- 1Fujian Provincial Key Laboratory of Marine Ecological Conservation and Restoration, Third Institute of Oceanography, Ministry of Natural Resources, Xiamen, China
- 2College of Environmental Science and Engineering, Ocean University of China, Qingdao, China
- 3Guangxi Key Laboratory of Polysaccharide Materials and Modification, School of Marine Sciences and Biotechnology, Guangxi Minzu University, Nanning, China
- 4Key Laboratory of Marine Environment and Ecology, Ocean University of China, Ministry of Education, Qingdao, China
- 5College of Fisheries and Life Science, Dalian Ocean University, Dalian, China
We conducted an annual monthly sampling in the aquaculture areas of Lianjiang and Zhangpu, Fujian province, in the west coast of the Taiwan Strait, to gain insight into the effects of shellfish aquaculture on total phytoplankton biomass and its distribution in phytoplankton size fractions. We observed that the total chlorophyll-a (TChl-a) concentration was higher in Zhangpu (annual mean 2.690 ± 2.375 μg/L) than in Lianjiang (annual mean 1.700 ± 1.500 μg/L) and that Lianjiang had a high N/P ratio during the study, which may have been the main reason for the differences in phytoplankton biomass between the two shellfish aquaculture areas. The response of phytoplankton to shellfish aquaculture was different in the northern versus southern areas. During the aquaculture period of Lianjiang, the TChl-a concentration of surface water was approximately 14% lower than that of bottom water, and such a decreasing trend of Chl-a concentration was consistently present in micro-, nano-, and pico-size fractions. Phytoplankton depletion was not observed during investigation in Zhangpu, which showed highly variable in environmental factors. The contribution of pico-sized Chl-a to TChl-a is evaluated by shellfish aquaculture to some extent in Lianjiang. The shift from the dominance of nano-phytoplankton to the dominance of micro- phytoplankton and pico-phytoplankton was caused mainly by seasonal variation in environmental conditions. In complex marine environments, the distribution of phytoplankton biomass in phytoplankton size fractions showed no significant response to shellfish aquaculture.
1 Introduction
Coastal ecosystems, as transitional zones for material and energy exchange at land-sea interface, are facing frequent anthropogenic disturbances that affect aquatic biota (Srichandan et al., 2019). As the environment deteriorates and pressure on natural fisheries, aquaculture is experiencing rapid development, with shellfish as the world’s largest aquaculture species in terms of production (FAO, 2020). Shellfish is capable of filtering plankton and particulate organic matters from the water for growth (Dame, 2011). The filtration of plankton by shellfish may exert a top-down control on the plankton community (Ward & Shumway, 2004). Meanwhile, the metabolism and excretion of shellfish affect the turnover rates and stoichiometry of nutrients (Souchu et al., 2001), thereby exerting bottom-up control on phytoplankton. Intensive coastal aquaculture may amplify these two processes, thereby exacerbating anthropogenic disturbances to offshore ecosystems.
Phytoplankton are sensitive indicators of changes in coastal ecosystems due to their high growth rate to a short-term anthropogenic disturbances or natural environmental variation (Paerl et al., 2006). For shellfish aquaculture system, a decrease in phytoplankton abundance and changes in phytoplankton community composition has been observed in poorly flushed waters as a result of high filtration rates of shellfish feeding (Ogilvie et al., 2000; Strohmeier et al., 2008; Jiang et al., 2016). Active feeding by shellfish affects production of the dominant phytoplankton species (Newell, 2004), meanwhile the feeding preferences can efficiently remove some certain type or class of phytoplankton (Rosa et al., 2017). Phytoplankton depletion was commonly found in micro- and nano-sized fraction, resulting in high abundance of picoplankton (Trottet et al., 2008; Smaal et al., 2013; Jiang et al., 2016). Moreover, the efficient recycle of nitrogen and phosphorus relative to silica through shellfish metabolism, may favors the growth of non-diatom microalgae (Newell, 2004; Lucas et al., 2016). These interaction between shellfish and phytoplankton are affected by system flushing rate (Newell et al, 2005). Short residence time precludes the accumulation of phytoplankton biomass, which affect food availability for shellfish, and plays a key role in the advection of nutrients and/or phytoplankton (Filgueira et al., 2016; Campbell & Hall, 2019). Hydrodynamics may complicate the impact of aquaculture on phytoplankton.
The Taiwan Strait (TWS) is a typical strait ecosystem, and is an important channel between the East China Sea (ECS) and the South China Sea (SCS). Circulations in the TWS are strongly influenced by the East Asian monsoon. The SCS Warm Current and Kuroshio Current intrude into the TWS in both monsoon seasons, with stronger invasion along the middle and eastern TWS during the northeast (NE) monsoon (September-March) (Zhong et al., 2020). Wind-driven with topographic forcing upwellings (e.g. Dongshan upwelling, Pingtan upwelling) occur during southwest (SW) monsoon (June-August) (Tang et al., 2002). Meanwhile, low-salinity water from the Min River, the Jiulong River, the Han River and even the Pearl River also extends into the TWS (Hong et al., 2011). Significantly different water masses produced great heterogeneity in the physics, chemistry and biology of the TWS (Hong et al., 2011; Zhong et al., 2020). Moreover, the human forces along the west coast of the TWS is rising up along with the development of socioeconomics (Dai et al., 2023). The eutrophication coupled with the recurrent of harmful algal blooms (HABs) are threatening the ecosystem stability, with increasing HABs occurring in the northern TWS (Peng et al., 2021).
Accumulating human impacts could pose a threat to coastal ecosystem, and an understanding of dynamic aquaculture-environment interactions is important for effective coastal management (Filgueira et al., 2016; Dai et al., 2023). The impact of aquaculture on coastal water provides essential information for a balance between production and protection. Control of phytoplankton by shellfish farming in the western TWS is not well studied. We hypothesized that the impact of shellfish aquaculture on phytoplankton differs from different hydrodynamics conditions. Annual surveys were conducted in two typical aquaculture areas in the northern and southern regions of the TWS to a) quantify the contribution of different phytoplankton size classes to the total biomass, and b) evaluate the impact of aquaculture on phytoplankton size class patterns and the surrounding ecosystem.
2 Materials and methods
2.1 Description of the study sites
The shellfish aquaculture farming of Lianjiang and Zhangpu are located in the north and south coastal areas of the western TWS, respectively, with water depth less than 10 m (Figure 1). The Zhangpu site is located in the seasonal upwelling-influenced area of Dongshan (Figure 1A), and the Lianjiang site is near the Min River estuary (Figure 1B) (Hong et al., 2011; Zhong et al., 2020). These sites are subjected to the SW monsoon from June to August and the NE monsoon from September to March, with April and May being the monsoon transition period (Jan et al., 2006). Except for its southern region, the TWS is dominated by regular semi-diurnal tides (Hong et al., 2011), with an average tidal current amplitude of 0.46 m/s and a maximum amplitude of 0.80 m/s at in the northeast and southeast of the strait entrance (Wang et al., 2003).
Fujian Province, which is on the west coast of the TWS, is the second-largest seawater aquaculture province in China, with oyster and abalone as the main shellfish aquaculture species, and the numbers of shellfish aquaculture farming increasing gradually from north to south (Peng et al., 2021; Liu et al., 2022; Ma et al., 2022). The combined investigation of oyster and abalone aquaculture can provide an overall understanding of phytoplankton biomass and size composition affected by shellfish aquaculture in the western TWS. The oyster aquaculture period in Lianjiang was February-August, while abalone aquaculture occurs only in December and from February to April. During the warm season, the abalone was transferred to the north of China. The aquaculture season in Zhangpu is longer. It takes place almost all year round, except July-August for oyster, and January-February for abalone. Both types of shellfish are cultured with rafts, with culture rope/cages placed at a depth of about 1 m underwater.
2.2 Sampling
Monthly surveys were conducted from October 2021 to September 2022. Three groups of sampling sites, including one reference station, two oyster aquaculture stations, and two abalone aquaculture stations, were set up in each of the Lianjiang and Zhangpu shellfish aquaculture farming. To assess the effects of seawater shellfish aquaculture on the total phytoplankton biomass and its distribution in phytoplankton size fractions, we delineated culture and non-culture periods for each of the two shellfish species according to its aquaculture periods.
Surface water and bottom water sampling was conducted using a Plexiglas water collector during the field investigation (the absent of bottom water in Lianjiang during March–April and June, and the absent of bottom water in Zhangpu in March). Temperature, salinity, dissolved oxygen (DO), and turbidity were in situ measured using YSI multiparameter EXO-3 (Yellow Springs Instrument, Yellow Springs, OH, USA). Nutrient concentrations were determined by colorimetry using a nutrient analyzer (QuaTro, Seal Analytical, Mequon, WI, USA) (Strickland and Parsons, 1972), and the limit of detection for NH4-N, NO3-N, NO2-N, soluble reactive phosphorus (SRP), and dissolved silicate (DSi) was 0.02, 0.01, 0.02, 0.004, and 0.005 μmol/L, respectively. Dissolved inorganic nitrogen (DIN) was determined as the sum of NO3-N, NO2-N, and NH4-N.
