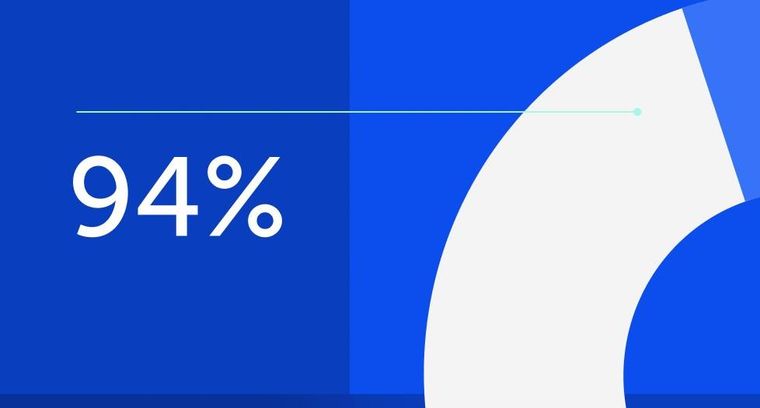
94% of researchers rate our articles as excellent or good
Learn more about the work of our research integrity team to safeguard the quality of each article we publish.
Find out more
ORIGINAL RESEARCH article
Front. Mar. Sci., 23 May 2023
Sec. Marine Conservation and Sustainability
Volume 10 - 2023 | https://doi.org/10.3389/fmars.2023.1180876
This article is part of the Research TopicSpatial Planning for Sustainable Use of Marine Ecosystem Services and ResourcesView all 9 articles
As the intensity of ocean spatial exploitation increases, the offshore island tourism has been thriving, and the utilization of uninhabited islands is attracting more and more attention. In order to achieve the sustainable development of offshore island tourism resources, it is important to evaluate population carrying capacity and conduct sustainability assessment for uninhabited islands. To this end, we proposed a quantitative evaluation method for the sustainable development of offshore coral reef islands based on the combination of scenario simulation and emergy analysis. This method was tested on a coral reef island in the South China Sea, namely Lingyang Reef. The dynamics in emergy related structures and indicators of Lingyang Reef system were revealed under different scenarios, providing decision-making references for the pre-development sustainability assessment of an uninhabited coral reef island. The evaluation results showed that (1) when accommodating 2000 persons, the island system was in a low-load state, with sustainable values in terms of renewable energy input percentage, environmental load ratio, and emergy sustainability index, except for the critical state of emergy yield ratio; (2) when accommodating 5000 persons, the emergy yield ratio was in an unsustainable state, with the emergy sustainability index in a critical state, and both of the renewable energy input percentage and environmental load ratio in sustainable states, indicating that socioeconomic activities were not beyond the carrying capacity, and the development and utilization intensity was moderate; (3) when accommodating 10000 persons, the renewable energy input percentage and emergy sustainability index were in critical states, and the emergy yield ratio and environmental load ratio were in unsustainable states, indicating that the corresponding human activities had exceeded the carrying capacity.
The 21st century is the oceanic century, and the vigorous development of the ocean economy has become a common goal for the world (Sun et al., 2022; Ji et al., 2023). Many countries and governments regard accelerating the utilization of ocean resources and the development of the ocean economy as a national strategy (Jiang et al., 2014; Kakazu, 2022; Wu and Li, 2022). With the increasing scale and intensity of ocean spatial exploitation, the development of offshore coral reef island tourism is thriving, and the development and utilization of uninhabited islands are also attracting much more attention (Kurniawan et al., 2019; Li et al., 2020; Wolf et al., 2021). The uninhabited islands have limited areas, inconvenient transportation, and fragile ecological environments. Their ecosystems are easily degraded and difficult to recover under external disturbances (Steibl and Laforsch, 2019; Zhao et al., 2022). At present, excessive pursuit of economic benefits, blind development of tourism resources, and extensive management of tourism activities have seriously damaged the ecological environment of offshore uninhabited islands, restricting the sustainable development of offshore uninhabited island tourism (Bolden and Johnston, 2020; Chi et al., 2020; Chen et al., 2022b). In order to achieve the sustainable development of offshore uninhabited island tourism and the scientific and reasonable utilization of natural resources, it is significantly important to evaluate the population carrying capacity and sustainable development level of offshore islands in advance (Walker et al., 2021).
Different scholars have varying views on the concept of sustainable development for offshore coral reef islands (Fuldauer et al., 2019; Zhang et al., 2020; Skene, 2021; Chen et al., 2022a; Quyet et al., 2022), but they all involve the same essence of planning and development based on the unique characteristics, so as to find a coordinated pathway between resources, environment, and human activities in the island system. Meaurio and Murray (2001) believed that the island tourism might bring many problems, such as weakening the traditional culture of local communities, causing unfair distribution of benefits, and resulting in environmental damages. Hsu et al. (2019) studied the relationship between factors affecting residents’ attitudes toward supporting sustainable tourism in island environments and found that community participation has a positive and direct impact on residents’ support for sustainable tourism. Chen (2019) studied the future direction of island tourism development from the perspective of far-reaching impacts of global warming on island ecological systems and believed that reducing tourism and employing professional guides could promote harmonious and sustainable development between human and nature in the islands. McElroy and De Albuquerque (1998) chose 20 small islands in the Caribbean Sea for study and proposed the concept of “tourism penetration index” and classified them. After that, management measures and suggestions were put forward for the 20 islands with different index levels to achieve sustainable development. Walker et al. (2021) proposed that slow tourism could be considered as an important component of sustainable islands, which would provide important support for business development, product sales, and tourism enhancement, and promote regional tourism development. The above studies primarily focus on the definition of island tourism, evaluation of benefits, sustainable development models and strategies for island tourism, and mostly adopt qualitative descriptions. The evaluation standards are relatively single-sided and lack comprehensive quantitative assessment.
