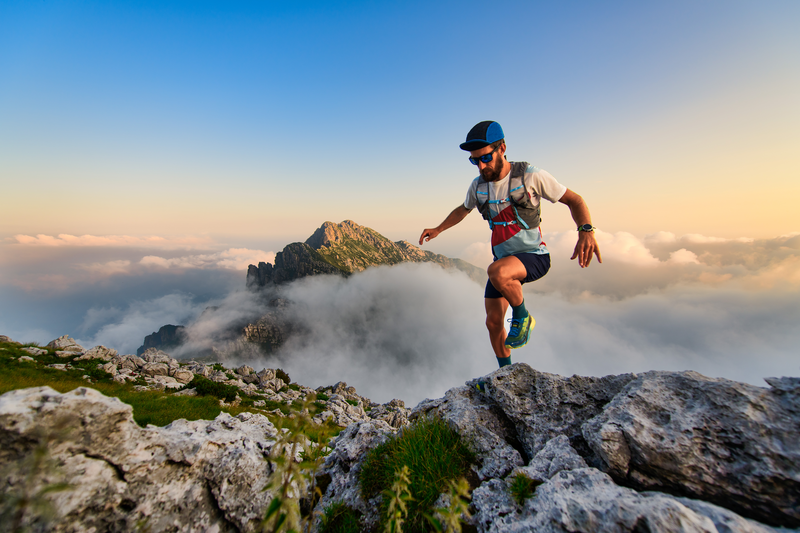
94% of researchers rate our articles as excellent or good
Learn more about the work of our research integrity team to safeguard the quality of each article we publish.
Find out more
REVIEW article
Front. Mar. Sci. , 07 July 2023
Sec. Marine Biotechnology and Bioproducts
Volume 10 - 2023 | https://doi.org/10.3389/fmars.2023.1232891
This article is part of the Research Topic The Discovery, Identification and Application of Marine Microorganisms Derived Natural Products View all 5 articles
The marine environment can harbor millions of macro- and micro-organisms. These habitats have gained more attention as it was found they produce a plethora of novel secondary metabolites. Recently, marine-derived fungi have become the hotspot in drug discovery and development. Deep-sea-associated fungi are an important group of marine fungi living in the ocean below 1000 m. The extreme living environment of high pressure and low temperature mean the deep-sea-associated fungi are difficult to collect but has resulted in the evolution of the special secondary metabolic genes in them. Although the vast majority of deep-sea fungi are undiscovered and very few natural products have been reported from them compared to the fungi derived from the near and shallow sea, they are still considered to be potential prolific sources of novel bioactive compounds that are of considerable interest for new drug leads. This review will expound on the sources of strains, chemical structures, and biological activity of 184 new natural products isolated from 46 deep-sea-associated fungi, which were reported from 2020-2022.
Oceans cover more than 70% of the Earth’s surface and provide 99% of the living space (Subramani and Sipkema, 2019). Animals, plants, and other organisms that live in the oceans, coastal areas, or brackish coastal water can harbor millions of bacteria, fungi, and many other organisms. Some of these marine species live in stressful habitats: high salinity, low temperature, high pressure, and low light conditions (Li et al., 2021; Wu et al., 2022b). Importantly, a large number of species with high diversity survive under such conditions and produce fascinating and structurally complex natural products that are not found in terrestrial organisms. This may be due to the bioaccumulation of metabolites in marine microorganisms, as they adapt and survive in harsh conditions that differ from other habitats (Bhakuni and Rawat, 2005; Debbab et al., 2010). Therefore, the associated microorganisms could produce bioactive substances with unique structural skeletons and bioactivity. These metabolites also act as chemical defense mechanisms for marine organisms. This is especially true for soft-bodied organisms which lack structural defense mechanisms (Jensen and Fenical, 1994). For example, the sequence of malate dehydrogenase in mollusks in the Conch is produced by the organisms to adapt to high temperatures, while the Mariana lionfish genome species undergoes mutations in genes that regulate bone development and ossification, leading to an early termination of the calcification process of its bones, making most of its bones cartilage, in response to deep-sea pressure. The chlorine-secreting cells of barracuda gills can be flexibly adjusted to adapt to different salinities (Kobayashi and Yuki, 1954; Skinner, 1982; Liao et al., 2017).
Many deep-sea organisms need to coexist with microorganisms to survive in these harsh environments, such as sulfide-oxidizing bacteria symbiosis with tubular worms near deep-sea cold springs. Thus, it is known that bioactive metabolites discovered from marine organisms such as sponges and sea slugs are the products of microbial metabolism (Gerwick and Moore, 2012).
Studies on marine-derived microorganisms were intensively initiated in the nineties. Marine microorganisms quickly proved to be prolific sources of novel bioactive compounds that are of considerable interest as new drug leads in the pharmaceutical industry (Marmann et al., 2014). Although studies on marine microbial natural products are fascinating, there are some limitations as well. For example, only 5% of the marine microbes can be cultured with normal laboratory microbiological conditions and techniques, taxonomy is very poorly defined, and fermentation yields are very low. Also, the complexity of the molecule makes it difficult to resynthesize (Jimenez et al., 2009; Ameri, 2014). Even with these disadvantages, the development of microbial natural products with good bioactivity is of great interest.
Recently published research articles showed that most of the marine microbial metabolites are potent anticancer compounds and most of them are clinically used or under clinical trials. Already, 23 marine-inspired agents are in clinical use, of which 16 are of anticancer antitumor interest (Ameri, 2014; Almaliti and Gerwick, 2023). The most famous example of a marine microbial compound is salinosporamide A (marizomib), which is isolated from marine Salinispora bacteria and is a proteasome inhibitor that is currently undergoing clinical studies as a potential new anticancer drug (Marmann et al., 2014). Didemnin B and thiocoraline are two drug candidates that were identified from marine microorganisms and show potent antitumor anticancer activities. They are used for the treatment of different cancers at present (Ameri, 2014). Recently, in 2018, the novel diketopiperazine derivative plinabulin was discovered in marine Aspergillus sp. and is now in a worldwide phase III clinical trial for non-small cell lung carcinoma (Blayney et al., 2016).
