- 1Laboratory of Antarctic and Sub-Antarctic Marine Ecosystems (LEMAS), Faculty of Sciences, University of Magellan, Punta Arenas, Chile
- 2Cape Horn International Center (CHIC), Omora Ethnobotanical Park, Universidad de Magellan, Puerto Williams, Chile
- 3PhD. Programme in Antarctic and Subantarctic Sciences, University of Magellan, Punta Arenas, Chile
- 4Millenium Institute Biodiversity of Antarctic and Subantarctic Ecosystems (MI-BASE), Santiago, Chile
- 5Research Centre Gaia- Antarctica, University of Magellan, Punta Arenas, Chile
- 6School of Environmental Studies, University of Victoria, Victoria, BC, Canada
- 7Limnology Institute, Austral University, Valdivia, Chile
- 8Marine Botany, University of Bremen, Bremen, Germany
These extensive kelps forest are among the most productive and diverse habitats on the planet, playing an important ecological role in marine ecosystems. These habitats have been affected by anthropogenic factors worldwide and directly by environmental variations resulting from climate change. The Magellan ecoregion has the southernmost kelp forests in the world, dominated by the species Macrocystis pyrifera. This species presents high ecophysiological plasticity being able to inhabit heterogeneous environments, characteristic of the fjord and channel systems of the region, and has high ecological, sociocultural, and economic importance for local coastal communities. To understand the ecophysiological acclimation strategies of M. pyrifera, samples from different blades were collected at different depths at four locations in the Magellan Ecoregion: Possession Bay, Skyring Sound, Otway Sound, and Puerto del Hambre seasonally. Abiotic measurements (salinity, temperature, and PAR light) were carried out for each location sampled. Measurements of photosynthetic parameters, Fv/Fm, rETRmax, Ek and α; pigment analysis of Chl a, Chl c, and fucoxanthin; and fecundity analysis of the sporophylls of each population studied were carried out on the M. pyrifera sporophytes. Significant differences were observed between seasons, locality, and depth of blades. Each population generally showed different photoacclimation processes, depending on the local conditions such as salinity values and probably tidal cycles. This is reflected in the photosynthetic, pigment, and fecundity values obtained during this study. The higher Fv/Fm values in all populations during the winter and autumn seasons and the differences in Chl c and fucoxanthin concentration during the winter period in Otway Sound and Puerto del Hambre population suggest the marked seasonal acclimation of M. pyrifera. In addition, the coastal environmental heterogeneity observed in the Magellan ecoregion related to salinity gradients (Skyring Sound) or wide tidal amplitudes (Possession Bay) influences the acclimation strategy of each population of M. pyrifera. Therefore, the characteristics of each population should be considered in order to promote its sustainability in times of social and climate change.
Introduction
Kelp forests are to extensive underwater habitats, occurring in 43% of the world’s coastal marine ecoregions (Krumhansl et al., 2016). These forests are among the most productive and diverse habitats on the planet (Reed and Brzezinski, 2009; Eger et al., 2022), playing an important ecological role in marine ecosystems (Friedlander et al., 2023). These kelps are capable of sequestering approximately 173 metric tons of atmospheric carbon per year, contributing to reducing the effects of global climate change in the oceans (Duarte et al., 2017). Despite their ecological, economic, cultural, and social importance, macroalga forests have been decimated by increasing anthropogenic activity in recent decades worldwide (Steneck et al., 2002). Some of the anthropogenic activities that stand out are overharvesting for the extraction of alginates, eutrophication of the oceans, and the effects of global climate change (e.g., increasing sea level rise, increased ocean temperatures, increased heat events, more frequent marine storms, among others) (Byrnes et al., 2011; Smale and Vance, 2015; Smale, 2020), even causing modifications in the food web within kelp forests (Ling et al., 2009).
Macroalgae have been used in Chile for more than 60 years mainly in the food, cosmetic, pharmaceutical, and biotechnology industries (Mansilla and Ávila, 2011). Currently, 13 species of macroalgae are extracted mainly from natural kelps forest (97.6%); only 2.4% come from cultivation (Camus et al., 2018; SERNAPESCA, 2023), to obtain components for human food use, aquaculture, and agriculture (Buschmann et al., 2008). SERNAPESCA (2023) describes how artisanal landings of Macrocystis pyrifera from natural stocks have increased in the last decade, reaching 42,882 tons in 2020, 45% higher than that recorded in 2011, making it the species with the second highest landings in recent years in Chile. Despite efforts to produce biomass from different culture systems to maintain natural populations (Westermeier et al., 2006; Vásquez et al., 2014; Westermeier et al., 2014), the biomass produced in cultivation did not exceed 2 tons in the latest data for 2019 (SERNAPESCA, 2023).
The Magellan subantarctic ecoregion (MSE) (Rozzi et. al., 2012) is the largest marine–terrestrial area in the world with sub-Antarctic environments at these latitudes. This region has a heterogeneous environment of fjords and channels (Vergara, 2003), formed by erosion during the Quaternary glaciation process (Clapperton, 1994). Due to its unique environmental heterogeneity, the Magellan ecoregion harbors a great diversity of marine ecosystems, dominated by extensive underwater kelp forests that have not yet been affected by anthropogenic activities (Friedlander et al., 2020). M. pyrifera kelp forests in the Magellan ecoregion are among the most pristine ecosystems in the world, which is why today they are considered true sentinels of climate change.
The extensive kelp forests of M. pyrifera inhabit environments in the Magellan ecoregion that are strongly subjected to oceanographic factors such as temperature and salinity gradients (Bahamonde et al., 2022). These changes in salinity and temperature within the ecoregion can be influenced by glacial melt and freshwater discharge, generating an estuarine system with marked vertical and horizontal gradients (Bahamonde et al., 2022). There are also extreme atmospheric factors: the dominance of strong winds, rainfall, large input of freshwater from rain and/or snow (Casiccia et al., 2003), and a marked seasonality with changes in light intensity (Ojeda et al., 2014; Ojeda et al., 2019). These atmospheric factors directly affect the photosynthetic performance of macroalgae (Hurd et al., 2014). These factors affect the internal processes of macroalgae and the growth and reproductive cycles (Falkowski and LaRoche, 1991), which may have an impact on the survival of natural populations.
Furthermore, this region is vulnerable to climate change (Mansilla et al., 2012; Wernberg et al., 2018; Qiu et al., 2019), as is M. pyrifera (Mansilla et al., 2012) kelp forest. Climate change and the environmental variations it causes directly affect M. pyrifera populations, mainly in its life cycle, photosynthetic capacity, external morphology, population structure, and size (Ríos et al., 2007; Marambio et al., 2017; Rodríguez et al., 2019), with direct implications for the sustainability of southern marine ecosystems (Hollarsmith et al., 2020; Davis et al., 2022.)
The kelp forest of M. pyrifera that inhabit the Magellan ecoregion have been studied extensively, including work on ecology (Adami and Gordillo, 1999; Mansilla et al., 2005; Plana et al., 2007; Ríos et al., 2007; Rosenfeld et al., 2014; Friedlander et al., 2023), bioactive compounds such as carotenoids and polyphenols (Mansilla and Ávila, 2011; Mansilla et al., 2012; Astorga-España and Mansilla, 2014), remote sensing (Mora-Soto et al., 2020; Mora-Soto et al., 2021), and reproduction (Palacios and Mansilla, 2003; Rodríguez et al., 2019; Camus et al., 2021). In an evolutionary context, it has been shown that M. pyrifera along the Chilean coast has a low haplotypic diversity but with marked phylogeographic disjunctions related to the biogeographic breaks of 33°S and 42°S (Macaya and Zuccarello, 2010). Therefore, the Magellanic populations would have been strongly affected by historical events such as the Last Glacial Maximum (LGM) (Macaya and Zuccarello, 2010). On the other hand, studies in ecophysiology have shown how M. pyrifera populations have physiological traits that allow them to respond to different scenarios it has also been observed that there are populations that have unique traits that allow them to survive certain environmental conditions (Marambio et al., 2017; Rodríguez et al., 2019; Ziemann dos Santos et al., 2019; Palacios et al., 2021). However, these studies have been focused on populations that inhabit a specific area of the MSE. More recently, a latitudinal study of thermal tolerance in M. pyrifera populations showed that individuals from this ecoregion would not be less resistant to heat compared to populations from Peru and central Chile (Becheler et al., 2022). Furthermore, these authors conclude that this wide range of thermal tolerance would support the idea that the gametophytic phase would be a resistance stage and that fecundity (proportion of egg cells successfully fertilized) would be more influenced by geographic origin than by temperature (Becheler et al., 2022). However, this study focused mainly on evaluating the effect of temperature on a regional scale and did not consider other variables such as salinity variations and seasonal changes, which are very characteristic of the Magellan ecoregion. Therefore, in the context of climate change and the future management of this species, it is very relevant to study how ecophysiological and reproductive processes vary between natural kelp forests and how the marked seasonality present in the ecoregion may influence the internal processes of the species.
The aim of this study is to obtain an approximation to the understanding of the seasonal ecophysiological and reproductive behavior of Macrocystis pyrifera in natural grasslands inhabiting heterogeneous environments, to contribute to a better understanding of the acclimatiz ation and adaptation capacity of this species to anthropogenic and climate change effects at high latitudes.
Materials and methods
Sampling sites
Samples of the brown seaweed M. pyrifera were collected seasonally (spring, summer, autumn, and winter) during 2014–2015 by scuba diving. The collection of material was carried out in four localities of the sub-Antarctic region of Magallanes (Figure 1): Possession Bay (52°19′54.68 ″S, 69°28′44.57 ″W) (Figure 1), Skyring Sound (52°33′39.89 ″S, 71°44′3.36 ″W) (Figure 1), Otway Sound (53°8′54.20 ″S, 71°30′36.80 ″W) (Figure 1), and Puerto del Hambre (53°36′51.32 ″S, 70°55′42.32 ″W) (Figure 1). Photosynthetic and pigment data for Puerto del Hambre population were reused from the publication Marambio et al. (2017).
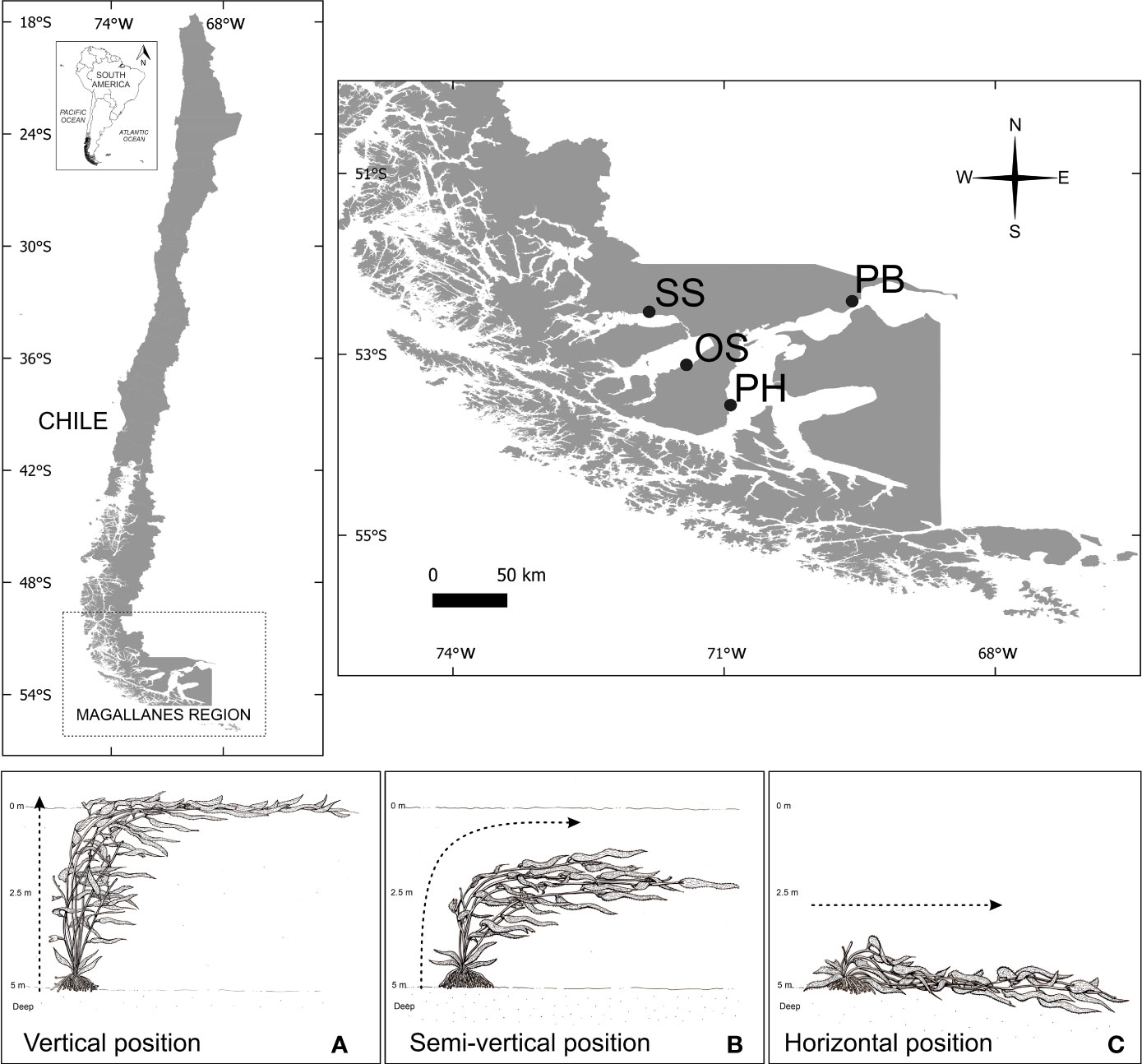
Figure 1 Populations of M. pyrifera studied during this study in the Magellan ecoregion are indicated by the abbreviations: (PB) Possession Bay, (SS) Skyring Sound, (OS) Otway Sound, and (PH) Puerto del Hambre. The position of M. pyrifera sporophytes in their natural environments, are shown in capital letters: (A) M. pyrifera positioned vertically in Otway Sound, Puerto del Hambre and high tide in Possession Bay; (B) semi-vertical position in population of Skyring Sound; and (C) horizontal position at low tide in Possession Bay.
