- 1New South Wales (NSW) Department of Primary Industries, Fisheries Conservation Technology Unit, National Marine Science Centre, Southern Cross University, Coffs Harbour, NSW, Australia
- 2Marine and Estuarine Ecology Unit, School of Biological Sciences, University of Queensland, Brisbane, QLD, Australia
- 3Fishwell Consulting, Queenscliff, VIC, Australia
- 4Department of Statistics, University of Auckland, Auckland, New Zealand
Increases in the size and amount of meshes turned 90° ('T90') in an Australian fish-trawl codend were investigated to improve the size selection of deepwater flathead Neoplatycephalus conatus and reduce discards. The conventional codend comprised 94-mm mesh throughout with normal orientation (diamond-shaped) in the posterior half but T90 in the anterior half ('half 94-mm T90' codend). Two new codends had 105-mm T90 mesh in the anterior section only ('half 105-mm T90') and throughout ('full 105-mm T90'). Both larger-meshed codends caught fewer immature deepwater flathead, but also lost some larger fish, especially the full 105-mm T90 codend, which had 80% more T90 meshes. The larger-meshed codends also allowed some discarded species to escape, but similarly affected other targets. Collected deepwater flathead morphological data support a T90 mesh size of ~100 mm in the anterior codend or ~94 mm throughout to maintain target sizes. However, irrespective of changes to codend meshes, owing to comparable inter-specific sizes and shapes, the discard percentage in this fishery will probably remain consistent at >75%, which is more than double the global average for fish trawls. Future efforts to improve selection in the fishery should consider modifications other than codend changes.
Introduction
Fish trawling is among the world’s most common fishing methods, producing ~25 million t of seafood each year or ~25% of global marine production (Pérez Roda et al., 2019). Notwithstanding their importance, most fisheries involve multiple targets of varying morphologies and sizes, which can debase trawls to less-than-ideal selection and cause large subsets of catches to be discarded.
Various options are available for improving fish-trawl selectivity, although >70% of efforts have focused on the codend and historically by legislating appropriate diamond-mesh ('T0') sizes (Kennelly and Broadhurst, 2021). Efforts at improving codend selectivities have also included regulating other factors affecting lateral-mesh openings (regardless of mesh size), including twine diameters (Lowry and Robertson, 1996), codend circumferences (Reeves et al., 1992), or different mesh orientations (Kennelly and Broadhurst, 2021). The latter initially involved turning meshes 45° ('T45'; Robertson and Stewart, 1988) but more recently 90° ('T90') (Moderhak, 1997; Digre et al., 2010). Regardless of mesh type, shortened lastridge ropes have also been used to increase openings (Ingólfsson and Brinkhof, 2020).
Most work to improve fish-trawl selectivity has occurred in Europe (Kennelly and Broadhurst, 2021). Fewer efforts have been made in the Southern Hemisphere, including Australia, where there are 14 separately managed fish-trawl fisheries and ~135 licences, but with most in the south. Among the least selective fisheries is in the Great Australian Bight, where deepwater flathead, Neoplatycephalus conatus is a key target (Broadhurst et al., 2022). However, >300 species are discarded, mostly latchet, Pterygotrigla polyommata and stingarees, Urolophidae (van Putten et al., 2019).
Recently, to reduce unwanted bycatches and following overseas success (Ingólfsson and Brinkhof, 2020), Great Australian Bight operators have been using legislated codends comprising anterior sections (50% of codend) of T90 mesh made from conventional meshes [~94-mm stretched mesh opening (SMO)]. We assessed the utility of this commercially fished 'half 94-mm T90' codend against a traditional diamond-mesh design (Broadhurst et al., 2022). The T90 codend did not affect selection for the key target, deepwater flathead, and other retained species (ocean jacket, Nelusetta ayraud; boarfish, Paristiopterus gallipavo; or red gurnard, Chelidonichthys kumu) or discarded species (latchets) (Broadhurst et al., 2022). These results were attributed to the mesh being too small, irrespective of orientation.
Deepwater flathead are regulated via a total catch (~1,200 t p.a), and while there is no size limit, they reach maturity at ~40-cm total length (TL), which is an appropriate minimum harvest size. The species is dorsally compressed, and theoretically, this body shape means that assuming their girth is less than the mesh perimeter, small fish should escape an appropriate-sized T90 mesh (Broadhurst et al., 2006). Owing to few fish trawlers in Australia, the range of available mesh sizes is limited and typically offered in 10-mm increments. Beyond 90- to 95-mm mesh, the next available material in similar twine diameters is ~105 mm.
