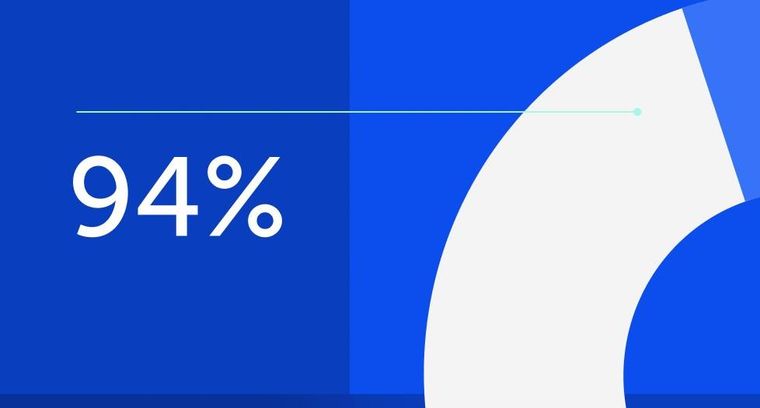
94% of researchers rate our articles as excellent or good
Learn more about the work of our research integrity team to safeguard the quality of each article we publish.
Find out more
ORIGINAL RESEARCH article
Front. Mar. Sci., 20 October 2023
Sec. Marine Biology
Volume 10 - 2023 | https://doi.org/10.3389/fmars.2023.1187249
In situ testing of candidate odorant cues directly in coral-reef communities has the advantage that, in principle, it permits the behavioral responses of all fish species to be evaluated in the species’ natural environments. The success of in situ testing depends critically, however, on a suitable method of odorant release. We provide here a proof-of-concept for a new method of release that (instead of using distilled water as an ejection medium) ejects odorant-containing local reef water remotely and silently over an extended time period using gravity as the motive force. The ejected water, being neutrally buoyant, remains in the reef community, dispersing in local currents. From our observations of fish behavior during ejection of candidate odorants in Curaçao reefs, we confirm that brown chromis (Chromis multilineata) are likely attracted to dimethylsulfoniopropionate (DMSP), and we obtain suggestive evidence that they are attracted to a mix of three betaines: glycine betaine, proline betaine, and trigonelline. Creole wrasse (Clepticus parrae) and yellowhead wrasse (Halichoeres garnoti) are likely repelled by DMSP. Many observed fish species gave no evidence of being affected by either DMSP or betaines.
Water-borne odorants can attract or repel coral reef animals, and sometimes play essential roles in their life cycles (Ben-Tzvi et al., 2010; Dixson and Hay, 2012; Paris et al., 2013; DeBose and Paul, 2014; Foretich et al., 2017). Thus knowledge of odorant effects may be important for understanding reef ecology, and odorant effects might potentially be harnessed in reef restoration programs.
Two chemical classes of potential odorants – often studied together because of their similarities in chemical structure (Rhodes and Hanson, 1993) – are the tertiary sulfonium compounds (e.g., dimethylsulfoniopropionate, DMSP) and quaternary ammonium compounds (e.g., glycine betaine). As part of our recent research program to enhance knowledge of these compounds in coral-reef animals (Hill et al., 1995; Hill et al., 2004; Hill et al., 2010; Frade et al., 2016; Hill et al., 2017; Hill, 2022), we wanted to survey the potential roles of these compounds as water-borne odorants affecting the behavior of reef fish swimming among coral structures in the benthic coral-reef community. Inspired by the study of DeBose et al. (2008), we therefore designed the present study in which we have released the compounds into a coral-reef community and monitored responses of all observable fish species. However, for our release mechanism, we wanted to avoid the clever but potentially problematic distilled-water method that DeBose et al. (2008) employed for odorant release because, as we explain later, when odorant is released in distilled water in a seawater system, fish sensory processes may respond in a confounded way to the odorant itself and to the low salinity of the release water. Thus we have devised an entirely novel method for odorant release in which the release medium, instead of being distilled water, is local seawater.
The behavioral effects of an odorant on coral reef animals can be tested in two principal ways: (1) laboratory testing and (2) field testing carried out in situ in natural coral reef communities. In the laboratory, captive animals in highly defined artificial environments can subjected to highly defined odorant response tests (DeBose et al., 2010; Foretich et al., 2017). Alternatively, during in situ testing, candidate odorant cues are released into natural communities where the responses of free-living animals are observed (DeBose et al., 2008). In situ testing has weaknesses compared to laboratory testing; for example, neither odorant concentration nor potential inter-animal interactions can be as accurately controlled. In situ testing, by contrast, has three notable advantages: (1) animals are studied under ecologically realistic conditions; (2) because all reef species are exposed to released odorants, the method is suited to discovery of responses in all species; and (3) information can be gathered on species or age classes that are intolerant of captivity. Already in 1995, in studies of anemonefish larval behavior, Elliott et al. (1995; see also Sale, 1990) compared laboratory and in situ testing and, despite the potential problems of the latter, argued that in situ testing is at least as valuable as laboratory testing.
Here we demonstrate a new, minimally disruptive procedure for in situ odorant release. The method employs freshly collected, local reef seawater as an ejection medium. Many liters of this water are collected in a Teflon® bag, to which odorant is added. Then, during a study period when behavioral effects on reef residents are observed, the odorant-containing seawater is ejected into the reef environment steadily, remotely and silently by use of gravity exerting a compressive force on the bag of odorant-containing seawater.
Applying this novel method in a Caribbean coral reef community, we carry out an exploratory in situ study of the potential responses of fish species to two candidate odorant cues: dimethylsulfoniopropionate (DMSP) and betaines (methylated amino acids). We demonstrate that odorant-containing seawater ejected by our method is neutrally buoyant and thus disperses within the benthic reef environment in the same way as natural seawater. We also confirm certain earlier findings on fish responses to odorants and identify responses previously unknown. DMSP and betaines were selected for study because they are abundant metabolites in the tissues of many scleractinian coral species. DMSP was first measured in coral tissues in a study of three Pacific species (Hill et al., 1995). Since then, it has been found in many Caribbean (Hill et al., 2010; Yost et al., 2012; Frade et al., 2016) and Indo-Pacific corals (Yancey et al., 2010; Jones and King, 2015; Swan et al., 2017), as well as in coral-associated bacteria (Kuek et al., 2022). Regarding betaines, in this paper, we use contemporary chemical terminology (Hill, 2022), in which, for example, “glycine betaine” or “trimethylglycine” is the correct name for the compound long called simply “betaine.” Betaines have recently been demonstrated to be abundant metabolites in the tissues of both Caribbean (Hill et al., 2010) and Indo-Pacific (Yancey et al., 2010; Hill et al., 2017; Hill, 2022) corals. Both DMSP and betaines are abundant also in tridacnid (giant) clams, which are important and often numerous members of Indo-Pacific coral reef communities (Hill et al., 2004; Hill et al., 2017). Inasmuch as DMSP and betaines are small molecules of low molecular weight that are highly soluble in seawater, it is plausible to hypothesize that fish species use them as odorant cues. For example, during corallivory (Cole et al., 2008; Rotjan and Lewis, 2008), the compounds could affect the gustatory (i.e., taste related) attractiveness of the coral tissues for the corallivores. The compounds could also exert water-borne behavioral effects in coral reef communities (e.g., attraction of early life stages to specific habitats; Ben-Tzvi et al., 2010; Paris et al., 2013) by leaking from the tissues of healthy corals into the ambient reef water (Masdeu-Navarro et al., 2022) or by being released from coral tissues during events that disrupt tissue integrity such as heat stress (Raina et al., 2013; Garren et al., 2014) or corallivory (Cole et al., 2009). Although our past research has been on DMSP and betaines produced in the tissues of corals and tridacnids (see also Guibert et al., 2020) – and our comments thus far emphasize that consideration – it is also germane to note that the fish in a coral-reef community can respond behaviorally to DMSP or betaines of other origins, such as that released from phytoplankton or seaweeds (Keller et al., 1999; DeBose et al., 2008) or from other invertebrates such as cnidarians (Van Alstyne et al., 2006).
