- 1One Health Initiative, University of Tennessee, Knoxville, TN, United States
- 2Department of Biomedical and Diagnostic Services, University of Tennessee College of Veterinary Medicine, Knoxville, TN, United States
- 3Department of Biological Sciences, Florida Atlantic University, Boca Raton, FL, United States
- 4Upwell, Monterey, CA, United States
- 5Department of Pathology & Laboratory Medicine, Division of Comparative Pathology, University of Miami Miller School of Medicine, Miami, FL, United States
- 6University of Tennessee Institute of Agriculture, Center for Wildlife Health, Knoxville, TN, United States
Introduction: Fungal diseases are a rising health problem globally, in humans, nonhuman animals, and plants. Emerging fungal diseases have been associated with mass mortality events. A recent example of fungal disease pathogenicity is sea turtle egg fusariosis (STEF). The pathogenicity of STEF has been linked to fungi within the Fusarium solani species complex (FSSC). This complex is composed of over 45 phylogenetically identifiable species commonly found in the environment. Species within the FSSC lineage have been isolated from the nests of multiple sea turtle species and are linked to decreased hatching success in all 7 of the extant sea turtle species. Fungi within this lineage are also known to cause cutaneous and subcutaneous infections. These fungi are not only a threat to sea turtles but also to other animals, including humans, that use coastal waters and beaches inhabited by Fusarium spp. The presence of Fusarium spp., in the context of sea turtle health, has not been investigated on southeastern Florida beaches which are fundamentally important for at least three sea turtle species that nest there in large numbers.
Methods: We performed a retrospective assessment of necropsy reports from dead captive leatherback neonates from 2010 to 2022 to assess the most common microscopic diagnoses and the presence of skin lesions associated with mycotic dermatitis. Additionally, live captive leatherbacks and dead-in-nest samples from the 2022 hatching season were used to assess the presence and effect of mycotic dermatitis in Juno Beach and Boca Raton, Florida, USA. This was accomplished by observing gross lesions, fungal cultures, and blood values.
Results: The retrospective analysis of dead captive neonates revealed that the diagnosis of mycotic dermatitis on histopathology has significantly increased since 2010. The assessment of gross skin lesions associated with mycotic dermatitis in dead and live captive leatherback neonates also revealed a similar increase. Investigations in live captive leatherbacks revealed fungal cultures positive for Fusarium spp. and significant differences in blood values at emergence between healthy turtles and those that later developed mycotic dermatitis.
Discussion: Positive dead-in-nest culture results suggest that Fusarium spp. are likely present in leatherback sea turtle nests in Boca Raton and Juno Beach, Florida, USA. Additionally, the occurrence of mycotic dermatitis in dead and live captive leatherback neonates suggests that the presence of Fusarium spp. in the nest likely affects leatherback neonates even after emergence.
Introduction
Fungal diseases are a rising health problem globally in wildlife (Fisher et al., 2012; Casadevall, 2018; Fisher et al., 2020; Thornton, 2020). Some fungal pathogens can even cause disease across large taxonomic groups (i.e., trans-kingdom pathogenicity), spanning vertebrates, invertebrates, and plants (Jain et al., 2011; Gauthier and Keller, 2013). Consequently, emerging fungal diseases are truly a One Health concern (Sáenz et al., 2020). A driving force worsening the growing problem of fungal diseases is climate change (Garcia-Solache and Casadevall, 2010; Casadevall, 2018; One Health, 2019). Changes in local weather patterns are increasing stress on both plants and animals, making them more susceptible to fungal infections (One Health, 2019). Additionally, with regional climatic shifts the thermal exclusion zone, which previously provided protection from fungal pathogens, is now shrinking (One Health, 2019). Fungal pathogens are now able to thrive in previously uninhabitable regions, increasing the risk of infection to naive animals and plants (One Health, 2019). Fungal diseases are not only a threat at the individual level, but they can also devastate whole populations (Fisher et al., 2012). To date, newly emerging fungal diseases have caused mass mortality events in animal and plant populations around the world (Berger et al., 1998; Anderson et al., 2004; Lötters et al., 2009; Sarmiento-Ramírez et al., 2010; Kwon et al., 2017; Yadav et al., 2020; Hoyt et al., 2021).
In 2020, sea turtle egg fusariosis (STEF) was identified as an emerging disease in sea turtles (Gleason et al., 2020). Sea turtle egg fusariosis is caused solely by fungi in the Fusarium solani species complex (FSSC) lineage (Gleason et al., 2020). The FSSC is composed of over 45 phylogenetically distinct species commonly found and isolated from soil and plant debris (O’Donnell, 2000; Zhang et al., 2006). As ubiquitous fungi, commonly found in the environment, species within the FSSC have been isolated from the nests of multiple sea turtle species (Phillott et al., 2001; Sarmiento-Ramírez et al., 2010; Sarmiento-Ramírez et al., 2014; Sarmiento-Ramírez et al., 2017; Sidique et al., 2017; Hoh et al., 2020; Candan et al., 2021). Furthermore, FSSC has been linked to decreased hatching success in all 7 of the extant sea turtle species (Sarmiento-Ramírez et al., 2014; Gleason et al., 2020).
