- 1Institute of Systematics and Ecology of Animals, Siberian Branch of the Russian Academy of Sciences, Novosibirsk, Russia
- 2Biological Institute, Tomsk State University, Tomsk, Russia
- 3A.N. Severtsov Institute of Ecology and Evolution, Russian Academy of Sciences, Moscow, Russia
- 4Institute of Biophysics Siberian Branch of the Russian Academy of Sciences, Federal Research Center “Krasnoyarsk Science Center” Siberian Branch of the Russian Academy of Sciences, Krasnoyarsk, Russia
- 5Instituto de Investigación y Tecnología Agroalimentarias, San Carlos de la Rapita, Tarragona, Spain
Introduction: The elemental composition and physical properties of fish bile is a very important marker for ecotoxicological and physiological studies as bile is able to accumulate elements from environmental compartments. In the present study we focused on the effects of long term (seasons and years) and spatial distance (different water bodies) as well as other biotic (feeding habits, fish species, gut morphology) and abiotic (water salinity) factors on element composition of bile of 429 individuals belonging to 22 forms/species of fishes.
Methods: Element composition was determined using an inductively coupled plasma optical emission spectrometry. The fishes were analyzed from one marine (the Kandalaksha Gulf of the White Sea), two freshwater oligotrophic (Teletskoye and Baunt lakes), and one freshwater eutrophic water body (Chany Lake). In parallel, we have estimated the volume and density of bile from the same fishes using an automatic pipette and electronic balance.
Results and discussion: Based on fish bile density and volume data, we hypothesize that gastric fish species (those fishes that have a stomach) require a low volume of bile, but with higher density if compared to agastric fish species (those lacking a stomach) that mainly possessed large volumes of bile, but with a lower density. We have found that the concentrations of the following Na, K, Ca, Mg, S, P (major elements) and Al, Cu, Fe, Sr, Zn (trace elements) were the highest among the 28 elements studied. There were significant findings to contribute to a better understanding of the physiology of bile. First, we have determined the conditions formed in a water body in a given year (season) are more important for element composition of fish bile rather than other tested factors (feeding habits, gut morphology, etc.). Second, the feeding habits of fishes (carnivorous compared to non-carnivorous) had a significant effect based on differences among several elements. Third, the clear distinction between marine and freshwater fishes was found only for K and Na. Understanding which elements are produced as part of waste elimination and those that are present as a consequence of vital biological functions could improve the ecotoxicological study of bile as a marker of heavy metal contamination.
1 Introduction
Digestion is a physiological process that specifically transforms ingested organic material in the animal gut into energy and vital components required for survival and development of the organism (Ugolev and Kuzmina, 1993). Due to fishes occupying high positions in aquatic food webs they are an important part of many aquatic ecosystems in transfer and transformation of material and energy (Kanaya et al., 2019). Moreover, in the case of migratory fish species, fishes also play a crucial role in the biogeochemical cycles of nutrients important for sustaining terrestrial ecological systems (McIntyre et al., 2008; Bianchi et al., 2021). Given their ranking in trophic webs it also places them in a position for bioaccumulation of different chemical substances including various ions. On the one hand, it is based on the fact that among different fishes, a huge range of food items are consumed including vertebrates, invertebrates, sediment, and plant materials (algae and macrophytes), which are different in proximal composition (Solovyev et al., 2014). On the other hand, fish inhabit water bodies that vary in terms of water conditions (temperature and gas regimes, chemical composition) that can directly and indirectly effect various physiological processes, including digestion. It is well known that dietary factors and many other various biotic and abiotic factors (such as parasite infestations, stage of ontogeny, etc.) can significantly affect different parameters of the digestive system and, as a result, on digestive efficiency (Solovyev et al., 2014; Izvekova and Solovyev, 2016; Solovyev et al., 2016). For instance, cestodes from perch have been documented to have a high capacity for bioaccumulation and storage of metals as they compete for essential elements with their host. This ability to remove toxic metal loads from host tissues has been seen as one of the “benefits of parasitism” (Brázová et al., 2015) and may influence the overall composition of bile. Since digestion is a complicated process requiring specific conditions for the proper activity of various digestive enzymes in the stomach and intestine, the physico-chemical composition of the inner media of the fish gut is an extremely important parameter influencing digestive efficiency (Izvekova et al., 2013; Solovyev et al., 2018). The composition of this inner media of the gut is formed and affected by external and internal factors, and important among these factors are multiple chemical compounds and metabolites (different ions, fatty and amino acids, sugars, etc.) acquired or extracted from environmental compartments (food items, water, sediments, etc.), then consumed and digested by fish. In particular are the proteins and fatty acids that interact with G protein coupled receptors that signal the production of сholecystokinin (CCK) which triggers the release of bile into the gut. Thereby, the components of this inner media are derived internally mainly from the chemical composition of different secretions of the liver (bile), gastric gland (hydrochloric acid), pancreatic gland (secretions), and metabolites of the gut microbial community (Clements and Raubenheimer, 2008). Unfortunately, the available data describing the physico-chemical composition of the fish gut is very limited especially for fish that inhabit natural water bodies.
The bile is synthesized by the fish liver and accumulated in the gallbladder before being released into the intestine. Bile is formed by water and different biochemical molecules e.g., bile acids, phosphatidylcholine, bilirubin, proteins, as well as different ions (Boyer, 2013). The main function of bile is emulsification of lipids ingested with the diet to facilitate hydrolysis by lipases (Olsen and Ringø, 1997). Moreover, bile facilitates the assimilation of the fat-soluble vitamins (A, D, Е, К, etc.), and elimination of toxic metabolites, including some trace elements from the fish body. It is well known that fish bile consists of various major (abundant) elements and trace elements (Boyer, 2013). In rat models it has been shown that different transporter molecules such as MDR1 and MDR3 can be involved in moving specific ions into the biliary fluid in an ATP-dependent manner, although details of the mechanism are still unclear (Boyer, 2013). As vertebrates, fishes can be assumed to have a similar diverse repertoire of transporters with varying degrees of ion specificity. Also, metals can be reabsorbed from bile in the form of amino acid complexes as needed (Boyer, 2013). In general, there are many studies focused on the element composition of fish liver as the main detoxification organ, whereas the constituent elements of fish bile are less studied (Olsson et al., 1998; Subotić et al., 2013; Gutiérrez-Ravelo et al., 2020). Since bile is one of the body fluids of fish which accumulate different ions, it can be a good biomarker for a broad range of biological, ecological, toxicological, or other study topics (Hauser-Davis et al., 2012). It was shown that such elements as Fe, Cu, Pb, Cd, Na, K, Ca, Zn and several others are typical components of fish bile (Hunn, 1976; Yamazaki et al., 1996; Baldisserotto et al., 2004; Hauser-Davis et al., 2012; Nabavi et al., 2012). These elements accumulate in fish bile via multiple physiological pathways and can be designated as specific biomarkers of water, sediments, and food items (Hauser-Davis et al., 2012). By extension these biomarkers are relevant for the evaluation of toxic pollutants on a regional basis. While some elements like Zn may be actively absorbed by specific transporters because of a requirement for proper nutrition (Andree et al., 2004), others can be accumulated in tissues in the same manner as Hg and Pb or be eliminated as waste products (Mason et al., 1995). Although the separation into classes of which elements of bile are functional and which are truly waste products for elimination is not a question easily answered.
Major and trace elements are also very important components of fish bile affecting the digestive process by alteration of the level of activity of digestive enzymes in the fish intestine. It is well known that many ions, depending on their concentrations, may positively or negatively affect enzymatic activity (Wang et al, 2010; Ben Khaled et al, 2011; Silva et al, 2011; Costa et al, 2013; Temiz et al, 2013; Zhou et al, 2013). On one hand, different digestive enzymes may possess metal ions (Co, Zn) in their active sites or require metal ions for the proper level of catalytic reactions (metalloproteases). On the other hand, many trace elements may significantly inhibit the activity of digestive enzymes reducing the digestive efficiency (Golovanova, 2008). During recent decades culture-independent methods of microbial analysis have shown that the fish gut is inhabited by a great number of taxonomically diverse microorganisms that form the gut microbiome, with varied and multiple functions including digestion (Ringø et al., 2015). At the same time, many elements of bile may limit the growth of microorganisms, thus making bile function as an additional filter for selecting which microorganisms may colonize the gut of the specific fish species, or preventing others (Xia et al., 2018: Meng et al., 2018; Wang et al., 2019; Kakade et al., 2020; Spilsbury et al., 2022). Thus, understanding which biotic and abiotic factors affect the element composition of bile may help to improve knowledge of the digestive features of fish, for instance, for developing species-specific artificial diets for aquaculture industry.
In the territory of Russia there are a number of water bodies characterized by various hydro-chemical conditions ranging from shallow eutrophic to deep mountain/volcanic oligotrophic lakes. These water bodies are inhabited by fish characterized by different feeding habits (omnivorous, zoobenthivorous, zooplanktivorous, carnivorous, etc.), variations of gut morphology (gastric, agastric, with or without pyloric caeca), feeding behavior (for example, constantly feeding or with one-two peaks of feeding activity per day), preferred water temperatures (cold or warm water), salinity (freshwater, brackish water, or marine), and the systematics describing their evolutionary time and place of origin (for example, burbot (Lota lota) is a unique representative of the family Lotidae colonizing freshwater areas), etc. (Reshetnikov et al., 1997; Izvekova et al., 2013). Such diversity of fish and habitats provides a good opportunity to study the effect that different biotic and abiotic factors have on the element composition of fish bile.
The main aim of the present study was to estimate the effect of biotic (gut morphology, gut fullness, fish species, and feeding habits) and abiotic (season, year, water body, and salinity) factors on the element composition of bile from various fish species. The purpose was to test the following hypotheses: 1) the element composition of bile, in general, will be determined by a particular water body rather than other factors due to specific hydro-chemical conditions in each of them; 2) additional determinants such as the season and year of sampling will affect the element composition of fish bile due to availability of different food items (season) and various specific conditions that are temporally discontinuous over longer time periods (years); and 3) the different trophic position of a fish species in the food web will affect the element composition of fish bile due to the primacy of different elements that will be consumed in their diet and bioaccumulation of some elements (for instance, lead or iron).
2 Materials and methods
2.1 Description of sampling sites
The Chany lake system located on the Baraba Lowland (the southeastern part of the West Siberian Plain) includes two main lakes – Bolshie Chany Lake and Malye Chany Lake as well as two main rivers flowing into the lake e.g., Kargat River and Chulym River (Bulatov et al., 2015). The surface area of the lake is about 1500-2000 km2 and depths around 2.0 m (Aladin and Plotnikov, 1993; Drost et al., 2002; Vasilyev et al., 2005) but these parameters may significantly fluctuate depending on season and year. The sediments of the basin are mainly represented by layers of loams and sands and some places overlapping with eolian formations. The upper part of the cross-sections includes mainly lacustrine clays, silts, deluviums, ancient eolian and subaerial deposits (Bulatov et al., 2015). Chany Lake is a shallow eutrophic lake characterized by water that is heterogeneous in terms of salinity and ionic composition that is related to basin morphology and low water exchange between different parts of the lake (Savkin et al., 2015). In general, the upper reaches of the Chulym and Kargat rivers are fresh, less mineralized, with a predominance of sodium bicarbonate, while the middle and lower reaches have the same composition, but with a high content of sulfates. The mineralization of the waters of the Malye Chany Lake is higher in comparison with the inflowing rivers, but lower in comparison to Bolshie Chany Lake (Savkin et al., 2015). The water in the lake is alkaline and typical pH values range from 8.8 to 9.0 (Savkin et al., 2015). Among higher aquatic plants, plant formations of common reed (Phragmites australis) are dominant (Korolyuk et al., 2015). Chany Lake is populated by 14 fish species dominated by cyprinids (Popov et al., 2005). The most common fishes in the lake are European perch (Perca fluviatilsis), pikeperch (Sander lucioperca), pike (Esox lucius), Prussian carp (Carassius gibelio), crucian carp (Carassius carassius), common carp (Cyprinus carpio), ide (Leuciscus idus), roach (Rutilus rutilus), common dace (Leuciscus leuciscus) and freshwater bream (Abramis brama). In the areas adjacent to Chany Lake, active agricultural activities are carried out, mainly related to crop production (Zherelina et al., 2015).
Baunt Lake is oligotrophic water body located in the western part of the Baunt basin (Tsipikanskaya depression, the northeast of the Republic of Buryatia) that is part of the Tsipo-Tsipikanskaya system of lakes belonging to the Vitim River basin (Solotchin et al., 2020). The main tributaries of the lake are two rivers - the Upper Tsipa and Tsipikan, while the lower one is the Lower Tsipa. The upper part of the lake sections is represented by diatomaceous silts, silty clays with sand fractions, and the lower part is predominantly clayey-silty material (Krainov et al., 2017). The mineral composition of the lake sediments is dominated by layered silicates, quartz, and feldspars. The water of Baunt Lake has low-mineralized (hydrocarbonate calcium class) fresh water with a nearly neutral pH (the average values of pH vary from 6.80 to 7.25). Intensive heating of surface waters and some excess of evaporation over inflow cause a greater mineralization of the upper layers of water and an obstacle to the immersion of more mineralized waters into the depths of the lake (Solotchin et al., 2020). The most common fishes in the lake are European perch, pike, Prussian carp, ruffe (Gymnocephalus cernua), roach, common dace, pidschian-like whitefishes (Coregonus lavaretus pidschian), cisco-like whitefish (Coregonus baunti sp. nova) and burbot. In the area of Baunt Lake, the gold mining industry is more actively developed, and currently includes approximately 13 enterprises.
Teletskoye Lake is a deep freshwater oligotrophic reservoir located in the upper reaches of the Ob River in the northeastern part of the Altai Mountains at the junction with the Western Sayan Mountains, in a basin of tectonic origin. About 70 rivers flow into the lake, among which the Chulyshman River is the most significant for the water supply of the lake, and only the Biya River flows out. In cross-section, a sandy lower layer is distinguished, underlain by clayey silts, and a silty-clayey upper layer, between which there is an intermediate turbidite-homogenite layer. The clastic component of Teletskoye Lake sediments is represented by feldspar, quartz, biotite, chlorite, hydromica, muscovite, amphibole, epidote, garnet, and ilmenite (Buslov et al., 2001). Teletskoye Lake is a low-mineralized lake (hydrocarbonate calcium class), but in the southern part the level of mineralization is a bit higher. The pH of the water is weakly alkaline, though pH values do not exceed 7.85 (Dolmatova, 2011). As a consequence of the small littoral area, the predominance of rocky soils, low water temperature and low concentrations of biogenic substances there is a low level of growth and development of hydrophilic vegetation in the lake (Zarubina and Sokolova, 2007). The most common fishes in the lake are European perch, pike, pidschian-like whitefishes, Siberian sculpin (Cottus sibiricus), and burbot. Teletskoye Lake is the largest touristic place in the Altai Mountains, which causes water pollution with household waste, as well as petroleum products due to an increase in the number of small boats (Zuykova et al., 2009).
The Kandalaksha Gulf is one of the largest bays of the White Sea, which belongs to the Arctic Ocean basin, and is an asymmetric trench with a complex bottom relief, as well as an abundance of small bays and islands (Rybalko et al., 2020). Sandy-argillaceous deposits, boulder loams and sandy loams lie directly on the crystalline rocks of the basement of the bay. The upper part of sediments is composed of layered clay silts, which pass higher into loamy and sandy silts and clayey silty sands (Rybalko et al., 2020). The water of the Kandalaksha Gulf has a relatively low salinity, which is somewhat stratified, so the salinity of surface waters averages 16-18 ‰, and bottom waters 26–27 ‰. Numerous freshwater streams play a key role in the formation of the salinity and chemical composition gradient. The water in the bay has a slightly alkaline reaction (maximum pH values are 8.03) (Mazukhina et al., 2020). The most common fishes in the gulf are Atlantic cod (Gadus morhua marisalbi), common dab (Limanda limanda), navaga (Eleginus nawaga), shorthorn sculpin (Myoxocephalus scorpius), Atlantic herring (Clupea harengus) and ninespine stickleback (Pungitius pungitius). River runoff polluted by emissions from mining, chemical and metallurgical enterprises can also serve as a source of technogenic pollution of the Kandalaksha Gulf (Rozanov and Volkov, 2009).
2.2 Fish sampling
Adult fishes used in the current study were obtained from the following Russian Federation water bodies: the estuarine area of the Chany Lake — the Kargat River including Fadikha Lake and Chulym River (54°61’N; 78°21’E), Baunt Lake (55°23’N; 112°04’E), Teletskoye Lake (51°79’N; 87°26’E), and Kandalaksha Gulf (White Sea) (Figure 1). Fish were sampled in: 1) Chany Lake area in May 2019 and 2020 on depth of 1–2 m (points С3, С4, С5, see Figure 1A); 2) Chany Lake area in the end June – July 2019 and 2020 at the same depth (points С3, С4, C5, see Figure 1B); 3) Baunt Lake in July 2020 and 2021 depth of 3–15 m (points B1, B2, see Figure 1C); 4) Teletskoye Lake in the end of August – beginning of September 2019 and 2020 on depth of 3–15 m (points T1, T2, see Figure 1D); 5) Kandalaksha Gulf in July 2021 on depth of 2–15 m (points K1, K2, see Figure 1E).