A growing literature points to DMSP as an important mediator of animal behavior (DeBose et al., 2008; DeBose et al., 2010; Nevitt, 2011; Ferrer and Zimmer, 2012). Although far less is known of the behavioral roles of betaines, both Moore and Huxley (1976) and Suenaga (2004) found evidence that glycine betaine affects the feeding behavior of the corallivorous seastar Acanthaster planci. Mackie and Mitchell (1982) reported that glycine betaine stimulates feeding in the (non-reef) flatfish Solea solea, and Danaceau and Lucero (1998) reported that it elicits aversive reactions in a variety of cephalopods. Additional suggestive evidence for betaine roles in behavior comes from general surveys of the taste preferences of fish (principally non-reef species have been studied), such as the survey by Kasumyan and Døving (2003) reporting that glycine betaine stimulates feeding in some species.
Tests were carried out at two sites on the fringing coral reefs of Curaçao (formerly Netherlands Antilles), with essential support from the research station of the CARMABI Foundation (Willemstad). We chose Curaçao because (1) earlier research by DeBose et al. (2008) on behavioral effects of DMSP on reef fish was completed there and (2) DMSP and multiple betaines have been demonstrated to be abundant metabolites in the tissues of Curaçao scleractinians (Hill et al., 2010).
Along a transect from land to sea, Curaçao fringing reefs typically slope gently but steadily downward to a reef drop-off at about 10-12 m depth, where the slope steepens dramatically. Both of our study sites were located just above the drop-off. Site 1 was near Carmabi Buoy 1 (12°7’29’’N, 68°58’20’’W; Frade et al., 2016), whereas Site 2 was about 0.5 km to the west near Carmabi Buoy 2 (12°7’35’’N, 68°58’34’’W). At each study site three observation arenas and two odorant release sites were established in a line paralleling the reef drop-off (Figure 1). Each arena consisted, at bottom, of a 4 m × 4 m area of reef, plus the water column above to a height of about 4 m. Bottom depth averaged 9.2 m at the landward edges of the arenas and 10.8 m at the seaward edges. The corners of the arenas were marked with small buoys floating 0.8 m above the reef bottom (landward) or pink surveyor tape tied to reef structures (seaward). Cover by hard and soft corals was about 25% at Site 1 and 50% at Site 2 – the principal scleractinians being Agaricia agaricites (Sites 1 & 2), Colpophyllia natans (1 & 2), Diploria strigosa (2), Madracis mirabilis (1 & 2), Montastraea cavernosa (2), Orbicella annularis (2), O. faveolata (1 & 2), and Siderastrea siderea (1 & 2). During periods of odorant ejection, observers maintained neutral buoyancy in the water column 1-3 m above the bottom and 2-3 m to the seaward side of the arenas (Figure 1).
Figure 1 Observation arenas and odorant release sites at a study site, as seen from above, reef drop-off at the bottom of the image. Diver silhouettes mark the locations where observers hovered, neutrally buoyant in the water column. Only one release site was used at a time, depending on the direction of the prevailing ambient water current: If the ambient current flowed from left to right, the release site at left was employed, whereas if the current flowed from right to left, the release site at right was used.
Two odorant release sites were established at each study site (Figure 1). Only one of these (determined by direction of current flow) was used at a time. Currents tended to flow parallel to the reef drop-off at all times. If the current flowed from Arena A to C, the left release site was used (Figure 1). Conversely, if the current flowed from Arena C to A, the right release site was used. Thus there was always one observation arena upstream of the release site and two observation arenas downstream.
The observers were authors of this paper. Two, termed Observer 1 (PRF) and Observer 2 (AMF) were provided no information on odorant identities until the day after all field tests had been completed. Using slates, they recorded numbers of individuals of all fish species observed. Observer 1 always observed the middle arena (Arena B, Figure 1), which was the downstream arena proximal to the release site. Observer 2 observed the downstream arena distal to the release site. The third observer (RWH), present principally to assist logistics, was positioned at the upstream site regardless of current direction to ensure a consistent visual environment, and carried out descriptive fish counts not used in statistical analyses.
A key attribute in the design of our odorant release apparatus is that seawater collected at the study site is employed as the release medium. In the present study, as this seawater was collected and dispersed, all materials in contact with it were composed of Teflon® or other fluoropolymers (named later) rated for use with human foods and beverages.
To prepare the apparatus, seawater was collected by SCUBA, shortly before an odorant release test, from 10 m depth next to the study site. The water was collected in Teflon MPTFE bags (Welch Fluorocarbon) and brought to a boat tethered (engine off) at an anchor buoy. While in the boat the seawater was shaded from exposure to sunlight at all times. In the boat, the freshly collected seawater was transferred to a 18-L-capacity Teflon MPTFE odorant release bag (custom manufactured from 0.064-mm-thick sheeting by Welch Fluorocarbon) nested in a modified Nalgene polypropylene carboy for structural support (Figure 2). Odorant was added immediately, and the water was aerated for 5 min, using a battery-operated air pump and air stone, to disperse the odorant evenly (the odorants used in our study are nonvolatile and thus not depleted by the airstream). The odorant release bag was then sealed and – supported in the carboy – taken by SCUBA to the sea bottom, where it was placed at the seaward side of the observation arenas, 3-4 m from the odorant release site. The time required in the boat to prepare the odorant release bag, from the time water arrived at the boat to the time the assembled carboy was removed from the boat to be taken to the sea bottom, averaged 12 min. Typically, < 20 min elapsed between collection of seawater next to the study site and return of the odorant release apparatus to the seafloor.
