- 1Instituto Politécnico Nacional, Centro Interdisciplinario de Ciencias Marinas (CICIMAR-IPN), La Paz, Baja California Sur, Mexico
- 2Marine Conservation Medicine and Ecosystem Health, Cientinela del Mar A.C., La Paz, Baja California Sur, Mexico
- 3Animal Health Center, Ministry of Agriculture and Food, Abbotsford, BC, Canada
- 4Veterinary Science Department, The Marine Mammal Center, Sausalito, CA, United States
Defining baseline mortality and trends in wildlife populations is imperative to understand natural and anthropogenic threats to overall population health and improve conservation measures for species, particularly in geographically confined habitats. The Guadalupe fur seal Arctocephalus townsendi (GFS) is a threatened pinniped that ranges throughout the west coast of Mexico with sporadic dispersion to higher latitudes. Their breeding habitat is restricted to Guadalupe Island, Mexico, which is vulnerable to periodic and cyclic warming of the Northeast Pacific Ocean. The impacts of environmental change on GFS health and reproductive success at Guadalupe Island are poorly defined and the aim of this study was to establish baseline pup (GFSn) mortality rates and primary causes of death during the 2013-2016 breeding seasons at Guadalupe Island. Interannual mortality rates and causes of death were compared by year, breeding seasons and by geographic location. The highest mortality rate in GFSn was in 2015 (14.7%), followed by 2014 (8.2%), 2016 (6.7%) and 2013 (5.6%). The presumptive causes of mortality of GFSn were consistent with other published long term otariids health surveys and included: emaciation (49%), trauma (24%), infectious disease (8%), drowning (4%) stillbirth/perinatal mortality (4%) and undetermined (11%). However, in 2015 and coinciding with northeast Pacific marine heatwave in 2014-2016, emaciation accounted for 54% of GFSn mortality in contrast to 9% in 2013. For GFSn, terrestrial habitat may influence mortality rates and causes of mortality but like other marine predators, marine habitat features, such as an increase in sea surface temperature are associated with changes in maternal care, nutritional status and pups survival. Monitoring mortality rate and causes in GFSn at Guadalupe Island is crucial to establish baseline health trends, document potential impacts on species demographics and recruitment during marine heatwaves and potential consequences in population recovery.
1 Introduction
Survival rates in wildlife populations, including marine mammals, is an important demographic parameter (Mills, 2012) that varies between and throughout species development (Barlow and Boveng, 1991). Reduced survival rates during early life stages (Clinton and Le Boeuf, 1993; Wickens and York, 1997) have been associated with maternal (e.g. experience) and environmental (e.g. colony) factors (Lunn et al, 1994; Georges and Guinet, 2000) and can result in significant seasonal and spatial differences in population demographics and health (Spraker and Lander, 2010; Seguel et al., 2013; Michael et al., 2019).
The Guadalupe fur seal (Arctocephalus townsendi; GFS) is classified as at “risk of extinction” by Mexican law (Norma Oficial Mexicana, NOM-059-SEMARNAT, 2010) and in the United States (U.S.), as “threatened” under the Endangered Species Act. Throughout their historic range within northeast Pacific Ocean, the population was severely impacted by commercial harvest during the 18th and late 19th centuries (Hubbs, 1956). GFSs were extirpated from California in the early 19th century and considered extinct by the late 19th century, when a few remnant GFSs were observed on Guadalupe Island, Mexico. The pre-exploitation GFS population was estimated up to 200,000 individuals (Hubbs, 1979) and recently (2019) between 57,199-72,631 individuals were recorded at Guadalupe Island with an annual increase of 8.1-8.8% (1991- 2019) (Juárez-Ruiz et al., 2022). Currently, Guadalupe Island is the only GFS breeding site designated as critical habitat, where some anthropogenic activities (e.g. sport fishery and shark cage diving) were permitted until 2020 (DOF, 2023).
The GFS is a pelagic specialist with a preference for jumbo squid (Dosidicus gigas) and opalescent squid (Doryteuthis opalescens), as well to myctophids (Gallo-Reynoso and Esperón-Rodríguez, 2013; Amador-Capitanachi et al., 2017). The breeding season is from June to August (Gallo-Reynoso, 1994), during which lactating females nurse pups on shore for 5.1 – 6.5 days, interspersed with foraging trips to sea for up to 10 ± 4 days (range: 4-14 days), transit distances of 444 ± 151 km (range: 222 - 589 km) from Guadalupe Island and dive to depths of 17 ± 10 m (range: 3-82 m). Foraging accounts for up 75% of the GFS adult female time energy budget (Gallo-Reynoso et al., 2008) and results in production and transfer of milk with a fat content of 43% to pups (GFSn) during their attendance. The high fat content allows GFSn to fast for prolonged periods (maximum 24 days), until they are weaned, between 9 and 11 months of age (lactation length) (Figueroa-Carranza, 1994).
Ecological threats to GFS population recovery, such as climate change and reduced prey availability are recognized (Weber et al., 2004; Hernández-Camacho and Trites, 2018); however, the impacts of these changes in GFSn mortality rates and causes Guadalupe Island are unknown. Furthermore, between 2015 and 2020 unusual sightings of GFSs were reported in Mexico (Villegas-Zurita., et al 2015; Ortega-Ortiz et al., 2019). Record numbers of emaciated individuals stranded (washed ashore) along the California, Oregon and Washington coasts (D'Agnese et al., 2020) prompting the declaration of an Unusual Mortality Event (UME) by the National Oceanic and Atmospheric Administration (NOAA) (2015 - 2020) (NOAA, 2019). The UME coincided with anomalous increases in ocean surface temperatures (up to 2.5°C) (SST) between 2014 and 2016, attributed to a Northeast Pacific Heatwave along the North Pacific Ocean (Bond et al., 2015). This event was characterized by a depressed thermocline, an increase in stratification in coastal and off-shore areas along Central and Southern and California Current System (CCS) (Zaba and Rudnick, 2016; Rudnick et al., 2017), affecting the nutrient flux and reducing phytoplankton and zooplankton biomass, as well as prey availability for secondary predators (e.g. squid). This had an impact on the distribution of foraging areas for top predators such as marine mammals and sea birds, including GFS (Leising et al., 2015; Cavole et al., 2016; Piatt et al., 2020), which ecological and biological response are linked to population or individual adaptations (Smith et al., 2023).
To improve our understanding of the impact of regional oceanic warming on marine megafauna demography, in relation to mortality, this study assessed the mortality rate (MR) and presumptive cause of dead (PCOD) of GFSn during the breeding seasons from 2013 to 2016 at Guadalupe Island. Based on anecdotal reports, field observations and published accounts of stranded GFS in suboptimal nutritional condition and increased strandings in more remote areas, we hypothesized that an increase of MR and PCOD in GFSn was associated with changes in prey availability for lactating females and maternal care particularly during breeding season of 2014 and 2015, coinciding with the highest positive SST anomalies generated by Northeast Pacific Heatwave around Guadalupe Island (Gálvez et al., 2020).
2 Materials and methods
2.1 Study site and GFS pup mortality monitoring
Guadalupe Island is a volcanic island located in the northeastern Pacific Ocean, approximately 300 km west of the Baja California Peninsula with a surface area of 250 km (Figure 1). Guadalupe Island has been influenced by the southern portion of CCS and is sensitive to interannual climate variability and major ocean circulation conditions (Durazo and Baumgartner, 2002; Ren and Rudnick, 2021). The CCS is characterized by low salinities and sea surface temperatures (SST) (Lynn and Simpson, 1987) of 17–20°C during summer (June – August) (Lynn and Simpson, 1987). Regional productivity around Guadalupe Island is also influenced by wind stress curls and Ekman transport, as well local mesoscale eddies that promotes important offshore upwellings and primary production, particularly in spring and summer (Barocio-León et al., 2007; McClatchie, 2014; Durazo, 2015). These anomalies coincide with the GFS breeding season (June to August) at Guadalupe Island (Gallo-Reynoso, 1994).
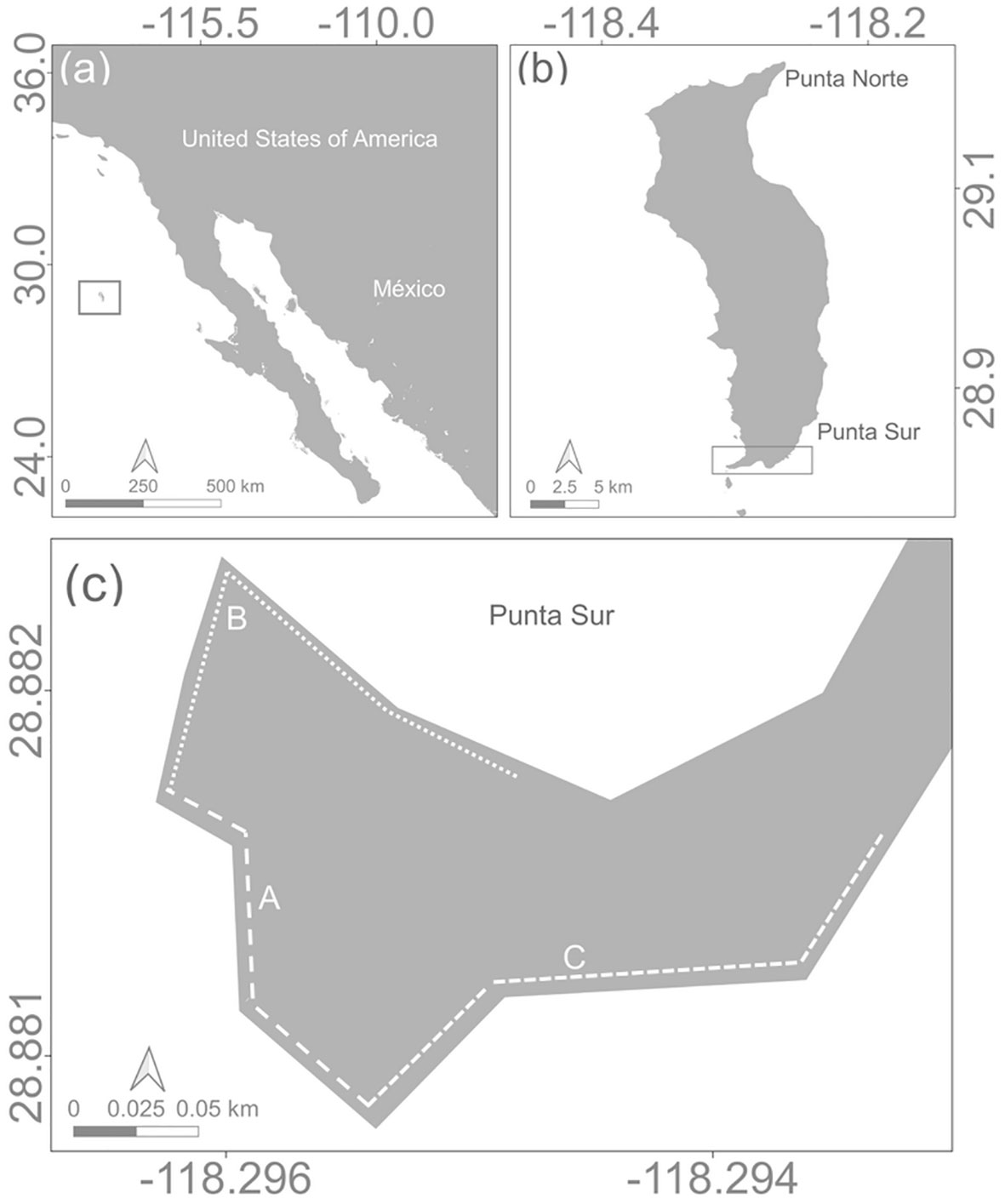
Figure 1 Study area. (A) Guadalupe Island (B) Punta Sur rookery and (C) locations of study areas at Punta Sur rookery: (A) La Lengüeta; (B) Las Pocitas; (C) El Muelle.
