- 1Guangdong Provincial Key Laboratory of Marine Biology, Shantou University, Shantou, China
- 2Institute of Marine Sciences, Shantou University, Shantou, China
- 3Department of Technical Service, Guangzhou Insighter Biotechnology Co., Ltd, Guangzhou, China
Introduction: The fungus Aspergillus niger is found to be predominant in the body of Pacific white shrimp (Penaeus vannamei); however, its role in shrimp’s health has not been explored. This study aimed to evaluate the influences of A. niger on growth, antioxidative activity, immune response, and gut microbiota of juvenile Pacific white shrimps under farming conditions.
Methods: Shrimps (2.30±1.05 g) were fed diets supplemented with 0 (control), 1.5 (A1.5), or 3.0 g/kg diet of A. niger (A3.0) for one month. At the end of the study, the growth, antioxidant enzyme activity and immune parameters, and gut microbiota in shrimps fed with different experimental diets were analyzed.
Results: The results showed a significant increase in weight gain, specific growth rate, and length gain of shrimps in A1.5, compared with other groups (P<0.05). With the exception of lysozyme in both A1.5 and A3.0, which were lower than in the control, the activity of superoxide dismutase (SOD), catalase, acid phosphatase, alkaline phosphatase, and phenol oxidase was higher than those in the control. Gene expressions of SOD, anti-lipopolysaccharide factor-ALF1, and ALF2 (in A1.5 and A3.0) and nitric oxide synthase, penaeidin-PEN2 (in A1.5) and PEN3 and PEN4 (in A3.0) were significantly up-regulated compared to the control (P<0.05). The addition of A. niger increased diversity and separated the microbial community in the gut of shrimps. At the phylum level, Firmicutes and Bacteroidota were dominant, and Proteobacteria was less abundant in the A1.5 and A3.0, as compared to the control. At the genus level, the relative abundance of Vibrio, Marivita, and Roseobacter was increased but Ruegeria was decreased in A1.5 and A3.0 when compared to the control. The genera Edwardsiella, Clostridium sensu stricto 1, and Shimia in the A1.5 were higher than in the control.
Discussion: These findings demonstrated the beneficial effects of A. niger on growth, health, and changes in the gut microbiota in shrimps under farming conditions. The recommended concentration of A. niger in the diet for shrimps is 1.5 g/kg diet.
1 Introduction
Aquaculture is one of the fastest-growing and largest food production sectors that play an important role in providing sustainable livelihood opportunities and food security for the world population (Dawood et al., 2019; Stentiford et al., 2020). The development of practice models that contributes to the outstanding growth of the aquaculture industry and promotes the increase in terms of production has been considered. Pacific white shrimp (Penaeus vannamei) is one of the most important marine aquaculture species globally, accounting for approximately 80% of the total annual production (Zheng et al., 2021b). Similar to the general situation of large-scale intensive aquaculture systems, the shrimp aquaculture industry faces to deteriorating farming environment and frequent disease outbreaks, which cause great economic loss (Deng et al., 2013; Zheng et al., 2021a). The developed methods of treatment that overcome growth performance and increase disease resistance of shrimps are needed (Deng et al., 2013). Several reports suggested that aquatic animals fed with a diet supplemented with prebiotics, probiotics, synbiotics, or postbiotics revealed an enhancement in growth performance, immune response, and disease resistance when compared to their non-added counterparts (Hai, 2015; Hoseinifar et al., 2016; Ringø, 2020; Tran et al., 2020; Tran and Li, 2022). In crustaceans, probiotics bacteria, such as the members of Bacillus, Enterococcus, Lactobacillus, and Pediococcus, that showed improving growth and digestive enzyme activity, modulating gut microbiota, and enhancing immune response and hematological parameters have been widely investigated (Ringø, 2020; Tran and Li, 2022). In Pacific white shrimp, for example, the mixed-species probiotics (Bacillus subtilis, Bacillus licheniformis, and Lactobacillus sp.) have shown benefits to their hosts, including enhancing growth and non-specific immunity, changing intestinal morphology, and modulating gut microbiota (Xie et al., 2019). Furthermore, the application of yeasts, yeast extract, and other fungal-origin compounds (such as β-glucan) showed beneficial effects on growth, antioxidant ability, immunity, disease resistance, and intestinal microbiota of Pacific white shrimp (Murthy et al., 2009; Deng et al., 2013; Boonanuntanasarn et al., 2016; Li et al., 2019; Ma et al., 2019; Ayiku et al., 2020; Zheng et al., 2021a).
The fungus Aspergillus niger has been reported as one of the predominant species of the genus Aspergillus harboring the body of Pacific white shrimp (Cruz da Silva et al., 2011). Previous studies have shown that A. niger used for the fermentation of groundnut oil cake, soybean meal, sunflower oil cake, and plant protein mix can enhance growth, feed efficiency, and hemolymph indices in Pacific white shrimp (Jannathulla et al., 2018a; Jannathulla et al., 2018b; Dayal et al., 2020; Jannathulla and Dayal, 2022). Recently, in common carp (Cyprinus carpio), supplementing A. niger in the diet increased survival rate, growth performance, feed utilization, immune responses, hematological indexes, digestive enzyme activity, and the load of the fungi in the intestine (Jasim et al., 2022). Therefore, results of previous studies have proven the potential use of A. niger as a probiotic organism conferring health benefits to aquatic animals. However, the influence of A. niger as the dietary probiotic supplementation in Pacific white shrimps has not been investigated. This study aimed to evaluate the influences of A. niger (Insighter Co. Ltd., Guangzhou, Guangdong, China) on growth, oxidative activity, immune response, and gut microbiota of juvenile Pacific white shrimps under farming conditions. The results of this study provided basic information on the potential use of A. niger as a probiotic organism in shrimp aquaculture.
2 Materials and methods
2.1 Shrimps, bacterial sources, and feeding trial
The study was conducted in a commercial shrimp farm in Lufeng, Shanwei, Guangdong Province, China (Latitude: 22°50’42.1” N; Longitude: 116°08’11.0” E). Healthy juvenile Pacific white shrimp (initial body weight: 2.30 ± 1.05 g; initial body length: 7.11 ± 1.31 cm) were cultured in earth ponds fulfilled with filtered aerated seawater (salinity 30‰, pH~8.0). The shrimps were reared in three ponds (pond area: 660 m2/pond; water depth: 1.8-2.0 m; stocking density: 350,000 post-larvae/pond) with aerators operated 24/24. All shrimps were fed with a commercial pelleted feed (≥43% crude protein, ≤15% crude ash, ≥5.0% crude lipid, ≤5.0% crude fiber, ≥2.6% lysine, ≤11% moisture, and 0.9-2.0% total phosphorus) (Fuzhou Haima Feed Co., Ltd., Fujian, China) four times per day. The culture water was exchanged twice daily.
The fungus A. niger (5×108 CFU/g) was made by Insighter Co. Ltd. (Guangzhou, Guangdong, China). The commercial pelleted feed mentioned above was used as a basal diet. Three diets, including control (basal diet supplemented with PBS), A1.5 (basal diet supplemented with A. niger at 1.5 g/kg diet), and A3.0 (basal diet supplemented with A. niger at 3.0 g/kg diet), were used for the feeding. The concentrations of A. niger used in this study were followed by recommendations from the manufacturer. The fungal powder was first mixed into PBS, and the suspension was sprayed uniformly onto the feed and dried at room temperature for 30 minutes before use. Shrimps in each pond were fed four times daily with corresponding diets, and the diets supplemented with A. niger were applied twice a day (at 10:00 and 14:00). During the experimental period, the temperature was about 30°C, pH was 7.5 to 8.5, salinity was about 30‰, and dissolved oxygen was higher than 5.5 mg/L. The water in culture ponds was exchanged eight times daily (water exchange rate about 5% each time). The feeding was conducted for one month.
2.2 Growth parameter analyses
At the beginning and end of the feeding trial (one month), 30 shrimps from each pond were collected and weighed to assess weight gain, specific growth rate, and length gain. The growth parameters were calculated as follows:
Weight gain rate (%) = [(Final body weight-Initial body weight)/Initial body weight]×100%
Specific growth rate (%/day) = [(ln Final body weight – ln Initial body weight)/t]×100%
Length gain (%) = [(Final body length - Initial body length)/Initial body length]×100%
2.3 Antioxidant enzyme activity and immune parameter analyses
At the end of the feeding trial, 30 shrimps from each pond were collected. Shrimps were chilled on ice and then used for collecting hepatopancreas and intestine samples. The 10 pooled samples were immediately frozen in liquid nitrogen and stored at −80°C until further analysis. The hepatopancreas samples (n=3) were homogenized with 10-fold ice-cold phosphate buffer separately. The homogenate was centrifuged at 4°C at 4000 ×g for 10 min to collect the supernatant, which was then used for analyzing of enzyme activities. The activities of superoxide dismutase (SOD), catalase, phenol oxidase (PO), lysozyme, acid phosphatase (ACP), and alkaline phosphatase (AKP) were measured using kits of Nanjing Jiancheng Bioengineering Institute (Nanjing, China) according to the manufacturer’s instructions.
