- 1Institute of Anthropic Impacts and Sustainability in Marine Environment, National Research Council (IAS-CNR), Palermo, Italy
- 2National Biodiversity Future Centre (NBFC), Palermo, Italy
- 3Istitute of Marine Sciences, National Research Council (ISMAR-CNR), Bologna, Italy
- 4Monolite UK Ltd., London, United Kingdom
Positive interactions among foundation species play a crucial role in achieving and maintaining a good state of the marine environment, enhancing the habitat stability, productivity, and the whole ecosystem functioning. In this regard, macroalgal canopies may affect the biotic and abiotic environmental features, improving the habitat quality for the establishment of other sedentary organisms. In this study, we investigated the effect of intertidal canopy-forming algae on the colonization success of the central-Mediterranean vermetid reef-builder Dendropoma cristatum (Biondi 1859) on artificial substrates produced for reef restoration purposes. An in situ experiment was carried out along the northwestern coast of Sicily during the breeding season of the vermetid snails, by using geopolymer concrete settlement discs with a topographic design to facilitate the vermetid settlement. The discs were placed on the seaward reef rim, underneath the macroalgal canopy, and on adjacent control bare reef areas. The canopy effect on the understory algal colonization and the environmental temperature at the vermetid settlement substrate were also surveyed. After 35 days of field exposure, the vermetid settlement increased by 1.7-fold on the discs underneath the macroalgal canopy compared with that on the bare settlement discs. Moreover, the understory algae showed a higher homogeneity and a lower percent cover on the settlement discs underneath the macroalgal canopy. The peaks of temperature were higher on the bare reef, and the macroalgal canopy also reduced temperature variability under maximum sun irradiance during the diurnal low tides. The vermetid settlement was positively correlated to the canopy cover, which, directly or indirectly, improved the success of colonization of the reef-builder snails on artificial substrates. The deployment of settlement discs where canopy-forming algae are naturally present may facilitate the gardening of vermetid clusters that may be translocated to restock the reef-builder density at degraded areas.
1 Introduction
Marine ecosystems are often formed by the association of multiple foundation species (sensu Dayton, 1972) with a conjunct impact on the physical and biological structure of the environment. This co-occurrence of foundation species is crucial for the creation and stability of habitats, as well as for their productivity and the whole ecosystem functioning, sustaining the ecosystem services (Angelini et al., 2011).
Where foundation species overlap, their interplay may generate positive interspecific interactions with mutual advantages for their stability, supporting the resilience and the resistance of the whole community against external drivers of changes (Angelini et al., 2015; Thomsen et al., 2018). A cordgrass meadow could facilitate the establishment of a mussel bed on the substrate (Altieri et al., 2007), a biogenic reef may kick-start seagrass and algae recovery (Reusch et al., 1994; Newell and Koch, 2004; Lang and Buschbaum, 2010; Guo and Pennings, 2012), whereas a macrophyte coverage may generate suitable abiotic conditions for the establishment of calcifying organisms (Hendriks et al., 2014; Wahl et al., 2018).
Understanding all these processes is crucial to boosting habitat conservation and restoration (Byers et al., 2006). The consideration of interspecific interactions in restoration plans could enhance the potential to recover lost ecological functions, accelerating ecosystem-structuring processes by a multi-habitat approach (e.g., Lang’at et al., 2013; Derksen-Hooijberg et al., 2018). Only recently restoration studies have incorporated the mechanisms of facilitation among species (Halpern et al., 2007; Renzi et al., 2019).
Designated as one of the most relevant biodiversity hotspots worldwide (Meyers et al., 2000), the Mediterranean Sea hosts many different mixed habitats originated by the overlap of multiple foundation species (e.g., gorgonians and erected sponges within the coralligenous outcrops, mussel beds within the P. oceanica meadows, hermatypic corals and other calcifying bioconstructors within forests of erected macroalgae). Among these bioconstructors, gregarious vermetids of the genus Dendropoma Mörch, 1861, in association with the crustose coralline alga (CCA) Neogoniolithon brassica-florida (Harvey), Setchell and Mason (1943) form ecologically important biogenic structures (called vermetid reef) along the central, the south, and the southeastern rocky coasts of the basin. These bioconstructions may co-create a complex three-dimensional framework with canopy-forming macroalgae (mainly belonging to the genus Cystoseira C. Agardh, 1820), which often fringe the seaward rim of the reef (Ingrosso et al., 2018).
The role of the vermetid reef in sustaining high levels of marine biodiversity, coastal processes, and ecosystems is widely recognized, because this bioconstruction magnifies the horizontal extension and three-dimensionality of intertidal rocky platforms, protects the coasts from physical erosion, regulates sediment transport, and is an important carbon sink, with subsidiary benefits also for humans (Chemello and Silenzi, 2011; Colombo et al., 2013; Milazzo et al., 2016; Ape et al., 2018).
The active protection of this habitat and bioengineering species is advocated in European legislation (Bern Convention European Council, 1979; Barcelona Convention Protocol concerning Specially Protected Areas and Biological Diversity in the Mediterranean, SPA/BD, 1995; Council Directive 92/42/EEC; the IUCN Red List of Mediterranean Habitats, Gubbay et al., 2016). However, during the last decade, living vermetid cover has drastically decreased throughout the Mediterranean rocky shores (Badreddine et al., 2019; Rilov et al., 2020; Bisanti et al., 2022). This collapse is hypothesized to be related to the global climate change and to intensive human coastal exploitation, with severe consequences for the bioconstruction maintenance and cascading effects on the whole rocky intertidal ecosystem (Galil, 2013; Rilov et al., 2020; Albano et al., 2021).
Experimental studies suggest that the future Mediterranean climatic scenario (i.e., the rapid increase of the sea surface temperature and extreme climatic events) will adversely affect the reef-builder Dendropoma sp. at different ontogenetic stages (embryos, early recruits, and adults) and the photosynthetic and calcification performance of the associated CCA, leading to the reef decline (Milazzo et al., 2014; Fine et al., 2016; Alessi et al., 2019; Milazzo et al., 2019). At the same time, the ocean CO2 enrichment should boost the reef-associated macroalgae with non-linear effects on the community (Celis-Plá et al., 2015; Cornwall et al., 2017; Milazzo et al., 2019).
Within this framework, the implementation of active and coordinated efforts for vermetid reef conservation should be an intergovernmental priority. Planning how to face local vermetid decline by successful restoration strategy should also consider biological interactions and the adoption of a multispecies approach (McAfee et al., 2022).
With this regard, canopy-forming macroalgae are known to influence the recruitment of sessile and reef-forming marine invertebrates in multiple ways (e.g., barnacles, Leonard (1999); mussels, Moreno (1995) and Bégin et al. (2004); oysters, Shelamoff et al. (2019) and McAfee et al. (2021)). Macroalgal canopies, indeed, represent valuable biogenic refugia for benthic organisms, especially in highly dynamic environments as the intertidal (Watt and Scrosati, 2013a; Watt and Scrosati, 2013b), allowing abiotic stress amelioration, the alteration of predator–prey interactions (Ware et al., 2019) and increasing the niche availability for the associated biota (Bulleri et al., 2016).
This study aims to assess the effect of canopy-forming algae on the settlement success of the central-Mediterranean vermetid reef-builder Dendropoma cristatum (Biondi 1859) on artificial discs that can be transferred and used to restore other vermetid reefs. We expect that vermetid settlers positively respond to the canopy presence, taking advantage of the macroalgal influence on the surrounding habitat. Furthermore, the influence of the macroalgal canopy on the temperature and understory algal colonization was also examined, to detect eventual features at the vermetid settlement surface that may co-vary with the canopy presence and affect the reef-builder settlement.
2 Materials and methods
2.1 Study area
A field-based experiment was conducted along the northwestern coast of Sicily, in the locality of “Addaura” (38°11′28″N; 13°20′55″E) during June and July 2022 (Figures 1A, B). Here, a continuous vermetid reef fringes the rocky coastline, covering an area of approximately 200 linear meters. During this period, the breeding season of Dendropoma cristatum was coincident with a dense belt of canopy-forming macroalgae mainly represented by the genus Cystoseira sp., covering the seaward rim of the reef and interrupted by patches of bare bioconstruction. The average vermetid density on this rim and the reef geomorphological details have been visually assessed and reported by La Marca et al. (2022).
