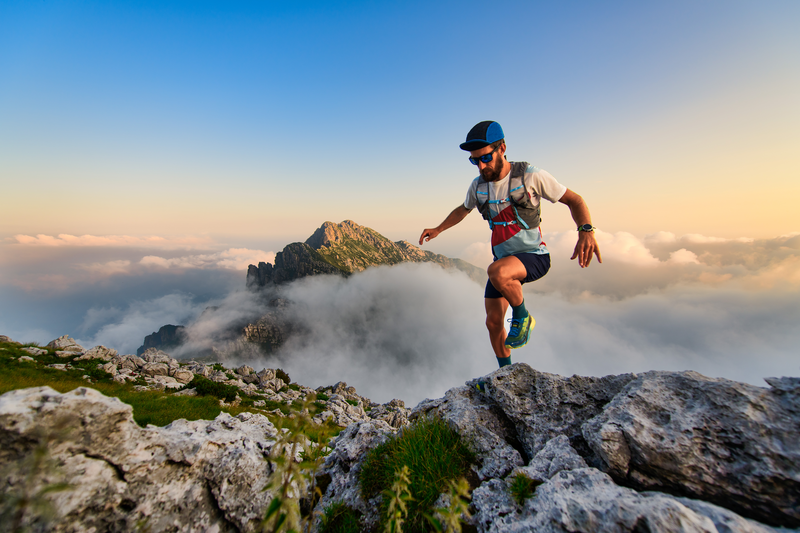
95% of researchers rate our articles as excellent or good
Learn more about the work of our research integrity team to safeguard the quality of each article we publish.
Find out more
ORIGINAL RESEARCH article
Front. Mar. Sci. , 17 May 2023
Sec. Marine Ecosystem Ecology
Volume 10 - 2023 | https://doi.org/10.3389/fmars.2023.1199048
This article is part of the Research Topic Restoration of Coastal Marine Ecosystems View all 22 articles
Marine heat waves (MHWs) are affecting corals populations, advocating their inclusion in restoration actions since conservation measures may be not sufficient. Cladocora caespitosa is a Mediterranean reef-building, long-living species, with low recruitment rate and high juvenile mortality, leading to the need for its inclusion in international and European legislations. The aim of this study, conducted in the southern Tyrrhenian Sea, was to test the translocation of several C. caespitosa colonies thriving on an artificial substrate intended for demolition, applying transplantation techniques. Thirty-four colonies were transplanted in May 2018, and monitored over 4 years, to check for their persistence and health status. The shaded position of the recipient site resulted adequate, considering that colony survival rates were as high as 82.4%, 70.6% and 55.9% in October 2018, January 2020 and October 2022, respectively. Colonies presented signs of suffering only after the high temperatures occurred during summer 2022, with a decreasing rate of -2.5 ± 0.4 corallite/month. To better interpret the documented survival rates, 40 fragments of C. caespitosa were reared in aquaria to test temperature and light effects on growth rates and resistance to thermal stress, simulating a MHW and exacerbating the dim-light natural conditions of the recipient site, exposing half of the fragments to complete darkness. Only bigger fragments produced new corallites, with a rate of 1.3 ± 0.3 corallites/month, like the natural growth rate obtained in the field before the thermal anomaly, highlighting the suitability of ex-situ rearing as a potential tool to supply restoration project. After 5-days at 28.5°C, all fragments survived, despite showing tissue retraction, shorter tentacles, lower responsiveness, and zooxanthellae density variation. Overall, our results highlighted a promising plasticity of C. caespitosa in the field, representing a good candidate for restoration purposes. In aquaria this adaptive potential has been tested on a single genotype and more tests are needed to assess the intraspecific variability of these responses. A first insight into the species-based siting selection was provided to ensure the success of a restoration action. Our results point out the importance of knowing life history traits and ecological optima to design proper management and restoration measures.
Cladocora caespitosa (Linnaeus, 1767) is a colonial zooxanthellate scleractinian, endemic to the Mediterranean Sea, where it is considered the most important reef-building species (Kružić and Benković, 2008), with a carbonate production up to 1.1–1.7 Kg CaCO3/m2/y (Peirano et al., 2001). This species can grow in a wide range of environmental conditions, from sheltered to exposed bottoms, and from the infralittoral plain, where it successfully competes with macroalgae, to the circalittoral one, where zooxanthellae can survive using indirect and diffuse light (Peirano et al., 1998; Peirano et al., 1999; Hoogenboom et al., 2010; Kersting et al., 2017a). Local hydrodynamic conditions, together with the typology and morphology of the substrate, influence colonies’ shape and growth patterns (Abel, 1959; Kersting and Linares, 2012). According to Peirano et al. (1998), C. caespitosa can be present as solitary colonies, beds (i.e., numerous and separate globose colonies) or banks (i.e., large frameworks of colonies reaching several square meters of extension), but also in mixed distributions, and it is also often found associated with rhodolits (Kersting et al., 2017b).
C. caespitosa is a long-living, slow growing species, with a very low recruitment rate and a high juvenile mortality (Kersting et al., 2014a). The longevity of this species (with a mean age of 50 years, up to 300 years for some colonies in the Columbretes Islands, Spain; Kersting and Linares, 2012), coupled with the production of a calcium carbonate skeleton, make C. caespitosa a suitable proxy to reconstruct past climate fluctuations on a decadal and centennial scale (Peirano and Kružić, 2004; Silenzi et al., 2005). At the same time, these same features make C. caespitosa extremely vulnerable to a variety of threats, like invasive algae (Kružić et al., 2008; Kersting et al., 2014b; Kersting et al., 2015), eutrophication (Kružić and Požar-Domac, 2007), pollution (El Kateb et al., 2016), trawling, dredging and related increase in sedimentation (Casado-Amezúa et al., 2015), limiting the distribution of extensive banks only to the Tunisian coast, the Gulf of Atalanta (Aegean Sea), the Ligurian Sea, the Columbretes Islands and Croatia (Laborel, 1961; Zibrowius, 1980; Peirano et al., 1998; Kersting and Linares, 2012). Despite these human pressures, the main reason for the ongoing regression of this coral over the last decades is the occurrence of mortality events, caused by the rapid increase of average sea temperatures and the increased frequency of marine heat waves (MHWs) (Rodolfo-Metalpa et al., 2005; Kružić et al., 2012; Jiménez et al., 2016; Garrabou et al., 2019; Garrabou et al., 2022). C. caespitosa is, in fact, well adapted to the seasonal temperature oscillations typical of the Mediterranean, but the extreme temperatures related to climate change (Lejeusne et al., 2010) affect its growth and health, causing bleaching and leading to colonies’ death (Jiménez et al., 2016). Considering its crucial role as ecosystem engineer (Jones et al., 1994) and its rapid decline and low recovery capability (Kersting et al., 2014a), C. caespitosa has been listed as Endangered in the IUCN Red List at Mediterranean regional level (Casado-Amezúa et al., 2015), and it is included in the Appendix II of the CITES, Annex II of the Protocol SPA/BD and in the Annex B of the EU Regulation Trade wild fauna and flora species (Otero et al., 2017). On the other hand, this coral has historically and recently shown unexpected rejuvenescence after warming-induced mortality events (i.e., the formation of a new polyp within an almost totally necrotic one) (Fedorowski, 1980; Kersting and Linares, 2019), suggesting an unexpected resilience and a high potential for successful translocation and restoration activities.
The endangered status of this species and its fundamental role in Mediterranean ecosystems advocate its inclusion in restoration actions, since conservation measures alone may be not sufficient (Swan et al., 2016; Fariñas-Franco et al., 2018). In fact, restoration, defined as “the process of assisting the recovery of an ecosystem that has been degraded, damaged, or destroyed” (SER, 2023), has been acknowledged as complementary to conservation and necessary to recover ecosystems’ functioning (Normile, 2009). Tropical scleractinians are among the marine organisms most involved in restoration projects, together with other invertebrates (mainly anthozoans and bivalves), vertebrates (fish and birds), algae and plants (mangroves, salt-marsh plants, seagrasses) (Rinkevich, 1995; Epstein et al., 2001; Rinkevich, 2005; Bayraktarov et al., 2016; Destro et al., 2018; Boström-Einarsson et al., 2020; Dehnert et al., 2022; Dehnert et al., 2023). One of the most implemented methods in tropical reef restoration is the translocation or transplantation of coral colonies (Kotb, 2016; Ferse et al., 2021), a technique that does not only benefit the translocated individuals, but also provides tangible conservation benefits at population, species or ecosystems levels (IUCN Species Survival Commission, 2012). These characteristics make restoration a powerful tool to preserve specimens of locally rare and threatened species when the primary habitat is destroyed (Butt et al., 2021).
