- 1Marine Science Institute, University of California Santa Barbara, Santa Barbara, CA, United States
- 2Department of Geography, University of California Los Angeles, Los Angeles, CA, United States
- 3Morro Bay National Estuary Program, Morro Bay, CA, United States
Coastal dunes are globally recognized as natural features that can be important adaptation approaches for climate change along urban and natural shores. We evaluated the recovery of coastal dunes on an intensively groomed urban beach in southern California over a six-year period after grooming was discontinued. Restoration actions were minimal and included installation of three sides of perimeter sand fencing, cessation of mechanical grooming and driving, and the addition of seeds of native dune plants. To track recovery, we conducted physical and biological surveys of the restoration site and an adjacent control site (groomed beach) using metrics including sand accretion, elevation, foredune and hummock formation, vegetation recovery, and wildlife use. Sediment accretion, elevation, and geomorphic complexity increased over time in the restoration site, largely in association with sand fencing and dune vegetation. A foredune ridge (maximum elevation increase of 0.9 m) and vegetated hummocks developed, along with a general increase in elevation across the restoration site (0.3 m). After six years, an estimated total volume of approximately 1,730 m3 of sand had accreted in the restoration site and 540 m3 of sand had accreted in the foredune ridge. Over the same period, more than a meter of sediment (vertical elevation change) accumulated along the perimeter sand fencing. Groomed control areas remained flat and uniform. The total cover of vegetation in the restoration site increased over time to a maximum of approximately 7% cover by the sixth year. No vegetation was observed on the groomed control site. Native plant species formed distinct zones across the restoration site beginning by the second year and increasing over time, with dune forming species aggregating closest to the ocean in association with the incipient foredune ridge. Ecological functions observed in the restoration area included presence of dune invertebrates, shorebird roosting, and use by a breeding federally threatened shorebird, the western snowy plover (Charadrius nivosus nivosus). Our findings on geomorphic and ecological responses of a pilot dune restoration on a heavily groomed urban beach provide new insights on the opportunities and expectations for restoring dunes as nature-based solutions for climate adaptation on urban shorelines.
1 Introduction
Sea level rise and coastal erosion have generated interest in improved understanding of the potential for nature-based approaches to enhance resilience on sandy shores. Sandy shores are globally recognized and highly valued as cultural and economic resources for coastal regions (Orams, 2003; King and Symes, 2004). However, their value as ecosystems is often far less appreciated (Schlacher et al., 2014). This is particularly apparent on urbanized coasts. Worldwide, beach and dune ecosystems and associated wildlife are highly impacted by a wide range of threats (Nordstrom et al., 2000, Schlacher et al., 2007; Defeo et al., 2009; Nordstrom and Jackson, 2021, Brown and McLaughlan, 2002), including extirpation of native species, erosion, non-natural sediment and sand transport through mechanical means or management activities, pollution, and loss of natural morphology due to daily disturbance with heavy equipment through driving (Houser et al., 2013), grooming, recreational activities, and other regular maintenance (Dugan et al., 2003; Defeo et al., 2009; Dugan and Hubbard, 2010; Schooler et al., 2019).
However, these dynamic sandy ecosystems can also offer a key element of defense for coastal areas in terms of nature-based protection from climate change (Newkirk et al., 2018; Griggs and Patsch, 2019). Beaches and dunes can provide a natural buffer from sea level rise (SLR), erosion, and storms (Brown et al., 2013; Bryant et al., 2019) that can protect homes, roads, and other infrastructure. Coastal sand dunes serve as a storage mechanism for sediment, thereby protecting the shoreline from erosion and aiding in recovery, with aeolian transport providing an opportunity for sand accretion (Davidson-Arnott et al., 2019; Walker, 2020; Walker et al., 2022). Plants act as traps for aeolian-driven sand (Suter-Burri et al., 2013) and can form a complex feedback relationship important to dune accretion (Stallins and Parker, 2003; Stallins, 2005; Miller, 2015). Dunes restored with appropriate native vegetation can show improved sediment retention, elevation increases, and exhibit resilience through disturbance recovery (Hilgendorf et al., 2022). Additional benefits can include wave break and attenuation, slowing and infiltrating water, reducing erosion, and sediment accumulation (Bridges et al., 2015).
California’s sandy beach and dune ecosystems are impacted by many threats, including erosion and sediment deficits (Patsch and Griggs, 2006; Vitousek et al., 2017), armoring (Runyan and Griggs, 2003; Dugan et al., 2008), driving (Schlacher et al., 2008), competing with recreation space, grooming (Dugan and Hubbard, 2010; Schooler et al., 2019), and coastal development. Soft sediment coastal systems have also been identified as nearing ‘tipping points’ with accelerated SLR, at which ecosystem function may be greatly diminished (Barnard et al., 2021). In the Los Angeles area of southern California, many beaches have been and are subjected to the continuous disturbance of natural features and removal of vegetation by beach maintenance (LACDBH, 2016), reducing their ability to buffer SLR and storm impacts. Widespread mechanized beach maintenance activities, such as grooming, filling with non-natural sediment, driving, and seasonal berm building have resulted in greatly reduced biodiversity and ecosystem functioning, highly altered morphology, removal of dunes, and decreased vegetation cover (Dugan et al., 2003; Dugan and Hubbard, 2010; Dugan and Hubbard, 2016; Schooler et al., 2019). Approximately ~45% (over 160 km) of southern California’s sandy beach shoreline is groomed at various frequencies (Dugan et al., 2003; Dugan and Hubbard, 2010), a practice that eliminates all dune vegetation and topography for many of these beaches. Beach driving and conflict with recreational activities have also reduced the ecological and geomorphological value of these systems (Anders and Leatherman, 1987; Schlacher et al., 2008). Additionally, these activities have caused the local extirpation of native and threatened species (USFWS, 2007; Hubbard et al., 2014). Many of these groomed beaches have broad, flat unvegetated zones that represent degraded coastal strand and dune habitat (Dugan and Hubbard, 2010). However, these highly impacted but often extensive urbanized beaches can offer exceptional opportunities for restoration of beaches and dunes (King et al., 2018; Myers et al., 2019) that have the potential to enhance coastal resilience.
There is an increasing need to use the best available science to inform coastal adaptation strategies to climate change (e.g., Aerts et al., 2018; Grifman et al., 2020; Lebbe et al., 2021). Nature-based projects can be cost-effective (Gonyo et al., 2023), sustainable, and resilient, providing a myriad of ecological benefits including habitat restoration and ecosystem services, compared with traditional coastal defense barriers (e.g., seawalls, jetties) (Bridges et al., 2015). Nature-based approaches can be used to enhance resilience (Arkema et al., 2013; Newkirk et al., 2018), restore and preserve soft sediment coastal ecosystems, including sandy beaches and dunes, to provide a buffer against SLR and other consequences of climate change and provide a range of ecosystem services (e.g., Nordstrom et al., 2000; Nordstrom and Jackson, 2021). For urban coastal communities, nature-based projects that restore beaches and dunes can provide an innovative adaptation strategy to increase the resilience of the coastline to SLR and episodic flooding by creating more natural dune features and geomorphology (Nordstrom et al., 2000; Nordstrom et al., 2012, Walker et al., 2013). These nature-based approaches provide an alternative to traditional methods of hardscaping or armoring, which can have substantial ecological impacts (Dugan et al., 2008; Dugan et al., 2018, Jaramillo et al., 2021) and economic costs (Griggs, 2005; Aerts et al., 2018; Griggs and Reguero, 2021; Schroder et al., 2022). By restoring natural processes and features to impacted urban beach and dune systems, we can improve their ecological and utilitarian functions. However, examples of nature-based approaches that incorporate the restoration of sandy shore ecosystems in California are few (Newkirk et al., 2018).
With the goal of informing coastal management, policy, and the design of coastal restoration and resilience projects, we assessed the recovery of an impacted urban beach in southern California’s Los Angeles region over six years. Another key objective of our study was to refine expectations for similar dune restoration projects in the region. As the project was the first of its kind in the region, there were no specified success criteria. We evaluated the recovery of the study site against an overarching goal of increasing sediment accumulation and establishing dune features and vegetation that could support ecosystem services and resilience. Our primary research question was to investigate how passive beach restoration could promote the recovery of key geomorphic and biological features on an urban beach. To assess recovery, we evaluated a set of response variables including sediment accumulation and mass balance, foredune formation, and the abundance of vegetation, wrack, trash, and intertidal invertebrates at the restoration site and a groomed control site. We hypothesized that the restoration site would diverge from the groomed control site and from initial conditions over time through the development of natural geomorphic and ecological features.
2 Materials and methods
2.1 Study area
Santa Monica Beach is located in the middle of the Santa Monica littoral cell on the mainland coast of southern California Located in a highly urbanized setting in the county of Los Angeles, Santa Monica Beach is owned by the California Department of Parks and Recreation and managed by the City of Santa Monica. The beach is a major tourist attraction that receives millions of visitors annually. Longshore sediment movement on the beach is largely downcoast (to the south) in this cell with limited littoral drift (Griggs and Patsch, 2018). Santa Monica Beach has a long history of beach nourishment (placement of sand dredged from other locations), with seven nourishment events occurring between 1939 and 1957 for a total volume of 13.4 million cubic meters of sediment (ASBPA, 2022). Prior to nourishment, the beach was narrower and wave run-up was much closer to developed upland areas. Beach maintenance activities include daily mechanical grooming/raking, and frequent driving on the beach by management agencies. The study beach has been subject to daily beach grooming using heavy equipment in the summer season and multiple times per week in the winter season for more than 70 years. Regular grooming regimes are common on urban beaches throughout the world (Defeo et al., 2009), and the practice is particularly intense in southern California (Dugan et al., 2003). Beach grooming is generally implemented to remove trash and promote recreational activities and tourism (Defeo et al., 2009; Martin et al., 2022).
The study area has relatively low potential for wind-driven sand movement. Wind and drift roses using data from the Santa Monica Airport Station (NOAA, accessed 10 November 2022), indicated a dominant west-southwest wind (~250°) for the duration of the study, with a low proportion of the winds likely to result in sand drift (winds above 6.5 m/s). Wind drift analyses resulted in similar findings, including a low drift potential (0.145 vector units) and with a drift direction of 67.8° (Fryberger and Dean, 1979; da Silva and Hesp, 2010). Most of the readings (93.6%) from the local wind station were under the transport threshold for sand movement. The strongest wind gusts occurred in the spring, and the lowest in late summer and fall. The average wind speed across all years was 2.0 m/s with an average gust speed of 9.5 m/s. However, the drift potential was likely substantially underestimated due to the use of the closest weather station, which was inland. The site also has a large fetch distance, due to the width of the beach (~ 120-170 m, from the water line to infrastructure development).
The study area has a Mediterranean climate that is periodically subject to disturbance from storms, and swell events, particularly in the winter season (Polade et al., 2017). Annual rainfall averaged 29.7 cm from 1944-2021 (Los Angeles Almanac). In the first six wet seasons after restoration was initiated, overall average rainfall was similar to the long-term average (27.6 cm), but the site experienced variable annual precipitation (41.4, 9.7, 42.9, 33.3, 12.7, and 25.8 cm) during those six years (NOAA Climate Data Online). The shoreline orientation is NW-SE (310-130°, shore normal 220°), nearly parallel to the wave approach (Griggs and Patsch, 2018). Data from 2018-2022 at the Santa Monica wave buoy showed a dominant wave direction from the southwest, with seasonal variability, and the potential for wave-driven sediment transport (Coastal Data Information Program). Significant wave heights over 1.8 m were infrequent (< 1% of readings), and the peak period was predominantly 14-16 seconds from ~200°, with infrequent longer periods (> 20 seconds, < 1% of readings). Erosion from swell events and storms reduced the beach width in the winter (occasionally by > 10 m), with sediment transport processes increasing the beach width in the summer.
2.2 Pilot project
The Santa Monica Beach Pilot Project used a novel approach to sandy shore restoration for southern California. The project, led by The Bay Foundation, City of Santa Monica, California Department of Parks and Recreation, and University of California Santa Barbara, restored a portion of the heavily groomed urban beach into a coastal strand and incipient foredune habitat complex with no sediment addition or use of heavy equipment (Figure 1, Johnston et al., 2021). The 1.2-hectare area of a heavily groomed beach was passively restored by installing sand fencing around three sides of the site, (leaving the oceanward edge open) and adding native dune plant seeds in December 2016. Sand fencing is known totrap and accrete sand (Itzkin et al., 2019; Liu et al., 2023). Grooming was restricted to approximately 4-5 m outside of the fenced area. The restoration site (~150 × 60 m) exceeded the threshold recommendations identified for “Natural Shoreline Infrastructure” for California in Newkirk et al. (2018), including a minimum alongshore distance of > 30 m (~150), minimum dune cross-shore length of > 15 m (~60 m), and beach buffer of 30 m (~60-95 m), with a total beach width of ~120-170 m.
