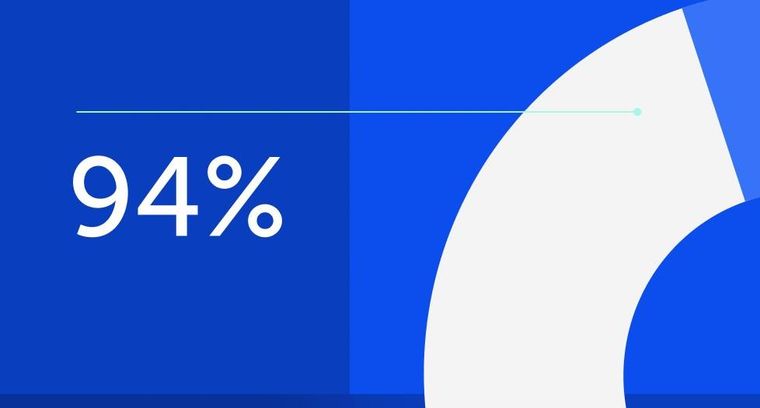
94% of researchers rate our articles as excellent or good
Learn more about the work of our research integrity team to safeguard the quality of each article we publish.
Find out more
ORIGINAL RESEARCH article
Front. Mar. Sci., 23 June 2023
Sec. Marine Megafauna
Volume 10 - 2023 | https://doi.org/10.3389/fmars.2023.1179236
Evaluating physiological responses in the context of a species’ life history, demographics, and ecology is essential to understanding the health of individuals and populations. Here, we measured the main mammalian glucocorticoid, cortisol, in an elusive Antarctic apex predator, the leopard seal (Hydrurga leptonyx). We also examined intraspecific variation in cortisol based on life history (sex), morphometrics (body mass, body condition), and ecological traits (δ15N, δ13C). To do this, blood samples, life history traits, and morphometric data were collected from 19 individual leopard seals off the Western Antarctic Peninsula. We found that adult leopard seals have remarkably high cortisol concentrations (100.35 ± 16.72 μg/dL), showing the highest circulating cortisol concentration ever reported for a pinniped: 147 μg/dL in an adult male. Leopard seal cortisol concentrations varied with sex, body mass, and diet. Large adult females had significantly lower cortisol (94.49 ± 10.12 μg/dL) than adult males (120.85 ± 6.20 μg/dL). Similarly, leopard seals with higher isotope values (i.e., adult females, δ15N: 11.35 ± 0.69‰) had lower cortisol concentrations than seals with lower isotope values (i.e., adult males, δ15N: 10.14 ± 1.65‰). Furthermore, we compared cortisol concentrations across 26 closely related Arctoid taxa (i.e., mustelids, bears, and pinnipeds) with comparable data. Leopard seals had the highest mean cortisol concentrations that were 1.25 to 50 times higher than other Arctoids. More broadly, Antarctic ice seals (Lobodontini: leopard seal, Ross seal, Weddell seal, crabeater seal) had higher cortisol concentrations compared to other pinnipeds and Arctoid species. Therefore, high cortisol is a characteristic of all lobodontines and may be a specialized adaptation within this Antarctic-dwelling clade. Together, our results highlight exceptionally high cortisol concentrations in leopard seals (and across lobodontines) and reveal high variability in cortisol concentrations among individuals from a single location. This information provides the context for understanding how leopard seal physiology changes with life history, ecology, and morphology and sets the foundation for assessing their physiology in the context of a rapidly changing Antarctic environment.
Cortisol, the main mammalian glucocorticoid, is an energy mobilization hormone that regulates a range of biological processes, including immune function, metabolism, and the stress response (Sapolsky, 1999; Palme et al., 2005; Reeder and Kramer, 2005; Wingfield and Romero, 2011; Haase et al., 2016). Cortisol levels can increase in response to a variety of intrinsic and extrinsic stressors (Kitaysky et al., 1999; Levine, 2002; Anestis et al., 2006; Wingfield, 2013a; Wingfield, 2013b; Aurich et al., 2015; Lafferty et al., 2015). Hence, it is commonly referred to as the “stress hormone” and has traditionally been measured in mammals as a means to evaluate stress levels (Creel, 2001; Abbott et al., 2003; Sheriff et al., 2011; Bryan et al., 2013; Kershaw and Hall, 2016; Beaulieu-McCoy et al., 2017). However, recent work has highlighted the diverse and complex functions that cortisol plays in mammals, many of which are not associated with stress (MacDougall-Shackleton et al., 2019; Romero and Beattie, 2022). As a result of its role in energy-mobilization, cortisol is an essential mammalian hormone that regulates key physiological processes; consequently, measuring and comparing cortisol concentrations within and between species provides important context for understanding the physiological responses of mammals to different abiotic and biotic stimuli.
Many factors affect baseline cortisol values, including life history stages (Kitaysky et al., 1999; Levine, 2002; Anestis et al., 2006; Aurich et al., 2015; Lafferty et al., 2015; Azevedo et al., 2019), interspecific interactions (Bartsh et al., 1992; Ashley et al., 2011; Creel et al., 2013; Levy et al., 2020), and environmental variables (Wingfield, 2013a; Wingfield, 2013b). For example, sex-specific life history events (i.e., reproductive status, lactation, competition for breeding) can lead to sex-based differences in cortisol, with animals showing sex-based changes in cortisol seasonally (Levine, 2002; Lafferty et al., 2015). Further, intraspecific differences in body size can lead to variation in cortisol due its influence on behavior interactions or dominance status (Creel, 2001; Abbott et al., 2003; Creel et al., 2013). Generally, larger and more dominant animals display lower cortisol concentrations compared to their smaller and subordinate counterparts (Creel, 2001; Abbott et al., 2003; Levy et al., 2020). Similarly, animals in poor body condition often have higher cortisol than animals in healthy body condition (Macbeth et al., 2012; Shero et al., 2015; Lemos et al., 2022), and this is often linked to dietary differences. In many animals, diet is measured with stable isotope analysis, where isotope ratios of nitrogen (δ15N) and carbon (δ13C) measured in animal tissues are used to indicate the trophic level and food sources (Gannes et al., 1998; Tykot, 2004). Animals with higher δ15N and δ13C values, indicating a more nutrient-enriched diet, can have lower cortisol concentrations than those with lower isotope values (Lafferty et al., 2015; Karpovich et al., 2019). Understanding how cortisol changes in response to life history, ecological, and physiological factors is important for interpreting the significance of high (or low) cortisol concentrations within and between taxa.
Cortisol is commonly measured in pinnipeds (seals, sea lions, and walrus) to evaluate the relationship between their physiology and other biological metrics (Constable et al., 2006; du Dot et al., 2009; Trumble et al., 2013; Keogh and Atkinson, 2015; Shero et al., 2015; Kershaw and Hall, 2016; Peck et al., 2016; Beaulieu-McCoy et al., 2017; Karpovich et al., 2019; Thompson and Romano, 2019; Keogh et al., 2020; Sperou, 2020; Ogloff et al., 2022). Among pinnipeds, Southern Ocean pinnipeds (six species: Ross seals, Ommatophoca rossii; Weddell seals, Leptonychotes weddellii; crabeater seals, Lobodon carcinophaga; leopard seals, Hydrurga leptonyx; Antarctic fur seals, Arctocephalus gazella; and southern elephant seals, Mirounga leonina) have some of the highest cortisol concentrations ever recorded (Liggins et al., 1979; Barrell and Montgomery, 1989; Bartsh et al., 1992; Liggins et al., 1993; Harcourt, 2001; Tryland et al., 2012). There is some evidence that cortisol concentrations in Southern Ocean pinnipeds vary as a function of reproductive status (Liggins et al., 1979; Bartsh et al., 1992; Shero et al., 2015), territoriality (Bartsh et al., 1992), age class (Liggins et al., 1979; Barrell and Montgomery, 1989; Bartsh et al., 1992; Tryland et al., 2012), size (Bartsh et al., 1992), and between species (Liggins et al., 1993; Tryland et al., 2012). However, many of these previous studies were conducted over 25 years ago, had small sizes, and provided little (or no) information about life history or body condition parameters; this limits our ability to compare and assess causes of intraspecific variation in cortisol in these pinnipeds.
Here, our study focuses on an Antarctic apex predator, the leopard seal. Only one study to date measured cortisol concentrations in leopard seals (Liggins et al., 1993). Like other Antarctic pinnipeds, Liggins et al. (1993) found that leopard seals had high cortisol concentrations only slightly lower than the closely related Weddell seal. However, interpretation of their results is limited by the small sample size (n=4) and the lack of data on life history covariates. Numerous factors likely influence intraspecific differences in leopard seal cortisol concentrations. For example, leopard seals show strong female-biased sexual dimorphism, where adult females are up to 50% larger than adult males (Laws, 1977; Bonner, 1994; Kienle et al., 2022). Leopard seals also feed across trophic levels with a diet ranging from large endothermic mesopredators (e.g., penguins, Antarctic fur seals) to small ectothermic prey (e.g., Antarctic fish, krill; (Øritsland, 1977; Boveng et al., 1998; Hall-Aspland and Rogers, 2004; Botta et al., 2018; Krause et al., 2020). Furthermore, leopard seal diet appears to be related to body size. Larger females often acquire larger, more energy-dense endothermic prey and have higher δ15N values than individuals of both sexes (Krause et al., 2020). However, it is currently unknown how differences in life history, body size, and diet affect leopard seal cortisol concentrations.
Therefore, the primary goals of this study were to measure cortisol in leopard seals from a single population and examine intraspecific variation in cortisol concentrations as a function of sex, body size (mass), body condition (scaling body mass index), and isotopic values associated with diet (δ15N and δ13C). We hypothesized that large bodied leopard seals, specifically females, would exhibit lower cortisol values than smaller leopard seals (e.g., adult males and smaller females). Additionally, we hypothesized that individuals feeding at higher trophic levels (higher δ15N) would exhibit lower cortisol concentrations and be in better body condition than those feeding at lower trophic levels. Finally, we compared cortisol concentrations measured in leopard seals with comparable data for related terrestrial and marine carnivorous mammals (i.e., Arctoids) to contextualize our findings.
