- 1Department of Marine Sciences, University of the Aegean, Mytilene, Greece
- 2School of Information Engineering, Zhejiang Ocean University, Zhoushan, Zhejiang, China
- 3Biological Field Station on Otsego Lake, State University of New York at Oneonta, Oneonta, NY, United States
- 4Department of Physics and Astronomy, University of Bologna, Bologna, Italy
- 5Research Department Transformation Pathways, Potsdam Institute for Climate Impact Research, Potsdam, Germany
- 6Department of Hydraulic Engineering, Faculty of Civil Engineering and Geosciences, Delft University of Technology, Delft, Netherlands
This contribution presents an assessment at a regional (island) scale of the beach erosion due to storm events under Climate Change. The approach adopted to assess beach erosion at the island scale consisted of three modules. First, the location, dimensions and other attributes of the Cypriot beaches were recorded on the basis of widely-available satellite imagery. Secondly, sea levels and waves were modeled along the coast under different climatic scenarios and dates in the 21st century. Finally, using these projections beach retreat due to the relative mean sea level rise (RSLR) and extreme sea levels (ESLs) was estimated using ensembles of analytical and numerical cross-shore morphodynamic models, respectively. Extreme sea levels (ESLs) were projected to (a) increase by up to 60% in 2100 from their baseline (2000) levels, and (b) vary along the coast, with the highest ESLs (and corresponding waves) projected for the southern and western coasts. The mostly narrow Cypriot beaches (91% recorded maximum widths of < 50 m) showed increased exposure to erosion. In 2100, about 47% and 72% (based on the median model estimates) of the 241 unprotected Cypriot beaches will be permanently eroded, due to mean sea level rise (SLR), to 50% of their present maximum width, depending on the scenario. In addition to the long-term erosion due to SLR, severe storm erosion is projected by 2050 even under the RCP4.5 scenario; the 100-year extreme sea level event (ESL100) may overwhelm (at least temporarily) 49% of the currently unprotected Cypriot beaches without effective adaptation responses, with the most exposed beaches located along the northern coast. As the beach carrying capacity and hedonic value will be severely compromised, effective adaptation policies and technical measures will be urgently required.
1 Introduction
Beaches (sandy shorelines) are critical coastal ecosystems. They constitute a substantial fraction of the global coastline (e.g. Luijendijk et al., 2018), are important habitats in their own right (e.g. Ackerman, 2017), have a high hedonic value (Boto-García and Leoni, 2022) and provide protection from coastal flooding to their backshore ecosystems, assets and infrastructure (e.g. Ciavola et al., 2018). At the same time, many beaches are under retreat/erosion (Wong et al., 2014), manifested as: (i) shoreline retreat due to relative mean sea level rise (RSLR) and negative sedimentary budgets (Nicholls and Cazenave, 2010); and/or (ii) short-term retreat caused by storm events, which although might not result in permanent erosion, it could be, nevertheless, devastating (Di Paola et al., 2023). Shoreline retreat in beaches backed by natural and/or artificial features, such as coastal cliffs and infrastructure/assets could result in beach ‘drowning’.
Assessments are determined by the spatial (global, regional, local) and temporal (short- or long-term) scales of the study and the available information and resources (Ranasinghe, 2016). These involve (a) evaluations of the current trends (e.g. Mentaschi et al., 2018), and/or (b) future projections using analytical, numerical and/or empirical models (e.g. Hinkel et al., 2013; de Winter and Ruessink, 2017; Vousdoukas et al., 2020). Assessments at the regional scale (100s of km of coastline) are particularly important for integrated adaptation policies and efficient allocation of the requisite resources (e.g. Monioudi et al., 2016; Toimil et al., 2017; Giardino et al., 2018).
Erosion can be particularly threatening for island beaches, as these commonly have limited dimensions and sediment supply (Monioudi et al., 2017). At the same time, in many cases, island beaches are major tourism destinations (e.g. Alvarez et al, 2022). Tourism, which has been increasingly associated with beach recreation according to the ‘Sun, Sea and Sand - 3S’ model (Monioudi and Velegrakis, 2022) accounts for about 33.5% (on average) of the Gross Domestic Product (GDP) of the Caribbean Small Island Developing States (SIDS) (ILO, 2020), with many Mediterranean islands being also dependent on beach tourism (UNWTO, 2019). In the period 2010 - 2021, the majority of the 246.5 million arrivals (20.1 million annual average) in Greece had a Greek island destination (SETE, 2023), whereas about 23% of the 71.6 million arrivals in Spain in 2022 had a Balearic destination (IBESTAT, 2023; INE, 2023).
In the future, the accelerating mean sea level rise, coupled with potential changes in the magnitude and recurrence frequency of storm events (e.g. Vitousek et al., 2017; IPCC, 2019) will inevitably Intensify beach erosion and coastal flooding, with severe impacts on the natural and human coastal systems (e.g. Bellard et al., 2014; Anderson et al., 2018; Toimil et al., 2018; Rodella et al., 2020). Therefore, although projecting the beach morphodynamics under a variable and changing climate is not an easy exercise due to inherent uncertainties in both forcing and the beach response (Le Cozannet et al., 2019), beach erosion assessment and mitigation should be decidedly important components in all sustainable development plans of the coastal zone (e.g. Summers et al., 2018).
The present contribution presents an approach for the assessment of beach retreat/erosion at a regional (island) scale under mean sea level and storm events and its application at the touristic island of Cyprus (Eastern Mediterranean). The spatial characteristics and other attributes of the Cypriot beaches were recorded from widely available satellite imagery. Projections of mean and extreme sea levels (ESLs) and corresponding waves under different climatic scenarios were then used to force cross-shore morphodynamic model ensembles to obtain estimates of beach erosion for the island beaches.
2 Cypriot beaches: environmental setting and socio-economic significance
Cyprus is the third largest Mediterranean island, with an area of 9,250 km2, a total population of about 1.25 million (2022) and a coastline length of about 740 km. The island is located in a tectonically active region and can be divided in 4 main geological zones (Figure 1): the northern Keryneia Terrane, comprising thrusted Cenozoic sedimentary sequences; (ii) the Troodos massif comprising uplifted, Cretaceous ophiolites; (iii) the Mesozoic sedimentary/basaltic Mamonia terrane in the west; and (iv) the Circum–Troodos sedimentary succession. Cyprus climate exhibits hot dry summers and changeable winters characterized by short-lived wet events that provide most of the annual precipitation (Price et al., 1999). The seasonal precipitation and the burgeoning summer water needs have prompted the construction of 108 river dams with a total capacity of about 332 million m3 (WDD, 2017) diminishing the sediment supply at their downstream beaches.
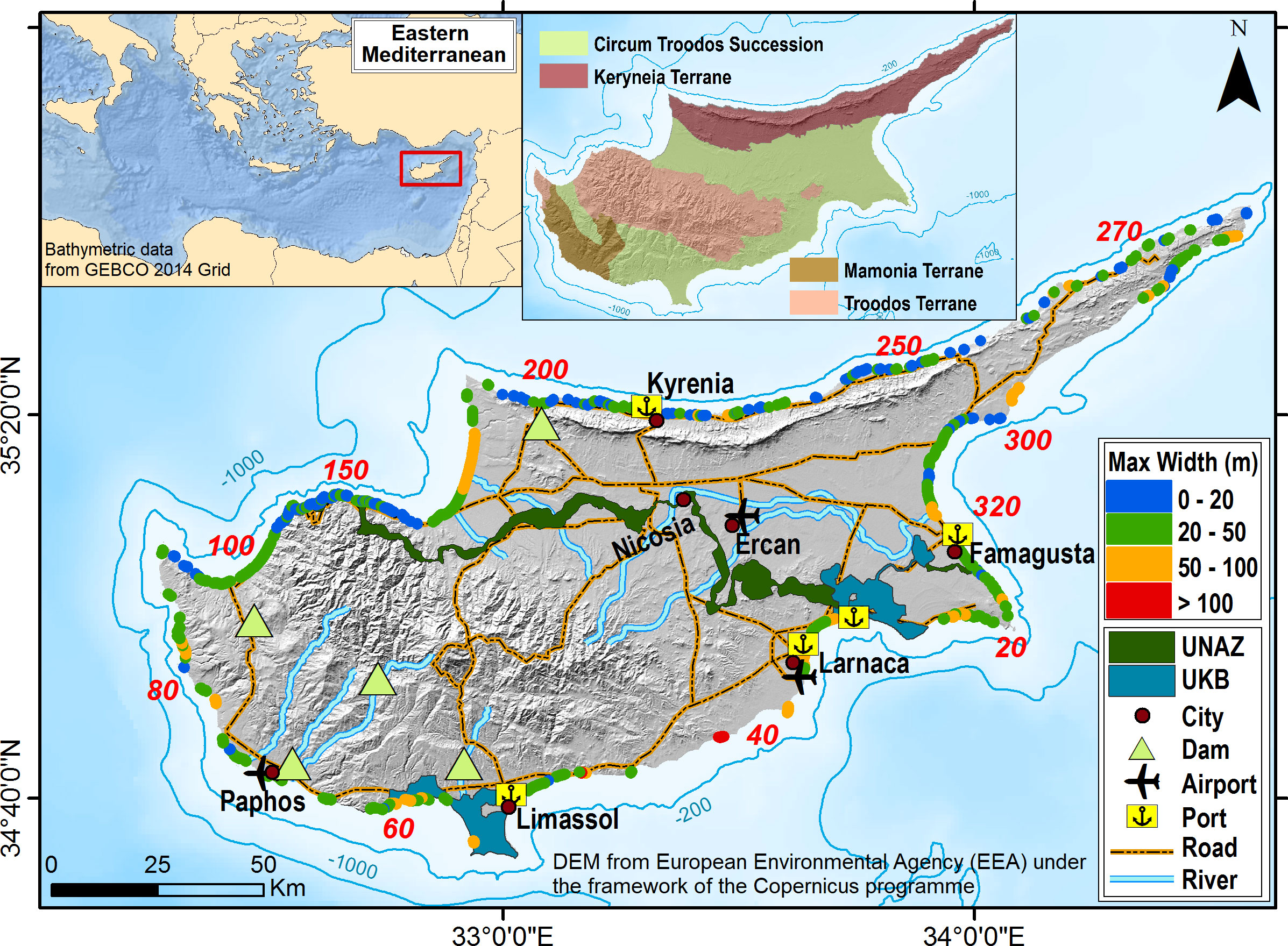
Figure 1 Cyprus: Major administrative boundaries, cities, transport network, rivers and major dams. The 322 beaches with their maximum widths recorded in this study are numbered clockwise from the eastern UNAZ border. Inset shows the generalized geology http://www.moa.gov.cy/moa/gsd/gsd.nsf/All/F9B6C7484AFDCADCC2258363003BE7C5?OpenDocument. Key: UNAZ, United Nations Administered Buffer Zone (https://unficyp.unmissions.org/); UKB, UK Sovereign Base Areas (https://www.army.mod.uk/deployments/cyprus/).
The coast of Cyprus is microtidal (Wolff et al., 2018) and its wave regime varies, with the most energetic waves observed along the western and southern coastline (Galanis et al., 2012). The regional sea level rise has accelerated to 2.4 – 3.8 mm/year since the late 1990s (Tsimplis et al., 2013). Regarding the extreme sea levels, their storm surge components (Section 3.2) have a seasonal footprint and heights that have rarely exceeded 0.4 m (e.g. Tsimplis and Shaw, 2010).
In recent decades, beach (3S) tourism has become a most significant economic activity in Cyprus that requires efficient and integrated coastal management. However, coastal governance, management and regulation in Cyprus are fragmented. The northern part of the island and about half of its coastline are administered by the self–declared Turkish Republic of Northern Cyprus (TRNC) (https://www.securitycouncilreport.org/un-documents/cyprus/). The Republic of Cyprus, an EU Member State since 2004, administers only the island’s southern and western coasts where there are also parts of the coast located in the UK Sovereign Base Areas (Akrotiri and Dhekelia) and the UN Buffer Zone (Figure 1).
In 2019, 4.0 million international tourists visited the Republic of Cyprus contributing about €2.7 billion to the economy, a contribution forecasted to reach up to about 27% of GDP by 2027 (CYSTAT, 2017; CYSTAT, 2019). Tourism infrastructure and activities are concentrated at the coast. In 2013, 94% of the hotel beds were located at the coast (Lemesios et al., 2016) whereas, in 2016, 91% of the international tourists stated that they had a coastal vacation (CYSTAT, 2017). The situation is similar in northern Cyprus (TRNC), where the vast majority of the tourism facilities/flows are also found at the coast (Farmaki et al., 2015; Safakli and Kutlay, 2016)
3 Methods
The approach adopted to assess beach erosion at the island scale consisted of three modules. First, the location, dimensions and other attributes of the Cypriot beaches were recorded on the basis of widely-available satellite imagery. Secondly, sea levels and waves were modeled along the coast under different climatic scenarios and dates in the 21st century. Finally, using these projections beach retreat due to the relative mean sea level rise (RSLR) and extreme sea levels (ESLs) was estimated using ensembles of analytical and numerical cross-shore morphodynamic models, respectively. A concise flow chart of the approach is presented as Figure 2.
