- 1Department of Marine Science and Convergence Engineering, College of Science and Convergence Technology, Hanyang University, Ansan, Republic of Korea
- 2Maritime Safety and Environmental Research Division, Korea Research Institute of Ships and Ocean Engineering, Daejeon, Republic of Korea
Although siloxanes are contaminants of emerging concerns, limited studies have been conducted on contamination and time trends in siloxanes from aquatic environments worldwide. To date, most previous studies have focused on the environmental relevance of cyclic siloxanes in coastal environments. In the present study, cyclic and linear siloxanes were measured in sediment collected from semi-enclosed bays in Korea in 2013 and 2021 to assess occurrence, spatial distribution, temporal trends, and ecological risks. Almost all siloxanes were detected in all sediment samples, indicating continuous contamination for the last decade. The concentrations of cyclic siloxanes in sediment were approximately two times higher than those of linear siloxanes. Decamethylcyclopentasiloxane (D5) and dodecamethylcyclohexasiloxane (D6) were predominantly detected in all sediment samples, possibly due to their high consumption and strong resistance. The overall concentrations of siloxanes in sediment gradually decreased from inner to outer parts of the bays, suggesting that industrial activities largely contributed to siloxane contamination. Moreover, the highest concentrations of siloxanes in both sampling years were observed in sediment near the outfall of wastewater treatment plant, implying a potential source in the coastal environment. Siloxane concentrations in sediment were significantly correlated with sedimentary organic carbon. The concentrations of siloxanes in sediment were not significantly different between 2013 and 2021, indicating ongoing contamination. The concentrations of D5 and D6 in our sediment samples showed a limited potential to pose a threat to benthic organisms.
1 Introduction
Siloxanes have been widely used in various industrial and consumer products, including electronics, furniture, medical products and equipment, cosmetics, personal care products, and kitchen appliances, due to their weak surface tension and excellent thermal stability (Horii et al., 2021; Xiang et al., 2021). Widespread consumption of siloxanes in a great variety of applications has resulted in their widespread detection in different environmental compartments, namely, atmosphere, water, soil, and deposited material (Wang et al., 2015; Lee et al., 2018a; Xiang et al., 2021; Horii et al., 2022a). Furthermore, even in environmental compartments from remote areas, cyclic siloxanes have been observed due to long-distance migration (Sanchís et al., 2015; Lee et al., 2019a; Panagopoulos et al., 2020; Gerhards et al., 2022). Although there is some debate on the biomagnification potentiality of siloxanes in food chains, they are certainly bioaccumulated in various species (Jia et al., 2015; Guo et al., 2021; Kim et al., 2022). Accumulated evidence indicates that several siloxanes have adverse health effects on the nervous, immune, endocrine, and reproductive systems of organisms as well as ecological risks (Wang et al., 2013a; Guo et al., 2021; Bernardo et al., 2022; Feng et al., 2022). Contamination and potential negative effects of siloxanes on organisms and humans are of increasing concern (Cheng et al., 2021; Horii et al., 2022a; Zhu et al., 2023).
Aquatic environments serve as the final reservoir of anthropogenic pollutants (He et al., 2021; Tong et al., 2022). The widespread addition of siloxanes in personal care products (PCPs) and other daily necessities leads to the enrichment of large amounts of siloxanes in domestic wastewater (Jin et al., 2016; Pelletier et al., 2022). Industrial wastewater discharge is also an important source of siloxanes in aquatic environments (He et al., 2021; Horii et al., 2022a). Although wastewater treatment plants (WWTPs) attempt to remove organic pollutants, including siloxanes, from wastewater, siloxanes in wastewater cannot be completely removed regardless of treatment methods applied (Lee et al., 2014a; Lee et al., 2014b; Guo et al., 2019; Horii et al., 2022a). Siloxanes are easily adsorbed on suspended particles and eventually deposit into underlying sediment (Wang et al., 2013b; Zhang et al., 2018; Pelletier et al., 2022). Thus, sediment acts as an important sink for siloxane contamination in the aquatic ecosystem (Mackay et al., 2015; Chen et al., 2022).
Higher siloxane concentrations have been observed in sediment from densely populated areas or industrialized coastal zones (Moon et al., 2008a; Lee et al., 2018a; He et al., 2021). However, limited studies have been conducted on the temporal trends of siloxanes in the aquatic sedimentary materials (Horii et al., 2022a; Liu et al., 2022). Since the 1970s, industrial complexes, such as petrochemicals, automobiles, chemical manufacturing, and ferrous and non-ferrous forging factory, have been constructed along the coastlines of Korea (Moon et al., 2008b; Lee et al., 2020a). Thus, it is essential to investigate the contamination, environmental behavior, and temporal trends of siloxanes in the coastal ecosystems related to industrial activities. In this study, linear and cyclic siloxanes were detected in sediment from industrialized bays and near the WWTP outfall to investigate pollutant profiles, source tracking, and time trends. The results could enhance our understanding for the key factors affecting the environmental distribution of siloxanes from sedimentary materials. Moreover, the results on time trends would support the necessity of implementing emission regulations as well as assessing future restrictions on siloxanes.
2 Materials and methods
2.1 Sampling
Sediment [0–4 cm depth, sedimentation rate was 0.54 cm/year (Lee et al., 2018a)] was collected at the same stations in Masan Bay (n=48), Haengam Bay (n=16), and the other outer parts of the bays (n=16), Korea in 2013 and 2021 (Figure 1). Sediment samples (n = 28) were additionally gathered around the outfall of WWTP to explore potential sources and the impact of effluents to the bay. A Van Veen grab sampler was used to collect sediment samples. Sediment samples were frozen during transportation to the laboratory, freeze-dried in the laboratory, and stored at –4°C.
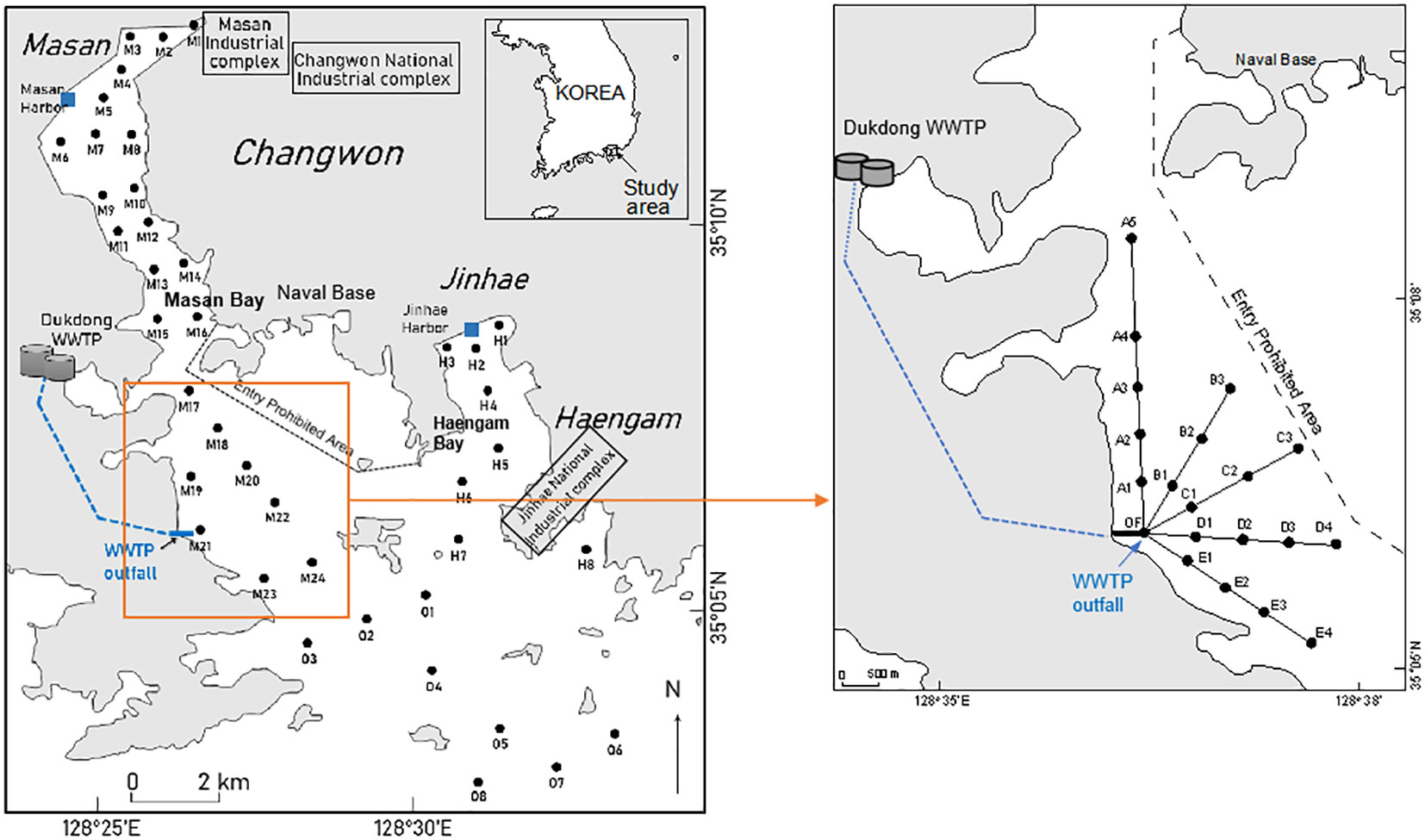
Figure 1 Sampling locations of sediment from Masan and Haengam Bays of Korea and around the submarine outfall of a wastewater treatment plant (enlarged map) collected in 2013 and 2021.
