- 1Tianjin Research Institute for Water Transport Engineering, Ministry of Transport, Tianjin, China
- 2Yangtze Institute for Conservation and Development, Hohai University, Nanjing, China
- 3Bureau of Hydrology Changjiang Water Resources Commission, Wuhan, China
Human activities have severely altered the Yangtze River (Changjiang) Estuary’s pattern, beach-trough shape, and delta in recent decades. Existing research has studied the sediment “source–sink” relationship between the Yangtze River mainstream and estuary using gauged suspended sediment data. This method overestimated the decline in the suspended sediment concentration in the estuary, as riverbed scouring or deposition have not filtered out amounts related to sand mining or waterway dredging. This study took the Yangtze River Basin as the research subject and used spot observed runoff, sediment, and riverbed topography data gathered between 1980 and 2020 to analyze the sediment trapping effect of the Three Gorges Reservoir (TGR) and study the “source-sink” relationship of suspended sediment transport in the middle-lower reaches of the Yangtze River. The Yangtze Estuary Delta is dominated by scouring, which shows an increasing trend. During the operation of the TGR (2003–2020), the suspended sediment load entering the Yangtze Estuary from the Yangtze River mainstream decreased by 68% compared to the amount prior to operation commencing (1960–2002). However, studies have found that there are still large amounts of bottom sand with riverbed load movement characteristics after the operation of the Three Gorges Project and that riverbed souring of the downstream dam has increased the degree of deposition recharge. The amount of sediment in the Yangtze Estuary decreased between 2003 and 2018 compared with the period 1981 to 2002, with an annual decrease of 16.9%. The cumulative deposition of the Yichang–Datong and Datong–Xuliujing reaches from 1981–2002 was 3.13×108 m3 and 1.60×108 m3, respectively, and cumulative scouring from 2002–2018 was 28.52×108 m3 and 16.01×108 m3. This study concluded that even if the suspended sediment load entering the estuary decreased significantly after the operation of the TGR, the riverbed in the middle-lower reaches of the Yangtze River has gradually transformed from the “sink” of sediment deposition to the “source” of sediment supply.
1 Introduction
Estuary deltas act as a “sink”, holding sediment from upstream river basins before they flow into the sea. In recent years many estuary deltas around the world have been shrinking, affected by a sharp decrease in the amount of sediment supplied by river basins (Manh et al., 2014) as a result of the construction of upstream reservoirs and dams, and the implementation of river control projects (Yang et al., 2011; Manh et al., 2014). Meanwhile, ground subsidence and rising sea levels have increased the degree of delta submergence and increased scouring power (Dai et al., 2018; Nienhuis et al., 2020; Paszkowski et al., 2021; Schmitt et al., 2021). Statistics on the connectivity of 1,200×104 km rivers globally found that only 37% of them flow freely, and 23% flow into the sea without being uninterrupted, with interruptions mainly due to waterway dredging and dam construction (Grill et al., 2019).
In recent decades, climate change and human activities have had a profound impact on the sediment loads and channel morphology of the Yangtze River in China (Li et al., 2018; Li et al., 2019; Guo et al., 2019; Zhou et al., 2020; Zhou et al., 2021). Waterway engineering construction and waterway dredging excavation have changed the shape of the natural shoal and troughs in the estuary delta (Pan et al., 2012; Dai et al., 2013; Wei et al., 2017; Zhao et al., 2018; Zhu et al., 2019). For example, the deep-water waterway project in the North Passage of the Yangtze Estuary prompted the front edge of the estuary to extend to the sea (Wang et al., 2017). Sediment contains life-nourishing resources for an estuary delta because it establishes a stable platform for vegetation growth (Nittrouer and Viparelli, 2014) and acts as a material source for urban land resource expansion (Zhang et al., 2018).
The construction of reservoirs and dams in the river basin blocks the continuous process of material transport from the river basin to the estuary (Wang et al., 2016), and affects material exchange and estuary resource sustainability (Liu et al., 2023). Some dams on small- and medium- sized rivers have been demolished (East et al., 2015; Warrick et al., 2015; Collins et al., 2017) to increase the material continuity of the rivers and alleviate the impact of sediment decrease in the river basin on estuary deltas. Even though there have been disputes regarding the construction of dams on rivers with abundant water resources, (Latrubesse et al., 2017), hydropower is perceived to be a clean energy source, and the operation of hydropower stations has had a positive effect on reducing global carbon emissions (Almeida et al., 2019; Bertassoli et al., 2021). The “source-sink” relationship of sediment traveling from the river basin to the estuary has always been a focus of research (Yang et al., 2015a; Gao et al., 2018; Gao et al., 2019). Existing studies have focused on the “source-sink” relationship of suspended sediment concentrations (Yang et al., 2014; Yang et al., 2015b; Yang et al., 2015c; Guo et al., 2019). River hydrographic stations have measured suspended sediment concentration, but have failed to observe the adjustment of riverbed shape and the sediments on the surface of river channels with load transfer characteristics. From the perspective of the “source-sink” relationship of suspended sediment concentration from the river basin to the estuary, the effect of bottom sand has not often been considered, and this has led to an overestimation of the reduction of suspended sediment concentration in the estuary (Li et al., 2012; Zou et al., 2012; Jing et al., 2013; Yang et al., 2014; Yang et al., 2020), promoting the belief that the scouring of the Yangtze Estuary will continue or worsen (Luan et al., 2016; Yang et al., 2017; Dai et al., 2018; Luan et al., 2021; Cheng et al., 2022; Tang et al., 2022; Xu et al., 2022). After the operation of the TGR, the middle-lower reaches of the Yangtze River (Xia et al., 2016; Zhou et al., 2018; Yang et al., 2019; Yang et al., 2021a), the tidal reach (Mei et al., 2021; Xie et al., 2021), and its South Branch (Wang et al., 2013; Luan et al., 2016; Zhao et al., 2018; Zhu et al., 2019; Guo et al., 2021a) as well as other areas were all scoured. Some of the fine particles from the riverbed replenished the suspended sediment and entered the estuary with the water flow, making estuarine suspended sediment concentration drops much lower than that in the river basin (Yang et al., 2014; Yang et al., 2015b). Other coarse sediment particles entered the estuary in the form of riverbed bottom sand and spread, causing partial estuary deposition (Guo et al., 2021b). In the calculation of riverbed scouring, full consideration should be given to sand mining (Brunier et al., 2014; Anthony et al., 2015; Bendixen et al., 2019; Xu et al., 2021; Yang et al., 2021a) and waterway dredging (Mossa and Chen, 2021; Yang et al., 2021a; Yang et al., 2021b) otherwise the riverbed scouring intensity is overestimated. Therefore, to scientifically assess the “source-sink” relationship of sediment from the Yangtze River Basin and the estuary, the impact of waterway dredging and land reclamation on riverbed scouring as well as suspended sediment and sand mining needs to be considered.
This study took the Yangtze River Basin as the research subject and used spot observed runoff, sediment, and riverbed topography data gathered between 1980 and 2020 to analyze the sediment trapping effect of the TGR and study the “source-sink” relationship of suspended sediment transport in the middle-lower reaches of the Yangtze River. Factors including riverbed scouring or deposition, sand mining, waterway dredging, and shoal reclamation are considered in this analysis of the impact of the bottom sand “source-sink” relationship on the estuary and future evolution trends of the Yangtze Estuary Delta are discussed.
2 Research area
The total length of the Yangtze River is approximately 6,397 km and its basin size is about 180×104 km2 (Figure 1A). The upper reaches (4,504 km) stretch above Yichang hydrographic station, the middle reaches (955 km) run from Yichang hydrographic station to Hukou hydrographic station, and the lower reaches (938 km) run below Hukou hydrographic station. The TGR was filled and opened in June 2003, and its impoundment level reached 175 m from 2009–2020, the total storage capacity is 39.3 billion cubic meters. The operation of the TGR does not change the total amount of runoff, but the regulation and storage effect reduce the peak flow and increase the minimum flow (Zheng, 2016). On the south bank of the downstream channel of the Three Gorges Dam, Dongting Lake, Poyang Lake, and the Yangtze River form a complex river-lake system. The Hanjiang River on the north bank merges into the Yangtze River from Wuhan City (Figures 1A, B). This study takes the section from the TGD to the Yangtze Estuary, the total length of the river’s channel is about 1,855 km (Figures 1B, C). Among them, the Yangtze Estuary has a “three-level branching and four outlets” river regime pattern, with Qidong shoal, Yiyuansha shoal, Hengsha shoal, Jiuduansha shoal, Jiangyanansha shoal, Nanhui shoal, and Deltas (Figure 1D).
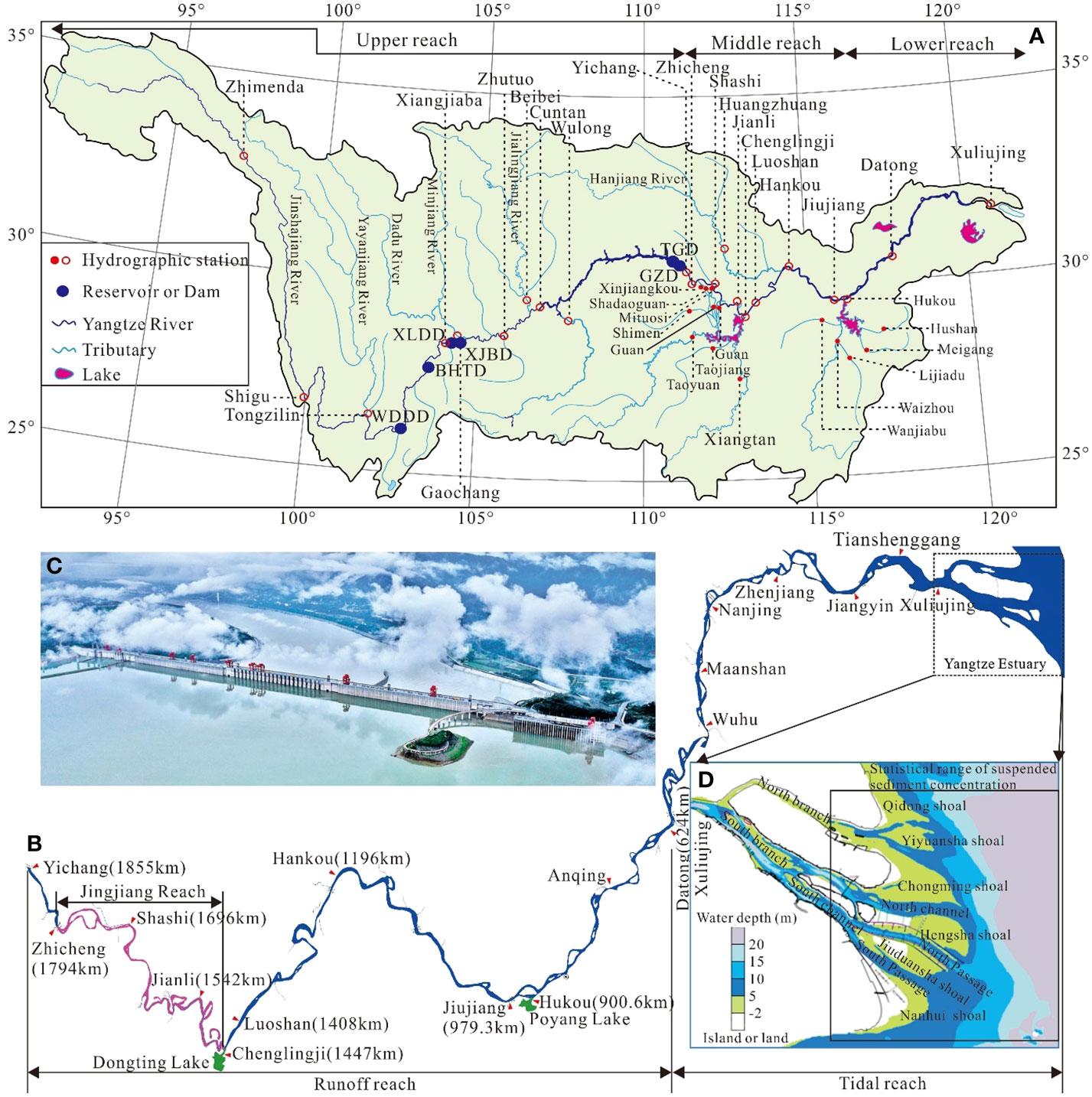
Figure 1 Basic information relating to the Yangtze River Basin; (A) Yangtze River Basin; (B) Three Gorges Dam to estuary; (C) Three Gorges Dam; (D) Yangtze Estuary. XLDD represents Xiluodu Dam, BHTD represents Baihetan Dam, WDDD represents Wudongde Dam, XJBD represents Xiangjiaba Dam, and GZBD represents Gezhouba Dam.
3 Data collection, and analytical methods
3.1 Data collection
The runoff and sediment load data of the Yangtze River mainstream and tributaries covering the period from 1980–2020 were collected, and the temporal and spatial characteristics of runoff and sediment load changes were analyzed. The riverbed topography data from Yichang hydrographic station to Yangtze Estuary in 1981, 2002, 2011, and 2018 were collected, with a measurement scale of 1:10,000, the vertical measurement error is less than 1.0 cm, and the riverbed scouring volume and intensity were analyzed; the waterway dredging volume, sand mining volume, and land reclamation data from 1984–2020 were collected to analyze the impact of human activities on the intensity of riverbed scouring and bottom sand discharge. The data on the amount of waterway dredging is formed from statistics of the amount of sediment in waterway dredging ships year by year from Changjiang Waterway Bureau. As there is a mixture of water and sediment in the ship, the ratio between the volume of the ship and the amount of excavation on the riverbed is 3:1, following the method from Yang et al. (2021b). The amount of sand mining is the planned amount (Changjiang Water Resources Commission (CWRC); 2004–2022). Due to the uncertainty of illegal sand mining, the planned amount of sand mining is used to analyze the influence on the riverbed scouring or deposition amount. Research data are shown in Table 1.
3.2 Analytical methods
3.2.1 Sediment exchange and “source-sink” relationship
Treating the discharge of the TGR, riverbed souring, tributaries, and lake exchange as the “source” of sediment and the Yangtze Estuary as the “sink” of sediment. The processes through which sediments travel from “source” to “sink” were analyzed, and the impact of the sediment “source-sink” relationship change on the estuary was studied.
