- 1Centre Agriculture Food Environment, University of Trento, San Michele all'Adige, Italy
- 2Department of Veterinary Medical Sciences, University of Bologna, Ozzano Emilia, Italy
- 3Institut de Recerca i Tecnologia Agroalimentàries (IRTA), Centre de la Ràpita, la Ràpita, Spain
- 4International Marine Centre - IMC Foundation, Oristano, Italy
The capacity of utilising a single cell protein (SCP) ingredient coming from Corynebacterium glutamicum was assessed on adult grey mullet (Mugil cephalus) reared in captive conditions. The experiment was carried out using triplicate groups of grey mullet of 68 g average initial body weight. Three diets, SCP0, SCP10 and SCP20 with increasing inclusion of SCP (0%, 10% and 20%) in substitution of soybean, poultry and fish meal were formulated to contain 30% protein, 10% fat and 18.5 Mj/kg feed of digestible energy. After 113 days, fish fed SCP diets presented significantly lower growth performance and a significant lower activity of the alkaline proteases and aminopeptidases compared to fish fed diet without SCP inclusion. Gut microbiota appeared modulated by SCP inclusion being dominated at the phylum level by Fusobacteria in fish fed SCP0 (51.1%), while in fish fed SCP10 (67.3%) and SCP20 (53.2%) Proteobacteria was dominant. Data evinces a deficiency in the protein utilisation as a cause of the poor growth performance in fish fed the SCP diets. A hypothesis has been proposed that an incomplete SCP cell-wall lysis accounts for this outcome because of the particular organisation of the digestive system of grey mullet (which lack of an acidic stomach digestion) and the failing in the development of a functional gizzard (no access to sand in captive conditions). Even though the outcomes of this research were quite unexpected, they will improve our knowledge on the digestive system of flathead grey mullet and provide some theoretical basis for an improved development of low FM and SBM aquafeed for the species.
Introduction
Flathead grey mullet (Mugil cephalus) is an omnivorous and detritivores fish species which has been selected as suitable for the diversification of Mediterranean aquaculture both in monoculture and polyculture systems due to its low trophic position (Vallainc et al., 2021; Solovyev and Gisbert, 2022). Grey mullet presents a rapid growth, a good adaptability to captivity and high market price of its salted and dried roe, known as “bottarga” (Whitfield et al., 2012). In addition, being a consumer of the lower trophic layers, its protein requirement is considered to be lower when compared to strict carnivorous farmed Mediterranean species. For instance, the protein content of standard aquafeed formulations for gilthead sea bream and European sea bass is normally around 45% (Busti et al., 2020a; Pelusio et al., 2022), while for flathead grey mullet can be as low as 30% (De Carvalho et al., 2010; Talukdar et al., 2020). Among the protein sources commonly used in aquafeeds, fishmeal (FM) is recognised as the most suitable in terms of nutritional value. However, the increased demand and the decline of the marine fishery stocks limit its application in aquafeeds (Glencross et al., 2016; Pereira et al., 2022). Alternatives to FM are conventionally represented by plant-based ingredients such as soybean meal (SBM), although with the presences of certain drawbacks (Colombo et al., 2022; Newton et al., 2023). As an example, SBM which is largely used into aquafeed formulation as a FM substitute, has a high carbon footprint if it is derived from crops grown on land converted from forest or savannah (Wilkinson and Young, 2020). In addition, its massive use into aquafeeds goes in direct competition with human consumption, as SBM is a key element in human diets.
In a circular economy, aquafeed ingredients derived from industry by-products such as single-cell ingredients are in the spotlight as a promising option for the sustainability of the aquaculture industry (Glencross et al., 2020; Colombo et al., 2022). Single-cell ingredients are a relatively broad class of materials including bacterial, fungal and microalgal-derived products, which have shown potential as a source of protein, lipids or specific nutritional components in aquafeed ingredients (Glencross et al., 2020; Pereira et al., 2022). Among such “circular” ingredients, bacterial single cell protein (SCP) presents a high percentage of crude protein with a favourable amino acid profile, along with vitamins and other functional compounds (Glencross et al., 2020; Jones et al., 2020). Bacteria may be produced using a wide range of substrates and nutrients ranging from industrial and agricultural residues to bioindustry by-products (Jones et al., 2020); thus, allowing the valorisation of waste streams and reducing the downstream costs related to industrial waste disposal (Pereira et al., 2022). Among different SCP sources, Corynebacterium glutamicum is a Gram-positive bacteria generally regarded as safe (GRAS organism) (Cheng et al., 2021; Liu et al., 2022), which is commonly used to produce amino acids (AA). After the AA production, filtered C. glutamicum cell mass, contains high protein content (>70%) and a suitable AA profile to be used as a replacement of conventional protein sources in livestock nutrition (Zhang et al., 2013). In aquaculture nutrition, its application has been successfully proven in gilthead seabream even at high inclusion level (Marchi et al., 2023). In general, digestibility and palatability of bacterial SCP is considered good; however, variable performances may appear between the tested bacteria genus (Glencross et al., 2020; Bratosin et al., 2021), the application of post-production cell lysis processes (e.g. autolysis or hydrolysis) (Schøyen et al., 2005; Teuling et al., 2019; Glencross et al., 2023) and the aquaculture species (Marit Berge et al., 2005; Hamidoghli et al., 2019; Zamani et al., 2020; Marchi et al., 2023).
To the best of our knowledge there is no literature to date concerning the application of a bacterial SCP in the diet of flathead grey mullet in captive conditions. In the present study, with the aim of providing a theoretical basis for the development of zero FM and SBM aquafeed for mullet, C. glutamicum was used to replace these conventional ingredients at three different inclusion levels. The effects of its inclusion in flathead grey mullet were evaluated on classical key performance indicators like growth performance, feed utilisation, and other variables like metabolic blood parameters, digestive enzyme activities and gut microbiota in order to evaluate holistically the effects of this new sustainable ingredient in fish nutrition.
Materials and methods
Experimental diets
C. glutamicum SCP was utilised as a replacement of the conventional ingredients soybean meal (SBM), poultry meal (PM) and fishmeal (FM) in the diet of flathead grey mullet. This ingredient consisted of bulk dried bacterial single cells composed of 73.5 ± 1.5 crude protein, 3.7 ± 0.6 crude lipid, 8.8 ± 0.4 moisture and 4.6 ± 0.3 ash (NT70, M.I. Gordini srl, Bologna, Italy). Three isonitrogenous, isolipidic and isoenergetic experimental diets were formulated to contain increasing inclusion levels of bacterial SCP: SCP0 (0% SCP), SCP10 (10% SCP) and SCP20 (20% SCP). In the SCP20 diet, both SBM and FM were completely replaced by the tested bacterial SCP, whereas PM was significantly reduced. Extruded diets (pellet size: 1.5 mm) were manufactured by SPAROS Lda (Olhão, Portugal). The contents of protein, lipid and energy in the diet formulations were decided on the basis of several research works (De et al., 2012; Talukdar et al., 2020). As observed by Ramos-Júdez and Duncan (2022), grey mullet requires small size pellet because of its particular feeding behaviour. The species do not chew and break the pellet into smaller particles but capture and keep it in the oral cavity or spit it out and recapture before ingestion. The ingredients and the proximate composition of the diets were shown in Table 1.
Fish rearing and feeding trial
Flathead grey mullet born in captivity at International Marine Centre (Oristano, Italy) were delivered to the Laboratory of Aquaculture at the University of Bologna (Cesenatico, Italy). At the beginning of the trial, fish (average body weight = 67.90 ± 15.46 g) were randomly distributed into 9 x 800 L fiberglass square tanks (45 fish/tank, each diet assigned in triplicate), connected to a closed recirculation unit. This unit consisted of a mechanical sand filter (PTK 1200; Astralpool, Barcelona, Spain), ultraviolet lights (UV PE 45; Sita Srl, Barcelona, Spain) and a biofilter (PTK 1200; Astralpool, Barcelona, Spain). Water consisted of a mixture of marine and freshwater (salinity 7 ± 2 ‰) (Cardona, 2006), the water exchange rate was kept at 100%/h in each tank and the overall water renewal in the system was at 5% daily. Temperature was kept at 26.8 ± 2.5°C, photoperiod was maintained at 12 h light/12 h dark through artificial light and oxygen saturation at 100% by an integrated control system. Nitrogen compounds (ammonia, nitrite and nitrate) and pH were daily monitored and kept at the optimum range for the fish welfare. Feed was provided to apparent satiation by oversupplying the feed (10% of the daily ration) by automatic feeders once a day for six days a week. Each meal lasted 6 hours to provide fish a continuous feed supply over daily hours. The uneaten pellets of each tank were collected three times daily at each meal (2, 4 and 6 hours after the beginning of meal), dried overnight at 105°C, and deducted for overall calculations following the method as described in (Bonvini et al., 2018).
Samplings
Before each sampling procedure, fish were either anaesthetised (100 mg L−1) or euthanised (300 mg L−1) by MS222. At the end of the experiment (day 113), all fish were anesthetised and individually weighted. The proximate composition of the carcasses was determined at the beginning of the experiment on a pooled sample of 10 fish and on a pooled sample of 3 fish per tank (9 fish per diet) at the end of the experiment, this information was later used for determining efficiency of nutrient (protein and lipid) uptake. Biometric indices [total body length, SL (cm); wet weight, BW (g); viscera and liver weight (g) and gut length (cm)] were recorded on 3 fish per tank (9 fish per diet). At the end of the experiment, fish were fed to satiety on a 6-hour meal and sampled for blood chemistry, gut microbiota and digestive enzyme’s activity. Blood was collected from the caudal vein from 6 fish per tank (18 fish per diet) and transferred to a clot activator tube (Vacutest Kima Srl, Padova, Italy). As soon as the blood clotted it was centrifuged at 3,000 x g for 10 min at 4°C, serum aliquots removed and stored at -80°C for chemical analysis. At the same time, 250 mg of hindgut content from 3 fish per tank (9 fish per diet) was collected aseptically by gently stripping the final 5 cm of the gut into a sterile tube and stored at -80°C for gut microbiota analysis. At the same time, samples for assessing the activity of digestive enzymes were also collected. Being that the duration of a meal was 6 hours, these samples were collected from fish over 0 to 6 hours after the end of the meal. To do so, the whole gastro-intestinal tract and stomach were dissected from 2 fish per tank (6 fish per diet) for the analysis of pancreatic enzymes and placed into resealable bags and snap frozen at −80 °C, until their analysis. All experimental procedures were evaluated and approved by the Ethical-Scientific Committee for Animal Experimentation of the University of Bologna, in accordance with European directive 2010/63/UE on the protection of animals used for scientific purposes.
Calculations
The formulae employed for evaluating somatic growth were as follows: Specific growth rate (SGR) (% day−1) = 100 * (ln FBW - ln IBW)/days (where FBW and IBW represent the final and the initial body weights, respectively). Feed intake (FI) (% ABW−1 day−1) = ((100 * total ingestion)/(ABW))/days)) (where ABW = (IBW + FBW)/2). Feed conversion rate (FCR) = feed intake/weight gain. Viscerosomatic index (VSI) (%) = 100 * (viscera weight/body weight). Hepatosomatic index (HSI) (%) = 100 * (liver weight/body weight). Relative gut length (RGL) = 100 * (gut length/body length). Fulton’s condition factor (K) = 100 * (FBW/body length3). Protein efficiency rate (PER) = (FBW–IBW)/protein intake. Lipid efficiency rate (LER) = (FBW–IBW)/lipid intake. Gross protein efficiency (GPE) (%) = 100 * [(% final body protein * FBW) - (% initial body protein * IBW)]/total protein intake fish. Gross lipid efficiency (GLE) (%) = 100 * [(% final body lipid * FBW) - (% initial body lipid * IBW)]/total lipid intake fish.
Analytical methods
Diets and whole carcasses were analysed for moisture, nitrogen (N), lipid and ash contents. Moisture was calculated by gravimetric analysis following oven drying at 105°C overnight. Protein level was determined as total nitrogen (N x 6.25) after performing the Kjeldahl method. Lipid level was determined according to Bligh and Dyer’s extraction method (Bligh and Dyer, 1959). Ash content was determined by gravimetric analysis following incineration of the sample in a muffle furnace at 450°C overnight. Diets’ buffering capacity was defined as the slope of the titration curve from the initial pH of the diet to a value below 3. The procedure consisted in suspending 1 g of the diet in 5 mL of distilled water, keeping it magnetically stirred, then adding successive aliquots of HCl and recording the pH 30 s after each addition of HCl as described in Márquez et al. (2012) and Parma et al. (2019). All pH measurements were performed by using a Hanna HI 991002 pH-meter (Hanna instruments, Woonsocket, USA). Metabolic blood parameters were determined in a 500 μL serum sample by using an automated analyser (AU 480; Beckman Coulter Inc., Brea, USA) with dedicated methods (Olympus system reagent, OSR). The type of reaction used in the assay and the OSR identification number were reported in brackets following the reported parameters. Metabolic blood parameters include glucose (hexokinase reaction, OSR6121), urea (urease reaction, OSR6134), uric acid (uricase reaction, OSR6198), bilirubin (colorimetric reaction, OSR6112), cholesterol (enzymatic method, OSR6116), high-density lipoprotein (HDL, enzymatic method, OSR6187), triglycerides (TG, enzymatic method, OSR61118), lactic acid (enzymatic method, OSR6193), total protein (biuret method, OSR6132), albumin (ALB, bromocresol green method, OSR6102), aspartate aminotransferase (AST), alanine transaminase (ALT), alkaline phosphatase (ALP), creatine kinase (CK) and lactate dehydrogenase (LDH) (enzymatic reaction, OSR6009, OSR6170, OSR6004, OSR6179 and OSR6128, respectively), total calcium (Ca total, oCPC colorimetric reaction, OSR6113), calcium ion (Ca2+, arsenazo colorimetric reaction), phosphorus (P, molybdate reaction, OSR6122), magnesium (Mg, xylidin blue reaction, OSR6189), iron (Fe), sodium (Na+), potassium (K+) and chloride (Cl) (ion selective electrode indirect method). The following ratios were also calculated: albumin (ALB)/globulin (GLOB) and Na/K, as well as the Ca × P.