Field work was conducted at the U.S. Antarctic Marine Living Resources (AMLR) Program research station on Cape Shirreff, Livingston Island, Antarctic Peninsula (62.47°S, 60.77°W). Nineteen individual leopard seals were chemically immobilized during two field seasons (January and April-May 2018; April-May 2019; Table 1). One female seal (#397) was captured and sampled in both 2018 and 2019, for a total of 20 samples. Immobilization and sample collection followed established protocols and are detailed in Charapata et al. (2023) and Kienle et al. (2022). In brief, leopard seals were sedated with a midazolam-butorphanol protocol administered through a jab stick as established in Pussini and Goebel, 2015; there was no manual restraint prior to capture. While sedated, morphometric data (e.g., lengths, mass), life history data (e.g., sex, age class), and blood samples were collected. Body mass (kg) was measured using a sling, digital scale (DynaLink Measurement Systems International, Rice Lake, WI), hand winch, and tripod. Standard length was recorded (cm; tip of nose to tip of tail along the dorsal side of the seal). Sex was visually assessed based on genitalia. Age was estimated based on the relationship between standard length and age (Laws, 1957); seals <260 cm in length were classified as juveniles (n=1) and seals >260 cm were classified as adults (n=19; including repeat sample). After blood samples were collected, they were immediately stored on ice until they were centrifuged in the lab within 3 hours of collection. Vacutainers were centrifuged at ~1,200 rpms for ~15 mins to separate serum, plasma, and red blood cells and stored at –80°C until analysis. Research was approved by the Institutional Animal Care and Use Committee (IACUC) at the University of California, Santa Cruz and conducted under federal authorizations for marine mammal research under National Marine Fisheries Service permit #19439 and Antarctic Conservation Act permit #2018-016.
We measured cortisol concentrations in individual leopard seals from the blood serum samples (n=20, including repeat individual and juvenile). Cortisol concentrations were assessed in duplicate using a commercially available radioimmunoassay (RIA, MP Biomedical). Samples were ran neat, and no lipid extraction was performed. Due to the high concentration of cortisol in the leopard seal samples, all serum samples were diluted 0.6x with zero calibrator solution to fit on the standard curve, and the dilution factor was applied to the raw concentrations. A pooled sample was serially diluted five times (1:2, 1:4, 1:8, 1:16, 1:32) and compared to the standard curve. Serially diluted samples yielded a curve that was parallel to the standard curves. To assess accuracy, samples were spiked with kit standards, and the percent recovery of added hormone was calculated (99.2 ± 11%). Mean intra-assay coefficient of variation was calculated for all samples (2.1 ± 1.5%). All samples were analyzed in one run.
Plasma samples were used for bulk carbon (δ13C) and nitrogen (δ15N) stable isotope analysis. The half-life of isotopes is typically short-lived in plasma, reflecting ~1 weeks of information prior to data collection as shown in bears and birds (Hobson and Clark, 1993; Hilderbrand et al., 1996). The relative abundance of δ13C and δ15N can provide information on individuals’ diet, where δ15N reflects trophic position (Hobson and Welch, 1992; Rau et al., 1992; Lesage et al., 2001) and δ13C is used for carbon resources exploited by consumers (Ramsay and Hobson, 1991).
Aliquots of plasma samples (500 μl) were separated into individual cryovials, freeze dried for ~24 hours, and homogenized with a metal spatula. Dried homogenized samples were packaged (~0.7 mg) into 5 x 9 mm tin capsules for stable isotope analysis. Lipids can affect δ13C values in animal tissues (DeNiro and Epstein, 1977). The typical atomic C/N ratios of lipid-extracted marine mammal prey ranges between 3 and 4 (Newsome et al., 2010). A previous study found carbon and nitrogen ratios from non-extracted leopard seal plasma were below 3.5 and thus were considered reliable indicator of the animal’s diet (Krause et al., 2020). Therefore, to directly compare our δ13C and δ15N values between these studies, we also did not perform a lipid extraction or correction (Folch et al., 1957; Krause et al., 2020).
Carbon and nitrogen stable isotope analysis was performed at Baylor University using Elemental Analyzer (EA) Costech 4010 Elemental Combustion System (ECS) paired with a Conflow IV interphase (Thermo Scientific) and Thermo Delta V Advantage continuous flow Isotope Ratio Mass Spectrometer (EA-IRMS). Plasma nitrogen (δ15N) and carbon (δ13C) isotope values are expressed in delta notation (δ) in units of per mil (‰). Additionally, isotope values are reported as the ratio of the heavy to light isotope relative to international standards; atmospheric nitrogen and Vienna Peedee Belemnite (VPDB), respectively, using the following equation:
where X is the 13C or 15N and R is the corresponding ratio of 13C/12C or 15N/14N. The Rstandard value is set by PeeDee Belemnite for δ13C and atmospheric N2 for δ15N.
We assessed both body size and body condition to ensure our analysis accounted for the possibility that individuals may be in healthy body condition even though they are small in size and vice versa (Traylor and Alisauskas, 2006; Macbeth et al., 2012). Body mass (kg) was used as a proxy for body size. Body condition was visually assessed in the field and from high-resolution photographs showing leopard seals with a full-body profile taken from a perpendicular angle. We followed a qualitative protocol evaluating bony protrusions for body condition assessment as described in Hupman et al., 2020. Individuals were classified as in severe, poor, good, or excellent body condition based on this evaluation method (Hupman et al., 2020). A body condition index was also calculated for each individual using the following scaled body mass (SBM) index equation (Peig and Green, 2009):
where Mi and Li are the mass and standard length of the individual, Lo is the mean standard length of the different age classes, bsma is the scaling exponent from plotting natural log transformed mass by standard length for all seals, and the
the individual when standardized to Lo. We calculated a SBM index for all individuals except for the one juvenile seal since there was only a single representative in this age class. This SBM index allows for quantitative comparison of body condition between individuals while accounting for differences in age class, length, and mass (Peig and Green, 2009; DeRango et al., 2019b; Charapata et al., 2023).
We conducted a metadata analysis of the peer-reviewed literature to compare cortisol concentrations among extant species of the mammalian clade Arctoidea for which comparable data were available. The Arctoidea clade includes three extant families of carnivorans: (1) Mustelidae (e.g., sea otters, ferrets, badgers), (2) Ursidae (e.g., bears), and (3) Pinnipedia. To ensure that we could directly compare absolute hormone concentrations across different studies, we implemented guidelines to obtain average cortisol values from studies that measured cortisol in the same tissue (blood) and similar assay type. Previous studies have demonstrated that cortisol concentrations measured from both serum and plasma samples are directly comparable but other tissue samples (e.g., whiskers, hair, blubber) are not (Foster and Dunn, 1974; Sheriff et al., 2011). Therefore, our literature search only included studies for species where cortisol concentrations were measured in serum and/or plasma. Cortisol concentrations can also vary based on the analytical method (e.g., liquid chromatography–mass spectrometry (LC/MS) vs. RIA; (Faupel-Badger et al., 2010); as a result, we narrowed our search to only include studies where cortisol concentrations in plasma and serum were measured with an immunoassay (e.g., radioimmunoassay, enzyme-linked immunosorbent assay, chemiluminescence immunoassay; Webster et al., 1990; Shimada et al., 1995; Graham et al., 2015; Franco-Martinez et al., 2019).
We performed a comprehensive literature review using Google Scholar and Web of Science, specifically using the words ‘serum’, ‘plasma’, and ‘cortisol’ in our search terms. Individuals of both sexes and any age class were included in the comparison. From each relevant paper, we extracted the cortisol mean and median values and ranges, if provided. If multiple cortisol means and median values were reported within a study, such as between sexes, locations, age classes, and seasons, we included all the values in our comparison, and then averaged all values from the literature to get one value for each species. Since some papers only included a mean value, we provide a range based on the lowest and highest mean values reported across all papers for each species (henceforth: “mean range”). All cortisol concentrations were converted to μg/dL for comparison. Studies that were conducted on individuals living in human care facilities (e.g., zoos, aquariums) were not included in this review due to significant hormonal differences in captive and wild individuals (Gardiner and Hall, 1997; Creel, 2001). Some studies report serial cortisol concentrations as part of an experimental design (e.g., ACTH administrations); in these cases, we only used values that were collected as baseline measurements prior to the start of the experiment. Studies where animals were chemically immobilized were included in this review; previous work on other carnivorans has shown that cortisol values are not influenced by immobilization (Champagne et al., 2012). For Ross seals, the only study with serum cortisol concentrations stated values were “above the threshold of cortisol analysis” (>1380nmol/L; Tryland et al., 2012), thus the threshold value (1380nmol/L = 50 μg/dL) was used in this analysis. Mean values from each publication were combined to calculate one average value for each species.
We conducted all data analyses in R version 1.4.1103 (R Core Team, 2022). Since there was only a single juvenile, age class was not assessed among this data and the juvenile was excluded from all analyses. Additionally, we only included one value chosen randomly from the one repeat individual (#397; season 2018). To reduce model complexity and account for our small sample size, we first ran univariate models to assess the associations between each predictor variable (sex, mass, δ15N, and δ13C, season, and SBM index) and the response variable (cortisol). Pearson’s correlation was used to measure the strength of the relationships and then we plotted and visualized the relationships (ggplot2, tidyverse; Wickham, 2016; Wickham et al., 2019). Variables that did not show significant associations were excluded from the multivariate model, specifically season and SBM index. We used variance inflation factors (VIF) to assess for multicollinearity among the predictor variables in our regression model. VIFs were calculated for each predictor variable, with values > 5 indicating multicollinearity (Hair et al., 2011). Given the imbalance between sexes and the multicollinearity observed between sex and mass (VIF > 5), sex was not included in the multivariate model. After removing sex as a predictor variable, no multicollinearity existed between the remaining variables (VIF< 5). We used analysis of variance (ANOVAs) to assess the relationship between sex and cortisol and evaluated sex-specific differences in SBM index, mass, δ15N, and δ13C.
We then ran linear models to determine relationships between cortisol and all other variables (i.e., δ15N, δ13C, mass). A previous leopard seal study identified a relationship between mass and δ15N (Krause et al., 2020), thus we tested for a relationship and a significant interaction between mass and δ15N with a linear regression and visualized the interaction plot (Figure S1). The full model included mass, δ13C, δ15N, and the biologically relevant interaction between mass and δ15N. We ran all relevant combinations of the full model to compare with the full model (Table S1). Each candidate model was compared to the null model using likelihood ratio tests of null and residual deviances. Model residuals were visually assessed for approximate normality and homoscedasticity (Winter, 2013). Models were ranked based on Akaike information criterion corrected for small sample sizes (AICc) using the aictab function in the AICcmodavg package (Burnham, 2011; Mazerolle, 2020). The model with the lowest AICc represented the model with the highest support. We then examined the contribution of each fixed effect of our top model by looking at the estimated coefficients and p-value. All reported values are derived from the linear models. For our metadata analysis, we assessed if cortisol varied between groups of Arctoid (i.e., mustelids, ursids, pinnipeds) and pinnipeds (lobodontines and other pinnipeds) using an ANOVA followed by a Tukey’s test to adjust the p-value for multiple comparisons.