2.2 Sample pretreatment and analysis
Sample pretreatment procedures, compounds, reagents, and instruments have referred to our published studies (Lee et al., 2018a; Chen et al., 2022). Briefly, we added mass-labelled D4, D5, and D6 (200 ng each) as internal standards to the sediment samples. Then, samples were extracted through accelerated solvent extraction (ASE) by dichloromethane under 1500 psi and 100°C. Sulfur in sediment samples was removed by adding copper activated by concentrated hydrochloric acid. Each extract was quantified to 1 mL of hexane through nitrogen stream for instrumental detection.
Siloxanes were analyzed (D4–D7 and L4–L16) by gas chromatography mass spectrometry (GC-MS; Agilent 7890/5975C, Wilmington, TX, USA). The instrument was installed with a DB-5MS capillary column (30 m, 0.25 mm i.d., 0.25 µm f.t.). Setting parameters for the analytical instrument have been referred to published literature (Lee et al., 2018a; Chen et al., 2022). Briefly, the instrument temperature rise procedure was as follows: 40°C for 2 min – 220°C at 20°C/min – 280°C at 5°C/min – 300°C for 5 min. The internal standard method was implemented for the quantification of D4–D6, while the external standard method was implemented for the quantification of D7 and linear siloxanes (L4–L16) due to the unavailability of commercial mass-labelled standards. Detailed MS parameters of quantification and confirmation of targeted siloxanes are included in Table S1.
In addition, the contents of total organic carbon (TOC) in sedimentary materials were measured by Elemental Analyzer.
2.3 Quality control
Due to the widespread consumption of siloxanes, certain levels of siloxanes inevitably exist in the indoor environment compartments (Lee et al., 2018a; Chen et al., 2022). To avoid background contamination, a strict quality control (QC) protocol was implemented throughout the experiment and the GC-MS instrumentation (Chen et al., 2022). Cosmetics and PCPs were prohibited from being contacted by laboratory personnel during the entire experimental procedure, and all experimental appliances were precleaned with acetone. Procedure blank samples (n=12) were prepared during the experiment to check for background contamination, and the range of siloxanes in the blank sample was from<LOQ (D7) to 15.7 (D5) ng/g dry weight (dw). The values in procedural blanks were subtracted from the concentrations measured in real sediment samples. Matrix-spiked sample test was performed on eight sediment samples to assess the matrix effect. The recoveries of matrix-spike samples were in the ranges of 73%–114% (mean: 101%) for cyclic siloxanes and 65%–70% (mean: 68%) for linear siloxanes. The limits of quantification (LOQs) ranged from 0.0002 (D4) to 2.77 (L15) ng/g dw. Details of the QC results implemented in this study are presented in Table S1.
2.4 Potential ecological risk assessment
The hazard quotients (HQ) were implemented to explore the ecological risk of siloxanes in sediment to benthos, using previous literature as references (Nika et al., 2020; Liu et al., 2022). Based on the scarce toxicity literature, only three cyclic siloxanes (D4, D5, and D6) in the sediment were assessed for ecological risk using following equation:
where the MEC (ng/g) represents the measured concentrations of siloxane in sedimentary materials, and the PNECsediment (ng/g) is the predicted no-effect concentration in sedimentary materials, which were obtained from previous studies (Redman et al., 2012; Homem et al., 2017; He et al., 2021; Liu et al., 2022). The PNECsediment cited in the present study for D4, D5 and D6 were 1.30 × 103, 6.90 × 103, 4.84 × 103 ng/g dw, respectively. An HQ ≥ 1.0 indicates the presence of ecological risk, while HQ< 1.0 indicates no ecological risk (Liu et al., 2022).
2.5 Statistical analysis
Statistical analysis was implemented for siloxanes with a detection rate greater than 50% in sediment samples. Quantitative results for individual congeners below LOQs were replaced by 0. Spearman correlation analysis was implemented to explore the relationships between siloxane congeners, as well as the relationships between siloxane levels and TOC contents. Mann Whitney U-test was used to compare statistical differences in siloxane levels caused by spatial and temporal trends. We carried out the statistical analyses with SPSS 23.0 software.
3 Results and discussion
3.1 Sedimentary concentrations of siloxanes
Siloxane concentrations in sediment from Masan and Haengam Bays in Korea are presented in Table 1. Regardless of the sampling locations and years, detectable levels of siloxanes were observed for all sediment samples, asserting continuous contamination in industrialized coastal bays (Chen et al., 2022; Horii et al., 2022a). Among the 17 congeners, D5, D6, L7, L8, L9, L10, L11, L12, and L13 showed higher detection rates (> 80% of total samples) for all sediment samples. D4, D7, L5, L6, and L14 showed moderate detection rates, ranging from 15% to 70%. Some linear siloxanes, such as L4, L15, and L16, were hardly detected (< 6%) for sediment samples, indicating they are rarely used in Korea.
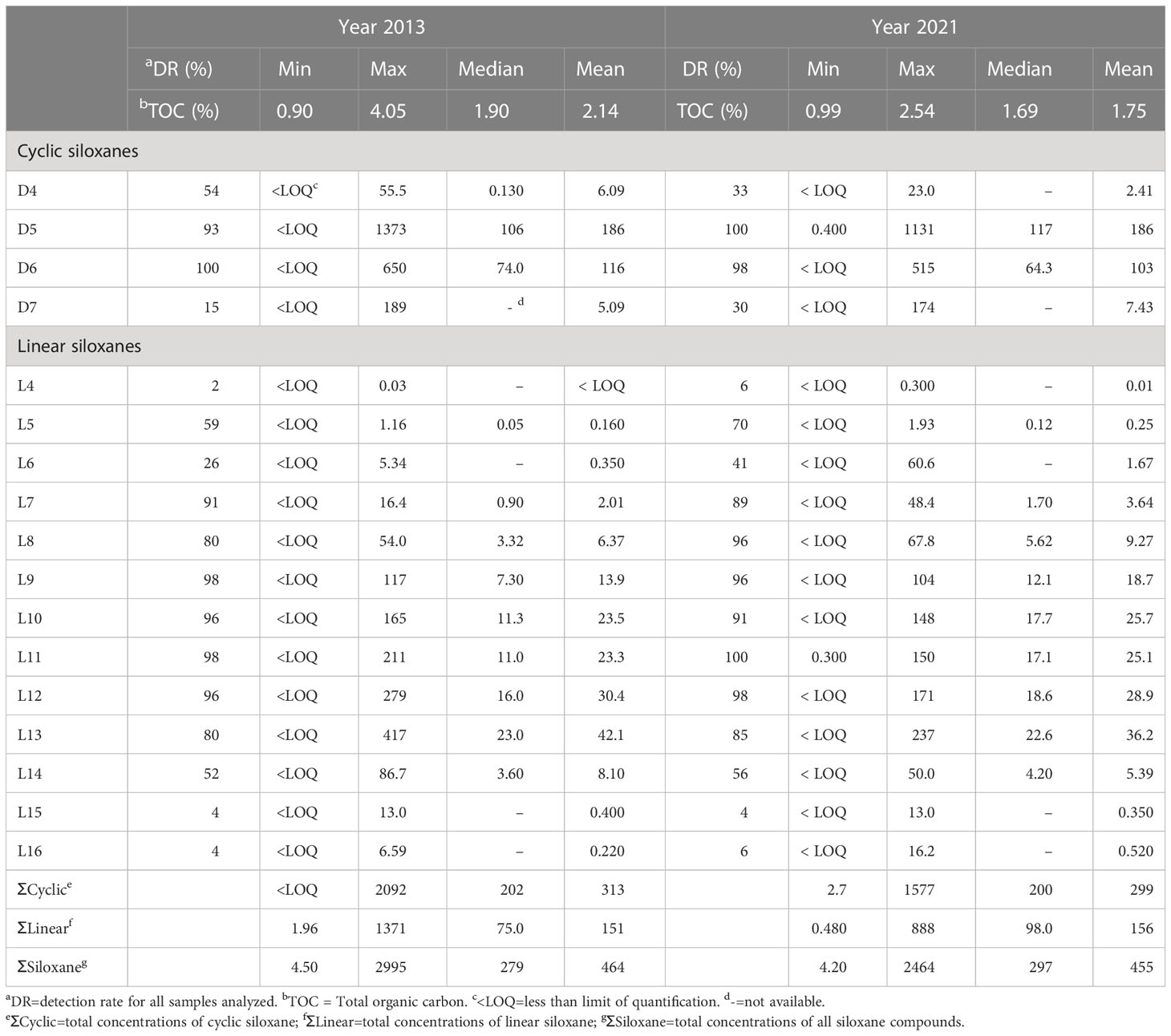
Table 1 Summary of concentrations (ng/g dry weight) of cyclic and linear siloxanes in sediment collected from Masan and Haengam Bays of Korean in 2013 and 2021.