Considering the above, the aims here were to compare the relative size and species selectivities of the half 94-mm T90 codend described by Broadhurst et al. (2022) and two new designs comprising 105-mm T90 mesh in the anterior section only and throughout. We sought to test the hypothesis that the new codends would retain deepwater flathead ≥40-cm TL and most ventrally compressed species, but allow small deepwater flathead and some unwanted catches to escape.
Materials and methods
The experiment was performed in the Great Australian Bight (33.12°S; 128.06°E to 33.27°S; 129.40°E) during 7 days in November 2022 using the FV “Explorer S” (35 m). The vessel had a conventional, single, two-seam trawl (Broadhurst et al., 2022). The posterior body (100 meshes in the transverse direction–T) was configured to alternately attach three extensions/codends (below). The vessel had a Scanmar trawl monitoring system to measure otter-board spread, a Lowrance global positioning system (GPS) to record distance and speed over the ground (SOG), and a Furuno echo sounder for depth.
Extensions and codends
Three four-panel extension/codend sections were constructed using up to four sheets of knotted polyethylene, braided netting. Each was measured for 20 replicate SMOs using a legislated purpose-built gauge comprising a weight (3 kg) attached below callipers. Twine diameters were recorded using vernier callipers. All extensions were made from a mean (± SE) SMO of 105.9 (0.18) mm (3-mm diameter–Ø twine) orientated as T0, measuring 24.5 meshes in the normal (N) direction and 100 T (Figure 1). Four lastridge ropes (24-mm Ø twisted polypropylene) were attached at the seams of the extension sections and measured the same stretched length (Figure 1).
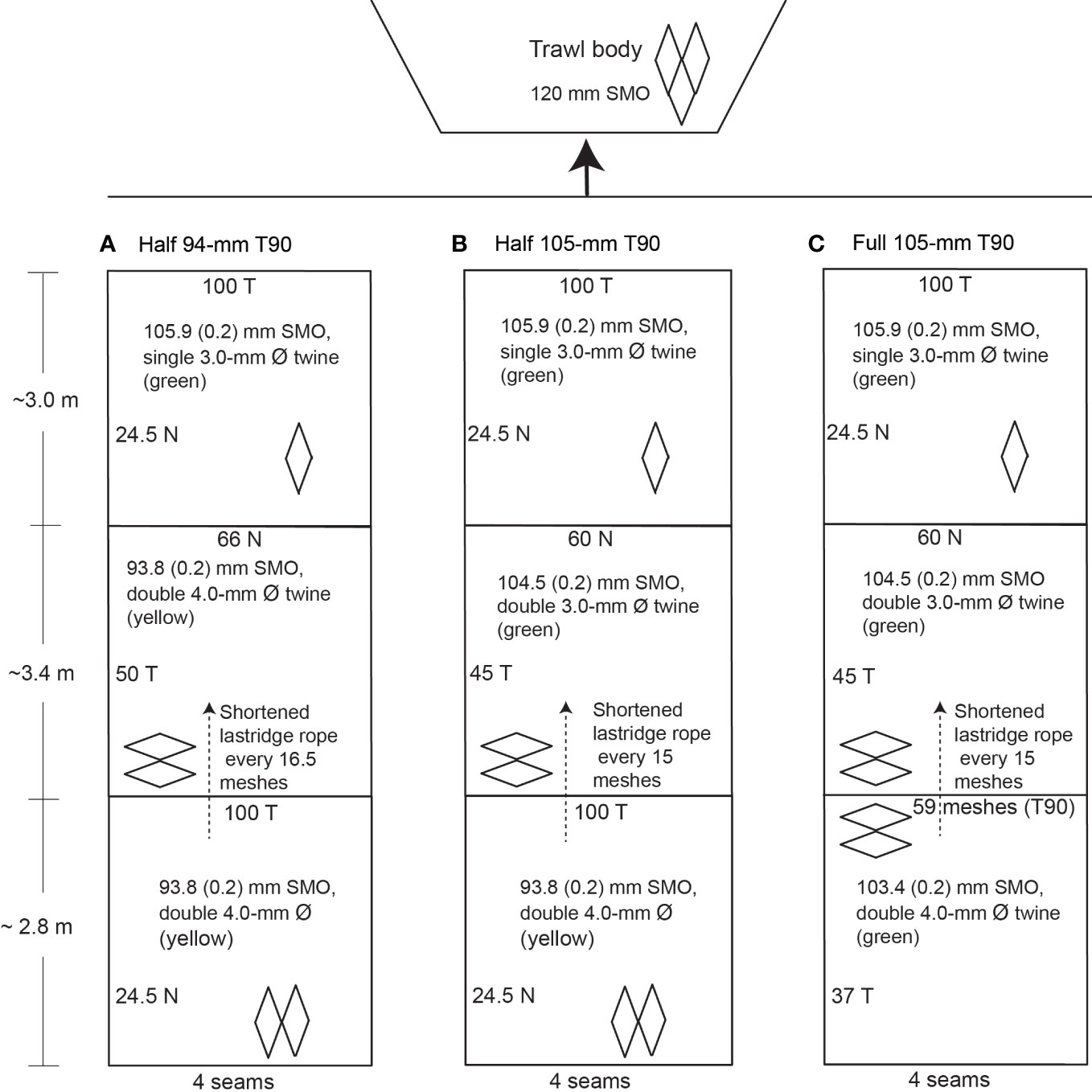
Figure 1 Schematic diagram of the treatment codends, including the traditional (A) half 94-mm T90 and new (B) half and (C) full 105-mm T90 codends. Ø, diameter; N, normal direction; T, transverse direction; SMO, stretched mesh opening. Standard errors in parentheses.
