- 1Geophysics Research Section l, National Institute of Oceanography and Applied Geophysics (OGS), Trieste, Italy
- 2Department of Mathematics and Geosciences, University of Trieste, Trieste, Italy
- 3Jean Monnet Centre of Excellence on Sustainable Blue Economy, Euro-Mediterranean University EMUNI, Piran, Slovenia
Among anthropogenic activities, marine seismic surveys are a fundamental tool for oil and gas explorations, geophysical research, environmental hazard, and risk analysis. This resulting noise may affect a range of species, eliciting masking, behavioral responses, and changes in acoustic repertoires. There is an urgency to understand in depth the potential effects of seismic surveys on marine ecosystems since the information available is still scarce. Using Scopus® and Web of Science™ 2023 Clarivate bibliographic databases, we systematically reviewed the scientific literature addressing seismic surveys’ effects on free-ranging marine fauna. The first selection of articles matching selected keywords yielded 680 articles from Scopus and 320 from Web of Science. Screening for research articles written in English investigating marine fauna in its natural environment and performing a quality assessment process resulted in selecting 31 articles since 2001. We found a trend of increasing research efforts in this field with a decrease after 2020 and a broad spectrum of journals that hosted the publications (31 papers published in 12 journals). Although several taxa are investigated, most studies focused on effects on marine mammals. There is a lack of research on diverse animal taxa, and no research papers compare the effects on different taxa along the food chain. Behavioral and physiological effects are the most found by authors in the field. However, observed behavioral changes cannot always be uniquely attributed to the exposure to seismic surveys, as many authors report the influence of other variables (e.g., environmental conditions) during the observations.
1 Introduction
Marine fauna depends on sound and needs a suitable acoustic environment to thrive (Au, 2000; Hawkins and Popper, 2017; Popper et al., 2020). Human-made noise is widely recognized as a pollutant and a key stressor that may have long-term detrimental effects on marine ecosystems (Commission Decision (EU) 2017/848; Kunc et al., 2016). Anthropogenic underwater sound sources have become ubiquitous, thus increasing background noise and soundscape complexity (Richardson et al., 1995; Duarte et al., 2021). The forecasted growth in the blue economy sector primarily involves emerging ocean-based industries (OECD, 2016) that will generate increased sound emissions in the marine environment (such as Industrial marine aquaculture, deep– and ultra-deepwater hydrocarbons, offshore wind energy, ocean renewable energy, and marine and seabed mining (Camerlenghi, 2021). Among anthropogenic activities, marine seismic surveys are a fundamental tool for mineral explorations, scientific research, environmental hazard assessments, and risk analysis (Gisiner, 2016). Marine seismic surveys employ artificially generated sounds for subsurface prospection designed to produce a single mainly downward-directed impulse that propagates through the water column and into the seabed. Impulse reflections, refractions, and diffractions can be detected from hydrophones or geophones in the water column and seabed. The data is used to understand subsurface sediments and rocks’ petrophysics, the geometry of the geological structure, and the nature of formation fluids (e.g., Gisiner, 2016). The so-called ‘airguns’ are the most commonly used sound sources, whose impulses are generated by expanding a specific volume of compressed air. Airguns are often used in arrays (e.g., Caldwell and Dragoset, 2000). Survey characteristics may vary drastically following the scientific or prospect targets. The volume of compressed air may vary from a fraction of a liter in the case of high-resolution, shallow-penetration targets to tens of liters in deep-penetrating, lower-resolution surveys. Survey duration and density of survey lines over a survey area may range from days to weeks (e.g., three-dimensional surveys) to a single pass (e.g., two-dimensional surveys). In addition to the downward propagating sound useful for prospection, some acoustic energy also radiates laterally from the source generating a complex radiation pattern. Echoes (sound reverberation between reflecting surfaces in the subsurface and with the water/air interface) may produce a longer sound residence in the water column than needed for the prospection. Multiple propagation paths increase the complexity of the received signal and can create long reverberant waveforms of several seconds at extended ranges (Greene and Richardson, 1988; Gordon et al., 2003).
Other lower energy sources that are not considered in this review are used for subsurface prospection in geophysics, such as sparkers or parametric sub-bottom profilers.
An increasing number of scientific studies suggest that the impulses generated during seismic surveys adversely impact marine animal species, eliciting masking, behavioral responses, and changes in acoustic repertoires (e.g., Weilgart, 2023). Consequently, procedures for mitigating the effects of seismic surveys are enforced by the governments with jurisdiction over the Continental Shelf on which the survey is planned. Mitigation measures and the process to release the necessary authorizations vary considerably between governments (also in the case of coastal countries of the same marine basin), at times resulting in lengthy procedures with uncertain results, conflicting relations with non-governmental organizations, loss of opportunities for operators and researchers as well as an increased environmental hazard.
There is an urgency to improve the understanding of the potential effects of seismic surveys on marine ecosystems (e.g., Carroll et al., 2017; Elliott et al., 2019; Affatati, 2020);. Knowledge gaps still exist due to scarce information available on species distribution, the use of habitats, and the lack of standardized measurements. The issue’s complexity requires the involvement of several stakeholders, such as the engagement of a diverse community of public, private, and non-governmental bodies and, often, citizens.
Building on the work of the Technical Group on Underwater Noise (Commission Decision (EU) 2017/848; Dekeling et al., 2020; Sigray et al., 2023) to guide the implementation of the Marine Strategy Framework Directive (MSFD) by EU Member States, we conduct a systematic review of the scientific literature of the peer-reviewed studies addressing the effect of seismic surveys on free-ranging marine fauna. Only field studies on wild animals are considered because they guarantee ecological realism and produce results more suitable to influence the regulatory process (e.g., Tougaard et al., 2015; Gomez et al., 2016; Carroll et al., 2017). It follows that tanks/labs experiments, theoretical, modeling-only studies, and/or research performed in freshwater habitats are not considered in this review.
Underwater noise experiments are frequently performed in tanks due to reduced cost, gain from logistical simplicity, and the possibility of working in a controlled environment. However, researchers need to be aware of the issues of transposing measurement methods from field- to laboratory-based studies since these conditions are not representative of marine ecosystems (Ellison et al., 2012; Rogers et al., 2016; Carroll et al., 2017). Sound propagation is very different from an acoustic free field in these conditions since it is constrained by tank boundaries and complex patterns made from interference at small spatial scale (Duncan et al., 2016; Gray et al., 2016: Jensen et al., 2011; Rogers et al., 2016; Slabbekoorn et al., 2019). In closed artificial environments, source playbacks need to be used instead of real sources, leading to issues in recreating the physical features of the source. On the other hand, fieldwork implies costs and logistical constraints (Rogers et al., 2016). Furthermore, experiments in extremely shallow water depths (e.g., Pearson et al., 1994; Payne et al., 2007) have to deal with challenges in overestimating the level of acoustic energy created by reflections on the water surface (McCauley et al., 2000).
The goal of this review is not to delve into quantitative relations among airgun sources, environmental parameters and effects on animal taxa. Rather, we aim at providing a synthesis of the knowledge through a systematic and repeatable literature analysis.
In this study, we adopt the definition of ‘effects’ of anthropogenic activities specifically on marine mammals proposed by Erbe et al. (2019) as follows: behavioral responses, acoustic interference (i.e., masking), temporary or permanent shifts in hearing threshold (TTS, PTS), and stress (e.g., Richardson et al., 1995; Nowacek et al., 2007; Erbe et al., 2018).
The volume of airgun sources is expressed in cubic inches, shortened with the symbol in3, where 1 in3 = 0.016387 1.
2 Materials and methods
Systematic reviews aim to assess the largest amount of relevant and available scientific literature and to minimize bias using explicit search methods. Whereas narrative literature reviews do not provide procedure details (e.g., databases searched, search terms used), such information is necessary for systematic reviews to replicate the study or validate the interpretation and examine the comprehensiveness of the systematic search. In this way, systematic reviews are a tool complementary to narrative reviews. We acknowledge that systematic reviews are widely applied in health research but are less common in environmental science (Greenhalgh and Peacock, 2005).
This systematic review has been implemented following the guidelines from Snyder (2019) and Mikolajewicz and Komarova (2019) to perform a comprehensive literature search and screening process. Keeping a consistent approach throughout the search allowed us to tackle a transdisciplinary subject in a structured manner. Figure 1 shows a flowchart of the systematic literature review process.
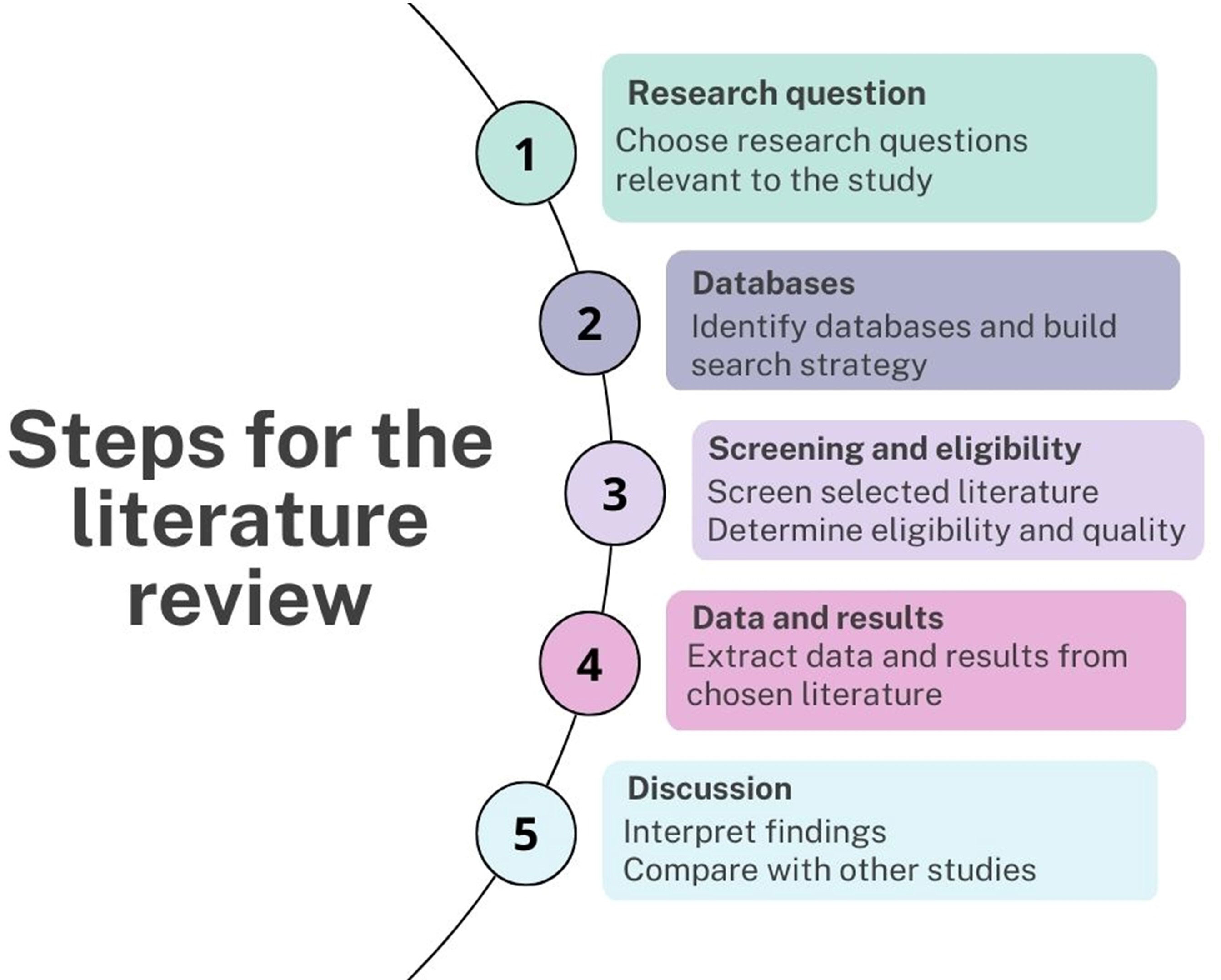
Figure 1 Flowchart showing the steps followed for this systematic literature review. Flowchart created with https://www.canva.com.
2.1 Keywords and search strategy
Following the process highlighted in Figure 1, we set out to address the first stage with the research question: “How do seismic airgun sources impact marine fauna?”.
In stage 2, the abstract and citation databases used in this analysis were Scopus® and Web of Science™ 2023 Clarivate (initial search February 7 - 11, 2022; validations performed on January 27, 2023, and July 16, 2023). Combining two databases ensured result validation since the Web of Science search engine is more selective than Web of Science. Before choosing the query string, the keywords and the Boolean operators were tested and paired. Through an iteration process, the results obtained were validated among the authors and through their comparison with a selection of expected relevant papers (e.g., Mikolajewicz and Komarova, 2019).