Digestive enzymes activity
The quantification of the activity of several digestive enzymes (total alkaline protease, trypsin, chymotrypsin, leucine aminopeptidase, α-amylase and bile salt-activated lipase) were conducted on independent samples coming from 2 fish per tank (6 fish per diet). Digestive enzymes activity was quantified according to the methods described in Gisbert et al. (2018) and samples handled according to Solovyev and Gisbert (2016) in order to prevent their degradation during storage and handling. Briefly, samples were homogenised by using an Ultra-Turrax T25 basic (IKA©-Werke, Germany). After, 0.50 g of sample were adjusted in 30 volumes (v/w) of ice-cold mannitol (50 mM mannitol, 2 mM Tris-HCl buffer; pH 7.0), following the addition of 0.1 M of CaCl2. Samples were re-homogenised for 30 seconds and sonicated for 90 seconds. The resultant crude tissue extract was centrifuged at 9,000 × g (10 min at 4°C) and then, 1 ml of supernatant was stored at −80°C in Eppendorf tube until enzyme quantification. Total alkaline proteases were quantified using 0.5% (w/v) azo-casein as substrate in 50 mM Tris-HCl buffer (pH 8.0). One unit of total alkaline proteases per mL (U) was defined as 1 μmol azocasein hydrolysed per min and mL of extract at λ = 366 nm (García-Carreño and Haard, 1993). Trypsin activity was assayed using BAPNA (N-α-benzoyl-DL-arginine p-nitroanilide) as substrate (Holm et al., 1988). Chymotrypsin activity was quantified using suc-AAPF-pNA (N-Succinyl-Ala-Ala-Pro-Phe p-nitroanilide) as substrate (Decker, 1977). The quantification of trypsin and chymotrypsin is achieved from the cytosolic extraction and following their kinetic curve in the spectrophotometer (λ = 405 nm, 5 min). The activity of α-amylase was determined using 0.3% starch dissolved in Na2HPO4 buffer (pH 7.4) as substrate and its activity (U) was defined as the mg of starch hydrolysed per min and mL of extract (λ = 580 nm) (Métais and Bieth, 1968). Bile salt-activated lipase activity was measured using p-nitrophenyl myristate as substrate in 0.25 mM Tris-HCl (pH 7.9), 0.25 mM 2-methoxyethanol and 5 mM sodium cholate buffer (Iijima et al., 1998). The activity of bile salt-activated lipase (U) was defined as the μmol of substrate hydrolysed per min and mL of extract (λ = 405 nm). Leucine aminopeptidase activity was determined using leucine–alanine as substrate in 50 mM Tris–HCl buffer (pH 8.0); one unit of enzyme activity (U) was defined as 1 nmol of the hydrolysed substrate per min per ml of tissue homogenate (λ = 530 nm) (Nicholson and Kim, 1975). All enzyme activities were expressed as total specific activity (U mg protein-1) and soluble protein in enzyme extracts was quantified by means of the Bradford’s method, using bovine serum albumin as standard (Bradford and Ward, 1976). The activity of all enzymes was determined at 25°C in triplicate per sample (methodological replicates).
Gut bacterial community DNA extraction, sequencing and bioinformatic analysis
For gut microbiota studies, the DNA was extracted from each sample as previously described in De Cesare et al. (2019). Briefly, 250 mg of hindgut contents were suspended in 1 mL lysis buffer (500 mM NaCl, 50 mM Tris-Cl, pH 8.0, 50 mM EDTA, 4% SDS) with steel beads and vigorously shaken using a MM400 mixer mill (Retsch GmbH, Haan, Germany) for 2 minutes at a frequency of 30 Hz. Samples were then heated at 70°C for 15 min and further centrifuged to separate the DNA from the bacterial cellular debris. This process was repeated on the remaining cellular debris with a second 0.3 mL aliquot of lysis buffer. Then, samples were subjected to 10 M v/v ammonium acetate precipitation, followed by isopropanol precipitation and a 70% ethanol wash and resuspended in 0.1 mL 1X Tris-EDTA (Sigma, Milan, Italy). Samples were treated with DNase-free RNase (Roche, Milan, Italy) and incubated overnight at 4°C, before being processed following the instructions of the QIAmp DNA Stool Mini Kit (Qiagen, Milan, Italy). DNA libraries were prepared following the Illumina 16S Library preparation protocol, amplifying the variable V3 and V4 regions of the 16S rRNA in order to obtain a single amplicon of approximately 460 bp. Sequencing was performed in 251 x 2 paired-end in the Illumina MiSeq with the MiSeq Reagent kit v2 500 cycles. Sequencing produced an average of 14.0 MB per sample. All the samples were analysed using a bioinformatics pipeline based on QIIME2 (http://qiime.org/) except two SCP0 samples (i.e. 6_SCP0 and 7_SCP0) with a low sequence output that were discarded. First, the sequences were imported as paired-end reads and processed with the dada2 algorithm in order to perform the denoising and to merge forward and reverse sequences per each pair. By this, we retained about 70% of input sequences per sample homogeneously along the sample groups (diets). The taxonomic classification of cleaned data was performed by applying the VSEARCH-based classifier implemented in QIIME and adopting the Greengenes 13_8 97% OUT dataset as reference. High variability among samples within treatment groups required that the data set be adjusted for this. To do so alpha diversity of all samples was calculated. Samples with an alpha diversity not respecting 25-percentile were not considered and are not present in the figure (i.e. 2_SCP0, 1_SCP10, 7_SCP10, 8_SCP10, 9_SCP10 and 4_SCP20).
Statistical analysis
Significant differences in growth performance, nutritional indices, metabolic serum parameters and digestive enzyme activities between dietary groups were determined using one way ANOVA (p < 0.05). The assumptions related to data normal distribution and homogeneity of variances were explored through Anderson Darling’s test and Levene’s test, respectively. Analyses were performed using Minitab software (Minitab 19.0.1; Pennsylvania State University, USA). For the microbiota analysis, mean values for the relative frequency of abundance of each taxonomic level in each sample were compared using the two-sided t-test on R software (package stats, function t.test). The R environment was also adopted to perform the alpha and beta diversity analysis using the package vegan and respectively the functions diversity and vegdist. For alpha diversity the indices Shannon, Simpson and Fisher were calculated. Beta diversity was explored by using a non-metric multi-dimensional scaling (NMDS). Significant separation between groups was observed with PERMANOVA using the package Adonis2 in R; data comply with the assumption related to data homogeneity of multivariate dispersion. On each statistical analysis the p-values ≤ 0.05 were considered statistically significant while 0.5 < p-value < 0.1 were considered borderline (tendency). Results are presented as mean ± standard deviation (SD), unless mentioned otherwise.
Results
Growth performance and feed utilisation
Growth performances of flathead grey mullet fed diets differing in their content in bacterial SCP are shown in Table 2. At the end of the experiment, grey mullet fed the experimental diets, presented significant differences in terms of growth performance and feed utilisation indicators (p < 0.05). Fish fed SCP0 showed significantly higher FBW and SGR compared to fish fed SCP10 and SCP20 diets. Fish fed the SCP20 diet showed significantly lower values for these two parameters in comparison to the other groups. Values of FI and FCR of fish fed the SCP0 diet were significantly lower in comparison to fish fed SCP10 and SCP20, whereas fish from the SCP20 feeding regimen showed significantly higher values for the two parameters (p < 0.05).
Biometric and nutritional indices, and body composition
Data on biometric indices, body composition and nutritional indices are shown in Table 3. At the end of the experiment, no significant differences among dietary groups were observed in terms of VSI, HSI and RGL values (p > 0.05). In contrast, K values from fish fed the SCP20 diet were significantly lower to those found in fish from the SCP0 group (p < 0.05), whereas fish fed the SCP10 diet showed intermediate values which are found to be non-statistically different from the groups SCP0 and SCP20. The content of protein, lipid and moisture was not significantly affected by the diet (p > 0.05). On the contrary, the ash carcass content was significantly different only between the groups SCP0 and SCP20 (p > 0.05). Nutritional indices like PER, LER, GPE and GLE from fish fed the SCP0 diet were significantly higher in comparison to fish fed SCP10 and SCP20 diets (p < 0.05), whereas fish fed the SCP20 diet showed significantly lower values for all nutritional indices.
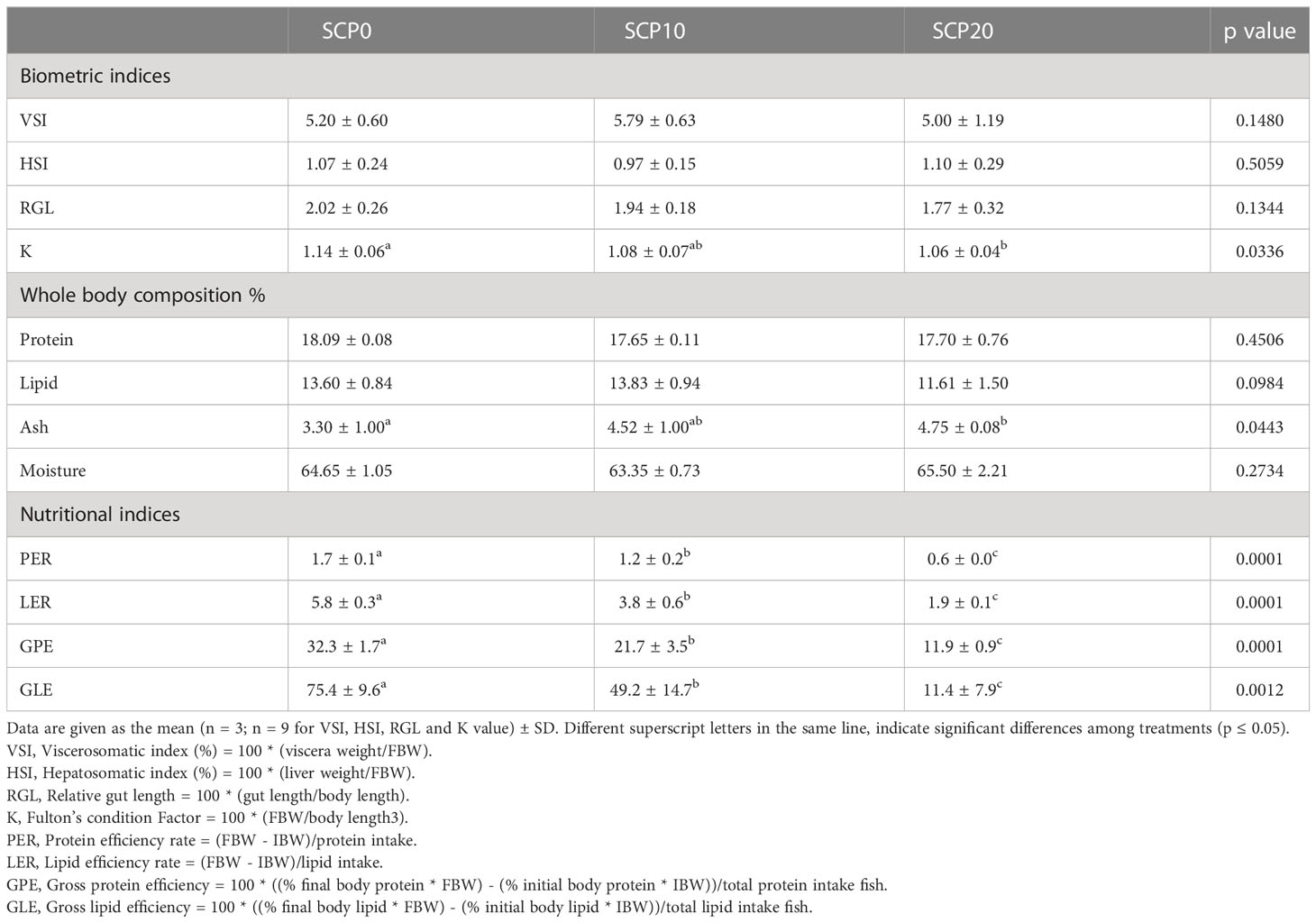
Table 3 Biometric indices, body composition and nutritional indices of flathead grey mullet fed the experimental diets.
Feed buffer capacity
Feed buffer capacity is shown in Figure 1. The three experimental diets SCP0, SCP10 and SCP20 presented a significant difference in pH of 6.00 ± 0.01, 5.62 ± 0.00 and 5.22 ± 0.00, respectively (p < 0.05). Similarly, the diet buffer capacity (as mmol of HCL to lower 1 g of the diet to pH 3) significantly decreased by the inclusion of the SCP ingredient being 0.64 ± 0.01, 0.59 ± 0.00 and 0.52 ± 0.01 mmol of HCl for SCP0, SCP10 and SCP20 diets, respectively.
