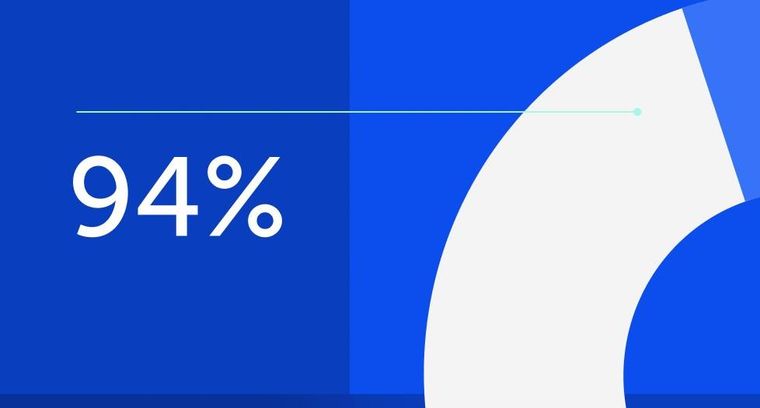
94% of researchers rate our articles as excellent or good
Learn more about the work of our research integrity team to safeguard the quality of each article we publish.
Find out more
ORIGINAL RESEARCH article
Front. Mar. Sci., 12 May 2023
Sec. Marine Fisheries, Aquaculture and Living Resources
Volume 10 - 2023 | https://doi.org/10.3389/fmars.2023.1172644
This article is part of the Research TopicMore Food From the Ocean - Blue is the New GreenView all 5 articles
Whiteleg shrimp Penaeus vannamei farming in clear water recirculating aquaculture systems (RAS) is relatively recent, and consequently, knowledge on the shrimp dietary demands is still insufficient, particularly in the initial developmental stages. This study aimed at assessing the dietary protein requirement of whiteleg shrimp post-larvae (PL) in a clear-water recirculating aquaculture system (RAS). Six microdiets were formulated to contain 34%, 44%, 49%, 54%, 58%, and 63% crude protein (P34, P44, P49, P54, P58 and P63, respectively) and were evaluated in triplicates. Whiteleg shrimp PL (3.2 mg wet weight) were reared for 21 days in a clear-water RAS at Riasearch Lda. At the end of the feeding period, the optimal protein requirement was estimated at 47.1%, 46.4%, 47.2%, and 44.0% for weight gain, relative growth rate (RGR), feed conversion ratio (FCR), and survival, respectively. PL fed the P54, P58, and P63 diets achieved significantly higher final body weights than those fed P34. PL fed P34 showed significantly lower RGR and survival and significantly higher FCR values than those fed the remaining diets, suggesting that low protein diets may not be adequate to be used in this stage of shrimp development and/or for the clear-water RAS husbandry conditions. Moreover, diet P34 seemingly reduced the overall antioxidant status of the PL when compared to P44, P49, and P54. However, the P34 diet seems to have stimulated the PL immune mechanisms when compared to P44, P49, and P54, possibly due to increased levels of fish and algae oil. Similarly, despite the good growth performances, a diet containing 63% of protein also seemed to have compromised the overall shrimp PL antioxidant status and stimulate their immune system. Shrimp fed diet P54 showed an apparent overall superior antioxidant status when compared to the remaining diets, evidencing that using protein inclusion levels up to 54% in aquafeeds not only potentiates growth performances and survival but also can potentially be beneficial to the health status of P. vannamei PL grown in a clear-water RAS. Hence, results from this study suggest that a minimum of approximately 47% of protein should be considered when tailoring microdiets for whiteleg shrimp PL grown in a clear-water RAS, but inclusion levels up to 54% can be used with benefits to the PL antioxidant status.
The whiteleg shrimp (Penaeus vannamei Boone, 1931) production has increased steadily over the last decade, and in 2020, a total of 5.8 million tonnes were reached, making it the most-produced animal species in aquaculture (FAO, 2022a). In fact, the culture of P. vannamei generates profits of over 33 billion USD/year worldwide (FAO, 2022b). Major producers are Asian and South American countries, and in 2019, imports of Penaeus shrimp to Europe reached 284.270 tonnes with a total value of 1.98 billion EUR (EUMOFA, 2020). Nonetheless, there has been an increase in overall consumer awareness of the potential negative socioecological impacts of traditional shrimp farming, and therefore, there is a growing preference for certified sustainable production. Accordingly, recent pilot projects in the USA and Europe have been focusing on growing P. vannamei in recirculating aquaculture system (RAS) using specific pathogen-free (SPF) or specific pathogen-resistant (SPR) individuals. These allow shrimp production to be biosecure and closer to consumption areas, reducing its ecological footprint while also contributing to the expansion and diversification of the aquaculture market in these geographical areas (Browdy et al., 2006; EUMOFA, 2020).