2.3 Chlorophyll-a
At each station, 0.1–2.0 L of water samples were vacuum filtered (vacuum pressure<0.02 MPa) using 25 mm diameter membranes with a pore size of 20 μm (Nylon membrane filters, Millipore, Burlington, MA, USA), 2.7 μm (GF/D, Whatman, Maidstone, UK), or 0.3 μm (GF-75, Advantec, Toyo Roshi Kaisha, Japan). The full-size chlorophyll-a (Chl-a) was acquired according to the GF-75 filter, and we modified the size-fractional Chl-a concentration for >20 μm, 2.7–20 μm, and 0.3–2.7 μm to represent micro-, nano-, and pico-phytoplankton, respectively (Sieburth et al., 1978). The collected filters were placed in 5 mL plastic centrifuge tubes and 90% acetone was added, followed by extraction in dark at −20°C for 24 h. Fluorometric analysis of Chl-a was performed using a Turner Designs Model fluorometer (Model No. Trilogy 040, San Jose, CA, USA) (Strickland and Parsons, 1972).
2.4 Definition of phytoplankton depletion index
A phytoplankton depletion index (PDI, %) was modified from Filgueira et al. (2014):
Phytoplankton depletion index. (PDL, %) , where [Chl-a]surf. and [Chl-a]bot. are the concentrations of a certain type of Chl-a at surface water and bottom water, respectively. Values of this ratio below 0% indicate depletion, whereas values above 0% indicate enrichment of a certain Chl-a in the surface water compared with that in the bottom water.
2.5 Statistic analysis
Data analyses were performed using Origin 2023. The nonparametric Wilcoxon test and Kruskal-wallis ANOVA were performed to evaluate the differences in surface and bottom water, culture period and non-culture period, respectively. The differences with p<0.05 were considered to be significant. Multiple correlations of Redundancy analysis (RDA) were used to understand the ecological linkage between physicochemical and biological variables of different seasons (Clarke and Warwick, 2001).
3 Results
3.1 Hydrological parameters
Seasonal changes in water temperature, salinity, DO, and turbidity are shown in Figures 2A–D. Surface water temperature varied from 10.3°C to 31.9°C in Lianjiang. Compared with other months in Lianjiang, salinity was significantly lower in June to August (p< 0.01) and varied from 2.5 to 19.6. Except for March, the salinity in all months was lower than 30. DO and turbidity distributed in a similar pattern, being high in the cold season and low in warm season.
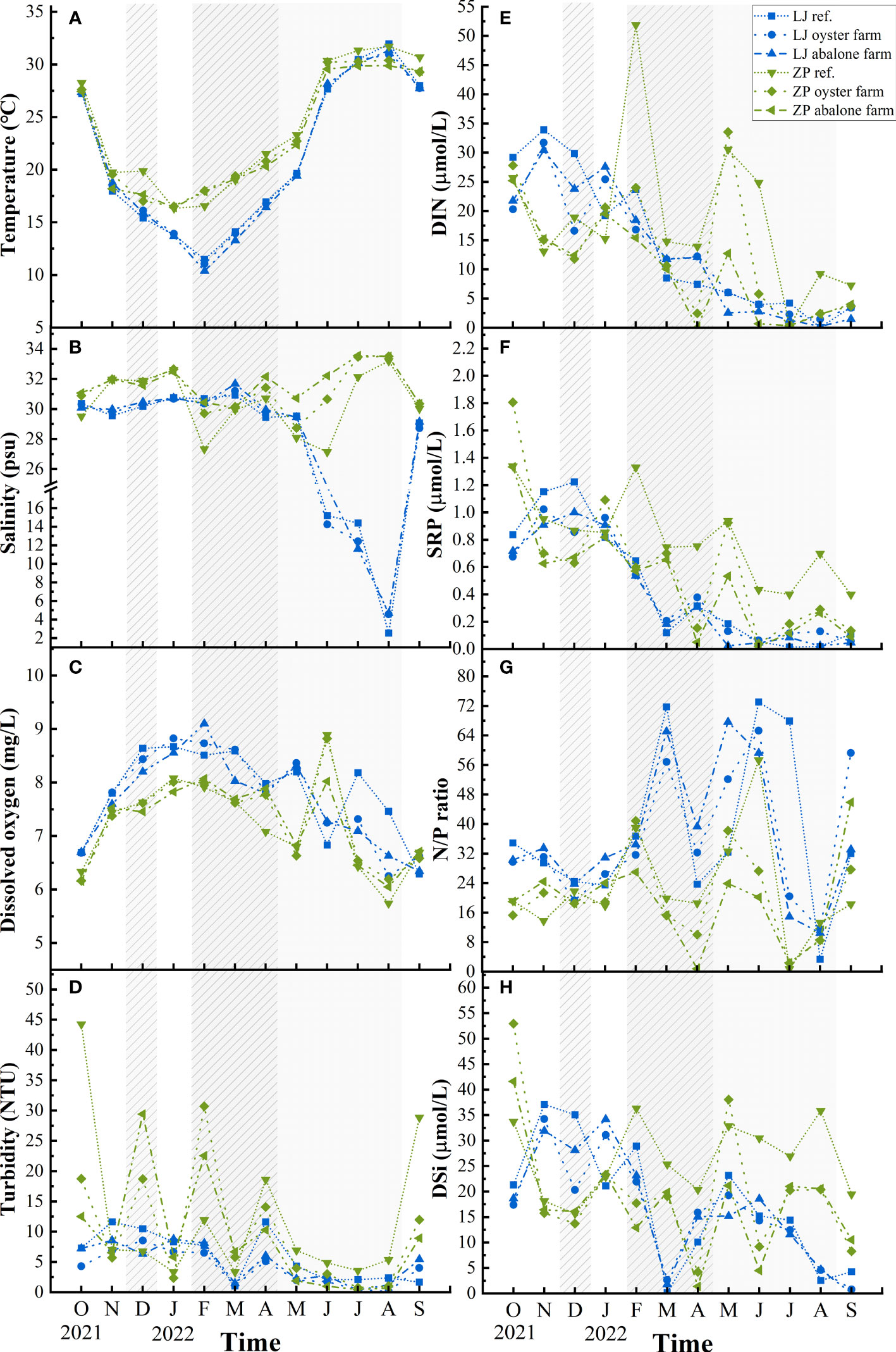
Figure 2 Temporal and spatial variations of surface environmental parameters in Lianjiang site and Zhangpu site. Grey and shaded areas represent oyster and abalone culture period in Lianjiang. Culture periods in Zhangpu cover a whole year, except Jul. and Aug. for oyster and Jan. and Feb. for abalone. (A) temperature; (B) salinity; (C) dissolved oxygen; (D) Turbidity; (E) dissolved inorganic nitrogen; (F) soluble reactive phosphate; (G) N/P ratio; (H) dissolved inorganic silicate.
Surface water temperature in Zhangpu varied from 16.3°C to 31.7°C. Salinity of the aquaculture stations increased to 33.6 in July and August. Compared with the aquaculture stations, salinity of the reference station varied to a smaller extent, being lower than 30 in February–May. DO peaked sharply in June, and turbidity showed great fluctuations in the cold season. Differences in water temperature, DO, and turbidity between surface and bottom waters was observed at both aquaculture areas (Table 1).
