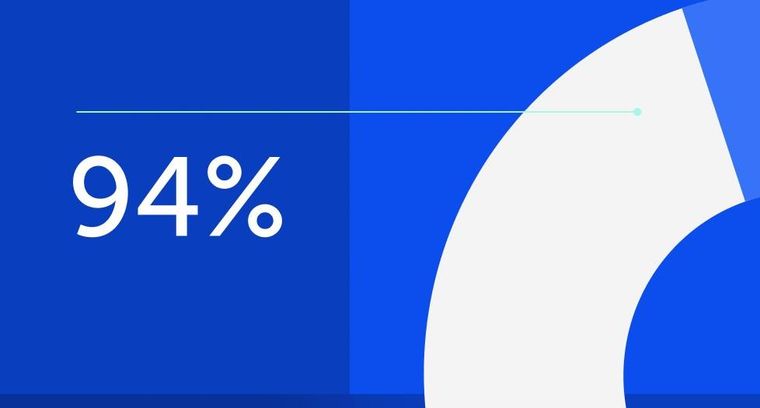
94% of researchers rate our articles as excellent or good
Learn more about the work of our research integrity team to safeguard the quality of each article we publish.
Find out more
ORIGINAL RESEARCH article
Front. Mar. Sci., 03 November 2023
Sec. Global Change and the Future Ocean
Volume 10 - 2023 | https://doi.org/10.3389/fmars.2023.1212412
In the Baltic Sea, cold-water adapted dinoflagellates and diatoms dominate the phytoplankton spring bloom of the Northern and Eastern Basins of the Baltic Sea. In the Central and Southern parts, where such species are less prominent, they cause occasional biomass peaks. We hypothesized that these dynamics correlate with ice cover, sea surface temperature (SST), and water transport processes, as the large Basins of the Central Baltic Sea are too deep to build-up blooms from their own seed banks. Long-term monitoring data from the past 40 and 20 years in the central and southern Baltic Sea, respectively, were analyzed here for biomass development of five cold-adapted taxa: the diatoms Pauliella taeniata, Thalassiosira baltica, Thalassiosira levanderi and Melosira spp. and the dinoflagellate Peridinella catenata. Results show that diatoms generally reached high biomass peaks in the 1980s and in shorter periods from 1995-1997, 2003- 2006, and 2010-2013 in all areas. We detected good correlations with the length of the ice cover period as well as low minimum and mean winter and spring SSTs. In contrast, biomass dynamics of the dinoflagellate P. catenata are more independent from these factors but have decreased strongly since the beginning of the 21st century. A numerical ocean model analysis confirmed the hypothesis that large blooms in the deep basins are seeded through water transport from adjacent shallow, ice-covered coastal areas such as the Gulf of Finland and the Gulf of Riga. Our results show that under ongoing climate warming, the common cold-water species may disappear from spring blooms in southern and central areas with unknown consequences for the ecosystem.
In the northern parts of the Baltic Sea, the phytoplankton spring bloom largely consists of cold-water adapted species of which some are cryophilic “ice-algae”. Typical representatives are the diatoms Pauliella taeniata, Chaetoceros wighamii, T. baltica, species of Melosira spp. like M. arctica. and one of the few cryophilic dinoflagellates Peridinella catenata. The diatom P. taeniata, one of the dominating species in the early spring bloom in the northern Baltic Sea (Hoglander et al., 2004) needs water temperatures close to 0°C for growth and thus represents psychrophilic species known from polar waters (Fiala and Oriol, 1990). Some cold-water phytoplankton grow in or directly below the ice. They thus seed the pelagic community, which is usually recruited directly from seed banks in the sediment, additionally during and after ice breakup (Norrmann and Andersson, 1994; Haecky et al., 1998). Melosira arctica, for instance, is a typical sub-ice colonial species that is free-floating or builds up loosely attached curtains at the ice subsurface (Poulin et al., 2011). Thus, the lack or the presence of sea ice as well as the timing of the break-up of the ice cover strongly affect the spring phytoplankton dynamics and composition in these areas (Kononen and Niemi, 1984; Wasmund et al., 1998; Hjerne et al., 2019).
However, little is known about the distribution and origin of cold-water phytoplankton in the central basins (Eastern- and Western Gotland Basin, Bornholm Basin, and Arkona Basin) and the southern parts of the Baltic Sea like the Mecklenburg Bight during spring blooms. In contrast to the northern parts, these areas often remain ice-free throughout the winter period with ice build-up only in the coastal regions (Schmelzer and Holfort, 2012). Until the mid-1980s, cold-water species regularly appeared in higher abundance in the spring blooms of these areas. P. taeniata, for instance, was a highly abundant species in the Eastern Gotland Basin during spring (HELCOM, 2006). It has remained unclear whether the respective populations originated from the central Basins and were transported south by ocean currents or whether - in cold winters - they were seeded under the ice by local coastal populations.
Bloom patterns of cold-water species have been disrupted in recent decades, and early spring blooms of strictly cold-water adapted and cryophilic species now seem to appear only occasionally (Wasmund et al., 2011; Wasmund et al., 2012). Since the abundances of Baltic cold-water phytoplankton depend on ice cover and low sea surface temperature (SST) during winter and spring, the general trend of climate warming is expected to affect cold-water adapted phytoplankton significantly. In the central and northern Basins of the Baltic Sea, annual SST had increased by 0.9°C in 2016 compared to the long-term average 1990-2016. (HELCOM, 2017). In the Bornholm Basin, average SST in March was even 1.5°C higher in the period 1989-2005 compared to the period 1969-1988 (Hinrichsen et al., 2007). In the southern Baltic Sea, SST in the winter period (December-February) has already increased by around 1.2°C from 1980 to 2020 (Stramska and Bialogrodzka, 2015). Latest climate models predict an overall increase in the annual mean SST of +3.5°C by the end of the century for the Baltic Sea (HELCOM, 2021; Meier et al., 2022). In the offshore areas of the western Baltic Sea and east of Bornholm, the extent of ice cover has already decreased to a minimum area over the past 30 years (von Storch et al., 2015) and is predicted to further decrease in the entire Baltic Sea area (HELCOM, 2021; Meier et al., 2022). In the northern Baltic Sea, a temperature-related shift in spring phytoplankton composition towards a weaker representation of sea ice-associated diatoms has already occurred in the past decades in conjunction with climate warming (Hjerne et al., 2019). Cold-water phytoplankton generally contribute a significant fraction of primary production during spring. Hence, changes in the spring phytoplankton composition and bloom magnitude are expected to significantly affect food web dynamics and energy transfer to higher trophic levels in pelagic and benthic ecosystems.
During the past decades, cold-water phytoplankton have incrementally disappeared also from spring blooms in the Central and Southern Baltic Sea (HELCOM, 2006). This has been suggested to result from the substantially reduced length of ice-covered periods (Schwegmann and Holfort, 2021) and the tendency towards ice-free or mild ice seasons (von Storch et al., 2015).
