- 1Environmental Defense Fund, Seattle, WA, United States
- 2Environmental Defense Fund, Santa Barbara, CA, United States
- 3Marine Science Institute, University of California, Santa Barbara, Santa Barbara, CA, United States
- 4Perry Institute for Marine Science, Waitsfield, VT, United States
- 5Gulf and Caribbean Fisheries Institute, Marathon, FL, United States
- 6Department of Marine Resources, Ministry of Agriculture, Marine Resources and Local Government, Nassau, Bahamas
- 7Ecosystem-Based Management Unit, Belize Fisheries Department, Belize City, Belize
- 8Environmental Defense Fund, San Francisco, CA, United States
- 9Institute of Marine Sciences, University of California, Santa Cruz, Santa Cruz, CA, United States
- 10Environmental Defense Fund, Boston, MA, United States
- 11Turneffe Atoll Sustainability Association, Belize City, Belize
- 12Environmental Defense Fund, Belize City, Belize
- 13Coastal Zone Management Authority and Institute, Belize City, Belize
- 14Fishing Guide, Sweetings Cay, Bahamas
- 15Environmental Defense Fund, Austin, TX, United States
Recreational fishing is a pillar of the multibillion-dollar tourism sector in the Caribbean, supporting economic development and community livelihoods. However, as climate change drives increased habitat degradation, key recreational target species may experience declines. To effectively prioritize adaptation and mitigation efforts it is critical to project climate change impacts on recreational species and the communities that depend on them. We conducted a comprehensive climate vulnerability assessment (CVA) for three recreationally important tidal flats species in Belize and The Bahamas: bonefish (Albula vulpes), tarpon (Megalops atlanticus), and permit (Trachinotus falcatus). Species vulnerability was assessed by coupling 1) a research-based CVA to evaluate the sensitivity and exposure of species to climate impacts with 2) a participatory workshop involving 17 fishing guides, resource managers, and science and policy experts working in fisheries systems in Belize and/or The Bahamas. The workshop elicited local expert knowledge to resolve and contextualize CVA scoring and to identify strategies to increase climate resilience. According to the research-based CVA, key climate factors in the Caribbean are expected to see a ‘very high’ magnitude of change by 2050. All three species exhibit ‘very high’ vulnerability to these changes based on life history traits and reliance on nearshore habitats that are exposed to rapid temperature increases and storm damage. The expert stakeholder group confirmed a ‘very high’ magnitude of expected climate impacts in the Caribbean region, to which bonefish, the most valuable species, is likely to have a ‘very high’ vulnerability. However, stakeholders perceived tarpon and permit to be less vulnerable to these impacts than the CVA predicted, based on “on the water” observations of their habitat flexibility and resilience to disturbance. The group identified strategies at the individual, community, national, and international levels to enhance climate resilience in the recreational fishing sector. Our work highlights how participatory CVA processes can support a stronger understanding of species’ vulnerability while building capacity and collaboration to increase climate change readiness.
Highlights
● Recreational fisheries are a key source of income and livelihoods in the Caribbean
● We assess climate vulnerability combining desktop analyses and local knowledge
● Climate change impacts are already apparent and are expectedto increase by 2050
● Three key recreational fishes have traits conferring high to very high vulnerability
● We suggest solutions to increase resilience in the Caribbean recreational sector
1 Introduction
Climate change is posing serious and accelerating threats to tropical marine ecosystems and fisheries. Many tropical species live close to their thermal limits, and a mass redistribution of marine life is predicted as water temperatures rise and suitable thermal regimes shift (Pinsky et al., 2020). This redistribution is expected to result in declines in stock biomass available to local tropical fisheries (Cheung et al., 2010; Lam et al., 2016), and the complete loss of some species from the exclusive economic zones (EEZs) of tropical nations (Oremus et al., 2020). Furthermore, ongoing and intensifying threats related to climate change, including more severe storms, sea-level rise, ocean acidification, and run-off associated with changes in patterns of precipitation will continue to degrade important habitats including coral reefs, mangroves and seagrass beds (Hoegh-Guldberg et al., 2007; Waycott et al., 2009; Sippo et al., 2018), which provide food and shelter for many species at key life stages. In the absence of effective mitigation and adaptation efforts, these cumulative threats layered on top of other human-induced stressors such as overfishing, coastal pollution, and shoreline development could severely impact the abundance and productivity of key species and result in losses of income, food security, and livelihoods for millions of people dependent on fisheries and marine resources in coastal and island communities throughout tropical regions (Lam et al., 2020; Golden et al., 2021; Teneva et al., 2023).
Recreational fisheries are vital components of the economic and social fabric of nations in the Caribbean, yet their future under climate change remains uncertain. Recreational fishing can create greater economic value from decreased fisheries biomass due to the added value that associated tourism activities bring to communities such as lodging, food, transport, and other provisions (Tufts et al., 2015; Fedler, 2019; Smith et al., 2022). When managed sustainably, recreational fisheries can have a lower impact on stock health than commercial fleets if catch-and-release requirements are in place and post-release mortality rates are low (Tufts et al., 2015). Recreational fishers can also be environmental stewards, lending powerful support to habitat conservation and restoration efforts (Cowx et al., 2010). For these reasons, enhancing the recreational sector may provide opportunities for communities to bolster fishing livelihoods, even under climate change (Uddin et al., 2021).
However, recreational fisheries rely on high-quality long-term fishing experiences. The Caribbean is home to some of the most picturesque fishing locations in the world, and opportunities to catch some of the largest and most exciting sport fish in tidal flats, blue water, and reef ecosystems (Fedler, 2010). Increased scarcity of fish due to a combination of climate change and unsustainable fishing practices, or habitat degradation arising from storms, dredging and development, could lead to a decline in the quality of fishing experiences and concomitant declines in angler participation and tourism revenue (Townhill et al., 2019). To maintain high quality recreational opportunities into the future, climate impacts need to be mitigated via targeted habitat restoration and carbon sequestration efforts and by implementing adaptive, climate-smart fisheries management. Additionally, developing demand and infrastructure to support sustainable fisheries tourism within communities, strengthening management and governance to increase sustainability (Arlinghaus and Cooke, 2009), and minimizing leakage of economic benefits via empowerment of local operators, lodges, and guides can help enhance resilience of the recreational fisheries sector under climate change (Butler et al., 2020; Sandbrook, 2010; Wood et al., 2013).
To guide mitigation and adaptation efforts, it is important to understand the magnitude of climate change impacts, and the relative risks that they pose to different marine species. Climate vulnerability assessments (CVAs) are a common tool to understand the relative vulnerability of species to climate change based on their functional traits (Hare et al., 2016). While desktop assessments of climate vulnerability can be useful, they are often hampered by an incomplete picture of local climate conditions and species’ ecology. Experts with local and experiential knowledge are likely to be among the first to notice climate change impacts “on the water” (Seara et al., 2020). Local knowledge can fill key gaps in data-limited systems by refining scientific understanding of climate change at local scales and identifying sensitivity and resilience attributes of marine systems to these perturbations (Gianelli et al., 2021; Mason et al., 2022; Mills et al., 2022). More importantly, involving local experts including the fishing community can help to build a community-of-practice and increase capacity for assessing and responding to climate change impacts (Samhouri et al., 2019; Eurich et al., 2023). This encourages an inclusive management approach that allows research and conservation priorities to be co-developed by stakeholders who are at the frontline of climate change, as opposed to management strategies that are imposed externally or strictly from management authorities (Karr et al., 2017). Ultimately, communities should be empowered to design and implement their own solutions to climate change that are in line with local stewardship practices and that manage the unique suite of tradeoffs relevant to a fishery’s social-ecological context.
We conducted a CVA for three important recreational fishery species in the Caribbean: bonefish (Albula vulpes), tarpon (Megalops atlanticus), and permit (Trachinotus falcatus). These tidal flats species have enormous economic and social importance in the Caribbean, supporting hundreds of millions of dollars in revenue annually and thousands of jobs (Fedler, 2014; Fedler, 2019). Recent work has begun to explore the complex, cascading impacts of climate change on tidal flats ecosystems and on the recreationally important species that depend on them (Murchie et al., 2011; Danylchuk et al., 2023). However, there remains much to learn regarding species-specific vulnerability to climate change, how stakeholders perceive climate threats, and how local communities can be empowered to address climate challenges (Danylchuk et al., 2023). We first undertook a comprehensive desktop analysis of available information on the expected magnitude of change in key climate factors, and an assessment of each species’ sensitivity to expected changes by conducting a review of their key functional traits. We then convened a participatory workshop with an expert multi-stakeholder group from Belize, The Bahamas, and the United States consisting of local fishing guides, fishery managers, and scientists (Table S1), with the aim of amplifying diverse perspectives within the recreational fishing and resource management sectors and fostering inclusive research practices in the Caribbean region (Halpern et al., 2023). This group discussed and refined vulnerability scores from the desktop analysis, identified socioeconomic implications of vulnerability in the fishery, and outlined a series of recommendations that could be undertaken by governments and communities in Belize and The Bahamas to increase climate resilience in the recreational sector.
Our study aims to assess species-specific vulnerability of bonefish, tarpon, and permit to climate change, examine how stakeholders perceive climate threats, and identify pathways for local fishing communities to address climate challenges. This is a first step toward developing stronger local capacity and preparedness to respond to climate change within the Caribbean recreational fishing sector.
2 Materials and methods
2.1 Geographic scope
The geographic scope of the study was the region encompassing the Caribbean Large Marine Ecosystem (CLME), extended to include the EEZs of Caribbean nations in the Atlantic, inclusive of the EEZs of The Bahamas and Belize (Figure 1). This regional approach was taken to encompass major ecosystems and oceanographic processes in the region. Despite this regional focus for assessing the physical and ecological impacts of climate change, the social and economic focus of the assessment was limited to Belize and The Bahamas. The two nations have different fisheries governance regimes, and these contexts are important for understanding and contrasting the ways in which species-level vulnerability to climate change may translate into outcomes for fisheries and fishery-dependent communities.
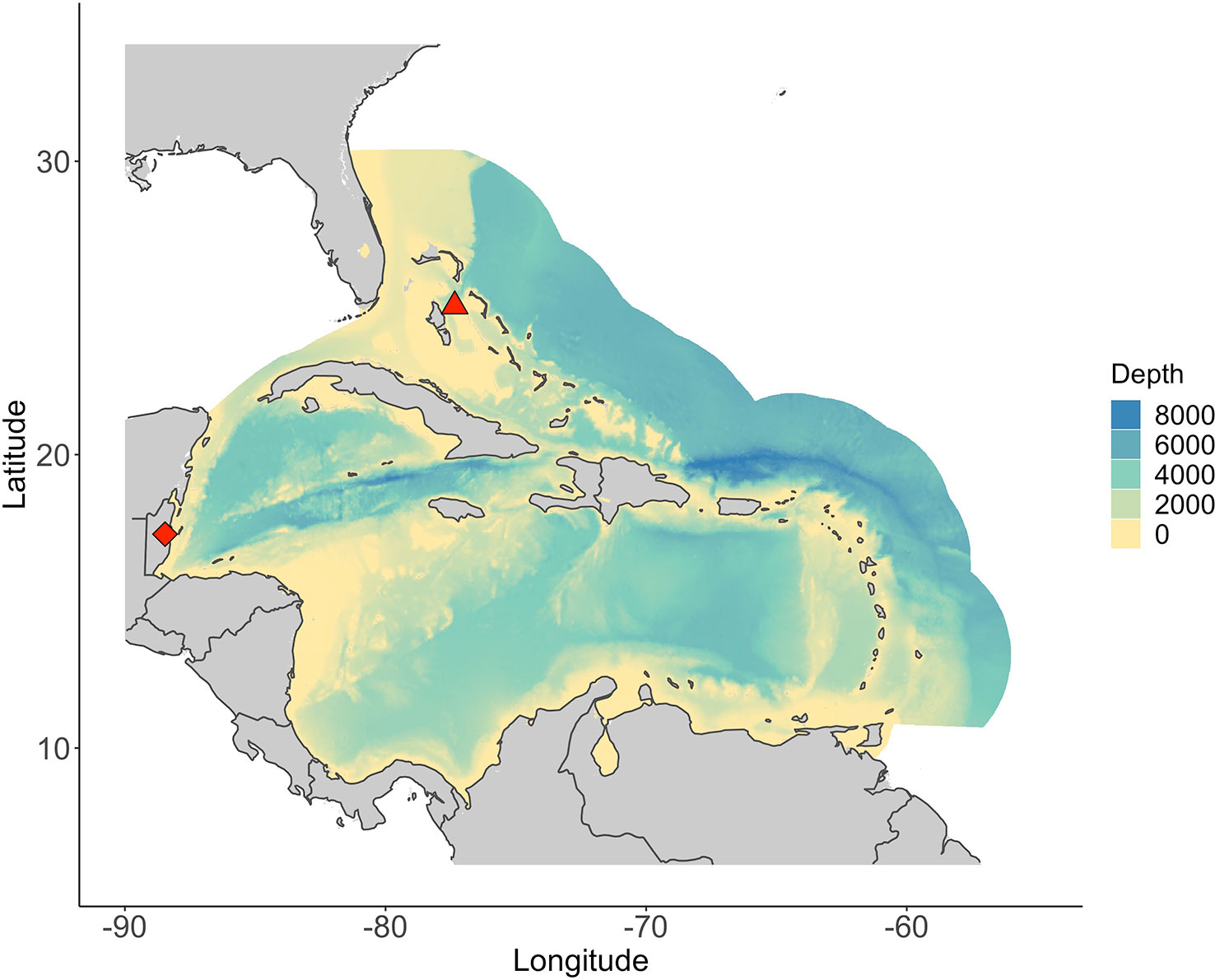
Figure 1 Map of the study area considered in analyses of climate vulnerability of recreational fishery species in the Caribbean. The study area comprises the Caribbean Sea large marine ecosystem extended out to the edge of the 200 nautical mile exclusive economic zones (EEZs) of Caribbean nations in the Atlantic Ocean. Seafloor depth (m) is shown, and the locations of the cities of Nassau (The Bahamas) and Belmopan (Belize) are denoted by a red triangle and diamond respectively.
