- 1Fisheries College, Guangdong Ocean University, Zhanjiang, China
- 2Guangdong Provincial Key Laboratory of Aquatic Animal Disease Control and Healthy Culture, Guangdong Ocean University, Zhanjiang, China
Heatwaves have become increasingly frequent and intense, posing a significant threat to the survival and health of marine bivalves. The temperature fluctuations associated with heatwaves can cause significant alterations in the composition and quantity of microbial communities in bivalves, resulting in changes to their immunological responses, gut microbiome, oxidative stress levels, and other physiological processes and eventually making them more susceptible to diseases and mass mortalities. This is particularly concerning because some of these bivalves are consumed raw, which could represent a risk to human health. This paper provides an overview of the current state of knowledge regarding the impact of marine heatwaves on bivalves and their microbial communities, demonstrating the intricate relationship between heatwaves, microbial ecosystems, and bivalve health. Our analysis highlights the need for additional research to establish the underlying mechanisms of these reactions and to develop appropriate conservation and management strategies to limit the impact of heatwaves on bivalves and their microbial ecosystems.
1 Introduction
Marine heatwaves have been widely recognized as one of the most significant drivers of change in the marine environment in recent years (Oliver et al., 2018). These heatwaves, defined as prolonged and intense periods of elevated water temperature, have become more frequent and intense with global warming, leading to severe impacts on the marine ecosystem (Frölicher et al., 2018; He et al., 2022a; Xu et al., 2022). One of the areas of particular concern is the effect of marine heatwaves on bivalve microbial communities, which are an essential component of the marine ecosystem and play a critical role in maintaining its stability and health. The diversity of microbial communities in bivalves has been extensively studied recently.
A growing body of literature demonstrates the presence of various bacteria, archaea, and eukaryotic microorganisms (Haygood et al., 1999; Fiore et al., 2010; Robledo et al., 2019). Bivalves harbor a diverse community of bacteria, including Proteobacteria, Firmicutes, and Bacteroidetes. Vibrionaceae, Pseudomonadaceae, and Flavobacteriaceae families (Leite et al., 2017; Akter et al., 2022). The structure of microbial communities in bivalves is highly variable, with different regions of the bivalve’s body harboring distinct microbial communities. For example, the gills of clams contained a different microbial community than the digestive tract, with the gills being dominated by Proteobacteria and the digestive tract being dominated by Firmicutes (Zhang et al., 2016). Similarly, the gut of mussels contained a different microbial community, with the gut being dominated by Bacteroidetes and the gills being dominated by Proteobacteria (Musella et al., 2020). The function of microbial communities in bivalves is not well understood. However, recent studies have suggested that they play essential roles in the survival and growth of these organisms. For example, the microbial communities Vibrio, Bacteroides, and Pseudomonas in oysters play a crucial role in the digestion of food, including the breakdown of complex carbohydrates and the production of essential amino acids (Pierce and Ward, 2019). Similarly, the microbial communities Gammaproteobacteria, and Alphaproteobacteria in mussels play crucial roles in detoxifying pollutants, including heavy metals and organochlorines (Milan et al., 2018; Wang et al., 2020). In recent years, the effects of marine heatwaves on the bivalve microbial communities have become a focus of scientific research. This is because the microbes present in these bivalve mollusks play an essential role in the health and survival of the host and are thought to play a significant role in mediating the effects of heat waves on the bivalve mollusks (Ertl et al., 2016).
Mortality events affecting adult and juvenile bivalves have been reported throughout history (Jones et al., 2017; Alfaro et al., 2019). These events have been observed across all ages and production stages (Lattos et al., 2020; Soon and Zheng, 2020), some attributed to pathogens favored by temperature increase. One such disease affecting farmed Pacific oysters on the West Coast is the Protistan parasite Mikrocytos mackini (Denman Island Disease), firstly reported in the 1960s on Vancouver Island and has resulted in mortality events (Sweet and Bateman, 2016; Garcia et al., 2018). In addition, another parasite, Haplosporidium nelsoni, was associated with mortality events in Crassostrea virginica in 2007 (Matt et al., 2020).
However, despite the growing interest in this area, a comprehensive review of the existing research is yet to be conducted. This review paper aims to provide a comprehensive overview of the current state of knowledge on the effects of marine heatwaves on bivalve microbial communities. To do this, we conducted a thorough literature search covering articles and studies published in peer-reviewed journals, conference proceedings, and technical reports. The review paper will provide valuable insights into the current understanding of the effects of heatwaves on the bivalve microbial communities. It will inform future research efforts and potential human health risks via bivalve consumption. The results of this review will also have important implications for the management and conservation of marine ecosystems, as well as for developing mitigation strategies to deal with the impacts of these heat waves on bivalves.
2 Bivalve microbiome
The microbiome of bivalves consists of a diverse community of microorganisms, including bacteria, viruses, and fungi (Rey-Campos et al., 2022). The bacterial community is the most well-studied component of the bivalve microbiome. It plays a critical role in host health and disease (Figure 1). The composition of the bivalve microbiome can vary significantly depending on several factors, including habitat, water quality, and host species (Paillard et al., 2022). Bivalves inhabit a wide range of aquatic habitats, from freshwater streams to deep-sea hydrothermal vents (Zgouridou et al., 2022). The microbiome of bivalves is known to vary depending on the habitat in which they are found. For example, bivalves that inhabit polluted waters may have a different microbiome than those found in unpolluted waters (Martinez-Colon et al., 2009). Similarly, bivalves that live in close proximity to hydrothermal vents may have a different microbiome, such as Methanoperedens and Endoriftia, found in the gill tissue of the hydrothermal vent mussel Bathymodiolus thermophilus, which cannot be found in other marine environments (German et al., 2011; Smith and Wrighton, 2019; Lee D. Y. et al., 2021).
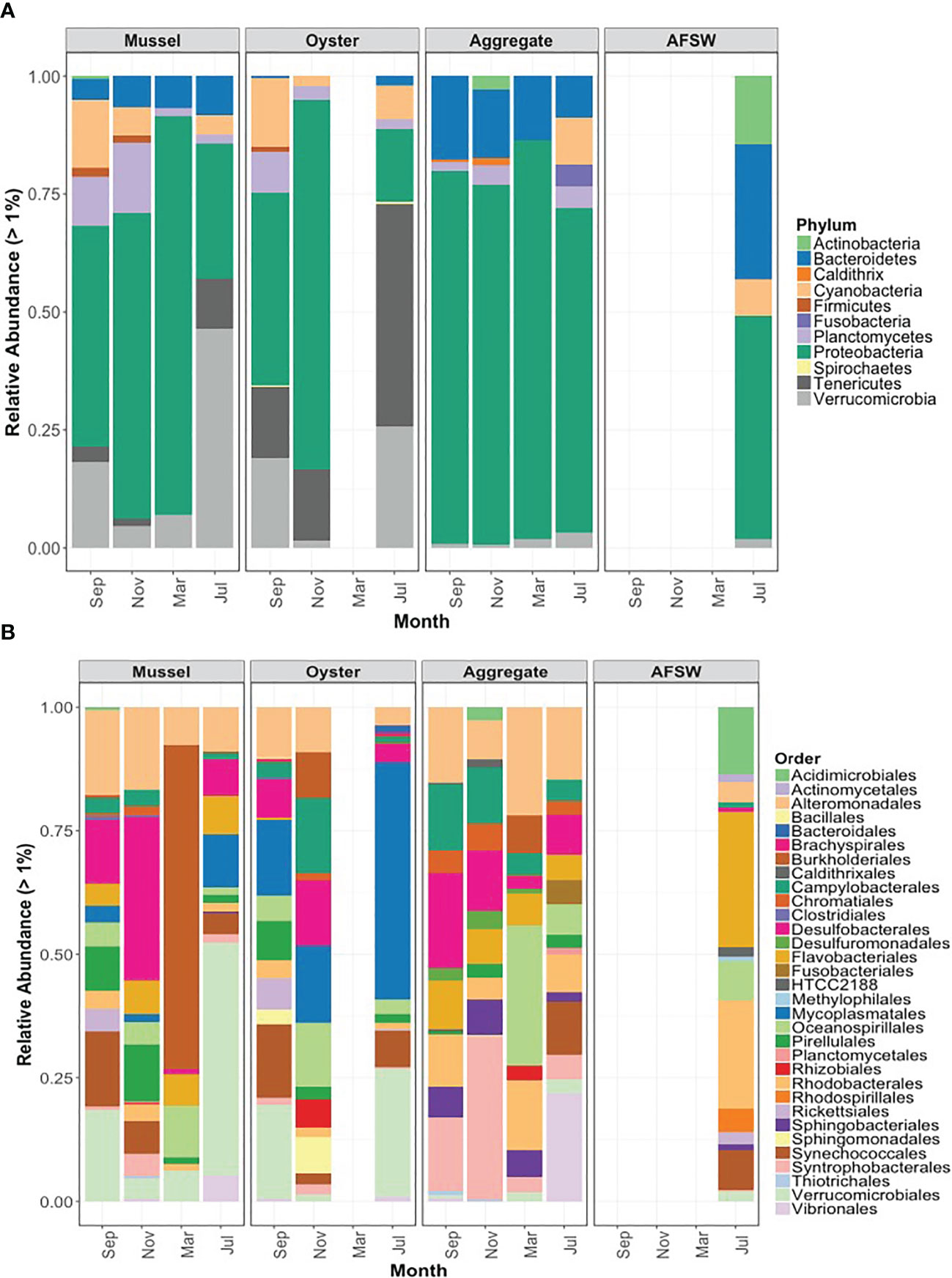
Figure 1 Microbial Community composition of each sample type by phylum and order. (A) Showing the relative abundance of each taxonomic group >1% of the total for mussel gut, oyster gut, aggregate, and aggregate-free seawater (AFSW) samples, categorized by phylum. Colors correspond to different phyla, as indicated in the legend. (B) Showing the relative abundance of each taxonomic group >1% of the total for each sample type, categorized by order. Colors correspond to different orders, as indicated in the legend. Data were obtained from (Pierce and Ward, 2019).
