- 1School of Biological and Marine Science, University of Plymouth, Plymouth, United Kingdom
- 2Institute for Coastal and Marine Research, Nelson Mandela University, Gqeberha, South Africa
- 3Marine programme, South African National Biodiversity Institute, Cape Town, South Africa
- 4Marine Ecosystems, Joint Nature Conservation Committee, Peterborough, United Kingdom
Despite its remoteness, human activity has impacted the deep sea and changes to the structure and function of deep-sea ecosystems are already noticeable. In terrestrial and shallow water marine environments, demonstrating how ecosystems support human well-being has been instrumental in setting policy and management objectives for sustainable resource use. Foundational to this approach is a framework of ecosystem service (ES) classification and a synthesis of the knowledge base, which can then be used to structure decision-support tools such as ecosystem accounts or Environmental Impact Assessments. At present, no such framework exists for the deep sea. There is thus an urgent need to determine and assess the ES provided by deep-sea habitats and species before (potentially irreversible) decisions are made about deep-sea habitat use and governance. As a first step towards the incorporation of ES in such decision-making, we undertake two systematic reviews of the scientific literature based on the principles of the Preferred Reporting Items for Systematic Reviews and Meta-analysis (PRISMA) systematic process. This was to define a comparative ES framework and synthesise the current evidence base for how deep-sea habitats support ecosystem services. Our framework proposes four supporting services, three regulating services, four provisioning services and three cultural services for which there is an established and growing body of evidence for the role of deep-sea habitats. The ES framework presented here provides a structure for deep-sea ecosystem services. In its next phase of development, this could provide the foundation for the development of habitat-ecosystem service matrices, which are a critical component for truly accounting for ES in decision-making, particularly spatial management. This framework has significant implications for deep-sea management, conservation and policy, as it provides an ecosystem services-based tool that can be used in any deep-sea ecosystems management across the planet, and it also shows how critical these data gaps are for today’s decisions and how seriously they should be considered in decision-making processes.
1 Introduction
Marine ecosystems are some of the most heavily exploited in the world (Barbier, 2017). Oceans are facing unprecedented threats from over-exploitation (Arora and Mishra, 2020; Winther et al., 2020), climate change (Armstrong et al., 2019; Morato et al., 2020), pollution and declining biodiversity (Danovaro et al., 2008; Rogers et al., 2020). Interest in understanding human effects, including cumulative impacts, on marine habitats is increasing rapidly in the eyes of both governing bodies and the public (Santos et al., 2019).
The deep sea supports one of the most biodiverse ecosystems on Earth (Hessler and Sanders, 1967; Sanders and Hessler, 1969; Snelgrove and Smith, 2002), maintaining important biological and mineral resources (Ramirez-Llodra et al., 2010). The development of marine technologies has allowed the exploration and consequently exploitation of several deep-sea regions (Ramirez-Llodra et al., 2010; Santos et al., 2019). Although many human activities in the deep sea are likely to increase in coming decades (Ramirez-Llodra et al., 2010), there is still much to learn about the full impacts of the exploitation of deep-sea resources (Ramirez-Llodra et al., 2010; Jobstvogt et al., 2014; Santos et al., 2019).
One of the considerations around human use of the marine environment is the potential impact of activities on ecosystem services (ES). Marine ES are processes, functions and structures of the marine environment that directly or indirectly contribute to social welfare, health and economic activities (Beaumont et al., 2007; Liquete et al., 2013). Thus, human well-being depends on such services in multiple ways. The Millennium Ecosystem Assessment (MEA) (MEA, 2005) described ‘ecosystem services’ as the linkage of ecosystems to human well-being, and classified them into four categories:
- Supporting services are the underlying ecosystem functions that are essential for the production of the other services. These are considered to influence human well-being indirectly. See Figure 1 for further details.
- Provisioning services are those obtained directly from the ecosystems and are used by humans, such as fish, oil and gas.
- Regulating services contribute to the natural production and resilience of habitats and ecosystem processes, such as climate regulation, carbon sequestration and storage.
- Cultural services are the non-material benefits people obtain from habitats through education, recreational and spiritual elements (Figure 1).
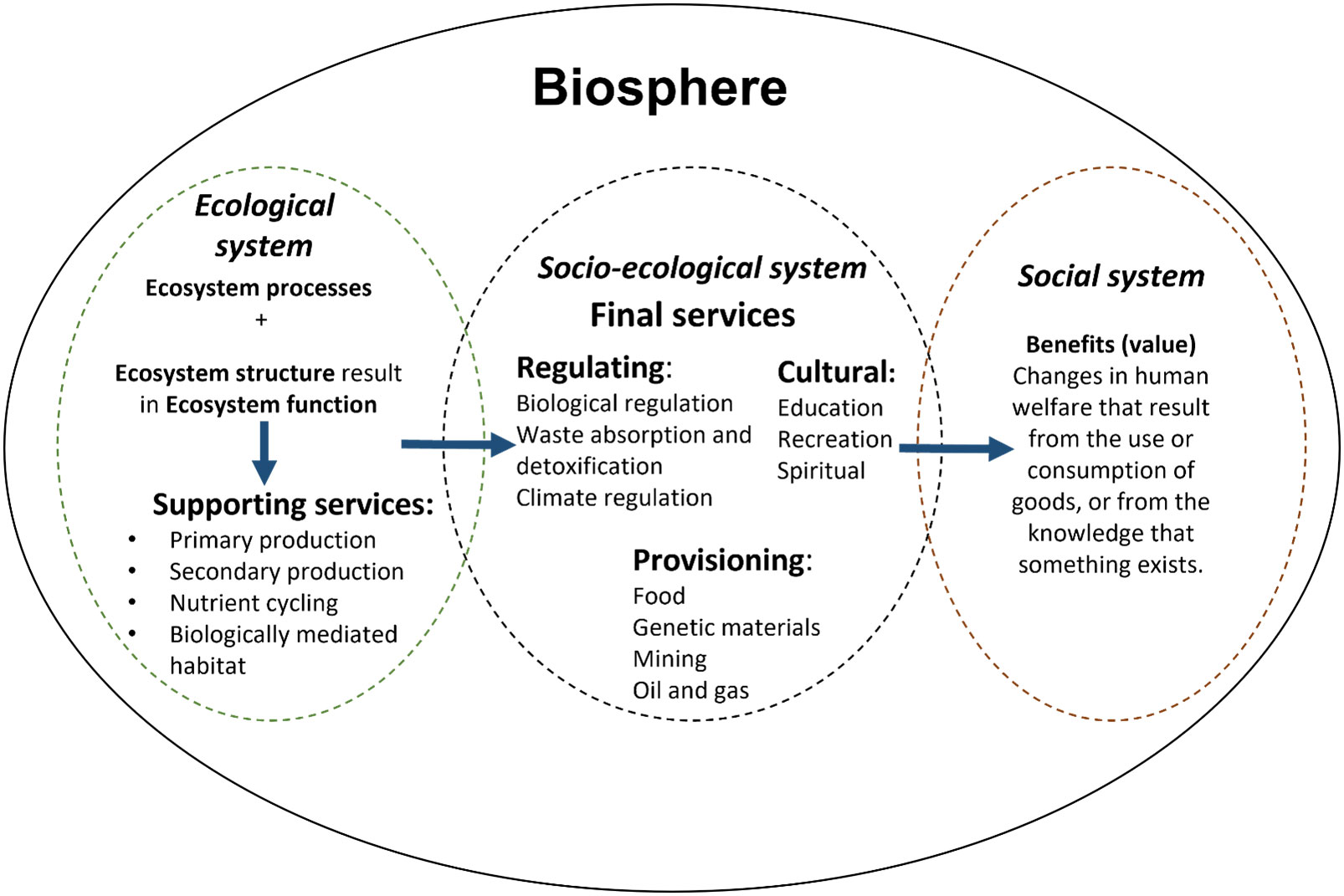
Figure 1 The biosphere captures natural processes, ecosystem services and human needs. The cascade framework of (Haines-Young and Potschin, 2010) demonstrated the conceptual flow between ecological and social systems. This framework establishes that ecosystem processes (abiotic components) and ecosystem structures (biotic components) link to ecosystem functions (e.g. primary production) to provide ecosystem services (e.g. fish) that can be realised as a good or benefit (e.g. food) (Rees et al., 2022).
For this study, we group natural processes, ecosystem services and human needs as part of the biosphere (Figure 1). This concept derives from the cascade framework theory ((Haines-Young and Potschin, 2010) by linking ecological systems and human needs. Supporting services, such as primary production or nutrient cycling, are distinguished from final services (regulating, provisioning, cultural) which generate the goods and benefits, such as the provisioning of fish and algae (Haines-Young and Potschin, 2010; Potts et al., 2014; Hooper et al., 2019b). However, the final services depend on the delivery of supporting services (Figure 1) (Haines-Young and Potschin, 2010).
Provisioning services are more readily understandable than supporting services. For example, food provisioning in the form of fisheries has been addressed extensively (Aanesen and Armstrong, 2016; Diz et al., 2017). However, the underlying ecological functions and processes supporting this provisioning service’s delivery are more problematic to quantify (Diz et al., 2017) and map. This dichotomy is reflected in the peer-reviewed literature. Whilst the number of studies on marine ES is increasing, the services considered are still largely limited to provisioning services that provide benefits to commercial fisheries, and communities; and infrastructure that benefit from coastal protection (Liquete et al., 2013; Mace et al., 2015; Culhane et al., 2018). Assessments of supporting services have fallen behind in the global agenda, as they sit behind those ES that more directly influence the realisation of benefits within the social system (Culhane et al., 2018). More recently, within the UK context, supporting services have been recently re-named as ‘output processes’ (van Rein, H, pers. comm.). This is a valuable description that places supporting services within the ecological system. However, for this research, we have used the term supporting services to maintain alignment with current international processes.
In marine systems, frameworks for describing ES that can be directly useful for integration into decision support tools have been developed (Beaumont et al., 2007; Liquete et al., 2013; Culhane et al., 2018). By making marine ES more explicit, the ES approach can promote better-informed discussions about ES trade-offs in different marine spatial planning scenarios and ultimately recognise the multiple interactions within ecosystems where humans are an integral part. The use of an ES approach is meant to inform the spatial distribution of existing and emerging seas uses, use-conflict reduction, ecosystem health and protection and sustainable use of ES (Galparsoro et al., 2021). Mapping and assessment of ES can become a framework that links different sectorial and environmental policies (Galparsoro et al., 2021).
The use of a habitat-service matrix approach to link ecological and economic systems has gained attention in the last decade (Potts et al., 2014; Campagne and Roche, 2018; La Bianca et al., 2018; Hattam et al., 2021). The matrix approach has been increasingly used to support natural resource management, particularly for terrestrial systems. Prior to the development of a habitat-service matrix that is project-specific for local and regional scale ES mapping and assessment, the most fit-for-purpose classification system for ES should be chosen (Heink et al., 2016). However, within these classification systems, which were designed for shallow water ecosystems, supporting services, represented in deep-sea ecosystems, were often not considered as these are accounted for as part of the final services whose benefits to human systems are realised closer to the coast. In these existing frameworks, concern has been raised that separating the role of supporting services from provisioning, regulating and cultural services could result in double counting and lead to error in economic assessments (Armstrong et al., 2012). Nevertheless, in the context of the deep sea, assessing these supporting services and their linkages to benefits is critical. Deep-sea supporting services represent extensive and complex biogeochemical cycles fundamental for terrestrial life, including humans (Dell’Anno and Danovaro, 2005). For example, the decomposition by viruses in deep-sea sediment undergo fast decay releasing ~37-50 megatons of carbon per year that represent an important source of labile organic compounds in deep-sea ecosystems (Dell’Anno et al., 2015). Thus, virus decomposition provides an important deep-sea ecosystem function that plays a crucial role in nutrient cycling within the largest ecosystem of the biosphere (Dell’Anno et al., 2015). Including supporting services, as in ecological functions and structures, in habitat-service matrices can help in explicitly recognising the ecosystem functions that underpin the rest of the services. Ultimately, this will present a more holistic approach where detailed picture of the interactions among species, and species and their habitats, are factored in, with the ambition to maintain the health and productivity of deep-sea systems.