2.2 Species selection
The focus of the assessment was the recreational ‘flats’ fisheries in Belize and The Bahamas, which are dominated by catches of bonefish (Albula vulpes), tarpon (Megalops atlanticus), and permit (Trachinotus falcatus). These fishes inhabit coastal tidal flats ecosystems including estuaries and mangroves, often school in large numbers during pre-spawning and spawning aggregations and are caught on light tackle in shallow water by recreational anglers facilitated by local sport fishing guides. This fishery represents an industry of significant economic and social importance in both Belize and The Bahamas (Fedler, 2014). For example, in The Bahamas, the recreational tidal flats fishing sector contributes $169 million USD to the Bahamian economy and supports 7,800 full-time equivalent jobs (Fedler, 2019), particularly in the “Family Islands” or outer islands where there are few economic opportunities outside of tourism centers (e.g., Nassau). In Belize, the recreational tidal flats fishing sector contributes upwards of $50 million USD to the economy and supports upwards of 2,000 full-time jobs (Fedler, 2014). All three species are protected in both nations by commercial fishing bans (all three species in “the flats” in The Bahamas and in all marine waters in Belize), net restrictions, and catch-and-release regulations to reduce fishing pressure on their populations. However, anglers fishing the flats with an appropriate license are allowed to retain one bonefish, tarpon, or permit for personal consumption at any given time in The Bahamas. Understanding the vulnerability of this fishery to climate change impacts, especially alongside other stressors including coastal development, dredging, pollution, and commercial fishing pressure, will be important to help the sector build resilience to change (Battista et al., 2017; Mason et al., 2022).
2.3 Desktop climate vulnerability assessment
To assess climate vulnerability in Caribbean recreational fisheries, we first conducted an extensive literature-based desktop analysis to score 1) climate exposure – the expected magnitude of changes in ocean climate likely to be experienced by each species over the coming 30 years, and 2) species sensitivity – biological traits that might increase species’ likelihood of responding negatively to climate exposure and that limit their ability to cope, resist, or recover from the effects of climate change (i.e., adaptive capacity; Hare et al., 2016). Taken together, exposure and sensitivity constitute the vulnerability of a species to climate change impacts.
The literature review was not systematic, but in all cases began with a Google Scholar search for the species or climate variable of interest with the keywords ‘Caribbean’ and ‘Caribbean + climate change’ as a starting point to find relevant published material to score each factor according to a range of criteria (details in Hare et al., 2016). In addition to primary literature for each species, the online database FishBase (www.fishbase.se) was used to identify numerical values and sources of biological information, and the IUCN Red List of Threatened Species (https://www.iucnredlist.org/) report for each species was used to identify additional primary sources. During the literature review stage, local experts were also invited to submit relevant material to score each factor.
2.3.1 Climate exposure
We scored the expected magnitude of change in 12 climate exposure factors on a Likert scale of 1 (‘low’ magnitude of change) to 4 (‘very high’ magnitude of change) following the published framework and associated scoring criteria found in Hare et al. (2016). We selected factors that are likely to impact ecosystems and fisheries in the Caribbean based on the IPCC report (Bindoff et al., 2019) with feedback from the expert stakeholder group: 1) storm frequency, 2) storm severity, 3) precipitation, 4) sea level rise, 5) coastal erosion, 6) sea surface temperature, 7) variability in sea surface temperature, 8) dissolved oxygen, 9) ocean acidity, 10) surface salinity, 11) ocean currents, and 12) upwelling intensity. Where possible, the expected magnitude of change in these factors in the Caribbean was determined using CMIP6 ensembled global climate models (https://psl.noaa.gov/ipcc/cmip6/) forced by a “fossil fuel-based development” scenario representing about a 4.5°C change in global surface temperature by year 2100 (SSP5-8.5). The magnitude of change in each factor was calculated as a standardized anomaly value indicating their change over the coming 30 years (2020–2049) relative to a baseline for the previous 30 years (1990–2019) (see Hare et al., 2016 for threshold anomaly values used for scoring the magnitude of change). To supplement these projections and to score factors that are not represented in global climate models, we conducted a literature review for studies that described alternative model projections, or historical trends to infer the likely direction and rate of change over the coming 30 years.
The magnitude of expected change in each climate factor was scored once for the whole region, then individual species’ spatial overlap with changes in each factor were also scored on a Likert scale of 1 (‘low’ overlap with expected climate change) to 4 (‘very high’ overlap with expected climate change) based on species distributions and habitat use. Regional scores were multiplied by overlap scores and rescaled between 1 – 4 to produce a final exposure score.
2.3.2 Species sensitivity
We scored 12 sensitivity attributes for each species following Hare et al. (2016): 1) stock status, 2) population growth, 3) habitat specificity, 4) prey specificity, 5) adult mobility, 6) reproductive complexity, 7) spawning cycle, 8) larval dispersal, 9) early life history requirements, 10) sensitivity to temperature, 11) sensitivity to ocean acidification, and 12) other stressors. Attributes were scored on a Likert scale of 1 (species trait confers low sensitivity to climate change) to 4 (species trait confers very high sensitivity to climate change) based on criteria developed in Hare et al. (2016).
2.3.3 Total vulnerability
Total vulnerability was calculated based on the framework in Hare et al. (2016). Overall exposure and sensitivity scores were calculated using a logic rule where three or more factors ≥4 resulted in a final score of ‘very high’, two or more scores ≥3 resulted in a score of ‘high’, and two or more scores ≥2 resulted in a score of ‘moderate’, while all other scores resulted in a score of ‘low’. An overall climate vulnerability score was calculated by multiplying the overall exposure and sensitivity scores. The product of the two component numeric scores results in a value between 1 and 16. The overall climate vulnerability rank is then classified as: 1–3 ‘low’, 4–6 ‘moderate’, 8–9 ‘high’, and 12–16 ‘very high’.
2.4 Participatory climate vulnerability assessment workshop
We assembled a group of 17 experts (14 stakeholders plus three facilitators) who have expertise in recreational fishing and guiding, fisheries management, and/or fisheries and climate science in Belize and/or The Bahamas (for a list of participants, their country/ies of expertise and job titles, see Appendix 1; Table S1). The group had a combination of scientific knowledge and local “on the water” experience, which helped develop a comprehensive and complementary picture of climate change and its likely impacts on fishery species in the Caribbean, and of the likely impacts of these changes on recreational fisheries and communities. Although this was a relatively small group and did not represent the full range of identities, experiences and perspectives of the recreational fisheries community across the two nations, participants were selected to achieve a balance between representatives from Belize and The Bahamas, and from different professional groups. Group size was constrained in order to allow each participant space to voice their concerns fully in the workshop. The smaller group size was also designed to facilitate the development of a “community of practice” to discuss climate change impacts in the region, and to foster increased communication and trust across national and professional boundaries.
Stakeholders were first engaged in discussions around study design (e.g., species selection) as well as community and capacity-building needs to ensure the workshop reflected the interests and priorities of the community. The group then convened over the course of a two-day virtual workshop in March 2022 to exchange information on observed climate change impacts, contextualize and refine desktop vulnerability scores for key species, and identify challenges and opportunities for responding to climate impacts in Belize and The Bahamas. During the workshop we used two complementary sessions to allow participants to provide their insights into climate exposure and species sensitivity: 1) a scientific knowledge session, and 2) a local “on the water” session to provide additional observations and context.
2.4.1 Scoring and contextualization of climate vulnerability
In the breakout group focused on capturing local scientific knowledge, we used Mentimeter, a web-based polling tool, to allow participants to score 1) each regional climate exposure variable, and 2) the sensitivity attributes of each species (Appendix 2). Participants were presented with a summary of the desktop research for each factor and asked to score the factor based on their interpretation of the scientific information and their own personal observations or insights using the same Likert scale as the desktop assessment (i.e., ‘low’ to ‘very high’). The Mentimeter tool was used to derive a mean and distribution of scores across all participants that could be compared with the original desktop score. A concurrent breakout group of local guides and managers focused on capturing “on the water” local knowledge. We elicited qualitative narratives from guides and managers about observed climate change impacts in local areas, and descriptions of the distribution, habitat selection, and behavior of primary target species (i.e., bonefish, tarpon, and permit). Participants in the “on the water” session were asked to reflect on the desktop scores, with a focus on identifying which were the most concerning climate change impacts in their system, and their opinions of the relative vulnerability of the three flats species.
These narratives and observations were presented alongside numerical scores in plenary to the broader workshop to provide additional context for understanding perceptions of climate change impacts, particularly by recreational fishing guides. To assess alignment of local knowledge and expectations with predictions from scientific literature and global climate models the group participated in a facilitated discussion to decide if any species scores needed to be altered based on the local knowledge from the “on the water” session with guides and managers.
2.4.2 Socio-economic implications of climate change impacts and strategies to increase resilience
Participants were divided into two groups representing Belize and The Bahamas respectively for a structured discussion of the socioeconomic implications of predicted ecological impacts and potential adaptation and mitigation measures. As part of this discussion, participants were asked a series of questions (Appendix 3) to define: 1) what a “good day” of fishing and guiding looks like, 2) what types of declines in the fishery would start to impact their livelihoods, and 3) ways in which climate change has already impacted livelihoods. Each group then identified strategies to increase resilience within the recreational fishery and associated communities. This discussion included immediate next steps and long-term strategies to mitigate the ecological and socioeconomic impacts and, where necessary, to adapt to a changing fishery.
3 Results
3.1 Desktop climate vulnerability assessment
3.1.1 Climate exposure
The desktop analysis highlighted expected changes in key climate variables in the Caribbean by 2050, based on interpretation of global climate model outputs and a literature review (Figure 2). However, while there are some climate factors for which there is a relatively strong evidence base and scientific consensus (e.g., temperature, precipitation, and sea level rise that are well-resolved by global climate models), much uncertainty remains regarding other dimensions of climate change (e.g., changes in ocean currents and upwelling dynamics). A summary of current knowledge regarding changes in climate exposure factors (numbered and italicized) is included below, along with information on observed or expected impacts of these changes on ocean ecosystems. Information for each climate exposure factor including Likert scores is described in Table 1. We present information on climate change for the whole study region, but scores for exposure of each individual species to these impacts weighted based on spatial overlap are presented in Table S2 (Appendix 4), along with information regarding data quality.
