- 1College of Life Science and Engineering, Southwest University of Science and Technology, Mianyang, Sichuan, China
- 2Department of Animal Science, Leshan Academy of Agriculture Science, Leshan, Sichuan, China
- 3Key Laboratory of Freshwater Fisheries and Germplasm Resources Utilization, Ministry of Agriculture and Rural Affairs, Freshwater Fisheries Research Center, Chinese Academy of Fishery Sciences, Wuxi, China
Introduction: This research aimed to assess the impact of Fermented Chinese herbs (FCHM) on the intestinal barrier, immunity, and protection against Aeromonas hydrophila (A. hydrophila) infection in largemouth bass (Micropterus salmoides).
Methods: Four experimental diets were formulated, including H0 (basal diet), H1, H2, and H3, which contained 1%, 3%, and 5% FCHM added to the basal diet, respectively. The fish were randomly allocated to four treatment groups, each with 3 parallel per treatment, consisting of 20 fish per replicate and were raised for 56 days.
Results and discussion: The experiment revealed that: compared with the control group, adding 1% FCHM significantly improved the weight gain rate (WGR) and specific growth rate (SGR) of the juvenile largemouth bass (P< 0.05). The ingestion of FCHM substantially elevated the activities of CAT, SOD, GSH-PX, APK, ACP, and LZM, and T-AOC level in the gut region of largemouth bass, while decreasing the MDA content in intestine (P< 0.05). Supplementation with FCHM enhanced the intestinal villus height and relative mRNA expression of intestinal barrier genes ZO-1, Claudin, and Occludin in juvenile largemouth bass. After injecting A.hydrophila, all groups of largemouth bass experienced mortality, but the consumption of FCHM resulted in a decrease in cumulative mortality. After infected with A.hydrophila, the antioxidant enzymes and immune enzymes activities of all test groups were enhanced compared to those before infection, and the antioxidant enzymes and immune enzymes activities of all groups were considerably higher than the control after feeding FCHM (P< 0.05). After infected with A.hydrophila, the intestinal MDA content of largemouth bass was higher compared with that before infection in all cases (P< 0.05), but after feeding FCHM, the MDA content was lower than the control (P< 0.05). Upon consuming FCHM, the mRNA relative expressions of pro-inflammatory biomarkers IL-1β, TNF-α, IL-15 and IL-8 in largemouth bass infected with A.hydrophila were decreased in comparison to the control group. In contrast, the mRNA expression of the anti-inflammatory biomarkers TGF-β and IL-10 were significantly elevated (P< 0.05). In summary, FCHM could improve the intestinal morphology, immunity, and antioxidant capacity of juvenile largemouth bass, and enhance it against A.hydrophila, with a better effect at 1% addition.
1 Introduction
China is a large aquaculture country, intensification of aquaculture is an important part of Chinese fisheries, it has become a major growth point for fishermen to increase their income and rural revitalization (Hou et al., 2021; Hu et al., 2021). Yet, the exacerbation of the aquaculture process is affected by factors such as parasites, bacteria, and fungi, which leads to outbreaks of diseases in aquatic animals and causes mass mortality, seriously threatening aquaculture industries (Valladão et al., 2015; Yarahmadi et al., 2016). Therefore, enhancing the immunity and disease resistance of fish in aquaculture has become increasingly crucial. The gut is the biggest immune organ of fish (Fan and Pedersen, 2021). And plays a vital role in various functions such as nutrient digestion and absorption, excretory functions, resistance to pathogenic microorganisms, participation in antimicrobial peptides and secretion of ingestion-regulating hormones. If the function of the intestine has abnormalities, it will affect the growth of the fish (Bischoff et al., 2014; Celi et al., 2019; Zhao et al., 2021). In traditional aquaculture, antibiotics commonly used to treatment disease in animals, but the misuse of antibiotics not only created the problem of drug resistance, but use of these antibiotics could have numerous adverse impacts on the water system, animals and humans, and this treatment method has been criticized (Sapkota et al., 2008; Milić et al., 2013; Kovalakova et al., 2020). Therefore, fermented herbs have a good prospect as a green feed additive after the era of antibiotics. Fermenting Chinese herbs involves the use of enzymes and microorganisms to increase their inherent properties and create new benefits (Hussain et al., 2016; Li et al., 2020a). The fermentation could degrade cellulose, lignin and other substances in the cell wall, the active ingredients were released, and the active ingredients in herbs were dismantled to small moieties to improve the effectiveness of the medicines (Mandebvu et al., 1999; Chang et al., 2012; Hussain et al., 2016; Wang et al., 2016). Studies have shown that fermented herbs are commonly employed in farming due to their antioxidative, antidiarrheal, antiviral, and hepatoprotective properties (Hussain et al., 2016). Feeding Bacillus subtilis fermented citrus by-products to juvenile olive flounder Could exert salutary influences on inborn immunity, thus improving the ability of juvenile olive flounder to survive and resist disease (Lee et al., 2013). In addition to enhancing the hematologic and physiological traits of catfish, fermented herbs have positively influenced the survival, specific growth, and feed conversion rates of catfish (Syawal et al., 2021). Incorporating probiotic fermented herbs into the diet of grouper may enhance their Growth capacity and anti-oxidation ability, while also decreasing foregut lipase levels (Nie et al., 2022). Furthermore, researchers have revealed that fermented herbs could modify the intestinal oxidative stress and innate immunity in fish by altering the gut microbiota, regulating the gut barrier, and inflammatory response (Zhang et al., 2015; Park et al., 2016; Wang et al., 2021b; Zhang et al., 2022).
Largemouth bass (Micropterus salmoides) is native to the United States and it’s a freshwater fish species. During the 20th century, it was introduced to China because of its rapid growth, adaptability, fresh and tender meat, and has since become a primary species for freshwater aquaculture in the country (Li et al., 2020b; Xu et al., 2021). In order to reduce usage of antibiotics and guarantee the quality and security of aquatic animals, fermented Chinese herbs have emerged as a viable solution for improving the gut health of largemouth bass and mitigating intestinal diseases. Our study aimed to explore the impact of incorporating fermented Chinese herbs into the diets of juvenile largemouth bass. Specifically, we examined the effects on their intestinal barrier function, immunity, and resistance to A.hydrophila. The goal was to offer effective strategies and insights for promoting the healthy farming of largemouth bass.
2 Materials and methods
2.1 Diets
We prepared four test diets, including the basal diet and three others with 1%, 3%, and 5% FCHM in the basal diets. The components of the basal diet can be found in Table 1. FCHM was made by reference to previous studies with slight improvements (Zhou et al., 2021). The Chinese herbs (hailing from Sichuan, China) were crushed and mixed with 35% distilled water 0.8% glucose, 4% cornstarch, 0.1% microorganisms, and enzymes. The mixture was then fermented for 72 hours at a temperature of 36°. The herbs used in the mixture included astragalus, cypress, scutellaria, honeysuckle and dandelion (in a ratio of 3:2:3:3:2). The microorganisms and enzymes were purchased from a market and met the Product Standard No. Q/12JX 4450-2019. The probiotics included Saccharomyces cerevisiae, Bacillus subtilis and Lactobacillus plantarum, and the enzymes included xylanase, β-mannanase, β-glucanase and cellulase.
2.2 Animals
The fish were acquired from a farm in Meishan, Sichuan, China, and had an average weight of around 5 grams. These fish were acclimated in the experimental environment for 14 days. After a 24-hour period of starvation, the fish were divided randomly into 4 groups. The control group, H0, was given the basal diet, while groups H1-H3 were given the basal diet added for FCHM at 1%, 3%, and 5%, respectively. Each group had 3 parallels, with 20 fish in each parallel, and the experiment was conducted in tanks measuring 1 m × 0.5 m × 1 m. The fish were fed once a day, at 8:30 am and 4:30 pm, with the amount of food adjusted based on the fry’s feeding and growth. Every 2 days, we replaced 1/5 - 3/5 of the water and used a pump to clearance feces from the bottom of the tank. The water temperature was kept at a natural level (around 18 ± 3.5°), with dissolved oxygen levels maintained at 6.0mg/L or higher, pH levels at 7.0 ± 0.2, and ammonia nitrogen levels ≤ 0.02 mg/L. The entire experiment was conducted over a period of 56-days.