Characteristic of the sampling sites
Skyring Sound and Otway Sound were proglaciar lakes, which went through a marine transgression during the deglaciation event some 14,000 years ago (Kilian et al., 2013). This event allowed entry of oceanic waters, rendering them marine fjords. Skyring Sound has a longitude of approximately 100 km, whereas Otway Sound extends some 151 km from West to East (Kilian et al., 2013). Both these fjords possess a predominantly rocky substrate composed of round pebbles, although some rocky platforms can also be found in Otway Sound (Kilian et al., 2013). The two fjords are connected via the Fitz-Roy Channel. Concomitantly, Skyring Sound is connected in the west with strait of Magallanes via the Gajardo Channel, whereas Otway Sound is connected in the east through the Jeronimo Channel (Valdenegro and Silva, 2003). The topography of Skyring Sound oceanic floor restricts the entry of oceanic waters (Pinilla et al., 2013). A constant salinity of approximately 15–20 psu can be found throughout the entire fjord’s water column (Pinilla et al., 2021). Nonetheless, salinity values remain stable at the surface in the external areas of the fjords but increase at lower depths, exceeding 30.0 psu (Pinilla et al., 2021). The sea surface temperature has a mean of 7.3°C (Pinilla et al., 2021). Meanwhile, mean salinity and temperature values in Otway Sound are found to be 30.1 psu and 7.4°C, respectively, due to the stronger oceanic association with Jeronimo Channel (Valdenegro and Silva, 2003).
Meanwhile, Possession Bay is located in the Eastern micro-basin of Magellan’s strait and is highly influenced by waters from the Atlantic Ocean (Antezana et al., 1992). This region’s seabed is characterized by its softness, with a few rocky fragments and small round-shaped pebbles (Aldea and Rosenfeld, 2011). The wide tidal range, reaching a maximum of 9 m, exposes horizontally M. pyrifera populations found in the subtidal region (Mora-Soto et al., 2020). There is little variation in salinity and temperature in the oceanic eastern micro-basin, with means of 32.5 psu and 5.8°C, respectively (Valdenegro and Silva, 2003). Finally, Puerto del Hambre area is located in the central micro-basin of Magellan’s strait and is distinguished by the presence of large rocky platforms (Rosenfeld et al., 2018). This central micro-basin is composed of estuarine waters as a result of the mixing of subantarctic currents from the Pacific Ocean and freshwater from the Western micro-basin (Valdenegro and Silva, 2003). The entirety of its water column is constituted of estuarine and saline water, with mean salinity and temperature values of 30.8 psu and 7.0°C (Valdenegro and Silva, 2003).
Sample collection
Vegetative blades were randomly extracted from (n=7) M. pyrifera sporophytes (sporophytes). A basal, mid-thallus, and apical frond was removed from each plant following the methodology of Marambio et al. (2017); the samples were collected seasonally (spring, summer, autumn, and winter). Reproductive blades or sporophylls of M. pyrifera were also collected at all sampling locations during the winter season (n=7). Three sporophylls were collected from each plant sampled. This stratification of the seaweed was carried out because this species can reach great length, up to 30 m (Plana et al., 2007). However, in this study, we worked with young sporophytes of 2.5–3 m length.
Environmental parameter measurements
The environmental parameters (temperature and salinity) were recorded using a multi-parameter YSI model 556 MPS. For each location, measurements were taken at the surface (0m) and at the bottom (3m). Eight measurements were made at each depth and sampling location during the summer and winter. PAR light measurements were performed using a PMA 2200 photometer radiometer (Solar Light) PAR sensor in the surface.
Photosynthetic performance
Photosynthetic measurements were performed in situ on vegetative and sporophyll blades; the optimum quantum yield of photosystem II (Fv/Fm) in M. pyrifera was measured in vivo after 15 min of dark adaption. Subsequently, photosynthesis–irradiance (P–E) curves were recorded up to an actinic light of 0–2,950 µmol photons m−2 s−1. We estimated the photosynthetic parameters as the maximum relative transport rate using the P–E curve (rETRmax), saturation irradiance (Ek), and photosynthetic efficiency (α, initial linear slope). The P–E curves were fitted to the equation of Platt et al. (1980) using KaleidaGraph version 4.5.4 (Synergy Software, Reading, PA, USA). All measurements were carried out with an amplitude-modulated chlorophyll fluorometer (Diving-PAM, Heinz Walz GmbH, Effeltrich, Germany).
Pigment concentrations
Pigment concentrations analysis of M. pyrifera was carried out following the methodology of Mansilla et al. (2016) and Marambio et al. (2017) for brown algae. A total of 0.125 g dry weight (DW) per sample was incubated in 1 mL of ≥99% dimethyl sulfoxide (DMSO) for 15 min. Subsequently, the main and accessory pigments were extracted using DMSO. Subsequently, spectrophotometry (Genesys UV) at room temperature was used to measure chlorophyll a (Chl a), chlorophyll c (Chl c), and fucoxanthin (Fucox). The absorbance at 750.0 nm was used as a correction factor for scattered light. For the quantification of Chl a, Chl c, and Fucox, the methodology and equations of Seely et al. (1972) were used; results were expressed in mg g−1 DW.
Reproductive potential
The sporophylls of M. pyrifera were stored in a cooler in the dark, with seawater at low temperature (<10°C), and were transported to the laboratory on the same day of collection, following the methodology of Rodríguez et al. (2019). The area of coverage and position of the sporangial sorus of the collected sporophylls was calculated by digital photography, using Acrobat Reader DC Version 2015 software. Spore release was performed using the method proposed by Ávila et al. (2010), in 0.45-μm-filtered seawater and Provasoli culture medium, under temperature conditions of 8°C, irradiance of 40 μmol photon m−2 s−1. Spore density was calculated using an OLYMPUS CX31 optical microscope and a Sedgewick Rafter Cell s50 camera, estimating the number of spores contained in 1 mL, measuring (n=15) for each site.
Statistical analysis
To evaluate the effect of the independent variables, which for this study are the temporal, spatial, bathymetric, and fecundity variability in the photosynthetic performance and pigment composition (dependent variables) of M. pyrifera, a multifactorial analysis was performed with Generalized Linear Models (GLM) using the R 4.1.0 statistical software and the “MASS” and “emmeans” packages (R Core Team, 2017). All ecophysiological variables were fitted to a Gamma distribution (Marambio et al., 2022). A comparison was made between vegetative basal blades and sporophylls to evaluate the variability between reproductive stages during winter. The first complete model included three factors: season (fixed, four levels), location (fixed, four levels), and depth (fixed, three levels), all crossed. The second model included two factors: location (fixed, four levels) and phenological stage (fixed, two levels), all crossed. Subsequently, we performed an analysis of deviance and Tukey’s post-hoc test for pairwise comparisons (e.g., Betancourtt et al., 2018).
To evaluate the differences in reproductive effort, sorus coverage and position, and spore density in sporophylls blades, data were analyzed using a Kruskal–Wallis non-parametric test for multiple comparisons of independent groups. Reproductive statistical analyses were performed using STATISTICA 7.1 software, using a 95% confidence interval (p ≤ 0.05).
Results
Environmental parameters
The abiotic factors temperature (°C) and PAR µmol photons m−2 s−1 light varied between seasons (winter and summer). It should be noted that photoperiods are also different depending on the season analyzed. Salinity and dissolved O2 (mg/L) varied among locations (Table 1). Skyring Sound had the lowest values of salinity with respect to Possession Bay and Puerto del Hambre that presented high values (Table 1). M. pyrifera kelp forest in the Magellan ecoregion showed differences in the position of the sporophytes (Figures 1A–C) among the studied localities. Sporophytes in vertical position (Figure 1A) were observed in the kelp forest of Puerto del Hambre, Otway Sound, and Possession Bay, the last at high tide. Sporophytes in semi-vertical position (Figure 1B) were observed in the Skyring Sound kelp forest, while sporophytes in horizontal position (Figure 1C) were recorded in the Possession Bay kelp forest at low tide.
Photosynthetic performance in vegetative blades
The ecophysiological differences between locations were found to be significant between the Possession Bay and Skyring Sound populations during winter (p ≤ 0.05, Figure 2; Supplementary Tables S1–S5).
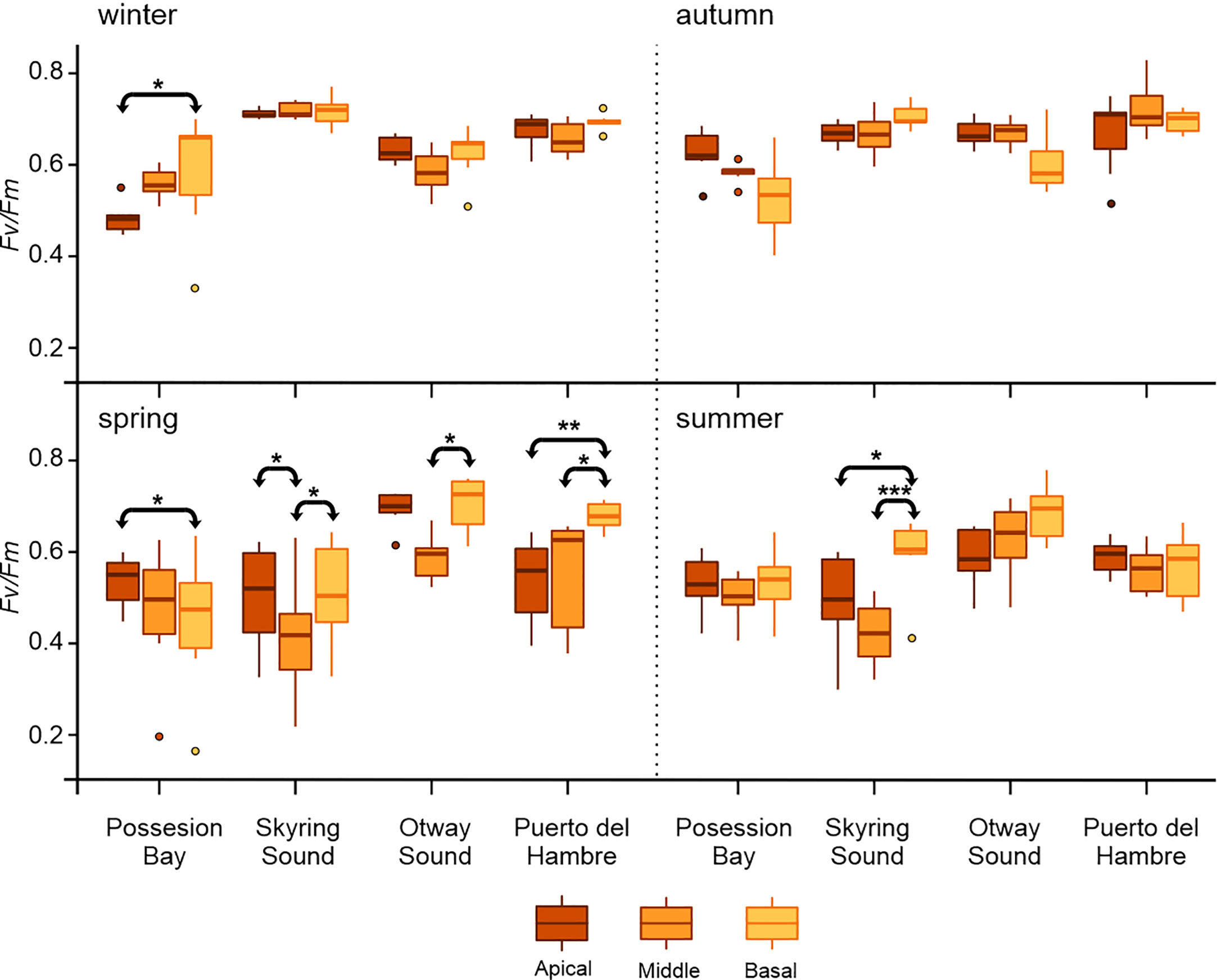
Figure 2 Mean ± SD for Fv/Fm (n=7) in vegetative blades of Macrocystis pyrifera. The figure shows the comparison between frond types (apical, middle, and basal), for each population sampled (Possession Bay, Skyring Sound, Otway Sound, and Puerto del Hambre) seasonally: winter, autumn, spring, and summer. The horizontal line with arrows indicates significant differences between kinds of blades. ***p<0.001, **p<0.01, *p<0.05.
