- 1Ocean BioGeosciences, National Oceanography Centre, Southampton, United Kingdom
- 2Marine Systems Modelling, Plymouth Marine Laboratory, Plymouth, United Kingdom
- 3Maldives Marine Research Institute, Ministry of Fisheries, Marine Resources and Agriculture, Male, Maldives
- 4School of GeoSciences, University of Edinburgh, Edinburgh, United Kingdom
- 5National Centre for Sustainable Coastal Management, Ministry of Environment, Forest and Climate Change, Anna University, Chennai, India
- 6The International Union for Conservation of Nature (IUCN), Sri Lanka Country Office, Horton Place, Colombo, Sri Lanka
- 7Department of Environmental and Natural Science, Faculty of Engineering, Science and Technology, The Maldives National University, Male, Maldives
Nitrogen pollution is a widespread and growing problem in the coastal waters of South Asia yet the ecological impacts on the region’s coral ecosystems are currently poorly known and understood. South Asia hosts just under 7% of global coral reef coverage but has experienced significant and widespread coral loss in recent decades. The extent to which this coral ecosystem decline at the regional scale can be attributed to the multiple threats posed by nitrogen pollution has been largely overlooked in the literature. Here, we assess the evidence for nitrogen pollution impacts on corals in the central Indian Ocean waters of India, Sri Lanka and the Maldives. We find that there is currently limited evidence with which to clearly demonstrate widespread impacts on coral reefs from nitrogen pollution, including from its interactions with other stressors such as seawater warming. However, this does not prove there are no significant impacts, but rather it reflects the paucity of appropriate observations and related understanding of the range of potential impacts of nitrogen pollution at individual, species and ecosystem levels. This situation presents significant research, management and conservation challenges given the wide acceptance that such pollution is problematic. Following from this, we recommend more systematic collection and sharing of robust observations, modelling and experimentation to provide the baseline on which to base prescient pollution control action.
1 Introduction
Occupying less than 0.1% of global ocean area coral reefs host around a quarter of all marine species and serve a variety of critical environmental, economic and cultural functions, valued at US$2.7 trillion per year (Birkeland, 1997; Hoegh-Guldberg, 1999; Moberg and Folke, 1999; Wilkinson, 2000; Harrison and Booth, 2007; Burke et al., 2011; Spalding et al., 2017; Souter et al., 2021). The high biodiversity associated with coral reefs supports fisheries and human livelihoods, whilst the reef structure provides key habitats, enhanced coastal protection, and a focus for tourism, which alone has been valued at US$36 billion in foreign currency exchange (Spalding et al., 2017; UNEP, 2019). Corals, however, face multiple and diverse threats at different spatial and temporal scales ranging from i) physical destruction of the reef structure as a result of fishing practices, coastal development, reef extraction or coral mining, ii) increased risk of mortality from pollution, disease, or competition with macroalgae, and iii) ocean-scale acidification and temperature-induced bleaching (Wilkinson et al., 2016; Souter et al., 2021). The long-term fate of coral reefs is predicted to be widespread ecosystem loss and reduced ecoservice provision with a recent global assessment of coral reef health describing a 14% reduction in coral reef coverage in the decade to 2018 (Souter et al., 2021). This ecosystem decline is driven by the combination of the global stressors of increased ocean temperature and ocean acidity resulting from rising atmospheric CO2 levels, coupled in impact with more localized anthropogenic stressors (van Hooidonk et al., 2016; IPCC, 2019; UNEP, 2020; Eddy et al., 2021).
Long-term global temperature trends are expected to push corals above their optimal thermal tolerances over the coming century (IPCC, 2019), yet there is considerable uncertainty over the timing and severity of impacts at more regional scales. This uncertainty includes factors around potential rates of adaptation and acclimation, and related to environmental heterogeneity, particularly in coastal environments (Hughes et al., 2003; Smale et al., 2019; Guan et al., 2020; McClanahan et al., 2020; Oliver et al., 2020; UNEP, 2020; Ziegler et al., 2021). The cumulative effects and timings of anthropogenic stressors on coral mortality rates remain difficult to generalize because such impacts are frequently region specific, though it is acknowledged that those coral ecosystems most heavily impacted by anthropogenic stressors are those typically found along urbanized coastlines (Halpern et al., 2008; Rosenberg et al., 2022). Geographic remoteness from human impacts, however, does not necessarily provide additional protection from the impacts of global stressors (Strona et al., 2021; Baumann et al., 2022).
In South Asia, which contains ~7% of global coral coverage (Spalding et al., 2001; Wilhelmsson, 2002; Rajasuriya et al., 2004; GEER, 2008; Tamelander and Rajasuriya, 2008; Bahuguna et al., 2013), local stressors such as fishing and anthropogenic pollution are recognized as serious and immediate threats to coral ecosystems and have been argued to contribute to, or accelerate, regional coral loss (Rajasuriya et al., 2000; Rajasuriya et al., 2004; Tamelander and Rajasuriya, 2008). In this context pollution is frequently a catch-all term representing multiple pollution types that can include chemical, heavy metal or biological pollutants with diverse transport pathways including atmospheric deposition, industrial effluents, maritime activities, sewage discharge, terrestrial runoff and groundwater seepage. Nutrient pollution, primarily in the form of excessive nitrogen and phosphorus inputs to coastal waters, is widespread across South Asia and significant at the regional scale (BOBLME, 2015; Raghuram et al., 2021). Yet, despite the socioeconomic and cultural significance of corals throughout the region (Price and Firaq, 1996; Townsley, 2004; MEE, 2017a), the ecological impacts of anthropogenic nutrient inputs on the region’s coral reef ecosystems remain widely alluded to but poorly studied (Selman et al., 2008; BOBLME, 2015; SACEP, 2019).
For the South Asian region in particular, quantitative information on coastal nutrient concentrations, how the nutrient seascape may be changing with time and how such changes impact coral ecosystems remains patchy. The aim of this study is to assess and review the impact of nutrient (nitrogen) pollution on coral reefs found in the central Indian Ocean waters of India, Sri Lanka and the Maldives with recommendations for future management and research directions. The paper consists of a short summary of reported nutrient impacts on corals (section 2), national assessments on water quality indicators, reported impacts of nutrient pollution on coral reefs and current legislative safeguards (section 3), a regional assessment of nutrient inputs from the identified dominant sources and recommendations for future research (section 4), and concludes with recommendations for improved regional monitoring (section 5).
2 Corals and their nutrient environment
2.1 Corals and anthropogenic nutrients
Corals grow best in shallow, optically clear, warm (>18-20°C), stratified, and nutrient poor waters of the tropics (Lewis, 1981; Lerman, 1986; Kleypas et al., 1999; Wilkinson et al., 2016), and are generally absent from regions receiving significant freshwater input or where nutrient concentrations, sediment loads and algal densities are high (Spalding et al., 2001; Spalding and Brown, 2015). The mean long-term environmental conditions experienced by healthy coral reefs are governed by the prevailing large-scale ocean circulation, but seasonality and physical mixing mechanisms are important for inducing short-term environmental variability around optimal growth conditions, which may include mild seasonal upwelling and changes to local nutrient fields (Doty and Oguri, 1956). Coral reefs therefore are dynamic environments that naturally experience fluctuations in temperature, salinity, light, turbidity, nutrients, wave action, weather, disease, and competition over multiple timescale (Hoegh-Guldberg, 1999; Burke et al., 2011).
Anthropogenic nutrient inputs arise primarily from land-use change, population growth and coastal development which lead to increased discharges of sewage, industrial or municipal effluents and terrestrial runoff (Robin et al., 2013; Karthik et al., 2020) but may also include changes to groundwater nutrient concentrations and subsequent seepage to coastal waters (Santos et al., 2021). Changes in the intensity of agricultural practices, the extent of land use change and the extent and speed of coastal developments generally influence the diffuse supply of nutrients from runoff to coastal waters whilst population growth and urbanization influence point-source discharges. Anthropogenic nutrient inputs can lead to elevated dissolved inorganic nitrogen (DIN; e.g. nitrate, nitrite and ammonium) or dissolved inorganic phosphorous (DIP; phosphate) concentrations in the coastal zone, may ultimately distort ambient nutrient fields and impact water quality (NRC, 2000; Cloern, 2001), and have become a focus of study for understanding human impacts on coastal habitats and coral health (e.g. (Dubinsky and Stambler, 1996; Fabricius, 2005; D’Angelo and Wiedenmann, 2014; Serrano et al., 2018)). The impacts on specific habitats such as coral reefs can be both direct and indirect (Risk, 2014; Fernandes de Barros Marangoni et al., 2020). Nitrogen pollution resulting from sewage discharge, terrestrial run-off and wastewater discharge is widely recognized (Wear and Thurber, 2015; Brodie et al., 2019; Tuholske et al., 2021) and has been linked to multiple impacts within coral ecosystems including i) increased macroalgal-coral competition (Dubinsky and Stambler, 1996; Karcher et al., 2020), ii) permanent shifts in coral-macroalgal dominance (phase shifts) (Bell, 1992; Done, 1992), iii) increased prevalence of coral disease (Antonius, 1985; Bruno et al., 2003), iv) impacts on coral growth and calcification rates (Ferrier-Pagès et al., 2000; Koop et al., 2001; Renegar and Riegl, 2005), v) alteration of the balance between calcification and bioerosion (Highsmith, 1980; Hallock, 1988), vi) impacts on coral reproduction, recruitment and larval success (Humanes et al., 2016; Liu et al., 2020), vii) reduced thresholds to bleaching (Wiedenmann et al., 2012; Donovan et al., 2020), viii) changes to coral-zooxanthella symbiosis (Schlöder and D’Croz, 2004), ix) impacts on coral larval performance (Serrano et al., 2018; Liu et al., 2020), x) changes to community species diversity (Fabricius, 2005) and xi) infestations of the corallivorous crown-of-thorns starfish (Acanthaster planci) (Brodie et al., 2005); Table 1. Nitrogen pollution poses a serious and complex threat to corals but with impacts that can be indirect, highly dispersed, variable in intensity and hard to quantify (Dubinsky and Stambler, 1996; Duce et al., 2008; Wilkinson et al., 2016), the range of reported impacts means that the subject of nitrogen enrichment has at times proven controversial and despite increased investigation much remains unclear (Bell, 1992; Szmant, 2002; Fabricius, 2005; D’Angelo and Wiedenmann, 2014; Risk, 2014; Lesser, 2021).
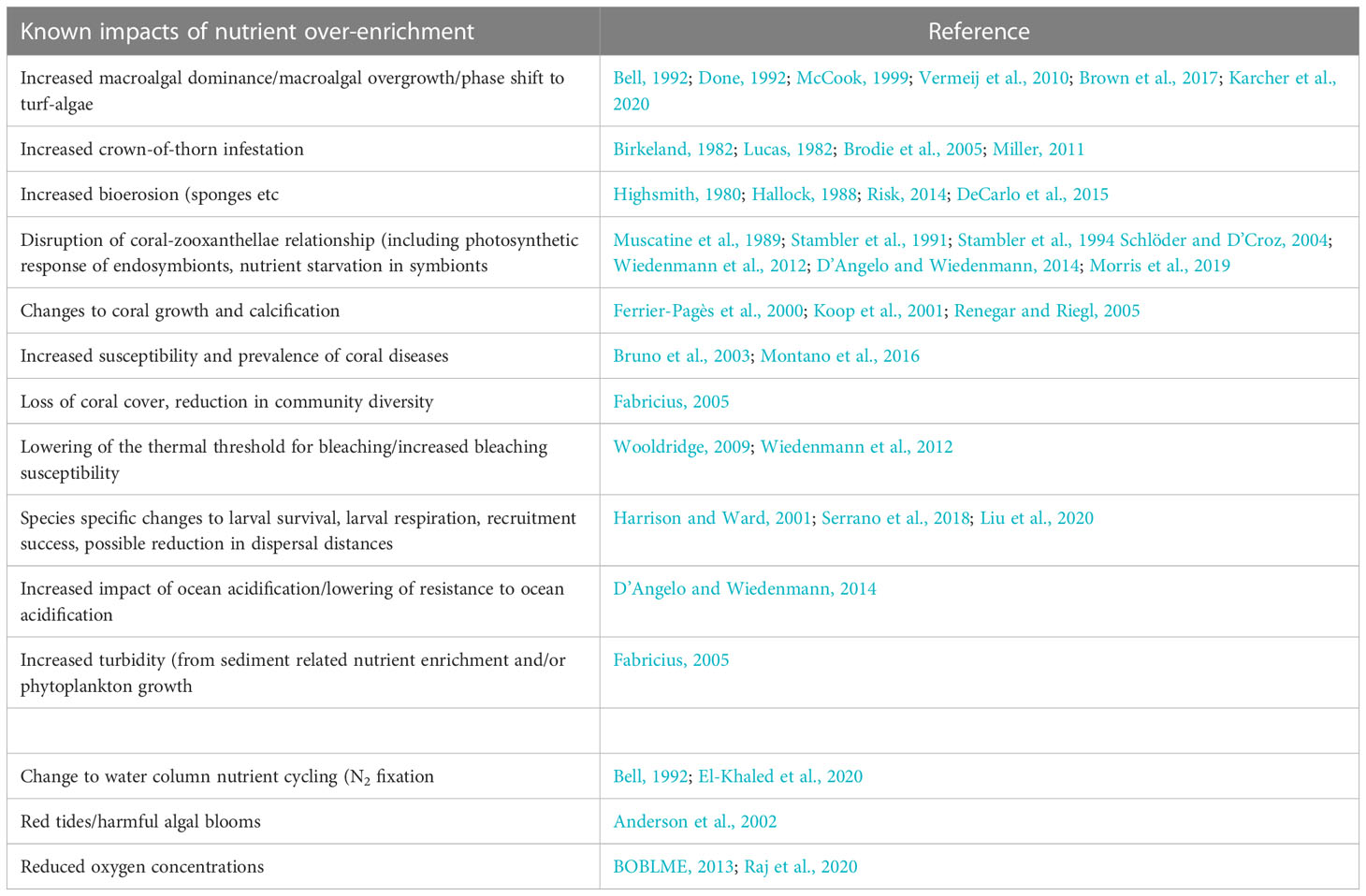
Table 1 Summary of nutrient enrichment impacts on corals or coral ecosystems and selected example studies.
In addition to the impacts reported on corals, anthropogenic nutrient inputs are also associated with increased phytoplankton productivity, algal biomass and abundance, fish kills and nuisance blooms, leading to increased water column turbidity, increased particulate setting rates, and in some instances decreased dissolved oxygen concentrations resulting from increased bacterial remineralization. These additional responses to nutrient inputs can all alter the environmental conditions that corals experience even if the corals themselves appear unaffected. Anthropogenic nutrient inputs have also been linked to other important ecosystem changes such as increased nitrogen fixation activity, which can impact nitrogen cycling through local pelagic ecosystems with the potential for secondary impacts on corals (Bell, 1992; El-Khaled et al., 2020). While the underlying mechanism linking ambient nutrient pools to coral health is still being debated (D’Angelo and Wiedenmann, 2014), there is general agreement that excessive and persistent increases in inorganic nutrient concentrations have a long-term detrimental impact via the promotion of macroalgal communities or stoichiometric imbalances impacting physiological processes (Wilkinson, 2008; Zhao et al., 2021; Buckingham et al., 2022). There is also growing acceptance that the impact of nutrient over-enrichment can be highly variable between sites (and species), which may partially explain some of the reported inconsistencies between studies (Szmant, 2002; Shantz and Burkepile, 2014; Lesser, 2021). Additional complexities arise due to coral species-specific responses to nutrient over-enrichment leading to both intra-regional and inter-regional differences in pollution impacts (Burkepile et al., 2020).
2.2 Nitrogen cycling in corals
Until quite recently a common understanding was that nutrient cycling within corals was largely determined by endosymbiont population dynamics. Under typical environmental conditions the autotrophic endosymbiont zooxanthellae (photosynthetic dinoflagellate of genera Symbiodinium), can provide around 30% of daily coral nitrogen requirements (Bythell, 1988), with the remaining 70% provided heterotrophically via particulate matter capture and ingestion (Bak et al., 1998). Under conditions of increased ambient nutrient concentrations, however, rapid growth of the algal symbionts can lead to competition between coral host and algae with the retention and increased usage of photosynthates by the algae at the expense of coral host metabolism (Ezzat et al., 2015; Morris et al., 2019). Corals, however, represent a complex assemblage of organisms living in symbiosis. This assemblage, referred to as the coral microbiome or coral holobiont, consists of the invertebrate coral and the endosymbiont zooxanthellae, but also includes associated bacteria, archaea, fungi and viruses. As each organism has its own physiological traits, the overall health of the holobiont can be disrupted by changes in the local environment impacting one or more of the individual component species. The significance of the holobiont model for understanding coral nitrogen demand lies in the emerging, yet poorly understood, relationship between the community of microorganisms contributing to the holobiont and how nitrogen is cycled and regulated within corals (Glaze et al., 2022; Mohamed et al., 2022). Recent studies indicate that the holobiont microbial community may be key to regulating fluctuations in environmental nitrogen availability and in maintaining the critical relationship between the coral host and its endosymbionts (Tilstra et al., 2021). More precisely, the holobiont makeup may be important for stabilizing nitrogen availability and maintaining nitrogen limitation in the endosymbiont via the relative actions of denitrifying and nitrogen-fixing bacteria. Whilst nitrogen fixation has been reported as a critical process supporting primary production by zooxanthellae endosymbionts during periods of low nutrient availability (Rädecker et al., 2015), it has also been reported that regulation of nitrate availability by microbial denitrification rates can occur when ambient nitrate concentrations are high thus potentially limiting the zooxanthellae response to elevated nutrient levels (Tilstra et al., 2021). Yet despite nitrogen fixation by free-living and colonial pelagic diazotrophs (Bell, 1992; El-Khaled et al., 2020), by benthic organisms (Cardini et al., 2014) and by bacterial symbionts (Lesser et al., 2007) being common within reef environments, nitrogen fixation is currently considered to be only a minor to moderate source of fixed nitrogen in the overall coral nitrogen budget, with estimates generally indicating a supply of <10%, but perhaps reaching as high as 20% under certain specific conditions (Rädecker et al., 2015; Benavides et al., 2017; Glaze et al., 2022; Moynihan et al., 2022).
The presence of highly efficient yet complex nitrogen cycling pathways within the holobiont not only supports the contradictory existence of productive coral reef ecosystems within otherwise nutrient-poor oligotrophic tropical waters but also highlights the challenges posed by anthropogenic nutrient inputs. As many of the pathways between bacteria, zooxanthellae and coral remain unclear there is considerable uncertainty over how and why increased ambient nutrient concentrations impact corals (Lesser, 2021). Multiple processes ranging from the acquisition of dissolved inorganic nitrogen, ingestion of particulate nitrogen, retention and intracellular translocation of dissolved organic nitrogen, conversion between nitrogen forms and inputs via nitrogen fixation are central to the productivity of coral reefs. Yet whilst elevated ambient nitrogen concentrations have been shown to broadly amplify coral bleaching impacts thus linking the impacts of nutrient inputs to global temperature trends (Donovan et al., 2020), studies have also shown that the form of the nitrogen substrate added is significant with greater sensitivity reported to elevated concentrations of nitrate than to ammonium or urea (Burkepile et al., 2020; Donovan et al., 2020; Fernandes de Barros Marangoni et al., 2020). Thus, whilst the overall health of the holobiont, and corals more generally, appears sensitive to chemical imbalances in the ambient environment (e.g. (Wiedenmann et al., 2012; D’Angelo and Wiedenmann, 2014)), there is still much to be understood particularly at regional scales. Nevertheless, long-term monitoring of the coral microbiome has been proposed as a useful means of obtaining early warning of the potential impacts of anthropogenic stressors on coral reefs due to the way in which microbial communities can change rapidly in response to environmental disturbances (Zaneveld et al., 2016; Glasl et al., 2019; Ziegler et al., 2019). Presently, however, there is a general lack of data on the temporal evolution of the microbiome in response to environmental (nutrient) stress, and insufficient understanding of the variability in bacterial communities between coral species to assess the usefulness of this approach for gauging the impact of specific stressors on corals or for understanding the implications for nitrogen cycling within the microbiome more generally (Glaze et al., 2022).
3 Coral reefs in the South Asia region
3.1 India
3.1.1 Coral reef extent, condition and environmental setting
India’s peninsula coastline and its offshore islands face three biogeochemically distinct oceanic environments. To the west is the productive Arabian Sea, to the south is the subtropical Indian Ocean, and to the east is the comparatively fresh Bay of Bengal. Coral reef distribution in Indian waters is primarily restricted to the southern and western coasts and includes i) the Gulf of Kachchh and parts of the west coast, ii) the Gulf of Mannar and Palk Bay along the southern coast, iii) the offshore Andaman and Nicobar Islands and iv) the offshore Lakshadweep Islands (Figure 1). These regions are located far from the major riverine inputs occurring in the northern Bay of Bengal, where strong salinity fluctuations and high sediment loadings largely prevent coral colonization. The mean annual nitrate concentrations for the surface waters of India’s Exclusive Economic Zone (EEZ) are typically 0-2.5 μmol L-1 (Arabian Sea), 0-3 μmol L-1 (Bay of Bengal) and 0-3.5 μmol L-1 (Andaman Sea) (Nair, 2010; Prema et al., 2017). Monsoon-driven seasonality is recognized as the dominant mode of environmental variability across the region with impacts that include the reversal of coastal currents (Shetye, 1998), seasonal coastal upwelling (Retnamma et al., 2020), substantial freshwater and nutrient discharge to Indian coastal waters (Singh and Ramesh, 2011; Krishna et al., 2016; Rao et al., 2017), and increased terrestrial runoff in the Andaman and Nicobar Islands (Raghuraman et al., 2013). Seasonal upwelling, particularly along the south-west Indian coastline, can increase surface nitrate concentrations 4-fold during the southwest monsoon months (Jun-Sep) while phosphate and silicate concentrations are reported to vary by less than 2-fold (Retnamma et al., 2020).
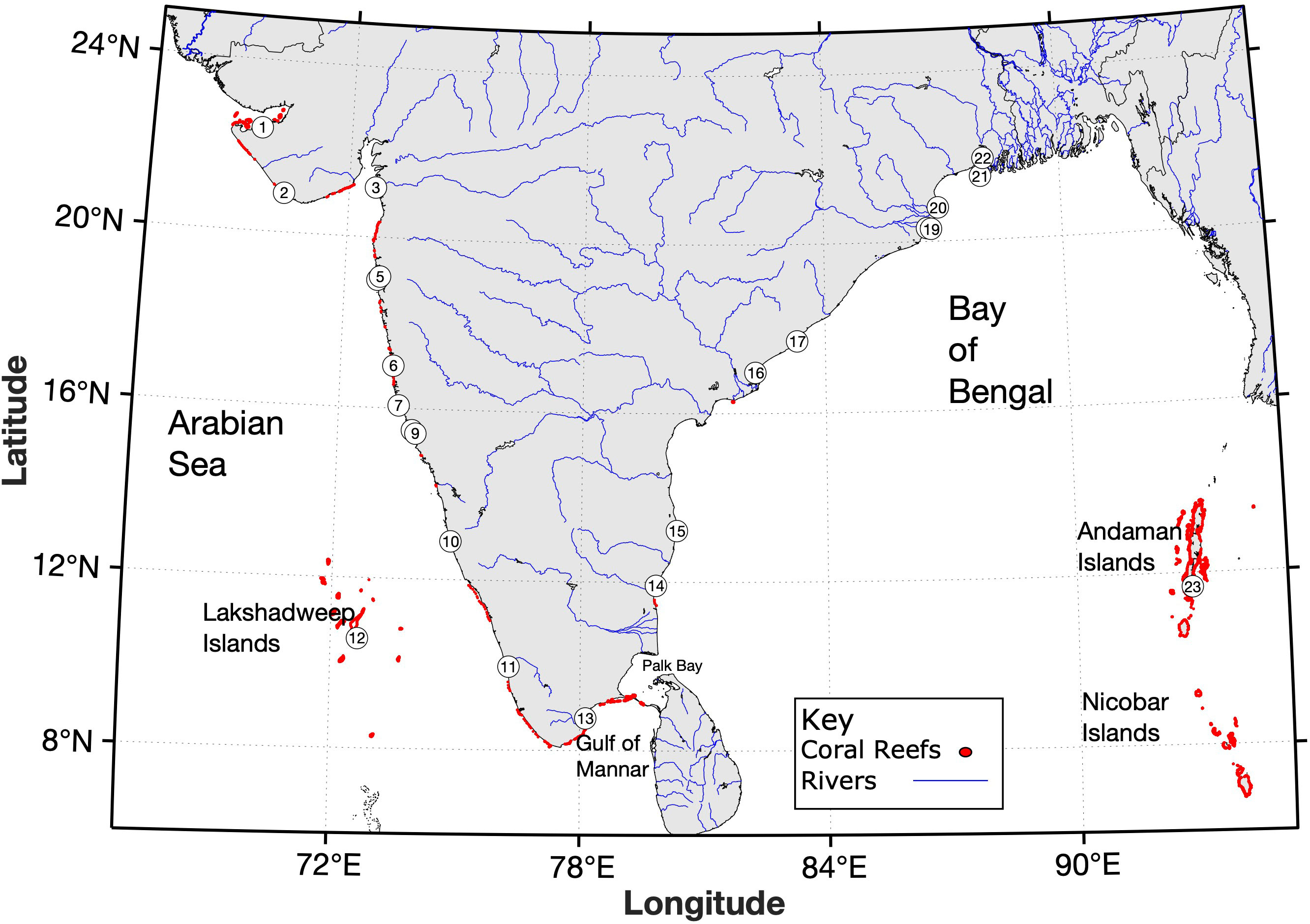
Figure 1 Distribution of corals in Indian waters. Coral distribution based on data compiled by UNEP-WCMC et al. (2018). Numeric indicators signify water quality monitoring locations shown in Figure 2.
Reef types include fringing, barrier, atoll and patch reefs (Table 2). Fringing reefs are found in the Andaman and Nicobar Islands, the Gulf of Kachchh, Palk Bay and the Gulf of Mannar. Barrier reefs are found in the Andaman and Nicobar Islands whilst coral atolls are found in the Lakshadweep archipelago (De et al., 2017). Patch reefs are observed in the intertidal regions of Ratnagiri, Malvan and Redi as well as on the continental shelf between Vengurla and Vijaydurg. Submerged reefs patch reefs are also found along the Maharashtra coast, on Angria Bank off Sindhudurg in Maharashtra, around Grande Island in Goa, around Netrani Island on the Karnataka coast, on Gaveshani Bank off the Malpe coast, near Quilon and Vizhinjam along the Kerala coast, and Enayem along the Tamil Nadu coast. Mesophotic coral ecosystems are situated off the Puducherry coast (Jasmine et al., 2009; De et al., 2017; Kumar et al., 2019; Laxmilatha et al., 2019).
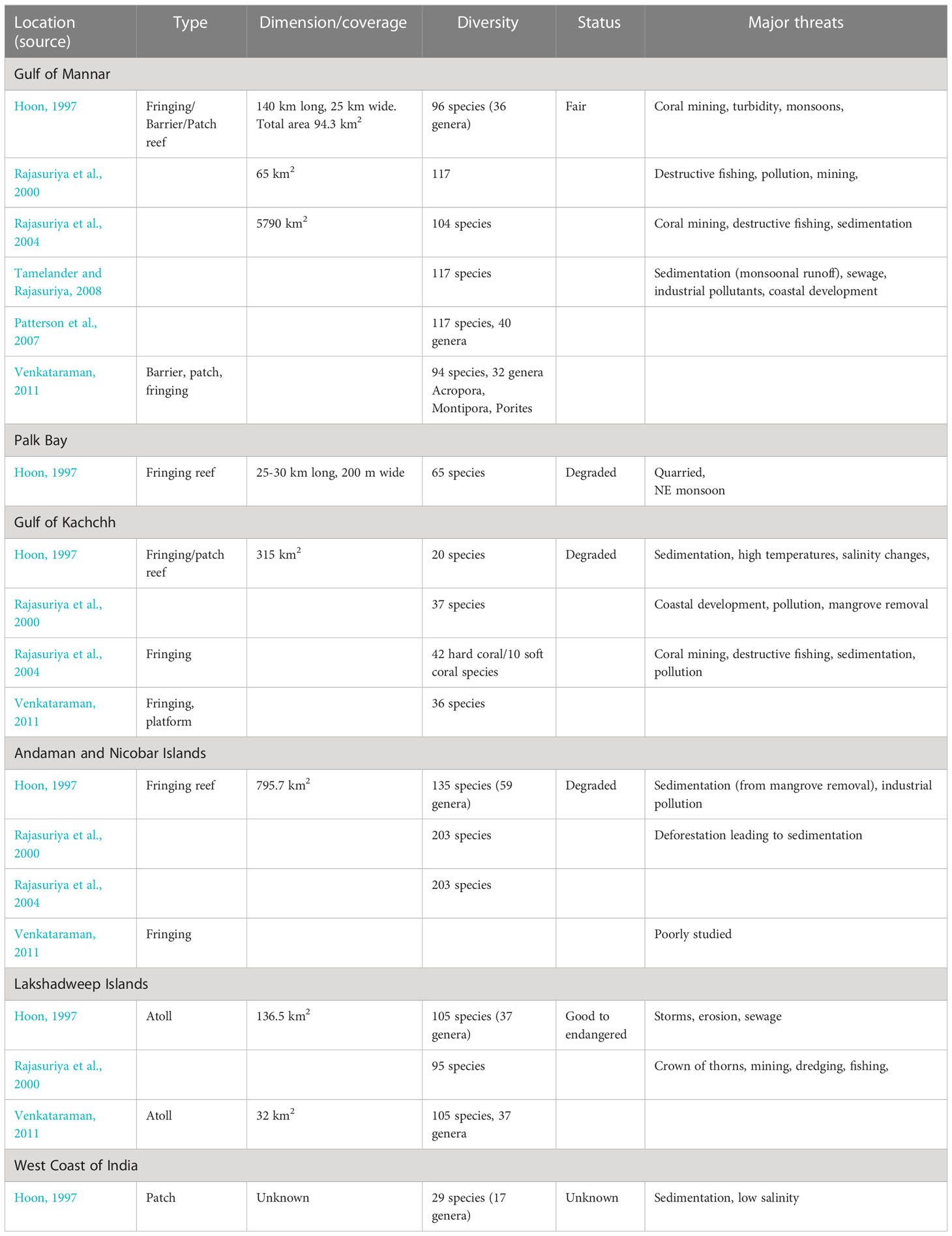
Table 2 Literature reports of coral reef type, spatial coverage, diversity and status for the major coral reef locations in Indian waters.
Coral species richness is variable between the main hosting regions and estimates vary significantly between studies due to occasional investigation. A latitudinal diversity gradient is recognized with species richness increasing from north to south. In the Gulf of Kachchh 36-68 species have been recorded (Venkataraman et al., 2003; MoEF, 2014; Marimuthu et al., 2018; Chandran et al., 2021), increasing to ~100 species in the Gulf of Mannar/Palk Bay (Bhatt et al., 2012b) and almost 150 species in the Lakshadweep Islands (Pillai and Jasmine, 1989; Government of India, 2012). Until recently around 200 species were thought to be present in Indian waters (Venkataraman et al., 2003; Venkataraman, 2011), though early diversity estimates remain uncertain (Bhatt et al., 2012b; Raghuraman et al., 2013). Species diversity estimates have now risen to 424 or even 588 species in more recent studies (Raghuraman et al., 2013; Majumdar et al., 2018; Jha et al., 2019) with the Andaman and Nicobar Islands alone potentially hosting a significant proportion of global coral diversity (Venkataraman et al., 2003; MoEF, 2009; Singh and Chaturvedi, 2017). Elsewhere however, a total of 560 species has been reported of which 478 are hermatypic (reef-building) representing around 60% of global species diversity (Raghuraman et al., 2013; MoEF, 2014).
Estimates of the total reef area in India also vary significantly between studies due to differences in the method of data compilation, method of analysis or resolution scale used (Garg, 2015). Based on remote sensing data published by different agencies during the periods 1992-1993, 2004-2007 and 2007-2008 estimates of total coral reef area fluctuated between 841 km2, 2384 km2 and 1420 km2 respectively (Garg et al., 1998; SAC, 2011; Bahuguna et al., 2013). Spalding et al. (2001) estimated a total reef coverage of 5790 km2 representing 2.04% of global coral area. More recent estimates indicate a reduced reef area of ~2375-2383 km2 (SoE, 2009; Venkataraman, 2011; Bahuguna et al., 2013) – equivalent to 0.92% of global reef area (Souter et al., 2021). Coral coverage is presently estimated to be ~352 km2 (Gulf of Kachchh), 76 km2 (the Gulf of Mannar/Palk Bay), 1021 km2 (the Andaman and Nicobar Islands) and 934 km2 (the Lakshadweep Islands), (Bahuguna et al., 2013). Both the Lakshadweep Islands and the Andaman and Nicobar Islands contribute ~40% each to India’s total reef area (Bahuguna et al., 2013; Saroj et al., 2016).