Here we examine trends in the timing and distribution of cold-water and ice-associated phytoplankton and their blooms over the past decades in the Central and the southern Baltic Sea in order to identify drivers and causal mechanisms of potential changes in the context of global warming and better understand the role of water mass transport in seeding and representation of cold-water species in the deep basins of the Central Baltic Sea. The biomass trends of five representative cold-water phytoplankton species during spring blooms were analyzed over the past 40 and 20 years in the Central Baltic Sea (Eastern Gotland Basin, Bornholm Basin, Arkona Basin) and the southern part of Mecklenburg Bight in relation to hydrographic parameters. We aimed to better understand the drivers of potentially changed patterns and the role of climate warming. We additionally used numerical ocean model analyses to examine the role of ocean currents in the potential transport of seed populations and subsequent bloom formation in the Central Baltic Sea.
We adopted the assessment units defined by (HELCOM, 2017) for our analyses. The analysis included the central and southern Baltic Sea area, specifically the German Belt Sea area (Mecklenburg Bight) and the Central Baltic Sea, except for the Western Gotland Basin (Figure 1). The Mecklenburg Bight was represented by a coastal data set (originating from the long-term monitoring station of the Leibniz-Institute for Baltic Sea Research Warnemuende (IOW): Heiligendamm) and data from the open sea area due to quite different environmental conditions and frequencies of sampling. The Central Baltic Sea included the Arkona Basin, the Bornholm Basin, and the Eastern Gotland Basin. For more detailed information on these areas, see (Wasmund et al., 1998).
Figure 1 Average surface current field (upper 15m) during winter period (December, January, February). The color coding indicates the current speed, the arrows the direction. Please note the non-linear color bar. We also indicated the individual basins. The insert shows the location of the southern Baltic Sea in northern Europe.
Data on biological (phytoplankton species biomass) and physical (temperature) parameters were obtained according to the guidelines for the Baltic Sea Phytoplankton Monitoring Program of HELCOM (HELCOM, 2017), which are based on the procedures described by (Utermöhl, 1958; Olenina et al., 2006). Phytoplankton data used here was based on wet weight biomass being the most reliably represented parameter of the database. In cases of lacking biomass data, biovolume data were transformed into wet weight (1 mm³ = 1 mg). All wet weight data were adjusted to mg m-3.
The data used in this analysis only included measurements from the upper 10m of the water column, which represents the standard HELCOM phytoplankton monitoring procedure and is assumed to reflect the upper mixed layer and the euphotic zone. Samples consist of equal volumes of water taken from discrete depth of 1, 2.5, 5, 7.5, and 10m. If discrete samples from only one depth within the upper 10 m were available, this data was used. Sampling events close to large river plumes (low salinity served as a criterion) such as those from the Oder, Vistula and the Klaipeda Strait with species composition and nutrient conditions strongly deviating from the ones in the open sea (Liu et al., 2017) have been excluded from the data set.
Taxa and species names often changed over the 40 year sampling period. Thus, all different synonyms were included in the data base analysis and transferred to the actually valid synonym: Pauliella taeniata = Achnanthes taeniata; Peridinella catenata = Gonyaulax catenata. As species of the taxa Melosira are hardly to differentiate in routine light microscopy, Melosira spp. was kept as taxa group. It included species identified as: Aulacoseira sp., Aulacoseira granulata, Aulacoseira islandica, Aulacoseira italica, Melosira sp., Melosira arctica, Melosira lineata, Melosira nummuloides, Melosira varians.
Overall, the data originated from four different sources: ICES database (https://www.ices.dk/), data of (Wasmund et al., 2011), ODIN database (https://odin2.io-warnemuende.de/), and the Baltic Environment Database (BED, https://balticnest.org). The HELCOM data are kept in the ICES database and were obtained for phytoplankton and abiotic parameters. The Baltic Environment Database (BED) was checked for additional abiotic data. From the IOW database (ODIN), biological and abiotic data of the Leibniz Institute for Baltic Sea Research, which were not part of the HELCOM monitoring and, therefore, not contained in international databases, were extracted. For the coastal region of Mecklenburg Bight, data originates from ODIN and includes mainly the IOW long-term monitoring station Heiligendamm. For the open sea area, data were additionally obtained from LUNG (Landesamt für Umwelt, Naturschutz & Geologie). Hydrographic and hydrochemical data originate from ICES, BED, and ODIN.
Sea ice data from the German Baltic was provided by BSH (Bundesamt für Seeschiffahrt and Hydrographie Rostock/GERMANY). We used “accumulated sea ice volume sum” (m) as a parameter describing changes in sea ice. It comprises changes in sea ice coverage as well as thickness (Schwegmann and Holfort, 2021). The accumulated sea ice volume of the German Baltic Sea coast was used to check for correlation with maximum spring species biomass in the Mecklenburg Bight.
The data set generated for this study, including information on sample station ID and geographical coordinates, is available with manuscript submission under IOW database DOI 10.12754/data-2023-0005. The time series of the numerical ocean model can be accessed here: DOI 10.5281/zenodo.7764362.
The analyses include data from 1979/80 to 2019 for all regions except the coastal part of the Mecklenburg Bight. Here measurements started 1995/96 at a pier west of Rostock-Warnemuende (LA: 45.1833, LO: 12.0583) and switched to Heiligendamm (LA:54.145, LO: 11.843), another pier, 14.5 km west of Warnemuende with comparable conditions (see also Wasmund et al., 2019). For 1997, the database included additional sampling events at Kühlungsborn (LA: 54.1558, LO: 11.763), a station located 5 km west of Heiligendamm, and in 2001 all spring samplings were taken here. Sampling frequency at Heiligendamm was three to four times per month, resulting in a high-resolution dataset at a depth of 1 m. Sampling frequency in Central Baltic Sea areas varied between years. The total number of sampling events for each sub-basin can be found in Supplementary Table 1. In 1983 and 1988, data was only available from the end of the spring bloom period (May) in Bornholm Basin due to unknown reasons. In such years the blooms of cold-water phytoplankton might have been missed and records thus have to be considered carefully. Nevertheless, Supplementary Figures 1, 2 show that high maximum biomass values have been represented by several similar high peaks of the same species in each year, which support strongly the robustness of the data set. The whole data set was checked for outliers before analyses.
In the Baltic Sea, the beginning of the annual spring bloom varies depending on the latitude. Bloom initiation starts in the South, progressing northward, and happening approximately four weeks later in the central areas. Hence, blooms typically start earlier in the Mecklenburg Bight than in the Central Baltic Sea (Groetsch et al., 2016) and spring season has to be defined separately for the different Basins: In Mecklenburg Bight it is defined as the period from February to April. In contrast, in the Central Baltic Sea, the spring period begins in early March and lasts to the end of May (HELCOM, 1996; Wasmund et al., 2011).