2.4 RNA extraction, cDNA library synthesis, and gene expression quantification
Total RNA was extracted from the intestine of shrimps using TRIzol® Reagent (Ambion, USA), which was followed by synthesis of the first-strand cDNA with PrimeScript™ RT Reagent Kit (TaKaRa, Dalian, China) according to the manufacturer’s instructions. RT-qPCR was performed with Premix Ex Taq (Probe qPCR) (TaKaRa, Dalian, China) to investigate the expression of SOD, nitric oxide synthase (NOS), prophenoloxidase (proPO), and antimicrobial peptides (AMPs, including crustin, anti-lipopolysaccharide factor-ALF1, ALF2, penaeidin-PEN2, PEN3, and PEN4) in the shrimp’s intestine. Primers used for the amplification of mRNA transcripts was listed in Table 1. The β-actin was used as the internal control. The RT-qPCR conditions were conducted at 95°C for 30 s, followed by 40 cycles at 95°C for 5 s and 60°C for 20 s, and 72°C for 15 s. The relative expression of genes was normalized to the β-actin gene expression, and calculated by the 2−ΔΔCt method (Livak and Schmittgen, 2001).
2.5 Intestinal microbiota analysis
The gut contents collected from 30 individuals in each experimental group were used for analyzing changes in the gut microbiota of shrimps fed with A. niger-containing diets as compared to the control. Gut contents from 10 shrimps were pooled for each biological replicate, and the pooled samples were used for DNA extraction using TIANamp Genomic DNA Kit (Tiangen Biotech Co., Ltd., Beijing, China) according to the manufacturer’s instructions. The extracted DNA was used as the template for amplifying the V3-V4 hypervariable region of 16S rRNA using universal primers 341F/806R with the barcode. PCR reactions (30 µL) contained 10 ng of DNA, 15 µL of Phusion R High-Fidelity PCR Master Mix with GC buffer (New England Biolabs, Ipswich, MA, USA), 0.2 µM of forward and reverse primers, and 2 µL of distilled water. The PCR program included an initial denaturation (at 98°C) for 1 min, 30 cycles of 98°C for 10 s, 56°C for 30 s, and 72°C for 30 s, and a final elongation step (at 72°C) for 5 min. PCR products were purified using DNA Gel Extraction Kit (Axygen, Hangzhou, China). Purified PCR products were used for synthesizing sequencing libraries and the libraries were sequenced using Illumina-based high-throughput sequencing (Illumina NovaSeq6000).
The unique barcode was used for assigning single-end reads to each sample. Reads were analyzed with the UCHIME algorithm (Edgar et al., 2011) and the reads were assigned to operational taxonomic units (OTUs) based on a similarity cutoff of 97% using UPARSE software (Version 7.0.1001) (Edgar, 2013). Representative sequences from each OTU were classified using the SILVA reference database (confidence threshold of 80%). The alpha and beta diversity parameters were analyzed using QIIME (Version 1.7.0) (Caporaso et al., 2010). Principal coordinates analysis (PCoA) used to visualize the Jaccard distances among samples was analyzed using VEGAN (Dixon, 2003). Raw sequencing reads have been deposited in GenBank under BioProject code PRJNA900677.
2.6 Data analyses
All data are shown as mean ± Std. Differences between groups were analyzed using an Independent-sample t-test on SPSS 16.0 (IBM Corporation, Armonk, NY, USA). Statistically significant differences were considered at P<0.05.
3 Results
3.1 Growth parameters
The growth performance of shrimps fed diets supplemented with or without A. niger is shown in Figure 1. Results showed that the weight gain, specific growth rate, and length gain of shrimps in the A1.5 were significantly higher than those in the A3.0 and control (P<0.05). The obtained data indicated that the supplementation of A. niger (at 1.5 g/kg diet) can improve the growth of shrimps under culture conditions.
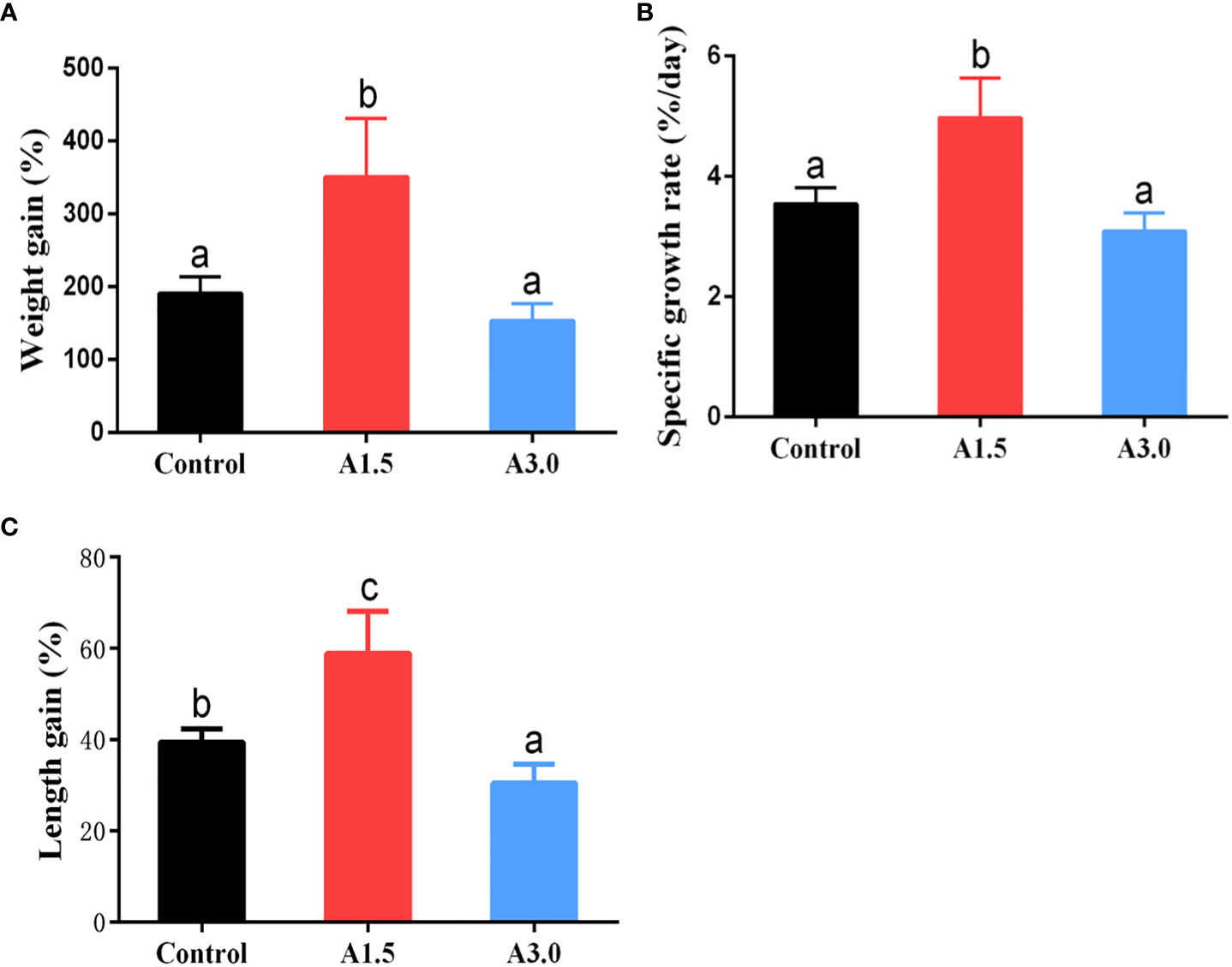
Figure 1 Growth parameters (as shown by weight grain (A), specific growth rate (B) and length gain (C)) of Pacific white shrimp fed diets supplemented with different Aspergillus niger concentrations for one month. Values are expressed as means ± Std from 30 shrimps in each group. Different superscript letters indicate significant differences among experimental groups (P<0.05).