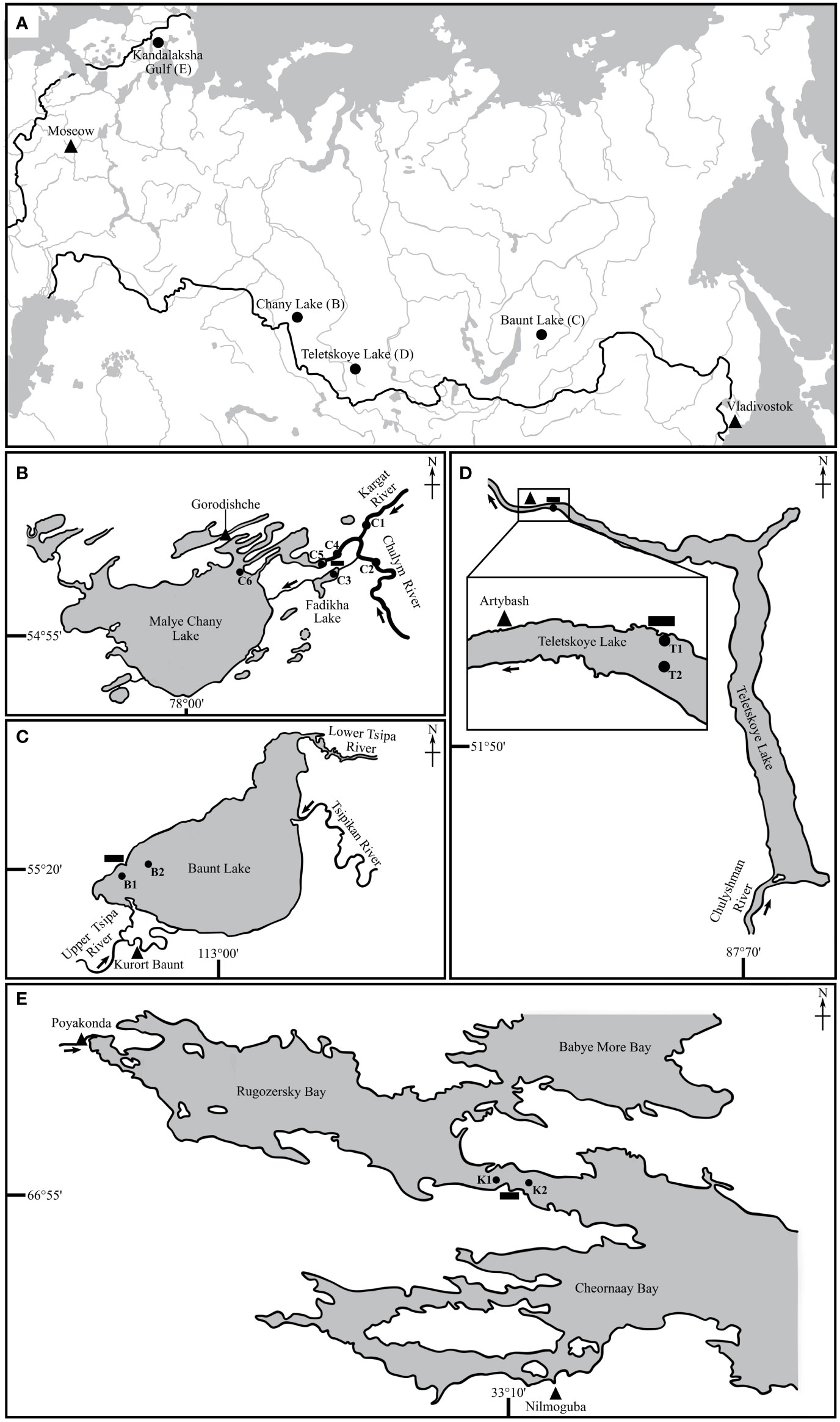
Figure 1 Maps indicating different sampling points (see text for detailed geographical coordinates for sampling sites): general map (A); Chany Lake area (B): C1 – Kargat River, C2 – Chulym River, C3 – Fadikha Lake, C4 – “Pier”, C5 – “Shilovskiy” Bay, C6 – Malye Chany Lake; Baunt Lake (C), B1 – net point 1, B2 – net point 2; Teletskoye Lake (D), T1 – net point 1, T2 – net point 2; Kandalaksha Gulf (White Sea) (E), K1 – net point 1, K2 – net point 2. Triangle – city/village, rectangle – field station.
All fish were caught using gill nets with mesh sizes that varied from 20 to 70 mm. Immediately after removing fish from the nets, they were transported alive in water-filled plastic containers with aeration (duration approximately 1 h), to the field station (Chany Lake, Teletskoye Lake, and Kandalaksha Gulf) or field laboratory (Baunt Lake) where fish were measured for their total body length (TL) and weight (BW) to the nearest mm and grams, respectively.
2.3 Water and sediment sampling
Water temperature was measured with HI 8314 portable pH meter (Hanna Instruments, USA) equipped with temperature electrode. In the present work, the studied area including the estuary of Malye Chany – Kargat River and Fadikha Lake will be designated as “Chany Lake”.
In order to study the distribution of some hydro-chemical characteristics and concentration of major and trace elements being studied in the water of the Chany Lake area the additional points such as Kargat River (point C1), Chulym River (point C2) (according to water flow direction), and Malye Chany Lake (point C6) were analyzed (Figure 1B). Since Chany Lake is a shallow lake with an average depth around 2.0 m, the water samples were taken from 0-0.5 m of depth. From the same points the samples of sediments were collected as well.
The samples of water from Baunt Lake were collected from point B1 at a depth of 0–0.5 m (upper horizon) located near to the shore and point B2 at a depth of 0–0.5 m (upper horizon) located around 500 m from the shore where the depth increased until 15–20 m. From the same point B1 the samples of sediments were also collected (Figure 1C).
For the same aims the samples of water were collected from Teletskoye Lake from point T1 at a depth of 0–0.5 m (upper horizon) located near to the shore and point T2 at a depth of 0–0.5 m (upper horizon) and of 15 m (near to the bottom) located near to the center of the studied part of the lake (Figure 1D). From the same point T1 the samples of sediments were also collected.
The samples of water from Kandalaksha Gulf (White Sea) were collected from points K1 and K2 at a depth of 0–0.5 m (upper horizon); a location situated near to the center of the studied part of the Gulf (around 500 m from the shore) where the depth was around 15–20 m (Figure 1E). Given the depth and lacking special equipment, we did not collect sediment samples from these sample points. All samples of water from the upper horizon of the corresponding water body were collected in 1.5 L plastic bottles, whereas samples of water from 15 m (Teletskoye Lake) were taken by bathometer and then transferred to 1.5 L plastic bottle. Sediment samples were collected in a total mass of 5 g of wet weight using a Petersen grab. All water and sediment samples were frozen and stored at -80°C until analysis.
2.4 Bile collection
All data regarding fish species, their number, total body length, sampling sites, etc. are given in Supplementary Table S1. The fishes were killed by a blow to the head and the digestive system was extracted. The gallbladder from each fish was carefully segregated then cleaned from the remains of liver, hepatopancreas, fat, or some blood. Further, the gallbladder was pierced by a disposable syringe, whereupon the whole volume of bile was collected by syringe. Afterwards, the bile from the syringe was dispensed into one or several 2.0 ml Eppendorf tube (depends on total volume of bile) and immediately frozen in liquid nitrogen until transported to the laboratory of the Institute of Systematics and Ecology of Animals, SB RAS (Novosibirsk, Russia), where the tubes were transferred to -80°C freezer and stored until analysis.
The total volume (ml) is the whole volume of bile in the gallbladder of the fish and was measured using an automatic pipette (Eppendorf, Germany). For large fish such as burbot, pike and common carp that had a total bile volume >2 mL, the level of accuracy was 0.01 ml while for other fish species that had a total bile volume <1 mL the level of accuracy was 0.001 ml. The wet (g) and dry mass (g) of bile was assayed using an electronic balance (Explorer Pro EP114C, Ohaus Corporation, USA) with accuracy equal to 0.1 mg. Here and later the wet mass of bile was measured before lyophilization while the dry mass of bile was estimated after 48 hours of lyophilization (the pressure and temperature in the freeze-dryer chamber were 400 mTorr and -55°C, respectively) using freeze-dryer TFD5503 (ilShinBioBase, Korea). The volume of bile per gram of fish was calculated as total volume divided by total body weight of the associated fish:
The relative wet (%) and dry mass of bile (%) were calculated as wet and dry mass of bile divided by total body weight of the associated fish and multiplied by 100%:
The density of bile () was calculated as the bile wet mass divided by its total volume:
2.5 Element analysis
Before running the element analysis, all bile and sediment samples were lyophilized during 48 hours (400 mTorr, -55°C) until their weight was constant. In preliminary experiments we have estimated that the dry weight of bile should range between 20–50 mg in order to conduct element analyses properly. In many cases the total volume of bile exceeded the required weight for element analysis, but in other cases when fish were quite small or the gallbladder was almost empty, we pooled bile from several fish of the same species (the same time, place, and level of gut fullness) together in order to reach the desired dry mass for element analysis.
2.5.1 ICP - OES analysis of bile, water and sediment
Sediment samples were homogenized in a mortar and pestle. Before the analyses, sediment and bile samples were dried at 105 ˚С in a drying oven, ED53 (Binder, Germany), until weight was constant.
Analysis of elements was conducted with inductively coupled plasma optical emission spectrometry (ICP – OES) using an iCAP 6300 Duo spectrometer (Thermo Scientific, U.K.). Bile and sediments (0.2 g of dry weight) were digested in a mixture of HNO3/HClO4 (1/1, v/v, high purity) in glass beakers on a hot plate, then were transferred into polypropylene test tubes and diluted with 18 MΩ cm−1 deionized water (DW) to 14 ml. Before ICP - OES analysis sediment samples were additionally diluted 10 times with DW. Water samples from the Kandalaksha Gulf were diluted five- and fifteen folds before analysis. All water samples were acidified with HCl (high purity) (0.1 ml acid/10 ml of water sample).
ICP spectrometer was calibrated using multi-element standard solutions for ICP (IV and IX (Merck, Germany), 43843-100ML (Fluka, Switzerland), 140-102-011 (SCP Science, Canada)), single element standards (Magnesium (N2-MG674973), Phosphorus (N2-P668818), Sulfur (M2-S658762), Iron (M2-FE659835) (Inorganic Ventures, USA)), CaO, KCl and Na2SO4 of analytical grade. Sc (5 mg l−1, Scandium Standard for ICP 92504, Sigma-Aldrich) was used as internal standard for water, bile and detection of As, S, P, Tl, Se in sediment analysis. Indium (100 mg l−1, In2O3 analytical grade) was used as internal standard for detection of metals in sediments.
For every type of sample – fresh and salt water, bile and sediments, corresponding calibration standards were used (Anishchenko et al., 2020). Trace element calibration standards for salt water analysis contained Na 600 mg l−1 (Na2SO4), which corresponded to the matrix of the studied water samples. The sediment samples were measured in duplicates. Limits of detection (LOD) for the studied elements are listed in Supplementary Table S2.
2.5.2 Hydrochemical features of water analysis
Specific conductivity of water was measured with Starter ST300C (Ohaus Instruments, China), рН was determined with a PB-11 рН-meter (Sartorius, Germany) in the laboratory. Cl- in water was detected argentometrically, and by titration with 0.1N or 0.01 N HCl until pH 4.3. The Nessler method was used for ammonium nitrogen in freshwater (Clesceri et al., 1989) and salt water with preliminary precipitation of Mg and Ca with 6N KOH (Kalacheva et al., 2002). Nitrates were converted to nitrites by means of a cadmium column, nitrite was determined by the colorimetric method after reacting with sulfanilamide and α-naphthylamine, soluble reactive phosphorus was assessed using the ascorbic acid and ammonium molybdate method (Clesceri et al., 1989). The optical density of solutions was determined with a KFK-2MP spectrophotometer (Zagorsk Optical and Mechanical Plant, Russia).
2.6 Statistics
The relationships of the relative wet weight of bile with factors “gut fullness”, “feeding habits”, and “gut morphology” (gastric by agastric species) were established using the Spearman’s rank correlation coefficient and one-way PERMANOVA. To estimate the effect of such factors as “gut fullness” on composition and concentration of studied elements we have analyzed the following fish species: Prussian carp, crucian carp, ide, and European perch from Chany Lake and pidschian-like whitefish from Teletskoye (whitefish TL) and Baunt lakes (whitefish BL), as well as Atlantic cod, navaga, common dab and shorthorn sculpin from Kandalaksha Gulf (Table 1). The relationships between “gut fullness” and concentrations of studied elements in bile were estimated using Spearman’s rank correlation coefficient. The effect of factor “gut fullness” on the same parameters of bile was assayed by one-way PERMANOVA in the case of stomach, and two-way PERMANOVA in the case of anterior and posterior intestines. The degree of fullness of the gut was assessed qualitatively using arbitrary units (AU) ranging from 0 (empty stomach or intestine) to 5 (full stomach or intestine).
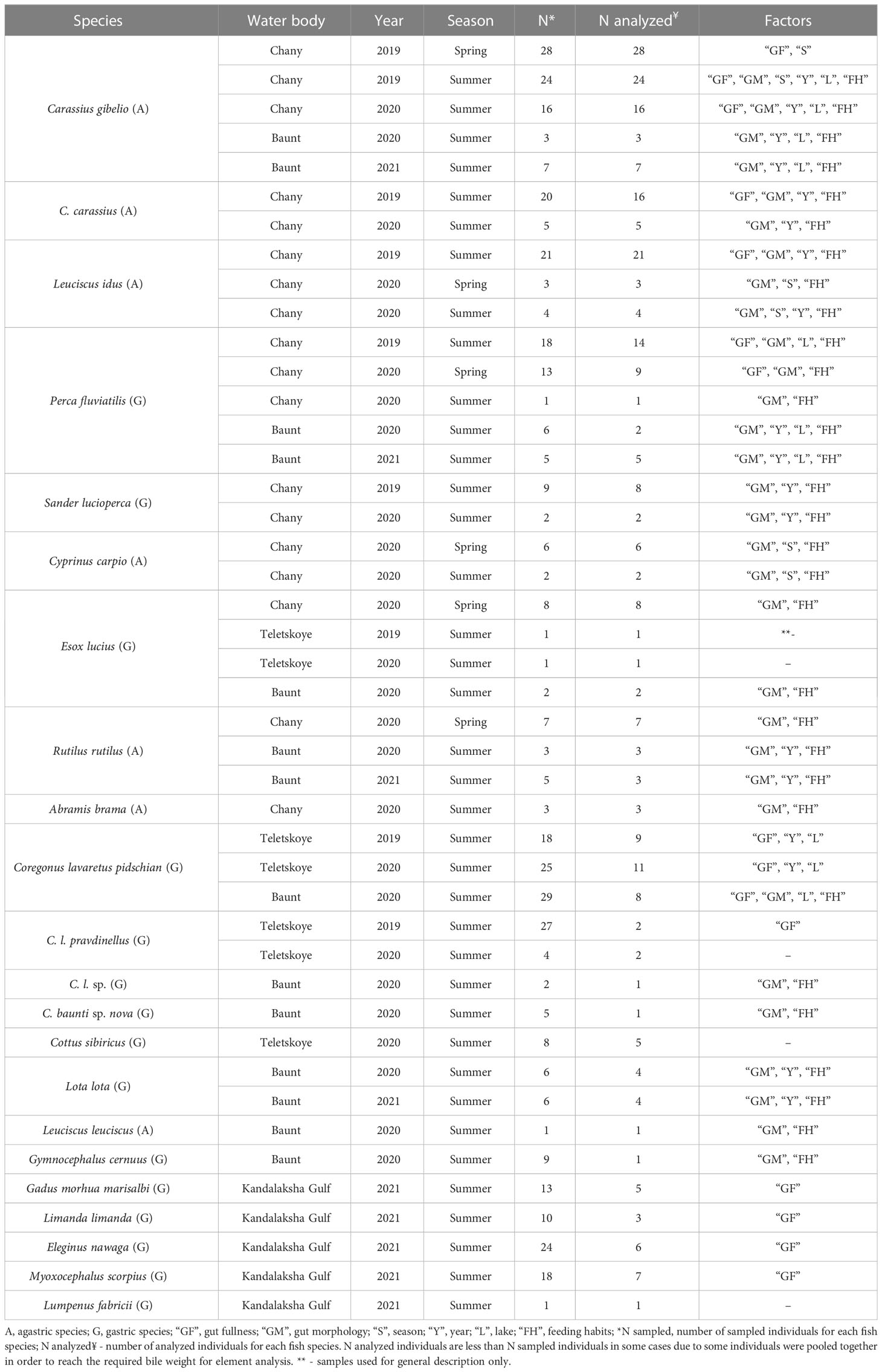
Table 1 Sample information for fish bile including studied fish species, water body, year and season of sample collection as well as the number of analyzed individuals and affected factors.
In order to estimate the effect of the factor “season” we have analyzed the same fish species obtained from different seasons from the same lake: Prussian carp, ide, and common carp from Chany Lake. In order to assay the effect of the factor “year” we have analyzed the same fish species obtained from different years, but from the same lake and season: Prussian carp, crucian carp, ide, and pikeperch from Chany Lake, whitefish TL from Teletskoye Lake, and roach, burbot, Prussian carp, and European perch from Baunt Lake. The effects of both factors “season” and “year” were estimated separately using one-way PERMANOVA. The combined effect of these two factors (“season” and “year”) on the element composition of bile was analyzed using a discriminant analysis carried out for all fish species obtained for all time points for each of the studied lakes (Chany Lake, Teletskoye Lake, and Baunt Lake).
In order to estimate the effect of factor “lake” we have used in the analysis the same species, but inhabiting different lakes: Prussian carp and European perch from Chany and Baunt lakes, whitefish (C. l. pidschian) from Teletskoye and Baunt lakes (Table 1). The effect of factor “lake” was estimated using a one-way PERMANOVA.
The effect of factor “feeding habits” (carnivorous by non-carnivorous) was estimated based on the composition and concentration of the studied elements in fish bile using a one-way PERMANOVA. For these comparisons the following fishes from the same lake, season, and year were chosen: non-carnivorous – Prussian carp, crucian carp, common carp, ide, roach, freshwater bream, and carnivorous – European perch, pikeperch, pike collected from Chany Lake. Additionally, we collected from Baunt Lake the non-carnivorous – Prussian carp, roach, whitefish BL, whitefish BL sp. (C. l. sp.), cisco-like whitefish (C. baunti sp. nova), common dace, ruffe, and carnivorous – European perch, pike, and burbot.
The effect of “gut morphology” (gastric/agastric fish species) was estimated as a factor contributing to composition and concentration of the studied elements in fish bile using a one-way PERMANOVA. For this comparison fish from the same lake, season, and year were studied in order to eliminate the effect of other factors (Table 1). These criteria fit for agastric Prussian carp, crucian carp, common carp, roach, freshwater bream, ide and gastric European perch, pikeperch, pike from Chany Lake as well as for agastric Prussian carp, roach and common dace, and gastric European perch, pike, burbot, ruffe, whitefish BL, whitefish BL sp., cisco-like whitefish from Baunt Lake.
In all cases when the effects of studied factors were significant the Forward Stepwise of General Discriminant Analysis Model (GDA) was applied in order to estimate which elements determine the effects that were found. In all tests, the significance level was set at P< 0.05. The concentrations of all studied elements were measured in duplicates. All data are given as a mean ± standard error of the mean.