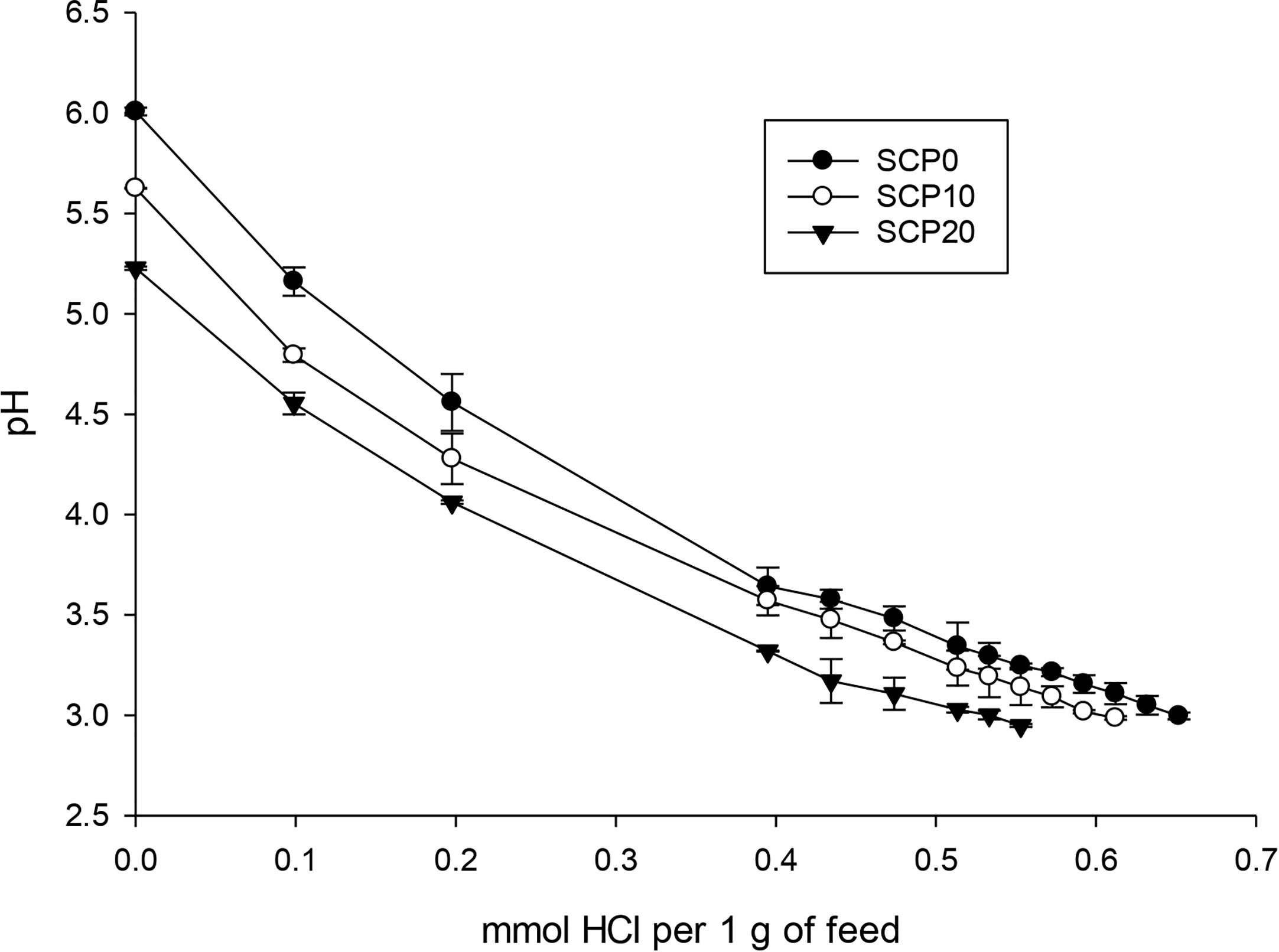
Figure 1 Plot showing changes in pH with the addition of HCL for the three diets considered (SCP0, SCP10 and SCP20). Data are shown as mean ± SD (N = 3).
Serum metabolic parameters
The results of serum metabolic parameters are shown in Table 4. The levels of glucose, total calcium, Ca2+, Fe, K+ and the result of Ca × P were significantly higher in fish fed the SCP0 diet compared to those fed with SCP10 and SCP20 diets (p < 0.05). By contrast, the ratio Na+/K+ was significantly lower in fish fed the SCP0 diet compared to those from SCP10 and SCP20 groups (p < 0.05). Urea tended to be higher (p = 0.0533) in fish fed SCP10 and SCP20 diet compared to the SCP0 group. On the contrary, the serum enzyme alkaline phosphatase (ALP) tended to be higher (p = 0.0652) in fish fed the SCP0 diet with respect to their congeners fed SCP10 and SCP20 diets. No significant differences between the treatments were observed for uric acid, total bilirubin, cholesterol, HDL, TG, lactic acid, TP, ALB, AST, ALT, CK, LDH, P, Mg, Na+, Cl and the ratio ALB/GLOB (p > 0.05).
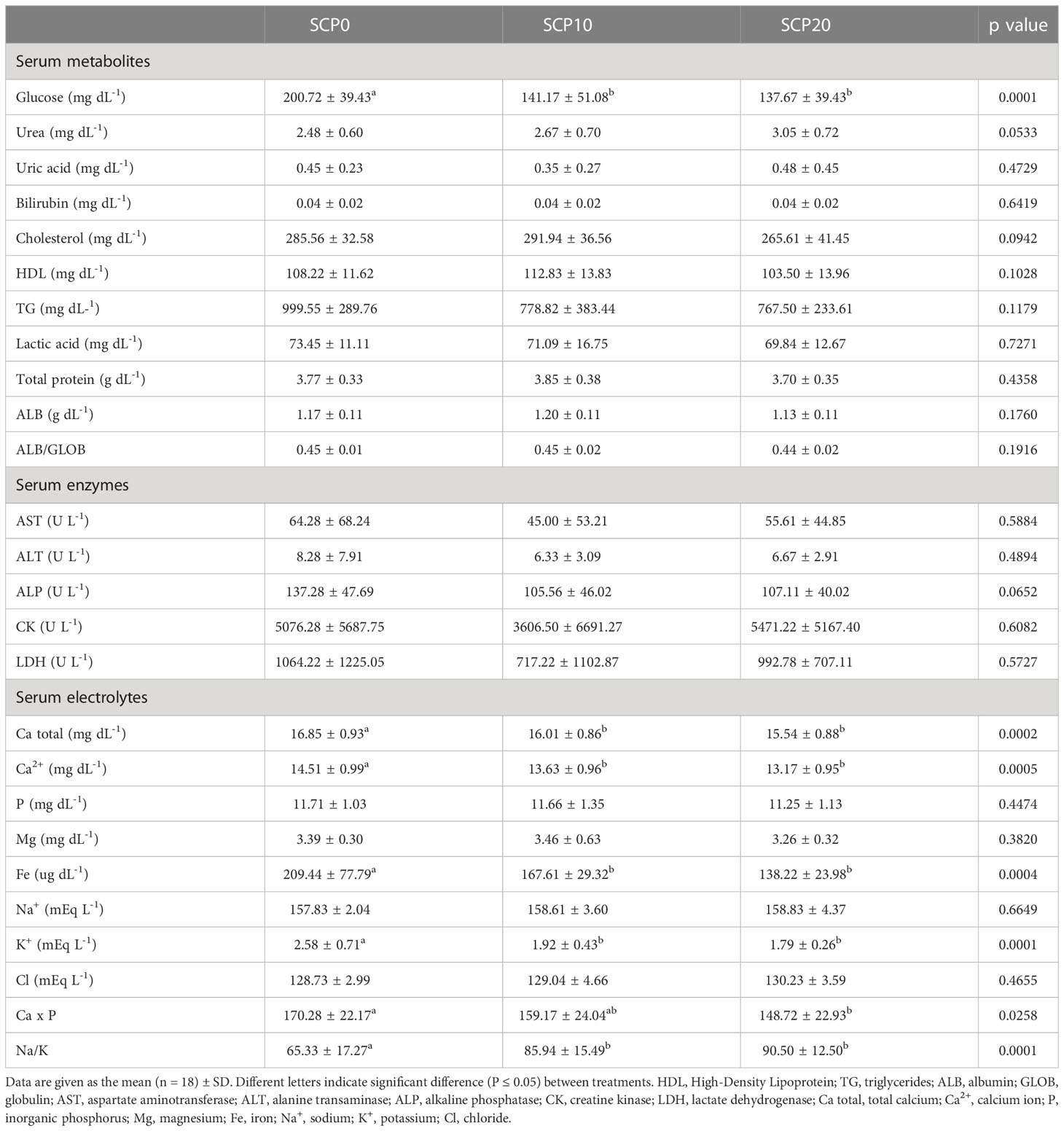
Table 4 Serum metabolites, enzymes and electrolytes values for flathead grey mullet fed the experimental diets.
Activity of digestive enzymes
The specific activity of total alkaline proteases, trypsin, chymotrypsin, leucine aminopeptidase, α-amylase and bile salt-activated lipase are shown in Figure 2. The activity of pancreatic and intestinal proteases decreased in fish fed SCP diets. Specifically, total alkaline proteases, trypsin, chymotrypsin and leucine aminopeptidase activities were significantly higher in fish fed the SCP0 diet compared to fish fed SCP10 and SCP20 diets (p = 0.0011, p = 0.009, p = 0.0034 and p = 0.0021 respectively). No differences were detected in the activity of both α-amylase and bile salt-activated lipase between the dietary treatments.
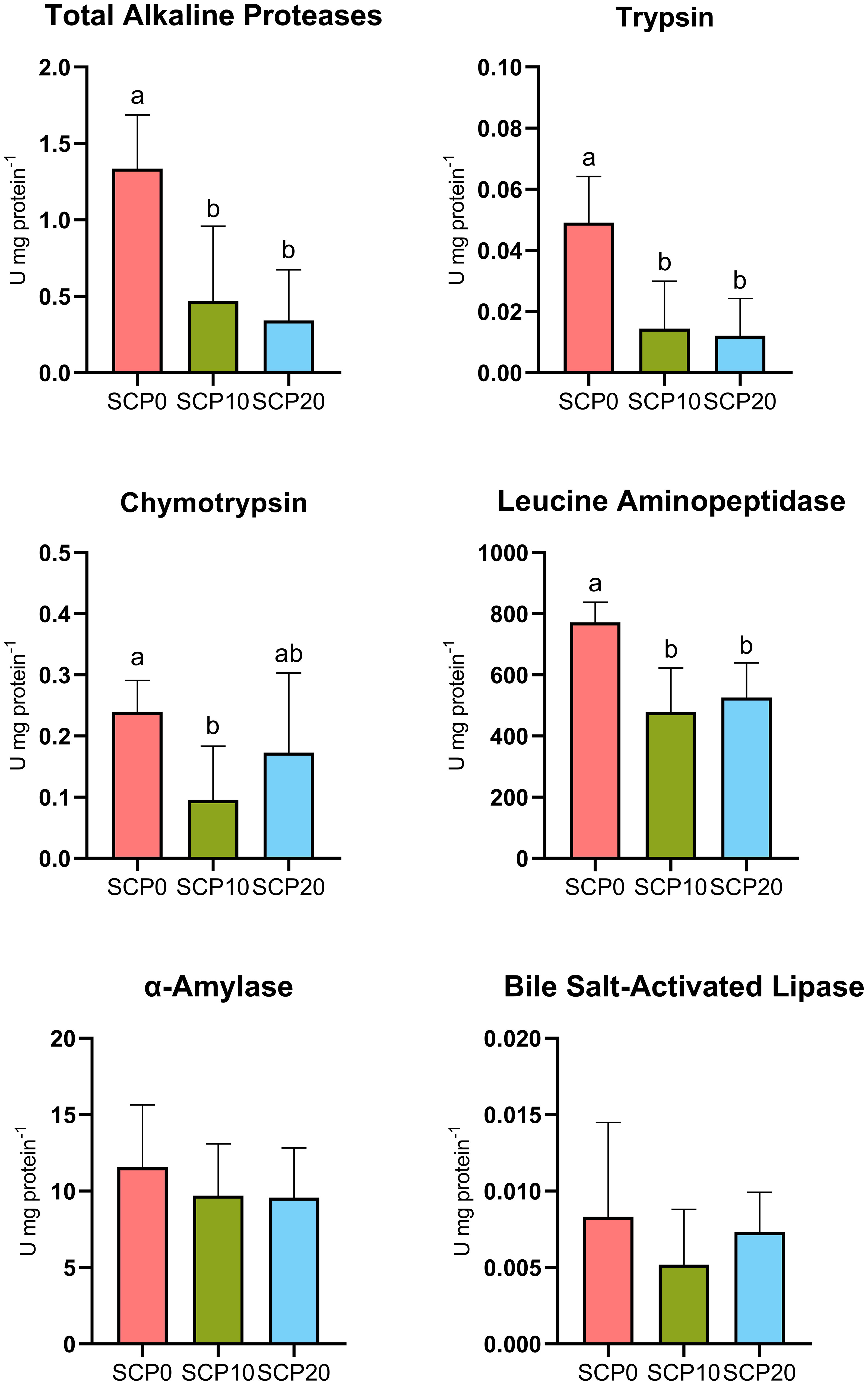
Figure 2 Digestive enzymes activity of flathead grey mullet fed experimental diets after 113 days. Enzymes activity was quantified on the whole stomach and intestine on 2 independent samples per tank (6 samples per diet). Different letters indicate significant difference (p ≤ 0.05) between treatments.
Gut bacterial community profiles
Gut bacterial community profiles at phylum and at genus level are shown in Figure 3. Because the variability of the samples was high even within the same group, data were adjusted based on the distribution of the alpha diversity. As shown in Table 5, after parametric t-test between the groups, some significant differences appeared in the composition of gut microbiota. At the phylum level, gut microbiota was dominated by Fusobacteria in fish fed the SCP0 diet (51.1 ± 24.1%) while the most abundant phylum was Proteobacteria in fish fed SCP10 (67.3 ± 16.6%) and SCP20 (53.2 ± 20.3%) diets. Firmicutes was present at a much lower relative abundance in all groups, and it was significantly higher in fish from the SCP10 group compared to their congeners fed the SCP0 diet (p = 0.0134), and almost significantly higher in fish fed SCP20 compared to SCP0 (p = 0.0852), but not between the groups fed both SCP diets (p = 0.2465). Samples 2_SCP10, 3_SCP10 and 6_SCP10, and 2_SCP20, 3_SCP20, 8_SCP20, and 9_SCP20 included to a greater or lesser amount Actinobacteria, which is the corresponding phylum for C. glutamicum (data not shown). For some samples this phylum was even a dominant as can be expected. The differences observed at the phylum level were maintained at the genus level, mainly due to the differences in the relative abundance of Cetobacterium spp. This genus appears to be more abundant in fish fed the diet without inclusion of bacterial SCP. In particular, as shown in Table 5, its abundance is significantly higher in fish fed SCP0 compared to SCP10 (p = 0.0055) and in fish fed SCP10 compared to SCP20 (p = 0.0382) and almost significantly higher in fish fed SCP0 compared to SCP20 (p = 0.0743). Other statistically significant differences observed at the genus level included Corynebacterium spp., which is higher in fish fed the SCP20 diet compared to those from the SCP0 group (p = 0.0222) and almost significantly higher in fish fed the SCP10 diet compared to the SCP0 group (p = 0.0991). Furthermore, Streptococcus spp. was statistically higher in fish fed the SCP10 diet compared to the control group (SCP0 diet) (p = 0.0502) and in fish fed the SCP20 diet compared to the SCP0 group (p = 0.0432). No significant differences were observed in the alpha diversity between fish fed different diets for the three diversity indices considered (i.e. Shannon, Simpson and Fisher diversity) (Figure 4). The gut microbiota variations between samples (beta-diversity) were plotted on a two-dimensional non-metric multidimensional scaling (NMDS) plot based on Bray-Curtis values. According to the findings, groups showed a significant variation in terms of overall gut microbiota composition (PERMANOVA, p = 0.012) (Figure 5).