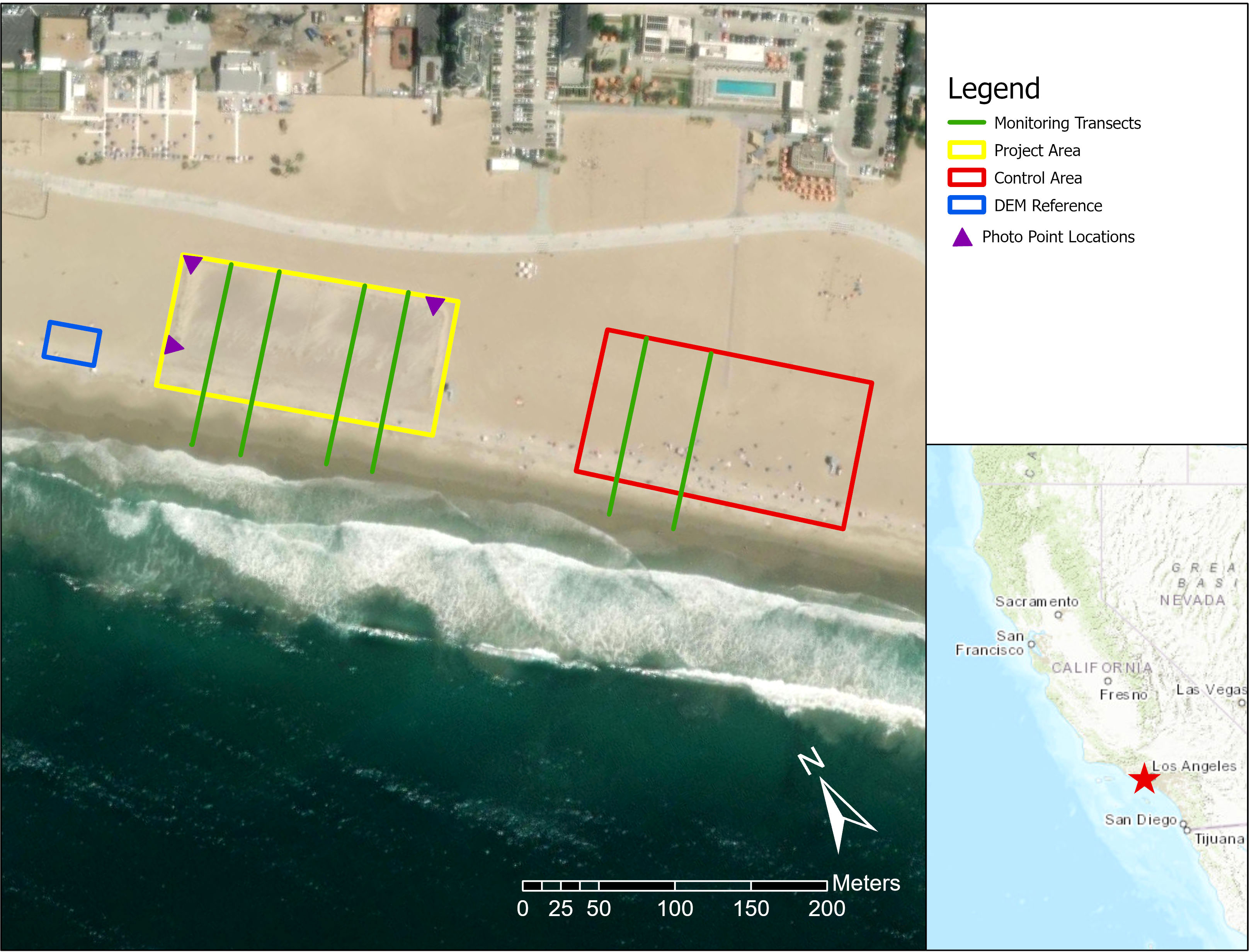
Figure 1 Map of the study area with the locations of the restoration project study site (yellow box) and groomed control site (red box) on Santa Monica Beach, California, fixed transects used in surveys (light green lines), georeferenced photo points (purple triangles), and reference area for drone-based elevation analyses (blue box). Red star on the inset map of California indicates the project location in Santa Monica Bay, southern California, USA.
The restoration used 1-meter wood slat sand fences, a central path through the restoration area, seeding of California native perennial dune plant species, two interpretive signs installed along pathways, and an unenclosed perimeter on the seaward edge. Four species of native coastal strand and foredune plants (including known ecosystem engineers) were seeded evenly across the site once. The seed addition rate was 23 kg per hectare. Plant species included Abronia maritima (red sand verbena), Ambrosia chamissonis (beach bur), Atriplex leucophylla (beach salt bush), and Camissoniopsis cheiranthifolia (beach evening primrose). No irrigation was used. Maintenance consisted of occasional removal of a non-native plant, Cakile maritima (sea rocket). A control site of approximately the same area was identified approximately 100 m south of the restoration site. The control site was not seeded or fenced and was subject to continued frequent mechanical grooming throughout the duration of the study.
2.3 Field and analysis methods
Surveys were conducted to assess sand accretion, geomorphic feature formation, vegetation development, and additional biological indicators over a six-year period. Results of surveys were used to assess changes at the restoration project site including comparisons to pre-project conditions (December 2016) and comparisons to a groomed control site approximately 100 m south of the restoration site (Figure 1).
Two methods were used to assess geomorphological variation at the restored and control sites over time: 1) repeated elevation profiles to assess the sediment accretion and erosion over time, and 2) uncrewed aerial system (UAS) surveys. Elevation profiles were used to assess changes in sand volume since project initiation; UAS surveys were used to assess total sand volume accretion, relative to a control area; and extrapolated UAS survey transects were used to calculate high resolution shore normal relative transect elevations.
Elevation profiles were conducted on fixed shore normal transects within the restoration site (N = 4) and within the control site (N = 2) on a quarterly basis for the first five years. The profiles started at the 0 m mark, which was permanently fixed at the eastern fence line, and progressed seaward. Elevation data were collected every 3 m along the transects using a Trimble Geo7X Global Positioning System (GPS) unit referencing the NAVD88 datum. Data were quality control corrected to retain only those data with a defined precision vertical accuracy level of ± 10 cm (latitude and longitude accuracy were ± 5 cm). Data points with poorer resolutions were not included in the analysis. Sand volumes were calculated from the cross-sections of the elevation profiles by integrating the area under the curves, then multiplying by 1 m beach width to estimate cubic meters of sand volume per meter of shoreline for each transect. Volumes were then averaged across the restoration site and control site for each survey. We then normalized the volumes as referenced to the pre-project condition (initial survey conducted prior to implementation in December 2016). Similarly, the control data were normalized relative to their pre-project transect conditions. Elevation profiles and volume change were analyzed to calculate the sediment budget (volume change over time). Additionally, georeferenced photographs using SolocatorTM (smart phone application) were collected from seventeen fixed locations and compass headings quarterly. Representative photographs from three locations were selected for evaluation of qualitative geomorphic change.
Six years after project initiation, a UAS survey was conducted on 10 November 2022 with a DJI Phantom 4 RTK Drone paired with a D-RTK 2 High Precision GNSS Mobile Station to generate a structure for motion digital elevation model (DEM). The survey was conducted using the Phantom 4 built-in 20 MP RGB camera angled at 90° and flown at 25 m above ground level with 80% horizontal and vertical image overlap. Horizontal position accuracy was 1.2 ± 0.02 cm and vertical position accuracy was 2.7 ± 0.2 cm. Collected imagery (996 photos) was imported to Agisoft Metashape Pro where photos were aligned at the highest accuracy with generic preselection, a key point limit of 128,000, tie point limit of 10,000, and adaptive camera model fitting. Next, a high quality dense point cloud was generated with mild depth filtering and then used to build the DEM. DEM raster analyses were conducted in ArcGIS Pro (ESRI). The DEM was used to estimate elevation within the restoration site relative to an adjacent section of beach outside of the restoration site. Sand volume accretion was estimated based on pixel-scale area (0.0147 x 0.0147 m) and elevation relative to the reference control box. Mean elevation of the un-restored reference control box was calculated and the restoration site was normalized to that value at the pixel level such that resulting positive elevation values reflect locations with elevations higher than the control box location and negative elevation values reflect locations with elevations lower than the reference control box location. We then estimated the volume of sand in the restoration site as the sum of each pixel volume where pixel size (i.e., area) was multiplied by each pixel’s relative elevation above or below the reference area. The same methods were applied to estimate foredune sand volume and the sand volume independent of the fence line, with a 10 m buffer. A repeat UAS survey was conducted in January 2023 using the same collection and processing steps described above. The DEM was used to calculate relative elevation across six shore normal transects throughout the project site. Profile graphs were generated for each of the six transects, and elevation values were normalized such that the lowest observation on each transect = 0.
To describe changes in plant cover over time, we conducted quarterly surveys of vegetation cover and composition using line intercept shore normal surveys along four fixed restoration site transects, and two fixed groomed control site transects for the first five years. The cover of marine wrack, terrestrial debris (e.g., leaves, wood), and trash were also measured using line intercept shore normal transect surveys along six randomly selected transects each in the restored and control sites six times during the study, with one additional survey conducted in December 2016 prior to restoration implementation (pre-project survey). The repeated surveys along fixed transects led to obvious trampling and disturbance, so we modified the survey method to place transects randomly. Vegetation cover and composition were assessed using the randomly placed shore-normal transects beginning in August 2021 (three surveys). Cover classes were quantified as the length and location of contact encountered on the transect, with a minimum cover distance of ≥ 1 cm. Data were summarized as percent cover ± standard error.
To describe the native plant species associated with geomorphic features and to compare patterns of plant zonation in the restoration site, we used plant distribution data from the vegetation surveys (shore normal line intercept transects) conducted in March 2019 and January 2023 (two and six years after implementation). Average cover was estimated for each species in 10 m zones beginning at the back fence of the study site (inland 0 m mark).
On six dates, beach invertebrates were surveyed in two zones along the seaward edge of the project: the upper intertidal or talitrid amphipod zone, and the high or supralittoral zone, using protocols comparable to Dugan et al. (2003). Quantitative sampling was conducted along the same six transects used for vegetation and wrack surveys on those dates. Ten uniformly spaced cores (10 cm diameter, 20 cm depth) were collected across each of the zones. Core spacing was adjusted to span each of the zones. The cores from each transect and zone were pooled and placed in a mesh bag with an aperture of 1.5 mm for sieving. Sediments were removed by sieving the mesh bag in the adjacent swash zone. Animals retained on the mesh bag were transferred to labeled plastic bags, chilled, and transported to the laboratory for freezing and later processing. Animals were identified to species, and abundance values were calculated as the number of individuals per meter of shoreline. Additional anecdotal information was collected on shorebird use of the site and other biological indicators such as dune beetle presence.
3 Results
The emergent features of the restoration site diverged substantially from pre-project conditions and from conditions at the control site over time and were dynamic across seasons and years (Figure 2). An oblique aerial view of the project site taken in November 2022 shows major features including the project boundary, perimeter fence, various habitat types, incipient foredune ridge, and groomed beach (including the control site). Dunes formed along the perimeter sand fence, distinctly visible along the northern fence line. Vegetated plant hummocks and the incipient foredune ridge are visible along the perimeter of the site. The restoration site formed shadow dunes where aeolian transport deposited finer grained sand as a “tail” of some dune features, in areas where wind velocity slowed due to vegetation and reductions in the shear stress, in alignment with the prevailing wind drift vector (Figure 2).
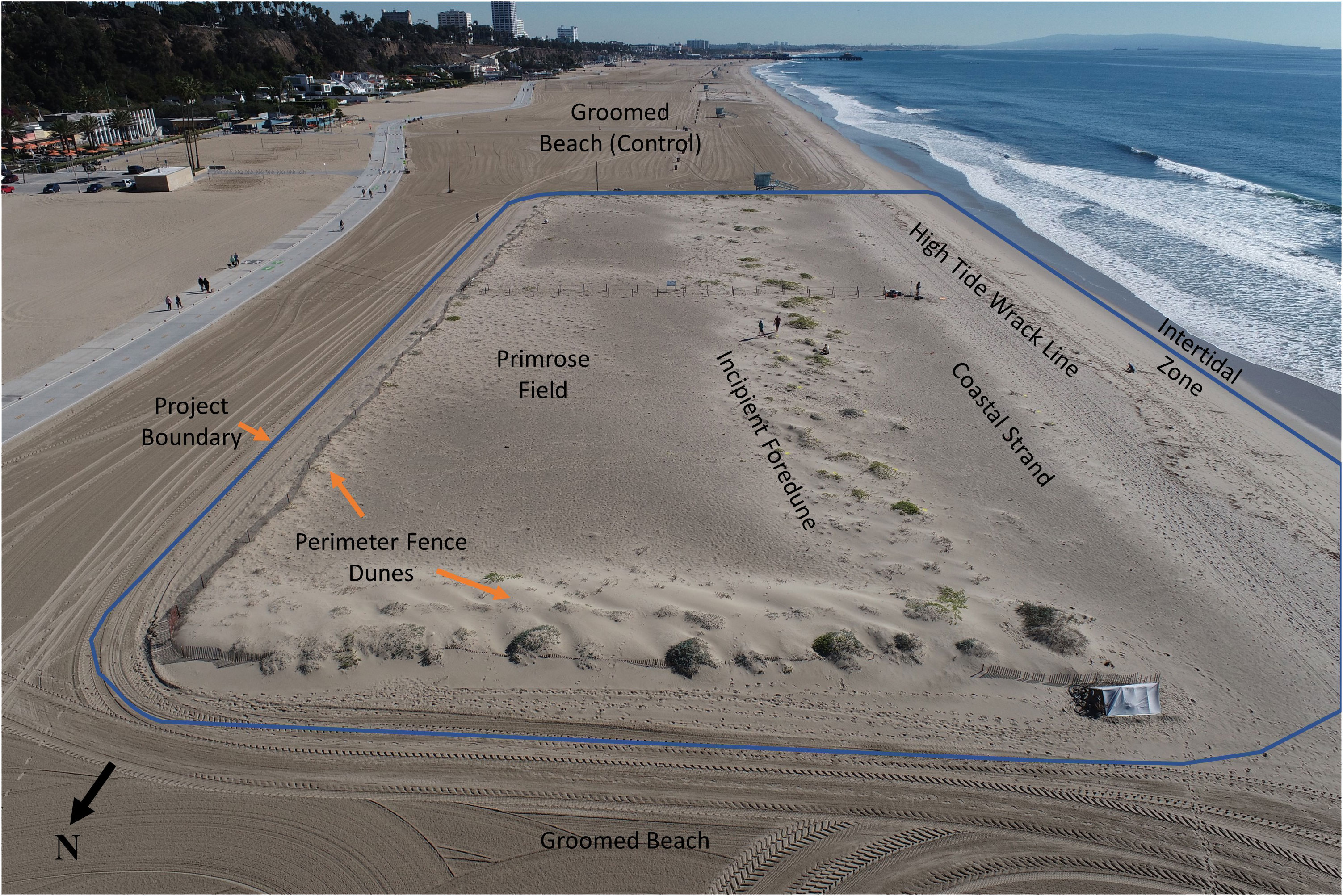
Figure 2 Oblique aerial photograph of the restoration project site from the 10 November 2022 UAS flight, with key elements labeled including the project boundary, perimeter fence, various habitat types, adjacent groomed beach (control site), and the incipient foredune ridge. Alongshore distance of the site was ~150 m, with a dune cross-shore length of ~60 m.