Starting from Yichang hydrographic station (SYichang), we considered the influence of Dongting Lake (SDongting Lake), Poyang Lake (SPoyang Lake), Hanjiang River (SHanjiang River), and the inward import relationship of the interval on the sediment amount and the amount of sediment scouring or deposition of the riverbed (S) recharge from Yichang to Datong reaches (Figure 2). Datong hydrographic station (SDatong) is the last hydrographic station of the Yangtze River mainstream. The calculation formula of riverbed recharge suspended sediment from Yichang to Datong reaches is as follows:
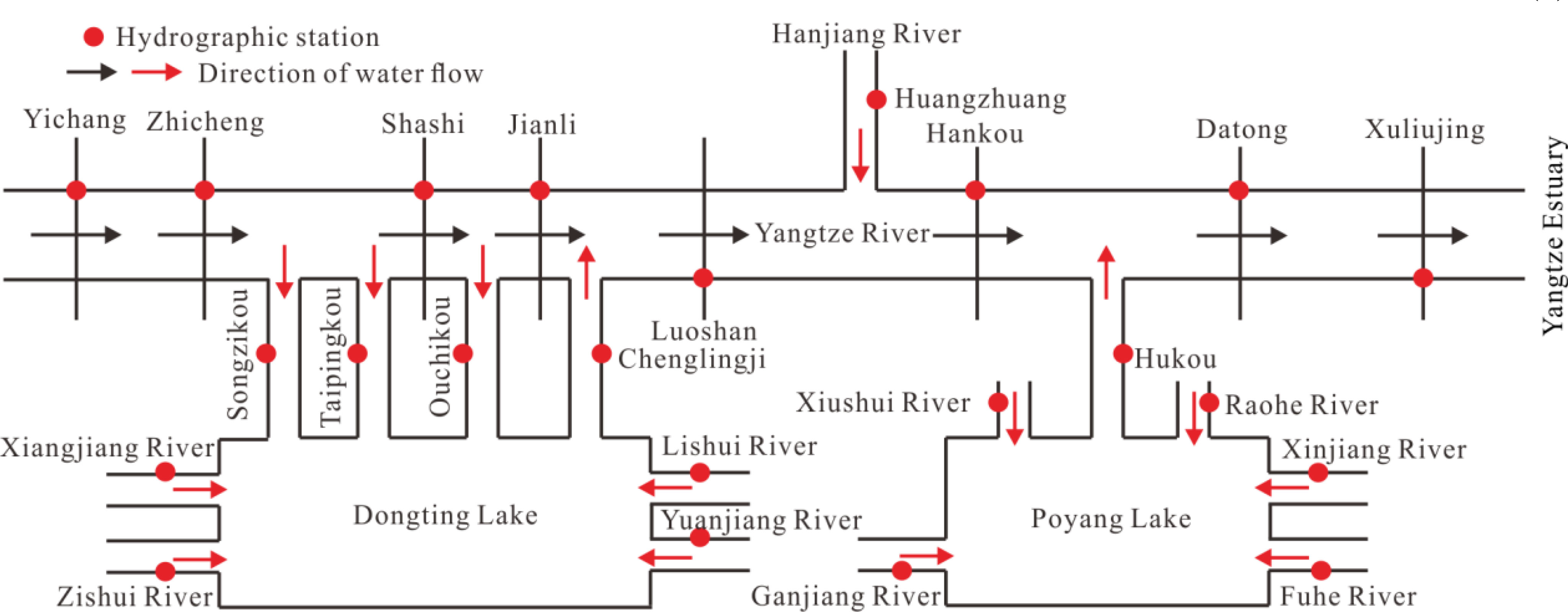
Figure 2 Key hydrographic stations and reaches for the calculation of suspended sediment load budget.
The sediment quantity of Dongting Lake (SDongting Lake), into the Yangtze River mainstream is the difference between Chenglingji hydrographic station (SChenglingji) and Three Outlets (SThree Outlets). The calculation formula is as follows:
The Three Outlets at which Dongting Lake diverts sediment from the Yangtze River are Songzikou (SSongzikou), Taipingkou (STaipingkou), and Ouchikou (SOuchikou). That is, the formula for calculating the amount of sediment at the three mouths of Dongting Lake is as follows:
The hydrographic station where Poyang Lake merges into the Yangtze River is the Hukou station (SHukou). Namely:
The hydrographic station where the Hanjiang River merges into the Yangtze River is the Huangzhuang station (SHuangzhuang). Namely:
There is no sediment monitoring station in Datong–Xuliujing reaches. A topographic method should be used to calculate the suspended sediment recharge in this section. The river sediment test primarily aimed to analyze the suspended sediments, and there was an unmeasured area at a certain thickness at the bottom. This part of the sediment has traction load movement characteristics, part of which exchanges with the riverbed sand in the suspended sediment, and most of which is transported downstream along the riverbed surface (Wang et al., 2020; Yang et al., 2021b). The bottom sand exchange was measured by the bottom-supported system at the Yangtze Estuary and was concentrated in the deep-water waterway of the North Passage (Liu et al., 2011). Meanwhile, according to the estimate using the Rousse formula, the sediment content of the unmeasured area at the bottom is significantly higher than the suspended sediment concentration (Wang et al., 2020). At the same time, there is a large amount of waterway dredging (Vwd), sand mining (Vsm), and land reclamation (Vlr) in the river channels, as well as riverbed scouring or deposition. The suspended sediment transport balance and the amount of sediment entering the representative section can be estimated using the following calculation formula:
Where Vriverbed-sand is the amount of sediment replenished riverbed scouring; VTotal is the total amount of riverbed scouring.
3.2.2 Calculation method of riverbed scouring or deposition
The cross-sectional areas are calculated from the topographic cross-sections along the river (Figure 3A), and the watercourses upstream and downstream of the river channels, according to Eq. (7):
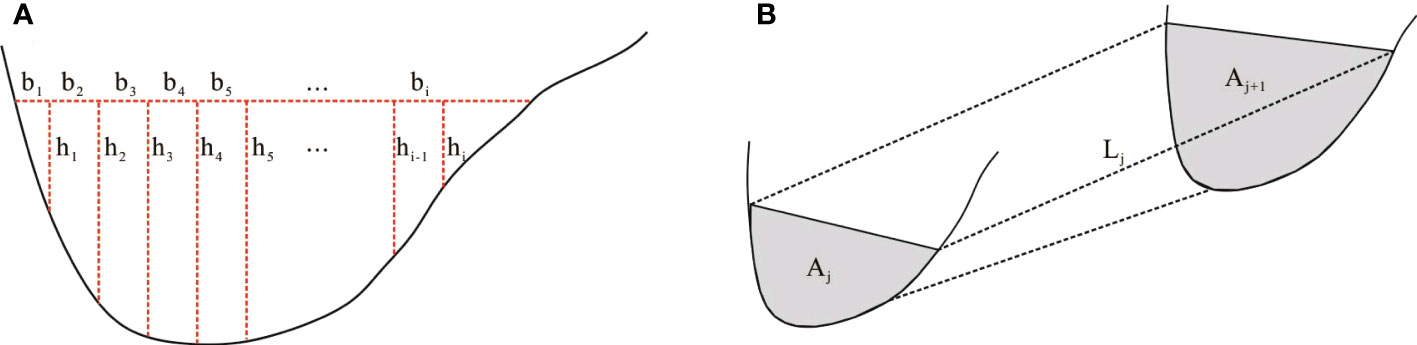
Figure 3 An anabraching river channel according to (A) areas of the cross-sectional, and (B) river channel capacity calculation models.
Where i is the number of points at which the elevation of a transverse of the river is measured; j is the number of rivers profiles; Ai is the cross-sectional area (m2); and are the water depths at two consecutive points of a section (m); and bi is the width at two consecutive points (m).
The volume of the rivers channel Vj (Figure 3B) between the up and downstream sections at the corresponding water level is calculated according to Eq. (8). Subsequently, the total river channel volume is obtained via Eq. (8):
Where Vj is the volume of the channel between adjacent sections (m3), Ai,j and Ai,j+1 are the areas of adjacent sections (m2), and Lj is the distance between adjacent sections (m).
Sand mining in the Yangtze River, which increases riverbed scouring, is mainly used to scour for construction materials. The sediment from waterway dredging of the Yangtze River channel is mainly used for reclamation of shoals or land, and will also increase riverbed scouring after waterway dredging. To facilitate the comparison between the amount of riverbed scouring or deposition, the measurement unit of the suspended sediment load from the river basin, the amount of riverbed scouring or deposition, the amount of sand mining, and the amount of waterway dredging are all converted to t/y (tons/year).
4 Results and discussion
4.1 The impact of the TGR’s sediment interception on the “source-sink” of sediments
In the period between 2003 and 2020, both the sediment inflow from the Jinshajiang, Minjiang, Yalongjiang, Wujiang, and Jialingjiang Rivers in the upper reaches of the Yangtze River to the TGR area and the sediment outflow from the TGR decreased, from 4.25×108 t/y in 1980–2002 to 1.45×108 t/y, a decrease of 65.9% (Figure 4A). From 2003 to 2020 sediment inflow to the TGR from the Jinshajiang, Minjiang, Yalongjiang, Wujiang, and Jialingjiang Rivers in the upper reaches of the Yangtze River was 26.15×108 t, the sediment outflow from the reservoir was 6.26×108 t, and the accumulated sediment in the reservoirs area was 19.89×108 t (Figure 4B). In other words, the TGR and upstream cascade reservoir, soil and water conservation, climate change, and sand mining intercepted 76% of the incoming sediment from the upper reaches of the Yangtze River Basin (Figure 4C).
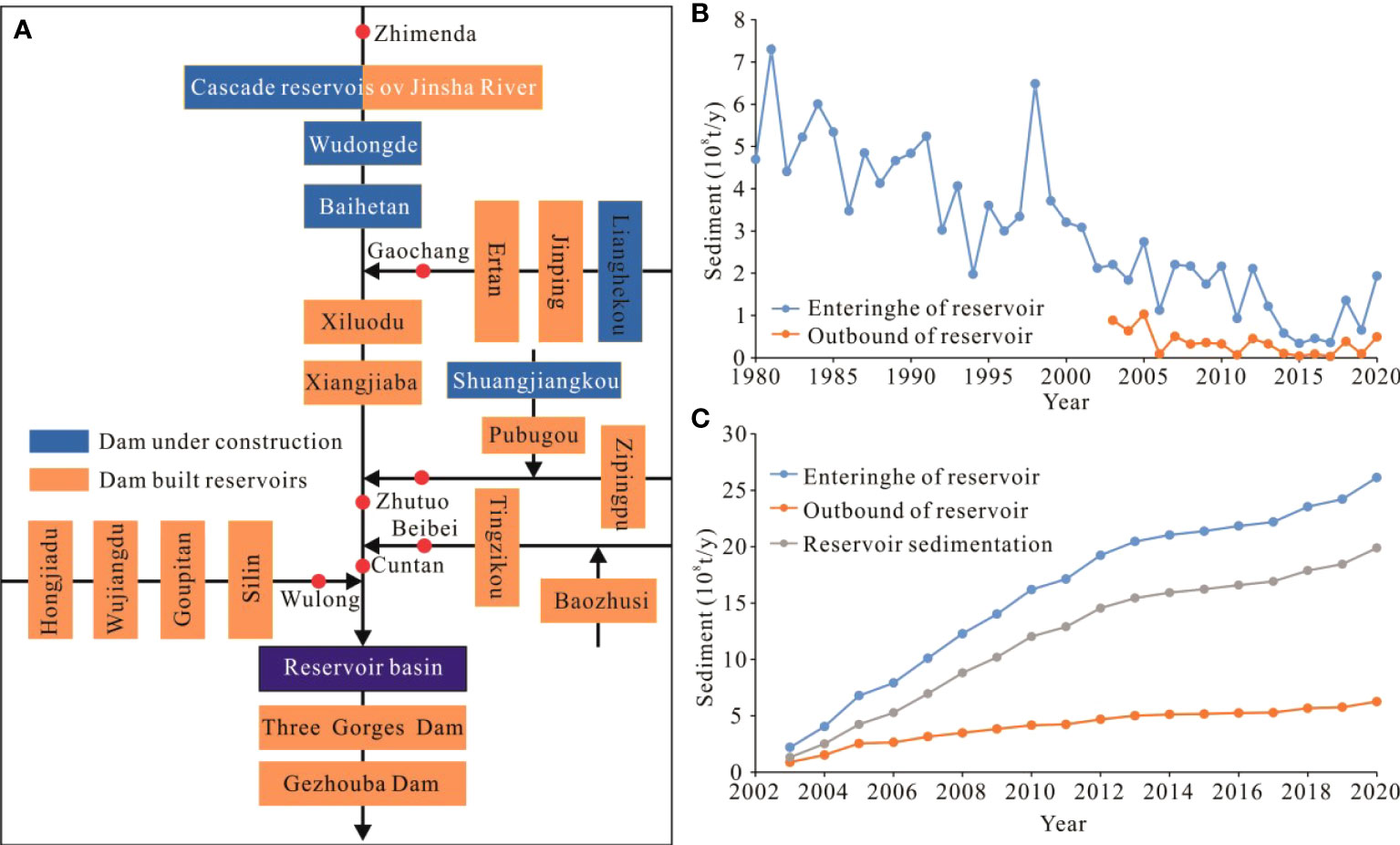
Figure 4 Relationship between sediment inflow and outflow of the TGR; (A) Distributions of the TGR and the upstream cascade reservoirs; (B) Chronological variations of sediment inflow and outflow from the TGR; (C) Cumulative variation of sediment inflow and outflow from the TGR.
4.2 The source–sink relationship sediment in the runoff control section of the middle–lower reaches Yangtze River
4.2.1 Composition of the runoff and sediments in the middle-lower reaches of the Yangtze River
According to the operation time of Gezhouba Dam, Three Gorges Dam, and cascade reservoirs in the upper reaches of the Yangtze River, the study period is divided into 1980–2002, 2003–2011, and 2012–2020.
Due to the diversion of Dongting Lake, the runoff at Yichang, Zhicheng, Shashi, and Jianli hydrographic stations decreased, and the rate of decrease slowed down from upstream to downstream. Affected by the inflow of Dongting Lake, Hanjiang River, and Poyang Lake, the runoff at Luoshan, Hankou, and Datong hydrographic stations increased. In general, the operation of the TGR and changes in the relationship between rivers and lakes in the lower reaches of the Yangtze River mainstream has not changed the characteristics of the runoff and had little impact on the total amount of runoff. Around 47% of the runoff entering the Yangtze Estuary from 1980–2020 came from the area above Yichang hydrographic stations (Figure 5A; Table 2). From 1980–2002, the amount of sediment at each hydrographic station first decreased and then increased, and from 2003–2020, it increased along the way (Figure 5B; Table 2). From 1980 until 2002, 2003–2011, and 2012–2020, the runoff and sediment of the three mouths of Dongting Lake all decreased, and the decrease of the sediment was significantly higher than that of the runoff (Figures 5C; D, Table 3). Both the runoff and sediments of the Dongting Lake discharged into the Yangtze River decreased first before increasing, and the amount was smaller after the TGR went into operation. The runoff of Poyang Lake discharged into the Yangtze River first decreased and then increased, the amount of sediment first increased and then decreased. Conversely, the amount of runoff and sediment from the Hanjiang River discharged into the Yangtze River decreased throughout this time.
