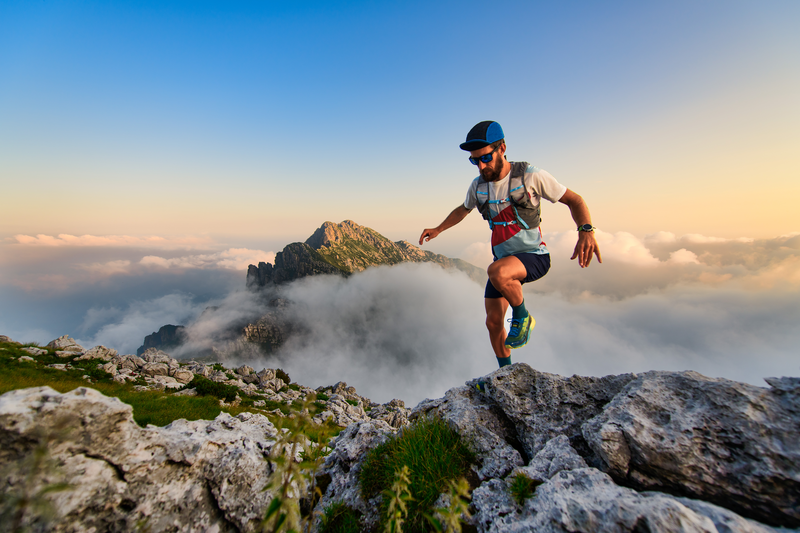
94% of researchers rate our articles as excellent or good
Learn more about the work of our research integrity team to safeguard the quality of each article we publish.
Find out more
ORIGINAL RESEARCH article
Front. Mar. Sci. , 23 June 2023
Sec. Coastal Ocean Processes
Volume 10 - 2023 | https://doi.org/10.3389/fmars.2023.1215883
This article is part of the Research Topic Fate and Transport of Terrestrial Materials to the Sea and Their Effect on Coastal Eutrophication, Deoxygenation and Acidification View all 7 articles
Anaerobic ammonium oxidation (anammox) plays an important role in nitrogen removal in coastal seas, and ladderanes, as specific biomarkers of anammox bacteria, can be used to indicate the anammox activity. However, the origins of ladderanes and their controlling factors in the coastal seas influenced by anthropogenic activities are still not well constrained. To address this, we reported ladderanes, long-chain n-alkanols (terrestrial biomarker) and sterols (marine biomarker) in suspended particulates from the estuaries and inner area of Laizhou Bay in North China, to study ladderane sources and its distribution patterns. This study proposed a novel index, Rlad, using ladderane ratio of ladderane III to ladderane IV, and by correlating this index with other biomarker distributions to evaluate the source of ladderanes. High Rlad values (> 0.9) indicated biosynthesis by terrestrial anammox bacteria Ca. Brocadia and Ca. Kuenenia, while low Rlad values (< 0.9) indicated biosynthesis by marine anammox bacteria Ca. Scalindua. High Rlad values and high ladderane concentrations in particulates from the estuaries and nearshore area of Laizhou Bay revealed sources from the terrestrial input via riverine inflow as well as in situ production in oxygen-depleted estuaries, supported by high concentrations of terrestrial biomarkers; Low Rlad values and low ladderane concentrations in particulates from offshore area indicated sources from marine environment via the cold hypoxia water input by the Bohai circulation. Comparison of ladderane concentrations of our study with previously published results from a wide range of environments with human influences from Chinese coastal area revealed that high ladderane concentrations synthesized by terrestrial anammox bacteria could contribute significantly to coastal seas, and the anammox process in river-estuary-bay system might be underestimated. This study provides new understandings about the evaluation of the source and distribution of ladderanes under anthropogenic influences in coastal seas.
Dissolved inorganic nitrogen (DIN) is a key limiting nutrient in ocean primary production (Arrigo, 2005; Rush and Sinninghe Damsté, 2017). But in recent decades, enhanced DIN inputs by anthropogenic sewage and agricultural wastewater caused a series of environmental problems like eutrophication and hypoxia in coastal areas (e.g., Jickells, 1998; Conley et al., 2009; Altieri and Gedan, 2015; Wang et al., 2019b), which required a reduction of nutrient discharge for a healty ecostal ecosystem. Denitrification process was the predominant natural pathway for nitrogen removal in the marine environment. However, the imbalance in marine nitrogen budget revealed that the significance of other nitrogen loss pathways could have been underestimated, such as anaerobic ammonium oxidation (anammox) (Kuypers et al., 2003; Dalsgaard et al., 2012; Thamdrup, 2012). Anammox process was mediated by anammox bacteria, which could uniquely convert directly to N2 in anammoxosome (Van de Graaf et al., 1995). Anammox was first found in sewage treatment plants (Mulder et al., 1995), and in the past decades this process has been proved to be an effective nitrogen removal pathway in natural environment (Kuypers et al., 2003; Kuypers et al., 2005; Karthäuser et al., 2021). In marine nitrogen cycle, anammox even could be predominant in nitrogen loss in marine hypoxia zones and OMZs (Lam et al., 2009; Ward, 2013). For example, the contribution of anammox process to nitrogen loss was up to ~40% in the Black Sea OMZ (Kuypers et al., 2003), ~50% in the Peruvian OMZ (Lam et al., 2009) and ~67% in the Baltic-North Sea OMZ (Thamdrup and Dalsgaard, 2002).
Therefore, the evaluation of anammox activity in marine environments is important for a better understanding of nitrogen biogeochemical process and ecosystem functions (Peeters and van Niftrik, 2019). Nitrogen isotope labelling was the first technique applied to trace the biological origin of anammox (Van de Graaf et al., 1997), which remains the most frequently used method to quantify anammox activity (e.g., Engström et al., 2005; Boumann et al., 2009; Li et al., 2021; Teng et al., 2022). Molecular techniques in gene level, including qPCR and high-throughput sequencing, were used to locate the certain segment of functional genes to further investigate community structure and abundances of anammox bacteria (Klotz and Stein, 2008; Hirsch et al., 2011; Li et al., 2013; Li et al., 2022). Using the above techniques, seven chemolithoautotrophic microbial genera were found (Pereira et al., 2017 and references therein), known as Ca. Brocadia, Ca. Kuenenia, Ca. Scalindua, Ca. Jettenia, Ca. Anammoxoglobus, Ca. Brasilis and Ca. Anammoximicrobium. Among these genera, Ca. Brocadia, Ca. Kuenenia and Ca. Scalindua were confirmed as three dominating genera of anammox bacteria found in natural environments (e.g., Gonzalez-Silva et al., 2017; Zhang et al., 2018; Fozia et al., 2020). Most species of Ca. Brocadia and Ca. Kuenenia were found in terrestrial environments while species of Ca. Scalindua mainly occurred in marine environments (e.g., Risgaard-Petersen et al., 2004; Hou et al., 2013; Fozia et al., 2020). The presence of anammox bacteria can also be inferred from the detection and quantification of ladderane lipids, which are membrane lipids uniquely biosynthesized by anammox bacteria with the core structure of 3–5 concatenated cyclobutane moieties (Figure 1) (Sinninghe Damsté et al., 2002; Rush and Sinninghe Damsté, 2017). Ladderane lipids have been proved to be the stable, specific, and easily-identified biomarkers to study the anammox activity (Brandsma et al., 2011; Pitcher et al., 2011; Rush et al., 2012a; Zhao et al., 2013; Rush and Sinninghe Damsté, 2017). Therefore, ladderanes provide a molecular indication for the nitrogen cycle. In the water columns, high ladderane concentrations and high anammox rates were simultaneously found in hypoxic zones with high nitrogen loss (e.g., Kuypers et al., 2005; Jaeschke et al., 2007). Besides, ladderane lipids in sedimentary records could be used to reconstruct past changes of anammox activity and to indicate the change of marine nitrogen cycle and hypoxia events (e.g., Jaeschke et al., 2009; Zhao et al., 2019).
Figure 1 The molecular structure of ladderane fatty acid methyl esters (ladderane FAMEs) used in this study (modified after Sinninghe Damsté et al., 2002).
The contributions of anammox to nitrogen loss in sediments have been well studied, e.g., up to 19% in the Jiaozhou Bay of the Yellow Sea (Wu et al., 2019), 22% in the Bohai Sea (Zhang et al., 2018) and 42% in southern New England Estuary and its shelf area (Brin et al., 2014). However, due to the difficulties of bacteria culture, studies of anammox were hardly carried out in particulates of the water column using isotope labelling or sequencing techniques. Ladderane biomarker could thus be a valuable proxy for anammox activity in water columns, but its distribution and origin in particulate were not well studied until now. The origin of ladderanes was generally considered as marine in situ production by anammox bacteria without allochthonous input in ocean (e.g., Jaeschke et al., 2007; Jaeschke et al., 2009; Rush et al., 2012a; Rush et al., 2012b; Rush and Sinninghe Damsté, 2017). However, ladderane sources in coastal waters might be more complicated due to the riverine input and anthropogenic influences, since anammox activities were universal in terrigenous environments such as rivers, sewage water discharge and soils (e.g., Hou et al., 2013; Shen et al., 2013; Zhang et al., 2017b). The origins of ladderanes in coastal seas influenced by the anthropogenic activities were still uncertain, limiting our understanding of the indication of ladderanes anammox studies.
Herein, we present ladderanes, marine and terrestrial biomarkers data in suspended particulates of the water column from Laizhou Bay to study the source and distribution of ladderanes and factors controlling anammox activities. Laizhou Bay is located at the south part of the Bohai Sea, China (Figure 2). As a typical bay with multiple small river inputs and complicated hydrological condition, the estuary-bay system here has been considered as an ideal study area influenced by anthropogenic activity. The main objectives of this study are: 1) to investigate the distribution pattern of ladderanes in the estuary-bay system of the Laizhou Bay, 2) to distinguish the origin of ladderanes by using biomarker index and 3) to evaluate the anammox activities in different coastal areas. This case study will be essential to understand the distribution and source of ladderanes in particulates from the coastal system, which can provide new insights into anammox activity and marine nitrogen cycle in coastal areas influenced by anthropogenic activities.