Sand accretion in the restoration site led to dune forms that increased in height and became more complex over time. Changes in vegetation and topography over time are illustrated in the photograph series (Figure 3). The series begins with the first time point (top photos) about a month after the restoration project was installed (January 2017), in May 2018 (middle photos), and ends in November 2022 (bottom photos) (Figure 3, see also Figure 2). The photograph series shows the gradual growth of plants, increases in elevation and changes in topography, including the formation of the incipient foredune ridge (Figure 3, Northwest Point). Plants were repeatedly buried or partially buried by sand, and then continued to grow above the newly deposited sand. The perimeter sand fence was buried over time (e.g., Figure 3, Northeast Point, 2022, and Figure 2).
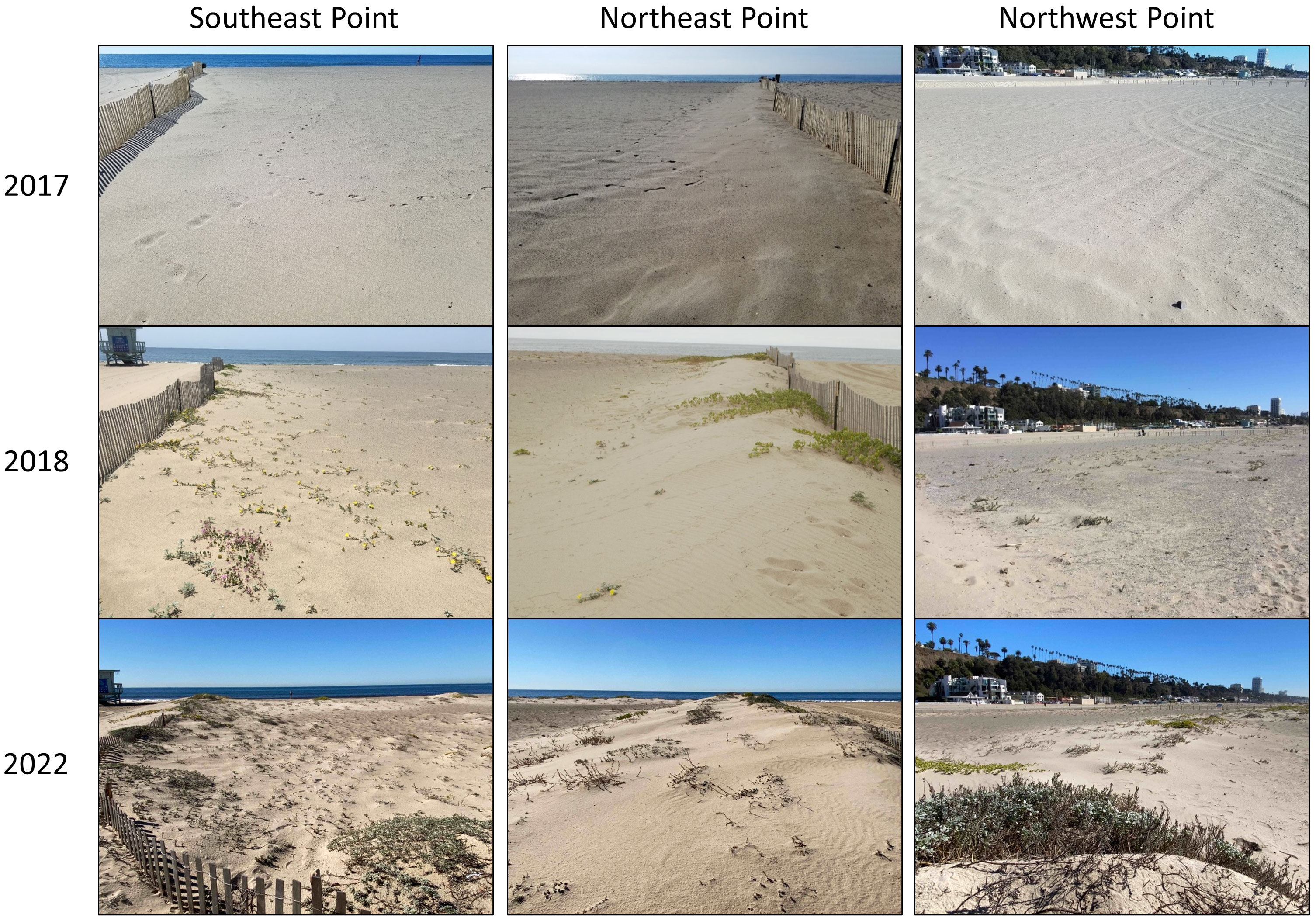
Figure 3 Combination panel of georeferenced photographs of the restoration site over time. Top photographs were taken in January 2017, middle in May 2018, and bottom in November 2022. Left series is the southeastern portion of the restoration area and fence line, middle is the northeastern point and fence line, and right is the northwest point facing south across the incipient foredune ridge.
Key vegetation establishment and geomorphic variation events are presented on a restoration timeline in Figure 4, including early sand capture along the fence (within one month of implementation), plant germination (within two months), and the formation of a lag surface with small pebbles and larger sand grain sizes (within three months). The formation of the incipient foredune ridge was initiated within 18 months of project implementation, beginning with small plants forming hummocks less than 0.5 m in width.
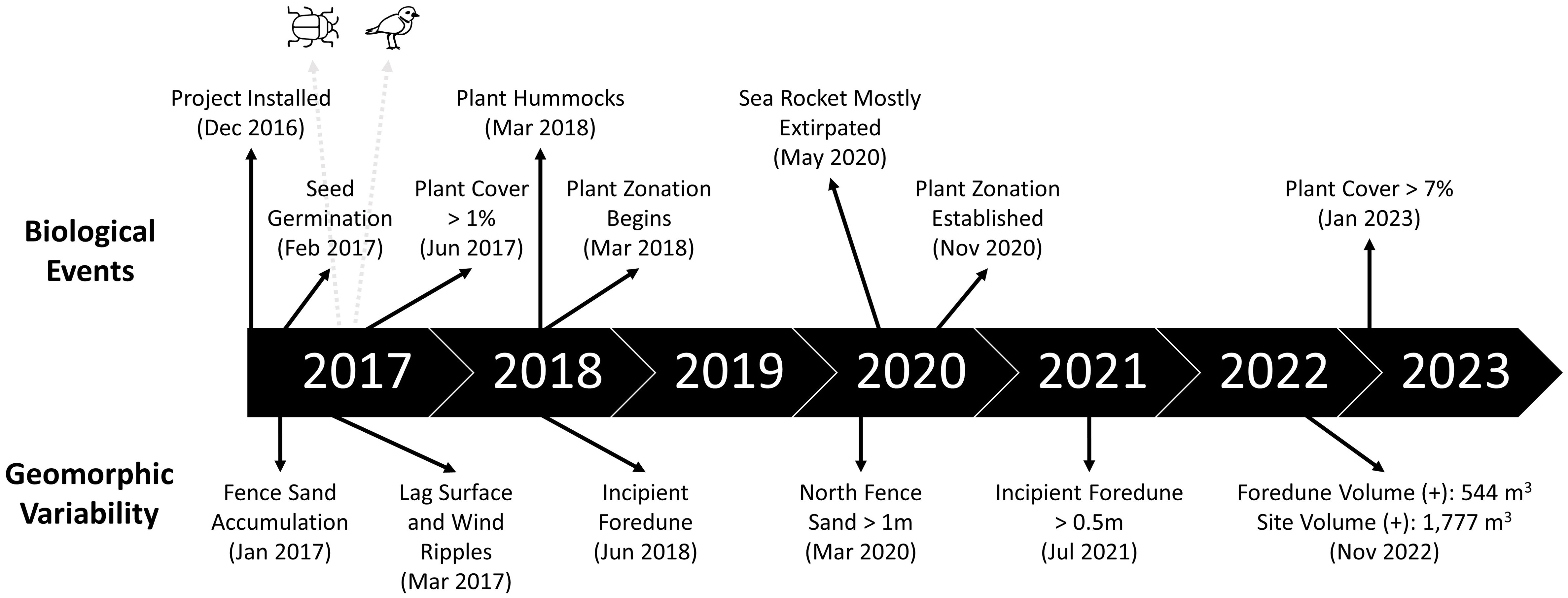
Figure 4 Timeline of key events for biological events (top) and geomorphic variability (bottom) at the restoration site during the study. Arrows indicate dates. Beetle icon indicates first observation of dune beetles (April 2017). Bird icon indicates nesting event for western snowy plover (April 2017).
At the restoration site, overall increases in elevation from the pre-project conditions indicating deposition and retention of sand were evident in all profiles from elevation surveys (Supplemental Data A). Sand accretion was greatest along the incipient foredune ridge, and along the northern and southern fence perimeters (see also Figure 2). The southeast corner of the restoration site showed a high relative buildup of sediment, which aligns with the predominant wind pattern moving sand in that direction. Survey data showed that the control site had less variability over time as compared to the restoration site (Supplemental Data A), with seasonal changes in the berm and beach face, elevation variability, and a generally flat and even beach profile, all likely due to mechanical grooming. Profiles at the restoration site showed a greater increase in elevation compared to control site profiles. The highest rate of sand accumulation was observed in association with the perimeter sand fencing at the restoration site, which accumulated more than 15 cm within the first six months. Additionally, profiles captured the formation of sand associated with plant hummocks across multiple seasons and increases in topographic variability at the restoration site. By the fourth year, the vegetated incipient foredune was clearly established within the site (see also Figures 2, 3, 4).
Estimated change in sand volume from profiles of the restoration site showed an overall increase with time that roughly followed an asymptotic curve (Figure 5), with variation in volume change relative to the pre-project conditions. By August 2021, the estimated increase in sand volume was 30 m3 per m of shoreline (Figure 5). At the control site, net sand volume change was lower and varied over time, with erosion in the first two years, and accretion in later years. The dynamics of the variation in the control transects are most likely artifacts of beach grooming maintenance activities rather than aeolian transport and accretion of sand.
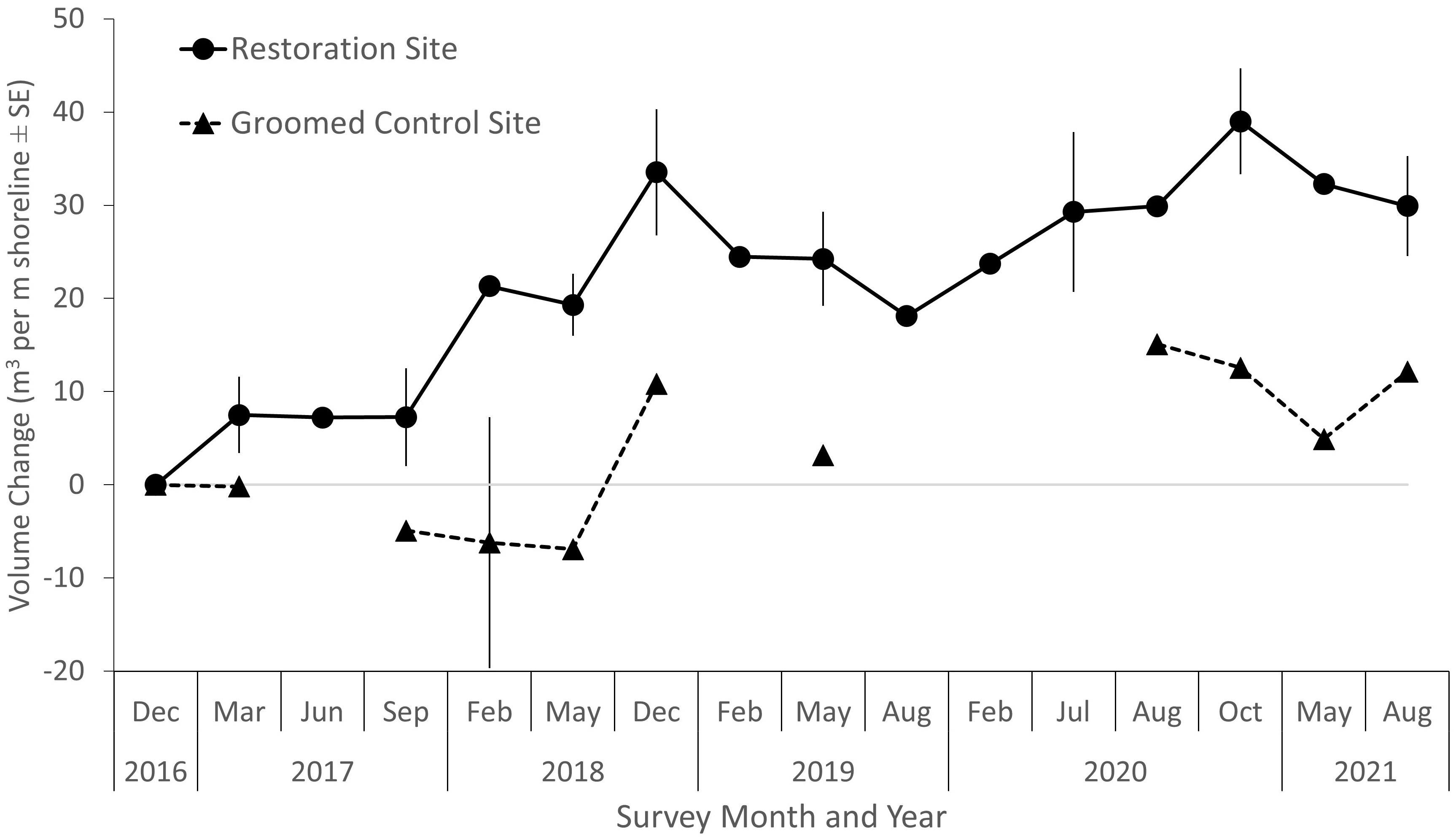
Figure 5 Sand volume change over time per meter of shoreline (± standard error) estimated from 50 m long elevation profiles at the restoration site and groomed control site. The control site had fewer estimates that met the quality control requirements. Light gray line indicates baseline of zero elevation change.