A different four-panel codend was attached to each extension. The first (half 94-mm T90) comprised an anterior section of 93.8 (± 0.17)-mm SMO (4-mm Ø double twine) turned 90° sewn across four panels for a total of 66 meshes around × 50 meshes deep (Figure 1A). Four 16-mm Ø Dynema lastridge ropes were attached at the junction of each panel and were 17% shorter than the stretched anterior codend length (Figure 1A). The T90 section was attached to a posterior T0 section measuring 24.5 N × 100 T and made from the same mesh as above, with lastridge ropes measuring the same length as the stretched meshes.
The second codend ('half 105-mm T90') had the same posterior section as the half 94-mm T90, but an anterior section made from four panels of 104.5 (± 0.19)-mm SMO T90 mesh (3-mm Ø double twine) totalling 60 meshes around × 45 meshes long (Figure 1B). Lastridge ropes were attached as above. The third codend ('full 105-mm T90') had the same T90 anterior section as the second, but the posterior section was also made from T90 mesh with an SMO of 103.4 (± 0.21) mm (4-mm Ø double twine) and was 59 meshes × 37 meshes long (i.e., 80% of the area of the anterior section; Figure 1C). Four 17% shorter lastridge ropes extended throughout both codend sections.
Experimental design
The codends were alternately attached to the trawl and deployed across the same locations over 7 days. Technical data were collected on the fished location, distance (km) and duration (h), otter-board spread (m), SOG (ms−1), and depth (m). The latter three variables were logged every ~15 min to provide an average deployment−1. After retrieval, the codend was emptied into an area with a measured volume, and the total catch weight was estimated. Retained catches were separated and boxed before weighing and counting. The total weight of discarded catch was estimated by subtracting the confirmed retained component from the total catch. Subsamples of discards were counted and weighed and extrapolated to the totals. Randomly selected subsamples of key species, but predominantly deepwater flathead and latchet (up to 130 deployment−1) were measured to the nearest 0.5 cm (TL for fish and disc width for stingarees).
During three randomly selected deployments, 102 deepwater flathead were collected and immediately underwent measurement of their TLs and maximum heights (MHs), widths (MWs), and girths (MGs; all to the nearest 1 mm). The MH and MW were recorded using vernier callipers, while MG was taken by wrapping a length of polyamide twine (~1 mm Ø) around the thickest part of each fish and then measuring this.
Statistical analyses
Data describing otter-board spread were analysed using linear mixed models (LMM) comprising the fixed effects of 'codend', 'depth', and 'SOG'. The random term was 'day'. Backward elimination was used with non-significant fixed terms removed until the rest were significant at the 5% level using Wald tests. Standardized (ha−1 trawled; i.e., using otter-board spreads) weights of key species and combined catches were analysed with the Tweedie distribution (which incorporates zero inflation in non-negative numeric data and is applied on the log scale) and fitted using a generalized linear mixed model (GLMM) with codend and day as fixed and random effects, respectively.
Generalized additive modelling (GAM) was used to fit relative selectivity curves to the TL-frequency data for deepwater flathead and latchet (Supplementary Material). Length frequencies were first scaled up by deployment subsampling fractions to estimate total frequencies. Relative selectivity was assessed for each of the three possible pairings of the three gears (following Broadhurst et al., 2022). This catch-comparison analysis was implemented using the SELECT R package, which includes bootstrap functionality to incorporate between-haul variability (Millar et al., 2004; Millar, 2021). A permutation test was also used (10,000 resamples) to assess for statistical significance of codend configuration (Broadhurst et al., 2022).
The morphometric data for deepwater flathead were pooled across deployments and fitted against TL using linear regression. All analyses were performed using the R programming language.
Results
The three codends were fished during 20 deployments (six for the half 94-mm T90 codend and seven for each larger-meshed T90 codends) across comparable mean (± SE) durations (5.02 ± 0.04 h), depths (145.2 ± 4.4 m), and SOGs (1.6 ± 0.0 ms−1). Otter-board spread (154.6 ± 1.0 m) was not affected by codend (LMM, p > 0.05) but did increase with depth (LMM, p < 0.001).
