- 1College of Marine Science and Environment, Dalian Ocean University, Dalian, China
- 2State Key Laboratory of Coastal and Offshore Engineering, Dalian University of Technology, Dalian, China
- 3Operational Oceanographic Institution, Dalian Ocean University, Dalian, China
- 4National Marine Environmental Monitoring Center, Dalian, China
Stock enhancement can effectively increase population sustainablility and improve fishery resources, making it crucial to discern the suitable habitats for stock enhancement based on efficiency considerations. In this paper, a comprehensive model was established to simulate environmental characteristics in the Liaodong Bay. A habitat suitability model was developed by considering the optimal growth conditions of the Portunus trituberculatus larvae (PTL). The coupled model showed that the optimal area for stock-enhancement with PTL occurs in late June, and the initial suitable habitat area identified represents 17.12% of the whole Liaodong Bay. Based on the larval migration model of PTL, the deviation between the larvae and the suitable habitat, as well as the actual available area for stock enhancement, were further determined after larval release in the initial suitable habitat. Only 33.67% of the larvae fulfilled the criteria of remaining within the suitable habitat for 95% of the time, and these larvae represented 6.19% of the whole area of Liaodong Bay. These findings means that the truly area available for stock enhancement is likely to be a very small portion of the entire bay, and more precise release of larvae will be necessary to ensure survival rates after release. Our study actually provides a methodological framework for the identification of suitable environment of stock enhancement. This methodology can provide technical guidance for the stock enhancement of marine larvae with same applicability for other bays, which in turn contributes to the sustainable use of marine ecosystem services and fisheries resources.
1 Introduction
As overfishing, environmental pollution, and habitat destruction become increasingly serious, marine resources around the world are facing significant challenges (Etiegni et al., 2011; Horodysky et al., 2016; Utomo et al., 2019). Many countries have adopted fisheries management measures to address the current decline of marine fisheries resources, including establishing fishing quotas, marine reserves, and stock enhancement (Roberts et al., 2005; Nielsen and Holm, 2007; Colloca et al., 2013; Green et al., 2014). Among them, stock enhancement programs, with their effective ecosystem restoration function and advantage in alleviating the scarcity of fishery resources, are considered to be an important measure for restoring fishery resources (Taylor et al., 2005; Purcell et al., 2012; Han et al., 2016; Wang Z. et al., 2022). Stock enhancement refers to the breeding and release of fish, shrimp, shellfish, algae, and other aquatic organisms into natural waters to allow for their natural growth, in order to increase the population size of marine species, rebuilt biomass of the fished stock, attempting to restore ecological balance (Lorenzen et al., 2010; Hilborn et al., 2020; Pilnick et al., 2021).
In the late 19th century, breakthroughs in artificial breeding technology for marine fish allowed countries such as the United States, Japan, and some European nations to establish marine fish hatcheries (Shelbourne, 1964; Yoshimura et al., 1996; Christou et al., 2013). Economically valuable species such as Gadus and Oncorhynchus keta were artificially propagated, and attempts were made to increase the wild populations in natural water bodies by releasing hatchery-reared fish (Blankenship and Leber, 1995; Kitada, 2014). Since the 1950s, China has been exploring stock enhancement activities in its nearshore fisheries (Dong et al., 2009). In the early 1980s, China’s efforts in stock enhancement and large-scale larva release experiments in its nearshore fisheries have continuously achieved success (Steady growth in catch and input ratios) (Hong and Zhang, 2002; Han et al., 2016). The Portunus trituberculatus, with its high reproductive potential, survival rates, high economic profitability, and the technology of artificial breeding, has been identified as a key organism for stock enhancement and release in China’s northern waters, particularly in the Liaodong Bay, China (Wang et al., 2020). However, the efficiency of stock enhancement has long been at a low level due to incomplete identification of oceanic environmental factors (such as tidal currents) (Wang et al., 2017). Some studies have suggested that larvae released during stock enhancement programs are vulnerable to extreme physical factors, such as typhoon waves, which can lead to high mortality rates (Gao et al., 2022). Ensuring the survival rate of released larvae in natural marine habitats has been challenging (Xie et al., 2014; Wang et al., 2018). Thus, it is crucial to efficiently describe tidal currents, temperature, salinity, and wave conditions in the releasing area, to determine suitable areas for larval survival.
In the turn of the century, the fishing industry and researchers made easier to identify environmental characteristics of fish culture and release areas by using remote monitoring systems with integrated temperature and salinity sensors (Laroche et al., 2016; Lee et al., 2022; Skålvik et al., 2023). However, when faced with the need to understand the environmental features of larger oceanic areas, this technology becomes increasingly difficult to implement, as larger areas require more equipment and personnel investment, leading to a significant growing in production costs. In recent years, with the continuous development and improvement of ocean mathematical models, an increasing number of researchers utilized numerical simulation techniques to estimate the environmental characteristics of large marine areas (Karydis and Kitsiou, 2013; Uzun et al., 2022; Ma et al., 2023). The advantages of mathematical models lie in their ability to provide rapid feedback of environmental features over a large area at a low cost. Therefore, this enabled the selection of suitable areas for stock enhancement through numerical modeling of basic environmental variables.
This study focused on the stock enhancement population, through the release of Portunus trituberculatus larvae (PTL) as a case of study. Firstly, a comprehensive numerical model of the water environment in the Liaodong Bay was developed, which took into account physical factors such as tidal currents, water temperature, salinity, and waves. Based on this, an environmental suitability model and migration model of PTL were established, aiming to identify through the assess suitable areas for the stock enhancement of PTL in the Liaodong Bay. The results of this study are expected to provide effective guidance for the selection of stock enhancement and release areas, thereby facilitating the management, conservation, and sustainability of regional fisheries resources.
2 Materials and methods
2.1 Study area
The study area is located in the Liaodong Bay, China (Figure 1A), one of the main areas for stock enhancement in the Bohai Sea, with a sea area of 18,784.53 km2. The Liaodong Bay is situated in the northern part of the Bohai Sea, with an average water depth of 18 m. The bay is influenced by irregular semidiurnal tides, with an average tidal range of 2.7 m. It is one of the most important spawning and breeding grounds for many commercially valuable marine species, such as Portunus trituberculatus and Penaeus orientalis (Xu et al., 2010; Wang et al., 2013). The average annual water temperature in the bay ranges from 8 to 10°C. The sea-ice season typically begins in December and ends in March of the following year (Hou et al., 2020; Wang et al., 2021). The main species for aquaculture release in Liaodong Bay are Portunus trituberculatus, Chinese shrimp and Paralichthys olivaceus. Taking the PTL as an example, the window for stock enhancement and release is from June to July each year. This is because the larvae are prone to freeze and die before this period, while later releases may not be harvested before the onset of the ice season. In addition, the stock enhancement and release is usually carried out during the neap tide period due to the relatively stable marine environment compared to the spring tide period. With this in mind, this study focuses primarily on four neap tide periods occurring in June and July of 2019, namely, early June, late June, early July, and late July.
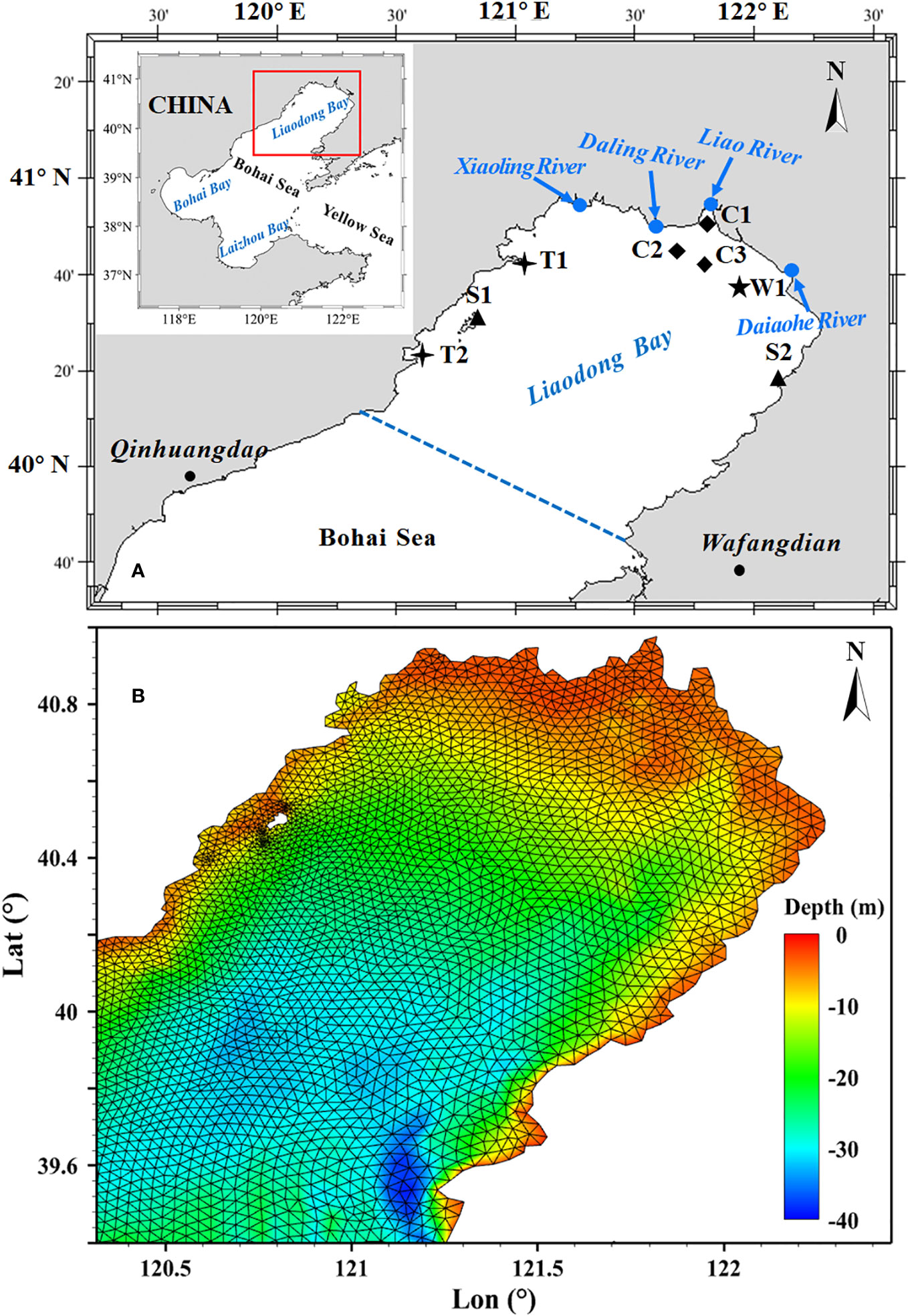
Figure 1 (A) Location of study area and distribution of observation stations. (B) The water depth and computational grids of the Liaodong Bay.