Fusarium keratoplasticum (Fk) and Fusarium falciforme (Ff) fall within the FSSC lineage and have been identified as causative agents of STEF (Smyth et al., 2019). However, Fk and Ff are also known to cause cutaneous and subcutaneous infections in both humans and marine animals (Higgins, 2000; Nucci and Anaissie, 2002; Tanaka et al., 2012; Smyth et al., 2019; Cafarchia et al., 2020). Mycotic dermatitis due to Fusarium spp. has been reported in many marine animals, including but not limited to: false killer whales, white sided dolphins, pygmy sperm whales, California sea lions, grey seals, sharks, seahorses, and loggerhead sea turtles (Higgins, 2000; Salter et al., 2012; Tanaka et al., 2012; Pirarat et al., 2016; Desoubeaux et al., 2018; Cafarchia et al., 2020). In marine animals Fusarium spp. are believed to be opportunistic pathogens normally found on the skin (Higgins, 2000; Tanaka et al., 2012). The pathogenesis of the disease is thought to be associated with decreased immunocompetence (Higgins, 2000; Tanaka et al., 2012; Smyth et al., 2019; Cafarchia et al., 2020). Immunosuppression may be due to multiple factors such as environmental stressors, physiologic stressors due to niche alterations or captivity, or illness (Higgins, 2000; Tanaka et al., 2012).
While STEF has been documented as an emerging disease that impacts hatchling production of multiple imperiled sea turtle species, to date, there is no information on the presence or effect of Fusarium spp. on sea turtle nests, eggs, or neonates from two well-studied nesting beaches along Florida’s east coast in Palm Beach County: Juno Beach and Boca Raton. In 2021, over 37,000 sea turtle nests were reported in this county, accounting for approximately 1/3 off all leatherback nests in the continental U.S.A. (Florida Statewide Nesting Beach Survey, 2021). Although previous research investigated the effect of STEF on hatchling condition after emergence (Phillott and Parmenter, 2014), no information exists on the potential long-term effects of STEF in neonatal sea turtles after emergence from affected nests. As Fusarium spp. are not only pathogens of sea turtle eggs but also have the potential to affect humans and other animals, it is important to document their presence and determine their potential effect(s) on the densely nested and highly touristic beaches of Boca Raton and Juno Beach, Florida (Palm Beach County, 2023). Addressing these data gaps in Florida and elsewhere could explain decreased hatching success in sea turtle nests and serve as a baseline for sea turtle conservation efforts on the beaches in this region. Additionally, understanding the presence and effect(s) of Fusarium spp. could help prevent downstream effects in turtles, other animals, and immunocompromised humans.
Here we focus on the health of an endangered species, the leatherback sea turtle (Dermochelys coriacea), that has a nesting “hot spot” spanning Juno Beach and Boca Raton, Florida. We hypothesized that Fusarium spp. are present in leatherback sea turtle nests and are responsible for downstream effects in neonatal leatherback sea turtles. The objectives of this study were to: (1) compare and identify retrospective and current trends of gross and microscopic diagnoses from all captive neonate leatherbacks that died across 12 nesting seasons, 2010 to 2022; (2) detect the presence of Fusarium spp. in dead-in-nest hatchlings, dead-in-nest eggs, live captive neonates, and dead captive neonates sampled from the 2022 season; (3) identify relationships between the detection of Fusarium spp. and nest hatching success in 2022; (4) identify relationships between blood values of live captive neonates and the development of mycotic dermatitis in 2022.
Materials and methods
Animals and study site
The leatherback sea turtle neonates involved in this investigation originated from naturally oviposited nests on Juno Beach and Boca Raton, Florida. Samples were collected from three sources. The first source was dead captive neonates. From 2010 to 2022 a total of 83 dead captive neonates from 57 different nests were included in this study. The second source was live captive neonates from 2021 and 2022. This included a total of 127 live captive neonates (77 and 50, respectively) from 27 different nests. All live captive neonates were maintained for 4-70 days in a research colony at the Florida Atlantic University (FAU) Marine Laboratory for an on-going unrelated study. The neonates were housed indoors in an open-flow sea water system with UVA-UVB fluorescent lighting 45 cm above the tanks. The lights were on a 12:12 hour cycle. The seawater used to house the neonates was Atlantic ocean water that was filtered through two (100 μm) filtration socks, cleaned via protein skimming, and then treated with ultraviolet light exposure sufficient to eliminate microbes. The water was also sent through a chiller to maintain the temperature at 23-25°C. Neonates were housed in tanks individually or in groups with other neonates from the same nest. If a neonate showed clinical signs of mycotic dermatitis, biosecurity measures were taken to prevent the spread of disease and the neonate was isolated. The third source was dead-in-nest hatchlings and eggs from 2022. A total of 14 individual dead-in-nest hatchlings or eggs from 9 nests were available for sampling. Dead-in-nest hatchlings successfully hatched from the egg but died prior to full emergence.