Traditionally, a semi-intensive strategy is practiced in shrimp farms, where fertilizers are frequently employed to stimulate the natural productivity of ponds, serving as a supplementary feed source for the shrimp. Additionally, the detrital matter that builds up in the bottom of the ponds is also a significant source of nutrients to the shrimp, supplying important amounts of protein from the associated bacterial communities (Nunes et al., 1997; Gamboa-Delgado et al., 2003). Inert feeds typically only constitute up to 20% of the stomachal contents of the shrimp but have a high relative contribution to growth in terms of carbon and nitrogen (Gamboa-Delgado, 2014). There is evidence that protein levels in inert diets can be as low as approximately 25% in semi-intensive systems without compromising the whiteleg shrimp growth performance, survival, feed conversion ratio, and pond productivity (kg of shrimp per pond m3) (Martinez-Cordova et al., 2002; Jatobá et al., 2014). Moreover, there has been an increase in intensive systems utilizing biofloc technology. This production method is characterized by purposely stimulating the growth of heterotrophic bacteria in the system to avoid the buildup of nitrogenous compounds in the water while also serving as a supplemental feed source for the shrimp (Crab et al., 2012; Bossier and Ekasari, 2017). Initial reports have shown that, like in semi-intensive systems, dietary protein level can be reduced to 25% in biofloc systems, without affecting P. vannamei growth, survival, feed conversion ratio, or the status of the immune response and antioxidant defense (Xu et al., 2012; Xu and Pan, 2014a; Xu and Pan, 2014b). However, several authors have recently suggested an optimal dietary protein level of approximately 35% when farming P. vannamei in bioflocs (Jatobá et al., 2014; Yun et al., 2016; Olier et al., 2020; Pinho and Emerenciano, 2021).
Shrimp farming in clear-water RAS is relatively distinct from the previously mentioned production methods, particularly regarding nutrition, since all the protein requirements of the shrimp need to be fulfilled by the protein content of inert feeds. This type of production method is relatively recent, and consequently, knowledge of the shrimp dietary demands is still insufficient, particularly in the initial stages of development. Despite the availability of several commercial diets for the whiteleg shrimp larval/post-larval phases, there is room for improvements in formulations, as these are often not tailored specifically for product type and can result in an under/overestimation of the dietary requirements of the animals. Therefore, determining the optimal protein levels to incorporate in diets for the whiteleg shrimp post-larvae (PL) produced in clear-water RAS is of extreme importance since it is the most essential nutrient for growth and also the costliest, greatly influencing the overall production expenditures. Doing so may strengthen knowledge in shrimp farming in clear-water RAS by improving feeding efficiency and reducing the overall feed-associated costs, reducing environmental impacts while potentially enhancing shrimp growth performances and health status. Hence, this study aimed at assessing the dietary protein requirement of P. vannamei PL in clear-water RAS by evaluating their growth performance, survival, and health status when fed diets containing graded crude protein levels ranging from 34% to 63%.
Six microdiets were formulated to contain 34%, 44%, 49%, 54%, 58%, and 63% crude protein (P34, P44, P49, P54, P58, and P63, respectively) and were evaluated in triplicates. Inclusion levels of the following ingredients were the same across all diets: fish meal, fish protein hydrolysate, wheat gluten, vitamin and mineral premix, and cholesterol. Different levels of squid meal, wheat meal, and fish oil were incorporated to have isolipidic diets. Feed formulation of the experimental diets and their proximate analysis are presented in Tables 1, 2, respectively. The proximate composition of the experimental diets was analyzed following the Association of Official Analytical Chemists procedures (AOAC, 2000). Briefly, feed samples were dried at 105°C to determine dry matter, crude protein () by the Kjeldahl method after acid digestion using a Kjeltec digestion and distillation unit, crude fat by petroleum ether extraction (Soxtec HT System), ashes by incineration at 450°C for 16 h in a muffle furnace, and gross energy by direct combustion in an adiabatic bomb calorimeter (PARR Instruments, Moline, IL, USA; PARR model 1261). Diets were produced at Sparos Lda (Olhão, Portugal) using extrusion at low temperatures, as described by Barreto et al. (2023). These were then crumbled (Neuero Farm, Germany) and sieved to achieve 400–600- and 600–800-µm feed particles.
Table 1 Feed formulation of the experimental microdiets with graded crude protein levels from 34% to 63% used to culture whiteleg shrimp (Penaeus vannamei) PL in clear-water RAS for 21 days.
Table 2 Proximal composition of the experimental microdiets with graded crude protein levels from 34% to 63% used to culture whiteleg shrimp (Penaeus vannamei) PL in clear-water RAS for 21 days.
Whiteleg shrimp post-larvae (PL18), obtained from Sea Product Development—Division of Global Blue Technologies (Texas, USA), were reared for 21 days at Riasearch Lda (Murtosa, Portugal). Trial duration was chosen considering that whiteleg shrimp PL of this size can increase their body weight by more than 10-fold in this timeframe (Barreto et al., 2023), which is sufficient to detect any differences between dietary treatments if they occur. A total of 4,500 PL were randomly allocated to 18 tanks with 50 L, which were part of a clear-water RAS. Each tank was equally stocked with 250 individuals (shrimp density of 5,000 m3) averaging 3.2 mg of wet weight. These were reared following the procedures described by Barreto et al. (2023). Environmental conditions maintained were as follows: temperature, 28.3°C ± 0.6°C; dissolved oxygen concentration, 7.23 ± 0.2 mg L−1; salinity, 21.5 ± 0.8 g L−1; pH, 8.05 ± 0.1; NH3, 0.02 ± 0.03 mg L−1; and NO2, 0.05 ± 0.05 mg L−1. The feed size given was 400–600 µm for the first 7 days and 600–800 µm from the eighth day onward.
