- 1Institute of Aquatic Ecology (IEA), Department of Environmental Sciences, Universitat de Girona (UdG), Girona, Spain
- 2Genetic Ichthyology Laboratory (LIG), Department of Biology, Universitat de Girona (UdG), Girona, Spain
Small pelagic fish are key elements of marine trophic networks and are of great importance to the total landing of marine species. Over the last decades, a decrease in biomass and catches of European sardine has been described, especially in the Mediterranean Sea, as an indication of a drop in stock condition. Multiple causes, including direct and indirect anthropogenic factors, seem to affect this ecologically and economically important species. To address this issue, it is important to identify the current variability in sardine status and understand the strategies for energy allocation, which are intimately related to reproductive potential and recruitment. We analyzed the somatic condition through tissue and mesenteric lipid measurements, relative condition (Kn) and hepatosomatic (HSI) indices, and reproductive condition with the gonadosomatic index (GSI) in four subareas of the Mediterranean (Northern Alboran, Northern Spain, the Northern Adriatic, and the Aegean Sea) and an outgroup from the Atlantic. The analyses were performed within the gonadal development cycle since translocation to reproduction may mask the state of actual energy reserves for comparison. The results revealed marked differences in health status among subareas, highlighting the low condition of Northern Spain sardines throughout the annual cycle. The uniform condition throughout gonadal development in the Northern Adriatic suggests that resource availability modulates the reproductive strategy since in this locality, sardines would have high dependence on immediate reserves, not behaving as strict capital breeders. Moreover, similarities between Alboran and Atlantic stocks are discussed, highlighting the marked energy replenishment of the latter after reproduction compared to the Mediterranean stocks. The Aegean stock was the fastest to reach its maximum after spawning, presenting low condition values from the developing to actively spawning period, but with the greatest recovery at the regressing phase, coinciding with a peak in productivity. Finally, the role of the liver in sardine energy storage is analyzed considering stock heterogeneity, potentially indicating that HSI is intimately related to feeding activity. Given the divergences among stocks, we propose concrete measures for managing this resource (i.e., the need for adapting the close seasons), which could be applied to other species in similar contexts.
1 Introduction
Growing human pressures, including climate change, are having profound and diverse consequences on marine ecosystems (Doney et al., 2012). Among the impacts throughout the food web, the effects of a changing environment include variations in the life history traits of fish, which are closely linked to population dynamics (De Roos et al., 2003; King and McFarlane, 2003; Liang et al., 2014). This includes changes in the growth rate and somatic condition, length and age at maturation, and fecundity (Devine et al., 2012; Lloret et al., 2013), which in turn are closely related to each other because of the trade-offs between the energy invested in growth, maintenance, and reproduction (Albo-Puigserver et al., 2021). Important collapses have been documented in pelagic fish in recent decades due to population alterations as a likely result of induced changes in life traits (Fréon et al., 2005). The Mediterranean is considered one of the most impacted seas in the world since climate change interacts synergistically with many other disturbances (Halpern et al., 2008; Lejeusne et al., 2010), such as fishing pressure. In fact, together with the Black Sea, it presented the highest percentage (62.5%) of stocks fished at unsustainable levels worldwide in 2017 (FAO, 2020), and the second highest in 2019 (63.4%) behind the Southeast Pacific (FAO, 2022). Moreover, it ranks among the fastest warming ocean regions (Marbà et al., 2015), favoring the periodicity of extreme events (Di Biagio et al., 2020) in combination with other drivers. In this context, small pelagic fish stocks in the Mediterranean have been experiencing population declines (Ramírez et al., 2021), and some of them have collapsed (Colloca et al., 2017). This has been mostly attributed to human activities, including intensive exploitation throughout the decades, and to the sensitivity of these species to environmental fluctuations (Martín et al., 2012; Van Beveren et al., 2014; Coll et al., 2019), especially related to the thermal factor that has been altered by anthropogenic effects at a global scale. Increasing temperature has a direct effect not only on the physiology and behavior of individuals, but also on plankton productivity and available resources (Brosset et al., 2017), making it a dominant environmental driver for predicting pelagic fishery health (Lloret et al., 2021). In addition to the potential economic consequences of this event, the decrease in small pelagics in ecosystems may affect all biological levels since they act on the higher and lower trophic levels, exerting wasp-waist control over these organisms in the system (Cury et al., 2000; Corrales et al., 2015).
The European sardine, Sardina pilchardus (Walbaum, 1792), is a neritic small pelagic fish species with cool-temperate water affinity that is widely distributed in the northeastern Atlantic areas from the North Sea to Senegal, including the Mediterranean and its adjacent seas (Parrish et al., 1989). It accounts for approximately 15-20% of the total Mediterranean marine-captured production (Tsikliras and Koutrakis, 2013). Its reproduction is characterized by early maturation, many eggs per body mass, and batch spawning, common strategies to compensate for the short lifetime fecundity of the small pelagics (Ganias et al., 2014). Furthermore, its reproductive cycle coincides with that of a capital breeder (McBride et al., 2015) since it stores energy mainly during a defined period, in this case, during the peak of production in spring-summer, and then allocates it to reproduction in autumn-winter, although the reproductive phenology of sardine stocks depends on the area (Caballero-Huertas et al., 2022b). Therefore, its reproductive strategy determines its condition throughout the annual reproductive cycle, which is also affected by environmental factors. Studies have reported a decrease in biomass and catches in several subregions of the Mediterranean basin, associated with slower growth, a decrease in body length, the disappearance of older individuals, and a worse body condition and health status of sardines (e.g., Sinovčić et al., 2008; Van Beveren et al., 2014; Brosset et al., 2017; Quattrocchi and Maynou, 2017; Şenbahar et al., 2020). To address these issues, understanding the variability in population condition and health constitutes a principal question in fisheries research and is of special importance to management. Condition and energy storage have important implications for life history traits, such as growth, mortality, or reproduction, affecting recruitment and population dynamics, which, ultimately, may alter pelagic ecosystem structures (Albo-Puigserver et al., 2017; Albo-Puigserver et al., 2020; Caballero-Huertas et al., 2022b).
Although the Mediterranean is, globally considered, an oligotrophic sea, it presents considerable heterogeneity (Estrada, 1996), so sardine stocks are affected by a differential set of climate and ocean conditions, mainly during larval development and recruitment (Leitão et al., 2014). In addition to the different productivities of the various ecosystems, the trophic status and the genetic distance among populations may be key factors in the potential diversity of body conditions reflected throughout the Mediterranean distribution of sardines (Dimarchopoulou and Tsikliras, 2022), which may also influence reproductive phenology and potential. In this sense, there are few works that simultaneously recorded the variability in sardine condition and reproduction along the Mediterranean Sea, since the studies that compared individuals from several locations involved a few Mediterranean subregions (e.g., Ganias et al., 2007; Albo-Puigserver et al., 2021; Caballero-Huertas et al., 2022c) and/or analyzed other biological traits such as sardine growth (e.g., Dimarchopoulou and Tsikliras, 2022) or length at maturity (e.g., Silva et al., 2006). An investigation by Brosset et al. (2017) on the spatiotemporal variability in the body status of sardine (also anchovy) in different subregions of the Mediterranean registered an asynchronous drop in body condition at various locations for both species with data collected over the period between 1975 and 2015; therefore, it makes sense to propose new research that explores the current condition and health status of sardine within its reproductive context and continues the time series. Thus, the scientific interest of this work lies in describing the present characteristics and peculiarities of each sardine stock associated with a specific area and speculating on the source and origin of the potential differences, taking into account the annual cycle of each stock due to its implication in energy status. To analyze the energy dynamics considering the actual gonadal stage of the individuals allows for a more accurate approximation of the true body condition and the factors that determine it than evaluating the individuals considering the sampling months, as has already been done for other species (e.g., Muñoz and Casadevall, 2002; Serrat and Muñoz, 2022). Furthermore, as fishing quotas/sustainable yields should be adapted to a regional scale because of the regional variability that conditions the status of the stocks (Leitão et al., 2014), updated knowledge of these aspects throughout the Mediterranean distribution of sardine is essential for the efficient management of this resource.
The main objective of this study was to characterize and compare the body condition and reproductive features of diverse Mediterranean stocks and the closest Atlantic population within the gonadal developmental cycle to globally document the current health status (quantified by indices of somatic condition and lipid content) of S. pilchardus throughout a considerable part of its distribution, in which a greater vulnerability has been documented. Hence, our research aims to answer the following questions: is there quantifiable variability in the current condition and energy storage of the Mediterranean sardine? If so, is this due to the different life strategies modulated by the environment? How should this fishing resource be managed, and how should the fishing policies be updated?
2 Materials and methods
2.1 Sample collection and area descriptions
Specimens of European sardine, Sardina pilchardus, (N = 3101) were collected seasonally (Supplementary Table 1) (seasons defined as winter: January, February, March; spring: April, May, June; summer: July, August, September; autumn: October, November, December) from 2019 to 2021 throughout four Mediterranean subareas (GSA), following the General Fisheries Commission for the Mediterranean (GFCM) delimitations established for stock assessments by commercial fisheries: GSA 1-Northern Alboran Sea, and GSA 6-Northern Spain (Western Mediterranean Sea); GSA 17-Northern Adriatic Sea (Central Mediterranean Sea); and GSA 22-Aegean Sea (Eastern Mediterranean Sea). An external point to the Mediterranean Sea was selected, located in the Northeast Atlantic Ocean, according to the subareas and divisions of FAO fishing areas (Portuguese Waters - East (FAO fishing area division 27.9.a)) (Figure 1). Immediately after purchase, samples were frozen at - 20 °C, which has been demonstrated to not affect somatic condition variables (Brosset et al., 2015). Except for 32 individuals out of the total (1.03%), all specimens in this study respected the minimum landing size for sardine (total length (LT) of ≥11 cm)) in the Mediterranean Sea (Popescu, 2018).
