- 1Olive Ridley Project, Clitheroe, Lancashire, United Kingdom
- 2Conservation Education Society, Diani, South Coast, Kenya
- 3Kenya Wildlife Service, Kisite Mpunguti Marine Park, Ukunda, Kenya
- 4Pwani University Biosciences Research Centre (PUBReC), Pwani University, Kilifi, Kenya
- 5School of Biodiversity, One Health & Veterinary Medicine (SBOHVM), University of Glasgow, Glasgow, United Kingdom
- 6Research and Conservation Support Society (RECOURSE), Kilifi, Kenya
Sea turtle in-water monitoring has been conducted in Diani-Chale National Marine Reserve (DCMR) in Kenya’s South Coast since 2018, where green sea turtles (Chelonia mydas) may be found resting or foraging. Underwater surveys are conducted at a regular basis to collect data on sea turtle occurrence, abundance, and distribution, incorporating photographic identification (Photo-ID) as a capture-mark-recapture method. Photo-ID is a non-invasive, low-cost, citizen-science-friendly approach that allows researchers to obtain discrete information about individuals’ locations and health status at a given time, which is essential knowledge for spatial planning and conservation management of endangered species. When visible in the photos collected on a single individual, the occurrence of external tumours is noted, and the turtle is flagged for fibropapilloma (FP) monitoring. From July 2018 to December 2022, 2757 green turtle encounters were recorded, resulting in the identification of 571 individuals. External tumours were observed in 75 individuals. The temporal progression of the tumour’s growth was monitored through subsequent re-sightings of 47 of the affected individuals, with evidence of tumour progression and regression. Documenting the incidence of this disease on sea turtles usually involves the manipulation of captured animals, and is quite possibly under-documented in foraging grounds. Tracking the evolution of this disease using Photo-ID can be a useful, non-invasive method to understand its extent in foraging aggregations where turtles can be easily observed underwater.
1 Introduction
Green turtles (Chelonia mydas) are marine reptiles with a complex life history, having a pelagic existence during their initial years, and recruiting into benthic inshore waters at the age of 3-5 years (Reich et al., 2007). While they are known to perform ontogenic and reproductive migrations in open- sea, green sea turtles are typically found in coral reefs, mangroves, and seagrass lagoons where they remain most of their lives (Bolten et al., 2003; Arthur et al., 2008b). Classified as Endangered throughout its global range (Seminoff, 2004), green sea turtles are a conservation priority. They are exposed to numerous threats in coastal habitats, including interaction with fisheries, habitat loss and degradation, and human consumption of their meat and eggs (Seminoff, 2004). The prevalence of disease is also a cause of additional stress to some populations of green sea turtles, and fibropapillomatosis (FP) is the most devastating epizootic disease affecting the species. FP is a neoplastic condition, possibly caused by the chelonid alphaherpesvirus 5 (ChHV-5) (Herbst, 1994, 1999; Quackenbush et al., 1998; Lackovich et al., 1999). It is thought to be transmitted through direct contact between infected and healthy turtles (Work et al., 2015; Chaves et al., 2017; Jones et al., 2020), indirectly via a diversity of potential vectors such as leeches (Greenblatt et al., 2004) or cleaner fish (Lu et al., 2000), or through exposure to contaminated water or food (Landsberg et al., 1999; Arthur et al., 2006; Arthur et al., 2008a; Van Houtan et al., 2010; Van Houtan et al., 2014). The disease may affect multiple organs (Herbst et al., 1999; Page-Karjian et al., 2017) and typically manifest as lesions with the appearance of wart-like growths on affected individuals. These lesions can occur cutaneously, ocularly and viscerally (Work et al., 2004), and while benign, their excessive growth can impair vision, feeding, and locomotion (Rodenbusch et al., 2014). Moreover, some studies suggest that affected turtles can become chronically stressed and immunosuppressed and are thus more prone to opportunistic infections (Cray et al., 2001; Work et al., 2001; Work et al., 2003; Page-Karjian et al., 2014; Perrault et al., 2021). In more advanced stages of the disease, the tumours can cover large areas of the body and interfere with the turtle’s ability to see, swim, and feed. The debilitated individual becomes more vulnerable to predation, starvation and boat-strike, and its chances of survival are reduced (Page-Karjian et al., 2019). The exact cause of the tumours is not fully understood, but it is believed to be a complex combination of viral, genetic, and environmental factors (García-Sastre and Sansonetti, 2010; Manes et al., 2022).
FP has been detected in all sea turtle species, although it is most observed in green sea turtles (Jones et al., 2016). FP is particularly prevalent in some areas, for example, over 80.0% of stranded turtles in the Hawaiian Islands were euthanized due to advanced FPs (Work et al., 2004), and roughly half of the wild population was affected during the 1990s (Chaloupka et al., 2009). The disease has also been documented in sea turtle populations in the Caribbean, Mediterranean, and in Australia, among other regions (Monteiro et al., 2021). In the Western Indian Ocean, information is scarce, but there have been reports of individual sea turtles with FP tumours observed in Kenyan waters, mostly from opportunistic observations of infected individuals, e.g. from bycatch or strandings (Jones et al., 2021). Given the widespread nature of FP in sea turtle populations, efforts are needed to better understand the disease and to develop effective strategies for managing it. The global distribution and prevalence of FP among sea turtle populations, as well as the factors that may influence its occurrence and severity in different regions, is a research priority in sea turtle biology and conservation (Hamann et al., 2010; Jones et al., 2016).
In this study, we present data on the monitoring of the prevalence of this disease in a population of foraging green turtles in Kenya’s south coast using photo identification (Photo-ID). This non-invasive and low-cost capture-mark-recapture method is commonly used to monitor any megafauna species with distinct skin pattern unique to each individual, such as manta rays, whale sharks and sea turtles (Holmberg et al., 2009; Jean et al., 2010; Kitchen-Wheeler, 2010; Hancock et al., 2020). The use of Photo-ID is fast becoming an alternative to traditional tagging programs, which are considered invasive, and potentially promote the horizontal transmission of the disease between tagged individuals (Balazs and Jacobson, 1990; Balazs, 1999).
We discuss how this method can assist in identifying FP lesions in early stages and monitor the disease over time in affected individuals in foraging aggregations.