Classifying and quantifying ES of the deep sea is difficult, due to the paucity of data, knowledge and understanding. In recent years, progress has been made in defining the potential linkages between humans and deep-sea ecosystems using existing ES classifications (Armstrong et al., 2012; Thurber et al., 2013) but this approach has yet to be applied in marine management (particularly in a spatial context). These authors linked deep-sea ES to the MEA classification by highlighting potential provisioning services and the importance of deep-sea supporting services. Through their work and others, these efforts show that the deep sea provides many valuable supporting services that are crucial to sustaining human well-being (Ramirez-Llodra et al., 2010; Folkersen et al., 2018; Levin et al., 2019). Although (Armstrong et al., 2012; Thurber et al., 2013) discussed the existence of deep-sea ES for the first time, we ought to recognise that these reviews report conceptual ecological systems and socio-ecological system knowledge that lacks an operational format aimed to support decision making that could be used in ecosystem accounts or Environmental Impact Assessments. To integrate deep-sea ES in local, regional and national accounting valuation, a standardised list of deep-sea ES can facilitate the flow of information between scientists, policy makers and other interested stakeholders.
ES, Economic valuation approaches, which favour geographical locations where an economic value is transferred into the human system (e.g. coastal), have potentially led to a systemic downgrading of the role of the deep sea in ‘supporting’ life-critical aspects of human well-being. In 2021, the System of Environmental Economic Accounting-Ecosystem Accounting (SEEA-EA) compiled an accounting framework at the regional level to economically value the functions of ecosystem assets and the ES they produce to inform policy development (Committee of Experts on Environmental- Economic Accounting, 2021). Remarkably, this framework considers broad categories within the deep sea (e.g. submarine canyons, abyssal plains) with no consideration of its faunal heterogeneity and biophysical processes that underpin the entire system, which eventually would diminish the spatial accountability of deep-sea ES and make it impossible to elaborate any meaningful Environmental Impact Assessment. The deep sea has lagged behind in the global, national and local economic accounts that could generate (potentially irreversible) decisions for deep-sea uses and governance.
Currently, studies have yet to discuss the practical application of ES frameworks in deep-sea ecosystem management and research. With the present study, we aim to develop a deep-sea ES framework that is compatible with existing marine ES frameworks, and update existing reviews on deep-sea ES to incorporate directly into current processes the role that deep-sea ES have in supporting human well-being. This will determine the sustainable (and equitable) use of deep-sea resources.
2 Methods
2.1 Literature search
We conducted two systematic reviews based on existing marine ES frameworks and deep-sea ES to develop a common language for deep-sea ES. These two systematic literature reviews were based on the main principles of the PRISMA framework (Preferred Reporting Items for Systematic Reviews and Meta-analysis) (Moher et al., 2010) and adapted to the scope of our reviews. The first literature review aimed to collate published marine ES frameworks, while the second sought to update reviews by Armstrong et al. (2012) and Thurber et al. (2014) on deep-sea ES. The bibliographic search was performed with the Web of Science engine; Scopus engine; Science Direct engine and Google Scholar. When the search provided more than 100 hits, we sorted the database according to relevance and exported the first 100 articles. The first screening of the literature was carried out by reading the title of each article, removing those not of relevance to this analysis and duplicates. Non-English publications were omitted from this review.
2.1.1 Marine ES frameworks
The first review aimed to collate relevant peer-reviewed evidence on existing marine ES frameworks (see supp. materials for a diagram of the systematic review process). The search included any paper or review ever published until 22/03/2022 with the following terms found in title, keywords, abstract or topic: (“ecosystem services framework”, “ecosystem approach” or “ecosystem services matrix”) and (“marine” or “deep sea”). This was the last date that we conducted our searches, however, we acknowledge there may a few relevant papers published after this date that are not included in our database.
2.1.2 Deep-sea ES frameworks
Our second systematic literature review focused on recent studies on deep-sea ES since the publication of Armstrong et al. (2012) and Thurber et al. (2014). We extracted ES relevant to deep-sea habitats from Armstrong et al. (2012) and Thurber et al. (2014), and adjusted the categories based on existing frameworks and services. Our aim was to search for deep-sea supporting, regulating, provisioning and cultural services, as these were the categories identified in the first review. For the second review, we used the existing ES terminology and integrated them around the main ecosystem processes in line with the selected literature. Second review included any paper or review published from 2014 until 23/03/2022 with the following terms found in the title, keywords, abstract or topic: (“nursery ground” or “nutrient cycling” or “chemosynthetic primary production” or “secondary production” or “habitat resilience” or “carbon sequestration” or “antibiotic” or “bioprospecting” or “pharmaceutical” or “genetic material” or “fossil fuels” or “fisher*” or “bioaccumulation” or “bioremediation” or “oil and gas extraction” or “climate regulation”) and (“marine” or “deep sea”).
2.2 Integration of deep-sea habitat complexity in ES frameworks
To develop an integrated framework, it is important to recognize the abiotic processes of an ecosystem. These components play a role in ecosystem processes in terms of the causal contributions of their parts to an ecosystem activity (Teixeira et al., 2019). Abiotic services derive from “non-living components of the ecosystems and include water for industrial use, energy and space and waterways (such as for military activity, shipping and for the transit and landfall of cables and pipelines)” (Hooper et al., 2019). That said, there has been a lack of consistency in whether abiotic services should be included within ES frameworks (Hooper et al., 2019). MEA IMEA, 2005) and The Economics of Ecosystems and Biodiversity (TEEB) (De Groot et al., 2010) exclude abiotic processes to prioritize the value of biodiversity, which implies the analysis of biotic components only. In some cases, the inclusion of abiotic components may be deemed essential. For example, when considering the application of ES frameworks for environmental impact assessments (EIA), integrating abiotic services should be adequate in treating the ecosystem as a whole and minimize potential damage towards less obvious ecological processes (Hooper et al., 2019). The Common International Classification for Ecosystem Services (CICES) includes abiotic services (Haines-Young and Potschin, 2018). For the scope of this study, the integration of abiotic services in deep-sea ES frameworks was considered important to recognize the existing ecological processes that help regulate the ecological balance as we know it. Herein, waste disposal in the deep sea is considered as a biotic service, within the description of 3.3.2.5 Mediation of wastes or toxic substances of anthropogenic origin by living processes. No review was conducted on the dumping, accumulation and burring of human waste in the deep sea. Using the deep sea as a dumping ground is not considered a service within this framework, as no biotic or abiotic processes degrade or ameliorate the problems associated with this type of waste (Thurber et al., 2014). It is also important to note that storage of gas, including CO₂, in the deep sea is also mentioned in the literature. This framework does not include this activity; its impacts may limit the expansion of CO₂ storage in the deep sea from experimentation to realized service (Thurber et al., 2014).
2.3 Developing a deep-sea ES framework
In summary, we gathered the information from the literature reviews, synthesized what applied to deep-sea ecosystems from current ES frameworks and integrated it with updated knowledge on deep-sea ES. Here we propose a ES framework tailored for deep sea.
3 Results
3.1 Literature reviews
Our first research question was to collate a list of marine ES frameworks potentially applicable to deep-sea habitats. For the first review, the results summed to 2444 papers. From this first sift, 194 papers were selected for the second screening. During the second screening attention was given to studies that mention marine ecosystem-based approach and marine ES. Studies were included for further eligibility assessment if they specifically used ES in the marine and deep-sea context. Studies were excluded if they investigated exclusively freshwater and transitional waters; economy orientated, blue economy, blue growth; policy and marine spatial planning; human activities and human impacts on coastal and marine ecosystems. From the 151 papers abstracts, only 12 papers fulfilled our scope and included at least a type of marine ES framework in their analysis.
Our second research questions were to update the existing deep-sea ES since Armstrong et al., 2012 and Thurber et al., 2014. For the second review, the results summed up to 3236 papers. Papers that were related to ecosystem economic valuation, terrestrial assessment, biodiversity indicators, and conservation management tools were omitted from the analysis. From this first sift, 555 papers were selected for the abstract’s eligibility. Of 555 paper abstracts, 182 papers discussed deep-sea ES to some extent and were considered relevant to our analysis.
3.2 Marine ES frameworks
The first revision of the selected studies allowed the comparison of all marine ES frameworks published up to 22/03/2022. Eight marine ES frameworks were found from our search, which recognised two types of ES classification: 1) supporting and final services and 2) final services only. The MEA (MEA, 2005), (Beaumont et al., 2007), TEEB (De Groot et al., 2010); Armstrong et al., 2012; Liquete et al., 2013; Thurber et al., 2014. included supporting/habitat services, regulating, provisioning and cultural services. While the Common International Classification for Ecosystem Services (CICES) is comprised of only final services (Haines-Young and Potschin, 2018). The proposed scheme is not a new classification, but an adaptation of existing published frameworks based on our reviews of the literature that combines the two types of ES classifications.
3.3 Deep-sea ES
Our proposed framework consists of four supporting services and three final services categories i.e. three regulating, four provisioning and three cultural services, based on our review of current ES frameworks and recent literature on deep-sea ES (Table 1). This study found that, since 2014, more papers have been published on some functions, processes and benefits (e.g., nitrogen cycles, genetic materials and deep-sea mining), than others (e.g., biological regulation, waste absorption and detoxification), which have lagged behind (Figure 2). For completeness, a full list of references per each service is included in the Supplementary Materials.
