- 1College of Fisheries and Life Science, Shanghai Ocean University, Shanghai, China
- 2Guangdong Provincial Key Laboratory of Fishery Ecology and Environment, Ministry of Agriculture and Rural Affairs of China, South China Sea Fisheries Research Institute, Chinese Academy of Fishery Science, Guangzhou, China
- 3Key Laboratory of Open-Sea Fishery Development, Ministry of Agriculture and Rural Affairs of China, South China Sea Fisheries Research Institute, Chinese Academy of Fishery Science, Guangzhou, China
Mariculture of bivalves (e.g., mussels, scallops, and oysters) is developing rapidly in coastal waters. This process releases shell debris that can accumulate on the seabed under farms. The ecological consequences of shell accumulation on the bioturbation of benthic macrofauna, which play a key role in the biogeochemical processes of sediment, are not yet fully understood. Thus, it is necessary to quantify the shell debris that accumulated under bivalves farms and evaluate whether the shell debris hinders the burrowing capacity of macrofauna. Oysters are common bivalve species cultured worldwide. However, no data are available on shell accumulation due to oyster farming and its possible effects on the burrowing capacity of polychaetes. Thus, we conducted a preliminary study on shells debris accumulation under an oyster farm in Daya Bay, southern China, and assessed the effects of shell accumulation on the burrowing activity of the polychaete Perinereis aibuhitensis in a mesocosm experiment for 32 days. The results showed that oyster farming significantly (p < 0.05) increased the accumulation of shell debris under the farm. The amount of shell debris under oyster farm (6.0 ± 1.3 kg (dry weight (DW)) m-2 or 130.0 ± 24.7 g (DW) kg-1 dry sediment) was approximately two times that in a non-culture area (3.5 ± 0.9 kg (DW) m-2 or 74.9 ± 10.5 g (DW) kg-1 dry sediment). The presence of shell debris did not affect the survival of P. aibuhitensis in experiment, but the animals in sediment with shell added exhibited greater losses of body weight compared with those without, indicating that the presence of shell causes animals to expend more energy in burrowing. The numbers of burrowing holes were lower in sediment with shell debris added compared with those in sediment without shell addition throughout the experiment. Whereas, this does not due to the P. aibuhitensis in +Shell treatment were less active in terms of their burrowing activities. The lower numbers of holes in +Shell treatment was mainly due to P. aibuhitensis were unable to use surface areas where shells were present, the amount of area occupied by shell material physically prevented P. aibuhitensis from forming burrows in these locations. The hindering effects of shells on the burrowing activity of P. aibuhitensis would decrease as the shells degraded into debris. Therefore, we recommend using small shells when conducting “shell addition” bioremediation strategies to avoid the possible negative effects of shells on burrowing by polychaetes.
Introduction
Mariculture is developing rapidly in coastal waters and it is one of the fastest-growing food-producing sectors in the world (FAO, 2022). Bivalves (e.g., mussels, scallops, and oysters) are important groups with an annual production of >17.7 million tons in 2020 (FAO, 2022). Suspended longline culture is a commonly used method for culturing bivalves in shallow coastal waters. Suspended culture increases the sedimentation of organic matter, such as biodeposition (e.g., feces and pseudofeces) into the bottom sediments under farms (Yu et al., 2017; Rosa et al., 2018; Gray and Langdon, 2019). Many studies have investigated remineralization, nutrient fluxes, and the biological succession associated with organic enrichment due to the suspended bivalves culture (Crawford et al., 2003; Zhou et al., 2006; Hargrave et al., 2008; Sanz-Lazaro and Marín, 2008).
Whereas, bivalve suspended culture also leads to another biodeposit, that of shells. Bivalve shells can accumulate on and in sediment for a long period before their degradation into shell-hash (Fitridge et al., 2012; Sanchez-Jerez et al., 2019) due to their low degradability (Rajagopal et al., 2003; Fitridge et al., 2012; Sanchez-Jerez et al., 2019). Shells integrated into sediment can have long-term effects on sediments. In particular, they can modify the physical topology and increase the habitat complexity of sediments (Gutiérrez et al., 2003; D’Amours et al., 2008), as well as simulating or inhibiting assemblage and bioturbation effects of macrofauna (Gutiérrez et al., 2003; Casado-Coy et al., 2017; Bergström et al., 2020; Casado-Coy et al., 2022). However, most previous studies have focused on the impact of organic sedimentation from aquaculture on the sediment environment, whereas, few have considered shell deposition and accumulation, and its effects on bioturbation capacity of macrofauna (Papageorgiou et al., 2009; Weise et al., 2009; Adámek and Maršálek, 2013). Moreover, most relevant studies investigated mussels (Wilding, 2012; Casado-Coy et al., 2017; Casado-Coy et al., 2022) and fish farms (Sanchez-Jerez et al., 2019). The oyster is a commonly farmed bivalve species in many countries including China, the USA, and France. The global production of oysters in 2020 was ~6 million tons (Mt), which was the highest among all bivalve species (FAO, 2022). Oysters do not have byssus, they attach to culture facilities (e.g., polyethylene ropes and cement columns) by secreting calcium carbonate in the early stage and only once in their lifetime. Oysters are readily lost from their supports and deposited on the seabed during harvesting, infrastructure cleaning, and because of typhoon storm damage, particularly in tropical and subtropical waters.
Polychaetes are a very important component of benthic community (Tomassetti and Porrello, 2005). They are common species of macrobenthos communities in Daya Bay (Du et al., 2011; Rao et al., 2021; Li et al., 2023) and many other areas of the world (Nasi et al., 2018; Jansen et al., 2019). Polychaetes are scavengers, they fed on particulate organic matters such as fish and bivalves faeces, so that to act as an extractive component in the ecosystem (Nederlof et al., 2020). In addition, polychaetes have a high ability of bioturbation. Therefore, they are assumed to have efficient bioremediation potential and can be used as bioremediation candidates for enriched environment such as fish and bivalves farms (Jansen et al., 2019). However, to the best of our knowledge, no previous studies have investigated the shell deposition and accumulation of shells on the seabed due to oyster farming and whether it affects the burrowing capacity of polychaetes.