2.3 A.hydrophila infection trial
Once the feeding trial concluded, the rest of the sampled fish underwent an injection of A.hydrophila. In the pre-experiment, we determined that the LC50 of A.hydrophila on the fish was 1.65 × 106 CFU/ml an injectable rate of 0.2 ml per fish. After being anesthetized with 50 ppm of MS-222 for 3-5 minutes, the test fish were injected slowly with 0.2 ml of A.hydrophila liquid, which had a concentration of 1.65×106 CFU/ml, into their abdominal cavity using a 1 ml injector. The farming conditions was maintained unchanged throughout the experiment, and daily mortality was monitored after A.hydrophila infection, with cumulative mortality being calculated.
“D1 refers to the total count of fish that have died, while D2 represents the complete number of fish that were tested.
2.4 Sample collection
At the end of the farming trial, ten fish were selected at random from each parallel group following a 24-hour period of fasting. Measure the weight and length of all fish to conduct a statistical analysis of their growth performance. Following anesthesia using 200 ppm MS-2222, dissection was performed. The viscera and livers of all fish were then weighed and recorded, and the midgut was chosen randomly from 3 fish in each duplicate to be used in 10% formaldehyde fixation for gut histological investigation. The guts of the remaining fish were cryogenically frozen in liquid nitrogen and refrigerated in -80°C. At day 3 following the hydrophilic toxicity study, all fish were fasted for 24 hours, anesthetized with 200 ppm MS-222, viscera were extracted and snap-frozen in liquid nitrogen (-196°C) for testing. Samples were subsequently stored at -80°C. Growth performance is calculated using the following formula:
Mt and M0 represent the initial and final weight data at the start and end of the experiment, respectively, and are measured in grams (g); M denotes the body weight per fish, Mv stands for the viscerosomatic weight of each fish, and Mh refers to the liver weight of each fish, all measured in grams (g). The variable t represents the number of experimental days in days (day), while L represents the length of the fish in centimeters (cm).
2.5 Histomorphology of the gut
The fish’s midgut was gently cleaned with physiological saline before being preserved with 4% paraformaldehyde. Hematoxylin-eosin was used to stain the 5 m slices after they had been made using a paraffin slicer (HE). The slices were next studied at low magnification, and using light microscopy, the appropriate parts were picked out to be photographed at high magnification. Using Image-Pro Plus software, the essential data were examined for muscle layer thickness, breadth, and villi height.
2.6 Analysis of intestinal biochemical indicators
To prepare the intestinal tissues for analysis, start by thawing the tissues (Both pre- and post-A.hydrophilic infection) that were stored at -80°C in refrigerator. Then, using the instructions provided in the kit, homogenize the tissues with the appropriate amount of tissue homogenizing solution. Next, the homogenized mixture should be Centrifugation for 10 minutes at 4°C and 2500r/min, after which the supernatant can be collected as the tissue homogenizer. Using an enzyme marker or spectrophotometer, the levels of acid phosphatase (ACP), glutathione peroxidase (GSH-Px), lysozyme (LZM), alkaline phosphatase (AKP), catalase (CAT), malondialdehyde (MDA), and superoxide dismutase (SOD) were measured in the intestine following the steps outlined in the instructions provided in the kit from Nanjing Jiancheng Institute of Biological Engineering.
2.7 Measurement of intestinal physical barrier gene and inflammatory factor expression
To extract total RNA from the intestinal tissues, they were first placed in RNAase-free centrifuge tubes after being stored at -80°C, and then ground using a microtissue homogenizer. The Trizol method (TaKaRa, Japan) was used for the extraction process. By using micro-UV spectroscopy and 1% gel polymeric electrophoresis, RNA quality and concentrations were measured. According the instructions, the kit (RR047A, TaKaRa) was used to create the first thread of the cDNA. β-actin was utilized as an inner gene of reference, and the primers used for qPCR testing were obtained from previous research. (Xv et al., 2021), and are displayed in Table 2. NovoStart SYBR qPCR Super Mix Plus was used to conduct quantitative real-time PCR (qPCR) on a Bio-Rad CFX96, following the manufacturer’s instructions and utilizing a total volume of 20 uL. The thermocycling amplified procedures consisted of denaturing at 95°C for 15 s, annealing at 56°C for 15 s, extending at 72°C for 30 s, and repeating for 40 cycles.
2.8 Statistical analysis
With IBM SPSS Statistics 23, a one-way analysis of variance (ANOVA) was used to assess significance levels. The Tukey multiple range test was used to compare numerous things. P < 0.05 was chosen as the cutoff for statistical significance. Data are presented in the table as mean and combined standard deviation (SD). GraphPad Prism6 was used to create images (GraphPad Software, USA).
3 Results
3.1 Growth performance
The growth performance of juvenile largemouth bass fed with FCHM for 56 days is shown in Table 3. The addition of FCHM did not have a significant effect on CF, HSI, and VSI of the juvenile largemouth bass (P > 0.05). Compared to the control group, adding 1% FCHM significantly improved the WGR and SGR of the juvenile largemouth bass (P< 0.05).
3.2 The cumulative mortality rate after infection with A.hydrophila
Following the culture trial, A.hydrophila was introduced to the largemouth bass, and mortality was monitored for 72 hours. Figure 1 indicates that all tested fish experienced mortality within 24 hours of the A.hydrophila injection. However, the cumulative mortality rate was higher in H0 than in H1, H2 and H3. The cumulative mortality rate was 43.33% in group H0, 30.00% in group H1,23.33% in group H2 and 26.67% in group H3 throughout A.hydrophila infection.
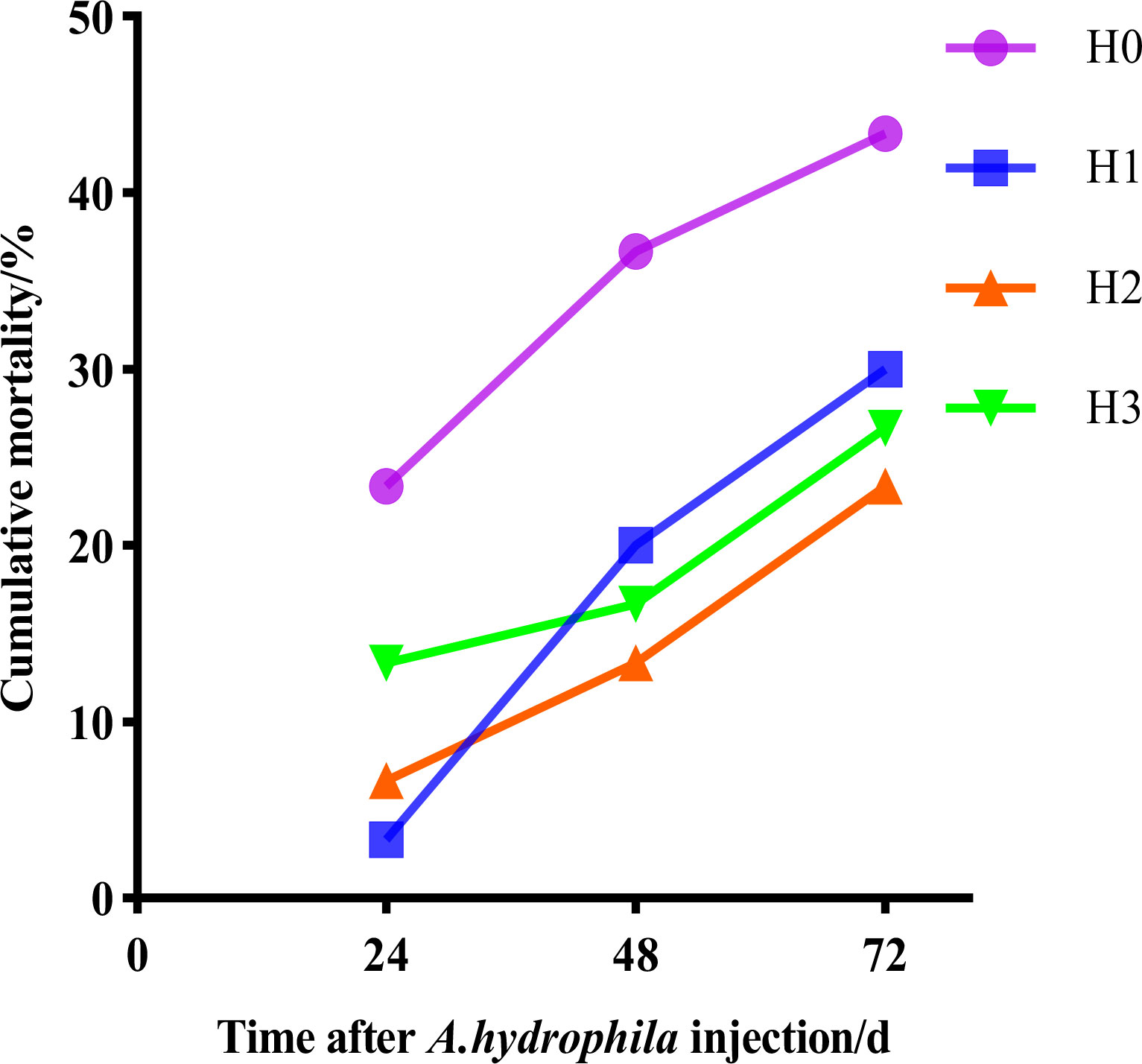
Figure 1 The cumulative mortality rates of largemouth bass at 72 hours following the injection of hydrophilic bacteria.