3.2 Antioxidant enzyme activity and immune response
The activity of antioxidant enzymes and immune responses of shrimps fed with different experimental diets are shown in Figure 2. The activities of SOD and catalase in shrimps in A1.5 and A3.0 were significantly higher than those of the control (P<0.05), with the highest activity in the A3.0. The lysozyme activity was significantly decreased in A1.5 and A3.0 as compared to the control (P<0.05). The activities of ACP and AKP in A. niger-fed shrimps were significantly higher than that in controls (P<0.05), with the exception of ACP in the A3.0, which was not significantly different from the control (P>0.05). The activity of PO of shrimps in A1.5 and A3.0 was significantly higher than in the control (P<0.05).
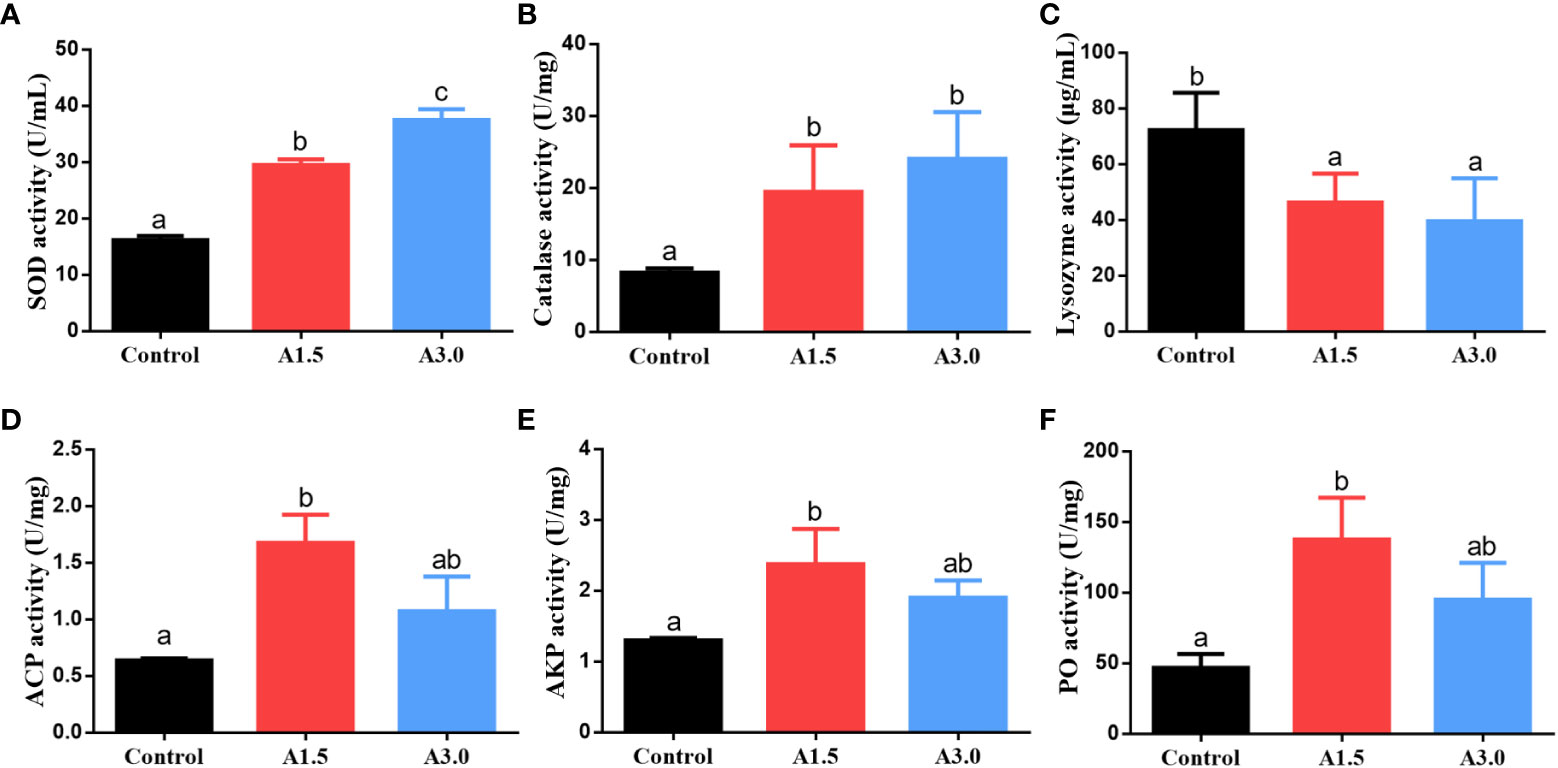
Figure 2 Activity of the enzymes (superoxide dismutase (SOD) (A), catalase (B), lysozyme (C), acid phosphatase (ACP) (D), alkaline phosphatase (AKP) (E), and phenol oxidase (PO) (F)) in the hepatopancreas of Pacific white shrimp fed diets supplemented with different Aspergillus niger concentrations for one month. Values are expressed as means ± Std from three samples. Different superscript letters indicate significant differences among experimental groups (P<0.05).
The expressions of NOS, SOD, crustin, ALF1, ALF2, PEN2, PEN3, PEN4, and proPO genes in the intestine of shrimps fed different diets were assessed using the RT-qPCR (Figure 3). Results revealed that the expression of SOD was significantly increased in A1.5 and A3.0; whereas those of NOS was significantly increased in the A3.0 only as compared to the control (P<0.05). Crustin gene expression in shrimps in A1.5 and A3.0 was significantly lower than in the control (P<0.05). Shrimps in A1.5 and A3.0 had significantly higher ALF1 and ALF2 gene expression when compared to their counterparts in the control (P<0.05). The significantly increased expression of PEN2 was only found in shrimps in the A1.5 and PEN3 and PEN4 were only in the A3.0 as compared to the control (P<0.05). There was an increase in the expression of proPO in the intestine of shrimps fed A. niger-containing diets but did not significantly differ from that of the controls (P>0.05).
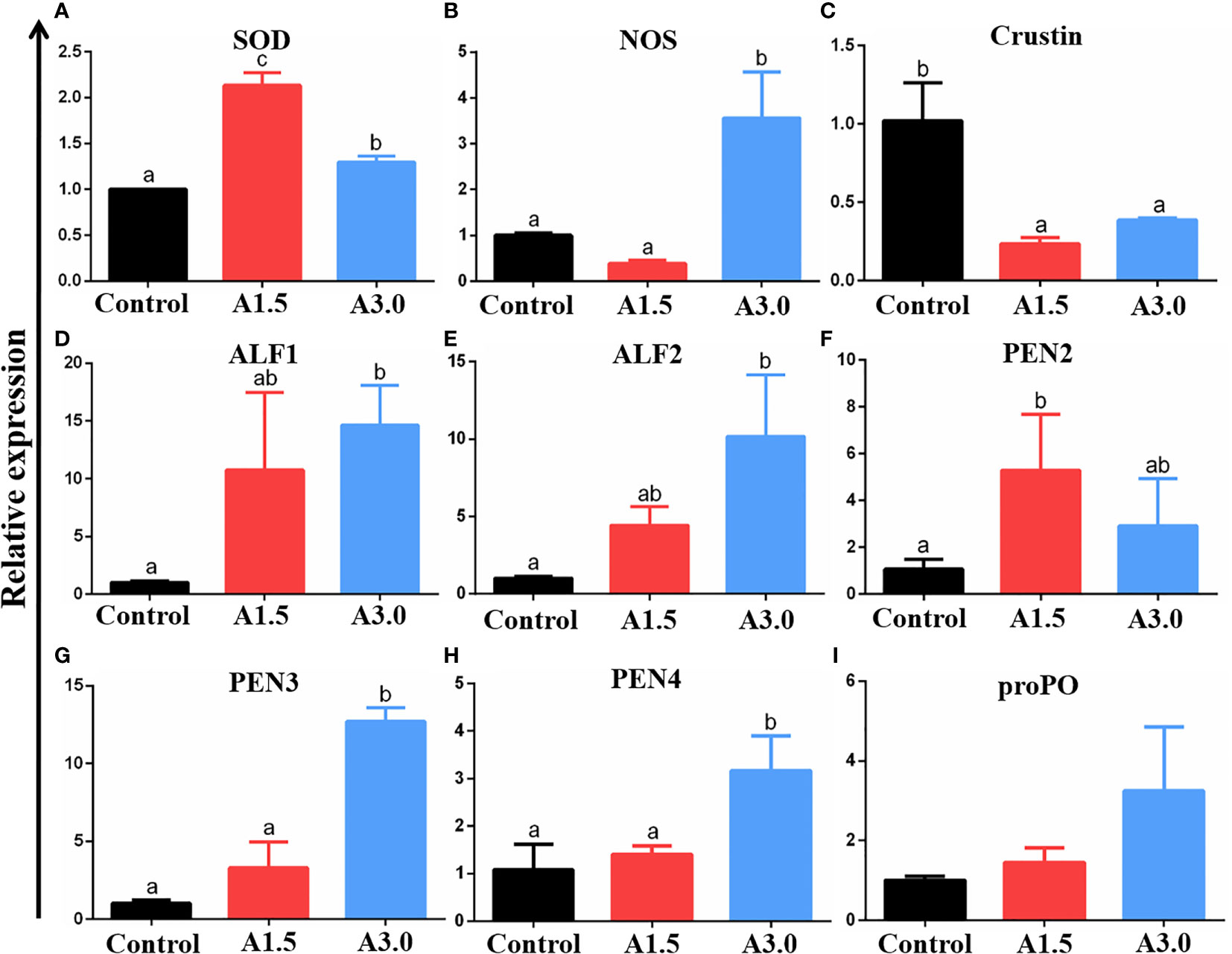
Figure 3 Relative mRNA expressions of genes (superoxide dismutase (SOD) (A), nitric oxide synthase (NOS) (B), crustin (C), anti-lipopolysaccharide factor (ALF)-1 (D), ALF-2 (E), Penaeidin(PEN)-2 (F), PEN3 (G), PEN4 (H), and prophenoloxidase (proPO) (I)) (as analyzed by RT-qPCR) in the intestine of Pacific white shrimp fed diets supplemented with different Aspergillus niger concentrations for one month. The values are expressed as means ± Std from three samples. Different superscript letters indicate significant differences among experimental groups (P<0.05).