Portunus trituberculatus is a significant economic crab species, classified under the phylum Crustacea, order Decapoda, and family Portunidae. It is primarily found in the coastal regions of China, Korea, and Japan. In the larval stage, the larvae generally acquire the ability to settle after six moults, and in this process, it takes about 25-30 days for the individuals to become larger and gradually acquire the ability to swim (Chen et al., 2023). Larvae can be released 5 days after hatching, when the cephalothoracic armor width needs to be greater than 6 mm (China, 2014).
2.2 Habitat environment model of Portunus trituberculatus larvae
Given the importance of the habitat characteristics of PTL in the context of its stock enhancement and release, a numerical model of the marine water environment in the Liaodong Bay was developed in this study. The general model consisted of a three-dimensional hydrodynamic model to reproduce the tidal characteristics of the Liaodong Bay, a three-dimensional temperature and salinity model to simulate the distribution of water temperature and salinity in the Liaodong Bay, and a wave model to reflect the wave propagation characteristics in the Liaodong Bay. The hydrodynamic model was based on the Reynolds-averaged Navier-Stokes equations (RANS) simplified under the hydrostatic assumption. The governing equations of the hydrodynamic model are as follows:
where t is the time; x, y are Cartesian coordinate system coordinates; η is the surface elevation; d is the still water depth; h = η + d is the total water depth; u, v and w are the velocity components in x, y and z directions respectively; f is the Coriolis force coefficient, f = 2ωsinφ (ω is the angular rate of revolution, φ is the local latitude); g is the acceleration of gravity; ρ is the density of water; Sxx, Sxy and Syx are components of the radiation stress tensor; is the vertical turbulent (or eddy) viscosity; is the atmospheric pressure; is the reference density of water. S is the magnitude of the discharge due to point sources and (, ) is the velocity by which the water is discharged into the ambient water.
The model presented in this study utilized a non-structured triangular mesh (Figure 1B), consisting of 14,002 grid elements with a minimum resolution of 100 meters for nearshore areas. The depth and shoreline data used in the model were obtained from the ETOPO1 database provided by the National Oceanic and Atmospheric Administration (NOAA). In addition, the model accounted for four major rivers (the Liao River, the Daliao River, the Daling River, and the Xiaoling River) flowing into the Liaodong Bay, with monthly discharge data provided for each. The predicted tidal level obtained through harmonic analysis was used to provide the open boundary tidal condition.
Apart from the influence of current, water temperature and salinity are also significant factors that cannot be ignored in the growth of PTL (Xu and Liu, 2011; Dai et al., 2014). Based on the validated hydrodynamic model, another model was established to simulate the temperature and salinity distribution characteristics of the Liaodong Bay. The temperature and salinity boundary conditions were provided by monthly average data from the Copernicus Marine Environment Monitoring Center website (https://marine.copernicus.eu/). The model control equations are as follows:
where is the vertical turbulent (eddy) diffusion coefficient. is a source term due to heat exchange with the atmosphere. and are the temperature and the salinity of the source.
On the basis of temperature and salinity, the food source depletion and flow field heterogeneity caused by wave breaking are potential factors affecting the migration and distribution of marine organisms (Hou et al., 2022). Therefore, the MIKE21-SW model was used to calculate the wave characteristics in the Liaodong Bay, which takes into account meteorological conditions such as wind speed and provides the distribution of the significant wave height in the Liaodong Bay, which is widely used for wave characteristics simulation. The background wind field used meteorological data from the Copernicus Marine Environment Monitoring Officer website (https://marine.copernicus.eu/). The model control equations are as follows:
where N (, σ, θ, t) is the action density, t is the time, = (x, y) is the Cartesian co-ordinates; = (cx, cy, cθ, cσ) is the propagation velocity of a wave group in the four-dimensional phase space , σ, θ and S is the source term for the energy balance equation.
The energy source term, S, represents the superposition of source functions describing various physical phenomena.
where represents the transmission of energy by wind, is the wave energy transfer due to non-linear wave-wave interaction, is the dissipation of wave energy due to whitecapping, is the dissipation due to the bottom friction and is the dissipation of wave energy due to the depth-induced breaking.
2.3 Habitat suitability model for Portunus trituberculatus larvae
Previous studies have shown that fluid shear stress caused by waves and tides affects the transport, attachment and recruitment of marine organism larvae (Shanks et al., 2003; Bolle et al., 2009; Reidenbach et al., 2009; Whitman and Reidenbach, 2012; Hou et al., 2022), while water temperature and salinity directly affect larval feeding and development (Zimmerman and Pechenik, 1991; Nurdiani and Zeng, 2007; McGeady et al., 2021). Existing research and the Chinese technical specifications for the stock enhancement indicate the appropriate growth conditions for PTL (China, 2014; Ge, 2019; Wang et al., 2022), as shown in the Table 1 below.
In the suitability model for the habitat of PTL, it is initially believed that flow velocity, water temperature, salinity, and significant wave height are the main limiting factors for the habitat of PTL. The single-factor Habitat Suitability Index (HSI) was used to quantify the suitability of the target organism in a particular factor. The HSI ranges from 0 (least suitable) to 1 (most suitable), indicating that the factor has no restriction on the survival of the target organism when the HSI index is 1. The Comprehensive Suitability Index (CSI) was calculated based on the HSI of each habitat factor and the main limiting factors for the survival of the organism. The CSI ranges from 0 to 1. In this assessment, it was considered that larvae could settle and survive when the CSI was greater than 0.95. The single-factor habitat suitability index (HSI) and the comprehensive suitability index (CSI) of the larvae on the day of stock enhancement can be expressed as:
where i is the i-th grid cell, is the total time in the i-th grid cell that a single suitable condition is met within a day; n is the number of habitat factors that affect the growth of individual organisms; , , and are the flow velocity, water temperature, salinity and significant wave height, respectively, which are environmental factors characterizing the unit i. These features are calculated by the habitat environment model. , , and are the habitat suitability index based on flow velocity, water temperature, salinity and significant wave height at unit i, respectively.
The Weighted Usable Area (WUA) is calculated by simulating the suitability of larvae to hydrological factors. Specifically, WUA is defined as the summation of the product of the area of each control unit () and the CSI within the study region.
2.4 Migration model of Portunus trituberculatus larvae
During the early stage of stock enhancement and release, the movement of crab larvae is largely determined by ocean currents, due to their small size and weak self-swimming ability (Jones et al., 2015; Giltz et al., 2020). To predict the migration and distribution of the larvae, we developed a particle tracking numerical model. This model considers the larvae as particles (600 in total) that move freely attached to water quality points. After being released within the initial suitable area identified by the habitat suitability model, the transport and diffusion of the larvae follows the following principles:
where is the drift term; is the diffusion term; is the random number. To compute the trajectory of Y for a given time discretization, we set the initial value as and recursively solve for using the following equation.
where n=1, 2, 3,...,the value of n is contingent upon the Euler drift coefficient and the diffusion coefficient . = − ∈N (μ = 0, σ2 = Δn) is within the continuous time period ( ≤ t ≤ ), based on the Gaussian increment of the Wiener process.
We utilized a particle tracking model to assess the migration characteristics of larvae and the likelihood of their persistence within suitable habitats during a specified duration following their release.
2.5 Error statistics
The root mean square error (RMSE) is a commonly used evaluation metric for testing the reliability of models.
where is the modeling series, is the observation series, and N is the total number of data in the series. Given that error measures may not always be indicative of optimal performance for numerical models that simulate natural phenomena such as atmospheric dynamics, ocean circulation, or wave generation and propagation, the concept of the skill model has been introduced to enhance evaluation (Hou et al., 2021).
where M is the modeling series, D is the observation series, is the average of the observation data, and N is the total number of data in the series. Performance levels are categorized as: larger than 0.65 excellent; between 0.65 and 0.5 very good; between 0.5 and 0.2 good; smaller than 0.2 poor.
3 Results
3.1 Model validation and results analysis
A series of field data were initially collected for the validation of the model reliability, which included tide gauge data from two stations (S1, and S2) obtained from continuous 25-hour measurements at 00:00 (UTC) on May 25, 2022, provided by the China National Marine Information Center; current data from three stations (C1, C2, and C3) measured using a current meter at 02:00 (UTC) on September 18, 2021; monthly averages of seawater temperature and salinity (T1 and T2) for 2021 were obtained from the Copernicus Marine Service website (https://marine.copernicus.eu/); and wave data from one station (W1), obtained from a fixed acoustic wave gauge over a 30-hour continuous observation period at 04:00 (UTC) on May 14, 2016. The measured data collected covered at least one full tidal cycle (semidiurnal tides) to minimize temporal heterogeneity and non-robustness of model predictions.
