- 1Faculty of Sustainable Design Engineering, University of Prince Edward Island, Charlottetown, PE, Canada
- 2Département de Génie Mécanique, École de Technologie Supérieure, Montréal, QC, Canada
- 3University of Montréal Hospital Research Centre (CRCHUM), Montréal, QC, Canada
This paper describes the development and testing of two compositions of biodegradable soft bait fishing lures. A water-soluble form of chitosan, known as carboxymethyl chitosan, was added to the biodegradable lure formula to investigate if the lure properties, such as tensile strength, swelling, and underwater performance, could be improved. A three-month shelf-life study was completed to compare the lure properties of two compositions of biodegradable baits: the first composition containing no carboxymethyl chitosan and the second composition containing 5 wt.% carboxymethyl chitosan. The baits manufactured with carboxymethyl chitosan showed increased tensile strength and underwater performance compared to the lures manufactured without this ingredient. Also, the lures manufactured with carboxymethyl chitosan showed increased swelling when submerged in fresh water, which is not desired; however, these lures stayed intact longer before beginning to degrade. When submerged in salt water, the lures manufactured with and without carboxymethyl chitosan showed similar characteristics. The following results will assist in completing further formula optimizations to improve other hindering properties of the current biodegradable lures. The development of more environmentally friendly fishing options is needed to preserve the world’s oceans and freshwater systems for the future generation of recreational anglers.
1 Introduction
Fishing is an everyday recreational activity, providing considerable social and economic benefits to many communities (Brownscombe et al., 2019; Post et al., 2022). Over the years, fisheries management has been mainly focused on the effects of commercial fisheries; however, with the rise of recreational angling, more focus is placed on recreational fishing and its potential environmental impacts (Lewin et al., 2019; Mcphee et al., 2002). It is critical to effectively manage oceans and freshwater ecosystems to reduce the potential consequences to future generations. Pollution from recreational angling occurs due to lost or discarded fishing tackle. This pollution includes lines, hooks, weight, nets, lures, and packages from these products. Typically, these items are manufactured of materials containing plastic or metal, which are non-degradable and can remain in the ecosystem indefinitely. This pollution source can also harm aquatic and terrestrial animals as they may consume these items, mistaking them as food (Franson et al., 2006; Ruxton and Hansell, 2010).
Soft plastic lures (SPLs) have become a significant contributor to this pollution due to their versatility and low cost compared to other bait options (Raison et al., 2014; Sanft et al., 2018). SPLs are typically manufactured of plastisol, a combination of polyvinyl chloride (PVC), plasticizers, and phthalates, which are harmful not only harmful to the environment but also to wildlife (Sanft et al., 2018; Perito et al., 2022). Phthalates are known endocrine disrupters and can percolate into the water, causing potentially harmful effects to fish (Mehta et al., 2002; Xu et al., 2009; Ustun et al., 2015). SPLs are also often infused with salts, which cause the lures to absorb water and swell, increasing the weight and length of the lures. If ingested, this could decrease the chance of fish passing the lures. Several studies have been completed on the ingestion of lost fishing tackle, specifically the ingestion of SPLs. If an aquatic species ingests an SPL, several issues may arise, including blocking of the digestive tract and the formation of gastric bezoars. In a paper by Danner et al., the ingestion of SPLs was studied on brook trout. The fish that were fed SPLs lost significant weight due to the accumulated mass of SPLs, which could not be digested (Danner et al., 2009). It should also be noted that the fish would consume SPLs at the bottom of the tank, confirming that lost or discarded fishing lures sitting motionless could be a hazard to fish (Sanft et al., 2018).
Several attempts have been made to develop more environmentally friendly fishing baits; however, the lure properties do not adequately compare to SPLs. To be accepted as a viable alternative to SPLs, the biodegradable baits must show similar properties, such as sufficient strength, flexibility, and the ability to withstand significant impacts during fishing actions such as casting, reeling, and trolling (Ollis et al., 2004). Sadly, most biodegradable alternatives must be improved in one or more of these areas, reducing their acceptance among anglers. It is hypothesized that adding water-soluble chitosan to biodegradable fishing lures can improve these properties.
Chitosan is a polysaccharide manufactured by the alkaline deacetylation of chitin, an abundant amino polysaccharide polymer found naturally in crustaceans, insects, and fungi (Pillai et al., 2009; Elieh-Ali-Komi and Hamblin, 2016). Chitosan is used in a wide variety of industries, including biomedical, pharmaceutical, biotechnology, wastewater treatment, food, and cosmetics, due to its advantageous characteristics such as being non-toxic, biodegradable, biocompatible, and bioadhesive (Goosen, 1997; Mourya et al., 2010). It is important to note that the uses of chitin and chitosan are restricted as both are insoluble in many organic solvents and water (Zargar et al., 2015; Mathew and Arumainathan, 2022). Chitosan, however, can be converted into a water-soluble form known as carboxymethyl chitosan, which is manufactured using depolymerization or chemical alterations, including carboxymethylation reactions (Butler et al., 2021; Raimunda de Abreu and Campana-Filho, 2008; Naseri et al., 2021). The main benefits of carboxymethyl chitosan include its solubility in many solvents and antimicrobial properties (Raimunda de Abreu and Campana-Filho, 2008). The use of chitosan has been investigated in the manufacturing of nanocomposites (Mathew and Arumainathan, 2022), edible food packaging (Stefanescu et al., 2022), and scaffolding (Kumar et al., 2017). Chitosan is known for its low mechanical strength; however, its properties can be enhanced when mixed with other polymers, such as gelatin. In a paper by Kumar et al., chitosan-gelatin scaffolds were prepared and showed higher tensile strength due to the potential of stronger hydrogen bonds being formed between the two polymers (Kumar et al., 2017).
This paper describes the development of a biodegradable fishing lure manufactured using carboxymethyl chitosan. Two compositions of biodegradable lures were manufactured for this study: no chitosan and 5.0% (wt.) chitosan. A three-month shelf-life study was completed to investigate the change in properties of the biodegradable lures over time when stored in three different types of packaging. Various monthly tests were completed to characterize the performance of the lure, including force testing after submerging the lures in water for an extended period, swelling characterization, and an underwater performance study to examine how long the lures can stay on a fishing hook before breaking. The results presented in this paper will aid in furthering the development of more environmentally fishing options. It is hypothesized that the baits manufactured with carboxymethyl chitosan will show increased tensile strength due to more durable hydrogen bonds being formed between the water-soluble chitosan and gelatin during the manufacturing process.
2 Materials and methods
2.1 Material preparation
The biodegradable soft bait fishing lures were manufactured using injection molding, similar to the production of SPLs (Nakajima and Harrell, 2001). A schematic of the manufacturing process is shown in Figure 1. As listed in Table 1, the main lure ingredients included garlic powder (Compliments, CA), beef gelatin (2837, Wholesale2go, CA), xanthan gum (Duinkerken, CA), carboxymethyl chitosan (Mark Nature, CN), deionized water, and vegetable glycerin (WS21823969, Ingredients Depot, CA). The weighed ingredients were mixed and stirred rigorously for two minutes prior to heating the mixture. Before heating, food coloring (Clubhouse, CA) and non-plastic glitter (Today Glitter, Miami, US) were added to the formula.
An electric stove was used to heat the biodegradable lure mixture by elevating a 600 mL stainless-steel beaker (4352T4, McMaster-Carr, US) in a boiling pot of water. As the formula was heated, the mixture was manually stirred to ensure a consistent formula throughout. After 10 minutes of heating, injection molding was used to manufacture the biodegradable baits. A 45 mL aluminum syringe (B07BH5ZL9N, Amazon) was used to inject the heated formula into a two-piece aluminum mold. Two molds were used to manufacture the biodegradable soft bait lures: Mold A – 3 ½” KT swim bait aluminum mold (5XLU-123, Lurecraft, US) and Mold B – 5 ½” paddle tail sink-o aluminum mold (5XK-SPT525-4, Lurecraft, US). After 10 minutes, the mold was separated, and the lures were removed. The aluminum molds can be manufactured into many different shapes and sizes depending on the lure configuration you wish to fabricate.