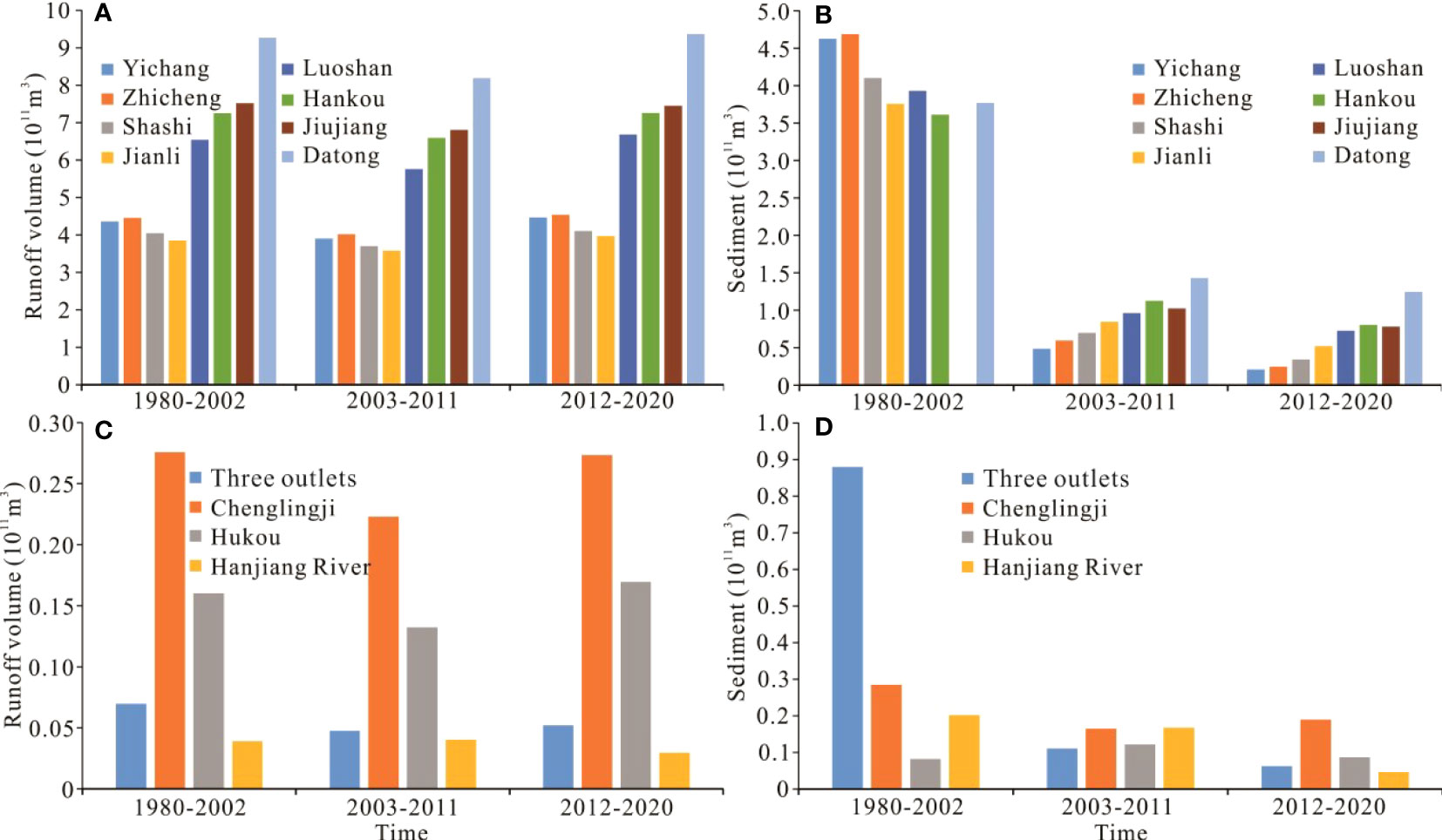
Figure 5 Runoff and sediment fluxes downstream of the TGR; (A) Runoff of the Yangtze River mainstream; (B) Sediment of the Yangtze River mainstream; (C) Runoff of the tributaries and lakes; (D) Sediment of the tributaries and lakes.
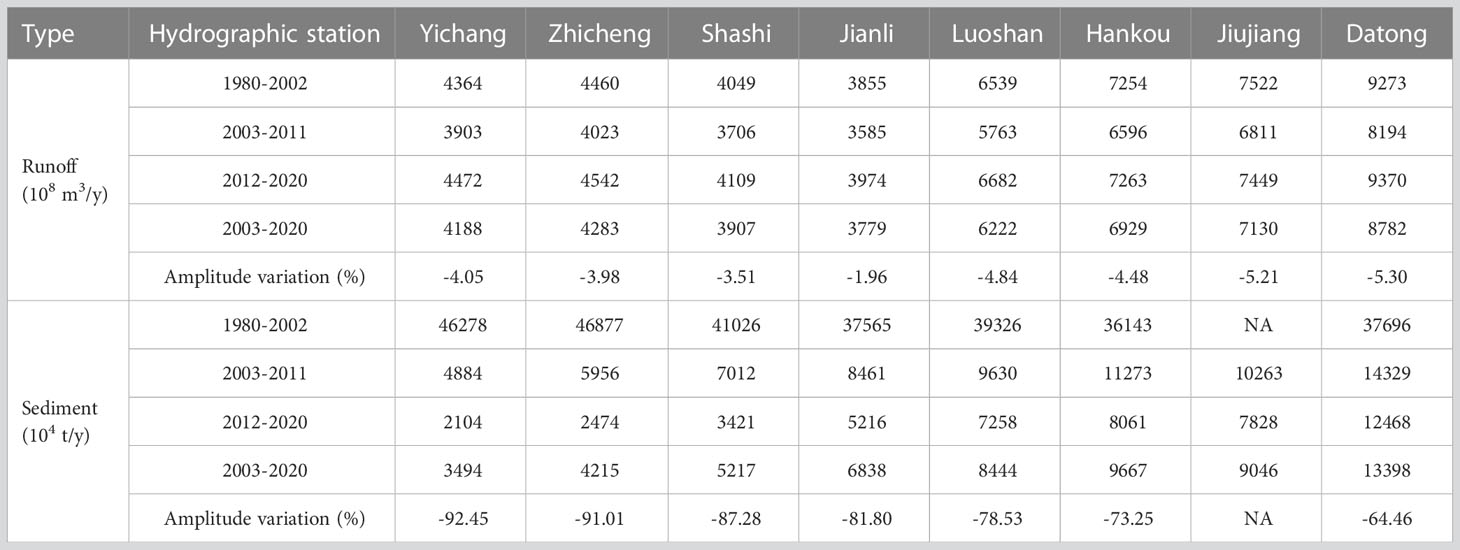
Table 2 Variation of runoff and sediment at the hydrographic station of the Yangtze River mainstream.
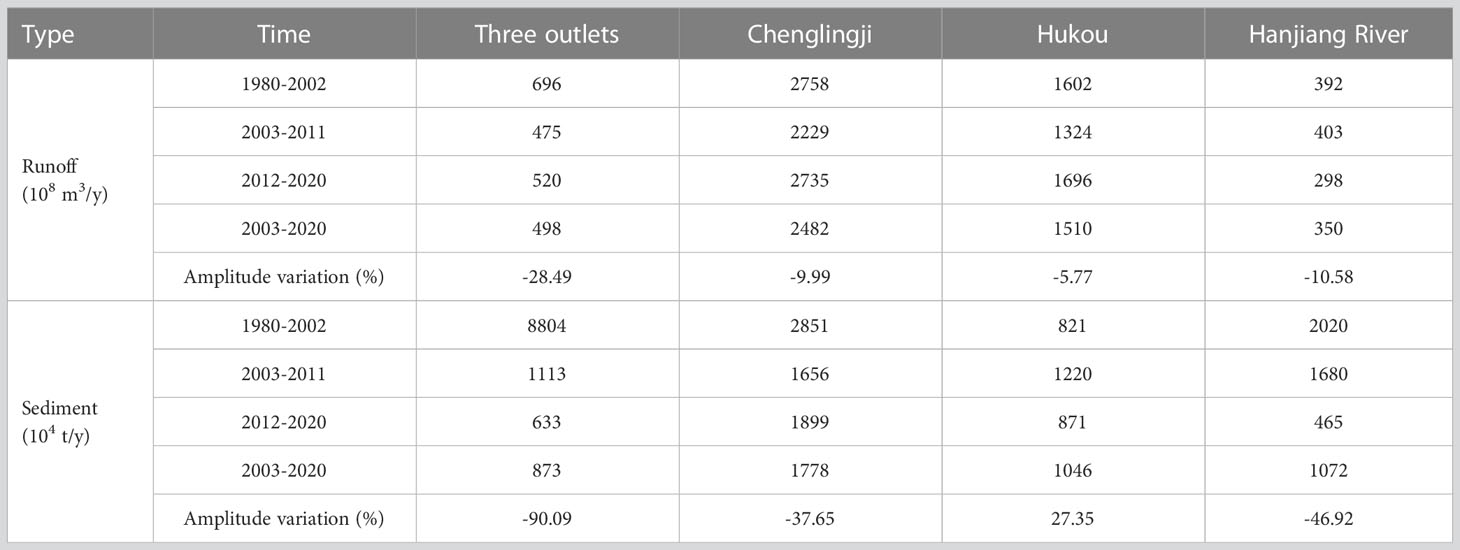
Table 3 Variation of runoff and sediment at the hydrographic station in the tributaries and lakes of the Yangtze River.
In the periods of 1980–2002, 2003–2011, and 2012–2020, the runoff at the Datong hydrographic station came from Yichang hydrographic station, Dongting Lake, Hanjiang River, and Poyang Lake; the confluence proportion was 47–48%, 21–24%, 3–5%, 16–18%, and 7–10% respectively, indicating that the composition of the runoff into the Yangtze Estuary before and after the operation of the TGR was stable (Figure 6A). From 1980–2002, the sediment amount at Yichang hydrographic station was higher than that at Datong hydrographic station; the riverbed at Dongting Lake and from Yichang–Datong reaches were all deposition, only 4% of the sediments at Datong hydrographic station came from Poyang Lake and Hanjiang River. From 2002–2011, the sediments at Datong hydrographic station came from Yichang, Dongting Lake, Hanjiang River, and Poyang Lake, with proportions of 34%, 4%, 12%, 8%, and 42%, respectively. These proportions changed in the period from 2012–2020 to 17%, 10%, 4%, 7%, and 62%. From 2012–2020 compared with 2003–2011, the proportion of suspended sediment in Datong hydrographic station in Yichang hydrographic station decreased by 17%, and the proportion of deposition recharge by riverbed scouring in Datong hydrographic station increased by 20% (Figure 6B).
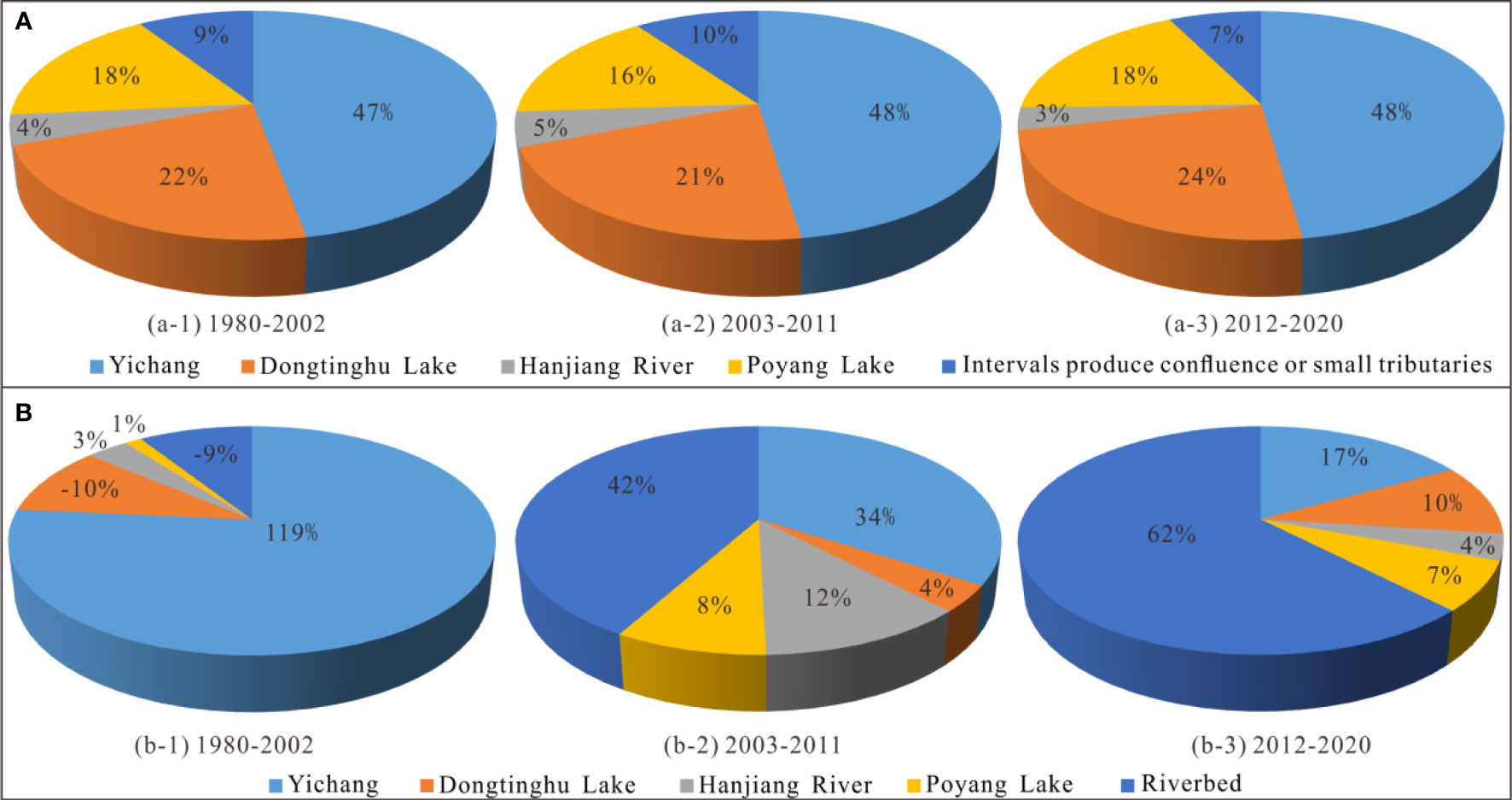
Figure 6 Composition of runoff and sediment at the Datong hydrographic station of the Yangtze River. (A) Composition of runoff; (B) Composition of sediment.
4.2.2 Exchange characteristics between suspended sediment and riverbed sand in the middle-lower reaches of the Yangtze River
The reach from Yichang–Dabujie (120 km) has a sandy pebble riverbed, transitioning to a purely sandy riverbed below Dabujie, with a great riverbed anti-scouring ability. Under the background of reduced sediment discharge from the TGR, the sediment scouring of the alluvial riverbed shows good exchange and sorting characteristics. The main characteristic is that the fine sediment in the riverbed first compensates for the suspended sediment and thus causes the riverbed to be in a scouring state, while the relatively coarse sediment will remain in the riverbed and only start to transport in a flood period. In the scouring process of the sandy riverbed in the middle-lower reaches of the Yangtze River, the fine sediment (d ≤ 0.125 mm) washed by the riverbed surface fed the suspended sediment, causing an increase in the amount of fine sediment in the suspended sediment (Figure 7A). Coarse sediment (d>0.125 mm) was recharged from the reaches between Yichang–Jianli reaches. The coarse sediment showed an increasing trend along the rivers from 2003–2021 (Figure 7B), which is also the area with the greatest scouring intensity in the riverbed downstream of the TGR (Yang et al., 2022a; Yang et al., 2023a; Yang et al., 2023b; Yang et al., 2023c). In the reach of the sandy and cobble-riverbed, due to the small thickness of the sandy overburden of the riverbed, the scouring of fine sediment in the riverbed is complete at present (Figure 7C), and the anti-scouring of the riverbed is in a quasi-equilibrium state due to the significant coarsening of the riverbed. The median particle size of suspended sediment at the Yichang hydrographic station during 1987–2002, 2003–2012, and 2013–2021 was 0.022 mm, 0.005 mm, and 0.009 mm, respectively, and that at Hankou hydrographic station was 0.018 mm, 0.014 mm, and 0.015mm. At Datong hydrographic station, the values are 0.017 mm, 0.010 mm, and 0.015 mm. The median particle size of suspended sediment decreases first and then increases (Figure 7D), that is, the coarse sediment scoured by the riverbed gradually recharges the suspended sediment. Therefore, under the background of riverbed scouring, the sediment on the riverbed surface near the dam section is significantly coarsened, while the sediment coarsening phenomenon is not significant when it enters the sandy riverbed.