Figure 2 The odorant release apparatus in sagittal section (not drawn exactly to scale). The Teflon® odorant release bag containing seawater was placed, for mechanical support, inside a polypropylene carboy (nominal volume: 5 US gallons) from which the top had been sawed off. The odorant release bag was filled through an integral filling sleeve at its top (not shown); after filling, the sleeve was rolled up within a Welch Fluorocarbon Clip-N-Seal®, sealing the bag. To pressurize the seawater in the bag, a side-walled acrylic plate filled with crushed rock was placed on top. The high sidewall was essential because it helped keep the base of the plate positioned approximately parallel to the carboy bottom. Another essential attribute was the use of small stones (e.g., crushed rock) evenly distributed over the plate bottom; dispersed mass (rather than a single massive object) was required for the apparatus to work reliably. The stones were enclosed in a plastic bag to prevent direct contact with the ambient seawater. Sizable gas bubbles must be avoided in the odorant release bag (bubbles will tend to coalescence and interfere with the exertion of even pressure by the mass of stones). Gas bubbles must also be avoided in the outflow tube, lest they cause airlocks. Whereas the rate of discharge from the odorant release bag depends on the mass of crushed rock and resistance posed by the outflow tube, it is independent of both the depth of the ambient water column and the height difference between the bag and release orifice.
After the odorant release apparatus was placed on the seafloor, a circular plate of 6.4-mm-thick acrylic sheet (ca. 12 mm smaller in diameter than the internal diameter of the carboy) was placed on top of the odorant release bag. As seen in Figure 2, this plate had a 12-cm-high side wall, forming a large bowl in which about 7 kg of crushed rock was placed. This negatively buoyant mass pressed on the top of the odorant release bag, pressurizing the seawater inside.
At its bottom, the odorant release bag was manufactured with a short Kynar fluoropolymer exit port, to which was attached an outflow tube, consisting of 6.4-mm-ID Tygon® SE-200 fluoropolymer-lined tubing (Figure 2). The tubing was initially clamped shut with a plastic external clamp. The tubing ran 3-4 m to the selected odorant release site (Figure 1), where it was connected to an orifice tethered 0.5 m above the seafloor, approximately equal to the heights of the highest nearby reef structures. When the time came to release odorant, the outflow tube was partially unclamped so that seawater pressurized in the release bag could flow to the release orifice and be ejected into the ambient water. The ejection rate depended on (1) the mass of crushed rock and (2) the extent to which the clamp on the outflow tube was opened. We had adjusted these during preliminary tests so that 12-16 L of water from the odorant release bag would be ejected over a period of 40-50 min (i.e., ca. 300 mL/min).
Observers assumed their positions in the water column after full assembly of the odorant release apparatus on the seafloor had been completed. At this time, the outflow tube remained fully clamped and no odorant-containing seawater was being released. After a waiting period of 5 min, Observers 1 and 2 recorded the presence and abundance of fish species in their observation arenas for a 5-min enumeration period. During this and subsequent enumeration periods, they recorded all fish species present, based on observations of individuals large enough to be visually identified at the distances entailed at our study sites. Adults and juveniles were counted separately when categorically distinguishable by size (e.g., chromis) or coloration (e.g., parrotfish). Fish names (common and scientific) used in this report adhere to the current recommendations (July, 2022) of FishBase (Froese and Pauly, 2022).
Following the initial 5-min enumeration period, the outflow tube was partially unclamped, permitting odorant-containing seawater to be steadily ejected into the ambient water via the release orifice at the odorant release site (Figure 1). During the subsequent uninterrupted ejection period, which lasted 50 min, Observers 1 and 2 recorded the presence and abundance of fish species in the observation arenas during the final 5 min of each 10-min interval. Thus, each odorant test consisted of six 5-min enumeration periods: one prior to the start of odorant ejection and five sequential periods following the start of ejection.
Three odorant types were investigated. One, and only one, of these was used in any particular test.
A. Control. The odorant release bag was filled with plain, local seawater.
B. Dimethylsulfoniopropionate (DMSP). The odorant release bag was filled with a solution of DMSP (Research Plus, Barnegat, NJ, USA) dissolved in local seawater at a concentration of 4 × 10-6 M. This concentration was chosen to create a minimum concentration of 1 × 10-9 M (the minimum target concentration defined by DeBose et al., 2008) when distributed in 64 m3 of water (volume in a 4 m × 4 m × 4 m arena).
C. Betaine mix. The odorant release bag was filled with a solution of three betaines – glycine betaine (Sigma), proline betaine (HDH Pharma, Morrisville, NC, USA), and trigonelline (Fluka) – each dissolved in local seawater at a concentration of 2 × 10-4 M (an exploratory concentration intended to create an approximate minimum concentration of about 1 × 10-7 M in arenas).
During testing, only one of us (RWH) knew the identity of odorants used. To prevent Observers 1 and 2 or anyone else (e.g., ship’s crew) from learning the identity, a vial containing 40 mL of distilled water was always employed on shipboard; this vial was emptied into the 18 L of freshly collected seawater in the release bag. For preparing the “control” odorant type, the 40 mL of distilled water contained no added solutes. For preparing the DMSP (or betaine) odorant type, the distilled water contained DMSP (or betaines) at a concentration that produced the target solute concentration when added to the seawater in the release bag. The solutions of solutes in distilled water were made within 24 h of use and refrigerated or frozen until use.
At each of the two study sites, only one odorant release test (using one odorant type) was carried out on any given day. Releases at Site 1 were initiated at 08:30 - 10:05 Atlantic Standard Time, whereas releases at Site 2 were started at 15:25 -16:50 AST; the study period was 30 June to 13 July 2011. Ocean water temperatures measured at the study sites were 27-29°C. The three odorant types were employed in random order at each study site, the two sites being randomized independently. A total of 20 odorant tests was completed (6 control tests, 8 tests using betaines, 6 tests using DMSP).
Prior to undertaking the tests with odorants, we carried out extensive tests near the study sites with dye to ascertain the behavior of water ejected from the odorant release apparatus. During these tests, the apparatus was set up to eject seawater as already described, except that the ejected seawater contained red food-coloring dye instead of an odorant.
For analysis of results, we categorized fish species as resident or nonresident based on the behavior we observed at the time, place, and spatial extent of our investigation. A species was considered resident if individuals exhibited site fidelity on the reef (e.g., bicolor damselfish, Stegastes partitus, and dusky damselfish, S. adustus). A species was classed as nonresident if individuals entered and exited our study sites without evident site fidelity (e.g., brown chromis, Chromis multilineata, and yellowhead wrasse, Halichoeres garnoti).
Throughout this paper, in describing fish counts, we refer to “numbers of fish enumerated” rather than “numbers of individuals” because we had no way to identify individuals other than by keeping track of them in our visual field. In any one count, the number “enumerated” was the same as the number of individuals observed. However, in repeat counts during a single test, those enumerated during one count could in principle be the same or different individuals from those enumerated during another count.
For each species, we calculated an index of abundance as a descriptive statistic not used for hypothesis testing, but useful for organizing the full dataset for analysis. This is the grand total of the numbers enumerated in all 360 enumeration periods during this research. [Each of the 20 odorant tests entailed 18 enumeration periods (6 periods at each of 3 observation arenas).]
For statistical analysis of a species, unless otherwise stated, the fish counts used were the totals of adults and juveniles observed. When adults and juveniles were readily distinguishable (see earlier), the two were separately tallied during field observations, then added.