Maximum species biomass was defined as the highest biomass value of all available measurements during one spring season for each species and sub-basin. Supplementary Figures 1, 2 represent the biomass data of every available measurement, separated for species and locations.
Minimum spring temperature was defined as the lowest temperature value of all available measurements during one spring season for each species and sub-basin. Unfortunately, no temperature data was available for 1992 from Mecklenburg Bay (open sea areas) and 1997 from the coastal station.
To test for the effects of minimum spring temperature and accumulated sea ice volume on the long term biomass trends of the cold-water species (result section 3.1), we used linear models. Statistical significance of the linear models was assessed using permutation tests as implemented in the R package lmPerm (R4.3.1), since even after log(x+1) transforming biomass, the residuals were not normally distributed in all models based on the Shapiro-Wilk test. The p-values of the individual linear models were further corrected for multiple testing using the false-discovery-rate (FDR) adjustment (Table 1; Supplementary Table 2).
Table 1 Significant effects (p-values of the slope) of the lm-model, tested for the effects of minimum spring temperature (°C, all investigated basins) and accumulated sea ice volume sum (m³; Mecklenburg Bight only) on the log-transformed maximum spring biomass (mg m-3, see also Figure 2) of the investigated cold water phytoplankton species P.taeniata, T. baltica, T. levanderi, and Melosira spp.
During winter and early spring, dissolved inorganic nutrient concentrations in the Baltic Sea are high and sufficiently available, offering good conditions for diatoms to start a bloom (Kremp et al., 2008; Nausch et al., 2008; Edwards et al., 2016; Andersson et al., 2017). Thus, there is no evidence for nutrient limitation during spring-bloom build-up (Kremp et al., 2008), particularly at spring bloom start for the early growing ice-algae. Hence, winter/early spring dissolved inorganic nutrient concentrations have not been checked as a parameter.
Data from a numerical ocean model of the entire Baltic Sea were analyzed to untangle underlying correlations of the phytoplankton data with hydrographic drivers (result section 3.2., 3.3). The data from the numerical model were used as a second independent data set (the first one is the observational data, see section 2.2). The advantage of the numerical model is that we have consistent time series that are not prone to undersampling, as regular monitoring cruises are. Moreover, the numerical model can provide 3D current fields which are difficult to measure. The model was integrated with a horizontal resolution of 1 nautical mile and 50 vertical terrain-following adaptive levels (Gräwe et al., 2019) and computed standard variables like temperature, salinity, currents, and ice coverage. The data was stored as daily mean fields along selected vertical transects, as daily mean surface fields of SST, salinity and currents, as well as full 3D monthly mean fields. Average winter time surface current field is shown in Figure 1. The model run covers 1961 to 2021, but only 1979-2019 was used here. From the stored output fields, we extracted the measures defined in Table 2. We computed the mean of those measures and the 95% confidence intervals to quantify significant changes between years with ice-algae presence (species biomass ≥0.1 mg m-3) and absence (biomass 0.00 mg m-3). We further applied a two sample t-test to test the hypothesis if the abiotic factors for presence/absence sample show equal/unequal means (p-values). Moreover, we calculated the Pearson’s Linear Correlation Coefficient (Matlab) between the biomass and the hydrographic drivers and tested for statistical significance (by computing the associated p-value). With these two measures, we detect four scenarios: 1. a correlation between abiotic factors and biomass, no correlation, a correlation and a change in mean state of the abiotic factors during presence and absence of species, and the lack of both. The presence of a species might not be correlated with the mean winter SST (they have no common temporal pattern). However, the presence of species can still be associated with a lower mean winter SST or a higher mean ice coverage.
To prepare the biomass data for the correlation analysis of each phytoplankton species at a given location, we transformed them into the natural logarithm (see section 2.3). An overview of all results can be found in the Supplementary Tables 3, 4. A summary of the main results is given in Tables 3, 4.
Table 3 Summary of the ocean model analyses results, representing conditions of Baltic Sea ice coverage (km2), winter minimum, mean SST (°C), and the number of days with an SST <3°C supporting or preventing bloom biomass build-up of P. taeniata, T. baltica, T.levanderi, Melosira spp., and P. catenata.
Table 4 Current flow speed, salinity and mixing depth in the Eastern Gotland Basin, Bornholm Basin, Arkona Basin, and adjacent areas like the Gulf of Riga and the Gulf of Finland, showing differences during bloom and no-bloom situations.
Eastern Gotland Basin: P. taeniata, and T. baltica occurred periodically with biomass peaks in the 1980s, 1995-1997, and between 2003-2006 (Figure 2A). The highest maximum spring biomass of P. taeniata was significantly reached in periods of low minimum spring SST (Figure 2A; Table 1). The maximum biomass of Melosira spp. was lower compared to the other species (Figure 2A). The maximum biomass of T. levanderi was very low during spring between 1979-1995 (<6 mg m-3; Figure 2A). Since 1996 T. levanderi has occurred more regularly. However, bloom biomass was low (<100 mg m-3, Figure 2A) with few higher peaks, which did not correlate with minimum spring temperature (Supplementary Table 2). P. catenata regularly appeared with maximum biomass values above 2500 mg m-3 (max: 29 000 mg m-3) in the 1990s. Since the beginning of the 21st century, biomass has decreased strongly to values of approximately 500 mg m-3 (Figure 2A).
Figure 2 Maximum spring biomass (mg m-3) of the cold-water species P. taeniata, T. baltica, T. levanderi, Melosira spp. and P. catenata in the: (A) Eastern Gotland Basin, (B) Bornholm Basin, (C) Arkona Basin, (D) Mecklenburg Bight: open sea area, (E) Mecklenburg Bight: coastal stations. For symbol attribution see legend. Trend lines show significant biomass trends over time. Grey bars in the back represent the overall four periods of high cold-water species biomass in the Central and Southern Baltic Sea.
Bornholm Basin: The overall maximum spring biomass was lower compared to the Eastern Gotland Basin for all diatom species (Figures 2A, B) and nearly negliglible in the 1980s. (Figure 2B). In the period from 1990-2006, both T. baltica and T. levanderi, regularly appeared during spring blooms (Figure 2B). The maximum spring biomass of P. taeniata, T. baltica, and T. levanderi significantly correlated with minimum spring SST (Figure 2B; Supplementary Figure 3; Table 1). Particularly during the last cold winter/spring period 2010-2013, spring biomass reached high maximum values between 250 and 600 mg m-3 (Figure 2B). Biomass of Melosira spp. remained low throughout the study period and did not correlate with minimum spring SST (Figure 2B; Supplementary Table 2). The dinoflagellate P. catenata produced high biomasses in the 1990s (max: >2000 mg m-3). Since 2003, biomass has decreased to values below 50 mg m-3, besides occasional peaks between 2010-2013 (Figure 2B). Maximum biomass correlated significantly with minimum spring temperature (Figure 2B; Supplementary Figure 3; Table 1).