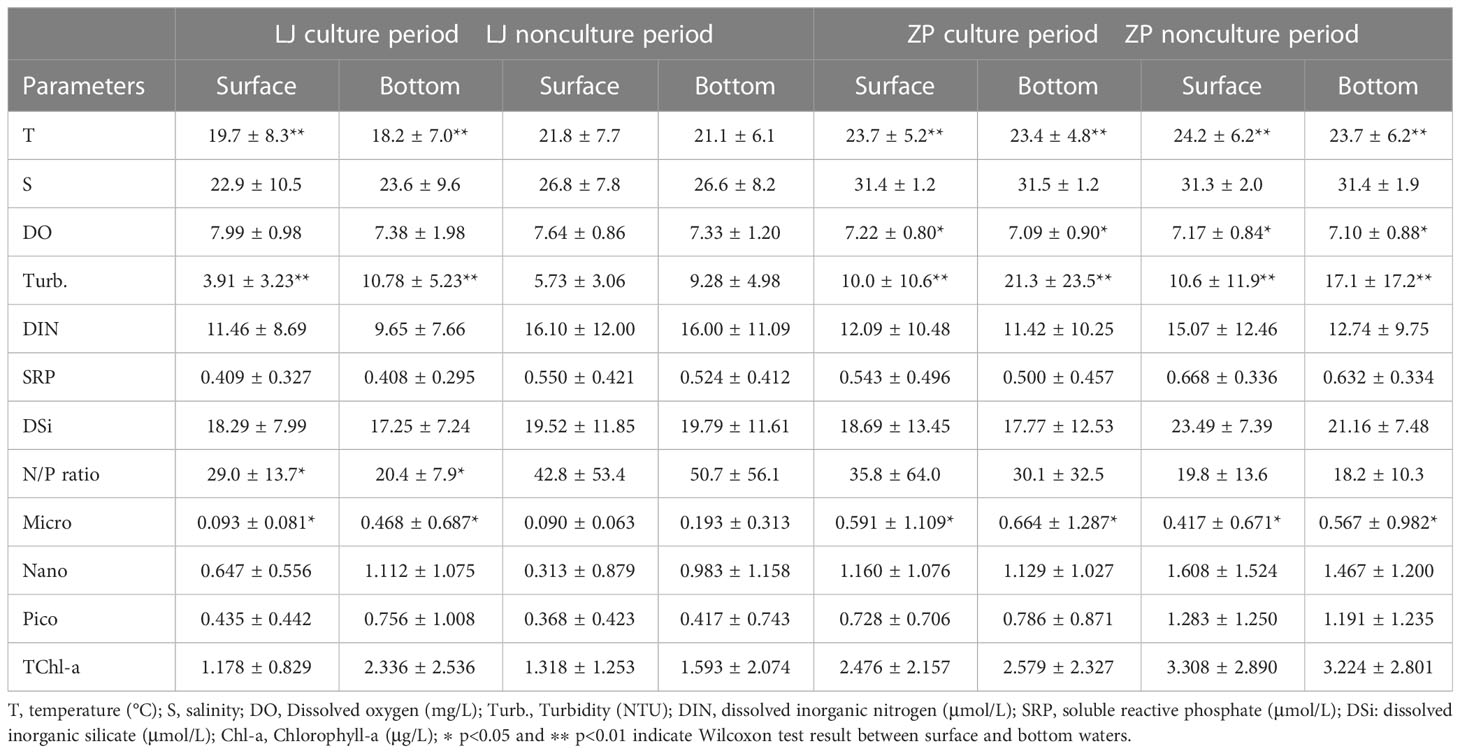
Table 1 Averages of environmental parameters and size-fractioned concentration of Chl-a in surface and bottom water of Lianjiang and Zhangpu sites during culture and nonculture period.
3.2 Nutrients
Surface DIN, SRP, and N/P ratio exhibited clear seasonality at both aquaculture areas (Figures 2E, F). Concentrations of DIN and SRP presented an annual cycle characterized by high concentrations from October to February. In Lianjiang, SRP was generally below 0.1 μmol/L since July. The N/P ratio increased dramatically from 32 during cold season to 75 during warm season, with an annual mean of 36.1 (Figure 2G). DSi in Lianjiang declined sharply in March, August, and September (Figure 2H). Concentrations of DIN, SRP and DSi at the Zhangpu reference station were generally higher than that at the aquaculture stations, with DIN depleted only in July and SRP remaining above 0.4 μmol/L. Except for May, DIN and SRP decreased significantly at the aquaculture stations in April–September. The annual mean of N/P ratio in Zhangpu was 20.7, and was constantly low in July–August. DSi at the Zhangpu aquaculture stations depleted in April and June. Significant difference in nutrient concentrations were not observed between the culture and non-culture periods (p > 0.05), nor between the surface and bottom waters (p > 0.05) (Table 1). Redfield ratio (N:P:Si ratio) was calculated and plotted (Figure 3) (Brzezinski, 1985). N/P ratio was above 16 except for July and August at both areas. Average of N/Si ratio in Lianjiang during March was 12.9, and was below 1.5 in the other months.
3.3 Spatial and temporal changes of size fractioned Chl-a concentration
Total chlorophyll-a (TChl-a) of Lianjiang and Zhangpu exhibited multi-peaks during March-August (Figure 4). Decrease of TChl-a, by 23% on average, was observed in Lianjiang aquaculture stations compared to the reference station during the culture period, with the maximum decline occurring in March-April. Micro-sized Chl-a at the aquaculture stations peaked in June (1.051–1.389 μg/L), which accounted for 36.7% of the TChl-a. Nano-sized Chl-a was dominant in October–May at the aquaculture stations, accounting for 69% of the TChl-a. Proportion of pico-sized Chl-a to TChl-a increased since May at the aquaculture stations, and accounted for more than 60% in September (Figures 4B, C). In Lianjiang, the nano-sized Chl-a comprised 73% of TChl-a on average at reference station in October-August.
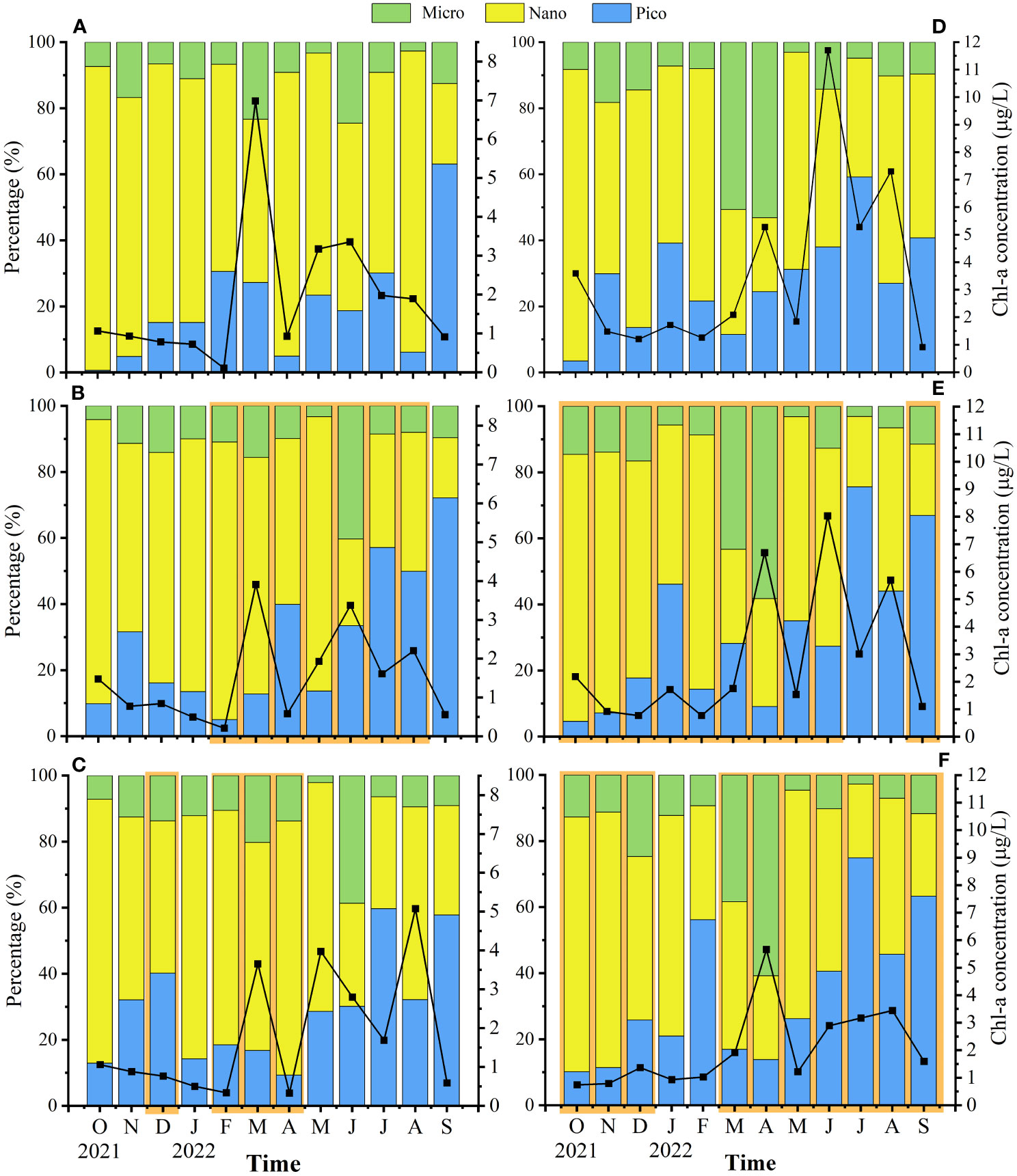
Figure 4 Temporal and spatial variations of surface total Chl-a concentration and percentage of size-fractioned Chl-a to Total Chl-a in Lianjiang site (A) reference station, (B) Oyster farm, (C) Abalone farm) and Zhangpu site (D) reference station, (E) Oyster farm, (F) Abalone farm). Orange areas indicate culture period.
The TChl-a at the Zhangpu aquaculture stations decreased compared to the reference station during investigation (Figures 4D–F), even decreasing by 32% on average during the non-culture period. The temporal changes of size composition were similar between aquaculture and reference station, with micro-sized Chl-a comprising 57% of TChl-a on average in March and April, and pico-sized Chl-a comprising 58% of TChl-a on average in July–September. Nano-sized Chl-a was dominant in other months.