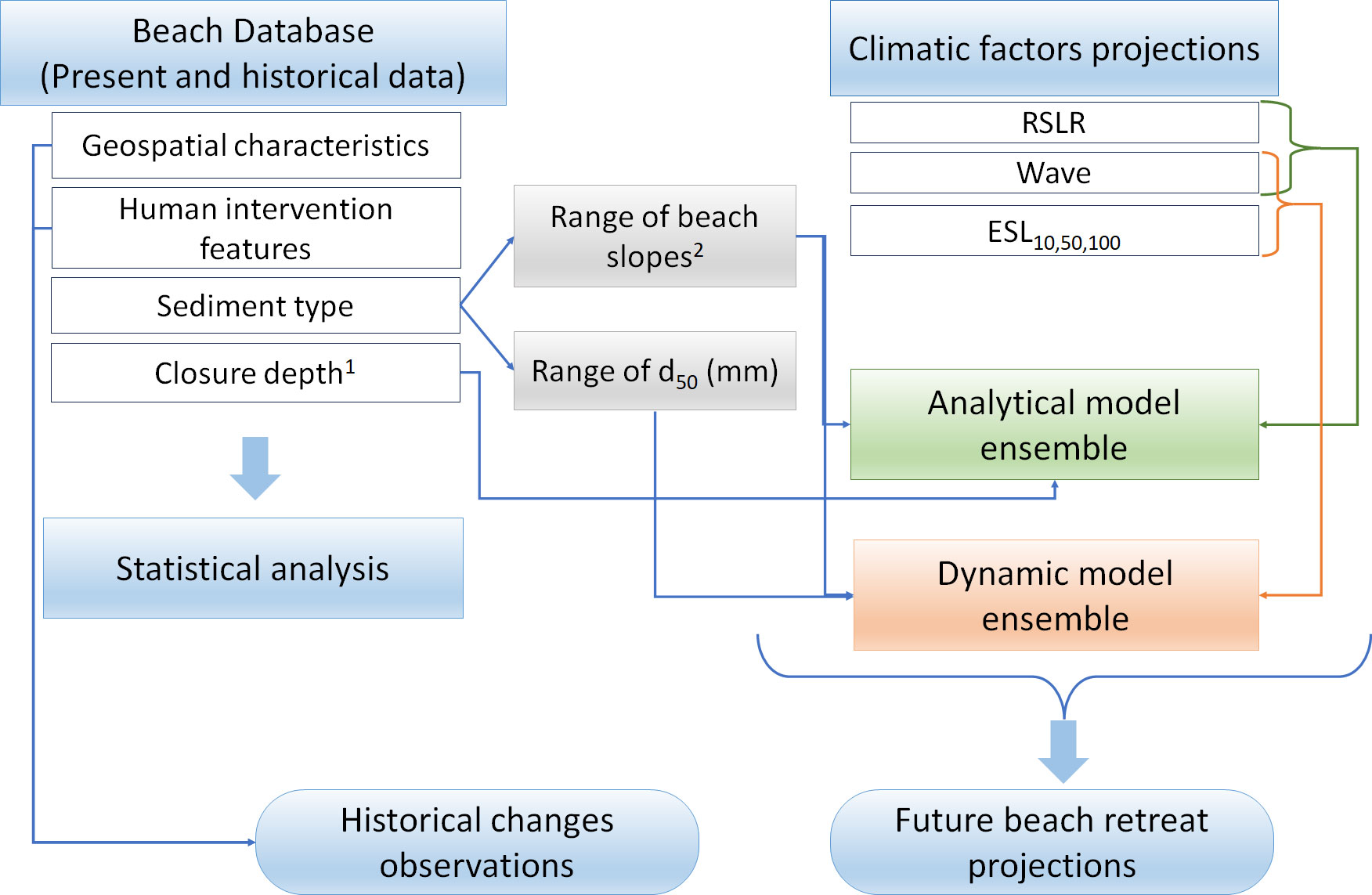
Figure 2 Flow chart of the methodology used in the present study. Key 1: Athanasiou (2019); 2: Bujan et al. (2019).
3.1 Characteristics of Cyprus beaches
The beach inventory was created using the satellite images and related optical information and tools available in the Google Earth Pro application. To facilitate consistency, a standard operating procedure (SOP) for capturing beaches from the images was used. The subaerial (‘dry’) beaches were digitized as polygons (Figure 3), with their: (i) landward boundaries defined by either backshore natural features (vegetated dunes and/or cliffs), or permanent artificial structures (e.g. coastal embankments, roads and buildings); (ii) lateral extent delimited by promontories, rocky coasts or coastal works; and (iii) seaward boundaries defined by the shoreline, i.e., the median line of the foaming swash zone in the imagery.
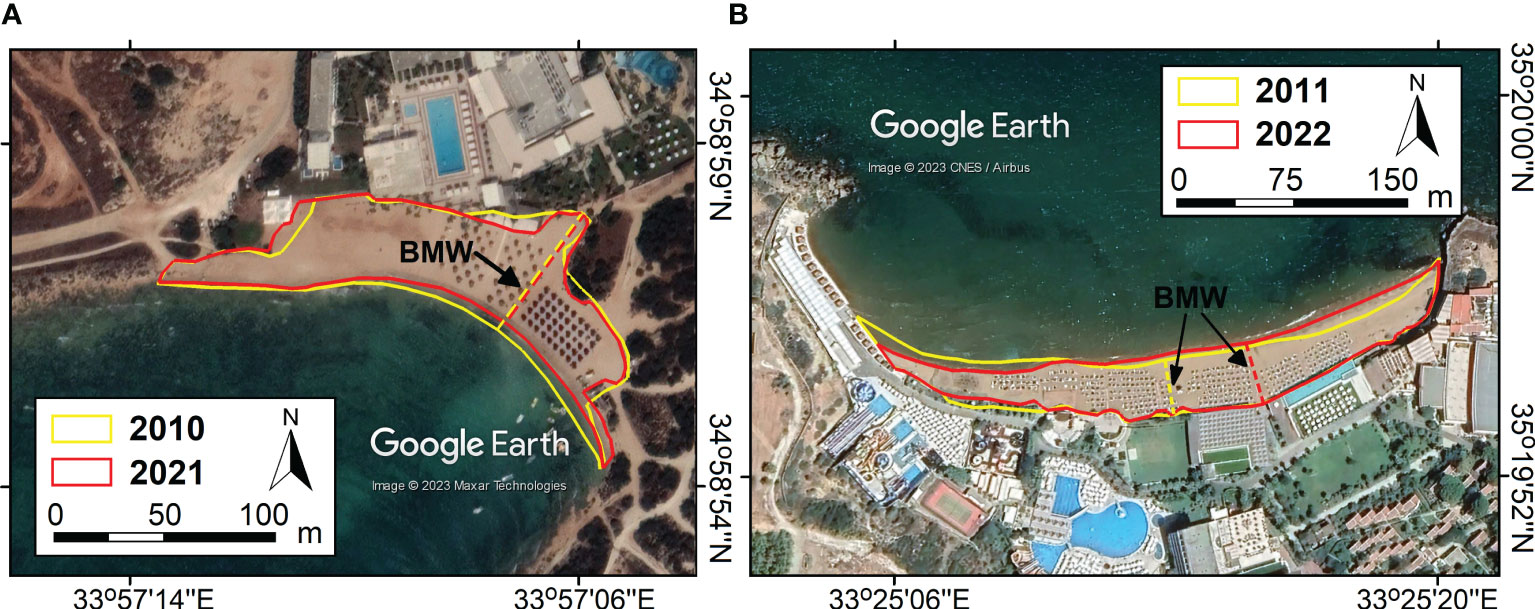
Figure 3 Beach delimitation in the historical imagery of (A) a southern beach (ID 27) and (B) a northern beach (ID 223) (for location see Figure 1). The differing landward boundary position in (A) is due to changes in vegetation coverage. Note that there are apparent area losses in both embayed beaches, but the maximum beach widths (BMWs, stippled lines) of the ‘dry’ beaches have not changed significantly.
Geo-spatial characteristics and other attributes of the beach database include: beach location, dimensions (e.g. area and beach maximum width, BMW) and orientation; imagery acquisition dates; the presence of natural and artificial features (backshore sand dunes and cliffs, coastal defenses); the coverage of the infrastructure/assets at the immediate backshore as a fraction of its length; road accessibility; and a qualitative classification of the beach sediment texture (e.g. gravel, sand) based on the available optical information (e.g. Wolff et al., 2018). Very small beaches (lengths< 30 m) were not recorded. Digitization was carried out by a unique analyst who followed consistently the SOP rules, whereas production of metadata from the digitized polygons was carried out in a GIS environment. The database includes characteristics of the 322 recorded beaches (beach location in Figure 1).
In order to assess historical changes, images from Google Earth historical image tool were used; in total 2363 images spanning the period 2003 – 2022 were examined. However, comparisons were carried out between images from the 2010 – 2014 and 2020 – 2022 periods for 317 beaches (no available images for beaches 284 – 288 from the period 2010 – 2014). This was due to the lack of concurrent images of appropriate quality covering the whole Cyprus coastline; thus, the time span of the comparisons differs along the coast. Since in an interannual scale longshore transport processes can affect beach morphology altering positions of accreted and eroded parts alongshore it can be assumed that a decrease in the recorded BMW implies erosion for the total beach area.
Constraints of the approach in terms of capturing the beach features and their dynamics can stem from the accuracy/resolution of the images from which the information was extracted. Moreover, the available satellite imagery has not been synoptic over the island, having been collected in different times and under different hydrodynamic conditions preceding image acquisition. Although tidal effects on the shoreline position in the microtidal Cyprus coast can be considered small and care was taken to compare images from the same season (when possible) and under low hydrodynamic conditions, recorded beach dimensions may not represent the ‘mean’ conditions as there could be considerable short-term variability in response to the nearshore hydrodynamics (e.g. Chatzipavlis et al., 2019). This, however, cannot be avoided in comparisons of historical coastal satellite imagery (e.g. Mentaschi et al., 2018).
3.2 Sea level and waves
The Projections for the 21st century under the IPCC representative concentration pathway scenarios RCP4.5 and RCP8.5 were obtained from the dataset presented in Vousdoukas et al. (2018b) for every 25 km along the coastline. Extreme sea levels at the coast are due to mean sea level, the astronomical tide (ηtide) and the episodic coastal water levels (ηCE) due to storm surges and wave set ups. Projections have been derived using dynamic models, namely the DFLOW-FM (Muis et al., 2016) for the storm surges and the WAVEWATCH III (Tolman, 2009) for the offshore wave characteristics while mean sea level (RSLR) projections are according to Jevrejeva et al. (2016). As detailed information on the nearshore bathymetry has not been available to provide a precise estimation of the wave set ups, these were assessed using the generic approximation (0.2 x Hs) of CEM (2002). The dataset combined all ESL components and their uncertainties in a probabilistic fashion through Monte Carlo simulations. https://www.aviso.altimetry.fr/en/data/products/auxiliary-products/global-tide-fes.html
In addition to the ESLs, beach response during the extreme sea level events is controlled by the corresponding waves (Toimil et al., 2017). This was assessed by bivariate copulas, i.e., joint distributions on the unit square with uniform [0,1] margins (e.g. Oswaldo et al., 2017; Li et al., 2018). In this study, the variables involved were transformed to [0,1] margins through their empirical margins and the correlation of total water level with the wave parameters (Hs, T and direction) was investigated with Spearman’s rank correlation coefficient. The pairs of transformed margins were then fitted to 7 copula types (Gaussian, Gumbel, Clayton, Frank, Plackett, t and Joe-Clayton BB7), representing various dependency structures (Joe, 2014). For each pair, the type best fitting the data was chosen by goodness-of-fit statistics (Genest et al., 2009):
where is the empirical copula, and is a parametric copula with parameter estimated from the sample of length .
For a given ESL value, the probability of exceedance on the marginal distribution of total water levels was computed. However, when this was lower than , indicating that the selected margin values could not include such an extreme case, the margins were amended using parametric distributions of extreme values for each margin (except wave direction); generalized Pareto distribution, fitted to values above the 98.5th percentile threshold, was employed for this purpose. Finally, the conditional marginal distribution of each wave parameter given P(ESL) was computed, providing both the expected (mean) value of the wave parameters and the uncertainty bounds. The copula dependency analysis, which was based on the reanalysis data, allowed to obtain for every ηCE the corresponding most likely significant wave height (Hs), period (T) and wave direction. These waves were used to force the morphodynamic models.
3.3 Beach erosion projections
Two ensembles of cross-shore (1-D) morphodynamic models were used: (a) an analytical ensemble consisting of the models Bruun (Bruun, 1988), Dean (Dean, 1991) and Edelman (Edelman, 1972) which was used to assess beach retreat due to mean sea level rise (RSLR) and (b) a numerical model ensemble comprising the models SBEACH (Larson and Kraus, 1989), Leont’yev (Leont’yev, 1996), Xbeach (Roelvink et al., 2010) and Boussinesq (Karambas and Koutitas, 2002) which was used to assess beach retreat under ESLs. Ensemble methods in coastal modelling have been found to perform generally better than individual models and their application have been predicted to increase in the future (Sherwood et al., 2022). As the sensitivity to different forcing factors varies among individual morphodynamic models, ensemble techniques exploit each model’s strength and mitigate its weaknesses (Simmons and Splinter, 2022). In the present case, a validation exercise involving the same model ensembles, the results of which were compared with results of physical experiments (Monioudi et al., 2017), showed that ensemble modeling improves considerably the comparisons between the projected beach retreats with those recorded in the physical experiments under both the mean sea level rise and extreme sea levels.