To design proper management and restoration measures, it is crucial to know life history traits, the ecological optima, and the potential threats compromising the survival of the target species. In this sense, the hermatypic coral C. caespitosa was here tested as a model species: considering its symbiosis with zooxanthellae, the species could be more sensitive to the effect of climate warming, as already demonstrated in tropical corals (e.g., Sammarco and Strychar, 2009; Brown and Cossins, 2011; Jones and Berkelmans, 2012; Pettay et al., 2015; Lough et al., 2018). Neverthless, autotrophic symbionts may also buffer the presence of multiple stressors (e.g., thermal stress and low food availability), as hypothesized for the temperate sea whip Eunicella singularis (Esper, 1791) (Fava et al., 2010).
Here we present the first attempt to apply the translocation technique, with restoration purposes, on C. caespitosa. A long-term monitoring on the transplantation site was conducted to assess the survival and status of relocated colonies. To complement and support the relocation activity, additional fragments of C. caespitosa were reared in aquaria as a potential source of corallites and colonies to supply future restoration actions. Additionally, considering the increasing impact of climate change on Mediterranean habitats, a MHW was simulated to test the resistance of C. caespitosa fragments to rising temperatures.
The transplantation was carried out in the Bagnoli Bay, located in the western part of the Gulf of Naples (Tyrrhenian Sea, Italy) (Figures 1A, B). Bagnoli was characterized by high levels of pollution in the sediments due to the historical presence, in the nearby area, of the dismissed industrial area of Bagnoli-Coroglio (Armiento et al., 2020). The National authorities identified the area as a Site of National Interest (SNI) and a priority area for habitat restoration (Dpr. 31/08/2001).
Figure 1 Map showing (A) the location of Bagnoli Bay (Naples, Italy) and (B) the detail of the donor and recipient sites of Cladocora caespitosa colonies. Picture of (C) the colony sampling with hammer and chisel and (D) the colony translocation using a two-component epoxy.
In the framework of the project ABBaCo (“Pilot experiments for the environmental restoration and balneability of the Bagnoli-Coroglio coastal area”), colonies of Cladocora caespitosa were translocated from two docks intended for demolition (depth ~ 9 m), (i.e., the donor site) to a site located in the north-western side of the Nisida Island (40°47’52.0”N, 14°09’32.6”E) (Figure 1B). The recipient site in Nisida Island was selected since its seabed presents the only natural hard substrates of the area (Gaglioti et al., 2020), the depth is comparable with the donor site and its north-western side is the most sheltered from the strong south-easterly winds (De Pippo et al., 2002). Additionally, the altitude of the island (~110 m) shaded the recipient site for most of the day. The presence of already existing colonies in the recipient area (Carlo Cerrano personal observation) together with the low genetic connectivity of the species (Casado-Amezúa et al., 2014) made the north-western side of the Nisida Island a good selection to avoid the isolation of the transplanted colonies.
Field activities started in May 2018 (T0) and performed by two scientific divers, after a pre-survey conducted in July 2017. Colonies of C. caespitosa were gently detached from the substrate with hammer and chisel (Figure 1C), stored in a tank avoiding air exposure, and brought to the recipient area. Colonies were attached through a two-component epoxy (Subcoat S, Veneziani Yachting; https://venezianiyachting.com/) on small portions of rock (Figure 1D), previously cleaned from epibionts with a metal brush. Colonies were transplanted in exposed horizontal substrates to avoid the potential detachment before the complete hardening of the epoxy.
The shape of C. caespitosa colonies at the donor site, belonged to the morphologies described by Abel (1959) for individuals exposed to high water movement and shaded conditions. In the recipient site, characterized by similar environmental features, a total of 34 colonies were monitored.
The condition of transplanted colonies was checked a first time one week after the transplantion (T1), where only the persistence and status of the colonies was evaluated, since the potential loss of the fragments for detachment usually occurs right after the transplantion (Guest et al., 2011). Afterwards, three subsequent monitoring events were conducted in October 2018 (T2), January 2020 (T3) and October 2022 (T4), during which the persistence of the colonies was evaluated through visual census, and a photo of each colony was taken to assess their health status and potential growth. Pictures were taken using a Canon G9X camera, at 34 cm distance from the substrate with the support of a 28 cm x 21 cm frame (0.0588 m2) (Roveta et al., 2022).
The effectiveness of the transplantion was evaluated monitoring the persistence of colonies at T1, T2, T3 and T4, compared to the colonies originally translocated at T0, by calculating the frequency (%) of healthy (i.e., attached and not bleached, with no evident sign of stress, such as tissue necrosis or tissue retraction), bleached (i.e., attached, with tissue still present but bleached) and detached (i.e., not in place, either lost or dead) colonies. Their potential growth was assessed by photo sampling, counting the number of healthy corallites of each colony at T2, T3 and T4 (reported as average ± standard error, SE). The change in the number of corallites per month (i.e., increase and/or decrease, reported as average ± SE) was calculated as the difference in the number of corallites between each sampling time and T0. Additionally, the size of the colonies was estimated through the software ImageJ (Rasband, 2012) and correlated to the number of corallites of each colony through time.
Potential differences in the number of corallites per sampling time were checked with the non-parametric Kruskal-Wallis analysis of variance. If statistically significant differences were found, the Dunn’s post-hoc comparison with the Bonferroni correction of the p-value was performed. The analyses were performed using the free software PAST (PAleontological STatistics), version 4.05 (Hammer et al., 2001), and a 95% confidence interval.
SSTs data were obtained from the National Oceanic and Atmospheric Administration (NOAA) Optimum Interpolation Sea Surface Temperature (OISST), a product with a global 1/4-degree gridded dataset of Advanced Very High Resolution Radiometer derived SSTs at a daily resolution. For the detection and description of MHWs, the time series from 01-01-1982 to 31-12-2022 was considered, using the standardized method developed in Hobday et al. (2016; 2018). Each MHW was individualized and characterized in terms of event duration, frequency, intensity, and cumulative intensity (Table S1), thanks to the R package heatwaveR (Schlegel and Smit, 2018), which also allowed to classify each event in four categories (I-moderate, II-strong, III-severe, and IV-extreme), as defined by Hobday et al. (2018).
Regarding MHWs, only the period from June 2018 to October 2022 was considered for the analyses. Differences in the maximum intensity, cumulative intensity, duration, and frequencies of MHWs of each year were investigated using the non parametric Kruskal–Wallis analysis of variance.
Conversely, for the analyses of the SSTs, only the summer periods (from the 21/06 to the 22/09; Table S2) of each year of monitoring (2018, 2019, 2020, 2021, 2022) were considered. Differences in the SSTs over the years were investigated using the Kruskal-Wallis test in PAST, version 4.05 (Hammer et al., 2001). The Dunn’s post-hoc comparison with the Bonferroni correction of the p-value was performed when significant differences were found.
An additional sample of C. caespitosa was collected in Alassio (Ligurian Sea, Italy) in August 2022 at 20 m depth and maintained in aquaria to perform two experiments testing the growth and budding rates and thermal stress resistance. The choice to collect samples from a different site (far from Bagnoli) was related to the literature available on the response of C. caespitosa under controlled conditions, that in the Italian seas mainly came from the Ligurian area.
Prior to the start of the experiment, the colony was divided in 40 fragments with a different number of corallites (10 fragments with 1, 2, 3, 4 corallites, respectively), and left to acclimate for two weeks, before the start of the experiment on December 19th, 2022. Eight 30 L aquaria were set up, each one equipped with a pump (NEWA® Maxi, 375 L/H) and a biological filter, and positioned inside a bigger tank of around 400 L to guarantee a continuous recirculation of the water. Temperature was maintained at 18°C with a heat exchanger (Askoll THERM XL 200W) and salinity was maintained constant at 35 PSU.