We measured cortisol concentrations, body mass, body condition (qualitative assessment and SBM index), and δ15N and δ13C in 19 individual leopard seals (Tables 1, 2). The mean cortisol concentration for adult leopard seals was 100.35 ± 16.72 μg/dL, with a range from 74.93 to 147.40 μg/dL.
Table 2 Leopard seal cortisol concentrations measured in blood serum and δ15N and δ13C values measured in blood plasma samples for the 20 leopard seals samples in this study.
We found sex-specific differences in cortisol, body mass, δ15N, and δ13C. Specifically, adult female leopard seals were significantly larger (445 ± 61 kg) than adult males (302 ± 21 kg; F1,16 = 20.03, p< 0.001). Adult males, the smaller sex, had significantly higher cortisol concentrations (120.86 ± 20.52 μg/dL) than adult females (94.49 ± 10.12 μg/dL; F1,16 = 13.32, p = 0.002; Figure 1A). Mean δ15N was 11.08 ± 1.05‰ and ranged from 8.56 to 12.62‰ and differed between sexes (F1,16 = 5.04, p = 0.03; Figure 1B). Females had higher δ15N values (11.35 ± 0.69‰) than males (10.14 ± 1.65‰; F1,16 = 5.04, p = 0.03). Additionally, there was a significant relationship between mass and δ15N; larger seals had higher δ15N than smaller seals (F1,16 = 6.90, p = 0.018; Figure 2A). Mean plasma δ13C was -24.13 ± 0.55‰ and ranged from -23.12‰ to -25.18‰ and varied by sex (F1,16 = 4.68, p = 0.04), where females had slightly higher δ13C (-24.00 ± 0.43‰) than males (-24.94 ± 0.73‰). We did not find sex-specific differences in body condition. Rather, all leopard seals were in ‘excellent’ body condition based on the qualitative assessment of bony protrusions (Hupman et al., 2020), and we found no differences based on the SBM index (p< 0.05).
Figure 1 Sex-specific differences in cortisol and δ15N values. (A) Violin plot comparing differences in mean cortisol concentrations between males and females (F1,16 = 13.31, p = 0.002). (B) Violin plot comparing in δ15N between males and females (F1,16 = 5.04, p = 0.03). In both plots, horizontal bars represent the mean concentrations for each sex and the ends represent the range. Females are shown in purple, and males in light blue.
Figure 2 Relationships between cortisol, body mass, and δ15N in leopard seals. (A) Linear regression between δ15N and body mass for adult leopard seals (y = 8.17 + 0.007x, R2 = 0.30, p = 0.01). (B) Linear regression of the relationship between cortisol and body mass for adult leopard seals (y = 152.49 – 0.12x, R2 = 0.39, p = 0.006). (C) Bubble plot showing the linear regression between cortisol and δ15N, with bubble size representing the mass of each seal and their sex (y = 201.7 – 9.14x, R2 =, 0.33, p = 0.01). For all graphs, females are shown in purple, and males are in light blue.
Intraspecific variability in cortisol concentrations was explained by body mass, δ15N, and the interaction of body mass and δ15N (F3,14 = 19.91, p< 0.001; Table S2) and explained the most variance in cortisol levels (R2 = 0.81, Adjusted R2 = 0.77). Cortisol varied by mass (t = -5.28, p< 0.001); smaller individuals exhibiting higher cortisol concentrations (Figure 2B). Similarly, cortisol varied by δ15N (t = -5.46, p< 0.001); seals with higher δ15N values had lower cortisol concentrations than seals with lower δ15N values. Finally, cortisol varied based on the interaction between mass and δ15N (t = 5.04, p< 0.001), indicating that body size and diet (δ15N) collectively influence cortisol levels (Figure 2C). Neither body condition (SBM index), season, nor plasma δ13C contributed to variability in cortisol concentrations.
We compared cortisol concentrations among 26 Arctoid species (pinnipeds, n=20; ursids, n=3; mustelids, n=3) from 41 peer-reviewed studies (Table 3; Figure 3). For pinnipeds, the mean cortisol concentration among the 20 species was 25.79 ± 30.49 μg/dL (mean range: 0.01 – 120.85 μg/dL). For ursids, the mean cortisol concentration among the three species was 8.94 ± 6.23 μg/dL (mean range: 1.55 – 19 μg/dL). For mustelids, the mean cortisol concentration among the three species was 4.72 ± 2.08 μg/dL (mean range: 2.17 – 9.34 μg/dL). Mean cortisol concentrations significantly differed among the three Arctoid groups (F2,113 = 5.0, p = 0.008, Figure 4A). Specifically, pinnipeds had significantly higher cortisol concentrations compared to mustelids (p = 0.02). However, pinniped cortisol concentrations did not differ from ursids (p = 0.09), nor between ursids and mustelids (p = 0.91). Across taxa, leopard seals had the highest cortisol concentration (mean: 93.01 ± 17.03 μg/dL; mean range: 61.95 – 120.86 μg/dL; this study, Liggins et al, 1993). More broadly, Antarctic seals, specifically the Lobodontini [Antarctic seals: crabeater, leopard, Ross, and Weddell seals] had the highest mean cortisol concentrations (76.94 ± 21.59 μg/dL, mean range: 38.82 – 120.86 μg/dL) compared to the other 16 pinniped taxa (mean: 11.17 ± 9.79 μg/dL; F1,88 = 17.95, p< 0.001; Figure 4B).
Table 3 Cortisol concentrations in 26 Arctoid species (e.g., pinnipeds, ursids, mustelids) from 41 peer-reviewed studies.
Figure 3 Comparison of mean circulating cortisol concentrations in pinnipeds (blue), ursids (green), and mustelids (yellow) calculated from peer-reviewed studies and this study. Bars represent the combined mean cortisol concentration for each species. Error bars represent the standard error of the mean. References for each species can be found in Table 3.
Figure 4 Circulating cortisol concentrations vary between pinnipeds (seals, sea lions, and walruses), ursids (bears), and mustelids (i.e., skunks, minks, ferrets). (Large plot) Violin plot comparing mean cortisol concentrations between the groups of Arctoidea (F2,113 = 5, p = 0.008). Horizontal bars represent the mean cortisol concentration for each group, and the ends of each violin plot represent the range. Pinniped (blue) cortisol concentrations differed from mustelids (yellow; p = 0.02) but not ursids (green; p>0.05), and ursids and mustelids did not significantly differ from each other. (Sub plot) Violin plot comparing mean cortisol concentrations between lobodontine, or Antarctic seals (light blue), to all other pinnipeds (dark blue; F1,88 = 17.95, p< 0.001). Horizontal bars represent the mean cortisol concentration for each group, and the ends of the violin plot represent the range.
Leopard seals have high cortisol concentrations compared to other related pinniped and Arctoid taxa. This finding aligns with the one previous study that measured cortisol concentrations in four leopard seals (Liggins et al., 1993). Our study expands our understanding of leopard seal physiology by measuring and comparing intraspecific variation in cortisol concentrations in one of the largest samples of leopard seals to date. As we predicted, leopard seal cortisol concentrations vary based on sex, morphometrics, and diet. Together, this study provides the foundation for evaluating the physiology of leopard seals in the context of a rapidly changing Antarctic environment.
Our first hypothesis was that larger leopard seals, specifically females, would have lower cortisol concentrations than smaller seals (e.g., adult males and smaller females), and our results support this hypothesis. We find that larger bodied leopard seals have lower cortisol values compared to smaller seals. In fact, one of the smallest adult seals (male, 285 kg) had cortisol concentrations 58% higher than one of the largest seals (female, 500 kg). These size-based differences are inherently linked to sex. Leopard seals exhibit female-biased sexual dimorphism, where adult female leopard seals are up to 1.5 times larger than males (Kienle et al., 2022; this study). As a result, adult female leopard seals, the larger sex, are the ones that have lower cortisol compared to adult males, the smaller sex.
Additionally, we hypothesized that leopard seals feeding at higher trophic levels (higher δ15N values) would have lower cortisol concentrations and be in better body condition than seals feeding at lower trophic levels. Our findings were consistent with our hypothesis to some extent; leopard seals that have higher δ15N isotopic values have lower cortisol values and are primarily large-bodied adult females. However, while there was a size-based difference in δ15N values, there was no difference in body condition between any of the seals. While we had hypothesized that seals in better body condition would have higher δ15N values, all of our seals were in excellent body condition and showed little variation. This finding may be location-specific, as leopard seals in other locations appear to show more variation in body condition (Hupman et al., 2020); in these areas, there may be a relationship between δ15N and body condition that was not seen in this study. Furthermore, although we did not see a complete shift in trophic position, we did observe difference in isotopic values. Together these results showcase the importance of the interplay of sex, body size, and diet (δ15N isotopic values) on cortisol regulation in leopard seals. These findings also align with our current knowledge on leopard seals’ foraging ecology and behavior.
Large-bodied female leopard seals have some of the highest δ15N values and lowest cortisol concentrations. Similarly, a recent study also reported higher δ15N values in adult female leopard seals at Cape Shirreff (individuals not included in this study) than adult males (Krause et al., 2020). The authors suggested that females’ higher nitrogen values reflected an increased consumption of prey with higher δ15N (or nitrogen-enriched prey), such as Antarctic fur seals and penguins, compared to males (Krause et al., 2020). At Cape Shirreff, female leopard seals routinely feed on penguins and Antarctic fur seal pups from December to March (Krause et al., 2015; Krause et al, 2020; Krause et al, 2022). Long-term studies at Cape Shirreff have documented that large adult female leopard seals haul-out more frequently and in higher concentrations near Antarctic fur seal and penguin breeding colonies than anywhere else in the region (Krause et al., 2015; Krause et al., 2016; Kienle et al., 2022). Further, large female leopard seals exclude males and less dominate females from these nutrient rich resources (Krause et al., 2015; Krause and Rogers, 2019). These large female leopard seals are so successful at predating on pups that they are driving the decline of Antarctic fur seals at Cape Shirreff (Hiruki et al., 1999; Krause et al., 2015; Krause et al, 2020). Similarly, behavioral observations during feeding events have shown that female leopard seals occasionally engage in kleptoparasitism and steal prey from conspecifics (Krause et al., 2015). Video footage confirms that large adult females are the ones that are successful when defending or stealing prey (Krause et al., 2015). Success during these behavioral interactions seems to be driven by the combination of both sex and body size, suggesting that females’ large body size confers a competitive advantage in these interspecific foraging interactions, especially when feeding on endothermic prey.