The concentrations of ƩSiloxane in sediment collected in 2013 and 2021 ranged from 19.1 to 2995 (mean: 464) ng/g dw and 4.23 to 2464 (mean: 455) ng/g dw, respectively. No statistical differences were observed for the ƩSiloxane concentrations in sediment between 2013 (median: 279 ng/g dw) and 2021 (median: 297 ng/g dw). The TOC contents of sediment samples excavated in 2013 and 2021 ranged from 0.90% to 4.05% (mean: 2.14%) and from 0.99% to 2.54% (mean: 1.75%), respectively. The TOC contents in sediment collected in 2021 were conspicuously (p< 0.01) lower than those collected in 2013, suggesting decreasing trends in land-based organic matters. This trend may be attributed to domestic regulatory actions (e.g., Total Pollution Load Management System) implemented by the Korean government for Masan and Haengam Bays (Jin et al., 2016; Shen et al., 2018; Kim et al., 2020). Despite a decreasing TOC trend in sediment between both years, siloxane concentrations in sediment were maintained during eight years. Considering the strong affinity of siloxanes with sedimentary TOC (Lee et al., 2018a; Horii et al., 2022a), the increased contamination by siloxanes could be suggested. Previous report has also confirmed that the pollution levels of siloxanes historically increased in an industrialized bay of Korea since the 1970s (Lee et al., 2018a). In contrast to siloxanes, concentrations of persistent organic pollutants (POPs), including brominated flame retardants, polychlorinated dibenzo-p-dioxins and dibenzofurans (PCDD/Fs), and polychlorinated naphthalenes (PCNs), declined in sediment from developed industrial bays of Korea during the last two decades due to global and domestic regulations (Moon et al., 2008a; Jin et al., 2016; Lee et al., 2022b).
The concentrations of ƩCyclic and ƩLinear in sediment collected in 2013 ranged from 8.59 to 2092 (mean: 313) ng/g dw and 1.58 to 1371 (mean: 151) ng/g dw, respectively. Similarly, the concentrations of ƩCyclic and ƩLinear in sediment collected in 2021 ranged from 2.69 to 1577 (mean: 299) ng/g dw and 0.478 to 888 (mean: 156) ng/g dw, respectively. ƩCyclic levels in sediment were approximately two times higher than those of ƩLinear, consistent with published literature (Lee et al., 2019b; Zhi et al., 2019; Chen et al., 2022). This suggests that cyclic siloxanes contribute to the majority of consumption market in the global and Korean markets. In fact, cyclic siloxanes (including D4, D5, and D6) are highly consumed chemicals worldwide and they have been listed as “High Production Volume Chemicals” (US EPA (United States Environmental Protection Agency), 2021; Xiang et al., 2021; Liu et al., 2022).
Among 17 siloxane congeners, the highest level was found in sediment for D5 (mean: 186 and 186 ng/g dw in 2013 and 2021, respectively), followed by D6 (mean: 116 and 103 ng/g dw in 2013 and 2021, respectively). The results are probably due to the extensive use and strong stability for environmental processes of cyclic siloxanes compared to others (Lee et al., 2018a; Horii et al., 2022a). Among linear congeners, the highest level in sediment was found for L13 (mean: 42.1 and 36.2 ng/g dw in 2013 and 2021, respectively), followed by L10–L12, which had relatively high concentrations. Published studies have also reported high concentrations of L10–L13 relative to other linear congeners in sediment from the Korean Industrial Bays (Lee et al., 2018a; Lee et al., 2019b; Chen et al., 2022).
Clear correlations existed among siloxanes in sedimentary materials from Masan and Haengam Bays in 2013 and 2021 (r = 0.321–0.996, p< 0.05) (Table S2). ƩCyclic concentrations were significantly correlated with those of ƩLinear (r = 0.935, p< 0.001 in 2013; r = 0.948, p< 0.001 in 2021), suggesting that cyclic and linear congeners have similar sources and environmental behaviors in aquatic environments. TOC contents were statistically correlated with ƩCyclic levels (r = 0.605, p< 0.001 in 2013; r = 0.654, p< 0.001 in 2021) and ƩLinear levels (r = 0.515, p< 0.001 in 2013; r = 0.653, p< 0.001 in 2021). Moreover, almost all siloxanes showed significant correlations with TOC (r = 0.330–0.679, p< 0.005).
3.2 Global pollution status of siloxanes in sedimentary materials
The levels of siloxanes in sediment were compared with those in published literature to assess the contamination status of siloxanes in sediment observed in this study (Table S3). We focused on siloxanes in sediment excavated after 2010 to ensure a purposeful investigation. Although the numbers of measured siloxanes in previous studies varied, we found that the dominant siloxanes in sediment were comparable to those detected in this study. The sedimentary levels of siloxanes in this study were located within the ranges presented in global research results. The ƩCyclic levels in our sediment samples (mean: 313 ng/g dw in 2013, 299 ng/g dw in 2021) were higher than those reported in rivers, lakes, industrial bays and oil fields in China (170–257 ng/g dw; Zhang et al., 2018; Zhi et al., 2018; Zhi et al., 2019; Liu et al., 2022) and Korean industrial bays (219–245 ng/g dw; Lee et al., 2018a; Lee et al., 2019a). The overall ƩCyclic concentrations in this study were 10 to 1000 times higher than those reported in rivers and along the Korean coasts (3.39–106 ng/g dw; Wang et al., 2021; Chen et al., 2022; Kim et al., 2022), freshwater from China (28.5–58.1 ng/g dw; Zhi et al., 2018; He et al., 2021; Jiang et al., 2022), rivers near Tokyo Bay in Japan (71.9 ng/g dw; Horii et al., 2022b), river water in Spain (3.39 ng/g dw; Sanchís et al., 2013), and river water in Canada (50.9 ng/g dw; Pelletier et al., 2022). In contrast, our results were lower than those observed in sediment from Tokyo Bay, Japan (905 ng/g dw; Horii et al., 2022b), waters in Zhangjiagang, Kunming, and Beijing, China (978–1035 ng/g dw; Jiang et al., 2022), Rubí Brook, Spain (2070 ng/g dw; Sanchís et al., 2013), and waters in northern Vietnam (2518 ng/g dw; Nguyen et al., 2022).
The levels of ƩLinear (mean: 151 ng/g dw in 2013, 156 ng/g dw in 2021) in sedimentary materials observed in this study were similar to or higher than those presented in rivers and Korean coasts (9.38–85.5 ng/g dw; Lee et al., 2019b; Wang et al., 2021; Chen et al., 2022; Kim et al., 2022), rivers and lakes in China (12.2–146 ng/g dw; Zhi et al., 2019; He et al., 2021; Jiang et al., 2022), and rivers near Tokyo Bay, Japan (117 ng/g dw; Horii et al., 2022b). By comparison, our results showed that the ƩLinear concentrations in this study were obviously lower than those detected in rivers and oil fields in China (165–1558 ng/g dw; Zhi et al., 2018; Zhi et al., 2019; Jiang et al., 2022), Korean industrial bays (467 ng/g dw; Lee et al., 2018a), and Tokyo Bay, Japan (1310 ng/g dw; Horii et al., 2022b). Pollution levels of siloxanes in the aquatic environment from different research areas has varied significantly, which may be affected by regional sources and/or industrial demand (Lee et al., 2018a; Chen et al., 2022).
3.3 Spatial distribution of siloxanes
The spatial distribution of siloxanes in sedimentary materials from the Masan and Haengam Bays, Korea collected in 2013 and 2021 is shown in Figure 2A. In 2013, sediment from Masan Bay (ƩCyclic: 192 ng/g dw; ƩLinear: 69.5 ng/g dw) and Haengam Bay (ƩCyclic: 162 ng/g dw; ƩLinear: 81.2 ng/g dw) showed similar siloxane levels, while offshore waters had the lowest concentrations (ƩCyclic: 19.7 ng/g dw; ƩLinear: 18.7 ng/g dw). The most severe siloxane contamination was observed in sediment near the outfall of a WWTP (ƩCyclic: 600 ng/g dw; ƩLinear: 312 ng/g dw). In 2021, the siloxane levels in sediment from Masan Bay (ƩCyclic: 228 ng/g dw; ƩLinear: 109 ng/g dw) and Haengam Bay (ƩCyclic: 246 ng/g dw; ƩLinear: 112 ng/g dw) were statistically higher than those measured in offshore waters (ƩCyclic: 28.2 ng/g dw; ƩLinear: 21.0 ng/g dw). By contrast, sediment near the WWTP outfall (ƩCyclic: 494 ng/g dw; ƩLinear: 277 ng/g dw) had the highest concentration. These results confirmed that the pollution sources of siloxanes are primarily originated from industrial and WWTP activities in the coastal environment, which is consistent with the distribution of other organic pollutants reported from the published literature (Moon et al., 2008a; Moon et al., 2008b; Jin et al., 2016; Kim et al., 2020).