Based on the comparison between the on-site observation data and the results obtained from model calculations (Figures 2, 3), it was concluded that the modeled tidal flow values were general agreement with the characteristics of tidal propagation under natural conditions. The simulated results of sea water temperature, salinity, and waves were also found to be in good agreement with the measured data.
The results of the error analysis (Table 2) indicated that the calculated root mean square error (RMSE) of the tidal level has an average value of 0.08 m, while the RMSE of the flow velocity and flow direction has an average value of 0.07 m/s and 15.61°, respectively. The RMSE of the water temperature and salinity are 1.05°C and 1.21‰, respectively. The RMSE of the significant wave height and period are 0.11 m and 0.08 s, respectively. Overall, the observed and simulated values showed good agreement. In addition, the evaluation of skill model for tidal level, flow velocity, temperature, salinity, significant wave height (SWH), and Significant wave period (SWP) were 0.93, 0.90, 0.90, 0.91, 0.88, and 0.90, respectively, indicating excellent performance of the established habitat environment model. Consequently, we concluded that the established numerical model was capable of describing the water environment characteristics (tidal flow, water temperature, salinity, and waves) in the Liaodong Bay.
3.2 Habitat characteristics of Portunus trituberculatus larvae
According to the results of the numerical model of water environment and the habitat suitability model in Liaodong Bay (Figure 4), the habitat suitability index of flow velocity () in the Liaodong Bay during the four neap tide periods showed a gradual increase from the sea to the land. The flow velocity suitability index of the coastal waters on the north side of Liaodong Bay was generally above 0.95, and other suitable areas were sporadically distributed in shallow waters on both sides of Liaodong Bay, where the flow velocity was relatively low due to the dumpening effect of topographical factors. In the southeastern waters of Liaodong Bay, the flow velocity suitability index was less than 0.20. This was mainly due to the high flow velocity caused by the water depth exceeding 30 meters and the complex coastline. The habitat environment model results showed that the maximum flow velocity in this area during the four periods was 1.87 m/s, which occurred in early June.
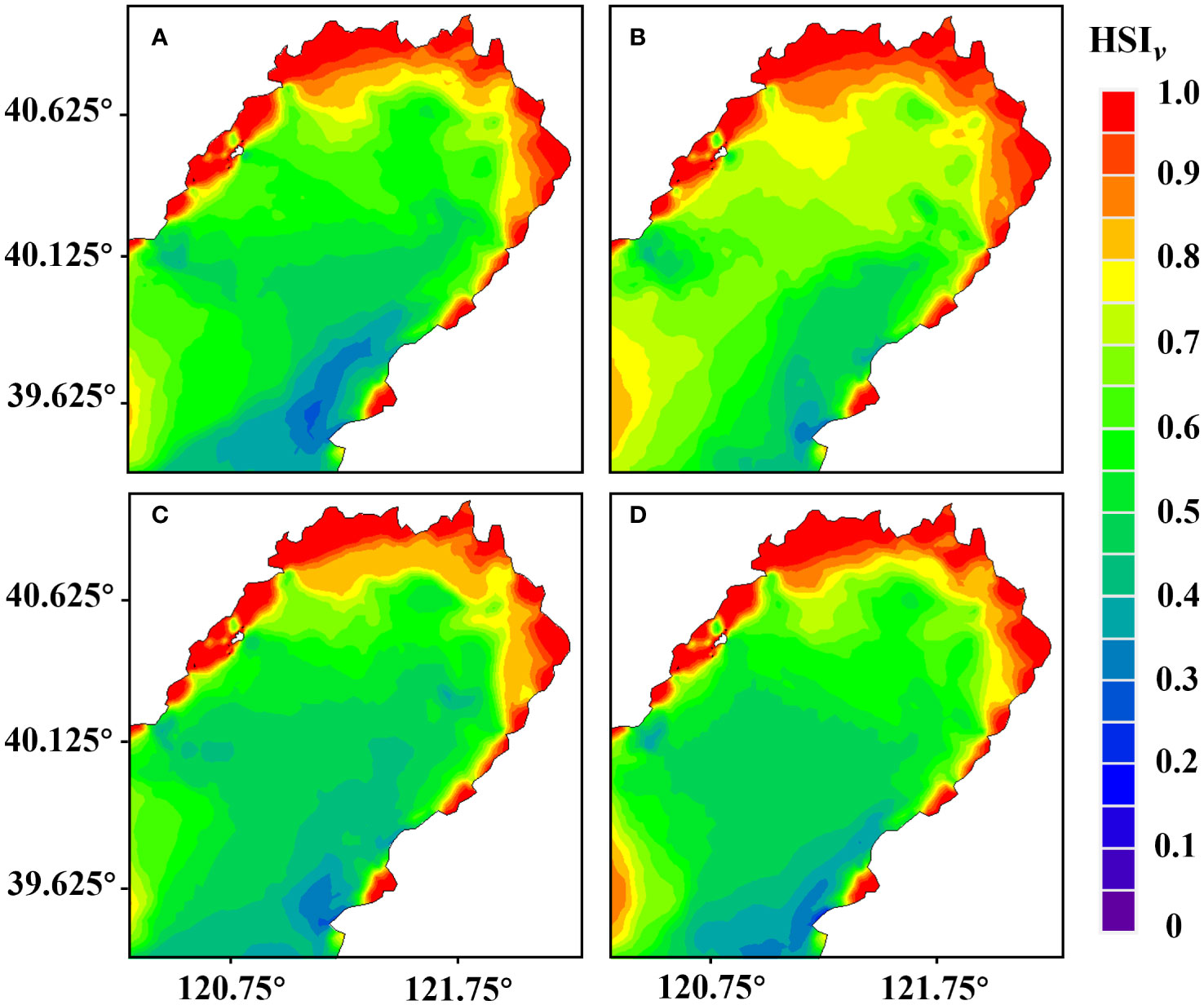
Figure 4 Distribution of the habitat suitability index for flow velocity () during various periods in the Liaodong Bay. (A) Early June. (B) Late June. (C) Early July. (D) Late July.
Under flow velocity constraints, the maximum extent of suitable habitat for PTL in late June was found to be the largest, approximately 1,488.06 km2, accounting for 7.92% of the total area of Liaodong Bay. Conversely, the optimal area was found to be the smallest in early June, at approximately 1,337.49 km2, accounting for 7.12% of the total area. The suitable area in early July was 1,371.96 km2 and in late July was 1,472.04 km2, and the flow rate suitable area accounted for about 7.30% and 7.84% of the whole Liaodong Bay area, respectively. It is evident that under the influence of tidal current stress, the habitat in late June is relatively more conducive to the growth of PTL.
The computational model results showed temporal variations in water temperature within the Liaodong Bay. Water temperature exhibited a significant increase from June to July. At the spatial scale, water temperature gradually decreased from the land towards the ocean. The suitability analysis results (Figure 5) indicated that there were unfavorable temperatures for the growth of PTL in the early June and late July, while the habitat suitability index of water temperature () for the entire Liaodong Bay was 1.0 in late June and early July, indicating that water temperature was highly suitable for the growth of PTL in late June and early July.
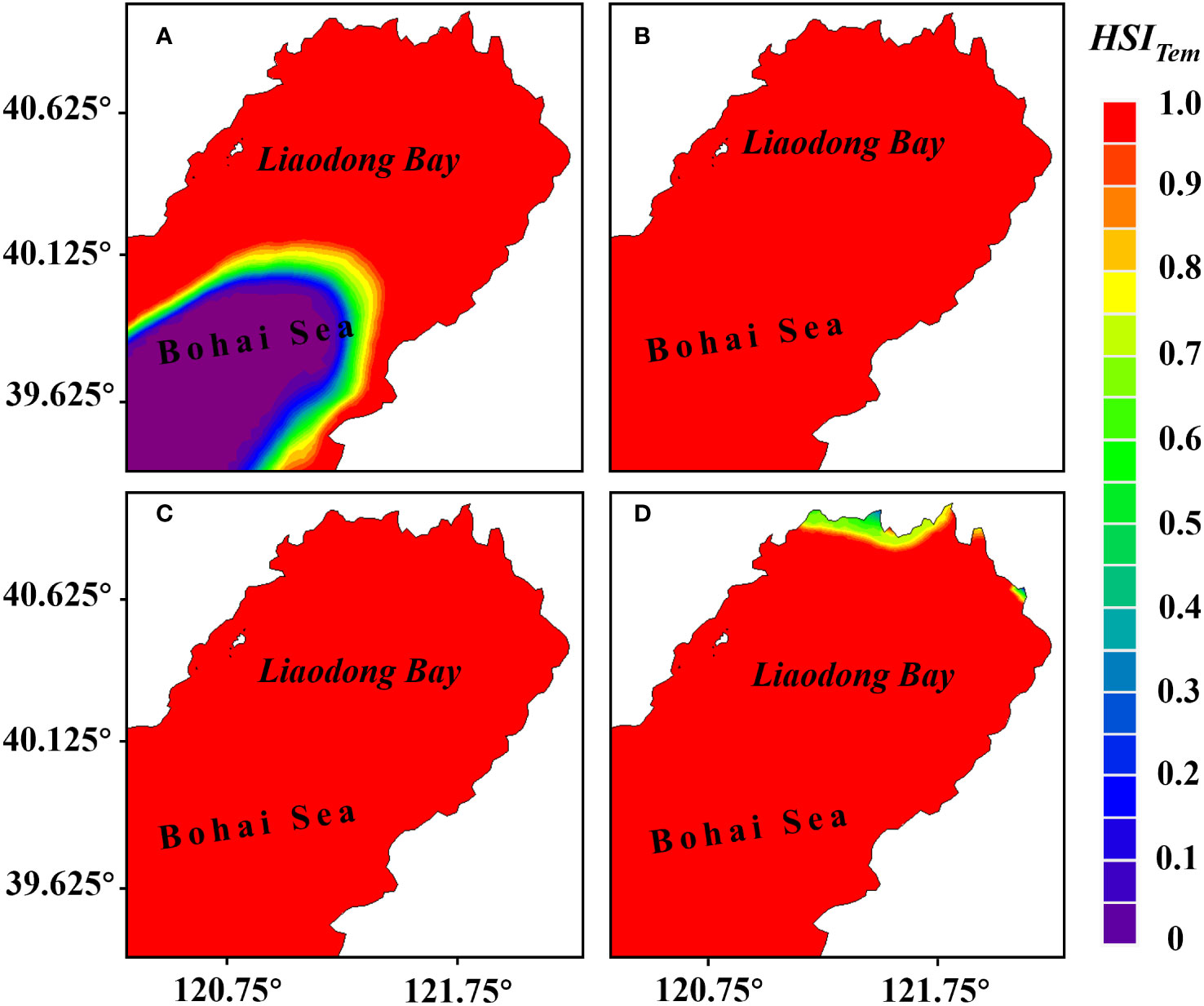
Figure 5 Distribution of the habitat suitability index for water temperature () during various periods in the Liaodong Bay. (A) Early June. (B) Late June. (C) Early July. (D) Late July.