3 Results
3.1 Volume, mass, and density of fish bile
3.1.1 Marine species
The inter- and intraspecies variability of total volume and volumes per gram of fish, relative, wet and dry mass, as well as density of bile of marine and freshwater fishes is given in Supplementary Table S1. Among all studied marine fish species, the total volume of bile varied from 0.05 ± 0.01 ml (navaga) to 0.10 ± 0.07 ml (Atlantic cod), whereas the volume of bile per gram of fish ranged from 0.0005 ± 0.0001 ml/g (Atlantic cod and navaga) to 0.001 ± 0.0002 ml/g (shorthorn sculpin). There were no significant correlations (the anterior intestine for navaga was an exception) between gut fullness and the volume of bile per gram of fish for navaga, Atlantic cod, shorthorn sculpin, and common dab (Supplementary Table S3, 3.1.1a). The relative wet mass of bile among all studied marine fishes had the lowest value for Atlantic cod (0.05 ± 0.01%), whereas the highest value was found for shorthorn sculpin (0.10 ± 0.016%). The correlation relationships between values of relative wet mass of bile and gut fullness were similar if compared to correlations between gut fullness and the volume of fish bile per gram of fish (Supplementary Table S3, 3.1.1b). The bile density of the studied marine fish was characterized by low levels of inter- and intraspecific variabilities in the range from 1.00 to 1.27 g/ml, (average of 1.1 g/ml). There were no significant differences in bile density among studied marine fish species (one-way PERMANOVA, P>0.05).
3.1.2 Freshwater species
Among all studied freshwater species, the total volume of bile ranged from 0.0036 ± 0.0003 ml for cisco-like whitefish (Baunt Lake, summer, 2020) to 1.28 ± 0.84 ml for burbot (Baunt Lake, summer, 2020), whereas the volume of bile per gram of fish varied from 0.00013 ± 0.00003 ml/g for ruffe (Baunt Lake, summer, 2020) to 0.0018 ± 0.0002 ml/g for Prussian carp (Chany Lake, summer, 2020).
Irrespectively to fish species, the correlation relationships between the volume of bile per gram of fish and fullness of anterior intestine were negative in many cases whereas for posterior intestine such relationships were found in fewer cases: crucian carp, Prussian carp, and whitefish TL. For stomach the correlation relationships between the volume of bile per gram of fish and fullness were almost absent with one exception such as whitefish TL (Supplementary Table S3, 3.1.2a).
The lowest value (0.011 ± 0.002%) of relative wet mass of bile was found for Pravdin’s whitefish (Coregonus lavaretus pravdinellus) (Teletskoye Lake, summer, 2019) whereas the highest value (0.18 ± 0.02%) was registered for Prussian carp (Chany Lake, summer, 2020). The correlation relationships between values of relative wet mass of bile and gut fullness were similar if compared to correlations between the gut fullness and the volume of bile per gram of fish. There were no significant correlations between the stomach fullness and relative wet mass of bile, with one exception that was found – whitefish TL (Teletskoye Lake, summer, 2020) (ρ=-0.70, Р=0.003). In contrast, the significant correlations between values of relative wet mass of bile and fullness of anterior intestine for the majority of freshwater fishes were found, whereas for the same fishes the significant correlations for posterior intestine were almost absent, but with the exceptions for ide, crucian carp, Prussian carp, and whitefish TL (Supplementary Table S3, 3.1.2b).
As was found for marine fishes, the bile density of studied freshwater fishes was characterized by low levels of inter- and intraspecific variabilities as well, and ranged from 1.00 to 1.40 g/ml, (in average 1.1 g/ml) (Supplementary Table S1). Based on one-way PERMANOVA the density of bile was significantly higher for such species as Siberian sculpin (1.17 ± 0.02 g/ml, P=0.0001–0.043, Teletskoye Lake, summer, 2020), ruffe (1.27 ± 0.03 g/ml, P=0.0001–0.025, Baunt Lake, summer, 2020), pike from Teletskoye Lake (1.25 g/ml, P=0.034–0.045, summer, 2020), pike from Baunt Lake (1.18 ± 0.09 g/ml, P=0.005–0.049, summer, 2020), burbot (1.18 ± 0.04 g/ml, P=0.0001–0.047 and 1.21 ± 0.03 g/ml, P=0.0001–0.037, Baunt Lake, summer, years 2020 and 2021, respectively), whitefish TL (1.10 ± 0.01 g/ml, P=0.0001–0.049, summer, 2020), whitefish BL (1.18 ± 0.02 g/ml, P=0.0001–0.046, summer, 2020), and whitefish BL sp. (1.27 ± 0.16 g/ml, P=0.005–0.045, summer, 2020) compared to other freshwater fishes (Figure 2; Supplementary Table S1). For a few fish species such as Prussian carp (anterior intestine), crucian carp (posterior intestine), and whitefish TL (stomach) significant positive correlations were found between the density of bile and gut fullness, whereas in all other cases there was no significant correlation (Supplementary Table S3, 3.1.2c).
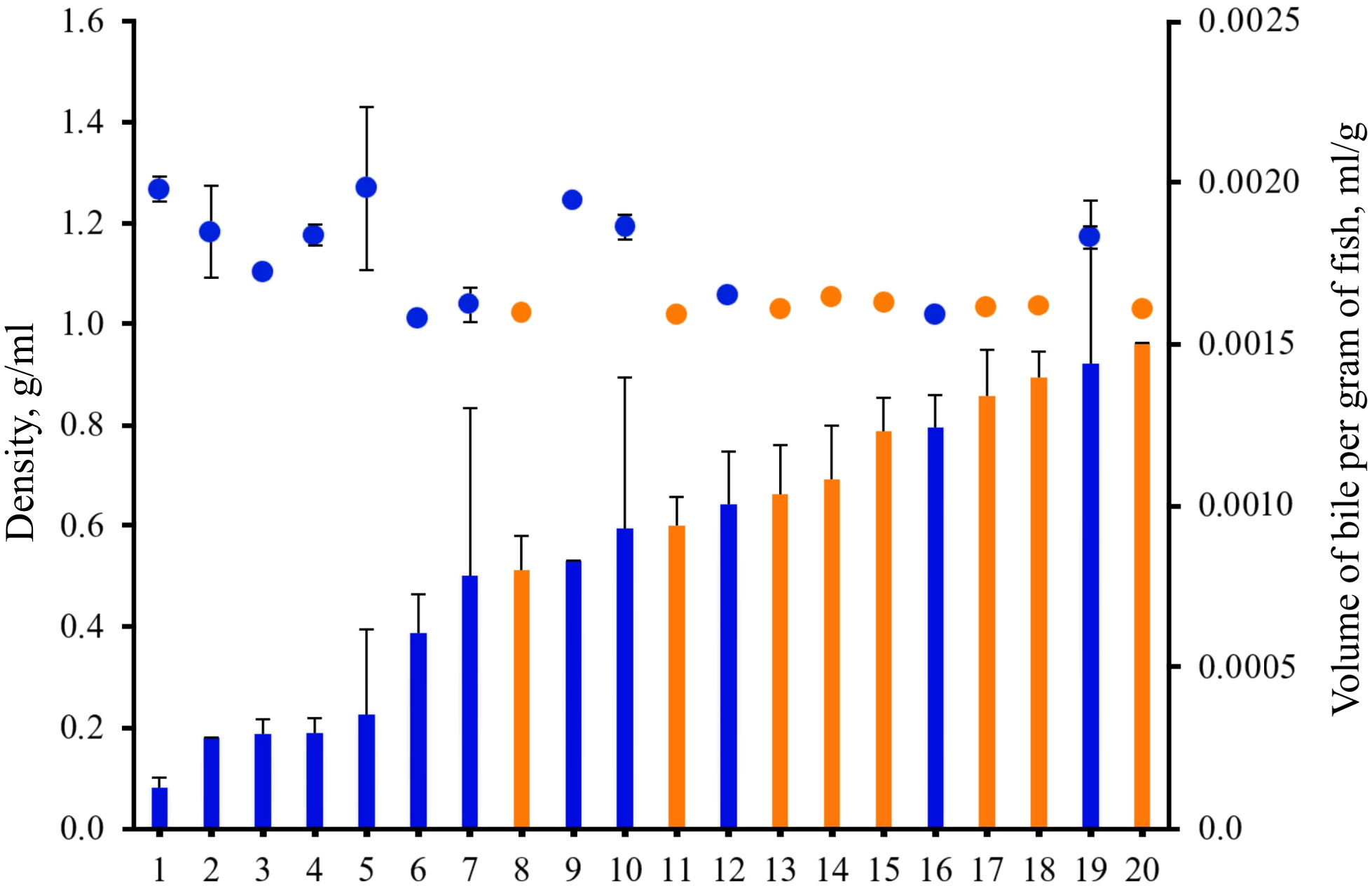
Figure 2 The volume of bile per gram of fish (bars) and density (colored circles) of bile of gastric (G) and agastric (A) freshwater fish species. Orange – agastric (without stomach). Blue – gastric (with stomach). The data are presented as a mean ± standard error (SE). 1 - Gymnocephalus cernuus, Baunt Lake (G); 2 - Esox lucius, Baunt Lake (G); 3 - Coregonus lavaretus pidschian, Teletskoye Lake (G); 4 - C. l. pidschian, Baunt Lake (G); 5 - C. l. sp. Baunt Lake (G); 6 - Sander lucioperca, Chany Lake (G); 7 - C. l. pravdinellus, Teletskoye Lake (G); 8 - Abramis brama, Chany Lake (A); 9 - E. lucius, Teletskoye Lake (G); 10 - Lota lota, Baunt Lake (G); 11 - Carassius carassius, Chany Lake (A); 12 - Perca fluviatilis, Baunt Lake (G); 13 - C. gibelio, Baunt Lake (A); 14 - Rutilus rutilus, Baunt Lake (A); 15 - Leuciscus idus, Chany Lake (A); 16 – P. fluviatilis, Chany Lake (G); 17 - Cyprinus carpio, Chany Lake (A); 18 – C. gibelio, Chany Lake (A); 19 - Cottus sibiricus, Teletskoye Lake (G); 20 - L. leuciscus, Baunt Lake (A).
There were significant differences for density (one-way PERMANOVA, P=0.0001, F=87.3) and the volume of bile per gram of fish (one-way PERMANOVA, P=0.0001, F=48.3) and between gastric and agastric fishes (factor is “gut morphology”) (Figure 2).
3.1.3 The effect of fullness, feeding habits, and gut morphology on bile mass
Significant effects of “gut morphology” and “feeding habits” (one-way PERMANOVA) on the relative wet mass of bile were found for almost all analyzed fish groups (Supplementary Table S3, 3.1.3a), (for the fishes from Baunt Lake in the year 2021 the effects of these factors were not significant, one-way PERMANOVA, P>0.05). It was found that the relationship between “gut fullness” and the relative wet mass of bile depended on such factors as “season”, “year”, and “fish species” (Supplementary Table S3, 3.1.3b). For example, the level of fullness of any part of the gut did not significantly affect the relative wet mass of bile of European perch. Although, in the case of whitefish BL, the level of fullness in the posterior intestine significantly affected the relative wet mass of bile, whereas in the stomach and in anterior intestine such effects were not found. At the same time, the level of fullness in both anterior and posterior intestines significantly affected the relative wet mass of bile of ide and crucian carp. In the case of Prussian carp, the relationships between these two variables (fullness and relative wet mass of bile) were affected by season and year, but in general was significant for both anterior and posterior intestines.
3.2 Composition of major and trace elements in fish bile
3.2.1 Marine species
3.2.1.1 Major elements
The concentrations of all studied elements are given in Supplementary Table S4. For all studied samples of fish bile, the highest concentrations were found for Na (37815 – 47316 ± 1178 mg/kg) and S (34041 – 46185 ± 2408 mg/kg). Moreover, the differences in concentrations between Na and S were absent for the majority of studied fish species (with the exception of Atlantic cod one-way PERMANOVA, P>0.05). Significantly lower concentrations were registered for Ca (493 – 2732 ± 137 mg/kg), K (2289 ± 84 – 4615 mg/kg), Mg (336 – 585 ± 81 mg/kg) and P (240 – 650 ± 75 mg/kg). According to one-way PERMANOVA, there are significant differences among the major elements studied, whereas between P and Mg the significant differences (P>0.05) were absent for all studied fishes. The interspecific variability in concentration of major elements was low and significant differences were almost absent (one-way PERMANOVA, P>0.05). However, based on one-way PERMANOVA analysis, significant difference was found for concentrations of Ca and K between Atlantic cod and common dab, as well as for concentrations of K, Na, and P between navaga and common dab, and for concentrations of Mg and Na between navaga and Atlantic cod, and for concentrations of Na between Atlantic cod and shorthorn sculpin (Supplementary Table S3, 3.2.1.1a).
Based on correlation analysis, there were no significant relationships among concentrations of different major elements with few exceptions. Thus, significant positive correlations were found between Mg and P as well as between Na and S in bile of Atlantic cod, and between K and P in bile of navaga, and between K and Na in bile of shorthorn sculpin (Supplementary Table S3, 3.2.1.1b).
In general, there were no significant correlations between the concentrations of major elements and the gut fullness, but there were significant correlations between the concentration of K in bile and fullness of anterior intestine of Atlantic cod, as well as between the concentration of Mg in bile and posterior intestine of shorthorn sculpin (Supplementary Table S3, 3.2.1.1c).
3.2.1.2 Trace elements
Among twenty-two studied trace elements, the elements As, Cu, Li, Se and Sr were found in the bile of all studied fishes irrespective of their diets. At the same time, Bi, Mo and Sb were absent or below detection limit for the majority of studied fishes (Supplementary Table S4).
The correlation analysis has shown significant relationships between the following trace elements: Al and Mn (Atlantic cod and shorthorn sculpin), Ba and Sr (navaga and shorthorn sculpin), Ba and Zn (Atlantic cod and navaga), Ga and Tl (navaga and shorthorn sculpin), Se and Ti (Atlantic cod and shorthorn sculpin) (Supplementary Table S3, 3.2.1.2a).
In most cases, significant correlations were absent between the levels of gut fullness and the concentrations of trace elements in fish bile. However, in several cases such significant correlations were found: stomach fullness and Ni in bile of Atlantic cod; fullness of anterior intestine and Ti, posterior intestine and both Ga and Tl in bile of navaga; the fullness of anterior intestine and Mn, posterior intestine and Ba, Sr, Ti, and V in bile of shorthorn sculpin (Supplementary Table S3, 3.2.1.2b). The factor of “gut fullness” has never shown any statistically significant effects on the concentrations of trace elements in the studied marine fish species (one-way PERMANOVA, P>0.05).
3.2.1.3 Correlations between major and trace elements
There were significant correlations found for navaga between the following trace elements: Ca and Ba, Ca and Sr, Mg and Li, Mg and Ni, Na and As, S and Al, S and Ba, whereas for the species shorthorn sculpin significant correlations were found between: K and Li, K and Ni, Mg and Ba, Mg and Sr, Na and Li, P and Co, P and Cr (Supplementary Table S3, 3.2.1.3).
3.2.2 Freshwater species
3.2.2.1 Major elements
For all studied fishes, the highest concentrations, among all assayed elements, were found for Na (32335 ± 613 – 120026 ± 33316 mg/kg) and S (24420 ± 1090 – 65813 ± 9278 mg/kg) (Supplementary Table S4). Significantly lower concentrations were registered for Ca (1973 ± 52 – 6031 ± 897 mg/kg), K (1896 ± 298 – 10148 ± 4589 mg/kg), Mg (243 – 1191 ± 467 mg/kg) and P (70 ± 17 – 3092 ± 959 mg/kg). According to one-way PERMANOVA, there were no significant differences (P>0.05) between concentrations of Na and S for 90% of the studied fish species (Prussian carp, Chany Lake, spring 2019 and whitefish TL, Teletskoye Lake, summer 2019). Moreover, the differences in concentrations between K and Ca, P and Mg were absent or below detection limit for 67% of the studied fish species (one-way PERMANOVA, P>0.05) (Supplementary Table S3, 3.2.2.1a).
Positive significant correlations were found between Ca and Mg, Ca and Na, and K and Р for the majority of studied fish species. The relations found were noted for all studied fishes irrespective of season, year or lake where the samples were collected (Supplementary Table S3, 3.2.2.1b).
In many cases, significant correlations were not found between the levels of fullness of the segment of the gut (stomach, or/and anterior, or/and posterior intestines) and concentrations of major elements in fish bile. The concentration of K was an exception. It was found that the concentrations of K in the bile of Prussian carp, crucian carp and ide (Chany Lake, summer, 2019) were significantly correlated with the levels of fullness in the anterior and posterior intestines, and also for Prussian carp (Chany Lake, summer, 2020) in the bile and the posterior intestine (Supplementary Table S3, 3.2.2.1c).
The effect of factor “gut morphology” on the major element concentrations was assayed using all gastric and agastric fishes from Chany (summer, years 2019 and 2020; spring, 2020) and Baunt lakes (summer, years 2020 and 2021). Significant differences between gastric and agastric fishes for the concentration of Na were found for fishes from Chany Lake (summer, years 2019 and 2020; spring, 2020) and Baunt Lake (summer, 2021). For fishes from Chany Lake (summer, 2019) and Baunt Lake (summer, 2021) the significant differences for concentrations of Ca and K were also found. For fishes from Chany Lake (summer, 2019 and spring, 2020) significant differences for the concentrations of Mg were detected. In all other cases, a significant effect was not registered for P, S, Са or K (Supplementary Table S3, 3.2.2.1d).
3.2.2.2 Trace elements
Among twenty-two studied trace elements, such elements as Cu, Li, and Sr were found in the bile of all studied fishes irrespective of their habits, gut morphology, or diets (Supplementary Table S4). At the same time, Cd, Mo, and Tl were absent or below detection limit for the majority of studied fishes (Supplementary Table S4).
Among different trace elements, Fe and Mn correlated between each other in the majority cases. Also, in many cases significant correlations were found between the following trace elements: Al and Cu, Al and Fe, Al and Sb, Al and Tl, Ba and Mn, Co and Sr, Cr and Mn, Cr and Sb, Ga and Sr, Li and Mn, Li and Sr, Mn and Ni, Mn and Sr, Ni and Pb (Supplementary Table S3, 3.2.2.2a).