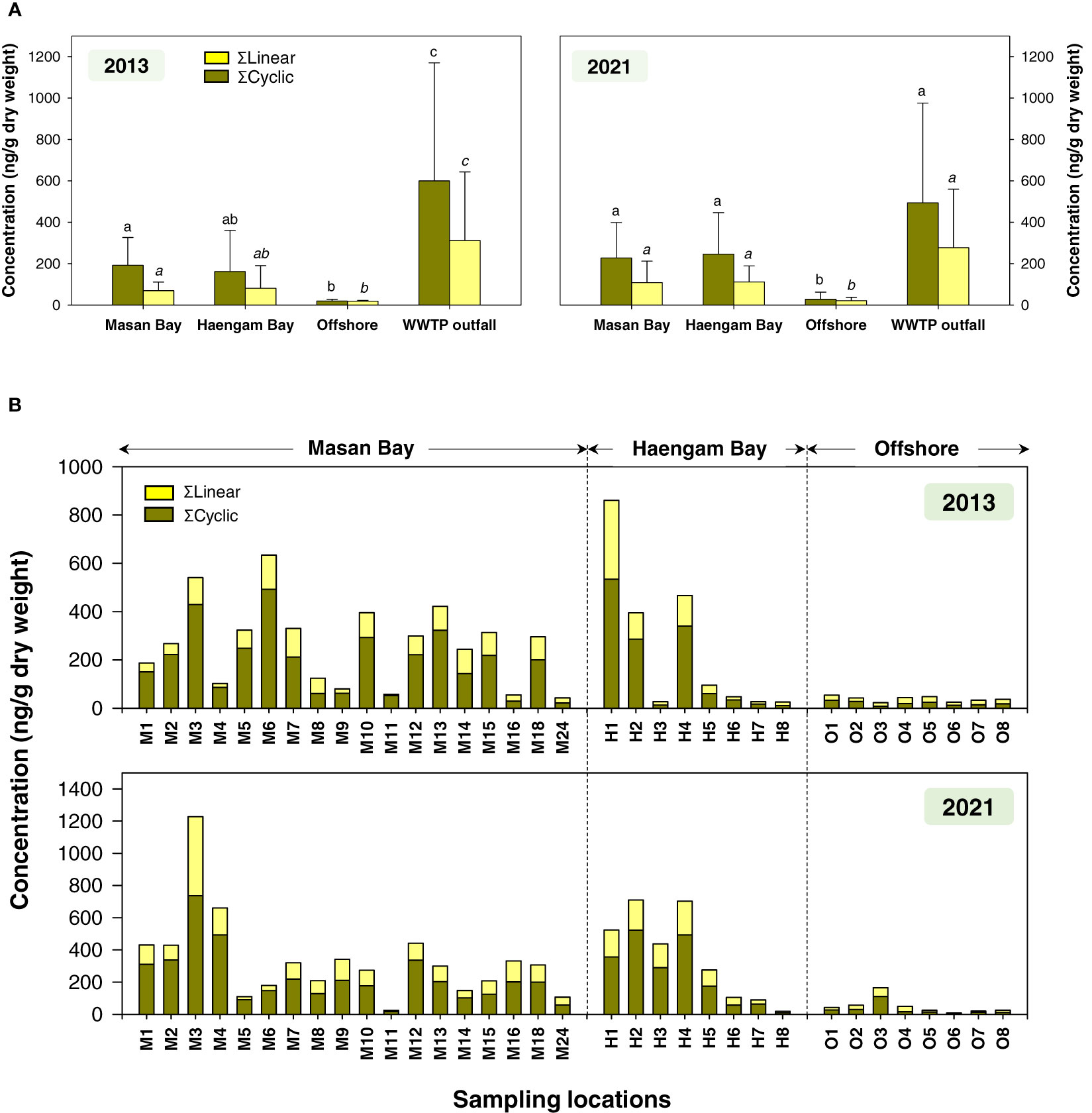
Figure 2 The spatial distribution of cyclic and linear siloxanes in sediment from Masan and Haengam Bays of Korea and around the submarine outfall of a wastewater treatment plant collected in 2013 and 2021. (A) Concentrations of cyclic and linear siloxanes in sediment from four coastal zones in our study collected in 2013 and 2021. (B) Concentrations of cyclic and linear siloxanes in sediment from each sampling locations collected in 2013 and 2021.
In this study, the ƩCyclic levels in sediment from Masan and Haengam Bays and near the WWTP outfall were approximately two times higher than those of ƩLinear in 2013 and 2021. This suggests that cyclic siloxanes have been primarily consumed by Korean industrial markets. The concentrations of ƩCyclic and ƩLinear in sediment from offshore waters were similar in 2013 and 2021. In comparison to Masan Bay (TOC = 2.42% and 2.01% in 2013 and 2021, respectively), Haengam Bay (TOC = 1.99% and 1.75% in 2013 and 2021, respectively) and the WWTP outfall (TOC = 2.67% and 1.68% in 2013 and 2021, respectively), the lowest TOC contents were observed in sediment from offshore waters (TOC = 1.26% and 1.31% in 2013 and 2021, respectively). Previous studies have reported significant correlations between siloxanes with TOC in sediment (Lee et al., 2014a; Lee et al., 2018a; Lee et al., 2019b). However, the inherent characteristics (log KOC and log KOW) of cyclic and linear congeners are incomplete (Lee et al., 2019b), which can lead to different binding abilities with TOC or different environmental behaviors. Therefore, the TOC content and environmental behavior of siloxanes may contribute to alterations in the distribution of cyclic and linear congeners between industrialized bays and offshore waters.
The highest siloxane levels in sediment from Masan Bay were observed at Stations M6 (634 ng/g dw) and M3 (541 ng/g dw) in 2013, and Stations M3 (1228 ng/g dw) and M4 (661 ng/g dw) in 2021 (Figure 2B). For Haengam Bay, the highest siloxane levels were observed at Stations H1 (861 ng/g dw) and H4 (466 ng/g dw) in 2013, and Stations H2 (710 ng/g dw) and H4 (702 ng/g dw) in 2021. These stations were particularly close to industrial complexes and harbor zones, indicating that industrial activities and shipping logistics have led to serious pollution of siloxanes in the coastal environment, which is consistent with published studies (Lee et al., 2018a; Chen et al., 2022).
3.4 Impact of WWTP effluents to sedimentary environment
To assess the contribution of the discharge of the WWTP for siloxane contamination in Masan Bay, Korea, the distribution of siloxanes was investigated in sedimentary materials from transect lines of the WWTP outfall (Figure 3). The highest concentration of ƩSiloxane was measured at the WWTP outfall (OF), which contained 2995 and 2467 ng/g dw of ƩSiloxane in 2013 and 2021, respectively. The overall concentrations of siloxanes appeared significant decreasing trends with increasing distances from the WWTP outfall, implying that the discharge of the WWTP was a reliable source of siloxanes in sedimentary materials. The previous investigation on POPs (including PBDEs, PCDD/Fs, PCBs, and perfluorinated compounds) in sediment near the outfall of a WWTP in Masan Bay also revealed that the discharge of WWTP effluents is a key source of these compounds in sediment (Moon et al., 2008b; Jin et al., 2016). Earlier studies have confirmed that WWTPs could not completely remove siloxanes in influents (Lee et al., 2014a; Horii et al., 2022a; Jiang et al., 2022).
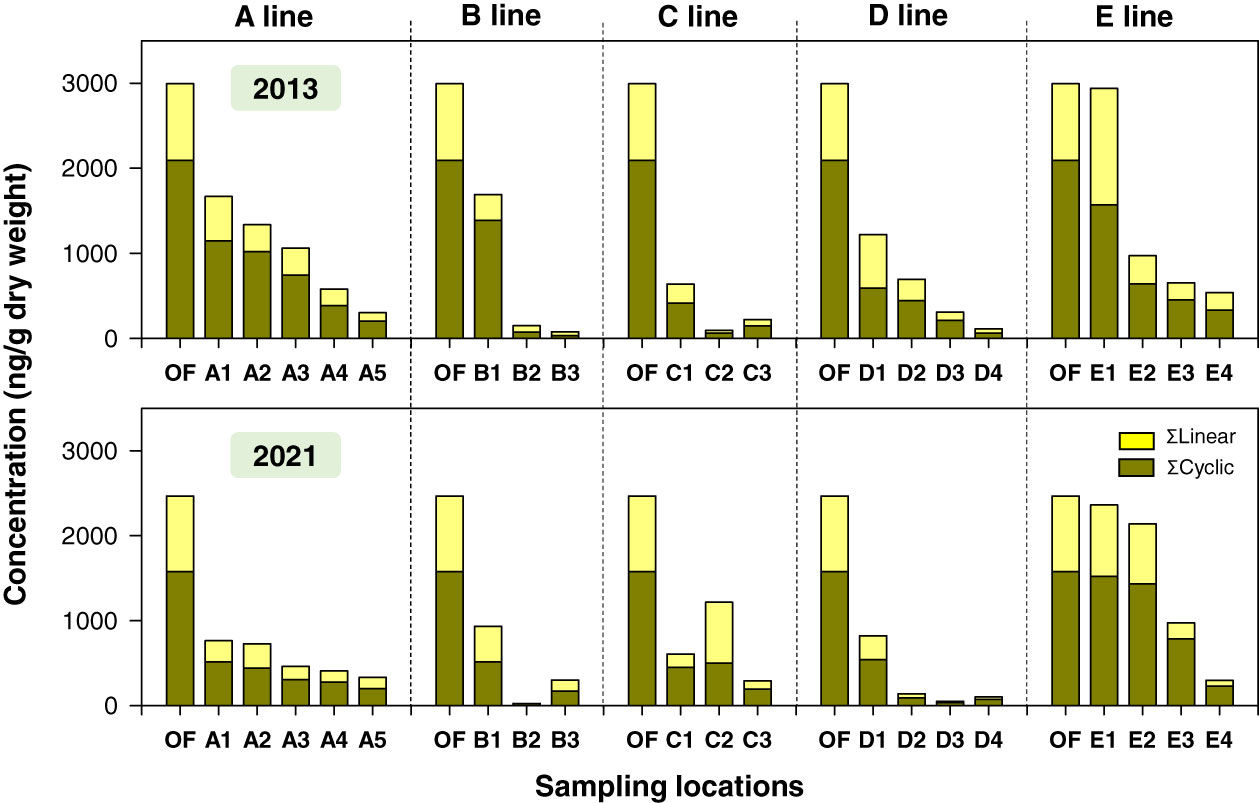
Figure 3 Concentrations of cyclic and linear siloxanes in sediment from the sampling locations of each transect line from the submarine outfall of a wastewater treatment plant in Masan Bay, Korea collected in 2013 and 2021.
