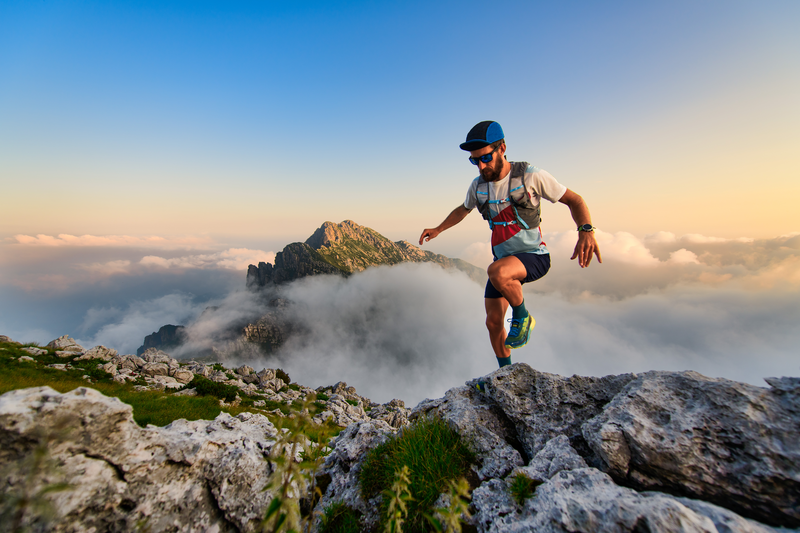
95% of researchers rate our articles as excellent or good
Learn more about the work of our research integrity team to safeguard the quality of each article we publish.
Find out more
ORIGINAL RESEARCH article
Front. Mar. Sci. , 21 June 2023
Sec. Marine Fisheries, Aquaculture and Living Resources
Volume 10 - 2023 | https://doi.org/10.3389/fmars.2023.1185898
This article is part of the Research Topic Offshore Aquacultural Engineering and Its Interaction with the Marine Environment View all 18 articles
Sebases schlegelii is an important economic reef fish in northern China. Its distribution in the reef area is affected by the reef structure and flow field. To evaluate the effects of differently shaped artificial reefs with distinct flow field properties on the distribution of adult S. schlegelii, four types of artificial reefs (cubic (CAR), pyramidal (PAR), star-shaped (SAR) and tubular (TAR) artificial reef) and the related flow regimes were experimentally examined. The mean gathering rate (MGR) and wavelet analysis were adopted to analyze the distribution patterns of S. schlegelii adults around reef bodies in three representative environmental conditions (static water (FE1), 40 Hz water flow environment (FE2), and 50 Hz water flow environment (FE3)). The significant gathering behaviors around the artificial reefs were depicted through the MGR of adult fish during physical observations. For FE1, the MGR of adult fish showed a decrease in the order PAR > CAR > SAR > TAR. With increasing incoming velocities, the MGR changed such that the quantity of adult fish in the CAR gradually increased more than that in the PAR, but no significant differences were observed between them (P>0.05). Wavelet analysis showed that the fluctuations in the gathering behaviors of adults decreased with increasing flow velocities. Based on the two-factor analysis of variance, the reef structural characteristics had a more significant impact than the flow velocities on the gathering behavior of adult fish. In summary, the PAR and CAR are recommended to restore the habitats of S. schlegelii in marine ranches given their dominant aggregation effects on S. schlegelii.
Marine life habitats have been destroyed by the negative effects of anthropogenic pressures, such as pollutant emissions and overfishing, leading to massive declines in natural fisheries (Wilson et al., 2010). Fish are the single most important source of high-quality protein for humans, providing 16% of the animal protein consumed by the world’s population (Tidwell and Allan, 2001). Therefore, marine habitat restoration is receiving increasing attention. Artificial reefs are widely used in the United States, Australia, Europe and Asia and are regarded as effective measures for marine habitat restoration and fishery resource conservation (Lima et al., 2019). Differently shaped artificial reefs released into the sea can provide shelter, refuge and additional ecosystem services for organisms in open environments and have been shown to be of great value to many fish and invertebrate species (Komyakova et al, 2013; Lima et al., 2019). Recent studies have shown that artificial reefs can create productive habitats in certain systems (Claisse et al., 2014; Layman et al., 2016; Smith et al., 2016). They can be effective at adding fish rather than just attracting nearby fish from rocky reefs (Folpp et al., 2020). However, Baine (2001) reports that only approximately 50% of studies have found evidence of successful outcomes after deployment of artificial reefs. This may be related to the coverage area of artificial reefs and the complexity of artificial reef structures (Lemoine et al., 2019). Studies have shown that artificial reefs have a larger number of sheltering holes and more abundance and diversity of fish (Hixon and Beets, 1989; Hackradt et al., 2011). The design of artificial reefs has a significant impact on their effectiveness (Vivier et al., 2021). Fully understanding the influence of artificial reef structure on the behavioral distribution of fish can help improve artificial reef design and marine ranch construction.