The factor “gut fullness” was almost never significant (one-way PERMANOVA, P>0.05) and there were no correlations (with few exceptions for Prussian carp and whitefish TL) with the concentrations of the studied trace elements (Supplementary Table S3, 3.2.2.2b).
3.2.2.3 Correlations between major and trace elements
In many cases, significant correlations were found between Sr (trace element) and such major elements as Ca and Mg. Moreover, in around 40% of the cases significant correlations were found between concentrations of P and Mn. Also, in around 30% of the cases significant correlations were found between Ca and As, K and Zn, Na and Sr, K and Li, Mg and Li, and S and Se. Also, in some cases we have registered significant correlations of Ca with Cd, Fe, Li, Mn, Zn; of K with Ba and Co; of Mg with As and Fe; and of Na with Bi and Li (Supplementary Table S3, 3.2.2.3).
3.2.3 Comparisons of Trace Elements between Freshwater and Marine Fishes
Statistically significant differences were established in the concentrations of both major elements and trace elements separately between the bile of marine and freshwater fish species, and when all elements were analyzed together (Supplementary Table S3, 3.2.3a). According to the discriminant analysis (Figure 3), the elements that made the greatest contributions to the separation of these two groups were: major elements – K, P, S and trace elements – Al, As, Cr, Li, Mo and Ti (Supplementary Table S3, 3.2.3b). In addition, among all the studied fish species, as a rule, the concentrations of K, Na, Al, As, Fe, Li, Mn, Se and Ti were higher by 50 percent or more in the bile of marine fish species than in the bile of freshwater ones. In contrast, the concentrations of Bi and Cu in the bile of freshwater fishes exceeded the concentrations of these elements in the bile of marine fishes by two folds or more.
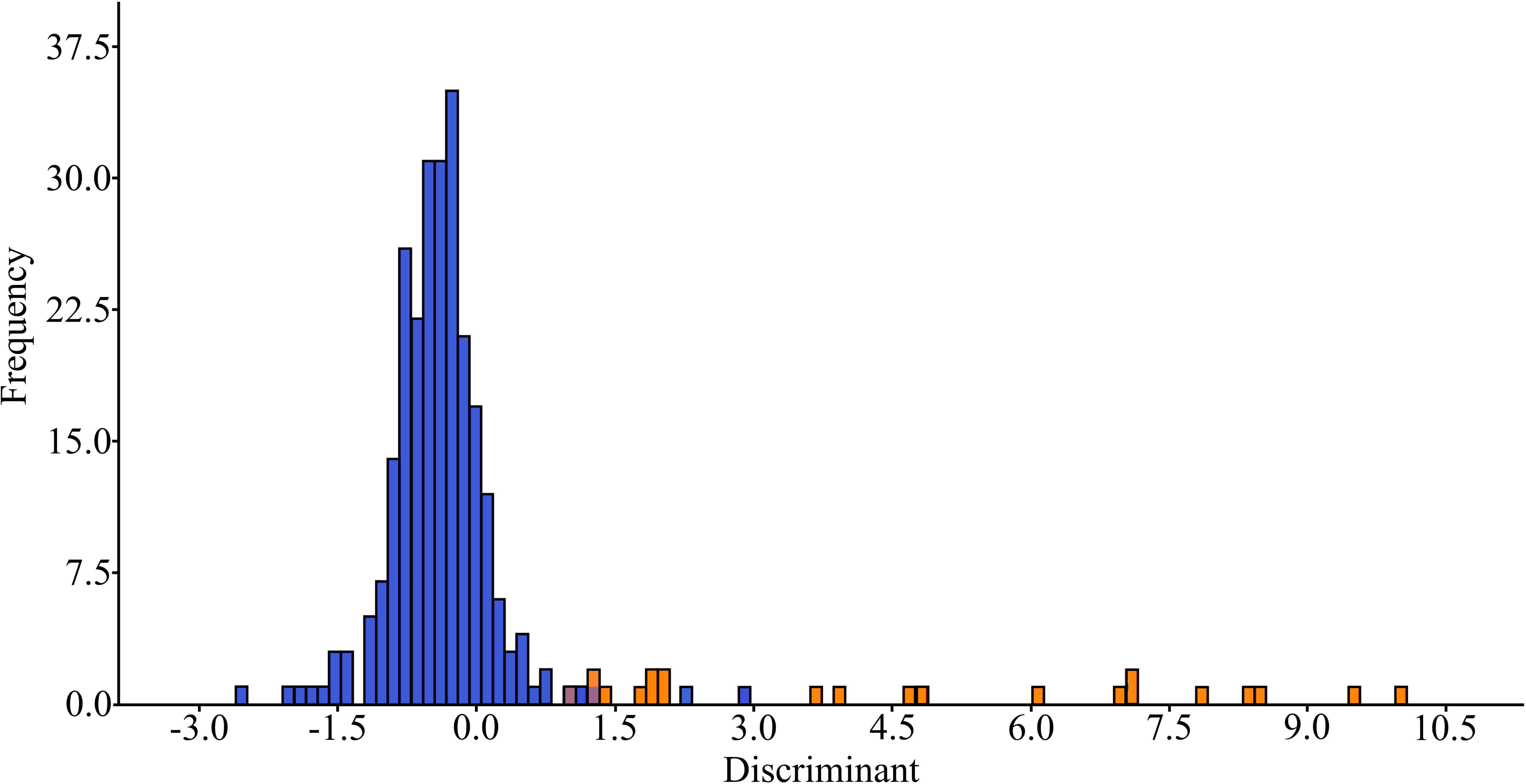
Figure 3 The discriminant analysis of the element composition of bile from marine (orange) and freshwater (blue) fish species.
3.3 Water and sediments
3.3.1 Major and trace elements in water
All concentrations of studied elements in water samples are given in Supplementary Table S5. Among all the analyzed elements in water sampled from different points on Chany Lake in the summer of the year 2020, the concentrations of the following elements were the highest: Na (153 ± 15 mg/kg), Ca (58 ± 2.3 mg/L), Mg (53 ± 5.4 mg/L), S (41 ± 11 mg/L), K (6.4 ± 0.6 mg/L), and Sr (0.86 ± 0.04 mg/L). Such elements as Mo, Pb, and Tl were absent or below detection limit in all samples of water from Chany Lake. Based on Principal Coordinate Analysis (PCoA) calculated with all studied major and trace elements, the samples № С4, С5, and С6 were grouped together. At the same time, the sample of water from point С3 (Fadikha Lake) was completely different from points С4, С5, and С6. The water samples from points С1 (Kargat River) and С2 (Chulym River) were similar to points С3, С4, С5, and C6 (Figure 4A).
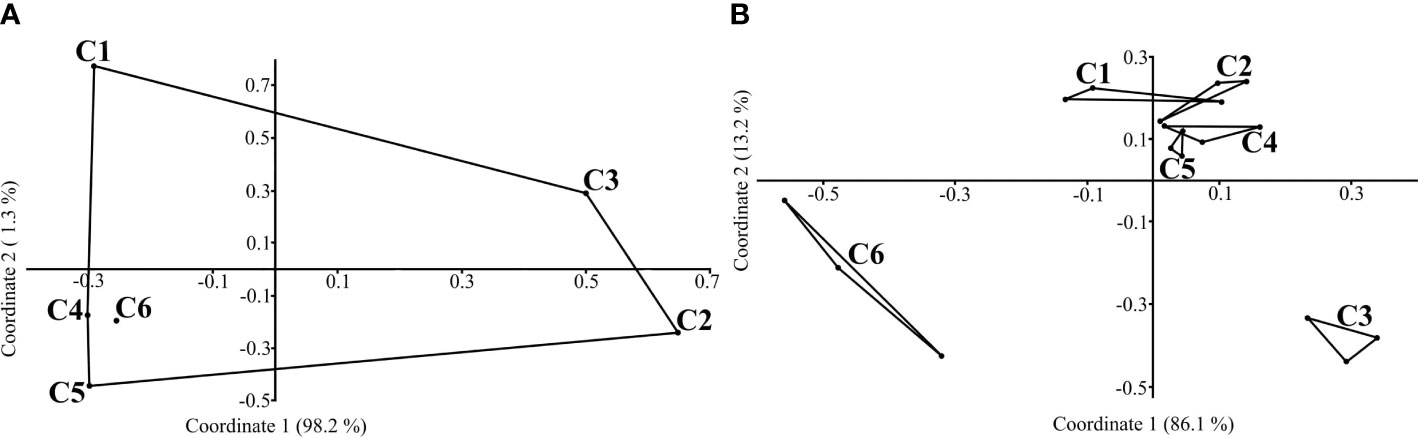
Figure 4 Principal Coordinate Analysis based on the element composition of water (A) and sediment (B) sampled in different points of Chany Lake (summer of 2020 year): C1 – Kargat River, C2 – Chulym River, C3 – Fadikha Lake, C4 – “Pier”, C5 – “Shilovskiy” Bay, C6 – Malye Chany Lake.
The concentrations of major and trace elements in water samples collected from Teletskoye and Baunt lakes were similar to each other, but more than five times lower if compared to water samples from Chany Lake. In the water samples from Teletskoye and Baunt lakes the highest concentrations were registered for Ca (14.3 ± 0.4 and 9.9 ± 0.9 mg/L, respectively), K (0.66 ± 0.02 and 0.55 ± 0.01 mg/L, respectively), Mg (2.50 ± 0.3 and 1.05 ± 0.06 mg/L, respectively), Na (1.51 ± 0.02 and 1.63 ± 0.18 mg/L, respectively), S (1.33 ± 0.05 and 1.71 ± 0.10 mg/L, respectively), Sr (0.06 ± 0.07 mg/L, Teletskoye Lake), and Fe (0.15 ± 0.01 mg/L, Baunt Lake). Such elements as As, Mo, Pb, Sb, Tl and Zn were absent or below detection limit in all water samples from both Teletskoye and Baunt lakes.
In the sample of water from Kandalaksha Gulf (White Sea), the highest concentrations were found for Na (7098 mg/L), Mg (1009 mg/L), S (682 mg/L), Ca (281 mg/L), K (239 mg/L), and Sr (5.4 mg/L). The concentration of these elements in water from Kandalaksha Gulf was the highest if compared to water from the studied lakes.
3.3.2 Other hydrochemical features of water
All studied hydro-chemical parameters of water samples are given in Supplementary Table S6. The highest pH values were measured in water sampled from Chany Lake and Kandalaksha Gulf (White Sea) – 8.89 ± 0.13 and 8.0 ± 0.1, respectively; whereas in water from Teletskoye Lake (7.81 ± 0.21) and Baunt Lake (7.26 ± 0.15) these values were lower. The highest levels of CO3- (20.5 ± 5.7 mg/L), HCO3- (313.5 ± 19.1 mg/L), specific conductivity (1228 ± 112 μS/cm), mineral P (0.058 ± 0.014 mg/L), and total P (0.069 ± 0.015 mg/L) were found in water from Chany Lake. The quantity of Cl- in water samples ranged from a minimum level in Teletskoye (2.24 ± 0.19 mg/L) and Baunt (2.21 ± 0.25 mg/L) lakes to the maximums in Chany Lake (189.0 ± 28.8 mg/L) and Kandalaksha Gulf (White Sea) (14135.8 ± 50.5 mg/L). The concentrations of NO2- were similar among water samples from Teletskoye Lake, Baunt Lake, and Kandalaksha Gulf with average values of 0.0081 ± 0.0042 mg/L; whereas in water from Chany Lake this value was lower and reached only 0.0013 ± 0.0008 mg/L. The highest values for NO3- were found in water samples from Teletskoye Lake (1.07 ± 0.12 mg/L) and Kandalaksha Gulf (1.10 mg/L), whereas for NH+4 the highest values were from water collected at Chany Lake (0.43 ± 0.16 mg/L).
3.3.3 Major and trace elements in sediments
All concentrations of studied elements in sediment samples are given in Supplementary Table S5. Among all the analyzed elements in sediments sampled from different points on Chany Lake in the summer of the year 2020, the concentrations of the following elements were the highest: Al (4909–47210 mg/kg), Ca (6625–52255 mg/kg), Fe (3966–39690 mg/kg), K (952–10684 mg/kg), Mg (1411–16016 mg/kg), S (619–21703 mg/kg), and Ti (582–2307 mg/kg). At the same time, such elements as Bi, Mo, Se, Sb, and Tl were almost absent or below detection limit in sediments. Based on the PCoA, the sample points С1, С2, С4, and С5 (all are parts of rivers) were grouped together whereas the sample points С3 (Fadikha Lake) and С6 (Chany Lake) were grouped separately (Figure 4B).
The average concentrations of different elements from Teletskoye Lake sediment samples were lower if compared to Chany Lake sediment samples, but higher than in Baunt Lake sediment samples. In both Teletskoye and Baunt lakes, such elements as Al (20719 ± 3779 and 3921 mg/kg, respectively), Ca (7500 ± 2154 and 3853 mg/kg, respectively), Fe (25901 ± 3031 and 3989 mg/kg, respectively), Mg (11364 ± 990 and 530 mg/kg, respectively), P (555 ± 47 and 182 mg/kg, respectively), and Ti (1420 ± 288 and 2026 mg/kg, respectively) had the highest concentrations, whereas such elements as Bi, Cd, Mo, Se, and Tl in sediments samples from these lakes were absent or below detection limit.
3.4 The effects of different biotic and abiotic factors on element composition of fish bile
3.4.1 The effect of sampling season and year
3.4.1.1 The effect of factor “season”
For crucian carp the factor “season” was significant for major elements, trace elements, and when all elements were analyzed together (Figure 5). At the same time, the factor “season” did not affect the concentrations of all elements analyzed together, as well major elements and trace elements separately, in bile of ide and common carp (Supplementary Table S3, 3.4.1.1a).
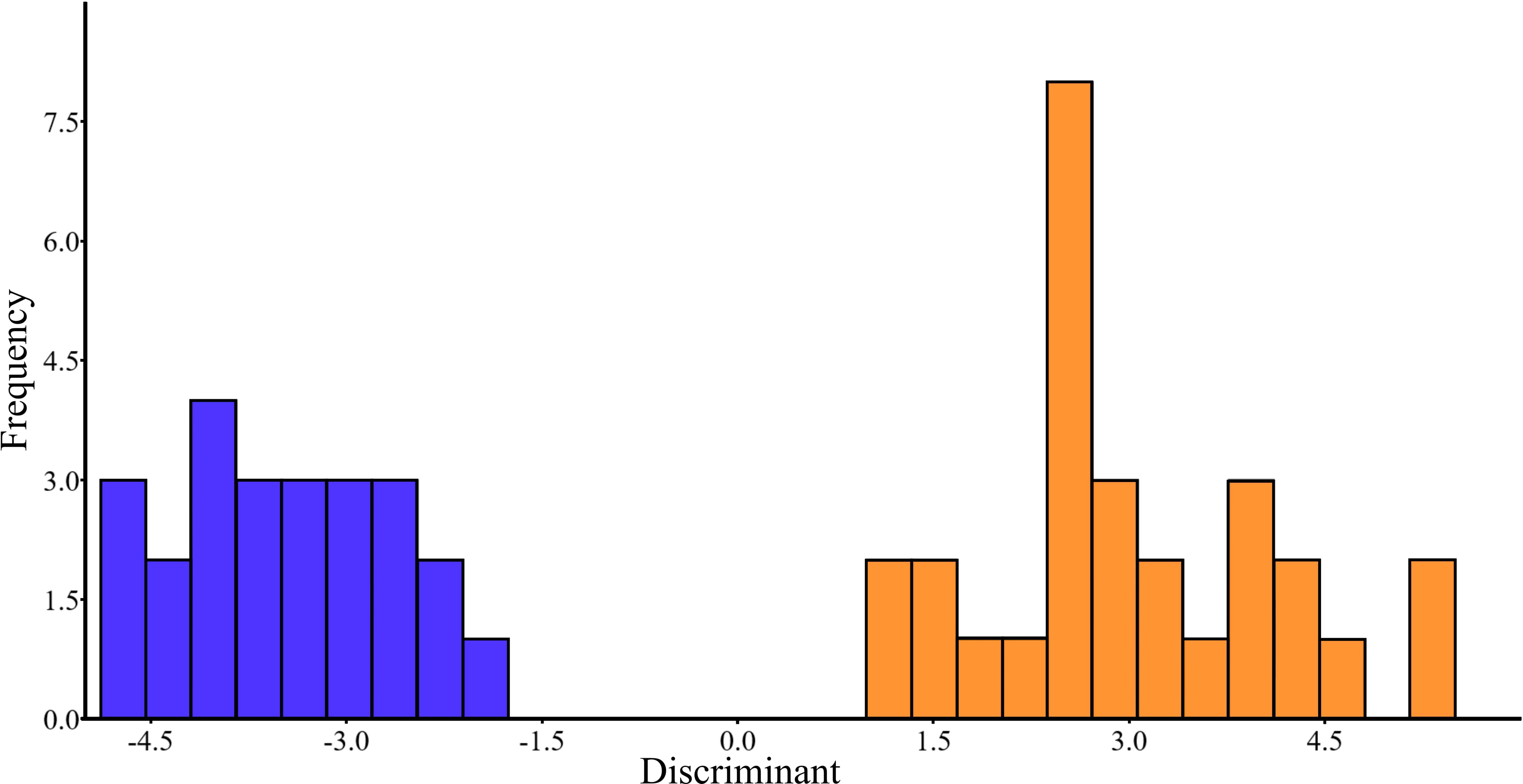
Figure 5 Seasonal changes in the element composition based on discriminant analysis of bile of C. gibelio obtained in spring (blue) and summer (orange) of 2019 year.
The Forward Stepwise of General Discriminant Analysis Model (GDA) permitted us to estimate which elements discriminate the studied fishes dependent on the factor “season”. The highest input in differentiation of elements for bile samples of Prussian carp collected during the spring and summer seasons was related to major elements such as Na and S, as well as to trace elements such as Ba, Bi, Co, Mn, and Zn (Supplementary Table S3, 3.4.1.1b).
3.4.1.2 The effect of factor “year”
Based on one-way PERMANOVA, significant interannual variability was found for trace elements of bile of Prussian carp, crucian carp, ide and European perch. Such significant interannual relationships found for all analyzed fishes from Chany Lake was never detected for fishes from Teletskoye Lake and Baunt Lake. At the same time, significant interannual variability was not found for major elements as well as when all elements were analyzed (major and trace elements together) in bile of any studied fishes with one exception: Prussian carp from Baunt Lake that demonstrated significant interannual variability for major elements (Supplementary Table S3, 3.4.1.2a).