A large amount of sand accumulated along the perimeter fence and the incipient foredune ridge by 2022, along with some erosion in the northern corner of the restoration site (lag surface), and sediment accumulation across the southern half of the site that was similar to the qualitative assessments from the georeferenced photographs (Figure 6, see also Figure 2). The area of the DEM as defined by the restoration project boundary was 7,777 m2. The mean elevation of the restoration site was 0.22 m above the reference control box located outside of the restoration area (Figure 1). The estimated net sand accretion in the restoration site was 1,726 m3. This volume of sand is the equivalent of 2,760 metric tons (using 1,600 kg per m3). The DEM evaluation yielded a volume of approximately 12 m3 of sand per m of shoreline; and an average annual rate of accretion of 2 m3 per meter of shoreline (3 metric tons) per year.
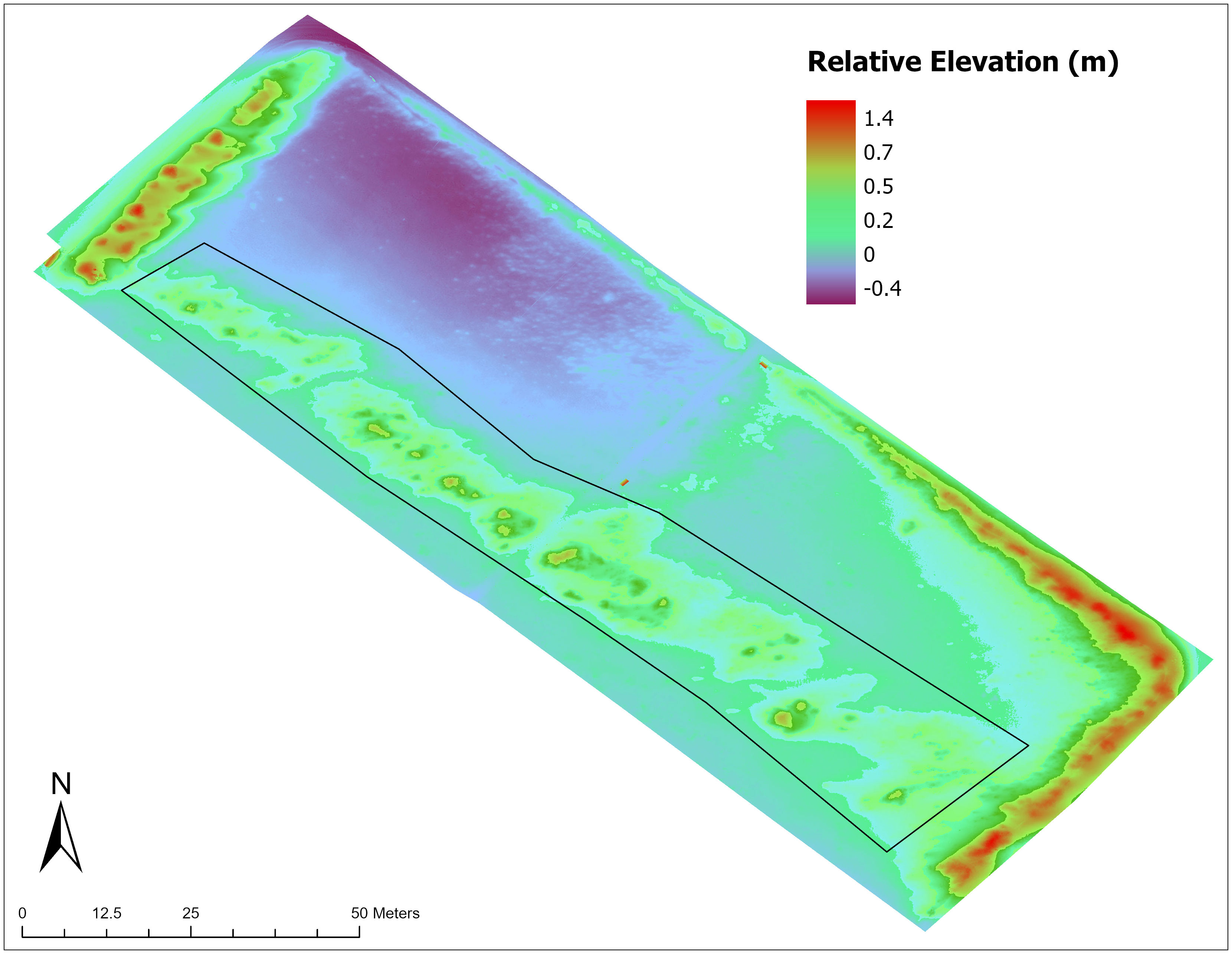
Figure 6 Topography of the restoration project site (Digital Elevation Model from November 2022 aerial survey). Black polygon outline indicates the evaluated foredune area. Purple colors indicate areas of lag surface deflation and blue-greens to reds indicate areas of sediment accretion.
The foredune area was evaluated separately, yielding an area of 2,178 m2, with a mean elevation 0.25 m higher than the reference control box outside of the restoration site (Figure 6). The maximum crest heights of the foredune ranged from 0.75 to 0.90 m above the reference control box elevation. We estimated an accreted sand volume of 544 m3 in the foredune. The foredune area contained roughly 32% of the site-wide accreted sand volume in 28% of the project area. An estimated 870 metric tons of sand was contained within the foredune footprint. The total foredune length evaluated was 125 m, yielding an approximate volume of 4.35 m3 of sand per m shoreline accumulated in the foredune and an estimated annual rate of accumulation of 0.73 m3 per meter of shoreline (1.2 metric tons). The remaining sand volume was accumulated largely within the north and south fence footprints where maximum crest heights ranged from 1.0 to 1.3 m above the reference control box elevation.
An additional evaluation of the interior of the restoration site was conducted by removing a 10 m buffer from the sand-fenced edges (i.e., north, east, and south project boundaries). This yielded a total interior area of 5,393 m2, covering approximately 69% of the total restoration project site, with a mean elevation of 0.16 m above the reference control box elevation. The pixel-scale area method estimated sand volume at 842 m3 (1,350 metric tons). Based on these analyses, we estimated that approximately half of the total sand volume of the restored site was accumulated in the perimeter fence dunes.
The cover of vegetation also increased over time in the restoration site. No native or non-native vegetation was detected in the restoration or control site at the initiation of the study in December 2016. Vegetation was not detected in any subsequent surveys of the control site. At the restoration site, native plants germinated from added seed within five weeks, following several rain events. Native plant cover increased slowly over time to a maximum of approximately 7% native cover by the sixth year (Figure 7, January 2023). Non-native plant cover remained very low across all surveys (<< 1%). Plant cover varied seasonally due primarily to dormancy of some species, the burial of vegetation by sand, and precipitation variability. Additionally, when plants were in a dormant phase, some of the cover was below the minimum resolution for recording (i.e., < 1 cm).
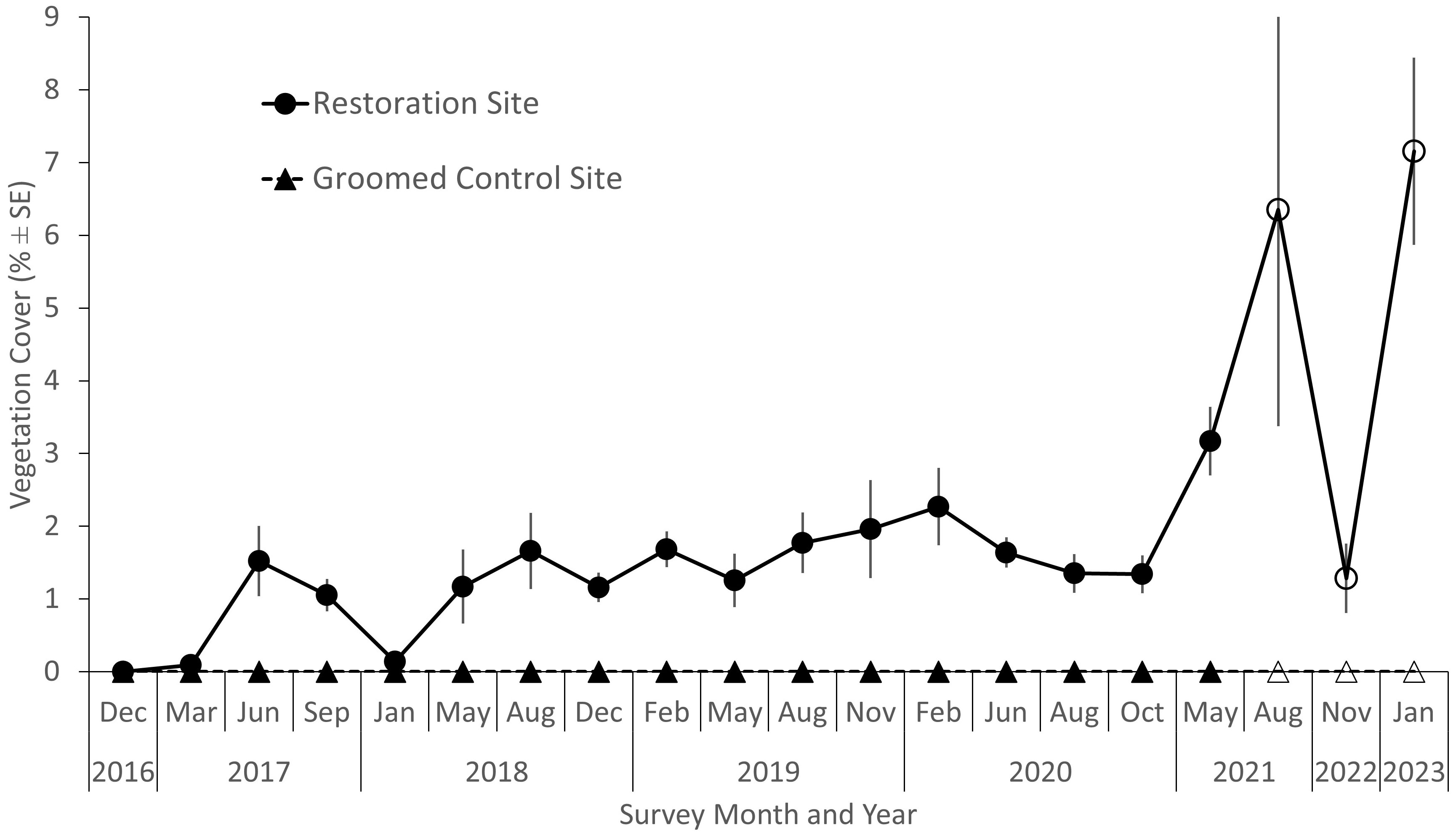
Figure 7 Average native vegetation cover over time at the restoration site and groomed control site (± standard error). Note: open circles and triangles in August 2021, November 2022, and January 2023 were collected with random transects instead of fixed and the time scale varies.
The four species of native plants (i.e., A. maritima, A. chamissonis, A. leucophylla, and C. cheiranthifolia) that were seeded at the restoration site germinated within and immediately adjacent to the perimeter fence and in the exterior buffer where the restoration site was not groomed. Three other plant species germinated within the restoration site, including two native species, Nemacaulis denudata (woolly heads) and Abronia umbellata (pink sand verbena) and one non-native species, C. maritima. The germination of these additional species suggests there was either an existing seed bank in the restoration site, or that they were transported into the site from source populations via wind, waves, birds, humans, or as unintentional inclusions in the seed mix. C. maritima was periodically removed, and decreased over the years as the seed bank was reduced. Little to no C. maritima was identified in the last two years of surveys (< 0.1%), and almost no site maintenance occurred in that time period.
Zonation of native plant species developed at the restoration site over time (Figure 8A, B). Although all plant species were seeded evenly across the site, zonation patterns developed rapidly (evident in mid-2018, approximately 18 months after project implementation, Figure 4). This early establishment was notable for C. cheiranthifolia (inland) and A. maritima (initial development of the incipient seaward foredune ridge in the 30-40 m zone) (March 2019, Figure 8A). These zones became more evident over time, leading to distinct zones for each species (January 2023, Figure 8B). By January 2023, A. maritima and A. chamissonis reached their highest cover estimates in the incipient foredune ridge (9.2 ± 7.6% and 4.4 ± 8.7%, respectively), and the cover of C. cheiranthifolia was highest further inland (5.4 ± 1.3%, eastern portion of the site). While the foredune contained all four seeded species, A. maritima and A. chamissonis were the dominant species of this zone.