Thirty-nine tonnes, comprising >55 species, was caught, of which 8.4 t (>24 species) was retained and 30.9 t or 79% was discarded (Supplementary Table 1). Eight species (similar-sized) had sufficient quantities for analyses: latchet (98.5% discarded), deepwater flathead (none discarded), ocean jacket (44.9% discarded), southern fiddler ray, Trygonorrhina dumerilii (100% discarded), yellow-spotted boarfish (0% discarded), Australian burrfish, Allomycterus pilatus (100% discarded), red gurnard (0.3% discarded), and ringed toadfish, Omegophora armilla (100% discarded) (Supplementary Table 1).
Species selectivity
The GLMMs detected significant codend effects for the weights of retained total catches and deepwater flathead (both ≥40- and <40-cm TL) and discarded latchet and ringed toadfish (p < 0.05; Table 1). The weights of retained yellow-spotted boarfish and red gurnard had p-values of 0.08 and 0.06, respectively (Table 1). Most variables, except for southern fiddler rays (larger than all other species), showed the same trend of progressively lower weights in the half and then full 105-mm T90 codends than the conventional half 94-mm T90 codend (Table 1).
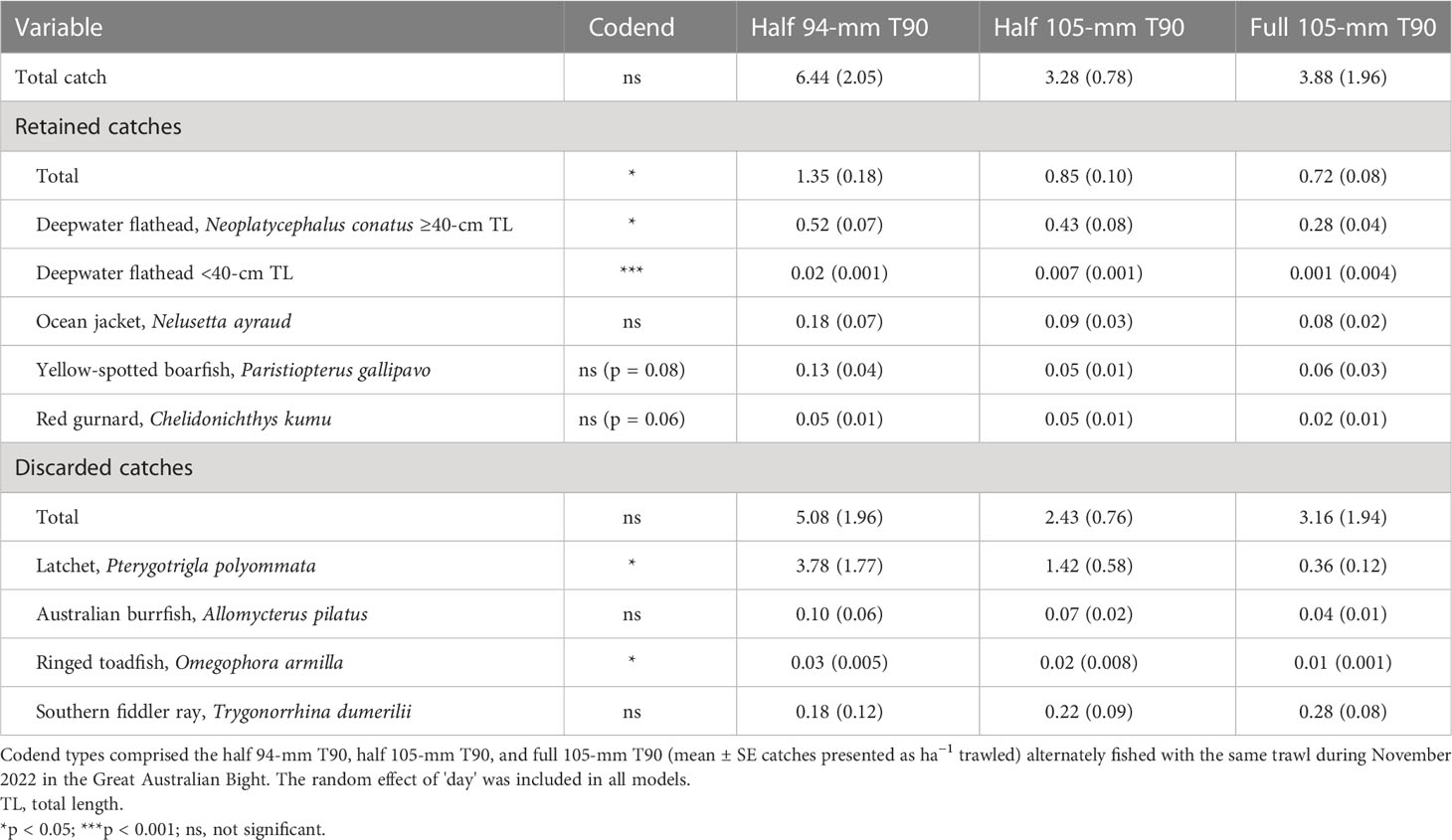
Table 1 Summaries of significance from Tweedie generalized linear mixed models for an effect of codend type on catch weights.
