- 1Fisheries College, Jimei University, Xiamen, China
- 2Fujian Provincial Key Laboratory of Marine Fishery Resources and Eco-environment, Fisheries College of Jimei University, Xiamen, China
Mulberry leaf extract (ELM) has the functions of promoting growth, antioxidant, improving intestinal microbial composition, thus providing a potential solution the occurrence of fish intestinal diseases. Therefore, this experiment was conducted to explore the effects of ELM on intestinal health of spotted sea bass Lateolabrax maculatus. A total of 360 spotted sea bass (9.00 ± 0.02 g) were selected and randomly divided into 6 groups. Fish in each group were given feed with varying ELM concentration (0, 3, 6, 9, 12, 15 g/kg) for 52 days, respectively. Results show, dietary intake of 9 g/kg ELM increased the weight gain, specific growth ratio and feed intake of the spotted sea bass (P<0.05). Meanwhile, dietary intake of 9 g/kg ELM increased the activity of enteric trypsin, amylase and lipase (P<0.05). The enteric catalase activity was improved in fish fed with 3 g/kg ELM (P<0.05), while a limited effect of ELM on the activity of enteric superoxide dismutase, glutathione, and content of malonaldehyde was observed (P>0.05). ELM improved the morphology of fish intestine, as manifested in significant improvement in the length of intestinal villi, thereby increasing the surface area of the intestinal tract (P<0.05). Compared with the control group, dietary intake of ELM significantly increased the intestinal microbial ACE, Chao1, and Shannon indexes of fish (P<0.05), indicated that the intestinal microbial composition and the abundance of the dominant flora of fish were improved. The above results suggested that the dietary supplementation of about 9 g/kg ELM can improve the growth performance, enteric antioxidant capacity, and intestinal morphology and microbial composition, therefore improving the intestinal health of spotted sea bass. The research results provide a theoretical basis for the application of ELM in improving the enteric health of spotted sea bass, and providing a potential solution the occurrence of fish intestinal diseases.
1 Introduction
Spotted sea bass (Lateolabrax maculatus), a carnivorous fish of the order Perciformes, has high food value and medicinal value. In 2021, the annual output of spotted sea bass will reach 199,106 tons, accounting for 10.79% of China’s total marine fish farming (Wang et al., 2022). However, there are many problems in the breeding process of spotted sea bass, such as the feeding of feed and the pollution of breeding water may lead to the occurrence of intestinal diseases or imbalance of intestinal microorganisms in spotted sea bass. On the other hand, the main source of protein in spotted sea bass feed is fishmeal. In recent years, the price of fishmeal has continued to rise. In order to pursue high efficiency and reduce production costs, farmers use plant protein raw materials to replace the amount of fishmeal added in feed. However, excessive addition of plant protein raw materials in feed can cause intestinal diseases and intestinal function damage (Refstie et al., 2000; Uran et al., 2009). These problems are also common problems in aquaculture, so it is particularly important to solve the problem of intestinal health of farmed fish.
As an important digestive organ of animals, the intestine is an important place for the absorption and metabolism of nutrients, and has important functions such as blocking intestinal endotoxins and bacterial invasion (Li et al., 2022a). Moreover, the microorganisms in the intestinal tract have a certain influence on the growth and immunity of the animal body (Langlois et al., 2021). In summary, the health status of the intestinal tract affects the metabolism, growth, immunity, and disease resistance of the animal body to a certain extent. Therefore, it is of great significance to explore the maintenance of gut health and improve its function.
Mulberry (Morus alba L) is a perennial plant that can be picked many times a year, with strong adaptability and wide distribution. Mulberry leaf resources are abundant, but the utilization of mulberry leaves is less, and most of the mulberry leaf resources are treated as waste. As a Chinese herbal medicine, mulberry leaves contain a large amount of bioactive substances such as flavonoids, alkaloids, and polysaccharides (Sun et al., 2013), which have the functions of anti-oxidation (Tu et al., 2019), growth promotion (Fan et al., 2020), immune enhancement (Tang et al., 2022), and improvement of intestinal flora (Song et al., 2023). Therefore, mulberry leaves can replace the application of antibiotics in aquaculture, thereby reducing the pollution of antibiotics to the environment and the drug residue in the body of farmed animals. At present, the application types of mulberry leaves in farming mainly include fresh mulberry leaves, mulberry leaf powder, fermented mulberry leaves and mulberry leaf extract (ELM) (Huang et al., 2020a). The content of anti-nutritional factors in the first three application types is higher, and the content of anti-nutritional factors in ELM is less (Huang et al., 2020a). And previous studies have shown that ELM can improve the activity of digestive enzymes and antioxidant capacity in the intestinal tract of animals, and improve the composition of intestinal microorganisms (Feng et al., 2021). But the effect of ELM on the gut health of spotted sea bass remains unknown. Therefore, it is of great significance to explore the effect of ELM on the intestinal health of spotted sea bass.
2 Materials and methods
All procedures of this study are subject to the Regulations on the Management of Experimental Animals (National Animal Control Regulations of China No. 11, Law and Regulation of The State Council No. 676 amended on March 1, 2017), which has been approved by the Ethics Committee of Animal Experiments of Jimei University (Xiamen, China).
2.1 Experiment material
The experiment used the spotted sea bass purchased from an aquaculture farm in Zhangzhou, Fujian, China. ELM (mainly containing 1.16% 1-deoxynojirimycin) was purchased from Nanjing Jingzhu Biotechnology Co., Ltd. (Jiangsu, China), and zinc oxide eugenol cement was purchased from Heshanfu Technology Co., Ltd. (Guangzhou, China). Fishmeal and soybean meals were the main protein sources, fish oil and soybean oil were the fat sources, and 0.0% (CC), 0.3% (ELM1), 0.6% (ELM2), 0.9% (ELM3), 1.2% (ELM4) and 1.6% (ELM5) of ELM were added to the diets to prepare 6 experimental diets. After feed preparation is complete, store it at -20°C. Basic feed formulation design refers to the nutritional requirements of spotted sea bass (Table 1) (Ai et al., 2004; Huang et al., 2020b).
2.2 Feeding and management
This experiment was conducted in the Aquaculture Research Institute of Jimei University, Xiamen, China. After two weeks of temporary breeding of spotted sea bass in the experiment, 360 spotted sea bass with good health and an initial average weight of 9.00 ± 0.02 g were selected and randomly divided into 6 groups, each group was repeated 3 times, and 20 fish were repeated each time. Carry out the breeding experiment with the breeding cycle of 52 days. Feed twice a day (9:00 am and 5:30 pm), stop feeding until the test fish are obviously full, and the dirt was sucked and replaced with water after 30 minutes. During the test, 40% of the fresh water was replaced every day to remove dissolved waste in the aquaculture water, and the water temperature was kept at about 25-28°C. The concentration of ammonia nitrogen was less than 0.20 mg/L, the concentration of nitrite was less than 0.025 mg/L, and the concentration of dissolved oxygen was greater than 5.00mg/L, pH around 8.0.
2.3 Sample collection and preparation
After the experimental spotted sea bass was fed for 52 days, it was fasted for 24 hours, and then it was anesthetized with eugenol cement (1:10000). After anesthesia, the number of fish in each tank was recorded for calculating the survival rate (SR), and the total weight of the experimental fish in each tank was weighed and recorded for calculating the weight gain (WG) and specific growth rate (SGR) of spotted sea bass, and respectively the body length and body weight of each fish were measured to calculate the condition factor (CF). The intestinal tracts of 6 fish were collected from each experimental cylinder in sterile cryopreservation tubes, immediately frozen in liquid nitrogen, and stored at -80°C for detection of intestinal microbial flora, digestive enzyme activity [trypsin (TRS), lipase (LPS) and amylase (AMS)], intestinal immunity [alkaline phosphatase (AKP) and acid phosphatase (ACP)] and oxidation capacity [catalase (CAT), superoxide dismutase (SOD), glutathione (GSH), and malonaldehyde (MDA)]. In addition, the intestines of two fish were taken from each experimental cylinder, and fixed in 4% paraformaldehyde solution for used in observation and analysis of intestinal tissue morphology.
2.4 Growth performance and survival rate analysis
Growth performance indicators mainly include WG, SGR, CF and feed intake (FI) (Chen et al., 2020).
Note: Wt is the final body weight of the fish (g), W0 is the initial weight of the fish (g), L is the final body length of the fish (cm), F is the feeding amount (g), and day is the number of days of the test (d). A is the number of fish at the end of the breeding test, and B is the number of fish at the beginning of the breeding test.
