- 1Wageningen Marine Research, Den Helder, Netherlands
- 2Marine Ecology, Waardenburg Ecology, Culemborg, Netherlands
- 3Data Science and Water Quality, Deltares, Delft, Netherlands
- 4Marine Animal Ecology, Wageningen University, Wageningen, Netherlands
Introduction: The European flat oyster (Ostrea edulis) is a biogenic reef former, internationally recognised as threatened and declining in the NE Atlantic by OSPAR and one of the focal species in nature inclusive designs in offshore windfarms in The Netherlands. Oyster reefs offer habitat to many other benthic hard substrate and fish species and provide ecosystem functions such as shelter and feeding grounds. European flat oyster reefs have disappeared from the Dutch North Sea in the early 1900s due to overfishing and diseases but are now subject of nature restoration under the Dutch Marine Strategy.
Method: Since 2018, pilot projects have started in the Dutch North Sea to restore European flat oysters at suitable locations, such as offshore windfarms or natural reefs, which are protected from bottom trawling. We compared European flat oyster performance in five pilot projects, using translocated adult oysters sourced from Ireland, Norway, and the Netherlands. The aim of this research was to assess the performance of translocated oysters between pilots, to assess the installation and monitoring techniques, and to come forward with recommendations for future pilot projects.
Results: We found that translocation of both foreign sourced flat oyster populations (Ireland and Norway in nearshore and offshore areas) and local oysters (in nearshore areas) result in good oyster performance. Oysters were able to grow (max 3.67 mm/month) and reproduce (larvae present) in their new environment. We found that growth rate was explained by origin and average water temperature, to a lesser extent by number of months, location and salinity and not to other environmental factors such as pH and O2. Correlations between growth and environmental conditions need to be considered with caution, since not all pilots were sampled just before and after the growing season. Oysters were Bonamia-negative at the start and end of the pilots, indicating that the offshore Dutch North Sea is still Bonamia-free.
Discussion, conclusions, recommendations: By the year 2050 more than ten new offshore farms will be constructed in the Dutch North Sea and some sites will be suitable for oyster restoration. We conclude that local and foreign sourced oysters performed well at all locations. Based on the success and failure of the different outplacement and monitoring techniques, we provide recommendations on good practice for the future, including developing standardized monitoring protocols. This will enable better inter-site comparisons in upcoming oyster restoration pilots.
1 Introduction
The European flat oyster (Ostrea edulis) was once one of the dominant species of the North Sea, covering an estimated 6.2% of the seafloor (Bennema et al., 2020; Merk et al., 2020). However, overharvesting, especially industrial trawling, led to the collapse of the North Sea population by the mid-20th century (Reise, 2005; Callaway et al., 2007; Smaal et al., 2015). This has had major consequences to the ecological functioning of the North Sea (Reise, 2005). The European oyster is internationally recognised as ‘threatened and declining’ in the NE Atlantic by the OSPAR Commission (OSPAR, 2008). At present European oyster beds are rare or absent in most of their natural range (OSPAR BDC, 2020). Several European nations have consequently adopted strategies for its conservation and restoration. In the Netherlands, one of the environmental targets is the “return and recovery of biogenic reefs including flat oyster beds” (Ministry of Infrastructure and Water Management (I&W) and ministry of Agriculture, Nature and Food Quality (LNV), 2018). The species is one of the focal species for nature inclusive building and restoration projects in offshore wind parks.
Flat oysters are sessile reef-building “ecosystem-engineers” (Smyth & Roberts, 2010; Rodriguez-Perez et al., 2019). Oysters provide key beneficial impacts to their surroundings (Cobacho et al., 2020). For instance, the physical characteristics of the oyster reef functions as a nursery for other species, which is a positive driver to the system’s biodiversity (Smaal et al., 2015; Kerckhof et al., 2018). This not only enhances the primary productivity and ecological functioning of the habitat, but also has a beneficial impact to commercial fish stocks (Gilby et al., 2018; Bureau Waardenburg, 2020).
In the months June to August gamete release (i.e. the spawning act) takes place, and sperm, organized in spermatozeugmata (O’Foighil, 1989), are released into the water column and drawn into the female mantle cavity. Here the fertilization takes place and the young larvae are brooded for 6 to 10 days (Korringa, 1940; O’Foighil and Taylor, 2000). Subsequently, O. edulis larvae are released into the water column and after a pelagic stage of about 10 days the larvae search for suitable hard substrate on which to settle and develop into oyster spat and grow to adult oysters (Korringa, 1940; Rodriguez-Perez et al., 2021).
Notably, oyster reef systems contain positive feedback loops (Maathuis et al., 2020) where oyster larvae typically settle on oyster shells (Smyth et al., 2016; Christianen et al., 2018) and adults provide chemical settlement cues (Tamburri et al., 2008). Therefore, trawling activities in the 19th and 20th century not only reduced the reproduction capacity of the North Sea population, but also drastically decreased the suitability of the North Sea to future populations by removing adults and available hard substrate and preventing reef formation (Smaal et al., 2015; Bennema et al., 2020). Since the 1950s, the flat oyster remains functionally extinct in the Dutch North Sea (Beck et al., 2011; Merk et al., 2020). This sequence of events resulting in the collapse of the population is not unique to the flat oyster (Beck et al., 2011). Oyster species around the world, such as the Crassostrea virginica and Ostrea lurida, have suffered a similar narrative (Kennedy et al., 2011; Cobacho et al., 2020; Ridlon et al., 2021).
Initial oyster restoration efforts began in the United States with the specific aim of restoring the commercial fisheries (Hargis and Haven, 1988; Anderson and Hedgecock, 2004). From the 1980s onwards, restoration aims expanded to include restoring the ecological functioning of the system (Kennedy et al., 2011; Cobacho et al., 2020). Compared to the United States, Europe is in its “infancy” regarding shellfish restoration (Pogoda et al., 2019). In recent years, several European Union directives and initiatives have helped to foster flat oyster restoration efforts (Beck et al., 2011; Pogoda et al., 2019). In the North Sea, offshore windfarms are seen as especially promising restoration sites because turbine foundations act as artificial reefs (Smaal et al., 2017) and the areas in-between the turbines are closed to trawling fisheries, resulting in minimal bottom disturbance (Kamermans et al., 2018a), which is one of the major requirements of oyster restoration (Sas et al., 2019).
Like for the US (Baggett et al., 2015), European oyster restoration efforts have been criticized for being ad hoc, and in need of a more formal monitoring protocol (Bromley et al., 2016; Pogoda et al., 2019). In light of these growing restoration efforts, the Native Oyster Restoration Alliance (NORA) released a handbook, outlining the optimal set up and monitoring procedures for flat oyster restoration projects (zu Ermgassen et al., 2021).
Because the flat oyster is often locally extinct, a key consideration for many restoration projects is the reliance on active restoration by reintroduction (Bromley et al., 2016; Kerckhof et al., 2018) often by translocation of oysters from a foreign population, either a natural bed, or a culture site, to the project site (Elliott et al., 2007; Kerckhof et al., 2018; zu Ermgassen et al., 2023). This has the risk of importing disease/pests and depletes the stock of the imported population (Smaal et al., 2015; Bromley et al., 2016; Pogoda et al., 2019). Therefore, it is important to assess how oysters of different origins respond to the environmental conditions of the site location (Pogoda et al., 2011; Bromley et al., 2016; Holbrook, 2021). Environmental conditions, such as temperature and salinity, play an important role in triggering spawning events (Maathuis et al., 2020) and different regional populations have different thresholds (Colsoul et al., 2021). To justify the translocation, it is important to ensure that the environmental conditions of the site can support the imported stock.
In the Netherlands, several nearshore and offshore oyster restoration pilots have been executed since 2016 (Sas et al., 2016; Didderen et al., 2018; Sas et al., 2018; Didderen et al., 2019a; Didderen et al., 2019b; Didderen et al., 2019c; Didderen et al., 2019d; Sas et al., 2019; Didderen et al., 2020; Kardinaal et al., 2020; Gheerardyn et al., 2021; Schutter et al., 2021). These pilots share the general goal to initiate European oyster reef development, first by assessing if the Dutch North Sea conditions are suitable to reintroduce and sustain flat oyster populations and in a second stage by translocating adult oysters in order to re-install a source of oyster larvae. The pilots are not aligned and differ in their design, using oysters from different origins in various experimental setups with variations in terms of oyster deployment method, sampling design, and number of oysters used. So far, an inter-site comparison of oyster performance and monitoring methods has been lacking.
This study is the first attempt to congregate and collectively analyse monitoring data these five individual pilots. The aim of this study was to compare the performance of translocated oysters between projects i.e. survival, growth, condition index, and larval production of oyster. Furthermore, we compared monitoring techniques and come forward with recommendations for future projects, based on the successes and failures of techniques used.
2 Materials and methods
2.1 Study area
In this study we analysed oyster performance in five oyster restoration pilots that were carried out in the period May 2018 to July 2021 (Figure 1 and Table 1).
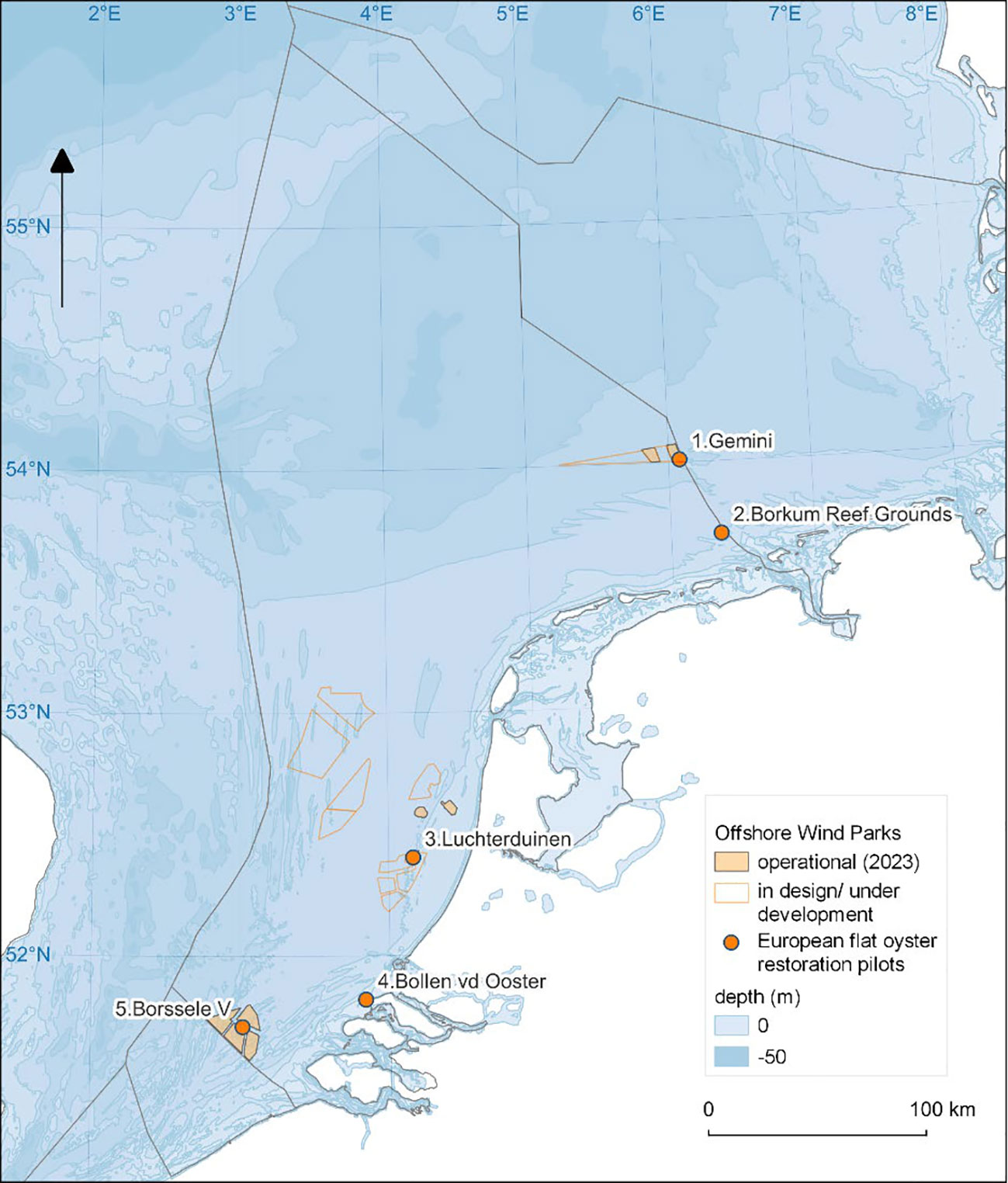
Figure 1 Ostrea edulis. The five oyster restoration pilots in the Dutch North Sea: (1) Gemini offshore windfarm ‘Buitengaats’, (2) Borkum Reef Grounds, (3) Luchterduinen offshore windfarm, (4) Bollen van de Ooster and (5) Borssele V offshore wind farm.
