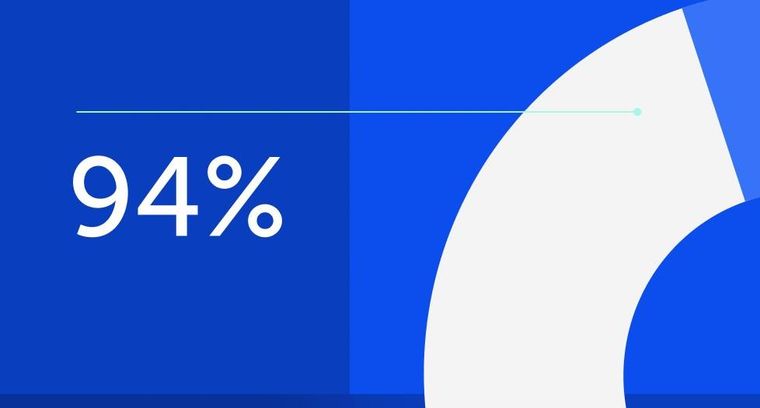
94% of researchers rate our articles as excellent or good
Learn more about the work of our research integrity team to safeguard the quality of each article we publish.
Find out more
ORIGINAL RESEARCH article
Front. Mar. Sci., 13 October 2023
Sec. Marine Megafauna
Volume 10 - 2023 | https://doi.org/10.3389/fmars.2023.1231337
The Australian fur seal (Arctocephalus pusillus doriferus, AUFS) population is still recovering from the over-exploitation of the commercial-sealing era (18th and 19th centuries). While the population is considered to be only < 47% of its pre-harvest size, it now represents the greatest resident marine predator biomass in the south-eastern Australian marine ecosystem. The region is experiencing rapid environmental change and, as a keystone predator species, the AUFS is an indicator of ecosystem health. In the present study, the body mass, standard length and body condition index (BCI) were analysed between 1997-2021 in adult female AUFS provisioning pups on Kanowna Island (northern Bass Strait), the third largest colony for the species. While substantial inter-annual fluctuations were observed, there was no temporal trend in standard length during the 23-year study period. In contrast, body mass and, consequently, BCI decreased significantly, suggesting the population is experiencing changing nutritional conditions. While these changes do not appear to be due to competition with commercial fisheries or population expansion, weak but significant negative relationships were observed between BCI and 1-year lagged sea surface temperature and summer zonal winds in the Bonney Upwelling region, and both current- and 2-year lagged Indian Ocean Dipole (IOD). These findings suggest the BCI of AUFS may continue to decline under predicted climate change conditions. While a lack of a concurrent decline in pup production could indicate a degree of nutritional tolerance or flexibility in energy allocation, further monitoring is required to assess decreases in reproductive parameters (e.g., birth mass, pre-weaning growth rates) or vital rates, which would be expected with continued nutritional stress.
In order to survive, animals must have sufficient nutritional resources for maintenance metabolism and behavioural activity, with resources in excess being available for growth, reproduction and storage (Karasov, 1992). Animals primarily store body reserves in the form of adipose tissue, a lipid-dense, easily accessible, physiological source of energy (Lindstedt and Boyce, 1985). Correspondingly, when nutritional intake is restricted, animals may draw on their adipose stores and shift to lipid catabolism for metabolic energy (Melvin and Andrews, 2009). In species facing regular periods of food scarcity or fasting, adipose stores play an essential role in survival and may also be the catalyst for successful reproduction (Testa and Adams, 1998; Rödel et al., 2016). In mammals, adipose stores are closely linked to lactation strategies, enabling mothers to spatially and temporally separate foraging and nursing activities (Oftedal, 2000). Consequently, the extent of adipose stores in female mammals can influence offspring growth and survival in addition to their own future reproductive output and survival (Thayer et al., 2020). Therefore, knowledge of factors affecting adipose stores and their dynamics in mammals can provide insights into sources of variation in reproductive success and survivorship (Wade and Schneider, 1992; Birsoy et al., 2013).
Measuring adipose stores in free-ranging animals can be logistically challenging, especially for large, cryptic species. Methods for obtaining measures of body adipose reserves include direct destructive estimates of body lipid mass (Hewison et al., 1996; Garroway and Broders, 2005) and indirect non-destructive approaches such as hydrogen isotope dilution (Reilly and Fedak, 1990; Arnould and Ramsay, 1994), bio-impedance (Hwang et al., 2005; Pitt et al., 2006) and body condition indices (Marker and Dickman, 2003; Castelblanco-Martínez et al., 2021). Body condition indices have been used widely due to their relative simplicity, combining measures of body mass and structural size (generally body mass scaled to a body dimension, such as length) (Schulte-Hostedde et al., 2005; Castelblanco-Martínez et al., 2021). Such methods are commonly used as these measurements can be obtained from live-captured animals using non-invasive techniques (Cattet et al., 2002; Stevenson and Woods, 2006) and provide a reliable index of body condition (reflective of nutritional status) within a population (Schulte-Hostedde et al., 2005).
Pinnipeds (seals, fur seals, sea lions and walruses) are large, long-lived marine mammals with high annual survival and low lifetime reproductive success (K-strategists (Pianka, 1970)) subject to the high temporal and spatial variability of the oceanic environment (Constable et al., 2003). Such variation can alter the abundance, distribution and quality of prey species (Parmesan, 2006; Thomas, 2010). Correspondingly, as their foraging range is largely limited by access to prey resources near suitable land or ice sites for mating and parturition (Estes, 1979; Boness et al., 2002; Younger et al., 2016), pinnipeds can experience substantial fluctuations in foraging conditions which impact their energy budgets. In particular, female otariid seals (fur seals and sea lions), which as income breeders adopt a central place foraging strategy during pup-rearing, are restricted in the distance they can travel in search of food by the fasting duration of their young (Boness and Bowen, 1996). Therefore, reductions in local prey resources can impact the condition and survivorship of nursing females (Houston et al., 2006), the offspring they are provisioning (Soto et al., 2006) and the viability of the foetus they are gestating (Soto et al., 2004).
The Australian fur seal (Arctocephalus pusillus doriferus; AUFS) is the largest of the fur seal species, with average adult female and male body masses of 76 kg and 279 kg, respectively (Warneke and Shaughnessy, 1985). It has a breeding distribution largely restricted to offshore islands within Bass Strait (Kirkman and Arnould, 2018), where it is still recovering from the over-exploitation of the commercial sealing era (c. 1792-1825 (Gill, 1967; Arnould et al., 2003). With a currently estimated population of 85,500 – 120,000 individuals (c. 28-47% of its pre-sealing level (McIntosh et al., 2018)), the species represents the largest resident marine predator biomass in south-eastern Australia (Kirkwood et al., 2010).
Bass Strait, a region of low marine primary productivity (Gibbs et al., 1986; Chambers et al., 2015), is influenced by numerous interacting oceanographic currents. Nutrient-poor warm water is delivered by the East Australian Current (EAC) in the east and the South Australian Current (SAC) in the west which mixes with cold Sub-Antarctic Surface Water (SASW) from the south (Newell, 1961; Sandery and Kämpf, 2007). In addition, the seasonal Bonney Upwelling brings cold, nutrient-rich waters from the western region in the summer months (Sandery and Kämpf, 2005). Previous studies have documented the influence of these oceanographic features on the foraging ecology and reproductive biology on a range of marine predators in Bass Strait (Afán et al., 2015; Berlincourt and Arnould, 2015; Rodríguez-Malagón et al., 2021). In addition, south-eastern Australia is considered one of the fastest warming oceanic regions (Hobday and Pecl, 2014), with expected changes to oceanographic currents already leading to changes in the abundance, diversity and distribution of marine prey species (Gervais et al., 2021).
While environmental influences on Australian fur seal foraging behaviour, habitat use and diet have previously been documented (Speakman et al., 2020; Speakman et al., 2021; Geeson et al., 2022; Kliska et al., 2022; McIntosh et al., 2022), little is known of factors impacting body condition over the long term in the species (Arnould and Warneke, 2002; Wall et al., 2023). Such information is crucial for predicting how the species, and the ecosystem they dominate, may respond to future environmental change. The aims of present study, therefore, were to investigate: 1) inter-annual variability in; and 2) potential environmental influences on body condition in adult female Australian fur seals.
The study was conducted on Kanowna Island (39°09’S, 146°18’E), central northern Bass Strait, in south-eastern Australia (Figure 1). The tussock-covered granite island (32.7 ha) hosts the third largest breeding colony of AUFS, with an annual pup production of c. 2400-3400 and approximately 15,000 individuals (Kirkwood et al., 2010; McIntosh et al., 2018; Geeson et al., 2022). The AUFS on Kanowna Island have been the focus of a long-term research program investigating the physiology, demography, reproductive biology and foraging ecology of the species (Arnould and Hindell, 2001; Arnould and Warneke, 2002; Gibbens and Arnould, 2009b; Speakman et al., 2020; Geeson et al., 2022). Data in the present study were collected as part of this ongoing long-term research program.
