- 1School of Forest, Fisheries, and Geomatics Sciences, University of Florida, Gainesville, FL, United States
- 2Department of Biology, University of Louisiana at Lafayette, Lafayette, LA, United States
- 3Southeast Fisheries Science Center, National Marine Fisheries Service, Panama City, FL, United States
- 4National Marine Fisheries Service, Southeast Fisheries Science Center, Pascagoula, MS, United States
- 5Nature Coast Biological Station, University of Florida, Cedar Key, FL, United States
- 6Department of Earth Ocean & Atmospheric, Florida State University, Tallahassee, FL, United States
- 7College of Marine Science, University of South Florida, St. Petersburg, FL, United States
- 8Department of Biology, Texas A&M University Corpus Christi, Corpus Christi, TX, United States
- 9United States Geological Survey, Columbia Environmental Research Center, Columbia, MO, United States
The goal of this paper was to review the evidence of population-level impacts of the Deepwater Horizon Oil Spill (DWH) on Gulf of Mexico (GOM) continental shelf taxa, as well as evidence of resiliency following the DWH. There is considerable environmental and biological evidence that GOM shelf taxa were exposed to and suffered direct and indirect impacts of the DWH. Numerous assessments, from mesocosm studies to analysis of biopsied tissue or tissue samples from necropsied animals, revealed a constellation of physiological effects related to DWH impacts on GOM biota, some of which clearly or likely resulted in mortality. While the estimated concentrations of hydrocarbons in shelf waters and sediments were orders of magnitude lower than measured in inshore or deep GOM environments, the level of mortality observed or predicted was substantial for many shelf taxa. In some cases, such as for zooplankton, community shifts following the spill were ephemeral, likely reflecting high rates of population turnover and productivity. In other taxa, such as GOM reef fishes, impacts of the spill are confounded with other stressors, such as fishing mortality or the appearance and rapid population growth of invasive lionfish (Pterois spp.). In yet others, such as cetaceans, modeling efforts to predict population-level effects of the DWH made conservative assumptions given the species’ protected status, which post-DWH population assessments either failed to detect or population increases were estimated. A persistent theme that emerged was the lack of precise population-level data or assessments prior to the DWH for many taxa, but even when data or assessments did exist, examining evidence of population resiliency was confounded by other stressors impacting GOM biota. Unless efforts are made to increase the resolution of the data or precision of population assessments, difficulties will likely remain in estimating the scale of population-level effects or resiliency in the case of future large-scale environmental catastrophes.
1 Introduction
The Gulf of Mexico (GOM) is a highly productive large marine ecosystem that generates numerous ecosystem services for coastal and inland human populations alike (NRC, 2013). Among the GOM’s various marine regions, none is more productive than the north central GOM (hereafter ncGOM) continental shelf. Nutrient-rich freshwater flowing onto the shelf from the Mississippi-Atchafalaya and Mobile River drainages fuels phytoplankton productivity that in turn supports shellfish and finfish fisheries that are among the more economically valuable in the US (Darnell, 2015). While this system supports other major industries, such as shipping and petroleum extraction, none are as tied to the health of the ecosystem as fisheries. However, other services provided by the ncGOM shelf ecosystem, from biogeochemical cycling to tourism, are also heavily dependent on ecosystem health.
Numerous anthropogenic stressors, such as climate change, algal blooms, hypoxia, overfishing, and release of toxic chemicals threaten the ncGOM shelf ecosystem and the services it provides, with many of these acting as chronic stressors on the system. It was against this backdrop of an altered, chronically stressed ecosystem that the Deepwater Horizon Oil Spill (DWH) occurred in spring and summer 2010. Oil poured from the Macondo Well at Mississippi Canyon Block 252 (MC252) wellhead for 87 days, with a total estimated volume of approximately 750 x 103 m3 (McNutt et al., 2012) being released over an area of nearly 150 thousand km2 of the ncGOM (MacDonald et al., 2015; Figure 1). In addition, approximately 250 x 103 mt of natural gas were released into the water column from the spill (Joye et al., 2016), and approximately 6.8 x 103 m3 of chemical dispersants were applied to keep oil from coming ashore along the ncGOM coast (Trustees, 2016; Murawski et al., 2019). The extent of the spill and desire to minimize human impacts instigated fishery closures that were in place from May 2 to November 24, 2010, except for a small (<3,000 km2) area around the wellhead that was closed until April 2011, with a maximum closed area of 225,290 km2 in late June 2010 (Gohlke et al., 2011; Ylitalo et al., 2012).
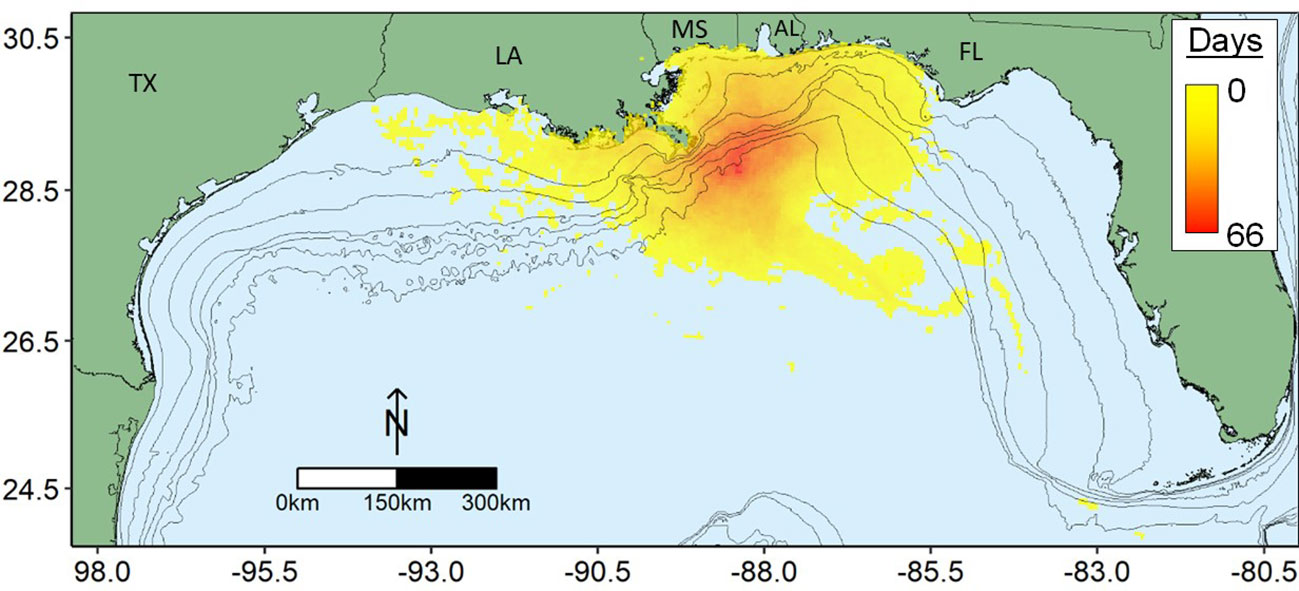
Figure 1 Map of the north central Gulf of Mexico showing spatial extent of the shelf and the estimated extent of surface oiling during the duration of the Deepwater Horizon Oil Spill. Legend indicates cumulative days oil observed via satellite imagery (MacDonald et al., 2015). Isobaths shown are 25, 50, 100, 200, 500, and 1000 m. State abbreviations: AL, Alabama; FL, Florida; LA, Louisiana; MS, Mississippi; and TX, Texas.
Considerable evidence was gathered and reported in the decade after the DWH about the direct and indirect effects of the spill on the ncGOM shelf ecosystem. Much of the early evidence of DWH ecosystem effects, in the days to weeks following the start of the spill, consisted of oiled megafauna, such as birds, turtles, and mammals, or oiled habitats, such as Sargassum, marshes, or beaches. More comprehensive analyses followed in the months or years after the spill, and indicated acute and chronic DWH effects occurred across multiple levels of organization, from sub-cellular to individuals to populations and communities. Despite compelling evidence of a constellation of impacts of the DWH on GOM biota, several authors have commented that detecting population-level effects has been elusive (Fodrie et al.,2014; Oken et al., 2022). Therefore, the overall goals of this paper were to examine population trends of ecologically or economically important species among a range of communities or continental shelf habitats, evaluate whether declining trends, when observed, were likely caused by the DWH, and then evaluate whether populations displayed resiliency in the decade following the DWH. Analysis, review, and assessment conducted herein we were not meant to be comprehensive or exhaustive, but instead were focused on representative taxa from key communities or habitats affected by the DWH. Taxa or communities examined include plankton, benthic invertebrates, demersal reef fishes, and higher vertebrates. We accessed previous analyses via searches in Google Scholar with key words “Deepwater Horizon Oil Spill” or “Macondo Oil Spill,” followed by names of taxa of interest, or via workgroup reports published online as part of the Natural Resources Damage Assessment (NRDA) process. In some cases, population or ecosystem models existed to examine post-DWH trends and the potential for resiliency. For others, new analyses were conducted to assess population trends with data collected by academic researchers and federal agencies, such as various fishery-independent NOAA Fisheries (National Oceanic and Atmospheric Administration National Marine Fisheries Service) surveys, or by the NRDA trustees. Important components of our review were an assessment whether there was evidence of direct or indirect effects of DWH oil or natural gas on taxa or populations of interest, what the likely population-level vulnerability of these taxa was to DWH effects, and whether taxa or populations showed signs of recovery in the decade following the DWH.
2 Zooplankton
Results of ncGOM zooplankton studies suggest a dichotomy of vulnerability, as well as resilience, existed among individuals, populations, and communities in response to the DWH. Empirical studies demonstrated individual zooplankters are vulnerable to the toxic effects of crude oil and oil dispersant chemical constituents (Almeda et al., 2013), and there is clear evidence DWH oil-derived carbon entered the planktonic food web on the ncGOM continental shelf through trophic transfer from bacteria to microzooplankton (Graham et al., 2010; Chanton et al., 2012). Yet, data from field studies tracking wild zooplankton populations and communities on the Alabama shelf indicate ephemeral shifts in abundance and assemblage structure occurred during May-June 2010 following exposure to DWH petroleum and dispersant (Carassou et al., 2014). Densities of some dominant taxa (e.g., calanoid and cyclopoid copepods, ostracods, bivalve larvae, and cladocerans) increased relative to previous years (2005-2009), but observed changes were no longer significant by July 2010. Increased densities of these taxa were attributed to increased microbial activity related to consumption of oil carbon (bacterial methanotrophy), which may have enhanced secondary production through microbial-zooplankton trophic linkages (Graham et al., 2010; Chanton et al., 2012; Carassou et al., 2014), with microzooplankton (<0.2 μm) providing a trophic linkage between microbial and metazoan food webs. However, the higher than average rainfall in spring of 2010 also increased nutrient-rich inputs onto the ncGOM, and human-induced freshwater diversions from Mississippi River may have also enhanced primary production in the system in 2010 (Hu et al., 2011).
Daly et al. (2021) also reported high nearshore zooplankton densities in 2010 on the northern Florida shelf east of DeSoto Canyon, where oil exposure was more limited. Not only were zooplankton densities high during August 2010, they remained elevated during May and September 2011 compared to abundances in other years of their 2005–2014 sampling period. Maximum nearshore zooplankton densities occurred near the surface during August 2010, with a second peak near the sea floor where chlorophyll concentrations were highest. Numerically abundant zooplankton taxa included copepods, larvaceans, chaetognaths, ostracods, and cladocerans. In all years, zooplankton abundances were highest near shore (<25 m depths) and decreased towards the outer shelf. Species composition during and after the oil spill (2010–2104) were similar. Abundance, biomass, species composition, and diversity indices for zooplankton collected between 2010–2014 (Daly et al., 2021) also were within the range of previously published values (e.g., Houde and Chitty, 1976; Iverson and Hopkins, 1981; Ortner et al., 1989).
It is difficult to compare zooplankton densities between Alabama and northern Florida shelf sites owing to differences in water column depths and sampling approaches (e.g., type and mesh size of nets, etc). Carassou et al. (2014) reported that during August 2010 calanoid copepod densities, collected with a BIONESS net rigged with a 202-µm mesh net, were approximately 8,500 individuals m-3 at a 20-m deep station. On the northern Florida shelf at a 25-m depth station, calanoid copepod mean densities were 1,823 individuals m-3, based on SIPPER (Shadowed Image Particle Profiling and Evaluation Recorder) towed camera images. In contrast, bongo tows (333-µm mesh net) produced lower calanoid copepod density estimates (ca. 853 individuals m-3; Daly, unpubl.). With respect to the net tows, unfortunately it is not possible to determine if differences in calanoid copepod densities were related to net extrusion of smaller individuals by the bongo net, local biological and oceanographic conditions (e.g., regional productivity, predator fields), or a combination of multiple factors.
Any effects of oil on zooplankton on the Alabama shelf appear to have been ephemeral, and there was no detectable impact of oil on the north Florida shelf zooplankton community. This may have been due to the physiological resilience of key mesozooplankton taxa to DWH chemical constituent exposure, the intersection of zooplankton life-histories and the biophysical conditions of the ncGOM that may confer population- and community-level resistance, enhanced primary or secondary production due to nutrient-rich freshwater inputs on the shelf or bacterial methanotrophy, or sampling limitations that possibly contribute to the apparent contradiction in the response among the ecological scales of zooplankton. Among potentially impacted mesozooplankton, copepods numerically dominate ncGOM shelf assemblages (Carassou et al., 2012; Roman et al., 2012; Daly et al., 2021). Lethal and sublethal impacts of polycyclic aromatic hydrocarbons (PAHs) on copepods include narcosis (Barata et al., 2005), changes in feeding (Saiz et al., 2009), swimming behavior (Cohen et al., 2014), reproduction (Suderman and Marcus, 2002; Bellas and Thor, 2007; Calbet et al., 2007), and development (Bejarano et al., 2006). Short-term PAH toxicity tests on Acartia tonsa, a common shelf species, indicated survival was 50% at 594, 2366 and >640 nM for fluoranthene, phenanthrene and pyrene, respectively (Bellas and Thor, 2007). While those levels are well above concentrations typically found in coastal marine waters, they can be found in chronically pollutated estuaries and even higher concentrations were measured in shelf waters after the Prestige off the northwest coast of Spain and the DWH in the north central GOM (Bellas and Thor, 2007). Egg production and recruitment rates were reduced by 60-88% with exposure to these three compounds. The effect of pyrene toxicity on egg production was amplified by ultraviolet light, decreasing recruitment and survival of females by 3–16 fold. These lethal and sublethal effects were also found when field-collected mesozooplankton were exposed to increasing oil concentrations and dispersant in mesocosm experiments (Almeda et al., 2013). Specifically, mesozooplankton survival decreased with increasing oil concentrations. And, at the 1∶20 ratio of dispersant to oil commonly used in the treatment of oil spills, dispersant and dispersant-treated oil were 2-3X more toxic to mesozooplankton than oil alone. As seen in the laboratory studies, ultraviolet light exacerbated lethal effects of crude oil in mesozooplankton communities, increasing mortality by 35%. Cohen et al. (2014) also showed that a common ncGOM shelf copepod, Labidocera aestiva, was particularly sensitive to Corexit EC9500A dispersant exposure, with greater acute effects on survival from dispersant alone, and effects on swimming behavior when dispersant was mixed with MC252 crude oil.
The outcomes of toxicity studies unequivocally indicate that ncGOM zooplankton were susceptible to mortality as a result of exposure to crude oil and oil dispersant from DWH. It is therefore expected that the synergism of these lethal and sublethal effects on individuals would have led to drastic reductions in the abundance and biomass of their populations and changes in community structure. Broadly, mesozooplankton have characteristics that would suggest populations would be sensitive to perturbations like oil spills. They are small (0.2 to 20 mm), unable to escape because they cannot swim against ocean currents, often have specialized modes of nutrition and feeding, have distributions that overlap with the area of exposure, have sensitive life stages, and have limited detoxifying capabilities, which is principally accomplished via metabolic and depuration processes (Hansen et al., 2008; Berrojalbiz et al., 2009). However, despite possessing characteristics that would imply a high degree of vulnerability at the individual level to oil spills, shifts between pre- and post-DWH mesozooplankton assemblages were either ephemeral or could not be detected (Carassou et al., 2014; Dubickas, 2019; Daly et al., 2021). Investigators have noted variability in ncGOM zooplankton production and community composition is primarily driven by environmental conditions (e.g., temperature and chlorophyll) and other related coastal processes, such as river discharge (Liu and Dagg, 2003; Daly et al., 2021), freshwater diversions (Hu et al., 2011), and upwelling. It is also possible that zooplankton in the ncGOM have become adapted to crude oil exposure. Lee et al. (2017) found evolutionary increases in crude oil tolerance in wild populations of a common estuarine copepod, Eurytemora affinis, after eight generations following the DWH. The post-oil spill population showed increased survival and rapid development time in the presence of crude oil. Using a 9-10-day generation time, this timescale of adaptation suggests copepod populations had the capacity to become more tolerant to petroleum compounds three months after the DWH (Lee et al., 2017).
Although direct evidence of zooplankton population declines after DWH are largely lacking, indirect evidence from trophic studies suggests some zooplankton taxa may have experienced localized declines. Condition of larval Spanish mackerel, Scomberomorus maculatus, which become increasingly piscivorous after 3 mm notochord length, was not significantly affected by the DWH (Ransom et al., 2016), while red snapper, Lutjanus campechanus, larvae, which generally feed on mesozooplankton throughout their larval stage, were in significantly worse condition after the DWH (Hernandez et al., 2016). Tarnecki and Patterson (2015) reported sub-adult and adult red snapper collected at ncGOM reef sites (within 10-100 m isobaths) experienced diet shifts from substantial contributions from zooplankton prior to the DWH, to a diet dominated by larger invertebrates (e.g., cephalopods, decapods, stomatopods) and fishes, and an absence of zooplankton, during the months following DWH. The authors suggested a post-DWH decline in zooplankton may have led to a shift in available prey for red snapper (a generalist predator). This hypothesis is in contrast to the zooplankton increases (notably copepods) reported in other studies (e.g., Carassou et al., 2014; Daly et al., 2021). However, the dominant zooplankton prey in red snapper diets (e.g., hyperiid amphipods, stomatopod larvae, pteropods) were largely unrepresented in the pelagic zooplankton surveys (Carassou et al., 2014; Daly et al., 2021). Therefore, it may be that certain zooplankton groups found closer to the benthos, and thus more available as prey for demersal red snapper, experienced a different population response to oil exposure. This community also was likely undersampled by net tows which generally do not extend to near-bottom waters.