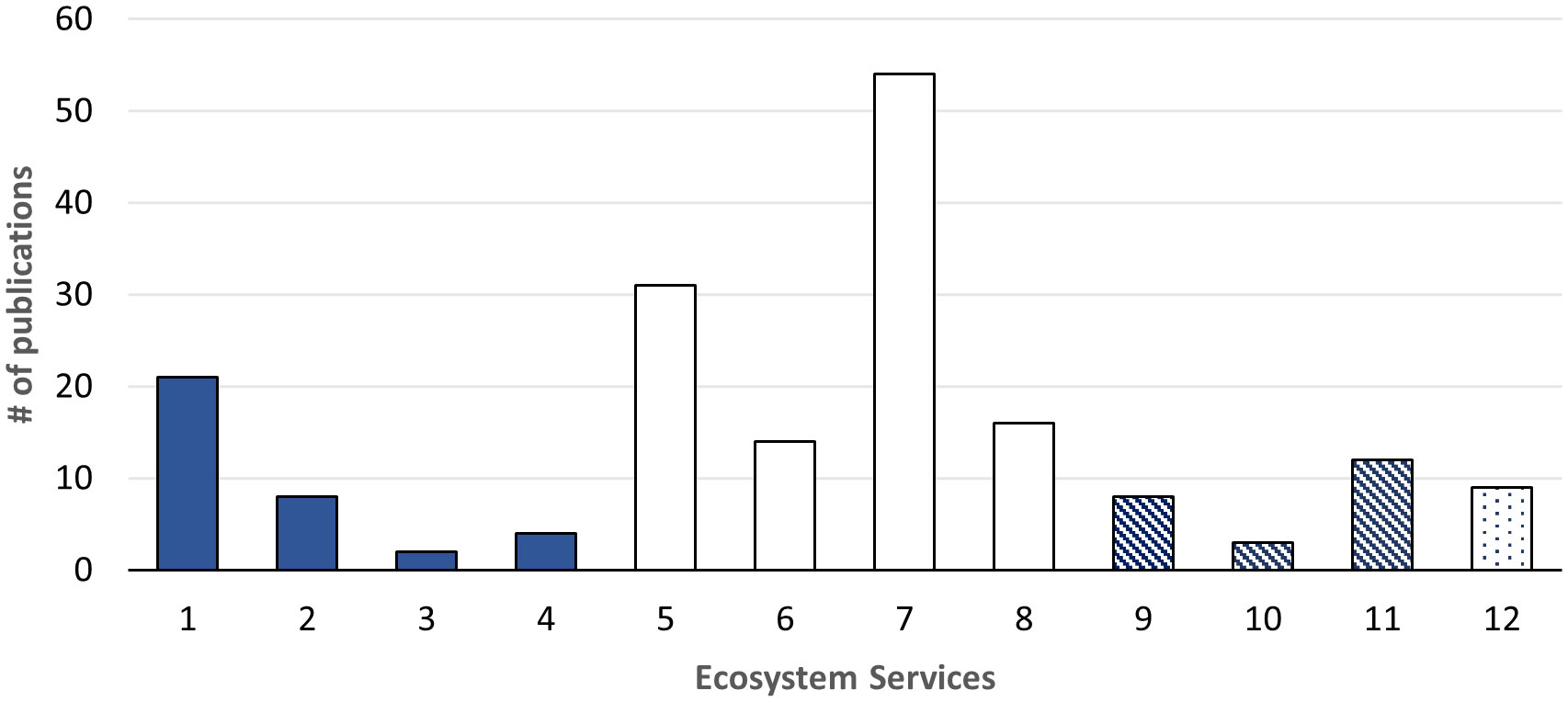
Figure 2 Services are pattern-coded according to type: full=supporting services; blank=provisioning services; diagonal lines=regulating services; dots=cultural services. The ecosystem services correspond to: 1=nutrient cycling; 2=chemosynthetic primary production; 3=secondary production; 4=biologically mediated habitat; 5=individual genes extracted from organisms for the design and construction of new biological entities;6=wild animals used for nutritional purposes; 7=mineral substances used for materials; 8= mineral substances used as an energy source; 9=mediation of wastes or toxic substances of anthropogenic origin by living processes; 10= pest and disease control; 11=regulation of chemical composition of atmosphere and oceans, atmospheric composition and condition; 12=cultural services.
3.3.1 Deep-sea supporting services
Our proposed framework consists of four supporting services (Table 2) based on the review of Armstrong et al. (2012) and Thurber et al. (2014).
3.3.1.1 Nutrient cycling
We include ‘Nutrient cycling’ as a supporting and regulating service, which is included in five out of six marine ES frameworks analysed in this review. In the last decade, an effort has been put into understanding the complexity of microbial processes in deep-sea habitats (Danovaro et al., 2014; Corinaldesi, 2015; Dombrowski et al., 2017; Lin et al., 2021); the role of viruses, protists and prokaryotes in nutrient cycling (Dell’Anno et al., 2015; Xu et al., 2017; Huang et al., 2019; Gooday et al., 2020; Langlet et al., 2020); and the role of sponges, which are identified as nutrient providers for the marine environment, recycling organic matter into various forms of bioavailable nutrients such as ammonium and nitrate (Li et al., 2016; Dunham et al., 2018; Rooks et al., 2020; Bart et al., 2021; Maldonado et al., 2021). Shallow and deep water system connection is an important aspect of ecosystem functionality (Vilas et al., 2020) and including ‘Nutrient cycling’ services can help in addressing the complexity of water system interactions.
3.3.1.2 Chemosynthetic primary production
‘Chemosynthetic primary production’ service is not considered in any existing marine ES framework. Both deep-sea ES reviews, Armstrong et al. (2012) and Thurber et al. (2014), highlight the importance of such services for deep-sea habitats. Chemosynthesis in the deep sea occurs everywhere but is perhaps best known from hydrothermal vent and cold seep ecosystems (Armstrong et al., 2012). In the last decade, scientists have hypothesised the potential links between chemosynthetic functionality, climate regulation and fishery resources (Grupe et al., 2015; Higgs et al., 2016). An important discovery found connections between chemosynthetic producers and lobster fisheries in shallow waters (Higgs et al., 2016). Higgs et al. (2016) show that commercial Caribbean spiny lobsters obtain 20% of their diet from seagrass beds clams. These clams host chemoautotrophic bacterial symbionts in their gills that synthesise organic matter using reduced sulfur compounds, providing nutrients to their hosts (Higgs et al., 2016). Although these linkages were established in shallow water, similar functional links between deep-sea habitats and human society are likely to occur. Although deep-sea chemosynthetic ecosystems are attracting the interest of researchers from an ES perspective (Grupe et al., 2015; Levin et al., 2016), substantial knowledge gaps exist in adequately measuring or even describing the ES of methane seeps and other deep-sea chemosynthetic ecosystems (Grupe et al., 2015).
3.3.1.3 Secondary production
Secondary production is the formation of biomass-fuelled by organic carbon degradation and assimilation, resulting in the provision of biomass for human consumption (Thurber et al., 2013). Recent evidence found that deep-sea fauna respond with greater sensitivity to food quality changes than shallow-water fauna, potentially reflecting the overall scarcity of food that makes this a limiting factor (Campanyà-Llovet et al., 2017) in the deep sea. In their study, (Campanyà-Llovet et al., 2017) recorded increased abundance of deep-sea benthic meiofauna with increasing lipids and proteins, while no such pattern was observed in shallow habitats. For benthic organisms, infaunal functions such as bioturbation significantly influence nutrient fluxes, mineral dissolution rates, pollutant, trace metal cycling and organic carbon preservation (Snelgrove et al., 2014). Therefore, (Campanyà-Llovet et al., 2017) suggest that food quality influences benthic food webs and affects key ecosystem functions such as bioturbation and nutrient cycling.
3.3.1.4 Biologically mediated habitat
Biologically mediated habitat service is the benefit derived from habitats formed by living marine organisms (Beaumont et al., 2007). The service represents the structure of living organisms such as reef formation and sponge grounds. These natural habitats provide nursery grounds, breeding spaces, hiding places from predators and surfaces for feeding (Beaumont et al., 2007). This service is the building block of many other services. Cold-water corals, and the reef structures they can form, are some of the best-known examples of biogenic habitat that forms complex structures and support biodiversity (Baillon et al., 2012) and ecosystem functioning in the deep sea (Bongiorni et al., 2010; Thurber et al., 2014), including commercially valuable fisheries in some regions (Armstrong et al., 2014; Henderson et al., 2020).
3.3.2 Deep-sea final services
We consider ‘final services’ as those that result from the interactions of functions, namely regulating, provisioning and cultural services. This selection was based on the reviews of Armstrong et al. (2012) and Thurber et al. (2014). For example, corals and sponges create habitats where fish and their prey aggregate (structure), generating trophic interactions and secondary production (function). This function is significant to humans as it may affect the fish harvest in a designated fishing zone (service). Combined with various degrees of human input, it can lead to socioeconomic benefits as food and livelihood provision (Haines-Young and Potschin, 2010; Le et al., 2017). All marine ES frameworks from our review included final services; however, we acknowledge significant gaps in knowledge of the flow from structure-function-service-benefit for deep-sea ecosystems.
Due to the several classifications found in our literature reviews, we compared existing classification schemes to understand the compatibility of the existing classes (Table 3). We adopted CICES v.5 scheme (Haines-Young and Potschin-Young, 2018), which is versatile and the most common ES classification now used internationally, to ensure that ES are defined in a consistent and common format. This allowed us to map the component parts and to input into decision support tools such as ecosystem accounting (Maes et al., 2015; Hooper et al., 2019a; Hooper et al., 2019b; Rees et al., 2022).
3.3.2.1 Medicinal, biochemical and genetic resources
Extraction molecules and genetic material alongside food is included in all existing ES frameworks. The role of natural products as a potential source of novel antibiotics has historically been of crucial importance. Although academic and industrial interests in deep-sea biotechnology have increased (Moloney, 2016; Harden-Davies, 2017; Tortorella et al., 2018; Collins et al., 2021; Jiang et al., 2021; Keeler et al., 2021; Ma et al., 2021; Quemener et al., 2021), the regulating and supporting ES provided by fauna of interest have often been overlooked in bioprospecting research (Ottaviani, 2020). Deep-sea sponges and their associated bacteria and fungi are some of the targeted fauna, as a potential novel source of bioactive metabolites for biotechnological applications such as anti-tumour, antibacterial, antiviral, toxin inhibitors and anti-inflammatory metabolites (Rateb and Ebel, 2011; Batista-García et al., 2017; Koch et al., 2021). For this review, it is important to highlight that the biodiscovery pipeline could generate monetary and non-monetary values. Data, information, scientific cooperation, training, capacity building and technology are all non-monetary benefits that occur at each stage of the pipeline, while monetary values are obtained towards the end of the production stages (Harden-Davies, 2017; Edrada-Ebel et al., 2018).
The field of bioprospecting is emerging globally and its development has posed management challenges to the Biodiversity of Areas Beyond National Jurisdiction (BBNJ) negotiations. A key issue of which relates to how to share the benefits of bioprospecting in Areas Beyond National Jurisdiction (ABNJ) fairly (Harden-Davies, 2017). Of interest is the recent effort in developing a qualitative evaluation of services provided by deep-sea sponges and pioneering the economic appraisal of these fauna using TEEB ((Ottaviani, 2020). From an ES perspective, this is the first attempt to qualitatively assess the functions and services provided by deep-sea sponges.