The dim-light natural conditions, characterizing the recipient site in Bagnoli, were here exacerbated, exposing fragments to a complete darkness. In fact, four aquaria were maintained in dark conditions, while the other four received a 12h:12h light:dark photoperiod by means of a POWER-GLO lighting system (1750 lumen, 40 W). Five fragments, with the same number of corallites, were placed in each aquaria, and fed twice a week with freshly hatched Artemia salina nauplii (Figure 2A). The quantity of food distributed per aquaria is reported in Figure 2A and calculated following Leal et al. (2014). During feeding periods, a small aquarium of 1.4 L was positioned over the fragments to maintain a high food concentration and optimize the possibility of polyps’ to feed; additionally, the continuous recirculation was stopped for an hour to avoid changes in the water parameters.
Figure 2 Experimental set-up. (A) Growth rate experiment: eight 30L aquaria (four with 12h:12h light:dark photoperiod and four under dark conditions) containing 5 fragments each of Cladocora caespitosa, with 1-, 2-, 3-, 4-corallites, respectively. (B) Thermal stress experiment: four 30L aquaria (two with 12h:12h light:dark photoperiod and two under dark conditions) containing three 2L beakers in a water bath, with one fragment of C. caespitosa each. In two aquaria (control, CTRL), the temperature was stable (18°C) and in other two the temperature was increased up to 28.5°C. The amount of food distributed per aquaria is also shown.
The experiment lasted for 2 months, until the 19th of February, during which fragments’ growth rate and the presence of new corallites were evaluated in both experimental conditions (light and dark) with a photographic sampling performed every 2 weeks. Pictures were taken orthogonally with a GoPro8 hero Black and a metric reference on the side of each fragment. Photos were analyzed using ImageJ (Rasband, 2012), and the surface area of fragments and of new corallites was measured. The increase in the number of corallites per month (reported as average ± SE) was calculated for each fragment as the difference in the number of corallites between the start and the end of the experiment. For samples maintained in the dark, the level of depigmentation was evaluated using the Australian CoralWatch Coral Health Chart (https://coralwatch.org). Additionally, at the end of the experiment, the zooxanthellae density (see paragraph below) was evaluated in both conditions to determine if the potential loss in color could be related with a lower number of zooxanthellae.
The measures of the areas were tested for normality and homogeneity using the Shapiro-Wilk and Levene tests, then data were analyzed with a repeated measure ANOVA using the free software PAST, version 4.05 (Hammer et al., 2001). The Tukey post-hoc comparison was used when significant differences were found.
Before and after the experiment, the number of zooxanthellae was determined in 6 corallites per treatment using the following protocol: a single corallite (including soft tissues and skeleton) was homogenized in a mortar using a pestle with the addition of 1.5 ml of artificial seawater. Then, the homogenate was centrifuged at 5000 rpm for 5 minutes, the supernatant removed, and the resulting pellet was resuspended in 1 ml of artificial seawater. Zooxanthellae were counted using an Improved Neubauer haemocytometer grid (bright line BRAND Tiefe Depth Profondeur 0.100 mm 0.0025) in a volume of 10 μl under a light microscope (Nikon model C-PS N).
During the counting, zooxanthellae (reported as average ± SE) were classified in two classes, single (i.e., single rounded cells) and doublets (i.e., mitotic cells with a cell plate and wall material clearly visible between cells) (Wilkerson et al., 1988). Doublets were counted and the mitotic index (%) calculated as follow:
To evaluate the response to thermal stress (survivorship and number of zooxanthellae) of C. caespitosa, only the fragments producing more buds (12) in the growth rate and budding experiment were used and were exposed to increasing temperatures, from 18°C to 28.5°C (i.e., maximum temperature recorded in Bagnoli; Table S2). To this end, four 30 L aquaria were set up, each one containing three 2 L beakers in water bath, with one fragment of C. caespitosa each. The beakers were not fully submerged, with no re-circulation among the beakers and the 30 L tank which was only used to maintain the temperature stable (Figure 2B). The water inside the beakers was changed every 2 days to avoid any shift in the water parameters (e.g. nitrates increase). Two 30 L aquaria were considered as control and maintained at 18°C, while the temperature in the other two 30 L aquaria was gradually increased (1°C per day) up to 28.5°C. This temperature was then maintained for 5 days, to simulate a MHW (see Hobday et al., 2016 for definition). One control and one experimental aquaria were maintained in dark conditions, while the other control and the other experimental aquaria with a 12h:12h light:dark photoperiod by means of a LED light BluBios (800 lumen, 14 W). Fragments were fed twice a week, as explained above (Figure 2B). At the end of the experiment, the zooxanthellae density was evaluated for all treatments (control and experimental) and conditions (light and dark) following protocol previously presented.
Also for this analysis, the free software PAST (version 4.05; Hammer et al., 2001) was used. The normality and homogeneity of the data were tested before running statistical analyses. A two-way analysis of variance (two-way ANOVA) with period (two levels: pre and post) and condition (two levels: light and dark) as factors was performed to test differences in the number of zooxanthellae and mitotic index among the control organisms. A second two-way ANOVA with treatment (two levels: control and experiment) and condition (two levels: light and dark) as factors was conducted to check for differences also between the control and the experiment. In both tests, the Tukey post hoc comparison was used when significant differences were found.
Of the 34 monitored colonies, after the first check on their status at T1, 5 colonies (14.7%) were found detached, decreasing the total number of healthy colonies to 29 (85.3%). Overall, a general decrease in the percentage of healthy colonies was recorded during years, with 28 colonies (82.4%) at T2, 24 (70.6%) at T3 and 19 (55.9%) at T4. At T2 and T3, one colony (2.9%) was completely bleached, while at T4 this number increased up to 5 (14.7%). The percentage of detached colonies after T1 was the same at T2 (14.7%) but increased with time reaching 9 colonies (26.5%) at T3 and 10 colonies (29.4%) at T4 (Figure 3A).
Figure 3 (A) Bar plot showing the percentage of healthy, detached, and bleached colonies in the five monitoring times (T0 = May 2018; T1 = one week after T0; T2= October 2018; T3 = January 2020; T4 = October 2022). (B) Linear correlation, with 95% confidence interval (represented by the grayish area), between the number of corallites and the colony areas.
The average number of corallites of transplanted colonies showed statistical differences during time (Kruskal-Wallis, p< 0.05), with a significant increase in T3 (181 ± 46 corallites) compared to T0 (102 ± 25 corallites) and T4 (92 ± 27 corallites) (Dunn’s post-hoc, p< 0.05), but not with T2 (125 ± 30 corallites) (Dunn’s post-hoc, p > 0.05). During this period, an increase in the number of corallites per month was recorded, being 1.9 ± 0.7 corallites/month, with a positive correlation (y = 0.2803x - 4.9844, R2 = 0.92) between the colony area and the number of corallites (Figure 3B). Conversely, a significant decrease in the average number of corallites was recorded in T4 compared to T3 (see above) (Dunn’s post-hoc, p< 0.05), with a rate of -2.5 ± 0.4 corallites/month.
Since 2018, a total of 43 MHWs occurred in the Bagnoli area (8 in 2018, 2020 and 2021, 9 in 2022 and 10 in 2019), with most of the event categorized as I-moderate, and only 3 as II-strong (1 during August 2018 and 2 in 2022, in July and October, respectively) (Table S1).
Considering the SSTs, the highest temperatures were recorded during July-August of 2018 and 2022, reaching almost 29°C (Table S2). Overall, the summer of 2022 can be considered the warmer one, with the mean temperature per month being the highest compared with previous years, especially in June (26.6 ± 1.0°C) and July (27.2 ± 0.8°C) (Table 1).
Table 1 Average sea surface temperatures (SST ± standard deviations) at Bagnoli Bay (Naples, Italy) recorded during the summer months of each year of monitoring (2018–2022).