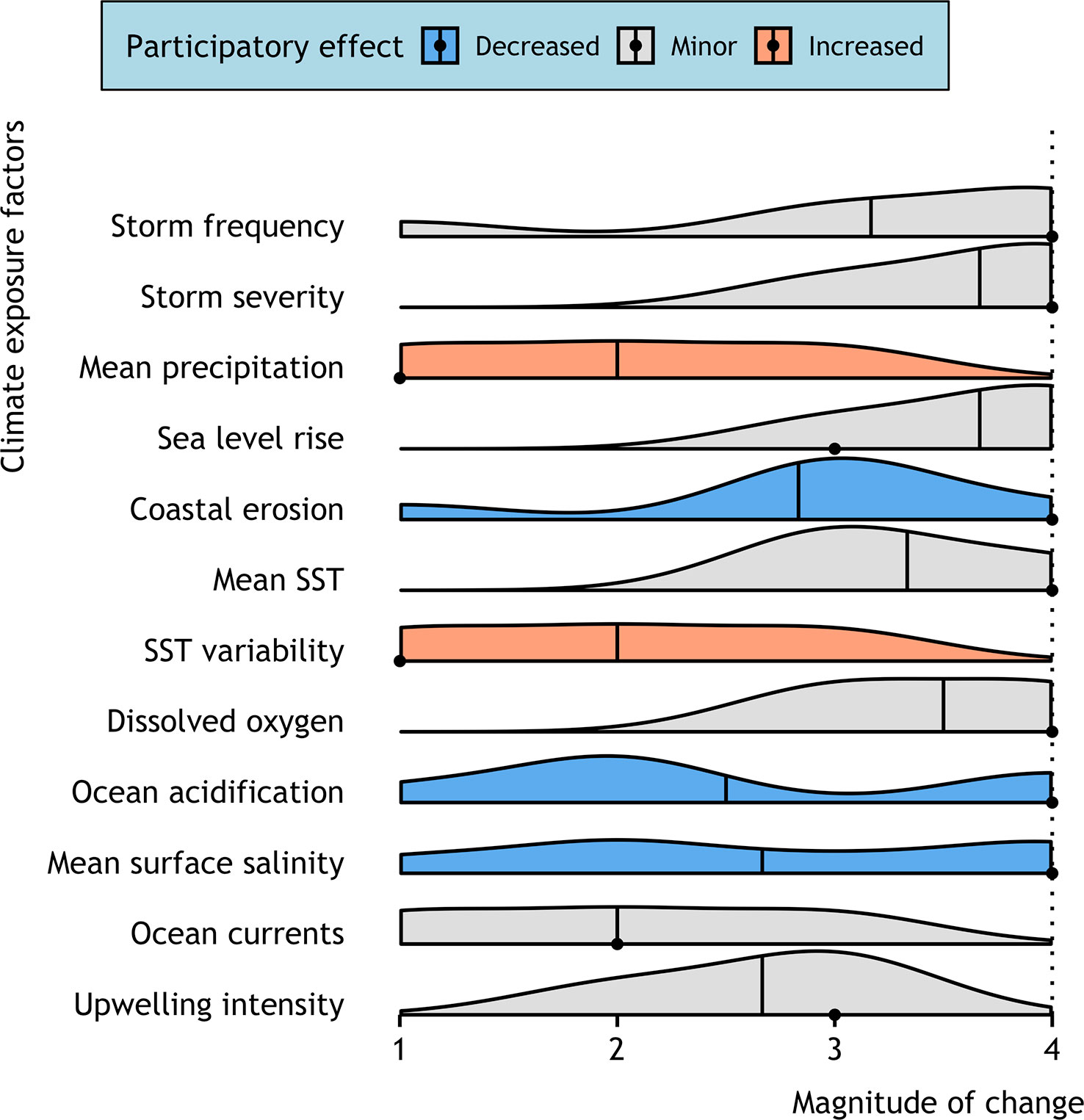
Figure 2 Predicted magnitude of change in ocean climate factors in the Caribbean in the 30 years from 2020–2049 compared to a 30-year baseline of 1990–2019. Smoothed density plots for each exposure factor represent the distribution of scores from the participatory workshop after stakeholders contextualized and refined the desktop analysis results. Participants independently scored each factor as having a 1) ‘low’, 2) ‘moderate’, 3) ‘high’, or 4) ‘very high’ magnitude of change. The mean responses are denoted by a solid line with a dashed line showing the final score. Exposure factor distributions are colored if the desktop analysis score (point) decreased (blue) or increased (orange) following the participatory exchange of information on local climate change impacts where the participant mean score would have resulted in a new category being chosen (i.e., change ≥1). If the stakeholder group confirmed the desktop vulnerability score or a score changed with minor directionality (i.e., change ≤1) no color is presented (gray).
3.1.1.1 Storm impacts, sea level rise, and coastal erosion
Storm damage already poses a significant threat to fisheries and communities in the Caribbean (Heck et al., 2021) and the region has been suggested to be a hotspot for increasing tropical storms, with warming waters providing more fuel to power destructive storms. Despite low certainty surrounding regional predictions of changes in storm activity in the future as a function of global warming (Vecchi et al., 2021), we assessed both 1) storm frequency and 2) storm severity to have a very high magnitude of change over the next 30 years due to expected increases in impacts of storm damage to habitats and fishing communities across the Caribbean. The effects of storm damage on coastal systems are likely to be magnified by increased flood risk due to changes in 3) precipitation and concurrent 4) sea level rise (Vosper et al., 2020) which are expected to cause increasing damage to assets and infrastructure in the future (Mendelsohn et al., 2012). The Bahamas is predicted to see a slight increase in average rainfall of approximately 20-30 mm per year by 2050, while most of the Caribbean Sea including Belize will see a slight to moderate decrease of approximately 70 mm per year based on the fossil fuel-based development scenario SSP5-8.5 from the CMIP6 ensemble of global climate models. However, changes in seasonal to interannual patterns of rainfall not captured by annual averages are already apparent, and drought and flooding at local to regional scales as a result of increased variability is likely (KS, pers. comm.). Any changes in precipitation will influence river flow, turbidity, agricultural runoff, and patterns of sedimentation. These climate impacts, paired with sea level rise and increases in 5) coastal erosion, are expected to severely impact seagrass beds, mangroves, and tidal flats (Albert et al., 2017), all critical nearshore habitats for bonefish, tarpon, and permit.
3.1.1.2 Sea surface temperature and ocean chemistry
Mean 6) sea surface temperature is predicted to increase significantly in the Caribbean over the next 30 years, despite a low projected magnitude of change in the 7) variability in sea surface temperature, based on CMIP6 SSP5-8.5. This continues a long-term warming trend in the Caribbean that is in line with recent increases in global sea surface temperatures (Glenn et al., 2015). In addition to temperature, 8) dissolved oxygen is predicted to decrease globally in association with ocean warming, and coastal, tropical reef systems such as those in the Caribbean are expected to be hotspots of decreased oxygen levels (Hughes et al., 2020). The Caribbean Sea has also already experienced increasing 9) ocean acidity (Friedrich et al., 2012), with an ongoing decline in oceanic pH resulting from the uptake of atmospheric CO2 that is expected to continue in coming decades. The cumulative impact of acidification has already been observed to compromise Caribbean corals and other marine calcifiers (Albright et al., 2010). Lastly, projected changes in ocean 10) surface salinity due to increased temperature, precipitation, and runoff are expected to impact seawater density, which in turn can influence ocean currents, circulation, and regional weather patterns, as well as species’ physiological responses.
3.1.1.3 Ocean currents and upwelling
There are few studies specifically looking at climate-driven changes in regional 11) ocean currents in the Caribbean. In the broader North Atlantic region, a decrease in velocity of the Gulf Stream has been projected under climate change, though evidence for an early signal in altimetry data or in situ measurements is mixed (Dong et al., 2019; Zhang et al., 2020). It remains unclear whether any slowdown will occur after Gulf Stream waters have already flowed north out of the Caribbean region, or how changes might affect the Caribbean. Conversely, 12) upwelling intensity has decreased over recent decades in the Cariaco basin, Venezuela, apparently due to a decline in upwelling-favorable wind stress linked to large-scale climate change (Taylor et al., 2012). This has led to increased stratification and reduced delivery of nutrients to surface waters in the coastal Caribbean, resulting in lower net primary production and reduced phytoplankton bloom intensity. However, evidence for changes in upwelling in other parts of the Caribbean is mixed, with some evidence for an increase in winter upwelling in La Guajira upwelling system off Colombia (Santos et al., 2016).
3.1.2 Species sensitivity
All species were found to have a ‘high’ to ‘very high’ sensitivity to climate exposure based on the desktop analysis of their biological traits. Tarpon was found to have ‘very high’ biological sensitivity, whereas biological sensitivity for bonefish and permit were scored as ‘high’ based on the 12 sensitivity attributes (Appendix 4). A description of key traits contributing to sensitivity are described below; scores for each sensitivity attribute are presented in Figure 3 (Table S3).
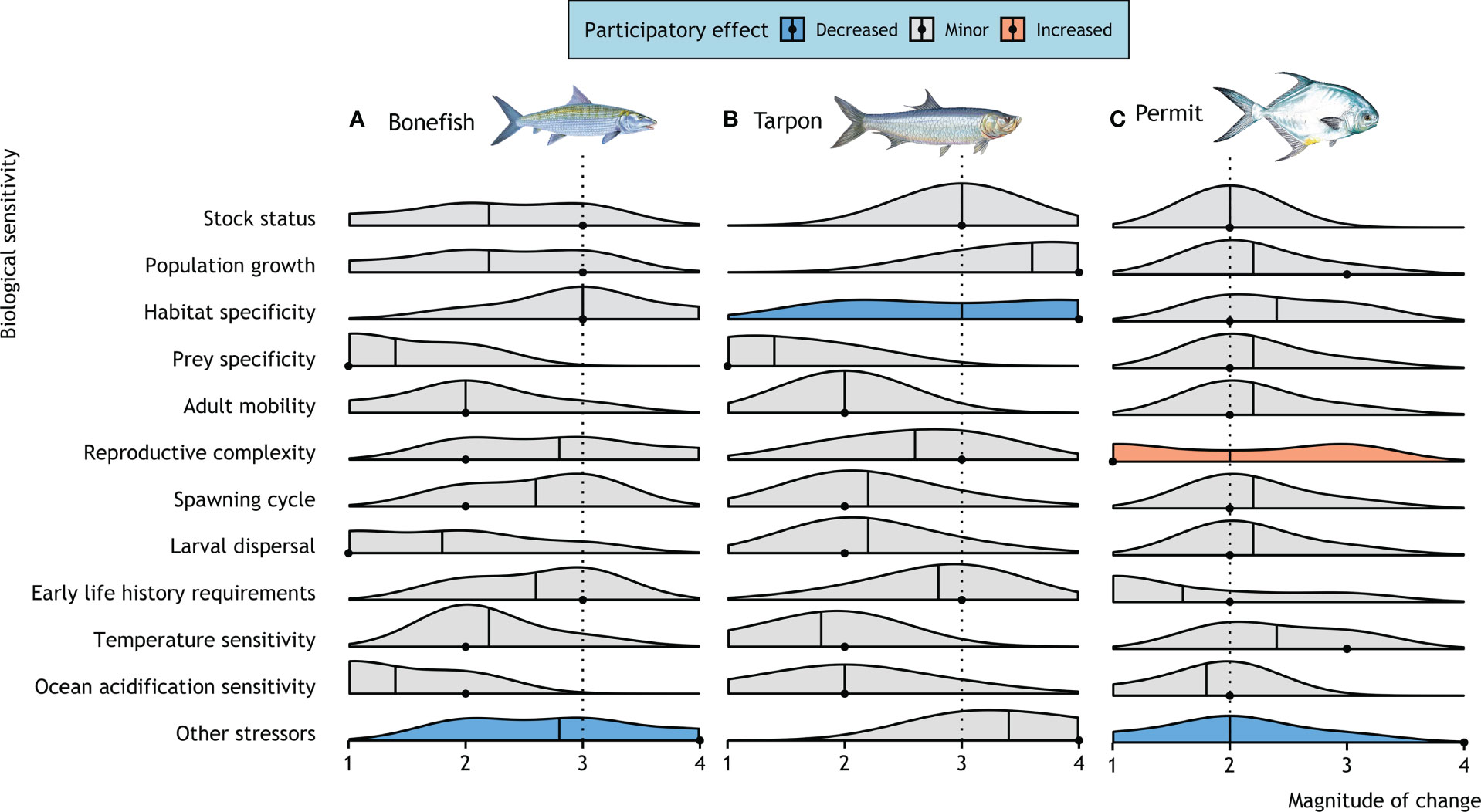
Figure 3 Biological sensitivity attributes of three recreationally important tidal flats species in the Caribbean: (A) bonefish (Albula vulpes), (B) tarpon (Megalops atlanticus), and (C) permit (Trachinotus falcatus). Smoothed density plots for each sensitivity attribute represent the distribution of scores from the participatory workshop. Participants independently scored each factor based on information from the desktop analysis contextualized by their own expert local knowledge. Scores are on a scale of 1) ‘low’, 2) ‘moderate’, 3) ‘high’, or 4) ‘very high’ sensitivity to climate change. The mean responses are denoted by a solid line with a dashed line showing the final score. The distributions are colored if the desktop analysis score (point) decreased (blue) or increased (orange) following the participatory exchange of information where the participant mean score would have resulted in a new category being chosen (i.e., change ≥1). If the stakeholder group confirmed the desktop vulnerability score or a score changed with minor directionality (i.e., change ≤1) no color is presented.
3.1.2.1 Bonefish
Bonefish stock status is listed as Near Threatened by the IUCN (Adams et al., 2012), with a 42% reduction in catch and large declines in catch-per-unit-effort in South Florida observed since 1980 (Santos et al., 2017; Klarenberg et al., 2019). No stock assessments or estimates of abundance are available for bonefish in Caribbean waters. However, bonefish have demographic characteristics that confer a moderate to high sensitivity to disturbance with ~20 year lifespans, low natural adult mortality, and relatively fast growth rates to their maximum size of 80 cm (Larkin, 2011).
Bonefish inhabit shallow tidal flat ecosystems including those comprising seagrasses, mangroves, sandy bottoms, and tidal creeks (Perez et al., 2019). Thus, bonefish are sensitive to broadscale reductions in the availability or quality of critical nearshore habitats. Due to their habitat specificity to extremely shallow (<2 m) nearshore waters, bonefish are exposed to large temperature fluctuations (>12°C) across daily tidal cycles, which may give them a physiological buffer to cope with the effects of ocean warming. However, upper critical thermal limits for bonefish have been determined to be 37°C, and under worst-case IPCC projections, waters in their preferred feeding habitat are likely to exceed this critical threshold 18% of the time by 2100 (Murchie et al., 2011).
Adult bonefish demonstrate high site fidelity throughout their life cycle, with daily foraging ranges of approximately 2 km across multiple habitats (Murchie et al., 2013). However, bonefish adult mobility is high (movements of >100 km), specifically during seasonal migrations to spawning grounds (Larkin et al., 2011; Murchie et al., 2013). Thus, healthy bonefish stocks rely on a mosaic of different interconnected habitats and corridors (Adams et al., 2023). Additionally, larval dispersal is high with dispersing distances of 100–1000s of km from spawning sites (Zeng et al., 2019). Mobility characteristics of both adults and early life history stages indicate the ability of bonefish to colonize suitable new areas if conditions on ‘home flats’ deteriorate (Boucek et al., 2019). However, new potential ‘home flats’ may not be easily discovered by bonefish if the habitat mosaic needed for bonefish throughout its lifecycle is insufficient. It is likely that spawning is optimized to environmental conditions, with adult bonefish being observed to test properties of the water column prior to releasing gametes (Lombardo et al., 2020).
