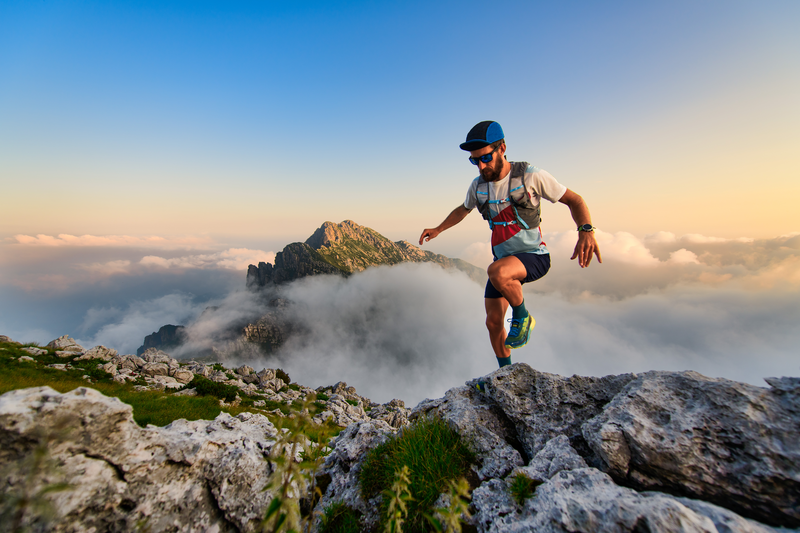
94% of researchers rate our articles as excellent or good
Learn more about the work of our research integrity team to safeguard the quality of each article we publish.
Find out more
ORIGINAL RESEARCH article
Front. Mar. Sci. , 26 September 2023
Sec. Marine Ecosystem Ecology
Volume 10 - 2023 | https://doi.org/10.3389/fmars.2023.1213184
This article is part of the Research Topic Restoration of Coastal Marine Ecosystems View all 22 articles
Introduction: Active restoration is the strategy needed to trigger or accelerate the recovery of degraded marine habitats, which provide the goods and services essential for preserving biodiversity and human wellbeing. Ecosystem engineers are generally the target species in the ecological restoration of marine ecosystems, and large-sized brown macroalgae (e.g., the Cystoseira complex) are a priority due to their crucial ecological importance and vulnerability in many regions of the Mediterranean Sea.
Methods: Here, we present the results of a successful intervention of ecological restoration of Gongolaria barbata.
Results and Discussion: One year after the restoration intervention, we observed the recovery of the canopy in terms of ca. 15m2 with a significant increase in faunal abundance and biodiversity compared with reference areas. However, despite the high restored macroalgal growth rates, the assemblage structure was still significantly different from that of reference pristine areas. Despite the fast faunal colonization of a new 3D habitat linked to the successful re-introduction of the ecosystem engineers (i.e., macroalgae), the complete restoration of the properties of a habitat can be a much longer process. We conclude that, after 1 year, active restoration of macroalgal forests still results in a partial recovery of ecosystem functions (i.e., rehabilitation) rather than a full ecosystem restoration, thus stressing the need for long-term monitoring of restoration interventions.
Coastal marine ecosystems provide crucially important ecosystem goods and services but are, at the same time, the most impacted by anthropogenic activities and episodic events (e.g., heatwaves) linked to global changes (Micheli et al., 2013; Halpern et al., 2015; Garrabou et al., 2022). Among the threats affecting coastal habitat, physical destruction and degradation of environmental quality (due to increased water turbidity and/or pollution) are the most serious direct causes of the loss of macroalgal forests and their biodiversity (Crain et al., 2009; Orfanidis et al., 2021). Despite the countermeasures taken, from the mitigation of human pressures to the implementation of conservation measures, the recovery of coastal ecosystems is rare and slow, even when the proximate drivers of loss are removed (Lotze et al., 2011; Colletti et al., 2020; Sala et al., 2021).
In this scenario, ecological restoration is increasingly acknowledged as the most convenient (or unique) strategy to actively trigger or accelerate the recovery of degraded coastal habitats (Abelson et al., 2020). This has been recently recognized internationally by the declaration of the UN Decade on Ecosystem Restoration (2021–2030). Although marine ecosystem restoration is still at a pioneer stage (with the unique exception of coral reef restoration) when compared to terrestrial ecosystems, significant signs of progress have been made recently for several coastal habitats, including seagrasses, saltmarshes, oyster reefs, mangroves, and kelp forests (Bayraktarov et al., 2016). However, to make restoration interventions successful and affordable at large spatial scales, a detailed census and mapping of the habitat loss, the distribution of the potential donor populations, and the identification of the stressors/impacts that caused their decline or loss are needed (Gann et al., 2019).
Ecosystem engineers are the most common target species of marine ecosystem restoration, as these species are best suited to create structural habitat features (Byers et al., 2006). These species have proven to be efficient in reversing species’ local declines (including those of commercial interest) and recovering ecosystem functions. However, recent investigations showed that only a minority of the restored marine species are considered of conservation interest (Swan et al., 2016). Moreover, baseline knowledge is generally very limited and insufficient to plan cost-effective restoration interventions (Bayraktarov et al., 2016). Macroalgal forests formed by fucalean algae (Cystoseira sensu lato, including the genera Cystoseira, Ericaria, and Gongolaria; Molinari-Novoa and Guiry, 2020) are critical habitats of the upper infralittoral of the Mediterranean Sea (Sala et al., 2012) and represent an excellent case study in terms of restoration interventions for their fragility and vulnerability to local and climate change impacts.
Cystoseira s.l. forests indeed host highly diverse communities, providing food and shelter for associated organisms and representing nursery habitats for fish assemblages of commercial interest (Cheminée et al., 2013; Cheminée et al., 2017) and many other benthic organisms (Bianchelli et al., 2016; Mancuso et al., 2021). Their presence enhances coastal primary productivity, and their role in maintaining high levels of biodiversity and the ecological functioning of rocky habitats has long been recognized. Consequently, several species (Ericaria sedoides Neiva & Serrão, E. amentacea (C. Agardh) Molinari & Guiry, E. mediterranea (Sauvageau) Molinari & Guiry, E. zosteroides (C. Agardh) Molinari & Guiry, and G. montagnei (J. Agardh) Kuntze) have been protected since 1982, with the enforcement of the Bern Convention (1979). In 2009, an amendment of the Mediterranean Action Plan (Annex IV, SPA/BD Protocol—United Nations Environment Programme) adopted within the framework of the Barcelona Convention (1976) identified the conservation of all species of the Cystoseira genus, with the only exception of C. compressa (Esper) Gerloff & Nizamuddin. Despite the robust legislative framework, specific conservation measures for the protection of these habitat-forming species have never been implemented (Fraschetti et al., 2011). For example, the selection of marine sites deserving protection under the Natura 2000 Sites network in the Mediterranean is generally based on the presence of Posidonia oceanica (Linnaeus) Delile meadows, while the presence of Cystoseira s.l. is only incidental, as these species are not listed in the Habitat Directive annexes (Directive 92/43 EEC) but only in the habitat that they colonize (1170 “Reefs”).