Histopathology
From 2010 to 2022, full necropsies were performed on 83 dead captive leatherback neonates within 72 hours of death. Prior to necropsy all neonates were stored in a refrigerator at 3-5°CC. Based on the pathologist’s knowledge and extensive experience with this species’ samples, the quality of all samples was sufficient for inclusion in this study. During necropsy sections of multiple tissues were placed into 10% buffered formalin for histopathology. Tissues examined included skin, muscle, brain, heart, lung, pancreas, stomach, intestines, liver, spleen, kidney, gonads, and adrenal glands. All formalin-fixed tissues were submitted to the University of Tennessee Knoxville Department of Biomedical and Diagnostic services for histologic examination. They were routinely processed and stained with hematoxylin and eosin (H&E). Additional special stains for fungal hyphae (Gomori methenamine silver, GMS) or bacteria (Gram; acid-fast) were used as indicated by the pathologist. The two most common microscopic diagnoses each year were converted into percent of occurrence per year to account for different numbers of available necropsy reports each year. Linear least squares regressions were used to assess trends of the percent of occurrence over time.
Gross skin lesions
Gross skin lesions associated with mycotic dermatitis were reported in both dead captive and live captive neonates. In dead captive neonates, gross skin lesions associated with mycotic dermatitis included white patches, tan areas, white films, brown discoloration, yellow discoloration, and ulceration. In live captive neonates, gross skin lesions associated with mycotic dermatitis included white-grey discoloration or patches, scale sloughing, yellow-tan discoloration, and ulceration (Figure 1). The occurrence of gross skin lesions in dead captive neonates from 2010-2022 and live captive neonates from 2021-2022 was converted to percent of occurrence each year. For dead captive neonates a linear least squares regression was used to identify trends in the change of percent of occurrence of gross skin lesions from 2010 to 2022.
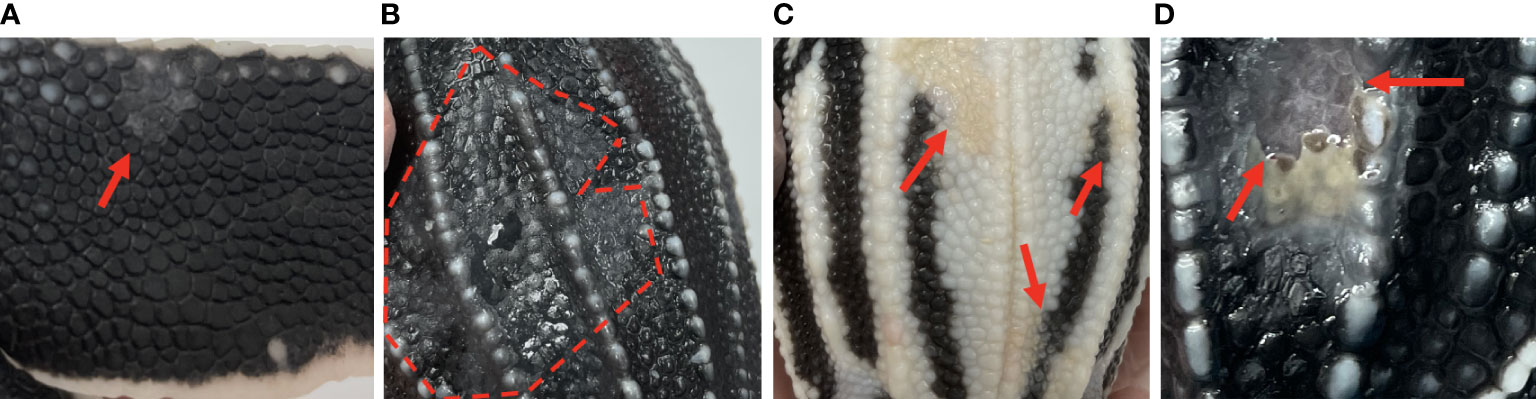
Figure 1 Gross skin lesions associated with mycotic dermatitis in live captive neonates. (A) small grey-white discoloration or patch with scale sloughing (arrow) (B) large region of grey-white discoloration with scale sloughing, shown as the dull black scales (encircled by the dashed line), (C) yellow-tan discoloration of the normally white scales (arrows) (D) ulceration (arrows).
Fungal culture
All dead-in-nest fungal samples (hatchlings and eggs) were collected during the 2022 season. Nest inventory occurred after the mass emergence event or between 70-80 days of incubation if there was no emergence as per our Marine Turtle Permit. All dead-in-nest hatchlings and eggs were stored in the refrigerator at 3-5°CC (<24 hours) prior to sampling. Using sterile technique, sterile swabs were used to sample multiple regions on and in dead-in-nest hatchlings and eggs (i.e., skin, inside the yolk sac, internal surface of the eggshell, external surface of the eggshell) for fungal culture. Prior to external surface swabs all external surfaces (i.e., skin or external surface of the eggshell) were first rinsed with 200 mL of sterile water. A total of 19 swabs were collected from 9 dead-in-nest hatchlings and 5 dead-in-nest eggs across 9 nests (Table 1).