Mesozooplankton in the ncGOM are strongly controlled by both large-scale climate drivers and regional environmental conditions that vary in space and time (Carassou et al., 2012; Roman et al., 2012; Gomez et al., 2019; Daly et al., 2021). Therefore, the high degree of environmental variability these populations and communities experience and the patchy distribution of oil across the ncGOM shelf during the spill may partly explain their apparent resilience to the DWH. These communities may also contain enough functional redundancy that a local, temporary reduction (or elimination) of a given taxon does not result in destabilization of the planktonic food web or disruption of key trophic interactions. While the DWH covered a large area, advection of mesozooplankton into local areas where zooplankton were negatively affected by the spill may have ameliorated oil spill-related declines in abundance and shifts in community composition (Daly et al., 2021). Hindcast simulations of passive particle movement using a GOM HYCOM model indicated that over a 30-day period during July 2010 currents carried particles to the oil spill region from beyond both the western and eastern outer reaches of the spill (Daly et al., 2020). Although zooplankton do not behave like wholly passive particles, these simulation results provide an estimate of transport by currents in this region and suggest connectivity with adjacent regions, which, in addition to short generation times, functional redundancies, and rapid evolution of petroleum tolerance, may explain the lack of evidence for sustained population-level impacts to ncGOM zooplankton communities.
3 Benthic invertebrates
There are rich invertebrate communities on the ncGOM shelf owing to the productivity of the system, the diversity of benthic habitats, and the semi-tropical to temperate climate. Among these taxa, there were numerous potential pathways of exposure to DWH petroleum compounds, including exposure across gills or skin for compounds in the water column, contact with the skin or exoskeletons for benthic taxa, or via consumption of contaminated prey. Furthermore, results of mesocosm and laboratory studies demonstrate lethal or sublethal toxic effects of petroleum compounds to invertebrates (Fern et al., 2015; Giltz and Taylor, 2017; Mitchelmore et al., 2020). However, while mechanisms of potential exposure are evident, and lethal and sublethal effects of exposure to petroleum are well-described, that did not necessarily translate to evident population- or community-level effects of the DWH on shelf invertebrate taxa. Furthermore, analysis of sediment samples from coastal, shelf, and continental slope habitats indicated chronic exposure to DWH petroleum compounds by benthic taxa on the shelf was likely orders of magnitude lower than in inshore or deepwater habitats (Murawski et al., 2016; Romero et al., 2017).
Much of the baseline data used to describe population density and community structure of ncGOM benthic invertebrates prior to the DWH were generated via fishery-independent Southeast Area Monitoring and Assessment (SEAMAP) summer and fall trawl surveys. Results of early analyses conducted to examine population- or community-level effects of the DWH on shelf invertebrates indicated no effects were apparent (Trustees, 2016). However, population-level impacts can take years to manifest, even after acute events like the DWH (Murawski et al., 2021). Therefore, we also analyzed data from SEAMAP surveys to test if post-DWH population declines became apparent in the years following the spill, and whether trends differed among GOM regions where oil was observed on the shelf versus other regions. We selected three geographically widespread invertebrate taxa that are frequently encountered in the SEAMAP summer trawl survey: brown shrimp, Farfantepenaeus aztecus, lesser blue crab, Callinectes similis, and squids, Doryteuthis spp., to examine population trends over time. While squids are not benthic as adults, they are often captured in trawls near the seabed and they have a benthic egg stage. For all three species, we obtained standardized trawl catch data from the SEAMAP fall surveys from 2001 to 2018 and computed generalized linear models to produce standardized indices of abundance for the ncGOM following standard methods (Pollack and Hanisko, 2022).
Brown shrimp standardized CPUE displayed no trend from 2001 to 2018 (Figure 2A). Brown shrimp is basically an annual species, with recruitment and productivity mostly driven by density-independent environmental factors (Haas et al., 2001). Murawski et al. (2021) reported little trend in CPUE of brown shrimp or white shrimp, Litopenaeus setiferus, in estuaries from Louisiana to northwest Florida, with those inshore habitats serving as the nursery groups for estuarine-dependent penaeids. In fact, in the first five years after the spill brown shrimp abundance in Louisiana waters increased, while the white shrimp abundance index was basically without trend (Murawski et al., 2021). Landings for brown shrimp, which are greatest in summer given their life cycle, declined in 2010 and 2011 (Figure 3A). However, much of that drop was due to fishery closures on the shelf that were instituted during the spill to guard against human consumption of contaminated seafood and mirrored the spatial distribution of observed surface oil (Figure 1).
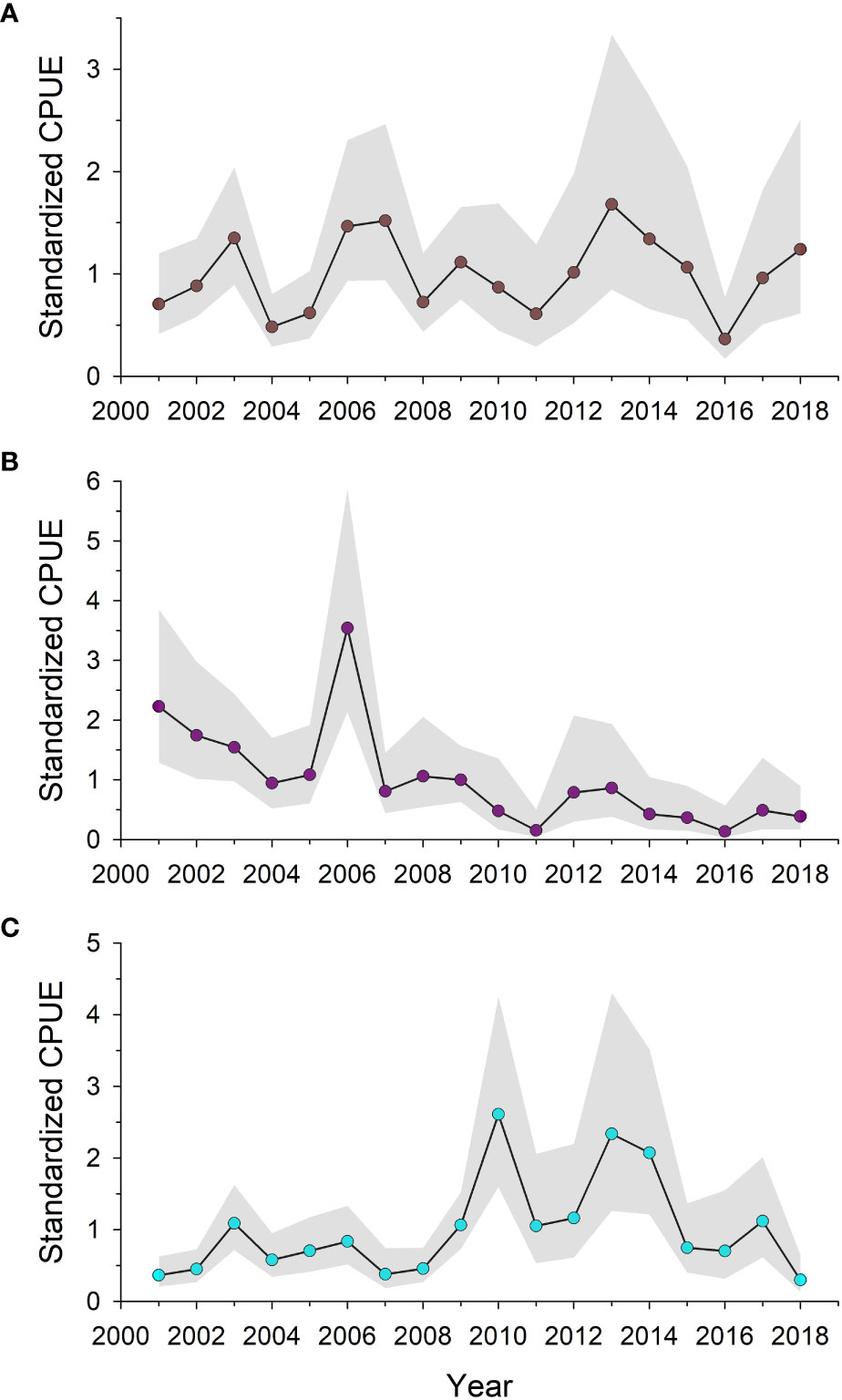
Figure 2 Standardized catch per unit effort for shelf taxa captured during SEAMAP fishery-independent trawl sampling during 2001-2018 in the north central Gulf of Mexico. Data were fitted with generalized linear models and normalized to CPUE = 1; shaded regions are 95% confidence intervals. (A) brown shrimp, Farfantepenaeus aztecus, (B) lesser blue crab, Callinectes similis, (C) squids, Doryteuthis spp.
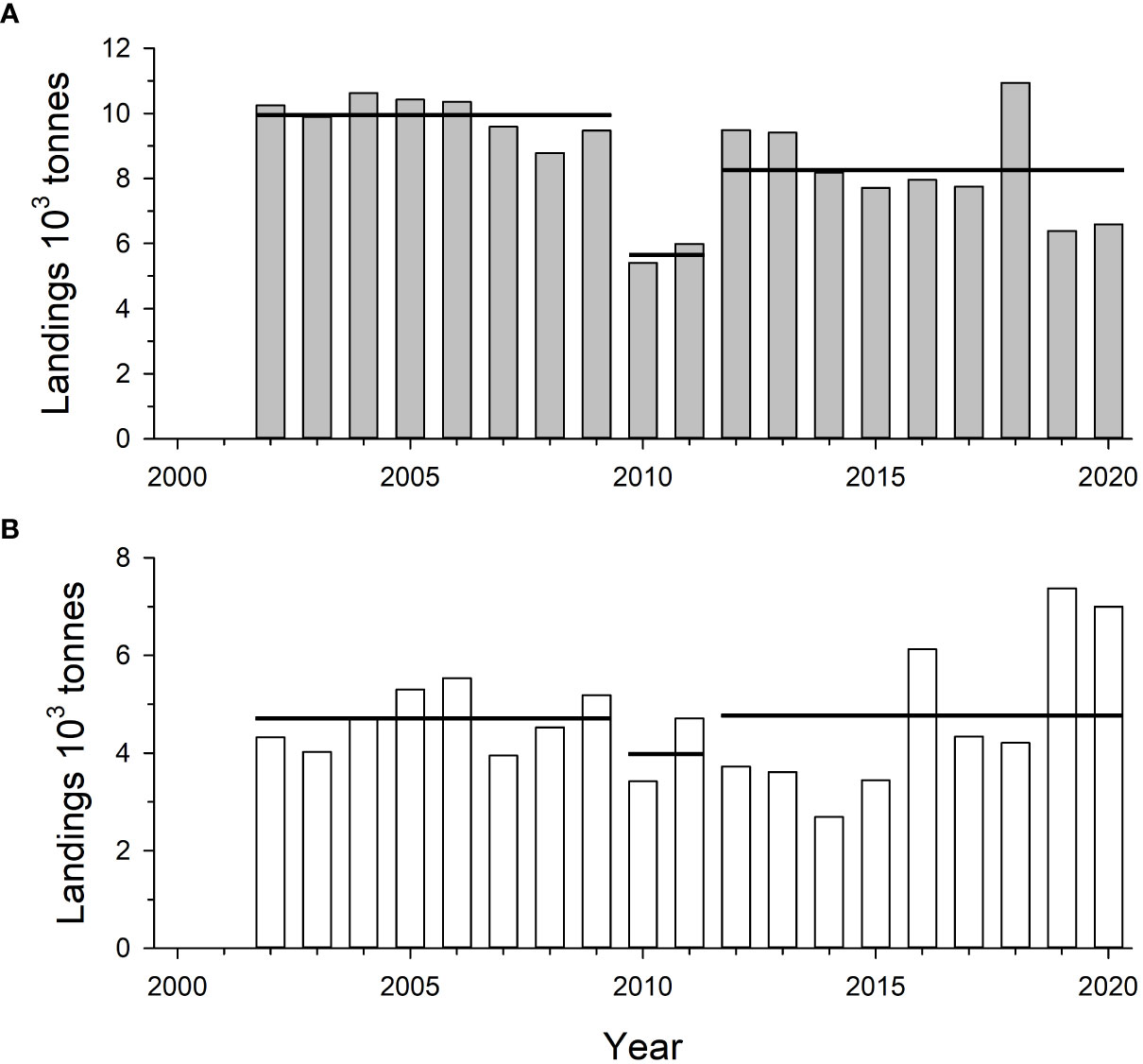
Figure 3 Estimated annual landings of (A) brown shrimp, Farfantepenaeus aztecus, and (B) white shrimp, Litopenaeus setiferus, captured in the eastern Gulf of Mexico off Mississippi, Alabama, and northwestern Florida during 2002-2020. Horizontal lines indicate mean values for the time periods shown.
Harvest of white and brown shrimps from ncGOM shelf waters has been variable over time (Figures 3A, B). There was a slight decline in mean ncGOM landings of brown shrimp in the decade after DWH, but the decline since 2015 was observed in the western GOM as well, where the bulk of brown shrimp landings are taken (Hart, 2017), and most likely reflects the >70% decline in shrimping effort during that time period (Gallaway et al., 2020). The opposite is apparent in the ncGOM white shrimp landings data where there has been an overall increase in landings since 2015. For both species, trends in landings have been within historical ranges, and population declines following the DWH are not apparent in fishery-independent survey data (Figure 2A). Jointly, fishery-dependent and -independent data do not suggest substantial population-level effects of DWH occurred for ncGOM peaenid shrimps.
Opposite trends were apparent in standardized CPUE for lesser blue crab and Doryteuthis squids (Figures 2B, C). Lesser blue crab abundance was declining in the ncGOM for the decade prior to the DWH, which follows a similar pattern observed for its congener, blue crab, Callinectes sapidus (Gallaway et al., 2016b). However, since 2010 lesser blue crab CPUE has had no apparent trend among ncGOM SEAMAP summer trawl survey stations. Gallaway et al. (2016b) speculated shelf hypoxia might be responsible for the long-term decline of GOM blue crab populations on the shelf, but that is less of an issue in the ncGOM than it is off western Louisiana and northeastern Texas (Bianchi et al., 2010). However, penaeid shrimp and squid abundance have not shown such declines, which might be expected given their overlapping habitats with Callinectes spp. The trend in squid abundance on the ncGOM shelf was relatively flat in the decade prior to the DWH, but then increased in the year of the spill and for a few years thereafter (Figure 2C). Doryteuthis squids typically feed on small fishes and crustaceans, which may have been more susceptible to predation following the DWH. Alternatively, top-down controls may have abated due to declines in some shelf fish taxa (see below) in the years following the DWH (Lewis et al., 2020). Regardless of the mechanism, any toxicological effects of the DWH on squids appears to have been more than offset either by enhanced feeding opportunities or lower mortality. Lastly, given the high productivity and short (months to a few year years) lifespan of many shelf invertebrate taxa, any negative effects of the DWH may have been short-lived and shelf populations were resilient given the lack of persistent exposure to petroleum compounds.
The shelf invertebrate taxa that displayed the most apparent injuries after the DWH spill were mesophotic (depth = 50-100 m), outer-shelf gorgonian and antipatharian soft corals. Silva et al. (2016) described a range of injuries, ranging from flocculent material (floc) or biofilms covering parts of colonies, to bare skeletons or broken or missing branches suffered by gorgonian colonies that were surveyed in 2011 with a remotely operated vehicle (ROV) near the shelf break south of Alabama and Mississippi in a region known as the Pinnacles (29.2N to 29.5N, 87.5W to 88.5W). Sediment samples revealed PAH concentrations in 2011 were above pre-spill levels but well below toxicity thresholds (Silva et al., 2016). Etnoyer et al. (2016) compared gorgonian pathologies observed in 2011 and 2014 among colonies at impacted sites to colonies at eastern GOM sites that occur at approximately the same latitude and depth range but just outside the extent of observed surface oil. They also examined images from historical (late 1980s to mid 2000s) fixed-camera or ROV surveys to assess pre-DWH injuries to corals in impacted versus reference areas. Coral condition was assessed with a qualitative injury scale that was developed to evaluate injury to deepwater gorgonians on the continental slope (White et al., 2012; Fisher et al., 2014). There was no significant difference in post- versus pre-DWH condition at reference sites. However, the odds of coral injury among the impacted Pinnacles reefs were nearly 11 times greater post-DWH, and odds of substantial (≥10% of colony) injury were seven times higher after the spill (Etnoyer et al., 2016). Apparently healthy Hypnogorgia and Swiftia colonies that were marked in 2011 also appeared to be healthy when revisited in 2014. However, colonies that demonstrated injury in 2011 were in worse condition in 2014 than when originally observed.
It is clear that mesophotic gorgonian and antipatharian corals on the ncGOM outer shelf suffered injuries due to the DWH, but estimating population-level effects is challenging because little is known about species-specific population sizes or dynamics throughout the region. Etnoyer et al. (2016) estimated the reefs they examined within the Pinnacles Trend constituted <3% of the mesophotic reef habitat in the northern GOM. Therefore, the full extent of DWH effects on mesophotic gorgonian communities remains unknown. In the deep GOM, ROV surveys conducted after the spill revealed previously unknown gorgonian communities, which suffered substantial but patchy impacts of the spill (White et al., 2012; Hsing et al., 2013; Fisher et al., 2014). The vast majority of GOM benthic habitats from the edge of the continental shelf to deepest parts of the basin remain unexplored, thus it is not possible to estimate population-level impacts of the DWH on deepwater corals. However, Girard et al. (2018) estimated a 10% reduction in deepwater coral biomass at the more impacted sites, with model projections indicating it would take up to three decades for colonies to recovery. To our knowledge, similar estimates have not been made for mesophotic corals on the outer shelf. However, the extent of injuries to individual colonies appears to have been less among mesophotic versus deeper corals (Fisher et al., 2014; Etnoyer et al., 2016), and gorgonian and antipatharian growth rates typically decline with depth (Goffredo and Lasker, 2006; Prouty et al., 2011; Benedetti et al., 2016), which is related to exported surface phytoplankton production diminishing with depth (Wei et al., 2010). A large-scale effort is underway to inventory and map ncGOM mesophotic and continental slope benthic communities, including soft corals, as part of DWH-related restoration efforts (Bassett et al., 2023). Greater understanding of their distribution, density, community structure, and population dynamics may facilitate revised estimates of DWH impacts and population-level recovery trajectories.