Figure 1 Location of the Kanowna Island breeding colony study site (●) within south-eastern Australia and the influencing major water bodies of Bass Strait and the Bonney Upwelling region. EAC: East Australian Current; SAC: South Australian Current; SASW: Sub-Antarctic Surface Waters. Inset map shows the positioning of the region relative to Australia. The shaded areas of the Bonney Upwelling and Bass Strait indicate the region from which the local environmental parameters were retrieved.
Sampling occurred between late-April and early-September (mostly May-July), corresponding to the period of peak nutritional demand for lactating females (Arnould and Hindell, 2001) each year from 1998-2021. Individuals from the main breeding colony on the island, which accounts for 65% of all pup production (Lourie et al., 2014), were the focus of the study. Adult females nursing a pup were selected at random and captured using a modified hoop-net before being sedated and/or anaesthetised for safe handling (Hoskins et al., 2015). Individuals were then transferred to a flat board for weighing, using a digital spring scale (± 0.1 kg), and measured for standard length (Mammals, A. S. O. M. C. O. M. 1967) using a metal tape measure (± 0.5cm). From these measurements, a body condition index (BCI) was calculated for each individual as the residual value from the power function regression of mass against length of all individuals sampled (Arnould and Warneke, 2002; Gibbens and Arnould, 2009a).
To investigate the effect of environment on BCI, standardised monthly means of local environmental parameters in central Bass Strait during May-July (winter), which corresponds to the period of peak milk yield in lactating females (Arnould and Hindell, 1999), and for the preceding summer upwelling period (January-March) in the Bonney Upwelling region (Figure 1) were used. The seasonally-active Bonney Upwelling intrudes nutrient-rich waters into Bass Strait and is a major driver of primary productivity for the region (Middleton and Bye, 2007). Seasonal means of sea surface temperature (SST) °C, zonal wind components m·s−1, and chlorophyll-a (Chl-a) mg·m−3 concentration were extracted at a 4-9 km resolution and analysed. Monthly means of SST were derived from CSIRO 3-d composite for the years 1998-2008 (http://www.marine.csiro.au/remotesensing) and from RAMSSA for the years 2009-2021 (Beggs et al., 2011). Monthly means of Chl-a were derived from SeaWiFS and MODIS NASA satellite-based ocean colour imagery for the years 1998-2010 and 2011-2021, respectively (NASA Goddard Space Flight Center, O. E. L., Ocean Biology Processing Group, 2018a; NASA Goddard Space Flight Center, O. E. L., Ocean Biology Processing Group, 2018b). In the study region, the values for the overlapping period are comparable when these reprocessed products are used. Zonal wind components were extracted as 6 hourly readings from the NCEP Reanalysis 2 dataset (http://ncdc.noaa.gov). The mean seasonal (summer and winter) zonal wind components were then calculated from these 6 hourly readings. These variables were selected as they have been shown to influence the foraging ecology, breeding biology and prey availability of AUFS (Kirkwood et al., 2008; Gibbens and Arnould, 2009b; Hoskins and Arnould, 2014; Speakman et al., 2020) and other marine predators (Ropert-Coudert et al., 2009; Berlincourt and Arnould, 2015) within Bass Strait.
The broad-scale environmental indices of the Indian Ocean Dipole (IOD) (Saji et al., 1999), the Southern Annular Mode (SAM) (Lovenduski and Gruber, 2005) and the Southern Oscillation Index (SOI) (Middleton et al., 2007) have been observed to influence the marine ecosystem of south-eastern Australia, including the foraging and reproductive ecologies of locally breeding predators (Bunce et al., 2002; Kliska, 2016; Speakman et al., 2021; Geeson et al., 2022). Correspondingly, relationships between the annual means of these indices and BCI were investigated. Monthly values for the broad-scale environmental indices were obtained from the National Oceanic and Atmospheric Administration (https://psl.noaa.gov) to generate annual calendar year mean values.
Local environmental parameters and broad-scale environmental indices were both analysed separately as conditions on a local-scale can be affected by broad-scale environmental indices (Cadby et al., 2010), and independent analyses allows the influences of various environmental parameters to be better disentangled while also reducing over-parametrisation of statistical models. Lagged influences of environmental responses have been previously reported as having significant effects on AUFS (Hoskins and Arnould, 2014; Speakman et al., 2020; Speakman et al., 2021; Geeson et al., 2022). These lagged effects have been suggested to occur as a result of changes in the recruitment or growth periods of prey species, causing a delayed influence on marine food chains (Poloczanska et al., 2013). Correspondingly, 1- and 2-year lagged conditions for both seasonal local variables and annual broad-scale indices were included in the analyses.
All statistical analysis were conducted in the R statistical environment (version 4.2.1, R Core Team (2022)). Tests for normality and data exploration were conducted using methods described by Zuur et al. (2010). Pearson correlation coefficients were used before analysis of environmental parameters and indices to determine correlations between predictor variables independently at each scale using the package corrplot version 0.84 (Wei et al., 2017). Where a pair of predictor variables had a strong correlation, defined as r ≥ 0.7 (Overholser and Sowinski, 2008), one variable from that pair was excluded from the candidate set. The variable to be excluded prior to analysis was selected based on which of the pair would exclude the lowest number of variables from the candidate set.
The relationships between selected dependent variables and BCI were investigated using linear models with the package nlme version 3.1-158 (Pinheiro et al., 2020). Further model selection was completed using Akaike’s Information Criterion, corrected for small sample sizes (AICc) (Burnham and Anderson, 2004). For multiple candidate models with a ΔAICc < 4 identified, a multi-model inference framework with model averaging was adopted to best describe the relationship between variables (Grueber et al., 2011; Symonds and Moussalli, 2011) using the package MuMIn version 1.46.0 (Barton, 2015). An adjusted r2 value was calculated to assess the model fit and, in addition, 95% confidence intervals were used to measure the significance of each variable. Unless otherwise stated, data are presented as mean ± SE.
Matched body mass and standard length data were obtained from a total of 482 adult female AUFS between 1998 and 2021. Throughout the study, average body mass was 76.3 ± 0.6 kg (range: 44.5 kg - 120.5). However, there was a negative significant temporal trend (r2 = 0.5, P < 0.001; Figure 2A), with annual mean body mass decreasing from 84.5 ± 2.7 kg in 1998 to 69.9 ± 3.7 kg in 2021. In contrast, there was substantial interannual variability in standard length, with high and low annual mean values generally corresponding to those of body mass (Figure 2B). However, there was no significant temporal trend in standard length (r2 = 0.01, P > 0.5) over the study period, with overall mean standard length being 151.8 ± 0.4 cm (range: 123.0– 174.0).
Figure 2 The relationships between the body mass (A), standard length (B), and body condition index (BCI) (C) of adult female Australian fur seals and year as linear models with 95% confidence intervals. For visual clarity only the annual means are presented, sample sizes for each year are provided in parentheses.
The relationship between body mass and standard length followed a power curve with the equation y = 0.0005·x2.4 (r2 = 0.7). The BCI calculated from the residuals of this relationship varied substantially (range: -22.6 to 41.1). There was a significant negative temporal trend in annual means of BCI throughout the study period (r2 = 0.7, P < 0.001), with annual means decreasing from 9.9 ± 1.8 in 1998 to -5.7 ± 1.8 in 2021 (Figure 2C). To account for this decrease when investigating the influence of environmental variables, a temporal linear regression of BCI was conducted and the residual value of each measurement was then used as a de-trended body condition index.
Analysis of local-scale variables on BCI was limited to data collected between 2000-2021 as lagged Chl-a values were not available for 1998-99. Local-scale variable selection included Chl-a, SST, and zonal wind (wind-u) (Table 1A). As there were multiple candidate models following AIC selection, model averaging was conducted (Burnham and Anderson, 2004). The results (Table 2A) indicated consistent negative influences on BCI of 1-year lagged SST (SSTsummerBULag1; Figure 3A) and zonal winds (Wind-usummerBULag1; Figure 3B) in the Bonney Upwelling region during summer.
Table 1 Candidate models, in descending order, to explain the relationship between fluctuations in female Australian fur seal body condition index (BCI) and interannual variation in local environmental parameters (A) and broad-scale environmental indices (B).
Table 2 The 95% Confidence Intervals for the parameter estimates of local environmental parameters (A) and broad-scale environmental indices (B) in their relationship with female Australian fur seal Body Condition Index (BCI) following model averaging.