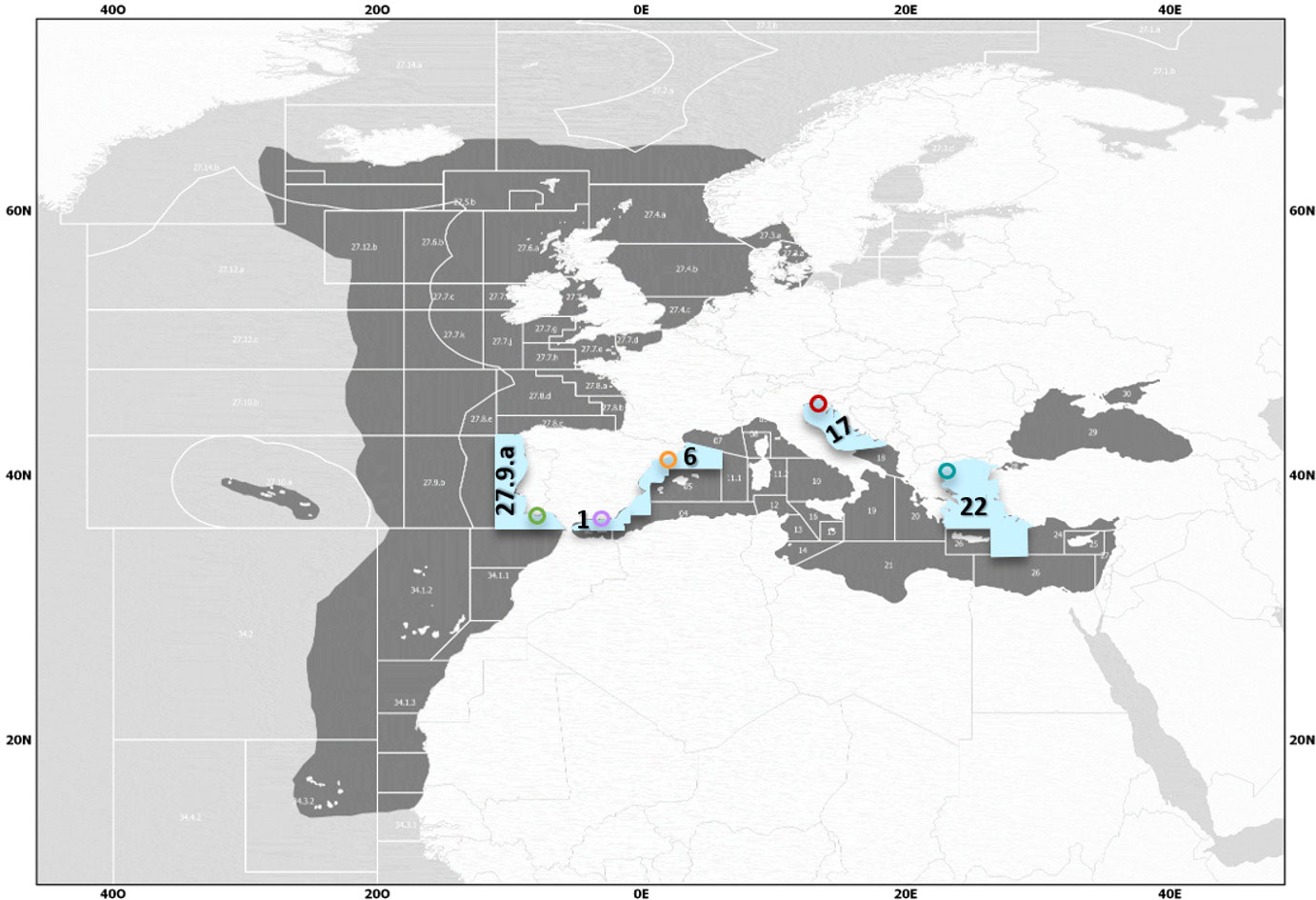
Figure 1 Geographical Subareas (GSAs) of the Mediterranean selected for the study (light blue) and approximate sample collection areas. Areas defined by the General Fisheries Commission for the Mediterranean (GFCM) as GSA 1 (violet): Northern Alboran Sea (Coast of Málaga), GSA 6 (orange): Northern Spain (Catalan Coast), GSA 17 (red): Northern Adriatic (Gulf of Trieste), and GSA 22 (turquoise): Aegean Sea (Thermaikos Gulf), and the external sampling point in the Atlantic, as FAO division 27.9.a: Portuguese Waters – East (Southern Portugal and Gulf of Cádiz) (green).
The Northern Alboran Sea subarea (GSA 1) is located in the southeastern Iberian Peninsula in the western Mediterranean Sea. It constitutes a transitional zone between Mediterranean and Atlantic waters, connected by the Strait of Gibraltar. This area does not present as much annual temperature variability as other Mediterranean areas (Figure 2A). There is a marked influence of the circulation, with a strong frontal area that divides a productive sector of intense upwelling in the coastal zone, and a more oligotrophic area far offshore (Vargas-Yáñez et al., 2017; García-Martínez et al., 2019; Vargas-Yáñez et al., 2019; Caballero-Huertas et al., 2022c). The Northern Spain subarea (GSA 6) is located in the northwestern (NW) Mediterranean and comprises the Catalan Sea, in which samples from this area were collected, and the Gulf of Valencia. Sea surface temperature (SST) varies significantly during the year: summers see the water exceed 26 °C while winters are temperate-cool (Figure 2A). Except for the areas near the mouth of the Ebro River, production remains at low and constant levels throughout the year (Figure 2B). The commercial exploitation of small pelagics in the area has been significant since the early 1940s, with catches dominated by sardines, probably due to their coastal distribution, constituting one of the most productive Mediterranean sardine stocks (Palomera et al., 2007; Silva et al., 2008). The Northern Adriatic Sea subarea (GSA 17) is located in the northernmost section of the most septentrional Mediterranean subbasin. It is one of the major chlorophyll hot spots in the Mediterranean Sea (Figure 2B), as it is influenced by the nutrient discharge from the Po River and a dozen small rivers that flow into the Adriatic Sea north of the Po River delta and in the Gulf of Trieste, accounting for approximately 40% of the chlorophyll production of the whole Adriatic (Rizzi et al., 2016). Small pelagic fishing by midwater trawlers and purse seiners (mainly focused on anchovy and sardine) is mostly concentrated in the northern part of the Adriatic Sea (Carpi et al., 2017). The North Aegean Sea subarea (GSA 22) shows variations in SST during the seasons, with hot summer months (Figure 2A). Although its production levels are low throughout the year, except for variable increases during some summer months (Figure 2B), it is more productive than the highly oligotrophic southern part, as it is influenced by Black Sea waters and large rivers, constituting one of the most important small pelagic fishing grounds in the eastern Mediterranean (Somarakis and Nikolioudakis, 2007). European sardines from the external sampling point (27.9.a) were mainly collected along the southern coast of Portugal and the Gulf of Cádiz. In this area, SST variations throughout the year are lower than those on the Mediterranean Coast (Figure 2A). It is characterized by notable and continuous productivity (Figure 2B) mainly due to river discharges (Caballero-Huertas et al., 2022c), since the effects of upwelling are reduced and occasionally occur under the influence of local westerly winds or when upwelled waters from the west Portuguese Coast intrude over the southern shelf break (Garrido et al., 2008). Sardina pilchardus is the main target species of the purse seine fleet, contributing approximately 98% of the landings in this division (Leitão et al., 2014).
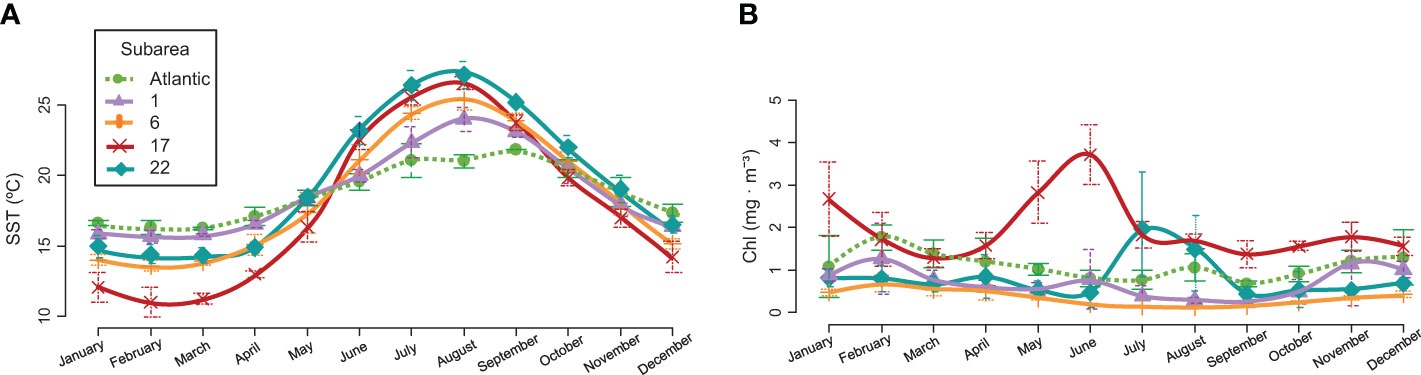
Figure 2 (A) Average sea surface temperature (SST, °C) and (B) chlorophyll concentration (Chl, mg · m-3) ± standard deviation by subarea in the period 2019 - 2021. Areas defined by the General Fisheries Commission for the Mediterranean (GFCM) as GSA 1 (violet): Northern Alboran Sea (Coast of Málaga), GSA 6 (orange): Northern Spain (Catalan Coast), GSA 17 (red): Northern Adriatic (Gulf of Trieste), and GSA 22 (turquoise): Aegean (Thermaikos Gulf), and the external sampling point in the Atlantic, as FAO division 27.9.a: Portuguese Waters – East (Southern Portugal and Gulf of Cádiz) (green and dotted). NOAA Optimum Interpolation (OI) SST V2 data provided by the NOAA PSL, Boulder, Colorado, USA, from their website at https://psl.noaa.gov, and NASA Goddard Space Flight Center, Ocean Ecology Laboratory, Ocean Biology Processing Group. Sea-Viewing Wide Fieldof-View Sensor (SeaWiFS) Ocean Color Data; In 2018 Reprocessing; NASA OB.DAAC: Greenbelt, MD, USA. Available online: https://oceancolor.gsfc.nasa.gov/, were used for SST and chlorophyll graphs, respectively.
2.2 Analysis of reproductive investment and phenology
The gonadosomatic index (, where WG ± 0.0001 g is the gonad weight, and WE ± 0.01 g is the eviscerated body weight) was calculated as an indirect method to indicate the reproductive investment (Somarakis et al., 2004; Brosset et al., 2016). Sex and reproductive developmental stage (Figure 3) were visually and macroscopically determined and confirmed by stereomicroscopy (Zeiss binocular magnifier) to classify the gonads according to the criteria developed by Brown-Peterson et al. (2011) into the following categories: immature (sexual maturity still not reached); developing (gametes begin to develop in gonads that start to grow); spawning capable (almost ready for reproduction); actively spawning (expelling the gametes); regressing (gonads almost empty of gametes); and regenerating (mature but reproductively inactive).