Water quality is an essential factor that can influence the microbiome of bivalves. A range of factors, including pollution, nutrient enrichment, and changes in temperature and salinity, can influence the quality of the water. Studies have shown that changes in water quality can lead to changes in the composition of the bivalve microbiome (Bentzon-Tilia et al., 2016; Michan et al., 2021). For example, bivalves living in nutrient-rich waters may have a different microbiome than those in nutrient-poor waters (Pusch et al., 1998; Bang et al., 2018). The microbiome of bivalves can also vary depending on the host species. Different bivalve species may have different requirements for their microbiome, which can result in differences in the microbiome composition (Vezzulli et al., 2018; Pierce and Ward, 2019). For example, some bivalve species may require specific bacteria to aid in the digestion of their food, such as Spirochaetes in clams; these bacteria are involved in the breakdown of complex carbohydrates (Harwood and Canale-Parola, 1984). In contrast, other species may require different bacteria to help with other aspects of their physiology.
The microbiome of bivalves is thought to play an essential role in host health and disease. The bacterial component of the bivalve microbiome is known to be involved in a range of functions, including digestion, nutrient cycling, and immune defense (Pierce and Ward, 2018; Timmins-Schiffman et al., 2021). Studies have shown that some bacteria in the bivalve microbiome produce antimicrobial compounds that help protect the host from pathogens (Destoumieux-Garzón et al., 2020; Balbi et al., 2021). Additionally, certain bacteria in the bivalve microbiome are associated with increased resistance to disease. Bacteroidetes and Rhizobiales exemplify this (Dubé et al., 2019). These bacteria produce enzymes that break down complex carbohydrates and have been shown to contribute to the immune response of bivalves. The microbiome of bivalves is also involved in nutrient cycling, an essential process for maintaining healthy ecosystems (Moruf et al., 2020). Additionally, the bivalve microbiome can play a role in transferring pathogens between different organisms in the ecosystem (Paillard et al., 2022).
3 Role of bivalve-microbe symbioses in mediating heatwave effect
Heatwaves are a frequent and growing problem in many parts of the world due to the effects of climate change (He et al., 2022b). These extreme weather events can devastate marine and freshwater ecosystems, leading to declines in biodiversity and changes in ecosystem function (Smale et al., 2019). In recent years, the study of bivalve-microbe symbioses has become increasingly important in understanding how these organisms can mediate the effects of heatwaves on aquatic ecosystems. The microbiome of bivalves has important implications for the ecology of aquatic ecosystems. Bivalves are filter feeders that can remove large amounts of organic matter from the water column, which can help to improve water quality. Bivalves and microbes are critical to water quality management as they are essential in maintaining the balance of dissolved oxygen, pH, and nutrient levels (Zhou et al., 2009; Mohapatra et al., 2013). This balance is essential for aquatic organisms’ survival and helps mitigate the adverse effects of heatwaves on these ecosystems (Table 1).
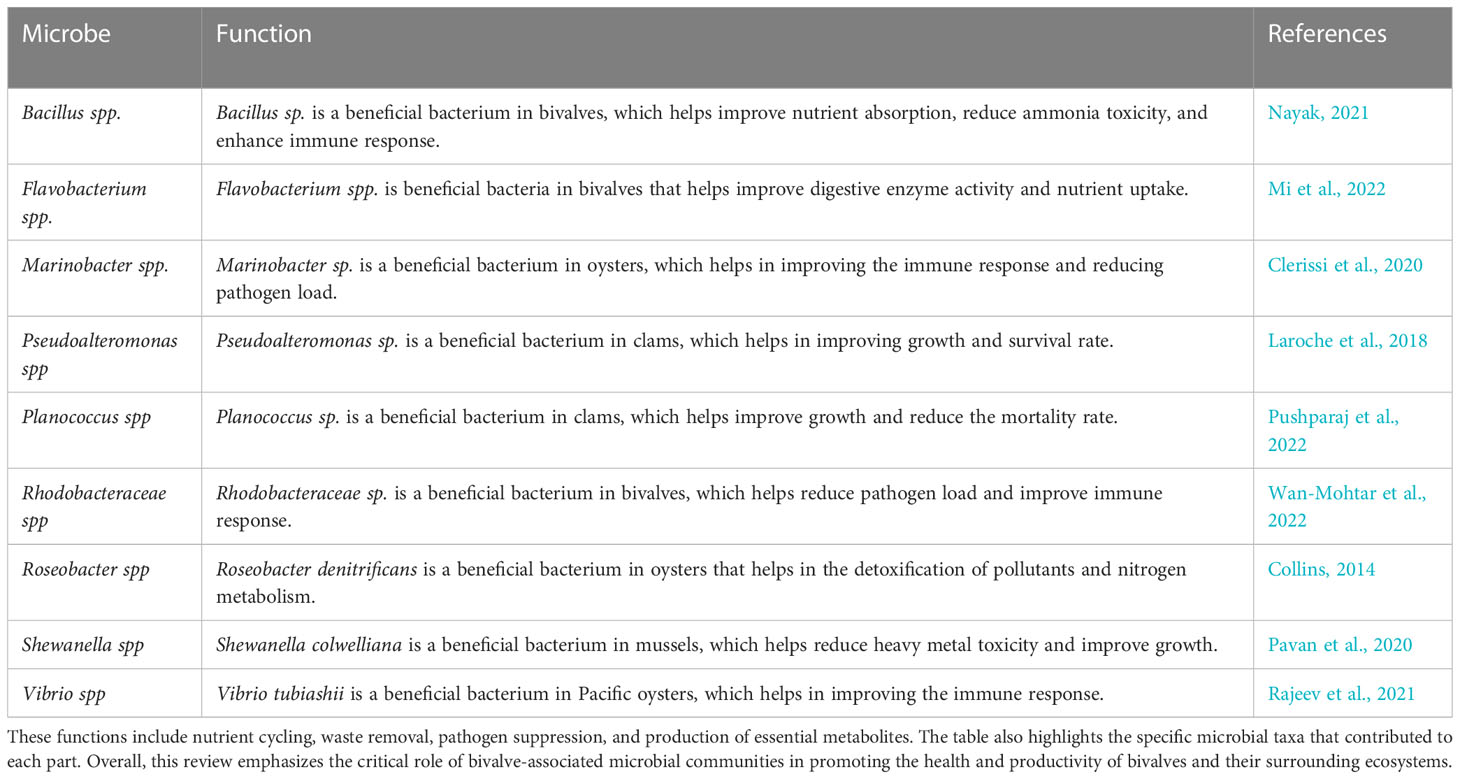
Table 1 Summarizes the diverse beneficial functions performed by microbial communities associated with bivalves.