Figure 3 The relationships between the body condition index (BCI) of adult female Australian fur seals at Kanowna Island and 1-year lagged summer SST (A) and 1-year lagged summer zonal wind (B) in the Bonney Upwelling region 2000-2021, current year (C) and 2-year lagged (D) Indian Ocean Dipole 1998-2021 and current year Southern Annular Mode 1998-2021 (E) as linear models with 95% confidence intervals.
Broad-scale environmental indices selected for analysis included the IOD, SAM, and SOI and both 1- and 2- year lagged values for each (Table 3). As with the seasonal local environmental variables, there were numerous candidate models with very low weight explaining the variation in BCI (Table 1B). Model averaging showed a consistent negative influence of the IOD (Figure 3E) and 2-year lagged IOD (Figure 3D) on BCI. In addition, the SAM (Figure 3E) was found to positively influence BCI.
Table 3 Local environmental parameters and broad-scale environmental indices used in the analysis to investigate the environmental influences on body condition in female Australian fur seal body condition index (BCI).
Analysis of the influence of local-scale variables on detrended BCI followed the same variable selection and timescale as for BCI. Multiple candidate models were derived for detrended BCI (Table 4A) and so model averaging was conducted (Table 5A). The results indicated a consistently positive influence of 1-year lagged sea-surface chlorophyll-a concentration during winter in Bass Strait on detrended BCI (Figure 4A). No influence of other local-scale environmental parameters was detected.
Table 4 Candidate models, in descending order, to explain the relationship between fluctuations in detrended adult female Australian fur seal body condition index (BCIdetrended) and interannual variation in local environmental parameters (A) and broad-scale environmental indices (B).
Table 5 The 95% Confidence Intervals for the parameter estimates of local environmental parameters (A) and broad-scale environmental indices (B) in their relationship with detrended adult female Australian fur seal body condition index (BCIdetrended) following model averaging.
Figure 4 Candidate models, in descending order, to explain the relationships between the detrended body condition index (BCIdetrended) of adult female Australian fur seals at Kanowna Island and 1-year lagged winter Chl-a from the Bass Strait region 2000-2021 (A), current year Southern Annular Mode 1998-2021 (B) and 1-year lagged Indian Ocean Dipole 1998-2021 (C) as linear models with 95% confidence intervals.
Similar to patterns observed with broad-scale environmental indices on BCI, there were multiple candidate models of low weight for relationships with detrended BCI (Table 4B). Model averaging (Table 5B) revealed 1-year lagged IOD and SAM consistently had a positive influence on detrended BCI (Figures 4B, C).
The waters of south-eastern Australia, including Bass Strait, are some of the fastest warming in the world (Hobday and Pecl, 2014). Anticipated oceanographic changes are already leading to alterations in the diversity, abundance and distribution of marine prey in the region (Gervais et al., 2021) and this is expected to continue for the century (Hobday et al., 2015). Knowledge of the potential impacts of these changes on marine predators is crucial for predicting their population responses. The results of the present study revealed inter-annual variation in body mass, standard length, and BCI in female AUFS from Kanowna Island, northern Bass Strait. In addition to a long-term decline in body mass and condition, linkages between BCI and both local-scale environmental variables and broad-scale indices were detected. While annual pup production has not decreased during this period (Geeson et al., 2022), a consistent decrease in body condition can be the first indicator that a population may experience a decline (Trites and Bigg, 1992; Trites and Donnelly, 2003; Wilcox et al., 2018).
Throughout the study period, inter-annual fluctuations in body mass broadly corresponded to those in standard length. This is consistent with body mass being greatly influenced by structural size in mammals (Gingerich, 1990; Trites and Bigg, 1996; Christiansen et al., 2019). While these fluctuations could be an artefact of small sample sizes in some years, this seems unlikely as sampling methods have remained consistent throughout the study period, there were no apparent trends between sample size and mean length or mass, and the fluctuations appeared broadly cyclical. An alternative explanation is that these fluctuations reflect changes in the population age-structure of breeding females.
As the skeletal size of female otariids increases after attainment of sexual maturity (Arnould and Hindell, 2002; McKenzie et al., 2007), albeit at a reduced rate, a greater proportion of older-aged individuals in the breeding population would be expected to result in greater average body lengths (Arnould and Warneke, 2002; Sweeney et al., 2015). Conversely, an increase in the recruitment of female juveniles into the proportion of the population provisioning pups could function to reduce the mean body size of animals available to be sampled (Caughley, 1966). Interestingly, the shorter body lengths observed between 2012-14 occurred 3-5 years after high annual pup production at the study colony (Geeson et al., 2022), which corresponds to when female Australian fur seals would commence breeding (Gibbens and Arnould, 2009b). Indeed, with inter-annual variations in pup production of up to 40% being recorded at the study colony (Geeson et al., 2022), and length at sexual maturity generally being 87% of asymptotic body length in pinnipeds (Laws, 1956), fluctuations in recruitment could have a substantial impact on mean adult body lengths.
In addition to the inter-annual fluctuations, there was an overall gradual ~17% decline in body mass during the 21-year study period (approximately -0.8% per year). While standard length remained relatively constant, the observed decrease in body mass has resulted in a significant decrease in body condition over the last two decades. Few studies of large mammals have documented significant declines in body mass over similar time frames, with most suggesting climate change-induced alterations to food availability to be the main cause (Calkins et al., 1998; Rode et al., 2010; Harwood et al., 2012; Mason et al., 2014; Boveng et al., 2020; Bush et al., 2020; Vermeulen et al., 2023). A decline in body mass and condition is the first indicator of sustained nutritional stress experienced by populations (Wilcox et al., 2018) and can portend negative effects on their reproductive output and trajectory (Trites and Bigg, 1992; Trites and Donnelly, 2003).
The results of the present study revealed weak relationships between female AUFS BCI and local-scale environmental variables. Lower BCI values were associated with warmer 1-year lagged sea surface temperatures and greater zonal U winds (weaker easterly winds) during summer in the Bonney Upwelling region. Lags in environmental effects have been previously observed on the foraging ecology of AUFS and has been suggested to reflect their influence on the recruitment of key prey species (Hoskins and Arnould, 2014; Speakman et al., 2020).
The long-term decline in BCI observed in the present study coincides with a general increase in summer SST in the Bonney Upwelling area over the last two decades (Beggs et al., 2011; Wijffels et al., 2018). This is consistent with the documented rapid oceanic warming in the south-eastern Australia region (Hobday and Pecl, 2014). Similarly, while zonal winds in the Bonney Upwelling region have not consistently increased throughout the study period, they have experienced two periods of gradual increase (1999-2006 and 2007-2019; NCEP Reanalysis 2 dataset (http://ncdc.noaa.gov)) over the period of observed decrease in adult female BCI. In addition, the seasonally active (summer) Bonney Upwelling has been shown to significantly influence the productivity of the region (Gibbs et al., 1986) and this upwelling is driven by south-easterly winds bringing cold, nutrient-rich waters to the surface through Ekman transport (leading to lower SST) which then cascade into Bass Strait with the eastward flow of the South Australian Current (Nieblas et al., 2009). Thus, in years of stronger south-easterly winds (reduced zonal U), greater marine productivity enters the region. A similar influence of increased easterly winds has been observed on pup production in AUFS at Kanowna Island, with weaker summer zonal winds in the Bonney Upwelling region leading to increased pup numbers in the following year (Geeson et al., 2022).
Weak but consistent relationships were also observed between adult female BCI and broad-scale environmental indices. Negative relationships were observed between BCI and both current-year and 2-year lagged IOD. The long-term decline in the BCI of adult female AUFS reported in the present study, therefore, is consistent with the IOD trending positive over the last few decades (Cai et al., 2021). However, the linkages between IOD and the factors influencing local prey availability in Bass Strait are not known, such that it is not possible to propose a causal relationship to AUFS body condition. Paradoxically, previous studies have found positive relationships between adult female AUFS foraging success (measured as the proportion of benthic dives during foraging trips that were successful) and current-year and 2-year lagged IOD (Speakman et al., 2020). These findings could indicate that adult female AUFS experience periods with a high abundance of low-quality prey which do not meet their nutritional needs, as has been suggested for other pinniped populations (Trites and Donnelly, 2003; Adame et al., 2020).
A weak positive influence of the SAM on adult female BCI values was also observed. As the SAM index has been trending to more positive phases during the current study (Fogt and Marshall, 2020), this relationship would appear contrary to the observed general decline in BCI of adult female AUFS. However, while BCI decreased significantly during the study period, the overall trend was not constant and there were phases of increasing condition which may account for the observed weak positive relationship with the SAM. Indeed, the detrended BCI values were also positively influenced by current year SAM. Positive SAM phases are associated with weaker zonal winds in southern Australia (Marshall et al., 2018) and a stronger Bonney Upwelling during summer driven by more easterly winds (Foo et al., 2021). The increased marine productivity cascading into Bass Strait could then provide more favourable foraging conditions for AUFS (Middleton and Bye, 2007), potentially leading to increased body condition.