3.1.2.2 Tarpon
Tarpon have population growth characteristics that are sensitive to climate disturbance. Tarpon are long-lived, with a maximum age of 55 years, typically maturing at 6-7 years old, and growing slowly to a maximum length of 250 cm (Schneider, 1990). Although there is no current estimate of stock status, there have been global declines of at least 30%, including major declines in previously well-described fishing areas, which are now almost devoid of tarpon (Ault, 2010). These declines in tarpon stocks have led to a listing of Vulnerable by the IUCN (Adams et al., 2019).
Tarpon occupy many habitats, requiring marshy mangrove or anoxic freshwater swamp habitats as juveniles (Ault, 2010; Elmo et al., 2021) and moving to coastal or up-river habitats as adults (Ault, 2010; Griffin et al., 2018; Kurth et al., 2019). Despite diverse adult habitat specificity, tarpon distribution may ultimately be limited by the highly specific and vulnerable habitat availability (e.g., wetlands) that are required for juveniles (Elmo et al., 2021). Although adult tarpon have fidelity to specific locations, they are capable of migrating large distances to warm oceanic waters where they release gametes in spawning aggregations (Griffin et al., 2018). Tracked tarpon experienced an ambient temperature range of 20–32°C and migrations appear to follow the 26°C isotherm, which may represent the optimal temperature for gonad development (Luo and Ault, 2012), indicating that tarpon may redistribute to follow this isotherm as thermal regimes shift, if habitat availability and connectivity allows.
3.1.2.3 Permit
There is no evidence that permit populations are in decline in the Caribbean, and the species is listed as Least Concern by the IUCN (Smith-Vaniz et al., 2015). Permit can live to ~25 years, mature at 2-3 years, and reach a maximum size of 120 cm (Crabtree et al., 2001). Juvenile permit are found in both estuary and open coastal sandy beach habitats (Adams and Blewett, 2004; Andrades et al., 2012), whereas adults move between shallow flat sandy areas (< 3 m) to forage and deeper reef areas (15–40 m) to spawn (Brownscombe et al., 2020). Stable isotope analyses indicate that permit may rely heavily on seagrass beds for foraging (Brownscombe et al., 2022). Permit remain within a home range of 100 km but move frequently among distinct habitat types within this area (Brownscombe et al., 2022), indicating some potential for redistribution by adults under unfavorable climate conditions.
Permit spawn from February to October depending on location, forming large aggregations with thousands of fish gathering on natural and artificial offshore structures where they swim in a spiral pattern while releasing gametes. The larvae produced by permit populations in Belize and Cuba have high local larval retention to specific spawning sites (Bryan et al., 2015), which may result in ecological ‘traps’ if conditions in these areas become less favorable under climate change.
3.1.2.4 Additional stressors for flats fishes
Bonefish, tarpon and permit are also exposed to additional stressors that could decrease their resilience to climate impacts. These other stressors encompass threats from coastal development including dredging, limestone mining, and reclamation of tidal flats for hotel and marina development. These impacts will degrade or destroy important nursery, spawning, and feeding habitat. Environmental degradation may also result in decreased connectivity among habitats, including impacts to corridors between juvenile and adult habitats. Although all three species are catch-and-release only in Belize and commercial harvest for bonefish, tarpon, and permit is not allowed in the flats in The Bahamas, all species are commercially harvested in other parts of the Caribbean including Cuba. Illegal fishing also occurs, and there is a recreational possession limit of one fish for these species in The Bahamas. In the recreational sector, post-release mortality is also a threat, with significant predation by lemon sharks on released bonefish (Cooke and Philipp, 2004; Danylchuk et al., 2007; Adams, 2017).
3.2 Participatory climate vulnerability assessment workshop
3.2.1 Climate exposure factors
The expert group highlighted several climate factors as likely to cause major threats to fisheries in the Caribbean in the future. After reviewing the desktop analysis and reflecting on their experience of recent storm events, participants agreed that storm activity was one of the most concerning climate change factors in the region (Figure 2). Poll results indicated a perception that storm severity (participatory mean magnitude of change score of 3.7; desktop analysis score of 4) and storm frequency (participatory 3.2; desktop 4) will show ‘high’ to ‘very high’ magnitudes of change over the next 30 years. Participants from The Bahamas highlighted their experience with Hurricane Dorian in 2019, one of the strongest Atlantic hurricanes on record and the strongest hurricane ever to hit The Bahamas. Hurricane Dorian reshaped the flats fishery around the islands of Abaco and Grand Bahama, with devastating impacts for important mangrove habitats. Although there remains some uncertainty surrounding the trajectory of storm severity under future climate change, there was a perception by stakeholders that any increase in the severity of the average storm, or the frequency of severe storms, would have strong, negative consequences for the recreational flats fishery.
Sea level rise was another factor that stakeholders agreed was a pressing issue in the Caribbean, with a ‘very high’ expected magnitude of change over the coming 30 years (participatory 3.7; desktop 3). In addition to sea level rise, coastal erosion was identified as a significant concern by participants from both countries. Although local experts decreased the desktop score significantly (participatory 2.8; desktop 4), several experts in the workshop noted that natural erosion is being compounded by erosion from coastal and inland development. Participants from Belize were especially concerned with dredging upstream in rivers leading to increased erosion and sedimentation on the coast. Concerns surrounding sea level rise and coastal erosion, in combination with storm activity, reflect the reliance of the recreational flats fishery on sensitive inshore ecosystems on sandy, low-lying islands, which are especially vulnerable to these climate impacts.
Participants also noted that mean sea surface temperature is likely to have a high magnitude of change (participatory 3.3; desktop 4), with stakeholders from The Bahamas citing personal observations that waters have already warmed. One guide highlighted that in Grand Bahama, particularly in the summer, bonefish are increasingly seeking refuge in cooler waters in the shade of mangroves. Dissolved oxygen was also predicted to show a ‘high’ to ‘very high’ magnitude of change in the region (participatory 3.5; desktop 4). In both countries, participants noted that while mean rainfall appeared to have a ‘low’ projected magnitude of change based on global climate models (participatory 2; desktop 1), their experiences of rainfall events are becoming more variable. These observations included longer periods without rain and then more intense rain events when they occur. In Belize, it was noted that yearly weather patterns seem to have changed, with dry spells during the rainy season and increased precipitation during the dry season. This suggests that variability in precipitation may be a more important consideration for shaping the experiences of locals in the Caribbean than average trends, and highlights potential impacts on coastal systems via runoff, erosion, salinity, sediment deposition, and turbidity.
Stakeholders did not expect any climate factors to show a ‘low’ magnitude of change (i.e., participatory score <2). However, there was considerably less consensus regarding the direction and magnitude of change in some ocean climate factors. For example, ocean currents (participatory 2; desktop 2) and upwelling intensity (participatory 2.6; desktop 3) both had very wide distributions around the mean, suggesting that changes in these factors were either more contentious or less understood by participants, in line with the paucity of scientific data that was available for these factors.
3.2.2 Species sensitivity
Expert stakeholders noted that the major threats to bonefish, tarpon, and permit result from their specificity to nearshore habitats at various life stages, including seagrass and mangroves associated with tidal flats. Participants scored habitat specificity as ‘moderate’ for permit (Figure 3) (participatory mean score of 2.4; desktop analysis score of 2) to ‘high’ for bonefish (participatory 3; desktop 3) and tarpon (participatory 3; desktop 4). Participants from Belize further highlighted that the sensitivity of all three flats species to changes in habitat corridors from both climate change and human activity was a primary concern in their country. There is also a strong need to better understand habitat use and connectivity in order to better manage threats to habitats of recreational fishes on the flats.
For bonefish, the other main traits that stakeholders identified as likely to confer ‘high’ sensitivity to climate change were reproductive complexity due to the formation of large pre-spawning and spawning aggregations (participatory 2.8; desktop 2), and spawning cycles which occur seasonally and appear to correspond to specific oceanographic conditions (participatory 2.6; desktop 2). Although participants scored bonefish stock size (participatory 2.2; desktop 3) and population growth (participatory 2.2; desktop 3) rates as conferring ‘moderate’ sensitivity, there was a high degree of uncertainty surrounding scores for these factors. Several participants from The Bahamas indicated concerns about bonefish stock declines following Hurricane Dorian in 2019, which destroyed important tidal habitats. Participants highlighted a ‘high’ sensitivity for bonefish resulting from additional stressors on top of recreational fishing and climate change (participatory 2.8; desktop 4), highlighting habitat degradation, subsistence fishing practices, and post-release mortality due to predation or poor handling protocols by inexperienced guides as potential stressors for bonefish populations. While bonefish have legal protection in Belize, the species are still caught and kept for consumption as bycatch from other fishing activities or during illegal and unreported fishing activities, remain a historically important food source for coastal communities, and are a symbol of cultural identity. Consequently, despite an overall participatory score of 2.3 the stakeholders agreed to maintain the desktop analysis output as ‘high’ for the biological sensitivity of bonefish (Table 2).
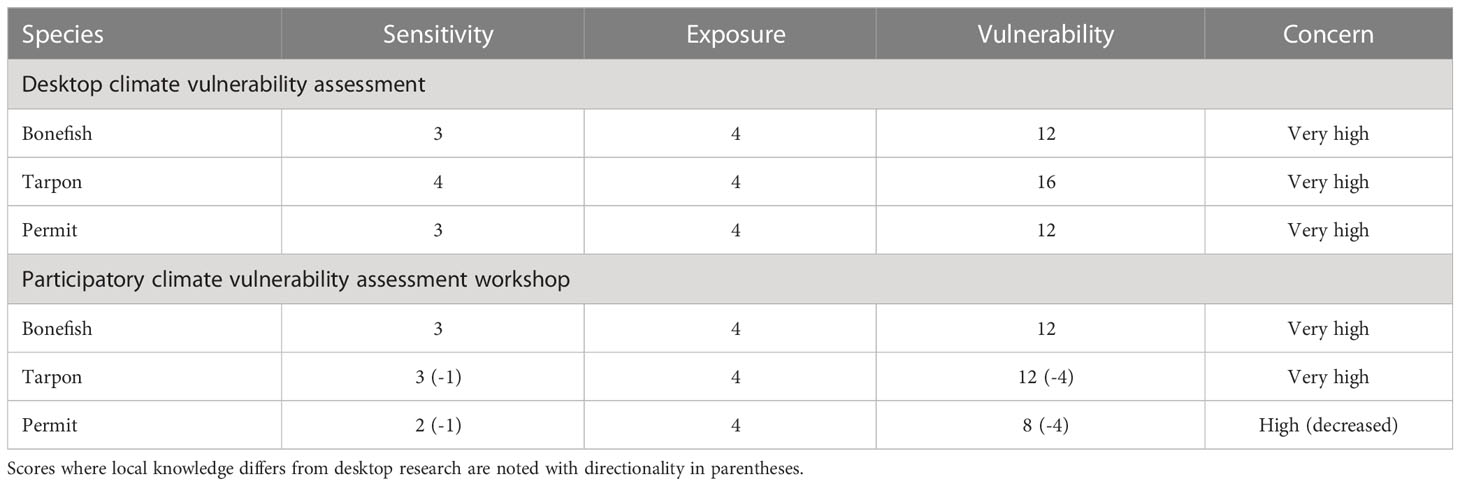
Table 2 Final vulnerability scores from the desktop climate vulnerability assessment and after the participatory workshop (sensitivity x exposure = vulnerability): 12-16 = ‘very high’ concern, 8-9 = ‘high’ concern, 4-6 = ‘moderate’ concern, and 1-3 = ‘low’ concern.
For tarpon, participants from both countries highlighted that, despite slow growth rates (participatory 3.6; desktop 4) and diminished stock size (participatory 3; desktop 3), there is anecdotal evidence that populations may be rebounding. Participants credited the perceived upward trajectory of these tarpon stocks to the 2020 Belize gill net bans (Belize, 2020) and the ban of commercial take of tarpon (in Belize since 2010 and in The Bahamas flats since 2017). However, while gill nets are banned in Belize’s marine waters, the nets can still be used in rivers which may lead to capture of tarpon within rivers as by-catch or targeted illegal fishing activities. There was less consensus surrounding habitat specificity scores for tarpon. Stakeholders scored habitat specificity as ‘high’ (participatory 3; desktop 4), but with a wide distribution in responses. Stakeholders noted that despite the ‘very high’ specificity of tarpon to marshy wetlands in the early juvenile phase, the wide range of habitats in which adult tarpon are caught in the Caribbean (i.e., from freshwater to estuarine to coastal environments) and ability to survive in anoxic environments may ultimately make them more resilient to habitat disturbances. In general guides were not highly concerned about climate change impacts to tarpon stocks based on their local experience. Thus, stakeholder-informed scores (mean of 2.5) reduced the biological sensitivity score for tarpon from ‘very high’ to ‘high’ (Table 2).
For permit, there was agreement from stakeholders that ecological information was relatively limited, but that the species may be less sensitive to climate change than bonefish or tarpon, based on their more ‘moderate’ sensitivities associated with habitat specificity (participatory 2.4; desktop 2), sensitivity to temperature (participatory 2.4; desktop 3); adult mobility (participatory 2.2; desktop 2), and larval dispersal (participatory 2.2; desktop 2). Participants maintained the species as ‘moderately’ sensitive based on stock status (participatory 2; desktop 2) and population growth (participatory 2.2; desktop 3). In the final scores, stakeholders reduced biological sensitivity from ‘high’ to ‘moderate’ (Table 2).