2 Method
The Diani-Chale National Marine Reserve (DCNMR) is a marine protected area located on the southern coast of Kenya, about 35 km south of Mombasa. It is situated in Kwale county, between the towns of Diani Beach and Msambweni, and covers an area of approximately 2,500 ha and approximately 18 Km of coastline (Figure 1). The Reserve includes a range of marine habitats, including coral reefs, mangrove forests and seagrass lagoons. About 3 Km of coral reef, split into 13 different sections (dive sites), have been monitored on a nearly daily basis since July 2018 for the presence of sea turtles (Figure 1). Surveys are conducted on whichever dive site is chosen by a partner dive centre on that specific day, and is dependent on weather conditions and guests. Data on sea turtle presence is obtained during targeted or opportunistic in-water transects by scuba diving performed by a trained diver along the coral reef or obtained via citizen science submissions. The transects are mostly conducted one-way along the coral reef slope at an average of 12 m depth (range 8-18 m) and are between 300-500 m long. The fringing reef protects an extensive shallow seagrass lagoon (1-5 m depth) that is mostly accessed by sea turtles at high tide or via reef channels located near Galu and Kongo sites. Sites such as Leopard Beach and Swahili Beach are two nearby sites that represent two sections of an extensive area of seagrass and seaweed patches at depths >13 m and are presumably mainly used as a foraging area. Galu dive site is the most important along the study area in terms of sea turtle density, being dominated by soft coral and possessing two cleaning stations, while other sites are dominated by hard corals. Mwanamochi and Kongo dive sites represent two different sections of the same long stretch of reef, the same occurring for Jadini, Kisima Mungu, Milele and Igloo.
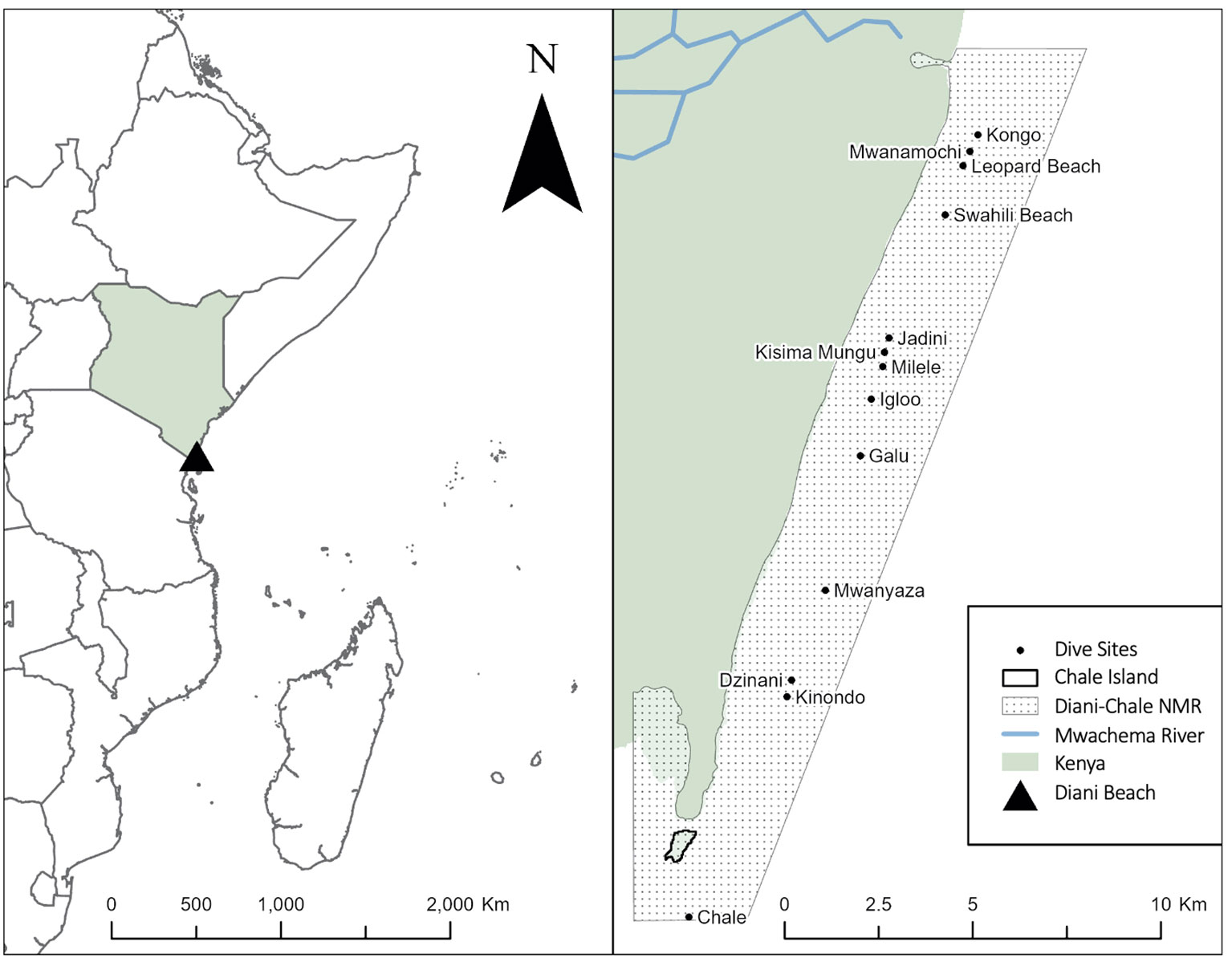
Figure 1 Location of Diani Beach, the Diani-Chale National Marine Reserve, and all dive sites monitored during this study.
Sea turtles were photographed whenever possible, with the quality of the photos being dependent on water visibility, depth of the encounter, camera used and the turtle’s collaboration. Photos submitted by citizen scientists were also included in the study. Each individual was identified by the unique pattern of facial scales on either side of their head (Carpentier et al., 2016). The identification process involved two of the authors visually matching images, which were subsequently uploaded to the online platform Internet of Turtles (iot.wildbook.org). Matches were confirmed using the Wildbook Image Analysis machine learning and computer vision stack. Individuals were grouped in different age groups based on gross estimates of curved carapace length (juveniles <65cm; sub-adults 65-90cm; adults >90cm) (Sanchez et al., 2023). The division between the two immature stages was based on the minimum size at which the appearance of male sexual characteristics was first observed in individuals at the study site (J. Hancock, pers. obs.).
When the full body of the sea turtle was visible in the photo, a general appraisal of the sea turtle’s health status was performed, including noting the presence of injuries, scars, parasites or epibionts. Moreover, the turtle (directly or through its photo) was carefully screened for the presence of external FP tumours, in particular the skin and eyes. After identifying a suspected case of FP, the existing photographs were analysed by at least two of the authors, including a sea turtle biologist and a veterinarian expert, to confirm the disease status. If the turtle was confirmed to be affected, it was marked for future monitoring in subsequent sightings to assess the progression of the disease.