Moreover, a previous study suggested that adding mollusk-shell debris to sediment could mitigate the deleterious effects of organic pollution on marine sediments, and thus it could be used as a remediation strategy (Casado-Coy et al., 2017). In this regard, it is necessary to firstly evaluate the possible effects of shell debris on the functions of benthic macrofauna. Burrowing activity allows macrofauna to implement their irrigation and bioturbation functions. However, it is still unclear whether shell debris affects the burrowing capacity of polychaetes.
In the present study, we conducted (1) a field survey of the shell density in the seabed in an oyster farming area and a non-culture area in Daya Bay, southern China. We aimed to provide quantitative information to further evaluate the effects of shells on the topological structure and habitat complexity of sediment; and (2) a 32-day mesocosm experiment to assess whether the presence of oyster shells negatively affected the burrowing capacity of the polychaete Perinereis aibuhitensis based on temporal variation in the number of holes as an indicator. Our findings help to understand the environmental effects of large-scale bivalve aquaculture.
Materials and methods
Study site
The in field study was conducted in Dapeng Cove, which is a ~14 km2 embayment in Daya Bay. Daya Bay is a ~650 km2 semi-enclosed subtropical embayment in the northern South China Sea, located in Guangdong Province, southern China. The minimum sea surface temperature was 15°C in the winter, and the maximum temperature was 30°C in summer and autumn (Han et al., 2020). Daya Bay is one of the most intensive mariculture areas in China. Dapeng Cove is in the southwest portion of Daya Bay. It is located about 30 km from Hong Kong and about 60 km from the Shenzhen Special Economic Zone. Suspended longline culture of the oyster Crassostrea angulata in Dapeng Cove and Daya Bay has been practiced since the 1980s, with annual production of ~ 6.6 × 104 tonnes in the bay (Qi et al., 2019). The longline ropes are about ~200 m in length and the width interval between ropes is ~2.5 m. Oyster spat are attached on strings (~3.0 m long) hung from the submerged ropes. Oyster are raised in two cycles per year, i.e., oyster spat are deployed during January or February (winter) and harvested after a growth period of ~6 months, before restocking from summer to winter.
Sediment collection and shell debris quantification
The experiment was conducted from November 2020 to January 2021. To determine the shell density, sediment samples were collected from three oyster farms, within each farm three samples were collected randomly from three sites, and three samples were randomly collected from closed non-culture areas located ~1.0 km from the oyster farms. The water depth was ~5 m. Sediment samples were collected using a Van-Veen grab bottom sampler. The surface area of sampler was ~340 cm2, and the depth of sediment collected was ~15 cm. To determine the shell density, sediment samples were sieved through a 2-mm mesh sieve, and the intact shells and shell-hash were collected and counted. The shells were sorted in the laboratory, dried at 65°C for 24 h, and weighed. One sediment subsample was used to estimate the moisture content by drying at 65 °C for 48 h, and the actual dry weights of sediments were calculated accordingly. Bottom seawater from 0.5 m above the seabed was also collected in situ and filtered through with an acetate membrane with a pore size of 0.45 μm to remove microalgae and other suspended particulate matter, and used in the subsequent incubation experiment.
Experimental design and incubation procedure
To assess whether the presence of shells would affect the burrowing activities of macrofauna, we conducted a mesocosm experiment using the polychaete P. aibuhitensis as the experimental model animal (Figure 1D). The experimental P. aibuhitensis individuals were obtained from a local supplier of fishing bait near Daya Bay, Shenzhen city, southern China.
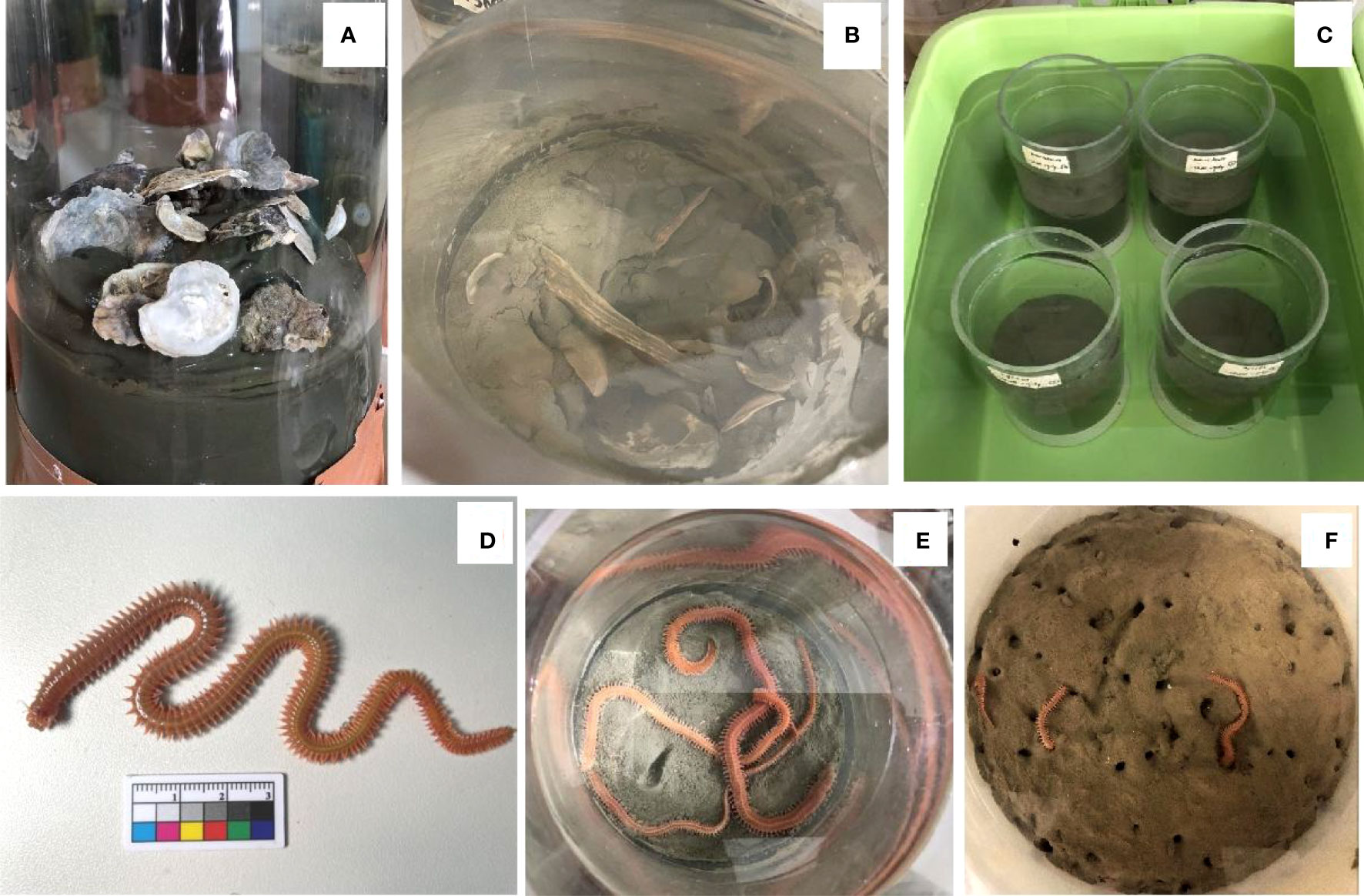
Figure 1 Images illustrating the experimental protocol: (A, B) oyster shell debris present in the middle and on the surface of sediment core, (C) sediment cores incubated in plastic box, (D, E) experimental polychaete Perinereis aibuhitensis, and (F) holes made by P. aibuhitensis burrowing.