3.2.3 Vulnerability
The desktop analysis of biological sensitivity and climate exposure suggested that vulnerability to climate change should be of ‘very high’ concern for all three species, bonefish, tarpon, and permit. However, the reduction in biological sensitivity for tarpon and permit resulted in a suggested change in vulnerability score for permit from ‘very high’ to ‘high’ (Figure 4; Table 2). The participatory approach and local expertise did not modify the overall vulnerability rating for bonefish and tarpon.
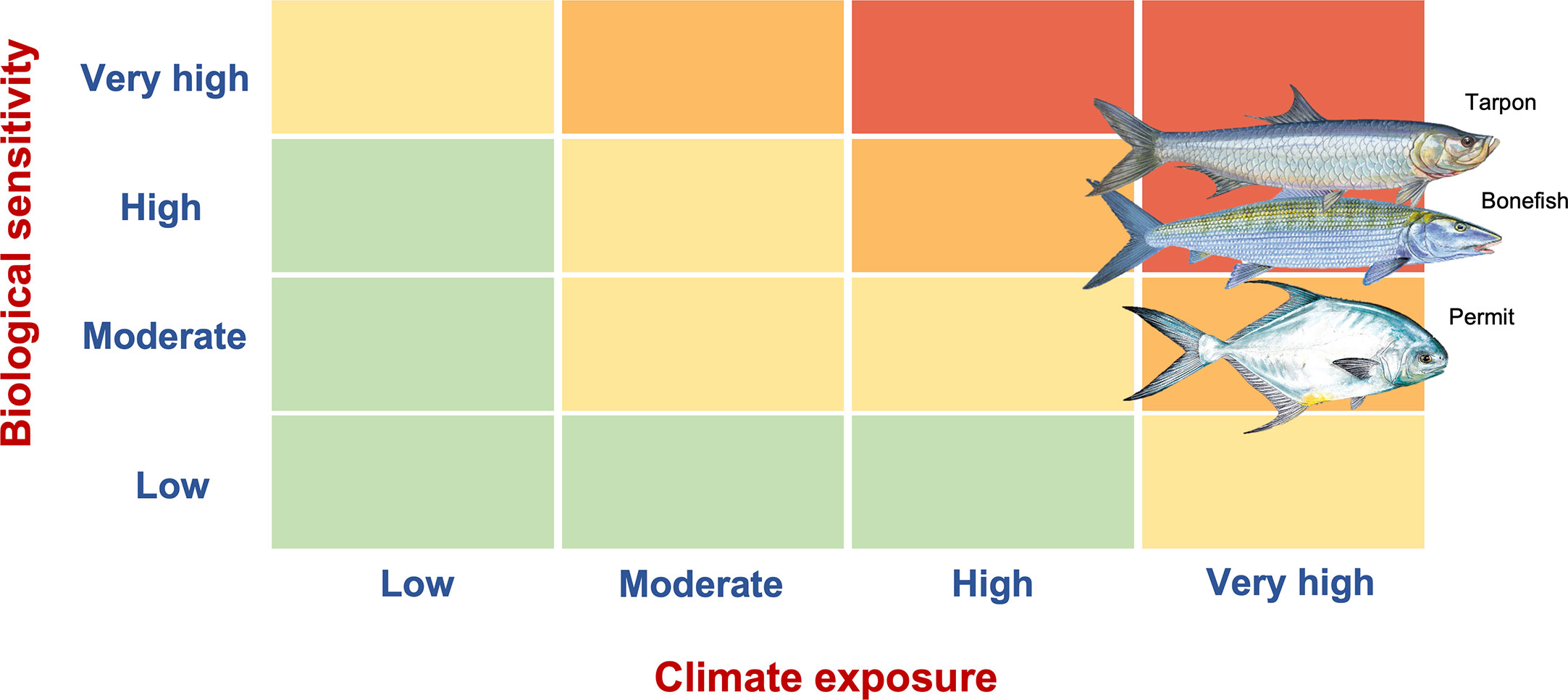
Figure 4 Final climate vulnerability scores for bonefish, tarpon, and permit (top to bottom, vertically) integrating the local knowledge of expert stakeholders.
3.2.4 Socioeconomic implications of climate change and resilience
The structured discussion of the socioeconomic implications of the CVA, ecological impacts, and potential adaptation measures was centered on three scales of mitigation: national, community, and individual strategies (Table 3). Both The Bahamas and Belize stakeholder groups agreed that, at the national level, stock assessments and monitoring programs for recreational stocks are the highest priority (see Table S4 for country specific responses). However, due to resource limitations (Table S5, both groups focused on increasing conservation through sustainable regulations of fishing and development, increased permitting, multilateral collaboration, enforcement, and compliance. At the community scale, both groups highlighted that to increase resilience to climate change, communities should focus on increasing science-based education, promoting open and inclusive communication, and supporting local restoration projects, and increased efforts to increase participation of the guiding community in restoration and monitoring. At the individual level, both groups agreed that increasing education and communication with fishers and guides on recreational fishing impacts, such as post-release mortality, would improve the fisheries’ sustainability.
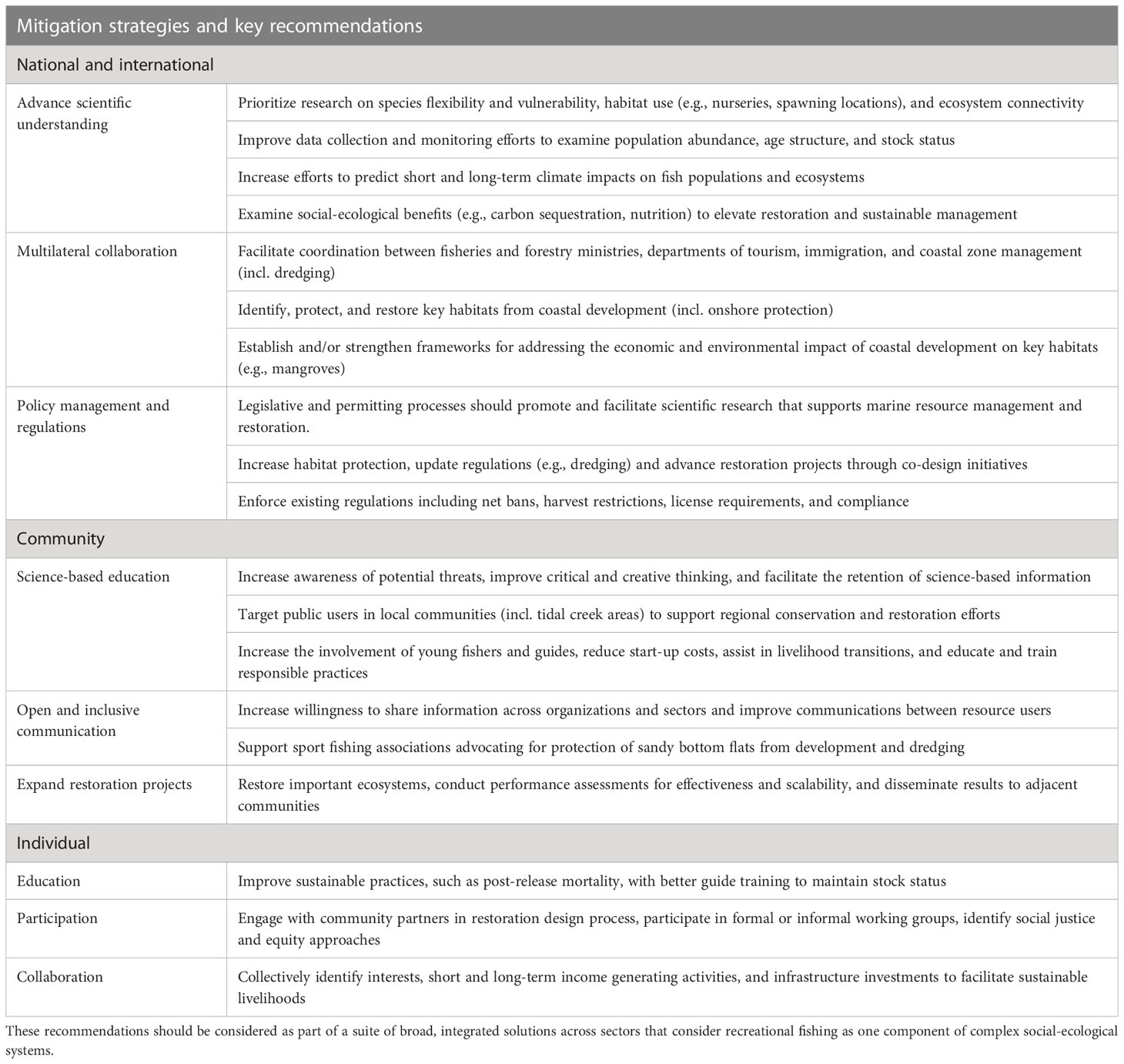
Table 3 Mitigation strategies identified by workshop participants to increase resilience to climate change in Caribbean flats fisheries and associated guiding communities.
4 Discussion
The desktop-based climate vulnerability assessment (CVA) and participatory workshop suggest strong effects from climate change on marine biota and habitats in the Caribbean by 2050, with implications for recreational fisheries. Our results support previous research that suggests ocean temperatures, sea levels, coastal erosion, ocean acidification, and storm damage are likely to increase significantly in the Caribbean in coming decades. These climate impacts coupled with anthropogenic factors will degrade sensitive nearshore ecosystems, with consequences for species like bonefish, tarpon and permit that rely on a mosaic of high-quality tidal flats habitats (Danylchuk et al., 2023). The desktop analysis found that bonefish, tarpon, and permit each exhibit ‘very high’ vulnerability, including specificity to sensitive coastal ecosystems, slow population growth rates, and high fishing pressure. Any declines in these species as a result of this vulnerability will likely result in significant socioeconomic impacts on fishing livelihoods, and the associated multibillion-dollar tourism industry. Our study demonstrates the power of coupling a desktop analysis with a participatory process to elicit local expertise, fill information gaps in data-limited fisheries, and evaluate areas of concern for stakeholders. By eliciting the local expert knowledge of fishing guides, resource managers, and scientists in the region, we identified individual, community, national, and international government relevant strategies to enhance climate resilience.
4.1 Climate vulnerability assessment
Stakeholder perceptions of both the magnitude of climate change in the Caribbean and the sensitivity of key recreational species to these changes were generally slightly lower than the desktop scores. Differences in exposure scores for physical climate change factors may reflect 1) local knowledge that provides more nuance than global studies of climate change impacts or 2) difficulties calibrating a science-based scoring framework and thresholds therein with human perceptions of change that may occur at different timescales. While stakeholder miscalibration or preconceptions are possible when conducting a CVA, and the relatively small number of participants precludes generalizing these results to the full range of experiences of those engaged in the recreational fishing system, the group identified that significant changes are occurring across a suite of climate variables, and that these changes will have negative impacts on stocks in the region. The final conclusions of the workshop align well with the many previous studies investigating perceptions and understanding of climate risk by fishery participants (e.g., Tiller et al., 2016; Sarkar et al., 2022).
Stakeholder-adjusted species’ sensitivity scores resulted in a down-weighting of sensitivity for both tarpon and permit from ‘very high’ to ‘high’ and ‘high’ to ‘moderate’, respectively. This resulted in a shift in the overall vulnerability of permit from ‘very high’ to ‘high’. The difference in stakeholder perceptions and the desktop analysis scores for species’ sensitivity may reflect differences between the ecology and status of Caribbean stocks and southeast United States stocks, where most published information for tarpon and permit were available. During discussions, stakeholders weighted some sensitivity factors as being more important than others for determining outcomes for species in the Caribbean, which may have influenced overall vulnerability (Seara et al., 2020). For example, stakeholders noted that the broad suite of habitats occupied by tarpon during adult life stages and the ability to migrate to suitable spawning grounds (Ault, 2010; Elmo et al., 2021) is likely to confer a relatively low vulnerability to climate change impacts and recommended adjusting the score to reflect a moderate sensitivity.
Importantly, stakeholders emphasized that climate change is likely to amplify existing anthropogenic threats to flats species and their ecosystems in Belize and The Bahamas. These include unsustainable coastal development including shoreline hardening, dredging, and limestone mining (Suchley and Alvarez-Filip, 2018); recreational fishing pressure including catch-and-release mortality in both countries and catch-and-eat practices for bonefish in The Bahamas (Adams, 2017); and illegal, unreported, and unregulated (IUU) fishing including netting of tarpon in inland freshwater habitats in Belize (Steinberg, 2015). In many cases, these local, anthropogenic threats may be more immediate than climate change, as well as more tractable to solve using existing conservation and management levers in the short term. Identifying strategies to mitigate threats to the recreational flats fishery is a priority, and there is a strong need to find integrated solutions that account for potential climate change impacts in the context of other stressors.
The relatively limited number of stakeholders involved in the participatory process cannot be considered representative of all practitioners working in recreational fisheries in the Caribbean. While the assessments of the local experts provide a foundation for future scientific investigation and climate-resilient management action, these should be considered preliminary, and robust participatory processes designed to elicit input and feedback from local communities should be an ongoing part of climate adaptation efforts at all stages.