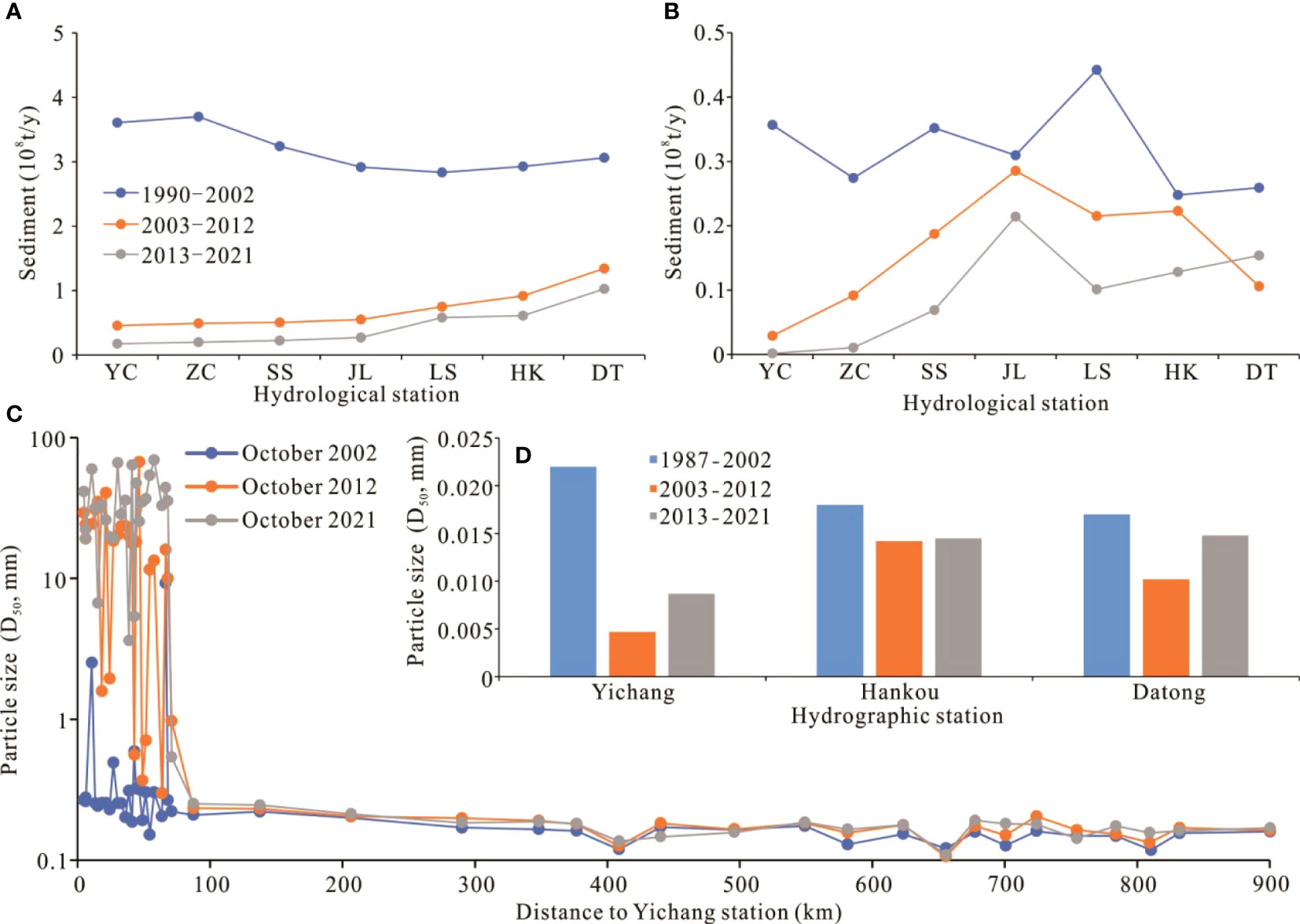
Figure 7 Condition of transport of particle size and grouped sediment in the middle–lower reaches of the Yangtze River. (A) Amount of suspended sediment d ≤ 0.125 mm; (B) Amount of suspended sediment d > 0.125 mm; (C) Median sediment particle size of the riverbed surface; (D) Median particle size of suspended sediment at the main hydrographic stations.
4.2.3 Calculation of sediment source–sink relationships of the Yichang–Datong reaches
After the operation of the TGR, the riverbeds between Yichang–Zhicheng, Jingjiang (Zhicheng–Chenglingji), Chenglingji–Hankou, Hankou–Hukou, Hukou–Datong reaches all experienced cumulative scouring. Since 2012, this scouring intensity has increased (Figure 8A). The cumulative deposition of the Yichang–Datong reaches from 1981–2002 was 3.13×108 m3, respectively (Figure 8B). The cumulative scouring of the Yichang–Datong reaches from 2002–2018 was 28.52×108 m3. In the periods of 1981–2002, 2003–2011, and 2012–2018, the amounts of sediments passing through the Datong section of the Yangtze River Basin decreased. Based on the topographic difference calculation, the amount of riverbed scouring increased (switching from deposition to scouring). The amount of scouring after sand mining and waterway dredging deducted also increased, indicating a switch from deposition to scouring (Figure 8C). In the 1981–2002, 2003–2011, and 2012–2018 periods, the amount of suspended sediment passing through the Datong section were 3.73×108 t/y, 1.43×108 t/y, and 1.22×108 t/y, respectively. From 1981–2002, 2003–2011 and 2012–2018, the riverbed scouring or deposition of the Yichang–Datong reaches were –0.25 × 108 t/y, 1.98 × 108 t/y, and 3.45 × 108 t/y, the amount of sand mining was 0.50 × 108 t/y, 0.40 × 108 t/y and 0.40 × 108 t/y, the waterway dredging amount was 0, 40 ×104 t/y and 0.13×108 t/y, respectively. The amount that entered the bottom sediment of the tidal reach of the Yangtze River through the Datong section was –0.20×108 t/y, (suspended sediments deposition in the riverbed and no bottom sand migrated downstream) 0.98×108 t/y, and 2.14×108 t/y, respectively (Figure 8D). The total amount of suspended and bottom sand that entered the Datong section was 3.56×108 t/y, 2.41×108 t/y, and 3.36×108 t/y, respectively, which first decreased and then increased, in the periods 1981–2002, 2003–2011, and 2012–2018, respectively (Figure 8D).
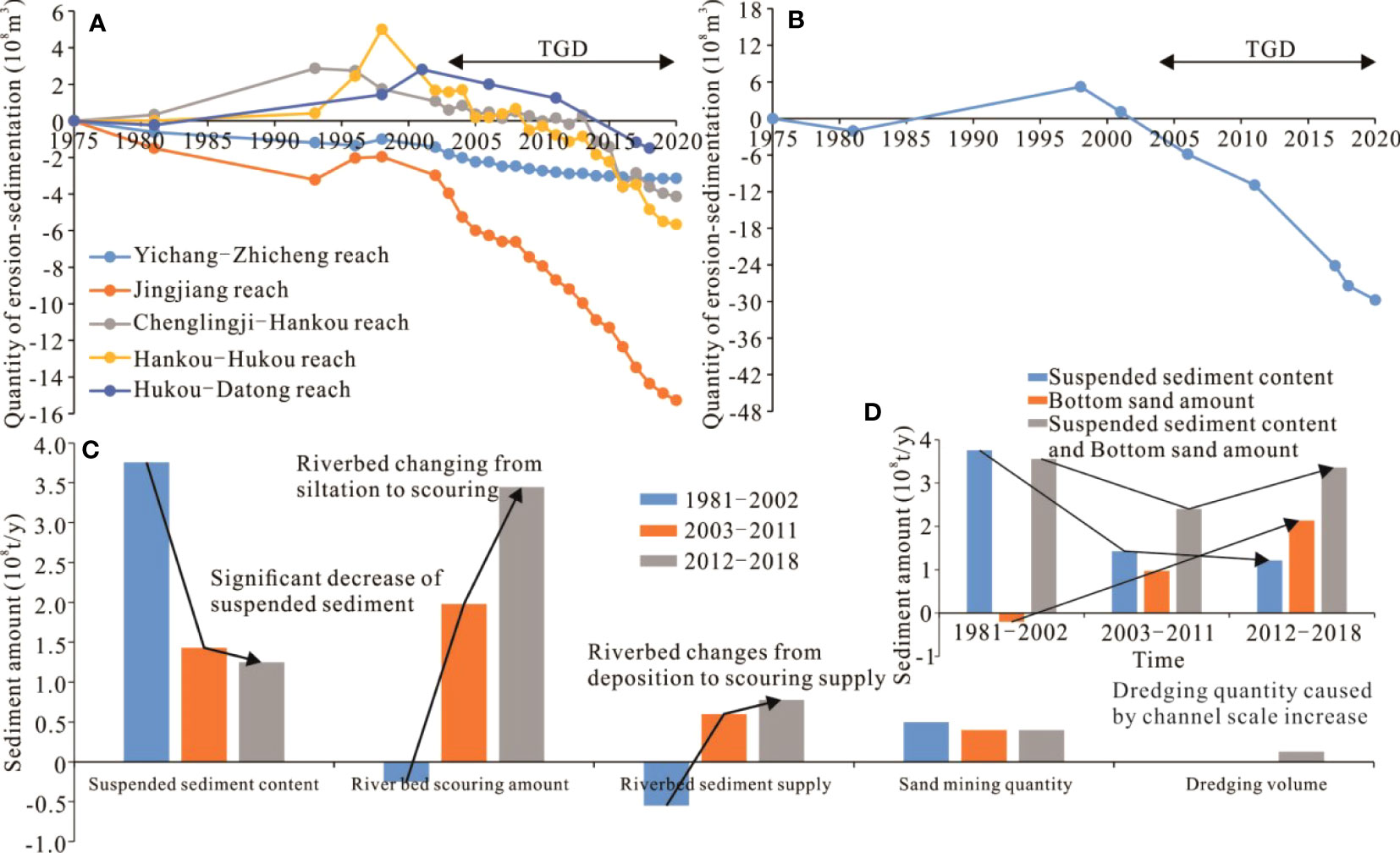
Figure 8 Changes in riverbed scouring or deposition along the Yichang–Datong reaches of the Yangtze River; (A) Cumulative scouring or deposition of riverbed sections based on river channel topography data; (B) Total amount of riverbed scouring or deposition; (C) Sediment source-sink relationship in the Yichang–Datong reaches.
4.3 Effect of riverbed scouring in tidal reach on estuary “source-sink”
4.3.1 Relationship of suspended sediment concentration in the tidal reach of the Yangtze River
Whether the suspended sediment concentration in the Yangtze Estuary will decrease or not is still under debate (Yang et al., 2014; Yang et al., 2015b). Most studies believe that the estuarine suspended sediment concentration will decrease (Li et al., 2012; Zou et al., 2012; Jing et al., 2013; Yang et al., 2014; Yang et al., 2015b), and some even overestimated the reduction in suspended sediment (Yao et al., 2015), as it provides direct evidence of delta scouring (Luan et al., 2016; Zhu et al., 2016; Yang et al., 2017; Dai et al., 2018; Luan et al., 2021);, front tidal flat scouring (Wei et al., 2015) and riverbed scouring (Pan et al., 2012; Dai et al., 2013; Wei et al., 2017). The suspended sediment concentrations at Xuliujing, Sheshan, and Hengsha stations did not reduce between 1998 and 2001, which can be attributed to the resuspension effect (Jin et al., 2006). The suspended sediment concentration in the flood and dry seasons of 2003 and 2004 did not decrease when compared with their corresponding annual averages (between 1950 and 2002 at the Datong hydrographic station) (Zhai, 2006). From 1980–2020, the runoff at the Xuliujing hydrographic section was equal to that at Datong hydrographic station (Figure 9A) and was related to the quasi-balanced runoff at the interval branch (Zhang et al., 2011). The sediment concentration in the Yangtze Estuary from 1980–2020 was similar to the value of the Datong hydrographic station, with a consistent decreasing trend (Figure 9B). In 2002–2011 and 2012–2018, the amount of sediment deposition on the inner edge was equivalent to the amount of sediment compensation in the riverbed along the way, indicating in a quasi-balanced state. Due to the decrease in the amount of sediment from the Yangtze River mainstream, the degree of unsaturation in the stream flow in the Datong–Xuliujing reaches increased, increasing the transport intensity of bottom sediments. Therefore, the sediment entering the Yangtze Estuary through the Xuliujing section was composed of suspended sediment in the drainage basin and bottom sand scouring from the riverbed in the hydrographic section between Datong and Xuliujing. Comparing 2003–2020 with 1980–2002, the vertical suspended sediment concentration from outside the Yangtze Estuary decreased by 21.4% (Figure 9C), which is consistent with existing research findings (Jing et al., 2013; Yang et al., 2014; Yang et al., 2015b). The peak area of horizontal suspended sediment has been gradually moving to the north and expanding to the deep-water waterway of the North Passage (Figure 9D). This is one of the reasons why the North Passage has required waterway dredging and maintenance in recent years (Dai et al., 2013; Luan et al., 2016; Luan et al., 2018).