Size selectivity
Permutation tests detected significant TL effects for deepwater flathead in both 105-mm T90 codends vs. the half 94-mm T90 and for latchet in the full vs. half 105-mm T90 codends (p < 0.05; Figures 2A, C, F). Based on the fitted GAM cubic splines, deepwater flathead significance manifested as proportionally smaller fish (<~55-cm TL; albeit with considerable variability) escaping from the 105-mm T90 codends and especially the full design (Figures 2A, C). Among latchet, proportionally more individuals <~40-cm TL escaped the full than the half 105-mm T90 codend (Figures 2B, D, E).
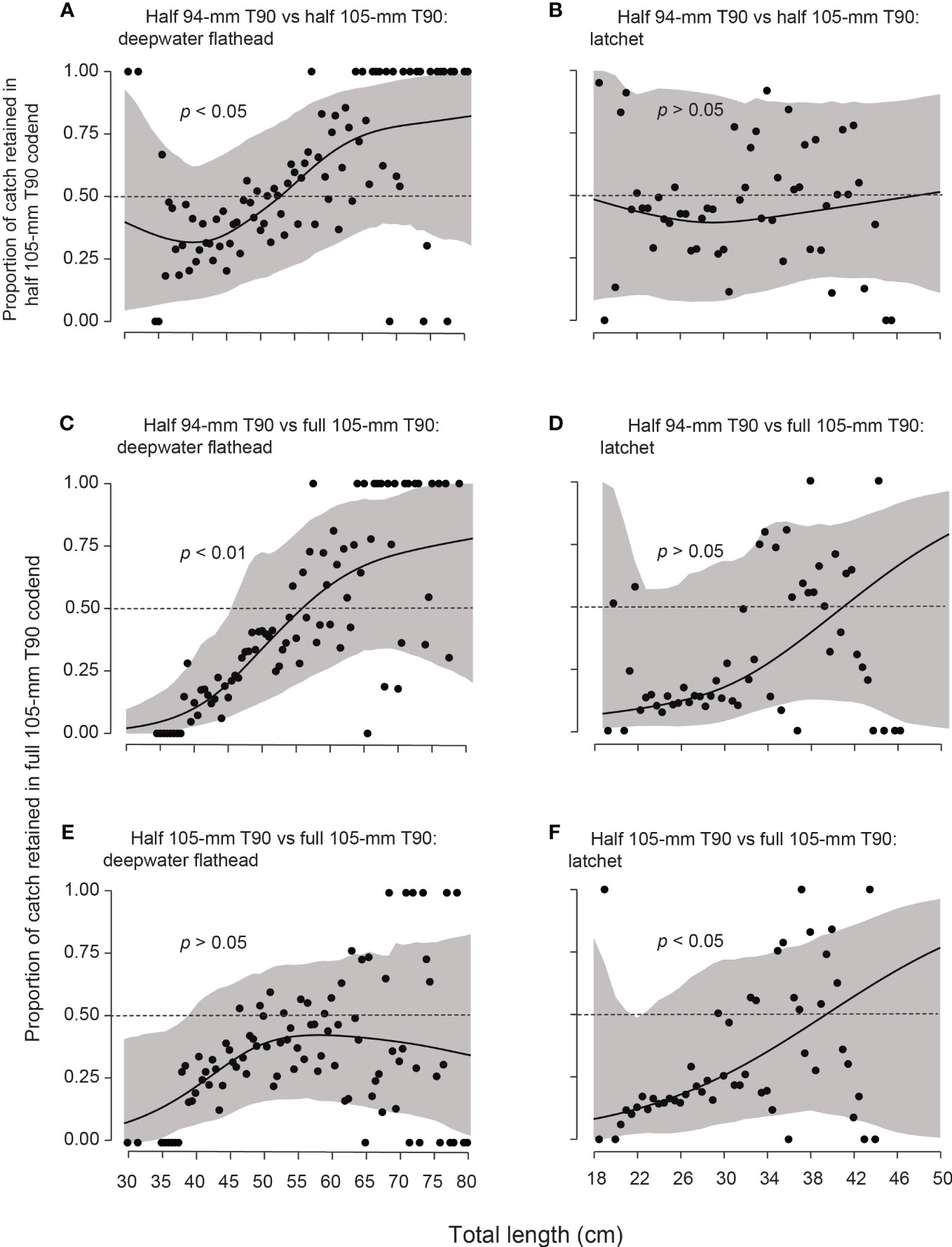
Figure 2 Cubic regression splines (solid lines) with 95% pointwise confidence intervals (grey bands) fitted to the proportions (black circles) of the combined catches of each total length (TL) class for deepwater flathead, Neoplatycephalus conatus and latchet, Pterygotrigla polyommata retained in the trawls with the (A, B) half 105-mm T90 and (C, D) full 105-mm T90 codends (from trawls with these codends and the half 94-mm T90), and the (E, F) full 105-mm codend (from trawls with this design and the half 105-mm T90 codend). The 0.5 proportions retained (i.e., baseline of equal efficiency between trawls) are marked with the narrow-dashed horizontal lines, and the p-values for effects of TL on relative selection (permutation test) are provided.