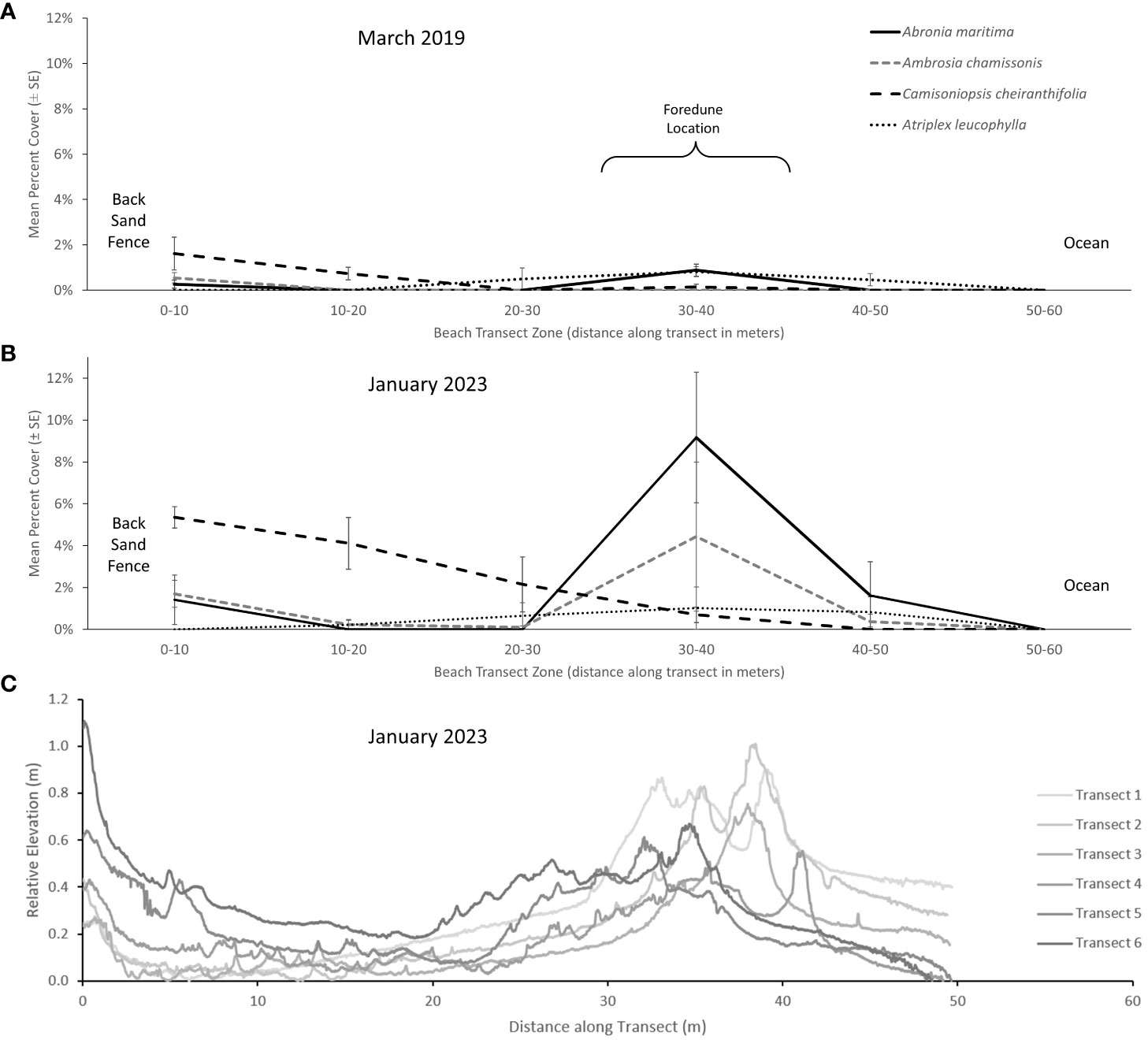
Figure 8 The distribution of mean values of cover (± standard error) for the three dominant species of native dune plants across 10 m zones extending from the landward (left) to the open ocean perimeters (right) of the restoration site for surveys in (A) March 2019 and (B) January 2023. The incipient foredune ridge occurs largely within the 30-40 m zone. (C) Topography of the restoration site as shown by transects of relative elevation profile extending from the back sand fence to the open ocean perimeter extracted from the January 2023 DEM survey.
Plant zonation was directly associated with areas of sand accumulation based on elevation profiles from the DEM (Figure 8C); areas with higher plant cover also had the highest relative elevation of sand (e.g., sand fencing and foredune areas, Figure 8B). Even with a low cover of dune-forming vegetation < 2% in the foredune (e.g., Figure 8A, March 2019), the foredune had already accumulated up to 30 cm of sand associated with individual plant hummocks such as A. maritima and A. chamissonis (see also Figure 4, Supplemental Data A). Accelerated sand accumulation was associated with the foredune plants; by May 2019, an incipient foredune could be identified in the elevation profiles (Supplemental Data A). As the vegetation established and the cover of key dune forming species increased, the resulting relative elevation of the foredune and sand fence showed the continued accretion of sand (Figure 8B, C). By January 2023, the foredune contained the highest plant cover observed and reached a relative maximum elevation of > 0.8 m in several places.
Elevations varied considerably across the restoration site in the recent surveys (Figure 8C). The DEM elevation profiles in the northern portion of the restoration site (e.g., Transects 1 and 2, Figure 8C) display the lower elevation lag surface areas (left-hand side of the graph). In contrast, the elevation profiles in the southern portion of the restoration site (e.g., Transects 5 and 6) display the areas with the highest back beach sand accumulation adjacent to the fence line.
Overall, the cover of marine wrack was very low (< 1% cover, range 0.0-0.6%) across all surveys at both the restoration and control sites (Figure 9A). The cover of marine wrack was slightly higher in the restoration site compared to the control site for four of the five surveys, with higher cover (> 15 times the control cover) recorded for only one survey (November 2022) (Figure 9A, Kruskall-Wallis (KW) test, H(5) = 2.76, p < 0.05). Marine wrack was dominated by Macrocystis pyrifera (giant kelp), Phyllospadix torreyi (surfgrass), and other species common to the study region. The cover of terrestrial debris was also low, averaging < 1% cover (range 0.0-0.7%) across all surveys (Figure 9B). This material was dominated by small bits of wood, leaves, feathers, and other biological debris washed ashore by waves. The cover of terrestrial debris was slightly higher in the restoration site compared to the control site in four surveys, with substantially higher cover recorded (3 and 30 times higher than control site) for two surveys: July 2017 (KW test, H(5) = 3.93, p < 0.05; Figure 9B) and March 2018 (KW test, H(5) = 3.16, p < 0.05). The mean cover of trash was low overall (< 0.1% cover, range 0.0 to 0.4%) but slightly higher in the control site than the restoration site; trash did not differ between the two sites in any of the surveys (Figure 9C). With the exception of the March 2019 survey of the control site (cover = 0.4 ± 0.3%), the rest of the surveys found < 0.1% cover of trash.
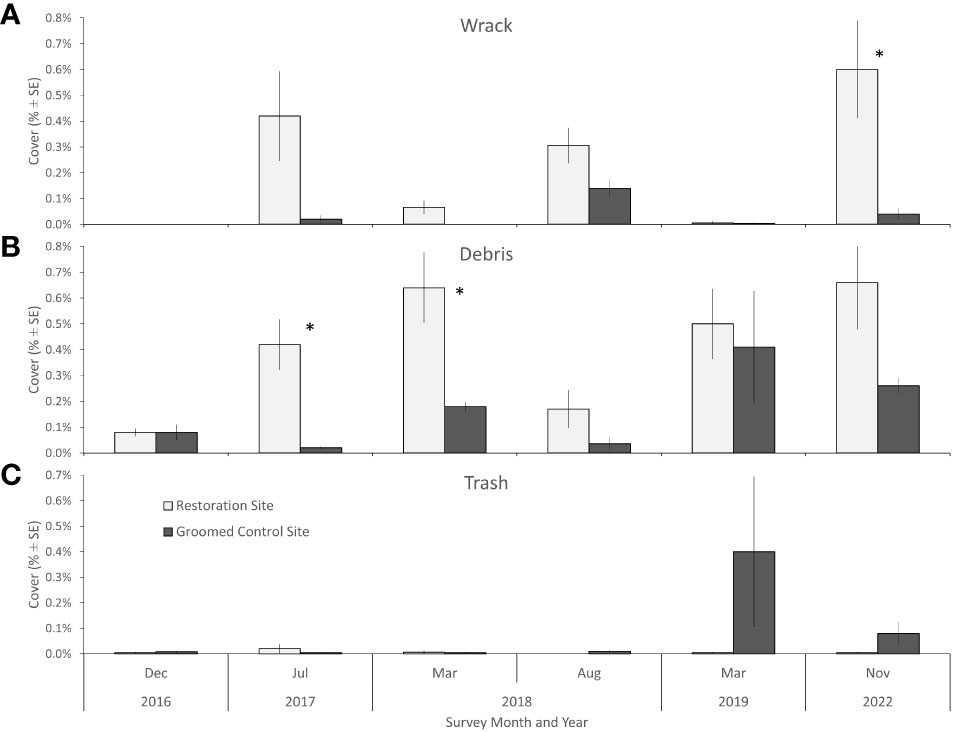
Figure 9 Marine wrack (A), wood and terrestrial debris (B) (e.g., leaves and feathers), and trash (C) cover (± standard error) at the restoration site (light gray) and control site (dark gray) for each survey. Single asterisk indicates p < 0.05.
For the upper intertidal invertebrates, which were primarily identified as talitrid amphipods (Megalorchestia spp.), no differences in abundance were detected between the restoration and control site overall when combining all survey data (KW test, H(6) = 1.18, p = 0.28). However, variation was high and the abundance of talitrid amphipods was higher in the restoration site than the control site for two surveys, July 2017 (four times higher, KW test, H(7) = 2.51, p < 0.05) and August 2018 (almost six times higher, KW test, H(6) = 6.16, p < 0.001) (Figure 10). Too few invertebrates were found in the supratidal cores for analyses.
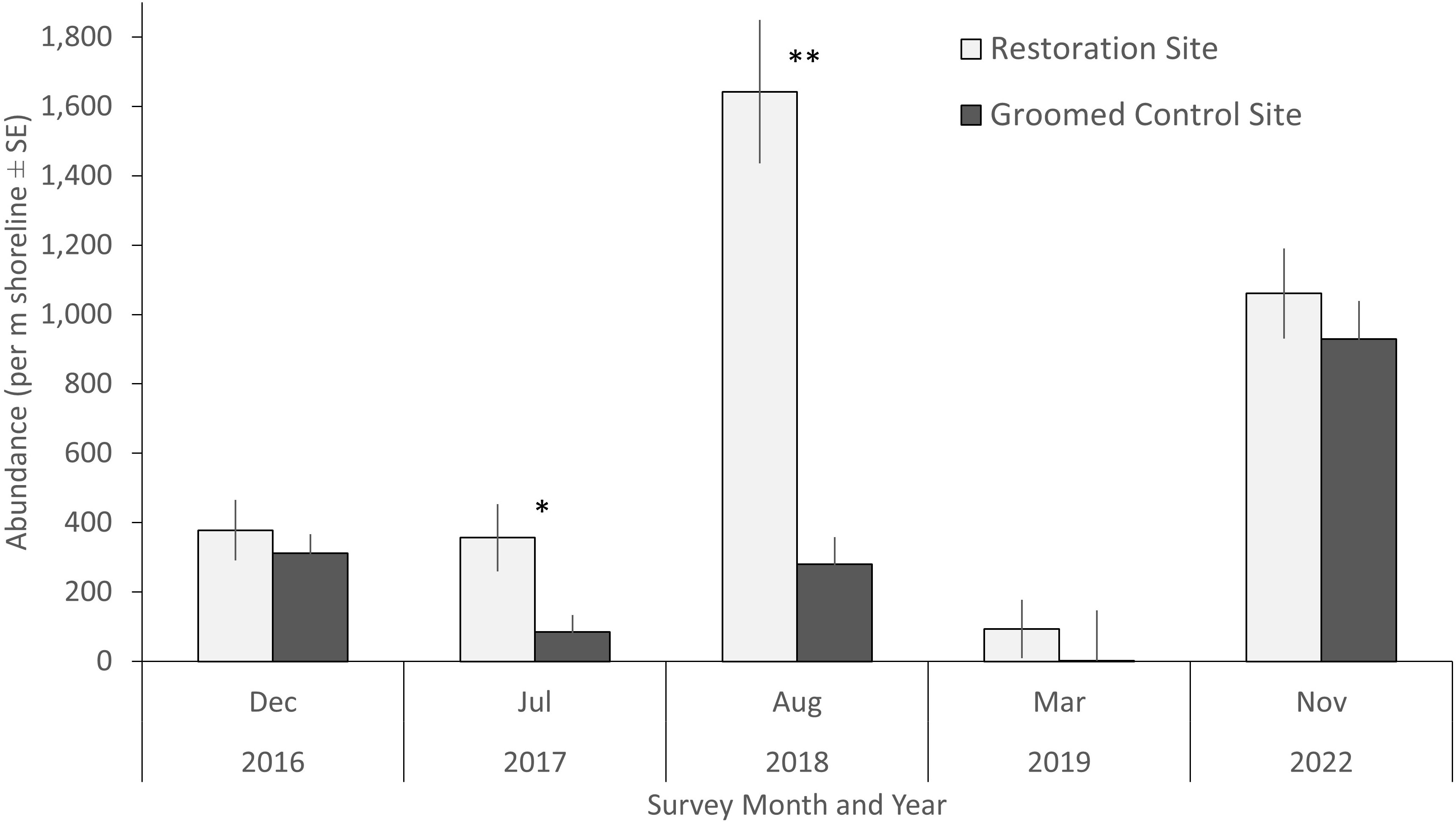
Figure 10 Talitrid amphipod (Megalorchestia spp.) abundance (± standard error) per meter of shoreline for each survey. Single asterisk indicates p < 0.05, double asterisk indicates p < 0.001.
Dune beetles (Coelus sp.) were observed in the restoration site within eight months of project implementation (see Figure 4). Dune beetles were not observed at the control site or in the pre-project surveys prior to the restoration implementation.
Two breeding shorebird species (plovers), including one pair of threatened western snowy plover (Charadrius nivosus nivosus; Figure 4) and one pair of killdeer (Charadrius vociferus) nested in the restoration site during our study. Santa Monica Bay Audubon Society has been monitoring the beach area for western snowy plover activity for more than a decade. No nesting attempts were observed prior to the restoration or at all in the control site. Western snowy plovers had never been recorded using the restoration site prior to implementation, but since the restoration, they were observed at the site across multiple seasons of all six years.