Emergy analysis is a quantitative methodology for assessing the energy and material resources used in a system (Odum et al., 2000), which is defined as the energy that has been used up directly and indirectly to create a product or service (Odum et al., 1987). This method converts resources, environment, economy, and energy factors in an ecological system into solar energy values, making it possible to compare various energy sources in a unified solar energy value, overcoming the limitations of traditional approaches, and providing a metric and scientific basis for the rational utilization of natural resources and evaluation of ecological system sustainability. Emergy analysis is useful for assessing the systematic sustainability and making comparisons among different systems (Fonseca et al., 2017; Alkhuzaim et al., 2021). In addition to the applications in regional sustainability assessment and ecological system evaluation (Su et al., 2020; Deymi-Dashtebayaz and Norani, 2021), it has been widely used to provide a new perspective for sustainable development research in offshore island tourism (Wei et al., 2020; Alkhuzaim et al., 2021; Zhu et al., 2022). Nam et al. (2010) employed the emergy analysis method to evaluate the carrying capacity of an uninhabited island located in the southwest waters of South Korea and predicted the number of people that could be sustained under a sustainable development scenario. Jung et al. (2018) used this method to evaluate the sustainable development state of Jeju Island between 2005 and 2015 and predicted its development status in 2030, providing important theoretical support for the formulation of future development strategies for the tourism-led island. Zhan et al. (2019) applied emergy analysis to evaluate the ecosystem service functions of Chongming Island and identified the service supply and flow between the ecosystem and the urban area, highlighting the necessity of promoting land use planning and maintaining ecosystem services. Yang et al. (2020) employed the emergy analysis framework to evaluate the sustainability in three island nations and found that the island ecosystems were more fragile and sensitive compared to other regions. Although the prior studies have effectively evaluated the sustainability of different islands, there is still a lack of research that uses emergy analysis and scenario simulation to conduct the sustainability assessment of a typical coral reef island ecosystem, especially for the pre-assessment of uninhabited island development.
The South China Sea is located in the tropical sea area and has distinct tourism resources, with a broad tourism market prospect. The islands in the South China Sea are far from the mainland of surrounding countries, making them highly attractive to urban people who are constantly under high levels of stress and fatigue from working in tall buildings. Therefore, it is particularly necessary to evaluate the tourism development sustainability of coral reef island the South China Sea. This study presents a quantitative evaluation method for the sustainable development of offshore island tourism based on a combination of scenario simulation and emergy analysis. The method is applied to a typical coral reef island in the South China Sea (i.e., Lingyang Reef) as a case study. The changes in emergy value structure of Lingyang Reef under different scenarios are simulated, and the resultant sustainability levels of Lingyang Reef under different scenarios are quantitatively analyzed, thereby providing scientific evidence and novel methodology for the pre-evaluation of the sustainable development of ecological tourism in offshore coral reef islands.
Lingyang Reef is located in the South China Sea with geographical coordinates of around 16°28’N and 111°35’E, as shown in Figure 1. It is a closed reef located in the southwest of the Yongle Atoll in the Xisha Islands. The reef is approximately triangular in shape, with a lagoon in the center. The reef is 12 km long from north to south and about 4 km wide from east to west. During low tides, large areas are exposed, and during typhoons and high tides, it is often submerged by sea water. The total area of Lingyang Reef is about 15 km2, with a reef platform area of approximately 9.5 km2, a lagoon area of approximately 5.5 km2, and the circumference of outer reef edge is about 15,000 m. Lingyang Reef is rich in fishing resources and qualifies as aquaculture and fishing area.
According to statistical data provided by Hainan Strait Shipping Co., Ltd., the average number of tourists received per cruise to the Xisha Islands is around 250. Since Lingyang Reef is a large atoll with unique coral coastlines and abundant marine resources, it has the potential to be dredged and developed for tourism purposes in the future, and can serve as a popular destination for underwater sightseeing and island leisure vacations for tourists on cruise ships.
In this study, we obtained the time series of meteorological data for Lingyang Reef from the Prediction of Worldwide Energy Resource (POWER) project at NASA Langley Research Center (https://power.larc.nasa.gov/data-access-viewer/). The data includes solar radiation, wind speed, precipitation, and temperature. The average yearly solar radiation at Lingyang Reef is 1947.74 KWh/m2. The air density is 1.1718 kg/m³. The average wind speed at a height of 10 meters is 5.81 m/s. The average yearly precipitation is 2745.38 mm. According to previous studies, the average tidal range in the surrounding sea area is 3.2 m (Zhao, 1996), and the yearly wave energy resource storage is 7.2E+10 joules per meter (Zheng, 2011).