The Bruun model is based on the equilibrium profile concept with its results controlled by the beach slope and the closure depth which was abstracted from the database of Athanasiou (2019). The Edelman model can deal with temporally variable sea level changes and realistic beach profiles, whereas the Dean model assigns a greater significance on wave hydrodynamics than the previous models. The Leont’yev (1996) model uses the energetic approach to simulate the hydrodynamic conditions and estimates sediment transport separately for the surf and swash zones, whereas the SBEACH model contains descriptions of the wave transformation in shoaling waters and estimates sediment transport through the coastal wave energy flux. The Xbeach model contains a time dependent, wave action balance equation and simulates sediment transport using a depth-averaged advection/diffusion equation. Finally, the Boussinesq model computes nonlinear wave transformation involving high-order equations (Karambas and Koutitas, 2002) and its sediment transport module estimates sheet flow as well as bed and suspended load over uneven sea beds (e.g. Karambas, 2006). The numerical models were run from 20 m depth to the beach (up to a 10 m elevation above the mean level), with the simulation duration controlled by beach profile stabilization. Further details on the models used and their comparison with experimental data can be found elsewhere (e.g. Vousdoukas et al., 2009; Monioudi et al., 2016; Monioudi et al., 2017).
For each of the 322 recorded beaches, the nearest mean sea level, ESL and corresponding waves (at 20 m water depth) were selected from their available projections along the coastline (Section 3.2) and were used to force the models. Given the spatial and temporal scales of the application, the input seabed slope and sediment data could not be based on observations or on open source nearshore bathymetric datasets (e.g. GEBCO or EMODnet products) due to their coarse resolution. Instead, the models, used in a stationary mode, were set up using a range of linear beach profiles; this was justified, since the validation of the models against physical experiments has shown that, at least for the conditions tested, the beach retreats projected from the model ensembles set up with equivalent linear profiles were reasonably close to those by the physical experiments with natural profiles (Monioudi et al., 2017).
Regarding the bed slopes and sediments, it has been widely suggested that beach face slopes and sediment size can be interdepended, with coarser sediment sizes associated with steeper slopes (e.g. Reis and Gama, 2010). Based on the slope-grain size relationship presented by Bujan et al. (2019) and on the recorded (qualitative) sediment texture (Section 3.1) a plausible range of beach slopes and median gain sizes were selected for each beach. Beaches were grouped in 3 categories based on their sediment texture: sandy, mixed (sandy gravels) and gravel beaches. The models were run for various combinations of bed slopes and median sediment sizes (d50): bed slopes between 1/20 – 1/30 and sediment sizes between 0.2 and 0.8 mm for sandy beaches; (ii) bed slopes between 1/10 – 1/15 and sediment sizes between 0.8 and 1 mm for mixed texture beaches; and (iii) bed slopes between of 1/10 and sediment sizes (d50) between 2 – 5 mm.
Cross-shore beach retreat was assessed for the 1-10, 1-50 and 1-100 year ESLs projected for the years 2050 and 2100 under the IPCC RCP4.5 (moderate) and RCP8.5 scenarios. Due to the different conditions used in the model set ups, each model produced a range of beach erosion projections. The minimum, maximum and median values of the projections of the ensembles were then calculated to project the minimum (most conservative), median and maximum beach retreat, respectively, for each beach (Supplementary Table 1). These retreats were then compared to the recorded beach maximum widths (BMWs) (Section 3.1) to assess the future impacts on each beach. The change in beach maximum width was selected as it is a conservative indicator regarding the impacts of the beach retreat on the safety of the (frontline) backshore infrastructure/assets and the beach carrying capacity, since a decrease in the recorded BMW implies even worse erosion and impacts for the rest of the beach. Projections are not provided for about 25% of the Cypriot beaches recorded as being ‘perched’ and/or protected by various coastal protection schemes (Velegrakis et al., 2016); nevertheless, these beaches will also experience impacts in the future, as most coastal defenses have not been designed to mitigate erosion under mean sea level rise (e.g. Arns et al., 2017). Finally, it is noted that beach retreats due to episodic extreme sea levels and waves may be reversible.
4 Results
4.1 Beach characteristics
In Cyprus, 322 beaches were recorded (Figure 1), with a total area of approximately about 2.9 km2 that indicates a total beach carrying capacity, i.e. the number of visitors that can be simultaneously hosted, of about 131,800 users (22 m2 per user, Chen and Teng 2016). Regarding coastal management, the Republic of Cyprus administers 142 beaches (with an area of 1.24 km2), whereas 163 (1.46 km2), 10 (0. 2 km2) and 7 beaches (0.01 km2) are located in northern Cyprus, the UK Bases and the UN Administered Zone, respectively.
Most Cypriot beaches are narrow: almost 42% recorded maximum ‘dry’ beach widths (BMWs) of less than 20 m and 91% of less than 50 m, with only 0.6% recording maximum widths exceeding 100 m in the most recent images. Regarding the sediment texture, the majority of the beaches have been classified as sandy beaches (166 beaches, 52% of the total); this finding is in broad agreement with that presented in the Mediterranean coastal database of (Wolff et al., 2018). Mixed texture (sand- gravel beaches) was allocated to 103 beaches (32%), whereas about 16% were classified as gravel beaches. Very few beaches were found associated with active river mouths, whereas about half of the Cypriot beaches are backed directly by cliffs and/or coastal infrastructure and assets; such beaches have a high ‘coastal squeeze’ risk from increasing sea levels (Nicholls and Cazenave, 2010).
Statistical tests (a Levene’s test followed by a Kruskal-Wallis test, p< 0.05) showed that beaches facing the northern sector (NW, N and NE, 53% of all beaches) recorded mean (per direction) BMWs of less than 20.5 m, whereas mean BMWs for the other directions were exceeding 36 m; the largest mean BMWs were found at the S and SW facing beaches (about 39 m). As the northern beaches are affected by less energetic waves (Section 4.2), these findings indicate that beach dimensions may have been controlled by the geology, antecedent topography and sediment supply rather than the wave energetics. For example, it appears that the northern beaches associated with the Troodos and Kyreneia terranes are generally narrower than those built in coastal areas associated with the other major geological units (Figure 1). A significant correlation (p< 0.05) was also found between BMWs and sediment texture: sandy beaches were found to be wider than those built on sand-gravel and, particularly, gravels. In addition to natural and geological factors, human interventions have been found to impact beach width (BMW). Beaches associated with coastal protection structures have an average BMW of 37.3 m, whereas unprotected beaches have an estimated average BMW of 24.6 m. This finding suggests that the presence of hard structures may have a positive effect on beach stability.
Most (63%) Cypriot beaches do not show any significant backshore development, which is an interesting finding, as Cyprus has a mature 3S tourism industry, with the majority of its beaches (85%) easily accessible by road (49% directly connected to the main road network) and there are also 45 ‘Blue Flag’ beaches. The remainder of the beaches (37%) were found to directly front coastal infrastructure/assets, without any construction line ‘set-back’; on these beaches there is a high/intense beach tourism during summer, manifested by the beach umbrellas and the presence of watersport facilities in many of them.
The density of the backshore frontline assets (where any) is variable, ranging from few houses observed in remote beaches to the heavy concentrations of public and private assets found behind the beaches of Larnaca, Limassol, Kyrenia and Famagusta (Figure 1). Frontline backshore asset coverage (expressed as a percentage of the beach length) is higher than 30% for about 13% of the Cyprus beaches, and higher than 80% for about 2% of the beaches. Finally, 77 beaches (24%) were observed to host coastal protection schemes, most of them (46) located in the Republic of Cyprus. Groynes were found to be the dominant schemes (14% of beaches) either alone or in mixed schemes that may also include offshore breakwaters or jetties. It should be noted that in the area of Limassol most beaches (no 29-54) are fronted by detached breakwaters that cover the entire beach length.
Analysis of the changes in BMWs (Figure 3) showed discernible decreases for 152 (48%) beaches and increases for 164 (52%) beaches (Figure 4A); interestingly these results appear comparable to those of the global assessment of Luijendijk et al. (2018). It is noted that in the present study manual mapping was carried out using high resolution Google Earth images, whereas in the global Luijendijk et al. (2018) assessment an automated mapping technique on coarser 30 m Landsat images was applied. Concerning the frontline backshore development (urbanization) (Figure 4B), 46 beaches showed discernible changes in the coverage of the frontline backshore assets (as a percentage of the beach length), with fast development detected mostly along the southern and the eastern coast.
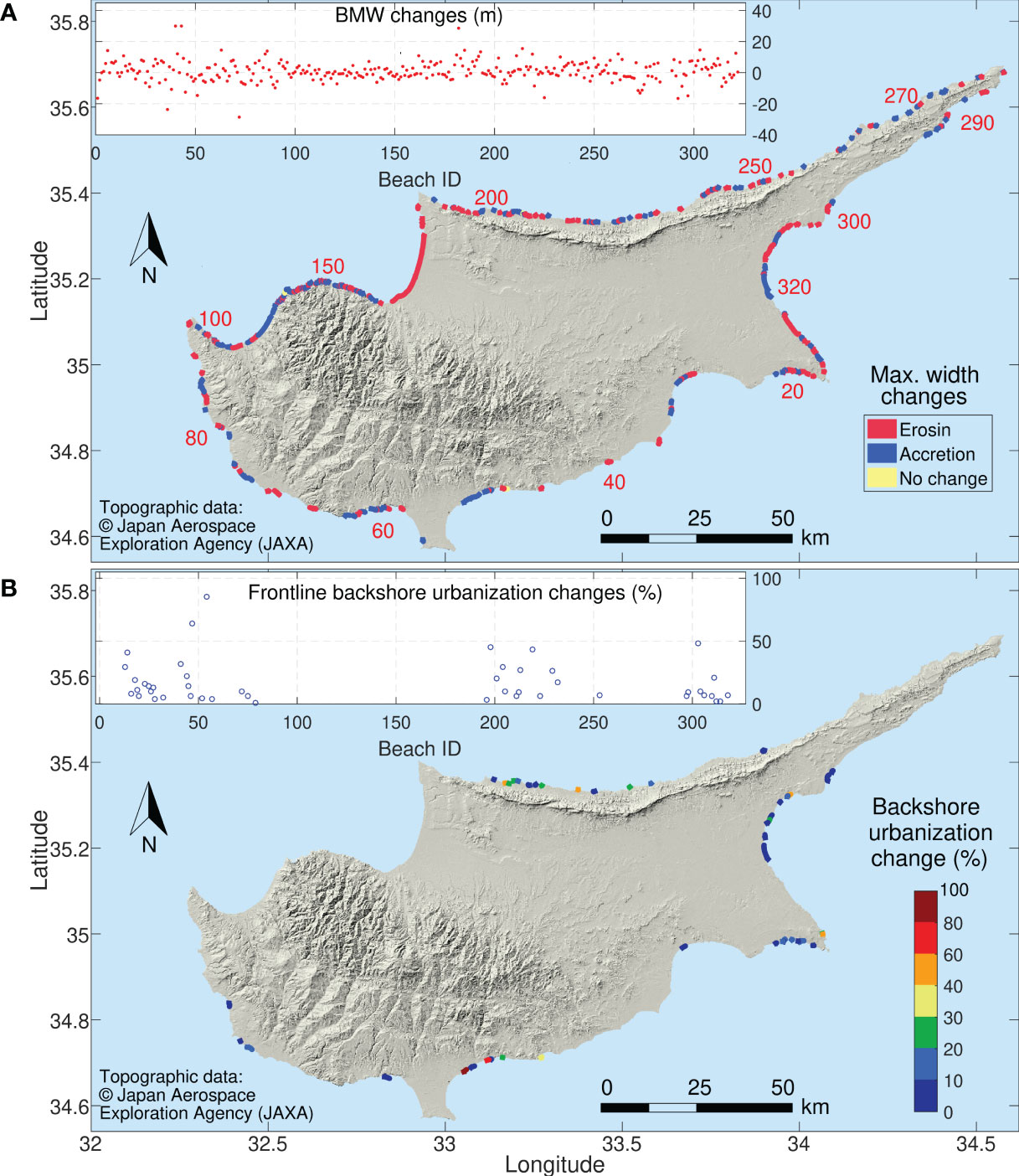
Figure 4 Historical changes in the (A) recorded BMWs and (B) frontline backshore urbanization, i.e. in the coverage of the frontline assets as a percentage of the beach length (only the 46 beaches recording changes are shown). Changes shown are between the periods 2010-2014 and 2020-2022.
4.2 Mean and extreme sea levels and waves
Mean sea levels are projected to rise considerably in the course of the century, with the rate of rise increasing with the time and the RCP scenario; small spatial RSLR variability (0.01-0.05 m) is also projected along the coastline (Table 1). In comparison, ESLs are projected to be more variable and consistently higher along the southern and western Cyprus coast (Table 1 and Figure 5). For the baseline (2000) year, the 1 in a 100 years extreme sea level (ESL100), was found to range along the coast between 0.83 and 1.29 m above the mean sea level. Future ESLs will also exhibit spatial variability, with the differentials between the highest and the lowest ESLs100 projected along the coastline (at beaches 73-86 and 225-235, respectively) varying between 23 and 35%, depending on the date and climatic scenario.