Arkona Basin: P. taeniata still occurred regularly in the 1980s in the Arkona Basin, but since the beginning of the 1990s, only occasionally present in the community, with sudden high biomass peaks (Figure 2C). Conspicuously, exceptionally high biomass values were recorded for P. taeniata in 2011 during the last cold winter/spring period (2010-2013), amounting to as much as 25 000 mg m-3. Maximum spring biomass of both P. taeniata, T. baltica, T. levanderi and Melosira spp. significantly correlated with minimum spring SST (Figure 2C; Supplementary Figure 3; Table 1). Whereas T. baltica occurred only sporadically in spring, T. levanderi has been a regular spring bloom constituent since the mid-1980s (Figure 2C). P. catenata occurred regularly during spring blooms in the 1980s and 1990s but without correlations to minimum spring SST (Table 2; Supplementary Table 2). Since the year 2000 this species has nearly disappeared from spring blooms (Figure 2C).
Open sea area of Mecklenburg Bight: Maximum spring biomass of P. taeniata significantly correlated with years of lowest minimum spring SST and years of high sea ice volume sum at the German Baltic Sea coast (Figure 2D; Supplementary Figure 3; Table 1). Both T. baltica and T. levanderi were quite typical during spring blooms in the 1980s and 1990s. However, since 2000, both these species have nearly disappeared (Figure 2D), as reflected by a significant decreasing trend of T. baltica (r²=0.19; p=0.005). Melosira spp. was rare in the 1980s and 1990s and has nearly disappeared since 2000 (Figure 2D). P. catenata rarely occurred in this area, and higher maximum biomass values >50 mg m-³ were only reached in the 1990s (Figure 2D).
Coastal stations of Mecklenburg Bight: The cold-water diatom species were rarely found in the spring blooms and had disappeared entirely since 2013 (Figure 2E). Biomass was comparably low to the other Baltic Sea areas (Figure 2E) and did not correlate with minimum spring SST (Supplementary Figure 3; Supplementary Table 2), besides for Melosira spp. (Table 1). The maximum biomass of Melosira spp. was significantly highest in periods of lowest minimum spring SST and high sea ice volume sum at the German Baltic Sea coast (Figure 2E; Table 1). However, maximum biomass constantly decreased (r²=0.18; p=0.029; Figure 2E) until total disappearance. The dinoflagellate P. catenata appeared regularly in low biomass values with a significant decreasing trend (r²=0.23; p=0.013; Figure 2E). The maximum biomass was significantly highest in periods of high sea ice volume sum at the German Baltic Sea coast (Table 1).
Eastern Gotland Basin: The presence of P. taeniata and Melosira spp. is associated with low mean winter SST, low minimum winter SST, a high number of days with SST <3°C and strong ice coverage (Table 3). The presence of T. baltica is significantly correlated with low mean winter SST and is associated with low minimum winter SST (Table 3). T. levanderi and P. catenata, instead, did not show any associations with different abiotic factors (Table 3; Supplementary Table 3).
Bornholm Basin: The presence of P. taeniata is associated with a high number of days with SST <3°C and low mean winter SST (Table 3). However, neither minimum winter SST nor ice cover affected the occurrence of any of the species (Supplementary Table 3).
Arkona Basin: The presence of the diatom species P. taeniata, T. baltica and Melosira spp. and the dinoflagellates P. catenata is associated or even significantly correlated with a high number of days with SST <3°C (Table 3). The presence of P. taeniata is further associated with low winter mean SST and ice coverage (Table 3). Minimum winter SST did not affect bloom build-up of any of the species (Supplementary Table 3).
Pooled data of all Central Baltic Sea areas (Eastern Gotland-, Bornholm-, Arkona Basin): The presence of P. taeniata and P. catenata is associated with Baltic Sea ice cover (Figure 3; Supplementary Table 5). However, the other tested factors (minimum SST, SST <3°C) showed no significant results (Figure 3; Supplementary Table 5).
Figure 3 Pooled data of the ocean model analyses for the species biomass (mg m-3), the number of days with the SST below 3°C, minimum SST (°C) and Baltic sea ice-cover (km²) of the Central Baltic Sea areas (Eastern Gotland Basin, Bornholm Basin, Arkona Basin). For symbol attribution see legend.
Open sea area of Mecklenburg Bight: The presence of both P. taeniata and Melosira spp. is associated with low minimum winter SST, a high number of days with SST <3°C and strong ice coverage (Table 3). Whereas the presence of P. taeniata is further significantly correlated with low mean winter SST (Table 3), this factor is associated just slightly with the presence of Melosira spp. (Supplementary Table 3). The presence of P. catenata predominantly occurred at low minimum winter SST (Table 3).
Eastern Gotland Basin(eGo): The presence of P. taeniata and Melosira spp. during spring blooms is associated with a stronger water column stratification in the Gulf of Finland (GoF), Gulf of Riga (GoR), and in the basin itself (GoF mld, eGo mld, GoR mld; Table 4). Additionally, the mean current flow speed in the Gulf of Finland (GoF mean flow) and the Gulf of Riga (GoR mean flow, only Melosira spp.) is enhanced (Table 4). The presence of T. baltica is associated with a low mean current speed within the Eastern Gotland Basin (eGo mean flow), i.e. reduced water flow from South to North (Table 4). The presence of T. levanderi coincided with mean current flow speed from the Gulf of Finland into the Gotland Basin (GoF mean flow), i.e., reduced water outflow from East to West spring blooms. (Table 4). In contrast, the mean current flow from the Gulf of Riga was slightly enhanced at times where T. levanderi was present (Table 4), leading to increased water transport from East to West (water outflow, GoR mean flow). The mean current speed (eGo mean flow) and the water circulation inside the Eastern Gotland Basin (eGo mean circ) were also reduced (Table 4).
Bornholm Basin (BB): During the presence of P. taeniata and T. baltica, the water circulation within the Bornholm Basin (BB mean circ) was enhanced compared to absence situations (Table 4). The presence of Melosira spp. is significantly correlated with an enhanced mean current flow speed (BB mean flow) and a lower mixing depth (BB mld, Table 4). The presence of the dinoflagellate P. catenata is associated with a lower mean water circulation (BB mean circ), and a reduced mean surface salinity (BB mean salinity) within the Bornholm Basin (Table 4). It is further slightly significantly correlated with a lower mixing depth (BB mld, Table 4).
Arkona Basin (AB): The presence of P. taeniata during spring bloom is associated with a lower mean current flow speed (AB mean flow) from the Arkona Basin (Table 4). The presence of T. baltica is associated with a reduced mean surface salinity (AB mean salinity; Table 4). This factor is further slightly associated with the presence of T. levanderi (Supplementary Table 4). The presence of the dinoflagellate P. catenata is associated with a weaker water circulation (AB mean circ, Table 4).