Deepwater flathead morphology
Significant linear regressions were derived between TL (n = 102) and MG (MG = 0.541TL–46.680; r2 = 0.92), MW (MW = 0.226TL–26.423; r2 = 0.91), and MH (MH = 0.145TL–23.162; r2 = 0.83) (p < 0.001). For deepwater flathead at 40-cm TL, the MG, MW and MH were ~170, 64, and 35 mm, respectively. The perimeter of a 105-mm SMO mesh (~210 mm) corresponded to a 47.5-mm TL individual.
Discussion
The data reiterate that slight increases in T90 mesh size and/or their amount in a codend can considerably affect species-specific size selection (Veiga-Malta et al., 2019; Cheng et al., 2020). Further, the changes to the codend were reasonably matched to deepwater flathead morphology, which supports maintained mesh openings throughout the new T90 codends, but with variability that appeared to increase with the amount of T90 meshes (i.e., the full 105-mm T90 had 80% more T90 meshes than the half 105-mm codend and allowed more fish across a greater size range to escape). Both larger-meshed T90 codends also caught fewer discards, although as for other multi-species fisheries (e.g., Cheng et al., 2020; Robert et al., 2020), there were concomitantly lower catches of desired species, cumulatively reducing retained total catch. The utility of T90 here can be discussed by considering the morphology of deepwater flathead (and their fishing mortality prioritization) along with similar-sized retained and discarded species. Ultimately, this information might guide other selection refinements to regional trawls.
Any fish escaping through a mesh requires sufficient contact (often repeated) and cross-sectional dimensions smaller than mesh openings or, ultimately, the mesh perimeter (Broadhurst et al., 2006). Deepwater flathead have an MH-to-MW ratio of ~0.5, which matches the shape of a T90 mesh when held open by lastridge ropes (Broadhurst et al., 2006). Given the data collected here and assuming sufficient contact, conspecifics up to ~48-mm TL had a girth matching the perimeter of the 105-mm mesh, and so these and slightly larger fish (depending on orientation and compression) could have passed through. This estimated TL range is supported by the modelled relative selectivity curves, but clearly was not the only influence on the probability of deepwater flathead escaping, given the greater reductions in catches of fish <40-cm TL by the full 105-mm T90 codend. Presumably, increasing the amount of T90 meshes at an area where fish had the greatest probability of repeatedly encountering meshes (anterior to the catch) increased their escape (Robertson and Stewart, 1988; Reeves et al., 1992; Broadhurst et al., 2006).
The above results imply that a full T90 codend (or possibly the posterior section only) with a slightly smaller SMO might be appropriate for better regulating deepwater flathead size selection. We showed no effects on the size selection of deepwater flathead for the conventionally fished codend comprising 94-mm T90 mesh in the anterior section only (Broadhurst et al., 2022). This mesh corresponds to the girth of a 39-cm TL deepwater flathead, and so extending 94-mm T90 mesh throughout the codend might allow at least some conspecifics <40-cm TL to escape. Alternatively, increasing the T90 mesh to ~100 mm in the anterior section only—corresponding to a 42-cm TL deepwater flathead but assuming less probability of contact—might have some benefit.