Studies on microbial natural products have become a famous research area due to their ability to produce biologically active, biosynthetically rare, structurally diverse, and similar metabolites as the host. The ability to produce metabolites on a large scale without damaging the hosts, consumption of minimal period, and reproducibility are also advantageous qualities in this field of study. Thus, the secondary metabolites produced by marine microorganisms have unique and diverse structures, and significant biological activities, which have great potential for traditional Chinese medicine (Yin et al., 2023).
As an important component of the deep-sea microbial community, deep-sea fungi have attracted much attention from researchers due to their rich types of secondary metabolites, high emergence rates, and significant biological activity (Gozari et al., 2020). More and more researchers are pinning their hopes for developing new drugs on the discovery of secondary metabolites from marine fungi. This article reviews the literature on deep-sea fungal active substances during the years 2020-2022 and describes 184 new compounds first reported from marine sources in 47 papers. In this review, compounds are classified into several broad categories based on their structure. This review also summarizes the biological activities of these compounds and the source of bacteria.
Cyclopeptides simplicilliumtides N and O (1 and 2) were isolated from the extract of the fermented mycelium of the deep-sea-derived Simplicillium obclavatum EIODSF 020. The configurations of 1 and 2 were determined by the advanced Marfeys method. Compounds 1 and 2 showed strong antifungal activity against Alternaria solani and Colletotricum asianum with MIC values of 0.195-6.250 μg/disc (Firmansyah et al., 2022). Indole diketopiperazine alkaloids chevalinulins A (3) and B (4) were obtained from Aspergillus chevalieri CS-122 (Yan et al., 2022) and displayed proangiogenic activity in transgenic zebrafish model at the concentrations of 40 and 80 μg/mL. Deep-sea-derived fungus Aspergillus sp. FS445 afforded aspechinulins A-D (5-8). Compounds 6 and 7 inhibit the production of NO at 20-90 μM (Liu et al., 2022b). A study of Mycosphaerella sp. SCSIO z05 led to the identification of a new iron (III) chelator, mycosphazine A (9), which was active against Bacillus amyloliquefaciens with a rate of about 249% at a concentration of 100 μg/mL (Huang et al., 2020). Western Atlantic deep-sea fungus Penicillium chrysogenum SCSIO 07007 yielded indolyl diketopiperazine, penilline C (10) (Han et al., 2020).
Investigation of Aspergillus sydowii MCCC 3A00324 fermented broth afforded acremolin D (11), which was cytotoxic toward K562, Hela-S3, A549, HepG2, and K562 cell lines at the concentration of 20 μM (Niu et al., 2022). Investigation of a South China Sea-derived fungus, Curvularia verruculosa, led to the discovery of vercytochalasins A (12) and B (13). Compound 12 exhibited angiotensin-I-converting enzyme inhibitory activity whereas 13 showed potent inhibitory activity against aquatic pathogenic bacteria Vibrio anguillarum, V. harveyi, and V. parahaemolyticus with MIC values 32, 8, and 4 μg/mL, respectively (the MIC values of positive control chloromycetin were 0.5, 1, and 2 μg/mL, respectively) (Hu et al., 2023). Fermentation of Aspergillus puniceus SCSIO z021 isolated from deep-sea sediment collected in Okinawa Trough yielded puniceusine O (14) and (±)-puniceusine P (15) (Liu et al., 2022a). A new quinoline alkaloid (16) was separated from Aspergillus sp. SCSIO06786 (Pang et al., 2020). Phomopsis lithocarpus FS508 yielded isoindolinone lactam lithocarlactam A (17), which was isolated from a deep-sea sediment sample collected at a depth of 3606 m in the Indian Ocean (Hu et al., 2022a). One new iron (III) chelator of coprogen-type siderophore mycosphamide A (18) was separated from a culture of Mycosphaerella sp. SCSIO z059 from deep-sea sediment from Okinawa Trough (Huang et al., 2020).
Three new usnic acid derivatives ochuscins C-E (19-21) were separated from Ochroconis sp. FS449, which was isolated from the sediment collected from the Indian Ocean (Chen et al., 2020d). Compounds 19 and 20 showed inhibitory activities against acetylcholinesterase with the IC50 values ranging from 49 to 80 μM (Chen et al., 2020d). A new paraherquamide, aculeaquamide A (22), was discovered from Aspergillus aculeatinus WHF0198 (Wu et al., 2022a). Diphenazine derivatives phenazostatins E-J (23-28) were separated from Cystobasidium laryngis, a strain isolated from deep-sea sediment collected from the Indian Ocean Ridge (Lee et al., 2022). Compound 28 was active against NUGC-3 with an IC50 of 7.7 nM. Verruculoid A (29), 12-nor-cytochalasin F (30), 22-methoxycytochalasin B6 (31), 19-hydroxycytochalasin B (32), and 20-deoxycytochalasin B (33) were obtained from Curvularia verruculosa CS-129, among which 29 showed anti-infective activity against Escherichia coli with an MIC of 2 μg/mL. Compound 32 was cytotoxic against MCF-7, HCT-116, and HepG-2, with IC50 values ranging from 5.2 to 12 μM (Hu et al., 2021). A study on the Aspergillus sp. derived from a Sinularia sp. soft coral resulted in (±)-17hydroxybrevianamide N (34) and (±)-N1-methyl-17-hydroxybrevianamide N (35) (Hu et al., 2021). The pyridone alkaloid arthpyrone L (36) was separated from the Arthrinium sp, and showed antiproliferative effects toward human A549, MG63, U2OS, MCF-7, and MDA-MB-231 cells (Zhang et al., 2021).