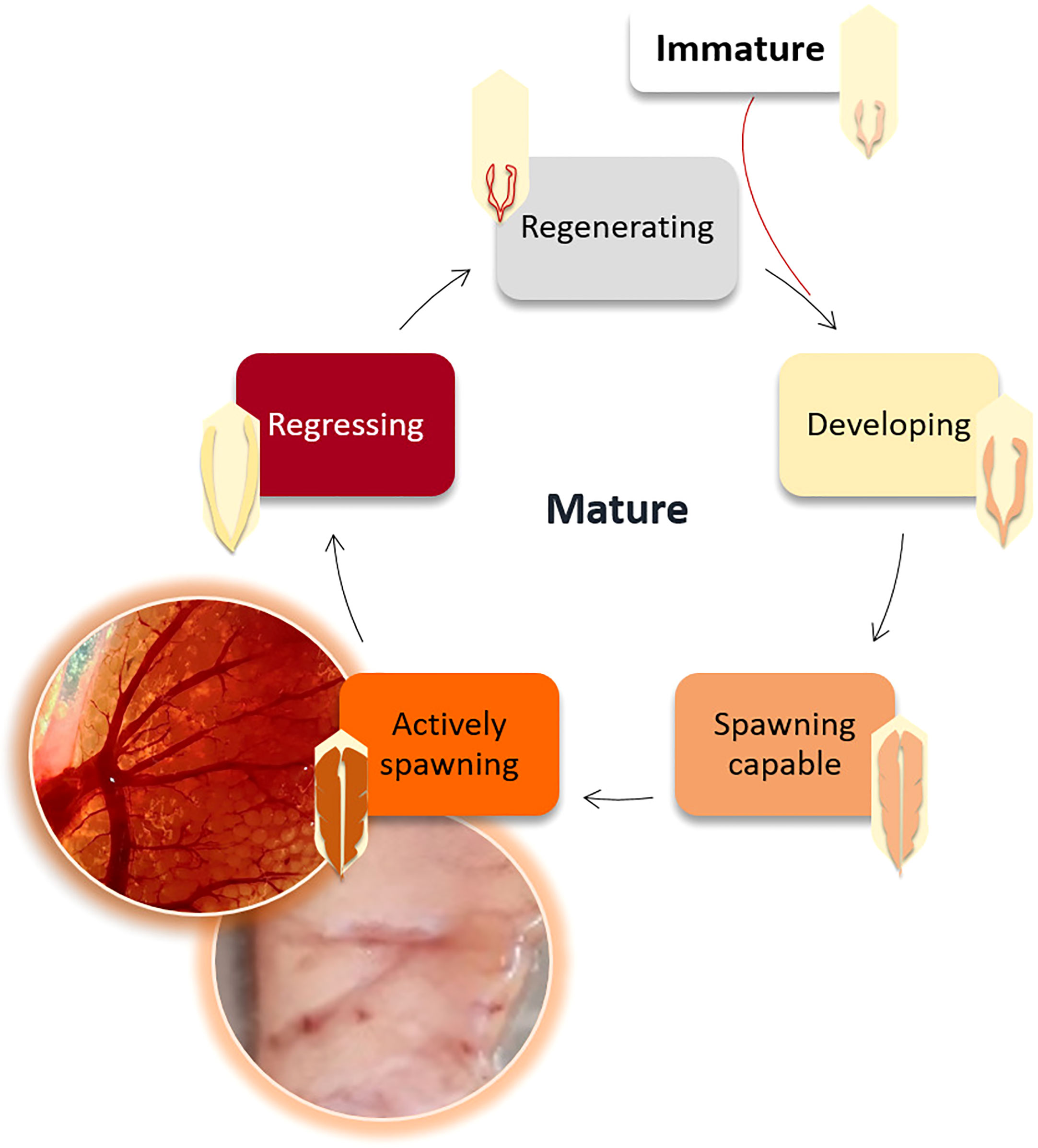
Figure 3 Reproductive cycle of the European sardine (Sardina pilchardus) according to the gonadal developmental stages defined by Brown-Peterson et al. (2011).
2.3 Somatic condition evaluation
Morphogravimetric values to estimate the body condition of sardines were collected by measuring length (total length, LT ± 0.1 cm) and weight (total body weight, WT ± 0.01 g; eviscerated body weight, WE ± 0.01 g). Liver (WL ± 0.0001 g) weight was also measured to calculate the hepatosomatic index ()
A morphophysiological approach for evaluating the general somatic condition was obtained by the calculation of the relative condition index (Kn) (Le Cren, 1951), considered higher-than-average when Kn exceeded 1 for a given individual and lower when it was below this value:
where Wr is defined as the predicted eviscerated weight of an individual of a given total length. α and β are coefficients obtained by the regression line of the logarithms of length and mass ( = 0.0037, = 3.2418). Moreover, total tissue fat content (i.e., muscle total lipids) was estimated by the average of both sides along the fish lateral line using a fish fat meter (Distell Model FM 992) (Kent, 1990) calibrated for the European sardine. In addition, a visual scale for fat mesenteric reserves (Van Der Lingen and Hutchings, 2005) was used.
2.4 Statistical analysis
Analyses were carried out using R software version 4.2.1 (R Development Core Team, 2018). Differences among categories were considered statistically significant if P < 0.05. Significance values are indicated as follows in the Results section: P < 0.05; < 0.01; < 0.001. When continuous dependent variables were involved, the Shapiro-Wilk test was applied to test the assumption of normality, and Levene’s test was executed to determine the homogeneity of variances (Zar, 1996) in all parameters. If both assumptions were met, a one-way analysis of variance (ANOVA) was performed. Conversely, when only the homoscedasticity assumption was violated, the data were analyzed with Welch’s t-test. For those parameters in which normal distribution was lacking but homoscedasticity was present, Kruskal-Wallis analysis of variance was applied. In the analyses of the indices without normal distribution and non-homogenous variances, and for which data transformation did not succeed in correcting the lack of normality or heteroscedasticity, a generalized linear model (GLM) with binomial error distribution and logit link function was carried out. When needed, multiple comparison or post-hoc tests (Tukey’s range test, Dunn’s method with Bonferroni adjustment, or Games-Howell test, when applicable) were applied to identify different categories. For qualitative dependent variables, Pearson’s chi-squared test was performed.
3 Results
3.1 Reproductive period analysis: spatial variability in reproductive phenology along the Mediterranean
The overall sex ratio by subarea (m/f) did not deviate significantly from the hypothetical 1/1 distribution at the Atlantic sampling point (X2 = 1.217, df = 1, P > 0.05), Northern Alboran (GSA 1) (X2 = 1.715, df = 1, P > 0.05), and Northern Spain (GSA 6) (X2 = 0.025, df = 1, P > 0.05) but differed in Northern Adriatic (GSA 17), with the highest number of females (292/411: X2 = 20.144, df = 1, P < 0.000), and Aegean Sea (GSA 22), with more males than females (335/281: X2 = 4.734, df = 1, P < 0.05). Spawning-capable sardines appeared during autumn in all the locations, with active spawners recorded in the Mediterranean subareas but not in the Atlantic in this season (samples from the early autumn) (Figure 4). The highest percentage of active spawners was observed during winter in all the subareas, with more than 80% of the individuals at this stage, although 87.56% of the spawners in the Northern Adriatic (GSA 17) were recorded in autumn. The spawning season was lengthened in time in the Atlantic and in the Atlantic-Mediterranean transition zone GSA 1, with a significant number of active individuals in both zones in spring (40.20 and 23.56%, respectively). Gonad maturation started to be observed in the summer in all the locations except in Alboran (GSA 1), with most individuals at the regenerating stage in this locality. Few immature individuals were recorded in winter in the Atlantic, in summer in Northern Alboran (GSA 1) and Northern Adriatic (GSA 17), and a larger number of them were obtained in spring and autumn in Northern Alboran (GSA 1) (5.28 and 5.94%, respectively), in spring and summer in Northern Spain (GSA 6) (17.27 and 9.52%, respectively), and in autumn in the Aegean Sea (GSA 22) (9.55%).
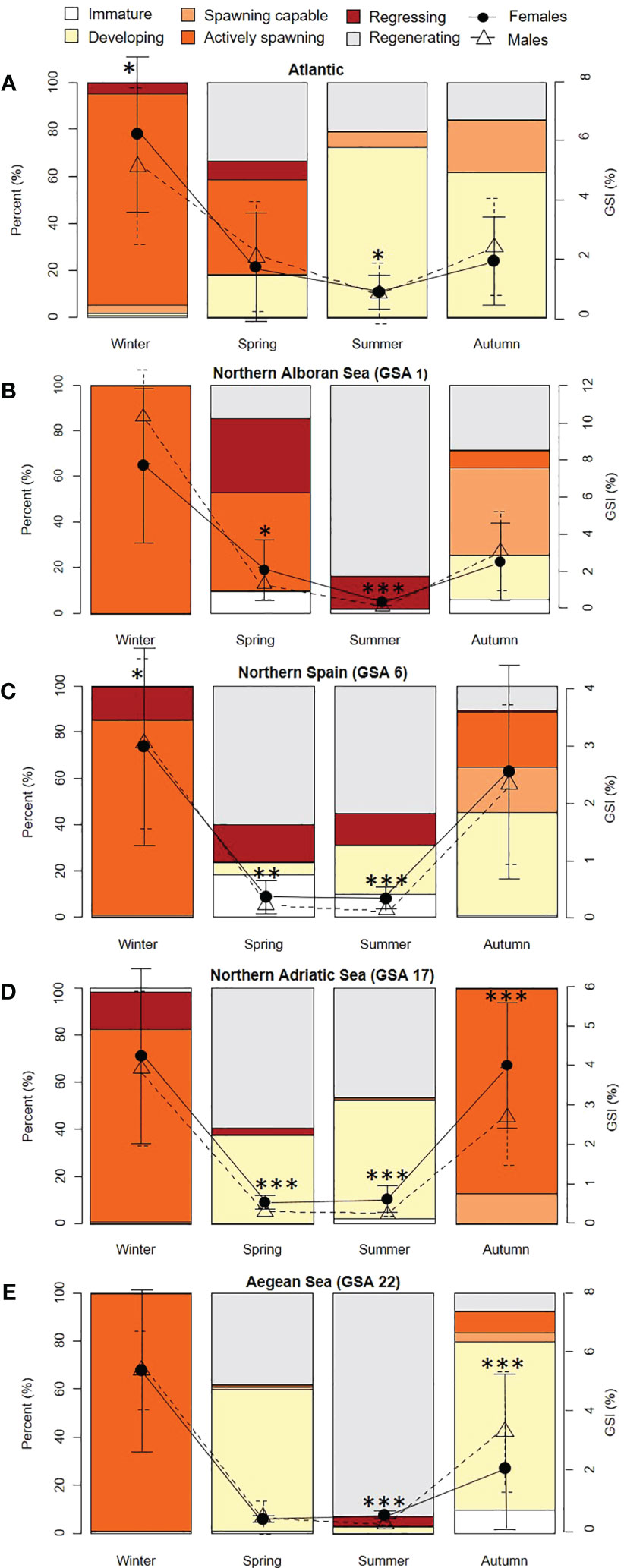
Figure 4 Seasonal reproductive analysis of European sardine (Sardina pilchardus) in the Atlantic stock (A) (FAO division 27.9.a: Portuguese Waters – East) and four Mediterranean GSAs ((B) Northern Alboran Sea (GSA 1); (C) Northern Spain (GSA 6); (D) Northern Adriatic Sea (GSA 17); (E) Aegean Sea (GSA 22)). Stages of the gonads are represented by the percentage (%) of individuals at each reproductive developmental phase (left y-axis), and mean GSI (%) ± SD (right y-axis). Females, solid line; males, dashed line. Significance values are indicated as *P < 0.05; **P < 0.01; ***P < 0.001. GSI data for each stock have been analyzed by sex to provide more information at the reproductive level by season.