The proposed coral reef island sustainability evaluation framework based on emergy value calculation includes the following steps: (1) determining the main sources of materials and energy in the island system to create an energy flow diagram; (2) converting each material and energy into solar energy value based on the emergy conversion rate in the island system to generate an emergy analysis table; (3) constructing an evaluation framework of coral reef island sustainability based on the corresponding analysis table and evaluating the future development and utilization of the island system accordingly.
Given that water, electricity, and food are the primary basic needs for island inhabitants following the development and utilization of offshore coral reef island, it is feasible to assume that freshwater, sea food, and vegetables can be provided through various methods such as local rainwater collection, seawater desalination, aquaculture or fishing, and soil planting. In order to ensure a stable electricity supply in the island system, electricity can be provided through both local renewable energy sources and diesel generators, although diesel is dependent on external supplies. Considering factors such as the balanced nutrition, staple foods (e.g., grains and potatoes, fruits, milk and beans, meat and eggs) are provided through supplies. As shown in Figure 2, the system energy value of the island and reef system is composed of five parts: renewable energy (RE), non-renewable resource (NR), indigenous renewable resource (IR), imports (IM), and exports (EX). According to the law of energy conservation, the formula for calculating the system energy value of islands and reefs is as follows:
where represents the total emergy output of the island system, represents the sub-item emergy value belonging to the category of renewable energy, represents the sub-item emergy value belonging to the category of non-renewable resources within the system, represents the sub-item emergy value belonging to the category of indigenous renewable resource, represents the emergy value imported from outside, and represents the emergy value exported to outside. Here, the units of emergy value are all solar emjoules (Sej), that is, the solar energy contained in the energy or material flow belonging to a certain category.
Correspondingly, we classified the five components of the island system into subcategories for a quantitative analysis of their emergy values. Renewable energy (RE) sources include solar, wind, precipitation, wave, and tidal energy. Non-renewable resources (NR) are limited to topsoil loss because all the soil needed for future development will come from outside the system and topsoil organic matter regenerates slowly. Indigenous renewable resources (IR) include self-sufficient resources such as fresh water use, seafood consumption, vegetable consumption, and renewable energy generation. It should be noted that the renewable energy generation component only contains the human labor related emergy for power generation facilities (e.g., manufacturing, installation, operation, and maintenance) without any double counting with the renewable energy component (e.g., sun, wind, wave, and tide). Imports (IM) refer to external inputs required for living and production on the island and include food such as potatoes, fruits, dairy and egg products, and diesel for power generation. There are no exports (EX) because this paper assumes that most waste and discarded materials can be processed locally and the island is located in a relatively resource-poor area.
In the context of emergy, the concept of hierarchy is used to describe the different levels of organization within a system, with each level being characterized by a specific degree of complexity, organization, and energy quality. The higher the level of organization, the greater the transformity and the higher the quality of energy. For example, solar energy has a very low transformity and is considered a high-quality energy source, whereas fossil fuels have a higher transformity and are considered lower-quality energy sources. In order to accurately calculate the emergy value of various components in the coral reef island system, the unit energy value (UEV) is used to convert various mass and energy in the island system into solar energy values. The UEV is the amount of energy contained in each unit of a certain category of energy or mass. The higher the UEV of energy or mass, the higher its emergy value and its higher level in the energy system. The calculation formula for the emergy value of a specific kind of energy or mass is expressed as follows:
where represents the emergy value of a certain energy, with units in solar emjoules. represents the conversion rate of the emergy value, with units in solar emjoules per joule. represents the embodied energy, with units in joules.
The energy conversion rates for each energy value sub-item in this article are based on previous studies, as shown in Table 1. Since the energy value sub-items such as aquatic products, vegetables, cereals and potatoes, fruits, dairy and beans, meat and eggs, and diesel are based on material consumption, measured in kilograms or tons, it is necessary to perform mass-to-energy conversion before calculating the energy value embodied in each material (Nam et al, 2010; Jung et al., 2018). The mass-to-energy conversion rates for different categories of materials are shown in Table 2.
Emergy analysis can unify the flow of all materials and energy in an island system into a single unit of measurement for accounting purposes. By calculating the sustainability assessment indicators of the island system, and referring to the evaluation standards of sustainable development status, the sustainability of the future development of the island system can be quantified. The sustainable development evaluation indicators of the island system calculated in this study mainly include the percentage of renewable energy input percentage (), emergy yield ratio (), environmental loading ratio (), and emergy sustainability index ().
According to the emergy analysis method in section 3.1, the corresponding emergy values of various components such as renewable energy (RE), non-renewable resources (NR), indigenous renewable resources (IR), and imports (IM) can be calculated in the island system. Thus, the total emergy yield of the island system can be obtained, and the calculation formula is outlined as follows:
where Y represents the total emergy yield of the island system, which is the total amount of emergy used in a year; R represents the total amount of renewable emergy, equal to the sum of the emergy values of the renewable energy and indigenous renewable resources in the island system, i.e., R = RE + IR; N represents the non-renewable emergy, equal to the emergy value of non-renewable resources in the island system, i.e., N = NR; F represents the emergy input from the outside into the island system, i.e., F = IM.