Behavioral interactions—such as aggressive and competitive interactions among conspecifics—can have severe physical and physiological consequences (Bronson and Eleftheriou, 1964; Manogue et al., 1975; Creel et al., 1997; Creel, 2001; Creel, 2005). During competitive interactions over resources, the ‘losers’ often have higher cortisol concentrations (Bronson and Eleftheriou, 1964; Manogue et al., 1975; Creel, 2001; Abbott et al., 2003), while the ‘winners’ typically have lower cortisol concentrations and are often the larger individuals (Clutton-Brock et al., 1988; Rutberg and Greenberg, 1990; Archie et al., 2006; Stockley and Bro-Jørgensen, 2011). Large adult females seem to outcompete smaller individuals of both sexes (e.g., adult males, smaller females, and juveniles), resulting in access to high quality, nitrogen-rich prey resources which is reflected in their high δ15N values [(Krause et al., 2020); this study]. Physiologically, this may lead to larger leopard seals, specifically females, having lower cortisol values than smaller conspecifics.
Similarly, animals that consume less food, lower-quality food, and/or have a less nutritious diet can exhibit nutritional stress, an imbalance of the intake of essential nutrients, that can lead to higher cortisol concentrations (McEwen and Lasley, 2002; Bryan et al., 2013); this pattern is well documented in terrestrial mammals (Barboza et al., 2004; Bryan et al., 2013; George et al., 2014; Lafferty et al., 2015) and birds (Kitaysky et al., 1999; Cockrem, 2007). In harbor (Phoca vitulina), ringed (Pusa hispida), and spotted (Phoca largha) seals, for example, studies have shown an inverse relationship between cortisol and diet (δ15N); as δ15N values increase, cortisol decreases ((Karpovich et al., 2019; Ogloff et al., 2022). Furthermore, in spotted seals, measurements of cortisol and δ15N along whiskers (which represents a longitudinal time-series) showed that cortisol increased concurrently with a decrease in δ15N; this shows that higher cortisol is associated with feeding at a lower trophic level (Karpovich et al., 2019). In this study, we see the same pattern. Adult leopard seals with higher δ15N had lower cortisol concentrations than leopard seals with lower δ15N; this suggests diet regulates cortisol differences in this species.
Together, leopard seals show intraspecific variation in cortisol concentrations based on the interplay of sex, body mass, and δ15N. Specifically, female leopard seals are larger, have lower cortisol concentrations, higher δ15N, and show behaviors consistent with foraging at the top of the food web. Conversely, males are smaller, have higher cortisol concentrations, lower δ15N, and likely feed lower on the food web. While our sample size is small, our results reveal high variability in life history, ecological, morphological, and physiological traits in leopard seals from a single location. We predict that future studies with increased sample sizes and geographic coverage will further confirm high variability in leopard seal ecophysiological traits, as well as drivers of cortisol differences between individuals and populations.
Adult leopard seals in our study had mean cortisol concentration ~100 μg/dL, which is 1.6 times higher than previously reported for the species (Liggins et al., 1993). Here, the highest measured cortisol value was 147 μg/dL in a male leopard seal; this is the highest circulating cortisol concentration ever reported for a pinniped. Previously, the highest measured pinniped cortisol value was 104.8 μg/dL in a Weddell seal (Bartsh et al., 1992). Nearly half of the leopard seals in this study (47%, n=9) had cortisol concentrations that exceeded 100 μg/dL. Leopard seals also had the highest cortisol concentrations among 26 related mammals (e.g., pinnipeds, ursids, and mustelids) with values that ranged from 1.25 (Weddell seals) to 50 times higher (Galapagos sea lion). These findings showcase just how remarkably high leopard seal cortisol concentrations are.
More broadly, we found that lobodontines (Antarctic seals; crabeater, leopard, Ross, and Weddell seals) had much higher cortisol concentrations than other pinnipeds, ursids, and mustelids. Lobodontines have cortisol concentrations up to 7 times higher than other pinnipeds. Previous studies have also noted high cortisol levels in Antarctic seals (Liggins et al., 1979; Bartsh et al., 1992; Hogg and Rogers, 2009; Tryland et al., 2012). One hypothesis for high cortisol levels in lobodontines is that most of these species are deep divers and that high cortisol helps protect against high pressure syndrome (Liggins et al., 1993; Hogg and Rogers, 2009). Weddell seals were used to support this hypothesis; the species is characterized by both high cortisol (mean: ~80 μg/dL) and relatively deep, long dives (mean dive depth: 100-350 m, mean dive duration: 15-20 min; Kooyman et al., 1981; Castellini et al., 1992; Liggins et al., 1993; Davis and Kanatous, 1999). However, southern elephant seals (Mirounga leonina), another Southern Ocean pinniped, are deeper, longer divers (mean dive depth: 300–600 m, mean dive duration: 25 mins; (Hindell et al., 1991; Jonker and Bester, 1998; Engelhard et al., 2002) but do not exhibit high cortisol (mean: ~16 μg/dL). Leopard seals also do not support this hypothesis; leopard seals are short, shallow divers (mean dive depth: 16-29 m, mean dive duration: 2-3 min; (Krause et al., 2016; Kienle et al., 2022), yet have the highest cortisol concentrations.
We suggest that high cortisol is a specialized adaption within the lobodontines clade. There are six species of Southern Ocean pinnipeds, which are split into phylogenetically distinct clades: lobodontines (4 species), southern elephant seals (Mirounga), and Antarctic fur seals (Otariinae; (Arnason et al., 2006). This clade of lobodontines diverged from elephant seals approximately 6.9 Ma (Berta et al., 2022). Southern elephant seals and Antarctic fur seals are often distributed farther north in subpolar habitats and rely on land, rather than ice, during the breeding season (Leboeuf et al., 1986; Siniff, 1991; Laws, 1994; Lunn et al., 1994). In contrast, lobodontines have a more southern distribution, inhabit sub-Antarctic and Antarctic waters, and breed on pack-ice (Siniff, 1991; Berta et al., 2022). These different life histories, distribution patterns, and evolutionary pressures may explain why lobodontines exhibit significantly higher cortisol compared to other pinnipeds.
One method that can be used to better assess the role of high cortisol in lobodontines is to measure the amount of free cortisol. Free cortisol is the active cortisol that can be utilized by tissues during presumably stressful events (Desantis et al., 2013). Cortisol is bound by a protein in the blood, the corticosteroid binding globulin (CBG), and when cortisol is bound by CBG, it becomes inactive and has no biological effect. Free cortisol is unbound, biologically active, and available for uptake and use by tissues. Under chronic stress, individuals will exhibit high levels of glucocorticoids, a reduction in CBG, and an increase in free cortisol (Mendel, 1989; Boonstra et al., 1998; Boonstra, 2005). Most studies, including this one, have analyzed cortisol concentrations in blood serum or plasma samples, thereby measuring total (free and bound). Only a handful of studies have compared free and bound cortisol separately in lobodontines. For example, Liggins et al. (1979) reported that more than 90% of cortisol was bound in Weddell seals and reported a similar result in a single leopard seal sample (Liggins et al., 1993). A recent study measured free glucocorticoids by assessing the maximum corticosteroid binding capacity (MCBC); the authors found that two lobodontines (crabeater and Weddell seals) had MCBC concentrations 4 to 15 times higher than other non-lobodontine pinnipeds (e.g., California sea lions, Antarctic and Australian fur seals; (Delehanty et al., 2020). These results suggest that lobodontines have a ‘reservoir’ of available, but not biologically active, cortisol. Based on these findings, we would expect that cortisol in leopard seals would be mostly bound and not active, suggesting leopard seals (as a species) are not physiologically stressed. Therefore, we suggest that high cortisol levels may be an evolutionary adaption that is specific to leopard seals and, more broadly, all lobodontine seals, rather than a reflection of high stress levels. However, additional research comparing free versus bound cortisol among pinnipeds and within lobodontines species is needed to elucidate the role of high cortisol in these Antarctic-dwelling predators.
The primary goal of our study was to analyze cortisol levels of leopard seals and evaluate how they compared to other Arctoid species. Additionally, we aimed to assess how cortisol varies within a population of leopard seals, based on the factors sex, body mass and condition, and isotopic values. We demonstrate that leopard seals have high cortisol concentrations that vary with body mass, sex, and diet (δ15N). This is the most comprehensive study of leopard seal cortisol concentrations and the first study to investigate the relationship between cortisol and ecophysiological traits. Leopard seals show high variability in ecological, morphological, and physiological traits, even from a single location, like Cape Shirreff.
Because leopard seals are difficult to study, our study’s sample size is relatively small, especially for males and juveniles. While this limits our ability to determine the mechanistic drivers of intraspecific variability in cortisol, future studies will likely build on this work to confirm and expand our understanding of leopard seal physiology. Future research should target leopard seals across their core and extended range throughout the Southern Ocean and increase sampling across different life history stages. Furthermore, our metanalysis showcased a comparison of cortisol concentrations across various carnivoran taxa. For our metadata analysis, we implemented stringent criteria for a study to be included in our analysis; however, we acknowledged that our metanalysis should not serve as a definitive benchmark for cortisol values across different species, as each study had its own limitations regarding sample sizes, collection and analysis methods, and results should be interpreted accordingly. Nevertheless, to our knowledge this is the first metanalysis to conduct inter-species comparisons in cortisol concentrations, and these types of comparisons are valuable for comparative studies investigating drivers and evolutionary patterns of physiological traits.
In conclusion, evaluating the physiology of leopard seals in regard to their life history and ecology provides crucial baseline knowledge on the health of individuals and the population. Leopard seals are one of the least studied apex predators on Earth but play a disproportionately large role in Antarctic ecosystem structure and function. Therefore, our study provides a strong foundation for assessing leopard seals’ physiology, which is fundamental for understanding their vulnerability to climate change.
The original contributions presented in the study are included in the article/Supplementary Material. Further inquiries can be directed to the corresponding author.
The animal study was reviewed and approved by Institutional Animal Care and Use Committee at the University of California, Santa Cruz, federal authorizations for marine mammal research under National Marine Fisheries Service permit #19439, and an Antarctic Conservation Act permit #2018-016.