Figure 2 Sampling sites and circulations in Laizhou Bay, Bohai Sea. The red star on the left map is the city Beijing of China. Yellow shadows in the left map represent the summer hypoxia zones in the Bohai Sea, modified after Wei et al. (2019). The surface circulations displayed by gray arrows were modified after Guan (1994), Qiao et al. (2010), Wei et al. (2019) and Zhang et al. (2022). Area A in brown texture fill represents the nearshore area which is significantly influenced by small river inputs. Area B in white texture fill represents the offshore area which represented the central and north area of Laizhou Bay. Blue dots in the right map represent sampling sites including estuarine sites and bay sites. YR, Yellow River; XQR, Xiaoqing River; JLR, Jiaolai River; NYR, Nanyang River; JR, Jie River; 1, Zimai River; 2, Mi River; 3, Bailang River; 4, Wei River.
The Laizhou bay is a semi-closed bay of the Bohai Sea, China, covering about ~6000 km2 area with around ~320 km coastline (Wang et al., 2020). The Laizhou Bay is generally shallow, with the average water depth of ~9 m (Jiang et al., 2018). Multiple rivers (Figure 2) discharge terrestrial materials and nutrients to the bay each year (Jiang et al., 2005; Guo et al., 2015; Wang et al., 2019b; Zhang et al., 2019; Peng et al., 2021). The highest nutrient concentrations normally occurred in summer due to the strong river runoff in wet season (Peng et al., 2021). Rivers flowing into Laizhou Bay, like the Xiaoqing River (XQR), were severely contaminated by industrial and agricultural sewage, which could further affect the coastal ecological environment and biogeochemical cycles in Laizhou Bay (He et al., 2018a; He et al., 2018b). These processes and nearshore marine aquaculture activities (Cui et al., 2005) have resulted in low subsurface dissolved oxygen (DO) concentration in the central Bohai Sea during summer (Song et al., 2016; Zhai et al., 2019; Zheng et al., 2020), which also influenced the distribution of hypoxic zone in adjacent regions via hydrodynamic processes (Wei et al., 2019). Hypoxia in sea water has been observed in the Xiaoqing River Estuary, which is strongly impacted by anthropogenic activities (He et al., 2018b). Moreover, the river discharge and the south Bohai anti-clock coastal current results in rather complicated hydrologic conditions for this small bay (Qiao et al., 2010; Zhou et al., 2017; Wei et al., 2019). The eutrophication area and hydrologic condition of Laizhou Bay were closely linked to the river input, which might reveal the potential differences of biogeochemical process in nearshore area and offshore area (Jiang et al., 2018; Zhang et al., 2019; Peng et al., 2021).
We divided our study area into the sub-regions as ‘estuary’, ‘nearshore areas’ closed to river mouths, and ‘offshore areas’ to discuss the source of ladderanes. The estuarine area includes the small river estuaries along the south and east bank of Laizhou Bay. This area was dominated by riverine lateral transportation from upper stream, showing brackish condition between river freshwater discharge and sea water intrusion. The biogeochemical process in these small rivers were less studied comparing with the Yellow River although they were also impacted by strong anthropogenic activity with the development of agriculture and industry in the northern Shandong Peninsula (Zhang et al., 2015; He et al., 2018a). The nearshore ‘area A’ (Figure 2) along the coast was intensely influenced by river input (Zhang et al., 2015; Jiang et al., 2018). The offshore ‘area B’ (Figure 2) was impacted by the Yellow River discharge and Bohai Sea circulations. Although the Yellow River contributed significantly to the hydrology and ecology condition in the northwestern bay (Liu et al., 2012; Peng et al., 2021), anammox activity in the Yellow River Estuary was proved to be very weak comparing with that of the Bohai Sea (Zhang et al., 2017b; Zhang et al., 2018).
Twenty-one suspended particulate samples (6 m depth) from Laizhou Bay were collected in August 2018, and eight suspended particulate samples from estuaries were collected in August 2020 (Figure 2). The sea waters for particulates collection were obtained by submerged pump to large High-Density Polyethylene (HDPE) bottles on board aboard a small vessel of LuChangYu 60003, and about 25 L of water at each station was filtered through pre-combusted glass fiber filters (Whatman GF/F, 150 mm) to get the suspended particulates. The collection of particulate samples from the inner bay in 2018 and from the estuaries in 2020 were both collected in summer, representing similar hydrodynamic conditions in wet season. Specifically, mean water temperature in Laizhou Bay were 27.2 °C and 26.9 °C in the summer of 2018 and 2020, respectively, and water salinity in Laizhou Bay were 30.9 and 30.0 in the summer of 2018 and 2020, respectively (Lu, 2022). Higher temperature and lower salinity both occurred in the western bay in 2018 and 2020 (Lu, 2022). Moreover, according to the monitoring data of water quality, biodiversity and pollution discharge in the Laizhou Bay, the river flows and sea water environments of 2018 and 2020 were similar, e.g., highest DIN concentration both occurred near the XQR estuary at 38.2 and 33.5 μmol/L in 2018 and 2020, respectively (Lu, 2022). We herein suggest our data of particulate samples in estuaries and embayment were comparable.
Water temperature and salinity were measured by a portable multiparameter probe (WTW Multi 3420). Dissolved oxygen (DO) was measured by an automatic potentiometric titrator (Metrohm 848 Titrino plus) after in situ water was additionally collected by Polymethyl Methacrylate (PMMA) hydrophore. Water samples for nutrient analysis were filtrated through GF/F filters (Whatman) and immediately frozen at –20°C. Dissolved inorganic nitrogen (DIN) in seawater including , and were quantified using flow injection analysis (QuAAtro, Seal, Germany) after thawing the samples at 4 °C. Precision of measurements above were 0.1 °C for water temperature, 0.1 for salinity, 0.1 mg/L for DO and 0.01 μmol/L for nutrients. The detection limits were 0.03 μmol/L for , 0.02 μmol/L for , 0.05 μmol/L for . All these parameters were used as the average value after triple measurement.
Measurement of bulk organic carbon in particulate samples followed the procedure of Hu et al. (2016). Suspended particulates on filters were first freeze-dried and then treated with 4 mol/L HCl to remove inorganic carbon. After drying in an oven at 55°C for 24 hours to volatilize the excess HCl, filters were wrapped in tin boat. Particulate organic carbon (POC) was analyzed with a CHN element analyzer (Thermo Flash EA2000). Atropine Standard (OC = 70.50 ± 0.24%) and Low Organic Content Soil Standard OAS (OC = 1.61 ± 0.09%) were used as standard samples, and the relative deviation was less than 5% (n = 6). The POC contents were reported as μg/L in seawater of this study, with the detection limit of 3 μg/L.
Sterol and n-alkanol extraction and purification followed the procedure of Zhao et al. (2006). Freeze-dried suspended particulate samples were extracted using the mixture of dichloromethane (DCM) and methanol (MeOH) (3:1, v/v) with internal standard C19 n-alkanol added. The extracts were dried under gentle nitrogen flow and then were hydrolyzed using 6% KOH in MeOH for 12 hours. The hydrolyzed extracts were further extracted with hexane, concentrated under gentle nitrogen flow, and subsequently separated using silica gel column chromatography. The hydrocarbon fraction was eluted with hexane. The alcohol fraction (containing sterols and n-alkanols) was eluted with DCM: MeOH (95:5 v/v) after the hydrocarbon fraction. Finally, the alcohol fraction was silylated with 80 μL BSTFA (N, O-bis(trimethylsilyl)-trifluoroacetamide) for further analysis. Agilent 7890N GC with flame ionization detector and HP-1 capillary column (50 m) was used for detecting the sterols and long-chain n-alkanols. H2 was applied as the carrier gas at 1.3 mL/min. The oven was kept initially at 80 °C for 1 min, programmed to 200°C at 25°C/min, followed at 4°C/min to 250°C, 1.8°C/min to 300°C, and finally holding at 300°C for 5 mins. The detection limits of sterols and n-alkanols were 10 ng/g particulate (about 1 ng/L water volume). The average standard deviation was below 10%.
Ladderane extraction and purification were modified after the procedure of Zhao et al. (2019). Freeze-dried suspended particulate samples were extracted using mixture of DCM and MeOH (2:1, v/v). The extracts were dried under gentle nitrogen flow and added with copper to remove the sulfur. The total extracts were hydrolyzed with 6% KOH in MeOH for 12 hours. Subsequently, the hydrolyzed extracts were further extracted with hexane. The residual liquids were acidified to pH of 1 and then were extracted with hexane for fatty acids fraction (containing ladderane lipids). After drying with anhydrous sodium sulfate, fatty acids were converted to FAMEs using BF3/MeOH solution. 2-hydroxy-4-methoxy benzophenone was spiked into the extracts as internal standard before instrumental analysis.