2.5 Detection of digestive enzyme activity, intestinal immunity, and antioxidant capacity
Intestinal digestive enzyme activity (Catalog No AMS: C016-1-1, LPS: A054-1-1, TRS: A080-2), intestinal immunity (Catalog No AKP: A059-2, ACP: A060-2) and antioxidant capacity (Catalog No CAT: A007-1-1, SOD: A001-3, GSH: A006-2-1, MDA: A003-1) were detected using commercial kits (Nanjing Jiancheng Institute of Bioengineering, Nanjing, China). In short, the sample tissue and 0.9% cold saline were ground in a tissue crusher at a ratio of 1:9 for 30s, then placed in a high-speed refrigerated centrifuge at 3000r/min for 10min, and the supernatant was taken, used perform biochemical index detection. The specific determination method is as follows. TRS can catalyze the hydrolysis of the ester chain of ethyl arginine and increase the absorbance value at 253nm. Therefore, TRS activity is determined by colorimetry (Sun et al., 2011). LPS can hydrolyze triglycerides, thereby reducing scattered light and turbidity, and then LPS activity is determined by colorimetry (Mckellar and Cholette, 1986). Starch can combine with iodine to form a blue complex, and AMS can hydrolyze starch, so the unhydrolyzed starch content is measured by colorimetry, and then the AMS activity is calculated (Bernfeld, 1955). AKP and ACP can decompose disodium phenyl phosphate to produce free phenol, and phenol reacts with 4-aminoantipyrine under alkaline conditions to generate red quinone derivatives, so activities of AKP and ACP were determined by colorimetry (Powell and Smith, 1954). H2O2 can combine with ammonium molybdate, so CAT activity is determined by the ammonium molybdate method (Hamza and Hadwan, 2020). GSH can react with dithiodinitrobenzoic acid to produce a yellow compound, so GSH content is determined by colorimetry (Harrap, 1967). MDA in lipid peroxidation degradation products can react with thiobarbituric acid (TBA) to form a red substance, so the TBA method was used to determine MDA content (Buege and Aust, 1978). Water-soluble tetrazolium salt (WST-1) can react with superoxide anion to form a water-soluble dye, and will not react with the reduced form of xanthine oxidase, so SOD activity is measured by WST-1 (McCord and Fridovich, 1969).
2.6 Preparation and observation of intestinal tissue slices
After samples were collected, they were fixed in 4% paraformaldehyde solution for 48 h. Take the tissue sample out of the fixative, trim the tissue flat with a scalpel, and rinse the trimmed sample with 75% ethanol. And put in 75% ethanol for 4h, 85% ethanol for 2h, 90% ethanol for 2h, 95% ethanol for 1h, absolute ethanol I for 30min, absolute ethanol II for 30min for dehydration. When replacing the dehydrating agent, the tissue needs to be left for 30 minutes to remove the residual solution and prevent the concentration of the dehydrating agent from changing. After the tissue is dehydrated, immerse it in the alcohol-benzene solution for 10 minutes, and then put it in the xylene solution for 10 minutes to ensure the transparency of the tissue section. After the tissue was dehydrated and transparent, it was soaked in a xylene-paraffin isoproportional mixture at 65°C for 30 minutes before embedding. Pour the paraffin solution preheated at 65°C into the embedding frame, and use heated forceps to immerse the tissue. After the paraffin solidified, tissues were sliced using a microtome (RM2016, Shanghai Leica Instrument Co., Ltd.) with thickness of 4 μm. After slicing the tissues, place the slices in warm water at 40°C for flattening, pick them up with a glass slide after flattening, and bake the slices in an oven at 60°C.
After the sections are dried, put the paraffin sections in xylene I for 20 minutes, xylene II for 20 minutes, absolute ethanol I for 5 minutes, absolute ethanol II for 5 minutes, and 75% alcohol for 5 minutes, rinse with water, and dewax the paraffin sections for hydration. Put the slices into the hematoxylin solution for 3-5 minutes, wash off the excess dye solution with tap water, differentiate with 1% hydrochloric acid aqueous solution for a few seconds, rinse with tap water, and then turn blue with 0.6%-0.7% ammonia aqueous solution, rinse with running water. Then, the paraffin sections were dehydrated in 85% ethanol and 95% ethanol, and stained in eosin staining solution for 5 min. After staining, put the paraffin sections in absolute ethanol I for 5 min, absolute ethanol II for 5 min, absolute ethanol III for 5 min, n-butanol for 5 min, xylene I for 5 min, and xylene II for 5 min for transparent treatment, then removed from the xylene solution to dry. After the sections are dry, use neutral glue to seal the slides. And use WT1000GM system to measure the height and width of intestinal villi, muscle layer thickness, and crypt depth in tissue sections.
2.7 Intestinal microbial flora detection and analysis
After the TGuide S96 magnetic bead method was used to extract the total bacterial genomic DNA of intestinal samples, the concentration of the extracted nucleic acid was detected by a synergy HT microplate reader, and the integrity of the extracted nucleic acid was detected by 1.5% agarose gel. The 16SrDNA V3-V4 regions were amplified by using forward primer 338F (5’- ACTCCTACGGGAGGCAGCA-3’) and the reverse primer 806R (5’- GGACTACHVGGGTWTCTAAT-3’). PCR amplicons were quantitatively detected using 1.8% agarose gel and Image J software, and the quality of PCR amplicons was detected by the Qsep-400 method. After passing the quality inspection, it was sequenced using Illumina Novaseq6000. The DATA2 (Callahan et al., 2016) method in QIIME2 2020.6 (Bolyen et al., 2019) was used to denoise the experiment data, and the venn figure was used to show the number of OTUs obtained (Chen and Boutros, 2011). The α diversity of intestinal microorganisms was analyzed using QIIME2 2020.6(Callahan et al., 2016). The feature sequence was classified and annotated using a naive Bayesian classifier, thus that the composition and abundance of the intestinal microbial community of each sample were counted at the taxonomic level of phylum and genus.
2.8 Data analysis
Statistical analysis of experimental data was done in SPSS 25.0 software. And use one-way ANOVA to analyze the differences between all experiment groups. If there were differences, Duncan’s method was used for multiple comparisons. All experimental results were expressed as mean values and standard deviation, and the significance threshold was set at P<0.05.
3 Result
3.1 The effects of ELM on growth performance and survival rate of spotted sea bass
The effects of ELM on spotted sea bass WG, SGR, CF, FI, and SR are shown in Table 2. Compared with the control group, ELM significantly increased the WG, SGR and FI of spotted sea bass (P<0.05). The WG, SGR and FI increased continuously with the increase of ELM content, and reached the highest when the ELM content was 9g/kg (P<0.05). ELM had no significant effect on the CF of spotted sea bass, but there was a certain increasing trend (P>0.05). ELM had no effect on the SR of spotted sea bass (P>0.05).
3.2 The effects of ELM on the activity of intestinal digestive enzymes
The effects of ELM on the activities of TRS, LPS and AMS in the intestinal tract of spotted sea bass are shown in Table 3. Compared with the blank group, the intestinal TRS activities in the experimental group were significantly increased (P<0.05). The activities of TRS increased continuously with the increase of the concentration of ELM and start to decrease after reaching the highest. And the activity of TRS reached the highest when the addition of ELM was 6 g/kg (P<0.05). Compared with the blank group, the intestinal AMS and LPS activity in the experimental group tended to increase, but not significantly. AMS and LPS activity reached the highest when 15g/kg ELM was added.
3.3 The effects of ELM on antioxidative capacity
The effects of ELM on intestinal CAT, SOD activity and GSH, MDA content are shown in Table 4. Compared with the blank group, the intestinal CAT activity in the experimental group was significantly increased, and the CAT activity reached the highest when the ELM was added at 3 g/kg (P<0.05). Compared with the blank group, the intestinal SOD activity and GSH content in the test group has a tendency to increase, and MDA has a tendency to decrease, but they are not significant, and the SOD activity reaches the highest when the ELM is added at 15g/kg, and the GSH content reached the highest when the ELM was added at 15 g/kg, and the MDA content is at 12g/kg ELM reached the minimum (P>0.05).
3.4 The effects of ELM on intestinal immunity
As shown in Table 5, compared with the control group, ELM increased the activities of AKP and ACP in the gut of spotted sea bass, but not significantly. The activities of AKP and ACP increased with the increase of ELM, ACP reached the highest when the addition of ELM was 6g/kg, and AKP reached the highest when the addition of ELM was 9g/kg. After reaching the highest level, AKP and ACP decreased continuously with the increase of ELM content (P>0.05).
3.5 The effects of ELM on intestinal tissue morphology
The effect of ELM on the intestinal morphology of spotted sea bass are shown in Table 6 and Figure 1. Compared with the blank group, ELM significantly increased the height of intestinal villi, and significantly decreased the width of intestinal villi (P<0.05). And the villus height was the highest when the addition of ELM was 6g/kg, and the villi width reached the lowest when the addition of ELM was 6g/kg. Compared with the blank group, ELM had no significant effect on the thickness of the intestinal muscular layer and the depth of crypts, but the intestinal muscular layer had a decreasing trend, and reached the lowest when the ELM addition amount was 15g/kg (P>0.05). The depth of crypts had an increasing trend, and crypt depth reached the highest when the addition of ELM was 6g/kg (P>0.05).