To design artificial reef structures that can provide better habitats for fish, extensive research has been conducted on flow field effects (Zheng et al., 2015; Jiao et al., 2017; Ma et al., 2022). The materials (Brown, 2005; Sempere-Valverde et al., 2018), shapes (Vivier et al., 2021) and distances (Froehlich et al., 2021) of artificial reefs have also been investigated aiming to improve their ecological effects. Fish behavior is a direct response to the environment (Martins et al., 2012), and by observing fish distribution around artificial reefs, understanding the positive and negative behaviors of fish in reef selection can help develop better artificial reef design and conservation strategies. Underwater camera observation is a common method to study the biological benefits of reefs, as it allows to collect data over long time scales while avoiding human disturbance factors. It is useful for collecting valuable information regarding the distribution, relative abundance, and species–habitat associations of various fish species (Easton et al., 2015). Parsons et al. (2016) used drop cameras to study the effects of temperate rocky reef habitats on fish abundance and species composition. Li et al. (2021) studied the effects of artificial reefs and the flow field environment on the habitat selection behavior of juvenile scorpionfish Sebastes schlegelii with an underwater camera system, and the results showed that there were significant differences in fish aggregation around different reef structures.
Sebastes schlegelii is an economically important rocky reef fish that is mainly distributed in China, Japan and the eastern and western coasts of the Korean Peninsula (Xi et al., 2017). artificial reefs have considerable effects on the aggregation of rocky reef fishes (Folpp et al., 2020). Adult S. schlegelii are sexually mature individuals in the population, with reproductive functions. In industrial fishing, a large number of adult fish will be caught, resulting in a sharp decrease in their numbers in the population. Stock enhancement is an effective way to protect fish biodiversity. In China, millions of juvenile fish are released every year to restore and increase the population. Releasing populations will cause a series of negative effects on wild populations (Lorenzen et al., 2010), which is not conducive to the sustainable development of fisheries. A comprehensive understanding of the influence of reef structure on the spatial behavior of S. schlegelii will indeed maximize the positive consequence of the conservation of S. schlegelii.
The goal of our study was to determine the attraction of artificial reefs with different structures to adult S. schlegelii and the influence of flow field environment on this attraction. This was achieved by placing artificial reefs with different structures in indoor deep concrete pools that can create different flow field environments and using underwater cameras to observe the distribution of adult S. schlegelii. These observed data were used to calculate the mean gathering rate and perform wavelet analysis. Furthermore, two-way ANOVA was used to analyze the influence of reef structure and flow field environment on S. schlegelii distribution. Our results may contribute to the design of artificial reefs for the conservation of specific fishes and the selection of reef types for marine ranch construction.
Adults S. schlegelii were obtained from a local commercial aquaculture farm and kept in a holding tank for 48 h. After temporary rearing, 48 adult fish with no apparent deformities or injuries and strong constitution were selected for the experiment. The average body length was 30 ± 0.5 cm, and the average weight was 565 ± 28 g. To reduce the influence of the external environment on fish behavior, no oxygenation and no baiting were used during the experiment or resting period.
In this experiment, four types of reefs were selected according to their structures from the commonly used reef types in the construction of marine ranches in China. They were cube-shaped artificial reef (CAR), pyramid-shaped artificial reef (PAR), star-shaped artificial reef (SAR) and tube-shaped artificial reef (TAR). The outer dimensions of the CAR was 50 cm×50 cm×50 cm (length×width×height), and the diameter of the surface hole was 30 cm (Figure 1A). The outer dimensions of the PAR was 63 cm×63 cm×58 cm (length×width×height), and the height of the pyramid structure was 50 cm (Figure 1B). The SAR had a height of 50 cm and a blade width of 21 cm (Figure 1C). The TAR was 50 cm in length and 50 cm in diameter (Figure 1D).
Figure 1 Detailed parameters for the artificial reef models tested in this study. (A) The cube-shaped artificial reef; (B) The pyramid-shaped artificial reef; (C) The star-shaped artificial reef; (D) The tube-shaped artificial reef.
The experiment was carried out in a disinfected indoor concrete pool. The pool size was 7 m×7 m×2 m (length×width×height), and the experimental depth was 1.2 m (Figure 2). To facilitate observation, the bottom of the pool was painted blue with environmentally friendly aquaculture paint. To facilitate statistics, the pool was divided into 196 square areas with environmentally friendly red aquaculture paint, where the side of each square area was 0.5 m, and each area was numbered as shown in Figure 2. Four submersible pumps for flow generation were installed at the bottom corners of the square pool (1-1,1-E, E-1 and E-E). The four reef placement positions are shown in Figure 2. Four floodlights were placed in the upper corner of the pool for illumination during observation. A panoramic camera was mounted on the roof beam approximately 2 m above the experimental pool, and 8 color underwater cameras were fixed near the reef fish in the pool. The video signal acquisition system comprised the camera, a router and a computer, which could monitor and record the behavior and distribution of experimental fish in the pool without interference (Figure 2).
