- 1Coastal and Offshore Resources Research Center, Fisheries Research Institute, Council of Agriculture, Kaohsiung City, Taiwan
- 2Department of Environment Biology and Fisheries Science, National Taiwan Ocean University, Keelung City, Taiwan
- 3Marine Fisheries Division, Fisheries Research Institute, Council of Agriculture, Keelung City, Taiwan
- 4Agriculture and Fishery Bureau Penghu County Government, Magong, Taiwan
Blue sprat (Spratelloides gracilis) is an economically valuable species that inhabits the central Taiwan Strait. However, despite the implementation of a local management scheme involving a closed season between 1 May and 1 June, the annual catch has rapidly decreased over the decades. Thus, the efficacy of the implemented regulations must be investigated. This study sought to clarify the reproductive biology, spawning ground, and behavior of this species in the waters of Penghu. In total, 6,549 specimens were collected between March 2021 and September 2022. Considering gonadosomatic index, group maturity, and oocyte diameter, we found that the spawning season (March–September) peaked in April; new cohorts were distinctly recruited after the spawning peak. The distribution of the spawning ground (latitude, 23°41′N to 23°43′N; longitude, 119°32′E to 119°39′E) was determined by considering the fishing area of mature females and DNA analysis of adhered eggs. We further noted shoals of fish darting in the bottom water without clear schooling movement, females’ abdomen frequently contacting substrata for eggs able to attach on during oviposition, and males releasing sperms making water milky white; these indicate that S. gracilis exhibits promiscuous spawning behavior. Our findings may facilitate the management and conservation of S. gracilis in this region.
1 Introduction
Blue sprat (Spratelloides gracilis) is a small, pelagic, schooling clupeid distributed in the tropical and temperate Indo-West Pacific region (Lewis et al., 1983; Whitehead, 1985; Randall, 1995; Weng et al., 2005). This species inhabits the relatively clear water of coastal, lagoon, and seaward reefs and feeds on planktonic species such as diatoms, copepods, and other invertebrates. S. gracilis is mainly used as baitfish for the tuna fishery in the waters of the Solomon Islands and Papua New Guinea (Daly and Richardson, 1980; Blaber et al., 1990; Milton et al., 1990a; Milton et al., 1990b; Milton et al., 1991); it is also commercially caught in southern Japan (Shirafuji et al., 2007). Likewise, in Taiwan, S. gracilis is an economically valuable species for fisheries using various implements: drag-nets, gill nets, Taiwanese Danish seines, and purse-seine nets. The annual catch of this fish increased from 639 metric tons in 2,000 to 1,022 metric tons in 2007 and then plummeted to 79 metric tons in 2021 (Fisheries Agency, 2022) as a consequence of high market demand for decades. Of the total annual catch, approximately 99% is obtained around the Penghu islands, central Taiwan Strait, which appears to be a major fishing ground for this species. The number of fishing vessels in situ (Penghu waters) decreased rapidly from 87 boats in 1988 to 9 boats currently, all operated with Taiwanese Danish seine.
Ecological studies have been conducted on S. gracilis in the waters of tropical regions, Japan, and Australia. Ecological traits in terms of fish growth, size at maturity, and spawning season are similar between the Solomon Islands and Papua New Guinea (Shirafuji et al., 2007); however, these markedly vary between the temperate waters of Australia, Japan, and the tropical region. Weng et al. (2005) reported that the spawning season of S. gracilis extended from February to September and peaked in March–April and July–August between 2001 and 2003 in the subtropical waters of Penghu.
For the sustainability of the traditional fishery in Penghu waters, a management scheme was implemented by the local government in 1995 for the conservation of S. gracilis, which involved a closed season between 1 May and 1 June. However, the regulation scheme was based on the experience of fishermen rather than precise scientific evidence, which limited the efficacy of the regulations. The reproductive period of this species reported by Weng et al. (2005) also suggests that the local management scheme failed to account for the reproductive behavior of S. gracilis, thus limiting the scheme’s efficacy in reducing the fishing pressure on spawners and recruits.
The increasing trend in global warming and extreme climate may interfere with spawning season (Shoji et al., 2011). S. gracilis has been studied in situ over the past decades. However, few studies have provided precise information on the spawning behavior of this species in the waters of Penghu. Therefore, we conducted this study to clarify the reproductive biology, spawning ground, spawning behavior, and spawning period time shift of S. gracilis. For this, we used data collected by sampling fishing vessels and performing underwater observation between 2021 and 2022. Our findings may provide useful information for the effective management of this species in the future.
2 Materials and methods
2.1 Collection of specimens
Specimens were collected every month between March 2021 and September 2022 (except May 2021) from fishery landings of specific Taiwanese Danish seine sampling vessels at Magong fish market and Chikan harbor, Penghu. The fishing ground was determined by considering the operation area recorded using the Voyage Data Recorder (VDR) and logbooks of sampling vessels as aforementioned (Figure 1).
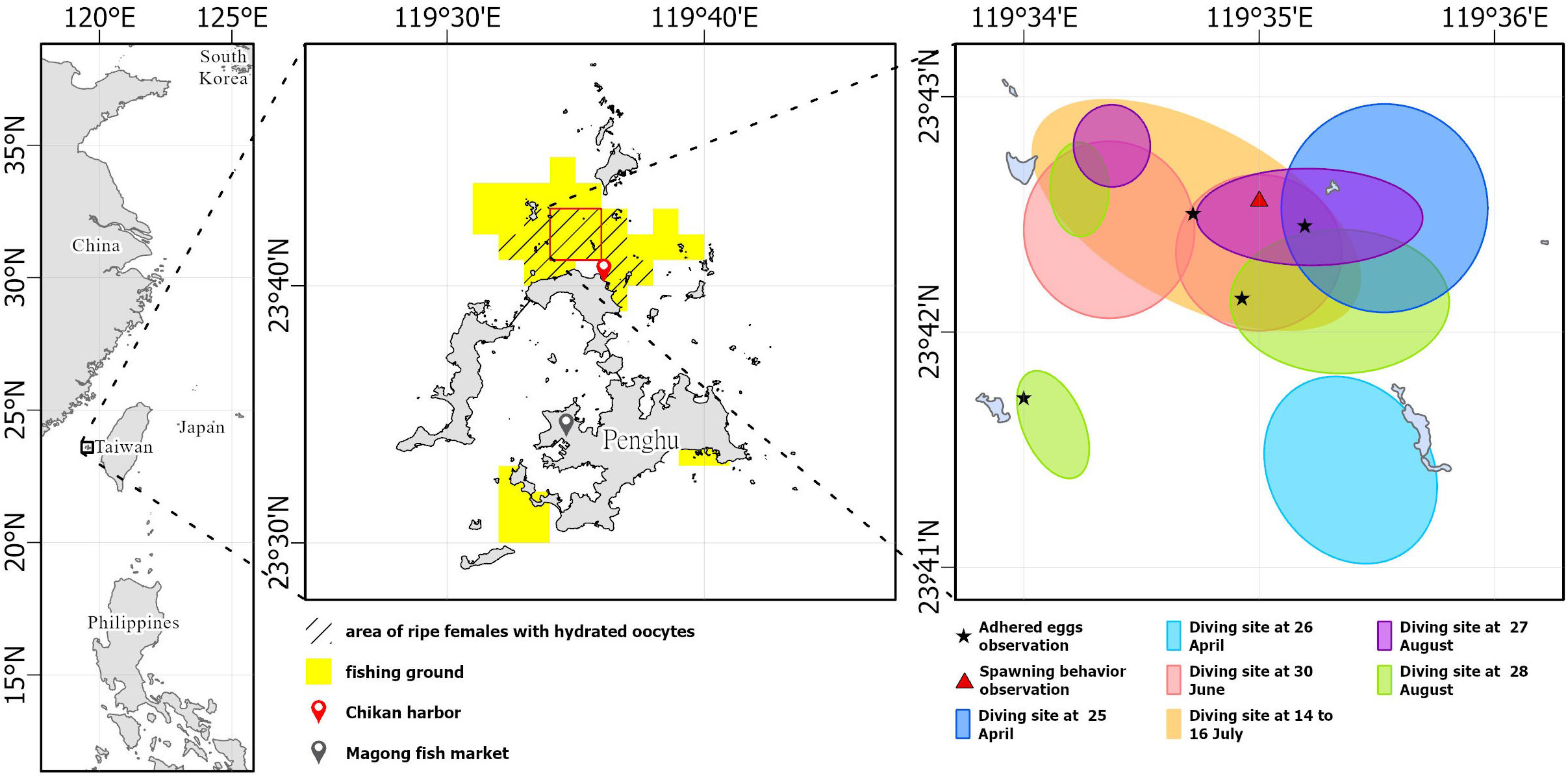
Figure 1 S. gracilis fishing area (yellow block), sampling area for mature females (black slash) and adhered eggs (black star), spawning behavior observation area (red square), and direct underwater observation area around Penghu waters.
