation
The spawning and egg-hatching process of the Maldives damselfish was observed from late February 2022 to early June 2022. The nesting depth ranges from 5 m to 25 m deep.
The spawning territory cleaning behavior of the Maldives damselfish was observed. The male selected the nesting site, which is frequently situated on the side of a rock or other hard substrate (e.g., we observed nesting on a discarded PVC pipe). Before spawning, the male cleaned the nest area with small bites and scrapes to clean a section of the substrate (Figures 3A–C). During spawning, the female led with a slow deliberate motion, dipped her genital pore on the nest area (Figure 3D), and left a trail of eggs that stuck to the bottom (Figure 3F). The male followed closely behind and shook his tail sideways while fertilizing the eggs (Figure 3E). After fertilizing the eggs, the male attentively guarded this nest and constantly remained in close proximity (within ~50 cm) to the nest until hatching occurred (Figure 3G). The male Maldives damselfish also checked and cleaned the nest with small bites (Figure 3H) and fanned seawater toward the eggs with his pectoral fins (Figure 3I).
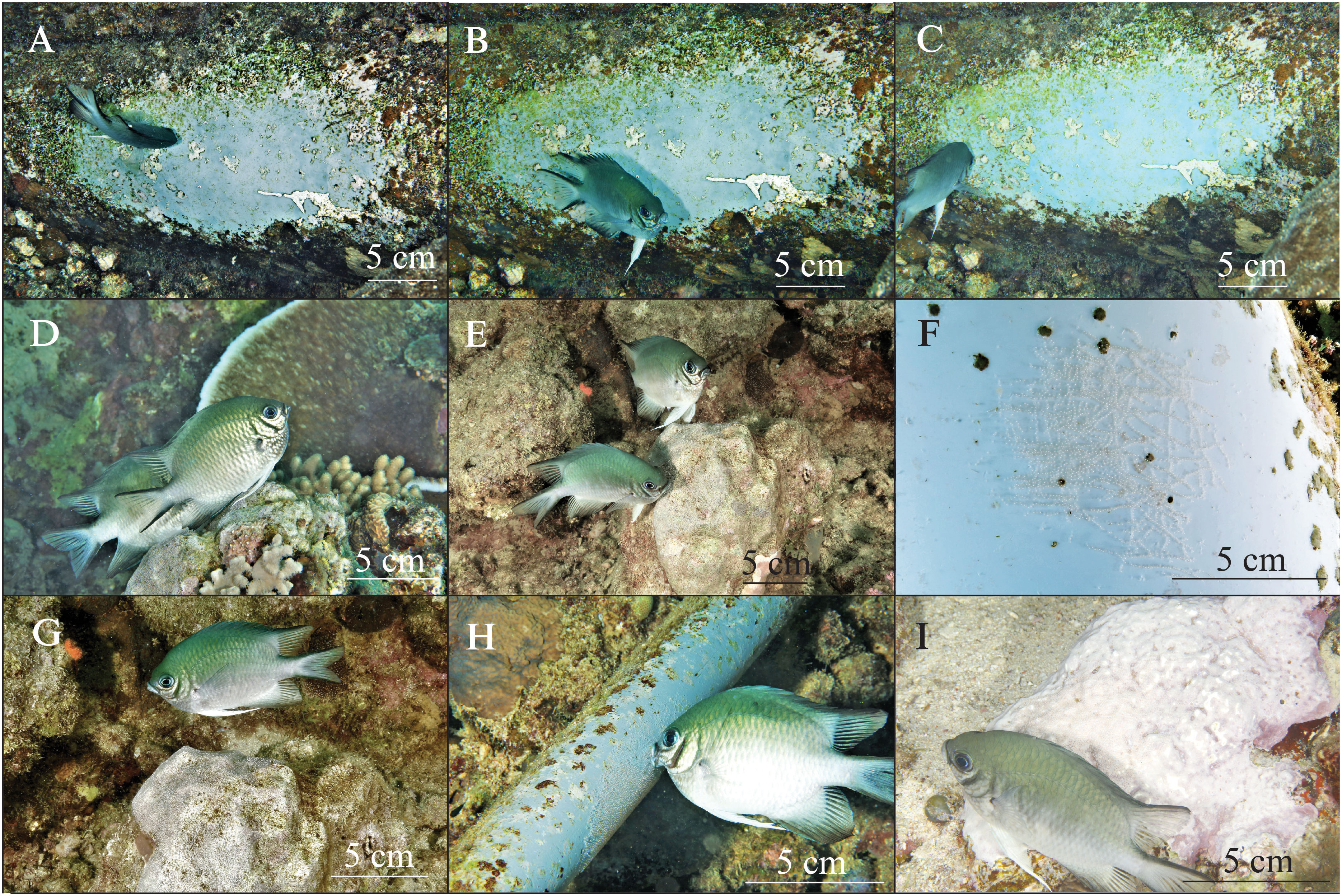
Figure 3 Reproductive behaviors of Maldives damselfish (Amblyglyphidodon indicus). Nest cleaning behavior (A–C) on a section of PVC pipe. Spawning behavior, with the female laying eggs and the male following close behind to fertilize the eggs (D, E). Trails of spawned eggs (F). Nest