Sediment collected from the oyster farm was transported to the laboratory. Any stones, shells, and living organisms were removed using a 2-mm mesh sieve. Sediment was mixed thoroughly to be homogenized before the experiment started. The median grain size and clay: sand: silt percentage (11.2%: 43.0%: 45.8%) of sediments from oyster farms and non-culture areas were similar. The homogenized sediment was incubated in 6 methyl methacrylate bottles (with an internal diameter of 12 cm and height of 32 cm). The bottles were filled with sediment to a depth of 10 cm. The bottom of each core was sealed with a rubber stopper, leaving 15 cm of water above the sediment. The bottles were evenly divided into two treatments, each with 3 replicates (bottles). In the first treatment, each sediment sample was mixed with ~68.3 g cleaned oyster shells and debris (referred as “+Shell”), which was corresponded to a realistic shell density in the natural environment. Shells were distributed on the surface and buried in the sediment (Figures 1A, B). In the second treatment, no shell was added (referred as “−Shell”). And then, eighteen healthy and active polychaetes P. aibuhitensis individuals (wet body weight 2.5 ± 0.84 g, body length 17.70 ± 0.87 cm) were randomly distributed to the six bottles, each with three animals (Figure 1E). The body length and body weight were measured for each P. aibuhitensis before and after the incubation experiment. The bottles were randomly placed in 63-L plastic tanks filled with 30 cm of flowing water at 20°C and thus all the cores were kept under the same temperature conditions (Figure 1C). The tanks were covered with a non-transparent cover to maintain relative darkness similar as that of the sea bottom. The sediment was left in the cores for 4 days to allow stratification before P. aibuhitensis individuals were added. The experiment lasted for 32 days. Each core received continuous moderate aeration by an air circulating pump, except the aeration was stopped for 30 min before counting the numbers of holes made by P. aibuhitensis burrowing (Figure 1F). The numbers of holes were counted on days 1, 3, 8, 15, 25, and 32 after the experiment began.
At the end of the incubation experiment, the survival rates were recorded for P. aibuhitensis. The body weights were measured. The increment rate of hole number was calculated as: 100% × (Nt –N(t–1)), where the Nt is the number of holes counted on a specific day, and N(t–1) is the number of holes counted previously.
Data analysis
All of the evaluated variables (shell density, number of holes, increment rate of hole number, and loss of body weight) were compared by an analysis of one-way analysis of variance (ANOVA) to determine any significant (P < 0.05) differences between the observed values. Conformance to the assumptions of normality and homoscedasticity was confirmed before conducting further statistical analysis. All tests were performed using SPSS 18.0 for Windows (IBM Corp., Armonk, N.Y., USA). Values were expressed as the mean ± SEM (standard error of the mean (SEM)) based on triplicate groups.
Results
Oyster shell debris accumulation
The amount of shell debris was significantly higher in the sediment collected under the oyster farm compared to the non-culture area close to the oyster farm. Under the oyster farm, except for shell debris, 36 intact oyster shells were found with upper and lower valves still attached together (Figure 2), and the largest shell length was 10.5 cm. By contrast, no oyster shells were found in the non-culture area, where only small shell debris (shell-hash) from non-farmed species was present. The amount of shell debris was significantly (p < 0.05) higher under the oyster farm (6.0 ± 1.3 kg (dry weight (DW)) m-2 or 130.0 ± 24.7 g (DW) kg-1 dry sediment) than the non-culture area (3.5 ± 0.9 kg (DW) m-2 or 74.9 ± 10.5 g (DW) kg-1 dry sediment), i.e., approximately two times greater.