The northernmost pilot ‘Gemini’ was located 85 km offshore, in windfarm Gemini Buitengaats, close to the German border. Here, flat oysters originating from a wild bed in Hafrsfjord in Norway were placed at 30 m depth on the seafloor in 2018 (14,000 oysters) and 2019 (11,000 oysters) by putting them overboard close to the scour protections of two wind turbines. A subsample of deployed oysters was kept in baskets so that their performance could be monitored and tracked. For this, five light weight research racks were used each containing one basket with 20 oysters (Figure 2). The monitoring racks had a dimension of 1x1x1m and were constructed of rebar. The baskets were BST baskets (12 mm mesh size) used to culture oysters (https://www.bstoysters.com) with the size of the basket adjusted to fit in the rack. The research racks were placed on the bottom in windfarm Gemini in May 2018 and one was retrieved in July 2018. The others were lost. In 2019 another three monitoring racks were deployed in April. Only one of the three was retrieved in August 2019 and the others were lost. In 2019, individual oysters were tagged with glue-on 4x8 mm polyethylene shellfish tags (www.hallprint.com) using an ethyl-based instant adhesive (Loctite 422) (Figure 2).
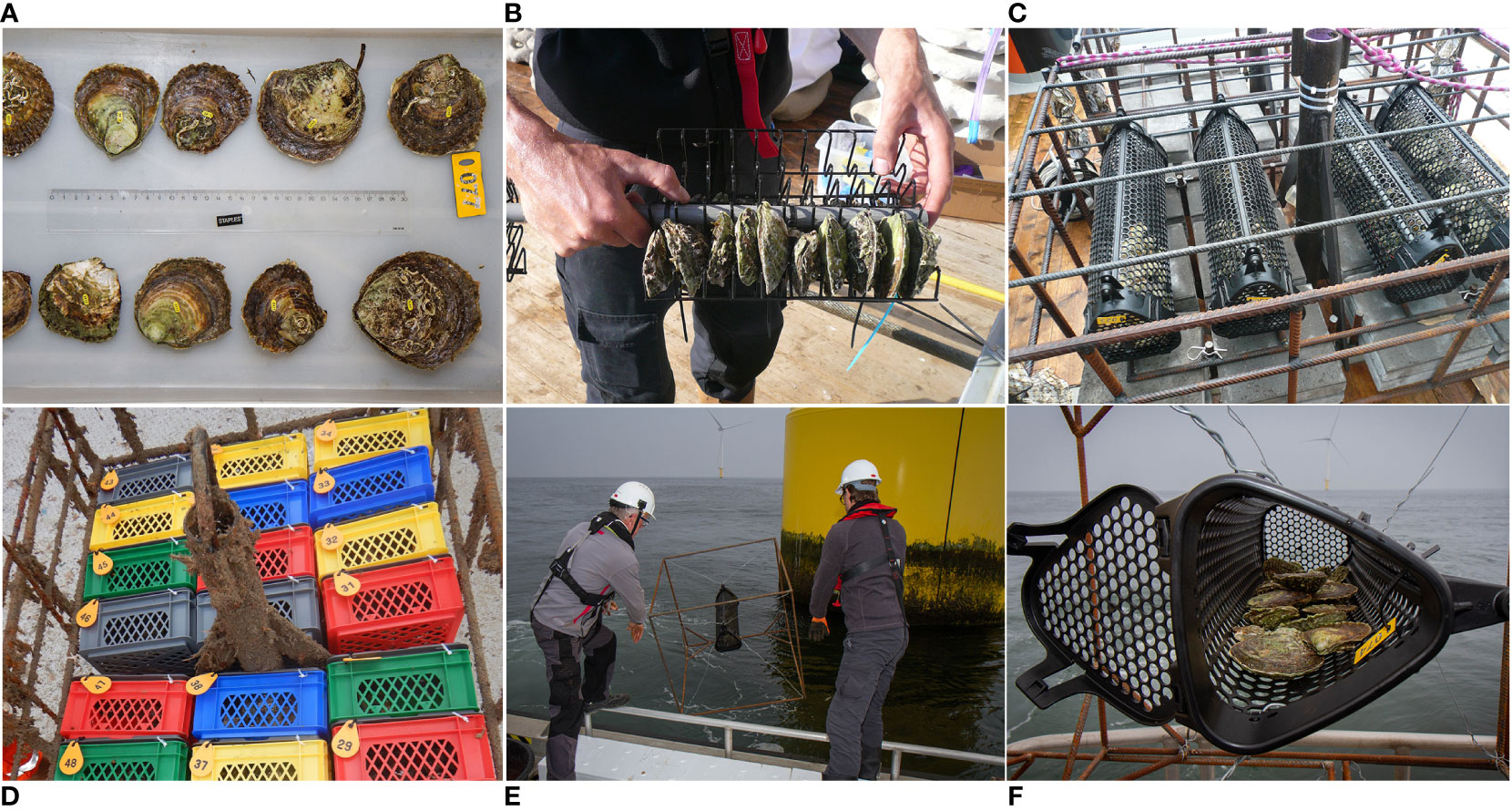
Figure 2 Ostrea edulis restoration pilot monitoring methods. (A) Individually numbered oysters. (B) Individually identifiable oysters in a holding tower as used in Borkum Reef Grounds and Bollen van de Ooster. (C) Heavy monitoring rack with 4 baskets as used in Borkum Reef Ground (2 layers of tiles), Luchterduinen (3 layers of tiles) and Bollen van de Ooster (no tiles). (D) Heavy monitoring rack in Borssele offshore windfarm. (E + F) Light monitoring rack with one basket as used in Gemini offshore wind farm.
Further south, but also close to the German border, the second pilot, ‘Borkum Reef Grounds’ was located 20 km offshore at Borkum Reef Grounds (Figure 1 and Table 1). Here 60,000 flat oysters from the same fjord in Norway were deployed on the seafloor in May 2018 at 25 m depth. In addition, four heavy weight monitoring racks with four BST baskets each (17 mm mesh size) were also placed in May at the seafloor (Figure 2). The racks were 1x1.2x0.4m and made of barbed wire. Each rack had extra weight from two layers of sidewalk tiles. Concrete sidewalk tiles are readily available. There was space for three layers and the number of layers was adjusted to the dynamics of the pilot site. Each basket contained 40 oysters. In one basket per rack, four holding towers with 10 oysters each were used to individually identify oysters (Figure 2). One monitoring rack was retrieved and placed back in July 2018. In September 2019 and in September 2020 eight baskets of two racks were sampled.
The third oyster restoration pilot, ‘Luchterduinen’, is southwestwards of ‘Borkum Reef Grounds’ and located in the offshore windfarm Luchterduinen (Figure 1 and Table 1). Here, 480 flat oysters from Hafrsfjord in Norway were deployed in BST baskets (17 mm mesh size) placed in heavy weight monitoring racks similar to those used in the Borkum Reef pilot. Only here the racks had three layers of tiles instead of two. Three racks with four baskets each holding 40 oysters were deployed in November 2018 at 20 m depth. No oysters were put on the seafloor and all racks were retrieved in July 2019.
The fourth pilot ‘Bollen van de Ooster’ was located in the southwestern part of the Netherlands, in the nearshore of the Voordelta (Figure 1 and Table 1). This is the only site with flat oysters from multiple origins: Hafrsfjord in Norway and nearby Oosterschelde as well as Lake Grevelingen in The Netherlands. Similar heavy weight monitoring racks as used in the Borkum Reef pilot were deployed here, but these racks had no layers of tiles. Four racks with four BST baskets (17 mm mesh size) each holding 40 oysters were deployed in May 2018 at 4 m depth. As in the Borkum reef pilot, one basket per rack contained four holding towers to individually identify oysters (Figure 2). Oyster baskets were retrieved in June and October 2018, April 2019 and June 2020. In addition, 22,000 oysters from culture plots in lake Grevelingen and Oosterschelde were deployed on the seafloor.
The fifth pilot ‘Borssele V’ was located 55 km offshore close to the Belgium border in the windfarm Borssele V (Figure 1 and Table 1). Here, flat oysters originating from fished beds in Tralee Bay in Ireland were used. The oysters were placed in baskets (crates) in heavy weight racks (Figure 2). These racks were weighted down with three layers of tiles, and each fitted 24 baskets of which 5 were used for oysters. Each basket contained 6 oysters. The racks were deployed in October 2020 at 30 m depth and retrieved in July 2021. In addition to these monitoring racks a total of 1000 oysters were glued onto four broodstock structures.
Prior to deployment oysters in all pilots were inspected according to the treatment protocol of Van den Brink & Magnesen (2018) to avoid translocation of invasive species. Further details concerning the different pilots can be found for Gemini in Didderen et al. (2018); for Borkum Reef Ground in Didderen et al. (2019c; 2020) and Kardinaal et al. (2020); for Luchterduinen in Didderen et al. (2019a); for Bollen van de Ooster in Didderen et al. (2019b; 2019d); and for Borssele V in Schutter et al. (2021).
2.2 Environmental parameters
2.2.1 Copernicus data
Environmental parameters considered are (1) salinity and (2) temperature, both of which influence the functioning of a marine ecosystem (Lenihan et al., 1999; zu Ermgassen et al., 2021); (3) chlorophyll concentration for approximate biomass of phytoplankton, describing the approximate food availably at each site (Kamermans et al., 2018b; Pogoda et al., 2020; Stechele et al., 2022), (4) dissolved oxygen, because certain areas of the North Sea are subject to stratification which can cause oxygen depletion, limiting habitat suitability (Kamermans et al., 2018a) and (5) pH because it has been correlated with low density oyster reefs in Australia and multiple meta-analyses have reported a decrease of pH to have a negative impact on growth rates of molluscs (Harvey et al., 2013; Catalán et al., 2019; Benthotage et al., 2022).
Daily-averaged model output was extracted for all of the above-mentioned environmental variables from the Atlantic-European North West Shelf-Ocean Biogeochemistry Reanalysis model (Copernicus Marine Service’s Data Portal https://marine.copernicus.eu). For each variable, the output has a spatial resolution of 7 km and is at a depth of 10 m below the sea surface (European Union-Copernicus Marine Service, 2020).
2.2.2 Comparison abiotic surface versus bottom
To assess whether the Copernicus output at 10 m depth is representative of the seafloor conditions, an analysis was carried out using the Deltares 3D Dutch Continental Shelf Model - Flexible Mesh (3D DCSM-FM. The Deltares model output used is the sixth generation of the 3D Dutch Continental Shelf Model - Flexible Mesh (3D DCSM-FM). Its domain covers the Northwest European Continental Shelf, including the North Sea as well as adjacent shallow seas and estuaries in the Netherlands (Zijl et al., 2023). The model has a z-sigma layer vertical grid. At each of the locations where output was extracted, the model only has sigma layers, i.e. the water column is divided into 20 layers of uniform thickness. Layer thickness can vary per location, depending on depth. In these coastal regions, the flexible mesh of the model has a horizontal resolution of 0.5 nautical miles (Zijl et al., 2023). This hydrodynamic model is coupled online with a water quality model (more information in van Leeuwen et al., 2023) which resolves: phytoplankton- and filter feeder processes (primary production, respiration, mortality, grazing and excretion), light extinction, the decomposition of particulate organic matter in the water and sediment, nitrification and denitrification, reaeration, settling, burial as well as the carbonate system equilibrium. These processes and their parameterization are further described by Blauw et al. (2009). For this study, only the weekly output of temperature, salinity, chlorophyll-a, pH and oxygen were extracted, once at the seabed and once at 10 m below the sea surface. The values at 10 m below the surface and at the seabed were comparable (Figure 3). Thus, it can be concluded that the use of the Copernicus output at 10 m below the surface is representative of conditions at the seafloor. The only exceptions are the temperature and chlorophyll at Gemini, which were repeatedly lower at the bottom than at the surface. This is probably a result of stratification. Temperature recordings carried out at the Gemini pilot in 2018 showed lower temperatures at the seafloor compared to the surface in June (Figure 4).