guarding behavior by a male damselfish (G). A male damselfish tends the eggs and uses his mouth to clean the nest (H). Males fan seawater toward the nest by using their pectoral fins (I). Size scales are indicated in each panel.
For the first 24 hours after the female Maldives damselfish finished egg laying (Figures 4A, B), each egg in the nest looked like a transparent capsule with a white dot on the top (Figure 4B). These newly laid eggs had no obvious embryo formed, with only a few divided cells that formed an obvious white dot of the immature embryo tissues. The yolk sack filled up the majority space of each egg and had almost no color at all (Figure 4B).
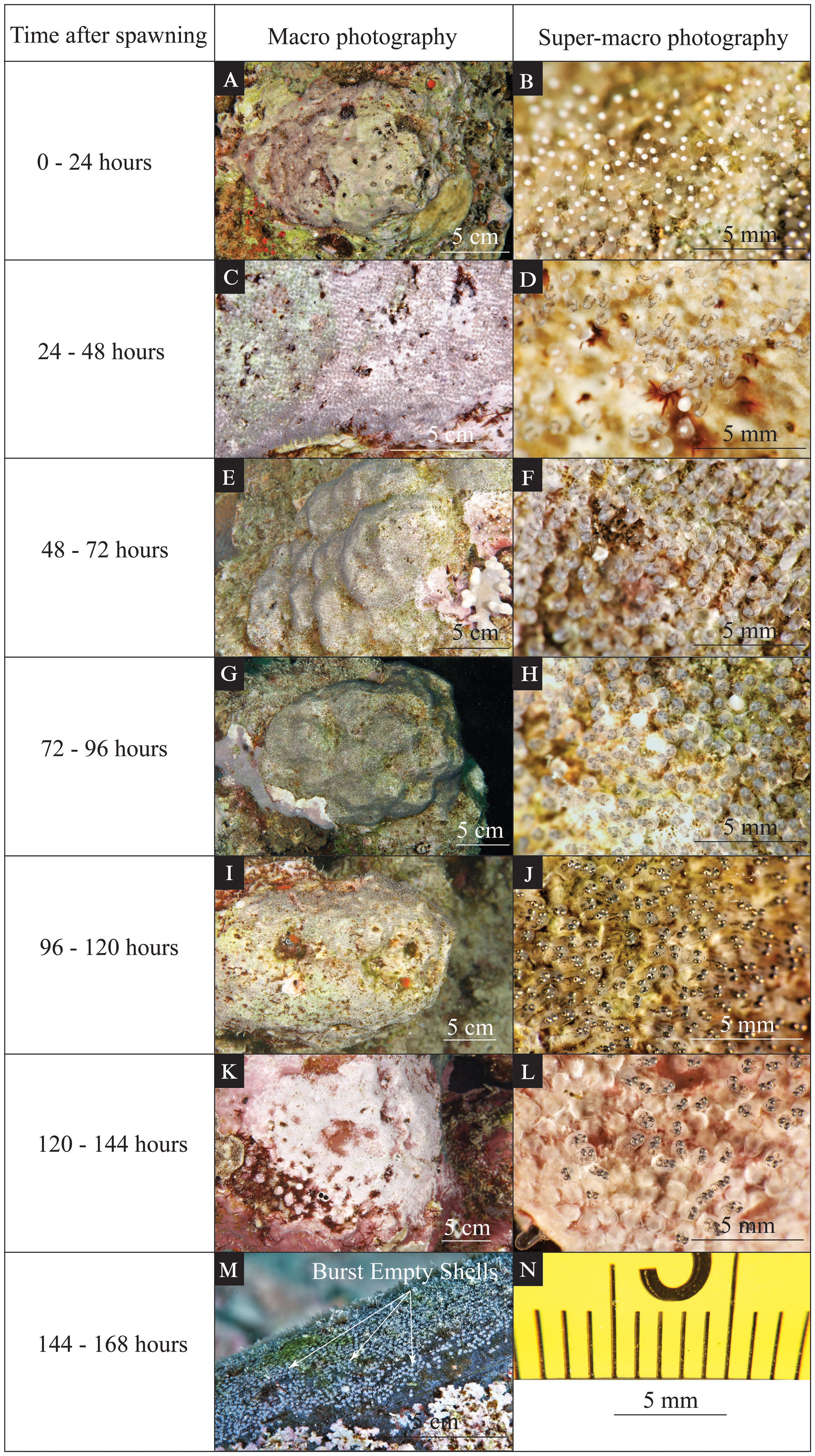
Figure 4 Time-series photographs of various visible changes during egg development for Maldives damselfish (Amblyglyphidodon indicus). The left column indicates the time after spawning. The middle column (A, C, E, G, I, K, M) shows an in situ macro photo highlighting the coloration and features of the eggs that can be seen by the naked eye. The right column (B, D, F, H, J, L) shows an in situ super-macro photo of the same clutch during the same dive. The scales for macro photos are indicated on each panel. Super-macro photos have minor image distortion at the periphery of the image (N); however, due to a fixed focal length, the central portion of the image has a reliable size scale.