Species of Cystoseira s.l. are regressing in many areas of the Mediterranean Sea (Thibaut et al., 2005; Thibaut et al., 2014; Iveša et al., 2016; Tamburello et al., 2022), and their natural recovery has rarely been observed (Perkol-Finkel and Airoldi, 2010; Blanfuné et al., 2016). Restoration has been proposed as a promising approach to halting their decline (Gianni et al., 2013), and several European projects (e.g., MERCES (http://www.merces-project.eu/) and AFRIMED (http://afrimed-project.eu/)) developed and tested new techniques to reintroduce Cystoseira s.l. after local extinction, regenerating self-sustaining populations (Verdura et al., 2018; Gran et al., 2022). A roadmap for a successful restoration of Mediterranean macroalgal forests was recently proposed to assist researchers and stakeholders in decision-making, considering the most effective methods, also in terms of cost and cost-effectiveness, starting from the selection of donor and restoration sites, target species, and techniques to the adoption of complementary actions (e.g., provisioning of supplementary substrates or herbivory management; Cebrian et al., 2021).
However, a standardized definition of success for Cystoseira s.l. restoration is still lacking and repeatedly claimed by the scientific community (Fraschetti et al., 2021). Following the existing roadmap (Cebrian et al., 2021), we carried out a study to select the most suitable sites for restoration intervention of Gongolaria barbata (Stackhouse) Kuntze along the Conero Riviera (Western-Central Adriatic Sea). We also compared two techniques of restoration based on in situ and ex situ recruitment. The success was measured by monitoring not only the survival of the restored populations but also the biodiversity recovery of the associated meio- and macrofauna.
In this study, we tested the hypothesis that different environmental and anthropogenic impact conditions affect the success of G. barbata transplants and that different methodologies (i.e., recruitment approach and substrate typology) applied in a restoration intervention affect its success, in terms of algal growth and associated diversity.
This study was carried out along the Conero Riviera (Central-Western Adriatic, Mediterranean Sea), characterized by a cliff forming a 15-km-long rocky shore, south of Ancona City (ca. 100,000 citizens). The area is located within two Sites of Community Importance (SCI IT5230005 “Coast between Ancona and Portonovo” and IT5320006 “Portonovo e falesia calcarea a mare”), included in the “Monte Conero” Special Protection Area (SPA IT5230007, sensu Bird Directive 79/409/EEC, 2009/147/EC), in the Natura 2000 Network. According to Habitat Directive 92/43/EEC, the area hosts at sea 1110 “sandbanks, which are slightly covered by sea water all the time”, 1160 “large shallow inlets and bays”, and 1170 “reefs” habitats.
Gongolaria barbata is distributed in the Mediterranean Sea and on the coasts of Atlantic regions close to the Mediterranean (Guiry and Guiry, 2021). In the Adriatic Sea, it is common in areas characterized by harsh seabed, such as the lagoon of Venice or along the Conero Riviera, where it can form real forests and oases of biodiversity, promoting spatial heterogeneity of the coastal rocky seabed, increasing the number of habitats, and producing three-dimensional complexity. Its distribution, which is usually in distinct patches measuring from 1 m to 10 m in diameter, is limited to the first meters of depth of the infra-coastal zone. The species prefers sheltered areas characterized by reduced hydrodynamism, a low rate of sedimentation, and intense irradiance. These factors are crucial to ensuring reproductive success and the settlement of zygotes (Irving et al., 2009). Moreover, G. barbata is one of a few Cystoseira s.l. species that are able to grow in eutrophicated bays and coastal lagoons, e.g., Venice lagoon (Iveša et al., 2022). In addition, the basal disc that adheres to the substrate can be easily detached during heavy storms, especially in juvenile thalli.
Along the coast, four G. barbata populations were identified at four sites: Piscinetta Passetto and Scalaccia rock pools, Sassi Neri, and Numana. Among these, Piscinetta Passetto and Scalaccia North were selected as donor sites for the purposes of this study. Both are natural inlets and are partially sheltered from emerging rocks; Piscinetta Passetto is located in the urban area of Ancona, whereas Scalaccia North is less accessible. Other sites were selected as possible receiving sites for transplants and further restoration interventions (Figure 1): Falconara, Marina Dorica, Grotta Azzurra, Scalaccia South (outside rock pools), and Vela Portonovo. These sites were selected as characterized by putatively different typology along a gradient of anthropogenic pressures: Falconara is located within a site of national interest (SNI), naturally recovered after historical pollution and hosts artificial breakwaters (Corinaldesi et al., 2022); Marina Dorica hosts a touristic harbor (Piva et al., 2011); Grotta Azzurra is an “urban beach”, characterized by the presence of artificial structures and subjected to intensive summer tourism and sewage discharge; moreover, it is located in short distance (approximately 1 km) of the Ancona commercial harbor (Pennesi and Danovaro, 2017). Scalaccia South site is a wave-exposed and less accessible site, reachable only via a small path or by boat, and Vela Portonovo is a highly wave-exposed site, subjected to seasonal touristic pressure (Perkol-Finkel and Airoldi, 2010). At Grotta Azzurra, Scalaccia South, and Vela Portonovo the historical presence of a few scattered individuals of G. barbata was previously reported (Perkol-Finkel and Airoldi, 2010).
Figure 1 Study area along the Conero Riviera (Central-Western Adriatic Sea). Dark dots are donor sites: Piscinetta Passetto and Scalaccia N rock pools. Light dots are receiving sites: site of national interest (Falconara), touristic harbor (Marina Dorica), city center (Grotta Azzurra), exposed-remote site (Scalaccia S), and exposed-touristic site (Vela Portonovo). The map was generated using Map data ©2019 Google.