3.3 The morphology of the gut
Figure 2 illustrates the midgut composition of juvenile largemouth bass, while Table 4 outlines the features of the midgut villi. The control group of largemouth bass had sparse and shorter midgut intestinal villi, a smaller number of villi and narrower muscle thickness. In contrast, the midgut villi of largemouth bass were tightly packed and neatly arranged after feeding FCHM. The height of the gut villi was notably greater in H2 and H3 when compared with H0. Similarly, the width of the villi was higher in H1 than in H0, and the thickness of the muscularis was notably higher in H2 than in H0 (P< 0.05).
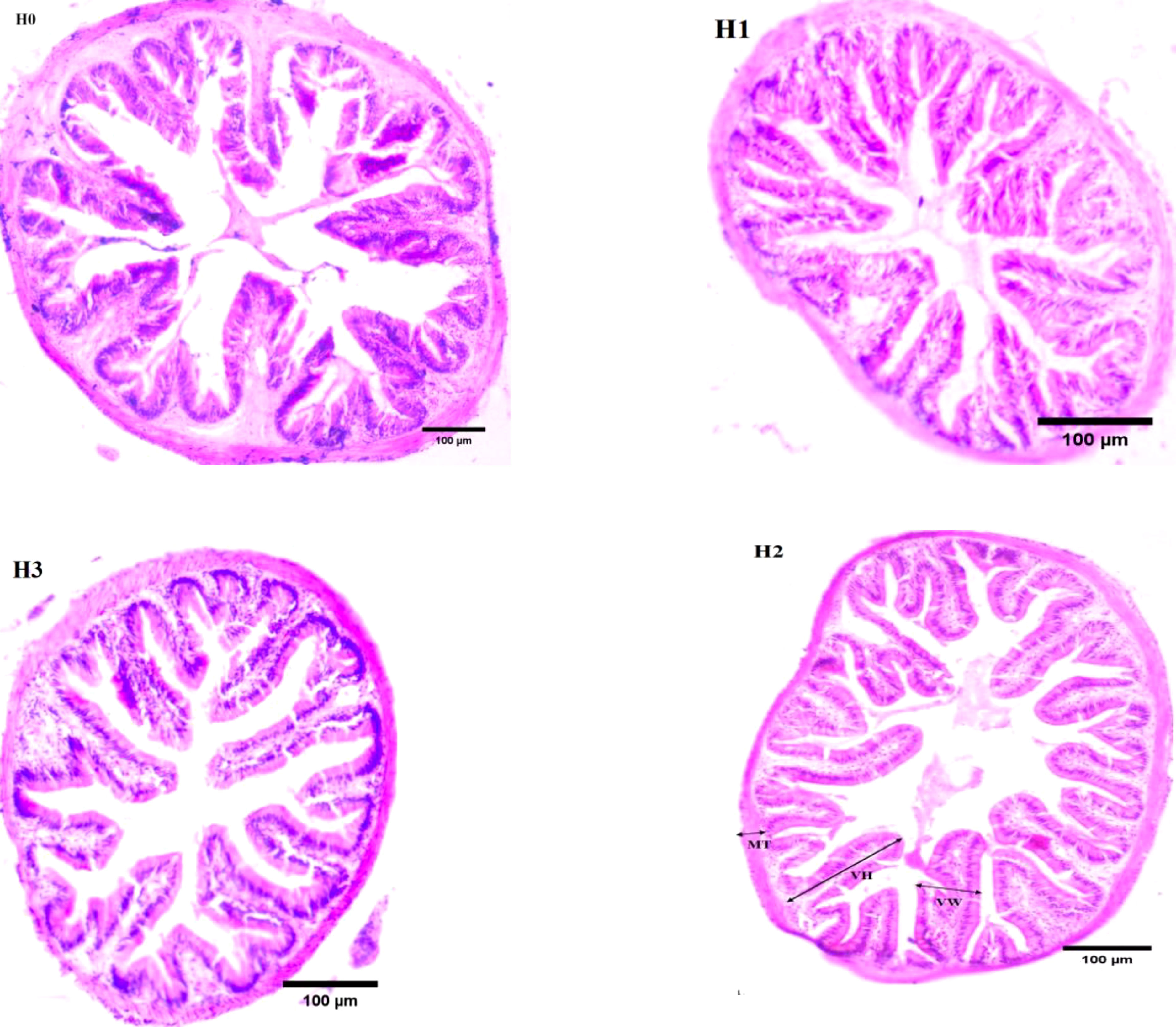
Figure 2 The mid-gut morphology of juvenile largemouth bass was evaluated under varying scales of FCHM treatment. The image was captured at X100 magnification and stained with H&E, with a scale bar of 100 μm; MT, muscular layer thickness; VH, villi height; VW, villi width.
3.4 Relative expression level of physical barrier genes in the largemouth bass intestine
Figure 3 displays the gene expression of the gut barrier in juvenile largemouth bass. Following the 64 days feeding trial, the inclusion of FCHM in their diet led to an increase in the relative mRNA expression of ZO-1, Claudin, and Occludin in the intestines of juvenile largemouth bass compared with H0 (p< 0.05). Additionally, the highest relative expression of Occludin, Claudin and ZO-1 mRNA was observed in H1. Furthermore, the relative mRNA expression of Claudin and ZO-1 in H1 was significantly higher compared to H2 and H3.
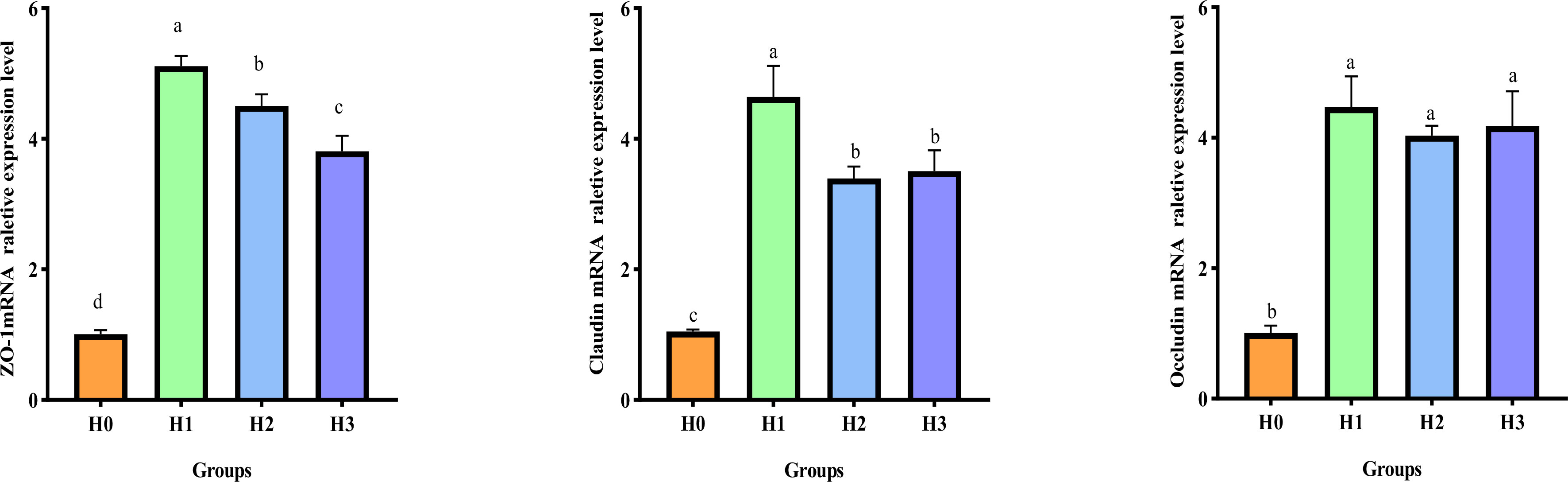
Figure 3 Effect of supplemental FCHM from the gut barrier gene relative expression in largemouth bass. ZO-1, zonula occluden-1. Different lowercase letters indicate significant differences (P< 0.05).