The fork length (FL) and body weight (BW) of the collected specimens were measured to the nearest 0.01 mm and 0.01 g, respectively. The gonads were weighed to the nearest 0.01 g. Before the macroscopic examination of specimens, gonads were excised to determine maturity status and preserved in 10% formalin until further processing. A potential regression was used to describe the length–weight relationship using the following equation: BW = a × FLb, where a and b are constants to be estimated. Sex-specific FL–BW relationships were analyzed using maximum likelihood ratio test (Kimura, 1980a), and all data are fitted using the statistical programming language R (R Development Core Team; www.r-project.org ).
2.2 Assessment of gonad development
The gonads were weighed before calculating the gonadosomatic index (GSI) value ([gonad weight (g)/BW (g)] × 100) and sex ratio ([number of females/total number of specimens] × 100). During the reproductive period, ovarian regions may develop differently. Therefore, data homogeneity was evaluated. Five ovaries obtained from different fish at different developmental stages were examined to ensure homogeneity in oocyte diameter and number. Each ovary was divided into six portions, and 0.05 g of tissue was sampled from each portion. The tissue samples were assessed using a Nikon profile projector at a magnification of 50×. Two-way analysis of variance indicated no significant difference in the number of oocytes between the portions collected from the same S. gracilis (p > 0.05; n = 30) but revealed significant differences across specimens (p < 0.05). Similar patterns were noted in the frequency distribution of the diameter of oocytes obtained from the same portion, which indicated homogeneity in oocyte diameter and number. For consistency, the middle portion of the left lobe of each ovary was assessed in this study.
In total, 49 ovaries of mature females obtained during spawning season (March to September) were used to detect their histological examination and oocyte diameters. The eggs per 0.05 g of ovaries were measured with a Nikon profile projector V-12 at a magnification of 50×, and the distribution of oocyte diameter in each stage was calculated. Reproductive activity was assessed by investigating the macroscopic characteristics and histological sections of specimens (Weng et al., 2005), and four stages were identified (Supplementary Table 1). In general, females are regarded as sexually mature if they have reached the tertiary yolk, migratory nucleus, or hydrated oocyte stage (Weng et al., 2005; Arocha and Bárrios, 2009). In males, the testis is slender and erythroid during the immature stage and becomes enlarged, opaque, and milky during the reproductive season. Specimens with secondary spermatocytes, spermatids, or spermatozoa were considered to be sexually mature (Weng et al., 2005). We assessed the monthly changes in the GSI values and the relative proportions of various gonad developmental stages, which indicate group maturity of S. gracilis to clarify their spawning season.
2.3 Assessment of spawning ground and reproductive behaviors
The spawning ground was determined on the basis of the location where mature female specimens with hydrated oocytes were captured. To confirm the brooding behavior of S. gracilis, three researchers performed scuba diving (8 days between April and August 2022) to directly observe the specimens in situ by using digital video cameras (Tough TG-6, Olympus, Tokyo, Japan and GoPro Hero7 Black, CA, USA; Table 1). The range of diving sites was determined using the coordinates of the entry and exit points of diving, which were recorded using a global positioning system. Eggs present on the substrata at the spawning ground were manually sampled, preserved in 95% ethanol, until entering the Chikan harbor. The samples transferred to our laboratory were kept frozen at −20°C for further analysis.
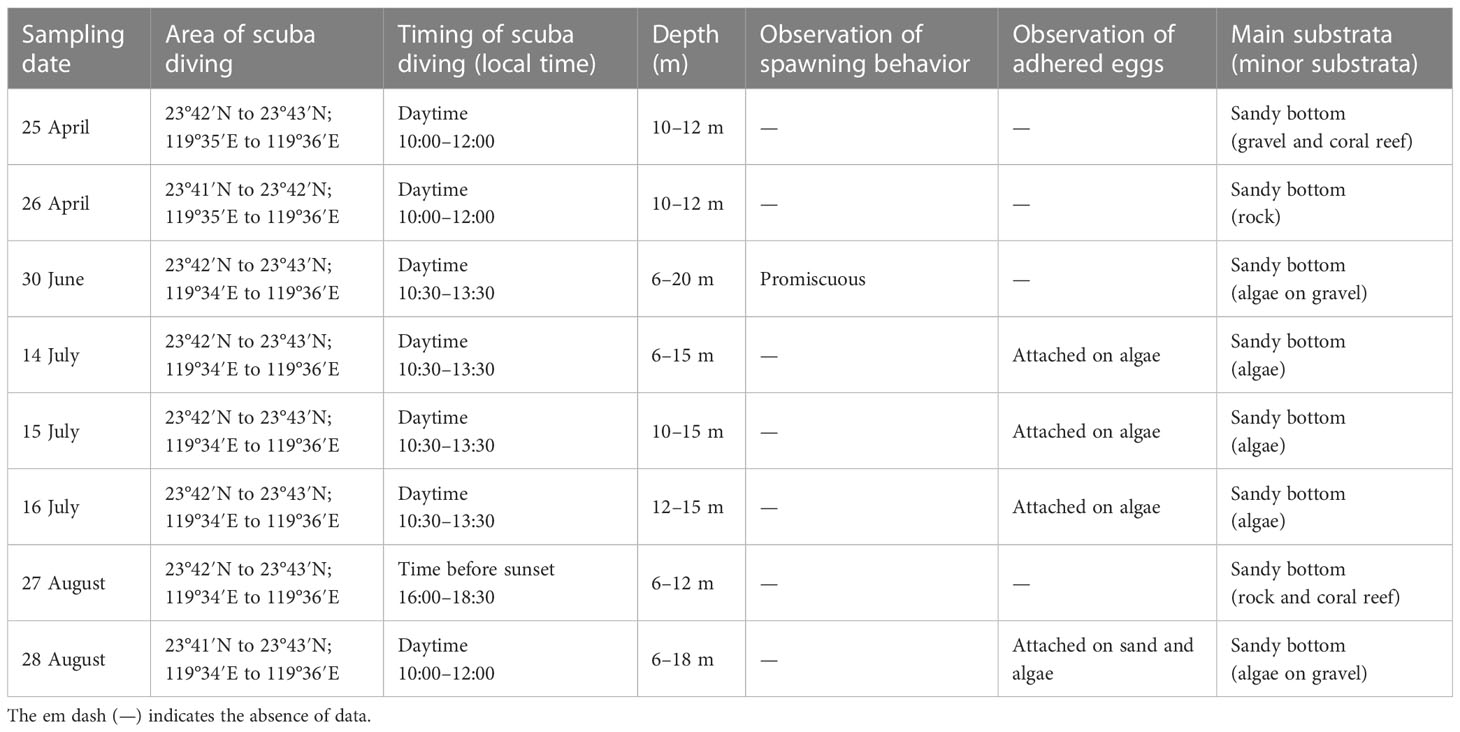
Table 1 Fishing area, substratum type, spawning behavior, and location of adhered eggs of S. gracilis at the scuba diving sites in Penghu waters.
The substrata comprised several types of sands and algae, which were identified by observing their phenotypic characteristics. Sands were sampled; the diameter of particle samples was measured using a Nikon profile projector V-12 at a magnification of 50×. All egg samples used for genomic analysis were fixed with 95% ethanol (−20°C) and preserved in the Molecular Phylogeny Laboratory, Marine Fisheries Division, Fisheries Research Institute, Council of Agriculture, Taiwan. Mitochondrial cytochrome c oxidase subunit I (COI) and 16S rRNA genes were used as molecular markers for species identification. Total genomic DNA was extracted from each egg using a commercial DNA isolation kit (Gentra, Minneapolis, MN, USA). The LCO-1490 (forward primer, 5′-GGT CAA CAA ATC ATA AAG ATA TTG G-3′) and HCO-2198 (reverse primer, 5′-TAA ACT TCA GGG TGA CCA AAA AAT CA-3′) primer set, which helps amplify COI, was used (Folmer et al., 1994).