During the culture period, concentrations of micro-sized Chl-a in Lianjiang was significantly lower in the surface water than in the bottom water (p< 0.05), and the difference was not observed during non-culture period (Table 1). Micro-sized Chl-a was significantly lower in the surface water than in the bottom water in Zhangpu during both culture and non-culture periods (p< 0.05, p< 0.05). Surface Chl-a concentration of each sized-fraction in Lianjiang decreased compared to that in the bottom water during the culture period (Figure 5A). The phytoplankton depletion index (PDI) of micro- and pico-phytoplankton in Lianjiang was significantly lower during the culture period than during the non-culture period (p< 0.05, p< 0.01), with a mean value of −38% and −44%, respectively. The PDI of total Chl-a and nanophytoplankton dropped to −14% and −16%, respectively. In Zhangpu, however, the PDI of each sized-fraction Chl-a in the culture period was generally consistent with that in the non-culture period (Figure 5B).
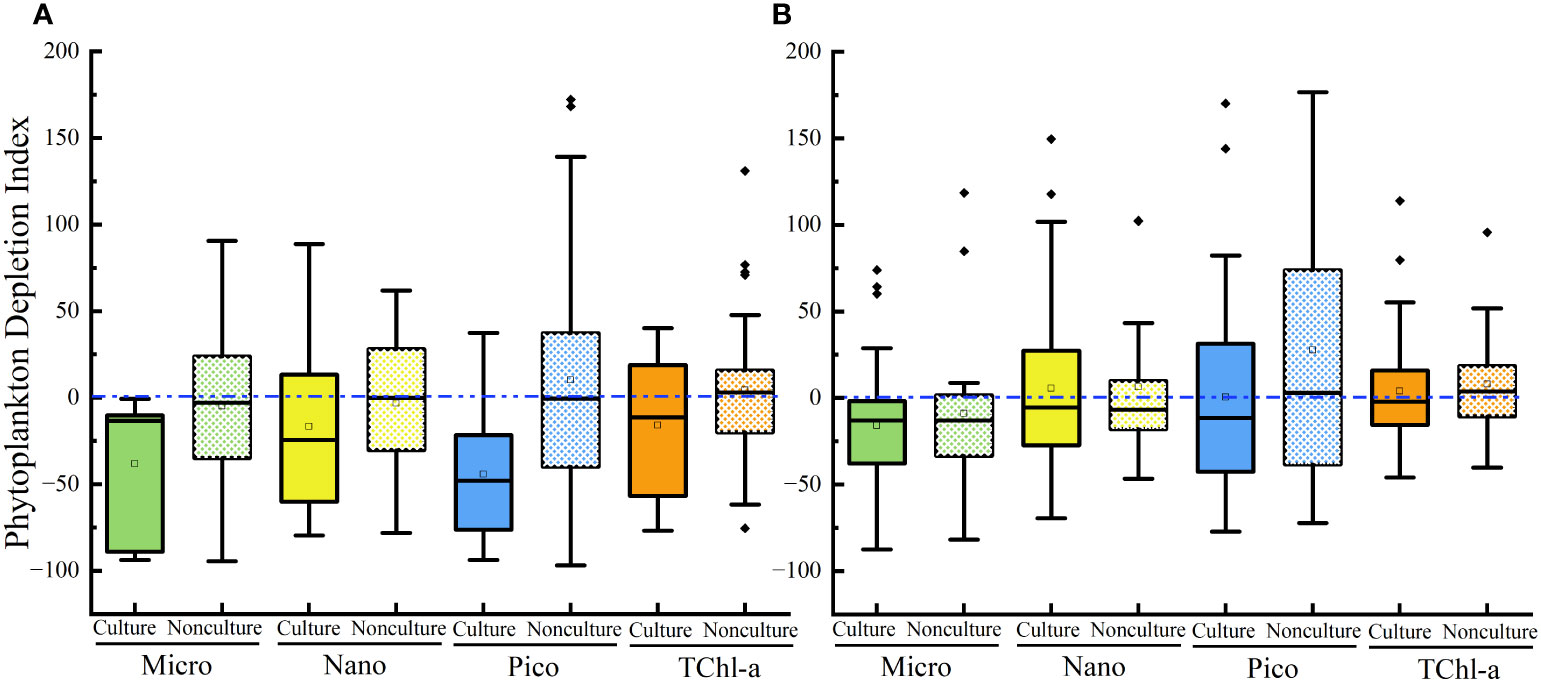
Figure 5 Phytoplankton depletion index of size-fractioned Chl-a and TChl-a in Lianjiang site (A) and Zhangpu site (B). Blue dash lines show depletion index of zero.
3.4 Size fractioned Chl-a concentration and environmental variables
The effects of environmental factors on the distribution of size-fractional Chl-a were explored using redundancy analysis (RDA). Base on the seasonal variability of environmental variables, we grouped water samples by season. It is evident that samples taken during spring and summer fell on the right side of RDA axis 1, while autumn and winter clustered on the left side (Figure 6). In Lianjiang, environmental factors accounted for 91.5% of the variance of size-fractional Chl-a. The dominant size-fraction was nano-phytoplankton during spring and converted to micro-, pico-phytoplankton during summer. The Chl-a concentrations of different size classes were negatively correlated with nutrient concentrations and positively correlated with the N/P ratio. In Zhangpu, environmental factors accounted for 86.9% of the variance of size-fractional Chl-a, with the micro- and picophytoplankton being the dominant fraction in spring and summer, respectively. The nano-sizedd Chl-a was the main contributor to the TChl-a. The pico-sized Chl-a was positively correlated with temperature and salinity, whereas the TChl-a concentration was negatively correlated with the N/P ratio.
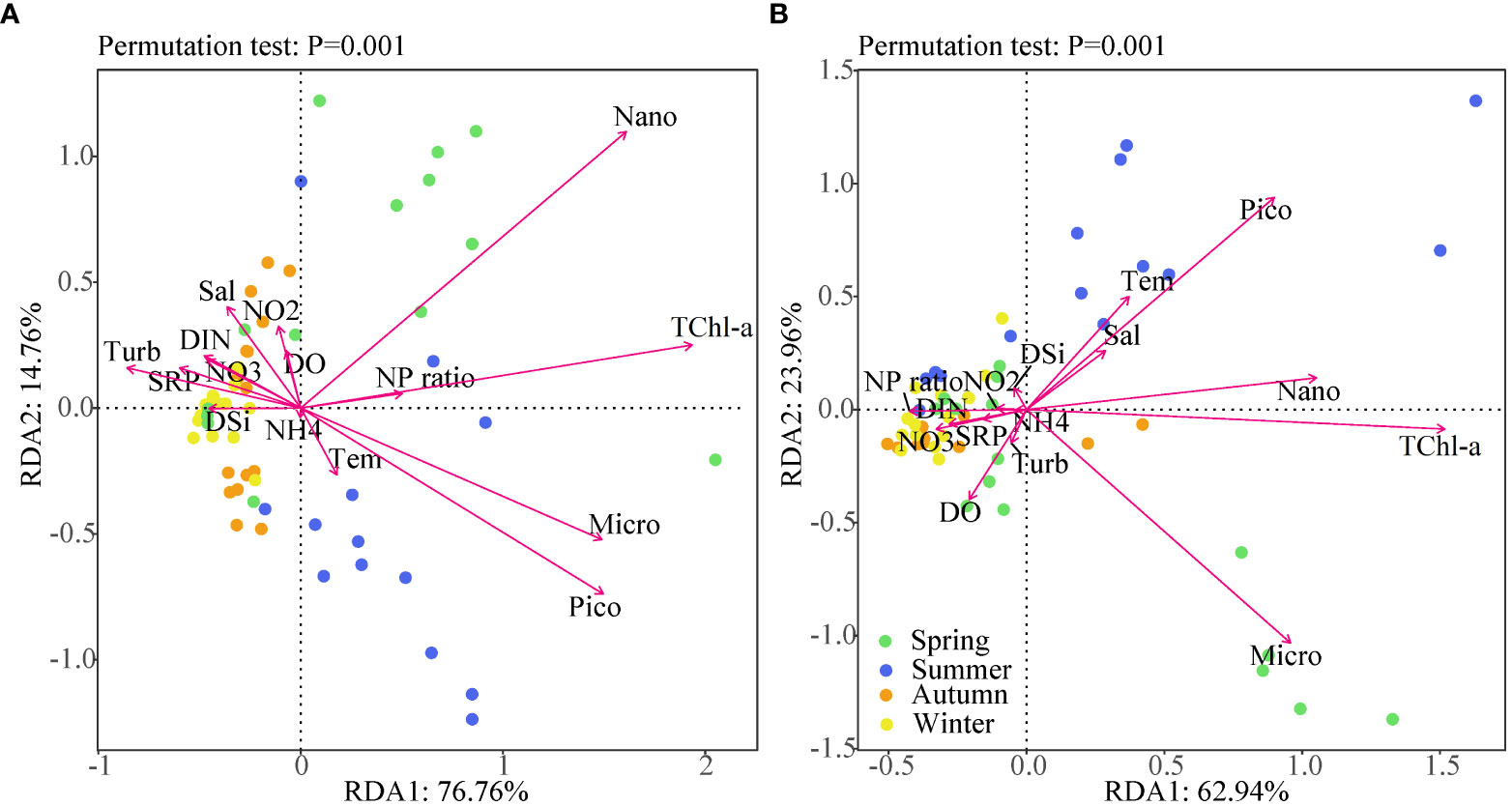
Figure 6 RDA t showing the interrelationship between physicochemical and biological variables in Lianjiang site (A) and Zhangpu site (B). Dots represent the different seasons. The arrow lengths are proportional to the influence of the individual variables in the ordination.