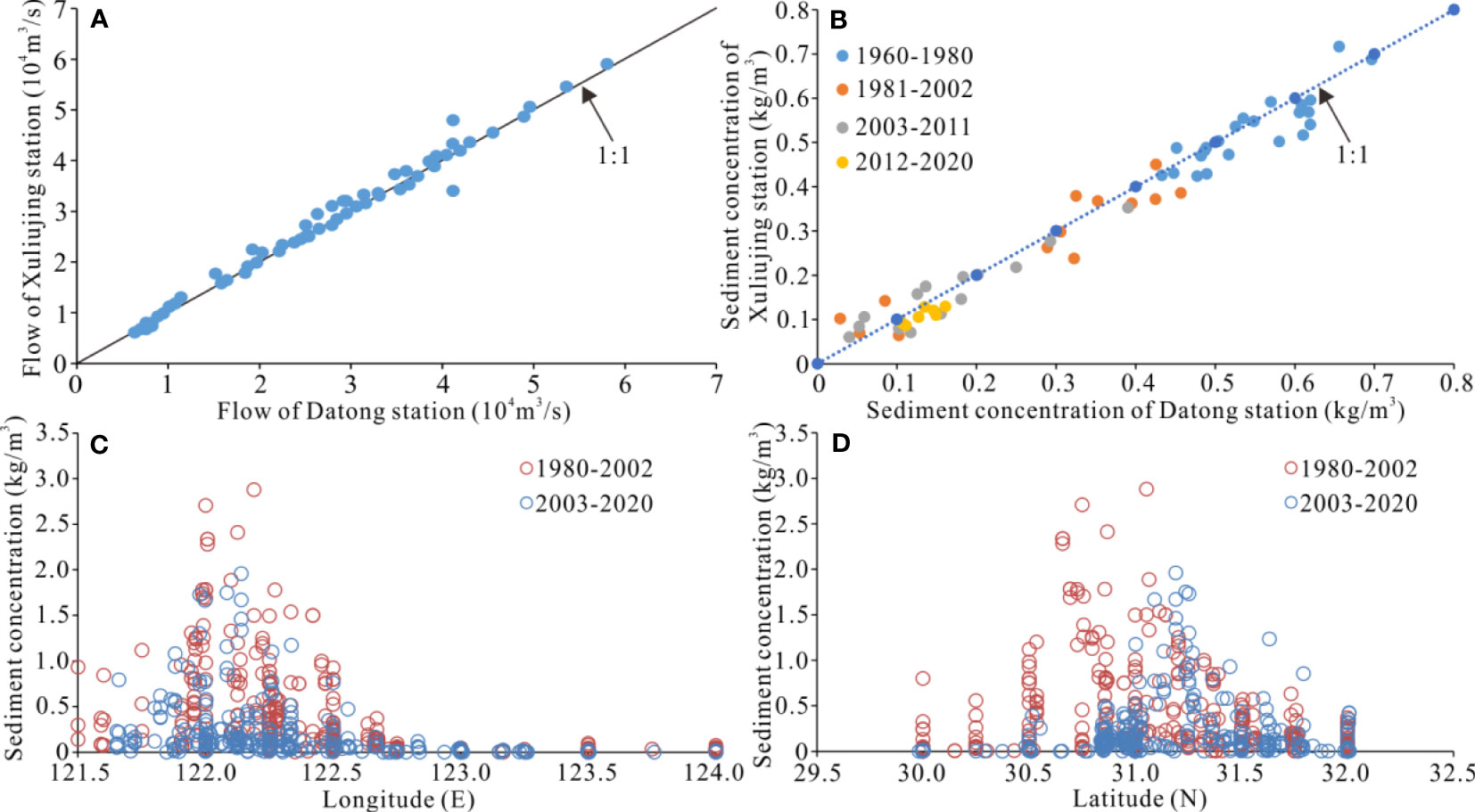
Figure 9 Changes in water and sediment conditions in the Yangtze Estuary; (A) Relationship between runoff at Xuliujing and Datong hydrographic stations of 1998–2020; (B) Relationship between sediment concentration at Xuliujing and Datong hydrographic stations of 1960–2020; (C) Longitudinal variations in the suspended sediment concentration in Yangtze Estuary of 1980–2020; (D) Latitude variation of suspended sediment concentration in Yangtze Estuary of 1980–2020.
4.3.2 Analysis of transport relationship between suspended sediment and riverbed sand in the tidal reach
According to Changjiang Sediment Bulletin (Changjiang Water Resources Commission (CWRC), 2004–2022), few stations test riverbed load sediment in the middle-lower reaches of the Yangtze River, and the amount of riverbed load sediment is much smaller than that of suspended sediment. In the middle–lower reaches of the Yangtze River, only the Yichang–Dabujie reaches have a sandy and cobbly bed with a certain amount of bedload. Below the Dabujie, the riverbed load sediment of the sandy riverbed is small (Yao et al., 2017), but the development of sediment wave is significant (Zheng et al., 2022). In July 2021, suspended sediment and bottom sand were monitored by way of sitting bottom-mounted observation (Figure 10A), in the straight reach of the Yangtze River tidal reach. The sediment concentration above the 1.0 m of the riverbed surface was significantly higher than that below the 1.0 m of the riverbed surface (Figure 10B), with an average ratio of 2.21 (Figure 10C).
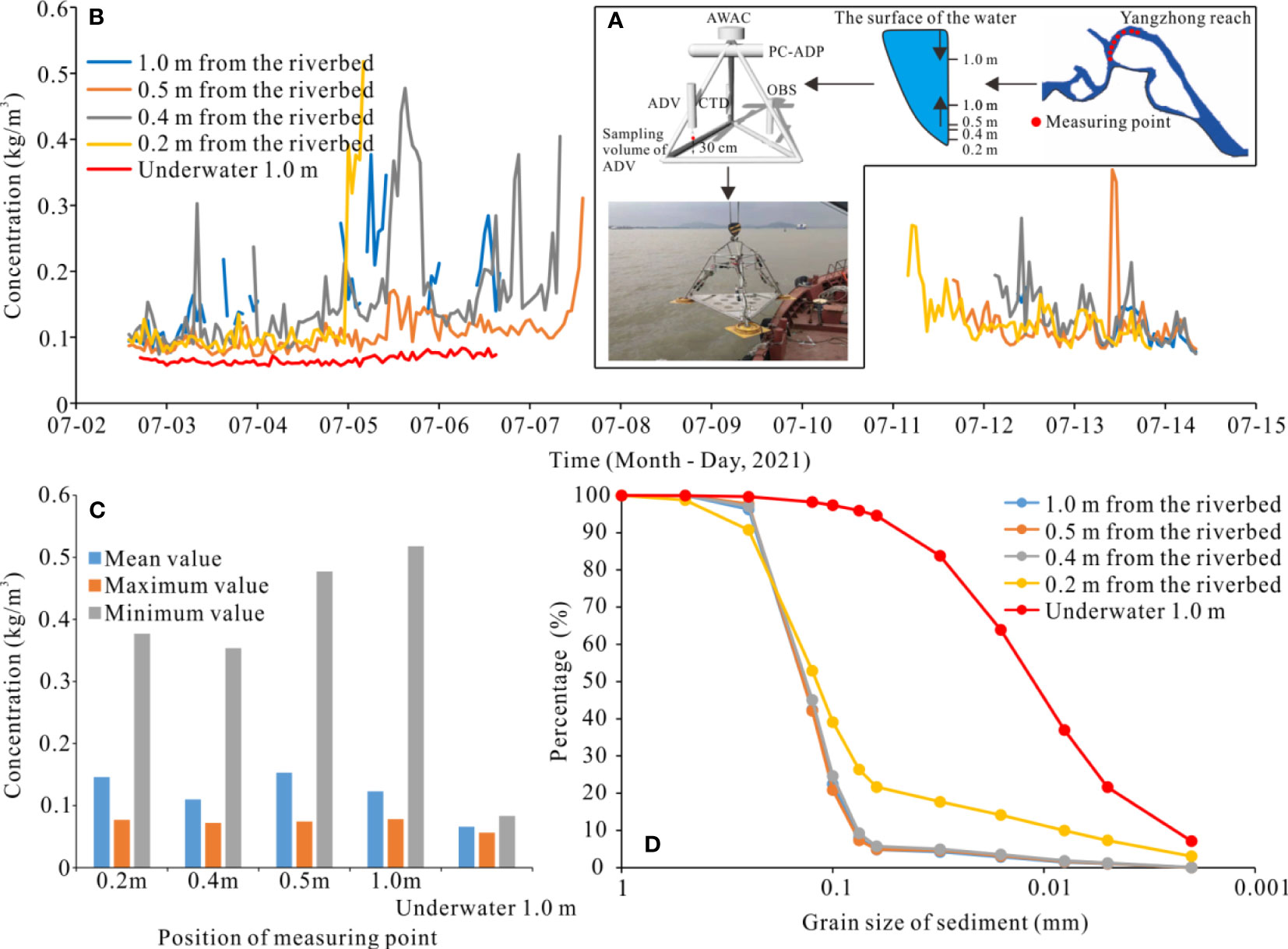
Figure 10 Monitoring results of suspended sediment and bottom sand in the tidal reach; (A) Placement of field monitoring points; (B) Changes in sediment concentrations monitored on site; (C) Comparison of monitoring results; (D) Variation of sediment particle size.
Under the influence of hydrodynamic environments such as runoff, tidal currents, water waves, and salt water in the Yangtze Estuary, the sediment deposited by saltwater action flocculation in the mouth bar area is fine particles. In the other areas, the surface sediment of the riverbed is the same as that in the tidal reach (Yan et al., 2009; Yan et al., 2011; Luo et al., 2012; Chen et al., 2021), and there is a good relationship between sediment particle changes and riverbed scouring or deposition (Chen et al., 2021). Therefore, the sediment from the Yangtze River Basin into the estuarine includes two parts, fine grained sediment in the river water body and bottom sand in the riverbed surface. The composition of deposition sediment in the estuary is different due to the differences in the hydrodynamic environment.
The high sand-bearing water (bottom sand) in the above 1.0 m of the riverbed surface links the areas of the suspended sediment and the areas of riverbed scouring or deposition, with the exchange of bed sediment between the riverbed and the water body. The grain size of the sediment in this area is significantly coarser than the suspended sediment in the water body (Figure 10D). After the operation of the TGR, the suspended sediment particles in the estuary were refined (Yang et al., 2018; Yang et al., 2022b), and the sediment in the riverbed was slightly coarse (Luo et al., 2012). The grain size characteristics of sediments in different areas of the Yangtze Estuary are significantly different. The surface sediment particles in the mouth groin dam area are fine, and the surface sediment particles in the south branch and South channel are the same as those in the tidal reach (Zhang and Zhao, 2016).
4.3.3 Calculation of sediment source–sink relationships of the Datong–Xuliujing reaches
After the operation of the TGR, the riverbeds between Datong–Jiangyin and Jiangyin–Xuliujing reaches all experienced cumulative scouring. Since 2012, this scouring intensity has increased (Figure 11A). The cumulative deposition of the Datong–Xuliujing reaches from 1981–2002 was 1.60×108 m3, respectively (Figure 10B). The cumulative scouring of the Datong–Xuliujing reaches from 2002–2018 was 16.01×108 m3, respectively (Figure 11B). The total amount of suspended and bottom sand that entered the Datong section were 3.56×108 t/y, 2.41×108 t/y, and 3.36×108 t/y, respectively, which first decreased and then increased. In the periods 1981–2002, 2003–2011, and 2012–2018, the total sediment passing through the Xuliujing section in the upper reaches of the river basin decreased. Based on topographic difference calculation, the amount of riverbed scouring increased (switching from deposition to scouring). The amount of scouring after sand mining and waterway dredging also increased (switching from deposition to scouring) (Figure 11C). Suspended sediment passing through the Xuliujing section in the periods from 1981–2002, 2003–2011, and 2012–2018 were 3.73×108 t/y, 1.43×108 t/y, and 1.22×108 t/y, respectively, showing decreasing trends; the amount of bottom sand entering the Yangtze Estuary through the Xuliujing section were –0.61×108 t/y (suspended sediments deposition in the riverbed and no bottom sand migrated downstream), 0.89×108 t/y, and 1.51×108 t/y (Figure 11D). From 1981–2002, 2003–2011, and 2012–2018, the riverbed scouring or deposition of the Datong–Xuliujing reaches were –0.11 × 108 t/y, 1.11 × 108 t/y and 1.98 × 108 t/y, the amount of sand mining was 0.50 × 108 t/y, 0.22 × 108 t/y, and 0.37 × 108 t/y, and the waterway dredging amount was 0, 20 ×104 t/y and 0.11×108 t/y, respectively. The total amount of suspended and bottom sand entering the Yangtze Estuary was 3.12×108t/y, 2.32×108t/y, and 2.73×108t/y, respectively, which first decreased and then increased. This study believes that the total sediment passing through the Datong–Xuliujing reaches from 2003–2020 did not show a continuous decreasing trend due to the reduction in suspended sediment load, while the supply from bottom sand volume exhibited an increasing trend and the “sink” of sediment scouring or deposition turned into the “source” of sediment replenishing.
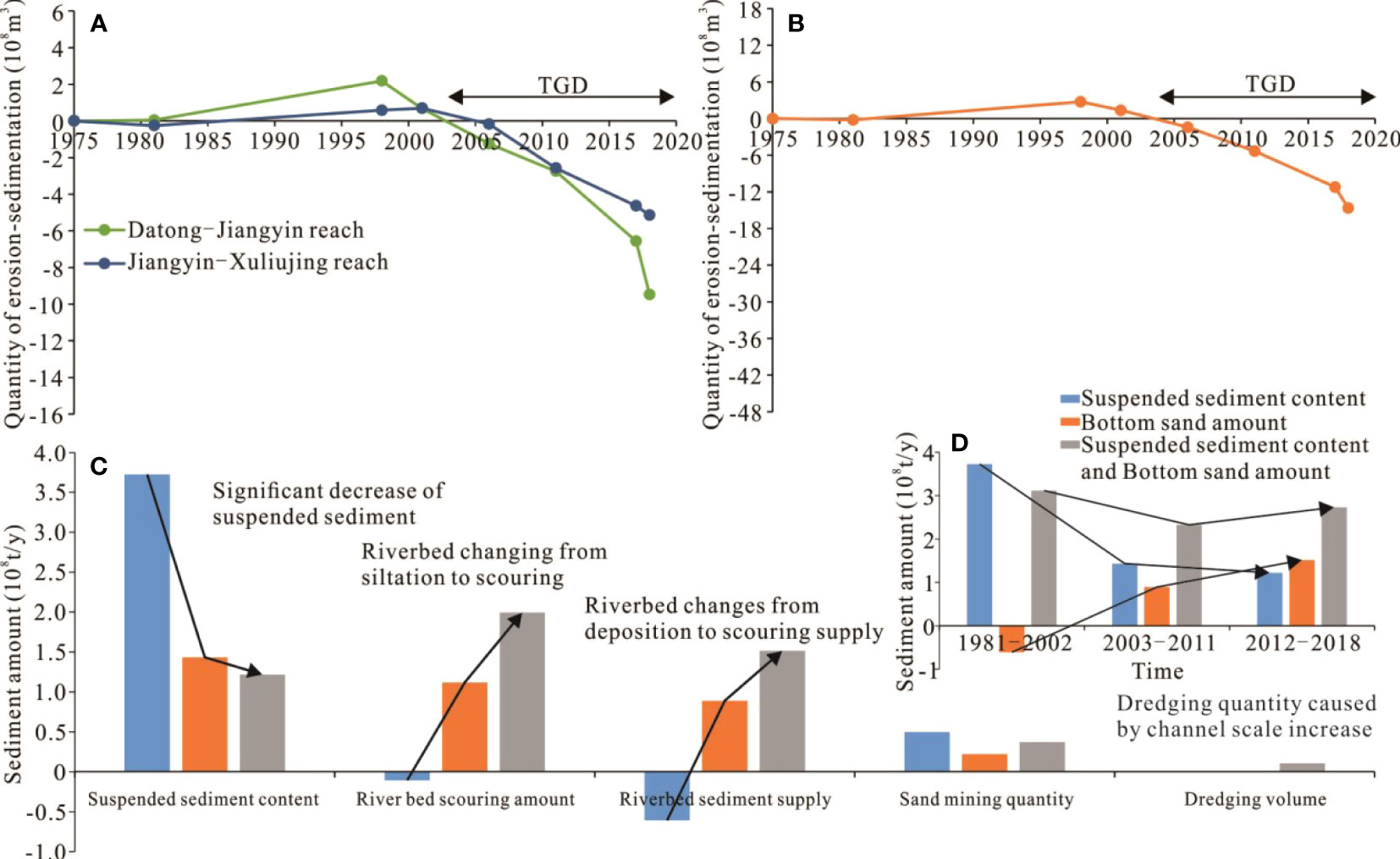
Figure 11 Changes in riverbed scouring and deposition along the Datong–Xuliujing reaches of the Yangtze River; (A) Cumulative scouring or deposition of riverbed sections based on rivers channel topography data; (B) Total amount of riverbed scouring or deposition; (C) Sediment source-sink relationship in the Datong–Xuliujing reaches.