In all tests with dye, we observed that the seawater ejected at the release orifice was metered out slowly and steadily over the 50 min following the start of ejection. Moreover, as the ejected seawater – flowing in the consistently present local currents – dispersed three dimensionally into the ambient seawater, it neither floated upward nor sank downward in any sort of consistent way. That is, the mean height above the reef bottom of the cloud of dispersing dye remained approximately equal to the height of the release orifice (0.5 m), indicating that the ejected seawater was neutrally buoyant. As others have observed (DeBose and Nevitt, 2008; Michaelis et al., 2020), the dye typically flowed away from the odorant release orifice in filaments of relatively high concentration separated by regions of lower concentration. The intensity of the dye color decreased as the dye moved away from the release orifice, indicating that the dye was gradually becoming thoroughly mixed with the ambient water. Carried in the along-shore current flowing parallel to the reef drop-off, dye typically moved far enough to cross the study arenas within a few minutes.
Before proceeding to our analysis of our data for nonresident species, we note that all of our original in situ observation data are presented in Supplementary Table 2 for seven of the most abundant species at our study site: blue chromis, bluehead wrasse, brown chromis, creole wrasse, redband parrotfish, yellow goatfish, and yellowhead wrasse.
Because different observers often differ in fish counts when observing the same fish in natural communities, we use only single-observer measures for statistical analysis. For fish of each nonresident species observed in a particular arena during a particular odorant test, we have calculated a “response statistic” as the difference in numbers counted before and after the start of odorant release. Because a single observer recorded all counts in any particular arena and odorant test, this statistic is ensured to not be confounded by observer heterogeneity. The response statistic was calculated as (n2 + n3 + n4)/3 – n0, where n0 is the number counted during the enumeration period prior to odorant release and n1, n2, n3, n4, and n5 are the numbers counted in the successive enumeration periods following the start of odorant release. We omitted n1 in the calculation because odorant might not yet have reached steady-state concentration in the ambient water at that time. We omitted n5 because, during our 20 odorant tests, we were concerned during 2 tests that odorant release might have stopped prior to the end of the final enumeration period, and thus we deemed it best to use counts only from prior to the final enumeration period.
For each fish species, values for the response statistic collected by both Observers 1 and 2 during our full set of odorant tests were analyzed in a blocked statistical design, blocking on observer. Recognizing that the enumeration data were often highly nonnormal in statistical distribution, we used a nonparametric statistical test, the two-factor Friedman test for multiple observations per experimental unit (Marascuilo and McSweeney, 1977), the same test used by DeBose et al. (2008). The two factors were the odorant ejected into the reef water (control, DMSP, or betaines) and observer.
The two most abundant nonresident species were the brown chromis (Chromis multilineata), for which the index of abundance for adults alone was 21808, and the blue chromis (C. cyanea), for which the index for adults and juveniles combined was 5321. We based our analysis of brown chromis on numbers of adults only; juveniles were not included because they were often present in uncountable numbers in the high hundreds or thousands. For the Friedman nonparametric test, the response statistics are rank ordered, and the analysis is based on the ranks. Figure 3 presents the median ranks and other descriptive statistics for the three odorant types. In the Friedman test, χ2 = 4.79 (df = 2). Although this value is not statistically significant at the p = 0.05 level (where the threshold for rejecting the null hypothesis is χ2 = 5.99), it is significant at the p = 0.10 level (threshold for null hypothesis rejection: χ2 = 4.61). We thus obtained marginally significant evidence for differences in adult brown chromis response to the three odorant types. Based on inspection (Figure 3), adult brown chromis are attracted to DMSP and betaines.
Figure 3 Box-whisker plots of ranks of the response statistics of adult brown chromis (Chromis multilineata) measured during odorant tests with control, DMSP, and betaines. Within data gathered by each observer, response statistics were ranked from lowest (assigned a rank of 1) to highest, as dictated by the method of the two-factor Friedman nonparametric test. Each plot is based on the combined data of the two observers and depicts median rank, lower boundary of 2nd quartile, upper boundary of 3rd quartile, and (whiskers) minimum and maximum. Data from one odorant test could not be used because the ambient water current switched direction as the test was in progress.
The blue chromis analysis was based (as usual) on total numbers observed, including both adults and juveniles. For this species (see Supplementary Figure 1), χ2 = 0.308 (df = 2), which is not statistically significant at either the p = 0.05 or 0.10 level. We thus obtained no evidence that DMSP or betaines affect the behavior of blue chromis.
The third most abundant nonresident species, considering both adults and juveniles, was the creole wrasse (Clepticus parrae). However, during some observation periods, juvenile creole wrasse were too numerous to count, compelling us to limit our analysis to just adults, abundance index = 407 (creole wrasse and brown chromis were the only species in this study in which juveniles were excluded for statistical analysis). For adult creole wrasse χ2 = 5.99 (df = 2), which is significant at the p = 0.05 level. Inspection shows that the response statistic was lower in the DMSP tests than in the control or betaine tests (Figure 4), indicating that adult creole wrasse are repelled by DMSP.
Figure 4 Box-whisker plots of ranks of the response statistics of adult creole wrasse (Clepticus parrae) during odorant tests with control, DMSP, and betaines. See caption of Figure 3 for explanation.
Among the 10 most abundant nonresident species, the remaining seven were all analyzed based on the sums of adult and juvenile numbers and are listed here in order of index of abundance (in parentheses): bluehead wrasse, Thalassoma bifasciatum (998), χ2 = 0.557 (df = 2); striped parrotfish, Scarus iseri (637), χ2 = 1.51; yellowhead wrasse, Halichoeres garnoti (605), χ2 = 4.95; princess parrotfish, Scarus taeniopterus (370), χ2 = 0.87; blue tang surgeonfish, Acanthurus coeruleus (364), χ2 = 2.60; redband parrotfish, Sparisoma aurofrenatum (334), χ2 = 1.15; and yellow goatfish, Mulloidichthys martinicus (305), χ2 = 0.092. Among these species, our evidence points to a significant difference among odorants only in the yellowhead wrasse (significant at the p = 0.10 level). For that species, inspection shows that the response statistic was lower in the DMSP tests than the control or betaine tests, suggesting that yellowhead wrasse are repelled by DMSP (Figure 5). Supplementary Figure 1 presents the results for the other six species.
Figure 5 Box-whisker plots of ranks of the response statistics of adult and juvenile yellowhead wrasse (Halichoeres garnoti) during odorant tests with control, DMSP, and betaines. See caption of Figure 3 for explanation.
We did not carry out statistical analysis for nonresident species that were less common than those already noted because of the sparseness of observations on such species. In species with index of abundance < 300, individuals were sufficiently uncommon that, typically, < 1 individual was observed in an observation arena during a 5-min observation period, suggesting that our behavioral observations would have little chance of detecting odorant responses. Supplementary Table 1 provides descriptive data for the 24 nonresident species with indices of abundance < 300 but > 30.
In regards our analyses of abundant nonresident species, Supplementary Table 3 presents a preliminary, post hoc analysis of trends over time in fish abundance in the cases in which our Friedman’s tests indicate a statistically significant response to an odorant. We think this analysis will be useful for future investigators designing follow-up studies.