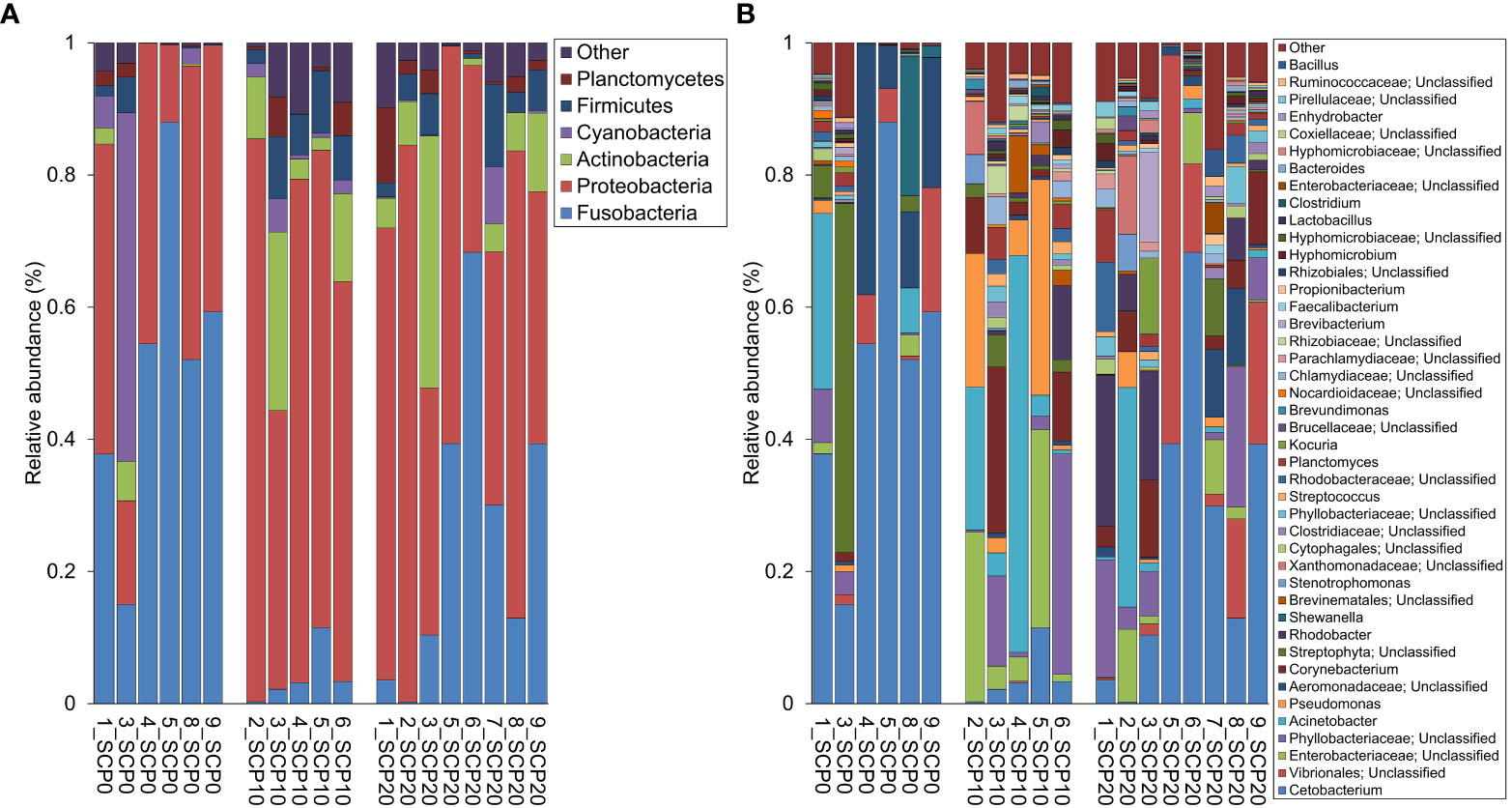
Figure 3 Relative abundance (%) of gut microbiota composition at phylum (A) and genus (B) levels measured on flathead grey mullet fed experimental diets after 113 days. Microbiota analysis were performed individually on a sample of hindgut content for each fish. Only taxa with relative abundance > 0.1% in at least 1 sample were included.
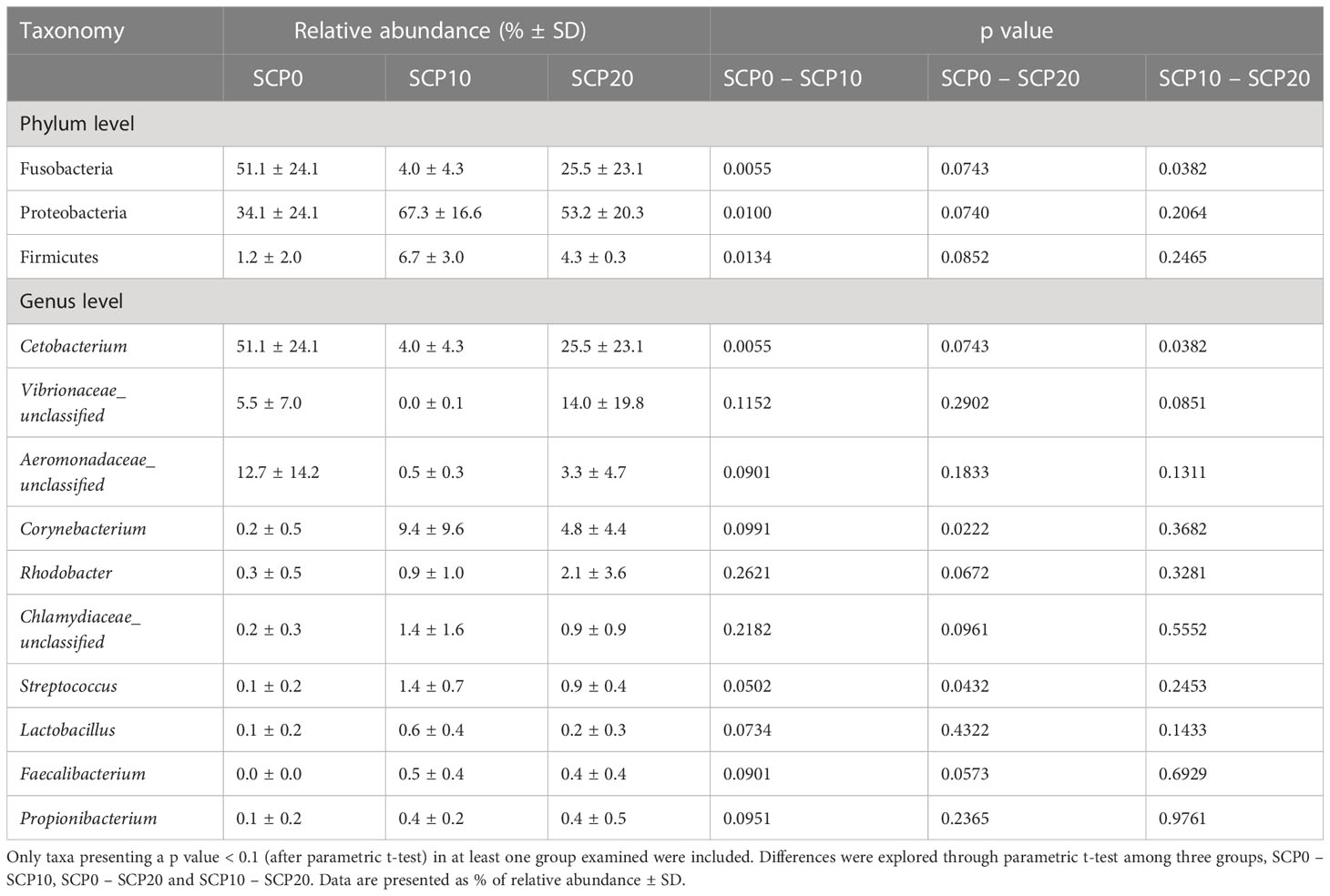
Table 5 Table showing the relative abundance of bacteria taxa at phylum and genus level found in the gut of flathead grey mullet fed experimental diets.
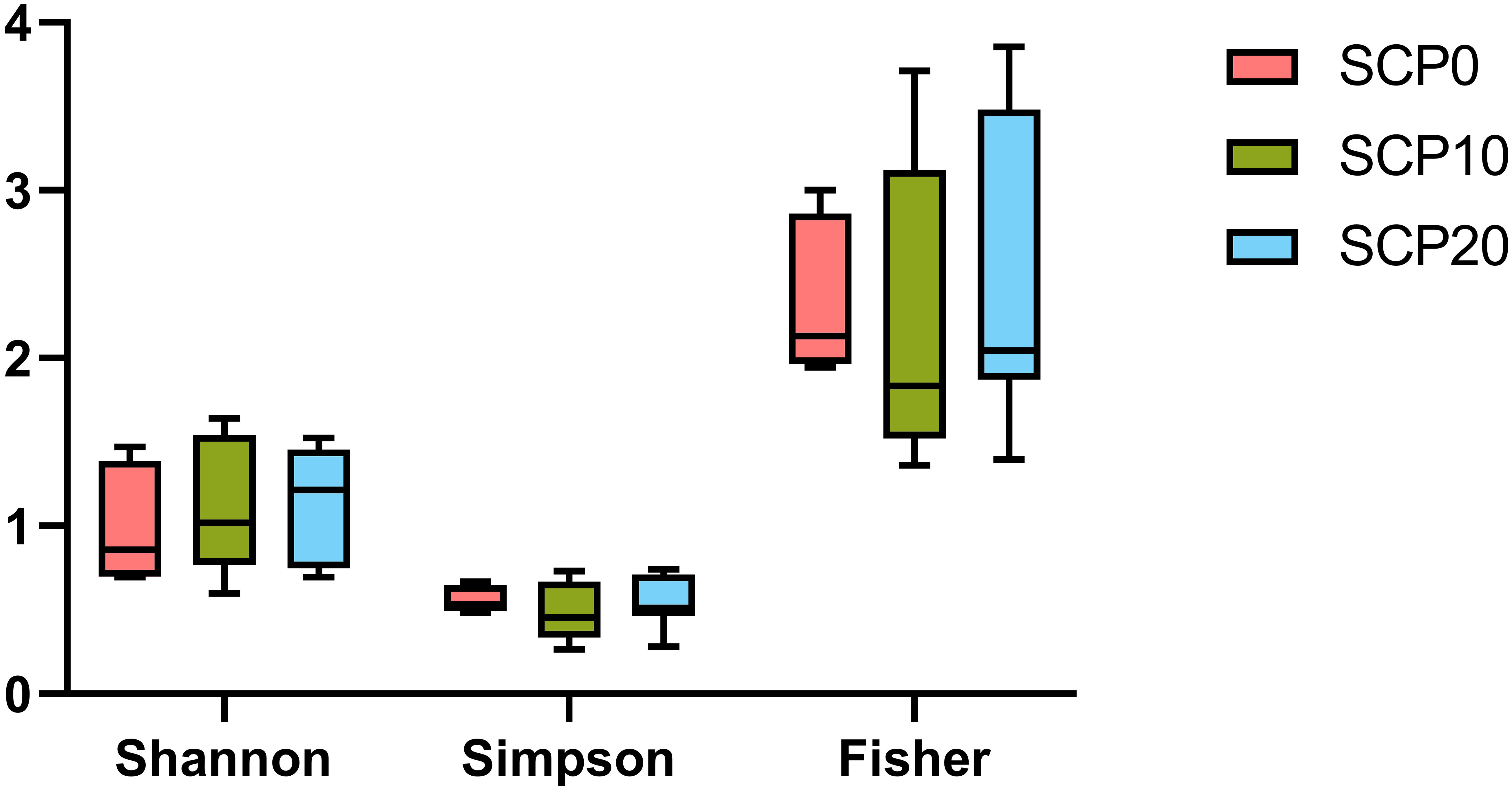
Figure 4 Boxplot showing alpha diversity in the grey mullet gut microbiota calculated with different indexes (i.e. Shannon diversity, Simpson diversity and Fisher diversity). No significant differences (p ≤ 0.05) were obtained after parametric t-test.