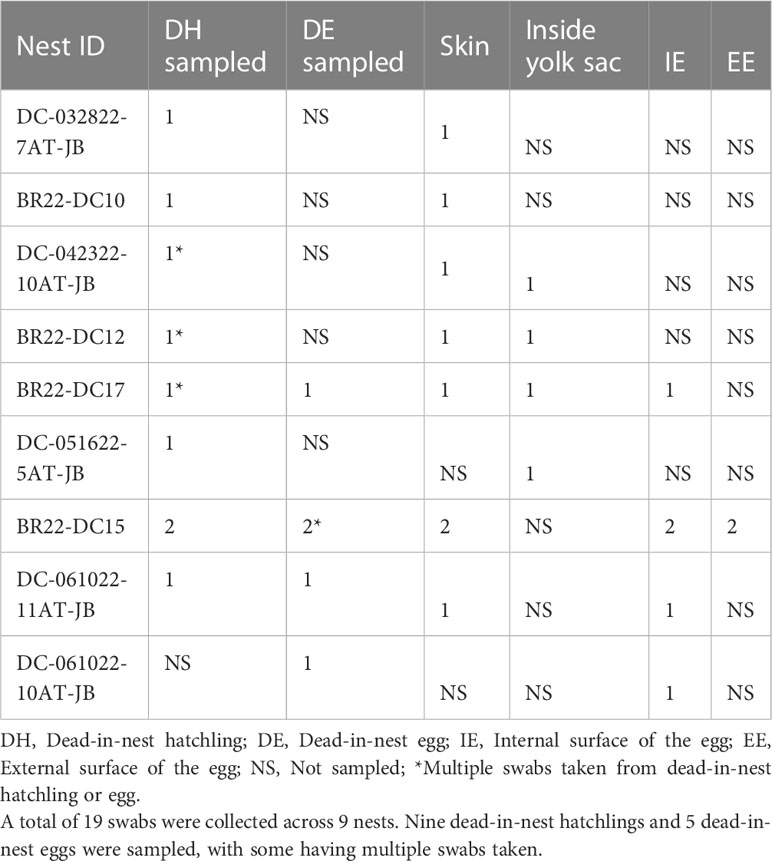
Table 1 Number and sample sites of dead-in-nest hatchling and egg swabs taken from each study nest in 2022.
Swabs for fungal culture were collected from live captive neonates during the 2022 season. Each week neonates were thoroughly examined for gross skin lesions. Diagnostic culture samples were collected when gross skin lesions associated with mycotic dermatitis were first seen on examination (Figure 1). Following rinsing with 200 mL of sterile water, a sterile swab was used to swab the leading edge of the lesion(s). A total of 20 swab samples were collected from symptomatic neonates originating from 6 different nests (Table 2). Additionally, one asymptomatic neonate was swabbed and cultured as a negative control for diagnostic comparison.
All fungal cultures were performed in-house. Samples were plated on Inhibitory Mold Agar (IMA) and grown at room temperature (22-24°CC) for 7-21 days (Scognamiglio et al., 2010). Fungal identification was performed using lactophenol aniline blue dye and the morphologic descriptions of Fusarium spp. in the Fusarium Laboratory Manual (Leslie et al., 2006). All live captive neonates with gross skin lesions and a positive fungal culture were diagnosed with mycotic dermatitis.
Hatching success
During nest inventory, the total number of eggs in each nest was estimated using the remaining eggshells plus remaining unhatched eggs. During the 2022 season, 13 different nests were included in this study, 6 from Boca Raton, Florida and 7 from Juno Beach, Florida. Nests included those from which dead-in-nest samples (hatchling or egg) were taken and those from which all live captive neonates emerged from. Hatching success of each nest was estimated by dividing the number of hatched eggs by the total number of eggs in the nest. Hatching success was then compared to the detection of Fusarium spp. in samples originating from each nest using a Wilcoxon Rank Sum test.
Blood analyses
During the 2022 hatching season, blood samples were collected from live captive neonates (n = 48) on the day of emergence. After a single application of 0.05% chlorhexidine solution and 70% isopropyl alcohol, blood was collected from the external jugular vein using a heparinized 26-gauge needle. Blood collection did not exceed 0.7% of the total body weight (g) of the animal or 0.2 mL. Packed cell volume (PCV) was determined immediately following blood collection. A microhematocrit capillary tube was filled, centrifuged (13,000 g) for 1 minute, and a microhematocrit reader was used to measure the PCV. The remaining blood, prior to clotting, was transferred into a 1.5 mL microcentrifuge tube and centrifuged at 6000 RPM for 1 minute. A small amount of the plasma was used to estimate total protein via refractive index using a Fisherbrand™ handheld analog clinical refractometer with automatic temperature compensation. The remaining plasma was then transferred to a 1.5 mL cryotube and stored at -80°CC. Frozen plasma was then submitted to the Avian & Wildlife Laboratory (University of Miami, Miami, Florida) for analysis by agarose gel protein electrophoresis within 28 days of collection. The methods described by Cray (2021) were used to perform agarose gel electrophoresis. All blood values were compared between mycotic dermatitis positive and negative neonates using the Wilcoxon Rank Sum test.