For the first time, the model analyses presented here confirm the hypothesis that high biomasses of cold-water phytoplankton in the central Baltic Sea largely originate in the Gulfs of Finland and/or Riga and are transported south by low salinity surface water currents into the Eastern Gotland Basin and further even into the Bornholm and Arkona Basin.
The long-term data analyses performed in this study showed that both in the Central Baltic Sea, and in the Southern region, the presence and/or even high maximum biomass peaks of the cold-water diatoms correlate with low winter and/or spring SST, a high number of days with SST <3°C and ice-cover. Instead, biomass values of the dinoflagellate P. catenata appeared to be less dependent on these factors. Since 2013, the general trend of increased winter/spring SST coincided with decreasing biomass of all investigated species and lack of typical bloom formation of cold-water phytoplankton.
Long-term analyses identified four periods of high cold-water diatom biomass in the Central (all 4 investigated Basins) and southern Baltic Sea: the 1980s, the periods from 1995 to 1997, from 2003 to 2006, and from 2010 to 2013. These coincided with winters that were generally cold and ice-rich, as also reported by Schmelzer and Holfort (2012). Even in the typically ice-free southern Baltic Sea areas below 56° North, winters of the 1980s were classified as “strong to medium” by the sea ice index with high sea ice volume and low SST, especially between 1985-1990 (von Storch et al., 2015).
Since the 1990s, in contrast, winters have been generally classified as “weak”, except for a few short periods of cold winter conditions in 1995/1996 and 2010-2012. Winters of 2008/2009 were even classified as very “weak” with sea ice only being formed in the northernmost basins, the Bothnian Bay, and coastal areas of the Gulf of Finland (von Storch et al., 2015). Our analyses revealed that since the early 2000s, the biomasses of cold-water and ice-adapted diatoms have significantly decreased, to the extent that these species have nearly disappeared from spring blooms in all basins of the Central Baltic Sea and the Mecklenburg Bight in the south, suggesting a direct impact of changing climate conditions (Wasmund et al., 1998; Hjerne et al., 2019).
The frequency of bloom events, together with maximum spring biomass trends of the dinoflagellate P. catenata, particularly in the Eastern Gotland and Bornholm Basins clearly reflected the period of dinoflagellate-dominated spring blooms in the 1990s in the Central Baltic Sea, e.g. (Wasmund et al., 2001) and the northern areas, such as the Gulfs of Bothnia and Finland (Klais et al., 2011). Different mechanisms have been suggested to explain the respective dinoflagellate dominance patterns at that time, which appear largely basin- and species-specific. Mild and ice-free/low-ice winters supposedly favor spring blooms dominated by the dinoflagellate P. catenata in the central basins, where deep circulation resuspending cells into the euphotic layer is suppressed under such conditions, and motile cold-water dinoflagellates are selected over non-motile diatoms (Kononen and Niemi, 1984; Wasmund et al., 1998; Sundström et al., 2009). Like other cold-water and ice-associated dinoflagellates (particularly Apocalathium malmogiense and Biecheleria baltica) they might benefit from locally specific weather and ice conditions relating to climate warming, which promote their recruitment from benthic seed banks and subsequent bloom formation (Klais et al., 2011; Klais et al., 2013).
The analyses of water transport by ocean currents provided evidence for their crucial role in the distribution of ice-algae and cold-water phytoplankton in the central and southern Baltic Sea. Particularly the presence of the diatoms P. taeniata, Melosira spp., and T. levanderi was associated strong with an enhanced current flow speed from the Gulf of Finland and/or the Gulf of Riga. This suggests that cold-adapted spring phytoplankton in the Eastern Gotland Basin likely originates from seed banks in shallower areas of the Northern Gulfs and is transported via near-surface out flowing water. Ice melting in spring, particularly after strong winters, enhances the nearly permanent outflow of low-salinity surface water from the northern areas southwards (Hordoir and Meier, 2010) and the concomitant transport of species. Our results suggest that even population of Melosira spp., T. baltica and P. catenata in the Arkona and Bornholm Basin originated rather from the Eastern Gotland Basin and northern areas than from seed banks of local populations. Higher biomass occurred under reduced salinity, reduced water circulation and/or a lower mixing depth - good conditions for establishing local blooms in these areas. This is in accordance with earlier reports (Klais et al., 2013), who found the spring bloom dinoflagellate Biecheleria baltica to be transported via clockwise circulation of the surface current (Team, 2008) from the Gulf of Finland to the Northern Baltic Proper and further south along the Swedish coast. Likewise, an unusual bloom of P. taeniata at the Swedish Baltic Proper coast in 1994 was related to water transport from the North (Norrmann and Andersson, 1994; Haecky et al., 1998). A recent correlation analysis (Godhe et al., 2016) showed a significant relationship between gene flow patterns and oceanographic connectivity within the Baltic Skeletonema marinoi spring bloom, supporting the conclusion that water transport is an important factor in the southward distribution of Northern Baltic cold-adapted phytoplankton in early spring. The S. marinoi population from the southern Baltic was, however, genetically distinct, suggesting that here the species is predominantly seeded locally.
Cold, ice-rich winters with late ice-breakup are generally assumed to favor diatom-dominated spring blooms in the Baltic Sea (Alheit et al., 2005; Klais et al., 2011; Wasmund et al., 2011). Sea ice-associated phytoplankton species often build up high biomasses already in or underneath the ice (Norrmann and Andersson, 1994; Haecky et al., 1998), which supports bloom formation via a large seed population after ice break-up (Hjerne et al., 2019) and enhances primary production.
Our analyses showed that diatom presence and/or maximum biomass is associated or even significantly correlated with low SST in winter and spring and/or a high number of days with SST <3°C, known as the main drivers for the build-up of sea ice in the Baltic Sea, additionally effected by surface salinity, wind speed and current (Schmelzer and Holfort, 2015). Especially the cold-water specialist P. taeniata, but also T. baltica, and Melosira spp. showed strong associations between presence and low SST in nearly all basins. P. taeniata and Melosira spp. account even as sea ice-specialists (Horner, 1985). Thus, their bloom formations (high cell concentrations and biomasses) related strong to ice-coverage in the Eastern Gotland Basin and Mecklenburg Bight.
Although mainly ice-free over the whole winter period, the hydrography of the Eastern Gotland Basin is strongly affected by water currents from the northern, often ice-covered Gulf of Finland and the Bothnian Sea, which can transport cold-water- and sea-ice-associated species here. Little is known about the origin of cold-adapted phytoplankton in the southern Baltic, specifically the Mecklenburg Bight. Due to the long distance from the northern areas, it is unlikely that populations originate from the Gulf of Finland or Riga. Despite, its`s generally low water depth (max depth: 35m), particularly in the surrounding coastal areas, the Mecklenburg Bight generally represents favorable conditions for recruitment from local seed banks, particularly in cold, ice-rich winters. Nevertheless, the overall elevated winter and spring temperatures of the last decade might interfere with recruitment: Seeding of cold-water phytoplankton is often regulated by a germination “window” at low temperatures (Rengefors and Anderson, 1998; Kremp and Anderson, 2000) and may be suppressed when such temperatures are not reached. Lack of coastal seeding would, subsequently, prevent growth and bloom formation in the southern Baltic Sea, and likely explain the overall strong decrease in the cold-water species biomass during the last decades in the Mecklenburg Bight.