From 24 to 48 hours after egg laying (Figures 4C, D), each egg capsule became light gray in the nest (Figure 4D). A half-transparent embryo was formed during this period, and some black pigmentation started to develop on both the yolk sack and embryo (Figure 4D). The light gray color resulted from these newly developed pigmentations (Figure 4D). At this stage, it is possible to see some differences in the developmental progress of some individual eggs. In addition to the eggs developing as described above, some of the eggs had no noticeable change from the previous period, and a few of the eggs seemed to have failed to develop an embryo and appeared to be filled with white tissues (Figure 4D).
From 48 to 72 hours after egg laying (Figures 4E, F), gray shades from the embryo and dark pigmentation were apparent on the tip of the transparent yolk sack (Figure 4F). Eye structures could be observed during this period but without obvious pigmentation on them (Figure 4F). The normally developed embryos were less transparent than during the previous period, and a few eggs with failed development were still visible within each nest (Figure 4F). This is a notable contrast to the Red Sea anemonefish, which seems to promptly remove any failed eggs.
From 72 to 96 hours after egg laying (Figures 4G, H), black shades from the eye pigmentation dominated the tip of the transparent yolk sack (Figure 4H). The eye pigmentation was more developed than in the previous period. Obvious pigmentation could be seen on the whole embryo, giving the whole egg a dark-gray appearance (Figure 4H).