3.3.2.2 Wild animals used for nutritional purposes
Fisheries is considered by both Armstrong et al. (2012) and Thurber et al. (2014) and it is classified in CICES as ‘wild animals used for nutritional purposes’. Despite the limited primary productivity, fishing in the deep sea has become a reality in the last 60 years and deep-sea fish are increasingly harvested for human consumption ((Pitcher et al., 2007; Armstrong et al., 2012). Deep water fisheries include species like orange roughy (Hoplostethus atlanticus), oreos (Allocyttus niger, Pseudocyttus maculatus), roundnose grenadier (Coryphaenoides rupestris), Bigeye grenadier (Macrourus holotrachys), blue ling (Molva dypterygia), black scabbardfish (Aphanopus carbo), beaked redfish (e.g. Sebastes mentella, S. marinus.), Greenland halibut (Reinhardtius hippoglossoides) and a number of deep-water dogfish (e.g. Portuguese shark Centroscymnus coelolepis) (Victorero et al., 2018). Since 1950 to 2015, at least 72 fish species or species groups were caught primarily with bottom trawls mostly at depths greater than 400 m (Victorero et al., 2018). Research on deep-sea fishing has shed light on the impact of some fishing methods upon benthic habitats (Thurber et al., 2014; Clark et al., 2016; Bueno-Pardo et al., 2017; Victorero et al., 2018). Since the 1970s, a decline in the abundance of commercial fish species in the northeast Atlantic, where much of the fish biomass is extracted, has been recorded (Clarke et al., 2015) and in part resulted in the introduction of the fishing ban using bottom trawling beyond 800 m in the European Exclusive Economic Zones (EEZ) and United Kingdom EEZ at present. Lessons from shallower marine ecosystems demonstrate that fishing activity across the ocean has expanded in line with the technological innovation and market demand for fish and shellfish protein. This demand and expansion has resulted in unsustainable fishing practices that have led to the collapse of stocks and loss of supporting ecosystems (e.g. nursery areas) (Pauly et al., 2003; Pauly, 2007; Clark et al., 2016). The complicated reality of commercial fishing exists also beyond national territories, and has been proven to be unprofitable without subsidies and low labour costs (Sala et al., 2018). At the global level, many fish stocks are already below the sustainable levels (FAO, 2018). The opportunity of integrating the ecosystem approach in deep-sea fisheries management would allow a holistic view of food provisioning and potential economic return of the sector and evaluation of the impact of different fishing strategies on other services (e.g. (Hooper et al., 2019b). It would also help in reducing the loss of potential ES that would result in high costs for society.
3.3.2.3 Mineral substances used for materials
Deep-sea mining is classified as a provisioning service in both (Thurber et al., 2014) and (Armstrong et al., 2012), and is classified in the ‘mineral substances used for materials’ class in CICES v.5 (Haines-Young and Potschin, 2018). Large areas of concentrated metals reserves exist on the abyssal floor, and at certain hydrothermal vents and seamounts there are reservoirs of rich crusts of minerals (Thurber et al., 2014). Interest and investment in deep-sea mining has increased since the 1970s and the International Seabed Authority (ISA) has issued 31 contracts (by March 2022) for the exploration of deep-sea polymetallic nodules, polymetallic sulphides and cobalt-rich ferromanganese crusts located in the Clarion-Clipperton Fracture Zone, Central Indian Ocean Basin, Western Pacific Ocean, South West Indian Ridge, Central Indian Ridge, Mid-Atlantic Ridge and Western Pacific Ocean. Environmental concerns over the consequences of mining such as direct impacts on marine fauna have generated a growing urgency for the development of regulations for commercial exploitation of such minerals (Gollner et al., 2017; Dunn et al., 2018; Niner et al., 2018; Santos et al., 2019; Boldy et al., 2021). Our review identified 52 papers that deal with deep-sea mining, of which 12 articles mention ‘ecosystem services’. The existing evidence that links deep-sea mining and ES advocates for the following priority actions: the identification of which ES are vulnerable to potential mining impacts; evaluation of the role of ecological functions in providing ES; development of ES indicators; valuation of ES, and implementation of ES concepts (Le et al., 2017; Durden et al., 2018).
3.3.2.4 Mineral substances used for as an energy source
Oil and gas activities are represented by ‘mineral substances used for as an energy source’ class in CICES v.5 (Haines-Young and Potschin, 2018). Up to today, drilling for oil and gas is performed in waters of greater than 200 m depth in offshore Australia, Africa, Southeast Asia, India, South America, the Gulf of Mexico, the Northern Atlantic Ocean and the Arctic Ocean (Cordes et al., 2016; Mejjad and Rovere, 2021). The growing demand for oil and gas already discussed in (Armstrong et al., 2012) and (Thurber et al., 2014) has posed difficult questions to environmental management approaches on the impacts of these activities (Ramirez-Llodra et al., 2011). Although we already know of the environmental consequences on deep-sea habitats caused by accidental oil and gas spills (Cordes et al., 2016; Joye et al., 2016) and the effects on other activities like offshore fishing industries (Pascoe and Innes, 2018), poor understanding of impacts in areas proposed for oil and gas extraction is often linked to paucity of ecosystem data and the lack of access to specific environmental impact assessment (EIA) data, which ultimately prevents a better description and recognition of biodiversity and spatial habitat distribution to interested stakeholders (Almada and Bernardino, 2017). Another issue linked to the extraction of oil and gas, as well as for other human activities in the deep sea, is the protection of habitats other than cold-water corals that provide important ES including sponge grounds, sea-pen fields and seeps. Extractable oil and gas resources are often associated with the presence of methane seepage and chemosynthetic communities, and studies have shown that cold seeps are often overlooked during the creation of marine protected areas (MPAs) in favour of other more charismatic habitats, such as cold-water corals, despite their ecological importance for associated faunal communities (Amon et al., 2017; Bendia et al., 2021). The integration of ES frameworks in comprehensive environmental assessments as part of baseline research should be considered to help the evaluation of status and changes in ecosystem functions and ES.
3.3.2.5 Mediation of wastes or toxic substances of anthropogenic origin by living processes
These two services are considered in (Armstrong et al., 2012) and (Thurber et al., 2014) as ‘waste absorption and detoxification’, a regulating service. Although CICES v.1 (Haines-Young and Potschin, 2018) consider ‘bioremediation’ and ‘filtration’ as two separate services, in this study we merge these two services into one, as bioremediation and filtration, storage and sequestration depend on similar marine biological processes that store, bury and transform many waste materials through assimilation and chemical transformation, directly and indirectly (Armstrong et al., 2012). Our review found one recent paper that provides some evidence of the benthic-pelagic coupling of deep-sea sponges and assessed the economic value associated with seawater filtration by sponges (Pham et al., 2019). It is noted that our knowledge of the ecological functions of sponges is mostly derived from shallow-water species due to operational constraints. Evidence shows that through water pumping, sponges are capable of filtering vast volumes of water to feed on bacteria and dissolved organic matter (DOM) (Ottaviani, 2020). DOM is the primary source of food for shallow water sponge species, which is often measured in dissolved organic carbon (DOC). (Pham et al., 2019) describe a few examples of shallow and deep sponges species and their ecological roles including shallow glass sponges Aphrocallistes vastus (Yahel et al., 2007; Kahn et al., 2015) and Rhabdocalyptus dawsoni (Yahel et al., 2007); deep demosponges Geodia barretti, G. atlantica, and G. macandrewii (Kutti et al., 2013; Cathalot et al., 2015; Leys et al., 2018). For some species, DOM uptake has been suggested (Leys et al., 2018), and for others it was not found (Yahel et al., 2007; Kahn et al., 2015). Only one deep-sea sponge species has shown DOC uptake, using laboratory-made stable-isotope enriched DOM (Rix et al., 2016). Direct evidence of ambient DOM uptake by deep-sea sponges is still not available at present (Bart et al., 2021). An increasing body of evidence shows that sponges with low microbial abundances are capable of consuming DOC. However there are still too few available data on sponge carbon fluxes to fully understand the key functional traits that determine the strategy of sponges to process dissolved organic matter (DOM) (Bart et al., 2021). Nevertheless, the efficiency of sponges in removing bacteria from the water column is extremely high and affects the turnover of several nitrogen nutrients; their removal would likely affect the delicate ecological balance of deep-sea benthic ecosystems (Pham et al., 2019; Ottaviani, 2020).
3.3.2.6 Pest and disease control
This service is the contribution of marine ecosystems to the maintenance of natural healthy population dynamics to support ecosystem resilience through maintaining food web structure and flows, interactions between species and genotypes including biological pest control (Thurber et al., 2014). This service stems from a ‘biologically mediated habitat’ and indicates the ability of the habitat to persist over time and to recover from pressures. One of the oldest examples of ‘biological regulation’ service from shallow water ecological studies is the interaction between herbivorous parrot fish and coral reefs. Experimental and observational studies have found that parrotfish grazing can exert top-down impacts on the cover of macroalgae on reefs (Mumby, 2009; Cramer et al., 2017). This grazing behaviour benefits corals by helping to limit algal competitors and minimise the intensification of coral disease and bleaching epidemics. These benefits have been studied since the 1960s, generating many controversial opinions on the natural and anthropogenic threats posed to coral reefs (Russ et al., 2015) including those linked to impacts from fisheries targeting parrot fish. Similar trophic cascade examples are lacking from the deep-sea literature, meaning that future research would need to address this valuable and important service provided by benthic trophic assemblages.
3.3.2.7 Regulation of chemical composition of atmosphere and oceans; atmospheric composition and conditions
‘Climate regulation’ is included in all existing marine ES frameworks as a regulating service. This service is linked to nutrient cycling and involves biological processes transporting organic material from the ocean surface to deeper layers. In the last decade, several aspects of the role of deep-sea habitats in sequestering carbon have been investigated (Dunlop et al., 2016; Boyd et al., 2019; Huang et al., 2019; Hilmi et al., 2021). Recent important findings for the deep-sea benthos include the role of dead coral framework and associated microbial communities in nutrient cycling, and potential implications for carbon storage (Maier et al., 2021); the role of bacteria in short-term carbon cycling (Sweetman et al., 2019) and the role of glass sponge reefs in carbon sequestration (Dunham et al., 2018).
3.3.2.8 Educational, spiritual and recreational (cultural) services
Defining cultural services has been extensively debated in the social sciences in the last two decades and, for the scope of this study, we adopt the original definition of cultural services published by MEA, which describes them as ‘the non-material benefits people obtain from ecosystems through spiritual enrichment, cognitive development, reflection, recreation and aesthetic experiences’ (MEA, 2005). Despite the profound scientific, spiritual and imaginative inspirations that the deep sea has evoked in humans (Armstrong et al., 2012; Thurber et al., 2014), integration of cultural services into any marine management protocols, and specifically into MSP, has been slow due to the non-spatial nature of many cultural services (Gee et al., 2017). Our review found a few papers that discuss the importance of cultural services linked to the deep sea. Some evidence exists on the educational and spiritual connections between humans and the deep sea. New ocean literacy media have pushed the boundaries of public engagement in deep-sea science by using simulated dives and deep-sea exploration through virtual learning (Salazar et al., 2019; Sánchez et al., 2020; Morais et al., 2022). Recently, another aspect that has raised interest is the spiritual dimension associated with deep-sea habitats, including links with religion, cultural traditions, art and literature (Turner et al., 2020). Exploration for mineral resources in the ABNJ has also raised many cultural concerns. Cultural, social and religious issues were raised against deep-sea mining in the Bismarck Sea of Papua New Guinea, the world’s first deep-sea mine site known as Solwara 1 (Childs, 2022). Another aspect is also the archaeological and historical heritage of the Middle Passage seascape in the Atlantic seabed (Turner et al., 2020). Thus, African diasporic cultural memory not only has raised awareness of the cultural heritage of the Area, but also pushes regulators, like the ISA, to consider ways to respect those who lost their lives and came to rest on the seabed prior to mineral exploitation (Turner et al., 2020). Contextualisation and local knowledge are recognised as important to robustly incorporate these values into decision-support tools (Knox, 2017). Effective participation is crucial to this and one such method is through participative mapping exercises (Gee et al., 2017). Although time-consuming and requiring expert capacity, the incorporation of a community-based narrative into socio-ecological frameworks is an opportunity to identify traditional knowledge linked to the deep sea that is particularly strong yet largely undocumented (Levin et al., 2021). This is demonstrated by the interest of deep-sea mining off Pacific Islands, where indigenous peoples and local communities’ insights has been brought to the knowledge of a wider public (Tilot et al., 2021; Childs, 2022). Recently, an interdict to stop seismic activity off the South African coast was successful, partly due to local communities’ cultural and ancestral connection the deep sea (Lombard, A., pers. comm.). Integrating cultural services in ecosystem assessments has a significant responsibility in securing the protection of indigenous peoples’ human rights and the human rights of other communities whose identity and livelihoods are intrinsically linked to marine biodiversity and humans (Knox, 2017).