In early June, the water temperature in the southern waters of the Liaodong Bay was below 16°C, which limited the growth of the PTL, resulting in of less than 0.1 in the region. However, in late July, the water temperature in the nearshore waters of the northern part of the Liaodong Bay exceeded 28°C, unconducive to the growth of the PTL. As a result, the suitability index for this area was lower. Under the constraint of water temperature, in early June, the suitable growth area for the PTL was 11,873.52 km2, accounting for 63.21% of the entire Liaodong Bay area. In late July, the suitable growth area for the PTL was 18,591.48 km2, accounting for 98.97% of the entire Liaodong Bay area. In late June and early July, the proportion of suitable area reached 100%. Therefore, it can be seen that under high temperatures, the entire Liaodong Bay is suitable for the growth of the PTL in late June and early July.
According to the distribution of the suitability index for salinity (), the majority of the areas in the Liaodong Bay were highly suitable for the growth of PTL. The areas with lower suitability were mainly located in the coastal waters on the north and south sides of the Liaodong Bay. In the nearshore areas on the northern side of the Liaodong Bay, the influx of freshwater from rivers resulted in localized areas of water with a salinity of less than 20‰. On a temporal scale, the was inversely proportional to the river discharge (Table 3). In early June, the relatively low river discharge led to relatively high salinity levels in the estuarine area. In early June, the relatively small river discharge resulted in relatively higher salinity in the estuarine area. However, with the onset of the rainy season, the increasing river discharge gradually reduced the salinity in the nearshore estuarine area, with the maximum river discharge in late July resulting in the lowest in the northern nearshore area of Liaodong Bay. In contrast, on the southern side of Liaodong Bay, due to the deeper water depth, the salinity exceeds 32‰, which limited the growth of PTL.
Under salinity influences, the optimal growth area of PTL was largest in late June (Figure 6), with an area of approximately 18775.53 km2, accounting for 99.95% of the entire Liaodong Bay. In early June, the optimal growth area was approximately 18,740.61 km2, while in early July, it was approximately 18,772.20 km2, and in late July, it was approximately 18,646.65 km2, accounting for 99.77%, 99.93%, and 99.27% of the entire Liaodong Bay, respectively. Overall, the salinity of water in Liaodong Bay had little effect on the growth of PTL in June and July.
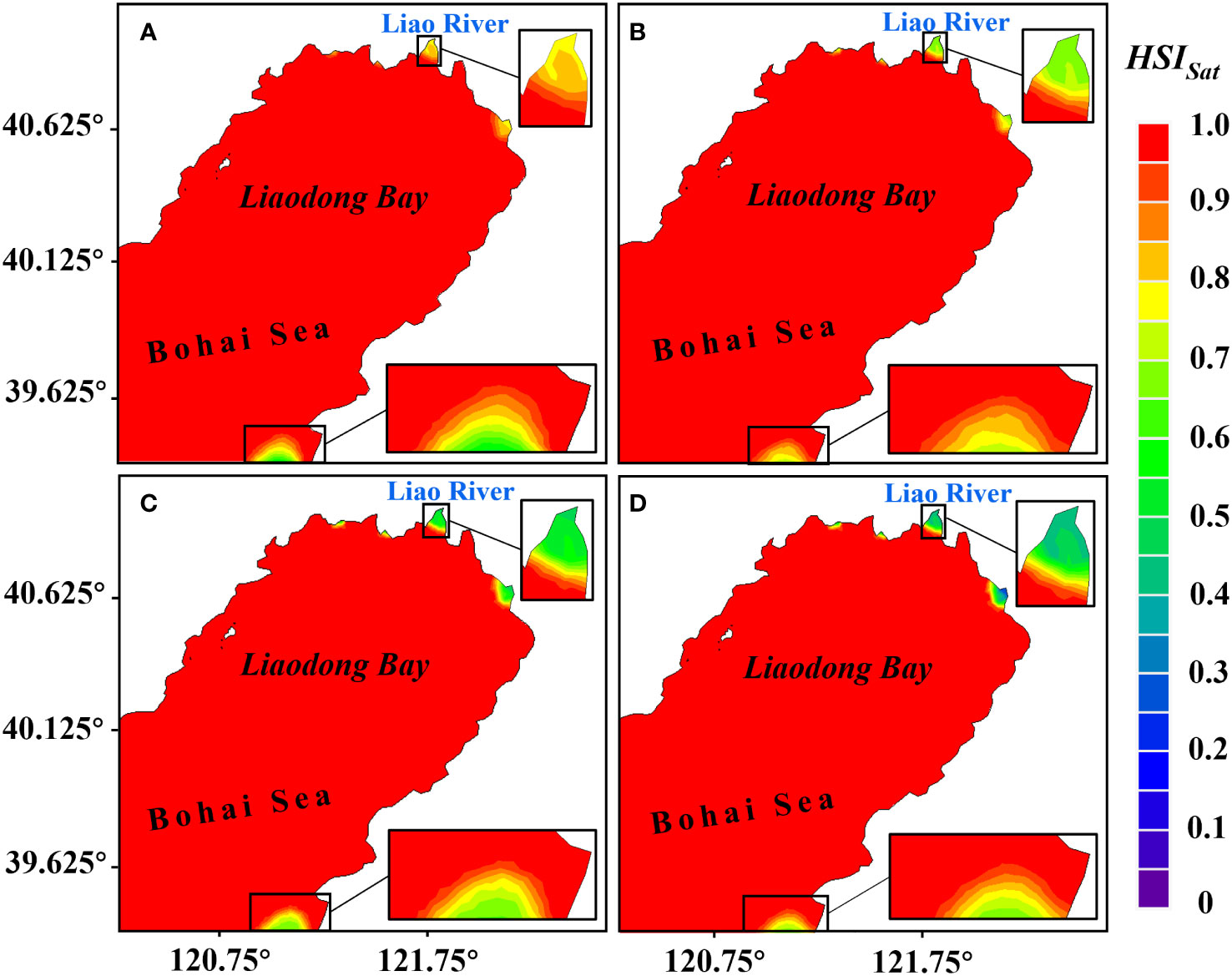
Figure 6 Distribution of the habitat suitability index for water salinity () during various periods in the Liaodong Bay. (A) Early June. (B) Late June. (C) Early July. (D) Late July.
When considering the growth restriction of PTL by waves alone, we found that the suitability index for waves () in the Liaodong Bay was 1 in all four periods, meaning that the significant wave height (SWH) was less than 0.5 m. This indicates that wave conditions in the entire Liaodong Bay are suitable for larval growth during these four periods. Therefore, we regarded waves as a non-primary limiting factor when calculating the comprehensive suitability index (CSI).
3.3 Suitable growth areas for Portunus trituberculatus larvae
The results of the habitat suitability model (Figure 7) showed that under the combined effects of flow velocity, water temperature, salinity, the suitable areas for the stock enhancement of PTL were mainly distributed in the north of Liaodong Bay. In both June and July, the of PTL showed a trend of gradually increase from sea to land. The of nearshore areas generally exceeded 0.85, indicating that the milder natural conditions in nearshore areas were adequate for the survival of PTL. Based on the results of the , the suitable growth area (CSI>0.95) for the PTL was more concentrated in late June, mainly in the northern waters of the Liaodong Bay.