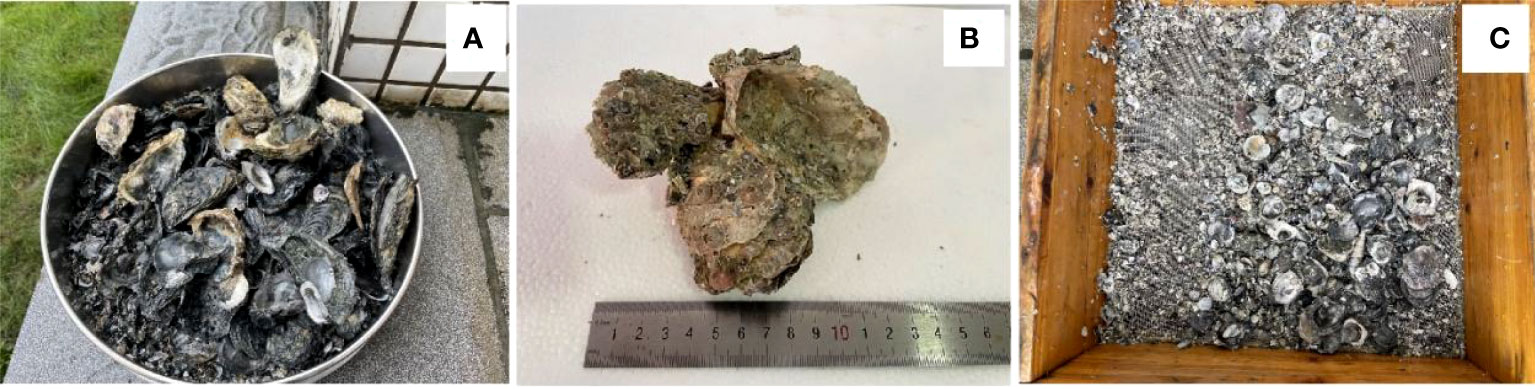
Figure 2 Shell debris in sediment under (A, B) oyster suspended longline culture farm and (C) adjacent non-culture area.
Performances of experimental P. aibuhitensis
After incubation for 32 days, the experimental P. aibuhitensis individuals were healthy and active, and their survival rates were 100% in all treatments. However, the wet body weights were lower compared with the initial body weights under all treatments. The polychaetes lost more body weight in +Shell sediments group (16.1 ± 0.13%) than the −Shell sediments (0.56 ± 0.01%) (p < 0.05, Figure 3).
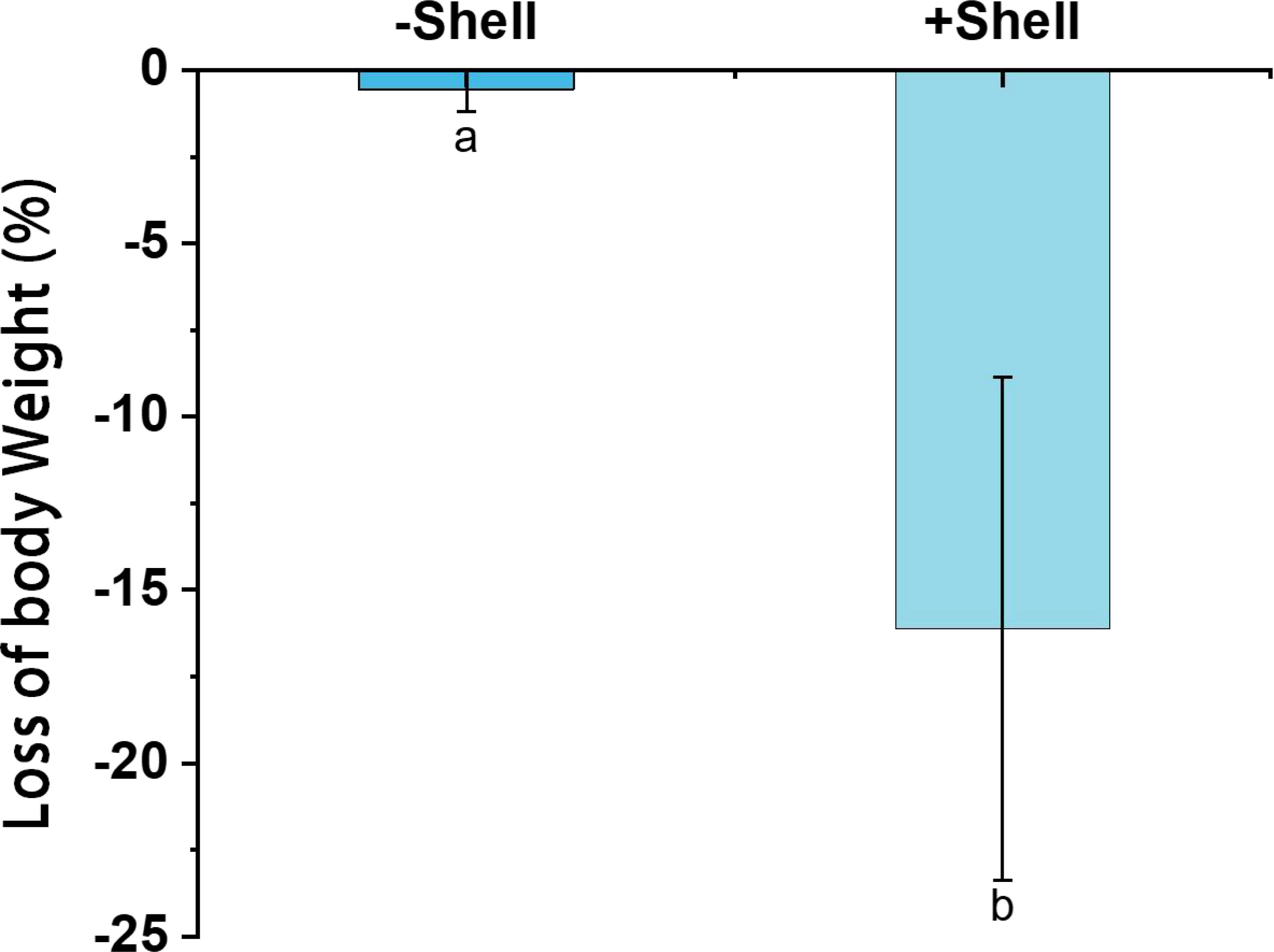
Figure 3 Losses of body weight by the polychaete Perinereis aibuhitensis in sediment with shells (+Shell) and without shell debris (−Shell). Different superscript letters indicate significant differences (P < 0.05). Values represent the mean ± SEM based on three replicate cores (n = 3).