Polymerase chain reaction (PCR) was performed in a total reaction volume of 25 μl, containing genomic DNA (100 ng), forward primer (5 pmol/L), reverse primer (10 pmol/L), and Taq DNA Polymerase 2X Master Mix Red (12.5 μl; Ampliqon, Odense, Denmark). A thermal cycler (Veriti; Applied Biosystems, Foster City, CA, USA) was used for PCR. The reaction conditions were as follows: denaturation (1 cycle) at 95°C for 4 min; 40 cycles at 95°C for 1 min, 48°C for 1 min, and 72°C for 3 min; and final extension at 72°C for 10 min. The products were preserved at 4°C.
PCR products were assessed through agarose gel (1%) electrolysis to confirm the accuracy of fragment lengths. Afterward, the products were eluted using the QIAquick Gel Extraction Kit (Qiagen, Hilden, Germany). Purified PCR products were sent to MB Mission Biotech (Taipei, Taiwan) for DNA sequencing.
Sequence data were manually edited and automatically assembled using BioEdit 7.0 (Hall, 1999) and Clustal X (Thompson et al., 1997). The sequences were deposited in GenBank under the accession numbers OP804318–OP804321. Alosa sapidissima (National Center for Biotechnology Information [NCBI] accession: MG570434) was used as an outgroup in phylogenetic analyses. Phylogenetic relationships were inferred using the neighbor-joining (NJ) and maximum parsimony (MP) methods. NJ trees were constructed using MEGA X (Kumar et al., 2018) and the Kimura two-parameter model of base substitution (Kimura, 1980b) with 1,000 bootstrap repetitions (Felsenstein, 1985). MP tree topology was estimated using the tree bisection–reconnection algorithm.
3 Results
3.1 Size composition
In total, 6,549 specimens (2,553 females, 3,739 males, and 257 sex unknown) were collected. FL and BW ranged from 17.66 to 83.25 mm and 0.02 to 4.75 g, respectively (Figure 2). The maximum-likelihood ratio test indicated that the length–weight relationship varied significantly between the sexes (p < 0.01; p = 4.76 × 10−8) and the following were the equations derived (Figure 2).
Female: BW = 6.949 × 10−6 FL3.031 (n = 2,553, range: 0.02–4.62 g BW, 22.39–82.20 mm FL);
Male: BW = 4.590 × 10−6 FL3.126 (n = 3,739, range: 0.02–4.75 g BW, 18.06–83.25 mm FL).
A single mode value was consistently observed in the distribution of FL frequency data obtained during the study period. Seasonally, the mean FL (range, 60–80 mm) was significantly larger in March–April 2021 and January–April 2022 (p < 0.05). The mean FL of juvenile ranged from 25 to 50 mm in June 2021, August–September 2021, May 2022, and July–September 2022 (Figure 3).
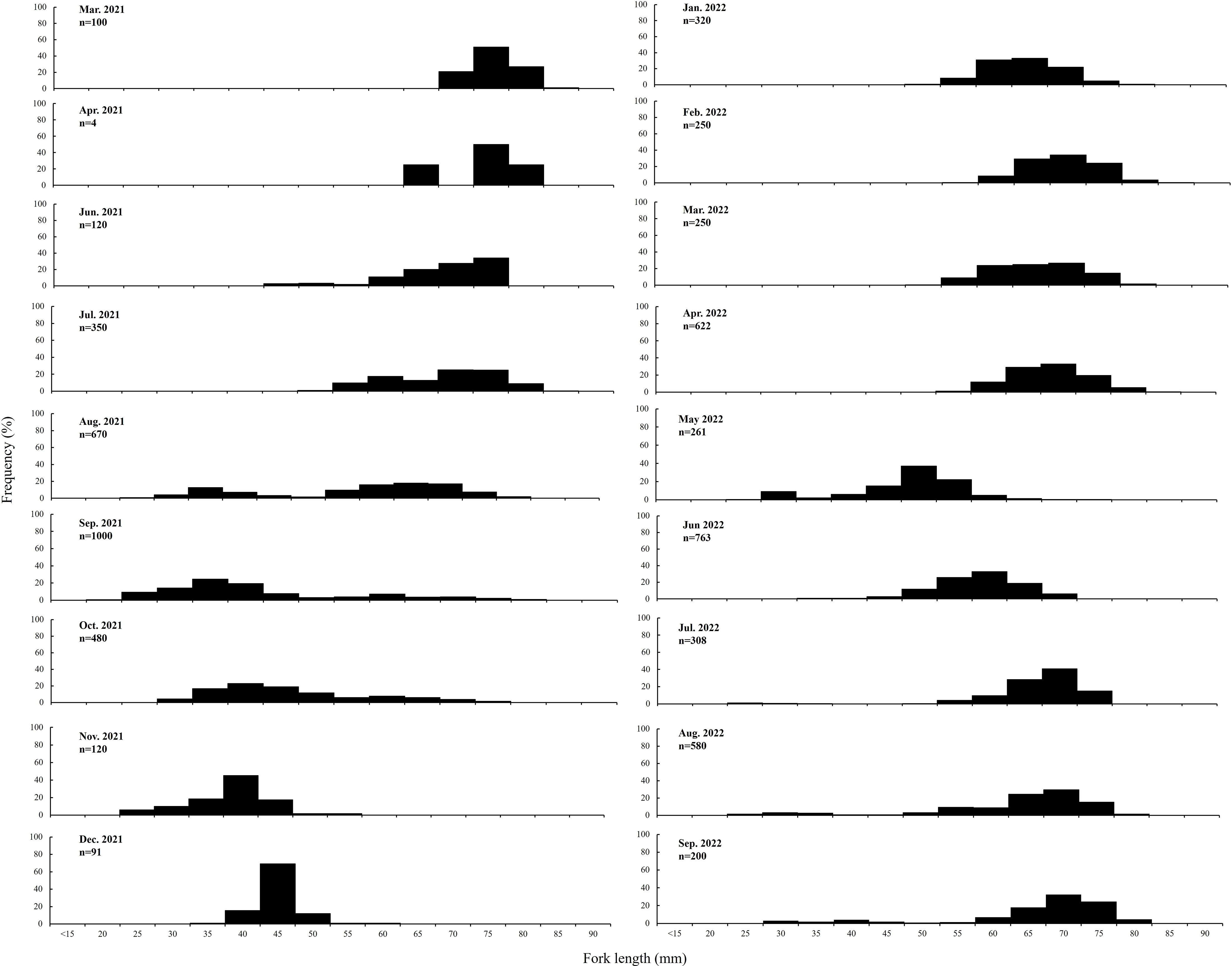
Figure 3 Distribution of the fork length of S. gracilis specimens collected in the waters of Penghu, central Taiwan Strait.
3.2 Monthly changes in GSI and group maturity
The sex ratio of S. gracilis was significantly different from 0.5 (p < 0.05; Supplementary Table 2). The sample size in April 2021 (n = 4) was too small to obtain statistically significant results. The sex ratio peaked in April 2022 (47.91%) and was lowest in June 2021 (27.93%). The chi-square test indicated that the sex ratio was significantly different from 0.5 in March 2021, June–November 2021, and May–July 2022 (p < 0.05; Supplementary Table 2).
The GSI of females ranged from 0.01 to 15.79 during the study period. In 2021, it increased from 7.6 in March to 15.79 (first peak) in April and then decreased in June but increased again to 6.57 (second peak) in July. From August 2021, a gradual decrease was noted in the aforementioned GSI value. The low value was maintained until March 2022. In 2022, the first peak (GSI value, 6.88) was observed in April, and the value decreased in May. A relatively high value (4.34–4.94) was maintained between June and September. However, no second peak was noted in 2022. Similar patterns were observed for the monthly changes in the GSI of males (Figure 4).
The monthly proportion of mature females, as the tendency of GSI, increased in March and peaked in April 2021. The proportion substantially decreased to 3.23% in June 2021 when 6.45% of the female specimens were in the spent stage. Nonetheless, in July 2021 (second peak), 40% of the female specimens were in the mature stage. This proportion slightly decreased in August and September 2021. Between October 2021 and March 2022, all specimens were in the immature stage. Mature females appeared late and peaked (41.61%) in April 2022. Their proportion rapidly decreased to 1% in May 2022 but increased to >15% between June and August 2022 and then peaked (second peak in 2022; 30%) in September. Similar patterns were observed for the monthly changes in the group maturity of males (Figure 5).
3.3 Spawning season and ground
The histological examination of S. gracilis gonads revealed mature oocytes between March and September. Both GSI and the proportion of mature S. gracilis showed a first peak in April 2021 and 2022 and a second peak in July 2021; higher values were maintained from June to August 2022. Monthly changes in length frequency distribution indicated that new recruits appeared between May and November. Thus, S. gracilis in the waters of Penghu spawned between March and September and exhibited a distinct peak in April in both years. The area for fishing mature females with hydrated oocytes indicated the northern inshore waters of Penghu as the spawning ground of S. gracilis (Figure 1).