A notable drop in GSI values was seen after winter, coinciding with the decrease in the number of active spawners, until reaching the minimum values in spring and summer and recovering afterward during autumn. When comparing GSI values among stocks, we observed marked differences throughout the cycle, except for immature individuals (NS) (observable in Supplementary Table 2 and in the scale of the right y-axes of Figure 4). In this regard, the lowest values observed in the actively reproductive stage in the Northern Spanish stock should be noted. Despite these significant differences among subareas along the reproductive cycle, differences between sexes in GSI by location were especially remarkable in the spring and summer, with significant female values above male values (Figure 4). Significant higher values for male individuals were only observed in autumn in the Aegean (GSA 22) (Kruskal-Wallis X2 = 19.906, df = 1, P < 0.001).
3.2 Energy storage and relative condition: differences along the Mediterranean distribution according to the reproductive cycle
Taking into consideration the adult and reproductive sardines (although the analysis of energy storage and relative condition was also performed for immature individuals, Figures 5A–C), the lowest tissue fat content values (Figures 5D, G, J, M, P) were generally found during active spawning (Figure 5J), accompanied by the lowest mesenteric fat values (Supplementary Table 2). In general, there was a continuous decrease in Kn from the developing stage to the actively spawning phase (Figures 5E, H, K). When individuals reached the regressing phase (Figure 5N), values started to increase, reaching the regenerating phase, as seen in the Kn medians by subarea, which exceeded the threshold value of 1. However, variations were detected among subareas throughout the reproductive cycle in relation to the time when individuals reached maximum somatic condition and their subsequent decline. The Atlantic sardines presented high condition values (especially reflected by the tissue fat content and Kn) in the developing and spawning capable stages. However, during the active spawning and regressing phases, these values were low (Supplementary Table 2 and Figures 5J–O) and, in particular, the lowest for Kn (F = 119.03, P < 0.001; and F = 7.946, P < 0.001, for both stages, respectively). Nonetheless, during gonad regeneration (Figures 5P–R), condition values in the Atlantic were the highest of the stocks analyzed for tissue fat content, Kn, and HSI. In the Alboran (GSA 1), the highest fat content value was recorded at the spawning capable stage, accompanied by a high Kn, reaching similar values to the Atlantic stock, despite having been lower during the previous gonadal phase (i.e., developing). During the active spawn and regression phases, GSA 1 individuals (together with the rest of the Mediterranean stocks in the latter phase) surpassed the Atlantic outgroup in body condition, but they were below this outgroup again during the regenerating phase. The Northern Spanish stock (GSA 6) presented the lowest Kn values along the whole reproductive cycle, accompanied by low tissue fat content values except in the developing and spawning capable stages. The Northern Adriatic (GSA 17) and the Aegean (GSA 22) individuals were similar regarding the Kn values (except in the developing stage, in which the Northern Adriatic had higher values). Nevertheless, despite the similarities in Kn during the spawning capable and active spawning phases (Figures 5H, K), tissue fat content differed between these two subareas in the regressing and regenerating phases, being higher in the Aegean but becoming lower in the developing phase (Figures 5G, J).
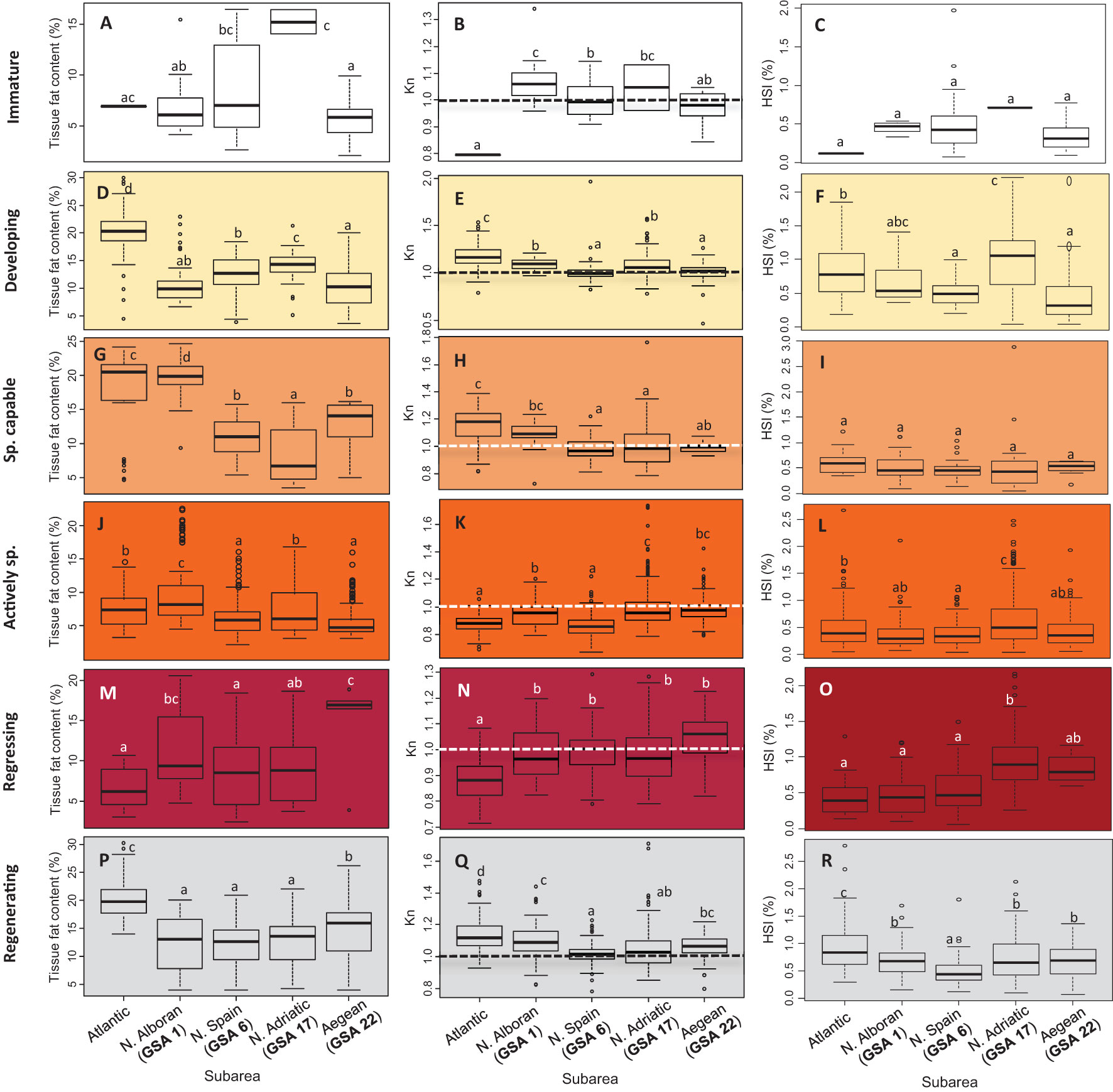
Figure 5 Tissue fat content (%) (A, D, G, J, M, P), relative condition factor (Kn) (B, E, H, K, N, Q), and hepatosomatic index (HSI, %) (C, F, I, L, O, R) by subregion (GSA) throughout the annual reproductive cycle. Different letters on the graphs indicate significant differences (P > 0.05) among stocks. Data of females and males were pooled due to the few differences between the sexes by stage in each stock. Dotted line indicated the threshold of higher-than-average condition in Kn. Atlantic stock (FAO division 27.9.a: Portuguese Waters – East); GSAs 1: Northern Alboran Sea, 6: Northern Spain, 17: Northern Adriatic Sea, 22: Aegean Sea.
3.3 Hepatosomatic index and its great divergence in the Northern Adriatic
The values of the hepatosomatic index (HSI) generally decreased immediately before the spawning capable phase and started to increase again at the regressing phase, although some differences were observed (Figure 6). The outgroup (Atlantic) and Alboran (GSA 1) individuals followed the same pattern, as HSI continued increasing at the regenerating phase (Figure 6), although significant differences in this increase from the regression to the regeneration phase were detected only in the Atlantic (F value = 30.48, P < 0.001) (Figures 5F, I, L, O, R). This trend was not recorded in the rest of the Mediterranean locations. The Atlantic and Alboran specimens differed only during the regenerating phase, with higher values in the Atlantic (Figure 5R). In contrast, sardines in the Northern Adriatic presented the highest significant HSI values during the active spawning phase (Figure 5L), surpassing even the Atlantic outgroup and the Alboran, as also observed at the subsequent regressing phase (Figure 5O). Moreover, a slight increase in HSI (despite not being significant) from the spawning capable to the active spawning stage was recorded in this location, which did not occur in the rest of the subareas (Figure 6). It is important to highlight these liver features in the Northern Adriatic because, even visually, the size of the livers relative to the fish was remarkable.