4 Discussion
Our study proposes a standardised deep-sea ES framework comprising four supporting services and ten final services including three regulating services, four provisioning services, and three cultural services. Our review shows that healthy deep-sea ecosystems provide at least 14 ES that contribute to human needs and well-being across the globe. These services include nutrient cycling, habitat provisioning for other species, climate change mitigation, food from fishing, genetic resources for medical purposes, and minerals for technological use and human cultural development. This framework is compatible with existing marine ES frameworks and represents an important step towards developing an applied framework for decision-support in deep-sea contexts. Armstrong et al. (2012) and Thurber et al. (2014) outlined and structured deep-sea ES based on the MEA (2005) framework, which is a theoretical framework that lacks direct applicability in ecosystem accounting such as the Common International Classification of Ecosystem Services (CICES) scheme (Haines-Young and Potschin, 2018). The framework provided in this study builds on their work to provide a standardised list of services using terminology from CICES (2018). A recent publication, Le et al. (2017), has proposed an ES framework based on TEEB (2010), a previous framework version of CICES. One of the advantages of using CICES, as shown in Table 3, provides additional details for each service unit. This results in ease of comparisons of ES trade-offs amongst geographically different areas and type of ecosystem.
Structured frameworks, modelling and ecological indicators are some tools that can help transfer ecological knowledge to ecosystem-based management approaches. Successful spatial planning uses scientific and geospatial information to address conflicts and allocate ocean space to human activities while maintaining ecosystem health, function and services (Ehler and Douvere, 2009). Our framework represents an important step towards operationalising area-based management approaches by providing a standardised classification of deep-sea ES applicable to the global deep-sea benthos. This is a prerequisite for developing a habitat-service matrix that enables researchers, local managers and decision-makers to represent ecosystem services spatially. The framework outlined in this study is part of a preparatory stage that considers those service types in the deep sea, making them explicit units that allow the integration of better-informed discussions in different deep-sea spatial planning scenarios where humans play an integral part. A second stage involves attributing services to different habitat types, and the final stage requires mapping of habitat types across a study region. This framework is an important tool to account for deep-sea ES provision within spatial planning.
Data deficiency in many marine areas has been described as the most significant obstacle in the advancement of this type of ES assessment (Eigenbrod et al., 2010) and the deep sea is widely recognised as being data-poor (Webb et al., 2010; Howell et al., 2020; Cordier et al., 2022). To overcome the data paucity challenge, limited funding and restricted project-specific timeframes, expert elicitation is often a complementary assessment stage and requires experts with extensive knowledge of the area and ES of interest. The lack of evidence in deep-sea ES provisioning may necessitate the use of shallow and coastal water examples to support some expert scores and identify potential ecological processes carried out by analogous deep-sea habitats. However this can also result in pushback and a lack of trust in output. As use of expert opinion is fraught with challenges, guidelines have been developed to encourage transparency and limit biases in evaluating of each service (Campagne and Roche, 2018). The framework outlined in our study establishes the foundations to develop a service matrix; however, it will require the adoption of a deep-sea habitat classification system to operationalise its use in local, national and international decision-making, and the development of habitat maps of regions of interest (e.g. Howell, 2010; McQuaid et al., 2020).
There remain, however, substantial knowledge gaps for deep-sea ES. Some fundamental questions on the mechanisms underpinning the role of deep-sea functional groups in service delivery remain entirely neglected (Danovaro et al., 2008; Leduc et al., 2013; Howell et al., 2020; Manea et al., 2020). While in principle there are already international guidelines and practices on applying the ecosystem approach to fisheries management (FAO, 2009; Kenny et al., 2018), we are lagging behind and in fact our study shows that we do not yet have enough understanding to this well. Additionally, a lack of practical guidelines on how best to incorporate cultural services into deep-sea spatial planning was evident. Deep-sea remoteness should not be an excuse for excluding cultural services, but we are at an early stage of considering ES in marine environmental management let alone in the environmental management of the less accessible deep sea. Although this is beyond the scope of this study, future research in this area should address the role of cultural services for deep-sea policy and decision-making and explore new ways by which to classify and value them. We advise on including different stakeholders from various disciplines and sectors in participative-based assessments to bridge the current knowledge gaps in assessing ES. Despite the data gaps identified in this review, our proposed framework also shows how important these data gaps are for today’s decisions and how seriously they should be considered in the decision-making processes. This study also resonates with the research needs outlined in Howell et al. (2020), and echoes a need for substantial research effort over the next decade on the links between organisms, their functions and ES provision.
5 Conclusions
Globally, the expansion of human activities has led to concerns over the health of marine ecosystems and the impact of poor health on the ES they provide to humans. Previous reviews set out a structure that links deep-sea ecosystems to human society, but these were conceptual. Our study builds on these foundations to operationalise these concepts into a practical framework consisting of four supporting services, three regulating services, four provisioning services, and three cultural services. Moving forward, we need to build knowledge on the flow from structure-function-service-benefit for deep-sea ecosystems. This will enable the scientific community to feed deep-sea ES information into different spatial and temporal scales required for specific projects and spatial planning. Relevant stakeholders at local, regional, national and global scales should be engaged in the formulation and implementation of management decisions. Ultimately, this would contribute to the maintenance of long-term benefits from deep-sea ecosystems.
Data availability statement
The original contributions presented in the study are included in the article/Supplementary Material. Further inquiries can be directed to the corresponding author.
Author contributions
GLB, KH, SR contributed to the conception and design of the analysis. GLB undertook the analysis, wrote the first draft and led on revisions. All authors contributed to the article and approved the submitted version.
Funding
This study and all authors (with the exception of HR), were funded by the GCRF One Ocean Hub. GLB was funded with in-kind support from the University of Plymouth. HR was funded by JNCC. KS also acknowledges the Deep Connections Project (NRF Grant129216) funded through the African Coelacanth Ecosystem Project. This work was supported by the United Kingdom Research and Innovation (UKRI), Global Challenges Research Fund (GCRF), One Ocean Hub (Grant Ref: NE/S008950/1).
Acknowledgments
We thank Elisa Morgera and Bernardette Snow for their advice during the revision of the original manuscript.
Conflict of interest
The authors declare that the research was conducted in the absence of any commercial or financial relationships that could be construed as a potential conflict of interest.
Publisher’s note
All claims expressed in this article are solely those of the authors and do not necessarily represent those of their affiliated organizations, or those of the publisher, the editors and the reviewers. Any product that may be evaluated in this article, or claim that may be made by its manufacturer, is not guaranteed or endorsed by the publisher.
Supplementary material
The Supplementary Material for this article can be found online at: https://www.frontiersin.org/articles/10.3389/fmars.2023.1176230/full#supplementary-material
References
Aanesen M., Armstrong C. W. (2016). The political game of European fisheries management. Environ. Resource Economics 63 (4), pp.745–pp.763. doi: 10.1007/s10640-015-9878-0
Almada G. V. de M. B., Bernardino A. F. (2017). Conservation of deep-sea ecosystems within offshore oil fields on the Brazilian margin, SW Atlantic. Biol. Conserv. 206, 92–101. doi: 10.1016/j.biocon.2016.12.026
Amon D. J., Gobin J., Van Dover C. L., Levin L. A., Marsh L., Raineault N. A. (2017). Characterization of methane-seep communities in a deep-Sea area designated for oil and natural gas exploitation off Trinidad and Tobago. Front. IN Mar. Sci. 4, 342. doi: 10.3389/fmars.2017.00342
Armstrong C. W., Foley N. S., Kahui V., Grehan A. (2014). Cold water coral reef management from an ecosystem service perspective. Mar. Policy 50 (PA), 126–134. doi: 10.1016/j.marpol.2014.05.016
Armstrong C. W., Foley N. S., Tinch R., van den Hove S. (2012). Services from the deep: steps towards valuation of deep sea goods and services. Ecosystem Serv. 2, 2–13. doi: 10.1016/j.ecoser.2012.07.001
Armstrong C. W., Vondolia G. K., Foley N. S., Henry L.-A. A., Needham K., Ressurreicao A. M. (2019). Expert assessment of risks posed by climate change and anthropogenic activities to ecosystem services in the deep north Atlantic. Front. Mar. Sci. 6 (MAR). doi: 10.3389/fmars.2019.00158
Arora N. K., Mishra I. (2020). Ocean sustainability: essential for blue planet. Environ. Sustainability 3 (1), 1–3. doi: 10.1007/s42398-020-00100-6
Baillon S., Hamel J. F., Wareham V. E., Mercier A. (2012). Deep cold-water corals as nurseries for fish larvae. Front. Ecol. Environ. 10 (7), pp.351–pp.356. doi: 10.1890/120022
Barbier E. B. (2017). Marine ecosystem services. Curr. Biol. 27 (11), pp.R507–R510. doi: 10.1016/j.cub.2017.03.020
Bart M. C., Mueller B., Rombouts T., van de Ven C., Tompkins G. J., Osinga R., et al. (2021). Dissolved organic carbon (DOC) is essential to balance the metabolic demands of four dominant north-Atlantic deep-sea sponges. Frontiers in Marine Science 66, 3, 925–938. doi: 10.1002/lno.11652
Batista-García R. A., Sutton T., Jackson S. A., Tovar-Herrera O. E., Balcázar-López E., Sánchez-Carbente M. D. R., et al. (2017). Characterization of lignocellulolytic activities from fungi isolated from the deep-sea sponge stelletta normani. PLoS One. 12 (3), e0173750. doi: 10.1371/journal.pone.0173750
Beaumont N. J., Austen M. C., Atkins J. P., Burdon D., Degraer S., Dentinho T. P., et al. (2007). Identification, definition and quantification of goods and services provided by marine biodiversity: implications for the ecosystem approach. Mar. pollut. Bull. 54 (3), 253–265. doi: 10.1016/j.marpolbul.2006.12.003
Bendia A. G., Signori C. N., Nakamura F. M., Butarelli A. C. D. A., Passos J. G., Ramos R. B., et al. (2021). Microbial perspective on the giant carbonate ridge alpha crucis (Southwestern Atlantic upper slope). FEMS Microbiol. Ecol. 97 (8), 1–14. doi: 10.1093/femsec/fiab110
Boldy R., Santini T., Annandale M., Erskine P. D., Sonter L. J. (2021). Understanding the impacts of mining on ecosystem services through a systematic review. Extractive Industries Soc. 8 (1), pp.457–pp.466. doi: 10.1016/j.exis.2020.12.005
Bongiorni L., Mea M., Gambi C., Pusceddu A., Taviani M., Danovaro R. (2010). Deep-water scleractinian corals promote higher biodiversity in deep-sea meiofaunal assemblages along continental margins. Biol. Conserv. 143 (7), pp.1687–1700. doi: 10.1016/j.biocon.2010.04.009
Boyd P. W., Claustre H., Levy M., Siegel D. A., Weber T. (2019). Multi-faceted particle pumps drive carbon sequestration in the ocean. Nature 568, 7752, 327–335. doi: 10.1038/s41586-019-1098-2
Bueno-Pardo J., Ramalho S. P., García-Alegre A., Morgado M., Vieira R. P., Cunha M. R., et al. (2017). Deep-sea crustacean trawling fisheries in Portugal: quantification of effort and assessment of landings per unit effort using a vessel monitoring system (VMS). Sci. Rep. 7 (December 2016), 1–10. doi: 10.1038/srep40795
Campagne S. C., Roche P. K. (2018). May the matrix be with you! guidelines for the application of expert-based matrix approach for ecosystem services assessment and mapping. One Ecosystem 3, e24134. doi: 10.3897/oneeco.3.e24134
Campanyà-Llovet N., Snelgrove P. V. R., Parrish C. C. (2017). Rethinking the importance of food quality in marine benthic food webs. Prog. Oceanography 156, pp.240–pp.251. doi: 10.1016/j.pocean.2017.07.006
Cathalot C., Van Oevelen D., Cox T. J. S., Kutti T., Lavaleye M., Duineveld G., et al. (2015). Cold-water coral reefs and adjacent sponge grounds: hotspots of benthic respiration and organic carbon cycling in the deep sea. Front. Mar. Sci 2. doi: 10.3389/fmars.2015.00037
Childs J. (2022). Geographies of deep sea mining: a critical review. Extractive Industries Soc. 9 (January), 101044. doi: 10.1016/j.exis.2022.101044
Clark M. R., Althaus F., Schlacher T. A., Williams A., Bowden D. A., Rowden A. A. (2016). The impacts of deep-sea fisheries on benthic communities: a review. ICES J. OF Mar. Sci. 73, pp.51–pp.69. doi: 10.1093/icesjms/fsv123
Clarke J., Milligan R. J., Bailey D. M., Neat F. C. (2015). A scientific basis for regulating deep-Sea fishing by depth. Curr. Biol. 25 (18), pp.2425–2429. doi: 10.1016/j.cub.2015.07.070
Collins F. W. J., Walsh C. J., Gomez-Sala B., Guijarro-García E., Stokes D., Jakobsdóttir K. B., et al. (2021). The microbiome of deep-sea fish reveals new microbial species and a sparsity of antibiotic resistance genes. Gut Microbes 13, 1, 1–13. doi: 10.1080/19490976.2021.1921924
Committee of Experts on Environmental- Economic Accounting (2021). System of environmental-economic accounting{{/amp]]mdash; ecosystem accounting (SEEA EA Vol. 5 (White cover publication, pre-edited text subject to official editing), 362.