Despite uncertainties over the total reef area it is widely assumed that the spatial extent has declined in recent decades due to increased human pressures (Latha and Prasad, 2010; Ramadas and Rajeswari, 2011; Saroj et al., 2016; Rebekah and Inamdar, 2018) and the effects of significant coral bleaching events (Arora et al., 2019b). Widespread coral bleaching in 1998 reduced coral coverage by 20-40% in the Gulf of Kachchh and Gulf of Mannar, by 20-30% in the Lakshadweep Islands and by<10% in the Andaman and Nicobar Islands (Venkataraman, 2011). In 2016, approximately 70% of corals in Palk Bay and 46% of corals across the coastal Thoothukkudi Islands in the Gulf of Mannar experienced bleaching due to high sea surface temperatures of 34.0°C (Krishnan et al., 2018). Coral bleaching events in 1998, 2010, 2016 have had a distinct impact on India’s corals, albeit with some notable variation attributed to species-specific resilience to increased temperatures (Krishnan et al., 2011; Jeevamani et al., 2013; Arora et al., 2019a; Arora et al., 2019b; Hussain and Ingole, 2020). Nevertheless, bleaching has led to widespread and significant mortality contributing to a general decline in coral reef coverage in India (Chandra et al., 2021).
The Andaman Islands suffered additional coral loss during the 2004 Indian Ocean tsunami which alone reduced coral coverage by 20-30% (Patterson et al., 2006; Majumdar et al., 2018). While the effects of bleaching can be severe, they may be temporary, and corals can recover over time. Repeated observations from Palk Bay, however, indicate a sustained decline in mean coral coverage which decreased by 37% between 2002 and 2018 (Marimuthu et al., 2020), and including an average decline of 2.5% per annum between 2007 and 2013 (Patterson et al., 2015). Anthropogenic factors (unspecified) have been implicated in the sustained deterioration of Palk Bay corals and as of 2018 live coral coverage in Palk Bay was only 17% (Marimuthu et al., 2020). Notably, whilst coral coverage has declined there has been a coincident increase in the prevalence of coral disease and of macroalgal coverage throughout Palk Bay which have been attributed to nutrient inputs (Patterson et al., 2015; Marimuthu et al., 2020). In the Gulf of Mannar coral coverage persists at around 30-37% (Magesh and Krishnakumar, 2019). Overall, the health of Indian coral reefs is generally reported as being poor (e.g. (Latha and Prasad, 2010; Ramadas and Rajeswari, 2011; Saroj et al., 2016; Rebekah and Inamdar, 2018) with pristine coral habitats described as now limited to a few areas of the Andaman and Nicobar Islands (Venkataraman, 2011; Bhatt et al., 2012b).
3.1.2 Water quality indicators
By the mid 1980’s it was estimated that India’s coastal population already discharged 4.1 x 109 m3 yr-1 of domestic sewage to the coastal zone, with a further contribution of 50 x 106 m3 yr-1 coming from inland communities via rivers (Qasim et al., 1988). By 2015, domestic sewage production in India had increased by over 350% reaching 22.5 x 109 m3 yr-1 of which ~8.4 x 109 m3 yr-1 (37%) was treated with the remaining ~15.1 x 109 m3 yr-1 (63%) discharged as untreated sewage to seas and inland water bodies (Sahasranaman and Ganguly, 2018). In addition, it is estimated that between 1981 and 2014 the national consumption of agricultural fertilizer increased by ~400% (Ramesh et al., 2017), with around 5 x 106 tons of fertilizer residue being discharged to the coastal ocean each year (Latha and Prasad, 2010). There are few studies that specifically address the consequences of these temporal trends in pollution loadings on Indian corals (Sampath, 2003; Bhatt et al., 2012b; Jameson, 2012; Samuel et al., 2012; De et al., 2017; Balachandar et al., 2023). The knowledge base for assessing nutrient impacts on the general status and trends of corals, including biodiversity and ecosystem services, is considered patchy (Bhatt et al., 2012b) and quantitative physiological studies examining the impacts remain limited (Sampath, 2003). As a necessary first step towards understanding the impact of nutrient inputs on corals quantification of the extent and severity of nutrient enrichment of coastal waters remains either unclear or unavailable for many coastal areas (Ramadas and Rajeswari, 2011; Bhatt et al., 2012a), and recent efforts to assess nutrient pollution in Indian coastal waters have highlighted difficulties caused by the lack of openness of appropriate datasets (Ramesh et al., 2017).
Nevertheless, water quality monitoring within the coastal zone has a multi-decade history in India and began in 1986 with an exploratory program that collected seasonal observations of biological and chemical variables from 120 estuarine and coastal locations to identify sources and levels of pollutants in marine waters (Madeswaran et al., 2018). In 1990 this sampling effort transferred to the Coastal Ocean Monitoring and Prediction System program (COMAPS) of the Ministry of Earth Sciences (MoES), which continued regular sampling at 84 coastal locations but with annual, bi-annual or seasonal frequency. Following a subsequent program review water quality monitoring was relaunched under the Sea Water Quality Monitoring programme (SWQM) in 2012 but financial and logistical constraints reduced sampling to 24 locations where clear signatures of reduced dissolved oxygen concentrations and increased nutrient concentrations had previously been identified (Madeswaran et al., 2018).
The underlying data of the COMAPS/SWQM programmes are not publicly available. Madeswaran et al. (2018) however presented summary results of this sampling effort with the general conclusion being that there has been a widespread decline in coastal water quality throughout India, with increased discharge of untreated sewage being the major contributory factor. Increased phosphate and ammonium concentrations dominated observed changes to nutrient concentrations and were coincident with increased faecal coliform counts. Other studies, meanwhile, highlight nutrient enrichment resulting from agricultural runoff, which is said to be restricted to coastal waters near the Hooghly, Mahanadhi, Subernarekha, and Krishna Rivers and the Godavari estuary on the east coast (Sampath, 2003). BOBLME (2015) also reported nutrient enrichment within the Mandovi-Zuari and Cochin estuaries on the west coast. Notably, these (west coast) estuaries are not close to prominent coral hosting sites but the downstream transport pathways and residence time of any inputs are not clear. In contrast, results derived from a recent global assessment of coastal eutrophication potential indicated that ~6% of coastal waters along the west and south Indian coastline exhibited eutrophication potential, compared to only 2% of waters along the east Indian coastline (de Raus Maure et al., 2021).
In the final analysis of water quality indices for India presented by Madeswaran et al. (2018), water quality in the Gulf of Kachchh (Vadinar) was deemed to be poor, in the Gulf of Mannar (Tuticorin) and the Andaman and Nicobar Islands (Port Blair) it was deemed moderate, and in the offshore location of the Lakshadweep Islands (Kavaratti) it was considered good. However, for individual water quality parameters (e.g. faecal coliform counts, total N, total P), all locations were classed as having very poor water quality based on high faecal coliform counts highlighting the widespread prevalence of sewage in coastal waters (Figure 2). Notably, Tuticorin, Kavaratti and Port Blair were deemed to have good water quality based on observed nutrient enrichment levels suggesting that the impacts of nutrient input should not be generalized but should be examined for each region separately.
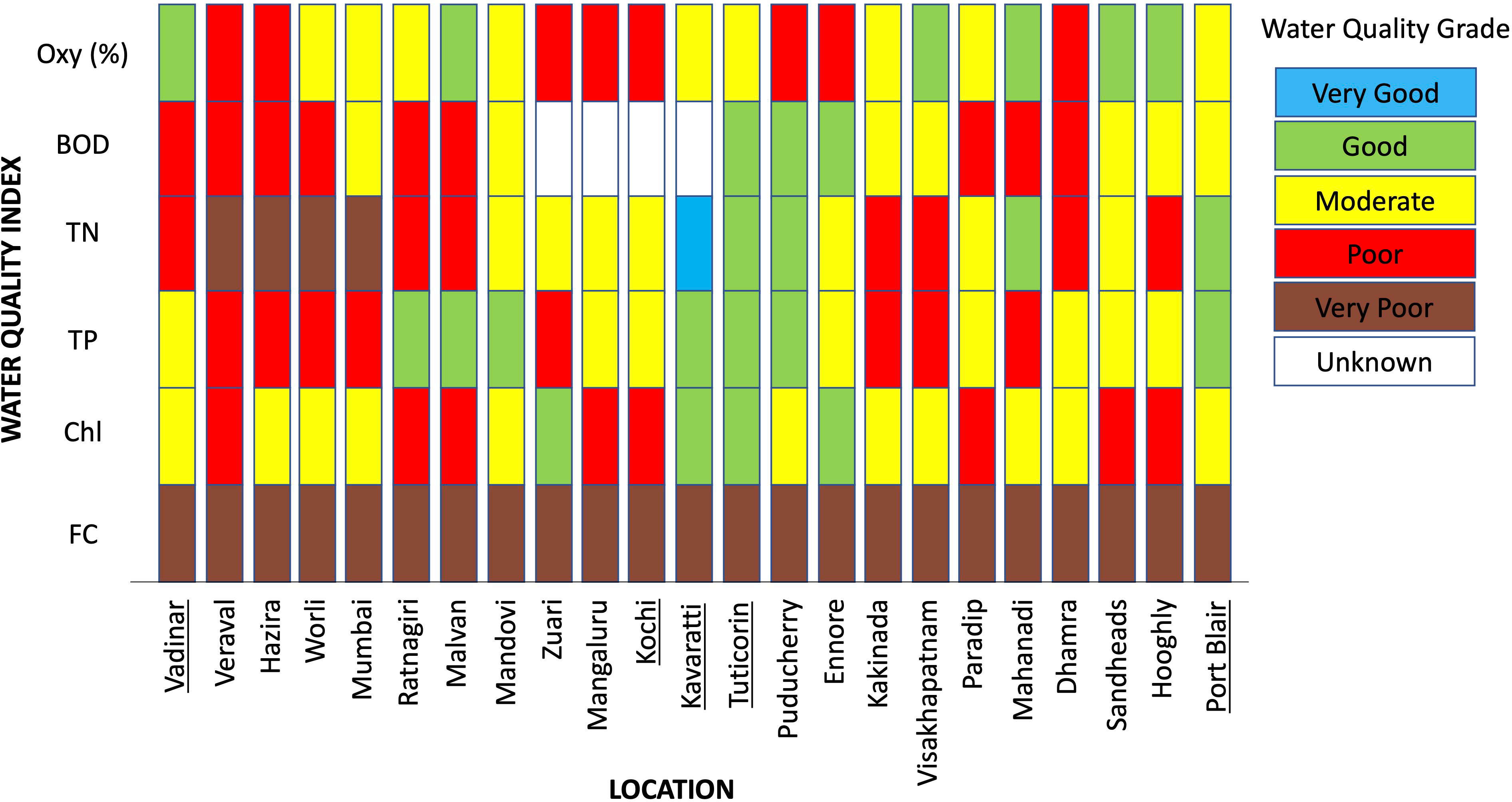
Figure 2 Water quality indicators at selected locations around India. Sampling sites close to major coral regions are underlined. All sampling sites are indicated on Figure 1 and numbered from left to right (Vadinar = 1,… Port Blair = 23). Indicators are dissolved oxygen saturation (Oxy %), Biological Oxygen Demand (BOD), Total Nitrogen (TN), Total Phosphates (TP), Chlorophyll-a (Chl) and Faecal Coliforms (FC). Figure modified from Figure 4.3.1 of Madeswaran et al. (2018).
The detrimental effects of marine litter on reef ecosystems have also received increased attention recently (Mueller and Schupp, 2020), and are briefly mentioned here due to some similarity in impacts on coral systems. Recent studies indicate that the consequences of marine litter, notably plastic waste, have grown in both scale and impact (Mulochau et al., 2020; Karthik et al., 2022), and that litter contamination can now be linked to coral death and the proliferation of invasive species (Lamb et al., 2018; Valderrama Ballesteros et al., 2018; Patterson et al., 2020).
3.1.2.1 Gulf of Kachchh
The Gulf of Kachchh experiences strong environmental variability including significant sediment and particulate loadings (Muley et al., 2000; Balasubramanian and Khan, 2001), and wide salinity ranges of 8.5 to 39 or higher (Nair et al., 1993; Mohandass et al., 2010). The sea surface temperature typically ranges from 24 to 30°C but can be higher in shallow waters. Recent water quality measurements are hard to find and can be inconsistent. Nair (2002) considered the water quality in the Gulf of Kachchh to be good, with limited potential for degradation around neighboring urban and industrial areas due to strong tidal flushing (Vethamony and Babu, 2010) and a residence time of approximately 1 day for the central Gulf (Patgaonkar et al., 2012). However, Patel et al. (2017) reported nitrate concentrations of 9.9 to 31.9 μmol L-1 and phosphate concentrations of 3.2 to 6.3 μmol L-1 specifically from reef waters in the Gulf, whilst Christian et al. (2019) reported even wider concentration ranges of 0.1 to 58.2 μmol L-1 for nitrate and 0.2 to 37.5 μmol L-1 for phosphate Saravanakumar et al. (2008), presented a two-year timeseries of nutrient concentrations that revealed nitrate and phosphate concentrations ranged from 0.23 to 7.26 μmol L-1 and 0.13 to 3.12 μmol L-1 respectively. It was further observed that nutrient concentrations were highest in August (the SE monsoon period), and lowest in March-May (summer). The maximum nutrient concentrations reported by Madeswaran et al. (2018) for the Vadinar monitoring location indicated DIN concentrations could reach ~18 μmol L-1 at inshore locations (0.5 km) and ~10 μmol DIN L-1 offshore (5 km), whilst DIP concentrations were less variable but elevated at 5.1 and 5.6 μmol L-1 for inshore and offshore waters respectively. Given the variability in reported nutrient concentrations and the absence of impact studies, the overall impact of nutrient inputs on corals is unclear though water quality indicators (Figure 2), suggest a degraded environment.
3.1.2.2 Gulf of Mannar/Palk Bay
The local circulation and ambient environmental conditions of the Gulf of Mannar and Palk Bay are strongly influenced by the changing monsoon seasons (Silas, 1968; Rao et al., 2011; Jagadeesan et al., 2013; Magesh and Krishnakumar, 2019). During the southwest monsoon, the prevailing current flow in the Gulf of Mannar is northwards towards Palk Bay, and a clockwise circulation may be determined throughout the Gulf. During the northeast monsoon, the West India Coastal Current transports low salinity waters from the Bay of Bengal into Palk Bay, but these waters are largely prevented from flowing into the Gulf of Mannar by the shallow bathymetry of Adam’s Bridge, an extensive series of limestone shoals (Rao et al., 2011). The monsoon seasons drive significant temporal and spatial variability in ambient environmental conditions (Kumar and Geetha, 2012). Coral reefs in the Gulf of Mannar are found along a string of 21 (2 submerged) coastal islands that are located around 8 km from the main shoreline and thus potentially insulated from the worst effects of pollution by the prevailing circulation. Across the region high-density seagrass ecosystems likely serve as important filters for excessive land-based nutrient input to coastal waters (Purvaja et al., 2018), though seagrass research remains rather limited (Patro et al., 2017). Nevertheless, whilst coral reefs in the Gulf of Mannar are generally considered to be better studied than elsewhere, information on the general oceanography of the Gulf and neighboring Palk Bay has been described as being limited (Rao et al., 2008; Jagadeesan et al., 2013; Jyothibabu et al., 2013). Consequently, there is uncertainty over both ambient nutrient concentrations and nutrient pollution impacts, with anthropogenic pollution effects on corals described as severe (Ramadas and Rajeswari, 2011) and negligible (Kumar and Geetha, 2012) by different studies.
Temperature, salinity, pH, and nutrient concentrations fluctuate seasonally within both the Gulf of Mannar and Palk Bay (Jagadeesan et al., 2013; Anand et al., 2015; Krishnan et al., 2018; Abhilash et al., 2019) though the timing of annual minima may vary spatially. Temperature, pH, and salinity typically reach their annual maxima in May-June, while nutrient concentrations generally reach their annual minima at this time, though some studies have reported annual minima during January-March, particularly in northwest Palk Bay (Sridhar et al., 2006; Sridhar et al., 2008; Anand et al., 2015). Reported nutrient concentrations vary widely between studies (Table 3) but in the Gulf of Mannar highest concentrations are typically measured in November-December during the northern monsoon. Annual maximum nitrate concentrations of 7 to 10 μmol L-1 and annual minimum concentrations of ~0.2 μmol L-1 are considered broadly typical for the Gulf of Mannar (Anand et al., 2015) yet several studies have reported higher annual maxima which may be indicative of localized pollution sources or terrestrial runoff (e.g. (Thangaradjou and Kannan, 2007; Pitchaikani and Lipton, 2016). Water quality monitoring activities at Tutichorin reported a maximum DIN concentration of ~60 μmol L-1 at an inshore (0.5 km) location and ~42 μmol L-1 at the offshore location (5km), while DIP concentrations reached maxima of 3.9 and 2.7 μmol L-1 respectively (Madeswaran et al., 2018). Despite the reported variability in nutrient concentrations recent near-synoptic nutrient measurements along the southeast Indian coastline during the southwest monsoon period indicate that nutrient concentrations within the Gulf of Mannar may be less variable and lower on average than concentrations found further north along the Kerala coastline (Anandavelu et al., 2020).
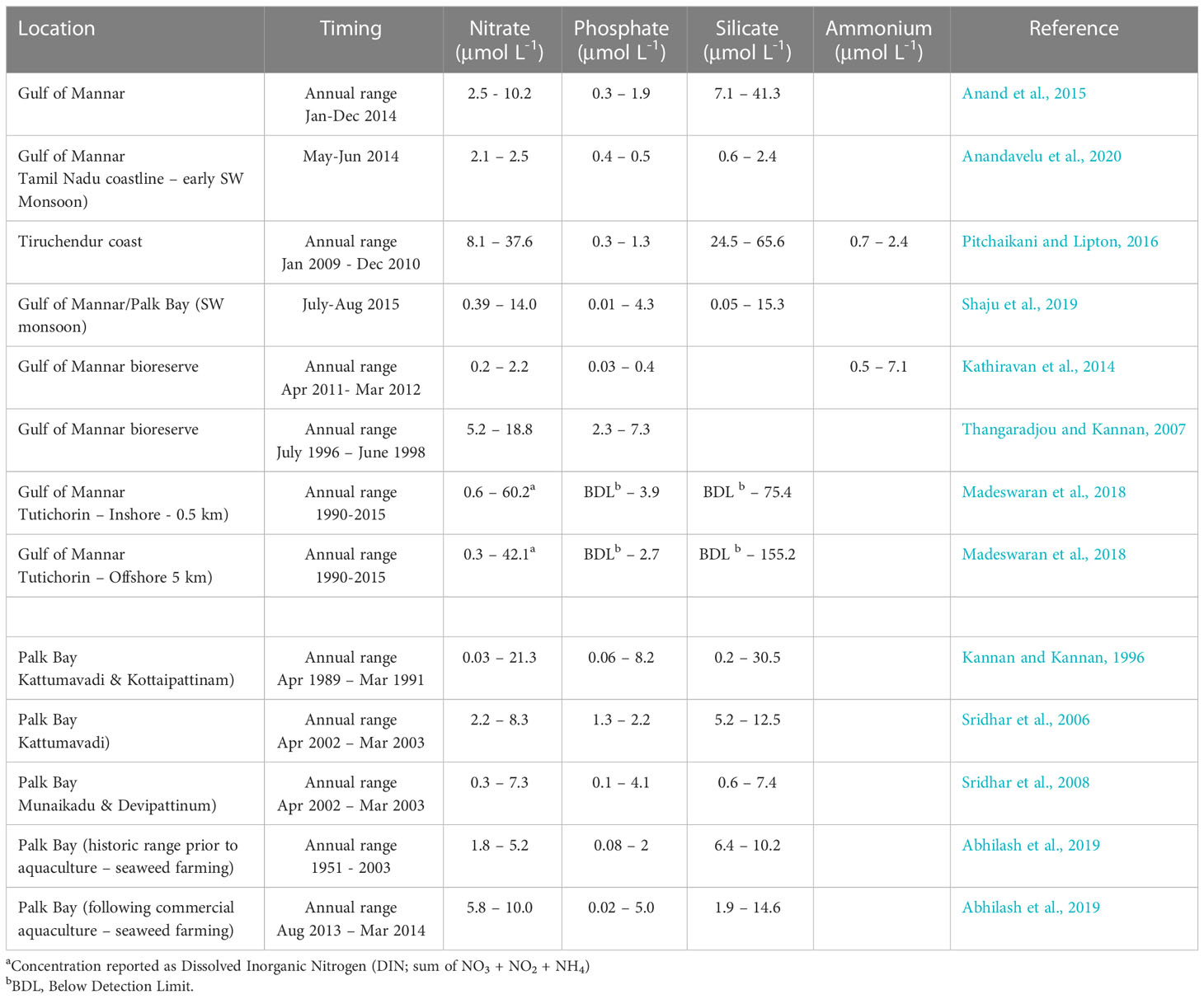
Table 3 Selected literature estimates of ambient nutrient concentrations within the Gulf of Mannar and Palk Bay.
Similar variability is reported in nutrient measurements from Palk Bay (Table 3) where significant spatial and temporal variability is evident (Sridhar et al., 2006; Sridhar et al., 2008; Purvaja et al., 2018). Along the northwest coastline of Palk Bay (Kattumavadi region), Sridhar et al. (2006) observed the highest concentrations of nitrate (8.3 μmol L-1), phosphate (2.2 μmol L-1), and silicate (12.5 μmol L-1) variously during the summer/premonsoon months (Apr-Sep) whilst the lowest concentrations were measured during the postmonsoon months (Jan-Mar). Based on a comparison with previous observations, this study reported no detrimental impacts on nutrient concentrations from aquaculture effluents, which are common in this region. However, previous observations collected between 1989 and 1991 observed a broader range of nutrient concentrations and a difference in the timing of annual nutrient maxima, which occurred during the post-monsoon season (Jan-Mar) (Kannan and Kannan, 1996). Notably, terrestrial runoff during and after the monsoon rains (Oct-Dec) was thought to have caused nutrient influx into coastal waters, resulting in a nitrate concentration of 21.3 μmol L-1.
3.1.2.3 Andaman and Nicobar Islands
The Andaman and Nicobar Islands contain 40-50% of all coral reefs in India (Jeyabaskaran et al., 2007; Jha et al., 2019) and are keenly observed for anthropogenic impacts (Majumdar et al., 2018). Despite their remoteness, human impacts on the islands reefs and marine ecosystems have increased as the human population has grown from ~25,000 in 1901 to ~380,000 in 2011 (Jha et al., 2019), with ~125,000 people living in the capital Port Blair (Jeyabaskaran et al., 2007). Anthropogenic impacts stemming from fishing, sewage discharge, fertilizer, and pesticide runoff have been reported and recent coastal development related to tourism and human population growth is said to have led to significant anthropogenic pollution of coastal waters (Majumdar et al., 2018). Outside of Port Blair, sand mining, which increases water column turbidity and sedimentation, is considered the main cause of coral demise on many islands (Jeyabaskaran et al., 2007). Sea surface temperature typically ranges from 25-29°C, and salinity is comparatively fresh (~32-33) (Varkey et al., 1996; Balasubramanian and Khan, 2001; Brown, 2007). Heavy monsoon rains and terrestrial runoff influence coastal water quality by increasing turbidity and reducing salinity, with near coastal salinities around the Andaman Islands of ~25 or lower (Sampath, 2003).
Water quality studies in the main urbanized areas of Port Blair Bay (South Andaman) and Rangat Bay (Central Andaman) reveal contrasting results, potentially as a result of environmental heterogeneity. Poor water quality conditions and higher average nitrate concentrations have been reported within both Port Blair Bay and Rangat Bay (0.5-0.9 μmol L-1) compared to less urbanized areas (<0.35 μmol L-1) (Jha et al., 2013; Jha et al., 2015a; Jha et al., 2015b). Muduli et al. (2011) found that inorganic nitrogen ( ) concentrations gradual increased from 1.3 μmol L-1 outside Port Blair Bay to 3.9 μmol L-1 at the head of the bay, which they attributed to anthropogenic factors (urban development). Similarly, phosphate concentrations increased along the gradient from 0.2 to 0.9 μmol L-1. Based on long-term nutrient measurements, however, Madeswaran et al. (2018), concluded that Port Blair Bay had good water quality (Figure 2). Despite these contradictory findings, sewage and fertilizer nutrient inputs are currently thought to be localized to waters around the major urban and agricultural areas. Indeed, several studies demonstrate significant yet very localized impacts within Port Blair Bay or Rangat Bay from runoff and sewage discharge in the form of high faecal coliform bacterial abundances or elevated nutrient concentrations (Sahu et al., 2013; Dheenan et al., 2014; Dheenan et al., 2016). Such studies also suggest rapid dilution of bacterial abundances within ~2 km of discharge points and/or spatially variable impacts from runoff, suggesting that anthropogenic impacts, whilst present, remain spatially localized.
In the South Andaman Islands, elevated nutrient concentrations of 0.2 to 21 μmol L-1 and 0.04 to 5 μmol L-1 have been reported in near coastal waters in response to land use change with significant seasonal variability in nutrient concentrations driven by monsoon rainfall (Ramesh et al., 2010). Coral reef degradation and coral loss have been reported from the South Andaman Islands, but this has been attributed to repeated temperature-induced bleaching and increased spatial competition with macroalgae and sponges though links to nutrient inputs remain unclear (Malakar et al., 2021).
Fertilizer usage in the Andaman Islands is reportedly below the national average for India (17-32 kg ha-1 vs 96.4 kg ha-1; (Jeyabaskaran et al., 2007; Government of India, 2013). During the period 2007-2012 total fertilizer imports to the Andaman Islands peaked at 1800 tons per year (Jha et al., 2019). The increase in fertilizer consumption over time may be linked to the appearance of periodic algal blooms within Port Blair Bay, which have been attributed to terrestrial nutrient runoff, though nutrient concentrations coincident with observed blooms appear only moderately enriched ( ~0.6 μmol L-1, 0.2-0.4 μmol L-1) (Goswami et al., 2020). In the less urbanized waters of Aerial Bay on North Andaman Island Jha et al. (2014) reported nutrient concentrations of<0.48 ± 0.34 μmol L-1 and<0.35 ± 0.21 μmol L-1, though they noted that nearshore concentrations were generally 50% higher than those recorded in the outer bay area due to the influence of agricultural runoff.
3.1.2.4 Lakshadweep Islands
Located in the Arabian Sea the Lakshadweep Islands, also known as the Laccadive, Minicoy, and Aminidivi Islands, are a collection of 32 islands (10 inhabited) located 200 to 440 kilometers off the southwest coast of India in the Laccadive Sea (Purvaja et al., 2019). The islands make up India’s smallest Union Territory, with a total surface area of 32 km2, a lagoon area of 4,200 km2, territorial waters of 20,000 km2, and an exclusive economic zone of 400,000 km2. Lakshadweep is the only atoll-formed reef in India and forms the northernmost segment of the Chagos-Maldive-Laccadive oceanic ridge. The fishing activities are mostly centered on the 11 islands and reef areas of Perumul Par, Valiyapani, and Cheriyapani, and several submerged banks and open reefs. The Lakshadweep atolls feature different morphological and ecological zones and are considered one of India’s most biodiverse places. The Lakshadweep Archipelago has been reported to host 148 species of corals, 91 species of sponges, 114 species of seaweed, 7 species of seagrass, 150 species of crustaceans, 424 species of molluscs, 4 species of lobsters, 225 species of echinoderms, 300 species of ornamental fishes, 601 species of finfishes, 4 species of turtles, 4 species of mammals, 101 species of birds, and many other groups of reef organisms (Government of India, 2012).
Average sea surface temperatures around the islands have increased by 0.2°C per decade since the 1980’s (Abhiya et al., 2015), and annual sea surface temperatures now range from ~28.1-31.4°C (Kumaresan et al., 2018). Salinity is typically 35-36 though seasonally can range from 34 to 38. Coral predation by Crown of Thorns starfish (A. planci) has increased in selected areas of the Lakshadweep Islands, though the cause remains unclear (Senthilnathan et al., 2014). Nutrient concentrations vary significantly between studies with strong seasonality driven by the monsoons and interannual variability associated with El Niño events likely to explain this (Arora et al., 2019a; Arora et al., 2019b). For the open waters of the central Lakshadweep Sea, Sengupta et al. (1979) reported average nutrient concentrations during October of 4.21 μmol L-1 and 0.97 μmol L-1 and concentrations of 0.29 μmol L-1 and 0.46 μmol L-1 during March/April, further noting the widespread seasonal appearance of nitrate depleted surface waters (0-75 m) and dense Trichodesmium blooms during April coincident with ammonium concentrations of up to 5.5 μmol L-1. In contrast, data reported by Kumaresan et al. (2018) indicated mean annual nutrient concentrations of 6.08 μmol L-1 and 0.93 μmol L-1 for the Lakshadweep Sea and strong seasonal and spatial variability, with concentrations varying from 0.29 to 14.8 μmol L-1, and from 0.29 to 2.1 μmol L-1 across the study period. Notably, average nutrient concentrations were highest during the summer months (Mar-May) in contrast to the results of Sengupta et al. (1979).
In more localized studies conducted around the inhabited Kavaratti atoll, nutrient concentrations appear more variable. Citing studies from the 1970’s, Balasubramanian and Khan (2001) inferred limited seasonality and low nutrient concentrations of<0.3 μmol L-1 and<0.4 μmol L-1 both within the lagoon and in the surrounding open sea. More recently, concentrations of 1.95 to 8.96 μmol L-1, 0.12 to 0.33 μmol L-1, and 2.47 to 5.93 μmol Si L-1 have been reported from within the extensive lagoon area (Antony et al., 2020). Notably, as measurements from two neighboring offshore sites indicated lower nutrient concentration ranges of 1.99 to 5.82 μmol L-1, 0.07 to 0.21 μmol L-1, and 2.50 to 4.40 μmol Si L-1, Antony et al. (2020) concluded that anthropogenic nutrient enrichment of Kavaratti lagoon waters was now evident. Whilst the observations reported by Antony et al. (2020) do appear substantially higher than those recorded from this lagoon previously (e.g. (Karati et al., 2017) (<0.5 μmol L-1) they remain broadly comparable to concentrations reported by Kumaresan et al. (2018) for the surrounding Lakshadweep Sea such that high variability in lagoonal nutrient concentrations due to water exchange cannot be fully ruled out. Karati et al. (2017) reported spatially extensive nutrient measurements across the Kavaratti lagoon during the peak of the 2016 El Niño event and obtained an average nitrate concentration of only 0.46 μmol L-1, with the average concentration reducing to 0.16 μmol L-1 as the El Niño weakened. Similarly, average phosphate concentrations were 0.26 μmol L-1 and 0.13 μmol L-1 during and after the El Niño event. High ammonium concentrations of 5.4 to 8.7 μmol L-1 were also recorded within the lagoon, which is noteworthy as recent estimates of sewage discharge volumes (50,000 to 120,000 liters per day (Purvaja et al., 2019)), have increased expectations of anthropogenic impacts. Yet in March 2006 Robin et al. (2012) measured concentrations of ~3.2 μmol L-1, 0.65 μmol Si L-1, ~0.25 μmol L-1 and 0.34 μmol L-1 thus, it is far from clear whether existing nutrient measurements from the Lakshadweep Islands are sufficient to document anthropogenic impacts here, with long-term observations suggesting an absence of nutrient impacts (Figure 2; Madeswaran et al., 2018).
According to climatology, the Arabian Sea generally sees only one cyclonic storm every year. However, cyclonic disturbances over the Arabian Sea have increased to nearly four per year on average between 2015 and 2020, with cyclones and severe cyclones increasing to two per year on average. A report published in 2015 by the National Disaster Management Authority identified a rise in the severity and frequency of cyclones on India’s western coast as a result of global warming (NDMA, 2015). Two recent cyclones (Ockhi in Nov 2017; Tauktae in May 2021) caused severe devastation across the islands with impacts observed up to a depth of 20 m in the eastern reef system of Kavaratti Island following cyclone Ockhi (Riyas et al., 2020).