At the end of the experiment, all shrimp from each tank were counted to determine survival. Additionally, 90 PL were randomly selected and weighed in groups of 10 larvae to determine final body weight, relative growth rate (RGR), and for analysis of feed conversion ratio (FCR). Afterward, 40 PL from each tank were randomly selected for oxidative and immune status analyses, 10 for gene expression analysis, and 30 for whole-body protein content analysis. PL were fasted for 12 h prior to samplings to avoid any potential interference of eaten feeds in the analyses.
A total of 10 whiteleg shrimp PL per tank sampled at the end of the trial were freeze-dried, and their total nitrogen (N), hydrogen (H), and carbon (C) contents were determined using an elemental analyzer (Elementar Vario EL III, Hanau, Germany). Protein contents were then calculated as .
Sample preparation and analyses of oxidative stress and immune parameters were performed following the procedures described by Barreto et al. (2023). Briefly, whole whiteleg shrimp PL from each tank were homogenized in sets of 10 individuals. Catalase (CAT; expressed as U mg protein−1), superoxide dismutase (SOD; expressed as U mg protein−1), total glutathione (tGSH; expressed as nmol mg protein−1), lipid peroxidation (LPO; expressed as nmol g weight−1), lysozyme (expressed as µg mg protein−1), and pro-phenoloxidase (expressed as U ml−1) activity levels were then determined in the homogenized samples.
PL sampled for gene expression analysis were individually homogenized in NZYol (NZYTech, w/v proportion according to the manufacturer’s instructions) using a Precellys 24 tissue homogenizer (Bertin Instruments, Montigny-le-Bretonneux, France). RNA extraction, analyses of its concentration and purity, attainment of cDNA strands, reverse transcription, and RT-PCR were performed for each sample as described by Barreto et al. (2023). The genes examined were chosen due to their association with antioxidant or immunity mechanisms (Table 3). The relative expression of target genes was determined according to the Pfaffl method (Pfaffl, 2001), using bactn and rpl-8 as housekeeping genes.
Table 3 Selected genes and specific primers used to evaluate the immune status of whiteleg shrimp (Penaeus vannamei) PL at the end of the experimental period.
RGR (% weight day−1) was calculated as follows: , where e = exponential, , Wf = final weight, and Wi = initial weight. The FCR was calculated as , where Fg = feed given and Wg = weight gain. Survival (%) was calculated as , where nf = final number of PL and ni = initial number of PL. To assess statistically significant dissimilarities in growth performance, FCR, survival, oxidative stress, immune condition, gene expression, and PL protein contents between dietary treatments, one-way ANOVAs followed by Tukey’s honestly significant difference (HSD) multiple comparison tests were performed. The estimation of the optimal dietary protein level was performed using broken-line models on linear regression analyses of the PL weight gain, RGR, FCR, and survival data. Based on the linear regression models for these parameters, new regression models were estimated as having broken-line relationships with protein levels in the diets, using the “segmented” package for R software (Muggeo, 2003). These produce a straight line with a positive or negative slope that joins the linear model line at a changepoint in the response variable, interpreted as the protein requirement above which there is no significant change. Multivariate linear discriminant analyses (LDAs) were performed for the antioxidant and immune status parameters and gene expression to evaluate how these contributed to the dissociation of the diets in the discriminant functions generated. A MANOVA was performed to assess discriminatory significance using Wilk’s λ test, after checking data compliance to the statistics assumptions. The distance between group centroids was measured by Mahalanobis distance, and its significance was inferred by one-way ANOVA statistics. Results are presented as mean ± standard deviation (SD). An arcsine transformation was carried out before any statistical test when results were expressed as percentages: . All statistical analyses were performed using the software R version 4.2.2, considering a significance level of p< 0.05.
Increasing levels of protein in the diets did not result in an increasing linear trend in the whole-body protein contents of shrimp PL. No significant differences between treatments were found (Table 4).
Table 4 Whole-body protein content of whiteleg shrimp (Penaeus vannamei) PL at the end of the experimental period.
Shrimp PL fed the P54, P58, and P63 diets achieved a significantly higher final body weight than those fed the P34 diet. The P34 dietary treatment resulted in significantly lower shrimp RGR and survival and significantly higher FCR values than all the remaining experimental diets (Table 5).
Table 5 Initial and final weight, relative growth rate (RGR), feed conversation ratio (FCR), and survival of whiteleg shrimp (Penaeus vannamei) PL during the experimental period.
The optimal protein requirement was estimated at 47.1%, 46.4%, 47.2%, and 44.0% for weight gain, RGR, FCR, and survival, respectively, according to broken-line models on linear regression analysis (Figures 1–4).
Figure 1 Relationship between dietary protein levels (%) and mean weight gain (mg) of whiteleg shrimp post-larvae. The optimal protein requirement estimated according to the broken-line model, which is given by the abscissa value at the breakpoint, was 47.1%.
Figure 2 Relationship between dietary protein levels (%) and RGR (% body weight day−1) of whiteleg shrimp post-larvae. The optimal protein requirement estimated according to the broken-line model, which is given by the abscissa value at the breakpoint, was 46.4%. RGR, relative growth rate.
Figure 3 Relationship between dietary protein levels (%) and FCR of whiteleg shrimp post-larvae. The optimal protein requirement estimated according to the broken-line model, which is given by the abscissa value at the breakpoint, was 47.2%. FCR, feed conversion ratio.