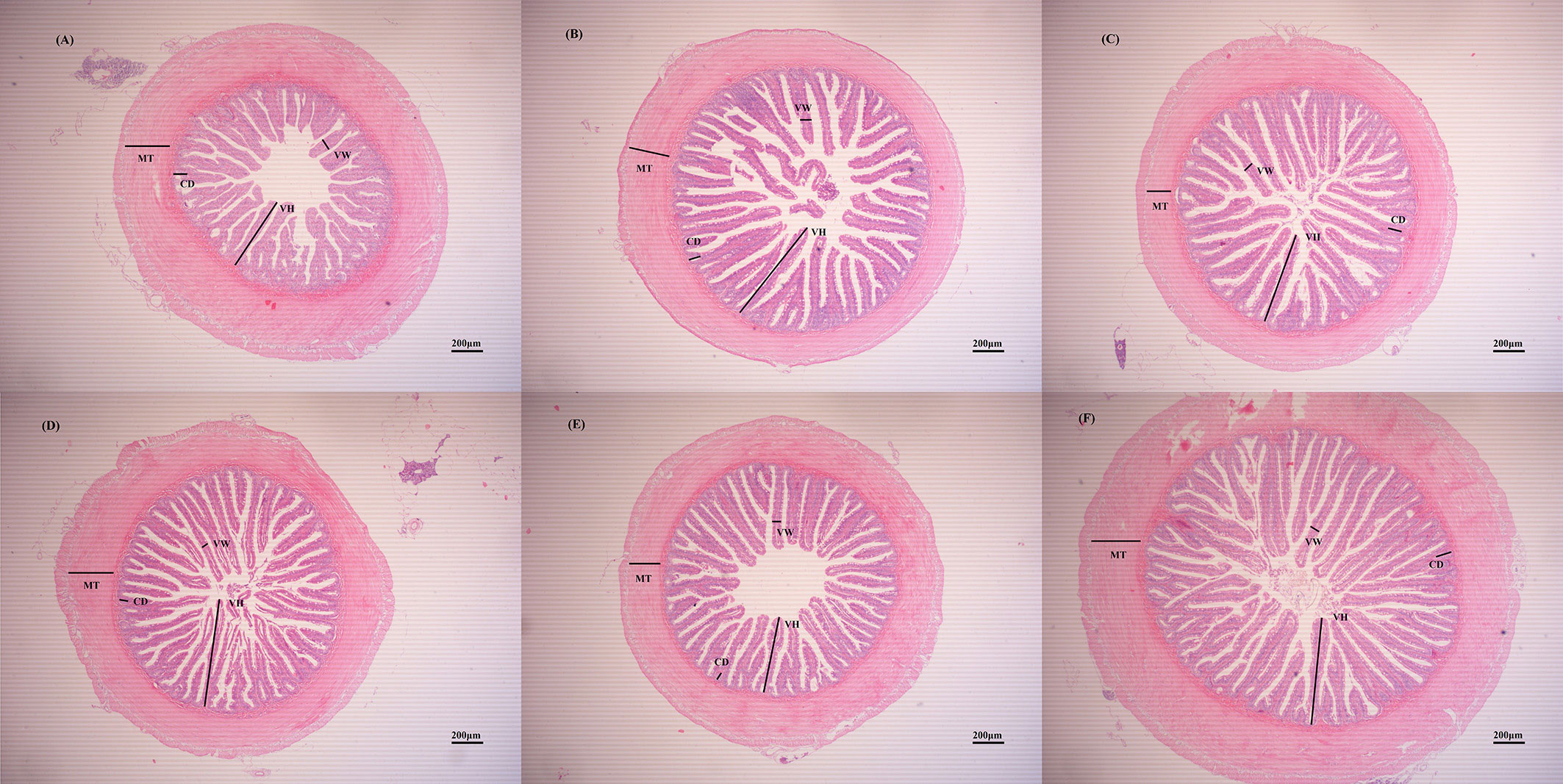
Figure 1 The effects of ELM on intestinal tissue morphology. Note: (A–F) were HE slices of spotted sea bass intestines of CC, ELM1, ELM2, ELM3, ELM4, ELM5 group under 40× magnification, respectively. VH, VW, MT, and CD in the picture represent villi height, villi width, muscle layer thickness, and crypt depth, respectively.
3.6 The effects of ELM on intestinal microbiota
3.6.1 The effects of ELM on intestinal microbial OTUs
A total of 2,322,945 sequences were obtained from the spotted sea bass intestinal flora. After being filtered, DADA2 denoised, and chimera removed, 2,316,565 high-quality sequences were finally obtained. Screening for uniquely representative sequences identified a total of 14,314 OTU homologous alignments. The Venn diagram shows the number of reciprocal and specific OTUs. As shown in Figure 2, 1444, 1831, 1270, 6919, 841, and 1033 OTUs were detected in CC, ELM1, ELM2, ELM3, ELM4, and ELM5, respectively. The specific OTUs of each group were 1392, 1779, 1218, 6867, 789, 981, respectively. The total of mutual OTUs is 52.
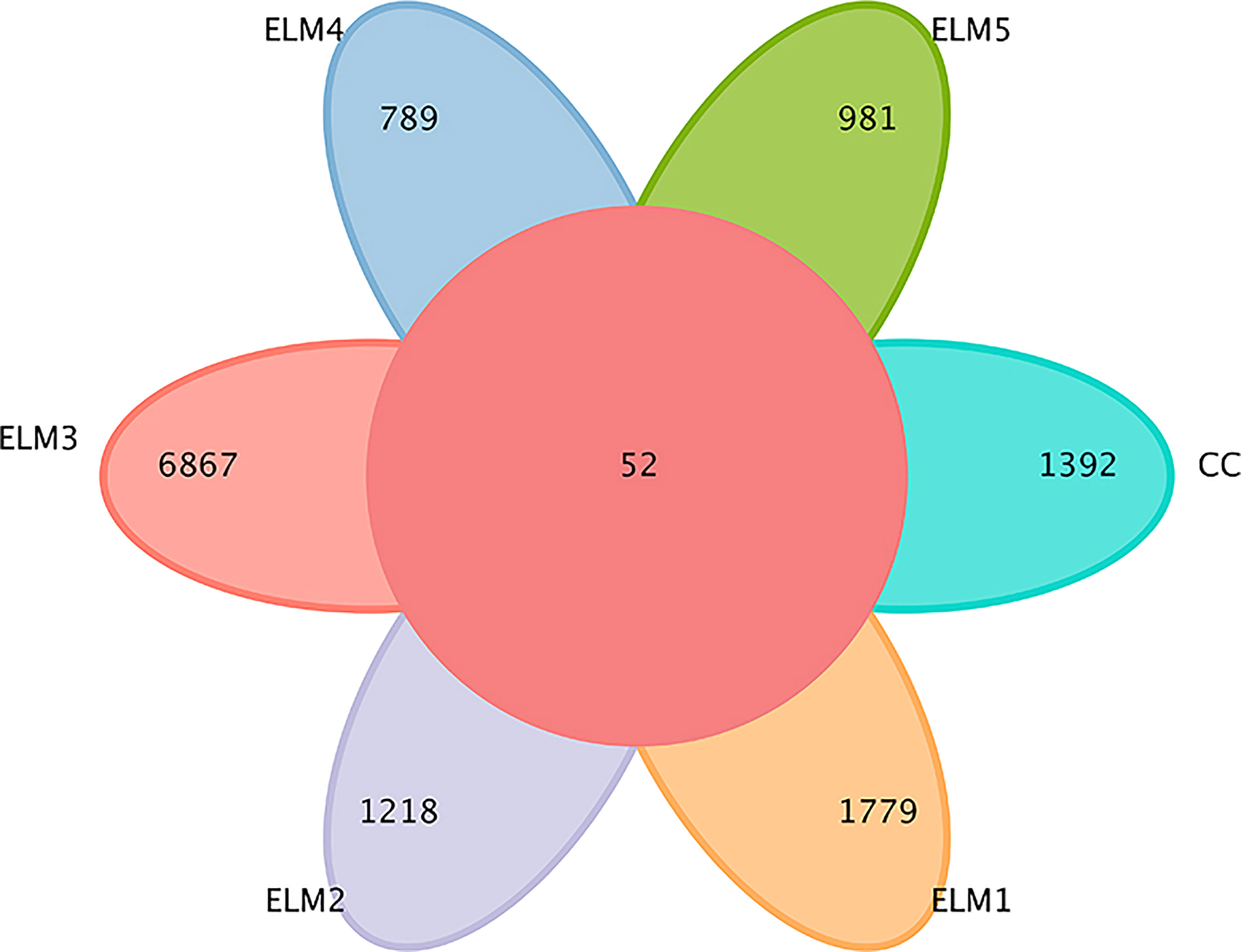
Figure 2 The effects of ELM on intestinal microbial OTUs. The circle in the middle represents the common number of OTUs, and each petal of a different color represents the number of OTUs unique to each group.