The weak positive influence of 1-year lagged IOD on detrended BCI appears contrary to the long-term decline of female body condition while the IOD has been trending positive. However, this relationship could be reflective of interactions or coupling between SAM and IOD (Cai et al., 2011). In contrast, the positive relationship between 1-year lagged Chl-a concentration during winter and detrended BCI in adult female AUFS is consistent with increased primary productivity leading to improved prey availability in subsequent years for Bass Strait marine predators (Berlincourt and Arnould, 2015; Speakman et al., 2020).
When individuals within a population are unable to maintain a healthy body condition, it indicates the population is experiencing nutritional stress (Rode et al., 2010), and sustained population-wide declines in body condition can lead to decreases in reproductive output and/or survival (Testa and Adams, 1998; Trites and Donnelly, 2003). Decreases in body condition may be a result of increased intra- or inter-specific competition for food resources and/or decreases in food availability due to environmental change or anthropogenic influences (Garroway and Broders, 2005; Øigård et al., 2013; Bogstad et al., 2015). Therefore, determining the cause of nutritional stress is important for predicting the trajectory of populations in response to such factors (Calkins et al., 1998). However, disentangling the factors influencing body condition changes in large mammals experiencing a variable environment, recovering from historical over-harvesting and susceptible to numerous anthropogenic factors is difficult (Mason et al., 2014; Vermeulen et al., 2023).
Like all otariid species, AUFS were severely over-exploited during the commercial sealing era of the 18th-19th centuries (Gill, 1967; Arnould et al., 2003). While the species has slowly recovered, the population is considered to still be at <50% of its pre-sealing level (Kirkwood et al., 2010; McIntosh et al., 2018). Consequently, it would seem unlikely that the observed decline in adult female BCI is due to the rebounding population having surpassed its original carrying capacity (Fowler, 1981). However, the recovery of the AUFS population over the last two centuries has coincided with a major increase in commercial fishing activity, both locally and globally (Goldsworthy et al., 2003). Such sustained increased fishing pressure could potentially have resulted in a reduction of prey resources for AUFS, either through direct or indirect competition, leading to a lower carrying capacity in the region (Hobday et al., 2011). However, commercial fishing catches have not increased in south-eastern Australia over the last two decades (Patterson et al., 2022), thus making this scenario improbable.
Reduction in the prey resources AUFS depend upon are likely due to ecosystem changes. Indeed, there has been sustained low biomass levels of several commercial fish species which have not recovered despite reduced fishing pressure (Edgar et al., 2018; Nitschke et al., 2022), suggesting wider ecosystem changes have occurred. While the exact linkages between environmental variables and prey diversity, abundance and distribution for the species are not known (Kirkwood et al., 2008; Kliska, 2016; Kliska et al., 2022), the results of the present study demonstrate local- and broad-scale influences on AUFS body condition over the last two decades. In addition, the south-eastern Australia oceanic region is rapidly warming (Hobday and Pecl, 2014) and major ecosystem changes are anticipated in the coming decades (Hobday and Lough, 2011; Pethybridge et al., 2020). Furthermore, globally, the average body mass of many marine predator prey species has been shown to be decreasing (Florko et al., 2021; Thoral et al., 2021; Gallagher et al., 2022). These decreases have been linked to increased foraging effort and lower foraging success in other otariid species (Osman et al., 2004).
Decreases in nutritional resources have been linked to a reduction in body condition with subsequent effects on fecundity, offspring growth rate, survival and, ultimately, population abundance in other otariids and mammals in general (Trillmich, 1990; Trillmich et al., 1991; Guinet et al., 1998; Testa and Adams, 1998; Garroway and Broders, 2005). Interestingly, while there have been some regional declines in annual pup production in AUFS over the last decade (McIntosh et al., 2022), average pup production at the Kanowna Island colony has not decreased during the study period (Geeson et al., 2022) and, thus, recruitment and colony population size is likely to have remained relatively stable (Kirkwood et al., 2005). While changes to vital rates in response to alterations in nutritional resources may be slow in large mammals (Bluhm and Gradinger, 2008), these findings could reflect a degree of nutritional tolerance or flexibility in energy allocation (Laidre et al., 2008; Champagne et al., 2012). Such a capacity, however, would be limited and continued decreases in body condition would be expected to lead to lower vital rates (Fowler, 1981). Alternatively, in response to reductions in nutritional resources and adult female body condition, the population could have experienced decreases in offspring birth mass and pre-weaning growth rate (Boyd and McCann, 1989; Arnbom et al., 1997; Boltnev and York, 2001). Indeed, recent studies have highlighted regional interannual variability in AUFS pup growth and condition in relation to environmental variables (Wall et al., 2023). Hence, further monitoring of these parameters is needed to assess whether they have changed at Kanowna Island since the start of the study period when they were last measured (Arnould and Hindell, 2002).
In summary, the results of the present study revealed a long-term decrease of both mass and body condition in adult female AUFS from the species’ third largest breeding colony (Kirkwood et al., 2010). These decreases are likely to be due to a combination of ecosystem changes and potentially density-dependence following population recovery and competition for declining prey with commercial fisheries. Weak but significant relationships between body condition and environmental variables suggest the population could continue to experience reduced nutritional resources under predicted climate change scenarios (Thompson et al., 2009; Hobday and Lough, 2011). While further investigations are urgently required to better understand the linkages between environmental forces and prey resource availability in AUFS, the results of the present study provide another clear signal of environmental change in this global warming hotspot (Cavole et al., 2016; Holt and Boersma, 2022).
The raw data supporting the conclusions of this article will be made available by the authors, without undue reservation.
The animal study was approved by Deakin University Animal Ethics committee (approval A33/2004, A16/2008, A14/2011, B16/2014, B04/2017), Macquarie University Animal Care and Ethics committee (approval 97001, 2000/004), University of Melbourne Animal Experimentation Ethics committee (approval 01146) and Department of Sustainability and Environment (Victoria, Australia) wildlife research permits (10000187, 10000706, 10001143, 10001672, 10002269, 10005362, 10007153, 10008286 and 10005848). The study was conducted in accordance with the local legislation and institutional requirements.
Conceived and designed the experiments: JJG, JPYA. Performed the experiments: JPYA, JJG. Acquired the data: JPYA, JJG, MAH, AJH. Analysed the data: JJG, JPYA, MAH, AJH, CNS. All authors contributed to manuscript preparation and gave final approval for publication.
Financial support was provided by research grants from the Australian Research Council, Sea World Research and Rescue Foundation, Winifred Violet Scott Charitable Trust and Holsworth Wildlife Research Endowment.
We thank the many staff, students and volunteers involved in the data collection over the years. The logistical support provided by Parks Victoria, Prom Adventurer Charters (Geoff Boyd) and Best Helicopters (Sean Best and Cameron Lang) is gratefully acknowledged. The assistance of Jason Hartog in extracting and providing the environmental data is much appreciated.
The authors declare that the research was conducted in the absence of any commercial or financial relationships that could be construed as a potential conflict of interest.
All claims expressed in this article are solely those of the authors and do not necessarily represent those of their affiliated organizations, or those of the publisher, the editors and the reviewers. Any product that may be evaluated in this article, or claim that may be made by its manufacturer, is not guaranteed or endorsed by the publisher.