Figure 4 Relationship between dietary protein levels (%) and survival (%) of whiteleg shrimp post-larvae. The optimal protein requirement estimated according to the broken-line model, which is given by the abscissa value at the breakpoint, was 44%.
CAT and LPO levels were similar across all treatments; tGSH levels were significantly higher in the P54 diet than in the P34 and P63 diets, with no significant differences between the remaining treatments; SOD levels were significantly higher in the P54 diet than in the P63 diet, with no significant differences among the remaining treatments. As for the immune parameters, no significant differences between treatments were observed (Table 6). The relative expression of hmc gene was significantly higher in the P34 and P63 dietary treatments than in P44 and P58; transcripts of gpx gene were significantly higher in whiteleg shrimp PL fed the P58 diet than in those fed the P44, P49, and P54; and no significant differences were found between treatments in the relative expression of the remaining genes (Table 7).
Table 6 Catalase (CAT), superoxidase dismutase (SOD), total glutathione (tGSH), lipid peroxidation (LPO), lysozyme, and pro-phenoloxidase levels in whiteleg shrimp (Penaeus vannamei) PL at the end of the experimental period.
Table 7 Relative expression to housekeeping (bactn and rpl-8) of target immune-related genes of whiteleg shrimp (Penaeus vannamei) PL fed the experimental diets.
The LDA of the antioxidant biomarkers (CAT, SOD, tGSH, and LPO) and transcripts of genes associated with the antioxidant defense (trd, gst, and gpx) resulted in five discriminant functions, with the first two accounting for 84.80% of the data variability (Wilks λ = 0.266, p< 0.001). In the first discriminant function (LDA1; score of 68.95%) (Figure 5), the analysis revealed a significant group separation between diets P34, P58, and P63 and the remaining diets and between diets P44 and P49 and diet P54. Group discrimination was mainly positively loaded by gpx and negatively by CAT and tGSH. In the second discriminant function (LDA2; score of 13.51%), a significant separation of diet P58 from diets P44, P49, and P63 is also shown (Figure 5). This discrimination was mainly positively loaded by gpx and negatively by LPO.
Figure 5 Linear discriminant analysis (LDA) of antioxidant biomarkers (CAT, SOD, tGSH, and LPO) and relative expression of genes associated with the antioxidant defense (trd, gst, and gpx) of whiteleg shrimp post-larvae fed the different experimental diets. Percentages indicate the main function (LDA1 and LDA2) discriminant score. Small circles represent diet data distribution, while big circles represent diet centroids. Variable loads for both discriminant functions are represented by red arrows. Wilk’s lambda test was significant (p< 0.001). In LDA1, P34, P58, and P63 diets were discriminated from the remaining diets, and diets P44 and P49 from diet P54, mainly due to lower levels of CAT and tGSH and higher transcripts of gpx. In LDA2, P58 was discriminated from P44, P49, and P63 mainly due to lower levels of LPO. CAT, catalase; SOD, superoxide dismutase; tGSH, total glutathione; LPO, lipid peroxidation.
The LDA of the immune parameters (lysozyme and pro-phenoloxidase) and transcripts of genes associated with the shrimp innate immune system (casp-3, pen-3, hmc, lys, and lect) resulted in five discriminant functions, with the first two accounting for 81.20% of the data variability (Wilks λ = 0.532, p< 0.001). In the first discriminant function (LDA1; score of 43.87%) (Figure 6), the discriminant analysis revealed a significant group separation between P34 and diets P44, P49, and P54 and between P58 and P54. Group discrimination was mainly positively loaded by lysozyme and pro-phenoloxidase. In the second discriminant function (LDA2; score of 37.33%), a significant separation of diet P58 from diets P34 and P63 is also shown (Figure 5). This discrimination was mainly positively loaded by lysozyme and negatively by pro-phenoloxidase and hmc.
Figure 6 Linear discriminant analysis (LDA) of immune parameters (lysozyme and pro-phenoloxidase) and relative expression of genes associated with the shrimp innate immune system (casp-3, pen-3, hmc, lys, and lect) of whiteleg shrimp post-larvae fed the different experimental diets. Percentages indicate the main function (LDA1 and LDA2) discriminant score. Small circles represent diet data distribution, while big circles represent diet centroids. Variable loads for both discriminant functions are represented by red arrows. Wilk’s lambda test was significant (p< 0.001). In LDA1, P34 was discriminated from diets P44, P49, and P54 and diet P58 from P54, mainly due to higher levels of lysozyme and pro-phenoloxidase. In LDA2, P58 was discriminated from P34 and P63 mainly due to lower levels of lysozyme, pro-phenoloxidase, and transcripts of hmc.