3.4 Spawning behavior
To validate the aforementioned findings regarding spawning period and ground, direct underwater observation was performed for 8 days (during the daytime) in 2022 (Table 1). The diving sites were the areas where mature females with hydrated eggs were caught by sampling vessels, and the water depth was 12–20 m. The spawning substrata consisted mainly of sand with a grain size of 0.196–1.319 mm (n = 30), and gravel, rocks, coral reef, seagrass, sponges, sea anemone, and various algae were distributed sporadically (Supplementary Figure 1).
The spawning behavior of S. gracilis was observed on 30 June (lunar date, 2 June; Table 1; Figure 1). Without any clear schooling movement, shoals of fish were found to dart around rapidly, circling near the bottom of the water column and passing close to potential spawning substrata singly or in a tiny shoal comprising two to four individuals. The abdomens, with pectoral fins, of mature females frequently contacted substrata surfaces for oviposition; males released sperms, making the water milky white. The caudal fin was deflected ventrally while the fish moved forward with rapid tail beats, blowing sand grains (Figure 6; Supplementary Video). This indicates that S. gracilis exhibit promiscuous spawning behaviors.
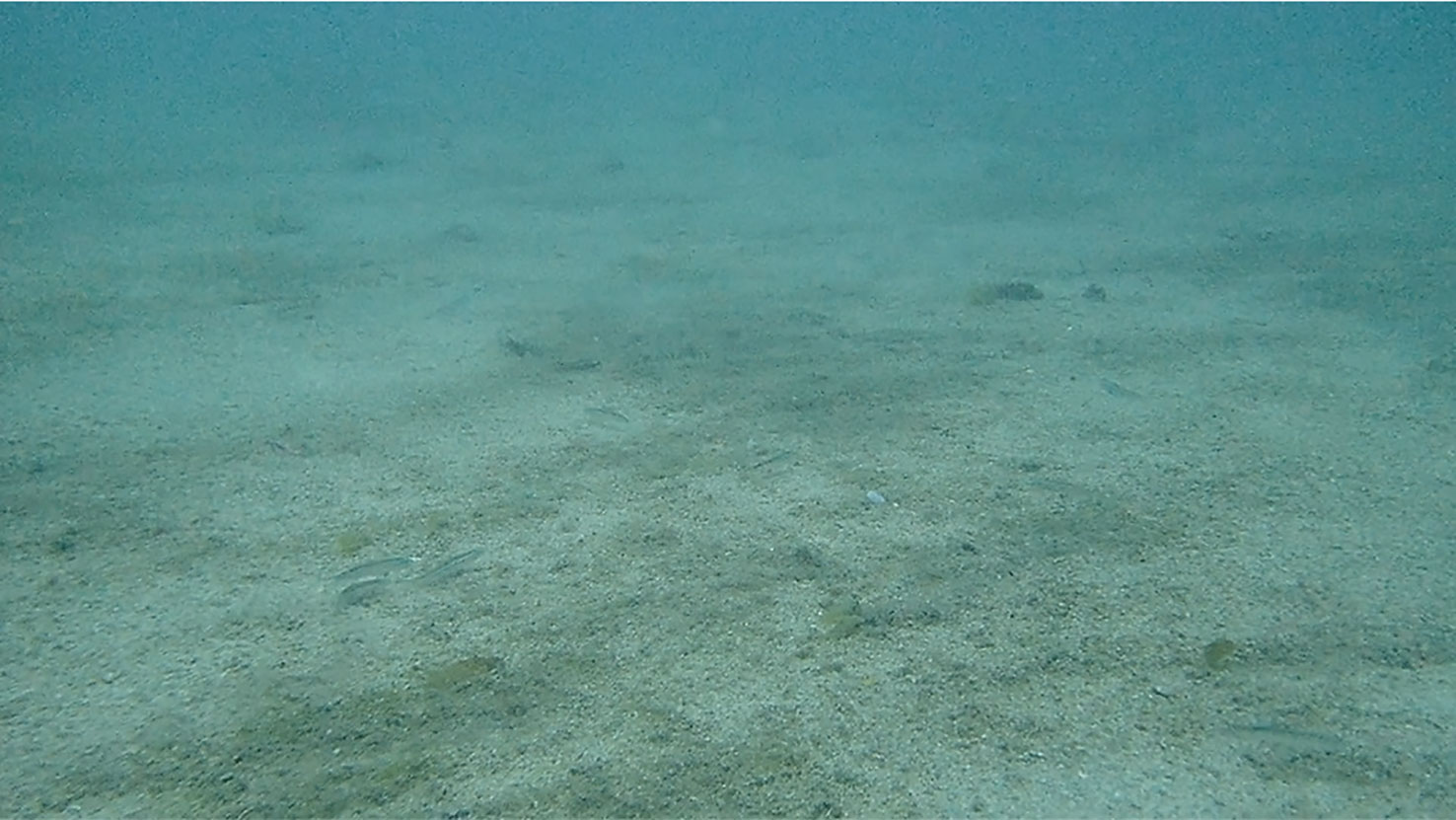
Figure 6 Area (between 23°42′N to 23°43′N and 119°34′E to 119°36′E) where the spawning behavior of S. gracilis was observed.
Eggs adhering to sand and red (Halymenia floresia subsp. harveyana) and brown (Ecklonia kurome) algae were sampled (Figures 1, 7; Table 1) on 14–16 July (lunar date, 16–18 June) and 28 August (lunar date, 2 August). Four sequences of the COI barcode were successfully retrieved from the egg clumps. No insertion, deletion, or stop codon was detected in the COI sequences. All nucleotide sequences were trimmed and aligned to a short 602-bp sequence. The analysis of nucleotide pair frequency revealed 214 variable sites, of which 176 were parsimony-informative sites and 38 were singleton variable sites. On the basis of COI sequence data, eggs (0714a, 0715a, 0716a, and 0828a) were identified to be laid by S. gracilis. The COI phylogenies constructed using NJ and MP methods exhibited identical topologies (Figure 8). The genetic distances between the eggs and S. gracilis sequences obtained from NCBI were very small (0–0.007). The analysis of the COI sequences revealed that the eggs formed a clade with S. gracilis, which was strongly supported by a bootstrap value of ≥99, and the clade was distinct from the other species of Spratelloides and Spratelloidinae. These findings show that the northern inshore waters of Penghu were a key spawning ground of S. gracilis.