High-performance liquid chromatography-mass spectrometer (HPLC-MS) with Zorbax Eclipse XDB-C8 column (3 mm × 250 mm, 5 µm, Agilent) was used for detecting ladderane FAMEs. The mobile phased was eluted with MeOH (0.3 mL/min, 15 mins), then brought to the mixture of MeOH: H2O (v/v=85%:15%, 2 mins) and held for 3mins. For MS parameters, corona was set up to 3 μA, cone voltage to 35 V, source temperature to 120°C and APCI probe temperature to 550°C. The cone gas flow was at 120 L/h, desolvation gas flow at 400 L/h. Ladderane FAMEs were measured under Selected Ion Recording (SIR) mode at [M+H]+ m/z = 289, 291, 317, and 319 for C18-[5]-ladderane FAME (I), C18-[3]-ladderane FAME (II), C20-[5]-ladderane FAME (III), and C20-[3]-ladderane FAME (IV), respectively. The chromatogram traces and MS spectra of ladderane core lipids were shown in Figure S1. The concentration of ladderanes were given as relative amounts because ladderane lipid standards are not available commercially, and ladderane concentration data were normalized by comparing the signals of ladderanes with the internal standard. The detection limit of ladderane lipids was 30 ng/g particulate (about 0.003 μg/L water volume). The average standard deviation was below 10%.
Terrestrial and marine biomarkers ratio, abbreviated as TMBR, has been used to evaluate the relative contribution of terrestrial organic carbon to the total organic carbon (Xing et al., 2011). TMBR′ has also been used by using n-alkanols in the calculation to replace the n-alkanes which are susceptible to pollution (Yuan et al., 2013). TMBR′ is calculated using the following equation:
where Σ(C26 + C28 + C30 n-alkanols) represents the terrestrial input. Σ(B + D + A) is the sum of marine biomarker concentrations, referring to brassicasterol (B), dinosterol (D) and C37 alkenones (A), respectively. Alkenones were below detection limit in all samples from Laizhou Bay, thus the sum of marine biomarker concentrations in this study was represented as Σ(B + D).
The NL5 ratio has been widely used to infer ladderane synthesis temperature. In order to keep the fluidity of anammoxosome membrane in different environment, the membrane lipid chain length of anammox bacteria is temperature dependent. NL5 is calculated using following equations (Rattray et al., 2010):
Distribution maps of sea water temperature, salinity, DO, DIN, POC, biomarker concentrations and biomarker indices were generated using software Ocean Data View (Schlitzer, 2021). Maps were created using weight-average gridding loaded by Ocean Data View version 5.6.3.
Statistical tests including t test and Pearson’s correlation analysis were performed with OriginPro 2018C software. A probability (p) of 0.05 was used to judge the statistical significance.
The water temperature, salinity and DO were measured both in estuaries and bay. The water temperature in estuaries was in the range of 27.5–29.2°C, slightly higher than that from the area A (26.0–29.2°C) and area B (25.2–29.1°C) (Figure 3A). Water temperature in the bay was the highest in the most southwestern site of area A nearby the XQR estuary (Figure 3A). The salinity ranged from 2.0 to 12.0 in waters from the estuaries (Figure 3B), showing a brackish condition between river freshwater discharge and sea water intrusion. In the aera A and area B, the salinity varied from 28.4–31.8 and 29.3–32.2, respectively, with lower salinity in the western bay (Figure 3B). DO concentrations in estuaries varied from 1.8 to 8.4 mg/L, with the lowest DO concentration in the XQR Estuary (1.8 mg/L) located at the southwest bank (Figure 3C). In the area A, the lowest DO concentration (5.3 mg/L) was found in the southwestern bay nearby the XQR estuary, while the highest value (6.3 mg/L) occurred in the southwestern bay nearby the Nanyang River (NYR) estuary and Jiaolai River (JLR) estuary. In the area B, the lowest DO concentration (4.1 mg/L) was found in the northwestern bay in the adjacent area of the Yellow River Estuary, while the highest value (7.0 mg/L) occurred in the most northeast site (Figure 3C). DIN was measured in the bottom water in Laizhou Bay. Generally, Total DIN and individual DIN (, and ) concentrations were generally higher in the western bay of both area A and B, especially in the southwestern sites of area A nearby the XQR Estuary (Figure S2).
Figure 3 Spatial distributions of (A) temperature, (B) salinity and (C) dissolved oxygen (DO) concentrations in estuaries and bay. The area A and area B was divided in different black polygon. See Figure 2 for abbreviations of the rivers and the explanations of area A and area B.
POC concentrations varied from 897 to 4279 μg/L in estuaries, which were much higher than those from the area A (260–767 μg/L) and area B (148–560 μg/L). Maximum POC concentrations were found in the JLR Estuary located at the southeast bank of Laizhou Bay (Figures 4A, S3A). In Laizhou Bay, the highest POC concentrations of 767 μg/L and 638 μg/L were detected at two nearshore sites in the area A (Figure 4A).
Figure 4 Spatial distributions of (A) particulate organic carbon (POC) concentrations in all sites, (B) long chain n-alkanol concentrations in all sites, (C) sterols (sum of brassicasterol and dinosterol) concentrations in all sites, (D) TMBR′ values in suspended particulates in all sites, (E) ladderane concentrations in estuaries, and (F) ladderane concentrations in bay. Concentrations of POC and biomarkers were measured from suspended particulates of each sampling site. See Figure 2 for abbreviations of the rivers and the explanations of area A and area B.
Concentrations of terrestrial biomarker long-chain (C26+C28+C30) n-alkanols were 80–930 ng/L in estuaries, 12–80 ng/L in the area A and 20–180 ng/L in the area B (Figures 4B, S3B). Concentrations of marine biomarker sterols (sum of brassicasterol and dinosterol) were 215–1500 ng/L in estuaries, 74–412 ng/L in the area A and 155–409 ng/L in the area B (Figures 4C, S3C). Long-chain n-alkanols concentrations were generally high in the western bay (Figure 4B), while marine sterols concentrations displayed relatively high values in the central bay (Figure 4C). Terrestrial and marine biomarker ratio TMBR′ values were higher in estuaries (0.19–0.61, average 0.33) than that in the area A (0.06–0.41, average 0.20) and area B (0.05–0.41, average 0.17) (Figure 4D), which displayed similar distribution pattern with that of n-alkanols (Figures 4B–D). Although average TMBR′ values were close between area A (0.20) and area B (0.17), OC sources were quite different. For terrestrial OC, area A was mainly influenced by small river inputs, while area B influenced by Yellow River input (Figure 4B). Besides, higher primary productivity as indicated by high sterols concentrations (Figure 4C) in offshore area B, resulting in the relative low TMBR′ values in the central and northern of Laizhou Bay.
Anammox biomarker ladderane lipids were detected in all suspended particulates from the estuaries and bay. In estuaries, total ladderane concentrations varied from 1.1 to 6.0 μg/L, with ladderane III and ladderane IV as the two dominating compositions (accounting for 50% and 45% of total ladderanes, respectively) (Figure 4E). For the bay, total ladderane concentrations varied from 0.01 to 2.5 μg/L, showing high values in the area A (Figure 4F). Ladderane III and ladderane IV were also the two most abundant ladderane in Laizhou Bay, accounting for 43% and 42% of the total ladderanes, respectively (Figure S4).
The distinct spatial differences of ladderane lipid concentrations and compositions were combined with the spatial characteristics of terrestrial and marine lipid biomarkers to discuss ladderane sources and processes for these spatial distributions. Our study area comprised Laizhou Bay and the estuaries of the surrounding small rivers (Figure 2).
Ladderanes in particulates and sediments from the open ocean were commonly considered as in situ production (Jaeschke et al., 2007; Jaeschke et al., 2009; Rush et al., 2012a; Rush et al., 2012b; Zhao et al., 2019), however, ladderane sources in coastal seas are more complicated due to riverine inputs and anthropogenic influences (Hou et al., 2013; Hu et al., 2016; Zhang et al., 2017b). In this study, we interpret the distinct differences of ladderane lipid compositions and concentrations from the estuaries (average 2.6 μg/L) to area A (average 0.9 μg/L) and then to area B (average 0.1 μg/L) as broadly reflecting ladderane sources. However, the biosynthetic origins of ladderanes from terrestrial and marine anammox bacteria were not well constrained, which limited our understanding of the controlling mechanism of ladderane sources in coastal area. We herein used a ladderane compound ratio in particulates, and the correlation between ladderanes and terrestrial-marine biomarkers (e.g., Hu et al., 2016; Zhao et al., 2019) to semi-quantitatively estimate the origins of ladderanes in Laizhou Bay. The proportion of individual ladderane compounds varied in different anammox bacteria genera (Rattray et al., 2008). The ladderane components were broadly similar in two terrestrial anammox bacteria (Ca. Brocadia and Ca. Kuenenia) while the marine anammox bacteria Ca. Scalindua showed a notably different ladderane proportion (Rattray et al., 2008). The absolute values of ladderane concentrations from different publications were difficult to be compared due to analytical different methods used. However, the relative proportion of each individual compounds in different environments were comparable according to the Rattray et al. (2010), in which another ladderane ratio index ‘NL5’ was proposed and been widely used in the following studies. We herein proposed a new index in terms of ladderane ratios, Rlad, to distinguish the biosynthetic origin of ladderanes:
Rlad was the ratio of ladderane lipids (ladderane III: C20-[5]-ladderane FAME and ladderane IV: C20-[3]-ladderane FAME), both occurred widely with higher concentration than lad I and lad II in natural environments (e.g. Hu et al., 2016; Zhao et al, 2019). In a few culture experiments, Rlad value was 1.1 ± 0.2 in terrestrial anammox bacteria Ca. Brocadia and Ca. Kuenenia, and 0.6 in marine anammox bacteria Ca. Scalindua (single measurement) (Rattray et al., 2008). By summarizing published ladderane data (41 samples) of both particulates and sediments, we found Rlad values varied significantly in different environments. Rlad values were generally above 0.9 in coastal areas, for example 1.0–1.8 (average 1.4) in the Senegal River Estuary (Jaeschke et al., 2010), 0.9–9.0 (average 2.3) in the Pearl River Estuary (Zhu, 2014) and 0.9–2.1 (average 1.5) in the Changjiang River Estuary (Hu et al., 2016). On the other, Rlad values were lower than 0.9 in more open ocean environments, such as 0.6–0.8 (average 0.7) in the Celtic Sea (Rattray, 2008; Jaeschke et al., 2009), 0.2–0.3 (average 0.3) in the Arabian Sea (Jaeschke et al., 2007; Rattray, 2008) and 0.1–0.4 (average 0.3) in the Black Sea (Kuypers et al., 2003; Rattray, 2008). Though the absolute ladderane contents might be different in these studies due to the different methods used, the ratio of individual ladderanes were more comparable in the application of Rlad ratio. Thus, we propose that the Rlad values could semi-quantitatively distinguish ladderane from terrestrial or marine anammox bacteria, with Rlad values higher than 0.9 indicating terrestrial source and Rlad values lower than 0.9 indicating marine source.