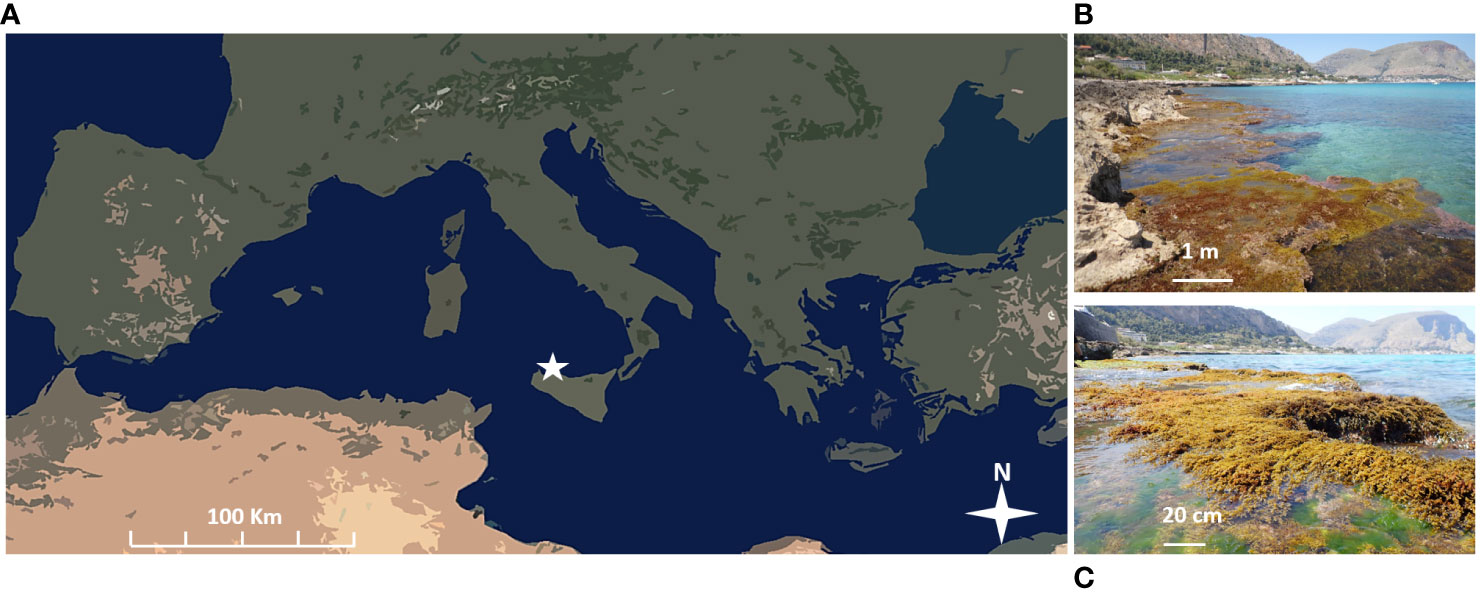
Figure 1 (A) Location of the study area; the white star in the map shows the experimental site; (B) vermetid reef where the experiment has been carried out; (C) macroalgal canopy covering the seaward rim of the reef (mainly represented by the genus Cystoseira sp.).
A settlement experiment was conducted across two different habitat types along the seaward part of this natural vermetid reef: the first habitat type was characterized by a well-developed canopy coverage (+C) (Figure 1C), whereas the second habitat was interspersed within the first type but was unaffected by the canopy since it was uncovered by erected macroalgae (−C).
2.2 Settlement experiment
Artificial settlement substrates were used to test the colonization success of vermetids in the presence (+C) and absence (−C) of the macroalgal canopy for 35 days. These substrates were discs made of geopolymer (La Marca et al., 2022) of 8 cm in diameter and 2 cm in thickness, offering on their top face roughly 50 cm2 as total settlement surface. The upper face of the discs was engineered with shelters 2-mm wide and 3-mm deep, improving the topographic complexity for crawling vermetid snails at the pre-settlement stage. These geopolymer discs were produced by casting the concrete mixture within a silicon mould obtained by three-dimensional printing (produced by Binder Jetting 3D Printing) of a computer-aided drafting (CAD) design (Figures 2A, B).
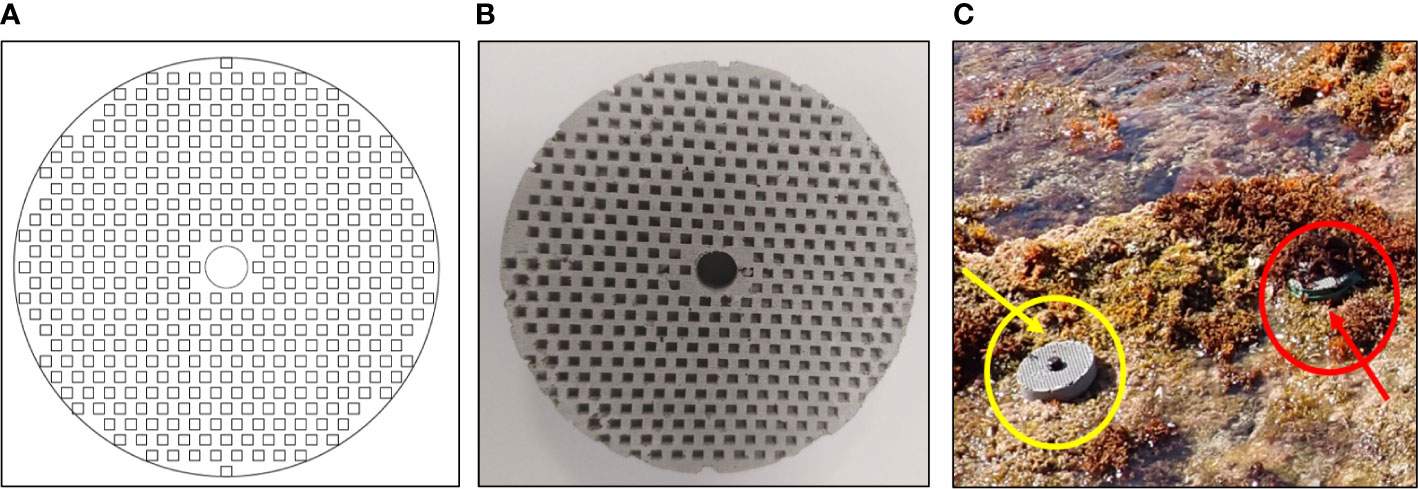
Figure 2 Discs of geopolymer concrete employed for the settlement experiment. (A) CAD design of the discs; (B) example of produced discs; (C) discs deployed across the two habitat types during the settlement experiment: under the canopy (in the red circle) and on the bare reef (in the yellow circle).
Overall, 24 settlement discs were secured to the seaward reef part by galvanized screws and were randomly distributed in pairs across the two habitat types (+C and −C), distanced from each other by more or less 30 cm, covering a total area of 50 m (Figure 2C).
Once collected, the geopolymer discs were photographed and stored at −20°C in the lab. The macroalgal canopy that covered each disc was sampled, and its biomass was calculated as dry weight after heater at 100°C for 24 h.
The number of vermetids settled on each disc was counted under the stereomicroscope (Leica MDG41), and only the settlers attached to the disc top face were considered for the analysis.
2.3 Understory algal colonization and environmental temperature at the vermetid settlement surface
To assess the influence of the macroalgal canopy on the understory algal colonization on the settlement discs, the percentage cover of the vegetation established on the top face of the geopolymer substrate was visually assessed by a photo analysis, through the open-source software ImageJ. A taxonomic description of the algal groups established on the settlement discs was also done.
To monitor the environmental temperature experienced by vermetids settled in the presence and the absence of the macroalgal canopy, temperature loggers (EnvLogger T2.4 version, Electricblue, Portugal) were installed in proximity of settlement discs: two underneath the macroalgal canopy and two on the bare reef. The environmental temperature was registered at intervals of 30 min, with a resolution of 0.1°C. Once the settlement experiment ended, temperatures were downloaded from each thermo-logger, and the whole dataset was analyzed and compared between the two habitat types (+C and −C). Because the algal canopy is supposed to exert a higher control over the substrate temperature when the reef rim is simultaneously emerged and exposed to the direct sun irradiation, the temperatures coinciding with the diurnal low tides were selected and compared across the two habitat types (+C and −C).
2.4 Statistical analysis
One geopolymer disc got lost during the experiment, and its replacement with a new one was not possible. This resulted in an unbalanced experimental design due to the different number of replicates among the two habitat types (n = 12 discs for +C and n = 11 discs for −C).
Firstly, a linear regression analysis was used to relate the vermetid density and the biomass of the macroalgal canopy cover on each disc, by using the software OriginPro 2022 (OriginLab Corporation, Northampton MA, United States).
The vermetid density and the understory algal percent cover on each disc were compared across the two habitat types by univariate distance–based permutational non-parametric analyses of variance (one fixed factor “Habitat type” with two levels: +C and –C; PERMANOVA; McArdle and Anderson, 2001; Anderson, 2017). For both response variables, raw data were ordinated using the Euclidean distance, and the analyses were set with 9,999 permutations of residuals under a restricted model, with a Type III (partial) sum of squares (Anderson, 2001; Anderson, 2017). The statistical analyses were performed with the Primer-E v7 statistical software package with the PERMANOVA+ extension (Plymouth Marine Laboratory; Clarke and Warwick, 1994; Clarke and Gorley, 2006).
3 Results
3.1 Settlement experiment
Overall, 2,221 settlers were counted on the top face of the discs, all inside the shelters of 2 mm × 2 mm × 3 mm. A total of 1,444 individuals were counted on the substrates covered by the macroalgal canopy (+C), whereas 777 settlers were found on the discs placed on the bare vermetid reef (−C).
