- 1Department of Ocean Sciences, University of California, Santa Cruz, Santa Cruz, CA, United States
- 2Fisheries Ecology Division, Southwest Fisheries Science Center, National Marine Fisheries Service, National Oceanic and Atmospheric Administration, Santa Cruz, CA, United States
- 3Department of Applied Mathematics, University of California, Santa Cruz, Santa Cruz, CA, United States
Through daily vertical movements, mesopelagic fishes contribute to global carbon export and, when eaten, link primary consumers to higher trophic level predators. Although the importance of mesopelagic fishes as prey to individual predator species has been explored, a comprehensive assessment of mesopelagic fishes as prey at the scale of a large marine ecosystem would advance our observing, modeling, and predicting of biodiversity and ecosystem function. We use diet samples from over 105,000 individual predators from 143 taxa in the California Current Ecosystem (CCE) to quantify and evaluate the role of mesopelagic fishes as prey. For 11 predator taxa, including protected mammal species, pelagic squids, a shelf-edge-associated rockfish and highly migratory species, mesopelagic fishes occurred in greater than 25% of all diet samples, likely comprising an important source of prey. Of the 143 taxa represented, individuals from 36 taxa, or 25% percent of all predator taxa in the database, consumed at least one mesopelagic fish species, including economically important fishery species such as Bluefin tuna (16% of all non-empty diet samples), Albacore (19%), Swordfish (50%), Humboldt squid (52%), and Pacific hake (4%). Compared with coastal pelagic fish species (essential prey in the CCE), mesopelagic fish were more frequently encountered in the diets of 21 predator taxa. Lanternfish (family Myctophidae) were the most common prey and consumed by the greatest diversity of predators (32 taxa), but an additional 16 families of mesopelagic fishes were also consumed by predators, highlighting the diversity of organisms inhabiting mesopelagic depths. Mesopelagic fishes were found in the diets of predators collected from shelf depths to well offshore, accentuating the role of mesopelagic fishes as prey across habitats, especially for predators foraging over the slope and further offshore. Our work illuminates the importance of mesopelagic fishes as prey to a diversity of economically valuable and protected species, underscoring the need to incorporate mesopelagic fishes more comprehensively into food web models, global carbon budgets and ultimately our understanding of ecosystem function.
Introduction
Mesopelagic fishes are among the most abundant vertebrates on earth, with a biomass likely exceeding that of total global fisheries landings (Beamish et al., 1999; Irigoien et al., 2014). Historically, mesopelagic fishes have been of little direct economic value due to their small size, waxy composition, and difficulty of capture in loose aggregations at deep depths. In recent years, however, there has been growing interest in developing industrial fisheries, particularly as potential fishmeal and fish oil for aquaculture operations that produce more desirable fish species (Standal and Grimaldo, 2020). Beyond economic value, mesopelagic organisms contribute to global carbon export through their vertical migration into surface waters to feed and their subsequent return to depth. In the California Current, mesopelagic fishes are estimated to account for 15–17% of all carbon exported from surface productivity (Davison et al., 2013). In addition to their role as consumers, mesopelagic fishes are vital as prey to higher trophic-level predators, including economically valuable fish species and protected marine mammals (Pauly, 1998; Brodeur and Yamamura, 2005; Duffy et al., 2017). While the importance of mesopelagic fishes as prey to individual predator species is regularly stated, the complex trophic interactions between diverse mesopelagic species and potential predators can be best examined at an ecosystem level. As a case study, we use a unique database of diet data from diverse predators within the California Current Ecosystem (CCE) to investigate the role of mesopelagic fishes as prey. This work benefits future biodiversity and ecosystem function modeling and provides context to inform ongoing and potential future fisheries management actions.
The mesopelagic zone, occurring at depths of ~200–1000 m, is one of the largest habitats on earth, and one of the least understood (St. John et al., 2016). Organisms inhabiting mesopelagic depths often form complex aggregations of diverse taxa referred to as “deep scattering layers”; their initial discovery was via strong acoustic backscatter (Eyring et al., 1948). The mesopelagic environment is connected to epipelagic processes such as upper thermal mixing and pycnocline layers that structure and concentrate primary production and secondary consumers in pelagic systems. Mesopelagic organisms typically depend on raining organic matter from the surface for food or interact with upper waters directly during a daily vertical migration to feed. During these nighttime sojourns to the surface, mesopelagic organisms are prone to predation by epipelagic nocturnal predators. While it is assumed that most mesopelagic fishes return to depth during the day to avoid predation by visually oriented predators (see review by Longhurst, 1976), which can vary by species and life-history (Benoit-Bird et al., 2017), some predators are capable of hunting mesopelagic prey during the daytime by foraging at mesopelagic depths. Thus, mesopelagic fishes are vulnerable to predation by both daytime diving predators as well as nocturnal predators foraging near the surface.
Mesopelagic fishes have been recorded in the diets of cephalopods, elasmobranchs, fishes (including tuna, salmon, and groundfish species), seabirds, pinnipeds, and cetaceans (Gjosaeter and Kawaguchi, 1980; Pauly, 1998; Brodeur and Yamamura, 2005; Spear et al., 2007), among others. Myctophids, one of the most abundant and diverse families of fish in the mesopelagic, are noted for their high energy density (Van de Putte et al., 2006), which makes them a particularly important prey for predators with high energy demands. While Myctophids are an important link between primary consumers and higher trophic level predators in epipelagic surface waters, they additionally connect surface production to deep-ocean food webs (Choy et al., 2017). While some predator species specifically target mesopelagic fishes (e.g., Northern elephant seal (Goetsch et al., 2018)), other predators may rely on mesopelagic prey during times of decreased coastal prey (e.g. Kittiwakes, Paredes et al., 2014). Myctophids are also important consumers of krill, and in their central role between primary consumers and higher trophic level predators, they may stabilize krill-dominated food webs, such as in the Southern Ocean, during periods of warming temperatures and declining krill (Saunders et al., 2019). In the CCE, mesopelagic fishes may likewise be important as prey for higher trophic level predators during periods of variable oceanographic conditions. Survey data from both the southern and central California Current indicates mesopelagic fishes may fluctuate out of phase with other forage species, with mesopelagic taxa tending to be more abundant, (Hsieh et al., 2009; Koslow et al., 2011; Ralston et al., 2015; Santora et al. 2021), and more diverse (Santora et al., 2017), in years defined by warmer ocean temperatures. Thus, during warm periods, when other forage species are likely to decline, mesopelagic fishes may provide similar resiliency for predators in the CCE.
The California Current is an eastern boundary current upwelling ecosystem that is characterized by seasonally strong upwelling-favorable conditions and associated high levels of primary productivity (Checkley and Barth, 2009). This productivity supports robust coastal pelagic species and economically important fisheries, considered indispensable to the CCE food web (Miller et al., 2010). The biomass of mesopelagic fish has been estimated to be approximately equivalent to that of the epipelagic forage community (Davison et al., 2015), but with far greater uncertainty regarding total abundance and species composition. Although previous work in the region has attempted to summarize trophic relationships between mesopelagic prey (and other forage species) and higher trophic level predators (Dufault et al., 2009; Szoboszlai et al., 2015), these studies were based on literature reviews rather than analysis of raw diet data and were consequently limited in their ability to evaluate the importance of mesopelagic prey to predators with fine-scale taxonomic resolution. Our study is the first to use a meta-synthesis of raw diet data to conduct a comprehensive examination of the role of the full suite of mesopelagic fishes as prey to the broader predator community of the CCE. The results should inform future ecosystem modeling efforts, by providing guidance on complex trophic interactions and aid ongoing management efforts, such as the current moratorium on new fisheries for mesopelagic fishes and other forage species in the California Current.
Materials and methods
Examining the diet of higher trophic level predators is an imperfect method for describing predator consumption patterns and trophodynamics. While there are limitations with sampling a snapshot in time, the inferences that can be made regarding the diet composition and relative importance of distinct prey items make gut content analysis nonetheless an indispensable method for understanding trophic interactions (Baker et al., 2014). In this study, we use the “California Current Trophic Database,” referred to as the CCTD (Bizzarro et al., 2023), available publicly via the NOAA Environmental Research Division’s Data Access Program (ERDDAP). The CCTD combines 24 separate datasets from 1967 to 2019 on food habit studies from predominately stomach, and some scat samples, from over 100,000 individual predators, including taxa of squids (n = 5), elasmobranchs (n = 13), bony fishes (n = 118), and marine mammals (n = 7).
We investigated and summarized the role of mesopelagic fishes in the diet of higher trophic level predators in the CCE. To identify which prey species could be categorized as “mesopelagic,” we reviewed all prey fish species from the CCTD database (total of 323 fish taxa). For each of these taxa, we searched Fishbase (https://www.fishbase.se/) for available depth range values. In those cases where data were missing for a given species, or for higher level taxonomic groupings (i.e., family or genus as opposed to species), we additionally looked up the taxon of question in “Pacific Coast Fishes,”(Eschmeyer et al., 1983) “Guide to the coastal marine fishes of California,” (Miller and Lea, 1972) and/or “Deep-sea fishes” (Priede, 2017), and in some cases the broader literature (see Supplementary Table S1 for additional references). We define a taxon as “mesopelagic” if its daytime adult distribution is pelagic and occurs at depths of ~200–1000 m. Many “mesopelagic” taxa have distributions that extend beyond 1000 m into the bathypelagic zone. In this study, what we consider to be “mesopelagic” also includes those prey species with distributions extending into the bathypelagic zone. For specifics, see Supplementary Table S1. For broader taxonomic categories, we chose depth criteria based on representative species within a given taxa in the prey list, or other representative species from those taxa available in Fishbase, and only included the broader grouping as “mesopelagic” if all available representatives were mesopelagic. In total, there were 62 taxa that we consider to be mesopelagic, or ~19% of all identified fish prey species within the database (62/323 fishes).