The study site was in the Punta Sur rookery located along the southern extremity of Guadalupe Island (29° 00’ N, 118°15 30 W) (Figure 1). The Punta Sur was subdivided in three topographic areas as follows: (a) La Lengüeta, which is sheltered with large rock outcroppings and shallow tide pools; (b) Las Pocitas, a rocky platform with boulders and deep tide pools, and (c) El Muelle (“dock” in Spanish): bound by a cliff and large rocks with cobblestone beaches (Figures 1; S1).
During the breeding seasons ‘s from 2013 to 2016 at Punta Sur, almost daily surveys were undertaken to count and collect GFSn carcasses (post mortem codes 1-2) (Geraci and Lounsbury, 2005). Human effort for GFS pups counting and carcasses access and collection depended on meteorological (e.g. tides, storms), biological (e.g. aggression), safety and ecological (e.g. births) factors during the breeding season at Guadalupe Island. Also, GFS mortality monitoring was avoided in crowded or high-density areas during nursing periods and when neonates were observed (presence of blood, or placenta). Therefore, results presented herein are an approximation of the GFS population dynamics of pups at Punta Sur and an overview of Guadalupe Island.
Each breeding season (early June to late August) was subdivided into three periods: early (15 June to 6 July), middle (July 7 to 27 July) and late (28 July to 13 August), based on the GFS breeding season length from early June to late August (Gallo-Reynoso, 1994). For this study, the Institutional Animal Care and Use Committee was consulted; however, an ethical review was not warranted.
2.2 Investigating presumptive causes of death in GFS pups
GFSn carcasses were collected and transferred to a secure site for necropsy according to conventional pinniped postmortem protocols (Dierauf and Pinniped Forensic, Necropsy and Tissue Collection Guide U.S, 1994). Signalment and morphometric data included date, sex, body weight (BW) (using a hanging scale, precise to 0.5 kg), linear standard body length (SBL) (from nose to tail tip), abdominal girth (ABG) (Gentry and Holt, 1982), and mid sternal blubber thickness ( ± 0.1 cm) (VBD). During a GFSn necropsy, the external and internal aspects of the carcasses were inspected and a presumptive cause of dead (PCOD) assigned according to the following case definitions:
Emaciation (EM) in GFSn was defined by reduced body mass below 2.5 standard deviations of the mean, diminished ventral sternal blubber thickness, volume and composition of stomach contents (e.g. milk, pica), presence or absence of gastric mucosal erosions or ulcers, and sparse or complete lack of feces in the descending colon and rectum. Trauma (TR) (crush and blunt) in GFSn was diagnosed in cases of bones and organ fractures with associated hemorrhage and edema (to differentiate ante- versus postmortem injuries), and other lesions consistent with blunt force trauma, crush injury or compression, and asphyxiation (Spraker and Lander, 2010; Spraker et al., 2014). Drowning by immersion (DR) in GFSn was considered in specific circumstances of habitat, such as recovery of a submerged carcasses and gross internal findings consistent with drowning (Spraker et al., 2014). Stillbirth and perinate mortality (STP) in GFSn were considered if full term neonates presented stillborn, or death occurred within the first hours of life, typically with a fresh umbilicus, portions of attached or expelled placenta, atelectatic or partially inflated lungs and meconium within the colon. Infectious Diseases (ID) in GFSn was established by localized abscessation or generalized septicemia, including evidence of lymphadenopathy, splenomegaly, pulmonary congestion, and edema, multisystemic hemorrhage, inflammation and necrosis or other attendant processes. With more acute stages of fulminant infection, animals showed moderate to good nutritional condition, whereas, with subacute and more chronic disorders, the animals tended to present in suboptimal body condition. Infectious Diseases and low body weight (IDLBW) in GFSn was designated for perinates and pups that were emaciated with gross evidence of an infectious or inflammatory process. Unknown (UNK) causes in GFSn included carcasses in advanced degree of decomposition, postmortem scavenging, and in an inaccessible site that precluded recovery. Undetermined (UND), where an animal was examined, but no conclusive death cause was identified. UNK and UND morphometric data were not included in the analyzed data. Due to the pathology consisting of gross findings with no ancillary diagnostic studies, pathological analysis GFSn deaths were assigned as presumptive (PCOD).
2.3 Statistical analysis
Statistical analyses were performed using software STATISTIC 7.0 (Statistic ft Inc., Tulsa, OK) to identify interannual differences in GFSn BW (kg), SBL (cm), ABG (cm) and VBD (cm), between sexes (F= female and M= male) and to assess potential relationships with PCOD during the 2013 to 2016 breeding season. Data were assessed for normality with Kolmogorov-Smirnov-Lilliefors test and Levene test for homogeneity (Zar, 1999) and differences across breeding season in PCOD were associated to morphometric data applying an Kruskal-Wallis test, as well as correlations using a Spearman Rank and Mann-Whitney– U-test for statistics differences. A linear regression was built to establish a relationship between morphometric variables (Zar, 1999). Frequencies of PCOD by breeding period (early, middle, and late) and area explored (A, B and C) in Punta Sur were evaluated by using Chi-square tests. Results were considered significant when value was P < 0.05. GFSn carcasses counted during surveys were estimates of the total number of dead pups (TD) by breeding season (2013-2016). To obtain the interannual MR (%), the total number of pups born (TB) were compiled through to the latter stage of each breeding season (August). The MR was obtained (TD/TB * 100) and interannual PCOD and MR were expressed as percentages (%).
3 Results
3.1 Mortality rate in GFS pups
A total of 5,112 GFS births and 439 GFSn carcasses were counted during breeding season of 2013 – 2016 in Punta Sur (Table 1). The mortality rate (MR) was estimated at 7.3% (quartile 25%-75% 6.0% – 11.4%) with differences in TD between breeding seasons. The highest GFSn recorded mortalities were in 2015 (14.7%), followed by 2014 (8.2%), 2016 (6.7%) and 2013 (5.6%), respectively (X2 = 14.819 df = 3 P < 0.05) (Table 1). Necropsy was performed on 63% (n= 277/439) of carcasses between 2013 and 2016, with no significant differences between sexes (F= 146 and M= 131) (Mann-Whitney – U-test; P > 0.05). Most of GFSn carcasses in Punta Sur, were identified in mid-season, 64% (n= 280), while 22% (n= 98) and 14% (n= 61) were recorded in the late and early breeding seasons, respectively (X2 = 187.865, df = 2, P < 0.05) with differences in their frequency by area A (61%, n= 266), B (34%, n= 151) and C (5%, n= 22) (X2 = 203.696, df = 2, P < 0.05).
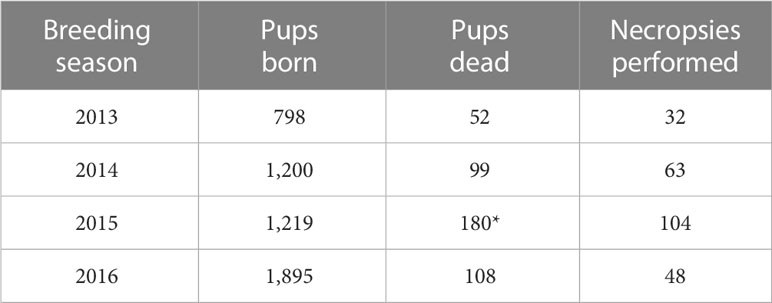
Table 1 Annual number of Guadalupe fur seal pups that were born alive, stillborn or found dead and total number of necropsies performed by breeding season from 2013 to 2016 at Punta Sur rookery, Guadalupe Island (chi-square, P < 0.05)*.
3.2 Morphometric findings in GFS pups
Morphometric data from 89% of GFSn (n= 247/277, F = 135 and M = 112) were analyzed (2013 - 2016) (Table 2). The cause of death in the remaining 11% could not be determined (UNK and UND) and were excluded from statistical analysis as the animals were inaccessible, exhibited advanced decomposition, or no conclusive PCOD was identified. GFSn BW (kg) was similar between sexes (median: 5 kg, min-max: 3 - 8 kg); however, males were longer (min-max: 58 - 80 cm) than females (min-max 54 -78) (Mann-Whitney – U-test; P < 0.05) (2013-2016). GFSn BW was positive and correlated with ABG (Spearman-Rank; 0.58, P < 0.05) and VBD (Spearman-Rank; 0.51, P < 0.05) in contrast to SBL (Spearman-Rank; 0.34, P < 0.05).