4 Reef fishes
No group among shelf taxa received greater sampling effort with respect to examining exposure to and effects of DWH petroleum compounds than reef fishes. This is likely due to a combination of factors, including their ecological and economic importance, the ability to conduct integrated sampling to assess petroleum exposure and effects across a variety of levels of organization, and the existence of pre-DWH databases to examine spill effects on individuals, populations, or communities. The extensive seafood sampling conducted on the shelf during fishery closures in summer and fall 2010 was primarily focused on fishes as well as crustaceans. This sampling produced only six (of 3,810, or 0.16%) instances in which human sensory analysis (i.e., expert analysis of smell or taste) suggested petroleum compounds might be present in a fish or crustacean sample (Ylitalo et al., 2012). However, concentrations of PAHs or the dispersant component, dioctyl sodium sulfosuccinate (DOSS), were estimated to be at least two orders of magnitude below limits of concern among all samples, which was similar to results reported for fish tissues tested during the 2019 northeastern Brazil oil spill (Magalhães et al., 2022). Perhaps the low occurrence of detected petroleum compounds in fish muscle during the DWH should not be surprising given testing only occurred once surface oil was no longer detected in a given area, hence it was being considered for re-opening to fishing. Furthermore, fishes have well-developed enzyme systems that enable them to clear toxicants via xenobiotic metabolism in the liver, thus preventing accumulation in muscle tissue (Koenig et al., 2013; Santana et al., 2018).
Despite the low incidence of positive detections of oil compounds in fish or crustacean muscle samples (Ylitalo et al., 2012), as well as total hydrocarbon concentrations being orders of magnitude lower in shelf versus inshore or continental slope sediment samples (Romero et al., 2017), there was substantial evidence of exposure of shelf reef fishes to petroleum compounds during the spill and thereafter. Murawski et al. (2014) reported low concentrations of PAHs in muscle tissue of shelf and upper slope reef fishes following the DWH, but high PAH metabolite concentrations in bile samples indicated high rates of PAH metabolism occurring via detoxifying hepatic enzyme systems (van der Oost et al., 2003). Biliary PAH metabolite concentrations dropped from 2011 to 2012, as did the incidence of skin lesions observed among demersal and benthic reef fishes. Snyder et al. (2015) reported PAH metabolites in bile samples of a suite of ncGOM outer shelf and upper slope reef fishes were approximately four times higher in golden tilefish, Lopholatilus chamaeleonticeps, which live in muddy burrows on the upper slope, versus red snapper, Lutjanus campechanus, a demersal reef fish that occurs across the shelf to depths of approximately 160 m. Among all species, metabolite concentrations declined from 2011 through 2013. Hepatic enzymes involved in PAH metabolism were elevated in ncGOM shelf reef fish liver samples following the DWH, but declined over a three-year post-spill period (Smeltz et al., 2017). Lastly, Pulster et al. (2020) reported median liver PAH concentrations were highest in the ncGOM among a comprehensive series of samples taken from 91 fish species throughout the Gulf of Mexico and northern Cuba. However, elevated concentrations also occurred in fish sampled in the northwest GOM, which the authors inferred suggests chronic exposure to PAHs likely occurs in that region as well as the ncGOM.
A constellation of physiological effects, many of which are sublethal but with significant impacts on condition, have been observed in fishes exposed to PAH concentrations in the range measured on the ncGOM shelf during the DWH. These include, but are not limited to, abnormal cardiac development and function (Hicken et al., 2011; Incardona et al., 2011), abnormal sensory organ function (Magnuson et al., 2018; Schlenker et al., 2019), endocrine and metabolic stress (Reddam et al., 2017), and poor swimming performance and behavioral shifts (Stieglitz et al., 2016; Johansen and Esbaugh, 2017). While assessing the likelihood of mortality, particularly for adult fishes, is important for evaluating population-level impacts of acute disturbance events like the DWH, sublethal effects, particularly in the case of chronic exposure, may have more substantial long-term effects on fish populations (Whitehead et al., 2012; Pasparakis et al., 2019). Furthermore, ecosystem impacts that alter predator-prey dynamics can also diminish fish condition and growth, hence affect mortality. With respect to the DWH, Tarnecki and Patterson (2015) reported diet analysis and muscle stable isotope ratios indicated adult red snapper switched to feeding on higher trophic position and more benthic prey in the year following the spill. Muscle stable isotope data presented by Patterson et al. (2020) demonstrated this phenomenon occurred in a suite of ncGOM reef fishes beyond red snapper, but that species-specific trophic positions had returned to pre-spill levels by 2012. Analysis of reef fish muscle δ14C clearly demonstrated that microbial consumption of petroleum compounds, chiefly methane (Graham et al., 2010), had transferred up the food web and was assimilated into reef fish muscle tissue (Patterson et al., 2020), thus confirming the link between changes in prey resources and the DWH. Diminished prey resources could lead to higher energetic costs in seeking prey, or insufficient resources to fuel growth as well as reproductive output. Consistent with that hypothesis, diminished growth rates were reported for ncGOM red snapper following the DWH (Herdter et al., 2017).
Myriad analyses provide compelling data demonstrating reef fish exposure to toxic petroleum components, as well as direct and indirect effects of exposure, occurred following the DWH, but what about population- or community-level impacts? Among standardized, fishery-independent bottom longline (BLL) sets made on the GOM shelf and upper slope (depth range: 16-632 m) during 2011-2017, Murawski et al. (2018) reported red snapper was the second most frequently captured fish, behind only Atlantic sharpnose shark, Rhizoprionodon terraenovae. Among their ncGOM (86W to 92W) sets (n = 144), mean red snapper CPUE (fish per set) declined linearly (linear regression R2 = 0.89) from 10.6 in 2011 to 2.5 in 2017. A similar pattern was observed for snappers and groupers overall, although there was no clear trend in CPUE among all species captured in BLL sets. Lewis et al. (2020) reported results of ROV sampling at a series of natural reef sites (n = 16) sampled quarterly during 2009-2017 across the ncGOM (86W to 88W; depth range: 17-72 m). There were significant declines in reef fish abundance at the species, trophic guild, and overall community levels between pre- and post-DWH samples (Figure 4). Declines in fish density ranged between 35.0–95.5% among seven trophic guilds of obligate reef or reef-associated species, which was accompanied by significant shifts in fish community structure, richness, and diversity. In the year following the DWH, red snapper abundance declined by 69% (Figure 4A), while demersal browsers and demersal invertivores declined by 87% and 82% (Figures 4B, C), respectively. Red snapper abundance further declined through 2017, while demersal browsers and invertivore abundance showed some recovery through 2015 and then declined again through 2017. Reef planktivore abundance, driven chiefly by vermilion snapper, Rhomboplites aurorubens, initially declined by 36% in fall 2010, but there was a net increase in their abundance through 2017 (Figure 4D).
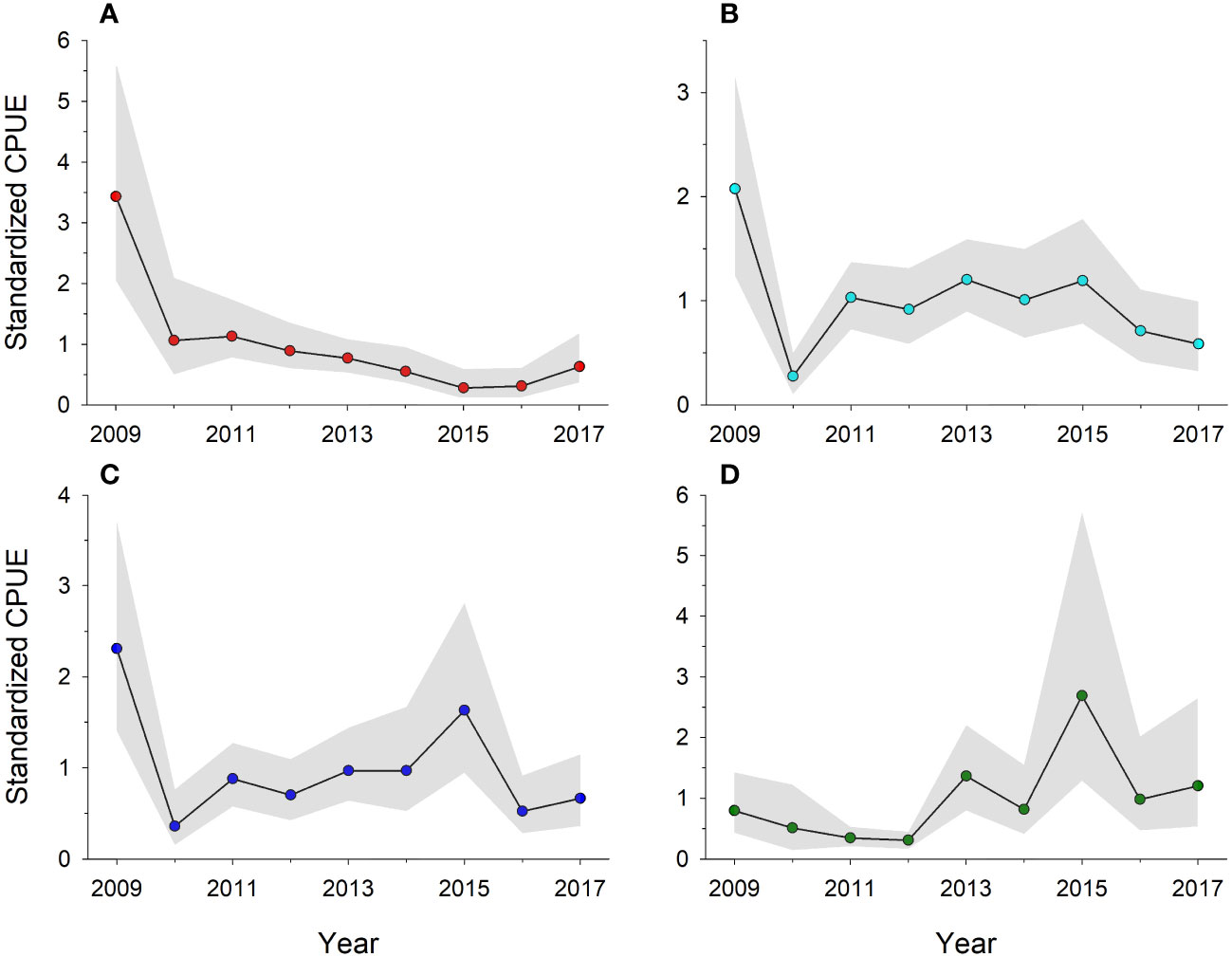
Figure 4 Standardized catch per unit effort for shelf reef fish taxa or feeding guilds observed during remotely operated vehicle sampling at north central Gulf of Mexico natural reef sites during 2009-2017 (Lewis et al., 2020). Data were fitted with generalized linear models and normalized to CPUE = 1; shaded regions are 95% confidence intervals. (A) red snapper, Lutjanus campechanus, (B) demersal browsers, (C) demersal invertivores, and (D) planktivores.
The reef fish that displayed the largest increase in abundance during the post-DWH period was invasive lionfish, Pterois spp. (Figure 5). These Indo-Pacific fish were introduced into US Atlantic waters off eastern Florida in the 1980s, first reported from the GOM in 2009 (Aquilar-Perera and Tuz-Sulub, 2010), and first observed on natural reefs in the ncGOM in summer 2010 (Dahl and Patterson, 2014). Their densities quickly grew on natural and artificial reefs (Figure 5), and by 2014 their densities on ncGOM artificial reefs were the highest in their invasive range (Dahl and Patterson, 2014; Dahl et al., 2019). Lionfish have had tremendous effects on native taxa in reef ecosystems throughout the western Atlantic (Côté et al., 2013), as they directly consume small reef or reef-associated fishes or compete with larger fishes for prey (Curtis et al., 2017; Smith and Côté, 2021). Their rapid population growth in the ncGOM represents a substantial confounding factor for examining reef fish population resiliency following the DWH (Chagaris et al., 2020). Other important confounding factors include intensive reef fish fisheries in the region, as well as the shelf fishery closures in place during 2010-2011.
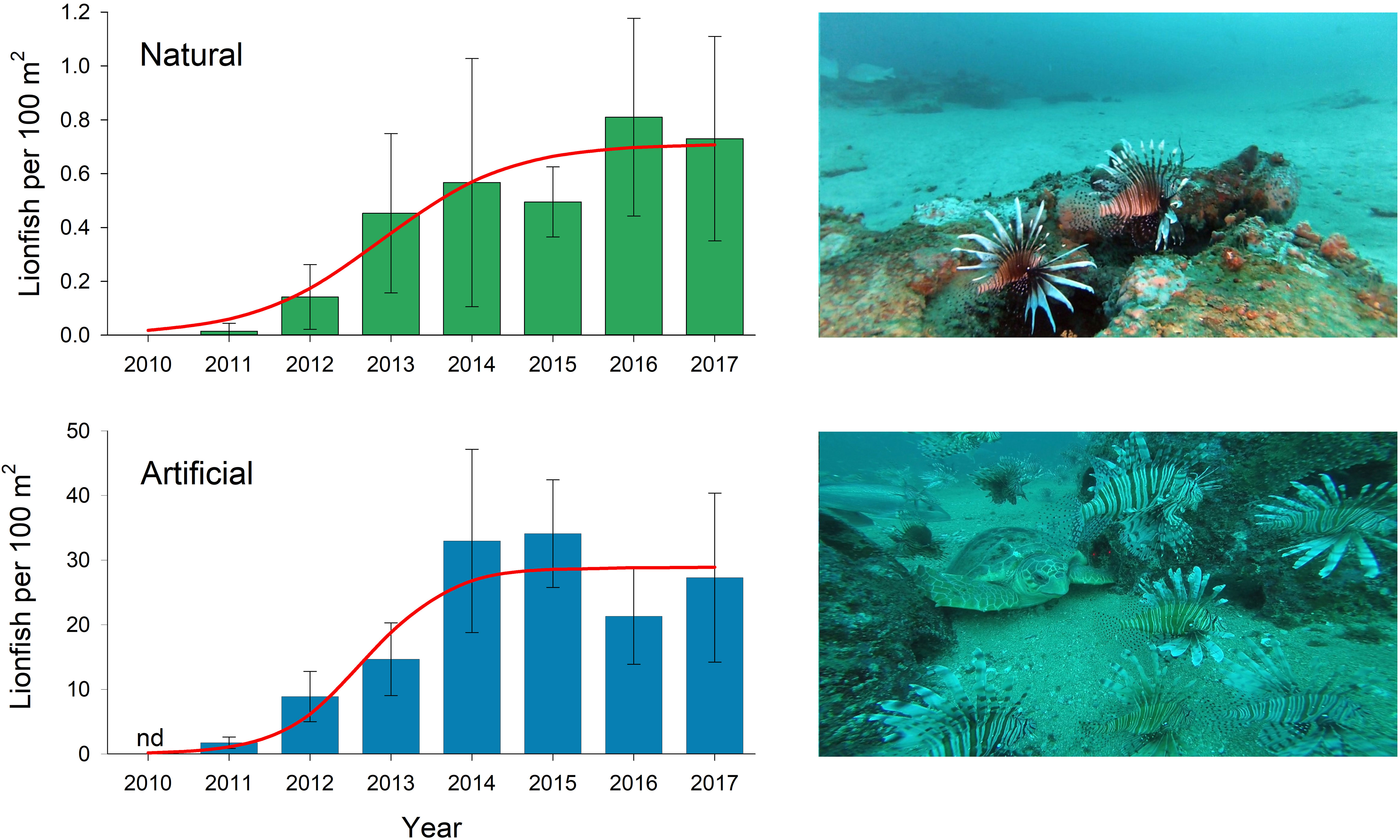
Figure 5 Trends in invasive lionfish, Pterois spp., density estimates (mean ± 95% confidence intervals) at natural and artificial reef sites in the north-central Gulf of Mexico estimated via remotely operated vehicle surveys during 2010-2017 (Dahl and Patterson, 2014; Dahl et al., 2019). Images of lionfish at a natural (top) and artificial (bottom) reef in 35 and 32 m of water, respectively, approximately 40 km south of Pensacola, Florida in August 2016.
Several single-species or ecosystem models exist to evaluate potential reef fish population or community effects of the DWH. Stock assessments are routinely conducted for red snapper in US waters of the GOM, with stock dynamics modeled separately for western (west of 89W) and eastern subunits of the stock in recent assessments. Red snapper has been estimated to be overfished in the US GOM since the 1980s (SEDAR, 2018), but the pace of stock recovery increased for both sub-units following the reauthorization of the Magnuson-Stevens Fishery Conservation and Management Act in 2006 due to its more conservative management requirements (Figure 6). The recovery trajectory for the western GOM red snapper sub-unit maintained a relatively constant slope from the mid-2000s until 2016, the terminal year of the data in the 2018 stock assessment. However, stock biomass plateaued in the eastern GOM after 2010 and remained flat from 2011 to 2016 (Figure 6). To date, ecosystem effects of the DWH have not been explicitly incorporated into red snapper stock assessments.