Notwithstanding improved deepwater-flathead size selection due to increasing either the size or area of T90 mesh, other similar-sized species mean there will be cumulative reductions in not only discards but also some targets (Cheng et al., 2020; Robert et al., 2020). The discard species most affected by the larger-meshed T90 codends were latchet and ringed toadfish. Both fish are approximately fusiform, and like for deepwater flathead, smaller individuals were able to escape, especially from the full 105-mm T90 codend. Among the retained species, red gurnard is similarly fusiform, and while there was no significant reduction in their catches by the larger-meshed T90 codends, fewer were retained in the full 105-mm T90 codend. Based on morphometric data collected by Broadhurst et al. (2006), red gurnard with a girth of 210 mm (matching the 105-mm mesh) might measure ~38-cm TL, but individuals are retained down to <25-cm TL, and so there would be lost catches. In contrast, the retained ocean jacket and yellow-spotted boarfish are ventrally compressed, which might have limited many escaping, although mean catches were lower in the larger-meshed T90 codends.
It is clear in terms of discards, and notwithstanding relatively few replicate tows, that T90 mesh will not be an effective selective modification beyond allowing some small deepwater flathead to escape. Potentially, simpler codend modifications within existing configurations could have comparable utility. For example, although not as novel as T90 mesh, larger T0 mesh with or without shortened lastridge ropes might have sufficient benefits. Certainly, while complex industry-developed modifications should be encouraged, these should be compared against simpler options. An example of the problems of not following this protocol involves size-sorting grids in the Barents Sea (with 135-mm T0 codends), which were mandated to improve selection for cod, Gadus morhua during the late 1990s. However, Jørgensen et al. (2006) subsequently concluded that simply increasing the conventional T0 mesh to 155 mm (without a grid) provided the same benefits at a lower cost and complexity.
Regardless of the appropriate mesh size or shape to regulate deepwater flathead fishing mortality, ongoing work is clearly required because the discard rate recorded here is at least twice the global average for fish trawls (~30%; Pérez Roda et al., 2019). Beyond codend changes, other options include modifying the anterior trawl and excluding unwanted species by exploiting either tactile (e.g., alternative ground gears or separator panels) or visual (e.g., lights) stimuli (McHugh et al., 2017; Kennelly and Broadhurst, 2021). Such options may warrant investigation in the Great Australian Bight fishery, but like all efforts at improving trawl selectivity, this work will require close collaboration with, and support from, the industry to realise effective changes.
Data availability statement
The raw data supporting the conclusions of this article will be made available by the authors, without undue reservation.
Author contributions
Conceptualization: MB, IK and RM; Data curation: MB; Formal analyses: RM; Funding acquisition: MB; Investigation: MB and IK; Methodology: MB and RM; Project administration: MB; Resources: MB; Software: MB and RM; Supervision: MB and IK; Validation: MB; Visualization: MB and RM; Roles/Writing – original draft: MB; Writing – review and editing: MB, IK and RM. All authors contributed to the article and approved the submitted version.
Funding
The NSW Department of Primary Industries and the Fisheries Research and Development Corporation provided funding via project 2019-027: “Improving and promoting fish-trawl selectivity in the Commonwealth Trawl Sector (CTS) and Great Australian Bight Trawl Sector (GABTS) of the Southern and Eastern Shark and Scalefish Fishery (SESSF)” on behalf of the Australian Government.
Acknowledgments
We thank Jim Raptis, Barney Williams, Bradley McKay, Russell Hudson, and Matt Koopman for advice and assistance; the Explorer S crew for their work at sea; and the Great Australian Bight Industry Association, South East Trawl Fishing Industry Association, and Australian Fisheries Management Authority for advice.
Conflict of interest
Author IA is a Director of Fishwell Consulting.
The remaining authors declare that the research was conducted in the absence of any commercial or financial relationships that could be construed as a potential conflict of interest.
Publisher’s note
All claims expressed in this article are solely those of the authors and do not necessarily represent those of their affiliated organizations, or those of the publisher, the editors and the reviewers. Any product that may be evaluated in this article, or claim that may be made by its manufacturer, is not guaranteed or endorsed by the publisher.