Microbes such as Pseudomonas and Aspergillus play a critical role in decomposing organic matter in water (Zhou et al., 2009), which releases nutrients essential for the growth of aquatic plants and animals. During heatwaves, the decomposition of organic matter can accelerate, increasing nutrient levels that can cause harmful algal blooms and other adverse effects on water quality. However, some types of bacteria and fungi can also help break down harmful substances in the water, such as pollutants and toxins, improving water quality and protecting aquatic life (Chaturvedi et al., 2015). By regulating nutrient and toxin levels, microbes help to maintain the delicate balance of water quality that supports healthy aquatic ecosystems (Hlordzi et al., 2020).
One of the most critical roles of bivalve-microbe symbioses in mediating the effects of heatwaves is heat stress protection (Turner et al., 2016). During heatwaves, bivalves can experience thermal stress that can damage their tissues and impair their physiological functions (Masanja et al., 2022; Liu et al., 2023). However, some species of bivalves have been found to host microbial communities that protect against heat stress. For example, the bivalve Crassostrea virginica and Mytilus coruscus have been found to harbor a diverse and stable microbial community such as Vibrio spp, Rhizobiales, Endozoicomonas that can protect the host from thermal stress by producing compounds that act as heat shock proteins, chaperones and antioxidants (Lokmer and Mathias Wegner, 2015; Li et al., 2018). Bivalves rely on microbes for the acquisition of essential nutrients, such as nitrogen and phosphorus, which are often limited in their environment. During heatwaves, the availability of these nutrients can be further reduced, which can have adverse effects on bivalve growth and survival. However, some species of bivalves have been found to host microbial communities that can increase the availability of nutrients during heatwaves. For example, the bivalve Mercenaria mercenaria has been found to host a diverse microbial community, Rhizobiales and Planctomycetes, that can increase nitrogen availability during heatwaves by fixing atmospheric nitrogen (King et al., 2019; Soon and Zheng, 2020).
Bivalves rely on their immune system to defend against pathogenic microbes and other harmful agents (Rahman et al., 2019). However, during heatwaves, the immune system can be impaired, increasing the susceptibility of bivalves to disease (Nascimento-Schulze et al., 2021). Some species of bivalves have been found to host microbial communities that can enhance the host’s immune defense during heatwaves (Allam and Espinosa, 2016). For example, bivalves have been found to harbor Vibrionaceae and Rhodobacteraceae that can produce antimicrobial compounds that can protect the host from pathogenic bacteria during heatwaves (Leite et al., 2017; Baden et al., 2021; Scanes et al., 2021b).
Bivalves are critical in carbon cycling in aquatic ecosystems (Hakenkamp and Palmer, 1999). However, their ability to do so can be influenced by heatwaves. During these extreme weather events, bivalves may experience changes in feeding behavior and metabolism (Liu et al., 2023), which can ultimately affect their contribution to carbon cycling. Despite these challenges, some species of bivalves have been found to host microbial communities that can enhance their ability to participate in carbon cycling during heatwaves. For instance, studies have shown that bivalves host a microbial community of planctomycetes that can enhance the host’s ability to assimilate and recycle organic matter during heatwaves (Doni et al., 2023). Another example of a bivalve species that can benefit from microbial communities is the giant clam Tridacna maxima. This bivalve has been found to host symbiotic algae, which can increase the clam’s photosynthetic capacity and carbon uptake even during periods of high-temperature stress (Soo and Todd, 2014).
Overall, understanding the complex relationships between bivalves, their microbial communities, and the carbon cycle is critical for accurately predicting the impacts of climate change on aquatic ecosystems. Bivalves are known for their ability to produce calcified structures, such as shells, that are important for their survival and ecology (Ysebaert et al., 2019). However, the biomineralization process can be affected by heatwaves (He et al., 2022a), which can alter these structures’ chemical and mechanical properties. Some species of bivalves have been found to host microbial communities that can enhance the process of biomineralization during heatwaves. The bivalve Crassostrea gigas, commonly known as the Pacific oyster, has been found to harbor a diverse and stable microbial community that can regulate the chemical composition of the shell and enhance its mechanical properties during heatwaves (Lokmer et al., 2016).
4 Bivalve microbial community changes due to heatwave events
Heatwaves have been found to alter the microbial diversity of bivalve communities (Green et al., 2019; Alma et al., 2020).
Changes in microbial interactions due to heatwaves have been found to alter the interactions between bivalve microbial communities and their hosts (Scanes et al., 2021). A decrease in symbiotic relationships and an increase in pathogenic relationships have been observed, suggesting a shift from a mutualistic to a parasitic interaction (Willing et al., 2011). This may be due to changes in the microbial community structure and function in response to heatwave events. Heatwaves have been found to alter the transport of nutrients and organic matter in bivalve microbial communities (Wetz and Yoskowitz, 2013). An increase in nutrient uptake and a decrease in organic matter export have been observed, suggesting a shift from a source to a sink (IPCC, 2018). This adaptation may be an attempt to maintain energy balance and avoid thermal stress. Heatwaves have been found to alter the production of toxins by bivalve microbial communities (Zgouridou et al., 2022). An increase in toxin production has been observed, potentially as a response to increased competition and changes in microbial interactions. This adaptation may threaten the health of the bivalve host and the surrounding ecosystem.
Heatwaves have been found to alter the aggregation of bivalve microbial communities (Neu et al., 2021). An increased aggregation has been observed, potentially as a response to increased competition and changes in microbial interactions. This adaptation may affect the transport and processing of nutrients and organic matter in the bivalve microbial community (Paillard et al., 2022).
5 Ocean warming and the emergence of bivalve food poisoning
Seawater and bivalve hosts may harbor pathogenic microorganisms that significantly threaten human health (Table 2). These microorganisms can survive and reproduce under specific physiochemical conditions (Chahouri et al., 2022). Bivalve shellfish is a popular food source worldwide, with consumption reported across all five continents, as documented by the United Nations Food and Agricultural Organization (FAO, 2000). Despite its popularity, only a few nations, precisely 12, have recorded instances of bivalve-associated illnesses. These cases are distributed evenly across the continents, with four countries from Europe, Asia, North America, and Australia each reporting outbreaks. Among these, the largest-ever recorded outbreak occurred in Shanghai, China, in 1988, affecting 290,000 individuals infected with hepatitis A after consuming clams (Tang et al., 1991). This outbreak is particularly noteworthy for the high number of deaths it caused, with 47 fatalities recorded. Additionally, three other significant outbreaks of bivalve-associated illnesses were documented in Australia in 1979, the United States in 1986, and Japan in 1991, involving over 800 patients in each case (Murphy et al., 1979; Morse et al., 1986; Otsu, 1999).