3.6.2 The effects of ELM on intestinal microbial alpha diversity
The effects of ELM on the intestinal microbial α-diversity of spotted sea bass is shown in Figure 3. Compared with the control group, ELM significantly increased the ACE, Chao1, and Simpson indices of the gut microbiota of spotted sea bass (P<0.05). ELM had no significant effect of the Shannon indices of the gut microbiota of spotted sea bass (P>0.05). The intestinal α-diversity index of spotted sea bass increased with the increase of ELM in the feed, and reached the highest when the amount of ELM was 9g/kg. And after the α-diversity reached the highest, it decreased continuously with the increase of ELM addition.
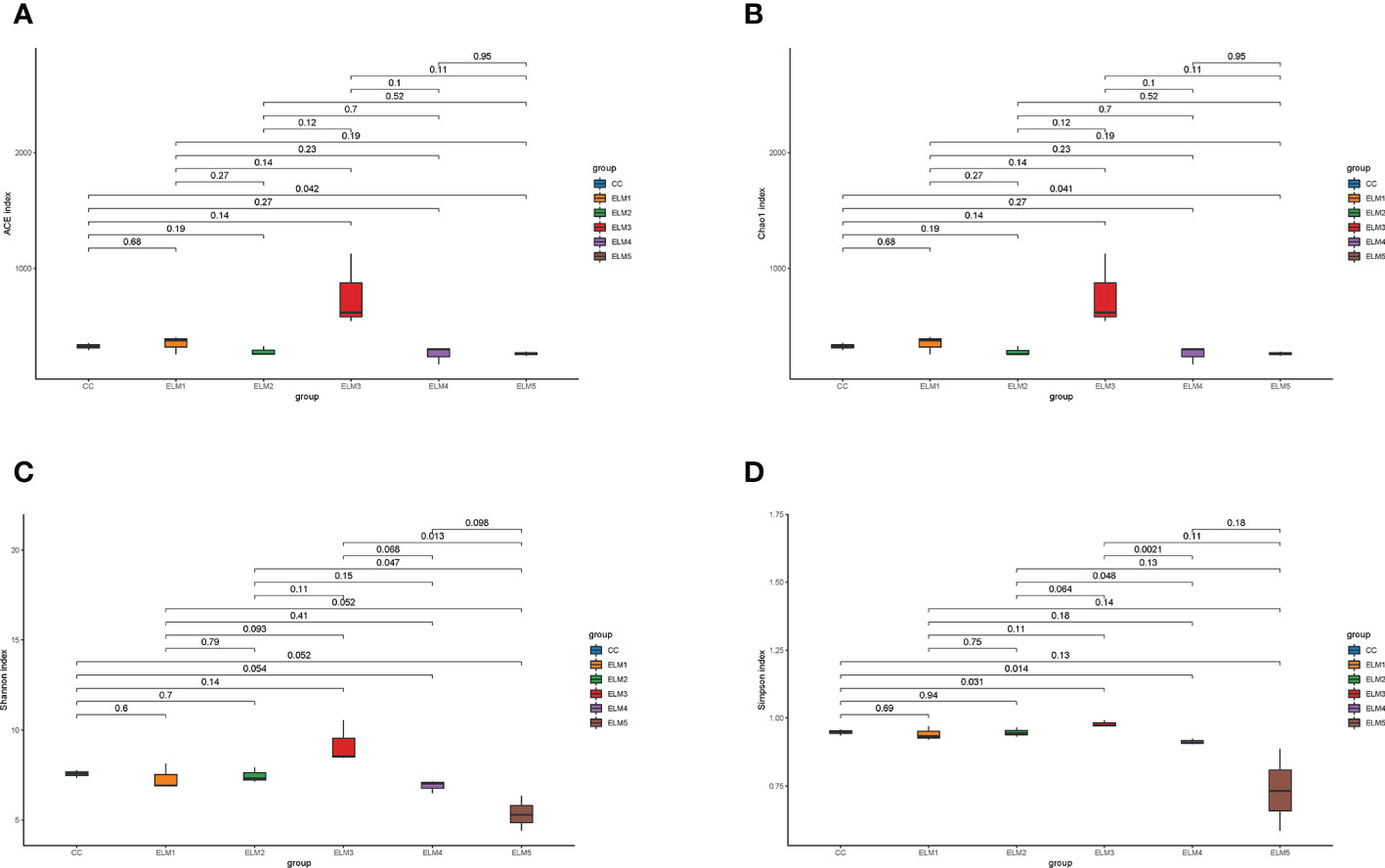
Figure 3 The effects of ELM on ACE index (A), Chao1 index (B), Simpson index (C) and Shanon index (D) of intestinal microorganisms of spotted sea bass. The abscissa is the group name, and the ordinate is the corresponding alpha diversity index value. The data are expressed as the mean and standard deviation from triplicate groups (n = 3), The number on the line between the columns is the P-value of the T-test (P<0.05).
3.6.3 The effects of ELM on species composition and abundance of intestinal microbes
There were 44 phyla, 117 classes, 327 orders, 688 families and 1580 genera in the intestinal microbiota of spotted sea bass. The top 10 phyla with relative abundance in each group were sorted, and the composition structure was analyzed (Figure 4A). Firmicutes, Proteobacteria, Fusobacteria, and Bacteroidetes are the dominant flora in the intestinal tract of spotted sea bass. Compared with the control group, ELM significantly increased the abundance of Firmicutes, Proteobacteria and Fusobacteria. At the genus level (Figure 4B), Cetobacterium and Lactobacillus are the dominant flora in the intestinal tract of spotted sea bass. Compared with the control group, ELM significantly increased the abundance of Cetobacterium and Lactobacillus in the gut of spotted sea bass.
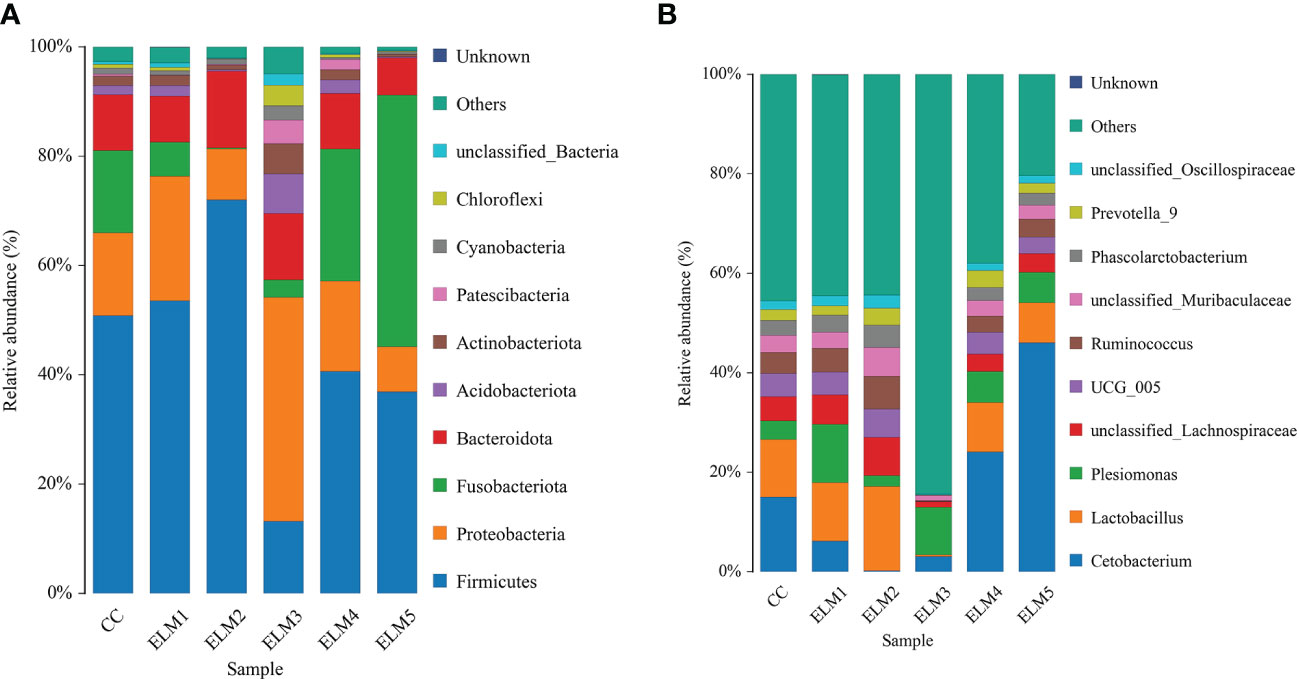
Figure 4 The effects of ELM on species composition and abundance at the phylum (A) and genus (B) levels of the gut microbiota of spotted sea bass, respectively.