Two query strings were chosen to interrogate each database (Table 1). The terms composing Query String 1 were intentionally selected as broad as possible (“ALL fields” option was used) as suggested by Ahadi et al. (2022). Query String 2 was formulated in order to validate the search with the previous string (“Title-Abstracts-Keywords” were searched in the databases). The terms used in our query strings address source (‘Seismic’, ‘Airgun’, ‘Geophysical prospecting’), impact (‘Effect’, Impact’, ‘Sound Exposure Level’, ‘Behavioral effect’, ‘Physical effect’, Indirect effects) and recipient fauna (‘Marine mammal’, ‘Cetacean’, ‘Whale’, ‘Dolphin’, Pinniped’, ‘Seal’, ‘Bird’, ‘Fish’, ‘Reptile’, ‘Invertebrate’, ‘Amphibian’) are shown in Table 1.
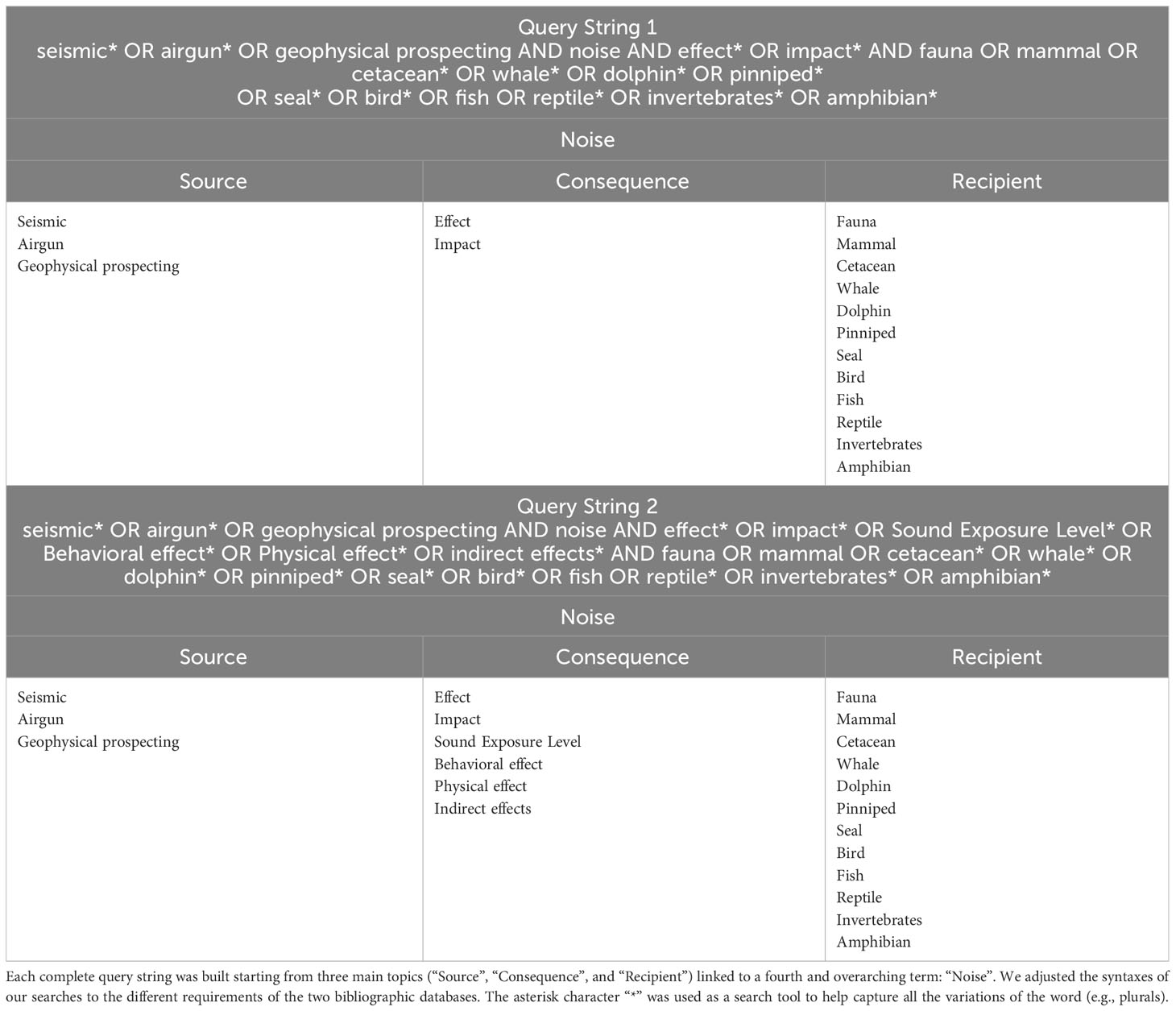
Table 1 Query strings used to perform the search on the two databases (Advanced search section in Scopus and Web of Science).
The search was not restricted to a time period, but we analyzed results up to and including 2022.
2.2 Screening process and quality assessment
In stage 3 (Figure 1), we manually screened the titles obtained from both database searches for the topic’s eligibility. A complete outline of the screening process is shown in the PRISMA (Preferred Reporting Items for Systematic Reviews and Meta-Analyses) flowchart in Figure 2. After screening the titles, we evaluated their abstracts, and, as a last step, we inspected the complete paper’s text. The resulting studies provided information relevant to the study aims, clearly stated the study context, their research objectives, and the results.
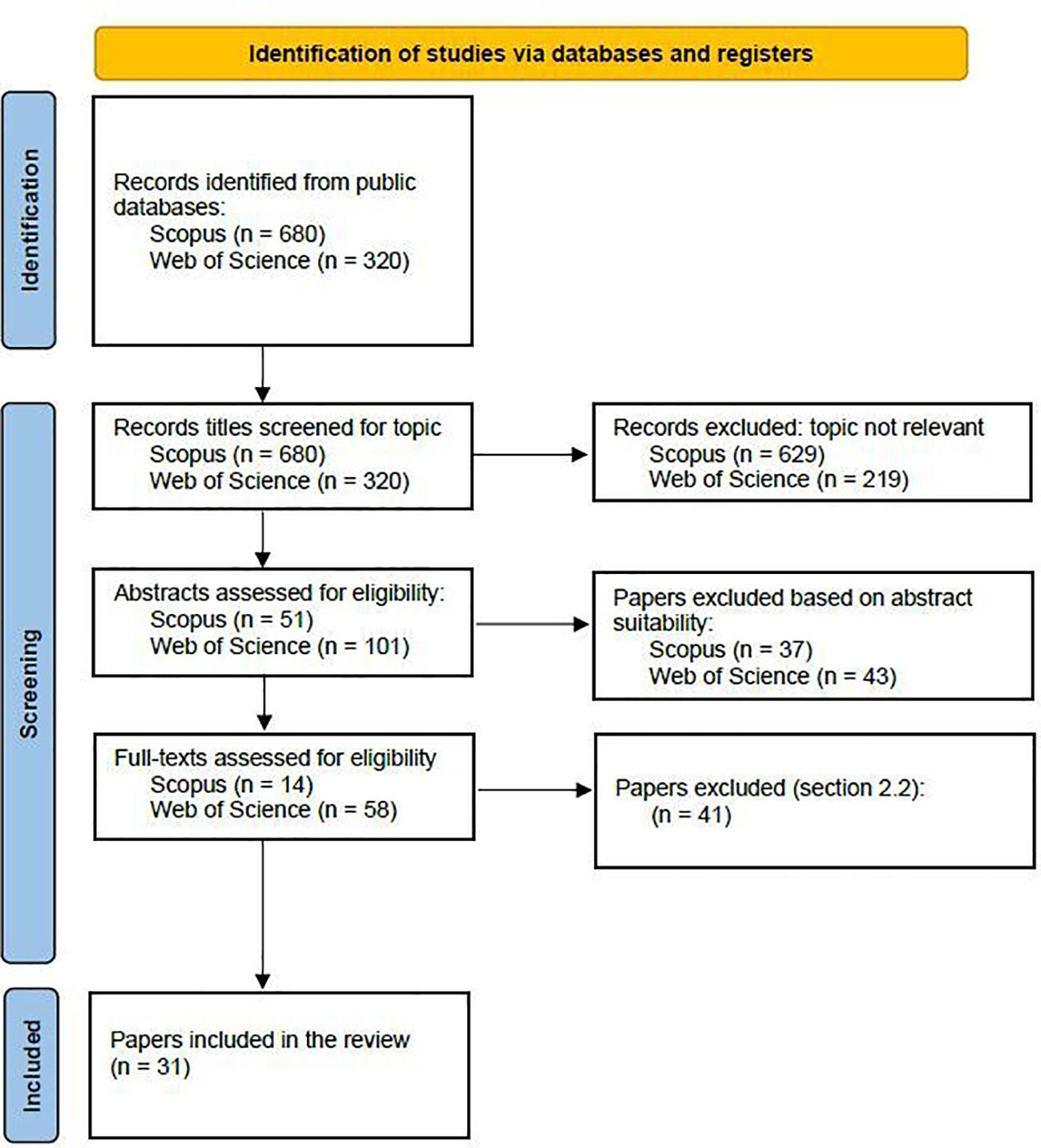
Figure 2 PRISMA 2020 flow diagram for new systematic reviews which included searches of databases and registers only modified from Page et al., 2021 (http://prisma-statement.org/prismastatement/flowdiagram.aspx).
Papers eligibility was screened following these inclusion criteria:
• Peer-reviewed original research articles dealing with effects (excluding peer-reviewed conference abstracts due to potential lack of detailed information and review papers, following Hackenbroich et al., 2022)
• Articles exclusively targeting responses to seismic surveys (therefore, excluding cumulative responses due to other anthropogenic stressors and papers studying responses to playbacks and ramp-ups)
• Articles addressing wild-ranging fauna
• Articles presenting fieldwork in the marine environment
• Articles written in English.
The Quartile of the scientific journal in which the articles were published has not been used as a criterion of inclusion/exclusion. When comparing the results from both strings, duplicates were removed.
A final Quality Assessment was conducted following the standard proposed by Dybå and Dingsøyr (2008).
2.3 Limits of the systematic search and methods used to reduce bias in the screening process
The two databases, Scopus® and Web of Science™, used to conduct the query string search were chosen to reduce potential bias in the study design. The Google Scholar database was not employed in our search because the automated database compilation (‘crawling’) provides no additional useful results considering our eligibility and quality assessment criteria.
The selection of research questions and keywords in the database search is crucial, although there is no entirely objective way for their choice (Pullin et al., 2018). Narrowing the spectrum with precise vocabularies may result in missing relevant papers. For this reason, keywords are kept general in order to include the largest amount of literature on the topic (Sierra-Correa and Cantera Kintz, 2015), and no arbitrary search limiters such as geographical regions or year of publication are included in the database search (e.g., Cumpston et al., 2019).
Quality assessment is a critical element of systematic versus narrative literature reviews. This step is mandatory since the broad search needed to avoid missing relevant papers also implies that many non-relevant papers are present in the search results (e.g., medical or engineering science papers). The term “quality” used in this context is not meant to describe the scientific value of a publication. Several papers within the broad aim of the review (i.e., the effects of seismic surveys on wild-ranging marine fauna) have been excluded from our analysis because they lack sufficient details necessary to answer the research question (Tran et al., 2021). In our case, the missing details causing exclusion of papers that may include good quality research in general terms published in Q1 journals are mainly on seismic sources used in the field experiment that do not satisfy the requirements set for our review (compilation of Table 2).
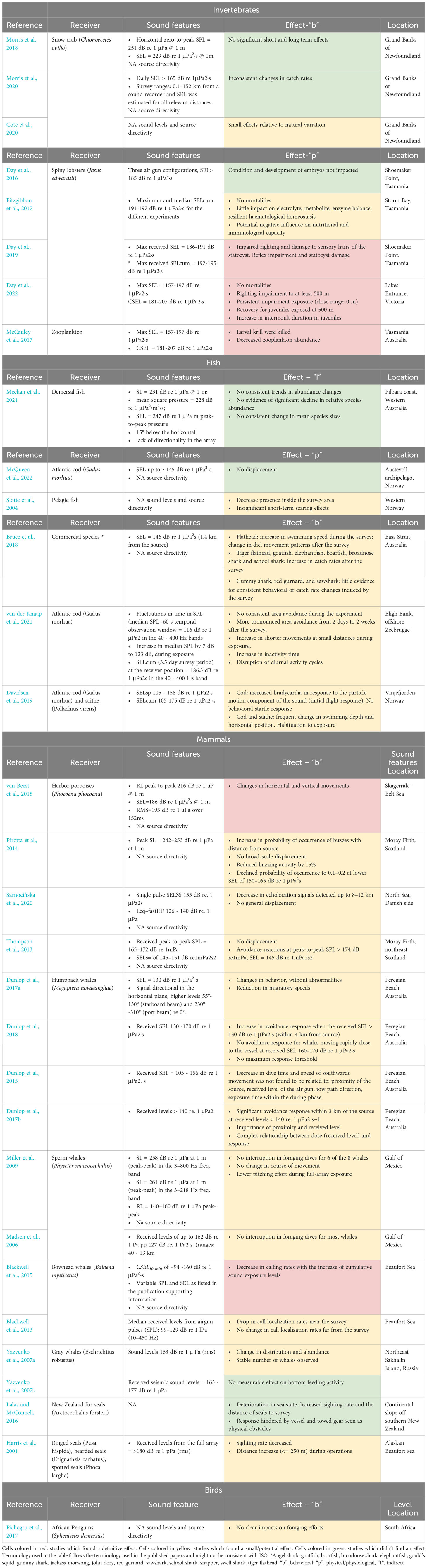
Table 2 Recipients, effects produced by seismic surveys, noise level, location, and reference of final studies are shown.