4 Discussion
4.1 The response of phytoplankton biomass to aquaculture in the western TWS
Shellfish are opportunistic filter feeders, relying on aquatic particulate matter, especially phytoplankton as their food source (Hulot et al., 2020). When the intensity of filter-feeding of phytoplankton by shellfish exceeds the recovery rate of phytoplankton, phytoplankton exhibit negative growth (Prins et al., 1997). The decrease in phytoplankton biomass as a result of filter-feeding in shellfish aquaculture areas has been widely reported (Ogilvie et al., 2000; Strohmeier et al., 2008; Lin et al., 2016; Jiang et al., 2016), with the Chl-a concentration of surface water during culture periods being 40–80% lower in aquaculture areas than in non-culture areas. However, phytoplankton biomass decreased in a small extent in both aquaculture areas of western TWS. The impact of shellfish aquaculture on phytoplankton community and biomass depends on culture density as well as phytoplankton biomass and hydrodynamic characteristics (Trottet et al., 2008). Aquaculture areas where phytoplankton biomass was known to decrease significantly are often located in poorly flushed bay with tidal current velocities of <10 cm/s (Ogilvie et al., 2000; Jiang et al., 2016). A weak water exchange rate is conducive to the accumulation of phytoplankton biomass, and an extension of water residence time increases the probability of filter-feeding by shellfish (Strohmeier et al., 2005; Sun et al., 2021). Decline of phytoplankton biomass in shellfish aquaculture areas decrease with increasing water flow velocity (Cerco & Noel, 2010). The TWS, as a key channel connecting the ECS and the SCS, has complex hydrodynamic conditions, with tidal current velocity about 40 cm/s on average (Wang et al., 2003; Hong et al., 2011). Tidal current propagating from ECS has been the dominant force in driving the hydrodynamics in the TWS, and it is amplified due to the shoaling topography, which strengthens on the west coast of TWS (Jan et al., 2004; Yu et al., 2015). In this study, the investigated stations were located in the west coast of TWS, with highly variable in environmental parameters, especially in Zhangpu area. The active water exchange between coastal water and open sea water may responsible for the slight decline of phytoplankton biomass in the aquaculture areas of western TWS.
Phytoplankton biomass showed no depletion in April at the Zhangpu aquaculture stations compared with the reference station, and exhibited a peak along with the massive consumption of nutrients. Notably, in April, the TChl-a concentration was slightly higher at the Zhangpu aquaculture stations than at the reference station, and the reference station had high levels of nutrients, especially phosphorous, while maintaining high biomass. These phenomena, when combined with the salinity changes at the reference station, suggest that the reference station received additional nutrient inputs. In a poor flushing bay, environmental parameters varied in a similar way for both reference stations and aquaculture stations (Jiang et al., 2016). In this study, the reference station was close to the aquaculture stations, but it still showed significant variation. Therefore, it was not a suitable reference station in open waters with complex hydrological changes to investigate changes in the grazing pressure on phytoplankton. During the survey period, the surface water and the bottom water at any sampling stations did not differ from each other in nutrient variation, although they differed from each other in temperature and turbidity. Therefore, we selected the Chl-a of bottom water as a reference to evaluate phytoplankton depletion.
4.2 The difference of phytoplankton depletion in north and south of the western TWS
Particles removal rate in turbid water is faster than that in clean waters, and residence time was shorter when the concentration of suspend particle matters was higher (Yang et al., 2006; Zhong et al., 2019). The southern shellfish aquaculture area, characterizing by high temperature, high salinity and high turbidity, showed faster particle removal rate than that in northern area with low temperature, low salinity and low turbidity (Wei et al., 2010). In Lianjiang, consumption of the TChl-a was observed as well as the different size-fractional Chl-a during the culture period. In contrast, no difference of depletion index in Zhangpu was observed between the culture and non-culture periods. Decline in phytoplankton biomass was supposed increase with increasing culture density (Jiang et al., 2016), however phytoplankton biomass in Zhangpu showed negligible reduction, despite a higher culture density in the Zhangpu compared with in Lianjiang (Ma et al., 2022). The obvious fluctuations in environmental parameters in the Zhangpu shellfish aquaculture farms may responsible for the complex interaction between aquaculture and surrounding water (Campbell & Hall, 2019). The growth of shellfish in aquaculture areas is achieved through feed input and filter-feeding on phytoplankton, which leads to increased release of nutrients and excreta debris (Bouwman et al., 2013). The released nutrients can promote the growth of phytoplankton, which offsets, to some extent, the reduction in phytoplankton biomass caused by filter-feeding. In some shellfish aquaculture areas, such as the Grande-Entrée Lagoon, phytoplankton depletion had not been observed (Trottet et al., 2008), and sometimes Chl-a in the aquaculture area was even higher than that in reference areas (Sonier et al., 2016). The filter-feeding efficiency of shellfish varied with its growth period, with oysters showing the highest growth rates in April and October, and lower growth rates in summer (Campbell & Hall, 2019). In the aquaculture area of Lianjiang, TChl-a decreased by 48% in March and April, but significant decreases did not occur in other months. Seasonal differences have also been observed in the mussel aquaculture area of Beatrix Bay (Ogilvie et al., 2000).
Dinoflagellates are reported to be more nutritious than diatoms (Menden-Deuer & Lessard, 2000), and a preference for dinoflagellate by shellfish was observed in filed studies (Ward & Shumway, 2004; Jiang et al., 2016). Diatom was the first major group in Dongshan-Zhangpu area, while dominant species shifted from dinoflagellate to diatom in Lianjiang during late spring (Wang et al., 2016; Zhang et al., 2023). The phosphorus deficient water in Lianjiang also favors the growth of dinoflagellate comparing with diatom (Ou et al., 2006). The relative lack of favorable food source in Zhangpu aquaculture area, which has a longer culture period than Lianjiang, may also explain the negligible phytoplankton depletion in Zhangpu. However, the contribution of diatoms to shellfish diet still requires further study in the western TWS. Overall, the difference of phytoplankton depletion in the northern and southern TWS suggests a more resilient system to shellfish aquaculture in Zhangpu than that in Lianjiang.
4.3 Phytoplankton size class dynamics in aquaculture areas of the western TWS
The filter-feeding process of shellfish exhibits selectivity toward particle size, and the filtration efficiency increases non-linearly with particle size (Ward & Shumway, 2004). Oysters can remove 50% of the 1.7-μm particles, and the filtration efficiency for particles >7 μm reaches 100% (Riisgård, 1988; Ward & Shumway, 2004). Remarkable decrease of micro-phytoplankton was observed during culture period in a high-density oyster aquaculture farm (Jiang et al., 2016). The filter-feeding of shellfish can also reduce the abundance of small plankton, such as ciliates and flagellates, which lowers the grazing pressure on small phytoplankton and leads to a shift in the phytoplankton community toward dominance of pico-size plankton (Tomaru et al., 2002). The dominance of pico-phytoplankton is considered to be an indicator of phytoplankton depletion in shellfish aquaculture areas (Safi & Gibbs, 2003; Jiang et al., 2016). In Lianjiang, the contribution of pico-phytoplankton to the total Chl-a concentration generally varied with season, but it occasionally increased during the culture period. The preferential filtration for micro-phytoplankton by shellfish aquaculture may enhance the dominance of smaller size-fractional phytoplankton. Nano- and pico-phytoplankton account for 60–80% of the total phytoplankton biomass in the TWS, with nano-phytoplankton accounting for up to 59% in the northern region and pico-phytoplankton accounting for 71% in the southern region (Wang et al., 1997). Although the impact of shellfish aquaculture on phytoplankton biomass is not obvious, the size composition of phytoplankton is affected by shellfish aquaculture to some extent.