4 Discussion
4.1 Growth performance of spotted sea bass
WG and SGR are the most intuitive indicators to measure the growth performance of the body. The growth results of this experiment showed that ELM significantly increased the WG and SGR of spotted sea bass, and promoted the growth of spotted sea bass. Previous studies have also found that ELM can increase the weight gain rate and daily weight gain rate of broilers, and has the function of promoting body growth (So-In and Sunthamala, 2022). In summary, ELM may prevent intestinal function damage by improving intestinal antioxidant capacity and immunity. Thereby increasing the activity of intestinal digestive enzymes and promoting the growth of spotted sea bass. On the other hand, ELM can also improve the growth performance of spotted sea bass by improving the abundance of dominant flora in the intestinal tract of spotted sea bass, improving intestinal immunity, and enhancing the digestion, utilization and absorption of nutrients in the intestine of spotted sea bass. Therefore, ELM has the function of promoting the growth of spotted sea bass.
4.2 Intestinal digestion and absorption capacity
The intestinal tract is an important place for the digestion and absorption of nutrients, and the activity of digestive enzymes secreted by the intestinal tract is an important criterion for measuring the digestive capacity of the body (Li et al., 2022b). Amylase can participate in the hydrolysis of starch and glycogen, which plays an important role in the digestion ability of fish (Zhu et al., 2020). Lipase can hydrolyze triglycerides, releasing free fatty acids and monoacylglycerols for tissue absorption or storage in adipose tissue (Young et al., 2019). Protease can catalyze the decomposition of protein in food into amino acids for cell absorption (Applebaum, 1985). Trypsin, as the most important hydrolytic protease, is a kind of serine protease, which hydrolyzes protein in the form of cleaving basic amino acid carboxyl protein chains, participates in various physiological and biochemical processes, and promotes the growth of the body (Cohen, 1998; Agusti and Cohen, 2000). Thus, activity of digestive enzymes in the gut is closely related to the body’s growth. The results of this experiment showed that ELM significantly increased the activity of TRS. increased the activities of LPS and AMS in the intestinal tract of spotted sea bass, but not significantly. Previous studies have shown that ELM can increase the activities of amylase, lipase and trypsin in the intestines of crucian carp and giant salamander, which is consistent with the results of this experiment (Li et al., 2020a; Li et al., 2020b). Intestinal digestive enzymes can decompose macromolecules such as protein, fat, carbohydrates into small molecules, and improve the absorption of nutrients in the intestine. Spotted sea bass is a carnivorous fish, and the activity of amylase in its intestinal tract is low, and the main nutrient in the feed of spotted sea bass is protein. Therefore, ELM mainly improves the digestion and utilization of nutrients in the intestine of spotted sea bass by increasing the activity of trypsin in the intestine of spotted sea bass, thereby promoting intestinal function. The above results indicated that ELM can improve the digestive and absorption capacity of the intestinal tract of spotted sea bass and promote the growth of spotted sea bass by increasing the activity of digestive enzymes.
4.3 Intestinal antioxidant capacity
Reactive oxygen species widely exist in organisms, mainly including oxygen free radicals and hydroxyl free radicals, which can participate in the immune process of the body (Nauseef, 2008), regulate signal transduction (Thannickal and Fanburg, 2000), control gene expression (Allen and Tresini, 2000), and then participate in the growth of body cells, differentiation and other functions (Valko et al., 2007). However, excessive reactive oxygen species can cause damage to cell structure, and in severe cases, it can lead to cell death (Gill and Tuteja, 2010). Biological organisms contain antioxidant enzymes such as SOD and non-enzymatic antioxidants such as GSH, which can improve the antioxidant capacity of animals by scavenging oxygen free radicals in biological organisms (Martinez-Alvarez et al., 2005; Ren et al., 2005), maintain the normal function of the body. MDA is the final product of lipid oxidation metabolism (Vohringer et al., 1998). The content of MDA in the body can measure the antioxidant capacity of the organism to a certain extent (Zhao et al., 2018a). The test results showed that the ELM significantly increased the intestinal CAT activity of spotted sea bass. The ELM increased the SOD activity and GSH level in the intestinal of the spotted sea bass, and decreased the content of MDA in the intestinal tract of the spotted sea bass, but not significantly. And studies have shown that ELM can increase the activity of CAT and reduce the content of MDA in largemouth bass (Tingsen et al., 2022). Adding mulberry leaf powder to the feed can increase the GSH content of tilapia (Miao et al., 2020). In summary, ELM can remove excessive oxygen free radicals, hydroxyl free radicals, hydrogen peroxide and other reactive oxygen species in the intestinal tract by increasing the CAT, SOD activity and GSH content in the intestinal tract of spotted sea bass, and prevent reactive oxygen species from causing damage intestinal cells. Maintain the normal function of intestinal cells, and improve the antioxidant capacity of the intestinal tract. The reduction of MDA content in the intestine also indicates to some extent that ELM reduces lipid oxidation in the intestine. The test results are consistent with the results of the study on the effect of mulberry leaf extract on the antioxidant capacity of largemouth bass (Tingsen et al., 2022). It is also consistent with the results of the study on the effect of mulberry leaf powder on the antioxidant capacity of juvenile tilapia (Miao et al., 2020). The above results indicated that the ELM could improve the intestinal antioxidant capacity of the spotted sea bass by increasing the activity of antioxidant enzymes and the content of non-enzymatic antioxidants in the gut of the spotted sea bass, thereby improving intestinal health.
4.4 Intestinal immunity
Intestinal AKP is the first barrier of the intestine, which can promote the elimination of endotoxin and bacterial lipopolysaccharide in the intestine and inhibit intestinal inflammation, and can also limit the systemic circulation of bacterial lipopolysaccharide. Intestinal AKP participates in the regulation of intestinal lipid absorption in addition to maintaining intestinal barrier function (Ghosh et al., 2021). ACP widely exists in biological organisms, and is a hydrolase that catalyzes the hydrolysis of phosphate monoesters to generate inorganic phosphate under acidic conditions. Hydrolase plays an important role in the process of removing and killing pathogenic microorganisms and parasites in the body (Pipe, 1990; Liu et al., 2003; Wu et al., 2017). Previous studies have shown that ELM can increase the activity of AKP and ACP in rats (Fallon et al., 2008), which is consistent with the results of this experiment that ELM can increase the activity of AKP and ACP in the intestinal tract of spotted sea bass. In summary, ELM can inhibit the occurrence of intestinal inflammation by increasing the activity of AKP and ACP in the intestinal tract of spotted sea bass, improve the intestinal barrier of spotted sea bass, and prevent the colonization and growth of harmful bacteria in the intestine, thereby reducing intestinal diseases of spotted sea bass occurrence and impairment of function. In summary, ELM can improve the intestinal health of spotted sea bass by improving its intestinal immune performance.
4.5 Intestinal tissue morphology
The intestinal tissue morphology affects the digestion and absorption of nutrients in the intestine to a certain extent (Biasato et al., 2018). Intestinal villi can increase the surface area of intestinal digestion and absorption and the residence time of nutrients in the intestine through the increase of height and width, so as to increase the digestion and absorption of nutrients in the intestine (Meng, 1987; Zhai et al., 2016). And the intestinal tract can also fully mix food and digestive juice through the contraction of the intestinal muscle layer, thereby improving the body’s digestion and absorption of nutrients (Yin et al., 2005). Crypt depth is also closely related to intestinal absorption of nutrients. The results of this experiment showed that the ELM significantly increased the length of the villi and the depth of the crypts in the intestinal, but had no significant effect on the width of the villi and the thickness of the intestinal muscle layer. And previous studies have also found that ELM flavonoids can increase the height of the intestinal villi and the thickness of the muscle layer of Litopenaeus vannamei, and improve intestinal health (Wang et al., 2020). Therefore, ELM promoted the digestion, absorption and utilization of nutrients in the intestine due to the improvement in intestinal structure, which indicated that ELM can improve intestinal normal function and health by improving intestinal tissue morphology.