The severity of FP was subjectively classified based on visual inspection of sea turtle’s photographs by a co-author with extensive experience treating clinical FP, considering tumour size, location, distribution and external morphology of tumours. The four-stage classification criteria was “Stage 0 - absent” (no obvious tumours), “Stage 1 - mild” (small corneal tumour), “Stage 2 - moderate” (evident tumours in eyes and/or body) or “Stage 3 - severe” (vision or movement impaired) (Balazs, 1991; Hirama and Ehrhart, 2007; Borrowman, 2008). Despite some degree of subjectivity, this tumour scoring strategy has been adopted widely by conservation organisations that monitor disease prevalence and survivorship in these species (e.g., Work and Balazs, 1999; Rossi et al., 2016; Page-Karjian et al., 2019). The disease’s evolution in each animal re-sighted at a later survey was classified as “Stable” (no evolution observed or assessed), “Progression” (clear increase in tumour size and/or number) or “Regression” (clear decrease in tumour size and/or number).
For spatial analysis, results were analysed per dive site, as well as per sections of the reef where sites are contiguous, share similar features and where sea turtle movement between them has been demonstrated by Photo-ID records. A total of seven sections were identified: Section 1 - Kongo and Mwanamochi; Section 2 - Leopard Beach and Swahili Beach; Section 3 - Jadini, Kisima Mungu, Milele and Igloo; Section 4 - Galu; Section 5 - Mwanyaza; Section 6 - Dzinani and Kinondo; and Section 7 - Chale Island. Sections 1 and 3 represent a single long reef which is monitored from each stated dive site as a starting point. Maps were created using ArcGIS Pro3.0.3; the .shp file for Kenya was sourced from the GADM database (www.gadm.org), version 2.5, July 2015. The rivers shp. file was sourced from RCMRD GeoPortal Africa GeoPortal. Diani-Chale MPA.shp file was accessed through Allen Coral Atlas and downloaded from ProtectedPlanet (UNEP-WCMC, 2023).
3 Results
A total of 894 surveys conducted between July 2018 and December 2022 in Diani-Chale National Marine Reserve resulted in 2757 green turtle sightings, that allowed the identification of 571 individual green turtles. The sites with most individuals identified were Galu (n=104), Kongo (n=101), Milele (n=90), Kisima Mungu (n=77) and Mwanamochi (n=70) as these correspond to the sites with a higher density of sea turtles, and are also the most visited by the dive centres. FP was detected in a total of 75 identified individuals, indicating a 13.0% prevalence of the disease across all surveyed sites. The disease was detected in all but three sites (Dzinani, Kinondo and Chale), and the spatial distribution of the FP affected individuals varied between the remaining sites (see Supplementary Table 1 for detailed data for each dive site). The highest disease prevalence was found in the seagrass and algae dominated sites of Swahili Beach and Leopard Beach, with 28.5% and 50.0% of affected individuals, respectively. These sites were followed by Galu, Milele and Mwanamochi, each with a similar percentage of affected individuals (17.0%). When looking at prevalence amongst reef sections, Section 2 (38.6%) was the highest, followed by Section 4 (17.3%), then Sections 1 and 3 (12.1% and 13.2%) respectively (Figure 2). There was no significant correlation between the severity of the identified FP cases and location (Kruskal-Wallis test, p>0.05; a chi-square test was also performed on the data but results were not reliable due to the small sample size recorded at some of the locations).
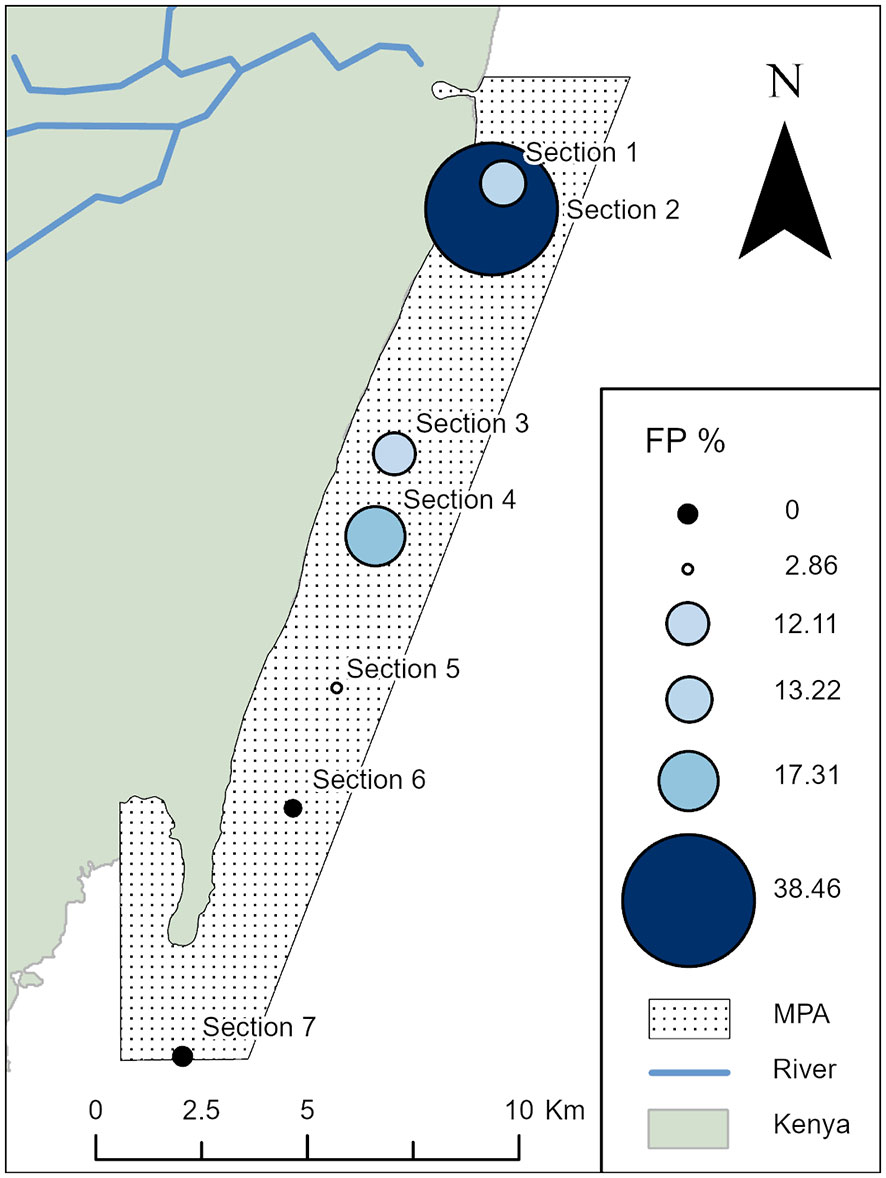
Figure 2 Spatial distribution of fibropapillomatosis (FP) prevalence in the Diani-Chale National Marine Reserve; showing the proportion of identified individual green sea turtles affected with FP per reef section. The percentage of FP per given section is displayed with graduated symbols, set to correlate to the percentage value of individual turtles encountered with FP at a given location. Total individuals encountered at each site Section 1, n=174, Section 2, n=13, Section 3, n=223, Section 4, n=104, Section 5, n=35, Section 6, n=21 and Section 7, n=1.