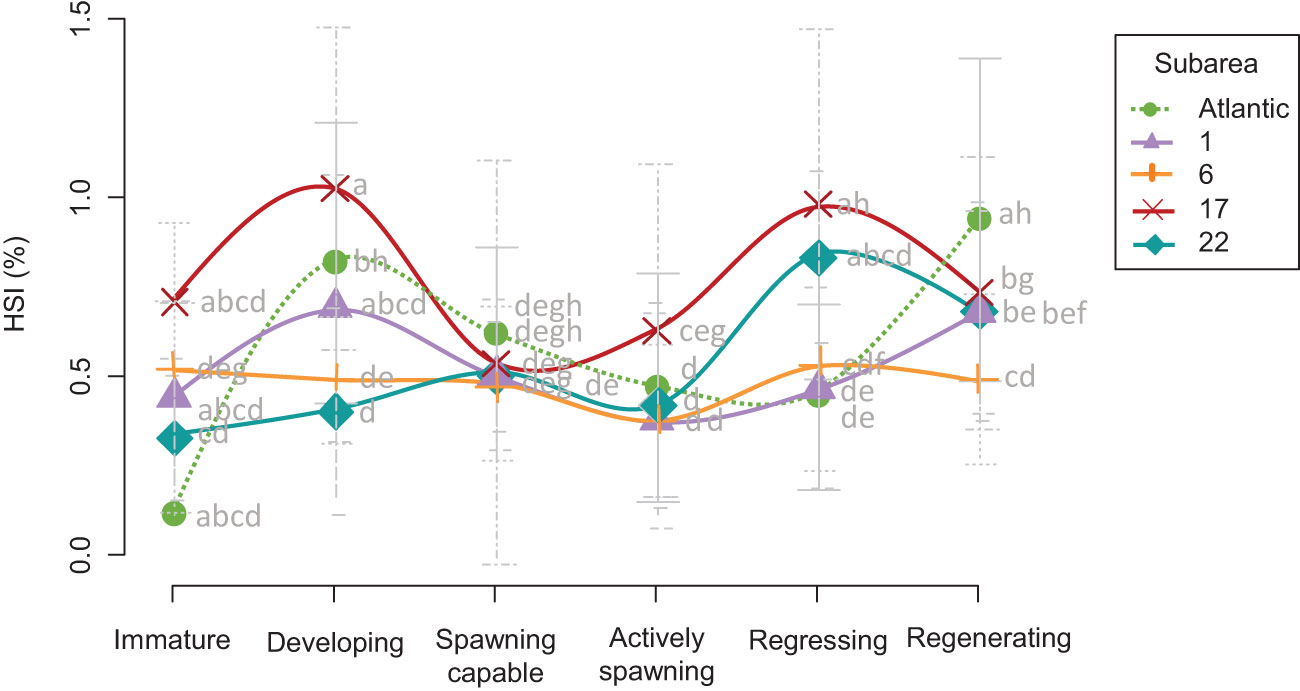
Figure 6 Hepatosomatic index (HSI) trend ± SD recorded in the five subareas analyzed in this study Atlantic (green and dotted); GSA 1 (violet): Northern Alboran; GSA 6 (orange): Northern Spain; GSA 17 (red): Northern Adriatic; GSA 22 (turquoise): Aegean Sea) throughout the reproductive cycle. Different letters on the graphs indicate significant differences (P > 0.05) among stocks. Atlantic stock (FAO division 27.9.a: Portuguese Waters – East); GSAs 1: Northern Alboran Sea, 6: Northern Spain, 17: Northern Adriatic Sea, 22: Aegean Sea.
4 Discussion
The life history traits of fish stocks are often modulated by unique environmental characteristics (i.e., combinations of physicochemical, biological factors, and human impacts that come together in different ways throughout the area of distribution), which influence the phenotypic plasticity of the species and different selective and adaptive processes. Focusing on somatic condition and energy storage, fish can exhibit substantial variation in energy density (energy per unit wet weight) within a species, mostly resulting from variation in the amount of stored lipids, with important ecological consequences (Martin et al., 2017). Environmental factors such as temperature variations may cause striking changes in internal biomass and energy allocation to permit the maintenance of a high level of biological activity, and the direction and magnitude of the effect vary substantially among populations (McManus and Travis, 1998). In this context, genetic variance in energy storage has been found among fish populations (Borowsky and Kallman, 1993). It is expected that these differences in body condition influence the transfer of energy to gonadal development, and therefore, different reproductive phenologies appear in species with marked reproductive seasons, as is the case with the European sardine.
In this regard, the present study shows the current spatial heterogeneity in the Mediterranean Sea in sardine reproduction and condition, as reflected by both the GSI and reproductive phenology, and the differences in energy storage in muscle, liver, and mesentery, as well as the relative condition index Kn according to the annual cycle. In this line, previous global analyses of the mean annual sardine body condition in the Mediterranean detected variability among locations during the period 1971-2016 (Brosset et al., 2017). Moreover, monthly local scale studies in this sea also revealed spatial variations for sardines in terms of body condition, fat content, reproduction indices and phenology, as well as stable isotopic values (Ganias et al., 2007; Frigola-Tepe et al., 2022; Lloret-Lloret et al., 2022).
In general, the stock with the lowest somatic condition parameters throughout the annual cycle was that from Northern Spain (GSA 6), which was also reflected in the reproductive potential (GSI does not exceed 3 in the actively spawning stage). Tissue fat content and Kn (only slightly surpassing 1 at the regenerating phase) were generally low before and after the breeding season, and HSI values were moderate throughout all the gonadal phases. In this area, located in the western Mediterranean, many studies have been carried out due to the changes that have been recorded in recent decades in sardine life-history traits and the fall in catches and biomass (e.g., Van Beveren et al., 2014; Albo-Puigserver et al., 2019). Studies point to changes in the marine environment, especially to the warming pattern in the entire Western Mediterranean Sea that occurred over the last 40 years at different depths and to a gradual salinity rise that might have substantial repercussions on the ocean currents and on the stability of the water column, with subsequent alterations of the nutrient supply from the deep layers to the photic zone (Quattrocchi, 2017). In addition, the effects of climate change on planktonic communities in the Western Mediterranean, including the Catalan Coast, are evidenced by the changes in the structure of the plankton communities and the metabolism of the ecosystem, favoring the smallest plankton (Calvo et al., 2011) and affecting forage species such as sardines. Furthermore, the disappearance of older individuals and truncation of the age structure have been observed in the area (Albo-Puigserver et al., 2021), limiting the ability of sardine to deal with environmental events and affecting the quantity and quality of sardine spawn (Brosset et al., 2016).
Conversely, the Northern Adriatic stock (GSA 17) shows a trend in which the body condition seems to be generally above the other stocks analyzed during the actively reproductive period. We speculate that this is because sardines in this area (obtained from the Gulf of Trieste, but results may be extrapolated to practically the entire north of the Adriatic basin due to the pelagic nature of the species (see Škrivanić and Zavodnik, 1973)) have accessible feeding resources all year round in the pelagic ecosystem (Conversi et al., 2009) (Figure 2B), mainly due to the geographical location and semi-enclosed basin (Brosset et al., 2017), in addition to the input of nutrients by rivers, making the area even more eutrophic at late winter-spring when sufficient inorganic nutrients are available to sustain the main diatom bloom of the year (Umani et al., 2012), and coinciding with the sardine reproductive season. In fact, the typical phytoplankton seasonal succession in the Northern Adriatic displays the following pattern: a late winter-early spring diatom bloom, followed by a nanophytoplankton bloom in late spring-early summer, a cyanobacteria peak in late summer-early autumn, and a second relative diatom maximum, usually in November (Cibic et al., 2018). Nevertheless, it must be considered that in the Gulf of Trieste, sardines feed mainly on copepods (56%), and phytoplankton never exceeds 10% of the prey (Borme et al., 2022), although the large primary production promotes the abundance of this zooplanktonic species. Furthermore, HSI showed the highest values and a different trend in the Northern Adriatic compared to the rest of the stocks. In the present study, we surprisingly observed large livers that started to grow before and during active reproduction, overlapping with this greater availability of food in the mid-autumn (spawning capable and active spawners) and winter-early spring seasons (active spawners). However, in the study of Ganias et al. (2007) performed in two highly oligotrophic seas (central Aegean and Ionian), the seasonality of spawning did not match the variations in HSI, although it was consistently higher in reproductively active females during the whole spawning period. In this sense, evidence indicates that the liver is not the main lipid storage compartment in sardines (Bandarra et al., 2018) but has some relation to body condition and feeding activity, playing a role in nutrient transfer between the different organs. The accumulation of fat around the gut (mesenteric fat) and within the muscle are the main lipid storage compartments to be allocated to reproduction (Nunes et al., 2011). This hypothesis may be reinforced by the fact that, in our study, it was observed that in stocks in which there is little availability of resources throughout the year, such as in the Aegean Sea, the HSI is higher during the regressing-regenerating phases, coinciding with a peak in production in this sea, and in Northern Spain, a flat trend in HSI is present during the whole annual cycle. In addition, in this area of the Northern Adriatic, the greatest temperature difference between the winter and summer months was recorded compared to other subareas, which can also alter the way energy is stored and allocated, as previously discussed, as well as trigger the reproductive period, reaching the spawning peak in autumn, the earliest of the analyzed times. Taking into account the relatively high body condition indices of the Adriatic stock in the reproductive season, we can conclude that the classification of the European sardine as a capital breeder would be conditioned by the population under study. Geographical location can determine changes in the feeding behavior of adult sardine (Rumolo et al., 2016), and as reported in the Adriatic, the lowest percentage of empty stomachs is detected in winter (Zorica et al., 2016) since the feeding intensity of sardine is higher when productivity is high (Nikolioudakis et al., 2011). Therefore, we propose that the storage period and mobilization of energy for reproduction in the Northern Adriatic are not as marked as they could be in other stocks. Thereby, our findings imply that, in addition to capitalized energy, sardine also uses current income to support reproduction (Ganias, 2009) and that the degree of dependence of reproduction on immediate reserves is closely linked to the availability of resources. These traits may be the result of local adaptation processes in the Adriatic population, which seems to differ genetically from other Mediterranean stocks (Caballero-Huertas et al., 2022a).
The Aegean stock (GSA 22) presented low values of somatic condition from the developing to active spawning period, but the recovery at the regressing phase was the largest of all the stocks analyzed in this study, as reflected by the tissue fat content and Kn values. In the regenerating phase, however, the Atlantic stock exceeded these values, although GSA 22 individuals showed the best condition parameters in the Mediterranean in this phase. In this aspect, a peak in primary productivity is recorded in the area during the summer, in which a large fraction of individuals in a state of regression were captured, so it is expected that these individuals are feeding intensely at this time in the area and, therefore, present better parameters, to which a high HSI value similar to that of the Adriatic (GSA 17) is added. The rising temperatures since spring, turning Aegean (GSA 22) into the subarea with the warmest summers and autumns analyzed, do not seem to affect the body condition of the sardine in that time window. In fact, sardines at the Thermaikos Gulf are further selective for warm waters (Giannoulaki et al., 2005), which shows the great plasticity of this species throughout its distribution range, although it may be associated with the greater availability of resources during this time period.