4.6 Intestinal microbiota
Intestinal microorganisms play an important role in intestinal development and nutrient digestion and absorption, thus maintaining intestinal health and intestinal homeostasis (Ouwehand et al., 2002). The species, abundance and proportion of intestinal microbes affect intestinal health and nutrient utilization, and the dominant intestinal flora plays a dominant role in improving intestinal function (Li et al., 2022a). Firmicutes and Bacteroidetes are both one of the dominant bacterial groups in the intestinal tract, it has the effect of improving the body’s metabolism of carbohydrates and have a mutually reinforcing symbiotic relationship, which can jointly promote the host to absorb or store energy. Therefore, Firmicutes and Bacteroidetes in the intestinal tract play a very important role in intestinal health (Chen et al., 2022). The dominant flora of intestinal microorganisms of perch were Firmicutes, Proteobacteria, Fusobacteria, and Bacteroidetes (Li et al., 2022a), which was consistent with the results of this experiment. The results of this experiment showed that the abundance of Firmicutes and Bacteroides in the intestinal tract increased with the addition of ELM. In summary, ELM can improve the digestion and metabolism of sugar in the intestine of spotted sea bass by increasing the abundance of the dominant flora in the intestine of spotted sea bass, and promote the absorption of nutrients in the intestine. The increase in the abundance of sugar-metabolizing flora in the intestinal tract of spotted sea bass may be due to the polysaccharide component in ELM, which increases the digestion and utilization of sugar in the intestine of spotted sea bass. Therefore, ELM can improve the intestinal health of spotted sea bass and promote the growth of spotted sea bass by increasing the abundance of species of intestinal microbial phylum.
When the intestinal microorganisms were analyzed at the level of genus classification, the dominant bacterial groups of the intestinal microorganisms were Cetobacterium and Lactobacillus. Cetobacterium belongs to the Fusobacterium phylum, and the increased abundance of Fusobacterium in this experiment may be due to the increased abundance of Cetobacterium in the gut. The main metabolite of Cetobacterium is acetic acid, which can regulate the expression of insulin and promote the utilization of sugar by the body (Wang et al., 2021). Cetobacterium can also improve the body’s metabolism of amino acids and promote the body’s utilization of protein (Suzuki et al., 2022; Zhang et al., 2022). The results of this experiment show that ELM can improve the digestion and utilization of sugar and protein in the intestinal tract of spotted sea bass by increasing the activity of intestinal amylase and trypsin. And ELM can also regulate the expression of intestinal insulin and improve the metabolism of amino acids by increasing the abundance of Cetobacterium bacterium in the gut, thereby promoting the digestion and utilization of sugar and protein in the gut of the spotted sea bass. The above results indicate that ELM can increase the activity of amylase and trypsin in the intestine by increasing the abundance of Cetobacterium, and promote the digestion and utilization of carbohydrates and proteins in the intestine of spotted sea bass. Therefore, regulating the abundance of Cetobacterium in the intestinal can improve the utilization of carbohydrates and proteins of spotted sea bass.
Lactobacillus belongs to Firmicutes, and the increase in the abundance of Firmicutes in this test may be due to the increase in the abundance of Lactobacillus. Studies have found that Lactobacillus, as a probiotic in the intestine, can regulate the balance of intestinal microbial flora, inhibit the colonization and growth of pathogenic bacteria, increase the barrier function of intestinal epithelial cells, regulate the immune capacity of the body, and improve the antioxidant capacity of the body (Gomez et al., 2016; Ma et al., 2020; Zhao et al., 2018b). The results of this experiment show that ELM can improve the intestinal immunity of spotted sea bass by increasing the activity of ACP and AKP in the intestine of spotted sea bass. And ELM can also improve the intestinal immunity of spotted sea bass by increasing the abundance of intestinal Lactobacillus. The above results indicated that ELM could increase the activity of ACP and AKP in the intestinal tract of spotted sea bass and enhance the intestinal immunity of the fish by increasing the abundance of Lactobacillus in the intestinal tract of the fish.
In summary, ELM increased the abundance of bacteria involved in intestinal glucose metabolism, further indicating that ELM can affect the utilization of intestinal sugar. Fish have low sugar utilization due to low insulin secretion, while ELM can increase the abundance of bacteria that regulate insulin expression and improve the sugar utilization of spotted sea bass. On the other hand, ELM can inhibit the colonization and growth of pathogenic microorganisms in the intestinal tract of spotted sea bass by regulating the abundance of Lactobacillus in the intestinal tract of spotted sea bass, and improve the intestinal immunity of spotted sea bass. Therefore, ELM can improve the utilization of nutrients by regulating the abundance of Cetobacterium and Lactobacillus in intestinal microorganisms, and prevent intestinal diseases caused by bacteria, thereby improving the intestinal health of spotted sea bass. Promotes the growth of spotted sea bass.
5 Conclusion
Enteric diseases are widespread in aquaculture. At present, fisherfolks use chemically synthesized drugs to treat diseases that occur in the breeding process, but the use of these chemically synthesized drugs will pollute the environment and can vestigital in the body. As a kind of Chinese herbal medicine, mulberry leaves are non-polluting to the environment compared with chemically synthesized drugs, and can be metabolized by biological organisms without residue in biological organisms. Therefore, with the development of the green ecological farming model, Chinese herbal feed additives will become the mainstream direction of aquaculture research in the future. In conclusion, this study investigated the effects of ELM on intestinal health of spotted sea bass. The results showed that the ELM could affect the structure and composition of intestinal microflora, intestinal tissue morphology and structure, intestinal immunity, intestinal digestibility and intestinal antioxidant capacity of spotted sea bass. ELM can improve the intestinal health of spotted sea bass. These results enrich the research on the application of ELM in spotted sea bass culture, and provided a theoretical basis for the application of Chinese herbal medicine feed additives in spotted sea bass culture. In summary, mulberry leaves have great application potential in aquaculture. But mulberry leaves as a Chinese herbal feed additive also have some limitations, such as its complex components and interactions, and its specific mechanism of action needs further exploration.
Data availability statement
The data presented in the study are deposited in the figshare repository, accession number 10.6084/m9.figshare.22770140.
Ethics statement
The animal study was reviewed and approved by Ethics Committee of Animal Experiments of Jimei University.
Author contributions
SZ and ZBL conceived and designed the experiments. SZ, ZH, HL, LK, JM, ZYL, HQ, LL, and YL performed the experiments. SZ performed the analyses. SZ analyzed the data, wrote the paper, and prepared figures and tables. SZ, ZH, and ZBL discussed the result together. ZBL reviewed drafts of the paper. All authors contributed to the article and approved the submitted version.
Funding
This experiment was funded by Science and Technology Planning Project in Fujian, China (Grant No. 2015N0010) and Science and Technology Planning Project in Xiamen, China (Grant No. 3502Z20143017).
Conflict of interest
The authors declare that the research was conducted in the absence of any commercial or financial relationships that could be construed as a potential conflict of interest.
Publisher’s note
All claims expressed in this article are solely those of the authors and do not necessarily represent those of their affiliated organizations, or those of the publisher, the editors and the reviewers. Any product that may be evaluated in this article, or claim that may be made by its manufacturer, is not guaranteed or endorsed by the publisher.
References
Agusti N., Cohen A. C. (2000). Lygus hesperus and l-lineolaris (Hemiptera: miridae), phytophages, zoophages, or omnivores: evidence of feeding adaptations suggested by the salivary and midgut digestive enzymes. J. Entomol. Sci. 35, 176–186. doi: 10.18474/0749-8004-35.2.176
Ai Q. H., Mai K. S., Li H. T., Zhang C. X., Zhang L., Duan Q. Y., et al. (2004). Effects of dietary protein to energy ratios on growth and body composition of juvenile Japanese seabass. Lateolabrax japonicus. Aquacult. 230, 507–516. doi: 10.1016/j.aquaculture.2003.09.040
Allen R. G., Tresini M. (2000). Oxidative stress and gene regulation. Free Radical Biol. Med. 28, 463–499. doi: 10.1016/S0891-5849(99)00242-7
Bernfeld P. (1955). “Amylase, α and β,” in Methods in enzymology. Eds. Colowick S. P., Kaplan N. O. (New York, NY: Academic Press), 149–158.