Tumours were observed in turtles of all size classes, with the majority of affected individuals being juvenile (n=59), followed by sub-adults (n=12), and adults (n=2) (Supplementary Table 1). All confirmed cases included at least ocular FP (n=75), but tumours found in other locations on the body were identified in several individuals (n=27). These tumours were most commonly found on the upper body of the turtle, around the neck, shoulders, and axillary area and very rarely on lower limbs; the presence of tumours on the underside of the body was difficult to evaluate due the difficulty to photograph this part of the body, as most turtles are found resting or swimming close to the reef. All stages of FP severity were identified, and as per the most recent sighting of the affected individuals, Stage 2 was more commonly observed (n=28) while Stage 1 and Stage 3 were similarly distributed (n=20 and n=21respectively). There was no significant correlation between the observed stage of the disease progression on first sighting and the size of the affected animal or the site it was spotted on (Kruskal-Wallis test, p>0.05).
A total of 291 identified individuals were re-sighted during the study period with at least 1-month interval, with an average of 10.4 ± 12.9 sightings per turtle (range 2-82 sightings). Individuals were monitored for relatively long periods of time, with an average of 559.8 ± 442.5 days between first and last sighting (range 31-1579 days). From our sightings data, we could observe that the vast majority of the identified turtles was unaffected at first sighting and remained FP free when resighted (n=431), while we were able to monitor the disease evolution in 47 individuals and assess either progression or regression in tumour size and/or load (n=26 and n=9 respectively), or no evident evolution of the disease (n=12) (Table 1). The minimum time interval between different stages of tumour severity varied from 6 days (Stage 0 to Stage 1), to 211 days (Stage 1 to Stage 2) and 147 days (Stage 2 to Stage 3). We were able to observe cases of regression (n=9), but some individuals showed cycles of regression followed by progression between Stage 0 and Stage 1 (n=6), and the evolution of the disease did not appear to be resolved, so we considered the evolution of the disease to remain stable at Stage 1 to account for potential biases due to the quality of the photo. Full recovery took an average of 471.5 days to occur. Two case studies are provided to illustrate the dynamics of FP evolution in the study population (Figure 3).
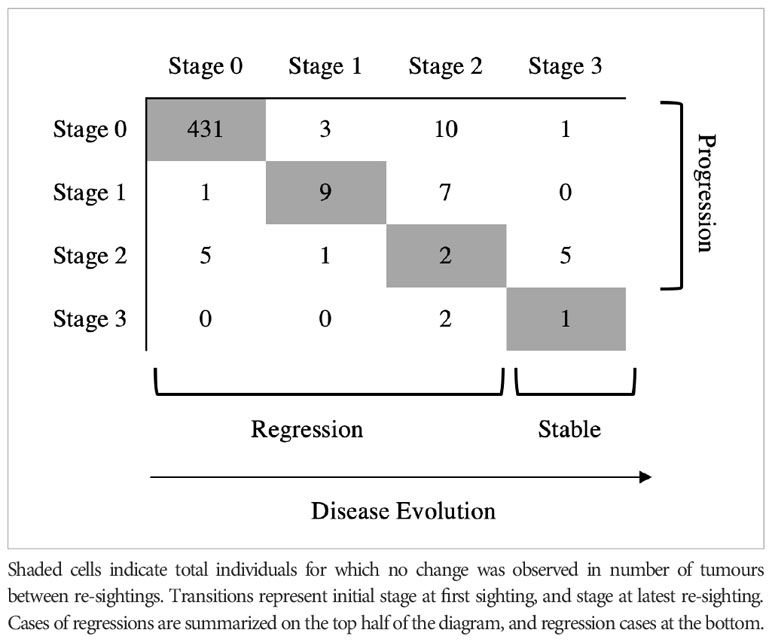
Table 1 Distinct transitions observed in re-sighted green turtles (n) affected with fibropapillomatosis in Diani-Chale National Marine Reserve between 2018-2022.
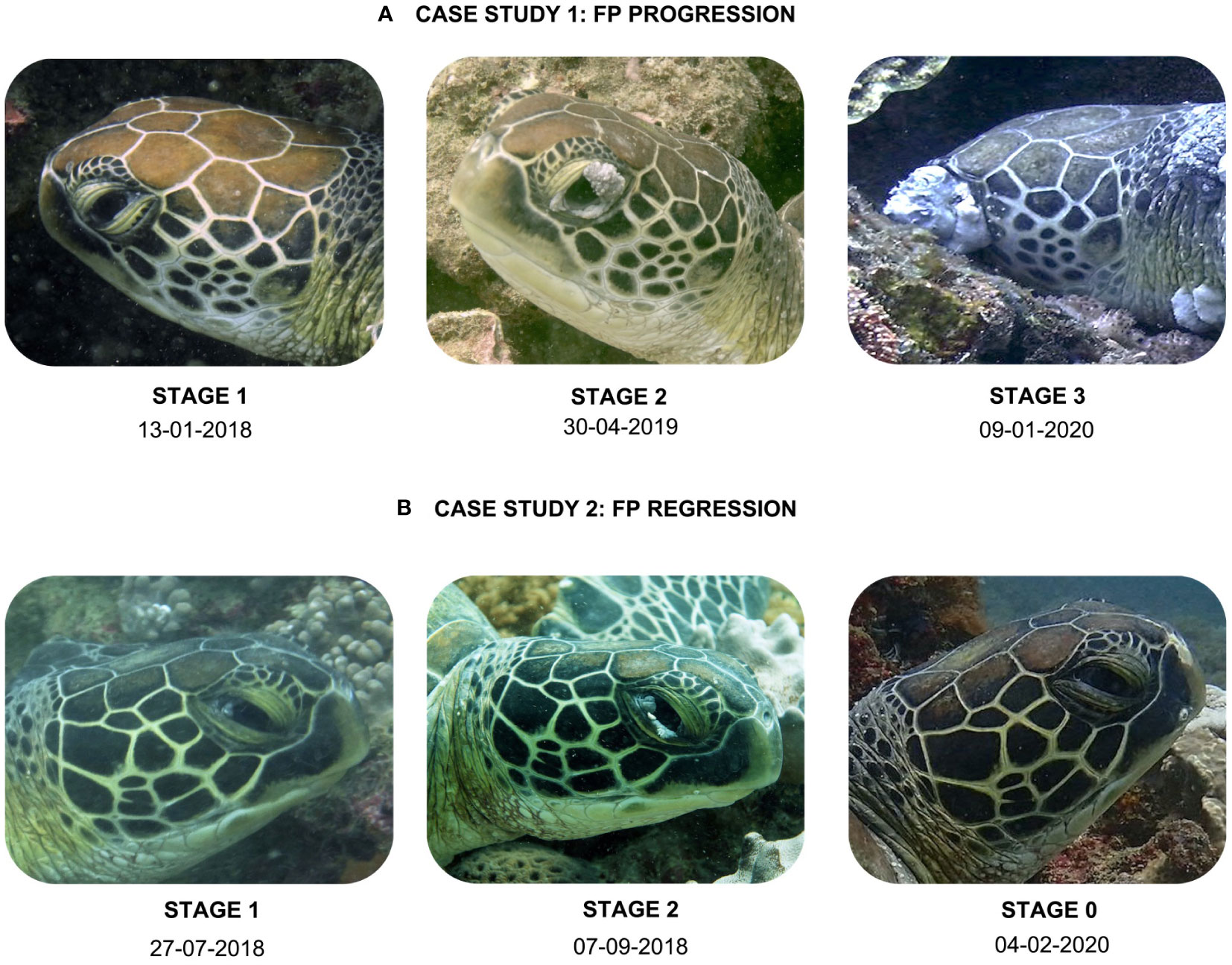
Figure 3 Presentation of two case studies in the study site that show the time interval between evolution of FP in distinct stages of severity. Case study A (turtle ID# G038, Mwanamochi site) shows an example of disease progression and Case study B (turtle ID# G006, Galu site) provides an example of disease regression.