Temporal variation in number of burrowing holes
To determine whether the presence of oyster shell debris negatively affected the burrowing activities of P. aibuhitensis, the numbers of holes between the +Shell and −Shell treatments were compared. We observed that P. aibuhitensis began to burrow holes very soon after settling down on the sediment cores. In the two treatments, the numbers of holes increased during the experiment, the numbers of holes were significantly (p < 0.05) higher on days 25 and 32 than on days 1, 3, and 8 (Figure 4A). The numbers of holes in the sediment were higher in the −Shell groups than the +Shell groups throughout the incubation process, although there was a significant (p < 0.05) difference between the two treatments only on day 25 (Figure 4A). The increment rates were highest at the start of incubation, but the increasing trend tended to level off after 8 days, the numbers of holes continually increased, but the numbers of holes did not differ significantly on each observation day subsequently (Figure 4B).
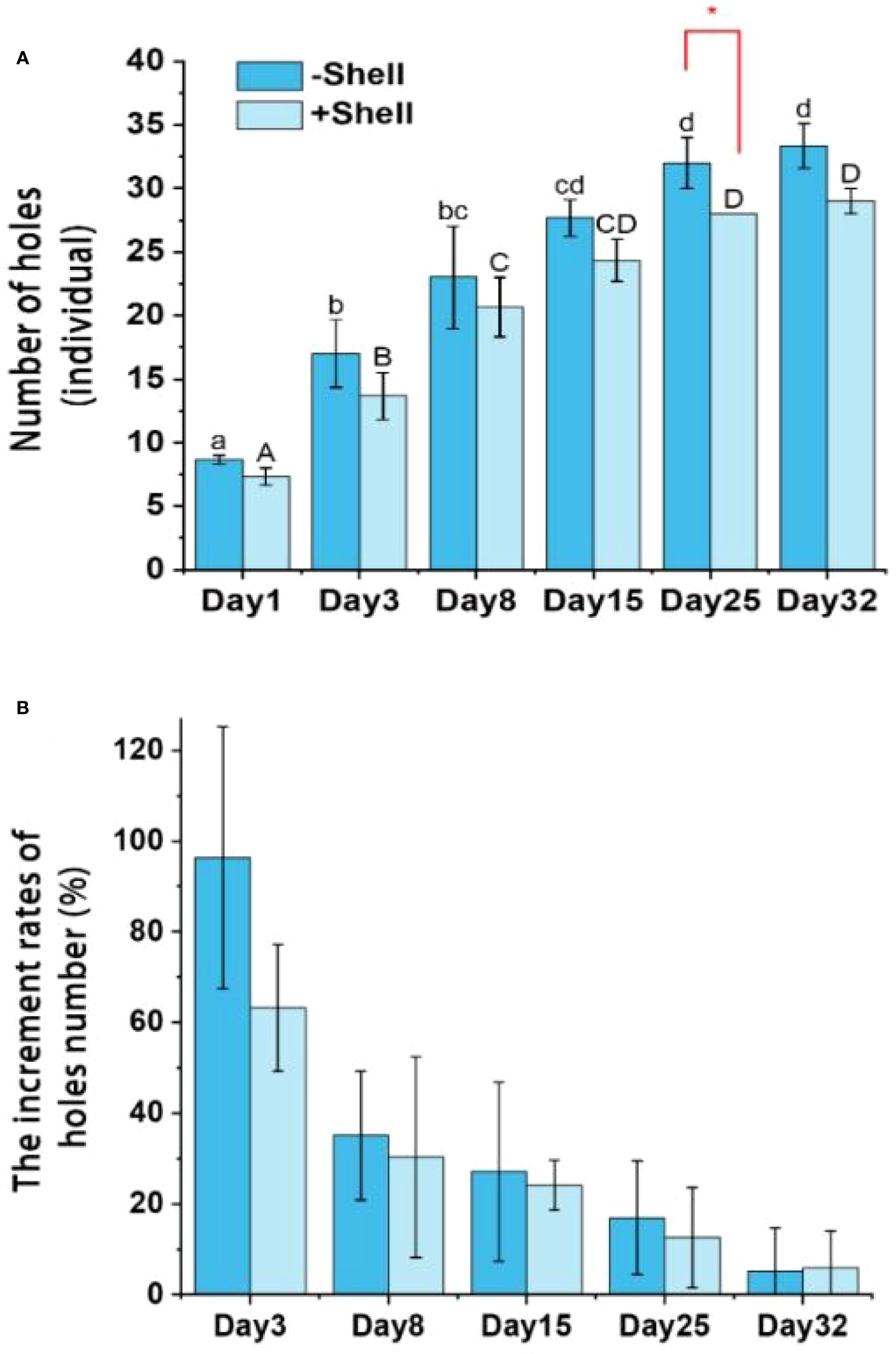
Figure 4 Temporal variations in number of holes (A) and increment rates of the number of holes (B) in the +Shell and −Shell groups. Different lowercase and uppercase letters above the columns represent statistically different results within +Shell and −Shell treatments, respectively. The red asterisk (*) indicates significant difference between the +Shell and −Shell treatments. Values represent the mean ± SEM based on three replicate cores (n = 3).
Discussion
Accumulation of shell debris under oyster farm
In suspended longline culture, the infrastructure (e.g., ropes and buoys) is usually artificially cleaned before farming to remove fouling organism such as oyster and mussel (e.g., Perna viridis in Daya Bay) shells, which are deposited on the seabed. In addition, typhoon storms are frequent during the summer season in waters in tropical and subtropical zones, such as Daya Bay. Cultivated oysters may fall from longlines due to the action of violent waves. Moreover, some oysters inevitably fall from longlines during harvesting operations. Many oysters also die during the summer season due to high temperatures, while some are abandoned and thrown to the bottom of farms during the harvest by workers. Thus, it is necessary to quantify the shells on and in the sediments under bivalve farms to assess their ecological consequences. However, no quantitative information has been reported previously regarding the accumulation of shells under oyster farms.