4.2 Climate resilience strategies identified by stakeholders
4.2.1 National and international level
The main threats to bonefish, tarpon, and permit populations are degradation of tidal flats, seagrass beds, mangroves, and other inshore habitats, primarily from coastal development, coastal erosion, rising sea levels, and storm damage (Steinberg, 2015). National protection of these habitats is therefore a critical strategy to build resilience (Albert et al., 2017). However, limited spatial research of essential habitats, such as nursery grounds and spawning locations, exists for flats species in the Caribbean. Increased understanding, comprehensive mapping, and data sharing with resource managers are needed to identify and proactively protect sensitive, ecologically, and recreationally important areas from development and climate change. Previous projects have highlighted habitat restoration as a key strategy to build ecological resilience of tidal flat species to climate change. For example, following Hurricane Dorian, Bonefish and Tarpon Trust and the Perry Institute for Marine Science undertook mangrove restoration projects in The Bahamas, and fishing guides noted during our workshop that bonefish are returning to restored areas. As efficacy and cost of restoration can vary by habitat type, location, and local labor market forces (Bayraktarov et al., 2016), ongoing and future restoration projects should include monitoring to ensure effective implementation. Bolstering fisheries management is another important step toward buffering target species and their ecosystems from the effects of climate change (Gaines et al., 2018; Free et al., 2020). In both Belize and The Bahamas, fisheries management measures are in place to protect recreational stocks, including a commercial fishing ban for bonefish in both countries (Sherman et al., 2018), and the ban of gill and lobster nets in marine waters in Belize that incidentally catch flats species. However, regulations need to be systematically reviewed to ensure they are meeting their goals, are responsive to climate impacts, and remain enforceable. Furthermore, existing fisheries management and risk may not be well understood by fishers and their communities. There is a need to improve communication of existing laws and their importance for protecting key stocks (Battista et al., 2017). An inclusive management approach that allows research and conservation priorities to be co-developed with stakeholders who are at the frontline of climate change should be adopted, as opposed to management strategies that are imposed externally or strictly from management authorities (Karr et al., 2017).
Better coordination between government agencies was suggested by stakeholders as a way to improve management and enforcement. In the case of illegal transboundary fishing, lack of coordination among coastguard or the national defense force, immigration, and fisheries departments was cited as a cause of decreased enforcement efficacy. Lack of coordination between government agencies has also been observed to hamper protection efforts, with mangroves falling between the jurisdictions of fisheries management and forestry agencies in The Bahamas. Creation of working groups and a national strategy to coordinate between associated agencies, while also strengthening fisheries governance is an important step in improving resilience in the system. For example, a coordinated managed access program in Belize, a form of Territorial Use Rights for Fishing (TURFs), for specific flats areas could reduce fishing pressure when weather concentrates effort to nearshore locations close to ports, while simultaneously empowering traditional fishers by ensuring greater participation in the decision-making process (Kleisner et al., 2021). Compliance could also be improved through a focus on behavior change interventions, such as leveraging social norms through persuasive communication (Bergseth and Roscher, 2018) to promote pro-environmental behaviors within these fisheries (Stern et al., 1999).
Enhanced international collaboration between Caribbean nations could further improve fisheries management and monitoring (Gaines et al., 2018; Palacios-Abrantes et al., 2020). Given the transboundary nature of bonefish, tarpon, and permit stocks (Adams et al., 2012; Smith-Vaniz et al., 2015; Adams et al., 2019), their migrations and larval connectivity (Bryan et al., 2015; Zeng et al., 2019), and their potential to redistribute across borders (Oremus et al., 2020), cooperation between nations to undertake stock assessments, set agreements on commercial fishing practices, and implement catch-and-release protocols could strengthen conservation and increase resilience. Existing international governance structures can provide mechanisms for collaboration between Caribbean nations. These include the Caribbean Regional Fisheries Mechanism (CRFM), which promotes sustainable fisheries through research, education, policy, and technical cooperation among 17 member states, and the Cartagena Convention, a legal framework under the United Nations Environment Program that contains the Specially Protected Areas for Wildlife (SPAW) Protocol, which obligates 26 member states to manage protected areas and conserve threatened species. These mechanisms could facilitate enhanced cross-jurisdictional research and management, and provide access to diverse sources of financing and support for fishing guides and their communities for infrastructure, training, and livelihood diversification.
4.2.2 Community level
Science-based education and knowledge access will increase awareness of potential threats, improve critical and creative thinking, facilitate the retention of science-based information, and increase learning capacity within communities in the Caribbean (Mason et al., 2022). In the guiding community, many experienced guides are reaching retirement age, and there are fewer younger guides entering the industry. This problem, referred to as “graying of the fleet”, indicates a loss of traditional knowledge and is a warning sign of declining livelihood opportunities within a fishery system (Cramer et al., 2018; Johnson and Mazur, 2018; Haugen et al., 2021). This has further implications for managing fisheries under climate change, as lost knowledge has the potential to shift baselines against which the health of resources are measured (Pauly, 1995). Efforts to facilitate learning opportunities from guides who have decades of experience on the water, and to involve them in participatory science and management efforts could prevent their wisdom from being lost.
Additionally, young guides who are entering the industry, often from the commercial sector, require training to understand key habitats, fish distributions, and best practice protocols for catching and releasing fish, which may help to reduce post-release mortality during recreational fishing (Adams, 2017). Knowledge co-production initiatives (e.g., incorporating traditional use with science to evaluate effective and equitable pathways; Mills et al., 2022) could improve compliance and enforcement with harvest restrictions, and existing regulations, and engagement with younger participants. More broadly, education campaigns targeting the public could be amplified, with the potential to enhance sustainable stewardship of marine resources and reduce illegal fishing. By improving ethical and inclusive communication between resource users, information will circulate across organizations and sectors to collectively identify interests and improve sustainability of the fishing sector (Bevitt et al., 2022).
4.2.3 Individual level
Recreational fisheries and guiding provide one strategy for commercial and small-scale fishers to diversify livelihoods, while leveraging their in-depth knowledge of the marine environment and maintaining personal cultural ties to fishing activities (Uddin et al., 2021). One consideration in planning for potential climate-induced declines in recreational flats fisheries is to assess the feasibility of shifting recreational fishing effort toward less vulnerable stocks (Wood et al., 2013). Livelihood diversification in fisheries is generally hypothesized to reduce fishing pressure and conserve ecological benefits while enabling people to maintain income-generating activities (Allison and Ellis, 2001). Belizean stakeholders suggested blue water sportfishing of pelagic species as an avenue to reduce fishing pressure on the flats. However, many pelagic species are data deficient in the Caribbean and further scientific research is required to ensure the sustainability of increasing recreational fishing effort. Future work could solicit input from recreational anglers who visit the Caribbean for sportfishing tournaments and vacations to understand some of the issues that impact their decision-making around where or when to visit, and which factors (e.g., species availability, season length, degradation of fishing sites, catch rates, etc.) would impact their choice to travel (Young et al., 2020). This could serve the dual purpose of understanding thresholds in viability of recreational flats fishing under climate change, and understanding customer preferences to direct any future diversification efforts (Wielgus et al., 2009).
The availability of economic and social resources represents a further barrier to meeting expected livelihood outcomes and diversifying to other recreational fisheries in the Caribbean (Roscher et al., 2022). For example, the stakeholders noted that targeting flats species requires a much smaller vessel than blue water sportfishing, and guides and other local community members often do not have access to the capital required to obtain larger vessels. Another key consideration in expanding livelihoods tied to recreational fisheries is to better understand the role of other members in the value chain. Further research is required to reduce the risk of exposing new vulnerabilities and adverse trade-offs.
4.3 Conclusions and recommendations
Climate change will have significant impacts on nearshore tropical habitats and ecosystems by 2050, compounding a host of ongoing anthropogenic stressors. Populations of recreationally important tidal flats species including bonefish, tarpon, and permit will be highly exposed to these impacts, and exhibit life history and behavioral traits that confer high to very high vulnerability. Without the implementation of measures to mitigate climate change and its impacts, reductions in population size and declines in the quality of recreational fishing opportunities are likely to occur, with serious consequences for the fishing and tourism industries in places like Belize and The Bahamas.
By incorporating local knowledge into a research-based analysis, we took a step towards better understanding local climate impacts, and potential repercussions for recreational fisheries systems in the Caribbean. We were also able to identify key measures that may enhance the resilience of ecosystems and dependent communities, building upon a strong foundation of scientific research and fisheries management that is emerging in the region. Future work to build on these discussions with more representatives from local communities would help to reduce scientific uncertainties, and continued engagement with communities throughout the process of designing and implementing conservation and management solutions will ensure that community perspectives and priorities shape climate adaptation efforts in the Caribbean region.
Data availability statement
The original contributions presented in the study are included in the article and Supplementary Material. Further inquiries can be directed to the corresponding author.
Ethics statement
Ethical review and approval was not required for the study on human participants in accordance with the local legislation and institutional requirements. Written informed consent to participate in this study was provided by the workshop participants. Written informed consent was obtained from the individuals for the publication of any potentially identifiable images or data included in this article.
Author contributions
The study was designed by SH, GC, KMK, KAK, SP, and NR. KS, RG, MB, KC, AC, CD, VB-P, VS, and ST participated in the workshop. GC, SH, and SP facilitated the workshop. GC and JE drafted the manuscript. All authors contributed to the article and approved the submitted version.
Funding
This paper resulted from a workshop generously funded by the Kingfisher Foundation (2021FIM04).
Acknowledgments
Parts of this manuscript have been released as an online report at the Environmental Defense Fund Fishery Solutions Center (Braynen et al., 2022). We extend great appreciation to all the workshop participants–collaborators, fishing guides, scientists, resource managers, and other experts–that contributed their knowledge, expertise, and time to the workshop (see Table S1 in the Supplementary material, Appendix 1 for full list). We specifically thank Dennis Garbutt, Fabian Green, Ryan Moore, and Lester Gittens for their contributions during the workshop, and we thank Katherine Mah for workshop preparation and assistance.
Conflict of interest
The authors declare that the research was conducted in the absence of any commercial or financial relationships that could be construed as a potential conflict of interest.
Publisher’s note
All claims expressed in this article are solely those of the authors and do not necessarily represent those of their affiliated organizations, or those of the publisher, the editors and the reviewers. Any product that may be evaluated in this article, or claim that may be made by its manufacturer, is not guaranteed or endorsed by the publisher.
Supplementary material
The Supplementary Material for this article can be found online at: https://www.frontiersin.org/articles/10.3389/fmars.2023.1177715/full#supplementary-material
References
Adams A. J. (2017). Guidelines for evaluating the suitability of catch and release fisheries: lessons learned from Caribbean flats fisheries. Fish. Res. 186, 672–680. doi: 10.1016/j.fishres.2016.09.027
Adams A. J., Blewett D. A. (2004). Spatial patterns of estuarine habitat type use and temporal patterns in abundance of juvenile permit, trachinotus falcatus, in Charlotte harbor, Florida. Gulf Caribb. Res. 16 (2), 129–139. doi: 10.18785/gcr.1602.01
Adams A., Danylchuk A. J., Cooke S. J. (2023). Conservation connections: incorporating connectivity into management and conservation of flats fishes and their habitats in a multi-stressor world. Environ. Biol. Fish. 106, 117–130. doi: 10.1007/s10641-023-01391-4
Adams A., Guindon K., Horodysky A., MacDonald T., McBride R., Shenker J., et al. (2012). Albula vulpes (The IUCN Red List of Threatened Species), e.T194303A2310733.
Adams A., Guindon K., Horodysky A., MacDonald T., McBride R., Shenker J., et al. (2019). Megalops atlanticus (errata version published in 2020) (The IUCN Red List of Threatened Species), e.T191823A174796143.
Albert S., Saunders M. I., Roelfsema C. M., Leon J. X., Johnstone E., Mackenzie J. R., et al. (2017). Winners and losers as mangrove, coral and seagrass ecosystems respond to sea-level rise in Solomon islands. Environ. Res. Lett. 12 (9), 094009. doi: 10.1088/1748-9326/aa7e68
Albright R., Mason B., Miller M., Langdon C. (2010). Ocean acidification compromises recruitment success of the threatened Caribbean coral acropora palmata. Proc. Natl. Acad. Sci. 107 (47), 20400–20404. doi: 10.1073/pnas.1007273107
Allison E. H., Ellis F. (2001). The livelihoods approach and management of small-scale fisheries. Mar. Pol. 25 (5), 377–388. doi: 10.1016/S0308-597X(01)00023-9
Altieri A. H., Harrison S. B., Seemann J., Collin R., Diaz R. J., Knowlton N. (2017). Tropical dead zones and mass mortalities on coral reefs. Proc. Natl. Acad. Sci. 114 (14), 3660–3665. doi: 10.1073/pnas.1621517114
Andrades R., Bolzan M. S., Contaifer L. S. (2012). Evidence of sandy beaches as growth grounds for commercial fish in the south-western Atlantic. Pan-Am. J. Aquat. Sci. 7 (2), 107–110.
Arlinghaus R., Cooke S. J. (2009). “Recreational fisheries: socioeconomic importance, conservation issues and management challenges,” in Recreational hunting, conservation and rural livelihoods. Eds. Dickson B., Hutton J., Adams W. M.. doi: 10.1002/9781444303179.ch3
Ault J. S. (2010). “Silver king: a most perfect and ancient sportfish–the biology, ecology, management of megalops atlanticus–and its precarious future,” in A passion for tarpon (Mill Creek, WA: Wild River Press), 266–292.