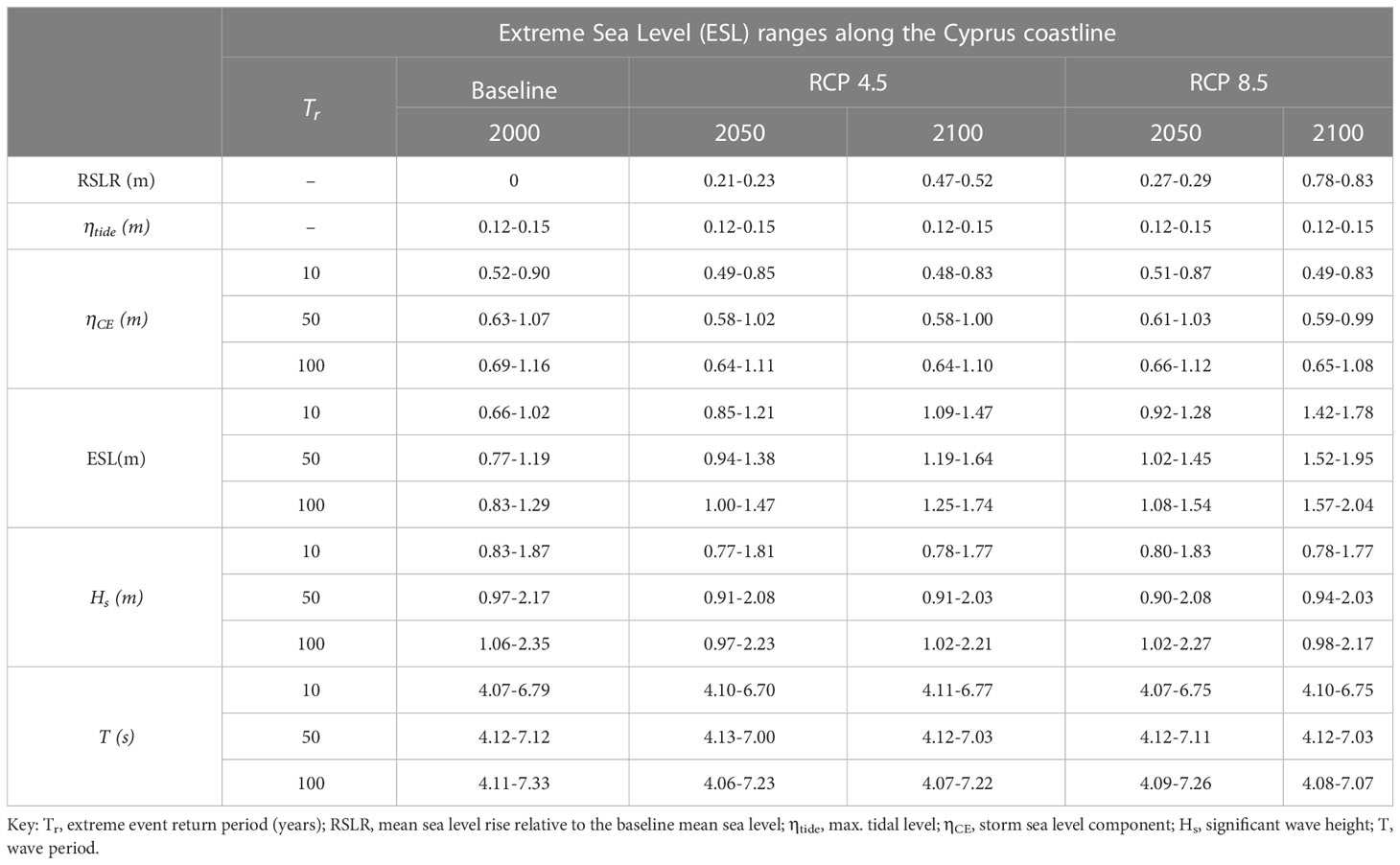
Table 1 Ranges of the 10-, 50- and 100-year extreme sea levels (ESLs10, ESLs50 and ESLs100) and their components (RSLR, ηtide, and ηCE) and corresponding wave conditions along the Cypriot coastline (Figure 4) for different dates (2000-baseline, 2050 and 2100) and under the IPCC RCP4.5 and RCP8.5 scenarios.
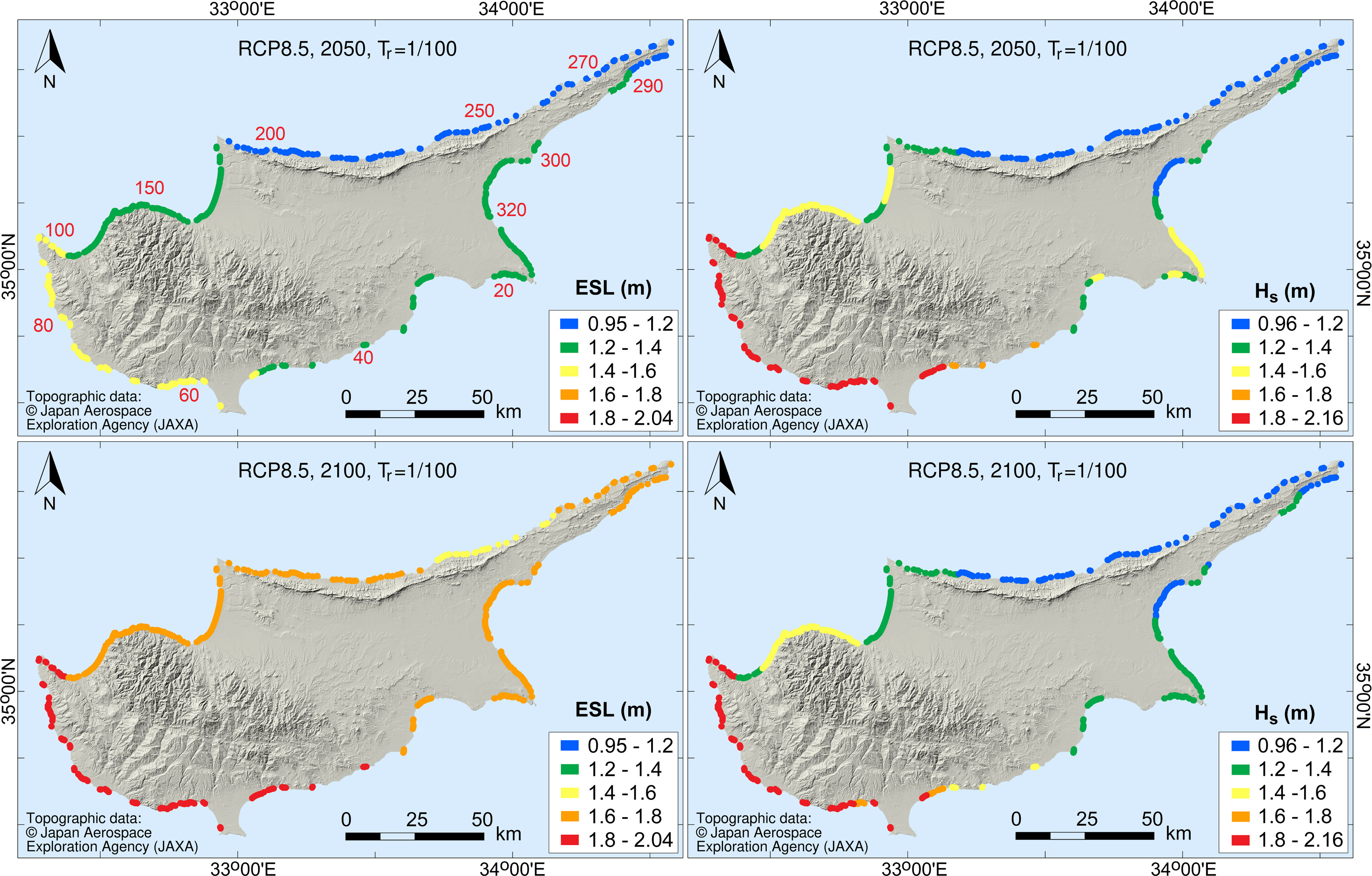
Figure 5 Extreme sea levels (Tr 1/100, ESLs100) and corresponding wave heights (Hs) at the Cypriot beaches for different dates under RCP8.5.
ESLs are projected to rise in the course of the century, being increasingly controlled by the emission scenario (Table 1). For example, the maximum ESL100 along the Cyprus coastline in 2050 is projected as 1.47 and 1.54 m higher than the baseline mean sea level under RCP4.5 and RCP8.5, respectively, whereas in 2100 the spread between the two climatic scenarios has increased threefold (1.74 - 2.04). It also appears that the ESL increases are controlled by the RSLR (Table 1). Considering the corresponding to the baseline ESL100 waves (Section 3.2), their heights and periods were projected to also differ along the coastline, varying between 1.1 and 2.35 m and 4.1 and 7.3 s, respectively (Table 1). More energetic waves were consistently projected along the western and southern coasts (Figure 5), but heights appear to be overall slightly decreasing during the century under most tested scenarios (see also Camus et al., 2017).
Regarding the contributions of the different ESL components and their temporal evolution (Table 1), no changes are projected for the tidal range/maxima. It also appears that although the episodic ηCE is the primary contributor of the baseline period, its dominance will decrease in the future as it will change little compared with the accelerating RSLR (e.g. Androulidakis et al., 2015).
The return periods of ESL events with particular magnitudes will significantly decrease over time (Table 1 and Figure 6); for example, depending on the location along the Cypriot coastline, the baseline (2000) ESL100 will be occurring in 2050 every about 9 – 27 (RCP4.5) and 2.5 – 13 years (RCP8.5). respectively. By 2100, and under the RCP8.5 scenario, the baseline ESL100 will occur many times annually. These projections may have significant implications for the design of coastal works.
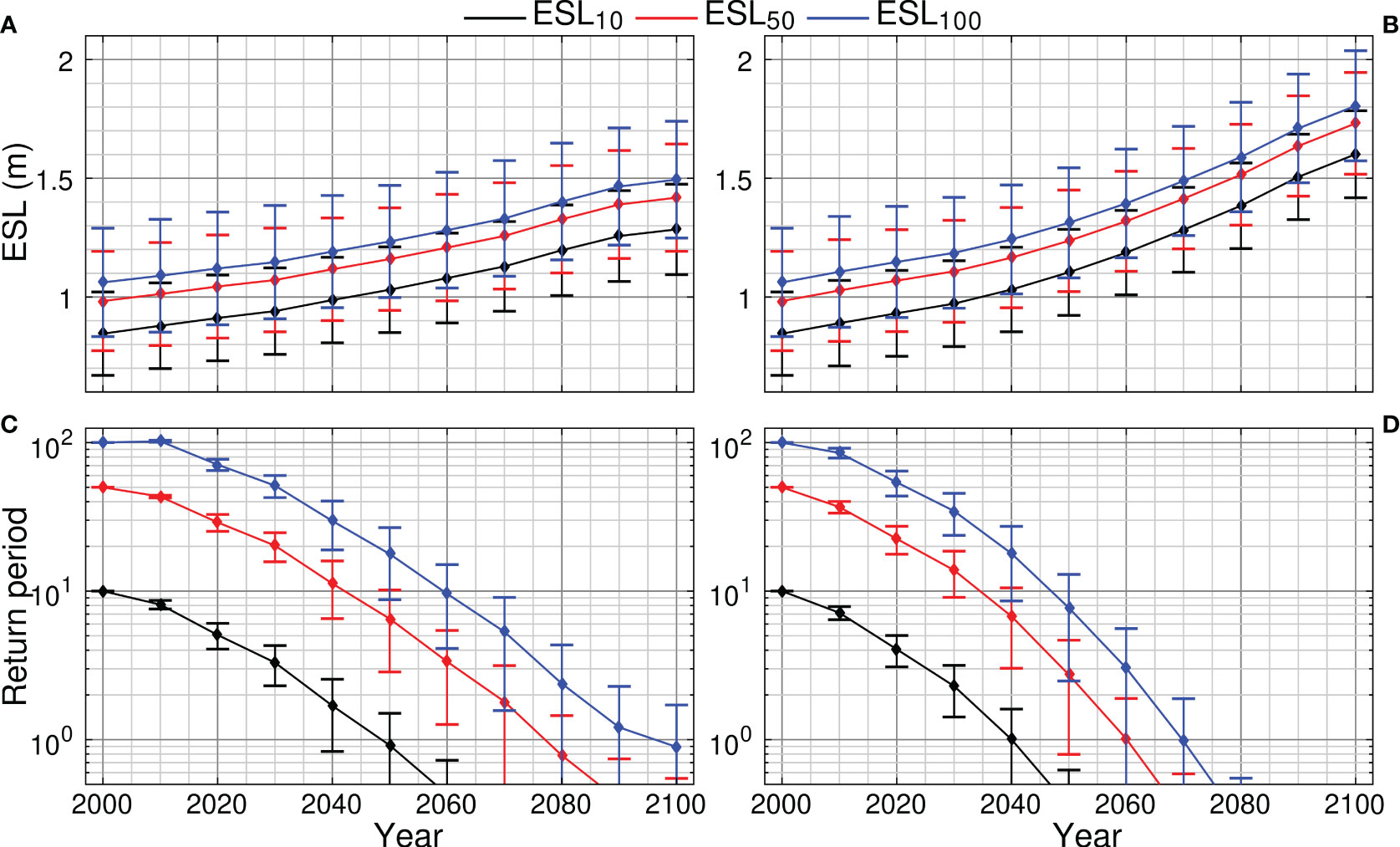
Figure 6 Time evolution of ESLs, i.e., the sum of the RSLR, the maximum astronomical tide and the episodic level rise due to storm surges and wave set ups (A, B) and of their corresponding return periods (C, D) compared to their baseline return periods for the 10- 50- and 100-year events, under RCP4.5 (A, C) and RCP8.5 (B, D). Predictions are for all beaches which explains the result spread.
Changes in the intensity/direction of (extreme) coastal wave energy fluxes (WEFs) under CV&C could also impact the Cypriot coastal natural and human environment. Global simulations for the extreme WEFs (Mentaschi, 2017), suggest that Cypriot coastline is currently characterized by relatively low to moderate extreme WEFs, with the highest intensities found in the western Cyprus. Our analysis shows that the 100-year significant wave height ranges between 0.95 and 2.16 m with higher waves observed at the W and SW coast of Cyprus and less energetic waves at the NE and E coast; no significant changes are observed through the course of the century (Figure 5)
4.3 Beach retreat
Models generally displayed differential behavior for almost all tested conditions, showing varying shoreline retreats and profile changes (Figure 7). At the same time, each model gave a range of results, reflecting the varying bed slopes and sediment sizes used; thus, the median value and minimum and maximum projections for each condition and scenario tested are considered (see also Section 3.3). The beach slope and hydrodynamics were found to represent major controls. Beach retreat projections decreased with increasing Iribarren number (ξ =(β/(H/L)1/2, where β the seabed slope and H and L the wave height and length, respectively), for the range of bed slopes and wave conditions considered.