Three new hybrid sorbicillinoids, bisorbicillchaetones A-C(37-39), were discovered from Penicillium sp. SCSIO06868. Compounds 37 and 38 showed inhibitory activity towards NO production in LPS-activated RAW264.7, with IC50 values of 80.3 ± 3.6 μM and 38.4 ± 3.3 μM, respectively (Pang et al., 2022). Purpureocillium sp. SCSIO 06693 produced (E)-2-(8,9-dihydroxy-6,8-dimethyldec-4-en2-yl)-methylfuran-3(2H)-one (40), which inhibits the pancreatic lipase enzyme at 50 μg/mL (Song et al., 2022).
Two new polyketides fischerins A (41) and B (42) were obtained from Aspergillus fischeri FS452, among which 42 showed cytotoxicity against A549, HepG-2, MCF-7, and SF-268 ranging from 7 to 10 μM (Liu et al., 2021). Aspergillus puniceus SCSIO z021 produced three new metabolites, (±)-puniceusone A (43), (±)-2,3-dihydroxypropyl 6-ethyl-2,4-dihydroxy3-methylbenzoate (44), and (5-ethyl-3-hydroxy-2-methyl) phenyl-b-D-glucoside (45)(Liu et al., 2022a). Phomalichenones E-G (46-48) were isolated from Alternaria sp. MCCC 3A00467, and 47 showed cytotoxic activity against U266 cells (Zhong et al., 2022). Two new macrolides cyclopiumolides A (49) and B (50) with a spiculisporic acidic moiety were isolated from Penicillium cyclopium SD-413 and showed cytotoxicity against TE-1, FaDu, and SF126 cells with IC50 values ranging from 5.86-17.05 µM (Li et al., 2022). A study of Paraconiothyrium hawaiiense FS482 resulted in the identification of hawatides A-G (51-57). Compound 54 inhibited the production of NO and cytotoxicity against HepG-2, A549, SF-268, and MCD-7 cell lines (Chen et al., 2021b). Ochroconis sp. FS449 yielded a series of usnic acid derivatives ochuscins A(58), B(59), F(60), and G (61), of which compounds 59 and 61 inhibited the action of acetylcholinesterase (Chen et al., 2020d). Lithocaldehydes A (62) and B (63), were isolated from Phomopsis lithocarpus FS508, which displayed antifungal and antibacterial activity at micromolar levels (Liu et al., 2020).
A series of polyketides asperochratides A-J (64-73) were produced by Aspergillus ochraceus, and compounds 68-71 displayed cytotoxicity toward BV-2 cells (Zou et al., 2020). Cultivation of Penicillium chrysogenum SCSIO 07007 produced new α-pyrone derivatives chrysopyrones A and B (74 and 75) which inhibit human protein tyrosine phosphatases (Han et al., 2020). A chemical investigation of a culture extract of Phomopsis tersa FS441 led to the discovery of chlorinated azaphilones tersaphilones A-E (76-80). Compounds 79-80 demonstrated cytotoxicity against cancer cell lines A549, MCF-7, and SF-268 (Chen et al., 2021a). Spiromastol L-N (81-83) and spiromastixin (84) were isolated from an extract of Spiromastix sp. SCSIO F190, which displayed inhibitory activity against methicillin-resistant bacterial strains of Staphylococcus aureus, Enterococcus faecalis ATCC 29212, and Bacillus subtilis BS01 (Cai et al., 2023).
Steckwaic acids A-D (85-88), 11-ketotanzawaic acid D (89), 6,15-dihydroxytanzawaic acid M (90), (15R)-methoxytanzawaic acid M (91), 15S-methoxytanzawaic acid M (92), 8-hydroxytanzawaic acid M (93), and 8-hydroxytanzawaic acid B (94) were obtained from Penicillium steckii AS-324, which was isolated from Acanthogorgiidae sp. coral in the Western Pacific Ocean. Compounds 85, 89, and 93 displayed antibacterial activity with Vibrio anguillarum, Micrococcus luteus, and Pseudomonas aeruginosa (Hu et al., 2022b). Peniterphenyls A-C (95-97) was discovered in Penicillium sp. SCSIO4103, which displayed activity against HSV-1/2 with EC50 values in the range of 1.4 ± 0.6 to 9.3 ± 3.7 μM (Chen et al., 2021c). Penithoketone (98) and penithochromones A-L (99-109) were produced by Penicillium thomii YPGA3. Compound 98 displayed cytotoxicity against MCF-7, MDA-MB-468, C4-2B, and C4-2B/ENZR (Li et al., 2020). Aspergillus terreus CC-S06-18 afforded a dihydrobenzofuran-phenyl acrylate hybrid compound, aspeterreurone A (110), which displayed cytotoxic activities against cell lines BGC823, MGC803, HGC27, and AGS and inhibited the STAT3 phosphorylation (Wang et al., 2020).
Hypoxone A (111), 4,8-dimethoxy-1-naphthol (112), and 1’-hydroxy-4’,8,8’-trimethoxy[2,2’]binaphthalenyl-1,4-dione (113) were produced by the fungus Hypoxylon rubiginosum FS521, and were cytotoxic against the tumor cell lines SF-268, MCF-7, HepG-2, and A549 (Zhang et al., 2020). The dibenzodioxocinone canescenin A (114) produced by the Penicillium canescens SCSIO z053 showed weak antibacterial and antibiofilm activities toward Bascillus amyloliquefaciens and Pseudomonas aeruginosa (Dasanayaka et al., 2020). Two phenols, insphenol A (115) and acetylpeniciphenol (116), were isolated from the cold sea-derived Aspergillus insuetus SD-512. Compound 116 inhibited Edwardsiella tarda, Vibrio alginolyticus, and Vibrio vulnificus (Chi et al., 2021a).