Figure 2 Schematic diagram showing the experimental pool, where the shaded part is the experimental area.
Four submersible pumps were used to create a flow field by adjusting the electric control system outside the pool to control the flow of seawater and then adjusting the different flow rates of seawater. Three types of flow field environment were tested in the experiment. When the working frequency of the submersible pump was 0 Hz, the hydrostatic environment was named FE1; when the working frequency of the submersible pump was 40 Hz, the flow field environment was named FE2; and when the working frequency of the submersible pump was 50 Hz, the higher flow field environment was named FE3. Before the experiment, 14 key points were measured by an electromagnetic current meter. The S1–S13 velocity measuring points are shown in Figure 2, and key point S14 was located inside the CAR reef.
Before the experiment, we used a multiparameter water quality detector to measure the water environment parameters. The measured results are temperature=11°C, dissolved oxygen=8.04 mg/L, pH=7.02 and salinity=31.83‰.
Throughout the experiment, there was no feeding, oxygenation or natural light to avoid interfering with the behavior of the fish. During the experiment, four lamps with a power of 15 W were used for lighting. The experiment was divided into three experimental conditions according to different flow field environments, namely, FE1, FE2 and FE3. The observation time of each experiment was from 8:00 in the morning to 18:00 in the evening. Continuous video signals were collected throughout the experiment, and photos were taken every 10 min.
The MGR refers to the ratio between the quantity of scorpionfish gathered around a reef at a fixed time point in the experiment and the total quantity of scorpionfish in the experiment: (Li et al., 2021)
where Mi is the quantity of fish aggregated at a fixed time point in hour i, M is the total quantity of S. schlegelii adults in the experiment, and n is the number of adult fish recorded.
In this study, python wavelet analysis was used to analyze the fluctuation in the quantity of adult fish attracted to the artificial reef at different times and obtain the wavelet power spectrum (Qin et al., 2016; Li et al., 2021).
All statistical analyses were performed using SPSS 20.0 for Windows. One-way analysis of variance (ANOVA) was used to distinguish significant differences among different structures and flow field environments, and differences were considered statistically significant at P<0.05. Two-way ANOVA was used to evaluate reef structure and flow field environment, which were the key environmental factors affecting habitat selection of adult S. schlegelii, and extremely significant factors were considered statistically significant at P<0.001.
According to the video observations, under the static water environment (FE1), adult fish swam quickly to the wall after being placed in the experimental pool. At this time, no adult fish entered the reef area, and they are all distributed near the wall and in the dark area. After 8 min, a few adult fish tentatively swam around the reef. After approximately 1 h of adaptation, the adult fish around the reef were frequently active, and most of the adult fish freely shuttled around the four types of reefs (Figure 3A). After 2 h, most of the adults began to swim freely in the boundary area between the four reefs and the pool and chased each other (Figure 3B). At the same time, the quantity of adults in the interior of the reef and the shaded area gradually tended to be stable.
Figure 3 Fish behavior captured by panoramic camera in experimental pool. (A) The state of the fish after 1h in the experimental pool under FE1; (B) The state of the fish after 2h in the experimental pool under FE1; (C) The fish gather in the middle blank area enclosed by the four reefs under FE3.
In the flow field environments FE2 and FE3, due to the influence of the flow generating equipment and flow field, the adult fish showed a significant stress response when the flow generating equipment was started, swimming rapidly from the pool wall to the vicinity of the reef and gathering in large numbers in the middle blank area enclosed by the four reefs(Figure 3C). After 1 h of adaptation, some adult fish began to frequently move around the four reefs and near the pool wall.
According to one-way ANOVA, in the static water environment (FE1), the MGR of adult S. schlegelii showed a decreasing trend in the order PAR (MGR=38.31 ± 6.02%) > CAR (MGR=35.03 ± 6.66%) > SAR (MGR=15.14 ± 4.60%) > TAR (MGR=11.52 ± 4.68%). Under the FE2 condition, the MGR showed the same trend as FE1. The MGR in the PAR was the highest at 42.53 ± 6.86% and it was the lowest in the SAR at 11.54 ± 5.35%. There was a significant difference between the MGR of adult fish of the PAR and CAR (P<0.05), while the MGR was not significantly different between the TAR and SAR (P>0.05). In the FE3 flow environment, there was no significant difference in MGR between the PAR and CAR (P>0.05), and there was a significant difference in MGR between the TAR and SAR (P<0.05). The MGRs of reefs with the same structure in the three different flow environments were also different (Figure 4). While the PAR showed no significant difference in MGR among the three flow environments (P>0.05), there were significant differences in MGR in the other three reef types between the standing water and flowing water environments (P<0.05). This indicates that the PAR has a significant advantage in attracting adult fish and has the strongest stability, while the attraction degree of the other reefs to adult fish is greatly affected by the flow field environment.