4.4 Contribution of watershed sediment to the Yangtze Estuary
4.4.1 Distribution of riverbed scouring or deposition and variation in the shoal rear of the Yangtze Estuary
The north branch of the Yangtze Estuary was dominated by deposition between 2002–2018, and the south branch, north channel, and south channel mainly scouring. The high shoal area of the front tidal flat mainly experienced deposition, and the front of the delta mainly experienced scouring (Figures 12A, B). In the Yangtze River Estuary, the continent body is at the condition of sediment deposition, which is caused by the “sink” of the sediment after entering the Yangtze Estuary. The waterway area is scouring due to the regulation of buildings, and the deltas area scouring (Figure 12C). Simultaneously, all the areas of the front tidal flat increased at first and then decreased. During 2002–2011, the areas at the water depths of 0 m, 2 m, and 5 m were 22.12%, 12.67%, and 9.35% respectively, while during 2011–2018 it became –7.13%, –17.58%, and –14.67% respectively (Figure 12D). During 2002–2018, the changes of the areas at the water depths of 0 m were –21.09%, +47.63%, –0.57%, +35.91%, and –7.23% for Yiyuansha shoal, Chongming shoal, Hengsha shoal, Jiuduansha shoal, and Nanhui shoal respectively, with an increased total area of 13.41% (Figure 12D).
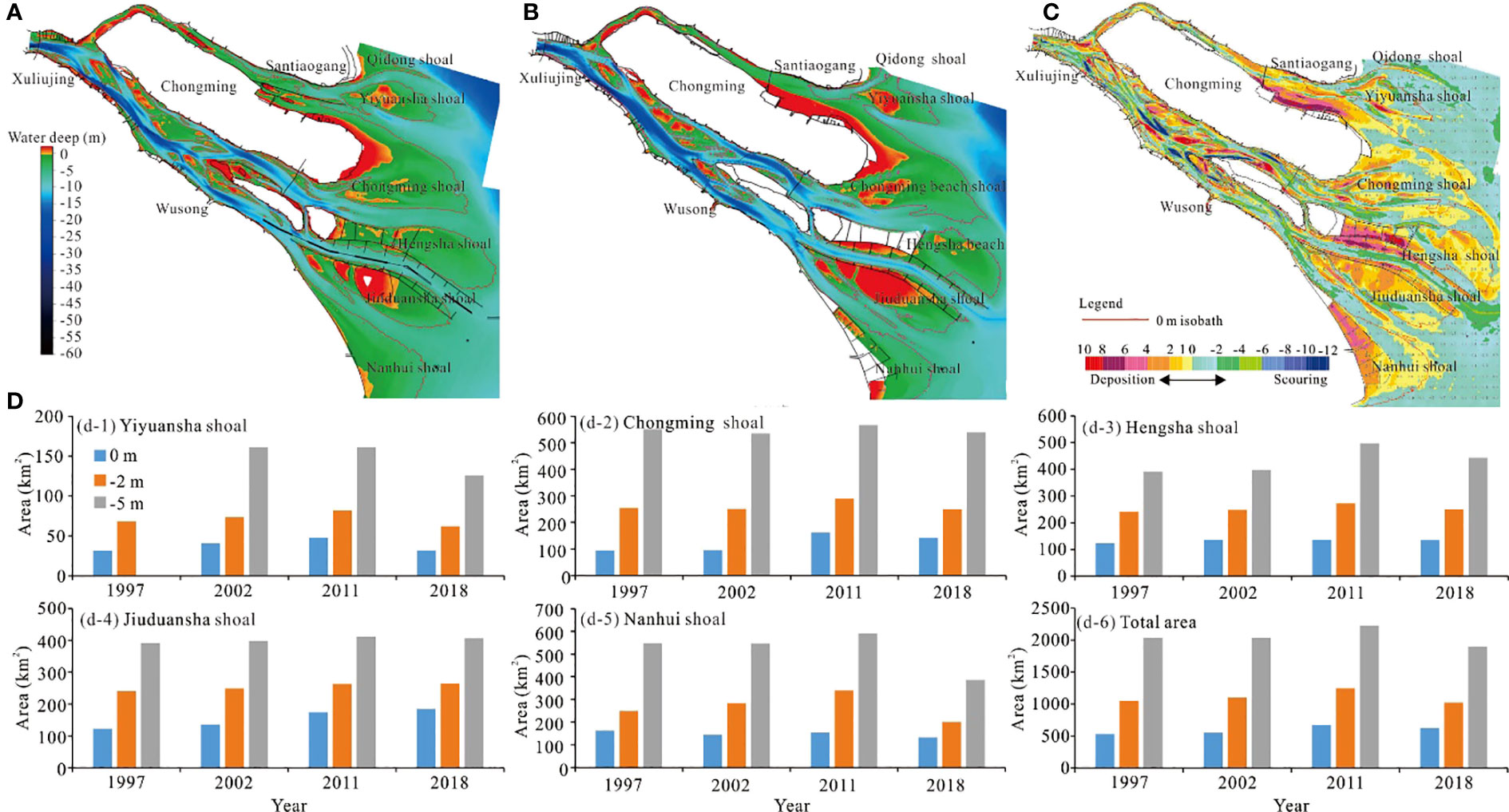
Figure 12 Distribution characteristics of riverbed scouring or deposition in the Yangtze Estuary; (A) Terrain in 2002, data from Yu and Zhang (2021); (B) Terrain in 2018, data from Yu and Zhang (2021); (C) Distribution of riverbed scouring or deposition; (D) Shoal area.
4.4.2 Calculation of sediment source–sink relationships in the Yangtze Estuary
From 1982–2018, both the north and south branches experienced cumulative scouring, but the total amount of riverbed sand in the Yangtze Estuary was relatively small, which means that without considering the impact of riverbed scouring or deposition in engineering areas such as land reclamation, waterway dredging, the Yangtze Estuary was in a quasi-balanced state between scouring or deposition (Figure 13A). The cumulative waterway dredging volume of the North Passage of the Yangtze Estuary between 1982 and 2018 was 11.07×108 m3 (ship volume/topographic volume=1.30, sediment wet bulk density/dry bulk density=1.65, converted to a weight of 14.05×108 t) (Figure 13B). During this period, the land reclamation area of the Yangtze Estuary was 820.57 km2, and the amount of deposition caused by the reclamation was 12.31×108 m3 (sediment wet bulk density/dry bulk density=1.65, and the average thickness of waterway dredging filling was calculated as 1.5 m (Figure 13B), and the converted sediment weight was 20.31×108 t) (Figures 13C, D). The total amount of suspended and bottom sand entering the estuary area through the Xuliujing section in the 1982–2002, 2003–2011, and 2012–2018 periods was 3.12×108 t/y, 2.32×108 t/y, and 2.73×108 t/y, a decrease of 16.9% in 2003–2018 compared with 1984–2002. In the above periods, after deducting the amount of riverbed scouring or deposition, land reclamation, and waterway dredging, through the Yangtze Estuary, the amount of dispersed sediment was 2.82×108 t/y, 1.81×108 t/y, and 1.28×108 t/y, respectively.
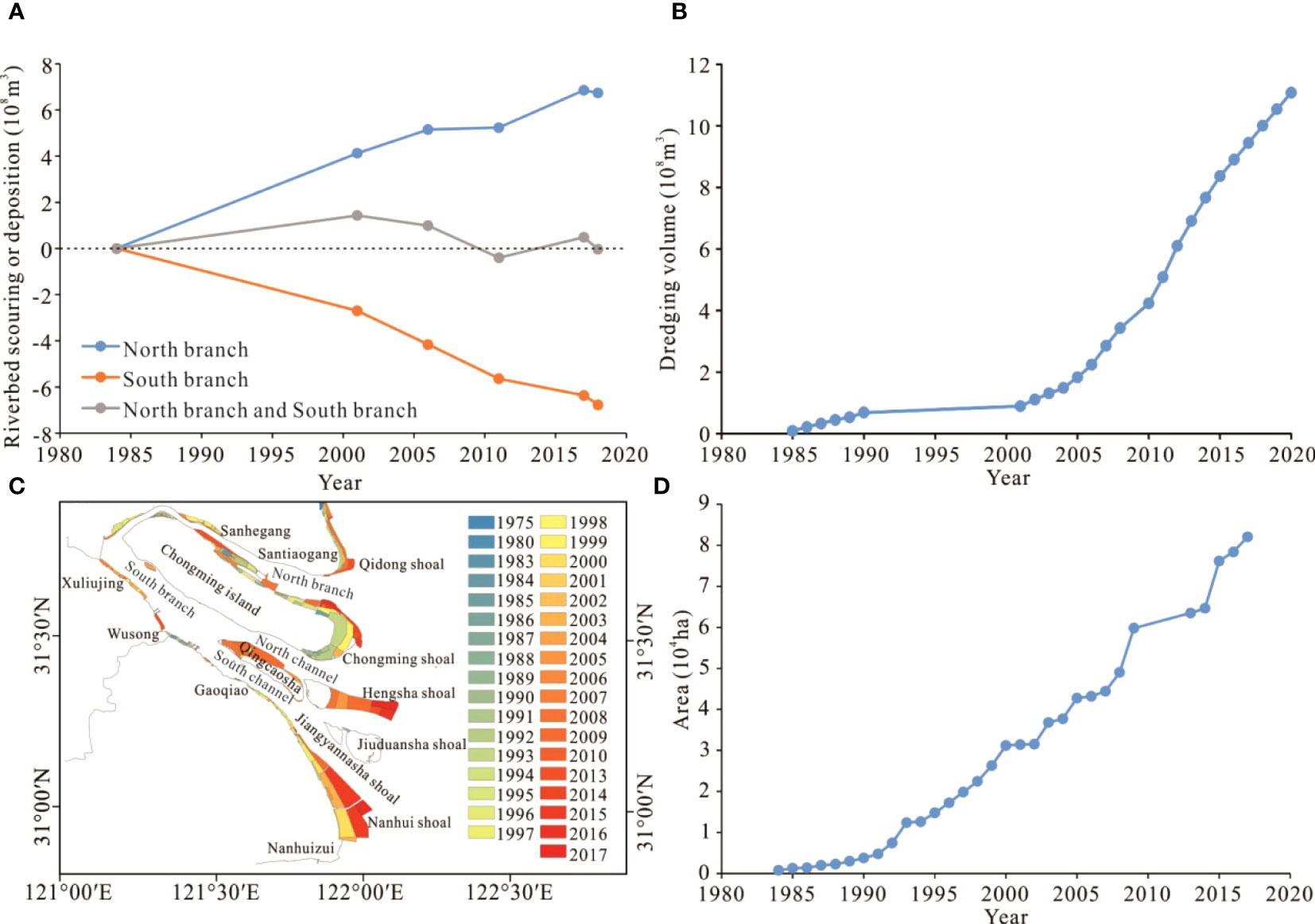
Figure 13 Sediment supply relationship in the Yangtze Estuary; (A) Cumulative amount of riverbed scouring or deposition; (B) Waterway dredging volume; (C) Reclamation area, data from Qiu et al. (2019); (D) Shoals area.
4.5 Sediment supplies from the riverbed as a new source to sustain the Yangtze Estuary Deltas
Calculated by the measured topographic data of 1958–2015 (The years are 1958, 1978, 1997, 2002, 2007, 2010, 2012, and 2015), the critical suspended sediment volume for the balance of scouring or deposition of the front tidal shoals and deltas at the Yangtze Estuary was calculated as 2.18×108 t/y (Luan et al., 2021). A large amount of waterway dredging has been carried out in the construction of the north channel of the Yangtze Estuary deep-water waterway, and the waterway regulation structures have interfered with the local geomorphic evolution characteristics, after deducting the impact of the estuary project from 1997–2010, the corresponding critical suspended sediment volume was 2.34×108 t/y (Luan et al., 2021). However, the amount of sediment at Datong hydrographic station from 2003–2011 was significantly lower than before the TGR began to operate, with an average value of 1.29×108 t/y, which was significantly lower than the critical amount of sediment for scouring or deposition balance. Many studies during this period believed that the deltas were dominated by scouring (Wei et al., 2015; Luan et al., 2016; Zhu et al., 2016; Yang et al., 2017; Dai et al., 2018; Luan et al., 2021). Simultaneously, the construction of dams on the upper reaches of the Yangtze River and lakes may cause the interruption of sediment supply to the lower reaches. It is possible that in the near future, the Yangtze Estuary landform system will experience large-scale scouring (Dai et al., 2018). Regarding the amount of sediment in the Yangtze Estuary, when only considering the balance of suspended sediment exchange, the amount of sediment at Datong hydrographic station in the 1980s and 2000s was calculated to be 4.51×108 t/y and 1.51×108 t/y, respectively, and the amount of suspended sediment entering the estuary through Xuliujing section were 4.06×108 t/y and 1.72×108 t/y respectively, and the amount entering the deltas area was 3.70×108 t/y and 1.75×108 t/y (Yang et al., 2015a; Xie et al., 2017a). This significantly reduced the suspended sediment concentration in the estuary, which will cause the delta area to switch to scouring. Taking human activities into account like sand mining and waterway dredging, through the comprehensive calculation of suspended sediment transport and riverbed scouring or deposition, the total amount of suspended and bottom sand in the Yangtze River Basin that entered the estuary through the Xuliujing section during the 2003–2011 and 2012–2018 periods were calculated as 2.32×108 t/y and 2.73×108 t/y, respectively. The riverbed scouring intensity of the reach from the Yichang–Xuliujing reaches was increasing, which was beneficial to safeguarding the sediment resources of the Yangtze Estuary. Recent studies have also proven that Jiuduansha shoal deposition is, significantly, in its natural state (Wei et al., 2016; Wei et al., 2017; Zhao et al., 2018; Zhu et al., 2019; Zhang et al., 2021). Meanwhile, in the deposition center of the Yangtze Estuary Delta, the deposition rate remained at 10 cm/y from 1958–2009 (Dai et al., 2014): between 1997–2015, only the net scouring depth from 2011–2013 was 10.76 cm/y, and the net scouring depth was 0.91–3.48 cm/y in other years (Sun, 2018). In 2021, the amount of river sediment outside of the Xinliuhesha and Qingcaosha Reservoirs did not decrease but instead started to increase, representing the first natural increase in recent years (https://view.inews.qq.com/a/20211114V02W9E00). In addition, the decrease in the amount of sediment entering the sea from the Yangtze River has no significant impact on the landform evolution of Hangzhou Bay, mainly due to the buffering effect of existing sediment outside the Yangtze Estuary (Xie et al., 2017b).
Human activities have put tremendous pressure on global rivers (Best, 2019). The overall water connectivity of the Yangtze River Basin is still maintained. However, several studies have proposed that blocking sediment through dams and relative sea level rise will result in the submersion of the estuary delta and front tidal flats. In nearly 1,000 deltas around the world, dam construction has led to a severe reduction of anthropogenic sediment flux (by more than 50%), forcing an annual loss of delta land of about 12 ± 3.5 km2. Not all estuary deltas lose land resources due to dams (Nienhuis et al., 2020). For example, the supply of sand from the Mississippi River is stable and sustainable (Edmonds and Slingerland, 2010; Nittrouer and Viparelli, 2014), and these sediments can form deltas through engineering measures capturing sediment from the Mississippi River. The thickness of the sand layer from the Dabujie section to the estuary of the Yangtze River is more than 100 m (Cao et al., 2012; Mei et al., 2021). The ancient river channel has a wide range of lateral swing space, and a large amount of fine-grained sediment remains (Song et al., 2013). In the future, regardless of whether the amount of suspended sediment in the Yangtze River Basin decreases, the riverbed scouring of the Yichang–Xuliujing reaches will have abundant bottom sand to replenish, and this amount will show an increasing trend due to the continuous decrease in the amount of sediment in the river basin, which can guarantee the amount of sediment entering the Yangtze Estuary from the river basin. Comprehensive analysis suggests that the Yichang–Datong and Datong–Xuliujing reaches have gradually transformed from the condition of sediment deposition to the condition of sediment scouring, which provide an important sediment source for the deposition of estuary shoals, and stability of deltas and land resources.