Frequency of occurrence (FO) is a robust metric in diet analysis studies for quantifying prey prevalence, while avoiding the pitfalls inherent in other gut content metrics (Baker et al., 2014), especially as methods of diet sample collection and processing varied across taxa and collections. FO is calculated as the total number of stomachs [or scats for marine mammals inhabiting onshore colonies, see (Lowry et al., 2022)], containing a prey taxon of interest, divided by the total number of non-empty stomachs for that given predator taxa. We first determined which of the predators in the CCTD database had consumed any mesopelagic fish prey (Figure 1A). The FO was then calculated as the number of predator diet samples containing mesopelagic fish prey divided by the total number of non-empty diet samples for that predator (Figure 1B). To compare the relative contribution of mesopelagic fishes to that of coastal pelagic fishes, as defined by the Pacific Fishery Management Council, and considered to be essential prey in the CCE, we calculated a combined FO for Pacific sardine Sardinops sagax, Pacific (chub) mackerel Scomber japonicus, Northern anchovy Engraulis mordax, Jack mackerel Trachurus symmetricus, Pacific herring Clupea pallasii pallasii, and Jacksmelt Atherinopsis californiensis. We then compared the relative contribution of mesopelagic fishes to that of the combined coastal pelagic fish species for predator taxa that consumed mesopelagic prey (Figure 1B).
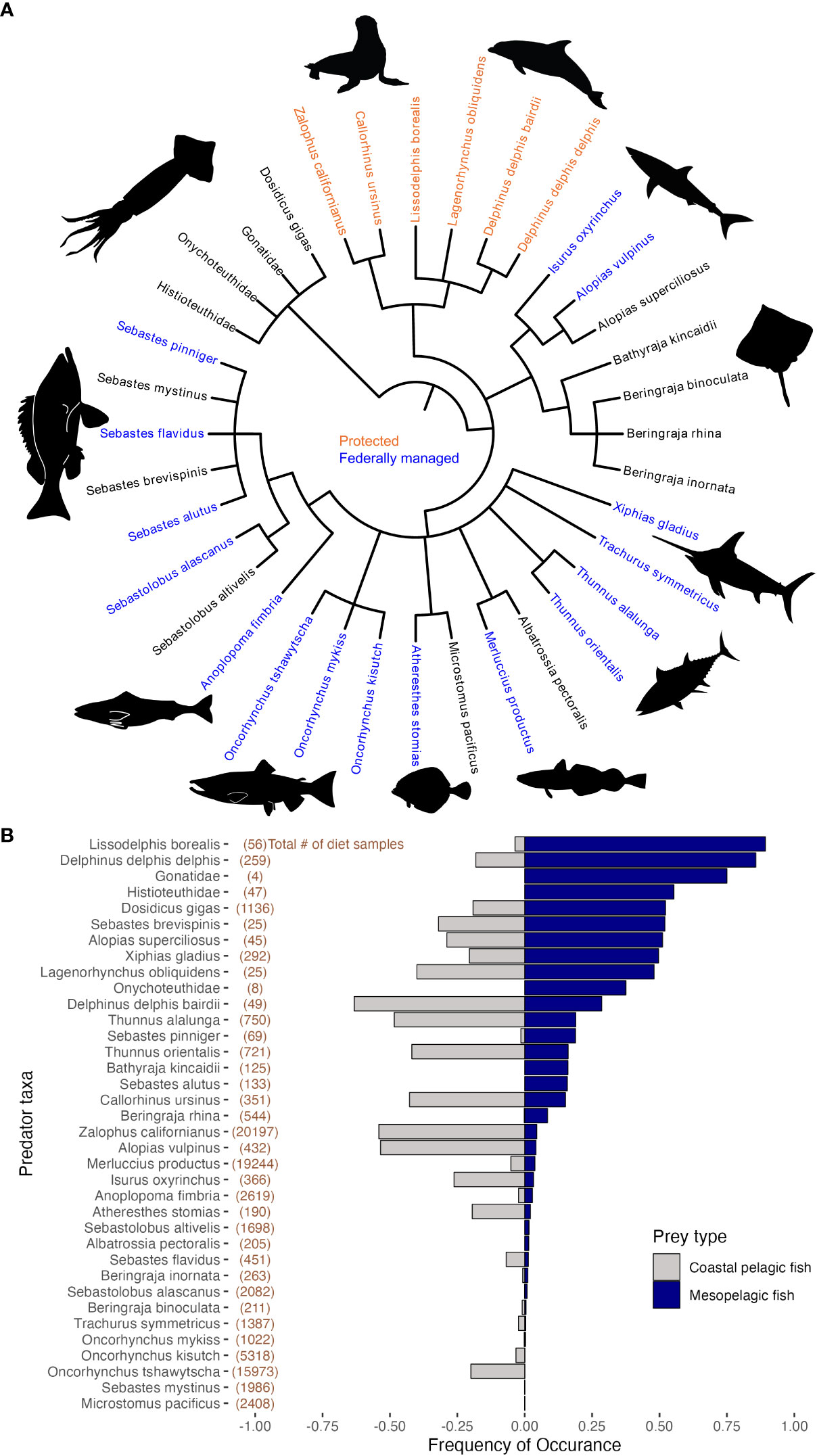
Figure 1 (A) Phylogeny of predator taxa that consumed a minimum of one mesopelagic fish prey. In total 36 predator taxa had mesopelagic fishes in their diet samples, equivalent to ~25% of all predator taxa within the California Current Trophic Database (CCTD). Of those predator species, 16 are federally managed or under international agreement (shown in blue), and six are protected marine mammal species (orange) or in the case of specific Salmonid stock/species both federally managed and protected. (B) FO of mesopelagic (blue) and coastal pelagic (gray) fish prey. The top 11 predator taxa had FO values greater than 0.25 (or 25% of all individuals within these taxa had consumed mesopelagic prey), indicating mesopelagic fish may be especially important for these predator taxa.
Of the mesopelagic fish consumed (Figures 2A, B), we were also interested in which mesopelagic fish taxa were most frequently encountered in predator diets, as this could indicate abundance and/or trophic importance of specific mesopelagic prey types. We chose to group by mesopelagic fish family as opposed to individual taxa due to challenges associated with reliably identifying gut/scat material to the species level between different collections. We calculated a FO per mesopelagic fish family as the number of unique predator stomachs containing prey from each family of mesopelagic fish, divided by the total # of non-empty stomachs per predator species. We then plotted this family level FO value for each predator taxa and examined trends in predator diet across mesopelagic fish families (Figure 3). Myctophids were the most frequently encountered mesopelagic fish prey, so we further examined the percentage occurrence of specific species of Myctophids contributing to this abundance (Figure 2C).
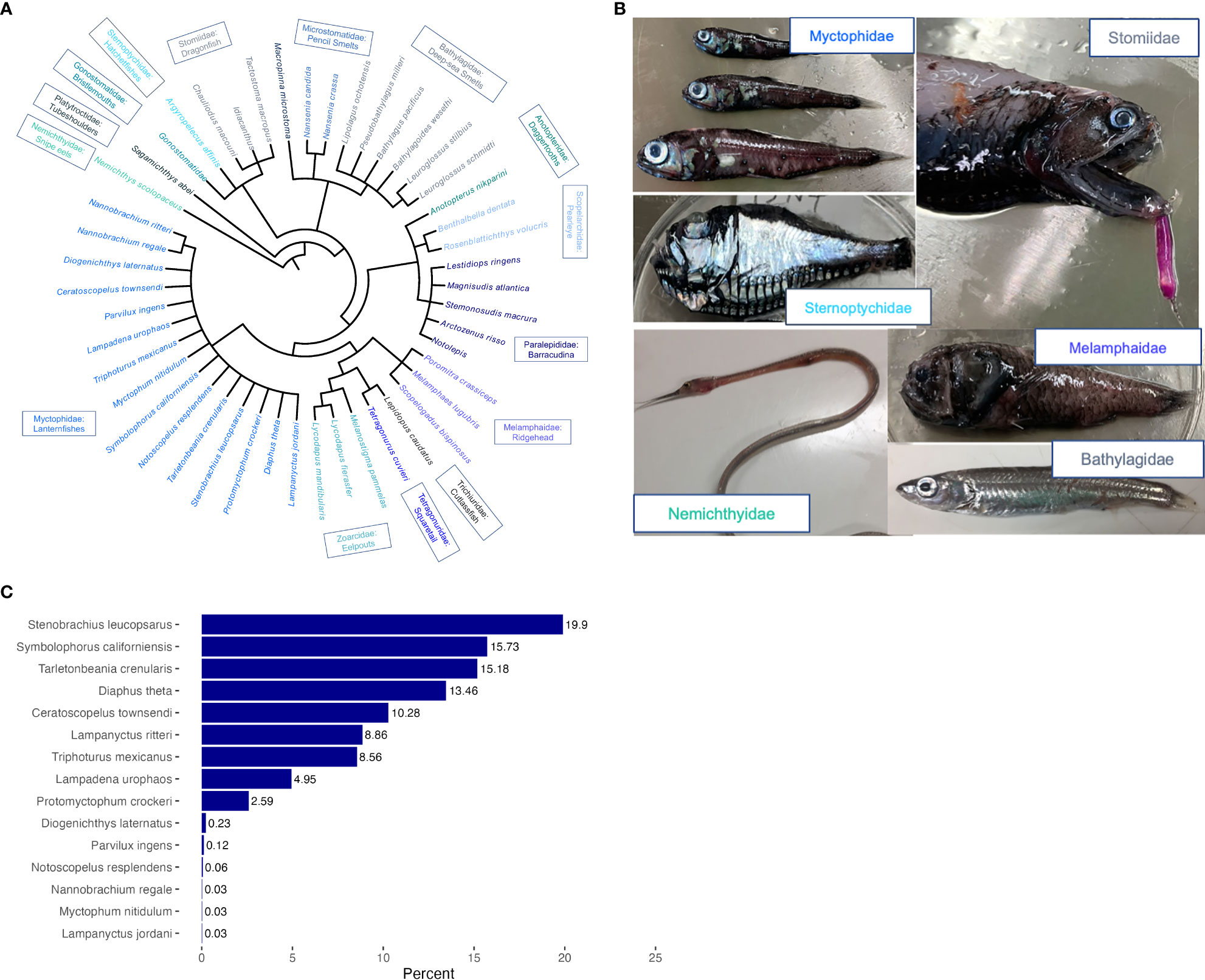
Figure 2 (A) Phylogeny representing the diversity of mesopelagic fish prey found in predator diet samples. (B) Pictures of representative families of mesopelagic families. (C) Of those fishes from the Myctophidae family that were identified to species, the most abundant Myctophid species as percent of total identified Myctophids.