In estuaries, the highest ladderane concentrations and highest TMBR′ values were found in the XQR and Jie River (JR) estuaries (Figures 4D, E, S3D). Thus, ladderane concentrations showed significant positive correlation with terrestrial TMBR′ index (Figure 5A, r = 0.82, p< 0.05), indicating terrestrial sources for ladderanes (Hu et al., 2016; Shi et al., 2021). The Rlad values were in the range of 0.9–1.9 in estuaries (Figures 6A, B) and provided strong evidence that ladderanes in these small river estuaries were produced by terrestrial anammox bacteria Ca. Brocadia and Ca. Kuenenia. By using molecular techniques, the abundances of anammox bacteria were found to be negatively correlated with salinity in Laizhou Bay (Zhang et al., 2014), which proved that brackish estuarine water was favorable for the growth of terrestrial anammox bacteria.
Figure 5 Relationships of ladderane concentrations with (A) TMBR′ values and with (B) DO concentrations in suspended particulates from the Laizhou Bay. Blue triangles in the plots represent sites in estuaries; Black squares and red dots in the plots represent sites in the area A and the area B of the inner bay, respectively.
Figure 6 (A) Spatial distribution and (B) box-and-whisker plot of Rlad values in suspended particulates. (C) Spatial distribution and (D) box-and-whisker plot of NL5 values of in suspended particulates. Black dots in the box plots represent the outliers of Rlad or NL5 values in different areas. See Figure 2 for abbreviations of the rivers and the explanations of area A and area B.
Specific river and estuarine conditions caused the different ladderane concentrations and compositions in the estuaries. Although the highest n-alkanols concentrations were found in the JLR Estuary (Figures 4B, S3B), its total ladderane concentration was lower than those of the JR Estuary and the XQR Estuary (Figure 4E). Therefore, additional factors could have contributed to the source and distribution of ladderanes in estuaries. Consistent with previous studies (e.g., Brandsma et al., 2011; Sáenz et al., 2012; Hu et al., 2016; Zhao et al., 2019), we found a significant negative correlation between ladderane and DO concentrations in estuaries (Figure 5B, r = − 0.89, p< 0.05). The DO concentrations were significantly low (under t-test, p< 0.05) in the XQR Estuary (1.8 mg/L) and the JR Estuary (2.4 mg/L). Thus, DO was an important factor for ladderane distribution. Besides, the relative contribution of anammox to N2 production were higher in low DO environment of estuaries (Zhang et al., 2014), further proved that hypoxia caused by anthropogenic influence could promote the activity of anammox in estuaries of Laizhou Bay, which was in accordance with our high ladderane concentrations at XQR and JR estuaries with hypoxic condition (Figure 7). We suggested that high ladderane concentration in the XQR was the combined contribution of in situ production under lower DO concentration and the lateral transportation from upper river reach (Figure 7). In contrast, the small JR received much less terrestrial input (Figure 4B), and in situ ladderane production under low DO conditions would be the main source of ladderanes in the estuary (Figure 7). As to the JLR Estuary, although terrestrial input was high as revealed by high n-alkanols concentrations (Figure 4B), high DO concentration (> 8mg/L; Figure 3C) might restrict the annamox activity and the in situ production of ladderanes (Liu et al., 2008). Therefore, ladderane concentration was just in moderate level in the JLR (Figures 4E, 6). In other small river estuaries of Laizhou Bay, the combination of low terrestrial input and high DO conditions resulted in lowed ladderane concentrations (Figure 7). Although all these river estuaries in Laizhou Bay were easily influenced by saltwater intrusion (Shi and Jiao, 2014; Zhang et al., 2017a), saltwater intrusion was not likely be the main origin of ladderanes because ladderanes in estuaries were mainly originated by freshwater anammox bacteria revealed by high Rlad values (Figure 6A). In addition, a study in the Changjiang River showed that dam could enhanced the anammox role in nitrogen loss (Chen et al., 2019), thus might influence the origin of ladderanes. However, comparing with the Changjiang River, river flows were much lower at the east and south bank of Laizhou Bay, with the highest runoff at 8.78×108 m3/a from the Xiaoqing River. The influence of front-dam to the source of ladderanes remained uncertain in Laizhou Bay and should be further explored in the future.
Figure 7 Schematic illustrations of ladderane distributions in Laizhou Bay. In the Xiaoqing River (XQR) Estuary, high terrestrial input and in situ production of ladderanes in the hypoxic water resulted in high ladderane concentrations. In the Jie River (JR) Estuary, low terrestrial input and in situ biosynthesis in the hypoxic water column also caused high ladderane concentrations. In the Jiaolai River (JLR) Estuary, ladderanes showed moderate values, accompanied with the abundant terrestrial input and the suppression of saturated DO (> 8mg/L). In other small river estuaries of Laizhou Bay, low terrestrial input and sufficient oxygen resulted in the relatively low ladderane concentrations. Although the Yellow River discharge was high, anammox rates in the Yellow River were very low (Zhang et al., 2017b), thus made very limited contribution to the ladderane concentrations in Laizhou Bay. Sewage plants and outlets were shown as factory icons along the rivers. Inside the bay, Area A and Area B was distinguished by different colors inside the bay, and ladderane concentrations in area A was higher than in area B because of the southern and eastern small river inputs. Ladderanes/anammox bacteria in area B could be transported via exogenous Bohai circulation.
The compositions of ladderanes were similar between estuaries and area A, with high proportions of Ladderane III and IV contributing 95% and 85% to total ladderanes in estuaries and area A, respectively (Figures 4E, S3). The spatial distributions of total ladderanes and individual ladderane in area A were broadly consistent with the corresponding estuaries. For example, high ladderane concentrations at the southwest and northeast sites from area A agreed with the high ladderane concentrations in the XQR and JR estuaries (Figures 4E, F). The extremely low ladderane concentration (< 0.01 μg/L) occurred at one station from the southeast of area A (Figure 4F), which was adjacent of the NYR Estuary with lowest ladderane concentration among all the estuaries (Figure 4E). These qualitative correlations suggested that particulate ladderane in area A were mainly biosynthesized in terrestrial environment and consequently transported to the bay by river discharges. This was further supported by the positive linear correlation between ladderane concentration and the TMBR′ index (r = 0.72, p< 0.05, Figure 5A). Furthermore, the average value of Rlad (1.1) in area A was comparable with those from estuaries (Figure 6B), confirming the terrestrial biosynthesis origin of ladderanes. It should be noted that the Rlad value in the southeast station in area A was much lower than that of the nearby NYR Estuary (Figure 6A), likely a result of less river influence in the southeast Laizhou Bay by the low river flow (in Figures 4B–D). The poor correlation between the ladderane and DO concentrations (r = − 0.10, p > 0.05) (Figure 5B) suggested that the ladderanes were not likely produced in situ in area A. The measured DO concentrations in the area A were all above 3 mg/L (the threshold of hypoxia), not favorable for the anammox growth (Kuypers et al., 2005; Hamersley et al., 2007; Dalsgaard et al., 2012) and supporting the above deduction. Overall, ladderanes area A originated from the similar terrestrial sources as in estuaries, with limited contribution from in situ production.
Ladderane concentrations had poor relationship with TMBR′ values (Figure 5A), indicating terrestrial input from rivers might not be the main source of ladderanes in area B. However, long-chain n-alkanol concentrations and TMBR′ values were both high in the northwestern bay (Figures 4B–D), because the Yellow River could transport huge amount of terrestrial OC to area B (Qiao et al., 2010). The anammox activity was proved to be very weak in the Yellow River (Zhang et al., 2017b) comparing with that of the Bohai Sea (Zhang et al., 2018), and ladderanes from the Yellow River were unlikely the main sources of ladderanes in Laizhou Bay (Figure 7). This conclusion was supported by the lower Rlad values in the area B (0.2–0.9, Figure 6B), which indicated a marine biosynthetic origin of ladderanes. On the other hand, the NL5 values (0.42–0.82) from the area B corresponded to a range of 14.8–19.8 °C for ladderane biosynthesis temperature, much lower than the in situ sea water temperature (25.2–29.4°C) in the area B (Figure 3A). Thus, ladderanes were not likely produced within area B, but could have originated from a lower temperature environment and then be transported to area B. One site at the boundary of the area B and the Bohai had relatively high ladderane concentration (0.58 μmol/L, Figure 4F), and this site was easily influenced by the anti-clockwise Bohai current which resulted in lower water temperature (Qiao et al., 2010; Zhou et al., 2017; Wei et al., 2019), suggesting a potential source of ladderane from this region. The DO concentration was also low in this site (4.1 mg/L, Figure 3C), providing a suitable environment for anammox bacteria. However, we suggest that in situ production here was not the main origin of ladderane lipids since the ladderane biosynthesis temperature revealed by NL5 as 19.2 °C was much lower than in situ temperature 26.3 °C. Indeed, previous studies had reported that there were bottom hypoxic zones in the northwest of Laizhou Bay (e.g., Zhou et al., 2017; Wei et al., 2019; Figure 2) with significant anammox rates (Zhang et al., 2018). We thus propose that ladderanes produced in the Bohai Sea and near the Bohai Sea-Laizhou Bay boundary could be transported to area B in summer by the anti-clockwise Bohai circulation (Wei et al., 2003; Qiao et al., 2010; Hu et al., 2013; Hu and Wang, 2015).