In summary, over the first six years of observations, the strongest responses at the restoration site were found in the geomorphic and vegetation characteristics (Table 1). Geomorphic complexity increased, especially with the formation of plant hummocks and the incipient foredune, sand accumulated, and topographic variability occurred across the restoration site. Vegetation increased over time, with distinct plant zonation forming over time. The growth of species, such as A. maritima and A. chamissonis, were associated with sand accumulation in the incipient foredune. In contrast, responses of wrack, debris, and trash cover at the restoration site were not apparent. Although dune beetles associated with vegetation appeared rapidly in the restoration site, the abundance of wrack-associated invertebrates did not differ from the groomed control site.
4 Discussion
Our study results illustrate the potential for the restoration of coastal dunes on groomed urban beaches, with the strongest responses observed in metrics that could enhance resilience to climate change including sand accumulation, formation and heterogeneity of geomorphic features, and native vegetation, including dune-building species. The formation of an incipient foredune ridge, associated with perennial native vegetation and aeolian processes, combined with sediment accumulation along the fence lines, resulted in a net increase in sediment volume. Our findings demonstrate the high potential for recovery of coastal dunes with minimal human intervention following the removal of key stressors and impacts (i.e., grooming and beach driving). Overall, our estimates of sand accretion rates from the DEM and profile data suggested an elevation increase that was higher than current projected SLR rates for the near term at 3.6-9.6 mm/yr for southern California (Griggs et al., 2017).
Reducing the disturbance (mechanized grooming and driving) at the restoration site and implementing a relatively passive dune restoration project using sand fencing and seeds of native dune-forming species allowed for the recovery of natural geomorphic processes and interactions with vegetation that accreted sand over time without any manual or mechanical sediment addition. Mechanized grooming and driving have been shown to significantly impact beach and dune plant communities (Dugan et al., 2003; Schlacher et al., 2008; Dugan and Hubbard, 2010; Houser et al., 2013; Schooler et al., 2019; Pinardo-Barco et al., 2023). Beach driving alone has also been shown to impact recovery of dune systems (Houser et al., 2013; Pinardo-Barco et al., 2023). However, few studies have evaluated the passive recovery of dunes following the cessation of beach grooming. Based on results for the control site, grooming is an especially effective maintenance activity at preventing the recruitment and growth of dune plants. Removing that stressor combined with eliminating most driving across the site allowed for the natural recovery of the dune vegetation community at the restoration site. Other stressors such as human activity, dog walking, and invasive mammals or birds were not evaluated as part of this study, though they may affect the restoration site. Additional stressors to dunes range from global, such as sea level rise (Seabloom et al., 2012), to local scales, such as coastal squeeze from development (Mendoza-González et al., 2013; Schlacher et al., 2014), armoring (Dugan et al., 2018), invasive plants (Walker et al., 2013) and off-road vehicle use (Defeo et al., 2009).
Strong edge effects are present at our restoration site with plant recruitment occurring outside the fenced site, but older perennial vegetation was limited to areas within a few meters of the fencing (limit of grooming restrictions). Nordstrom et al. (2012) also found that removing the disturbance of raking allowed for recovery of the dune system and increased sand accumulation in a similar study in New Jersey, USA. They identified the use of sand fencing as an initial method to provide increased berm height for flood protection but suggested that allowing for natural recovery could improve the long-term return of these systems to more natural coastal dune types. Nordstrom and Jackson (2021) provide detailed discussions and examples of various types of dune restoration and strategies for implementation, including the effectiveness of sand fencing to trap wind-driven sand.
Key geomorphic features and sediment dynamics of the restoration site diverged strongly both from pre-project conditions and the groomed control site over time. These features included lag surfaces, vegetated hummocks and an incipient foredune ridge as well as dunes associated with the perimeter sand fencing (Figures 2, 3, 6). Along with increased geomorphic heterogeneity, the dynamic changes and responses at the restoration site included increasing sand retention over time, and increased surface elevation. The finding of an overall positive change in sand volumetric balance, represents a net gain (surplus) for sediment. Similar studies calculating sediment budgets, such as Walker et al., 2022, have shown that a positive sediment budget and altering management activities that prevent sediment accumulation leads to dune formation.
Sand accretion varied spatially across the restoration site due to a number of factors, including wind direction, a lag surface, and the effects of the perimeter fencing. This resulted in the formation of heterogeneous topography and a range of geomorphic features across the site. The net accumulation of sand we observed was largely associated with the perimeter fence and the incipient foredune ridge. Sand fencing is a common restoration method of accreting wind-blown sand (Itzkin et al., 2019; Liu et al., 2023). The DEM also showed accumulation of sand across the southern half of the site, trapped by the sand fence, which conformed with the predominant wind direction. However, the incipient foredune and its maximum crest height (0.75 to 0.9 m) illustrated a natural accumulation of sand associated with vegetation establishment, independent of any added physical elements, such as the sand fencing. Notably, at the restoration site, the sand accumulation began within the first six months, more than a year before the establishment of mature vegetation. In the Netherlands, beach surface characteristics, such as a lag surface, influenced aeolian transport and sediment flux (Van der Wal, 1998). Within a few months, we identified a deflated lag surface in the northern half of the site, formed by the wind-driven removal of fine sand and retention of coarser grained sand and pebbles, which likely contributed to the observed spatial variation in sand accretion.
We saw substantial sediment accumulation at the restoration site, especially in association with the perimeter fence and in the incipient foredune ridge, even with low average vegetation percent cover and low aeolian processes. This is likely a result of the sufficient sand supply and aeolian processes, combined with adequate fetch distance of the study site. Jackson and Cooper (1999) found sand supply is more important than fetch distance. However, in sediment-limited systems, fetch distance has been identified as an important geomorphic element (Van der Wal, 1998). Nordstrom et al. (2011) found that aeolian sand transport functions across short distances on the New Jersey coast, if the surface is relatively dry. For comparison, at a coastal dune location with higher and more sustained wind and greater sand supply in central California (Oceano Dunes), both Walker et al. (2022) and Bowen and Inman (1966) reported sand accumulation rates of approximately 5 m3 per m shoreline per year. The lower rate for foredune accretion (0.73 m3 per m shoreline per year) we observed at the restoration site was expected, because the driving aeolian processes are much weaker in Santa Monica Bay and southern California than in central California. The good agreement of the orientation and shape of dunes and dune shadows that formed at the restoration site indicated the importance of aeolian sand transport as a key mechanism of geomorphic processes.
Our study results demonstrate that limited restoration actions (native plant seeding one time with no irrigation and no container stock) were sufficient for perennial, dune-forming vegetation to become established at the restoration site. Within two years, all the native dune plants were flowering, producing seeds, and recruiting or growing at a rate that resulted in continued expansion of native vegetation over time without additional restoration or maintenance activities. In particular, A. maritima and A. chamissonis, native species that are recognized as ecosystem engineers in early successional dunes (Barbour et al., 1976; Johnson, 1977) were prominent at the restoration site. Although the cover and composition of the vegetation community in the restoration site varied seasonally, it diverged greatly from the control site (no vegetation) and itself over time (pre-project vegetation was zero).
The appearance of plant zonation we observed, which formed in association and interaction with geomorphic elements, such as the foredune ridge, became more distinct as time progressed. The important (Stallins, 2005; Miller, 2015) and complex (Stallins and Parker, 2003) role of plants in dune sand accretion is well recognized, and characteristics of accretion or erosion are often species-dependent (Moreno-Casasola, 1986). Physical site-specific gradients, in characteristics such as salt spray and moisture, can influence plant zonation on coastal dunes (Stallins and Parker, 2003). Our plant zonation results agree with salinity tolerances for these species reported in Barbour and DeJong (1977) and with results from Fink and Zedler (1990), who found C. cheiranthifolia to be less tolerant of saltwater inundation and sand burial closer to the ocean, whereas A. maritima and A. chamissonis were tolerant of both saltwater and sand burial events. At our restoration site, the more salt-tolerant A. maritima and A. chamissonis were predominantly associated with the incipient foredune ridge at the seaward edge and along the sand fencing, and the more salt-sensitive C. cheiranthifolia was distributed across the lag surface at the back of the restoration site (Figure 2); salt-tolerant A. leucophylla was found in low densities throughout the site. Our results suggest interactions between the salt-tolerant dune plants accreting sand, growing above the new surface, and then repeating this sequence, enhanced sand accumulation and contributed to the development of key geomorphic features associated with higher elevation, especially the incipient foredune and the dunes along the sand fencing.
While sand accumulation and vegetation cover showed clear divergence between the restoration and control sites, other characteristics did not, including cover of wrack, terrestrial debris (such as leaves), and trash. The grooming practices for the study beach included a seasonal effort to maintain wrack at and below the high tide line across the whole beach (including both the restoration and control sites) during the California grunion (Leuresthes tenuis) spawning season (March-August annually). Thus, our finding of similar wrack cover between the restoration and control sites is likely due to the similarity of grooming practices below the high tide line (no grooming) between March and August. The only survey conducted outside of the grunion season (November 2022) recorded higher wrack cover in the restoration site. The oceanward edge of the restoration site remained unfenced and experienced periodic driving and occasional grooming. Similarly, in comparing the abundance of trash at the restoration site and control site, results indicated that grooming had little effect on the overall abundance of trash between the two sites. Trash was an important factor to evaluate because it was a potential concern from management agencies prior to implementation.
The abundance of selected taxa of wrack-associated intertidal invertebrates that are highly sensitive to beach grooming showed a lack of recovery, and no consistent differences in abundance or composition were found between the restoration and control sites over the six years of our study. The continued occasional disturbances in this zone (driving), low wrack availability as a food source, lack of nearby source populations, and the small and confined ‘island’ effect of the restoration site (McLaughlan and Brown, 2006; Schlacher et al., 2012) may underlie this finding. Invertebrate recovery after disturbance has been shown to be highly variable and dependent on many factors such as physical site characteristics and biological characteristics of individual species (Bejarano and Michel, 2016). In addition, although the restoration area is largely protected, it only covers approximately 150 m total length of shoreline edge, and it may not be large enough to support the recovery of these wrack-dependent invertebrate populations (Schooler et al., 2017, 2019). The restoration site is also isolated from source populations of these taxa by large stretches of intensely groomed beach.
Dynamic beach and dune restoration projects to conserve habitat and increase protection and resilience of urban shorelines and their adjacent coastal infrastructure can be identified along coastlines throughout the world. Dune restoration can also provide many additional ecosystem services, including socio-economic benefits to coastal communities (Pérez-Maqueo et al., 2013; Van der Biest et al., 2017). Recovery and restoration of dune systems are largely dependent on appropriate time and spatial scales (Walker et al., 2017; Toft et al., 2021), with some metrics, such as sand accumulation responding more rapidly than vegetation or invertebrate community metrics. Coastal dune restoration projects span the globe, including Brazil (Manes et al., 2023), Pacific Island nations (Kiddle et al., 2021), Australia (Morris et al., 2020), and New Zealand (Hesp and Hilton, 2013) in the southern hemisphere; and Ireland (Lawlor and Jackson, 2022), Netherlands (Konlechner et al., 2014), Canada (Walker et al., 2013), and the United States in the northern hemisphere. Examples of dune restoration are found on all coasts of the United States including the Gulf of Mexico (Gittman et al., 2014; Houser et al., 2015; Kelly and Jose, 2021); New York, New Jersey, Virginia, and the Chesapeake Bay (Nordstrom et al., 2000; Nordstrom et al., 2011; Psuty and Silveira, 2013); Pacific Northwest (Zarnetske et al., 2015), and several in northern California (Hilgendorf et al., 2022; Walker et al., 2022). However, examples from southern California are extremely limited.
While resilience through reduced potential for flooding can be inferred for the restoration site based on sand accumulation over time, the site did not experience a significant erosion event during our study period; therefore, recovery or self-repair after a major disturbance event remains to be tested. Examples of dune recovery after hurricane events on the east and Gulf coasts suggest varying short and long-term recovery trajectories, depending on site-specific conditions and metrics evaluated (e.g., Miller et al., 2010; Houser et al., 2015). However, no impacts or significant erosion were evident at the restoration site after a strong storm event combined with swell and high tides occurred in January 2023. This was the only major disturbance event occurring within the study period, and while it caused substantial erosion at several beaches in the Los Angeles region, it did not appear to strongly affect the restoration site. In the restoration site, wave runup stopped at the foredune, whereas at the control site, wave runup extended approximately 20-30 m further up the beach. Future data collection from the study site, including responses to disturbance events and other stressors, will assess the long-term performance of this restoration project and inform its potential for implementation at a larger scale.
5 Conclusions
The need for coastal communities to plan for adaptation and enhance resilience in the face of sea level rise is urgent, as many communities face escalating challenges with respect to coastal flooding and hazards (Hanak and Moreno, 2011; Brown et al., 2013; Finkl, 2013; Griggs and Reguero, 2021). Decreased overall precipitation and increases in extreme precipitation and storm events that are likely to cause erosion are two additional likely outcomes of climate change in California (Polade et al., 2017). Recent studies suggest that diverse solutions, including nature-based strategies, are vital to improve coastal resilience (Kabisch et al., 2017; Morris et al., 2020; Xuan et al., 2022; Manes et al., 2023). Socio-economic benefits such as recreation, cultural values, and aesthetics are also recognized as ecosystem services provided by dunes (Pérez-Maqueo et al., 2013).
Our study findings suggest that passive restoration of dynamic beach and dune ecosystems could be used to increase protection and resilience of urban shorelines and their adjacent coastal infrastructure. This restoration project was the first of its kind for the region; it was implemented in a cost-effective manner compared to traditional hardscape alternatives (Aerts et al., 2018; Schroder et al., 2022, Gonyo et al., 2023). The applicability of dune restoration in urban areas requires careful consideration of opportunities and constraints, such as balancing with existing recreational uses, including site specific aspects of feasibility and scalability (Van der Biest et al., 2017). Studies, such as those by Nordstrom et al. (2000) and Nordstrom and Jackson (2021) provide, a pathway for removing stressors and scaling dune restoration projects. Our assessment of results of this restoration project over six years suggests that replication of a dune nature-based adaptation strategy could be a successful approach to improve coastal resilience in appropriate geomorphic conditions, e.g., wide beaches with sufficient aeolian sand transport and fetch distance (Nordstrom et al., 2011; Manes et al., 2023).