Figure 4 Significant difference analysis of Sebastes schlegelii adults attracted by four different ARs under the same flow field environment. *Different letters (a, b, c & ab) over the bar for the same reef type indicate significant differences among flow field environments (P<0.05).
By comparing the MGR of adult fish inside and outside the four types of reefs, it was found that the aggregation of adult fish inside and outside the reefs was different in the three types of flow field environments. In the static water environment (FE1), there were significant differences in the MGR of adult fish in and out of all reefs except the CAR (P<0.05). The MGR outside the PAR was higher than that inside the PAR, while the opposite was true for the TAR and SAR. Compared with the static water environment (FE1), in the flow environments (FE2 and FE3), the MGR inside the CAR gradually decreased and that outside of it gradually increased, while the MGR inside the PAR increased significantly and that outside of it decreased. The MGRs of the adult fish inside and outside the reefs were significantly different (P<0.05). The MGRs inside the TAR and SAR decreased significantly, but there was no significant difference in the distribution of adult fish in and out of the TAR and SAR in FE3 (P>0.05). Overall, as the flow rate increased, the adults tended to cluster outside the CAR and inside the PAR (Figure 5).
Figure 5 Comparison of differences in the distribution of Sebastes schlegelii adults inside and outside the reefs. *Different letters (a, b, c & ab) over the bars for the same reef type indicate significant differences in the mean gathering rate (MGR) inside and outside the reef (P<0.05).
The amount of aggregation of S. schlegelii adults in different reef structures was analyzed by wavelet analysis, and the variation characteristics of aggregation were studied (Figure 6). The results of the wavelet analysis show that in FE1, the number of adult fish in the CAR had three significant periods, from 8:00 to 12:00, 12:00 to 14:00 and 16:00 to 18:00. At 12:00, the continuity of the small wave spectrum was the strongest and fluctuated greatly. During 16:00~18:00, the continuity of the small wave spectrum was poor; that is, the number of adult fish changed little and tended to be stable during this period. The population of adults around the PAR showed the strongest continuity at 12:00, and the population of adult fish changed significantly. From 14:00 to 18:00, the continuity of the small wave spectrum was the worst; that is, the distribution of adult fish in the reef area fluctuated the least. In the TAR, the continuity of the wavelet spectrum was relatively strong at 12:00, and it was poor at the rest time, so the stability of adult fish aggregation was the strongest throughout the day. The continuity of the SAR wavelet spectrum was weak throughout the day, and the population of adult fish changed most stably during 8:00~10:00 and 14:00~18:00. In FE2, the CAR had the strongest wavelet continuity at 14:00. The PAR had poor continuity of the wavelet spectrum throughout the day. The TAR had the worst spectral continuity throughout the day, with little change. In FE3, the spectral continuity of the CAR was strong and fluctuated significantly from 14:00 to 16:00, and it was the worst from 12:00 to 14:00; that is, the population of adult fish fluctuated the least during this period. In the PAR, the spectrum continuity was good during 14:00~16:00, that is, the fluctuation was relatively large. The distribution of adult fish in the TAR was the least and the most stable. The continuity of the SAR wavelet spectrum was strong during 12:00~16:00. The wavelet spectrum analysis showed that the continuity of the adult fish spectrum in different reef areas in the flow field environment was weaker than that in the standing water environment, and the aggregation of adult fish was more stable compared to the standing water environment.
Figure 6 Time-frequency and frequency-domain change periods in the quantity of Sebastes schlegelii adults in different artificial reefs *: The conical range represents the effective value; the closed region of the black solid line is the power spectrum value tested by the standard background spectrum with 95% confidence; the color bar on the right represents the wavelet power spectrum; the larger the value, the stronger the continuity of the wavelet spectrum.
The effects of reef structure and flow field environment on the attraction of S. schlegelii adults were studied by two-factor ANOVA (Table 1). The effects of the reef structure and flow field environment factors and their interaction were all extremely significant (P<0.001). These results indicate that the two factors have significant effects on the MGR of S. schlegelii adults, and the reef structure had a stronger effect than the flow field environment, indicating that reef structure has the strongest effect on the aggregation of S. schlegelii adults.
Table 1 Analysis results of the influence of flow field environment and reef structure on MGR of S. schlegelii adults.