Differences in plant stratification were observed among the studied M. pyrifera populations. In Possession Bay, the apical blades exhibited a decrease in Fv/Fm during winter, while the basal blades tended to increase their value (Figure 2; Supplementary Table S1). Conversely, during spring in Possession Bay, the apical blades displayed a higher Fv/Fm value compared to the basal blades. Significant differences (p ≤ 0.05) were found between blades in both spring and summer in Skyring Sound, with a decrease in Fv/Fm values observed in the middle blades. This resulted in significant differences (p ≤ 0.05) between the middle blades and the apical and basal blades (Figure 2; Supplementary Table S1). The middle blades of the Otway Sound population exhibited low Fv/Fm values, while the basal blades displayed higher values during spring differentiated significantly from Seno Skyring and Possession Bay (p ≤ 0.05, Figure 2; Supplementary Table S2). A significant difference (p ≤ 0.05) was observed between the middle and basal blades of the Otway Sound population (Figure 2). In the Puerto del Hambre population, the basal frond values showed an increase in spring, leading to significant differences (p ≤ 0.05) between the blades (Figure 2).
Significant seasonal differences (p≤ 0.05, Figure 3; Supplementary Table S1) were observed in the Ek parameter of M. pyrifera (Supplementary Table S3). Puerto del Hambre population presented high Ek values across all analyzed seasons, resulting in significant differences from the other populations (p≤ 0.05, Supplementary Table S3). Similarly, Possession Bay population showed high Ek values during winter and spring. These elevated Ek values in Possession Bay led to significant differences compared to the other analyzed populations (p≤ 0.05, Figure 3; Supplementary Table S3). In Possession Bay, there was an increase in Ek values during the winter and spring seasons. Specifically, during spring, the middle fronds exhibited the highest Ek value, which significantly differed (p≤ 0.05) from both the apical and basal blades (Figure 3; Supplementary Table S1). On the other hand, Skyring Sound did not exhibit significant differences between blades (p≤ 0.05, Figure 3) during the winter, autumn, and summer seasons. However, in spring, a low Ek value was observed in the apical blades, while the middle blades showed an increase in Ek, resulting in a significant difference (p≤ 0.05, Figure 3). The Otway Sound population did not show a significant difference in blade types during the winter and autumn seasons. However, the apical blades exhibited a low Ek value in spring, which increased significantly during the summer (p≤ 0.05, Figure 3; Supplementary Table S3).
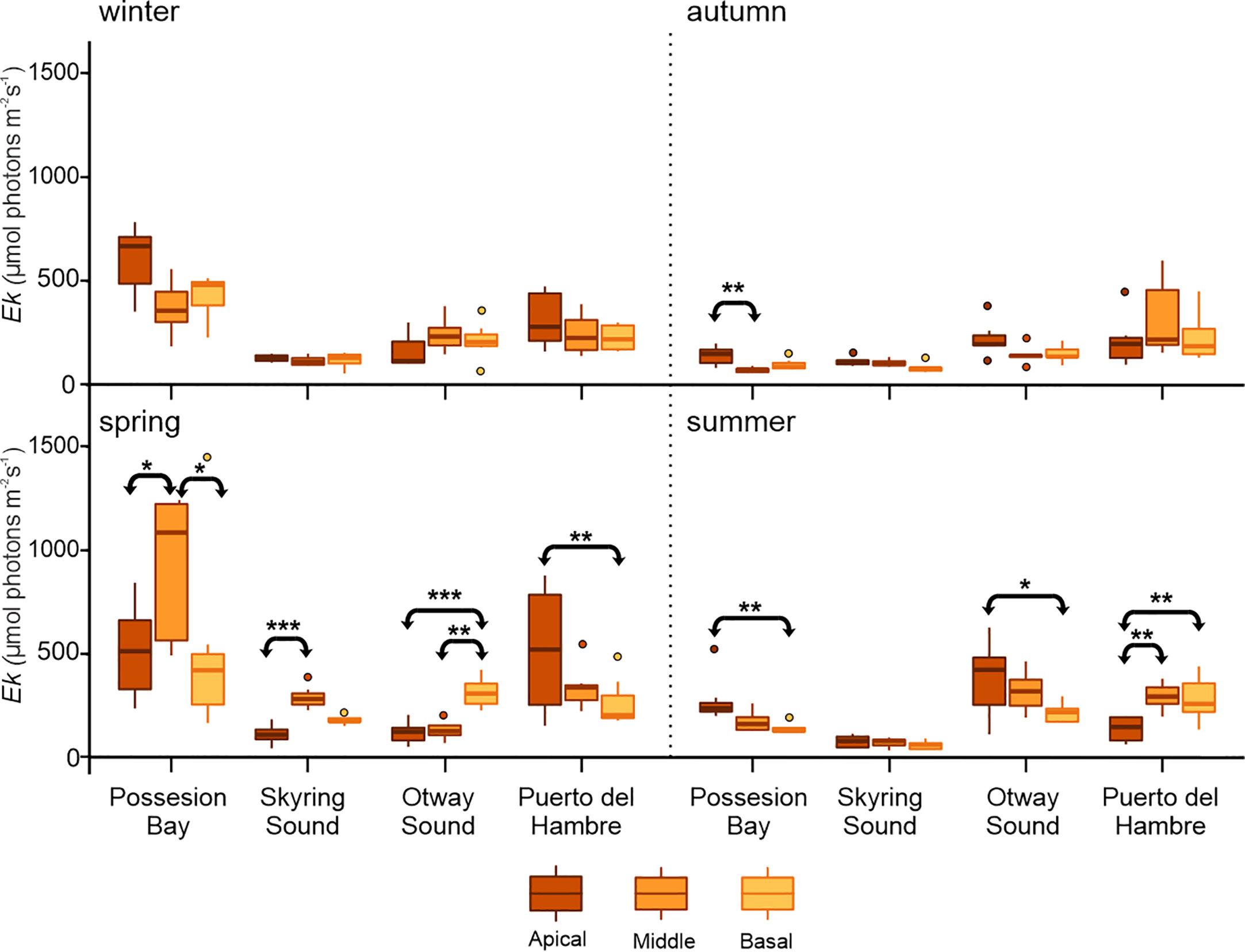
Figure 3 Mean ± SD for Ek (n=7) in vegetative blades of Macrocystis pyrifera. The figure shows the comparison between frond types (apical, middle, and basal), for each population sampled (Possession Bay, Skyring Sound, Otway Sound, and Puerto del Hambre) seasonally: winter, autumn, spring, and summer. The horizontal line with arrows indicates significant differences between kinds of blades. ***p<0.001, ** p<0.01, * p<0.05.
The spring season exhibited the greatest variability in the photosynthetic efficiency α response between the frond types of Skyring Sound and Otway Sound (Supplementary Figure S1). Furthermore, differences were observed between apical blades and the other blade types, with significant differences observed in autumn and spring (Supplementary Figure S1; Supplementary Tables S1, S4). In Puerto del Hambre population, there were significant differences in photosynthetic efficiency between the apical blades and the middle and basal blades (Supplementary Figure 1; Supplementary Table 1). The rETRmax followed a similar trend to Ek, where the Possession Bay population exhibited the highest values during winter and spring (Supplementary Figure S2; Supplementary Table S5).
Photosynthetic performance in sporophyll blades
During winter, the Fv/Fm values in the basal blades and sporophylls of M. pyrifera exhibited the following patterns: Possession Bay displayed lower values compared to the other populations, resulting in significant differences between Possession Bay and Skyring Sound and Puerto del Hambre populations (p≤ 0.05, Figure 4A; Supplementary Table 3). Regarding the blade types, it was observed that in all sampled localities, the basal blades of M. pyrifera tended to have higher Fv/Fm values compared to the sporophyll blades. However, this difference did not generate significant differences between basal and sporophyll blades in any population (Figure 4A).
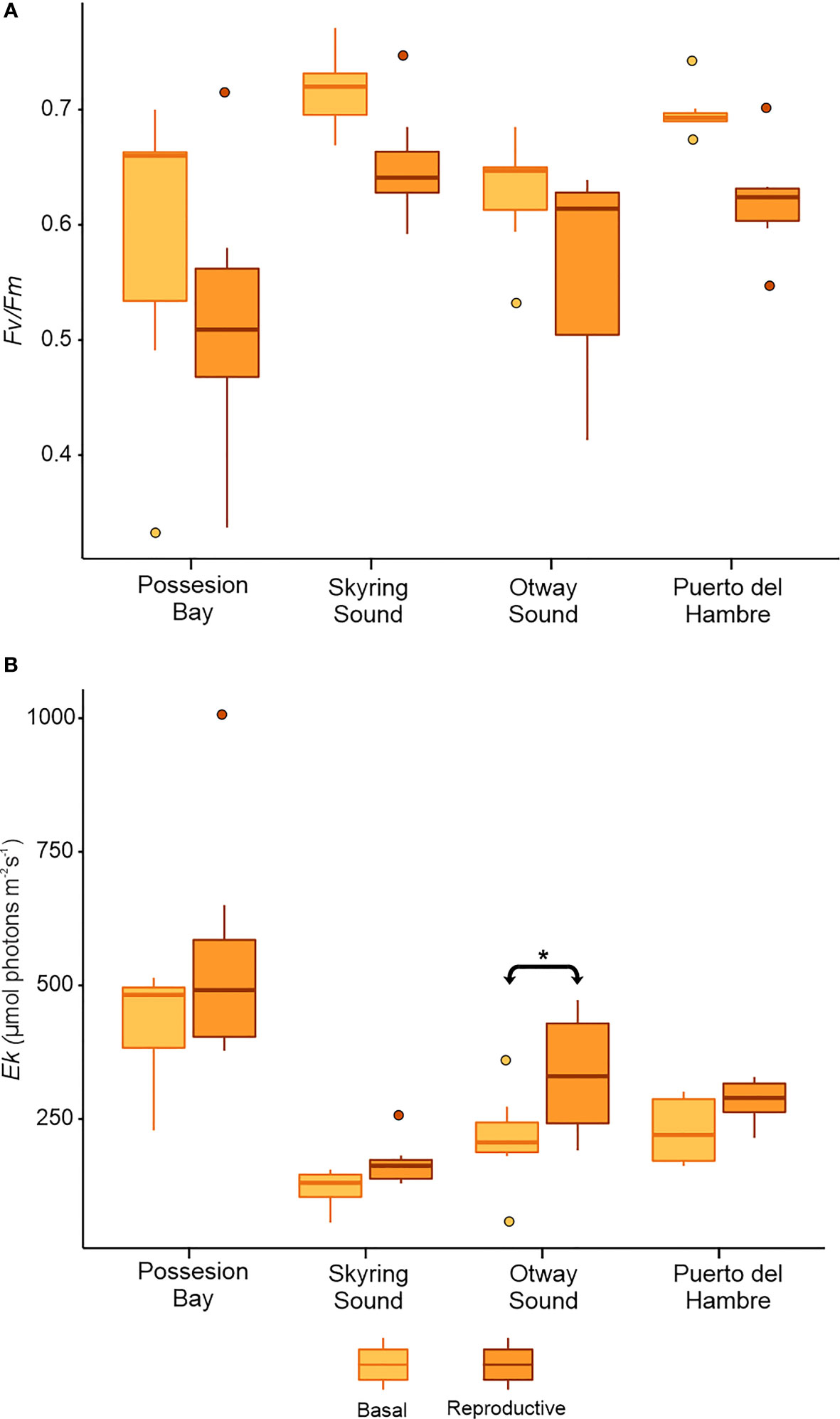
Figure 4 Mean±SD for Fv/Fm and Ek (n=7) in basal and sporophyll blades of Macrocystis pyrifera. The figure shows the comparison for each population sampled (Possesion Bay, Skyring Sound, Otway Sound, and Puerto del Hambre) in winter. (A) parameter optimum quantum yield - Fv/Fm, and (B) parameter saturation point -Ek. The horizontal line with arrows indicates significant differences between kinds of blades. *p<0.05.