Given that many beaches and much of California’s sandy shoreline are eroding (Vitousek et al., 2017; Myers et al., 2019), evaluating expectations for nature-based coastal resilience projects, such as dune restoration, is increasingly important. The results of our study of a small dune restoration project on an urban beach demonstrates the potential use of dune restoration with limited intervention, cost, and maintenance as an adaptation approach that not only restores coastal habitat and function but could improve resilience to sea level rise and coastal erosion compared to traditional hardscape measures. Overall, our study highlights how restoration of dunes on urban shorelines can serve as a nature-based solution that may enhance resilience to sea level rise and could be used as a basis for a scalable model for the southern California coastline and beyond.
Data availability statement
The raw data supporting the conclusions of this article will be made available by the authors, without undue reservation.
Author contributions
KKJ, DMH, JED, and MWG made substantial contributions to concept, design, and implementation of the pilot project and research design. Acquisition of data and analyses were conducted by all authors. KAE led the UAS survey and analyses. KKJ was the lead author, and all authors provided substantial edits, text, and important intellectual content. All authors approved publication of the final version of this paper.
Funding
U.S. Environmental Protection Agency and the Metabolic Studio (Annenberg Foundation; Grant 15-541) funded the initial implementation of the pilot restoration project. Research funding came from the University of Southern California Sea Grant Program (NOAA: NA22OAR4170104). KAE was funded by the National Science Foundation (OCE #2126607). The National Science Foundation also provided support to JED, DMH, and KAE including OCE #1458845 and the Santa Barbara Coastal Long Term Ecological Research project (OCE #1232779 and OCE#1831937).
Acknowledgments
We would like to thank our partners at City of Santa Monica, California Department of Parks and Recreation, and The Bay Foundation (TBF) for their long-term support for this project and research. We also thank the students at Loyola Marymount University Coastal Research Institute, University of California Santa Barbara, and TBF staff who contributed many hours to implementation, monitoring, and research. We also thank Dr. Ian Walker who provided feedback on the geomorphic evaluations in the draft manuscript and the journal reviewers who provided helpful feedback in the revision process. This project is located on unceded Gabrielino-Tongva Tribal land, and we pay our respects and honor to the Tribal members past and present and thank them for their support. We would also like to thank the many supporters of this project and members of the public, without whom it would not have happened.
Conflict of interest
The authors declare that the research was conducted in the absence of any commercial or financial relationships that could be construed as a potential conflict of interest.
Publisher’s note
All claims expressed in this article are solely those of the authors and do not necessarily represent those of their affiliated organizations, or those of the publisher, the editors and the reviewers. Any product that may be evaluated in this article, or claim that may be made by its manufacturer, is not guaranteed or endorsed by the publisher.
Supplementary material
The Supplementary Material for this article can be found online at: https://www.frontiersin.org/articles/10.3389/fmars.2023.1187488/full#supplementary-material
References
Aerts J. C. J. H., Barnard P. L., Botzen W., Grifman P., Finzi Hart J., De Moel H., et al. (2018). Pathways to resilience: adapting to sea level rise in Los Angeles. Ann. N.Y. Acad. Sci. 1427, 1–90. doi: 10.1111/nyas.13917
American Shore and Beach Preservation Association (ASBPA) (2022). Available at: https://asbpa.org/national-beach-nourishment-database/ (Accessed 10 January 2023).
Anders F. J., Leatherman S. P. (1987). Disturbance of beach sediment by off-road vehicles. Environ. Ged Water Sci. 9 (3), 183–189. doi: 10.1007/BF02449950
Arkema K. K., Guannel G., Verutes G., Wood S. A., Guerry A., Ruckelshaus M., et al. (2013). Coastal habitats shield people and property from sea-level rise and storms. Nat. Climate Change 3, 913–918. doi: 10.1038/NCLIMATE1944
Barbour M. G., DeJong T. M. (1977). Response of west coast beach taxa to salt spray, seawater inundation, and soil salinity. Bull. Torrey Botanical Club 104 (1), 29–34. doi: 10.2307/2484662
Barbour M. G., DeJong T. M., Johnson A. F. (1976). Synecology of beach vegetation along the pacific coast of the united states of America: a first approximation. J Biogeogr. 3 (1), 55–69. doi: 10.2307/3038099
Barnard P. L., Dugan J. E., Page H. M., Wood N. J., Finzi Hart J. A., Cayan D. R., et al. (2021). Multiple climate change-driven tipping points for coastal systems. Sci. Rep. 11, 15560. doi: 10.1038/s41598-021-94942-7
Bejarano A. C., Michel J. (2016). Oil spills and their impacts on sand beach invertebrate communities: a literature review. Environ. pollut. 218, 709–722. doi: 10.1016/j.envpol.2016.07.065
Bowen A. J., Inman D. L. (1966). Budget of littoral sands in the vicinity of point arguello, California (No. 19) (Scripps Institution of Oceanography, University of California San Diego: US Department of the Army, Corps of Engineers), 41.
Bridges T., Wagner P. W., Burks-Copes K. A., Bates M. E., Collier Z. A., Fischenich C. J., et al. (2015). Use of natural and nature-based features for coastal resilience (US Army Engineer Research and Development Center), 480. Final Report.
Brown A. C., McLachlan A. (2002). Sandy shore ecosystems and the threats facing them: some predictions for the year 2025. Environ. Conserv. 29 (1), 62–77. doi: 10.1017/S037689290200005X
Brown S., Nicholls R. J., Woodroffe C. D., Hanson S., Hinkel J., Kebede A. S., et al. (2013). Chapter 5: Sea-level rise impacts and responses: a global perspective in Coastal Hazards. Ed. Finkl C. W. Coastal Research Library, Vol 1000. Springer, Dordrecht. doi: 10.1007/978-94-007-5234-4_5
Bryant D. B., Anderson Bryant M., Sharp J. A., Bell G. L., Moore C. (2019). The response of vegetated dunes to wave attack. Coast. Eng. 152, 103506. doi: 10.1016/j.coastaleng.2019.103506
Coastal Data Information Program Santa Monica Buoy wave data. Available at: https://cdip.ucsd.edu/ (Accessed 12 November 2022).
da Silva G. M., Hesp P. (2010). Coastline orientation, aeolian sediment transport and foredune and dunefield dynamics of moçambique beach, southern Brazil. Geomorphology 120 (3-4), 258–278. doi: 10.1016/j.geomorph.2010.03.039
Davidson-Arnott R., Bauer B., Houser C. (2019). Introduction to coastal processes and geomorphology. 2nd ed (Cambridge: Cambridge University Press). doi: 10.1017/9781108546126
Defeo O., McLachlan A., Schoeman D., Schlacher T., Dugan J., Jones A., et al. (2009). Threats to sandy beach ecosystems: a review. Estuar. Coast. Shelf Sci. 81, 1–12. doi: 10.1016/j.ecss.2008.09.022
Dugan J. E., Emery K. A., Alber M., Alexander C. R., Byers J. E., Gehman A. M., et al. (2018). Generalizing ecological effects of shoreline armoring across soft sediment environments. Estuaries coasts 41, 180–196. doi: 10.1007/s12237-017-0254-x
Dugan J. E., Hubbard D. M. (2010). Loss of coastal strand habitat in southern California: the role of beach grooming. Estuaries Coasts 33 (1), 67–77. doi: 10.1007/s12237-009-9239-8
Dugan J., Hubbard D. (2016). “Sandy beaches,” in Ecosystems of California. Eds. Mooney H., Zavaleta E., Chapin M. (Oakland, California: University of California Press), 389–408. doi: 10.1525/j.ctv1xxzp6.25
Dugan J. E., Hubbard D. M., McCrary M., Pierson M. (2003). The response of macrofauna communities and shorebirds to macrophyte wrack subsidies on exposed sandy beaches of southern California. Estuarine Coast. Shelf Sci. 58S, 25–40. doi: 10.1016/S0272-7714(03)00045-3
Dugan J. E., Hubbard D. M., Rodil I. F., Revell D. L., Schroeter S. (2008). Ecological effects of coastal armoring on sandy beaches. Mar. Ecol. 29 (1), 160–170. doi: 10.1111/j.1439-0485.2008.00231.x
Fink B. H., Zedler J. B. (1990). Maritime stress tolerance studies of California dune perennials. Madroño 37 (3), 200–213.
Finkl C. W. (2013). Coastal hazards Coastal Research Library (Florida Atlantic University, FL, USA.: Springer). doi: 10.1007/978-94-007-5234-4
Fryberger S. G., Dean G. (1979). “Dune forms and wind regime,” in ed. McKee E. D. A study of global sand seas, 137–169.
Gittman R. K., Popowich A. M., Bruno J. F., Peterson C. H. (2014). Marshes with and without sills protect estuarine shorelines from erosion better than bulkheads during a category 1 hurricane. Ocean Coast. Manage. 102, 94–102. doi: 10.1016/j.ocecoaman.2014.09.016
Gonyo S. B., Zito B., Burkart H. (2023). The cost of shoreline protection: A comparison of approaches in coastal New England and the Mid-Atlantic. Coast. Manage. 51 (2), 1–14. doi: 10.1080/08920753.2023.2186091
Grifman P. M., Grubbs M., Johnston K. K. (2020). Planning to adaptation: informing regional nature-based adaptation to improve coastal resiliency in Santa Monica bay. Shore Beach 88 (3), 39–52. doi: 10.34237/1008834
Griggs G. B., Cayan D., Tebaldi C., Fricker H. A., Arvai J., DeConto R., et al. (2017). Rising seas in California - an update on sea-level rise science. World Scientific Encyclopedia of Climate Change: Case Studies of Climate Risk, Action, and Opportunity 3, 105–111. doi: 10.1142/9789811213960_0016
Griggs G. B., Patsch K. (2019). The protection/hardening of california’s coast: times are changing. J. Coast. Res. 35 (5), 1051–1061. doi: 10.2112/JCOASTRES-D-19A-00007.1
Griggs G. B., Reguero B. G. (2021). Coastal adaptation to climate change and sea-level rise. Water 13 (16), 2051. doi: 10.3390/w13162151
Griggs G. B., Patsch K. (2018). Natural changes and human impacts on the sand budgets of the Zuma and Santa Monica littoral cells, Southern California. Shore & Beach 86 (1), 3–16.
Hanak E., Moreno G. (2011). California Coastal management with a changing climate. Climate Change 111, 45–73. doi: 10.1007/s10584-011-0295-2
Hesp P. A., Hilton M. J. (2013). “Restoration of foredunes and transgressive dunefields: case studies from new Zealand,” in Restoration of coastal dunes. Eds. Martinez M., Gallego-Fernández J., Hesp P. Springer Series on Environmental Management. Springer, Berlin, Heidelberg. doi: 10.1007/978-3-642-33445-0_5
Hilgendorf Z., Marvin M. C., Turner C. M., Walker I. J. (2022). Assessing geomorphic change in restored coastal dune ecosystems using a multi-platform aerial approach. Remote Sens. 13 (354), 1–34. doi: 10.3390/rs13030354
Houser C., Labude B., Haider L., Weymer B. (2013). Impacts of driving on the beach: case studies from assateague island and padre island national seashores. Ocean Coast. Manage. 71, 33–45. doi: 10.1016/j.ocecoaman.2012.09.012
Houser C., Wernette P., Rentschlar E., Jones H., Hammond B., Trimble S. (2015). Post-storm beach and dune recovery: implications for barrier island resilience. Geomorphology 234, 54–63. doi: 10.1016/j.geomorph.2014.12.044
Hubbard D. M., Dugan J. E., Schooler N. K., Viola S. M. (2014). Local extirpations and regional declines of endemic upper beach invertebrates in southern California. Estuarine Coast. Shelf Sci. 150 (A), 67–75. doi: 10.1016/j.ecss.2013.06.017
Itzkin M., Moore L. J., Ruggiero P., Hacker S. D. (2019). The effect of sand fencing on the morphology of natural dune systems. Geomorphology 352, 106995. doi: 10.1016/j.geomorph.2019.106995
Jackson D., Cooper A. (1999). Beach fetch distance and aeolian sediment transport. Sedimentology 46 (3), 517–522. doi: 10.1046/j.1365-3091.1999.00228.x
Jaramillo E., Dugan J., Hubbard D., Manzano M., Duarte C. (2021). Ranking the ecological effects of coastal armoring on mobile macroinvertebrates across intertidal zones on sandy beaches. Sci Total Environ. 755 (2), 142573. doi: 10.1016/j.scitotenv.2020.142573
Johnson A. (1977). A survey of the strand and dune vegetation along the pacific and southern gulf coasts of Baja California, Mexico. J. Biogeogr. 4 (1), 83–99. doi: 10.2307/3038131
Johnston K. K., Enyart C., Jenkins M., Lazarus D., Cuadra S. (2021). Santa Monica Beach restoration pilot project: year 5 annual report (Los Angeles, CA, USA: Technical Report prepared by The Bay Foundation for City of Santa Monica, California Coastal Commission, US Environmental Protection Agency, and California Department of Parks and Recreation), 118.
Kabisch N., Korn H., Stadler J., Bonn A. (2017). Nature-based solutions to climate change adaptation in urban areas (Cham, Switzerland: Springer International Publishing). doi: 10.1007/978-3-319-56091-5
Kelly E., Jose F. (2021). Geomorphologic recovery of north captiva island from the landfall of hurricane Charley in 2004. Geosciences 11, 358. doi: 10.3390/geosciences11090358
Kiddle G. L., Bakineti T., Latai-Niusulu A., Missack W., Pedersen Zari M. P., Kiddle R., et al. (2021). Nature-based solutions for urban climate change adaptation and wellbeing: evidence and opportunities from Kiribati, Samoa, and Vanuatu. Front. Environ. Sci. 2021 (9). doi: 10.3389/fenvs.2021.723166
King P. G., Nelsen C., Dugan J. E., Hubbard D. M., Martin K. L. (2018). Valuing beach ecosystems in an age of retreat. Shore Beach 86 (4), 45–59.