Two compositions of biodegradable fishing lures were manufactured: the first composition containing no carboxymethyl chitosan and the second containing 5.0% (wt.) carboxymethyl chitosan (Table 1). A three-month shelf-life study was completed for both lure compositions, which is described in section 2.2.
2.2 Rheological characterization
The rheological characterization study explored the shear viscosity of four compositions of biodegradable lures and two SPL samples using a rotational rheometer (Kinexus, Ulta +, Netzsch, Germany). The results of the biodegradable baits were compared to the SPL samples which included the Yum dinger and the Berkley Powerbait ripple shad. All trials were completed over a temperature range of 20 to 100°C using the parallel plate geometry where deionized water was added to the solvent trap to prevent sample evaporation. A trial was also completed for a biodegradable bait containing carboxymethyl chitosan as the only polymer in the formula. For this trial, the same formulation as the 0% chitosan lure described in Table 1 was used, however, the amount of beef gelatin was substituted for carboxymethyl chitosan, leading to a bait containing 28.8% chitosan.
2.3 Shelf-life study
A three-month shelf-life study was completed to investigate the storage life of the biodegradable lures. Monthly testing was completed to investigate whether the properties of the baits change over time which included force testing after the lures were submerged in water, lure swelling, and underwater testing to observe how long the lures could stay on a hook before breaking. Several different types of packages were tested during the shelf-life study (Figure 2). The packaging options included a clear zip bag (HZB64, Crystal Clear Bags, CA) composed of polyethylene terephthalate (PET) and cast unoriented polypropylene (CPP), a kraft stand bag (S-21232KRFT, ULINE, CA) composed of paper, PET, and linear low-density polyethylene (LLDPE), and a ziplock compostable bag (B07XKWHFMT, Amazon) made of polyester and starch. Lastly, a clamshell tray (CLM4, Clear Bags, CA) was tested using the clear zip bag and ziplock compostable bag to see if it helped preserve the properties and shape of the baits.
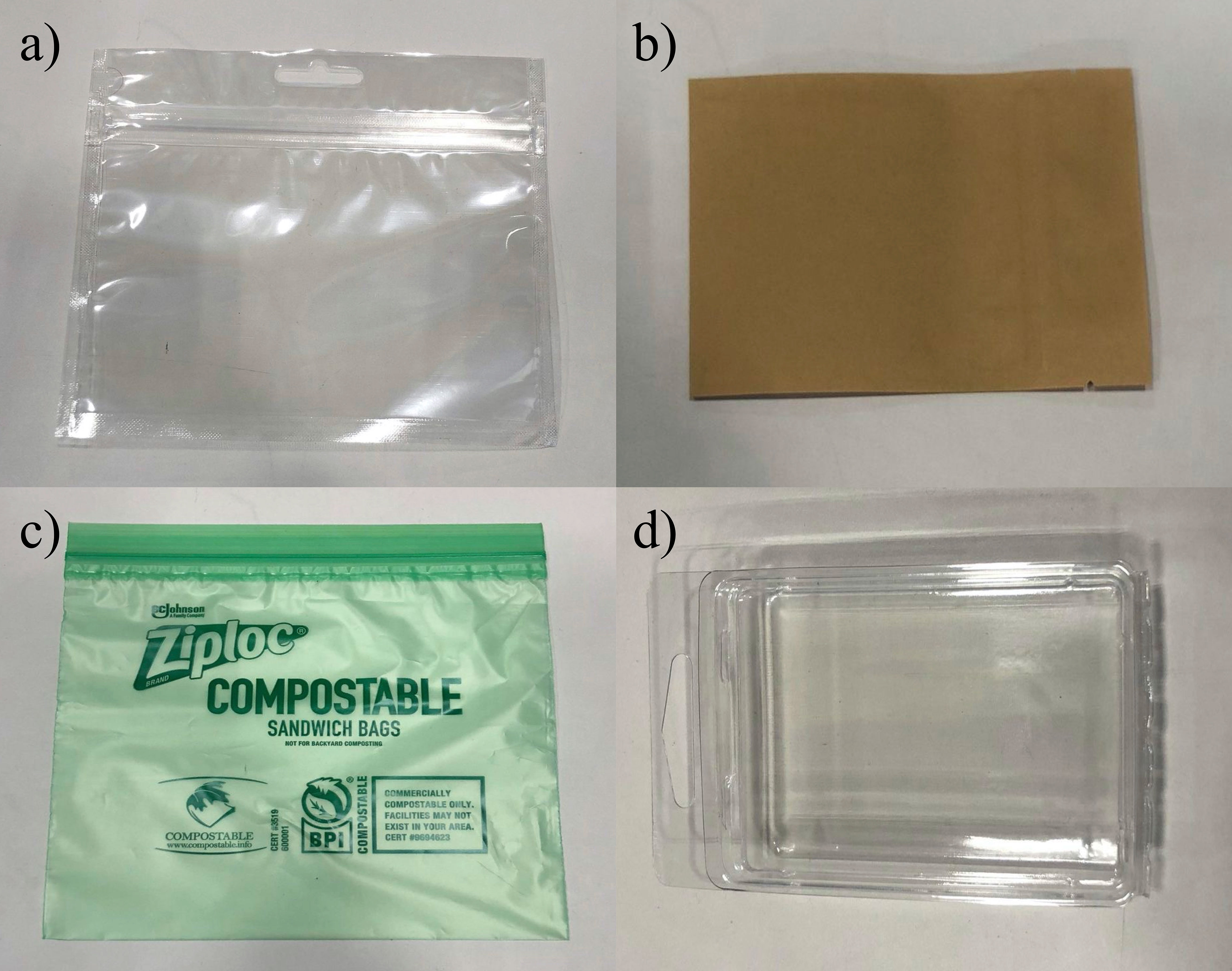
Figure 2 Packaging options tested during the shelf-life study, (A) Clear zip bag, (B) Kraft stand bag, (C) Ziplock compostable bag, (D) Clamshell package.
The packaged baits were stored in the same room where the temperature varied between 18.2°C to 28.0°C, while the relative humidity varied between 18-71% over the entire study. The reason for the large variation in temperature and relative humidity is due to the change in environmental conditions between the summer and fall months. In total, 324 biodegradable lures were tested throughout the shelf-life study. Lastly, the lures were packaged in batches of six, leading to a total of 54 bags (18 of each bag type) and 36 clamshells being evaluated.
2.4 Force testing
The force test characterized the tensile strength of the biodegradable lures where readings were taken using a custom-built testing track to achieve consistent and accurate force measurements (Figure 3A). A 3-D printed attachment (Figure 3B) was fabricated to attach the lure to the force gauge. Two force gauges were used for the force readings; the Mark-10 force gauge with a maximum load of 5lbF (RK-25302-73, Cole Parmer, CA) and CNYST with a max load of 110 lbF (B08HM6S22Q, Amazon).
The biodegradable lures were submerged in 20°C fresh water for one hour, two-hour, and four-hour periods prior to completing the force measurements. The reason for submerging the lures prior to the force testing was to examine how the strength of the lure was affected after being submerged in water for an extended period. The lures were manufactured using the 5 ½” paddle tail sink-o aluminum mold where the paddle tail was removed so that the lure was symmetrical and had the same thickness along the entire lure body.
2.5 Swelling
The swelling study investigated the change in weight and length of the lures after being submerged in fresh water and salt water for two days. The biodegradable baits were placed in 600 mL jars of water and weight and length readings were taken using a balance and caliper, respectively. The lures were manufactured using the 3 ½” KT swim bait aluminum mold.