Spearman correlation analysis was performed to further explore the contamination source and environmental behavior of siloxanes in sediment collected near the WWTP outfall (Table S4). Significant correlations were found between TOC and all siloxanes (except for D7), with r = 0.446–0.727 (p< 0.05), implying that TOC plays an important role in retaining siloxanes in sediment contaminated by the WWTP discharge. These findings are consistent with previous reports for other POPs, including PBDEs, PCBs, PCDD/Fs, and PFCs, in the sediment of WWTP discharge (Moon et al., 2008b; Jin et al., 2016). Regarding individual siloxanes, significant correlations were observed between almost all siloxanes, with r = 0.547–0.931 (p< 0.05) and r = 0.456–0.994 (p< 0.05) for cyclic and linear siloxanes, respectively. Taken together with our other findings, the WWTP discharge is a common source of organic contaminants including the POPs and siloxanes. More evidence is needed to confirm the environmental burden of organic contaminants from WWTP activities into the coastal environment.
3.5 Time trends of siloxanes
Temporal comparison of siloxane contamination in sediment collected in 2013 and 2021 are presented in Figure 4. The ƩSiloxane concentrations in sediment from Masan Bay (increase rate = 28%), Haengam Bay (47%), and offshore (28%) increased from 2013 to 2021, but the increase was not significant (p > 0.05). These results imply that the consumption of siloxanes in industrial markets of Korea has been expanding in the past decade. As a matter of fact, the global production of siloxanes increased by 25% from 2017 to 2022 (He et al., 2021). The levels of D5 and D6, which were dominant in the sediment core from Tokyo Bay, Japan, reached the highest value in 2006–2007, and then remained steady from 2007 to 2013 (Horii et al., 2022a). Siloxane pollution in the sediment core from Ulsan Bay, Korea, increased rapidly from the 1970s to 2015 (Lee et al., 2018a). These results emphasize the importance of continuous monitoring and investigation of the siloxane consumption market.
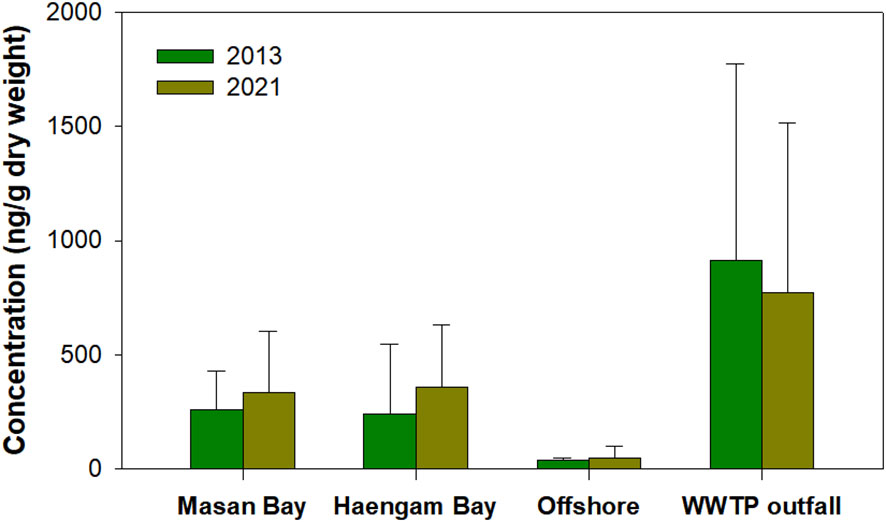
Figure 4 Time trends in the concentrations of siloxanes in sediment from Masan and Haengam Bays, offshore, and the submarine outfall of a wastewater treatment plant collected between 2013 and 2021.
Note that the increase rate of linear siloxanes (increase rate = 56%) in the sediment from Masan Bay between 2013 and 2021 was greater than that of cyclic siloxanes (increase rate = 18%). Conversely, the increase rate of cyclic siloxanes (increase rate = 52%) in sediment from Haengam Bay was greater than that of linear siloxanes (increase rate = 38%). This suggests that the consumption pattern of siloxanes, depending on the industrial types, has been changing. However, the concentration of siloxanes in the sediment from offshore may be affected by the comprehensive long-distance effect from the WWTP and industrial activities, where the increase rates of cyclic and linear congeners were 43% and 12%, respectively. Moreover, the ƩSiloxane levels in sediment from the WWTP outfall decreased by 15% from 2013 to 2021, of which the decrease rates of ƩCyclic and ƩLinear were 18% and 11%, respectively. This is likely due to the improved treatment capacity of the WWTP operation for organic contaminants in influents.
3.6 Composition profiles and pollution sources of siloxanes
The composition pattern for individual siloxanes in sediment from Masan Bay, Haengam Bay, offshore and the WWTP outfall collected in 2013 and 2021 is presented in Figure 5. D5 and D6 were found to be the highest contributors to the sediment across all the sampling locations from 2013 to 2021. The contribution of D5 of the ƩSiloxane concentrations increased from 31% in 2013 to 38% in 2021, while the contribution of D6 decreased from 34% in 2013 to 27% in 2021. D5 and D6 are widely added to commercial detergents for various uses (Horii and Kannan, 2008; Wang et al., 2013a), and are also widely added to consumer products, such as toothpaste, hair conditioners, skin care products, cosmetics, and sunscreen products (Lu et al., 2011; Gobas et al., 2015; Zhang et al., 2018). The higher contributions of D5 and D6 observed in our study may be due to intensive industrial activities and rapid urban development in the coastal areas (Lee et al., 2018b). The contributions of individual linear siloxanes (L8–L13) in sediment ranged from 1% to 9% of the ƩSiloxane concentrations, without any significant changes depending on the sampling years. The contributions of remaining siloxanes, such as D4, D7, L3–L7, and L14–L17, were less than 1%. Linear siloxanes, such as L6–L13, are commonly used in cosmetics and PCPs, toys, cooking utensils, and household consumer goods (Horii and Kannan, 2008; Li et al., 2016; Xu et al., 2017; Zha et al., 2018; He et al., 2021). The contributions of L8–L13 found in our study may come from personal care products and consumer products.
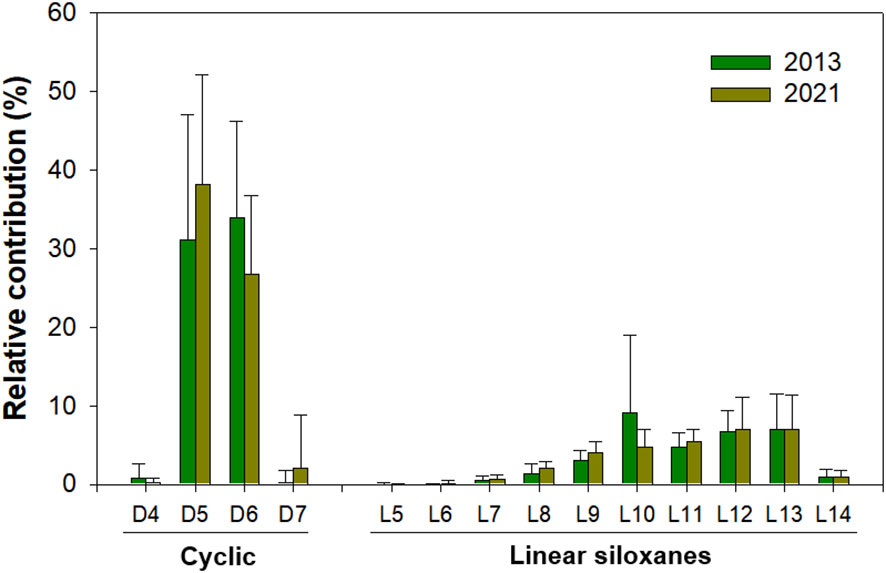
Figure 5 Compositional profiles of individual siloxanes in sediment from Masan and Haengam Bays, offshore, and the submarine outfall of a wastewater treatment plant collected between 2013 and 2021.