3 Results of the search and analysis of the final studies
3.1 General overview
This section analyzes the number of papers published in time, the recipients targeted, the scientific journals, and the locations of the final studies (Figures 3–7).
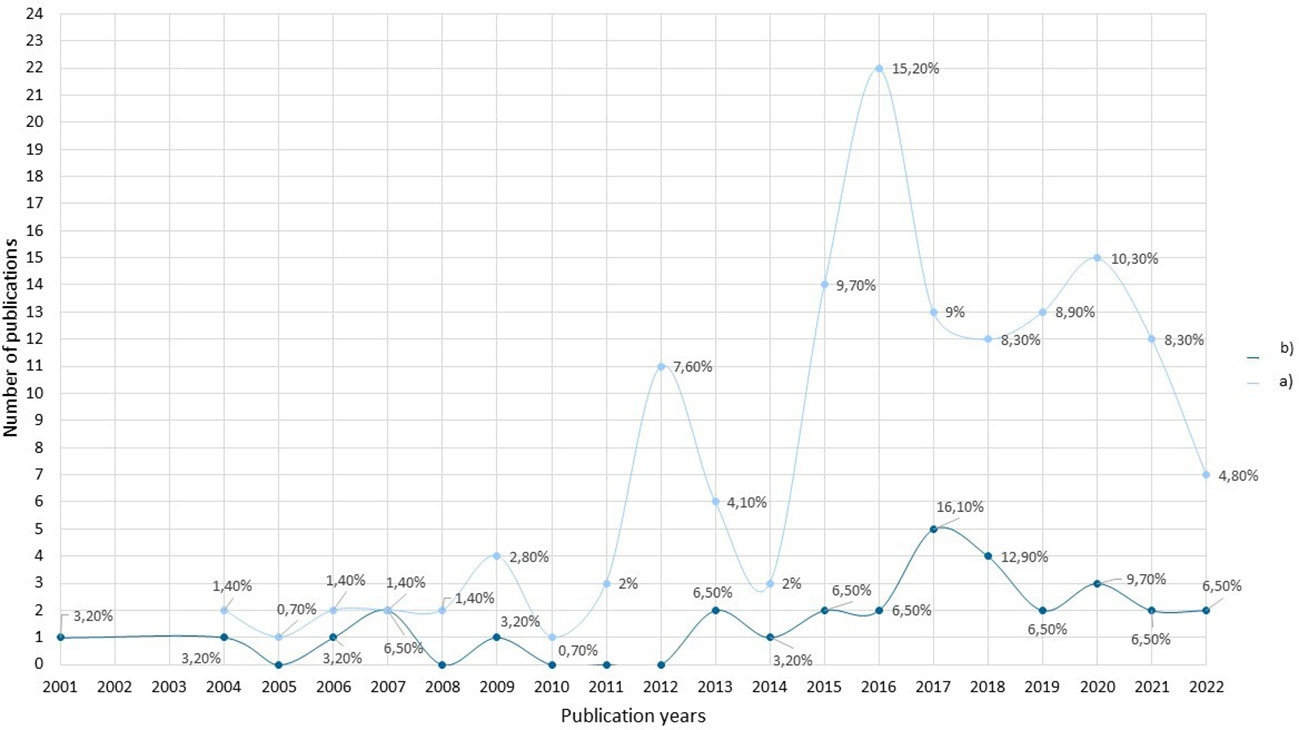
Figure 3 Trend in publication year of analyzed papers. (a) papers resulting from the first search with the query strings before the screening (n= 145); (b) Resulting papers (n=31). Percentages are relative to the total papers in each category.
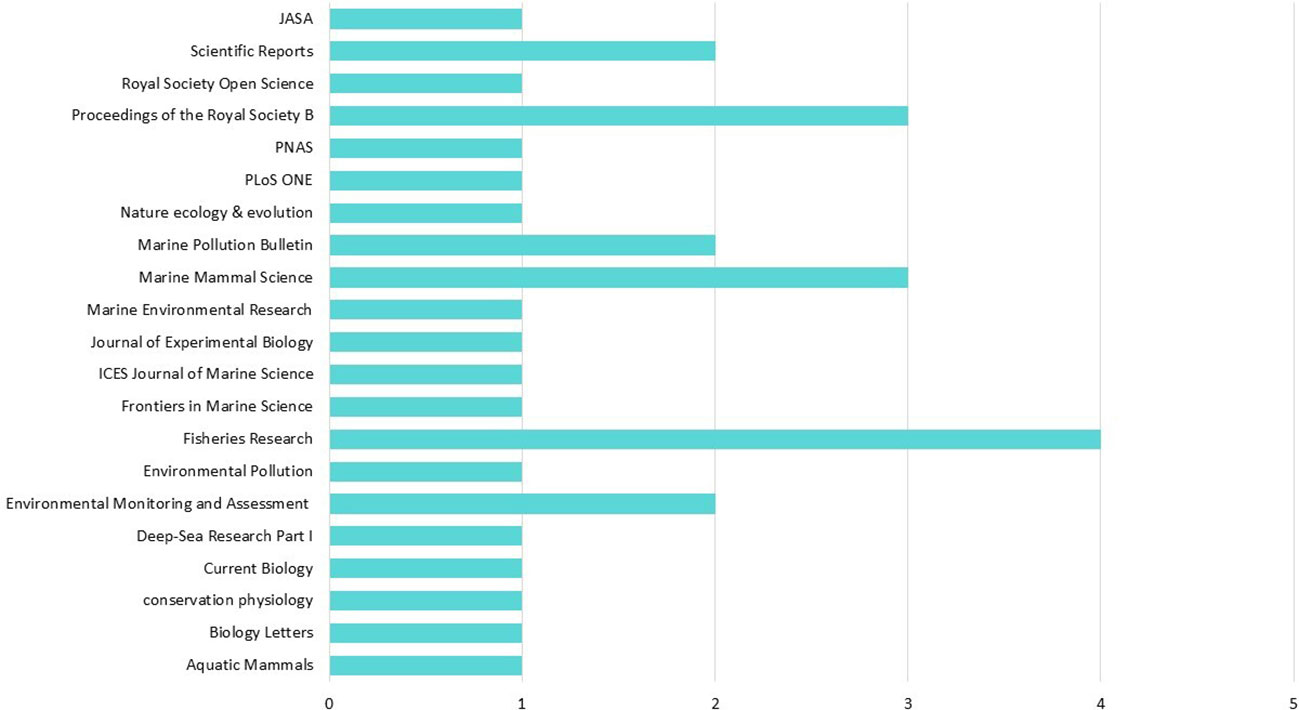
Figure 4 Scientific peer-reviewed journals in which the papers that passed the screening step are published. Complete Journal names that are shortened in the Figure: JASA, The Journal of the Acoustical Society of America; Proceedings of the Royal Society B, Proceedings of the Royal Society B-Biological Sciences; PNAS, Proceedings of the National Academy of Sciences of the United States of America, Deep-Sea Research Part I -oceanographic research papers.
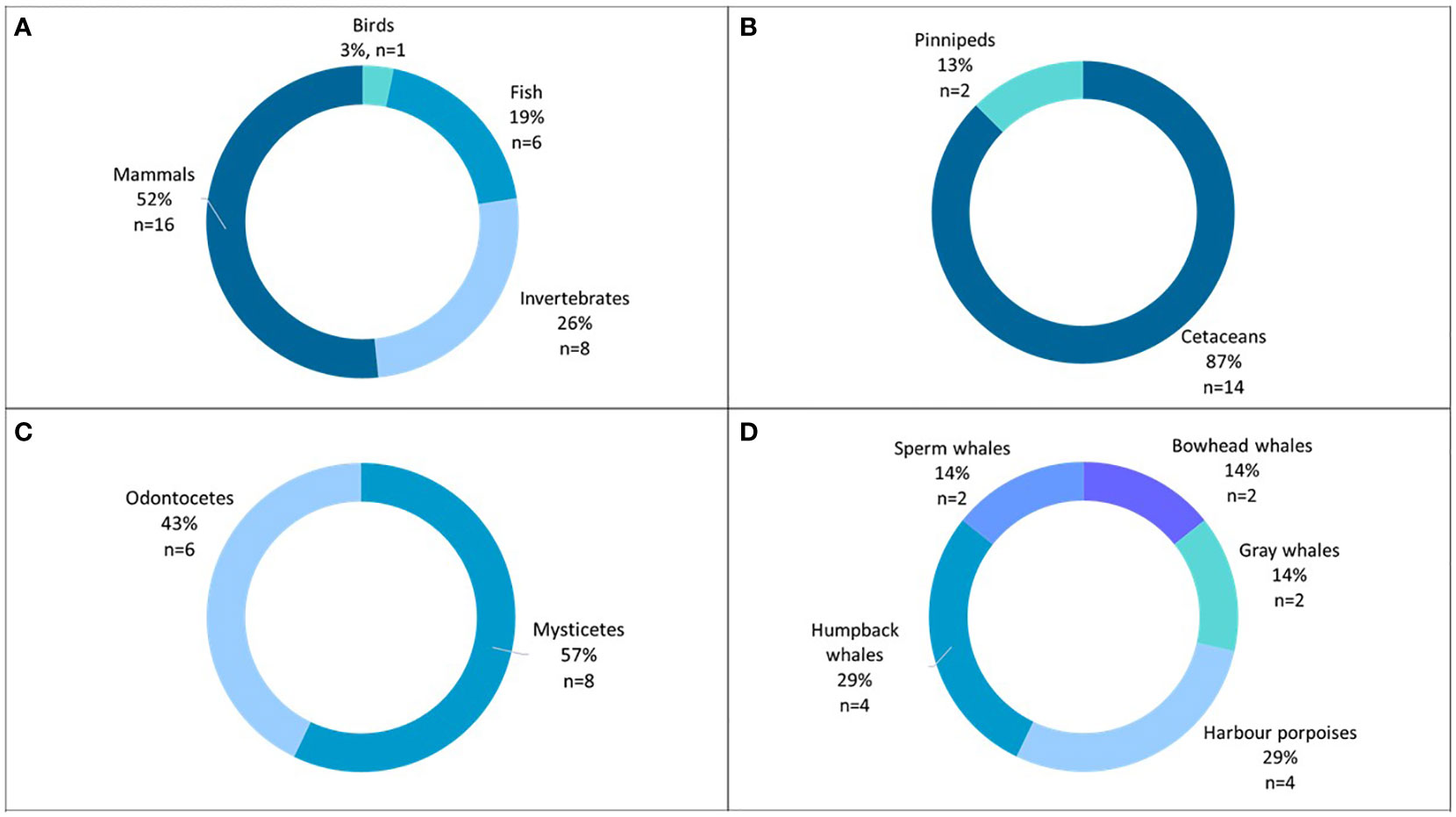
Figure 5 Animals studied in the 31 resulting papers (A) animal classes; (B) taxonomic groups, (C) cetacean parvorders, (D) cetacean species.
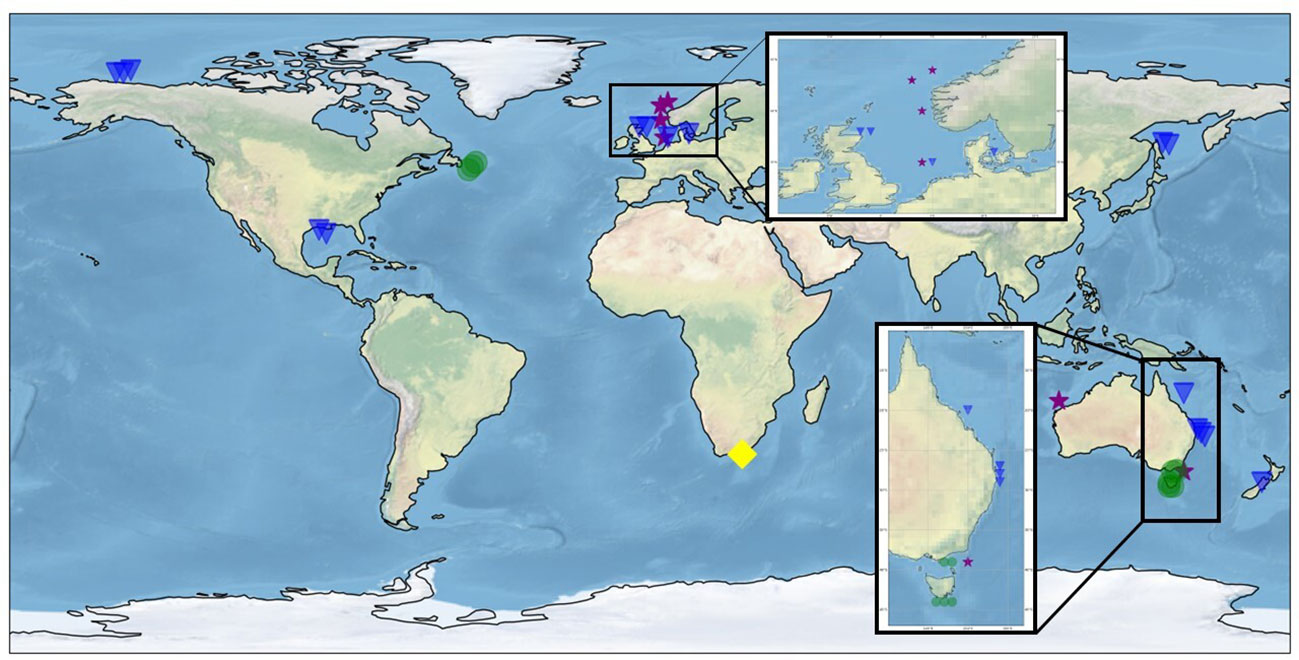
Figure 6 World map with locations of final studies on mammals (blue triangles), fish (purple stars), birds (yellow diamond), and invertebrates (green dots). The inserts show the main studies clustered in Eastern Australia and Northern Europe.