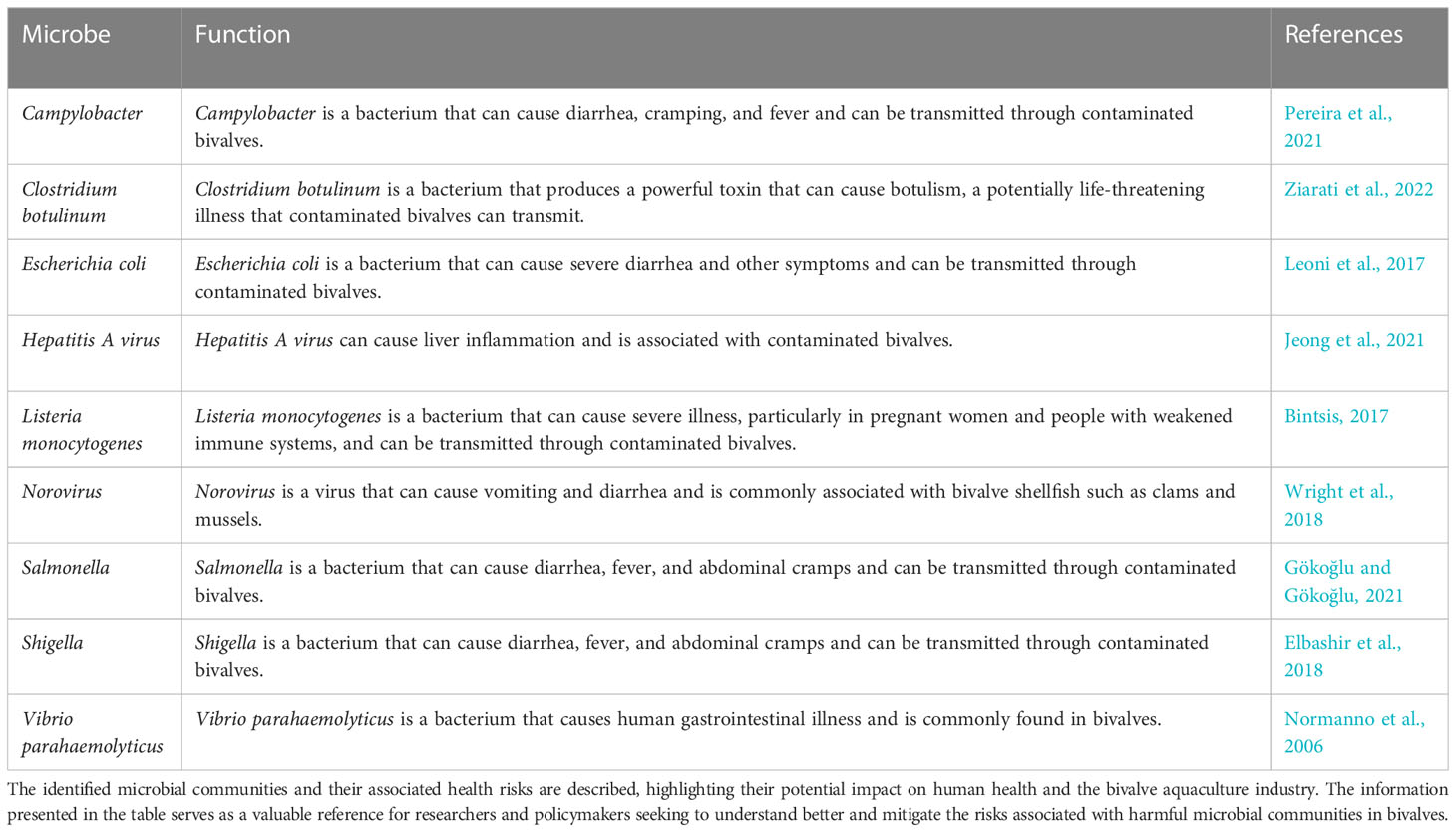
Table 2 Provides a summary of harmful microbial communities identified in various bivalve species, including bacteria, viruses, and parasites.
Escherichia coli strains, including Shiga toxin-producing ones, can cause severe symptoms, such as stomach cramps, vomiting, and bloody diarrhea (Cabrera-Sosa and Ochoa, 2020; Vishram et al., 2021; Butt et al., 2022). Research shows that increased temperatures can modify E. coli gene expression, leading to the emergence of more hazardous strains (Kinghorn et al., 2002; Chung et al., 2006; Kumar and Libchaber, 2013). Studies on temperature adaptation indicate that mutations in genes that affect cellular processes can enhance E. coli’s fitness at elevated temperatures (Hirota et al., 1970; Rudolph et al., 2010). Salmonella is another pathogen that can cause gastroenteritis, leading to severe dehydration, especially in children and the elderly (Dennehy, 2005; Barrett and Fhogartaigh, 2017). While large outbreaks of Salmonella make headlines, most cases go undiagnosed and are not part of any known outbreak. Salmonella infections result in an estimated 93.8 million cases of gastroenteritis and 155,000 deaths globally every year (Moura et al., 2018; Gong et al., 2022).
It is vital to comprehend the factors that can influence the emergence and spread of these pathogenic microorganisms to prevent future outbreaks and safeguard public health. Studies consistently show that the incidence of Salmonella infection is positively associated with higher ambient temperatures (Yun et al., 2016; Wang et al., 2018). This is likely because warmer temperatures facilitate more rapid bacterial replication, increasing infection rates. In South Korea, 17,638 cases of the Hepatitis A virus were reported (Lee D. Y.et al., 2021; Jeong et al., 2021); the primary source was Jogaejeot, seasoned Venerupis philippinarum, a traditional fermented food of Korea, and Jogaejeot is eaten raw. According to the Korean Centers for Disease Control and Prevention (KCDC), the Hepatitis A virus resists high temperatures.
Therefore, it is associated with considerable risk during times of ocean warming. Ocean warming has been identified as a significant factor in the emergence of bivalve food poisoning caused by harmful microbes. As ocean temperatures continue to rise, the distribution and abundance of these harmful microbes in bivalve populations may increase, leading to a greater risk of foodborne illness for humans who consume contaminated seafood. Therefore, continued monitoring of ocean temperatures and the presence of harmful microbes in bivalve populations is necessary to mitigate the potential health risks associated with consuming contaminated seafood.
6 Conclusion and implications for bivalve aquaculture and coastal ecosystem health in a changing climate
In conclusion, this scientific review paper highlights the significant impacts of heatwaves on bivalve microbial communities and their implications for both bivalve and human health. The review demonstrates that heatwaves have the potential to alter the composition and diversity of microbial communities within bivalves, leading to changes in nutrient cycling, metabolism, and immune function. These alterations can ultimately impact bivalve health and may have implications for human health through the consumption of contaminated shellfish. The evidence presented in this review suggests that heatwaves may increase harmful bacteria and toxins in bivalves, including Vibrio spp. and norovirus, which have been associated with foodborne illness outbreaks. Furthermore, the impacts of heatwaves on bivalve microbial communities may exacerbate existing stressors on bivalve populations, including pollution and habitat destruction, further threatening bivalve health and ecosystem resilience.
Therefore, further research is needed to understand better the complex interactions between heatwaves, bivalve microbial communities, and human health. This research should improve our understanding of the underlying mechanisms driving changes in microbial community composition and function and the implications for bivalve and human health. Overall, this review emphasizes the urgent need for action to address the impacts of heatwaves on bivalves and their associated microbial communities. This includes efforts to mitigate the effects of climate change, reduce pollution, and protect critical bivalve habitats. Such actions are critical not only for the health of bivalve populations and the ecosystems they support but also for the health and well-being of human populations that rely on these valuable shellfish as a source of food and nutrition.
Author contributions
FM: Writing – original draft, data curation, investigation, validation. KY: Data curation, writing – review & editing, validation. YX: Writing – review & editing, validation, data curation. GH: Data curation, writing – review & editing, validation. XLL: Writing – review & editing, validation, data curation. XX: Data curation, writing – review & editing, validation. XJ: Writing – review & editing, validation, data curation. XL: Writing – review & editing, validation. RM: Writing – review & editing. YD: Conceptualization, resources. LZ: Conceptualization, writing-original draft preparation, project administration. All authors contributed to the article and approved the submitted version.
Funding
The present study has been made possible by research grants from the National Science Foundation of China (42076121, M-0163, 42211530423), the Guangdong Zhujiang Talents Program (2021QN02H665), the Department of Education of Guangdong Province (2020KTSCX050 and 2022ZDZX4012), the earmarked fund for Modern Agro-industry Technology Research System (CARS-49), and the program for Scientific Research Start-up Funds of Guangdong Ocean University.