Northern Alboran sardines followed a similar reproductive seasonality as Atlantic individuals, coinciding with their closeness in space to this area. However, an earlier maturation of the gonads was recorded in summer in the Atlantic, although no active spawners were present in autumn, as was observed in the Alboran in this season. As proposed in a recent work, we hypothesize that Atlantic individuals enter Mediterranean waters when they are able to spawn and are triggered by a drop in temperature along the Alboran coast, which could favor the establishment of the important sardine nursery grounds along the northern coast of the Alboran Sea, Málaga, and Almería Bay (Caballero-Huertas et al., 2022c), resulting in the overlapping of stocks in GSA 1. Despite their similarities regarding reproductive phenology, we could observe differences regarding energy storage and condition along the reproductive cycle. During the spawning capable phase, the relative condition (Kn) was similar in both stocks, although the energy stored in the muscles was slightly higher in the Alboran stock. From this time forward in the reproductive cycle, better somatic condition was observed in the Alboran zone in terms of both indices, although in the state of regeneration, the Atlantic individuals were able to recover better and more quickly. In addition, a common HSI pattern was shared, with no significant differences between stocks except in the regeneration state, which was higher in the Atlantic. This relatively low body condition during the actively spawning phase in the Atlantic is possibly linked to a higher allocation of resources for reproduction (one of the highest GSI values recorded in this study, 5.23 ± 2.46, Supplementary Table 2). Moreover, the fastest recovery during regeneration may be due to the early energy acquisition and reserve recruitment facilitated in an area with a continued nutrient input (Caballero-Huertas et al., 2022c). These reasons could be key to understanding why sardine abundance is generally lower in the Mediterranean than in Atlantic waters (Silva et al., 2008). The spatiotemporal analysis of sardine health status for different Mediterranean areas (Brosset et al., 2017) revealed a drastic decrease in the body condition and maximum size of the specimens captured between 1975 and 2015 in most areas analyzed, with the exception of the Northern Alboran Sea (GSA 1), where the body condition notably improved from 2010 on, although Albo-Puigserver et al. (2021) began to see signs of decline. Our results showed that Alboran is the Mediterranean subarea in which the highest condition values are generally recorded throughout the annual cycle. This could be due to the potential genetic variability and plasticity induced by the overlapping of Atlantic and Alboran individuals in this locality (Albo-Puigserver et al., 2021; Caballero-Huertas et al., 2022c). Moreover, the temperatures in this Mediterranean subarea are more favorable for this species with a cool-temperate affinity, with less marked seasonal variations than in the other localities.
4.1 Some applications to fisheries management
Due to the importance of body condition in the health status of sardine stocks and its direct influence on recruitment, the monitoring of this species from the reproductive framework (related to its annual cycle) is essential as a way to contextualize its status from a biologically relevant perspective. Moreover, the different reproductive phenologies among stocks suggest that the closed periods during the reproductive season must be established according to the features of each stock. In several subareas, closed seasons do not completely overlap with the spawning capable-actively spawning phases, which can be problematic for the recruitment and health of the stocks. This occurs, for example, in the Northern Adriatic (GSA 17), as the closed season in August (Farrugio & Soldo, 2014) does not protect the spawning peak of sardines in the area according to our results, from November to February. In this context, the Recommendation GFCM/42/2018/8 (GFCM, 2018) on further emergency measures in 2019-2021 for small pelagic stocks in the Adriatic Sea called for considering these temporary restrictions according to the spawning season. Likewise, this study evidences the heterogeneity in stocks of the same species, which calls for management measures to be established respecting the particularities of each stock but taking into account the potential connectivity of some of them (Atlantic stocks of the Gulf of Cadiz-South of Portugal and Alboran). In this regard, our proposal is to assess and manage the southern area of Portugal - Southwestern Spain [Southern European Stock, ICES Subareas 27.8.c and 27.9.a, fished by Spain and Portugal (ICES, 2017)] as a co-shared stock with the GFCM, considering GSAs 1, 2, and 3 combined. This would even be potentially useful for other species in which connectivity may play a fundamental role, such as the European hake (Merluccius merluccius) and the blackspot seabream (Pagellus bogaraveo) (García-Lafuente et al., 2021). Furthermore, the status of sardines in Northern Spain (GSA 6) must be monitored, and the establishment of protection measures for this stock is of particular interest. In addition to the changes in the planktonic composition in this area of the Western Mediterranean related to warming (Calvo et al., 2011), excessive fishing pressure would have contributed to the decrease in their size and condition, following the line of the individuals from the Gulf of Lion (GSA 7) (Saraux et al., 2019), located northward, with the practically total closure of the sardine fisheries currently. In this sense, Ramírez et al. (2021) noted a lower sensitivity of the southernmost small pelagic community to the simultaneous impacts of climate and fisheries compared to the northernmost locations, although that study already mentioned the possible early warning sign for future declines southward, which may be currently reflected by our results.
Due to the dynamism of environmental conditions accentuated by global change, especially in the Mediterranean region, the recurrence of this type of analysis with environmental discussion is essential. In fact, life history traits that rapidly respond to environmental changes, such as body condition, are good indicators to foresee future population declines (Lloret et al., 2012; Albo-Puigserver et al., 2021). Including reproductive and body condition data into stock or ecosystem assessment approaches and models is a step that has begun to be taken in the field of fisheries and marine management (“next-generation forecasting in marine ecosystems”), as it provides valuable insight (Lloret et al., 2012; Bolin et al., 2021). In this way, the assessment approaches are more comprehensive, as they are also based on information related to the physiology of the fish rather than information and projections on distribution alone (Bolin et al., 2021). In this sense, the data presented in this study could be useful in an ecosystem-based management framework and in all new assessment methods that can contribute to sustainable catches. Furthermore, it is important to incorporate new stocks to understand the status of the species in a global way. Future studies should evaluate stocks in areas such as the North Sea, Mediterranean and Atlantic African coasts, and the eastern-most Mediterranean Sea, to continue building knowledge on the status of this important fishery resource.
Data availability statement
The raw data supporting the conclusions of this article will be made available by the authors, without undue reservation.
Ethics statement
Ethical review and approval was not required for the animal study because we only analyze dead individuals from commercial fishing.
Author contributions
MC-H analyzed the samples and results and wrote the original manuscript, XF-T contributed to sardines’ analysis and reviewed the manuscript, and JV and MM helped in the design, supervised the research, and directed the project under which this study is framed. All authors contributed to the article and approved the submitted version.
Funding
We want to express our gratitude to the funding support of the Spanish Ministry of Science, Innovation and Universities (RTI2018-097544-B-I00, I+D+i Retos de Investigación; TED2021-132521-B-I00 and ‘Nex GenerationEU’/PRTR), and the Agency for Management of University and Research Grants (AGAUR) of the Generalitat de Catalunya (Ajuts per a la contractacio de personal investigador novell (FI-2020)).
Acknowledgments
Thank you to Sònia Lara, Carla Fernández, and Clara Bautista for their help in the analysis of the samples.
Conflict of interest
The authors declare that the research was conducted in the absence of any commercial or financial relationships that could be construed as a potential conflict of interest.
Publisher’s note
All claims expressed in this article are solely those of the authors and do not necessarily represent those of their affiliated organizations, or those of the publisher, the editors and the reviewers. Any product that may be evaluated in this article, or claim that may be made by its manufacturer, is not guaranteed or endorsed by the publisher.
Supplementary material
The Supplementary Material for this article can be found online at: https://www.frontiersin.org/articles/10.3389/fmars.2023.1187071/full#supplementary-material
References
Albo-Puigserver M., Bellido J. M., Colmenero A. I., Giráldez A., Hidalgo M., Pennino M. G., et al. (2019). Historical changes in life-history traits of small pelagic fish in the western Mediterranean Sea along a latitudinal gradient. Available at: http://hdl.handle.net/10261/205320.
Albo-Puigserver M., Muñoz A., Navarro J., Coll M., Pethybridge H., Sánchez S., et al. (2017). Ecological energetics of forage fish from the Mediterranean Sea: seasonal dynamics and interspecific differences. Deep Sea Res. Part II: Topical Stud. Oceanography 140, 74–82. doi: 10.1016/j.dsr2.2017.03.002
Albo-Puigserver M., Pennino M. G., Bellido J. M., Colmenero A. I., Giráldez A., Hidalgo M., et al. (2021). Changes in life history traits of small pelagic fish in the western Mediterranean Sea. Front. Mar. Sci. 8. doi: 10.3389/fmars.2021.57035
Albo-Puigserver M., Sánchez S., Coll M., Bernal M., Sáez-Liante R., Navarro J., et al. (2020). Year-round energy dynamics of sardine and anchovy in the north-western Mediterranean Sea. Mar. Environ. Res. 159, 105021. doi: 10.1016/j.marenvres.2020.105021
Bandarra N. M., Marçalo A., Cordeiro A. R., Pousão-Ferreira P. (2018). Sardine (Sardina pilchardus) lipid composition: does it change after one year in captivity? Food Chem. 244, 408–413. doi: 10.1016/j.foodchem.2017.09.147
Bolin J. A., Schoeman D. S., Evans K. J., Cummins S. F., Scales K. L. (2021). Achieving sustainable and climate-resilient fisheries requires marine ecosystem forecasts to include fish condition. Fish Fisheries 22 (5), 1067–1084. doi: 10.1111/faf.12569
Borme D., Legovini S., de Olazabal A., Tirelli V. (2022). Diet of adult sardine sardina pilchardus in the gulf of Trieste, northern Adriatic Sea. J. Mar. Sci. Eng. 10 (8), 1012. doi: 10.3390/jmse10081012
Borowsky R., Kallman K. (1993). Genetic variation of fat and glycogen storage in xiphophorus variatus (Pisces; poeciliidae). Comp. Biochem. Physiology–Part A: Physiol. 105 (3), 579–586. doi: 10.1016/0300-9629(93)90437-9
Brosset P., Fromentin J. M., Ménard F., Pernet F., Bourdeix J. H., Bigot J. L., et al. (2015). Measurement and analysis of small pelagic fish condition: a suitable method for rapid evaluation in the field. J. Exp. Mar. Biol. Ecol. 462, 90–97. doi: 10.1016/j.jembe.2014.10.016
Brosset P., Fromentin J. M., Van Beveren E., Lloret J., Marques V., Basilone G., et al. (2017). Spatio-temporal patterns and environmental controls of small pelagic fish body condition from contrasted Mediterranean areas. Prog. oceanography 151, 149–162. doi: 10.1016/j.pocean.2016.12.002
Brosset P., Lloret J., Muñoz M., Fauvel C., Van Beveren E., Marques V., et al. (2016). Body reserves mediate trade-offs between life-history traits: new insights from small pelagic fish reproduction. R. Soc. Open Sci. 3 (10), 160202. doi: 10.1098/rsos.160202
Brown-Peterson N. J., Wyanski D. M., Saborido-Rey F., Macewicz B. J., Lowerre-Barbieri S. K. (2011). A standardized terminology for describing reproductive development in fishes. Mar. Coastal Fisheries 3 (1), 52–70.