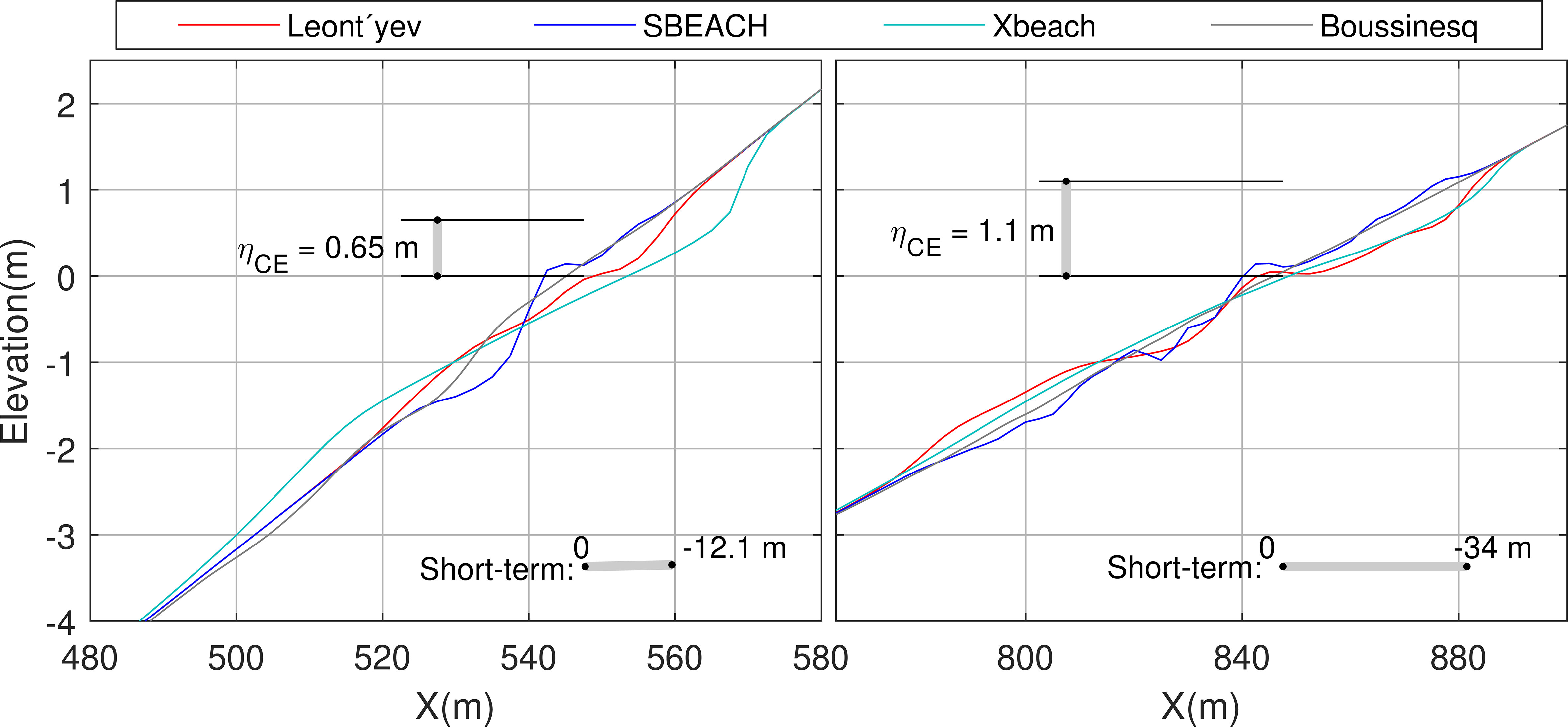
Figure 7 Morphodynamic changes in the upper beach profile of a northern beach (left, ID 238, equivalent linear bed slope: 1/15, d50: 1 mm) and a southern beach (right, ID 73, 1/30, 0.5 mm) projected by the numerical (short-term) model ensemble. Projections are for the ESL100. Models are forced by waves (at 20 m water depth, origin of x axis) with heights of 1 and 2 m and periods of 6 and 7 s, respectively. Mean shoreline retreats for 2050 (RCP4.5) are shown as horizontal bars. Key: ηCE, episodic level rise.
The most sensitive models were found to be the Edelman and the SBEACH models and the least sensitive the XBeach model. Regarding the sediment texture, a weak decreasing trend in beach retreat was observed (for the numerical models) with increases in the sediment size. Generally, Xbeach provided the most ‘pessimistic’ predictions (Figure 7), whereas the Leont’yev and SBEACH models projected similar retreats. With regard to the analytical models, the Dean model gave the highest erosion predictions, whereas the Bruun and Edelman models projected mostly similar retreats.
RSLR was projected by the analytical model ensemble to lead to beach retreats (Table 2). By 2050, according to the projection median values, about 2% of the tested beaches will retreat by more than 100% and about 11 and 16% by 50% of the current BMW under the RCP4.5 (Figure 8) and RCP8.5, respectively.
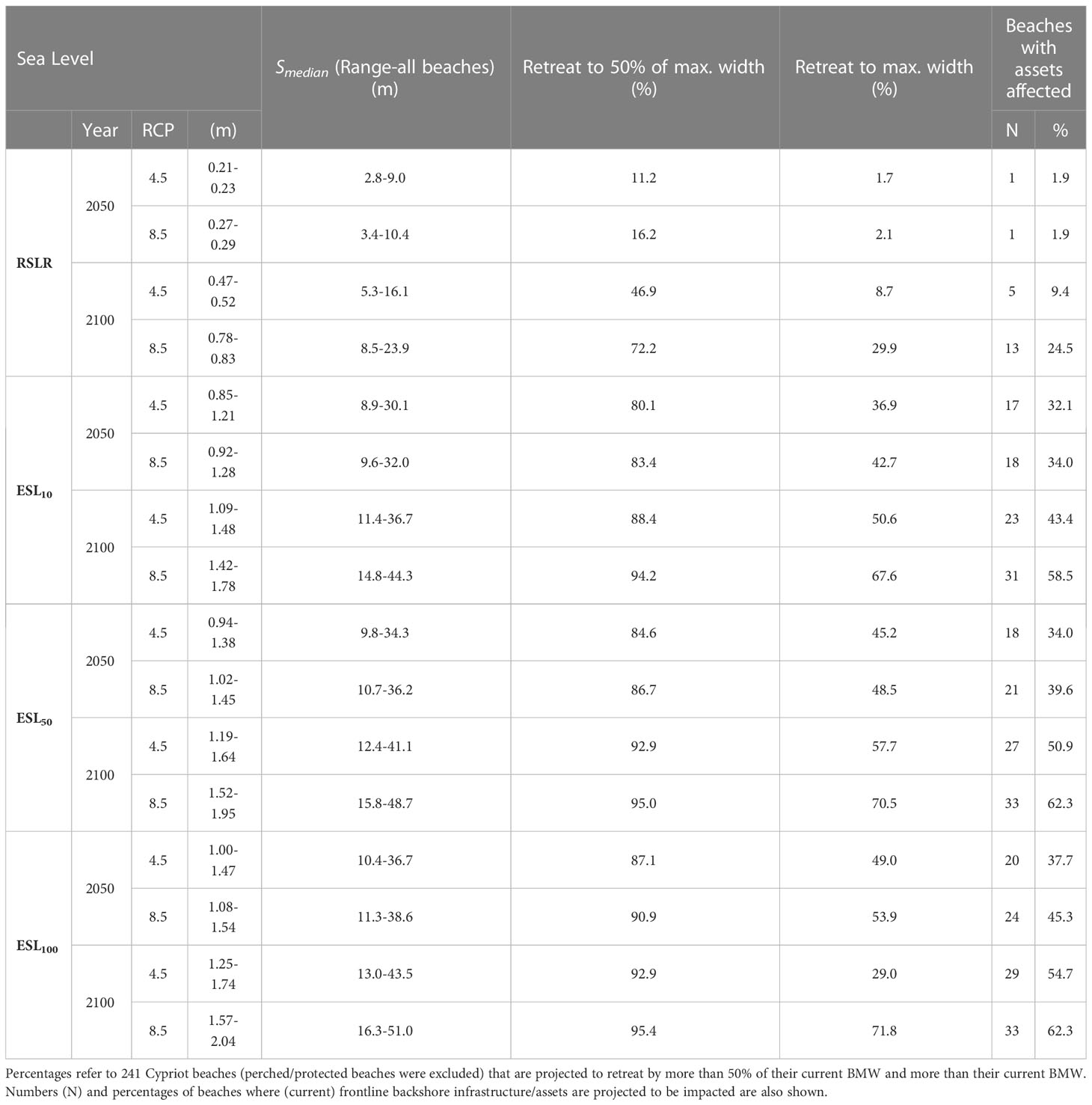
Table 2 Model ensembles’ estimates of the range of median beach retreat (Smedian) along the Cyprus coast under mean sea level rise and extreme events.
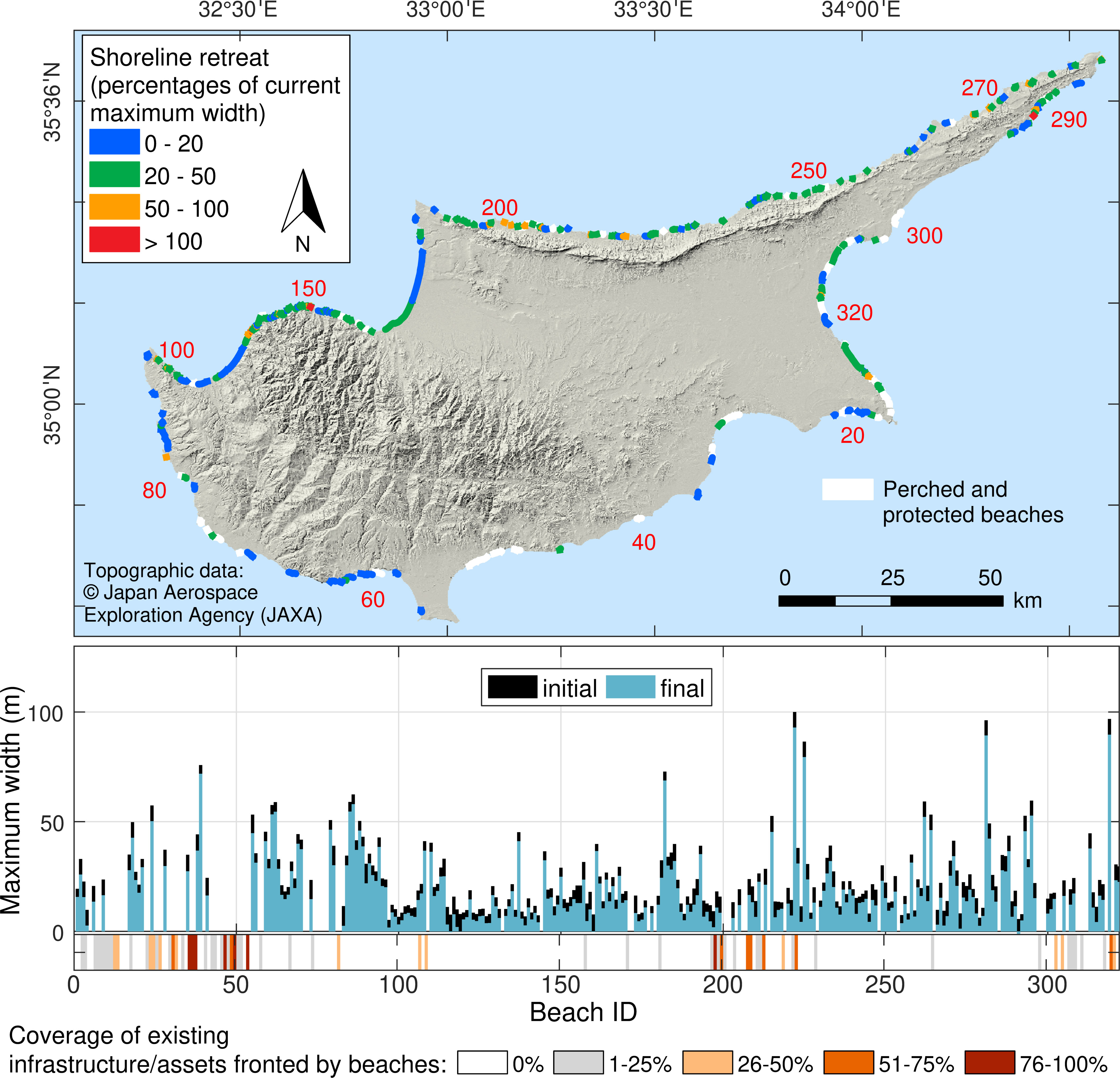
Figure 8 Projection of the impacts of the RSLR under RCP4.5 on the 241 currently unprotected Cypriot beaches in 2050. The impacts are shown on the map as reductions (%) in the current BMW due to the median beach retreats projected by the analytical model ensemble (Section 3.3). In the lower panels, the projected median retreats are shown together with the recorded coverage of frontline backshore assets (as a percentage of the beach length); the current (initial) BMWs (black bars) are compared with those resulting from the beach retreats (blue bars).