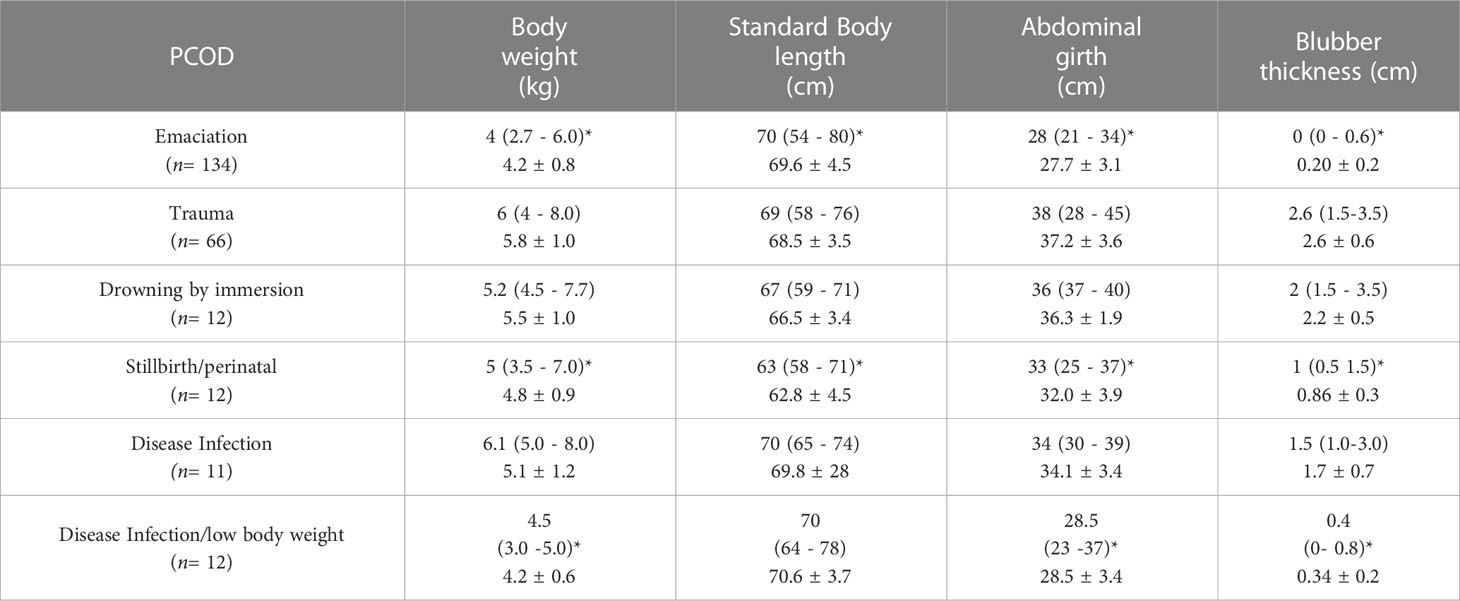
Table 2 Morphometric data collected for Guadalupe fur seal pups (N= 247) (median, min-max and mean ± SD) by Presumptive Cause of Dead (PCOD) during the breeding seasons between 2013 and 2016 at Punta Sur rookery, Guadalupe Island (Kruskal-Wallis, P < 0.05)*.
3.3 Presumptive causes of death in GFS pups
Presumptive PCOD was confirmed in 277/439 GFSn at Punta Sur (2013-2016). PCOD prevalence during the GFS breeding season were: EM 49% (n= 134/277); TR (crush/blunt) 24% (n= 66/277); UNK 11% (n= 30/277); ID and ID/LBW 8% (n= 23/277); DR 4% (n= 12/277) and STP 4% (n= 12/277).The highest percentage of PCOD (not include UNK/UND cases) by breeding season (n= 247) from the highest to lowest values were observed in 2015 (42%), followed by 2014 (25%), 2016 (20%) and 2013 (13%) (X2 = 44.283, df = 3, P < 0.05) (Figure 2). Statistical differences were identified by PCOD (X2 = 221.198, df = 4, P < 0.05) and morphology in GFSn (Kruskal-Wallis test, P < 0.05) (Table 2).
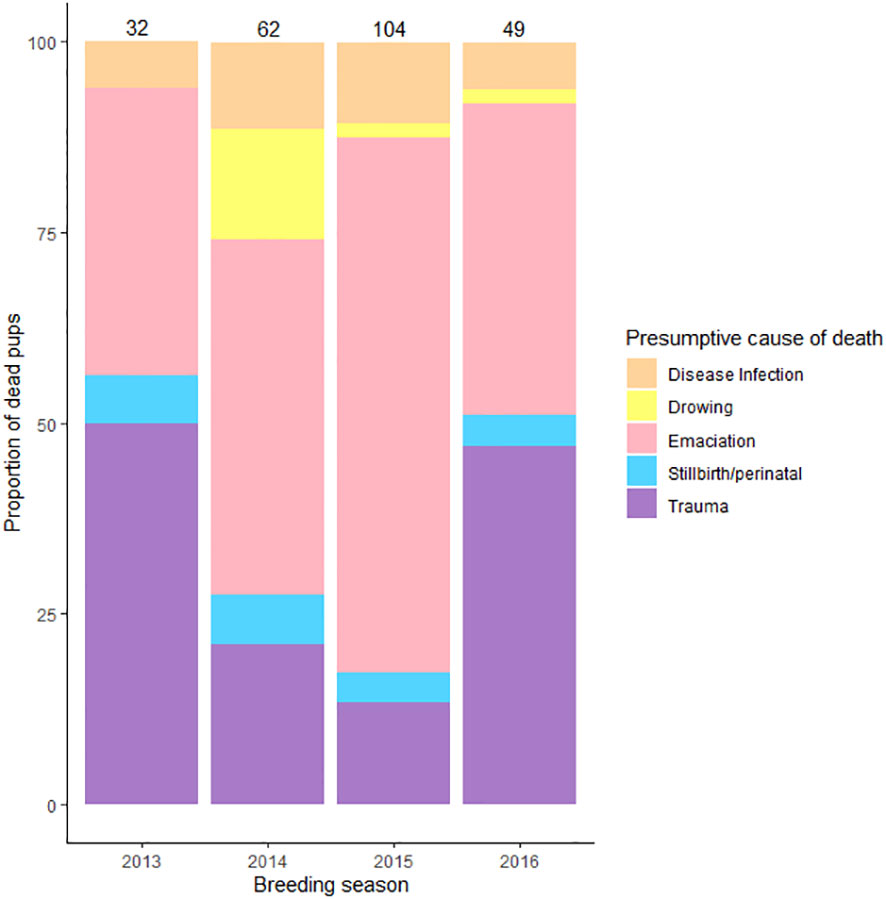
Figure 2 Incidence of Presumptive Causes of Dead in Guadalupe fur seal pups between breeding seasons 2013 and 2016 at Punta Sur rookery, Guadalupe Island. Each number represents total # pup deaths by year. Data not included for pups assigned as Unknown and Undetermined.
Macroscopic and morphologic findings in GFSn by breeding season, time and study area at Punta Sur are described below.
3.3.1 Emaciation
EM represented 49% of PCOD in GFSn (n= 134/277, F = 71, M = 63) during the breeding season (2013 -2016) at Punta Sur. Percentage differences in EM by breeding season ranged from 54%, (n= 73/134) in 2015 to 22% (n= 29/134) in 2014, 15% (n= 20/134) in 2016 and 9% (n= 12/134) in 2013 (X2 = 66.418, df = 3, P < 0.05) (Figure 2) with a preponderance of cases detected during middle time interval (62%, n= 83/134) with fewer in late (31%, n= 41/134) and early breeding season (7%, n= 10/134) (X2 = 60.195, df = 2, P < 0.05) (Figure 3), particularly in area A (60%, n= 80/134) and B (40%, n= 51/134), with a few cases observed in C (5%, n= 3/134) (X2 = 67.818, df = 2, P < 0.05).
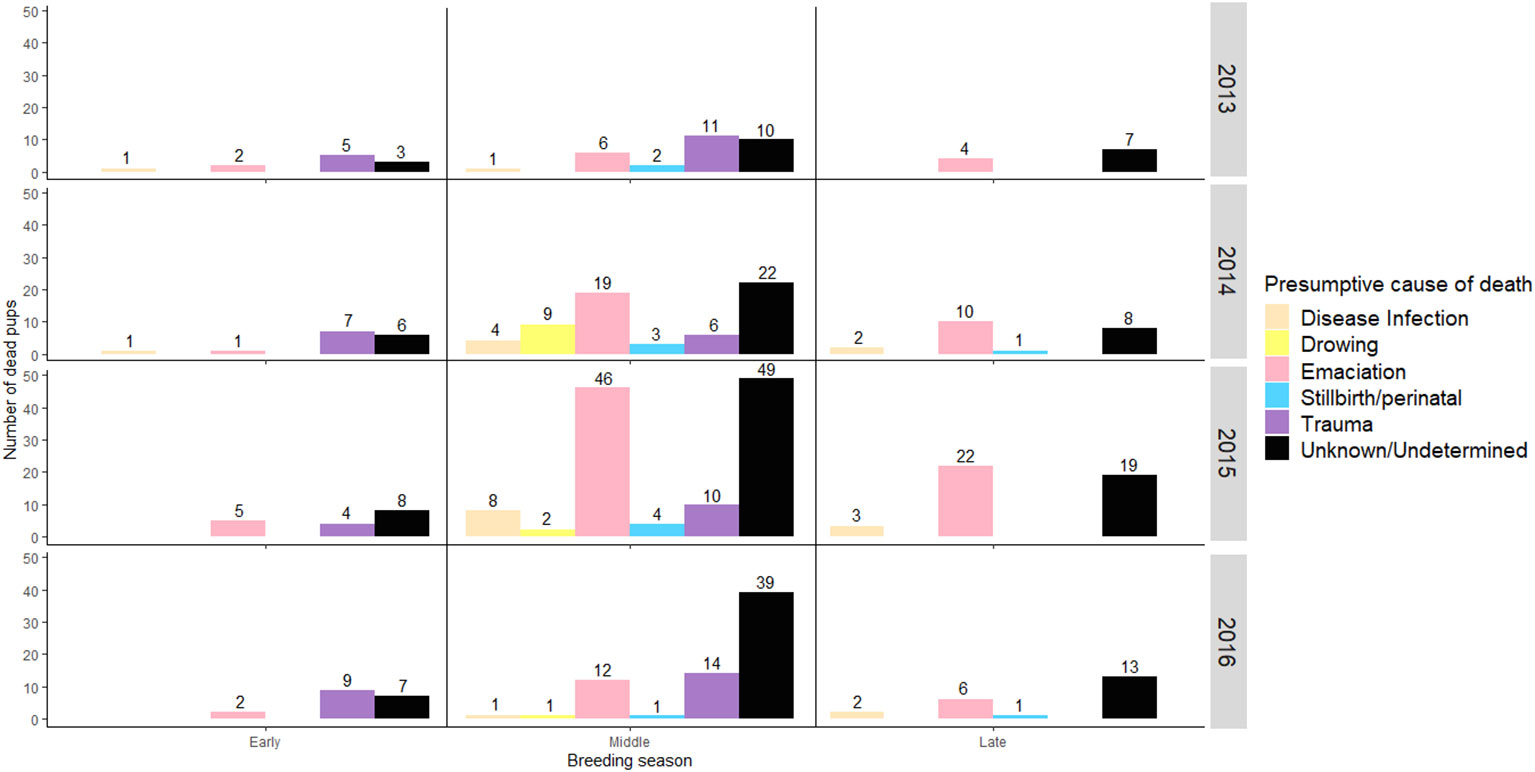
Figure 3 Temporal trends of Presumptive Causes of Dead (PCOD) in Guadalupe fur seal pups during the breeding seasons 2013 to 2016 at Punta Sur rookery, Guadalupe Island. Each number represent total of recovered and necropsied pups by PCOD, including cases per year. All PCOD including Unknown and Undetermined causes were included. In contrast to early and late times of the season, 64% of the cases were Unknown or Undetermined.