The first selection of keywords yielded 680 and 320 titles for Scopus and Web of Science, respectively. The screening and quality assessment processes resulted in the selection of 31 papers that were analyzed in terms of years of publication, journals, and study locations (Figures 3–6).
The trend of the resulting publications through time (Figure 3, trend b) shows an increase of the research effort from 2012 to 2017, while there is a decline in publications from 2017 to 2022. The trend a) in Figure 3 shows the yearly number of publications identified generically targeting seismic surveys (n=145).
The spectrum of scientific peer-reviewed journals in which the 31 papers that passed the screening titles were published is wide (31 papers published in 21 journals), with the largest number (n=4) published in the journal Fisheries Research, (Figure 4).
The most studied animal class (52%) is marine mammals, of which 87% are cetaceans, of which mysticetes are the most studied parvorders (Figure 5).
Most marine mammal studies were conducted in Northern European and Eastern Australia seas (Figure 6). Hotspots for invertebrate studies were identified in the Newfoundland and Tasmania continental shelves. Clustered studies appear to be parts of the same research project, frequently supported by the same funding agencies.
The word cloud of the keywords gathered from the abstracts of the 31analyzed papers if shown in Figure 7. The most frequently used words are: seismic, seismic survey, response, effect.
All the results presented in the following sections refer to Table 2 in which species, sound exposure levels and observed effects are described relative to each analyzed paper. Some of the articles describe experiments conducted in the framework of the same project and will be shown grouped under the same project umbrella. Since the studies referring to a common project benefit from a detailed description of the source used, all the papers were kept in this review.
3.2 Mammals
3.2.1 Cetaceans - Harbor Porpoise (Phocoena phocoena)
A ten-day commercial 2D seismic survey with a 470-in3 airgun array (number of airguns not mentioned) was conducted in 2011 by Thompson and colleagues (2013). Acoustic and visual comparison of detection rates of porpoises indicated avoidance responses, showing an increase in relative density at greater distances from the source. However, the disturbance effect was lower than natural variability, and the authors did not find a correlation between prolonged surveys and animal displacement.
Pirotta and colleagues (2014) used the same seismic survey to investigate additional effects. The authors found a 15% reduction in buzz inter-click intervals during the survey. Although there was a high natural variability in the detection of buzzes, the probability of occurrence of the buzzes increased significantly with increasing distance from the source.
During a 3D seismic survey lasting 103 days, Sarnocińska and colleagues (2020) studied two alternating airgun arrays made up of three subarrays of 21 elements with a volume of 3,570 in3. Over a short timescale (minutes), porpoise acoustic activity varied non-linearly with distance from the seismic source. Three indicators of porpoise acoustic activity were investigated: clicks per minute (CPM); porpoise positive minutes (PPM); and the ratio between minutes with buzzes and minutes with any click train calculated per hour: BPM/PPM). They did not differ between the control and the impact stations. The authors observed a dose-response effect with the lowest activity closest to the source vessel, increasing to an 8–12 km range.
Echolocation indexes decreased as a single airgun shot’s broadband sound exposure level increased. Large-scale responses (before, during, and after the survey) showed that before the seismic survey started, the mean CPM, PPM, and BPM/PPM did not differ between control and impact stations. Their mean values increased at both the control and impact stations during the seismic survey. By contrast, the mean BPM/PPM was lower during the seismic survey than before.
Van Beest and colleagues (2018) studied the exposure to a single 10 in3 airgun (reduced volume sleeve gun-I 40 in3). Effects on 5 tagged porpoises (2 females and 3 males) were evaluated. Porpoise ID3 (male) endured the highest noise exposure level of all individuals and showed abrupt changes in horizontal and vertical movement patterns during exposure. The animal altered its vertical movement following noise exposure with temporary declines in dive duration, maximum dive depth, and post-dive surface duration compared to baseline behavior. However, Porpoise ID 5 (female) showed no apparent airgun-related effects in vertical movement patterns. Porpoises with IDs 1,2,4 showed behavioral reactions unlikely to be airgun-related responses.
3.2.2 Cetaceans - Sperm whales (Physeter macrocephalus)
The papers related to the effects on Sperm whales (Physeter macrocephalus) acknowledged the same funding source (Minerals Management Service Cooperative Agreements 1435-01-02-CA-85186 and NA87RJ0445, the Office of Naval Research grants N00014-99-1-0819 and N00014-02-10187, and the Strategic Environmental Research and Development Program grant D8CA7201C0011) and investigated the effects on eight sperm whales in the Gulf of Mexico.
Miller et al. (2009) studied the effects of a dedicated seismic vessel towing an industry-standard airgun array. In 2002, the vessel towed a 20-airgun array (total volume of 1,680 in3). A year later, the vessel towed a 31-airgun array (total volume of 3,090 in3). In both years, the ramp-up procedure was used. Uninterrupted foraging dives were observed throughout the exposure. One of the whales, sw253a, performed typical deep dives alternated by shallower ones; this did not indicate a link between the change of dive pattern and the seismic source sounds. The whale named sw173b showed a bigger effect due to exposure. In this case, the foraging dive performed in the post-exposure condition appeared typical of such species. However, this whale produced a longer-than-average resting bout. The whales did not make strong turns away from the vessel during the survey periods, and the direction of movement during exposure matched movement in previous conditions. Apart from sw173b, the remaining whales exhibited lower pitch during exposure.
Sound exposure levels recorded by acoustic tags (ranges 1.4-12.6 km) from controlled airgun array sources were analyzed by Madsen et al. (2006). The analysis focused on a seismic campaign in 2002 (20 airgun array with a volume of 1680 in3) and in 2003, the seismic vessel used 28 airguns with a total volume of 2590 in3. Due to multipath propagation, the animals were exposed to complex propagation patterns during seismic operations, and high energy pulses (0.3-3kHz) when close to the surface.
3.2.3 Cetaceans - Humpback whales (Megaptera novaeangliae)
The papers analyzed in this subsection are dedicated to effects on southward migrating humpback whales (Megaptera novaeangliae) along the east coast of Australia. Experiments related to the Behavioral Response of Australian Humpback whales to Seismic Surveys (BRAHSS) funded by the Joint Industry Program on E&P Sound and Marine Life (JIP) and by the United States Bureau of Ocean Energy Management (BOEM), (https://www.brahss.org.au/content/project.html). The airgun source used had a volume of 20, 140, and 3,130 in3.
Dunlop et al. (2015) investigated humpback whale (Megaptera novaeangliae) groups’ behavioral response to a 20 in3 air gun. The source vessel moved across or into the migratory flow leading humpback whales to decrease dive time and to move quicker. Results did not show a difference in behavioral response between the control trials and the experimental groups; therefore, the authors conclude that there was evident impact related to the seismic vessel. However, the response magnitude did not depend on the distance from the source, the received level or the exposure time, or the tow direction.
Dunlop et al. (2017a) used the effects of an airgun array of 3,130 in3 (number of airguns not mentioned) during the southward migration of the Eastern Australian humpback whales. Acquisition was preceded by a 30- minute ramp-up phase of the source with volume steps of 40, 250, 500, 1440 in3. Focal and control groups of humpback whales were composed of males, females, and calves. All control groups approached the vessel to a minimum distance of 5.5 km. They significantly reduced their dive time (by 45 and almost 60 seconds, respectively), but returned to their usual diving time after the vessel stopped the survey. Individuals had an elevated blow rate (20% increase) that remained significantly elevated after the survey, suggesting a prolonged effect of the airgun stimulus. Focal groups containing a calf and multiple adult groups tended to have shorter dive times than other adult-only cohorts, as did socially interacting groups. The deviance of the group from their prior course and speed was significantly dependent on the combination of source characteristics, environment variables, and water depth. The general behavioral response to the survey was not considered outside the usual behavior repertoire.
In another study, Dunlop and colleagues (2017b) investigated the magnitude of the behavioral response of humpback whales to noise using a 20 and a 140 in3 airgun array in order to determine the existence of a dose-response relationship. The authors found that whales avoided the surveys inside an area of 3 km from the source if noise levels exceeded 140 re. 1 µPa2 s−1.
Building on the previous studies, Dunlop and colleagues (2018) developed a dose-response model in order to account for the survey vessel and the context of the exposure investigated and study whales’ behavioral responses to an air gun, a small clustered seismic array and a commercial array. In this case, whales showed a response at a 4 km distance from the source and when the received level was above 130 dB re 1 μPa2·s. However, estimating a maximum response threshold was challenging due to the variable behavior exhibited by the whales.
3.2.4 Cetaceans - Bowhead whales (Balaena mysticetus)
The studies by Blackwell et al. (2013) and Blackwell et al. (2015) were funded by Greeneridge Sciences, Inc. and Western EcoSystems Technology, Inc. and investigated the effect of seismic survey exposure on Bowhead whales (Balaena mysticetus) in the Alaskan Beaufort sea.
In the study by Blackwell et al. (2015) bowhead whales were exposed to seismic surveys from 2007 to 2010 using variable size airgun arrays (total volume 3,147 in3 for 3D surveys with 24 guns and from 20 to 880 in3 for shallow hazards surveys). Throughout the study, bowhead whale calls were localized at five sites. On average, the peak calling rate at the site farther away from the survey, was up to 5–6 times higher than that at other sites. The study shows an unexpectedly complex change in bowhead whale calling behavior to received levels of large volume, 3D airgun surveys, characterized by increase, plateaus, and decrease patterns. However, for remote seismic operations or low-level shallow hazard surveys, the increase in calling was considered not to be significant.
Blackwell and colleagues (2013) investigated bowhead whale call localization rates in sites close and far to the seismic operations before, during, and after airgun use. Once the airguns were operating, call localization rates decreased near the survey, but stayed unchanged at a distance. The decrease might result from cessation of vocalizations or avoidance of the activities, but the authors did not have enough information to pick one of the two hypotheses.
3.2.5 Cetaceans - Gray whales (Eschrichtius robustus)
The results by Yazvenko et al. (2007a); Yazvenko et al. (2007b) were in the framework of oil and gas operations by Exxon Neftegas Limited offshore Sakhalin Island, Russia. DalMorNefteGeofizika conducted a 3D seismic survey from August 17 to September 2001. The data was collected with aerial surveys from July 19 to November 19. The volumes used were 100, 370, 1,640, and 3,090 in3.
Yazvenko et al. (2007a) collected data on the distribution and abundance of gray whales after the 3D seismic survey and in relation to environmental factors. Date and proxies of depth, sea state, visibility, and the received sound energy accumulated over 3 days significantly affected distribution and abundance; however, the number of whales remained unchanged during the survey.
Frequency of mud plumes observed on the surface were used by Yazvenko et al. (2007b) as proxies of gray whales feeding activity. The results highlighted that seismic surveys did not hinder feeding activities; on the other hand, the transect number (used as proxy for water depth) and swell height (used as proxy for sea state) could be correlated with an impact from surveys.
3.2.6 Pinnipeds - New Zealand fur seals (Arctocephalus forsteri), ringed seals (Pusa hispida), bearded seals (Erignathus barbatus), and spotted seals (Phoca largha)
Lalas and McConnell (2016) recorded the responses of New Zealand fur seals (Arctocephalus forsteri) during daylight hours during a 3D seismic survey (two identical airgun arrays with a volume of 3,090 in3) offshore southern New Zealand. Results were not conclusive since the sighting rate and the distance also decreased with deteriorating sea state and the survey vessel and the towed instruments created obstacles that elicited a response.
Numbers, distance, and behavior of ringed seals, bearded seals, and spotted seals were investigated during seismic operations offshore northern Alaska (July-September 1996; 11 Bolt 1900LX airguns with a total array volume of 1,320 in3; Harris et al., 2001). About 79% were first seen within 250 m of the seismic vessel, and the sighting rate declined rapidly at lateral distances >50 m. Seals tended to stay farther away during full-array seismic. There was partial avoidance of the zone <150 m from the boat during full-array seismic, but seals apparently did not move much beyond 250 m.
3.3 Invertebrates
3.3.1 Crustaceans
The projects whose main results are described in this subsection belong to the same funding framework (Environmental Studies Research Fund and Fisheries and Oceans Canada) and target the effects on snow crabs (Chionoecetes opilio). In this project, total volumes of airgun arrays of 4880 in3f or the 2 D and of 4,130 in3 for the 3D were used.