4 Discussion
Obtaining information on the probable disease state for individual turtles, such as healthy, afflicted with FP, or recovered from FP, is key to advance our understanding of FP dynamics. These health assessments rely on a variety of quantification and qualification methods to study the differences in the clinical manifestations of FP (Rossi et al., 2016). A combination of diagnostic tools, including visual inspection, histopathology, PCR assay, and serology, may be needed to correctly diagnose and study FP in sea turtles (e.g., Monteiro et al., 2021). However, in many places where sea turtles occur, laboratory-based tools are difficult to access, either logistically or due to financial restraints, hindering efforts to understand the dynamics of this complex disease. In this study, we show that the combination of targeted underwater surveys, citizen science and the use of Photo-ID can be a low cost, non-invasive method useful in documenting the prevalence of the disease in neritic environments where sea turtles are relatively friendly and can be easily approached, or good environmental conditions prevail.
Our results showed that the disease is prevalent in Diani-Chale National Marine Reserve, with a small percentage of individuals affected by the disease (~13.0%). This percentage is comparable with values detected in other populations in various locations around the globe (Shaver et al., 2019; Silva-Júnior et al., 2019). When compared to the values obtained of stranded green turtles studied at Watamu National Marine Park and Reserve in Kenya, the prevalence is lower (13.0% versus 24.0%, Jones et al., 2021).
The size-class distribution of green sea turtles with FP aligns with the current understanding that this condition predominantly affects juvenile turtles after they have migrated to near-shore habitats (Kelley et al., 2022). According to a recent study, in some green turtle populations most turtles are carriers of the FP virus and may quickly develop FP tumours if they are recruited to the inshore habitats. Upon migration to the juvenile foraging grounds, they will completely recover before emigrating (Kelley et al., 2022). Our assessment is limited in the timescale, but the re-sighting of some individuals allowed the observation of disease evolution over time in several cases, with both cases of progression and regression detected. In our study, we observed regression in turtles with Stage 1 ocular tumour, but in some cases, the tumours continued to progress into Stage 2 and 3 tumours in a matter of months in a single individual. Recovery in the study population can take over one year to occur, a time period similar to recorded elsewhere (see Patrício et al., 2016; Page-Karjian et al., 2019). These observations would benefit from a larger timescale data collection for a better understanding of the dynamics of the disease, including survivorship. Studies indicate that individuals with ocular FP are estimated to have lower survivorship than those affected with plaque-like FP lesions, which have a higher regression rate (Page-Karjian et al., 2014). Individuals observed with Stage 3 tumours appeared lethargic, often unresponsive to divers’ presence and in poor body conditions (J. Hancock, pers. obs.). While we observed regression from Stage 2 to Stage 0 in some cases, some turtles affected by Stage 2 showed lower rates of recovery, with some cases rapidly evolving to Stage 3, which is likely a result of increased immunosuppression observed in more advanced stages of the disease (Cray et al., 2001; Work et al., 2001; Work et al., 2003). It is possible that once a turtle passes a certain threshold of the disease, it loses the ability to recover. This is very likely to be related to the impaired vision that initiates in Stage 3, and culminates in the last stage of the disease.
The prevalence of the FP disease has been suggested to likely be a result of the significant time juvenile green turtles spend in neritic environments, which are heavily impacted by anthropogenic activities and degradation (e.g. Van Houtan et al., 2010). A global study looking at various risk factors of FP concluded that the disease is more prevalent in sites exposed to toxic phytoplankton blooms, likely as a result of higher nutrient load (Arthur et al., 2006; Arthur et al., 2008a; Dujon et al., 2021). And sea turtles can be regarded as sentinels to ecosystem health (Aguirre and Lutz, 2004). Our results hint at a higher prevalence of the disease on turtles occurring in feeding areas on the outer reef (Leopard Beach, Swahili Beach) close to the river mouth of Mwachema river, or close to channels connecting the outer reef to the seagrass lagoon (Section 4 – Galu), but these results were not statistically significant, likely due to the small sample size at Sections 2 and 7 of the reef (Leopard Beach and Swahili Beach, as well as Chale Island). It is clear that more surveys are needed to obtain a more reliable estimate of the prevalence of the disease at these sites. Moreover, these observations have not been correlated with environmental variables, but as the reef studied is located within a marine protected area, biochemical assessments could be conducted to understand the potential impacts of contaminants and disturbance in this important marine ecosystem.