Even though no differences were found in the maximum intensity, cumulative intensity, frequency and duration of MHWs among years, significant differences were detected among the SSTs recorded during the summer period of each year of monitoring (Kruskal-Wallis, p< 0.001), with the SSTs registered in 2022 (26.9 ± 1.0°C) being significantly higher than all the past years, and the ones registered in 2020 (25.9 ± 1.1°C) and 2021 (25.9 ± 1.0°C) significantly lower (Dunn’s post-hoc, p< 0.001) (Figure 4; Table 1).
Figure 4 Box plot showing the sea surface temperatures (SSTs) recorded in Bagnoli Bay during the summer period (21/06-22/09) of each year of monitoring, from 2018 to 2022. Solid lines represent the median values; the rhombuses indicate the average values.
During the entire duration of the experiment, all fragments were observed healthy in both conditions (dark and light). Fragments exposed to light did not show any signs of bleaching (Tables 2, 3A), keeping a tone of E6-5 of the Australian CoralWatch Coral Health Chart for all the duration of the experiment. Conversely, all fragments placed in dark conditions started from a tone of E6-5 and, already after 3 weeks, 8 out of 20 fragments started to bleach, while, at the end of the experiment, except for one 1-corallite fragment with a tone of E5, all the others bleached reaching tone E2 (Table 2). Related to this, also the content of zooxanthellae significantly varied (Table 3A) between light and dark conditions (Tukey post-hoc, p<0.05), with higher densities recorded in light-exposed fragments (Table 2).
Table 2 Coral tissue tones, average zooxanthellae contents (± standard error) and average mitotic index (± standard error) measured in fragments of Cladocora caespitosa in different periods (pre- and post-thermal stress experiment) and exposed to different treatments (control, CTRL, and experimental, EXP) and conditions (light, 12h:12h light:dark photoperiod, and dark).
Table 3 Two-way analysis of variance (ANOVA) to test differences in the: (A) zooxanthellae density between periods (pre, post) and conditions (light, dark); (B) zooxanthellae density between treatments (control, experimental) and conditions (light, dark); (C) mitotic index between periods (pre, post) and conditions (light, dark); (D) mitotic index between treatments (control, experimental) and conditions (light, dark).
For fragments under light conditions, three 3-corallites fragments and all 4-corallites fragments showed the presence of new corallites already after three weeks (1-4 new corallites each), with an average increase of 1.3 ± 0.3 corallites/month. Smaller fragments (1-corallite and two 2-corallites) produced only 1 new corallite each. Under dark conditions, only two 3-corallites fragments showed 1 new corallite.
Apart from three colonies maintained in light conditions, which showed a significant increment in the total area already after one month (Tukey post-hoc, p<0.05), no significant changes were found for any of the other fragments during the entire duration of the experiment, being the area of the new corallites generally very low (from 0.003 mm² to 0.011 mm²).
Overall, all colonies survived the thermal stress, some of them also displaying new corallites. As a matter of fact, one fragment maintained under dark conditions and subjected to the simulated MHW showed 2 new corallites, while one control and two experimental fragments under light conditions showed 1 and 2 new corallites, respectively.
However, observing fragments under the stereomicroscope, control fragments presented expanded polyps and tentacles (Figures 5A, B), while experimental ones displayed visible shorter tentacles, and polyps never fully opened (Figures 5C, D). Only control fragments maintained under light conditions kept an intact tissue over the skeleton (Figure 5E), while a clear reduction of the tissue coverage was observed in the control dark and both experimental conditions (Figures 5F-H). Additionally, fragments exposed to thermal stress showed a reduced response with a slower closure of the polyps, especially for the ones kept under the light.
Figure 5 Pictures of Cladocora caespitosa fragments exposed to control temperature (CRTL, 18°C) and thermal stress (EXP, 28.5°C). Top view of control corallites maintained under (A) light and (B) dark conditions presenting expanded polyps and tentacles, and of experimental corallites maintained under (C) light and (D) dark conditions with visible shorter tentacles, and less extended polyps. Lateral view of control corallites maintained under (E) light and (F) dark conditions showing intact and retracted tissue respectively, and of experimental corallites maintained under (G) light and (H) dark conditions displaying a clear reduction of the tissue coverage. A pale color characterizes all the fragments maintained in dark conditions. Scale bars = 2 cm.
The two-way ANOVAs showed statistically significant differences in the total number of zooxanthellae per cm2 (Table 3B), especially between light and dark conditions in all periods and under any treatment (Tukey post-hoc, p<0.05), with higher values found in the fragments exposed to light (Table 2). Additionally, under light conditions, the number of zooxanthellae resulted significantly higher in the fragments exposed to thermal stress (13.71 ± 0.52 ×107 cell/cm2) compared to the control ones (12.37 ± 0.11 ×107 cell/cm2). Also for the mitotic index, significant differences were found (Tables 3C, D), with generally higher values in fragments under light conditions (Table 2) (Tukey post-hoc, p<0.05). However, in fragments maintained in dark conditions and exposed to thermal stress, an increase in the mitotic index was recorded (4.3 ± 0.6%), reaching values similar to the ones recorded in fragments exposed to the light (4.9 ± 0.2% and 5.7 ± 0.4% for control and thermal stress, respectively), and resulting significantly different from the control dark (3.0 ± 0.2%; Table 2) (Tukey post-hoc, p<0.05).
Ecosystem restoration is essential to recover degraded habitats worldwide impacted by a synergy of anthropogenic and natural stressors (SER, 2023). In this sense, the United Nations has declared the Ecosystem Restoration Decade (2021-2030) to boost global-scale initiatives engaging not just the academic or scientific environments but also non-governmental organizations, managers and stakeholders (UNEP, 2021). However, the scarcity of knowledge on species ecology and their response to environmental and anthropogenic stressors might impair the success of any restoration attempt.
Cladocora caespitosa is a well-studied species that, over the last years, experienced mass mortality events at Mediterranean scale (Kersting et al., 2013; Garrabou et al., 2019; Garrabou et al., 2022). Even though it is one of the most important reef-building species of the basin (Kružić and Benković, 2008), no restoration effort has been made so far. In this study, several colonies of C. caespitosa were translocated to avoid their damage due to demolition activities, following their fate for 4 consecutive years. This study represents one of the few records of long-term monitoring, since most of the studies usually report less than 18 months of survey (Boström-Einarsson et al., 2020), and one of the few focusing on a temperate coral (Steinberg et al., 2020).
The survival rate recorded in this study after 19 months is higher (82.4%) than the average survival reported for tropical species (60-70%, Boström-Einarsson et al., 2020). However, in the long-term, we observed a decrease of survival percentage, reaching a value of 55.9%, still in line with previous short-term studies (Boström-Einarsson et al., 2020). This decline might be related with the extremely high temperatures recorded during summer 2022, which caused stress conditions and mortality events also in other cnidarians, such as the red coral Corallium rubrum (Linnaeus, 1758) (Roveta et al., 2023), and other gorgonian species, such as Paramuricea clavata (Risso, 1826) a and Eunicella cavolini (Kock, 1887) (Gómez-Gras et al., 2022). Despite the thermal anomalies experienced in the study area (Table S1, S2), more than half of the colonies survived, resisting these extreme conditions. This resilience might be related to the peculiar characteristics of the recipient site, shaded during most of the day, thus, reducing the direct exposure of the coral to sunlight, potentially limiting the damages of an excessive irradiance (Hoogenboom et al., 2006). As a matter of fact, a high irradiance coupled to an increased temperature might be detrimental for the fitness of the coral, affecting the photosynthetic rates and inducing bleaching (Ban et al., 2014). For future application of this translocation method, an accurate measurement of the irradiance should be performed both in the donor and recipient sites to ensure the survival of the transplanted colonies. Furthermore, it is recommended to apply respirometry and pulse amplitude modulation fluorimetry to monitor the photosynthetic potential of the transplanted colonies over time.