Chemical analysis of Paraconiothyrium hawaiiense FS482 isolated from the sediment of the Indian Ocean afforded four cyclized diterpenoids hawanoids A-D (117-120), which displayed inhibitory activities against PAF-induced platelet aggregation (Chen et al., 2023). Penicillium polonicum CS-252 yielded new verrucosidin derivatives poloncosidins G-K (121–125). 121 was active against aquatic (Micrococcus luteus, Edwardsiella ictarda) and human pathogenic (Pseudomonas aeruginosa and Escherichia coli) bacteria (Li et al., 2023). A fermentation broth of Aspergillus sp. SCSIO06786 afforded sesquiterpene derivatives compound 126-127 and compound 128 (Pang et al., 2020). Photeroids A (129) and B (130), two structurally fascinating meroterpenoids, together with phomeroids A (132) and B (132), were isolated from the deep-sea-derived fungus Phomopsis tersa FS441, which showed strong cytotoxicity (Chen et al., 2020b; Chen et al., 2020c);. Phenolic bisabolanes Asperbisabolanes A-N (133-149), were purified from Aspergillus sydowii MCCC 3A0032. Compound 138 exhibited inhibitory activity against NO secretion in LPS-activated BV-2 microglia cells. Compound 144 also showed anti-inflammatory activity (Niu et al., 2020). Asperal acids A-E (150-154) were produced by Aspergillus alabamensis and showed diverse antimicrobial activity. For example, 153 displayed antimicrobial activity against Triticum aestivum L. and 150-153 and 155 showed antimicrobial activities against Staphylococcus aureus, Bacillus subtilis, Penicillium italicum, Fusarium graminearum, and Fusarium oxysporum (Hu et al., 2022c).
Phomactin diterpenes neocucurbols A-D (156-159) produced by marine fungus Neocucurbitaria unguis-hominis FS685 has a structurally complex polycyclic ring system, while neocucurbols E-H (160-163) has a tricyclic ring system (Cai et al., 2020). Aspergillus insuetus SD-512 collected cold seep sediments produced new ophiobolin sesterterpenoids: (6R)-16,17,21,21-O-tetrahydroophiobolin G (164), (6R)-16,17-dihydroophiobolin H (165), (5S, 6S)-16,17-dihydroophiobolin H (166), and three phthalide derivatives farnesylemefuranones D-F (167-169). Compounds 166 and 167-169 had a broad spectrum of antibacterial activity against a panel of pathogenic bacteria including Escherichia coli QDIO-1, Pseudomonas aeruginosa QDIO-2, Aeromonas hydrophilia QDIO-10, Edwardsiella tarda QDIO-8, Vibrio alginolyticus QDIO-7, V. anguillarum QDIO-9, V. parahemolyticus QDIO-5, and V. vulnificus QDIO-4 (Chi et al., 2020). Pyran-3,5-dione derivative, canescenin B (170), was isolated from Penicillium canescens SCSIO z053, and showed weak antibacterial and antibiofilm activities toward Bascillus amyloliquefaciens and Pseudomonas aeruginosa at 100 μM (Dasanayaka et al., 2020).
Punicesterones A-G (171-177) were discovered from Aspergillus puniceus SCSIO z021. 172 and 173 displayed antibacterial activity and the ability to reduce triglyceride production (Huang et al., 2022). A new steroidal compound, solitumergosterol A (178), was isolated from Penicillium solitum MCCC 3A00215; 178 exhibited anticancer activity against MB231 (He et al., 2021). A culture of Phomopsis tersa FS441 produced phosteoid A (179) (Chen et al., 2020a). A marine-derived fungus, Aspergillus unguis IV17-109, produced aspersterols A-D (180-183). Compound 180 showed growth inhibition against six cancer cell lines. Compounds 181-183 displayed anti-inflammatory activity as well (Cao et al., 2022). The steroid 7b,8b-epoxy-(22E,24R)-24-methylcholesta-4,22-diene-3,6-dione (184), was isolated from the fungus Aspergillus penicillioides SD-311. Compound 184 showed antibacterial activity against Vibrio anguillarum with an MIC value of 32 μg/mL (Chi et al., 2021b).
In summary, we reported 184 new compounds derived from 46 deep-sea-associated fungi during 2020-2022 (Table 1). The categories of the 184 new compounds discovered during the review period is shown in the following Figures 1, 2. In the past three years, researchers have discovered 80 polyketides, 68 terpenoids, and 36 Nitrogen-containing compounds (Figure 1). It can be hypothesized that the content of polyketides and terpenoids in the metabolites of deep-sea fungi is higher than that of Nitrogen-containing compounds, but it remains to be verified. According to the biological activity profile of these new compounds (Figure 1), only 70 new compounds have detected biological activity. Of the 70 active new compounds, 20 were associated with cytotoxicity. Almost half of the compounds have anti-infective activity. Meanwhile, 11 compounds have shown enzyme inhibitory activity, and a few have anti-inflammatory activity. These activities will lead the research culture to develop new drug leads for crucial diseases like cancer. Analysis from the environmental source of strains (Figure 2) suggested that mud was the main source of the deep-sea fungi, contributing 34 out of all 46 strains. Analysis of the microbial origin of these compounds found that Aspergillus fungi were the main contributors to these new compounds, contributing a total of 82 new compounds derived from 17 different strains. In terms of culture medium (Figure 3), 36 out of 46 strains were cultured using the rice medium and 10 strains using liquid culture medium. All of these media are rich in nutrients and differ significantly from the oligotrophic environment in the deep sea. No habitat-based cultivation strategies according to the deep-sea environmental factors, such as low oxygen and high pressure, were explored and applied to activate the silent gene cluster of deep-sea fungi, although these strategies have been successfully applied to other deep-sea microorganisms.