Although visual inspection is the most used method for identifying FP, it has limitations that should be considered. One of the major challenges in identifying FP visually is that the disease can manifest in various ways, such as tumours varying in size, shape, location, and colour. Detecting early-stage skin tumours is particularly difficult, as they may be hidden in skin folds and can be difficult to distinguish from normal skin features such as scars or barnacles. This can lead to under-identification or misidentification of FP. Additionally, turtles in early stages of the disease or those with internal tumours may not exhibit any visible external tumours, making it impossible to identify them through visual inspection alone (Monteiro et al., 2021). Such turtles may serve as potential carriers of the disease, spreading the FP virus to other turtles. Another problem with relying solely on visual inspection is the subjective nature of identifying the disease based on tumour severity, which may affect the reliability of the identification. For the reasons described above, we recognise that our study is limited to subjective visual assessment of the expression of a disease that is considered challenging to study and understand. Additionally, our study relies heavily on photos that are taken underwater by researchers and citizen scientists, often biased towards the head or the face, and with their quality often influenced by underwater conditions. Finally, we recognise and suggest that tumour evolution analyzed photogrammetrically can give a much better picture of true tumour burden and rates of tumour severity transitions and this approach could complement this study well.
More research needs to be done to better understand the prevalence and impact of FP on sea turtle populations in Kenya. Questions related to dynamics of the disease, such as why some turtles show clinical signs of FP but not others, and what factors impact the severity and duration of individual FP affliction were not addressed in this study. However, there are ongoing efforts being conducted in collaboration with relevant research groups in Kenya to address these, including genetic studies and evaluation of habitat quality. Despite the limitations discussed, our study shows that photo identification is a valuable tool for low-cost and non-invasive monitoring of the FP disease in wild sea turtle populations. With time, this method, when coupled with long-term monitoring effort, may help to understand the impacts of FP on sea turtle health and population dynamics.
Data availability statement
The datasets presented in this study can be found in online repositories. The names of the repository/repositories and accession number(s) can be found below: https://iot.wildbook.org/.
Ethics statement
Ethical approval was not required for the studies involving humans because no human research was involved. The studies were conducted in accordance with the local legislation and institutional requirements. Written informed consent for participation was not required from the participants or the participants’ legal guardians/next of kin in accordance with the national legislation and institutional requirements because no human research was involved. Ethical approval was not required for the study involving animals in accordance with the local legislation and institutional requirements because there was no handling of animals during the study, method was 100% non-invasive.
Author contributions
JH and SK contributed to the conception and design of the study. JH, LM, and JC collected and managed all data and performed the preliminary data analysis. MP and SK reviewed and confirmed all health assessments. JH and SK wrote the first draft of the manuscript. MP and MS wrote sections of the manuscript. All remaining authors contributed to manuscript revision and read and approved the submitted version on behalf of partnering institutions.
Funding
Funding for this project was provided by The Sands at Nomad Boutique Hotel and Marine Life Protectors, Germany, and numerous individual donors who have supported our activities through the Sea Turtle Adoption Program.
Acknowledgments
The authors would like to acknowledge the invaluable logistic support provided by the team at The Crab Diving Centre and Denis Moser/D. M. Tours. While most photos are collected by our team, many were submitted by members of local conservation groups such as Diani Turtle Watch and Conservation Education Society, or via our citizen science program. Maps were produced by Jane Lloyd. This project is developed under a collaborative research agreement with Pwani University Biosciences Research Centre (PUBReC) in Kenya, with knowledge of the Kenya Wildlife Service.
Conflict of interest
The authors declare that the research was conducted in the absence of any commercial or financial relationships that could be construed as a potential conflict of interest.
Publisher’s note
All claims expressed in this article are solely those of the authors and do not necessarily represent those of their affiliated organizations, or those of the publisher, the editors and the reviewers. Any product that may be evaluated in this article, or claim that may be made by its manufacturer, is not guaranteed or endorsed by the publisher.
Supplementary material
The Supplementary Material for this article can be found online at: https://www.frontiersin.org/articles/10.3389/fmars.2023.1217683/full#supplementary-material
References
Aguirre A.A., Lutz P. L. (2004). Marine turtles as sentinels of ecosystem health: is fibropapillomatosis an indicator? EcoHealth 1, 3. doi: 10.1007/s10393-004-0097-3
Arthur K. E., Boyle M. C., Limpus C. J. (2008b). Ontogenetic changes in diet and habitat use in green sea turtle (Chelonia mydas) life history. Mar. Ecol. Prog. Ser. 362, 303–311. doi: 10.3354/meps07440
Arthur K. E., Limpus C. J., Balazs G. H., Capper A., Udy J., Shaw G. R., et al. (2008a). The exposure of green turtles (Chelonia mydas) to tumour promoting compounds produced by the cyanobacterium Lyngbya majuscula and their potential role in the aetiology of fibropapillomatosis. Harmful Algae 7 (1), 114–125. doi: 10.1016/j.hal.2007.06.001
Arthur K., Shaw G., Limpus C., Udy J. (2006). A review of the potential role of tumour-promoting compounds produced by Lyngbya majuscula in marine turtle fibropapillomatosis. Afr. J. Mar. Sci. 28 (2), 441–446. doi: 10.2989/18142320609504194
Balazs G. H. (1991). “Current status of fibropapillomas in the Hawaiian green turtle, Chelonia mydas,” in Research plan for marine turtle fibropapilloma (NOAATM-NMFS-SWFSC 156). Eds. Balazs G. H., Pooley S. G. (Honolulu: U.S: Department of Commerce, National Oceanographic and Atmospheric Administration, National Marine Fisheries Service), 47–57.
Balazs G. H. (1999). “Factors to consider in the tagging of sea turtles. Research and management techniques for the conservation of sea turtles,” in Research and management techniques for the conservation of sea turtles. Eds. Eckert K. L., Bjorndal K. A., Abreu-Grobois F. A., Donelly M. (Washington DC: IUCN/Species Survival Commission Marine Turtle Specialist Group), 1–9.
Balazs G., Jacobson E. (1990). Health advisory for fibropapilloma disease. Marine Turtle Newsletter 49 (27).
Bolten A. B., Lutz P. L., Musick J. A., Wyneken J. (2003). “Variation in sea turtle life history patterns: neritic vs. oceanic developmental stages,” in The biology of sea turtles, v.2. Eds. Lutz P. L., Musick J. A., Wyneken J. (Boca Raton: CRC Press), 243–257.
Borrowman K. M. (2008). Prevalence and severity of fibropapillomatosis in juvenile green turtles (Chelonia mydas) in three habitats on Florida’s East coast. Masters dissertation (Orlando, Florida: University of Central Florida).