In the summer of 2020, natural pebbles (ca. 20–30 cm diameter) with juvenile thalli of G. barbata (1–4 cm height) were collected from two donor sites (two rock inlets, Piscinetta Passetto and Scalaccia North) by snorkeling at depths between 0.5 m and 1.5 m. This approach exploits the natural in situ recruitment of G. barbata and assumes that pebbles of this size would be easily moved by autumn-winter sea storms, which would cause their overturning and rolling, thus causing the death of many young thalli recruited (Perkol-Finkel et al., 2012; Strain et al., 2015). Three to five pebbles were fixed with epoxy putty (Perkol-Finkel et al., 2012) inside the two donor sites (Piscinetta and Scalaccia N), whereas others were transplanted in receiving sites (Falconara, Marina Dorica, Grotta Azzurra, Scalaccia South, and Vela Portonovo). This allowed us to compare the algal growth in the receiving sites with that occurring in suitable habitats (already hosting donor populations). The receiving sites were selected as being putatively characterized by different anthropogenic impacts: a site of national interest (SNI, Falconara), a touristic harbor (Marina Dorica), a site near the city center characterized by pressure from local users (Grotta Azzurra), an exposed-remote site, and an exposed-remote site (Scalaccia South), site characterized by touristic pressure (Vela Portonovo). After transplantation was carried out in June 2020, all sites were monitored monthly to assess the growth of algal juvenile thalli and the response of the associated meio- and macrofaunal benthic assemblages. At Falconara and Marina Dorica, all algae on pebbles disappeared after 48 hours from the transplant, probably due to grazing pressure, so they were excluded from monitoring and analyses.
After selecting a suitable site, we compared in situ vs. ex situ restoration techniques. The approach based on in situ recruitment foresaw the use of (i) natural pebbles and (ii) round clay tiles (7 cm in diameter and 1.5 cm thick, with a rough surface) fixed on artificial structures consisting of aluminum bars 50 cm long positioned beside the canopies of donor populations during the reproductive period at Piscinetta Passetto and Scalaccia North donor sites. Ex situ recruitment was obtained by using the same artificial structures and the application of the modified protocol reported in Verdura et al. (2018). Two 190-L tanks were prepared in the aquaria facility, each filled with 150 L of synthetic water enriched with 450 mL/L of Von Stosch solution and equipped with Askoll Fluval pumps sp2 (3,600 L/h). Four artificial structures of 19 tiles were distributed between the two tanks. Each tank was equipped with LED lights (GNC Silver Moon Marine), a timer to reproduce the 14 h L:10 h D photoperiod, and Teko tk 2000 and chillers were used to avoid temperature variations. During the whole experiment, salinity (up to 35 psu), temperature (initially set to 16°C), light intensity, and exposure time have been monitored and regulated. Receptacles were collected from donor sites (Piscinetta del Passetto and Scalaccia North) on 10th, and 20th–28th May 2021 (for tanks 1 and 2, respectively), after assessing the degree of apical frond maturation. After a thermal shock, receptacles were inserted in mesh pockets, suspended over the structures, and removed after a week. In both tanks, the temperature was raised to 18°C in early June.
On 1 June 2021, all the recruits obtained in the field and in the laboratory were transplanted to the selected site for restoration over an area of ca. 15 m2. The pebbles were fixed with epoxy putty, and the artificial structures (used for both in situ and ex situ recruitment procedures) were anchored to the seabed by self-tapping dowels (Fischer), screwed with the use of a Nemo V2 HD underwater drill. The monitoring of all variables was conducted monthly, from June 2021 to June 2022. We used the adult donor populations living in the Piscinetta and Scalaccia N as reference sites.
The growth of G. barbata after in situ and ex situ recruitment was monitored by assessing the height of the algal thalli, the percentage of surface area covered by the canopy, and the average number of individuals (the latter only for ex situ treatment, during the recruitment phase at a laboratory with a stereomicroscope). On each sampling date, three height measurements were taken for each stone or tile (nine to 12 replicates per sampling site and time). The canopy was expressed as a cover percentage on a standard surface (50 cm × 50 cm frame quadrat).
During the selection of sites and the restoration intervention (where both in situ and ex situ recruitment techniques were applied), faunal samples were collected by means of a modified manual corer (inner diameter, 9 cm), allowing the scratching of the rocky substrate (Danovaro and Fraschetti, 2002). For each sampling site and time, three replicates were collected for the analysis of meio- and macrofaunal variables. Once in the lab, samples were immediately sieved through meshes of 20 µm and 500 µm to separate meio- and macrofaunal organisms. Meiofauna was extracted by centrifugation in a density gradient from a solution of Ludox HS40 (Heip et al., 1985; Danovaro, 2010). Both meio- and macrofaunal samples were then analyzed in terms of abundance and identified at a higher taxonomic level to assess the richness of taxa and the taxonomic composition.
The datasets on algae growth, meio- and macrofaunal abundance, and richness of taxa regarding the selection of suitable sites for restoration are reported in Supplementary Tables S1, S2, respectively. We applied an experimental design with two factors: condition (fixed, five levels: donor Piscinetta, donor Scalaccia N, city center, exposed-remote, and exposed-touristic) and time (fixed, four to 10 levels for G. barbata height, four to six levels for meio- and macrofauna variables, depending on the condition, see Supplementary Tables S1, S2, respectively, for details).
For the restoration intervention, to compare reference and restored populations, we applied an experimental design with two factors: population (fixed, three levels: donor Piscinetta, donor Scalaccia N, and restored) and time (fixed, five levels, for donor populations and the restored one, respectively).
For the restored G. barbata population, it was impossible to compare the two recruitment approaches (ex situ vs. in situ) since the algae recruited with the ex situ technique disappeared completely after 1 month. In this case, we could compare only the substrate typology used for the in situ recruitment (natural pebbles vs. artificial clay structure). To do this, we applied an experimental design with two factors: substrate (fixed, two levels: natural pebbles and artificial structure) and time (fixed, 12 levels for G. barbata).
For benthic diversity assemblages, we compared abundance, diversity, and taxonomic composition under the growing algae (recruited with the in situ approach) and under the structures where the algae did not grow (as a reference). To do this, we applied an experimental design considering two factors: algal growth (fixed, two levels: growth G+, no growth G−) and time (fixed, five levels).
The experimental designs were applied to permutational analyses of variance (PERMANOVA), in a univariate (for algal heights, total meio- and macrofaunal abundance) and multivariate context (for taxonomic composition of the meio- and macrofaunal assemblages) and based on Euclidean distance and Bray–Curtis similarity matrices, respectively. When significant differences were observed, pair-wise tests were performed to establish between which levels significant differences were present. To visualize differences in the taxonomic composition, multi-dimensional scaling (MDS) or resemblance-based discriminant analysis via canonical analysis of principal coordinates (CAP) biplots were also produced. Similarity percentage (SIMPER) tests were also performed to quantify the dissimilarity between the donor and the restored site in the meio- and macrofaunal taxonomic composition. To assess the temporal variability after the restoration intervention in meio- and macrofaunal taxonomic composition, we analyze the component of variation across monitoring times after multivariate PERMANOVA tests. PERDISP tests were also applied to ascertain the homogeneity of data distribution for both experiments in the investigated variables (results are reported in Supplementary Table S3). All statistical analyses were carried out using the PRIMER7 software package (Clarke and Gorley, 2015).