Results
Histopathology
The most common microscopic diagnoses in dead captive neonates across all years were pneumonia (granulocytic and granulomatous; 38/83), mycotic dermatitis (27/83), pneumonitis (11/83), and nephrosis (14/83). Twenty dead captive neonates had more than one of these microscopic diagnoses. From 2010 to 2019, pneumonia (Figure 2A) was the most common microscopic diagnosis, but from 2020 to 2022 the most common microscopic diagnosis shifted to mycotic dermatitis (Figures 2B, 3). The percent occurrence of mycotic dermatitis significantly increased from 2010 to 2022 (r2 = 0.65; p = 0.02; Figure 4). No other microscopic diagnoses had statistically significant changes in percent of occurrence from 2010 to 2022.
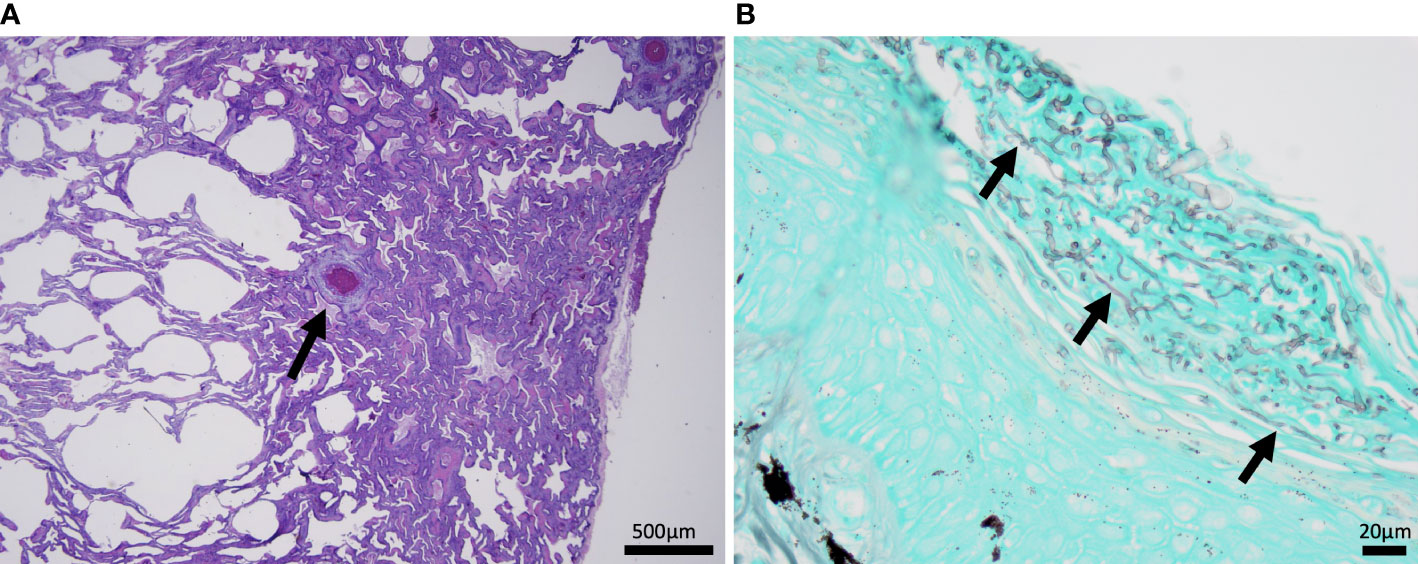
Figure 2 Histomicrographs from dead captive neonates. (A) H&E stained section of lung from 2019 with granulomatous pneumonia. Within the lung there is a small granuloma (arrow) with a central area of cellular necrosis that is hypereosinophilic and surrounded by macrophages and few lymphocytes and granulocytes. (B) A gomori methenamine silver (GMS) stained section of the plastron from 2022 with mycotic dermatitis. The keratin layer is expanded and disrupted by fungal hyphae represented as linear grey structures (arrows).