Carpentier A. S., Jean C., Barret M., Chassagneux A., Ciccione S. (2016). Stability of facial scale patterns on green sea turtles Chelonia mydas over time: a validation for the use of a photo-identification method. J. Exp. Mar. Biol. Eco. 476, 15–21. doi: 10.1016/j.jembe.2015.12.003
Chaloupka M., Balazs G. H., Work T. M. (2009). Rise and fall over 26 years of a marine epizootic in Hawaiian green sea turtles. J. Wildl. Dis. 45 (4), 1138–1142. doi: 10.7589/0090-3558-45.4.1138
Chaves A., Aguirre A. A., Blanco-Peña K., Moreira-Soto A., Monge O., Torres A. M., et al. (2017). Examining the role of transmission of cheloid alphaherpesvirus 5. Ecohealth 14 (3), 530–541. doi: 10.1007/s10393-017-1248-7
Cray C., Varella R., Bossart G. D., Lutz P. (2001). Altered in vitro immune responses in green turtles (Chelonia mydas) with fibropapillomatosis. J. Zoo. Wildl. Med. 32, 436–440. doi: 10.1638/1042-7260
Dujon A. M., Schofield G., Venegas R. M., Thomas F., Ujvari B. (2021). Sea turtles in the cancer risk landscape: a global meta-analysis of fibropapillomatosis prevalence and associated risk factors. Pathogens 10 (10), 1295. doi: 10.3390/pathogens10101295
García-Sastre A., Sansonetti P. J. (2010). Host-pathogen interactions. Curr. Opin. Immunol. 22, 425–427. doi: 10.1016/j.coi.2010.06.005
Greenblatt R. J., Work T. M., Balazs G. H., Sutton C. A., Casey R. N., Casey J. W. (2004). The Ozobranchus leech is a candidate mechanical vector for the fibropapilloma-associated turtle herpesvirus found latently infecting skin tumors on Hawaiian green turtles (Chelonia mydas). Virol. J. 321 (1), 101–110. doi: 10.1016/j.virol.2003.12.026
Hamann M., Godfrey M. H., Seminoff J. A., Arthur K., Barata P. C. R., Bjorndal K. A., et al. (2010). Global research priorities for sea turtles: informing management and conservation in the 21st century. Endanger Species Res. 11 (3), 245–269. doi: 10.3354/esr00279
Hancock J., Choma J., Mainye L., Stelfox M., Hudgins J. (2020). Photo identification as a tool to study sea turtle populations in Kenyan marine protected areas. Available at: https://conbio.org/groups/sections/africa/act/photo-identification-as-a-tool-to-study-sea-turtle-populations-in-Kenyan-ma (Accessed 29 Apr. 2023). conbio.org.
Herbst L. H. (1994). Fibropapillomatosis of marine turtles. Annu. Rev. Fish. Dis. 4, 389–425. doi: 10.1016/0959-8030(94)90037-X
Herbst L. H., Jacobson E. R., Klein P. A., Balazs G. H., Moretti R., Brown T., et al. (1999). Comparative pathology and pathogenesis of spontaneous and experimentally induced fibropapillomas of green turtles (Chelonia mydas). Vet. Pathol. 36, 551–564. doi: 10.1354/vp.36-6-551
Hirama S., Ehrhart L. (2007). Description, prevalence and severity of green turtle fibropapillomatosis in three developmental habitats on the east coast of Florida. Florida Sci. 70, 435–448.
Holmberg J., Norman B., Arzoumanian Z. (2009). Estimating population size, structure, and residency time for whale sharks Rhincodon typus through collaborative photo-identification. Endanger Species Res. 7 (1), 39–53. doi: 10.3354/esr00186
Jean C., Ciccione S., Talma E., Ballorain K., Bourjea J. (2010). Photo-identification method for green and hawksbill turtles: first results from Reunion. IOTN 11, 8–13.
Jones K., Ariel E., Burgess G., Read M. (2016). A review of fibropapillomatosis in green turtles (Chelonia mydas). Vet. J. 212, 48–57. doi: 10.1016/j.tvjl.2015.10.041
Jones K., Burgess G., Budd A. M., Huerlimann R., Mashkour N., Ariel E. (2020). Molecular evidence for horizontal transmission of chelonid alphaherpesvirus 5 at green turtle (Chelonia mydas) foraging grounds in Queensland, Australia. PloS One 15 (1), e0227268. doi: 10.1371/journal.pone.0227268
Jones S. M., Caspi I., Lucas C. (2021). Fibropapillomatosis infection in a population of green turtles at Watamu Bay, Kenya. WIOJMS 20 (1), 111–123. doi: 10.4314/wiojms.v20i1.10
Kelley J. R., Kelley K. L., Savage A. E., Mansfield K. L. (2022). Novel disease state model finds most juvenile green turtles develop and recover from fibropapillomatosis. Ecosphere 13 (3), e4000. doi: 10.1002/ecs2.4000
Kitchen-Wheeler A. M. (2010). Visual identification of individual manta ray (Manta alfredi) in the Maldives Islands, Western Indian Ocean. Mar. Biol. Res. 6 (4), 351–363. doi: 10.1080/17451000903233763
Lackovich J. K., Brown D. R., Homer B. L., Garber R. L., Mader D. R., Moretti R. H., et al. (1999). Association of herpesvirus with fibropapillomatosis of the green turtle Chelonia mydas and the loggerhead turtle Caretta caretta in Florida. Dis. Aquat. Organ. 37, 89–97. doi: 10.3354/dao037089
Landsberg J. H., Balazs G. H., Steidinger K. A., Baden D. G., Work T. M., Russell D. J. (1999). The potential role of natural tumor promoters in marine turtle fibropapillomatosis. J. Aquat. Anim. Health 11 (3), 199–210. doi: 10.1577/1548-8667(1999)011<0199:tpront>2.0.co;2
Lu Y., Yu Q., Zamzow J. P., Wang Y., Losey G. S., Balazs G. H., et al. (2000). Detection of green turtle herpesviral sequence in saddleback wrasse Thalassoma duperrey: a possible mode of transmission of green turtle fibropapilloma. J. Aquat. Anim. Health 12 (1), 58–63. doi: 10.1577/1548-8667(2000)012<0058:dogths>2.0.co;2
Manes C., Pinton D., Canestrelli A., Capua I. (2022). Occurrence of fibropapillomatosis in green turtles (Chelonia mydas) in relation to environmental changes in coastal ecosystems in Texas and Florida: a retrospective study. Animals 12 (10), 1236. doi: 10.3390/ani12101236
Monteiro J., Duarte M., Amadou K., Barbosa C., El Bar N., Madeira F. M., et al. (2021). Fibropapillomatosis and the chelonid alphaherpesvirus 5 in green turtles from West Africa. EcoHealth 18 (2), 229–240. doi: 10.1007/s10393-021-01526-y