Biasato I., Gasco L., De Marco M., Renna M., Rotolo L., Dabbou S., et al. (2018). Yellow mealworm larvae (Tenebrio molitor) inclusion in diets for male broiler chickens: effects on growth performance, gut morphology, and histological findings. Poultry Sci. 97, 540–548. doi: 10.3382/ps/pex308
Bolyen E., Rideout J. R., Dillon M. R., Bokulich N. A., Abnet C. C., Al-Ghalith G. A., et al. (2019). Reproducible, interactive, scalable and extensible microbiome data science using QIIME 2 (vol 37, pg 852, 2019). Nat. Biotechnol. 3, 1091. doi: 10.1038/s41587-019-0252-6
Buege J. A., Aust S. D. (1978). Microsomal lipid peroxidation. Methods Enzymol. 52, 302–310. doi: 10.1016/S0076-6879(78)52032-6
Callahan B. J., McMurdie P. J., Rosen M. J., Han A. W., Johnson A., Holmes S. P. (2016). DADA2: high-resolution sample inference from illumina amplicon data. Nat. Methods 13, 581. doi: 10.1038/NMETH.3869
Chen H., Boutros P. C. (2011). VennDiagram: a package for the generation of highly-customizable Venn and Euler diagrams in r. BMC Bioinf. 12, 35. doi: 10.1186/1471-2105-12-35
Chen S. J., Liu P., Jia Y. M., Liao H. P., Zhu S. X., Zhou L. L., et al. (2020). Dietary lipid concentrations influence growth, body composition, morphology of the liver and mid-intestine, and antioxidant status of marbled eel (Anguilla marmorata). Aquacult. Int. 28, 2287–2302. doi: 10.1007/s10499-020-00590-5
Chen S., Zhang F., Fan Y. F., Peng W. Q., Hong X. Y., Wu P. X. (2022). Dietary bile acid improves intestinal inflammation in nutritionally obese rats and its effect on the ratio of bacteroidetes and firmicutes in the intestinal flora. J. Gastroenterol. Hepatol. 31, 1126–1131. doi: 10.3969/j.issn.1006-5709.2022.10.009 (in Chinese)
Cohen A. C. (1998). Biochemical and morphological dynamics and predatory feeding habits in terrestrial heteroptera (Lanham MD: Entomological Society of America).
Fallon E., Zhong L. T., Furne J. K., Levitt M. D. (2008). A mixture of extracts of black and green teas and mulberry leaf did not reduce weight gain in rats fed a high-fat diet. Altern. Med. Rev. 13, 43–49. doi: 10.1111/j.1365-2036.2008.03613.x
Fan L. J., Peng Y., Wu D., Hu J. H., Shi X. E., Yang G. S., et al. (2020). Morus nigra l. leaves improve the meat quality in finishing pigs. J. Anim. Physiol. Anim. Nutr. 10, 1904–1911. doi: 10.1111/jpn.13439
Feng Q. F., Li Z. F., Hang X. Z., Li H., Zhai X. L., Xue Y., et al. (2021). Effects of dietary supplementation of mulberry leaf extract and 1-deoxynojiri mycin on growth, digestion, immune ability and intestinal flora of Andrias davidianus. Acta Hydrobiol. Sin. 45, 582–559. doi: 10.7541/2021.2020.116 (in Chinese)
Ghosh S. S., Wang J., Yannie P. J., Cooper R. C., Sandhu Y. K., Kakiyama G., et al. (2021). Over-expression of intestinal alkaline phosphatase attenuates atherosclerosis. Circ. Res. 128, 1646–1659. doi: 10.1161/CIRCRESAHA.120.317144
Gill S. S., Tuteja N. (2010). Reactive oxygen species and antioxidant machinery in abiotic stress tolerance in crop plants. Plant Physiol. Biochem. 48, 909–930. doi: 10.1016/j.plaphy.2010.08.016
Gomez N. C., Ramiro J., Quecan B., de Melo Franco B. (2016). Use of potential probiotic lactic acid bacteria (LAB) biofilms for the control of listeria monocytogenes, salmonella typhimurium, and escherichia coil O157:H7 biofilms formation. Front. Microbiol. 7. doi: 10.3389/fmicb.2016.00863
Hamza T. A., Hadwan M. H. (2020). New spectrophotometric method for the assessment of catalase enzyme activity in biological tissues. Curr. Anal. Chem. 16 (8), 1054–1062. doi: 10.2174/1573411016666200116091238
Harrap K. R. (1967). The application of 5,5' dithiobis-2-nitrobenzoic acid to the determination of the acid-soluble disulphide content of blood. Biochem. Pharmacol. 16 (4), 725–731. doi: 10.1016/0006-2952(67)90086-X
Huang Z. F., Li Z. B., Xu A. L., Zheng D. J., Ye Y. L., Wang Z. (2020b). Effects of exogenous multienzyme complex supplementation in diets on growth performance, digestive enzyme activity and non-specific immunity of the Japanese seabass. Lateolabrax japonicus. Aquacult. Nutr. 26, 306–315. doi: 10.1111/anu.12991
Huang J., Liao Y. X., Wei J. T., Wu H. (2020a). Application status and development mode of mulberry leaf in aquaculture. North. Sericult. 41, 45–49. doi: 10.19443/j.cnki.1673-9922.2020.04.011 (in Chinese)
Langlois L., Akhtar N., Tam K. C., Dixon B., Reid G. (2021). Fishing for the right probiotic: host-microbe interactions at the interface of effective aquaculture strategies. FEMS Microbiol. Rev. 45, 1–16. doi: 10.1093/femsre/fuab030
Li Z. F., Chen X. C., Chen Y. J., Li W. L., Feng Q. F., Zhang H. D., et al. (2020b). Effects of dietary mulberry leaf extract on the growth, gastrointestinal, hepatic functions of Chinese giant salamander (Andrias davidianus). Aquacult. Res. 51, 2613–2623. doi: 10.1111/are.14639
Li J. N., Fan Z., Wu D., Wang L. S. (2022b). Effects of probiotics in high sugar diet on growth performance, intestinal digestive enzyme and antioxidant enzyme activities and immune gene expression of songpu mirror carp. Chin. J. Anim. Nutr. 35, 1–1. doi: 10.12418/CJAN2023.106 (in Chinese)
Li L. K., Liu X. J., Wang Y., Huang Y. Q., Wang C. F. (2022a). Effects of alternate feeding between fish meal and novel protein diets on the intestinal health of juvenile largemouth bass (Micropterus salmoides). Aquacult. Rep. 23, 101023. doi: 10.1016/j.aqrep.2022.101023
Li H. T., Lu L., Wu M., Xiong X. Q., Luo L., Ma Y. T., et al. (2020a). The effects of dietary extract of mulberry leaf on growth performance, hypoxia-reoxygenation stress and biochemical parameters in various organs of fish. Aquacult. Rep. 18, 100494. doi: 10.1016/j.aqrep.2020.100494
Liu Z. H., Meng H. J., Wang Q. Y. (2003). Research progress of immune related enzymes in mollusca. Mar. Fish. Res. 24, 86–90. doi: 10.3969/j.issn.1000-7075.2003.03.016 (in Chinese)
Ma J. G., Wang W., Sun C. B., Gu L. Y., Liu Z. J., Yu W., et al. (2020). Effects of environmental stresses on the physiological characteristics, adhesion ability and pathogen adhesion inhibition of lactobacillus plantarum KLDS 1.0328. Process Biochem. 92, 426–436. doi: 10.1016/j.procbio.2020.02.001
Martinez-Alvarez R. M., Morales A. E., Sanz A. (2005). Antioxidant defenses in fish: biotic and abiotic factors. Rev. Fish Biol. Fish. 15, 75–88. doi: 10.1007/s11160-005-7846-4
McCord J. M., Fridovich I. (1969). The utility of superoxide dismutase in studying free radical reactions. i. radicals generated by the interaction of sulfite, dimethyl sulfoxide, and oxygen. J. Biol. Chem. 244 (22), 6056–6063. doi: 10.1016/S0021-9258(18)63505-7
Mckellar R. C., Cholette H. (1986). Determination of the extracellular lipases of pseudomonas-fluorescens spp in skim milk with the beta-naphthyl caprylate assay. J. Of Dairy Res. 53 (2), 301–312. doi: 10.1017/S0022029900024900
Miao L. H., Charles O., Lin Y., Gong Y. T., Zhu W. B., Wang L. M., et al. (2020). Interactive effects of mulberry leaf meal and bamboo charcoal additive on growth performance, anti-oxidant capacity, and disease resistance of genetically improved farmed tilapia (GIFT) juvenile (Oreochromis niloticus). Aquacult. Rep. 18, 100483. doi: 10.1016/j.aqrep.2020.100483
Nauseef W. M. (2008). Nox enzymes in immune cells. Semin. Immunopathol. 30 (3), 195–208. doi: 10.1007/s00281-008-0117-4
Ouwehand A., Isolauri E., Salminen S. (2002). The role of the intestinal microflora for the development of the immune system in early childhood. Eur. J. Nutr. 41 (Suppl 1), I32–I37. doi: 10.1007/s00394-002-1105-4