Figure 1 Structural and bioactive categories of deep-sea-associated fungi natural products (left: structures, right: bioactivities).
Figure 2 Source categories of deep-sea-associated fungi and their natural products (left: environmental samples, right: microbial origin).
In summary, these structurally novel active compounds reveal an astonishing unique secondary metabolic system of deep-sea fungi. However, deep-sea fungi are still a special resource with infinite potential of biologically active and structurally new compounds that has not been fully recognized, explored, and utilized. In the cultivation of deep-sea fungi, it is necessary to try to activate their silent gene clusters using the deep-sea habitat-simulating strategy, so as to further tap their secondary metabolic potential. This requires the joint efforts of researchers dedicating their efforts to deep-sea-derived fungi.
ZW drafted the manuscript. MQ reviewed and corrected the manuscript. CW, YW, and FK conceived, guided, and revised. QW revised the manuscript. All authors have read and agreed to the published version of the manuscript.
This work was supported by the Natural Science Foundation of Hainan Province (822QN488), Guangxi Natural Science Foundation under Grant (2021GXNSFBA075036), 2021 University-Level Scientific Research Projects of Guangxi Minzu University (2021 MDKJ003), the Talent Scientific Research Initiation Project of Guangxi Minzu University (2021KJQD09), the Guangxi Scholarship Fund of Guangxi Education Department, the Xiangsi Lake Youth Innovation Team Project of Guangxi Minzu University (2021RSCXSHQN01) and the Hainan Province Clinical Medical Center (QWYH202175).
The authors declare that the research was conducted in the absence of any commercial or financial relationships that could be construed as a potential conflict of interest.
All claims expressed in this article are solely those of the authors and do not necessarily represent those of their affiliated organizations, or those of the publisher, the editors and the reviewers. Any product that may be evaluated in this article, or claim that may be made by its manufacturer, is not guaranteed or endorsed by the publisher.
Almaliti J., Gerwick W. H. (2023). Methods in marine natural product drug discovery: what’s new? Expert Opin. Drug Discovery 18, 687–691. doi: 10.1080/17460441.2023.2214360
Ameri A. (2014). Marine microbial natural products. Jundishapur J. Natural Pharm. Prod. 9, e24716. doi: 10.17795/jjnpp-24716
Bhakuni D. S., Rawat D. S. (2005). Bioactive marine natural products (New Delhi, India: Anamaya Publishers).
Blayney D. W., Bazhenova L., Lloyd G. K., Huang L., Mohanlal R. (2016). Plinabulin, a novel small molecule that ameliorates chemotherapy-induced neutropenia, is administered on the same day of chemotherapy and has anticancer efficacy. Blood: Amer Soc. Hematol. 128, 2508–2508. doi: 10.1182/blood.V128.22.2508.2508
Cai C. L., Chen Y. Y., Zhou L., Gong N. Y., Zhang H., Sun C. L., et al. (2023). Antimicrobial polyketides from the marine-derived fungus spiromastix sp. SCSIO F190. J. Nat. Prod. 86, 589–595. doi: 10.1021/acs.jnatprod.2c00900
Cai S. Y., He J. L., Bai K. K., Niu S. W., Hong B. H. (2020). Secondary metabolites of deep-sea fungi and their biological activities: research advances. J. Int. Pharm. Res. 1674-0440, 12–1033-15. doi: 10.13220/j.cnki.jipr.2020.12.003
Cao V. A., Kwon J. H., Kang J. S., Lee H. S., Heo C. S., Shin H. J. (2022). Aspersterols a-d, ergostane-type sterols with an unusual unsaturated side chain from the deep-Sea-Derived fungus Aspergillus unguis. J. Nat. Prod. 85, 2177–2183. doi: 10.1021/acs.jnatprod.2c00398
Chen S. S., Chen Y. C., Li S. N., Liu H. X., Li D. L., Liu Z. M., et al. (2021b). Hawatides a-G, new polyketides from the deep-sea-derived fungus Paraconiothyrium hawaiiense FS482. Tetrahedron 93, 132303. doi: 10.1016/j.tet.2021.132303
Chen S. C., Liu Z. M., Chen Y. C., Tan H. B., Liu H. X., Zhang W. M. (2021a). Tersaphilones a-e, cytotoxic chlorinated azaphilones from the deep-sea-derived fungus Phomopsis tersa FS441. Tetrahedron 78, 131806. doi: 10.1016/j.tet.2020.131806
Chen S. C., Liu Z. M., Chen Y. C., Tan H. B., Zhu S., Liu H. X., et al. (2020a). Phosteoid a, a highly oxygenated norsteroid from the deep-sea-derived fungus Phomopsis tersa FS441. Tetrahedron Lett. 61, 151555. doi: 10.1016/j.tetlet.2019.151555
Chen S. S., Liu H. X., Li S. N., Chen Y. C., Ye W., Li H. H., et al. (2023). Hawanoids a-e, unprecedented diterpenoids with PAF-induced platelet aggregation inhibitory activities from the deep-sea-derived fungus Paraconiothyrium hawaiiense. Chin. Chem. Lett. 34, 107513. doi: 10.1016/j.cclet.2022.05.027
Chen S. C., Liu Z. M., Tan H. B., Chen Y. C., Li S. N., Li H. H., et al. (2020b). Phomeroids a and b: two novel cytotoxic meroterpenoids from the deep-sea-derived fungus Phomopsis tersa FS441. Organic Chem. Frontiers. 7, 557–562. doi: 10.1039/C9QO01365B
Chen S. C., Liu Z. M., Tan H. B., Chen Y. C., Zhu S., Liu H. X., et al. (2020c). Photeroids a and b, unique phenol-sesquiterpene meroterpenoids from the deep-sea-derived fungus Phomopsis tersa. Org. Biomol. Chem. 18, 642–645. doi: 10.1039/c9ob02625h