By 2100, beach retreat has been projected to be very significant: between 9 and 30% of the (currently unprotected) Cypriot beaches will retreat by > 100% of their current BMW under RCP4.5 and RCP8.5, respectively, whereas between 47 and 72% will retreat by 50% of their current BMW. As many of these beaches lack the accommodation space to retreat landwards (Table 2), such retreats may translate to coastal squeeze and permanent erosion (Nicholls and Cazenave, 2010). In this case, appropriate beach replenishment schemes would be required to avoid damage to the backshore assets/infrastructure and maintain the beach carrying capacity (e.g. Monioudi and Velegrakis, 2022).
Cypriot beaches are also threatened by the extreme events (Table 2). According to the median projections of the numerical model ensemble, the 10-, 50- and 100-year events (ESL10, ESL50 and ESL100) in 2050 (RCP4.5) will result in storm beach retreats of up to about 30, 34 and 37 m, respectively; under RCP8.5, retreat is projected to be slightly greater (up to about 32, 36 and 39 m); the difference in the projections between the two RCP scenarios will increase by 2100 (Table 2). Although these retreats can be temporary and the beaches could in time partially or totally recover, there could be very significant impacts on the backshore frontline infrastructure/assets. By 2050 and under RCP4.5, about 37, 45 and 47% of the beaches tested are projected to temporarily retreat by more than 100% of their current BMW, under the ESL10, ESL50, and ESL100, respectively, whereas under RCP 8.5, more beaches (43, 49 and 54%, respectively) will retreat more than their current BMWs. By 2100, shoreline retreats under extreme events could be devastating for the backshore assets/infrastructure under both tested scenarios (Table 2). Concerning the spatial distribution of the projections, the most impacted beaches will be also along the northern and eastern Cyprus coast (Figure 9).
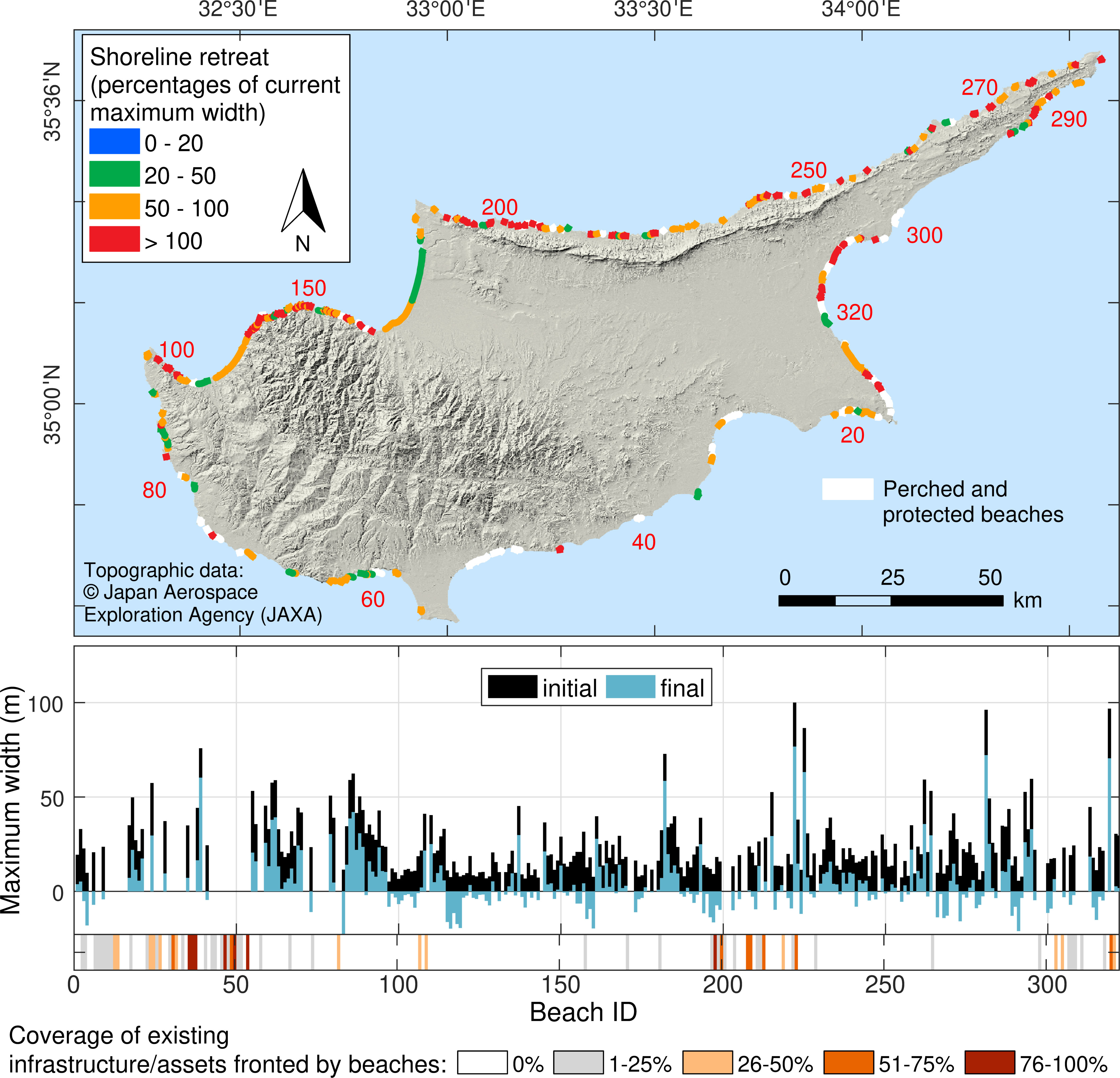
Figure 9 Projection (RCP4.5) of the ESL100 impacts on the 241 currently unprotected Cypriot beaches in 2050, shown as decreases (%) in the current BMW due to the median projections by the numerical model ensemble (Section 3.3). In the lower panels, the projected median retreats are shown together with the recorded coverage of the frontline backshore assets (as a percentage of the beach length); the current (initial) BMWs (black bars) are compared with those resulting from the beach retreat projections (blue bars); negative values indicate beach retreat more than the current BMW.
It appears that in the next decades, many Cyprus beaches and their backshore infrastructure/assets will be seriously impacted by extreme events. It should be noted that these projections are considered conservative as: a) they refer to retreats relative to the recorded beach maximum widths; and b) they do not account for other beach retreat/erosion contributing factors such as potential beach sediment losses from the extreme events (and their sequencing) during the intervening time and the diminishing river sediment supply due to the many Cyprus riverine dams (Section 2).
5 Discussion
Most approaches for regional and large-scale assessments of shoreline retreats under climate change have limitations stemming from, amongst others, their requirements for detailed coastal topographic information (e.g. Rueda et al., 2017). In the present approach, ranges of shoreline retreats under the mean and extreme sea levels (and corresponding waves) are projected for different plausible bed slopes and sediment sizes; thus, it can account for the lack of specific information on the slope of the active beach profile (Ranasinghe et al., 2012) and sediments when such information is not available which is commonly the case for the present and, certainly, for the future beaches. Nevertheless, there are also several constraints.
Regarding the historical trends, in addition to the difficulty to extract probabilistic estimates of shoreline retreat required in coastal management/planning frameworks (Ranasinghe, 2016), there are also geo-referencing issues in the imagery comparisons, as well as issues associated with the varying hydrodynamic conditions during the image collection that can affect shoreline delimitation (e.g. Chatzipavlis et al., 2019). Although the former is not a large issue as the ‘dry’ beach maximum widths are used in the comparisons, the latter may introduce uncertainties which, however, cannot be avoided in large-scale studies (e.g. Mentaschi et al., 2018).
Some processes are inevitably neglected in the modeling. These include, amongst others, cumulative storm impacts, the effects of storm duration/spacing, as well as the potential effects of a changing wave regime on multi-decadal mean sea level changes (Callaghan et al., 2008; Melet et al., 2018). Moreover, predictions are based on the assumption that the Cyprus (mostly ‘pocket’) beaches comprise sediment reservoirs with no lateral sediment exchanges and/or other sediment sinks/sources (e.g. Dean and Houston, 2016). In addition, although perched/protected beaches were excluded from the beach retreat projections to avoid uncertainties from the influence of the natural and/or artificial coastal protection structures (e.g. Velegrakis et al., 2016; Stripling et al., 2017), the implications of potential nearshore benthic ecosystems (e.g. seagrasses) on wave attenuation (e.g. Twomey et al., 2020; Peduzzi et al., 2022) have not been considered. Finally, the results are based on cross-shore modelling which cannot account for longshore sedimentary processes/transport; this issue, however, cannot be resolved without incorporating full 2D (or 3D) modeling that requires detailed topographic/sedimentary information for meaningful set up and high computational resources, which are prohibitively expensive endeavors for other than local scale assessments (Le Cozannet et al., 2014; Toimil et al., 2017).
Notwithstanding these constraints, the study provides the first comprehensive assessment of the Cypriot beach retreat/erosion hazard under climate change on the basis of easily obtainable information and modest investment in resources. Results could be fine-tuned, if high resolution coastal topographies/bathymetries (DEMs) were to become available. In any case, the aim of the exercise has not been to replace detailed local studies for individual beaches (Ranasinghe, 2016).
Under the RSLR alone, beach retreat/erosion has been projected to become an issue after 2050. By comparison, the projected increases in ESLs combined with the corresponding storm waves will have severe impacts on Cyprus beaches; by 2100, impacts could be devastating (Table 2). It is noted that the present projections refer to shoreline retreat/erosion and cannot deal with the implications of the potential increases in coastal flooding and/or wave runup. Regarding the former, a study on the European coastal flood risk has estimated that the Expected Annual Damages (EADs) in Cyprus will range from 0.6 to 0.8% and 1.7 to 8.3% of Cyprus GDP in 2050 and 2100, respectively, depending on the scenario (Vousdoukas et al., 2018a). Concerning the latter, although wave runup (i.e., the wave incursion length (or height) onto the dry beach under extreme waves) is a critical factor for shoreline change (e.g. Chatzipavlis et al., 2019) as well as a very significant boundary for the legal demarcation of the beach (Velegrakis et al., 2021), its assessment requires detailed/accurate nearshore bathymetric information (e,g. Gomes da Silva et al., 2020; Almar et al., 2021).
The findings of the present study strongly indicate that plans to respond effectively to projected beach erosion/retreat should be urgently drawn up in Cyprus with different adaptation options considered. First, effective policies and regulation should be introduced. The allocation of buffer (‘set-back’) zones, such as those prescribed in Art. 8.2 of the 2008 ICZM Protocol to the Barcelona Convention (ICZM, 2008), behind retreating shorelines where no future development shall be allowed, could be a critical regulatory development (e.g. Summers et al., 2018). Nevertheless, since many Cypriot beaches show minimal (or no) backshore development (Section 4.1), both sustainable policies and coastal development might still be possible.
Secondly, several adaptation options should be considered, although given the dire nature of the problem costly ‘hard’ engineering schemes might be, in many cases, inevitable (e.g. Narayan et al., 2016). Projections on the future magnitude and recurrence frequency of ESLs and waves along the island’s coast can provide vital information for the selection, design, timing, costs and benefits of the requisite technical responses (Arns et al., 2017); in any case, such assessments are prescribed by the European Directive 2014/52/EU for all future coastal projects in the EU Members States.
The importance of the beaches as socio-economic resources and the low effectiveness of the hard coastal defenses (groynes, offshore breakwaters and seawalls/revetments) to protect them under increasing mean sea levels (Semeoshenkova and Newton, 2015; Beuzen et al., 2018), suggest that beach replenishment schemes will be required, at least for beaches which function as “armors” to valuable backshore infrastructure and assets, serve as important environments of leisure and/or have particular environmental significance (e.g. marine turtle nesting beaches, Mavris, 2011). The results of our analysis indicate that the presence of hard structures may have a positive effect on beach stability. However, it is important to note that the effectiveness of these coastal protection structures cannot be definitively concluded due to the fact that beach nourishment projects are also commonly implemented in the most touristic beaches of the southern sector, where the majority of coastal works have been implemented. As marine aggregates form the most suitable (but often scarce) material for beach replenishment (Velegrakis et al., 2010), the sustainability of such deposits, should be ensured in the marine spatial planning of Cyprus (see also EU Directive 2014/89/EU), as a matter of priority. Moreover, and considering that the intense dam construction in Cyprus have been found in past studies to cause recession of adjacent beaches and delta areas through sediment trapping (Toumazis et al., 2008), efficient management of terrestrial sediments is required.
6 Conclusion
This study represents the first comprehensive effort to record the characteristics of the beaches of the island of Cyprus and provide an assessment of their exposure to erosion due to Climate Change. Cypriot beaches were found to be mostly sandy and narrow with 91% recording maximum widths of less than 50 m and only 0.6% of more than 100 m. Analysis of the historical changes showed (a) discernible erosion and accretion in 152 and 164 beaches, respectively and (b) increases in backshore urbanization at 46 beaches, which are mostly located along the southern and eastern coast. Substantial increases in the magnitude of RSLR and ESLs were projected for the 21st century, which will vary along the Cyprus coast with the highest ESLs (and waves) projected for its western and southern sectors.
In terms of beach erosion, even under a moderate emission scenario (RCP4.5) and as early as 2050, the one in 100-year extreme sea level event (ESL100) could completely (at least temporarily) overwhelm 49% of the 241 unprotected Cyprus beaches in the absence of effective technical responses. In 2100 impacts will be devastating, with 72% of (currently unprotected) beaches projected to be completely (at least temporarily) eroded under the business as usual (i.e. RCP8.5) scenario, with the most exposed beaches located along the western and northern coast. These results suggest that the primary natural resource of the island’s booming 3S tourism is under a clear and increasing risk. Plans to respond effectively to the projected risk should be urgently drawn up with different regulatory (shoreline development) and technical adaptation (coastal fortification) options examined.