2.6 Underwater testing
To characterize the underwater ability of the lures, a lure testing tank was constructed to examine how long the lures can stay on the hook before breaking (Figure 4). The lure testing tank was developed using a 60 L fish tank where a high-lift submersible water pump rated up to 800 gal/hr (PP80006, Amazon) was used to create an underwater current. All underwater testing was performed using fresh water where the temperature ranged between 19 to 22°C. An acrylic channel was manufactured to allow the lure to stay within the pump current. To ensure the acrylic channel remained in place throughout the trials, a 1 kg weight was placed on the base of the channel to prevent it from moving. The lures were manufactured using the 3 ½” KT swim bait aluminum mold. The trial was stopped after 12 hours to simulate a full day of fishing.
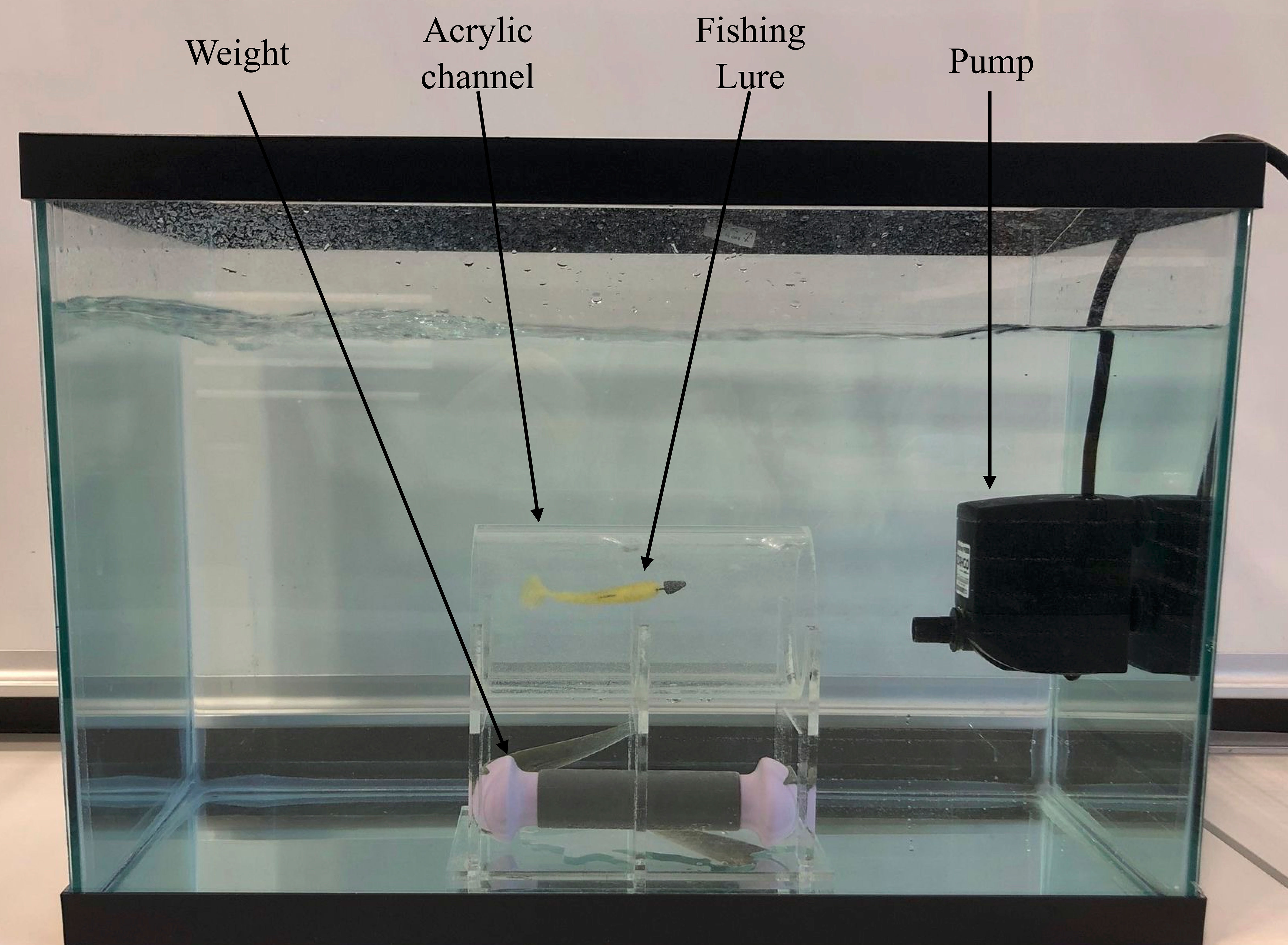
Figure 4 Lure testing tank used to characterize the underwater performance of the biodegradable lures.
2.7 Statistical analysis
All shelf-life testing was completed in triplicate with the mean values and standard deviations being reported. The Tukey test was used to determine if there was a significant difference between the two compositions of biodegradable lures and to compare the monthly testing results of the individual packages.
3 Results and discussion
3.1 Rheological characterization
The results of the rheological study are presented in Figure 5 where the shear viscosity of the biodegradable lures was investigated over a temperature range of 20 to 100°C. Four compositions of biodegradable baits and two SPL samples were tested using the rotational rheometer.
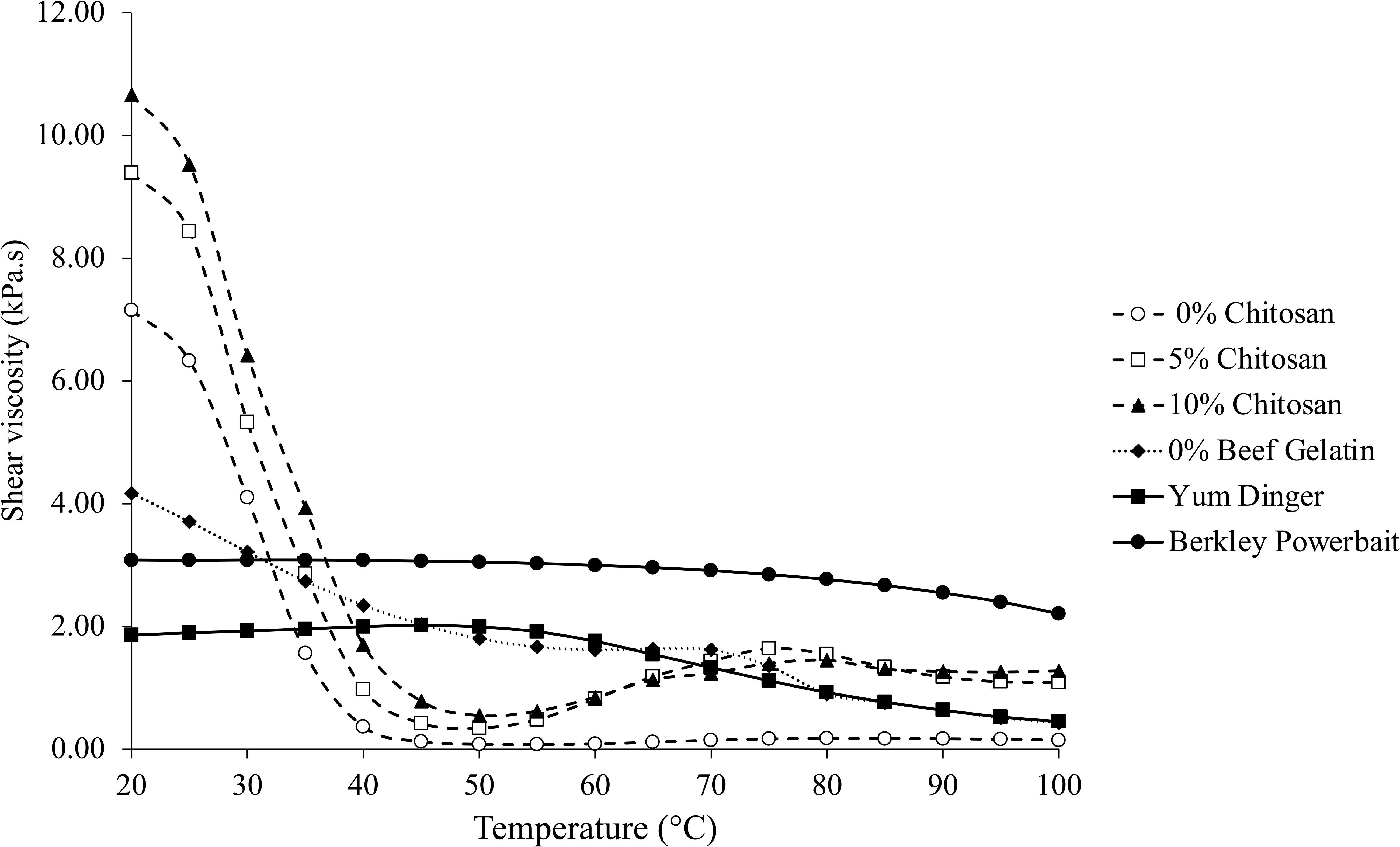
Figure 5 Rheological characterization for the four compositions of biodegradable lures and two SPL samples.