Morris et al. (2018) reported on Before-After-Control-Impact experiments in 2015 and 2016 to study prolonged exposure to a 2D commercial seismic survey. The median daily sound exposure level was higher in 2015 than in 2016 (13 dB higher at the control site and 11 dB higher at the treatment site). The seismic source did not generate interface waves that would increase particle motion at the seabed. Catch rates during the survey varied with water depth, but no correlation could be found between catch rates and exposure to the seismic survey.
In a later work, Morris and colleagues (2020) found a consistent difference in SEL between the new and previously studied 2D and 3D seismic surveys in the same area in 2017 and 2018. 2D seismic surveys increased the daily SEL by −30 dB in the survey area on the day of the survey. In comparison, 3D seismic surveys similarly increased the daily SEL in each ship pass, while multiple passes generated an overall larger increase in daily SEL. As in the 2018 study, the conclusion was that the significant factor affecting catch variability was water depth and not exposure to the 2D seismic survey. In relation to the 3D seismic surveys, catch rates were found to be greater two weeks after than during exposure. In 2017, the authors found a 95% reduction in catch rates during exposure compared to two weeks after the exposure. In 2018, an increase of 204% occurred after several weeks of exposure, followed by a decrease of 43% two weeks after the end of the survey.
Cote and colleagues (2020) studied telemetry tracks for 245 snow crabs (78% of individuals tagged) in relation to a 2D commercial survey vessel. Crab velocity in movements outside the seismic exposure was influenced by time since release, the hour of the day, and water temperature. These invertebrates tend to increase their velocity at night and in warm waters, especially following release and until they stabilize after 60 h. The authors studied the behavioral states by analyzing step lengths and turning angles during seismic exposure. Although snow crabs changed three behavior states, their behavior and movement direction did not show correlations with seismic exposure. The average movement direction was consistent after exposure but not before and after.
The results of the first three studies described below belong to the Fisheries Research and Development Corporation (FRDC Grant 2012/008). The four manuscripts describe effects of seismic surveys on the spiny lobster (Jasus edwardsii). A Sercel G Gun II with 45 in3 or 150 in3 chamber was used.
Day et al. (2016) studied the exposure of egg-bearing female spiny lobsters to signals from three airgun configurations. Based on the conditions of the hatched larvae, the authors maintained that there were no indications of impacts of seismic surveys on larvae fecundity, morphology, and competency.
Four controlled field experiments were conducted by Fitzgibbon et al. (2017) to examine the effects of seismic acoustic signals on spiny lobster. The results of the total haemocyte count and haemolymph refractive index tests performed after exposure showed that exposure to seismic sources can impact lobsters’ immunological capacity and hamper nutritional conditions. On the other hand, the investigation of 24 haemolymph biochemical parameters indicated that no impact was found after exposure.
Results following exposure equivalent to a full-scale commercial array (100–500 m distance), exposure to air gun signals caused morphological damage to the statocyst of lobsters. J. edwardsii showed reflex impairment and statocyst damage persistent up to 365 days post-exposure and did not improve after moulting (Day et al., 2019).
Day et al. (2022) investigated mortality rates, dorsoventral righting reflex, and progression through moult cycle of J. edwardsii juveniles and puerulus lobster exposed to seismic surveys. Exposure did not result in mortality, although it caused righting impairment in adults and juveniles. The authors found a significant increase in intermoult duration in juveniles very close to the source; the change in this parameter might indicate the potential for hindered development, growth, and an increase in stress response.
3.3.2 Zooplankton
In March 2015, during a 2-day seismic survey with a single 150 in3 airgun, McCauley and colleagues (2017) studied thirty-four plankton taxa in net tows with 189 taxa/tow combinations. The received sound exposure levels were: 156 dB re 1 μPa2s−1 (183 dB re 1 μPa peak-to-peak) at 509–658 m range, and 153 dB re 1 μPa2 s−1 (178 dB re 1 μPa peak-to-peak) at 1.1–1.2 km range. After air gun exposure, there was a statistically significant lower zooplankton abundance (reduced by ≥50% of the number of individuals per m3 216 of water). Abundance reductions after exposure of no-change, 25%, and 50%, compared with control values, occurred at ranges of 808, 639, and 409 m from the seismic source, respectively. There were two to three times more dead zooplankton specimens after exposure compared with control treatments, and all krill larvae were dead following exposure.
3.4 Fish
3.4.1 Demersal fish
Meekan et al. (2021) investigated the impacts of a 5-day long commercial 3D seismic survey using two airgun arrays (10 Sercel G Gun II) of 2,600 in3. The researchers did not find evidence of major changes in the fish assemblage structure or species richness. No change in the mean size of L. punctulatus, L. sebae, L. vitta, Epinephelus areolatus, Epinephelus multinotatus, Plectropomus maculatus and in the time of first feeding for Nemipterus furcosus, A. stellatus, L. punctulatus, L. sebae, L. vitta, E. areolatus, E. multinotatus, Argyrops notialis, P. maculatus was found. The authors argue that there was no change after exposure in the likelihood of feeding on the bait for L. vitta, E. areolatus, E. multinotatus, A. notialis.
3.4.2 Pelagic fish
The spatial distribution and abundance of pelagic fish before, during, and after three 3D seismic surveys (2 arrays of 20 airguns each with a total volume of 61800 in3) were investigated by Slotte and colleagues (2004). Abundance increased moving away from the center of the seismic survey area during the first two surveys but not during the third one. Fish vertical migration was influenced by the time of day and the seismic activity in both blue whiting (Micromesistius poutassou, 10 m deeper during shooting periods) and mesopelagic fish (50 m deeper). The conclusion is that with the exception of a possible influence on vertical movement, there is no evidence of substantial short-term effects.
McQueen et al. (2022) investigated potential cod (Gadus morhua) displacement caused by seismic surveys from spawning grounds. The tagging fieldwork spanned from 2019 to 2021; 136 mature cod in the test site and 45 in the reference site were equipped with acoustic transmitters. The seismic survey lasted for 1 week during the spawning seasons in 2020 and 2021 and was conducted with two 40-in3 airguns. Movement variations varied between 2020 and 2021, but were similar between test and reference sites, indicating no significant cod displacement due to seismic exposure (received SEL up to 145 dB re 1 μPa2 244 · s).
In another study focused on the movements of free-swimming Atlantic cod (Gadus morhua), van der Knaap and colleagues (2021) analyzed the effect of a full-scale seismic survey. The results showed that while cods did not avoid the area during the survey, they did from 2 days to 2 weeks after the survey. However, during the experiments, G. morhua exhibited disruptions of diurnal feeding activities, unraveling an issue that could potentially hinder energy budgets leading to consequences at the population level.
Davidsen et al. (2019) performed a short-term field experiment using a Bolt Longlife airgun (40 in3) to investigate the effects on Atlantic cod (Gadus morhua) and saithe (Pollachius virens). Biologgers and acoustic transmitters recording heart rate and body temperature and acoustic transmitters recording acceleration and depth were used to monitor free-swimming individuals and the behavior of cod and saithe was also monitored using video recordings. The authors stated that cod suffering from bradycardia due to particle motion might be an indication of an initial flight response. Although no startle response was observed, cod and saithe increase unpredictability of swimming depth and horizontal position. Results show fish habituation when exposed to repeated sounds, and airgun signals used in the study are unlikely to cause long-term physiological or behavioral effects.
In the experiments performed by Bruce et al. (2018), a 2D marine seismic survey was undertaken over part of the western Gippsland Basin with a single 2,530-in3 airgun array (16 airguns). During the seismic survey, no tagged swell sharks (Cephaloscyllium ventriosum) were found near the array. Environmental variables were also investigated and were found to be crucial. Where the water temperature was high, the swell sharks covered less distance, and sharks tagged during the control experiment moved more with respect to the experimental array. Average daily temperature and hour of the day were the most significant variables for gummy sharks (Mustelus antarcticus) and swell sharks, respectively. On the other hand, the combination of hour of day, temperature, and period with respect to the survey was found to be essential variables in connection with flathead sharks (Apristurus macrorhynchus) movements; these animals were more sedentary before, during, and after the survey.
During the six months following the survey, six species (tiger flathead, goatfish, elephant fish, boarfish, broadnose shark and school shark) showed an increase in catch rate, and three species (gummy shark, red gurnard, and sawshark) showed reductions. Little consistency in behavioral responses or catch rate changes was found in connection with the seismic survey.
3.5 Birds
3.5.1 African Penguins (Spheniscus demersus)
The impact of seismic surveys on penguins was investigated by Pichegru and colleagues (2017). 2D surveys were made with four arrays with a total volume of 4,230 in3. No information is available on sound levels. During the surveys, penguins showed a marked avoidance of foraging areas and a preference for foraging areas farther away from the vessel. Foraging behavior reverted to normal after the end of the survey. However, a group of penguins stationed in St. Croix Island preferred foraging areas closer to the seismic survey vessel’s location in 2013. By contrast, Bird Island penguins consistently traveled away from their colonies, regardless of seismic activities. The authors stated it was not possible to distinguish if penguins’ avoidance reaction was due to a potential change in prey distribution or by a disturbance caused by noise.
Table 3 shows how many of the resulting studies investigate effects from large-scale oil and gas surveys or smaller studies that can be compared to research-style surveys.
4 Critical discussion on the main results from final studies
4.1 Lack of results on a broad variety of animal taxa
The analyzed bibliography confirms that the marine organisms that are mostly studied in the natural environment to assess the effects of seismic surveys are mammals and, more specifically, mysticetes (Figure 4). The reason for this focus is that these apex predators rely on sound for most of their biological functions and that sound can influence the presence of other predators or their prey (Glen and Dickman, 2014). However, odontocetes represent the first trophic level in marine ecosystems, and a first important consideration drawn from this review is that little knowledge exists on species belonging to other levels of the trophic chain that play an important ecological role.
Even if still scarce, the number of studies on fish and invertebrates has proliferated in recent years (e.g., de Jong et al., 2020; Solé et al., 2023), allowing for an improved understanding of the possible consequences of marine seismic surveys on ecosystem dynamics (Williams et al., 2015). Particular attention should be paid to the fact that fish and invertebrates are sensitive to the particle motion component of sound. Only a few studies reported values for particle motion (e.g., Table 2; Popper and Fay, 2011; Morley et al., 2014), and the use of sound pressure to derive particle motion is not commonly feasible (Day et al., 2022).
Long-term impacts data on fish is often missing (Carroll et al., 2017; Hawkins and Popper, 2017). McQueen et al. (2022) and Slotte et al. (2004) did not find evidence of any specific short-term effects on cod and shark behavior. However, because tagged cods were not displaced from their spawning grounds in this study, it cannot be excluded that the vertical movement of mesopelagic species indicate a potential short-term reaction to anthropogenic noise. Regarding shark behavior, Bruce et al. (2018) did not find consistencies among observed impacts, and their survey revealed insignificant short-term effects. Nevertheless, despite the scarce data on the physical and behavioral effects of seismic surveys on fish, with behavioral effects being the most studied (Hawkins and Popper, 2017; Popper et al., 2014), the American National Standards Institute has provided peer-reviewed recommendations for setting criteria for fish. Sound exposure criteria need to be provided for non-hearing taxa (e.g., invertebrates, Hawkins and Popper, 2017), while the development of criteria has been focused on protected species of marine mammals. In addition, there is still an incomplete understanding of impacts on invertebrates (Day et al., 2016). More specifically, there is a lack of studies focussing on effects on marine invertebrate embryos, and some authors (e.g., Day et al., 2016) highlight the potential disputableness of some of the methods used to obtain the published results.
The only resulting study investigating marine birds offers some explanations for potential disturbances by seismic surveys observed in penguins. Due to the lack of data, Pichegru et al. (2017) discuss potential general effects on marine mammals and state that some information is available only on the underwater hearing capacities of cormorants. However, it is unclear whether penguins’ avoidance is a direct disturbance from the noise or a consequence of prey limitation (Pichegru et al., 2017), and the authors do not have enough definitive results.
4.2 Focus on single Vs. multi-species studies
Most of the research analyzed focus on the effects of seismic surveys on one species in a specific area. The exceptions that tackle more than one species are 4 studies analyzing fish (Table 3; Bruce et al., 2018; Davidsen et al., 2019; Slotte et al., 2004; Meekan et al., 2021).
We note a general interest in analyzing fine-scale movements and individual animal behavior in response to exposure. For example, van Beest et al. (2018) investigated individual harbor porpoises’ fine-scale movement responses, concluding that inter-individual variability is frequent, with reactions varying by gender, age, sexual condition, and other physical factors such as habitat use. While detailed knowledge is important, pursuing a detailed-oriented approach might lead to a lack of a comprehensive view. Impact assessments are often not transferable nor generalizable, and there is a strong need for a standard procedure that can be applied, under given hypotheses, to different groups of individuals in different areas (Przeslawski et al., 2018). Day et al. (2019); Day et al. (2022) compared impacts on lobsters in cages produced by a commercial seismic source (MV Geo Coral 2820 in3) with those produced by a single 150-in3 airgun source claiming that the exposure can be scaled from the single source to a large array such as those used for commercial geophysical surveys. In contrast, Gomez et al. (2016) argue that theoretical values of sound exposure, or values obtained in experiments, cannot be extrapolated among different groups of individuals or species.