The macroalgal canopy dry biomass that covered each settlement disc was 0 for the disc placed on the bare reef and varied between a minimum of 4.23 g and a maximum of 8.05 g for the discs placed under the canopy. A positive relationship was found between the settlement success of the vermetid snails and this macroalgal canopy dry biomass (r= 0.6571; p<0.01; n= 23, Figure 3).
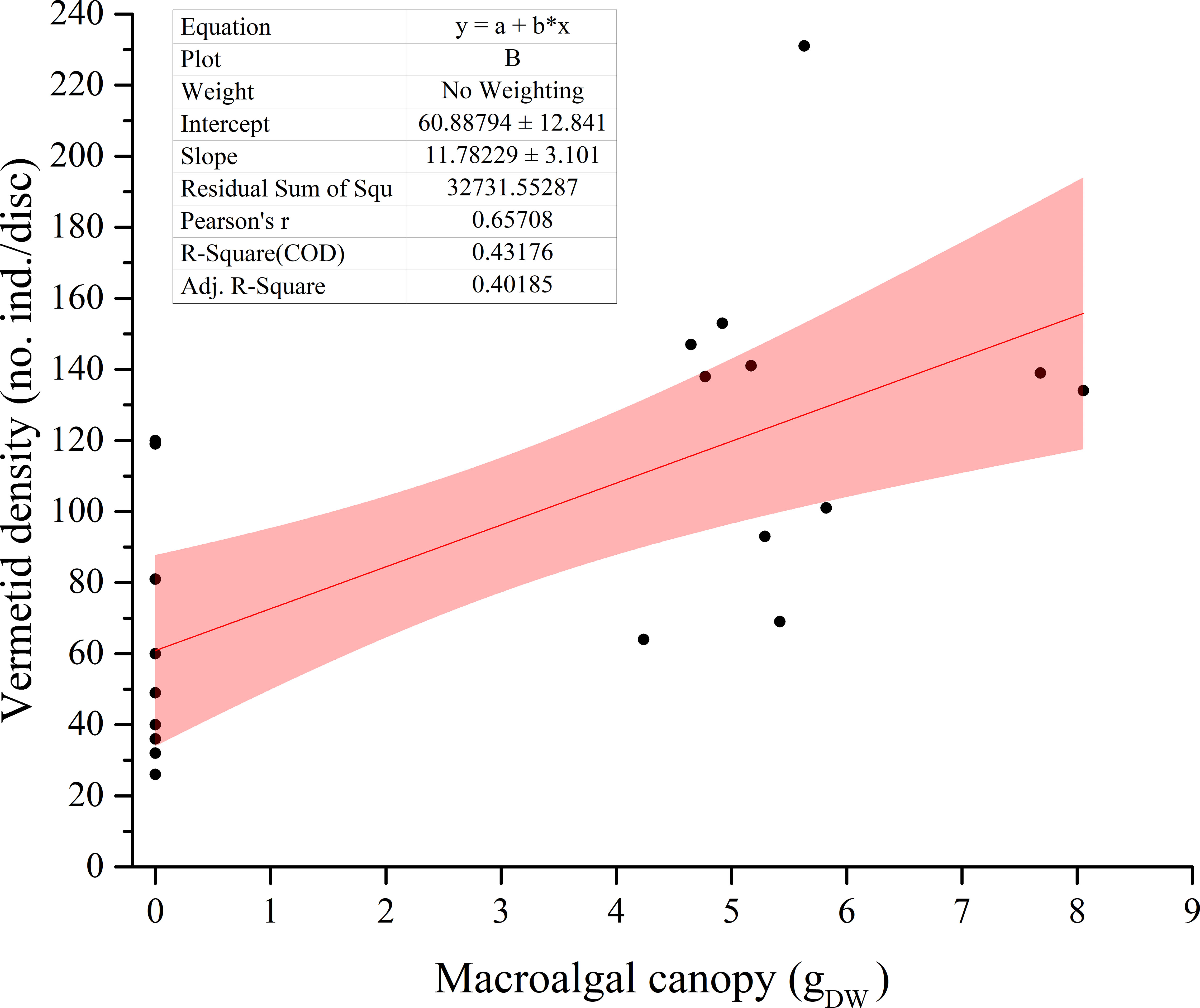
Figure 3 Linear regression between the number of vermetids and the macroalgal canopy biomass on each disc. r = 0.6571 (p < 0.01); n = 23.
The vermetid settler density significantly differed among the two habitat types (+C and −C) (p < 0.05, Table 1). On average, 120.33 (± 15 S.E.) ind./disc and 70.63 (± 15.07 S.E.) ind./disc were found, respectively, on the settlement substrates placed under the macroalgal canopy (+C) and on the bare reef (−C) (Figure 4).

Table 1 Results of the univariate PERMANOVA analysis comparing the total number of vermetid settlers (no. ind./disc) among the two habitat types (+C and −C).
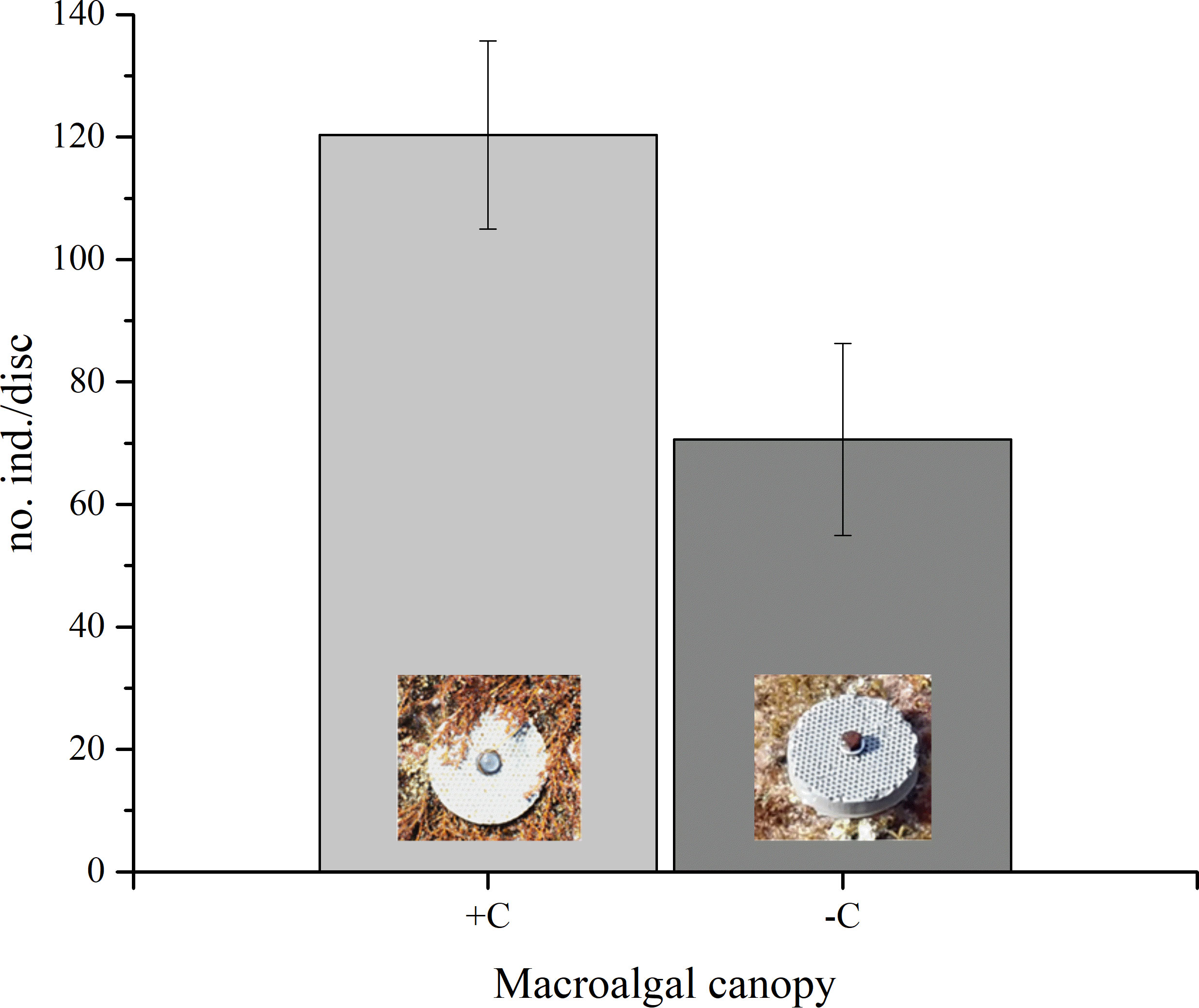
Figure 4 Average number (± S.E.) of vermetids settled on artificial geopolymer discs placed under the macroalgal canopy (+C, n = 12) and on the bare reef (−C, n = 11) (p < 0.05).