Few studies have focused on the effects of dietary protein requirements of P. vannamei PL during the nursery stage of production in clear-water systems devoid of natural productivity, and studies available have divergent results. Some authors suggest optimal dietary protein levels for whiteleg shrimp PL in clear-water RAS to be as low as between 21.4% and 24.5% (Velasco et al., 2000) or 34% (Hu et al., 2008). However, Otoshi et al. (2001) described increases in whiteleg shrimp PL weight gain when fed a diet with 52% protein over a 45% protein diet during the nursery phase. Moreover, Xie et al. (2020) evaluated several diets with gradient levels of protein (46%, 47%, 49%, 50%, and 51%) and estimated 45.6% and 50.0% to be optimal, based on the shrimp PL weight gain and survival, respectively. In the current study, six experimental diets were formulated to contain 34%, 44%, 49%, 54%, 58%, and 63% of crude protein, covering a wide range of inclusion levels to contemplate several scenarios. The optimal protein level estimated was 47.1%, 46.4%, 47.2%, and 44.0% for weight gain, RGR, FCR, and survival, respectively. These results suggest that when tailoring microdiets for whiteleg shrimp PL grown in clear-water RAS, a minimum of approximately 47% of protein should be considered for optimal growth performances and survival. There have been reports of detrimental effects on whiteleg shrimp growth when levels of dietary protein were higher than 48% (Kureshy and Davis, 2002), which was not verified in the present study. Although not statistically significant, shrimp PL fed diets containing over the previously stated protein level tended to have higher mean weight gain and RGR values. Moreover, the multivariate analysis performed showed an apparent overall superior antioxidant status of shrimp PL fed the P54 diet compared to the remaining diets, evidencing that using protein inclusion levels up to 54% in aquafeeds not only potentiate growth performances and survival but also can potentially be beneficial to the health status of P. vannamei PL grown in clear-water RAS devoid of natural productivity.
A diet containing 34% protein showed detrimental effects, leading to a decrease in the PL growth performances and survival, when compared with diets with higher protein contents (P54, P58, and P63), suggesting that low protein diets may not be adequate to be used in this stage of shrimp development and/or for the clear-water RAS husbandry conditions. Furthermore, tGSH levels were lower in shrimp fed the P34 diet compared with those fed the P54 dietary treatment, indicating a potential decline in their protection against oxidative damage, as tGSH plays a key role in the antioxidant defense of the shrimp. These neutralize hydroxyl radicals (OH) against which there is no enzymatic protection (Cardona et al., 2016). In fact, according to the multivariate analysis performed, the P34 diet seemingly reduced the overall antioxidant status of the whiteleg shrimp PL when compared to the P44, P49, and P54 dietary treatments due to not only lower levels of tGSH but also CAT activity, an antioxidant enzyme that converts H2O2 into molecular oxygen and water (Rodrigues et al., 2017). However, shrimp PL fed P34 showed higher transcripts of the gene gpx, responsible for the encoding of glutathione peroxidase, which has an important role in antioxidant protection by removing lipids and hydrogen peroxides formed during immune and physiological processes (Liu et al., 2007). Although shrimp were reared under standard rearing conditions that should not promote oxidative stress, the accelerated development P. vannamei undergo in the larval/PL stages may result in oxidative damage, as fast growth is likely to produce excess ROS, damaging key physiological structures (Monaghan et al., 2009). Despite having similar LPO levels to the remaining treatments, the apparently compromised antioxidant mechanisms of shrimp fed the P34 dietary treatment may have contributed to the lower survival observed when compared to diets with higher protein contents. However, the multivariate analysis performed showed that the P34 diet seems to have stimulated the shrimp PL immune system when compared to the P44, P49, and P54 dietary treatments. PL fed P34 showed higher activity levels of lysozyme, an important hemocyte-specific protein with antibacterial properties (de-la-Re-Vega et al., 2006), and pro-phenoloxidase, an enzyme involved in the innate immune system of invertebrates (Kim et al., 2014). These results may be related to the increased proportions of wheat meal in the P34 diet when compared to the higher protein diets. Although wheat is often not the most problematic plant ingredient, it contains anti-nutritional factors that can have adverse impacts on the overall health status of the cultured animals (Francis et al., 2001), and the increased levels of this ingredient may have caused an innate immune response in shrimp PL. Parallelly, the increment of fish and algae oil quantities in diet P34 may have positively influenced the shrimp PL immune mechanisms, as both ingredients have been shown to enhance the health condition of whiteleg shrimp PL (Nonwachai et al., 2010; Xie et al., 2019). The gene expression analysis also showed an increase in transcripts of hmc in the P34 dietary treatment when compared to the P44 and P58 diets. It should be noted that hemocyanin is a multifunctional protein associated with various important physiological processes apart from innate immunity like oxygen transportation, protein storage, osmoregulation, molt cycle, and exoskeleton formation. Therefore, higher expressions of this gene may not be exclusively related to changes in the whiteleg shrimp PL immune status (Zhang et al., 2009; Zheng et al., 2016).
Similarly, a diet containing 63% of protein also seemed to compromise the overall shrimp PL antioxidant status. The multivariate analysis performed showed a significant separation of P63 from P44, P48, and P54, mainly due to lower levels of CAT and tGSH and higher transcripts of gpx gene. These results suggest a lower dependency on these antioxidant molecules to sustain good growth performances. Nevertheless, reduced activity levels of the latter can indicate a decreased antioxidant capacity, particularly important in more challenging husbandry conditions than those maintained in this study. Still, contrary to what was observed in the P34 dietary treatment, growth performance and survival were not compromised in P63. Additionally, just like P34, P63 was significantly segregated from P58 due to higher activity levels of lysozyme and pro-phenoloxidase and higher transcripts of the hmc gene, suggesting that protein levels as high as 63% may have originated an innate immune response in the shrimp PL. However, it is unclear what may have caused this response. Hence, additional research should be conducted to clarify the potential detrimental and beneficial effects of microdiets with the highest protein levels tested in the current study since increases in protein inclusion quantities represent increments in diet prices. These are of extreme importance in large-scale industrial settings, as feeds have a high preponderance on the overall production expenditures, and higher-cost diets could compromise the economic feasibility of shrimp farms.