In the present study, for the first time, we quantified the shells under an oyster farm in Daya Bay, South China Sea. Our results clearly showed that suspended longline oyster farming activities led to a local increase in the accumulation of shells under farms. Previously, Sanchez-Jerez et al. (2019) measured the mollusk shell debris that accumulated in the seabed under coastal fish farming, and they also found a significant increase in the amount of shells in the sediment under fish farms compared with zones close to fish farm cages. The average shell density under the fish cage farm was 53 g kg–1, which is only half of that found in the present study (130 g kg–1). According to Wilding and Nickell (2013), the shell hash content at seven sites sampled under mussel farms ranged from 46 to 2640 g m–2, which was also much lower than the value determined in the present study (3465 g m–2). The higher density in the present study could be explained by the fact that oyster shells are much larger and heavier than mussel shell debris. Also, as mentioned above, in contrast to mussels, oysters do not have byssus and are more readily fall down on bottom seabed.
It should be noted that the structure of oyster shell is relatively less rigid and more readily be broken compared with scallop and mussel shells (personal empirical observation). During sediment sampling, we found that many oyster shells were thin and loose, particularly at the margins, and they were easily broken with our bare hands. Moreover, the shells had many holes, and some of the holes penetrated the shells (Figure S1). We found many oyster shell fragments in the sediment. Previous studies showed that the accumulation of shell debris in sediment could modify the sediment structure, e.g., increase the habitat complexity and heterogeneity of the sediment (Cardinale et al., 2002; Zaiko et al., 2009). Suspended longline oyster culture has been practiced for more than 40 years in Daya Bay. It is possible that considerable amounts of shells had been buried in the sediment, and some parts of these shells may have degraded into hash or debris and incorporated in the sediment. This process can increase the size (Martinez-Garcia et al., 2015) and porosity of sediment (Martinez-Garcia et al., 2015), and change its redox status (Wilding, 2012). Casado-Coy et al. (2017) found that adding shell hash debris to sediment at a density of 1900 g m–2 reduced the amount of toxic by-products (e.g., sulfides and ammonium) produced by organic pollution stimulating anaerobic metabolic pathways. Thus, they recommended adding shell hash debris to the seabed as a potential remediation tool to mitigate the effects of organic enrichment on marine sediments in coastal waters that receive organic inputs. This finding has positive implications for alleviating the eutrophication stress in Daya Bay, because it is surrounded by highly urbanized areas (Shenzhen city and Huizhou city), and it receives inputs from river sewage. However, before implementing this strategy, it is necessary to assess whether the presence of shells would affect the bioturbation capacity of benthic macrofauna.
Whether the presence of oyster shells hindered the burrowing activities of polychaetes?
Shells that accumulate on and in sediment have complex effects on the activities of benthic macrofauna. Casado-Coy et al. (2022) tested the effects of mollusk shell hash on the bioturbation capacity of macrofaunal assemblages within sediments affected by either fish or mussel farms. They found that mollusk shell has promoted macrofaunal assemblages with a high bioturbation potential, regardless of the influence of aquaculture. P. aibuhitensis is a typical benthic polychaete with a high level of mobility and reworking potential. It plays a key role in the structure and functioning of sediments, and could be an ecosystem regenerator. In the present study, we observed that P. aibuhitensis individuals were very active and with a high capacity for burrowing holes, where they dug continuously and maintained burrows. The numbers of holes in the sediments were higher in −Shell treatment than the +Shell treatment throughout the experiment (Figure 4). Whereas, we actually observed that the P. aibuhitensis in +Shell treatment were not less active in terms of their burrowing activities compared to that in the −Shell treatment. The lower numbers of holes in +Shell treatment could be mainly due to P. aibuhitensis were unable to use surface areas where shells were present, the amount of area occupied by shell material physically prevented P. aibuhitensis from forming burrows in these locations. Some shells were positioned horizontally in the sediment, it was observed that when P. aibuhitensis individuals encountered these shells while digging, they tended to change their direction of digging or abandoned a hole that was already half-dug. By contrast, P. aibuhitensis individuals in the −Shell group could dig holes from the surface all the way down to the bottom of the sediment. Nevertheless, the difference in the numbers of holes was generally not significant, so we might able to say that the negative effect of shells on burrowing was not that great. Moreover, it should be noted that we added relatively large oyster shells in the present study. It is reasonable to expect that smaller shells or debris (shell-hash) would hinder the burrowing activities of polychaetes less. During sediment collection, we found that large shells were mainly present on the surface of the sediment, whereas only shell fragments were found in the deeper layers, with few large shells, which suggests that large shells gradually degrade into debris during the burial process. Thus, the hindering effect of shells in the deeper layers was lower than that in the surface. Casado-Coy et al. (2017) suggested adding mollusk-shell debris to sediment as a remediation strategy to mitigate the deleterious effects of organic pollution on marine sediments induced by aquaculture. Based on the results from our study, in combination with the influence of shells on burrowing capacity of polychaetes, we recommend using small shells when implementing this strategy thereby avoiding the possible negative effect of shells on the bioturbation of macrofauna.
In the present study, all of the experimental P. aibuhitensis individuals survived in a very healthy and active state. However, all of them lost some of their body weight. P. aibuhitensis is usually considered a carnivorous species that predates benthic macrofauna for food. However, in the present study, the incubated sediments used were sieved and any macrofauna was removed before use. We observed abundant fecal particles produced by P. aibuhitensis on the surface of the sediment as well as in the holes (Figure S2). We found that P. aibuhitensis individuals lost more weight in the +Shell group than the −Shell group, possibly because the presence of shells led to P. aibuhitensis consuming more energy in the burrowing process. Mussel shells were used in the study of Casado-Coy et al. (2017) and the valves were broken into fragments, so the shell hash debris was much smaller in size, which may have prevented it from negatively affecting the burrowing activities of Lumbrineris latreilli. Our results indicate that the size of the shell added could be an important factor, and thus shells should be broken into small hash fragments before using for bioremediation purposes.
Conclusion
In conclusion, we quantified the number of shells in the sediment under an oyster farm for the first time. Our results showed that suspended oyster farming led to a local increase in the accumulation of shells under the farm. The presence of oyster shells hindered the burrowing activities of P. aibuhitensis to some degree, but it is possible that this negative effect could be alleviated with degrading of big shells into shell hash. Further studies are needed to investigate the interactions between the accumulation of shells and the bioturbation of macrofauna, as well as the related ecological consequences, such as effects on the porosity of sediment, organic matter remineralization, and nutrient fluxes.