The main results of this study show that (1) all four types of artificial reef can attract S. schlegelii adults, but there are significant differences in their effects. The PAR and CAR, with relatively complex structures and more holes, have stronger attracting effects on fish, while the SAR and TAR, with relatively simple structures and fewer holes, have poor attracting effects. (2) The flow field has a significant influence on the fish-attracting effect of the four reef types. The MGR trend of the four reef types in the standing water environment (FE1) was PAR > CAR > SAR > TAR. The MGRs of the CAR and PAR increased, while the MGRs of the SAR and TAR decreased with increasing incoming flow velocity. Affected by the flow field, the adult fish aggregated around the SAR and TAR were driven to the PAR and CAR. (3) The flow field also has a significant effect on the MGRs inside and outside of the reefs. In FE1, there was no significant difference in the MGRs inside and outside of the CAR, and the MGR outside of the PAR was higher than that inside of it. Due to the influence of the flow field, the MGR inside of the CAR decreased and that outside increased, while the PAR showed the opposite trend, that is, the MGR inside increased while that outside MGR decreased. (4) In contrast with the SAR and TAR, the quantity of adult fish in the PAR and CAR showed higher wavelet spectrum continuity and more considerable fluctuations, and their diurnal variation trend was higher. Wavelet analysis showed that the fluctuations in the gathering behaviors of adults decreased with increasing flow velocities. (5) Based on the two-factor ANOVA, reef structure and flow field environment have significant effects on the MGR, and the reef structure had a more significant impact than the flow field environment on the gathering behavior of adult fish. Since fish behavior around artificial reefs can be affected by dissolved oxygen levels, light conditions, unsuitable temperatures, forced proximity to humans and feeding opportunities (Martins et al., 2012), to ensure the reliability of our results, we did not provide food, oxygenation, or natural light throughout the experiment to avoid disturbing fish behavior.
Countershading refers to a type of body color with a dark back and light belly, which can prevent biological detection by predators or bait to achieve camouflage and stealth (Ruxton et al., 2004). Sebastes schlegelii have a blackish brown back, irregular dark black patches and a grayish white belly. This coloration is conducive to enemy avoidance and feeding. Through video observation, we noticed an interesting phenomenon in which S. schlegelii adults were mainly distributed at the bottom of the PAR, inside and outside of the CAR and SAR, and inside and on both sides of the TAR, which are all shaded areas, because the shadows match the body color, allowing them to hide. Many marine organisms exhibit behaviors in which they actively select backgrounds that match their species, morphology, or individual horizontal appearance (Ryer et al., 2008; Stevens and Merilaita, 2009; Morgans and Ord, 2013), and some even change their body color and brightness to quickly match the background (Johnsen et al., 2004; Smithers et al., 2018). Sebastes schlegelii cannot quickly change its color to match the background environment (Leclercq et al., 2010), so it can only achieve shading by actively selecting the shaded area, which is more conducive to hiding. In the experiment, we also found that S. schlegelii moved rapidly to the shaded area of the pool wall when transferred to the experimental pool. This behavior is also observed in juvenile fish (Li et al., 2021). Therefore, the shaded area is an important factor affecting the distribution behavior of S. schlegelii. Both juvenile (Li et al., 2021) and adult S. schlegelii exhibit background matching behavior, and appropriate shading and material color should be considered in reef design.
All four reef types had aggregation effects on adult and juvenile S. Schlegelii (Table 2), but the aggregation effects are quite different in standing water environments. The PAR had the best aggregation effect on adults but a poor aggregation effect on juveniles, while the CAR had a good aggregation effect on both adults and juveniles. This is related to the area of the artificial reef, the number of holes, the size of the holes and fish living habits. Species richness and H’ diversity are higher in large-area artificial reefs, and the number of holes is the most important factor for reef fish (Hackradt et al., 2011). In addition, the size of the hole is related to the shelter effect provided, and relatively large holes are less effective in protecting small fish from predators (Jordan et al, 2005). Juvenile scorpionfish tend to be social, large in number and occupy a large space, while adult scorpionfish tend to be solitary, but the individuals are large, so the CAR, with a good shelter effect and large internal holes, had a good aggregation effect on both juvenile and adult scorpionfish. Compared with the other reefs, the PAR has more holes but less space, which is not conducive to the movement of young fish but can provide a individual shelter for adult fish. Although the TAR also has large holes, it had a poor aggregate effect on adults and juveniles (Table 2) because its holes are a straight-through structure with easy penetration, which is not conducive to hiding and shading S. schlegelii. Moreover, S. schlegelii will mark their territories and attack other fish entering their field of view (Xu et al., 2021). Visual barriers provided by artificial reefs can reduce aggressive behavior (Zhang et al., 2023). A simple structure such as the TAR cannot provide visual shelter, while a complex structure such as the PAR can provide more shelter space. Sebastes schlegelii adults preferred this structure, which is relatively enclosed and has enough room to fit their bodies.
Table 2 Average MGR of Sebastes schlegelii adults and juveniles (Li et al, 2021) in different reef areas under three flow rates.