The percentage of detached colonies was lower (14.7%) than reported for tropical massive species (e.g., Diploastrea heliopora (Lamark, 1816)) after 6 months of transplantation (40%, Bongiorni et al., 2011), even though it increased during the following years of monitoring. Despite the selection of a sheltered recipient site, this higher detachment might be related to the abundant mucus production typical of the species (Herndl and Velimirov, 1986; Peirano et al., 1999), which has been demonstrated to be a factor favoring the observed phenomenon (Bongiorni et al., 2011). Moreover, the lack of a frequent cleaning and maintenance of the transplanted colonies could have been an additional factor promoting detachment (Bongiorni et al., 2011). Since the beginning, bleaching was observed in a single colony (2.9%), right after the summer of 2018. Nonetheless, this number increased at an alarming rate (14.7%) during our last monitoring in October 2022 (T4). The increase in duration of episodes of anomalously high SSTs in the western Mediterranean, as well as in the recipient site (up to 28.5°C; Table S2), might have induced a stronger stress in the translocated colonies. Additionally, a significant reduction of the total number of healthy corallites per colony was observed in T4, with an overall decreasing rate (-2.5 ± 0.4 corallites/month) higher than the increasing rate observed in the time interval between T0 and T3 (1.9 ± 0.7 corallites/month). Bleaching as well as tissue necrosis were the main causes of this trend, possibly related to the high temperatures (over 28°C) experienced in our site for more than 45 days (Table S2), as already demonstrated in controlled conditions by Rodolfo-Metalpa et al. (2006). A general reduction in colony size with the increase of temperature seems to be a common condition along the Italian coasts, being also observed in the Ligurian Sea since the ‘90s (Rodolfo-Metalpa et al., 2005; Azzola et al., 2022).
Compared to the field observations, a similar value of new corallites (1.3 ± 0.3 corallites/months) was obtained in the growth rate experiment, supporting the feasibility of aquaria as a potential ex-situ nursery also for this species (Ng et al., 2012; Merck et al., 2022). Budding of new polyps was observed mostly on fragments with multiple corallites both under light and dark, but with a lower rate in the last condition. Asexual reproduction through budding requires high energy expenditure and it is normally observed under favorable conditions and high availability of zooplankton (Rodolfo-Metalpa et al., 2008). Although fragments in dark conditions received the same amount of food, the scarce autotrophic contribution might explain this difference in the number of new polyps. This positive effect of light is further supported by the fact that in smaller fragments the production of new polyps was observed only under light conditions. Nonetheless, this imbalance between small and big fragments (both exposed to light) might be related: (i) on the one hand, to the experimental set-up, since following Leal et al. (2014), the quantity of food given was estimated per corallite, thus making the quantity of food available for 1-corallite fragments more diluted than for the ones with more corallites; (ii) on the other hand, to the fact that a fragment with more corallites presents a higher tentacle density, increasing the ‘encounter zone’ (i.e., by the extension of the polyps’ tentacles, in which prey are likely to be caught) (Madin, 1988), which likely renders the total energy available to be relatively higher in bigger colonies (Sakai et al., 2019).
An increase in the number of corallites was also recorded in some fragments exposed to the simulated MHW (both in light and dark conditions), suggesting temperature as a triggering factor for asexual reproduction through budding, a condition also common in other Mediterranean cnidarians (Di Camillo et al., 2021) as well as in tropical scleractinians (e.g., Pocillopora damicornis (Linnaeus, 1758), Jiang et al., 2017). Nonetheless, all experimental fragments showed clear signs of stress, displaying tissue retraction as well as a reduction in the tentacles size and reactivity under stimuli (Rodolfo-Metalpa et al., 2006; Bellis and Denver, 2017) (Figure 5).
The variation of the color of the tissue from E6-5 to E2 in the colonies kept in the dark was related to the lower content of zooxanthellae, always being lower in dark than in light conditions (Table 2) (Hill and Ralph, 2007), a result observed in both experiments. Surprisingly, in heat-treated fragments exposed to light, an increase in zooxanthellae density was recorded after 5 days compared to the control. Conversely, Rodolfo-Metalpa et al. (2006) encountered a reduction in their number after 34 days in nubbins exposed to 28°C. Considering the duration of our experiment, this observation might be a short-term response of the zooxanthellae to high temperatures, potentially decreasing in density after a mid-term exposure. Regardless of the exposure to light, our fragments presented at least 1 degree of magnitude higher concentrations of zooxanthellae compared not only to tropical scleractinians (Stimson et al., 2002), but also to other studies on C. caespitosa collected in different Mediterranean locations (Rodolfo-Metalpa et al., 2006; Hoogenboom et al., 2010; Rodolfo-Metalpa et al., 2010). This higher concentration is considered an adaptation of temperate corals to more shaded and cooler environments but might also represent a species adaptation to particular local conditions (Lasker, 2003; Eagleson et al., 2023). Future studies focused on the evaluation of the zooxanthellae content of Bagnoli transplanted colonies (constantly thriving in a shaded environment) will help to confirm this hypothesis.
The percentage of mitotic index recorded for C. caespitosa was comparable to the one recorded for other massive scleractinians, such as Siderastrea stellata Verril, 1878 from Brazil (Costa et al., 2013), and its increase with temperature was in line with Caroselli et al. (2015). While no difference in the mitotic index was recorded in the fragments exposed to light and higher temperature, the experimental fragments kept in the dark showed an increment of this parameter. However, this was not followed by an increase in zooxanthellae density (Table 2), a situation potentially explained by the capability of this coral to rely more on heterotrophic feeding, digesting its symbionts under thermal stress, as observed in various Acropora spp. (Fujise et al., 2014).
Overall, our results obtained in the field highlight the high capability of C. caespitosa to adapt to adverse environmental conditions, thus rendering this species a good candidate for future restoration actions. The aquaria experiments gave a first insight into the potential adaptability of this species at individual level. A higher number of genotypes is needed to assess intraspecific variability in the response to thermal stress and light exposure.
Despite the crucial roles played by this species as ecosystem engineer and in the Mediterranean shallow waters carbon budget (Rodolfo-Metalpa et al., 2010) and being protected by the European and international legislations, restoration efforts are still lacking. In this sense, our study represents the first insight into the species-based siting selection, highlighting for C. caespitosa the importance of considering the “exposure to light” criteria and the potential shading of colonies to ensure the survival and, later, the success of a restoration action of zooxanthellate species. Finally, considering the similar increase in corallites per month obtained in the field and in the laboratory, our study points out the suitability of aquaria rearing as a potential tool to supply coral fragments for restoration purposes.
The original contributions presented in the study are included in the article/Supplementary Material. Further inquiries can be directed to the corresponding author.
CC conceived the idea. CC and FT conducted the field activities. BC, MC, CR and SP performed the aquaria experiments. MC, CGC, TM, TPM and CR processed the data and run the statistical analyses. MC, CR, FT and LV wrote the original draft. All authors contributed to editing and reviewing the manuscript. All authors contributed to the article and approved the submitted version.
This study was supported by the project ABBaCo funded by the Italian Ministry for Education, University and Research (grant number C62F16000170001).
Authors would like to thank the Stazione Zoologica Anton Dohrn (Naples) diving staff, Marco Cannavacciuolo, Francesco Terlizzi, Gianluca Zazo and Giovanni De Martino, for its support during field activities.
The authors declare that the research was conducted in the absence of any commercial or financial relationships that could be construed as a potential conflict of interest.
All claims expressed in this article are solely those of the authors and do not necessarily represent those of their affiliated organizations, or those of the publisher, the editors and the reviewers. Any product that may be evaluated in this article, or claim that may be made by its manufacturer, is not guaranteed or endorsed by the publisher.
The Supplementary Material for this article can be found online at: https://www.frontiersin.org/articles/10.3389/fmars.2023.1199048/full#supplementary-material
Abel E. F. (1959). Zur kenntnis der marinen höhlenfauna unter besonderer berücksichtigung der anthozoen. Pubblic. Della. Stazione. Zool. di. Napoli. 30, 1–94.