Patterns of algal growth in the donor and receiving sites are shown in Figure 2, and data are reported in Supplementary Table S1. Among transplanted juveniles in different conditions, similar heights were observed from T0 to T3 (June to September 2020), whereas higher heights were observed in the exposed-remote or donor condition (Scalaccia South and Piscinetta) from T7 to T12 (January to July 2021; PERMANOVA, p < 0.05). In the city center (Grotta Azzurra), the lowest values were observed. For each condition, differences were observed across time (i.e., from May 2020 to July 2021; PERMANOVA, p < 0.05). In the exposed-touristic condition (Vela), the algae grew during the first 3 months (until September 2020), but then the transplanted algae were detached by a strong storm occurring at the beginning of October 2020.
Figure 2 G. barbata growth on natural pebbles transplanted in the donor (Piscinetta) and in different tested conditions. T0 = June 2020. Reported are data as average ± standard error (Avg ± SE).
The total meiofaunal abundance and richness of taxa are reported in Figures 3A, B. In exposed-remote conditions (Scalaccia South), the highest values of both variables were observed (PERMANOVA, p < 0.05). In each condition, temporal variation of both variables was observed (PERMANOVA, p < 0.05). One of the donor sites (Piscinetta Passetto), the exposed-remote condition (Scalaccia South), and the exposed-touristic condition (Vela) showed an increasing pattern of total abundance across time (PERMANOVA, p < 0.05) and an increasing pattern of richness of taxa in the first 3 months after the transplants (from June to August 2020). In the second donor site and in the city center (Scalaccia North and Grotta Azzurra), no clear patterns were observed.
Figure 3 Total meiofaunal abundance (A), richness of taxa (B), and MDS show the differences in the taxonomic composition over time (C) in the different tested conditions and donor sites. In (A), reported are data as average ± standard deviation (Avg ± SD). In (C), the arrows indicate the temporal variation from T0 to the final T in each condition; stress = 0.16. T0 = June 2020.
The MDS plots showed that, among the tested conditions, in the exposed-remote one (Scalaccia South), the temporal changes in the taxonomic composition of meiofaunal assemblages were the closest to the donor sites (Figure 3C).
Total macrofaunal abundance and richness of higher taxa are shown in Figures 4A, B. For both variables, the highest values were observed in the exposed-remote condition (Scalaccia South) (PERMANOVA, p < 0.05). In the two donor sites (Piscinetta and Scalaccia North), an increasing pattern and a decrease in the last sampling time were observed. In the city center (Grotta Azzurra), exposed-remote condition (Scalaccia South), and exposed-touristic condition (Vela), no significant pattern was observed.
Figure 4 Total macrofaunal abundance (A), richness of taxa (B), and MDS show the differences in the taxonomic composition over time (C) in the putative sites for restoration and donor sites. In (A), reported are data as average ± standard deviation (Avg ± SD). In (C), the arrows indicate the temporal variation from T0 to the final T in each condition; stress = 0.14. T0 = June 2020.
The MDS plots showed that, among the tested conditions, in the exposed-remote one (Scalaccia South) the taxonomic composition and the end of the experiment were the most similar to the donor sites (Figure 4C).
According to the data resulting from the selection of the site, the exposed and remote conditions (corresponding to the Scalaccia South site) were the most suitable for the restoration intervention (over an area of ca. 15 m2). The algal growth at this receiving site is reported in Figure 5A. The results indicated a significant growth over time of algae recruited with the in situ approach, up to 32.6 cm ± 2.1 cm and 30.5 cm ± 6.8 cm, respectively, on natural pebbles and artificial structures (PERMANOVA, p < 0.05). No significant differences were observed in the growth of algae recruited on natural pebbles and artificial structures in the field (PERMANOVA, p = ns). Conversely, the algae recruited with the ex situ approach significantly grew in the first month after transplants (PERMANOVA, p < 0.05) but then completely disappeared. The algal cover due to the algal growth constantly increased over time (Figure 5A).
Figure 5 G. barbata growth on natural pebbles, artificial structure with in situ and ex situ recruitment and % cover, in selected site for restoration, Scalaccia South (A) and G. barbata adults’ heights in the reference ecosystems (Piscinetta and Scalaccia North donor sites) (B). Reported are data as average ± standard error (Avg ± SE).
In the same period, in the reference ecosystem (donor populations), algae adults’ heights ranged from 40.8 cm ± 14.2 cm to 69.7 cm ± 13.5 cm, at Piscinetta, and from 29.4 cm ± 11.1 cm to 51.5 cm ± 10.1 cm at Scalaccia N sites (Figure 5B). The observed temporal pattern followed the seasonal changes of G. barbata individuals, with the highest values in late winter–spring and the lowest in late summer.
Regarding the benthic assemblages, meiofaunal abundance and richness of taxa in the restored site and in the reference ecosystem (donor sites, under G. barbata adult individuals) are reported in Figures 6A–D. In the restored site, meiofaunal abundance increased over time. At the end of the experiment, it resulted higher where G. barbata grew than where G. barbata did not grow, and in both cases, even higher than that observed in the donor sites (PERMANOVA, p < 0.05). At the end of the experiment, the richness of taxa was similar where G. barbata grew and did not grow (15 and 16, respectively), with similar values observed in the donor sites (14 taxa at Scalaccia N donor site).
Figure 6 Total meiofaunal abundance (A, B), richness of taxa (C, D), and CAP output show the difference in the taxonomic composition (E) in the restored site and reference ecosystems. Beg, beginning of the experiment, when algae canopy is not yet present; G−, G. barbata not growing; G+, G. barbata growing. T0 = June 2021. Reported are data as average ± standard deviation (Avg ± SD).
The analyses on meiofaunal taxonomic composition (Figure 6E) revealed significant differences between the donor and the restored sites 12 months after the transplant (PERMANOVA, p < 0.01). No significant differences were observed between sites where G. barbata grew and did not grow. The SIMPER indicated that the overall dissimilarity between donor and restored sites was about 55%. This dissimilarity was due to the exclusive presence of Priapulida and their larvae, as well as crustaceans’ larvae, in the donor site, whereas Tardigrada and Rotifera were in the restored site. Moreover, several taxa, comprising those belonging to temporary meiofauna (i.e., Polychatea, Oligochaeta, Isopoda, Cumacea, and Gasteropoda) were represented in higher percentages in the donor site than in the restored one. Other taxa (such as Copepoda, Amphipoda, Bivalvia, Ostracoda, Acarina, Tanaidacea, Hydrozoa, and Turbellaria) showed the opposite pattern.