From 96 to 120 hours after egg laying (Figures 4I, J), the glistening eyes and the developed embryo were obvious on the tip of the yolk sack (Figure 4J). Eye structure and pigmentation were well-developed (Figure 4J). The jaw/mouth structure could be observed easily (Figure 4J).
From 120 to 144 hours after egg laying (Figures 4K, L), some eggs had already hatched and left empty egg shells, while slower-developing eggs had well-developed embryos that would presumably hatch within the next 24 hours (Figure 4L). The failed eggs, matured eggs, and hatched eggs could be observed together in the same nest during this period (Figure 4L). Empty shells could be observed shortly after the hatching event (see white arrows in Figure 4M).
In the nests of the Maldives damselfish, substantial algal development was apparent in the spaces between eggs, but little algae was visible on the surface of the eggs themselves. This is in contrast to the observations described above for the Red Sea anemonefish, which had visible algal filaments on individual eggs.
4 Discussion
The current study produced the first in situ underwater observations on the stages of egg development and hatching for Red Sea anemonefish (A. bicinctus) and Maldives damselfish (A. indicus). This information will be valuable for future studies linking environmental conditions to the reproductive cycles of these fishes.
The Red Sea anemonefish egg stage duration (144 to 168 hours) is similar to previous studies using lab observations on other Amphiprion spp. (Allen, 1991; Gordon and Bok, 2001; Ignatius et al., 2001; Kumar and Balasubramanian, 2009; Ghosh et al., 2012; Uthayasiva et al., 2014). Biofouling of fungus on the clownfish eggs was documented in aquarium conditions (Allen, 1991) but the algal growth on anemonefish eggs in their natural habitat is documented here for the first time (Figure 2). The observation record of the marine whelks (Pisania sp.) grazing algae growing on the tip of Red Sea anemonefish eggs suggests a temporary mutual beneficial interaction between marine whelks and anemonefish. This potentially novel symbiosis was only observed 2–3 days after spawning, at which time the biofouling algae likely reached a certain biomass fit for grazing. Given the typical intensive parental care provided by Red Sea anemonefish and the aggressive nest-guarding behavior of most damselfishes (Allen, 1991), we speculate that the parents are actively allowing the whelk access to the eggs. Potentially, the parents only permit certain sizes of the whelk, as larger individuals could damage or predate on the eggs. The whelks may be assisting with delicate “housekeeping” at a stage when the usual mouthing cleaning of eggs by the parents might compromise the integrity of the egg.
The Maldives damselfish have a shorter egg development period than Red Sea anemonefish nesting in the same area but are still within the range of 85 hours to 384 hours of duration found in other Pomacentridae damselfishes (Muñoz-Cordovez et al., 2019). In our observations, some Amblyglyphidodon eggs began hatching as early as 120 hours post-spawning, whereas Amphiprion eggs never hatched until at least 144 hours post-spawning. There was a turnover stage observed in the final hours of Chromis crusma egg development, in which the head was repositioned from the bottom of the egg to the top of the egg before the hatching. We did not observe turnover in the present study; the head of the embryo was positioned on the top of the egg throughout the development period. Variations in certain traits (e.g., pigmentation pattern, development of movement, and visual structures) could serve as potential indicators of differences among Pomacentridae genera and subfamilies (Kavanagh and Alford, 2003). The presence and absence of the turnover stage by the end of egg development might be one of the traits that could be used for species differentiation.
Some caution must be applied, however, since fluctuations in temperature could influence the developmental rate in damselfishes (Muñoz-Cordovez et al., 2019). In our study, we did not have in situ temperature data available. Although satellite-derived sea surface temperature (SST) data for the period of study were available, we did not think SST data were sufficiently resolved or accurate enough to understand the potential impact of temperature on the developing eggs in our study. Future studies seeking to calibrate developmental rate would benefit from in situ temperature measurements at the scale of each nest, particularly if comparing observations several months apart when general water temperatures may have consistent differences.