Page-Karjian A., Gottdenker N. L., Whitfield J., Herbst L., Norton T. M., Ritchie B. (2017). Potential noncutaneous sites of chelonid herpesvirus 5 persistence and shedding in green sea turtles Chelonia mydas. J. Aquat. Anim. Health 29 (3), 136–142. doi: 10.1080/08997659.2017.1321590
Page-Karjian A., Norton T. M., Krimer P., Groner M., Nelson S. E., Gottdenker N. L. (2014). Factors influencing survivorship of rehabilitating green sea turtles (Chelonia mydas) with fibropapillomatosis. J. Zoo. Wildl. Med. 45 (3), 507–519. doi: 10.1638/2013-0132r1.1
Page-Karjian A., Perrault J. R., Zirkelbach B., Pescatore J., Riley R., Stadler M., et al. (2019). Tumor re-growth, case outcome, and tumor scoring systems in rehabilitated green turtles with fibropapillomatosis. Dis. Aquat. Org. 137, 101–108. doi: 10.3354/dao03426
Patrício A. R., Diez C. E., van Dam R. P., Godley B. J. (2016). Novel insights into the dynamics of green turtle fibropapillomatosis. Mar. Ecol. Prog. Ser. 547, 247–255. doi: 10.3354/meps11644
Perrault J. R., Levin M., Mott C. R., Bovery C. M., Bresette M. J., Chabot R. M., et al. (2021). Insights on immune function in free-ranging green sea turtles (Chelonia mydas) with and without fibropapillomatosis. Animals 11 (3), 861. doi: 10.3390/ani11030861
Quackenbush S. L., Work T. M., Balazs G. H., Casey R. N., Rovnak J., Chaves A., et al. (1998). Three closely related herpesviruses are associated with fibropapillomatosis in marine turtles. Virology 246 (2), 392–399. doi: 10.1006/viro.1998.9207
Reich K. J., Bjorndal K. A., Bolten A. B. (2007). The ‘lost years’ of green turtles: using stable isotopes to study cryptic lifestages. Biol. Lett. 3 (6), 712–714. doi: 10.1098/rsbl.2007.0394
Rodenbusch C. R., Baptistotte C., Werneck M. R., Pires T. T., Melo M. T. D., de Ataíde M. W., et al. (2014). Fibropapillomatosis in green turtles Chelonia mydas in Brazil: Characteristics of tumors and virus. Dis. Aquat. Org. 111, 207–217. doi: 10.3354/dao02782
Rossi S., Sanchez-Sarmiento A. M., Vanstreels R. E. T., Dos Santos R. G., Prioste F. E. S., Gattamorta M. A., et al. (2016). Challenges in evaluating the severity of fibropapillomatosis: a proposal for objective index and score system for green sea turtles (Chelonia mydas) in Brazil. PloS one 11 (12), e0167632. doi: 10.1371/journal.pone.0167632
Sanchez C. L., Bunbury N., Mortimer J. A., A’Bear L., Betts M., von Brandis R., et al. (2023). Growth rate and projected age at sexual maturity for immature hawksbill turtles and green turtles foraging in the remote marine protected area of Aldabra Atoll, Seychelles. Mar. Biol. 170 (4), 49. doi: 10.1007/s00227-023-04197-1
Seminoff J. A. (2004) Chelonia mydas. IUCN red list of threatened species (Southwest Fisheries Science Center, U.S) (Accessed May 3, 2023).
Shaver D. J., Walker J. S., Backof T. F. (2019). Fibropapillomatosis prevalence and distribution in green turtles Chelonia mydas in Texas (USA). Dis. Aquat. Organ 136 (2), 175–182. doi: 10.3354/dao03403
Silva-Júnior E. S. D., de Farias D. S. D., Costa Bomfim A. D., Boaviagem Freire A. C. D., Revorêdo R. Â., Rossi S., et al. (2019). Stranded marine turtles in northeastern Brazil: incidence and spatial–temporal distribution of fibropapillomatosis. Chel. Conserv. Biol. 18 (2), 249–258. doi: 10.2744/ccb-1359.1
UNEP-WCMC. (2023). Protected area profile for diani chale marine from the world database on protected areas, may 2023. Available at: www.protectedplanet.net.
Van Houtan K. S., Hargrove S. K., Balazs G. H. (2010). Land use, macroalgae, and a tumour-forming disease in marine turtles. PloS One 5 (9), e12900. doi: 10.1371/journal.pone.0012900
Van Houtan K. S., Smith C. M., Dailer M. L., Kawachi M. (2014). Eutrophication and the dietary promotion of sea turtle tumors. PeerJ 2, e602. doi: 10.7717/peerj.602
Work T. M., Balazs G. H. (1999). Relating tumor score to hematology in green turtles with fibropapillomatosis in Hawaii. J. Wild. Dis. 35 (4), 804–807. doi: 10.7589/0090-3558-35.4.804
Work T. M., Balazs G. H., Rameyer R. A., Morris R. A. (2004). Retrospective pathology survey of green turtles Chelonia mydas with fibropapillomatosis in the Hawaiian Islands 1993-2003. Dis. Aquat. Org. 62 (1-2), 163–176. doi: 10.3354/dao062163
Work T. M., Balazs G. H., Wolcott M., Morris R. (2003). Bacteraemia in free-ranging Hawaiian green turtles Chelonia mydas with fibropapillomatosis. Dis. Aquat. Org. 53 (1), 41–46. doi: 10.3354/dao053041
Work T. M., Dagenais J., Balazs G. H., Schettle N., Ackermann M. (2015). Dynamics of virus shedding and in situ confirmation of chelonid herpesvirus 5 in Hawaiian green turtles with fibropapillomatosis. Vet. Pathol. 52 (6), 1195–1201. doi: 10.1177/0300985814560236
Keywords: fibropapillomatosis, Chelonia mydas, monitoring, Indian Ocean, citizen science
Citation: Hancock JM, Choma J, Mainye L, Wambi P, Stelfox MR, Polyak MMR, Wambua S and Köhnk S (2023) Using Photo-ID to document and monitor the prevalence of fibropapilloma tumours in a foraging aggregation of green turtles. Front. Mar. Sci. 10:1217683. doi: 10.3389/fmars.2023.1217683
Received: 05 May 2023; Accepted: 29 August 2023;
Published: 21 September 2023.
Edited by:
Andrea D. Phillott, Flame University, IndiaReviewed by:
Hideaki Nishizawa, Kyoto University, JapanThierry Work, United States Department of the Interior, United States
Copyright © 2023 Hancock, Choma, Mainye, Wambi, Stelfox, Polyak, Wambua and Köhnk. This is an open-access article distributed under the terms of the Creative Commons Attribution License (CC BY). The use, distribution or reproduction in other forums is permitted, provided the original author(s) and the copyright owner(s) are credited and that the original publication in this journal is cited, in accordance with accepted academic practice. No use, distribution or reproduction is permitted which does not comply with these terms.
*Correspondence: Joana M. Hancock, am9hbmEuaGFuY29ja0BvbGl2ZXJpZGxleXByb2plY3Qub3Jn