There is a need for more comparison of effects among animal classes (e.g., Erbe et al., 2019; Williams et al., 2015) and for comprehensive studies that bridge the gap among disciplines and integrate visual observations, passive acoustic monitoring, tagging studies, environmental variables, and numerical simulations (Kellet et al., 2014).
Furthermore, all studies on fish (e.g., Cod (Gadus morhua)) and the seven studies on invertebrates (i.e., the Southern Rock Lobster (Jasus edwardsii) and the snow crab (Chionoecetes opilio)) investigate commercially important organisms. The focus on commercial species, however, is not as common as it may appear from this review’s systematic selection (Edmonds et al., 2016; Carroll et al., 2017).
4.3 Changes in behavior and physiological effects
Observed changes in movement behavior (e.g., swimming speed) showed extensive variability, depending on the taxa, exposure conditions, specific activity, and context (e.g., Davidsen et al., 2019).
As mentioned by Yazvenko et al. (2007b), studying the effects on animal activity patterns (e.g., foraging) might be particularly challenging also due to a lack of related available literature.
In the experiments conducted by Dunlop et al. (2017a), the behavior of migrating humpback whales did not depend on the presence of the seismic vessel but only on the presence of other whales and the engaged behavioral activity. Group composition influence movement patterns and dive parameters. Female–calf pairs were reported to be performing consistent course deviations even after the end of the survey or when exposed to low received levels. Even if the seismic source characteristics were not reported, Cerchio et al. (2014) reported significant decrease in the number of humpback whale singers with increasing received levels of the surveys. From this observation, authors infer a disruption in breading display.
Heide-Jørgensen et al. (2021) argued that narwhals were affected by airgun pulses and tried to avoid the source by changing their horizontal swimming direction. On the other hand, Miller et al. (2009) reported that sperm whales did not diverge from their course of travel to avoid the seismic vessel inside an exclusion zone of 13 km. Satellite observations on tagged sperm whales during multi-year seismic prospecting activities in the Gulf of Mexico suggests that distances and orientations between whales and active seismic vessel airgun arrays at this scale appear to be randomly distributed with no evidence of horizontal avoidance (Winsor et al., 2017).
However, as mentioned by Southall et al. (2021), the absence of behavioral responses does not equate to an absence of impact. Miller and colleagues (2009) further noted that the whales reduced their foraging rate even at moderate received levels and large distances from the sound source. A rare study about the seismic survey effect on penguins inside a survey exclusion zone of 100 km showed a change of foraging direction away from the vessel (Pichegru et al., 2017). It can be argued that in specific circumstances (e.g., foraging, mating), animals need to trade-off between exposure to noise and nutritional needs or reproductive opportunities (Lusseau, 2014). Although animals might be exposed to airgun noise regularly depending on their habitat, habituation cannot be considered a solution to the problem of underwater noise.
Biologically significant behavioral changes might affect one or more life functions given the importance of the animals’ energetic status (van Beest et al., 2018). Blow rates of humpback whales increased by ~ 20% during and after exposure to airgun pulses (Dunlop et al., 2017a). Displacements of individuals harbor porpoises or groups from feeding areas or disruption of natural behaviors implies an increase in energy consumption (van Beest et al., 2018; Wisniewska et al., 2018; Sarnocińska et al., 2020). Different marine environments may stir animals’ energetic demands. For example, cetaceans living in cold environments might have high energy demand or may be vulnerable to energetic migration costs resulting in implications for the growth potential of calves (Dunlop et al., 2017a; Sarnocińska et al., 2020).
Among behavioral effects, changes in vocalizations are often observed. When discussing vocalization changes due to noise, the available literature usually refers to the Lombard response - a change in vocal amplitude or acoustic frequency due to noise (Gomez et al., 2016; Thode et al., 2020). The Lombard effect has an energetic cost because “vocal performance affects metabolic rate” (Holt et al., 2015).
Observed changes in porpoises’ buzzing patterns could be linked to disruption of foraging and may be the result of an indirect effect of noise (Pirotta et al., 2014). A reduction in vocal activity of harbor porpoises during exposure to a seismic survey was highlighted by Pirotta and colleagues (2014). According to Blackwell et al. (2015) “Cessation of calling or vocalization variability is likely one of the first measurable behavioral changes”. The authors maintained that whales resumed calling very rapidly after shut-down of the seismic sources.
Dunlop et al. (2015); Dunlop et al. 2017a; Dunlop et al. 2017b; Dunlop et al., 2018) conducted their experiments on the effects of seismic surveys on humpback whales in the framework of the BRAHSS project. During the years, the authors found a high variability in behavioral response which, although more likely to occur within the avoidance zone, was inconsistent among groups in terms of onset, duration, and received level. Dunlop et al., (2017a) and colleagues did not find evidence of threat perception (no fight-or-flight response), changes in dive behavior or respiratory rates.
Physiological effects were reported in studies investigating invertebrates (mostly lobsters) and fish and showed variability depending on taxa and growth stage (Table 2). Seismic surveys did not result in invertebrates mortalities with the exception of larval krill (McCauley et al., 2017). Negative influences on lobster nutritional and immunological capacity, impaired righting and damage to sensory hairs of the statocyst were identified in the fieldwork performed by Day et al. (2019); Day et al. (2022). The authors also found persistent righting impairment in adult individuals at close range from the source, and an increase in intermoult duration in juveniles. Slotte and colleagues (2004) did not find “convincing evidence of short-term scaring effects” on cod.
4.4 Problems in standardization of experiments and in choosing metrics
Research studies on behavioral reactions of cetaceans to anthropogenic sounds report a wide variety of responses (Blackwell et al., 2013). When dealing with the effects of seismic surveys on marine fauna there is a need for common metrics (Ainslie et al., 2017; de Jong et al., 2021). This need is being stressed particularly in the work of the ISO groups. Metrics should be reported according to the International Organization for Standardization, ISO 18405:2017.
Differences in behavioral and social conditions limit the potential for comparisons among taxa, species, and individuals (Gomez et al., 2016). Moreover, a high variability in vocabulary is used in studies to describe behavioral activities (Ainslie et al., 2021). In addition, most studies lack complete information on seismic sources and make use of dissimilar methods of measuring responses, and received levels definitions (e.g., peak-to-peak, RMS, bandwidth; ISO 18405:2017, Table 2). Moreover, sound propagation metrics (e.g., water depth, bathymetry, seabed, and subsurface composition) are rarely reported from measurements or assumptions. Sound exposure criteria have been adopted for marine mammals (Southall et al., 2007; National Marine Fisheries Service, 2018; Southall et al., 2019). Southall et al. (2007) published a set of recommended metrics that should consider the species, the exposure context, and the specific metrics. Nevertheless, criteria differ worldwide, and researchers and stakeholders are aiming to implement common and complete metrics (Hawkins and Popper, 2017).
We believe that there is a focus on the effects on small size groups of individuals, and a general need for more information, shared methodologies and data, and standardization. As an example, following the new threshold values for impulsive noise that should be implemented in all EU regions (Sigray et al., 2023), Juretzek and colleagues (2023) presented three regional case studies that allowed them to evaluate noise registries data availability, quality, and accuracy.
Bridging the gap of disciplines through interdisciplinary collaborations is needed to advance scientific knowledge, as suggested also by Kellet et al. (2014) in the framework of the effects of ship radiated noise.
Finally, it is worth noting that some authors focus on studies whose objective is oil and gas exploration surveys. As an example, Meekan et al. (2021) used measurements of SEL, keeping the exposure as close as possible to industry surveys operating in similar water depths. In 2016, Day and colleagues performed their experiments using three different survey configurations suited to intermediate resolution shallow geological investigations, or shallow gas surveys, exploring the dose dependency and the environmental contribution. The resulting SEL (referred to lobsters in this case) was tentatively compared to that produced by large-scale industrial seismic surveys. We note a paucity of studies (Table 2) aimed at assessing the impacts of the typical source parameters used for academic marine geophysical research commonly employed by research vessel fleets worldwide.
4.5 Context variability
Context plays a role in determining response variability, in terms of the immediate social environment of the animals and their behavioral state (Ellison and Frankel, 2012; Ellison et al., 2012). Other aspects, such as proximity of the source (not just received levels) need to be factored in (Dunlop et al., 2018). In addition, the animal may be avoiding not just the seismic source but also the survey vessel (Dunlop et al., 2017a). In their review of the effect of underwater noise on marine mammals, Gomez et al. (2016) assessed the importance of coupling a specific Received Level (RL) with contextual information. The authors argued that a more severe behavioral response does not necessarily correspond to a higher received level, and additional information is needed to establish a relationship dose-response (see also Dunlop et al., 2017b). For example, different cetaceans belong to different functional hearing groups, and their response profiles depend on the source type (Gomez et al., 2016, Table 4). Intrinsic characteristics of each sound source may be important in predicting the severity of behavioral responses. McCauley and Duncan (2017) went a little further and speculated that signal sharpness is the feature that causes physiological effects in marine invertebrates.
5 Conclusions
We conducted a systematic review of the scientific literature of the peer-reviewed studies addressing the effects of seismic surveys on free-ranging marine fauna. The advanced search was performed in Scopus® and Web of Science™ 2023, yielding a total of 1000 peer-reviewed papers which were screened using various eligibility criteria. This systematic literature review addresses the resulting 31 relevant papers spanning in time from 2001 to 2022.
The main conclusions of this literature review are:
• The relevant animal classes in the research studies are: mammals (n = 16), invertebrates (n=8), fish (n=6), and birds (n=1). There is still a disparity in the number of papers focusing on mammals with respect to other classes. There is a lack of studies that analyze more than one taxon. Most of the studies address a single species (e.g., Harbor Porpoises); when addressing more than one species, these belong to the same class (e.g., fish). Frequently, several papers are published using the same data of one experiment funded within one research project. This situation might lead to the creation of hotspots where the majority of studies are clustered.
• A variety of responses is linked to seismic surveys effects. For example, observed behavioral changes cannot be always uniquely attributed to the exposure to seismic surveys. Sometimes, authors reported the influence of other factors (e.g., movement patterns also influenced by prey availability, vertical migrations also influenced by time of day) on behavioral changes during the observations. Moreover, the majority of the studies exploit independently-planned surveys (n=25); therefore noise produced by vessels should be also taken into account, leading to further variability and complexity of responses. In a dedicated survey, sound produced by vessels might be reduced by temporarily reducing or stopping the propellers.
• Research on the effects of marine seismic surveys on marine fauna suffers from a lack of common metrics and standardization of measurements. Further studies are needed to explore and assess disturbance generated by sources used for seismic surveys, specifically those planned for academic research, through field observations that include long-term consequences, ecosystem-level implications, and indirect effects over diverse animal classes.
Data availability statement
The original contributions presented in the study are included in the article/Supplementary material. Further inquiries can be directed to the corresponding author.
Author contributions
AA and AC conceived the study. AA designed the study, wrote the first draft of the manuscript, and performed the data analysis. All authors reviewed the manuscript, read, and approved the submitted version.
Funding
AA is funded by the National Institute of Oceanography and Applied Sciences - OGS, the University of Trieste, and JASCO Applied Sciences. This research is conducted within the activities of projects funded by the Italian Ministry of University and Research (MUR): BlueSkills: Blue Jobs and Responsible Growth in the Mediterranean, labeled by the UfM and PNRR iNEST. We acknowledge the funding by the Jean Monnet Centre of Excellence on Sustainable Blue Economy granted by the European Commission to the Euro-Mediterranean University EMUNI (grant number 101048038) in financially supporting this publication.
Acknowledgments
The ECORD Science Support and Advisory Committee (ESSAC) Office has supported the early phase of the literature review. We gratefully acknowledge Dr. Federica Pace (JASCO Applied Sciences) for her insightful comments that led to a significant improvement in the quality of the manuscript. This review is an effort aiming at the implementation of the Institutional Position of the National Institute of Oceanography and Experimental Geophysics - OGS regarding anthropogenic noise in the marine environment (https://bgo.ogs.it/pdf/full/vol_61_supp1_complete.pdf). We appreciate the reviewers' valuable comments and suggestions, which helped us in improving the quality of the manuscript.
Conflict of interest
The authors declare that the research was conducted in the absence of any commercial or financial relationships that could be construed as a potential conflict of interest.
Publisher’s note
All claims expressed in this article are solely those of the authors and do not necessarily represent those of their affiliated organizations, or those of the publisher, the editors and the reviewers. Any product that may be evaluated in this article, or claim that may be made by its manufacturer, is not guaranteed or endorsed by the publisher.
Supplementary material
The Supplementary Material for this article can be found online at: https://www.frontiersin.org/articles/10.3389/fmars.2023.1222523/full#supplementary-material
References
Affatati A. (2020). Underwater noise in the marine environment: Sources, effects on fauna and mitigation measures | Rumore subacqueo in ambiente marino: Fonti, effetti sulla fauna e misure di mitigazione. Bollettino di Geofisica Teorica ed Applicata 61, s3–s108. doi: 10.4430/bgta0323
Ahadi A., Singh A., Bower M., Garrett M. (2022). Text mining in education—A bibliometrics-based systematic review. Educ. Sci. 12 (3), 210. doi: 10.3390/educsci12030210
Ainslie M. A., de Jong C. A., Halvorsen M. B., Ketten D. R., Prior M. K. (2017). Standards for processing and reporting metrics of underwater sound for use in risk assessment. J. Acoustical Soc. America 141 (5), 3846–3846. doi: 10.1121/1.4988575
Ainslie M. A., Halvorsen M. B., Robinson S. P. (2021). A terminology standard for underwater acoustics and the benefits of international standardization. IEEE J. Oceanic Eng. 47 (1), 179–200. doi: 10.1109/JOE.2021.3085947
Au W.W.L. (2000). “Hearing in whales and dolphins: An overview,” in Hearing by Whales and Dolphins, edited by Au W.W.L., Popper A.N., Fay R.R.. Springer-Verlag, New York, pp. 1–42.