Our study of the sources and spatial distributions of ladderanes in Laizhou Bay region provides an example of using ladderanes as an indicator to evaluate human influences on anammox activities in the river-coastal water environments. Laizhou Bay has been considered as a typical eutrophic bay since the 1980s (Jiang et al., 2005), with DIN concentration increased of 10 folds from the 1980s to the 2000s followed by gradual reduction since 2010 (Zhu et al., 2019). Nutrients and OC into Laizhou Bay were transported by rivers mostly flow through the Shandong Province, where the population and economy had been growing fast in North China (National Bureau of Statistics of China, 1999–2020). According to the official statistical results of pollution source published by the Shandong Provincial Department of Ecological Environment (http://117.73.254.13:8801/wryfb/MapMainT.html), ~ 100 key sewage outlets and ~ 50 key sewage plants existed in the drainage area of rivers entering Laizhou Bay. Most of these outlets were located in the Xiaoqing River basin and the Jiaolai River basin (Figure 7). The Xiaoqing River, for example, was severely contaminated by anthropogenic discharge especially in wet season. A recent study reported that the Xiaoqing River displayed high discharge flux to Laizhou Bay with 2649.2 t/a for DIN, 89.94 t/a for phosphate and 4495.6 t/a for COD (chemical oxygen demand) (Lu, 2022). Besides, multiple wastewater outlets and sewage treatment plants were located along the river as reported by Shandong Provincial Department of Ecological Environment (Figure 7). The eutrophication and organic matter pollution triggered oxygen depletion along the river and in the estuary (Meng et al., 2005; He et al., 2018a; He et al., 2018b), providing favorable conditions for anammox growth (e.g., Dang et al., 2010; Zhao et al., 2019; Li et al., 2021). The point sources of sewage discharge could be also an important origin of ladderanes in estuaries because anammox bacteria and ladderanes were generally detected in sewage water/sludge (e.g., Ren et al., 2022) and nearby the wastewater outlets (e.g., Shi et al., 2021). Therefore, the ladderane contribution to the river estuaries was closely linked to the contaminated condition of rivers under anthropogenic influences. Inside Laizhou Bay, the distribution of ladderanes was less influenced by the in situ water condition but rather being mostly affected by river inputs or by bay circulation transport.
In order to further evaluate the anammox activity affected by the terrestrial and anthropogenic influences in a wider context, we compared the ladderane concentrations in particulates from Laizhou Bay with published data (Figure 8). Since that ladderane concentrations could reflected the intense of anammox activity (e.g., Pitcher et al., 2011; Rush et al., 2012a; Zhao et al., 2019), we could compare the anammox activity under different source mechanisms in different sea area. It should be noted that the previous studies only focused on the anammox process in sediments of Chinese marginal seas by using molecular techniques and isotope labelling technique (e.g., Dang et al., 2010; Hou et al., 2013; Zhang et al., 2019; Teng et al., 2022), but did not include the potential anammox activity in water particulates. We only compared ladderane concentrations from the China marginal seas because these data were analyzed using same procedures including ladderane extraction, purification, detection and quantification (Zhu, 2014; Hu, 2016; Hu et al., 2016; Shi et al., 2021). Generally, ladderane concentrations at hypoxia sites were higher than other sites in the same study area (Figure 8), which further proved that oxygen level (human influences) was the main controlling mechanism of anammox activity. By comparing ladderane data between different studies, the highest ladderane concentrations occurred in the estuaries of Laizhou Bay (average 2.6 μg/L, this study, Figure 8), where ladderanes mainly originated from terrestrial environment under severe anthropogenic influence. Highest ladderane concentrations in Laizhou Bay estuaries indicated the more intense anammox activity in small river estuaries comparing with other coastal areas. Ladderane concentrations in Jiaozhou Bay were also high (average 1.5 μg/L) though no obvious hypoxia occurred (Shi et al., 2021). As a semi-closed small bay in North China, Jiaozhou Bay was also strongly affected by anthropogenic discharge. Ladderanes distribution in Jiaozhou Bay was affected by river flow and wastewater discharge and their concentrations could reflect the bottom water quality condition (Shi et al., 2021). However, comparing with these small-scale systems above, ladderane concentrations were low in large rivers such as the Changjiang River and the Pearl River (Figure 8), which are two of the biggest rivers in China with large terrestrial input (Gao et al., 2002; Sun et al., 2020; Zhou et al., 2022) and occasional hypoxia events in estuaries (Dai et al., 2006; Li et al., 2020). Moreover, anammox activities had been observed in both two rivers and provided evidence for the terrestrial input of ladderanes in estuaries (Hou et al., 2018; Wang et al., 2019a), we thus deduced that the concentrations of terrestrial ladderanes might be diluted by the huge riverine flow. As to the East China Sea (ECS) adjacent to the Changjiang Estuary, ladderanes in the bottom water were proved to be produced in situ rather than terrestrial input (Hu et al., 2016), generally lower than in bays and most estuaries (Figure 8). However, ladderane concentrations in the hypoxic area of the ECS were much higher than other sites of the ECS and comparable with the hypoxic area of the Changjiang Estuary (Figure 8). The bottom hypoxia in the ECS has been occurring since the 1950s (Wei et al., 2007; Zhu et al., 2011; Liu et al., 2020). Increasing DIN discharge from the Changjiang River was the main reason of the occurrence and increasing of hypoxia zone in the East China Sea (Zhang et al., 2007; Wang et al., 2016). In conclusion, high ladderane concentrations in particulates were more likely occurred in small bays and hypoxic areas, furthermore indicated that the anammox activity could be enhanced by anthropogenic influence like sewage discharge, eutrophication and hypoxia. Nitrogen loss by anammox process might be underestimated in such small-scale system. In addition, the main terrestrial source of ladderanes in estuaries could prove key evidence for the importance of terrestrial contribution to coastal anammox process. Therefore, by identifying the sources of the ladderanes in human-influenced bays, we can better evaluate the intensity of anammox activity in these small but vital river and estuarine systems and further estimate the anammox contribution in the nitrogen cycle from coastal seas.
Figure 8 Comparisons of ladderane concentrations in suspended particulates from China marginal seas. Black bars in the plot represent the average ladderane concentrations. The red stars represent the ladderane concentrations at oxygen-depleted sites in each region. The ladderane data were obtained from Shi et al. (2021) in the Jiaozhou Bay, Hu et al. (2016) in the Changjiang Estuary, Hu (2016) in the East China Sea, and Zhu (2014) in the Pearl River Estuary.
This study reported new data of anammox biomarker ladderanes and other lipid biomarkers in suspended particulates from Laizhou Bay and surrounding river estuaries, Bohai Sea in North China. Based on ladderane distributions, compositions, and ratios, as well as correlations between ladderanes and terrestrial index and DO concentrations, our conclusions were as follows: (1) The ladderane ratio of Rlad was proposed to distinguish ladderane biosynthetic origins by terrestrial anammox bacteria (Rlad > 0.9) and marine anammox bacteria (Rlad< 0.9) of ladderanes. (2) Ladderanes in particulates from the estuaries and nearshore area of Laizhou Bay mainly originated from the terrestrial input via riverine inflow as well as in situ production in oxygen-depleted estuaries, while ladderanes in offshore area were possibly from ex situ marine environment, such as the cold hypoxia water input by the Bohai circulation. (3) By comparing our ladderane data in particulates with previously published data from China marginal seas under different human influences, we found that high ladderane concentrations synthesized by terrestrial anammox bacteria could contribute significantly in coastal seas. In bays and estuaries, the role of anammox in nitrogen cycle, like the rate and contribution of N2 production, should be further evaluated for the nitrogen budget influenced by enhanced anthropogenic activities.
The raw data supporting the conclusions of this article will be made available by the authors, without undue reservation.
MS: Conceptualization, Investigation, Writing - Original Draft. XX: Conceptualization, Writing - Review & Editing, Supervision. HM: Resources, Investigation. LL: Validation, Resources. YW: Validation, Resources. CD: Validation, Resources. YD: Validation, Resources. MZ: Conceptualization, Writing - Review & Editing, Funding acquisition. All authors contributed to the article and approved the submitted version.
This study is supported by the National Natural Science Foundation of China (42230412, U1706219).
We thank Zicheng Wang, Weiqiang Zhao and Zhong Cao for their help to collect the samples, and colleagues in Shandong Marine Resources and Environment Research Institute for providing CTD data. We also thank Hailong Zhang and Gui’e Jin for the technical support, Julian P. Sachs for constructive comments on the manuscript. This is MCTL contribution No. 313.
The authors declare that the research was conducted in the absence of any commercial or financial relationships that could be construed as a potential conflict of interest.
All claims expressed in this article are solely those of the authors and do not necessarily represent those of their affiliated organizations, or those of the publisher, the editors and the reviewers. Any product that may be evaluated in this article, or claim that may be made by its manufacturer, is not guaranteed or endorsed by the publisher.