3.3 Gut microbiota analysis
Microbial alpha diversity in samples was evaluated based on the Shannon and Simpson indexes, and results showed a significant increase in the microbial diversity (as calculated by Shannon and Simpson indexes) in both A1.5 and A3.0 over the control (P<0.05) (Figures 4A, B). The PCoA analysis revealed a distinct separation in the microbial community among the experimental groups (Figure 4C). The findings suggest changes in gut microbiota of shrimps after feeding with A. niger-containing diets.
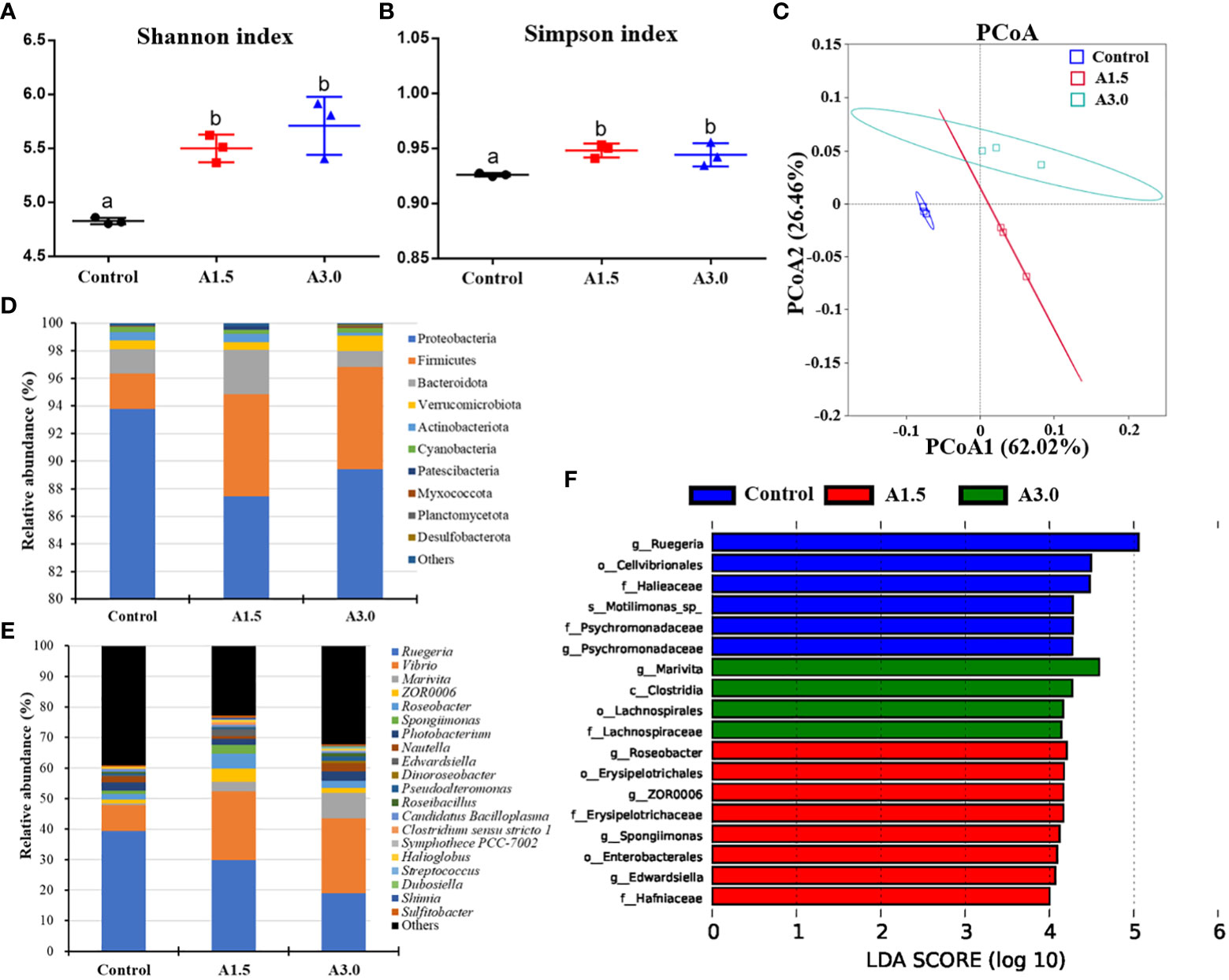
Figure 4 Modulation of gut microbiota of Pacific white shrimp fed diets supplemented with different Aspergillus niger concentrations for one month. (A) and (B) Alpha diversity indices. (C) Principal coordinates analysis (PCoA) (PCoA1: 62.02% and PCoA2: 26.46% of the explained variance). (D) Predominant phyla and (E) genera of microbiota in the intestine. (F) Linear discriminant analysis effect size (LEfSe) (LDA>4, P<0.05). Values are expressed as means ± Std from three samples. Different superscript letters indicate significant differences among experimental groups (P<0.05).
Differences in the major taxonomical profiles of the gut microbiota in the shrimps fed with A. niger-containing diets and the controls were analyzed. The top 10 phyla are illustrated in Figure 4D. Relative abundance of the phylum Proteobacteria in the A1.5 (87.45 ± 4.46%, t=2.41, P=0.13), A3.0 (89.43 ± 1.86%, t=3.59, P=0.02) were lower than that in the control (93.8 ± 0.99%). In contrast, the abundance of Firmicutes was enriched in the A1.5 (7.4 ± 1.84%, t=-4.48, P=0.01) and A3.0 (7.4 ± 1.25%, t=-6.46, P=0.00) as compared to the control (2.55 ± 0.37%). A higher relative abundance of Bacteroidota was observed in the A1.5 (t=-1.24, P=0.34) and A3.0 (t=2.01, P=0.12) in comparison to the control group. At the genus level, results showed that several bacterial genera shifted their relative abundance in the gut of shrimps after feeding with A. niger-containing diets. The most abundant genera are shown in Figure 4E. There was a significant increase in the relative abundance of the genera Vibrio (t=-30.0, P=0.00, and t=-3.64, P=0.07), Marivita (t=-6.03, P=0.00, and t=-7.49, P=0.02), and Roseobacter (t=-5.49, P=0.03, and t=-2.36, P=0.08) in A1.5 and A3.0, respectively, when compared to the control. However, the relative abundance of Ruegeria was decreased in the A1.5 (t=5.99, P=0.03) and A3.0 (t=17.26, P=0.00) as compared to the control. The genera Edwardsiella (t=-5.93, P=0.00), Clostridium sensu stricto 1 (t=-5.87, P=0.03), and Shimia (t=-25.63, P=0.00) were significantly increased in the A1.5 as compared with such in the control.
The LEfSe analysis (LDA>4.0, P<0.05) identified the bacterial taxa related to the experimental groups (Figure 4F). The results revealed that the taxa Clostridia, Lachnospiraceae, Lachnospirales, and Marivita were enriched in the A3.0 group, whereas, Erysipelotrichales, Erysipelotrichaceae, Enterobacterales, Hafniaceae, Roseobacter, ZOR0006, Spongiimonas, and Edwardsiella were predominant in the A1.5, as well as, Cellvibrionales, Halieaceae, Psychromonadaceae, Ruegeria, Motilimonas, were in the control.