ES: conceptualization, investigation, methodology, data visualization, formal analysis, writing (original, review, and editing). DEC: conceptualization, investigation, methodology, sampling acquisition, funding, writing (review and editing editing). RB-C: sample acquisition, formal analysis, writing (review and editing). DK: formal analysis, writing (review and editing). DPC, MG, SBK, and ST: conceptualization, sample acquisition, funding, project administration, writing (review and editing). SSK: conceptualization, sample acquisition, project administration, funding, supervision, writing (review and editing). All authors contributed to the article and approved the submitted version.
This work was funded by NSF #1644256 and the ANID PIA/BASAL FB0002.
We thank the US Antarctic Program, the crew of the Lawrence M. Gould, and NOAA Antarctic Marine Living Resources Program and the Chilean Antarctic Institute (INACH) for logistic support during data collection. We thank K. J. Smith, B. Morris, P. Cunningham, A. Kirkpatrick, A. Leahy, and P. Charapata at Baylor University for support during data analysis and manuscript preparation. A special thanks to Dr. R. Zhang at the Baylor Stable Isotope Facility for his expertise and help running samples.
The authors declare that the research was conducted in the absence of any commercial or financial relationships that could be construed as a potential conflict of interest.
All claims expressed in this article are solely those of the authors and do not necessarily represent those of their affiliated organizations, or those of the publisher, the editors and the reviewers. Any product that may be evaluated in this article, or claim that may be made by its manufacturer, is not guaranteed or endorsed by the publisher.
The Supplementary Material for this article can be found online at: https://www.frontiersin.org/articles/10.3389/fmars.2023.1179236/full#supplementary-material
Abbott D. H., Keverne E. B., Bercovitch F. B., Shively C. A., Mendoza S. P., Saltzman W., et al. (2003). Are subordinates always stressed? a comparative analysis of rank differences in cortisol levels among primates. Horm. Behav. 43, 67–82. doi: 10.1016/s0018-506x(02)00037-5
Anestis S. F., Bribiescas R. G., Hasselschwert D. L. (2006). Age, rank, and personality effects on the cortisol sedation stress response in young chimpanzees. Physiol. Behav. 89, 287–294. doi: 10.1016/j.physbeh.2006.06.010
Archie E. A., Morrison T. A., Foley C. A. H., Moss C. J., Alberts S. C. (2006). Dominance rank relationships among wild female African elephants, loxodonta africana. Anim. Behav. 71, 117–127. doi: 10.1016/j.anbehav.2005.03.023
Arnason U., Gullberg A., Janke A., Kullberg M., Lehman N., Petrov E. A., et al. (2006). Pinniped phylogeny and a new hypothesis for their origin and dispersal. Mol. Phylogenet. Evol. 41, 345–354. doi: 10.1016/j.ympev.2006.05.022
Ashley N. T., Wingfield J. C., Demas G. E., Nelson R. J. (2011). Sickness behavior in vertebrates. Ecoimmunology (New York, NY:Oxford University Press) 296, 45–91.
Atkinson S., Arnould J. P. Y., Mashburn K. L. (2011). Plasma cortisol and thyroid hormone concentrations in pre-weaning Australian fur seal pups. Gen. Comp. Endocrinol. 172, 277–281. doi: 10.1016/j.ygcen.2011.03.014
Aurich J., Wulf M., Ille N., Erber R., von Lewinski M., Palme R., et al. (2015). Effects of season, age, sex, and housing on salivary cortisol concentrations in horses. Domest. Anim. Endocrinol. 52, 11–16. doi: 10.1016/j.domaniend.2015.01.003
Azevedo A., Bailey L., Bandeira V., Dehnhard M., Fonseca C., de Sousa L., et al. (2019). Correction: age, sex and storage time influence hair cortisol levels in a wild mammal population. PloS One 14, e0222963. doi: 10.1371/journal.pone.0222963
Barboza P. S., Hartbauer D. W., Hauer W. E., Blake J. E. (2004). Polygynous mating impairs body condition and homeostasis in male reindeer (Rangifer tarandus tarandus). J. Comp. Physiol. B 174, 309–317. doi: 10.1007/s00360-004-0416-6
Barrell G. K., Montgomery G. W. (1989). Absence of circadian patterns of secretion of melatonin or cortisol in weddell seals under continuous natural daylight. J. Endocrinol. 122, 445–449. doi: 10.1677/joe.0.1220445
Bartsh S. S., Johnston S. D., Siniff D. B. (1992). Territorial behavior and breeding frequency of male weddell seals (Leptonychotes weddelli) in relation to age, size, and concentrations of serum testosterone and cortisol. Can. J. Zool. 70, 680–692. doi: 10.1139/z92-102
Beaulieu-McCoy N. E., Sherman K. K., Trego M. L., Crocker D. E., Kellar N. M. (2017). Initial validation of blubber cortisol and progesterone as indicators of stress response and maturity in an otariid; the California sea lion (Zalophus californianus). Gen. Comp. Endocrinol. 252, 1–11. doi: 10.1016/j.ygcen.2017.07.003
Bennett K. A., Moss S. E. W., Pomeroy P., Speakman J. R., Fedak M. A. (2012). Effects of handling regime and sex on changes in cortisol, thyroid hormones and body mass in fasting grey seal pups. Comp. Biochem. Physiol. A Mol. Integr. Physiol. 161, 69–76. doi: 10.1016/j.cbpa.2011.09.003
Berta A., Churchill M., Boessenecker R. W. (2022). “The origin of phocid seals and evolution of key behavioral character traits,” in Ethology and behavioral ecology of phocids. Eds. Costa D. P., McHuron E. A. (Cham: Springer International Publishing), 3–30. doi: 10.1007/978-3-030-88923-4_1
Boonstra R. (2005). Equipped for life: the adaptive role of the stress axis in Male mammals. J. Mammal. 86, 236–247. doi: 10.1644/BHE-001.1
Boonstra R., Dušek A., Flynn R. W. (2018). DHEA and territoriality during the nonbreeding season in male American martens (Martes americana). J. Mammal. 99, 826–835.
Boonstra R., Hik D., Singleton G. R., Tinnikov A. (1998). The impact of predator-induced stress on the snowshoe hare cycle. Ecol. Monogr. 68, 371–394. doi: 10.1890/0012-9615(1998)068[0371:tiopis]2.0.co;2
Botta S., Secchi E. R., Rogers T. L., Prado J. H. F., de Lima R. C., Carlini P., et al. (2018). Isotopic niche overlap and partition among three Antarctic seals from the Western Antarctic peninsula. Deep Sea Res. Part 2 Top. Stud. Oceanogr. 149, 240–249. doi: 10.1016/j.dsr2.2017.11.005
Boveng P. L., Hiruki L. M., Schwartz M. K., Bengtson J. L. (1998). Population growth of antarctic fur seals: limitation by a top predator, the leopard seal? Ecology 79, 2863–2877. doi: 10.1890/0012-9658(1998)079[2863:pgoafs]2.0.co;2
Brannon R. D. (1985). Serum chemistry of central and northern Alaska grizzly bears. J. Wildl. Manage. 49, 893–900. doi: 10.2307/3801365
Bronson F. H., Eleftheriou B. E. (1964). Chronic physiological effects of fighting in mice. Gen. Comp. Endocrinol. 4, 9–14. doi: 10.1016/0016-6480(64)90033-4
Bryan H. M., Darimont C. T., Paquet P. C., Wynne-Edwards K. E., Smits J. E. G. (2013). Stress and reproductive hormones in grizzly bears reflect nutritional benefits and social consequences of a salmon foraging niche. PloS One 8, e80537. doi: 10.1371/journal.pone.0080537
Burnham K. P., Anderson D. R., Huyvaert K. P. (2011). AIC model selection and multimodel inference in behavioral ecology: some background, observations, and comparisons. Behav. Ecol. Sociobiol. 65, 23–35. doi: 10.1007/s00265-010-1029-6
Castellini M. A., Kooyman G. L., Ponganis P. J. (1992). Metabolic rates of freely diving weddell seals: correlations with oxygen stores, swim velocity and diving duration. J. Exp. Biol. 165, 181–194. doi: 10.1242/jeb.165.1.181
Champagne C. D., Houser D. S., Costa D. P., Crocker D. E. (2012). The effects of handling and anesthetic agents on the stress response and carbohydrate metabolism in northern elephant seals. PloS One 7, e38442. doi: 10.1371/journal.pone.0038442
Champagne C. D., Houser D. S., Crocker D. E. (2005). Glucose production and substrate cycle activity in a fasting adapted animal, the northern elephant seal. J. Exp. Biol. 208, 859–868. doi: 10.1242/jeb.01476
Charapata P., Clark C. T., Miller N., Kienle S. S., Costa D. P., Goebel M. E., et al. (2023). Whiskers provide time-series of toxic and essential trace elements, Se:Hg molar ratios, and stable isotope values of an apex Antarctic predator, the leopard seal. Sci. Total Environ. 854, 158651. doi: 10.1016/j.scitotenv.2022.158651
Chow B. A. (2013). Stress physiology of bears: cortisol dynamics and identification of novel serum proteins. (University of Waterloo)
Clutton-Brock T. H., Green D., Hiraiwa-Hasegawa M., Albon S. D. (1988). Passing the buck: resource defence, lek breeding and mate choice in fallow deer. Behav. Ecol. Sociobiol. 23, 281–296. doi: 10.1007/bf00300575
Cockrem J. F. (2007). Stress, corticosterone responses and avian personalities. J. fur Ornithol. 148, 169–178. doi: 10.1007/s10336-007-0175-8
Constable S., Parslow A., Dutton G., Rogers T., Hogg C. (2006). Urinary cortisol sampling: a non-invasive technique for examining cortisol concentrations in the weddell seal,Leptonychotes weddellii. Zoo Biol. 25, 137–144. doi: 10.1002/zoo.20088
Creel S. (2001). Social dominance and stress hormones. Trends Ecol. Evol. 16, 491–497. doi: 10.1016/S0169-5347(01)02227-3