As in the analysis of nonresident species, for analyzing our data on resident species we have used only single-observer statistical comparisons. We have used a different analysis, however, because we assume that, to a first approximation, individual resident fish were present at reef locales all the time. Our field tests therefore primarily provided insight into the extent to which steadily present individuals emerged into locations where we could see them and count them, as opposed to sheltering in locations where we could not see them. Accordingly, only within-arena comparisons could be informative in the study of resident species.
For resident species, we analyzed numbers counted during odorant release, calculated as (n2 + n3 + n4)/3. We compared the numbers counted during DMSP or betaine trials with the numbers counted by the same observer in the same arena during control trials. Observer 1 always enumerated fish in the same arena, the middle arena (Arena B, Figure 1), whether at Site 1 or Site 2. We analyzed the data of Observer 1 with the Friedman two-factor test already specified, one factor being odorant (control, DMSP, or betaines), the other being site (i.e., blocking on site). Regarding Observer 2, because of shifts in the ambient water current, there were four odorant tests during which Observer 2 was assigned to a different arena than usual. We analyzed the data of Observer 2 with the Friedman two-factor test as just described, but using data only for the Observer’s usual arena [that at the left (Arena A) in Figure 1], which was used a total of 16 times.
One resident species, the bicolor damselfish, Stegastes partitus, with an index of abundance of 12806, was far more abundant than any other. For Observer 1, χ2 = 0.58 (df = 2), and for Observer 2, χ2 = 2.84 (df = 2), neither of which is statistically significant at the p = 0.05 or 0.10 level. Thus, based on data for large numbers of individual bicolor damselfish observed, we obtained no evidence that DMSP or betaines affect the behavior of the species.
Two additional resident species were common enough to have an index of abundance > 400 (after them, the next most abundant species had an index of only 204). These species were the threespot damselfish (Stegastes planifrons) and dusky damselfish (S. adustus), which had abundance indices of 457 and 403, respectively. A flaw (misidentification) during a few early field trials in recording juvenile threespot damselfish prevented us from completing a rigorous evaluation of the species, although in general our data provided no evidence that DMSP or betaines affect the behavior of threespot damselfish.
Regarding the dusky damselfish, a noteworthy observation was that at Site 1, not even one individual was ever observed in the middle arena (Arena B, Figure 1; monitored by Observer 1) throughout our research, nor were dusky damselfish ever observed at Site 1 in the arena to the right of that one (Arena C, Figure 1), although they were observed by Observer 2 in the arena to the left (Arena A, Observer 2’s usual arena). Both observers recorded numerous dusky damselfish in all arenas at Site 2. The “spotty” occurrence of dusky damselfish (never seen at some places while consistently seen at others) provides support for the concept that the species functioned as a resident species at the time and place of our study.
Statistically, Observer 1 obtained data on dusky damselfish only at Site 2 because of the patterns just described. We analyzed those data with the nonparametric Kruskal-Wallace test, obtaining χ2 = 0.60 (df = 2). Because Observer 2 obtained data at both sites, we analyzed that observer’s data in the usual way using Friedman’s two-factor test, one factor being odorant, the other being site. In this case χ2 = 0.13 (df = 2). We thus obtained no evidence that DMSP or betaines affect the behavior of dusky damselfish.
As addressed in detail in the Introduction, DMSP and betaines are highly soluble metabolites, often abundant in the tissues of scleractinian corals (and sometimes other reef inhabitants, e.g., tridacnid clams), that could function as important signaling odorants, or infochemicals (Nevitt, 2011), in coral-reef communities, by affecting the behavior of fish species present. For understanding the effects of candidate odorant cues on fish, in situ testing is not without flaws, as noted in the Introduction. The responses of any one species, for example, could be affected by the presence or absence of other species, e.g., predators. Moreover, unlike in a laboratory study where odorant can be stirred into the ambient water to create a uniform study concentration, in an in situ study – whether carried out with the distilled-water method or an alternative odorant-release method – released odorant flows outward in filaments of relatively high concentration that gradually disperse and mix with the ambient water, meaning that fish can experience a range of odorant concentrations (DeBose and Nevitt, 2008; Michaelis et al., 2020). Also, in an in situ study, as released odorant becomes mixed into the ambient seawater, ambient microbes can potentially metabolize odorant, changing its concentration. In situ testing nonetheless also has major advantages, notably that, in principle, it permits the responses of all species and life stages present to be evaluated in their natural environments (see Introduction; Elliott et al., 1995). The study we report here is an in situ study, and as such cannot be made as unambiguous as a lab study but can provide insights that are potentially unattainable in a lab study.
The success of in situ testing depends critically on a suitable method of in situ odorant release. The present research is in part a proof-of-concept for a new method of in situ odorant release. We have designed, and demonstrated the practical utility of, an apparatus (Figure 2) that ejects local reef water steadily, remotely, and silently over an extended period of time. As our tests with dye document, the ejected water – because it is recently collected, local reef water – is neutrally buoyant in the local environment, meaning that it simply flows with local currents after ejection. Candidate odorant cues can be added to the water prior to ejection for studies of odorant effects on the behavior of fish in the reef.
The distilled-water method of DeBose et al. (2008) is a clever, already-used, alternative method for in situ odorant release. In that method, odorant is added to distilled water contained in a vessel (e.g., carboy) tethered at a fixed depth in the water column. Removing the cap of the vessel permits the distilled water to exit and ascend in the water column because of its low density in comparison with the density of seawater. In this way, the odorant is dispersed remotely and silently into the natural environment.
Both methods of odorant release share virtues noted in the Introduction: They permit discovery of odorant effects in all reef species and life stages, regardless of whether the species and life stages can be successfully studied in captivity. Both methods also operate without requiring a human-made power source and – once activated – silently and automatically release their full payload of odorant. We designed our apparatus so that only food-grade fluoropolymer materials (i.e., materials rated for use with human foods and beverages) were in contact with the ejection medium (seawater) from the time of collection to the time of release. That is not, however, a distinguishing feature of our method because food-grade materials are commercially available that would permit a similar refinement in the application of the distilled-water method.
The key distinguishing advantage of our method is that it does not depend on the buoyancy of distilled water. In the abstract, there are at least two concerns with a distilled-water mechanism for odorant ejection in an ocean setting. First, as ejection occurs, it seems inevitable that in the water column, regions of elevated odorant concentration will co-occur with regions of reduced salinity. Thus fish sensory processes may respond in a confounded way to simultaneous chemosensory signals and osmotic signals (McInerney, 1964; Fritz and Garside, 1974; Herbert-Read et al., 2010; Surrano et al., 2010; Kȕltz, 2012). Second, with a distilled-water ejection mechanism, ejection occurs asymmetrically upward, toward the water surface. Even if the ejection site is within the benthic structural boundaries of the reef, ejected odorant tends to rise out of those boundaries, and species of fish closely associated with reef structure may not fully experience odorant presence (in fact, strictly resident species may not experience the odorant at all). Our new method avoids these potential shortcomings by using gravitational force to eject local seawater as the ejection medium, thereby avoiding not only the artificiality of distilled water but also all other possible unintended (but potentially confounding) differences between the ejection medium and the local seawater.