Chen S. S., Liu H. X., Ye W., Li S. N., Li D. L., Liu Z. M., et al. (2020d). Ochuscins a-G, highly oxygenated usnic acid derivatives from the deep-sea-derived fungus Ochroconis sp. FS449. Tetrahedron 76, 131066. doi: 10.1016/j.tet.2020.131066
Chen W. H., Zhang J. W., Qi X., Zhao K., Pang X. Y., Lin X. P., et al. (2021c). P-terphenyls as anti-HSV-1/2 agents from a deep-Sea-Derived penicillium sp. J. Nat. Prod. 84, 2822–2831. doi: 10.1021/acs.jnatprod.1c00400
Chi L. P., Li X. M., Wan Y. P., Li Y. H., Li X., Wang B. G. (2021a). Two new phenol derivatives from the cold seep-derived fungus Aspergillus insuetus SD-512. Chem. Biodivers. 18, e2100512. doi: 10.1002/cbdv.202100512
Chi L. P., Li X. M., Wan Y. P., Li X., Wang B. G. (2020). Ophiobolin sesterterpenoids and farnesylated phthalide derivatives from the deep Sea cold-Seep-Derived fungus Aspergillus insuetus SD-512. J. Nat. Prod. 83, 3652–3660. doi: 10.1021/acs.jnatprod.0c00860
Chi L. P., Yang S. Q., Li X. M., Li X. D., Wang B. G., Li X. (2021b). A new steroid with 7β, 8β-epoxidation from the deep sea-derived fungus Aspergillus penicillioides SD-311. J. Asian Nat. PROD Res. 23, 884–891. doi: 10.1080/10286020.2020.1791096
Dasanayaka S. A. H. K., Nong X. H., Liang X., Liang J. Q., Amin M., Qi S. H. (2020). New dibenzodioxocinone and pyran-3, 5-dione derivatives from the deep-sea-derived fungus Penicillium canescens SCSIO z053. J. Asian Nat. PROD Res. 22, 338–345. doi: 10.1080/10286020.2019.1575819
Debbab A., Aly A. H., Lin W. H., Proksch P. (2010). Bioactive compounds from marine bacteria and fungi. Microbial. Biotechnol. 3, 544–563. doi: 10.1111/j.1751-7915.2010.00179.x
Firmansyah K., Xiao L., Qi S. H. (2022). Bioassay-guided isolation of antifungal cyclopeptides from the deep-sea-derived fungus Simplicillium obclavatum EIODSF 020. Phytochem. Letters. 48, 1874–3900. doi: 10.1016/j.phytol.2022.01.017
Gerwick W. H., Moore B. S. (2012). Lessons from the past and charting the future of marine natural products drug discovery and chemical biology. Chem. Biol. 19, 85–98. doi: 10.1016/j.chembiol.2011.12.014
Gozari M., Alborz M., El-Seedi H. R., Jassbi A. R. (2020). Chemistry, biosynthesis, and biological activity of terpenoids and meroterpenoids in bacteria and fungi isolated from different marine habitats. Eur. J. Med. Chem. 210, 112957. doi: 10.1016/j.ejmech.2020.112957
Han W. R., Cai J., Zhong W. M., Xu G. M., Wang F. Z., Tian X. P., et al. (2020). Protein tyrosine phosphatase 1B (PTP1B) inhibitors from the deep-sea fungus Penicillium chrysogenum SCSIO 07007. Bioorg. Chem. 96, 103646. doi: 10.1016/j.bioorg.2020.103646
He Z. H., Xie C. L., Hao Y. J., Xu L., Wang C. F., Hu M. Y., et al. (2021). Solitumergosterol a, a unique 6/6/6/6/5 steroid from the deep-sea-derived Penicillium solitum MCCC 3A00215. Org. Biomol. Chem. 19, 9369–9372. doi: 10.1039/d1ob01392k
Hu X. Y., Li X. M., Wang B. G., Meng L. H. (2022b). Tanzawaic acid derivatives: fungal polyketides from the deep-Sea coral-derived endozoic Penicillium steckii AS-324. J. Nat. Prod. 85, 1398–1406. doi: 10.1021/acs.jnatprod.2c00211
Hu X. Y., Li X. M., Yang S. Q., Li X., Wang B. G., Meng L. H. (2023). Vercytochalasins a and b: two unprecedented biosynthetically related cytochalasins from the deep-sea-sourced endozoic fungus Curvularia verruculosa. Chin. Chem. Lett. 34, 107516. doi: 10.1016/j.cclet.2022.05.030
Hu X. Y., Wang C. Y., Li X. M., Yang S. Q., Li X., Wang B. G., et al. (2021). Cytochalasin derivatives from the endozoic Curvularia verruculosa CS-129, a fungus isolated from the deep-Sea squat lobster Shinkaia crosnieri living in the cold seep environment. J. Nat. Prod. 84, 3122–3130. doi: 10.1021/acs.jnatprod.1c00907
Hu J. H., Wang N. Y., Liu H. X., Li S. N., Liu Z. M., Zhang W. M., et al. (2022a). Secondary metabolites from a deep-sea derived fungal strain of Phomopsis lithocarpus FS508. Nat. Prod Res. 27, 1–8. doi: 10.1080/14786419.2022.2044810
Hu Z. B., Zhu Y. J., Chen J., Chen J. J., Li C. Y., Gao Z. Z., et al. (2022c). Sesquiterpenoids with phytotoxic and antifungal activities from a pathogenic fungus aspergillus alabamensis. J. Agric. Food Chem. 70, 12065–12073. doi: 10.1021/acs.jafc.2c05703
Huang Z. H., Liang X., Gu Q., Ma X., Qi S. H. (2022). Punicesterones a-G, polyhydroxylated mycoecdysteroids from the deep-sea-derived fungus Aspergillus puniceus SCSIO z021. Phytochemistry 205, 113511. doi: 10.1016/j.phytochem.2022.113511
Huang Z. H., Liang X., Qi S. H. (2020). A new iron(III) chelator of coprogen-type siderophore from the deep-sea-derived fungus Mycosphaerella sp. SCSIO z059. Chin. J. Nat. Med. 18, 243–249. doi: 10.1016/S1875-5364(20)30029-7
Jensen P. R., Fenical W. (1994). Strategies for the discovery of secondary metabolites from marine bacteria: ecological perspective. Annu. Rev. Microbiol. 48, 559–584. doi: 10.1146/annurev.mi.48.100194.003015
Jimenez J. T., Sturdikova M., Sturdik E. (2009). Natural products of marine origin and their perspective in the discovery of new anticancer drugs. Acta Chimica Slovaca. 2, 63–74.