3.5 Changes in gut antioxidant indices of juvenile largemouth bass
Figure 4 displays the alterations in intestinal antioxidant indices of juvenile largemouth bass. After64-days feeding trial, the inclusion of FCHM in the diets led to a significant increase in the activities of intestinal SOD, CAT, and GSH-Px, and T-AOC levels, and a significant reduction in intestinal MDA content compared with H0 (P< 0.05). Among all groups, H1 exhibited significantly higher SOD and GSH-Px activities, T-AOC level, and significantly lower MDA content (P< 0.05). After being infected by A.hydrophila, the intestinal SOD, CAT, and GSH-Px activities, and T-AOC content, were increased, and the MDA content was significantly reduced in H1, H2, and H3 groups compared with H0 (P< 0.05). In all groups, the activities of SOD, CAT, and GSH-Px, and the contents of T-AOC and MDA, were significantly higher after A.hydrophila infection than before infection (P< 0.05), but the contents of MDA in H1, H2, and H3 were lower than those in H0 (P< 0.05).
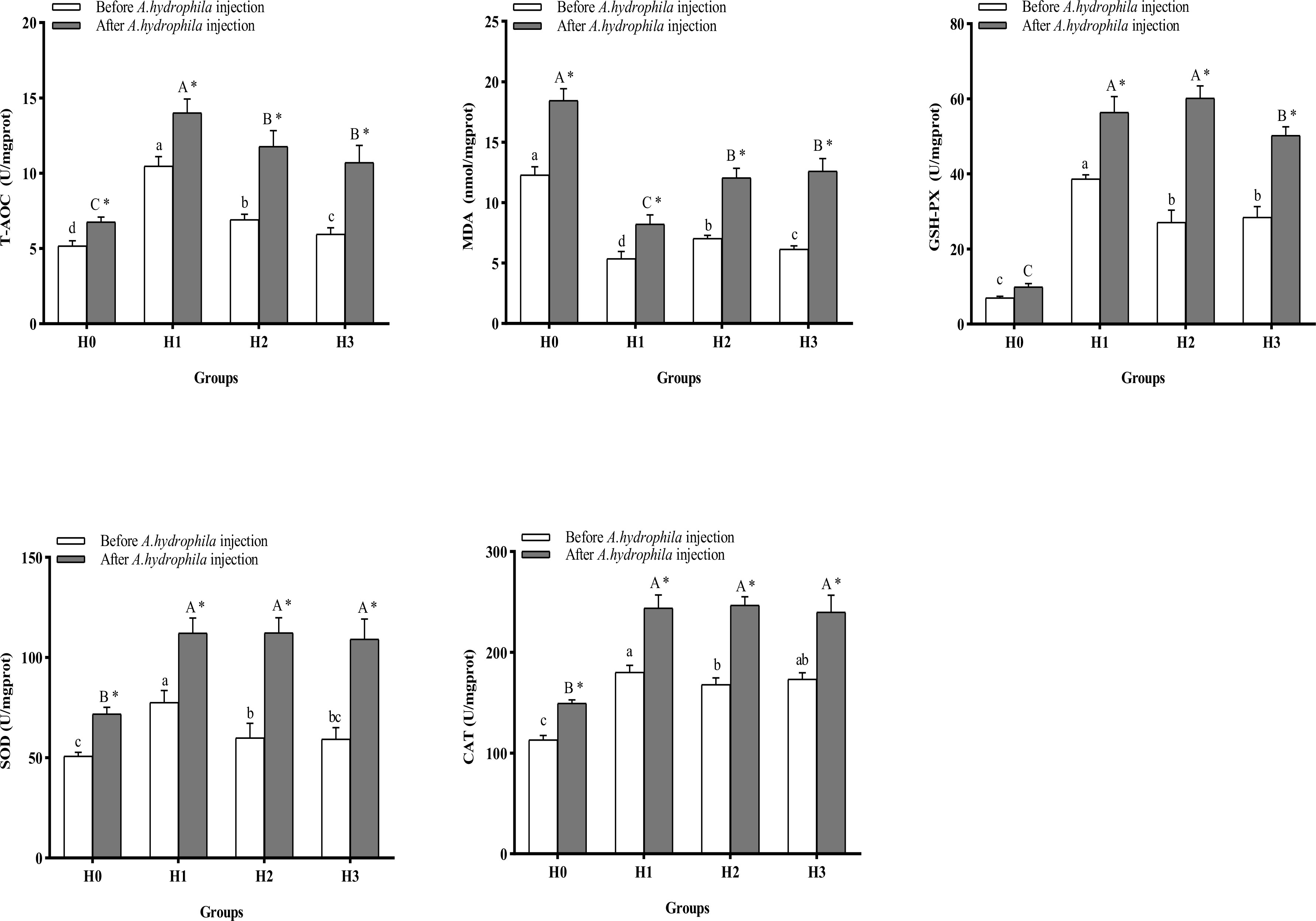
Figure 4 The alterations in intestinal anti-oxidation ability of largemouth bass were examined before and after A.hydrophila infection. Value columns with distinct small letters indicate significant differences among different groups before A.hydrophila injection (P< 0.05), and significant differences between groups after injection of A.hydrophila are indicated by different capital letters. (P< 0.05). An asterisk (*) indicates a significant difference between before and after A.hydrophila injection in the same group (P< 0.05). SOD, superoxide dismutase; CAT, catalase; MDA, malondialdehyde; T-AOC, total antioxidant capacity; GSH-PX, glutathione peroxidase.
3.6 Changes in intestinal immune enzyme activity of largemouth bass
Figure 5 displays the alterations in intestinal immune enzyme activity of largemouth bass. Supplementation with FCHM in the diet enhanced the activities of intestinal ACP, AKP, and LZM of juvenile largemouth bass compared to H0 at the end of the 8-weeks breeding experiment, with the highest activities in H1 (P< 0.05). Following A.hydrophila infection, the activities of intestinal ACP, LZM, and AKP were higher in all groups than in H0 (P< 0.05). Moreover, the activity of intestinal ACP, LZM, and AKP was higher in all four groups of largemouth bass after A.hydrophila infection than before the infection (P< 0.05).
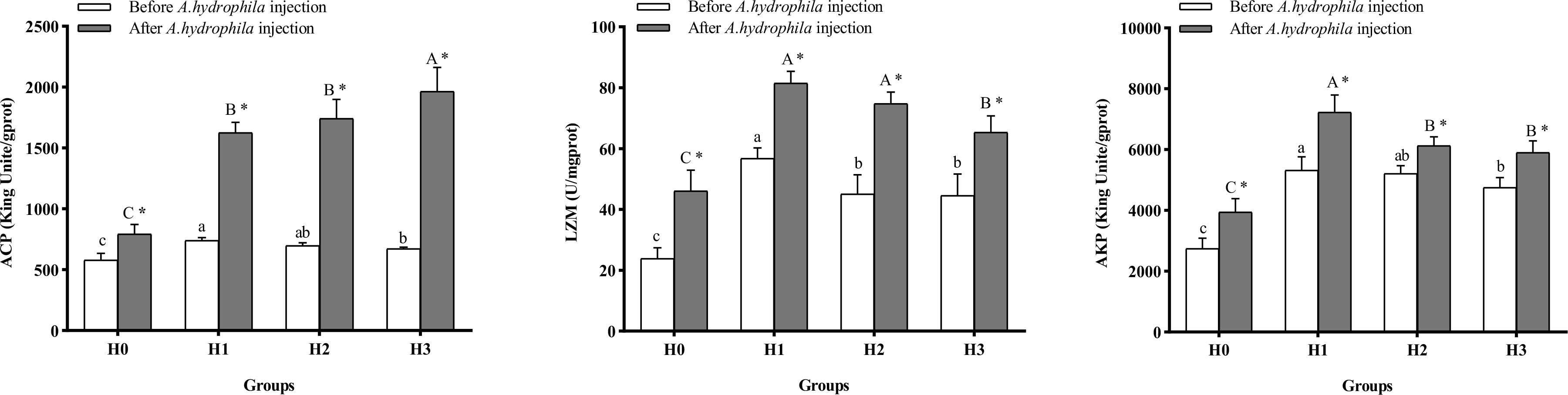
Figure 5 The alterations in intestinal immunoenzyme activity of largemouth bass were analyzed before and after A.hydrophila infection. Value columns with distinct small letters indicate significant differences among different groups before A.hydrophila injection (P< 0.05), and significant differences between groups after injection of A.hydrophila are indicated by different capital letters. (P< 0.05). An asterisk (*) indicates a significant difference between before and after A.hydrophila injection in the same group (P< 0.05). ACP, acid phosphatase; LZM, lysozyme, AKP, alkaline phosphatase.