Creel S. (2005). Dominance, aggression, and glucocorticoid levels in social carnivores. J. Mammal. 86, 255–264. doi: 10.1644/BHE-002.1
Creel S., Creel N. M., Mills M. G. L., Monfort S. L. (1997). Rank and reproduction in cooperatively breeding African wild dogs: behavioral and endocrine correlates. Behav. Ecol. 8, 298–306. doi: 10.1093/beheco/8.3.298
Creel S., Dantzer B., Goymann W., Rubenstein D. R. (2013). The ecology of stress: effects of the social environment. Funct. Ecol. 27, 66–80. doi: 10.1111/j.1365-2435.2012.02029.x
Crocker D. E., Ortiz R. M., Houser D. S., Webb P. M., Costa D. P. (2012). Hormone and metabolite changes associated with extended breeding fasts in male northern elephant seals (Mirounga angustirostris). Comp. Biochem. Physiol. A Mol. Integr. Physiol. 161, 388–394. doi: 10.1016/j.cbpa.2011.12.013
Davis R. W., Kanatous S. B. (1999). Convective oxygen transport and tissue oxygen consumption in weddell seals during aerobic dives. J. Exp. Biol. 202, 1091–1113. doi: 10.1242/jeb.202.9.1091
Delehanty B., Bossart G. D., Champagne C., Crocker D. E., Elliott K. H., Fair P. A., et al. (2020). Measurement of free glucocorticoids: quantifying corticosteroid binding capacity and its variation within and among mammal and bird species. Conserv. Physiol. 8, coaa057. doi: 10.1093/conphys/coaa057
DeNiro M. J., Epstein S. (1977). Mechanism of carbon isotope fractionation associated with lipid synthesis. Science 197, 261–263. doi: 10.1126/science.327543
DeRango E. J., Greig D. J., Gálvez C., Norris T. A., Barbosa L., Elorriaga-Verplancken F. R., et al. (2019a). Response to capture stress involves multiple corticosteroids and is associated with serum thyroid hormone concentrations in Guadalupe fur seals ( arctocephalus philippii townsendi ). Mar. Mamm. Sci. 35, 72–92. doi: 10.1111/mms.12517
DeRango E. J., Prager K. C., Greig D. J., Hooper A. W., Crocker D. E. (2019b). Climate variability and life history impact stress, thyroid, and immune markers in California sea lions (Zalophus californianus) during El niño conditions. Conserv. Physiol. 7, coz010. doi: 10.1093/conphys/coz010
DeRango E. J., Schwarz J. F. L., Piedrahita P., Páez-Rosas D., Crocker D. E., Krüger O. (2021). Hormone-mediated foraging strategies in an uncertain environment: insights into the at-sea behavior of a marine predator. Ecol. Evol. 11, 7579–7590. doi: 10.1002/ece3.7590
Desantis L. M., Delehanty B., Weir J. T., Boonstra R. (2013). Mediating free glucocorticoid levels in the blood of vertebrates: are corticosteroid-binding proteins always necessary? Funct. Ecol. 27, 107–119. doi: 10.1111/1365-2435.12038
Di Poi C., Atkinson S., Hoover-Miller A., Blundell G. (2015). Maternal buffering of stress response in free-ranging pacific harbor seal pups in Alaska. Mar. Mamm. Sci. 31, 1098–1117. doi: 10.1111/mms.12217
du Dot T. J., Rosen D. A. S., Richmond J. P., Kitaysky A. S., Zinn S. A., Trites A. W. (2009). Changes in glucocorticoids, IGF-I and thyroid hormones as indicators of nutritional stress and subsequent refeeding in steller sea lions (Eumetopias jubatus). Comp. Biochem. Physiol. A Mol. Integr. Physiol. 152, 524–534. doi: 10.1016/j.cbpa.2008.12.010
Engelhard G. H., Brasseur S. M. J. M., Hall A. J., Burton H. R., Reijnders P. J. H. (2002). Adrenocortical responsiveness in southern elephant seal mothers and pups during lactation and the effect of scientific handling. J. Comp. Physiol. B 172, 315–328. doi: 10.1007/s00360-002-0257-0
Engelhardt F. R., Ferguson J. M. (1980). Adaptive hormone changes in harp seals, phoca groenlandica, and gray seals, halichoerus grypus, during the postnatal period. Gen. Comp. Endocrinol. 40, 434–445. doi: 10.1016/0016-6480(80)90006-4
Ensminger D. C., Somo D. A., Houser D. S., Crocker D. E. (2014). Metabolic responses to adrenocorticotropic hormone (ACTH) vary with life-history stage in adult male northern elephant seals. Gen. Comp. Endocrinol. 204, 150–157. doi: 10.1016/j.ygcen.2014.04.024
Faupel-Badger J. M., Fuhrman B. J., Xu X., Falk R. T., Keefer L. K., Veenstra T. D., et al. (2010). Comparison of liquid chromatography-tandem mass spectrometry, RIA, and ELISA methods for measurement of urinary estrogens. Cancer Epidemiol. Biomarkers Prev. 19, 292–300. doi: 10.1158/1055-9965.EPI-09-0643
Ferreira A. P. S., Martínez P. E., Colares E. P., Robaldo R. B., Berne M. E. A., Miranda Filho K. C., et al. (2005). Serum immunoglobulin G concentration in southern elephant seal, mirounga leonina (Linnaeu ), from elephant island (Antarctica): sexual and adrenal steroid hormones effects. Veterinary Immunol. Immunopathology 106, 239–245. doi: 10.1016/j.vetimm.2005.02.024
Folch J., Lees M., Sloane Stanley G. H. (1957). A simple method for the isolation and purification of total lipids from animal tissues. J. Biol. Chem. 226, 497–509. doi: 10.1016/S0021-9258(18)64849-5
Foster L. B., Dunn R. T. (1974). Single-antibody technique for radioimmunoassay of cortisol in unextracted serum or plasma. Clin. Chem. 20, 365–368. doi: 10.1093/clinchem/20.3.365
Franco-Martinez L., Tvarijonaviciute A., Martinez-Subiela S., Teles M., Tort L. (2019). Chemiluminescent assay as an alternative to radioimmunoassay for the measurement of cortisol in plasma and skin mucus of oncorhynchus mykiss. Ecol. Indic. 98, 634–640. doi: 10.1016/j.ecolind.2018.11.046
Gannes L. Z., Martínez del Rio C., Koch P. (1998). Natural abundance variations in stable isotopes and their potential uses in animal physiological ecology. Comp. Biochem. Physiol. A Mol. Integr. Physiol. 119, 725–737. doi: 10.1016/s1095-6433(98)01016-2
Gardiner K. J., Hall A. J. (1997). Diel and annual variation in plasma cortisol concentrations among wild and captive harbor seals (Phoca vitulina). Can. J. Zool. 75, 1773–1780. doi: 10.1139/z97-806
George S. C., Smith T. E., Mac Cana P. S. S., Coleman R., Montgomery W. I. (2014). Physiological stress in the Eurasian badger (Meles meles): effects of host, disease and environment. Gen. Comp. Endocrinol. 200, 54–60. doi: 10.1016/j.ygcen.2014.02.017
Graham M. L., Gresch S. C., Hardy S. K., Mutch L. A., Janecek J. L., Hegstad-Davies R. L. (2015). Evaluation of commercial ELISA and RIA for measuring porcine c-peptide: implications for research. Xenotransplantation 22, 62–69. doi: 10.1111/xen.12143
Guinet C., Servera N., Mangin S., Georges J.-Y., Lacroix A. (2004). Change in plasma cortisol and metabolites during the attendance period ashore in fasting lactating subantarctic fur seals. Comp. Biochem. Physiol. A Mol. Integr. Physiol. 137, 523–531. doi: 10.1016/j.cbpb.2003.11.006
Gulland F. M. D., Haulena M., Lowenstine L. J., Munro C., Graham P. A., Bauman J., et al. (1999). Adrenal function in wild and rehabilitated pacific harbor seals (Phoca vitulina richardii) and in seals with phocine herpesvirus-associated adrenal necrosis. Mar. Mamm. Sci. 15, 810–827. doi: 10.1111/j.1748-7692.1999.tb00844.x
Haase C. G., Long A. K., Gillooly J. F. (2016). Energetics of stress: linking plasma cortisol levels to metabolic rate in mammals. Biol. Lett. 12, 20150867. doi: 10.1098/rsbl.2015.0867
Haave M., Ropstad E., Derocher A. E., Lie E., Dahl E., Wiig Ø., et al. (2003). Polychlorinated biphenyls and reproductive hormones in female polar bears at Svalbard. Environ. Health Perspect. 111, 431–436. doi: 10.1289/ehp.5553
Hair J. F., Ringle C. M., Sarstedt M. (2011). PLS-SEM: indeed a silver bullet. J. Marketing Theory Pract. 19, 139–152. doi: 10.2753/MTP1069-6679190202
Hall-Aspland S. A., Rogers T. L. (2004). Summer diet of leopard seals (Hydrurga leptonyx) in prydz bay, Eastern Antarctica. Polar Biol. 27, 729–734. doi: 10.1007/s00300-004-0662-9
Harcourt R. G. (2001). Advances in new Zealand mammalogy 1990–2000: pinnipeds. J. R. Soc N. Z. 31, 135–160. doi: 10.1080/03014223.2001.9517644
Harlow H. J., Beck T. D. I., Walters L. M., Greenhouse S. S. (1990). Seasonal serum glucose, progesterone, and cortisol levels of black bears (Ursus americanus). Can. J. Zool. 68, 183–187. doi: 10.1139/z90-025
Hilderbrand G. V., Farley S. D., Robbins C. T., Hanley T. A., Titus K., Servheen C. (1996). Use of stable isotopes to determine diets of living and extinct bears. Can. J. Zool. 74, 2080–2088. doi: 10.1139/z96-236
Hindell M. A., Slip D. J., Burton H. R. (1991). The diving behavior of adult Male and female southern elephant seals, mirounga-leonina (Pinnipedia, phocidae). Aust. J. Zool. 39, 595–619. doi: 10.1071/zo9910595
Hiruki L. M., Schwartz M. K., Boveng P. L. (1999). Hunting and social behaviour of leopard seals (Hydrurga leptonyx) at seal island, south Shetland islands, Antarctica. J. Zool. 249, 97–109. doi: 10.1111/j.1469-7998.1999.tb01063.x
Hobson K. A., Clark R. G. (1993). Turnover of 13 c in cellular and plasma fractions of blood: implications for nondestructive sampling in avian dietary studies. Auk 110, 638–641. doi: 10.2307/4088430
Hobson K. A., Welch H. E. (1992). Determination of trophic relationships within a high Arctic marine food web using δ 13 c and δ 15 n analysis. Mar. Ecol. Prog. Ser. 9–18.