Armiento G., Caprioli R., Cerbone A., Chiavarini S., Crovato C., Cassan De, et al. (2020). Current status of coastal sediments contamination in the former industrial area of bagnoli-coroglio (Naples, Italy). Chem. Ecol. 36 (6), 579–597. doi: 10.1080/02757540.2020.1747448
Azzola A., Bianchi C. N., Morri C., Oprandi A., Peirano A., Montefalcone M. (2022). Population structure change in a temperate reef coral after a quarter of century. Estuar. Coast. Shelf. S. 270, 107851. doi: 10.1016/j.ecss.2022.107851
Ban S. S., Graham N. A., Connolly S. R. (2014). Evidence for multiple stressor interactions and effects on coral reefs. Glob. Change Biol. 20 (3), 681–697. doi: 10.1111/gcb.12453
Bayraktarov E., Saunders M. I., Abdullah S., Mills M., Beher J., Possingham, et al. (2016). The cost and feasibility of marine coastal restoration. Ecol. Appl. 26 (4), 1055–1074. doi: 10.1890/15-1077
Bellis E. S., Denver D. R. (2017). Natural variation in responses to acute heat and cold stress in a sea anemone model system for coral bleaching. Biol. Bull. 233 (2), 168–181. doi: 10.1086/694890
Bongiorni L., Giovanelli D., Rinkevich B., Pusceddu A., Chou L. M., Danovaro R. (2011). First step in the restoration of a highly degraded coral reef (Singapore) by in situ coral intensive farming. Aquaculture 322, 191–200. doi: 10.1016/j.aquaculture.2011.09.024
Boström-Einarsson L., Babcock R. C., Bayraktarov E., Ceccarelli D., Cook N., Ferse S. C., et al. (2020). Coral restoration–a systematic review of current methods, successes, failures and future directions. PloS One 15 (1), e0226631. doi: 10.1371/journal.pone.0226631
Brown B. E., Cossins A. R. (2011). “The potential for temperature acclimatisation of reef corals in the face of climate change,” in Coral reefs: an ecosystem in transition. Eds. Dubinsky Z., Stambler N. (Berlin, Germany: Springer), 421–433. doi: 10.1007/978-94-007-0114-4_24
Butt N., Chauvenet A. L., Adams V. M., Beger M., Gallagher R. V., Shanahan D. F., et al. (2021). Importance of species translocations under rapid climate change. Conserv. Biol. 35 (3), 775–783. doi: 10.1111/cobi.13643
Caroselli E., Falini G., Goffredo S., Dubinsky Z., Levy O. (2015). Negative response of photosynthesis to natural and projected high seawater temperatures estimated by pulse amplitude modulation fluorometry in a temperate coral. Front. Physiol. 6. doi: 10.3389/fphys.2015.00317
Casado-Amezúa P., Kersting D., Linares C., Bo M., Caroselli E., Garrabou J., et al. (2015). Cladocora caespitosa. the IUCN red list of threatened species 2015 e.T133142A75872554. doi: 10.2305/IUCN.UK.2015-2.RLTS.T133142A75872554.en
Casado-Amezúa P., Kersting D. K., Templado J., Machordom A. (2014). Regional genetic differentiation among populations of Cladocora caespitosa in the Western Mediterranean. Coral. Reefs. 33 (4), 1031–1040. doi: 10.1007/s00338-014-1195-5
Costa C. F., Sassi R., Gorlach-Lira K. (2013). Diversity and seasonal fluctuations of microsymbionts associated with some scleractinian corals of the picãozinho reefs of paraíba state, Brazil. Pan-Am. J. Aquat. Sci. 8(4), 240–252.
Dehnert I., Galli P., Montano S. (2023). Ecological impacts of coral gardening outplanting in the Maldives. Restor. Ecol. 31 (1), e13783. doi: 10.1111/rec.13783
Dehnert I., Saponari L., Isa V., Seveso D., Galli P., Montano S. (2022). Exploring the performance of mid-water lagoon nurseries for coral restoration in the Maldives. Restor. Ecol. 30 (7), e13600. doi: 10.1111/rec.13600
De Pippo T., Donadio C., Pennetta M., Terlizzi F., Vecchione C., Vegliante M. (2002). Seabed morphology and pollution along the bagnoli coast (Naples, italy): a hypothesis for environmental restoration. Mar. Ecol. 23, 154–168. doi: 10.1111/j.1439-0485.2002.tb00015.x
Destro G. F. G., De Marco P., Terribile L. C. (2018). Threats for bird population restoration: a systematic review. Perspect. Ecol. Conserv. 16 (2), 68–73. doi: 10.1016/j.pecon.2018.03.003
Di Camillo G. C., Arossa S., Pica D., Azzurra B., Torsani F., Cerrano C. (2021). Phenology of Anemonia viridis and Exaiptasia diaphana (Cnidaria: anthozoa) from marine temperate ecosystems. Mediterr. Mar. Sci. 22 (1), 40–50. doi: 10.12681/mms.24600
Eagleson R. G., Álvarez-Filip L., Lumsden J. S. (2023). A review of research on the mustard hill coral, Porites astreoides. Diversity 15 (3), 462. doi: 10.3390/d15030462
El Kateb A., Stalder C., Neururer C., Pisapia C., Spezzaferri S. (2016). Correlation between pollution and decline of scleractinian Cladocora caespitosa (Linnaeus 1758) in the gulf of gabes. Heliyon 2, e00195. doi: 10.1016/j.heliyon.2016.e00195
Epstein N., Bak R. P. M., Rinkevich B. (2001). Strategies for gardening denuded coral reef areas: the applicability of using different types of coral material for reef restoration. Restor. Ecol. 9 (4), 432–442. doi: 10.1046/j.1526-100X.2001.94012.x
Fariñas-Franco J. M., Allcock A. L., Roberts D. (2018). Protection alone may not promote natural recovery of biogenic habitats of high biodiversity damaged by mobile fishing gears. Mar. Environ. Res. 135, 18–28. doi: 10.1016/j.marenvres.2018.01.009
Fava F., Bavestrello G., Valisano L., Cerrano C. (2010). Survival, growth and regeneration in explants of four temperate gorgonian species in the Mediterranean Sea. Ital. J. Zool. 77 (1), 44–52. doi: 10.1080/11250000902769680
Ferse S. C., Hein M. Y., Rölfer L. (2021). A survey of current trends and suggested future directions in coral transplantation for reef restoration. PloS One 16 (5), e0249966. doi: 10.1371/journal.pone.0249966
Fujise L., Yamashita H., Suzuki G., Sasaki K., Liao L. M., Koike K. (2014). Moderate thermal stress causes active and immediate expulsion of photosynthetically damaged zooxanthellae (Symbiodinium) from corals. PloS One 9 (12), e114321. doi: 10.1371/journal.pone.0114321
Gaglioti M., Fernández T. V., Musco L., Gambi M. C. (2020). Habitat and benthic diversity in the bay of bagnoli and surrounding areas (Gulf of Naples, italy): a historical baseline for environmental restoration. Mar. Environ. Res. 157, 104925. doi: 10.1016/j.marenvres.2020.104925
Garrabou J., Gomez-Gras D., Ledoux J. B., Linares C., Bensoussan N., Lopez-Sendino P., et al. (2019). Collaborative database to track mass mortality events in the Mediterranean Sea. Front. Mar. Sci. 6. doi: 10.3389/fmars.2019.00707
Garrabou J., Gómez-Gras D., Medrano A., Cerrano C., Ponti M., Schlegel R., et al. (2022). Marine heatwaves drive recurrent mass mortalities in the Mediterranean Sea. Glob. Change Biol. 28 (19), 5708–5725. doi: 10.1111/gcb.16301
Gómez-Gras D., Bensoussan N., Ledoux J. B., López-Sendino P., Cerrano C., Ferretti E., et al. (2022). Exploring the response of a key Mediterranean gorgonian to heat stress across biological and spatial scales. Sci. Rep. 12 (1), 21064. doi: 10.1038/s41598-022-25565-9
Guest J. R., Dizon R. M., Edwards A. J., Franco C., Gomez E. D. (2011). How quickly do fragments of coral “self-attach” after transplantation? Restor. Ecol. 19 (2), 234–242. doi: 10.1111/j.1526-100X.2009.00562.x
Hammer Ø., Harper D. A. T., Ryan P. D. (2001). PAST: paleontological statistics software package for education and data analysis. Palaeontol. Electron. 4 (1), 1–9.