3.2 Understory algal colonization and environmental temperature at the vermetid settlement surface
3.2.1 Understory algal colonization
Under the macroalgal canopy, the discs were consistently less vegetated compared to those placed on the bare reef (p < 0.001, Table 2). On average, the vegetation percentage cover was 15.19% (± 1.78 S.E.) on the discs placed under the canopy (+C) and 31.09% (± 3.02 S.E.) on the bare reef discs (−C) (Figure 5).

Table 2 Results of the univariate PERMANOVA analysis comparing the understory vegetation percentage cover/disc among the two habitat types (+C and −C).
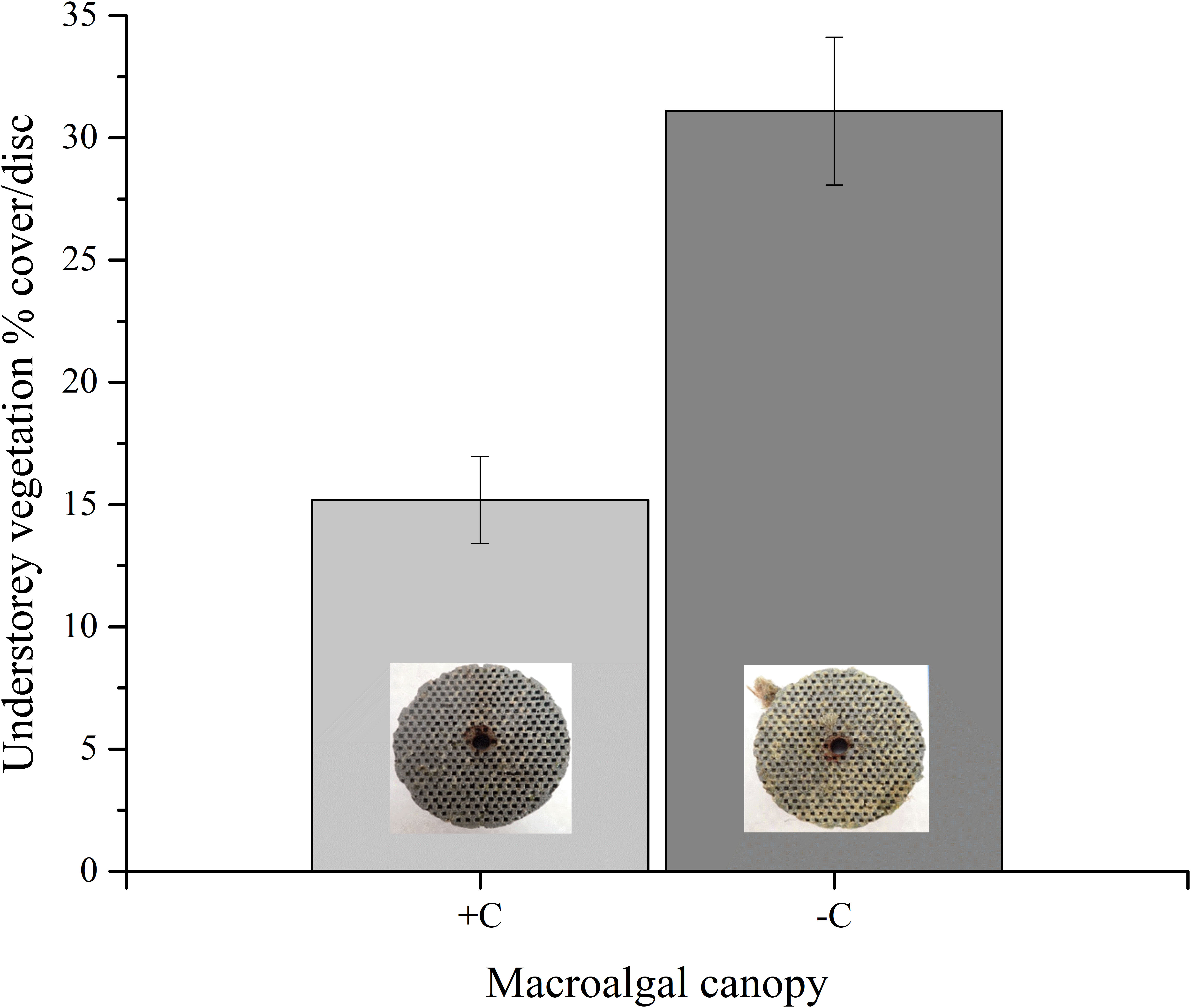
Figure 5 Average understory algal percentage cover (± S.E.) on settlement discs placed under the macroalgal canopy (+C, n = 12) and on the bare reef (−C, n = 11) (p < 0.001).
Specifically, the algal assemblage on the settlement discs was represented by a layer of epilithic biofilm, crustose coralline algae (CCA), small patches of erected algae (Jania rubens, Laurencia sp., and Corallina sp.), and algal turf. These last two taxonomic groups were more abundant on the discs placed on the bare reef, whereas, under the macroalgal canopy, the assemblage was mainly represented by CCA and epilithic biofilm.
Furthermore, the erected algae settled within the refuges of 2 mm × mm 2 × 3 mm and their small frond shaded the disc surface, whereas the CCA encrusted the disc surface and covered the boundaries of the refuges (Figure 6).
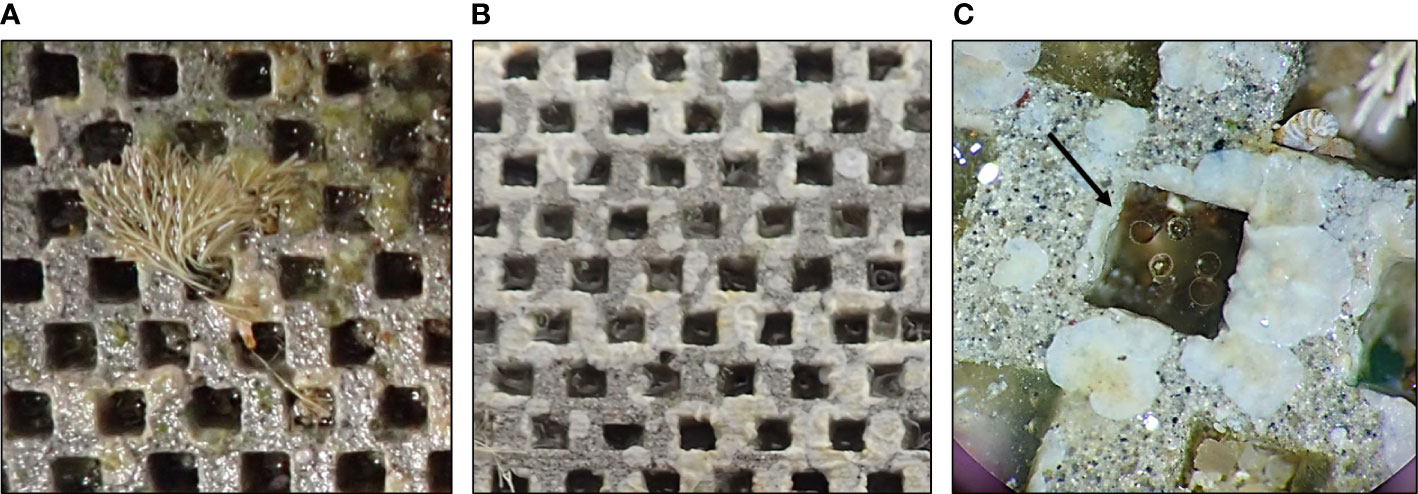
Figure 6 Close-up view of the assemblage on the topside of the geopolymer discs. (A) J. rubens frond settled within a refuge of 2 mm × 2 mm; (B) crustose coralline algae encrusting the disc surface and the refuge edges; (C) five D. cristatum settlers within a refuge of 2 mm × 2 mm (pointed out by the black arrow).
3.2.2 Environmental temperature at the vermetid settlement surface
The temperatures at the settlement substrate ranged between 20.50°C and 30.30°C under the macroalgal canopy (average temperature: 25.73°C ± 0.046 S.E.) and between 20.60°C and 32.75°C on the bare vermetid reef (average temperature: 25.86°C ± 0.047 S.E.) (Figure 7A).