We confirm the finding of DeBose et al. (2008) that DMSP is likely an attractant for brown chromis (C. multilineata), one of the most abundant fish species in many Caribbean reefs. Working in the same Curaçao reef system as we studied, DeBose et al. (2008) dispersed DMSP using the distilled-water method from carboys tethered at a depth of 7-12 m, rated as “midwater” at their study sites. Divers hovering at midwater depths downstream from the carboys then observed potential fish responses on a far larger spatial scale than in our study. In our research, DMSP-containing seawater was released among benthic reef structures about 0.5 meter above the local bottom, and observers monitored potential fish responses on a spatial scale of about 4 m in all dimensions. Certainly it is striking that two such different methods – which target fish at different elevations in the water column – converge in pointing to DMSP as an attractant for brown chromis. In addition, our results on brown chromis (Figure 3) suggest an attractant role for betaines (studied as a mix of glycine betaine, proline betaine, and trigonelline).
Among the 10 nonresident species for which we obtained sufficient data for statistical analysis, we obtained no evidence for odorant roles of DMSP or betaines in 7 species. The two species, in addition to brown chromis, in which we observed significant odorant effects were the creole wrasse (Clepticus parrae) and yellowhead wrasse (Halichoeres garnoti), in which we observed potential repellent effects of DMSP (Figures 4, 5). DeBose et al. (2008), in addition to the effects on brown chromis already noted, obtained data indicating an attractant role for DMSP in creole wrasse and boga (Haemulon vittatum). Our results on creole wrasse are for adults only, whereas DeBose et al. (2008) counted both adults and juveniles. Possibly this distinction helps explain differences in our results. Another possible explanation is difference of ecological context; DeBose et al. investigated DMSP as a long-range, planktonic foraging cue, whereas we investigated DMSP as a possible cue for entry into the benthic habitat immediately surrounded by coral structures. We did not obtain useful new information on boga because we observed only low numbers (abundance index = 161).
Turning now to resident species of fish, feasibility of study is the first point to emphasize: Our new odorant-ejection method makes the in situ study of odorant responses in resident species possible. The distilled-water method ejects odorant-containing water up and away from the coral-reef structures with which resident individuals are associated, helping to explain why DeBose et al. (2008) focused on nonresident fish species in relatively open water. As our tests with dye document, water ejected by our new method 0.5 m above the seafloor in the midst of benthic coral structures tends to remain near that level and disperse among the structures. We can realistically assume, therefore, that the damselfish resident fish we studied were exposed to the ejected odorants. Accordingly, our data indicate that when bicolor damselfish (Stegastes partitus) are exposed to DMSP or betaines, they do not alter their position in the water column. With weaker evidence, we can say the same about threespot damselfish (S. planifrons) and dusky damselfish (S. adustus). All this said, we must also acknowledge that we would have been unable to detect changes of fish position too restricted to change the number of individuals countable with our observational method.
We call attention to the fact that the odorant-release apparatus we have invented could be used on much longer time scales than that employed in the present study. For example, researchers such as Sweatman (1988) and Elliott et al. (1995) designed experiments requiring odorant release in natural habitats for up to 12.5 h. They employed electrically powered, submerged bilge pumps to pump the odorant-release medium. The sounds of such pumps, which could confound odorant research (Kunc et al., 2016), could be avoided by using an odorant-release apparatus such as ours, by adjusting the volume and rate of release of the odorant-release medium.
We also stress our use of food-grade fluoropolymers. As noted by Sweatman (1988), when random plastics or other materials are used to assemble apparatuses for odorant release, there is always the concern that the plastics could release unspecified compounds with odorant effects, confounding studies of target odorants. With advances in fluoropolymer commercialization, it is now feasible to reduce such risks dramatically. We demonstrate here for the first time in such research that, by use of off-the-shelf and custom made fluoropolymer tubing and bags (at relatively low expense), a useful odorant-release apparatus can be designed in which all surfaces contacted by the odorant release medium are composed of relatively inert, food-grade fluoropolymers.
Although our study was demanding in both time and resources, it was in certain ways a pilot study intended to help pinpoint questions of particular concern for the design of future investigations of this kind. Accordingly, we highlight three methodological considerations that, based on our experience, deserve particular attention in future studies.
a. We conclude that the statistical identification of firm conclusions will be facilitated by devoting increased attention to the definition of fish numbers present prior to odorant release. In a study using SCUBA, the total number of enumeration periods during an odorant test is more or less fixed by the observers’ oxygen supply. Within that constraint, we recommend increased investment of effort in the pre-odorant-release phase. To illustrate, in our case, using single tanks of compressed air, we were limited to six enumeration periods at 10-min intervals. In retrospect, we would recommend that, under that constraint, 2-3 of the observation periods would be best devoted to pre-odorant enumerations, and 3-4 to enumerations following the start of odorant ejection (rather than, respectively, 1 and 5 as in the present study). Especially in nonresident species, the numbers of fish enumerated exhibit sufficiently high variability to justify such increased attention to the pre-odorant-release phase.
b. The urge to assess odorant responses in as many species as possible during an in situ study should be tempered by realistic expectations regarding species abundance and its implications. Among the 70 species of fish we observed, the index of abundance ranged from 1 to >12,000, with the distribution of indices being strongly skewed toward low values. For example, the index was >300 in only 13 species. Conversely, 32 species had an index < 30. For species with such low abundance as the latter, zero counts (enumeration periods in which no members of the species are observed) are common, rendering any sort of rigorous statistical analysis (parametric or nonparametric) almost impossible. Effort needs to be focused on species that are sufficiently abundant for statistical rigor to be feasible.
c. Sample sizes are a potential central challenge even when focusing on just the most abundant 15-20% of fish species. In our statistical analysis phase, for obtaining firm statistical conclusions we found ourselves desiring a database of at least twice the size we obtained (e.g., 40 field odorant tests instead of 20). Options for enlarging the database are constrained by the reality of observer inhomogeneity; increasing the number of observers would not necessarily be a cure-all because of nonuniformity in the ways observers count fish. Yet small numbers of highly qualified observers cannot realistically be expected often to sign up for a month of days filled with repetitive observation periods. Possibly the full realization of the potential of in situ testing will not be achieved until automated methods for fish enumeration (e.g., video identification) are developed.
The original contributions presented in the study are included in the article/Supplementary Material. Further inquiries can be directed to the corresponding author.
RH designed the odorant release apparatus. RH, PF, and AF designed and executed all aspects of the field research. RH, PF, MV, and AF contributed equally to the analysis of results and the preparation of this manuscript. All authors contributed to the article and approved the submitted version.
This research was funded in part through the 2020-2021 BiodivERsA and Water JPI COFUND call for research proposals, with the national funders Austrian Science Fund (FWF) (project I 5838-B). For the purpose of open access, the author has applied a CC-BY public copyright license to any Author Accepted Manuscript version arising from this submission. In addition to the funding from the Austrian Science Fund, RH provided funding for this research.