Data availability statement
The original contributions presented in the study are included in the article/Supplementary Material. Further inquiries can be directed to the corresponding author.
Ethics statement
The studies involving animals were reviewed and approved by Committee on Laboratory Animal Welfare and Ethics of South China Sea Fisheries Research Institute, Chinese Academy of Fishery Sciences (nhdf 2022-01).
Author contributions
YC and RS: Conceptualization, Methodology, Writing‐;Original draft preparation. TH and HL: Investigation, Formal analysis. ZQ: Funding acquisition, Supervision, Writing–Reviewing and Editing. HH: Funding acquisition, Formal analysis. All authors contributed to the article and approved the submitted version.
Funding
This work was supported by the National Natural Science Foundation of China (31900094, 41976149), Guangdong Basic and Applied Basic Research Foundation (2021A1515011377), Central Public-interest Scientific Institution Basal Research Fund, South China Sea Fisheries Research Institute, CAFS (2021SD03), Central Public-interest Scientific Institution Basal Research Fund, CAFS (2020TD15), Financial Fund of the Ministry of Agriculture and Rural Affairs, China (NFZX2021). Natural resources affairs special fund project of Guangdong Province (2023-199001).
Conflict of interest
The authors declare that the research was conducted in the absence of any commercial or financial relationships that could be construed as a potential conflict of interest.
Publisher’s note
All claims expressed in this article are solely those of the authors and do not necessarily represent those of their affiliated organizations, or those of the publisher, the editors and the reviewers. Any product that may be evaluated in this article, or claim that may be made by its manufacturer, is not guaranteed or endorsed by the publisher.
Supplementary material
The Supplementary Material for this article can be found online at: https://www.frontiersin.org/articles/10.3389/fmars.2023.1219184/full#supplementary-material
Supplementary Figure 1 | Oyster shells deposited on sediment under the suspended longline oyster culture farm in Daya Bay, southern China.
Supplementary Figure 2 | Feces deposited by the experimental polychaete Perinereis aibuhitensis (A) on sediment’s surface and (B) in the burrowed holes.
References
Adámek Z., Maršálek B. (2013). Bioturbation of sediments by benthic macroinvertebrates and fish and its implication for pond ecosystems: a review. Aquac. Int. 21, 1–17. doi: 10.1007/s10499-012-9527-3
Bergström P., Durland Y., Lindegarth M. (2020). Deposition of shells modify nutrient fluxes in marine sediments: effects of nutrient enrichment and mitigation by bioturbation below mussel farms. Aquacult. Environ. Interact. 12, 315–325. doi: 10.3354/aei00366
Cardinale B., Palmer M., Swan C., Brooks S., LeRoy Poff N. (2002). The influence of substrate heterogeneity on biofilm metabolism in a stream ecosystem. Ecology 83, 412–422. doi: 10.1890/0012-9658(2002)083[0412:TIOSHO]2.0.CO;2
Casado-Coy N., Martínez-García E., Sánchez-Jerez P., Sanz-Lázaro C. (2017). Mollusc-shell debris can mitigate the deleterious effects of organic pollution on marine sediments. J. Appl. Ecol. 54, 547–556. doi: 10.1111/1365-2664.12748
Casado-Coy N., Sánchez-Jerez P., Troncoso J. S., Sanz-Lazaro C. (2022). Mollusc-shell debris derived from aquaculture can promote macrofaunal communities with a high bioturbation capacity. Aquaculture 548, 737642. doi: 10.1016/j.aquaculture.2021.737642
Crawford C., Macleod C., Mitchell I. (2003). Effects of shellfish farming on the benthic environment. Aquaculture 224, 117–140. doi: 10.1016/S0044-8486(03)00210-2
D’Amours O., Archambault P., McKindsey C., Johnson L. (2008). Local enhancement of epibenthic macrofauna by aquaculture activities. Mar. Ecol. Prog. Ser. 371, 73–84. doi: 10.3354/meps07672
Du F., Wang X., Jia X., Yang S., Ma S., Chen H., et al. (2011). Species composition and characteristics of macrobenthic fauna in Daya Bay, south China Sea. J. Fish. Sci. China. 18 (4), 877–892. doi: 10.3724/SP.J.1118.2011.00877
FAO (2022). “The state of world fisheries and aquaculture 2022,” in Towards blue transformation (Rome: FAO). doi: 10.4060/cc0461en
Fitridge I., Dempster T., Guenther J., De Nys R. (2012). The impact and control of biofouling in marine aquaculture: a review. Biofouling 28, 649–669. doi: 10.1080/08927014.2012.700478
Gray M., Langdon C. (2019). Particle processing by olympia oysters Ostrea lurida and pacific oysters Crassostrea Gigas. Estuar. Coast. 42, 779–791. doi: 10.1007/s12237-018-0480-x
Gutiérrez J., Jones C., Strayer D., Iribarne O. (2003). Mollusks as ecosystem engineers: the role of shell production in aquatic habitats. Oikos 101, 79–90. doi: 10.1034/j.1600-0706.2003.12322.x
Han T., Shi R., Qi Z., Huang H., Wu F., Gong X. (2020). Biogenic acidification of Portuguese oyster Magallana angulata mariculture can be mediated through introducing brown seaweed Sargassum hemiphyllum Aquaculture 520, 734972. doi: 10.1016/j.aquaculture.2020.734972
Hargrave B., Holmer M., Newcombe C. (2008). Towards a classification of organic enrichment in marine sediments based on biogeochemical indicators. Mar. pollut. Bull. 56 (5), 810–824. doi: 10.1016/j.marpolbul.2008.02.006