3.7 The relative expression of gut inflammatory genes in juvenile largemouth bass
A.hydrophila-induced intestinal inflammation caused an upregulation of pro-inflammatory factors and a downregulation of anti-inflammatory factors. The mRNA relative expression of inflammatory biomarkers in the intestines of juvenile largemouth bass was evaluated in this study following A.hydrophila-induced intestinal inflammation, as illustrated in Figure 6. Compared with H0, the mRNA relative expressions of intestinal anti-inflammatory biomarkers IL-10 and TGF-β significantly enhanced (P< 0.05), while the mRNA relative expressions of pro-inflammatory factors IL-1β, IL-15, TNF-α, and IL-8 significantly decreased (P< 0.05) after feeding FCHM to largemouth bass for 8-weeks. These findings suggest that FCHM can effectively regulate the expression of inflammatory factors following A.hydrophila-induced enteritis.
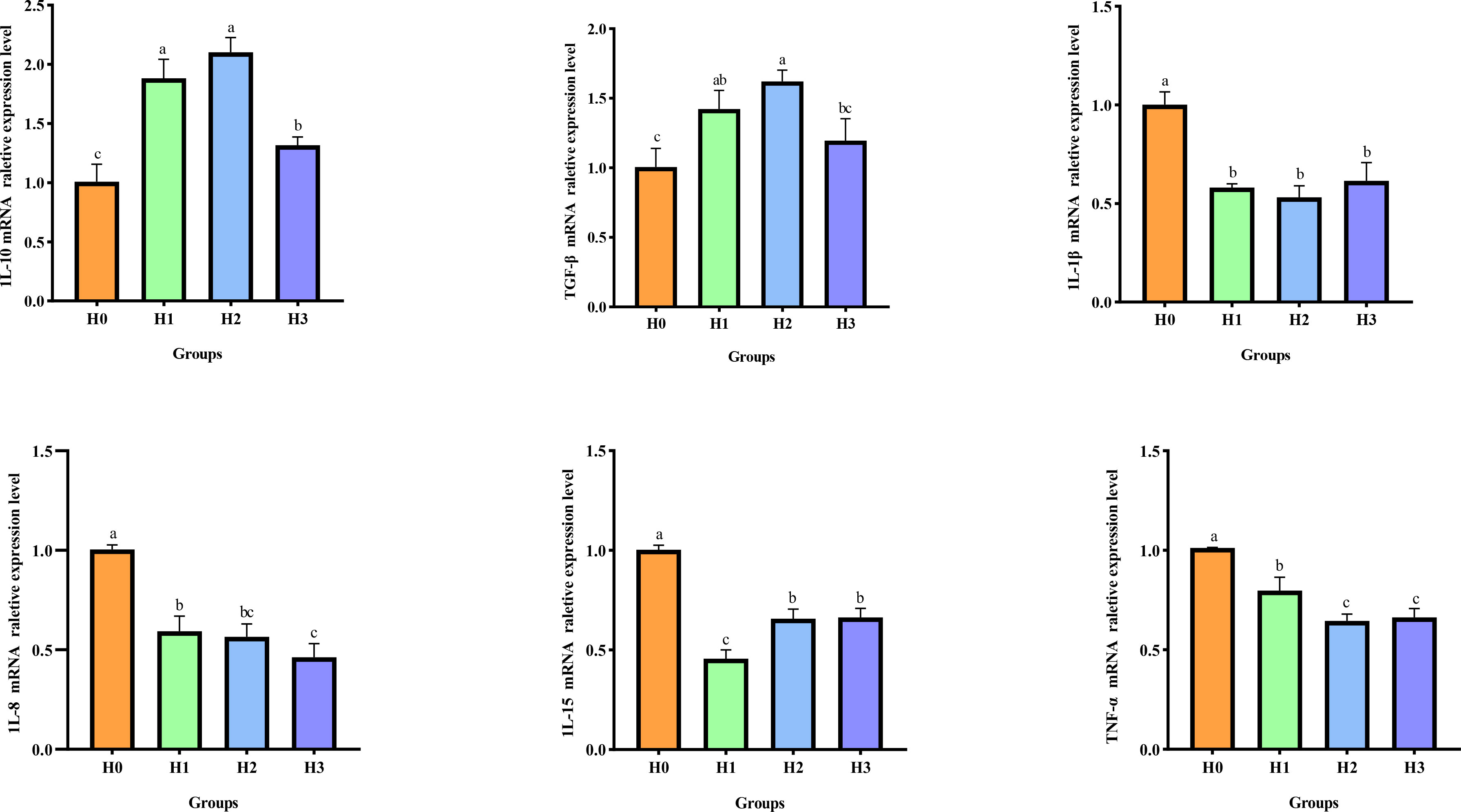
Figure 6 After being infected with A.hydrophila, the expression of inflammatory biomarkers in the gut of largemouth bass was examined to determine the effect of feeding FCHM. TGF-β, transforming growth factor-β1; TNF-α, tumor necrosis factor-α; IL, interleukin; Different lowercase letters indicate significant differences (P< 0.05).
4 Discussion
Chinese herbal medicine contained many biologically active ingredients, which could stimulate the appetite or digestive function of animals, increase feed intake and growth performance (Awad and Awaad, 2017; Pu et al., 2017). In addition, fermented Chinese herbal medicine also contained many probiotics, which could improve the intestinal health and morphology of animals, increase the digestibility and absorption rate of feed in the intestine, promote the metabolism of nutrients, and improve the production performance of animals (Melara et al., 2022; Chen et al., 2023). Our research found that supplementing FCHM improved the WGR and SGR of juvenile largemouth bass, possibly because FCHM promoted their digestion and absorption of nutrients, thereby promoting growth.
Bacterial infection was frequently used as a fish health indicator at the end of culture trials (Shan et al., 2018). Freshwater fish commonly suffer from bacterial infections in their intestines, and A. hydrophila is often considered a primary pathogen among the various disease-causing bacteria (Maulu et al., 2021; Xv et al., 2021). Our study revealed that largemouth bass fed with FCHM displayed a greater ability to survive after being infected with A.hydrophila. This indicates that FCHM could potentially improve the immunity of largemouth bass and enhance their resistance to A.hydrophila.
When fish undergo metabolic processes during their life activities, oxygen radicals are generated. To counteract their harmful effects, antioxidant enzymes like CAT, GSH-Px, and SOD form the fish’s antioxidant defense system. The SOD can convert the excess reactive oxygen radicals in the body into hydrogen peroxide, while the CAT can cooperate with the SOD to convert the hydrogen peroxide into water, thus slowing down the oxidative stress (Harikrishnan and Balasundaram, 2005; Hongling Zhang et al., 2020a). T-AOC level is a crucial measure of the anti-oxidant capacity in animals and is directly indicative of the anti-oxidant ability of fish (Ge et al., 2021). When the body exposed to adverse conditions will produce a lot of oxygen radicals, which can attack the cell membrane and produce lipid peroxidation reactions, converted into MDA, thus causing oxidative damage to the body (Hoseinifar et al., 2021). When the body is exposed to adverse conditions will produce many oxygen radicals, which can attack the cell membrane and produce lipid peroxidation reactions, converted into MDA, thus causing oxidative damage to the body (Wei Zhao et al., 2022). Hydrogen peroxide and lipid peroxides can also be scavenged by GSH-Px, while GSH can synergize with GSH-Px to scavenge free radicals (Taso et al., 2019). Therefore, the increase in antioxidant enzyme activity could improve the disease resistance of fish. Feeding fermented moringa oleifera lam could improve the serum SOD and CAT activity, reduce the content of serum MDA and improve the resistance to A.hydrophila of crucian carp (Liu et al., 2019). Lactobacillus fermented Artemia could improve antioxidant capacity and antibacterial ability of fish and has potential as a feed additive (Li et al., 2021). Our study revealed that administering FCHM to juvenile largemouth bass resulted in heightened activity of SOD, GSH-PX, and CAT while reducing MDA concentration in their intestines. The inclusion of FCHM in their diet significantly enhanced the antioxidant capacity of the fish. This can be attributed to FCHM’s ability to scavenge reactive oxygen radicals in animals and minimize lipid peroxidation reactions.