Supplementary material
The Supplementary Material for this article can be found online at: https://www.frontiersin.org/articles/10.3389/fmars.2023.1196660/full#supplementary-material
References
Broadhurst M. K., Dijkstra K. K. P., Reid D. D., Gray C. A. (2006). Utility of morphological data for key fish species in southeastern Australian beach-seine and otter-trawl fisheries: predicting mesh size and configuration. NZ. J. Mar. Freshw. Res. 40, 259–272. doi: 10.1080/00288330.2006.9517419
Broadhurst M. K., Knuckey I. A., Millar R. B. (2022). Benefits of conventional anterior codend meshes turned 90o in an Australian trawl fishery are limited to an improved quality of Neoplatycephalus conatus. Fron. Mar. Sci 9, 951549. doi: 10.3389/fmars.2022.951549
Cheng Z. H., Winger P. D., Bayse S. M., Kebede G. E., DeLouche H., Einarsson H. A., et al. (2020). Out with the old and in with the new: T90 codends improve size selectivity in the Canadian redfish (Sebastes mentella) trawl fishery. Can. J. Fish. Aquat. Sci. 77, 1711–1720. doi: 10.1139/cjfas-2020-0063
Digre H., Hansen U. J., Erikson U. (2010). Effect of trawling with traditional and 'T90' trawl codends on fish size and on different quality parameters of cod Gadus morhua and haddock Melanogrammus aeglefinus. Fish. Sci. 76, 549–559. doi: 10.1007/s12562-010-0254-2
Ingoílfsson Ó. A., Brinkholf J. (2020). Relative size selectivity of a four-panel codend with short lastridge ropes compared to a flexigrid with a regular codend in the barents Sea. Fish. Res. 232 (105724), 1–5. doi: 10.1016/j.fishres.2020.105724
Jørgensen T., Ingólfsson O. A., Graham N., Isaksen B. (2006). Size selection of cod by rigid grids - is anything gained compared to diamond mesh codends only? Fish Res. 79, 337–348. doi: 10.1016/j.fishres.2006.01.017
Kennelly S. J., Broadhurst M. K. (2021). A review of bycatch reduction in demersal fish trawls. Rev. Fish Biol. Fish. 31, 289–318. doi: 10.1007/s11160-021-09644-0
Lowry N., Robertson J. H. B. (1996). The effect of twine thickness on cod-end selectivity of trawls for haddock in the north Sea. Fish. Res. 26, 353–363. doi: 10.1016/0165-7836(95)00418-1
McHugh M. J., Broadhurst M. K., Sterling D. J. (2017). Choosing anterior-gear modifications to reduce the global environmental impacts of penaeid trawls. Rev. Fish Biol. Fish. 27, 111–134. doi: 10.1007/s11160-016-9459-5
Millar R. B. (2021) R package SELECT for estimation of the size selectivity of fishing gears. Available at: https://github.com/rbmillar/SELECT.
Millar R. B., Broadhurst M. K., Macbeth W. G. (2004). Modelling between-haul variability in the size selectivity of trawls. Fish. Res. 67, 171–181. doi: 10.1016/j.fishres.2003.09.040
Moderhak W. (1997). Determination of selectivity of cod codends made of netting turned through 90o bull. Sea Fish. Ins. 1, 3–14.
Pérez Roda M. A., Gilman E., Huntington T., Kennelly S. J., Suuronen P., Chaloupka M., et al. (2019). A third assessment of global marine fisheries discards (Rome: FAO), 78.
Reeves S. A., Armstrong D. W., Fryer R. J., Coull K. A. (1992). The effects of mesh size, cod-end extension length and cod-end diameter on the selectivity of Scottish trawls and seines. ICES J. Mar. Sci. 49, 279–288. doi: 10.1093/icesjms/49.3.279
Robert M., Morandeau F., Scavinner M., Fiche M., Larnaud P. (2020). Toward elimination of unwanted catches using a 100 mm T90 extension and codend in demersal mixed fisheries. PloS One 15 (7), e0235368. doi: 10.1371/journal.pone.0235368
Robertson J. H. B., Stewart P. A. M. (1988). A comparison of size selection of haddock and whiting by square and diamond mesh codends. J. du. Cons. 44, 148–161. doi: 10.1093/icesjms/44.2.148
van Putten I., Koopman M., Hobday A. J., Knuckey I., Zhou S. (2019). Fresh eyes on an old issue: demand-side barriers to a discard problem. Fish. Res. 209, 14–23. doi: 10.1016/j.fishres.2018.09.007
Keywords: T90 codend, selectivity, bycatch and discards, fish trawl, lastridge rope
Citation: Broadhurst MK, Knuckey IA and Millar RB (2023) Multi-specific effects of increasing T90 mesh size and amount in an Australian fish trawl. Front. Mar. Sci. 10:1196660. doi: 10.3389/fmars.2023.1196660
Received: 30 March 2023; Accepted: 31 May 2023;
Published: 20 June 2023.
Edited by:
Francois Bastardie, Technical University of Denmark, DenmarkReviewed by:
Eduardo Grimaldo, SINTEF Ocean, NorwayAlessandro Lucchetti, National Research Council (CNR), Italy
René Holst, University of Oslo, Norway
Copyright © 2023 Broadhurst, Knuckey and Millar. This is an open-access article distributed under the terms of the Creative Commons Attribution License (CC BY). The use, distribution or reproduction in other forums is permitted, provided the original author(s) and the copyright owner(s) are credited and that the original publication in this journal is cited, in accordance with accepted academic practice. No use, distribution or reproduction is permitted which does not comply with these terms.
*Correspondence: Matt K. Broadhurst, bWF0dC5icm9hZGh1cnN0QGRwaS5uc3cuZ292LmF1