GFSn with EM featured the lowest BW, VBD and ABG. These findings were similar to IDLBW with the lowest SBL and STP in contrast to other PCOD (Kruskal-Wallis test, P < 0.05) (Table 2). The most important external findings were prominent ribs, dorsal and lateral spinal processes, and pelvic prominences (100%, n= 134/134). The primary internal lesions were either absence (70%, n= 95/134) or significative decrease of VBD (30%, n= 40/134), lack of perirenal and retroperitoneal fat infiltration (100%, n= 134/134), scant feces (100%, n= 134/134) and gastric erosive lesion (27%, n= 37/134) with generalized atrophy of the skeletal musculature and liver (100%, n= 134/134) (Figure S2).
3.3.2 Trauma (crush and blunt)
TR was a major cause of GFSn mortality with an incidence of 24% (n= 66/277, F= 37, M = 29) during breeding season (2013 – 2016) at Punta Sur. TR in GFSn were identified as compression/crushing (66%, n= 44/66) and blunt type (34%, n= 22/66) The peak incidence of TR was observed in 2016 (35%, n= 23/66), with reduced numbers in 2013 (24%, n= 16/66), 2015 (21%, n= 14/66) and 2014 (20%, n= 13/66) (X2 = 3.69, df = 2, P < 0.05) breeding seasons (Figure 2). Most carcasses with TR were located between and under rocks, especially in area A (60%, n= 37/66), B (40%, n= 26/66) and C (10%, n= 3/66) (X2 = 27.364, df = 2, P < 0.05), within (60%, n= 40/66) and outside of male territories (40%, n= 25/66) with the highest percentages during the early (62%, n= 41/66) and middle (38%, n= 25/66)breeding season (X2 = 38.818, df = 2, P < 0.05) at Punta Sur (Figure 3). GFSn with TR exhibited the widest ranges of BW, VBD and ABG; however, the STB was consistent with other PCOD (Table 2).
Fatal TR lesions differed by anatomic location including the abdominal cavity (41%, n= 18/44) and involved the liver (40%, n= 13/18) or kidney (1%, n= 2/18) and consistently featured rents or fractures with associated hemorrhage and hemoperitoneum (83%, n= 15/18). Cranial and thoracic crush/compression lesions (59%, n= 26/44) presented with subconjunctival congestion, epistaxis, subcutaneous petechiae, hematoma, edema, and dissecting hemorrhage of the hypodermis in the neck (31%, n= 8/26) and torso (69%, n= 18/26). Affected lungs featured subpleural to parenchymal contusions and atelectasis (50%, n= 13/26) with hemothorax (30%, n= 8/26). In few GFSn, regurgitated milk was noted in oropharynx and larynx and frequently was observed in lower respiratory arcade (29%, n= 13/44) (Figure S3) consistent with agonal regurgitation and aspiration. In the cranium, blunt TR was associated with simple to comminuted closed radial fractures with variable displacement of bone margins and periosteal, epidural and intracranial hemorrhage (95%, n= 21/22). One GFSn from area C had a laryngeal fracture (5%, n= 1/22), with epistaxis, subcutaneous cervical hemorrhage, edema, and segmental emphysema (Figure S4).
3.3.3 Drowning by immersion
DR diagnosis was rendered in 4% of GFSn (n= 12/277, F= 8 and M= 4) during breeding season at Punta Sur (2013 – 2016). The highest proportion of DR were in 2014 (75%, n= 9/12), with fewer cases in 2015 (17%, n= 2/12) and 2016 (8%, n= 1/12) (X2 = 13.66, df = 2, P < 0.05) Figure 2) in middle breeding season (100%, n= 12/12) (Figure 3) at area A (60%, n= 7/12) and B (40%, n= 5/12) (X2 = 9.562, df = 2, P < 0.05). After days of strong wave action, live GFSn were observed in deep rockpools (> 2 m) in area B and were unable to haul out. In these cases, metabolic exhaustion, dehydration and possible hypoglycemia were considered the immediate cause of death. GFSn with DR had higher BW, ABG and VBD than EM and IDLBW (Kruskal-Wallis test, P < 0.05). However, SBL was similar to other PCOD (Table 2).
At necropsy, fresh cutaneous lacerations were identified in fore-flippers (100%, n= 12/12) and chin (90%, n= 11/12) (Figure S5). Stable tracheobronchial froth and pulmonary edema were also evident in affected animals (80%, n= 10/12). Lungs were expanded with prominent, interlobular septae (100%, n= 12/12). A similar number of DR animals were observed with puncture wounds consistent with seal dentition (80%, n= 10/12).
3.3.4 Stillbirth and perinatal mortality
STP mortalities were diagnosed in 3.9% of GFSn (n= 12/277, F = 6 and M = 6) during breeding season (2013 - 2016) at Punta Sur. A similar percentage of STP e cases were reported in 2015 (31%, n= 4/11), 2014 (31%, n= 4/12), 2013 (12%, n= 2/12) and 2016 (12%, n= 2/12) (X2 = 1.33, df = 3, P > 0.05) (Figure 2). in the middle (90%, n= 10/11) interval with fewer in the early (10%, n= 2/12) (X2 = 14, df = 2, P < 0.05) breeding season (Figure 3). The affected animals were recovered only in areas A (60%, n= 8/11) and B (40%, n= 4/11) (X2 = 8, df = 2, P < 0.05). GFSn with STP exhibited similar BW, VBD and ABG to IDLBW and EM, but had lower SBL in contrast to other PCOD (Kruskal-Wallis test, P < 0.05) (Table 2).
The most common gross findings of STP included: uninflated lungs, meconium in the colon and the presence of placenta and fresh umbilical cord (40%, n= 7/11) (Figure S6). Additional gross lesions associated with other examined perinates with STL (died within 24 hours postpartum) consisted of hepatic parenchymal lacerations and hemoperitoneum (30%, n= 3/11) and aspirated colostrum (30%, n= 3/11). In one case, there was focal linear rupture of the intestine with meconium dispersed throughout the abdominal cavity (9%, n= 1/11).
3.3.5 Infectious diseases and low body weight
ID and IDLBW was confirmed in 8% of GFSn (n= 23/277, F = 13 and M = 10) during the breeding seasons of 2013 - 2016 at Punta Sur. The highest percentage of ID and IDLBW cases was recorded in 2015 (48%, n= 11/23), with reduced numbers of cases in 2014 (30%, n= 7/23), 2016 (13%, n= 3/23) and 2013 breeding season (9%, n= 2/23) (X2 = 10.727, df = 3, P < 0.05) (Figure 2). The incidence of ID was most prominent in the middle of the breeding season (61%, n= 14/23) with fewer in the late (30%, n= 7/23), and early (9%, n= 2/23) stages (X2 = 9.487, df = 2, P < 0.05) (Figure 3).
ID/IDLBW cases were recovered from all study areas in similar percentages, A (56%, n= 13/23), B (30%, n= 7/23) and C (14%, n= 3/23) (X2 = 3.836, df = 2, P > 0.05) at Punta Sur. GFSn with IDLBW (n = 12) exhibited a lower BW, ABG, VBD in contrast to ID (n= 11) and other PCOD but SBL was similar to ID (Kruskal-Wallis test, P < 0.05) (Table 2). During necropsy of ID/IDLBW, the most salient gross lesions were identified in lungs (69%, n= 16/23) and were characterized by pulmonary consolidation (56%, n= 9/16), edema (25%, n= 4/16), hemorrhage (12%, n= 2/23) and emphysema (44%, n=7/23) (Figure S7). GSFn with IDLBW lacked gastrointestinal contents (100%, n= 12/12), but in contrast to ID, had gastric erosions (50%, n= 6/12), fibrinous peritonitis (19%, n= 2/11), and intestinal distension with distended pale-yellow segments of bowel, suggestive of enteritis (19%, n= 2/11).
3.3.6 Unknown and undetermined
Cases designated as UNK were rendered in 11% of examined GFSn (n= 30/277, F = 11 and M = 19) during the breeding seasons of 2013 - 2016 at Punta Sur. Considering UNK (n = 30) and UND (n = 162) cases during study (2013-2016) at PS, the PCOD was not determined in 44% of observed GFSn (n = 191/439). The highest UNK/UND incidence was recorded in 2015 (39%, n= 76) with fewer in 2016 (31%, n= 60), 2014 (19%, n= 35), and 2013 (11%, n= 20) breeding season (X2 = 900.96, df = 3, P < 0.05) (Figure 2) with the highest values registered in the middle (62%, n= 120) and late (25%, n= 47) season followed by the early (13%, n= 24) period (X2 = 78.93, df = 2, P < 0.05) (Figure 3), especially in area A (63%, n= 120), B (31%, n= 58), and C (6%, n= 13) (X2 = 90.68, df = 2, P < 0.05) at Punta Sur (Figure S6).
4 Discussion
Our results reinforce the importance of high offspring production and neonate/perinate survival in the GFS population recovery process, particularly related to marine environmental changes around Guadalupe Island (Weber et al., 2004; Gálvez et al., 2020; Juárez-Ruiz et al., 2022). Review of published data (Spraker and Lander, 2010; Seguel et al., 2013) and ongoing field observations prompted further investigation of dead pups at Guadalupe Island to characterize MR and PCOD and better inform current management and conservation schemes in Mexico. Data from this study indicate that perinate and neonate loss was multifactorial and associated with marine environmental changes (i.e., marine heatwaves).