4 Discussion
Sharma et al. (2022) indicated that A. niger is a potential probiotic, which can increase egg production and quality, and decrease the cecal microbial load of pathogenic bacteria (such as Clostridium perfringens, Salmonella spp., and Escherichia coli) in laying hens. In aquaculture, A. niger has been demonstrated to be a potential probiotic that confers health benefits on common carp (including the enhancement of growth performance, immunity, hematology, the load of the gut fungi, and digestive enzymes) (Jasim et al., 2022). However, the probiotic potential of A. niger on shrimp has not been evaluated yet. In this study, the effects of A. niger on growth performance, antioxidative activity, immune response, and changes in the gut microbiota of juvenile Pacific white shrimp under farming conditions were investigated for the first time. Results showed that the inclusion of A. niger can enhance the weight gain, specific growth rate, and length gain of shrimps, especially in the A1.5, indicating the growth promotion of A. niger (at 1.5 g/kg diet) shrimps under culture conditions. This similar to the previous findings in common carp fed a diet supplemented with A. niger (at 1×103 or 1×106 CFU/g diet) (Jasim et al., 2022). Jasim et al. (2022) showed an improvement in the final length and weight, weight gain, thermal growth coefficient, protein efficiency ratio, lipid efficiency ratio, feed conversion ratio, immune response, and activity of digestive enzymes of fish fed with diets supplemented with A. niger. Also, in another study, supplementation of Aspergillus oryzae singly or in combination with β-glucan can increase the growth, feed efficiency, antioxidant status, and immunomodulation of Nile tilapia (Dawood et al., 2020). The improvement in the growth of fish by A. niger supplementation has been discussed to relate to the positive benefits on feed digestibility, activity of digestive enzymes (protease, amylase, lipase, alkaline phosphatase, and trypsin), and feed absorbance efficiency with an increase in the absorptive area, microvilli density and height (Dawood et al., 2020; Jasim et al., 2022). Our study observed that A. niger added to the diet of shrimps (at 1.5 g/kg diet, A1.5) showed the best effectiveness in the growth performance of shrimps; however, the mechanisms of action were lacking, which merits further investigations. Collectively, the outcomes of this current study indicate that the low dose of A. niger (1.5 g/kg diet) is more suitable for use in shrimp aquaculture.
The antioxidant system plays an important role in the physiological response of hosts in responding to effects of noninfectious or infectious agents. SOD and catalase are the main components in the antioxidant system that protects hosts against oxidative stress, specific to the overproduction and residuals of reactive oxygen species during inflammatory responses (Parrilla-Taylor et al., 2013). Our results revealed that the activities of SOD and catalase of shrimps were increased in A1.5 and A3.0 as compared to the control, indicating that A. niger-containing diets can enhance the antioxidant capacity in juvenile Pacific white shrimp. Moreover, NOS, which is one of the oxidation-derived compounds, has been proven to have antimicrobial effects on the innate immunity of crustaceans (Li et al., 2012b). The result herein showed the increased expression of the NOS gene in shrimps fed the A. niger-containing diet (A3.0), suggesting the stimulation of the probiotic to the induction of NOS. A similar observation was found in mouse macrophagocytes that showed the expression of inducible NOS after being treated with β-glucan derived from Aureobasidum pullans SM-2001 (Choi et al., 2016). Thus, it is speculated that A. niger can activate the non-specific defense of shrimps by stimulating NOS production.
Lysozyme activity is important in innate immunity, which is responsible for attacking and causing damage to the cell structures of invading bacteria (Saurabh and Sahoo, 2008). The lysozyme activity was found to increase in Nile tilapia (Oreochromis niloticus) fed with probiotic-or synbiotic-supplemented diets (Sîrbu et al., 2022), Chinese drum (Miichthys miiuy) fed with live or dead cells of Clostridium butyrium CB2-supplemented diets (Pan et al., 2008), and red sea bream (Pagrus major) fed with β-glucan-supplemented diets (Dawood et al., 2017) but to remain unchanged in grouper (Epinephelus coioides) fed with Bacillus pumilus or B. clausii (Sun et al., 2010). However, our findings found that dietary A. niger supplement significantly decreased the lysozyme activity in shrimps, referring to that the probiotic showed negative effects on the activity of lysozyme in the innate immunity of shrimps. Differences in results among studies may be due to differences in probiotic organisms, dosage, host species, experimental designs, and nutritional and environmental factors. Furthermore, acid phosphatase (ACP) and alkaline phosphatase (AKP) are important in the immune system of crustaceans, where the former is responsible for digesting invading pathogens and the latter is for detoxifying during the normal living and phagolysis and digesting and absorbing nutrients (Xie et al., 2019). The findings herein showed an increase in activities of ACP and AKP in the shrimps fed A. niger-containing diets. Similar results were observed in the Pacific white shrimp fed with a probiotic mixture (Xie et al., 2019) and ridgetail white prawn (Exopalaemon carinicauda) fed with Psychrobacter sp. B6 (Lai et al., 2022).
Antimicrobial peptides (AMPs) contribute to the innate immune system as the first line of defense against invading pathogens. The current study found that crustin, which showed antibacterial activity against Gram-positive and Gram-negative bacteria, and virus (Du et al., 2019), was down-regulated in the shrimps fed with A. niger. This is consistent with findings of the giant tiger shrimp crustin-like, which was down-regulated in the Candida haemulonii S27-fed shrimps, and then up-regulated in these animals after challenge with WSSV (Antony et al., 2011). Furthermore, ALFs and PENs are important in the immune defense of shrimps, showing broad-spectrum antimicrobial activities against bacteria, fungi, parasites, and viruses (Gu et al., 2018; Wu et al., 2019). The increase of gene expression of ALF1, ALF2, PEN2, PEN3, and PEN4 in shrimps fed with A. niger-containing diets suggested that the probiotic can promote the production of these AMPs and confer health benefits on shrimps. Therefore, our results indicated the role of A. niger in improving the immune response of shrimps.
In crustaceans, the proPO system, which is activated by the pattern recognition receptor family (e.g., β-1, 3-glucanase related protein), is vital in the humoral immune response. The binding of β-1, 3-glucan to these proteins activates the immune signaling pathway and enhances the PO activity (Li et al., 2019). Herein, the increase of proPO mRNA expression and PO activity in shrimp fed with A. niger-containing diets suggests interactions between A. niger and the proPO system regulation in promoting shrimp humoral immune response. A similar finding was previously reported in black tiger shrimp where β-glucan participates in the activation of the proPO system and increase of PO activity, resulting in the protection of hosts against WSSV (Chang et al., 2003; Thitamadee et al., 2014). Collectively, dietary A. niger supplement shows an effectiveness in stimulating immune responses in shrimps, which may help hosts in resisting invading pathogens during the culture period.