A distinction of biomass and size composition of phytoplankton between two shellfish aquaculture areas was showed in the conceptual graph (Figure 7). Studies have shown that the composition of phytoplankton size fractions is more influenced by season and geographic location than by the aquaculture process (Pitta et al., 1998; Jiang et al., 2016). The general pattern of biomass was low during NE monsoon and high during SW monsoon, with phytoplankton community shifting from nano-phytoplankton during NE monsoon to micro- and pico-phytoplankton during SW monsoon in this study. Phytoplankton bloom was basically coincident with an apparent decline of silica in both Lianjiang and Zhangpu. Small diatoms with fast growth rate benefit from nutrient absorption and are dominant in temperate sea waters (Terseleer et al., 2014; Leblanc et al., 2018). It is suggested that nanoplankton diatoms thrive and lead to N/Si ratio exceeding 2 in March in Lianjiang. Different from Lianjiang, microplankton diatoms prevailed during spring in Zhangpu, where nutrients and temperature were suitable for the growth of centric and chain-forming diatoms (Wang et al., 2016; Rajaneesh et al., 2018). As nutrient decreasing during summer, picoplankton (e.g., Prochlorococcus, Synechococcus) with high-temperature and high-salinity and low-nutrient niches takes advantage to growth (Zhong et al., 2020). Considering the relatively broad niche breadths for each environmental variable of Synechococcus, together with the N-deficient waters during summer in this study, it could conclude that Synechococcus constitutes most part of picoplankton in Zhangpu and Lianjiang (Wen et al., 2017; Zhong et al., 2020).
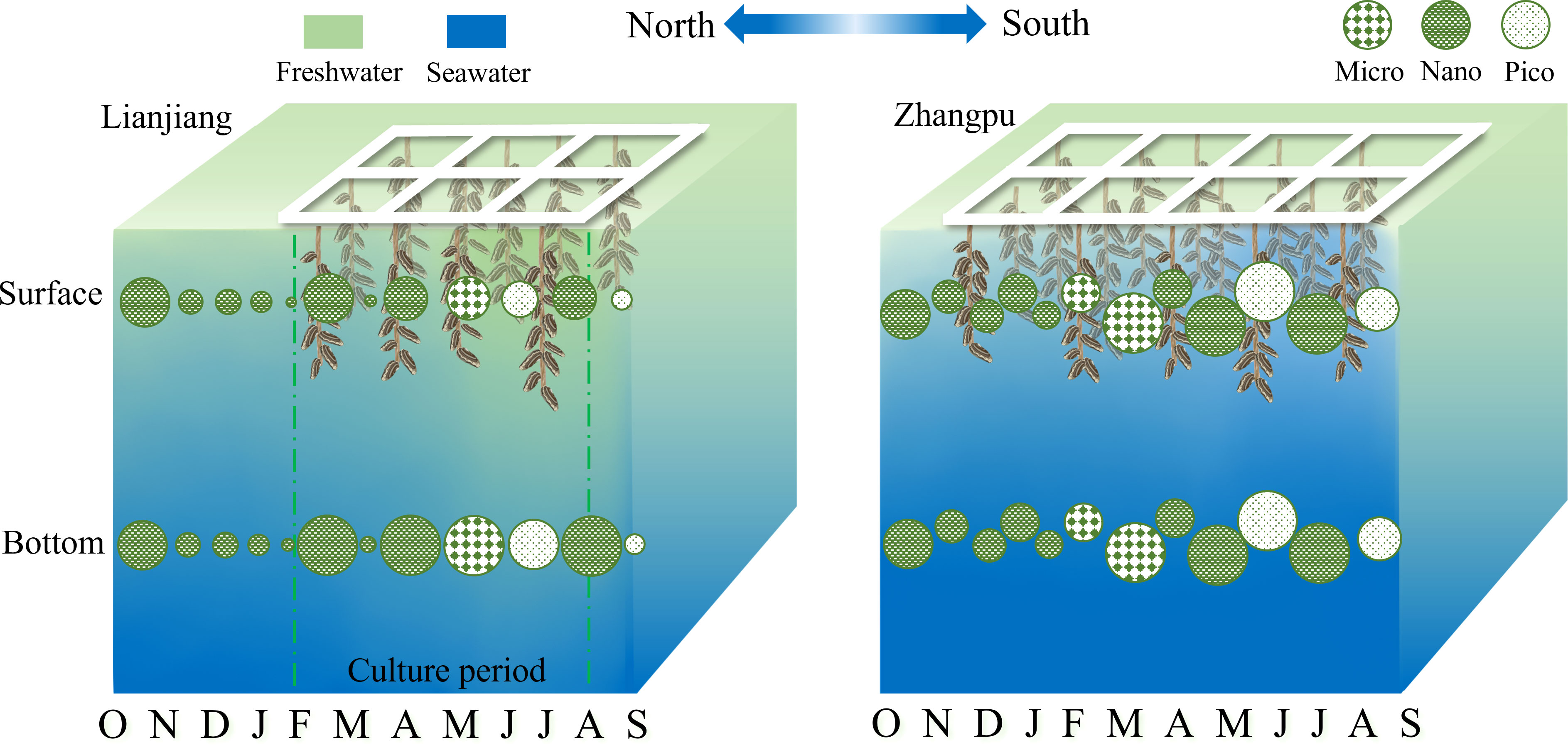
Figure 7 Schematic representation of the temporal variations of dominant fraction Chl-a in Lianjiang and Zhangpu aquaculture areas.
4.4 Nutrient inputs in the aquaculture areas of the western TWS
Fundamentally, nutrient availability or assimilate deviations from N:Si:P ratio of 16:15:1 suggest the possibility of nutrient-limited (Brzezinski, 1985). The nutrient ratios considered in the present study show that phosphorus is the main limited nutrient in the western TWS, especially in Lianjiang. Phytoplankton growth is usually limited by phosphorus in the TWS (Ou et al., 2006; Wang et al., 2016; Wen et al., 2017). Nutrient input from vertical mixing and upwelling is primary supply for phytoplankton in the southern TWS, and phosphorus limitation can be abated during upwelling events (Ou et al., 2006; Hong et al., 2011). Although we are lack of direct evidence to demonstrate vertical mixing in Zhangpu area, the fluctuations of high turbidity and high silica concentration indicate a turbulent water to some extent (Wen et al., 2017; Rajaneesh et al., 2018). Upwelling occurs in the Taiwan Bank in the summer, which was characterized by low temperature and high salinity (T< 25°C, S > 33.9) (Hu et al., 2015). Accordingly, in this study, we observed that the aquaculture station with a salinity of 33.6 in Zhangpu in July and August. It is supposed that the continuous nutrient supply maintains the high phytoplankton biomass in Zhangpu. In contract, nutrient concentrations in Lianjiang are constantly low during warm season. Nutrient inputs from submarine groundwater discharge (SGD) are supposed to be the predominant regulator in Lianjiang aquaculture area, with DIN and SRP fluxes contributing by SGD over total nutrient inputs approximately 58% and 73%, respectively in July (Peng et al., 2021). An obvious elevation in nitrogen concentration was not observed despite the dramatic decrease of salinity occurred in this study. Due to the changing environment such as tides, precipitation and assimilation of phytoplankton, it is not adequate for illustrating the low nutrient concentration with data presented in this study (Jiang et al., 2016; Zhang et al., 2023).
5 Conclusion
In this study, we assessed the phytoplankton community structure variation under the influence of marine shellfish aquaculture in the west coast of the TWS in terms of the Chl-a concentration and the size fractions. A weak change of phytoplankton biomass and size structure in response to shellfish aquaculture was observed. Phytoplankton depletion was different between the northern and southern shellfish aquaculture areas. During the culture period in the northern aquaculture area (Lianjiang), the TChl-a concentration of surface water at aquaculture stations was approximately 14% lower than that of bottom water, and this decreasing trend was consistently present in micro-, nano-, and pico-size fractions. Phytoplankton biomass showed negligible depletion during culture period in the southern aquaculture area (Zhangpu), where environmental parameters varied greatly during the survey period. The shift from a dominance of nano-phytoplankton to the dominance of micro- and pico-phytoplankton was caused mainly by seasonal changes in environmental conditions. In aquaculture areas with complex hydrological conditions, the Chl-a of bottom water may serve as an appropriate reference for evaluating phytoplankton depletion.
Data availability statement
The raw data supporting the conclusions of this article will be made available by the authors, without undue reservation.
Author contributions
Conceptualization, LW and ZL; methodology, LW; software, YM; validation, YM; formal analysis, YM, CJ and XC; investigation, RO and JQ; writing—original draft preparation, YM; writing—review and editing, LW; visualization, YM; supervision, AL and HH; funding acquisition, LW. All authors have read and agreed to the published version of the manuscript.
Funding
This study was funded by the National Key Research and Development Program of China No. 2022YFF0802204, the Scientific Research Foundation of Third Institute of Oceanography, M.N.R., No. 2018017, 2019017 & 2019018.
Acknowledgments
We are grateful to the measurements and analyses of samples by Ms. Lulu Pei and Dr. Jianhua Kang. We also thank the graphic processing software of Ocean Data View (version 4.5.7) contributed by Schlitzer, R., http://odv.awi.de, and OriginPro 8.5 (OriginLab Corporation©, Northampton, MA 01060 USA). We do appreciate the comments and suggestions on the manuscript from reviewers.