Possession Bay exhibited high Ek values, resulting in significant differences compared to other localities (p≤ 0.05, Figure 4B). Sporophylls tended to have higher Ek values compared to other frond types, but significant differences were observed only in Otway Sound. A similar trend was observed in the comparison of basal blades between localities (Figure 4B; Supplementary Table S3).
The photosynthetic efficiency α demonstrated significant differences between locations (p≤ 0.05; Supplementary Table S4 and Supplementary Figure S3A), with increased values in the basal blades and sporophylls) of Skyring Sound. The rETRmax also exhibited a similar pattern to Ek, with increased values in the Possession Bay population for basal vegetative and sporophyll blades (Supplementary Table S5; Supplementary Figure S3B). Differences among different frond types were observed in Possession Bay and Otway Sound (Supplementary Figure S3B).
Pigment concentrations in vegetative blades
The pigment concentrations of M. pyrifera exhibited seasonal variations (Table 2; Supplementary Table S6). Chl a had higher concentration compared to Chl c and Fucox. Chl a displayed seasonal variation in all populations, characterized by a decrease in concentration during winter. Significant differences were observed between seasons (p≤ 0.05, Table 2; Supplementary Table S6). Chl c concentration increased in autumn, resulting in significant differences between autumn and the other seasons (p≤ 0.05, Table 2; Supplementary Table S2). Fucox concentration tended to decrease during winter in Possession Bay and Puerto del Hambre, leading to significant differences with the other seasons (p≤ 0.05, Table 2; Supplementary Table S6). On the other hand, in Skyring Sound, there was an increase in Fucox concentration during winter, generating significant differences (p≤ 0.05, Table 2; Supplementary Table S6) compared to the other seasons.
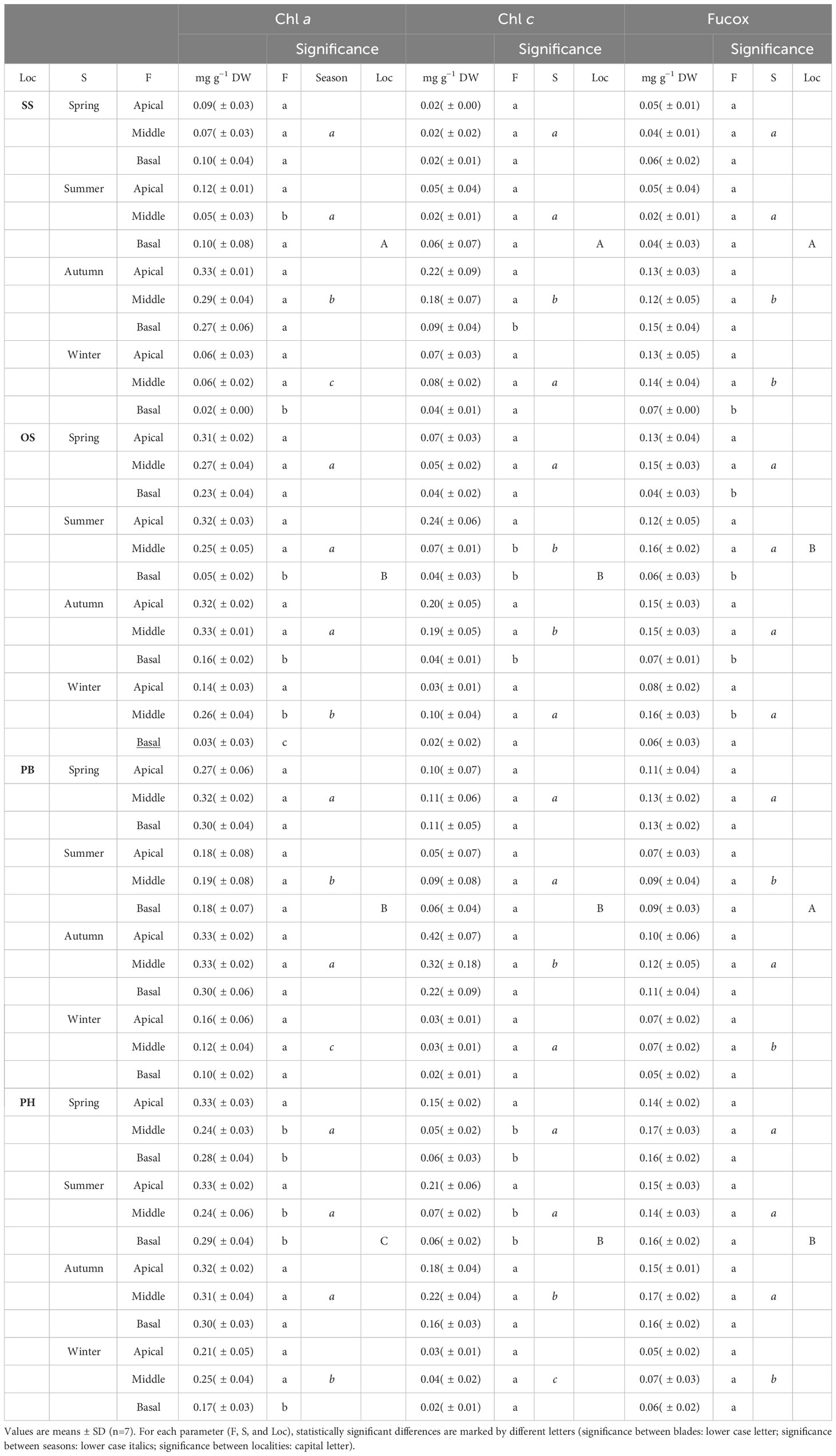
Table 2 Concentration of main pigments [mg g−1 dry weight (DW)] of Macrocystis pyrifera: Chlorophyll a (Chl a), Chlorophyll c (Chl c), Fucoxanthin (Fucox), Locality (Loc), Possession Bay (PB), Skyring Sound (SS), Otway Sound (OS), Puerto del Hambre (PH), Season (S) and Frond (F).
The concentration of Chl a exhibited significant difference between populations (p≤ 0.05, Table 2; Supplementary Table S6). Skyring Sound displayed low Chl a value, while Puerto del Hambre exhibited high values. Both populations showed significant differences compared to the other populations (p≤ 0.05, Table 2; Supplementary Table S6). The lowest Chl c values were found in Skyring Sound, resulting in significant differences (p≤ 0.05, Table 2; Supplementary Table S6) compared to the other populations (Table 2; Supplementary Table S6). The concentration of Fucox tended to be lower in Skyring Sound and Possession Bay, while it was higher in Otway Sound and Puerto del Hambre, generating significant differences between populations (p≤ 0.05, Table 2; Supplementary Table S6).
A more specific analysis of photosynthetic pigments in the blade’s stratification of M. pyrifera (Table 2) revealed the following observations: for Chl a, the concentration in different blades types of the Possession Bay population remained unchanged. However, variations in Chl a concentration were observed among the blade’s types of Skyring Sound, Otway Sound, and Puerto del Hambre populations. The apical and basal blades types showed significant differences compared to the other blades types (p≤ 0.05, Table 2; Supplementary Table S6). In the case of Chl c, there were no variations between the different blade’s types in the Possession Bay population. However, the basal and middle blades types of Puerto del Hambre population showed variations compared to the apical blades type. Additionally, significant differences were observed only between the basal blades type and the middle and apical in Skyring Sound during autumn (p≤ 0.05, Table 2; Supplementary Table S6). Regarding Fucox, there were no significant differences between the blade’s types in Possession Bay and Puerto del Hambre populations. However, significant differences (p≤ 0.05) were observed between the basal blade type and the middle and apical blades types in both Skyring Sound and Otway Sound populations (Table 2; Supplementary Table S6).
Pigment concentrations in sporophyll blades
Pigment concentration in basal blades and sporophylls of M. pyrifera in winter showed the following: Chl a showed no significant differences (p≤ 0.05, Table 3; Supplementary Table S6) between localities, while it did show differences between basal blades and sporophylls in Skyring Sound. Chl c showed a high value in the sporophylls of Otway Sound, which generated significant differences (p≤ 0.05, Table 3; Supplementary Table S6) between localities. The concentration of Chl c did not show significant differences between blades. The concentration of Fucox was low in sporophylls of Possession Bay, which generated significant differences between localities. However, no significant differences were observed for Fucox (Table 3; Supplementary Table S6).
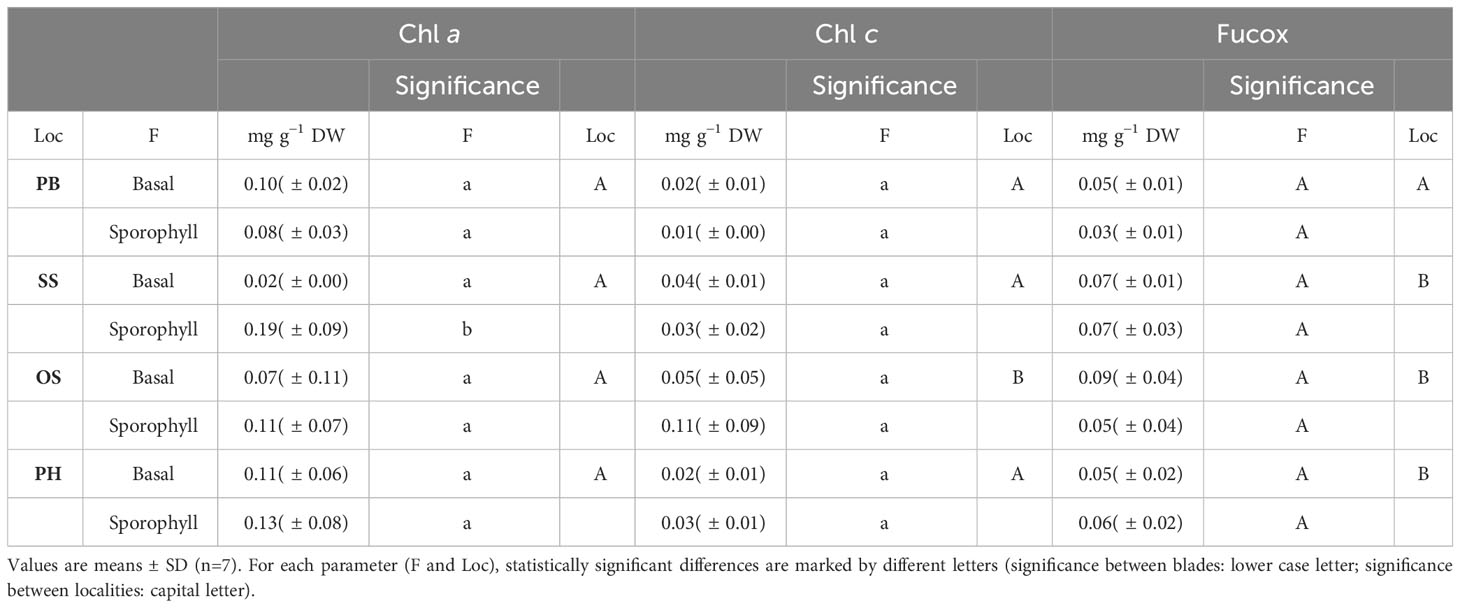
Table 3 Concentrations of main pigments [mg g−1 dry weight (DW)] of sporophylls of Macrocystis pyrifera: Chlorophyll a (Chl a), Chlorophyll c (Chl c), Fucoxanthin (Fucox), Locality (Loc), Possession Bay (PB), Skyring Sound (SS), Otway Sound (OS), Puerto del Hambre (PH), and Frond (F).