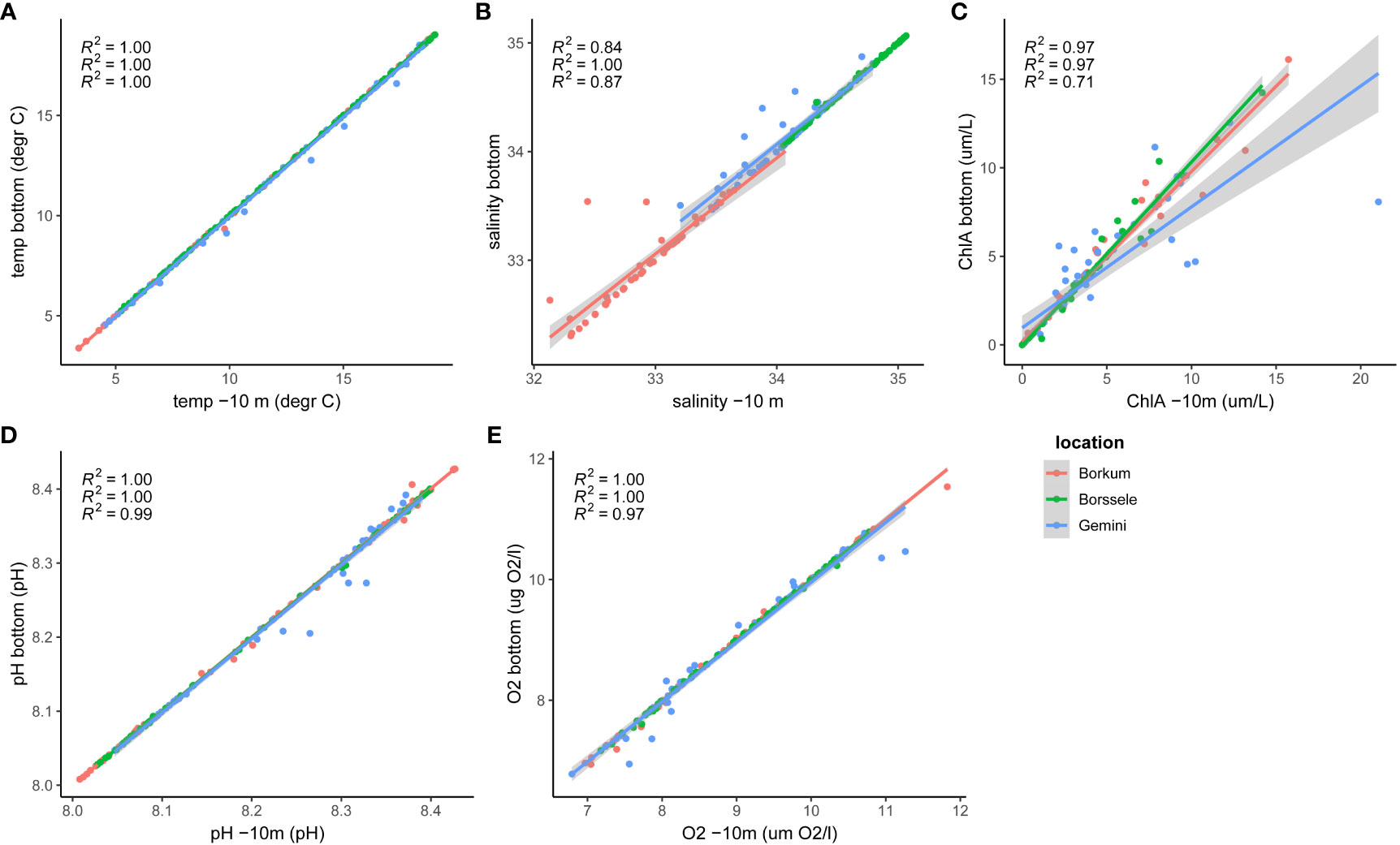
Figure 3 Comparison of abiotic parameters at 10m below the surface (x-axis) (data: Copernicus) versus at the seafloor (y-axis) (A) temperature (B) salinity (C) Chlorophyll-a (D) pH and (E) Dissolved oxygen (O2) (data: 3D-DCSM model, Deltares).
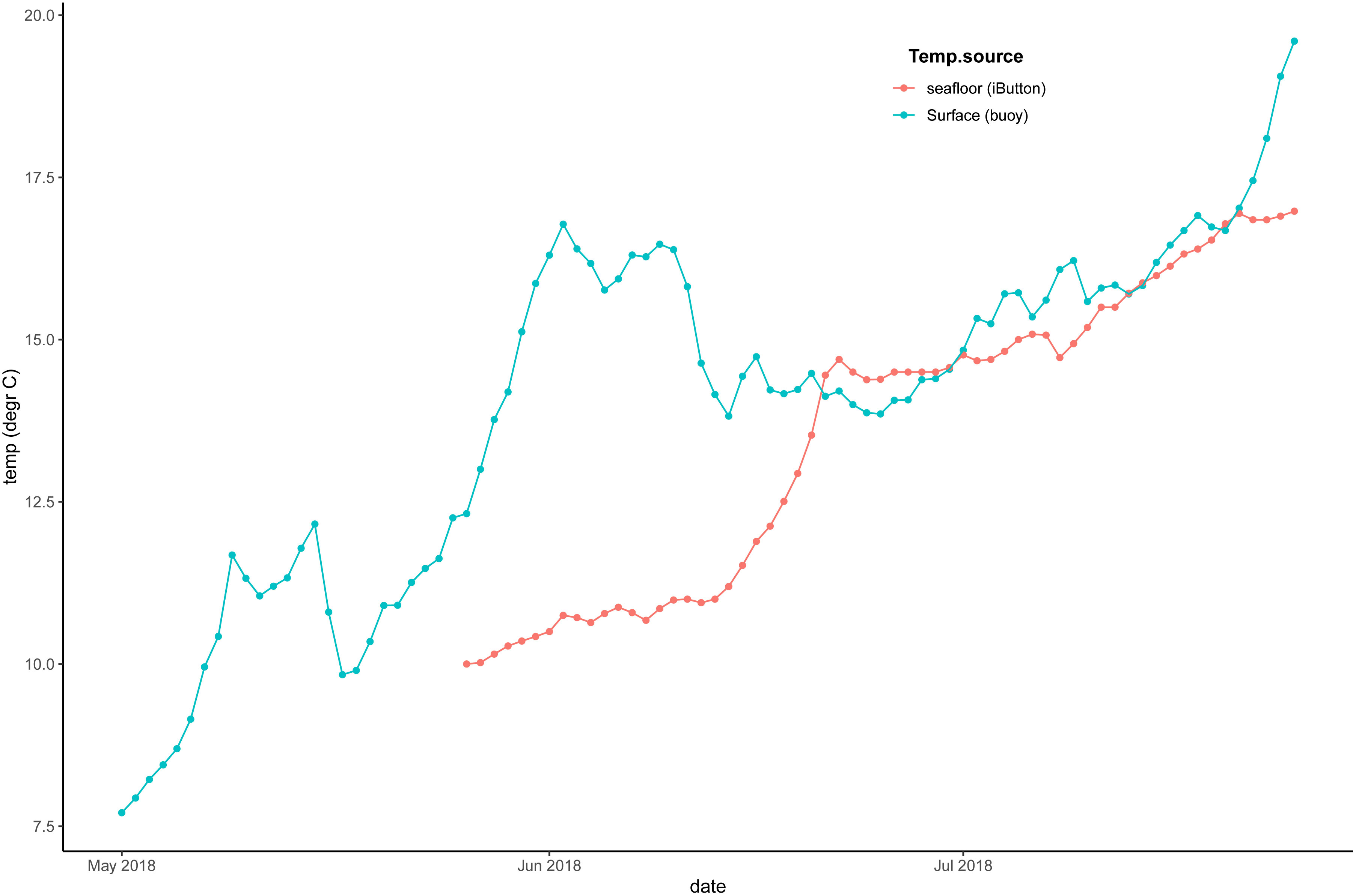
Figure 4 Gemini offshore windfarm Buitengaats. Temperature measured at the seafloor in Gemini by an i-Button attached to the oyster monitoring rack, versus temperature recorded at the surface by a weather buoy in the same wind farm.
Since the shallow pilot location ‘Bollen van de Ooster’ (-7 m) had no output at -10 m, the deepest available Copernicus output was already representative of the seabed at that location. For the other locations (Borkum Reef Ground, Gemini windfarm and Borssele windfarm), relevant environmental parameters of Copernicus and Deltares models were compared at -10 m in 2017 as the Deltares model did not cover the period 2018-2021.
2.3 Monitoring oyster performance
2.3.1 Growth and survival
All oysters were counted and measured at T0 (time of installation). Shell width was measured with callipers to the nearest mm. Shell width is the distance between the two shell edges perpendicular to the line from the hinge to the edge. Monitoring racks were retrieved at different time intervals (T1, T2 or T3) according to the schedule presented in Table 1. All oysters were retrieved from the baskets. Dead oysters were separated from live specimen and both groups were counted and measured. For individually marked oysters, either by number tags or holding towers, growth rates were calculated as increase in shell width according to (LTx – LT0)/t, where LTx = final shell width in mm; LT0 = initial shell width in mm; t = time in months in which one month is 30 days. When individuals were not marked (see Table 1), the average shell width of all live oysters in one basket was calculated for T0 and T1 and, when applicable T2 or T3, and the increase in shell width was calculated as above. Survival was calculated per basket, as a percentage per month:[(final number of oysters/initial number) *100]/t, where t = time in months in which one month is 30 days. For growth analysis, only the oysters in baskets that had high survival rates were included. This means that the oysters from Luchterduinen were not used.
2.3.2 Condition index, Bonamia presence and breeding
During most of the samplings, a subsample of oysters was sacrificed to determine the condition index, the presence of larvae in the mantle cavity and the presence of the Bonamia parasite. Oysters were opened with an oyster knife. When oysters were sampled in July, the presence of larvae was noted. O. edulis is a brooding species which means that the eggs are fertilised inside the mantle cavity and remain there for about 10 days before being released. Recently fertilised eggs have a white colour, further developed larvae are grey and fully developed larvae are black and ready to be released (Korringa, 1940). Thereafter, a small piece of gill was sampled and stored in 96% ethanol for Bonamia PCR analysis, as described in Kamermans et al. (2023). Then, the meat was separated from the shell and the two components per individual were dried at 70°C until no further weight loss was observed. The weight of both, dried meat and dried shell, was determined with a scale in 0.00 g. Condition Index was calculated according to Walne & Mann (1975) as the ratio between dry weight (DW) of the oyster meat and the dry weight of the oyster shell: (DW meat/DW shell)*100. In general, condition of the oysters is lower in July, during larval release, and higher in the remainder of the year (Walne, 1970).
2.3.3 Larval abundance
Larvae were sampled by filtering 200 L (2 x 100 L) of seawater per sample near the seafloor using a 35 m long hose and a pump, over a 100 µm plankton net. The residue of one 200 L sample was stored in 4% formaldehyde or 96% ethanol and used for microscopic counts. In the lab the samples of larvae were filtered using a 30 µm plankton gauze. The volume of the samples was reduced to 20 – 60 mL, depending on the amount of suspended matter. From the concentrated samples subsamples were taken for counting numbers of larvae. A Hensen plunger-sampling pipette was used to take subsamples. Bivalve larvae were identified and counted using an inverted microscope. Three subsamples of each sample were analysed. Depending on the density of the samples, subsamples of 1 to 2.5 mL were counted. Larvae were identified according to Loosanoff et al. (1966) and Hendriks et al. (2005) combined with data obtained from cultured larvae.