Carlos Winterdaal was our boat captain, without whose steadfast commitment to excellence this study could not have been completed. RWH thanks Woods Hole Oceanographic Institution and Mark Hahn for an outstanding writing venue for composing the initial draft of this manuscript.
The authors declare that the research was conducted in the absence of any commercial or financial relationships that could be construed as a potential conflict of interest.
All claims expressed in this article are solely those of the authors and do not necessarily represent those of their affiliated organizations, or those of the publisher, the editors and the reviewers. Any product that may be evaluated in this article, or claim that may be made by its manufacturer, is not guaranteed or endorsed by the publisher.
The Supplementary Material for this article can be found online at: https://www.frontiersin.org/articles/10.3389/fmars.2023.1187249/full#supplementary-material
Supplementary Figure 1 | Box-whisker plots of ranks of the response statistics of the adults and juveniles of seven species during odorant tests with control, DMSP, and betaines: blue chromis (Chromis cyanea); blue tang surgeonfish (Acanthurus coeruleus); bluehead wrasse (Thalassoma bifasciatum); princess parrotfish (Scarus taeniopterus); redband parrotfish (Sparisoma aurofrenatum): striped parrotfish (Scarus iseri); and yellow goatfish (Mulloidichthys martinicus). Statistically there were no significant differences among the odorant types for any of these species. See caption of Figure 3 for explanation.
Ben-Tzvi O., Tchernov D., Kiflawi M. (2010). Role of coral-derived chemical cues in microhabitat selection by settling Chromis viridis. Mar. Ecol. Progr. Ser. 409, 181–187. doi: 10.3354/meps08627
Cole A. J., Pratchett M. S., Jones G. P. (2008). Diversity and functional importance of coral-feeding fishes on tropical coral reefs. Fish Fish. 9, 286–307. doi: 10.1111/j.1467-2979.2008.00290.x
Cole A. J., Pratchett M. S., Jones G. P. (2009). Effects of coral bleaching on the feeding response of two species of coral-feeding fish. J. Exp. Mar. Biol. Ecol. 373, 11–15. doi: 10.1016/j.jembe.2009.02.016
Danaceau J. P., Lucero M. T. (1998). Betaine activates a hyperpolarizing chloride conductance in squid olfactory receptor neurons. J. Comp. Physiol. A 183, 225–235. doi: 10.1007/s003590050250
DeBose J. L., Lema S. C., Nevitt G. A. (2008). Dimethylsulfoniopropionate as a foraging cue for reef fishes. Science 319, 1356. doi: 10.1126/science.1151109
DeBose J. L., Nevitt G. A. (2008). The use of odors at different spatial scales: comparing birds with fish. J. Chem. Ecol. 34, 867–881. doi: 10.1007/s10886-008-9493-4
DeBose J. L., Nevitt G. A., Dittman A. H. (2010). Rapid communication: Experimental evidence that juvenile pelagic jacks (Carangidae) respond behaviorally to DMSP. J. Chem. Ecol. 36, 326–328. doi: 10.1007/s10886-010-9755-9
DeBose J. L., Paul V. J. (2014). Chemical signatures of multi-species foraging aggregations are attractive to fish. Mar. Ecol. Prog. Ser. 498, 243–248. doi: 10.3354/meps10617
Dixson D. L., Hay M. E. (2012). Corals chemically cue mutualistic fishes to remove competing seaweeds. Science 338, 804–807. doi: 10.1126/science.1225748
Elliott J. K., Elliott J. M., Mariscal R. N. (1995). Host selection, location, and association behaviors of anemonefishes in field settlement experiments. Mar. Biol. 122, 377–389. doi: 10.1007/BF00350870
Ferrer R. P., Zimmer R. K. (2012). Community ecology and the evolution of molecules of keystone significance. Biol. Bull. 223, 167–177. doi: 10.1086/BBLv223n2p167
Foretich M. A., Paris C. B., Grosell M., Stieglitz J. D., Benetti D. D. (2017). Dimethyl sulfide is a chemical attractant for reef fish larvae. Sci. Rep. 7, 2498. doi: 10.1038/s41598-017-02675-3
Frade P. R., Schwaninger V., Glasl B., Sintes E., Hill R. W., Simó R., et al. (2016). Dimethylsulfoniopropionate in corals and its interactions with bacterial assemblages in coral surface mucus. Environ. Chem. 13, 252–265. doi: 10.1071/EN15023
Fritz E. S., Garside E. T. (1974). Salinity preferences of Fundulus heteroclitus and F. diaphanous (Pisces: Cyprinodontidae): their role in geographic distribution. Can. J. Zool. 52, 997–1003. doi: 10.1139/z74-133
Froese R., Pauly D. (Eds.) (2022). FishBase. (Calamba, Philippines: World Wide Web electronic publication). Available at: www.fishbase.org.
Garren M., Son K., Raina J.-B., Rusconi R., Menolascina F., Shapiro O. H., et al. (2014). A bacterial pathogen uses dimethylsulfoniopropionate as a cue to target heat-stressed corals. ISME J. 8, 999–1007. doi: 10.1038/ismej.2013.210
Guibert I., Bourdreaux F., Bonnard I., Pochon X., Dubousquet V., Raharivelomanana P., et al. (2020). Dimethylsulfoniopropionate concentration in coral reef invertebrates varies according to species assemblages. Sci. Rep. 10, 9922. doi: 10.1038/s41598-020-66290-5
Herbert-Read J. E., Logendran D., Ward A. J. W. (2010). Sensory ecology in a changing world: salinity alters conspecific recognition in an amphidromous fish, Pseudomugil signifier. Behav. Ecol. Sociobiol. 64, 1107–1115. doi: 10.1007/s00265-010-0925-0
Hill R. W. (2022). Quaternary ammonium compounds as candidate photoprotective compounds in reef-building corals. Front. Mar. Sci. 9, 869739. doi: 10.3389/fmars.2022.869739
Hill R. W., Armstrong E. J., Florn A. M., Li C., Walquist R. W., Edward A. (2017). Abundant betaines in giant clams (Tridacnidae) and Western Pacific reef corals, including study of coral betaine acclimatization. Mar. Ecol. Prog. Ser. 576, 27–41. doi: 10.3354/meps12181
Hill R. W., Dacey J. W. H., Hill S. D., Edward A., Hicks W. A. (2004). Dimethylsulfoniopropionate in six species of giant clams and the evolution of dimethylsulfide after death. Can. J. Fish. Aquat. Sci. 61, 758–764. doi: 10.1139/f04-029
Hill R. W., Dacey J. W. H., Krupp D. A. (1995). Dimethylsulfoniopropionate in reef corals. Bull. Mar. Sci. 57, 489–494.