MR and PCOD in GFSn were similar to reports in other otariids during the first three months of life (Castinel et al., 2007; Spraker and Lander, 2010; Seguel et al., 2013; Spraker et al., 2014) and consistent with a previous study performed during GFS breeding season on Guadalupe Island (9%) (Gallo-Reynoso, 1994). Differences in MR and PCOD in otariid species are associated with intrinsic (e.g. aggressiveness) and extrinsic (e.g. substrate) characteristics. Hence, temporal and spatial differences among populations of otariids and pinnipeds are expected (Mattlin, 1978; Castinel et al., 2007; Maniscalco et al., 2008; Spraker and Lander, 2010; Michael et al., 2019).
The primary PCOD of GFSn was EM. In otariids pups, EM is considered a natural PCOD related to maternal (e.g. inexperience) and environmental factors (e.g. prey availability) that may compromise pups nutritional status and survival. A wide range of EM prevalence has been reported among different otariid species (8%-23%) (Doidge et al, 1984; Maniscalco et al., 2008; McIntosh and Kennedy, 2013; Seguel et al., 2013 Castinel et al., 2007; Michael et al., 2019) and this variability in incidence is consistent with our observations of GFSn. Based on the number of GFSn in suboptimal nutritional condition and secondary or opportunistic ID, the possibility of mid to late trimester maternal EM, may be a contributing factor in EM prevalence of GFSn. A lack or insufficient colostral consumption may also predispose pups to opportunistic ID.
In pinnipeds, detection of EM increased significatively (47% - 100%) during ocean warming (e.g. El Niño) and was attributed to prey shortages and changes in maternal care and behavior (Trillmich et al., 1991; Soto et al., 2004; Reid and Forcada, 2005; Soto et al., 2006). The highest incidence of EM in GFSn was in 2015 and accounted for 54% of total pup deaths. In 2015, stable isotope values of GFSn were quantified in fur and prey in feces collected at Punta Sur and revealed expanded foraging areas of GFS lactating females (Amador-Capitanachi et al., 2020). This phenomenon coincided with the lowest BW and survivability of GFSn associated to alterations in maternal care and prey availability due to the Northeast Pacific Heatwave (Gálvez et al., 2020). For example, opalescent squid in SCC (Chasco et al., 2022; Suca et al., 2022) has a poorer nutritional content linked to a decrease in BW of California sea lion pups (Zalophus californianus) at San Benito Archipelago, Mexico (GFS non-reproductive colony) in 2015 (Elorriaga-Verplancken et al., 2016a). On San Miguel Island, California, similar impacts of limited prey availability have been reported in California sea lion pups (McClatchie et al., 2016) as well as with increased strandings of emaciated seabirds along the California coast (Piatt et al., 2020).
Based on the average duration of GFS females foraging trips and the lack of gastric contents in GFSn with EM, observed declines of VBD indicated, that in severely affected GFSn, milk may not have been consumed for at least 23 days prior to death (Gallo-Reynoso et al., 2008). This extended fasting, particularly during 2014 and 2015 breeding season, coincided with highest positive SST anomalies recorded within the vicinity of Guadalupe Island and GFS females foraging grounds (Gálvez et al., 2020). A similar phenomenon linked to prolonged inappetence, or anorexia was proposed for emaciated Steller sea lion pups (Eumetopias jubatus) (Trites and Jonker, 2000) and was subsequently modeled (McHuron et al., 2023). However, it is also necessary to interpret observations in the context of maternal characteristics (e.g. primiparous versus multiparous) and other processes involved in EM cases.
The second most common PCOD in GFSn was TR. TR incidence was consistent with other published accounts of mortality in otariid pups (9.9% - 35%) (Castinel et al., 2007; Spraker and Lander, 2010; McIntosh and Kennedy, 2013; Seguel et al., 2013; Michael et al., 2019). In pinnipeds, colony dynamics such as demographics, density, male abundance, intraspecific aggression, birth peaks, topography and limited locomotion of pups are linked to variations in spatial and temporal TR incidence (Doidge et al., 1984; Georges and Guinet, 2000; Maloney et al., 2009; Michael et al., 2019). At Punta Sur, areas A and B feature a rocky substrate that in contrast to area C, which provided shelter and protection for GFSn against waves (Arias-del-Razo et al, 2016; García-Capitanachi et al., 2017). The highest TR in GFSn by crush injury was reported in areas A and B; whereas, blunt force type injuries were most frequent in area C and attributed to impact from falls.
GFS is an aggressive species during the breeding season and peak parturition occurs between early June and mid-July at Guadalupe Island (Gallo-Reynoso, 1994). In pinnipeds, TR injuries associated with impact from falls or crushing was inferred by gross findings of cranial fractures asphyxiation and suffocation, and correlated statistically with topography of the colony, age-limited mobility and aggression. Stampedes and abrupt movements that resulted in TR were particularly pronounced in territorial males and females such as northern fur seals (Callorhinus ursinus) and New Zealand sea lions (Phocarctos hookeri) (Gazo et al., 2000; Spraker and Lander, 2010; Seguel et al., 2013; Michael et al., 2019). In our study, TR coincided with the peak in births (early and middle breeding season) reported for this species (Gallo-Reynoso, 1994) and in areas with the highest GFS crowding (A and B), which is considered an optimal characteristic during GFS breeding season (Arias-del-Razo et al, 2016). Additionally, a subadult male was observed harassing a GFS pup at Punta Sur. Therefore, intraspecific aggression cannot be dismissed as a cause of TR in GFSn during the breeding season. TR cases were associated with the widest variation in BW, SBL and VBD among PCOD. This finding differs with other published accounts, where lower BW was associated with greater susceptibility to TR (Calambokidis and Gentry, 1985; Castinel et al., 2007). Considering that PCOD was not determined in all GFSn, the relationship of TR to nutritional condition may be underestimated.
ID/IDLBW was the third highest PCOD in GFSn. In pinnipeds pups, ID rate is highly variable (3%-28.2%) and animals typically present with localized abscessation or generalized septicemia. Infection may be secondary to in utero or post-partum malnutrition, insufficient colostrum consumption, prolonged maternal separation, or exposure to infectious agents. Clinical signs and gross findings may be associated with pneumonia, enterocolitis, osteoarthritis, or omphalophlebitis (Castinel et al., 2007; Spraker and Lander, 2010; Seguel et al., 2011; Michael et al., 2019). ID may present as localized abscessation or systemic infection. In some cases, bacterial involvement may be the primary agent or possibly exacerbated by parasitism or environmental change. For example, Uncinaria spp. infection New Zealand sea lion pups (Castinel et al., 2007; Michael et al., 2019), and enteritis and septicemia in South American fur seal pups (Arctocephalus australis gracilis) (Seguel et al., 2011), are associated with increased SST, decreased growth rates, malnutrition and impaired immune function (Seguel et al., 2018). The highest incidences of ID and IDLBW in GFSn were in 2015 and 2014 and coincided with northeast Pacific heatwave around Guadalupe Island, thus are likely attributed to changes in GFS feeding habits and nutritional condition (Amador-Capitanachi et al, 2020; Gálvez et al., 2020). Based on immune function studies in a variety of mammal species (Li et al., 2007; Acevedo-Whitehouse and Duffus, 2009) a suboptimal nutritional status of GFSn (BW and VBD) presumably affected immune function and contributed to increased IDLBW/ID observed in 2014 and 2015. A connection between climatic anomalies and immune competence has been found in the California sea lion (Banuet-Martínez et al., 2017) during the same period, and further studies are required to explore potential associations and mechanisms in GFS.
DR accounted for 4% of GFSn mortality in this case series. This incidence is lower than findings in other otariid pup case studies (16.7% - 31.9%) and may reflect environmental, tidal, topographic, and climactic conditions (Doidge et al., 1984; Maniscalco et al., 2008; Seguel et al., 2013). During Hurricane Darby in the summer of 1992, up to 33% of GFSn died during or shortly after the event (Gallo-Reynoso, 1994). In pinnipeds, DR diagnosis is related to macroscopic and microscopic lesions, circumstances associated with recovery of the carcass, environmental and climatic conditions, and physical limitations of neonates and weanling (Maniscalco et al., 2008; Seguel et al., 2011; Seguel et al., 2013). During the summer of 2014, tropical storms “Douglas” and “Hernan” occurred in late July in the Northwest Pacific Ocean and the peninsula of Baja California (NOAA, 2014). Subsequent to these meteorologic events, increased numbers of GFSn carcasses were recovered submerged in, or hauled out around rocky pools in areas A and B. Rocky pools and GFS births close to wave break areas may predispose pups to displacement into confined rocky recesses or deep pools by surging waves. These events, coupled with physical limitation to escape or evade the incidents may contribute in part to spatial and temporal differences in the incidence of DR.
STP was the fifth highest PCOD in GFSn and the incidence ranged from 2.3% - 15.4%. The pathogenesis of this condition is likely multifactorial (Soto et al., 2004; Castinel et al., 2007; Maniscalco et al., 2008; Spraker and Lander, 2010; Seguel et al., 2013) and may be related to maternal and neonate characteristics (e.g. feto-maternal disproportion), ID (e.g. placentitis or pneumonia) (Spraker and Lander, 2010 Soto et al., 2004) and environmental and population factors, such as colony density and demographics (e.g. TR) (Doidge et al., 1984). In this study, VBD in GFSn was lower than other PCOD and the presence of a fresh umbilicus and placenta, pulmonary atelectasis and meconium suggested dystocia or fetal distress. These findings have previously been reported in New Zealand sea lions and other pinniped species (Spraker and Lander, 2010; Michael et al., 2019). In NFS dystocia has been linked to larger pups (fetomaternal disproportion) (Spraker and Lander, 2010), whereas, in our case series, STL was more consistent with IDLBW and EM cases. However, in otariid populations during ocean warming and prey shortage resulted in increased abortion rates (Trillmich et al., 1991), which could not be confirmed in this study. During fieldwork at Guadalupe Island, a dead full term pregnant GFS was identified in area A (June 2015) and necropsy revealed a breach presentation. It is unknown whether the fetal position, size, maternal or other factors may have contributed to the dystocia and loss of this animal.