Research has demonstrated that AKP, ACP, and LZM are crucial enzymes in the fish immune defense system, indicating the organism’s immune condition (Zhang et al., 2020b). The utilization of LZM can serve as a crucial measure for assessing non-specific immunity in fish, and it holds a significant function in regulating inflammatory processes, repairing, and regenerating tissues, as well as exterminating bacteria within the organism (Chen et al., 2021). AKP and ACP are crucial hydrolytic enzymes in the immune defense system of fish and are also important metabolic regulatory enzymes, which mainly catalyze the hydrolysis of phosphate esters to form inorganic phosphate and thus promote the production of ATP during the immune reaction. Meanwhile, phosphatase activity in aquatic animals can be used as an indicator to determine the immunity of animals (Shi et al., 2021; Wang et al., 2022). The conclusions of this study indicate that administering FCHM to juvenile largemouth bass led to an increase in AKP, ACP, and LZM levels in their intestines both pre-infection and post-infection with A.hydrophila. This indicates that FCHM has the potential to enhance non-specific immunity and bolster the largemouth bass’s ability to resist diseases. The overall health of fish intestines is influenced by factors like the morphology of the gut villi, physical barriers, and the activity of digestive enzymes. By improving the morphology of gut tissues in fish, including the width and length of villi and thickness of the muscle layer, the digestibility of nutrients can be enhanced, ultimately contributing to the maintenance of fish health (Yu et al., 2020; Dawood, 2021; Wang et al., 2021a). Fermented herbs improve the physical barrier of the gut, mainly in the height of the gut villus and the enhanced muscle layer thickness (Nawaz et al., 2018; Abdel-Latif et al., 2020). Adding fermented astragalus to the diets could enhance the height of gut villi, thus increasing the digestive and absorption capacity of carp for nutrients and improving growth performance (Chen et al., 2023). It was found that fermented lemon peel could improve the intestinal morphology of Lates calcarifer and can be utilized as a functional feed supplement to maintain intestinal health (Shi et al., 2022). In line with prior research, FCHM sustained the gut health of largemouth bass by enhancing their gut morphology.
We assessed the expression levels of gut barrier genes in largemouth bass to gain more insight into how FCHM controls intestinal health in largemouth bass. In the intestine, the intestinal tight junctions are a dynamic, changing structure that allows for the reabsorption of water, ions, and nutrients to regulate intercellular permeability, thus preventing external pathogens and toxins from entering the intestinal lumen (Zhuo et al., 2021). Claudin mainly constitutes a tight junction protein that forms intercellular pores in intestinal epithelial and endothelial cells that affect intestinal permeability (Assimakopoulos et al., 2011). Occludin is another cell adhesive component and a part of tight junction proteins, its main role is to participate in the closure of the paracellular gap and to regulate the signals generated in tight junctions, the increased or decreased adhesion proteins will be directly reflected in the intestinal permeability, resulting in changes in intestinal permeability (Günzel and Yu, 2013). ZO-1 is a crucial component of tight junction proteins that serve to safeguard and uphold the integrity of the intestinal mucosa (Schneeberger and Lynch, 2004). Assessing the expressed of these 3 tight junction proteins provides a clearer picture of how FCHM supports the gut mucosal integrity. As such, we discovered that the expression of Occludin, Claudin and ZO-1 in the intestines of young largemouth bass was boosted after an 8-weeks FCHM diet. This suggests that FCHM is advantageous in preserving the integrity of the gut mucosa and improving the physical barrier function of the gut of largemouth bass. Inflammatory responses mediated by cytokines are critical to the immunity of fish organisms and can regulate immune responses by increasing or decreasing cytokine production (Coyne et al., 2002). Cytokines in fish are divided into anti-inflammatory biomarkers (e.g., TGF-β and IL-10) and pro-inflammatory biomarkers (e.g., IL-8, IL-15, TNF-α, and IL-1β) that are crucial in immune responses. Enteritis could reduce the expression of anti-inflammatory biomarkers and increase pro-inflammatory biomarkers, which enable them to react to molecular-level inflammatory damage (Safari et al., 2016; Fehrmann-Cartes et al., 2019). Research has shown that the innate immunity of fish intestines can be regulated by herbs and probiotics, which can boost the production of anti-inflammatory biomarkers and reduce the expression of pro-inflammatory biomarkers. This enhances the fish’s resilience against pathogenic bacteria, thereby reduce inflammation (Gil, 2002; Song et al., 2014; Giri et al., 2015; Safari et al., 2016). The research demonstrated that feeding FCHM to juvenile largemouth bass effectively modulated the expression of gut inflammatory factors following infection with A.hydrophila. FCHM was observed to alter the inflammatory response in the fish’s gut, thereby decreasing the incidence of gut disease caused by A.hydrophila.
5 Conclusion
The study revealed that supplementing a specific quantity of FCHM enhanced the resistance of juvenile largemouth bass to A.hydrophila and growth performers by improving the physical barrier, immune enzyme activity, anti-oxidant capacity, and inflammatory response of the intestines. The most effective concentration was observed to be 1%.
Data availability statement
The raw data supporting the conclusions of this article will be made available by the authors, without undue reservation.
Ethics statement
The animal study was reviewed and approved by College of Life Science and Engineering, Southwest University of Science and Technology.
Author contributions
LJ: Supervision, Methodology, Conceptualization, Investigation, Writing – original draft. XZ: Conceptualization, Methodology, Data curation, Formal analysis, Writing – original draft. SB: Data curation, Investigation, Formal analysis. QW: Data curation, Investigation. JL: Investigation. YW: Supervision, Methodology, Investigation, BL: Supervision, Writing – review and editing. All authors contributed to the article and approved the submitted version.
Funding
This work was supported by the Central Public interest Scientific Institution Basal Research Fund, CAFS (2020TD59), Project of National Key R&D Program of China (2019YFD09002).
Conflict of interest
The authors declare that the research was conducted in the absence of any commercial or financial relationships that could be construed as a potential conflict of interest.
Publisher’s note
All claims expressed in this article are solely those of the authors and do not necessarily represent those of their affiliated organizations, or those of the publisher, the editors and the reviewers. Any product that may be evaluated in this article, or claim that may be made by its manufacturer, is not guaranteed or endorsed by the publisher.