Jansen H. M., Hansen P. K., Brennan N., Dahlgren T. G., Fang J., Nederlof M. A. J., et al. (2019). Enhancing opportunistic polychaete communities under fish farms: an alternative concept for integrated aquaculture. Aquacult. Environ. Interact. 11, 331–336. doi: 10.3354/aei00318
Li X., Li Y., Chen Q., Ning J., Wang L., Xu L., et al. (2023). Structural characteristics and relative influencing factors of macrobenthos community in intertidal zone of daya bay. South China Fish. Sci. 19 (3), 42–50. doi: 10.12131/20220202
Martinez-Garcia E., Carlsson M., Sanchez-Jerez P., Sánchez-Lizaso L., Sanz-Lazaro C., Holmer M. (2015). Effect of sediment grain size and bioturbation on descomposition of organic matter from aquaculture. Biogeochemistry 125, 133–148. doi: 10.1007/s10533-015-0119-y
Nasi F., Nordström M. C., Bonsdorff E., Auriemma R., Cibic T., Del Negro P. (2018). Functional biodiversity of marine soft-sediment polychaetes from two Mediterranean coastal areas in relation to environmental stress. Mar. Environ. Res. 137, 121–132. doi: 10.1016/j.marenvres.2018.03.002
Nederlof M., Fang J., Dahlgren T., Rastrick S., Smaal A., Strand Ø., et al. (2020). Application of polychaetes in (de)coupled integrated aquaculture: an approach for fish waste bioremediation. Aquacult. Environ. Interact. 12, 385–399. doi: 10.3354/aei00371
Papageorgiou N., Sigala K., Karakassis I. (2009). Changes of macrofaunal functional composition at sedimentary habitats in the vicinity of fish farms. Estuar. Coast. Shelf S. 83, 561–568. doi: 10.1016/j.ecss.2009.05.002
Qi Z., Shi R., Yu Z., Han T., Li C., Xu S., et al. (2019). Nutrient release from fish cage aquaculture and mitigation strategies in Daya Bay, Southern China. Mar. pollut. Bull. 146, 399–407. doi: 10.1016/j.marpolbul.2019.06.079
Rajagopal S., van der Velde G., van der Gaag M., Jenner H. (2003). How effective is intermittent chlorination to control adult mussel fouling in cooling water systems? Water Res. 37, 329–338. doi: 10.1016/S0043-1354(02)00270-1
Rao Y., Cai L., Chen X., Zhou X., Fu S., Huang H. (2021). Responses of functional traits of macrobenthic communities to human activities in Daya Bay (A subtropical semi-enclosed bay), China. Front. Environ. Sci. 9, 766580. doi: 10.3389/fenvs.2021.766580
Rosa M., Ward J., Shumway S. (2018). Selective capture and ingestion of particles by suspension-feeding bivalve molluscs: a review. J. Shellfish Res. 37 (4), 727–746. doi: 10.2983/035.037.0405
Sanchez-Jerez P., Krüger L., Casado-Coy N., Valle C., Sanz-Lazaro C. (2019). Mollusk shell debris accumulation in the seabed derived from coastal fish farming. J. Mar. Sci. Eng. 7, 335. doi: 10.3390/jmse7100335
Sanz-Lazaro C., Marín A. (2008). Assessment of finfish aquaculture impact on the benthic communities in the Mediterranean Sea. Dyn. Biochem. Process Biotechnol. Mol. Biol. 2, 21–32.
Tomassetti P., Porrello S. (2005). Polychaetes as indicators of marine fish farm organic enrichment. Aquacul. Int. 13, 109–128. doi: 10.1007/s10499-004-9026-2
Weise A., Cromey C., Callier M., Archambault P., Chamberlain J., McKindsey C. (2009). Shellfish-DEPOMOD: modelling the biodeposition from suspended shellfish aquaculture and assessing benthic effects. Aquaculture 288, 239–253. doi: 10.1016/j.aquaculture.2008.12.001
Wilding T. (2012). Changes in sedimentary redox associated with mussel (Mytilus edulis l.) farms on the west-coast of Scotland. PloS One 7 (9), e45159. doi: 10.1371/journal.pone.0045159
Wilding T., Nickell T. (2013). Changes in benthos associated with mussel (Mytilus edulis l.) farms on the West-Coast of Scotland. PloS One 8 (7), e68313. doi: 10.1371/journal.pone.0068313
Yu Z., Zhou Y., Qi Z. H., Luo P., Hu C. (2017). Temporal dynamics in clearance rate of the Portuguese oyster Crassostrea angulata cultivated in Dapeng Cove, Southern China. Aquaculture 479, 824–828. doi: 10.1016/j.aquaculture.2017.07.030
Zaiko A., Daunys D., Olenin S. (2009). Habitat engineering by the invasive zebra mussel Dreissena polymorpha (pallas) in a boreal coastal lagoon: impact on biodiversity. Helgoland Mar. Res. 63 (1), 85–94. doi: 10.1007/s10152-008-0135-6
Keywords: aquaculture, oyster, shell, polychaetes, Perinereis aibuhitensis, bioturbation
Citation: Cao Y, Shi R, Han T, Liu H, Huang H and Qi Z (2023) Shell accumulation on seabed due to suspended coastal oyster farming and effects on burrowing capacity of the polychaete Perinereis aibuhitensis. Front. Mar. Sci. 10:1219184. doi: 10.3389/fmars.2023.1219184
Received: 08 May 2023; Accepted: 19 June 2023;
Published: 29 June 2023.
Edited by:
Jinghui Fang, Chinese Academy of Fishery Sciences (CAFS), ChinaReviewed by:
Wenguang Liu, Chinese Academy of Sciences (CAS), ChinaTao Jiang, Yantai University, China
Copyright © 2023 Cao, Shi, Han, Liu, Huang and Qi. This is an open-access article distributed under the terms of the Creative Commons Attribution License (CC BY). The use, distribution or reproduction in other forums is permitted, provided the original author(s) and the copyright owner(s) are credited and that the original publication in this journal is cited, in accordance with accepted academic practice. No use, distribution or reproduction is permitted which does not comply with these terms.
*Correspondence: Zhanhui Qi, qizhanhui@scsfri.ac.cn
†These authors have contributed equally to this work
‡Present address: Honghui Huang, Sanya Tropical Fisheries Research Institute, Sanya, China