Water flow will affect the distribution of fish around artificial reefs by increasing their swimming energy consumption and feeding patterns (Clarke et al., 2009). The biological responses of fish to water flow and turbulence are species-specific (Wang et al., 2021). This is related to the size, swimming ability and swimming pattern of the fish (Finelli et al., 2009). Bottom-dwelling fish will move closer to the sidewall to reduce energy costs when velocity increases (Wiegleb et al., 2020). In this study, increased flow rates were found to drive adults aggregating in the SAR and TAR to the PAR and CAR. This is due to the low flow rate around the PAR and CAR (Table 3), which is conducive to lower swimming energy expenditure. The current not only affects the MGR of adult fish among reef types but also has a significant effect on the MGR inside and outside the reefs. Upwelling and eddies will occur outside the CAR (Ma et al., 2022), and the flow field characteristics inside the PAR are more complex due to the presence of disks. It is possible that the flow field characteristics in these ARs are more conducive to attracting S. schlegelii adults. Although studies have shown that the wake regions generated by artificial reefs may have a positive impact on fish aggregation (Song et al., 2022). However, it is necessary to further study which flow field characteristics are more favorable to S. schlegelii. Some studies have found that fish reefs with complex structures are more attractive to fish aggregation (Lemoine et al., 2019), which is consistent with our research results. The PAR and CAR, with relatively complex structures, showed better aggregation effects on S. schlegelii. This may be because reefs with complex structures can provide more holes and habitat space and have more complex flow field environment.
In conclusion, there are differences in reef type selection between juvenile and adult S. schlegelii, but the PAR and CAR have good aggregation effects and flow field environment, which makes them suitable for placing in northern coastal areas of China. The shaded area, number and size of holes, flow field environment and complexity of the artificial reef structure are important factors determining the distribution of S. schlegelii, which should be taken into account in the design of reefs. The results of our study can provide guidance for managers and conservation planners for reef type selection when installing artificial reefs to restore natural habitats and protect reef fish.
The raw data supporting the conclusions of this article will be made available by the authors, without undue reservation.
The animal study was reviewed and approved by Institutional Animal Care and Use Committee of the Yellow Sea Fisheries Research Institute (Qingdao, China).
PG participated in the experimental design, data processing and manuscript writing. JL participated in the experimental design, project administration and funding acquisition. GW and CG contributed to subsequent revisions. ZM and YJ contributed to the experiment and data analysis. All authors contributed to the article and approved the submitted version.
This study is supported by the National Natural Science Foundation of China (32002442) and the National Key R&D Program (2019YFD0902101).
The authors declare that the study was conducted in the absence of any commercial or financial relationships that could be construed as a potential conflict of interest.
All claims expressed in this article are solely those of the authors and do not necessarily represent those of their affiliated organizations, or those of the publisher, the editors and the reviewers. Any product that may be evaluated in this article, or claim that may be made by its manufacturer, is not guaranteed or endorsed by the publisher.
Baine M. (2001). Artificial reefs: a review of their design, application, management and performance. Ocean Coast. Manage. 44 (3-4), 241–259. doi: 10.1016/s0964-5691(01)00048-5
Brown C. J. (2005). Epifaunal colonization of the loch linnhe artificial reef: influence of substratum on epifaunal assemblage structure. Biofouling 21 (2), 73–85. doi: 10.1080/08927010512331344197
Claisse J.T., Pondella D.J. II, Love M., Zahn L.A., Williams C.M., et al. (2014). Oil platforms off California are among the most productive marine fish habitats globallyle. Proc. Natl. Acad. Sci. USA 111(43), 15462-15467. doi: 10.1073/pnas.1411477111
Clarke R. D., Finelli C. M., Buskey E. J. (2009). Water flow controls distribution and feeding behavior of two co-occurring coral reef fishes: II. laboratory experiments. Coral Reefs 28 (2), 475–488. doi: 10.1007/s00338-009-0479-7
Easton R. R., Heppell S. S., Hannah R. W. (2015). Quantification of habitat and community relationships among nearshore temperate fishes through analysis of drop camera video. Mar. Coast. Fisheries 7 (1), 87–102. doi: 10.1080/19425120.2015.1007184
Finelli C. M., Clarke R. D., Robinson H. E., Buskey E. J. (2009). Water flow controls distribution and feeding behavior of two co-occurring coral reef fishes: i. field measurements. Coral Reefs 28 (2), 461–473. doi: 10.1007/s00338-009-0481-0
Folpp H. R., Schilling H. T., Clark G. F., Lowry M. B., Maslen B., Gregson M., et al. (2020). Artificial reefs increase fish abundance in habitat-limited estuaries. J. Appl. Ecol. 57 (9), 1752–1761. doi: 10.1111/1365-2664.13666
Froehlich C. Y. M., Garcia A., Cintra-Buenrostro C. E., Hicks D. W., Kline R. J. (2021). Structural differences alter residency and depth activity of red snapper (Lutjanus campechanus) at two artificial reefs. Fisheries Res. 242, 106043. doi: 10.1016/j.fishres.2021.106043
Hackradt C. W., Felix-Hackradt F. C., Garcia-Charton J. A. (2011). Influence of habitat structure on fish assemblage of an artificial reef in southern Brazil. Mar. Environ. Res. 72 (5), 235–247. doi: 10.1016/j.marenvres.2011.09.006
Hixon M. A., Beets J. P. (1989). Shelter characteristics and Caribbean fish assemblages: experiments with artificial reefs. Bull. Mar. Sci. 44(2), 666-680.