The Supplementary Material for this article can be found online at: https://www.frontiersin.org/articles/10.3389/fmars.2023.1215883/full#supplementary-material
Altieri A. H., Gedan K. B. (2015). Climate change and dead zones. Glob. Change Biol. 21, 1395–1406. doi: 10.1111/gcb.12754
Arrigo K. R. (2005). Marine microorganisms and global nutrient cycles. Nature 437, 349–355. doi: 10.1038/nature04159
Boumann H. A., Longo M. L., Stroeve P., Poolman B., Hopmans E. C., Stuart M. C. A., et al. (2009). Biophysical properties of membrane lipids of anammox bacteria: i. ladderane phospholipids form highly organized fluid membranes. Biochim. Biophys. Acta - Biomembr. 1788, 1444–1451. doi: 10.1016/j.bbamem.2009.04.008
Brandsma J., van de Vossenberg J., Risgaard-Petersen N., Schmid M. C., Engström P., Eurenius K., et al. (2011). A multi-proxy study of anaerobic ammonium oxidation in marine sediments of the gullmar fjord, Sweden. Environ. Microbiol. Rep. 3, 360–366. doi: 10.1111/j.1758-2229.2010.00233.x
Brin L. D., Giblin A. E., Rich J. J. (2014). Environmental controls of anammox and denitrification in southern new England estuarine and shelf sediments. Limnol. Oceanogr. 59, 851–860. doi: 10.4319/lo.2014.59.3.0851
Chen L., Liu S., Chen Q., Zhu G., Wu X., Wang J., et al. (2019). Anammox response to natural and anthropogenic impacts over the Yangtze river. Sci. Total Environ. 665, 171–180. doi: 10.1016/j.scitotenv.2019.02.096
Conley D. J., Paerl H. W., Howarth R. W., Boesch D. F., Seitzinger S. P., Havens K. E., et al. (2009). Controlling eutrophication: nitrogen and phosphorus. Science 323, 1014–1015. doi: 10.1126/science.1167755
Cui Y., Chen B., Chen J. (2005). Evaluation on self-pollution of marine culture in the yellow Sea and bohai Sea. Chin. J. Appl. Ecol. 16, 180–185.
Dai M., Guo X., Zhai W., Yuan L., Wang B., Wang L., et al. (2006). Oxygen depletion in the upper reach of the pearl river estuary during a winter drought. Mar. Chem. 102, 159–169. doi: 10.1016/j.marchem.2005.09.020
Dalsgaard T., Thamdrup B., Farías L., Revsbech N. P. (2012). Anammox and denitrification in the oxygen minimum zone of the eastern south pacific. Limnol. Oceanogr. 57, 1331–1346. doi: 10.4319/lo.2012.57.5.1331
Dang H., Chen R., Wang L., Guo L., Chen P., Tang Z., et al. (2010). Environmental factors shape sediment anammox bacterial communities in hypernutrified jiaozhou bay, China. Appl. Environ. Microbiol. 76, 7036–7047. doi: 10.1128/AEM.01264-10
Engström P., Dalsgaard T., Hulth S., Aller R. C. (2005). Anaerobic ammonium oxidation by nitrite (anammox): implications for N2 production in coastal marine sediments. Geochim. Cosmochim. Acta 69, 2057–2065. doi: 10.1016/j.gca.2004.09.032
Fozia Z., Hou L., Zhang Z., Chen F., Gao D., et al. (2020). Anaerobic ammonium oxidation (anammox) bacterial diversity, abundance, and activity in sediments of the indus estuary. Estuar. Coast. Shelf Sci. 243. doi: 10.1016/j.ecss.2020.106925
Gao Q., Tao Z., Shen C., Sun Y., Yi W., Xing C. (2002). Riverine organic carbon in the xijiang river (South china): seasonal variation in content and flux budget. Environ. Geol. 41, 826–832. doi: 10.1007/s00254-001-0460-4
Gonzalez-Silva B. M., Rønning A. J., Andreassen I. K., Bakke I., Cervantes F. J., Østgaard K., et al. (2017). Changes in the microbial community of an anammox consortium during adaptation to marine conditions revealed by 454 pyrosequencing. Appl. Microbiol. Biotechnol. 101, 5149–5162. doi: 10.1007/s00253-017-8160-5
Guan X. (1994). Patterns and structures of the currents in bohai, huanghai and East China seas. Oceanology China Sea , 17–26. doi: 10.1007/978-94-011-0862-1_3
Guo F., Liu. S., Wang F., Hou G. (2015). Distribution of nutrients in the summer of laizhou bay and influence factors. Mar. Geology Front. 32, 38–44. doi: 10.16028/j.1009-2722.2016.02006
Hamersley M. R., Lavik G., Woebken D., Rattray J. E., Lam P., Hopmans E. C., et al. (2007). Anaerobic ammonium oxidation in the Peruvian oxygen minimum zone. Limnol. Oceanogr. 52, 923–933. doi: 10.4319/lo.2007.52.3.0923
He D., Zhang K., Cui X., Tang J., Sun Y. (2018a). Spatiotemporal variability of hydrocarbons in surface sediments from an intensively human-impacted xiaoqing river-laizhou bay system in the eastern China: occurrence, compositional profile and source apportionment. Sci. Total Environ. 645, 1172–1182. doi: 10.1016/j.scitotenv.2018.07.193
He D., Zhang K., Tang J., Cui X., Sun Y. (2018b). Using fecal sterols to assess dynamics of sewage input in sediments along a human-impacted river-estuary system in eastern China. Sci. Total Environ. 636, 787–797. doi: 10.1016/j.scitotenv.2018.04.314
Hirsch M. D., Long Z. T., Song B. (2011). Anammox bacterial diversity in various aquatic ecosystems based on the detection of hydrazine oxidase genes (hzoA/hzoB). Microb. Ecol. 61, 264–276. doi: 10.1007/s00248-010-9743-1
Hou L., Xie X., Wan X., Kao S. J., Jiao N., Zhang Y. (2018). Niche differentiation of ammonia and nitrite oxidizers along a salinity gradient from the pearl river estuary to the south China Sea. Biogeosciences 15, 5169–5187. doi: 10.5194/bg-15-5169-2018
Hou L., Zheng Y., Liu M., Gong J., Zhang X., Yin G., et al. (2013). Anaerobic ammonium oxidation (anammox) bacterial diversity, abundance, and activity in marsh sediments of the Yangtze estuary. J. Geophys. Res. Biogeosciences 118, 1237–1246. doi: 10.1002/jgrg.20108
Hu J. (2016). Spatial and temporal distribution of anammox activity in the changjiang estuary deduced from multi-biomarkers in suspended particulates (China: Ocean Univ).
Hu L., Shi X., Guo Z., Wang H., Yang Z. (2013). Sources, dispersal and preservation of sedimentary organic matter in the yellow Sea: the importance of depositional hydrodynamic forcing. Mar. Geol. 335, 52–63. doi: 10.1016/j.margeo.2012.10.008
Hu J., Wang X. (2015). Progress on upwelling studies in the China seas. Riview Geophys. 54, 653–673. doi: 10.1002/2015RG000505
Hu J., Zhang H., Li L., Wang Y., Zhao M. (2016). Seasonal changes of organic matter origins and anammox activity in the changjiang estuary deduced from multi-biomarkers in suspended particulates. Sci. China Earth Sci. 59, 1339–1352. doi: 10.1007/s11430-016-5286-8
Jaeschke A., Abbas B., Zabel M., Hopmans E. C., Schouten S., Sinninghe Damsté J. S. (2010). Molecular evidence for anaerobic ammonium-oxidizing (anammox) bacteria in continental shelf and slope sediments off northwest Africa. Limnol. Oceanogr. 55, 365–376. doi: 10.4319/lo.2010.55.1.0365
Jaeschke A., Hopmans E. C., Wakeham S. G., Schouten S., Damsté J. S. S. (2007). The presence of ladderane lipids in the oxygen minimum zone of the Arabian Sea indicates nitrogen loss through anammox. Limnol. Oceanogr. 52, 780–786. doi: 10.4319/lo.2007.52.2.0780
Jaeschke A., Rooks C., Trimmer M., Nicholls J. C., Hopmans E. C., Schouten S., et al. (2009). Comparison of ladderane phospholipid and core lipids as indicators for anaerobic ammonium oxidation (anammox) in marine sediments. Geochim. Cosmochim. Acta 73, 2077–2088. doi: 10.1016/j.gca.2009.01.013
Jiang H., Cui Y., Chen B. J., Chen J. F., Song Y. L. (2005). The variation trend of nutrient salts in the bohai Sea. Mar. Fish. Res. 26, 61–67. doi: 10.3969/j.issn.1000-7075.2005.06.012