2.4 Statistical analysis
Growth per month was calculated as the average growth of oysters per basket per location:
where Tx is T1, T2 or T3, and T = time in months, in which one month is 30 days. Data for Luchterduinen offshore windfarm were not considered, since most oysters had died due to sand waves covering the experimental set-up. In addition, the average shell width of the remaining oysters showed a decrease compared to T0. The resulting dataset of average growth consisted of 56 observations (baskets). Values for abiotic factors (Temp, Chla, O2, pH, Salinity) were calculated for each oyster basket as the average value for the monitoring period (T0 to Tx).
Data were analyzed using Rstudio version 2022.07.0 (R Core Team, 2023) and figures were made using packages ‘ggplot2’, ‘ggpmisc’ and ‘patchwork’. The relations between growth per month and environmental factors were explored following the steps recommended by Zuur et al. (2010), including boxplots for outliers and plots per variable to test for collinearity between explanatory variables. The relation between growth rate and environmental parameters was modelled using a Generalized Linear Model (GLM) of the form:
where location = Gemini, Borkum Reef Grounds, BorsseleV or Bollen van de Ooster, origin = Norway, Ireland, Grevelingen (GV) or Oosterschelde (OS), and Nmonths is time between Tx and T0 (months).
For the other performance metrics, a Kruskal-Wallis test was performed to test for differences in the averages.
3 Results
The complete dataset is available as Supplementary material.
3.1 Growth and survival
Survival of the oysters ranged from 66-100% per month (Figure 5 and Supplementary Table S1). A comparison between pilots is difficult as the periods in between sampling the oysters differed. The shortest interval was 4.5 months and the longest 28 months. Within a location there were no significant differences in survival between large or small oysters (BvO: Kruskal-Wallis rank sum test chi-squared = 5.1652, df = 2, p-value = 0.07558; Bork: Kruskal-Wallis chi-squared = 0.10666, df = 1, p-value = 0.744), or between oysters of different origin (BvO: Kruskal-Wallis chi-squared = 0.10761, df = 1, p-value = 0.7429; Bork: Kruskal-Wallis chi-squared = 0.81911, df = 1, p-value = 0.3654). Survival was visibly lower in some subsamples at Luchterduinen compared to the other locations. At Luchterduinen, ROV images showed that the research racks were partially buried in the sand, explaining the low survival. The oysters that did not survive, did show some increase in size, as observed in their length frequency histograms comparing their size at deployment and after 8 months (Figure 6). The average size of the survivors decreased (Figure 6).
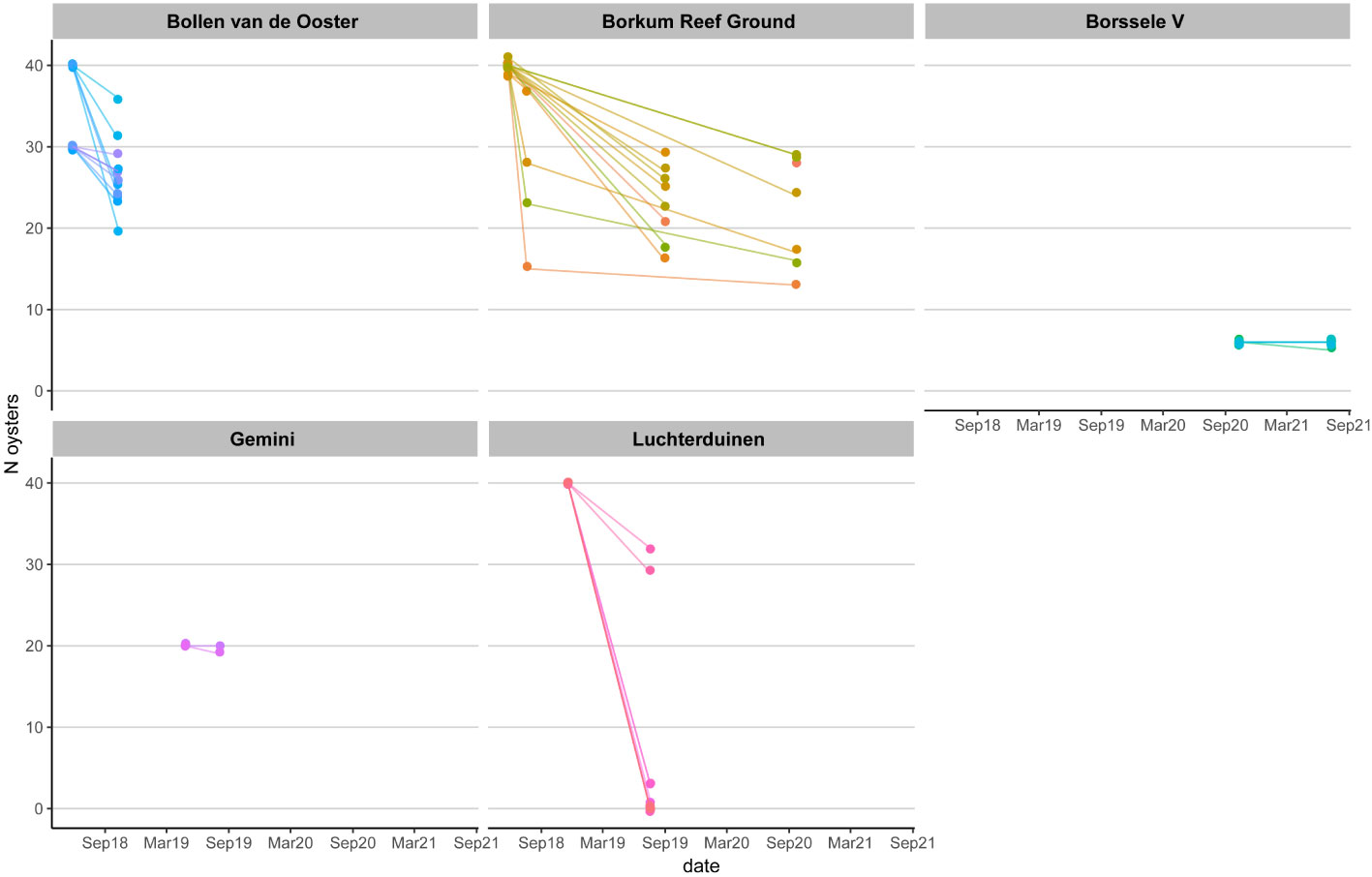
Figure 5 Ostrea edulis. Survival of adult oysters per location per basket (N per basket at T0, T1, and/or T2 and/or T3).
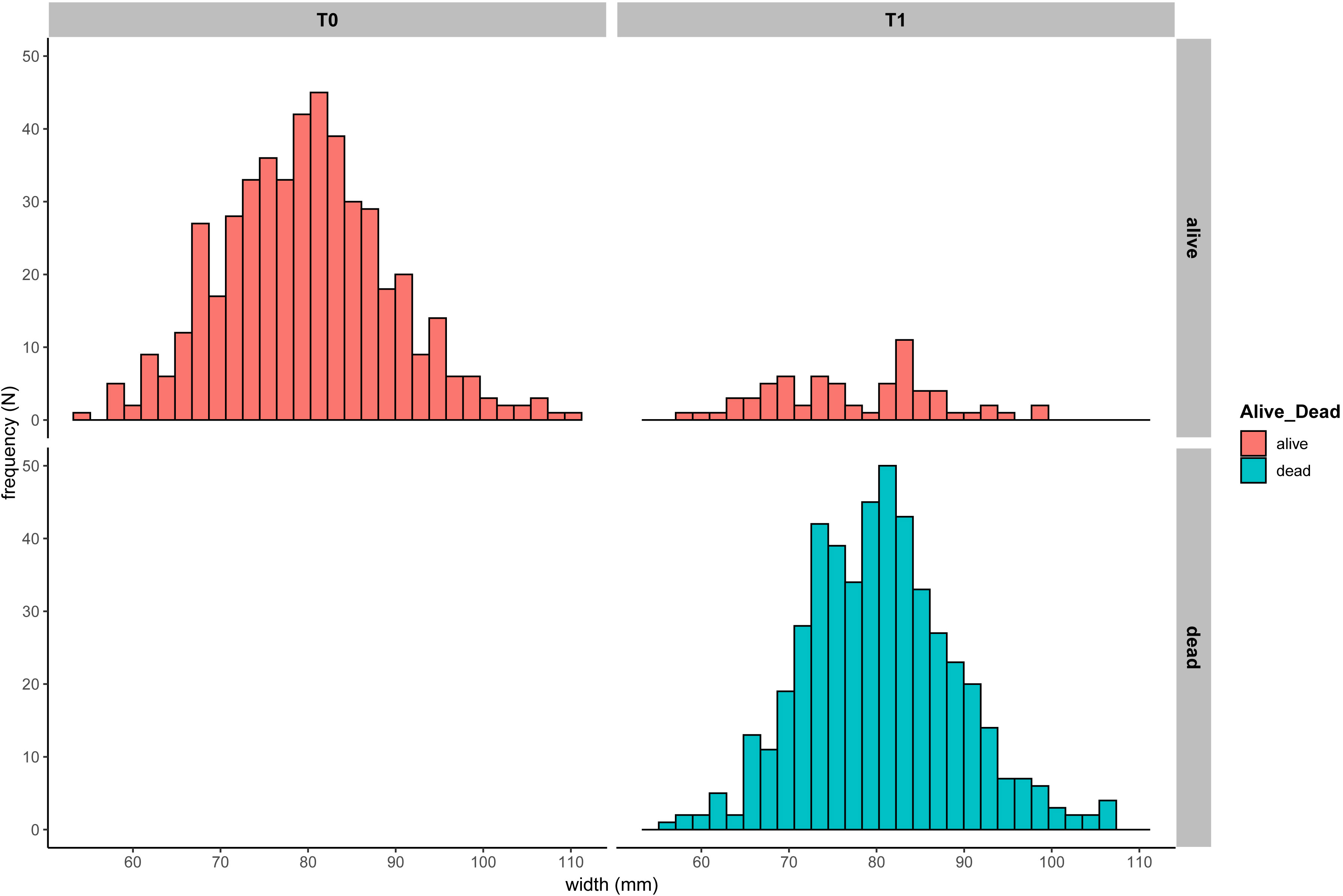
Figure 6 Ostrea edulis. Size-frequency plot (width, mm) of Luchterduinen oysters at deployment (T0: Nov 2018) and after 8 months (T1: Jul 2019).
Identifying individual oysters with holding towers caused extra mortality at Borkum Reef Grounds (Supplementary Table S1). At Borkum Reef Grounds average survival in the holding towers was 89% per month as opposed to 94% for oysters that were kept loose inside the baskets (Supplementary Table S1). However, only one of the two baskets with holding towers at Bollen van de Ooster showed lower survival. The tagging method using glued-on tags, tested at Gemini, was more effective. After 3 months in the oyster baskets in the field, tags were still attached to the oysters and had no impact on mortality rate.
The shell width of the oysters increased over time (Figure 7). Highest growth rates (3.67 mm/month) were observed at Bollen van de Ooster and lowest at Borssele. However, the period over which growth was calculated showed large differences between pilots. At Bollen van de Ooster the period from May to October coincided with the seasons in which most growth takes place, while at Borssele, the period from October to July started in the winter where no growth is expected and ended halfway during the growing season. Thus, the growth rate expressed per month underestimates the growth rate that can be achieved, because it is calculated as an average over growth and non-growth seasons.