According to GDA only the following trace elements were important: Bi, Cd, Ga and Li for Prussian carp; Bi and Li for crucian carp; Al, Co, Pb and Zn for ide; Bi, Cd, Cr, Ga, Mn, and Ti for European perch (Supplementary Table S3, 3.4.1.2b).
3.4.1.3 The effect of factors “season” and “year”
Based on discriminant analysis of element composition of bile, all fish species inhabiting Teletskoye, Chany, and Baunt lakes were grouped according to their lake if these fishes were obtained in the same time (season and/or year) (Figure 6). Moreover, according to the same analysis, the “season” was a grouping factor for different species caught in different years, but in the same season in Chany Lake (Figure 6C). According to two-way PERMANOVA, the factors «year» and «season» significantly affected the composition of all major elements and trace elements together as well as separately in bile of fishes from Chany Lake. According to the discriminant analysis, for the fish species caught in Teletskoye (trace elements) and Baunt (both trace and major elements) lakes the grouping factor was the “year” of sampling (Figures 6A, B). According to the one-way PERMANOVA, for the same fish species from Teletskoye and Baunt lakes the factor “year” of sampling was also significant (Supplementary Table S3, 3.4.1.3).
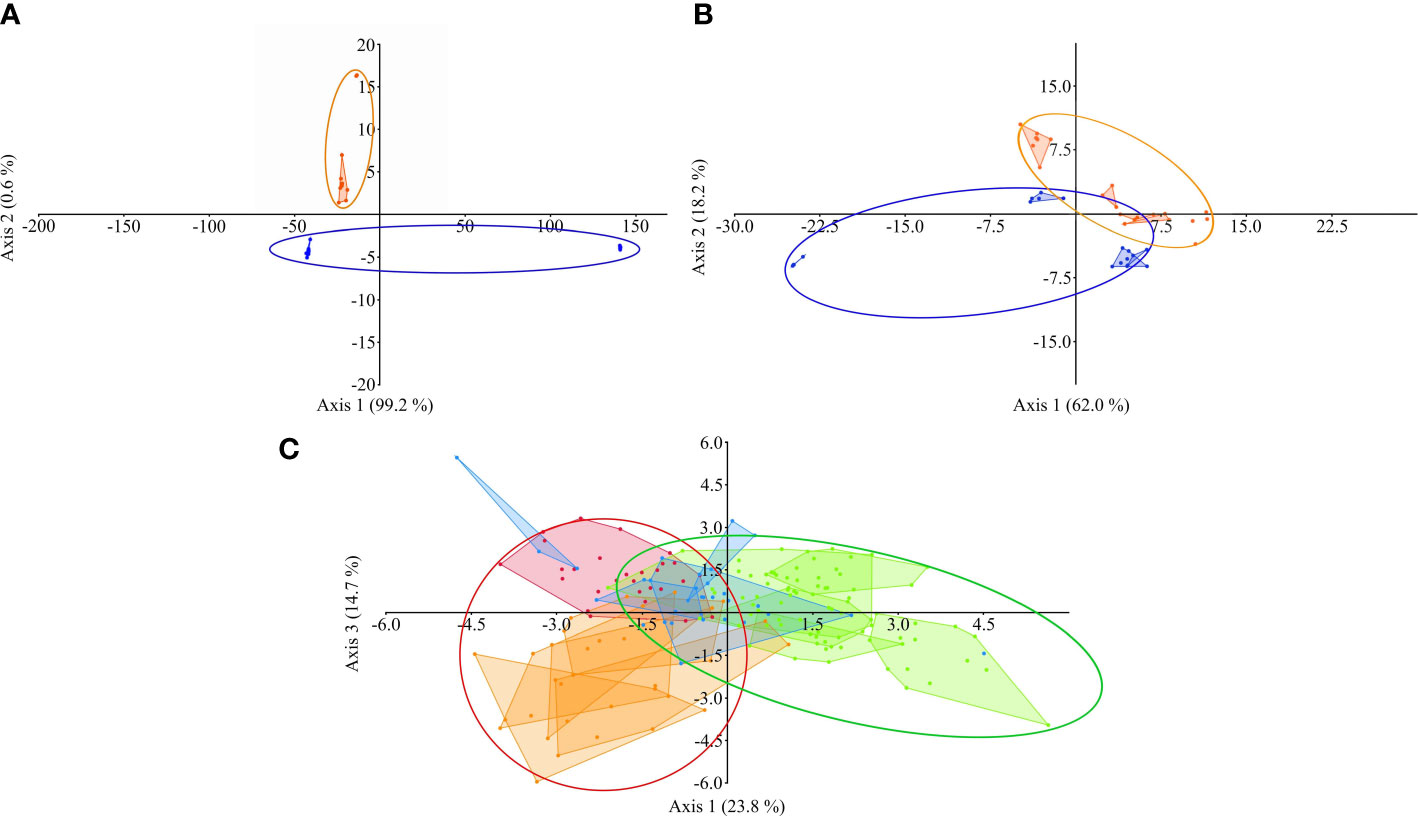
Figure 6 The discriminant analysis of the element composition of bile from all studied fish species obtained during the entire period of observations from Teletskoye Lake (A), blue figures – fishes obtained in 2019, orange figures – fishes obtained in 2020; Baunt Lake (B), blue figures – fishes obtained in 2020, orange figures – fishes obtained in 2021; and Chany Lake (C), red figures – fishes obtained in spring of 2019, orange figures – fishes obtained in spring of 2020, green figures – fishes obtained in summer of 2019, blue figures – fishes obtained in summer of 2020. For Teletskoye and Baunt lakes the ellipses designate the grouping effect of factor “year”. For Chany Lake the ellipses designate the grouping effect of the factor “season” for fish species obtained in the same season, but in different years (red – spring, green – summer).
3.4.2 The effect of factor “lake”
In contrast to the interannual variability, the factor “lake” significantly affected the variability for both major and trace elements. Thus, this significant effect was found for concentrations of trace and major elements as well as when all elements were analyzed together in bile of Prussian carp (Chany Lake, summer, 2019 by Baunt Lake, summer, 2020; Chany Lake, summer, 2019 by Baunt Lake, summer, 2021; Chany Lake, summer, 2020 by Baunt Lake, summer, 2020) and European perch (Chany Lake, summer, 2019 by Baunt Lake, summer, 2020; Chany Lake, summer, 2019 by Baunt Lake, summer, 2021). For whitefish (C. l. pidschian), significant differences between lakes were found only for trace elements (Teletskoye Lake, summer, 2019 by Baunt Lake, summer, 2020), whereas for whitefish from summer of year 2020 of the same lakes the differences were not significant (Supplementary Table S3, 3.4.2a).
According to GDA, the discriminant elements for Prussian carp were the following: major elements – Ca, Na, Mg, and P and trace elements – As, Ba, Ga, Li, and Sr. Ca, Li, and Sr were the elements with maximum weight in differentiation of Prussian carp between Chany Lake and Baunt Lake. In contrast, for European perch and whitefish the discriminant elements were trace elements only and represented by As, Ba, Li, Se and Bi, Cd, Pb, V, and Zn, respectively. Li, Ba, As and Pb, V, Bi were the elements with maximum weight in differentiation of European perch and whitefish TL and BL, respectively (Supplementary Table S3, 3.4.2b).
3.4.3 The effect of factor “feeding habits”
Based on one-way PERMANOVA, the factor “feeding habits” (carnivorous by non-carnivorous) significantly affected the concentrations of the studied major elements and trace elements, as well as when all elements were analyzed together in bile of fish from Chany Lake (summer, 2019) (Figure 7). In all other cases, the factor “feeding habits” either did not affect the concentrations of elements at all (Chany Lake, spring, 2020; Baunt Lake, summer, 2020), or only affected major elements, and when all elements were analyzed together, but not for trace elements (Chany Lake, summer, 2020; Baunt Lake, summer, 2021) (Supplementary Table S3, 3.4.3a).
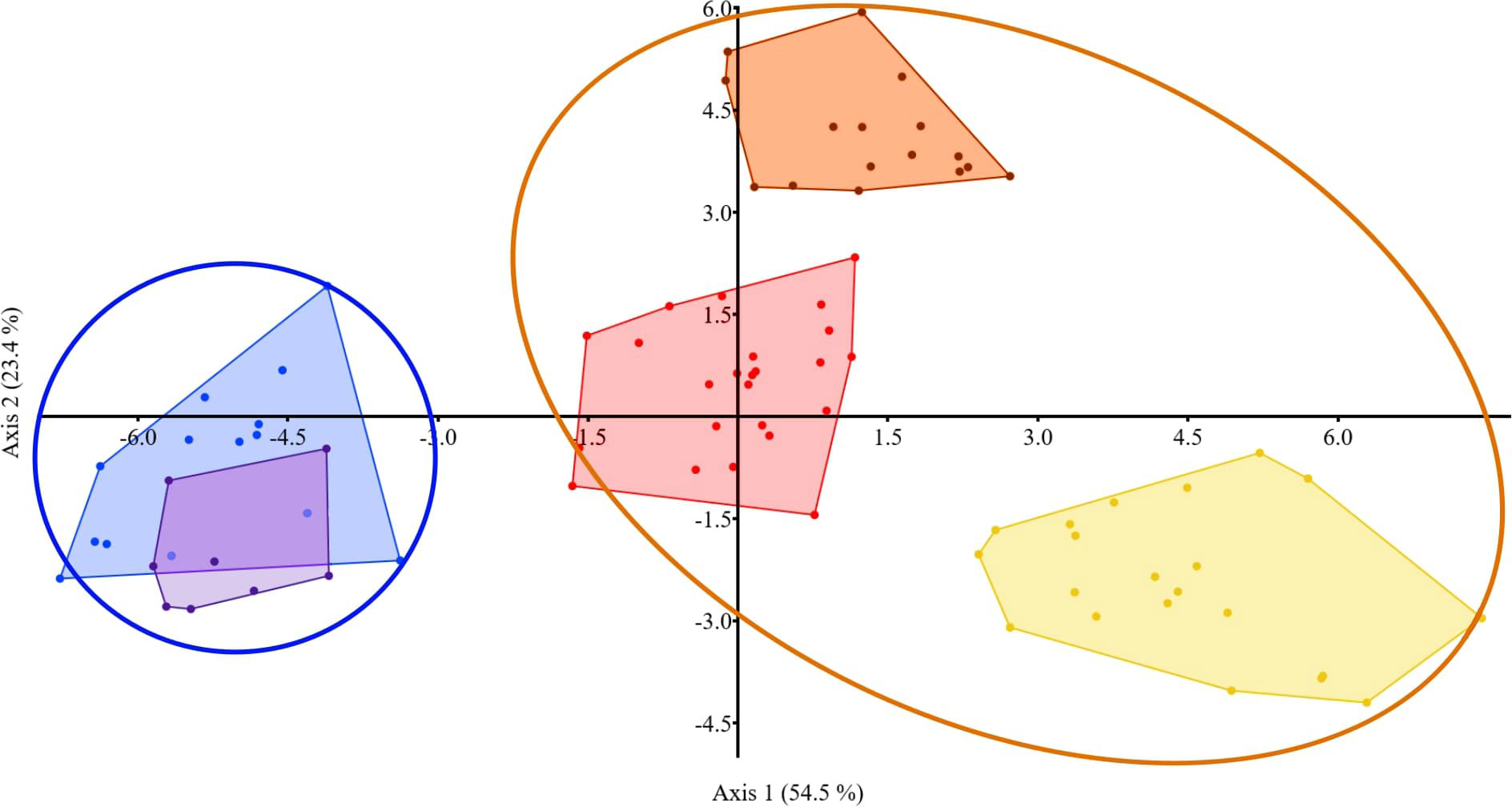
Figure 7 The discriminant analysis of element composition of bile from carnivorous and non-carnivorous fish species obtained from Chany Lake (summer of 2019). The ellipses show carnivorous (blue) and non-carnivorous (orange) fishes. Non-carnivorous fishes: orange – C. carassius, red – C. auratus, yellow – L. idus; carnivorous: violet – S. lucioperca, blue – P. fluviatilis.
According to the GDA, such major elements as Ca, Na, P, and S and trace elements such as Ba, Cr, Cu, Sr, and V were elements that discriminated between “carnivorous” and “non-carnivorous” fishes (Chany Lake, summer, 2019) (Supplementary Table S3, 3.4.3b).
4 Discussion
4.1 Volume, mass and density of bile
The bile of fishes, like that of most vertebrates, is a complex liver secretion consisting of water with dissolved organic and inorganic substances, which performs the function of binding lipids in the intestine for further hydrolysis by lipase (Boyer, 2013). In the present study we have found that the bile volume varies in a wide range among different fish species as well as among different individuals of the same species. The intraspecific variability is mainly explained by differences in body mass of fish. Indeed, the factors that determine the relative volume of fish bile include fish size, age, and gut fullness (Vethaak et al., 2016). We have found that the volume of bile per gram of fish was significantly and negatively correlated with gut fullness of fish. The similar negative correlation between gut fullness and bile volume were obtained for rainbow trout under experimental conditions (Grosell et al., 2000). Since during periods of starvation the bile is accumulated in the gallbladder and then released into the intestine after fish resume to feed, thus leading to a negative correlation between gut fullness and bile volume (Aldman and Holmgren, 1987).
The density of fish bile has been characterized with a low level of inter- and intraspecific variabilities. The majority of studied marine and freshwater fishes had a density of bile around 1.1 g/ml. The available information associated with density of fish bile is nearly absent. For other vertebrates the values of bile density were similar with results obtained for fishes in the present study: pigs - 1.015-1.033 g/ml (Nguyen and Obara, 2020) and humans – around 1.00 g/ml (Mushtak Al-Atabi Ooi et al., 2012) and 1.007-1.035 g/ml (Sablin et al., 2002). At the same time, for several gastric non-carnivorous (Siberian sculpin, ruff, whitefish BL, whitefish TL) and carnivorous fishes (pike and burbot) the density of bile was higher and ranged from 1.17 to 1.27 g/ml. It was shown that the bile density is apparently dependent on the feeding status of fish, since some chemical compounds like proteins are accumulated in bile during periods of starvation that may increase the bile density (Collier and Varanasi, 1991). In the present study, we did not find relationships between the levels of gut fullness and bile density due to the differences in individual feeding activity among those collected. Moreover, under natural conditions we are unable to control the exact time when fish feed, number feeding acts, and total amount of food consumed, whereas under experimental condition all these variables are strongly controlled. Moreover, fish may regurgitate some part of their food from the stomach or anterior intestine (agastric species) in fish nets. Hence, these limitations may mask the possible presentation of relationships between bile density and gut fullness.
In general, based on obtained results, we hypothesize that gastric fishes required relatively low volume of bile, but with higher density if compared to agastric fishes that mainly possessed relatively large volumes of bile, but with a lower level of density (Figure 2). It has been shown that the relative length of intestine is higher for agastric and non-carnivorous fish if compared to gastric and carnivorous ones. Thus, for gastric fish it is apparently more important to secrete relatively low volume, but high density bile in a shorter intestine, by comparison to agastric fish with a relatively longer intestine for which a larger volume of bile with lower density is more physiologically appropriate. This relation between gut length and bile volume helps to fill the space within the gut with a proportionate bile volume along its entire length. At the same time, we did not find significant relationships between the volume of bile and body weight of fish with higher density of bile within the group of gastric fishes; whereas for agastric fishes such positive significant correlations were found. Perhaps, large agastric fishes require more volume of bile with higher density than small ones. In order to clarify these relationships further studies are needed.
4.2 Element composition of bile
4.2.1 Major elements
We have estimated the concentration of major elements in bile of 429 specimens of marine and freshwater fishes. While the concentration of studied major elements had been characterized by inter- and intraspecific variations, the ratios of these elements were stable. Thus, the concentration of S and Na was similar and formed more than 90% from total concentration of all elements for all studied fish species. If the relatively high concentration of S is related to function, the concentrations of this element could be due to S forming covalent, hydrogen, and disulfide bonds in the protein molecules that could be present in fish bile, while the relatively high concentration of Na is apparently related to osmoregulatory function of the fish gallbladder (Hirano and Bern, 1972). Studying the concentration of major elements in bile of 25 fish species Hunn (1976) has found that Na had the highest concentration when compared to concentrations of Ca, K, and Mg. Higher concentration of Na, if compared to K and Ca, have also been obtained for 23 fish species from the Amazonian region (Baldisserotto et al., 2004). A similar relationship was found in the bile of the Japanese silver carp (Carassius auratus langsdorfii), while the concentration of Na in the gallbladder was more than five times higher than in different analyzed tissues and organs (Yamazaki et al., 1996). Due to the concentration of Na in the gallbladder it is also significantly higher than in liver and plasma of blood; the main process of Na accumulation is related to the gallbladder (Hirano and Bern, 1972; Yamazaki et al., 1996; Grosell et al., 2000). Regarding to other major elements, we have found that the sum of concentrations of Ca and K as well as Mg and Fe for studied fish species was around 8 and 2% respectively. Similar results for Ca, K, and Mg were also supported by other studies (Hunn, 1969; Hunn, 1976; Hunn and Christenson, 1977; Grosell et al., 2000; Baldisserotto et al., 2004). Moreover, the concentration of Na, K, and Mg was significantly higher in bile from gallbladder if compared to hepatic bile (Grosell et al., 2000).