3.1.3 Reported impacts of nutrient pollution
Despite Indian corals having been widely studied, particularly since the 1960’s (Saroj et al., 2016; De et al., 2017), it remains difficult to quantify or satisfactorily attribute nutrient pollution impacts to long-term changes in coral coverage or coral health in most cases. Indeed, most time-series observations of Indian coral ecosystems post-date the first global bleaching event in 1998, with the duration of many studies limited to only a few years or less (Chandra et al., 2021). Algal overgrowth is identified as a threat to Indian corals generally (MoEF, 2014), with exotic seaweed cultivation considered a particular concern (Patterson et al., 2015). Indeed, whilst coral overgrowth by green algae is reported along the northern (Keelakarai) coastline in the Gulf of Mannar and is generally attributed to nutrient inputs (Rajasuriya et al., 2000), with increased macroalgal coverage subsequently linked to reductions in reef-associated faunal biodiversity (Ramesh et al., 2020), the cause for such changes is not always clear. Effluent discharge from several major industries, including copper smelting, chemical manufacture, and salt panning, contribute to broader pollution impacts in this region likely obscuring impacts from nutrient inputs alone (Samuel et al., 2012; Anand et al., 2015). In addition, ash residue from a regional thermal power station (at Thoothukudi) is also released directly into the coastal zone, likely increasing water column turbidity or smothering localized benthic habitats (Anand et al., 2015). In neighboring Palk Bay, the appearance of epiphytes within seagrass meadows has been attributed to nutrient inputs though such appearances do not yet appear to be widespread (Purvaja et al., 2018). Abhilash et al. (2019) found no evidence of any negative impacts on water quality from commercial-scale seaweed cultivation activities in Palk Bay, though their data appear to show a significant temporal (decadal) increase in ambient nitrate and phosphate concentrations, which may be indicative of longer-term changes in seasonal nutrient inputs to Palk Bay from the Bay of Bengal (mainly riverine in origin) and neighboring coastal waters. In the Andaman and Nicobar Islands deforestation has increased sediment (and likely nutrient) loadings to nearshore reefs (Rajasuriya et al., 2000), while a growing tourism industry has increased sewage release within the Andaman Islands (Majumdar et al., 2018). Information on nutrient pollution impacts on coral reefs in the Gulf of Kachchh and along the west Indian coast remains limited, though in the Gulf of Kachchh corals are now considered exposed to significant anthropogenic disturbances (Raghuraman et al., 2013; Madeswaran et al., 2018) in addition to strong environmental fluctuations (Muley et al., 2000; Chandran et al., 2021).
Quantified impacts on corals and other related habitats from agricultural runoff remain limited, but river and sewage discharges have been linked to an increase in the frequency and distribution of phytoplankton blooms in Indian coastal waters over recent decades (Jameson, 2012; Padmakumar et al., 2012; Samuel et al., 2012; BOBLME, 2015; Ramesh et al., 2017; Karthik et al., 2020). Between 1908-2009, 101 algal blooms were reported along the western and eastern coastlines, and in particular along the southwest coastline of India, with an increase in frequency after 1950 (D’Silva et al., 2012). These algal blooms were found primarily in waters off the coasts of Goa, Mangalore, Kozhikode, and Kerala (west coast) and attributed to seasonal upwelling and/or high riverine discharge following the southwest monsoon rains. Padmakumar et al. (2012), reported a far greater frequency of blooms identifying 80 algal blooms around India between 1997 and 2010 alone, with a strong geographical focus around the southwest coast between Goa and Kollam. Historically, algal blooms within the main coral regions have been infrequent with only 3 cyanobacterial blooms reported in the Lakshadweep Islands, 2 dinoflagellate blooms in the Andaman and Nicobar Islands, 2 cyanobacterial blooms in Palk Bay, and a single dinoflagellate bloom in the Gulf of Mannar, nevertheless, almost all occurred after 1950 (D’Silva et al., 2012; Padmakumar et al., 2012). No blooms have been reported from the Gulf of Kachchh. More recently, Shaju et al. (2019) argued that aquaculture activities in the Gulf of Mannar were very likely linked to increased nutrient concentrations, decreased water quality conditions, and the appearance of a Trichodesmium dominated algal bloom. While unusual phytoplankton blooms can be indicative of long-term changes to nutrient conditions, recently reported blooms of the bioluminescent dinoflagellate Noctiluca scintillans within the Gulf of Mannar (Gopakumar et al., 2009; D’Silva et al., 2012; Samuel et al., 2012; Shunmugaraj et al., 2020) and their role in creating temporarily hypoxic conditions leading to increased coral mortality (Raj et al., 2020) should be interpreted cautiously. N. scintillans is not only widespread in the Arabian Sea (Gomes et al., 2008; Gomes et al., 2014) but its geographic distribution has changed significantly in recent years with these changes linked to freshwater inputs and snow loss over the Himalayan-Tibetan plateau (Goes et al., 2020). The presence of N. scintillans in Indian waters is also common and multiple blooms of this species have been recorded (D’Silva et al., 2012). Recent changes in the distribution of N. scintillans towards coral regions may therefore reflect wider ocean warming trends and the spread of hypoxic conditions throughout the Indian Ocean (Gomes et al., 2014) rather than the impact of local nutrient inputs alone.
3.1.4 Current protections and safeguards
Across India, around 35 million people live within 30 km of a coral reef, with many coastal communities dependent upon reefs for livelihoods and for the provision of edible protein (Burke et al., 2011; Balaji et al., 2012; Raghuraman et al., 2013). Ecosystem services rendered by coral reefs include fisheries, tourism and recreation, maintenance of biodiversity, construction materials for Islanders, and protection of coastal areas against erosion, flooding and salinity ingression (Hoon and Tamelander, 2005; Dixit et al., 2010). Despite legal safeguards and other conservation initiatives to protect India’s reefs, localized anthropogenic factors continue to have significant detrimental impacts on the coastal zone and associated ecosystems, which are considered to be substantially damaged due to human pressures (Latha and Prasad, 2010). While destructive fishing practices, lime production and coral mining were once thought to be the most serious threats facing corals (Patterson, 2002), with the visual impacts of these activities perhaps explaining why reports of their negative impacts are more widespread and appeared earlier in the literature, the list of threats has grown. Damage due to bottom trawling, siltation due to coastal developmental, coral disease due to pollution and sewage discharge, exotic algal invasion, and increased coral mortality due to pelagic algal blooms are increasingly common (Wilson et al., 2005; Chandrasekaran et al., 2008; Thinesh et al., 2013; Machendiranathan et al., 2016; Asir et al., 2020; Raj et al., 2020). Growing tourist inflow and recreational diving have led to increased physical damage with subsequent impacts on the ecological dynamics of the reef (Purvaja et al., 2019). In more recent years, heavy industry, rapid coastal development, and anthropogenic shoreline change have added additional stress to the marine environment in the form of increased sedimentation, turbidity and industrial and municipal discharges (Patterson, 2002; MoEF, 2014; Magesh and Krishnakumar, 2019; Jinoj et al., 2020; Jinoj et al., 2021). Proposed major infrastructure projects, such as the long-planned Sethusamudram Shipping Canal, threaten to deliberately dredge the centre of the Gulf of Mannar and through the limestone shoals of Adam’s Bridge to create a navigable sea route, with the full impacts on the surrounding marine habitats poorly known (Rao et al., 2008).
The negative effects of marine nutrient pollution are recognized regionally but quantification of long-term impacts on the coastal zone remain difficult to obtain due to the limitations of existing data (Kaly, 2004; BOBLME, 2010; BOBLME, 2015). There has been only limited specific study of the links between the discharge of dissolved nutrients and coral health in India. At the same time, various measures, both scientific and managerial, already exist to minimize pollution impacts particularly on human health or on fisheries but there remains a broader need to protect reef ecosystems for the benefit of communities that depend upon the services they provide. Such communities are at high risk and vulnerable to factors that degrade the reef environment. Coral transplantation involving local communities has been carried out in attempts to promote reef recovery and restoration in degraded areas (Mathews, 2008; Melkani, 2008; Subburaman et al., 2014; Kumar et al., 2017; Nanajkar et al., 2019). Such efforts, however, do not on their own address underlying pollution issues.
Proper management of coral reefs within Marine Protected Areas (MPAs) has a beneficial influence on the resilience and functioning of reefs (Roberts et al., 2017; Topor et al., 2019) and provides additional benefits to coastal communities. The mix of National Parks, Sanctuaries and Biosphere Reserves in India comprises 25 MPAs around the mainland and 106 MPAs across the island territories. In the MPA network, the Marine National Park and Marine Sanctuary in the Gulf of Kachchh, the Malvan Marine Sanctuary in Maharashtra, the Gulf of Mannar Marine National Park in Tamil Nadu (part of the Gulf of Mannar Biosphere Reserve), the many national parks, sanctuaries, and biosphere reserves in the Andaman and Nicobar Islands, and the Pitti Bird Sanctuary in the Lakshadweep Islands provide legal protection for coral reefs and their biodiversity, in some cases since the 1970s. In addition to location-specific protection status through MPAs, species-specific protection is also provided for corals in India. The Wild Life (Protection) Act, 1972 includes reef-building corals, black corals, organ pipe corals, fire corals, and sea fans as Schedule-I species and provides legal protection against harvesting them in India. Under the Coastal Regulation Zone (CRZ) Notifications of 1991, 2011 and 2019, corals, coral reefs, and associated biodiversity are also designated as Ecologically Sensitive Areas (ESA) - Coastal Regulation Zone-I (CRZ-I) areas. The ecological sensitivity of reef habitats is further specified under the Environment (Protection) Act, 1986, which both prohibits and regulates coastal development activities in and around reef ecosystems (MoEF, 1991; MoEF, 2011; MoEFCC, 2019). The CRZ Notification, 2011 and 2019, also established effluent standards under the Environment (Protection) Act, 1986, and specifically prohibits the discharge of untreated wastewater and effluents onto the coast.
In principle, therefore, coral reef ecosystems are accorded the highest legal protection status to ensure their long-term protection and conservation. As part of this protected status, detailed Coastal Zone Management Plans (CZMP) are required by the respective coastal state or union territory to monitor and implement the provisions of the CRZ Notification for conserving coastal habitats and to aid the sustainable development of coastal stretches along the mainland coast of India. Special attention is paid to the protection and conservation of ESA during the preparation of developmental plans.
Coral reefs are classified as CRZ 1A (ii), and the conservation, protection and management framework for ecologically sensitive areas is provided in Annexure 1, 1.2 – iv a & b which reads,
a. Active and live corals and coral reefs identified and delineated shall be declared and notified as an ESA under the Environment (Protection) Act 1986 (29 of 1986);
b. It shall be ensured that no activities that are detrimental to the health of corals, coral reefs and their associated biodiversity, such as mining, effluent and sewage discharge, dredging, ballast water discharge, ship washings, fishing other than traditional non-destructive fisheries, construction activities and the like, are taken up in and around the coral areas.
The CRZ protects coastal areas along the coastline of India. Amendments to the CRZ Notification introduced simultaneously with the Island Protection Zone (IPZ) legislation in 2011 and 2019 resulted in a division of the protective legislation. Currently, the CRZ applies only to the mainland coast of India, while the IPZ applies only to the islands of Andaman and Nicobar and Lakshadweep. While the islands of a large geographical area are managed as per the Island Coastal Regulation Zone (ICRZ) Plan, the rest of the islands in Andaman and Nicobar and all the islands in Lakshadweep are to be managed in accordance with the Integrated Island Management (IIM) Plan, as provided under the IPZ Notification. The ICRZ shall ensure that no activities that are detrimental to the health of corals, coral reefs and their associated biodiversity, such as mining, effluent and sewage discharge, dredging, ballast water discharge, ship washings, fishing other than traditional non-destructive fisheries, construction activities and the like, are taken up in and around the coral areas.
That there exist clear requirements to consider the impact of effluent and sewage discharge on coral ecosystems and that the evidence to assess the impact of such discharges is currently limited argues strongly for improvements and broadening of coral monitoring activities and coordinated efforts within existing water quality monitoring programmes to ensure corals are fully considered going forward.
3.2 Sri Lanka
3.2.1 Coral reef extent, condition and environmental setting
Coral reefs are found discontinuously around the Sri Lankan coastline (Figure 3). Estimates of total coral reef coverage vary widely from 680 km2 or 0.24% of the global coral area (Spalding et al., 2001) to more recent estimates of 475 km2 (0.18%; (Bahuguna et al., 2013)), though the latter estimate may be inaccurate as it omits an important area between Mannar Island and the Kalpitiya peninsula where the largest shallow water coral reef patches are found. Modelled projections of coral conducive habitat area however reach 2739 km2 (1.05%; (Ellepola et al., 2021)). Coral reef types consist of fringing, patch and barrier reefs with the near-shore distribution of corals along approximately 2% of the Sri Lankan coastline (Rajasuriya and White, 1995; Kumara et al., 2008). Major coral habitats are found in the north/northwest, east, and southwest of the country, with the distribution said to be strongly influenced by ambient nutrient concentrations (Ellepola et al., 2021). Coral diversity is currently estimated to be ~245 species (Rajasuriya et al., 2004; Rajasuriya, 2012; Weerakoon et al., 2018; Arulananthan et al., 2021), rising from earlier estimates of ~170 species in the early 1990’s (Rajasuriya and White, 1995). The full diversity is likely higher due to new species reports emerging from the poorly studied Jaffna Peninsula (Arulananthan et al., 2021).
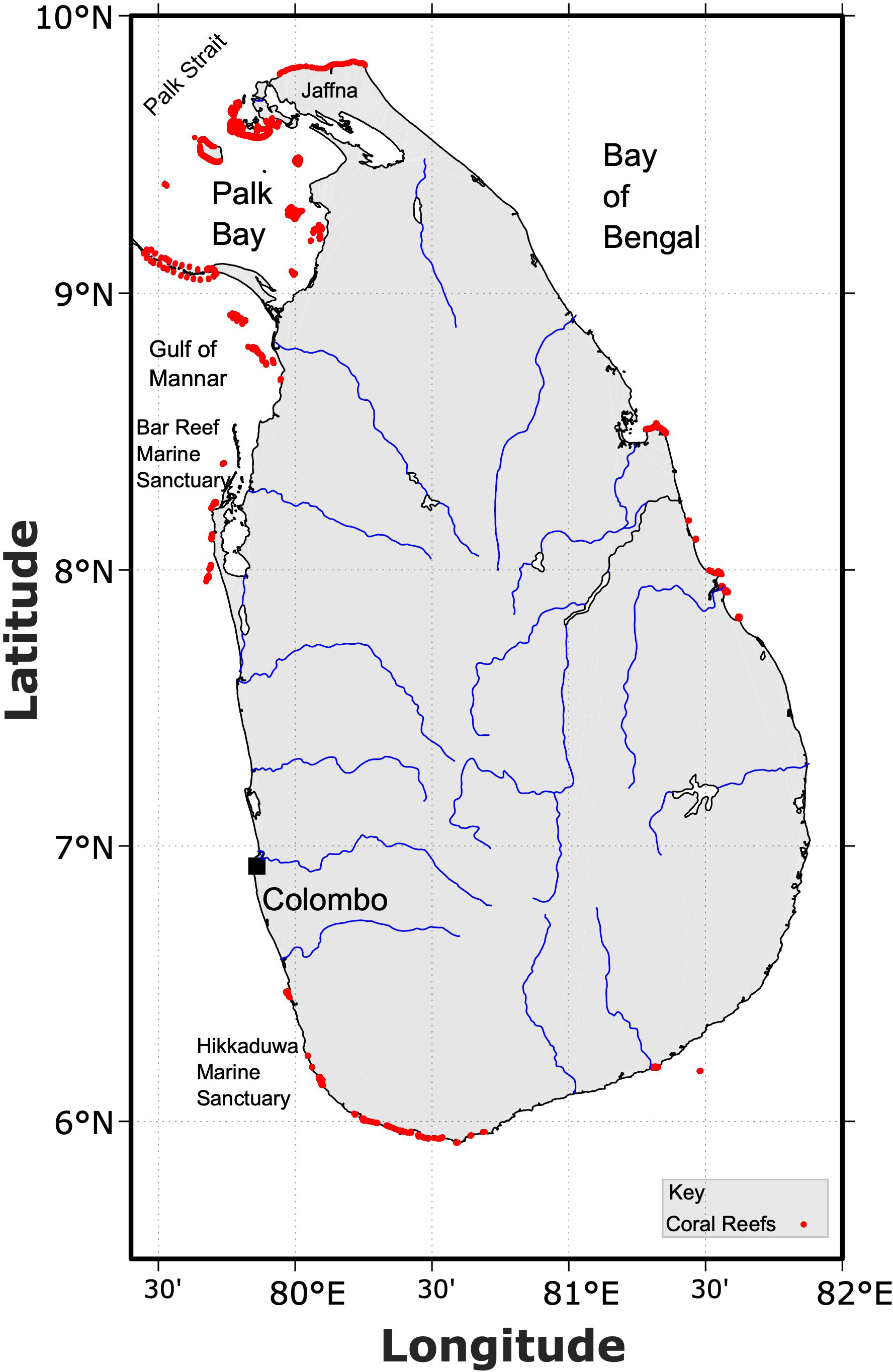
Figure 3 Map showing the distribution of coral reefs in Sri Lankan waters and placement of major river systems. Coral distribution based on data compiled by UNEP-WCMC et al. (2018).
Sri Lanka’s climate is tropical, with two main seasons namely the southwest monsoon (May-Sep) and the NE monsoon (Oct-Feb) periods, both of which bring heavy rain. The 103 rivers in Sri Lanka discharge considerable river-borne sediment loads creating a particularly turbid coastal environment. Mean sediment loads within Sri Lankan rivers are above average compared to other Asian rivers (Silva et al., 2005). The continental shelf around Sri Lanka covers approximately 30,000 km2, has an average width of 22 km, and typical water depths of 20-65 m, which increase rapidly beyond the shelf break to depths of 3500 m (UNEP, 1986; BOBLME, 2013). Sea surface temperatures typically range from 26-30°C over the year, and like the prevailing surface currents, are heavily influenced by the monsoon seasons (Rajasuriya, 2002; Survey Department of Sri Lanka, 2007). Ocean currents reverse seasonally along the eastern, western and southern coasts due to the monsoons (Schott and McCreary, 2001; Shankar et al., 2002; de Vos et al., 2014) whilst upwelling occurs along the southern and southeastern coastlines during the southwest monsoon period (Vinayachandran, 2004; Yapa, 2009; de Vos et al., 2014).
Reefs on the west coast of Sri Lanka are mainly based on sandstone/limestone foundations and typically have very low coral coverage of less than 5%, an exception being Bar Reef which is a comparatively biodiversity rich coral habitat. The majority of coral species belong to the family Faviidae. Small colonies of tabulate Acroporids are found occasionally. However, the reefs support high fish diversity as well as many species of invertebrates, including 5 species of spiny lobsters (Panulirus Homarus, P. longipes, P. pencillatus, P. ornatus, and P. polyphagus). Isolated coral patches with an extent of about 1 hectare occur 15 to 20 km offshore on the west coast at a depth of about 20 m (Survey Department of Sri Lanka, 2007). Many coral species live in these coral patches, mainly in the families of Faviidae, Poritidae, Mussidae and Pocilloporidae, but due to the water depth, they lack the branching Acroporids like Acropora formosa, tabulate A. hyacinthus, A. cytherea and the foliose Montipora spp. that are common on shallow coral reefs.
Coral reefs in northern Sri Lanka, particularly around the Jaffna Peninsula and in the Gulf of Mannar, are relatively undamaged and minimally impacted by human activities (Rajasuriya, 2008). In contrast, coral reefs found elsewhere around Sri Lanka have suffered extensive degradation, and few, if any, can be considered pristine. Rapid deterioration due variously to mining, blast fishing, A. planci infestation, and sedimentation was noted almost four decades ago (UNEP, 1986). Since then, repeated bleaching events, tsunami damage, and poor fishing practices have continued to significantly degrade Sri Lanka’s reefs (Rajasuriya, 2005; Rajasuriya, 2008; Souter et al., 2021). While the overall condition of corals in Sri Lanka is considered poor (Tamelander and Rajasuriya, 2008), resident coral populations have recovered well following bleaching events, indicating that environmental conditions remain favourable (Chandra et al., 2021). Coral reefs along parts of eastern Sri Lanka, particularly within Pigeon Island National Park (off Trincomalee), have also experienced and recovered from A. planci infestations including a notable outbreak in the late 1960s and early 1970s that led to the localised loss of coral cover (De Bruin, 1972; Rajasuriya et al., 2005). While this outbreak was ultimately short lived, with coral recovery noted over subsequent decades (Rajasuriya et al., 2005), ongoing local efforts to locate and remove A. planci occasionally return large numbers of this corallivorous starfish.
The major coral regions include notable formations at Bar Reef, Silavathurai, and Arippu and Vankalai in the Gulf of Mannar, in the northern islands around Jaffna Peninsula, and along the southern and eastern coastlines (Rajasuriya, 2002). Current reef locations, status, and major threats are summarized in Table 4. Coral reefs around Sri Lanka have long been recognized for their rich biodiversity, yet almost all reef formations have endured threats from destructive fishing practices, overfishing, coastal development (sedimentation and alteration of nearshore currents), mining, and pollution for at least the last 20 years and very often longer (Rajasuriya et al., 2000; Rajasuriya et al., 2004).
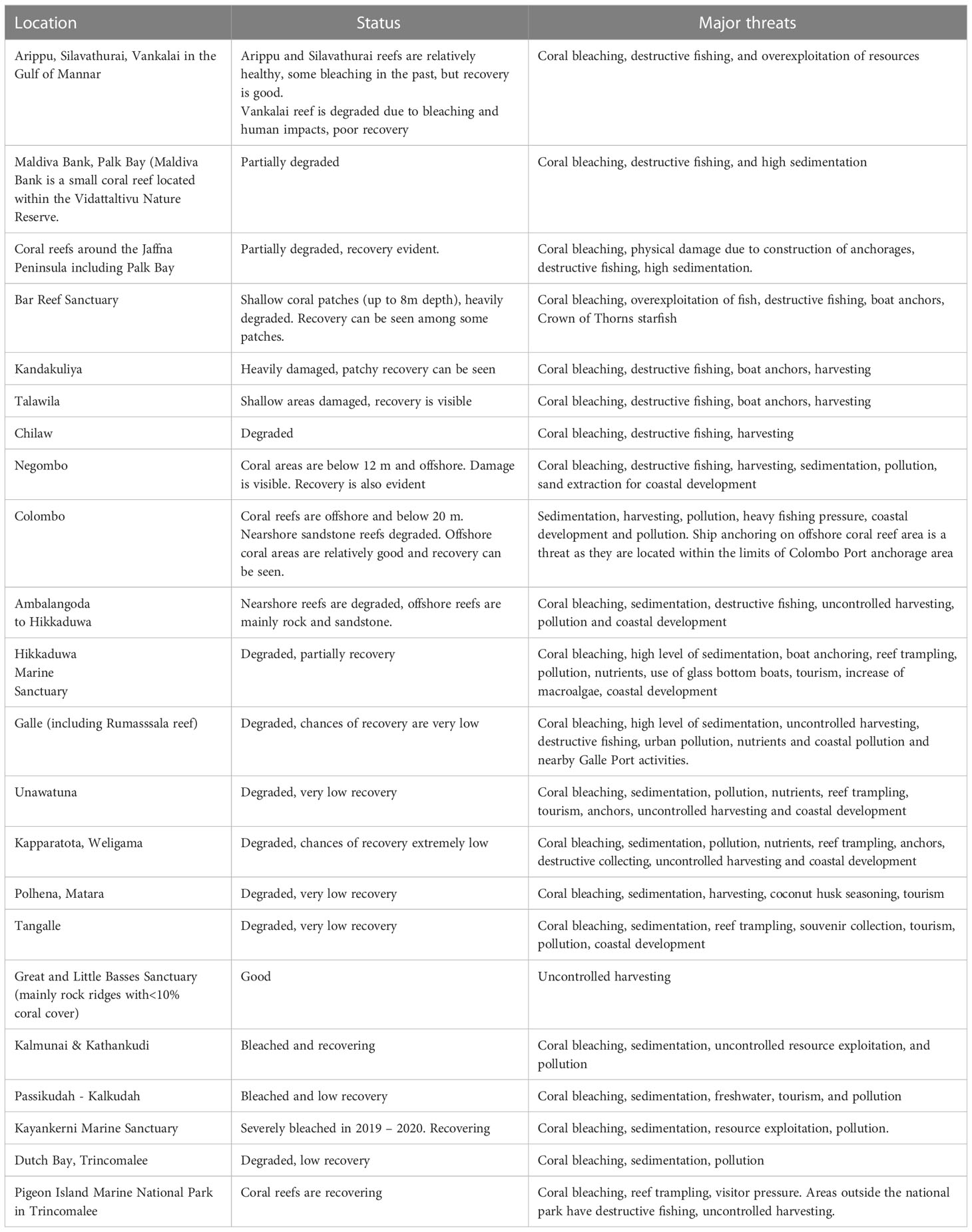
Table 4 Major coral reef locations in Sri Lanka, their status and major threats facing their long-term future.
3.2.2 Water quality indicators
Pollution of inland and coastal waters has been a noted problem in Sri Lanka for several decades and arises largely from agricultural runoff, industrial activities, coastal development, sewage, and maritime activities (UNEP, 1986; Bandara, 2003; Joseph, 2003; BOBLME, 2013; BOBLME, 2015). Despite the coastal zone hosting 4.6 million people, or around 25% of the country’s population, and over 60% of industrial activity (Rajarathna and Nianthi, 2019), studies on coastal water quality remain very limited (Ileperuma, 2000; Joseph, 2003; BOBLME, 2013; Niroshana et al., 2013; BOBLME, 2015; Manage et al., 2022). Many industries continue to openly discharge effluents without pre-treatment directly into fresh and marine waters (UNEP, 1986; Bandara, 2003; Geekiyanage et al., 2015). Riverine habitats have undergone a rapid environmental change in response to anthropogenic activities (Amarathunga et al., 2013), whilst estuaries and coastal lagoons experience notable pollution (Gammanpila, 2010). Historically, there has been heavy national usage of fertilizers in Sri Lanka compared to other South Asian countries, with fertilizer application increasing over 30-fold between 1950 and 2000 reaching over 600,000 tons per annum (Joseph, 2003). Recent Government efforts since 2015 have decreased fertilizer consumption significantly (World Bank, 2021). Widespread contamination of coastal aquifers (Jayasingha et al., 2011; Jayasingha et al., 2012), coastal lagoons (e.g. (Gammanpila, 2010)) and the occurrence of coastal algal blooms have been linked to nitrate pollution of coastal waters from agricultural runoff, though the evidence base to assess the breadth and severity of pollution impacts on the marine environment remains small, and many impacts are largely unknown (Ileperuma, 2000; Joseph, 2003; Geekiyanage et al., 2015). In recent years, high levels of organic and inorganic nutrient pollution have been reported in the coastal waters along parts of the west coast, south of Negombo (Hettige et al., 2014), inferred along parts of the eastern coast (Perera, 2019), or measured in coastal lagoons (Gammanpila, 2010; Harris and Vinobaba, 2013), while oil and organic nutrient pollution has been reported within fishing areas along the southern coast (Niroshana et al., 2013; Weerasekara et al., 2015). Common indicators of coastal nutrient enrichment, such as increased chlorophyll concentrations or decreased dissolved oxygen levels, have not been widely recorded from Sri Lankan coastal waters (Bandara, 2003; BOBLME, 2013), and only rarely within surface water and estuarine settings (Silva, 1996; Dahanayaka et al., 2013). Recent observations of high biological oxygen demand in coastal harbor settings may have multiple origins, including fish processing, oil and grease discharge, and sewage input (Bandara, 2003; Niroshana et al., 2013; Weerasekara et al., 2015; Manage et al., 2022). In some coastal areas, eutrophication effects may be masked by seasonal upwelling, particularly along the southeastern and southern coastlines during the southwest monsoon months, where reduced sea surface temperatures and elevated chlorophyll concentrations may be advected and overlap with known coral formations (Vinayachandran, 2004; Yapa, 2009).
Industries like agriculture, aquaculture, and fisheries remain central to the Sri Lankan economy, yet with approximately one-third of the land area in Sri Lanka cultivated, including 17% of the coastal zone, widespread and heavy usage of fertilizers, pesticides, and herbicides result in high nutrient discharge to the coastal environment (BOBLME, 2013). Despite existing detailed summaries of the major pollution sources, the magnitude of pollution inputs to coastal waters and the impacts on specific habitats like coral ecosystems remain poorly documented. A general lack of observational data, particularly time-series data, with which to study water quality, ascertain temporal trends, or identify pollution hotspots, makes quantification of pollution impacts difficult. Consequently, Joseph (2003) ranked pollution as fourth on the list of causes of reef damage and degradation in Sri Lanka, after coral mining, coral bleaching, and destructive fishing practices. Coral mining, however, stopped after the 2004 Indian Ocean tsunami due to the strict enforcement of a national ban on the use of lime kilns and the prohibition of using coral lime for construction purposes (Rajasuriya, 2005). The degradation of Sri Lankan coral reefs due specifically to nitrogen inputs to coastal waters has not been adequately ascertained, and the present evidence base is insufficient for quantifying the extent of nutrient over-enrichment of coastal waters. There are currently no studies that investigate the physiological impacts of nutrient enrichment on Sri Lankan corals, and past studies on coral reef habitats are now considered to be outdated (Ellepola et al., 2021).
Nutrient observations for the coastal zone are sparse. Along southern Sri Lanka, Ekanayaka et al. (2016) reported mean concentrations of 7.07 ± 5.9 μmol L-1 for nitrate, and 1.42 ± 0.78 μmol L-1 for phosphate during the southwest monsoon and inter-monsoon months (August to October). This study included sites with known coral coverage, including Rumassala Marine Sanctuary and Polhena Reef Fishery Managed Area (Perera and Vos, 2007), suggesting that relatively high ambient nutrient concentrations may occur seasonally, possibly in response to upwelling. Such concentrations are comparable to typical nutrient concentrations reported for Sri Lankan rivers of ~10 μmol N L-1 and 1.6 μmol P L-1 (Silva et al., 2005), but higher than those reported along the western coastline (0.3-3 μmol L-1; (Hettige et al., 2014)). Historic nutrient data in the World Ocean Database (WOD 2018) for the region between 5 and 10°N and 79 and 82.5°E, consists of only 27 observations of nitrate and 37 observations of phosphate for the period 1960 to 1995, with observations mainly from waters southwest of Sri Lanka. The available data, however, is useful for providing an order of magnitude estimate of ambient nutrient concentrations in offshore waters and indicates near surface (5 m) nitrate concentrations of 0.01–2.2 μmol L-1 (mean ± stdev 0.25 ± 0.47 μmol L-1) and phosphate concentrations of 0.04 –0.86 μmol L-1 (mean ± stdev 0.23 ± 0.19 μmol L-1). Near surface N:P molar ratios range from 0.01 to 5.44 (1.18 ± 1.1) indicating a nitrogen limited environment.
During a year-long study of the coastal Batticaloa lagoon (east coast), Harris and Vinobaba (2013) observed significant nitrate concentrations of 12 to 82 μmol L-1 and phosphate concentrations of ~0.9 to ~11 μmol L-1. The lagoon itself has a limited and intermittent exchange with the open ocean, but does receive water from the surrounding land, so the reported nutrient concentrations are likely elevated in the lagoon due to a lack of flushing. Weerasekara et al. (2015) meanwhile, investigated water pollution in several fishing harbors along the south and southwest coasts that do exchange freely with the open ocean and observed typical nitrate concentrations of ~7 to ~9 μmol L-1 and phosphate concentrations of ~4 to 10 μmol L-1. A similar study from another fishing harbor reported comparable maximum phosphate concentrations of ~9 μmol L-1 (Niroshana et al., 2013). Both studies confirmed severe oil pollution, chemical pollution, and microbial contamination of harbor waters. Perhaps the most extensive study of coastal water quality to date is that of Manage et al. (2022), who surveyed conditions around the southern and western coastlines, a region home to over 40% of Sri Lanka’s population and a region receiving considerable anthropogenic inputs. Though the overall conclusion was that environmental conditions had been degraded by anthropogenic activities, with widespread observations of faecal coliform and oil and grease contamination, the reported nutrient observations broadly suggested the presence of nitrogen limitation within coastal waters and of limited nitrogen enrichment. Mean nitrate concentrations along both the western and southern coastlines were only 0.7 μmol L-1, and despite sampling a range of locations including fishing harbors, exposed beach front areas, tributaries, river plumes, coastal lagoons and sea canals, no enrichment was observed. Ammonium concentrations were more variable reaching ~2.5 μmol L-1 along the western coastline and 4.4 μmol L-1 along the southern coastline but in both regions the mean concentrations were comparable at 0.23 and 0.27 μmol L-1, respectively. Phosphate concentrations meanwhile reached ~13.6 μmol L-1 and 7.2 μmol L-1, along the western and southern coastlines, and were generally elevated averaging 5.3 μmol L-1 and 4.5 μmol L-1.