Jiao L., Yan-Xuan Z., Pi-Hai G., Chang-Tao G. (2017). Numerical simulation and PIV experimental study of the effect of flow fields around tube artificial reefs. Ocean Eng. 134, 96–104. doi: 10.1016/j.oceaneng.2017.02.016
Johnsen S., Widder E. A., Mobley C. D. (2004). Propagation and perception of bioluminescence: factors affecting counterillumination as a cryptic strategy. Biol. Bull. 207 (1), 1–16. doi: 10.2307/1543624
Jordan L. K. B., Gilliam D. S., Spieler R. E. (2005). Reef fish assemblage structure affected by small-scale spacing and size variations of artificial patch reefs. J. Exp. Mar. Biol. Ecol. 326 (2), 170–186. doi: 10.1016/j.jembe.2005.05.023
Komyakova V., Munday P. L., Jones G. P. (2013). Relative importance of coral cover, habitat complexity and diversity in determining the structure of reef fish communities. PloS One 8 (12), e83178. doi: 10.1371/journal.pone.0083178
Layman C. A., Allgeier J. E., Montaña C. G. (2016). Mechanistic evidence of enhanced production on artificial reefs: a case study in a Bahamian seagrass ecosystem. Ecol. Eng. 95, 574–579. doi: 10.1016/j.ecoleng.2016.06.109
Leclercq E., Taylor J. F., Migaud H. (2010). Morphological skin colour changes in teleosts. Fish Fisheries 11 (2), 159–193. doi: 10.1111/j.1467-2979.2009.00346.x
Lemoine H. R., Paxton A. B., Anisfeld S. C., Rosemond R. C., Peterson C. H. (2019). Selecting the optimal artificial reefs to achieve fish habitat enhancement goals. Biol. Conserv. 238, 108200. doi: 10.1016/j.biocon.2019.108200
Li J., Li J., Gong P., Guan C. (2021). Effects of the artificial reef and flow field environment on the habitat selection behavior of sebastes schlegelii juveniles. Appl. Anim. Behav. Sci. 245. doi: 10.1016/j.applanim.2021.105492
Lima J. S., Zalmon I. R., Love M. (2019). Overview and trends of ecological and socioeconomic research on artificial reefs. Mar. Environ. Res. 145, 81–96. doi: 10.1016/j.marenvres.2019.01.010
Lorenzen K., Leber K. M., Blankenship H. L. (2010). Responsible approach to marine stock enhancement: an update. Rev. Fisheries Sci. 18 (2), 189–210. doi: 10.1080/10641262.2010.491564
Ma Q., Ding J., Xi Y., Song J., Liang S., Zhang R. (2022). An evaluation method for determining the optimal structure of artificial reefs based on their flow field effects. Front. Mar. Sci. 9, 962821. doi: 10.3389/fmars.2022.962821
Martins C. I., Galhardo L., Noble C., Damsgard B., Spedicato M. T., Zupa W., et al. (2012). Behavioural indicators of welfare in farmed fish. Fish Physiol. Biochem. 38 (1), 17–41. doi: 10.1007/s10695-011-9518-8
Morgans C. L., Ord T. J. (2013). Natural selection in novel environments: predation selects for background matching in the body colour of a land fish. Anim. Behav. 86 (6), 1241–1249. doi: 10.1016/j.anbehav.2013.09.027
Parsons D. F., Suthers I. M., Cruz D. O., Smith J. A. (2016). Effects of habitat on fish abundance and species composition on temperate rocky reefs. Mar. Ecol. Prog. Ser. 561, 155–171. doi: 10.3354/meps11927
Qin Y., Xing J., Mao Y. (2016). Weak transient fault feature extraction based on an optimized morlet wavelet and kurtosis. Measurement Sci. Technol. 27 (8), 085003. doi: 10.1088/0957-0233/27/8/085003
Ruxton G. D., Speed M. P., Kelly D. J. (2004). What, if anything, is the adaptive function of countershading? Anim. Behav. 68 (3), 445–451. doi: 10.1016/j.anbehav.2003.12.009
Ryer C. H., Lemke J. L., Boersma K., Levas S. (2008). Adaptive coloration, behavior and predation vulnerability in three juvenile north pacific flatfishes. J. Exp. Mar. Biol. Ecol. 359 (1), 62–66. doi: 10.1016/j.jembe.2008.02.017
Sempere-Valverde J., Ostale-Valriberas E., Farfan G. M., Espinosa F. (2018). Substratum type affects recruitment and development of marine assemblages over artificial substrata: a case study in the alboran Sea. Estuar. Coast. Shelf Sci. 204, 56–65. doi: 10.1016/j.ecss.2018.02.017
Smith J. A., Lowry M. B., Champion C., Suthers I. M. (2016). A designed artificial reef is among the most productive marine fish habitats: new metrics to address ‘production versus attraction’. Mar. Biol. 163, 1–8. doi: 10.1007/s00227-016-2967-y