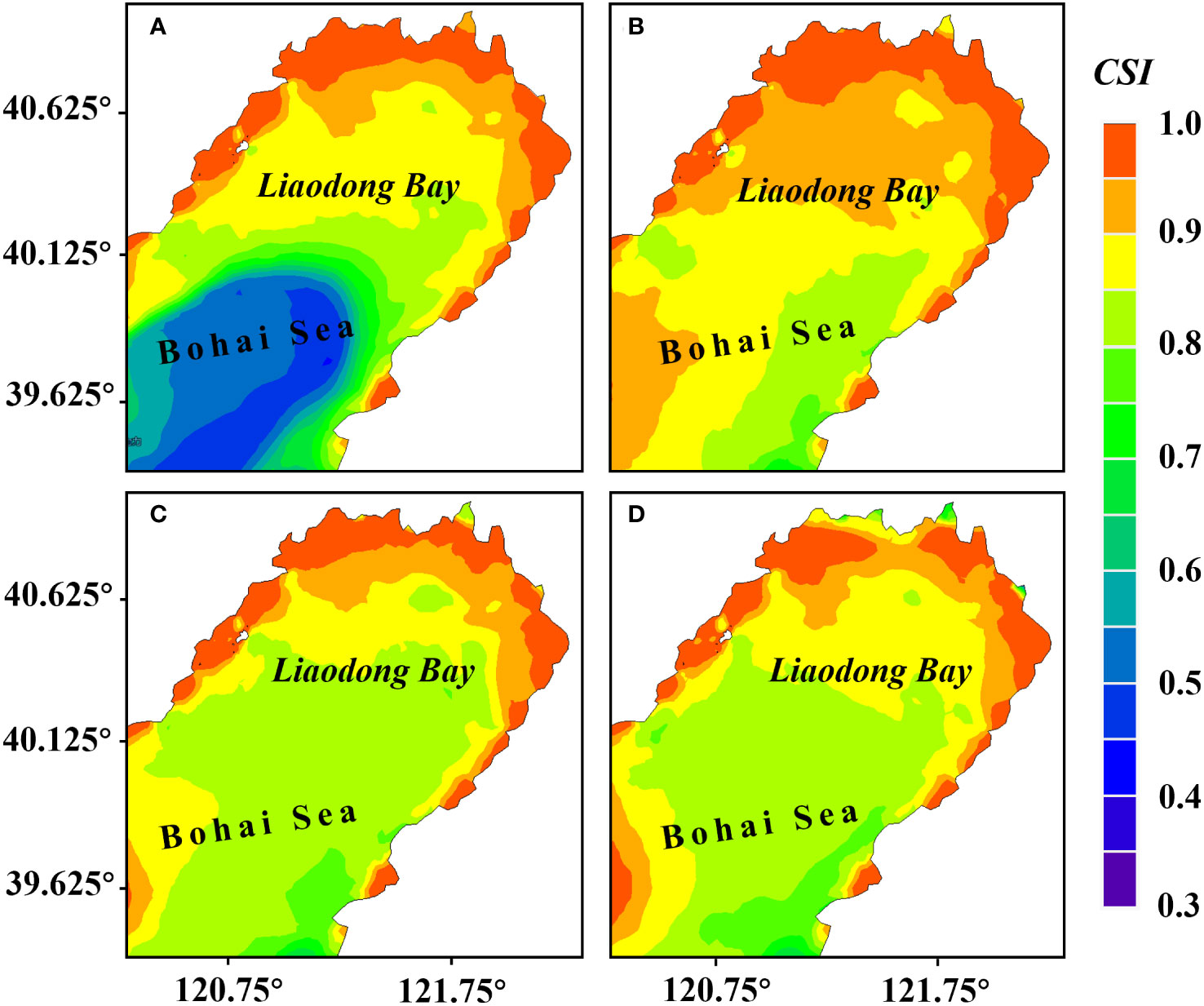
Figure 7 Distribution of the comprehensive suitability index () for stock enhancement of PTL during various periods in the Liaodong Bay. (A) Early June. (B) Late June. (C) Early July. (D) Late July.
In terms of suitable PTL growth area, it was largest in late June, reaching 3,217.77 km2, while the suitable areas in early June, early July, and late July were 2,503.89 km2, 2,253.51 km2, and 2,121.73 km2, respectively. Therefore, it can be inferred, based on the consideration of the operational space for stock enhancement, the best period for stock enhancement is during the neap tide of late June because it presents the largest suitable area for such a purpose. Subsequently, we initially identified suitable area by determining those with a greater than 0.95 in late June.
3.4 Migration characteristics of Portunus trituberculatus larvae
After determining the initial suitable area, our interest was in ascertaining the true survival rate of the larvae placed post-release. This was because the distribution of suitable areas in real environments is subject to dynamic changes in environmental factors. Thus, it is crucial to determine whether the larvae will detach from the suitable area during the process of migration along with currents after being released.
Within the initial suitable area (Figures 7B, 8A identified by the habitat suitability model, larvae (600 in total) were released at even intervals of 2400 meters. The migration trajectory model subsequently predicted the migration positions of these larvae on the 10th, 20th, and 30th days (Figures 8B-D). It should be noted that each larva represents the migratory characteristics within a range of 2400m x 2400m around its initial location. The results indicate that 10 days after release, the suitable area decreased, with 26.35% of PTL modelled to be outside the suitable area. The overall depth of larvae decreased compared to the initial time, with an average depth of about -2.12 m (below the surface). After 20 days of release, PTL tended to aggregate in the nearshore area, with an average depth of about -0.89 m; approximately 32.67% of the larvae were located outside the suitable area at this time. When the larvae were released for 30 days, with the reduction of the suitable area, more larvae were detached from it, accounting for approximately 46.81% of the total number of larvae.
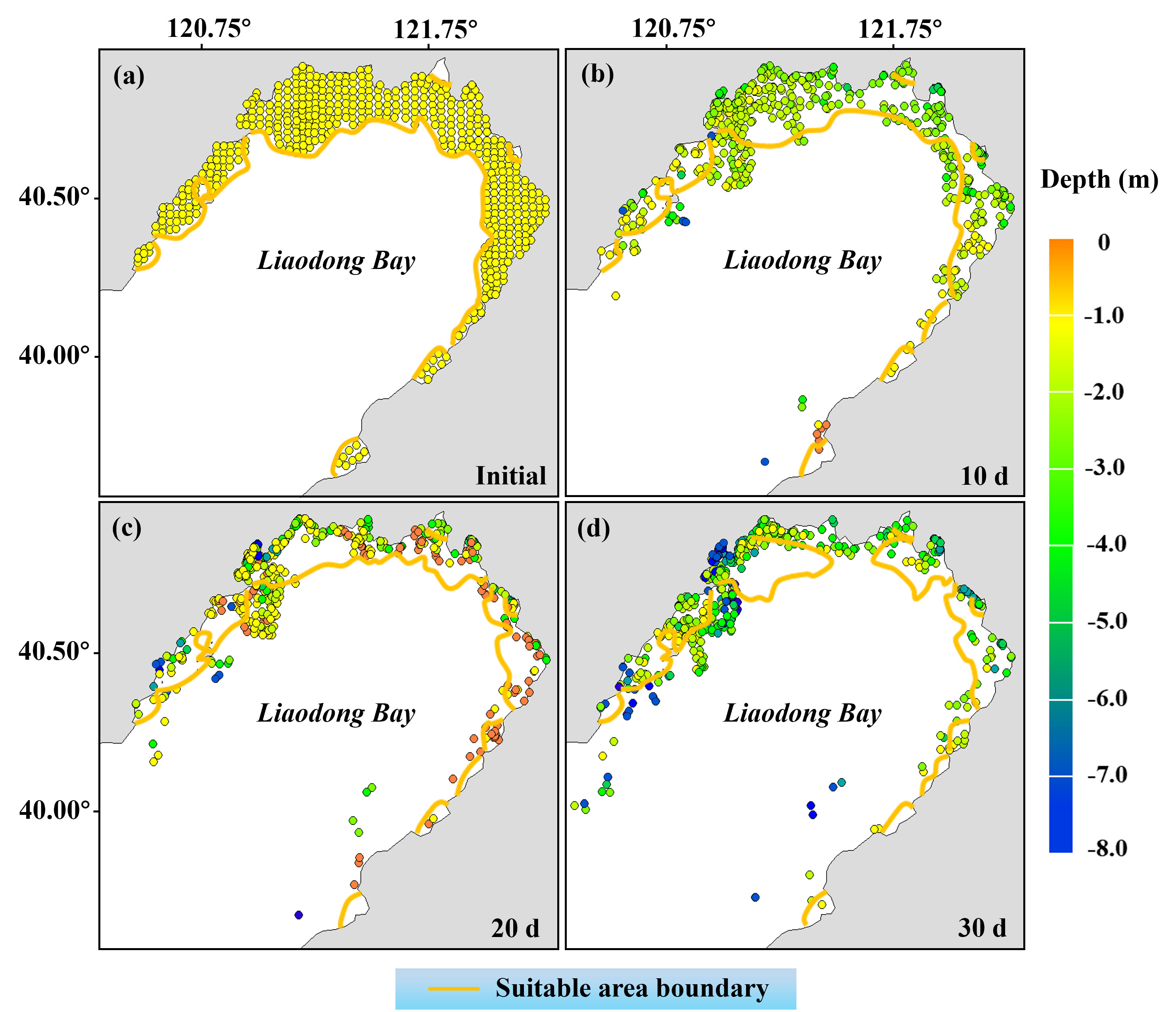
Figure 8 Migration location and distribution depth of PTL within 30 days after release. (A) Early June. (B) Late June. (C) Early July. (D) Late July. The yellow line indicates the boundary of the initially identified suitable area.
The observation implies that despite having selected regions with superior environmental suitability for stock enhancement and initial release, dynamic fluctuations in environmental conditions may cause the PTL to struggle to survive if they move/are moved outside the favorable area. Therefore, our initial estimation of the suitable area may have been overestimated. In the light of this, we evaluated the actual available area for stock enhancement of PTL.
3.5 The actual available area for stock enhancement of Portunus trituberculatus larvae
In reality, the survival probability of the PTL is only high when they stay within the suitable habitat for a sufficiently long period of time. Specifically, the probability of larvae escaping from the suitable area after initial release indicates whether the surrounding area of 2400m×2400m around the initial location of the larvae is truly suitable for stock enhancement. If the larvae stay in the suitable area for more than 95% of the time within 30 days after release, the area of 5.76 km2 (2400m×2400m) represented by the initial release location is considered as the true available area for natural proliferation of larvae and release of additionally reared ones.
The larvae are released within the initially designated suitable area five days after hatching, with settlement typically requiring 25 to 30 days. We assumed that larvae do not have the ability to swim autonomously prior to settlement in this study. For the purpose of quantification, we tabulated the total initial areas that can be utilized for larval stock enhancement when the proportion of time that larvae spend within the suitable area during a 30-day period reaches 50%, 75%, 95%, and 100%, respectively (Figure 9). The results indicate that a total of 424 larvae satisfied the condition of spending 50% of their time in the suitable area, corresponding to a viable area for growth and release of approximately 2,442.24 km2, which represents 75.89% of the initial suitable area and 13.00% of the entire Liaodong Bay area. When the percentage of time spent in the suitable area was 75%, the available area for stock enhancement decreased to about 2004.48 km2, accounting for 62.29% of the initial suitable area and 10.67% of the entire Liaodong Bay. Nevertheless, when the percentage of time spent in the suitable area reached 95%, only 33.67% of larvae (202 individuals) met the conditions, and the actual available area for stock enhancement decreased to 1163.52 km2, which accounted for 36.16% of the initial suitable area and 6.19% of the entire Liaodong Bay. After being released at their initial area, 147 larvae remained within the suitable area throughout the entire observation period. The actual available area for stock enhancement was only 846.72 km2, accounting for 26.31% of the initial suitable area and 4.51% of the entire Liaodong Bay.