Figure 5 displays the results for the four biodegradable samples and two SPL samples including the Yum dinger and the Berkley Powerbait ripple shad. At 20°C, the shear viscosity of the biodegradable baits containing 0.0 to 10% chitosan ranged from 7.16 to 10.66 kPa.s, while at 100°C, the shear viscosity reduced between the range of 0.15 to 1.28 kPa.s. Comparing the results to the two commercial SPL samples, the shear viscosity at 20°C ranged between 1.86 to 3.08 kPa.s and decreased between the range of 0.45 to 2.21 kPa.s at 100°C.
A common trend is observed for the biodegradable lure samples containing 0, 5 and 10% chitosan where the shear viscosity begins to decrease rapidly as the temperature is increased. Compared to the SPL samples, the shear viscosity stays consistent between 20 to 55°C, before decreasing from 55 to 100°C. The reason for the significant difference in shear viscosity between the biodegradable baits and the SPL samples is due to the use of a heat stabilizer in the SPLs. Soft plastic lures manufactured of PVC require a heat stabilizer to function properly. Without a heat stabilizer SPLs composed of PVC would not be as effective in terms of strength, durability, elasticity, and ability to withstand high temperatures. Typically, heat stabilizers are composed of harmful materials such as organic compounds, metallic soaps, or complex organometallic compounds which could have a negative effect on the environment and aquatic species (Othmer, 1994).
Another trend is observed for the 5 and 10% chitosan samples where the viscosity begins to increase suddenly from 50 to 75°C before plateauing from 80 to 100°C. It is hypothesized that this is due to the melting point of the polymers. The melting point of gelatin is approximately 35°C, while the melting point of chitosan is greater than 225°C (“C181153” Toronto research chemicals, 2017; Calvarro et al., 2016). Another possible hypothesis for this result is a change in the polymer structure as the formula is heated, leading to new bonds being formed (Kumar et al., 2017).
Due to the trend observed in the 5 and 10% chitosan trials, a trial was completed using chitosan as the only polymer in the biodegradable bait to examine how the shear viscosity compares over the same temperature range. The shear viscosity of the chitosan only sample decreased from 4.17 kPa.s at 20°C to 1.67 kPa.s at 55°C before plateauing from 55 to 70°C. After 70°C, the shear viscosity of the chitosan only sample decreases from 1.62 to 0.43 kPa.s at 100°C, very similarly to the Yum sample. It is evident that the choice of polymer has a clear effect on the shear viscosity. It should also be noted that the manufacturing of the baits becomes significantly more difficult as the formula becomes very thick and adhesive when the amount of carboxymethyl chitosan is increased in the lure formula.
3.2 Force testing
The force testing results for the two compositions of biodegradable lures are displayed in Figure 6 where the lures were submerged for one hour, two-hour, and four-hour periods prior to completing the force measurements. A similar trend is observed among all trials where the 5% chitosan lures showed higher force readings compared to the 0% chitosan lures. Using the Tukey method, the 5% chitosan lures showed a significant difference compared to the 0% chitosan for their respective packaging.
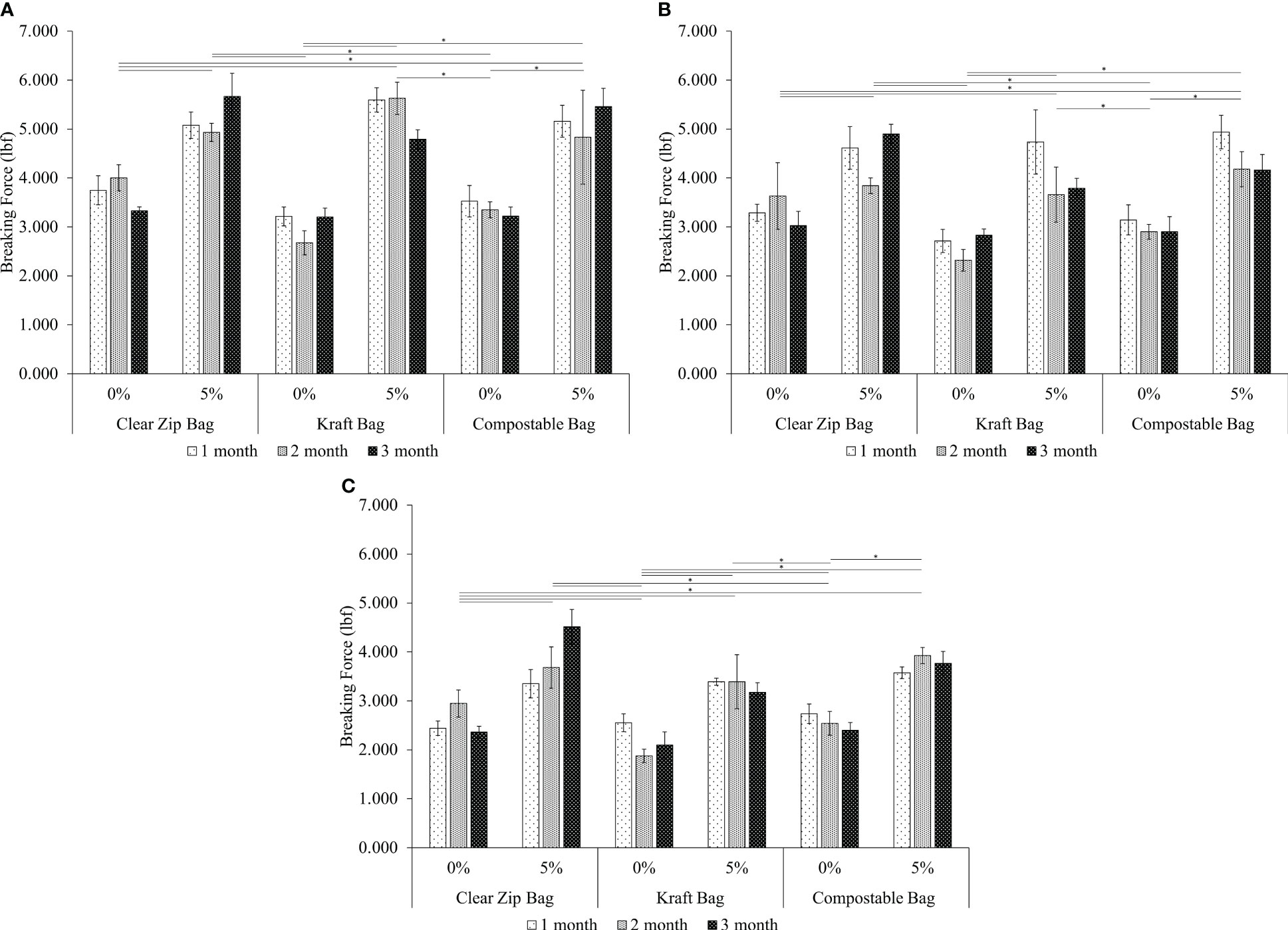
Figure 6 Breaking force of the biodegradable lures after submerging in water, (A) 1 hr submersion, (B) 2 hr submersion, (C) 4 hr submersion. The * symbol is used above the tukey lines to detail the significant difference in results for an individual group.