Pipe R. K. (1990). Hydrolytic enzymes associated with the antigranulocytes hemocytes of the marine mussel mytilus-edulis. Histochem. J. 22, 595–603. doi: 10.1007/BF01072941
Powell M. E., Smith M. J. (1954). The determination of serum acid and alkaline phosphatase activity with 4-aminoantipyrine (A.A.P.). J. Clin. Pathol. 7 (3), 245–248. doi: 10.1136/jcp.7.3.245
Refstie S., Korsoen O. J., Storebakken T., Baeverfjord G., Lein I., Roem A. J. (2000). Differing nutritional responses to dietary soybean meal in rainbow trout (Oncorhynchus mykiss) and Atlantic salmon (Salmo salar). Aquaculture 190, 49–63. doi: 10.1016/S0044-8486(00)00382-3
Ren S. C., Ding X. L., Shi X. H. (2005). Antioxidant activiy of ax-5"-methane-3'-methoxymaysin and ax-4"-oh-3'-methoxymaysin from corn silk. J. Zhengzhou Instit. Technol. 26, 5–8. doi: 10.3969/j.issn.1673-2383.2005.06.002 (in Chinese)
So-In C., Sunthamala N. (2022). The effects of mulberry (Morus alba linn.) leaf supplementation on growth performance, blood parameter, and antioxidant status of broiler chickens under high stocking density. Vet. World 15, 2715–2724. doi: 10.14202/vetworld.2022.2715-2724
Song M., Wang C. P., Yu M., Deng D., Liu Z. C., Cui Y. Y., et al. (2023). Mulberry leaf extract improves intestinal barrier function and displays beneficial effects on colonic microbiota and microbial metabolism in weaned piglets. J. Sci. Food Agric. 103, 1561–1568. doi: 10.1002/jsfa.12254
Sun G. X., Liang Y., Wang J., Wu F. (2013). Fermentation process optimization of mulberry black tea by RSM. Adv. Mater. Res. 2203, 634–638. doi: 10.4028/www.scientific.net/AMR.634-638.1481
Sun Y. Z., Yang H. L., Ma R. L., Zhang C. X., Lin W. Y. (2011). Effect of dietary administration of psychrobacter sp. on the growth, feed utilization, digestive enzymes and immune responses of grouper epinephelus coioides. Aquacult. Nutr. 17 (3), E733–E740. doi: 10.1111/j.1365-2095.2010.00837.x
Suzuki A., Shirakata C., Anzai H., Sumiyama D., Suzuki M. (2022). Vitamin B12 biosynthesis of Cetobacterium ceti isolated from the intestinal content of captive common bottlenose dolphins (Tursiops truncatus). Microbiol. (Reading England) 168, 001244. doi: 10.1099/mic.0.001244
Tang X. L., Fu J. H., Gao Q. N., Liu G. F., Ye J. W., Guan W. T., et al. (2022). Effects of mulberry (Morus alba l.) leaf extracts on growth, immune response, and antioxidant functions in nile tilapia (Oreochromis niloticus). Ann. Anim. Sci. 22, 349–369. doi: 10.2478/aoas-2021-0038
Thannickal V. J., Fanburg B. L. (2000). Reactive oxygen species in cell signaling. Am. J. Physiology-lung Cell. Mol. Physiol. 279, L1005–L1028. doi: 10.1152/ajplung.2000.279.6.L1005
Tingsen J., Hui L., Junwa H., Zhe L., Yu L., Honghao J., et al. (2022). Mulberry leaf extract improves non-specific immunity and antioxidant capacity of largemouth bass (Micropterus salmoides) fed a high-starch diet. Front. Mar. Sci. 2022. doi: 10.3389/fmars.2022.1029360
Tu J. M., Shi D. D., Wen L. R., Jiang Y. M., Zhao Y. P., Yang J. L., et al. (2019). Identification of moracin n in mulberry leaf and evaluation of antioxidant activity. Food Chem. Toxicol. 132, 110730. doi: 10.1016/j.fct.2019.110730
Uran P. A., Schrama J. W., Jaafari S., Baardsen G., Rombout J., Koppe W., et al. (2009). Variation in commercial sources of soybean meal influences the severity of enteritis in Atlantic salmon (Salmo salar l.). Aquacult. Nutr. 15, 492–499. doi: 10.1111/j.1365-2095.2008.00615.x
Valko M., Leibfritz D., Moncol J., Cronin M., Mazur M., Telser J. (2007). Free radicals and antioxidants in normal physiological functions and human disease. Int. J. Biochem. Cell Biol. 39, 44–84. doi: 10.1016/j.biocel.2006.07.001
Vohringer M. L., Becker T. W., Krieger G., Jacobi H., Witte I. (1998). Synergistic DNA damaging effects of malondialdehyde/Cu (II) in PM2 DNA and in human fibroblasts. Toxicol. Lett. 94, 159–166. doi: 10.1016/S0378-4274(98)00002-2
Wang Y. M., Chen B., Cao J. M., Hang Y. H., Wang G. X., Peng K., et al. (2020). Effects of mulberry leaf flavone on intestinal mucosal morphology and intestinal flora of Litopenaeus vannamei. Chin. J. Anim. Nutr. 32, 1817–1825. doi: 10.3969/j.issn.1006-267x.2020.04.040 (in Chinese)
Wang D., Wu F. X., Song D. D., Gao H. Q. (2022). China Fisheries yearbook (Beijing, China: China Agricultural Press).
Wang A. R., Zhang Z., Ding Q. W., Yang Y. L., Bindelle J., Ran C., et al. (2021). Intestinal cetobacterium and acetate modify glucose homeostasis via parasympathetic activation in zebrafish. Gut Microbes 13, 1–15. doi: 10.1080/19490976.2021.1900996
Wu L., Chen M. Z., Wang S. F. (2017). Research progress of shellfish immune mechanism. Pharm. Biotechnol. 24, 68–71. doi: 10.19526/j.cnki.1005-8915.20170115 (in Chinese)
Yin J. X., Zhang Y. G., Li P., Tuo Y. (2005). Preliminary study on the histology and histochemistry of the digestive tract of Sinilabeo rendahli kimurai. Freshw. Fish. 35, 7–10. doi: 10.3969/j.issn.1000-6907.2005.06.002 (in Chinese)
Young S. G., Fong L. G., Beigneux A. P., Allan C. M., He C. W., Jiang H. B., et al. (2019). GPIHBP1 and lipoprotein lipase, partners in plasma triglyceride metabolism. Cell Metab. 30, 51–65. doi: 10.1016/j.cmet.2019.05.023
Zhai S. W., Shi Q. C., Chen X. H. (2016). Effect of dietary antimicrobial peptides-surfactin supplementation on parameters of intestinal health indices of genetically improved farmed tilapia (gift, oreochromis niloticus). Acta Hydrobiol. Sin. 40, 823–829. doi: 10.7541/2016.106 (in Chinese)
Zhang Z. Y., Fan Z. J., Yi M. M., Liu Z. G., Ke X. L., Gao F. Y., et al. (2022). Characterization of the core gut microbiota of Nile tilapia (Oreochromis niloticus): indication of a putative novel Cetobacterium species and analysis of its potential function on nutrition. Arch. Microbiol. 204, 690. doi: 10.1007/s00203-022-03301-1
Zhao J. C., Tian F. W., Yan S., Zhai Q. X., Zhang H., Chen W. (2018b). Lactobacillus plantarum CCFM10 alleviating oxidative stress and restoring the gut microbiota in d-galactose-induced aging mice. Food Funct. 9, 917–924. doi: 10.1039/c7fo01574g
Zhao T., Wang G. J., Peng X. K., Zhang E. P. (2018a). Effects of ammonia and hydrogen sulfide stress on immunity and antioxidant function of goats. Acta Vet. Zootech. Sin. 49, 2191–2204. doi: 10.11843/j.issn.0366-6964.2018.10.015 (in Chinese)
Keywords: mulberry leaf extract, spotted sea bass, digestive enzyme activity, antioxidant ability, intestinal tissue morphology, intestinal microorganism
Citation: Zhou S, Huang Z, Lin H, Kong L, Ma J, Long Z, Qin H, Liu L, Lin Y and Li Z (2023) Effects of mulberry leaf extract on the intestinal health of spotted sea bass (Lateolabrax maculatus). Front. Mar. Sci. 10:1185795. doi: 10.3389/fmars.2023.1185795
Received: 14 March 2023; Accepted: 02 May 2023;
Published: 17 May 2023.
Edited by:
Amina Zuberi, Quaid-i-Azam University, PakistanReviewed by:
Sehrish Taj, Hainan University, ChinaQin Zhang, Guangxi University for Nationalities, China
Copyright © 2023 Zhou, Huang, Lin, Kong, Ma, Long, Qin, Liu, Lin and Li. This is an open-access article distributed under the terms of the Creative Commons Attribution License (CC BY). The use, distribution or reproduction in other forums is permitted, provided the original author(s) and the copyright owner(s) are credited and that the original publication in this journal is cited, in accordance with accepted academic practice. No use, distribution or reproduction is permitted which does not comply with these terms.
*Correspondence: Zhongbao Li, bGl6aG9uZ2Jhb0BqbXUuZWR1LmNu