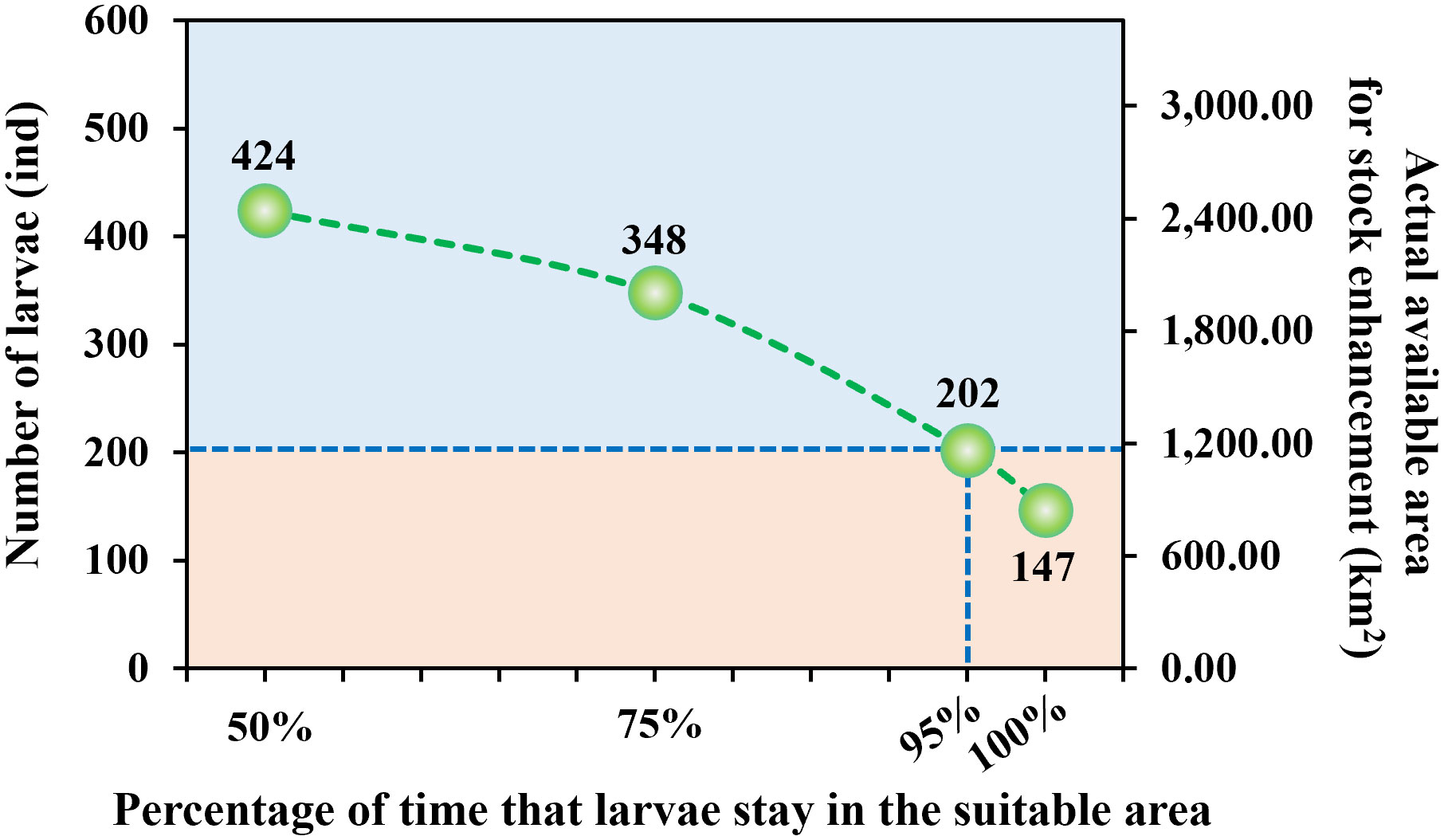
Figure 9 The relationship between the percentage of time that larvae stay in the suitable area and the number of larvae as well as the actual available area for stock enhancement.
4 Discussion
In view of the importance of the marine environment for carrying out stock enhancement, it was developed a comprehensive aquatic environmental model of the Liaodong Bay. This model can simulate the Bay’s temperature, salinity, tides, currents, and wave characteristics. Based on a habitat suitability model, this study evaluated the environmental suitability of the Liaodong Bay during the neap tide in June and July 2019, and identified suitable areas for the stock enhancement of PTL. A combined analysis of habitat suitability and environmental models demonstrates that flow velocity and water temperature in the Liaodong Bay have significant effects on the growth of the PTL, while the effects of wave and salinity are relatively minor. Previous studies have shown that there are significant differences in water temperature among different seasons, which have also constrained larvae distribution, and the implementation of stock enhancement and release (Gilbert et al., 2010; Nozawa, 2012; Han et al., 2016; Teodosio et al., 2016; Lin and Nozawa, 2023). Generally, water temperature in summer is significantly higher near the coast than in the open sea (Yang et al., 2004; Han and Wang, 2022). This study also confirms that suitability of water temperature in June and July exhibits different spatial distributions, with relatively higher temperature near the coast. Meanwhile, tidal and ocean currents are affected by the coastline and seafloor topography, showing considerable spatial variations.
Based on considerations of the success rate of the stock enhancement, this study employed a combination model to identify the environmental suitability of PTL. Specifically, the study provides a reference for the suitable area and time for proliferation and release activities in the Liaodong Bay. In accordance with the results of this study, the optimal time for stock enhancement was found to be late June, when the largest area for implementation was available. The recommended stock enhancement sites were primarily concentrated in the northern waters of the Liaodong Bay and the coastal waters, with the areas near Jinzhou, Panjin, and Yingkou were identified as the best release locations within the study area (Wang et al., 2013; Ge, 2019). Additionally, it should be noted that different species have varying degrees of adaptability to environmental factors, thus suitable stock enhancement timing and locations should be selected based on the specific species in question.
Hydrodynamic models based on individual behavioral characteristics are widely used to predict larval transport (Christensen et al., 2008; Metaxas and Saunders, 2009; Kim et al., 2010; Zhong et al., 2023). The results of the migration model for PTL suggested that during their migration process, they move out of suitable areas and do not survive. This migration loss is inferred based on the assumption that the larvae lack autonomous swimming ability. As the larvae grow, they gradually acquire the ability to swim autonomously. Swimming behaviors could contribute significantly to the overall larval transport potential since they are always responding to the stimuli provided by changes in depth (Gallager et al., 1996; Silva et al., 2016; Baptista et al., 2019). Therefore, when considering the growth model of the larvae, it is necessary to take into account a certain period of time after they are released, which is one of the reasons why our migration trajectory model does not simulate a longer period of time. In addition, considering the characteristics of the larvae behavior in pursuing food sources (Fenaux et al., 1994; Feehan et al., 2018), the abundance of food in the marine environment is a factor worth to be refined some time after the larvae were released. This study did not take into account the potential effects of food limitation on larvae, as food is generally abundant during the early stages of larval development (Chen et al., 2021).
Rational stock enhancement of aquatic organisms facilitate rebuilding conservation of fishery resources. The results of this study held significant implications for the management of offshore aquatic resources and ecological conservation. Furthermore, the proposed model and evaluation methods presented in this paper provided valuable references for the research of stock enhancement in other marine areas, and the selection of suitable habitats for marine organisms in general. These results contribute to the achievement of sustainable utilization of aquatic resources, and ecological conservation.
5 Conclusions
The focus of this study identified the suitable environment stock enhancement of PTL in the Liaodong Bay. Firstly, the HSI of the basic environment factors (including tidal current, temperature, salinity and waves), was identified by combining the habitat environmental model and the habitat suitability model. The results indicated that the main limiting factors for the stock enhancement of PTL were flow velocity and water temperature, while waves were considered as a non-primary limiting factor and were not considered in the calculation of the CSI. Secondly, the habitat suitability model showed that late June was the most suitable time to carry out the stock enhancement of PTL when considering multiple factors. The preliminary estimated suitable habitat area was 3,217.77 km2. Finally, based on the migration model of PTL, the deviation position of larvae leaving the suitable area and the actual available area for stock enhancement were further determined after releasing larvae within the initial suitable area. Only 33.67% of the larvae fulfilled the criteria of remaining within the suitable habitat for 95% of the time, resulting in an effective available area of 1,163.52 km2 for stock enhancement purposes. This area accounts for 36.16% of the initial suitable habitat and 6.19% of the entire Liaodong Bay. This means that the actual area available for stock enhancement is likely to be a very small portion of the entire bay, and more precise release of larvae will be necessary to ensure survival rates. Based on these results, our study actually provides a methodological framework for the identification of suitable environment of stock enhancement. This methodology can provide technical guidance in stock enhancement of marine larvae with analogous applicability in other, similar bays, thereby contributing to the sustainable exploitation of fish stocks.
Data availability statement
The original contributions presented in the study are included in the article/supplementary material. Further inquiries can be directed to the corresponding authors.
Ethics statement
The manuscript presents research on animals that do not require ethical approval for their study.