The force results after submerging the lures for one hour are displayed in Figure 6A. When packaged in the clear zip bag, the strength of the 0% chitosan lures ranged between 3.332 ± 0.078 to 4.003 ± 0.296 lbf, while the strength of the 5% chitosan lures ranged between 4.933 ± 0.185 to 5.695 ± 0.441 lbf. When packaged in the kraft bag, the strength of the 0% chitosan lures ranged between 2.677 ± 0.247 to 3.215 ± 0.193 lbf, while the strength of the 5% chitosan lures ranged between 4.790 ± 0.195 to 5.627 ± 0.330 lbf. Lastly, when packaged in the compostable bag, the strength of the 0% chitosan lures ranged between 3.223 ± 0.184 to 3.525 ± 0.321 lbf, while 5% chitosan lures ranged between 4.833 ± 0.960 to 5.462 ± 0.370 lbf.
The force results for the lures submerged for two hours are shown in Figure 6B. The strength of the 0% chitosan lures packaged in the clear zip bag ranged from 3.027 ± 0.291 to 3.632 ± 0.680 lbf, while the 5% chitosan lures ranged between 3.840 ± 0.160 to 4.902 ± 0.194 lbf. When packaged in the kraft bag, the strength of the 0% chitosan lure ranged from 2.317 ± 0.222 to 2.833 ± 0.123 lbf, while the strength of the 5% chitosan lures ranged between 3.660 ± 0.562 to 4.735 ± 0.657 lbf. Lastly, when packaged in the compostable bag, the strength of the 0% chitosan lures ranged from 2.900 ± 0.309 to 3.143 ± 0.309 lbf, while the strength of the 5% chitosan lures ranged between 4.160 ± 0.319 to 4.938 ± 0.343 lbf.
The force results for the lures submerged for four hours are presented in Figure 6C. Looking at the results for the clear zip bag, the strength of the 0% chitosan lures ranged from 2.365 ± 0.116 to 2.947 ± 0.277 lbf, while the strength of the 5% chitosan lures ranged from 3.351 ± 0.289 to 4.513 ± 0.356 lbf. When packaged in the kraft bag, the force results for the 0% chitosan lures ranged from 1.877 ± 0.138 to 2.553 ± 0.183 lbf, while the force measurements of the 5% chitosan lures ranged between 3.175 ± 0.197 to 3.392 ± 0.552 lbf. Finally, when packaged in the compostable bag, the force results for the 0% chitosan lures ranged from 2.400 ± 0.158 to 2.737 ± 0.200 lbf, while the strength of the 5% chitosan lures ranged from 3.575 ± 0.120 to 3.927 ± 0.165 lbf.
Review of the force testing results show no significant difference between the monthly testing results for the specific bag type and composition of biodegradable lures, concluding that the packages aided in preserving the lures and keeping the strength consistent over time. As mentioned, the 5% chitosan lures showed higher strength characteristics compared to the 0% chitosan lures for all three submersion times. It is also important to note that the strength of the lures decreases as the submersion time is increased. When manufacturing the 5% chitosan lures, the formula was much thicker compared to the 0% chitosan lures. It is hypothesized that the addition of carboxymethyl chitosan is acting as a binder within the formula, allowing the ingredients to strongly adhere to one another. Based on the rheological characterization, it is hypothesized that when the formula is heated during the manufacturing process, new bonds are being formed, leading to a stronger lure.
3.3 Swelling
The swelling properties of the biodegradable lures were studied by measuring the change in weight and length of the lures after being submerged in both fresh water and salt water for two days. The results of the weight and length swelling are shown in Figures 7, 8, respectively.
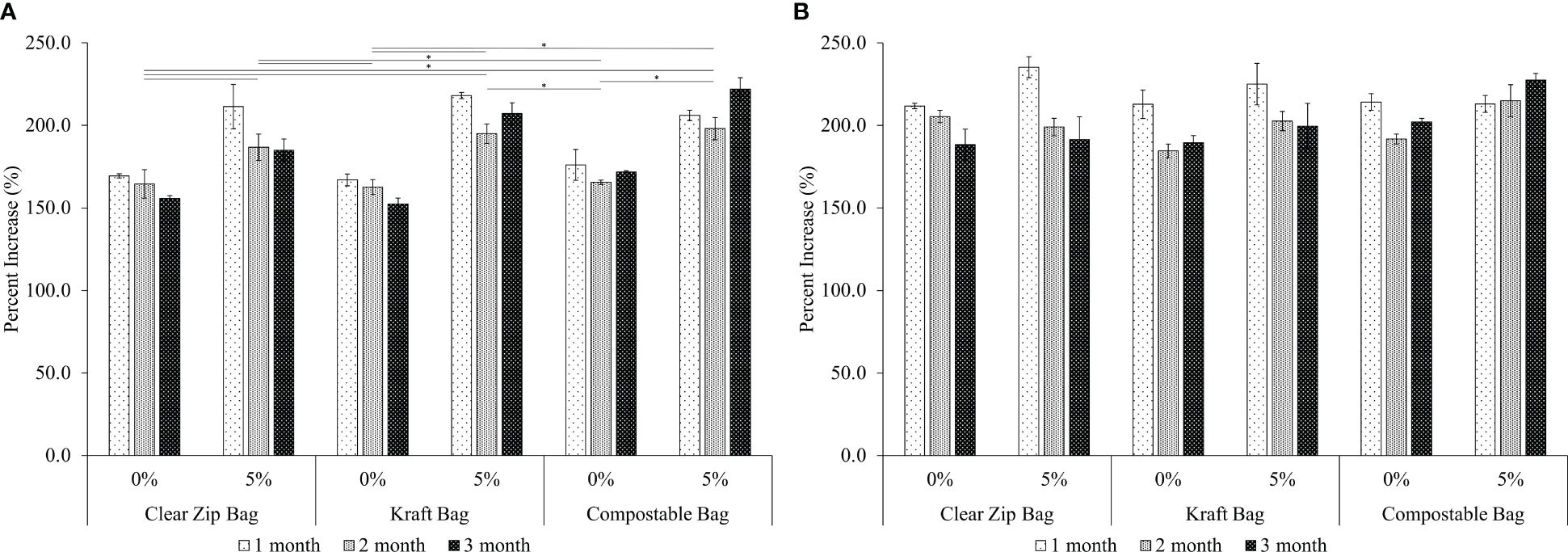
Figure 7 (A) Weight increase in fresh water, (B) weight increase in salt water. The * symbol is used above the tukey lines to detail the significant difference in results for an individual group.