Conflict of interest
The authors declare that the research was conducted in the absence of any commercial or financial relationships that could be construed as a potential conflict of interest.
Publisher’s note
All claims expressed in this article are solely those of the authors and do not necessarily represent those of their affiliated organizations, or those of the publisher, the editors and the reviewers. Any product that may be evaluated in this article, or claim that may be made by its manufacturer, is not guaranteed or endorsed by the publisher.
References
Akter S., Wos-Oxley M. L., Catalano S. R., Hassan M. M., Li X., Qin J. G., et al. (2022). Host species and environment shape the gut microbiota of cohabiting marine bivalves. doi: 10.21203/rs.3.rs-1921789/v1
Alfaro A. C., Nguyen T. V., Merien F. (2019). The complex interactions of ostreid herpesvirus 1, Vibrio bacteria, environment and host factors in mass mortality outbreaks of Crassostrea gigas. Rev. Aquacult. 11 (4), 1148–1168. doi: 10.1111/raq.12284
Allam B., Espinosa E. P. (2016). Bivalve immunity and response to infections: are we looking at the right place? Fish. Shellfish. Immunol. 53, 4–12. doi: 10.1016/j.fsi.2016.03.037
Alma L., Kram K. E., Holtgrieve G. W., Barbarino A., Fiamengo C. J., Padilla-Gamiño J. L. (2020). Ocean acidification and warming affects the physiology, skeletal properties, and microbiome of the purple-hinge rock scallop. Comp. Biochem. Physiol. Part A.: Mol. Integr. Physiol. 240, 110579. doi: 10.1016/j.cbpa.2019.110579
Baden S., Hernroth B., Lindahl O. (2021). Declining populations of mytilus spp. in north Atlantic coastal waters–a Swedish perspective. J. Shellfish. Res. 40 (2), 269–296. doi: 10.2983/035.040.0207
Balbi T., Auguste M., Ciacci C., Canesi L. (2021). Immunological responses of marine bivalves to contaminant exposure: contribution of the-omics approach. Front. Immunol. 12, 618726. doi: 10.3389/fimmu.2021.618726
Bang C., Dagan T., Deines P., Dubilier N., Duschl W. J., Fraune S., et al. (2018). Metaorganisms in extreme environments: do microbes play a role in organismal adaptation? Zoology 127, 1–19. doi: 10.1016/j.zool.2018.02.004
Barrett J., Fhogartaigh C. N. (2017). Bacterial gastroenteritis. Medicine 45 (11), 683–689. doi: 10.1016/j.mpmed.2017.08.002
Bentzon-Tilia M., Sonnenschein E. C., Gram L. (2016). Monitoring and managing microbes in aquaculture–towards a sustainable industry. Microbial. Biotechnol. 9 (5), 576–584. doi: 10.1111/1751-7915.12392
Bintsis T. (2017). Foodborne pathogens. AIMS. Microbiol. 3 (3), 529. doi: 10.3934/microbiol.2017.3.529
Butt S., Jenkins C., Godbole G., Byrne L. (2022). The epidemiology of shiga toxin-producing escherichia coli serogroup O157 in England 2009–2019. Epidemiol. Infect., 150. doi: 10.1017/S0950268822000206
Cabrera-Sosa L., Ochoa T. J. (2020). “Escherichia coli diarrhea,” in Hunter’s tropical medicine and emerging infectious diseases (Elsevier: Amsterdam, Netherlands), 481–485. doi: 10.1016/B978-0-323-55512-8.00046-6
Chahouri A., Radouane N., Yacoubi B., Moukrim A., Banaoui A. (2022). Microbiological assessment of marine and estuarine ecosystems using fecal indicator bacteria, salmonella, vibrio and antibiotic resistance pattern. Mar. pollut. Bull. 180, 113824. doi: 10.1016/j.marpolbul.2022.113824
Chapter I. P. C. C. (2019). Changing ocean, marine ecosystems, and dependent communities. In: IPCC Special Report on the Ocean and Cryosphere in a Changing Climate Pörtner H.-O., Roberts D.C., Masson-Delmotte V., Zhai P., Tignor M., Poloczanska E., et al (eds.) (Cambridge, UK and New York, NY, USA: Cambridge University Press), 447–587. doi: 10.1017/9781009157964.007
Chaturvedi A. D., Pal D., Penta S., Kumar A. (2015). Ecotoxic heavy metals transformation by bacteria and fungi in aquatic ecosystem. World J. Microbiol. Biotechnol. 31 (10), 1595–1603. doi: 10.1007/s11274-015-1911-5
Chung H. J., Bang W., Drake M. A. (2006). Stress response of escherichia coli. Compr. Rev. Food Sci. Food Saf. 5 (3), 52–64. doi: 10.1111/j.1541-4337.2006.00002.x
Collins A. J. (2014). Bacterial symbioses and the innate immune response of the model host: euprymna scolopes.
Clerissi C., Luo X., Lucasson A., Mortaza S., de Lorgeril J., Toulza E., et al (2014). A core of functional complementary bacteria infects oysters in Pacific Oyster Mortality Syndrome. doi: 10.1101/2020.11.16.384644
Dennehy P. H. (2005). Acute diarrheal disease in children: epidemiology, prevention, and treatment. Infect. Dis. Clinics 19 (3), 585–602. doi: 10.1016/j.idc.2005.05.003
Destoumieux-Garzón D., Canesi L., Oyanedel D., Travers M. A., Charrière G. M., Pruzzo C., et al. (2020). Vibrio–bivalve interactions in health and disease. Environ. Microbiol. 22 (10), 4323–4341. doi: 10.1111/1462-2920.15055
Doni L., Oliveri C., Lasa A., Di Cesare A., Petrin S., Martinez-Urtaza J., et al. (2023). Large-Scale impact of the 2016 marine heatwave on the plankton-associated microbial communities of the great barrier reef (Australia). Mar. pollut. Bull. 188, 114685. doi: 10.1016/j.marpolbul.2023.114685
Dubé C. E., Ky C. L., Planes S. (2019). Microbiome of the black-lipped pearl oyster pinctada margaritifera, a multi-tissue description with functional profiling. Front. Microbiol. 10, 1548. doi: 10.3389/fmicb.2019.01548
Elbashir S., Parveen S., Schwarz J., Rippen T., Jahncke M., DePaola A. (2018). Seafood pathogens and information on antimicrobial resistance: a review. Food Microbiol. 70, 85–93. doi: 10.1016/j.fm.2017.09.011
Ertl N. G., O’Connor W. A., Papanicolaou A., Wiegand A. N., Elizur A. (2016). Transcriptome analysis of the Sydney rock oyster, saccostrea glomerata: insights into molluscan immunity. PloS One 11 (6), e0156649. doi: 10.1371/journal.pone.0156649
FAO (2000). Food and agriculture organization of the united nations statistical database (Rome) 200.