Gut microbiota is important in dietary polysaccharide digestion, nutrient absorption, physiological homeostasis, and immune stimulation in their hosts. The gut microbiota in aquatic animals has been known to be affected by several factors, such as host species, nutrient supplementation, aquatic environment, and geolocation (Tran et al., 2017). In this study, the supplementation of A. niger significantly increased the alpha diversity and distinctly separated the microbial community structure in shrimps, referring to the great contribution of probiotics in shaping the gut microbiota of shrimps. This is in agreement with the previous findings in Pacific white shrimps fed with the probiotic mixture of B. subtilis, B. licheniformis, and Lactobacillus sp. (Xie et al., 2019). However, different results were observed in another study which found a decrease in the richness and diversity, but unchanged relative abundance (at the phylum level) of gut microbiota in Pacific white shrimps fed with β-glucan (Li et al., 2019). The difference herein may be explained by the difference in water salinity used for the culture of experimental animals between the studies. Our results found that the bacterial phyla Proteobacteria, Firmicutes, Bacteroidota, Verrucomicrobiota, Actinobacteriota, and Cyanobacteria are the core bacteria in the gut of shrimp, which is similar to the previous findings (Duan et al., 2018; Li et al., 2019). Bacteroidetes and Firmicutes are responsible for the metabolism of undigested food remnants (Tran et al., 2018a). The members of Bacteroidetes are known as producers of short-chain fatty acids (especially propionate) during the anaerobic fermentation, which has been demonstrated to confer health benefits on their hosts (Rios-Covian et al., 2017; Tran et al., 2020). The abundance of Firmicutes gives a good parameter concerning the health status of the gut, of which many members, such as Lachnospiraceae, Clostridium, Bacillus, Lachmoclostridium, and Lactobacillus, are beneficial bacteria (Duan et al., 2018). Among them, the members of the family Lachnospiraceae are capable of producing butyrate via the butyryl-CoA or acetate CoA transferase pathways or the butyrate kinase pathway by using lactate and acetate (Zhang et al., 2019a). Our findings showed that the abundance of members of Lachnospiraceae was increased in both A1.5 and A3.0 as compared to that in the control (data not shown), indicating the positive effects of the A. niger supplementation on the gut health of shrimps. Proteobacteria is related to energy disequilibrium and unstable gut microbiota (Shin et al., 2015), which was enriched in grass carp (Ctenopharyngodon idellus) with intestinal disease (Tran et al., 2018b) and humans with Crohn’s disease and inflammatory bowel disease (Li et al., 2012a; Zhu et al., 2014). Our results showed that the relative abundance of Firmicutes and Bacteroidota was increased, but such of Proteobacteria was decreased in the shrimps fed A. niger-supplemented diets. Several bacterial genera, such as Vibrio, Marivita, ZOR0006, Roseobacter, Ruegeria, Psychromonadaceae, Edwardsiella, Clostridium sensu stricto 1, Halioglobus, and Shimia, were predominated in the gut of shrimps. Of these genera, Vibrio, Marivita, and Roseobacter were found to be increased in the A. niger-fed shrimps, which is almost consistent with the previous findings (Li et al., 2019; Liao et al., 2022). Roseobacter 27-4 is a probiotic bacterium for protecting turbot (Scophthalmus maximus) larvae against Vibrio anguillarum (Planas et al., 2006). Vibrio and Edwardsiella are known as opportunistic pathogens causing diseases in both cultured and wild aquatic animals (Park et al., 2012; Li et al., 2018). Vibrio spp. may cause an outbreak of vibriosis in cultured shrimps under the deterioration of the environment, but the pathogenic effect of Vibrio spp. is species-specific (Intaraprasong et al., 2009; Li et al., 2019). Intaraprasong et al. (2009) found that the extracellular products of Vibrio harveyi cause high toxicity to black tiger shrimp (P. monodon) but not Pacific white shrimp. Also, the previous study has demonstrated that the susceptibility of Pacific white shrimp is depended on the Vibrio species and doses (Aguirre-Guzmán et al., 2001). The study showed that V. alginolyticus showed a high survival of shrimp larvae, while V. harveyi and V. parahaemolyticus induced high mortality only at high doses (105 and 107 cfu/mL), and V. penaeicida caused high mortality and only at low dose (103 cfu/mL) in postlarvae 1 (Aguirre-Guzmán et al., 2001). An increase in the abundance of Vibrio in shrimps fed A. niger (in the A1.5 and A3.0) may speculate that these microorganisms are non-pathogenic species causing infections in shrimps. Also, the overgrowth of Vibrio spp. in A. niger-fed shrimps may indicate their beneficial role to the host. This is supported by the findings that Vibrio alginolyticus UTM 102 can enhance feed utilization and disease resistance against V. parahaemolyticus in Pacific white shrimp (Balcázar et al., 2007). Similarly, Edwardsiella sp. 34HN showed an effectiveness in promoting growth, immunity, and expression of myostatin and pituitary adenylate cyclase-activating polypeptide in African catfish (Clarias gariepinus) (Selim et al., 2019). Thus, A. niger can promote beneficial bacteria and inhibit opportunistic pathogens colonizing the gut of shrimps. Similar findings were observed in Pacific white shrimps fed with beer yeast and yeast extract (Zheng et al., 2021a).
5 Conclusions
This study proved the beneficial effects of A. niger in promoting weight gain, specific growth rate, and length gain, as well as, improving antioxidant activity and immune response of juvenile Pacific white shrimps. Also, the addition of A. niger can promote the selective growth of gut microbiota (with an increase in the abundance of beneficial bacteria and a decrease in that of opportunistic pathogens). Therefore, A. niger can be used as a probiotic in shrimp aquaculture. Based on growth promotion, the recommended supplementation of A. niger is a 1.5 g/kg diet. However, the limitation of this study is lacking an evaluation of the germination ability of fungal conidia in the gut of shrimps. Also, whether the positive impact of A. niger on shrimp health status is through the composition of fungal conidia or through the mycelium after germination and colonization of shrimp gut need to be further evaluated in the future.
Data availability statement
The datasets presented in this study can be found in online repositories. The names of the repository/repositories and accession number(s) can be found below: https://www.ncbi.nlm.nih.gov/, PRJNA900677.
Ethics statement
The animals were processed according to “the Regulations for the Administration of Affairs Concerning Experimental Animals” established by Guangdong Provincial Department of Science and Technology on the Use and Care of Animals, and the experiments were approved by the Institutional Animal Care and Use Committee of Shantou University.
Author contributions
MZ and HL: Investigation, Data curation. YL, YZ, ZT and WC: Data curation. SL and XP: Conceptualization, Project administration, Funding acquisition, Writing- review and editing. NT: Conceptualization, Investigation, Writing- original draft, review and editing. All authors contributed to the article and approved the submitted version.
Funding
This work was supported by grants from National Natural Science Foundation of China (42076125, 41876152, 31850410487), STU Scientific Research Foundation for Talents (NTF21027), Guangdong Provincial Project of Science and Technology (2017B020204003), and Guangdong Provincial Special Fund for Modern Agriculture Industry Technology Innovation Teams (2019KJ141).
Conflict of interest
Authors ZT, WC and XP were employed by Guangzhou Insighter Biotechnology Co., Ltd. Guangzhou, China.
The remaining authors declare that the research was conducted in the absence of any commercial or financial relationships that could be construed as a potential conflict of interest.
Publisher’s note
All claims expressed in this article are solely those of the authors and do not necessarily represent those of their affiliated organizations, or those of the publisher, the editors and the reviewers. Any product that may be evaluated in this article, or claim that may be made by its manufacturer, is not guaranteed or endorsed by the publisher.