4.2.2 Trace elements
The concentrations and ratios of different trace elements are characterized by much broader ranges of inter- and intraspecific variations if compared to major elements in fish bile. In several studies it was shown that such high levels of variability are related to a number of internal (biochemical pathways for accumulation and elimination of different elements) and external factors (ratios and concentrations of elements in water, sediments, and food items) affecting fishes (Allen, 1995; Andréasson and Dave, 1995; Nigussie et al., 2010). It has been shown that the concentration of trace elements (Pb, Mn, Cu, Hg) depends on the length of time passed after these elements were consumed (Klaassen, 1976). This relationship, caused by the constant renewal of bile and the subsequent elimination of the metal with faeces, may also affect the concentrations of trace elements in the bile among individuals of different fish species. Further, in the case of Hg it has been shown that the inorganic form of elemental Hg and methyl-Hg are absorbed in tissues differentially from water or phytoplankton since the partitioning of these two forms occurs first in the phytoplankton themselves. Thus, grazing on phytoplankton can be a determinant in whether elemental Hg is present or there is accumulation of methyl-Hg in the tissues (Mason et al., 1995). However, despite these differences in concentrations of elements, in the present study the bile of most of the marine and freshwater fish species were dominated by Al, Cu, Fe, Sr, and Zn, when compared to other analyzed trace elements. It is known that the secretion of bile is a key physiological mechanism for elimination of divalent metals such as Cu, Fe, and Zn (Boyer, 2013). This fact explains the accumulation these trace elements in fish bile in the present study. For instance, the accumulation of Cu in the bile by active transport was shown for rainbow trout (Oncorhynchus mykiss) (Andréasson and Dave, 1995), Lebranche mullet (Mugil liza) (Hauser-Davis et al., 2012) and pikeperch (Nabavi et al., 2012). It was also shown that Zn is accumulated in bile of rainbow trout (Andréasson and Dave, 1995), and pikeperch (Nabavi et al., 2012). Moreover, in the bile of pikeperch Zn was found in high concentrations, second only after Fe (Nabavi et al., 2012), and being an essential element for health and homeostasis there are several isoforms of transporters for its active intracellular accumulation (Andree et al., 2004); this is also the case for various ion-specific transporters. Regarding the biochemical pathways of accumulation of Al and Sr in fish bile there are only a limited number of studies. Thus, it has been shown that both elements are accumulated by fish gill and intestine (Sánchez-Aceves et al., 2021), although, significant levels of these elements have also been registered in fish bile (Yamazaki et al., 1996). In the present study, we have found the relatively high concentrations of Al, Fe, and Sr in water and sediments of the lakes. Thus, we may assume that the water and sediments are possible sources of these elements accumulated by fish via active transport and/or feeding.
At the same time, Mn, Cr and Pb, although they were found in concentrations lower than a number of the aforementioned trace elements, also tend to accumulate in bile through active transport. Thus, the rapid accumulation and elimination of Mn in the bile was previously described in several studies conducted under experimental conditions on rats and cats (Klaassen, 1976). But despite the fact that the bile excretion of Mn is the main route of its elimination from the body (Aschner and Aschner, 2005), this element is not able to accumulate in the food webs of aquatic ecosystems (Suedel et al., 1994), which may cause its reduced concentrations in the bile of the studied fishes. The accumulation of Cr in bile for its further elimination from the body is also related to bile to a great extent (Norseth et al., 1982), which was previously shown for fish (Seymore et al., 1996). However, as for Mn and Cr accumulation with increasing trophic levels is not observed in aquatic ecosystems, which explains the low concentrations of these elements in fish (Suedel et al., 1994). Bile excretion of Pb was previously established under experimental conditions with intravenous administration into the body of sheep and rats (Klaassen, 1976). In a number of studies, relatively high concentrations of Pb were found in blood and tissues in comparison with the concentration of this element in the bile of the studied fish (Andréasson and Dave, 1995; Nigussie et al., 2010; Nabavi et al., 2012). However, in aquatic ecosystems, the accumulation of Pb in hydrobionts is more associated with the absorption of this element from water and bottom sediments than from food items (Suedel et al., 1994). Thus, despite the fact that the bile of fish is likely to have a mechanism for the active accumulation of Pb, its concentrations in the environment (water and sediments from the studied water bodies) were quite low to be detectable in the bile of studied fishes.
On the other hand, low concentrations of some other trace elements found in the present study are most likely associated with the peculiarities of the mechanism of their transfer into bile. So, although Ba is able to accumulate in the bile of rats in a small amount, further, the concentration of this element rapidly decreases even immediately after its introduction into the body (Edel et al., 1991). This may be a reason for the low concentrations of Ba in fish bile found both in the present and previous studies (Yamazaki et al., 1996).
The main role in the accumulation and elimination of Co, V and Ni in vertebrates (humans, rats, sharks) is played by the kidneys, while bile, although it can serve as a pathway for the elimination of these elements, accumulates them only in negligible amounts (Sabbioni et al., 1981; Susnea and Weiskirchen, 2016; González-Montaña et al., 2020; Squadrone et al., 2022). In previous studies aimed at establishing the elemental composition of fish bile, as well as in the present work, reduced concentrations of Co were found (Yamazaki et al., 1996; Nigussie et al., 2010), which confirms the low ability of fish to accumulate this element in their bile. The same pattern can also be seen with V and Ni, whose concentrations in the bile of the studied fishes were low. In addition, lower concentrations of Li, in comparison with the concentrations of this element in other organs and tissues (muscles, kidneys, gills and brain), were previously found in the liver of several fish species (Thibon et al., 2021), while the concentration of Ga was not detected in shark liver (Squadrone et al., 2022). These data may indicate a low degree of accumulation of these elements in fish bile as seen in the present study for concentrations of Li and V.
In bile of the majority of freshwater fish species we did not find Cd, Mo, and Tl, whereas in bile of marine fishes such elements as Bi, Mo, and Sb were also not detected. Under experimental conditions, it was shown that a significant increase of concentration of Cd in the bile of tilapia (Oreochromis aureus) could be found after being subjected to exposure to a high concentration of this element in water during one week (Allen, 1995). Similar results were found for Cd in the bile of rainbow trout when concentration of Cd was progressively increased to a high concentration in water (Andréasson and Dave, 1995). In water and sediments from Chany, Teletskoye, and Baunt lakes we have registered Cd only in very low concentrations that are likely to not be enough for accumulation in fish bile in detectable amounts. There are no available data for fish regarding the accumulation of Mo, Bi, and Tl.
The information regarding the role of bile in the accumulation of Se and Ti in the body of fish is absent while it is known that Se is able to be transported in aquatic ecosystems through food webs (Suedel et al., 1994). In addition, the absorption of this element by fish is possible from water and bottom sediments (Suedel et al., 1994; Barwick and Maher, 2003). Thus, the reduced concentrations of Se in the bile of the studied fish may be due to the low concentration of this element in environmental compartments found in the present study. The concentrations of Ti, although low in water, in bottom sediments exceeded the concentrations of many other elements, but this trace element had not accumulated in the bile of most of the studied fish. This may be due to many factors such as the lack of mechanisms for the transfer of Ti to the liver, and/or the low ability to accumulate in the body from bottom sediments or through food webs.
4.3 Biotic and abiotic factors affecting element composition of bile
4.3.1 Marine by freshwater
The hypertonic nature of marine waters means that marine fish will actively drink water to equilibrate the loss of water to the environment (Evans, 2010). The active consumption of water by marine fishes would necessarily lead to some accumulation of the ions dissolved therein. The non-essential elements would be eliminated as waste in the urine and also the bile. As seen in Figure 3, there is a clear distinction between the concentrations of K and Na that were higher in bile of marine fish species when compared to freshwater ones. The difference seen has significant correlation to the quality of the waters of the respective fish habitats and also the amount of water that is ingested. This assumption is also supported by the fact that the absorption/accumulation ability of gallbladders of marine and freshwater fish species are similar, thus, in turn, the differences found in concentrations between marine and freshwater fish species have to be related to external factors such as ion concentrations in marine and fresh waters (Hirano and Bern, 1972).
In bile of marine fishes, we have also found a relatively high concentration of As whereas this trace element was almost absent or below detection limit in many freshwater fish species. For rats injected intravenously under experimental conditions it has been shown that the concentration of As in bile (transported from liver) positively correlates with increased concentration of the As dose injected (Klaassen, 1976) marking this as a route of elimination for As. With regards to fish, it was found for green sunfish (Lepomis cyanellus) that concentration of As in bile increased with increased time of exposure in tanks with high concentration of As in the water (Sorensen et al., 1979). Similar results were found for fish under natural conditions when concentration of As in bile was significantly higher in rough dab (Hippoglossoides platessoides) from areas contaminated by As when compared to areas free from this element (Westerlund et al., 1998). However, in the present study, we did not find a significant concentration of As in the water of Kandalaksha Gulf and fish bile, thus, we assume that fishes may accumulate this trace element from sediments or food items. At the same time the local contamination of water by As may have occurred in the past and been retained in different components of the biota like fish until such time as we collected the samples of fish bile. The latter assumption is also supported by the fact that different rivers that flow into Kandalaksha Gulf may be contaminated by As from mining, chemical, and metallurgical factories located in that area (Rozanov and Volkov, 2009).
4.3.2 Temporal changes in bile composition
In the present study we have found seasonal and annual variations of element composition of fish bile. Seasonal availability of food items and temporal changes in water parameters can influence uptake of nutrients (i.e. - elements) by resident aquatic species. There are obvious changes between marine and freshwater environments and more subtle, but no less significant, seasonal differences within a freshwater body. For instance, the amount of rainfall influences terrestrial run-off. Day length and temperature regime influence the spawning of insects, the growth and blooming of plants, and the overall nutrient loading of waters driven by natural biogeochemical cycles. In this study, collective data from Prussian carp demonstrates that there are seasonal differences in the element composition of bile (Figure 5). The spring condition appears somewhat more or less stable, though there is more variability in the summer samples. The increased variability could be attributed to competition for food resources during the warmer season where rising temperatures would have accelerated growth of both prey items and also resource competitors; the greater abundance and diversity of food items together with competition creates rapid changes in abundance/ingestion (Figure 5).
These seasonal differences are also evident in the composition of the bile at the level of chemical elements as made evident in samples obtained from Teletskoye and Baunt lakes. It was shown for Teletskoye and Baunt lakes that different fish species are grouping together according to the sampling year (Figures 6A, B). Here we see somewhat inverted patterns of correlation across axis 2 (Figures 6A, B) that likely relate to the abundance of food items and incidence of ingestion. Some of the ingestion can include primary or incidental ingestion of sediments. Due to the geophysical differences of each water body, chemical constituents of the water can differ and some minerals obtained from food items would also be distinct within each water body. While Teletskoye Lake is rockier with a narrow limiting littoral zone, Baunt Lake has a more extensive littoral area and less rocky substrata. The differences in pH and mineral solubility also will contribute. This effect of seasons is seen more clearly when all fish are included in the analysis (Figure 6C).
We may assume that this observation explained by similarities in conditions formed in a water body in a given year or season, affected all fish species inhabiting the water bodies in a similar manner. Thus, all fish species for Chany Lake were grouped together based on sampling season or year. Moreover, the factor “season” also significantly affected element composition of bile in a similar manner in different years of sampling. Thus, cyclic seasonal changes in natural conditions in a lake and associated physiological processes in the fish body affected the element composition in fish bile in similar ways for different fish species and was repeated from year to year. Similar interannual changes are also found for concentrations of several elements in fish bile from the same lake. For instance, the concentration of Cr in bile of all studied fish species from Chany Lake was relatively low for spring of both years of sampling (2019 year 0.33 ± 0.06 mg/kg and 2020 year 0.19 ± 0.04 mg/kg), whereas during the summer season the concentration of Cr was significantly increased (2019 year 1.68 ± 0.11 mg/kg and 2020 year 1.79 ± 0.92 mg/kg). For Co we have found the inverse relationships between its concentration in fish bile and the season of both years of sampling. Similarly, there were seasonal variations in concentration of such elements as Cd, Cu, and Zn in muscle, gill, and liver that were registered for eel (Anguilla anguilla), freshwater bream and pikeperch, inhabiting the same lake (Farkas et al., 2000). The concentration of such elements as Al, Fe, As, Zn, Ni, Cu, and Pb in muscle of tench (Tinca tinca) was significantly changed from season to season (Aktumsek and Gezgin, 2011). Such seasonal variations in concentrations of different elements in fish bile are apparently related to changes in metabolic processes in the fish body that are season-dependent, as well as with alterations in fish diets. We assume that all biotic and abiotic factors that are changed annually in a lake (water temperature, food supply, chemical conditions in water such as pH, hardness, conductivity, etc.) may affect all fish species inhabiting a given lake in a similar manner.
4.3.3 Water and sediments in different water bodies and element composition of bile
There are differences between the studied water bodies in the underlying rocks, degree of salinity, ion composition, average pH values, as well as the degree of anthropogenic load. Teletskoye Lake (hydrocarbonate-calcium and low-salinity waters) is a deep lake with a narrow coastal zone and a predominance of rocky soils. Baunt Lake, although it has a number of similarities with Teletskoye Lake, for example, in the ionic composition and degree of water salinity, is a less deep-water body with a larger littoral area and less rocky substrate. According to our results, both of these lakes have a relatively high concentrations of Ca, K, Mg, Na, S (major elements), Sr and Fe (trace elements) in water and Ca, K, Mg (major elements), and Al, Ti, Fe (trace elements) in sediments. In the bile of different fishes inhabiting these lakes we have found Na, Ca, K, P, Mg, S (major elements), and Fe, Cu, Zn, Al (trace elements). At the same time, Chany Lake is sharply different from both of the above-mentioned lakes because it is a complex lacustrine area with many inlets and a shallow profile overall, which has sandy and silty sediments, the water of which is characterized by a brackish sodium chloride composition. With regard to the sampling sites, sites C3 and C6 are areas likely to have lower overall flow rates leading to sedimentation of colloidal particles containing elements of interest to this study. Areas more aligned with the main channel flows and river canals would likely carry colloidal material until low velocities lead to settlement. This may partially explain some of the differences seen among the sediment and water samples from Chany Lake (Figure 4). For both types of analyzed samples, sites C3 and C6 stand somewhat apart. According to the present results, Chany Lake has higher concentration but similar profile with Teletskoye and Baunt lakes i.e. Ca, K, Mg, Na, S (major elements), and Sr (trace element) in water and Ca, K, Mg, S, Na (major elements), and Al, Ti, Fe (trace elements) in sediments. In bile of different fishes from Chany Lake we have also found Ca, K, Mg, Na, S, P (major elements), and Fe, Cu, Zn, Al, Sr (trace elements). In Kandalaksha Gulf the dominant elements in water were similar with the lakes but the concentration was significantly higher i.e. Na, Mg, S, Ca, K, and Sr. In fish bile from the Gulf some of the elements have also shown high concentrations i.e. Na, Ca, K (major elements) and As, Cu, Li, Se, and Sr. We cannot make conclusions regarding to all elements due to a lack of data associated with elemental composition of sediments from the Gulf.
These and many other differences associated with the hydrochemical, geographic, and geophysical characteristics of the water bodies will contribute to the specifics of the input of elements, as well as their bioavailability for fish species inhabiting the lake. In addition, bottom sediments are known to accumulate various elements, especially heavy metals, which can be remobilized into the aquatic environment through interactions between sediments and water. The concentrations of some elements in fish bile are directly related to the elemental composition of bottom sediments, which was demonstrated both in natural populations (Nigussie et al., 2010) and under experimental conditions (Andréasson and Dave, 1995). In general, we may summarize that the main part of both major and trace elements is similar between environmental compartments (water and sediments) and fish bile. It is explained by direct accumulation and/or bioaccumulation from ingestion of these elements by fishes from diet, sediments, and water or/and indirectly through gills and skin from the water.
4.3.4 Feeding habits and diet
4.3.4.1 Carnivorous by non-carnivorous fishes
According to our results, diet is another important factor that significantly affects the element composition of fish bile. It is well known that different components of the fish diet (crustaceans, mollusks, insects, plants, sediments, etc.) may have different ratios of elements. Hence, the compositional elements of the fish body, including the bile, reflect a number of different components collected from distinct environmental sources including diets with different ratios of elements. The concentration of elements in different organs and tissues such as gill, muscle, and liver is determined by diet (Watanabe et al., 2003). Thus, carnivorous fish species accumulate more Fe, Ba, Mn (Subotić et al., 2013) and Se (Pourang, 1995) if compared to fish species that occupy a lower trophic position in the food web and accumulate more Cu, Zn, Cd, Pb, Cr, and Co (Pourang, 1995; Farkas et al., 2000; Andreji et al., 2006; Kenšová et al., 2010; Subotić et al., 2013). However, these patterns of accumulation by fish with different feeding habits will vary according to different organs and tissues and we cannot extrapolate them to fish bile fully.
Physiologically, the retention time of bile in gallbladder is related to fish feeding activity during one feeding cycle. Indeed, Grosell et al. (2000) showed the significant positive relationships between concentrations of Na, K, and Mg in bile of rainbow trout and time after feeding under experimental conditions due to reabsorption of these elements by the epithelium of the gallbladder. In the present study we have also found significant relationship between the levels of gut fullness and concentrations of K in bile of Prussian carp, crucian carp, ide, perch, and whitefish (benthivorous forms from Teletskoye and Baunt lakes), whereas for other major elements such relationships were absent. Baldisserotto et al. (2004), expected significant relationships between levels of gut fullness and the concentrations of Na, Ca, and Mg in hepatic bile when compared to gallbladder bile due to the change in ion concentrations that occur after each cycle of gallbladder refilling when food enters the gut. However, this assumption was confirmed only for two out of 23 fish species obtained from natural populations (Baldisserotto et al., 2004).
In the present study, we have found that carnivorous fish (perch and pikeperch) have more similarities in element composition than non-carnivorous fish (Prussian carp, crucian carp, and ide). Indeed, it was previously shown, based on analysis of the diets that Prussian carp and crucian carp should be considered as omnivorous fish whereas ide belongs to a zoobenthivorous-zooplanktivorous group (Kashinskaya et al., 2018). Similar results were also obtained by studying the element composition in the liver of fish with different feeding habits (Subotić et al., 2013). Thus, carnivorous fish species (wels catfish (Silurus glanis) and pikeperch) were grouped together, while burbot (preferred fish and insects) and common carp (omnivorous) formed two other groups. Given the differences in biochemical composition of diets for carnivorous and non-carnivorous fishes it can be expected that some elements that are incorporated into the fish body are also distinct. Some elements are readily incorporated into tissues for growth and cellular differentiation; while non-essential elements, those that are toxic, or elements provided in super-abundances for which there is no specific storage mechanism become waste that is filtered from the blood and eliminated. These differences are evident in our results (Figure 7) and raise additional questions about the difference between those elements that are important for the processes of digestion versus those that are merely excretory waste products.
One aspect that was outside the scope of the present study was the presence of parasites as a biotic influence on bile composition. While cestodes may compete for essential elements with their host by accumulating and storing ions (Brázová et al., 2015) there was no focus in the sampling protocol during this present study, but this may present another area for focus in future studies on bile composition.