Fiore C. L., Jarett J. K., Olson N. D., Lesser M. P. (2010). Nitrogen fixation and nitrogen transformations in marine symbioses. Trends Microbiol. 18 (10), 455–463. doi: 10.1016/j.tim.2010.07.001
Frölicher T. L., Fischer E. M., Gruber N. (2018). Marine heatwaves under global warming. Nature 560 (7718), 360–364. doi: 10.1038/s41586-018-0383-9
Garcia C., Haond C., Chollet B., Nerac M., Omnes E., Joly J. P., et al. (2018). Descriptions of mikrocytos veneroïdes n. sp. and Mikrocytos donaxi n. sp. (Ascetosporea: Mikrocytida: mikrocytiidae), detected during important mortality events of the wedge clam Donax trunculus linnaeus (Veneroida: donacidae), in France between 2008 and 2011. Parasites Vectors 11 (1), 1–16. doi: 10.1186/s13071-018-2692-0
German C. R., Ramirez-Llodra E., Baker M. C., Tyler P. A., ChEss Scientific Steering Committee (2011). Deep-water chemosynthetic ecosystem research during the census of marine life decade and beyond: a proposed deep-ocean road map. PloS One 6 (8), e23259. doi: 10.1371/journal.pone.0023259
Gökoğlu N., Gökoğlu N. (2021). Shellfish safety. Shellfish. Process. Preserv. (Springer, Cham) 281–312. doi: 10.1007/978-3-030-60303-8_5
Gong B., Li H., Feng Y., Zeng S., Zhuo Z., Luo J., et al. (2022). Prevalence, serotype distribution and antimicrobial resistance of non-typhoidal salmonella in hospitalized patients in conghua district of guangzhou, China. Front. Cell. Infect. Microbiol. 12, 805384. doi: 10.3389/fcimb.2022.805384
Green T. J., Siboni N., King W. L., Labbate M., Seymour J. R., Raftos D. (2019). Simulated marine heat wave alters abundance and structure of Vibrio populations associated with the pacific oyster resulting in a mass mortality event. Microbial. Ecol. pp. 77, 736–747. doi: 10.1007/s00248-018-1242-9
Hakenkamp C. C., Palmer M. A. (1999). Introduced bivalves in freshwater ecosystems: the impact of corbicula on organic matter dynamics in a sandy stream. Oecologia 119, 445–451. doi: 10.1007/s004420050806
Harwood C. S., Canale-Parola E. (1984). Ecology of spirochetes. Annu. Rev. Microbiol. 38 (1), 161–192. doi: 10.1146/annurev.mi.38.100184.001113
Haygood M. G., Schmidt E. W., Davidson S. K., Faulkner D. J. (1999). Microbial symbionts of marine invertebrates: opportunities for microbial biotechnology. J. Mol. Microbiol. Biotechnol. 1 (1), 33–43.
He G., Peng Y., Liu X., Liu Y., Liang J., Xu X., et al. (2022b). Post-responses of intertidal bivalves to recurrent heatwaves. Mar. pollut. Bull. 184, 114223. doi: 10.1016/j.marpolbul.2022.114223
He G., Zou J., Liu X., Liang F., Liang J., Yang K., et al. (2022a). Assessing the impact of atmospheric heatwaves on intertidal clams. Sci. Total. Environ. 841, 156744. doi: 10.1016/j.scitotenv.2022.156744
Hirota Y., Mordoh J., Jacob F. (1970). On the process of cellular division in Escherichia coli: III. thermosensitive mutants of Escherichia coli altered in the process of DNA initiation. J. Mol. Biol. 53 (3), 369–387. doi: 10.1016/0022-2836(70)90072-0
Hlordzi V., Kuebutornye F. K. A., Afriyie G., Abarike E. D., Lu Y., Chi S., et al. (2020). The use of bacillus species in maintenance of water quality in aquaculture: a review. Aquacult. Rep. 18, 100503. doi: 10.1016/j.aqrep.2020.100503
IPCC. (2018). Global warming of 1.5°C. An IPCC Special Report on the impacts of global warming of 1.5°C above pre-industrial levels and related global greenhouse gas emission pathways, in the context of strengthening the global response to the threat of climate change, sustainable development, and efforts to eradicate poverty Masson-Delmotte V., Zhai P., Pörtner H.O., Roberts D., Skea J., Shukla P.R., et al (eds.) In press.
Jeong H. W., Kim M. K., Yi H. J., Kim D. M., Jeon S. J., Lee H. K., et al. (2021). Hepatitis a virus strains identified in jogaejeot associated with outbreaks in Seoul, south Korea. Lett. Appl. Microbiol. 73 (1), 107–112. doi: 10.1111/lam.13482
Jones H. F., Pilditch C. A., Hamilton D. P., Bryan K. R. (2017). Impacts of a bivalve mass mortality event on an estuarine food web and bivalve grazing pressure. New Z. J. Mar. Freshw. Res. 51 (3), 370–392. doi: 10.1080/00288330.2016.1245200
King W. L., Jenkins C., Seymour J. R., Labbate M. (2019). Oyster disease in a changing environment: decrypting the link between pathogen, microbiome, and environment. Mar. Environ. Res. 143, 124–140. doi: 10.1016/j.marenvres.2018.11.007
Kinghorn S. M., O’Byrne C. P., Booth I. R., Stansfield I. (2002). Physiological analysis of the role of truB in Escherichia coli: a role for tRNA modification in extreme temperature resistance. Microbiology 148 (11), 3511–3520. doi: 10.1099/00221287-148-11-3511
Kumar P., Libchaber A. (2013). Pressure and temperature dependence of growth and morphology of Escherichia coli: experiments and stochastic model. Biophys. J. 105 (3), 783–793. doi: 10.1016/j.bpj.2013.06.029
Laroche O., Symonds J. E., Smith K. F., Banks J. C., Mae H., Bowman J. P., et al. (2018). Understanding bacterial communities for informed biosecurity and improved larval survival in pacific oysters. Aquaculture 497, 164–173. doi: 10.1016/j.aquaculture.2018.07.052
Lattos A., Giantsis I. A., Karagiannis D., Michaelidis B. (2020). First detection of the invasive Haplosporidian and mycobacteria parasites hosting the endangered bivalve Pinna nobilis in thermaikos gulf, north Greece. Mar. Environ. Res. 155, 104889. doi: 10.1016/j.marenvres.2020.104889
Lee D. Y., Chae S. J., Cho S. R., Choi W., Kim C. K., Han M. G. (2021). National seroprevalence of hepatitis a in south Korea from 2009 to 2019. PloS One 16 (2), e0245162.
Lee W. K., Juniper S. K., Perez M., Ju S. J., Kim S. J. (2021). Diversity and characterization of bacterial communities of five co-occurring species at a hydrothermal vent on the Tonga arc. Ecol. Evol. 11 (9), 4481–4493. doi: 10.1002/ece3.7343
Leite L., Jude-Lemeilleur F., Raymond N., Henriques I., Garabetian F., Alves A. (2017). Phylogenetic diversity and functional characterization of the Manila clam microbiota: a culture-based approach. Environ. Sci. pollut. Res. 24, 21721–21732. doi: 10.1007/s11356-017-9838-z
Leoni F., Chierichetti S., Santarelli S., Talevi G., Masini L., Bartolini C., et al. (2017). Occurrence of arcobacter spp. and correlation with the bacterial indicator of faecal contamination Escherichia coli in bivalve molluscs from the central Adriatic, Italy. Int. J. Food Microbiol. 245, 6–12. doi: 10.1016/j.ijfoodmicro.2017.01.006
Li Y. F., Yang N., Liang X., Yoshida A., Osatomi K., Power D., et al. (2018). Elevated seawater temperatures decrease microbial diversity in the gut of Mytilus coruscus. Front. Physiol. 9, 839. doi: 10.3389/fphys.2018.00839