The approach adopted in the present study can provide a framework for regional assessments of the beach erosion under climate change and produce vital information for the selection, design and timing of the requisite technical adaptation options. In this context, it may assist managers and policy makers at island settings to identify beaches under increased risk, estimate losses in beach carrying and hedonic value, and plan and prioritize effective adaptation responses.
Data availability statement
The raw data supporting the conclusions of this article will be made available by the authors, without undue reservation.
Author contributions
IM, AV, and TH contributed to the conception, scope and design of the study. IM assembled the models, performed the simulations to assess beach erosion under mean and extreme sea levels and wrote sections of the manuscript. AV contributed to the results interpretation and discussion and wrote sections of the manuscript. MV and LM performed the dynamic simulations for the projections of the extreme sea levels, their components and the waves. DP and OM-N performed the bivariate copula statistics to obtain the corresponding most likely wave characteristics for the episodic sea level rise. DC and CS studied/selected the satellite images to be analyzed, and assembled the beach inventory. AC performed the statistical analysis of the database and wrote the relevant sections of the manuscript. DW, EM, and GB collated the information regarding the environmental setting, wrote the corresponding sections of the manuscript and prepared the relevant figures. All authors contributed to the article and approved the submitted version.
Funding
This work was supported by the research project BEACHTECH, co-funded by the European Union (ERDF) and national funds of Greece and Cyprus.
Acknowledgments
The authors acknowledge the support of Erasmus+ International Credit Mobility Program that facilitate exchanges between the Universities of the Aegean (Greece) and Nanjing (China), as well as of the Constantinides Foundation that supported exchanges between the Universities of the Aegean and Rhode Island (USA). Both exchanges were instrumental for the development and implementation of the study.
Conflict of interest
The authors declare that the research was conducted in the absence of any commercial or financial relationships that could be construed as a potential conflict of interest.
Publisher’s note
All claims expressed in this article are solely those of the authors and do not necessarily represent those of their affiliated organizations, or those of the publisher, the editors and the reviewers. Any product that may be evaluated in this article, or claim that may be made by its manufacturer, is not guaranteed or endorsed by the publisher.
Supplementary material
The Supplementary Material for this article can be found online at: https://www.frontiersin.org/articles/10.3389/fmars.2023.1188896/full#supplementary-material
References
Ackerman R. A. (2017). “The nest environment and the embryonic development of sea turtles,” in The biology of Sea turtles. Eds. Lutz P. L., Musick J. A. (Boca Raton: CRC Press), 83–106.
Almar R., Ranasinghe R., Bergsma E. W. J., Diaz H., Melet A., Papa F., et al. (2021). A global analysis of extreme coastal water levels with implications for potential coastal overtopping. Nat. Commun. 12, 3775. doi: 10.1038/s41467-021-24008-9
Alvarez S., Bahja F., Fyall A. (2022). A framework to identify destination vulnerability to hazards. Tour. Manag. 90, 104469. doi: 10.1016/j.tourman.2021.104469
Anderson T. R., Fletcher C. H., Barbee M. M., Romine B. M., Lemmo S., Delevaux J. M. S. M. S. (2018). Modeling multiple sea level rise stresses reveals up to twice the land at risk compared to strictly passive flooding methods. Sci. Rep. 8, 14484. doi: 10.1038/s41598-018-32658-x
Androulidakis Y. S., Kombiadou K. D., Makris C. V., Baltikas V. N., Krestenitis Y. N. (2015). Storm surges in the Mediterranean Sea: variability and trends under future climatic conditions. Dynamics. Atmospheres. Oceans. 71, 56–82. doi: 10.1016/j.dynatmoce.2015.06.001
Arns A., Dangendorf S., Jensen J., Talke S., Bender J., Pattiaratchi C. (2017). Sea-Level rise induced amplification of coastal protection design heights. Sci. Rep. 7, 40171. doi: 10.1038/srep40171
Athanasiou P. (2019). Global distribution of nearshore slopes. Version 1, dataset. Internet: 4TU.Centre for Research Data. doi: 10.4121/uuid:a8297dcd-c34e-4e6d-bf66-9fb8913d983d
Bellard C., Leclerc C., Courchamp F. (2014). Impact of sea level rise on the 10 insular biodiversity hotspots. Glob. Ecol. Biogeogr. 23, 203–212. doi: 10.1111/geb.12093
Beuzen T., Turner I. L., Blenkinsopp C. E., Atkinson A., Flocard F., Baldock T. E. (2018). Physical model study of beach profile evolution by sea level rise in the presence of seawalls. Coast. Eng. 136, 172–182. doi: 10.1016/j.coastaleng.2017.12.002
Boto-García D., Leoni V. (2022). The hedonic value of coastal amenities in peer-to-peer markets (DEA WP no.94. Palma, Ballearic Islands, Palma, Ballearic Islands: Departament d’Economia Aplicada, Universitat de Les Illes Balears).
Bruun P. (1988). The bruun rule of erosion by Sea-level rise: a discussion on Large-scale two- and three-dimensional usages. J. Coast. Res. 4, 627–648.
Bujan N., Cox R., Masselink G. (2019). From fine sand to boulders: examining the relationship between beach-face slope and sediment size. Mar. Geol. 417, 106012. doi: 10.1016/j.margeo.2019.106012
Callaghan D. P., Nielsen P., Short A., Ranasinghe R. (2008). Statistical simulation of wave climate and extreme beach erosion. Coast. Eng. 55, 375–390. doi: 10.1016/j.coastaleng.2007.12.003
Camus P., Losada I. J., Izaguirre C., Espejo A., Menéndez M., Pérez J. (2017). Statistical wave climate projections for coastal impact assessments. Earths. Future 5, 918–933. doi: 10.1002/2017EF000609
Chatzipavlis A., Tsekouras G. E., Trygonis V., Velegrakis A. F., Tsimikas J., Rigos A., et al. (2019). Modeling beach realignment using a neuro-fuzzy network optimized by a novel backtracking search algorithm. Neural Comput. Appl. 31 (6), 1747–1763. doi: 10.1007/s00521-018-3809-2
Chen C.-L., Teng N. (2016). Management priorities and carrying capacity at a high-use beach from tourists’ perspectives: a way towards sustainable beach tourism. Mar. Policy 74, 213–219. doi: 10.1016/j.marpol.2016.09.030
Ciavola P., Harley M. D., den Heijer C. (2018). The RISC-KIT storm impact database: a new tool in support of DRR. Coast. Eng. 134, 24–32. doi: 10.1016/j.coastaleng.2017.08.016
CYSTAT (2017) Tourism statistics 2016 (Nicosia, Cyprus: Statistical Service of Cyprus). Available at: https://library.cystat.gov.cy/Documents/Publication/TOURISM_STATISTICS-2016-141117.pdf (Accessed February 2, 2023).
CYSTAT (2019) Tourism statistics 2017-2019 (Nicosia, Cyprus: Statistical Service of Cyprus). Available at: https://library.cystat.gov.cy/Documents/Publication/TOURISM_%20STATISTICS-2017_19-EN-120820.pdf (Accessed February 2, 2023).
Dean R. G. (1991). Equilibrium beach profiles: characteristics and applications. J. Coast. Res. 7, 53–84.
Dean R. G., Houston J. R. (2016). Determining shoreline response to sea level rise. Coast. Eng. 114, 1–8. doi: 10.1016/j.coastaleng.2016.03.009
de Winter R. C., Ruessink B. G. (2017). Sensitivity analysis of climate change impacts on dune erosion: case study for the Dutch Holland coast. Clim. Change 141, 685–701. doi: 10.1007/s10584-017-1922-3
Di Paola G., Rodríguez G., Rosskopf C. M. (2023). Shoreline dynamics and beach erosion. Geosciences 13 (3), 74. doi: 10.3390/geosciences13030074
Edelman T. (1972). “Dune erosion during storm conditions,” in 13th conference on coastal engineering(Vancouver, Canada: ASCE), 1305–1312. doi: 10.1061/9780872620490.073
Farmaki A., Altinay L., Botterill D., Hilke S. (2015). Politics and sustainable tourism: the case of Cyprus. Tour. Manage. 47, 178–190. doi: 10.1016/j.tourman.2014.09.019
Galanis G., Hayes D., Zodiatis G., Chu P. C., Kuo Y.-H., Kallos G. (2012). Wave height characteristics in the Mediterranean Sea by means of numerical modeling, satellite data, statistical and geometrical techniques. Mar. Geophys. Res. 33, 1–15. doi: 10.1007/s11001-011-9142-0
Genest C., Rémillard B., Beaudoin D. (2009). Goodness-of-fit tests for copulas: a review and a power study. Insur. Math. Econ. 44, 199–213. doi: 10.1016/j.insmatheco.2007.10.005
Giardino A., Schrijvershof R., Nederhoff C. M., de Vroeg H., Brière C., Tonnon P.-K., et al. (2018). A quantitative assessment of human interventions and climate change on the West African sediment budget. Ocean. Coast. Manage. 156, 249–265. doi: 10.1016/j.ocecoaman.2017.11.008
Gomes da Silva P., Coco G., Garnier R., Klein A. H. F. (2020). On the prediction of runup, setup and swash on beaches. Earth Sci. Rev. 204, 103148. doi: 10.1016/j.earscirev.2020.103148
Hinkel J., Nicholls R. J., Tol R. S. J., Wang Z. B., Hamilton J. M., Boot G., et al. (2013). A global analysis of erosion of sandy beaches and sea-level rise: an application of DIVA. Glob. Planet. Change 111, 150–158. doi: 10.1016/j.gloplacha.2013.09.002
IBESTAT (2023) Turismo. flujo de turistas (FRONTUR). Available at: https://www.caib.es/ibestat/estadistiques/043d7774-cd6c-4363-929a-703aaa0cb9e0/ed5d4d88-cb17-46bd-b7b0-29fbaa5dba19/es/I208002_n101.px (Accessed February 25, 2023).
ICZM (2008) Protocol to the Barcelona convention on integrated coastal zone management (ICZM) in the Mediterranean. Available at: https://www.unep.org/unepmap/who-we-are/contracting-parties/iczm-protocol (Accessed February 25, 2023).
ILO (2020) Tourism sector in the English- and Dutch speaking Caribbean: an overview and the impact of COVID-19 on growth and employment (Port of Spain: International Labour Organization, Office for the Caribbean). Available at: http://www.ilo.int/wcmsp5/groups/public/—americas/—ro-lima/—sro-port_of_spain/documents/publication/wcms_753077.pdf (Accessed February 15, 2023).
INE (2023). Tourist movements at borders (FRONTUR) December 2022 and 2022 year. provisional data (Madrid, Spain: Instituto Nacional de Estadística. Madrid, Spain).
IPCC (2019). “Summary for policymakers,” in IPCC special report on the ocean and cryosphere in a changing climate. Eds. Pörtner H.-O., Roberts D. C., Masson-Delmotte V., Zhai P., Tignor M., Poloczanska E., et al. (Cambridge, UK and New York, NY, USA: Cambridge University Press), 3–35. doi: 10.1017/9781009157964.001
Jevrejeva S., Jackson L. P., Riva R. E. M., Grinsted A., Moore J. C. (2016). Coastal sea level rise with warming above 2 °C. Proc. Natl. Acad. Sci. 113, 13342–13347. doi: 10.1073/pnas.1605312113
Joe H. (2014). Dependence modeling with copulas. 1st ed (New York: Chapman and Hall/CRC). doi: 10.1201/b17116
Karambas T. V. (2006). Prediction of sediment transport in the swash-zone by using a nonlinear wave model. Cont. Shelf. Res. 26, 599–609. doi: 10.1016/j.csr.2006.01.014
Karambas T. V., Koutitas C. (2002). Surf and swash zone morphology evolution induced by nonlinear waves. J. Waterw. Port. Coast. Ocean. Eng. 128, 102–113. doi: 10.1061/(ASCE)0733-950X(2002)128:3(102
Larson M., Kraus N. C. (1989). SBEACH: numerical model for simulating storm-induced beach change; report 1: empirical foundation and model development (Washington, DC: US Army Coastal Engineering Research Center).