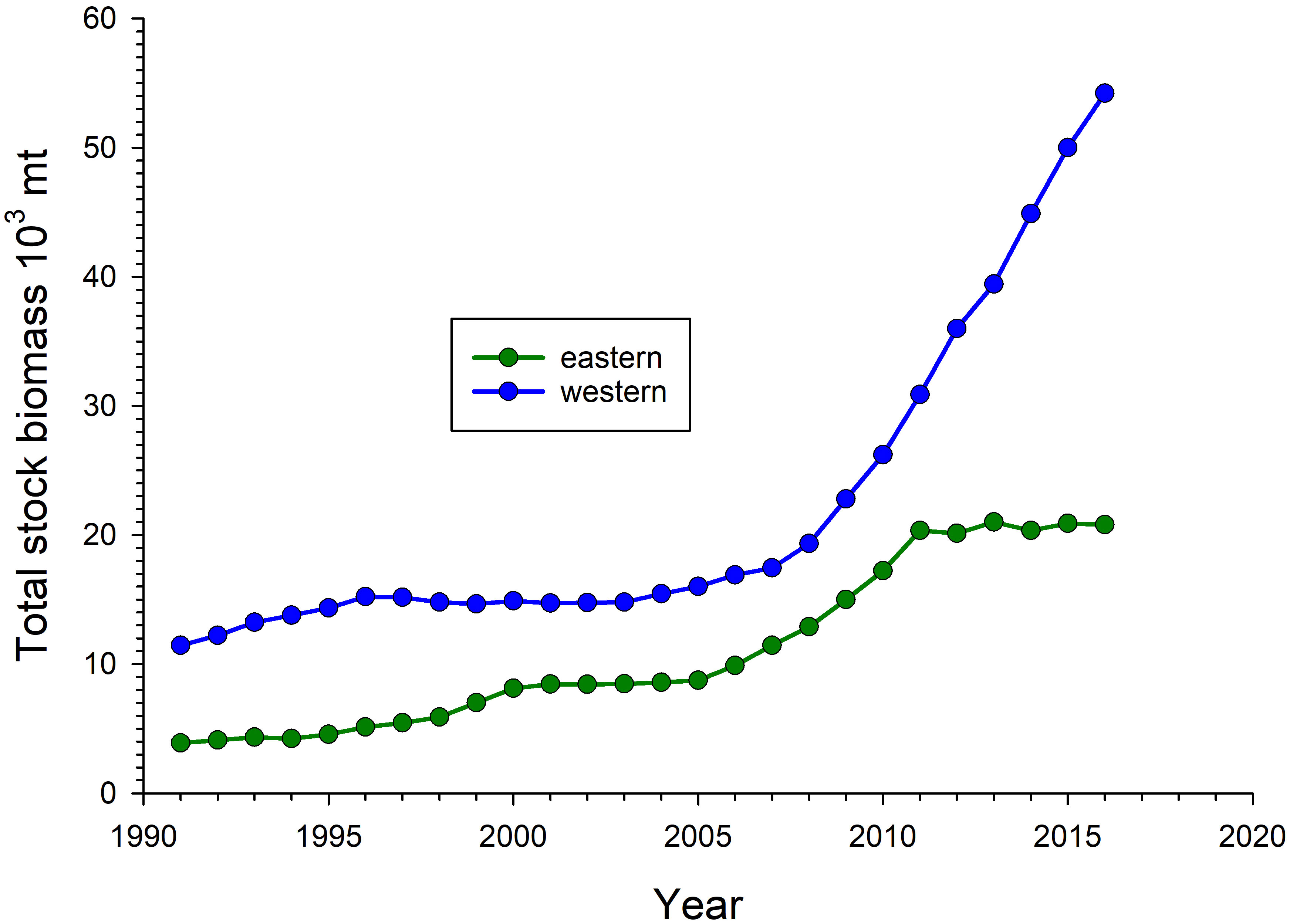
Figure 6 Estimated total stock biomass for eastern and western subunits of the US Gulf of Mexico red snapper, Lutjanus campechanus, stock. Estimates were produced with an integrated statistical catch-at-age model with fishery-dependent, fishery-independent, and life history data as model inputs (SEDAR, 2018).
Ainsworth et al. (2018) evaluated ecosystem impacts of the DWH on ncGOM fish guilds with a spatially-explicit Atlantis model parameterized to examine GOM ecosystem dynamics. They estimated 40-85% declines in biomass for a range of reef fish taxa, including snappers (Family: Lutjanidae), groupers (Family: Serranidae), and small demersal reef fishes (Families: Blenniidae, Labridae, Pomacanthidae, Pomacentridae, and others). Model projections indicated it would take 30 years or more for populations of larger, slower growing and later maturing fishes to recover to pre-DWH levels. For higher productivity species, such as short-lived small demersal reef fishes, projections indicated recovery in ten years or fewer. However, the Atlantis model did not have lionfish as an ecosystem component, thus the potential for confounding effects of lionfish on population recovery could not be assessed. Model simulations suggested the loss of fish larvae due to toxicological effects of the DWH had only minor impacts on fish population biomass or recovery, likely due to the fact that most marine fish larvae die each year regardless of perturbation, and due to the fact that recruitment is often highly variable among years (Ainsworth et al., 2018). Similarly, fishery closures on the shelf were estimated to have little impact on population or community dynamics given that areas were closed only for months and most fishery species in the system have potential lifespans of decades. The temporal scale of closures was simply too short to have substantial impacts, especially on long-lived reef fishes like snappers and groupers.
Chagaris et al. (2020) constructed an Ecopath model of the ncGOM reef ecosystem to model the relative impacts of the DWH, invasive lionfish, and fishery exploitation on reef fishes, and then conducted Ecopath with Ecosim (EwE) simulations to predict recovery of reef fishes following the DWH. The Ecopath model was composed of 63 biomass groups calibrated to time series of abundance from 2009 to 2016, as well as diet matrices derived from site-specific data and other studies. Biomass trajectories following the DWH were simulated to examine projected impacts of single stressors (i.e., DWH, invasive lionfish, and fishery exploitation), as well as cumulative impacts of these stressors and direct and indirect food web effects. Larger, exploited species, such as snappers and groupers, were most affected by fishing mortality, which was projected to delay recovery from observed declines following the DWH. Small demersal reef fishes, which showed the largest declines following the DWH (Lewis et al., 2020), received some predator release from native piscivores, but this was more than offset by invasive lionfish. Lastly, EwE simulations demonstrated the trophic guild that rebounded quickest following the DWH, reef-associated planktivores (Lewis et al., 2020), did so because of predator release due to declines in larger piscivores. The model attributed those declines due to DWH-related mortality. However, declines in piscivore biomass could have also been due to movement as reef fishes that typically display moderate site fidelity can move 10s or 100s of km due to large-scale stressors (Patterson et al., 2001; Wiernicki et al., 2020)
The substantial (>90%) declines observed in small demersal reef fishes following the DWH occurred when only a few lionfish had been observed in the ncGOM (Dahl and Patterson, 2014). Otolith ageing later revealed that at least some lionfish were present in the system as early as 2008 (Dahl et al., 2019), but densities were low enough that fish were not detected until summer 2010. Given their population growth from 2010 to 2017, model simulations projected lionfish would exert strong top-down controls on small demersal reef fishes in the ncGOM well into the future, which has been observed in other regions of their invasive range (Côté et al., 2013). It is unclear, however, why invasive lionfish displayed such rapid population growth in the ncGOM to begin with. Thermal tolerance modeling predicted lionfish could survive across the shelf throughout the year in the region (Kimball et al., 2004), but lionfish populations did not increase as rapidly on the west Florida shelf or the northwestern GOM off Louisiana and Texas (Campbell et al., 2022). Chagaris et al. (2017) modeled the interaction between fishery species on the west Florida shelf and invasive lionfish and demonstrated that higher native mesopredator biomass inhibited lionfish population growth, even without direct consumption of lionfish, which is supported by empirical data in other systems (O’Farrell et al., 2014; Smith and Côté, 2021). If native piscivores directly consume lionfish (e.g., Maljković et al., 2008; Mumby et al., 2011; Dahl and Patterson, 2020), then their presence on a given reef or region would likely exert direct in addition to indirect controls on lionfish production and biomass.
Overall, reef fish populations in the ncGOM displayed perhaps the clearest population declines following the DWH, with ample evidence that fish were exposed to petroleum compounds that likely persisted in the food web for years after the spill. Furthermore, stomach content and stable isotope data suggest lower trophic position prey of mesopredators or higher-level consumers were also affected by the DWH due to the switch to higher trophic position and more benthic prey items. One might have expected for short-lived small demersal reef fishes, such as wrasses, damselfishes, or butterflyfishes, to have recovered in the decade after the spill. However, the rapid population growth of lionfish, which may have resulted from reduced biotic resistance due to depleted higher trophic position fish biomass, has added a confounding effect that may suppress population sizes of their native reef fish prey into the future. Lionfish may prefer small demersal reef fishes as prey, but they are actually quite generalist in their feeding ecology (Dahl and Patterson, 2014; Dahl et al., 2017), thus compete with native mesopredators in the ncGOM for prey resources. Ecosystem modeling has demonstrated this has the potential to suppress population recovery of mesopredators and higher level predators in the ncGOM shelf ecosystem, but the effect of lionfish on fishery species is not as great as fishing mortality, which further impacted post-DWH population recovery (Chagaris et al., 2020). With the sunset of GOMRI funding, and with it the ROV and BLL surveys that provided so much information on reef fish exposure to petroleum compounds, population trends, and rapidly increasing lionfish populations in the decade after the DWH, the opportunity to measure potential recovery and changing community dynamics over time has been greatly diminished. Therefore, despite the vast amount of data collected to demonstrate the impact of the DWH on reef fish populations, there may be considerable uncertainty moving forward about their resiliency.
5 Higher vertebrates
Images of oiled birds, turtles, and cetaceans were some of the clearest examples of direct DWH impacts on ncGOM biota. Tens of thousands of sea birds, sea turtles, and marine mammals were estimated to have died due to exposure to DWH petroleum, with further population impacts estimated due to likely effects on reproductive output (Trustees, 2016). Takeshita et al. (2021) reviewed the effects of oil exposure on vertebrates and noted distinct commonalities in impacts, from impairment of stress responses and adrenal gland function, to cardiotoxicity and blood cell disruption, to immune system and oxidative damage. They also noted a progression of pathologies from molecular and subcellular to cellular and organ effects, and eventually to organ systems, was commonly seen across a diversity of vertebrate taxa. Once petroleum effects became systemic, exposure had the potential to compromise growth, reproduction, and survival. Despite the persistent presence of these effects in the data across taxa, population-level impacts were less obvious and, estimated population-level impacts of the spill were only partially based on observed mortalities. Instead, analyses often relied on observed mortalities, tissue or organ pathologies detected during necropsies, and the overlap in species distribution and oil maps to compute likely oil exposure, and then those individual impacts were extrapolated to population level effects (Schwacke et al., 2017). However, population estimates are uncertain for many higher vertebrate taxa because abundance data are sparse and surveys to estimate population size, when they do exist, are often conducted infrequently on the shelf, including in the decade following the DWH.
Much of the observed oil exposure suffered by higher vertebrates was observed in the coastal zone (Murawski et al., 2016; Trustees, 2016; Murawski et al., 2021), the deep benthic (Schwing et al., 2020), and the mesopelagic water column (Sutton et al., 2022), not necessarily on the shelf. This translated to the majority of estimated higher vertebrate mortalities occurring in the coastal zone. For example, there were an estimated 79,919 birds killed due to the DWH spill, with approximately 97% of that mortality occurring to birds in the coastal zone (Trustees, 2016). Given most seabird surveys occur in nesting areas versus on the water, and the fact that rookeries for many ncGOM offshore bird species [e.g., boobies (Family: Sulidae), shearwaters and storm-petrels (Family: Procellariidae), and terns (Family: Laridae)] occur outside the region (Burger, 2017), it would be exceedingly difficult to directly estimate population-level impacts and resiliency in offshore bird populations. Tracking population trends for marine mammals is also difficult, but there are periodic surveys conducted by NOAA Fisheries to estimate abundance for marine mammal populations in US waters of the GOM. Sea turtles, especially nesting females, are the least difficult higher vertebrate taxa to assess population trends over time due to extensive annual efforts to estimate the numbers of nests and protect them on GOM beaches. However, among the five sea turtle species that occur in the GOM only Kemp’s ridley turtles, Lepidochelys kempii, nest nearly exclusively in the GOM, with 95% of Kemp’s ridley nests occurring in the Mexican state of Tamaulipas (Márquez, 1994; Caillouet et al., 2018).
5.1 Sea turtles
The ncGOM shelf constitutes important foraging grounds for Kemp’s ridley, loggerhead, Caretta caretta, and green, Chelonia mydas, sea turtles, while hawksbill, Eretmochelys imbricata, and leatherback, Dermochelys coriacea, turtles also occur in this region. Potential pathways for their exposure to DWH petroleum compounds in the shelf environment included direct oiling at the surface, ingesting prey that had been oiled or had consumed contaminated prey themselves, or inhalation of vaporized petroleum compounds at the sea surface (Trustees, 2016; McDonald et al., 2017; Wallace et al., 2017). The most obvious direct evidence of exposure was the observation of oiled turtles. In total, 574 turtles were captured at sea during the spill, with 81% of these animals displaying evidence of external oiling, 20% displaying moderate to heavy oiling, and the extent of oiling dissipating with distance from the MC252 wellhead (Wallace et al., 2017). Approximately half of oiled turtles were Kemp’s ridleys, which translated to approximately 206,000 small (<age-3) juveniles and 22,000 larger juveniles and adults estimated to have been oiled during the spill given the extent of surface oil and the estimated density of Kemp’s ridley turtles in the ncGOM, (McDonald et al., 2017; Wallace et al., 2017). Estimates of 100% mortality for heavily oiled turtles and 30% mortality for otherwise oiled turtles (Mitchelmore et al., 2017) translated to an estimated 86,500 <age-3 and 3,110 age-3+ Kemp’s ridley deaths due to DWH (Trustees, 2016).
The habitat utilization, nesting behavior, and restricted geographic range of Kemp’s ridley turtles make them well-suited to examine population-level DWH effects. Most juvenile and adult Kemp’s ridleys remain within the GOM throughout life, and 82% of satellite-tagged individuals utilized the shelf of Alabama, Mississippi, and Louisiana as foraging habitat (Gredzens and Shaver, 2020). Moreover, 95% of annually observed Kemp’s ridley nesting occurs on a geographically confined series of beaches in the Mexican state of Tamaulipas, just south of Texas (Márquez, 1994). Historical Tamaulipas nesting data indicate the Kemp’s ridley population had been reduced by approximately 99% by 1985 due to habitat destruction, direct harvest of adults and eggs, and fisheries bycatch (Bevan et al., 2016). Extensive conservation efforts began in Mexico in the 1980s and in 1987 turtle excluder devices became required for shrimp trawls in US federal waters of the GOM. The Kemp’s ridley population responded quickly, with annual nest counts increasing approximately 15% or more per year through 2009 (Gallaway et al., 2016a; Gallaway et al., 2016b). However, the exponential increase in Kemp’s ridley nests came to a halt in 2010, coincident with the DWH spill (Caillouet, 2011). There was a nearly 40% decline in Tamaulipas nests in 2010, which was especially concerning given the prior two decades of rapid population growth (Figure 7). The conservation status of Kemp’s ridley turtles, the magnitude of the DWH event, and the estimated exposure of turtles to oil rightly prompted the question whether the DWH drove the decline in turtle nesting, especially given the exponential increase in nests prior to 2010 (Caillouet, 2011).
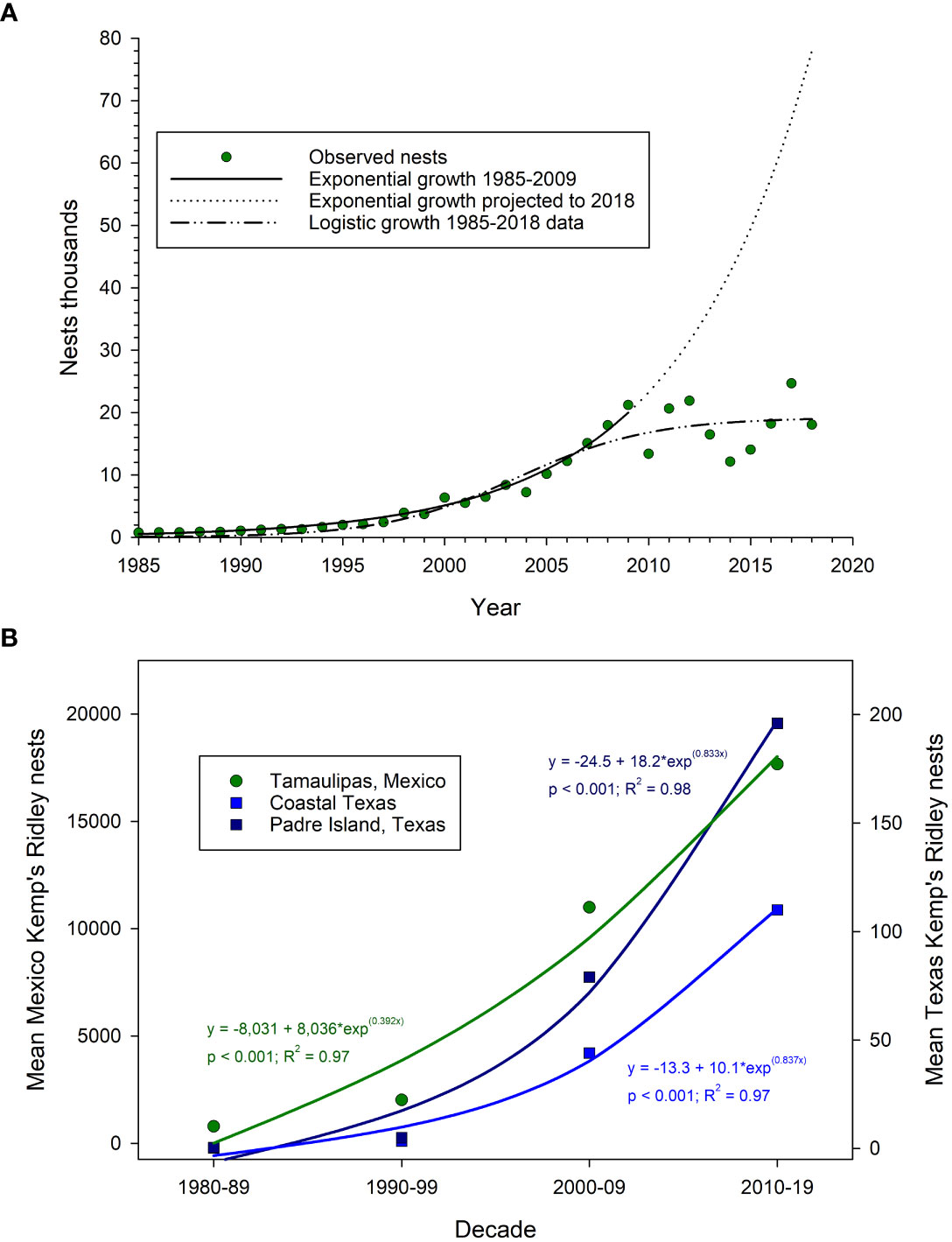
Figure 7 (A) Kemp’s ridley sea turtle, Lepidochelys kempii, nest counts (circles) in Tamaulipas, Mexico during 1985-2018. Solid line is exponential population growth model fit to the data through 2009, with dotted line indicating forward projection of exponential growth to 2018. Dash-dotted line indicates a logistic growth model fit to the data (Data: Gallaway et al., 2020). Models are extensions of the Caillouet et al. (2018) models fit to nest count data. (B) Mean Kemp’s ridley sea turtle nest counts by decade in Tamaulipas, Mexico, coastal Texas, and South Padre Island, Texas during 1980-2019 (Data: NPS, 2020). Solid lines are exponential functions fit to each data series, with functions shown on the panel.