The proportion of renewable energy input is the ratio of the total amount of renewable energy in the island system to the total energy output of the island system. The calculation formula is used to reflect the status and role of RE and IR in the island system, and to measure the development level of the island system. The higher the value, the greater the contribution of RE and IR to the island system, the higher the dependence of the island system on RE and IR, and the lower the development level of the island system. This also indicates that the island system has abundant natural resources and low environmental pressure.
The emergy yield ratio is the ratio of the total emergy output of the island system to the emergy input from outside the island system. The calculation formula is used to measure the efficiency of the island system’s output with a certain economic input. The higher the emergy yield ratio, the higher the emergy value obtained by the island system, and the higher the efficiency of the island system’s output.
The environmental loading ratio represents the ratio of the sum of non-renewable energy and energy input from outside the island system to the total amount of renewable energy in the island system. The calculation formula is used to characterize the environmental pressure on the island system under a certain level of economic activity. The higher the environmental loading ratio, the more the development relies on the consumption of non-renewable resources within the island system and the support from outside the island system, and the greater the environmental pressure; while the lower the environmental loading ratio, the more the development relies on renewable energy and indigenous renewable resources, and the smaller the environmental pressure.
The emergy sustainability index (ESI) is defined as the ratio of EYR to ELR. It is used to measure the efficiency of social and economic output under a certain level of environmental pressure. The higher the ESI, the higher the efficiency and the stronger the ability of the island-reef system to achieve sustainable development. However, a higher ESI value does not necessarily indicate a higher degree of sustainability. If the ESI value is between 1 to 10, it indicates the vitality and development potential of the coral reef island system. If the ESI is greater than 10, it means that local social and economic development is severely lagged. If the ESI is less than 1, it means that the resources in the island system are being over-consumed (Ulgiati et al., 1994).
Table 3 shows the change intervals of each indicator and their corresponding sustainable development status, according to the calculation results of , , and , which is based on the prior research of Jung et al. (2018).
This article uses scenario simulation method to set up three scenarios in advance: low carrying capacity scenario, moderate carrying capacity scenario, and high carrying capacity scenario, to simulate the water, electricity, and food consumption of the future permanent population on the island within one year. Referring to Jeju Island in South Korea, which has an area of 1833.2 square kilometers and a population of about 680,000 (Jung et al., 2018), after land reclamation, the land area of Lingyang Reef could reach 9.5 square kilometers, with a potential population of at least 35,000. Considering the natural environment, geographical location, marine weather, and other factors of Lingyang Reef, the estimated population capacities for the low, moderate, and high carrying capacity scenarios are set at 2,000, 5,000, and 10,000 respectively.
Due to the different functional orientations for future development of the coral reef island, the areal and structural differences of various land use types within the island system are enormous. Therefore, we adopted the per capita quota method for simplification. By referring to relevant national urban planning standards and guidelines, resident dietary guides, and taking into account the actual situation of the harsh natural environment of the offshore coral reef island, such as increasing the intake of starchy foods and legumes under the high loading scenario and reducing the intake of fruits and high-quality proteins that are difficult to preserve and eat, to ensure that food should at least meet the minimum energy needs. This study has also revised the per capita domestic water and electricity consumption, and food intake such as seafood, vegetables, starchy foods, fruits, dairy and legumes, eggs and meat, for the population that can be sustained on the coral reef island under different scenarios, as shown in Table 4.
Based on the population that can be sustained on the coral reef island in the future under the low, moderate, and high loading scenarios, and the per capita quota of resource consumption, the total amount of water, electricity, and food consumption during a year in the island system under different scenarios were calculated, as shown in Table 5. In this study, we assumed that the treated wastewater can be directly used as ecological water and other miscellaneous water with a conversion rate of 80%. Due to the stability of diesel power generation and the high electricity consumption in the high loading scenario, it is assumed that in low-load, moderate-load, and high-load scenarios, the island’s renewable energy power generation accounts for 80%, 40%, and 0% of total electricity consumption, respectively. Correspondingly, diesel for power generation accounts for 20%, 60%, and 100% of the total electricity consumption. Based on the power generation per cubic meter of diesel (2658.55 kWh/m3) and the diesel density (850 kg/m3), the diesel power generation can be converted into diesel consumption.
There is no direct relationship between the emergy values contained in renewable and non-renewable resources and the population that an island can sustain. The calculation is only related to parameters such as the land area, shoreline length, wind speed, precipitation, tidal range, etc. Therefore, the emergy values contained in the renewable and non-renewable resources of the island do not change under different scenarios. To ensure the integrity of the energy calculation, the non-renewable emergy values of the island system includes the emergy values of the island terrestrial system and the island marine system (island inner lagoon and adjacent sea areas), as shown in Table 6. The specific calculation formulas for the solar, wind, precipitation chemical, wave, and tidal energy of the island terrestrial and marine systems, as well as the emergy caused by the topsoil loss in non-renewable resources, can be found in previous studies (Nam et al., 2010; Liu et al., 2011).
Based on water, electricity, and food consumptions of the island system under different scenarios (Table 5) and emergy conversion rates (Table 1), this paper calculates emergy values of different components of the island system under respective scenarios,as shown in Table 7, and aggregates the corresponding emergy values and their proportions for the five components including renewable energy, non-renewable resources, indigenous renewable resources, imports, and exports, as shown in Figure 3.