Kobayashi S., Yuki R. (1954). On the specificity of kidney catalase activity in salmonid fishes. Bull. Faculty Fish. Hokkaido University 5, 137–148.
Lee H. S., Kang J. S., Cho D. Y., Choi D. K., Shin H. J. (2022). Isolation, structure determination, and semisynthesis of diphenazine compounds from a deep-Sea-Derived strain of the fungus Cystobasidium laryngis and their biological activities. J. Nat. Prod. 85, 857–865. doi: 10.1021/acs.jnatprod.1c00985
Li H. H., Du X. Y., Chen C. Y., Qi J. F., Wang Y. (2021). Current research on natural products and biological activities of Aspergillus fungi from marine sources. Pharm. Inform. 10, 92. doi: 10.12677/pi.2021.103013
Li Q., Xu W., Fan R. Z., Zhang J., Li Y. L., Wang X. W., et al. (2020). Penithoketone and penithochromones a-l, polyketides from the deep-Sea-Derived fungus Penicillium thomii YPGA3. J. Nat. Prod. 83, 2679–2685. doi: 10.1021/acs.jnatprod.0c00571
Li Y. H., Yang S. Q., Li X. M., Li X., Wang B. G., Li H. L. (2022). Cyclopiumolides a and b, unusual 13-membered macrolides from the deep sea-sourced fungus Penicillium cyclopium SD-413 with antiproliferative activities. Bioorg. Chem. 128, 106104. doi: 10.1016/j.bioorg.2022.106104
Li Y. H., Yang S. Q., Li X. M., Li X., Wang B. G., Li H. L. (2023). Five new verrucosidin derivatives from Penicillium polonicum, deep-sea cold-seep sediment isolated fungus. Fitoterapia 165, 105387. doi: 10.1016/j.fitote.2022.105387
Liao M. L., Zhang S., Zhang G. Y., Chu Y. M., Somero G. N., Dong Y. w. (2017). Heat-resistant cytosolic malate dehydrogenases (cMDHs) of thermophilic intertidal snails (genusEchinolittorina): protein underpinnings of tolerance to body temperatures reaching 55°C. J. Exp. Biol. 220, 2066–2075. doi: 10.1242/jeb.156935
Liu Z. M., Chen Y. C., Li S. N., Hu C. Y., Liu H. X., Zhang W. M. (2022b). Indole diketopiperazine alkaloids from the deep-sea-derived fungus aspergillus sp. FS445. Nat. Prod Res. 36, 5213–5221. doi: 10.1080/14786419.2021.1925271
Liu Z. M., Li S. N., Chen Y. C., Li M. Q., Liu H. X., Zhang W. M. (2021). Cytotoxic polyketides from the deep-sea-derived fungus Aspergillus fischeri FS452. Nat. Prod Res. 36, 5701–5707. doi: 10.1080/14786419.2021.2015595
Liu C. M., Liang X., Yao F. H., Qi S. (2022a). Five new aromatic polyketides and isoquinoline alkaloids from the deep-sea-derived fungus Aspergillus puniceus SCSIO z021. Tetrahedron 126, 133067. doi: 10.1016/j.tet.2022.133067
Liu H. B., Liu Z. M., Li H. H., Tan H. B., Zhang Q. B., Li D. L., et al. (2020). Lithocaldehydes a and b, polyketides from the deep sea-derived fungus Phomopsis lithocarpus FS508. Org. Biomol. Chem. 18, 7326–7329. doi: 10.1039/d0ob01674h
Marmann A., Aly A. H., Lin W., Wang B., Proksch P. (2014). Co-Cultivation: a powerful emerging tool for enhancing the chemical diversity of microorganisms. Mar. Drugs 12, 1043–1065. doi: 10.3390/md12021043
Niu S. W., Chen Z. M., Pei S. X., Shao Z. Z., Zhang G. Y., Hong B. H. (2022). Acremolin d, a new acremolin alkaloid from the deep-sea sediment-derived Aspergillus sydowii fungus. Nat. Prod Res. 36, 4936–4942. doi: 10.1080/14786419.2021.1913587
Niu S. W., Yang L. H., Zhang G. Y., Chen T. T., Hong B. H., Pei S. X., et al. (2020). Phenolic bisabolane and cuparene sesquiterpenoids with anti-inflammatory activities from the deep-sea-derived Aspergillus sydowii MCCC 3A00324 fungus. Bioorg. Chem. 105, 104420. doi: 10.1016/j.bioorg.2020.104420
Pang X. Y., Lin X. P., Zhou X. F., Yang B., Tian X. P., Wang J. F., et al. (2020). New quinoline alkaloid and bisabolane-type sesquiterpenoid derivatives from the deep-sea-derived fungus aspergillus sp. SCSIO06786. Fitoterapia 140, 104406. doi: 10.1016/j.fitote.2019.104406
Pang X. Y., Wang P., Liao S. R., Zhou X. F., Lin X. P., Yang B., et al. (2022). Three unusual hybrid sorbicillinoids with anti-inflammatory activities from the deep-sea derived fungus penicillium sp. SCSIO06868. Phytochemistry 202, 113311. doi: 10.1016/j.phytochem.2022.113311
Skinner R. H. (1982). The interrelation of water quality, external parasites and gill pathology of some fishes from Biscayne Bay, Florida. Fishery Bulletin. 80. doi: 10.1577/1548-8446(1982)007<0020:EVOLMF>2.0.CO;2