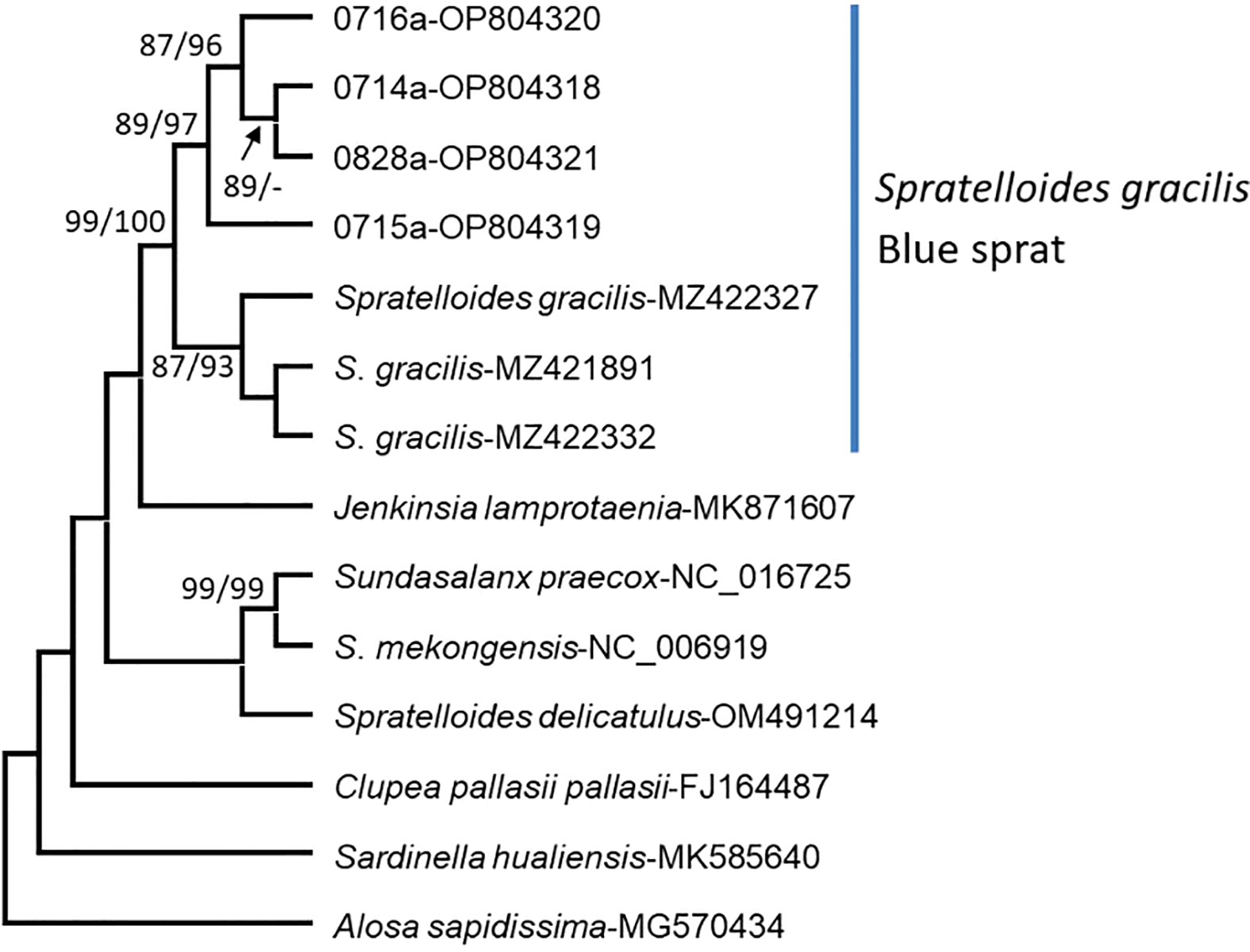
Figure 8 Phylogenetic trees constructed for the eggs of S. gracilis. Other species of Spratelloides and Spratelloidinae were used as reference sequences. The numbers 0714a–0716a and 0828a indicate eggs collected in this study. The other numbers indicate the sequences of Spratelloidinae obtained from the National Center for Biotechnology Information. Branch support was estimated by analyzing the bootstrap pseudoreplicates of each branch of trees constructed using the neighbor-joining and maximum parsimony methods. A minus sign (−) indicates a bootstrap value of <85%.
4 Discussion
Fish employ diverse reproductive strategies to increase the production of offspring and the survival rate of larvae till adulthood; successful reproduction depends on spawning frequency, spawner type, mating practice, fertilization, egg type, and spawning time, which are influenced by physiological and environmental factors (Johannes, 1978; Potts and Wootton, 1984; Gross, 1991; Fleming, 1996; LePage and Cury, 1997; D’Onghia et al., 1999; Murua and Saborido-Rey, 2003; Melvin et al., 2009). A major factor that influences the spawning period of herrings is temperature (Lambert, 1987). The spawning season of S. gracilis may be June–October (Mizuta et al., 2000), May–October (Matsumura, 1987), April–November (Shirafuji et al., 2007), or even the entire year (Dalzell, 1985; Milton et al., 1991) in the regions located north of Japan and south of tropical waters (Table 2). Weng et al. (2005) reported that S. gracilis spawned between February and September in the Penghu region in 2001–2003. On the basis of the macroscopic ovary appearance, GSI value, and group maturity of S. gracilis in our study, their spawning period was determined to be March–September 2021 and April–September 2022, which was delayed compared with the period indicated by Weng et al. (2005). As otolith (sagitta) is deposited daily in S. gracilis (Milton et al., 1990a; Milton et al., 1990b; Milton et al., 1991; Shirafuji et al., 2007), larvae and juveniles have an FL of 20–60 mm, daily age was 23–53 days, the hatching date was between April and early October, and new recruits appeared between May and November. With the incubation period of S. gracilis being approximately 1 week (Shirafuji, 2004; Shirafuji et al., 2007), the spawning date fell between early April and late September in 2021 and 2022, which is consistent with the spawning season determined in the present study.
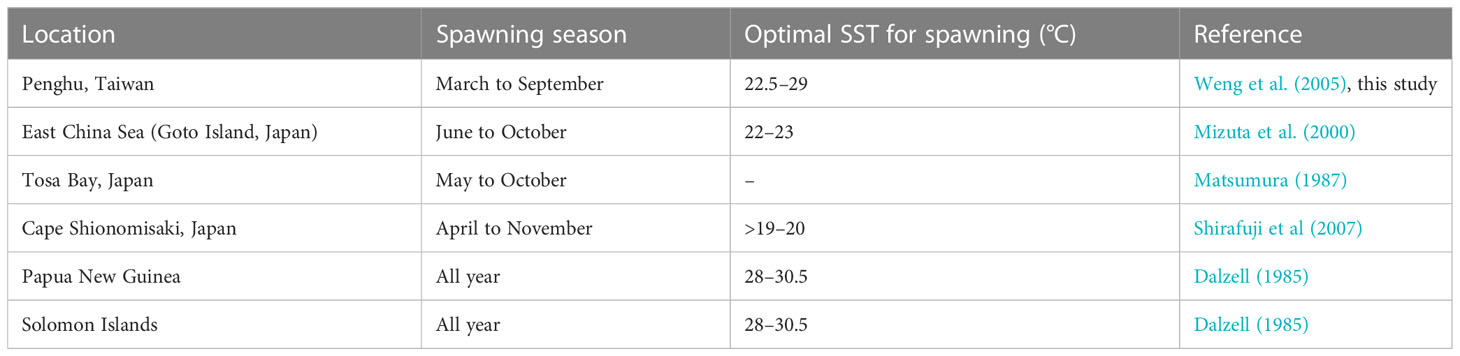
Table 2 Spawning seasons and optimal sea surface temperatures for the spawning of S. gracilis reported by studies conducted in various regions.
Climate and environmental changes affect various features of fish spawning (e.g., spawning period); their reproductive characteristics are regulated by several hydrographic parameters (Gillet and Dubois, 2007; Paumier et al., 2019). In this study, the sea surface temperature (SST) around Penghu was the lowest in March 2021 (21.5°C) and February 2022 (19°C); in both years, the temperature increased to >24°C between April and November (Supplementary Table 2). Thus, the spawning season of S. gracilis began with an increase in SST and ended with a substantial decrease in SST. In our study, the second spawning peak was not apparent in 2022, unlike in July 2021; this might be because the monthly SST in 2022 was higher (29°C) than that in 2021 and exceeded the optimal spawning temperature (22.5°C–27.3°C) reported by Weng et al. (2005). This high temperature persisted for more than 2 months (July and August), thus delaying spawning and reducing egg numbers (Brown et al., 2006). The development of S. gracilis ovaries is strongly correlated with ambient water temperature (Weng et al., 2005). A study conducted in Papua New Guinea (Dalzell, 1985) indicated no significant correlation between spawning intensity and environmental factors, such as SST and salinity.
Herring are highly migratory species. The extent of annual migration for feeding, overwintering, and spawning is <10 km for Atlantic resident stocks but >3,000 km for migratory Atlanto-Scandian herrings (Haegele and Schweigert, 1985; Lambert, 1987). They have similar migration routes across years (Stobo, 1982; Frost and Diele, 2022). Atlantic herring, which are generally classified as spring and autumn spawners, exhibit high levels of fidelity to their spawning seasons and grounds (Lambert, 1987; Brophy et al., 2006; Berg et al., 2017; Frost and Diele, 2022). Similar patterns are noted in S. gracilis found in Penghu waters. Although these S. gracilis generally spawn between March and September, they can be divided into two classes with different primary reproductive strategies on the basis of spawning period. Spring spawners exhibit a breeding peak between April and May, grow rapidly into adults (the size close to the first maturity length is attained within 3 to 4 months at a relatively warm water temperature), and reproduce between late summer and early autumn, thus producing distinct (in terms of the daily age of otolith and monthly changes in length) larvae recruits between late August and November. SST markedly decreases between late autumn and winter; therefore, spawners school southward for feeding and overwintering. They spend more than 4 to 5 months in relatively cold water before attaining sexual maturity and migrating north toward their spawning ground in early spring, when SST begins to increase. The maximum daily age of breeding adults (FL > 80 mm) was approximately 216 days. No spawning stock was found after the appearance of breeding peaks in spring and autumn. Considering the monthly changes in the GSI value, group maturity, and FL distribution of S. gracilis in our study, we believe that this species is a semelparous spawner with a lifespan of <1 year in the study area (Figure 9).