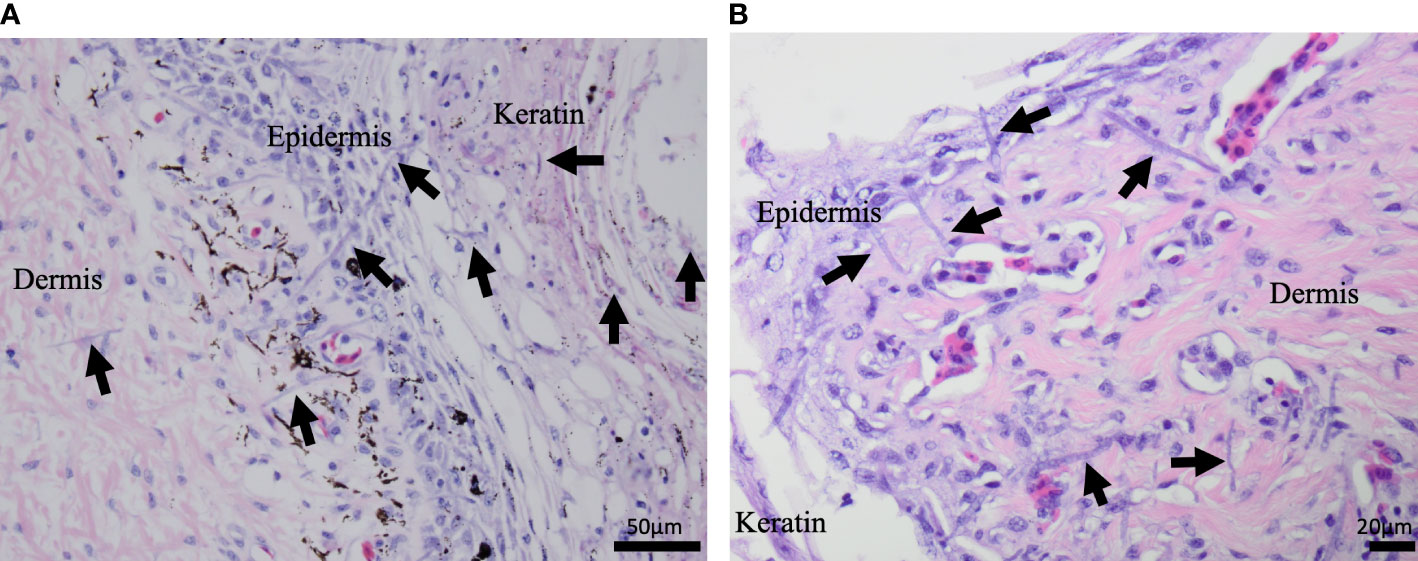
Figure 3 H&E stained microscopic images from dead captive leatherback neonate samples. (A) A cross section of neonate skin in 2015. Fungal hyphae (purple linear structures; arrows) are infiltrating the keratin, epidermis, and dermis. (B) A cross section of neonate skin from 2022. Fungal hyphae (purple linear structures; arrows) are infiltrating the keratin, epidermis, and extend into the underlying dermis.
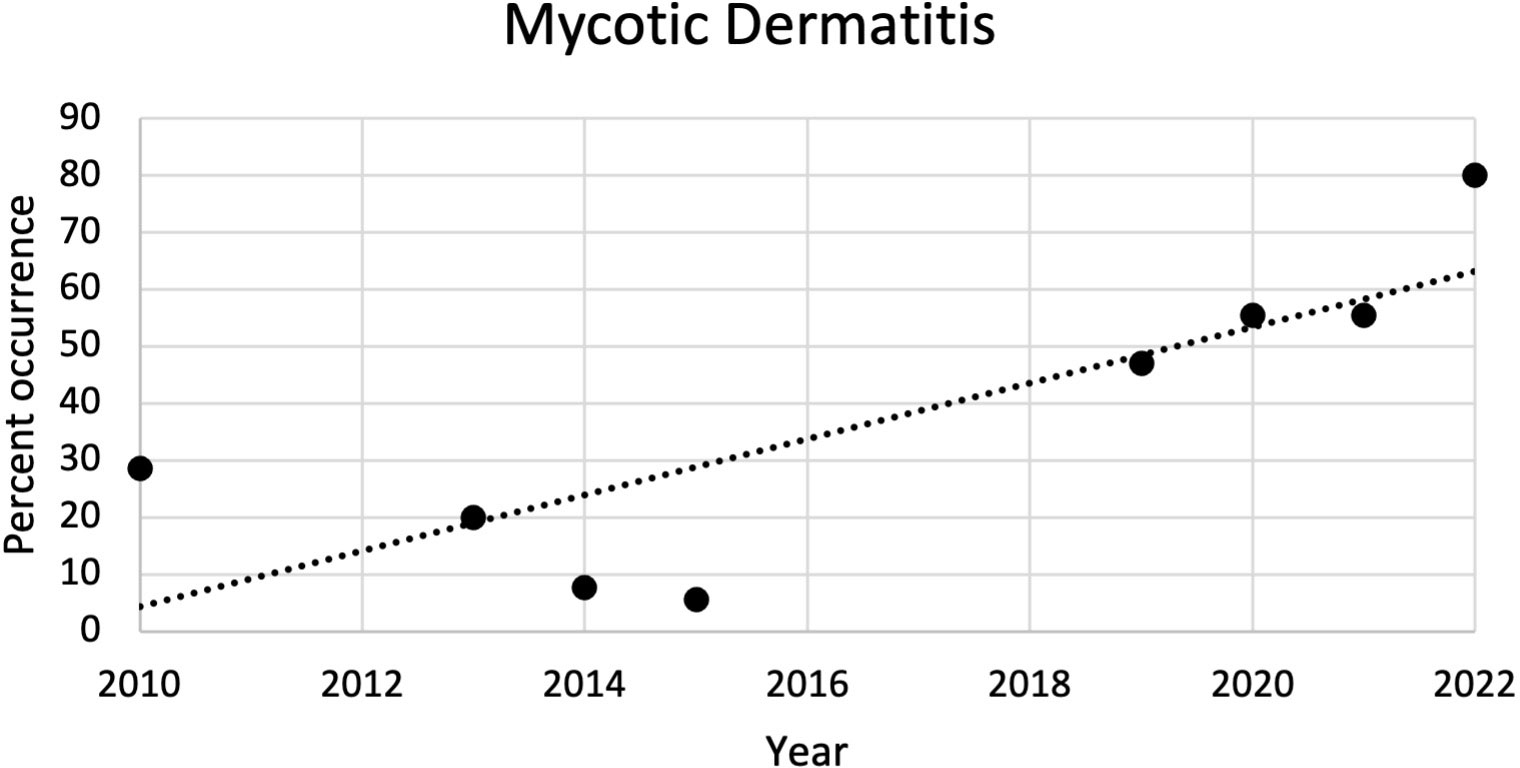
Figure 4 Plot of the percent of occurrence of mycotic dermatitis in dead captive leatherback neonates from 2010 to 2022. The percent of occurrence significantly increased over time (r2 = 0.65; p = 0.02).
Gross skin lesions