Sorus position, coverage, and spore density in sporophylls blades
In the Skyring Sound, Otway Sound, and Puerto del Hambre, over 60% of the sporophylls analyzed exhibited a distribution of sori in the central zone of the blade (Figure 5). On the other hand, in Puerto del Hambre, approximately 75% of the blades analyzed showed a distribution of sori in the apical zone.
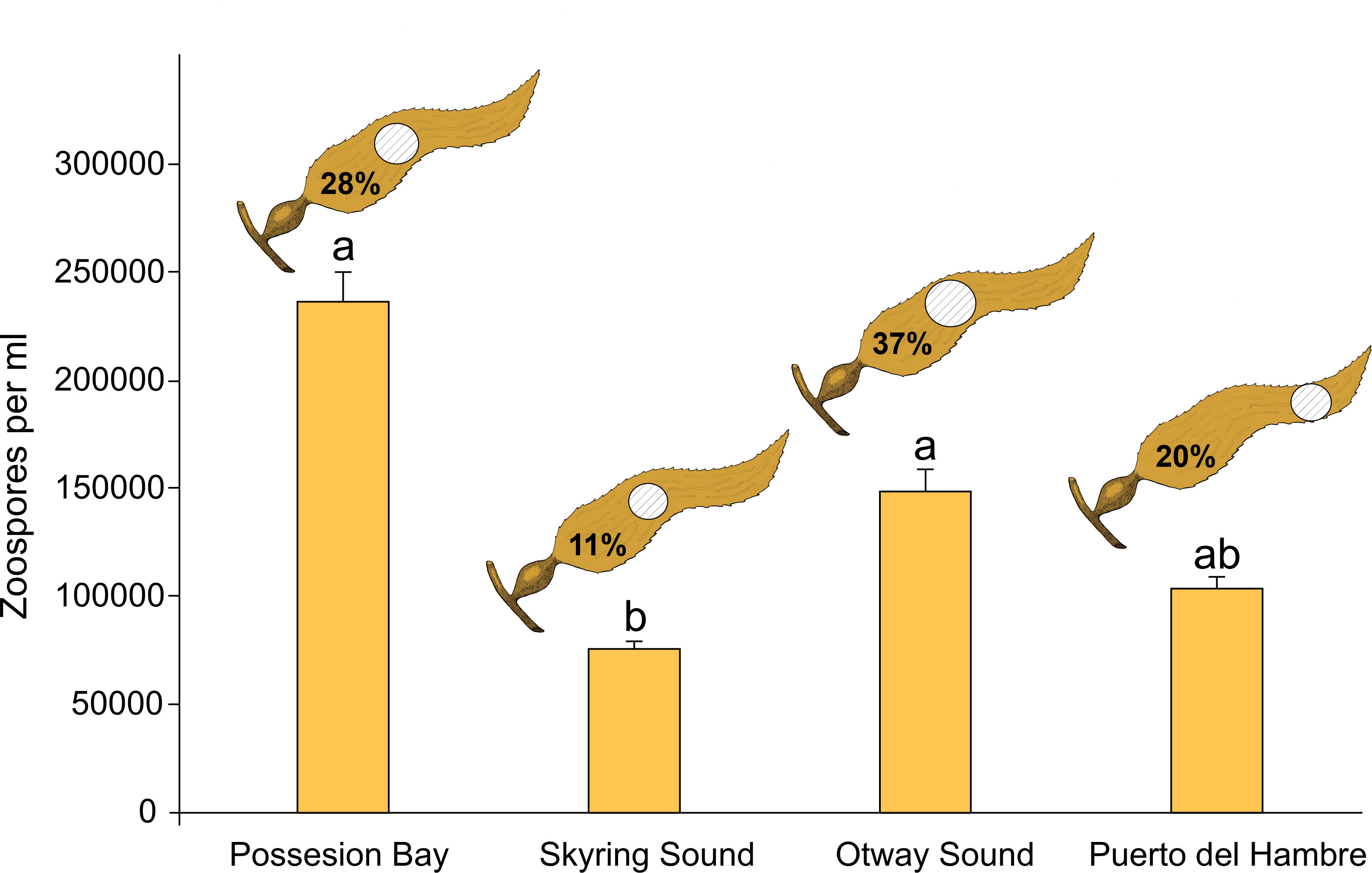
Figure 5 The figure shows the percentage of sorus area and the circle represents the distribution of sori (>65%) in the apical, middle and basal zones of the sporophylls (n=20 per location), and the zoospore density in sporophylls of Macrocystis pyrifera, mean and ±1 standard error (n=15 per location). Different letters indicate statistically significant differences between zoospore density of sporophylls per population (p≤ 0.05.).
Skyring Sound had the lowest percentage of sorus coverage (11%) in the sporophylls, while Otway Sound had the highest percentage (37%); this difference was significant (p≤ 0.05; Figure 5). Possession Bay had a sorus cover percentage of 29% (p =0.019), while in Puerto del Hambre it was 20% (p =0.007).
Spore density was highest at Possession Bay (236.733 ± 13.716 zoospores/mL) and lowest at Skyring Sound (75.933 ± 3.499 zoospores/mL). Significant differences were observed between Skyring Sound and Otway Sound (p =0.00009), Skyring Sound and Possession Bay (p ≤0.05), and Possession Bay and Puerto del Hambre (p ≤0.05; Figure 5; Supplementary Table S7).
Discussion
Unlike other kelp species around the world, such as Laminaria solidungula, Saccharina latissima, and Alaria esculenta in the Canadian Arctic, which rely on both the rigidity of their stipes and currents to maintain an upright position and capture light (Bluhm et al., 2022), M. pyrifera stands out for its fronds incorporating aerocysts or pneumatocysts in their morphological structure. These gas-filled aerocysts allow the kelp to float and remain in a vertical position for light capture (Salavarría et al., 2014). However, populations in the Magallanes ecoregion exhibit differences in the position of the sporophyte plants observed in situ. Three different types of positions were observed in the four populations sampled: two related to the buoyancy of the pneumatocysts and one associated with tidal amplitude. The first type is the vertical position, where the canopy of M. pyrifera floats at 0 m, exposed to the air; this type of kelp forest was observed in Otway Sound and Puerto del Hambre. This has been previously reported for the population in Puerto del Hambre (Marambio et al., 2017). The second type is the semi-vertical position, where the canopy of M. pyrifera does not float at 0 m, remaining submerged without reaching the surface; this type of kelp forest was observed in Skyring Sound, without being previously documented. The third type is the horizontal position, where M. pyrifera populations during low tide periods remain completely settled on the rocky seabed. This type of kelp forest was observed in Possession Bay, previously described by Aldea and Rosenfeld et al. (2011). Thus far, the effect of algae position related to its buoyancy capacity has not been evaluated in these M. pyrifera populations. Nevertheless, it has been observed in other kelp species that buoyancy is influenced by various factors, such as the hydrostatic pressure gradient, pneumatocyst volume, seawater density, and depth (Liggan and Martone, 2018; Liggan and Martone, 2020). Liggan and Martone et al. (2018) demonstrated that under environmental stress conditions (e.g., changes in seawater density and depth), the cells of the pneumatocyst wall in bull kelp Nereocystis luetkeana produce more gas to maintain internal pressure and, thus, buoyancy. However, this may have physiological consequences for the algae, as it increases energy expenditure and hampers growth (Liggan and Martone, 2020). It is likely that similar mechanisms regulate the buoyancy of M. pyrifera aerocysts in environmentally stressed conditions in the Magallanes ecoregion, such as in populations in Skyring Sound and sites influenced by glaciers, affecting their ecophysiological capacity.
These results from in situ observation are key to understanding the ecophysiological ranges of this species, and the acclimatization strategies depending on the habitat they inhabit. The M. pyrifera populations of Puerto del Hambre and Otway Sound showed similar levels of Fv/Fm during this study. These two populations have an upright meadow type, which means that only the apical blades are exposed to environmental variables at 0 m. Possibly due to the type of position of the Puerto del Hambre population, a stratified photosynthetic efficiency was recorded in autumn and summer where the apical frond showed high α values, but both populations showed an increase in Fv/Fm values in the basal blades during spring. This kind of stratification variation is in agreement with Colombo-Pallotta et al. (2006) for M. pyrifera at different depths from 0 to 6 m. Ek at Puerto del Hambre only showed seasonal variations, with high values during autumn and spring, while the Otway Sound site showed high Ek values in basal blades during spring and apical blades in summer. The high Ek values recorded during this study agree with those observed by Koch et al. (2016), where in warm months Ek values are 286.98 ± 19.06, while in the cooler season, Ek values decrease to 121.60 ± 18.12. It is worth noting that α values were low in both populations during winter. This is related to the low concentration of Chl a recorded in this season for both populations. Colombo-Pallotta et al. (2006) and Koch et al. (2016) showed similar trends for α and Chl a, which demonstrates the high intraspecific variation of M. pyrifera, since the parameter α behaves as an indicator of photosynthetic efficiency. Chl a was the pigment with the highest concentration compared to Chl c and Fucox in M. pyrifera for Puerto del Hambre and Otway Sound. There were seasonal differences during this study in the populations of Puerto del Hambre and Otway Sound; low pigment values of Chl c and Fucox were recorded in winter. Hallerud (2014) described how in brown algae such as Laminaria digitata, high irradiance and increased temperature during warmer seasons generate higher pigment concentration in algal tissue, calling these variations “seasonal acclimation.” This seasonal acclimation reduces irradiance uptake and avoids overexcitation of photosystems and the occurrence of chronic photoinhibition (Marambio and Bischof, 2021).
Skyring Sound presented a semi-vertical position where the plant is always covered with water, not reaching the surface. This population, like those mentioned above, showed high variability in the warmer seasons for the photosynthetic parameter Fv/Fm between the apical and middle blades. The increase in Fv/Fm values in the basal blades of Skyring Sound during spring shows that sporophytes generate greater photosynthetic activity in deeper blades, possibly due to the low irradiance reaching the basal blades during this time of the year. Edwards and Kim (2010) described how the photoacclimation of M. pyrifera blades depends on the depth at which they are located. Ek values in the Skyring Sound are generally low and do not differ between frond types and may be attributed to a shade-adapted population as described by Palacios et al. (2021), who reported Ek values ranging from 75 μmol photon m−2 s−1 to 120–140 μmol photon m−2 s−1, depending on the study site. However, the spring season showed a different pattern; M. pyrifera sporophytes achieved an ecophysiological stratification, which is observed not only in the increase in Ek values in the middle blades but also in the high values observed in rETRmax in middle and basal blades. α in Skyring Sound showed an increase during autumn–winter; this increase in photosynthetic efficiency is supported by the increase in photosynthetic pigments Chl a, Chl c, and Fucox in the colder seasons. Kilian et al. (2013) reported that Skyring Sound has a surface layer of approximately 80 m of low salinity. This surface layer is interfered by the increase in winds in the area, which occurs mainly in spring, causing waters with higher salinity to rise from the bottom (Kilian et al., 2007). This variation in salinity could explain why during the year M. pyrifera in Skyring Sound needs to generate more photosynthetic activity to maintain plant regulation. Karsten (2007) describe how algae synthesize low molecular weight molecules in response to changes in salinity and rapidly exchange water with the surrounding medium, balancing the internal osmotic pressure. This process of internal osmotic regulation may generate a higher energy expenditure in algal species, which could affect the growth and development of the sporophyte M. pyrifera in natural populations such as Skyring sound.