Jiang H., Liu D., Song X., Ma Y., Wang Y., Liu A., et al. (2018). Response of phytoplankton assemblages to nitrogen reduction in the laizhou bay, China. Mar. pollut. Bull. 136, 524–532. doi: 10.1016/j.marpolbul.2018.09.042
Jickells T. D. (1998). Nutrient biogeochemistry of the coastal zone. Science 281, 217–222. doi: 10.1126/science.281.5374.217
Klotz M. G., Stein L. Y. (2008). Nitrifier genomics and evolution of the nitrogen cycle. FEMS Microbiol. Lett. 278, 146–156. doi: 10.1111/j.1574-6968.2007.00970.x
Karthäuser C., Ahmerkamp S., Marchant H. K., Bristow L. A., Hauss H., Iversen M. H., et al. (2021). Small sinking particles control anammox rates in the Peruvian oxygen minimum zone. Nat. Commun. 12, 1–12. doi: 10.1038/s41467-021-23340-4
Kuypers M. M. M., Lavik G., Woebken D., Schmid M. C., Fuchs B. M., Amann R., et al. (2005). Massive nitrogen loss from the benguela upwelling system through anaerobic ammonium oxidation. Proc. Natl. Acad. Sci. U. S. A. 102, 6478–6483. doi: 10.1073/pnas.0502088102
Kuypers M. M. M., Silekers A. O., Lavik G., Schmid M., Jøorgensen B. B., Kuenen J. G., et al. (2003). Anaerobic ammonium oxidation by anammox bacteria in the black Sea. Nature 422, 608–611. doi: 10.1038/nature01472
Lam P., Lavik G., Jensen M. M., van de Vossenberg J., Schmid M., Woebken D., et al. (2009). Revising the nitrogen cycle in the Peruvian oxygen minimum zone. Proc. Natl. Acad. Sci. U. S. A 106 (12), 4752–4757. doi: 10.1073/pnas.0812444106
Li D., Gan J., Hui R., Liu Z., Yu L., Lu Z., et al. (2020). Vortex and biogeochemical dynamics for the hypoxia formation within the coastal transition zone off the pearl river estuary. J. Geophys. Res. Ocean. 125, 1–16. doi: 10.1029/2020JC016178
Li M., Hong Y., Cao H., Gu J. (2013). Community structures and distribution of anaerobic ammonium oxidizing and nirS-encoding nitrite-reducing bacteria in surface sediments of the south China Sea. Microb. Ecol. 66, 281–296. doi: 10.1007/s00248-012-0175-y
Li J., Li J., Zhang Y., Lu H. (2022). The responses of marine anammox bacteria-based microbiome to multi-antibiotic stress in mariculture wastewater treatment. Water Res. 224, 119050. doi: 10.1016/j.watres.2022.119050
Li X., Qian W., Hou L., Liu M., Chen Z., Tong C. (2021). Human activity intensity controls the relative importance of denitrification and anaerobic ammonium oxidation across subtropical estuaries. Catena 202, 105260. doi: 10.1016/j.catena.2021.105260
Liu J., Krom M. D., Ran X., Zang J., Liu J., Yao Q., et al. (2020). Sedimentary phosphorus cycling and budget in the seasonally hypoxic coastal area of changjiang estuary. Sci. Total Environ. 713, 136389. doi: 10.1016/j.scitotenv.2019.136389
Liu S., Li L., Zhang G., Liu Z., Yu Z., Ren J. (2012). Impacts of human activities on nutrient transports in the huanghe (Yellow river) estuary. J. Hydrol. 430–431, 103–110. doi: 10.1016/j.jhydrol.2012.02.005
Liu S., Yang F., Xue Y., Gong Z., Chen H., Wang T. (2008). Evaluation of oxygen adaptation and identification of functional bacteria composition for anammox consortium in non-woven biological rotating contactor. Bioresour. Technol. 99, 8273–8279. doi: 10.1016/j.biortech.2008.03.006
Lu Q. (2022). Study on the fluxes and temporal and spatial evolution characteristics of major chemical pollutants in laizhou bay based on sea-land collaborative survey (Shanghai Ocean Univ). doi: 10.27314/d.cnki.gsscu.2022.000803
Meng C., Deng C., Yao P., Zhang X., Mi T., Chen H., et al. (2005). Dissolved oxygen in the xiaoqinghe estuary and adjacent waters. Mar. Environ. Sci. 24, 25–28. doi: 10.16028/j.1009-2722.2019.04001
Mulder A., Vandegraaf A. A., Robertson L. A., Kuenen J. G. (1995). Anaerobic ammonium oxidation discovered in a denitrifying fluidized-bed reactor. FEMS Microbiol. Ecol. 16 (3), 177–183. doi: 10.1111/j.1574-6941.1995.tb00281.x
National Bureau of Statistics of China (2020) China Statistic yearbook. Available at: http://www.stats.gov.cn/sj/ndsj/.
Peeters S. H., van Niftrik L. (2019). Trending topics and open questions in anaerobic ammonium oxidation. Curr. Opin. Chem. Biol. 49, 45–52. doi: 10.1016/j.cbpa.2018.09.022
Peng H., Geng H., Mao X., Shi J., Lv X. (2021). Study on total control of total nitrogen in the laizhou bay. Water (Switzerland) 13, 2439. doi: 10.3390/w13172439
Pereira A. D., Cabezas A., Etchebehere C., Chernicharo C. A., de L., de Araújo J. C. (2017). Microbial communities in anammox reactors: a review. Environ. Technol. Rev. 6, 74–93. doi: 10.1080/21622515.2017.1304457
Pitcher A., Villanueva L., Hopmans E. C., Schouten S., Sinninghe Damsté J. S. (2011). Niche segregation of ammonia-oxidizing archaea and anammox bacteria in the Arabian Sea oxygen minimum zone. ISME J. 5, 1896–1904. doi: 10.1038/ismej.2011.60
Qiao S., Shi X., Zhu A., Liu Y., Bi N., Fang X., et al. (2010). Distribution and transport of suspended sediments off the yellow river (Huanghe) mouth and the nearby bohai Sea. Estuar. Coast. Shelf Sci. 86, 337–344. doi: 10.1016/j.ecss.2009.07.019
Rattray J. E. (2008). Ladderane lipids in anammox bacteria: occurrence, biosynthesis and application as environmental markers (Utrecht, Netherlands: Utrecht University. Available at: https://www.researchgate.net/publication/27709903_Ladderane_Lipids_in_Anammox_Bacteria_Occurence_Biosynthesis_and_Application_as_Environmental_Markers.
Rattray J. E., Van Vossenberg J., Hopmans E. C., Kartal B., Van Niftrik L., Rijpstra W. I. C., et al. (2008). Ladderane lipid distribution in four genera of anammox bacteria. Arch. Microbiol. 190, 51–66. doi: 10.1007/s00203-008-0364-8
Rattray J. E., Van Vossenberg J., Jaeschke A., Hopmans E. C., Wakeham S. G., Lavik G., et al. (2010). Impact of temperature on ladderane lipid distribution in anammox bacteria. Appl. Environ. Microbiol. 76, 1596–1603. doi: 10.1128/AEM.01796-09
Ren Z., Wang H., Zhang L., Du X., Huang B., Jin R.. (2022). A review of anammox-based nitrogen removal technology: from microbial diversity to engineering applications. Bioresour. Technol. 363, 127896. doi: 10.1016/j.biortech.2022.127896
Risgaard-Petersen N., Meyer R. L., Schmid M., Jetten M. S. M., Enrich-Prast A., Rysgaard S., et al. (2004). Anaerobic ammonium oxidation in an estuarine sediment. Aquat. Microb. Ecol. 36, 293–304. doi: 10.3354/ame036293
Rush D., Hopmans E. C., Wakeham S. G., Schouten S., Sinninghe Damsté J. S. (2012a). Occurrence and distribution of ladderane oxidation products in different oceanic regimes. Biogeosciences 9, 2407–2418. doi: 10.5194/bg-9-2407-2012
Rush D., Sinninghe Damsté J. S. (2017). Lipids as paleomarkers to constrain the marine nitrogen cycle. Environ. Microbiol. 19, 2119–2132. doi: 10.1111/1462-2920.13682
Rush D., Wakeham S. G., Hopmans E. C., Schouten S., Sinninghe Damsté J. S. (2012b). Biomarker evidence for anammox in the oxygen minimum zone of the Eastern tropical north pacific. Org. Geochem. 53, 80–87. doi: 10.1016/j.orggeochem.2012.02.005
Sáenz J. P., Hopmans E. C., Rogers D., Henderson P. B., Charette M. A., Schouten S., et al. (2012). Distribution of anaerobic ammonia-oxidizing bacteria in a subterranean estuary. Mar. Chem. 136–137, 7–13. doi: 10.1016/j.marchem.2012.04.004
Schlitzer R. (2021) Ocean data view. Available at: https://odv.awi.de/.
Shandong Provincial Department of Ecological Environment (2018) Bulletin of ecological environment in Shandong province. Available at: http://xxgk.sdein.gov.cn/xxgkml/.