Blackwell S. B., Nations C. S., McDonald T. L., Thode A. M., Mathias D., Kim K. H., et al. (2015). Effects of airgun sounds on bowhead whale calling rates: evidence for two behavioral thresholds. PloS One 10 (6), e0125720. doi: 10.1371/journal.pone.0125720
Blackwell S. B., Nations C. S., McDonald T. L., Greene C. R. Jr, Thode A. M., Guerra M., et al. (2013). Effects of airgun sounds on bowhead whale calling rates in the Alaskan Beaufort Sea. Marine Mammal Science 29(4), E342–E365.
Bruce B., Bradford R., Foster S., Lee K., Lansdell M., Cooper S., et al. (2018). Quantifying fish behaviour and commercial catch rates in relation to a marine seismic survey. Mar. Environ. Res. 140, 18–30. doi: 10.1016/j.marenvres.2018.05.005
Caldwell J., Dragoset W. (2000). A brief overview of seismic air-gun arrays. leading edge 19 (8), 898–902. doi: 10.1190/1.1438744
Camerlenghi A. (2021). The future challenge of decreasing underwater acoustic pollution. Bull. Geophysics Oceanography 91, 91–96.
Carroll A. G., Przeslawski R., Duncan A., Gunning M., Bruce B. (2017). A critical review of the potential impacts of marine seismic surveys on fish & invertebrates. Mar. pollut. Bull. 114 (1), 9–24. doi: 10.1016/j.marpolbul.2016.11.038
Cerchio S., Strindberg S., Collins T., Bennett C., Rosenbaum H. (2014). Seismic surveys negatively affect humpback whale singing activity off northern Angola. PloS One 9 (3), e86464. doi: 10.1371/journal.pone.0086464
Commission Decision (EU). (2017). Available at: https://eur-lex.europa.eu/legal-content/EN/TXT/?uri=CELEX%3A32017D0848.
Cote D., Morris C. J., Regular P. M., Piersiak M. G. (2020). Effects of 2D Seismic on snow crab movement behavior. Fisheries Res. 230, 105661. doi: 10.1016/j.fishres.2020.105661
Cumpston M., Li T., Page M. J., Chandler J., Welch V. A., Higgins J. P., et al. (2019). Updated guidance for trusted systematic reviews: a new edition of the Cochrane Handbook for Systematic Reviews of Interventions. Cochrane Database systematic Rev. 2019 (10), ED000142. doi: 10.1002/14651858.ED000142
Davidsen J. G., Dong H., Linné M., Andersson M. H., Piper A., Prystay T. S., et al. (2019). Effects of sound exposure from a seismic airgun on heart rate, acceleration and depth use in free-swimming Atlantic cod and saithe. Conservation Physiology 7(1), coz020.
Day R. D., Fitzgibbon Q. P., McCauley R. D., Baker K. B., Semmens J. M. (2022). The impact of seismic survey exposure on the righting reflex and moult cycle of Southern Rock Lobster (Jasus edwardsii) puerulus larvae and juveniles. Environ. pollut. 309, 119699. doi: 10.1016/j.envpol.2022.119699
Day R. D., McCauley R. D., Fitzgibbon Q. P., Hartmann K., Semmens J. M. (2019). Seismic air guns damage rock lobster mechanosensory organs and impair righting reflex. Proc. R. Soc. B 286 (1907), 20191424. doi: 10.1098/rspb.2019.1424
Day R. D., McCauley R. D., Fitzgibbon Q. P., Semmens J. M. (2016). Seismic air gun exposure during early-stage embryonic development does not negatively affect spiny lobster Jasus edwardsii larvae (Decapoda: Palinuridae). Sci. Rep. 6 (1), 1–9. doi: 10.1016/j.envpol.2022.119699
de Jong K., Forland T. N., Amorim M. C. P., Rieucau G., Slabbekoorn H., Sivle L. D. (2020). Predicting the effects of anthropogenic noise on fish reproduction. Rev. Fish Biol. Fisheries 30, 245–268. doi: 10.1007/s11160-020-09598-9
de Jong C. A. F., Jansen H. W. J., Halvorsen M. B., Hannay D. E., Ainslie M. A., Racca R. G. (2021). Measurement procedures for underwater sound sources associated with oil and gas exploration and production activities (TNO, Den Haag, The Netherlands: Prepared for E&P Sound and Marine Life Joint Industry Programme), 68. TNO 2021 R11210-A. Contract: JIP22 III-15-13, Schedule No: 04 (III-17).
Dekeling R. P. A., Ainslie M. A., Anderson M., Borsani J. B., Le Courtois F., Hedgeland D., et al. (2020). Towards threshold values for underwater noise - Common methodology for assessment of impulsive noise, TG Noise Technical Advice report DL, Vol. 1.
Duarte C. M., Chapuis L., Collin S. P., Costa D. P., Devassy R. P., Eguiluz V. M., et al. (2021). The soundscape of the Anthropocene ocean. Science 371 (6529), eaba4658. doi: 10.1126/science.aba4658
Duncan A. J., Lucke K., Erbe C., McCauley R. D. (2016). “Issues associated with sound exposure experiments in tanks,” in Proceedings of meetings on acoustics 4enal, vol. 27. (Acoustical Society of America), 070008.
Dunlop R. A., Noad M. J., McCauley R. D., Kniest E., Paton D., Cato D. H. (2015). The behavioural response of humpback whales (Megaptera novaeangliae) to a 20 cubic inch air gun. Aquat. Mammals 41 (4), 412. doi: 10.1578/AM.41.4.2015.412
Dunlop R. A., Noad M. J., McCauley R. D., Kniest E., Slade R., Paton D., et al. (2017a). The behavioural response of migrating humpback whales to a full seismic airgun array. Proc. R. Soc. B: Biol. Sci. 284 (1869), 20171901. doi: 10.1098/rspb.2017.1901
Dunlop R. A., Noad M. J., McCauley R. D., Kniest E., Slade R., Paton D., et al. (2018). A behavioural dose-response model for migrating humpback whales and seismic air gun noise. Mar. pollut. Bull. 133, 506–516. doi: 10.1016/j.marpolbul.2018.06.009
Dunlop R. A., Noad M. J., McCauley R. D., Scott-Hayward L., Kniest E., Slade R., et al. (2017b). Determining the behavioural dose–response relationship of marine mammals to air gun noise and source proximity. J. Exp. Biol. 220 (16), 2878–2886. doi: 10.1242/jeb.160192
Dybå T., Dingsøyr T. (2008). Empirical studies of agile software development: A systematic review. Inf. software Technol. 50 (9-10), 833–859. doi: 10.1016/j.infsof.2008.01.006
Edmonds N. J., Firmin C. J., Goldsmith D., Faulkner R. C., Wood D. T. (2016). A review of crustacean sensitivity to high amplitude underwater noise: data needs for effective risk assessment in relation to UK commercial species. Mar. pollut. Bull. 108 (1-2), 5–11. doi: 10.1016/j.marpolbul.2016.05.006
Elliott B. W., Read A. J., Godley B. J., Nelms S. E., Nowacek D. P. (2019). Critical information gaps remain in understanding impacts of industrial seismic surveys on marine vertebrates. Endangered Species Res. 39, 247–254. doi: 10.3354/esr00968
Ellison E. A., Frankel W. T. (2012). “A common sense approach to source metrics,” in The effects of noise on aquatic life. Eds. Popper A. N., Hawkins A. D. (New York: Springer Science + Business Media, LLC), 443–448.
Ellison W. T., Southall B. L., Clark C. W., Frankel A. S. (2012). A new context-based approach to assess marine mammal behavioral responses to anthropogenic sounds. Conserv. Biol. 26 (1), 21–28. doi: 10.1111/j.1523-1739.2011.01803.x
Erbe C., Dunlop R., Dolman S. (2018). “Effects of noise on marine mammals,” in Effects of anthropogenic noise on animals. Eds. Slabbekoorn H., Dooling R. J., Popper A. N., Fay R. R. (New York, NY: Springer), 277–309. doi: 10.1007/978-1-4939-8574-6_10
Erbe C., Marley S. A., Schoeman R. P., Smith J. N., Trigg L. E., Embling C. B. (2019). The effects of ship noise on marine mammals—A review. Front. Mar. Sci. 6. doi: 10.3389/fmars.2019.00606
Fitzgibbon Q. P., Day R. D., McCauley R. D., Simon C. J., Semmens J. M. (2017). The impact of seismic air gun exposure on the haemolymph physiology and nutritional condition of spiny lobster, Jasus edwardsii. Mar. pollut. Bull. 125 (1-2), 146–156. doi: 10.1016/j.marpolbul.2017.08.004
Glen A. S., Dickman C. R. (2014). “The importance of predators,” in Carnivores of Australia: past, present and future (Collingwood, Australia: CSIRO Publishing), 1–12.
Gomez C., Lawson J. W., Wright A. J., Buren A. D., Tollit D., Lesage V. (2016). A systematic review on the behavioural responses of wild marine mammals to noise: the disparity between science and policy. Can. J. Zoology 94 (12), 801–819. doi: 10.1139/cjz-2016-0098
Gordon J., Gillespie D., Potter J., Frantzis A., Simmonds M. P., Swift R., et al. (2003). A review of the effects of seismic surveys on marine mammals. Mar. Technol. Soc. J. 37 (4), 16–34. doi: 10.4031/002533203787536998
Gray M. D., Rogers P. H., Popper A. N., Hawkins A. D., Fay R. R. (2016). “Large” tank acoustics: how big is big enough?,” in The effects of noise on aquatic life II (New York: Springer), 363–369.
Greene C. R. Jr., Richardson W. J. (1988). Characteristics of marine seismic survey sounds in the Beaufort Sea. J. Acoustical Soc. America 83 (6), 2246–2254. doi: 10.1121/1.396354
Greenhalgh T., Peacock R. (2005). Effectiveness and efficiency of search methods in systematic reviews of complex evidence: audit of primary sources. Bmj 331 (7524), 1064–1065. doi: 10.1136/bmj.38636.593461.68
Hackenbroich S., Kranke P., Meybohm P., Weibel S. (2022). Include or not to include conference abstracts in systematic reviews? Lessons learned large Cochrane network meta-analysis including 585 trials. Systematic Rev. 11 (1), 1–10. doi: 10.1186/s13643-022-02048-6
Harris R. E., Miller G. W., Richardson W. J. (2001). Seal responses to airgun sounds during summer seismic surveys in the Alaskan Beaufort Sea. Mar. Mammal Sci. 17 (4), 795–812. doi: 10.1111/j.1748-7692.2001.tb01299.x
Hawkins A. D., Popper A. N. (2017). A sound approach to assessing the impact of underwater noise on marine fishes and invertebrates. ICES J. Mar. Sci. 74 (3), 635–651. doi: 10.1093/icesjms/fsw205
Heide-Jørgensen M. P., Blackwell S. B., Tervo O. M., Samson A. L., Garde E., Hansen R. G., et al. (2021). Behavioral response study on seismic airgun and vessel exposures in narwhals. Front. Mar. Sci. 8. doi: 10.3389/fmars.2021.658173
Holt M. M., Noren D. P., Dunkin R. C., Williams T. M. (2015). Vocal performance affects metabolic rate in dolphins: implications for animals communicating in noisy environments. J. Exp. Biol. 218 (11), 1647–1654. doi: 10.1080/09524622.2017.1359670
Jensen F. B., Kuperman W. A., Porter M. B., Schmidt H., Tolstoy A. (2011). Computational ocean acoustics Vol. 2011 (New York, NY: Springer New York).
Kellet P., Turan O., Incecik A. (2014). Underwater noise and marine wildlife: current and future implication and assessment. Proc. 1st International Meeting-Propeller noise and Vibrations. Istambul, Turkey, 63–70.
Kunc H. P., McLaughlin K. E., Schmidt R. (2016). Aquatic noise pollution: implications for individuals, populations, and ecosystems. Proc. R. Soc. B: Biol. Sci. 283 (1836), 20160839. doi: 10.1098/rspb.2016.0839
Lalas C., McConnell H. (2016). Effects of seismic surveys on New Zealand fur seals during daylight hours: Do fur seals respond to obstacles rather than airgun noise? Mar. Mammal Sci. 32 (2), 643–663. doi: 10.1111/mms.12293
Madsen P. T., Johnson M., Miller P. J. O., Aguilar Soto N., Lynch J., Tyack P. (2006). Quantitative measures of air-gun pulses recorded on sperm whales (Physeter macrocephalus) using acoustic tags during controlled exposure experiments. J. Acoustical Soc. America 120 (4), 2366–2379. doi: 10.1121/1.2229287
McCauley R. D., Day R. D., Swadling K. M., Fitzgibbon Q. P., Watson R. A., Semmens J. M. (2017). Widely used marine seismic survey air gun operations negatively impact zooplankton. Nat. Ecol. Evol. 1 (7), 1–8. doi: 10.1038/s41559-017-0195
McCauley R. D., Duncan A. J. (2017). How do impulsive marine seismic surveys impact marine fauna and how can we reduce such impacts? (Acoustical Society of America: Acoustics, Perth).