Non-parametric multidimensional scaling (nMDS) analysis was performed to identify the pollution sources of siloxanes in sediment collected in this study area in 2021 (Figure 6). All sampling stations were divided into four sets with a stress value of 0.03. Group A included sampling locations near the WWTP outfall (OF, C2, E1, and E2), and the industrial complex of Masan Bay (M3). Group B included most sampling locations in Masan Bay (M1, M2, M4, M7–M10, M12, M13, M15, M16, and M18) and Haengam Bay (H1–H5), as well as most locations near the WWTP outfall (A1–A5, B1, B3, C1, C3, D1, E3, and E4). Group C included some sampling locations from Masan Bay (M5, M6, M14, and M24), Haengam Bay (H6 and H7), the WWTP outfall (D2–D4), and offshore (O1–O4), most of which were located in transitional zones between bay and offshore. Group D included the central part of Masan Bay (M11) and offshore sampling locations (O5–O8 and H8) far away from the bays. All groups were dominated by D5 and D6, but the contribution of siloxanes in each group differed. The contribution of D5 in Group D (23%) was lower than that in Group B (42%), Group A (36%) and Group C (33%), whereas the contribution of D6 in Group D (36%) was higher than that in Group B (23%), Group A (21%) and Group C (33%), For linear siloxanes, the contributions of L5–L9, L11, and L13 were similar within clustered groups. The contributions of dominant siloxanes, D5, D6, and L10, varied among different groups. In particular, D5 in Group D, the area far away from the contamination source, had a significantly lower contribution than other areas.
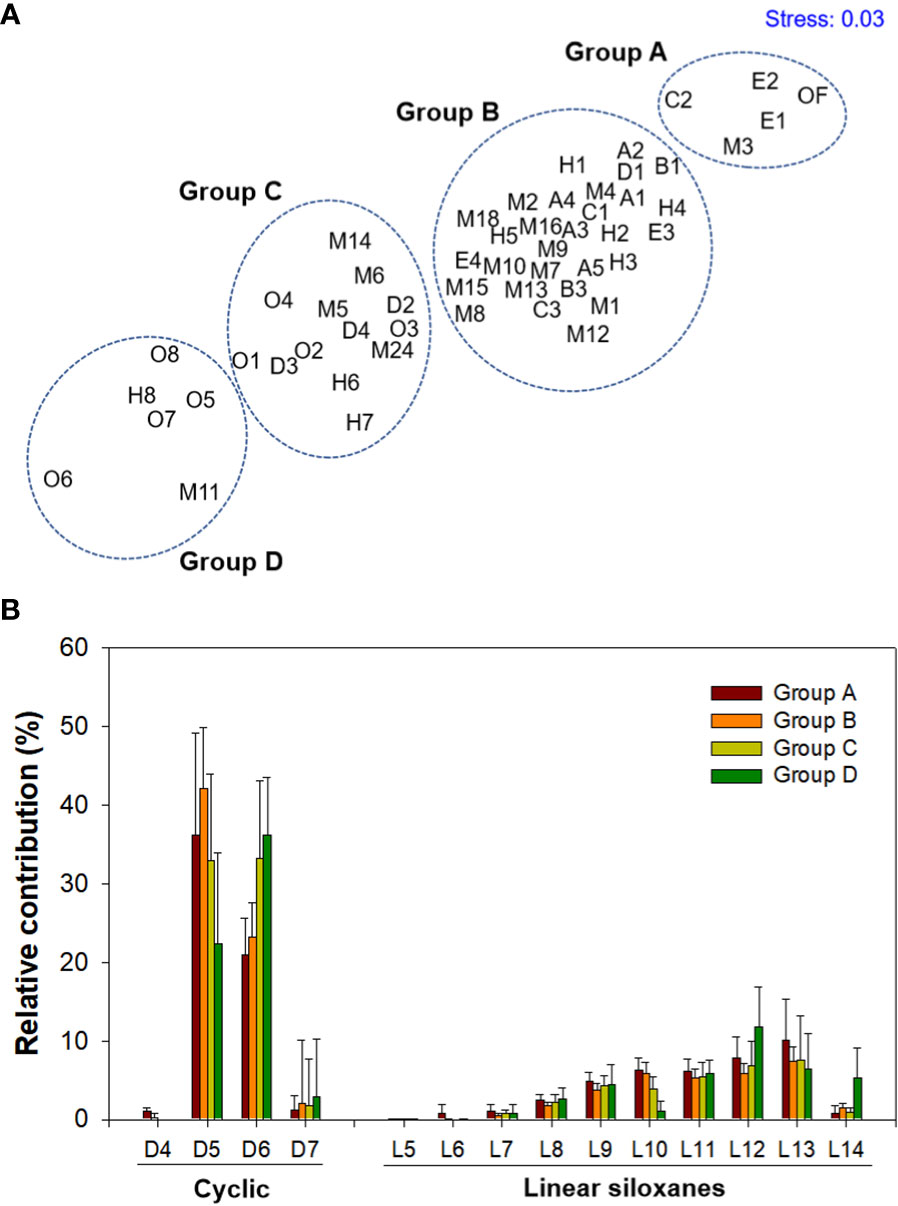
Figure 6 Non-parametric multidimensional scaling (nMDS) ordination plot (A) and their respective compositional profiles (B) of siloxanes in sediment from Masan and Haengam Bays, offshore, and the submarine outfall of a wastewater treatment plant collected in 2021.
3.7 Ecological risk assessment of sedimentary siloxanes
The levels of D4, D5, and D6 in all sedimentary materials measured in this study were lower than PNECsediment (HQs< 1), indicating that they have no significant ecological risk to benthos (Table S5). These results are consistent with published literature, such as in Daqing Oilfield, Bohai Bay and Lake Chaohu in China, industrial bays in Korea, and Tokyo Bay in Japan (Zhi et al., 2018; Lee et al., 2019b; He et al., 2021; Horii et al., 2022b; Liu et al., 2022). Although it is currently believed that these three cyclic siloxanes pose inappreciable risks to benthos (Woodburn et al., 2018), He et al. (2021) reported the ecological risks of D4 and D5 to fish in Chinese rivers (He et al., 2021). It should be noted that the results from all earlier studies are affected by the different PNECs employed for ecological risks to siloxanes. Additionally, the potential synergy caused by the co-existence of siloxanes and other organic contaminants, such as POPs, has not been considered and the risks posed by linear siloxanes have been ignored. This may lead to an underestimation of potential health risks. Considering the continuous increase in siloxane consumption, the ecological risk of siloxanes to the aquatic environment may be unpredicted. Therefore, further investigations are necessary to keep us alert for ecological risks of siloxanes.
4 Conclusions
This study presents an investigation into the spatial distribution and temporal trend in siloxane contamination in sedimentary materials from industrial bays of Korea over the period 2013–2021. Our results indicate that siloxanes were present in all sediments collected, suggesting continuous contamination for the past decade. The higher concentrations of D5 and D6 observed in our samples are likely due to their high consumption and resistance to environmental processes. Industrial and WWTP activities were found to be the primary sources of siloxane contamination in the coastal environment, with TOC playing a key role in the migration and transformation of siloxanes. There were no significant time trends observed in sedimentary siloxane concentrations between 2013 and 2021 despite increased global and domestic markets. Our study found that D4, D5, and D6 concentrations in all sedimentary materials were limited in their potential to pose a threat to benthos. However, given the increasing demand and limitations of risk assessment, exposure to siloxanes by aquatic organisms cannot be ignored in the future.
Data availability statement
The raw data supporting the conclusions of this article will be made available by the authors, without undue reservation.
Author contributions
WC conducted all statistical analysis and wrote the draft of the manuscript. YK, H-KL, and J-EL collected the sediment samples, developed and validated the analytical methods of target contaminants. ML processed and analyzed chemical data and critically reviewed the draft of the manuscript. H-BM processed all analytical data and edited the manuscript for journal submission. All authors contributed to the article and approved the submitted version.
Funding
This study was supported by the projects entitled “Development of technology for impact assessment and management of HNS discharged from marine industrial facilities (KIMST-20210660)” and “Development of source identification and apportionment methods for toxic substances in marine environments (KIMST-20220534)” of Korea institute of Marine Science & Technology Promotion funded by the Ministry of Oceans and Fisheries, Korea.
Conflict of interest
The authors declare that the research was conducted in the absence of any commercial or financial relationships that could be construed as a potential conflict of interest.
Publisher’s note
All claims expressed in this article are solely those of the authors and do not necessarily represent those of their affiliated organizations, or those of the publisher, the editors and the reviewers. Any product that may be evaluated in this article, or claim that may be made by its manufacturer, is not guaranteed or endorsed by the publisher.