References
Aguirre-Guzmán G., Vázquez-Juárez R., Ascencio F. (2001). Differences in the susceptibility of American white shrimp larval substages (Litopenaeus vannamei) to four vibrio species. J. Invertebr. Pathol. 78 (4), 215–219. doi: 10.1006/jipa.2001.5073
Antony S. P., Singh I. S. B., Sudheer N. S., Vrinda S., Priyaja P., Philip R. (2011). Molecular characterization of a crustin-like antimicrobial peptide in the giant tiger shrimp, Penaeus monodon, and its expression profile in response to various immunostimulants and challenge with WSSV. Immunobiology 216 (1), 184–194. doi: 10.1016/j.imbio.2010.05.030
Ayiku S., Shen J., Tan B.-P., Dong X.-H., Liu H.-Y. (2020). Effects of reducing dietary fishmeal with yeast supplementations on Litopenaeus vannamei growth, immune response and disease resistance against Vibrio harveyi. Microbiol. Res. 239, 126554. doi: 10.1016/j.micres.2020.126554
Balcázar J. L., Rojas-Luna T., Cunningham D. P. (2007). Effect of the addition of four potential probiotic strains on the survival of pacific white shrimp (Litopenaeus vannamei) following immersion challenge with Vibrio parahaemolyticus. J. Invertebr. Pathol. 96 (2), 147–150. doi: 10.1016/j.jip.2007.04.008
Boonanuntanasarn S., Wongsasak U., Pitaksong T., Chaijamrus S. (2016). Effects of dietary supplementation with β-glucan and synbiotics on growth, haemolymph chemistry, and intestinal microbiota and morphology in the pacific white shrimp. Aquac. Nutr. 22 (4), 837–845. doi: 10.1111/anu.12302
Caporaso J. G., Kuczynski J., Stombaugh J., Bittinger K., Bushman F. D., Costello E. K., et al. (2010). QIIME allows analysis of high-throughput community sequencing data. Nat. Methods 7 (5), 335–336. doi: 10.1038/nmeth.f.303
Chang C.-F., Su M.-S., Chen H.-Y., Liao I. C. (2003). Dietary β-1,3-glucan effectively improves immunity and survival of Penaeus monodon challenged with white spot syndrome virus. Fish Shellfish Immunol. 15 (4), 297–310. doi: 10.1016/S1050-4648(02)00167-5
Choi E. Y., Lee S. S., Hyeon J. Y., Choe S. H., Keum B. R., Lim J. M., et al. (2016). Effects of β-glucan on the release of nitric oxide by macrophages stimulated with lipopolysaccharide. Asian-Australas. J. Anim. Sci. 29 (11), 1664–1674. doi: 10.5713/ajas.16.0418
Cruz da Silva L. R., Camilo de Souza O., Dos Santos Fernandes M. J., Massa Lima D. M., Rodrigues Coelho R. R., Souza-Motta C. M. (2011). Culturable fungal diversity of shrimp Litopenaeus vannamei boone from breeding farms in Brazil. Braz. J. Microbiol. 42 (1), 49–56. doi: 10.1590/s1517-83822011000100007
Dawood M. A. O., Eweedah N. M., Moustafa E. M., Shahin M.G. (2020). Synbiotic effects of Aspergillus oryzae and β-glucan on growth and oxidative and immune responses of Nile tilapia, Oreochromis niloticus. Probiotics Antimicrob. Proteins 12 (1), 172–183. doi: 10.1007/s12602-018-9513-9
Dawood M. A. O., Koshio S., Abdel-Daim M. M., Doan H. V. (2019). Probiotic application for sustainable aquaculture. Rev. Aquac. 11 (3), 907–924. doi: 10.1111/raq.12272
Dawood M. A. O., Koshio S., Ishikawa M., Yokoyama S., El Basuini M. F., Hossain M. S., et al. (2017). Dietary supplementation of β-glucan improves growth performance, the innate immune response and stress resistance of red sea bream, Pagrus major. Aquacult. Nutr. 23 (1), 148–159. doi: 10.1111/anu.12376
Dayal J. S., Jannathulla R., Ambasankar K., Muralidhar M. (2020). Aspergillus niger fermented plant protein mix as a potential substitute for fishmeal in the diet of Penaeus vannamei (Boone 1931). Aquac. Nutr. 26 (3), 853–865. doi: 10.1111/anu.13044
Deng D., Mei C., Mai K., Tan B.-P., Ai Q., Ma H. (2013). Effects of a yeast-based additive on growth and immune responses of white shrimp, Litopenaeus vannamei (Boone 1931), and aquaculture environment. Aquac. Res. 44 (9), 1348–1357. doi: 10.1111/j.1365-2109.2012.03139.x
Dixon P. (2003). VEGAN, a package of r functions for community ecology. J. Veg. Sci. 14 (6), 927–930. doi: 10.1111/j.1654-1103.2003.tb02228.x
Du Z.-Q., Wang Y., Ma H.-Y., Shen X.-L., Wang K., Du J., et al. (2019). A new crustin homologue (SpCrus6) involved in the antimicrobial and antiviral innate immunity in mud crab, Scylla paramamosain. Fish Shellfish Immunol. 84, 733–743. doi: 10.1016/j.fsi.2018.10.072
Duan Y., Wang Y., Dong H., Ding X., Liu Q., Li H., et al. (2018). Changes in the intestine microbial, digestive, and immune-related genes of Litopenaeus vannamei in response to dietary probiotic Clostridium butyricum supplementation. Front. Microbiol. 9. doi: 10.3389/fmicb.2018.02191
Edgar R. C. (2013). UPARSE: highly accurate OTU sequences from microbial amplicon reads. Nat. Methods 10 (10), 996–998. doi: 10.1038/nmeth.2604
Edgar R. C., Haas B. J., Clemente J. C., Quince C., Knight R. (2011). UCHIME improves sensitivity and speed of chimera detection. Bioinformatics 27 (16), 2194–2200. doi: 10.1093/bioinformatics/btr381
Gu H.-J., Sun Q.-L., Jiang S., Zhang J., Sun L. (2018). First characterization of an anti-lipopolysaccharide factor (ALF) from hydrothermal vent shrimp: insights into the immune function of deep-sea crustacean ALF. Dev. Comp. Immunol. 84, 382–395. doi: 10.1016/j.dci.2018.03.016
Hai N. V. (2015). The use of probiotics in aquaculture. J. Appl. Microbiol. 119 (4), 917–935. doi: 10.1111/jam.12886
Hoseinifar S. H., Ringø E., Shenavar Masouleh A., Esteban M.Á. (2016). Probiotic, prebiotic and synbiotic supplements in sturgeon aquaculture: a review. Rev. Aquacult. 8, 89–102. doi: 10.1111/raq.12082
Intaraprasong A., Khemayan K., Pasharawipas T., Flegel T. W. (2009). Species-specific virulence of Vibrio harveyi for black tiger shrimp is associated with bacteriophage-mediated hemocyte agglutination. Aquaculture 296 (3), 185–192. doi: 10.1016/j.aquaculture.2009.08.005
Jannathulla R., Dayal J. S. (2022). Beneficial effects, challenges and opportunities of the filamentous fungus, Aspergillus niger with special reference to the shrimp feed industry-a review. Rev. Aquac. doi: 10.1111/raq.12775
Jannathulla R., Dayal J. S., Ambasankar K., Eugine A. C., Muralidhar M. (2018b). Fungus, Aspergillus niger, fermented groundnut oil cake as a fishmeal alternative in the diet of Penaeus vannamei. Aquac. Res. 49 (8), 2891–2902. doi: 10.1111/are.13756
Jannathulla R., Dayal J. S., Ambasankar K., Muralidhar M. (2018a). Effect of Aspergillus niger fermented soybean meal and sunflower oil cake on growth, carcass composition and haemolymph indices in Penaeus vannamei boon. Aquaculture 486, 1–8. doi: 10.1016/j.aquaculture.2017.12.005
Jasim S. A., Abdelbasset W. K., Shichiyakh R. A., Al-Shawi S. G., Yasin G., Jalil A. T., et al. (2022). Probiotic effects of the fungi, Aspergillus niger on growth, immunity, haematology, intestine fungal load and digestive enzymes of the common carp, Cyprinus carpio. Aquac. Res. 53 (10), 3828–3840. doi: 10.1111/are.15890
Lai X., Chen J., Liang S., Chen H., Liu S., Gao H. (2022). Effects of the probiotic Psychrobacter sp. B6 on the growth, digestive enzymes, antioxidant capacity, immunity, and resistance of Exopalaemon carinicauda to Aeromonas hydrophila. Probiotics Antimicrob. Proteins. doi: 10.1007/s12602-022-09919-3
Li Q., Wang C., Tang C., Li N., Li J. (2012a). Molecular-phylogenetic characterization of the microbiota in ulcerated and non-ulcerated regions in the patients with crohn's disease. PLoS One 7 (4), e34939. doi: 10.1371/journal.pone.0034939
Li E., Xu C., Wang X., Wang S., Zhao Q., Zhang M., et al. (2018). Gut microbiota and its modulation for healthy farming of pacific white shrimp Litopenaeus vannamei. Rev. Fish. Sci. Aquac. 26 (3), 381–399. doi: 10.1080/23308249.2018.1440530
Li H., Xu C., Zhou L., Dong Y., Su Y., Wang X., et al. (2019). Beneficial effects of dietary β-glucan on growth and health status of pacific white shrimp Litopenaeus vannamei at low salinity. Fish Shellfish Immunol. 91, 315–324. doi: 10.1016/j.fsi.2019.05.052
Li S., Zhang Z., Li C., Zhou L., Liu W., Li Y., et al. (2012b). Molecular cloning and expression profiles of nitric oxide synthase (NOS) in mud crab Scylla paramamosain. Fish Shellfish Immunol. 32 (4), 503–512. doi: 10.1016/j.fsi.2011.12.002
Liao W., Lin Z., Liao M., Xue Y., Zhou J., Wang Y., et al. (2022). Effects of sodium humate and probiotics on growth performance enzyme activity and microbial environment of Litopenaeus vannamei in high-density zero-water exchange systems. Front. Mar. Sci. 9. doi: 10.3389/fmars.2022.989325