Caballero-Huertas M., Frigola-Tepe X., Coll M., Muñoz M., Viñas J. (2022a). The current knowledge status of the genetic population structure of the European sardine (Sardina pilchardus): uncertainties to be solved for an appropriate fishery management. Rev. Fish Biol. Fisheries 1-19. doi: 10.1007/s11160-022-09704-z
Caballero-Huertas M., Frigola-Tepe X., Viñas J., Muñoz M. (2022b). Somatic condition and reproductive potential as a tandem in European sardine: an analysis with an environmental perspective in the northern Adriatic (Gulf of Trieste). Fishes 7 (3), 105. doi: 10.3390/fishes7030105
Caballero-Huertas M., Vargas-Yánez M., Frigola-Tepe X., Viñas J., Muñoz M. (2022c). Unravelling the drivers of variability in body condition and reproduction of the European sardine along the Atlantic-Mediterranean transition. Mar. Environ. Res., 105697. doi: 10.1016/j.marenvres.2022.105697
Calvo E., Simó R., Coma R., Ribes M., Pascual J., Sabatés A., et al. (2011). Effects of climate change on Mediterranean marine ecosystems: the case of the Catalan Sea. Climate Res. 50 (1), 1–29. doi: 10.3354/cr01040
Carpi P., Scarcella G., Cardinale M. (2017). The saga of the management of fisheries in the Adriatic Sea: history, flaws, difficulties, and successes toward the application of the common fisheries policy in the Mediterranean. Front. Mar. Sci. 4. doi: 10.3389/fmars.2017.00423
Cibic T., Cerino F., Karuza A., Fornasaro D., Comici C., Cabrini M. (2018). Structural and functional response of phytoplankton to reduced river inputs and anomalous physical-chemical conditions in the gulf of Trieste (northern Adriatic Sea). Sci. Total Environ. 636, 838–853. doi: 10.1016/j.scitotenv.2018.04.205
Coll M., Albo-Puigserver M., Navarro J., Palomera I., Dambacher J. M. (2019). Who is to blame? plausible pressures on small pelagic fish population changes in the northwestern Mediterranean Sea. Mar. Ecol. Prog. Ser. 617, 277–294. doi: 10.3354/meps12591
Colloca F., Scarcella G., Libralato S. (2017). Recent trends and impacts of fisheries exploitation on Mediterranean stocks and ecosystems. Front. Mar. Sci. 4. doi: 10.3389/fmars.2017.00244
Conversi A., Peluso T., Fonda-Umani S. (2009). Gulf of Trieste: a changing ecosystem. J. Geophysical Research: Oceans 114 (C3). doi: 10.1029/2008JC004763
Corrales X., Coll M., Tecchio S., Bellido J. M., Fernández Á.M., Palomera I. (2015). Ecosystem structure and fishing impacts in the northwestern Mediterranean Sea using a food web model within a comparative approach. J. Mar. Syst. 148, 183–199. doi: 10.1016/j.jmarsys.2015.03.006
Cury P., Bakun A., Crawford R. J., Jarre A., Quinones R. A., Shannon L. J., et al. (2000). Small pelagics in upwelling systems: patterns of interaction and structural changes in “wasp-waist“ ecosystems. ICES J. Mar. Sci. 57 (3), 603–618. doi: 10.1006/jmsc.2000.0712
De Roos A. M., Persson L., McCauley E. (2003). The influence of size-dependent life-history traits on the structure and dynamics of populations and communities. Ecol. Lett. 6 (5), 473–487. doi: 10.1046/j.1461-0248.2003.00458.x
Devine J. A., Wright P. J., Pardoe H. E., Heino M. (2012). Comparing rates of contemporary evolution in life-history traits for exploited fish stocks. Can. J. Fisheries Aquat. Sci. 69 (6), 1105–1120. doi: 10.1139/f2012-047
Di Biagio V., Cossarini G., Salon S., Solidoro C. (2020). Extreme event waves in marine ecosystems: an application to Mediterranean Sea surface chlorophyll. Biogeosciences 17 (23), 5967–5988. doi: 10.5194/bg-17-5967-2020
Dimarchopoulou D., Tsikliras A. C. (2022). Linking growth patterns to sea temperature and oxygen levels across European sardine (Sardina pilchardus) populations. Environ. Biol. Fishes 1-11. doi: 10.1007/s10641-022-01229-5
Doney S. C., Ruckelshaus M., Emmett Duffy J., Barry J. P., Chan F., English C. A., et al. (2012). Climate change impacts on marine ecosystems. Annu. Rev. Mar. Sci. 4, 11–37. doi: 10.1146/annurev-marine-041911-111611
Estrada M. (1996). Primary production in the northwestern Mediterranean. Scientia Marina 60 (S2), 55–64.
FAO (2020). “The state of world fisheries and aquaculture 2020,” in Sustainability in action (Rome: European Union). doi: 10.4060/ca9229en
FAO (2022). “The state of world fisheries and aquaculture 2022,” in Towards blue transformation. doi: 10.4060/cc0461en
Farrugio H., Soldo A. (2014). “Status and conservation of fisheries in the Adriatic Sea,” in Draft internal report for the purposes of the Mediterranean regional workshop to facilitate the 7-11 April 2014 (UNEP-MAP-RAC/SPA 2014) (Malaga, Spain: UNEP.Description of Ecologically or Biologically Significant Marine Areas).
Fréon P., Cury P., Shannon L., Roy C. (2005). Sustainable exploitation of small pelagic fish stocks challenged by environmental and ecosystem changes: a review. Bull. Mar. Sci. 76 (2), 385–462.
Frigola-Tepe X., Caballero-Huertas M., Viñas J., Muñoz M. (2022). Influence of thermal regimes on the relationship between parasitic load and body condition in European sardine along the Catalan coast. Fishes 7 (6), 358. doi: 10.3390/fishes7060358
Ganias K. (2009). Linking sardine spawning dynamics to environmental variability. Estuarine Coast. Shelf Sci. 84 (3), 402–408. doi: 10.1016/j.ecss.2009.07.004
Ganias K., Somarakis S., Koutsikopoulos C., Machias A. (2007). Factors affecting the spawning period of sardine in two highly oligotrophic seas. Mar. Biol. 151 (4), 1559–1569. doi: 10.1007/s00227-006-0601-0
Ganias K., Somarakis S., Nunes C. (2014). “Chapter 3 reproductive potential,” in Biology and ecology of sardines and anchovies. Ed. Ganias K. (Boca Raton, FL, USA: CRC Press), 79–121.
García-Lafuente J., Sánchez-Garrido J. C., García A., Hidalgo M., Sammartino S., Laiz R. (2021). “Biophysical processes determining the connectivity of the alboran Sea fish populations,” in Alboran Sea-ecosystems and marine resources. Eds. Báez J. C., Vázquez J. T., Camiñas J. A., Malouli Idrissi M. (Cham: Springer International Publishing), 459–487. doi: 10.1007/978-3-030-65516-7_12
García-Martínez M. C., Vargas-Yáñez M., Moya F., Santiago R., Reul A., Muñoz M., et al. (2019). Spatial and temporal long-term patterns of phyto and zooplankton in the w-mediterranean: radmed project. Water 11, 534. doi: 10.3390/w11030534
Garrido S., Ben-Hamadou R., Oliveira P. B., Cunha M. E., Chícharo M. A., van der Lingen C. D. (2008). Diet and feeding intensity of sardine sardina pilchardus: correlation with satellite-derived chlorophyll data. Mar. Ecol. Prog. Ser. 354, 245–256. doi: 10.3354/meps07201
GFCM (2018). Recommendation GFCM/42/2018/8 on further emergency measures in 2019-2021 for small pelagic stocks in the Adriatic Sea (geographical subareas 17 and 18) (GFCM) (Brussels (Belgium): European Union).
Giannoulaki M., Machias A., Somarakis S., Tsimenides N. (2005). The spatial distribution of anchovy and sardine in the northern Aegean Sea in relation to hydrographic regimes. Belgian J. Zoology 135 (2), 151.
Halpern B. S., Walbridge S., Selkoe K. A., Kappel C. V., Micheli F., d'Agrosa C., et al. (2008). A global map of human impact on marine ecosystems. Science 319 (5865), 948–952. doi: 10.1126/science.1149345
ICES. (2017). Report of the working group on southern horse mackerel, anchovy and sardine (WGHANSA). (Ref. ICES CM 2017/ACOM:17). ICES/CIEM—International council for the exploration of the sea/conseil international pour l’Exploration de la Mer. Available at: https://archimer.ifremer.fr/doc/00415/52665/.
Kent M. (1990). Hand-held instrument for fat/water determination in whole fish. Food Control 1 (1), 47–53.
King J. R., McFarlane G. A. (2003). Marine fish life history strategies: applications to fishery management. Fisheries Manage. Ecol. 10 (4), 249–264. doi: 10.1046/j.1365-2400.2003.00359.x
Le Cren E. D. (1951). The length-weight relationship and seasonal cycle in gonad weight and condition in the perch (Perca fluviatilis). J. Animal Ecol., 201–219.
Leitão F., Alms V., Erzini K. (2014). A multi-model approach to evaluate the role of environmental variability and fishing pressure in sardine fisheries. J. Mar. Syst. 139, 128–138. doi: 10.1016/j.jmarsys.2014.05.013
Lejeusne C., Chevaldonné P., Pergent-Martini C., Boudouresque C. F., Pérez T. (2010). Climate change effects on a miniature ocean: the highly diverse, highly impacted Mediterranean Sea. Trends Ecol. Evol. 25 (4), 250–260. doi: 10.1016/j.tree.2009.10.009
Liang Z., Sun P., Yan W., Huang L., Tang Y. (2014). Significant effects of fishing gear selectivity on fish life history. J. Ocean Univ. China 13, 467–471. doi: 10.1007/s11802-014-2167-7
Lloret J., Faliex E., Shulman G. E., Raga J. A., Sasal P., Muñoz M., et al. (2012). Fish health and fisheries, implications for stock assessment and management: the Mediterranean example. Rev. Fisheries Sci. 20 (3), 165–180. doi: 10.1080/10641262.2012.695817
Lloret J., Serrat A., Thordarson G., Helle K., Jadaud A., Bruno I., et al. (2021). The poor health of deep-water species in the context of fishing activity and a warming climate: will populations of molva species rebuild or collapse? J. Fish Biol. 98 (6), 1572–1584. doi: 10.1111/jfb.14347
Lloret J., Shulman G., Love R. M. (2013). Condition and health indicators of exploited marine fishes (Oxford, UK: John Wiley & Sons).