In other STL cases, mobility limitations of the peri- or neonate may have contributed to TR. These cases typically presented within a few days post-partum, and on necropsy, featured regurgitation and aspiration of milk and ruptured internal viscera with associated hemorrhage (Spraker and Lander, 2010). Punta Sur exhibit the highest GFS density during early and mid-breeding season and coincided with the highest TR incidence. GFS behavior (e.g. aggression) and substrate topography may have contributed to temporal and spatial differences in STL and TR at Punta Sur in this case series. However, the biological consequences of increased SST between 2013 and 2016 throughout the northeast Pacific Ocean due to the Northeast Pacific Heatwave (Cavole et al., 2016) impacted GFS female foraging habits in the vicinity of Guadalupe Island (Amador-Capitanachi et al., 2020) and the San Benito Archipelago (Elorriaga-Verplancken et al., 2016b), decreased BW of live GFSn (Gálvez et al., 2020) and coincided with the lowest recorded BW and VBD in GFSn with STL and EM cases during breeding season 2014 and 2015 at Punta Sur. Similar reports in other otariid species during ocean warming and prey shortage (Trillmich et al, 1991; Lunn et al., 1994) have been linked to reduced fat assimilation and subcutaneous blubber deposition (Riet-Sapriza et al., 2012).
The UNK and UND cases accounted for 44% of total GFSn deaths during study at Punta Sur and occurred predominantly during middle and latter stages of the breeding season of 2015 and 2016 in areas A and B. These areas and seasons are suitable terrestrial habitats for GFS adults and pups with highest abundances during the breeding season in Guadalupe Island (Arias-del-Razo et al, 2016; García-Capitanachi et al., 2017). In the 2015 breeding season, the highest percentage of PCOD were due to EM, and TR. However, the lack of complementary diagnostic analysis of UNK cases and potential impact of other factors could have been underestimated.
Nonetheless, our results from GFSn examinations with a PCOD demonstrate a susceptibility and document impacts associated with The Northeast Pacific heatwave, and the relevance of terrestrial and marine environmental characteristics during post-partum development. This species appears to be vulnerable to marine heatwaves and hence, to prey availability in the foraging grounds of adult females. However, long-term consequences in the species, in this regard, are unknown.
Although PCOD in GFSn are considered natural events, the contribution of anthropogenic activities (e.g. biological and chemical pollutants) on GFS population health and mortality dynamics at Punta Sur, particularly during lactation periods and maternal care, cannot be dismissed. Future efforts to incorporate histopathology and ancillary diagnostic studies (e.g. toxin and pathogen exposure and nutritional analysis) to differentiate impacts of non-human and human activities (e.g. pollution) on GFS mortality at Guadalupe Island are warranted. Inclusion of other age classes, such as juveniles and subadults, as well as a more long-term study may provide additional information that could be integrated with demographic, nutritional and health studies with the reemergence of GFSs in U.S., and habitat expansion in Mexico.
Finally, a decreased body size and survival of vulnerable species has been suggested as an important developments associated with global warming and an increase in marine heatwaves frequency (Gardner et al., 2011 Frölicher et al., 2018). In marine mammals, marine heatwaves have led to habitat compression and changes in prey distribution and biodiversity with negative consequences in foraging availability and individuals’ welfare (Santora et al., 2020; Maniscalco, 2023). However, overall biological and ecological long-term consequences in most marine megafauna are unknown (Gulland et al., 2022; Smith et al., 2023). The GFS is sensitive to the regional warming of the northeast Pacific Ocean due to marine heatwaves (Gálvez et al., 2020; Juárez-Ruiz et al., 2022) decreasing perinate and neonatal survival, which is a key stage in the population recovery of this species and its habitat expansion. This mortality research in its critical habitat should better inform GFS response to ocean warming, as well as biological factors involved in its demography and health. Our findings provide a tool to detect and prevent potential risks to GFS as well as information management and conservation schemes for the survival and recovery of this species in critical habitats.
Data availability statement
The original contributions presented in the study are included in the article/Supplementary Material. Further inquiries can be directed to the corresponding author.
Ethics statement
The animal study was reviewed and approved by CICIMAR-IPN. Ethics approval was not required.
Author contributions
CG: Conceptualization, Data collection, Data analysis, Methodology, Manuscript Writing. FE-V: Manuscript reviewing, Funds acquisition. SR: Manuscript Writing, Manuscript reviewing. FG-M: Manuscript reviewing. CF: Manuscript reviewing. All authors contributed to manuscript revision, read, and approved the submitted version.
Acknowledgments
We would like to thank Dr. Wendy Roe and Msc. Larissa Chávez-Soriano for review of this work and volunteers who contributed to data collection. We are grateful to the Secretaría de Marina Armada de México and Comisión Nacional de Áreas Naturales Protegidas-Isla Guadalupe and Dirección General de Vida Silvestre for providing research permits (SGPA/DGVS/01847/12, 11744/13, 00195/15, and DGVS/00050/16) and FE-V and FG-M thank the Instituto Politécnico Nacional for fellowships (COFAA, EDI) with SIP projects (20130944, 20140277 and 20160164) and to Consejo Nacional de Ciencia y Tecnología (Conacyt) for CG fellowship (2013-2015) (CVU: 511077).
Conflict of interest
The authors declare that the research was conducted in the absence of any commercial or financial relationships that could be construed as a potential conflict of interest.
Publisher’s note
All claims expressed in this article are solely those of the authors and do not necessarily represent those of their affiliated organizations, or those of the publisher, the editors and the reviewers. Any product that may be evaluated in this article, or claim that may be made by its manufacturer, is not guaranteed or endorsed by the publisher.
Supplementary material
The Supplementary Material for this article can be found online at: https://www.frontiersin.org/articles/10.3389/fmars.2023.1202533/full#supplementary-material
Supplementary Figure 1 | Areas explored for collection and quantification of Guadalupe fur seal pup carcasses in Punta Sur rookery at Guadalupe Island, B.C. (A) area A, rocky and sheltered large platform with multiple territories, newborns, and a pup carcass (arrow) classified as unknown; (B) area B, rocky platform with large rocks and deep tide pools; (C) area C, site with cliffs and large rocks holding adult males and pups (arrow).
Supplementary Figure 2 | Gross findings in Guadalupe fur seal pups with suboptimal nutritional condition (emaciation, images A and B) and normal adipose stores (images C and D) found in Punta Sur rookery at Guadalupe Island, B.C. (A) pup with generalized atrophy of the subcutaneous blubber and musculature; (B) lack of peri-renal and retroperitoneal fat; (C) well-conditioned pup that died of trauma with a normal blubber layer; (D) prominent normal peri-renal and retroperitoneal fat stores.
Supplementary Figure 3 | Gross findings in Guadalupe fur seal pups with evidence of crush trauma observed in Punta Sur rookery at Guadalupe Island, B.C. (A) in situ carcass in area A, presumably crushed. Note presence of milk draining from the oral and nasal cavities; (B) subcutaneous hematoma in neck subcutis and muscularus; (C) aspirated milk in lumen and overlying the mucosa of the trachea; (D) hemoperitoneum due to liver rupture; (E) severe subscapular renal hematoma attributed to abdominal compression.
Supplementary Figure 4 | Gross findings of blunt force trauma in a Guadalupe fur seal pup found in Punta Sur rookery at Guadalupe Island, B.C. (A) subcutaneous swelling of the intermandibular region; (B) incision of the area depicted in plate A with severe focally extensive acute edema, hemorrhage and laceration of subcutaneous fascia and muscle; (C) multifocal hypodermal petechiae with extensive subcutaneous emphysema. Note, overall fluffy appearance during examination.
Supplementary Figure 5 | Gross findings in Guadalupe fur seal pups recovered from a deep tidal pool with abrupt high walls in Punta Sur rookery at Guadalupe Island, B.C. The cause of death of this pup was drowning by immersion (A) pups carcasses trapped inside a deep rooky pools located in B area after storm in 2014 breeding season; (B) circular abrasion in chin; (C) multiple fresh abrasions with hemorrhage along the leading edge of a foreflipper (left).
Supplementary Figure 6 | Gross findings in a stillborn Guadalupe fur seal pup located in Punta Sur rookery at Guadalupe Island, B.C. (A) typical rocky substrate with an adult female and fresh dead neonate, the umbilicus and placenta are under female foreflippers; (B) atelectic lungs; (C) abundant meconium in the colon of stillborn pup.
Supplementary Figure 7 | Gross finding in a Guadalupe fur seal pup dead by infection found in Punta Sur rookery at Guadalupe Island, B.C. (A, B) pup lung with diffuse consolidation.