Adame K., Elorriaga-Verplancken F. R., Beier E., Acevedo-Whitehouse K., Pardo M. A. (2020). The demographic decline of a sea lion population followed multi-decadal sea surface warming. Sci. Rep. 10 (1), 1–15. doi: 10.1038/s41598-020-67534-0
Afán I., Chiaradia A., Forero M. G., Dann P., Ramírez F. (2015). A novel spatio-temporal scale based on ocean currents unravels environmental drivers of reproductive timing in a marine predator. Proc. R. Soc. B: Biol. Sci. 282 (1810), 20150721. doi: 10.1098/rspb.2015.0721
Arnbom T., Fedak M. A., Boyd I. L. (1997). Factors affecting maternal expenditure in Southern elephant seals during lactation. Ecology 78 (2), 471–483. doi: 10.1890/0012-9658(1997)078[0471:FAMEIS]2.0.CO;2
Arnould J. P. Y., Boyd I. L., Warneke R. M. (2003). Historical dynamics of the Australian fur seal population: evidence of regulation by man? Can. J. Zoology 81 (8), 1428–1436. doi: 10.1139/z03-134
Arnould J. P. Y., Hindell M. A. (1999). The composition of Australian fur seal (Arctocephalus pusillus doriferus) milk throughout lactation. Physiol. Biochem. Zoology 72 (5), 605–612. doi: 10.1086/316702
Arnould J. P. Y., Hindell M. A. (2001). Dive behaviour, foraging locations, and maternal-attendance patterns of Australian fur seals (Arctocephalus pusillus doriferus). Can. J. Zoology 79 (1), 35–48. doi: 10.1139/z00-178
Arnould J. P. Y., Hindell M. A. (2002). Milk consumption, body composition and pre-weaning growth rates of Australian fur seal (Arctocephalus pusillus doriferus) pups. J. Zoology 256 (3), 351–359. doi: 10.1017/S0952836902000389
Arnould J. P. Y., Ramsay M. A. (1994). Milk production and milk consumption in polar bears during the ice-free period in western Hudson Bay. Can. J. Zoology 72 (8), 1365–1370. doi: 10.1139/z94-180
Arnould J. P. Y., Warneke R. M. (2002). Growth and condition in Australian fur seals Arctocephalus pusillus doriferus (Carnivora : Pinnipedia). Aust. J. Zoology 50 (1), 53–66. doi: 10.1071/ZO01077
Barton K. (2015). Package ‘MuMIn’. R package version 1.10.0. 1. Available at: https://CRAN.R-project.org/package=MuMIn
Beggs H., Zhong A., Warren G., Alves O., Brassington G., Pugh T. (2011). RAMSSA—An operational, high-resolution, regional Australian multi-sensor sea surface temperature analysis over the Australian region. Aust. Meteorological Oceanographic J. 61 (1), 1–22. doi: 10.22499/2.6101.001
Berlincourt M., Arnould J. P. Y. (2015). Influence of environmental conditions on foraging behaviour and its consequences on reproductive performance in little penguins. Mar. Biol. 162 (7), 1485–1501. doi: 10.1007/s00227-015-2685-x
Birsoy K., Festuccia W. T., Laplante M. (2013). A comparative perspective on lipid storage in animals. J. Cell Sci. 126 (7), 1541–1552. doi: 10.1242/jcs.104992
Bluhm B. A., Gradinger R. (2008). Regional variability in food availability for Arctic marine mammals. Ecol. Appl. 18 (sp2), S77–S96. doi: 10.1890/06-0562.1
Bogstad B., Gjøsæter H., Haug T., Lindstrøm U. (2015). A review of the battle for food in the Barents Sea: cod vs. marine mammals. Front. Ecol. Evol. 3, 29. doi: 10.3389/fevo.2015.00029
Boltnev A. I., York A. E. (2001). Maternal investment in northern fur seals (Callorhinus ursinus): interrelationships among mothers' age, size, parturition date, offspring size and sex ratios. J. Zoology 254 (2), 219–228. doi: 10.1017/S0952836901000735
Boness D. L. J., Bowen D. W. (1996). The Evolution of Maternal Care in Pinnipeds: New findings raise questions about the evolution of maternal feeding strategies. BioScience 46 (9), 645–654. doi: 10.2307/1312894
Boness D. L. J., Clapham P. J., Mesnick S. L. (2002). Life history and reproductive strategies. Mar. mammal biology: an evolutionary approach, 278–324. doi: 10.2307/1312894
Boveng P. L., Ziel H. L., McClintock B. T., Cameron M. F. (2020). Body condition of phocid seals during a period of rapid environmental change in the Bering Sea and Aleutian Islands, Alaska. Deep Sea Res. Part II: Topical Stud. Oceanography 181, 104904. doi: 10.1016/j.dsr2.2020.104904
Boyd I. L., McCann T. S. (1989). Pre-natal investment in reproduction by female Antarctic fur seals. Behav. Ecol. Sociobiology 24 (6), 377–385. doi: 10.1007/BF00293265
Bunce A., Norman F., Brothers N., Gales R. (2002). Long-term trends in the Australasian gannet (Morus serrator) population in Australia: the effect of climate change and commercial fisheries. Mar. Biol. 141 (2), 263–269. doi: 10.1007/s00227-002-0838-1
Burnham K. P., Anderson D. R. (2004). Multimodel inference: understanding AIC and BIC in model selection. Sociological Methods Res. 33 (2), 261–304. doi: 10.1177/0049124104268644
Bush E. R., Whytock R. C., Bahaa-El-Din L., Bourgeois S., Bunnefeld N., Cardoso A. W., et al. (2020 6521). Long-term collapse in fruit availability threatens Central African forest megafauna. Science 370, 1219–1222. doi: 10.1126/science.abc7791
Cadby C. D., While G. M., Hobday A. J., Uller T., Wapstra E. (2010). Multi-scale approach to understanding climate effects on offspring size at birth and date of birth in a reptile. Integr. Zoology 5 (2), 164–175. doi: 10.1111/j.1749-4877.2010.00201.x
Cai W., Sullivan A., Cowan T. (2011). Interactions of ENSO, the IOD, and the SAM in CMIP3 models. J. Climate 24 (6), 1688–1704. doi: 10.1175/2010JCLI3744.1
Cai W., Yang K., Wu L., Huang G., Santoso A., Ng B., et al. (2021). Opposite response of strong and moderate positive Indian Ocean Dipole to global warming. Nat. Climate Change 11 (1), 27–32. doi: 10.1038/s41558-020-00943-1
Calkins D. G., Becker E. F., Pitcher K. W. (1998). Reduced body size of female Steller sea lions from a declining population in the Gulf of Alaska. Mar. Mammal Sci. 14 (2), 232–244. doi: 10.1111/j.1748-7692.1998.tb00713.x
Castelblanco-Martínez D. N., Slone D. H., Landeo-Yauri S. S., Ramos E. A., Alvarez-Alemán A., Attademo F. L. N., et al. (2021). Analysis of body condition indices reveals different ecotypes of the Antillean manatee. Sci. Rep. 11 (1), 19451. doi: 10.1038/s41598-021-98890-0
Cattet M. R. L., Caulkett N. A., Obbard M. E., Stenhouse G. B. (2002). A body-condition index for ursids. Can. J. Zoology 80 (7), 1156–1161. doi: 10.1139/z02-103
Cavole L. M., Demko A. M., Diner R. E., Giddings A., Koester I., Pagniello C. M., et al. (2016). Biological impacts of the 2013–2015 warm-water anomaly in the Northeast Pacific: winners, losers, and the future. Oceanography 29 (2), 273–285. Available at: https://www.jstor.org/stable/24862690.
Chambers L. E., Patterson T., Hobday A. J., Arnould J. P. Y., Tuck G. N., Wilcox C., et al. (2015). Determining trends and environmental drivers from long-term marine mammal and seabird data: examples from Southern Australia. Regional Environ. Change 15 (1), 197–209. doi: 10.1007/s10113-014-0634-8
Champagne C. D., Crocker D. E., Fowler M. A., Houser D. S. (2012). “Fasting physiology of the pinnipeds: The challenges of fasting while maintaining high energy expenditure and nutrient delivery for lactation,” in Comparative Physiology of Fasting, Starvation, and Food Limitation. Ed. McCue M. D. (Berlin, Heidelberg: Springer Berlin Heidelberg), 309–336.