McCauley R. D., Fewtrell J., Duncan A. J., Jenner C., Jenner M. N., Penrose J. D., et al. (2000). Marine seismic surveys—a study of environmental implications. APPEA J. 40 (1), 692–708. doi: 10.1071/AJ99048
McQueen K., Meager J. J., Nyqvist D., Skjæraasen J. E., Olsen E. M., Karlsen Ø., et al. (2022). Spawning Atlantic cod (Gadus morhua L.) exposed to noise from seismic airguns do not abandon their spawning site. ICES J. Mar. Sci. 79 (10), 2697–2708. doi: 10.1093/icesjms/fsac203
Meekan M. G., Speed C. W., McCauley R. D., Fisher R., Birt M. J., Currey-Randall L. M., et al. (2021). A large-scale experiment finds no evidence that a seismic survey impacts a demersal fish fauna. Proc. Natl. Acad. Sci. 118 (30), e2100869118. doi: 10.1073/pnas.2100869118
Mikolajewicz N., Komarova S. V. (2019). Meta-analytic methodology for basic research: a practical guide. Front. Physiol. 10. doi: 10.3389/fphys.2019.00203
Miller P. J., Johnson M. P., Madsen P. T., Biassoni N., Quero M., Tyack P. L. (2009). Using at-sea experiments to study the effects of airguns on the foraging behavior of sperm whales in the Gulf of Mexico. Deep Sea Res. Part I: Oceanographic Res. Papers 56 (7), 1168–1181. doi: 10.1016/j.dsr.2009.02.008
Morley E. L., Jones G., Radford A. N. (2014). The importance of invertebrates when considering the impacts of anthropogenic noise. Proc. R. Soc. B: Biol. Sci. 281 (1776), 20132683. doi: 10.1098/rspb.2013.2683
Morris C. J., Cote D., Martin B., Kehler D. (2018). Effects of 2D seismic on the snow crab fishery. Fisheries Res. 197, 67–77. doi: 10.1016/j.fishres.2017.09.012
Morris C. J., Cote D., Martin S. B., Mullowney D. (2020). Effects of 3D seismic surveying on snow crab fishery. Fisheries Res. 232, 105719. doi: 10.1016/j.fishres.2020.105719
National Marine Fisheries Service. (2018). Revision to: technical guidance for assessing the effects of anthropogenic sound on marine mammal hearing (Version 2.0): underwater thresholds for onset of permanent and temporary threshold shifts, tech. Rep. NMFS-OPR-59 (Silver Spring, MD: National Marine Fisheries Service).
Nowacek D. P., Thorne L. H., Johnston D. W., Tyack P. L. (2007). Responses of cetaceans to anthropogenic noise. Mamm. Rev. 37, 81–115. doi: 10.1111/j.1365-2907.2007.00104.x
Page M. J., McKenzie J. E., Bossuyt P. M., Boutron I., Hoffmann T. C., Mulrow C. D., et al. (2021). Updating guidance for reporting systematic reviews: development of the PRISMA 2020 statement. J. Clin. Epidemiol. 134, 103–112. doi: 10.1016/j.jclinepi.2021.02.003
Payne J. F., Andrews C. A., Fancey L. L., Cook A. L., Christian J. R. (2007). “Pilot study on the effects of seismic air gun noise on lobster (Homarus americanus),” in Canadian technical report of fisheries and aquatic sciences no. 2712. 1–53 (Science Branch, Fisheries and Oceans Canada).
Pearson W. H., Skalski J. R., Sulkin S. D., Malme C. I. (1994). Effects of seismic energy releases on the survival and development of zoeal larvae of Dungeness crab (Cancer magister). Mar. Environ. Res. 38 (2), 93–113. doi: 10.1016/0141-1136(94)90003-5
Pichegru L., Nyengera R., McInnes A. M., Pistorius P. (2017). Avoidance of seismic survey activities by penguins. Sci. Rep. 7 (1), 1–8. doi: 10.1038/s41598-017-16569-x
Pirotta E., Brookes K. L., Graham I. M., Thompson P. M. (2014). Variation in harbour porpoise activity in response to seismic survey noise. Biol. Lett. 10 (5), 20131090. doi: 10.1098/rsbl.2013.1090
Popper A. N., Fay R. R. (2011). Rethinking sound detection by fishes. Hearing Res. 273 (1- 2), 25–36. doi: 10.1016/j.heares.2009.12.023
Popper A. N., Hawkins A. D., Jacobs F., Jacobson P. T., Johnson P., Krebs J. (2020). Use of sound to guide the movement of eels and other fishes within rivers: a critical review. Rev. Fish Biol. Fisheries 30, 605–622. doi: 10.1007/s11160-020-09620-0
Popper A.N., Hawkins A.D., Fay R.R., Mann D.A., Bartol S., Carlson T.J., et al (2014). ASA S3/SC1.4 TR‐2014 Sound exposure guidelines for fishes and sea turtles: A technical report prepared by ANSI Accredited Standards Committee S3/SC1 and registered with ANSI. American National Standards Institute.
Przeslawski R., Brooke B., Carroll A. G., Fellows M. (2018). An integrated approach to assessing marine seismic impacts: lessons learnt from the Gippsland Marine Environmental Monitoring project. Ocean Coast. Manage. 160, 117–123. doi: 10.1016/j.ocecoaman.2018.04.011
Richardson W. J., Greene C. R. Jr., Malme C. I., Thomson D. H. (1995). Marine mammals and noise (Academic, London: Academic press).
Rogers P. H., Hawkins A. D., Popper A. N., Fay R. R., Gray M. D. (2016). “Parvulescu revisited: small tank acoustics for bioacousticians,” in The effects of noise on aquatic life II (Springer, New York), 933–941.
Sarnocińska J., Teilmann J., Balle J. D., van Beest F. M., Delefosse M., Tougaard J. (2020). Harbor porpoise (Phocoena phocoena) reaction to a 3D seismic airgun survey in the North Sea. Front. Mar. Sci. 6. doi: 10.3389/fmars.2019.00824
Sierra-Correa P. C., Cantera Kintz J. R. (2015). Ecosystem-based adaptation for improving coastal planning for sea-level rise: A systematic review for mangrove coasts. Mar. Policy 51, 385–393. doi: 10.1016/j.marpol.2014.09.013
Sigray P., Andersson M., André M., Azzellino A., Borsani J. F., Bou M., et al. (2023). MSFD common implementation strategy. Eds. Druon Jean-Noël, Hanke G., Casier M. (Luxembourg: Publications Office of the European Union). doi: 10.2760/60215,JRC133477
Slabbekoorn H., Dalen J., de Haan D., Winter H. V., Radford C., Ainslie M. A., et al. (2019). Population-level consequences of seismic surveys on fishes: An interdisciplinary challenge. Fish Fisheries 20 (4), 653–685. doi: 10.1111/faf.12367
Slotte A., Hansen K., Dalen J., Ona E. (2004). Acoustic mapping of pelagic fish distribution and abundance in relation to a seismic shooting area off the Norwegian west coast. Fisheries Res. 67 (2), 143–150. doi: 10.1016/j.fishres.2003.09.046
Snyder H. (2019). Literature review as a research methodology: An overview and guidelines. J. business Res. 104, 333–339. doi: 10.1016/j.jbusres.2019.07.039
Solé M., Kaifu K., Mooney T. A., Nedelec S. L., Olivier F., Radford A. N., et al. (2023). Marine invertebrates and noise. Front. Mar. Sci. 10, 185. doi: 10.3389/fmars.2023.1129057
Southall B. L., Bowles A. E., Ellison W. T., Finneran J. J., Gentry R. L., Greene C. R. Jr., et al. (2007). Marine mammal noise exposure criteria: initial scientific recommendations. Aquat. Mammals 33, I–521. doi: 10.1080/09524622.2008.9753846
Southall B. L., Finneran J. J., Reichmuth C., Nachtigall P. E., Ketten D. R., Bowles A. E., et al. (2019). Marine mammal noise exposure criteria: Updated scientific recommendations for residual hearing effects. Aquat. Mammals 45 (2), 125–232. 2. doi: 10.1578/AM.45.2.2019.125
Southall B. L., Nowacek D. P., Bowles A. E., Senigaglia V., Bejder L., Tyack P. L. (2021). Marine mammal noise exposure criteria: assessing the severity of marine mammal behavioral responses to human noise. Aquat. Mammals 47 (5), 421–464. doi: 10.1578/AM.47.5.2021.421
Thode A. M., Blackwell S. B., Conrad A. S., Kim K. H., Marques T., Thomas L., et al. (2020). Roaring and repetition: How bowhead whales adjust their call density and source level (Lombard effect) in the presence of natural and seismic airgun survey noise. J. Acoustical Soc. America 147 (3), 2061–2080. doi: 10.1121/10.0000935
Thompson P. M., Brookes K. L., Graham I. M., Barton T. R., Needham K., Bradbury G., et al. (2013). Short-term disturbance by a commercial two-dimensional seismic survey does not lead to long-term displacement of harbour porpoises. Proc. R. Soc. B: Biol. Sci. 280 (1771), 20132001. doi: 10.1098/rspb.2013.2001
Tougaard J., Wright A., Madsen P. (2015). Cetacean noise criteria revisited in the light of proposed exposure limits for harbour porpoises. Mar. Poll. Bull. 90 (1–2), 196–208. doi: 10.1016/j.marpolbul.2014.10.051
Tran L., Tam D. N. H., Elshafay A., Dang T., Hirayama K., Huy N. T. (2021). Quality assessment tools used in systematic reviews of in vitro studies: A systematic review. BMC Med. Res. Method. 21 (1), 101. doi: 10.1186/s12874-021-01295-w
van Beest F. M., Teilmann J., Hermannsen L., Galatius A., Mikkelsen L., Sveegaard S., et al. (2018). Fine-scale movement responses of free-ranging harbour porpoises to capture, tagging and short-term noise pulses from a single airgun. R. Soc. Open Sci. 5 (1), 170110. doi: 10.1098/rsos.170110
van der Knaap I., Reubens J., Thomas L., Ainslie M. A., Winter H. V., Hubert J., et al. (2021). Effects of a seismic survey on movement of free-ranging Atlantic cod. Curr. Biol. 31 (7), 1555–1562. doi: 10.1016/j.cub.2021.01.050
Weilgart L. (2023). "Ocean Noise Pollution." in The Ocean and Us. (Springer International Punlishing), 153-160.
Williams R., Wright A.J., Ashe E., Blight L.K., Bruintjes R., Canessa R., et al. (2015). Impacts of anthropogenic noise on marine life: Publication patterns, new discoveries, and future directions in research and management. Ocean & Coastal Management 115, 17–24.
Winsor M. H., Irvine L. M., Mate B. R. (2017). Analysis of the spatial distribution of satellite-tagged sperm whales (Physeter macrocephalus) in close proximity to seismic surveys in the gulf of Mexico. Aquat. Mammals 43 (4), 438–446. doi: 10.1578/AM.43.4.2017.439
Wisniewska D. M., Johnson M., Teilmann J., Rojano-Doñate L., Shearer J., Sveegaard S., et al. (2018). Response to “Resilience of harbor porpoises to anthropogenic disturbance: must they really feed continuously?” Mar. Mammal Sci. 34 (1), 265–270. doi: 10.1111/mms.12463
Yazvenko S. B., McDonald T. L., Blokhin S. A., Johnson S. R., Meier S. K., Melton H. R., et al. (2007a). Distribution and abundance of western gray whales during a seismic survey near Sakhalin Island, Russia. Environ. Monit. Assess. 134, 45–73. doi: 10.1007/s10661-007-9810-3
Keywords: systematic review, seismic surveys, marine fauna, marine mammals, fish, invertebrates, marine birds, seals
Citation: Affatati A and Camerlenghi A (2023) Effects of marine seismic surveys on free-ranging fauna: a systematic literature review. Front. Mar. Sci. 10:1222523. doi: 10.3389/fmars.2023.1222523
Received: 14 May 2023; Accepted: 01 September 2023;
Published: 20 September 2023.
Edited by:
Salvatore Siciliano, Fundação Oswaldo Cruz (Fiocruz), BrazilReviewed by:
Aristides M. Prospathopoulos, Hellenic Centre for Marine Research (HCMR), GreeceGiorgio Riccobene, Laboratori Nazionali del Sud (INFN), Italy
Copyright © 2023 Affatati and Camerlenghi. This is an open-access article distributed under the terms of the Creative Commons Attribution License (CC BY). The use, distribution or reproduction in other forums is permitted, provided the original author(s) and the copyright owner(s) are credited and that the original publication in this journal is cited, in accordance with accepted academic practice. No use, distribution or reproduction is permitted which does not comply with these terms.
*Correspondence: Alice Affatati, YWFmZmF0YXRpQG9ncy5pdA==