Figure 3 Emergy proportions for different components of the coral reef island system under three scenarios: (A) Low loading scenario; (B) Moderate loading scenario; (C) High loading scenario.
The total amount of emergy from renewable sources in the low loading scenario, as shown in Figure 3A, is 7.74E+19 Sej/year. The total amount of emergy from indigenous renewable resources is 1.45E+19 Sej/year, while the total amount of emergy from external imports is 9.18E+18 Sej/year. The total amount of emergy from IR is greater than that from IM, indicating a reasonable balance between the efficient development and utilization of local renewable resources and minimizing dependence on external inputs. The overall system performance is good.
As shown in Figure 3B under the moderate loading scenario, the total emergy value of the renewable emergy is 7.74E+19 Sej/year, the total emergy value of the indigenous renewable resources is 2.06E+19 Sej/year, and the total emergy value of the external imports is 4.52E+19 Sej/year. The total emergy value of the external input is more than twice the total emergy value of the local renewable resources, indicating that the island system is running stably at this time. It is suggested to reduce dependence on external material inputs, especially diesel, and to further increase the proportion of power generation of renewable energy and actively promote the application of new energy storage technology, so as to efficiently develop and utilize local renewable resources.
Under the high loading scenario, as shown in Figure 3C, the total emergy value of the renewable energy part is 7.74E+19 Sej/year, the total emergy value of the indigenous renewable resources part is 1.04E+19 Sej/year, and the total emergy value of the external input part is 1.33E+20 Sej/year. The total emergy value of IM is more than ten times the total emergy value of IR, indicating that the island system is in an unstable high-load operating state at present, with high dependence on diesel imports from outside, resulting in great environmental pressure. At the same time, local renewable resources are not fully utilized, and resource waste is relatively severe.
The sustainability of the island system can be evaluated by calculating various indicators, such as the renewable energy input percentage, emergy yield ratio, environmental loading ratio, and emergy sustainability index. This study used the method of emergy analysis and scenario simulation to calculate multiple indicators of island development under different scenarios. The results are shown in Table 8 and the comparison and analysis of different scenarios can reflect the operation status of the island system, thereby determining the reasonable development pathways favorable for the construction of island ecological civilization and sustainable development.
As shown in Table 8, in the low loading scenario, the island system is in a truly low-load state with critical EYR, and the indicators of %Renew, ELR and ESI are all in sustainable states. In the moderate loading scenario, the emergy yield ratio of the island system has reached an unsustainable state, and the emergy sustainability index, the percentage of renewable energy inputs and environmental loading ratio are in sustainable states, which indicates that the current island socioeconomic activities are within the system’s capacity and the development and utilization of resources are moderate. In the high loading scenario, the percentage of renewable energy inputs and emergy sustainability index are in critical states, while the emergy yield ratio is in an unsustainable state and only the environmental loading ratio remains sustainable, which indicates that the current island socioeconomic activities have exceeded the system’s carrying capacity.
Comparing the three development scenarios of Lingyang Reef comprehensively, it can be seen that: with the increase of the existing population, the island system gradually transitions from a sustainable state to an unsustainable state, and the indicator of EYR first changes from a critical state to an unsustainable state, indicating that it is still an unstable man-made system that is highly dependent on external material supply at this stage, while the values of ELR and %Renew are still sustainable and critical, indicating that the local renewable energy and resources are relatively abundant, under all the three scenarios, it is not fully utilized.
Since the emergy of renewable energy power generation and diesel fuel accounts for the largest proportion of the total emergy of the island and reef system, and considering that the sea area is rich in solar energy and wind energy resources, it is possible to install solar power generation devices on the roofs of buildings, and build wind farms on reefs in shallow waters, so as to promote the sustainable development of coral reef artificial islands. Only in this way, can we gradually increase the proportion of renewable energy power output, continuously reduce the consumption of fossil energy such as diesel, and build ideal ecological coral reef island as soon as possible.
The ecological fragility of offshore coral reef islands is one of the main factors restricting their sustainable development. Although countries have enacted laws and regulations to protect the ecological environment and develop natural resources of these uninhabited islands, such as formulating development plans, implementing classified development strategies, and conducting environmental impact assessments, the quantitative research on the development potential of uninhabited islands has not been carried out, which cannot ensure the sustainability of their development and utilization. Nam (2010) viewed uninhabited islands as natural closed systems, focusing on the carrying capacity of islands through the self-sufficient way and ignoring the carrying capacity of islands under external supply conditions, resulting in significant differences between theoretical calculations and actual results. This study regards uninhabited islands as semi-natural and semi-closed system, comprehensively considering the most basic needs such as water, electricity, and food consumption, and uses scenario simulation to evaluate the sustainable development capacity of islands under different population sizes, which will provide a reference for the sustainable development and utilization of uninhabited islands.