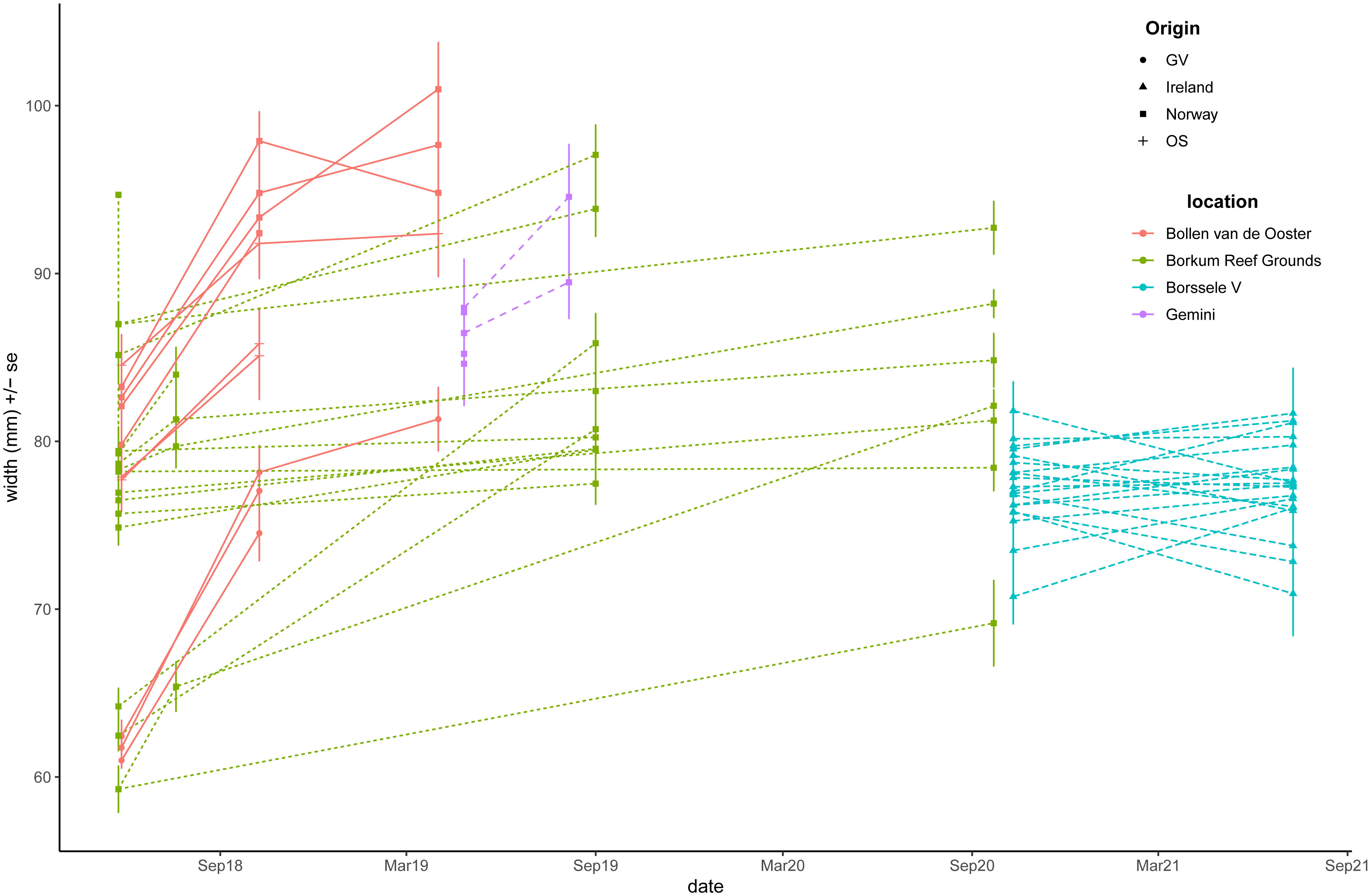
Figure 7 Ostrea edulis. Average width (mm) per cage per location. Oyster origin (GV=Grevelingen, OS=Oosterschelde, Ireland, Norway) is indicated by the symbols, oyster pilot location by colour (red: Bollen van de Ooster, green: Borkum Reef Grounds, aqua: Borssele V, blue: Gemini).
Data exploration (Figure 8 and Table 2) showed that both the abiotic factors O2 and pH did not explain growth. The GLM model was adapted to Growth (mm/month) ~ factor(location) + factor(origin)+ Nmonths + Temp + Salinity + e. The model showed that variation in growth rates could significantly be attributed to oyster origin and temperature, and to a lesser extent to time (number of months between Tx and T0) and to location and salinity.
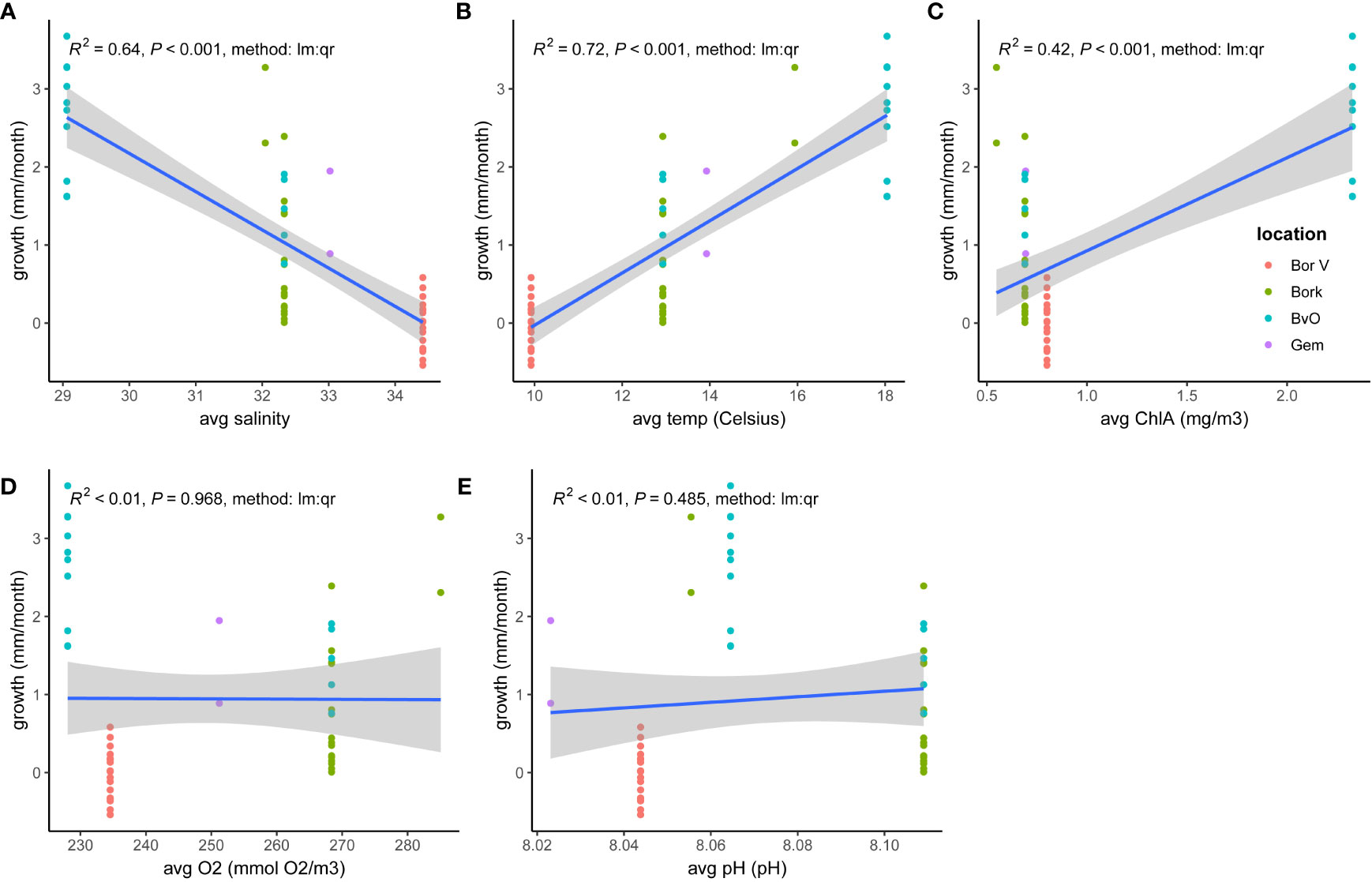
Figure 8 Ostrea edulis. Growth (mm/month, for T0-Tx) versus abiotic variables: (A) salinity, (B) average temp, (C) average Chla (mg/m3), (D) O2 (mmol/m3) and (E) pH.
3.2 Condition index, brooding oysters and Bonamia presence
The condition index of the oysters was determined at several points throughout the season and showed large variation among individuals (Figure 9). The highest observed value was 7.6 at Bollen van de Ooster and the lowest was 1.0 at Borkum Reef Ground.
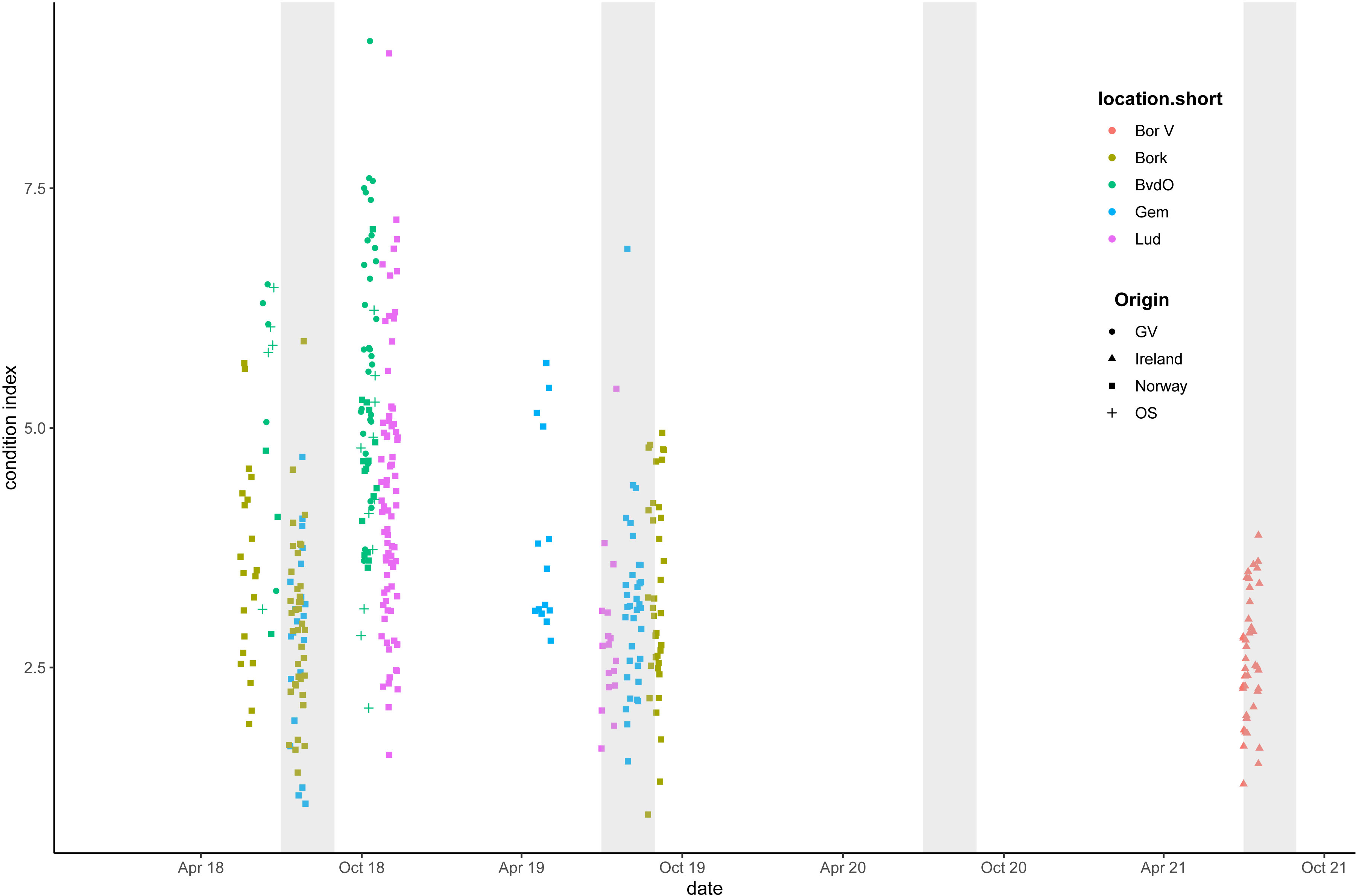
Figure 9 Ostrea edulis. Condition index for individual oysters. Oyster origin is indicated by the symbols, pilot location by colour. Grey areas show the reproduction months (July and August).