Smithers S. P., Rooney R., Wilson A., Stevens M. (2018). Rock pool fish use a combination of colour change and substrate choice to improve camouflage. Anim. Behav. 144, 53–65. doi: 10.1016/j.anbehav.2018.08.004
Song M., Wang J., Nie Z., Wang L., Wang J., Zhang J., et al. (2022). Evaluation of artificial reef habitats as reconstruction or enhancement tools of benthic fish communities in northern yellow Sea. Mar. pollut. Bull. 182, 113968. doi: 10.1016/j.marpolbul.2022.113968
Stevens M., Merilaita S. (2009). Animal camouflage: current issues and new perspectives. Philos. Trans. R. Soc. B-Biological Sci. 364 (1516), 423–427. doi: 10.1098/rstb.2008.0217
Tidwell J. H., Allan G. L. (2001). Fish as food: aquaculture's contribution - ecological and economic impacts and contributions of fish farming and capture fisheries. EMBO Rep. 2 (11), 958–963. doi: 10.1093/embo-reports/kve236
Vivier B., Dauvin J.-C., Navon M., Rusig A.-M., Mussio I., Orvain F., et al. (2021). Marine artificial reefs, a meta-analysis of their design, objectives and effectiveness. Global Ecol. Conserv. 27, e01538. doi: 10.1016/j.gecco.2021.e01538
Wang Y., Wai O. W. H., Chen Q. (2021). Laboratory study on fish behavioral response to meandering flow and riffle-pool sequence driven by deflectors in straight concrete flood channels. J. Hydrology 598, 125736. doi: 10.1016/j.jhydrol.2020.125736
Wiegleb J., Hirsch P. E., Egger B., Seidel F., Burkhardt-Holm P. (2020). Flow field-induced drag forces and swimming behavior of three benthic fish species. Limnologica 84, 125812. doi: 10.1016/j.limno.2020.125812
Wilson S. K., Fisher R., Pratchett M. S., Graham N. A., Dulvy N. K., Turner R. A., et al. (2010). Habitat degradation and fishing effects on the size structure of coral reef fish communities. Ecol. Appl. 20 (2), 442–451. doi: 10.1890/08-2205.1
Xi D., Zhang X., Lü H., Zhang Z. (2017). Cannibalism in juvenile black rockfish, sebastes schlegelii (Hilgendor), reared under controlled conditions. Aquaculture 479, 682–689. doi: 10.1016/j.aquaculture.2017.07.007
Xu X., Guo H., Zhang Z., Wang Y., Qin J., Zhang X. (2021). Impact of pre-aggressive experience on behavior and physiology of black rockfish (Sebastes schlegelii). Aquaculture 536, 736416. doi: 10.1016/j.aquaculture.2021.736416
Zhang Z., Chen Q., Guan X., Gong M., Zhang J., Cheng F., et al. (2023). Physical and social enrichment influences the adaptability-related behaviors of black rockfish sebastes schlegelii: an effect mediated by social behaviors, HPI axis and neurogenesis. Aquaculture 564, 739056 doi: 10.1016/j.aquaculture.2022.739056
Keywords: flow field environment, artificial reef, Sebastes schlegelii, adult fish, behavioral response, habitat selection
Citation: Gong P, Li J, Wang G, Guan C, Meng Z and Jia Y (2023) Influence of reef structure and its flow field effect on the spatial behavior of Sebastes schlegelii adults. Front. Mar. Sci. 10:1185898. doi: 10.3389/fmars.2023.1185898
Received: 14 March 2023; Accepted: 06 June 2023;
Published: 21 June 2023.
Edited by:
Edgar Mendoza, National Autonomous University of Mexico, MexicoReviewed by:
Hung-Jie Tang, National Cheng Kung University, TaiwanCopyright © 2023 Gong, Li, Wang, Guan, Meng and Jia. This is an open-access article distributed under the terms of the Creative Commons Attribution License (CC BY). The use, distribution or reproduction in other forums is permitted, provided the original author(s) and the copyright owner(s) are credited and that the original publication in this journal is cited, in accordance with accepted academic practice. No use, distribution or reproduction is permitted which does not comply with these terms.
*Correspondence: Jiao Li, bGlqaWFvQHlzZnJpLmFjLmNu
Disclaimer: All claims expressed in this article are solely those of the authors and do not necessarily represent those of their affiliated organizations, or those of the publisher, the editors and the reviewers. Any product that may be evaluated in this article or claim that may be made by its manufacturer is not guaranteed or endorsed by the publisher.
Research integrity at Frontiers
Learn more about the work of our research integrity team to safeguard the quality of each article we publish.