Song Y. Y., Song M. M., Chen W. H., Pang X. Y., Wang F. Z., Tian X. P., et al. (2022). One new furanone analog from the deep-sea fungus purpureocillium sp. SCSIO 06693. Nat. Prod Res. 18, 1–7. doi: 10.1080/14786419.2022.2089671
Subramani R., Sipkema D. (2019). Marine rare actinomycetes: a promising source of structurally diverse and unique novel natural products. Mar. Drugs 17, 249. doi: 10.3390/md17050249
Wang W. Y., Yang J., Liao Y. Y., Cheng G., Chen J., Mo S. W., et al. (2020). Aspeterreurone a, a cytotoxic dihydrobenzofuran-phenyl acrylate hybrid from the deep-Sea-Derived fungus Aspergillus terreus CC-S06-18. J. Nat. Prod. 83, 1998–2003. doi: 10.1021/acs.jnatprod.0c00189
Wu J., Wang F. Q., He L. M., Zhou S. Y., Wang S. B., Jia J., et al. (2022a). Aculeaquamide a, cytotoxic paraherquamide from the marine fungus Aspergillus aculeatinus WHUF0198. Nat. Prod Res. 36, 4388–4393. doi: 10.1080/14786419.2021.1998047
Wu Y. Z., Zhang H. M., An F. F., Zheng Y. B. (2022b). Research progress on secondary metabolites of aspergillus versicolor from different habitats. Natural Product Res. Dev. 35, 342–352. doi: 10.16333/j.1001-6880.2023.2.018
Xu W. F., Chao R., Hai Y., Guo Y. Y., Wei M. Y., Wang C. Y., et al. (2021). 17-hydroxybrevianamide n and its N1-methyl derivative, quinazolinones from a soft-Coral-Derived aspergillus sp. fungus: 13S enantiomers as the true natural products. J. Nat. Prod. 84, 1353–1358. doi: 10.1021/acs.jnatprod.1c00098
Yan L. H., Li P. H., Li X. M., Yang S. Q., Liu K. C., Wang B. G., et al. (2022). Chevalinulins a and b, proangiogenic alkaloids with a Spiro[bicyclo[2.2.2]octane-diketopiperazine] skeleton from deep-Sea cold-Seep-Derived fungus Aspergillus chevalieri CS-122. Org. Lett. 24, 2684–2688. doi: 10.1021/acs.orglett.2c00781
Yin Q. Z., Liu X. W., Zhang Z. J., Lei H. X., Wu B. (2023). Chemistry and bioactivities of alkaloids isolated from marine fungi (covering 2016-2022). Fitoterapia 164, 105377. doi: 10.1016/j.fitote.2022.105377
Zhang J., Chen Y. C., Liu Z. M., Guo B. C., Gao X. X., Liu H. X., et al. (2020). Cytotoxic secondary metabolites from a Sea-derived fungal strain of Hypoxylon rubiginosum FS521. Chin. J. Org. Chem. 40, 1367. doi: 10.6023/cjoc201912012
Zhang Y. Y., Li M. R., Zhang Q. Q., Wang Z. Y., Li X. X., Bao J., et al. (2021). Arthpyrone l, a new pyridone alkaloid from a deep-Sea arthrinium sp., inhibits proliferation of MG63 osteosarcoma cells by inducing G0/G1 arrest and apoptosis. Chem. Biodivers., 18, e2000639. doi: 10.1002/cbdv.202000639
Zhong T. H., Zeng X. M., Feng S. B., Zhang H. T., Zhang Y. H., Luo Z. H., et al. (2022). Three new phomalone derivatives from a deep-sea-derived fungus alternaria sp. MCCC 3A00467. Nat. Prod Res. 36, 414–418. doi: 10.1080/14786419.2020.1771706
Keywords: deep-sea fungi, chemical structures, marine natural products, new compounds, bioactivities
Citation: Wang Z, Qader M, Wang Y, Kong F, Wang Q and Wang C (2023) Progress in the discovery of new bioactive substances from deep-sea associated fungi during 2020-2022. Front. Mar. Sci. 10:1232891. doi: 10.3389/fmars.2023.1232891
Received: 01 June 2023; Accepted: 21 June 2023;
Published: 07 July 2023.
Edited by:
Dong-Lin Zhao, Chinese Academy of Agricultural Sciences, ChinaCopyright © 2023 Wang, Qader, Wang, Kong, Wang and Wang. This is an open-access article distributed under the terms of the Creative Commons Attribution License (CC BY). The use, distribution or reproduction in other forums is permitted, provided the original author(s) and the copyright owner(s) are credited and that the original publication in this journal is cited, in accordance with accepted academic practice. No use, distribution or reproduction is permitted which does not comply with these terms.
*Correspondence: Fandong Kong, a29uZ2ZhbmRvbmcwMTI3QDEyNi5jb20=; Cong Wang, d2FuZ2NvbmcxMjMyMDZAMTYzLmNvbQ==; Qi Wang, d3FpMTIyMEAxNjMuY29t
Disclaimer: All claims expressed in this article are solely those of the authors and do not necessarily represent those of their affiliated organizations, or those of the publisher, the editors and the reviewers. Any product that may be evaluated in this article or claim that may be made by its manufacturer is not guaranteed or endorsed by the publisher.
Research integrity at Frontiers
Learn more about the work of our research integrity team to safeguard the quality of each article we publish.