Cordes E. E., Jones D. O. B., Schlacher T. A., Amon D. J., Bernardino A. F., Brooke S., et al. (2016). Environmental impacts of the deep-water oil and gas industry: a review to guide management strategies (Frontiers in Environmental Science), Vol. 4. doi: 10.3389/fenvs.2016.00058
Cordier T., Angeles I. B., Henry N., Lejzerowicz F., Berney C., Morard R., et al. (2022). Patterns of eukaryotic diversity from the surface to the deep-ocean sediment. Sci. Adv. 8 (5), eabj9309. doi: 10.1126/sciadv.abj9309
Corinaldesi C. (2015). New perspectives in benthic deep-sea microbial ecology. Front. Mar. Sci. 2 (MAR). doi: 10.3389/fmars.2015.00017
Cramer K. L., O’Dea A., Clark T. R., Zhao J. X., Norris R. D. (2017). Prehistorical and historical declines in Caribbean coral reef accretion rates driven by loss of parrotfish. Nat. Commun. 8, 14160. doi: 10.1038/ncomms14160
Culhane F. E., Frid C. L. J., Royo Gelabert E., White L., Robinson L. A. (2018). Linking marine ecosystems with the services they supply: what are the relevant service providing units? Ecol. Appl. 28 (7), 1740–1751. doi: 10.1002/eap.1779
Danovaro R., Gambi C., Dell’Anno A., Corinaldesi C., Fraschetti S., Vanreusel A., et al. (2008). Exponential decline of deep-Sea ecosystem functioning linked to benthic biodiversity loss. Curr. Biol. 18 (1), 1–8. doi: 10.1016/j.cub.2007.11.056
Danovaro R., Snelgrove P. V. R., Tyler P. (2014). Challenging the paradigms of deep-sea ecology. Trends Ecol. Evol. 29 (8), pp.465–pp.475. doi: 10.1016/j.tree.2014.06.002
De Groot R., Fisher B., Christie M., Aronson J., Braat L., Gowdy J., et al. (2010). “Integrating the ecological and economic dimensions in biodiversity and ecosystem service valuation,” in The economics of ecosystems and biodiversity: ecological and economic foundations (Earthscan, Routledge) 9–40. doi: 10.4324/9781849775489
Dell’Anno A., Corinaldesi C., Danovaro R. (2015). Virus decomposition provides an important contribution to benthic deep-sea ecosystem functioning. Proceedings of the National Academy of Sciences 112, 16, E2014–E2019. doi: 10.1073/pnas.1422234112
Dell’Anno A., Danovaro R. (2005). Extracellular {DNA} plays a key role in deep-sea ecosystem functioning. Science 309, p.2179. doi: 10.1126/science.1117475
Diaz S., Demissew S., Carabias J., Joly C., Lonsdale M., Ash N., et al. (2015). The IPBES conceptual framework - connecting nature and people. Curr. Opin. IN Environ. SUSTAINABILITY 14, pp.1–pp16. doi: 10.1016/j.cosust.2014.11.002
Diz D., Morgera E., Wilson M. (2017). Sharing the benefits of sustainable fisheries: from global to local legal approaches to marine ecosystem services for poverty alleviation (Science policy analysis). SSRN Electronic J. doi: 10.2139/ssrn.2944330
Dombrowski N., Seitz K. W., Teske A. P., Baker B. J. (2017). Genomic insights into potential interdependencies in microbial hydrocarbon and nutrient cycling in hydrothermal sediments. Microbiome 5, 1, 106. doi: 10.1186/s40168-017-0322-2
Dunham A., Archer S. K., Davies S. C., Burke L. A., Mossman J., Pegg J. R., et al. (2018). Assessing condition and ecological role of deep-water biogenic habitats: glass sponge reefs in the salish sea. Marine environmental research 141, 88-99. doi: 10.1016/j.marenvres.2018.08.002
Dunlop K. M., van Oevelen D., Ruhl H. A., Huffard C. L., Kuhnz L. A., Smith K. L. (2016). Carbon cycling in the deep eastern north pacific benthic food web: investigating the effect of organic carbon input. Limnology Oceanography 61 (6), pp.1956–1968. doi: 10.1002/lno.10345
Dunn D. C., Van Dover C. L., Etter R. J., Smith C. R., Levin L. A., Morato T., et al. (2018). A strategy for the conservation of biodiversity on mid-ocean ridges from deep-sea mining 4, 7. doi: 10.1126/sciadv.aar4313
Durden J. M., Lallier L. E., Murphy K., Jaeckel A., Gjerde K., Jones D. O. B. (2018). Environmental impact assessment process for deep-sea mining in ‘the area’. Mar. Policy 87, 194–202. doi: 10.1016/j.marpol.2017.10.013
Edrada-Ebel R. A., Ævarsson A., Polymenakou P., Hentschel U., Carettoni D., Day J., et al. (2018). SeaBioTech: from seabed to test-bed: harvesting the potential of marine biodiversity for industrial biotechnology. doi: 10.1007/978-3-319-69075-9_12
Ehler C. N., Douvere F. (2009). Marine spatial planning. Offshore Energy Mar. Spatial Plann., 6–17. doi: 10.4324/9781315666877-2
Eigenbrod F., Armsworth P. R., Anderson B. J., Heinemeyer A., Gillings S., Roy D. B., et al. (2010). The impact of proxy-based methods on mapping the distribution of ecosystem services. J. Appl. Ecol. 47 (2), 377–385. doi: 10.1111/j.1365-2664.2010.01777.x
FAO (2009). International guidelines for the management of deep-sea fisheries in the high seas. 1–72.
FAO (2018). General situation of world fish stocks. united nations food and agriculture organization (FAO). 6–10. doi: 10.4060/ca9229en
Folkersen M. V., Fleming C. M., Hasan S. (2018). The economic value of the deep sea: a systematic review and meta-analysis. Mar. Policy 94 (May), 71–80. doi: 10.1016/j.marpol.2018.05.003
Galparsoro I., Pınarbaşı K., Gissi E., Culhane F., Gacutan J., Kotta J., et al. (2021). Operationalisation of ecosystem services in support of ecosystem-based marine spatial planning: insights into needs and recommendations. Mar. Policy 131 (May), 104609. doi: 10.1016/j.marpol.2021.104609
Gee K., Kannen A., Adlam R., Brooks C., Chapman M., Cormier R., et al. (2017). Identifying culturally significant areas for marine spatial planning. Ocean Coast. Manage. 136, 139–147. doi: 10.1016/j.ocecoaman.2016.11.026
Gollner S., Kaiser S., Menzel L., Jones D. O. B., Brown A., Mestre N. C., et al. (2017). Resilience of benthic deep-sea fauna to mining activities. Marine Environmental Research 129, 76-101. doi: 10.1016/j.marenvres.2017.04.010
Gooday A. J., Schoenle A., Dolan J. R., Arndt H. (2020). Protist diversity and function in the dark ocean – challenging the paradigms of deep-sea ecology with special emphasis on foraminiferans and naked protists. Eur. J. Protistology 75, p.125721. doi: 10.1016/j.ejop.2020.125721
Grupe B. M., Krach M. L., Pasulka A. L., Maloney J. M., Levin L. A., Frieder C. A. (2015). Methane seep ecosystem functions and services from a recently discovered southern California seep. Mar. Ecol. 36 (S1), pp.91–pp108. doi: 10.1111/maec.12243
Haines-Young R., Potschin M. (2010). The links between biodiversity, ecosystem services and human well-being. Ecosystem Ecol. 1, pp.110–pp.139. doi: 10.1029/JB087iB10p08501
Haines-Young R., Potschin M. (2018). “CICES V5. 1. guidance on the application of the revised structure,” in Common international classification of ecosystem services (CICES), 53.