Liu X., Peng Y., Xu Y., He G., Liang J., Masanja F., et al. (2023). Responses of digestive metabolism to marine heatwaves in pearl oysters. Mar. pollut. Bull. 186, 114395. doi: 10.1016/j.marpolbul.2022.114395
Lokmer A., Kuenzel S., Baines J. F., Wegner K. M. (2016). The role of tissue-specific microbiota in initial establishment success of pacific oysters. Environ. Microbiol. 18 (3), 970–987. doi: 10.1111/1462-2920.13163
Lokmer A., Mathias Wegner K. (2015). Hemolymph microbiome of pacific oysters in response to temperature, temperature stress and infection. ISME. J. 9 (3), 670–682. doi: 10.1038/ismej.2014.160
Martinez-Colon M., Hallock P., Green-Ruiz C. (2009). Strategies for using shallow-water benthic foraminifers as bioindicators of potentially toxic elements: a review. J. Foraminiferal. Res. 39 (4), 278–299. doi: 10.2113/gsjfr.39.4.278
Masanja F., Xu Y., He G., Liang F., Liu X., Yang K., et al. (2022). Exploring HSP90 as a biomarker for marine heatwaves in pinctada maxima. Front. Mar. Sci. 9. doi: 10.3389/fmars.2022.913920
Matt J. L., Guévélou E., Small J. M., Allen S. K. Jr. (2020). A field test investigating the influence of brood stock origin and ploidy on the susceptibility of Crassostrea virginica to “triploid mortality” in the Chesapeake bay. Aquaculture 526, 735375. doi: 10.1016/j.aquaculture.2020.735375
Mi R., Li X., Sun Y., Wang Q., Tian B., Ma S., et al. (2022). Effects of microbial community and disease resistance against Vibrio splendidus of yesso scallop (Patinopecten yessoensis) fed supplementary diets of tussah immunoreactive substances and antimicrobial peptides. Fish. Shellfish. Immunol. 121, 446–455. doi: 10.1016/j.fsi.2021.10.006
Michan C., Blasco J., Alhama J. (2021). High-throughput molecular analyses of microbiomes as a tool to monitor the well-being of aquatic environments. Microbial. Biotechnol. 14 (3), 870–885. doi: 10.1111/1751-7915.13763
Milan M., Carraro L., Fariselli P., Martino M. E., Cavalieri D., Vitali F., et al. (2018). Microbiota and environmental stress: how pollution affects microbial communities in Manila clams. Aquat. Toxicol. 194, 195–207. doi: 10.1016/j.aquatox.2017.11.019
Mohapatra S., Chakraborty T., Kumar V., DeBoeck G., Mohanta K. N. (2013). Aquaculture and stress management: a review of probiotic intervention. J. Anim. Physiol. Anim. Nutr. 97 (3), 405–430. doi: 10.1111/j.1439-0396.2012.01301.x
Morse D. L., Guzewich J. J., Hanrahan J. P., Stricof R., Shayegani M., Deibel R., et al. (1986). Widespread outbreaks of clam- and oyster-associated gastroenteritis: the role of Norwalk virus. N. Engl. J. Med. 314, 678–681. doi: 10.1056/NEJM198603133141103
Moruf R. O., Okunade G. F., Elegbeleye O. W. (2020). Bivalve mariculture in two–way interaction with phytoplankton: a review of feeding mechanism and nutrient recycling. Bull. Univ. Agric. Sci. Vet. Med. Cluj-Napoca. Anim. Sci. Biotechnol. 77 (2), 1. doi: 10.15835/buasvmcn-asb:2020.0010
Moura Q., Fernandes M. R., Silva K. C., Monte D. F., Esposito F., Dropa M., et al. (2018). Virulent nontyphoidal salmonella producing CTX-m and CMY-2 β-lactamases from livestock, food, and human infection, Brazil. Virulence 9 (1), 281–286. doi: 10.1080/21505594.2017.1279779
Murphy A., Grohmann G., Christopher P., Lopez W., Davey G., Millsom R. (1979). An Australia-wide outbreak of gastroenteritis from oysters caused by Norwalk virus. Med. J. Aust. 2, 329–333. doi: 10.5694/j.1326-5377.1979.tb104133.x
Musella M., Wathsala R., Tavella T., Rampelli S., Barone M., Palladino G., et al. (2020). Tissue-scale microbiota of the Mediterranean mussel (Mytilus galloprovincialis) and its relationship with the environment. Sci. Total. Environ. 717, 137209. doi: 10.1016/j.scitotenv.2020.137209
Nascimento-Schulze J. C., Bean T. P., Houston R. D., Santos E. M., Sanders M. B., Lewis C., et al. (2021). Optimizing hatchery practices for genetic improvement of marine bivalves. Rev. Aquacult. 13 (4), 2289–2304. doi: 10.1111/raq.12568
Nayak S. K. (2021). Multifaceted applications of probiotic bacillus species in aquaculture with special reference to Bacillus subtilis. Rev. Aquacult. 13 (2), 862–906. doi: 10.1111/raq.12503
Neu A. T., Hughes I. V., Allen E. E., Roy K. (2021). Decade-scale stability and change in a marine bivalve microbiome. Mol. Ecol. 30 (5), 1237–1250. doi: 10.1111/mec.15796
Normanno G., Parisi A., Addante N., Quaglia N. C., Dambrosio A., Montagna C., et al. (2006). Vibrio parahaemolyticus, Vibrio vulnificus, and microorganisms of fecal origin in mussels (Mytilus galloprovincialis) sold in the puglia region (Italy). Int. J. Food Microbiol. 106 (2), 219–222. doi: 10.1016/j.ijfoodmicro.2005.05.020
Oliver E. C., Donat M. G., Burrows M. T., Moore P. J., Smale D. A., Alexander L. V., et al. (2018). Longer and more frequent marine heatwaves over the past century. Nat. Commun. 9 (1), 1–12. doi: 10.1038/s41467-018-03732-9
Otsu R. (1999). Outbreaks of gastroenteritis caused by SRSVs from 1987 to 1992 in Kyushu, Japan: four outbreaks associated with oyster consumption. Eur. J. Epidemiol. 15, 175–180. doi: 10.1023/A:1007543924543
Paillard C., Gueguen Y., Wegner K. M., Bass D., Pallavicini A., Vezzulli L., et al. (2022). Recent advances in bivalve-microbiota interactions for disease prevention in aquaculture. Curr. Opin. Biotechnol. 73, 225–232. doi: 10.1016/j.copbio.2021.07.026
Pavan M. E., López N. I., Pettinari M. J. (2020). Melanin biosynthesis in bacteria, regulation, and production perspectives. Appl. Microbiol. Biotechnol. 104, 1357–1370. doi: 10.1007/s00253-019-10245-y
Pereira C., Costa P., Duarte J., Balcão V. M., Almeida A. (2021). Phage therapy as a potential approach in the biocontrol of pathogenic bacteria associated with shellfish consumption. Int. J. Food Microbiol. 338, 108995. doi: 10.1016/j.ijfoodmicro.2020.108995
Pierce M. L., Ward J. E. (2018). Microbial ecology of the bivalvia, with an emphasis on the family Ostreidae. J. Shellfish. Res. 37 (4), 793–806. doi: 10.2983/035.037.0410
Pierce M. L., Ward J. E. (2019). Gut microbiomes of the eastern oyster (Crassostrea virginica) and the blue mussel (mytilus edulis): temporal variation and the influence of marine aggregate-associated microbial communities. Msphere 4 (6), e00730–e00719. doi: 10.1128/mSphere.00730-19
Pusch M., Fiebig D., Brettar I., Eisenmann H., Ellis B. K., Kaplan L. A., et al. (1998). The role of micro-organisms in the ecological connectivity of running waters. Freshw. Biol. 40 (3), 453–495. doi: 10.1046/j.1365-2427.1998.00372.x
Pushparaj K., Kuchi Bhotla H., Pappuswamy M., Issara U., Balasubramanian B., Al-Dhabi N. A., et al. (2022). “Perspectives and implications of probiotics as beneficial mediators in aquaculture industry,” in Aquaculture science and engineering (Singapore: Springer Nature Singapore), 79–97.