The strong reduction in periods with coastal ice cover (Schwegmann and Holfort, 2021), and the decreasing trend of total ice volume since 1985 (1985-2015 more than 10% per decade in many regions like the Gulfs of Finland, Riga and Bothnia; HELCOM, 2021; Schwegmann and Holfort, 2021) likely further minimized the growth and distribution of ice-associated phytoplankton. The lack of ice denotes a strong reduction of their first habitat and a minimizing in their bloom start population biomass. The reduction in ice-coverage further reduces the volume of surface freshwater flow with the ice-melt, potentially transporting fewer species less far into the Central Baltic Sea areas. This is reflected in their overall strong decrease in biomass or even disappearance from spring blooms in all analyzed basins of the Central Baltic Sea and the Mecklenburg Bight. As P. taeniata is even used as an paleo-indicator species for climate cooling in the Baltic Sea (Tuovinen et al., 2008), its strong reduction clearly mirrors the effects of climate warming. Even T. levanderi, which showed the least correlations with SST, and which occurred quite frequently in the 1980 and 1990s, nearly disappeared during the last decade.
Cold-water dinoflagellates in the Baltic Sea are supposed to be favored by climate warming and have been generally related to mild winters, exhibiting the above-mentioned specific sequence of physical conditions, giving dinoflagellates a head start and thus supporting their bloom formation and dominance (Spilling et al., 2018). Likewise, P. catenata presence and/or maximum biomass was just minimally associated with SST or ice cover in this study, but were favored by short winter periods, i.e. lower number of days >3°C, in the Arkona Basin. Despite this, P. catenata biomasses have decreased strongly in the Eastern Gotland Basin and the Bornholm Basin since the beginning of the 21st century and nearly disappeared in all southern areas during the last decade. Also in the Gulf of Finland the proportion of P. catenata in the spring bloom community has decreased continuously (Klais et al., 2013). In contrast, other dinoflagellates, such as Biecheleria baltica, or Gymnodinium corollarium have continually increased in different Northern Baltic Sea basins over the past decades (Klais et al., 2013).
Several studies of the Baltic Sea have shown that changes in spring bloom species composition and species biomass are related to climate warming (e.g. Lewandowska and Sommer, 2010; Klais et al., 2011; Sommer and Lewandowska, 2011; Hjerne et al., 2019). The results of this study suggest that the ongoing trend of climate warming represents a threat to an ecologically specialized and functionally important component of the Baltic phytoplankton community. The loss of cold-water and ice-associated phytoplankton species in the southern and central parts of the Baltic Sea as important primary producers have already changed spring community composition, as shown here, with potential consequences for food webs and ecosystem function. Cold-water and under-ice blooming phytoplankton, specifically their lipids, can support zooplankton survival during winter (Grosbois et al., 2017). Loss of diatoms, for instance, is supposed to decrease the transport of organic matter to the benthic food web, potentially reducing secondary production (Tamelander and Heiskanen, 2004). In the deep areas of the Eastern Gotland and Bornholm Basin with minor or even no benthic community, decreased settling of organic material potentially partly reduces anoxic regions (Klais et al., 2013).
As the biomass of dinoflagellates is predominantly regenerated in the productive surface layer (Heiskanen, 1998), the loss of P. catenata could lead to decreased disintegration of organic material in the upper water column, which potentially reduce food availability for higher trophic levels in the pelagic system.
The ongoing increase in winter and spring SST (Hinrichsen et al., 2007, Stramska and Bialogrodzka 2015), concomitant with an ongoing reduction in ice cover (Meier et al., 2022) will lead to a decrease in low-salinity melt water and slower out-flowing surface waters and modulated overturning circulation (Burchard et al., 2018). Such will strongly reduce the seeding of Central Baltic Sea cold-water phytoplankton blooms from adjacent shallow Basins such as the Gulfs of Finland and Riga. The results of this study manifest that under such unfavorable conditions, cold-water phytoplankton and particularly the ice-associated diatoms will onward disappear from spring blooms in the southern and central areas of the Baltic Sea, with potential consequences for the ecosystem, the food web and the biogeochemical cycle. The future of the dinoflagellate P. catenata is less predictable.
However, the localisation of potential seed banks combined with genetic population analyses in the future would help to enhance the understanding of the origin of cold-water species in the Southern and Central Baltic Sea areas.
Publicly available datasets were analyzed in this study. This data can be found here: http://doi.io-warnemuende.de/10.12754/data-2023-0005. The time series of the numerical ocean model can be accessed here: https://zenodo.org/doi/10.5281/zenodo.7764361.
CP conducted the analyses of the phytoplankton data set, generated the graphs and conducted the statistical analyses. UG conducted the numerical ocean model analyses. CP wrote the manuscript together with all co-authors. All authors contributed to the article and approved the submitted version.
This research was funded by the DFG (German Research Foundation) with the project number: 426620852.
The author thanks the DFG for funding the project. The authors are grateful to Norbert Wasmund for his work on the data set collection. The authors further thank Christiane Hassenrück for the great support in statistical analyses. The BSH is thanked for providing the sea ice data of the German Baltic Sea coast. The authors thank the HELCOM, the IOW, LUNG, BED and particularly the data contributors for providing the monitoring data.
The authors declare that the research was conducted in the absence of any commercial or financial relationships that could be construed as a potential conflict of interest.
All claims expressed in this article are solely those of the authors and do not necessarily represent those of their affiliated organizations, or those of the publisher, the editors and the reviewers. Any product that may be evaluated in this article, or claim that may be made by its manufacturer, is not guaranteed or endorsed by the publisher.