The mean nesting date for Kemp’s ridleys in Tamaulipas ranges from May 1st to May 15th, and the 2010 peak in nesting occurred on May 15th (Gallaway et al., 2016b). The maximum swimming rate for the species is approximately 10 km h-1 (Renaud, 1995) and the straight-line distance between the MC252 wellhead and the nesting beaches in Tamaulipas is just over 1,000 km. Therefore, it does not seem plausible that adult Kemp’s ridley turtles that nested in Tamaulipas in 2010 had substantial exposure to DWH oil. Instead, perhaps the lower nest count in 2010 had more to do with January-March water temperatures being approximately 3°C above mean temperatures, which delayed nesting and may have had metabolic effects on turtles that affected reproductive output (Gallaway et al., 2016b). A rebound to 2009 nesting levels occurred in 2011 and 2012 as water temperatures returned to the decadal mean, but then nest counts dropped again in 2013 and 2014 with warmer than average temperatures (Gallaway et al., 2016b).
In the decade after 2010, Kemp’s ridley nest counts, hence population estimates, stabilized. There was no trend (linear regression, p = 0.89) in Tamaulipas nest counts from 2009 to 2018, and a logistic rather than exponential model best fits the nest trend time series (Caillouet et al., 2018; Figure 7A). Overall, decadal mean nest counts in Mexico show an exponential increase from the 1980s to the 2010s, and the same pattern is evident for Texas nesting beaches as well, albeit nest counts in Texas are two orders of magnitude lower than in Mexico (Figure 7B). Still, the Texas data are intriguing given they suggest Kemp's ridley populations have been expanding at similar rates outside of Mexico over the past two decades. Furthermore, there is a strong linear relationship (linear regression, R2 = 0.95) between nest counts in south Texas and those in Tamaulipas (Shaver et al., 2016), and the Texas data clearly show an increase in nesting season length and a decline in the number of neophyte nest-building females from 1978 to present (Shaver et al., 2016). Perhaps the expansion of nesting in Texas is related to climate change, as mean water temperature rose approximately 3°C in the northern GOM over the time period of the nesting data (Fodrie et al., 2010; Purtlebaugh et al., 2020), or perhaps density-dependence is more of a driving factor explaining this phenomenon (Gallaway et al., 2016b; Caillouet et al., 2018). As the population size of Kemp’s ridleys increased over recent decades, turtle growth rates declined (Avens et al., 2020), as did the number of eggs per nest and the number of nests per adult female (Gallaway et al., 2016b). Among a suite of population model scenarios examined by Gallaway et al. (2016b), the model which included density-dependent nesting fit the data best, thus providing further evidence for density-dependent population regulation is affecting Kemp’s ridley population growth.
Questions remain, however, about what impact the DWH had on the Kemp’s ridley turtle population, and what the implications of the spill are for the species’ long-term recovery potential. Via population modeling, Gallaway et al. (2016b) estimated the instantaneous mortality rate for small juvenile turtles was 1.32 y-1, while the rate due to the acute effects of the DWH was 0.31 y-1 in 2010. That translates to finite annual mortality rates of 73.3% in a typical year and 80.4% in 2010, with the relatively small increase in 2010 mortality attributable to DWH. Although not reported by the authors, the DWH mortality rate for age-3+ animals would have been even lower given the considerably lower death estimates for larger turtles (Mitchelmore et al., 2017). Still, the population size of Kemp’s ridley turtles in 2014 was estimated to be approximately half of the estimated historical carrying capacity (Gallaway et al., 2016b), although carry capacity in the GOM itself appears to have declined substantially since the first half of the 20th century (Bevan et al., 2016). Perhaps that is due to declines in habitat quality in important northern GOM foraging areas owing to hypoxia or other anthropogenic factors, or perhaps to declines in blue crab, an important Kemp’s ridley prey resource (Gallaway et al., 2016b; Caillouet et al., 2018). Evidence from reef fish tissue samples indicates petroleum compounds persisted on the shelf for three or four years following the DWH (Snyder et al., 2015; Smeltz et al., 2017), although stable isotope data, including from sea turtle samples, suggest the most substantial food web effects had mostly dissipated by the second year post-spill (Tarnecki and Patterson, 2015; Vander Zanden et al., 2016; Patterson et al., 2020). Therefore, it may be unlikely that the DWH had lasting effects on Kemp’s ridley prey resources.
Most of the exposure to oil and related mortality was estimated to occur in turtles less than age-3, but even if the estimated 86,000 deaths of small juvenile Kemp’s ridley turtles due to DWH oil exposure is close to accurate, approximately 1.5 x 106 animals within those year classes were estimated to be in the population in 2010 (Gallaway et al., 2016b). Not until approximately 2020 would females in those age classes begin to reach maturity and come ashore to nest (Avens et al., 2017). Given the 20+ year reproductive life span of females, it is unclear that the level of estimated impacts on juvenile turtles in 2010 would translate to long-term demographic shifts in the population. However, given the endangered status of Kemp’s ridley turtles and the historical investments made to recover their population from approximately 1% of carrying capacity in 1985, we echo calls to continue allocating resources to monitor and assess population trends, including through population models and more holistic ecosystem modeling (Shaver et al., 2016; Caillouet et al., 2018).
5.2 Marine mammals
There are 21 species of cetaceans and one sirenian, the West Indian manatee, Trichechus manatus, that occur in the GOM (Würsig, 2017). However, the GOM manatee population is distributed in estuarine to freshwater systems along the west Florida shelf. The recently recognized and rare species, Rice’s whale, Balaenoptera ricei, is also occasionally spotted on the outer shelf in the region (Rosel and Wilcox, 2014; Rosel et al., 2021). The bottlenose dolphin is by far the most prominent marine mammal in ncGOM inshore and shelf habitats, while the pantropical spotted dolphin, Stenella attenuata, may be more abundant in the region when including its predominantly oceanic habitat. The cosmopolitan bottlenose dolphin occurs in distinct inshore and shelf populations throughout its range (Wells et al., 1987), and the ncGOM is no exception. Population genetics indicate at least some reproductive mixing occurs among BSE (bay, sound, and estuary) populations and between BSE and coastal (shelf) populations in the ncGOM (Sellas et al., 2005; Rosel et al., 2017), but tagging studies have shown there is strong fidelity of the groups to inshore versus shelf habitats (Balmer et al., 2016; Wells et al., 2017).
Bottlenose dolphins, particularly BSE populations in coastal Louisiana, suffered the greatest exposure to DWH oil among marine mammals (Schwacke et al., 2014; Aichinger Dias et al., 2017). Hundreds of dolphins were observed to be directly oiled (Trustees, 2016), while other exposure pathways included ingestion of oil while feeding, or inhalation or aspiration of petroleum compounds directly into the lungs (Takeshita et al., 2017). Skin biopsies, blood samples, and pulmonary ultrasounds on live animals, as well as various tissues examined during necropsies of dead animals, clearly demonstrated petroleum exposure and resulting physiological stress among bottlenose and other delphinids, with bottlenose dolphin BSE populations, particularly those found in Louisiana estuaries, displaying much greater exposure to DWH petroleum compounds than animals on the shelf (Schwacke et al., 2014; Venn-Watson et al., 2015; Schwacke et al., 2017). Sub-lethal effects of DWH exposure included mortalities of near-term bottlenose dolphin fetuses, as well as greatly lowered reproductive success in BSE populations from coastal Louisiana to Alabama (Carmichael et al., 2012; Venn-Watson et al., 2015; Kellar et al., 2017). Other observed sub-lethal health effects included anemia, tooth loss, and liver damage (Schwacke et al., 2014).
The substantial exposure of cetaceans to DWH petroleum was the driving factor causing an unusual mortality event (UME) during 2010-2014 (NOAA, 2023), with a UME defined by the US Marine Mammal Protection Act (1972) as “a stranding that is unexpected; involves a significant die-off of any marine mammal population; and demands immediate response.” Inshore (BSE) bottlenose dolphin populations are particularly susceptible to UMEs (Würsig, 2017), and the 2010-2014 event actually started in late winter 2010, just prior to the DWH (NOAA, 2023). Carmichael et al. (2012) suggested stress due to an unusually cold winter in 2010, as well as abnormally high freshwater input into coastal estuaries in spring 2010, likely contributed to the observed mortalities in BSE bottlenose dolphins. Regardless, of the 1,141 cetaceans that stranded in the ncGOM during 2010-2014, all but 89 animals stranded following the DWH, with the peak in strandings occurring in winter through spring in 2011 (Trustees, 2016; NOAA, 2023). Most (85%) of the stranded animals were bottlenose dolphins, with 86% of stranded bottlenose dolphins in Alabama, Mississippi, and eastern to central Louisiana being estimated to be members of BSE versus coastal (shelf) populations based on skin stable isotope signatures (Hohn et al., 2017). Therefore, higher rates and degrees of exposure to DWH petroleum compounds led to greater incidence of stranding for BSE versus coastal bottlenose dolphins.
A mark-recapture study based on photo identification was utilized to estimate the percentage of bottlenose dolphins that died as a result of the DWH within Barataria Bay, Louisiana and Mississippi Sound (McDonald et al., 2017), and then results were extrapolated to other BSE and coastal populations based on the estimated proportion of strandings from those populations versus those in Louisiana (Trustees, 2016). Mortality estimates ranged from 12% for the Mobile Bay BSE to 59% for the Mississippi Delta BSE, among inshore populations, and were 1% and 38% for western (west of 89.5W longitude) coastal and northern (84W to 89.5W longitude) coastal populations, respectively (McDonald et al., 2017). Schwacke et al. (2017) utilized empirical data on bottlenose dolphin exposure, sub-lethal effects of exposure, including reproductive failure and changes in life history parameters, and estimated mortality to construct a matrix population model to predict the total time to recovery for each bottlenose population impacted by the DWH. This modeling produced estimates of 31 to 52 y to recovery for the four impacted BSE populations, but the northern coastal population on the shelf, which had much lower rates of exposure and stranding than any of the BSE populations, was also estimated to require 39 y to fully recover (Trustees, 2016; Schwacke et al., 2017). It seems counterintuitive that the northern coastal population would be predicted to take as long to fully recover as the BSE populations, but Schwacke et al. (2017) acknowledged that uncertainties existed in the shape of density dependence functions and estimates of the years required for populations to return to pre-DWH numbers.
For other cetacean populations that occur at least partially over the shelf, the percentage of each population exposed to surface oil was estimated based on satellite imagery of surface oil and estimates of population distributions from ship- or plane-based line transect surveys (DWH MMIQT, 2015). Estimates were 13% for Atlantic spotted dolphins, Stenella frontalis, 20% for pantropical spotted dolphins, 47% for spinner dolphins, S. longirostris, and 48% for Rice’s whales. Mortality due to the DWH for each of these populations was estimated by multiplying the percent population exposure by the estimated excess mortality due to DWH for Barataria Bay bottlenose dolphins, which was 35%. Additional population impacts were calculated by adding estimated reproductive failure and sub-lethal effects, again scaled using the Barataria Bay population data, and producing a sum of lost cetacean years for each population. This resulted in a negligible population reduction for Atlantic spotted dolphins, 9% for pantropical spotted dolphins, 23% for spinner dolphins, and 22% for Rice’s whales. Projected times for population recovery were 39 y for pantropical dolphins, 105 y for spinner dolphins, and 69 y for Rice’s whales (DWH MMIQT, 2015).
The DWH Marine Mammal Injury Quantification Team (DWH MMIQT, 2015) and Schwacke et al. (2017) suggested the approaches taken to estimate injury and time to recovery for GOM cetacean populations were conservative. By scaling injury estimates for shelf and oceanic cetaceans to those observed for Barataria Bay BSE bottlenose dolphins, the implicit assumption was the degree of oiling and oil exposure experienced in Barataria Bay, hence the severity of effects, was similar in shelf or oceanic cetaceans when oil was present. There may not have been an efficacious method to estimate the difference in cetacean exposure or exposure effects of patchy oil in a much thinner layer at the surface of a water column 10-200 m deep on the shelf versus what animals were exposed to in Barataria Bay. However, the worst-case scenarios, as modeled, may also have produced unrealistically high mortality and time to recovery estimates. For example, coastal bottlenose dolphins populations across the US GOM were collectively estimated to increase by 24% between 2012 and 2018, while the northern coastal population, whose ncGOM range is shelf waters from 84W to 89.5W longitude, was estimated to increase 367% between 2007 and 2018 (NOAA, 2023; Figures 8A, B). Pantropical and Atlantic spotted dolphins populations were each estimated to decline by approximately 25% between 2004 and 2018 (Figures 8C, D). However, the estimated sizes of those two populations have fluctuated over time, which may reflect their predominately oceanic distributions or changes in habitat use or detectability over time, and the observed declines are also within the statistical error of the estimates. Population estimates of spinner dolphins fluctuated the most among the three stenellid populations that occur at least partially on the ncGOM shelf, with coefficients of variation (CVs) approaching 1.0 in years when spikes in population estimates occurred over the 1994-2018 time period (Figure 8E). Population estimates for Rice’s whales have increased since 2005, but the high CVs (mean 1.1) on population estimates in all survey years means there is no statistical difference among them (Figure 8F).
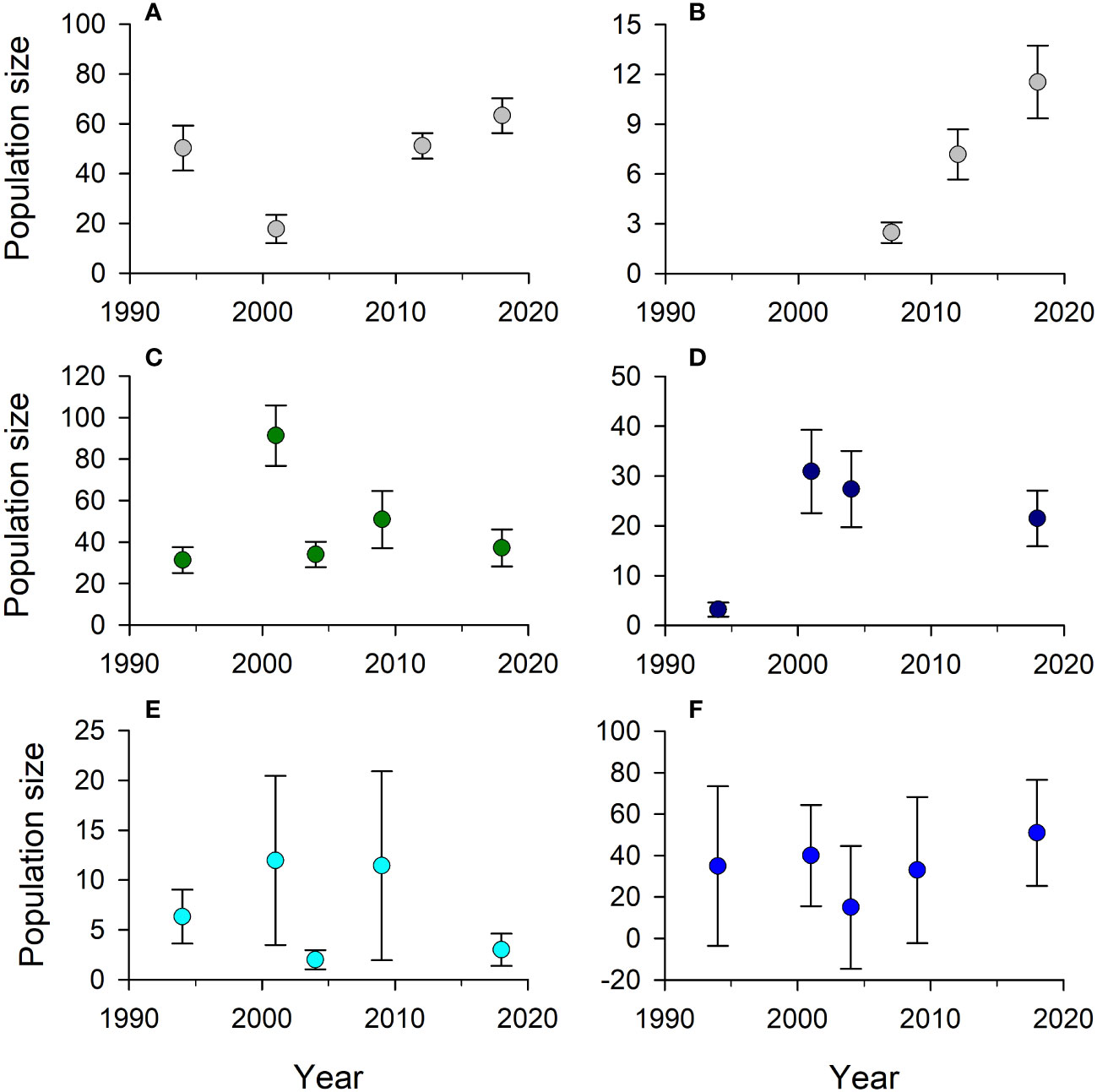
Figure 8 Estimated population size ( ± SD) over time for (A) coastal bottlenose dolphins, Tursiops truncatus, (B) northern (-84W to -89.5W) coastal bottlenose dolphins, (C) pantropical spotted dolphins, Stenella attenuata, (D) Atlantic spotted dolphins, Stenella frontalis, (E) spinner dolphins, Stenella longirostris, and (F) Rice’s whale, Balaenoptera ricei, in the US Gulf of Mexico. Population estimates are 103 for A-E, but not (F) Estimates are derived from plane- or ship-based line-transect surveys conducted by NOAA Fisheries and reported in US marine mammal stock assessment reports (NOAA, 2023).