Livak K. J., Schmittgen T. D. (2001). Analysis of relative gene expression data using real-time quantitative PCR and the 2–ΔΔCT method. Methods 25 (4), 402–408. doi: 10.1006/meth.2001.1262
Ma S., Wang X., Gao W., Zhang W., Mai K. (2019). Supplementation of yeast extract to practical diet improves the growth, anti-oxidative capacity and intestinal morphology of shrimp Litopenaeus vannamei. J. Ocean Univ. 18 (4), 933–938. doi: 10.1007/s11802-019-3970-y
Murthy H. S., Li P., Lawrence A. L., Gatlin D. M. (2009). Dietary β-glucan and nucleotide effects on growth, survival and immune responses of pacific white shrimp, Litopenaeus vannamei. J. Appl. Aquac. 21 (3), 160–168. doi: 10.1080/10454430903113644
Pan X., Wu T., Song Z., Tang H., Zhao Z. (2008). Immune responses and enhanced disease resistance in Chinese drum, Miichthys miiuy (Basilewsky), after oral administration of live or dead cells of Clostridium butyrium CB2. J. Fish Dis. 31 (9), 679–686. doi: 10.1111/j.1365-2761.2008.00955.x
Park S. B., Aoki T., Jung T. S. (2012). Pathogenesis of and strategies for preventing Edwardsiella tarda infection in fish. Vet. Res. 43 (1), 67. doi: 10.1186/1297-9716-43-67
Parrilla-Taylor D. P., Zenteno-Savín T., Magallón-Barajas F. J. (2013). Antioxidant enzyme activity in pacific whiteleg shrimp (Litopenaeus vannamei) in response to infection with white spot syndrome virus. Aquaculture 380, 41–46. doi: 10.1016/j.aquaculture.2012.11.031
Planas M., Pérez-Lorenzo M., Hjelm M., Gram L., Uglenes Fiksdal I., Bergh Ø., et al. (2006). Probiotic effect in vivo of Roseobacter strain 27-4 against Vibrio (Listonella) anguillarum infections in turbot (Scophthalmus maximus l.) larvae. Aquaculture 255 (1), 323–333. doi: 10.1016/j.aquaculture.2005.11.039
Ringø E. (2020). Probiotics in shellfish aquaculture. Aquac. Fish. 5 (1), 1–27. doi: 10.1016/j.aaf.2019.12.001
Rios-Covian D., Salazar N., Gueimonde M., de los Reyes-Gavilan C. G. (2017). Shaping the metabolism of intestinal Bacteroides population through diet to improve human health. Front. Microbiol. 8. doi: 10.3389/fmicb.2017.00376
Saurabh S., Sahoo P. K. (2008). Lysozyme: an important defence molecule of fish innate immune system. Aquac. Res. 39 (3), 223–239. doi: 10.1111/j.1365-2109.2007.01883.x
Selim K. M., El-Sayed H. M., El-Hady M. A., Reda R. M. (2019). In vitro evaluation of the probiotic candidates isolated from the gut of Clarias gariepinus with special reference to the in vivo assessment of live and heat-inactivated Leuconostoc mesenteroides and Edwardsiella sp. Aquacult. Int. 27 (1), 33–51. doi: 10.1007/s10499-018-0297-4
Sharma M. K., White D. L., Singh A. K., Liu H., Tan Z., Peng X., et al. (2022). Effect of dietary supplementation of probiotic Aspergillus niger on performance and cecal microbiota in hy-line W-36 laying hens. Animals 12 (18), 2406. doi: 10.3390/ani12182406
Shin N.-R., Whon T. W., Bae J.-W. (2015). Proteobacteria: microbial signature of dysbiosis in gut microbiota. Trends Biotechnol. 33 (9), 496–503. doi: 10.1016/j.tibtech.2015.06.011
Sîrbu E., Dima M. F., Tenciu M., Cretu M., Coadă M. T., Țoțoiu A., et al. (2022). Effects of dietary supplementation with probiotics and prebiotics on growth, physiological condition, and resistance to pathogens challenge in Nile tilapia (Oreochromis niloticus). Fishes 7 (5), 273. doi: 10.3390/fishes7050273
Stentiford G. D., Bateman I. J., Hinchliffe S. J., Bass D., Hartnell R., Santos E. M., et al. (2020). Sustainable aquaculture through the one health lens. Nat. Food. 1 (8), 468–474. doi: 10.1038/s43016-020-0127-5
Sun Y. Z., Yang H. L., Ma R. L., Lin W. Y. (2010). Probiotic applications of two dominant gut Bacillus strains with antagonistic activity improved the growth performance and immune responses of grouper Epinephelus coioides. Fish Shellfish Immunol. 29 (5), 803–809. doi: 10.1016/j.fsi.2010.07.018
Thitamadee S., Srisala J., Taengchaiyaphum S., Sritunyalucksana K. (2014). Double-dose β-glucan treatment in WSSV-challenged shrimp reduces viral replication but causes mortality possibly due to excessive ROS production. Fish Shellfish Immunol. 40 (2), 478–484. doi: 10.1016/j.fsi.2014.07.033
Tran N. T., Li S. (2022). Potential role of prebiotics and probiotics in conferring health benefits in economically important crabs. Fish Shellfish Immunol. Rep. 3, 100041. doi: 10.1016/j.fsirep.2021.100041
Tran N. T., Li Z., Wang S., Zheng H., Aweya J. J., Wen X., et al. (2020). Progress and perspectives of short-chain fatty acids in aquaculture. Rev. Aquac. 12 (1), 283–298. doi: 10.1111/raq.12317
Tran N. T., Wang G. T., Wu S. G. (2017). A review of intestinal microbes in grass carp Ctenopharyngodon idellus (Valenciennes). Aquac. Res. 48 (7), 3287–3297. doi: 10.1111/are.13367
Tran N. T., Xiong F., Hao Y. T., Zhang J., Wu S. G., Wang G. T. (2018a). Starvation influences the microbiota assembly and expression of immunity-related genes in the intestine of grass carp (Ctenopharyngodon idellus). Aquaculture 489, 121–129. doi: 10.1016/j.aquaculture.2018.02.016
Tran N. T., Zhang J., Xiong F., Wang G. T., Li W. X., Wu S. G. (2018b). Altered gut microbiota associated with intestinal disease in grass carp (Ctenopharyngodon idellus). World J. Microbiol. Biotechnol. 34 (6), 1–9. doi: 10.1007/s11274-018-2447-2
Wang P.-H., Gu Z.-H., Wan D.-H., Zhu W.-B., Qiu W., Chen Y.-G., et al. (2013). Litopenaeus vannamei toll-interacting protein (LvTollip) is a potential negative regulator of the shrimp toll pathway involved in the regulation of the shrimp antimicrobial peptide gene penaeidin-4 (PEN4). Dev. Comp. Immunol. 40 (3-4), 266–277. doi: 10.1016/j.dci.2013.02.011
Wu B., Zhang C., Qin X., Shi L., Zhao M. (2019). Identification and function of penaeidin 3 and penaeidin 5 in Fenneropenaeus merguiensis. Fish Shellfish Immunol. 89, 623–631. doi: 10.1016/j.fsi.2019.04.032
Xie J. J., Liu Q. Q., Liao S., Fang H. H., Yin P., Xie S. W., et al. (2019). Effects of dietary mixed probiotics on growth, non-specific immunity, intestinal morphology and microbiota of juvenile pacific white shrimp, Litopenaeus vannamei. Fish Shellfish Immunol. 90, 456–465. doi: 10.1016/j.fsi.2019.04.301
Zhang Y.-S., Li F.-X., Yao C.-L. (2019b). Glycogen phosphorylase of shrimp (Litopenaeus vannamei): structure, expression and anti-WSSV function. Fish Shellfish Immunol. 91, 275–283. doi: 10.1016/j.fsi.2019.05.043
Zhang J., Song L., Wang Y., Liu C., Zhang L., Zhu S., et al. (2019a). Beneficial effect of butyrate-producing lachnospiraceae on stress-induced visceral hypersensitivity in rats. JGH 34 (8), 1368–1376. doi: 10.1111/jgh.14536
Zheng Z., Li R., Aweya J. J., Yao D., Wang F., Li S., et al. (2021b). The PirB toxin protein from Vibrio parahaemolyticus induces apoptosis in hemocytes of Penaeus vannamei: PirB induces shrimp hemocytes apoptosis. Virulence 12 (1), 481–492. doi: 10.1080/21505594.2021.1872171
Zheng L., Xie S., Zhuang Z., Liu Y., Tian L., Niu J. (2021a). Effects of yeast and yeast extract on growth performance, antioxidant ability and intestinal microbiota of juvenile pacific white shrimp (Litopenaeus vannamei). Aquaculture 530, 735941. doi: 10.1016/j.aquaculture.2020.735941
Keywords: Aspergillus niger, Penaeus vannamei, growth, antioxidant activity, immune response, gut microbiota
Citation: Zhang M, Liang H, Lei Y, Zhang Y, Tan Z, Chen W, Li S, Peng X and Tran NT (2023) Aspergillus niger confers health benefits and modulates the gut microbiota of juvenile Pacific white shrimp (Penaeus vannamei) under farming conditions. Front. Mar. Sci. 10:1211993. doi: 10.3389/fmars.2023.1211993
Received: 25 April 2023; Accepted: 29 May 2023;
Published: 09 June 2023.
Edited by:
Yafei Duan, South China Sea Fisheries Research Institute, ChinaCopyright © 2023 Zhang, Liang, Lei, Zhang, Tan, Chen, Li, Peng and Tran. This is an open-access article distributed under the terms of the Creative Commons Attribution License (CC BY). The use, distribution or reproduction in other forums is permitted, provided the original author(s) and the copyright owner(s) are credited and that the original publication in this journal is cited, in accordance with accepted academic practice. No use, distribution or reproduction is permitted which does not comply with these terms.
*Correspondence: Ngoc Tuan Tran, tranntts@gmail.com; Xianfeng Peng, pengist@hotmail.com
†These authors have contributed equally to this work