Macrofaunal abundance and richness of taxa in the restored site and in the reference ecosystem (donor sites, under G. barbata adult individuals) are reported in Figures 7A–D. Macrofaunal abundance and richness of taxa showed similar patterns to those observed for meiofaunal assemblages, showing high temporal variability and an overall increase over time. Thus, 12 months after the transplant, the richness of macrofaunal taxa was similar between donor and restored sites, where G. barbata grew (16 and 15 taxa, respectively), whereas it was lower where G. barbata did not grow (12 taxa).
Figure 7 Total macrofaunal abundance (A, B), richness of taxa (C, D), and CAP output show the difference in the taxonomic composition (E) in the restored site and reference ecosystems. Beg, beginning of the experiment, when algae canopy is not yet evident; G−, G. barbata not growing; G+, G. barbata growing. T0 = June 2021. Reported are data as average ± standard deviation (Avg ± SD).
The analyses on macrofaunal taxonomic composition revealed significant temporal variation only in the restored site (PERMANOVA, p < 0.05), both where G. barbata grew and where did not. The same analysis revealed significant differences between donor and restored sites (Figure 7E). Moreover, no significant differences were observed between where G. barbata grew and did not grow. The SIMPER indicated that the overall dissimilarity between the donor and the restored site was 73.3%.
This dissimilarity was due to the exclusive presence of Caprellidae, Cladocera, Decapoda, Hydrozoa, Heterobranchia, Pycnogonida, and Polyplacophora in the restored site, while Echinoidea, Crustacea larvae, Ostracoda, and Pycnogonida were present only in the donor site.
The analyses of the components of variation across monitoring times after multivariate PERMANOVA test revealed high levels of temporal variability for both meio- and macrofauna. This variability was higher in the restored site than in the donor sites for both benthic components (Figure 8).
Figure 8 Component of variation across monitoring times (after multivariate PERMANOVA), indicating the temporal variation in the taxonomic composition of meio- and macrofaunal assemblages in restored and reference (donor) sites. Reported are data as average ± standard deviation (Avg ± SD).
In the last few years, the results of marine ecological restoration interventions have indicated that “where” the restoration activity is undertaken is of greater relevance to a successful outcome than “how” (method) the restoration is carried out (Fraschetti et al., 2021). The results of the present study confirm that this is particularly true for the restoration of G. barbata. Our preliminary experiment indeed allowed us to identify the most suitable conditions and the specific site for restoration intervention, considering that this area did not show evident signs of natural recovery after a significant loss of macroalgal canopy. Scientific literature (Perkol-Finkel and Airoldi, 2010) reports that in the 1940s, the canopy forests of fucoid algae in the area were highly diversified, including seven species of Cystoseira s.l. Only four species still existed in the 1960s, and since the 1990s, only two species (G. barbata and C. compressa) have been found. In early 2006, canopy forests were observed only at two localities along the Conero Riviera; therefore, canopies of Cystoseira should have been lost rapidly between 2002 and 2005 (Perkol-Finkel and Airoldi, 2010).
Our results indicate the importance of planning exploratory analysis to prioritize sites to be restored, which is necessary to guarantee the success of the G. barbata transplants. This analysis can be critical even at a very small spatial scale (15-km-long rocky coasts, such as the Conero Riviera) along a stretch of coast showing coexisting natural and anthropogenic pressures.
Specifically, the most suitable site for the restoration implementation was characterized by the lowest putative anthropogenic pressure, even if exposed to high hydrodinamisms (Scalaccia South). We observed the persistent survival of the transplanted individuals over the entire duration of the monitoring postintervention, the highest values of algal growth (both in terms of individual heights and canopy cover), coupled with the highest level of biodiversity of the associated meio- and macrobenthic assemblages (in terms of abundance and richness of taxa). The values observed at Scalaccia South, in terms of algal growth and biodiversity levels, were similar to those obtained when the transplants of juveniles were performed in the donor sites, assuming that these present the most suitable habitat for G. barbata since they host healthy populations of adults.
We compared the feasibility and success of transplants of juveniles obtained with in situ and ex situ recruitment/cultivation techniques, avoiding any impact on adult individuals from the donor populations (Perkol-Finkel and Airoldi, 2010; Verdura et al., 2018). Contrary to previous studies (Verdura et al., 2018), in our case, the transplants of juveniles obtained with ex situ recruitment failed due to the lack of survival of the recruits 1 month after the transplant. This could be related to the small size of the juveniles (a few millimeters in height), which were possibly completely covered by the high sediment loads or by blooms of microphytobenthos, typically occurring in summertime (and indeed documented by the Regional Agency for the Environmental Protection) in the study area (e.g., the toxic dinoflagellate Ostreopsis ovata; Totti et al., 2010). Also, the high temperatures could have impaired the growth of small thalli; in this regard, it has been demonstrated that 2-month-old thalli can survive up to 29°C, whereas older thalli can survive at 30°C–31°C (Orfanidis, 1991).
We report here that the transplants of juveniles obtained with in situ recruitment were successful and characterized by significant growth (both in terms of heights and canopy cover) of individuals over time, reaching heights of the same order of magnitude measured in adults after 1 year from the implementation of the intervention. This restoration was successful regardless of the substrates used (i.e., both with natural pebbles and clay tiles fixed on artificial structures; Figure 5A). Such a fast growth in the natural environment was rather unexpected, especially when compared to previous studies conducted in the Adriatic Sea (Orlando-Bonaca et al., 2021).
Overall, our results suggest that in areas characterized by donor populations living in “natural nurseries”, such as inlets like those occurring along the Conero Riviera, restoration interventions could be implemented by prioritizing in situ recruitment approaches. In this regard, the inlets could serve as natural nurseries. From an intervention upscaling perspective, to obtain large amounts of recruits, protocols based on ex situ approaches need to be optimized and synchronized to processes naturally occurring in the area (e.g., in our case, avoiding transplants of too small juveniles immediately before or concurrently with toxic microphytobenthos blooms).
According to the UN and the UN Decade of Ecosystem Restoration declared for 2021–2030 (https://www.decadeonrestoration.org/), “Ecosystem restoration means assisting in the recovery of ecosystems that have been degraded or destroyed, as well as conserving the ecosystems that are still intact”. This implies that the mere success of a species transplant cannot be considered a success of a restoration intervention. On the other hand, the limits of assessing restoration effectiveness in the absence of a standardized definition of success have been repeatedly claimed by the scientific community working on marine ecological restoration (Fraschetti et al., 2021). For this reason, besides the success of the G. barbata transplant, we used the diversity of associated meio- and macrobenthic assemblages over 1 year after the algae transplant.