Hogg C. J., Rogers T. L. (2009). “Measuring stress in Antarctic seals,” in Health of Antarctic wildlife: a challenge for science and policy. Eds. Kerry K. R., Riddle M. (Berlin, Heidelberg: Springer Berlin Heidelberg), 263–270. doi: 10.1007/978-3-540-93923-8_15
Hupman K., Visser I. N., Fyfe J., Cawthorn M., Forbes G., Grabham A. A., et al. (2020). From vagrant to resident: occurrence, residency and births of leopard seals (Hydrurga leptonyx) in new Zealand waters. N. Z. J. Mar. Freshw. Res. 54, 1–23. doi: 10.1080/00288330.2019.1619598
Jonker F. C., Bester M. N. (1998). Seasonal movements and foraging areas of adult southern female elephant seals, mirounga leonina, from Marion island. Antarct. Sci. 10, 21–30. doi: 10.1017/S0954102098000042
Karpovich S. A., Skinner J. P., Kapronczai L. A., Smith J. A., Janz D. M. (2019). Examination of relationships between stable isotopes and cortisol concentrations along the length of phocid whiskers. Mar. Mamm. Sci. 35, 395–415. doi: 10.1111/mms.12546
Keogh M. J., Atkinson S. (2015). Endocrine and immunological responses to adrenocorticotrophic hormone (ACTH) administration in juvenile harbor seals (Phoca vitulina) during winter and summer. Comp. Biochem. Physiol. A Mol. Integr. Physiol. 188, 22–31. doi: 10.1016/j.cbpa.2015.06.011
Keogh M. J., Atkinson S., Maniscalco J. M. (2013). Body condition and endocrine profiles of steller sea lion (Eumetopias jubatus) pups during the early postnatal period. Gen. Comp. Endocrinol. 184, 42–50. doi: 10.1016/j.ygcen.2012.12.016
Keogh M. J., Gastaldi A., Charapata P., Melin S., Fadely B. S. (2020). Stress-related and reproductive hormones in hair from three north pacific otariid species: steller sea lions, California sea lions and northern fur seals. Conserv. Physiol. 8, coaa069. doi: 10.1093/conphys/coaa069
Kershaw J. L., Hall A. J. (2016). Seasonal variation in harbour seal (Phoca vitulina) blubber cortisol - a novel indicator of physiological state? Sci. Rep. 6, 21889. doi: 10.1038/srep21889
Kienle S. S., Goebel M. E., LaBrecque E., Borras-Chavez R., Trumble S. J., Kanatous S. B., et al. (2022). Plasticity in the morphometrics and movements of an Antarctic apex predator, the leopard seal. Front. Mar. Sci. 9. doi: 10.3389/fmars.2022.976019
Kitaysky A. S., Wingfield J. C., Piatt J. F. (1999). Dynamics of food availability, body condition and physiological stress response in breeding black-legged kittiwakes. Funct. Ecol. 13, 577–584. doi: 10.1046/j.1365-2435.1999.00352.x
Kooyman G. L., Castellini M. A., Davis R. W. (1981). Physiology of diving in marine mammals. Annu. Rev. Physiol. 43, 343–356. doi: 10.1146/annurev.ph.43.030181.002015
Krause D. J., Goebel M. E., Marshall G. J., Abernathy K. (2016). Summer diving and haul-out behavior of leopard seals (Hydrurga leptonyx) near mesopredator breeding colonies at Livingston Island, Antarctic Peninsula. Mar Mam Sci. 32, 839–867. doi: 10.1111/mms.12309
Krause D. J., Bonin C. A., Goebel M. E. (2022). The rapid population collapse of a key marine predator in the northern Antarctic peninsula endangers genetic diversity and resilience to climate change. Front. Marine. doi: 10.3389/fmars.2021.796488
Krause D. J., Goebel M. E., Kurle C. M. (2020). Leopard seal diets in a rapidly warming polar region vary by year, season, sex, and body size. BMC Ecol. 20, 32. doi: 10.1186/s12898-020-00300-y
Krause D. J., Goebel M. E., Marshall G. J., Abernathy K. (2015). Novel foraging strategies observed in a growing leopard seal (Hydrurga leptonyx) population at Livingston island, Antarctic peninsula. Anim. Biotelemetry 3, 24. doi: 10.1186/s40317-015-0059-2
Krause D. J., Rogers T. L. (2019). Food caching by a marine apex predator, the leopard seal (Hydrurga leptonyx). Can. J. Zool. 97, 573–578. doi: 10.1139/cjz-2018-0203
Lafferty D. J. R., Laudenslager M. L., Mowat G., Heard D., Belant J. L. (2015). Sex, diet, and the social environment: factors influencing hair cortisol concentration in free-ranging black bears (Ursus americanus). PloS One 10, e0141489. doi: 10.1371/journal.pone.0141489
Larson S., Monson D., Ballachey B., Jameson R., Wasser S. K. (2009). Stress-related hormones and genetic diversity in sea otters (Enhydra lutris). Mar. Mamm. Sci. 25, 351–372. doi: 10.1111/j.1748-7692.2008.00260.x
Laws R. M. (1957). On the growth rates of the leopard seal, hydrurga leptonyx Vol. 1820 (De Blainville:Saugetierkundliche Mitt).
Laws R. M. (1977)The significance of vertebrates in the Antarctic marine ecosystem (Accessed November 7, 2022).
Laws R. M. (1994). “History and present status of southern elephant seal populations,” in Elephant seals: population ecology, behavior, and physiology (Berkeley: University of California Press), 49–65.
Leboeuf B. J., Costa D. P., Huntley A. C., Kooyman G. L., Davis R. W. (1986). Pattern and depth of dives in northern elephant seals, mirounga angustirostris. J. Zool. 208, 1–7. doi: 10.1111/j.1469-7998.1986.tb04705.x
Lemos L. S., Olsen A., Smith A., Burnett J. D., Chandler T. E., Larson S., et al. (2022). Stressed and slim or relaxed and chubby? a simultaneous assessment of gray whale body condition and hormone variability. Mar. Mamm. Sci. 38, 801–811. doi: 10.1111/mms.12877
Lesage V., Hammill M. O., Kovacs K. M. (2001). Marine mammals and the community structure of the estuary and gulf of St Lawrence, Canada: evidence from stable isotope analysis. Mar. Ecol. Prog. Ser. 210, 203–221. doi: 10.3354/meps210203
Levine J. E. (2002). Editorial: stressing the importance of sex. Endocrinology 143, 4502–4504. doi: 10.1210/en.2002-221041
Levy E. J., Gesquiere L. R., McLean E., Franz M., Warutere J. K., Sayialel S. N., et al. (2020). Higher dominance rank is associated with lower glucocorticoids in wild female baboons: a rank metric comparison. Horm. Behav. 125, 104826. doi: 10.1016/j.yhbeh.2020.104826
Lidgard D. C., Boness D. J., Bowen W. D., McMillan J. I. (2008). The implications of stress on male mating behavior and success in a sexually dimorphic polygynous mammal, the grey seal. Horm. Behav. 53, 241–248. doi: 10.1016/j.yhbeh.2007.10.003
Liggins G. C., France J. T., Knox B. S., Zapol W. M. (1979). High corticosteroid levels in plasma of adult and foetal weddell seals (Leptonychotes weddelli). Acta Endocrinol. 90, 718–726. doi: 10.1530/acta.0.0900718
Liggins G. C., France J. T., Schneider R. C., Knox B. S., Zapol W. M. (1993). Concentrations, metabolic clearance rates, production rates and plasma binding of cortisol in Antarctic phocid seals. Acta Endocrinol. 129, 356–359. doi: 10.1530/acta.0.1290356
Lunn N. J., Boyd I. L., Croxall J. P. (1994). Reproductive performance of female Antarctic fur seals: the influence of age, breeding experience, environmental variation and individual quality. J. Anim. Ecol. 63, 827–840. doi: 10.2307/5260
Macbeth B. J., Cattet M. R. L., Obbard M. E., Middel K., Janz D. M. (2012). Evaluation of hair cortisol concentration as a biomarker of long-term stress in free-ranging polar bears. Wildl. Soc Bull. 36, 747–758. doi: 10.1002/wsb.219
Macbeth B. J., Cattet M. R. L., Stenhouse G. B., Gibeau M. L., Janz D. M. (2010). Hair cortisol concentration as a noninvasive measure of long-term stress in free-ranging grizzly bears (Ursus arctos): considerations with implications for other wildlife. Can. J. Zool. 88, 935–949. doi: 10.1139/z10-057
MacDougall-Shackleton S. A., Bonier F., Romero L. M., Moore I. T. (2019). Glucocorticoids and “Stress” are not synonymous. Integr. Org Biol. 1, obz017. doi: 10.1093/iob/obz017
Manogue K. R., Leshner A. I., Candland D. K. (1975). Dominance status and adrenocortical reactivity to stress in squirrel monkeys (Saimiri sciureus). Primates 16, 457–463. doi: 10.1007/bf02382742
Mazerolle M. J. (2020). AICcmodavg: Model selection and multimodel inference based on (Q)AIC(c). r package version 2.3-1. Available at: https://cran.r-project.org/package=AICcmodavg.
McEwen B. S., Lasley E. (2002). The end of stress as we know it (Dana Press). Available at: https://psycnet.apa.org/fulltext/2002-06297-000.pdf.