The Supplementary Material for this article can be found online at: https://www.frontiersin.org/articles/10.3389/fmars.2023.1212412/full#supplementary-material
Alheit J., Mollmann C., Dutz J., Kornilovs G., Loewe P., Mohrholz V., et al. (2005). Synchronous ecological regime shifts in the central Baltic and the North Sea in the late 1980s. Ices J. Mar. Sci. 62, 1205–1215. doi: 10.1016/j.icesjms.2005.04.024
Andersson A., Tamminen T., Lehtinen S., Juergens K., Labrenz M., Viitasalo M. (2017). “The pelagic food web,” in Biological Oceanography of the Baltic Sea. Eds. Snoeijs-Leijonmalm P., Schubert H., Radziejewska T. (Dordrecht, The Netherlands: Springer Science and Business Media), 281–332. doi: 10.1007/978-94-007-0668-2
Burchard H., Bolding K., Feistel R., Gräwe U., Klingbeil K., MacCready P., et al. (2018). The Knudsen theorem and the Total Exchange Flow analysis framework applied to the Baltic Sea. Prog. Oceanogr. 165, 268–286. doi: 10.1016/j.pocean.2018.04.004
Edwards K. F., Thomas M. K., Klausmeier C. A., Litchman E. (2016). Phytoplankton growth and the interaction of light and temperature: a synthesis at the species and community level. Limnol. Oceanogr. 61, 1232–1244. doi: 10.1002/lno.10282
Fiala M., Oriol L. (1990). Light-temperature interactions in the growth on Antarctic diatoms. Pol. Biol. 10, 629–636. doi: 10.1007/BF00239374
Godhe A., Sjoeqvist C., Sildever S., Sefbom J., Hardardottir S., Bertos-Fortis M., et al. (2016). Physical barriers and environmental gradients cause spatial and temporal genetic differentiation of an extensive algal bloom. J. Biogeogr. 43.6, 1130–1142. doi: 10.1111/jbi.12722
Gräwe U., Klingbeil K., Kelln J., Dangendorf S. (2019). Decomposing mean sea level rise in a semi-enclosed basin, the Baltic Sea. J. Clim. 32, 3089–3108. doi: 10.1175/jcli-d-18-0174.1
Groetsch P. M. M., Simis S. G. H., Eleveld M. A., Peters S. W. M. (2016). Spring Blooms in the Baltic Sea have weakened but lengthened from 2000 to 2014. Biogeosci. Discus. 13, 4959–4973. doi: 10.5194/bg-2015-636
Grosbois G., Mariash H., Schneider T., Rautio M. (2017). Under-ice availability of phytoplankton lipids is key to freshwater zooplankton winter survival. Sci. Rep. 7, 11543. doi: 10.1038/s41598-017-10956-0
Haecky P., Jonsson S., Andersson A. (1998). Influence of sea ice on the composition of the spring phytoplankton bloom in the northern Baltic Sea. Pol. Biol. 20, 1–8. doi: 10.1007/s003000050270
Heiskanen A. S. (1998). Factors governing sedimentation and pelagic nutrient cycles in the Northern Baltic Sea. Monogr. Boreal. Environ. Res. 8, 1–80. Available at: http://urn.fi/URN:NBN:fi:bib:me:I00537326600
HELCOM (1996). Third periodic assessment of the state of the marine environment of the Baltic Sea 1989-1993; background document. Balt Sea Environ. Proc. 64 B, 252pp.
HELCOM (2006). Development of tools for assessment of eutrophication in the Baltic Sea, HELCOM Balt. Sea Environ. Proc. 104, 62pp.
HELCOM (2017) Manual for Marine Monitoring in the COMBINE Programme of HELCOM. Available at: http://helcomfi/Documents/Action%20areas/Monitoring%20and%20assessment/Manuals%20and%20Guidelines/Manual%20for%20Marine%20Monitoring%20in%20the%20COMBINE%20Programme%20of%20HELCOMpdf.
HELCOM (2021). Climate change in the Baltic sea. 2021 fact sheet., HELCOM balt. Sea Environ. Proc. n° 180. HELCOM/Baltic Earth 2021.
Hinrichsen H. H., Lehmann A., Petereit C., Schmidt J. (2007). Correlation analyses of Baltic Sea winter water mass formation and its impact on secondary and tertiary production. Oceanologica 49 (3), 381–395. Available at: https://www.researchgate.net/publication/26472645
Hjerne O., Hajdu S., Larsson U., Downing A. S., Winder M. (2019). Climate driven changes in timing, composition and magnitude of the baltic sea phytoplankton spring bloom. Front. Mar. Sci. 6. doi: 10.3389/fmars.2019.00482
Hordoir R., Meier H. E. M.. (2010). Freshwater fluxes in the Baltic Sea: A model study. J. Geophys. Res. 115. doi: 10.1029/2009JC005604
Hoglander H., Larsson U., Hajdu S. (2004). Vertical distribution and settling of spring phytoplankton in the offshore NW Baltic Sea proper. Mar. Ecol. Prog. Ser. 283, 15–27. doi: 10.3354/meps283015
Kara A. B., Rochford P. A., Hurlburt H. E. (2000). An optimal definition for Ocean Mixed Layer Depth. J. Geophys. Res. 105 (C7), 803–821. doi: 10.1029/2000JC900072
Klais R., Tamminen T., Kremp A., Spilling K., An B. W., Hajdu S., et al. (2013). Spring phytoplankton communities shaped by interannual weather variability and dispersal limitation: Mechanisms of climate change effects on key coastal primary producers. Limnol. Oceanogr. 58, 753–762. doi: 10.4319/lo.2013.58.2.0753
Klais R., Tamminen T., Kremp A., Spilling K., Olli K. (2011). Decadal-scale changes of dinoflagellates and diatoms in the anomalous Baltic Sea spring bloom. PloS One. 6 (6), e21567 doi: 10.1371/journal.pone.0021567
Kononen K., Niemi A. (1984). Long-term variation of the phytoplankton composition at the entrance to the Gulf of Finland. Ophelia, 101–110.
Kremp A., Anderson D. M. (2000). Factors regulating germination of resting cysts of the spring bloom dinoflagellate Scrippsiella hangoei from the northern Baltic Sea. J. Plankton Res. 22, 1311–1327. doi: 10.1093/plankt/22.7.1311
Kremp A., Tamminen T., Spilling K. (2008). Dinoflagellate bloom formation in natural assemblages with diatoms: nutrient competition and growth strategies in Baltic spring phytoplankton. Aqua Microb. Biol. 50, 181–196. doi: 10.3354/ame01163
Lewandowska A., Sommer U. (2010). Climate change and the spring bloom: a mesocosm study on the influence of light and temperature on phytoplankton and mesozooplankton. Mar. Ecol. Prog. Ser. 405, 101–111. doi: 10.3354/meps08520
Liu Y., Meier H. E. M., Eilola K. (2017). Nutrient transports in the Baltic Sea - results from a 30-year physical-biogeochemical reanalysis. Biogeosci> 14, 2113–2131. doi: 10.5194/bg-14-2113.2017
Meier H. E. M., Dieterich C., Groger M., Dutheil C., Borgel F., Safonova K., et al. (2022). Oceanographic regional climate projections for the Baltic Sea until 2100. Earth Syst. Dyn. 13, 159–199. doi: 10.5194/esd-13-159-2022
Nausch G., Nehring D., Nagel K. (2008). Nutrient concentrations, trends and their relation to eutrophication. in: State and evolution of the Baltic Sea 1952-2005. Eds. Feistel R., Nausch G., Wasmund N. (Hoboken NJ: John Wiley & Sons, Inc), 337–366.