Haines-Young R., Potschin-Young M. B. (2018). Revision of the common international classification for ecosystem services (CICES V5.1): a policy brief. One Ecosystem 3, 1–6. doi: 10.3897/oneeco.3.e27108
Harden-Davies H. (2017). Deep-sea genetic resources: new frontiers for science and stewardship in areas beyond national jurisdiction. Deep Sea Res. Part II: Topical Stud. Oceanography 137, 504–513. doi: 10.1016/j.dsr2.2016.05.005
Hattam C., Broszeit S., Langmead O., Praptiwi R. A., Lim V. C., Creencia L. A., et al. (2021). A matrix approach to tropical marine ecosystem service assessments in south east Asia. Ecosystem Serv. 51 (June), 101346. doi: 10.1016/j.ecoser.2021.101346
Heink U., Hauck J., Jax K., Sukopp U. (2016). Requirements for the selection of ecosystem service indicators - the case of MAES indicators. Ecol. Indic. 61, pp.18–pp.26. doi: 10.1016/j.ecolind.2015.09.031
Henderson M. J., Huff D. D., Yoklavich M. M. (2020). Deep-Sea coral and sponge taxa increase demersal fish diversity and the probability of fish presence. Front. IN Mar. Sci. 7. doi: 10.3389/fmars.2020.593844
Hessler R. R., Sanders H. L. (1967). Faunal diversity in the deep sea. Deep-Sea Res. 14, pp.65–pp.78. doi: 10.1016/0011-7471(67)90029-0
Higgs N. D., Newton J., Attrill M. J. (2016). Caribbean Spiny lobster fishery is underpinned by trophic subsidies from chemosynthetic primary production. Curr. Biol. 26 (24), pp.3393–3398. doi: 10.1016/j.cub.2016.10.034
Hilmi N., Chami R., Sutherland M. D., Hall-Spencer J. M., Lebleu L., Benitez M. B., et al. (2021). The role of blue carbon in climate change mitigation and carbon stock conservation. Front. Climate 3 (September). doi: 10.3389/fclim.2021.710546
Hooper T., Börger T., Langmead O., Marcone O., Rees S. E., Rendon O., et al. (2019). Applying the natural capital approach to decision making for the marine environment. Ecosystem Serv. 38 (June), 100947. doi: 10.1016/j.ecoser.2019.100947
Howell K. L. (2010). A benthic classification system to aid in the implementation of marine protected area networks in the deep/high seas of the NE Atlantic. Biological Conservation 143(5), 1041–1056. doi: 10.1016/j.biocon.2010.02.001
Howell K. L., Hilário A., Allcock A. L., Bailey D. M., Baker M., Clark M. R., et al. (2020). A blueprint for an inclusive, global deep-Sea ocean decade field program. Front. Mar. Sci. 7 (November). doi: 10.3389/fmars.2020.584861
Huang J.-M., Baker B. J., Li J.-T., Wang Y. (2019). New microbial lineages capable of carbon fixation and nutrient cycling in deepsea sediments of the northern south China Sea. Applied and environmental microbiology. 85 (15), e00523-19. doi: 10.1128/AEM.00523-19
Jiang T., Pu H., Duan Y. W., Yan X. H., Huang Y. (2021). New natural products of streptomyces sourced from deep-Sea, desert, volcanic, and polar regions from 2009 to 2020. Chin. J. OF ORGANIC Chem. 41 (5), pp.1804–1820. doi: 10.6023/cjoc202010004
Jobstvogt N., Townsend M., Witte U., Hanley N. (2014). How can we identify and communicate the ecological value of deep-sea ecosystem services? PloS One 9 (7), pp.1–pp11. doi: 10.1371/journal.pone.0100646
Joye S. B., Bracco A., Özgökmen T. M., Chanton J. P., Grosell M., MacDonald I. R., et al. (2016). The gulf of Mexico ecosystem, six years after the macondo oil well blowout. Deep Sea Res. Part II: Topical Stud. Oceanography 129, pp.4–pp19. doi: 10.1016/j.dsr2.2016.04.018
Kahn A. S., Yahel G., Chu J. W. F., Tunnicliffe V., Leys S. P. (2015). Benthic grazing and carbon sequestration by deep-water glass sponge reefs. Limnology Oceanography 60 (1), 78–88. doi: 10.1002/lno.10002
Keeler E., Burgaud G., Teske A., Beaudoin D., Mehiri M., Dayras M., et al. (2021). Deep-sea hydrothermal vent sediments reveal diverse fungi with antibacterial activities. FEMS Microbiol. Ecol. 97 (8), pp.1–pp20. doi: 10.1093/femsec/fiab103
Kenny A. J., Campbell N., Koen-Alonso M., Pepin P., Diz D. (2018). Delivering sustainable fisheries through adoption of a risk-based framework as part of an ecosystem approach to fisheries management. Mar. Policy 93 (October 2016), 232–240. doi: 10.1016/j.marpol.2017.05.018
Knox J. H. (2017). Report of the special rapporteur on the issue of human rights obligations relating to the enjoyment of a safe, clean, healthy and sustainable environment: biodiversity report (United Nations Human Rights Council, Wake Forest Univ), 189–212. doi: 10.1162/itgg.2008.3.2.189
Koch M. J., Hesketh-Best P. J., Smerdon G., Warburton P. J., Howell K., Upton M. (2021). Impact of growth media and pressure on the diversity and antimicrobial activity of isolates from two species of hexactinellid sponge. Microbiol. (United Kingdom) 167 (12). doi: 10.1099/MIC.0.001123
Kutti T., Bannister R. J., Fosså J. H. (2013). Community structure and ecological function of deep-water sponge grounds in the traenadypet MPA–northern Norwegian continental shelf. Continental Shelf Res. 69, pp.21–pp.30. doi: 10.1016/j.csr.2013.09.011
La Bianca G., Tillin H., Hodgson B., Erni-cassola G. (2018). Ascension island- natural capital assessment.
Langlet D., Bouchet V. M. P., Riso R., Matsui Y., Suga H., Fujiwara Y., et al. (2020). Foraminiferal ecology and role in nitrogen benthic cycle in the hypoxic southeastern Bering Sea. Front. IN Mar. Sci. 7, 582818. doi: 10.3389/fmars.2020.582818
Le J. T., Levin L. A., Carson R. T. (2017). Incorporating ecosystem services into environmental management of deep-seabed mining. Deep-Sea Res. Part II: Topical Stud. Oceanography 137, 486–503. doi: 10.1016/j.dsr2.2016.08.007
Leduc D., Rowden A. A., Pilditch C. A., Maas E. W., Probert P. K. (2013). Is there a link between deep-sea biodiversity and ecosystem function? Mar. Ecol. 34 (3), 334–344. doi: 10.1111/maec.12019
Levin L. A., Baco A. R., Bowden D. A., Colaco A., Cordes E. E., Cunha M. R., et al. (2016). Hydrothermal vents and methane seeps: rethinking the sphere of influence. Front. Mar. Sci. 3 (MAY). doi: 10.3389/fmars.2016.00072
Levin L. A., Bett B. J., Gates A. R., Heimbach P., Howe B. M., Janssen F., et al. (2019). Global observing needs in the deep ocean. Front. Mar. Sci. 6 (May). doi: 10.3389/fmars.2019.00241
Levin L., Escobar E., Willaert K., Turner P., Guilloux B. (2021). The necessity of traditional knowledge for management of deep-seabed mining. 1–6.
Leys S. P., Kahn A. S., Fang J. K. H., Kutti T., Bannister R. J. (2018). Phagocytosis of microbial symbionts balances the carbon and nitrogen budget for the deep-water boreal sponge geodia barretti. Limnology Oceanography 63 (1), pp.187–pp.202. doi: 10.1002/lno.10623
Li Z., Wang Y., Li J., Liu F., He L., He Y., et al. (2016). Metagenomic analysis of genes encoding nutrient cycling pathways in the microbiota of deep-Sea and shallow-water sponges. Marine Biotechnology 18, 6, 659–671. doi: 10.1007/s10126-016-9725-5
Lin G., Huang J., Lu J., Su M., Hu B., Lin X. (2021). Geochemical and microbial insights into vertical distributions of genetic potential of n-cycling processes in deep-sea sediments. Ecol. Indic. 125, p.107461. doi: 10.1016/j.ecolind.2021.107461
Liquete C., Piroddi C., Drakou E. G., Gurney L., Katsanevakis S., Charef A., et al. (2013). Current status and future prospects for the assessment of marine and coastal ecosystem services: a systematic review. PloS One 8 (7), e67737. doi: 10.1371/journal.pone.0067737
Ma L., Zhang W. J., Liu Z. W., Huang Y. B., Zhang Q. B., Tian X. P., et al. (2021). Complete genome sequence of streptomyces sp. SCSIO 03032 isolated from Indian ocean sediment, producing diverse bioactive natural products. Mar. Genomics 55, 100803. doi: 10.1016/j.margen.2020.100803
Mace G. M., Hails R. S., Cryle P., Harlow J., Clarke S. J. (2015). Towards a risk register for natural capital. J. Appl. Ecol. 52 (3), pp.641–pp.653. doi: 10.1111/1365-2664.12431
Maes J., Fabrega N., Zulian G., Barbosa A., Ivits E., Polce C., et al. (2015). Mapping and assessment of ecosystems and their services trends in ecosystems and ecosystem - JRC report number JRC94889 (Luxembourg: Publications Office of the European Union). doi: 10.2788/341839
Maier S. R., Mienis F., de Froe E., Soetaert K., Lavaleye M., Duineveld G., et al. (2021). Reef communities associated with ‘dead’ cold-water coral framework drive resource retention and recycling in the deep sea. Deep Sea Res. Part I: Oceanographic Res. Papers 175 (May), 103574. doi: 10.1016/j.dsr.2021.103574
Maldonado M., López-Acosta M., Busch K., Slaby B. M., Bayer K., Beazley L., et al. (2021). A microbial nitrogen engine modulated by bacteriosyncytia in hexactinellid sponges: ecological implications for deep-Sea communities (Frontiers in Marine Science.). doi: 10.3389/fmars.2021.638505
Manea E., Bianchelli S., Fanelli E., Danovaro R., Gissi E. (2020). Towards an ecosystem-based marine spatial planning in the deep Mediterranean Sea. Sci. Total Environ. 715, p.136884. doi: 10.1016/j.scitotenv.2020.136884
McQuaid K. A., Attrill M. J., Clark M. R., Cobley A., Glover A. G., Smith C. R., et al. (2020). Using Habitat Classification to Assess Representativity of a Protected Area Network in a Large, Data-Poor Area Targeted for Deep-Sea Mining. Frontiers in Marine Science 7, p.558860. doi: 10.3389/fmars.2020.558860
MEA (2005). Millennium ecosystem assessment. in: ecosystems and human well-being: synthesis (Washington, DC: Island Press), 137. doi: 10.5822/978-1-61091-484-0_1
Mejjad N., Rovere M. (2021). Understanding the impacts of blue economy growth on deep-Sea ecosystem services. Sustainability 13 (22), p.12478. doi: 10.3390/su132212478
Moher D., Liberati A., Tetzlaff J., Altman D. G. (2010). Preferred reporting items for systematic reviews and meta-analyses: the PRISMA statement. Int. J. Surg. 8 (5), pp.336–pp.341. doi: 10.1016/j.ijsu.2010.02.007
Moloney M. G. (2016). Natural products as a source for novel antibiotics. Trends Pharmacol. Sci. 37 (8), pp.689–pp.701. doi: 10.1016/j.tips.2016.05.001
Morais C., Moreira L., Teixeira A. S., Aguiar T. (2022). No waves from surface knowledge: diving into the social representations of the deep sea. Int. J. Sci. Education Part B: Communication Public Engagement 12 (1), pp.22–pp.41. doi: 10.1080/21548455.2021.2017507