Christiansen F., Sironi M., Moore M. J., Di Martino M., Ricciardi M., Warick H. A., et al. (2019). Estimating body mass of free-living whales using aerial photogrammetry and 3D volumetrics. Methods Ecol. Evol. 10 (12), 2034–2044. doi: 10.1111/2041-210X.13298
Constable A. J., Nicol S., Strutton P. G. (2003). Southern Ocean productivity in relation to spatial and temporal variation in the physical environment. J. Geophysical Research: Oceans 108 (C4), 8079. doi: 10.1029/2001JC001270
Edgar G. J., Ward T. J., Stuart-Smith R. D. (2018). Rapid declines across Australian fishery stocks indicate global sustainability targets will not be achieved without an expanded network of ‘no-fishing’reserves. Aquat. Conservation: Mar. Freshw. Ecosyst. 28 (6), 1337–1350. doi: 10.1002/aqc.2934
Estes J. A. (1979). Exploitation of marine mammals: r-selection of K-strategists? J. Fisheries Board Canada 36 (8), 1009–1017. doi: 10.1139/f79-140
Florko K. R., Tai T. C., Cheung W. W., Ferguson S. H., Sumaila U. R., Yurkowski D. J., et al. (2021). Predicting how climate change threatens the prey base of Arctic marine predators. Ecol. Lett. 24 (12), 2563–2575. doi: 10.1111/ele.13866
Fogt R. L., Marshall G. J. (2020). The Southern Annular Mode: variability, trends, and climate impacts across the Southern Hemisphere. Wiley Interdiscip. Reviews: Climate Change 11 (4), e652. doi: 10.1002/wcc.652
Foo D., McMahon C., Hindell M. A., Goldsworthy S. (2021). Shelf–oceanic dynamics of surface environmental parameters in the Kangaroo Island–Bonney Coast region. Mar. Freshw. Res. 72 (5), 679–692. doi: 10.1071/MF20100
Fowler C. W. (1981). Density dependence as related to life history strategy. Ecology 62 (3), 602–610. doi: 10.2307/1937727
Gallagher C. A., Chimienti M., Grimm V., Nabe-Nielsen J. (2022). Energy-mediated responses to changing prey size and distribution in marine top predator movements and population dynamics. J. Anim. Ecol. 91 (1), 241–254. doi: 10.1111/1365-2656.13627
Garroway C. J., Broders H. G. (2005). The quantitative effects of population density and winter weather on the body condition of white-tailed deer (Odocoileus virginianus) in Nova Scotia, Canada. Can. J. Zoology 83 (9), 1246–1256. doi: 10.1139/z05-118
Geeson J. J., Hobday A. J., Speakman C. N., Arnould J. P. Y. (2022). Environmental influences on breeding biology and pup production in Australian fur seals. R. Soc. Open Sci. 9 (4), 211399. doi: 10.1098/rsos.211399
Gervais C. R., Champion C., Pecl G. T. (2021). Species on the move around the Australian coastline: A continental-scale review of climate-driven species redistribution in marine systems. Global Change Biol. 27 (14), 3200–3217. doi: 10.1111/gcb.15634
Gibbens J., Arnould J. P. Y. (2009a). Age-specific growth, survival, and population dynamics of female Australian fur seals. Can. J. Zoology 87 (10), 902–911. doi: 10.1139/Z09-080
Gibbens J., Arnould J. P. Y. (2009b). Interannual variation in pup production and the timing of breeding in benthic foraging Australian fur seals. Mar. Mammal Sci. 25 (3), 573–587. doi: 10.1111/j.1748-7692.2008.00270.x
Gibbs C. F., Tomczak M. Jr., Longmore A. (1986). The nutrient regime of Bass Strait. Mar. Freshw. Res. 37 (4), 451–466. doi: 10.1071/MF9860451
Gill J. C. H. (1967). Notes on the sealing industry of early Australia. J. R. Historical Soc. Queensland 8 (2), 218–245.
Gingerich P. D. (1990). Prediction of body mass in mammalian species from long bone lengths and diameters. Contributions from the Museum of Paleontology 28 (4), 79–92. Available at: http://hdl.handle.net/2027.42/48540.
Goldsworthy S. D., Bulman C. M., He X., Larcombe J., Littnan C. L. (2003). Trophic interactions between marine mammals and Australian fisheries: an ecosystem approach (Hobart, Tasmania, Australia: Marine Mammals and Humans: Fisheries, Tourism and Management, CSIRO Publications), 62–99.
Grueber C. E., Nakagawa S., Laws R. J., Jamieson I. G. (2011). Multimodel inference in ecology and evolution: challenges and solutions. J. Evolutionary Biol. 24 (4), 699–711. doi: 10.1111/j.1420-9101.2010.02210.x
Guinet C., Roux J. P., Bonnet M., Mison V. (1998). Effect of body size, body mass, and body condition on reproduction of female South African fur seals (Arctocephalus pusillus) in Namibia. Can. J. Zoology 76 (8), 1418–1424. doi: 10.1139/z98-082
Harwood L. A., Smith T. G., Melling H., Alikamik J., Kingsley M. C. (2012). Ringed seals and sea ice in Canada's Western Arctic: Harvest-based monitoring 1992-2011. Arctic 65, 377–390. doi: 10.14430/arctic4236
Hewison A. J. M., Angibault J. M., Bideau E., Vincent J. P., Boutin J., Sempéré A. (1996). Annual variation in body composition of roe deer (Capreolus capreolus) in moderate environmental conditions. Can. J. Zoology 74 (2), 245–253. doi: 10.1139/z96-031
Hobday A. J., Arrizabalaga H., Evans K., Nicol S., Young J. W., Weng K. C. (2015). Impacts of climate change on marine top predators: advances and future challenges. Deep-Sea Res. Part II: Topical Stud. Oceanography 113, 1–8. doi: 10.1016/j.dsr2.2015.01.013
Hobday A. J., Lough J. M. (2011). Projected climate change in Australian marine and freshwater environments. Mar. Freshw. Res. 62 (9), 1000–1014. doi: 10.1071/MF10302
Hobday A. J., Pecl G. T. (2014). Identification of global marine hotspots: sentinels for change and vanguards for adaptation action. Rev. Fish Biol. Fisheries 24 (2), 415–425. doi: 10.1007/s11160-013-9326-6
Hobday A. J., Smith A. D. M., Stobutzki I. C., Bulman C., Daley R., Dambacher J. M., et al. (2011). Ecological risk assessment for the effects of fishing. Fisheries Res. 108 (2-3), 372–384. doi: 10.1016/j.fishres.2011.01.013
Holt K. A., Boersma P. D. (2022). Unprecedented heat mortality of Magellanic Penguins. Condor 124 (1), duab052. doi: 10.1093/ornithapp/duab052
Hoskins A. J., Arnould J. P. Y. (2014). Relationship between long-term environmental fluctuations and diving effort of female Australian fur seals. Mar. Ecol. Prog. Ser. 511, 285–295. doi: 10.3354/meps10935
Hoskins A. J., Costa D. P., Arnould J. P. Y. (2015). Utilisation of intensive foraging zones by female Australian fur seals. PloS One 10 (2), e0117997. doi: 10.1371/journal.pone.0117997
Houston A. I., Stephens P. A., Boyd I. L., Harding K. C., Mcnamara J. M. (2006). Capital or income breeding? A theoretical model of female reproductive strategies. Behav. Ecol. 18 (1), 241–250. doi: 10.1093/beheco/arl080
Hwang T. Y., Larivière S., Messier F. (2005). Evaluating body condition of striped skunks using non-invasive morphometric indices and bioelectrical impedance analysis. Wildlife Soc. Bull. 33 (1), 195–203. doi: 10.2193/0091-7648(2005)33[195:EBCOSS]2.0.CO;2
Karasov W. H. (1992). Daily energy expenditure and the cost of activity in mammals. Am. Zoologist 32 (2), 238–248. doi: 10.1093/icb/32.2.238
Kirkman S. P., Arnould J. P. Y. (2018). Cape and Australian fur seals: Arctocephalus pusillus pusillus and A. p. doriferus. Encyclopedia of Marine Mammals. 3rd ed. Eds. Würsig B., Thewissen J. G. M., Kovacs K. M. (Amsterdam, The Netherlands: Academic Press), 158–161.
Kirkwood R., Gales R., Terauds A., Arnould J. P. Y., Pemberton D., Shaughnessy P. D., et al. (2005). Pup production and population trends of the Australian fur seal (Arctocephalus pusillus doriferus). Mar. Mammal Sci. 21 (2), 260–282. doi: 10.1111/j.1748-7692.2005.tb01227.x
Kirkwood R., Hume F., Hindell M. (2008). Sea temperature variations mediate annual changes in the diet of Australian fur seals in Bass Strait. Mar. Ecol. Prog. Ser. 369, 297–309. doi: 10.3354/meps07633
Kirkwood R., Pemberton D., Gales R., Hoskins A. J., Arnould J. P. Y., Mitchell T., et al. (2010). Continued population recovery by Australian fur seals. Mar. Freshw. Res. 61 (6), 695–701. doi: 10.1071/MF09213
Kliska K. (2016). Environmental correlates of temporal variation in the diet of Australian fur seals (Masters of Research, Macquarie University, Faculty of Science and Engineering, Department of Biological Sciences).