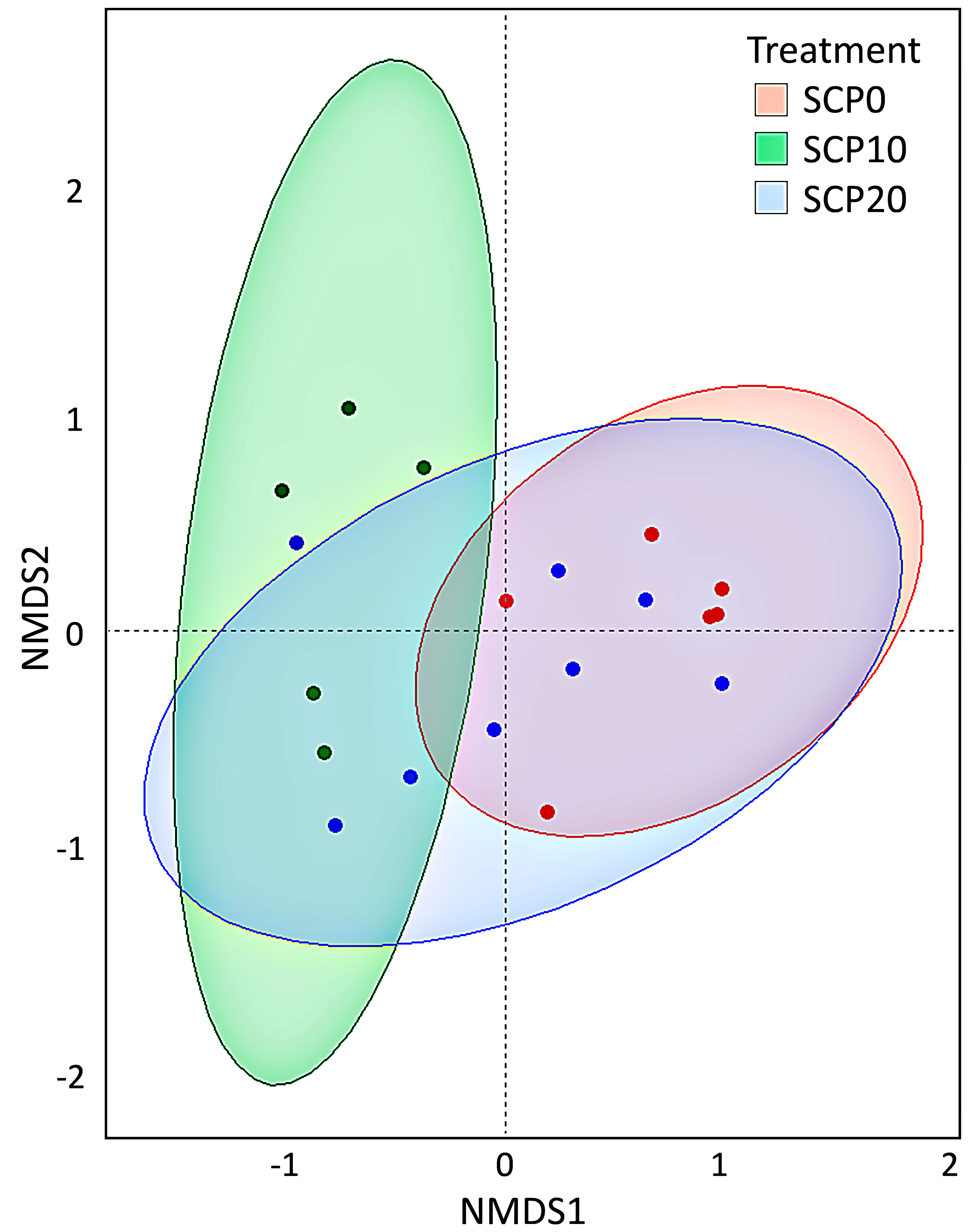
Figure 5 Two-dimension non-metric multidimensional scaling (NMDS) plot based on Bray-Curtis distance of grey mullet gut microbiota fed experimental diets after 113 days. Red dots are for gut microbiota communities found in fish fed SCP0, green dots for communities in fish fed SCP10 and blue dots for communities in fish fed SCP20. A significant separation among groups was observed [(p value = 0.012; Permutational Multivariate Analysis of Variance (PERMANOVA) (Adonis2)]. Data comply with the assumption related to homogeneity of multivariate dispersion (p value = 0.3544).
Discussion
The present study sought to define the capacity of flathead grey mullet, reared in captive conditions, in utilising a SCP ingredient derived from C. glutamicum in substitution of SBM, FM and PM. By using diets with equivalent levels of protein, lipid and digestible energy but different levels of bacterial SCP inclusion, a clear effect was seen at the end of the experiment. In particular, fish fed the SCP diets presented significantly lower growth performances, and feed and protein utilisation in comparison with fish fed control diet. To the best of our knowledge, there is no literature to date investigating the effects of such an ingredient in the diet of flathead grey mullet. Despite of this, the findings of this trial were quite unexpected, since representatives from the family mugilidae are able to utilise a variety of ingredients in captive conditions such as plant meals (Luzzana et al., 2005; Gisbert et al., 2016; Liu et al., 2021), algae (Rosas et al., 2019) and yeast (Wassef et al., 2001; Luzzana et al., 2005). In addition, considering the assumption that wild fish represent a reference for the development of formulated diets, and it has been reported previously that bacteria account for 15–30% of the organic carbon in the stomach content of this species (Moriarty, 1976; Crosetti and Blaber, 2015), the results from the current nutritional study were puzzling.
In our experiment, feed intake was higher in fish fed diets containing bacterial SCP inclusion. Published studies have revealed contradictory effects of SCP sources on feed intake in fish (Aas et al., 2006; Glencross et al., 2020). Those that found a positive correlation of SCP inclusion on feed intake, suggested that the high content of nucleotides (e.g. inosinic acid and inosine) presented in bacterial SCP may act as an attractant enhancing the palatability of the diet (Gamboa-Delgado and Márquez-Reyes, 2018; Hossain et al., 2020). However, our results were in agreement with previous studies conducted on rainbow trout (Perera et al., 1995; Zamani et al., 2020), Atlantic salmon (Marit Berge et al., 2005) and Pacific white shrimp (Hamidoghli et al., 2019), in which inclusion of dietary bacterial SCP did not affect or enhance feed intake, but lead to a poorer growth performance. This suggests that in our case the issue with SCP from C. glutamicum was not due to its palatability, but rather to the utilisation potential of the ingredient.
Across a range of SCP sources, bacteria are generally recognised as relatively digestible and their inclusion has been successfully demonstrated in several major finfish species of commercial interest without affecting growth or fish health (Glencross et al., 2020; Jones et al., 2020). In particular, the same SCP ingredient was tested by Marchi et al. (2023) on gilthead seabream, reporting no negative effects on the growth performance and an interesting probiotic effect on the gut microbiota, even at a high substitution rate. However, still some inconsistency appeared in the data being perceived as a limiting factor for their broader application (Glencross et al., 2023). Some works suggest that the thickness of the cell-wall may be a constraint for the digestibility of a number of SCP sources (Ugalde and Castrillo, 2002; Yamada and Sgarbieri, 2005; Ritala et al., 2017; Tibbetts et al., 2017; Teuling et al., 2019). C. glutamicum is gram-positive bacteria with a thick cell wall made out of a complex structure of peptidoglycan and arabinogalactan (Eggeling and Sahm, 2001; Cheng et al., 2021). In general, finfish can cope well with cell-wall lysis by producing acidic secretion of HCl and thus decreasing the stomach pH to values below 3 (Lobel, 1981). By observing the digestive system of grey mullet, the species presented a stomach with an alkaline pH (approx. 8.5), the same as the intestine (Payne, 1978). In the absence of a specific acid secretion of HCl in the stomach, the species has evolved relying on a mechanical trituration of feed particles carried out in the pyloric stomach, called the gizzard (Payne, 1978; Lobel, 1981; Crosetti and Blaber, 2015). A gizzard-like pyloric stomach is frequent in those species that eat detritus and flocculant benthic biofilm (e.g. algae, bacteria and non-living organic matter) such as P. lineatus (Bowen, 2022), H. niloticus (Agbugui et al., 2021), S. stomachicus (Wilson and Castro, 2010) and D. cepedianum (Watson et al., 2003). The wall of the gizzard is made of rings of smooth, non-striated muscle fibres and often contains sand or small debris to assist in grinding plant and fibrous material (Crosetti and Blaber, 2015). A work conducted by Sánchez et al. (1993) hypothesised that the poor growth that occurred in grey mullet fed a microalgae-based diet may have been due to the lack of access to sand in captive conditions, therefore preventing the development of a functional gizzard. To the best of our knowledge, no other scientific publications dealt with the possible effects of a non-functional gizzard on diet digestion in finfish species. On the other hand, this phenomena is well described in poultry nutrition in which it is known that the access to structural components such as whole cereals or coarse fibres, improve growth and nutrient absorption in chickens by enhancing gizzard functionality (Svihus, 2014; Idan et al., 2023). Flathead grey mullet used in this experiment were raised in a captive condition in fiberglass tanks, therefore having no access to sand or coarse material and possibly impairing the proper development of a functional gizzard. Thus, the lower growth performance observed in fish fed SCP may be related to poor nutrient digestion caused by an incomplete lysis of the C. glutamicum cell-wall, as described previously (Sánchez et al., 1993). The hypothesis of a deficiency in nutrient digestion appears to be reasonably acceptable after a look to the digestive enzyme activity. SCP inclusions lead to a reduction in the specific activity of the pancreatic and intestinal proteases (e.g. trypsin, chymotrypsin and leucine aminopeptidase), which is well correlated with an impaired protein absorption and low growth performance (Silva et al., 2010; Gisbert et al., 2016). A similar reduction in the activity of digestive proteases has been observed in carnivorous fish when fed too high inclusion of plant ingredients, and associated with poor growth and histopathological changes (e.g. enteritis) (Silva et al., 2010; Rodiles et al., 2012; Chikwati et al., 2013; Willora et al., 2022). How was suggested in a work conducted by Pujante et al. (2016) on thick-lipped grey mullet (Chelon labrosus) the enzymatic digestion of proteins mainly takes place in the anterior intestine by the action of alkaline proteases. A functional evidence for this is evinced from the lack of acid proteases activity (e.g. pepsin) in flathead grey mullet (Gisbert et al., 2016). Alkaline proteases are most active at a pH range from 8 to 10 (Nasri et al., 2011; Liew et al., 2013; Sanatan et al., 2013). Among them, trypsin activates other enzymes initially generated as inactive zymogens, such as chymotrypsin and therefore is considered a key enzyme in protein digestion (Asgeirsson et al., 1989). Trypsin and chymotrypsin catalyse the hydrolysis of the bonds of peptides into smaller dipeptides and oligopeptides which are split by the action of leucine aminopeptidase into free amino acids that can be absorbed into the enterocytes (Willora et al., 2022). In marine finfish with a functional gastric stomach, the acidity of the chyme entering from the stomach into anterior intestine is buffered by the secretion of HCO3 from the pancreas, which create the optimal pH condition for the activation of the alkaline proteases (Wilson et al., 2002; Márquez et al., 2012; Parma et al., 2019). It is interesting to note that in our trial, we have observed a decreased pH and buffer capacity in the diets containing the SCP from C. glutamicum. These findings are in line with a previous study conducted by Prodanovic and Sredanovic (2005) reporting that the buffer capacity of yeast SCP is significantly lower than that of SBM (9.15 and 21.15, respectively), as well as the pH (5.84 and 6.58, respectively) probably due to the higher content of nucleic acids, as suggested in a work conducted by Ritala et al. (2017). We do not know if this could have contributed to an inhibitory effect on the activity of alkaline proteases; however, it is likely that in our experiment the intestinal pH of grey mullet decreased as a function of bacterial SCP inclusion. The activity of the alkaline proteases is mediated by a complex cascade process involving pH, temperature and the concentration of ions. In fish fed SCP diets, we have also found significantly lower concentrations of ions of calcium (Ca2+) and potassium (K+) in the blood. Ca2+ is recognised as a trypsin activator, since its presence strengthens the interactions inside protein molecules and enhances thermal stabilisation of this pancreatic enzyme (Bezerra et al., 2005), while K+ has an effect on the activation of leucine aminopeptidase (Liew et al., 2013). No differences were observed in both α-amylase and bile salt-activated lipase activity between fish fed the different diets. If compared to carnivorous species, the higher α-amylase activity found in flathead grey mullet, broadly hints that carbohydrates may be preferred as a protein sparing substrate as it has been previously postulated by several authors (Pujante et al., 2016; Calixto da Silva et al., 2020; Solovyev and Gisbert, 2022).
If we consider the other performance indicators, we observed that fish fed the diet with the highest inclusion of SCP also showed a significantly lower Fulton’s condition factor (K). This length-weight relationship can be correlated with the wellness conditions of the fish, and its fluctuations with the interaction between feeding condition and energy reserves (Robinson et al., 2008; Datta et al., 2013; Yulianto et al., 2020). Because of the issue with nutrient digestion in fish fed SCP diets, flathead grey mullet belonging to these groups appeared underfed with lower energy reserves and therefore with a lower flesh/skeleton ratio, which also explain the increased content of ash in the body of this group of fish. In terms of nutritional indices, which are used for comparing the nutritional values of different ingredient sources, they appeared to be significantly decreased by SCP inclusion, which is reflected by the feed conversion rate and the growth. On the other hand, gross protein and lipid efficiency (32.3 ± 1.7 and 75.4 ± 9.6%, respectively) of fish fed the SBM diet were encouraging in order to further design compound feeds for this low trophic species. With a focus on the protein utilisation, grey mullet fed a diet with only 3% fishmeal, were able to convert almost 1/3 of the dietary protein into edible fillet. This ratio appears similar to that found for gilthead seabream (Busti et al., 2020a) and rainbow trout (Pelusio et al., 2020) and it is somewhat higher to previous findings with European seabass (Bonvini et al., 2018; Parma et al., 2020; Pelusio et al., 2022). These results may indicate that is possible to further decrease the level of fishmeal in aquafeeds for this species.