In the pilots where Bonamia-free oysters were introduced, oysters were sampled for Bonamia analysis. In all cases the oysters were not infected with the parasite (Table 3).
Oyster samples collected in July revealed several brooding individuals (Table 4). Most breeders were found in Irish oysters placed in Borssele, but breeding oysters were also found at Borkum reef Ground and Luchterduinen. This indicates that the introduced oysters were able to breed in the Dutch North Sea.

Table 4 Ostrea edulis. Number of brooding oysters. Recently fertilised eggs have a white colour, further developed larvae are grey and fully developed larvae are black.
3.3 Larval abundance
At all locations where oysters were deployed and flat oyster larvae were sampled in summer (Figure 10), larvae were detected. Larval densities varied between 3 and 125 flat oyster larvae per 100 L. Assuming that mainly locally produced larvae are collected, it was surprising that highest concentrations were observed at Luchterduinen, a location where only 480 flat oysters were deployed and where high mortality was detected in July. The next highest larval density was found at Borkum Reef Grounds. In this latter area most larvae were observed in the year that the oysters were deployed. The following years had lower larval densities.
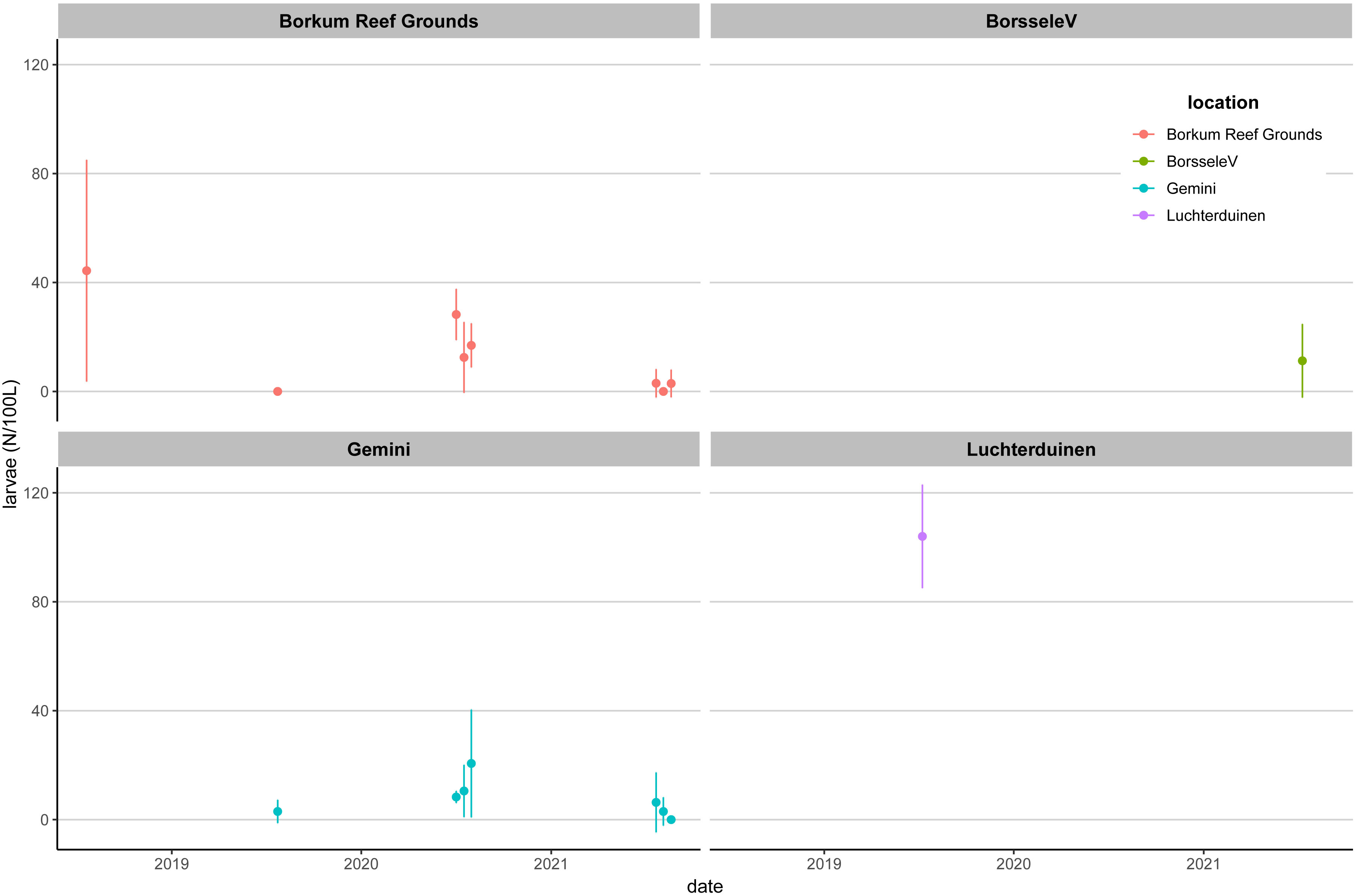
Figure 10 Ostrea edulis. Average oyster larval abundance (N larvae/100L +-SD) at 4 pilot oyster restoration sites: Borkum Reef Grounds (red), Borssele V (green), Gemini offshore wind farm Buitengaats (aqua) and Luchterduinen offshore windfarm (blue).
4 Discussion
As there is no longer a natural population of flat oysters present in the offshore North Sea, translocated oysters had to be sourced from elsewhere. Both foreign flat oyster populations (Ireland and Norway, deployed in nearshore and offshore areas) and local oysters (in a nearshore area) performed well in the Dutch North Sea. Survival was high except for conditions where the monitoring racks became buried in sand, or where oysters were restricted in movement by a monitoring device (holding tower). Growth rates were generally lower than those reported elsewhere for flat oysters (Robert et al., 1991; Da Silva et al., 2005; Pogoda et al., 2011; Merk et al., 2020). This has two explanations: Firstly, most oysters were large adults with a shell width of around 85 mm. Detecting growth is more difficult in large oysters as increase in size reduces with age (von Bertalanffy, 1934). The smallest oysters were deployed at Bollen van de Ooster (Grevelingen) and Borkum Reef Grounds (‘Norway-small’). The Grevelingen oysters increased in shell width from 65 mm to 75 mm in one growing season. The small oysters from Norway showed an increase in shell width from 65 mm to 80 mm in two growing seasons. Secondly, monitoring dates did not always coincide with the beginning and end of the growing season. For example, at Borssele oysters were collected in the middle of the growing season, thereby missing information on the total growth of that season. Thus, the growth rate at that location is underestimated. This may explain the statistical observation of the GLM model that ‘origin’ and the number of months were significantly contributing to the growth rate. Hence, correlations between growth and environmental conditions need to considered with caution, since not all pilots were sampled just before and after the growing season. The condition index of the oysters of the Dutch pilots falls within the range in condition indices observed offshore in the German Bight (Pogoda et al., 2011; Merk et al., 2020).
The finding of brooding oysters and larvae in the water column suggests that the oysters were able to reproduce in the first summer after deployment. O. edulis first matures as a male which is later followed by a female phase (Cole, 1941; Walne & Mann, 1975). The first production of larvae usually takes place in the second or third year (Cole, 1941). The deployment of larger oysters enhanced the likelihood of presence of females and subsequently successful fertilisation.
In two pilots, Borkum Reef Grounds and Borssele, spatfall was investigated using spat collectors (not reported here). In the Borkum Reef Grounds pilot a few smallest saddle oysters Heteranomia squamula were detected, but no flat oyster spat (Didderen et al., 2020). In the Borssele pilot, also no flat oyster spat was found (Schutter et al., 2021). Flat oyster spat may have been present on adult flat oysters at the seafloor and in the baskets in the Borkum Reef Grounds pilot (Didderen et al., 2020), however we cannot exclude that this spat was already present at the time of deployment.
The monitoring also revealed that the oysters were Bonamia-free after 2-9 months at the pilots, indicating that the offshore Dutch North Sea is still Bonamia-free. Bonamia is present however in the inshore and nearshore Delta area where European oysters have settled naturally in recent years (Christianen et al., 2018). The use of oysters from areas where Bonamia is not present (such as Hafrsfjord in Norway and Tralee Bay in Ireland) comes however with a risk. These oysters are susceptible to the disease and may show mortality in case Bonamia happens to arrive at the pilot location. A recent study by Kamermans et al. (2023) proposes an alternative source for oyster restoration projects. Flat oyster seed was produced in hatcheries from brood stock collected in an infected area. Through non-destructive pre-screening of the brood stock the produced oysters were disease free and potentially also tolerant to the disease.
The five oyster pilot restoration projects have each tested and developed monitoring methods in a parallel process of ‘learning by doing’. The pilots were organised by various parties (Table 1) that exchanged knowledge, but also developed methods themselves. The heavy weight monitoring racks were used in most studies (Table 1) and proved to function well overall, except at Luchterduinen, where most oysters died because of a sand wave that partly covered the oyster rack. This indicates that sediment dynamics should be taken into account when deciding where to place the oysters. In the Borssele pilot, racks were deployed on the scour protection. Installation and retrieval of heavy weight racks requires large and expensive offshore vessels. Therefore, as an alternative, light weight monitoring racks were used in Gemini offshore windfarm. They required a small vessel, but only few could be retrieved. The missing light weight racks were most probably moved away from the area by currents.
5 Conclusions and recommendations
Flat oyster restoration efforts in Europe continue to increase. In this study, we have synthesized data collected at five different oyster restoration pilot projects in the Dutch North Sea. Overall, oyster performance in terms of oyster survival, growth, condition and reproduction (production of larvae) was successful across all restoration pilots, except for survival at the Luchterduinen offshore wind farm pilot. Growth rate was significantly affected by the source of the oysters (Ireland, Norway, and the Dutch delta), negatively to the duration of the pilot and positively related to water temperature. However, other factors such as the initial size and timing of sampling may be the underlying causes for these observations. Data collection in terms of, for example, oyster length measurements, condition index determination, survival and larval production should be standardised to allow better comparisons. As an example, oysters should be individually identifiable and sampling across pilots should occur during the same time period.
The different experimental set-ups were part of a ‘learning by doing’ process since offshore oyster restoration is still under development. To allow for inter-site comparisons, it would be best to use standardized monitoring methodologies. To standardise monitoring, we advise developing a data collecting protocol to make all restoration projects in Europe comparable. An example of such a protocol is presented by zu Ermgassen et al. (2021).
The results indicate that many sites, from nearshore to far offshore, in the Dutch North Sea are suitable for European oyster growth. A next step is to test if recruitment in these pilots will lead to a self-sustaining source of oyster larvae.
To be able to relate abiotic parameters to oyster performance, we advise collecting of data in situ, using CTDs or other equipment, to have more accurate environmental data than the ones derived from models with large grid sizes. Furthermore, we recommend using standardized monitoring racks, and to tag oysters individually with labels so individual growth, mortality or the condition index can be derived, as an alternative to stacking oysters in a tower (Figure 2B) which hindered growth and movement. We also recommend harmonising the timing of sampling across pilot studies, because oysters do not grow or reproduce continuously, but only during their growing season or reproduction season, respectively. In addition, growth rates are best monitored with younger (smaller) oysters, as these grow faster than older ones.
Data availability statement
The original contributions presented in the study are included in the article/Supplementary Material. Further inquiries can be directed to the corresponding author.
Author contributions
OB and PK designed the Gemini pilot. KD, JB and PK were involved in designing, fieldwork, data analysis and reporting of the other pilots. OB, JB and PK collected the Gemini samples. PK and SD-P collected data for across pilot comparisons. OB, SD-P and PK analyzed data and performed statistical analyses and wrote the first draft of the manuscript. SH collected abiotic model parameters. All authors contributed substantially to the revisions. All authors contributed to the article and approved the submitted version.