Conflict of interest
The authors declare that the research was conducted in the absence of any commercial or financial relationships that could be construed as a potential conflict of interest.
Publisher’s note
All claims expressed in this article are solely those of the authors and do not necessarily represent those of their affiliated organizations, or those of the publisher, the editors and the reviewers. Any product that may be evaluated in this article, or claim that may be made by its manufacturer, is not guaranteed or endorsed by the publisher.
References
Bouwman L., Beusen A., Glibert P. M., Overbeek C., Pawlowski M., Herrera J., et al. (2013). Mariculture: significant and expanding cause of coastal nutrient enrichment. Environ. Res. Lett. 8 (4), 44026. doi: 10.1088/1748-9326/8/4/044026
Brzezinski M. A. (1985). The Si:C:N ratio of marine diatoms: interspecific variability and the effecct of some environmental variables. J. Phycol. 21 (3), 347–357. doi: 10.1111/j.0022-3646.1985.00347.x
Campbell M. D., Hall S. G. (2019). Hydrodynamic effects on oyster aquaculture systems: a review. Rev. Aquac. 11 (3), 896–906. doi: 10.1111/raq.12271
Cerco C. F., Noel M. R. (2010). Monitoring, modeling, and management impacts of bivalve filter feeders in the oligohaline and tidal fresh regions of the Chesapeake Bay system. Eco. Modell. 221 (7), 1054–1064. doi: 10.1016/j.ecolmodel.2009.07.024
Clarke K. R., Warwick R. M. (2001). A further biodiversity index applicable to species lists: variation in taxonomic distinctness. Mar. Ecol. Prog. Ser. 216, 265–278. doi: 10.3354/MEPS216265
Dai M. H., Zhao Y. Y., Chai F., Chen M. R., Chen N. ,. W., Chen Y. ,. M., et al. (2023). Persistent eutrophication and hypoxia in the coastal ocean. Cambridge Prisms: Coast. Futur. 1, 1–28, e19. doi: 10.1017/cft.2023.7
Dame R. F. (2011). Ecology of Marine Bivalves: An Ecosystem Approach. 2nd edn (Boca Raton, USA: CRC Press). doi: 10.1201/b11220
FAO (2020). The state of world fisheries and aquaculture (Rome: Food and Aquaculture Organization of the United Nations), 29–31. Available at: https://www.fao.org/publications/sofia/2022/en/.
Filgueira R., Guyondet T., Comeau L. A., Grant J. (2014). Physiological indices as indicators of ecosystem status in shellfish aquaculture sites. Ecol. Indic. 39, 134–143. doi: 10.1016/j.ecolind.2013.12.006
Filgueira R., Guyondet T., Comeau L. A., Tremblay R. (2016). Bivalve aquaculture-environment interactions in the context of climate change. Glob. Change Biol. 22, 3901–3913. doi: 10.1111/gcb.13346
Hong H., Chai F., Zhang C., Huang B., Jiang Y., Hu J. (2011). An overview of physical and biogeochemical processes and ecosystem dynamics in the Taiwan Strait. Cont. Shelf Res. 31 (6), S3–S12. doi: 10.1016/j.csr.2011.02.002
Hu J., Lan W., Huang B., Chiang K.-P., Hong H. (2015). Low nutrient and high chlorophyll a coastal upwelling system – A case study in the southern Taiwan Strait. Estuarine Coast. Shelf Sci. 166, 170–177. doi: 10.1016/j.ecss.2015.05.020
Hulot V., Saulnier D., Lafabrie C., Gaertner-Mazouni N. (2020). Shellfish culture: a complex driver of planktonic communities. Rev. Aquac. 12 (1), 33–46. doi: 10.1111/raq.12303
Jan S., Chern C. S., Wang J., Chao S. Y. (2004). The anomalous amplification of M2 tide in the Taiwan Strait. Geophy. Res. Lett. 31, L07308. doi: 10.1029/2003GL019373
Jan S., Sheu D. D., Kuo H. M. (2006). Water mass and throughflow transport variability in the Taiwan Strait. J. Geophys. Res. 111, (C12). doi: 10.1029/2006jc003656
Jiang T., Chen F., Yu Z., Lu L., Wang Z. (2016). Size-dependent depletion and community disturbance of phytoplankton under intensive oyster mariculture based on HPLC pigment analysis in Daya Bay, South China Sea. Environ. pollut. 219, 804–814. doi: 10.1016/j.envpol.2016.07.058
Leblanc K., Qu´eguiner B., Diaz F., Cornet V., Michel-Rodriguez M., Durrieu de Madron X., et al. (2018). Nanoplanktonic diatoms are globally overlooked but play a role in spring blooms and carbon export. Nat. Commun. 9, 953. doi: 10.1038/s41467-018-03376-9
Lin J., Li C., Zhang S. (2016). Hydrodynamic effect of a large offshore mussel suspended aquaculture farm. Aquaculture 451, 147–155. doi: 10.1016/j.aquaculture.2015.08.039
Liu X., Wang Z., Yang X., Liu Y., Liu B., Zhang J., et al. (2022). Mapping China’s offshore mariculture based on dense time-series optical and radar data. Int. J. Digit. Earth 15 (1), 1326–1349. doi: 10.1080/17538947.2022.2108923
Lucas L. V., Thompson J. K., Stacey M. T., Koseff J. R. (2016). Bivalve grazing can shape phytoplankton communities. Front. Mar. Sci. 3. doi: 10.3389/fmars.2016.00014
Ma Y., Qu X., Feng D., Zhang P., Huang H., Zhang Z., et al. (2022). Recognition and statistical analysis of coastal marine aquacultural cages based on R3Det single-stage detector: A case study of Fujian Province, China. Ocean Coast. Manage. 225, 106244. doi: 10.1016/j.ocecoaman.2022.106244
Menden-Deuer S., Lessard E. J. (2000). Carbon to volume relationships for dinoflagellates, diatoms, and other protist plankton. Limnol. Oceanogr. 45, 569–579. doi: 10.4319/lo.2000.45.3.0569
Newell R. I. E. (2004). Ecosystem influences of natural and cultivated populations of suspension-feeding bivalve molluscs: a review. J. Shellfish Res. 23 (1), 51–62. Available at: https://link.gale.com/apps/doc/A118543914/AONE?u=anon~1220bc4e&sid=bookmark-AONE&xid=63f7edd4.
Newell R. I. E., Fisher T. R., Holyoke R. R., Cornwell J. C. (2005). “Influence of eastern oysters on nitrogen and phosphorus regeneration in Chesapeake Bay, USA,” in The Comparative Roles of Suspension - Feeders in Ecosystems. Eds. Dame R. F., Olenin S. (Dordrecht, The Netherlands: Springer), 93–120. doi: 10.1007/1-4020-3030-4_6
Ogilvie S. C., Ross A. H., Schiel D. R. (2000). Phytoplankton biomass associated with mussel farms in Beatrix Bay, New Zealand. Aquaculture 181 (1), 71–80. doi: 10.1016/S0044-8486(99)00219-7
Ou L. J., Huang B. Q., Lin L. Z., Hong H. S. (2006). Phosphorus stress of phytoplankton in the Taiwan Strait determined by bulk and single-cell alkaline phosphatase activity assays. Mar. Ecol. Prog. Ser. 327, 95–106. doi: 10.3354/meps327095
Paerl H. W., Valdes L. M., Peierls B. L., Adolf J. E., Harding L. (2006). Anthropogenic and climatic influences on the eutrophication of large estuarine ecosystems. Limnol. Oceanogr. 51, 448–462. doi: 10.4319/lo.2006.51.1_part_2.0448
Peng T., Zhu Z. Y., Du J. Z., Liu J. N. (2021). Effects of nutrient-rich submarine groundwater discharge on marine aquaculture: A case in Lianjiang, East China Sea. Sci. Total Environ. 786, 147388. doi: 10.1016/j.scitotenv.2021.147388
Pitta P., Karakassis I., Tsapakis M., Zivanovic S. (1998). Natural vs. mariculture induced variability in nutrients and plankton in the eastern Mediterranean. Hydrobiologia 391 (1), 179–192. doi: 10.1023/A:1003501832069
Prins T. C., Smaal A. C., Dame R. F. (1997). A review of the feedbacks between bivalve grazing and ecosystem processes. Aquat. Ecol. 31 (4), 349–359. doi: 10.1023/A:1009924624259
Rajaneesh K. M., Mitbavkar S., Anil A. C. (2018). Dynamics of size-fractionated phytoplankton biomass in a monsoonal estuary: Patterns and drivers for seasonal and spatial variability. Estuar. Coast. Shelf Sci. 207, 325–337. doi: 10.1016/j.ecss.2018.04.026
Riisgård H. U. (1988). Efficiency of particle retention and filtration rate in 6 species of Northeast American bivalves. Mar. Ecol. Prog. Ser. 45, 217–223. doi: 10.3354/MEPS045217
Rosa M., Ward J. E., Holohan B. A., Shumway S. E., Wikfors G. H. (2017). Physicochemical surface properties of microalgae and their combined effects on particle selection by suspension-feeding bivalve molluscs. J. Exper. Mar. Bio. Eco. 486, 59–68. doi: 10.1016/j.jembe.2016.09.007
Safi K. A., Gibbs M. M. (2003). Importance of different size classes of phytoplankton in Beatrix Bay, Marlborough Sounds, New Zealand, and the potential implications for the aquaculture of the mussel, Perna canaliculus. N. Z. J. Mar. Freshw. Res. 37 (2), 267–272. doi: 10.1080/00288330.2003.9517164
Sieburth J. M., Smetacek V., Lenz J. (1978). Pelagic ecosystem structure: Heterotrophic compartments of the plankton and their relationship to plankton size fractions 1. Limnol. Oceanogr. 23 (6), 1256–1263. doi: 10.4319/lo.1978.23.6.1256
Smaal A. C., Schellekens T., van Stralen M. R., Kromkamp J. C. (2013). Decrease of the carrying capacity of the Oosterschelde estuary (SW Delta, NL) for bivalve filter feeders due to overgrazing? Aquaculture 404–405, 28–34. doi: 10.1016/j.aquaculture.2013.04.008
Sonier R., Filgueira R., Guyondet T., Tremblay R., Olivier F., Meziane T., et al. (2016). Picophytoplankton contribution to Mytilus edulis growth in an intensive culture environment. Mar. Biol. 163 (4), 73. doi: 10.1007/s00227-016-2845-7
Souchu P., Vaquer A., Collos Y., Landrein S., Deslous-Paoli J.-M., Bibent B. (2001). Influence of shellfish farming activities on the biogeochemical composition of the water column in Thau lagoon. Mar. Ecol. Prog. Ser. 218, 141–152. doi: 10.3354/meps218141
Srichandan S., Baliarsingh S. A.-O. X., Prakash S., Lotliker A. A., Parida C., Sahu K. C. (2019). Seasonal dynamics of phytoplankton in response to environmental variables in contrasting coastal ecosystems. Environ. Sci. pollut. Res. 26, 12025–12041. doi: 10.1007/s11356-019-04569-5
Strickland J. D. H., Parsons T. R. (1972). A Practical Handbook of Seawater Analysis. 2nd ed Vol. 167 (Ottawa, Canada: Fisheries Research Board of Canada Press).