In situ ecology observations, descriptions, and studies of damselfishes not only contribute to a better understanding of animal behavior, adaptation, and species evolution but can also be appreciated by the general diving public. By referencing the time scale provided by the current study, a quick observation by the naked eye, one could roughly tell the development stage of the eggs in the nest and approximate how much time is remaining before hatching. This would also help to decrease the underwater observation time of divers and minimize interference to nesting damselfishes but still enable the back-calculation of spawning times. The incorporation of this information with longer-term environmental data can provide important insight into factors driving reproductive cycles.
Data availability statement
The original contributions presented in the study are included in the article/supplementary materials, further inquiries can be directed to the corresponding author/s. The original photos generated during the current study are available from the corresponding author upon reasonable request.
Ethics statement
Ethical approval was not required for the study involving animals in accordance with the local legislation and institutional requirements because no live animal testing was performed during the study. Only underwater observation was conducted.
Author contributions
SH conceived and designed the study. SH conducted the field surveys. SH and MLB analyzed photo data. SH drafted the manuscript and MLB contributed to the writing and editing. Both authors read and approved the submitted version of the manuscript.
Funding
Financial support was provided by KAUST to MLB (baseline research funds) and Weida Sci. & Tech. Development Fund to SH.
Acknowledgments
We thank Associate Geological Engineer Mr. Kadir Suat for sharing his observation and photo records on the damselfish spawning process. We would also like to thank Italian Navy Capt. Paolo Bocci and the KAUST Red Sea Research Center Field Operations Coordinator Ms. Christine Nelson for their fieldwork assistance.
Conflict of interest
The authors declare that the research was conducted in the absence of any commercial or financial relationships that could be construed as a potential conflict of interest.
Publisher’s note
All claims expressed in this article are solely those of the authors and do not necessarily represent those of their affiliated organizations, or those of the publisher, the editors and the reviewers. Any product that may be evaluated in this article, or claim that may be made by its manufacturer, is not guaranteed or endorsed by the publisher.
References
Allen G. R. (1991). “Damselfishes of the world,” in Natural history and pet books(Melle, Germany: Mergus).
Anzeer F. M., Aneesh K., Abraham M. V., Darsana S., Santhosh B., Anil M., et al. (2019). Breeding, early development and larval rearing of cloudy damsel, Dascyllus carneus Fischer 1885. Aquaculture 505, 374–385. doi: 10.1016/j.aquaculture.2019.03.001
Beldade R., Bernardi G., Mills S. C. (2022). Anemonefish behavior and reproduction evolution, development and ecology of anemonefishes (Boca Raton, FL, USA: CRC Press), 129–142.
Fautin D. G., Allen G. R. (1992). Anemone fishes and their host sea anemones: a guide for aquarists and divers (Monterey, CA, USA: Sea Challengers).
Fricke R., Eschmeyer W. N., van der Laan R. (2023) Catalog of fishes: genera, species, references. Available at: http://researcharchivecalacademyorg/research/ichthyology/catalog/fishcatmainasp (Accessed May 2023).
Ghosh S., Kumar T. T. A., Balasubramanian T. (2012). Embryology of Maldives clownfish, Amphiprion nigripes (Amphiprioninae). J. Ocean Univ China 11, 174–180. doi: 10.1007/s11802-012-1885-y
Giglio V. J., Blumstein D. T., Motta F. S., Pereira-Filho G. H. (2022). Diver presence increases egg predation on a nesting damselfish. J. Exp. Mar. Biol. Ecol. 549, 151694. doi: 10.1016/j.jembe.2022.151694
Gopakumar G., Sreeraj T., Ajith Kumar T., Sukumaran T., Raju B., Unnikrishnan C., et al. (2002). Breeding and larval rearing of three species of damselfishes (Family: Pomacentridae). Mar. Fish Inf Serv Tech Ext Ser. 171, 3–5.