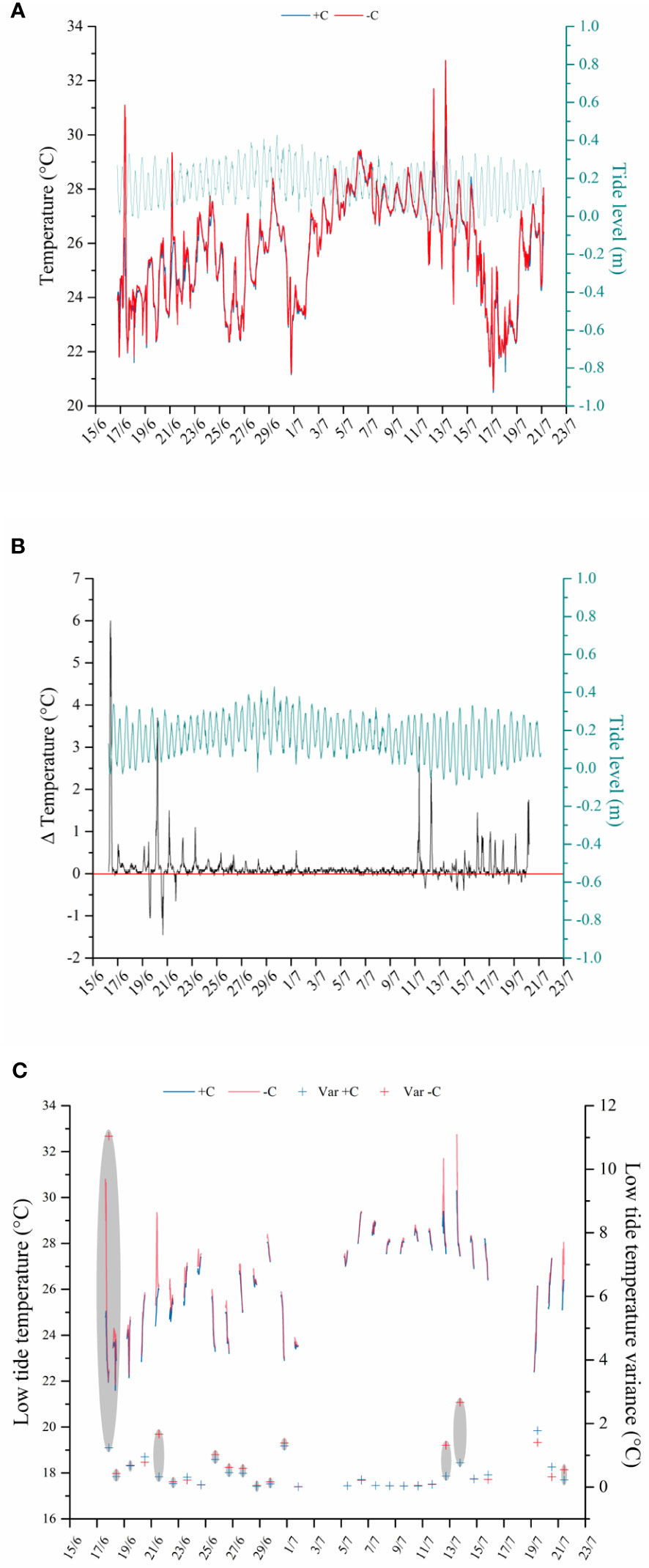
Figure 7 Temperatures (°C) recorded during the experiment by the thermo-loggers underneath the algal canopy in blue (+C) and on the bare vermetid reef in red (−C). (A) Temperatures during the whole experimental period; the green line shows the tide level (m) reported on the secondary Y-axis. (B) The difference among the temperature (Δ Temperature among −C and +C treatments) recorded on the bare vermetid reef and under the macroalgal canopy during the whole experimental period, with the tide level (m) indicated by the green line. The red line marks the 0 value when no differences among −C and +C treatments occur. (C) Temperatures recorded during the diurnal low-tide periods under the macroalgal canopy in blue (+C) and on the bare reef in red (−C) and variance among temperatures for each tidal series represented by the red and blue crosses. The gray circles point out when the variance is higher for the temperatures recorded on the bare substrate compared to the measurements collected under the canopy.
The difference across temperatures registered at the same time on the bare reef and under the canopy (measured as Δ Temperature) reached the full extent of 6°C, and, most of the time, it was higher than zero, reflecting a general cooler canopy temperature (Figure 7B). These temperature differences across the two habitat types were recorded during both the high- and low-tide peaks, and they were more pronounced during the low-tide periods (Figures 7A, B). This could be explained by a thermal buffering due to the seawater presence over the substrate during the high tide. Moreover, a zoom on the temperature regimes during the diurnal low tides (i.e., when the reef is exposed to the direct sun irradiation) was made to assess the thermal differences between the two canopy treatments under maximum irradiance (Figure 7C).
During diurnal low-tide periods, the temperature ranged between 21.60°C and 30.30°C under the macroalgal canopy and between 21.85°C and 32.75°C on the bare reef, with average values of 26.22°C (± 0.10 S.E.) and 26.54°C (± 0.10 S.E.), respectively. Furthermore, a higher temperature variation has been mostly found on the substrate missing the canopy, expressed by the variance among the values registered during each tidal period in each habitat type (Figure 7C).
4 Discussion
In our study, the intertidal macroalgal canopy does not obstruct the vermetid input on the artificial discs and it seems to positively affect the settlement of the considered reef-forming species.
Indeed, Dendropoma cristatum settlers were found on all the artificial substrates employed for the experiment, with increasing abundance in the presence of a coverage of macroalgal canopy, where they resulted 1.70-fold more abundant compared to the uncovered settlement discs. This biological facilitation may be explained by a habitat improvement by the canopy instead of the occurrence of a spatial variability in the vermetid post-larvae supply. Indeed, the experiment was conducted within an area where the D. cristatum adult density was homogeneous, guaranteeing a comparable local output of settlers among the sites where the geopolymer discs were deployed.
Peculiar sub-canopy conditions may regulate the mechanism by which vermetids positively respond to the erect macroalgae presence. In particular, this study highlights a negative control by the canopy on the understory algal colonization on the artificial settlement discs and on the environmental temperature in the proximity of the reef surface where D. cristatum settlement naturally occurs.
Macroalgal canopies are documented to inhibit the understory vegetation by a multiplicity of dynamics (e.g., by reducing the understory light, Connell (2003); by frond abrasion, Irving and Connell (2006); by altering the local hydrodynamic regime, O’Brien and Scheibling (2018)), and the reduction of the understory algal coverage is positively associated with the increase in settlement and recruitment of sessile invertebrates (Bégin et al., 2004; Shelamoff et al., 2019; McAfee and Bishop, 2019; McAfee et al., 2021). Interestingly, a negative association between the understory algal colonization and the vermetid settlement emerged also from our study. On the geopolymer discs, erected algae and turf settled within the same vermetid shelters (with a likely antagonistic role against the vermetid settlement), and their abundance was suppressed by the macroalgal canopy, increasing the availability of uncolonized refuges for the reef-builder attachment. By contrast, the coralline algae encrustation was well-developed also under the macroalgal canopy, where it colonized the disc surface, remaining outside the vermetid burrows.
Furthermore, according to Catalán et al. (2023), the algal assemblage under the canopy seems to be highly homogeneous, being mainly dominated by the encrusting red algae and the epilithic biofilm.
The slightly cooler temperature measured at the settlement surface under the macroalgal canopy is positively associated with the vermetid settlement. Changes in the temperature may significantly affect the survival of organisms living in the intertidal (Somero, 2010), especially if sedentary and sessile as reef-builder vermetids. Furthermore, thermal stress dampening by the macroalgal canopy has been recognized as one of the major benefits for the intertidal biota, generating a more favorable habitat for the community establishment and functioning (Bertness et al., 1999; Leonard, 1999; Coombes et al., 2013; Umanzor et al., 2017; Ørberg et al., 2018; Sarà et al., 2021).
At the reef scale, the temperature has been hypothesized as a factor that may control the settlement of D. cristatum (Franzitta et al., 2016), although a gap of knowledge persists about the thermal sensitivity of this reef-builder over its life cycle. This information would be essential to forecast the consequences of the expected temperature rise on this species and on the reef persistence at the Mediterranean scale.
Differences up to 6°C were revealed among the temperatures registered at the same time across the two habitat types. During the whole experiment, the highest temperature on the bare reef was 2.45°C higher than the highest temperature recorded under the macroalgal canopy. During the diurnal low tides, the canopy coverage reduced the average environmental temperature of 0.32°C and modulated the temperature oscillation when the reef was exposed to the air. This narrow temperature difference among habitat types may plausibly deter the vermetid settlement. A similar temperature variation, indeed, affects the D. cristatum reproductive performance in terms of the size and the number of embryos per egg capsule (Alessi et al., 2019).
The macroalgal canopy might buffer the thermal stress for settling vermetids when the reef is under direct sun irradiance, lowering temperature fluctuations and peaks at the substrate, as in our study. However, this is just an aspect of the influence of canopy-forming algae on microclimatic conditions along the intertidal vermetid reef and additional studies should focus on other near-surface features associated with heat. Extreme desiccation conditions documented along the Sicilian coasts during the summer 2022, due to prolonged reef aerial exposure, are suggested as possible triggers for vermetid mortality (Bisanti et al., 2022). The study of macroalgal canopy as biogenic refugia against these prolonged periods of physiological stress needs a higher concern, weighting the full potential of natural canopy-forming algae to ameliorate the rising climatic stress at the reef-scale.
Further studies on the physiology of the juvenile vermetid snails may clarify if their settlement competence is affected by the environmental temperature, if they undergo higher post-settlement mortality in the hotter environment or if they selectively discriminate among settlement sites, confirming that, in addition to biological cues (e.g., the presence of CCA, Spotorno-Oliveira et al. (2015), and the epilithic biofilm, La Marca et al. (2018)), also physical stress might drive the settlement of reef-builder vermetids.