Herndl G. J., Velimirov B. (1986). Microheterotrophic utilization of mucus released by the Mediterranean coral Cladocora caespitosa. Mar. Biol. 90, 363–369. doi: 10.1007/bf00428560
Hill R., Ralph P. J. (2007). Post-bleaching viability of expelled zooxanthellae from the scleractinian coral Pocillopora damicornis. Mar. Ecol. Progr. Ser. 352, 137–144. doi: 10.3354/meps07159
Hobday A. J., Alexander L. V., Perkins S. E., Smale D. A., Straub S. C., Oliver E. C., et al. (2016). A hierarchical approach to defining marine heatwaves. Prog. Oceanogr. 141, 227–238. doi: 10.1016/j.pocean.2015.12.014
Hobday A. J., Oliver E. C. J., Sen Gupta A., Benthuysen J. A., Burrow s M. T., Donat M. G., et al. (2018). Categorising and naming marine heatwaves. Oceanogr 31 (2), 162–173. doi: 10.5670/oceanog.2018.205
Hoogenboom M. O., Anthony K. R., Connolly S. R. (2006). Energetic cost of photoinhibition in corals. Mar. Ecol. Progr. Ser. 313, 1–12. doi: 10.3354/meps313001
Hoogenboom M., Beraud E., Ferrier-Pagès C. (2010). Relationship between symbiont density and photosynthetic carbon acquisition in the temperate coral Cladocora caespitosa. Coral. Reefs. 29, 21–29. doi: 10.1007/s00338-009-0558-9
IUCN Species Survival Commission (2012). IUCN guidelines for reintroductions and other conservation translocations Gland, Switzerland (Reintroduction Specialist Group, Invasive Species Specialist Group, Gland, Switzerland). 1–16.
Jiang L., Sun Y. F., Zhang Y. Y., Zhou G. W., Li X. B., McCook L. J., et al. (2017). Impact of diurnal temperature fluctuations on larval settlement and growth of the reef coral Pocillopora damicornis. Biogeosciences 14 (24), 5741–5752. doi: 10.5194/bg-14-5741-2017
Jiménez C., Hadjioannou L., Petrou A., Nikolaidis A., Evriviadou M., Lange M. A. (2016). Mortality of the scleractinian coral Cladocora caespitosa during a warming event in the levantine Sea (Cyprus). Reg. Environ. Change 16, 1963–1973. doi: 10.1007/s10113-014-0729-2
Jones A., Berkelmans R. (2012). The photokinetics of thermo-tolerance in Symbiodinium. Mar. Ecol. 33 (4), 490–498. doi: 10.1111/j.1439-0485.2012.00514.x
Jones C. G., Lawton J. H., Shachak M. (1994). Organisms as ecosystem engineers. Oikos 69, 373–386. doi: 10.2307/3545850
Kersting D. K., Ballesteros E., De Caralt S., Linares C. (2014b). Invasive macrophytes in a marine reserve (Columbretes islands, NW mediterranean): spread dynamics and interactions with the endemic scleractinian coral Cladocora caespitosa. Biol. Invasions. 16, 1599–1610. doi: 10.1007/s10530-013-0594-9
Kersting D. K., Bensoussan N., Linares C. (2013). Long-term responses of the endemic reef-builder Cladocora caespitosa to Mediterranean warming. PloS One 8 (8), e70820. doi: 10.1371/journal.pone.0070820
Kersting D. K., Cebrian E., Casado C., Teixidó N., Garrabou J., Linares C. (2015). Experimental evidence of the synergistic effects of warming and invasive algae on a temperate reef-builder coral. Sci. Rep. 5, 18635. doi: 10.1038/srep18635
Kersting D., Cebrian E., Verdura J., Ballesteros E. (2017a). A new Cladocora caespitosa population with unique ecological traits. Medit. Mar. Sci. 18 (1), 38–42. doi: 10.12681/mms.1955
Kersting D. K., Cebrian E., Verdura J., Ballesteros E. (2017b). Rolling corals in the Mediterranean Sea. Coral. Reefs. 36, 245–245. doi: 10.1007/s00338-016-1498-9
Kersting D. K., Linares C. (2012). Cladocora caespitosa bioconstructions in the columbretes islands marine reserve (Spain, NW mediterranean): distribution, size structure and growth. Mar. Ecol. 33 (4), 427–436. doi: 10.1111/j.1439-0485.2011.00508.x
Kersting D. K., Linares C. (2019). Living evidence of a fossil survival strategy raises hope for warming-affected corals. Sci. Adv. 5 (10), eaax2950. doi: 10.1126/sciadv.aax295
Kersting D. K., Teixidó N., Linares C. (2014a). Recruitment and mortality of the temperate coral Cladocora caespitosa: implications for the recovery of endangered populations. Coral. Reefs. 33, 403–407. doi: 10.1007/s00338-014-1144-3
Kotb M. M. (2016). Coral translocation and farming as mitigation and conservation measures for coastal development in the red Sea: aqaba case study, Jordan. Environ. Earth Sci. 75 (5), 439. doi: 10.1007/s12665-016-5304-3
Kružić P., Benković L. (2008). Bioconstructional features of the coral Cladocora caespitosa (Anthozoa, scleractinia) in the Adriatic Sea (Croatia). Mar. Ecol. 29 (1), 125–139. doi: 10.1111/j.1439-0485.2008.00220.x
Kružić P., Požar-Domac A. (2007). Impact of tuna farming on the banks of the coral Cladocora caespitosa in the Adriatic Sea. Coral. Reefs. 26, 665. doi: 10.1007/s00338-007-0237-7
Kružić P., Sršen P., Benković L. (2012). The impact of seawater temperature on coral growth parameters of the colonial coral Cladocora caespitosa (Anthozoa, scleractinia) in the eastern Adriatic Sea. Facies 58, 477–491. doi: 10.1007/s10347-012-0306-4
Kružić P., Žuljević A., Nikolić V. (2008). The highly invasive alga Caulerpa racemosa var. cylindracea poses a new threat to the banks of the coral Cladocora caespitosa in the Adriatic Sea. Coral. Reefs. 27, 441–441. doi: 10.1007/s00338-008-0358-7
Laborel J. (1961). Sur un cas particulier de concrétionnement animal. concrétionnement à Cladocora caespitosa (L.) dans le golfe de talante. Rapport. Commission. Int. Pour. L’exploration. Sci. La. Mer. Méditerranée. 16, 429–432.
Lasker H. R. (2003). Zooxanthellae densities within a Caribbean octocoral during bleaching and non-bleaching years. Coral. Reefs. 22 (1), 23–26. doi: 10.1007/s00338-003-0276-7
Leal M. C., Nejstgaard J. C., Calado R., Thompson M. E., Frischer M. E. (2014). Molecular assessment of heterotrophy and prey digestion in zooxanthellate cnidarians. Mol. Ecol. 23 (15), 3838–3848. doi: 10.1111/mec.12496
Lejeusne C., Chevaldonné P., Pergent-Martini C., Boudouresque C. F., Pérez T. (2010). Climate change effects on a miniature ocean: the highly diverse, highly impacted Mediterranean Sea. Trends Ecol. Evol. 25, 250–260. doi: 10.1016/j.tree.2009.10.009
Lough J. M., Anderson K. D., Hughes T. P. (2018). Increasing thermal stress for tropical coral reefs: 1871–2017. Sci. Rep. 8 (1), 6079. doi: 10.1038/s41598-018-24530-9
Madin L. P. (1988). Feeding behavior of tentaculate predators: in situ observations and a conceptual model. Bull. Mar. Sci. 43 (3), 413–429.
Merck D. E., Petrik C. G., Manfroy A. A., Muller E. M. (2022). Optimizing seawater temperature conditions to increase the productivity of ex situ coral nurseries. PeerJ 10, e13017. doi: 10.7717/peerj.13017
Ng C. S. L., Ng S. Z., Chou L. M. (2012). Does an ex situ coral nursery facilitate reef restoration in singapore’s waters? Contrib. Mar. Sci., 95–100.