The Possession Bay population shows a mixed type position due to the large tidal range of the zone. Fv/Fm during this study showed seasonal variations, in winter and spring, and variation in photosynthetic activity between frond types was observed in these seasons. These results are supported by the parameter α, which decreased in winter and reached maximal values during summer and autumn. Exposure to low tide can directly influence the pigment composition and photosynthetic parameters of seagrasses (Hanelt et al., 1997; Wiencke and Bischof, 2012). The variation in Fv/Fm and the low α values during this study show that M. pyrifera is highly sensitive to the high irradiance to which it is exposed during summer. For this reason, M. pyrifera of Possession Bay is not able to regulate its internal photosynthetic activity in a stratified way, but its ecophysiological strategy is to act as a single plant, not being able to regulate its photosynthetic activity until the winter season. In winter and spring M. pyrifera showed an increase in photosynthetic efficiency α and an increase in Ek values, in agreement with Colombo-Pallotta et al. (2006) and Edwards and Kim (2010) for kelp forest of the same species. High Ek values in winter–spring during this study are supported by the increase in Chl a and Chl c in autumn–spring. These parameters generate higher photosynthetic efficiency, as described by Koch et al. (2016) for M. pyrifera—Chl a concentration in summer reached 1.88 ± 0.25 µg mg−1 DW, while in winter, it increased to 2.24 ± 0.36 µg mg−1 DW. The pigments in seaweeds are highly sensitive to light, and in the presence of high irradiance, seaweeds are able to regulate their pigment content and composition, depending on the situation (Hanelt et al., 2003; Hurd et al., 2014.)
It is important to mention that our results differ from those of Becheler et al. (2022); these authors stated that M. pyrifera does not show local adaptation to different environments, but rather the ability to colonize distant and diverse habitats. Probably, M. pyrifera in terms of thermal tolerance may perform as a more plastic and not so selective species (Becheler et al., 2022). However, the ecophysiological pattern detected by Rodríguez et al. (2019) in four natural populations of M. pyrifera where it was evidenced that at the end of the reproductive cycle (e.g., spore germination, sex ratio, gametogenesis), each population has the ability to develop better depending on its local conditions. In this sense, from a reproductive and developmental point of view, these results are in concordance with this study. In addition, they show an important effect of salinity and temperature on the development of the different populations (Rodríguez et al., 2019). Therefore, our results, like Rodríguez et al. (2019), would suggest that M. pyrifera populations would perform better in their local conditions and that other variables besides temperature such as salinity, light regimes, and tidal cycles could play an important role in the life cycle of each population.
Photosynthetic activity in basal vegetative and sporophyll blades of M. pyrifera varied between locations sampled. Sporophylls from Possession Bay had low Fv/Fm values compared to the other reproductive blades, while Ek of basal vegetative and reproductive blades was higher compared to the other populations. This is supported by a decrease in Fucox in sporophylls in Possession Bay. Fucoxanthin is mainly considered as a protective and antioxidant agent, actively participating in light stress processes (PAR and UV light) (Dambeck and Sandmann, 2014), so the decrease in fucoxanthin and the low values found during this study are consistent with a mainly photosynthetic activity. The results presented in this study were measured during winter, a season of the year with a decrease in irradiance. Hurd et al. (2014) described how seasonal stress directly influences the ecophysiology of macroalgae, and therefore, low irradiance in cold seasons directly influences the increase in some photosynthetic parameters such as Ek. However, no differences in Fv/Fm and Ek were observed between basal vegetative and sporophylls blades. This is in agreement with Ziemann dos Santos et al. (2019), who did not observe photosynthetic variation between vegetative and sporophyll blades of M. pyrifera from Puerto del Hambre, Magellan ecoregion.
We did find not differences between basal vegetative and sporophyll blades of M. pyrifera in photosynthetic efficiency α; this is not in agreement with the report of Ziemann dos Santos et al. (2019), who found differences in photosynthetic efficiency between sporophylls 0.26 ± 0.04 (μmol photons m−2 s−1)−1 and basal vegetative blades 0.33 ± 0.03 (μmol photons m−2 s−1)−1.
To date, studies focusing on reproductive aspects in populations of M. pyrifera in sub-Antarctic environments are scarce. Buschmann et al. (2004); Buschmann et al. (2006) indicated that M. pyrifera populations in southern Chile develop reproductive strategies that maintain their survival in the face of changing environmental conditions. The results obtained in this study suggest that there are significant differences in the percentage of sorus area and the number of zoospores released among the populations studied, possibly associated with the effect of local environmental conditions. The lowest and highest values were obtained at Skyring Sound and Possession Bay, respectively. In Skyring Sound, low salinity could be an important factor determining the development and survival of M. pyrifera populations. Rodríguez et al. (2019) described how these populations are adapted to grow and develop in conditions of low salinity, 17 psu. However, acclimation to low salinity is reflected in a small population size (in situ observation) and a related reproductive strategy. Neushul and Harger (1985) described how reproductive performance increases in populations with a low density of individuals with blades with low spore density. Fecundity tends to be high in populations with this type of strategy (Reed, 1987), thus ensuring their survival.
Possession Bay had the highest zoospore release values (236.733 zoospores mL−1), even higher than those reported by Palacios and Mansilla (2003) for a population present in Tierra del Fuego (120.000 zoospores mL−1). High values of zoospore concentration in Possession Bay may be related to the need to release as many zoospores as possible into the environment. This may be related to the kind of substrate on which the Possession Bay sporophytes inhabit, as this locality is mostly composed of soft bottoms and small rocky patches (Aldea and Rosenfeld, 2011), so the possibility of finding a suitable substrate for spore attachment could be decreased. The soft sediments concentrations of 10 mg cm−2 are sufficient to have an impact on the spore settlement of M. pyrifera and affect their survival (Devinny and Volse, 1978; Airoldi, 2003; Geange et al., 2014; Lind and Konar, 2017)
Finally, one of the most relevant results observed in this study is the acclimatization capacity that the different populations of M. pyrifera have throughout the four localities analyzed. These localities represent marked environmental gradients, a characteristic of the MSE. In this work, we observed populations that tolerate low salinities and populations that inhabit areas with wide tidal cycles and high turbidity. These marked environmental differences mean that M. pyrifera plants present different ecophysiological strategies to acclimatization to different environments. For example, the high concentration of accessory pigments presents in some populations could suggest a strategy to optimize the photosynthetic efficiency of M. pyrifera to compensate for the low irradiance resulting from environmental conditions such as high turbidity generated by sandy substrates or the semi-vertical position of the plants due to low salinity (Gómez et al., 1997; Mansilla et al., 2016). In this sense, not only spatial differences were evidenced among the different populations of M. pyrifera, but also temporal differences were detected. During one year of sampling, we observed physiological and biochemical acclimation strategies depending on the characteristics of each locality. Different studies show how M. pyrifera is able to have a high degree of acclimation to environmental variables (Cabello-Pasini et al., 2011; Marambio et al., 2017; Mora-Soto et al., 2020; Palacios et al., 2021; this study). An interesting pattern observed in this study is related to measurements of optimum quantum yield of photosystem II (Fv/Fm) and the Ek where the variation was mainly seasonally dependent, with higher values in winter and autumn and the opposite in warm seasons. This seasonal change could be a relevant physiological trait that M. pyrifera individuals have to compensate for the low irradiance during the winter season (Hurd et al., 2014), which could be further enhanced by local site conditions (e.g. salinity, turbidity, and tidal cycles) and by the position of the individuals (e.g. semi-vertical and horizontal). Likewise, this same pattern was detected among the fronds of the different depths analyzed, where the Fv/Fm and Ek measurements showed greater variability between frond types in the warmer months of spring and summer, possibly due to an increase in algal biomass that limits the entry of irradiance into M. pyrifera forests, causing blades at different depths to require a higher effort to capture irradiance (Plana et al., 2007; Hurd et al., 2014).
Conclusion
The results obtained in this study suggest that M. pyrifera populations present in the Magellan ecoregion could be undergoing local acclimatization processes that allow them to thrive under a wide range of environmental conditions. Data obtained on physiological and reproductive parameters are key to understanding the spatial and temporal dynamics in which M. pyrifera populations develop. Each of the populations studied seems to use acclimation strategies that allow them to survive environmental variations, e.g., to increase their photosynthetic efficiency and reproductive capacity, among others. Increasing overfishing of brown algae along the Chilean coast and its southward movement is a constant threat to these large southern underwater macroalgal forests. For this reason, the Ministry of Economy, Development and Tourism and the Undersecretariat of Fisheries and Aquaculture (SUBPESCA) established for the first-time exempt decree No. 42 that establishes a 2-year (2022–2024) harvesting ban for M. pyrifera in the Magellan region. Therefore, it is important to provide baseline information that can be used in decision-making when lifting the ban and support the sustainability of these important marine ecosystems that act as sentinels of climate change. Based on the results obtained in this study, we propose that the physiological and reproductive acclimatization of each population of the kelp M. pyrifera to its local environmental conditions should be considered when making decisions. In addition, alternatives to the extraction of natural kelp forest should be sought, possibly through sustainable aquaculture within the reach of coastal communities.
Data availability statement
The original contributions presented in the study are included in the article/Supplementary Material. Further inquiries can be directed to the corresponding authors.
Author contributions
JM: conceptualization, research, methodology, formal analysis, writing—original draft and review. JR: research, methodology, and original draft and review. SR: research, statistical analysis, and original draft and review. FM: investigation. JO: research and methodology. PO: research. AM: validation, supervision, funding acquisition, and writing—review and editing. All authors contributed to the article and approved the submitted version.
Funding
This work was supported by the project FONDECYT 1140940 “Macroalgal adaptive radiation: potential links to ecological niche diversity in the ecoregion of Magellan and Chilean Antarctic.”
Acknowledgments
The authors wish to thank Project FONDEF IDEA ID23I10288 “Determination of the spectral footprint of kelp forests and associated flora to quantify and assess carbon pools and fluxes in marine ecosystems in the sub-Antarctic region of Magallanes” and the project Funding from the Cape Horn International Center (CHIC) Project ANID/BASAL FB210018. SR would like to thank the Project ANID–Millennium Science Initiative Program–ICN2021_002 and the INACH Project DG-10_22. The present study was part of the Master thesis presented by the first author to the Graduate Program of Science in Management and Conservation of Natural Resources in Sub-Antarctic Environments, Punta Arenas, Chile.
Conflict of interest
The authors declare that the research was conducted in the absence of any commercial or financial relationships that could be construed as a potential conflict of interest.
Publisher’s note
All claims expressed in this article are solely those of the authors and do not necessarily represent those of their affiliated organizations, or those of the publisher, the editors and the reviewers. Any product that may be evaluated in this article, or claim that may be made by its manufacturer, is not guaranteed or endorsed by the publisher.
Supplementary material
The Supplementary Material for this article can be found online at: https://www.frontiersin.org/articles/10.3389/fmars.2023.1222178/full#supplementary-material
References
Adami M. L., Gordillo S. (1999). Structure and dynamics of the biota associated with Macrocystis pyrifera (Phaeophyta) from the Beagle Channel, Tierra del Fuego. Sci. Mar. 63, 183–191. doi: 10.3989/scimar.1999.63s1183
Airoldi L. (2003). The effects of sedimentation on rocky coast assemblages. Oceanogr. Mar. Biol. Annu. Rev. 41, 169–171.
Aldea C., Rosenfeld S. (2011). Macromoluscos intermareales de sustratos rocosos de la playa Buque Quemado, Estrecho de Magallanes, sur de Chile. Rev. Biol. Mar. Oceanogr. 46 (2), 115–124. doi: 10.4067/S0718-19572011000200002
Antezana T., Guglielmo. L., Ghirardelli E. (1992). “Microbasins within the Strait of Magellan affecting zooplancton distribution,” in Oceanografia in antartide. Eds. Gallardo V. A., Ferretti O., Moyano H. (Rome: ENEA-PNRA), 453–458.
Astorga-España M. S., Mansilla A. (2014). Sub-Antarctic macroalgae: opportunities for gastronomic tourism and local fisheries in the Region of Magallanes and Chilean Antarctic Territory. J. Appl. Phycol. 26, 973–978. doi: 10.1007/s10811-013-0141-1
Ávila M., Merino C., Guissen K., Piel M. I. (2010). Manual de cultivo de macroalgas pardas. Desde el Laboratorio al océano (Iquique: Universidad Arturo Prat Press).