Supplementary material
The Supplementary Material for this article can be found online at: https://www.frontiersin.org/articles/10.3389/fmars.2023.1185314/full#supplementary-material
References
Bernardo F., Alves A., Homem V. (2022). A review of bioaccumulation of volatile methylsiloxanes in aquatic systems. Sci. Total Environ. 824, 153821. doi: 10.1016/j.scitotenv.2022.153821
Chen W., Kang Y.-J., Lee H.-K., Lee M., Moon H.-B. (2022). Nationwide monitoring of cyclic and linear siloxanes in sediment and bivalves from Korean coastal waters: occurrence, geographical distribution, and bioaccumulation potential. Mar. pollut. Bull. 185, 114201. doi: 10.1016/j.marpolbul.2022.114201
Cheng J. L., Tang Z. W., Ma Y., Yin H. M., Meng T., Sun J. Z. (2021). Methyl siloxanes in soils from a large silicone-manufacturing site, China: concentrations, distributions and potential human exposure. Environ. Geochem. Health 43, 3871–3881. doi: 10.1007/s10653-021-00873-7
Feng D., Zhang X. R., Yuan H., Li X. Y., Fan X. J. (2022). Identification, migration, and childhood exposure of methylsiloxanes in silicone infant bottle nipples marketed in China. Sci. Total Environ. 829, 154449. doi: 10.1016/j.scitotenv.2022.154449
Gerhards R., Seston R. M., Kozerski G. E., McNett D. A., Boehmer T., Durham J. A., et al. (2022). Basic considerations to minimize bias in collection and analysis of volatile methyl siloxanes in environmental samples. Sci. Total Environ. 851, 158275. doi: 10.1016/j.scitotenv.2022.158275
Gobas F., Xu S., Kozerski G., Powell D. E., Woodburn K. B., Mackay D., et al. (2015). Fugacity and activity analysis of the bioaccumulation and environmental risks of decamethylcyclopentasiloxane (D5). Environ. Toxicol. Chem. 34, 1–9. doi: 10.1002/etc.2942
Guo W. J., Dai Y., Chu X. T., Cui S., Sun Y. P., Li Y. F., et al. (2021). Assessment of bioaccumulation factor (BAF) of methyl siloxanes in crucian carp (Carassius auratus) around a siloxane production factory. Ecotoxicol. Environ. Saf. 213, 111983. doi: 10.1016/j.ecoenv.2021.111983
Guo J. Y., Zhou Y., Zhang B. Y., Zhang J. B. (2019). Distribution and evaluation of the fate of cyclic volatile methyl siloxanes in the largest lake of southwest China. Sci. Total Environ. 657, 87–95. doi: 10.1016/j.scitotenv.2018.11.454
He Y., Shuai S. C., Yang L., Tang Z. W. (2021). Occurrence of methylsiloxanes in sediments from a subtropical river-lake system in eastern China and its implication for ecological risks. Ecotoxicol. Environ. Saf. 223, 112627. doi: 10.1016/j.ecoenv.2021.112627
Homem V., Capela D., Silva J., Cincinelli A., Santos L., Alves A., et al. (2017). An approach to the environmental prioritisation of volatile methylsiloxanes in several matrices. Sci. Total Environ. 579, 506–513. doi: 10.1016/j.scitotenv.2016.11.068
Horii Y., Kannan K. (2008). Survey of organosilicone compounds, including cyclic and linear siloxanes, in personal-care and household products. Arch. Environ. Contam. Toxicol. 55, 701–710. doi: 10.1007/s00244-008-9172-z
Horii Y., Minomo K., Lam J. C. W., Yamashita N. (2022a). Spatial distribution and accumulation profiles of volatile methylsiloxanes in Tokyo bay, Japan: mass loadings and historical trends. Sci. Total Environ. 806, 150821. doi: 10.1016/j.scitotenv.2021.150821
Horii Y., Ohtsuka N., Minomo K., Motegi M., Takemine S., Motegi M., et al. (2021). Distribution characteristics of methylsiloxanes in atmospheric environment of saitama, Japan: diurnal and seasonal variations and emission source apportionment. Sci. Total Environ. 754, 142399. doi: 10.1016/j.scitotenv.2020.142399
Horii Y., Ohtsuka N., Nishino T., Kroda K., Imaizumi Y., Sakurai T. (2022b). Spatial distribution, mass balance, and benthic risk assessment of cyclic, linear, and modified methylsiloxanes in sediments from the Tokyo bay catchment basin, Japan: mass balance in extractable organosilicon. Sci. Total Environ. 838, 155956. doi: 10.1016/j.scitotenv.2022.155956
Jia H. L., Zhang Z. F., Wang C. Q., Hong W. J., Sun Y. Q., Li Y.-F. (2015). Trophic transfer of methyl siloxanes in the marine food web from coastal area of northern China. Environ. Sci. Technol. 49, 2833–2840. doi: 10.1021/es505445e
Jiang Y., Guo J., Zhou Y., Zhang B., Zhang J. (2022). Occurrence and behavior of methylsiloxanes in urban environment in four cities of China. Int. J. Environ. Res. Public Health 19, 13869. doi: 10.3390/ijerph192113869
Jin X., Lee H.-K., Badejo A. C., Lee S.-Y., Shen A., Lee S., et al. (2016). Decline in sediment contamination by persistent toxic substances from the outfall of wastewater treatment plant: effectiveness of legislative actions in Korea. Chemosphere 453, 426–435. doi: 10.1016/j.chemosphere.2016.03.075
Kim D., Cho H.-E., Won E.-J., Kim H.-J., Lee S., An K.-G., et al. (2022). Environmental fate and trophic transfer of synthetic musk compounds and siloxanes in geum river, Korea: compound-specific nitrogen isotope analysis of amino acids for accurate trophic position estimation. Environ. Int. 161, 107123. doi: 10.1016/j.envint.2022.107123
Kim S., Lee Y.-S., Moon H.-B. (2020). Occurrence, distribution, and sources of phthalates and non-phthalate plasticizers in sediment from semi-enclosed bays of Korea. Mar. pollut. Bull. 151, 110824. doi: 10.1016/j.marpolbul.2019.110824
Lee J. H., Hong S. J., Kwon B.-O., Cha S. A., Jeong H.-D., Chang W. K., et al. (2018b). Integrated assessment of persistent toxic substances in sediments from masan bay, south Korea: comparison between 1998 and 2014. Environ. pollut. 238, 317–325. doi: 10.1016/j.envpol.2018.02.064
Lee S., Kim K., Jeon J., Moon H.-B. (2019a). Optimization of suspect and non-target analytical methods using GC/TOF for prioritization of emerging contaminants in the Arctic environment. Ecotoxicol. Environ. Saf. 181, 11–17. doi: 10.1016/j.ecoenv.2019.05.070
Lee S.-Y., Lee. S., Choi M., Kannan K., Moon H.-B. (2018a). An optimized method for the analysis of cyclic and linear siloxanes and their distribution in surface and core sediments from industrialized bays in Korea. Environ. pollut. 236, 111–118. doi: 10.1016/j.envpol.2018.01.051
Lee H.-H., Lee S., Lee M. J., Moon H.-B. (2022). Spatial and temporal trends in polychlorinated naphthalenes in sediment from industrialized coastal environments of Korea: potential sources and ecotoxicological concerns. Environ. Geochem. Health. doi: 10.1007/s10653-022-01395-6
Lee H.-K., Lee S. Y., Lim J.-E., Moon H.-B. (2020a). Legacy and novel flame retardants in water and sediment from highly industrialized bays of Korea: occurrence, source tracking, decadal time trend, and ecological risks. Mar. pollut. Bull. 160, 111639. doi: 10.1016/j.marpolbul.2020.111639
Lee Y.-S., Lim J.-E., Lee S., Moon H.-B. (2020b). Phthalates and non-phthalate plasticizers in sediment from Korean coastal waters: occurrence, spatial distribution, and ecological risks. Mar. pollut. Bull. 154, 111119. doi: 10.1016/j.marpolbul.2020.111119
Lee S., Moon H.-B., Song G.-J., Ra K., Lee W.-C., Kannan K. (2014a). A nationwide survey and emission estimates of cyclic and linear siloxanes through sludge from wastewater treatment plants in Korea. Sci. Total Environ. 497–498, 106–112. doi: 10.1016/j.scitotenv.2014.07.083
Lee D., Park M.-K., Lee I.-S., Choi S.-D. (2019b). Contamination characteristics of siloxanes in coastal sediment collected from industrialized bays in south Korea. Ecotoxicol. Environ. Saf. 182, 109457. doi: 10.1016/j.ecoenv.2019.109457
Lee S., Song G. J., Kannan K., Moon H.-B. (2014b). Occurrence of PBDEs and other alternative brominated flame retardants in sludge from wastewater treatment plants in Korea. Sci. Total Environ. 470–471, 1422–1429. doi: 10.1016/j.scitotenv.2013.07.118
Li B., Li W., Sun S., Qi H., Ma W., Liu L., et al. (2016). The occurrence and fate of siloxanes in wastewater treatment plant in Harbin, China. Environ. Sci. pollut. Res. 23, 13200–13209. doi: 10.1007/s11356-016-6481-z