Mendel C. M. (1989). The free hormone hypothesis: a physiologically based mathematical model. Endocr. Rev. 10, 232–274. doi: 10.1210/edrv-10-3-232
Myers M. J., Litz B., Atkinson S. (2010). The effects of age, sex, season and geographic region on circulating serum cortisol concentrations in threatened and endangered steller sea lions (Eumetopias jubatus). Gen. Comp. Endocrinol. 165, 72–77. doi: 10.1016/j.ygcen.2009.06.006
Newsome S. D., Ralls K., Van Horn Job C., Fogel M. L., Cypher B. L. (2010). Stable isotopes evaluate exploitation of anthropogenic foods by the endangered San Joaquin kit fox (Vulpes macrotis mutica). J. Mammal. 91, 1313–1321. doi: 10.1111/j.1748-7692.2009.00354.x
Nordøy E. S., Aakvaag A., Larsen T. S. (1993). Metabolic adaptations to fasting in harp seal pups. Physiol. Zool. 66, 926–945. doi: 10.1086/physzool.66.6.30163747
Nordøy E. S., Ingebretsen O. C., Blix A. S. (1990). Depressed metabolism and low protein catabolism in fasting grey seal pups. Acta Physiol. Scand. 139, 361–369. doi: 10.1111/j.1748-1716.1990.tb08935.x
Ogloff W. R., Anderson R. A., Yurkowski D. J., Debets C. D., Anderson W. G., Ferguson S. H. (2022). Spatiotemporal variation of ringed seal blubber cortisol levels in the Canadian Arctic. J. Mammal. 103, 1208–1220. doi: 10.1093/jmammal/gyac047
Øritsland T. (1977). Food consumption of seals in the Antarctic pack ice. In: Llano GA (ed) Adaptations within Antarctic ecosystems. Proc 3rd Symp Antarct Biol. (Washington DC:Smithsonian Institution)
Palme R., Rettenbacher S., Touma C., El-Bahr S. M., Möstl E. (2005). Stress hormones in mammals and birds: comparative aspects regarding metabolism, excretion, and noninvasive measurement in fecal samples. Ann. N. Y. Acad. Sci. 1040, 162–171. doi: 10.1196/annals.1327.021
Peck H. E., Costa D. P., Crocker D. E. (2016). Body reserves influence allocation to immune responses in capital breeding female northern elephant seals. Funct. Ecol. 30, 389–397. doi: 10.1111/1365-2435.12504
Pedernera-Romano C., Aurioles-Gamboa D. (2010). Serum cortisol in California sea lion pups (Zalophus californianus californianus). Animal 19.3(2010), 275–280. doi: 10.1017/S0962728600001652
Peig J., Green A. J. (2009). New perspectives for estimating body condition from mass/length data: the scaled mass index as an alternative method. Oikos 118, 1883–1891. doi: 10.1111/j.1600-0706.2009.17643.x
Pussini N., Goebel M. E. (2015). A safer protocol for field immobilization of leopard seals ( hydrurga leptonyx ). Mar. Mamm. Sci. 31, 1549–1558. doi: 10.1111/mms.12232
Rainer Engelhardt F. (1982). Hydrocarbon metabolism and cortisol balance in oil-exposed ringed seals, phoca hispzda. Camp. Biochem. Physiol. 72C, 133–136. doi: 10.1016/0306-4492(82)90219-2
Ramsay M. A., Hobson K. A. (1991). Polar bears make little use of terrestrial food webs: evidence from stable-carbon isotope analysis. Oecologia 86, 598–600. doi: 10.1007/BF00318328
Rau G. H., Takahashi T., Des Marais D. J., Repeta D. J., Martin J. H. (1992). The relationship between δ13C of organic matter and [CO2(aq)] in ocean surface water: data from a JGOFS site in the northeast Atlantic ocean and a model. Geochim. Cosmochim. Acta 56, 1413–1419. doi: 10.1016/0016-7037(92)90073-R
R Core Team (2022) R: a language and environment for statistical computing. r foundation for statistical computing. Available at: https://www.R-project.org/.
Reeder D. M., Kramer K. M. (2005). Stress in free-ranging mammals: integrating physiology, ecology, and natural history. J. Mammal. 86, 225–235. doi: 10.1644/BHE-003.1
Romero L. M., Beattie U. K. (2022). Common myths of glucocorticoid function in ecology and conservation. J. Exp. Zool A Ecol. Integr. Physiol. 337, 7–14. doi: 10.1002/jez.2459
Rutberg A. T., Greenberg S. A. (1990). Dominance, aggression frequencies and modes of aggressive competition in feral pony mares. Anim. Behav. 40, 322–331. doi: 10.1016/S0003-3472(05)80927-3
Sapolsky R. M. (1999). Glucocorticoids, stress, and their adverse neurological effects: relevance to aging. Exp. Gerontol. 34, 721–732. doi: 10.1016/s0531-5565(99)00047-9
Seguel M., Perez-Venegas D., Gutierrez J., Crocker D. E., DeRango E. J. (2019). Parasitism elicits a stress response that allocates resources for immune function in south American fur seals (Arctocephalus australis). Physiol. Biochem. Zool. 92, 326–338. doi: 10.1086/702960
Sheriff M. J., Dantzer B., Delehanty B., Palme R., Boonstra R. (2011). Measuring stress in wildlife: techniques for quantifying glucocorticoids. Oecologia 166, 869–887. doi: 10.1007/s00442-011-1943-y
Shero M. R., Krotz R. T., Costa D. P., Avery J. P., Burns J. M. (2015). How do overwinter changes in body condition and hormone profiles influence W eddell seal reproductive success? Funct. Ecol. 29, 1278–1291. doi: 10.1111/1365-2435.12434
Shimada M., Takahashi K., Ohkawa T., Segawa M., Higurashi M. (1995). Determination of salivary cortisol by ELISA and its application to the assessment of the circadian rhythm in children. Horm. Res. 44, 213–217. doi: 10.1159/000184628
Siniff D. B. (1991). An overview of the ecology of Antarctic seals. Am. Zool. 31, 143–149. doi: 10.1093/icb/31.1.143
Sperou E. S. (2020). A comprehensive analysis of sex hormones and their role in reproductive suppression in female northern elephant seals (Mirounga angustirostris). scholarworks.calstate.edu
Stockley P., Bro-Jørgensen J. (2011). Female competition and its evolutionary consequences in mammals. Biol. Rev. Camb. Philos. Soc 86, 341–366. doi: 10.1111/j.1469-185X.2010.00149.x
Thompson L. A., Romano T. A. (2019). Effects of health status on pressure-induced changes in phocid immune function and implications for dive ability. J. Comp. Physiol. B 189, 637–657. doi: 10.1007/s00360-019-01228-6
Traylor J. J., Alisauskas R. T. (2006). Effects of intrinsic and extrinsic factors on survival of white-winged scoter (Melanitta fusca deglandi) ducklings. Auk 123, 67–81. doi: 10.1093/auk/123.1.67
Trumble S. J., O’Neil D., Cornick L. A., Gulland F. M. D., Castellini M. A., Atkinson S. (2013). Endocrine changes in harbor seal (Phoca vitulina) pups undergoing rehabilitation. Zoo Biol. 32, 134–141. doi: 10.1002/zoo.21036
Tryland M., Krafft B. A., Lydersen C., Kovacs K. M., Thoresen S. I. (2006). Serum chemistry values for free-ranging ringed seals (Pusa hispida) in Svalbard. Vet. Clin. Pathol. 35, 405–412. doi: 10.1111/j.1939-165x.2006.tb00156.x
Tryland M., Lydersen C., Kovacs K. M., Rafter E., Thoresen S. I. (2021). Serum biochemistry and haematology in wild and captive bearded seals (Erignathus barbatus) from Svalbard, Norway. Acta Vet. Scand. 63, 33. doi: 10.1186/s13028-021-00598-8
Tryland M., Lydersen C., Kovacs K. M., Thoresen S. I. (2009). Serum chemistry reference values in free-ranging north Atlantic male walruses (Odobenus rosmarus rosmarus) from the Svalbard archipelago. Vet. Clin. Pathol. 38, 501–506. doi: 10.1111/j.1939-165X.2009.00154.x
Tryland M., Nymo I. H., Nielsen O., Nordøy E. S., Kovacs K. M., Krafft B. A., et al. (2012). Serum chemistry and antibodies against pathogens in antarctic fur seals, weddell seals, crabeater seals, and Ross seals. J. Wildl. Dis. 48, 632–645. doi: 10.7589/0090-3558-48.3.632
Tykot R. H. (2004). “Stable isotopes and diet: you are what you eat,” in Physics methods in archaeometry (IOS press), 433–444. doi: 10.3254/978-1-61499-010-9-433
Verrier D., Atkinson S., Guinet C., Groscolas R., Arnould J. P. Y. (2012). Hormonal responses to extreme fasting in subantarctic fur seal (Arctocephalus tropicalis) pups. Am. J. Physiol. Regul. Integr. Comp. Physiol. 302, R929–R940. doi: 10.1152/ajpregu.00370.2011
Webster H. V., Bone A. J., Webster K. A., Wilkin T. J. (1990). Comparison of an enzyme-linked immunosorbent assay (ELISA) with a radioimmunoassay (RIA) for the measurement of rat insulin. J. Immunol. Methods 134, 95–100. doi: 10.1016/0022-1759(90)90116-d
Wickham H., Averick M., Bryan J., Chang W., McGowan L., François R., et al. (2019). Welcome to the tidyverse. J. Open Source Software 4, 1686. doi: 10.21105/joss.01686
Wingfield J. C. (2013a). Ecological processes and the ecology of stress: the impacts of abiotic environmental factors. Funct. Ecol. 27, 37–44. doi: 10.1111/1365-2435.12039
Wingfield J. C. (2013b). The comparative biology of environmental stress: behavioural endocrinology and variation in ability to cope with novel, changing environments. Anim. Behav. 85, 1127–1133. doi: 10.1016/j.anbehav.2013.02.018
Wingfield J. C., Romero L. M. (2011). Adrenocortical responses to stress and their modulation in free-living vertebrates. Compr. Physiol. doi: 10.1002/cphy.cp070411
Winter B. (2013) Linear models and linear mixed effects models in r with linguistic applications. Available at: http://arxiv.org/abs/1308.5499.
Keywords: leopard seal, marine mammals, intraspecific variation, pinniped, cortisol, physiology, body size, stable isotope
Citation: Sperou ES, Crocker DE, Borras-Chavez R, Costa DP, Goebel ME, Kanatous SB, Krause DJ, Trumble SJ and Kienle SS (2023) Large and in charge: cortisol levels vary with sex, diet, and body mass in an Antarctic predator, the leopard seal. Front. Mar. Sci. 10:1179236. doi: 10.3389/fmars.2023.1179236
Received: 03 March 2023; Accepted: 01 June 2023;
Published: 23 June 2023.
Edited by:
Todd Atwood, U.S. Geological Survey, United StatesReviewed by:
Emily Virgin, Cincinnati Zoo and Botanical Garden, United StatesCopyright © 2023 Sperou, Crocker, Borras-Chavez, Costa, Goebel, Kanatous, Krause, Trumble and Kienle. This is an open-access article distributed under the terms of the Creative Commons Attribution License (CC BY). The use, distribution or reproduction in other forums is permitted, provided the original author(s) and the copyright owner(s) are credited and that the original publication in this journal is cited, in accordance with accepted academic practice. No use, distribution or reproduction is permitted which does not comply with these terms.
*Correspondence: Emily S. Sperou, ZW1pbHlfc3Blcm91MUBiYXlsb3IuZWR1
Disclaimer: All claims expressed in this article are solely those of the authors and do not necessarily represent those of their affiliated organizations, or those of the publisher, the editors and the reviewers. Any product that may be evaluated in this article or claim that may be made by its manufacturer is not guaranteed or endorsed by the publisher.
Research integrity at Frontiers
Learn more about the work of our research integrity team to safeguard the quality of each article we publish.