In summary, results from this study confirm the importance of using a high-protein aquafeed in the nursery phase if P. vannamei PL are grown in clear-water RAS devoid of natural productivity since inert diets are the exclusive source of nutrients in this type of production system. They also suggest that feeds that work well in semi-intensive or biofloc systems may not fulfill the shrimp PL protein requirements in clear-water farming. A minimum of approximately 47% of the protein was estimated as optimal for weight gain and survival and is recommended when tailoring microdiets for the nursery phase of whiteleg shrimp PL grown in clear-water RAS. Moreover, results indicate that protein inclusion levels up to 54% can also seemingly improve the overall antioxidant status of whiteleg shrimp PL. A diet containing only 34% protein impaired PL growth performances and survival when compared to higher protein diets. Meanwhile, results also demonstrate that both extreme levels of dietary protein inclusion (34% and 63%) impaired the antioxidant mechanisms of PL but stimulated the production of immunity-related molecules. Despite the excellent shrimp PL growth performances obtained, using a diet with protein levels as high as 63% should be considered cautiously, as the current study suggests some putative negative effects on shrimp health, along with predictable higher nitrogen discharges into the environment and higher feed costs.
The raw data supporting the conclusions of this article will be made available by the authors, without undue reservation.
Ethical review and approval were not required for the study on animals in accordance with the local legislation and institutional requirements.
Conceptualization, LC and BC; data curation, AB; formal analysis, AB; funding acquisition, RR, LC, and BC; investigation, AB, DP, and CF; methodology, AB, WP, LC, and BC; project administration, RR, LC, and BC; resources, RR, LC, and BC; supervision, RR, LC, and BC; writing-original draft, AB; writing-review and editing, DP, CF, WP, RR, LC, and BC. All authors have read and agreed to the published version of the manuscript and agree to be accountable for all aspects of the work.
This work was part of the project FA_05_2017_005 SHELLWIN, financed by the Blue Fund program of the Ministry of the Sea, Portuguese Republic. This study was also supported by national funds through FCT - Foundation for Science and Technology within the scope of UIDB/04423/2020 and UIDP/04423/2020. DP and BC were supported by FCT through grants UI/BD/150900/2021 and 2020.00290.CEECIND, respectively. CF was supported by the Ministry of Universities of the Government of Spain through the European Recovery Instrument ¨Next Generation-EU¨ (Margarita Salas Program).
Special thanks to Renata Serradeiro. The authors also would like to acknowledge technical support from Adriana Laranjeira and Bruna Silva during shrimp rearing and sampling at Riasearch; André Santos, and Ana Gonçalves and Tomé Silva, at Sparos, for laboratory and statistical analysis, respectively; and Jorge Dias and the Sparos feed production team for manufacturing the tested microdiets.
Authors AB and RR were employed by Riasearch Lda. AS, WP and LC worked for Sparos Lda during this study.
The remaining authors declare that the research was conducted in the absence of any commercial or financial relationships that could be construed as a potential conflict of interest.
All claims expressed in this article are solely those of the authors and do not necessarily represent those of their affiliated organizations, or those of the publisher, the editors and the reviewers. Any product that may be evaluated in this article, or claim that may be made by its manufacturer, is not guaranteed or endorsed by the publisher.
Barreto A., Peixoto D., Fajardo C., Pinto W., Rocha R. J. M., Conceição L. E. C., et al. (2023). Health-promoting additives supplemented in inert microdiets for whiteleg shrimp (Penaeus vannamei) post-larvae: effects on growth, survival, and health status. Animals 13 (4), 726. doi: 10.3390/ani13040726
Boone L. (1931). A collection of anomuran and macruran Crustacea from the Bay of Panama and the fresh waters of the Canal Zone. Bulletin of the American Museum of Natural History 63, 137–189
Bossier P., Ekasari J. (2017). Biofloc technology application in aquaculture to support sustainable development goals. Microb. Biotechnol. 10, 1012–1016. doi: 10.1111/1751-7915.12836
Browdy C., Seaborn G., Atwood H., Davis D. A., Bullis R. A., Samocha T. M., et al. (2006). Comparison of pond production efficiency, fatty acid profiles, and contaminants in Litopenaeus vannamei fed organic plant-based and fish-meal-based diets. J. World Aquac Soc. 37, 437–451. doi: 10.1111/j.1749-7345.2006.00057.x
Cardona E., Lorgeoux B., Chim L., Goguenheim J., le Delliou H., Cahu C. (2016). Biofloc contribution to antioxidant defence status, lipid nutrition and reproductive performance of broodstock of the shrimp Litopenaeus stylirostris: consequences for the quality of eggs and larvae. Aquaculture 452, 252–262. doi: 10.1016/j.aquaculture.2015.08.003
Crab R., Defoirdt T., Bossier P., Verstraete W. (2012). Biofloc technology in aquaculture: beneficial effects and future challenges. Aquaculture 356-357, 351–356. doi: 10.1016/j.aquaculture.2012.04.046
de-la-Re-Vega E., García-Galaz A., Díaz-Cinco M. E., Sotelo-Mundo R. R. (2006). White shrimp (Litopenaeus vannamei) recombinant lysozyme has antibacterial activity against gram negative bacteria: Vibrio alginolyticus, Vibrio parahemolyticus and Vibrio cholerae. Fish Shellfish Immunol. 20, 405–408. doi: 10.1016/j.fsi.2005.06.005
EUMOFA (2020). “Species analyses – 2020 edition,” in European Market observatory for fisheries and aquaculture products. maritime affairs and fisheries (Luxembourg: Publications Office of the European Union). doi: 10.2771/790742
FAO (2022a). The state of world fisheries and aquaculture 2022. towards blue transformation (Rome: FAO). doi: 10.4060/cc0461en
FAO (2022b). Top 10 species groups in global, regional and national aquaculture 2020. supplementary materials to the factsheet on top 10 species groups in global aquaculture 2020 (Rome: FAO).