Funding
This cross-comparison of pilot studies and the Gem pilot study were supported by the Top Sector Energy Subsidy from the Ministry of Economic Affairs, project JIP ECO-FRIEND (RVO reference number TEWZ118017) and Gemini. The other pilot studies were supported by WWF Netherlands (Bork: project Borkum Reef Oyster Pilot), Eneco (Luch: Oesterproject Luchterduinen), ARK Rewilding NL (BvO: project Droomfonds Haringvliet) and Van Oord (Bor V: project EcoScour).
Acknowledgments
We are grateful to WWF Netherlands, ARK Rewilding NL, Van Oord, Gemini and Eneco for permission to use the data of their pilot restoration sites. We thank Jan Groot (Esmelda), Luuk Folkerts (Gemini), the Gemini reps, and the crews of the CTV ‘BMS Vrijheid’ (Bos Marine Services) and CTV ‘Vrijheid III’ (Ubels Offshore) for their kind assistance during preparation and execution of the ECO-FRIEND fieldwork at Gemini. We would like to thank the crew of VOS Star for their kind assistance during fieldwork at Luchterduinen, and the crews of the Panda, ZK 47 and MS Tender for their kind assistance during fieldwork at Borkum Reef Ground. We thank the following people for preparation and execution of fieldwork Wageningen Marine Research: Jimmy van Rijn (pilot Gem), Arnold Bakker (Gem), Linda Tonk (pilots Gem and BorV), Emiel Brummelhuis (BorV), Joop Coolen (Bork); Waardenburg Ecology: JB, Wouter Lengkeek, Tom van der Have (Gem, Bork, Luch, BvO, BorV), Edwin Kardinaal (Gem, Bork, BorV), Mirjam Schutter (Gem, BorV), Margot Maathuis (Gem, BvO), Rebecca Bakker (Luch, BorV), Udo van Dongen (Gem, Bork and BorV), Floor Driessen (Bork); Van Oord: Remment ter Hofstede (Luch, BorV), Rick Bosscher (BorV), Jesper Elzinga (Luch, BorV), Willemijn Foursoff (BorV), Frank Krassenburg (BorV), Nathalie Strookman (BorV), Stichting de Noordzee: Nathalie Strookman (Luch), Guido Schild (Luch), Christiaan van Sluis (Luch), Eneco: Sytske van den Akker (Luch); WWF: Emilie Reuchlin-Hugenholtz (Gem, Bork), Brenda van Doorn-Deden (Bork), S. Verbeek (Bork); Waterproof: Roelant Snoek (Bork); Scalmarin: Knut Magnus Persson (Bork), Sas Consultancy: Hein Sas (BvO, Luch). We thank Pim van Dalen (WMR) for identifying and counting oyster larvae and Ad van Gool (WMR) for determination of condition index. The Bonamia analyses were carried out by Wageningen Bioveterinary Research. We thank Luca van Duren (Deltares) and Brecht Sechele (UGent) for their time and expertise regarding environmental data and Marinka van Puijenbroek (WMR) for help with graphics. We thank Thijs Bosker of Leiden University for his role in supervising the BSc thesis of SD-P.
Conflict of interest
The authors declare that the research was conducted in the absence of any commercial or financial relationships that could be construed as a potential conflict of interest.
Publisher’s note
All claims expressed in this article are solely those of the authors and do not necessarily represent those of their affiliated organizations, or those of the publisher, the editors and the reviewers. Any product that may be evaluated in this article, or claim that may be made by its manufacturer, is not guaranteed or endorsed by the publisher.
Supplementary material
The Supplementary Material for this article can be found online at: https://www.frontiersin.org/articles/10.3389/fmars.2023.1233744/full#supplementary-material
Supplementary Table 1 | Survival of oysters in flat oyster restoration pilots. * indicates that oysters were placed in a holding tower.
References
Anderson J., Hedgecock D. (2004). “History and current status of restoring native oyster reefs in the chesapeake bay,” in Nonnative oysters in the chesapeake bay (National Academies Press), 10796). doi: 10.17226/10796
Baggett L. P., Powers S. P., Brumbaugh R. D., Coen L. D., DeAngelis B. M., Greene J. K., et al. (2015). Guidelines for evaluating performance of oyster habitat restoration. Restor. Ecol. 23 (6), 737–745. doi: 10.1111/rec.12262
Beck M. W., Brumbaugh R. D., Airoldi L., Carranza A., Coen L. D., Crawford C., et al. (2011). Oyster reefs at risk and recommendations for conservation, restoration, and management. BioSci. 61 (2), 107–116. doi: 10.1525/bio.2011.61.2.5
Bennema F. P., Engelhard G. H., Lindeboom H. (2020). Ostrea edulis beds in the central North Sea: Delineation, ecology, and restoration. ICES J. Mar. Sci. 77 (7–8), 2694–2705. doi: 10.1093/icesjms/fsaa134
Benthotage C., Schulz K. G., Cole V. J., Benkendorff K. (2022). Water quality and the health of remnant leaf oyster (Isognomon ephippium) populations in four Australian estuaries. Sci. Total Environ. 826, 154061. doi: 10.1016/j.scitotenv.2022.154061
Blauw A. N., Los H. F., Bokhorst M., Erftemeijer P. L. (2009). GEM: a generic ecological model for estuaries and coastal waters. Hydrobiologia 618, 175–198. doi: 10.1007/s10750-008-9575-x
Bromley C., McGonigle C., Ashton E. C., Roberts D. (2016). Bad moves: Pros and cons of moving oysters – A case study of global translocations of Ostrea edulis Linnaeus 1758 (Mollusca: Bivalvia). Ocean Coast. Manage. 122, 103–115. doi: 10.1016/j.ocecoaman.2015.12.012
Bureau Waardenburg (2020). Options for biodiversity enhancement in offshore wind farms: Knowledge base for the implementation of the Rich North Sea Programme. Report 19–0153 (Culemborg: Bureau Waardenburg).
Callaway R., Engelhard G. H., Dann J., Cotter J and Rumohr H. (2007). A century of North Sea epibenthos and trawling: Comparison between 1902–1912, 1982–1985 and 2000. Mar. Ecol. Prog. Ser. 346, 27–43. doi: 10.3354/meps07038
Catalán I. A., Auch D., Kamermans P., Morales-Nin B., Angelopoulos N. V., Reglero P., et al. (2019). Critically examining the knowledge base required to mechanistically project climate impacts: A case study of Europe’s fish and shellfish. Fish Fish. 20 (3), 501–517. doi: 10.1111/faf.12359
Christianen M. J. A., Lengkeek W., Bergsma J. H., Coolen J. W. P., Didderen K., Dorenbosch M., et al. (2018). Return of the native facilitated by the invasive? Population composition, substrate preferences and epibenthic species richness of a recently discovered shellfish reef with native European flat oysters (Ostrea edulis) in the North Sea. Mar. Biol. Res. 14 (6), 590–597. doi: 10.1080/17451000.2018.1498520
Cobacho S. P., Wanke S., Konstantinou Z., El Serafy G. (2020). Impacts of shellfish reef management on the provision of ecosystem services resulting from climate change in the Dutch Wadden Sea. Mar. Policy 119, 104058. doi: 10.1016/j.marpol.2020.104058
Cole H. A. (1941). The fecundity of Ostrea edulis. J. Mar. Biol. Assoc. UK 25 (2), 243–260. doi: 10.1017/s0025315400054710
Colsoul B., Boudry P., Pérez-Parallé M. L., Bratoš Cetinić A., Hugh-Jones T., Arzul I., et al. (2021). Sustainable large-scale production of European flat oyster (Ostrea edulis) seed for ecological restoration and aquaculture: A review. Rev. Aquacult. 13 (3), 1423–1468. doi: 10.1111/raq.12529
Da Silva P. M., Fuentes J., Villalba A. (2005). Growth, mortality and disease susceptibility of oyster Ostrea edulis families obtained from brood stocks of different geographical origins, through on-growing in the Ría de Arousa (Galicia, NW Spain). Mar. Biol. 147, 965–977. doi: 10.1007/s00227-005-1627-4
Didderen K., Bergsma J. H., Kamermans P. (2019a). Offshore flat oyster pilot Luchterduinen wind farm. Results campaign 2 (July 2019) and lessons learned. Report 19-184 (Culemborg: Bureau Waardenburg).
Didderen K., Kamermans P., Bergsma J., Maathuis M. (2019b). Verslag monitoring oesterpilot Voordelta 2019. Notitie 19-0171 (Culemborg: Bureau Waardenburg).
Didderen K., Kamermans P., Lengkeek W. (2018). Gemini wind farm oyster pilot. Results 2018 (Culemborg: Bureau Waardenburg).
Didderen K., Lengkeek W., Bergsma J. H., van Dongen U., Driessen F. M. F., Kamermans P. (2020). WWF & ARK Borkum Reef Ground oyster pilot: Active restoration of native oysters in the North Sea - Monitoring September 2019. Report 19-227 (Culemborg: Bureau Waardenburg).
Didderen K., Lengkeek W., Kamermans P., Deden B., Reuchlin-Hugenholtz E. (2019c). Pilot to actively restore native oyster reefs in the North Sea. Comprehensive report to share lessons learned in 2018. Report 19-013 (Culemborg: Bureau Waardenburg).
Didderen K., van der Have T. M., Bergsma J. H., van der Jagt H., Lengkeek W., Kamermans P., et al. (2019d). Shellfish bed restoration pilots Voordelta, Netherlands Annual report 2018, ARK Report.
Elliott M., Burdon D., Hemingway K. L., Apitz S. E. (2007). Estuarine, coastal and marine ecosystem restoration: Confusing management and science – A revision of concepts. Estuar. Coast. Shelf S. 74 (3), 349–366. doi: 10.1016/j.ecss.2007.05.034
European Union-Copernicus Marine Service (2020). Atlantic-european north west shelf- ocean biogeochemistry reanalysis (Mercator Ocean International). doi: 10.48670/MOI-00058
Gheerardyn H., Engelberts A., Meulenbroek K., Schellekens T., Verduin E. (2021). Scientific work performed in OWF Borssele 3/4, T-1, 1-5 July 2021. Field Report J00002898 (Amsterdam-Duivendrecht: Eurofins AquaSense).
Gilby B. L., Olds A. D., Peterson C. H., Connolly R. M., Voss C. M., Bishop M. J., et al. (2018). Maximizing the benefits of oyster reef restoration for finfish and their fisheries. Fish Fish. 19 (5), 931–947. doi: 10.1111/faf.12301
Hargis W. J., Haven D. S. (1988). The imperilled oyster industry of virginia: A critical analysis with recommendations for restoration. Special reports in applied marine science and ocean engineering (SRAMSOE) no. 290 (Williamsburg, Virginia Institute of Marine Science, College of William and Mary). doi: 10.21220/V5JF2K
Harvey B. P., Gwynn-Jones D., Moore P. J. (2013). Meta-analysis reveals complex marine biological responses to the interactive effects of ocean acidification and warming. Ecol. Evol. 3 (4), 1016–1030. doi: 10.1002/ece3.516
Hendriks I. E., Van Duren L. A., Herman P. M. J. (2005). Image analysis techniques: a tool for the identification of bivalve larvae? J. Sea Res. 54 (2), 151–162. doi: 10.1016/j.seares.2005.03.001
Holbrook Z. (2021). Restocking the European Flat Oyster Ostrea edulis in the Solent – A Model for Ecosystem Service Restoration Across Europe. Thesis for Doctor of Philosophy (University of Southampton). Available at: http://eprints.soton.ac.uk/id/eprint/450587.
Kamermans P., Blanco A., van Dalen P., Engelsma M., Bakker N., Jacobs P., et al. (2023). Bonamia-free flat oyster (Ostrea edulis L.) seed for restoration projects: non-destructive screening of broodstock, hatchery production and test for Bonamia-tolerance. Aquat. Living Resour. 36, 11. doi: 10.1051/alr/2023005
Kamermans P., van Duren L., Kleissen F. (2018a). European flat oysters on offshore wind farms: Additional locations : opportunities for the development of European flat oyster (Ostrea edulis) populations on planned wind farms and additional locations in the Dutch section of the North Sea Report C053/18 (Yerseke: Wageningen Marine Research). doi: 10.18174/456358
Kamermans P., Walles B., Kraan M., Duren, van Luca, Kleissen F., et al. (2018b). Offshore wind farms as potential locations for flat oyster (Ostrea edulis) restoration in the Dutch North Sea. Sustainability-Basel 10 (11), 3942. doi: 10.3390/su10113942
Kardinaal W. E. A., Bergsma J. H., van Dongen L. G. J. M., Driessen F. M. (2020). WWf & ARK Nature Borkum Reef Ground oyster pilot. Active restoration of native oysters in the North Sea. Monitoring September 2020 (Culemborg: Bureau Waardenburg).