Battista W., Karr K., Sarto N., Fujita R. (2017). Comprehensive assessment of risk to ecosystems (CARE): a cumulative ecosystem risk assessment tool. Fish Res. 185, 115–129. doi: 10.1016/j.fishres.2016.09.017
Bayraktarov E., Saunders M. I., Abdullah S., Mills M., Beher J., Possingham H. P., et al. (2016). The cost and feasibility of marine coastal restoration. Ecol. Appl. 26 (4), 1055–1074. doi: 10.1890/15-1077
Belize (2020). Statutory Instrument Fisheries Resources Regulations. Belize City, Belize: Ministry of Fisheries, Forestry, the Environment and Sustainable Development.
Bergseth B. J., Roscher M. (2018). Discerning the culture of compliance through recreational fisher’s perceptions of poaching. Mar. Pol. 89, 132–141. doi: 10.1016/j.marpol.2017.12.022
Bevitt K., Cohen P. J., Diver R., Kutub Uddin M., Lukanga E., Patel A., et al. (2022). A good practice guide for ethical and inclusive communications involving small-scale fisheries (Penang, Malaysia: WorldFish and Rome, FAO).
Bhatia K. T., Vecchi G. A., Knutson T. R., Murakami H., Kossin J., Dixon K. W., et al. (2019). Recent increases in tropical cyclone intensification rates. Nat. Commun. 10 (1), 1–9. doi: 10.1038/s41467-019-08471-z
Bindoff N. L., Cheung W. W. L., Kairo J. G., Arístegui J., Guinder V. A., Hallberg R., et al. (2019). “Changing ocean, marine ecosystems, and dependent communities,” in IPCC special report on the ocean and cryosphere in a changing climate. Eds. Poürtner H.-O., Roberts D. C., Masson-Delmotte V., Zhai P., Tignor M., Poloczanska E., Mintenbeck K., Alegría A., Nicolai M., Okem A., Petzold J., Rama B., Weyer N. M. (New York, NY, USA: Cambridge University Press), 447–587. doi: 10.1017/9781009157964.007
Boucek R. E., Lewis J. P., Stewart B. D., Jud Z. R., Carey E., Adams A. J. (2019). Measuring site fidelity and homesite-to-pre-spawning site connectivity of bonefish (Albula vulpes): using mark-recapture to inform habitat conservation. Environ. Biol. Fish. 102, 185–119. doi: 10.1007/s10641-018-0827-y
Braynen M. T., Burns-Perez V., Callwood K., Carroll C., Castañeda A., Dahlgren C., et al. (2022). A climate vulnerability assessment for Caribbean recreational fisheries (Fishery Solutions Center, Environmental Defense Fund). Available at: https://fisherysolutionscenter.edf.org/resources/climate-vulnerability-assessment-caribbean-recreational-fisheries.
Brownscombe J. W., Griffin L. P., Morley D., Acosta A., Hunt J., Lowerre-Barbieri S. K., et al. (2020). Seasonal occupancy and connectivity amongst nearshore flats and reef habitats by permit trachinotus falcatus: considerations for fisheries management. J. Fish Biol. 96 (2), 469–479. doi: 10.1111/jfb.14227
Brownscombe J. W., Shipley O. N., Griffin L. P., Morley D., Acosta A., Adams A. J., et al. (2022). Application of telemetry and stable isotope analyses to inform the resource ecology and management of a marine fish. J. Appl. Ecol. 59 (4), 1110–1121. doi: 10.1111/1365-2664.14123
Bryan D. R., Luo J., Ault J. S., McClellan D. B., Smith S. G., Snodgrass D., et al. (2015). Transport and connectivity modeling of larval permit from an observed spawning aggregation in the dry tortugas, Florida. Environ. Biol. Fishes. 98 (11), 2263–2276. doi: 10.1007/s10641-015-0445-x
Butler E., Childs A. R., Saayman A., Potts W. (2020). Can fishing tourism contribute to conservation and sustainability via ecotourism? a case study of the fishery for giant African threadfin polydactylus quadrifilis on the kwanza estuary, Angola. Sustainability. 12, 4221. doi: 10.3390/su12104221
Cashman A., Nurse L., John C. (2010). Climate change in the Caribbean: the water management implications. J. Environ. Dev. 19 (1), 42–67. doi: 10.1177/1070496509347088
Cheung W. W., Lam V. W., Sarmiento J. L., Kearney K., Watson R. E. G., Zeller D., et al. (2010). Large-Scale redistribution of maximum fisheries catch potential in the global ocean under climate change. Glob. Chan. Biol. 16 (1), 24–35. doi: 10.1111/j.1365-2486.2009.01995.x
Cooke S. J., Philipp D. P. (2004). Behavior and mortality of caught-and-released bonefish (Albula spp.) in Bahamian waters with implications for a sustainable recreational fishery. Biol. Conserv. 118 (5), 599–607. doi: 10.1016/j.biocon.2003.10.009
Cowx I. G., Arlinghaus R., Cooke S. J. (2010). Harmonizing recreational fisheries and conservation objectives for aquatic biodiversity in inland waters. J. Fish. Biol. 76 (9), 2194–2215. doi: 10.1111/j.1095-8649.2010.02686.x
Crabtree R. E., Peter B., Snodgrass D. (2001). Age, growth, and reproduction of permit (Trachinotus falcatus) in Florida water. J. Fish. Bull. 100, 26–34.
Cramer L. A., Flathers C., Caracciolo D., Russell S. M., Conway F. (2018). Graying of the fleet: perceived impacts on coastal resilience and local policy. Mar. Pol. 96, 27–35. doi: 10.1016/j.marpol.2018.07.012
Danylchuk A. J., Danylchuk S. E., Cooke S. J., Goldberg T. L., Koppelman J. B., Philipp D. P. (2007). Post-release mortality of bonefish, albula vulpes, exposed to different handling practices during catch-and-release angling in eleuthera, the Bahamas. Fish. Manage. Ecol. 14 (2), 149–154. doi: 10.1111/j.1365-2400.2007.00535.x
Danylchuk A. J., Griffin L. P., Ahrens R., Allen M. S., Boucek R. E., Brownscombe J. W., et al. (2023). Cascading effects of climate change on recreational marine flats fishes and fisheries. Environ. Biol. Fish 106, 381–416. doi: 10.1007/s10641-022-01333-6
Dong S., Baringer M. O., Goni G. J. (2019). Slow down of the gulf stream during 1993–2016. Sci. Rep. 9 (1), 1–10. doi: 10.1038/s41598-019-42820-8
Elmo G. M., Crane D. P., Kimball M. E. (2021). Juvenile tarpon megalops atlanticus use of natural and managed marsh habitats in coastal south Carolina. Environ. Biol. Fish. 104 (12), 1655–1664. doi: 10.1007/s10641-021-01193-6
Eurich J. G., Tekiau A., Seto K. L., Aram E., Beiateuea T., Golden C. D., et al. (2023). Resilience of a giant clam subsistence fishery in Kiribati to climate change. Pac. Conserv. Biol. PC22050. doi: 10.1071/PC22050
Fedler T. (2010). The economic impact of flats fishing in the Bahamas (Vero Beach, FL: The Bahamian Flats Fishing Alliance).
Fedler A. J. (2014). 2013 economic impact of flats fishing in Belize (Vero Beach, FL: Bonefish and Tarpon Trust).
Fedler T. (2019). The 2018 economic impact of flats fishing in the Bahamas (Miami, FL: Report to the Bonefish and Tarpon Trust).
Free C. M., Mangin T., Molinos J. G., Ojea E., Burden M., Costello C., et al. (2020). Realistic fisheries management reforms could mitigate the impacts of climate change in most countries. PloS One 15 (3), e0224347. doi: 10.1371/journal.pone.0224347
Friedrich T., Timmermann A., Abe-Ouchi A., Bates N. R., Chikamoto M. O., Church M. J., et al. (2012). Detecting regional anthropogenic trends in ocean acidification against natural variability. Nat. Clim. Change. 2 (3), 167–171. doi: 10.1038/nclimate1372
Gable F. J. (1997). Climate change impacts on Caribbean coastal areas and tourism. J. Coast. Res., 49–69.
Gaines S. D., Costello C., Owashi B., Mangin T., Bone J., Molinos J. G., et al. (2018). Improved fisheries management could offset many negative effects of climate change. Sci. Adv. 4 (8), eaao1378. doi: 10.1126/sciadv.aao1378
Gianelli I., Ortega L., Pittman J., Vasconcellos M., Defeo O. (2021). Harnessing scientific and local knowledge to face climate change in small-scale fisheries. Glob. Environ. Change. 68, 102253. doi: 10.1016/j.gloenvcha.2021.102253
Glenn E., Comarazamy D., González J. E., Smith T. (2015). Detection of recent regional sea surface temperature warming in the Caribbean and surrounding region. Geophys. Res. Lett. 42 (16), 6785–6792. doi: 10.1002/2015GL065002
Golden C. D., Koehn J. Z., Shepon A., Passarelli S., Free C. M., Viana D. F., et al. (2021). Aquatic foods to nourish nations. Nature. 598, 315–320. doi: 10.1038/s41586-021-03917-1
Griffin L. P., Brownscombe J. W., Adams A. J., Boucek R. E., Finn J. T., Heithaus M. R., et al. (2018). Keeping up with the silver king: using cooperative acoustic telemetry networks to quantify the movements of Atlantic tarpon (Megalops atlanticus) in the coastal waters of the southeastern united states. Fish. Res. 205, 65–76. doi: 10.1016/j.fishres.2018.04.008
Halpern B., Boettiger C., Dietze M. C., Gephart J. A., Gonzalez P., Grimm N. B., et al. (2023). Priorities for synthesis in ecology and environmental science. Front. Ecol. Environ. 14 (1), e4342. doi: 10.1002/ecs2.4342
Hare J. A., Morrison W. E., Nelson M. W., Stachura M. M., Teeters E. J., Griffis R. B., et al. (2016). A vulnerability assessment of fish and invertebrates to climate change on the northeast U.S. continental shelf. PloS One 11 (2), e0146756. doi: 10.1371/journal.pone.0146756
Haugen B. I., Cramer L. A., Waldbusser G. G., Conway F. D. (2021). Resilience and adaptive capacity of oregon’s fishing community: cumulative impacts of climate change and the graying of the fleet. Mar. Policy 126, 104424.
Heck N., Beck M. B., Reguero B. (2021). Storm risk and marine fisheries: a global assessment. Mar. Pol. 132, 104698. doi: 10.1016/j.marpol.2021.104698
Hoegh-Guldberg O., Mumby P. J., Hooten A. J., Steneck R. S., Greenfield P., Gomez E., et al. (2007). Coral reefs under rapid climate change and ocean acidification. Science. 318 (5857), 1737–1742. doi: 10.1126/science.1152509
Hughes D. J., Alderdice R., Cooney C., Kühl M., Pernice M., Voolstra C. R., et al. (2020). Coral reef survival under accelerating ocean deoxygenation. Nat. Clim. Change. 10 (4), 296–307. doi: 10.1038/s41558-020-0737-9
Johnson T. R., Mazur M. D. (2018). A mixed method approach to understanding the graying of maine's lobster fleet. Bull. Mar. Sci. 94 (3), 1185–1199. doi: 10.5343/bms.2017.1108
Karr K. A., Fujita R., Carcamo R., Epstein L., Foley J. R., Fraire-Cervantes J. A., et al. (2017). Integrating science-based co-management, partnerships, participatory processes and stewardship incentives to improve the performance of small-scale fisheries. Front. Mar. Sci. 4. doi: 10.3389/fmars.2017.00345
Klarenberg G., Ahrens R., Shaw S., Allen M. (2019). Use of a dynamic population model to estimate mortality and recruitment trends for bonefish in Florida bay. Environ. Biol. Fish. 102 (2), 349–363. doi: 10.1007/s10641-018-0805-4
Kleisner K. M., Ojea E., Amoros S., Battista W., Burden M., Cunningham E., et al. (2021). Identifying policy approaches to build social-ecological resilience in marine fisheries with differing capacities and contexts. ICES J. Mar. Sci., fsab080. doi: 10.1093/icesjms/fsab080
Kossin J. P., Knapp K. R., Olander T. L., Velden C. S. (2020). Global increase in major tropical cyclone exceedance probability over the past four decades. reefs. Proc. Natl. Acad. Sci. 117 (22), 11975–11980. doi: 10.1073/pnas.1920849117
Kurth B. N., Peebles E. B., Stallings C. D. (2019). Atlantic Tarpon (Megalops atlanticus) exhibit upper estuarine habitat dependence followed by foraging system fidelity after ontogenetic habitat shifts. Estuar. Coast. Shelf Sci. 225, 106248. doi: 10.1016/j.ecss.2019.106248
Lam V. W., Allison E. H., Bell J. D., Blythe J., Cheung W. W., Frölicher T. L., et al. (2020). Climate change, tropical fisheries and prospects for sustainable development. Nat. Rev. Earth Environ. 1 (9), 440–454. doi: 10.1038/s43017-020-0071-9
Lam V., Cheung W., Reygondeau G., Sumaila U. R. (2016). Projected change in global fisheries revenues under climate change. Sci. Rep. 6, 32607. doi: 10.1038/srep32607
Lewis J. (2021) Large Scale mangrove restoration project begins in the northern Bahamas. Available at: https://www.bonefishtarpontrust.org/blog/2021-01-26-large-scale-mangrove-restoration-project-begins-in-the-northern-bahamas/ (Accessed 1, 2022).