Gordon A. K., Bok A. W. (2001). Frequency and periodicity of spawning in the clownfish Amphiprion akallopisos under aquarium conditions. Aquarium Sci. Conserv. 3, 293–299. doi: 10.1023/A:1013127714071
Ignatius B., Rathore G., Jagadis I., Kandasamy D., Victor A. (2001). Spawning and larval rearing technique for tropical clown fish Amphiprion sebae under captive condition. J. Trop. Agric. 16, 241–249.
Kavanagh K. D., Alford R. A. (2003). Sensory and skeletal development and growth in relation to the duration of the embryonic and larval stages in damselfishes (Pomacentridae). Biol. J. Linn Soc. 80, 187–206. doi: 10.1046/j.1095-8312.2003.00229.x
Krishna R., Anil M., Neethu Raj P., Santhosh B. (2016). Seed production and growth of Neopomacentrus cyanomos (Bleeker 1856) in captivity. Indian J. Fish 61, 50–56. doi: 10.21077/ijf.2016.63.3.55058-06
Kumar T. A., Balasubramanian T. (2009). Broodstock development, spawning and larval rearing of the false clown FIsh, Amphiprion ocellaris in captivity using estuarine water. Curr. Sci. 97, 1483–1486.
Laudet V., Ravasi T. (2022). Evolution, development and ecology of anemonefishes: model organisms for marine science (Boca Raton, FL, USA: CRC Press).
Marais A., Seccombe A. D. (2010). Identification guide to the seashells of South Africa (Groenkloof, South Africa: Centre for Molluscan Studies).
Muñoz-Cordovez R., de la Maza L., Pérez-Matus A., Carrasco S. A. (2019). Embryonic and larval traits of the temperate damselfish Chromis crusma reveal important similarities with other Pomacentridae throughout the family’s thermal range. J. Fish Biol. 95, 613–623. doi: 10.1111/jfb.14055
Nanninga G. B., Saenz-Agudelo P., Zhan P., Hoteit I., Berumen M. L. (2015). Not finding Nemo: limited reef-scale retention in a coral reef fish. Coral Reefs 34, 383–392. doi: 10.1007/s00338-015-1266-2
Parenti P. (2021). An annotated checklist of damselfishes, Family Pomacentridae Bonaparte 1831. J. Anim. Divers. 3, 37–109. doi: 10.52547/JAD.2021.3.1.6
Pressley P. H. (1980). Lunar periodicity in the spawning of yellowtail damselfish, Microspathodon chrysurus. Environ. Biol. Fishes 5, 155–159. doi: 10.1007/BF02391622
Robitzch V., Saenz-Agudelo P., Berumen M. L. (2020). Travel with your kin ship! Insights from genetic sibship among settlers of a coral damselfish. Ecol. Evol. 10, 8265–8278. doi: 10.1002/ece3.6533
Keywords: underwater observations, damselfishes, Red Sea, reproductive behavior, super-macro photography, fish embryo, parental care
Citation: He S and Berumen ML (2023) Egg development and hatching in two Red Sea damselfishes. Front. Mar. Sci. 10:1235919. doi: 10.3389/fmars.2023.1235919
Received: 06 June 2023; Accepted: 30 August 2023;
Published: 29 September 2023.
Edited by:
Reuven Yosef, Ben-Gurion University of the Negev, IsraelReviewed by:
Michael J. Childress, Clemson University, United StatesAugusto Flores, University of São Paulo, Brazil
Copyright © 2023 He and Berumen. This is an open-access article distributed under the terms of the Creative Commons Attribution License (CC BY). The use, distribution or reproduction in other forums is permitted, provided the original author(s) and the copyright owner(s) are credited and that the original publication in this journal is cited, in accordance with accepted academic practice. No use, distribution or reproduction is permitted which does not comply with these terms.
*Correspondence: Song He, c29uZy5oZUBrYXVzdC5lZHUuc2E=