References
Abdel-Latif H. M. R., Abdel-Tawwab M., Dawood M. A. O., Menanteau-Ledouble S., El-Matbouli M. (2020). Benefits of dietary butyric acid, sodium butyrate, and their protected forms in aquafeeds: a review. Rev. Fisheries Sci. Aquaculture 28, 421–448. doi: 10.1080/23308249.2020.1758899
Assimakopoulos S. F., Papageorgiou I., Charonis A. (2011). Enterocytes' tight junctions: from molecules to diseases. World J. Gastrointest Pathophysiol 2, 123–137. doi: 10.4291/wjgp.v2.i6.123
Awad E., Awaad A. (2017). Role of medicinal plants on growth performance and immune status in fish. Fish Shellfish Immunol. 67, 40–54.doi:10.1016/j.fsi.2017.05.034. doi: 10.1016/j.fsi.2017.05.034
Bischoff S. C., Barbara G., Buurman W., Ockhuizen T., Schulzke J.-D., Serino M., et al. (2014). Intestinal permeability – a new target for disease prevention and therapy. BMC Gastroenterol. 14, 189. doi: 10.1186/s12876-014-0189-7
Celi P., Verlhac V., Pérez Calvo E., Schmeisser J., Kluenter A.-M. (2019). Biomarkers of gastrointestinal functionality in animal nutrition and health. Anim. Feed Sci. Technol. 250, 9–31. doi: 10.1016/j.anifeedsci.2018.07.012
Chang J., Cheng W., Yin Q., Zuo R., Song A., Zheng Q., et al. (2012). Effect of steam explosion and microbial fermentation on cellulose and lignin degradation of corn stover. Bioresource Technol. 104, 587–592. doi: 10.1016/j.biortech.2011.10.070
Chen G., Li Z., Liu S., Tang T., Chen Q., Yan Z., et al. (2023). Fermented chinese herbal medicine promoted growth performance, intestinal health, and regulated bacterial microbiota of weaned piglets. Anim. (Basel) 13 (3), 476. doi: 10.3390/ani13030476
Chen J., Liu N., Li B., Zhang H., Zhao Y., Cao X. (2021). The effects of fipronil exposure on oxidative stress, non-specific immunity, autophagy, and apoptosis in the common carp. Environ. Sci. pollut. Res. 28, 27799–27810. doi: 10.1007/s11356-021-12573-x
Coyne C. B., Vanhook M. K., Gambling T. M., Carson J. L., Boucher R. C., Johnson L. G. (2002). Regulation of airway tight junctions by proinflammatory cytokines. Mol. Biol. Cell 13, 3218–3234. doi: 10.1091/mbc.e02-03-0134
Dawood M. A. O. (2021). Nutritional immunity of fish intestines: important insights for sustainable aquaculture. Rev. Aquaculture 13, 642–663. doi: 10.1111/raq.12492
Fan Y., Pedersen O. (2021). Gut microbiota in human metabolic health and disease. Nat. Rev. Microbiol. 19, 55–71. doi: 10.1038/s41579-020-0433-9
Fehrmann-Cartes K., Coronado M., Hernández A. J., Allende M. L., Feijoo C. G. (2019). Anti-inflammatory effects of aloe vera on soy meal-induced intestinal inflammation in zebrafish. Fish Shellfish Immunol. 95, 564–573. doi: 10.1016/j.fsi.2019.10.075
Ge Q., Yang B., Liu R., Jiang D., Yu H., Wu M., et al. (2021). Antioxidant activity of lactobacillus plantarum NJAU-01 in an animal model of aging. BMC Microbiol. 21, 182. doi: 10.1186/s12866-021-02248-5
Gil A. (2002). Modulation of the immune response mediated by dietary nucleotides. Eur. J. Clin. Nutr. 56 Suppl 3, S1–S4. doi: 10.1038/sj.ejcn.1601475
Giri S. S., Sen S. S., Chi C., Kim H. J., Yun S., Park S. C., et al. (2015). Effect of guava leaves on the growth performance and cytokine gene expression of labeo rohita and its susceptibility to Aeromonas hydrophila infection. Fish Shellfish Immunol. 46, 217–224. doi: 10.1016/j.fsi.2015.05.051
Günzel D., Yu A. S. (2013). Claudins and the modulation of tight junction permeability. Physiol. Rev. 93, 525–569. doi: 10.1152/physrev.00019.2012
Harikrishnan R., Balasundaram C. (2005). Modern trends in aeromonas hydrophila disease management with fish. Rev. Fisheries Sci. 13, 281–320. doi: 10.1080/10641260500320845
Hoseinifar S. H., Yousefi S., Van Doan H., Ashouri G., Gioacchini G., Maradonna F., et al. (2021). Oxidative stress and antioxidant defense in fish: the implications of probiotic, prebiotic, and synbiotics. Rev. Fisheries Sci. Aquaculture 29, 198–217. doi: 10.1080/23308249.2020.1795616
Hou N., Zhu Q., Yang J., Zhang D., Liu W., Chang H. (2021). The impact of environmental governance on the development of fishery economy–the intermediary role of technological innovation. Sustainability 13 (20), 11378. doi: 10.3390/su132011378
Hu F., Zhong H., Wu C., Wang S., Guo Z., Tao M., et al. (2021). Development of fisheries in China. Reprod. Breed. 1, 64–79. doi: 10.1016/j.repbre.2021.03.003
Hussain A., Bose S., Wang J.-H., Yadav M. K., Mahajan G. B., Kim H. (2016). Fermentation, a feasible strategy for enhancing bioactivity of herbal medicines. Food Res. Int. 81, 1–16. doi: 10.1016/j.foodres.2015.12.026
Kovalakova P., Cizmas L., McDonald T. J., Marsalek B., Feng M., Sharma V. K. (2020). Occurrence and toxicity of antibiotics in the aquatic environment: a review. Chemosphere 251, 126351. doi: 10.1016/j.chemosphere.2020.126351
Lee B. J., Kim S. S., Song J. W., Oh D. H., Cha J. H., Jeong J. B., et al. (2013). Effects of dietary supplementation of citrus by-products fermented with a probiotic microbe on growth performance, innate immunity and disease resistance against edwardsiella tarda in juvenile olive flounder, paralichthys olivaceus (Temminck & schlegel). J. Fish Dis. 36, 617–628. doi: 10.1111/jfd.12035
Li L., Wang L., Fan W., Jiang Y., Zhang C., Li J., et al. (2020a). The application of fermentation technology in traditional chinese medicine: a review. Am. J. Chin. Med. 48, 899–921. doi: 10.1142/S0192415X20500433
Li X., Wei X., Guo X., Mi S., Hua X., Li N., et al. (2020b). Enhanced growth performance, muscle quality and liver health of largemouth bass (Micropterus salmoides) were related to dietary small peptides supplementation. Aquaculture Nutr. 26, 2169–2177. doi: 10.1111/anu.13155
Li G., Zhan J., Hu L., Yuan C., Takaki K., Ying X., et al. (2021). Identification of a new antioxidant peptide from porcine plasma by in vitro digestion and its cytoprotective effect on H2O2 induced HepG2 model. J. Funct. Foods 86, 104679. doi: 10.1016/j.jff.2021.104679
Liu K., Liu Y., Chen F. (2019). Effect of storage temperature on lipid oxidation and changes in nutrient contents in peanuts. Food Sci. Nutr. 7, 2280–2290. doi: 10.1002/fsn3.1069
Mandebvu P., West J. W., Froetschel M. A., Hatfield R. D., Gates R. N., Hill G. M. (1999). Effect of enzyme or microbial treatment of bermudagrass forages before ensiling on cell wall composition, end products of silage fermentation and in situ digestion kinetics. Anim. Feed Sci. Technol. 77, 317–329. doi: 10.1016/S0377-8401(98)00247-8
Maulu S., Hasimuna O. J., Mphande J., Munang’andu H. M. (2021). Prevention and control of streptococcosis in tilapia culture: a systematic review. J. Aquat. Anim. Health 33, 162–177. doi: 10.1002/aah.10132
Melara E. G., Avellaneda M. C., Valdivié M., García-Hernández Y., Aroche R., Martínez Y. (2022). Probiotics: symbiotic relationship with the animal host. Animals 12 (6), 719. doi: 10.3390/ani12060719
Milić N., Milanović M., Letić N. G., Sekulić M. T., Radonić J., Mihajlović I., et al. (2013). Occurrence of antibiotics as emerging contaminant substances in aquatic environment. Int. J. Environ. Health Res. 23, 296–310. doi: 10.1080/09603123.2012.733934
Nawaz A., Bakhsh javaid A., Irshad S., Hoseinifar S. H., Xiong H. (2018). The functionality of prebiotics as immunostimulant: evidences from trials on terrestrial and aquatic animals. Fish Shellfish Immunol. 76, 272–278. doi: 10.1016/j.fsi.2018.03.004
Nie H., Liang Q., Xie X. (2022). Effects of a probiotic-fermented herbal medicines mixture on the growth performance, immune and antioxidative function of acanthopagrus schlegelii): research square. PPR511688, 1–20. doi: 10.21203/rs.3.rs-1714834/v1
Park K. I., Kim D. G., Lee B. H., Ma J. Y. (2016). Fermented herbal formulas kiom-ma128 ameliorate il-6-induced intestinal barrier dysfunction in colon cancer cell line. Mediators Inflammation 2016, 6189590. doi: 10.1155/2016/6189590
Pu H., Li X., Du Q., Cui H., Xu Y. (2017). Research progress in the application of chinese herbal medicines in aquaculture: a review. Engineering 3, 731–737. doi: 10.1016/J.ENG.2017.03.017