Author contributions
XH: Conceptualization, modeling, calculation, validation, writing–original draft. WH: Methodology, visualization, formal analysis, writing–original draft. ZS: Data curation, calculation. YX: Data curation, formal analysis. JY: Data curation, formal analysis. RZ: Methodology, supervision, funding acquisition, writing–review & editing. All authors contributed to the article and approved the submitted version.
Funding
The author(s) declare financial support was received for the research, authorship, and/or publication of this article. The work was funded by the National Natural Science Foundation of China (Grant No. 31302232) and National Key Research and Development Program of China (2019YFC1407700).
Acknowledgments
We thank the two reviewers for their valuable suggestions for this study.
Conflict of interest
The authors declare that the research was conducted in the absence of any commercial or financial relationships that could be construed as a potential conflict of interest.
Publisher’s note
All claims expressed in this article are solely those of the authors and do not necessarily represent those of their affiliated organizations, or those of the publisher, the editors and the reviewers. Any product that may be evaluated in this article, or claim that may be made by its manufacturer, is not guaranteed or endorsed by the publisher.
References
Baptista V., Morais P., Cruz J., Castanho S., Ribeiro L., Pousao-Ferreira P., et al. (2019). Swimming abilities of temperate pelagic fish larvae prove that they may control their dispersion in coastal areas. Diversity-Basel 11, (10). doi: 10.3390/d11100185
Bolle L. J., Dickey-Collas M., van Beek J. K. L., Erftemeijer P. L. A., Witte J. I. J., van der Veer H. W., et al. (2009). Variability in transport of fish eggs and larvae. Iii. Effects of hydrodynamics and larval behaviour on recruitment in plaice. Mar. Ecol. Prog. Ser. 390, 195–211. doi: 10.3354/meps08177
Chen J., Zhang W., Jian J., Zhang K., Chen Q., Li R. (2023). Growth characteristics of portunus trituberculatus cultured in ponds. J. Aquat. Sci. 47 (03), 133–144.
Chen C., Zhao L., Gallager S., Ji R., He P., Davis C., et al. (2021). Impact of larval behaviors on dispersal and connectivity of sea scallop larvae over the northeast us shelf. Prog. Oceanogr. 195, 102604. doi: 10.1016/j.pocean.2021.102604
China M. O. A. (2014). Technical specifications for the stock enhancement of hydrobios—portunus trituberculatus. Industry Standard
Christensen A., Jensen H., Mosegaard H., John M. S., Schrum C. (2008). Sandeel (ammodytes marinus) larval transport patterns in the north sea from an individual-based hydrodynamic egg and larval model. Can. J. Fish Aquat. Sci. 65 (7), 1498–1511. doi: 10.1139/F08-073
Christou P., Savin R., Costa-Pierce B. A., Misztal I., Whitelaw C. B. A. (2013). “Marine fisheries enhancement: coming of age in the new millennium,” in Sustainable Food Production. Eds. Christou P., Savin R., Costa-Pierce B. A., Misztal I., Whitelaw C. B. A. (Springer Science).
Colloca F., Cardinale M., Maynou F., Giannoulaki M., Scarcella G., Jenko K., et al. (2013). Rebuilding mediterranean fisheries: a new paradigm for ecological sustainability. Fish Fish 14 (1), 89–109. doi: 10.1111/j.1467-2979.2011.00453.x
Dai C., Wang F., Fang Z., Dong S. (2014). Effects of temperature on the respiratory metabolism and activities of related enzymes of swimming crab portunus trituberculatus. Prog. FISH. Sci. 35 (2), 90–96. doi: 10.3969/j.issn.1000-7075.2014.02.013
Dong J., Jiang L. X., Tan K. F., Liu H. Y., Purcell J. E., Ye L. (2009). Stock enhancement of the edible jellyfish (rhopilema esculentum kishinouye) in Liaodong bay, China: a review. Hydrobiologia 1 (616), 113–118. doi: 10.1007/s10750-008-9592-9
Etiegni C. A., Ostrovskaya E., Leentvaar J., Eizinga F. (2011). Mitigation of illegal fishing activities: enhancing compliance with fisheries regulation in lake Victoria (Kenya). Reg. Environ. Change 11 (2), 323–334. doi: 10.1007/s10113-010-0134-4
Feehan C. J., Grauman-Boss B. C., Strathmann R. R., Dethier M. N., Duggins D. O. (2018). Kelp detritus provides high-quality food for sea urchin larvae. Limnol Oceanogr. 63, S299–S306. doi: 10.1002/lno.10740
Fenaux L., Strathmann M. F., Strathmann R. R. (1994). 5 tests of food-limited growth of larvae in coastal waters by comparisons of rates of development and form of echinoplutei. Limnol Oceanogr. 39 (1), 84–98. doi: 10.4319/lo.1994.39.1.0084
Gallager S. M., Manuel J. L., Manning D. A., Odor R. (1996). Ontogenetic changes in the vertical distribution of giant scallop larvae, placopecten magellanicus, in 9-m deep mesocosms as a function of light, food, and temperature stratification. Mar. Biol. 124 (4), 679–692. doi: 10.1007/BF00351049
Gao L., Bai X., Wang Y. (2022). Dynamic prediction of the ricker-type model of portunus trituberculatus on the basis of marine environmental factors. Front. Mar. Sci. 9. doi: 10.3389/fmars.2022.850317
Ge Y. (2019). Study on the stocking and release of portunus trituberculatus in panjin area of northern Liaodong bay. Dalian Ocean Univ. doi: 10.27821/d.cnki.gdlhy.2019.000049
Gilbert C. S., Gentleman W. C., Johnson C. L., Dibacco C., Pringle J. M., Chen C. (2010). Modelling dispersal of sea scallop (placopecten magellanicus) larvae on georges bank: the influence of depth-distribution, planktonic duration and spawning seasonality. Prog. Oceanogr. 87 (1-4), 37–48. doi: 10.1016/j.pocean.2010.09.021
Giltz S. M., Grey E. K., Gyory J., Ko D. S., Nero R. W., Taylor C. M. (2020). Estimating blue crab (callinectes sapidus) larval release sites in the gulf of Mexico using an oceanographic particle-tracking model. B Mar. Sci. 96 (4), 563–575. doi: 10.5343/bms.2018.0075
Green A. L., Fernandes L., Almany G., Abesamis R., Mcleod E., Alino P. M., et al. (2014). Designing marine reserves for fisheries management, biodiversity conservation, and climate change adaptation. Coast. Manage. 42 (2), 143–159. doi: 10.1080/08920753.2014.877763
Han Q. X., Keesing J. K., Liu D. Y. (2016). A review of sea cucumber aquaculture, ranching, and stock enhancement in China. Rev. Fish Sci. Aquac 24 (4), 326–341. doi: 10.1080/23308249.2016.1193472
Han S., Wang Z. (2022). Simulation of seasonal variation characteristics of offshore water temperature based on roms model 2022 Global Reliability and Prognostics and Health Management (PHM-Yantai). Technical Manual. doi: 10.1109/PHM-Yantai55411.2022.9942034
Hilborn R., Amoroso R. O., Anderson C. M., Baum J. K., Branch T. A., Costello C., et al. (2020). Effective fisheries management instrumental in improving fish stock status. P Natl. Acad. Sci. U.S.A. 117 (4), 2218–2224. doi: 10.1073/pnas.1909726116
Hong W., Zhang Q. (2002). Artificial propagation and breeding of marine fish in China. Chin. J. Oceanol. Limnol. 20 (1), 41–51.