4.3.4.2 Possible effects on digestive enzymes and gut microbiota
The gut microbiota of fish is a very diverse community in terms of taxonomy and physiological functions, such as producing different bioactive substances that may be involved in various processes including digestion. The taxonomical composition of fish gut microbiota depends on numerous different biotic and abiotic factors among which the diet is one of the most important (Ringø et al., 2015). Thus, the chemical elements are also part of the diet that may alter the gut microbial community. As numerous studies have shown that different elements may inhibit or stimulate the growth of different groups of bacteria in fish gut (Meng et al., 2018; Wang et al., 2019; Kakade et al., 2020; Spilsbury et al., 2022). For instance, high concentration of Cu in water increased the ratio of Firmicutes in the intestine of Japanese pufferfish (Takifugu fasciatus) (Wang et al., 2019) as well as Fusobacteria, Bacteroidetes, Cyanobacteria, and Verrucomicrobia in the intestine of common carp (Meng et al., 2018). Kakade et al. (2020) showed that increasing the concentrations of Cu, Cd, and Cr in water increased the ratios of Firmicutes to Fusobacteria, Proteobacteria until there was full domination of Firmicutes in the fish gut microbiome. A similar effect was shown for Pb when high concentrations of this element in water induced higher ratios of Firmicutes and Bacteroidetes in the intestine of Zebra fish (Danio rerio) (Xia et al., 2018). High concentrations of Pb, Cd, and Zn in water have also increased the ratios of Bacteroides, Akkermansia, and Shinella in the intestine of Prussian carp (Wang et al., 2020). The alteration of bacterial community structure of the fish gut was also found when such elements as V, Ni, and Fe were added to the fish diet. Thus, it was shown that such phyla as Proteobacteria, Firmicutes, and Bacteroidetes were the most sensitive to tested elements under experimental conditions using juveniles of barramundi (Lates calcarifer) (Spilsbury et al., 2022). Similar effects on the bacterial community of fish intestine may be expected from bile as this is one of the primary sources of different elements in the fish intestinal lumen. Herein, we propose that the element composition of bile provides a specific contribution to the inner media of the intestine that is additive to the physico-chemical composition determining indirect influences on the gut microbial community, and this is induced by the fish diet (including water ingested).
It is well known that many digestive enzymes are also sensitive to different concentrations of various elements. For instance, the activity of trypsins originated from fish and bacteria are affected by major elements such as Na, K, Mg, and Ca and trace elements such as Al, Cd, Zn, Cu, Pb, Co, Li, Ba, Mn, and Fe (Krishnan and Chandra, 1983; Orhan et al, 2005; Wang et al, 2010; Annamalai et al, 2011; Ben Khaled et al, 2011; Silva et al, 2011; Costa et al, 2013; Temiz et al, 2013; Zhou et al, 2013; Gong et al, 2015; Lakshmi et al, 2018; Alonazi et al, 2021). In the present study, the concentrations of such major elements as Na, K, Mg, and Ca are comparable to concentrations in the above-mentioned studies where significant effects of these elements on trypsin activity were found. At the same time, the concentrations of trace elements were much lower in the bile of studied fish (though for some individuals the concentrations were relatively high) if compared to studies on trypsin activity where significant effects of these elements were found. But the sum of concentrations of trace elements will probably be enough for inhibition or activation of trypsin or some other digestive enzymes in the fish intestine. Hence, we may expect significant pleotropic effects of the element composition of bile on different parts of the digestive process, such as the activity of digestive enzymes of different origins (host gut, host gut microbiota, and in some instances also food items), and the composition of gut microbiota, and, consequently, on digestive efficiency of the host fish species.
The emphasis of this study was not on the toxicity of particular heavy metals, but rather to understand their sources and potential for inclusion in digestive processes versus primary excretion as waste. The associations among different heavy metals have been ascribed in some instances as protective for the host against the toxicity of some heavy metals (Land et al., 2018). The values for ratios among the elements described herein may serve the same purpose. The interactive effects of elemental pairs have been evaluated previously, and these data contribute to understanding further how bile element composition relates to both homeostasis and biological functions, as well as concerning potential toxic effects. The use of bile as a biomarker of heavy metal exposure in fish has been described previously (Hauser-Davis et al., 2012), but in the context of the current study, data concerning the health status of the fish was not collected thus preventing any interpretation of associations of elements identified to possible acute or chronic toxic effects due to exposures.
5 Conclusions
Given that bile is an integral part of the digestive processing of food and it can also serve as an eliminatory waste product, the findings herein demonstrate there are significant changes in the elemental composition of bile that vary among dietary habits, geophysical conditions, and temporally. This may be related to either a need by the fish to access different elements for proper bile formulation and function in the gut; or it may reflect different waste products that accumulate seasonally due to changes in feeding habits. The composition of the basal condition of bile where bile lacks any eliminatory waste elements is still a somewhat open question. Gaining a deeper understanding of this difference is key to improving the utilization of the element composition of bile as a biomarker for ecotoxicology. However, given that the composition of bile can change as bile is synthesized and the gallbladder refills during feeding cycles, from wild fish that are not on a regular feeding regime there is a need to keep careful records of data on gut fullness, diet, season, presence of parasites, etc. of fish sampled to understand potential sources of this variance.
Data availability statement
The original contributions presented in the study are included in the article/Supplementary Material. Further inquiries can be directed to the corresponding author.
Ethics statement
The animal study was reviewed and approved by Ethical Committee of Biological Institute of Tomsk State University (approval certificate # 44).
Author contributions
Supervision: MS. Conceptualization: MS, AS, KA. Methodology: MS. Sample collection: MS, AS, PV, EK. Writing Original draft preparation: MS, AS, KA. Writing-Reviewing and Editing: MS, AS, OA, PV, EK, KA. Data curation: AS, OA. Investigation: OA. Software: AS. Visualization: AS, MS. Statistical analysis: AS, MS. All authors contributed to the article and approved the submitted version.
Funding
The sample collection, expedition, and chemical analyses (for all fish except pike, perch, roach, and ide) were paid by the Russian Science Foundation (grant number 19-74-10054). The sample chemical analyses for pike and perch was supported by the Russian Foundation for Basic Research (grant number 19-34-60028) whereas for roach and ide was paid by Russian international scientific collaboration program Mega-grant (mega-grant № 075-15-2022-1134).
Conflict of interest
The authors declare that the research was conducted in the absence of any commercial or financial relationships that could be construed as a potential conflict of interest.
Publisher’s note
All claims expressed in this article are solely those of the authors and do not necessarily represent those of their affiliated organizations, or those of the publisher, the editors and the reviewers. Any product that may be evaluated in this article, or claim that may be made by its manufacturer, is not guaranteed or endorsed by the publisher.
Supplementary material
The Supplementary Material for this article can be found online at: https://www.frontiersin.org/articles/10.3389/fmars.2023.1217787/full#supplementary-material
References
Aktumsek A., Gezgin S. (2011). Seasonal variations of metal concentrations in muscle tissue of tench (Tinca tinca), water and sediment in Beysehir Lake (Turkey). Environ. Technol. 32 (13), 1479–1485. doi: 10.1080/09593330.2010.540717
Aladin N. V., Plotnikov I. S. (1993). Large saline lakes of former USSR: a summary review. Hydrobiologia. 267, 1–12. doi: 10.1007/BF00018787
Aldman G., Holmgren S. (1987). Control of gallbladder motility in the rainbow trout, Salmo gairdneri. Fish Physiol. Biochem. 4, 143–155. doi: 10.1007/BF02110881
Allen P. (1995). Accumulation profiles of lead and cadmium in the edible tissues of Oreochromis aureus during acute exposure. J. Fish Biol. 47, 559–568. doi: 10.1111/j.1095-8649.1995.tb01922.x
Alonazi M., Karray A., Badjah-Hadj-Ahmed A. Y., Ben Bacha A. (2021). Alpha Amylase from Bacillus pacificus Associated with Brown Algae Turbinaria ornata: Cultural Conditions, Purification, and Biochemical Characterization. Process. 9 (1), 16. doi: 10.3390/pr9010016
Andréasson M., Dave G. (1995). Transfer of heavy metals from sediment to fish, and their biliary excretion. J. Aquat. Ecosyst. Health 4, 221–230. doi: 10.1007/BF00118002
Andree K. B., Kim J., Kirschke C. P., Gregg J. P., Paik H. Y., Joung H., et al. (2004). Investigation of lymphocyte gene expression for use as biomarkers for zinc status in humans. J. of Nut. 134, 1716–1723. doi: 10.1093/jn/134.7.1716
Andreji J., Stránai I., Massányi P., Valent M. (2006). Accumulation of some metals in muscles of five fish species from lower Nitra river. J. Environ. Sci. Health A. 41 (11), 2607–2622. doi: 10.1080/10934520600928003
Anishchenko O. V., Tolomeev A. P., Ivanova E. A., Drobotov A. V., Kolmakova A. A., Zuev I. V., et al. (2020). Accumulation of elements by submerged (Stuckenia pectinata (L.) Börner) and emergent (Phragmites australis (Cav.) Trin. ex Steud.) macrophytes under different salinity levels. Plant Physiol. Biochem. 154, 328–340. doi: 10.1016/j.plaphy.2020.05.019
Annamalai N., Thavasi R., Vijayalakshmi S., Balasubramanian T. (2011). Extraction, purification and characterization of thermostable, alkaline tolerant α-amylase from Bacillus cereus. Indian. J. Microbiol. 51 (4), 424–429. doi: 10.1007/s12088-011-0160-z
Aschner J. L., Aschner M. (2005). Nutritional aspects of manganese homeostasis. Mol. Aspects Med. 26, 353–362. doi: 10.1016/j.mam.2005.07.003
Baldisserotto B., Lopez-Vasquez K., Silva L. V. F., Golombieski J. I., Val A. L. (2004). Ionic levels of the gallbladder bile of some teleosts from the Rio Negro, Amazon. J. Fish Biol. 65, 287–292. doi: 10.1111/j.0022-1112.2004.00438.x
Barwick M., Maher W. (2003). Biotransference and biomagnification of selenium copper, cadmium, zinc, arsenic and lead in a temperate seagrass ecosystem from Lake Macquarie Estuary, NSW, Australia. Mar. Environ. Res. 56 (4), 471–502. doi: 10.1016/S0141-1136(03)00028-X
Ben Khaled H., Jellouli K., Souissi N., Ghorbel S., Barkia A., Nasri M. (2011). Purification and characterization of three trypsin isoforms from viscera of sardinelle (Sardinella aurita). Fish Physiol. Biochem. 37 (1), 123–133. doi: 10.1007/s10695-010-9424-5
Bianchi D., Carozza D. A., Galbraith E. D., Guiet J., DeVries T. (2021). Estimating global biomass and biogeochemical cycling of marine fish with and without fishing. Sci. Adv. 41, eabd7554. doi: 10.1126/sciadv.abd7554
Boyer J. L. (2013). Bile formation and secretion. Compr. Physiol. 3 (3), 1035–1078. doi: 10.1002/cphy.c120027
Brázová T., Hanzelová V., Miklisová D., Šalamún P., Vidal-Martínez V. M. (2015). Host-parasite relationships as determinants of heavy metal concentrations in perch (Perca fluviatilis) and its intestinal parasite infection. Ecotox. Environ. Safety. 122, 551–556. doi: 10.1016/j.ecoenv.2015.09.032
Bulatov V. I., Rotanova I. N., Kazantsev V. A., Kurepina N. (2015). “Geographical position, geology, geomorphology and relief” in Chany Lake (West Siberia) Environmental Profile. Eds. Vasiliev O. F., Veen J. (Novosibirsk: Academic Publishing House “GEO” Inc.), 9–18.
Buslov M. M., Smirnova L. V., Theunissen K. (2001). “Geological structure and basement evolution of the region of Lake Teletskoye” in Physical and geological environment of Lake Teletskoye. Eds. Selegei V., Dehandschutter B., Klerkx J., Vysotsky E. (Belgium: Department of geology and mineralogy Royal Museum of Central Africa Inc.), 183–204.
Clements K., Raubenheimer D. (2008). “Feeding and Nutritional,” in The Physiology of Fishes., 3rd ed. Eds. Evans D. H., Claiborne J. B. (Boca Raton, London, New York: CRC Press Taylor & Francis Group), 47–83.
Clesceri L. S., Greenberg A. E., Trussell R. R. (1989). Standard methods for the examination of water and wastewater. 17th ed (Washington, DC: American Public Health Association).
Collier T. K., Varanasi U. (1991). Hepatic activities of xenobiotic metabolizing enzymes and biliary levels of xenobiotics in English sole (Parophrys vetulus) exposed to environmental contaminants. Arch. Environ. Contam. Toxicol. 20 (4), 462–473. doi: 10.1007/BF01065834
Costa H. M., Freitas Júnior A. C., Amaral I. P., Hirata I. Y., Paiva P. M., Carvalho L. B. Jr., et al. (2013). Metal-sensitive and thermostable trypsin from the crevalle jack (Caranx hippos) pyloric caeca: purification and characterization. Chem. Cent. J. 7, 166. doi: 10.1186/1752-153X-7-166
Dolmatova L. A. (2011). Osobennosti gidrohimicheskogo rezhima Teleckogo ozera v period osennego ohlazhdeniya. [The peculiarities of hydrochemical regime of Lake Teletskoye its autumn cooling]. Mir nauki, kul'tury, obrazovaniya 6 (31), 418–422. (In Russian)
Drost H. J., Kipriyanova L. M., Kovalevskaya N. M., Savkin V. M., Dvurechenskaya S. Y., Popov P. A., et al (2002). Environmental profile Chany Lake (West Siberia). Sci. Ann. Danube Delta Institute Res. Dev. 2000–2001, 49–54.
Edel J., Di Nucci A., Sabbioni E., Manzo L., Tonini M., Minnoia C., et al. (1991). Biliary excretion of barium in the rat. Biol. Trace. Elem. Res. 30 (3), 267–276. doi: 10.1007/BF02991421
Evans D. H. (2010). A brief history of the study of fish osmoregulation: the central role of the Mt. Desert Island Biological Laboratory. Front. Physiol. 13. doi: 10.3389/fphys.2010.00013
Farkas A., Salánki J., Varanka I. (2000). Heavy metal concentrations in fish of Lake Balaton. Lakes Reserv.: Res. Manage. 5 (4), 271–279. doi: 10.1046/j.1440-1770.2000.00127.x
Golovanova I. L. (2008). Effects of heavy metals on the physiological and biochemical status of fishes and aquatic invertebrates. Inland Water Biol. 1 (1), 93–101. doi: 10.1007/s12212-008-1014-1
Gong J.-S., Li W., Zhang D.-D., Xie M.-F., Yang B., Zhang R.-X., et al. (2015). Biochemical characterization of an arginine-specific alkaline trypsin from Bacillus licheniformis. Int. J. Mol. Sci. 16 (12), 30061–30074. doi: 10.3390/ijms161226200
González-Montaña J. R., Escalera-Valente F., Alonso A. J., Lomillos J. M., Robles R., Alonso M. E. (2020). Relationship between vitamin B12 and cobalt metabolism in domestic ruminant: An update. Anim. (Basel). 10 (10), 1855. doi: 10.3390/ani10101855
Grosell M., O’donnell M. J., Wood C. M. (2000). Hepatic versus gallbladder bile composition: in vivo transport physiology of the gallbladder in rainbow trout. Am. J. Physiol. Regul. Integr. Comp. Physiol. 278 (6), 1674–1684. doi: 10.1152/ajpregu.2000.278.6.R1674
Gutiérrez-Ravelo A., Gutiérrez Á.J., Paz S., Carrascosa-Iruzubieta C., González-Weller D., Caballero J. M., et al. (2020). Toxic metals (Al, Cd, Pb) and trace element (B, Ba, Co, Cu, Cr, Fe, Li, Mn, Mo, Ni, Sr., V, Zn) levels in Sarpa salpa from the North-Eastern Atlantic ocean region. Int. J. Environ. Res. Public Health 17 (19), 7212. doi: 10.3390/ijerph17197212
Hauser-Davis R. A., Bastos F. F., Oliveira T. F., Ziolli R. L., Campos R. C. (2012). Fish bile as a biomarker for metal exposure. Mar. Pollut. Bull. 64, 1589–1595. doi: 10.1016/j.marpolbul.2012.05.017
Hirano T., Bern H. A. (1972). The teleost gall bladder as an osmoregulatory organ. Endocrinol. Jpn. 19 (1), 41–46. doi: 10.1507/endocrj1954.19.41
Hunn J. B. (1969). Inorganic composition of gallbladder bile from fasted rainbow trout. Prog. Fish C. 31 (4), 221–222. doi: 10.1577/1548-8640(1969)31[221:ICOGBF]2.0.CO;2
Hunn J. B. (1976). Inorganic composition of gallbladder bile from freshwater fishes. ASIH. 3, 602–605. doi: 10.2307/1443389
Hunn J. B., Christenson L. M. (1977). Chemical composition of blood and bile of the shovelnose sturgeon. Prog. Fish-Cult. 39 (2), 59–61. doi: 10.1577/1548-8659(1977)39[59:CCOBAB]2.0.CO;2
Izvekova G. I., Solovyev M. M. (2016). Characteristics of the effect of cestodes parasitizing the fish intestine on the activity of the host proteinases. Biol. Bull. 2, 182–187. doi: 10.1134/S1062359016010076
Izvekova G. I., Solovyev M. M., Kashinskaya E. N., Izvekov E. I. (2013). Variations in the activity of digestive enzymes along the intestine of the burbot Lota lota expressed by different methods. Fish Physiol. Biochem. 5, 1181–1193. doi: 10.1007/s10695-013-9773-y
Kakade A., Salama E.-S., Pengya F., Liu P., Li X. (2020). Long-term exposure of high concentration heavy metals induced toxicity, fatality, and gut microbial dysbiosis in common carp, Cyprinus carpio. Environ. Pollut. 160, 257–264. doi: 10.1016/j.ecoenv.2018.05.050
Kalacheva G. S., Gubanov V. G., Gribovskaya I. V., Gladchenko I. A., Zinenko G. K., Savitsky S. V. (2002). Chemical analysis of Lake Shira water (1997–2000). Aquat. Ecol. 36, 123–141. doi: 10.1023/A:1015695813280
Kanaya G., Solovyev M. M., Shikano S., Okano J.-i., Ponomareva N. M., Yurlova N. (2019). Application of stable isotopic analyses for fish host–parasite systems: an evaluation tool for parasite-mediated material flow in aquatic ecosystems. Aquat. Ecol. 2, 217–232. doi: 10.1007/s10452-019-09684-6
Kashinskaya E. N., Simonov E. P., Kabilov M. R., Izvekova G. I., Andree K. B., Solovyev M. M. (2018). Diet and other environmental factors shape the bacterial communities of fish gut in an eutrophic lake. J. Appl. Microbiol. 125, 1626–1641. doi: 10.1111/jam.14064
Kenšová R., Čelechovská O., Doubravová J., Svobodová Z. (2010). Concentrations of metals in tissues of fish from the Věstonice Reservoir. Acta Veterinaria Brno. 79 (2), 335–345. doi: 10.2754/avb201079020335
Klaassen C. D. (1976). Biliary excretion of metals. Drug Metab. Rev. 5 (2), 165–196. doi: 10.3109/03602537609029977
Korolyuk A., Kipriyanova L. M., Drost H. J., Kovalevskaya N. M., Hanganu J., Grigoras I. (2015). Vegetation of Chany Lake region. Eds. Vasiliev O. F., Veen J. (Novosibirsk: Chany Lake (West Siberia) Environmental Profile. Academic Publishing House “GEO” Inc.), 117–135.