Francis G., Makkar P. S., Becker K. (2001). Antinutritional factors present in plant-derived alternate fish feed ingredients and their effects in fish. Aquaculture 199 (3-4), 197–227. doi: 10.1016/S0044-8486(01)00526-9
Gamboa-Delgado J. (2014). Nutritional role of natural productivity and formulated feed in semi-intensive shrimp farming as indicated by natural stable isotopes. Rev. Aquac 6 (1), 36–47. doi: 10.1111/raq.12023
Gamboa-Delgado J., Molina-Poveda C., Cahu C. (2003). Digestive enzyme activity and food ingesta in juvenile shrimp Litopenaeus vannamei (Boone 1931) as a function of body weight. Aquac Res. 34, 1403–1411. doi: 10.1111/j.1365-2109.2003.00959.x
Hu Y., Tan B., Mai K., Ai Q., Zheng S., Cheng K. (2008). Growth and body composition of juvenile white shrimp, Litopenaeus vannamei, fed different ratios of dietary protein to energy. Aquac Nutr. 14, 499–506. doi: 10.1111/j.1365-2095.2007.00555.x
Jatobá A., da Silva B. C., da Silva J. S., Vieira F., do N., Mouriño J. L. P., et al. (2014). Protein levels for Litopenaeus vannamei in semi-intensive and biofloc systems. Aquaculture 432, 365–371. doi: 10.1016/j.aquaculture.2014.05.005
Kim S. K., Pang Z., Seo H. C., Cho Y. R., Samocha T., Jang I. K. (2014). Effect of bioflocs on growth and immune activity of pacific white shrimp, Litopenaeus vannamei postlarvae. Aquac Res. 45, 362–371. doi: 10.1111/are.12319
Kureshy N., Davis D. A. (2002). Protein requirement for maintenance and maximum weight gain for the pacific white shrimp, Litopenaeus vannamei. Aquaculture 204 (1-2), 125–143. doi: 10.1016/S0044-8486(01)00649-4
Liu C. H., Tseng M. C., Cheng W. (2007). Identification and cloning of the antioxidant enzyme, glutathione peroxidase, of white shrimp, Litopenaeus vannamei, and its expression following Vibrio alginolyticus infection. Fish Shellfish Immunol. 23, 34–45. doi: 10.1016/j.fsi.2006.09.002
Martinez-Cordova L. R., Campaña-Torres A., Porchas-Cornejo M. A. (2002). The effects of variation in feed protein level on the culture of white shrimp, Litopenaeus vannamei (Boone) in low-water exchange experimental ponds. Aquac Res. 33, 995–998. doi: 10.1046/j.1365-2109.2002.00752.x
Monaghan P., Metcalfe N. B., Torres R. (2009). Oxidative stress as a mediator of life history trade-offs: mechanisms, measurements and interpretation. Ecol. Lett 12, 75–92. doi: 10.1111/j.1461-0248.2008.01258.x
Muggeo V. M. R. (2003). Estimating regression models with unknown break-points. Stat. Med. 22, 3055–3071. doi: 10.1002/sim.1545
Nonwachai T., Purivirojkul W., Limsuwan C., Chuchird N., Velasco M., Dhar A. K. (2010). Growth, nonspecific immune characteristics, and survival upon challenge with Vibrio harveyi in pacific white shrimp (Litopenaeus vannamei) raised on diets containing algal meal. Fish Shellfish Immunol. 29, 298–304. doi: 10.1016/j.fsi.2010.04.009
Nunes A. J. P., Gesteira T. C., Goddard S. (1997). Food ingestion and assimilation by the southern brown shrimp Penaeus subtilis under semi-intensive culture in NE Brazil. Aquaculture 149 (1-2), 121–136. doi: 10.1016/S0044-8486(96)01433-0
Olier B. S., Tubin J. S. B., de Mello G. L., Martínez-Porchas M., Emerenciano M. G. C. (2020). Does vertical substrate could influence the dietary protein level and zootechnical performance of the pacific white shrimp Litopenaeus vannamei reared in a biofloc system? Aquaculture Int. 28, 1227–1241. doi: 10.1007/s10499-020-00521-4
Otoshi C. A., Montgomery A. D., Look A. M., Moss S. M. (2001). Effects of diet and water source on the nursery production of pacific white shrimp Litopenaeus vannamei. J. World Aquac Soc. 32, 243–249. doi: 10.1111/j.1749-7345.2001.tb01102.x
Pfaffl M. W. (2001). A new mathematical model for relative quantification in real-time RT-PCR. Nucleic Acids Res. 29 (9), 45. doi: 10.1093/nar/29.9.e45
Pinho S. M., Emerenciano M. G. C. (2021). Sensorial attributes and growth performance of whiteleg shrimp (Litopenaeus vannamei) cultured in biofloc technology with varying water salinity and dietary protein content. Aquaculture 540, 736727. doi: 10.1016/j.aquaculture.2021.736727
Rodrigues A. C. M., Gravato C., Quintaneiro C., Bordalo M. D., Barata C., Soares A. M. V. M., et al. (2017). Energetic costs and biochemical biomarkers associated with esfenvalerate exposure in Sericostoma vittatum. Chemosphere 189, 445–453. doi: 10.1016/j.chemosphere.2017.09.057
Velasco M., Lawrence A. L., Castille F. L., Obaldo L. G. (2000). “Dietary protein requirement for litopenaeus vannamei,” in Avances En Nutrición Acuícola V. Eds. Cruz-Suárez L. E., Ricque-Marie D., Tapia-Salazar M., Olvera-Novoa M. A., Civera-Cerecedo R. (Memorias del V Simposium Internacional de Nutrición Acuícola: Mérida, Yucatán, México) 19–22.