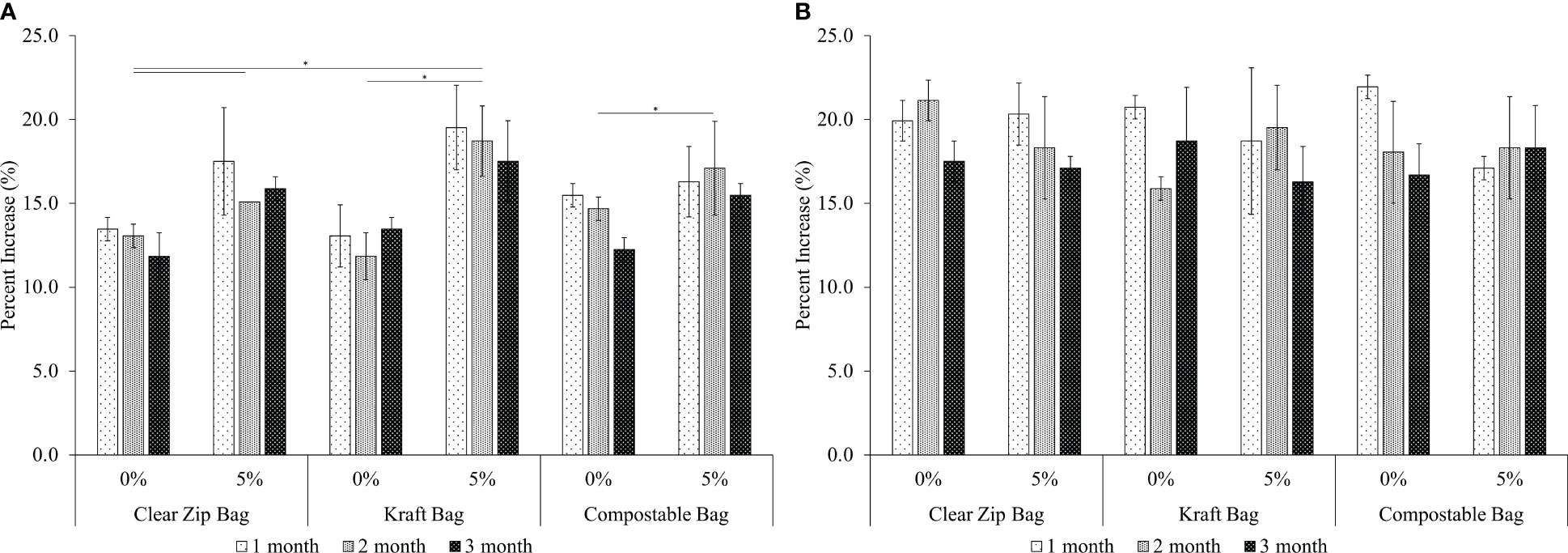
Figure 8 (A) Length increase in fresh water, (B) length increase in salt water. The * symbol is used above the tukey lines to detail the significant difference in results for an individual group.
The swelling results for the weight increase when submerged in fresh and salt water are displayed in Figure 7. The results for the weight increase when submerged in fresh water for two-days are shown in Figure 7A. A common trend is observed for the freshwater weight swelling where the 5% chitosan lures showed a higher increase in weight compared to the 0% chitosan lures. The 0% chitosan lures packaged in the clear zip bag increased in weight by 155.8 ± 1.7 to 169.4 ± 1.3% over the three testing dates, while the 5% chitosan lures increased in weight by 184.9 ± 6.8 to 211.3 ± 13.5%. Similarly, for the kraft bag, the 0% chitosan lures increased in weight by 152.3 ± 3.7 to 167.0 ± 3.6%, while the 5% chitosan lures increased in weight by 194.9 ± 5.9 to 218.0 ± 1.9%. Lastly for the compostable packaging, the 0% chitosan lures increase in weight by 165.4 ± 1.3 to 176.0 ± 9.4%, while the 5% chitosan lures increased in weight by 198.0 ± 6.7 to 222.0 ± 6.8%.
The results for the weight increase when submerged in salt water for two-days are presented in Figure 7B. It should be noted that the lures submerged in salt water showed a slightly higher weight increase compared to the lures submerged in fresh water. Unlike the fresh water weight swelling, the 5% chitosan lures do not show a significant different in weight swelling compared to the 0% chitosan lures. Looking at the results for the clear zip bag, the 0% chitosan lures increased in weight by 188.4 ± 9.3 to 211.8 ± 1.75%, while the 5% chitosan lures increased in weight by 191.4 + 13.9 to 235.3 ± 6.4%. When packaged in the kraft bag, the 0% chitosan lures increased in weight by 184.5 ± 4.1 to 212.8 ± 8.6%, while the 5% chitosan lures increased in weight by 199.6 ± 13.7 to 225.0 ± 12.6%. Lastly for the compostable packaging, the 0% chitosan lures increased in weight by 191.8 ± 3.0 to 214.2 ± 5.0%, while the 5% chitosan lures increased in weight by 213.0 ± 5.1 to 227.5 ± 4.0%.
The swelling results for the length increase when submerged in fresh and salt water are shown in Figure 8. The results for the length increase in fresh water are shown in Figure 8A where the 5% chitosan lures all showed increased swelling compared to the 0% chitosan lures. When packaged in the clear zip bag, the 0% chitosan lures increased in length by 11.9 ± 1.4 to 13.5 ± 0.7%, while the 5% chitosan lures increased in length by 15.1 ± 0.0 to 17.5 ± 3.2%. When packaged in the kraft bag, the 0% chitosan lures increased in length by 11.9 ± 1.4 to 13.5 ± 0.7%, while the 5% chitosan lures increased in length by 17.5 ± 2.4 to 19.5 ± 2.5%. Lastly, when packaged in the compostable bag, the 0% chitosan lures increased in length by 12.3 ± 0.7 to 15.5 ± 0.7, while the 5% chitosan lures increased in length by 15.5 ± 0.7 to 17.1 ± 2.8%.
The swelling results for the length increase in salt water are presented in Figure 8B. Comparing the swelling results of the 0% and 5% chitosan lures, the length increase is very similar between the two compositions and shows no significant difference between the results. The 0% chitosan lures packaged in the clear zip bag increased in length by 17.5 ± 1.2 to 21.1 ± 1.2%, while the 5% chitosan lures increased in length by 17.1 ± 0.7 to 20.3 ± 1.9%. When packaged in the kraft bag, the 0% chitosan lures increased in length by 15.9 ± 0.7 to 20.7 ± 0.7%, while the 5% chitosan lures increased in length by 16.3 ± 2.1 to 19.5 ± 2.5%. Lastly, when packaged in the compostable bag, the 0% chitosan lures increased in length by 16.7 ± 1.9 to 21.9 ± 0.7%, while the 5% chitosan lures increased in length by 17.1 ± 0.7 to 18.3 ± 3.0%.
Using the Tukey method, the monthly testing results for the respective biodegradable lure compositions and packages show no significant difference between one another, confirming again that lures are staying well preserved over time. A common trend is observed among all weight swelling trials where the 5% chitosan lures showed a higher increase in weight compared to the 0% chitosan lures. Carboxymethyl chitosan increases the moisture absorption and retention capabilities, and therefore more water is absorbed by the lure (Mourya et al., 2010). Another trend that was observed is that the lures submerged in salt water show a higher increase in both weight and length compared to the lures submerged in fresh water. It is hypothesized that this is due to the higher density of the salt water compared to fresh water due to the dissolved salts and minerals (Powell, 2018).
It should also be noted that the lures were left in the jars of water for an extended period after the two-day study to investigate when the baits begin to break down. When submerged in freshwater, the 0% chitosan lures began breaking down after four days in the water as the lures became slimy. Only a few of the 5% chitosan lures became slimy after four days in the water. After seven days in freshwater, both the 0 and 5% chitosan lures began breaking down as small pieces began flaking off. When submerged in salt water, both the 0 and 5% lures stayed fully intact up to four days before begin breaking down after ten days. If the biodegradable lures were ingested by fish, it is hypothesized that the fish would have a greater chance to digest the lures compared to SPLs as they breakdown relatively quick.
3.4 Underwater testing
The underwater ability of the biodegradable lures was studied by allowing the baits to flow in a current of water, like the action of reeling or trolling, to determine how long the lures can stay on the hook before breaking. The results of the underwater testing are shown in Figure 9.