Kliska K., McIntosh R. R., Jonsen I., Hume F., Dann P., Kirkwood R., et al. (2022). Environmental correlates of temporal variation in the prey species of Australian fur seals inferred from scat analysis. R. Soc. Open Sci. 9 (10), 211723. doi: 10.1098/rsos.211723
Laidre K. L., Stirling I., Lowry L. F., Wiig Ø., Heide-Jørgensen M. P., Ferguson S. H. (2008). Quantifying the sensitivity of Arctic marine mammals to climate-induced habitat change. Ecol. Appl. 18 (sp2), S97–S125. doi: 10.1890/06-0546.1
Laws R. M. (1956). Growth and sexual maturity in aquatic mammals. Nature 178, 193–194. doi: 10.1038/178193a0
Lindstedt S. L., Boyce M. S. (1985). Seasonality, fasting endurance, and body size in mammals. Am. Nat. 125 (6), 873–878. doi: 10.1086/284385
Lourie H. J., Hoskins A. J., Arnould J. P. Y. (2014). Big boys get big girls: Factors influencing pupping site and territory location in Australian fur seals. Mar. Mammal Sci. 30 (2), 544–561. doi: 10.1111/mms.12056
Lovenduski N. S., Gruber N. (2005). Impact of the Southern Annular Mode on Southern Ocean circulation and biology. Geophysical Res. Lett. 32 (11). doi: 10.1029/2005GL022727
Mammals, A. S. O. M. C. O. M. (1967). Standard measurements of seals. J. Mammalogy 48 (3), 459–462. doi: 10.2307/1377778
Marker L. L., Dickman A. J. (2003). Morphology, physical condition, and growth of the cheetah (Acinonyx jubatus jubatus). J. Mammalogy 84 (3), 840–850. doi: 10.1644/BRB-036
Marshall A. G., Hemer M. A., Hendon H. H., McInnes K. L. (2018). Southern annular mode impacts on global ocean surface waves. Ocean Model. 129, 58–74. doi: 10.1016/j.ocemod.2018.07.007
Mason T. H. E., Apollonio M., Chirichella R., Willis S. G., Stephens P. A. (2014). Environmental change and long-term body mass declines in an alpine mammal. Front. Zoology 11 (1), 69. doi: 10.1186/s12983-014-0069-6
McIntosh R. R., Kirkman S. P., Thalmann S., Sutherland D. R., Mitchell A., Arnould J. P. Y., et al. (2018). Understanding meta-population trends of the Australian fur seal, with insights for adaptive monitoring. PloS One 13 (9), e0200253. doi: 10.1371/journal.pone.0200253
McIntosh R. R., Sorrell K. J., Thalmann S., Mitchell A., Gray R., SChinagl H., et al. (2022). Sustained reduction in numbers of Australian fur seal pups: Implications for future population monitoring. PloS One 17 (3), e0265610. doi: 10.1371/journal.pone.0265610
McKenzie J., Page B., Goldsworthy S. D., Hindell M. A. (2007). Growth strategies of New Zealand fur seals in southern Australia. J. Zoology 272 (4), 377–389. doi: 10.1111/j.1469-7998.2006.00278.x
Melvin R. G., Andrews M. T. (2009). Torpor induction in mammals: recent discoveries fueling new ideas. Trends Endocrinol. Metab. 20 (10), 490–498. doi: 10.1016/j.tem.2009.09.005
Middleton J. F., Arthur C., Van Ruth P., Ward T. M., McClean J. L., Maltrud M. E., et al. (2007). El Niño effects and upwelling off South Australia. J. Phys. Oceanography 37 (10), 2458–2477. doi: 10.1175/JPO3119.1
Middleton J. F., Bye J. (2007). A review of the shelf-slope circulation along Australia’s southern shelves: Cape Leeuwin to Portland. Prog. Oceanography 75 (1), 1–41. doi: 10.1016/j.pocean.2007.07.001
NASA Goddard Space Flight Center, O. E. L., Ocean Biology Processing Group (2018a). Moderate-resolution Imaging Spectroradiometer (MODIS) Aqua Chlorophyll Data. Ed. N. OB.DAAC (Greenbelt, MD, USA).
NASA Goddard Space Flight Center, O. E. L., Ocean Biology Processing Group (2018b). Sea-viewing Wide Field-of-view Sensor (SeaWiFS) Chlorophyll Data. Ed. N. OB.DAAC (Greenbelt, MD, USA).
Newell B. (1961). Hydrology of south-east Australian waters: Bass Strait and New South Wales tuna fishing area. Technical paper (CSIRO. Division of Fisheries and Oceanography); no.10. doi: 10.25919/zmj6-ga07
Nieblas A.-E., Sloyan B. M., Hobday A. J., Coleman R., Richardsone A. J. (2009). Variability of biological production in low wind-forced regional upwelling systems: A case study off southeastern Australia. Limnology Oceanography 54 (5), 1548–1558. doi: 10.4319/lo.2009.54.5.1548
Nitschke J., Knuckey I., Koopman M., Hudson R., Huveneers C., Grammer G., et al. (2022). Spatio-temporal variability in the demersal fish assemblage on the outer continental shelf of the Great Australian Bight. Estuarine Coast. Shelf Sci. 271, 107852. doi: 10.1016/j.ecss.2022.107852
Oftedal O. T. (2000). Use of maternal reserves as a lactation strategy in large mammals. Proc. Nutr. Soc. 59 (1), 99–106. doi: 10.1017/S0029665100000124
Øigård T., Lindstrøm U., Haug T., Nilssen K., Smout S. (2013). Fuctional relationship between harp seal body condition and available prey in the Barents Sea. Mar. Ecol. Prog. Ser. 482, 287–301. doi: 10.3354/meps10272
Osman L., Hucke-Gaete R., Moreno C., Torres D. (2004). Feeding ecology of Antarctic fur seals at Cape Shirreff, South Shetlands, Antarctica. Polar Biol. 27, 92–98. doi: 10.1007/s00300-003-0555-3
Overholser B. R., Sowinski K. M. (2008). Biostatistics primer: part 2. Nutr. Clin. Pract. 23 (1), 76–84. doi: 10.1177/011542650802300176
Parmesan C. (2006). Ecological and evolutionary responses to recent climate change. Annu. Rev. Ecology Evolution Systematics 37 (1), 637–669. doi: 10.1146/annurev.ecolsys.37.091305.110100
Patterson H. B., Galeano D., Larcombe J., Timmiss T., Woodhams J., Curtotti R. (2022). Fishery status reports 2022. A. B. A. R. E. Sci. Canberra.
Pethybridge H. R., Fulton E. A., Hobday A. J., Blanchard J., Bulman C. M., Butler I. R., et al. (2020). Contrasting futures for Australia’s fisheries stocks under IPCC RCP8. 5 emissions–a multi-ecosystem model approach. Front. Mar. Sci. 7, 577964. doi: 10.3389/fmars.2020.577964
Pinheiro J., Bates D., Debroy S., Sarkar D. (2020). “R Core Team. nlme: linear and nonlinear mixed effects models,” in R package version 3, 1–147. https://CRAN.R-project.org/package=nlme
Pitt J. A., Larivière S., Messier F. (2006). Condition indices and bioelectrical impedance analysis to predict body condition of small carnivores. J. Mammalogy 87 (4), 717–722. doi: 10.1644/05-MAMM-A-264R1.1
Poloczanska E. S., Brown C. J., Sydeman W. J., Kiessling W., Schoeman D. S., Moore P. J., et al. (2013). Global imprint of climate change on marine life. Nat. Climate Change 3 (10), 919–925. doi: 10.1038/nclimate1958
R Core Team (2022). R: A language and environment for statistical computing (Vienna, Austria: R Foundation for Statistical Computing). Available at: https://www.R-project.org/.