Xie S., Wei D., Fang W., Wan M., Guo T., Liu Y., et al. (2019). Optimal dietary lipid requirement of postlarval white shrimp, Litopenaeus vannamei in relation to growth performance, stress tolerance and immune response. Aquac Nutr. 25, 1231–1240. doi: 10.1111/anu.12937
Xie S., Wei D., Fang W., Yin P., Liu Y., Niu J., et al. (2020). Survival and protein synthesis of post-larval white shrimp, Litopenaeus vannamei were affected by dietary protein level. Anim. Feed Sci. Technol. 263:114462. doi: 10.1016/j.anifeedsci.2020.114462
Xu W. J., Pan L. Q. (2014a). Dietary protein level and C/N ratio manipulation in zero-exchange culture of Litopenaeus vannamei: evaluation of inorganic nitrogen control, biofloc composition and shrimp performance. Aquac Res. 45, 1842–1851. doi: 10.1111/are.12126
Xu W. J., Pan L. Q. (2014b). Evaluation of dietary protein level on selected parameters of immune and antioxidant systems, and growth performance of juvenile Litopenaeus vannamei reared in zero-water exchange biofloc-based culture tanks. Aquaculture 426–427, 181–188. doi: 10.1016/j.aquaculture.2014.02.003
Xu W. J., Pan L. Q., Zhao D. H., Huang J. (2012). Preliminary investigation into the contribution of bioflocs on protein nutrition of Litopenaeus vannamei fed with different dietary protein levels in zero-water exchange culture tanks. Aquaculture 350–353, 147–153. doi: 10.1016/j.aquaculture.2012.04.003
Yun H., Shahkar E., Katya K., Jang I. K., Kim S., Bai S. C. (2016). Effects of bioflocs on dietary protein requirement in juvenile whiteleg shrimp, Litopenaeus vannamei. Aquac Res. 47, 3203–3214. doi: 10.1111/are.12772
Zhang Y., Yan F., Hu Z., Zhao X., Min S., Du Z., et al. (2009). Hemocyanin from shrimp Litopenaeus vannamei shows hemolytic activity. Fish Shellfish Immunol. 27, 330–335. doi: 10.1016/j.fsi.2009.05.017
Keywords: Penaeus vannamei, whiteleg shrimp, post-larvae, protein requirements, inert microdiets, clear water recirculating aquaculture system
Citation: Barreto A, Silva A, Peixoto D, Fajardo C, Pinto W, Rocha RJM, Conceição LEC and Costas B (2023) Dietary protein requirements of whiteleg shrimp (Penaeus vannamei) post-larvae during nursery phase in clear-water recirculating aquaculture systems. Front. Mar. Sci. 10:1172644. doi: 10.3389/fmars.2023.1172644
Received: 23 February 2023; Accepted: 28 April 2023;
Published: 12 May 2023.
Edited by:
Yngvar Olsen, Norwegian University of Science and Technology, NorwayReviewed by:
Luis Poersch, Federal University of Rio Grande, BrazilCopyright © 2023 Barreto, Silva, Peixoto, Fajardo, Pinto, Rocha, Conceição and Costas. This is an open-access article distributed under the terms of the Creative Commons Attribution License (CC BY). The use, distribution or reproduction in other forums is permitted, provided the original author(s) and the copyright owner(s) are credited and that the original publication in this journal is cited, in accordance with accepted academic practice. No use, distribution or reproduction is permitted which does not comply with these terms.
*Correspondence: André Barreto, YW5kcmViYXJyZXRvQHJpYXNlYXJjaC5wdA==
Disclaimer: All claims expressed in this article are solely those of the authors and do not necessarily represent those of their affiliated organizations, or those of the publisher, the editors and the reviewers. Any product that may be evaluated in this article or claim that may be made by its manufacturer is not guaranteed or endorsed by the publisher.
Research integrity at Frontiers
Learn more about the work of our research integrity team to safeguard the quality of each article we publish.