Norrmann B., Andersson A. (1994). Development of ice biota in a temperate sea area (Gulf of Bothnia). Pol. Biol., 531–537. doi: 10.1007/BF00238222
Olenina I., Hajdu S., Andersson A., Edler L., Wasmund N., Busch S., et al. (2006). Biovolumes and size-classes of phytoplankton in the Baltic Sea. HELCOM Balt. Sea Environ. Proc. 106, 144pp.
Poulin M., Daugbjerg N., Gradinger R., Ilyash L., Ratkova T., von Quillfeldt C. (2011). The pan-Arctic biodiversity of marine pelagic and sea-ice unicellular eukaryotes: a first-attempt assessment. Mar. Biodiv. 41, 13–28. doi: 10.1007/s12526-010-0058-8
Rengefors K., Anderson D. M. (1998). Environmental and endogenous regulation of cyst germination in two freshwater dinoflagellates. J. Phyco. 34, 568–577. doi: 10.1046/j.1529-8817.1998.340568.x
Schmelzer N., Holfort J. (2012). Klimatologischer Eisatlas für die westliche und südliche Ostsee, (1961-2010), Bundesamt für Seeschifffahrt und Hydrographie (BSH) Hamburg und Rostock, Germany, 6–9.
Schmelzer N., Holfort J. (2015). “Das Eis in der Nord- und Ostsee,” in Warnsignal Klima: Das Eis der Erde. Eds. Lozán J. L., Grassl H., Kasang D., Notz D., Escher-Vetter (Hrsg.) H., 210–217. Available at: https://www.klima-warnsignale.uni-hamburg.de. doi: 10.2312/warnsignal.klima.eis-der-erde.32
Schwegmann S., Holfort J. (2021). Regional distributed trends of sea ice volume in the Baltic Sea for the 30-year period 1982 to 2019. Meteorologische Zeitschrift (Contrib. Atm. Sci) 30, 1–11. doi: 10.1127/metz/2020/0986
Sommer U., Lewandowska A. (2011). Climate change and the phytoplankton spring bloom: warming and overwintering zooplankton have similar effects on phytoplankton. Global Change Biol. 17, 154–162. doi: 10.1111/j.1365-2486.2010.02182
Spilling K., Olli K., Lehtoranta J., Kremp A., Tedesco L., Tamelander T., et al. (2018). Shifting diatom-dinoflagellate dominance during spring bloom in the Baltic Sea and its potential effects on biogeochemical cycling. Front. Mar. Sci. 5. doi: 10.3389/fmars.2018.00327
Stramska M., Bialogrodzka J.. (2015). Spatial and temporal variability of sea surface temperature in the Baltic Sea based on 32-years (1982-2013) of satellite data. Oceanologia 57, 223–235. doi: 10.1016/j.oceano.2015.04.004
Sundström A. M., Kremp A., Daugbjerg N., Moestrup Ø., Ellegaard M., Hansen R., et al. (2009). Gymnodinium corollarium sp. nov. (dinophyceae) - a new cold water dinoflagellate responsible for cyst sedimentation events in the Baltic Sea. J. Phycol. 45, 938–952. doi: 10.1111/j.1529-8817.2009.00712.x
Tamelander T., Heiskanen A. S. (2004). Effects of spring bloom phytoplankton dynamics and hydrography on the composition of settling material in the coastal northern Baltic Sea. J. Mar. Syst. 52, 217–234. doi: 10.1016/j.jmarsys.2004.02.001
Team B. A. (2008). Assessment of Climate Change for the Baltic Sea Basin. Regional Climate Studies (Berlin, Heidelberg: Springer).
Tuovinen N., Virtasalo J. J., Kotilainen A. T. (2008). Holocene diatom stratigraphy in the Archipelago Sea, northern Baltic Sea. J. Paleolimnol. 40, 793–807. doi: 10.1007/s10933-008-9199-1
Utermöhl H. (1958). Zur Vervollkommnung der quantitativen Phytoplankton-Methodik. Mitt. d. Internat. Vereinig. f. Limnol. 9, 1–38.
von Storch H., Omstedt A., Pawlak J., Reckemann M. (2015). Second Assessment of Climate Change for the Baltic Sea Basin (Berlin, Heidelberg: Springer).
Wasmund N., AndrusHaitis A., Lysiak-Pastuszak E., Müller-Karulis B., Nausch G., Neumann T., et al. (2001). Trophic status of the south-eastern Baltic Sea: a comparison of coastal and open areas. Estuar. Coast. Shelf Sci. 53, 849–864. doi: 10.1006/ecss.2001.0828
Wasmund N., Nausch G., Matthaus W. (1998). Phytoplankton spring blooms in the southern Baltic Sea - spatio-temporal development and long-term trends. J. Plankton Res. 20, 1099–1117. doi: 10.1093/plankt/20.6.1099
Wasmund N., Pollehne F., Postel L., Siegel H., Zettler M. L. (2012). Biologische Zustandseinschätzung der Ostsee im Jahre 2011. Mar. Sci. Rep. 98, 1–87. Available at: http://www.io-warnemuende.de/meereswissenschaftliche-berichte.htm
Wasmund N., Tuimala J., Suikkanen S., Vandepitte L., Kraberg A. (2011). Long-term trends in phytoplankton composition in the western and central Baltic Sea. J. Mar. Syst. 87, 145–159. doi: 10.1016/j.jmarsys.2011.03.010
Keywords: ice algae, cold-water phytoplankton, Baltic Sea, climate change, warming
Citation: Paul C, Gräwe U and Kremp A (2023) Long-term changes in bloom dynamics of Southern and Central Baltic cold-water phytoplankton. Front. Mar. Sci. 10:1212412. doi: 10.3389/fmars.2023.1212412
Received: 26 April 2023; Accepted: 13 October 2023;
Published: 03 November 2023.
Edited by:
Patrick J. Neale, Smithsonian Environmental Research Center (SI), United StatesReviewed by:
Jeanette Gann, National Oceanic and Atmospheric Administration (NOAA), United StatesCopyright © 2023 Paul, Gräwe and Kremp. This is an open-access article distributed under the terms of the Creative Commons Attribution License (CC BY). The use, distribution or reproduction in other forums is permitted, provided the original author(s) and the copyright owner(s) are credited and that the original publication in this journal is cited, in accordance with accepted academic practice. No use, distribution or reproduction is permitted which does not comply with these terms.
*Correspondence: Carolin Paul, Y2Fyb2xpbi5wYXVsQGlvLXdhcm5lbXVlbmRlLmRl
Disclaimer: All claims expressed in this article are solely those of the authors and do not necessarily represent those of their affiliated organizations, or those of the publisher, the editors and the reviewers. Any product that may be evaluated in this article or claim that may be made by its manufacturer is not guaranteed or endorsed by the publisher.
Research integrity at Frontiers
Learn more about the work of our research integrity team to safeguard the quality of each article we publish.