Reilly J. J., Fedak M. A. (1990). Measurement of the body composition of living gray seals by hydrogen isotope dilution. J. Appl. Physiol. 69 (3), 885–891. doi: 10.1152/jappl.1990.69.3.885
Rode K. D., Amstrup S. C., Regehr E. V. (2010). Reduced body size and cub recruitment in polar bears associated with sea ice decline. Ecol. Appl. 20 (3), 768–782. doi: 10.1890/08-1036.1
Rödel H. G., Valencak T. G., Handrek A., Monclús R. (2016). Paying the energetic costs of reproduction: reliance on postpartum foraging and stored reserves. Behav. Ecol. 27 (3), 748–756. doi: 10.1093/beheco/arv217
Rodríguez-Malagón M. A., Speakman C. N., Sutton G. J., Angel L. P., Arnould J. P. Y. (2021). Temporal and spatial isotopic variability of marine prey species in south-eastern Australia: Potential implications for predator diet studies. PloS One 16 (11), e0259961. doi: 10.1371/journal.pone.0259961
Ropert-Coudert Y., Kato A., Chiaradia A. (2009). Impact of small-scale environmental perturbations on local marine food resources: a case study of a predator, the little penguin. Proc. R. Soc. B: Biol. Sci. 276 (1676), 4105–4109. doi: 10.1098/rspb.2009.1399
Saji N. H., Goswami B. N., Vinayachandran P., Yamagata T. (1999). A dipole mode in the tropical Indian Ocean. Nature 401 (6751), 360–363. doi: 10.1038/43854
Sandery P. A., Kämpf J. (2005). Winter-spring flushing of Bass Strait, south-eastern Australia: a numerical modelling study. Estuarine Coast. Shelf Sci. 63 (1-2), 23–31. doi: 10.1016/j.ecss.2004.10.009
Sandery P. A., Kämpf J. (2007). Transport timescales for identifying seasonal variation in Bass Strait, south-eastern Australia. Estuarine Coast. Shelf Sci. 74 (4), 684–696. doi: 10.1016/j.ecss.2007.05.011
Schulte-Hostedde A. I., Zinner B., Millar J. S., Hickling G. J. (2005). Restitution of mass–size residuals: validating body condition indices. Ecology 86 (1), 155–163. doi: 10.1890/04-0232
Soto K. H., Trites A. W., Arias-Schreiber M. (2004). The effects of prey availability on pup mortality and the timing of birth of South American sea lions (Otaria flavescens) in Peru. J. Zoology 264 (4), 419–428. doi: 10.1017/S0952836904005965
Soto K. H., Trites A. W., Arias-Schreiber M. (2006). Changes in diet and maternal attendance of South American sea lions indicate changes in the marine environment and prey abundance. Mar. Ecol. Prog. Ser. 312, 277–290. doi: 10.3354/meps312277
Speakman C. N., Hoskins A. J., Hindell M. A., Costa D. P., Hartog J. R., Hobday A. J., et al. (2020). Environmental influences on foraging effort, success and efficiency in female Australian fur seals. Sci. Rep. 10 (1), 17710. doi: 10.1038/s41598-020-73579-y
Speakman C. N., Hoskins A. J., Hindell M. A., Costa D. P., Hartog J. R., Hobday A. J., et al. (2021). Influence of environmental variation on spatial distribution and habitat-use in a benthic foraging marine predator. R. Soc. Open Sci. 8 (10), 211052. doi: 10.1098/rsos.211052
Stevenson R. D., Woods W. A. J. R. (2006). Condition indices for conservation: new uses for evolving tools. Integr. Comp. Biol. 46 (6), 1169–1190. doi: 10.1093/icb/icl052
Sweeney K. L., Shertzer K. W., Fritz L. W., Read A. J. (2015). A novel approach to compare pinniped populations across a broad geographic range. Can. J. Fisheries Aquat. Sci. 72 (2), 175–185. doi: 10.1139/cjfas-2014-0070
Symonds M. R. E., Moussalli A. (2011). A brief guide to model selection, multimodel inference and model averaging in behavioural ecology using Akaike’s information criterion. Behav. Ecol. Sociobiology 65 (1), 13–21. doi: 10.1007/s00265-010-1037-6
Testa J. W., Adams G. P. (1998). Body condition and adjustments to reproductive effort in female moose (Alces alces). J. Mammalogy 79 (4), 1345–1354. doi: 10.2307/1383026
Thayer Z. M., Rutherford J., Kuzawa C. W. (2020). The maternal nutritional buffering model: an evolutionary framework for pregnancy nutritional intervention. Evolution Medicine Public Health 2020 (1), 14–27. doi: 10.1093/emph/eoz037
Thomalla S. J., Fauchereau N., Swart S., Monteiro P. M. S. (2011). Regional scale characteristics of the seasonal cycle of chlorophyll in the Southern Ocean. Biogeosciences 8 (10), 2849–2866. doi: 10.5194/bg-8-2849-2011
Thomas C. D. (2010). Climate, climate change and range boundaries. Diversity Distributions 16 (3), 488–495. doi: 10.1111/j.1472-4642.2010.00642.x
Thompson P., Baird M., Ingleton T., Doblin M. (2009). Long-term changes in temperate Australian coastal waters: implications for phytoplankton. Mar. Ecol. Prog. Ser. 394, 1–19. doi: 10.3354/meps08297
Thoral E., Queiros Q., Roussel D., Dutto G., Gasset E., McKenzie D. J., et al. (2021). Changes in foraging mode caused by a decline in prey size have major bioenergetic consequences for a small pelagic fish. J. Anim. Ecol. 90 (10), 2289–2301. doi: 10.1111/1365-2656.13535
Trillmich F. (1990). The behavioral ecology of maternal effort in fur seals and sea lions. Behaviour 114 (1-4), 3–20. doi: 10.1163/156853990X00022
Trillmich F., Ono K. A., Costa D. P., Delong R. L., Feldkamp S. D., Francis J. M., et al. (1991). “The effects of El Niño on pinniped populations in the eastern Pacific,” in Pinnipeds and El Niño, vol. 88. Eds. Trillmich F., Ono K. A. (Berlin, Heidelberg: Springer), 247–270.
Trites A. W., Bigg M. A. (1992). Changes in body growth of northern fur seals from 1958 to 1974: density effects or changes in the ecosystem? Fisheries Oceanography 1 (2), 127–136. doi: 10.1111/j.1365-2419.1992.tb00031.x
Trites A. W., Bigg M. A. (1996). Physical growth of northern fur seals (Callorhinus ursinus): seasonal fluctuations and migratory influences. J. Zoology 238 (3), 459–482. doi: 10.1111/j.1469-7998.1996.tb05406.x
Trites A. W., Donnelly C. P. (2003). The decline of Steller sea lions Eumetopias jubatus in Alaska: a review of the nutritional stress hypothesis. Mammal Rev. 33 (1), 3–28. doi: 10.1046/j.1365-2907.2003.00009.x
Vermeulen E., Thavar T., Glarou M., Ganswindt A., Christiansen F. (2023). Decadal decline in maternal body condition of a Southern Ocean capital breeder. Sci. Rep. 13, 3228. doi: 10.1038/s41598-023-30238-2
Wade G. N., Schneider J. E. (1992). Metabolic fuels and reproduction in female mammals. Neurosci. Biobehav. Rev. 16 (2), 235–272. doi: 10.1016/S0149-7634(05)80183-6
Wall D., Thalmann S., Wotherspoon S., Lea M.-A. (2023). Is regional variability in environmental conditions driving differences in the early body condition of endemic Australian fur seal pups? Wildlife Res. doi: 10.1071/WR22113
Warneke R. M., Shaughnessy P. D. (1985). “Arctocephalus pusillus, the South African and Australian fur seal: Taxonomy, evolution, biogeography, and life history,” in Studies of Sea Mammals in South Latitude. Eds. Ling J. K., Bryden M. M. (Adelaide, South Australia, Australia: South Australian Museum), 54–77.
Wei T., Simko V., Levy M., Xie Y., Jin Y., Zemla J. (2017). Package ‘corrplot. Statistician 56 (316) e24.
Wijffels S. E., Beggs H., Griffin C., Middleton J. F., Cahill M., King E., et al. (2018). A fine spatial-scale sea surface temperature atlas of the Australian regional seas (SSTAARS): Seasonal variability and trends around Australasia and New Zealand revisited. J. Mar. Syst. 187, 156–196. doi: 10.1016/j.jmarsys.2018.07.005
Wilcox C., Hobday A. J., Chambers L. E. (2018). Using expert elicitation to rank ecological indicators for detecting climate impacts on Australian seabirds and pinnipeds. Ecol. Indic. 95, 637–644. doi: 10.1016/j.ecolind.2018.07.019
Younger J. L., Emmerson L. M., Miller K. J. (2016). The influence of historical climate changes on Southern Ocean marine predator populations: a comparative analysis. Global Change Biol. 22 (2), 474–493. doi: 10.1111/gcb.13104
Keywords: Australian fur seal, Arctocephalus, pinniped, otariid, body condition, Bass Strait, marine predator, climate variability
Citation: Geeson JJ, Hindell MA, Hobday AJ, Speakman CN and Arnould JPY (2023) Long-term decline in body condition of female Australian fur seals: potential causes and implications. Front. Mar. Sci. 10:1231337. doi: 10.3389/fmars.2023.1231337
Received: 30 May 2023; Accepted: 19 September 2023;
Published: 13 October 2023.
Edited by:
Nuno Queiroz, Centro de Investigacao em Biodiversidade e Recursos Geneticos (CIBIO-InBIO), PortugalReviewed by:
Emilio Sperone, University of Calabria, ItalyCopyright © 2023 Geeson, Hindell, Hobday, Speakman and Arnould. This is an open-access article distributed under the terms of the Creative Commons Attribution License (CC BY). The use, distribution or reproduction in other forums is permitted, provided the original author(s) and the copyright owner(s) are credited and that the original publication in this journal is cited, in accordance with accepted academic practice. No use, distribution or reproduction is permitted which does not comply with these terms.
*Correspondence: Johanna J. Geeson, Z2Vlc29uaEBkZWFraW4uZWR1LmF1
Disclaimer: All claims expressed in this article are solely those of the authors and do not necessarily represent those of their affiliated organizations, or those of the publisher, the editors and the reviewers. Any product that may be evaluated in this article or claim that may be made by its manufacturer is not guaranteed or endorsed by the publisher.
Research integrity at Frontiers
Learn more about the work of our research integrity team to safeguard the quality of each article we publish.