Although our experiment has been carried out in the field under multiple sources of variability and did not directly test the effect of the temperature on the settlement dynamics of D. cristatum, our results might corroborate the role of the environmental temperature as a limiting factor during the early life stages of different benthic invertebrates and also foundation species (Storch et al., 2011; Webster et al., 2011; Lathlean et al., 2013; Byrne et al., 2017; Huggett et al., 2018; Lang et al., 2023).
The macroalgal canopy may have a control on further abiotic and biotic factors that have not been considered at this step of our research. It is also plausible that it protects the newly settled snails against physical disturbances, contextually enhancing the environmental quality for the reef-builder vermetids in multiple ways (e.g., by shading the substrate and contrasting the development of sediment-entrapping understory turf assemblage, Connell et al. (2014); by reducing the competition with other benthic organisms removed by the mechanical canopy whiplash and benthic sweeping, Beermann et al. (2013) and Shelamoff et al. (2019)). Furthermore, extending the spatial-temporal setup of our investigation may also reveal if the facilitative effect provided by the canopy may depend on the local environmental context or if it is generalizable to other reef areas and if other biological interactions may succeed during the following stages of the vermetid colony development.
From a practical perspective, the use of modular and relatively small substrates (i.e., the geopolymer discs) is instrumental in deploying these artificial settlement units where the environmental context fits the initial step of the vermetid colonization (e.g., close to the macroalgal canopy at their settlement stage), avoiding more expensive and alternative solutions to artificially enhance the early vermetid recruitment. Moreover, the implementation of these rock-like D. cristatum colonized units is central for moving a vermetid group to selected sites and for rebuilding a self-sustaining population of reef-forming organisms, avoiding the transplantation of adults and overcoming the limited dispersal ability of these reef-builder gastropods (Calvo et al., 1998; Galil, 2013).
The efforts for the protection of the co-occurring vermetid reefs and canopy-forming macroalgae may, therefore, represent an opportunity to increase the effectiveness of actions focused on one single habitat, contributing to the conservation of the intertidal ecosystem at a wider scale. This multi-habitat approach (McAfee et al., 2022), moreover, is fundamental to strengthening the framework for the achievement of the UN Decade on Ecosystem Restoration goals (UN Resolution 73/284; Waltham et al., 2020) and is in line with the recent Nature Restoration Law (European Commission, 2022), which aims at implementing national restoration plans through measures that support the connectivity between habitats.
Data availability statement
The raw data supporting the conclusions of this article will be made available by the authors, without undue reservation.
Ethics statement
The manuscript presents research on animals that do not require ethical approval for their study.
Author contributions
EM, FA, AR, VM, and SM conceived this research; EM wrote the manuscript, with a contribution from AR, VM, and FA. ED provided the technical support for the geopolymer disc production. EM, AR, VM, MS, FA, and MM conducted the fieldwork; EM conducted the laboratory analysis; EM and AR performed the data analysis; AR, VM, FA, and SM contributed to the manuscript revision and discussion. SM provided funds to support the experiment. All authors commented on the first draft of the manuscript. All authors contributed to the article and approved the submitted version.
Funding
The author(s) declare financial support was received for the research, authorship, and/or publication of this article. This research received the financial support of the Italian Ministry of the University and Research (MUR), within the framework of the PRIN project MARHES - Marine habitats restoration in a climate change-impaired Mediterranean Sea (PRIN - cod. 2017MHHWBN_003 Linea C). This research also contributes to the “National Biodiversity Future Center - NBFC” goals, under the National Recovery and Resilience Plan (NRRP), Mission 4 Component 2 Investment 1.4, funded by the European Union – NextGenerationEU (Award Number: Project code CN_00000033, Concession Decree No. 1034 of 17 June 2022 adopted by the Italian Ministry of University and Research).
Acknowledgments
The authors are grateful to Dr. Evelyn Scicchigno for the English revision of the manuscript and, also to Maria Luisa Carelli, for technical and administrative support to this research. The authors are also grateful to the Vela Club staff for the facilitation provided during the fieldwork.
Conflict of interest
One of the authors ED is employed by the company producing the settlement substrates Monolite UK LTD, but the research did not receive any financial support from Monolite UK, and the author employed in the company did not influence the research outcomes.
The remaining authors declare that the research was conducted in the absence of any commercial or financial relationships that could be construed as a potential conflict of interest.
Publisher’s note
All claims expressed in this article are solely those of the authors and do not necessarily represent those of their affiliated organizations, or those of the publisher, the editors and the reviewers. Any product that may be evaluated in this article, or claim that may be made by its manufacturer, is not guaranteed or endorsed by the publisher.
References
Agardh C. A. (1820 ‘1821’). Species algarum rite cognitae, cum synonymis, differentiis specificis et descriptionibus succinctis. Volumen primum. Pars prima. pp. [i–iv], –[1]–168. Lundae [Lund]: ex officina Berlingiana
Albano P. G., Steger J., Boˇsnjak M., Dunne B., Guifarro Z., Turapova E., et al. (2021). Native biodiversity collapse in the eastern Mediterranean. Proc. R. Soc B 288, 20202469. doi: 10.1098/rspb.2020.2469
Alessi C., Giomi F., Furnari F., Sarà G., Chemello R., Milazzo M. (2019). Ocean acidification and elevated temperature negatively affect recruitment, oxygen consumption and calcification of the reef-building Dendropoma cristatum early life stages: Evidence from a manipulative field study. Sci. Total Environ. 693, 133476. doi: 10.1016/j.scitotenv.2019.07.282
Altieri A. H., Silliman B. R., Bertness M. D. (2007). Hierarchical organization via a facilitation cascade in intertidal cordgrass bed communities. Am. Nat. 169, 195–206. doi: 10.1086/510603
Anderson M. J. (2001). A new method for non-parametric multivariate analysis of variance. Austral Ecol. 26, 2–46. doi: 10.1111/j.1442-9993.2001.01070.pp.x
Anderson M. J. (2017). Permutational multivariate analysis of variance (PERMANOVA). Wiley statsRef: statistics reference online, © 2014–2017 (Hoboken, New Jersey, USA: John Wiley & Sons, Ltd). doi: 10.1002/9781118445112.stat07841
Angelini C., Altieri A. H., Silliman B. R., Bertness M. D. (2011). Interactions among foundation species and their consequences for community organization, biodiversity, and conservation. BioScience 61, 782–789. doi: 10.1525/bio.2011.61.10.8
Angelini C., Heide T., Griffin J. N., Morton J. P., Derksen-Hooijberg M., Lamers L. P., et al. (2015). Foundation species’ overlap enhances biodiversity and multifunctionality from the patch to landscape scale in southeastern United States salt marshes. Proc. Biol. Sci. 282 (1811), 20150421. doi: 10.1098/rspb.2015.0421
Ape F., Gristina M., Chemello R., Sarà G., Mirto S. (2018). Meiofauna associated with vermetid reefs: The role of macroalgae in increasing habitat size and complexity. Coral Reefs 37 (3), 875–889. doi: 10.1007/s00338-018-1714-x
Badreddine A., Milazzo M., Abboud-Abi Saab M., Bitar G., Mangialajo L. (2019). Threatened biogenic formations of the Mediterranean: current status and assessment of the vermetid reefs along the Lebanese coastline (Levant basin). Ocean Coast. Manage. 169, 137–146. doi: 10.1016/j.ocecoaman.2018.12.019
Beermann A. J., Ellrich J. A., Molis M., Scrosati R. A. (2013). Effects of seaweed canopies and adult barnacles on barnacle recruitment: The interplay of positive and negative influences. J. Exp. Mar. Biol. Ecol. 448, 162–170. doi: 10.1016/j.jembe.2013.07.001
Bégin C., Johnson L. E., Himmelman J. H. (2004). Macroalgal canopies: distribution and diversity of associated invertebrates and effects on the recruitment and growth of mussels. Mar. Ecol. Prog. Ser. 271, 121–132. doi: 10.3354/meps271121
Bertness M. D., Leonard G. H., Levine J. M., Schmidt P. R., Ingraham A. O. (1999). Testing the relative contribution of positive and negative interactions in rocky intertidal communities. Ecology 80, 2711–2726. doi: 10.1890/0012-9658(1999)080[2711:TTRCOP]2.0.CO;2
Bisanti L., Visconti G., Scotti G., Chemello R. (2022). Signals of loss: Local collapse of neglected vermetid reefs in the western Mediterranean Sea. Mar. pollut. Bull. 185, 114383. doi: 10.1016/j.marpolbul.2022.114383
Bulleri F., Bruno J. F., Silliman B. R., Stachowicz J. J. (2016). Facilitation and the niche: implications for coexistence, range shifts and ecosystem functioning. Funct. Ecol. 30, 70–78. doi: 10.1111/1365-2435.12528
Byers J. E., Cuddington K., Jones C. G., Talley T. S., Hastings A., Lambrinos J. G., et al. (2006). Using ecosystem engineers to restore ecological systems. Trends Ecol. Evol. 21 (9), 493–500. doi: 10.1016/j.tree.2006.06.002
Byrne M., Ross P. M., Dworjanyn S. A., Parker L. (2017). “Larval ecology in the face of changing climate—Impacts of ocean warming and ocean acidification,” in Evolutionary ecology of marine invertebrate larvae. Eds. Carrier T., Reitzel A., Heyland A. (Oxford: Oxford Academic). doi: 10.1093/oso/9780198786962.003.0017
Calvo M., Templado J., Penchaszadeh P. (1998). Reproductive biology of the gregarious mediterranean vermetid gastropod Dendropoma petraeum. J. Mar. Biol. Ass. 78 (2), 525–549. doi: 10.1017/S0025315400041606
Catalán A. M., López D. N., Fica-Rojas E., Broitman B. R., Valdivia N., Scrosati R. A. (2023). Foundation species canopies affect understory beta diversity differently depending on species mobility. Ecology 104 (4), e3999. doi: 10.1002/ecy.3999
Celis-Plá P. S. M., Hall-Spencer J. M., Horta P. A., Milazzo M., Korbee N., Cornwall C. E., et al. (2015). Macroalgal responses to ocean acidification depend on nutrient and light levels. Front. Mar. Sci. 2. doi: 10.3389/fmars.2015.00026
Chemello R., Silenzi S. (2011). Vermetid reefs in the Mediterranean Sea as archives of sea-level and surface temperature changes. Chem. Ecol. 27 (2), 121–127. doi: 10.1080/02757540.2011.554405
Clarke K. R., Warwick R. M. (1994). Change in marine communities: An approach to statistical analysis and interpretation. 2nd ed (Plymouth, U.K: Primer-e Ltd, Plymouth Marine Laboratory).