3.2.3 Reported impacts of nutrient pollution
Sri Lankan reefs have been extensively damaged by human activities related to coral mining, sedimentation, destructive fishing practices, boat anchors, and latterly by various chemical pollutants, though the effects and significance of any particular factor vary regionally (Öhman et al., 1993; Rajasuriya, 1997; Perera et al., 2002; Rajasuriya, 2002; CC & CRMD, 2018; Weerakoon et al., 2018). Degradation of corals due to pollution from discarded plastics and oil, deliberate discharge of sewage or industrial effluents, and agricultural runoff was noted almost 30 years ago (Öhman et al., 1993), but more recent assessments suggest that the impacts of sewage and other pollutants, including oil, may now be acute in some regions (BOBLME, 2013; Niroshana et al., 2013; MoMD&E, 2016; CC & CRMD, 2018). Currently, the impact of destructive fishing practices, sedimentation and bleaching is generally more evident and considered more directly connected to the long-term decline of Sri Lankan coral reef habitats than pollution (Rajasuriya and White, 1995; Joseph, 2003; MoMD&E, 2016; CC & CRMD, 2018). Recent maritime disasters, such as the sinking of the X-Press Pearl container ship in June 2021 (UNEP, 2021; Rubesinghe et al., 2022), have raised public awareness of the environmental and human health consequences of marine pollution, which may yet lead to longer-term and more widespread monitoring of coastal waters.
Nevertheless, extensive coral bleaching due to increased ocean temperatures with little or no post-bleaching recovery of coral communities in regions where local stress factors are evident represents a major existential threat to Sri Lankan corals (Perera et al., 2002), and suggests that that recovery from bleaching may be weaker in those regions where local stress (e.g. pollution) is high. However, monitoring efforts to quantify coral bleaching impacts on biodiversity, coral reef health and the contribution to the loss of fisheries do not specifically consider the additional pressures represented by nutrient pollution. Similarly, while overfishing and destructive fishing practices (including the use of explosives) are acknowledged challenges facing coral reef management, overfishing persists due to lack of enforcement, despite decreased fishery catches (Silva et al., 2018).
3.2.4 Current protections and safeguards
Until recent maritime accidents such as the X-Press Pearl sinking caused financial hardship for fishers and their dependents, many coastal communities were said to be unaware of the links between marine ecosystem health and their livelihoods and subsequently would not engage in management efforts (Joseph, 2003). The financial benefits provided to the Sri Lankan economy by healthy coral reefs, specifically through tourism, fisheries and coastal protection, are significant (Berg et al., 1998). Growth in the tourism sector, which before the COVID-19 pandemic represented 4.9% of the national Gross Domestic Product (GDP) and contributed almost US$4.4B in foreign exchange earnings (SLTDA, 2018), is partly linked to successful advertising of Sri Lanka’s coral reefs.
Management practices and local Sri Lankan laws intended to preserve and protect coral ecosystems are described as adequate but also as being largely unenforced (Rajasuriya and White, 1995; Weerakoon et al., 2018). Presently, however, multiple agencies oversee environmental protection. Management of the natural environment is the responsibility of the Ministry of Environment and Renewable Energy, with marine pollution matters expressly handled by the Marine Environmental Protection Agency (MEPA). The conservation of the aquatic environment is the responsibility of the Ministry of Fisheries and Aquatic Resources. Marine protected areas declared under the Fauna and Flora Protection Act (1972, 1993) meanwhile, are managed by the Department of Wildlife Conservation, which sits within the Ministry of Wildlife and Forestry Conservation.
The first marine protected area targeting coral reef protection was declared at Hikkaduwa in 1979. Since then, several further areas have been declared as marine protected areas. All MPAs are declared under the Fauna and Flora Protection Ordinance (FFPO) administered by the Department of Wildlife Conservation. The three MPA categories are: Marine National Park (MNP), Nature Reserve (NR) and Sanctuary. The MNP has the highest level of protection, and the sanctuary has the lowest level of protection under the FFPO. Although several protected areas have been declared only three of them have management plans, and relevant details are given in Table 5.
The Coastal Zone Management Plan (CZMP) addresses mainly the development, planning, and permitting systems for state and private development activities. The CZMP is reviewed and updated periodically, and the current plan is the CZMP of 2018. Adoption of the CZMP in 2004 strengthened many aspects of coastal management including the introduction of Special Coastal Management Areas (SCMA), which included community involvement in the comprehensive management of marine natural resources (Rajarathna and Nianthi, 2019). Co-management of the marine environment had been attempted previously in some coastal areas by the Department of Fisheries and Aquatic Resources, with limited success due to a lack of inclusiveness in engaging fishers in the planning and management of fisheries. The Marine Environment Protection Authority (MEPA) is the mandated government organization addressing pollution in the coastal and marine environment. However, water quality monitoring in coastal and marine waters lies primarily with both the National Aquatic Resources Research and Development Agency (NARA) and MEPA. Furthermore, while universities have conducted water quality studies as part of various projects, there is no centralized collation of that data due to the lack of a national database for water quality in the coastal and marine environment. Coordination between the various agencies has also proven challenging, with historic data scattered in hard-to-find reports and publications. Many data gaps prevent appropriate management decision-making and implementation of actions, particularly concerning pollution from agrochemicals and fertilizer use.
As a result of the complex management arena, the complications in aggregating historic measurements, and the apparent sparsity of appropriate observations it is presently difficult to synthesize a credible impact statement on the role that nitrogen pollution has played in the long-term degradation of coral habitats in Sri Lanka.
3.3 Maldives
3.3.1 Coral reef extent, condition and environmental setting
The Republic of the Maldives is a low-lying archipelago consisting of 1,192 islands within 26 natural atolls that stretch from 0.5°S to 7°N in the central Indian Ocean, (Figure 4). The total land area is approximately 227 km2 with most islands lying at an average height of 1 m above sea level (MEE, 2015b). Many islands are less than 0.5 km2 in area and are covered with nutrient-poor, carbonate sand saline soils that are highly porous and prone to leaching (Herzog and Konrad, 1992; UNEP, 2005). Coral reef coverage varies significantly between studies with estimates ranging from 14,533 km2 (Bahuguna et al., 2013), 8920 km2 (Spalding et al., 2001), 6372 km2 (Souter et al., 2021), to ~4,500 km2 (Naseer and Hatcher, 2004). Tropical conditions have contributed towards the growth of the Maldivian atoll reef system which is the seventh largest system in the world (Spalding et al., 2001). The reef comprises a combination of oceanic faros, oceanic platform reefs, patch reefs, and fringing reefs, all as part of the atoll system (Naseer, 1997), that support over 250 species of hermatypic corals (Pichon and Benzoni, 2007), 36 species of sponges, 285 species of algae, and over a 1000 species of fish (MEE, 2015b; MEE, 2017a).
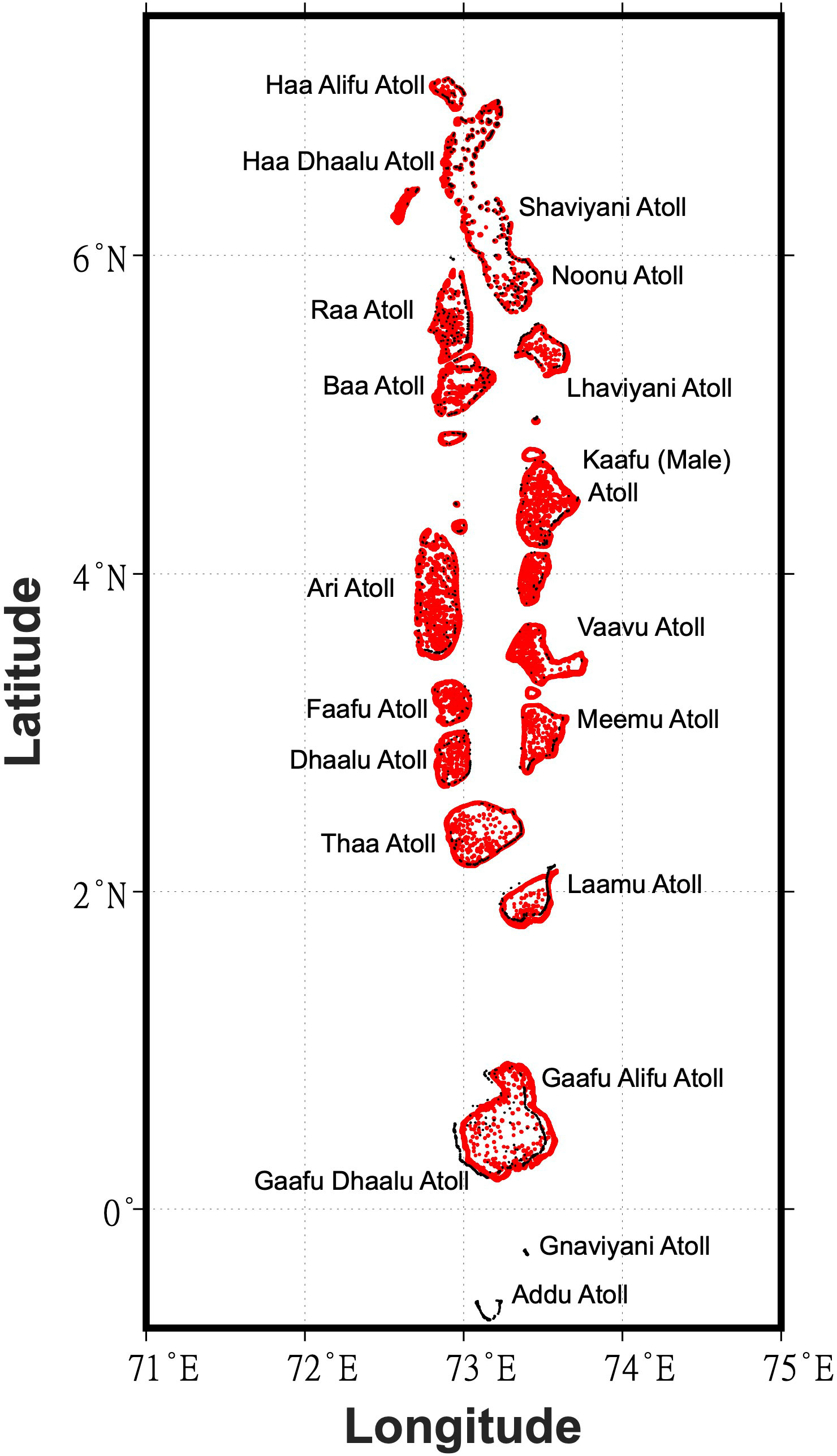
Figure 4 Map showing the distribution of coral reefs in Maldivian waters. Coral distribution based on data compiled by UNEP-WCMC et al. (2018).
The country experiences two monsoon seasons (southwest and northeast), has an average annual temperature of 30.7 °C and receives 2,124 mm of rainfall. The influence of the monsoon winds on local ocean circulation is significant and induces an appreciable island mass effect around the Maldives enhancing surface chlorophyll concentrations (Radice et al., 2019; Su et al., 2021). A recent numerical study of the island mass effect around the Maldives found both upwelling and downwelling favorable conditions during both monsoon seasons, but it was discovered that the seasonal development of downstream island wakes was limited to atolls above 4°N, with atolls further south being more heavily influenced by the zonal movement of equatorial currents (Su et al., 2021).
Existing nutrient measurements from the oceanic waters around the Maldives are sparse. For the region from -0.26 to 7.65°N, 69.5 to 77.5°E, WOD2018 indicates 22 observations of nitrate and 102 observations of phosphate. Near-surface (5 m) nitrate concentrations range from 0.27 – 2.6 μmol L-1 (mean ± stdev 0.46 ± 0.6 μmol L-1) with phosphate concentrations ranging from 0.01 – 0.8 μmol L-1 (mean ± stdev 0.25 ± 0.14 μmol L-1). Surface waters of the surrounding tropical ocean are nutrient-poor and naturally depleted in N relative to P (mean N:P of 2.39 ± 1.58) compared to the Redfield ratio, a measure of both average deep water nutrient ratios and mean phytoplankton composition (Redfield, 1958; Redfield et al., 1963; Tyrrell and Laws, 1997; Tyrrell, 1999). The range in observed nutrient concentrations is large compared to the mean annual climatological conditions derived from the same dataset for the Maldives archipelago (~0.15-0.2 μmol L-1; ~0.2 μmol L-1) (Garcia et al., 2019).
Coral bleaching events have devastated the Maldivian reef system over the last 20 years, and the 1998 global bleaching event alone killed >98% of branching corals species locally and reduced average live coral cover from >40% to close to 0% in some places (Edwards et al., 2001; Loch et al., 2002; Zahir, 2002; Pisapia et al., 2016). Much of the structure and coral diversity lost during the 1998 bleaching event was rediscovered in subsequent years, though recovery was not consistent across the archipelago (Zahir, 2002; Zahir et al., 2002; Lasagna et al., 2008; Morri et al., 2010; Morri et al., 2015). The 2009 survey of Maldivian National Coral Reef Monitoring (NCRM) sites, conducted a decade after the 1998 global bleaching event, indicated mean coral coverage varied between 7.5% and 59.4% (Zahir et al., 2010). However, bleaching again in 2016 resulted in live coral cover dropping close to or below 20% at studied sites, with associated mortality of ~80% (Ibrahim et al., 2017). Recent surveys by the Maldives Marine Research Institute (MMRI) at the NCRM sites indicate current live coral coverage continues to persist at these reduced levels with dead coral cover exceeding 70% in many areas, though a number of recruits were observed at many sites suggesting reefs have a capacity for resilience and recovery. This is supported by a recent nationwide assessment that reported recruitment densities averaging 14.3 individuals per m2, densities that exceed other similar reef systems in the region (Noo Raajje, 2021). Additionally, several other notable events occurred between 1998 and 2016 that affected the Maldivian reefs although their impacts were minimal in comparison to these two major bleaching events. These other events include the 2004 tsunami (Government of Australia and Marine Research Centre, 2005), localized bleaching events in 2003, 2007 and 2010, and outbreaks of the corallivorous crown-of-thorns starfish Acanthaster planci across Ari Atoll (Saponari et al., 2014; Pisapia et al., 2016; Saponari et al., 2018), though the extent and severity of A. planci infestations across the wider Maldivian atolls remains unclear.
3.3.2 Water quality indicators
There is little information available about the water quality in Maldivian waters. As ocean atolls experience considerable advective flushing there has long been an assumption that land-derived nutrient inputs have only minimal impact being either rapidly advected away from the atolls or perhaps being of smaller magnitude than any upwelling resulting from the island mass effect (e.g. (Szmant, 2002; Radice et al., 2019; Su et al., 2021)). For example, the 2011 State of the Environment Report issued by the Government of the Maldives (MEE, 2011) concluded that nutrient over-enrichment was not a significant problem for corals, though the evidence base was acknowledged as being slight. Subsequent studies by Jaleel (2013) and Radice et al. (2019) either reiterated or found little or no evidence for land-derived nutrients impacting coral reefs. Nevertheless, the 2016 State of the Environment Report (MEE, 2017a) concluded that land-derived nitrogen and phosphorous inputs may pose a more serious threat to Maldivian coral ecosystems than previously realized. Increased nutrient fluxes from expanding agricultural activities and from larger sewage and food waste discharges were highlighted as causes for concern. However, while fertilizer imports into the Maldives more than doubled between 2010 and 2015, corresponding with increased fertilizer usage across the country (MEE, 2015a), evidence for an impact on the marine environment remains limited. Key examples cited as potentially illustrating the impacts of nutrient inputs include i) increased seagrass coverage around agricultural islands (Laamu Atoll) (Miller and Sluka, 1999; BOBLME, 2010; MEE, 2017a), ii) the occurrence of “red tide” algal blooms, iii) several massive fish kills, and iv) the recognition that farmers may not be using fertilizers in accordance with manufacturer guidelines, leading to overuse and/or increased leaching (MEE, 2017a). In a specific example, coral survey results reported by Ibrahim (2019) from Huvadhu Atoll demonstrated that the benthic community structure of reef flat areas was negatively impacted by proximity to inhabited islands, with nutrient input derived from tuna fish processing, sewage and sediment input from development activities implicated as drivers of change.
Despite growing concerns about nutrient pollution impacts, a broad-scale isotopic survey of corals across the central Maldivian atolls demonstrated that deep ocean nitrate remains the dominant source of nitrogen for corals, supplemented with particulate organic matter (POM) filtered from seawater (Radice et al., 2019). Based on this approach, the impacts from land-derived nutrients are either spatially limited and not yet fully quantified or entirely minimized by strong oceanic flushing through the atolls by the monsoon winds. The novel use of benthic foraminifera to assess water quality and coral health across North Ari Atoll indicates that water quality generally remains suitable for healthy coral development, yet around inhabited islands detectable changes in the benthic foraminiferal assemblage indicate the onset of water quality degradation, though not yet sufficient to inhibit coral development (Pisapia et al., 2017). Miller and Sluka (1999) meanwhile, suggested that nutrient leaching may be driving long-term growth in sea grass coverage around agricultural atolls. While changes in seagrass coverage remain a potentially useful indicator of nutrient seascape changes, there are important subtleties between the impacts of moderate and excess nutrient enrichment that should be considered in addition to changes in seagrass spatial coverage (Mazarrasa et al., 2018). In particular, a comparable study from southern Indian waters concluded that (excessive) nutrient enrichment was likely driving a reduction in seagrass coverage (Purvaja et al., 2018).
More traditional indicators of water quality and nutrient enrichment, such as increased phytoplankton productivity or biomass, are difficult to distinguish as anthropogenic or natural in origin due to the island mass effect. A recent analysis of satellite surface chlorophyll concentrations revealed the substantial impact monsoon-driven upwelling has on the spatial and temporal distributions of chlorophyll concentrations around the Maldives (Radice et al., 2019). Whilst more intensive analyses related to the phytoplankton community, particularly concerning sporadic accounts of red tides or other harmful blooms (MEE, 2017a), may become an important future monitoring activity, not least because of the public health perspective (e.g. (Tamele et al., 2019)), they are not yet undertaken within the context of coral monitoring or water quality surveys. Furthermore, increased occurrences of red tides and fish kills across the wider Indian Ocean have been reported in recent years, though the cause is often uncertain (Amla and Uranie, 2014; Uranie and Vannier, 2014; Uranie, 2015; Uranie and Meriton-Jean, 2015), and a wider shift in phytoplankton community structure is increasingly evident across the much of the western and central Indian Ocean (Goes et al., 2020). An investigation into multiple fish kills within the Maldives concluded that only one such event could be linked to an algal bloom, though limitations in infrastructure and research capacity prohibited further investigation while alternative fish kill events lacked a clear connection to algal blooms (Naeem and Sattar, 2007).
3.3.3 Reported impacts of nutrient pollution
Studies on nitrogen pollution impacts are currently very limited for the Maldives. Regular national reports on bleaching (e.g. (Zahir et al., 2010; Ibrahim et al., 2017) acknowledge that pollution impacts, mainly from sewage and wastewater, are likely to be problematic, but no quantitative investigations have yet been undertaken. Long-term monitoring of Maldivian coral reefs implemented following significant bleaching in 1998 and now under the auspices of the MMRI, does not yet include monitoring of water quality specifically for nitrogen loadings, and the significance of terrestrial nitrogen transfer remains unclear.
Furthermore, whilst Environmental Impact Assessments assess and evaluate coral reefs near project developments (commonly harbor development and land reclamation projects) and numerous parties investigate coral reefs in various capacities, these efforts often capture similar aspects of known major reef stressors and focus on coral reef status and health only. The disparity between what is recorded and what needs to be recorded is pressing, not only because chemical fertilizer management has been identified as poor (MEE, 2017a), but also because the country has a growing agricultural sector dependent on increasing usage of various combinations of organic and inorganic fertilizers (Mohamed, 2018; FAO, 2020). In addition, the need to improve sewage facilities is widely recognized (MEE, 2017b), particularly where there are known instances of point source pollution from poor, insufficient, or failing sewage systems with the capital city of Male’ being a notable example (FAO, 2011). As the impacts of nutrient over-enrichment in the Maldives archipelago may become apparent at relatively minor levels of enrichment compared to ambient nutrient concentrations (~10%; as derived from the results of Guan et al. (2020)), proper management measures and enforcement of those measures are crucial.
Though the available evidence is limited, there are indications that proximity to human populations and the effectiveness of associated management regimes do significantly affect coral reefs in the Maldives. For example, Heikoop et al. (2000) investigated the difference in nitrogen content in corals between the sewage-affected Male’ reef and reference corals in Addu Atoll in the far south and found that nitrogen content, linked to nitrogen loading from untreated sewage, was significantly higher in the sewage-affected reef samples. Such results therefore differ from the findings reported by Radice et al. (2019). Separately, dead coral cover and filamentous algae cover were found to be higher close to areas with denser human populations (Brown et al., 2017), with human population density also shown to impact coral community composition during post-bleaching recovery periods (McClanahan and Muthiga, 2014). Both the population of the Maldives and the tourism sector have continued to grow in recent decades (Montefalcone et al., 2020), leading to increased anthropogenic pressures on the marine environment and increasing the demand for agricultural produce. Oil pollution stemming from the maritime sector is also problematic, largely due to the lack of appropriate disposal options (MEE, 2017a), and the high reliance on maritime craft for movement between atolls.
Nutrients, chemicals, and sediments released from tourist resort activities generally have a negative impact on resort reefs, even though resort or house reefs are considered a pseudo- marine managed area within the country. The overall negative impacts may still therefore outweigh the benefits of protection (Cowburn et al., 2018), though this is far from clear for all reef-associated organisms (Moritz et al., 2017), while the severity of anthropogenic impacts may also differ with depth (Nepote et al., 2016).
Coral diseases have been little studied in the Maldives but the general prevalence rate is estimated to be around 1.5% (Montano et al., 2016). Spatial variability in disease prevalence throughout the central region of the Maldives may potentially be linked to human population densities though regions with low coral densities and/or larger colonies were found to be more susceptible to infection than elsewhere (Montano et al., 2016). The incidence of disease amongst northern and southern atolls has not yet been sufficiently well investigated to allow assessment of disease prevalence.
3.3.4 Current protections and safeguards
Environmental degradation resulting from coastal development is recognized as a major anthropogenic threat to coastal ecosystems in the Maldives (Nepote et al., 2016; Stevens and Froman, 2019). Islands within the central Ari Atoll have undergone significant development, including the construction of an airport, which increased sedimentation on the surrounding reefs (Nepote et al., 2016). While coastal development is generally detrimental to coral reef management, it has responded to and created growth in the tourism sector, which benefits the Maldives economy as a whole.
Aside from the effectiveness of legislative and regulatory mechanisms, community attitudes can sometimes be a challenge in addressing marine threats (Nickerson and Maniku, 1997). There is a distinct lack of opportunities in terms of income, growth, and development within islands which forces people to pursue a limited range of professions and which hinders the willingness, capacity, and capability to address marine threats (Mancini et al., 2017). This is exacerbated by the fact that, while several Marine Protected Areas (MPA) exist within the country, capacity and resource constraints mean that many MPAs lack effective management mechanisms. However, these limitations can be addressed through an improved understanding of the relationship between local human populations and coral reef areas and reef conditions across the country (Agardy et al., 2017), which can enable the effective utilization of locally managed marine areas.
Successful management of the marine environment is central to the tourism sector, and regulations exist to manage the development of tourism successfully alongside the desire to protect the coral reef environment (MEE, 2017a). Nevertheless, it has been shown that reef conditions generally improve with distance from the capital, Malè, and from other community islands, whereas reef conditions can deteriorate with resort age (Price and Firaq, 1996). There is high public awareness of the need to conserve the marine environment and reef ecosystems, which is said to have resulted in considerate waste disposal by many resorts (MEE, 2017a).
4 The challenge of nutrient enrichment
Global stressors (e.g. ocean temperature rise, pH decline) and local stressors (e.g. fishing, pollution) both contribute to the degradation of coral habitats. While both type of stressors are ultimately anthropogenic in origin, local stressors are more important in the short-term because they contribute more to coral reef loss and habitat decline. Land-based pollution is already recognized as the top issue for marine ecosystem management both globally and for South Asian countries in particular, with sewage discharge, agricultural runoff, and industrial effluents recognized as the major pollution sources (Qasim et al., 1988; GESAMP, 2001; Kaly, 2004). Globally, 20-25% of anthropogenic nitrogen inputs to coastal watersheds are exported to coastal ecosystems primarily via rivers and atmospheric transportation pathways (Malone and Newton, 2020), though in some settings submarine groundwater discharge may also be a significant additional pathway (Santos et al., 2021; Selvam et al., 2022; Sajeev et al., 2023). Across South Asia, there is wide variation in the sources, nutrient loadings, and ability to monitor for marine pollution impacts (BOBLME, 2010; BOBLME, 2011; BOBLME, 2013; BOBLME, 2015). Sewage is considered the most significant source of marine pollution in the Maldives, while effluents from agriculture, aquaculture, and sewage are the most problematic in Sri Lanka and industrial effluents and sewage are the most significant in India (BOBLME, 2011; BOBLME, 2015). With few exceptions however, there is generally insufficient publicly available data to adequately assess the magnitude, severity, and trend of nutrient pollution impacts on coastal waters let alone on regional coral ecosystems. In this regard research focused on South Asian corals is not unique for there is arguably a general lack of research globally on the topic of nutrient enrichment and coral health (Li et al., 2022). Yet, wide variations in reported nutrient concentrations between studies, as well as the scope and duration of water quality monitoring programmes for the coastal zone in general, impede regional efforts to understand and quantify such impacts (BOBLME, 2015). Consequently, pollution impacts on corals have generally received less attention than the impacts from fishing or other (visually) destructive activities.
In the broader discussion, however, pollution (of all origins) is already recognized as a leading factor inhibiting coral recovery following bleaching events (Wilkinson, 2008; Wilkinson et al., 2016) and for the South Asian region marine pollution in conjunction with increased sedimentation due to land-use change and in some areas unregulated or destructive exploitation of marine resources (fishing) has been suggested as the major anthropogenic threat to the region’s corals (Wilhelmsson, 2002). Indeed, differences in pollution intensity across the region were previously suggested as a factor obscuring a clear and consistent regional trend in coral reef recovery following widespread coral bleaching (Tamelander and Rajasuriya, 2008). Yet despite this awareness quantitative studies linking nutrient pollution to the degradation of coral habitats across South Asia remain limited (Chandra et al., 2021), which raises the question - is it possible to infer the scale of the potential problem in the absence of sufficient experimental data to quantitatively demonstrate this impact?
4.1 Fertilizer inputs
Regional average fertilizer consumption across South Asia rose by over 7000% from ~2.4 kg ha-1 yr-1 in 1961 to ~170.1 kg ha-1 yr-1 by 2018 (World Bank, 2021). Fertilizer consumption across South Asia is 24% higher than the global average rate of 137 kg ha-1 yr-1 of arable land (World Bank, 2021). Within the region, average national consumption rates for all fertilizer types vary significantly, and for 2018 were 175 kg ha-1, 138 kg ha-1 and 115 kg ha-1 for India, Sri Lanka and the Maldives respectively (Figure 5; note that the total fertilizer consumption rate includes nitrogen, phosphate and potash-based fertilizers). Consumption rates in Sri Lanka have historically been higher than elsewhere in South Asia and peaked at over 300 kg ha-1 in 2008. Due to growing concerns about soil health (FAO and ITPS, 2015), ground-water contamination (Imbulana et al., 2006; Prabagar et al., 2020) and negative impacts on human health, which includes high rates of chronic kidney disease (e.g. (Abraham et al., 2016; Kafle et al., 2019), fertilizer consumption rates in Sri Lanka have rapidly declined in recent years due to Government initiatives to control fertilizer pollution even extending to a short-lived ban on the use of chemical fertilizers imposed by the Sri Lankan Government in 2021. Meanwhile, consumption rates in India continue to rise, increasing by 5-6% per year (Sutton et al., 2017), while reported consumption rates in the Maldives have increased by nearly 2000% since 2002.
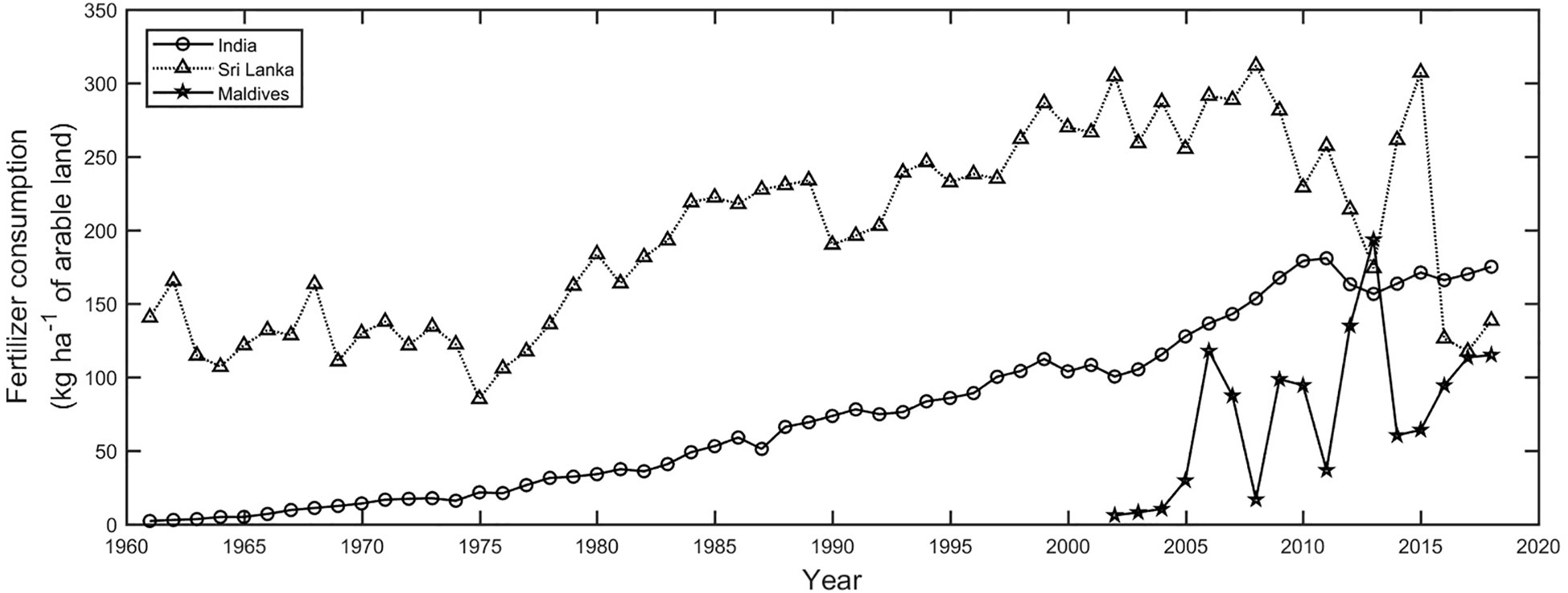
Figure 5 Annual fertilizer consumption rates for India, Sri Lanka and the Maldives for the period 1960-2018. Note that the estimates of fertilizer consumption are based on products that include nitrogen, phosphate and potash-based fertilizers. Total fertilizer-N consumption is thus lower than indicated here. Data from World Bank database (https://data.worldbank.org/indicator/AG.CON.FERT.ZS).