Strohmeier T., Aure J., Duinker A., Castberg T., Svardal A., Strand Ø. (2005). Flow reduction, seston depletion, meat content and distribution of diarrhetic shellfish toxins in a long-line blue mussel (Mytilus edulis) farm. J. Shellfish Res. 24 (1), 15–23. doi: 10.2983/0730-8000(2005)24[15:FRSDMC]2.0.CO;2
Strohmeier T., Duinker A., Strand Ø., Aure J. (2008). Temporal and spatial variation in food availability and meat ratio in a longline mussel farm (Mytilus edulis). Aquaculture 276 (1-4), 83–90. doi: 10.1016/j.aquaculture.2008.01.043
Sun K., Zhang J., Lin F., Ren J. S., Zhao Y., Wu W., et al. (2021). Evaluating the growth potential of a typical bivalve-seaweed integrated mariculture system - a numerical study of Sungo Bay, China. Aquaculture 532, 736037. doi: 10.1016/j.aquaculture.2020.736037
Tang D., Kester D. R., Ni I. H., Kawamura H., Hong H. (2002). Upwelling in the Taiwan Strait during the summer monsoon detected by satellite and shipboard measurements. Remote Sens. Environ. 83 (3), 457–471. doi: 10.1016/S0034-4257(02)00062-7
Terseleer N., Bruggeman J., Lancelot C., Gypens N. (2014). Trait-based representation of diatom functional diversity in a plankton functional type model of the eutrophied southern North Sea. Limnol. Oceanogr. 59, 1958–1972. doi: 10.4319/lo.2014.59.6.1958
Tomaru Y., Udaka N., Kawabata Z., Nakano S.i. (2002). Seasonal change of seston size distribution and phytoplankton composition in bivalve pearl oyster Pinctada fucata martensii culture farm. Hydrobiologia 481 (1), 181–185. doi: 10.1023/A:1021232832390
Trottet A., Roy S., Tamigneaux E., Lovejoy C., Tremblay R. (2008). Impact of suspended mussels (Mytilus edulis L.) on plankton communities in a Magdalen Islands lagoon (Québec, Canada): A mesocosm approach. J. Exp. Mar. Biol. Ecol. 365 (2), 103–115. doi: 10.1016/j.jembe.2008.08.001
Wang H., Huang B., Hong H. (1997). Size-fractionated productivity and nutrient dynamics of phytoplankton in subtropical coastal environments. Hydrobiologia 352 (1), 97–106. doi: 10.1023/A:1003017726533
Wang Y. H., Jan S., Wang D. P. (2003). Transports and tidal current estimates in the Taiwan Strait from shipboard ADCP observations, (1999–2001). Estuar. Coast. Shelf Sci. 57 (1), 193–199. doi: 10.1016/S0272-7714(02)00344-X
Wang Y., Kang J. H., Ye Y. Y., Lin G. M., Yang Q. L., Lin M. (2016). Phytoplankton community and environmental correlates in a coastal upwelling zone along western Taiwan Strait. J. Mar. Syst. 154, 252–263. doi: 10.1016/j.jmarsys.2015.10.015
Ward E. J., Shumway S. E. (2004). Separating the grain from the chaff: particle selection in suspension- and deposit-feeding bivalves. J. Exp. Mar. Biol. Ecol. 300 (1), 83–130. doi: 10.1016/j.jembe.2004.03.002
Wei C. L., Tsai J. R., Hou Y. R., Wen L. S., Sheu D. D., Chou W. C. (2010). Scavenging phenomenon elucidated from 234Th/238U disequilibrium in surface water of the Taiwan Strait. Terr. Atmos. Ocean. Sci. 21 (4), 713–726. doi: 10.3319/TAO.2009.08.26.01(Oc
Wen Z. Z., Lin W. F., Shen R., Hong H. Z., Kao S. J., Shi D. L. (2017). Nitrogen fixation in two coastal upwelling regions of the Taiwan Strait. Sci. Rep. 7, 17601. doi: 10.1038/s41598-017-18006-5
Yang W. F., Huang Y. P., Chen M., Zhang L., Liu H. B., Liu G. S., et al. (2006). Disequilibria between 210Po and 210Pb in surface waters of the southern South China Sea and their implications. Sci. China Earth Sci. 49, 103–112. doi: 10.1007/s11430-004-5233-y
Yu H. Q., Yu H. M., Ding Y., Wang L., Kuang L. (2015). On M2 tidal amplitude enhancement in the Taiwan Strait and its asymmetry in the cross-strait direcction. Continent. Shelf Res. 109 (15), 198–209. doi: 10.1016/j.csr.2015.09.005
Zhang X. S., Zhen G. M., Cui X. R., Zeng Y. L., Gao W. M., Yu K. L., et al. (2023). Effect of dissolved organic nutrients on the bloom of Prorocentrum donghaiense in the East China Sea coastal waters. Mar. Environ. Res. 183, 105841. doi: 10.1016/j.marenvres.2022.105841
Zhong Y. P., Liu X., Xiao W. P., Laws E. A., Chen J. X., Wang L., et al. (2020). Phytoplankton community patterns in the Taiwan Strait match the characteristics of their realized niches. Progr. Oceanogr. 186, 102366. doi: 10.1016/j.pocean.2020.102366
Keywords: phytoplankton, size structure, aquaculture, hydrodynamic, Taiwan strait
Citation: Mo Y, Li A, Luo Z, Jia C, Ou R, Qiu J, Chang X, Huang H and Wang L (2023) Inapparent decrease in phytoplankton biomass accompanied by significant size composition succession in the shellfish aquaculture farm in the western Taiwan strait. Front. Mar. Sci. 10:1204508. doi: 10.3389/fmars.2023.1204508
Received: 12 April 2023; Accepted: 05 July 2023;
Published: 21 July 2023.
Edited by:
Susana Carvalho, King Abdullah University of Science and Technology, Saudi ArabiaReviewed by:
Solomon Dan, Beibu Gulf University, ChinaJiaxing Liu, Chinese Academy of Sciences (CAS), China
Copyright © 2023 Mo, Li, Luo, Jia, Ou, Qiu, Chang, Huang and Wang. This is an open-access article distributed under the terms of the Creative Commons Attribution License (CC BY). The use, distribution or reproduction in other forums is permitted, provided the original author(s) and the copyright owner(s) are credited and that the original publication in this journal is cited, in accordance with accepted academic practice. No use, distribution or reproduction is permitted which does not comply with these terms.
*Correspondence: Lei Wang, wanglei@tio.org.cn