References
Acevedo-Whitehouse K., Duffus A. L. (2009). Effects of environmental change on wildlife health. Philos. Trans. R. Soc B Biol. Sci. 364, 3429–3438. doi: 10.1098/rstb.2009.0128
Amador-Capitanachi M. J., Moreno-Sánchez X. G., Juárez-Ruiz A., Ferretto G., Elorriaga-Verplancken F. R. (2017). ). Trophic variation between two existing Guadalupe fur seal colonies on Guadalupe Island and the San Benito Archipelago, Mexico. J. Aqua. Mamm. 43, 14–25. doi: 10.1578/AM.43.1.2017.14
Amador-Capitanachi M. J., Moreno-Sánchez X. G., Ventura-Domínguez P. D., Juárez-Ruiz A., González-Rodríguez E., Gálvez C., et al. (2020). Ecological implications of unprecedented warm water anoMalies on interannual prey preferences and foraging areas of Guadalupe fur seals. Mar. Mamm. Sci. 36, 1254–1270. doi: 10.1111/mms.12718
Arias-del-Razo A., Heckel G., Schramm Y., Pardo M. A. (2016). Terrestrial habitat preferences and segregation of four pinniped species on the islands off the western coast of the Baja California Peninsula, Mexico. Mar. Mamm. Sci. 32, 1416–1432. doi: 10.1111/mms.12339
Banuet-Martínez M., Espinoza-de-Aquino W., Elorriaga-Verplancken F. R., Flores-Morán A., García O., Camacho M., et al. (2017). Climatic anomaly affects the immune competence of California sea lions. PloS One 12, e0179359. doi: 10.1371/journal.pone.0179359
Barlow J., Boveng P. (1991). Modeling age-specific mortality for marine mammal populations. Mar. Mamm. Sci. 7, 50–65. doi: 10.1111/j.1748-7692.1991.tb00550
Barocio-León O., Millán-Núñez R., Santamaría-del-Ángel E., González-Silvera ,. A. (2007). Phytoplankton primary productivity in the euphotic zone of the California Current System estimated from CZCS imagery. C. Mar. 33, 59–72. doi: 10.7773/cm.v33i1.1037
Bond N. A., Cronin M. F., Freeland H., Mantua N. (2015). Causes and impacts of the 2014 warm anomaly in the NE Pacific. Geophys. Res. Let. 42, 3414–3420. doi: 10.1002/2015GL063306
Calambokidis J., Gentry R. L. (1985). Mortality of northern fur seal pups in relation to growth and birth weights. J. Wildl. Dis. 21, 327–330. doi: 10.7589/0090-3558-21.3.327
Castinel A., Duignan P. J., Pomroy W. E., Lopez-Villalobos N., Gibbs N. J., Chilvers B. L., et al. (2007). Neonatal mortality in New Zealand sea lions (Phocarctos hookeri) at Sandy Bay, Enderby Island, Auckland Islands from 1998 to 2005. J. Wildl. Dis. 43, 461–474. doi: 10.7589/0090-3558-43.3.461
Cavole L. M., Demko A. M., Diner R. E., Giddings A., Koester I., Pagniello C. M., et al. (2016). Biological impacts of the 2013–2015 warm-water anomaly in the Northeast Pacific: winners, losers, and the future. Oceanology. 29, 273–285. doi: 10.5670/oceanog.2016.32
Chasco B. E., Hunsicker M. E., Jacobson K. C., Welch O. T., Morgan C. A., Muhling B. A., et al. (2022). Evidence of temperature-driven shifts in market squid doryteuthis opalescens densities and distribution in the california current ecosystem. Mar. Coast. Fish. 14, e10190. doi: 10.1002/mcf2.10190
Clinton W. L., Le Boeuf B. J. (1993). Sexual selection's effects on male life history and the pattern of male mortality. J. Ecol. 74, 1884–1892. doi: 10.2307/1939945
D'Agnese E., Lambourn D., Rice J., Duffield D., Huggins J., Spraker T., et al. (2020). Reemergence of Guadalupe fur seals in the US Pacific Northwest: The epidemiology of stranding events during 2005–2016. Mar. Mamm. Sci. 36, 1–18. doi: 10.1111/mms.12678
Dierauf L. A., Pinniped Forensic, Necropsy and Tissue Collection Guide U.S (1994) Department of commerce, national oceanic and atmospheric administration and national marine fisheries service. Available at: https://repository.library.noaa.gov/view/noaa/26867.
DOF (2023) Reglamento Interior de la Secretaría de Medio Ambiente y Recursos Naturales. Programa de Manejo de la Reserva de la Biosfera Isla Guadalupe. Diario Oficial de la Federación. Available at: https://www.dof.gob.mx/nota_detalle.php?codigo=5676590&fecha=09/01/2023#gsc.tab=0.
Doidge D. W., Croxall J. P., Baker J. R. (1984). Density-dependent pup mortality in the Antarctic fur seal Arctocephalus gazella at South Georgia. J. of Zool. 202, 449–460. doi: 10.1111/j.1469-7998.1984.tb05095.x
Durazo R. (2015). Seasonality of the transitional region of the California Current System off Baja California. J. Geophys. Res. Oceans. 120, 1173–1196. doi: 10.1002/2014JC010405
Durazo R., Baumgartner T. R. (2002). Evolution of oceanographic conditions off Baja California: 1997–1999. Prog. Oceanogr. 54, 7–31. doi: 10.1016/S0079-6611(02)00041-1
Elorriaga-Verplancken F. R., Rosales-Nanduca H., Robles-Hernández R. (2016a). Unprecedented records of Guadalupe fur seals in La Paz Bay, southern gulf of California, Mexico, as a possible result of warming conditions in the Northeastern Pacific. J. Aqua. Mamm. 42, 261–267. doi: 10.1578/AM.42.3.2016.261
Elorriaga-Verplancken F. R., Sierra-Rodríguez G. E., Rosales-Nanduca H., Acevedo-Whitehouse K., Sandoval-Sierra J. (2016b). Impact of the 2015 El Niño-Southern Oscillation on the abundance and foraging habits of Guadalupe fur seals and California sea lions from the San Benito Archipelago, Mexico. PloS One 11, e0155034. doi: 10.1371/journal.pone.0155034
Figueroa-Carranza A. L. (1994). Early lactation and attendance behavior of the Guadalupe fur seal females (Arctocephalus townsendi). Master in Science Thesis. (Santa Cruz, USA: University of California), 99.
Frölicher T. L., Fischer E. M., Gruber N. (2018). Marine heatwaves under global warming. Nature. 560, 360–364. doi: 10.1038/s41586-018-0383-9
Gallo-Reynoso J. P. (1994). Factors affecting the population status of Guadalupe fur seal, Arctocephalus townsendi (Merriam 1897), at Isla de Guadalupe, Baja California, México. Doctoral Dissertation (Santa Cruz., USA: University of California), 199.
Gallo-Reynoso J. P., Esperón-Rodríguez M. (2013). Diet composition of the Guadalupe fur seal (Arctocephalus townsendi). Where and what do they eat? Mar. Freshw. Behav. Physiol. 46, 455–467. doi: 10.1080/10236244.2013.849400
Gallo-Reynoso J. P., Figueroa-Carranza A. L., Le Boeuf B. J. (2008). “Foraging behavior of lactating Guadalupe fur seal females,” in Avances en el Estudio de los Mamíferos de México, vol. 2 . Eds. Lorenzo C., Espinoza E., Ortega J. (Asociación Mexicana de Mastozoología Marina: Publicaciones Especiales), 505–614.
Gálvez C., Pardo M. A., Elorriaga-Verplancken F. R. (2020). Impacts of extreme ocean warming on the early development of a marine top predator: The Guadalupe fur seal. Prog. Oceanogr. 180, 102220. doi: 10.1016/j.pocean.2019.102220
García-Capitanachi B., Schramm Y., Heckel G. (2017). Population fluctuations of Guadalupe fur seals (Arctocephalus philippii townsendi) between the San Benito Islands and Guadalupe Island, Mexico, during 2009 and 2010. Aqua. Mamm. 43, 492–500. doi: 10.1578/AM.43.5.2017.492
Gardner J. L., Peters A., Kearney M. R., Joseph L., Heinsohn R. (2011). Declining body size: a third universal response to warming? Trends Ecol. Evol. 26, 285–291. doi: 10.1016/j.tree.2011.03.005
Gazo M., Aparicio F., Cedenilla M. A., Layna J. F., González L. M. (2000). ). Pup survival in the Mediterranean monk seal (Monachus monachus) colony at Cabo Blanco peninsula (Western Sahara-Mauritania). Mar. Mamm. Sci. 16, 158–168. doi: 10.1111/j.1748-7692.2000.tb00910.x
Gentry R. L., Holt J. R. (1982). “Equipment and techniques for handling northern fur seals.” in NOAA technical report NMFS SSRF; 758. United States: National Marine Fisheries Service. Available at: https://repository.library.noaa.gov/view/noaa/15407.
Georges J. Y., Guinet C. (2000). Early mortality and perinatal growth in the subantarctic fur seal (Arctocephalus tropicalis) on Amsterdam Island. J. of Zool. 251, 277–287. doi: 10.1111/j.1469-7998.2000.tb01078.x
Geraci J. R., Lounsbury V. J. (2005). National aquarium in baltimore (Galveston, Texas, USA: Texas A&M Sea Grant Publication), i–xi+305pp.
Gulland F. M., Baker J. D., Howe M., LaBrecque E., Leach L., Moore S. E., et al. (2022). A review of climate change effects on marine mammals in United States waters: Past predictions, observed impacts, current research and conservation imperatives. Climate Change Ecol. 3, 100054. doi: 10.1016/j.ecochg.2022.100054
Hernández-Camacho C. J., Trites A. W. (2018). Population viability analysis of Guadalupe fur seals Arctocephalus townsendi. End. Species R. 37, 255–267. doi: 10.3354/esr00925
Hubbs C. L. (1956). Back from oblivion. Guadalupe fur seal: still a living species Pac. Discov. 9, 14–21.
Hubbs C. L. (1979). Guadalupe fur seal. Advisory Committee on Mar. Res. Research. Working Party on Marine Mammals, FAO Fisheries Series 5, 24–27.
Juárez-Ruiz A., Pardo M. A., Hernández-Montoya J. C., Elorriaga-Verplancken F. R., Milanés-Salinas M.D.L.Á., Norris T., et al. (2022). Guadalupe fur seal pup production predicted from annual variations of sea surface temperature in the southern California Current Ecosystem. ICES J. of Mar Scie. 79, 1637–1648. doi: 10.1093/icesjms/fsac097
Leising A. W., Schroeder I. D., Bograd S. J., Abell J., Durazo R., Gaxiola-Castro G., et al. (2015). State of the california current 2014–15: impacts of the warm- water “Blob”. Calif. Coop. Ocean. Fish. Invest. Rep. 56, 31–68. Available at: http://calcofi.org/ccpublications/ccreports/calcofi-reports-pdf.html.
Li P., Yin Y. L., Li D., Kim S. W., Wu G. (2007). Amino acids and immune function. Br. J. Nutr. 98, 237–252. doi: 10.1017/S000711450769936X
Lunn N. J., Boyd I. L., Croxall J. P. (1994). Reproductive performance of female Antarctic fur seals: the influence of age, breeding experience, environmental variation and individual quality. J. Anim. Ecol. 63, 827–840. doi: 10.2307/5260
Lynn R. J., Simpson J. J. (1987). The California Current System: The seasonal variability of its physical characteristics. J. of Geophy. Res. Oceans 92, 12947–12966. doi: 10.1029/JC092iC12p12947
Maloney A., Chilvers B. L., Haley M., Muller C. G., Roe W., Debski I. (2009). Distribution, pup production and mortality of New Zealand sea lion (Phocarctos hookeri) on Campbell Island/Motu Ihupuku 2008. New Z. J. Ecol. 33, 97–105.
Maniscalco J. M. (2023). Changes in the overwintering diet of Steller sea lions (Eumetopias jubatus) in relation to the 2014–2016 northeast Pacific marine heatwave. Global Ecol. Conserv. 43, e02427. doi: 10.1016/j.gecco.2023.e02427
Maniscalco J. M., Calkins D. G., Parker P., Atkinson S. (2008). Causes and extent of natural mortality among Steller sea lion (Eumetopias jubatus) pups. J. Aqua. Mamm. 34, 277. doi: 10.1578/AM.34.3.2008.277
Mattlin R. H. (1978). Pup mortality of the New Zealand fur seal (Arctocephalus forsteri Lesson). New Z. J. Ecol. 1, 138–144.