Previous studies conducted on seagrasses (Cymodocea nodosa) revealed that the abundance and biodiversity of meiofaunal assemblages associated with the restored seagrasses were still lower than in donor seagrass meadows 1 year after the transplant (Da Ros et al., 2020). Conversely, in the present study, we observed an increase in meio- and macrofaunal abundance and richness of higher taxa that was indistinguishable from those observed in the reference ecosystems, despite a significantly different taxonomic composition.
Recent studies showed positive and additive effects of habitat heterogeneity: habitat ecological volume, its structural complexity, and its capacity to provide different resources within and between co-occurring foundation species (Thomsen et al., 2022). These findings highlighted the importance of habitat heterogeneity in promoting biodiversity via cascades of facilitative interactions. This is the case of the habitat-forming species Cystoseira s.l., which represents a foundation species. However, the direction and effects of facilitative cascades are still unclear, so predicting the composition of assemblages after a restoration intervention is difficult. Therefore, these aspects could be taken into consideration in restoration planning and expectations.
Overall, our data suggest that 1 year after the intervention, the effects of restoration became evident. In the present study, high levels of temporal variability after the intervention were observed for both meiofauna and macrofauna, particularly in the restored site. This could reflect the fast growth of G. barbata in the restored site and its capability to create a rehabilitated 3D habitat, thus promoting fast colonization of meio- and macrofaunal organisms.
However, although meio- and macrofaunal colonization of new substrates can be relatively fast (Mirto and Danovaro, 2004; Fonsêca-Genevois et al., 2006), the complete restoration of the properties of a macroalgal habitat can be a much longer process. Similar results were observed for restoration interventions in other ecosystems. In seagrass restoration that occurred in the same geographic area (Central Adriatic), indeed, meiofaunal taxa in transplanted seagrass plots did not recover completely after 13 months from transplantation if compared to the donor seagrass assemblages, thus suggesting that meiofauna may also take longer to fully recover the original conditions (Da Ros et al., 2020).
The European Nature Restoration Law in preparation will require the restoration of degraded marine habitats, among which macroalgal forests will represent a significant portion. The future restoration intervention must be effective in promoting biodiversity and conducted at large spatial scales.
This study confirms that the restoration interventions need to be carefully planned regarding the siting, timing, and methods used after the target species selection. Siting, approach, and timing should also be tailored site by site, as the restoration success may depend upon spatial and temporal variability of each area as well as ecological processes occurring in specific periods of the year, which could affect the success of the transplants at sea (i.e., the bloom of toxic microalgae impairing the success of the transplants based on the ex situ recruitment).
According to the Society for Ecological Restoration, to be successful, ecological restoration should sustain biodiversity and improve ecosystems’ resilience (Hallett et al., 2013). The determination of the “success” of an intervention in restoration will thus depend on the adoption of appropriate and standardized indicators.
The raw data supporting the conclusions of this article will be made available by the authors, without undue reservation.
The manuscript presents research on animals that do not require ethical approval for their study.
SB, SF, and RD conceived the work. SB and SF wrote the manuscript. FR performed the analyses on G. barbata. FM, MLM, EN, and DI performed the experiment and the laboratory analyses. All authors revised the manuscript and agreed to be accountable for the content of the work. All authors contributed to the article and approved the submitted version.
This study was conducted in the framework of the EASME-EMFF (Sustainable Blue Economy) Project AFRIMED (http://afrimed-project.eu/, grant agreement No. 789059), supported by the European Community, and National Recovery and Resilience Plan (NRRP), Mission 4 Component 2 Investment 1.4—Call for tender No. 3138 of 16 December 2021, rectified by Decree No. 3175 of 18 December 2021 of the Italian Ministry of University and Research funded by the European Union—NextGenerationEU; award number: Project code CN_00000033, Concession Decree No. 1034 of 17 June 2022 adopted by the Italian Ministry of University and Research, Project title: “National Biodiversity Future Center - NBFC”.
Francesca Cariccia, Melissa Orsini, Marika D’Apolito, Virginia Rossi, and Giulia Morello are acknowledged for their help during the field and lab activities.
The authors declare that the research was conducted in the absence of any commercial or financial relationships that could be construed as a potential conflict of interest.
The reviewer SO declared a past co-authorship with the author RD to the handling editor.
All claims expressed in this article are solely those of the authors and do not necessarily represent those of their affiliated organizations, or those of the publisher, the editors and the reviewers. Any product that may be evaluated in this article, or claim that may be made by its manufacturer, is not guaranteed or endorsed by the publisher.
The Supplementary Material for this article can be found online at: https://www.frontiersin.org/articles/10.3389/fmars.2023.1213184/full#supplementary-material
Abelson A., Reed D., Edgar G. J., Smith C. S., Kendrick G. A., Orth R. J., et al. (2020). Challenges for restoration of coastal marine ecosystems in the anthropocene. Front. Mar. Sci. 7. doi: 10.3389/fmars.2020.544105
Bayraktarov E., Saunders M.I., Abdullah S., Mills M., Beher J., Possingham H.P., et al. (2016). The cost and feasibility of marine coastal restoration. Ecologial Appl. 26 (4), 1055–1074. doi: 10.1890/15-1077
Bianchelli S., Buschi E., Danovaro R., Pusceddu A. (2016). Biodiversity loss and turnover in alternative states in the Mediterranean Sea: a case study on meiofauna. Sci. Rep. 6, 34544. doi: 10.1038/srep34544
Blanfuné A., Boudouresque C. F., Verlaque M., Thibaut T. (2016). The fate of Cystoseira crinita, a forest-forming Fucale (Phaeophyceae, Stramenopiles), in France (North Western Mediterranean Sea). Estuar. Coast. Shelf Sci. 181, 196–208. doi: 10.1016/j.ecss.2016.08.049
Byers J. E., Cuddington K., Jones C. G., Talley T. S., Hastings A., Lambrinos J. G., et al. (2006). Using ecosystem engineers to restore ecological systems. Trends Ecol. Evol. 21, 9. doi: 10.1016/j.tree.2006.06.002
Cebrian E., Tamburello L., Verdura J., Guarnieri G., Medrano A., Linares C., et al. (2021). A Roadmap for the restoration of Mediterranean macroalgal forests. Front. Mar. Sci. 8. doi: 10.3389/fmars.2021.709219
Cheminée A., Sala E., Pastor J., Bodilis P., Thiriet P., Mangialajo L., et al. (2013). Nursery value of Cystoseira forests for Mediterranean rocky reef fishes. J. Exp. Mar. Biol. Ecol. 442, 70–79. doi: 10.1016/j.jembe.2013.02.003
Cheminée A., Rider M., Lenfant P., Zawadzki A., Mercière A., Crec'hriou R., et al. (2017). Shallow rocky nursery habitat for fish: spatial variability of juvenile fishes among this poorly protected essential habitat. Mar. pollut. Bull. 119 (1), 245–254. doi: 10.1016/j.marpolbul.2017.03.051
Clarke K. R., Gorley R. N. (2015). PRIMER v7: User Manual/Tutorial (Plymouth, UK: PRIMER-EPlymouth).