Le Cozannet G., Bulteau T., Castelle B., Ranasinghe R., Wöppelmann G., Rohmer J., et al. (2019). Quantifying uncertainties of sandy shoreline change projections as sea level rises. Sci. Rep. 9, 42. doi: 10.1038/s41598-018-37017-4
Le Cozannet G., Garcin M., Yates M., Idier D., Meyssignac B. (2014). Approaches to evaluate the recent impacts of sea-level rise on shoreline changes. Earth Sci. Rev. 138, 47–60. doi: 10.1016/j.earscirev.2014.08.005
Lemesios G., Giannakopoulos C., Papadaskalopoulou C., Karali A., Varotsos K. V., Moustakas K., et al. (2016). Future heat-related climate change impacts on tourism industry in Cyprus. Reg. Environ. Change 16, 1915–1927. doi: 10.1007/s10113-016-0997-0
Leont’yev I. O. (1996). Numerical modelling of beach erosion during storm event. Coast. Eng. 29, 187–200. doi: 10.1016/S0378-3839(96)00029-4
Li F., Zhou J., Liu C. (2018). Statistical modelling of extreme storms using copulas: a comparison study. Coast. Eng. 142, 52–61. doi: 10.1016/j.coastaleng.2018.09.007
Luijendijk A., Hagenaars G., Ranasinghe R., Baart F., Donchyts G., Aarninkhof S. (2018). The state of the world’s beaches. Sci. Rep. 8, 6641. doi: 10.1038/s41598-018-24630-6
Mavris C. (2011). Sustainable environmental tourism and insular coastal area risk management in Cyprus and the Mediterranean. J. Coast. Res. 61, 317–327. doi: 10.2112/SI61-001.32
Melet A., Meyssignac B., Almar R., Le Cozannet G. (2018). Under-estimated wave contribution to coastal sea-level rise. Nat. Clim. Change 8, 234–239. doi: 10.1038/s41558-018-0088-y
Mentaschi L., Vousdoukas M. I., Voukouvalas E., Dosio A., Feyen L., Lionello P., et al. (2017). Global changes of extreme coastal wave energy fluxes triggered by intensified teleconnection patterns. Geophys Res Lett 44, 2416–2426. doi: 10.1002/2016GL072488
Mentaschi L., Vousdoukas M. I., Pekel J.-F., Voukouvalas E., Feyen L. (2018). Global long-term observations of coastal erosion and accretion. Sci. Rep. 8, 12876. doi: 10.1038/s41598-018-30904-w
Monioudi I. N., Karditsa A., Chatzipavlis A., Alexandrakis G., Andreadis O. P., Velegrakis A. F., et al. (2016). Assessment of vulnerability of the eastern Cretan beaches (Greece) to sea level rise. Reg. Environ. Change 16, 1951–1962. doi: 10.1007/s10113-014-0730-9
Monioudi I. N., Velegrakis A. F. (2022). Beach carrying capacity at touristic 3S destinations: its significance, projected decreases and adaptation options under climate change. J. Tourism. Hospit. 11, 500. doi: 10.35248/2167-0269.22.11.500
Monioudi I. N., Velegrakis A. F., Chatzipavlis A. E., Rigos A., Karambas T., Vousdoukas M. I., et al. (2017). Assessment of island beach erosion due to sea level rise: the case of the Aegean archipelago (Eastern Mediterranean). Nat. Hazards. Earth Syst. Sci. 17, 449–466. doi: 10.5194/nhess-17-449-2017
Muis S., Verlaan M., Winsemius H. C., Aerts J. C. J. H., Ward P. J. (2016). A global reanalysis of storm surges and extreme sea levels. Nat. Commun. 7, 11969. doi: 10.1038/ncomms11969
Narayan S., Beck M. W., Reguero B. G., Losada I. J., van Wesenbeeck B., Pontee N., et al. (2016). The effectiveness, costs and coastal protection benefits of natural and nature-based defences. PloS One 11, e0154735. doi: 10.1371/journal.pone.0154735
Nicholls R. J., Cazenave A. (2010). Sea-Level rise and its impact on coastal zones. Science 328, 1517–1520. doi: 10.1126/science.1185782
Oswaldo M.-N., Dominik P., Daniël W., Linda A.-B., Wim C. (2017). Characterization of precipitation through copulas and expert judgement for risk assessment of infrastructure. ASCE. ASME. J. Risk Uncertain. Eng. Syst. A. Civ. Eng. 3, 04017012. doi: 10.1061/AJRUA6.0000914
Peduzzi P., Velegrakis A., Chatenoux B., Estrella M., Karambas T. (2022). Assessment of the role of nearshore marine ecosystems to mitigate beach erosion: the case of Negril (Jamaica). Environments 9 (5), 62. doi: 10.3390/environments9050062
Price C., Michaelides S., Pashiardis S., Alpert P. (1999). Long term changes in diurnal temperature range in Cyprus. Atmos. Res. 51, 85–98. doi: 10.1016/S0169-8095(99)00022-8
Ranasinghe R. (2016). Assessing climate change impacts on open sandy coasts: a review. Earth Sci. Rev. 160, 320–332. doi: 10.1016/j.earscirev.2016.07.011
Ranasinghe R., Callaghan D., Stive M. J. F. (2012). Estimating coastal recession due to sea level rise: beyond the bruun rule. Clim. Change 110, 561–574. doi: 10.1007/s10584-011-0107-8
Reis A. H., Gama C. (2010). Sand size versus beachface slope [[/amp]]mdash; an explanation based on the constructal law. Geomorphology 114, 276–283. doi: 10.1016/j.geomorph.2009.07.008
Rodella I., Madau F., Mazzanti M., Corbau C., Carboni D., Simeoni U., et al. (2020). Carrying capacity as tool for beach economic value assessment (case studies of Italian beaches). Ocean. Coast. Manage. 189, 105130. doi: 10.1016/j.ocecoaman.2020.105130
Roelvink D., Reniers A., Van Dongeren A., Van Thiel De Vries J., Lescinski J., Report R. M. (2010). XBeach model description and manual (Delft: Unesco-IHE Institute for Water Education, Deltares and Delft University of Technology).
Rueda A., Vitousek S., Camus P., Tomás A., Espejo A., Losada I. J., et al. (2017). A global classification of coastal flood hazard climates associated with large-scale oceanographic forcing. Sci. Rep. 7, 5038. doi: 10.1038/s41598-017-05090-w
Safakli O. V., Kutlay K. (2016). Potential multiplier effect of tourism sector in northern Cyprus. Int. J. Acad. Res. Accounting. Financ. Manage. Sci. 6, 44–51. doi: 10.6007/IJARAFMS/v6-i4/2293
Semeoshenkova V., Newton A. (2015). Overview of erosion and beach quality issues in three southern European countries: Portugal, Spain and Italy. Ocean. Coast. Manag. 118, 12–21. doi: 10.1016/j.ocecoaman.2015.08.013
SETE (2023) Key figures of incoming tourism in greece. Greek tourism confederation. Available at: https://insete.gr/districts/?lang=en (Accessed February 24, 2023).
Sherwood C. R., van Dongeren A., Doyle J., Hegermiller C. A., Hsu T.-J., Kalra T. S., et al. (2022). Modeling the morphodynamics of coastal responses to extreme events: what shape are we in? Ann. Rev. Mar. Sci. 14, 457–492. doi: 10.1146/annurev-marine-032221-090215
Simmons J. A., Splinter K. D. (2022). A multi-model ensemble approach to coastal storm erosion prediction. Environ. Model. Software. 150, 105356. doi: 10.1016/j.envsoft.2022.105356
Stripling S., Panzeri M., Blanco B., Rossington K., Sayers P., Borthwick A. (2017). Regional-scale probabilistic shoreline evolution modelling for flood-risk assessment. Coast. Eng. 121, 129–144. doi: 10.1016/j.coastaleng.2016.12.002
Summers A., Fletcher C. H., Spirandelli D., McDonald K., Over J.-S., Anderson T., et al. (2018). Failure to protect beaches under slowly rising sea level. Clim. Change 151, 427–443. doi: 10.1007/s10584-018-2327-7
Toimil A., Díaz-Simal P., Losada I. J., Camus P. (2018). Estimating the risk of loss of beach recreation value under climate change. Tour. Manage. 68, 387–400. doi: 10.1016/j.tourman.2018.03.024
Toimil A., Losada I. J., Camus P., Díaz-Simal P. (2017). Managing coastal erosion under climate change at the regional scale. Coast. Eng. 128, 106–122. doi: 10.1016/j.coastaleng.2017.08.004
Tolman H. L. (2009). User manual and system documentation of WAVEWATCH III version 3.14 (Camp Springs, MD: U. S. Department of Commerce National Oceanic and Atmospheric Administration, National Weather Service, National Centers for Environmental Prediction).
Toumazis A., Kyrou K., Iacovou N., Sophos J., Zervos S., Anastasakis G. (2008). Pre – reservoirs: a sustainable solution for eroding beaches/deltas of dammed rivers. WIT. Trans. Built. Environ. 99, 179–188. doi: 10.2495/CENV080171
Tsimplis M. N., Calafat F. M., Marcos M., Jordà G., Gomis D., Fenoglio-Marc L., et al. (2013). The effect of the NAO on sea level and on mass changes in the Mediterranean Sea. J. Geophys. Res. Oceans. 118, 944–952. doi: 10.1002/jgrc.20078
Tsimplis M. N., Shaw A. G. P. (2010). Seasonal sea level extremes in the Mediterranean Sea and at the Atlantic European coasts. Nat. Hazards. Earth Syst. Sci. 10, 1457–1475. doi: 10.5194/nhess-10-1457-2010
Twomey A. J., O’Brien K. R., Callaghan D. P., Saunders M. I. (2020). Synthesising wave attenuation for seagrass: drag coefficient as a unifying indicator. Mar. pollut. Bull. 160, 111661. doi: 10.1016/j.marpolbul.2020.111661
UNWTO (2019). International tourism highlights (Madrid: United Nations World Tourism Organisation). doi: 10.18111/9789284421152
Velegrakis A. F., Ballay A., Poulos S., Radzevicius R., Bellec V., Manso F. (2010). European Marine aggregates resources: origins, usage, prospecting and dredging techniques. J. Coast. Res. 51, 1–14. doi: 10.2112/si51-002.1
Velegrakis A. F., Hasiotis T., Papadatou K. (2021). Coastal climate resilience: review of policies and regulations. deliverable 2.2 – ECFAS project (GA 101004211). Zenodo. doi: 10.5281/zenodo.6538546
Velegrakis A. F., Trygonis V., Chatzipavlis A. E., Karambas Th., Vousdoukas M. I., Ghionis G., et al. (2016). Shoreline variability of an urban beach fronted by a beachrock reef from video imagery. Nat. Hazards. 83, 201–222. doi: 10.1007/s11069-016-2415-9
Vitousek S., Barnard P. L., Fletcher C. H., Frazer N., Erikson L., Storlazzi C. D. (2017). Doubling of coastal flooding frequency within decades due to sea-level rise. Sci. Rep. 7, 1–9. doi: 10.1038/s41598-017-01362-7
Vousdoukas M. I., Mentaschi L., Voukouvalas E., Bianchi A., Dottori F., Feyen L. (2018a). Climatic and socioeconomic controls of future coastal flood risk in Europe. Nat. Clim. Change 8, 776–780. doi: 10.1038/s41558-018-0260-4
Vousdoukas M. I., Mentaschi L., Voukouvalas E., Verlaan M., Jevrejeva S., Jackson L. P., et al. (2018b). Global probabilistic projections of extreme sea levels show intensification of coastal flood hazard. Nat. Commun. 9, 2360. doi: 10.1038/s41467-018-04692-w
Vousdoukas M. I., Ranasinghe R., Mentaschi L., Plomaritis T. A., Athanasiou P., Luijendijk A., et al. (2020). Sandy coastlines under threat of erosion. Nat. Clim. Change 10, 260–263. doi: 10.1038/s41558-020-0697-0
Vousdoukas M. I., Velegrakis A. F., Karambas T. V. (2009). Morphology and sedimentology of a microtidal beach with beachrocks: vatera, Lesbos, NE Mediterranean. Cont. Shelf. Res. 29, 1937–1947. doi: 10.1016/j.csr.2009.04.003
WDD (2017)Catalogue of reservoirs, list of water reservoirs (dams). In: Water development department (WDD), Cyprus ministry of agriculture. Available at: http://www.moa.gov.cy/moa/wdd/wdd.nsf/All/25041A3BAC14BC48C22583D800396FF5?OpenDocument (Accessed February 25, 2023).
Wolff C., Vafeidis A. T., Muis S., Lincke D., Satta A., Lionello P., et al. (2018). A Mediterranean coastal database for assessing the impacts of sea-level rise and associated hazards. Sci. Data 5, 180044. doi: 10.1038/sdata.2018.44
Wong P. P., Losada I. J., Gattuso J.-P., Hinkel J., Khattabi A., McInnes K. L., et al. (2014). “Coastal systems and low-lying areas,” in Climate change 2014: impacts, adaptation, and vulnerability. part a: global and sectoral aspects. contribution of working group II to the fifth assessment report of the intergovernmental panel on climate. Eds. Field C. B., Barros V. R., Dokken D. J., Mach K. J., Mastrandrea M. D., Bilir T. E., et al (Cambridge, United Kingdom and New York, NY, USA: Cambridge University Press), 361–409.
Keywords: beach erosion, shoreline retreat, island beaches, extreme sea levels, sea level rise, climate change impacts
Citation: Monioudi IN, Velegrakis AF, Chatzistratis D, Vousdoukas MI, Savva C, Wang D, Bove G, Mentaschi L, Paprotny D, Morales-Nápoles O, Chatzipavlis AE, Hasiotis T and Manoutsoglou E (2023) Climate change - induced hazards on touristic island beaches: Cyprus, Eastern Mediterranean. Front. Mar. Sci. 10:1188896. doi: 10.3389/fmars.2023.1188896
Received: 18 March 2023; Accepted: 19 June 2023;
Published: 13 July 2023.
Edited by:
Achilleas G. Samaras, Democritus University of Thrace, GreeceReviewed by:
Dimitris Stagonas, University of Cyprus, CyprusGiandomenico Foti, Mediterranea University of Reggio Calabria, Italy
Copyright © 2023 Monioudi, Velegrakis, Chatzistratis, Vousdoukas, Savva, Wang, Bove, Mentaschi, Paprotny, Morales-Nápoles, Chatzipavlis, Hasiotis and Manoutsoglou. This is an open-access article distributed under the terms of the Creative Commons Attribution License (CC BY). The use, distribution or reproduction in other forums is permitted, provided the original author(s) and the copyright owner(s) are credited and that the original publication in this journal is cited, in accordance with accepted academic practice. No use, distribution or reproduction is permitted which does not comply with these terms.
*Correspondence: Isavela N. Monioudi, imonioudi@marine.aegean.gr