As mentioned previously, the SCP additive contains a high level of nucleic acids, mainly RNAs (Ritala et al., 2017). In our trial, we have seen an increasing concentration of urea in the blood of the fish fed SCP diets. This phenomena has been described in previous works and is ascribed to the catabolism of pyrimidines, which are presents in nucleic acid molecules (Agboola et al., 2021; Lin et al., 2022; Marchi et al., 2023). High concentrations of ammonia metabolites in the blood may also interfere with protein, fat and carbohydrate metabolism (Rumsey et al., 1992), which may also explain the lower performance of flathead grey mullet fed bacterial SCP diets in comparison to the control group. In our trial, the blood glucose concentration found in the fish appears higher than values found in literature for the same species (Wanshu, 1992; Calixto da Silva et al., 2020). Despite this, the significantly lower concentration of blood glucose in fish fed SCP diets is likely to support the hypothesis of there being a problem with the digestion of this ingredient rather than a pathological condition. Starvation or underfeeding conditions are generally associated with a decreased blood glucose level in many fish species (Polakof et al., 2012). The enzyme activity of the marker enzymes in the blood associated to liver damage (aspartate transaminase and alanine transaminase) and tissue damage (creatine kinase and lactate dehydrogenase) did not show any difference among the fish groups in our trial. Interestingly, the activity of the enzyme alkaline phosphatase showed a trend and appeared to be higher in fish fed the diet with no inclusion of SCP. This difference may be related to the higher nutrient absorption in this group of fish, since the enzyme is involved in the absorption and transport of lipid and carbohydrates from the intestine, and its activity is positively correlated with food ingestion and growth rates (Lemieux et al., 1999; Lallès, 2020).
The gut microbiota of fish plays an important role in regulating nutrient digestion, immune responses, disease resistance, and the colonisation of potential pathogens (Parma et al., 2016; Gu et al., 2017; Busti et al., 2020b; Pelusio et al., 2020; Xie et al., 2021). The composition of bacterial communities is strongly determined by the environment conditions both internal and external such as salinity (Kivistik et al., 2020) and temperature (Thompson et al., 2017; Sepulveda and Moeller, 2020), the immune/health status (Kashinskaya et al., 2019; Parshukov et al., 2019), the host species considered and their dietary components (Kashinskaya et al., 2018). In particular, the composition of flathead grey mullet microbiota in response to different dietary ingredients is a relatively unknown topic. While there is a published study conducted by Le and Wang (2020) aimed at the characterisation of the microbiota from wild flathead grey mullet, information is lacking for fish reared in captive conditions fed formulated diets. Our finding displayed Fusobacteria and Proteobacteria as the most abundant phyla, accounting for more than 70% of the total variability in all groups. Inclusion of C. glutamicum in the diet of flathead grey mullet promoted a shifting between the two phyla, increasing the amount of Proteobacteria over the Fusobacteria (with presence of Actinobacteria expected, particularly in the SCP10 and SCP20 groups). Using as a reference the gut microbiota observed in wild fish, with Proteobacteria and Firmicutes as the most abundant phyla (Le and Wang, 2020), it is interesting to note that this appears more similar to fish fed the SCP diets even though these groups displayed severely reduced growth performances. Proteobacteria are found to be dominant in the gut microbiota of several fish species fed marine-derived ingredients (Gu et al., 2017; Kim et al., 2021). The phylum Firmicutes accounts for only a minor part of the variability of the gut microbiota in all groups, even though it was more abundant in fish fed SCP supplemented diets. This phylum, and also especially the lactic acid bacteria, often dominate the composition of the microbiome of fish fed plant-based diets (Busti et al., 2020b; Pelusio et al., 2020), and it is normally associated with improved nutrient digestion (Parma et al., 2016; Parma et al., 2020; Marchi et al., 2023). In our trial, fish fed the diet without inclusion of SCP displayed an increased dominance of the phylum Fusobacteria, which is totally represented by the genus Cetobacterium. This genus has been found to be dominant in the gut of many freshwater species and its presence is associated with a positive impact on the digestion and metabolic efficiency of the host (Xie et al., 2021). At the genus level, we have seen an increased abundance of Streptococcus in fish fed SCP diets. Some species of streptococci, as potential spore forming species, may represent carry-over of bacteria from the SCP feed additive (Karlsen et al., 2022), as some feed components are now shown to bias the interpretation of microbiome analyses by acting as the vectors for introduction of species to the intestinal microbiome of the host. Moreover, several bacterial species within the genus Streptococcus spp. have been reported as important pathogens of fish species (Ringø et al., 2018; Halimi et al., 2020). Although without a significant difference, in fish fed SCP diets we have also seen an increasing trend for the family Vibrionaceae, a family associated with important pathogens of fish (Zhang et al., 2020; Sepúlveda et al., 2022). On the other hand, fish fed diets with inclusion of SCP showed in their gut microbiota a decreasing trend in the family Aeromonadaceae, while there was a trend toward increasing in the relative abundances of Rhodobacter, Corynebacterium and Lactobacillus. Different species of Aeromonas are described as important fish pathogens (Zmysłowska et al., 2009; Soto-Dávila et al., 2022). Rhodobacter and Lactobacillus are considered among the most promising probiotics used in aquaculture (Ringø et al., 2018; El-Saadony et al., 2021; Cao et al., 2022). Corynebacterium, as expected, was found significantly higher in fish fed SCP diets and the presence of some species has been associated with a probiotic effect (Horn et al., 2022; Marchi et al., 2023). Along these same lines of investigation of probiotics are studies that evince the quorum sensing interactions that bacterial food components can have (Davares et al., 2022). Quorum sensing utilises small molecules like acyl-homoserine lactones and oligopeptides for intercellular signalling. An unrefined product like SCP may include bioactive molecules with potential for interspecies bacterial communication. When using quorum sensing bacteria not only communicate with members of the same species but may also “eavesdrop” on the “conversation” of other species and modulate their behaviour in response to signal molecules they do not synthesise (Federle and Bassler, 2003). This can have unexpected consequences on diverse assemblages of bacteria from unique hosts, perhaps even some degree of dysbiosis and poor fitness of the microbiota, which could lead to some of the poor performance indicators found in this study. Overall the resulting microbiota data will contribute to improved characterisation of the taxonomic diversity of the gut bacterial community from grey mullet, which is essential for understanding the dynamics of the diet-host-gut-microbiota axis.
Conclusion
These results indicated that flathead grey mullet reared under captive conditions demonstrate incomplete utilisation of a protein source derived from C. glutamicum SCP. Probably due to the particular organisation of the digestive system of flathead grey mullet there was an incomplete protein digestion of SCP due to poor lysis of cell-wall components (this being due to lack of an acidic stomach digestion) and also associated with the captive conditions (failure in the development of a functional gizzard). Based on our assessment, the diet had significant negative effects on individual variables in analysis of both digestive enzymes and gut microbiota.
Data availability statement
The datasets presented in this study can be found in online repositories. The name of the repository and accession number can be found below: NCBI; PRJNA954609.
Ethics statement
The animal study was reviewed and approved by Ethical-Scientific Committee for Animal Experimentation of the University of Bologna.
Author contributions
ABe, PG, ABo and LP conceived and designed the experiment. ABe wrote the first draft of the manuscript. ABe, SN, EG, KA, DC, ABo and LP reviewed and edited the manuscript. ABe, SN, DC and LP carried out fish maintenance and sample collection. SN, EG and KA carried out digestive enzyme activity analysis and data processing. ABe, AD and VI carried out the microbiota analysis and data processing. FD carried out plasma biochemistry analysis. All authors reviewed, improved the writing, and approved the final manuscript. All authors contributed to the article and approved the submitted version.
Funding
This research was undertaken as a part of the FEAMP 2014-2020 project, action 3A. Pilot project for the application of techniques and methods aimed at the enhancement and diversification of fish products from Valliculture financed by Emilia Romagna region. Analyses of digestive enzymes conducted at IRTA were supported by the ACUISOST project funded by the Ministerio de Agricultura, Pesca y Alimentación from the Spanish Government and EU Next Generation funds (PRTR).
Conflict of interest
The authors declare that the research was conducted in the absence of any commercial or financial relationships that could be construed as a potential conflict of interest.
Publisher’s note
All claims expressed in this article are solely those of the authors and do not necessarily represent those of their affiliated organizations, or those of the publisher, the editors and the reviewers. Any product that may be evaluated in this article, or claim that may be made by its manufacturer, is not guaranteed or endorsed by the publisher.
References
Aas T. S., Grisdale-Helland B., Terjesen B. F., Helland S. J. (2006). Improved growth and nutrient utilisation in Atlantic salmon (Salmo salar) fed diets containing a bacterial protein meal. Aquaculture 259, 365–376. doi: 10.1016/j.aquaculture.2006.05.032
Agboola J. O., Øverland M., Skrede A., Hansen J.Ø. (2021). Yeast as major protein-rich ingredient in aquafeeds: a review of the implications for aquaculture production. Rev. Aquac. 13, 949–970. doi: 10.1111/raq.12507
Agbugui M. O., Egbo H. O., Abhulimen F. E. (2021). The biology of the African bonytongue Heterotis niloticus (Cuvier 1829) from the lower Niger river at agenebode in edo state, Nigeria. Int. J. Zool. 2021, e1748736. doi: 10.1155/2021/1748736
Asgeirsson B., Fox J. W., Bjarnason J. B. (1989). Purification and characterization of trypsin from the poikilotherm Gadus morhua. Eur. J. Biochem. 180, 85–94. doi: 10.1111/j.1432-1033.1989.tb14618.x
Bezerra R. S., Lins E. J. F., Alencar R. B., Paiva P. M. G., Chaves M. E. C., Coelho L. C. B. B., et al. (2005). Alkaline proteinase from intestine of Nile tilapia (Oreochromis niloticus). Process Biochem. 40, 1829–1834. doi: 10.1016/j.procbio.2004.06.066
Bligh E. G., Dyer W. J. (1959). A rapid method of total lipid extraction and purification. Can. J. Biochem. Physiol. 37, 911–917. doi: 10.1139/o59-099
Bonvini E., Bonaldo A., Parma L., Mandrioli L., Sirri R., Grandi M., et al. (2018). Feeding European sea bass with increasing dietary fibre levels: impact on growth, blood biochemistry, gut histology, gut evacuation. Aquaculture 494, 1–9. doi: 10.1016/j.aquaculture.2018.05.017
Bowen S. H. (2022). Digestion and assimilation of benthic biofilm by the sábalo, Prochilodus lineatus. J. Fish. Biol. 100, 107–116. doi: 10.1111/jfb.14924
Bradford H. F., Ward H. K. (1976). On glutaminase activity in mammalian synaptosomes. Brain Res. 110, 115–125. doi: 10.1016/0006-8993(76)90212-2
Bratosin B. C., Darjan S., Vodnar D. C. (2021). Single cell protein: a potential substitute in human and animal nutrition. Sustainability 13, 9284. doi: 10.3390/su13169284
Busti S., Bonaldo A., Dondi F., Cavallini D., Yúfera M., Gilannejad N., et al. (2020a). Effects of different feeding frequencies on growth, feed utilisation, digestive enzyme activities and plasma biochemistry of gilthead sea bream (Sparus aurata) fed with different fishmeal and fish oil dietary levels. Aquaculture 529, 735616. doi: 10.1016/j.aquaculture.2020.735616
Busti S., Rossi B., Volpe E., Ciulli S., Piva A., D’Amico F., et al. (2020b). Effects of dietary organic acids and nature identical compounds on growth, immune parameters and gut microbiota of European sea bass. Sci. Rep. 10, 21321. doi: 10.1038/s41598-020-78441-9
Calixto da Silva E., Sterzelecki F. C., Alves Musialak L., Sugai J. K., Castro J., de J. P., et al. (2020). Effect of feeding frequency on growth performance, blood metabolites, proximate composition and digestive enzymes of lebranche mullet (Mugil liza) juveniles. Aquac. Res. 51, 1162–1169. doi: 10.1111/are.14466
Cao H., Zhang S., An J., Diao J., Xu L., Gai C. (2022). Rhodobacter azotoformans supplementation improves defense ability of Chinese mitten crab Eriocheir sinensis against citrobacteriosis. Fish. Shellfish Immunol. 131, 991–998. doi: 10.1016/j.fsi.2022.11.012
Cardona L. (2006). Habitat selection by grey mullets (Osteichthyes: mugilidae) in Mediterranean estuaries: the role of salinity. Sci. Mar. 70, 443–455. doi: 10.3989/scimar.2006.70n3443
Cheng Y.-C., Duarte M. E., Kim S. W. (2021). Nutritional and functional values of lysed corynebacterium glutamicum cell mass for intestinal health and growth of nursery pigs. J. Anim. Sci. 99, skab331. doi: 10.1093/jas/skab331
Chikwati E. M., Sahlmann C., Holm H., Penn M. H., Krogdahl Å., Bakke A. M. (2013). Alterations in digestive enzyme activities during the development of diet-induced enteritis in Atlantic salmon, salmo salar l. Aquaculture 402–403, 28–37. doi: 10.1016/j.aquaculture.2013.03.023
Colombo S., Roy K., Mraz J., Wan A., Davies S., Tibbetts S., et al. (2022). Towards achieving circularity and sustainability in feeds for farmed blue foods. Rev. Aquac. 15 (3), 1115–1141. doi: 10.1111/raq.12766
Crosetti D., Blaber S. J. M. (2015). Biology, ecology and culture of grey mullets (Mugilidae) (CRC Press).
Datta S. N., Kaur V. I., Dhawan A., Jassal G. (2013). Estimation of length-weight relationship and condition factor of spotted snakehead Channa punctata (Bloch) under different feeding regimes. SpringerPlus 2, 436. doi: 10.1186/2193-1801-2-436
Davares A. K. L., Arsene M. M. J., Viktorovna P. I., Vyacheslavovna Y. N., Vladimirovna Z. A., Aleksandrovna V. E., et al. (2022). Quorum-sensing inhibitors from probiotics as a strategy to combat bacterial cell-to-Cell communication involved in food spoilage and food safety. Fermentation 8, 711. doi: 10.3390/fermentation8120711
De D., Ghoshal T. K., Kundu J. (2012). Effect of feeding different levels of protein on growth performance, feed utilization and digestive enzyme of grey mullet (Mugil cephalus l). Anim. Nutr. Feed Technol. 12, 179–186.