Uncertainty in estimated size of cetacean populations that occur at least partly on the ncGOM shelf makes evaluating the magnitude of DWH population effects difficult, and it also is problematic for evaluating population resiliency. Marine mammal surveys have not been conducted regularly on the shelf by NOAA Fisheries, and airplane- or ship-based surveys have changed over time (DWH MMIQT, 2015). Population estimates have been produced via distance sampling, hence detectability is estimated and accounted for in population estimates (Buckland et al., 2001; Laake and Borchers, 2004; Garrison et al., 2020), but the spatial coverage of surveys changed over time and there could be differences in cetacean avoidance or detectability between plane-based versus ship-based surveys. Survey methods did become more standardized over time, especially in the period just before the DWH and surveys conducted in the late 2010s (NOAA, 2023), thus relative abundance trends should still be informative with respect to species’ resiliency following the DWH.
Perhaps the cetacean of greatest conservation concern in the region is Rice’s whale. Its small population (<100 individuals) and estimated threats to its persistence in the GOM, such as fishing gear interactions, ship strikes, or environmental stressors like the DWH, led to its listing under the US Endangered Species Act (USA) in 2019, when still known as Bryde’s whale, Balaenoptera edeni. It is unclear what the species’ historical population size was in the GOM, or the current carrying capacity for it in the system. In fact, its presence in the GOM was first noted only in 1965 (Rice, 1965), and Rice's whales are rarely encountered given their low population size and outer shelf to upper continental slope distribution. They have been observed and counted during marine mammal surveys since the mid-1990s (Figure 8F), but their population size estimates are highly uncertain due to the low encounter rates (NOAA, 2023).
There is substantial uncertainty overall in the estimated population-level effects of the DWH on shelf cetaceans. Precautionary assumptions made during modeling efforts to estimate cetacean mortality and recovery timelines likely produced worst-case assessments. That may have been justified given the conservation status of marine mammals in the US, and in particular the recent listing of Rice’s whale under the ESA, but it makes tracking population recovery and resiliency difficult. For the species with the greatest shelf abundance and most precise population estimates over time, bottlenose dolphin, shelf population estimates have been increasing over the past 20 years, with the DWH event occurring in the middle of that time period (Figures 8A, B). Clearly, those data differ from the estimated DWH effects on coastal bottlenose dolphin populations on the shelf. For the other cetacean species that occur on the GOM shelf, estimated population trends are either not consistent with estimated levels of population decline, or the statistical uncertainty is too great to draw much inference from observed patterns (Figure 8). One way to reduce the uncertainty in estimated population trends of GOM marine mammals, which is also consistent with the resource recovery mandates of the DWH settlement, would be the implementation of standardized GOM-wide marine mammal surveys that occur at a prescribed frequency. It may not be feasible to conduct these surveys annually, and that may not be necessary given the longevity and life history of the species, but conducting standardized surveys at a set frequency should enhance the ability to track population trends over time.
6 Conclusions
Overall, there is substantial evidence of direct exposure to toxic petroleum compounds on the ncGOM shelf in the months to years following the DWH across of a broad range of taxa, from plankton to cetaceans. Direct observations of mortality were rare on the shelf versus inshore environments, which was likely affected by the sinking or transport of larger animals, the inability to directly observe mortality in smaller animals, such as plankton, and the fact that affected animals are often subjected to higher rates of predation. However, exposure experiments, organ biopsies, or health measures indicated significant sub-lethal physiological impairment resulting from exposure, and, when available, data indicate diminished condition and growth existed in many taxa as a result of the spill. In general, the more intense the sampling for a given taxon, the more apparent population-level effects were. For example, long-term surveys and datasets on the shallow shelf off Alabama enabled the detection of shifts in community structure and abundance increases in zooplankton, while pre-spill data on reef fish communities on reefs across the ncGOM enabled the detection of community and trophic structure shifts, as well as the appearance and rapid population growth of invasive lionfish populations, in the years after the DWH. In the case of shelf-edge soft corals, the sessile nature of colonies and geoposition data associated with pre-spill video or still camera images facilitated assessment of post-spill impacts to individual colonies in the north central versus eastern GOM. However, the lack of knowledge of species-specific population sizes and distributions precluded an assessment of population-level impacts or resiliency. Among higher vertebrates, substantial post-spill population declines were predicted with matrix models for cetacean populations that occur on the ncGOM shelf, but post-spill population assessments either were too variable to confirm those predictions or actually indicated population increases, such as observed for the northern coastal or overall coastal bottlenose dolphin stocks. It is understandable that conservative estimates of exposure and impacts to shelf cetaceans would be undertaken to estimate population-level DWH impacts, but the post-spill data do not match those predictions.
When examining resiliency to population-level impacts, predictions from life history theory match the recovery seen in some taxa, while confounding effects or lack of data hinder our ability to discern residency in others. Short-lived, highly productive zooplankton populations appear to have quickly recovered from any spill-related impacts due to ecosystem connectivity (transport of zooplankton by currents into the oil spill area) in the months following the DWH (Daly et al., 2021). Among reef fishes, small demersal species displayed the most substantial population declines following the DWH, but also would be predicted to recover the quickest given their life history. However, the appearance and rapid growth of invasive lionfish populations in the ncGOM appears to have precluded their recovery. Larger reef fishes, such as snappers and groupers, were predicted to take longer to recover, given longer generation times, slow growth, and later maturity. However, the population recovery for the most data-rich stock in the US GOM, and perhaps among all US regions, red snapper, continued on the pre-spill trajectory of biomass increase in the western GOM, while the population plateaued in the eastern GOM. Ecosystem modeling suggests this pattern in the eastern GOM was affected by acute effects of the DWH, but then recovery has been hampered by robust recreational fisheries in the region (Cowan et al., 2011; Putman and Gallaway, 2020), as well as competitive interactions with invasive lionfish (Chagaris et al., 2020).
Part of the uncertainty about population declines and resiliency among shelf taxa with respect to the DWH are the various environmental and anthropogenic stressors that already existed in the system prior to the spill. Ecosystem modeling revealed challenges in parsing effects of different stressors on reef fish population and community shifts following the DWH, but population modeling also suggested that what first appeared to be readily apparent effects of the spill on the Kemp’s ridley sea turtle population is more complicated due to estimated shifts in carrying capacity in the ecosystem. The DWH was estimated to have a substantial, acute effect via 86,000 juvenile Kemp’s ridley deaths, but population modeling revealed that only constituted an approximately 9% increase in mortality for that naturally vulnerable life stage. Concern was expressed by declines in nesting females in Tamaulipas, Mexico in 2010, but juveniles most affected by the spill would not become mature for nearly a decade thereafter. Additional years of data demonstrated that numbers of nesting females had actually plateaued, not declined, and modeling demonstrated this was likely due to the Kemp’s ridley population reaching a diminished carrying capacity in the ecosystem. It is possible that chronic DWH effects could have diminished productivity of the ecosystem, including for Kemp’s ridley turtles, but there is no indication of persistent food web impacts on the shelf over the past decade (Patterson et al., 2020), and GOM-wide fisheries landings, which could be seen as integrated signal of overall ecosystem health, have not diminished (Carroll et al., 2016; Swinea and Fodrie, 2021).
In the end, while there were clear impacts of the DWH on shelf taxa, including at the population level, the shelf ecosystem overall has proven to be remarkably resilient to one of the largest marine oil spills in history. It may be that the legacy environmental impacts of the spill will exist for some time in inshore or deep GOM environments, but that does not appear to be the case in general for shelf habitats or taxa, albeit uncertainty remains with respect to population-level impacts for some taxa, such as mesophotic soft corals. Substantial restoration funds ($42 x 106) have been allocated to restoration efforts for mesophotic and deepwater corals, with a portion of those funds focused on high-resolution mapping and video surveys of those habitats (Bassett et al., 2023). This may enable a more robust assessment of likely population-level impacts of the DWH on these slow-growing sessile invertebrates, but it will also form an important baseline in the event of another large-scale environmental disaster such as DWH. For many taxa, an emerging focus of RESTORE funding has been improved knowledge of life history, ecology, and distribution. However, an important lesson from the DWH is that existing NOAA Fisheries or SEAMAP surveys for a variety of taxa (e.g., plankton, benthic and demersal invertebrates, fishes, cetaceans), which were designed to assess population trends across the US GOM, in many cases produced variances that were too great to be of practical use to assess population-level impacts at spatial scales affected by the spill, or in estimating resiliency following the spill (Trustees, 2016). In the case of the Prince William Sound Oil Spill in 1989, a portion of fine monies was set aside as a corpus, with the interest earned on those funds utilized to fund science and monitoring efforts well into the future. That has not been the case with fine monies in case of the DWH disaster, which may seem like a missed opportunity if another spill occurs and the resolution of population-level data and assessment has not improved beyond what existed in 2010 on the eve of the DWH.
Author contributions
All authors contributed equally to the design, analysis, evaluation, and publication writing leading to this contribution. All authors contributed to the article and approved the submitted version.
Funding
Funding for the project was primarily provided by the Gulf of Mexico Research Initiative through several of its research centers including: the Center for Integrated Modeling and Analysis of Gulf Ecosystems (C-IMAGE), Deep Pelagic Nekton Dynamics of the Gulf of Mexico (DEEPEND) and Ecosystem Impacts of Oil & Gas Inputs to the Gulf (ECOGIG).
Acknowledgments
We thank the Gulf of Mexico Research Initiative (GoMRI) Research Board and the American Institute of Biological Sciences, including Chuck Wilson, Michael Feldman, Callan Yanoff, and Jennifer Pettit, for travel and logistical support for these and similar synthesis activities. We also thank GoMRI Research Board members Rick Shaw, Margaret Leinen, Dick Dodge, Bill Hogarth, Bob Shipp, John Farrington, and Ken Halanych, who participated in discussions that culminated in this paper. Specific data sources mentioned in the article that were collected with GOMRI funding are available on the GRIIDC website at: https://data.gulfresearchinitiative.org/. Any use of trade, firm, or product names is for descriptive purposes only and does not imply endorsement by the U.S. Government.
Conflict of interest
The authors declare that the research was conducted in the absence of any commercial or financial relationships that could be construed as a potential conflict of interest.
Publisher’s note
All claims expressed in this article are solely those of the authors and do not necessarily represent those of their affiliated organizations, or those of the publisher, the editors and the reviewers. Any product that may be evaluated in this article, or claim that may be made by its manufacturer, is not guaranteed or endorsed by the publisher.
Author disclaimer
The scientific results and conclusions, as well as any views or opinions expressed herein, are those of the author(s) and do not necessarily reflect those of NOAA or the Department of Commerce.
References
Aichinger Dias L., Litz J., Garrison L., Martinez A., Barry K., Speakman T. (2017). Exposure of cetaceans to petroleum products following the Deepwater Horizon oil spill in the Gulf of Mexico. Endang. Species Res. 33, 119–125. doi: 10.3354/esr00770
Ainsworth C. H., Paris C., Perlin N., Dornberger L. N., Patterson W. F III, Chancellor E., et al. (2018). Impacts of the Deepwater Horizon oil spill evaluated using an end-to-end ecosystem model. PloS One 13:e0190840. doi: 10.1371/journal.pone.0190840
Almeda R., Wambaugh Z., Wang Z., Hyatt C., Liu Z., Buskey E. J. (2013). Interactions between zooplankton, and crude oil: toxic effects and bioaccumulation of polycyclic aromatic hydrocarbons. PloS One 12:e0173999. doi: 10.1371/journal.pone.0067212
Aquilar-Perera A., Tuz-Sulub A. (2010). Non-native, invasive red lionfish (Pterois volitans [Linnaeus 1758]: scorpaenidae]), is first recorded in the southern Gulf of Mexico, off the northern Yucatan Peninsula, Mexico. Aquat. Invasions 5, S1–S12. doi: 10.3391/ai.2010.5.S1.003
Avens L., Goshe L. R., Coggins L., Shaver D. J., Higgins B., Landry AM Jr, et al. (2020). Variability in age and size at maturation, reproductive longevity, and long-term growth dynamics for Kemp’s ridley sea turtles in the Gulf of Mexico. PloS One 8, e67212. doi: 10.1371/journal.pone.0173999
Avens L., Goshe L. R., Coggins L., Shaver D. J., Higgins B., Landry A. M. Jr., et al. (2017). Variability in age and size at maturation, reproductive longevity, and long-term growth dynamics for Kemp's ridley sea turtles in the Gulf of Mexico. PLoS ONE 12:e0173999. doi: 10.1371/journal.pone.0173999
Balmer B., Sinclair C., Speakman T., Quigley B., Barry K. (2016). Extended movements of common bottlenose dolphins (Tursiops truncatus) along the northern Gulf of Mexico central coast. Gulf Mex. Sci. 33, 1–5. doi: 10.18785/goms.3301.08
Barata C., Calbet A., Saiz E., Ortiz L., Bayona J. M. (2005). Predicting single and mixture toxicity of petrogenic polycyclic aromatic hydrocarbons to the copepod Oithona davisae. Environ. Toxicol. Chem. 24, 2992–2999. doi: 10.1897/05-189r.1
Bassett R., Herting J., Frometa J., Sharuga S., Howell J., Siceloff L., et al. (2023). Comprehensive review of habitat assessment and evaluation datasets to support Deepwater Horizon mesophotic and deep benthic communities Vol. 68 (DWH MDBC Data Report DR-23-01. Silver Spring). doi: 10.25923/kz7t-4674
Bejarano A. C., Chandler G. T., He L., Coull B. C. (2006). Individual to population level effects of south Louisiana crude oil water accommodated fraction (WAF) on a marine meiobenthic copepod. J. Exp. Mar. Biol. Ecol. 332, 49–59. doi: 10.1016/j.jembe.2005.11.006
Bellas J., Thor P. (2007). Effects of selected PAHs on reproduction and survival of the calanoid copepod Acartia tonsa. Ecotoxicology 16, 465–474. doi: 10.1007/s10646-007-0152-2
Benedetti M. C., Priori C., Erra F., Santangelo G. (2016). Growth patterns in mesophotic octocorals: timing the branching process in the highly-valuable Mediterranean Corallium rubrum. Estuar. Coast. Shelf Sci. 171, 106–110. doi: 10.1016/j.ecss.2015.12.026
Berrojalbiz N., Lacorte S., Calbet E. S., Barata C., Dachs J. (2009). Accumulation and cycling of polycyclic aromatic hydrocarbons in zooplankton. Environ. Sci. Technol. 43, 2295–2301. doi: 10.1021/es8018226
Bevan E., Wibbels T., Najera B. M. Z., Sarti L., Martinez F. I., Cuevas J. M., et al. (2016). Estimating the historic size and current status of the Kemp’s ridley sea turtle (Lepidochelyskempii) population. Ecosphere 7:e01244. doi: 10.1002/ecs2.1244
Bianchi T. S., DiMarco S. F., Cowan J. H. Jr., Hetland R. D., Chapman P., Day J. W., et al. (2010). The science of hypoxia in the northern Gulf of Mexico: a review. Sci. Tot. Environ. 408, 1471–1784. doi: 10.1016/j.scitotenv.2009.11.047
Buckland S. T., Anderson D. R., Burnham K. P., Laake J. L., Borchers D. L., Thomas L. (2001). Introduction to distance sampling (Oxford: Oxford University Press).
Burger J. (2017). “Avian resources of the northern Gulf of Mexico,” in Habitats and biota of the Gulf of Mexico: before the Deepwater Horizon oil spill. vol. 2: fish resources, fisheries, sea turtles, avian resources, marine mammals, diseases and mortalities. Ed. Ward C. H. (New York: Springer), 1353–1488.
Caillouet J. C.W. (2011). Guest editorial: did the BP Deepwater Horizon-macondo oil spill change the age structure of the Kemp’s ridley population? Mar. Turtle Newslett. 130, 1–2.
Caillouet C. W., Raborn S. W., Shaver D. J., Putman N. F., Gallaway B. J., Mansfield K. L. (2018). Did declining carrying capacity for the Kemp’s ridley sea turtle population within the Gulf of Mexico contribute to the nesting setback in 2010-2017? Chelonian Conserv. Biol. 17, 123–133. doi: 10.2744/CCB-1283.1
Calbet A., Saiz E., Barata C. (2007). Lethal and sublethal effects of naphthalene and 1,2 dimethylnaphthalene on the marine copepod Paracartia grani. Mar. Biol. 151, 195–204. doi: 10.1007/s00227-006-0468-0
Campbell M. D., Pollack A. G., Thompson K., Switzer T., Driggers W. B., Hoffmayer E. R. (2022). Rapid spatial expansion and population increase of invasive lionfish (Pterois spp.) observed on natural habitats in the northern Gulf of Mexico. Biol. Invasions 24, 93–105. doi: 10.1007/s10530-021-02625-1
Carassou L., Hernandez F. J., Graham W. M. (2014). Change and recovery of coastal mesozooplankton community structure during the Deepwater Horizon oil spill. Env. Res. Lett. 9:124003. doi: 10.1088/1748-9326/9/12/124003
Carassou L., Hernandez F. J., Powers S. P., Graham W. M. (2012). Cross-shore, seasonal, and depth-related structure of ichthyoplankton assemblages in coastal Alabama. Trans. Amer. Fish. Soc 141, 1137–1150. doi: 10.1080/00028487.2012.675920
Carmichael R. H., Graham W. M., Aven A., Worthy G., Howden S. (2012). Were multiple stressors a ‘perfect storm’ for northern Gulf of Mexico bottlenose dolphins (Tursiops truncatus) in 2011? PloS One 7:e41155. doi: 10.1371/journal.pone.0041155
Carroll M., Genter B., Larkin S., Quigley K., Perlot N., Dehner L., et al. (2016). An analysis of the impacts of the Deepwater Horizon oil spill on the Gulf of Mexico seafood industry (New Orleans, LA: U. S. Dept. of the Interior. Bureau of Ocean Energy Management, Gulf of Mexico OCS Region). Available at: https://espis.boem.gov/final%20reports/5518.PDF.