Lloret-Lloret E., Albo-Puigserver M., Giménez J., Navarro J., Pennino M. G., Steenbeek J., et al. (2022). Small pelagic fish fitness relates to local environmental conditions and trophic variables. Prog. Oceanography 202, 102745. doi: 10.1016/j.pocean.2022.102745
Marbà N., Jordà G., Agustí S., Girard C., Duarte C. M. (2015). Footprints of climate change on Mediterranean Sea biota. Front. Mar. Sci. 2. doi: 10.3389/fmars.2015.00056
Martin B. T., Heintz R., Danner E. M., Nisbet R. M. (2017). Integrating lipid storage into general representations of fish energetics. J. Anim. Ecol. 86 (4), 812–825. doi: 10.1111/1365-2656.12667
Martín P., Sabatés A., Lloret J., Martin-Vide J. (2012). Climate modulation of fish populations: the role of the Western Mediterranean oscillation (WeMO) in sardine (Sardina pilchardus) and anchovy (Engraulis encrasicolus) production in the north-western Mediterranean. Climatic Change 110 (3), 925–939. doi: 10.1007/s10584-011-0091-z
McBride R. S., Somarakis S., Fitzhugh G. R., Albert A., Yaragina N. A., Wuenschel M. J., et al. (2015). Energy acquisition and allocation to egg production in relation to fish reproductive strategies. Fish Fisheries 16, 23–57. doi: 10.1111/faf.12043
McManus M. G., Travis J. (1998). Effects of temperature and salinity on the life history of the sailfin molly (Pisces: poeciliidae): lipid storage and reproductive allocation. Oecologia 114 (3), 317–325. doi: 10.1007/s004420050453
Muñoz M., Casadevall M. (2002). Reproductive indices and fecundity of helicolenus dactylopterus dactylopterus (Teleostei: scorpaenidae) in the Catalan Sea (western Mediterranean). J. Mar. Biol. Assoc. U. K. 82 (6), 995–1000. doi: 10.1017/S0025315402006513
Nikolioudakis N., Palomera I., Machias A., Somarakis S. (2011). Diel feeding intensity and daily ration of the sardine sardina pilchardus. Mar. Ecol. Prog. Ser. 437, 215–228. doi: 10.3354/meps09275
Nunes C., Silva A., Soares E., Ganias K. (2011). The use of hepatic and somatic indices and histological information to characterize the reproductive dynamics of Atlantic sardine sardina pilchardus from the Portuguese coast. Mar. Coast. Fisheries 3 (1), 127–144. doi: 10.1080/19425120.2011.556911
Palomera I., Olivar M. P., Salat J., Sabatés A., Coll M., García A., et al. (2007). Small pelagic fish in the NW Mediterranean Sea: an ecological review. Prog. Oceanography 74 (2-3), 377–396. doi: 10.1016/j.pocean.2007.04.012
Parrish R. H., Serra R., Grant W. S. (1989). The monotypic sardines, sardina and sardinops: their taxonomy, distribution, stock structure, and zoogeography. Can. J. Fisheries Aquat. Sci. 46 (11), 2019–2036. doi: 10.1139/f89-251
Popescu I. (2018). “Multiannual plan for small pelagic fish stocks in the Adriatic Sea,” in EPRS European parliamentary research service(Brussels, Belgium), 1–8.
Quattrocchi F. (2017). Modelling the relationships of medium and long-term variations of the anchovy and sardine catches in the Catalan Sea (NW Mediterranean) with the environmental drivers. Dissertation/thesis. Editor: Universitat Politècnica de Catalunya.
Quattrocchi F., Maynou F. (2017). Environmental drivers of sardine (Sardina pilchardus) in the Catalan Sea (NW Mediterranean Sea). Mar. Biol. Res. 13 (9), 1003–1014. doi: 10.1080/17451000.2017.1331039
Ramírez F., Pennino M. G., Albo-Puigserver M., Steenbeek J., Bellido J. M., Coll M. (2021). SOS Small pelagics: a safe operating space for small pelagic fish in the Western Mediterranean Sea. Sci. Total Environ. 756, 144002. doi: 10.1016/j.scitotenv.2020.144002
R Development Core Team (2018). R: a language and environment for statistical computing (Vienna, Austria: R Found. Stat. Comput.), 3–900051-07-0.
Rizzi J., Torresan S., Critto A., Zabeo A., Brigolin D., Carniel S., et al. (2016). Climate change impacts on marine water quality: the case study of the northern Adriatic Sea. Mar. Pollut. Bull. 102 (2), 271–282. doi: 10.1016/j.marpolbul.2015.06.037
Rumolo P., Bonanno A., Barra M., Fanelli E., Calabrò M., Genovese S., et al. (2016). Spatial variations in feeding habits and trophic levels of two small pelagic fish species in the central Mediterranean Sea. Mar. Environ. Res. 115, 65–77. doi: 10.1016/j.marenvres.2016.02.004
Şenbahar A. M., Güleç Ö., Tosunoğlu Z., Özaydin O. (2020). Length-weight relationship of the most landed pelagic fish species European pilchard (Sardina pilchardus walbau) and European anchovy (Engraulis encrasicolus linnaeu) in the izmir bay (Aegean Sea, Turkey) purse seine fishery. Mar. Sci. Technol. Bull. 9 (1), 32–37. doi: 10.33714/masteb.673318
Saraux C., Van Beveren E., Brosset P., Queiros Q., Bourdeix J. H., Dutto G., et al. (2019). Small pelagic fish dynamics: a review of mechanisms in the gulf of lions. Deep Sea Res. Part II: Topical Stud. Oceanography 159, 52–61. doi: 10.1016/j.dsr2.2018.02.010
Serrat A., Muñoz M. (2022). Can the life history trait divergence of two extremes of a cold-water genus distribution offer evidence for their vulnerability to Sea warming? Fishes 7 (4), 143. doi: 10.3390/fishes7040143
Silva A., Carrera P., Massé J., Uriarte A., Santos M. B., Oliveira P. B., et al. (2008). Geographic variability of sardine growth across the northeastern Atlantic and the Mediterranean Sea. Fisheries Res. 90 (1-3), 56–69. doi: 10.1016/j.fishres.2007.09.011
Silva A., Santos M. B., Caneco B., Pestana G., Porteiro C., Carrera P., et al. (2006). Temporal and geographic variability of sardine maturity at length in the northeastern Atlantic and the western Mediterranean. ICES J. Mar. Sci. 63 (4), 663–676. doi: 10.1016/j.icesjms.2006.01.005
Sinovčić G., Keč V.Č., Zorica B. (2008). Population structure, size at maturity and condition of sardine, sardina pilchardus (Walb), in the nursery ground of the eastern Adriatic Sea (Krka river estuary, Croatia). Estuarine Coast. Shelf Sci. 76 (4), 739–744. doi: 10.1016/j.ecss.2007.07.037
Škrivanić A., Zavodnik D. (1973). Migrations of the sardine (Sardina pilchardus) in relation to hydrographical conditions of the Adriatic Sea. Netherlands J. Sea Res. 7, 7–18. doi: 10.1016/0077-7579(73)90028-8
Somarakis S., Nikolioudakis N. (2007). Oceanographic habitat, growth and mortality of larval anchovy (Engraulis encrasicolus) in the northern Aegean Sea (eastern Mediterranean). Mar. Biol. 152 (5), 1143–1158. doi: 10.1007/s00227-007-0761-6
Somarakis S., Ganias K., Tserpes G., Koutsikopoulos C. (2004). Ovarian allometry and the use of the gonosomatic index: a case study in the Mediterranean sardine, Sardina pilchardus. Mar. Biol. 146, 181–189.
Tsikliras A. C., Koutrakis E. T. (2013). Growth and reproduction of European sardine, sardina pilchardus (Pisces: clupeidae), in northeastern Mediterranean. Cahiers Biologie Mar. 54 (3), 365–374
Umani S. F., Malfatti F., Del Negro P. (2012). Carbon fluxes in the pelagic ecosystem of the gulf of Trieste (Northern Adriatic Sea). Estuarine Coast. Shelf Sci. 115, 170–185. doi: 10.1016/j.ecss.2012.04.006
Van Beveren E., Bonhommeau S., Fromentin J. M., Bigot J. L., Bourdeix J. H., Brosset P., et al. (2014). Rapid changes in growth, condition, size and age of small pelagic fish in the Mediterranean. Mar. Biol. 161 (8), 1809–1822. doi: 10.1007/s00227-014-2463-1
Van Der Lingen C. D., Hutchings L. (2005). Estimating the lipid content of pelagic fish in the southern benguela by visual assessment of their mesenteric fat. Afr. J. Mar. Sci. 27 (1), 45–53. doi: 10.2989/18142320509504067
Vargas-Yáñez M., García-Martínez M. C., Moya F., Balbín R., López-Jurado J. L., Serra M., et al. (2017). Updating temperature and salinity mean values and trends in the Western Mediterranean: the RADMED project. Prog. Oceanography 157, 27–46. doi: 10.1016/j.pocean.2017.09.004
Vargas-Yáñez M., García-Martínez M. C., Moya F., López-Jurado J. L., Serra M., Santiago R., et al. (2019). The present state of marine ecosystems in the Spanish Mediterranean in a climate change context. Grupo Mediterráneo Cambio Climático pp, 1–185).
Keywords: capital breeder, European sardine, fisheries, gonadal stage, health status, HSI, small pelagic
Citation: Caballero-Huertas M, Frigola-Tepe X, Viñas J and Muñoz M (2023) From west to east: Heterogeneity in the life history traits of a small pelagic fish (Sardina pilchardus) throughout the Mediterranean. Front. Mar. Sci. 10:1187071. doi: 10.3389/fmars.2023.1187071
Received: 15 March 2023; Accepted: 08 June 2023;
Published: 05 July 2023.
Edited by:
Yunyan Deng, Chinese Academy of Sciences (CAS), ChinaReviewed by:
Olav Sigurd Kjesbu, Institute of the Marine Research, NorwayAlberto Santojanni, National Research Council (CNR), Italy
Copyright © 2023 Caballero-Huertas, Frigola-Tepe, Viñas and Muñoz. This is an open-access article distributed under the terms of the Creative Commons Attribution License (CC BY). The use, distribution or reproduction in other forums is permitted, provided the original author(s) and the copyright owner(s) are credited and that the original publication in this journal is cited, in accordance with accepted academic practice. No use, distribution or reproduction is permitted which does not comply with these terms.
*Correspondence: Marta Muñoz, bWFydGEubXVueW96QHVkZy5lZHU=
†Present addresses: Marta Caballero-Huertas, CIRAD, UMR ISEM, Montpellier, France;ISEM, Université de Montpellier, CIRAD, CNRS, IRD, EPHE, Montpellier, France