Colletti A., Savinelli B., Di Muzio G., Rizzo L., Tamburello L., Fraschetti S., et al. (2020). The date mussel Lithophaga lithophaga: Biology, ecology and the multiple impacts of its illegal fishery. Sci. Total Environ. 744, 140866. doi: 10.1016/j.scitotenv.2020.140866
Corinaldesi C., Bianchelli S., Rastelli E., Varrella S., Canensi S., Gambi C., et al. (2022). The paradox of an unpolluted coastal site facing a chronically contaminated industrial area. Front. Mar. Sci. 8. doi: 10.3389/fmars.2021.813887
Crain C. M., Halpern B. S., Beck M. W., Kappel C. V. (2009). Understanding and managing human threats to the coastal marine environment. Year Ecol. Conserv. Biol. 1162 (1), 39–62. doi: 10.1111/j.1749-6632.2009.04496.x
Danovaro R. (2010). Methods for the Study of Deep-Sea Sediments, Their Functioning and Biodiversity (Boca Raton, FL: CRC Press).
Danovaro R., Fraschetti S. (2002). Meiofaunal vertical zonation on hard-bottoms: comparison with soft-bottom meiofauna. Mar. Ecol. Prog. Ser. 230, 159–169. doi: 10.3354/meps230159
Da Ros Z., Corinaldesi C., Dell'Anno A., Gambi C., Torsani F., Danovaro R. (2020). Restoration of Cymodocea nodosa seagrass meadows: efficiency and ecological implications. Restor. Ecol. 29 (S2), e13313. doi: 10.1111/rec.13313
Fonsêca-Genevois V. D., Somerfield P. J., Neves M. H. B., Coutinho R., Moens T. (2006). Colonization and early succession on artificial hard substrata by meiofauna. Mar. Biol. 148, 1039–1050. doi: 10.1007/s00227-005-0145-8
Fraschetti S., Guarnieri G., Bevilacqua S., Terlizzi A., Claudet J., Russo G.F., et al. (2011). Conservation of Mediterranean habitats and biodiversity countdowns: what information do we really need? Aquat. Conservation: Mar. Freshw. Ecosyst. 21, 299–306. doi: 10.1002/aqc.1185
Fraschetti S., McOwen C., Papa L., Papadopoulou N., Bilan M., Boström C., et al. (2021). Where is more important than how in coastal and marine ecosystems restoration. Front. Mar. Sci. 8, 626843. doi: 10.3389/fmars.2021.626843
Gann G. D., McDonald T., Walder B., Aronson J., Nelson C.R., Jonson J., et al. (2019). International principles and standards for the practice of ecological restoration. Second edition Restor. Ecol. 27 (S1), S1–S46. doi: 10.1111/rec.13035
Garrabou J., Gómez-Gras D., Medrano A., Cerrano C., Ponti M., Schlegel R., et al. (2022). Marine heatwaves drive recurrent mass mortalities in the Mediterranean Sea. Global Change Biol. 28, 5708–5725. doi: 10.1111/gcb.16301
Gianni F., Bartolini F., Airoldi L., Ballesteros E., Francour P., Guidetti P., et al. (2013). Conservation and restoration of marine forests in the Mediterranean Sea and the potential role of Marine Protected Areas. Adv. Oceanogr. Limnol. 4 (2), 83–101. doi: 10.1080/19475721.2013.845604
Gran A., Movilla J., Ballesteros E., Sales M., Bolado I., Galobart C., et al. (2022). Assessing the expansion and success of a restored population of Gongolaria barbata (Stackhouse) Kuntze (Fucales, Phaeophyceae) using high-precision positioning tools and size distribution frequencies. Mediterr. Mar. Sci. 23 (4), 907–916. doi: 10.12681/mms.30500
Guiry M. D., Guiry G. M. (2021). AlgaeBase (National University of Ireland, Galway: World-wide electronic publication). Available at: https://www.algaebase.org.
Hallett L. M., Diver S., Eitzel M. V., Olson J. J., Ramage B. S., Sardinas H., et al. (2013). Do we practice what we preach? Goal setting for ecological restoration. Restor. Ecol. 21 (3), 312–319. doi: 10.1111/rec.12007
Halpern B. S., Frazier M., Potapenko J., Casey K.S., Koenig K., Longo C., et al. (2015). Spatial and temporal changes in cumulative human impacts on the world’s ocean. Nat. Communication 6, 7615. doi: 10.1038/ncomms8615
Heip C., Vincx M., Vranken G. (1985). The ecology of marine nematodes. Oceanogr. Mar. Biol.: Annu. Rev. 23, 399–489.
Iveša L., Bilajac A., Gljušćić E., Najdek M. (2022). Gongolaria barbata forest in the shallow lagoon on the southern Istrian Coast (northern Adriatic Sea). Botanica Marina 65 (4), 255–268. doi: 10.1515/bot-2022-0021
Iveša L., Djakovac T., Devescovi M. (2016). Long-term fluctuations in Cystoseira populations along the west Istrian Coast (Croatia) related to eutrophication patterns in the northern Adriatic Sea. Mar. pollut. Bull. 106 (1–2), 162–173. doi: 10.1016/j.marpolbul.2016.03.010
Irving A. D., Balata D., Colosio F., Ferrando G. A., Airoldi L. (2009). Light, sediment, temperature, and the early life-history of the habitat-forming alga cystoseira barbata. Mar. Biol. 156, 1223–1231. doi: 10.1007/s00227-009-1164-7
Lotze H. K., Coll M., Magera A.M., Ward-Paige C., Airoldi L. (2011). Recovery of marine animal populations and ecosystems. Trends Ecol. Evol. 26, 595–605. doi: 10.1016/j.tree.2011.07.008
Mancuso F. P., Milazzo M., Chemello R. (2021). Decreasing in patch-size of Cystoseira forests reduces the diversity of their associated molluscan assemblage in Mediterranean rocky reefs. Estuarine Coast. Shelf Sci. 250 (5), 107163. doi: 10.1016/j.ecss.2020.107163
Micheli F., Halpern B.S., Walbridge S., Ciriaco S., Ferretti F., Fraschetti S., et al. (2013). Cumulative human impacts on Mediterranean and Black Sea marine ecosystems: assessing current pressures and opportunities. PLoS One 8 (12), e79889. doi: 10.1371/journal.pone.0079889
Mirto S., Danovaro R. (2004). Meiofaunal colonisation on artificial substrates: a tool for biomonitoring the environmental quality on coastal marine systems. Mar. pollut. Bull. 48, 919–926. doi: 10.1016/j.marpolbul.2003.11.016
Molinari-Novoa E. A., Guiry M. D. (2020). Reinstatement of the genera Gongolaria boehmer and Ericaria stackhouse (Sargassaceae, Phaeophyceae). Notulae Algarum. 171, 1–10.