Hill R. W., Li C., Jones A. D., Gunn J. P., Frade P. R. (2010). Abundant betaines in reef-building corals and ecological indicators of a photoprotective role. Coral Reefs 29, 869–880. doi: 10.1007/s00338-010-0662-x
Jones G. B., King S. (2015). Dimethylsulphoniopropionate (DMSP) as an indicator of bleaching tolerance in scleractinian corals. J. Mar. Sci. Eng. 3, 444–465. doi: 10.3390/jmse3020444
Kasumyan A. O., Døving K. B. (2003). Taste preferences in fishes. Fish Fish. 4, 289–347. doi: 10.1046/j.1467-2979.2003.00121.x
Keller M. D., Kiene R. P., Matrai P. A., Bellows W. K. (1999). Production of glycine betaine and dimethylsulfoniopropionate in marine phytoplankston. I. Batch cultures. Mar. Biol. 135, 237–248. doi: 10.1007/s002270050621
Kuek F. W. I., Motti C. A., Zhang J., Cooke I. R., Todd J. D., Miller D. J., et al. (2022). DMSP production by coral-associated bacteria. Front. Mar. Sci. 9, 869574. doi: 10.3389/fmars.2022.869574
Kȕltz D. (2012). The combinatorial nature of osmosensing in fishes. Physiology 27, 259–275. doi: 10.1152/physiol.00014.2012
Kunc H. P., McLaughlin K. E., Schmidt R. (2016). Aquatic noise pollution: implications for individuals, populations, and ecosystems. Proc. R. Soc B 283, 20160839. doi: 10.1098/rspb.2016.0839
Mackie A. M., Mitchell A. I. (1982). Further studies on the chemical control of feeding behaviour in the Dover sole, Solea solea. Comp. Biochem. Physiol. A 73, 89–93. doi: 10.1016/0300-9629(82)90097-4
Marascuilo L. A., McSweeney M. (1977). Nonparametric and distribution-free methods for the social sciences. (Monterey: Blooks/Cole).
Masdeu-Navarro M., Mangot J.-F., Xue L., Cabrera-Brufau M., Gardner S. G., Kieber D. J., et al. (2022). Spatial and diel patterns of volatile organic compounds, DMSP-derived compounds, and planktonic microorganisms around a tropical scleractinian coral colony. Front. Mar. Sci. 9, 944141. doi: 10.3389/fmars.2022.944141
McInerney J. E. (1964). Salinity preference: an orientation mechanism in salmon migration. J. Fish. Res. Bd. Canada 21, 995–1018. doi: 10.1139/f64-092
Michaelis B. T., Leathers K. W., Bobkov Y. V., Ache B. W., Principe J. C., Baharloo R., et al. (2020). Odor tracking in aquatic organisms: the importance of temporal and spatial intermittency of the turbulent plume. Sci. Rep. 10, 7961. doi: 10.1038/s41598-020-64766-y
Moore R. J., Huxley C. J. (1976). Aversive behaviour of crown-of-thorns starfish to coral evoked by food-related chemicals. Nature 263, 407–409. doi: 10.1038/263407a0
Nevitt G. A. (2011). The neuroecology of dimethyl sulfide: a global-climate regulator turned marine infochemical. Integr. Comp. Biol. 51, 819–825. doi: 10.1093/icb/icr093
Paris C. B., Atema J., Irisson J.-O., Kingsford M., Gerlach G., Guigand C. M. (2013). Reef odor: A wake up call for navigation in reef fish larvae. PloS One 8, e72808. doi: 10.1371/journal.pone.0072808
Raina J.-P., Tapiolas D. M., Forêt S., Lutz A., Abrego D., Ceh J., et al. (2013). DMSP biosynthesis by an animal and its role in coral thermal stress response. Nature 502, 677–680. doi: 10.1038/nature12677
Rhodes D., Hanson A. D. (1993). Quaternary ammonium and tertiary sulfonium compounds in higher plants. Annu. Rev. Plant Physiol. Plant Mol. Biol. 44, 357–384. doi: 10.1146/annurev.pp.44.060193.002041
Rotjan R. D., Lewis S. M. (2008). Impact of coral predators on tropical reefs. Mar. Ecol. Progr. Ser. 367, 73–91. doi: 10.3354/meps07531
Sale P. F. (1990). Recruitment of marine species: Is the bandwagon rolling in the right direction? Trends Ecol. Evo. 5, 25–27. doi: 10.1016/0169-5347(90)90009-3
Suenaga K. (2004). Bioorganic studies on marine natural products with bioactivity, such as antitumor activity and feeding attractance. Bull. Chem. Soc. Japan 77, 443–451. doi: 10.1246/bcsj.77.443
Surrano X., Grosell M., Serafy J. E. (2010). Salinity selection and preference of the grey snapper Lutjanus griseus: field and laboratory observations. J. Fish Biol. 76, 1592–1608. doi: 10.1111/j.1095-8649.2010.02585.x
Swan H. B., Deschaseaux E. S. M., Jones G. B., Eyre B. D. (2017). The relative abundance of dimethylsulfoniopropionate (DMSP) among other zwitterions in branching coral at Heron Island, southern Great Barrier Reef. Anal. Bioanal. Chem. 409, 4409–4423. doi: 10.1007/s00216-017-0385-8
Sweatman H. (1988). Field evidence that settling coral reef fish larvae detect resident fishes using dissolved chemical cues. J. Exp. Mar. Biol. Ecol. 124, 163–174. doi: 10.1016/0022-0981(88)90170-0
Van Alstyne K. L., Schupp P., Slattery M. (2006). The distribution of dimethylsulfoniopropionate in tropical Pacific coral reef invertebrates. Coral Reefs 25, 321–327. doi: 10.1007/s00338-006-0114-9
Yancey P. H., Heppenstall M., Ly S., Andrell R. M., Gates R. D., Carter V. L., et al. (2010). Betaines and dimethylsulfoniopropionate as major osmolytes in cnidaria with endosymbiotic dinoflagellates. Physiol. Biochem. Zool. 83, 167–173. doi: 10.1086/644625
Keywords: Caribbean, chromis, Curaçao, DMSP, glycine betaine, proline betaine, sensory biology, trigonelline
Citation: Hill RW, Florn AM, Vermeij MJA and Frade PR (2023) In situ testing of candidate odorant cues in coral-reef fish: a new method with tests of dimethylsulfoniopropionate and betaines. Front. Mar. Sci. 10:1187249. doi: 10.3389/fmars.2023.1187249
Received: 15 March 2023; Accepted: 07 September 2023;
Published: 20 October 2023.
Edited by:
Dennis Higgs, University of Windsor, CanadaReviewed by:
Gabrielle Nevitt, University of California, Davis, United StatesCopyright © 2023 Hill, Florn, Vermeij and Frade. This is an open-access article distributed under the terms of the Creative Commons Attribution License (CC BY). The use, distribution or reproduction in other forums is permitted, provided the original author(s) and the copyright owner(s) are credited and that the original publication in this journal is cited, in accordance with accepted academic practice. No use, distribution or reproduction is permitted which does not comply with these terms.
*Correspondence: Richard W. Hill, aGlsbHJAbXN1LmVkdQ==
Disclaimer: All claims expressed in this article are solely those of the authors and do not necessarily represent those of their affiliated organizations, or those of the publisher, the editors and the reviewers. Any product that may be evaluated in this article or claim that may be made by its manufacturer is not guaranteed or endorsed by the publisher.
Research integrity at Frontiers
Learn more about the work of our research integrity team to safeguard the quality of each article we publish.