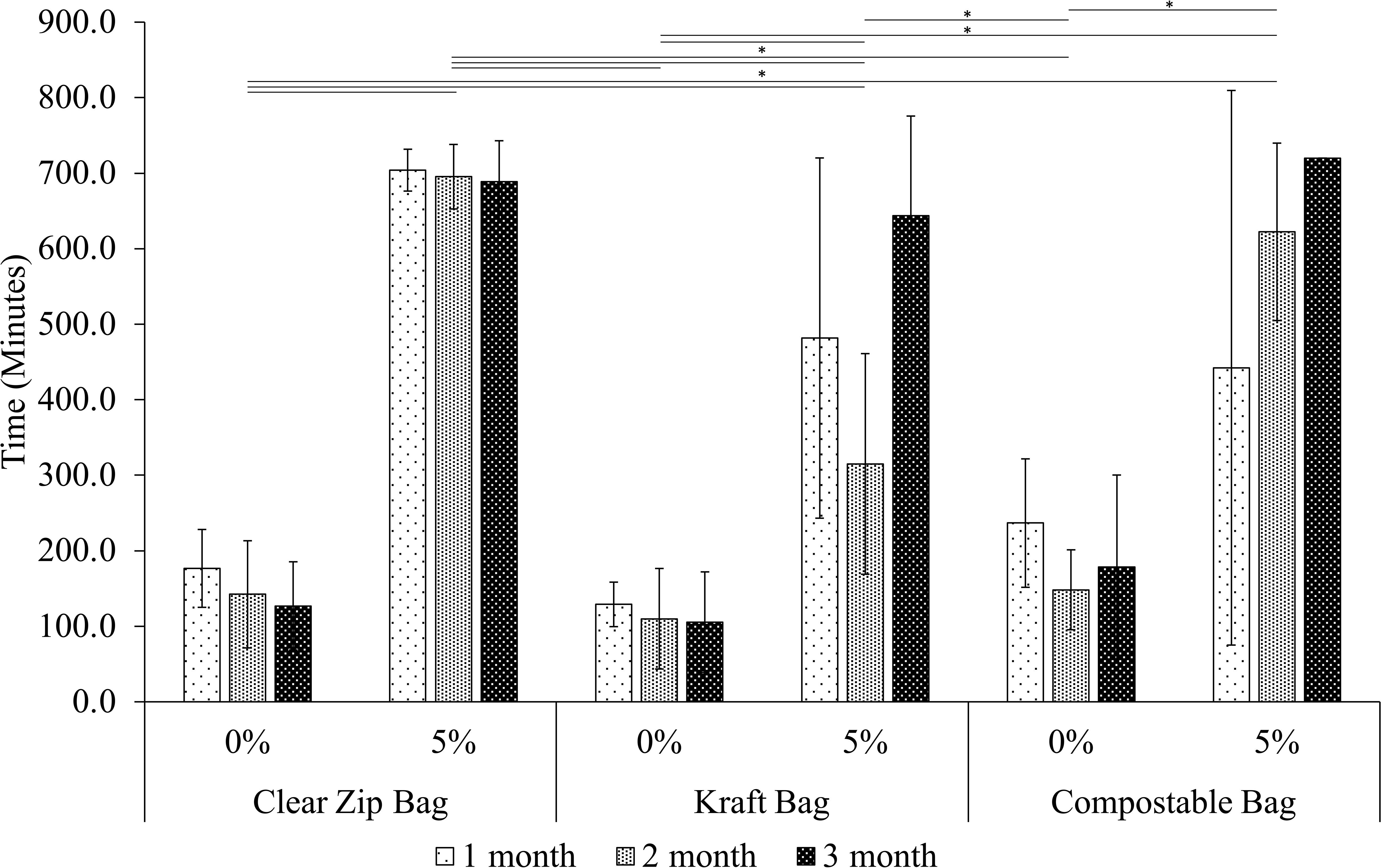
Figure 9 Total time the lure stayed on the hook before breaking. The * symbol is used above the tukey lines to detail the significant difference in results for an individual group.
The underwater testing results for the 0% and 5% chitosan compositions are displayed in Figure 9 based on their respective packaging. A similar trend is observed between all packaging options where the 5% chitosan lures lasted longer on the hook before breaking compared to the 0% chitosan lures. When packaged in the clear zip bag, the 0% chitosan baits lasted between 127.0 ± 58.4 to 176.7 ± 51.5 minutes before breaking, while the 5% chitosan baits lasted between 688.7 ± 54.2 to 704.0 ± 27.7 minutes before breaking. The 0% chitosan lures packaged in the kraft bag remained on the hook between 105.3 ± 66.7 to 129.0 ± 29.5 minutes before fracturing, while the 5% chitosan lures lasted between 315.0 ± 146.1 to 644.0 ± 131.6 minutes. Lastly, the 0% chitosan lures packaged in the compostable bag remained on the hook for 178.7 ± 121.6 to 236.7 ± 85.0 minutes before breaking, while the 5% lures lasted between 442.3 ± 367.2 to 720.0 ± 0.0 minutes prior to failure. Of the three packaging options, the baits packaged in the kraft stand bag remained on the hook for the least amount of time due to the baits becoming deformed in the packaging.
In total, 54 lures were tested for the underwater ability of the biodegradable baits. Of the 54 lures, only 13 of the lures were able to last 12 hours without breaking which were all the 5% chitosan composition. Of the other 41 lures which broke, 36 lures broke at the tail end of the lure, while the other five lures broke at the hooking points where the hook entered the head and/or body of the lure.
Using the Tukey method, the 5% chitosan lures showed a significant difference compared to the 0% chitosan lures for the time in which the lures could stay on the hook before breaking. A comparison of the monthly testing results for the individual packaging and the respective lure composition shows no significant difference between results, confirming that lure properties remain consistent over time. It is hypothesized that the 5% chitosan lures lasted longer on the hook compared to the 0% chitosan lures as the carboxymethyl chitosan is working as a binder within the formula, allowing the ingredients to adhere together much better.
A few conclusions can also be made regarding the packaging options. All packages showed similar properties, however, the clear zip bag is considered the most reliable option for several reasons. When using the kraft bag, the lures became deformed as the packaging was smaller compared to other options, leading to the lures breaking easier. When using the compostable bag, a clamshell must be used or else the lures dry-out and shrink in size. It should also be noted that some lures began growing mold after the packages were opened. This occurred for both the 0 and 5% compositions, and it is hypothesized that this is due to moisture entering the package, which could occur when touching or opening the lures with wet hands.
4 Conclusions
In this study, the comparison of two compositions of biodegradable soft bait fishing lures is described by investigating the important lure properties including force testing after submerging the lures in water, lure swelling, and the underwater ability of the lures. The results of the force testing after submerging the lures revealed that the biodegradable lures manufactured with 5% chitosan showed increased strength compared to the lures containing 0% chitosan. The results of the swelling study in fresh water indicated that the 5% chitosan increased in weight and length more compared to the 0% chitosan lures. When submerged in salt water, the results showed no significant difference between the two compositions of biodegradable baits. Lastly, the underwater performance testing revealed that the 5% chitosan lures lasted longer on the hook before breaking compared to the 0% chitosan lures. The addition of carboxymethyl chitosan to the biodegradable lures displayed increased tensile strength after the lures were submerged in water and improved underwater performance compared to the lures manufactured without the ingredient. Although the lures fabricated with carboxymethyl chitosan revealed increased swelling, these lures stayed intact longer before degrading. Of the three packaging options investigated, the clear zip bag is considered the most reliable option for several reasons. Compared to the other bag options, the clear zip bag allows the lures to keep their proper shape during storage, while also maintaining appropriate hardness after the bags were opened. The results presented in this study will aid in further formula optimizations to improve the other hindering properties of the biodegradable baits such as the durability and heat resistance of the baits.
Data availability statement
The raw data supporting the conclusions of this article will be made available by the authors, without undue reservation.
Author contributions
RL contributed to conceptualization, methodology, validation, investigation, formal analysis, writing – original draft, writing – review and editing. AA contributed to conceptualization, methodology, investigation, writing – review and editing, resources, supervision, project administration, funding acquisition. All authors contributed to the article and approved the submitted version.
Funding
The work was funded through the Mitacs Accelerate Program (IT23608).
Conflict of interest
The authors declare that the research was conducted in the absence of any commercial or financial relationships that could be construed as a potential conflict of interest.
Publisher’s note
All claims expressed in this article are solely those of the authors and do not necessarily represent those of their affiliated organizations, or those of the publisher, the editors and the reviewers. Any product that may be evaluated in this article, or claim that may be made by its manufacturer, is not guaranteed or endorsed by the publisher.
References
(2017). “C181153” Toronto research chemicals. Available at: https://www.trc-canada.com/product-detail/?C181153 (Accessed 20 01 2023).