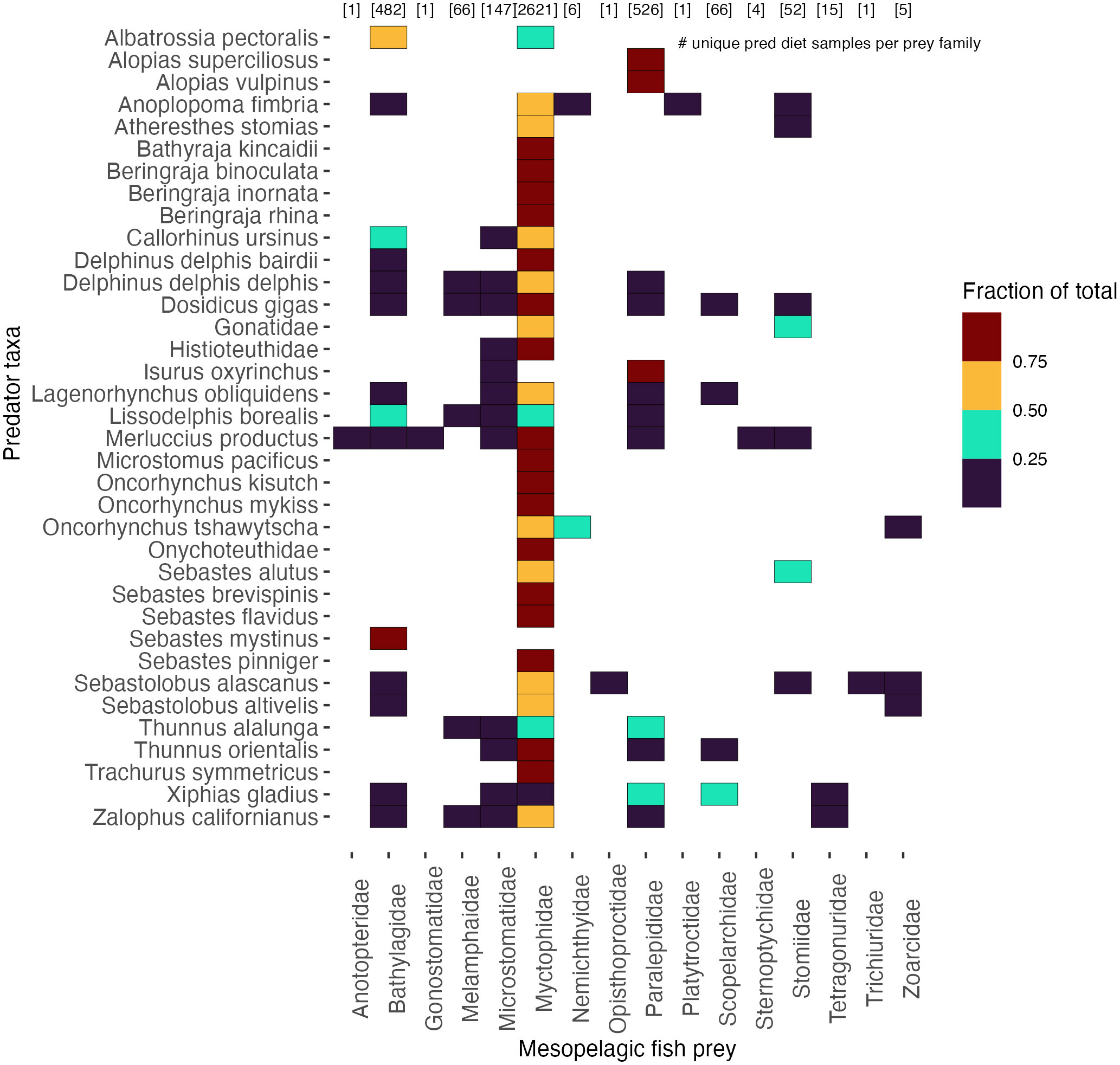
Figure 3 Diversity of mesopelagic fish prey consumed by predators within the CCTD depicted as the proportion of mesopelagic prey consumed for a given predator taxa. Myctophids had the greatest number of predators (32), followed by Bathylagids (14), Paralepididae and Microstomatidae (12 each), Melamphidae (5), Scopelarchidae (Pearleye) (4), and Stomiidae (7). Myctophids were also consumed by the greatest number of unique predators (2,621) followed by Paralepididae (526) and Bathylagidae (482) among others.
The CCTD database contains information on the method and location of predator diet sample collections. Predator collections were identified with a collection id that was unique in time and space, although the number of predator samples collected in any given collection was variable. Although collection information is not necessarily unique to an individual predator, the position of each collection provides valuable information on predator foraging habits and the proportion of predators at a given location that consumed mesopelagic prey. Location information was available for > 90% of mesopelagic predator collection records. For each predator collection location record in the database, we plotted the proportion of predators that had consumed mesopelagic fishes (Figure 4). For each collection record, we then assigned “habitat” type based on bottom depth: shelf (0–200 m), slope (200–1000 m), deep (> 1000 m), or scat samples from onshore marine mammal colonies (for California sea lion and Northern fur seal). We removed records for diet samples that were collected from strandings, as these samples did not accurately reflect foraging position. We used NOAA bathymetry data from the R package {marmap} (Pante and Simon-Bouhet, 2013) to extract information about the underlying bottom depth for each collection record. Although diet samples in the CCTD were not sampled evenly across habitat types, and we did not have sufficient data available to compare the actual depth of predator capture; we examined predator foraging habitat trends by comparing the number of collection locations that captured predators that had consumed mesopelagic prey (Figure 4 inset) and the composition of predator taxa that had consumed mesopelagic prey by habitat type (Figure 5). All quantitative analyses were conducted using the R programming language (R Core Team, 2022) in Rstudio (RStudio Team, 2022).
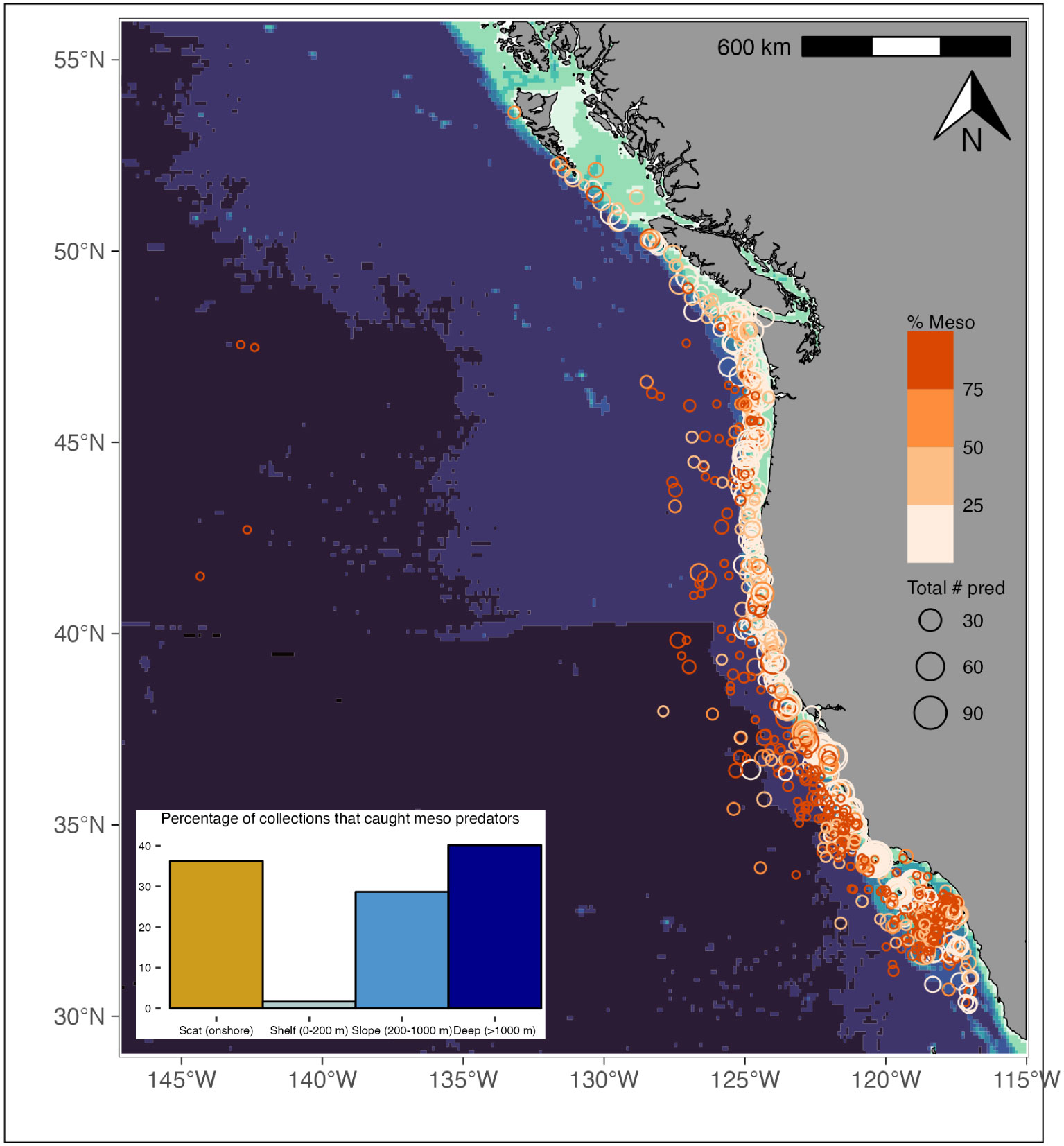
Figure 4 Map describing the location where predator collections occurred. Circle size corresponds to the number of predators captured at a given location while color describes the proportion of those predators that had consumed mesopelagic prey. Figure 4 inset: Depth distribution of predator collections that had consumed mesopelagic prey. Depths correspond to bottom depths.