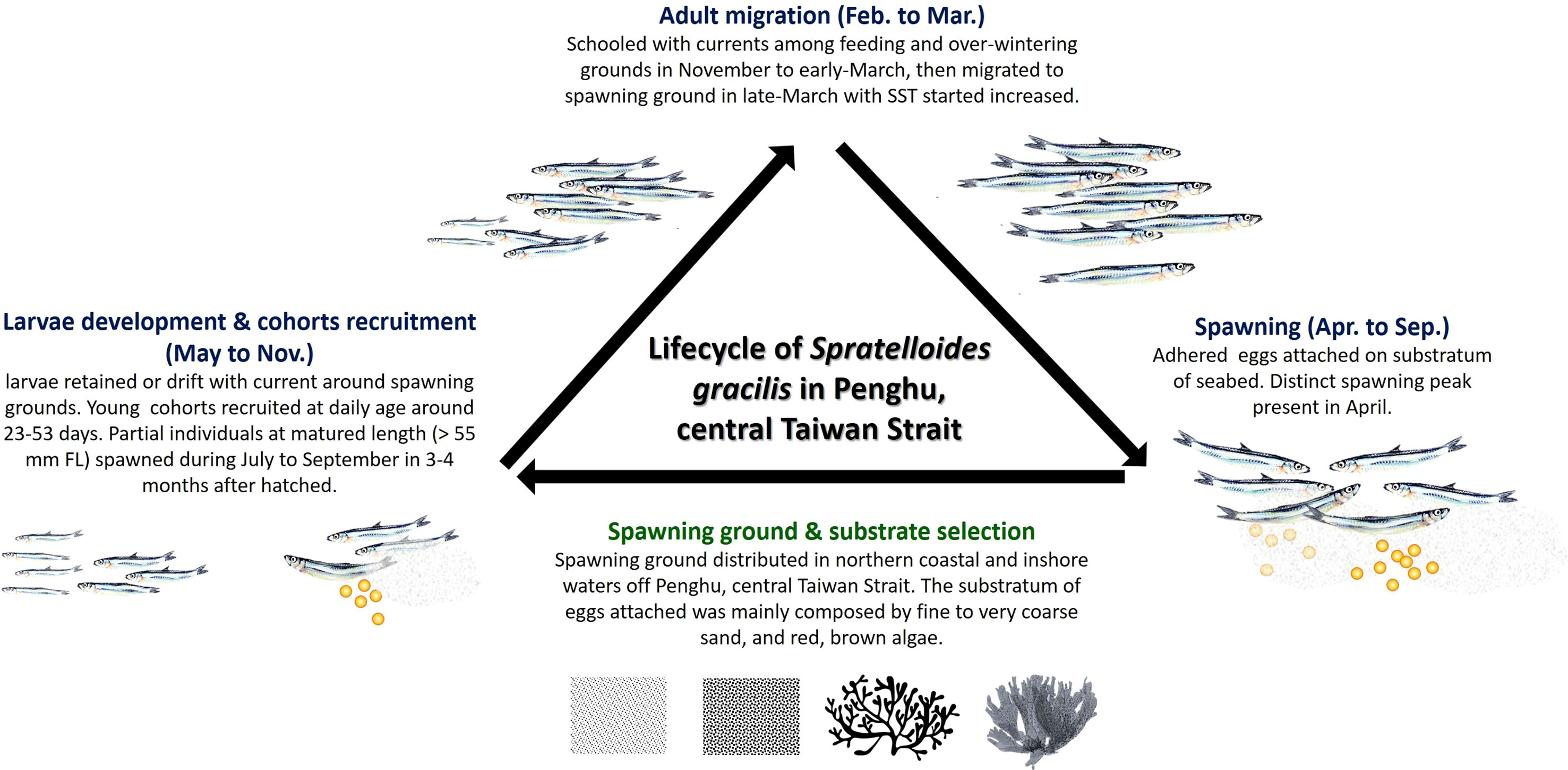
Figure 9 Life cycle of S. gracilis and the features of their spawning ground in the waters of Penghu, central Taiwan Strait.
Herring are marine clupeoids that lay demersal eggs, which adhere to benthic substrata, leading to environmental changes and habitat destruction, which eventually alter their reproduction (Maravelias et al., 2000; von Nordheim et al., 2018). The structural complexity of spawning ground substrata, which provide shelter, enhance food availability, reduce predation risk, and affect egg aggregation pattern, is generally considered to be the primary factor for the successful reproduction and egg development of S. gracilis (von Nordheim et al., 2018; Frost and Diele, 2022). Within the genus of clupeids, the spawning strategies of Pacific and Atlantic herrings are well documented. Pacific herring (Clupea pallasii) tends to spawn on submerged aquatic vegetation along the shallow littoral zone during spring (Hay, 1985). By contrast, Atlantic herring (C. harengus) does not exhibit any specific affinity for a spawning substratum; the predominant types of substrata (macrophytes, gravels, coarse sand, bivalve shells, kelp, and noncoralline macroalgae) may change gradually during the spring spawning period (Aneer and Nellbring, 1982; von Nordheim et al., 2018; Frost and Diele, 2022). Autumn-spawning stocks primarily spawn on gravel present in deeper coastal shelves (Maravelias et al., 2000; von Nordheim et al., 2018). Similar to the aforementioned herrings, S. gracilis tend to select substrata distributed in areas where the water flows close to the sea bottom rather than exclusively favoring a specific type of substratum (Higo and Terada, 1985; Takeuchi and Gushima, 2006). We noted that S. gracilis oviposited primarily on macroalgae and sand grains (fine to coarse sand) in the coastal and inshore regions (depth, 12–20 m) in the northern waters of Penghu. The predominant living species selected as spawning substrata were red and brown macroalgae with high structural complexity, which resulted in the scattering of the deposited eggs (Figure 9). The egg surface was in contact with the surrounding waters for sufficient oxygen supply and metabolic product disposal, which prevented the autointoxication of the embryo (Parrish et al., 1959; von Nordheim et al., 2018). Spawning substrata and time have been reported to vary across S. gracilis populations found in the waters of Japan. In Katanosaka, Kaimon, Wakimoto, Imuta Seto, and Arahito Saki, S. gracilis oviposited primarily on gravel and very coarse sand in coastal regions (Higo and Terada, 1985). However, the fish was likely to oviposit on intertidal or subtidal algae attached on rocks in the inlet of Kuchierabu-jima (Table 3) (Takeuchi and Gushima, 2006).
The spawning ground of S. gracilis in Penghu has strong water currents due to tidal exchanges and topographic features, similar to the situation in Arahito Saki (Higo and Terada, 1985). Atlantic herring are distributed in the Baltic Sea, North Sea, and the waters of Scotland (Haegele and Schweigert, 1985; von Nordheim et al., 2018; Frost and Diele, 2022). The North Sea autumn-spawning herring and Scotland stocks typically spawn in offshore banks under strong currents with a stratified water column; by contrast, the spring-spawning stocks primarily spawn in shallower inshore environment with aquatic vegetation, which protects against wave and tidal effects (Parrish, 1965; Haegele and Schweigert, 1985; von Nordheim et al., 2018; Frost and Diele, 2022). Herring spawn in high-energy environments with strong water currents, wave activity or tidal activity, and structurally complex substrata, including benthos-like macrophytes and coarse grains such as gravel and sand. Sediment buildup may hinder the development of eggs; hence, favorable circulation and oxygenation in water columns may improve the survival rate of eggs (von Nordheim et al., 2018; Frost and Diele, 2022). However, storm events such as extremely strong wind and tidal force negatively affect spawning and egg survival in shallow waters and nearshore environments; this phenomenon has been reported in the North Atlantic during the poor weather conditions in spring and autumn (Lambert, 1987; Moll et al., 2018).
The spawning time of S. gracilis in Penghu is dependent on the tidal cycle. We noted daily changes in the proportions of mature females with hydrated oocytes and spent females between 11 and 25 April 2022, and 1 June and 1 July 2022 (Supplementary Figure 2). Most S. gracilis spawn during the new and full moon periods, when the tidal range is high and plankton biomass is abundant; a similar observation has been reported by a study conducted in the Solomon Islands (Milton et al., 1991). In Kuchierabu-jima and Okino shima, S. gracilis consistently spawn at predawn (before sunrise), consistent with diurnal periodicity but not the tidal cycle (Takeuchi and Gushima, 2006). In Okaga-jima (Japan) and the waters around Penghu, spawning was barely observed during the daytime. No significant correlation was found between herrings’ spawning activity and lunar cycle (neap tide) in the North Atlantic (Lambert, 1987; Frost and Diele, 2022). Further studies should be conducted on the spawning behavior of S. gracilis and the changes in the environmental factors associated with their spawning grounds.