Morato T., González-Irusta J. M., Dominguez-Carrió C., Wei C. L., Davies A., Sweetman A. K., et al. (2020). Climate-induced changes in the suitable habitat of cold-water corals and commercially important deep-sea fishes in the north Atlantic. Global Change Biol. 26 (4), 2181–2202. doi: 10.1111/gcb.14996
Mumby P. J. (2009). Herbivory versus corallivory: are parrotfish good or bad for Caribbean coral reefs? Coral Reefs 28 (3), 683–690. doi: 10.1007/s00338-009-0501-0
Niner H. J., Ardron J. A., Escobar E. G., Gianni M., Jaeckel A., Jones D. O. B., et al. (2018). Deep-Sea mining with no net loss of biodiversity-an impossible aim. Front. IN Mar. Sci. 5, 53. doi: mars.2018.00195
Ottaviani D. (2020). Economic valuation of ecosystem services provided by deep-sea sponges (Rome). doi: 10.4060/cb2331en
Pascoe S., Innes J. P. (2018). Economic impacts of the development of an offshore oil and gas industry on fishing industries: a review of experiences and assessment methods. Rev. IN FISHERIES Sci. \& AQUACULTURE 26 (3), 350–370. doi: 10.1080/23308249.2018.1436521
Pauly D. (2007). The sea around us project: documenting and communicating global fisheries impacts on marine ecosystems. Ambio 36 (4), 290–295. doi: 10.1579/0044-7447(2007)36[290:TSAUPD]2.0.CO;2
Pauly D., Alder J., Bennett E., Christensen V., Tyedmers P., Watson R. (2003). The future for fisheries. Science 302 (5649), 1359–1361. doi: 10.1126/science.1088667
Pham C. K., Murillo F. J., Lirette C., Maldonado M., Colaço A., Ottaviani D., et al. (2019). Removal of deep-sea sponges by bottom trawling in the Flemish cap area: conservation, ecology and economic assessment. Sci. Rep. 9 (1), pp.1–pp13. doi: 10.1038/s41598-019-52250-1
Pitcher T. J., Morato T., Hart P. J., Clark M. R., Haggan N., Santos R. S. (2008). Seamounts: ecology, fisheries and conservation. (John Wiley & Sons). doi: 10.1002/9780470691953
Potts T., Burdon D., Jackson E., Atkins J., Saunders J., Hastings E., et al. (2014). Do marine protected areas deliver flows of ecosystem services to support human welfare? Mar. Policy 44, pp.139–pp.148. doi: 10.1016/j.marpol.2013.08.011
Quemener M., Dayras M., Frotté N., Debaets S., Le Meur C., Barbier G., et al. (2021). Highlighting the biotechnological potential of deep oceanic crust fungi through the prism of their antimicrobial activity. Mar. Drugs 19 (8), pp.1–pp15. doi: 10.3390/md19080411
Ramirez-Llodra E., Brandt A., Danovaro R., De Mol B., Escobar E., German C. R., et al. (2010). Deep, diverse and definitely different: unique attributes of the world’s largest ecosystem. Biogeosciences 7 (9), pp.2851–2899. doi: 10.5194/bg-7-2851-2010
Ramirez-Llodra E., Tyler P. A., Baker M. C., Bergstad O. A., Clark M. R., Escobar E., et al. (2011). Man and the last great wilderness: human impact on the deep sea. PloS One 6 (7), 1–25. doi: 10.1371/journal.pone.0022588
Rateb M. E., Ebel R. (2011). Secondary metabolites of fungi from marine habitats. Natural Product Rep. 28 (2), pp.290–pp.344. doi: 10.1039/c0np00061b
Rees S. E., Ashley M., Cameron A., Mullier T., Ingle C., Oates J., et al. (2022). A marine natural capital asset and risk register–towards securing the benefits from marine systems and linked ecosystem services. J. Appl. Ecol. 59 (4), pp.1098–1109. doi: 10.1111/1365-2664.14121
Rix L., de Goeij J. M., Mueller C. E., Struck U., Middelburg J. J., van Duyl F. C., et al. (2016). Coral mucus fuels the sponge loop in warm- and cold-water coral reef ecosystems. Sci. Rep. 6, 18715. doi: 10.1038/srep18715
Rogers A., Aburto-Oropeza O., Appeltans W., Assis J., Ballance L. T., Cury P., et al. (2020). Critical habitats and Biodiversity : inventory, thresholds and governance (Washington, DC: World Resources Institute), 1–91. Available at: www.oceanpanel.org/blue-papers/critical-habitats-and-biodiversity-inventory-thresholds-and-governance.
Rooks C., Fang J. K. H., Morkyed P. T., Zhao R., Rapp H. T., Xayier J. R., et al. (2020). Deep-sea sponge grounds as nutrient sinks: denitrification is common in boreo-Arctic sponges. BIOGEOSCIENCES 17 (5), pp.1231–1245. doi: 10.5194/bg-17-1231-2020
Russ G. R., Questel S. L. A., Rizzari J. R., Alcala A. C. (2015). The parrotfish–coral relationship: refuting the ubiquity of a prevailing paradigm. Mar. Biol. 162 (10), 2029–2045. doi: 10.1007/s00227-015-2728-3
Sala E., Mayorga J., Costello C., Kroodsma D., Palomares M. L. D., Pauly D., et al. (2018). The economics of fishing the high seas. Sci. Adv. 4 (6), 1–14. doi: 10.1126/sciadv.aat2504
Salazar J., Dominguez-Carrio C., Gili J.-M., Ambroso S., Grinyo J., Vendrell-Simon B. (2019). Building a new ocean literacy approach based on a simulated dive in a submarine: a multisensory workshop to bring the deep Sea closer to people. Front. IN Mar. Sci. 6, 576. doi: 10.3389/fmars.2019.00576
Sánchez J. A., Neira-Ramírez L., Rodríguez-Bermúdez A., Quattrini A. (2020). Role-playing to foster ‘Deep-Sea exploration’ through active and virtual learning: a class-design for Colombian higher education. Current: J. Mar. Educ. 34 (3), p.9. doi: 10.5334/cjme.55
Sanders H. L., Hessler R. R. (1969). Ecology of the deep-Sea benthos: More detailed recent sampling has altered our concepts about the animals living on the deep-ocean floor. Science 163 (3874), 1419-1424.
Santos R. S., Pham C. K., Ingels J. (2019). Anthropogenic disturbances in the deep Sea. Front. Res. Topics. doi: 10.3389/978-2-88963-288-6
Snelgrove P. V. R., Smith C. R. (2002). “A riot of species in an environmental calm: the paradox of the species-rich deep-sea floor,” in Gibson, RN and Barnes, m and Atkinson, RJA, ed. OCEANOGRAPHY AND MARINE BIOLOGY, vol. VOL 40. (Oceanography and Marine Biology), pp.311–pp.342.
Snelgrove P. V. R., Thrush S. F., Wall D. H., Norkko A. (2014). Real world biodiversity-ecosystem functioning: a seafloor perspective. Trends Ecol. Evol. 29 (7), 398–405. doi: 10.1016/j.tree.2014.05.002
Sweetman A. K., Smith C. R., Shulse C. N., Maillot B., Lindh M., Church M. J., et al. (2019). Key role of bacteria in the short-term cycling of carbon at the abyssal seafloor in a low particulate organic carbon flux region of the eastern pacific ocean. Limnology Oceanography 64 (2), 694–713. doi: 10.1002/lno.11069
Teixeira H., Lillebo A. I., Culhane F., Robinson L., Trauner D., Borgwardt F., et al. (2019). Linking biodiversity to ecosystem services supply: patterns across aquatic ecosystems. Sci. OF THE TOTAL Environ. 657, 517–534. doi: 10.1016/j.scitotenv.2018.11.440
Thurber A. R., Sweetman A. K., Narayanaswamy B. E., Jones D. O. B., Ingels J., Hansman R. L. (2013). Ecosystem function and services provided by the deep sea. Biogeosciences Discussions 10 (11), 18193–18240. doi: 10.5194/bgd-10-18193-2013
Thurber A. R., Sweetman A. K., Narayanaswamy B. E., Jones D. O. B., Ingels J., Hansman R. L. (2014). Ecosystem function and services provided by the deep sea. Biogeosciences 11 (14), 3941–3963. doi: 10.5194/bg-11-3941-2014
Tilot V., Willaert K., Guilloux B., Chen W., Mulalap C. Y., Gaulme F., et al. (2021). Traditional dimensions of seabed resource management in the context of deep Sea mining in the pacific: learning from the socio-ecological interconnectivity between island communities and the ocean realm. Front. Mar. Sci. 8 (April). doi: 10.3389/fmars.2021.637938
Tortorella E., Tedesco P., Esposito F. P., January G. G., Fani R., Jaspars M., et al. (2018). Antibiotics from deep-Sea microorganisms: current discoveries and perspectives. Mar. Drugs 16 (10), 355. doi: 10.3390/md16100355
Turner P. J., Cannon S., DeLand S., Delgado J. P., Eltis D., Halpin P. N., et al. (2020). Memorializing the middle passage on the Atlantic seabed in areas beyond national jurisdiction. Mar. Policy 122, 104254. doi: 10.1016/j.marpol.2020.104254
Victorero L., Watling L., Palomares M. L. D., Nouvian C. (2018). Out of sight, but within reach: a global history of bottom-trawled deep-Sea fisheries from > 400 m depth. Front. IN Mar. Sci. 5, 98. doi: 10.3389/fmars.2018.00098
Vilas D., Coll M., Pedersen T., Corrales X., Filbee-Dexter K., Pedersen M. F., et al. (2020). Kelp-carbon uptake by Arctic deep-sea food webs plays a noticeable role in maintaining ecosystem structural and functional traits. J. OF Mar. Syst. 203, 103268. doi: 10.1016/j.jmarsys.2019.103268
Webb T. J., vanden Berghe E., O’Dor R. (2010). Biodiversity’s big wet secret: the global distribution of marine biological records reveals chronic under-exploration of the deep pelagic ocean. PloS One 5 (8), 1–6. doi: 10.1371/journal.pone.0010223
Winther J. G., Dai M., Rist T., Hoel A. H., Li Y., Trice A., et al. (2020). Integrated ocean management for a sustainable ocean economy. Nat. Ecol. Evol. 4 (11), 1451–1458. doi: 10.1038/s41559-020-1259-6
Xu Z., Liu S., Xiang R., Song G. (2017). Live benthic foraminifera in the yellow Sea and the East China Sea: vertical distribution, nitrate storage, and potential denitrification. Mar. Ecol. Prog. Ser. 571, 65–81. doi: 10.3354/meps12135
Keywords: deep sea, millennium ecosystem assessment, supporting services, CICES, final services, nature’s benefits
Citation: La Bianca G, Rees S, Attrill MJ, Lombard AT, McQuaid KA, Niner HJ, van Rein H, Sink KJ and Howell KL (2023) A standardised ecosystem services framework for the deep sea. Front. Mar. Sci. 10:1176230. doi: 10.3389/fmars.2023.1176230
Received: 28 February 2023; Accepted: 02 May 2023;
Published: 22 May 2023.
Edited by:
Miriam Von Thenen, Leibniz Institute for Baltic Sea Research (LG), GermanyReviewed by:
Elva G. Escobar-Briones, National Autonomous University of Mexico, MexicoRicardo Serrão Santos, University of the Azores, Portugal
Copyright © 2023 La Bianca, Rees, Attrill, Lombard, McQuaid, Niner, van Rein, Sink and Howell. This is an open-access article distributed under the terms of the Creative Commons Attribution License (CC BY). The use, distribution or reproduction in other forums is permitted, provided the original author(s) and the copyright owner(s) are credited and that the original publication in this journal is cited, in accordance with accepted academic practice. No use, distribution or reproduction is permitted which does not comply with these terms.
*Correspondence: Giulia La Bianca, giulia.labianca@plymouth.ac.uk
†Present address: Henk van Rein, Howell Marine Consulting, Northumberland, United Kingdom