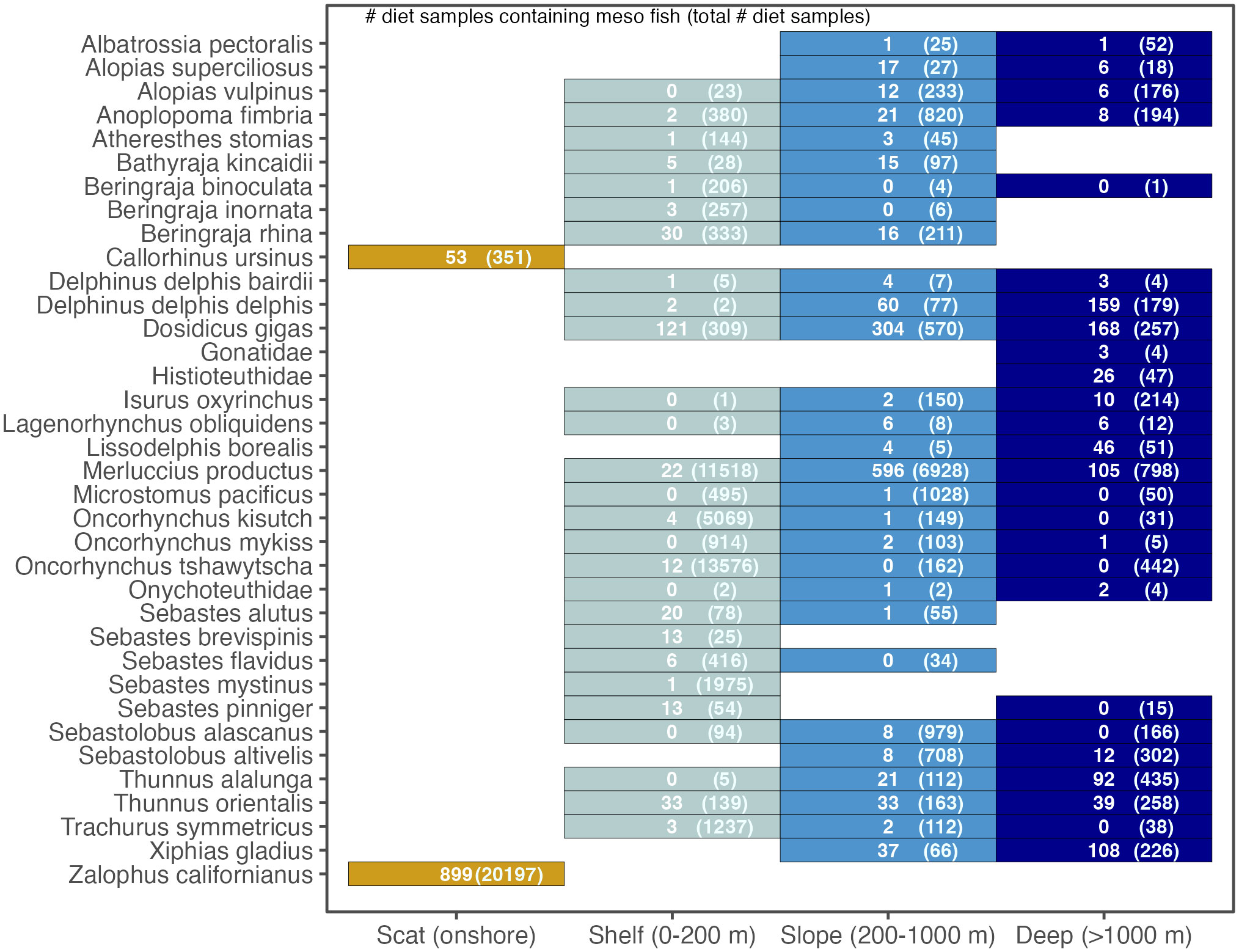
Figure 5 Comparison of predator composition (for those predator taxa that consumed mesopelagic fish in our database), across habitat types defined by bottom depth. For each habitat type, the total number of diet samples that contained mesopelagic fish prey and the total number of diet samples collected for that predator taxa (in parentheses) are displayed. Note that these values do not necessarily reflect the total number of diet samples collected, just those that had collection position information available in the database.
Prey length can be an important determinant of predation susceptibility. Most mesopelagic fish lengths were measured as standard length (SL) values, but total length (TL), fork length (FL), and in some cases back calculated SL measurements were also available. We used SL or back calculated SL measurements to compare the length frequency distribution of mesopelagic fish prey between families and compared SL of mesopelagic fish to coastal pelagic fish prey. For those prey records that lacked SL information, but had FL or TL measurements, we converted to SL using length to length conversion values available from fishbase.org. In total, we had length information for 3,267 mesopelagic fish prey, which were plotted for those mesopelagic fish families with at least 10 length records available (Figure 6A). We also compared the length frequency distribution of mesopelagic fishes with that of coastal pelagic prey for those predator species that consumed mesopelagic fishes within the database, to assess whether mesopelagic fishes had overlapping or distinct length distributions to coastal pelagic fishes (Figure 6B).
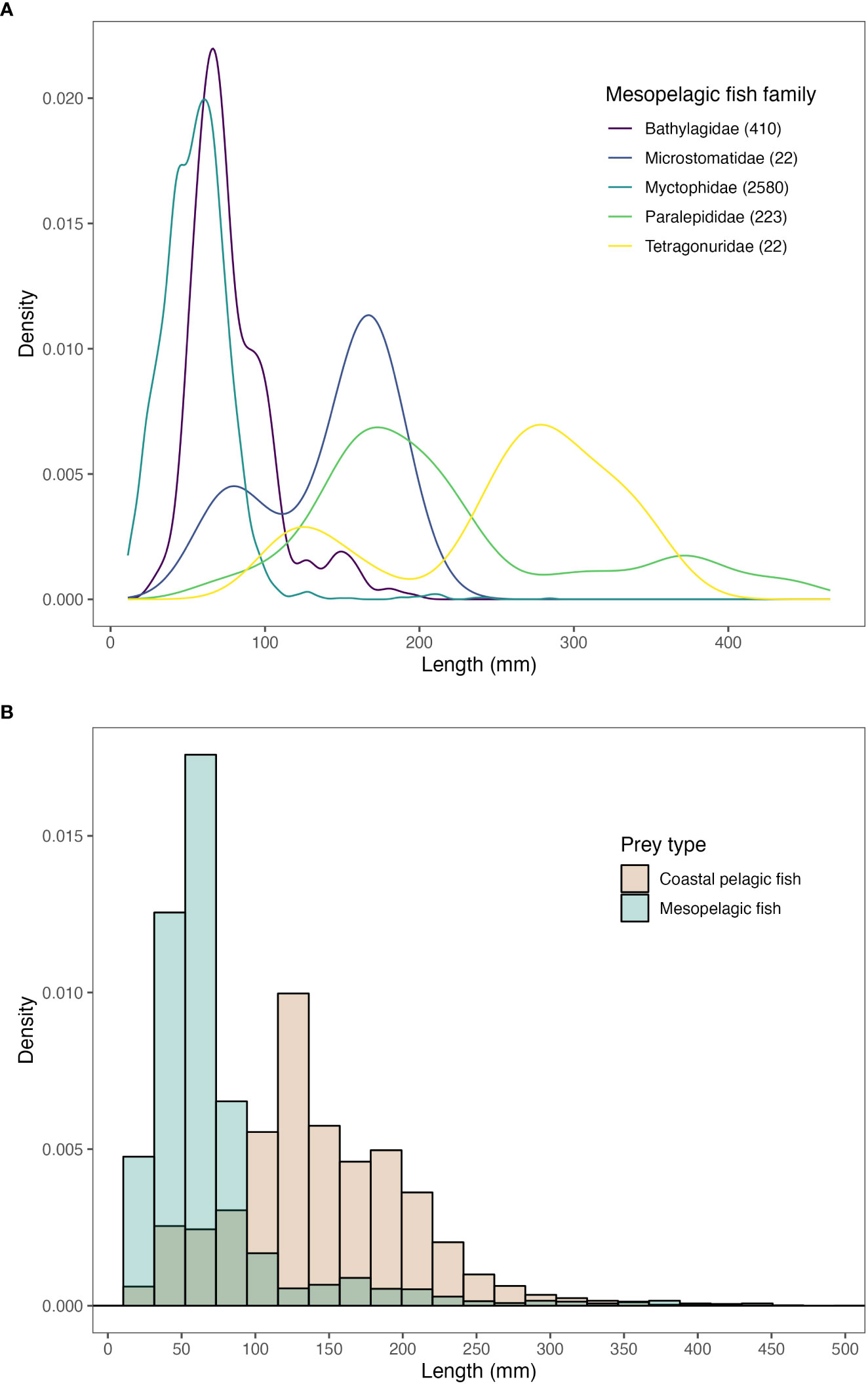
Figure 6 (A) Mesopelagic fish length values by family, for those families with at least 10 length measurements available. (B) A comparison of length frequencies for mesopelagic and coastal pelagic fish prey for those predator species that consumed mesopelagic prey. All length values are reported in mm from standard lengths and were either measured directly, back-calculated, or estimated from another either TL or FL measurements.