Kennedy V. S., Breitburg D. L., Christman M. C., Luckenbach M. W., Paynter K., Kramer J., et al. (2011). Lessons learned from efforts to restore oyster populations in Maryland and Virginia 1990 to 2007. J. Shellfish Res. 30 (3), 719–731. doi: 10.2983/035.030.0312
Kerckhof F., Coolen J. W. P., Rumes B., Degraer S. (2018). Recent findings of wild European flat oysters Ostrea edulis (Linnaeus 1758) in Belgian and Dutch offshore waters: New perspectives for offshore oyster reef restoration in the southern North Sea. Belg. J. Zool. 148 (1), 13–24. doi: 10.26496/bjz.2018.16
Korringa P. (1940). “Experiments and observations on swarming, pelagic life and setting in the European flat oyster Ostrea edulis L,” in Archives néerlandaises de zoologie 5, 1–249.
Lenihan H. S., Micheli F., Shelton S. W., Peterson C. H. (1999). The influence of multiple environmental stressors on susceptibility to parasites: An experimental determination with oysters. Limnol. Oceanogr. 44 (3part2), 910–924. doi: 10.4319/lo.1999.44.3_part_2.0910
Loosanoff V., Davis H. C., Chanley P. (1966). Dimensions and shapes of larvae of some marine bivalve molluscs. Malacologia 4 (2), 351–435.
Maathuis M. A. M., Coolen J. W. P., van der Have T., Kamermans P. (2020). Factors determining the timing of swarming of European flat oyster (Ostrea edulis L.) larvae in the Dutch Delta area: Implications for flat oyster restoration. J. Sea Res. 156, 101828. doi: 10.1016/j.seares.2019.101828
Merk V., Colsoul B., Pogoda B. (2020). Return of the native: Survival, growth and condition of European oysters reintroduced to German offshore waters. Aquat. Conserv. 30 (11), 2180–2190. doi: 10.1002/aqc.3426
Ministry of Infrastructure and Water Management (I&W), ministry of Agriculture, Nature and Food Quality (LNV) (2018) Marine Strategy (part 1). Update of current environmental status, good environmental status, environmental targets and indicators. 2018-2024. Available at: https://www.noordzeeloket.nl/publish/pages/158924/marine_strategy_part_1_main_document_2018_-_2024.pdf.
O’Foighil D. (1989). Role of spermatozeugmata in the spawning ecology of the brooding oyster Ostrea edulis. Gamete Res. 24, 219–228. doi: 10.1002/mrd.1120240209
O’Foighil D. O., Taylor D. J. (2000). Evolution of parental care and ovulation behavior in oysters. Mol. Phylogenet. Evol. 15 (2), 301–313. doi: 10.1006/mpev.1999.0755
OSPAR (2008) OSPAR List of Threatened and/or Declining Species and Habitats. OSPAR Agreement 2008-06. Available at: https://www.ospar.org/work-areas/bdc/species-habitats/list-of-threatened-declining-species-habitats.
OSPAR BDC (2020) Status Assessment 2020 - European flat oyster and Ostrea edulis beds. Available at: https://oap.ospar.org/en/ospar-assessments/committee-assessments/biodiversity-committee/status-assesments/european-flat-oyster/.
Pogoda B., Buck B. H., Hagen W. (2011). Growth performance and condition of oysters (Crassostrea gigas and Ostrea edulis) farmed in an offshore environment (North Sea, Germany). Aquaculture 319 (3–4), 484–492. doi: 10.1016/j.aquaculture.2011.07.017
Pogoda B., Brown J., Hancock B., Preston J., Pouvreau S., Kamermans P., et al. (2019). The Native Oyster Restoration Alliance (NORA) and the Berlin Oyster Recommendation: Bringing back a key ecosystem engineer by developing and supporting best practice in Europe. Aquat. Living Resour 32, 19. doi: 10.1051/alr/2019012
Pogoda B., Merk V., Colsoul B., Hausen T., Peter C., Pesch R., et al. (2020). Site selection for biogenic reef restoration in offshore environments: The Natura 2000 area Borkum Reef Ground as a case study for native oyster restoration. Aquat. Conserv. 30 (11), 2163–2179. doi: 10.1002/aqc.3405
R Core Team (2023). R: A language and environment for statistical computing (Vienna, Austria: R Foundation for Statistical Computing). Available at: https://www.R-project.org/.
Reise K. (2005). Coast of change: Habitat loss and transformations in the Wadden Sea. Helgoland Mar. Res. 59 (1), 9–21. doi: 10.1007/s10152-004-0202-6
Ridlon A. D., Marks A., Zabin C. J., Zacherl D., Allen B., Crooks J., et al. (2021). Conservation of marine foundation species: learning from native oyster restoration from california to british columbia. Estuar. Coast. 44 (7), 1723–1743. doi: 10.1007/s12237-021-00920-7
Robert R., Borel M., Pichot Y., Trut G. (1991). Growth and mortality of the European oyster Ostrea edulis in the Bay of Arcachon (France). Aquat. Liv. Res. 4, 265–274. doi: 10.1051/alr:1991028
Rodriguez-Perez A., James M., Donnan D. W., Henry T. B., Møller L. F., Sanderson W. G. (2019). Conservation and restoration of a keystone species: Understanding the settlement preferences of the European oyster (Ostrea edulis). Mar. Pollut. Bull. 138, 312–321. doi: 10.1016/j.marpolbul.2018.11.032
Rodriguez-Perez A., James M. A., Sanderson W. G. (2021). A small step or a giant leap: Accounting for settlement delay and dispersal in restoration planning. PloS One 16 (8), e0256369. doi: 10.1371/journal.pone.0256369
Sas H., Didderen K., van der Have T., Kamermans P., van den Wijngaard K., Reuchlin E. (2019). Recommendations for flat oyster restoration in the North Sea (SASCON). Available at: https://www.ark.eu/sites/default/files/media/Schelpdierbanken/Recommendations_for_flat_oyster_restoration_in_the_North_Sea.pdf
Sas H., Kamermans P., van der Have T. M., Christianen M. J. A., Coolen J. W. P., Lengkeek W., et al. (2018). Shellfish bed restoration pilots (Voordelta The Netherlands: SASCON). Annual report 2017 (Amsterdam: SASCON).
Sas H., Kamermans P., van der Have T., Lengkeek W., Smaal A. (2016). Shellfish reef restoration pilots Voordelta. The Netherlands. Annual report 2016 (Amsterdam: SASCON).
Schutter M., Tonk L., Kamermans P., Kardinaal E., ter Hofstede R. (2021). EcoScour project Borssele V: Outplacement methods of European flat oysters (Culemborg: Bureau Waardenburg), 21–328.
Smaal A., Kamermans P., Kleissen F., van Duren L., van der Have T. (2017). Flat Oysters on Offshore Wind Farms: Opportunities for the development of flat oyster populations on existing and planned wind farms in the Dutch section of the North Sea. Report C052/17 (Yerseke: Wageningen Marine Research). doi: 10.18174/418092
Smaal A., Kamermans P., van der Have T. M., Engelsma M., Sas H. J. W. (2015). Feasibility of Flat Oyster (Ostrea edulis L.) restoration in the Dutch part of the North Sea. Report C028/15 (IMARES Wageningen UR). Available at: https://edepot.wur.nl/335033.
Smyth D., Kregting L., Elsäßer B., Kennedy R., Roberts D. (2016). Using particle dispersal models to assist in the conservation and recovery of the overexploited native oyster (Ostrea edulis) in an enclosed sea lough. J. Sea Res. 108, 50–59. doi: 10.1016/j.seares.2015.12.009
Smyth D., Roberts D. (2010). The European oyster (Ostrea edulis) and its epibiotic succession. Hydrobiologia 655 (1), 25–36. doi: 10.1007/s10750-010-0401-x
Stechele B., Maar M., Wijsman J., van der Zande D., Degraer S., Bossier P., et al. (2022). Comparing life history traits and tolerance to changing environments of two oyster species (Ostrea edulis and Crassostrea gigas) through Dynamic Energy Budget theory. Conserv. Physiol. 10 (1), coac034. doi: 10.1093/conphys/coac034
Tamburri M. N., Luckenbach M. W., Breitburg D. L., Bonniwell S. M. (2008). Settlement of Crassostrea ariakensis larvae: Effects of substrate, biofilms, sediment and adult chemical cues. J. Shellfish Res. 27, 601–608. doi: 10.2983/0730-8000(2008)27[601:SOCALE]2.0.CO;2
Van den Brink A., Magnesen T. (2018). Follow-up test “treatment protocol flat oysters” with Norwegian oysters (Yerseke: Wageningen Marine Research).
van Leeuwen S. M., Lenhart H.-J., Prins T. C., Blauw A., Desmit X., Fernand L., et al. (2023). Deriving pre-eutrophic conditions from an ensemble model approach for the North-West European Seas. Front. Mar. Sci. 10. doi: 10.3389/fmars.2023.1129951
von Bertalanffy L. (1934). Untersuchungen über die Gesetzlichkeit des Wachstums. Wilhelm Roux’Archiv für Entwicklungsmechanik der Organismen 131 (4), 613–652. doi: 10.1007/BF00650112
Walne P. R. (1970). The seasonal variation of meat and glycogen content of seven populations of oysters Ostrea edulis L. and a review of the literature. Fishery Invest. Lond. Ser. 26 (3), 35.
Walne P. R., Mann R. (1975). “Growth and Biochemical Composition in Ostrea edulis and Crassostrea gigas,” in Proceedings of the Ninth European Marine Biology Symposium, 587–607 (Scotland: Aberdeen University Press).
Zijl F., Zijlker T., Laan S., Groenenboom J. (2023) 3D DCSM FM: a sixth-generation model for the NW European Shelf. Technical report, Deltares 2023. Available at: https://publications.deltares.nl/11208054_004_0003.pdf.
zu Ermgassen P., Bos O., Debney A., Gamble C., Glover A., Pogoda B., et al. (2021). European native oyster habitat restoration monitoring handbook (UK: The Zoological Society of London). Available at: https://noraeurope.eu/nora-publications/.
zu Ermgassen P. S. E., Strand Å., Bakker N., Blanco A., Bonačić K., Boudry P., et al. (2023). Overcoming Ostrea edulis seed production limitations to meet ecosystem restoration demands in the UN decade on restoration. Aquat. Living Resour 36, 16. doi: 10.1051/alr/2023012
Keywords: oyster restoration, offshore wind, Ostrea edulis, nature inclusive design, OSPAR, biogenic reef
Citation: Bos OG, Duarte-Pedrosa S, Didderen K, Bergsma JH, Heye S and Kamermans P (2023) Performance of European oysters (Ostrea edulis L.) in the Dutch North Sea, across five restoration pilots. Front. Mar. Sci. 10:1233744. doi: 10.3389/fmars.2023.1233744
Received: 02 June 2023; Accepted: 08 August 2023;
Published: 21 September 2023.
Edited by:
Rochelle Diane Seitz, College of William & Mary, United StatesReviewed by:
David Bushek, Rutgers, The State University of New Jersey, United StatesTanja Segvic-Bubic, Institute of Oceanography and Fisheries (IZOR), Croatia
Copyright © 2023 Bos, Duarte-Pedrosa, Didderen, Bergsma, Heye and Kamermans. This is an open-access article distributed under the terms of the Creative Commons Attribution License (CC BY). The use, distribution or reproduction in other forums is permitted, provided the original author(s) and the copyright owner(s) are credited and that the original publication in this journal is cited, in accordance with accepted academic practice. No use, distribution or reproduction is permitted which does not comply with these terms.
*Correspondence: Oscar G. Bos, oscar.bos@wur.nl