Although the supply of suspended sediments from the Yangtze Estuary has been significantly reduced, the amount of bottom sand in the riverbed is still abundant, with its replenishment capacity and supply increasing. A comprehensive analysis has shown that even if the amount of suspended sediment entering the estuary after the TGR went into operation are significantly reduced, the riverbed will gradually transform from a “sink” of deposition to a “source” of sediment supply, which will still provide a rich scouring of sediments for the Yangtze Estuary. Therefore, the Yangtze Estuary Delta, front tidal flats, and urban land resources are likely to be sustained.
5 Conclusions
Since the TGR began operating, the “source-sink” relationship of sediments from the middle-lower reaches of the Yangtze River has been significantly adjusted, which has directly affected the sustainability of the estuary. Based on the analysis of in-situ observed runoff, sediment load, and riverbed topography from 1981 to 2018, the present study examined the influence of the adjustment of the Yangtze River sediment “source-sink” relationship of the Yangtze River Basin on the estuary delta.
The TGR stored 76% of the sediment in the upper reaches of the Yangtze River and caused a decline of 68–97% in sediment load in the middle-lower reaches. These sharp declines in sediment supplies resulted in a decrease of 21.4% in the suspended sediment concentration in the estuary delta. From 2003–2020, cumulative river channel scouring occurred in the Yichang–Xuliujing reaches of the Yangtze River, and the riverbed transformed from being the “sink” of sediment to the “source” of sediment transport into the sea. The river basin to the estuary also experienced an adjustment in the sediment “source-sink” relationship, which released more sediment “sources” for those entering the sea. From 2012 to 2018, the amount of sediment at the Xuliujing section of the upper reaches of the river mouth exhibited an increasing trend. In the periods of 1980–2002, 2003–2011, and 2012–2018, the total amount of suspended and bottom sand entering the delta in the Yangtze River Basin first decreased and then increased. During 2012–2018, the total amount of suspended sediment and riverbed sediment entering the estuary of the Yangtze River Basin was equivalent to the critical sediment amount of the delta from deposition to flushing, but the total sediment entering the estuary of the Yangtze River Basin did not decrease significantly.
Data availability statement
The original contributions presented in the study are included in the article/supplementary material. Further inquiries can be directed to the corresponding authors.
Author contributions
YY and MZ conceived the full text, and wrote and completed the drawing of the graph. JZ provided the revision and discussion of the whole paper. LZ was responsible for the processing of the Yangtze Estuary topography and maintenance data. All authors contributed to the article and approved the submitted version.
Funding
This study was funded by the National Natural Science Foundation (52279066; 51979132), Fundamental Research Funds for Central Welfare Research Institutes (TKS20230105), Jiangsu Water Conservancy Science and Technology Project (2020001) and the Hydrographic Bureau of Yangtze River, and Yangtze River waterway bureau.
Conflict of interest
The authors declare that the research was conducted in the absence of any commercial or financial relationships that could be construed as a potential conflict of interest.
Publisher’s note
All claims expressed in this article are solely those of the authors and do not necessarily represent those of their affiliated organizations, or those of the publisher, the editors and the reviewers. Any product that may be evaluated in this article, or claim that may be made by its manufacturer, is not guaranteed or endorsed by the publisher.
References
Almeida R. M., Shi Q. R., Gomes-Selman J. M., Wu X. J., Xue Y. X., Angarita H., et al. (2019). Reducing greenhouse gas emissions of Amazon hydropower with strategic dam planning. Nat. Commun. 10, 4281. doi: 10.1038/s41467-019-12179-5
Anthony E., Brunier G., Besset M., Goichot M., Dussouillez P., Nguyen V. L. (2015). Linking rapid erosion of the Mekong river delta to human activities. Sci. Rep. 5, 14745. doi: 10.1038/srep14745
Bendixen M., Best J. L., Hackney C., Iversen L. L. (2019). Time is running out for sand. Nature 571 (7763), 29–31. doi: 10.1038/d41586-019-02042-4
Bertassoli D. J. J., Sawakuchi H. O., Araújo K. R. D., Camargo M. G. P. D., Alem V. A. T., Pereira T. S., et al. (2021). How green can Amazon hydropower be? net carbon emission from the largest hydropower plant in Amazonia. Sci. Adv. 7 (26), eabe1470. doi: 10.1126/SCIADV.ABE1470
Best J. (2019). Anthropogenic stresses on the world's big rivers. Nat. Geosci. 12 (1), 7–21. doi: 10.1038/s41561-018-0262-x
Brunier G., Anthony E. J., Goichot M., Provansal M., Dussouillez P. (2014). Recent morphological changes in the Mekong and bassac river channels, Mekong delta: the marked impact of river-bed mining and implications for delta destabilization. Geomorphology 224 (1), 177–191. doi: 10.1016/j.geomorph.2014.07.009
Cao G. J., Cuan J. B., Li Y. Y. (2012). Characteristics of the Yangtze river paleo-valley in jiangsu province in the last glacial maximum. Scientia Geographica Sin. 32 (8), 986–992. doi: 10.1007/s11783-011-0280-z
Changjiang Water Resources Commission (CWRC) (2004–2022). Changjiang sediment bulletin. 2003–2021. Available at: http://www.cjw.gov.cn/zwzc/bmgb/nsgb/.
Chen Y. W., Sheng H., Xu Q. H., Qu Y. B., Xing F., Li Z. H., et al. (2021). Analysis of sediment grain size change and its response to erosion and deposition pattern within the Yangtze river estuary for the past 40 years. Hydro-Science Eng. 189 (5), 8–18.
Cheng H. Q., Chen W., Li J. F., Jiang Y. H., Hu X., Zhang X. L., et al. (2022). Morphodynamic changes in the Yangtze estuary under the impact of the three gorges dam, estuarine engineering interventions and climate-induced sea level rise. Earth Planetary Sci. Lett. 580, 117385. doi: 10.1016/j.epsl.2022.117385
Collins M. J., Snyder N. P., Boardman G., Banks W. S. L., Andrews M., Baker M. E., et al. (2017). Channel response to sediment release: insights from a paired analysis of dam removal. Earth Surface Processes Landforms 42 (11), 1636–1651. doi: 10.1002/esp.4108
Dai Z. J., Liu J. T., Fu G., Xie H. L. (2013). A thirteen-year record of bathymetric changes in the north passage, changjiang (Yangtze) estuary. Geomorphology 187, 1010–1107. doi: 10.1016/j.geomorph.2013.01.004
Dai Z. J., Liu J. T., Wei W., Chen J. Y. (2014). Detection of the three gorges dam influence on the changjiang (Yangtze river) submerged delta. Sci. Rep. 4, 6600. doi: 10.1038/srep06600
Dai Z. J., Mei X. F., Darby S. E., Lou Y. Y., Li W. H. (2018). Fluvial sediment transfer in the changjiang (Yangtze) river-estuary depositional system. J. Hydrology 566, 719–734. doi: 10.1016/j.jhydrol.2018.09.019
East A. E., Pess G. R., Bountry J. A., Magirl C. S., Ritchie A. C., Logan J. B., et al. (2015). Large-Scale dam removal on the elwha river, Washington, USA: river channel and floodplain geomorphic change. Geomorphology 228, 765–786. doi: 10.1016/j.geomorph.2014.08.028
Edmonds D. A., Slingerland R. L. (2010). Significant effect of sediment cohesion on delta morphology. Nat. Geosci. 3 (2), 105–109. doi: 10.1038/ngeo730
Gao J. H., Jia J. J., Kettner A. J., Xing F., Wang Y. P., Li J., et al. (2018). Reservoir-induced changes to fluvial fluxes and their downstream impacts on sedimentary processes: the changjiang (Yangtze) river, China. Quaternary Int. 493, 187–197. doi: 10.1016/j.quaint.2015.03.015
Gao J. H., Shi Y., Shen H., Kettner A. J., Yang Y., Jia J., et al. (2019). Rapid response of the changjiang (Yangtze) river and East China Sea source-to-sink conveying system to human induced catchment perturbations. Mar. Geology 414, 1–17. doi: 10.1016/j.margeo.2019.05.003
Grill G., Lehner B., Thieme M., Geenen B., Tickner D., Antonelli F., et al. (2019). Mapping the world’s free-flowing rivers. Nature 569 (7755), 215–221. doi: 10.1038/s41586-019-1111-9
Guo X. J., Fan D. D., Zheng S. W., Wang H. M., Zhao B. C., Qin C. J. (2021a). Revisited sediment budget with latest bathymetric data in the highly altered Yangtze (Changjiang) estuary. Geomorphology 391, 107873. doi: 10.1016/j.geomorph.2021.107873
Guo L. C., Su N., Townend I., Wang Z. B., Zhu C. Y., Wang X. Y., et al. (2019). From the headwater to the delta: a synthesis of the basin-scale sediment load regime in the changjiang river. Earth-Science Rev. 197, 102900. doi: 10.1016/j.earscirev.2019.102900
Guo L. C., Xie W. M., Xu F., Wang X. Y., Zhu C. Y., Meng Y., et al. (2021b). A historical review of sediment export–import shift in the north branch of changjiang estuary. Earth Surface Processes Landforms 47 (1), 5–16. doi: 10.1002/esp.5084
Jin M., Yu Z. Y., He Q. (2006). On the relationship between maintenance conditions of Yangtze estuary deep-water channel and water & sediment transport from the valley. Port Waterway Eng. 386 (3), 46–51. doi: 10.3969/j.issn.1002-4972.2009.01.014
Jing X. Z., Lu B., He Y. H. (2013). Response of the turbidity maximum zone to fluctuations in sediment discharge from river to estuary in the changjiang estuary (China). Estuarine Coast. Shelf Sci. 131, 24–30. doi: 10.1016/j.ecss.2013.07.003
Latrubesse E. M., Arima E. Y., Dunne T., Park E., Baker V. R., d’Horta F. M., et al. (2017). Damming the rivers of the Amazon basin. Nature 546 (7658), 363–369. doi: 10.1038/nature22333
Li D. F., Lu X. X., Chen L., Wasson R. J. (2019). Downstream geomorphic impact of the three gorges dam: with special reference to the channel bars in the middle Yangtze river. Earth Surface Processes Landforms 44 (13), 2660–2670. doi: 10.1002/esp.4691
Li D. F., Lu X. X., Yang X. K., Chen L., Lin L. (2018). Sediment load responses to climate variation and cascade reservoirs in the Yangtze river: a case study of the jinsha river. Geomorphology 322, 41–52. doi: 10.1016/j.geomorph.2018.08.038
Li P., Yang S. L., Milliman J. D., Xu K. H., Qin W. H., Wu C. S., et al. (2012). Spatial, temporal, and human-induced variations in suspended sediment concentration in the surface waters of the Yangtze estuary and adjacent coastal areas. Estuaries Coasts 35 (5), 1316–1327. doi: 10.1007/s12237-012-9523-x
Liu X. B., Chen J., Yue W., Wang Q., Zhan C., Zeng L., et al. (2023). Tracing the source-sink process of fluvio-clastic materials: magnetic records of surface sediments in the Yangtze river basin. Front. Mar. Sci. 9, 1002335. doi: 10.3389/fmars.2022.1002335
Liu G. F., Zhu J. R., Wang Y. Y., Wu H., Wu J. X. (2011). Tripod measured residual currents and sediment flux: impacts on the silting of the deepwater navigation channel in the changjiang estuary. Estuar. Coast. Shelf Sci. 93 (3), 192–201. doi: 10.1016/j.ecss.2010.08.008
Luan H. L., Ding P. X., Wang Z. B., Ge J. Z., Yang S. L. (2016). Decadal morphological evolution of the Yangtze estuary in response to river input changes and estuarine engineering projects. Geomorphology 265, 12–23. doi: 10.1016/j.geomorph.2016.04.022
Luan H. L., Ding P. X., Wang Z. B., Yang S. L., Lu J. Y. (2018). Morphodynamic impacts of large-scale engineering projects in the Yangtze river delta. Coast. Eng. 141, 1–11. doi: 10.1016/j.coastaleng.2018.08.013
Luan H. L., Ding P. X., Yang S. L., Wang Z. B. (2021). Accretion-erosion conversion in the subaqueous Yangtze delta in response to fluvial sediment decline. Geomorphology 382, 107680. doi: 10.1016/j.geomorph.2021.107680
Luo X. X., Yang S. L., Zhang J. (2012). The impact of the three gorges dam on the downstream distribution and texture of sediments along the middle and lower Yangtze river (Changjiang) and its estuary, and subsequent sediment dispersal in the East China Sea. Geomorphology 179, 126–140. doi: 10.1016/j.geomorph.2012.05.034
Manh N. V., Dung N. V., Hung N. N., Merz B., Apel H. (2014). Large-Scale suspended sediment transport and sediment deposition in the Mekong delta. Hydrology Earth System Sci. 18 (8), 3033–3053. doi: 10.5194/hess-18-3033-2014
Mei X. F., Dai Z. J., Darby S. E., Zhang M., Cai H. Y., Wang J., et al. (2021). Landward shifts of the maximum accretion zone in the tidal reach of the changjiang estuary following construction of the three gorges dam. J. Hydrology 592, 125789. doi: 10.1016/j.jhydrol.2020.125789
Mossa J., Chen Y. H. (2021). Geomorphic insights from eroding dredge spoil mounds impacting channel morphology. Geomorphology 376, 107571. doi: 10.1016/j.geomorph.2020.107571
Nienhuis J. H., Ashton A. D., Edmonds D. A., Hoitink A. J. F., Kettner A. J., Rowland J. C., et al. (2020). Global-scale human impact on delta morphology has led to net land area gain. Nature 577 (7791), 514–518. doi: 10.1038/s41586-019-1905-9
Nittrouer J. A., Viparelli E. (2014). Sand as a stable and sustainable resource for nourishing the Mississippi river delta. Nat. Geosci. 7 (5), 350–354. doi: 10.1038/ngeo2142
Pan L. Z., Ding P. X., Ge J. Z. (2012). Impacts of deep waterway project on morphological changes within the north passage of the changjiang estuary, China. J. Coast. Res. 284 (5), 1165–1176. doi: 10.2112/JCOASTRES-D-11-00129.1
Paszkowski A., Goodbred S., Borgomeo E., Khan M., Hall J. W. (2021). Geomorphic change in the Ganges–Brahmaputra–Meghna delta. Nat. Rev. Earth Environ. 2 (11), 763–780. doi: 10.1038/s43017-021-00213-4
Qiu C. Y., Li X., Liu S. A., Chen D. (2019). Monitoring tidal flats in the Yangtze river delta using landsat images. J. Geo-information Sci. 21 (2), 269–278. doi: 10.12082/dqxxkx.2019.180344
Schmitt R. J. P., Giuliani M., Bizzi S., Kondolf G. M., Daily G. C., Castelletti A. (2021). Strategic basin and delta planning increases the resilience of the Mekong delta under future uncertainty. Proc. Natl. Acad. Sci. 118 (36), e2026127118. doi: 10.1073/pnas.2026127118
Song B., Li Z., Saito Y., Okuno J., Li Z., Lu A. Q., et al. (2013). Initiation of the changjiang (Yangtze) delta and its response to the mid-Holocene sea level change. Palaeogeography Palaeoclimatology Palaeoecol. 388, 81–97. doi: 10.1016/j.palaeo.2013.07.026
Sun G. (2018). Accretion-erosion evolution and its driving mechanisms of Yangtze underwater delta over the past nearly 20 years (Chengdu: Chengdu University of Technology).