Results
The FO analysis revealed mesopelagic fishes to be important prey for multiple phyla of higher trophic level predators. For 11 predator taxa, greater than 25% of all diet samples contained mesopelagic fish prey. Although there was variability in the total number of samples examined, we classify a FO value greater than 25% as an “important” prey item. For four dolphin species, Northern right whale dolphin Lissodelphis borealis, short-beaked common dolphin Delphinus delphis delphis, Pacific white-sided dolphin Lagenorhynchus obliquidens, and long-beaked common dolphin Delphinus delphis bairdii, 89, 86, 48, and 29% of samples, respectively, contained mesopelagic fish prey. Pelagic squids also appear to rely on mesopelagic fishes as prey, with four of the five squid taxa in the database containing percentages of mesopelagic fish in diet samples greater than 25%: Armhook squid Gonatidae spp., (75%) Cock-eyed squid Histioteuthis spp. (55%), Humboldt squid Dosidicus gigas (52%), and Hooked squid Onychoteuthidae spp. (38%). Additionally, Silvergray rockfish Sebastes brevispinis (52%), Bigeye thresher shark Alopias superciliosus (51%), and Broadbill swordfish Xiphias gladius (50%) all had a prevalence of mesopelagic fishes of 50% of samples or greater (Figure 1B and Supplementary Table S2). Just below our 25% criteria, Albacore Thunnus alalunga (19%) and Canary Rockfish Sebastes pinniger (19%) had a relatively high frequency of mesopelagic prey in their diet samples followed by the Sandpaper skate Bathyraja kincaidii, Pacific Ocean perch Sebastes alutus and Bluefin Tuna Thunnus orientalis, which each had a percent FO of 16% followed by Northern fur seal Callorhinus ursinus, 15% FO.
In addition, 36 total predator taxa in the CCTD database had a minimum of one record of a mesopelagic fish in their diet. This included six of the seven marine mammal species, 19 bony fishes including: swordfish, tuna, and salmon species, seven elasmobranchs and four of the five squid taxa, indicating that mesopelagic fish may be an occasional prey source for an even greater diversity of predators in the California Current. When we compare the contribution of mesopelagic fish with that of coastal pelagic fish species in the diet of predators, there were 21 taxa for which mesopelagic fishes were more frequently encountered in the diet than all coastal pelagic fish species combined and included: Northern right whale dolphin Lissodelphis borealis, short-beaked common dolphin Delphinus delphis, Armhook squid Gonatidae, Cock-eyed squid Histioteuthidae, Humboldt squid Dosidicus gigas, Silvergray rockfish Sebastes brevispinis, Bigeye thresher shark Alopias superciliosus, Broadbill swordfish Xiphias gladius, Pacific white-sided dolphin Lagenorphynchus obliquidens, and others; see Supplementary Table S3 for full list.
Myctophids were the most common family of prey, eaten by the greatest diversity of predator taxa, 32 in total (Figure 3), although we are not able to resolve whether this is a result of their abundance within the mesopelagic zone or predator selectivity. Myctophids comprised the top 8 most frequently encountered prey items according to the highest resolution taxon categories, and when aggregated by family, Myctophids were the dominant mesopelagic prey (Figure 3), occurring in the diets of 2,621 unique predators and not considering the actual count of individuals per predator or multiple species of Myctophid per diet sample. It was not possible to identify all Myctophids to species, such that 939 of a total of 4,372 Myctophid records were only identified to family. Of those Myctophids that were identified to 15 species, the following occurred in the greatest number of unique diet samples (Figure 2C): Northern lampfish Stenobrachius leucopsarus (~20%), Bigfin lanternfish Symbolophorus californiensis (16%), Blue lanternfish Tarletonbeania crenularis (15%), California headlightfish Diaphus theta (13%), Dogtooth lampfish Ceratoscopelus townsendi (10%), Broadfin lampfish Lampanyctus ritteri (9%), Mexican lampfish Triphoturus mexicanus (9%), Sunbeam lampfish Lampadena urophaos (5%), and California flashlightfish Protomyctophum crockeri (3%). The remaining taxa comprising less than 1% of the identified Myctophid samples.
While Myctophids had the greatest number of predator species and FO, likely attributable to their abundance, our work also highlights the prevalence of additional mesopelagic fish families (Figure 3): Paralepididae (Barracudina) occurred in 526 individual diet samples from 12 predator taxa, followed by Bathylagidae (Deep-sea smelts), 482 individual samples from 14 predator taxa, Microstomatidae (Pencil smelts), 147 samples from 12 predator taxa, Melamphaidae (Ridgehead), 66 samples from five predator taxa, Scopelarchidae (Pearleye), 66 samples from four predator taxa and Stomiidae (Dragonfishes), 52 samples from seven predator taxa. The remaining families represented less than 1% of the total number of diet samples. While some predator taxa consumed a limited number of mesopelagic fish taxa, other predator species consumed a wide diversity of mesopelagic taxa. Of the predators in our database, Humboldt squid Dosidicus gigas consumed the greatest number of mesopelagic taxa (30), followed by California Sea lion Zalophus californianus and short-beaked common dolphin Delphinus delphis delphis (22 taxa each), Pacific hake Merluccius productus (21), Northern right whale dolphin Lissodelphis borealis (19), and Sablefish Anoplopoma fimbria, Albacore Thunnus alalunga and Bluefin tuna Thunnus orientalis (13 each), (see Supplementary Table S4 for complete list), indicating that while Myctophids were the dominant mesopelagic fish prey encountered, additional mesopelagic fish families were also represented in predator diets.
Mesopelagic predator collection records occurred over a similar geographic extent as those of all predator collections, ranging from British Columbia south to Baja and out into the North Pacific Gyre (see Figure 4). The proportion of collection records with predators that had consumed mesopelagic prey was greatest in deep waters, 40% (bottom depths > 1000 m), followed by 29% for slope collections (bottom depths of 200–1000 m). These values were similar to the 36% observed from scat sample records collected onshore at marine mammal colonies. Contrastingly, but not surprising, given the deeper distribution of mesopelagic fishes, was the relatively small percentage (2%) of predator collection records that had consumed mesopelagic fish from bottom depths associated with the shelf (0–200 m); see Figure 4 inset.
The species composition of mesopelagic predators varied across habitats. The collection location of predators that had consumed mesopelagic fish was greater in deep habitat (bottom depths > 1000 m) for short-beaked common dolphin Delphinus delphis delphis, cock-eyed squid Histioteuthidae, Shortfin mako shark Isurus oxyrinchus, Northern right whale dolphin Lissodelphis borealis, Longspine thornyhead Sebastolobus altivelis, Albacore Thunnus alalunga, Pacific bluefin tuna Thunnus orientalis, and Broadbill swordfish Xiphias gladius (for those taxa with at least 10 diet samples collected, see Figure 5). This contrasted to predator taxa whose greatest number of collections containing mesopelagic fish prey occurred over the slope (bottoms depths from 200 to 1000 m): Bigeye thresher shark Alopias superciliosus, Common thresher shark Alopias vulpinus, Sablefish Anoplopoma fimbria, Sandpaper skate Bathyraja kincaidii, Humboldt squid Dosidicus gigas, and Pacific hake Merluccius productus. Longnose Skate Beringraja rhina, Chinook Salmon Oncorhynchus tshawytscha, Pacific Ocean perch Sebastes alutus, Silvergray Rockfish Sebastes brevispinis, and Canary Rockfish Sebastes pinniger were unique in having the greatest number of collections with mesopelagic prey occur over shelf depths (bottom depths from 0 to 200 m), shallower than the distribution of most mesopelagic fish species.
The comparison of length frequencies between mesopelagic and coastal pelagic fish prey revealed substantial overlap but with a mesopelagic fish length peak occurring at shorter lengths than coastal pelagic prey (Figure 6B); mesopelagic fish lengths were centered around ~50 mm (5 cm), while coastal pelagic species around ~125 mm (12.5 cm). In general, the range of length values for coastal pelagic and mesopelagic fishes were similar (11–466 mm for mesopelagic fish and 2–505 mm for coastal pelagic fish), but most mesopelagic fishes that were consumed by predators in our database were smaller than coastal pelagic fish species. When we compared length frequencies by mesopelagic fish family (for those families with at least 10 length samples available) (Figure 6A), there were disparities among families, with fishes from Bathylagidae and Myctophidae peaking around 50 mm (5 cm), and Microstomatidae, Paralepidiade, and Tetragonuridae peaking at greater lengths: ~175 mm (17.5 cm) for Microstomatidae/Paralepidade and ~300 mm (30 cm) for Tetragonuridae, indicating that distinct mesopelagic fish families cannot be easily categorized into one size class. For a full breakdown of mean SL per taxa, see Supplementary Table S5.
Discussion
Our study represents an important step in the use of compiled diet data from numerous existing predator diet studies to evaluate the importance of mesopelagic fish as prey at the scale of a large marine ecosystem. In total, 25% of all 143 predator taxa in the CCTD consumed a minimum of one mesopelagic fish, including economically important fishery species such as bluefin tuna (16% of samples), albacore (19%), swordfish (50%), Humboldt squid (52%), and Pacific hake (4%). Mesopelagic fishes were also prevalent in the diet of marine mammals, occurring in four species greater than 25% of the time (Dolphins and Pinnipeds). For 11 of these predator taxa, mesopelagic fishes occurred in more than 25% of all individuals examined, and thus likely represent an important source of prey. The diversity of evolutionary and ecological niches represented among these 11 predator taxa [four pelagic squid, a shelf-edge associated rockfish, Sebastes brevispinis, two vertically migrating pelagic predators (the Bigeye thresher shark Alopias superciliosus and Broadbill swordfish Xiphias gladius), and four species of dolphins], suggest that mesopelagic fishes are important across a variety of trophic levels and habitats. The CCTD includes data for a limited number of predator species and does not currently account for many deep water predator species, especially mesopelagic dwelling predators, who are known to prey on mesopelagic fishes (Dufault et al., 2009; Choy et al., 2013). The CCTD does not yet include data from seabirds, which are also significant global predators of mesopelagic fishes (Watanuki and Thiebot, 2018), so our assessment is still incomplete for the California Current. Finally, although invertebrate prey were accounted for in the CCTD database and there is evidence that mesopelagic invertebrates (e.g., Gonatidae squid and Sergestidae shrimp) are important components of the forage base for higher trophic level predators in the California Current (Szoboszlai et al., 2015), we did not include them in our study. Thus, although our results demonstrate the value of mesopelagic fishes as prey to higher level predators, the true contribution of mesopelagic organisms to the ecosystem is likely underestimated.