De Carvalho C. V. A., Bianchini A., Tesser M. B., Sampaio L. A. (2010). The effect of protein levels on growth, postprandial excretion and tryptic activity of juvenile mullet Mugil platanus (Günther). Aquac. Res. 41, 511–518. doi: 10.1111/j.1365-2109.2009.02340.x
De Cesare A., Faria do Valle Ì., Sala C., Sirri F., Astolfi A., Castellani G., et al. (2019). Effect of a low protein diet on chicken ceca microbiome and productive performances. Poult. Sci. 98, 3963–3976. doi: 10.3382/ps/pez132
Eggeling L., Sahm H. (2001). The cell wall barrier of Corynebacterium glutamicum and amino acid efflux. J. Biosci. Bioeng. 92, 201–213. doi: 10.1016/S1389-1723(01)80251-6
El-Saadony M. T., Alagawany M., Patra A. K., Kar I., Tiwari R., Dawood M. A. O., et al. (2021). The functionality of probiotics in aquaculture: an overview. Fish. Shellfish Immunol. 117, 36–52. doi: 10.1016/j.fsi.2021.07.007
Federle M. J., Bassler B. L. (2003). Interspecies communication in bacteria. J. Clin. Invest. 112, 1291–1299. doi: 10.1172/JCI20195
Gamboa-Delgado J., Márquez-Reyes J. M. (2018). Potential of microbial-derived nutrients for aquaculture development. Rev. Aquac. 10, 224–246. doi: 10.1111/raq.12157
García-Carreño F. L., Haard N. F. (1993). Characterization of proteinase classes in langostilla (pleuroncodes planipes) and crayfish (pacifastacus astacus) extracts. J. Food Biochem. 17, 97–113. doi: 10.1111/j.1745-4514.1993.tb00864.x
Gisbert E., Mozanzadeh M. T., Kotzamanis Y., Estévez A. (2016). Weaning wild flathead grey mullet (Mugil cephalus) fry with diets with different levels of fish meal substitution. Aquaculture 462, 92–100. doi: 10.1016/j.aquaculture.2016.04.035
Gisbert E., Nolasco H., Solovyev M. (2018). Towards the standardization of brush border purification and intestinal alkaline phosphatase quantification in fish with notes on other digestive enzymes. Aquaculture 487, 102–108. doi: 10.1016/j.aquaculture.2018.01.004
Glencross B., Blyth D., Irvin S., Bourne N., Campet M., Boisot P., et al. (2016). An evaluation of the complete replacement of both fishmeal and fish oil in diets for juvenile Asian seabass, Lates calcarifer. Aquaculture 451, 298–309. doi: 10.1016/j.aquaculture.2015.09.012
Glencross B., Huyben D., Schrama J. (2020). The application of single-cell ingredients in aquaculture feeds–a review. Fishes 5, 22. doi: 10.3390/fishes5030022
Glencross B., Muñoz-Lopez P., Matthew C., MacKenzie S., Powell A., Longshaw M., et al. (2023). Digestibility of bacterial protein by Atlantic salmon (Salmo salar) is affected by both inclusion level and acclimation time. Aquaculture 565, 739137. doi: 10.1016/j.aquaculture.2022.739137
Gu M., Bai N., Xu B., Xu X., Jia Q., Zhang Z. (2017). Protective effect of glutamine and arginine against soybean meal-induced enteritis in the juvenile turbot (Scophthalmus maximus). Fish. Shellfish Immunol. 70, 95–105. doi: 10.1016/j.fsi.2017.08.048
Halimi M., Alishahi M., Abbaspour M. R., Ghorbanpoor M., Tabandeh M. R. (2020). High efficacy and economical procedure of oral vaccination against Lactococcus garvieae/Streptococcus iniae in rainbow trout (Oncorhynchus mykiss). Fish. Shellfish Immunol. 99, 505–513. doi: 10.1016/j.fsi.2020.02.033
Hamidoghli A., Yun H., Won S., Kim S., Farris N. W., Bai S. C. (2019). Evaluation of a single-cell protein as a dietary fish meal substitute for whiteleg shrimp Litopenaeus vannamei. Fish. Sci. 85, 147–155. doi: 10.1007/s12562-018-1275-5
Holm H., Hanssen L. E., Krogdahl A., Florholmen J. (1988). High and low inhibitor soybean meals affect human duodenal proteinase activity differently: In vivo comparison with bovine serum albumin. J. Nutr. 118, 515–520. doi: 10.1093/jn/118.4.515
Horn K. J., Jaberi Vivar A. C., Arenas V., Andani S., Janoff E. N., Clark S. E. (2022). Corynebacterium species inhibit streptococcus pneumoniae colonization and infection of the mouse airway. Front. Microbiol. 12. doi: 10.3389/fmicb.2021.804935
Hossain M., Koshio S., Kestemont P. (2020). Recent advances of nucleotide nutrition research in aquaculture: a review. Rev. Aquac. 12, 1028–1053. doi: 10.1111/raq.12370
Idan F., Paulk C., Beyer S., Stark C. (2023). Effects of pellet diameter and crumble size on the growth performance and relative gizzard weight of broilers. J. Appl. Poult. Res. 32 (2), 100331. doi: 10.1016/j.japr.2023.100331
Iijima N., Tanaka S., Ota Y. (1998). Purification and characterization of bile salt-activated lipase from the hepatopancreas of red sea bream, Pagrus major. Fish. Physiol. Biochem. 18, 59–69. doi: 10.1023/A:1007725513389
Jones S. W., Karpol A., Friedman S., Maru B. T., Tracy B. P. (2020). Recent advances in single cell protein use as a feed ingredient in aquaculture. Curr. Opin. Biotechnol. 61, 189–197. doi: 10.1016/j.copbio.2019.12.026
Karlsen C., Tzimorotas D., Robertsen E. M., Kirste K. H., Bogevik A. S., Rud I. (2022). Feed microbiome: confounding factor affecting fish gut microbiome studies. ISME Commun. 2, 14. doi: 10.1038/s43705-022-00096-6
Kashinskaya E. N., Simonov E. P., Izvekova G. I., Parshukov A. N., Andree K. B., Solovyev M. M. (2019). Composition of the microbial communities in the gastrointestinal tract of perch (Perca fluviatilis l. 1758) and cestodes parasitizing the perch digestive tract. J. Fish. Dis. 43 (1), 23–38. doi: 10.1111/jfd.13096
Kashinskaya E. N., Simonov E. P., Kabilov M. R., Izvekova G. I., Andree K. B., Solovyev M. M. (2018). Diet and other environmental factors shape the bacterial communities of fish gut in a eutrophic lake. J. Appl. Microbiol. 125 (6), 1–16. doi: 10.1111/jam.14064
Kim P. S., Shin N.-R., Lee J.-B., Kim M.-S., Whon T. W., Hyun D.-W., et al. (2021). Host habitat is the major determinant of the gut microbiome of fish. Microbiome 9, 166. doi: 10.1186/s40168-021-01113-x
Kivistik C., Knobloch J., Käiro K., Tammert H., Kisand V., Hildebrandt J.-P., et al. (2020). Impact of salinity on the gastrointestinal bacterial community of Theodoxus fluviatilis. Front. Microbiol. 11. doi: 10.3389/fmicb.2020.00683
Lallès J.-P. (2020). Intestinal alkaline phosphatase in the gastrointestinal tract of fish: biology, ontogeny, and environmental and nutritional modulation. Rev. Aquac. 12, 555–581. doi: 10.1111/raq.12340
Le M. H., Wang D. (2020). Structure and membership of gut microbial communities in multiple fish cryptic species under potential migratory effects. Sci. Rep. 10, 7547. doi: 10.1038/s41598-020-64570-8
Lemieux H., Blier P., Dutil J.-D. (1999). Do digestive enzymes set a physiological limit on growth rate and food conversion efficiency in the Atlantic cod (Gadus morhua)? Fish. Physiol. Biochem. 20, 293–303. doi: 10.1023/A:1007791019523
Liew S. M., Tay S. T., Puthucheary S. D. (2013). Enzymatic and molecular characterisation of leucine aminopeptidase of Burkholderia pseudomallei. BMC Microbiol. 13, 110. doi: 10.1186/1471-2180-13-110
Lin Z., Yoshikawa S., Hamasaki M., Koyama T., Kikuchi K., Hosoya S. (2022). Effects of low fishmeal diets on growth performance, blood chemical composition, parasite resistance, and gene expression in the tiger pufferfish, Takifugu rubripes. Aquaculture 560, 738484. doi: 10.1016/j.aquaculture.2022.738484
Liu T., Han T., Wang J., Liu T., Bian P., Wang Y., et al. (2021). Effects of replacing fish meal with soybean meal on growth performance, feed utilization and physiological status of juvenile redlip mullet Liza haematocheila. Aquac. Rep. 20, 100756. doi: 10.1016/j.aqrep.2021.100756
Liu J., Xu J.-Z., Rao Z.-M., Zhang W.-G. (2022). Industrial production of l-lysine in Corynebacterium glutamicum: progress and prospects. Microbiol. Res. 262, 127101. doi: 10.1016/j.micres.2022.127101
Lobel P. S. (1981). Trophic biology of herbivorous reef fishes: alimentary pH and digestive capabilities. J. Fish. Biol. 19, 365–397. doi: 10.1111/j.1095-8649.1981.tb05842.x
Luzzana U., Valfrè F., Mangiarotti M., Domeneghini C., Radaelli G., Moretti V. M., et al. (2005). Evaluation of different protein sources in fingerling grey mullet Mugil cephalus practical diets. Aquac. Int. 13, 291–303. doi: 10.1007/s10499-004-3099-9
Marchi A., Bonaldo A., Scicchitano D., Candela M., De Marco A., Falciglia S., et al. (2023). Feeding gilthead sea bream with increasing dietary bacterial single cell protein level: implication on growth, plasma biochemistry, gut histology, and gut microbiota. Aquaculture 565, 739132. doi: 10.1016/j.aquaculture.2022.739132
Marit Berge G., Baeverfjord G., Skrede A., Storebakken T. (2005). Bacterial protein grown on natural gas as protein source in diets for Atlantic salmon, Salmo salar, in saltwater. Aquaculture 244, 233–240. doi: 10.1016/j.aquaculture.2004.11.017
Márquez L., Robles R., Morales G. A., Moyano F. J. (2012). Gut pH as a limiting factor for digestive proteolysis in cultured juveniles of the gilthead sea bream (Sparus aurata). Fish. Physiol. Biochem. 38, 859–869. doi: 10.1007/s10695-011-9573-1
Métais P., Bieth J. (1968). De´termination de l’a-amylase par une microtechnique. Ann. Biol. Clin. 26, 133–142.
Moriarty D. J. W. (1976). Quantitative studies on bacteria and algae in the food of the mullet Mugil cephalus l. and the prawn metapenaeus bennettae (Racek & dall). J. Exp. Mar. Biol. Ecol. 22, 131–143. doi: 10.1016/0022-0981(76)90090-3
Nasri R., Younes I., Lassoued I., Ghorbel S., Ghorbel-Bellaaj O., Nasri M. (2011). Digestive alkaline proteases from zosterisessor ophiocephalus, raja clavata, and Scorpaena scrofa: characteristics and application in chitin extraction. J. Amino Acids 2011, 913616. doi: 10.4061/2011/913616
Newton R. W., Maiolo S., Malcorps W., Little D. C. (2023). Life cycle inventories of marine ingredients. Aquaculture 565, 739096. doi: 10.1016/j.aquaculture.2022.739096
Nicholson J. A., Kim Y. S. (1975). A one-step l-amino acid oxidase assay for intestinal peptide hydrolase activity. Analytical Biochem. 63, 110–117. doi: 10.1016/0003-2697(75)90194-3
Parma L., Candela M., Soverini M., Turroni S., Consolandi C., Brigidi P., et al. (2016). Next-generation sequencing characterization of the gut bacterial community of gilthead sea bream (Sparus aurata, l.) fed low fishmeal-based diets with increasing soybean meal levels. Anim. Feed Sci. Technol. 222, 204–216. doi: 10.1016/j.anifeedsci.2016.10.022
Parma L., Pelusio N. F., Gisbert E., Esteban M. A., D’Amico F., Soverini M., et al. (2020). Effects of rearing density on growth, digestive conditions, welfare indicators and gut bacterial community of gilthead sea bream (Sparus aurata, l. 1758) fed different fishmeal and fish oil dietary levels. Aquaculture 518, 734854. doi: 10.1016/j.aquaculture.2019.734854
Parma L., Yúfera M., Navarro-Guillén C., Moyano F. J., Soverini M., D’Amico F., et al. (2019). Effects of calcium carbonate inclusion in low fishmeal diets on growth, gastrointestinal pH, digestive enzyme activity and gut bacterial community of European sea bass (Dicentrarchus labrax l.) juveniles. Aquaculture 510, 283–292. doi: 10.1016/j.aquaculture.2019.05.064
Parshukov A. N., Kashinskaya E. N., Simonov E. P., Hlunov O. V., Izvekova G. I., Andree K. B., et al. (2019). Variations of the intestinal gut microbiota of farmed rainbow trout, Oncorhynchus mykiss (Walbaum), depending on the infection status of the fish. J. Appl. Micro. 126 (6), 1–17. doi: 10.1111/jam.14302
Payne A. I. (1978). Gut ph and digestive strategies in estuarine grey mullet (Mugilidae) and tilapia (Cichlidae). J. Fish. Biol. 13, 627–629. doi: 10.1111/j.1095-8649.1978.tb03476.x
Pelusio N. F., Bonaldo A., Gisbert E., Andree K. B., Esteban M. A., Dondi F., et al. (2022). Different fish meal and fish oil dietary levels in European Sea bass: welfare implications after acute confinement stress. Front. Mar. Sci. 8. doi: 10.3389/fmars.2021.779053
Pelusio N. F., Rossi B., Parma L., Volpe E., Ciulli S., Piva A., et al. (2020). Effects of increasing dietary level of organic acids and nature-identical compounds on growth, intestinal cytokine gene expression and gut microbiota of rainbow trout (Oncorhynchus mykiss) reared at normal and high temperature. Fish. Shellfish Immunol. 107, 324–335. doi: 10.1016/j.fsi.2020.10.021
Pereira A. G., Fraga-Corral M., Garcia-Oliveira P., Otero P., Soria-Lopez A., Cassani L., et al. (2022). Single-cell proteins obtained by circular economy intended as a feed ingredient in aquaculture. Foods 11 (18), 2831. doi: 10.3390/foods11182831
Perera W., Carter C., Houlihan D. F. (1995). Apparent absorption efficiencies of amino acids in rainbow trout, Oncorhynchus mykiss (Walbaum), fed diets containing bacterial single-cell protein. Aquac. Nutr. 1, 95–103. doi: 10.1111/j.1365-2095.1995.tb00024.x
Polakof S., Panserat S., Soengas J. L., Moon T. W. (2012). Glucose metabolism in fish: a review. J. Comp. Physiol. B. 182, 1015–1045. doi: 10.1007/s00360-012-0658-7
Prodanovic O., Sredanovic S. (2005). Understanding the buffering capacity in feedstuffs. Biotechnol. Anim. Husb. 21, 309–313. doi: 10.2298/BAH0506309L
Pujante I. M., Díaz-López M., Mancera J., Moyano F. (2016). Characterization of digestive enzymes protease and alpha-amylase activities in the thick-lipped grey mullet (Chelon labrosus, risso 1827). Aquac. Res., 367–376 48. doi: 10.1111/are.13038
Ramos-Júdez S., Duncan N. (2022). Feeding habits and the influence of pellet diameter on the feeding responses of the flathead grey mullet (Mugil cephalus) in captivity. Anim. Feed Sci. Technol. 290, 115368. doi: 10.1016/j.anifeedsci.2022.115368
Ringø E., Hoseinifar S. H., Ghosh K., Doan H. V., Beck B. R., Song S. K. (2018). Lactic acid bacteria in finfish–an update. Front. Microbiol. 9. doi: 10.3389/fmicb.2018.01818
Ritala A., Häkkinen S. T., Toivari M., Wiebe M. G. (2017). Single cell protein–State-of-the-Art, industrial landscape and patents 2001–2016. Front. Microbiol. 8. doi: 10.3389/fmicb.2017.02009
Robinson M. L., Gomez-Raya L., Rauw W. M., Peacock M. M. (2008). Fulton’s body condition factor K correlates with survival time in a thermal challenge experiment in juvenile lahontan cutthroat trout (Oncorhynchus clarki henshawi). J. Therm. Biol. 33, 363–368. doi: 10.1016/j.jtherbio.2008.05.004
Rodiles A., Santigosa E., Herrera M., Hachero-Cruzado I., Cordero M. L., Martínez-Llorens S., et al. (2012). Effect of dietary protein level and source on digestive proteolytic enzyme activity in juvenile Senegalese sole, Solea senegalensis kaup 1850. Aquac. Int. 20, 1053–1070. doi: 10.1007/s10499-012-9508-6
Rosas V. T., Monserrat J. M., Bessonart M., Magnone L., Romano L. A., Tesser M. B. (2019). Fish oil and meal replacement in mullet (Mugil liza) diet with spirulina (Arthrospira platensis) and linseed oil. Comp. Biochem. Physiol. Part C. Toxicol. Pharmacol. 218, 46–54. doi: 10.1016/j.cbpc.2018.12.009
Rumsey G. L., Winfree R. A., Hughes S. G. (1992). Nutritional value of dietary nucleic acids and purine bases to rainbow trout (Oncorhynchus mykiss). Aquaculture 108, 97–110. doi: 10.1016/0044-8486(92)90321-B
Sanatan P. T., Lomate P. R., Giri A. P., Hivrale V. K. (2013). Characterization of a chemostable serine alkaline protease from Periplaneta americana. BMC Biochem. 14, 32. doi: 10.1186/1471-2091-14-32
Sánchez A., Cardona L., Castelló F. (1993). Crecimiento de alevines de Liza ramada (Osteichthyes, mugilidae) en agua dulce: efectos de la alimentación. Actas IV Conres Nac. Acuicult., 91–96.