Due to the lack of detailed data on the development and utilization of uninhabited islands, this study combines scenario simulation with emergy analysis to carry out the sustainability assessment of offshore coral reef islands. The proposed method is theoretically feasible. However, in practical applications, there remain several uncertainties, including: (1) the renewable energy and resources in different scenarios are provided locally by the island system, but this requires the establishment of corresponding infrastructure, such as vegetable planting bases, rainwater collection facilities, wastewater treatment facilities, wind turbines, etc.; (2) the scenarios assume that staple foods such as potatoes, fruits, milk and beans, eggs and meat, and diesel are imported from outside, without considering the anchorage capacity of coral reef island harbors. In addition, external inputs are easily affected by meteorological disasters such as typhoons; (3) this study uses the per capita comprehensive index method and sets the proportion of renewable energy in electricity consumption to simulate and calculate water, electricity, and food consumption under different scenarios, which has a certain subjectivity and may affect the accuracy of the results. In addition, the emergy analysis method also has limitations to some extent. In the calculation of emergy conversion rates, an accurate and detailed analysis of the island system is required. This study refers to the emergy conversion rates in relevant literature as the calculation data, subjectively ignoring the heterogeneity that generally exists among different systems, and the calculated results may not be very accurate. Finally, the sustainable development of the ecosystem on an offshore coral reef island is also restricted by various factors such as policies, culture, and markets, and it is still difficult to carry out quantitative analysis and provide respective explanations for these factors.
In terms of the future development and utilization of offshore coral reef islands, we have also tried to propose policy recommendations from the perspective of sustainable development, including but not limited to:
(1) Improving the related basic supporting facilities and enhancing the output efficiency of local renewable energy and resources. To improve the self-sufficiency capacity of local renewable resources, we should make a plan in advance for the construction of infrastructure such as vegetable planting bases, rainwater collection facilities, ports, etc.
(2) Using clean energy and optimizing the energy structure. To fully tap into the marine energy advantages of offshore coral reef islands, it is suggested that we should pay more attention to developing renewable clean energy, e.g., tidal energy, wind energy, solar energy, and wave energy.
(3) Strengthening efforts to promote awareness of environmental protection and increase per capita utilization efficiency. Freshwater and electricity resources are extremely scarce in natural islands, and it is necessary to focus on energy and water conservation, so as to promote high-quality development of offshore coral reef islands.
In this study, we presented three potential future development scenarios for Lingyang Reef based on different carrying capacities: low-load scenario (2000 people), moderate-load scenario (5000 people), and high-load scenario (10000 people). We also simulated the utilization and consumption of major resources, such as water, electricity, and food, under different scenario conditions. By combining the emergy analysis method with sustainable development evaluation indicators, we further carried out the supply-demand structure analysis and sustainability assessment of Lingyang Reef under each scenario. The main research conclusions are as follows:
Regarding the two parts of renewable energy and non-renewable resources, the total emergy value is 7.74E+19 solar emjoules per year, which accounts for a significant proportion of the entire system of Lingyang Reef. The renewable energy part includes solar energy, wind energy, chemical energy from precipitation, wave energy, and tidal energy. Comparatively, the non-renewable resources only include topsoil erosion on land surface, with a total emergy value of only 1.2E+16 solar emjoules per year, which can be ignored. This indicates that local renewable energy is abundant and suitable for large-scale and high-intensity renewable energy development and utilization activities.
Regarding the comparative analysis of the three different development scenarios (low-load, moderate-load, and high-load), as the actual population carrying capacity of the island increases, the island system gradually transitions from sustainable to unsustainable state. The emergy yield ratio is the first to transition from critical to unsustainable state, indicating that the island system is still highly dependent on external material supply and is an unstable artificial system. However, the environmental loading ratio and renewable energy input percentage still maintain a sustainable or critical state, indicating that local renewable energy and resources are relatively abundant and have not been fully utilized under all the three scenarios.
Regarding the development and utilization of local renewable energy and resources, the emergy values of renewable energy power generation and diesel power generation accounts for the largest proportion of the total emergy values in the island system. It is recommended to gradually increase the proportion of renewable energy output, especially solar and wind power generation, and continuously reduce the consumption of fossil fuels such as diesel, ultimately achieving a power supply-demand balance completely relying on renewable energy and resources to promote local sustainable development.
The original contributions presented in the study are included in the article/supplementary material. Further inquiries can be directed to the corresponding author.
Conceptualization: WW, HJ. Methodology: WW, HJ. Formal analysis and investigation: WW, PZ, HJ. Writing - original draft preparation: WW, PZ, HJ. Writing - review and editing: WW, PZ, HJ, FS. Funding acquisition: FS. Resources: FS, WW. Supervision: FS. All authors contributed to the article and approved the submitted version.
This work was supported by the National Key Research and Development Program of China (Grant No. 2022YFC3103105 and 2022YFC3103100), and the National Natural Science Foundation of China (Grant No. 42006171).
The authors declare that the research was conducted in the absence of any commercial or financial relationships that could be construed as a potential conflict of interest.
All claims expressed in this article are solely those of the authors and do not necessarily represent those of their affiliated organizations, or those of the publisher, the editors and the reviewers. Any product that may be evaluated in this article, or claim that may be made by its manufacturer, is not guaranteed or endorsed by the publisher.
Alkhuzaim L., Zhu Q., Sarkis J. (2021). Evaluating emergy analysis at the nexus of circular economy and sustainable supply chain management. Sustain. Production Consumption 25, 413–424. doi: 10.1016/j.spc.2020.11.022
Bastianoni S., Campbell D., Ridolfi R., Pulselli F. (2009). The solar transformity of petroleum fuels. Ecol. Model. 220 (1), 40–50. doi: 10.1016/j.ecolmodel.2008.09.003
Bolden A., Johnston M. (2020). Eradication of feral pigs on quail island to protect and restore ecological values. Victorian Nat. 137 (6), 219–227.