Mesopelagic fishes inhabit daytime depths of ~200–1000 m, so we would expect predators of mesopelagic fish to forage over bottom depths at least this great. Indeed, we found the proportion of collection records with predators that had consumed mesopelagic prey to be greatest in deep waters; 40% in waters with bottom depths greater than 1000 m and 29% over the slope (bottom depths between 200 and 1000 m). As neritic prey decreases with increasing distance offshore, mesopelagic fishes may play an increasing role as prey to predators foraging further offshore. Of the 11 predator taxa with FO values of mesopelagic fish greater than 25% (i.e., those species for which we consider mesopelagic prey to be important), six of these 11 predator taxa were most often collected having consumed mesopelagic prey over deeper waters (bottom depths > 1000 m). This total increases to nine of the 11 predator taxa if we include predators that were most often collected with mesopelagic prey over the slope (bottom depth 200–1000 m). This contrasts to only one predator taxa, Sebastes brevispinis, that was captured exclusively over shelf depths, but still had a high incidence of mesopelagic prey. The 11th species, Pacific white-sided dolphin Lagenorhynchus obliquidens was collected with mesopelagic prey in similar proportions over the shelf and slope. Our comparison of predator taxa across collection habitats thus provides evidence that mesopelagic fishes are increasingly important as prey to predator taxa with increasing distance offshore.
Our results also revealed, however, that mesopelagic species were being consumed by predators collected onshore (in scat samples collected from marine mammal rookeries) and over the shelf (bottom depths 0–200 m). Nineteen predator taxa that had consumed mesopelagic prey were collected over shelf depths (Figure 5), which raises the question of how do inshore predators find mesopelagic prey? In the Main Hawaiian Islands, mesopelagic organisms can migrate up to 1.8-km onshore each night to feed (Benoit-Bird et al., 2001), during which time they are vulnerable to nocturnal, shallow predators. Predator taxa that were exclusively collected over shelf depths, indicating an unlikeliness to forage offshore in deep regions, such as three rockfish species in our analysis (Silvergray rockfish Sebastes brevispinis, Blue rockfish Sebastes mystinus, and Canary rockfish Sebastes pinniger), may be specifically targeting locations where mesopelagic fishes are transported. Yellowtail rockfish Sebastes flavidus, which were collected in both shelf and slope habitats, but that had only consumed mesopelagic fishes over shelf depths, for example, have been observed preying upon large numbers of Myctophids transported from adjacent submarine canyons (Pereyra et al., 1969). Submarine canyons are a common feature of the U.S. west coast shelfbreak where they facilitate the concentration and persistence of krill abundance hotspots (Santora et al., 2018). Myctophids, many of which prey upon krill, are known to aggregate along submarine canyon walls (Bosley et al., 2004). Research has shown that abrupt topographic features, including canyons, but also including banks, pinnacles, and other shelf features, can aggregate and trap mesopelagic zooplankton and fishes, making them more susceptible to predation (Isaacs and Schwartzlose, 1965; Genin et al., 1988; Genin, 2004) and provide opportunities for predators around these features to encounter mesopelagic prey. Predators collected over the shelf break such as Pacific hake Merluccius productus in our study (Figure 5), where there is a high diversity and abundance of mesopelagic fishes (Pearcy, 1964), may likewise be intercepting mesopelagic fishes trapped after getting transported during vertical migration from deeper depths, as was documented for European hake Merluccius merluccius residing along shelf breaks in the Western Mediterranean (Cartes et al., 2009). In the Southern Ocean, during high wind periods, Myctophids have been advected onto shelf regions where they are likewise consumed by land-based predators (Perissinotto and McQuaid, 1992). It is likely that a similar mechanism is responsible for conveying mesopelagic prey to shelf associated predators in our study, although more research in this area would be beneficial.
So far, we have discussed mechanisms whereby mesopelagic fish and predator interactions are facilitated by the daily vertical migration of mesopelagic fishes into the upper water column. Also referred to as “running the gauntlet” of predators (Robison et al., 2020), the daily movement of mesopelagic organisms into the upper water column over distances upwards of hundreds of meters is assumed to be a tradeoff between increased prey availability in surface waters and avoiding predation by visual predators during the day. While it is estimated globally that ~50% of mesopelagic organisms undergo vertical migration, up to 90% are estimated to migrate in the Eastern Pacific where our study takes place (Klevjer et al., 2016). Many species of Myctophids (Lanternfishes), the most frequently encountered mesopelagic prey in our study, migrate vertically (Watanabe et al., 1999; Brodeur and Yamamura, 2005), although there is considerable variability among species and life stages. For those groups of mesopelagic fishes that do not migrate vertically or for migrating species at mesopelagic depths during the daytime, there is still the risk of predation by deep-diving predator taxa. In the CCTD, we did not have sufficient data about the capture depth of predators to infer foraging depths, but for some predator taxa, we know that individuals forage consistently at mesopelagic depths. Broadbill swordfish Xiphius gladius, for example, which have a FO of mesopelagic fishes in their diet of ~50% are known to target deep-scattering layers when foraging (Dewar et al., 2011) and can dive to depths of up to 2878 m (fishbase.org). One limitation of our study is the bias within the CCTD toward nearshore and epipelagic predators (Figure 4), which likely leads to an underestimation of the role of non-migrating mesopelagic fishes in predator diets. For example, Gonostomatidae (Bristlemouths), considered one of the most abundant families of mesopelagic fishes in the world, were only represented in our database by a single predator record. While this could be attributed to the inherent difficulty in identification, due to the fragile nature of these fish, more likely Gonostomatidae play an increasingly important role as prey to deeper water predator communities that were not included in the diet database. Future work would benefit from the inclusion of diet data from deeper dwelling predatory species.
While Myctophids dominated the mesopelagic prey in our study in terms of FO and diversity of predators that consumed them, it was beyond the scope of this study to examine whether this was a function of predator selectivity or the abundance of Myctophids within the mesopelagic zone. Research indicates that Myctophids likely dominate the biomass of mesopelagic fishes within the Southern California Current (Davison et al., 2015), so their prevalence in the diets of predators in our study is likely due to this abundance more than any predator selectivity. While our results highlight the importance of Myctophids to predator diets, we additionally described 16 families of mesopelagic fishes encountered in predator diets, highlighting the diversity of species inhabiting mesopelagic depths and the need to account for the complexity of the mesopelagic community more appropriately when evaluating the role of mesopelagic fishes in the broader ecosystem. Previous work to characterize predator-prey interactions within the California Current, for example, have exclusively considered Myctophids (Szoboszlai et al., 2015), or Myctophids and Viperfish (Dufault et al., 2009) to describe mesopelagic forage. Likewise, ecosystem models for the California Current, which can be used to evaluate the dependence of top predator populations on their forage base (Smith et al., 2011), often include a single functional group to describe mesopelagic fishes (Field et al., 2006; Horne et al., 2010; Kaplan et al., 2013). As the 16 mesopelagic families in our study inhabitant distinct depths and likely respond to oceanographic variability in distinct ways, reducing this complexity to a single functional group could reduce our ability to interpret the impact of any changes to the mesopelagic zone on higher trophic level predators. Furthermore, for taxa with consistent diet data available over decadal timescales, it could be possible to identify shifts in the mesopelagic community in response to ocean climate variability and even develop a standardized mesopelagic indicator index for ecosystem assessment and function. There is considerable uncertainty in current ecosystem-based models regarding the effect of changing mesopelagic fish populations on higher trophic level predators. For example, when Kaplan et al. (2013) estimated the effect of reducing mesopelagic fish on Albacore populations using two different models, declining mesopelagic fish either had no effect or reduced Albacore populations by over 20% depending on the dependence of Albacore on mesopelagic fishes. By incorporating a diversity of mesopelagic fish species previously not considered and refining our understanding of the importance of mesopelagic prey to specific predator taxa, our study expands our collective understanding of trophic interactions between mesopelagic fishes and their predators, thereby supporting basic needs for the monitoring and modeling of ecosystem function now and into the future.
The U.S. National Marine Fisheries Service protects unfished forage fish along the U.S. West Coast, including mesopelagic fishes from the families: Myctophidae, (Lanternfish) Bathylagidae (Deep-sea smelt), Paralepididae (Barracudina), and Gonostomatidae (Bristlemouths) (50 CFR §660.5-.6), members of which were found in the diets of predators in our study. Similar to a ban on fishing krill in federal waters [50 CFR §660.505(o)], this move recognizes the value of protecting a diverse forage base for higher trophic predators, specifically salmon, groundfish and highly migratory species. Our study provides evidence that this decision is justified, given the presence of mesopelagic fishes in the diets of each of these management units (salmon, groundfish, and highly migratory species). Our results further indicate that this action would protect a considerable amount of mesopelagic biomass, as 96.6% of all mesopelagic fish taxa counted from unique predator diet samples were from protected families. However, only ~56% of mesopelagic species identified in the database are from protected mesopelagic fish families, meaning there are many species still within the mesoepelagic zone that are not named for prohibitions on future fisheries. The families Microstomatidae, Melamphaidae, Scopelarchidae, and Stomiidae, for example, are not currently included in the ban, although they account for some of the most frequently encountered mesopelagic fish families after Myctophids, Paralepididae, and Bathylagidae. These species are protected, however, by a more general ban on the use of the type of net gear that would be needed to catch mesopelagic fish [50 CFR §600.725(v)]. Furthermore, while National Marine Fisheries Service regulations safeguard mesopelagic fishes within federal waters (3-200 nautical miles from shore), most mesopelagic habitat lies outside national jurisdiction in the high seas. Although these areas beyond national jurisdiction are overseen by the UN Convention on the Law of the Sea, currently there are inadequate provisions for governing potential emerging mesopelagic fisheries (Wright et al., 2020; Gjerde et al., 2021).