Normile D. (2009). Bringing coral reefs back from the living dead. Science 325 (5940), 559–561. doi: 10.1126/science.325_559
Otero M. D. M., Numa C., Bo M., Orejas C., Garrabou J., Cerrano C., et al. (2017). Overview of the conservation status of Mediterranean anthozoans. Malaga. Spain. pp, 1–71. doi: 10.2305/IUCN.CH.2017.RA.2.en
Peirano A., Kružić P. (2004). Growth comparison between ligurian and Adriatic samples of the coral cladocora caespitosa: first results. Biol. Mar. Medit. 11, 166–168.
Peirano A., Morri C., Bianchi C. (1999). Skeleton growth and density pattern of the temperate, zooxanthellate scleractinian Cladocora caespitosa from the ligurian Sea (NW Mediterranean). Mar. Ecol. Progr. Ser. 185, 195–201. doi: 10.3354/meps185195
Peirano A., Morri C., Bianchi C. N., Rodolfo-Metalpa R. (2001). Biomass, carbonate standing stock and production of the Mediterranean coral Cladocora caespitosa (L.). Facies 44, 75–80. doi: 10.1007/BF02668168
Peirano A., Morri C., Mastronuzzi G., Bianchi C. (1998). The coral Cladocora caespitosa (Anthozoa, scleractinia) as a bioherm builder in the Mediterranean Sea. Mem. Descr. Carta. Geol. Ita. 52, 59–74.
Pettay D. T., Wham D. C., Smith R. T., Iglesias-Prieto R., LaJeunesse T. C. (2015). Microbial invasion of the Caribbean by an indo-pacific coral zooxanthella. PNAS 112 (24), 7513–7518. doi: 10.1073/pnas.1502283112
Rasband W. S. (2012). ImageJ: image processing and analysis in Java (Astrophysics Source Code Library), ascl–1206.
Rinkevich B. (1995). Restoration strategies for coral reefs damaged by recreational activities: the use of sexual and asexual recruits. Restor. Ecol. 3 (4), 241–251. doi: 10.1111/j.1526-100X.1995.tb00091.x
Rinkevich B. (2005). Conservation of coral reefs through active restoration measures: recent approaches and last decade progress. Environ. Sci. Technol. 39 (12), 4333–4342. doi: 10.1021/es0482583
Rodolfo-Metalpa R., Bianchi C. N., Peirano A., Morri C. (2005). Tissue necrosis and mortality of the temperate coral Cladocora caespitosa. Ital. J. Zool. 72 (4), 271–276. doi: 10.1080/11250000509356685
Rodolfo-Metalpa R., Martin S., Ferrier-Pagès C., Gattuso J. P. (2010). Response of the temperate coral Cladocora caespitosa to mid-and long-term exposure to pCO2 and temperature levels projected for the year 2100 AD. Biogeosciences 7 (1), 289–300. doi: 10.5194/bg-7-289-2010
Rodolfo-Metalpa R., Peirano A., Houlbrèque F., Abbate M., Ferrier-Pagès C. (2008). Effects of temperature, light and heterotrophy on the growth rate and budding of the temperate coral Cladocora caespitosa. Coral. Reefs. 27, 17–25. doi: 10.1007/s00338-007-0283-1
Rodolfo-Metalpa R., Richard C., Allemand D., Ferrier-Pagès C. (2006). Growth and photosynthesis of two Mediterranean corals, Cladocora caespitosa and Oculina patagonica, under normal and elevated temperatures. J. Exp. Biol. 209 (22), 4546–4556. doi: 10.1242/jeb.02550
Roveta C., Marrocco T., Pica D., Pulido Mantas T., Rindi F., Musco L., et al. (2022). The effect of substrate and depth on hydroid assemblages: a comparison between two islands of the Tuscan archipelago (Tyrrhenian Sea). Mar. Biodivers. 52 (1), 9. doi: 10.1007/s12526-021-01254-0
Roveta C., Pulido Mantas T., Bierwirth J., Calcinai B., Coppari M., Di Camillo C. G., et al. (2023). Can colony resizing represent a strategy for octocorals to face climate warming? the case of the precious red coral Corallium rubrum. Coral Reefs 42, 535–549. doi: 10.1007/s00338-023-02365-9
Sakai K., Singh T., Iguchi A. (2019). Bleaching and post-bleaching mortality of Acropora corals on a heat-susceptible reef in 2016. PeerJ 7, e8138. doi: 10.7717/peerj.8138
Sammarco P. W., Strychar K. B. (2009). Effects of climate change/global warming on coral reefs: adaptation/exaptation in corals, evolution in zooxanthellae, and biogeographic shifts. Environ. Bioindic. 4 (1), 9–45. doi: 10.1080/15555270902905377
Schlegel R. W., Smit A. J. (2018). heatwaveR: a central algorithm for the detection of heatwaves and cold-spells. J. Open Sour. Softw. 3 (27), 821. doi: 10.21105/joss.00821
Silenzi S., Bard E., Montagna P., Antonioli F. (2005). Isotopic and elemental records in a non-tropical coral (Cladocora caespitosa): discovery of a new high-resolution climate archive for the Mediterranean Sea. Glob. Planet. Change 49, 94–120. doi: 10.1016/j.gloplacha.2005.05.005
Society for Ecological Restoration (SER) (2023). Available at: www.ser.org (Accessed Accessed: 22nd February 2023).
Steinberg R. K., Dafforn K. A., Ainsworth T., Johnston E. L. (2020). Know thy anemone: a review of threats to octocorals and anemones and opportunities for their restoration. Front. Mar. Sci. 7. doi: 10.3389/fmars.2020.00590
Stimson J., Sakai K., Sembali H. (2002). Interspecific comparison of the symbiotic relationship in corals with high and low rates of bleaching- induced mortality. Coral. Reefs. 21, 409–421. doi: 10.1007/s00338-002-0264-3
Swan K. D., McPherson J. M., Seddon P. J., Moehrenschlager A. (2016). Managing marine biodiversity: the rising diversity and prevalence of marine conservation translocations. Conserv. Lett. 9 (4), 239–251. doi: 10.1111/conl.12217
United Nations Environment Programme (UNEP) (2021) Becoming #GenerationRestoration: ecosystem restoration for people, nature and climate. Available at: https://www.unep.org/resources/ecosystem-restoration-people-nature-climate.
Wilkerson F. P., Kobayashi D., Muscatine L. (1988). Mitotic index and size of symbiotic algae in Caribbean reef corals. Coral. Reefs. 7, 29–36. doi: 10.1007/BF00301979
Keywords: restoration, temperate seas, ecosystem engineer, hexacorals, marine heat waves, aquaria
Citation: Roveta C, Coppari M, Calcinai B, Di Camillo CG, Marrocco T, Pulido Mantas T, Puce S, Torsani F, Valisano L and Cerrano C (2023) What’s the key for success? Translocation, growth and thermal stress mitigation in the Mediterranean coral Cladocora caespitosa (Linnaeus, 1767). Front. Mar. Sci. 10:1199048. doi: 10.3389/fmars.2023.1199048
Received: 02 April 2023; Accepted: 02 May 2023;
Published: 17 May 2023.
Edited by:
Ronald Osinga, Wageningen University and Research, NetherlandsReviewed by:
Davide Seveso, University of Milano-Bicocca, ItalyCopyright © 2023 Roveta, Coppari, Calcinai, Di Camillo, Marrocco, Pulido Mantas, Puce, Torsani, Valisano and Cerrano. This is an open-access article distributed under the terms of the Creative Commons Attribution License (CC BY). The use, distribution or reproduction in other forums is permitted, provided the original author(s) and the copyright owner(s) are credited and that the original publication in this journal is cited, in accordance with accepted academic practice. No use, distribution or reproduction is permitted which does not comply with these terms.
*Correspondence: Martina Coppari, bS5jb3BwYXJpQHN0YWZmLnVuaXZwbS5pdA==
Disclaimer: All claims expressed in this article are solely those of the authors and do not necessarily represent those of their affiliated organizations, or those of the publisher, the editors and the reviewers. Any product that may be evaluated in this article or claim that may be made by its manufacturer is not guaranteed or endorsed by the publisher.
Research integrity at Frontiers
Learn more about the work of our research integrity team to safeguard the quality of each article we publish.