Brownscombe J. W., Hyder K., Potts W., Wilson K. L., Pope K. L., Danylchuk A. J., et al. (2019). The future of recreational fisheries: advances in science, monitoring, management, and practice. Fish. Res. 211, 247–255. doi: 10.1016/j.fishres.2018.10.019
Butler H. M., Naseri E., MacDonald D. S., Tasker and A. Ahmadi R. A. (2021)Investigation of rheology, printability, and biocompatibility of N,O-carboxymethyl chitosan and agarose bioinks for 3D bioprinting of neuron cells. Materialia 18. doi: 10.1016/j.mtla.2021.101169
Calvarro J., Perez-Palacios T., Ruiz J. (2016). Modification of gelatin functionality for culinary applications by using transglutaminase. Int. J. Gastron. Food Sci. 5, 27–32. doi: 10.1016/j.ijgfs.2016.11.001
Danner G. R., Chacko J., Brautigam F. (2009). Voluntary ingestion of soft plastic fishing lures affects brook trout growth in the laboratory. North Am. J. Fish. Manage. 29, 352–360. doi: 10.1577/M08-085.1
Elieh-Ali-Komi D., Hamblin M. R. (2016). Chitin and chitosan: production and applications of versatile biomedical nanomaterials. Int. J. Adv. Res. 4, 411–427.
Franson J. C., Hansen S. P., Creekmore T. E., Brand C. J., Evers D. C., Duerr A. E., et al. (2006). Lead fishing weights and other fishing tackle in selected waterbirds. Waterbirds 26, 345–352. doi: 10.1675/1524-4695(2003)026[0345:LFWAOF]2.0.CO;2
Kumar P., Dehiya B. S., Sindhu A. (2017). Comparitive study of chitosan and chitosan-gelatin scaffold for tissue engineering. Int. Nano Lett. 7, 285–290. doi: 10.1007/s40089-017-0222-2
Lewin W.-C., Weltersbach M. S., Ferter K., Hyder K., Mugerza E., Prellezo R., et al. (2019). Potential environmental impacts of recreational fishing on marine fish stocks and ecosystems. Rev. Fish. Sci. Aquac. 27 (3), 287–330. doi: 10.1080/23308249.2019.1586829
Mathew S. A., Arumainathan S. (2022). Crosslinked chitosan-gelatin biocompatible nanocomposite as a neuro drug carrier. ACS Omega 7, 18732–18744. doi: 10.1021/acsomega.2c01443
Mcphee D. P., Leadbitter D., Skilleter G. A. (2002). Swallowing the bait: is recreational fishing in Australia ecologically sustainable? Pacific Conserv. Biol. 8, 40–51.
Mehta V., Ghorpade N., Khare M., Sinkar P., Krishman S. (2002). Toxicity study of diethyl phthalate on freshwater fish cirrhina mrigala. Ecotoxicol. Environ. Saf. 53 (2), 255–258. doi: 10.1006/eesa.2002.2212
Mourya V. K., Inamdar N. N., Tiwari A. (2010). Carboxymethyl chitosan and its applications. Adv. Mater. Lett. 1 (1), 11–33. doi: 10.5185/amlett.2010.3108
Nakajima N., Harrell E. R. (2001). Rheology of PVC plastisol: particle size distribution and viscoelastic properties. J. Colloid Interface Sci. 238 (1), 105–115. doi: 10.1006/jcis.2001.7468
Naseri E., Cartmell C., Saab M., Kerr and A. Ahmadi R. G. (2021). Development of N,O-carboxymethyl chitosan-starch biomaterial inks for 3D printed wound dressing applications. Macromol. Biosci. 21 (12), no. doi: 10.1002/mabi.202100368
Ollis H. D., Diaz-Verson S., Bell L. N., Weese J. O., Wei C. I., Wright R. A. (2004). Biodegradable fishing lure and material (United States of America Patent US 6,753,004 B2).
Othmer K. (1994). “Heat stabilizers,” in Encyclopedia of chemical technology (United States:John Wiley & Sons).
Perito E. D., Guerra N. B., Giovanela M., Machado G., da Silva Crespo J. (2022). Chemical, thermal and mechanical evaluation of poly(vinyl chloride) plastisol with different plasticizers. J. Elastomers Plastics 54 (8), 1277–1294. doi: 10.1177/00952443221135001
Pillai C., Paul W., Sharma C. P. (2009). Chitin and chitosan polymers: chemistry, solubility and fiber formation. Prog. Polymer Sci. 34 (7), 641–678. doi: 10.1016/j.progpolymsci.2009.04.001
Post J. R., Sullivan M., Cox S., Lester N. P., Walters C. J., Parkinson E. A., et al. (2022). Canada’s recreational fisheries: the invisible collapse? Fisheries 27 (1), 1–17. doi: 10.1577/1548-8446(2002)027<0006:CRF>2.0.CO;2
Powell J. (2018) Four biggest differences between the ocean & fresh water (Sciencing). Available at: https://sciencing.com/four-between-ocean-fresh-water-8519973.html (Accessed 09 02 2022).
Raimunda de Abreu F., Campana-Filho S. P. (2008). Characteristics and properties of carboxymethylchitosan. Carbohydr. Polymers 75, 214–221. doi: 10.1016/j.carbpol.2008.06.009
Raison T., Nagrodski A., Suski C. D., Cooke S. J. (2014). Exploring the potential effects of lost or discarded soft plastic fishing lures on fish and the environment. Water Air Soil Pollut. 225, 1869. doi: 10.1007/s11270-014-1869-1
Ruxton G. D., Hansell M. H. (2010). Fishing with a bait or lure: a brief review of the cognitive issues. Ethology 117 (1), 1–9. doi: 10.1111/J.1439-0310.2010.01848.X
Sanft E. J., Porreca A. P., Parkos J. J. III, Detmer T. M., Wahl D. H. (2018). Effects of ingestion of soft plastic fishing lures on largemouth bass. North Am. J. Fish. Manage. 38, 718–724. doi: 10.1002/nafm.10067
Stefanescu B. E., Socaciu C., Vodnar D. C. (2022). Recent progress in functional edible food packaging based on gelatin and chitosan. Coatings 12 (12), 1–26. doi: 10.3390/coatings12121815
Ustun I., Sungur S., Okur R., Sumbul A. T., Oktar S., Yilmaz N., et al. (2015). Determination of phthalates migrating from plastic containers into beverages. Food Anal. Methods 8, 222–228. doi: 10.1007/s12161-014-9896-5
Xu B., Gao N.-y., Cheng H., Xia S.-j., Rui M., Zhao D.-d. (2009). Oxidative degradation of dimethyl phthalate (DMP) by UV/H2O2 process. J. Hazard. Mater. 162 (2-3), 954–959. doi: 10.1016/j.jhazmat.2008.05.122
Keywords: biodegradable lures, carboxymethyl chitosan, injection molding, sustainability, recreational fishing
Citation: Legault R and Ahmadi A (2023) Investigation of carboxymethyl chitosan in the development of biodegradable soft bait fishing lures. Front. Mar. Sci. 10:1182395. doi: 10.3389/fmars.2023.1182395
Received: 09 March 2023; Accepted: 25 May 2023;
Published: 19 June 2023.
Edited by:
Edison Barbieri, Agência de Agronegócio e Tecnologia de São Paulo (APTA), BrazilReviewed by:
Marcelo Henriques, Agência de Agronegócio e Tecnologia de São Paulo (APTA), BrazilKarina Fernandes Oliveira Rezende, University of São Paulo, Brazil
Copyright © 2023 Legault and Ahmadi. This is an open-access article distributed under the terms of the Creative Commons Attribution License (CC BY). The use, distribution or reproduction in other forums is permitted, provided the original author(s) and the copyright owner(s) are credited and that the original publication in this journal is cited, in accordance with accepted academic practice. No use, distribution or reproduction is permitted which does not comply with these terms.
*Correspondence: Ali Ahmadi, ali.ahmadi@etsmtl.ca