In summary, the spawning season of S. gracilis in the waters of Penghu was between March and September. New recruits were noted after the spawning peak in April, which suggests the effects on the abundance of yearly recruits. The northern coastal and inshore waters of Penghu appear to be an important spawning ground for this species. The current local management scheme may be inefficient in reducing the fishing pressure on spawners. As S. gracilis have a short lifespan as indicated by the daily age determination through otolith, we suggest to adjust the closed fishing season from April to May. The area with a latitude of 23°42′N–23°43′N and a longitude of 119°34′E–119°36′E may be marked as a conservation area for this species (Figure 1) to provide larvae and brooding stocks with further breeding opportunities during the spawning season.
5 Conclusions
The spawning season of S. gracilis in central Taiwan Strait is between March and September, and a distinct peak is noted in April. The spawning period of this species begins and ends with an increase and a decrease in SST, respectively. The spawning ground appears to be the northern coastal and inshore waters of Penghu. S. gracilis exhibited fidelity to spawning grounds, and eggs were mainly deposited onto sand (fine to coarse grains), red algae (Halymenia floresia subsp. harveyana), and brown algae (Ecklonia kurome). Our findings may facilitate the development of effective fishery management strategies for S. gracilis.
Data availability statement
The original contributions presented in the study are included in the article/Supplementary Material. Further inquiries can be directed to the corresponding author.
Ethics statement
The specimens used in this study were cadavers from legal commercial fishery landings in Penghu, and the records of reproductive behavior were observing animals from a distance. Therefore, the approval of animal ethics is not required.
Author contributions
Conceptualization, LCC, JSW and KWL; methodology, LCC, YCL, JSW and STH; software, LCC, STH, YSW and HHH; validation, LCC, YCL and YSW; formal analysis, LCC, STH, YSW; investigation, LCC, YCL, JSW, YKC, JSH and YSW; resources, LCC, JSW, KWL and HAC; data curation, LCC, JSW and KWL; writing—original draft preparation, LCC.; writing—review and editing, LCC, JSW, KWL and STH; visualization, LCC; supervision, JSW, KWL; project administration, JSW, KWL; funding acquisition, JSW, KWL and HAC. All authors have read and agreed to the published version of the manuscript.
Funding
This research was supported by Fishery Agency, Taiwan, and Agriculture and Fishery Bureau Penghu County Government, Taiwan.
Acknowledgments
We would like to thank Tai-Kuai Zhang and Zhi-Jie Yang, the skippers of Taiwanese-Danish-Seines fishery in Penghu, who shared real-time fishing conditions and suggestions for us. We also acknowledge the following for provision of information: Yi-Le Xian (Penghu Marine Biology Research Center, Taiwan Fisheries Research Institute) helped identify the species of algae; Yun-Lin Qiu (Penghu Marine Biology Research Center, Taiwan Fisheries Research Institute) acted as diving buddy during direct underwater observation; and Chiu-Yueh Chen, Wan-Chi Huang, Jin-Hui Yang, and Po-Cheng Tsai assisted us with sampling, bioassay, and experimental preparation in this study.
Conflict of interest
The authors declare that the research was conducted in the absence of any commercial or financial relationships that could be construed as a potential conflict of interest.
Publisher’s note
All claims expressed in this article are solely those of the authors and do not necessarily represent those of their affiliated organizations, or those of the publisher, the editors and the reviewers. Any product that may be evaluated in this article, or claim that may be made by its manufacturer, is not guaranteed or endorsed by the publisher.
Supplementary material
The Supplementary Material for this article can be found online at: https://www.frontiersin.org/articles/10.3389/fmars.2023.1217897/full#supplementary-material
References
Aneer G., Nellbring S. (1982). A SCUBA-diving investigation of Baltic herring (Clupea harengus membras L.) spawning grounds in the Askö-Landsort area, northern Baltic proper. J. Fish Biol. 21, 433–442. doi: 10.1111/j.1095-8649.1982.tb02849.x
Arocha F., Bárrios A. (2009). Sex ratios, spawning seasonality, sexual maturity, and fecundity of white marlin (Tetrapturus albidus) from the western central Atlantic. Fish. Res. 95, 98–111. doi: 10.1016/j.fishres.2008.08.010
Berg F., Husebø Å., Godiksen J. A., Slotte A., Folkvord A. (2017). Spawning time of Atlantic herring (Clupea harengus) populations within a restricted area reflects their otolith growth at the larval stage. Fish. Res. 194, 68–75. doi: 10.1016/j.fishres.2017.05.009
Blaber S. J. M., Milton D. A., Rawlinson N. J. F., Tiroba G., Nichols P. V. (1990). Diets of lagoon fishes of the Solomon Islands: predators of tuna baitfish and trophic effects of baitfishing on the subsistence fishery. Fish. Res. 8, 263–286. doi: 10.1016/0165-7836(90)90027-S
Brophy D., Danilowicz B. S., King P. A. (2006). Spawning season fidelity in sympatric populations of Atlantic herring (Clupea harengus). Can. J. Fish. Aquat. Sci. 63, 607–616. doi: 10.1139/f05-235
Brown N. P., Shields R. J., Bromage N. R. (2006). The influence of water temperature on spawning patterns and egg quality in the Atlantic halibut (Hippoglossus L.). Aquaculture 261, 993–1002. doi: 10.1016/j.aquaculture.2006.08.025
Daly J. C., Richardson B. J. (1980). Allozyme variation between populations of baitfish species Stolephorus heterolobus and St. devisi (Pisces: Engraulidae) and Spratelloides gracilis (Pisces: Dussumieriidae) from Papua New Guinea waters. Mar. Freshw. Res. 31 (5), 701–711. doi: 10.1071/MF9800701
Dalzell P. (1985). Some aspects of the reproductive biology of Spratelloides gracilis (Schlegel) in the Ysabel Passage, Papua New Guinea. J. Fish Biol. 27 (3), 229–237. doi: 10.1111/j.1095-8649.1985.tb04023.x
D’Onghia G., Basanisi M., Matarrese A., Megli F. (1999). Reproductive strategies in macrourid fish: seasonality or not? Mar. Ecol. Prog. Ser. 184, 189–196. doi: 10.3354/meps184189
Felsenstein J. (1985). Confidence limits on phylogenies: an approach using the bootstrap. Evolution 39 (4), 783–791. doi: 10.1111/j.1558-5646.1985.tb00420.x
Fisheries Agency (2022). Fisheries statistical yearbook Taiwan, Kinmen and Matsu (Taiwan: Fisheries Agency, Council of Agriculture, Executive Yuan). Available at: https://en.fa.gov.tw/list.php?theme=Taiwan_Fisheries_Yearbook&subtheme.
Fleming I. A. (1996). Reproductive strategies of Atlantic salmon: ecology and evolution. Rev. Fish Biol. Fish. 6, 379–416. doi: 10.1007/BF00164323
Folmer O., Black M., Hoeh W., Lutz R., Vrijenhoek R. (1994). DNA primers for amplification of mitochondrial cytochrome c oxidase subunit I from diverse metazoan invertebrates. Mol. Mar. Biol. Biotechnol. 3, 294–299.
Frost M., Diele K. (2022). Essential spawning grounds of Scottish herring: current knowledge and future challenges. Rev. Fish Biol. Fish. 32, 721–744. doi: 10.1007/s11160-022-09703-0
Gillet C., Dubois J. P. (2007). Effect of water temperature and size of females on the timing of spawning of perch Perca fluviatilis L. @ in Lake Geneva from 1984 to 2003. J. Fish Biol. 70 (4), 1001–1014. doi: 10.1111/j.1095-8649.2007.01359.x
Gross M. R. (1991). Evolution of alternative reproductive strategies: frequency-dependent sexual selection in male bluegill sunfish. Philos. Trans. R. Soc Lond. B Biol. Sci. 332, 59–66. doi: 10.1098/rstb.1991.0033
Haegele C. W., Schweigert J. F. (1985). Distribution and characteristics of herring spawning grounds and description of spawning behavior. Can. J. Fish. Aquat. Sci. 42 (S1), s39–s55. doi: 10.1139/f85-261
Hall T. A. (1999). BioEdit: A user-friendly biological sequence alignment editor and analysis program for windows 95/98/NT. Nucleic Acids Symp. Ser. 41, 95–98.