Colombo F., Costa V., Dubois S. F., Gianguzza P., Mazzola A., Vizzini S. (2013). Trophic structure of vermetid reef community: High trophic diversity at small spatial scale. J. Sea Res. 77, 93–99. doi: 10.1016/j.seares.2012.10.005
Connell S. D. (2003). The monopolization of understorey habitat by subtidal encrusting coralline algae: A test of the combined effects of canopy-mediated light and sedimentation. Mar. Biol. 142, 1065–1071. doi: 10.1007/s00227-003-1021-z
Connell S. D., Foster M. S., Airoldi L. (2014). What are algal turfs? Towards a better description of turfs. Mar. Ecol. Prog. Ser. 495, 299–307. doi: 10.3354/meps10513
Coombes M. A., Naylor L. A., Viles H. A., Thompson R. C. (2013). Bioprotection and disturbance: Seaweed, microclimatic stability and conditions for mechanical weathering in the intertidal zone. Geomorphology 202, 4–14. doi: 10.1016/j.geomorph.2012.09.014
Cornwall C. E., Revill A. T., Hall-Spencer J. M., Milazzo M., Raven J. A., Hurd C. L. (2017). Inorganic carbon physiology underpins macroalgal responses to elevated CO2. Sci. Rep. 7, 46297. doi: 10.1038/srep46297
Council Directive 92/43/EEC of 21 May 1992 on the conservation of natural habitats and of wild fauna and flora (Eur-Lex).
Dayton P. K. (1972). “Toward an understanding of community resilience and the potential effects of enrichments to the benthos at McMurdo Sound, Antarctica,” in Proceedings of the colloquium on conservation problems in Antarctica. Ed. Parker B. C. (Lawrence, Kansas, USA: Allen Press), 81–96.
Derksen-Hooijberg M., Angelini C., Lamers L. P., Borst A., Smolders A., Hoogveld J. R., et al. (2018). Mutualistic interactions amplify saltmarsh restoration success. J. Appl. Ecol. 55, 405–414. doi: 10.1111/1365-2664.12960
European Commission (2022). Nature restoration law – Proposal for a Regulation of the European Parliament and of the Council on Nature Restoration (Brussels, Belgium: E. Commission). Available at: https://environment.ec.europa.eu/publications/nature-restoration-law_en.
European Council (1979). Bern convention - convention on the conservation of European wildlife and natural habitats. 10. (Bern, 19.9.1979: Council of Europe).
Fine M., Tsadok R., Meron D., Cohen S., Milazzo M. (2016). Environmental sensitivity of Neogoniolithon brassica-florida associated with vermetid reefs in the Mediterranean Sea. ICES J. Mar. Sci. 74 (4), 1074–1082. doi: 10.1093/icesjms/fsw167
Franzitta G., Capruzzi E., La Marca E. C., Milazzo M., Chemello R. (2016). Recruitment patterns in an intertidal species with low dispersal ability: the reef-building Dendropoma cristatum (Biondi 1859) (Mollusca: gastropoda). Ital. J. Zool. 83 (3), 400–407. doi: 10.1080/11250003.2016.1205152
Galil B. S. (2013). Going going gone: the loss of a reef building gastropod (Mollusca: caenogastropoda: Vermetidae) in the Southeast Mediterranean Sea. Zool. Middle East 59 (2), 179–182. doi: 10.1080/09397140.2013.810885
Gubbay S., Sanders N., Haynes T., Janssen J. A. M., Rodwell J. R., Nieto A., et al. (2016). European red list of habitats part 1 (Brussels, Belgium: Marine Habitats (European Union).
Guo H., Pennings S. C. (2012). Post-mortem ecosystem engineering by oysters creates habitat for a rare marsh plant. Oecologia 170, 789–798. doi: 10.1007/s00442-012-2356-2
Halpern B. S., Silliman B. R., Olden J. D., Bruno J. P., Bertness M. D. (2007). Incorporating positive interactions in aquatic restoration and conservation. Front. Ecol. Environ. 5, 153–160. doi: 10.1890/1540-9295(2007)5[153:IPIIAR]2.0.CO;2
Hendriks I. E., Olsen Y. S., Ramajo L., Basso L., Steckbauer A., Moore T. S., et al. (2014). Photosynthetic activity buffers ocean acidification in seagrass meadows. Biogeosci 11, 333–346. doi: 10.5194/bg-11-333-2014
Huggett M. J., McMahon K., Bernasconi R. (2018). Future warming and acidification result in multiple ecological impacts to a temperate coralline alga. Environ. Microbiol. 20, 2769–2782. doi: 10.1111/1462-2920.14113
Ingrosso G., Abbiati M., Badalamenti F., Bavestrello G., Belmonte G., Cannas R., et al. (2018). Mediterranean bioconstructions along the Italian coast. Adv. Mar. Biol. 79, 61–136. doi: 10.1016/bs.amb.2018.05.001
Irving A. D., Connell S. D. (2006). Physical disturbance by kelp abrades erect algae from the understorey. Mar. Ecol. Prog. Ser. 324, 127–137. doi: 10.3354/meps324127
La Marca E. C., Ape F., Martinez M., Rinaldi A., Montalto V., Scicchigno E., et al. (2022). Implementation of artificial substrates for Dendropoma cristatum (Biondi 1859) reef restoration: Testing different materials and topographic designs. Ecol. Eng. 183, 106765. doi: 10.1016/j.ecoleng.2022.106765
La Marca E. C., Catania V., Quatrini P., Milazzo M., Chemello R. (2018). Settlement performance of the Mediterranean reef-builders Dendropoma cristatum (Biondi 1859) in response to natural bacterial films. Mar. Environ. Res. 137, 149–157. doi: 10.1016/j.marenvres.2018.03.005
Lang A. C., Buschbaum C. (2010). Facilitative effects of introduced Pacific oysters on native macroalgae are limited by a secondary invader, the seaweed Sargassum muticum. J. Sea Res. 63, 119–128. doi: 10.1016/j.seares.2009.11.002
Lang B. J., Caballes C. F., Uthicke S., Doll P. C., Donelson M. J., Pratchett M. S. (2023). Impacts of ocean warming on the settlement success and post-settlement survival of Pacific crown-of-thorns starfish (Acanthaster cf. solaris). Coral Reefs 42, 143–155. doi: 10.1007/s00338-022-02314-y
Lang’at J. K. S., Kirui B. K., Skov M. W., Kairo J. G., Mencuccini M., Huxham M. (2013). Species mixing boosts root yield in mangrove trees. Oecologia 172, 271–278. doi: 10.1007/s00442-012-2490-x
Lathlean J. A., Ayre D. J., Minchinton T. E. (2013). Temperature variability at the larval scale affects early survival and growth of an intertidal barnacle. Mar. Ecol. Prog. Ser. 475, 155–166. doi: 10.3354/meps10105
Leonard G. H. (1999). Positive and negative effects of intertidal algal canopies on recruitment and survival of barnacles. Mar. Ecol. Prog. Ser. 178, 241–249. doi: 10.3354/meps178241
McAfee D., Bishop M. J. (2019). The mechanisms by which oysters facilitate invertebrates vary across environmental gradients. Oecologia 189, 1095–1106. doi: 10.1007/s00442-019-04359-3
McAfee D., Larkin C., Connell S. D. (2021). Multi-species restoration accelerates recovery of extinguished oyster reefs. J. Appl. Ecol. 58, 286–294. doi: 10.1111/1365-2664.13719
McAfee D., Reis-Santos P., Jones A. R., Gillanders B. M., Mellin C., Nagelkerken I., et al. (2022). Multi-habitat seascape restoration: optimising marine restoration for coastal repair and social benefit. Front. Mar. Sci. 9. doi: 10.3389/fmars.2022.910467
McArdle B. H., Anderson M. J. (2001). Fitting multivariate models to community data: a comment on distancebased redundancy analysis. Ecology 82, 290–297. doi: 10.1890/0012-9658(2001)082[0290:FMMTCD]2.0.CO;2
Meyers N., Mittermeier R. A., Mittermeier C. G., da Fonseca G. A. B., Kent J. (2000). Biodiversity hotspots for conservation priorities. Nature 403, 853–858. doi: 10.1038/35002501