Chagaris D., Binion-Rock S., Bogdanoff A., Dahl K., Granneman J., Harris H., et al. (2017). An ecosystem-based approach to evaluating impacts and management of invasive lionfish. Fisheries 42, 421–431. doi: 10.1080/03632415.2017.1340273
Chagaris D. D., Patterson W. F III, Allen M. S. (2020). Relative effects of multiple stressors on reef food webs in the northern Gulf of Mexico revealed via ecosystem modeling. Front. Mar. Sci. 7:513. doi: 10.3389/fmars.2020.00513
Chanton J. P., Cherrier J., Wilson R. M., Sarkodee-Adoo J., Bosman S., Mickle A., et al. (2012). Radiocarbon evidence that carbon from the Deepwater Horizon spill entered the planktonic food web of the Gulf of Mexico. Environ. Res. Lett. 7:45303. doi: 10.1088/1748-9326/7/4/045303
Cohen J. H., McCormick L. R., Burkhardt S. M. (2014). Effects of dispersant and oil on survival and swimming activity in a marine copepod. Bull. Environ. Contam. Toxicol. 92, 381–387. doi: 10.1007/s00128-013-1191-4
Côté I. M., Green S. J., Hixon M. A. (2013). Predatory fish invaders: insights from Indo-Pacific lionfish in the western Atlantic and Caribbean. Biol. Conserv. 164, 50–61. doi: 10.1016/j.biocon.2013.04.014
Cowan J. H. Jr., Grimes C. B., Patterson W. F III, Walters C. J., Jones A. C., Lindberg W. J., et al. (2011). Red snapper management in the Gulf of Mexico: science- or faith-based? Rev. Fish. Biol. Fisheries 21, 187–204. doi: 10.1007/s11160-010-9165-7
Curtis J. S., Wall K. R., Albins M. A., Stallings C. D. (2017). Diet shifts in a native mesopredator across a range of invasive lionfish biomass. Mar. Ecol. Prog. Ser. 573, 215–228. doi: 10.3354/meps12164
Dahl K. A., Edwards M. A., Patterson W. F. III. (2019). Density-dependent condition and growth of invasive lionfish in the northern Gulf of Mexico. Mar. Ecol. Prog. Ser. 623, 145–159. doi: 10.3354/meps13028
Dahl K. A., Patterson W. F. III. (2014). Habitat-specific diet and density of rapidly expanding invasive lionfish, Pterois volitans, populations in the northern Gulf of Mexico 9:e105852. doi: 10.1371/journal.pone.0105852
Dahl K. A., Patterson W. F. III. (2020). Movement, home range, and depredation of invasive lionfish revealed by fine-scale acoustic telemetry in the northern Gulf of Mexico. Mar. Biol. 167, 111. doi: 10.1007/s00227-020-03728-4
Dahl K. A., Patterson W. F III, Robertson A., Ortmann A. C. (2017). DNA Barcoding significantly improves resolution of invasive lionfish diet in the northern Gulf of Mexico. Biol. Invasions 19, 1917–1933. doi: 10.1007/s10530-017-1407-3
Daly K. L., Remsen A., Outram D., Broadbent H., Kramer K., Dubikas K. (2021) Resilience of the zooplankton community during and after the Deepwater Horizon oil spill. Mar. Pollut. Bull. 163:111882. doi: 10.1016/j.marpolbul.2020.111882
Daly K. L., Vaz A. C., Paris C. B. (2020). “Physical processes influencing the sedimentation and lateral transport of MOSSFA in the NE Gulf of Mexico,” in Scenarios and responses to future deep oil spills. Eds. Murawski S., Hollander D., Aimsworth C., Paris C. B., Schluter M., Wetzel D. (New York, NY: Springer Science), 300–314. doi: 10.1007/978-3-030-12963-7_18
Darnell R. (2015). The American Sea: a natural history of the Gulf of Mexico (Texas A&M Press, College Station), 554.
Dubickas K. (2019). Zooplankton community structure in the NE Gulf of Mexico: impacts of environmental variability and the Deepwater Horizon oil spill (St. Petersburg (FL: University of South Florida).
DWH MMIQT. (2015). Models and analyses for the quantification of injury to Gulf of Mexico cetaceans from the Deepwater Horizon oil spill. Available at: www.fws.gov/doiddata/dwh-ar-documents/876/DWH-AR0105866.pdf.
Etnoyer P. J., Wickes L. N., Silva M., Dubick J. D., Balthis L., Salgado E., et al. (2016). Decline in condition of gorgonian octocorals on mesophotic reefs in the northern Gulf of Mexico: before and after the Deepwater Horizon oil spill. Coral Reefs 35, 77–90. doi: 10.1007/s00338-015-1363-2
Fern R., Withers K., Zimba P., Wood T., Schoech L. (2015). Toxicity of three dispersants alone and in combination with crude oil on blue crab Callinectes sapidus megalopae. Southeast. Nat. 14, G82–G92. doi: 10.1656/058.014.0413
Fisher C. R., Demopoulos A. W. J., Cordes E. E., Baums I. B., White H. K., Bourque J. R. (2014). Coral communities as indicators or ecosystem-level impacts of the Deepwater Horizon spill. Bioscience 64, 796–807. doi: 10.1093/biosci/biu129
Fodrie F. J., Able K. W., Galvez F., Heck K. L. Jr., Jensen O. P., López-Duarte P. C., et al. (2014). Integrating organismal and population responses of estuarine fishes in Macondo spill research. Bioscience 64, 778–788. doi: 10.1093/biosci/biu123
Fodrie F. J., Heck Ken Jr, Powers S. P., Graham W. M., Robinson K. L. (2010). Climate-related, decadal-scale assemblage changes of seagrass-associated fishes in the northern Gulf of Mexico. Global Change Biol. 16, 48–59. doi: 10.1111/j.1365-2486.2009.01889.x
Gallaway B. J., Gazey W. J., Caillouet J. C.W., Plotkin P. T., Grobois F. A., Amos A. F., et al. (2016a). Development of a Kemp’s ridley sea turtle stock assessment model. Gulf Mex. Sci. 33, 138–157. doi: 10.18785/goms.3302.03
Gallaway B. J., Gazey W. J., Wibbels T., Bevan E., Shaver D. J., George J. (2016b). Evaluation of the status of the Kemp’s ridley sea turtle after the 2010 Deepwater Horizon oil spill. Gulf Mex. Sci. 33, 192–205. doi: 10.18785/goms.3302.06
Gallaway B. J., Raborn S. W., Picariello L., Putman N. F. (2020). Changes in shrimping effort in the Gulf of Mexico and the impacts to red snapper. iScience 23:101111. doi: 10.1016/j.isci.2020.101111
Garrison L. P., Ortega-Ortiz J., Rappucci G. (2020) Abundance of marine mammals in the waters of the U.S. Gulf of Mexico in the summer of 2017 and 2018. southeast fisheries science center, protected resources and biodiversity division, 75 Virginia beach dr., Miami, Florida 33140. PRBD contribution # PRBD-2020-07. 55pp. Available at: https://repository.library.noaa.gov/view/noaa/26505.
Giltz S. M., Taylor C. M. (2017). Sublethal toxicity of crude oil exposure in the blue crab, Callinectes sapidus, at two life stages. Bull. Environ. Contam. Toxicol. 98, 178–182. doi: 10.1007/s00128-016-2000-7
Girard F., Shea K., Fisher C. R. (2018). Projecting the recovery of a long-lived deep-sea coral species after the Deepwater Horizon oil spill using state-structured models. J. App. Ecol. 55, 1812–1822. doi: 10.1111/1365-2664.13141
Goffredo S., Lasker H. R. (2006). Modular growth of a gorgonian coral can generate predictable patterns of colony growth. J. Exp. Mar. Biol. Ecol. 336, 221–229. doi: 10.1016/j.jembe.2006.05.012
Gohlke J. M., Doke D., Tipre M., Leader M., Fitzgerald T. (2011). A review of seafood safety after the Deepwater Horizon blowout. Environ. Health Perspect. 119, 1062–1069. doi: 10.1289/ehp.1103507
Gomez F., Lee S.-K., Hernandez F. J. Jr., Chiaverano L. M., Muller-Karger F. E., Lamkin Y. L., et al. (2019). ENSO-induced co-variability of salinity, plankton biomass and coastal currents in the northern Gulf of Mexico. Sci. Rep. 9, 178. doi: 10.1038/s41598-018-36655-y
Graham W. M., Condon R. H., Carmichael R. H., D’Ambra I., Patterson H. K., Linn L. J., et al. (2010). Oil carbon entered the coastal planktonic food web during the Deepwater Horizon oil spill. Eviron. Res. Lett. 5:45301. doi: 10.1088/1748-9326/5/4/045301
Gredzens C., Shaver D. J. (2020). Satellite tracking can inform population-level dispersal to foraging grounds of post-nesting Kemp’s ridley sea turtles. Front. Mar. Sci. 7:559. doi: 10.3389/fmars.2020.00559
Haas H. L., Lamon III, E. C., Rose K. A., Shaw R. F. (2001). Environmental and biological factors associated with stage-specific abundance of brown shrimp (Penaeus aztecus) in Louisiana: applying a new combination of statistical techniques to long-term monitoring data. Can. J. Fish. Aquat. Sci. 58, 2258–2270. doi: 10.1139/cjfas-58-11-2258
Hansen B. H., Altin D., Vang S.-H., Nordtug T., Olsen A. J. (2008). Effects of naphthalene on gene transcription in calanus finmarchicus (Crustacea: copepoda). Aquat. Toxicol. 86, 157–165. doi: 10.1016/j.aquatox.2007.10.009
Hart R. A. (2017) Stock assessment for brown shrimp (Farfantepenaeus aztecus) in the U.S. Gulf of Mexico for the 2016 fishing year. Available at: https://gulfcouncil.org/wp-content/uploads/D-4a-Brown-Assess_Rpt_2017-CPT.pdf.
Herdter E. S., Chambers D. P., Stallings C. D., Murawski S. A. (2017). Did the Deepwater Horizon oil spill affect growth of red snapper in the Gulf of Mexico? Fish. Res. 191, 60–68. doi: 10.1016/j.fishres.2017.03.005
Hernandez F.J. Jr, Filbrun F. E., Fang J., Ransom J. T. (2016). Condition of larval red snapper (Lutjanus campechanus) relative to environmental variability and the Deepwater Horizon oil spill. Environ. Res. Lett. 11:94019. doi: 10.1088/1748-9326/11/9/094019
Hicken C. E., Linbo T. L., Baldwin D. H., Incardona J. P. (2011). Sublethal exposure to crude oil during embryonic development alters cardiac morphology and reduces aerobic capacity in adult fish. Proc. Nat. Acad. Sci. 108, 7086–7090. doi: 10.1073/pnas.1019031108
Hohn A. A., Thomas L., Carmichael R. H., Litz J., Clemons-Chevis C., Shippee S. F., et al. (2017). Assigning stranded bottlenose dolphins to source stocks using stable isotope ratios following the Deepwater Horizon oil spill. Endang. Species Res. 33, 235–252. doi: 10.3354/esr00783
Houde E. D., Chitty N. (1976) Seasonal abundance and distribution of zooplankton, fish eggs, and fish larvae in the eastern Gulf of Mexico 1972-74. NOAA technical report NMFS SSRF-701. Available at: https://spo.nmfs.noaa.gov/sites/default/files/legacy-pdfs/SSRF701.pdf.
Hsing P.-Y., Fu B., Larcom E. A., Berlet S. P., Shank T. M., Govindarajan A. F., et al. (2013). Evidence of lasting impact of the Deepwater Horizon oil spill on a deep Gulf of Mexico coral community. Elementa 1, 12. doi: 10.12952/journal.elementa.000012
Hu C., Weisberg R. H., Liu Y., Zheng L., Daly K. L., English D. C., et al. (2011). Did the northeastern Gulf of Mexico become greener after the Deepwater Horizon oil spill? Geophys. Res. Lett. 38, L09601. doi: 10.1029/2011GL047184
Incardona J. P., Collier T. K., Scholz N. L. (2011). Oil spills and fish health: exposing the heart of the matter. J. Expos. Sci. Environ. Epidem. 21, 3–4. doi: 10.1038/jes.2010.51
Iverson R. L., Hopkins T. L. (1981). A summary of knowledge of plankton production in the Gulf of Mexico: recent phytoplankton and zooplankton research in proceedings of a symposium of environmental research needs in the Gulf of Mexico, key Biscayne, Florida (Miami, FL: Atlantic Oceanographic and Meteorological Laboratories), 147–211.
Johansen J. L., Esbaugh A. J. (2017). Sustained impairment of respiratory function and swim performance following acute oil exposure in a coastal marine fish. Aquat. Toxicol. 187, 82–89. doi: 10.1016/j.aquatox.2017.04.002
Joye S. B., Bracco A., Ozgokmen T. M., Chanton J. P., Grosell M., MacDonald I. R., et al. (2016). The Gulf of Mexico ecosystem, six years after the Macondo oil well blowout. Deep-Sea Res. II 129, 4–19. doi: 10.1016/j.dsr2.2016.04.018
Kellar N. M., Speakman T. R., Smith C. R., Lane S. M., Balmer B. C., Trego M. L., et al. (2017). Low reproductive success rates of common bottlenose dolphins Tursiops truncatus in the northern Gulf of Mexico following the Deepwater Horizon disaster, 2010–2015). Endang. Species. Res. 33, 143–158. doi: 10.3354/esr00775
Kimball M. E., Miller J. M., Whitfield P. E., Hare J. A. (2004). Thermal tolerance and potential distribution of invasive lionfish (Pterois volitans/miles complex) on the east coast of the United States. Mar. Ecol. Prog. Ser. 283, 269–278. doi: 10.3354/meps283269
Koenig S., Fernández P., Monserrat S. (2013). Differences in cytochrome P450 enzyme activities between fish and crustacea: relationship with the bioaccumulation patterns of polychlorobiphenyls (PCBs). Aquat. Toxicol. 108, 11–17. doi: 10.1016/j.aquatox.2011.10.016
Laake J. L., Borchers D. L. (2004). “Methods for incomplete detection at distance zero. pages 108–189,” in Advanced distance sampling. Eds. Buckland S. T., Andersen D. R., Burnham K. P., Laake J. L., Thomas L. (New York: Oxford University Press).
Lee C. E., Remfert J. L., Opgenorth T., Lee K. M., Stanford E., Connolly J. W., et al. (2017). Evolutionary responses to crude oil from the Deepwater Horizon oil spill by the copepod Eurytemora affinis. Evol. Appl. 10, 813–828. doi: 10.1111/eva.12502
Lewis J. P., Tarnecki J. H., Garner S. B., Chagaris D. D., Patterson W. F III (2020). Changes in reef fish community structure following the Deepwater Horizon oil spill. Sci. Rep. 10, 5621. doi: 10.1038/s41598-020-62574-y
Liu H., Dagg M. (2003). Interactions between nutrients, phytoplankton growth, and micro- and mesozooplankton grazing in the plume of the Mississippi River. Mar. Ecol. Prog. Ser. 248, 31–42. doi: 10.3354/meps258031
MacDonald I. R., Daneshgar S., Beet A. R., Feng L. (2015). Natural and unnatural oil slicks in the Gulf of Mexico. J. Geophys. Res.: Oceans 120, 1–17. doi: 10.1002/2015JC011062
Magalhães K. M., Carreira R. S., Filho J. S. R., Rocha P. P., Santana F. M., Yogui G. T. (2022). Polycyclic aromatic hydrocarbons (PAHs) in fishery resources affected by the 2019 oil spill in Brazil: short-term environmental health and seafood safety. Mar. Poll. Bull. 175:113334. doi: 10.1016/j.marpolbul.2022.113334
Magnuson J. T., Khursigara A. J., Allmon E. B., Esbaugh A. J., Roberts A. P. (2018). Effects of Deepwater Horizon crude oil on ocular development in two estuarine fish species, red drum (Sciaenops ocellatus) and sheepshead minnow (Cyprinodon variegatus). Ecotoxicol. Environ. Saf. 166, 186–191. doi: 10.1016/j.ecoenv.2018.09.087
Maljković A., Van Leeuwen T. E., Cove S. N. (2008). Predation on the invasive red lionfish, Pterois volitans (Pisces: scorpaenidae), by native groupers in The Bahamas. Coral Reefs. 27, 501. doi: 10.1007/s00338–008–0372–9
Márquez M. R. (1994). Synopsis of biological data on the Kemp’s ridley turtle Lepidochelys kempi (Garman 1880). NOAA Tech Memo NMFS-SEFSC 343, 1–91.
McDonald T. L., Schroeder B. A., Stacy B. A., Wallace B. P., Starcevich L. A., Gorham J. (2017). Density and exposure of surface-pelagic juvenile sea turtles to Deepwater Horizon oil. Endang. Species Res. 33, 69–82. doi: 10.3354/esr00771
McNutt M. K., Camilli R., Crone T. J., Guthrie G. D., Heish P. A., Ryerson T. B., et al. (2012). Review of flow rate estimates of the Deepwater Horizon oil spill. Proc. Nat. Acad. Sci. 109, 20260–20267. doi: 10.1073/pnas.1112139108
Mitchelmore C. A., Bejarano A. C., Wetzel D. L. (2020). “A synthesis of DWH oil: chemical dispersant and chemically dispersed oil aquatic standard acute and chronic toxicity studies,” in Deep oil spills: facts, fate, and effects. Eds. Murawski S. A., Ainsworth C. H., Gilbert S., Hollander D. J., Paris C. B., Schlüter M., Wetzel D. L. (New York, NY: Springer Science), 480–496. doi: 10.1007/978-3-030-11605-728
Mitchelmore C. L., Bishop C. A., Collier T. K. (2017). Toxicological estimation of mortality of oceanic sea turtles oiled during the Deepwater Horizon oil spill. Endang. Species Res. 33, 39–50. doi: 10.3354/esr00758
Mumby P. J., Harbone A. R., Brumbaugh D. R. (2011). Grouper as a natural biocontrol of invasive lionfish. PloS One 6:e21510. doi: 10.1371/journal.pone.0021510
Murawski S. A., Fleeger J. W., Patterson W. F III, Hu C., Daly K., Romero I., et al. (2016). How did the Deepwater Horizon oil spill affect coastal and continental shelf ecosystems of the Gulf of Mexico? Oceanography 29, 160–173. doi: 10.5670/oceanog.2016.80
Murawski S. A., Hogarth W. T., Peebles E. B., Barbieri L. (2014). Prevalence of external skin lesions and polycyclic aromatic hydrocarbon concentrations in Gulf of Mexico fishes post-Deepwater Horizon. Trans. Amer. Fish. Soc 143, 1084–1097. doi: 10.1080/00028487.2014.911205
Murawski S. A., Kilborn J. P., Bejarano A. C., Chagaris D., Donaldson D., Hernandez J. F. Jr, et al. (2021). A synthesis of Deepwater Horizon impacts on coastal and nearshore living marine resources. Front. Mar. Sci. 7:594862. doi: 10.3389/fmars.2020.594862
Murawski S. A., Peebles E. B., Gracia A., Tunnell J. W. Jr., Armenteros M. (2018). Comparative abundance, species composition, and demographics of continental shelf fish assemblages throughout the Gulf of Mexico. Mar. Coast. Fish. 10, 325–346. doi: 10.1002/mcf2.10033
Murawski S. A., Schlüter M., Paris C. B., Aman Z. A. (2019). Resolving the dilemma of dispersant use for deep oil spill response. Environ. Res. Lett. 14, 091002. doi: 10.1088/1748-9326/ab3aa0
NOAA. (2023). Marine mammal stock assessments. Available at: https://www.fisheries.noaa.gov/national/marine-mammal-protection/marine-mammal-stock-assessments.