Our results demonstrate that the contribution of mesopelagic fishes to the diets of top predators is more diverse than what was previously known. Mesopelagic fishes are important prey to a diversity of higher trophic level predators, including 11 taxa, mostly caught over bottom depths > 200 m, which had mesopelagic fishes present in over 25% of all diet samples examined. Mesopelagic fishes were also found in diet samples of predator taxa collected over shallower depths (onshore to 200 m), highlighting the interconnectedness of mesopelagic fishes to nearshore and epipelagic habitats and predators. Changes in the availability of mesopelagic prey to higher trophic level predators, especially as a result of climate change, could have bioenergetic consequences, impacting economically valuable fisheries and protected species. Mesopelagic ecosystems are chronically under studied (St. John et al., 2016; Martin et al., 2020) and the response of mesopelagic fishes to oceanographic variability is still uncertain, especially for many less studied species and at different life stages [as most mesopelagic fishes inhabit epipelagic depths as larvae (Ahlstrom, 1969)]. Our study utilized a publicly available database to provide an ecosystem-level assessment of the importance of mesopelagic fishes to the predator community of the California Current and highlights the need to incorporate mesopelagic fishes into future management.
Data availability statement
The original contributions presented in the study are included in the article/Supplementary Material. Further inquiries can be directed to the corresponding author. R code available at : https://github.com/ilyiglesias/Meso_diet.
Author contributions
All authors contributed to this effort. II performed the analysis and writing. JS originally suggested the use of the CCTD database for exploring the mesopelagic and contributed ideas for analysis, provided figures, and substantial insights to the structure, organization, and flow of text. JCF contributed relevant research in the field and provided topics and direction for the discussion. JF provided feedback on figures, layout and text. All authors contributed to the article and approved the submitted version.
Funding
Fellowship funding for II was generously provided by the University of California, Cota Robles Fellowship. Additional support was provided by the Cooperative Institute for Marine, Earth and Atmospheric Systems; a cooperative institute between the Southwest Fisheries Science Center of the National Marine Fisheries Service and the University of California, Santa Cruz. Grant/Award Number: NA20OAR4320278-13.
Acknowledgments
We would like to thank the many contributors that provided diet composition data to the CCTD and to Joe Bizzaro and Lynn Dewitt for compiling these data and making them publicly available. We are grateful for the marine icons available from PhyloPic https://www.phylopic.org. This draft benefited from valuable feedback during NOAA internal review by Yvonne deReynier and Steve Lindley. We would finally like to thank our independent reviewers who provided valuable feedback on previous versions of our manuscript.
Conflict of interest
The authors declare that the research was conducted in the absence of any commercial or financial relationships that could be construed as a potential conflict of interest.
Publisher’s note
All claims expressed in this article are solely those of the authors and do not necessarily represent those of their affiliated organizations, or those of the publisher, the editors and the reviewers. Any product that may be evaluated in this article, or claim that may be made by its manufacturer, is not guaranteed or endorsed by the publisher.
Supplementary material
The Supplementary Material for this article can be found online at: https://www.frontiersin.org/articles/10.3389/fmars.2023.1220088/full#supplementary-material
References
Ahlstrom E. H. (1969). Mesopelagics and Bathypelagics Fishes in the California Current Region Vol. 13 (California: Calif. Coop. Ocean. Fish. Investig), 39–44. Available at: https://calcofi.com/publications/calcofireports/v13/Vol_13_Ahlstrom.pdf
Baker R., Buckland A., Sheaves M. (2014). Fish gut content analysis: robust measures of diet composition. Fish. Fish. 15, 170–177. doi: 10.1111/faf.12026
Beamish R. J., Leask K. D., Ivanov O. A., Balanov A. A., Orlov A. M., Sinclair B. (1999). The ecology, distribution, and abundance of midwater fishes of the Subarctic Pacific gyres. Prog. Oceanogr. 43, 399–442. doi: 10.1016/S0079-6611(99)00017-8
Benoit-Bird K. J., Au W. W. L., Brainard R. E., Lammers M. O. (2001). Diel horizontal migration of the Hawaiian mesopelagic boundary community observed acoustically. Mar. Ecol. Prog. Ser. 217, 1–14. doi: 10.3354/meps217001
Benoit-Bird K. J., Moline M. A., Southall B. L. (2017). Prey in oceanic sound scattering layers organize to get a little help from their friends. Limnol. Oceanogr. 62, 2788–2798. doi: 10.1002/lno.10606
Bizzarro J. J., Dewitt L., Curtis K. A., Santora J. A., Wells B. K., Field J. C. (in-press). A multipredator trophic database for the California Current Large Marine Ecosystem. Sci. Data.
Bosley K. L., Lavelle J. W., Brodeur R. D., Wakefield W. W., Emmett R. L., Baker E. T., et al. (2004). Biological and physical processes in and around Astoria submarine Canyon, Oregon, USA. J. Mar. Syst. 50, 21–37. doi: 10.1016/j.jmarsys.2003.06.006
Brodeur R., Yamamura O. (2005). Micronekton of the North Pacific. PICES working group 14 final report (B.C. Canada: North Pacific Marine Science Organization (PICES).
Cartes J. E., Hidalgo M., Papiolr V., Massutí E., Moranta J. (2009). Changes in the diet and feeding of the hake Merluccius merluccius at the shelf-break of the Balearic Islands: Influence of the mesopelagic-boundary community. Deep-Sea Res. Pap. 56, 344–365. doi: 10.1016/j.dsr.2008.09.009
Checkley D. M., Barth J. A. (2009). Patterns and processes in the California current system. Prog. Oceanogr. 83, 49–64. doi: 10.1016/j.pocean.2009.07.028
Choy C. A., Haddock S. H. D., Robison B. H. (2017). Deep pelagic food web structure as revealed by in situ feeding observations. Proc. R. Soc B Biol. Sci. 284, 20172116. doi: 10.1098/rspb.2017.2116
Choy C. A., Portner E., Iwane M., Drazen J. C. (2013). Diets of five important predatory mesopelagic fishes of the central North Pacific. Mar. Ecol. Prog. Ser. 492, 169–184. doi: 10.3354/meps10518
Davison P. C., Checkley D. M., Koslow J. A., Barlow J. (2013). Carbon export mediated by mesopelagic fishes in the northeast Pacific Ocean. Prog. Oceanogr. 116, 14–30. doi: 10.1016/j.pocean.2013.05.013
Davison P., Lara-Lopez A., Anthony Koslow J. (2015). Mesopelagic fish biomass in the southern California current ecosystem. Deep-Sea Res. Part II Top. Stud. Oceanogr. 112, 129–142. doi: 10.1016/j.dsr2.2014.10.007
Dewar H., Prince E. D., Musyl M. K., Brill R. W., Sepulveda C., Luo J., et al. (2011). Movements and behaviors of swordfish in the Atlantic and Pacific Oceans examined using pop-up satellite archival tags: Swordfish movements in the Atlantic and Pacific Oceans. Fish. Oceanogr. 20, 219–241. doi: 10.1111/j.1365-2419.2011.00581.x
Dufault A., Marshall K., Kaplan I. (2009). A Synthesis of Diets and Trophic Overlap of Marine Species in the California Current (U.S. Dept. Commer., NOAA Tech. Memo. NMFS-NWFSC-103), 81 p.
Duffy L. M., Kuhnert P. M., Pethybridge H. R., Young J. W., Olson R. J., Logan J. M., et al. (2017). Global trophic ecology of yellowfin, bigeye, and albacore tunas: Understanding predation on micronekton communities at ocean-basin scales. Deep Sea Res. Part II Top. Stud. Oceanogr. 140, 55–73. doi: 10.1016/j.dsr2.2017.03.003
Eschmeyer W., Herald E., Hammann H. (1983). Pacific coast fishes. (Boston New York: Houghton Mifflin Company)
Eyring C. F., Christensen R. J., Raitt R. W. (1948). Reverberation in the sea. J. Acoust. Soc Am. 20, 462–475. doi: 10.1121/1.1906399
Field J. C., Francis R. C., Aydin K. (2006). Top-down modeling and bottom-up dynamics: Linking a fisheries-based ecosystem model with climate hypotheses in the Northern California Current. Prog. Oceanogr. 68, 238–270. doi: 10.1016/j.pocean.2006.02.010
Genin A. (2004). Bio-physical coupling in the formation of zooplankton and fish aggregations over abrupt topographies. J. Mar. Syst. 50, 3–20. doi: 10.1016/j.jmarsys.2003.10.008
Genin A., Haury L., Greenblatt P. (1988). Interactions of migrating zooplankton with shallow topography: predation by rockfishes and intensification of patchiness. Deep Sea Res. Part Oceanogr. Res. Pap. 35, 151–175. doi: 10.1016/0198-0149(88)90034-9
Gjerde K. M., Wright G., Durussel C. (2021). Strengthening high seas governance through enhanced environmental assessment processes: A case study of mesopelagic fisheries and options for a future BBNJ treaty. STRONG High Seas Project. doi: 10.48440/iass.2021.001
Gjosaeter J., Kawaguchi K. (1980). A review of the world resources of mesopelagic fish. FAO Fish. Tech. Pap (Rome: Food and Agriculture organization of the United Nations) Vol. 193.