Brown M. T., McClanahan T. (1996). Emergy analysis perspectives of Thailand and Mekong river dam proposals. Ecol. Model. 91 (1-3), 105–130. doi: 10.1016/0304-3800(95)00183-2
Chen H. S. (2019). Establishment and application of an evaluation model for orchid island sustainable tourism development. Int. J. Environ. Res. Public Health 16 (5), 755. doi: 10.3390/ijerph16050755
Chen Q., Lin Y., Zhang Y., Wang C., Cai A. (2022a). Evaluation framework for determining the developmental suitability and sustainability of uninhabited islands. J. Coast. Res. 38 (4), 816–827. doi: 10.2112/JCOASTRES-D-21-00121.1
Chen S., Liu N., Xiao L., Gong Y., Xiao Y. (2022b). Comprehensive evaluation of environmental dimension reduction of multi-type islands: a sustainable development perspective. Environ. Sci. Pollut. Res. 29, 20947–20962. doi: 10.1007/s11356-021-17240-9
Chi Y., Zhang Z., Xie Z., Wang J. (2020). How human activities influence the island ecosystem through damaging the natural ecosystem and supporting the social ecosystem? J. Cleaner Production 248, 119203. doi: 10.1016/j.jclepro.2019.119203
Deymi-Dashtebayaz M., Norani M. (2021). Sustainability assessment and emergy analysis of employing the CCHP system under two different scenarios in a data center. Renewable Sustain. Energy Rev. 150, 111511. doi: 10.1016/j.rser.2021.111511
Fonseca C. R., Díaz-Delgado C., Esteller M. V., García-Pulido D. (2017). Geoinformatics tool with an emergy accounting approach for evaluating the sustainability of water systems: case study of the lerma river, Mexico. Ecol. Eng. 99, 436–453. doi: 10.1016/j.ecoleng.2016.11.034
Fuldauer L. I., Ives M. C., Adshead D., Thacker S., Hall J. W. (2019). Participatory planning of the future of waste management in small island developing states to deliver on the sustainable development goals. J. cleaner production 223, 147–162. doi: 10.1016/j.jclepro.2019.02.269
Hsu C. Y., Chen M. Y., Yang S. C. (2019). Residents’ attitudes toward support for island sustainable tourism. Sustainability 11 (18), 5051. doi: 10.3390/su11185051
Ji J., Liu H., Yin X. (2023). Evaluation and regional differences analysis of the marine industry development level: the case of China. Mar. Policy 148, 105445. doi: 10.1016/j.marpol.2022.105445
Jiang X. Z., Liu T. Y., Su C. W. (2014). China's marine economy and regional development. Mar. Policy 50, 227–237. doi: 10.1016/j.marpol.2014.06.008
Jung C., Kim C., Kim S., Suh K. (2018). Analysis of environmental carrying capacity with emergy perspective of jeju island. Sustainability 10 (5), 1681. doi: 10.3390/su10051681
Kakazu H. (2022). “Economy, technology and policy for future sustainable development of seascapes” 468. (London: Routledge)
Kurniawan F., Adrianto L., Bengen D. G., Prasetyo L. B. (2019). The social-ecological status of small islands: an evaluation of island tourism destination management in Indonesia. Tourism Manage. Perspect. 31, 136–144. doi: 10.1016/j.tmp.2019.04.004
Li B., Chen W., Liu J., Li J., Wang S., Xing H. (2020). Construction and application of nearshore hydrodynamic monitoring system for uninhabited islands. J. Coast. Res. 99 (SI), 131–136. doi: 10.2112/SI99-019.1
Liu G., Yang Z., Chen B., Ulgiati S. (2011). Monitoring trends of urban development and environmental impact of beijing 1999–2006. Sci. Total Environ. 409 (18), 3295–3308. doi: 10.1016/j.scitotenv.2011.05.045
McElroy J. L., De Albuquerque K. (1998). Tourism penetration index in small Caribbean islands. Ann. tourism Res. 25 (1), 145–168. doi: 10.1016/S0160-7383(97)00068-6
Meaurio A., Murray I. (2001). “Indicators of sustainable development in tourism: the case of the Balearic islands,” in Paper presented at the Conference on Sustainable Development and Management of Ecotourism in Small Island Developing States (SIDS) and Other Small Islands, Seychelles.
Nam J., Chang W., Kang D. (2010). Carrying capacity of an uninhabited island off the southwestern coast of Korea. Ecol. Model. 221 (17), 2102–2107. doi: 10.1016/j.ecolmodel.2010.05.016
Odum H. T. (1996). Environmental accounting: emergy and environmental decision making (New York: John Wiley and Sons).
Odum H. T., Brown M., Brandt-Williams S. (2000). Handbook of emergy evaluation. center for environmental policy (FL, USA: Center for Environmental Policy, University of Florida).
Odum H. T., Diamond C., Brown M. (1987). Emergy analysis and public policy in texas, policy research project report. Ecol. Economics 12 (5), 54–65.