NPS. (2020). Review of the Sea turtle science and recovery program, padre island national seashore. Available at: https://www.nps.gov/pais/learn/management/upload/PAIS-STSR-Review-Report_20210507_FINALamended_508.pdf.
NRC, National Research Council. (2013). Report of the committee on the effects of the Deepwater Horizon Mississippi canyon-252 oil spill on ecosystem services in the Gulf of Mexico (Washington, DC: The National Academies Press). Available at: https://www.nap.edu/download/18387.
O’Farrell S., Bearhop S., McGill R. A.R., Dahlgren C. P., Brumbaugh D. R., Mumby P. J. (2014). Habitat and body size effects on the isotopic niche space of invasive lionfish and endangered Nassau grouper. Ecosphere 5, 1–11. doi: 10.1890/ES14-00126.1
Oken K. L., Able K. W., de Mutsert K., Fodrie J., Lopez-Duarte P. C., Martin C. W., et al. (2022). Fishery closures, more predator release, increase persistence of nearshore fishes and invertebrates to the Deepwater Horizon oil spill. OSF. doi: 10.31219/osf.io/csfqn
Ortner P. B., Hill L. C., Cummings S. R. (1989). Zooplankton community structure and copepod species composition in the northern Gulf of Mexico. Cont. Shelf Res. 9, 387–402. doi: 10.1016/0278-4343(89)90040-X
Pasparakis C., Esbaugh A. J., Burggren W., Grosell M. (2019). Physiological impacts of Deepwater Horizon oil on fish. Comp. Biochem. Physiol. Part C 224, 108558. doi: 10.1016/j.cbpc.2019.06.002
Patterson W. F III, Chanton J. P., Hollander D. J., Goddard E. A., Barnett B. K., Tarnecki J. H. (2020). “The utility of stable and radioisotopes in fish tissues as biogeochemical tracers of marine oil spill food web effects,” in Scenarios and responses to future deep oil spills - fighting the next war. Eds. Murawski S. A., Hollander D., C. Ainsworth C., Gilbert S., Paris C. B., Schlüter M., Wetzel D. (New York, NY: Springer Science), 513–530. doi: 10.1007/978-3-030-12963-7_13
Patterson W. F III, Watterson J. C., Cowan J. H. Jr., Shipp R. L. (2001). Movement of tagged red snapper in the northern Gulf of Mexico. Trans. Amer. Fish. Soc 130, 533–545. doi: 10.1577/1548-8659(2001)130<0533:MOTRSI>2.0.CO;2
Pollack A. G., Hanisko D. S. (2022). “Red snapper abundance indices from groundfish surveys in the northern Gulf of mexico. SEDAR74-DW-30,”, vol. 74. (North Charleston, SC: SEDAR). Available at: https://sedarweb.org/assessments/sedar-74/.
Prouty N. G., Roark E. B., Buster N. A., Ross S. W. (2011). Growth rate and age distribution of deep-sea black corals in the Gulf of Mexico. Mar. Ecol. Prog. Ser. 423, 101–115. doi: 10.3354/meps08953
Pulster E. L., Gracia A., Armenteros M., Carr B. E., Mrowicki J., Murawski S. A. (2020). Chronic PAH exposures and associated declines in fish health indices observed for ten grouper species in the Gulf of Mexico. Sci. Tot. Environ. 703:135551. doi: 10.1016/j.scitotenv.2019.135551
Purtlebaugh C. H., Martin C. W., Allen M. S. (2020). Poleward expansion of common snook Centropomus undecimalis in the northeastern Gulf of Mexico and future research needs. PloS One 15:e0234083. doi: 10.1371/journal.pone.0234083
Putman N. F., Gallaway B. J. (2020). Using common age units to communicate the relative catch of red snapper in recreational, commercial, and shrimp fisheries in the Gulf of Mexico. N. Amer. J. Fish. Manage. 40, 232–241. doi: 10.1002/nafm.10404
Ransom J. T., Filbrun J. E., Hernandez J. F. Jr (2016). Condition of larval Spanish mackerel Scomberomorus maculatus in relation to the Deepwater Horizon oil spill. Mar. Ecol. Prog. Ser. 55, 143–152. doi: 10.3354/meps11880
Reddam A., Mager E. M., Grosell M., McDonald M. D. (2017). The acute impact of PAH exposure on toadfish glucocorticoid stress response. Aquat. Toxicol. 192, 89–96. doi: 10.1016/j.aquatox.2017.08.014
Renaud M. L. (1995). Movements and submergence patterns of Kemp’s ridley turtles (Lepidochelys kempii). J. Herpetol. 29, 370–374. doi: 10.2307/1564986
Roman M. R., Pierson J. J., Kimmel D. G., Boicourt W. C., Zhang X. (2012). Impacts of hypoxia on zooplankton spatial distributions in the northern Gulf of Mexico. Estuaries Coasts 35, 1261–1269. doi: 10.1007/s12237-012-9531-x
Romero I. C., Toro-Farmer G., Diercks A.-R., Schwing P., Muller-Karger F., Murawski S. A., et al. (2017). Large-Scale deposition of weathered oil in the Gulf of Mexico following a deep-water oil spill. Environ. Pollut. 228, 179–189. doi: 10.1016/j.envpol.2017.05.019
Rosel P. E., Wilcox L. A. (2014). Genetic evidence reveals a unique lineage of bryde’s whales in the northern Gulf of Mexico. Mar. Ecol. Prog. Ser. 25, 19–34. doi: 10.3354/esr00606
Rosel P. E., Wilcox L. A., Sinclair C., Speakman T. R., Tumlin M. C., Litz J. A., et al. (2017). Genetic assignment to stock of stranded common bottlenose dolphins in southeastern Louisiana after the Deepwater Horizon oil spill. Endang. Species Res. 33, 221–234. doi: 10.3354/esr00780
Rosel P. E., Wilcox L. A., Yamada T. K., Mullin K. D. (2021). A new species of baleen whale (Balaenoptera) from the Gulf of Mexico, with a review of its geographic distribution. Mar. Mamm. Sci. 37, 577–610. doi: 10.1111/mms.12776
Saiz E., Movilla J., Yebra L., Barata C., Calbet A. (2009). Lethal and sublethal effects of naphthalene and 1,2-dimethylnaphthalene on naupliar and adult stages of the marine cyclopoid copepod Oithona davisae. Environ. Poll. 157, 1219–1226. doi: 10.1016/j.envpol.2008.12.011
Santana M. S., Sandrini-Neto L., Neto F. F., Oliveira Ribeiro C. A., Di Domenico M., Prodocimo M. M. (2018). Biomarker responses in fish exposed to polycyclic aromatic hydrocarbons (PAHs): systematic review and meta-analysis. Environ. Pollut. 242, 449–461. doi: 10.1016/j.envpol.2018.07.004
Schlenker L. S., Welch M. J., Meredith T. L., Mager E. M., Lari E., Babcock E., et al. (2019). Damsels in distress: oil exposure modifies behavior and olfaction in bicolor damselfish (Stegastes partitus). Environ. Sci. Tech. 53, 10993–11001. doi: 10.1021/acs.est.9b03915
Schwacke L. H., Smith C. R., Townsend F. I., Wells R. S., Hart L. B., Balmer B. C., et al. (2014). Health of common bottlenose dolphins (Tursiops truncatus) in Barataria Bay, Louisiana following the Deepwater Horizon oil spill. Environ. Sci. Tech. 48, 93–103. doi: 10.1021/es403610f
Schwacke L. H., Thomas L., Wells R. S., McFee W. E., Hohn A. A., Mullin K. D., et al. (2017). Quantifying injury to common bottlenose dolphins from the Deepwater Horizon oil spill using an age-, sex- and class-structured population model. Endang. Species Res. 33, 265–279. doi: 10.3354/esr00777
Schwing P. T., Montagna P. A., Joye S. B., Paris C. B., Cordes E. E., McClain C. R., et al. (2020). A synthesis of deep benthic faunal impacts and resilience following the Deepwater Horizon oil spill. Front. Mar. Sci. 7:560012. doi: 10.3389/fmars.2020.56001
SEDAR. (2018). SEDAR 52 stock assessment report for Gulf of Mexico red snapperNorth Charleston, South Carolina. Available at: https://sedarweb.org/documents/sedar-52-gulf-of-mexico-red-snapper-final-stock-assessment-report/.
Sellas A. B., Wells R. S., Rosel P. E. (2005). Mitochondrial and nuclear DNA analyses reveal fine scale geographic structure in bottlenose dolphins (Tursiops truncatus) in the Gulf of Mexico. Conserv. Genet. 6, 715–728. doi: 10.1007/s10592-005-9031-7
Shaver D. J., Rubio C., Walker J. S., George J., Amos A. F., Reich K., et al. (2016). Kemp’s ridley sea turtle (Lepidochelys kempii) nesting on the Texas coast: geographic, temporal, and demographic trends through 2014. Gulf Mex. Sci. 33, 158–178. doi: 10.18785/goms.3302.04
Silva M., Etnoyer P. J., MacDonald I. R. (2016). Coral injuries observed at mesophotic reefs after the Deepwater Horizon oil discharge. Deep-Sea Res. II 129, 96–107. doi: 10.1016/j.dsr2.2015.05.013
Smeltz M., Rowland-Faux L., Ghiran C., Patterson W. F III, Garner S. G., Beers A., et al. (2017). A multi-year study of hepatic biomarkers in coastal fishes from the Gulf of Mexico after the Deepwater Horizon oil spill. Mar. Environ. Res. 129, 57–67. doi: 10.1016/j.marenvres.2017.04.015
Smith N. S., Côté I. M. (2021). Biotic resistance on coral reefs? direct and indirect effects of native predators and competitors on invasive lionfish. Coral Reefs 40, 1127–1136. doi: 10.1007/s00338-021-02117-7
Snyder S. M., Pulster E. L., Wetzel D. L., Murawski S. A. (2015). PAH exposure in Gulf of Mexico demersal fishes, post-Deepwater Horizon. Environ. Sci. Tech. 49, 8786–8795. doi: 10.1021/acs.est.5b01870
Stieglitz J. D., Mager E. M., Hoenig R. H., Beneti D. D., Grosell M. (2016). Impacts of Deepwater Horizon crude oil exposure on adult mahi-mahi (Coryphaena hippurus) swim performance. Environ. Toxicol. Chem. 35, 2613–2622. doi: 10.1002/etc.3436
Suderman B. L., Marcus N. H. (2002). The effects of orimulsion and fuel oil 6 on the hatching success of copepod resting eggs in the seabed of Tampa Bay, Florida. Environ. Pollut. 120, 787–795. doi: 10.1016/S0269-7491(02)00169-0
Sutton T. T., Milligan R. J., Daly K., Boswell K. M., Cook A. B., Cornic M., et al. (2022). The open-ocean Gulf of Mexico after Deepwater Horizon: synthesis of a decade of research. Front. Mar. Sci. 9. doi: 10.3389/fmars.2022.753391
Swinea S. H., Fodrie F. J. (2021). Gulf fisheries supported resilience in the decade following unparalleled oiling. Ecosphere 12:e03801. doi: 10.1002/ecs2.3801
Takeshita R., Burian S. J., Colegrove K. M., Collier T. K., Deak K., Dean K. M. (2021). A review of the toxicology of oil in vertebrates: what we have learned following the Deepwater Horizon oil spill. J. Toxicol. Envir. Health-B 24, 355–394. doi: 10.1080/10937404.2021.1975182
Takeshita R., Sullivan L., Smith C., Collier T., Hall A., Brosnan T., et al. (2017). The Deepwater Horizon oil spill marine mammal injury assessment. Endang. Species Res. 33, 95–106. doi: 10.3354/esr00808
Tarnecki J. H., Patterson W. F. III (2015). Changes in red snapper diet and trophic ecology following the Deepwater Horizon oil spill. Mar. Coast. Fish 7, 135–147. doi: 10.1080/19425120.2015.1020402
Trustees (Deepwater Horizon Natural Resource Damage Assessment Trustees). (2016). Deepwater Horizon oil spill: final programmatic damage assessment and restoration plan and final programmatic environmental impact statement. Available at: http://www.gulfspillrestoration.noaa.gov/restoration-planning/gulf-plan.
van der Oost R., Beyer J., Vermeulen N. P. E. (2003). Fish bioaccumulation and biomarkers in environmental risk assessment: a review. Eviron. Toxicol. Pharmcol. 13, 57–149. doi: 10.1016/S1382-6689(02)00126-6
Vander Zanden H. B., Bolten A. B., Tucker A. D., Hart K. M., LaMont M. M., Fujisaki I., et al. (2016). Biomarkers reveal sea turtles remained in oiled areas following the Deepwater Horizon oil spill. Ecol. Appl. 26, 2145–2155. doi: 10.1002/eap.1366
Venn-Watson S., Colegrove K. M., Litz J., Kinsel M., Terio K., Saliki J., et al. (2015). Adrenal gland and lung lesions in Gulf of Mexico common bottlenose dolphins (Tursiops truncatus) found dead following the Deepwater Horizon oil spill. PloS One 10:e0126538. doi: 10.1371/journal.pone.0126538
Wallace B. P., Stacy B. A., Rissing M., Cacela D., Garrison L. P., Graettinger G. D., et al. (2017). Estimating sea turtle exposures to Deepwater Horizon oil. Endang. Species Res. 33, 51–67. doi: 10.3354/esr00728
Wei C.-L., Rowe G. T., Hubbard G. F., Scheltema A. H., Wilson G. D. F., Petrescu I., et al. (2010). Bathymetric zonation of deep-sea macrofauna in relation to export of surface phytoplankton production. Mar. Ecol. Prog. Ser. 399, 1–14. doi: 10.3354/meps08388
Wells R. S., Schwacke L. H., Rowles T. K., Balmer B. C., Zolman E., Speakman T., et al. (2017). Ranging patterns of common bottlenose dolphins Tursiops truncatus in barataria bay, Louisiana, following the Deepwater Horizon oil spill. Endang. Species Res. 33, 301–310. doi: 10.3354/esr00732
Wells R. S., Scott M. D., Irvine A. B. (1987). “The social structure of free-ranging bottlenose dolphins,” in Current mammalogy, vol. 1 . Ed. Genoways H. H. (New York: Plenum Press), 247–305.
White H. K., Hsing P.-Y., Cho W., Shank T. M., Cordes E. E., Quanttrini A. M. (2012). Impact of the Deepwater Horizon oil spill on a deep-water coral community in the Gulf of Mexico. Proc. Nat. Acad. Sci. 109, 20303–20308. doi: 10.1073/pnas.1118029109
Whitehead A., Dubansky B., Bodinier C., Garcia T. I., Miles S., Pilley C., et al. (2012). Genomic and physiological footprint of the Deepwater Horizon oil spill on resident marsh fishes. Proc. Nat. Acad. Sci. 109, 20298–20302. doi: 10.1073/pnas.1109545108
Wiernicki C. J., O’Brien M. H. P., Zhang F., Lyubchich V., Li M., Secor D. H. (2020). The recurring impact of storm disturbance on black sea bass (Centropristis striata) movement behaviors in the mid-Atlantic bight. PloS One 15:e0239919. doi: 10.1371/journal.pone.0239919
Würsig B. (2017). “Marine mammals of the Gulf of Mexico,” in Habitats and biota of the Gulf of Mexico: before the Deepwater Horizon oil spill, volume 2: fish resources, fisheries, Sea turtles, avian resources, marine mammals, diseases and mortalities. Ed. Ward C. H. (New York, NY: Springer Science), 1489–1587. doi: 10.1007/978-1-4939-3456-0_5
Keywords: Deepwater Horizon, Gulf of Mexico, continental shelf, resiliency, population effects
Citation: Patterson WF III, Robinson KL, Barnett BK, Campbell MD, Chagaris DC, Chanton JP, Daly KL, Hanisko DS, Hernandez FJ Jr., Murawski SA, Pollack AG, Portnoy DS and Pulster EL (2023) Evidence of population-level impacts and resiliency for Gulf of Mexico shelf taxa following the Deepwater Horizon oil spill. Front. Mar. Sci. 10:1198163. doi: 10.3389/fmars.2023.1198163
Received: 31 March 2023; Accepted: 22 June 2023;
Published: 02 August 2023.
Edited by:
Justin Marshall, The University of Queensland, AustraliaReviewed by:
David Hala, Texas A&M University at Galveston, United StatesDaniel Pech, El Colegio de la Frontera Sur, Mexico
Copyright © 2023 Patterson, Robinson, Barnett, Campbell, Chagaris, Chanton, Daly, Hanisko, Hernandez, Murawski, Pollack, Portnoy and Pulster. This is an open-access article distributed under the terms of the Creative Commons Attribution License (CC BY). The use, distribution or reproduction in other forums is permitted, provided the original author(s) and the copyright owner(s) are credited and that the original publication in this journal is cited, in accordance with accepted academic practice. No use, distribution or reproduction is permitted which does not comply with these terms.
*Correspondence: William F. Patterson III, d2lsbC5wYXR0ZXJzb25AdWZsLmVkdQ==