King P., Symes D. (2004). Potential loss in GNP and GSP from a failure to maintain california's beaches. Shore Beach 72 (1), 3–8.
Konlechner T. M., Hilton M. J., Arens S. M. (2014). Transgressive dune development following deliberate de-vegetation for dune restoration in the Netherlands and new Zealand. Dynamiques Environnementales 33, 141–151.
Lawlor P., Jackson D. W. T. (2022). A nature-based solution for coastal foredune restoration: the case study of maghery, county Donegal, Ireland. in: Eds. Misiune I., Depellegrin D., Vigl L. E. Human-nature interactions: exploring nature’s values across landscapes (Cham, Switzerland: Springer), 417–429.
Lebbe T. B., Rey-Valette H., Chaumillon É., Camus G., Almar R., Cazenave A., et al. (2021). Designing coastal adaptation strategies to tackle sea level rise. Front. Mar. Sci. 2021 (8). doi: 10.3389/fmars.2021.740602
Liu J., Wu J., Kimura R. (2023). Evaluating the sand-trapping efficiency of sand fences using a combination of wind-blown sand measurements and UAV photogrammetry at tottori sand dunes, Japan. Remote Sens. 15 (4), 1098. doi: 10.3390/rs15041098
Los Angeles Almanac Precipitation data. Available at: https://www.laalmanac.com/index (Accessed 10 January 2023).
Los Angeles County Department of Beaches and Harbors Seasonal Sand Berm Protection Program (2016). Technical report prepared by noble consultants for LA county department of beaches and harbors. 41.
Manes S., Gama-Maia D., Vaz S., Pires A. P. F., Tardin R. H., Maricato G., et al. (2023). Nature as a solution for shoreline protection against coastal risks associated with ongoing sea-level rise. Ocean Coast. Manage. 235, 106487. doi: 10.1016/j.ocecoaman.2023.106487
Martin K. L. M., Reed D. R., Simmons D. J., Steers J. E., Studer M. (2022). Chapter 10: the beach ecology coalition: enhancing ecosystem conservation and beach management to balance natural resource protection and recreational use. in Sandy beaches as endangered ecosystems (Boca Raton, FL, USA: CRC Press).
McLachlan A., Brown A. C. (2006). The ecology of sandy shores. 2nd ed. (Burlington, MA, USA: Elsevier Academic Press).
Mendoza-González G., Martínez M. L., Rojas-Soto O. R., Vázquez G., Gallego-Fernández J. B. (2013). Ecological niche modeling of coastal dune plants and future potential distribution in response to climate change and sea level rise. Global Change Biol. 19 (8), 2524–2535. doi: 10.1111/gcb.12236
Miller T. E. (2015). Effects of disturbance on vegetation by sand accretion and erosion across coastal dune habitats on a barrier island. AoB Plants 7, plv003. doi: 10.1093/aobpla/plv003
Miller T. E., Gornish E. S., Buckley H. L. (2010). Climate and coastal dune vegetation: disturbance, recovery, and succession. Plant Ecol. 206, 97–104. doi: 10.1007/s11258-009-9626-z
Moreno-Casasola P. (1986). Sand movement as a factor in the distribution of plant communities in a coastal dune system. Vegetation 65, 67–76. doi: 10.1007/BF00044876
Morris R. L., Boxshall A., Swearer S. E. (2020). Climate-resilient coasts require diverse defence solutions. Nat. Climate Change 10, 485–487. doi: 10.1038/s41558-020-0798-9
Myers M. R., Barnard P. L., Beighley E., Cayan D. R., Dugan J. E., Feng D., et al. (2019). A multidisciplinary coastal vulnerability assessment for local government focused on ecosystems. Ocean Coast. Mgmt. 182, 104921. doi: 10.1016/j.ocecoaman.2019.104921
Newkirk S., Veloz S., Hayden M., Heady W., Leo K., Judge J., et al. (2018). Toward natural infrastructure to manage shoreline change in California (California, USA: California’s Fourth Climate Change Assessment, California Natural Resources Agency).
NOAA Climate Data Online. Available at: https://www.ncei.noaa.gov/cdo-web/ (Accessed 10 January 2023).
Nordstrom K. F., Jackson N. L. (2021). “Chapter 4 – restoring processes, structure, and functions,” in Beach and dune restoration (Cambridge University Press), 88–122. doi: 10.1017/9781108866453.005
Nordstrom K. F., Jackson N. L., Freestone A. L., Korotky K. H., Puleo J. (2012). Effects of beach raking and sand fences on dune dimensions and morphology. Geomorphology 179, 106–115. doi: 10.1016/j.geomorph.2012.07.032
Nordstrom K. F., Jackson N. L., Korotky K. H., Puleo J. (2011). Aeolian transport rates across raked and unraked beaches on a developed coast. Earth Surfaces Processes Landforms. 36, 779–789. doi: 10.1002/esp.2105
Nordstrom K. F., Lampe R., Vandemark L. M. (2000). Reestablishing naturally functioning dunes on developed coasts. Environ. Manage. 25 (1), 37–51. doi: 10.1007/s002679910004
Orams M. B. (2003). Sandy beaches as a tourism attraction: a management challenge for the 21st century. J. Coast. Res. 35, 74–84. http://www.jstor.org/stable/40928751.
Patsch K., Griggs G. B. (2006). Littoral cells, sand budgets, and beaches: understanding california’s shoreline (California, USA: University of California Santa Cruz), 40.
Pérez-Maqueo O., Martínez M. L., Lithgow D., Mendoza-González G., Feagin R. A., Gallego- Fernández J. B. (2013). The coasts and their costs. in: Martínez M. L., Gallego- Fernández J. B., Hesp P. A. Eds. Restoration of Coastal Dunes. Springer-Verlag, 289–304. doi: 10.1007/978-3-642-33445-0_18
Pinardo-Barco S., Sanromualdo-Collado A., García-Romero L. (2023). Can the long-term effects of beach cleaning heavy duty machinery on aeolian sedimentary dynamics be detected by monitoring of vehicle tracks? an applied and methodological approach. J. Environ. Manage. 325, 116645. doi: 10.1016/j.jenvman.2022.116645
Polade S. D., Gershunov A., Cayan D. R., Dettinger M. D., Pierce D. W. (2017). Precipitation in a warming world: assessing projected hydro-climate changes in California and other Mediterranean climate regions. Sci. Rep. 7, 10783. doi: 10.1038/s41598-017-11285-y
Psuty N. P., Silveira T. M. (2013). “Restoration of coastal foredunes, a geomorphological perspective: examples from new York and from new Jersey, USA,” in Restoration of coastal dunes. Eds. Martinez M. L., Gallego-Fernández J. B., Hesp P. A. Springer-Verlag, 33–47. doi: 10.1007/978-3-642-33445-0_18
Runyan K., Griggs G. B. (2003). The effects of armoring seacliffs on the natural sand supply to the beaches of California. J. Coast. Res. 19 (2), 336–347. Available at: https://www.jstor.org/stable/4299175.
Schlacher T. A., Dugan J. E., Schoeman D. S., Lastra M., Jones A., Scapini F., et al. (2007). Sandy beaches on the brink. Diversity Distributions 13, 556–560. doi: 10.1111/j.1472-4642.2007.00363.x
Schlacher T. A., Noriega R., Jones A., Dye T. (2012). The effects of beach nourishment on benthic invertebrates in eastern Australia: impacts and variable recovery. Sci. Total Environ. 435, 411–417. doi: 10.1016/j.scitotenv.2012.06.071
Schlacher T., Schoeman D. S., Jones A. R., Dugan J. E., Hubbard D. M., Defeo O., et al. (2014). Metrics to assess ecological condition, change, and impacts in sandy beach ecosystems. J. Environ. Manage. 144 (2014), 322–335. doi: 10.1016/j.jenvman.2014.05.036
Schlacher T. A., Thompson L. M. C., Walker S. J. (2008). Mortalities caused by off-road vehicles (ORVs) to a key member of sandy beach assemblages, the surf clam donax deltoides. Hydrobiologia 610, 345–350. doi: 10.1007/s10750-008-9426-9
Schooler N. K., Dugan J. E., Hubbard D. M. (2019). No lines in the sand: impacts of coastal urbanization on sandy beach ecosystems span the intertidal zone. Ecol. Indic. 106, 105457. doi: 10.1016/j.ecolind.2019.105457
Schooler N. K., Dugan J. E., Hubbard D. M., Straughan D. (2017). Local scale processes drive long-term change in biodiversity of sandy beach ecosystems. Ecol. Evol. 7 (13), 4822–4834. doi: 10.1002/ece3.3064
Schroder K., Hummel M. A., Befus K. M., Barnard P. L. (2022). An integrated approach for physical, economic, and demographic evaluation of coastal flood hazard adaptation in Santa Monica bay, California. Front. Mar. Sci. 9. doi: 10.3389/fmars.2022.1052373
Seabloom E. W., Ruggiero P., Hacker S. D., Mull J., Zarnetske P. (2012). Invasive grasses, climate change, and exposure to storm-wave overtopping in coastal dune ecosystems. Global Change Biol. 19 (3), 824–832. doi: 10.1111/gcb.12078
Stallins J. A. (2005). Stability domains in barrier island dune systems. Ecol. Complexity 2 (2015), 410–430. doi: 10.1016/j.ecocom.2005.04.011
Stallins J. A., Parker A. J. (2003). The influence of complex systems interactions on barrier island dune vegetation pattern and process. Ann. Assoc. Am. Geographers 93 (1), 13–29. doi: 10.1111/1467-8306.93102
Suter-Burri C., Leonard K. C., Graf F. (2013). Spatial patterns of aeolian sediment deposition in vegetation canopies: observations from wind tunnel experiments using colored sand. Aeolian Res. 8 (2013), 65–73. doi: 10.1016/j.aeolia.2012.11.002
Toft J. D., Dethier M. N., Howe E. R., Buckner E. V., Cordell J. R. (2021). Effectiveness of living shorelines in the salish Sea. Ecol. Eng. 167, 106255. doi: 10.1016/j.ecoleng.2021.106255
United States Fish and Wildlife Service (USFWS) (2007). Recovery plan for the pacific coast population of the Western snowy plover (Charadrius alexandrinus nivosus) Vol. 2 (Sacramento, California: US Fish and Wildlife Service), 751.
Van der Biest K., De Nocker L., Provoost S., Boerema A., Staes J., Meire P. (2017). Dune dynamics safeguard ecosystem services. Ocean Coast. Manage. 149, 148–158. doi: 10.1016/j.ocecoaman.2017.10.005
Van der Wal D. (1998). Effects of fetch and surface texture on aeolian sand transport on two nourished beaches. J. Arid. Environments 39, 533–547. doi: 10.1006/jare.1997.0364
Vitousek S., Barnard P. L., Limber P. (2017). Can beaches survive climate change? J. Geophys. Res. Earth Surf. 122, 1060–1067. doi: 10.1002/2017JF004308
Walker I. J. (2020). “Aeolian (windblown) sand transport over beaches,” in Sandy beach morphodynamics Elsevier 2020, 213–253. doi: 10.1016/B978-0-08-102927-5.00010-2
Walker I. J., Davidson-Arnott R. G. D., Bauer B. O., Hesp P. A., Delgado-Fernandez I., Ollerhead J., et al. (2017). Scale-dependent perspectives on the geomorphology and evolution of beach-dune systems. Earth-Sci. Rev. 171, 220–253. doi: 10.1016/j.earscirev.2017.04.011
Walker I. J., Eamer J. B. R., Darke I. B. (2013). Assessing significant geomorphic changes and effectiveness of dynamic restoration in a coastal dune ecosystem. Geomorphology 199 (2013), 192–204. doi: 10.1016/j.geomorph.2013.04.023
Walker I. J., Hilgendorf Z., Gillies J. A., Turner C. M., Furtak-Cole E., Nikolich G. (2022). Assessing performance of a “nature-based” foredune restoration project, oceano dunes, California, USA. Earth Surf. Process. Landforms 2022, 1–20. doi: 10.1002/esp.5478
Xuan T. L., Ba H. T., Thanh V. Q., Wright D. P., Tanim A. H., Anh D. T. (2022). Evaluation of coastal protection strategies and proposing multiple lines of defense under climate change in the Mekong delta for sustainable shoreline protection. Ocean Coast. Manage. 228, 106301. doi: 10.1016/j.ocecoaman.2022.106301
Keywords: beach grooming, foredune, remote sensing, vegetation zonation, sea level rise resilience, coastal adaptation, sediment dynamics
Citation: Johnston KK, Dugan JE, Hubbard DM, Emery KA and Grubbs MW (2023) Using dune restoration on an urban beach as a coastal resilience approach. Front. Mar. Sci. 10:1187488. doi: 10.3389/fmars.2023.1187488
Received: 16 March 2023; Accepted: 19 May 2023;
Published: 19 June 2023.
Edited by:
Carlos Alberto De Moura Barboza, Federal University of Rio de Janeiro, BrazilReviewed by:
Eduardo Bulhoes, Fluminense Federal University, BrazilFlavia Lins De Barros, Federal University of Rio de Janeiro, Brazil
Mariano Lastra, University of Vigo, Spain
Copyright © 2023 Johnston, Dugan, Hubbard, Emery and Grubbs. This is an open-access article distributed under the terms of the Creative Commons Attribution License (CC BY). The use, distribution or reproduction in other forums is permitted, provided the original author(s) and the copyright owner(s) are credited and that the original publication in this journal is cited, in accordance with accepted academic practice. No use, distribution or reproduction is permitted which does not comply with these terms.
*Correspondence: Karina K. Johnston, S2FyaW5hSm9obnN0b25AdWNzYi5lZHU=