Quyet L., Duy P., Toan V. (2022). “Sustainable development of tourism economy in phu quoc island, kien giang province, Vietnam: current situation and prospects,” in Paper presented at the IOP Conference Series: Earth and Environmental Science.
Skene K. R. (2021). No goal is an island: the implications of systems theory for the sustainable development goals. Environment Dev. Sustainability 23 (7), 9993–10012. doi: 10.1007/s10668-020-01043-y
Steibl S., Laforsch C. (2019). Disentangling the environmental impact of different human disturbances: a case study on islands. Sci. Rep. 9 (1), 13712. doi: 10.1038/s41598-019-49555-6
Su Y., He S., Wang K., Shahtahmassebi A. R., Zhang L., Zhang J., et al. (2020). Quantifying the sustainability of three types of agricultural production in China: an emergy analysis with the integration of environmental pollution. J. Cleaner Production 252, 119650. doi: 10.1016/j.jclepro.2019.119650
Sun J., Miao J., Mu H., Xu J., Zhai N. (2022). Sustainable development in marine economy: assessing carrying capacity of Shandong province in China. Ocean Coast. Manage. 216, 105981. doi: 10.1016/j.ocecoaman.2021.105981
Ulgiati S., Odum H. T., Bastianoni S. (1994). Emergy use, environmental loading and sustainability an emergy analysis of Italy. Ecol. Model. 73 (3-4), 215–268. doi: 10.1016/0304-3800(94)90064-7
Walker T. B., Lee T. J., Li X. (2021). Sustainable development for small island tourism: developing slow tourism in the Caribbean. J. Travel Tourism Marketing 38 (1), 1–15. doi: 10.1080/10548408.2020.1842289
Wei Y., Li Y., Liu X., Wu M. (2020). Sustainable development and green gross domestic product assessments in megacities based on the emergy analysis method–a case study of wuhan. Sustain. Dev. 28 (1), 294–307. doi: 10.1002/sd.2003
Wolf F., Filho W. L., Singh P., Scherle N., Reiser D., Telesford J., et al. (2021). Influences of climate change on tourism development in small pacific island states. Sustainability 13 (8), 4223. doi: 10.3390/su13084223
Wu J., Li B. (2022). Spatio-temporal evolutionary characteristics and type classification of marine economy resilience in China. Ocean Coast. Manage. 217, 106016. doi: 10.1016/j.ocecoaman.2021.106016
Yan M., Odum H. T. (1998). A study on emergy evaluation and sustainable development of Tibetan eco-economic system. J. Natural Resour. 13 (2), 116–125. doi: 10.11849/zrzyxb.1998.02.004
Yang L., Wang C., Yu H., Yang M., Wang S. (2020). Can an island economy be more sustainable? A comparative study of Indonesia, Malaysia, and the Philippines. J. Cleaner Production 242, 118752. doi: 10.1016/j.jclepro.2019.118572
Zhang K., Zhu J., Li Z., Wang H., Zhang W., Xu C. (2020). “Analysis and research on island development model and experience,” in Paper presented at the 2020 International Conference on Social Science, Economics and Education Research (SSEER 2020).
Zhan J., Zhang F., Chu X., Liu W., Zhang Y. (2019). Ecosystem services assessment based on emergy accounting in Chongming Island, Eastern China. Ecol. Indic. 105, 464–473. doi: 10.1016/j.ecolind.2018.04.015
Zhao D. Y., Ma Y. Y., Lin H. L. (2022). Using the entropy and TOPSIS models to evaluate sustainable development of islands: a case in China. Sustainability 14 (6), 3707. doi: 10.3390/su14063707
Zheng C. W. (2011). Wave energy and other renewable energy resources in south China Sea: advantages and disadvantages. J. Subtropical Resour. Environ. 6 (3), 76–81. doi: 10.19687/j.cnki.1673-7105.2011.03.011
Keywords: sustainability assessment, emergy analysis, scenario simulation, offshore coral reef island, carrying capacity
Citation: Wu W, Zhang P, Jiang H and Su F (2023) Sustainability assessment for an offshore coral reef island based on scenario simulation and emergy analysis. Front. Mar. Sci. 10:1180876. doi: 10.3389/fmars.2023.1180876
Received: 06 March 2023; Accepted: 09 May 2023;
Published: 23 May 2023.
Edited by:
Henning Sten Hansen, Aalborg University Copenhagen, DenmarkReviewed by:
Wenjia Hu, State Oceanic Administration, ChinaCopyright © 2023 Wu, Zhang, Jiang and Su. This is an open-access article distributed under the terms of the Creative Commons Attribution License (CC BY). The use, distribution or reproduction in other forums is permitted, provided the original author(s) and the copyright owner(s) are credited and that the original publication in this journal is cited, in accordance with accepted academic practice. No use, distribution or reproduction is permitted which does not comply with these terms.
*Correspondence: Huiping Jiang, amlhbmdocEBpZ3NucnIuYWMuY24=
Disclaimer: All claims expressed in this article are solely those of the authors and do not necessarily represent those of their affiliated organizations, or those of the publisher, the editors and the reviewers. Any product that may be evaluated in this article or claim that may be made by its manufacturer is not guaranteed or endorsed by the publisher.
Research integrity at Frontiers
Learn more about the work of our research integrity team to safeguard the quality of each article we publish.