Liu N. N., Zhao X. S., Xu L., Cai Y. Q. (2022). Temporal and spatial variation, input fluxes and risk assessment of cyclic methylsiloxanes in rivers-bohai Sea system. Ecotox. Environ. Saf. 231, 113169. doi: 10.1016/j.ecoenv.2022.113169
Lu Y., Yuan T., Wang W. H., Kannan K. (2011). Concentrations and assessment of exposure to siloxanes and synthetic musks in personal care products from China. Environ. pollut. 159, 3522–3528. doi: 10.1016/j.envpol.2011.08.015
Mackay D., Cowan-Ellsberry C. E., Powell D. E., Woodburn K. B., Xu S., Kozerski G. E., et al. (2015). Decamethylcyclopentasiloxane (D5) environmental sources, fate, transport, and routes of exposure. Environ. Toxicol. Chem. 34, 2689–2702. doi: 10.1002/etc.2941
Moon H.-B., Choi H.-G., Lee P.-Y., Ok G. (2008a). Congener-specific characterization and sources of polychlorinated dibenzo-p-dioxins, dibenzofurans, and dioxin-like polychlorinated biphenyls in marine sediments from industrialized bays of Korea. Environ. Toxicol. Chem. 27, 323–333. doi: 10.1897/07-337R.1
Moon H.-B., Yoon S.-P., Jung R.-H., Choi M. (2008b). Wastewater treatment plants (WWTPs) as a source of sediment contamination by toxic organic pollutants and fecal sterols in a semi-enclosed bay in Korea. Chemosphere 73, 880–889. doi: 10.1016/j.chemosphere.2008.07.038
Nguyen H. M. N., Khieu H. T., Le H. Q., Duong T. T., Do T. Q., Minh T. B., et al. (2022). Assessment of distributional characteristics and ecological risks of cyclic volatile methylsiloxanes in sediments from urban rivers in northern Vietnam. Environ. Sci. pollut. Res. 29, 29917–29926. doi: 10.1007/s11356-021-18487-y
Nika M. C., Ntaiou K., Elytis K., Thomaidi V. S., Gatidou G., Kalantzi O. I., et al. (2020). Wide-scope target analysis of emerging contaminants in landfill leachates and risk assessment using risk quotient methodology. J. Hazard. Mater. 394, 1–10. doi: 10.1016/j.jhazmat.2020.122493
Panagopoulos D., Warner N. A., Jantunen L., Jahnnke A., Wong F., MacLeod M. (2020). Investigating the presence and persistence of volatile methylsiloxanes in Arctic sediments. Environ. Sci.: Processes Impacts. 22, 908–917. doi: 10.1039/C9EM00455F
Pelletier M., Isabel L., Armellin A., McDaniel T., Martin P., McGoldrick D., et al. (2022). Influence of wastewater effluents on the bioaccumulation of volatile methylsiloxanes in the St. Lawrence River. Sci. Total Environ. 806, 151267. doi: 10.1016/j.scitotenv.2021.151267
Redman A. D., Mihaich E., Woodburn K., Paquin P., Powell D., Mcgrath J. A., et al. (2012). Tissue-based risk assessment of cyclic volatile methyl siloxanes. Environ. Toxicol. Chem. 31, 1911–1919. doi: 10.1002/etc.1900
Sanchís J., Cabrerizo A., Galban-Malagon C., Barcelo D., Farre M., Dachs J. (2015). Unexpected occurrence of volatile dimethylsiloxanes in Antarctic soils, vegetation, phytoplankton, and krill. Environ. Sci. Technol. 49, 4415–4424. doi: 10.1021/es503697t
Sanchís J., Martínez E., Ginebreda A., Farré M., Barceló D. (2013). Occurrence of linear and cyclic volatile methylsiloxanes in wastewater, surface water and sediments from Catalonia. Sci. Total Environ. 443, 30–38. doi: 10.1016/j.scitotenv.2012.10.047
Shen A., Lee S., Ra K., Suk D., Moon H.-B. (2018). Historical trends of perfluoroalkyl substances (PFASs) in dated sediments from semi-enclosed bays of Korea. Mar. pollut. Bull. 128, 287–294. doi: 10.1016/j.marpolbul.2018.01.039
Tong X., Mohapatra S., Zhang J., Tran N. H., You L., He Y., et al. (2022). Source, fate, transport and modelling of selected emerging contaminants in the aquatic environment: current status and future perspectives. Water Res. 217, 118418. doi: 10.1016/j.watres.2022.118418
US EPA (United States Environmental Protection Agency) (2021) EPA: High production volume list. Available at: https://comptox.epa.gov/dashboard/chemical_lists/EPAHPV/ (Accessed 2 May 2021).
Wang D. G., Aggarwal M., Tait T., Brimble S., Pacepavicius G., Kinsman L., et al. (2015). Fate of anthropogenic cyclic volatile methylsiloxanes in a wastewater treatment plant. Wat. Res. 72, 209–217. doi: 10.1016/j.watres.2014.10.007
Wang W. T., Cho H.-S., Kim K., Park K.-H., Oh J.-E. (2021). Tissue-specific distribution and bioaccumulation of cyclic and linear siloxanes in south Korean crucian carp (Carassius carassius). environ. Pollut. 288, 117789. doi: 10.1016/j.envpol.2021.117789
Wang D. G., Norwood W., Alaee M., Byer J. D., Brimble S. (2013a). Review of recent advances in research on the toxicity, detection, occurrence and fate of cyclic volatile methyl siloxanes in the environment. Chemosphere 93, 711–725. doi: 10.1016/j.chemosphere.2012.10.041
Wang D. G., Steer H., Tait T., Williams Z., Pacepavicius G., Young T., et al. (2013b). Concentrations of cyclic volatile methylsiloxanes in biosolid amended soil, influent, effluent, receiving water, and sediment of wastewater treatment plants in Canada. Chemosphere 93, 766–773. doi: 10.1016/j.chemosphere.2012.10.047
Woodburn K. B., Seston R. M., Kim J., Powell D. E. (2018). Benthic invertebrate exposure and chronic toxicity risk analysis for cyclic volatile methylsiloxanes: comparison of hazard quotient and probabilistic risk assessment approaches. Chemosphere 192, 337–347. doi: 10.1016/j.chemosphere.2017.10.140
Xiang X. L., Liu N. N., Xu L., Cai Y. Q. (2021). Review of recent findings on occurrence and fates of siloxanes in environmental compartments. Ecotoxicol. Environ. Saf. 224, 112631. doi: 10.1016/j.ecoenv.2021.112631
Xu L., Zhi L., Cai Y. (2017). Methylsiloxanes in children silicone-containing products from China: profiles, leaching, and children exposure. Environ. Int. 101, 165–172. doi: 10.1016/j.envint.2017.01.022
Zha C., Wang C., Li A. (2018). Toxicities of selected essential oils, silicone oils, and paraffin oil against the common bed bug (Hemiptera: Cimicidae). J. Econ. Entomol. 111, 170–177. doi: 10.1093/jee/tox285
Zhang Y. X., Shen M. C., Tian Y., Zeng G. M. (2018). Cyclic volatile methylsiloxanes in sediment, soil, and surface water from dongting lake, China. J. Soil. Sediment 18, 2063–2071. doi: 10.1007/s11368-018-1948-9
Zhi L. Q., Xu L., He X. D., Zhang C. H., Cai Y. Q. (2018). Occurrence and profiles of methylsiloxanes and their hydrolysis product in aqueous matrices from the daqing oilfield in China. Sci. Total Environ. 631-632, 879–886. doi: 10.1016/j.scitotenv.2018.03.098
Zhi L. Q., Xu L., He X. D., Zhang C. H., Cai Y. Q. (2019). Distribution of methylsiloxanes in benthic mollusks from the Chinese bohai Sea. J. Environ. Sci. 76, 199–207. doi: 10.1016/j.jes.2018.04.026
Keywords: siloxanes, temporal trend, source, benthic organism, ecological risk
Citation: Chen W, Kang Y, Lee H-K, Lim J-E, Lee M and Moon H-B (2023) Spatial distribution and temporal trends of cyclic and linear siloxanes in sediment from semi-enclosed and industrialized bays of Korea, in 2013 and 2021. Front. Mar. Sci. 10:1185314. doi: 10.3389/fmars.2023.1185314
Received: 13 March 2023; Accepted: 03 April 2023;
Published: 19 April 2023.
Edited by:
Junghyun Lee, Seoul National University, Republic of KoreaReviewed by:
Yunqiao Zhou, Chinese Academy of Sciences, ChinaMin-Kyu Park, Jeju National University, Republic of Korea
Copyright © 2023 Chen, Kang, Lee, Lim, Lee and Moon. This is an open-access article distributed under the terms of the Creative Commons Attribution License (CC BY). The use, distribution or reproduction in other forums is permitted, provided the original author(s) and the copyright owner(s) are credited and that the original publication in this journal is cited, in accordance with accepted academic practice. No use, distribution or reproduction is permitted which does not comply with these terms.
*Correspondence: Hyo-Bang Moon, aGJtb29uQGhhbnlhbmcuYWMua3I=