Goetsch C., Conners M. G., Budge S. M., Mitani Y., Walker W. A., Bromaghin J. F., et al. (2018). Energy-rich mesopelagic fishes revealed as a critical prey resource for a deep-diving predator using quantitative fatty acid signature analysis. Front. Mar. Sci. 5. doi: 10.3389/fmars.2018.00430
Horne P., Kaplan I., Marshall K., Levin P., Harvey C., Hermann A., et al. (2010). Design and Parameterization of a Spatially Explicit Ecosystem Model of the Central California Current. NOAA Technical Memorandum NMFS-NWFSC-104. (2725 Montlake Boulevard East Seattle, Washington 98112: Northwest Fisheries Science Center Conservation Biology Division)
Hsieh C. H., Kim H. J., Watson W., Di Lorenzo E., Sugihara G. (2009). Climate-driven changes in abundance and distribution of larvae of oceanic fishes in the southern California region. Glob. Change Biol. 15, 2137–2152. doi: 10.1111/j.1365-2486.2009.01875.x
Irigoien X., Klevjer T. A., Røstad A., Martinez U., Boyra G., Acuña J. L., et al. (2014). Large mesopelagic fishes biomass and trophic efficiency in the open ocean. Nat. Commun. 5, 3271. doi: 10.1038/ncomms4271
Isaacs J. D., Schwartzlose R. A. (1965). Migrant sound scatterers: interaction with the sea floor. Science 150, 1810–1813. doi: 10.1126/science.150.3705.1810
Kaplan I. C., Brown C. J., Fulton E. A., Gray I. A., Field J. C., Smith A. D. M. (2013). Impacts of depleting forage species in the California Current. Environ. Conserv. 40, 380–393. doi: 10.1017/S0376892913000052
Klevjer T. A., Irigoien X., Røstad A., Fraile-Nuez E., Benítez-Barrios V. M., Kaartvedt. S. (2016). Large scale patterns in vertical distribution and behaviour of mesopelagic scattering layers. Sci. Rep. 6, 1–11. doi: 10.1038/srep19873
Koslow J., Goericke R., Lara-Lopez A., Watson W. (2011). Impact of declining intermediate-water oxygen on deepwater fishes in the California Current. Mar. Ecol. Prog. Ser. 436, 207–218. doi: 10.3354/meps09270
Longhurst A. R. (1976). “Vertical Migration,” In Cushing D. H., Walsh J. J.. The Ecology of the Seas. (Philadelphia Toronto: WB Saunders Company), 116–137.
Lowry M., Nehasil S., Moore J. (2022). Spatio-temporal diet variability of the California sea lion Zalophus californianus in the southern California Current Ecosystem. Mar. Ecol. Prog. Ser. 692, 1–21. doi: 10.3354/meps14096
Martin A., Boyd P., Buesseler K., Cetinic I., Claustre H., Giering S., et al. (2020). Study the twilight zone before it is too late. Nature 580, 26–28. doi: 10.1177/002190966600100401
Miller T. W., Brodeur R. D., Rau G., Omori K. (2010). Prey dominance shapes trophic structure of the northern California Current pelagic food web : evidence from stable isotopes and diet analysis. Mar. Ecol. Prog. Ser. 420, 15–26. doi: 10.3354/meps08876
Miller D. J., Lea R. N. (1972). Guide to the coastal marine fishes of California (Sacramento, CA: Marine Resources Region, California Department of Fish and Game).
Pante E., Simon-Bouhet B. (2013). marmap: A package for importing, plotting and analyzing bathymetric and topographic data in R. PloS One 8 (9), e73051. doi: 10.1371/journal.pone.0073051
Paredes R., Orben R. A., Suryan R. M., Irons D. B., Roby D. D., Harding A. M. A., et al. (2014). Foraging responses of black-legged kittiwakes to prolonged food-shortages around colonies on the Bering Sea shelf. PloS One 9(3), e92520. doi: 10.1371/journal.pone.0092520
Pauly D. (1998). Diet composition and trophic levels of marine mammals. ICES J. Mar. Sci. 55, 467–481. doi: 10.1006/jmsc.1997.0280
Pearcy W. (1964). Some distributional features of mesopelagic fishes off Oregon. J. Mar. Res. 22, 83–102.
Pereyra W. T., Carvey F. E. Jr., Pearcy W. G. (1969). Sebastodes flavidus, a shelf rockfish feeding on mesopelagic fauna, with consideration of the ecological implications. J. Fish. Res. Board Can. 26, 2211–2215. doi: 10.1139/f69-205
Perissinotto R., McQuaid C. (1992). Land-based predator impact on vertically migrating zooplankton and micronekton advected to a Southern Ocean archipelago. Mar. Ecol. Prog. Ser. 80, 15–27. doi: 10.3354/meps080015
Priede I. (2017). Deep-sea Fishes: biology, diversity, ecology and fisheries. 1st ed (Cambridge: Cambridge University Press). doi: 10.1017/9781316018330
Ralston S., Field J. C., Sakuma K. M. (2015). Long-term variation in a central California pelagic forage assemblage. J. Mar. Syst. 146, 26–37. doi: 10.1016/j.jmarsys.2014.06.013
R Core Team (2022). R: A language and environment for statistical computing. R Foundation for Statistical Computing, Vienna, Austria. Available at: https://www.R-project.org/. R version 4.2.3 (2023-03-15)
Robison B. H., Sherlock R. E., Reisenbichler K. R., McGill P. R. (2020). Running the gauntlet: assessing the threats to vertical migrators. Front. Mar. Sci. 7. doi: 10.3389/fmars.2020.00064
RStudio Team (2022). RStudio: Integrated Development Environment for R. Posit Software, PBC, Boston, MA. Available at: http://www.posit.co/. Version 2023.6.0.421
Santora J. A., Hazen E. L., Schroeder I. D., Bograd S. J., Sakuma K. M., Field J. C. (2017). Impacts of ocean climate variability on biodiversity of pelagic forage species in an upwelling ecosystem. Mar. Ecol. Prog. Ser. 580, 205–220. doi: 10.3354/meps12278
Santora J. A., Zeno R., Dorman J. G., Sydeman W. J. (2018). Submarine canyons represent an essential habitat network for krill hotspots in a Large Marine Ecosystem. Sci. Rep. 8, 7579. doi: 10.1038/s41598-018-25742-9
Santora J. A., Schroeder I. D., Bograd S. J., Chavez F. P., Cimino M. A., Fiechter J., et al. (2021). Pelagic biodiversity, ecosystem function, and services: an integrated observing and modeling approach. Oceanography 34, 16–37.
Saunders R. A., Hill S. L., Tarling G. A., Murphy E. J. (2019). Myctophid Fish (Family Myctophidae) are central consumers in the food web of the Scotia Sea (Southern Ocean). Front. Mar. Sci. 6. doi: 10.3389/fmars.2019.00530
Smith A. D. M., Brown C. J., Bulman C. M., Fulton E. A., Johnson P., Kaplan I. C., et al. (2011). Impacts of fishing low–trophic level species on marine ecosystems. Science 333, 1147–1150. doi: 10.1126/science.1209395
Spear L. B., Ainley D. G., Walker W. A. (2007). Foraging dynamics of seabirds in the eastern tropical Pacific Ocean (Camarillo, CA: Cooper Ornithological Society).
Standal D., Grimaldo E. (2020). Institutional nuts and bolts for a mesopelagic fishery in Norway. Mar. Policy 119, 104043. doi: 10.1016/j.marpol.2020.104043
St. John M. A., Borja A., Chust G., Heath M., Grigorov I., Mariani P., et al. (2016). A dark hole in our understanding of marine ecosystems and their services: Perspectives from the mesopelagic community. Front. Mar. Sci. 3. doi: 10.3389/fmars.2016.00031
Szoboszlai A. I., Thayer J. A., Wood S. A., Sydeman W. J., Koehn L. E. (2015). Forage species in predator diets: Synthesis of data from the California Current. Ecol. Inform. 29, 45–56. doi: 10.1016/j.ecoinf.2015.07.003
Van de Putte A., Flores H., Volckaert F., van Franeker J. A. (2006). Energy content of antarctic mesopelagic fishes: implications for the marine food web. Polar Biol. 29, 1045–1051. doi: 10.1007/s00300-006-0148-z
Watanabe H., Moku M., Kawaguchi K., Ishimaru K., Ohno A. (1999). Diel vertical migration of myctophid fishes (Family myctophidae) in the transitional waters of the western North Pacific. Fish. Oceanogr. 8, 115–127. doi: 10.1046/j.1365-2419.1999.00103.x
Watanuki Y., Thiebot J.-B. (2018). Factors affecting the importance of myctophids in the diet of the world’s seabirds. Mar. Biol. 165, 79. doi: 10.1007/s00227-018-3334-y
Keywords: mesopelagic fishes, deep-sea prey, foraging ecology, forage fish, twilight zone, California Current Ecosystem, fisheries management
Citation: Iglesias IS, Santora JA, Fiechter J and Field JC (2023) Mesopelagic fishes are important prey for a diversity of predators. Front. Mar. Sci. 10:1220088. doi: 10.3389/fmars.2023.1220088
Received: 11 May 2023; Accepted: 12 July 2023;
Published: 28 July 2023.
Edited by:
Tracey T. Sutton, Nova Southeastern University, United StatesReviewed by:
Peter J. Auster, Mystic Aquarium, United StatesM. Pilar Olivar, Spanish National Research Council (CSIC), Spain
Julian Anthony Koslow, University of California, San Diego, United States
Copyright © 2023 Iglesias, Santora, Fiechter and Field. This is an open-access article distributed under the terms of the Creative Commons Attribution License (CC BY). The use, distribution or reproduction in other forums is permitted, provided the original author(s) and the copyright owner(s) are credited and that the original publication in this journal is cited, in accordance with accepted academic practice. No use, distribution or reproduction is permitted which does not comply with these terms.
*Correspondence: Ilysa S. Iglesias, iiglesia@ucsc.edu