Shen L., Liu S., Lou L., Liu W., Xu X., Zheng P., et al. (2013). Broad distribution of diverse anaerobic ammonium-oxidizing bacteria in Chinese agricultural soils. Appl. Environ. Microbiol. 79, 6167e6172. doi: 10.1128/AEM.00884-13
Shi L., Jiao J. J. (2014). Seawater intrusion and coastal aquifer management in China: a review. Environ. Earth Sci. 72, 2811–2819. doi: 10.1007/s12665-014-3186-9
Shi M., Xiao X., Liu K., Li L., Wang Y., Zhao M. (2021). Ladderanes indicate anammox activity in the jiaozhou bay. J. Ocean Univ. China 51, 058–071. doi: 10.16441/j.cnki.hdxb.20210044
Sinninghe Damsté J. S., Strous M., Rijpstra W. I. C., Hopmans E. C., Geenevasen J. A. J., van Duin A. C. T., et al. (2002). Linearly concatenated cyclobutane lipids form a dense bacterial membrane. Nature 419, 708–712. doi: 10.1038/nature01128
Song N., Wang N., Lu Y., Zhang J. (2016). Temporal and spatial characteristics of harmful algal blooms in the bohai Sea during 1952–2014. Cont. Shelf Res. 122, 77–84. doi: 10.1016/j.csr.2016.04.006
Sun X., Fan D., Liao H., Tian Y. (2020). Fate of organic carbon burial in modern sediment within Yangtze river estuary. J. Geophys. Res. Biogeosci. 125, 1–20. doi: 10.1029/2019JG005379
Teng Z., Zhen Y., Yu Z., Mi T., Cai T. (2022). Nitrogen-loss and associated microbial communities in sediments from the Yangtze estuary and adjacent sea. Front. Mar. Sci. 9, 1–17. doi: 10.3389/fmars.2022.1078653
Thamdrup B. (2012). New pathways and processes in the global nitrogen cycle. Annu. Rev. Ecol. Evol. Syst. 43, 407–428. doi: 10.1146/annurev-ecolsys-102710-145048
Thamdrup B., Dalsgaard T. (2002). Production of N2 through anaerobic ammonium oxidation coupled to nitrate reduction in marine sediments. Appl. Environ. Microbiol. 68, 1312–1318. doi: 10.1128/AEM.68.3.1312-1318.2002
Van de Graaf A. A., De Bruijn P., Robertson L. A., Jetten M. S. M., Kuenen J. G. (1997). Metabolic pathway of anaerobic ammonium oxidation on the basis of 15N studies in a fluidized bed reactor. Microbiology 143, 2415–2421. doi: 10.1099/00221287-143-7-2415
Van de Graaf A. A., Mulder A., De Bruijn P., Jetten M. S. M., Robertson L. A., Kuenen J. G. (1995). Anaerobic oxidation of ammonium is a biologically mediated process. Appl. Environ. Microbiol. 61, 1246–1251. doi: 10.1128/aem.61.4.1246-1251.1995
Wang H., Dai M., Liu J., Kao S. J., Zhang C., Cai W. J., et al. (2016). Eutrophication-driven hypoxia in the East China Sea off the changjiang estuary. Environ. Sci. Technol. 50, 2255–2263. doi: 10.1021/acs.est.5b06211
Wang X., Li H., Zhang Y., Zheng C., Gao M. (2020). Investigation of submarine groundwater discharge and associated nutrient inputs into laizhou bay (China) using radium quartet. Mar. pollut. Bull. 157, 111359. doi: 10.1016/j.marpolbul.2020.111359
Wang H., Pan R., Liu S., Zhao Y., Dang C., Ni J. (2019a). Relative abundance of anammox bacterial marker genes in the lower Yangtze river and their affecting factors. Acta Sci. Nat. Univ. Pekin. 55, 519–525. doi: 10.13209/j.0479-8023.2019.021
Wang J., Yu Z., Wei Q., Yao Q. (2019). Long-term nutrient variations in the bohai Sea over the past 40 years. J. Geophys. Res. Ocean. 124, 703–722. doi: 10.1029/2018JC014765
Wei H., He Y., Li Q., Liu Z., Wang H. (2007). Summer hypoxia adjacent to the changjiang estuary. J. Mar. Syst. 67, 292e303. doi: 10.1016/j.jmarsys.2006.04.014
Wei Z., Li C., Fang G., Wang X. (2003). Numerical diagnostic study of the summertime circulation in the bohai Sea and the water transport in the bohai strait. Adv. Mar. Sci. 21, 454–464. doi: 10.3969/j.issn.1671-6647.2003.04.013
Wei Q., Wang B., Yao Q., Xue L., Sun J., Xin M., et al. (2019). Spatiotemporal variations in the summer hypoxia in the bohai Sea (China) and controlling mechanisms. Mar. pollut. Bull. 138, 125–134. doi: 10.1016/j.marpolbul.2018.11.041
Wu J., Hong Y., Ye J., Li Y., Liu X., Jiao L., et al. (2019). Diversity of anammox bacteria and contribution to the nitrogen loss in surface sediment. Int. Biodeterior. Biodegrad. 142, 227–234. doi: 10.1016/j.ibiod.2019.05.018
Xing L., Zhang H., Yuan Z., Sun Y., Zhao M. (2011). Terrestrial and marine biomarker estimates of organic matter sources and distributions in surface sediments from the East China Sea shelf. Cont. Shelf Res. 31, 1106–1115. doi: 10.1016/j.csr.2011.04.003
Yuan Z., Xing L., Li L., Zhang H., Xiang R., Zhao M. (2013). Biomarker records of phytoplankton productivity and community structure changes during the last 14000 years in the mud area southwest off cheju island, East China sea. J. Ocean Univ. China 12, 611–618. doi: 10.1007/s11802-013-2230-9
Zhai W., Zhao H., Su J., Liu P., Li Y., Zheng N. (2019). Emergence of summertime hypoxia and concurrent carbonate mineral suppression in the central bohai Sea, china. J. Geophys. Res. Biogeosciences 124, 2768–2785. doi: 10.1029/2019JG005120
Zhang Y., Galy V., Yu M., Zhang H., Zhao M. (2022). Terrestrial organic carbon age and reactivity in the yellow river fueling efficient preservation in marine sediments. Earth Planet. Sci. Lett. 585, 117515. doi: 10.1016/j.epsl.2022.117515
Zhang J., Zhou J., Han Y., Zhang X. (2014). Start-up and bacterial communities of single-stage nitrogen removal using anammox and partial nitritation (SNAP) for treatment of high strength ammonia wastewater. Bioresour. Technol. 169C, 652–657. doi: 10.1016/j.biortech.2014.07.042
Zhang J., Gao X., Li P., Zhuang W., Zhou F. (2015). Nutrient distribution characteristics and long-term trends in the southwest of the laizhou bay and its adjacant rivers. Mar. Sci. Bulletin. 34, 222–232. doi: 10.11840/j.issn.1001-6392.2015.02.015
Zhang X., Miao J., Hu B., Liu H., Zhang H., Ma Z. (2017b). Hydrogeochemical characterization and groundwater quality assessment in intruded coastal brine aquifers (Laizhou bay, China). Environ. Sci. pollut. Res. 24, 21073–21090. doi: 10.1007/s11356-017-9641-x
Zhang S., Xia X., Liu T., Xia L., Zhang L., Jia Z. (2017a). Potential roles of anaerobic ammonium oxidation (anammox) in overlying water of rivers with suspended sediments. Biogeochemistry 132, 237–249. doi: 10.1007/s10533-017-0297-x
Zhang X., Zhang Q., Yang A., Hou L., Zheng Y., Zhai W., et al. (2018). Incorporation of microbial functional traits in biogeochemistry models provides better estimations of benthic denitrification and anammox rates in coastal oceans. J. Geophys. Res. Biogeosciences 123, 3331–3352. doi: 10.1029/2018JG004682
Zhang J., Zhang M., Zhang S., Xu Q., Liu X., Zhang Z. (2019). Nutrient distribution and structure affect the behavior and speciation of arsenic in coastal waters: a case study in southwestern coast of the laizhou bay, China. Mar. pollut. Bull. 146, 377–386. doi: 10.1016/j.marpolbul.2019.06.085
Zhang Y., Zhang J., Wu Y., Zhu Z. (2007). Characteristics of dissolved oxygen and its affecting factors in the Yangtze estuary. Environ. Sci. 28 (8), 1649–1654. doi: 10.3321/j.issn:0250-3301.2007.08.001
Zhao Z., Cao Y., Fan Y., Yang H., Feng X., Li L., et al. (2019). Ladderane records over the last century in the East China sea: proxies for anammox and eutrophication changes. Water Res. 156, 297–304. doi: 10.1016/j.watres.2019.03.046
Zhao Z., Cao Y., Li L., Song G., Yang H., Liu S., et al. (2013). Sedimentary ladderane core lipids as potential indicators of hypoxia in the East China Sea. Chin. J. Oceanol. Limnol. 31, 237–244. doi: 10.1007/s00343-013-1308-y
Zhao M., Mercer J. L., Eglinton G., Higginson M. J., Huang C. (2006). Comparative molecular biomarker assessment of phytoplankton paleoproductivity for the last 160 kyr off cap blanc, NW Africa. Org. Geochem. 37, 72–97. doi: 10.1016/j.orggeochem.2005.08.022
Zheng L., Zhai W., Wang L., Huang T. (2020). Improving the understanding of central bohai Sea eutrophication based on wintertime dissolved inorganic nutrient budgets: roles of north yellow Sea water intrusion and atmospheric nitrogen deposition. Environ. pollut. 267, 115626. doi: 10.1016/j.envpol.2020.115626
Zhou F., Huang D., Xue H., Xuan J., Yan T., Ni X., et al. (2017). Circulations associated with cold pools in the bohai Sea on the Chinese continental shelf. Cont. Shelf Res. 137, 25–38. doi: 10.1016/j.csr.2017.02.005
Zhou L., Yang Y., Shi Y., Xu X., Wang Y. P., Jia J., et al. (2022). Changes in organic carbon delivery to the Yangtze river delta over the last 2000 years. Front. Mar. Sci. 9. doi: 10.3389/fmars.2022.867820
Zhu M. (2014). The study on the spatial and temporal distribution of anammox in the pearl river estuary by ladderane lipids (China: Ocean Univ).
Zhu Y., Pei S., Zhang H., Ye S., Yuan H., Guo R. (2019). Characteristics and research progress of nutreints and eutrophication in laizhou bay, China. Mar. Geol. Front. 35, 1–9. doi: 10.16028/j.1009-2722.2019.04001
Keywords: biomarker, ladderane, particulate, anammox, anthropogenic influence
Citation: Shi M, Xiao X, Ma H, Li L, Wang Y, Dong C, Ding Y and Zhao M (2023) Ladderane and lipid biomarker estimate of the sources and distributions of anammox activities in Laizhou Bay of the Bohai Sea, China. Front. Mar. Sci. 10:1215883. doi: 10.3389/fmars.2023.1215883
Received: 02 May 2023; Accepted: 08 June 2023;
Published: 23 June 2023.
Edited by:
Xiangbin Ran, Ministry of Natural Resources, ChinaReviewed by:
Meilin Wu, South China Sea Institute of Oceanology (CAS), ChinaCopyright © 2023 Shi, Xiao, Ma, Li, Wang, Dong, Ding and Zhao. This is an open-access article distributed under the terms of the Creative Commons Attribution License (CC BY). The use, distribution or reproduction in other forums is permitted, provided the original author(s) and the copyright owner(s) are credited and that the original publication in this journal is cited, in accordance with accepted academic practice. No use, distribution or reproduction is permitted which does not comply with these terms.
*Correspondence: Xiaotong Xiao, eHR4aWFvQG91Yy5lZHUuY24=; Meixun Zhao, bWF4emhhb0BvdWMuZWR1LmNu
Disclaimer: All claims expressed in this article are solely those of the authors and do not necessarily represent those of their affiliated organizations, or those of the publisher, the editors and the reviewers. Any product that may be evaluated in this article or claim that may be made by its manufacturer is not guaranteed or endorsed by the publisher.
Research integrity at Frontiers
Learn more about the work of our research integrity team to safeguard the quality of each article we publish.