From 2010 to 2022, the most common external gross necropsy findings in dead captive neonates were skin lesions (Figure 5). Since 2010, the percent of occurrence of skin lesions significantly increased (r2 = 0.67; p = 0.01; Figure 6). In 2021, gross skin lesions were reported in 31 of the 77 (40%) live captive neonates maintained at the FAU Marine Laboratory. In 2022, 23 of the 50 (46%) live captive neonates maintained at the FAU Marine Laboratory developed gross skin lesions.
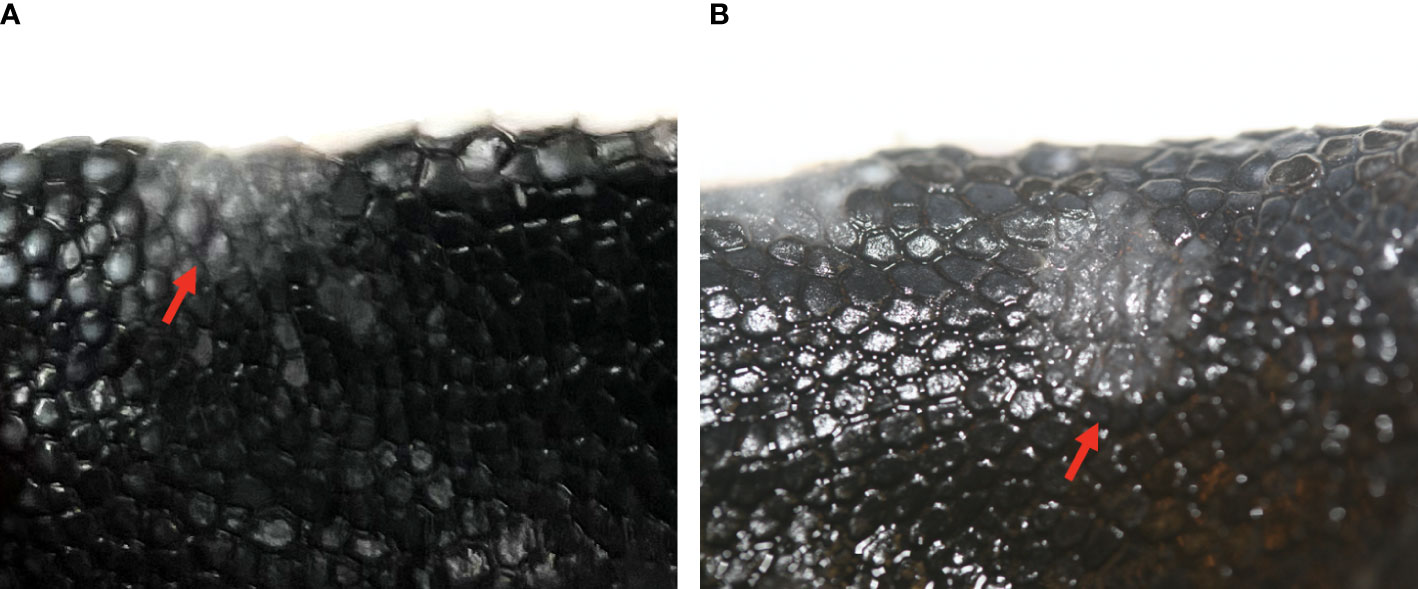
Figure 5 Gross skin lesions from dead captive leatherback neonates in 2010 and 2022. (A) Gross grey-white skin lesion on a front limb from 2022 (arrow) (B) Gross grey-white skin lesion on a front limb from 2010 (arrow).