Lombardo S. M., Adams A. J., Danylchuk A. J., Luck C. A., Ajemian M. J. (2020). Novel deep-water spawning patterns of bonefish (Albula vulpes), a shallow water fish. Mar. Biol. 167 (12), 1–11. doi: 10.1007/s00227-020-03799-3
Luo J., Ault J. S. (2012). Vertical movement rates and habitat use of Atlantic tarpon. Mar. Ecol. Prog. Ser. 467, 167–180. doi: 10.3354/meps09957
Mason J. G., Eurich J. G., Lau J. D., Battista W., Free C. M., Mills K. E., et al. (2022). Attributes of climate resilience in fisheries: from theory to practice. Fish Fish. 23, 522–544. doi: 10.1111/faf.12630
Mendelsohn R., Emanuel K., Chonabayashi S., Bakkensen L. (2012). The impact of climate change on global tropical cyclone damage. Nat. Clim. Change. 2 (3), 205–209. doi: 10.1038/nclimate1357
Mills K. E., Armitage D., Eurich J. G., Kleisner K. M., Pecl G., Tokunaga K. (2022). Co-Production of knowledge and strategies to support climate resilient fisheries. ICES J. Mar. Sci., Fsac110. doi: 10.1093/icesjms/fsac110
Monnereau I., Oxenford H. A. (2017). Impacts of climate change on fisheries in the coastal and marine environments of caribbean small island developing states (SIDS). Caribbean Mar. Climate Change Rep. card: Sci. Rev. 2017, 124–154.
Murakami H., Delworth T. L., Cooke W. F., Zhao M., Xiang B., Hsu P. C. (2020). Detected climatic change in global distribution of tropical cyclones. Proc. Nat. Acad. Sci. 117 (20), 10706–10714. doi: 10.1073/pnas.1922500117
Murchie K. J., Cooke S. J., Danylchuk A. J., Danylchuk S. E., Goldberg T. L., Suski C. D., et al. (2011). Thermal biology of bonefish (Albula vulpes) in Bahamian coastal waters and tidal creeks: an integrated laboratory and field study. J. Therm. Biol. 36 (1), 38–48. doi: 10.1016/j.jtherbio.2010.10.005
Murchie K. J., Cooke S. J., Danylchuk A. J., Danylchuk S. E., Goldberg T. L., Suski C. D., et al. (2013). Movement patterns of bonefish (Albula vulpes) in tidal creeks and coastal waters of eleuthera, the Bahamas. Fish. Res. 147, 404–412. doi: 10.1016/j.fishres.2013.03.019
Oremus K. L., Bone J., Costello C., Molinos J. G., Lee A., Mangin T., et al. (2020). Governance challenges for tropical nations losing fish species due to climate change. Nat. Sustain. 3, 277–280. doi: 10.1038/s41893-020-0476-y
Palacios-Abrantes J., Reygondeau G., Wabnitz C. C. C., Cheung W. W. (2020). The transboundary nature of the world’s exploited marine species. Sci. Rep. 10, 17668. doi: 10.1038/s41598-020-74644-2
Pauly D. (1995). Anecdotes and the shifting baseline syndrome of fisheries. Trends Ecol. Evol. 10 (10), 430. doi: 10.1016/S0169-5347(00)89171-5
Perez A. U., Schmitter-Soto J. J., Adams A. J., Heyman W. D. (2019). Connectivity mediated by seasonal bonefish (Albula vulpes) migration between the Caribbean Sea and a tropical estuary of Belize and Mexico. Environ. Biol. Fishes. 102 (2), 197–207. doi: 10.1007/s10641-018-0834-z
Pinsky M. L., Selden R. L., Kitchel Z. J. (2020). Climate-driven shifts in marine species ranges: scaling from organisms to communities. Ann. Rev. Mar. Sci. 12 (1), 153–179. doi: 10.1146/annurev-marine-010419-010916
Roscher M. B., Allison E. H., Mills D. J., Eriksson H., Hellebrandt D., Andrew N. L. (2022). Sustainable development outcomes of livelihood diversification in small-scale fisheries. Fish Fish. 23, 910–925. doi: 10.1111/faf.12662
Samhouri J. F., Ramanujam E., Bizzarro J. J., Carter H., Sayce K., Shen S. (2019). An ecosystem-based risk assessment for California fisheries co-developed by scientists, managers, and stakeholders. Biol. Conserv. 231, 103–121. doi: 10.1016/j.biocon.2018.12.027
Sandbrook C. G. (2010). Local economic impact of different forms of nature-based tourism. Conserv. Lett. 3 (1), 21–28. doi: 10.1111/j.1755-263X.2009.00085.x
Santos F., Gómez-Gesteira M., Varela R., Ruiz-Ochoa M., Días J. M. (2016). Influence of upwelling on SST trends in la guajira system. J. Geophys. Res. Oceans. 121 (4), 2469–2480. doi: 10.1002/2015JC011420
Santos R. O., Rehage J. S., Adams A. J., Black B. D., Osborne J., Kroloff E. K. (2017). Quantitative assessment of a data-limited recreational bonefish fishery using a time-series of fishing guides reports. PloS One 12 (9), e0184776. doi: 10.1371/journal.pone.0184776
Sarkar U. K., Karnatak G., Lianthuamluaia L., Puthiyottil M., Das Ghosh B., Johnson C., et al. (2022). Combining stakeholder perception and ecological approaches for assessing vulnerability of floodplain wetlands in changing climate: a regional study. Int. J. Biometeorol. 66 (7), 1415–1427. doi: 10.1007/s00484-022-02286-2
Schneider W. (1990). “FAO species identification sheets for fishery purposes,” in Field guide to the commercial marine resources of the gulf of Guinea (Rome: FAO Regional Office for Africa).
Seara T., Pollnac R., Jakubowski K. (2020). “Fishers’ perceptions of environmental and climate change in Puerto Rico: implications for adaptation and sustainability,” in Changing climate, changing worlds (Cham: Springer), 15–34.
Sherman K. D., Shultz A. D., Dahlgren C. P., Thomas C., Brooks E., Brooks, et al. (2018). Contemporary and emerging fisheries in the Bahamas – conservation and management challenges, achievements and future directions. Fish. Manage. Ecol. 25, 319–331. doi: 10.1111/fme.12299
Sippo J. Z., Lovelock C. E., Santos I. R., Sanders C. J., Maher D. T. (2018). Mangrove mortality in a changing climate: an overview. Estuar. Coast. Shelf Sci. 215, 241–249. doi: 10.1016/j.ecss.2018.10.011
Smith M., Fedler A. J., Adams A. J. (2022). Economic assessments of recreational flats fisheries provide leverage for conservation. Environ. Biol. Fish. 106, 131–145. doi: 10.1007/s10641-022-01375-w
Smith-Vaniz W. F., Williams J. T., Pina Amargos F., Curtis M., Brown J. (2015)Trachinotus falcatus. In: The IUCN red list of threatened species 2015. Available at: https://dx.doi.org/10.2305/IUCN.UK.2015-4.RLTS.T190407A16510662.en (Accessed 23, 2022).
Steinberg M. K. (2015). A nationwide assessment of threats to bonefish, tarpon, and permit stocks and habitat in Belize. Environ. Biol. Fish. 98 (11), 2277–2285. doi: 10.1007/s10641-015-0429-x
Stern P. C., Dietz T., Abel T. D., Guagnano G. A., Kalof L. (1999). A value-belief-norm theory of support for social movements: the case of environmentalism. Hum. Ecol. Rev. 6 (2), 81–97.
Strauss B., Kulp S. (2018). Sea-Level rise threats in the Caribbean: data, tools, and analysis for a more resilient future (Princeton, NJ: Climate Central).
Suchley A., Alvarez-Filip L. (2018). Local human activities limit marine protection efficacy on Caribbean coral reefs. Conserv. Lett.11 5), e12571. doi: 10.1111/conl.12571
Taylor G. T., Muller-Karger F. E., Thunell R. C., Scranton M. I., Astor Y., Varela R., et al. (2012). Ecosystem responses in the southern Caribbean Sea to global climate change. Proc.Nat. Acad. Sci. 109 (47), 19315–19320. doi: 10.1073/pnas.1207514109
Teneva L., Free C. M., Hume A., Agostini V. N., Klein C. J., Watson R. A., et al. (2023). Small island nations can achieve food security benefits through climate-adaptive blue food governance by 2050. Mar. Pol. 151, 105577. doi: 10.1016/j.marpol.2023.105577
Tiller R., De Kok J. L., Vermeiren K., Richards R., Ardelan M. V., Bailey J. (2016). Stakeholder perceptions of links between environmental changes to their socio-ecological system and their adaptive capacity in the region of troms, Norway. Front. Mar. Sci. 3. doi: 10.3389/fmars.2016.00267
Townhill B. L., Radford Z., Pecl G., van Putten I., Pinnegar J. K., Hyder K. (2019). Marine recreational fishing and the implications of climate change. Fish Fish. 20 (5), 977–992. doi: 10.1111/faf.12392
Tufts B. L., Holden J., DeMille M. (2015). Benefits arising from sustainable use of north america’s fishery resources: economic and conservation impacts of recreational angling. Int. J. Environ. Stud. 72 (5), 850–868. doi: 10.1080/00207233.2015.1022987
Uddin M. M., Schneider P., Asif M., Islam R., Rahman M. S., Mozumder M. M. H. (2021). Fishery-based ecotourism in developing countries can enhance the social-ecological resilience of coastal fishers–a case study of Bangladesh. Water. 13 (3), 292. doi: 10.3390/w13030292
Vecchi G. A., Landsea C., Zhang W., Villarini G., Knutson T. (2021). Changes in Atlantic major hurricane frequency since the late-19th century. Nat. Commun. 12 (1), 1–9. doi: 10.1038/s41467-021-24268-5
Vosper E. L., Mitchell D. M., Emanuel K. (2020). Extreme hurricane rainfall affecting the Caribbean mitigated by the Paris agreement goals. Environ. Res. Lett. 15 (10), 104053. doi: 10.1088/1748-9326/ab9794
Waycott M., Duarte C. M., Carruthers T. J., Orth R. J., Dennison W. C., Olyarnik S., et al. (2009). Accelerating loss of seagrasses across the globe threatens coastal ecosystems. Proc. Nat. Acad. Sci. 106 (30), 12377–12381. doi: 10.1073/pnas.0905620106
Wielgus J., Gerber L. R., Sala E., Bennett J. (2009). Including risk in stated-preference economic valuations: experiments on choices for marine recreation. J. Environ. Manage. 90 (11), 3401–3409. doi: 10.1016/j.jenvman.2009.05.010
Wood A. L., Butler J. R., Sheaves M., Wani J. (2013). Sport fisheries: opportunities and challenges for diversifying coastal livelihoods in the pacific. Mar. Pol. 42, 305–314. doi: 10.1016/j.marpol.2013.03.005
Young E. G., Melnychuk M. C., Anderson L. E., Hilborn R. (2020). The importance of fishing opportunity to angler utility analysis in marine recreational fisheries. ICES J. Mar. Sci. 77 (6), 2344–2353. doi: 10.1093/icesjms/fsz234
Zeng X., Adams A., Roffer M., He R. (2019). Potential connectivity among spatially distinct management zones for bonefish (Albula vulpes) via larval dispersal. Environ. Biol. Fish. 102 (2), 233–252. doi: 10.1007/s10641-018-0826-z
Keywords: The Bahamas, Belize, climate change, recreational fisheries, resilience, tidal flats, tourism, sportfishing
Citation: Carroll G, Eurich JG, Sherman KD, Glazer R, Braynen MT, Callwood KA, Castañeda A, Dahlgren C, Karr KA, Kleisner KM, Burns-Perez V, Poon SE, Requena N, Sho V, Tate SN and Haukebo S (2023) A participatory climate vulnerability assessment for recreational tidal flats fisheries in Belize and The Bahamas. Front. Mar. Sci. 10:1177715. doi: 10.3389/fmars.2023.1177715
Received: 01 March 2023; Accepted: 24 April 2023;
Published: 17 May 2023.
Edited by:
D. Shallin Busch, National Oceanic and Atmospheric Administration (NOAA), United StatesReviewed by:
Karma Crispian Norman, National Oceanic and Atmospheric Administration (NOAA), United StatesAlfonso Aguilar-Perera, Universidad Autónoma de Yucatán, Mexico
Copyright © 2023 Carroll, Eurich, Sherman, Glazer, Braynen, Callwood, Castañeda, Dahlgren, Karr, Kleisner, Burns-Perez, Poon, Requena, Sho, Tate and Haukebo. This is an open-access article distributed under the terms of the Creative Commons Attribution License (CC BY). The use, distribution or reproduction in other forums is permitted, provided the original author(s) and the copyright owner(s) are credited and that the original publication in this journal is cited, in accordance with accepted academic practice. No use, distribution or reproduction is permitted which does not comply with these terms.
*Correspondence: Jacob G. Eurich, amV1cmljaEBlZGYub3Jn
†These authors have contributed equally to this work and share first authorship