Schøyen H. F., Frøyland J. R. K., Sahlström S., Knutsen S. H., Skrede A. (2005). Effects of autolysis and hydrolysis of bacterial protein meal grown on natural gas on chemical characterization and amino acid digestibility. Aquacult. Fish. Nutr. Feeding 248, 27–33. doi: 10.1016/j.aquaculture.2005.04.017
Sepúlveda D., Hansen M. J., Dalsgaard I., Skov J., Lorenzen N. (2022). Antigenic variability of vibrio anguillarum serotype O2a: a hurdle for vaccine efficacy against vibriosis in rainbow trout (Oncorhynchus mykiss). Fish. Shellfish Immunol. 131, 300–311. doi: 10.1016/j.fsi.2022.09.072
Sepulveda J., Moeller A. H. (2020). The effects of temperature on animal gut microbiomes. Front. Microbiol. 11. doi: 10.3389/fmicb.2020.00384
Silva F. C. P., Nicoli J. R., Zambonino-Infante J. L., Le Gall M.-M., Kaushik S., Gatesoupe F.-J. (2010). Influence of partial substitution of dietary fish meal on the activity of digestive enzymes in the intestinal brush border membrane of gilthead sea bream, Sparus aurata and goldfish, Carassius auratus. Aquaculture 306, 233–237. doi: 10.1016/j.aquaculture.2010.05.018
Solovyev M., Gisbert E. (2016). Influence of time, storage temperature and freeze/thaw cycles on the activity of digestive enzymes from gilthead sea bream (Sparus aurata). Fish Physiol. Biochem. 42, 1383–1394. doi: 10.1007/s10695-016-0226-2
Solovyev M., Gisbert E. (2022). Feeding regimes affected the circadian rhythms of pancreatic digestive enzymes and somatic growth in flathead grey mullet (Mugil cephalus) fry. Comp. Biochem. Physiol. A. Mol. Integr. Physiol. 264, 111116. doi: 10.1016/j.cbpa.2021.111116
Soto-Dávila M., Chakraborty S., Santander J. (2022). Relative expression and validation of aeromonas salmonicida subsp. salmonicida reference genes during ex vivo and in vivo fish infection. Infect. Genet. Evol. 103, 105320. doi: 10.1016/j.meegid.2022.105320
Svihus B. (2014). “Function of the digestive system,” in Presented as a part of the Informal Nutrition Symposium “From Research Measurements to Application: Bridging the Gap” at the Poultry Science Association’s annual meeting in San Diego, California, July 22–25, 2013. doi: 10.3382/japr.2014-00937
Talukdar A., Deo A. D., Sahu N. P., Sardar P., Aklakur M., Prakash S., et al. (2020). Effects of dietary protein on growth performance, nutrient utilization, digestive enzymes and physiological status of grey mullet, Mugil cephalus l. fingerlings reared in inland saline water. Aquac. Nutr. 26, 921–935. doi: 10.1111/anu.13050
Teuling E., Wierenga P. A., Agboola J. O., Gruppen H., Schrama J. W. (2019). Cell wall disruption increases bioavailability of nannochloropsis gaditana nutrients for juvenile Nile tilapia (Oreochromis niloticus). Aquaculture 499, 269–282. doi: 10.1016/j.aquaculture.2018.09.047
Thompson L. R., Sanders J. G., McDonald D., Amir A., Ladau J., Locey K. J., et al. (2017). A communal catalogue reveals earth’s multiscale microbial diversity. Nature 551, 457–463. doi: 10.1038/nature24621
Tibbetts S. M., Mann J., Dumas A. (2017). Apparent digestibility of nutrients, energy, essential amino acids and fatty acids of juvenile Atlantic salmon (Salmo salar l.) diets containing whole-cell or cell-ruptured chlorella vulgaris meals at five dietary inclusion levels. Aquaculture 481, 25–39. doi: 10.1016/j.aquaculture.2017.08.018
Ugalde U. O., Castrillo J. I. (2002). “Single cell proteins from fungi and yeasts,” in Applied mycology and biotechnology, agriculture and food production. Eds. Khachatourians G. G., Arora D. K. (Elsevier), 123–149. doi: 10.1016/S1874-5334(02)80008-9
Vallainc D., Concu D., Papiol G. G., Loi B., Leggieri F., Brundu G., et al. (2021). Producing flat-head grey mullet Mugil cephalus (Linnaeus 1758) fries in captivity from sexually mature adults collected in sardinian lagoons. Aquac. Rep. 21, 100844. doi: 10.1016/j.aqrep.2021.100844
Wanshu H. (1992). Plasma cortisol and glucose concentrations in the striped mullet (Mugil cephalus l.) subjected to intense handling stress. Chin. J. Oceanol. Limnol. 10, 40–43. doi: 10.1007/BF02844298
Wassef E. A., El Masry M. H., Mikhail F. R. (2001). Growth enhancement and muscle structure of striped mullet, Mugil cephalus l., fingerlings by feeding algal meal-based diets. Aquac. Res. 32, 315–322. doi: 10.1046/j.1355-557x.2001.00043.x
Watson D. L., Bayne D. R., DeVries D. R., Williams J. (2003). Influence of gizzard shad on phytoplankton size and primary productivity in mesocosms and earthen ponds in the southeastern U.S. Hydrobiologia 495, 17–32. doi: 10.1023/A:1025459422455
Whitfield A. K., Panfili J., Durand J.-D. (2012). A global review of the cosmopolitan flathead mullet Mugil cephalus Linnaeus 1758 (Teleostei: mugilidae), with emphasis on the biology, genetics, ecology and fisheries aspects of this apparent species complex. Rev. Fish. Biol. Fish. 22, 641–681. doi: 10.1007/s11160-012-9263-9
Wilkinson J. M., Young R. H. (2020). Strategies to reduce reliance on soya bean meal and palm kernel meal in livestock nutrition. J. Appl. Anim. Nutr. 8, 75–82. doi: 10.3920/JAAN2020.0007
Willora F. P., Vatsos I. N., Mallioris P., Bordignon F., Keizer S., Martınez-Llorens S., et al. (2022). Replacement of fishmeal with plant protein in the diets of juvenile lumpfish (Cyclopterus lumpus, l. 1758): effects on digestive enzymes and microscopic structure of the digestive tract. Aquaculture 561, 738601. doi: 10.1016/j.aquaculture.2022.738601
Wilson J. M., Castro L. F. C. (2010). “1 - morphological diversity of the gastrointestinal tract in fishes,” in Fish physiology, the multifunctional gut of fish. Eds. Grosell M., Farrell A. P., Brauner C. J. (Academic Press), 1–55. doi: 10.1016/S1546-5098(10)03001-3
Wilson R. W., Wilson J. M., Grosell M. (2002). Intestinal bicarbonate secretion by marine teleost fish–why and how? Biochim. Biophys. Acta BBA Biomembr. Epithelial Ion Transport-A Tribute Hans H. Ussing 1566, 182–193. doi: 10.1016/S0005-2736(02)00600-4
Xie M., Zhou W., Xie Y., Li Y., Zhang Z., Yang Y., et al. (2021). Effects of cetobacterium somerae fermentation product on gut and liver health of common carp (Cyprinus carpio) fed diet supplemented with ultra-micro ground mixed plant proteins. Aquaculture 543, 736943. doi: 10.1016/j.aquaculture.2021.736943
Yamada E. A., Sgarbieri V. C. (2005). Yeast (Saccharomyces cerevisiae) protein concentrate: preparation, chemical composition, and nutritional and functional properties. J. Agric. Food Chem. 53, 3931–3936. doi: 10.1021/jf0400821
Yulianto D., Indra I., Batubara A. S., Efizon D., Nur F. M., Rizal S., et al. (2020). Length–weight relationships and condition factors of mullets Liza macrolepis and Moolgarda engeli (Pisces: mugilidae) harvested from lambada lhok waters in aceh besar, Indonesia. F1000Research 9, 259. doi: 10.12688/f1000research.22562.2
Zamani A., Khajavi M., Nazarpak M. H., Gisbert E. (2020). Evaluation of a bacterial single-cell protein in compound diets for rainbow trout (Oncorhynchus mykiss) fry as an alternative protein source. Anim. Open Access J. MDPI 10, 1676. doi: 10.3390/ani10091676
Zhang X.-H., He X., Austin B. (2020). Vibrio harveyi: a serious pathogen of fish and invertebrates in mariculture. Mar. Life Sci. Technol. 2, 231–245. doi: 10.1007/s42995-020-00037-z
Zhang H. Y., Piao X. S., Li P., Yi J. Q., Zhang Q., Li Q. Y., et al. (2013). Effects of single cell protein replacing fish meal in diet on growth performance, nutrient digestibility and intestinal morphology in weaned pigs. Asian-Australas. J. Anim. Sci. 26, 1320–1328. doi: 10.5713/ajas.2013.13200
Keywords: single cell protein, novel species, digestive enzyme activity, Corynebacterium glutamicum, Mugil cephalus
Citation: Bertini A, Natale S, Gisbert E, Andrée KB, Concu D, Dondi F, De Cesare A, Indio V, Gatta PP, Bonaldo A and Parma L (2023) Exploring the application of Corynebacterium glutamicum single cell protein in the diet of flathead grey mullet (Mugil cephalus): effects on growth performance, digestive enzymes activity and gut microbiota. Front. Mar. Sci. 10:1172505. doi: 10.3389/fmars.2023.1172505
Received: 23 February 2023; Accepted: 31 May 2023;
Published: 15 June 2023.
Edited by:
Katerina Kousoulaki, Norwegian Institute of Food, Fisheries and Aquaculture Research (Nofima), NorwayReviewed by:
Francisco Javier Moyano, University of Almeria, SpainPatricia Diaz-Rosales, Centro de Investigación en Sanidad Animal, CISA (INIA-CSIC), Spain
Copyright © 2023 Bertini, Natale, Gisbert, Andrée, Concu, Dondi, De Cesare, Indio, Gatta, Bonaldo and Parma. This is an open-access article distributed under the terms of the Creative Commons Attribution License (CC BY). The use, distribution or reproduction in other forums is permitted, provided the original author(s) and the copyright owner(s) are credited and that the original publication in this journal is cited, in accordance with accepted academic practice. No use, distribution or reproduction is permitted which does not comply with these terms.
*Correspondence: Alessio Bonaldo, YWxlc3Npby5ib25hbGRvQHVuaWJvLml0