Hay D. E. (1985). Reproductive biology of Pacific herring (Clupea harengus pallasi). Can. J. Fish. Aquat. Sci. 42 (S1), s111–s126. doi: 10.1139/f85-267
Higo N., Terada K. (1985) A research on the blue sprat [Spratelloides japonicus] fishery around the Koshiki Islands [Japan], 1: On the adhere of blue sprat eggs to the substratum in the spawing ground off the coast of Arahito Saki. Memoirs Faculty Fisheries-Kagoshima Univ. (Japan) 34 (1), 97–109.
Johannes R. E. (1978). Reproductive strategies of coastal marine fishes in the tropics. Environ. Biol. Fishes 3, 65–84. doi: 10.1007/BF00006309
Kimura D. K. (1980a). Likelihood methods for the von Bertalanffy growth curve. Fish. Bull. 77 (4), 765.
Kimura M. (1980b). A simple method for estimating evolutionary rates of base substitutions through comparative studies of nucleotide sequences. J. Mol. Evol. 16, 111–120. doi: 10.1007/BF01731581
Kumar S., Stecher G., Li M., Knyaz C., Tamura K. (2018). MEGA X: molecular evolutionary genetics analysis across computing platforms. Mol. Biol. Evol. 35 (6), 1547–1549. doi: 10.1093/molbev/msy096
Lambert T. C. (1987). Duration and intensity of spawning in herring Clupea harengus as related to the age structure of the mature population. Mar. Ecol. Prog. Ser. 39 (3), 209–220. doi: 10.3354/meps039209
LePage C., Cury P. (1997). Population viability and spatial fish reproductive strategies in constant and changing environments: an individual-based modelling approach. Can. J. Fish. Aquat. Sci. 54 (10), 2235–2246. doi: 10.1139/f97-132
Lewis A. D., Smith B. R., Ellway C. P. (1983). A guide to the common tuna baitfishes of the South Pacific Commission area. Noumea, New Caledonia: South Pacific Commission. iv, 82 p.
Maravelias C. D., Reid D. G., Swartzman G. (2000). Seabed substrate, water depth and zooplankton as determinants of the prespawning spatial aggregation of North Atlantic herring. Mar. Ecol. Prog. Ser. 195, 249–259. doi: 10.3354/meps195249
Matsumura H. (1987). Biology of Spratelloides gracilis in the waters of Tosa Bay. Fish. Biol. Ocean. South-Western Waters Jpn 3, 9–17.
Melvin G. D., Stephenson R. L., Power M. J. (2009). Oscillating reproductive strategies of herring in the western Atlantic in response to changing environmental conditions. ICES J. Mar. Sci. 66 (8), 1784–1792. doi: 10.1093/icesjms/fsp173
Milton D. A., Blaber S. J. M., Rawlinson N. J. F. (1990a). Diet and prey selection of six species of tuna baitfish in three coral reef lagoons in the Solomon Islands. J. Fish Biol. 37 (2), 205–224. doi: 10.1111/j.1095-8649.1990.tb05853.x
Milton D. A., Blaber S. J. M., Rawlinson N. J. F. (1991). Age and growth of three species of tuna baitfish (genus: Spratelloides) in the tropical Indo-Pacific. J. Fish Biol. 39 (6), 849–866. doi: 10.1111/j.1095-8649.1991.tb04414.x
Milton D. A., Blaber S. J. M., Rawlinson N. J. F., Hafiz A., Tiroba G. (1990b). Age and growth of major baitfish species in Solomon Islands and Maldives. Tuna Baitfish Indo-Pacific Region. ACIAR Proc. 30, 134–140.
Mizuta K., Nakata M., Ichimaru T., Soyano K. (2000). “Gonadal maturation of the female blue sprat, Spratelloides gracilis, in the waters adjacent to the Goto islands [Japan],” in npif.
Moll D., Kotterba P., von Nordheim L., Polte P. (2018). Storm-induced Atlantic herring (Clupea harengus) egg mortality in Baltic Sea inshore spawning areas. Estuaries Coasts 41, 1–12. doi: 10.1007/s12237-017-0259-5
Murua H., Saborido-Rey F. (2003). Female reproductive strategies of marine fish species of the North Atlantic. J. Northwest Atl. Fish. Sci. 33, 23–31. doi: 10.2960/J.v33.a2
Parrish B. B. (1965). The biology of northern Atlantic herring populations. Oceanogr. Mar. Biol. - Annu. Rev. 3, 323–373.
Parrish B. B., Saville A., Craig R. E., Baxter I. G., Priestley R. (1959). Observations on herring spawning and larval distribution in the Firth of Clyde in 1958. J. Mar. Biolog. Assoc. U.K. 38 (3), 445–453. doi: 10.1017/S002531540000686X
Paumier A., Drouineau H., Boutry S., Sillero N., Lambert P. (2019). Assessing the relative importance of temperature, discharge, and day length on the reproduction of an anadromous fish (Alosa alosa). Freshw. Biol. 65 (2), 253–263. doi: 10.1111/fwb.13418
Potts G. W., Wootton R. J. (1984). Fish reproduction: strategies and tactics (London (UK): Academic Press).
Randall J. E. (1995). Coastal fishes of oman. University of hawaii press (Honolulu: University of Hawaii Press).
Shirafuji N. (2004). Life history and recruitment process of Spratelloides gracilis in the waters off Kushimoto (Tokyo: PhD Thesis, University of Tokyo).
Shirafuji N., Watanabe Y., Takeda Y., Kawamura T. (2007). Maturation and spawning of Spratelloides gracilis Clupeidae in temperate waters off Cape Shionomisaki, central Japan. Fish. Res. 73, 623–632. doi: 10.1111/j.1444-2906.2007.01375.x
Shoji J., Toshito S. I., Mizuno K. I., Kamimura Y., Hori M., Hirakawa K. (2011). Possible effects of global warming on fish recruitment: shifts in spawning season and latitudinal distribution can alter growth of fish early life stages through changes in daylength. ICES J. Mar. Sci. 68 (6), 1165–1169. doi: 10.1093/icesjms/fsr059
Stobo W. T. (1982). “Tagging studies on Scotian shelf herring,” in NAFO SCR doc. Available at: https://www.nafo.int/Portals/0/PDFs/sc/1982/scr-82-108.pdf.
Takeuchi N., Gushima K. (2006). Promiscuous spawning behaviour of the tropical herring Spratelloides gracilis. J. Fish Biol. 68 (1), 310–317. doi: 10.1111/j.0022-1112.2006.00900.x
Thompson J. D., Gibson T. J., Plewniak F., Jeanmougin F., Higgins D. G. (1997). The CLUSTAL_X windows interface: flexible strategies for multiple sequence alignment aided by quality analysis tools. Nucleic Acids Res. 25 (24), 4876–4882. doi: 10.1093/nar/25.24.4876
von Nordheim L., Kotterba P., Moll D., Polte P. (2018). Impact of spawning substrate complexity on egg survival of Atlantic herring (Clupea harengus, L.) in the Baltic Sea. Estuaries Coasts 41, 549–559. doi: 10.1007/s12237-017-0283-5
Weng J. S., Liu K. M., Lee S. C., Tsai W. S. (2005). Reproductive biology of the blue sprat Spratelloides gracilis in the waters around Penghu, central Taiwan Strait. Zool. Res. 44 (4), 475–486.
Keywords: reproductive biology, spawning season, spawning ground, spawning behavior, fishery management
Citation: Cheng LC, Lan YC, Lan KW, Weng JS, Chen YK, Hsiao ST, Wu YS, He JS, Huang HH, Huang YC and Cheng HA (2023) Spawning characteristics of blue sprat (Spratelloides gracilis) in the waters of Penghu, central Taiwan Strait. Front. Mar. Sci. 10:1217897. doi: 10.3389/fmars.2023.1217897
Received: 06 May 2023; Accepted: 10 July 2023;
Published: 27 July 2023.
Edited by:
S.M. Sharifuzzaman, University of Chittagong, BangladeshReviewed by:
Xiujuan Shan, Chinese Academy of Fishery Sciences (CAFS), ChinaTaner Yildiz, Istanbul University, Türkiye
Copyright © 2023 Cheng, Lan, Lan, Weng, Chen, Hsiao, Wu, He, Huang, Huang and Cheng. This is an open-access article distributed under the terms of the Creative Commons Attribution License (CC BY). The use, distribution or reproduction in other forums is permitted, provided the original author(s) and the copyright owner(s) are credited and that the original publication in this journal is cited, in accordance with accepted academic practice. No use, distribution or reproduction is permitted which does not comply with these terms.
*Correspondence: Jinn Shing Weng, ai1zLnVlbmdAbWFpbC50ZnJpbi5nb3YudHc=
†These authors have contributed equally to this work and share first authorship