Despite significant recent efforts to quantify nitrogen fluxes across South Asia (e.g. (Kaly, 2004; BOBLME, 2010; BOBLME, 2014; BOBLME, 2015)), and particularly within India via national nitrogen assessments (Abrol et al., 2017), much remains unknown about the fate and impact of anthropogenic nitrogen on coastal environments. For example, the Indian Nitrogen Assessment (Abrol et al., 2017), highlighted both an 11-fold increase in fertilizer N consumption within India between 1970 and 2011 and a 4-fold increase in the loss of fixed nitrogen to the environment (Abrol and Adhya, 2017) but concluded that the evidence to document an impact on the marine environment was currently patchy and incomplete (Prema et al., 2017; Ramesh et al., 2017). Consumption of fertilizer nitrogen in India now exceeds 18.8 Mt N per year (as of 2019-2020; (The Fertilizer Association of India, 2022)), with over 80% of this fertilizer nitrogen represented by urea-based products (Tewatia and Chandra, 2017). Detailed studies suggest an average consumption rate of ~89 kg N ha-1 yr-1, but strong regional variability can see this range from 5 to >200 kg N ha-1 yr-1 at the district level (Tewatia and Chandra, 2017). In contrast, total nitrogen inputs to agricultural lands, which in addition to synthetic fertilizer application also considers biological nitrogen fixation, atmospheric deposition, and organic manure application, are higher at around 135 kg N ha-1 yr-1 (Bijay-Singh, 2017). Estimates indicate that 65-68% of applied N (equivalent to around 93 kg of total N applied ha-1 yr-1) is subsequently lost to the environment (Abrol and Adhya, 2017; Bijay-Singh, 2017), with a significant proportion entering ground and surface water systems with the potential for export to coastal waters (Prakasa Rao et al., 2017). Yet, despite improved efforts to quantify and track fertilizer nitrogen application and loss across agricultural lands and through the land-ocean continuum, the lack of comprehensive monitoring of coastal waters severely limits efforts to quantify the export of applied nitrogen to the ocean (Kaly, 2004; BOBLME, 2010; BOBLME, 2014; BOBLME, 2015; Abrol and Adhya, 2017). Prema et al. (2017) estimated that India’s rivers export 1.6-1.8 Tg N yr-1, an amount equivalent to around 9% of applied fertilizer nitrogen. On this basis, they argued that the remaining 91% of nitrogen fertilizer was converted to crops, lost to the atmosphere, or recycled and retained within rivers and estuaries. This exported nitrogen remains significant, however, and was estimated to support ~13% of coastal new production (Prema et al., 2017). Fertilizer application and coastal discharge are not, however, uniform with 73% of fertilizer N applied to catchments that drain to the east coast (Bay of Bengal), and the remaining 27% draining to the west coast (Arabian Sea) (Swaney et al., 2015; Ramesh et al., 2017). This may signify limited potential for impact on coral ecosystems due to the broad absence of coral reefs in the Bay of Bengal (e.g. Figure 1). Yet, when fertilizer consumption rates are normalized to river catchment area it appears that west coast rivers export 4-times more nitrogen per unit area than east coast rivers, particularly during the monsoon months (west coast: 607 ± 700 kg N km-2 yr-1 vs east coast:161 ± 369 kg N km-2 yr-1; (Krishna et al., 2016; Ramesh et al., 2017). More detailed studies of fertilizer consumption show that >50% of fertilizer consumption within India occurs within just five states, (Uttar Pradesh, Maharashtra, Punjab, Gujarat, Madhya Pradesh), two of which have an Arabian Sea coastline (Bhattacharya et al., 2017).
While efforts to quantify riverine dissolved inorganic nitrogen fluxes to the coastal ocean and the contribution attributed to fertilizer application are improving, there is still a chance that a significant proportion of applied nitrogen that is later relevant to coral habitats is being overlooked in export estimates. Ramesh et al. (2017) noted that the ratio of inorganic to organic nitrogen exported by Indian rivers strongly favored organic forms with typically around 10% of riverine nitrogen exported as inorganic nitrogen and 90% as organic nitrogen compounds. Given India’s reliance on organic nitrogen-based fertilizers (Tewatia and Chandra, 2017), and the fact that the coral holobiont is sensitive to both inorganic and organic nutrient concentrations (e.g. (Rädecker et al., 2015), it appears critical that all nitrogen forms be captured in coastal water quality monitoring activities.
Comparable information on fertilizer inputs to the coastal regions of Sri Lanka or the Maldives is harder to find and currently less well understood than for India. The historically high fertilizer consumption rates for Sri Lanka and the increasing consumption rates for the Maldives (Figure 5), suggest high or increased inputs of fixed N to the coastal regions but as with India, the observational data needed to document impacts on the marine environment is limited (Manage et al., 2022).
4.2 Wastewater inputs
Nutrient losses from agriculture and wastewater discharge from households and industry represent the two most important sources of nutrient input to freshwater systems (Bouwman et al., 2005). By extension, they are also critical to assessing nutrient pollution inputs to coastal seas though a considerable reduction in aquatic nutrient loadings, by some estimates ~70% (Billen et al., 2013), occurs along the land-ocean continuum ultimately limiting the impact of nutrient inputs to freshwater systems on the coastal zone. Global raw sewage production is currently estimated to be around 19 Tg N yr-1, with wastewater treatment reducing net sewage inputs to ~6 Tg N yr-1 (Billen et al., 2013). By 2050, it is estimated that the nitrogen input to surface waters from sewage could reach 12-18 Tg N yr-1 (Van Drecht et al., 2009; van Puijenbroek et al., 2019) representing a significant and growing environmental perturbation.
The availability and accessibility of data relating to sewage generation, wastewater treatment, and discharge vary by country and are not always readily accessible. Summary statistics on access to different sanitation services from the WHO/UNICEF Joint Management Programme (WHO/UNICEF, 2021) are presented in Table 6 to illustrate the regional variability in access to different sanitation services. The data show, for example, that access to sewer connections by urban populations ranges from ~9% (Sri Lanka) to 100% (Maldives), or that at the national level use of latrines dominates over septic tanks in Sri Lanka (94.3% vs 1.5%), whilst in India use of latrines and septic tanks is more equal (34.6% vs 36.2%).
For India, as of June 2021, domestic sewage generation by urban populations was reportedly 72,368 million liters per day (MLD; (CPCB, 2021)). Total sewage treatment capacity is less than the generation rate and can handle only 31,841 MLD, so on average only 44% of India’s daily urban sewage generation is treatable. Operational treatment capacity however is lower at 20,236 MLD, indicating that on average only 28% of daily urban sewage generation is treated. The remaining 72% of daily urban sewage generation (~52,105 MLD) is released untreated. Based on the latest national survey results approximately 36% of treatment capacity is under-utilized or non-operational (CPCB, 2021), but even if treatment facilities were operating at full capacity less than half of the daily urban sewage generation can be treated. Sewage generation and treatment capacity also vary significantly at the state level (CPCB, 2021). Summary information for the offshore islands and west coast states, which are contiguous with major coral localities, is presented in Table 7. At best, a maximum of 54% of daily sewage generation can be treated (Gujarat). At worst, there is zero capacity to treat sewage (Andaman and Nicobar, Lakshadweep Islands), with septic tanks or direct discharge to the ocean the only option. Provisionally, around one-third of the daily sewage generation from the island and (west) coastal states is treated, with the remainder discharged untreated.
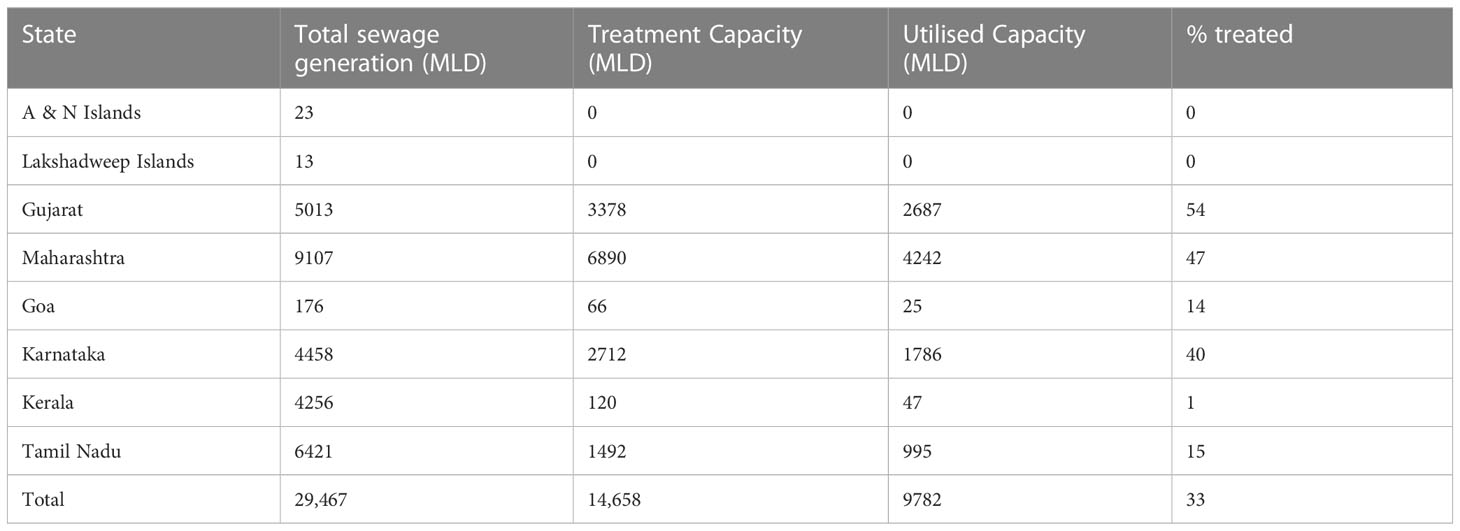
Table 7 Summary estimates of urban sewage generation and treatment in India (CPCB, 2021). MLD = million litres per day.
The current National Water Assessment for the Maldives (UNESCAP, 2021), indicates that sanitation services in the form of sewage treatment plants or septic tanks are available for 48% of the population covering 66 out of 187 inhabited islands. There are, however, many unknowns with regards to sewer outfall operations due to limited data availability, which makes it harder to assess any subsequent environmental impact. For example, flow data from various industries and households to the sewerage system (i.e. inputs) is insufficient and, in some cases, not measured, whereas total discharge from the sewerage system to the environment (i.e. outputs) is unquantified or unavailable (UNESCAP, 2021). The National Water Assessment highlights that discharges to the environment, which include island groundwater aquifers and the ocean, include brine from desalination processes, generator cooling waters, waste seawater, treated wastewater (from septic tanks or sewage treatment plants), and minimally or untreated wastewater from the sewerage system. The sewerage system’s treated and untreated wastewater is preferentially discharged to the ocean. Given current data availability, it is not possible to obtain an order of magnitude estimate of wastewater input to the ocean for the Maldives, but the presumption is that this is growing due to population increases and resort development and expansion. The uncertainty in discharge volumes is already a noted concern (UNESCAP, 2021), but it is also clear from the National Water Assessment that current wastewater discharges include significant thermal, saline, faecal, and chemical loadings with the potential for wide-ranging negative impacts on coral ecosystems.
The situation in Sri Lanka is more complex as data on wastewater production is neither readily available nor compiled by a single agency. Wastewater regulations stipulate that wastewater must undergo secondary level treatment and conform to strict water quality standards before discharge, particularly as treated wastewater is widely returned to inland surface waters and internal irrigation networks for subsequent reuse within the agricultural sector (Jayalal and Niroshani, 2012). Data from the WHO/UNICEF Joint Monitoring Programme (Table 6) indicates that only 9% of urban populations are connected to a sewerage network. Around Colombo, however, the combination of an aging sewer network unsuitable for modern demands and the cost of treatment means that sewage is discharged without even preliminary level treatment via two 1.5 km long offshore outfalls with the potential for subsequent impact on the marine environment (Mysan and Ranasinghe, 2014).
4.3 But what is the true impact of nitrogen pollution on South Asian coral ecosystems?
In examining the role of reactive nitrogen pollution in Indian coastal waters Prema et al. (2017) noted that whilst there are many generalized statements about pollution impacts it remains rather difficult to find quantitative evidence or even qualitative observations to support such statements in practice. Similar concerns have been raised elsewhere and stem from significant regional differences in water quality or habitat monitoring activities (BOBLME, 2015; Abrol and Adhya, 2017; Ramesh et al., 2017). Collectively, there is recognition that the South Asian region is at increased risk of pollution-induced eutrophication and that some areas may already be experiencing such impacts, but also that quantitative evidence to determine, track, and inform on these developments remains limited (BOBLME, 2015; Abrol and Adhya, 2017; Ramesh et al., 2017). In reviewing the existing literature for known nitrogen pollution impacts on coral ecosystems in the South Asia region it has become clear that there is significant variation in the extent of research between countries and consequently at the regional level there is inadequate information with which to diagnose impacts, trends, or confidently attribute specific changes to nutrient inputs (Table 8). In most cases no evidence exists to definitively link nutrient inputs to known impacts.
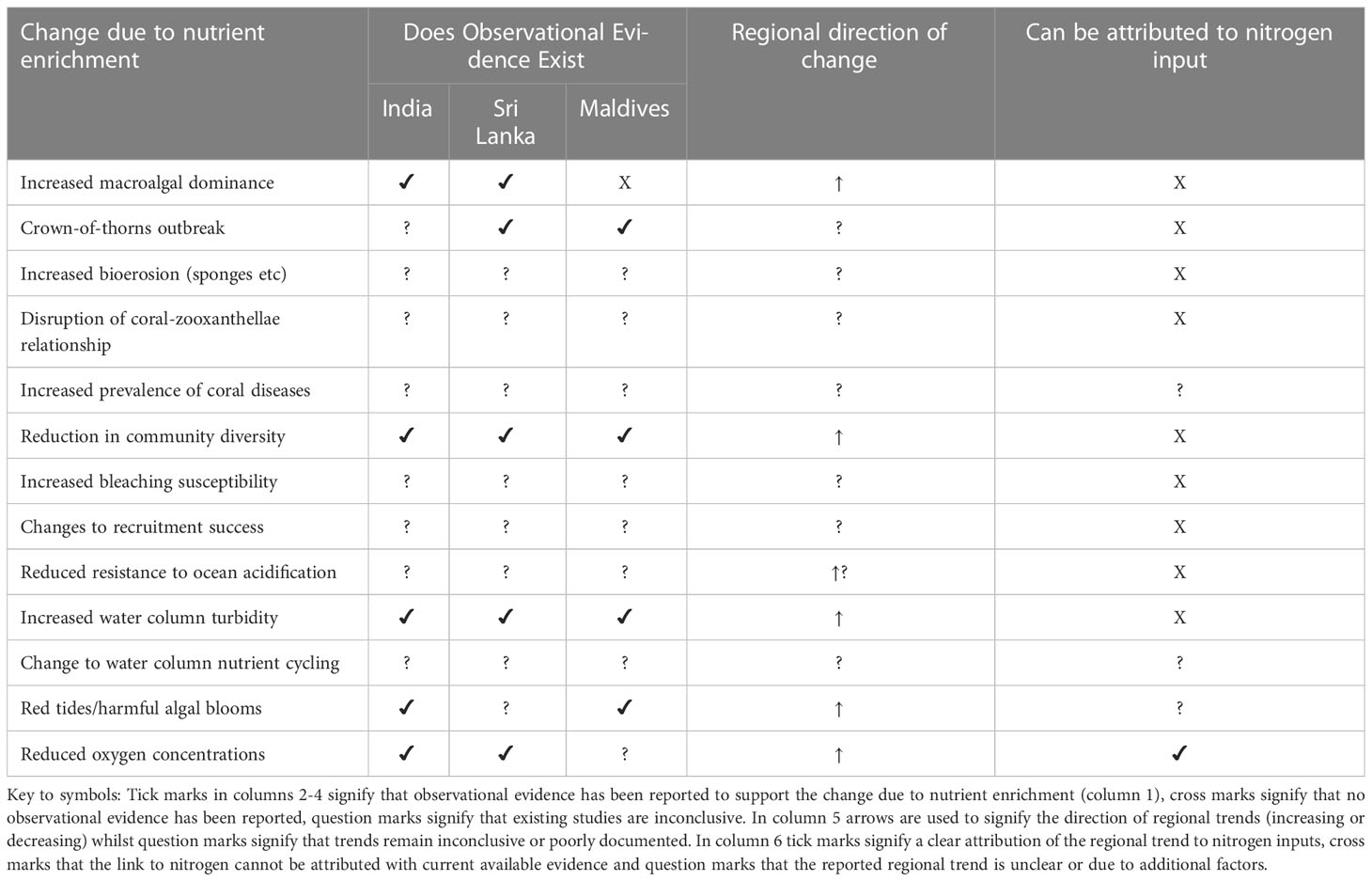
Table 8 Assessment matrix summarizing existing literature evidence for known nitrogen pollution impacts on coral reefs in India, Sri Lanka and the Maldives, the direction of change (if possible to determine) for the South Asia region, and whether such changes can clearly be attributed to nitrogen pollution.
There remains considerable difficulty in translating national fertilizer consumption rates or sewage trends into a nitrogen flux to the coastal environment, and thereafter to a specific habitat such as corals. The difficulty in assessing the impacts of nutrient pollution on the marine environment is also compounded by the complexities of identifying coastal transport pathways, national and transboundary pollution sources, managing rapidly growing human populations, enforcing existing legislation (Edeson, 2004), the financial cost of initiating and maintaining appropriate monitoring programmes, the wide range of pollution impacts on corals (Table 1), and the far more visible impacts from fishing or bleaching which garner greater attention. While the effects of bleaching have been better studied in South Asia in recent decades, more focused studies on pollution impacts have rarely been included. Generally, coral reef monitoring has not been undertaken with nutrient pollution in mind. Consequently, general statements regarding the impact of nutrient pollution are widespread but are rarely supported with definitive experimental observations, or even with qualitative observations covering a sufficient temporal period to support the validity of such statements (Bhatt et al., 2012a). The situation is however changing, driven in part by recognition that nutrient enrichment of coastal waters is a global and growing problem (NRC, 2000; Selman et al., 2008; Rabalais et al., 2009; Selman and Greenhalgh, 2009a; Selman and Greenhalgh 2009b; BOBLME, 2015; Malone and Newton, 2020), but also from greater understanding and concern about the consequences of high faecal coliform loads for human health and fisheries (Shuval, 2003; Islam and Tanaka, 2004). Even so, quantitative information on nutrient inputs, and movement within the coastal zone is patchy, and while efforts to evaluate the magnitude of national nutrient inputs to the coastal zone are growing (Mayorga et al., 2010; Seitzinger et al., 2010), it remains difficult to assess the environmental and ecological consequences of those inputs, particularly for the regions corals (Table 8).
Recent studies suggest that it may now be prudent to rapidly address deficiencies in data collection for a variety of reasons. Eutrophic and hypoxic conditions have been reported for coastal sites around India and Sri Lanka (Diaz et al., 2011), whilst model studies show that corals in the Maldives, and possibly more generally across the South Asian region, appear highly sensitive to low levels of nutrient enrichment (Guan et al., 2020). This latter study is particularly concerning as it indicated detrimental impacts on Maldivian coral reefs following an increase of only 0.02 μmol L-1 above local optimum conditions. Nutrient concentration increases of this magnitude relative to the regional mean are currently difficult to identify from extant nutrient observations (Boyer et al., 2018) and thus require more frequent targeted sampling efforts. Attributing nutrient pollution as a contributory and possibly even causative factor behind coral loss across the South Asian region in recent decades is compounded by the limited quantitative information available. Such knowledge gaps place significant limitations upon coral management and conservation decision making and do little to highlight the true scale of the nutrient problem or the severity of its impact on marine ecosystems.
To address this, six key research topics for future research activities have been identified as the most critical for improving abilities to quantify and manage nutrient enrichment impacts on South Asian coral habitats.
4.3.1 What is the magnitude of anthropogenic nutrient inputs to South Asian coral reefs?
Quantification of this most basic of requirements remains extremely difficult. Efforts to assess regional and national nutrient inputs to the ocean have developed rapidly in recent decades but as yet, the ability to quantify nutrient inputs to specific marine habitats remains challenging. Identifying, quantifying and evaluating nutrient inputs to coral habitats is a key objective of further study. Linked to this is a clear need to incorporate marine biogeochemical measurements within coral monitoring activities.
4.3.2 What are the regional environmental optimum conditions for coral reef growth and are anthropogenic nutrient inputs distorting this optimum?
Identifying baseline conditions against which to assess anthropogenic inputs is essential. What are those baseline conditions?, are they changing?, and if so how rapidly? are questions that need answering. Only once such baseline conditions are understood, can impacts and the severity of those impacts be identified and addressed. Presently, anthropogenic nutrient inputs are typically viewed in a qualitative or semi-quantitative sense, and additional quantitative measures are urgently required.
4.3.3 What is the regional connectivity between different coral sites and pollution sources?
Nutrient inputs from riverine inputs, contaminated groundwater, and terrestrial runoff are key variables to quantify and model when assessing nutrient inputs to coastal waters, but once nutrients have reached the ocean, how does the local circulation retain or disperse nutrient inputs to increase or decrease the risk to specific habitats and ecosystems? Presently, the pathways linking nutrient sources and coral habitats are poorly known, with even some aspects of local oceanography and the regional circulation considered incomplete. Improved understanding of regional connectivity, particularly via numerical models, may help identify regions more prone to enrichment problems due to water retention or low flushing rates, as well as those areas potentially less prone to advective problems.
4.3.4 To what extent is it possible to predict nutrient enrichment impacts?
There is growing awareness that inadequate knowledge of how environmental factors influence the symbiotic relationship between coral and zooxanthellae hinders the ability to predict how that relationship may break-down or change when environmental conditions themselves change. While much effort has been put into understanding the effects of heat stress on the symbiotic relationship, more effort should be put into understanding how nutrients influence that relationship. Separately, efforts to identify low cost indicator tools that can be widely distributed to aid monitoring efforts, including the use of indicator species, should be considered within preventative management plans.
4.3.5 Do coral growth metrics (calcification, linear extension, and bulk density) evidence nutrient enrichment problems?
Recent research suggests that coral skeletal formation may be measurably impacted by nutrient enrichment (e.g. Iijima et al., 2021; Buckingham et al., 2022). Though still an emerging field of research it follows that if coral growth metrics such as calcification rate or bulk density can be easily measured in the field, that they may provide additional indications, or even early warning, of nutrient enrichment problems and inform mitigation actions. Presently such growth metrics remain poorly documented for the South Asian region but with further research may represent a valuable source of new information on nutrient enrichment impacts.
4.3.6 What management actions are needed?
The need to preserve and protect coral reef habitats must be handled within appropriate management and legislative structures. The imposition of marine protected areas may protect corals from physical destruction, but how effective are such protections from dissolved nutrient pollution? Are nutrient dispersal pathways adequately considered? Could more be done to enforce existing legislation to minimize or remove the impacts of pollution? Such questions link to wider aspects of socioeconomic development, raising the prospect of difficult decision-making while balancing coral protection and economic prosperity, but it is precisely these connected issues that must be tackled.
5 A way forward
Addressing the identified research gaps will require coordinated regional efforts due to the transboundary nature of pollutant dispersal and the variability in regional research infrastructure and capability. Yet precisely this coordinated effort is already handled under the auspices of the South Asia Co-operative Environment Programme (SACEP), a regional inter-governmental organization that promotes the protection, management, and enhancement of the regional environment. In particular, the problems of marine nutrient pollution and coral habitat loss are already recognized and actively addressed (SACEP, 2008; SACEP, 2017; SACEP, 2019). However, the two problems of nutrient pollution and coral habitat loss appear to be currently handled separately, so coral reef monitoring has not generally been undertaken with nutrient pollution in mind, nor do water quality monitoring activities fully consider impacts on all aquatic habitats or biomes. Small changes or additions to existing activities including measurement of additional environmental parameters such as ambient inorganic and organic nutrient concentrations or more frequent monitoring of water quality in sensitive habits such as coral reefs may therefore be a cost-effective means of resolving the true impact of anthropogenic nutrient inputs on the region’s coral reefs, whilst also strengthening the protection and management of the region’s marine environment without resorting to the establishment of bespoke sampling networks or surveys.
Author contributions
SP and AT conceived the study, SP coordinated contributions from all authors and wrote the first draft. All authors contributed to manuscript revision, read and approved the submitted version.
Funding
This study is an output from the South Asian Nitrogen Hub (SANH), a five-year (2019-2024) collaborative project funded by the UK Research and Innovation Global Challenges Research Fund (GCRF) under NERC grant (NE/S009019/1).
Conflict of interest
The authors declare that the research was conducted in the absence of any commercial or financial relationships that could be construed as a potential conflict of interest.
Publisher’s note
All claims expressed in this article are solely those of the authors and do not necessarily represent those of their affiliated organizations, or those of the publisher, the editors and the reviewers. Any product that may be evaluated in this article, or claim that may be made by its manufacturer, is not guaranteed or endorsed by the publisher.
References
Abhilash K. R., Sankar R., Purvaja R., Samuel V. D., Sreeraj C. R., Krishnan P., et al. (2019). Impact of long-term seaweed farming on water quality: a case study from Palk Bay, India. J. Coast. Conserv. 23 (2), 485–499. doi: 10.1007/s11852-018-00678-4
Abhiya A. M., Minu S., Ramachandrankizhur (2015). Salient long-term observations of SST along Kerala coast and its comparative variation with Lakshadweep coast. Aquat. Proc. 4, 556–562. doi: 10.1016/j.aqpro.2015.02.072
Abraham G., Varughese S., Thandavan T., Iyengar A., Fernando E., Naqvi S. A., et al. (2016). Chronic kidney disease hotspots in developing countries in south Asia. Clin. Kidney J. 9 (1), 135–141. doi: 10.1093/ckj/sfv109
Abrol Y. P., Adhya T. K. (2017). “Technical summary,” in The Indian Nitrogen Assessment. Eds. Abrol Y. P., Adhya T. K., Aneja V. P., Raghuram N., Pathak H., Kulshrestha U., Sharma C., Singh B. (Elsevier), 1–5. Available at: https://www.sciencedirect.com/book/9780128118368/the-indian-nitrogen-assessment
Abrol Y. P., Adhya T. K., Aneja V. P., Raghuram N., Pathak H., Kulshrestha U., Sharma C., Singh B. (Eds.) (2017). The Indian Nitrogen Assessment: sources of reactive nitrogen, environmental and climate effects, management options and policies (Elsevier), 568. Available at: https://www.sciencedirect.com/book/9780128118368/the-indian-nitrogen-assessment
Agardy T., Hicks F., Nistharan F., Fisam A., Abdulla A., Schmidt A., et al. (2017). Ecosystem services assessment of north Ari atoll, Maldives (Gland, Switzerland: IUCN), 59. Available at: https://portals.iucn.org/library/sites/library/files/documents/2017-003_0.pdf.
Amarathunga A. A. D., Jinadasa S. U. P., Azmy S. A. M. (2013). Sedimentary characteristics and status of water quality in Polwatta river and weligama bay in Sri Lanka. J. Environ. Professionals Sri Lanka 2 (1), 38–51. doi: 10.4038/jepsl.v2i1.5745
Amla H., Uranie S. (2014) A ‘harmless’ reddish-brown coloured organisms caused ‘red tide’ in the Seychelles port of Victoria (Seychelles News Agency) (Accessed 24/08/2020).
Anand J. B., Kala M. J., Kumar V. (2015). Monthly variation of water quality along south east coast of India. Internal J. Geol. Agric. Environ. Sci. 3 (3), 10–15.
Anandavelu I., Robin R. S., Purvaja R., Ganguly D., Hariharan G., Raghuraman R., et al. (2020). Spatial heterogeneity of mesozooplankton along the tropical coastal waters. Continent. Shelf Res. 206, 104193. doi: 10.1016/j.csr.2020.104193
Anderson D. M., Glibert P. M., Burkholder J. M. (2002). Harmful algal blooms and eutrophication: nutrient sources, composition, and consequences. Estuaries 25, 704–726. doi: 10.1007/BF02804901
Antonius A. (1985). Coral diseases in the indo-pacific: a first record. Mar. Ecol. 6 (3), 197–218. doi: 10.1111/j.1439-0485.1985.tb00322.x
Antony S., Ajayan A., Dev V. V., Mahadevan H., Kaliraj S., Krishnan K. A. (2020). Environmental influences on zooplankton diversity in the Kavaratti lagoon and offshore, Lakshadweep archipelago, India. Reg. Stud. Mar. Sci. 37, 101330. doi: 10.1016/j.rsma.2020.101330
Arora M., Chaudhury N. R., Gujrati A., Patel R. C. (2019b). Bleaching stress on Indian coral reef regions during mass coral bleaching years using NOAA OISST data. Curr. Sci. 117 (2), 242–250. doi: 10.18520/cs/v117/i2/242-250
Arora M., Gujrati A., Chaudhury N. R., Chauhan P., Patel R. C. (2019a). Assessment of coral reef thermal stress over India based on remotely sensed sea surface temperature. Geocarto International 36, 1–18. doi: 10.1080/10106049.10102019.11624983
Arulananthan A., Herath V., Kuganathan S., Upasanta A., Harishchandra A. (2021). The status of the coral reefs of the Jaffna peninsula (Northern Sri Lanka), with 36 coral species new to Sri Lanka confirmed by DNA bar-coding. Oceans 2 (3), 509–529. doi: 10.3390/oceans2030029
Asir N. G. G., Kumar P. D., Arasamuthu A., Mathews G., Raj K. D., Kumar T. K. A., et al. (2020). Eroding islands of Gulf of Mannar, southeast India: a consequence of long-term impact of coral mining and climate change. Natural Haz. 103, 103–119. doi: 10.1007/s11069-020-03961-6
Bahuguna A., Chaudhury R. N., Bhattji N., Ajai and R. R., Navalgund (2013). Spatial inventory and ecological status of coral reefs of the central Indian Ocean. Indian J. Geo-Mar. Sci. 42 (6), 684–696.
Bak R. P. M., Joenje M., de Jong I., Lambrechts D. Y. M., Nieuwland G. (1998). Bacterial suspension feeding by coral reef benthic organisms. Mar. Ecol. Prog. Ser. 175, 285–288. doi: 10.3354/meps175285
Balachandar K., Viswanathan C., Robin R. S., Abhilash K. R., Sankar R., Deepak Samuel V., et al. (2023). Benthic foraminifera as an environmental proxy for pollutants along the coast of chennai, India. Chemosphere 310, 136824. doi: 10.1016/j.chemosphere.2022.136824
Balaji Jr. S., Patterson Edward J. K., Deepak Samuel V. (2012). Coastal and marine biodiversity of Gulf of Mannar, southeastern India - a comprehensive updated species list. Gulf of Mannar Biosphere Reserve Trust (Tamil Nadu, India: Gulf of Mannar Biosphere Reserve Trust, Ramanathapuram). 13 (22), 128. Available at: https://www.forests.tn.gov.in/app/webroot/img/document/publications/gotn/Publication%20No_22.pdf.
Balasubramanian T., Khan S. A. (2001). Coral reefs of India. Tamil Nadu, India, environmental information system centre (Environmental Information System Centre), 109.
Bandara N. J. G. J. (2003). Water and wastewater related issues in Sri Lanka. Water Sci. Technol. 47, 305–312. doi: 10.2166/wst.2003.0661
Baumann J. H., Zhao L. Z., Stier A. C., Bruno J. F. (2022). Remoteness does not enhance coral reef resilience. Global Change Biol. 28 (2), 417–428. doi: 10.1111/gcb.15904
Bell P. R. F. (1992). Eutrophication and coral reefs - some examples in the Great Barrier reef lagoon. Water Res. 26 (5), 553–568. doi: 10.1016/0043-1354(92)90228-V
Benavides M., Bednarz V. N., Ferrier-Pagès C. (2017). Diazotrophs: overlooked key players within the coral symbiosis and tropical reef ecosystems? Front. Mar. Sci. 4. doi: 10.3389/fmars.2017.00010
Berg H., Ohman M. C., Troeng S., Linden O. (1998). Environmental econmics of coral reef destruction in Sri Lanka. Ambio 27 (8), 627–634.
Bhatt J. R., Edward J. K. P., Macintosh D. J., Nilaratna B. P. (2012a). Coral reefs in India - status, threats and conservation measures (New Dehli, India: IUCN), 323.
Bhatt J. R., Kumar R., Edward J. K. P. (2012b). “Conservation and management of coral reefs in India,” in Coral reefs in India - status, threats and conservation measures. Eds. Bhatt J. R., Edward J. K. P., Macintosh D. J., Nilaratna B. P. (Dehli, India: IUCN), 1–17.