Bahamonde F., Rodríguez J. P., Rosenfeld S., Méndez F., López Z., Gerard K., et al. (2022). Variability at multiple spatial scales in intertidal and subtidal macrobenthic communities in a fjord with glaciers, Magellanic Subantarctic ecoregion, Chile. Prog. Oceanogr. 208, 102879. doi: 10.1016/j.pocean.2022.102879
Becheler R., Haverbeck D., Clerc C., Montecinos G., Valero M., Mansilla A., et al. (2022). Variation in thermal tolerance of the giant kelp´ s gametophytes: suitability of habitat, population quality or local adaptation? Front. Mar. Sci. 1176. doi: 10.3389/fmars.2022.802535
Betancourtt C., Zapata J., Latorre N., Anguita C., Castañeda F., Meynard A., et al. (2018). Spatio-temporal variation in the composition of the macroalgae assemblage of the intertidal rocky zone from Maitencillo, Valparaíso, central coast of Chile. Rev. Biol. Mar. Oceanogr. 53, 105–117. doi: 10.4067/S0718-19572018000100105
Bluhm B. A., Brown K., Rotermund L., Williams W., Danielsen S., Carmack E. C. (2022). New distribution records of kelp in the Kitikmeot Region, Northwest Passage, Canada, fill a pan-Arctic gap. Polar. Biol. 45 (4), 719–736. doi: 10.1007/s00300-022-03007-6
Buschmann A. H., Moreno C., Vásquez J. A., Hernández-González M. C. (2006). Reproduction strategies of Macrocystis pyrifera (Phaeophyta) in Southern Chile: the importance of population dynamics. J. Appl. Phycol. 18, 575–582. doi: 10.1007/s10811-006-9063-5
Buschmann A. H., Varela D. A., Hernández-González M. C., Huovinen P. (2008). Opportunities and challenges for the development of an integrated seaweed-based aquaculture activity in Chile: determining the physiological capabilities of Macrocystis and Gracilaria as biofilters. J. Appl. Phycol. 5 (20), 571–577. doi: 10.1007/s10811-007-9297-x
Buschmann A. H., Vásquez J. A., Osorio P., Reyes E., Filún L., Hernández-González M. C., et al. (2004). The effect of water movement, temperature and salinity on abundance and reproductive patterns of Macrocystis spp. (Phaeophyta) at different latitudes in Chile. Mar. Biol. 145, 849–862. doi: 10.1007/s00227-004-1393-8
Byrnes J. E., Reed D. C., Cardinale B. J., Cavanaugh K. C., Holbrook S. J., Schmitt R. J. (2011). Climate-driven increases in storm frequency simplify kelp forest food webs. Glob. Change Biol. 17 (8), 2513–2524. doi: 10.1111/j.1365-2486.2011.02409.x
Cabello-Pasini A., Macías-Carranza V., Abdala R., Korbee N., Figueroa F. L. (2011). Effect of nitrate concentration and UVR on photosynthesis, respiration, nitrate reductase activity, and phenolic compounds in Ulva rigida (Chlorophyta). J. Appl. Phyco. 23, 363–369. doi: 10.1007/s10811-010-9548-0
Camus C., Infante J., Buschmann A. H. (2018). Overview of 3 years precommercial seafarming of Macrocystis pyrifera along the Chilean coast. Rev. Aquac. 10 (3), 543–559. doi: 10.1111/raq.12185
Camus C., Solas M., Martínez C., Vargas J., Garcés C., Gil-Kodaka P., et al. (2021). Mates matter: gametophyte kinship recognition and inbreeding in the giant kelp, Macrocystis pyrifera (Laminariales, Phaeophyceae). J. Phycol. 57 (3), 711–725. doi: 10.1111/jpy.13146
Casiccia C., Kirchhoff V. W. J. H., Torres A. (2003). Simultaneous measurements of ozone and ultraviolet radiation: Spring 2000, Punta Arenas, Chile. Atmospheric Environ. 37 (3), 383–389. doi: 10.1016/S1352-2310(02)00890-7
Clapperton C. M. (1994). The quaternary glaciation of Chile: a review. Rev. Chil. Hist. Nat. 67 (4), 369–383.
Colombo-Pallotta M. F., García-Mendoza E., Ladah L. B. (2006). Photosynthetic performance, light absorption, and pigment composition of Macrocystis pyrifera (laminariales, phaeophyceae) blades from different depths. J. Phyco. 42 (6), 1225–1234. doi: 10.1111/j.1529-8817.2006.00287.x
Dambeck M., Sandmann G. (2014). Antioxidative activities of algal keto carotenoids acting as antioxidative protectants in the chloroplast. Photochem. Photobiol. 90 (4), 814–819. doi: 10.1111/php.12249
Davis T. R., Champion C., Coleman M. A. (2022). Ecological interactions mediate projected loss of kelp biomass under climate change. Divers. Distrib. 28 (2), 306–317. doi: 10.1111/ddi.13462
Devinny J. S., Volse L. A. (1978). Effects of sediments on the development of Macrocystis pyrifera gametophytes. Mar. Biol. 48, 343–348. doi: 10.1007/BF00391638
Duarte C. M., Wu J., Xiao X., Bruhn A., Krause-Jensen D. (2017). Can seaweed farming play a role in climate change mitigation and adaptation? Front. Mar. Sci. 4, 100. doi: 10.3389/fmars.2017.00100
Edwards M. S., Kim K. Y. (2010). Diurnal variation in relative photosynthetic performance in giant kelp Macrocystis pyrifera (Phaeophyceae, Laminariales) at different depths as estimated using PAM fluorometry. Aquat. Bot. 92 (2), 119–128. doi: 10.1016/j.aquabot.2009.10.017
Eger A. M., Marzinelli E. M., Christie H., Fagerli C. W., Fujita D., Gonzalez A. P., et al. (2022). Global kelp forest restoration: Past lessons, present status, and future directions. Biol. Rev. 97 (4), 1449–1475. doi: 10.1111/brv.12850
Falkowski P. G., LaRoche J. (1991). Acclimation to spectral irradiance in algae. J. Phycol. 27 (1), 8–14. doi: 10.1111/j.0022-3646.1991.00008.x
Friedlander A. M., Ballesteros E., Bell T. W., Caselle J. E., Campagna C., Goodell W., et al. (2020). Kelp forests at the end of the earth: 45 years later. PloS One 15 (3), e0229259. doi: 10.1371/journal.pone.0229259
Friedlander A. M., Ballesteros E., Caselle J. E., Hüne M., Adler A. M., Sala E. (2023). Patterns and drivers of benthic macroinvertebrate assemblages in the kelp forests of southern Patagonia. PloS One 18 (1), e0279200. doi: 10.1371/journal.pone.0279200
Geange S. W., Powell A., Clemens-Seely K., Cárdenas C. A. (2014). Sediment load and timing of sedimentation affect spore establishment in Macrocystis pyrifera and Undaria pinnatifida. Mar. Biol. 161, 1583–1592. doi: 10.1007/s00227-014-2442-6
Gómez I., Weykam G., Klöser H., Wiencke C. (1997). Photosynthetic light requirements, metabolic carbon balance and zonation of sublittoral macroalgae from King George Island (Antarctica). Mar. Ecol. Prog. Ser. 148, 281293. doi: 10.3354/meps148281
Hallerud C. B. (2014). Pigment composition of macroalgae from a Norwegian Kelp forest (Master’s thesis, Institutt for biologi). Norway: Norwegian University of Science and Technology.
Hanelt D., Melchersmann B., Wiencke C., Nultsch W. (1997). Effects of high light stress on photosynthesis of polar macroalgae in relation to depth distribution. Mar. Ecol. Prog. Ser. 149, 255–266. doi: 10.3354/meps149255
Hanelt D., Wiencke C., Bischof K. (2003). “Photosynthesis in marine macroalgae,” in Photosynthesis in algae, vol. 14 . Eds. Larkum W. A., Douglas S. E., Raven J. A. (Dordrecht: Springer Netherlands), 413–435.
Hollarsmith J. A., Buschmann A. H., Camus C., Grosholz E. D. (2020). Varying reproductive success under ocean warming and acidification across giant kelp (Macrocystis pyrifera) populations. J. Exp. Mar. Biol. Ecol. 522, 151247. doi: 10.1016/j.jembe.2019.151247
Hurd C. L., Harrison P. J., Bishof K., Lobban C. S. (2014). Seaweed ecology and physiology (Cambridge, United Kingdom: Cambridge University Press).
Karsten U. (2007). Research note: salinity tolerance of Arctic kelps from Spitsbergen. Phycol Res. 55, 257–262.
Kilian R., Baeza O., Breuer S., Ríos F., Arz H., Lamy F., et al. (2013). Late glacial and holocene paleogeographical and paleoecological evolution of the seno skyring and otway fjord systems in the Magellan region. An. Inst. Patagon. 41 (2), 5–26. doi: 10.4067/S0718-686X2013000200001
Kilian R., Baeza O., Steinke T., Arévalo M., Ríos C., Schneider C. (2007). Late Pleistocene to Holocene marine transgression and thermohaline control on sediment transport in the western Magellanes fjord system of Chile (53°S). Quat. Int. 161 (1), 90–107. doi: 10.1016/j.quaint.2006.10.043
Koch K., Thiel M., Hagen W., Graeve M., Gómez I., Jofre D., et al. (2016). Short-and long-term acclimation patterns of the giant kelp Macrocystis pyrifera (Laminariales, Phaeophyceae) along a depth gradient. J. Phycol. 52 (2), 260–273. doi: 10.1111/jpy.12394
Krumhansl K. A., Okamoto D. K., Rassweiler A., Novak M., Bolton J. J., Cavanaugh K. C., et al. (2016). Global patterns of kelp forest change over the past half-century. Proc. Natl. Acad. Sci. 113 (48), 13785–13790. doi: 10.1073/pnas.1606102113
Liggan L. M., Martone P. T. (2018). Under pressure: biomechanical limitations of developing pneumatocysts in the bull kelp (Nereocystis luetkeana, Phaeophyceae). J. Phycol. 54 (5), 608–615. doi: 10.1111/jpy.12776
Liggan L. M., Martone P. T. (2020). Gas composition of developing pneumatocysts in bull kelp Nereocystis luetkeana (Phaeophyceae). J. Phycol. 56 (5), 1367–1372. doi: 10.1111/jpy.13037
Lind A. C., Konar B. (2017). Effects of abiotic stressors on kelp early life-history stages. Algae 32 (3), 223–233. doi: 10.4490/algae.2017.32.8.7
Ling S. D., Johnson C. R., Frusher S. D., Ridgway K. (2009). Overfishing reduces resilience of kelp beds to climate-driven catastrophic phase shift. Proc. Natl. Acad. Sci. 106 (52), 22341–22345. doi: 10.1073/pnas.0907529106
Macaya E. C., Zuccarello G. C. (2010). Genetic structure of the giant kelp Macrocystis pyrifera along the southeastern Pacific. Mar. Ecol. Prog. Ser. 420, 103–112. doi: 10.3354/meps08893
Mansilla A., Ávila M. (2011). Using Macrocystis pyrifera (L.) C. Agardh from southern Chile as a source of applied biological compounds. Rev. Bras. Farmacogn. 21, 262–267. doi: 10.1590/S0102-695X2011005000072
Mansilla A., Ávila M., Yokoya N. S. (2012). Current knowledge on biotechnological interesting seaweeds from the Magellan Region, Chile. Rev. Bras. Farmacogn. 22, 760–767. doi: 10.1590/S0102-695X2012005000074
Mansilla A., Méndez F., Murcia S., Rodríguez J. P., Marambio J., Rosenfeld S., et al. (2016). Adjustment of pigment composition in Desmarestia (Desmarestiaceae) species along a sub-Antarctic to Antarctic latitudinal gradient. Polar Res. 35 (1), 29383. doi: 10.3402/polar.v35.29383
Mansilla A., Palacios M., Navarro N., Riquelme V. (2005). Studies of the populations of Macrocystis pyrifera (L) C. Agardh in Tierra del Fuego, Chile. Rep. Polar. Mar. Res. 507, 160–162. doi: 10.4067/S0717-65382007000100007
Marambio J., Bischof K. (2021). Differential acclimation responses to irradiance and temperature in two co-occurring seaweed species in Arctic fjords. Polar Res. 40, 5702. doi: 10.33265/polar.v40.5702
Marambio J., Rodriguez J. P., Mendez F., Ocaranza P., Rosenfeld S., Ojeda J., et al. (2017). Photosynthetic performance and pigment composition of Macrocystis pyrifera (Laminariales, Phaeophyceae) along a gradient of depth and seasonality in the ecoregion of Magellan, Chile. J. Appl. Phycol. 29, 2575–2585. doi: 10.1007/s10811-017-1136-0
Marambio J., Rosenfeld S., Bischof K. (2022). Hyposalinity affects diurnal photoacclimation patterns in the rhodophyte Palmaria palmata under mimicked Arctic summer conditions. J. Photochem. Photobiol. 11, 100124. doi: 10.1016/j.jpap.2022.100124
Mora-Soto A., Capsey A., Friedlander A. M., Palacios M., Brewin P. E., Golding N., et al. (2021). One of the least disturbed marine coastal ecosystems on Earth: Spatial and temporal persistence of Darwin’s sub-Antarctic giant kelp forests. J. Biogeogr. 48 (10), 2562–2577. doi: 10.1111/jbi.14221
Mora-Soto A., Palacios M., Macaya E. C., Gómez I., Huovinen P., Pérez-Matus A., et al. (2020). A high-resolution global map of giant kelp (Macrocystis pyrifera) forests and intertidal green algae (Ulvophyceae) with Sentinel-2 imagery. Remote Sens. 12 (4), 694. doi: 10.3390/rs12040694
Neushul M., Harger B. W. (1985). Studies of biomass yield from a near-shore macroalgal test farm. J. Sol. Energy Eng. 107 (1). doi: 10.1115/1.3267661
Ojeda J., Marambio J., Rosenfeld S., Contador T., Rozzi R., Mansilla A. (2019). Seasonal changes of macroalgae assemblages on the rocky shores of the Cape Horn Biosphere Reserve, Sub-Antarctic Channels, Chile. Aquat. Bot. 157, 33–41. doi: 10.1016/j.aquabot.2019.06.001
Ojeda J., Rosenfeld S., Marambio J., Rozzi R., Mansilla A. (2014). Patrones estacionales y espaciales de la diversidad de moluscos intermareales de bahía Róbalo, canal Beagle, Reserva de la Biosfera Cabo de Hornos, Chile. Rev. Biol. Mar. Oceanogr. 49 (3), 493–509. doi: 10.4067/S0718-19572014000300007
Palacios M., Mansilla A. (2003). Desarrollo de Gametófitos y Esporófitos de Macrocystis Pyrifera (L.) Agardh (Laminariales: Lessoniaceae) de la Región de Magallanes en Condiciones de Laboratorio. An. Instit. Patagon. 31, 43–53.