Milazzo M., Alessi C., Quattrocchi F., Chemello R., D’Agostaro R., Gil J., et al. (2019). Biogenic habitat shifts under long-term ocean acidification show nonlinear community responses and unbalanced functions of associated invertebrates. Sci. Total Environ. 667, 41–48. doi: 10.1016/j.scitotenv.2019.02.391
Milazzo M., Fine M., La Marca E. C., Alessi C., Chemello R. (2016). “Drawing the line at neglected marine ecosystems: ecology of vermetid reefs in a changing ocean,” in Marine animal forests, the ecology of benthic biodiversity hotspots. Eds. Rossi S., Bramanti L., Gori A., Orejas Saco del Valle C. (Springer International Publishing), 345–367. doi: 10.1007/978-3-319-17001-5_9-1
Milazzo M., Rodolfo-Metalpa R., Bin San Chan V., Fine M., Alessi C., Thiyagarajan V., et al. (2014). Ocean acidification impairs vermetid reef recruitment. Sci. Rep. 4, 4189. doi: 10.1038/srep04189
Moreno C. A. (1995). Macroalgae as a refuge from predation for recruits of the mussel Choromytilus chorus (Molina 1782) in southern Chile. J. Exp. Mar. Biol. Ecol. 191 (2), 181–193. doi: 10.1016/0022-0981(95)00050-2
Newell R. I., Koch E. W. (2004). Modeling seagrass density and distribution in response to changes in turbidity stemming from bivalve filtration and seagrass sediment stabilization. Estuaries 27, 793–806. doi: 10.1007/BF02912041
O’Brien J. M., Scheibling R. E. (2018). Turf wars: Competition between foundation and turf-forming species on temperate and tropical reefs and its role in regime shifts. Mar. Ecol. Prog. Ser. 590, 1–7. doi: 10.3354/meps12530
Ørberg S. B., Krause-Jensen D., Mouritsen K. N., Olesen B., Marbà N., Larsen M. H., et al. (2018). Canopy-forming macroalgae facilitate recolonization of sub-arctic intertidal fauna and reduce temperature extremes. Front. Mar. Sci. 5. doi: 10.3389/fmars.2018.00332
Renzi J. J., He Q., Silliman B. R. (2019). Harnessing positive species interactions to enhance coastal wetland restoration. Front. Ecol. Evol. 7. doi: 10.3389/fevo.2019.00131
Reusch T. B., Chapman A. R., Gröger J. P. (1994). Blue mussels Mytilus edulis do not interfere with eelgrass Zostera marina but fertilize shoot growth through biodeposition. Mar. Ecol. Prog. Ser. 108, 265–282. doi: 10.3354/MEPS108265
Rilov G., Peleg O., Guy-Haim T., Yeruham E. (2020). Community dynamics and ecological shifts on Mediterranean vermetid reefs. Mar. Environ. Res. 160, 105045. doi: 10.1016/j.marenvres.2020.105045
Sarà G., Milisenda G., Mangano M. C., Bosch-Belmar M. (2021). The buffer effect of canopy-forming algae on vermetid reefs’ functioning: A multiple stressor case study. Mar. pollut. Bull. 171, 112713. doi: 10.1016/j.marpolbul.2021.112713
Setchell W. A., Mason L. R. (1943). Goniolithon and Neogoniolithon: two genera of crustaceous coralline algae. Proceedings of the National Academy of Science of the United States of America 29, 87–92.
Shelamoff V., Layton C., Tatsumi M., Cameron M. J., Wright J. T., Johnson C. R. (2019). Ecosystem engineering by a canopy-forming kelp facilitates the recruitment of native oysters. Restor. Ecol. 27, 1442–1451. doi: 10.1111/rec.13019
Somero G. N. (2010). The physiology of climate change: how potentials for acclimatization and genetic adaptation will determine ‘winners’ and ‘losers’. J. Exp. Biol. 213 (6), 912–920. doi: 10.1242/jeb.037473
SPA/BD (1995). The Barcelona convention: protocol concerning specially protected areas and biological diversity in the Mediterranean (Brussels, Belgium: European Commission), 47.
Spotorno-Oliveira P., Figueiredo M. A. O., Tâmega F. T. S. (2015). Coralline algae enhance the settlement of the vermetid gastropod Dendropoma irregulare (d’Orbigny 1842) in the southwestern Atlantic. J. Exp. Mar. Biol. Ecol. 471, 137–145. doi: 10.1016/j.jembe.2015.05.021
Storch D., Fernández M., Navarrete S. A., Pörtner H. O. (2011). Thermal tolerance of larval stages of the Chilean kelp crab Taliepus dentatus. Mar. Ecol. Prog. Ser. 429, 157–167. doi: 10.3354/meps09059
Thomsen M. S., Altieri A. H., Angelini C., Bishop M. J., Gribben P. E., Lear G., et al. (2018). Secondary foundation species enhance biodiversity. Nat. Ecol. Evol. 2, 634–639. doi: 10.1038/s41559-018-0487-5
Umanzor S., Ladah L., Calderon-Aguilera L. E., Zertuche-González J. A. (2017). Intertidal macroalgae influence macroinvertebrate distribution across stress scenarios. Mar. Ecol. Prog. Ser. 584, 67–77. doi: 10.3354/meps12355
Wahl M., Schneider Covachã S., Saderne V., Hiebenthal C., Müller J. D., Pansch C., et al. (2018). Macroalgae may mitigate ocean acidification effects on mussel calcification by increasing pH and its fluctuations. Limnol. Oceanogr. 63, 3–21. doi: 10.1002/lno.10608
Waltham N. J., Elliott M., Lee S. Y., Lovelock C., Duarte C. M., Buelow C., et al. (2020). UN decade on ecosystem restoration 2021–2030—What chance for success in restoring coastal ecosystems? Front. Mar. Sci. 7. doi: 10.3389/fmars.2020.00071
Ware C., Dijkstra J. A., Mello K., Stevens A., O’Brien B., Ikedo W. (2019). A novel three dimensional analysis of functional-architecture that describes the properties of macroalgae as a refuge. Mar. Ecol. Prog. Ser. 608, 93–103. doi: 10.3354/MEPS12800
Watt C. A., Scrosati R. A. (2013a). Bioengineer effects on understory species richness, diversity, and composition change along an environmental stress gradient: experimental and mensurative evidence. Estuar. Coast. Shelf Sci. 123, 10–18. doi: 10.1016/j.ecss.2013.02.006
Watt C. A., Scrosati R. A. (2013b). Regional consistency of intertidal elevation as a mediator of seaweed canopy effects on benthic species richness, diversity, and composition. Mar. Ecol. Prog. Ser. 491, 91–99. doi: 10.3354/meps10521
Keywords: foundation species, macroalgal canopy, biogenic reefs, settlement, restoration ecology, eco-engineering, Mediterranean Sea
Citation: La Marca EC, Ape F, Rinaldi A, Spoto M, Martinez M, Dini E, Mirto S and Montalto V (2024) Canopy-forming algae improve the colonization success of the vermetid reef-builder Dendropoma cristatum (Biondi 1859) on artificial substrates. Front. Mar. Sci. 10:1214252. doi: 10.3389/fmars.2023.1214252
Received: 29 April 2023; Accepted: 29 November 2023;
Published: 15 January 2024.
Edited by:
Heliana Teixeira, University of Aveiro, PortugalReviewed by:
Augusto Flores, University of São Paulo, BrazilEdson A. Vieira, Federal University of Rio Grande do Norte, Brazil
Mats Westerbom, Natural Resources Institute Finland (Luke), Finland
Camilla Bertolini, Ca’ Foscari University of Venice, Italy
Copyright © 2024 La Marca, Ape, Rinaldi, Spoto, Martinez, Dini, Mirto and Montalto. This is an open-access article distributed under the terms of the Creative Commons Attribution License (CC BY). The use, distribution or reproduction in other forums is permitted, provided the original author(s) and the copyright owner(s) are credited and that the original publication in this journal is cited, in accordance with accepted academic practice. No use, distribution or reproduction is permitted which does not comply with these terms.
*Correspondence: Emanuela Claudia La Marca, claudia.lamarca@ias.cnr.it; emanuelaclaudia.lamarca@cnr.it