Bhattacharya S., Adhya T. K., Pathak H., Raghuram N., Sharma C. (2017). “Issues and policies for reactive nitrogen management in the Indian region,” in The Indian Nitrogen Assessment. Eds. Abrol Y. P., Adhya T. K., Aneja V. P., Raghuram N., Pathak H., Kulshrestha U., Sharma C., Singh B. (Elsevier), 491–513. Available at: https://www.sciencedirect.com/book/9780128118368/the-indian-nitrogen-assessment
Bijay-Singh (2017). “Management and use efficiency of fertilizer nitrogen in production of cereals in India - issues and strategies,” in The Indian Nitrogen Assessment. Eds. Abrol Y. P., Adhya T. K., Aneja V. P., Raghuram N., Pathak H., Kulshrestha U., Sharma C., Singh B. (Elsevier), 149–162. Available at: https://www.sciencedirect.com/book/9780128118368/the-indian-nitrogen-assessment
Billen G., Garnier J., Lassaletta L. (2013). The nitrogen cascade from agricultural soils to the sea: modelling nitrogen transfers at regional watershed and global scales. Philos. Trans. R. Soc. B 368, 20130123. doi: 10.20131098/rstb.20132013.20130123
Birkeland C. (1982). Terrestrial runoff as a cause of outbreaks of Acanthaster planci (Echinodermata: asteroidea). Mar. Biol. 69, 175–185. doi: 10.1007/BF00396897
Birkeland C. (1997). Life and death of coral reefs (Springer), 536. Available at: https://link.springer.com/book/9780412035418
BOBLME (2010). “Coastal pollution loading and water quality criteria,” in BOBLME-2010-Ecology-06 (Male, Maldives: BOBLME), 34.
BOBLME (2011). “Country report on pollution - India,” in BOBLME-2011-Ecology-07 (BOBLME), 200 pages. Available at: https://www.boblme.org
BOBLME (2013). “Country report on pollution - Sri Lanka,” in BOBLME-2011-Ecology-14 (BOBLME), 107 pages. Available at: https://www.boblme.org
BOBLME (2014). “Understanding nutrient loading and sources in the bay of Bay of Bengal Large Marine Ecosystem,” in BOBLME-2014-Ecology-18 (BOBLME), 40 pages. Available at: https://www.boblme.org
BOBLME (2015). “Nutrient loading and eutrophication of coastal waters of the south Asian seas – a scoping study,” in BOBLME-2015-Ecology-02 (BOBLME), 57 pages. Available at: https://www.boblme.org
Bouwman A. F., Van Drecht G., Knoop J. M., Beusen A. H. W., Meinardi C. R. (2005). Exploring changes in river nitrogen export to the world’s oceans. Global Biogeochem. Cycles 19 (1), GB1002. doi: 10.1029/2004GB002314
Boyer T. P., Baranova O. K., Coleman C., Garcia H. E., Grodsky A., Locarnini R. A., et al. (2018). World ocean database 2018 Vol. 87. Ed. Mishonov A. V. (NOAA Atlas NESDIS), 207. Available at: https://www.ncei.noaa.gov/products/world-ocean-database
Brodie J., Fabricius K., De’ath G., Okaji K. (2005). Are increased nutrient inputs responsible for more outbreaks of crown-of-thorns starfish? An appraisal of the evidence? Mar. Pollut. Bull. 51, 266–278. doi: 10.1016/j.marpolbul.2004.10.035
Brodie J., Johnson J. E., Waterhouse J., Erdmann S. (2019). Wastewater pollution and coral reefs: supporting science. [Townsville, Australia: C2O (Coasts Climate Oceans)].
Brown B. E. (2007). Coral reefs of the Andaman Sea - an integrated perspective. Oceanog. Mar. Biol.: Annu. Rev. 45, 173–194. doi: 10.1201/9781420050943.ch5
Brown K. T., Bender-Champ D., Bryant D. E. P., Dove S., Hoegh-Guldberg O. (2017). Human activities influence benthic community structure and the composition of the coral-algal interactions in the central Maldives. J. Exp. Mar. Biol. Ecol. 497, 33–40. doi: 10.1016/j.jembe.2017.09.006
Bruno J. F., Petes L. E., Harvell C. D., Hettinger A. (2003). Nutrient enrichment can increase the severity of coral diseases. Ecol. Lett. 6 (12), 1056–1061. doi: 10.1046/j.1461-0248.2003.00544.x
Buckingham M. C., D’Angelo C., Chalk T. B., Foster G. L., Johnson K. G., Connelly Z., et al. (2022). Impact of nitrogen (N) and phosphorus (P) enrichment and skewed N:P stoichiometry on the skeletal formation and microstructure of symbiotic reef corals. Coral Reefs 41, 1147–1159. doi: 10.1007/s00338-022-02223-0
Burke L., Reytar K., Spalding M., Perry A. (2011). Reefs at risk revisited (Washington, DC: World Resources Institute). 131 pages
Burkepile D. E., Shantz A. A., Adam T. C., Munsterman K. S., Speare K. E., Ladd M. C., et al. (2020). Nitrogen identity drives differential impacts of nutrients on coral bleaching and mortality. Ecosystems 23 (4), 798–811. doi: 10.1007/s10021-019-00433-2
Bythell J. C. (1988). A total nitrogen and carbon budget for the elkhorn coral Acropora palmata (Lamarck). in Proceedings of the 6th International Coral Reef Symposium. 6, 535–540.
Cardini U., Bednarz V. N., Fosterm R. A., Wild C. (2014). Benthic N2 fixation in coral reefs and the potential effects of human-induced environmental change. Ecol. Evol. 4 (9), 1706–1727. doi: 10.1002/ece3.1050
CC & CRMD (2018). Sri Lanka Coastal zone and coastal resources management plan 2018 (Colombo, Sri Lanka: Coast Conservation and Coastal Resources Management Department). Available at: http://www.coastal.gov.lk/index.php?option=com_content&view=article&id=26&Itemid=162&lang=en
Chandra K., Fairoz M., Freiwald J., Gallimore S., Hana Amir F., Hardman N., et al. (2021). “Status and trends of coral reefs of the south Asia region. in status of coral reefs of the world: 2020” in Global coral reef monitoring network (GCRMN) & international coral reef initiative (ICRI) Eds. Souter D., Planes S., Wicquart J., Logan M., Obura D., Staub F., 7. Available at: http://gcrmn.net/2020-report-v1-2023/.
Chandran R., Senthil Kumaran R., Vasavada D. T., Joshi N. N., Husen O. G. (2021). New distribution record of a true coral species, Psammocora contigua (Esper 1794) from Gulf of Kachchh marine national park and sanctuary, India. J. Threatened Taxa 13 (14), 20266–20271. doi: 10.11609/jott.26851.20213.20214.20266-20271
Chandrasekaran S., Nagendran N. A., Pandiaraja D., Krishnankutty N., Kamalakannan B. (2008). Bioinvasion of Kappaphycus alvarezii on corals in the Gulf of Mannar, India. Curr. Sci. 94, 1167–1172.
Christian L., Patel R., Bhagat R., Salvi H., Kamboj R. D. (2019). Assessment of seasonal variation in water quality at important coral and mangrove areas of Gulf of Kachchh, Gujarat. Res. Rev.: J. Ecol. 8 (3), 32–43.
Cloern J. E. (2001). Our evolving conceptual model of the coastal eutrophication problem. Mar. Ecol. Prog. Ser. 210, 223–253. doi: 10.3354/meps210223
Cowburn B., Moritz C., Birrell C., Grimsditch G., Abdulla A. (2018). Can luxury and environmental sustainability co-exist? Assessing the environmental impact of resort tourism on coral reefs in the Maldives. Ocean Coast. Manage. 158, 120–127. doi: 10.1016/j.ocecoaman.2018.1003.1025
CPCB (2021). National inventory of sewage treatment plants (Delhi, India: Central Pollution Control Board), 187.
Dahanayaka D. D. G. L., Tonooka H., Wijeyaratne M. J. S., Minato A., Ozawa S. (2013). Two decadal trends of surface chlorophyll-a concentrations in tropical lagoon environments in Sri Lanka using satellite and in-situ data. Asian J. Geoinform. 13 (3), 7–16.
D’Angelo C., Wiedenmann J. (2014). Impacts of nutrient enrichment on coral reefs: new perspectives and implications for coastal management and reef survival. Curr. Opin. Environ. Sustain. 7, 82–93. doi: 10.1016/j.cosust.2013.11.029
De K., Venkataraman K., Ingole B. (2017). Current status and scope of coral reef research in India: a bio-ecological perspective. Indian J. Geo-Mar. Sci. 46 (4), 647–662.
De Bruin G. H. P. (1972). The Crown-of-Thorns Starfish Acanthaster planci (Linne) in Ceylon. Bull. Fish. Res. Stn. (Sri Lanka (Ceylon) 23 (1 & 2), 37–41.
DeCarlo T. M., Cohen A. L., Barkley H. C., Cobban Q., Young C., Shamberger K. E., et al. (2015). Coral macrobioerosion is accelerated by ocean acidification and nutrients. Geology 43, 7–10. doi: 10.1130/G36147.1
de Raus Maure E., Terauchi G., Ishizaka J., Clinton N., DeWitt M. (2021). Globally consistent assessment of coastal eutrophication. Nat. Commun. 12 (1), 6142. doi: 10.1038/s41467-021-26391-9
de Vos A., Pattiaratchi C. B., Wijeratne E. M. S. (2014). Surface circulation and upwelling patterns around Sri Lanka. Biogeosciences 11 (20), 5909–5930. doi: 10.5194/bg-11-5909-2014
Dheenan P. S., Jha D. K., Das A. K., Vinithkumar N. V., Devi M. P., Kirubagaran R. (2016). Geographic information systems and multivariate analysis to evaluate fecal bacterial pollution in coastal waters of Andaman, India. Environ. pollut. 214, 45–53. doi: 10.1016/j.envpol.2016.03.065
Dheenan P. S., Jha D. K., Vinithkumar N. V., Ponmalar A. A., Venkateshwaran P., Kirubagaran R. (2014). Spatial variation of physicochemical and bacteriological parameters elucidation with GIS in Rangat Bay, middle Andaman, India. J. Sea Res. 85, 534–541. doi: 10.1016/j.seares.2013.09.001
Diaz R., Selman M., Chique C. (2011). Global eutrophic and hypoxic coastal systems. World Resources Institute (Eutrophication and Hypoxia: Nutrient Pollution in Coastal Waters). Available at: https://www.wri.org/data/world-hypoxic-and-eutrophic-coastal-areas
Dixit A. M., Kumar P., Kumar L., Pathak K. D., Patel M. I. (2010). Economic valuation of coral reef systems in Gulf of Kachchh (Gujarat: World Bank aided Integrated Coastal Zone Management (ICZM) Project), 158.
Done T. J. (1992). Phase shift in coral reef communitieis and their ecological significance. Hydrobiologia 247, 121–132. doi: 10.1007/BF00008211
Donovan M. K., Adam T. C., Shantz A. A., Speare K. E., Munsterman K. S., Rice M. M., et al. (2020). Nitrogen pollution interacts with heat stress to increase coral bleaching across the seascape. Proc. Natl. Acad. Sci. U.S.A. 117 (10), 5351–5357. doi: 10.1073/pnas.1915395117
Doty M. S., Oguri M. (1956). The island mass effect. ICES J. Mar. Sci. 22 (1), 33–37. doi: 10.1093/icesjms/22.1.33
D’Silva M. S., Anil A. C., Naik R. K. (2012). Algal blooms: a perspective from the coasts of India. Natural Haz. 63, 1225–1253. doi: 10.1007/s11069-012-0190-9
Dubinsky Z., Stambler N. (1996). Marine pollution and coral reefs. Global Change Biol. 2, 511–526. doi: 10.1111/j.1365-2486.1996.tb00064.x
Duce R. A., LaRoche J., Altieri K., Arrigo K. R., Baker A. R., Capone D. G., et al. (2008). Impacts of atmospheric anthropogenic nitrogen on the open ocean. Science 320, 893–897. doi: 10.1126/science.1150369
Eddy T. D., Lam V. W. Y., Reygondeau G., Cisneros-Montemayor A. M., Greer K., Palomares M. L. D., et al. (2021). Global decline in capacity of coral reefs to provide ecosystem services. One Earth 4 (9), 1278–1285. doi: 10.1016/j.oneear.2021.08.016
Edeson W. (2004). Review of legal and enforcement mechanisms in the BOBLME region. Bay of Bengal Large Marine Ecosystem (BOBLME) theme report (FAO-BOBLME Programme), 88. Available at: https://www.boblme.org
Edwards A. J., Clark S., Zahir H., Rajasuriya A., Naseer A., Rubens J. (2001). Coral bleaching and mortality on artificial and natural reefs in Maldives in 1998, sea surface temperature anomalies and initial recovery. Mar. pollut. Bull. 41 (1), 7–15. doi: 10.1016/S0025-1326X(1000)00200-00209
Ekanayaka K.B.S.S.J., Jayasiri H. B., Ranasinghe P. N. (2016). Phytoplankton abundance in relation to nutrient dynamics during southwest monsoon, southern coast of Sri Lanka. Proc. Natl. Aquat. Resour. Res. Dev. Agency (NARA) 2016, 71–75.
El-Khaled Y. C., Roth F., Tilstra A., Rädecker N., Karcher D. B., Kürten B., et al. (2020). In situ eutrophication stimulates dinitrogen fixation, denitrification, and productivity in Red Sea coral reefs. Mar. Ecol. Prog. Ser. 645, 55–66. doi: 10.3354/meps13352
Ellepola G., Harischandra S., Ranawana K. B. (2021). Spatial analysis of geophysical and environmental factors characterize distinct coral reef habitats around Sri Lanka; implications for management. Ocean Coast. Manage. 210, 105667. doi: 10.1016/j.ocecoaman.2021.105667
Ezzat L., Maguer J.-F., Grover R., Ferrier-Pages C. (2015). New insights into carbon acquisition and exchanges within the coral–dinoflagellate symbiosis under NH4 and NO3 supply. Proc. R. Soc. B 282, 20150610. doi: 10.20151098/rspb.20152015.20150610
Fabricius K. E. (2005). Effects of terrestrial runoff on the ecology of corals and coral reefs: review and synthesis. Mar. pollut. Bull. 50 (2), 125–146. doi: 10.1016/j.marpolbul.2004.11.028
FAO (2011). Aquastat profile – Maldives. (Rome, Italy: FAO), 12. Available at: https://www.fao.org/aquastat/en/countries-and-basins/country-profiles/country/MDV
FAO (2020) Maldives Country profile. Available at: http://www.fao.org/faostat/en/#country/132.
FAO, ITPS (2015). Status of the world’s soil resources (SWSR) – main report (Rome, Italy: Food and Agriculture Organization of the United Nations and Intergovernmental Technical Panel on Soils).
Fernandes de Barros Marangoni L., Ferrier-Pages C., Rottier C., Bianchini A., Grover R. (2020). Unravelling the different causes of nitrate and ammonium effects on coral bleaching. Sci. Rep. 10 (1), 11975. doi: 10.1038/s41598-020-68916-0
Ferrier-Pagès C., Gattuso J. P., Dallot S., Jaubert J. (2000). Effect of nutrient enrichment on growth and photosynthesis of the zooxanthellate coral Stylophora pistillata. Coral Reefs 19, 103–113. doi: 10.1007/s003380000078
Gammanpila M. (2010). Hydrography, nutrients and abundance and distribution of zooplankton in Negombo lagoon, Sri Lanka. Sri Lanka J. Aquat. Sci. 15, 13–24. doi: 10.4038/sljas.v15i0.5447
Garcia H. E., Weathers K. W., Paver C. R., Smolyar I. V., Boyer T. P., Locarnini R. A., et al. (2019). “World ocean atlas 2018, volume 4: dissolved inorganic nutrients (phosphate, nitrate and nitrate+nitrite, silicate),” in NOAA Atlas, vol. 84 . Ed. Mishonov A. (NESDIS), 35. Available at: https://www.ncei.noaa.gov/products/world-ocean-atlas
Garg J. K. (2015). Wetland assessment, monitoring and management in India using geospatial techniques. J. Environ. Manage. 148, 112–123. doi: 10.1016/j.jenvman.2013.12.018
Garg J. K., Singh T. S., Murthy T. V. R. (1998). Wetlands of India. project report: RSAM/SAC/RESA/PR/01/98 (Ahmedabad: Space Applications Centre (ISRO), 239.
Geekiyanage N., Vithanage M., Wijesekara H., Pushpakumara G. (2015). “State of the environment, environmental challenges and governance in Sri Lanka,” in Environmental challenges and governance. Eds. Mukherjee S., Chakraborty D. (London: Routledge), 92–108. doi: 10.4324/9781315717081
GEER (2008). Regional strategy for coral reef managment in south Asia (Gandhinagar, India: Gujarat Ecological Education and Research Foundation), 48.
GESAMP (IMO/FAO/UNESCO-IOC/WMO/WHO/IAEA/UN/UNEP Joint Group of Experts on the Scientific Aspects of Marine Environmental Protection), Advisory Committee on Protection of the Sea (2001). Protecting the oceans from land-based activities - Land-based sources and activities affecting the quality and uses of the marine, coastal and associated freshwater environment. Rep. Stud. (Nairobi, Kenya: GESAMP) 71, 162.
Glasl B., Bourne D. G., Frade P. R., Thomas T., Schaffelke B., Webster N. S. (2019). Microbial indicators of environmental perturbations in coral reef ecosystems. Microbiome 7 (1), 94. doi: 10.1186/s40168-019-0705-7
Glaze T. D., Erler D. V., Siljanen H. M. P. (2022). Microbially facilitated nitrogen cycling in tropical corals. ISME J. 16 (1), 68–77. doi: 10.1038/s41396-021-01038-1
Goes J. I., Tian H., Gomes H. D. R., Anderson O. R., Al-Hashmi K., deRada S., et al. (2020). Ecosystem state change in the Arabian Sea fuelled by the recent loss of snow over the Himalayan-Tibetan plateau region. Sci. Rep. 10 (1), 7422. doi: 10.1038/s41598-020-64360-2
Gomes H., Goes J. I., Matondkar S. G. P., Buskey E. J., Basu S., Parab S., et al. (2014). Massive outbreaks of Noctiluca Scintillans blooms in the Arabian Sea due to spread of hypoxia. Nat. Commun. 5, 4862. doi: 10.1038/ncomms5862
Gomes H., Goes J. I., Prabhu Matondkar S. G., Parab S. G., Al-Azri A. R. N., Thoppil P. G. (2008). Blooms of noctiluca miliaris in the Arabian Sea - an in situ and satellite study. Deep Sea Res. Part I 55 (6), 751–765. doi: 10.1016/j.dsr.2008.1003.1003
Gopakumar G., Sulochanan B., Venkatesan V. (2009). Bloom of Noctiluca Scintillans (Macartney) in Gulf of Mannar, southeast coast of India. J. Mar. Biol. Assoc. India 51 (1), 75–80.
Goswami P., Gupta S., Das A. K., Vinithkumar N. V., Dharani G., Kirubagaran R. (2020). Impact of a dinoflagellate bloom on the marine plankton community structure of Port Blair Bay, Andaman island. Reg. Stud. Mar. Sci. 37, 101320. doi: 101310.101016/j.rsma.102020.101320
Government of Australia and Marine Research Centre (2005) An assessment of damage to maldivian coral reefs and baitfish populations from the Indian Ocean tsunami. Available at: https://reliefweb.int/report/Maldives/assessment-damage-maldivian-coral-reefs-and-baitfish-populations-indian-ocean.
Government of India (2012). Lakshadweep cction plan on climate change (New Delhi, India), 274. Available at: https://moef.gov.in/en/division/environment-divisions/climate-changecc-2/state-action-plan-on-climatechange/.
Government of India (2013). Andaman and Nicobar Islands state action plan on climate change (New Delhi, India: Government of India), 177. Available at: https://moef.gov.in/en/division/environment-divisions/climate-changecc-2/state-action-plan-on-climate-change/.
Guan Y., Hohn S., Wild C., Merico A. (2020). Vulnerability of global coral reef habitat suitability to ocean warming, acidification and eutrophication. Glob Chang Biol 26, 5646–5660. doi: 10.1111/gcb.15293
Hallock P. (1988). The role of nutrient availability in bioerosion: consequences to carbonate buildups. Palaeogeog. Palaeoclimatol. Palaeoecol. 63, 275–291. doi: 10.1016/0031-0182(88)90100-9
Halpern B. S., Walbridge S., Selkoe K. A., Kappel C. V., Micheli F., D’Agrosa C., et al. (2008). A global map of human impact on marine ecosystems. Science 319, 948–952. doi: 10.1126/science.1149345
Harris J. M., Vinobaba P. (2013). Assessment the present status of batticaloa lagoon, Sri Lanka by means of water quality, fish diversity indices and pollution indicating planktons. J. Biodiversity Endangered Species 1 (2), 10000105. doi: 10.10004172/10002332-10002543.11000105
Harrison P., Booth D. J. (2007). “Coral reefs: naturally dynamic and increasingly disturbed ecosystems,” in Marine Ecology (Oxford: Oxford University Press), 316–377.
Harrison P. L., Ward S. (2001). Elevated levels of nitrogen and phosphorus reduce fertilization sucess of gametes from scleractinian reef corals. Mar. Biol. 139, 1057–1068. doi: 10.1007/s002270100668
Heikoop J. M., Risk M. J., Lazier A. V., Edinger E. N., Jompa J., Limmon G. V., et al. (2000). Nitrogen-15 signals of anthropogenic nutrient loading in reef corals. Mar. pollut. Bull. 40 (7), 628–636. doi: 10.1016/S0025-1326X(1000)00006-00000
Herzog H., Konrad R. (1992). Nitrogen movement in an arable sandy soil and ways of reducing nitrogen losses — preliminary results. J. Agron. Crop Sci. 169 (1-2), 135–143. doi: 10.1111/j.1439-1037X.1992.tb01192.x
Hettige N. D., Weerasekara K. A. W. S., Azmy S. A. M., Jinadasa S. U. P. (2014). Water pollution in selected coastal areas in western province, Sri Lanka: a baseline survey. J. Environ. Professionals Sri Lanka 3 (2), 12–24. doi: 10.4038/jepsl.v3i2.7843
Highsmith R. C. (1980). Geographical patterns of coral bioerosion: a productivity hypothesis. J. Exp. Mar. Biol. Ecol. 46, 177–196. doi: 10.1016/0022-0981(80)90030-1
Hoegh-Guldberg O. (1999). Climate change, coral bleaching and the future of the worlds coral reefs. Mar. Freshw. Res. 50, 839–866. doi: 10.1071/MF99078
Hoon V. (1997). “Coral reefs of India: review of their extent, condition, research and management status,” in Proceedings of the regional workshop on the conservation and sustainable management of coral reefs. Ed. Hoon V. (Madras: CRSARD).
Hoon V., Tamelander J. (2005). “Community-based monitoring of coral reef resource use in agatti island, union territory of Lakshadweep, India,” in Coral reef degradation in the Indian Ocean: status report. Eds. Souter D., Lindén O. (Sweden: CORDIO), 205–218.
Hughes T. P., Baird A. H., Bellwood D. R., Card M., Connolly S. R., Folke C., et al. (2003). Climate change, human impacts and the resilience of coral reefs. Science 301, 929–933. doi: 10.1126/science.1085046
Humanes A., Noonan S. H., Willis B. L., Fabricius K. E., Negri A. P. (2016). Cumulative effects of nutrient enrichment and elevated temperature compromise the early life history stages of the coral acropora tenuis. PloS One 11 (8), e0161616. doi: 10.1371/journal.pone.0161616
Hussain A., Ingole B. (2020). Massive coral bleaching in the patchy reef of grande island, along the eastern Arabian Sea during the 2015/16 global bleaching event. Reg. Stud. Mar. Sci. 39, 101410. doi: 10.1016/j.rsma.2020.101410
Ibrahim N. (2019). “Anthropogenic influence on the benthic community structure of reef flats in south Huvadhu atoll of the Maldives,” in Marine Ecology research centre (Coffs Harbour, Australia: The Southern Cross University), 43.
Ibrahim N., Mohamed M., Basheer A., Haleem I., Nistharan F., Naeem R., et al. (2017). Status of coral bleaching in the Maldives in 2016 (Malé, Maldives: Marine Research Centre), 47.
Iijima M., Yasumoto J., Iguchi A., Koiso K., Ushigome S., Nakajima N., et al. (2021). Phosphate bound to calcareous sediments hampers skeletal development of juvenile coral. R Soc. Open Sci. 8 (3), 201214. doi: 10.1098/rsos.201214
Ileperuma O. A. (2000). Environmental pollution in Sri Lanka: a review. J. Natl. Sci. Foundation Sri Lanka 28 (4), 301–325. doi: 10.4038/jnsfsr.v28i4.2644
Imbulana K. A. U. S., Wijesekara N. T. S., Neupane B. R. (2006). “Sri Lanka National water development report,” in UN-Water/WWAP/2006/11 (Colombella, Italy: MAI&MD, UN-WWAP, UNESCO and University of Moratuwa), 150.
IPCC (2019). IPCC special report on the ocean and cryosphere in a changing climate. Eds. Poürtner H.-O., Roberts D. C., Masson-Delmotte V., Zhai P., Tignor M., Poloczanska E., Mintenbeck K., Alegriía A., Nicolai M., Okem A., Petzold J., Rama B., Weyer N. M. (Cambridge, UK and New York, NY, USA: Cambridge University Press). Available at: https://www.ipcc.ch/srocc/
Islam M. S., Tanaka M. (2004). Impacts of pollution on coastal and marine ecosystems including coastal and marine fisheries and approach for management: a review and synthesis. Mar. pollut. Bull. 48 (7-8), 624–649. doi: 10.1016/j.marpolbul.2003.12.004
Jagadeesan L., Jyothibabu R., Anjusha A., Mohan A. P., Madhu N. V., Muraleedharan K. R., et al. (2013). Ocean currents structuring the mesozooplankton in the Gulf of Mannar and the Palk Bay, southeast coast of India. Prog. Oceanog. 110, 27–48. doi: 10.1016/j.pocean.2012.12.002
Jaleel A. (2013). The status of the coral reefs and the management approaches: the case of the Maldives. Ocean Coast. Manage. 82, 104–118. doi: 10.1016/j.ocecoaman.2013.05.009
Jameson J. D. (2012). “Issues relating to water quality changes in the coral reef environment,” in Coral reefs in India - status, threats and conservation measures. Eds. Bhatt J. R., Edward J. K. P., Macintosh D. J., Nilaratna B. P. (New Dehli, India: IUCN), 273–280.
Jasmine S., George R. M., Manisseri M. K., Kingsly J. (2009). Hard coral diversity along southwest coast of India. J. Mar. Biol. Assoc. India 51, 189–193.
Jayalal T. B. A., Niroshani S. L. N. (2012). “Wastewater production, treatment, and use in Sri Lanka,” in 2nd regional workshop for South, West and Central Asia. New Delhi, India (UN Water), 7. Available at: https://www.ais.unwater.org/ais/pluginfile.php/232/mod_page/content/116/SriLanka_Report.pdf.
Jayasingha P., Pitawala A., Dharmagunawardhane H. A. (2011). Vulverability of coastal aquifers due to nutrient pollution from agriculture: Kalpitiya, Sri Lanka. Water Air Soil Polution 219, 563–577. doi: 10.1007/s11270-010-0728-y
Jayasingha P., Pitawala A., Dharmagunawardhane H. A. (2012). “A review on nutrient pollution of coastal aquifers of Sri Lanka,” in Coastal environments: focus on Asian regions. Ed. Subramanian V. (Dordrecht: Springer), 66–77.
Jeevamani J. J. J., Kamalakannan B., Nagendran N. A., Chandrasekaran S. (2013). “Climate change induced coral bleaching and algal phase shift in reefs of the Gulf of Mannar, India,” in Knowledge systems of societies for adaptation and mitigation of impacts of climate change. Ed. Nautiyal S., Rao K. S., Kaechele H., Raju K. V., Schaldach R. (Berlin: Springer-Verlag), 87–94.
Jeyabaskaran R., Venkataraman K., Alfred J. R. B. (2007). “Implications for conservation of coral reefs in the Andman and Nicobar islands, India,” in The third international tropical marine ecosystem management symposium (ITMEMS3) (Cozumel, Mexico: ITMEMS (International Tropical Marine Ecosystem Management Symposium)).
Jha D. K., Devi M. P., Vidyalakshmi R., Brindha B., Vinithkumar N. V., Kirubagaran R. (2015a). Water quality assessment using water quality index and geographical information system methods in the coastal waters of Andaman Sea, India. Mar. pollut. Bull. 100 (1), 555–561. doi: 10.1016/j.marpolbul.2015.08.032
Jha D. K., Kirubagaran R., Vinithkumar N. V., Dharani G., Madeswaran P. (2019). “The Andaman and Nicobar Islands,” in World seas: an environmental evaluation (Academic Press), 185–209. doi: 110.1016/B1978-1010-1008-100853-100859.100013-100850
Jha D. K., Prashanthi Devi M., Vinithkumar N. V., Das A. K., Dheenan P. S., Venkateshwaran P., et al. (2013). Comparative investigation of water quality parameters of aerial & Rangat Bay, Andaman islands using In-situ measurements and spatial modelling techniques. Water Quality Exposure Health 5 (2), 57–67. doi: 10.1007/s12403-013-0086-2
Jha D. K., Vinithkumar N. V., Sahu B. K., Das A. K., Dheenan P. S., Venkateshwaran P., et al. (2014). Multivariate statistical approach to identify significant sources influencing the physico-chemical variables in Aerial Bay, north Andaman, India. Mar. pollut. Bull. 85 (1), 261–267. doi: 10.1016/j.marpolbul.2014.06.007
Jha D. K., Vinithkumar N. V., Sahu B. K., Dheenan P. S., Das A. K., Begum M., et al. (2015b). Multivariate and geo-spatial approach for seawater quality of Chidiyatappu Bay, South Andaman Islands, India. Mar. pollut. Bull. 96 (1-2), 463–470. doi: 10.1016/j.marpolbul.2015.05.004
Jinoj T. P. S., Bonthu S., Robin R. S., Babu K. K. I., Arumugam K., Purvaja R., et al. (2021). Numerical modelling approach for the feasibility of shore protection measures along the coast of Kavaratti Island, Lakshadweep archipelago. J. Earth System Sci. 130 (3), 165. doi: 10.1007/s12040-021-01665-4
Jinoj T. P. S., Bonthu S. R., Robin R. S., Idrees Babu K. K., Purvaja R., Ramesh R. (2020). Nearshore sediment dynamics of Kavaratti Island, Lakshadweep archipelago using integrated modelling system. Indian J. Geo-Mar. Sci. 49 (5), 845–857.
Joseph L. (2003). “National report of Sri Lanka on the formulation of a transboundary diagnostic analysis and strategic action plan for the Bay of Bengal Large Marine Ecosystem programme,” in BOBLME (Colombo, Sri Lanka), 125. Available at: https://docslib.org/doc/2936266/national-report-of-sri-lanka
Jyothibabu R., Mohan A. P., Jagadeesan L., Anjusha A., Muraleedharan K. R., Lallu K. R., et al. (2013). Ecology and trophic preference of picoplankton and nanoplankton in the Gulf of Mannar and the Palk Bay, southeast coast of India. J. Mar. Syst. 111-112, 29–44. doi: 10.1016/j.jmarsys.2012.09.006
Kafle K., Balasubramanya S., Horbulyk T. (2019). Prevalence of chronic kideny disease in Sri Lanka: a profile of affected districts reliant on groundwater. Sci. Total Environ. 694, 133767. doi: 10.1016/j.scitotenv.2019.133767
Kaly U. L. (2004). “Review of land-based sources of pollution to the coastal and marine environments in the BOBLME region,” in Bay of Bengal Large marine ecosystem (BOBLME) theme report (BOBLME), 108. Available at: https://www.boblme.org.
Kannan R., Kannan L. (1996). Physico-chemical characteristics of seaweed beds of the Palk Bay, southeast coast of India. Indian J. Mar. Sci. 25, 358–362.