McClatchie S. (2014). “Oceanography of the Southern California current system relevant to fisheries,” in In Regional Fisheries oceanography of the California Current system (Dordrecht: Springer), 13–60.
McClatchie S., Goericke R., Leising A., Auth T. D., Al E. (2016). State of the California current 2015–16: comparisons with the 1997–98 El niño. Calif. Coop. Ocean. Fish. Invest. Rep. 57, 5–61. Available at: https://par.nsf.gov/servlets/purl/10025363.
McHuron E. A., Jeremy T., Mangel M. (2023). The influence of prey availability on behavioral decisions and reproductive success of a central-place forager during lactation. J. of Theor. Biol. 560, 111392. doi: 10.1016/j.jtbi.2022.111392
McIntosh R. R., Kennedy C. W. (2013). Morphology, sex ratio and cause of death in Australian sea lion (Neophoca cinerea) pups. Aust. Mammalogy. 35, 93–100. doi: 10.1071/AM12037
Michael S. A., Hayman D. T., Gray R., Zhang J., Rogers L., Roe W. D. (2019). Pup mortality in New Zealand sea lions (Phocarctos hookeri) at Enderby Island, Auckland Islands 2013-18. PloS One 14, e0225461. doi: 10.1371/journal.pone.0225461
Mills L. S. (2012). Conservation of wildlife populations: demography, genetics, and management (Malden, Massachusetts, USA: Wiley-Blackwell), 352p.
NOAA. (2014) (National centers for environmental information), state of the climate: tropical cyclones for annual 2014. Available at: https://www.ncdc.noaa.gov/sotc/tropical-cyclones/201413 (Accessed January 2015).
NOAA. (2019) (National oceanic and atmospheric administration fisheries), guadalupe fur seal unusual mortality event in california 2013–2016. Available at: https://www.fisheries.noaa.gov/national/marine-life-distress/2013-2016-california-sea-lion-unusual-mortality-event-cali.
Norma Oficial Mexicana, NOM-059-SEMARNAT. (2010). Protección ambiental-Especies nativas de México de flora y fauna silvestres-Categorías de riesgo y especificaciones para su inclusión, exclusión o cambio. Lista de especies en riesgo. Secretaría de Medio Ambiente y Recursos Naturales (Diario Oficial de la Federación). Retriever from: NORMA Oficial Mexicana NOM-059-SEMARNAT-2010, Protección ambiental-Especies nativas de México de flora y fauna silvestres-Categorías de riesgo y especificaciones para su inclusión, exclusión o cambio-Lista de especies en riesgo (dof.gob.mx).
Ortega-Ortiz C. D., Vargas-Bravo M. H., Olivos-Ortiz A., Zapata M. G. V., Elorriaga-Verplancken F. R. (2019). Short note guadalupe fur seal encounters in the mexican central pacific. J. Aqua. Mamm. 45, 244–252. doi: 10.1578/AM.45.2.2019.24
Piatt J. F., Parrish J. K., Renner H. M., Schoen S. K., Jones T. T., Arimitsu M. L., et al. (2020). Extreme mortality and reproductive failure of common murres resulting from the northeast Pacific marine heatwave of 2014-2016. PloS One 15, e0226087. doi: 10.1371/journal.pone.0226087
Reid K., Forcada J. (2005). Causes of offspring mortality in the Antarctic fur seal, Arctocephalus gazella: the interaction of density dependence and ecosystem variability. Can. J. of Zool. 83, 604–609. doi: 10.1139/z05-045
Ren A. S., Rudnick D. L. (2021). Temperature and salinity extremes from 2014-2019 in the California Current System and its source waters. Commun. Earth Envir. 2, 1–9. doi: 10.1038/s43247-021-00131-9
Riet-Sapriza F. G., Duignan P. J., Chilvers B. L., Wilkinson I. S., Lopez-Villalobos N., Mackenzie D. D., et al. (2012). Interannual and individual variation in milk composition of New Zealand sea lions (Phocarctos hookeri). J. of Mamm. 93, 1006–1016. doi: 10.1644/11-MAMM-A-220.2
Rudnick D. L., Zaba K. D., Todd R. E., Davis R. E. (2017). A climatology of the California Current System from a network of underwater gliders. Prog. Oceanogr. 154, 64–106. doi: 10.1016/j.pocean.2017.03.002
Santora J. A., Mantua N. J., Schroeder I. D., Field J. C., Hazen E. L., Bograd S. J., et al. (2020). Habitat compression and ecosystem shifts as potential links between marine heatwave and record whale entanglements. Nat. Commun. 11 (1), 536. doi: 10.1038/s41467-019-14215-w
Seguel M., Montalva F., Perez-Venegas D., Gutierrez J., Paves H. J., Müller A., et al. (2018). Immune-mediated hookworm clearance and survival of a marine mammal decrease with warmer ocean temperatures. Elife. 7, e38432. doi: 10.7554/eLife.38432
Seguel M., Paredes E., Pavés H., Molina R., Henríquez F., De Groote F., et al. (2011). Pathological findings in South American fur seal pups (Arctocephalus australis gracilis) found dead at Guafo Island, Chile. J. of Comp. path. 145, 308–317. doi: 10.1016/j.jcpa.2011.01.006
Seguel M., Paves H., Paredes E., Schlatter R. (2013). ). Causes of mortality in South American fur seal pups (Arctophoca australis gracilis) at Guafo Island, southern Chile, (2004–2008). Mar. Mamm. Sci. 29, 36–47. doi: 10.1111/j.1748-7692.2011.00534.x
Smith K. E., Burrows M. T., Hobday A. J., King N. G., Moore P. J., Sen Gupta A., et al. (2023). Biological impacts of marine heatwaves. Annu. Rev. Mar. Sci. 15, 119–145. doi: 10.1146/annurev-marine-032122-121437
Soto K. H., Trites A. W., Arias-Schreiber M. (2004). The effects of prey availability on pup mortality and the timing of birth of South American sea lions (Otaria flavescens) in Peru. J. of Zool. 264, 419–428. doi: 10.1017/S0952836904005965
Soto K. H., Trites A. W., Arias-Schreiber M. (2006). Changes in diet and maternal attendance of South American sea lions indicate changes in the marine environment and prey abundance. Mar. Ecol. Prog. Ser. 312, 277–290. doi: 10.3354/meps312277
Spraker T. R., Lander M. E. (2010). Causes of mortality in Northern fur seals (Callorhinus ursinus), St. Paul Island, Pribilof Islands, Alaska 1986–2006. J. Wildl. Dis. 46, 450–473. doi: 10.7589/0090-3558-46.2.450
Spraker T. R., Lyons E. T., Kuzmina T. A., Tift M. S., Raverty S., Jaggi N., et al. (2014). Causes of death in preweaned northern elephant seal pups (Mirounga angustirostris, Gill 1866), Año Nuevo State Reserve, California 2012. J. of Vet. Diag. Inv. 26, 320–326. doi: 10.1177/1040638714523427
Suca J. J., Santora J. A., Field J. C., Curtis K. A., Muhling B. A., Cimino M. A., et al. (2022). Temperature and upwelling dynamics drive market squid (Doryteuthis opalescens) distribution and abundance in the California Current. ICES J. of Mar. Sci. 79, 2489–2509. doi: 10.1093/icesjms/fsac186
Trillmich F., Ono K. A., Costa D. P., DeLong R. L., Feldkamp S. D., Francis J. M., et al. (1991). “The effects of El Nino on pinniped populations in the eastern Pacific” in Pinnipeds and El Nino: responses to environmental stress. Eds. Trillmich F., Ono K. (Springer-Verlag, Berlin, Germany: Springer), 247–270. doi: 10.1007/978-3-642-76398-4
Trites A. W., Jonker R. A. (2000). Morphometric measurements and body condition of healthy and starveling Steller sea lion pups (Eumetopias jubatus). Aqua Mamm. 26, 151–157.
Villegas-Zurita F., Castillejos-Moguel F., Elorriaga-Verplancken F. R. (2015). Southernmost presence of the Guadalupe fur seal (Arctocephalus townsendi) in the Mexican South Pacific. Rev. mexicana biodiversidad 86, 1107–1109. doi: 10.1016/j.rmb.2015.09.011
Weber D. S., Stewart B. S., Lehman N. (2004). Genetic consequences of a severe population bottleneck in the Guadalupe Fur Seal (Arctocephalus townsendi). J. of Her. 95, 144–153. doi: 10.1093/jhered/esh018
Wickens P., York A. E. (1997). Comparative population dynamics of fur seals. Mar. Mamm. Sci. 13, 241–292. doi: 10.1111/j.1748-7692.1997.tb00631.x
Zaba K. D., Rudnick D. L. (2016). The 2014–2015 warming anomaly in the Southern California Current System observed by underwater gliders. Geophysical Res. Lett. 43 (3), 1241–1248. doi: 10.1002/2015GL067550
Keywords: ocean warming, marine mammal, vital demographic rates, pinniped breeding rookeries, pathology, marine mammal conservation, health
Citation: Gálvez C, Raverty S, Galván-Magaña F, Field CL and Elorriaga-Verplancken FR (2023) Mortality in an off-shore predator critical habitat during the Northeast Pacific marine heatwave. Front. Mar. Sci. 10:1202533. doi: 10.3389/fmars.2023.1202533
Received: 08 April 2023; Accepted: 20 July 2023;
Published: 11 August 2023.
Edited by:
Jose Carlos Báez, Spanish Institute of Oceanography (IEO), SpainReviewed by:
Daniel H. Monson, U.S. Geological Survey, Alaska, United StatesDavid Ainley, H.T. Harvey & Associates, United States
Copyright © 2023 Gálvez, Raverty, Galván-Magaña, Field and Elorriaga-Verplancken. This is an open-access article distributed under the terms of the Creative Commons Attribution License (CC BY). The use, distribution or reproduction in other forums is permitted, provided the original author(s) and the copyright owner(s) are credited and that the original publication in this journal is cited, in accordance with accepted academic practice. No use, distribution or reproduction is permitted which does not comply with these terms.
*Correspondence: Fernando R. Elorriaga-Verplancken, ZmVsb3JyaWFnYUBpcG4ubXg=