Tang M., Cheng H. Q., Xu Y., Hu H., Zheng S. W., Wang B., et al. (2022). Channel bed adjustment of the lowermost Yangtze river estuary from 1983 to 2018: causes and implications. Water 14 (24), 4135. doi: 10.3390/w14244135
Wang Y. H., Dong P., Oguchi T., Chen S. L., Shen H. T. (2013). Long-term, (1842–2006) morphological change and equilibrium state of the changjiang (Yangtze) estuary, China. Continental Shelf Res. 56, 71–81. doi: 10.1016/j.csr.2013.02.006
Wang S., Fu B. J., Piao S. L., Lü Y. H., Ciais P., Feng X. M., et al. (2016). Reduced sediment transport in the yellow river due to anthropogenic changes. Nat. Geosci. 9 (1), 38–41. doi: 10.1038/ngeo2602
Wang J. J., Yang Y. P., Shen X., Ying H. H., Qiao H. Q. (2020). Study on the deformation of the Point/Channel bar of the variable section of the tidal current limit of Yangtze river and its influence on the scouring and silting of dredged channel. J. Basic Sci. Eng. 28 (4), 751–762. doi: 10.16058/j.issn.1005-0930.2020.04.001
Wang C. L., Zhao Y. F., Zou X. Q., Xu X. W. H., Ge C. D. (2017). Recent changing patterns of the changjiang (Yangtze river) estuary caused by human activities. Acta Oceanologica Sin. 36 (4), 87–96. doi: 10.1007/s13131-017-1017-z
Warrick J. A., Bountry J. A., East A. E., Magirl C. S., Randle T. J., Gelfenbaum G., et al. (2015). Large-Scale dam removal on the elwha river, Washington, USA: source-to-sink sediment budget and synthesis. Geomorphology 246, 729–750. doi: 10.1016/j.geomorph.2015.01.010
Wei W., Dai Z. J., Mei X. F., Liu J. P., Gao S., Li S. S. (2017). Shoal morphodynamics of the changjiang (Yangtze) estuary: influences from river damming, estuarine hydraulic engineering and reclamation projects. Mar. Geology 386, 32–43. doi: 10.1016/j.margeo.2017.02.013
Wei W., Mei X. F., Dai Z. J., Tang Z. H. (2016). Recent morphodynamic evolution of the largest uninhibited island in the Yangtze (Changjiang) estuary during 1998–2014: influence of the anthropogenic interference. Continental Shelf Res. 124, 83–94. doi: 10.1016/j.csr.2016.05.011
Wei W., Tang Z. H., Dai Z. J., Lin Y. F., Ge Z. P., Gao J. (2015). Variations in tidal flats of the changjiang (Yangtze) estuary during 1950s–2010s: future crisis and policy implication. Ocean Coast. Manage. 108, 89–96. doi: 10.1016/j.ocecoaman.2014.05.018
Xia J. Q., Deng S. S., Lu J. Y., Xu Q. X., Zong Q. L., Tan G. M. (2016). Dynamic channel adjustments in the jingjiang reach of the middle Yangtze river. Sci. Rep. 6, 22802. doi: 10.1038/srep22802
Xie D. F., Pan C. H., Wu X. G., Gao S., Wang Z. B. (2017a). The variations of sediment transport patterns in the outer changjiang estuary and hangzhou bay over the last 30 years. J. Geophysical Research: Oceans 122 (4), 2999–3020. doi: 10.1002/2016JC012264
Xie D. F., Pan C., Wu X. G., Gao S., Wang Z. B. (2017b). Local human activities overwhelm decreased sediment supply from the changjiang river: continued rapid accumulation in the hangzhou bay qiantang estuary system. Mar. Geology 392, 66–77. doi: 10.1016/j.margeo.2017.08.013
Xie Q. C., Yang J., Lundström T. S. (2021). Sediment and morphological changes along Yangtze river’s 500 km between datong and xuliujing before and after three gorges dam commissioning. Sci. Rep. 11, 13662. doi: 10.1038/s41598-021-93004-2
Xu W., Cheng H. Q., Zheng S. W., Jiang Y. H., Zhou Q. P., Yang G. Q., et al. (2022). Riverbed deformation and its response to human intervention on the lower reaches of the Yangtze river. River Res. Appl. 38 (2), 222–234. doi: 10.1002/rra.3899
Xu Q. X., Li S. X., Yuan J., Yang C. G. (2021). Analysis of equilibrium sediment transport in the middle and lower reaches of the Yangtze river after the impoundment of the three gorges reservoir. J. Lake Sci. 33 (3), 806–818. doi: 10.18307/2021.0316
Yan H., Dai Z. J., Li J. F., Zhao J. C., Zhang X. L. (2009). Distribution of surficial tidal flat sediments in the Yangtze estuary. Acta Geographica Sin. 64 (5), 629–637. doi: 10.3321/j.issn:0375-5444.2009.05.012
Yan H., Dai Z. J., Li J. F., Zhao J. C., Zhang X. L., Zhao J. K. (2011). Distributions of sediments of the tidal flats in response to dynamic actions, Yangtze (Changjiang) estuary. J. Geographical Sci. 21 (4), 719–732. doi: 10.1007/s11442-011-0875-0
Yang Y. P., Deng J. Y., Zhang M. J., Li Y. T., Liu W. L. (2015b). The synchronicity and difference in the change of suspended sediment concentration in the Yangtze river estuary. J. Geographical Sci. 25 (4), 399–416. doi: 10.1007/s11442-015-1176-9
Yang Y. P., Li M., Liu W. L., Chai Y. F., Zhang J., Yu W. J. (2023b). Relationship between potential waterway depth improvement and evolution of the jingjiang reach of the Yangtze river in China. J. Geographical Sci. 33 (3), 547–575. doi: 10.1007/s11442-023-2096-8
Yang Y. P., Li Y. T., Sun Z. H., Fan Y. Y. (2014). Suspended sediment load in the turbidity maximum zone at the Yangtze river estuary: the trends and causes. J. Geographical Sci. 24 (1), 129–142. doi: 10.1007/s11442-014-1077-3
Yang H. F., Li B. C., Zhang C. Y., Qiao H. J., Liu Y. T., Bi J. F., et al. (2020). Recent spatio-temporal variations of suspended sediment concentrations in the Yangtze estuary. Water 12 (3), 818. doi: 10.3390/w12030818
Yang S. L., Milliman J. D., Li P., Xu K. H. (2011). 50,000 dams later: erosion of the Yangtze river and its delta. Global Planetary Change 75 (1–2), 14–20. doi: 10.1016/j.gloplacha.2010.09.006
Yang S. L., Xu K. H., Milliman J. D., Yang H. F., Wu C. S. (2015c). Decline of Yangtze river water and sediment discharge: impact from natural and anthropogenic changes. Sci. Rep. 5, 12581. doi: 10.1038/srep12581
Yang H. F., Yang S. L., Xu K. H., Wu H., Shi B. W., Zhu Q., et al. (2017). Erosion potential of the Yangtze delta under sediment starvation and climate change. Sci. Rep. 7, 10535. doi: 10.1038/s41598-017-10958-y
Yang Y. P., Yin H., Li M., Liu W. L., Li K. Y., Yu W. J. (2023c). Effect of water depth and waterway obstructions on the divergence and confluence areas of dongting lake and the Yangtze river after the operation of the three gorges project. River 2 (1), 88–108. doi: 10.1002/rvr2.31
Yang Y. P., Zhang M. J., Fan Y. Y., Li Y. T., Liu W. L. (2018). Variation trend and causes of suspended sediment characteristic in Yangtze estuary. J. Basic Sci. Eng. 24 (6), 1203–1218. doi: 10.16058/j.issn.1005-0930.2016.06.012
Yang Y. P., Zhang M. J., Li Y. T., Zhang W. (2015a). The variations of suspended sediment concentration in Yangtze river estuary. J. Hydrodynamics 27 (6), 845–856. doi: 10.1016/S1001-6058(15)60547-9
Yang Y. P., Zhang M. J., Liu W. L., Wang J. J., Li X. X. (2019). Relationship between waterway depth and low-flow water levels in reaches below the three gorges dam. J. Waterway Port Coastal Ocean Eng. 145 (1), 04018032. doi: 10.1061/(ASCE)WW.1943-5460.0000482
Yang Y. P., Zheng J. H., Zhang H. Q., Chai Y. F., Zhu Y. D., Wang C. Y. (2022a). Impact of the three gorges dam on riverbed scour and siltation of the middle reaches of the Yangtze river. Earth Surface Processes Landforms 47 (6), 1514–1531. doi: 10.1002/esp.5332
Yang Y. P., Zheng J. H., Zhang W., Zhu Y. D., Chai Y. F., Wang J. J., et al. (2021b). Quantitative relationship between channels and bars in a tidal reach of the lower Yangtze river: implications for river management. J. Geographical Sci. 31 (12), 1837–1851. doi: 10.1007/s11442-021-1925-x
Yang Y. P., Zheng J. H., Zhang M. J., Zhu L. L., Zhu Y. D., Wang J. J., et al. (2021a). Sandy riverbed shoal under anthropogenic activities: the sandy reach of the Yangtze river, China. J. Hydrology 603, 126861. doi: 10.1016/j.jhydrol.2021.126861
Yang Y. P., Zheng J. H., Zhu L. L., Zhang H. Q., Wang J. J. (2022b). Influence of the three gorges dam on the transport and sorting of course and fine sediments downstream of the dam. J. Hydrology 615, 128654. doi: 10.1016/j.jhydrol.2022.128654
Yang Y. P., Zhou L. P., Zhu L. L., Liu W. L., Wang J. J. (2023a). Impact of upstream reservoirs on geomorphic evolution in the middle and lower reaches of the Yangtze river. Earth Surface Processes Landforms 48 (3), 582–595. doi: 10.1002/esp.5504
Yao J., Shi Y., Zhang G. A. (2015). The change process of water and sediment volume of branching channel in the Yangtze river during 1958–2013. Acta Geographica Sin. 70 (5), 828–836. doi: 10.11821/dlxb201505013
Yao S. M., Wang H. Y., Mao B. P. (2017). Research on bed-load transport formula in the Cheng-han reach of the middle Yangtze river. Adv. Water Sci. 28 (3), 329–337. doi: 10.14042/j.cnki.32.1309.2017.03.002
Yu W. C., Zhang Z. L. (2021). Evolution trend of basic channel scour and morphological adjustment in Yangtze river estuary from 2002 to 2018. J. Yangtze River Sci. Res. Institute 38(8), 1–8, 13. doi: 10.11988/ckyyb.20201057
Zhai X. M. (2006). Elementray discussion of hydrodynamic and suspended sediment distribution characteristic in the Yangtze estuary [D] (Shanghai: East China Normal University).
Zhang E. F., Savenije H. G., Chen S. L., Chen J. Y. (2011). Water abstraction along the lower Yangtze river, China, and its impact on water discharge into the estuary. Phys. Chem. Earth 47–48, 76–85. doi: 10.1016/j.pce.2011.05.002
Zhang X. D., Xie R., Fan D. D., Yang Z. S., Wang H. M., Wu C., et al. (2021). Sustained growth of the largest uninhabited alluvial island in the changjiang estuary under the drastic reduction of river discharged sediment. Sci. China Earth Sci. 64 (10), 1687–4697. doi: 10.1007/s11430-020-9746-3
Zhang X. B., Zhang Y. X., Zhu L. H., Chi W. Q., Yang Z. S., Wang B. Y., et al. (2018). Spatial-temporal evolution of the eastern nanhui mudflat in the changjiang (Yangtze river) estuary under intensified human activities. Geomorphology 309, 38–50. doi: 10.1016/j.geomorph.2018.02.023
Zhang J. Y., Zhao D. Z. (2016). Analysis of temporal and spatial distribution characteristics of bedload in south chanel and north passage, Yangtze estuary. Resour. Environ. Yangtze Basin 25 (10), 1520–1527. doi: 10.11870/cjlyzyyhj20161006
Zhao J., Guo L. C., He Q., Wang Z. B., van Maren D. S., Wang X. Y. (2018). An analysis on half century morphological changes in the changjiang estuary: spatial variability under natural processes and human intervention. J. Mar. Syst. 181, 25–36. doi: 10.1016/j.jmarsys.2018.01.007
Zheng S. R. (2016). Reflections on the three gorges project since its operation. Engineering 2 (4), 389–397. doi: 10.1016/J.ENG.2016.04.002
Zheng S. W., Hu H., Xu S. J., Cheng H. Q., Li Z. J., Liu E. F. (2022). Spatial distribution and response of dunes to anthropogenic factors in the lower Yangtze river. Catena 212, 106045. doi: 10.1016/j.catena.2022.106045
Zhou Y. J., Li D. F., Lu J. Y., Yao S. M., Yan X., Jin Z. W., et al. (2020). Distinguishing the multiple controls on the decreased sediment flux in the jialing river basin of the Yangtze river, southwestern China. Catena 193, 104593. doi: 10.1016/j.catena.2020.104593
Zhou L., Shi Y., Zhao Y. Q., Yang Y., Jia J. J., Gao J. H., et al. (2021). Extreme floods of the changjiang river over the past two millennia: contributions of climate change and human activity. Mar. Geology 433, 106418. doi: 10.1016/j.margeo.2020.106418
Zhou M. R., Xia J. Q., Deng S. S., Lin F. F. (2018). Channel adjustments in a gravel-sand bed reach owing to upstream damming. Global Planetary Change 170, 213–220. doi: 10.1016/j.gloplacha.2018.08.014
Zhu C. Y., Guo L. C., van Maren D. S., Tian B., Wang X. Y., He Q., et al. (2019). Decadal morphological evolution of the mouth zone of the Yangtze estuary in response to human interventions. Earth Surface Processes Landforms 44 (12), 2319–2332. doi: 10.1002/esp.4647
Zhu L., He Q., Shen J., Wang Y. (2016). The influence of human activities on morphodynamics and alteration of sediment source and sink in the changjiang estuary. Geomorphology 273, 52–62. doi: 10.1016/j.geomorph.2016.07.025
Keywords: source-sink effect, sediment transport, riverbed adjustment, estuary delta, Yangtze estuary
Citation: Yang Y, Zheng J, Zhang M and Zhu L (2023) Sediment sink-source transitions in the middle and lower reaches of the Yangtze River estuary. Front. Mar. Sci. 10:1201533. doi: 10.3389/fmars.2023.1201533
Received: 06 April 2023; Accepted: 21 June 2023;
Published: 12 July 2023.
Edited by:
Xiuzhen Li, East China Normal University, ChinaCopyright © 2023 Yang, Zheng, Zhang and Zhu. This is an open-access article distributed under the terms of the Creative Commons Attribution License (CC BY). The use, distribution or reproduction in other forums is permitted, provided the original author(s) and the copyright owner(s) are credited and that the original publication in this journal is cited, in accordance with accepted academic practice. No use, distribution or reproduction is permitted which does not comply with these terms.
*Correspondence: Jinhai Zheng, jhzheng@hhu.edu.cn; Mingjin Zhang, zhangmingjin@tiwte.ac.cn