Karati K. K., Vineetha G., Madhu N. V., Anil P., Dayana M., Shihab B. K., et al. (2017). Variability in the phytoplankton community of Kavaratti reef ecosystem (northern Indian Ocean) during peak and waning periods of El nino 2016. Environ. Monit Assess. 189 (12), 653. doi: 10.1007/s10661-017-6369-5
Karcher D. B., Roth F., Carvalho S., El-Khaled Y. C., Tilstra A., Kurten B., et al. (2020). Nitrogen eutrophication particularly promotes turf algae in coral reefs of the central Red Sea. PeerJ 8, e8737. doi: 10.7717/peerj.8737
Karthik R., Robin R. S., Anandavelu I., Purvaja R., Singh G., Mugilarasan M., et al. (2020). Diatom bloom in the Amba River, west coast of India: a nutrient-enriched tropical river-fed estuary. Reg. Stud. Mar. Sci. 35, 101244. doi: 101210.101016/j.rsma.102020.101244
Karthik R., Robin R. S., Purvaja R., Karthikeyan V., Subbareddy B., Balachandar K., et al. (2022). Microplastic pollution in fragile coastal ecosystems with special reference to the X-Press Pearl maritime disaster, southeast coast of India. Environ. pollut. 305, 119297. doi: 10.1016/j.envpol.2022.119297
Kathiravan K., Natesan U., Vishnunath R. (2014). Spatio-temporal variability of hydro-chemical characteristics of coastal waters of Gulf of Mannar marine biosphere reserve (GoMMBR), south India. Appl. Water Sci. 7 (1), 361–373. doi: 10.1007/s13201-014-0251-1
Kleypas J. A., McManus J. W., Menez L. A. B. (1999). Environmental limits to coral reef development: where do we draw the line? Am. Zool. 39, 146–159. doi: 10.1093/icb/39.1.146
Koop K., Booth D., Broadbents A., Brodie J., Bucher D., Capone D., et al. (2001). ENCORE: the effect of nutrient enrichment on coral reefs. Synthesis Res. Conclusions. Mar. pollut. Bull. 42 (2), 91–120. doi: 10.1016/S0025-326X(00)00181-8
Krishna M. S., Prasad M. H. K., Rao D. B., Viswanadham R., Sarma V. V. S. S., Reddy N. P. C. (2016). Export of dissolved inorganic nutrients to the northern Indian Ocean from the Indian monsoonal rivers during discharge period. Geochimica Cosmochim. Acta 172, 430–443. doi: 10.1016/j.gca.2015.10.013
Krishnan P., Purvaja R., Sreeraj C. R., Raghuraman R., Robin R. S., Abhilash K. R., et al. (2018). Differential bleaching patterns in corals of Palk Bay and the Gulf of Mannar. Curr. Sci. 114 (3), 679–685. doi: 10.18520/cs/v114/i03/679-685
Krishnan P., Roy S. D., George G., Srivastava R. C., Anand A., Murugesan S., et al. (2011). Elevated sea surface temperature during may 2010 induces mass bleaching of corals in the Andaman. Curr. Sci. 100 (1), 111–117.
Kumar J. S. Y., Geetha S. (2012). Seasonal changes of hydrographic properties in sea water of coral reef islands, Gulf of Mannar, India. Int. J. Plant Anim. Environ. Sci. 2 (2), 135–159.
Kumar P., Mishra A., Pitchika V. K., Kumar S., Dubey K. M., Singh D., et al. (2019). Integrated geophysical appraisal of crustal structure and tectonic evolution of the Angria Bank, western continental margin of India. Mar. Geophysical Res. 40, 433–449. doi: 10.1007/s11001-019-09383-9
Kumar J. S. Y., Satyanarayana C., Venkataraman K., Chandra K. (2017). Studies on survival and growth rate of transplanted acroporidae in Gulf of Kachchh marine national park, India. J. Coast. Conserv. 21, 23–34. doi: 10.1007/s11852-016-0465-5
Kumara P. B. T. P., Kumara W. A. A. U., Sandaruwan H. A. A., Iroshanie R. G. A., Upendra H. B. L., Cumaranatunga P. R. T. (2008). “Impacts of reef related resource exploitation on coral reefs: some cases from southern Sri Lanka,” in Ten years after bleaching - facing the consequences of climate change on the Indian Ocean. CORDIO status report 2008. Eds. Obura D., Tamelander J., Linden O. (Mombassa: CORDIO (Coastal Oceans Research and Development in the Indian Ocean)/Sida-SAREC), 23–37.
Kumaresan S., Shekhar S., Chakraborty S., Sundaramanickam A., Kuly N. (2018). Environmental variables and nutrients in selected islands of Lakshadweep sea; addressing coral bleaching. Reg. Stud. Mar. Sci. 22, 38–48. doi: 10.1016/j.rsma.2018.05.004
Lamb J. B., Willis B. L., Fiorenza E. A., Couch C. S., Howard R., Rader D. N., et al. (2018). Plastic waste associated with disease on coral reefs. Science 359, 460–462. doi: 10.1126/science.aar3320
Lasagna R., Albertelli G., Giovannetti E., Grondona M., Milani A., Morri C., et al. (2008). Status of maldivian reefs eight years after the 1998 coral mass mortality. Chem. Ecol. 24(SUPPL 1.), 67–72. doi: 10.1080/02757540801966454
Latha S. S., Prasad M. B. K. (2010). “Current status of coastal zone management practices in India,” in Management and sustainable development of coastal zone environments. Eds. Ramanathan A., Bhattacharya P., Dittmar T., Prasad M. B. K., Neupane B. R. (New Delhi, India: Springer), 42–57.
Laxmilatha P., Jasmine S., Sreeram M. P., Rengaiyan P. (2019). Benthic communities of mesophotic coral ecosystem off Puducherry, east coast of India. Curr. Sci. 116, 982–987. doi: 10.18520/cs/v116/i6/982-987
Lerman M. (1986). Marine biology: environment, diversity, and ecology (Addison Wesley Publishing), 534.
Lesser M. P. (2021). Eutrophication on coral reefs: what is the evidence for phase shifts, nutrient limitation and coral bleaching. BioScience 71 (12), 1216–1233. doi: 10.1093/biosci/biab101
Lesser M. P., Falcón L., Rodríguez-Román A., Enríquez S., Hoegh-Guldberg O., Iglesias-Prieto R. (2007). Nitrogen fixation by symbiotic cyanobacteria provides a source of nitrogen for the scleractinian coral Montastraea cavernosa. Mar. Ecol. Prog. Ser. 346, 143–152. doi: 10.3354/meps07008
Lewis J. B. (1981). “Coral reef ecosystems,” in Analysis of marine ecosystems. Ed. Longhurst A. R. (London: Academic Press), 127–158.
Li T., Huang J., Du H., Liu X., Zhong C., Lin S. (2022). Coral bleaching from a nutrient perspective is understudied: a bibliometric survey. Front. Mar. Sci. 9. doi: 10.3389/fmars.2022.926783
Liu C.-Y., Zhang F., Sun Y.-F., Yu X.-L., Huang H. (2020). Effects of nitrate enrichment on respiration, photosynthesis, and fatty acid composition of reef coral pocillopora damicornis larvae. Front. Mar. Sci. 7. doi: 10.3389/fmars.2020.00531
Loch K., Loch W., Schuhmacher H., See W. R. (2002). Coral recruitment and regeneration on a Maldivian reef 21 months after the coral bleaching event of 1998. Mar. Ecol. 23 (3), 219–236. doi: 10.1046/j.1439-0485.2002.02773.x
Lucas J. S. (1982). Quantitative studies of feeding and nutrition during larval development of the coral reef asteroid Acanthaster planci. J. Exp. Mar. Biol. Ecol. 65, 173–194. doi: 10.1016/0022-0981(82)90043-0
Machendiranathan M., Senthilnathan L., Ranith R., A.Saravanakumar T., Thangaradjou S. B., Choudhry, et al. (2016). Trend in coral-algal phase shift in the Mandapam group of islands, Gulf of Mannar marine biosphere reserve, India. J. Ocean Univ. China 15, 1080–1086. doi: 10.1007/s11802-016-2606-8
Madeswaran P., Ramana Murthy M. V., Rajeevan M., Ramanathan V., Patra S., Sanitha K., et al. (2018). “Status report: seawater quality at selected locations along Indian coast,” in National centre for coastal research (Chennai, India: NCCR Publication), 177.
Magesh N. S., Krishnakumar S. (2019). “The Gulf of Mannar marine biosphere reserve, southern India,” in World Seas: an Environmental EvaluationSheppard C. (Elsevier), 169–184. doi: 10.1016/B978-0-08-100853-9.00012-9
Majumdar S. D., Hazra S., Giri S., Chanda A., Gupta K., Mukhopadhyay A., et al. (2018). Threats to coral reef diversity of Andaman islands, India: a review. Reg. Stud. Mar. Sci. 24, 237–250. doi: 10.1016/j.rsma.2018.08.011
Malakar B., Venu S., Samuel V. D., Abhilash K. R. (2021). Increasing signs of degradation of shallow water coral reefs due to repeated bleaching and spatial competition among benthic substrates. Wetlands Ecol. Manage. 29 (5), 669–675. doi: 10.1007/s11273-020-09744-x
Malone T. C., Newton A. (2020). The globalization of cultural eutrophication in the coastal ocean: causes and consequences. Front. Mar. Sci. 7. doi: 10.3389/fmars.2020.00670
Manage P. M., Liyanage G. Y., Abinaiyan I., Madusanka D. A. T., Bandara K. R. V. (2022). Pollution levels in Sri Lanka’s west-south coastal waters: making progress toward a cleaner environment. Reg. Stud. Mar. Sci. 51, 102193. doi: 102110.101016/j.rsma.102022.102193
Mancini A., Ibrahim M., Nistharan F., Afau A., Fisam A., Rasheedh R., et al. (2017) Resource dependence and social resilience in north Ari atoll, Maldives. Available at: https://portals.iucn.org/library/sites/library/files/documents/2017-032.pdf.
Marimuthu N., Verma A. K., Kumar J. S. Y., Adhavan D., Satyanarayana C. (2018). Integrated coastal zone management project funded coral restoration processes in the Gulf of Kachchh - a present scenario. Indian J. Geo-Mar. Sci. 47 (1), 15–19.
Marimuthu N., Wilson J. J., Kumaraguru A. K. (2020). Tracking decadal changes in Palk Bay coral community structure: a multivariate approach. J. Coast. Conserv. 24 (3), 32. doi: 10.1007/s11852-020-00752-w
Mathews G. (2008). Studies on the coral reefs restoration in Tuticorin region of the Gulf of Mannar southeast coast of India (Tamil Nadu, India: Manonmaniam Sundaranar University).
Mayorga E., Seitzinger S. P., Harrison J. A., Dumont E., Beusen A. H. W., Bouwman A. F., et al. (2010). Global nutrient export from WaterSheds 2 (NEWS 2): model development and implementation. Environ. Model. Soft. 25 (7), 837–853. doi: 10.1016/j.envsoft.2010.01.007
Mazarrasa I., Samper-Villarreal J., Serrano O., Lavery P. S., Lovelock C. E., Marba N., et al. (2018). Habitat characteristics provide insights of carbon storage in seagrass meadows. Mar. pollut. Bull. 134, 106–117. doi: 10.1016/j.marpolbul.2018.01.059
McClanahan T. R., Darling E. S., Maina J. M., Muthiga N. A., D’agata S., Leblond J., et al. (2020). Highly variable taxa-specific coral bleaching responses to thermal stresses. Mar. Ecol. Prog. Ser. 648, 135–151. doi: 10.3354/meps13402
McClanahan T. R., Muthiga N. A. (2014). Community change and evidence for variable warm-water temperature adaptation of corals in northern Male atoll, Maldives. Mar. pollut. Bull. 80 (1-2), 107–113. doi: 10.1016/j.marpolbul.2014.01.035
McCook L. (1999). Macroalgae, nutrients and phase shifts on coral reefs: scientific issues and management consequences for the Great Barrier reef. Coral Reefs 18, 357–367. doi: 10.1007/s003380050213
MEE (2011). State of the environment 2011 (Male, Republic of Maldives.: Ministry of Environment and Energy), 237.
MEE (2015a). Maldives National chemical profile (Male, Maldives: Ministry of Environment and Energy), 108. Available at: http://www.environment.gov.mv/v2/en/download/4307.
MEE (2015b). Fifth national report of Maldives to the convention on biological diversity (Male, Maldives.: Ministry of Environment and Energy). Available at: http://www.environment.gov.mv/biodiversity/archives/1195.
MEE (2017a). State of the environment 2016 (Male, Republic of Maldives.: Ministry of Environment and Energy), 216. Available at: http://www.environment.gov.mv/v2/wp-content/files/publications/20170202-pub-soe-2016.pdf.
MEE (2017b). National water and sewerage policy (Male, Maldives: Ministry of Environment and Energy). Available at: https://www.environment.gov.mv/v2/en/download/5880
Melkani V. K. (2008). Status of coral reefs in the Gulf of Mannar region, Tamil nadu, southeast India (Tamil Nadu: Gulf of Mannar Biosphere Reserve Trust), 15.
Miller I. (2011). “Acanthaster planci,” in Encycopedia of modern coral reefs. Ed. Hopley D. (Dordecht: Springer). doi: 10.1007/1978-1090-1481-2639-1002
Miller M. W., Sluka R. D. (1999). Patterns of seagrass and sediment nutrient distribution suggest anthropogenic enrichment in Laamu atoll, republic of Maldives. Mar. pollut. Bull. 38 (12), 1152–1156. doi: 10.1016/S0025-326X(99)00147-2
Moberg F., Folke C. (1999). Ecological goods and services of coral reef ecosystems. Ecol. Econom. 29, 215–233. doi: 10.1016/S0921-8009(99)00009-9
MoEF (1991). “Notification under section 3(1) and section 3(2)(v) of the environment (Protection) act 1986 and rule 5(3)(d) of environment (Protection) rules 1986, declaring coastal stretches as coastal regulation zone (CRZ) and regulating activities in the CRZ,” in Department of environment forests and wildlife (New Delhi: Ministry of Environment and Forests).
MoEF (2009). India’s fourth national report to the convention on biological diversity (Government of India: Ministry of Environment and Forests).
MoEF (2011). “Coastal regulation zone notification,” in Department of environment forests and wildlife (New Delhi: Ministry of Environment and Forests).
MoEF (2014). “India’s fifth national report to the convention on biological diversity,” in Ministry of environment and forests, government of India (New Delhi, India), 142. Available at: https://www.cbd.int/reports/nr5/.
Mohamed G. (2018). “Agroecological analysis of maldivian agricultural system to improve nutrient management,” in Faculty of landscape architecture, horticulture and crop production science (Alnarp: SLU, Swedish University of Agricultural Sciences), 66.
Mohamed H. F., Chen Y., Abd-Elgawad A., Rongshuo C. A. I., Xu C. (2022). The unseen drivers of coral health; coral microbiome; the hope for effective coral restoration. Polish J. Environ. Stud. 31 (2), 1–18. doi: 10.15244/pjoes/141044
Mohandass C., Kumar S. J., Ramaiah N., Vethamony P. (2010). Dispersion and retrievability of water quality indicators during tidal cycles in coastal Salaya, Gulf of Kachchh (West coast of India). Environ. Monit. Assess. 169, 639–645. doi: 10.1007/s10661-009-1203-3
MoMD&E (2016). National biodiversity strategic action plan 2016-2022, biodiversity secretariat (Ministry of Mahaweli Development and Environment), xxi + 284. Available at: https://www.cbd.int/nbsap/search/
Montano S., Strona G., Seveso D., Maggioni D., Galli P. (2016). Widespread occurrence of coral diseases in the central madives. Mrine Freshw. Res. 67, 1253–1262. doi: 10.1071/MF14373
Montefalcone M., Morri C., Bianchi C. N. (2020). Influence of local pressures on maldivian coral reef resilience following repeated bleaching events, and recovery perspectives. Front. Mar. Sci. 7. doi: 10.3389/fmars.2020.00587
Moritz C., Ducarme F., Sweet M. J., Fox M. D., Zgliczynski B., Ibrahim N., et al. (2017). The “resort effect”: can tourist islands act as refuges for coral reef species? Diversity Distribut. 23 (11), 1301–1312. doi: 10.1111/ddi.12627
Morri C., Aliani S., Bianchi C. N. (2010). Reef status in the rasfari region (North malé atoll, Maldives) five years before the mass mortality event of 1998. Estuar. Coast. Shelf Sci. 86 (2), 258–264. doi: 10.1016/j.ecss.2009.11.021
Morri C., Montefalcone M., Lasagna R., Gatti G., Rovere A., Parravicini V., et al. (2015). Through bleaching and tsunami: coral reef recovery in the Maldives. Mar. pollut. Bull. 98 (1), 188–200. doi: 10.1016/j.marpolbul.2015.06.050
Morris L. A., Voolstra C. R., Quigley K. M., Bourne D. G., Bay L. K. (2019). Nutrient availability and metabolism affect the stability of coral-symbiodiniaceae symbioses. Trends Microbiol. 27 (8), 678–689. doi: 10.1016/j.tim.2019.03.004
Moynihan M. A., Goodkin N. F., Morgan K. M., Kho P. Y. Y., Lopes Dos Santos A., Lauro F. M., et al. (2022). Coral-associated nitrogen fixation rates and diazotrophic diversity on a nutrient-replete equatorial reef. ISME J. 16 (1), 233–246. doi: 10.1038/s41396-021-01054-1
Muduli P. R., Vinithkumar N. V., Begum M., Robin R. S., Vardhan K. V., Venkatesan R., et al. (2011). Spatial variation of hydrochemical characteristics in and around Port Blair Bay Andaman and Nicobar Islands, India. World Appl. Sci. J. 13 (3), 564–571.
Mueller J. S., Schupp P. J. (2020). Shading by marine litter impairs the health of the two indo-pacific scleractinian corals porites rus and pavona cactus. Mar. pollut. Bull. 158, 111429. doi: 10.1016/j.marpolbul.2020.111429
Muley E. V., Venkataraman K., Alfred J. R. B., Wafar M. V. M. (2000). Status of coral reefs of India (Accessed Proceedsing of the 9th International Coral Reef Symposium).
Mulochau T., Lelabousse C., Sere M. (2020). Estimations of densities of marine litter on the fringing reefs of Mayotte (France - south Western Indian Ocean) - impacts on coral communities. Mar. pollut. Bull. 160, 111643. doi: 10.1016/j.marpolbul.2020.111643
Muscatine L., Falkowski P. G., Dubinsky Z., Cook P. A., McCloskey L. R. (1989). The effect of external nutrient resources on the population dynamics of zooxanthellae in a coral reef. Proc. R. Soc. B 236, 311–324.
Mysan S. M., Ranasinghe A. (2014). Design of sewerage system in Kirulapone for Colombo municipality. Engineer: J. Institution Engineers Sri Lanka 47 (4), 87–94. doi: 10.4038/engineer.v4047i4034.6887
Naeem S., Sattar S. (2007). A compilation of reported fish kills in the Maldives (Male, Maldives: Marine Research Centre), 27.
Nair V. (2002). Status of the flora and fauna of Gulf of Kachchh, India (Goa, India: National Institute of Oceanography), 27.
Nair K. K. C. (2010). Atlas on environmental parameters and productivity (Primary and secondary) on the Indian EEZ and adjoining areas (Kochi, India: Centre for Marine Living Resources and Ecology, Ministry of Earth Sciences), 296.
Nair V. R., Govindan K., Ramaiah N., Gajbhiye S. N. (1993). Fishery potential of the Gulf of Kachchh. J. Indian Fishe. Assoc. 23, 91–103.
Nanajkar M., De K., Ingole B. (2019). Coral reef restoration - a way forward to offset the coastal development impacts on Indian coral reefs. Mar. pollut. Bull. 149, 110504. doi: 10.1016/j.marpolbul.2019.110504
Naseer A. (1997). “Profile and status of coral reefs in Maldives and approaches to its management,” in Proceedings of the regional workshop on the conservation and sustainable management of coral reefs. Ed. Hoon V. (Madras: CRSARD).
Naseer A., Hatcher B. G. (2004). Inventory of the Maldives’ coral reefs using morphometrics generated from landsat ETM+ imagery. Coral Reefs 23 (1), 161–168. doi: 10.1007/s00338-003-0366-6
NDMA (2015). Strategies and lessons for preparing better and stengthening risk resilience in coastal regions of India (New Delhi, India: National Disaster Management Authority), 58.
Nepote E., Bianchi C. N., Chiantore M., Morri C., Montefalcone M. (2016). Pattern and intensity of human impact on coral reefs depend on depth along the reef profile and on the descriptor adopted. Estuar. Coast. Shelf Sci. 178, 86–91. doi: 10.1016/j.ecss.2016.05.021
Nickerson D. J., Maniku M. H. (1997). Workshop on integrated reef resources management in the Maldives - Bay of Bengal Programme (Madras, India: Bay of Bengal Programme (BOBP/REP/76).
Niroshana K. H. H., Asanthi H. B., Kumara P.B.T.P.K. (2013). An assessment of water quality and pollution in puranawella fishery harbour, Dewinuwara, Sri Lanka. J. Univ. Ruhuna 1 (1), 23–30. doi: 10.4038/jur.v1i1.6157
NRC (2000). Clean coastal waters: understanding and reducing the effects of nutrient pollution (Washington, DC: The National Academies Press), 405. doi: 10.17226/9812
Öhman M. C., Rajasuriya A., Lindén O. (1993). Human disturbances on coral reefs in Sri Lanka: a case study. Ambio 22 (7), 474–480.
Oliver E. C. J., Wernberg T., Benthuysen J., Chen K. (2020). Advances in understanding marine heatwaves and their impacts (Frontiers, Lausanne: Frontiers Media, SA). doi: 10.3389/3978-3382-88963-88697-88966
Padmakumar K. B., Menon N. R., Sanjeevan V. N. (2012). Is occurrence of harmful algal bloom in the exclusive economic zone of India on the rise? Int. J. Oceanog. 2012, 263946. doi: 10.261155/262012/263946
Patel R., Salvi H., Bhagat R., Kamboj R. D. (2017). Zooplankton diversity at important coral and mangrove areas of Gulf of Kachchh, Gujarat, India. Bull. Environ. Sci. Res. 6 (3-4), 1–7.
Patgaonkar R. S., Vethamony P., Lokesh K. S., Babu M. T. (2012). Residence time of pollutants discharged in the Gulf of Kachchh, northwestern Arabian Sea. Mar. pollut. Bull. 64 (8), 1659–1666. doi: 10.1016/j.marpolbul.2012.05.033
Patro S., Krishnan P., Samuel V. D., Purvaja R., Ramesh R. (2017). “Seagrass and salt marsh ecosystems in south Asia: an overview of diversity, distribution, threats and conservation status,” in Wetland science: perspectives from south Asia. Eds. Prusty B. A. K., Chandra R. (New Delhi, India: Springer), 87–104.
Patterson E. J. K. (2002). “The coral reefs of the tuticorin coast of the Gulf of Mannar, south-east India,” in Coral reef degradation in the Indian Ocean: status report 2002. Eds. Linden O., Souter D., Wilhelmsson D., Obura D. (Sweden, CORDIO: CORDIO Status Report 2002), 114–118.
Patterson E. J. K., Kulkanri S., Jeyabaskaran R., Lazarus S., Mary A., Venkataraman K., et al. (2006). “The effects of the 2004 tsunami on mainland India and the Andaman and Nicobar Islands. in status of coral reefs in tsunami affected countries: 2005,”. Eds. Wilkinson C., Souter D., Goldberg J. (Queensland: Global Coral Reef Monitoring Network, Australian Institute of Marine Science), 85–97.
Patterson E. J. K., Mathews G., Diraviya Raj K., Rajesh S. (2015). Study on the status of corals in Palk Bay, southeast coast of India during 2007-2013. Indian J. Geo-Mar. Sci. 44 (9), 1449–1462.
Patterson E. J. K., Mathews G., Patterson J., Wilhelmsson D., Tamelander J., Linden O. (2007). Coral reefs of the Gulf of Mannar, southeastern India–distribution, diversity and status (SDMRI Special Research Publication No.12), 113 pages
Patterson E. J. K., Mathews G., Raj K. D., Laju R. L., Bharath M. S., Kumar P. D., et al. (2020). Marine debris - an emerging threat to the reef areas of Gulf of Mannar, India. Mar. pollut. Bull. 151, 110793. doi: 10.1016/j.marpolbul.2019.110793
Perera N. (2019). A preliminary report on the status of Kavankerni coral reef (Sri Lanka: SACEP), 21.
Perera N., Vos A.d. (2007). Marine protected areas in Sri Lanka: a review. Environ. Manage. 40, 727–738. doi: 10.1007/s00267-005-0154-x
Perera C., Wilhelmsson D., Rajasuriya A. (2002). “Reef fisheries and coral reef degradation in Sri Lanka,” in Coral reef degradation in the Indian Ocean: status report 2002. Eds. Linden O., Souter D., Wilhelmsson D., Obura D. (Sweden, CORDIO: CORDIO Status Report 2002), 149–157.
Pichon M., Benzoni F. (2007). Taxonomic re-appraisal of zooxanthellate scleractinian corals in the Maldive archipelago. Zootaxa 1441 (1), 21–33. doi: 10.11646/zootaxa.1441.1.2
Pisapia C., Burn D., Yoosuf R., Najeeb A., Anderson K. D., Pratchett M. S. (2016). Coral recovery in the central Maldives archipelago since the last major mass-bleaching, in 1998. Sci. Rep. 6, 34720. doi: 10.31038/srep34720
Pisapia C., El Kateb A., Hallock P., Spezzaferri S. (2017). Assessing coral reef health in the north Ari atoll (Maldives) using the FoRAM index. Mar. Micropaleontol. 133, 50–57. doi: 10.1016/j.marmicro.2017.06.001
Pitchaikani J. S., Lipton A. P. (2016). Nutrients and phytoplankton dynamics in the fishing grounds off Tiruchendur coastal waters, Gulf of Mannar, India. Springerplus 5 (1), 1405. doi: 10.1186/s40064-016-3058-8
Prabagar J., Prabagar S., Thuraisingam S. (2020). Nitrate contamination of groundwater: an issue for livelihood in Jaffna peninsula, Sri Lanka. Afr. J. Agric. Res. 16 (7), 1025–1032. doi: 10.5897/ajar2020.14740
Prakasa Rao E. V. S., Puttanna K., Sooryanarayana K. R., Biswas A. K., Arunkumar J. S. (2017). “Assessment of nitrate threat to water quality in India,” in The Indian Nitrogen Assesment. Eds. Abrol Y. P., Adhya T. K., Aneja V. P., Raghuram N., Pathak H., Kulshrestha U., Sharma C., Singh B. (Elsevier), 323–333. Available at: https://www.sciencedirect.com/book/9780128118368/the-indian-nitrogen-assessment
Prema D., Singh V. V., Jeyabaskaran R., Kripa V. (2017). “Reactive nitrogen in coastal and marine waters of India and its relationship with marine aquaculture,” in The Indian Nitrogen Assessment. Eds. Abrol Y. P., Adhya T. K., Aneja V. P., Raghuram N., Pathak H., Kulshrestha U., Sharma C., Singh B. (Elsevier), 305–320. Available at: https://www.sciencedirect.com/book/9780128118368/the-indian-nitrogen-assessment
Price A. R. G., Firaq I. (1996). The environmental status of reefs on Maldivian resport islands: a prelininary assessment for tourism planning. Aquat. conservat.: Mar. Freshw. Ecosyst. 6, 93–106.
Purvaja R., Robin R. S., Ganguly D., Hariharan G., Singh G., Raghuraman R., et al. (2018). Seagrass meadows as proxy for assessment of ecosystem health. Ocean Coast. Manage. 159, 34–45. doi: 10.1016/j.ocecoaman.2017.11.026
Purvaja R., Yogeswari S., Debasis T., Hariharan G., Raghuraman R., Muruganandam R., et al. (2019). “Challenges and opportunities in the management of coral islands of Lakshadweep, India,” in Coasts and estuaries: the future. Eds. Wolanski E., Day J. W., Elliott M., Ramesh R. (Netherlands: Elsevier), 461–476.
Qasim S. Z., Sen Gupta R., Kureishy T. W. (1988). Pollution of the seas around India. Proc. Indian Natl. Sci. Acad. 97 (2), 117–131. doi: 10.1007/BF03179939
Rabalais N. N., Turner R. E., Díaz R. J., Justić D. (2009). Global change and eutrophication of coastal waters. ICES J. Mar. Sci. 66 (7), 1528–1537. doi: 10.1093/icesjms/fsp047
Rädecker N., Pogoreutz C., Voolstra C. R., Wiedenmann J., Wild C. (2015). Nitrogen cycling in corals: the key to understanding holobiont functioning? Trends Microbiol. 23 (8), 490–497. doi: 10.1016/j.tim.2015.03.008
Radice V. Z., Hoegh-Guldberg O., Fry B., Fox M. D., Dove S. G., Dorrepaal E. (2019). Upwelling as the major source of nitrogen for shallow and deep reef-building corals across an oceanic atoll system. Funct. Ecol. 33 (6), 1120–1134. doi: 10.1111/1365-2435.13314
Raghuram N., Sutton M. A., Jeffery R., Ramachandran R., Adhya T. K. (2021). From south Asia to the world: embracing the challenge of global sustainable nitrogen management. One Earth 4 (1), 22–27. doi: 10.1016/j.oneear.2020.12.017
Raghuraman R., Raghunathan C., Venkataraman K. (2013). “Present status of coral reefs in India,” in Ecology and conservation of tropical marine faunal communities. Eds. Venkataraman K., Sivaperuman C., Raghunathan C. (Berlin: Springer-Verlag), 351–380.
Raj K. D., Mathews G., Obura D. O., Laju R. L., Bharath M. S., Kumar P. D., et al. (2020). Low oxygen levels caused by Noctiluca Scintillans bloom kills corals in Gulf of Mannar, India. Sci. Rep. 10 (1), 22133. doi: 10.1038/s41598-020-79152-x
Rajarathna W. A., Nianthi K. W. G. (2019). “Special coastal management area concept experience in Sri Lanka,” in Coastal zone managment: global challenges and innovations. Eds. Krishnamurthy R. R., Jonathan M. P., Srinivasalu S., Glaeser B. (Academic Press), 5–20. Available at: https://www.sciencedirect.com/book/9780128104736/coastal-management
Rajasuriya A. (1997). “Coral reefs of Sri Lanka: current status and resource management,” in Proceedings of the regional workshop on the conservation and sustainable management of coral reefs. Ed. Hoon V. (Madras: CRSARD).
Rajasuriya A. (2002). “Status report on the condition of reef habitats in Sri lanka,” in Coral reef degradation in the Indian Ocean: status report 2002. Eds. Linden O., Souter D., Wilhelmsson D., Obura D. (Sweden, CORDIO: CORDIO Status Report 2002), 139–148.
Rajasuriya A. (2005). “The status of coral reefs in Sri Lanka in the aftermath of the 1998 coral bleaching event and the 2004 tsunami,” in Coral reef degradation in the Indian Ocean: status report 2005. Eds. Souter D., Linden O. (Kalmar, Sweden: CORDIO), 83–96.
Rajasuriya A. (2008). “Status of coral reefs in northern, western and southern coastal waters of Sri Lanka,” in Ten years after bleaching - facing the consequences of climate change on the Indian Ocean. CORDIO status report 2008. Eds. Obura D., Tamelander J., Linden O. (Mombassa, CORDIO: Coastal Oceans Research and Development in the Indian Ocean)/Sida-SAREC), 11–21.
Rajasuriya A. (2012). “Provisional checklist of corals in Sri Lanka,” in The national red list 2012 of Sri Lanka: conservation status of fauna and flora. Eds. Weerakoon D. K., Wijesundara S. (Colombo, Sri Lanka: Ministry of Environment), 376–383.
Rajasuriya A., Perera N., Fernando M. (2005). “Status of coral reefs in trincomalee, Sri Lanka,” in Coral reef degradation in the Indian Ocean: status report 2005. Eds. Souter D., Linden O. (Kalmar, Sweden: CORDIO), 97–103.
Rajasuriya A., White A. T. (1995). Coral-reefs of Sri Lanka - review of their extent, conditions and management status. Coast. Manage. 23 (1), 77–90. doi: 10.1080/08920759509362257
Rajasuriya A., Zahir H., Muley E. V., Subramanian B. R., Venkataraman K., Wafar M. V. M., et al. (2000). “Status of coral reefs in south Asia: Bangladesh, India, Maldives and Sri Lanka,” in Status of coral reefs of the world: 2000. Ed. Wilkinson C. (Townsville, Australia: Australian Institute of Marine Science), 95–115.
Rajasuriya A., Zahir H., Venkataraman K., Islam Z., Tamelander J. (2004). “Status of coral reefs in south Asia: Bangladesh, Chagos, India, Maldives and Sri Lanka,” in Status of coral reefs of the world: 2004, vol. 1 . Ed. Wilkinson C. (Townsville, Australia: Australian Institute of Marine Scien), 213–233.
Ramadas V., Rajeswari K. R. (2011). The effect of oil pollution and disposal of domestic sewage on the vitality and diversity of coral reefs of Palk Bay, Mandapam Region, south-east coast of India. IPCBEE 6, 165–169.