Palacios M., Osman D., Ramírez J., Huovinen P., Gómez I. (2021). Photobiology of the giant kelp Macrocystis pyrifera in the land-terminating glacier fjord Yendegaia (Tierra del Fuego): A look into the future? Sci. Total. Environ. 751, 141810. doi: 10.1016/j.scitotenv.2020.141810
Pinilla E., Arriagada M., Soto G., Ruiz C., Reche P. (2013). Informe final. Caracterización y modelación de patrones de circulación marina en la región de Magallanes (Proyecto ASIPA – MINECOM). Valparaíso: Instituto de Fomento Pesquero.
Pinilla E., Soto G., Valdebenito P., Soto C., Venegas O., San Martin J. (2021). Informe final. Determinación de las escalas de intercambio de agua en seno Skyring y canales aledaños, región de Magallanes y Antártica Chilena (Subsecretaria de Economía y Empresas de Menor Tamaño). Valparaíso: Instituto de Fomento Pesquero.
Plana J., Mansilla A., Palacios M., Navarro N. P. (2007). Estudio poblacional de Macrocystis pyrifera (L.) C. Agardh (Laminariales: Phaeophyta) en ambientes protegido y expuesto al oleaje en Tierra del Fuego. Gayana. 71 (1), 66–75. doi: 10.4067/S0717-65382007000100007
Platt T., Gallegos C. L., Harrison W. G. (1980). Photoinhibition of photosynthesis in natural assemblages of marine phytoplankton. J. Mar. Res. 38 (4), 687–701.
Qiu Z., Coleman M. A., Provost E., Campbell A. H., Kelaher B. P., Dalton S. J., et al. (2019). Future climate change is predicted to affect the microbiome and condition of habitat-forming kelp. Proc. R. Soc 286 (1896), 20181887. doi: 10.1098/rspb.2018.1887
R Core Team. (2017). R: A Language and Environment for Statistical Computing. https://www.R-project.org/.
Reed D. C. (1987). Factors affecting the production of sporophylls in the giant kelp Macrocystis pyrifera (L.) C. Ag. J. Exp. Mar. Biol. Ecol. 113 (1), 61–69. doi: 10.1016/0022-0981(87)90082-7
Reed D. C., Brzezinski M. A. (2009). Kelp forests. In The Management of Natural Coastal Carbon Sinks. (Gland, Switzerland: IUCN), pp. 31–37.
Ríos C., Arntz W. E., Gerdes D., Mutschke E., Montiel A. (2007). Spatial and temporal variability of the benthic assemblages associated to the holdfasts of the kelp Macrocystis pyrifera in the Straits of Magellan, Chile. Polar Biol. 31, 89–100. doi: 10.1007/s00300-007-0337-4
Rodríguez J. P., Terrados J., Rosenfeld S., Méndez F., Ojeda J., Mansilla A. (2019). Effects of temperature and salinity on the reproductive phases of Macrocystis pyrifera (L.) C. Agardh (Phaeophyceae) in the Magellan region. J. Appl. Phycol. 31, 915–928. doi: 10.1007/s10811-018-1693-x
Rosenfeld S., Marambio J., Ojeda J., Rodríguez J., González-Wevar C., Gerard K., et al. (2018). Trophic ecology of two co-existing Sub-Antarctic limpets of the genus Nacella: spatio-temporal variation in food availability and diet composition of Nacella magellanica and N. deaurata. Zookeys. 738, 1–25. doi: 10.3897/zookeys.738.21175
Rosenfeld S., Ojeda J., Hüne M., Mansilla A., Contador T. (2014). Egg masses of the Patagonian squid Doryteuthis (Amerigo) gahi attached to giant kelp (Macrocystis pyrifera) in the sub-Antarctic ecoregion. Polar Res. 33 (1), 21636. doi: 10.3402/polar.v33.21636
Rozzi R., Armesto J. J., Gutíerrez J., Massardo F., Likens G., Anderson C. B., et al. (2012). Integrating ecology and environmental ethics: Earth stewardship in the southern end of the Americas. Bioscience. 62 (3), 226–236. doi: 10.1525/bio.2012.62.3.4
Salavarría E., Benavente M., Kodaka P. G. (2014). Histología de Macrocystis pyrifera (Linnaeus) C. Agardh 1820 (Phaeophyceae: Laminariales) en la costa centro del Perú. Arnaldoa. 21 (1), 69–80.
Seely G. R., Duncan M. J., Vidaver W. E. (1972). Preparative and Analytical extraction of pigments from brown algae with Dymethil Sulfoxide. Mar. Biol. 12, 184–188. doi: 10.1007/BF00350754
SERNAPESCA (2023) Servicio Nacional de Pesca. Anuario Estadístico de Pesca y Acuicultura 2021. Available at: http://www.sernapesca.cl/informacion-utilidad/anuarios-estadisticos-de-pesca-y-acuicultura. (Accessed 5 May 2023).
Smale D. A. (2020). Impacts of ocean warming on kelp forest ecosystems. New Phytol. 225 (4), 1447–1454. doi: 10.1111/nph.16107
Smale D. A., Vance T. (2015). Climate-driven shifts in species’ distributions may exacerbate the impacts of storm disturbances on North-east Atlantic kelp forests. Mar. Freshw. Res. 67 (1), 65–74. doi: 10.1071/MF14155
Steneck R. S., Graham M. H., Bourque B. J., Corbett D., Erlandson J. M., Estes J. A., et al. (2002). Kelp forest ecosystems: biodiversity, stability, resilience and future. Environ. Conserv. 29 (4), 436–459. doi: 10.1017/S0376892902000322
Valdenegro A., Silva N. (2003). Caracterización oceanográfica física y química de la zona de canales y fiordos australes de Chile entre el estrecho de Magallanes y cabo de Hornos (CIMAR 3 Fiordos). Cienc. Tecnol. Mar. 26 (2), 19–60.
Vásquez J. A., Zuñiga S., Tala F., Piaget N., Rodríguez D. C., Vega J. A. (2014). Economic valuation of kelp forests in northern Chile: values of goods and services of the ecosystem. J. Appl. Phycol. 26, 1081–1088. doi: 10.1007/s10811-013-0173-6
Vergara J. F. A. (2003). Tipología y clasificación de fiordos y piedmonts submarinos de Magallanes, Chile. Investig. Geog. 37, 21. doi: 10.5354/0719-5370.2003.27746
Wernberg T., Coleman M. A., Bennett S., Thomsen M. S., Tuya F., Kelaher B. P. (2018). Genetic diversity and kelp forest vulnerability to climatic stress. Sci. Rep. 8 (1), 1851. doi: 10.1038/s41598-018-20009-9
Westermeier R., Murúa P., Patiño D. J., Muñoz L., Müller D. G. (2014). Giant kelp (Macrocystis) fishery in Atacama (Northern Chile): biological basis for management of the integrifolia morph. J. Appl. Phycol. 26, 1071–1079. doi: 10.1007/s10811-013-0176-3
Westermeier R., Patiño D., Piel M. I., Maier I., Mueller D. G. (2006). A new approach to kelp mariculture in Chile: production of free-floating sporophyte seedlings from gametophyte cultures of Lessonia trabeculata and Macrocystis pyrifera. Aquac. Res. 37 (2), 164–171. doi: 10.1111/j.1365-2109.2005.01414.x
Wiencke C., Bischof K. (2012). Seaweed biology. Ecol. Stud. 219, 3–495. doi: 10.1007/978-3-642-28451-9
Ziemann dos Santos M. A., Coelho de Freitas S., Moraes Berneira L., Mansilla A., Astorga España M. S., Colepicolo P., et al. (2019). Pigment concentration, photosynthetic performance, and fatty acid profile of sub-Antarctic brown macroalgae in different phases of development from the Magellan region, Chile. J. Appl. Phycol. 31, 2629–2642. doi: 10.1007/s10811-019-01777-x
Keywords: depth, photosynthesis, pigments, seasonality, sporophylls, vegetative blades
Citation: Marambio J, Rodríguez JP, Rosenfeld S, Méndez F, Ojeda J, Ocaranza P, Bischof K and Mansilla A (2023) New ecophysiological perspectives on the kelp Macrocystis pyrifera: generating a basis for sustainability in the sub-Antarctic region. Front. Mar. Sci. 10:1222178. doi: 10.3389/fmars.2023.1222178
Received: 13 May 2023; Accepted: 07 August 2023;
Published: 01 September 2023.
Edited by:
Paola Gabriela Scodelaro Bilbao, CONICET Centro de Recursos Naturales Renovables de la Zona Semiárida (CERZOS), ArgentinaReviewed by:
Ailen Melisa Poza, Instituto de Investigaciones Bioquímicas de Bahía Blanca (INIBIBB), ArgentinaKathryn Schoenrock, University of Galway, Ireland
Copyright © 2023 Marambio, Rodríguez, Rosenfeld, Méndez, Ojeda, Ocaranza, Bischof and Mansilla. This is an open-access article distributed under the terms of the Creative Commons Attribution License (CC BY). The use, distribution or reproduction in other forums is permitted, provided the original author(s) and the copyright owner(s) are credited and that the original publication in this journal is cited, in accordance with accepted academic practice. No use, distribution or reproduction is permitted which does not comply with these terms.
*Correspondence: Sebastián Rosenfeld, c2ViYXN0aWFuLnJvc2VuZmVsZEB1bWFnLmNs; Juan Pablo Rodríguez, anVyb2RyaWdAdW1hZy5jbA==