Horodysky A. Z., Cooke S. J., Graves J. E., Brill R. W. (2016). Themed issue article: conservation physiology of marine fishes fisheries conservation on the high seas: linking conservation physiology and fisheries ecology for the management of large pelagic fishes. Conserv. Physiol. 4. doi: 10.1093/conphys/cov059
Hou W., Liang S., Hu X., Li C., Sun Z., Ma Q. (2022). Ecological characteristics of a typical coastal artificial shoreline considering the key drivers involved. Estuar. Coast. Shelf 277, 108069. doi: 10.1016/j.ecss.2022.108069
Hou W., Zhang R., Xi Y., Liang S., Sun Z. (2020). The role of waterlogging stress on the distribution of salt marsh plants in the liao river estuary wetland. Glob Ecol. Conserv. 23. doi: 10.1016/j.gecco.2020.e01100
Hou W., Zhang R., Zhang P., Xi Y., Ma Q. (2021). Wave characteristics and berthing capacity evaluation of the offshore fishing port under the influence of typhoons. Appl. Ocean Res. 106. doi: 10.1016/j.apor.2020.102447
Jones B. T., Gyory J., Grey E. K., Bartlein M., Ko D. S., Nero R. W., et al. (2015). Transport of blue crab larvae in the northern gulf of Mexico during the deepwater horizon oil spill. Mar. Ecol. Prog. Ser. 527, 143–156. doi: 10.3354/meps11238
Karydis M., Kitsiou D. (2013). Marine water quality monitoring: a review. Mar. pollut. Bull. 77 (1-2), 23–36. doi: 10.1016/j.marpolbul.2013.09.012
Kim C., Park K., Powers S. P., Graham W. M., Bayha K. M. (2010). Oyster larval transport in coastal alabama: dominance of physical transport over biological behavior in a shallow estuary. J. Geophys. Res-Oceans 115, C10019. doi: 10.1029/2010JC006115
Kitada S. (2014). Japanese chum salmon stock enhancement: current perspective and future challenges. (Special features: social-ecological systems on walleye pollock under changing environment: inter-disciplinary approach.). Fish. Sci. 80 (2), 237–249. doi: 10.1007/s12562-013-0692-8
Laroche J., Boudry P., Charrier G., Morvezen R. (2016). Stock enhancement or sea ranching? Insights from monitoring the genetic diversity, relatedness and effective population size in a seeded great scallop population (pecten maximus). Heredity: Int. J. Genet 117, 142–148. doi: 10.1038/hdy.2016.42
Lee H., Chang Y., Liao C., Hsu T. (2022). Development of integrated multitrophic aquaculture-based cage rearing system in an underutilized fishing port and its application in marine stock enhancement. Front. Mar. Sci. 9. doi: 10.3389/fmars.2022.998198
Lin C., Nozawa Y. (2023). The influence of seawater temperature on the timing of coral spawning. Coral Reefs 42, 417–426. doi: 10.1007/s00338-023-02349-9
Lorenzen K., Leber K. M., Blankenship H. L. (2010). Responsible approach to marine stock enhancement: an update. Rev. In Fish. Sci. 18 (2), 189–210. doi: 10.1080/10641262.2010.491564
Ma Q., Liang S., Sun Z., Zhang R., Wang P. (2023). Development and evaluation of a gpu-based coupled three-dimensional hydrodynamic and water quality model. Mar. pollut. Bull. 187, 14494. doi: 10.1016/j.marpolbul.2022.114494
McGeady R., Lordan C., Power A. M. (2021). Shift in the larval phenology of a marine ectotherm due to ocean warming with consequences for larval transport. Limnol Oceanogr. 66 (2), 543–557. doi: 10.1002/lno.11622
Metaxas A., Saunders M. (2009). Quantifying the "bio-" components in biophysical models of larval transport in marine benthic invertebrates: advances and pitfalls. Biol. Bull-Us 216 (3), 257–272. doi: 10.1086/BBLv216n3p257
Nielsen K. N., Holm P. (2007). A brief catalogue of failures: framing evaluation and learning in fisheries resource management. Mar. Policy 31 (6), 669–680. doi: 10.1016/j.marpol.2007.03.014
Nozawa Y. (2012). Annual variation in the timing of coral spawning in a high-latitude environment: influence of temperature. Biol. Bull-Us 222 (3), 192–202. doi: 10.1086/BBLv222n3p192
Nurdiani R., Zeng C. (2007). Effects of temperature and salinity on the survival and development of mud crab, scylla serrata (forsskal), larvae. Aquac Res. 38 (14), 1529–1538. doi: 10.1111/j.1365-2109.2007.01810.x
Pilnick A. R., O'Neil K. L., Moe M., Patterson J. T. (2021). A novel system for intensive diadema antillarum propagation as a step towards population enhancement. Sci. Rep-Uk 11, (1). doi: 10.1038/s41598-021-90564-1
Purcell S. W., Hair C. A., Mills D. J. (2012). Sea cucumber culture, farming and sea ranching in the tropics: progress, problems and opportunities. Aquaculture 368-369 (Complete), 68–81. doi: 10.1016/j.aquaculture.2012.08.053
Reidenbach M. A., Koseff J. R., Koehl M. A. R. (2009). Hydrodynamic forces on larvae affect their settlement on coral reefs in turbulent, wave-driven flow. Limnol Oceanogr. 54 (1), 318–330. doi: 10.4319/lo.2009.54.1.0318
Roberts C. M., Hawkins J. P., Gell F. R. (2005). The role of marine reserves in achieving sustainable fisheries. Philos. T R Soc. B 360 (1453), 123–132. doi: 10.1098/rstb.2004.1578
Shanks A. L., Largier J., Brubaker J. (2003). Observations on the distribution of meroplankton during an upwelling event. J. Plankton Res. 25 (6), 645–667. doi: 10.1093/plankt/25.6.645
Shelbourne J. E. (1964). The artificial propagation of marine fish. Adv. Mar. Biol. 2, 1–83. doi: 10.1016/S0065-2881(08)60030-9
Silva C. S. E., Novais S. C., Lemos M. F. L., Mendes S., Oliveira A. P., Goncalves E. J., et al. (2016). Effects of ocean acidification on the swimming ability, development and biochemical responses of sand smelt larvae. Sci. Total Environ. 563, 89–98. doi: 10.1016/j.scitotenv.2016.04.091
Skålvik A. M., Saetre C., Frøysa K., Bjørk R., Tengberg A. (2023). Challenges, limitations, and measurement strategies to ensure data quality in deep-sea sensors. Front. Mar. Sci. doi: 10.3389/fmars.2023.1152236
Taylor M. D., Palmer P. J., Fielder D. S., Suthers I. M. (2005). Responsible estuarine finfish stock enhancement: an Australian perspective. J. Fish Biol. 67 (2), 299–331. doi: 10.1111/j.0022-1112.2005.00809.x
Teodosio M. A., Paris C. B., Wolanski E., Morais P. (2016). Biophysical processes leading to the ingress of temperate fish larvae into estuarine nursery areas: a review. Estuar. Coast. Shelf S 183, 187–202. doi: 10.1016/j.ecss.2016.10.022
Utomo A. D., Wibowo A., Suhaimi R. A., Atminarso D., Baumgartner L. J. (2019). Challenges balancing fisheries resource management and river development in Indonesia. Mar. Freshw. Res. 70 (9), 1265–1273. doi: 10.1071/MF19160
Uzun P., Farazande S., Guven B. (2022). Mathematical modeling of microplastic abundance, distribution, and transport in water environments: a review. Chemosphere 288. doi: 10.1016/j.chemosphere.2021.132517
Wang Z., Feng J., Lozano-Montes H. M., Loneragan N. R., Zhang X., Tian T., et al. (2022). Estimating ecological carrying capacity for stock enhancement in marine ranching ecosystems of northern China. Front. Mar. Sci. 9. doi: 10.3389/fmars.2022.936028
Wang Y., Gao L., Chen Y. (2018). Assessment of portunus (portunus) trituberculatus (miers 1876) stock in the northern east China sea. Indian J. Fish 65, 4. doi: 10.21077/ijf.2018.65.4.81039-03
Wang B., Liu X., Li Y., Dong J., Wang A., Yu X., et al. (2020). Stocking effectiveness of hatchery-raised swimming crabs (portunus trituberculatus) released into Liaodong bay. J. Fish. China 44 (8), 11. doi: 10.11964/jfc.20190811903
Wang B., Qin Y., Dong J., Li Y., Wang W., Li Y., et al. (2013). Dynamic distribution of nemopilema nomurai in inshore waters of the northern Liaodong bay, Bohai sea. Acta Ecol. Sin. 33 (6), 1701–1712. doi: 10.5846/stxb201112081878
Wang Z., Sun P., Wang L., Zhang M., Wang Z. (2021). Monitoring sea ice in Liaodong bay of Bohai sea during the freezing period of 2017/2018 using sentinel-2 remote sensing data. J. Spectrosc 2021, 9974845. doi: 10.1155/2021/9974845
Wang Y., Wang X., Ye T., Chen L., Zhou C. (2017). Spawner-recruit analysis of portunus (portunus) trituberculatus (miers 1876) in the case of stock enhancement implementation: a case study in Zhejiang sea area, China. Turk J. Fish Aquat. Sc 17 (2), 293–299.
Whitman E. R., Reidenbach M. A. (2012). Benthic flow environments affect recruitment of crassostrea virginica larvae to an intertidal oyster reef. Mar. Ecol. Prog. Ser. 463, 177–191. doi: 10.3354/meps09882
Xie Z., Qiu S., Hou C., Jin X. (2014). Recapture rates of swimming crabs(portunus trituberculatus) released in the waters off southern Shandong peninsula. J. Fish. Sci. China 21 (5), 10.
Xu Q., Liu Y. (2011). Gene expression profiles of the swimming crab portunus trituberculatus exposed to salinity stress. Mar. Biol. 158 (10), 2161–2172. doi: 10.1007/s00227-011-1721-8
Xu S., Song J., Duan L., Wu X., Xu Y. (2010). Structural changes of major fishery resources in the Bohai sea. Mar. Sci. 34 (6), 59–65.
Yang D., Gao Z., Chen Y., Wang P., Sun P. (2004). Influence of seawater temperature on phytoplankton growth in Jiaozhou bay, China. Chin. J. Oceanol. Limnol. 22 (2), 166–175.
Yoshimura K., Hagiwara A., Yoshimatsu T., Kitajima C. (1996). Culture technology of marine rotifers and the implications for intensive culture of marine fish in Japan. Mar.Freshwater Res. 47 (2), 217–222. doi: 10.1071/MF9960217
Zhong Y., Zhang J., Song D., Zhao Y., Liu Y., Wu W., et al. (2023). Numerical study of hydrodynamic effects on manila clam population distribution and transport in the southwest Laizhou bay, China. Sci. Total Environ. 865, 161214. doi: 10.1016/j.scitotenv.2022.161214
Keywords: habitat suitability model, numerical simulation, migration trajectory, particle tracking, Portunus trituberculatus
Citation: Hu X, Hou W, Sheng Z, Xi Y, Yu J and Zhang R (2023) Numerical models reveal the suitable habitat area for the stock enhancement and release of marine organism larvae in Liaodong Bay. Front. Mar. Sci. 10:1220429. doi: 10.3389/fmars.2023.1220429
Received: 10 May 2023; Accepted: 01 November 2023;
Published: 16 November 2023.
Edited by:
Henning Sten Hansen, Aalborg University Copenhagen, DenmarkReviewed by:
Tomas Vega Fernandez, Anton Dohrn Zoological Station Naples, ItalyBrett W. Molony, Oceans and Atmosphere (CSIRO), Australia
Copyright © 2023 Hu, Hou, Sheng, Xi, Yu and Zhang. This is an open-access article distributed under the terms of the Creative Commons Attribution License (CC BY). The use, distribution or reproduction in other forums is permitted, provided the original author(s) and the copyright owner(s) are credited and that the original publication in this journal is cited, in accordance with accepted academic practice. No use, distribution or reproduction is permitted which does not comply with these terms.
*Correspondence: Wenhao Hou, aHdoYW8wNjM2QDE2My5jb20=; Ruijin Zhang, cnVpamluekBkbG91LmVkdS5jbg==
†These authors have contributed equally to this work and share first authorship