Rahman M. A., Henderson S., Miller-Ezzy P., Li X. X., Qin J. G. (2019). Immune response to temperature stress in three bivalve species: pacific oyster Crassostrea gigas, Mediterranean mussel Mytilus galloprovincialis, and mud cockle Katelysia rhytiphora. Fish. Shellfish. Immunol. 86, 868–874. doi: 10.1016/j.fsi.2018.12.017
Rajeev R., Adithya K. K., Kiran G. S., Selvin J. (2021). Healthy microbiome: a key to successful and sustainable shrimp aquaculture. Rev. Aquacult. 13 (1), 238–258. doi: 10.1111/raq.12471
Rey-Campos M., Ríos-Castro R., Gallardo-Escárate C., Novoa B., Figueras A. (2022). Exploring the potential of metatranscriptomics to describe microbial communities and their effects in molluscs. Int. J. Mol. Sci. 23 (24), 16029. doi: 10.3390/ijms232416029
Robledo J. A. F., Yadavalli R., Allam B., Espinosa E. P., Gerdol M., Greco S., et al. (2019). From the raw bar to the bench: bivalves as models for human health. Dev. Comp. Immunol. 92, 260–282. doi: 10.1016/j.dci.2018.11.020
Rudolph B., Gebendorfer K. M., Buchner J., Winter J. (2010). Evolution of Escherichia coli for growth at high temperatures. J. Biol. Chem. 285 (25), 19029–19034. doi: 10.1074/jbc.M110.103374
Scanes E., Parker L. M., Seymour J. R., Siboni N., Dove M. C., O’Connor W. A., et al. (2021b). Microbiomes of an oyster are shaped by metabolism and environment. Sci. Rep. 11 (1), 21112. doi: 10.1038/s41598-021-00590-2
Scanes E., Parker L. M., Seymour J. R., Siboni N., King W. L., Danckert N. P., et al. (2021). Climate change alters the haemolymph microbiome of oysters. Mar. pollut. Bull. 164, 111991. doi: 10.1016/j.marpolbul.2021.111991
Smale D. A., Wernberg T., Oliver E. C. J., Thomsen M., Harvey B. P., Straub S. C., et al. (2019). Marine heatwaves threaten global biodiversity and the provision of ecosystem services. Nat. Climate Change 9 (4), 306–312. doi: 10.1038/s41558-019-0412-1
Smith G. J., Wrighton K. C. (2019). Metagenomic approaches unearth methanotroph phylogenetic and metabolic diversity. Curr. Issues Mol. Biol. 33 (1), 57–84. doi: 10.21775/cimb.033.057
Soo P., Todd P. A. (2014). The behaviour of giant clams (Bivalvia: cardiidae: tridacninae). Mar. Biol. 161, 2699–2717. doi: 10.1007/s00227-014-2545-0
Soon T. K., Zheng H. (2020). Climate change and bivalve mass mortality in temperate regions. Rev. Environ. Contamination. Toxicol. volume. 251, 109–129. doi: 10.1007/398_2019_31
Sweet M. J., Bateman K. S. (2016). Reprint of ‘Diseases in marine invertebrates associated with mariculture and commercial fisheries. J. Sea. Res. 113, 28–44. doi: 10.1016/j.seares.2016.06.001
Tang Y. W., Wang J. X., Xu Z. Y., Guo Y. F., Qian W. H., Xu J. X. (1991). A serologically confirmed, case-control study, of a large outbreak of hepatitis a in China, associated with consumption of clams. Epidemiol. Infect. 107, 651–657. doi: 10.1017/S0950268800049347
Timmins-Schiffman E., White S. J., Thompson R. E., Vadopalas B., Eudeline B., Nunn B. L., et al. (2021). Coupled microbiome analyses highlights relative functional roles of bacteria in a bivalve hatchery. Environ. Microbiome. 16, 1–12. doi: 10.1186/s40793-021-00376-z
Turner L. M., Alsterberg C., Turner A. D., Girisha S. K., Rai A., Havenhand J. N., et al. (2016). Pathogenic marine microbes influence the effects of climate change on a commercially important tropical bivalve. Sci. Rep. 6 (1). doi: 10.1038/srep32413
Vezzulli L., Stagnaro L., Grande C., Tassistro G., Canesi L., Pruzzo C. (2018). Comparative 16SrDNA gene-based microbiota profiles of the pacific oyster (Crassostrea gigas) and the Mediterranean mussel (Mytilus galloprovincialis) from a shellfish farm (Ligurian Sea, Italy). Microbial. Ecol. 75, 495–504. doi: 10.1007/s00248-017-1051-6
Vishram B., Jenkins C., Greig D. R., Godbole G., Carroll K., Balasegaram S., et al. (2021). The emerging importance of shiga toxin-producing Escherichia coli other than serogroup O157 in England. J. Med. Microbiol. 70, 7. doi: 10.1099/jmm.0.001375
Wang P., Goggins W. B., Chan E. Y. (2018). Associations of salmonella hospitalizations with ambient temperature, humidity and rainfall in Hong Kong. Environ. Int. 120, 223–230. doi: 10.1016/j.envint.2018.08.014
Wang S., Yan Z., Wang P., Zheng X., Fan J. (2020). Comparative metagenomics reveals the microbial diversity and metabolic potentials in the sediments and surrounding seawaters of qinhuangdao mariculture area. PloS One 15 (6), e0234128. doi: 10.1371/journal.pone.0234128
Wan-Mohtar W. A. A. Q. I., Ibrahim M. F., Rasdi N. W., Zainorahim N., Taufek N. M. (2022). Microorganisms as a sustainable aquafeed ingredient: a review. Aquacult. Res. 53 (3), 746–766. doi: 10.1111/are.15627
Wetz M. S., Yoskowitz D. W. (2013). An ‘extreme future for estuaries? effects of extreme climatic events on estuarine water quality and ecology. Mar. pollut. Bull. 69 (1-2), 7–18. doi: 10.1016/j.marpolbul.2013.01.020
Willing B. P., Russell S. L., Finlay B. B. (2011). Shifting the balance: antibiotic effects on host–microbiota mutualism. Nat. Rev. Microbiol. 9 (4), 233–243. doi: 10.1038/nrmicro2536
Wright A. C., Fan Y., Baker G. L. (2018). Nutritional value and food safety of bivalve molluscan shellfish. J. Shellfish. Res. 37 (4), 695–708. doi: 10.2983/035.037.0403
Xu Y., Liang J., He G., Liu X., Yang K., Masanja F., et al. (2022) Responses of pearl oysters to marine heatwaves as indicated by HSP70. Front. Mar. Sci. 9. doi: 10.3389/fmars.2022.847585
Ysebaert T., Walles B., Haner J., Hancock B. (2019). Habitat modification and coastal protection by ecosystem-engineering reef-building bivalves. Goods. Serv. Mar. Bivalves., 253–273. doi: 10.1007/978-3-319-96776-9_13
Yun J., Greiner M., Höller C., Messelhäusser U., Rampp A., Klein G. (2016). Association between the ambient temperature and the occurrence of human salmonella and campylobacter infections. Sci. Rep. 6 (1), 28442. doi: 10.1038/srep28442
Zgouridou A., Tripidaki E., Giantsis I. A., Theodorou J. A., Kalaitzidou M., Raitsos D. E., et al. (2022). The current situation and potential effects of climate change on the microbial load of marine bivalves of the Greek coastlines: an integrative review. Environ. Microbiol. 24 (3), 1012–1034. doi: 10.1111/1462-2920.15765
Zhang M., Sun Y., Chen L., Cai C., Qiao F., Du Z., et al. (2016). Symbiotic bacteria in gills and guts of Chinese mitten crab (Eriocheir sinensis) differ from the free-living bacteria in water. PloS One 11 (1), e0148135. doi: 10.1371/journal.pone.0148135
Zhou Q., Li K., Jun X., Bo L. (2009). Role and functions of beneficial microorganisms in sustainable aquaculture. Bioresource. Technol. 100 (16), 3780–3786. doi: 10.1016/j.biortech.2008.12.037
Keywords: bivalve, microbial communities, climate change, heatwaves, human health
Citation: Masanja F, Yang K, Xu Y, He G, Liu X, Xu X, Jiang X, Luo X, Mkuye R, Deng Y and Zhao L (2023) Bivalves and microbes: a mini-review of their relationship and potential implications for human health in a rapidly warming ocean. Front. Mar. Sci. 10:1182438. doi: 10.3389/fmars.2023.1182438
Received: 08 March 2023; Accepted: 15 May 2023;
Published: 02 June 2023.
Edited by:
Xiao Liang, Shanghai Ocean University, ChinaReviewed by:
Xiaoting Huang, Ocean University of China, ChinaCopyright © 2023 Masanja, Yang, Xu, He, Liu, Xu, Jiang, Luo, Mkuye, Deng and Zhao. This is an open-access article distributed under the terms of the Creative Commons Attribution License (CC BY). The use, distribution or reproduction in other forums is permitted, provided the original author(s) and the copyright owner(s) are credited and that the original publication in this journal is cited, in accordance with accepted academic practice. No use, distribution or reproduction is permitted which does not comply with these terms.
*Correspondence: Liqiang Zhao, bHpoYW9AZ2RvdS5lZHUuY24=