Ramesh C. H., Koushik S., Shunmugaraj T., Murthy M. V. R. (2020). Current biodiversity of mandapam group of islands in Gulf of Mannar marine biosphere reserve, southeast coast of Tamil nadu, India. Reg. Stud. Mar. Sci. 39, 101429. doi: 101410.101016/j.rsma.102020.101429
Ramesh R., Paneer Selvam A., Robin R. S., Ganguly D., Singh G., Purvaja R. (2017). “Nitrogen assessment in Indian coastal systems,” in The Indian Nitrogen Assessment. Eds. Abrol Y. P., Adhya T. K., Aneja V. P., Raghuram N., Pathak H., Kulshrestha U., Sharma C., Singh B. (Elsevier), 361–379. Available at: https://www.sciencedirect.com/book/9780128118368/the-indian-nitrogen-assessment.
Ramesh R., Senthilkumar B., Divia J. I., Purvaja R. (2010). “Influence of terrestrial inputs on mangrove and coral reef: primary productivity of the Andaman islands,” in Management and sustainable development of coastal zone environments. Eds. Ramanathan A., Bhattacharya P., Dittmar T., Prasad M. B. K., Neupane B. R. (New Delhi, India: Springer), 194–211.
Rao R. R., Girishkumar M. S., Ravichandran M., Gopalakrishna V. V., Thadathil P. (2011). Do cold, low salinity waters pass through the indo-Sri Lanka channel during winter? Int. J. Remote Sens. 32 (22), 7383–7398. doi: 10.1080/01431161.01432010.01523728
Rao D. G., Kanuri V. V., Kumaraswami M., Ezhilarasan P., Rao V. D., Patra S., et al. (2017). Dissolved nutrient dynamics along the southwest coastal waters of India during northeast monsoon: a case study. Chem. Ecol. 33 (3), 229–246. doi: 10.1080/02757540.2017.1287903
Rao D. V., Rao K. S., Iyer C. S., Chittibabu P. (2008). Possible ecological consequences from the Sethu Samudram canal project, India. Mar. pollut. Bull. 56 (2), 170–186. doi: 10.1016/j.marpolbul.2007.10.018
Rebekah S., Inamdar A. B. (2018). Mapping the distribution of coral reef extent and its temporal variation in Gulf of Mannar - comparison of pixel and object based approach. Indian J. Geo-Mar. Sci. 47 (3), 549–557.
Redfield A. C. (1958). The biological control of chemical factors in the environment. Am. Scientist 46 (3), 205–221.
Redfield A. C., Ketchum B. H., Richards F. A. (1963). “The influence of organisms on the composition of sea-water,” in The Sea: vol 2: composition of seawater comparative and descriptive oceanography. Ed. Hill M. N. (London: Interscience), 26–77.
Renegar D. A., Riegl B. M. (2005). Effect of nutrient enrichment and elevated CO2 partial pressure on growth rate of Atlantic scleractinian coral acropora cervicornis. Mar. Ecol. Prog. Ser. 293, 69–76. doi: 10.3354/meps293069
Retnamma J., Chinnadurai K., Loganathan J., Nagarathinam A., Singaram P., Jose A. K. (2020). Ecological responses of autotrophic microplankton to the eutrophication of the coastal upwelling along the southwest coast of India. Environ. Sci. pollut. Res. Int 28, 11401–11414. doi: 10.1007/s11356-020-11354-2
Risk M. J. (2014). Assessing the effects of sediments and nutrients on coral reefs. Curr. Opin. Environ. Sustain. 7, 108–117. doi: 10.1016/j.cosust.2014.01.003
Riyas C. A., Idreesbabu K. K., Marimuthu N., Sureshkumar S. (2020). Impact of the tropical cyclone ockhi on ecological and geomorphological structures of the small low-lying islands in the central Indian Ocean. Reg. Stud. Mar. Sci. 33, 100963. doi: 10.1016/j.rsma.2019.100963
Roberts C. M., O’Leary B. C., McCauley D. J., Cury P. M., Duarte C. M., Lubchenco J., et al. (2017). Marine reserves can mitigate and promote adaptation to climate change. Proc. Natl. Acadademy Sci. 114 (24), 6167–6175. doi: 10.1073/pnas.1701262114
Robin R. S., Kanuri V. V., Muduli P. R., Mishra R. K., Jaikumar M., Karthikeyan P., et al. (2013). Dinoflagellate bloom of Karenia Mikimotoi along the southeast Arabian Sea, bordering Western India. J. Ecosyst. 2013, 1–11. doi: 10.1155/2013/463720
Robin R. S., Muduli P. R., Vishnu Vardhan K., Nagarjuna A., Nallathambi T., Mishra R. K., et al. (2012). Planktonic communities and trophic interactions in the Kavaratti waters, Lakshadweep archipelago, India. Int. J. Ecosystem 2 (2), 5–18. doi: 10.5923/j.ije.20120202.02
Rosenberg Y., Simon-Blecher N., Lalzar M., Yam R., Shemesh A., Alon S., et al. (2022). Urbanization comprehensively impairs biological rhythms in coral holobionts. Glob Chang Biol. 28 (10), 3349–3364. doi: 10.1111/gcb.16144
Rubesinghe C., Brosche S., Withanage H., Pathragoda D., Karlsson T. (2022). X-Press Pearl, a ‘new kind of oil spill’ consisting of a toxic mix of plastics and invisible chemicals (Wahlund, Sweden: International Pollutants Elimination Network (IPEN), 37.
SAC (2011). “National wetland atlas,” in SAC/EPSA/ABHG/NWIA/ATLAS/34/2011 (Ahmedabad, India.: Space Applications Centre (ISRO).
SACEP (2008). Regional strategy for coral reef management in south Asia (Gandhinagar, India: Geer Foundation), 48.
SACEP (2017). Marine environment pollution: challenges and opportunities in south Asian seas region (Colombo, Sri Lanka: South Asian Co-operative Environment Programme), 19.
SACEP (2019). Marine and coastal biodiversity strategy for the south Asian seas regions: living in harmony with our oceans and coasts (Colombo, Sri Lanka: SACEP), 104.
Sahasranaman M., Ganguly A. (2018). Wastewater treatment for water security in India (Hyderabad, India: Institute for Resource Analysis and Policy), 36.
Sahu B. K., Begum M., Khadanga M. K., Jha D. K., Vinithkumar N. V., Kirubagaran R. (2013). Evaluation of significant sources influencing the variation of physico-chemical parameters in Port Blair Bay, south Andaman, India by using multivariate statistics. Mar. pollut. Bull. 66 (1-2), 246–251. doi: 10.1016/j.marpolbul.2012.09.021
Sajeev S., Muthukumar P., Selvam S. (2023). Submarine groundwater discharge: an Asian overview. Chemosphere 325, 138261. doi: 10.1016/j.chemosphere.2023.138261
Sampath V. (2003). National report on the status and development potential of the coastal and marine environment of the East Coast of India and its Living Resources. Bay of Bengal Large Marine Ecosystem Programme, BOBLME/FAO (BOBLME), 295 pages. Available at: https://www.boblme.org.
Samuel D., Sivaramakrishnan T., Mathews G., Edward J. K. P. (2012). “Threats to coral reefs of Gulf of Mannar marine national park,” in Coral reefs in India - status, threats and conservation measures. Eds. Bhatt J. R., Edward J. K. P., Macintosh D. J., Nilaratna B. P. (New Dehli, India: IUCN), 265–272.
Santos I. R., Chen X., Lecher A. L., Sawyer A. H., Moosdorf N., Rodellas V., et al. (2021). Submarine groundwater discharge impacts on coastal nutrient biogeochemistry. Nat. Rev. Earth Environ. 2 (5), 307–323. doi: 10.1038/s43017-021-00152-0
Saponari L., Montalbetti E., Galli P., Strona G., Seveso D., Dehnert I., et al. (2018). Monitoring and assessing a 2-year outbreak of the corallivorous seastar acanthaster planci in Ari atoll, republic of Maldives (Environmental Monitoring and Assessment), 190. doi: 10.1007/s10661-10018-16661-z
Saponari L., Montano S., Seveso D., Galli P. (2014). The occurrence of an acanthaster planci outbreak in Ari atoll, Maldives. Mar. Biodiversity 45 (4), 599–600. doi: 10.1007/s12526-014-0276-6
Saravanakumar A., Rajkumar M., Serebiah J. S., Thivakaran G. A. (2008). Seasonal variations in physico-chemical characteristics of water, sediment and soil texture in arid zone mangroves of Kachchh-Gujarat. J. Environ. Biol. 29 (5), 725–732.
Saroj J., Gautam R. K., Joshi A., Tehseen P. (2016). Review of coral reefs of India: distribution, status, research and management. Int. J. Sci. Environ. Technol. 5 (5), 3088–3098.
Schlöder C., D’Croz L. (2004). Responses of massive and branching coral species to the combined effects of water temperature and nitrate enrichment. J. Exp. Mar. Biol. Ecol. 313 (2), 255–268. doi: 10.1016/j.jembe.2004.08.012
Schott F. A., McCreary Jr. J. P. (2001). The monsoon circulation of the Indian Ocean. Prog. Oceanog. 51, 1–123. doi: 10.1016/S0079-6611(01)00083-0
Seitzinger S. P., Mayorga E., Bouwman A. F., Kroeze C., Beusen A. H. W., Billen G., et al. (2010). Global river nutrient export: a scenario analysis of past and future trends. Global Biogeochem. Cycles 24, GB0A08. doi: 10.1029/2009GB003587
Selman M., Greenhalgh S. (2009a). Eutrophication: sources and drivers of nutrient pollution (Washington, DC: World Resources Institute), 8.
Selman M., Greenhalgh S. (2009b). Eutrophication: policies, actions, and strategies to address nutrrient pollution (Washington, DC: World Resources Institute), 16.
Selman M., Greenhalgh S., Diaz R., Sugg Z. (2008). Eutrophication and hypoxia in coastal areas: a global assessment of the state of knowledge (Washington, DC: World Resources Institute), 6.
Selvam S., Muthukumar P., Roy P. D., Venkatramanan S., Chung S. Y., Elzain H. E., et al. (2022). Submarine groundwater discharge and associated nutrient influx in surroundings of the estuary region at Gulf of Mannar coast, Indian Ocean. Chemosphere 305, 135271. doi: 10.1016/j.chemosphere.2022.135271
Sengupta R., Moraes C., Kureishy T. W., Sankaranarayanan V. N., Jana T. K., Naqvi S. W. A., et al. (1979). Chemical oceanography of the Arabian Sea: part IV - Laccadive Sea. Indian J. Mar. Sci. 8, 215–221.
Senthilnathan L., Ranith R., Machendiranathan M., Thangaradjou T., Babu I., Choudhury S. B., et al. (2014). Are Lakshadweep corals heading toward COT outbreak? Atoll Res. Bull. 606, 1–11.
Serrano X. M., Miller M. W., Hendee J. C., Jensen B. A., Gapayao J. Z., Pasparakis C., et al. (2018). Effects of thermal stress and nitrate enrichment on the larval performance of two Caribbean reef corals. Coral Reefs 37 (1), 173–182. doi: 10.1007/s00338-017-1645-y
Shaju S. S., Prasad S. C., Vishnu P. S., Samal A. K., Menon N., N. M., et al. (2019). Effect of mariculture on bio-optical properties and water quality of Gulf of Mannar and Palk Bay. Reg. Stud. Mar. Sci. 29,100618. doi: 10.1016/j.rsma.2019.100618
Shankar D., Vinayachandran P. N., Unnikrishnan A. S. (2002). The monsoon current in the north Indian Ocean. Prog. Oceanog. 52, 63–120. doi: 10.1016/S0079-6611(02)00024-1
Shantz A. A., Burkepile D. E. (2014). Context-dependent effects of nutrient loading on the coral-algal mutualism. Ecology 95 (7), 1995–2005. doi: 10.1890/13-1407.1
Shetye S. R. (1998). West India Coastal current and Lakshadweep High/Low. Sadhana 23 (5-6), 637–651. doi: 10.1007/BF02744586
Shunmugaraj T., Sadhukhan K., Chatragadda R., Murthy M. V. R. (2020). Impact of Noctiluca Scintillans bloom on coastal fauna of Mandapam Region in Gulf of Mannar marine biosphere reserve, southeast coast of Tamil nadu, India. Int. J. Ecol. Environ. Sci. 46 (2), 135–140.
Shuval H. (2003). Estimating the global burden of thalassogenic diseases: human infectious diseases caused by wastewater pollution of the marine environment. J. Water Health 2, 53–64. doi: 10.2166/wh.2003.0007
Silas E. G. (1968). Tuna fishery of the Tinnevelly coast, Gulf of Mannar. Proc. symposium Scombroid fishes Part 3 Mar. Biol. Assoc. India. Part 3, 1083–1120.
Silva E. I. L. (1996). Water quality of Sri Lanka: a review of twelve water bodies (Kandy, Sri Lanka: Institute of Fundamental Studies), 152.
Silva E. I. L., Jennerjahn T. C., Ittekkot V. (2005). Nutrient fluxes into coastal waters via Sri Lankan rivers: a comparison with other Asian rivers. Int. J. Ecol. Environ. Sci. 31 (3), 213–221.
Silva E. N. S., Rajasuriya A., Sosai S. (2018). “Testing the attitude of fishers towards conservation: a snapshot,” in International union for conservation of nature (GEF Dugong and Seagrass Conservations Project).
Singh J. S., Chaturvedi R. K. (2017). Diversity of ecosystem types in India: a review. Proc. Indian Natl. Sci. Acad. 83 (3), 569–594. doi: 10.16943/ptinsa/12017/49027
Singh A., Ramesh R. (2011). Contribution of riverine dissolved inorganic nitrogen flux to new production in the coastal northern Indian Ocean: an assessment. Int. J. Oceanog. 2011, 983561. doi: 10.1155/2011/983561
SLTDA (2018). Annual statistical report of the Sri Lanka tourism development authority 2018 (Colombo, Sri Lanka: Sri Lanka Tourism Development Authority), 115.
Smale D. A., Wernberg T., Oliver E. C. J., Thomsen M., Harvey B. P., Straub S. C., et al. (2019). Marine heatwaves threaten global biodiversity and the provision of ecosystem services. Nat. Climate Change 9, 306-312. doi: 10.1038/s41558-41019-40412-41551
SoE (2009). State of environment report India-2009, ministry of environment and forests, government of India (New Delhi, India). 194. Available at: https://www.unep.org/resources/report/state-environment-report-india.
Souter D., Planes S., Wicquart J., Logan M., Obura D., Staub F. (2021). Tatus of coral reefs of the world: 2020 report (Global Coral Reef Monitoring Network (GCRMN)/International Coral Reef Initiative (ICRI). Available at: https://gcrmn.net/2020-report/.
Spalding M. D., Brown B. E. (2015). Warm-water coral reefs and climate change. Science 350, 769–771. doi: 10.1126/science.aad0349
Spalding M., Burke L., Wood S. A., Ashpole J., Hutchison J. (2017). Mapping the global value and distribution of coral reef tourism. Mar. Policy 82, 104–113. doi: 10.1016/j.marpol.2017.05.014
Spalding M. D., Ravilious C., Green E. P. (2001). World atlas of coral reefs (Berkeley, CA: University of Califrornia Press), 436.
Sridhar R., Thangaradjou T., Kannan L. (2008). Comparative investigation on physico-chemical properties of the coral reef and seagrass ecosystems of the Palk Bay. Indian J. Mar. Sci. 37 (2), 207–213.
Sridhar R., Thangaradjou T., Senthil Kumar S., Kannan L. (2006). Water quality and phytoplankton characteristics in the Palk Bay, southeast coast of India. J. Environ. Biol. 27 (3), 561–566.
Stambler N., Cox E. F., Vago R. (1994). Effect of ammonium enrichment on respiration, zooxanthellae densities, and pigment concentrations in two species in Hawaiian corals. Pacific Sci. 48, 284–290.
Stambler N., Popper N., Dubinsky Z., Stimson J. (1991). Effects of nutrient enrichment and water motion on the coral Pocillopora damicornis. Pacific Sci. 45, 299–307.
Stevens G. M. W., Froman N. (2019). “The Maldives archipelago,” in World seas: an environmental evaluation Sheppard C. (Ed.,) (Academic Press: Elsevier), 211–236. doi: 10.1016/B978-0-08-100853-9.00013-0. Available at: https://www.sciencedirect.com/book/9780081008539/world-seas-an-environmental-evaluation.
Strona G., Beck P. S. A., Cabeza M., Fattorini S., Guilhaumon F., Micheli F., et al. (2021). Ecological dependencies make remote reef fish communities most vulnerable to coral loss. Nat. Commun. 12 (1), 7282. doi: 10.1038/s41467-021-27440-z
Su D., Wijeratne S., Pattiaratchi C. B. (2021). Monsoon influence on the island mass effect around the Maldives and Sri Lanka. Front. Mar. Sci. 8. doi: 10.3389/fmars.2021.645672
Subburaman S., Goutham S., Joshi D., Venkatesh M., Praveenkumar B. M., Matwal M., et al. (2014). Daring to restore – coral reef recovery in mithapur (Uttar Pradesh, India: Wildlife Trust of India).
Survey Department of Sri Lanka (2007). “The national atlas of Sri Lanka, 2nd edition,” in The survey department, government of Sri Lanka (Colombo, Sri Lanka: Survey Department of Sri Lanka), 243.
Sutton M. A., Drewer J., Moring A., Adhya T. K., Ahmed A., Bhatia A., et al. (2017). “The Indian Nitrogen challenge in a global perspective,” in The Indian Nitrogen Assessment. Eds. Abrol Y. P., Adhya T. K., Aneja V. P., Raghuram N., Pathak H., Kulshrestha U., Sharma C., Singh B. (Elsevier), 9–28. Available at: https://www.sciencedirect.com/book/9780128118368/the-indian-nitrogen-assessment.
Swaney D. P., Hong B., Paneer Selvam A., Howarth R. W., Ramesh R., Purvaja R. (2015). Net anthropogenic nitrogen inputs and nitrogen fluxes from Indian watersheds: an initial assessment. J. Mar. Syst. 141, 45–58. doi: 10.1016/j.jmarsys.2014.09.004
Szmant A. M. (2002). Nutrient enrichment on coral reefs: is it a major cause of coral reef decline? Estuaries 25 (4b), 743–766. doi: 10.1007/BF02804903
Tamelander J., Rajasuriya A. (2008). “Status of coral reefs in south Asia: Bangladesh, Chagos, India, Maldives and Sri Lanka,” in Status of coral reefs of the world: 2008. Ed. Wilkinson C. (Townsville, Australia: Global Coral Reef Monitoring Network and Reef and Rainforest Research Centre), 119–130.
Tamele I. J., Silva M., Vasconcelos V. (2019). The incidence of marine toxins and the associated seafood poisoning episodes in the African countries of the Indian Ocean and the Red Sea. Toxins 11, 58. doi: 10.3390/toxins11010058
Tewatia R. K., Chandra T. K. (2017). “Trends in fertilizer nitrogen production and consumption in India,” in The Indian Nitrogen Assessment. Eds. Abrol Y. P., Adhya T. K., Aneja V. P., Raghuram N., Pathak H., Kulshrestha U., Sharma C., Singh B. (Elsevier), 45–56. Available at: https://www.sciencedirect.com/book/9780128118368/the-indian-nitrogen-assessment.
Thangaradjou T., Kannan L. (2007). Nutrient characteristics and sediment texture of the seagrass beds of the Gulf of Mannar. J. Environ. Biol. 28 (1), 29–33.
The Fertilizer Association of India (2022) All-India consumption of fertiliser nutrients - 1950-51 to 2019-20. Available at: https://www.faidelhi.org/statistics/statistical-database (Accessed 22/02/2022).
Thinesh T., Raj K. D., Mathews G., Edward J. K. P. (2013). Coral diseases are major contributors to coral mortality in shingle island, Gulf of Mannar, southeastern India. Dis. Aquat. Organisms 106, 69–77. doi: 10.3354/dao02634
Tilstra A., Roth F., El-Khaled Y. C., Pogoreutz C., Radecker N., Voolstra C. R., et al. (2021). Relative abundance of nitrogen cycling microbes in coral holobionts reflects environmental nitrate availability. R. Soc. Open Sci. 8, 201835. doi: 10.1098/rsos.201835
Topor Z. M., Rasher D. B., Duffy J. E., Brandl S. J. (2019). Marine protected areas enhance coral reef functioning by promoting fish biodiversity. Conserv. Lett. 12, e12638. doi: 10.1111/conl.12638
Townsley P. (2004). Review of coastal and marine livelihoods and food security in the Bay of Bengal Large Marine Ecosystem Region. Bay of Bengal Large Marine Ecosystem Theme Report, BOBLME/FAO (BOBLME), 131 pages. Available at: https://www.boblme.org.
Tuholske C., Halpern B. S., Blasco G., Villasenor J. C., Frazier M., Caylor K. (2021). Mapping global inputs and impacts from of human sewage in coastal ecosystems. PloS One 16 (11), e0258898. doi: 10.1371/journal.pone.0258898
Tyrrell T. (1999). The relative influences of nitrogen and phosphorous on oceanic primary production. Nature 400, 525–531. doi: 10.1038/22941
Tyrrell T., Laws C. S. (1997). Low nitrate:phosphate ratios in the global ocean. Nature 387, 793–796. doi: 10.1038/42915
UNEP (1986). “Environmental problems of the marine and coastal area of Sri Lanka,” in National report (Nairobi, Kenya: UNEP Regional Seas Resports and Studies No. 74), 106. Available at: https://www.unep.org/resources/report/regional-seas-reports-and-studies-no-074-environmental-problems-marine-and-coastal.
UNEP (2005). Maldives Post-tsunami environmental assessment (Nairobi, Kenya). 100. Available at: https://www.unep.org/resources/report/Maldives-post-tsunami-environmental-assessment.
UNEP (2019). “Global environment outlook – GEO-6,” in Healthy planet, healthy people(Nairobi, Kenya: UNEP), 745. doi: 10.1017/9781108627146
UNEP (2020). “Projections of future coral bleaching conditions using IPCC CMIP6 models,” in Climate policy implications, management applications, and regional seas summaries (Nairobi, Kenya: United Nations Environment Programme), 102.
UNEP (2021). “X-Press Pearl maritime disaster Sri Lanka,” in Report of the UN environmental advisory mission (July 2021(Nairobi, Kenya: UNEP), 70.
UNEP-WCMC, WorldFish Centre, WRI and TNC (2018). Global distribution of warm-water coral reefs, compiled from multiple sources including the Millennium Coral Reef Mapping Project. Version 4.0. Includes contributions from IMaRS-USF and IRD (2005), IMaRS-USF (2005) and Spalding et al. (2001). Cambridge (UK): UN Environment World Conservation Monitoring Centre.
UNESCAP (2021). Maldives National water accounts 2018 & 2019, united nations economic and social commission for Asia and the pacific (UN-ESCAP) (Bangkok, Thailand), 52. Available at: https://www.unescap.org/kp/2021/Maldives-national-waste-accounts-2018-2019-final-report
Uranie S. (2015) Toxic plankton is responsible for fish deaths in Seychelles - more tests needed to determine whether it is harmful to human (Seychelles News Agency) (Accessed 24/08/2020).
Uranie S., Meriton-Jean S. (2015) Toxic algal bloom or lack of oxygen? test needed to determine abnormal fish deaths in Seychelles (Seychelles News Agency) (Accessed 24/08/2020).
Uranie S., Vannier R. (2014) Possible algal bloom caused by nutrient excess at port Victoria (Seychelles News Agency) (Accessed 24/08/2020).
Valderrama Ballesteros L., Matthews J. L., Hoeksema B. W. (2018). Pollution and coral damage caused by derelict fishing gear on coral reefs around Koh Tao, gulf of Thailand. Mar. pollut. Bull. 135, 1107–1116. doi: 10.1016/j.marpolbul.2018.08.033
Van Drecht G., Bouwman A. F., Harrison J., Knoop J. M. (2009). Global nitrogen and phosphate in urban wastewater for the period 1970 to 2050. Global Biogeochem. Cycles 23, GB0A03. doi: 10.1029/2009GB003458
van Hooidonk R., Maynard J., Tamelander J., Gove J., Ahmadia G., Raymundo L., et al. (2016). Local-scale projections of coral reef futures and implications of the Paris agreement. Sci. Rep. 6, 39666. doi: 10.1038/srep39666
van Puijenbroek P., Beusen A. H. W., Bouwman A. F. (2019). Global nitrogen and phosphorus in urban waste water based on the shared socio-economic pathways. J. Environ. Manag. 231, 446–456. doi: 10.1016/j.jenvman.2018.10.048
Varkey M. J., Murty V. S. N., Suryanarayana A. (1996). Physical oceanography of the Bay of Bengal and the Andaman Sea. Oceanog. Mar. Biol.: Annu. Rev. 34, 1–70.
Venkataraman K. (2011). “Coral reefs of India,” in Encyclopedia of modern coral reefs. Ed. Hopley D. (Dordrecht: Springer). doi: 10.1007/1978-1090-1481-2639-1002_1064
Venkataraman K., Satyanarayana Ch., Alfred J. R. B., Wolstenholme J. (2003). Handbook on Hard Corals of India (Kolkata, India: Zoological Survey of India), 266.
Vermeij M. J., Van Moorselaar I., Engelhard S., Hörnlein C., Vonk S. M., Visser P. M. (2010). The effects of nutrient enrichment and herbivore abundance on the ability of turf algae to overgrow coral in the Caribbean. PloS One 5, e14312. doi: 10.1371/journal.pone.0014312
Vethamony P., Babu M. T. (2010). Physical processes in the Gulf of Kachchh: a review. Indian J. Geo-Mar. Sci. 39 (4), 497–503.
Vinayachandran P. N. (2004). Biological response of the sea around Sri Lanka to summer monsoon. Geophysical Res. Lett. 31 (1), L01302. doi: 10.1029/2003GL018533
Wear S. L., Thurber R. V. (2015). Sewage pollution: mitigation is key for coral reef stewardship. Ann. N Y Acad. Sci. 1355, 15–30. doi: 10.1111/nyas.12785
Weerakoon D., De A S., Wijewickrema T., Rajasuriya A., Perera N., Kumara T. P., et al. (2018). Conservation and sustainable use of biodiversity in the islands and lagoons of northern Sri Lanka (Colombo, Sri Lanka: International Union for Conservation of Nature), xxvii + 296.
Weerasekara K. A. W. S., Jayampathi O. M. M. D., Hettige N. D., Azmy S. A. M., Amarathunga A. A. D., Wickramaarachchi W. D. N., et al. (2015). Assessment of water pollution status of selected fishery harbours located in the southern province of Sri Lanka. J. Environ. Professionals Sri Lanka 4 (2), 36–46. doi: 10.4038/jepsl.v4i2.7861
WHO/UNICEF (2021) Joint management programme. Available at: https://washdata.org/ (Accessed 07/03/2022).
Wiedenmann J., D’Angelo C., Smith E. G., Hunt A. N., Legiret F.-E., Postle A. D., et al. (2012). Nutrient enrichment can increase the susceptibility of reef corals to bleaching. Nat. Climate Change 3 (2), 160–164. doi: 10.1038/nclimate1661
Wilhelmsson D. (2002). “Coral reef degradation in south Asia,” in Coral reef degradation in the Indian Ocean: status report 2002, vol. 2002 . Eds. Linden O., Souter D., Wilhelmsson D., Obura D. (Sweden, CORDIO: CORDIO Status Report), 93–102.
Wilkinson C. (2000). “Status of coral reefs of the world: 2000,”. Ed. Wilkinson C. (Townsville, Australia: Global Coral Reef Monitoring Network and Reef and Rainforest Research Centre), 363.
Wilkinson C. (2008). “Status of coral reefs of the world: 2008,”. Ed. Wilkinson C. (Townsville, Australia: Global Coral Reef Monitoring Network and Reef and Rainforest Research Centre), 296.
Wilkinson C., Salvat B., Eakin C. M., Brathwaite A., Francini-Filho R., Webster N., et al. (2016). “Tropical and sub-tropical coral reefs” in The first global integrated marine assessment: world ocean assessment Eds. Simcock I. A., Innis L. (Cambridge, UK: Cambridge University Press) Available at: http://www.un.org/depts/los/global_reporting/WOA_RPROC/Chapter_43.pdf.
Wilson J. J., Marimuthu N., Kumaraguru A. K. (2005). Sedimentation of silt in the coral reef environment of Palk Bay, India. J. Mar. Biol. Assoc. India 47, 83–87.
Wooldridge S. A. (2009). Water quality and coral bleaching thresholds: formalising the linkage for the inshore reefs of the Great Barrier Reef, Australia. Mar. pollut. Bull. 58 (5), 745–751. doi: 10.1016/j.marpolbul.2008.12.013
World Bank (2021) Fertilizer consumption. world development indicators. Available at: https://data.worldbank.org/indicator/AG.CON.FERT.ZS.
Yapa K. K. A. S. (2009). Upwelling phenomena in the southern coastal waters of Sri Lanka during southwest monsoon period as seen from MODIS. Sri Lankan J. Phys. 10, 7–15. doi: 10.4038/sljp.v10i0.3831
Zahir H. (2002). “Status of the coral reefs of Maldives,” in Coral reef degradation in the Indian Ocean: status report 2002. Eds. Linden O., Souter D., Wilhelmsson D., Obura D. (Sweden, CORDIO: CORDIO Status Report 2002), 119–124.
Zahir H., Clark S., Ajla R., Saleem M. (2002). “Spatial and temporal patterns of coral recruitment following a severe bleaching event in the Maldives,” in Coral reef degradation in the Indian Ocean: status report 2002. Eds. Linden O., Souter D., Wilhelmsson D., Obura D. (Sweden, CORDIO: CORDIO Status Report 2002), 125–134.
Zahir H., Quinn N., Cargilia N. (2010). Assessment of Maldivian coral reefs in 2009 after natural disasters (Male, Maldives: Marine Research Centre. Ministry of Fisheries and Agriculture), 64.
Zaneveld J. R., Burkepile D. E., Shantz A. A., Pritchard C. E., McMinds R., Payet J. P., et al. (2016). Overfishing and nutrient pollution interact with temperature to disrupt coral reefs down to microbial scales. Nat. Commun. 7, 11833. doi: 10.1038/ncomms11833
Zhao H., Yuan M., Strokal M., Wu H. C., Liu X., Murk A., et al. (2021). Impacts of nitrogen pollution on corals in the context of global climate change and potential strategies to conserve coral reefs. Science of the Total Environment, 145017. doi: 145010.141016/j.scitotenv.142021.145017
Ziegler M., Anton A., Klein S. G., Radecker N., Geraldi N. R., Schmidt-Roach S., et al. (2021). Integrating environmental variability to broaden the research on coral responses to future ocean conditions. Glob Chang Biol. 27 (21), 5532–5546. doi: 10.1111/gcb.15840
Keywords: corals, Indian Ocean, South Asia, nitrogen pollution, anthropogenic impacts
Citation: Painter SC, Artioli Y, Amir FH, Arnull J, Ganeshram RS, Ibrahim N, Samuel VD, Robin RS, Raghuraman R, Purvaja R, Ramesh R, Rajasuriya A, Rendon OR, Shazly A, Wilson AMW and Tudhope AW (2023) Anthropogenic nitrogen pollution threats and challenges to the health of South Asian coral reefs. Front. Mar. Sci. 10:1187804. doi: 10.3389/fmars.2023.1187804
Received: 16 March 2023; Accepted: 25 April 2023;
Published: 24 May 2023.
Edited by:
Hajime Kayanne, The University of Tokyo, JapanReviewed by:
Sutinee Sinutok, Prince of Songkla University, ThailandYu Umezawa, Tokyo University of Agriculture and Technology, Japan
Copyright © 2023 Painter, Artioli, Amir, Arnull, Ganeshram, Ibrahim, Samuel, Robin, Raghuraman, Purvaja, Ramesh, Rajasuriya, Rendon, Shazly, Wilson and Tudhope. This is an open-access article distributed under the terms of the Creative Commons Attribution License (CC BY). The use, distribution or reproduction in other forums is permitted, provided the original author(s) and the copyright owner(s) are credited and that the original publication in this journal is cited, in accordance with accepted academic practice. No use, distribution or reproduction is permitted which does not comply with these terms.
*Correspondence: Stuart C. Painter, c3R1YXJ0LnBhaW50ZXJAbm9jLmFjLnVr