Safari R., Hoseinifar S. H., Nejadmoghadam S., Jafar A. (2016). Transciptomic study of mucosal immune, antioxidant and growth related genes and non-specific immune response of common carp (Cyprinus carpio) fed dietary ferula (Ferula assafoetida). Fish Shellfish Immunol. 55, 242–248. doi: 10.1016/j.fsi.2016.05.038
Sapkota A., Sapkota A. R., Kucharski M., Burke J., McKenzie S., Walker P., et al. (2008). Aquaculture practices and potential human health risks: current knowledge and future priorities. Environ. Int. 34, 1215–1226. doi: 10.1016/j.envint.2008.04.009
Schneeberger E. E., Lynch R. D. (2004). The tight junction: a multifunctional complex. Am. J. Physiol. Cell Physiol. 286, C1213–C1228. doi: 10.1152/ajpcell.00558.2003
Shan C.-H., Guo J., Sun X., Li N., Yang X., Gao Y., et al. (2018). Effects of fermented Chinese herbal medicines on milk performance and immune function in late-lactation cows under heat stress conditions. J. Anim. Sci. 96, 4444–4457. doi: 10.1093/jas/sky270
Shi L., Wei L., Zhai H., Ren T., Han Y. (2021). An evaluation on betaine and trimethylammonium hydrochloride in the diet of carassius auratus: growth, immunity, and fat metabolism gene expression. Aquaculture Rep. 19, 100627. doi: 10.1016/j.aqrep.2021.100627
Shi H.-T., Zhao S.-Z., Wang K.-L., Fan M.-X., Han Y.-Q., Wang H.-L. (2022). Effects of dietary astragalus membranaceus supplementation on growth performance, and intestinal morphology, microbiota and metabolism in common carp (Cyprinus carpio). Aquaculture Rep. 22, 100955. doi: 10.1016/j.aqrep.2021.100955
Song X., Zhao J., Bo Y., Liu Z., Wu K., Gong C. (2014). Aeromonas hydrophila induces intestinal inflammation in grass carp (Ctenopharyngodon idella): an experimental model. Aquaculture 434, 171–178. doi: 10.1016/j.aquaculture.2014.08.015
Syawal H., Kurniawan R., Effendi I., Austin B. (2021). Fermented medicinal herbs improve hematological and physiological profile of striped catfish (Pangasianodonhypophthalmus). F1000Res 10, 466. doi: 10.12688/f1000research.52640.3
Taso O. V., Philippou A., Moustogiannis A., Zevolis E., Koutsilieris M. (2019). Lipid peroxidation products and their role in neurodegenerative diseases. Annals of research hospitals; vol 3 (January 2019): annals of research hospitals. 3, 1–10. doi: 10.21037/arh.2018.12.02
Valladão G. M. R., Gallani S. U., Pilarski F. (2015). Phytotherapy as an alternative for treating fish disease. J. Veterinary Pharmacol. Ther. 38, 417–428. doi: 10.1111/jvp.12202
Wang Q., Ayiku S., Liu H., Tan B., Dong X., Chi S., et al. (2022). Effects of dietary ESTAQUA® yeast culture supplementation on growth, immunity, intestinal microbiota and disease-resistance against vibrio harveyi in hybrid grouper (♀Epinephelus fuscoguttatus×♂E. lanceolatus). Aquaculture Rep. 22, 100922. doi: 10.1016/j.aqrep.2021.100922
Wang G.-H., Chen C.-Y., Lin C.-P., Huang C.-L., Lin C.-h., Cheng C.-Y., et al. (2016). Tyrosinase inhibitory and antioxidant activities of three bifidobacterium bifidum-fermented herb extracts. Ind. Crops Products 89, 376–382.doi:10.1016/j.indcrop.2016.05.037. doi: 10.1016/j.indcrop.2016.05.037
Wang Y., Li J., Xie Y., Zhang H., Jin J., Xiong L., et al. (2021b). Effects of a probiotic-fermented herbal blend on the growth performance, intestinal flora and immune function of chicks infected with salmonella pullorum. Poultry Sci. 100, 101196. doi: 10.1016/j.psj.2021.101196
Wang W., Wang C., Chen W., Ding S. (2021a). Advances in immunological research of amphioxus. Dev. Comp. Immunol. 118, 103992. doi: 10.1016/j.dci.2020.103992
Xu X., Li X., Xu Z., Yao W., Leng X. (2021). Dietary azomite, a natural trace mineral complex, improved the growth, immunity response, intestine health and resistance against bacterial infection in largemouth bass (Micropterus salmoides). Fish Shellfish Immunol. 108, 53–62. doi: 10.1016/j.fsi.2020.11.016
Xv Z., Zhong Y., Wei Y., Zhang T., Zhou W., Jiang Y., et al. (2021). Yeast culture supplementation alters the performance and health status of juvenile largemouth bass (Micropterus salmoides) fed a high-plant protein diet. Aquaculture Nutr. 27, 2637–2650. doi: 10.1111/anu.13391
Yarahmadi P., Kolangi Miandare H., Hoseinifar S. H. (2016). Haemato-immunological and serum biochemical parameters, intestinal histomorphology and growth performance of rainbow trout (Oncorhynchus mykiss) fed dietary fermentable fibre (Vitacel®). Aquaculture Nutr. 22, 1134–1142. doi: 10.1111/anu.12388
Yu Z., Li L., Zhu R., Li M., Duan J., Wang J.-Y., et al. (2020). Monitoring of growth, digestive enzyme activity, immune response and water quality parameters of golden crucian carp (Carassius auratus) in zero-water exchange tanks of biofloc systems. Aquaculture Rep. 16, 100283. doi: 10.1016/j.aqrep.2020.100283
Zhang H., Ran C., Teame T., Ding Q., Hoseinifar S. H., Xie M., et al. (2020a). Research progress on gut health of farmers teleost fish: a viewpoint concerning the intestinal mucosal barrier and the impact of its damage. Rev. Fish Biol. Fisheries 30, 569–586. doi: 10.1007/s11160-020-09614-y
Zhang X., Sun Z., Cai J., Wang J., Wang G., Zhu Z., et al. (2020b). Effects of dietary fish meal replacement by fermented moringa (Moringa oleifera lam.) leaves on growth performance, nonspecific immunity and disease resistance against Aeromonas hydrophila in juvenile gibel carp (Carassius auratus gibelio var. CAS III). Fish Shellfish Immunol. 102, 430–439. doi: 10.1016/j.fsi.2020.04.051
Zhang X. H., Sun Z. Y., Cao F. L., Ahmad H., Yang X. H., Zhao L. G., et al. (2015). Effects of dietary supplementation with fermented ginkgo leaves on antioxidant capacity, intestinal morphology and microbial ecology in broiler chicks. Br. Poultry Sci. 56, 370–380. doi: 10.1080/00071668.2015.1030590
Zhang X., Zhang B., Li L., Li X., Zhang J., Chen G. (2022). Fermented noni (Morinda citrifolia l.) fruit juice improved oxidative stress and insulin resistance under the synergistic effect of Nrf2/ARE pathway and gut flora in db/db mice and HepG2 cells. Food Funct. 13, 8254–8273. doi: 10.1039/d2fo00595f
Zhao W., Cui X., Wang Z.-Q., Yao R., Chen M.-D., Gao B.-Y., et al. (2022). Effects of barranca yajiagengensis powder in the diet of trachinotus ovatus on the growth performance, antioxidant capacity, immunity and morphology of the liver and intestine. Antioxidants 11 (7), 1220. doi: 10.3390/antiox11071220
Zhao L., Tang G., Xiong C., Han S., Yang C., He K., et al. (2021). Chronic chlorpyrifos exposure induces oxidative stress, apoptosis and immune dysfunction in largemouth bass (Micropterus salmoides). Environ. pollut. 282, 117010. doi: 10.1016/j.envpol.2021.117010
Zhou Y.-L., He G.-L., Jin T., Chen Y.-J., Dai F.-Y., Luo L., et al. (2021). High dietary starch impairs intestinal health and microbiota of largemouth bass, micropterus salmoides. Aquaculture 534, 736261. doi: 10.1016/j.aquaculture.2020.736261
Keywords: fermented Chinese herbs, largemouth bass, intestinal health, immunity parameters, A.ydrophila
Citation: Jiang L, Zhou X, Bao S, Wu Q, Li J, Wang Y and Liu B (2023) The consumption of fermented Chinese herbs has resulted in better intestinal health and increased resistance to Aeromonas hydrophila in juvenile largemouth bass (Micropterus salmoides). Front. Mar. Sci. 10:1199910. doi: 10.3389/fmars.2023.1199910
Received: 04 April 2023; Accepted: 02 May 2023;
Published: 12 May 2023.
Edited by:
Amina Zuberi, Quaid-i-Azam University, PakistanReviewed by:
Chang’an Wang, Chinese Academy of Fishery Sciences, ChinaGang Yang, Nanchang University, China
Copyright © 2023 Jiang, Zhou, Bao, Wu, Li, Wang and Liu. This is an open-access article distributed under the terms of the Creative Commons Attribution License (CC BY). The use, distribution or reproduction in other forums is permitted, provided the original author(s) and the copyright owner(s) are credited and that the original publication in this journal is cited, in accordance with accepted academic practice. No use, distribution or reproduction is permitted which does not comply with these terms.
*Correspondence: Yachao Wang, d2FuZ3lhY2hhbzE2ODhAMTI2LmNvbQ==; Bo Liu, bGl1YkBmZnJjLmNu
†These authors have contributed equally to this work and share first authorship