Orfanidis S. (1991). Temperature responses and distribution of macroalgae belonging to the warm-temperate Mediterranean-Atlantic Distribution Group. Botanica Marina 34 (6), 541–552. doi: 10.1515/botm.1991.34.6.541
Orfanidis S., Rindi F., Cebrian E., Fraschetti S., Nasto I., Taskin E., et al. (2021). Effects of natural and anthropogenic stressors on fucalean brown seaweeds across different spatial scales in the mediterranean sea. Front. Mar. Sci. 8, 658417. doi: 10.3389/fmars.2021.658417
Orlando-Bonaca M., Pitacco V., Lipej L. (2021). Loss of canopy-forming algal richness and coverage in the northern Adriatic Sea. Ecol. Indic. 125, 107501. doi: 10.1016/j.ecolind.2021.107501
Pennesi C., Danovaro R. (2017). Assessing marine environmental status through microphytobenthos assemblages colonizing the Autonomous Reef Monitoring Structures (ARMS) and their potential in coastal marine restoration. Mar. pollut. Bull. 125 (1–2), 56–65. doi: 10.1016/j.marpolbul.2017.08.001
Perkol-Finkel S., Airoldi L. (2010). Loss and recovery potential of marine habitats: an experimental study of factors maintaining resilience in subtidal algal forests at the Adriatic Sea. PLoSONE 5 (5), e10791. doi: 10.1371/journal.pone.0010791
Perkol-Finkel S., Ferrario F., Nicotera V., Airoldi L. (2012). Conservation challenges in urban seascapes: promoting the growth of threatened species on coastal infrastructures. J. Appl. Ecol. 49, 1457–1466. doi: 10.1111/j.1365-2664.2012.02204.x
Piva F., Ciaprini F., Onorati F., Benedetti M., Fattorini D., Ausili A., et al. (2011). Assessing sediment hazard through a weight of evidence approach with bioindicator organisms: A practical model to elaborate data from sediment chemistry, bioavailability, biomarkers and ecotoxicological bioassays. Chemosphere 83 (4), 475–485. doi: 10.1016/j.chemosphere.2010.12.064
Sala E., Mayorga J., Bradley D., Cabral R.B., Atwood T.B., Auber A., et al. (2021). Protecting the global ocean for biodiversity, food and climate. Nature 592, 397–402. doi: 10.1038/s41586-021-03371-z
Sala E., Ballesteros E., Dendrinos P., Di Franco A., Ferretti F., Foley D., et al. (2012). The structure of mediterranean rocky reef ecosystems across environmental and human gradients, and conservation implications. PloS One 7, e32742. doi: 10.1371/journal.pone.0032742
Strain E. M. A., van Belzen J., van Dalen J., Bouma T. J., Airoldi L. (2015). Management of local stressors can improve the resilience of marine canopy algae to global stressors. PLoS One 10 (3), e0120837. doi: 10.1371/journal.pone.0120837
Swan K. D., McPherson J. M., Seddon P. J., Moehrenschlager A. (2016). Managing marine biodiversity: the rising diversity and prevalence of marine conservation translocations. Conserv. Lett. 9 (4), 239–251. doi: 10.1111/conl.12217
Tamburello L., Chiarore A., Fabbrizzi E., Colletti A., Franzitta G., Grech D., et al. (2022). Can we preserve and restore overlooked macroalgal forests? Sci. Total Environ. 806, 150855. doi: 10.1016/j.scitotenv.2021.150855
Thibaut T., Blanfuné A., Markovic L., Verlaque M., Boudouresque C.F., Perret-Boudouresque M., et al. (2014). Unexpected abundance and long-term relative stability of the brown alga Cystoseira amentacea, hitherto regarded as a threatened species, in the north-western Mediterranean Sea. Mar. pollut. Bull. 89, 305–323. doi: 10.1016/j.marpolbul.2014.09.043
Thibaut T., Pinedo S., Torras X., Ballesteros E. (2005). Long-term decline of the populations of Fucales (Cystoseira spp. and Sargassum spp.) in the Albères coast (France, North-western Mediterranean). Mar. pollut. Bull. 50, 1472–1489. doi: 10.1016/j.marpolbul.2005.06.014
Thomsen M. S., Altieri A.H., Angelini C., Bishop M.J., Bulleri F., Farhan R., et al. (2022). Heterogeneity within and among co-occurring foundation species increases biodiversity. Nat. Commun. 13, 581. doi: 10.1038/s41467-022-28194-y
Totti C., Accoroni S., Cerino F., Cucchiari E., Romagnoli T. (2010). Ostreopsis ovata bloom along the Conero Riviera (northern Adriatic Sea): Relationships with environmental conditions and substrata. Harmful Algae 9 (2), 233–239. doi: 10.1016/j.hal.2009.10.006
Keywords: active restoration, Gongolaria barbata, indicators of success, associated biodiversity, meiofauna, macrofauna
Citation: Bianchelli S, Fraschetti S, Martini F, Lo Martire M, Nepote E, Ippoliti D, Rindi F and Danovaro R (2023) Macroalgal forest restoration: the effect of the foundation species. Front. Mar. Sci. 10:1213184. doi: 10.3389/fmars.2023.1213184
Received: 27 April 2023; Accepted: 07 September 2023;
Published: 26 September 2023.
Edited by:
Chiara Piroddi, Joint Research Centre, ItalyReviewed by:
Sotiris Orfanidis, Institute of Fisheries Research, GreeceCopyright © 2023 Bianchelli, Fraschetti, Martini, Lo Martire, Nepote, Ippoliti, Rindi and Danovaro. This is an open-access article distributed under the terms of the Creative Commons Attribution License (CC BY). The use, distribution or reproduction in other forums is permitted, provided the original author(s) and the copyright owner(s) are credited and that the original publication in this journal is cited, in accordance with accepted academic practice. No use, distribution or reproduction is permitted which does not comply with these terms.
*Correspondence: Silvia Bianchelli, c2lsdmlhLmJpYW5jaGVsbGlAdW5pdnBtLml0
Disclaimer: All claims expressed in this article are solely those of the authors and do not necessarily represent those of their affiliated organizations, or those of the publisher, the editors and the reviewers. Any product that may be evaluated in this article or claim that may be made by its manufacturer is not guaranteed or endorsed by the publisher.
Research integrity at Frontiers
Learn more about the work of our research integrity team to safeguard the quality of each article we publish.