Krainov M. A., Bezrukova E. V., Kerber E. V., Levina O. V., Ivanov E. V., Shchetnikov A. A., et al. (2017). First results of study of Lake Baunt bottom sediments (northern Transbaikalia). Russ. Geol. Geophys. 58 (11), 1764–1776. doi: 10.15372/GiG20171110
Krishnan T., Chandra A. K. (1983). Purification and Characterization of alpha-Amylase from Bacillus licheniformis CUMC305. Appl. Environ. Microbiol. 46 (2), 430–437. doi: 10.1128/aem.46.2.430-437.1983
Lakshmi B. K. M., Muni Kumar D., Hemalatha K. P. J. (2018). Purification and characterization of alkaline protease with novel properties from Bacillus cereus strain S8. J. Genet. Eng. Biotechnol. 16 (2), 295–304. doi: 10.1016/j.jgeb.2018.05.009
Land S. N., Rocha R. C. C., Bordon I. C., Saint’Pierre T. D., Ziolli R. L., Hauser-Davis R. A. (2018). Biliary and hepatic metallothionein, metals and trace elements in environmentally exposed neotropical cichlids Geophagus brasiliensis. J. of Trace Elements Med. Biol. 50, 347–355. doi: 10.1016/j.jtemb.2018.07.023
Mason R. P., Reinfelder J. R., Morel F. M. M. (1995). Bioaccumulation of mercury and methylmercury. Water Air Soil Pollut. 80, 915–921. doi: 10.1007/BF01189744
Mazukhina S. I., Maksimova V. V., Chudnenko K. V., Masloboev V. A., Sandimirov S. S., Drogobuzhskaya S. V., et al. (2020). Kachestvo vod Arkticheskoj zony Rossijskoj Federacii: fizikohimicheskoe modelirovanie formirovaniya vod, formy migracii elementov, vliyanie na organizm cheloveka. [Water quality of the Arctic zone of the Russian Federation: physicochemical modeling of water formation, forms of migration of elements, impact on the human body], Ed. E.N. Yeremeyeva, Publishing House FIC KNC RAN, Apatity. 97–106 (In Russian). doi: 10.37614/978.5.91137.437.2
McIntyre P. B., Flecker A. S., Vanni M. J., Hood J. M., Taylor B. W., Thomas S. A. (2008). Fish distributions and nutrient cycling in streams: can fish create biogeochemical hotspots? Ecology 89 (8), 2335–2346. doi: 10.1890/07-1552.1
Meng X.-L., Li S., Qin C.-B., Zhu Z.-X., Hu W.-P., Yang L.-P., et al. (2018). Intestinal microbiota and lipid metabolism responses in the common carp (Cyprinus carpio L.) following copper exposure. Ecotoxicol. Environ. Saf. 160, 257–264. doi: 10.1016/j.ecoenv.2018.05.050
Mushtak Al-Atabi Ooi R. C., Luo X. Y., Chin S. B., Bird N. C. (2012). Computational analysis of the flow of bile in human cystic duct. Med. Eng. Phys. 34 (8), 1177–1183. doi: 10.1016/j.medengphy.2011.12.006
Nabavi S. F., Nabavi S. M., Latifi A. M., Eslami S., Ebrahimzadeh M. A. (2012). Determination of trace elements level of pikeperch collected from the Caspian Sea. Bull. Environ. Contam. Toxicol. 88 (3), 401–405. doi: 10.1007/s00128-011-0513-7
Nguyen N. M., Obara H. (2020). Effect of pH on the viscosity and viscoelastic properties of bile. J. Biorheol. 34 (1), 30–37. doi: 10.17106/jbr.34.30
Nigussie K., Chandravanshi B. S., Wondimu T. (2010). Correlation among trace metals in Tilapia (Oreochromis niloticus), sediment and water samples of lakes Awassa and Ziway, Ethiopia. Int. J. Biol. Chem. Sci. 4 (5), 1641–1656. doi: 10.4314/ijbcs.v4i5.65544
Norseth T., Alexander J., Aaseth J., Langård S. (1982). Biliary excretion of chromium in the rat: a role of glutathione. Acta Pharmacol. Toxicol. (Copenh). 51 (5), 450–455. doi: 10.1111/j.1600-0773.1982.tb01052.x
Olsen R. E., Ringø E. (1997). Lipid digestibility in fish: A review. Rec Res. Devel Lip Res. 1, 199–265.
Olsson P. E., Kling P., Hogstrand C. (1998). “Mechanisms of heavy metal accumulation and toxicity in fish” in Metal Metabolism in Aquatic Environments. Eds. Langston W. J., Bebianno M. J. (Boston, MA: Springer). doi: 10.1007/978-1-4757-2761-6_10
Orhan E., Omay D., Güvenilir Y. (2005). Partial purification and characterization of protease enzyme from Bacillus subtilis and Bacillus cereus. Appl. Biochem. Biotechnol. 121–124, 183–194. doi: 10.1385/ABAB:121:1-3:0183
Popov P. A., Voskoboynikov V. A., Shchenev V. A. (2005). Ryby ozera Chany. [Fishes of the Lake Chany]. Sibirskii Ecologicheskii Jurnal. 12, 279–293 (In Russian).
Pourang N. (1995). Heavy metal bioaccumulation in different tissues of two fish species with regards to their feeding habits and trophic levels. Environ. Monit Assess. 35 (3), 207–219. doi: 10.1007/BF00547632
Reshetnikov Y. S., Bogutskaya N. G., Vasileva E. D., Dorofeeva E. A., Naseka A. M., Popova O. A., et al. (1997). An annotated check-list of the freshwater fishes of Russia. J. Ichthyol. 37, 687–736.
Ringø E., Zhou Z., Vecino J. L. G., Wadsworth S., Romero J., Krogdahl Å., et al. (2015). Effect of dietary components on the gut microbiota of aquatic animals. A never-ending story? Aquac. Nutr. 22 (2), 219–282. doi: 10.1111/anu.12346
Rozanov A. G., Volkov I. I. (2009). Bottom sediments of Kandalaksha Bay in the White Sea: manganese phenomenon. Geochemistry Int. 47 (10), 1004–1020. doi: 10.1134/S001670290910005X
Rybalko А.Е., Barymova А.А., Tokarev M., Repkina Т.Yu. (2020). Chetvertichnye otlozheniya i rel'ef Kandalakshskogo zaliva: istoriya izucheniya i sovremennye dannye ob ih formirovanii. [Quaternary sediments and the relief of the Kandalaksha Bay: the history of the study andmodern data on their formation]. Trudy Fersmanovskoj nauchnoj sessii GI KNC RAN. 17, 465–469. (In Russian)
Sabbioni E., Marafante E., Rade J., Gregotti C., Di Nucci A., Manzo L. (1981). Biliary excretion of vanadium in rats. Toxicol. Eur. Res. 3 (2), 93–98 In Russian).
Sablin O., Grinevich V., Uspensky Y., Ratnikov V. (2002). Funktsional’naya diagnostika v gastroenetrologii. [Functional diagnostics in gastroenterology]. In Educational and methodological manual. Rossijskaya voenno-medicinskaya akademiya Publishing House. Saint, 88. (In Russian)
Sánchez-Aceves L., Pérez-Alvarez I., Gómez-Oliván L. M., Islas-Flores H., Barceló D. (2021). Long-term exposure to environmentally relevant concentrations of ibuprofen and aluminum alters oxidative stress status on Danio rerio. Comp. Biochem. Physiol. C Toxicol. Pharmacol. 248, 109071. doi: 10.1016/j.cbpc.2021.109071
Savkin V. M., Orlova G. A., Vasiliev O. F., Dvurechenskaya S., Kondakova O. V., Saprykina Y. V., et al. (2015). Hydrology. Eds. Vasiliev O. F., Veen J. (Novosibirsk: Chany Lake (West Siberia) Environmental Profile. Academic Publishing House “GEO” Inc.), 42–77.
Seymore T., Preez H. H., Vuren J. H. J. (1996). Bioaccumulation of chromium and nickel in the tissues of Barbus marequensis A. Smith, 1841 from the Lower Olifants River, Mpumalanga. S. Afr. J. Zool. 31 (3), 101–109. doi: 10.1080/02541858.1996.11448401
Silva J. F., Espósito T. S., Marcuschi M., Ribeiro K., Cavalli R. O., Oliveira V., et al. (2011). Purification and partial characterisation of a trypsin from the processing waste of the silver mojarra (Diapterus rhombeus). Food Chem. 129 (3), 777–782. doi: 10.1016/j.foodchem.2011.05.019
Solotchin P. A., Solotchina E. P., Bezrukova E. V., Zhdanova A. N. (2020). Climate signals in the late quaternary bottom sediments of Lake Baunt (northern Transbaikalia). Russ. Geol. Geophys. 61 (10), 1397–1408. doi: 10.15372/GiG2020117
Solovyev M. M., Campoverde C., Öztürk S., Moreira C., Diaz M., Moyano F. J., et al. (2016). Morphological and functional description of the development of the digestive system in meagre (Argyrosomus regius): An integrative approach. Aquaculture. 464, 381–391. doi: 10.1016/j.aquaculture.2016.07.008
Solovyev M. M., Izvekova G. I., Kashinskaya E. N., Gisbert E. (2018). Dependence of pH values in the digestive tract of freshwater fishes on some abiotic and biotic factors. Hydrobiologia. 807, 67–85. doi: 10.1007/s10750-017-3383-0
Solovyev M. M., Kashinskaya E. N., Izvekova G. I., Gisbert E., Glupov V. V. (2014). Feeding habits and ontogenic changes in digestive enzyme patterns in five freshwater teleosts. J. Fish Biol. 85 (5), 1395–1412. doi: 10.1111/jfb.12489
Sorensen E. M. B., Henry R. E., Ramirez-Mitchell R. (1979). Arsenic accumulation, tissue distribution and cytotoxicity in teleosts following indirect aqueous exposures. Bull. Environ. Contam. Toxicol. 21, 162–169. doi: 10.1007/BF01685405
Spilsbury F., Foysal M. J., Tay A., Gagnon M. M. (2022). Gut microbiome as a potential biomarker in fish: Dietary exposure to petroleum hydrocarbons and metals, metabolic functions and cytokine expression in juvenile Lates calcarifer. Front. Microbiol. 13. doi: 10.3389/fmicb.2022.827371
Squadrone S., Biancani B., Da Rugna C., Favaro L., Pederiva S., Abete M. C. (2022). Trace and rare earth element bioaccumulation in the spotted dogfish (Scyliorhinus stellaris). Environ. Sci. Pollut. Res. 29, 70262–70268. doi: 10.1007/s11356-022-20886-8
Subotić S., Spasić S., Višnjić-Jeftić Z., Hegediš A., Krpo-Ćetković J., Mićković B., et al. (2013). Heavy metal and trace element bioaccumulation in target tissues of four edible fish species from the Danube River (Serbia). Ecotoxicol. Environ. Saf. 98, 196–202. doi: 10.1016/j.ecoenv.2013.08.020
Suedel B. C., Boraczek J. A., Peddicord R. K., Clifford P. A., Dillon T. M. (1994). “Trophic Transfer and Biomagnification Potential of Contaminants in Aquatic Ecosystems” in Reviews of Environmental Contamination and Toxicology. Reviews of Environmental Contamination and Toxicology. Ed. Ware G. W. (New York, NY: Springer), 136. doi: 10.1007/978-1-4612-2656-7_2
Susnea I., Weiskirchen R. (2016). Trace metal imaging in diagnostic of hepatic metal disease. Mass Spectrom. Rev. 35 (6), 666–686. doi: 10.1002/mas.21454
Temiz H., Ustun N. S., Turhan S., Aykut U. (2013). Partial purification and characterization of alkaline proteases from the Black Sea anchovy (Engraulis encrasicholus) digestive tract. Afr. J. Biotechnol. 12 (1), 56–63. doi: 10.5897/AJB12.2900
Thibon F., Weppe L., Vigier N., Churlaud C., Lacoue-Labarthe T., Metian M., et al. (2021). Large-scale survey of lithium concentrations in marine organisms. Sci. Total Environ. 751, 141453. doi: 10.1016/j.scitotenv.2020.141453
Ugolev A. M., Kuzmina V. V. (1993). In book: Digestion processes and adaptations in fish. Ed. Poddubny A. G.. Publishing House Gidrometeoizdat. Saint Petersburg, 238 (In Russian).
Vasilyev O. F., Kazantsev V. A., Popov P. A., Kirillov V. V. (2005). Obshchaya prirodnaya harakteristika i ekologicheskie problemy Chanovskoj i Kulundinskoj ozernyh sistem i ih bassejnov. [General natural characteristics and ecological problems of the Chany-Kulunda lacustrine system and basin of these lakes]. Sibirskii Ecologicheskii Jurnal. 2, 167–173 (In Russian).
Vethaak A. D., Baggelaar P. K., Lieverloo J. H. M., Ariese F. (2016). Decadal trends in polycyclic aromatic hydrocarbon (PAH) contamination assessed by 1-hydroxypyrene in fish bile fluid in the Netherlands: Declining in marine waters but still a concern in estuaries. Front. Mar. Sci. 3. doi: 10.3389/fmars.2016.00215
Wang Q., Gao Z.-X., Zhang N., Shi Y., Xie X.-L., Chen Q.-X. (2010). Purification and characterization of trypsin from the intestine of hybrid tilapia (Oreochromis niloticus × O. aureus). J. Agric. Food Chem. 58 (1), 655–659. doi: 10.1021/jf903052s
Wang T., Wei X., Chen T., Wang W., Xia X., Miao J., et al. (2019). Studies of the mechanism of fatty liver formation in Takifugu fasciatus following copper exposure. Ecotoxicol. Environ. Saf. 181, 351–361. doi: 10.1016/j.ecoenv.2019.06.013
Wang N., Guo Z., Zhang Y., Zhang P., Liu J., Cheng Y., et al (2020). Effect on intestinal microbiota, bioaccumulation, and oxidative stress of Carassius auratus gibelio under waterborne cadmium exposure. Fish Physiol Biochem. 46, 2299–2309. doi: 10.1007/s10695-020-00870-0
Watanabe K. H., Desimone F. W., Thiyagarajah A., Hartley W. R., Hindrichs A. E. (2003). Fish tissue quality in the lower Mississippi River and health risks from fish consumption. Sci. Total Environ. 302 (1-3), 109–126. doi: 10.1016/S0048-9697(02)00396-0
Westerlund S., Aas E., Andersen O. K. (1998). The use of bile in fish in a screening method for trace metal exposure. Mar. Environ. Res. 46 (1-5), 601–604. doi: 10.1016/S0141-1136(98)00017-8
Xia J., Lu L., Jin C., Wang S., Zhou J., Ni Y., et al. (2018). Effects of short term lead exposure on gut microbiota and hepatic metabolism in adult zebrafish. Comp. Biochem. Physiol. Part - C: Toxicol. Pharmacol. 209, 1–8. doi: 10.1016/j.cbpc.2018.03.007
Yamazaki M., Tanizaki Y., Shimokawa T. (1996). Silver and other trace elements in a freshwater fish, Carasius auratus langsdorfii, from the Asakawa River in Tokyo, Japan. Environ. Pollut. 94 (1), 83–90. doi: 10.1016/S0269-7491(96)00053-X
Zarubina E., Sokolova M. I. (2007). High aquatic vegetation in the North-West shallow water of Lake Teletskoye and factors her building. [Vysshaya vodnaya rastitel'nost' Severo-Zapadnogo melkovod'ya Teleckogo ozera i faktory ee formirovaniya]. Mir nauki, kul'tury, obrazovaniya. 3 (6), 28–31 (In Russian).
Zherelina I. V., Vasilenko V. A., Voskoboinikov V. A., Kurepina N., Pestova L. V., Popov P. A., et al. (2015). Use of natural resources. Eds. Vasiliev O. F., Veen J. (Novosibirsk: Chany Lake (West Siberia) Environmental Profile. Academic Publishing House “GEO” Inc.), 204–228.
Zhou A., Yin F., Zhao L., Gong C., Benjakul S., Liu X., et al. (2013). Purification and characterization of trypsin from the intestine of genetically improved Nile tilapia (Oreochromis niloticus). J. Aquat. Food Prod. Technol. 22, 421–433. doi: 10.1080/10498850.2012.658528
Keywords: feeding habits, gut morphology, bile density, bile volume, seasonal and annual changes
Citation: Solovyev MM, Shokurova AV, Anishchenko OV, Vlasenko PG, Kashinskaya EN and Andree KB (2023) Dependence of element composition of bile of freshwater and marine fishes on some abiotic and biotic factors. Front. Mar. Sci. 10:1217787. doi: 10.3389/fmars.2023.1217787
Received: 05 May 2023; Accepted: 18 July 2023;
Published: 18 October 2023.
Edited by:
Mariana Palma, University of Coimbra, PortugalReviewed by:
Moslem Sharifinia, Iranian Fisheries Science Research Institute, IranMarthe Monique Gagnon, Curtin University, Australia
Copyright © 2023 Solovyev, Shokurova, Anishchenko, Vlasenko, Kashinskaya and Andree. This is an open-access article distributed under the terms of the Creative Commons Attribution License (CC BY). The use, distribution or reproduction in other forums is permitted, provided the original author(s) and the copyright owner(s) are credited and that the original publication in this journal is cited, in accordance with accepted academic practice. No use, distribution or reproduction is permitted which does not comply with these terms.
*Correspondence: Mikhail M. Solovyev, yarmak85@mail.ru