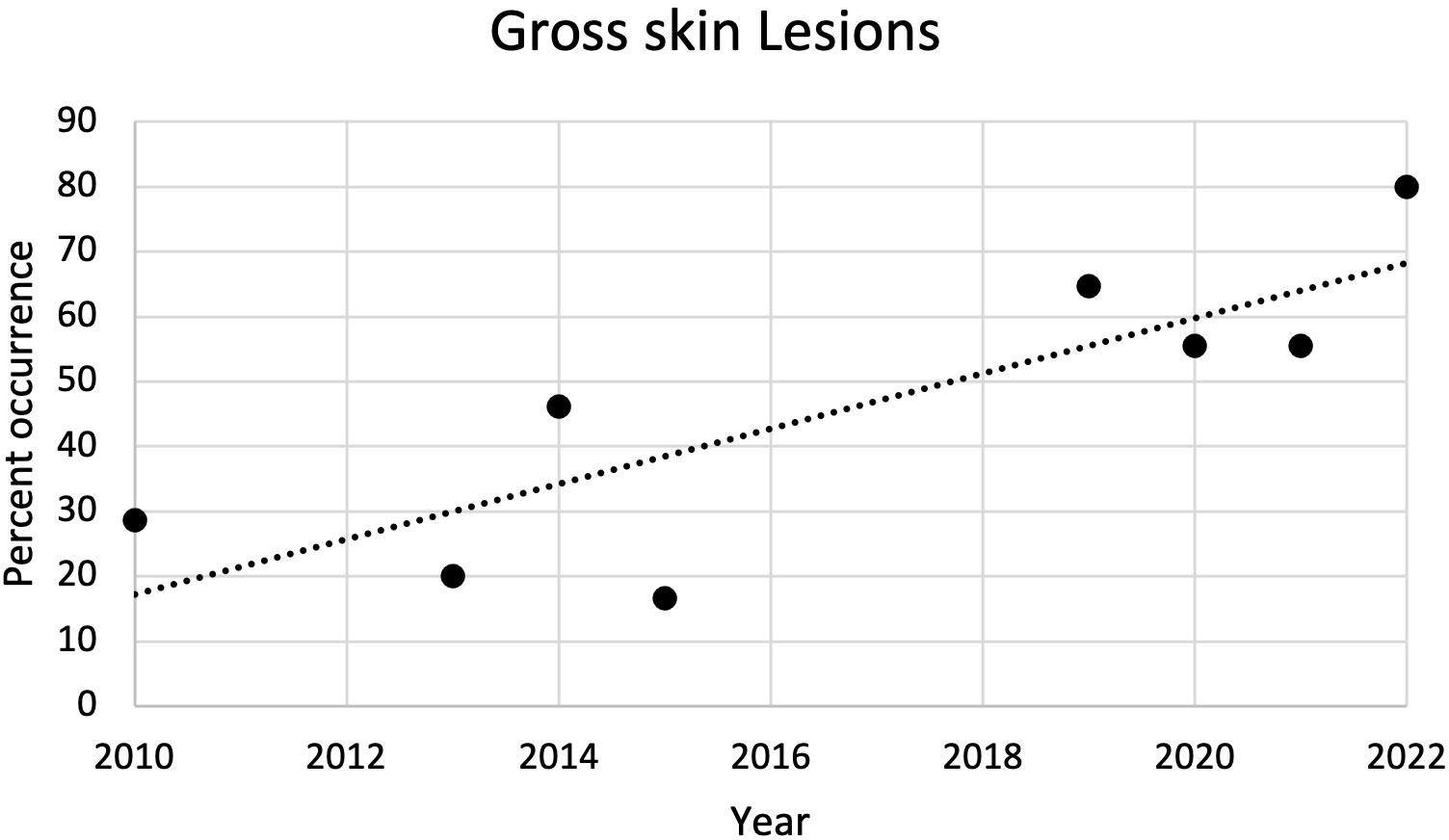
Figure 6 Plot of the percent of occurrence of gross skin lesions in dead captive leatherback neonates from 2010 to 2022. The percent of occurrence significantly increased over time (r2 = 0.67; p = 0.01).
Fungal culture
Fusarium spp. were isolated from 9 of the 19 swabs taken from dead-in-nest hatchlings and eggs (Table 3). Locations swabbed included the internal and external surfaces of intact eggshells, skin, and the inside of the yolk sac. Multiple individuals were sampled from 3 of the 5 nests that were positive for Fusarium spp. However, not all samples from each Fusarium-positive nest were positive for Fusarium spp. One sample from each of these 3 Fusarium-positive nests was negative via fungal culture. In 2022, a total of 23 live captive neonates developed gross signs of mycotic dermatitis. Of those, 20 were swabbed for fungal culture and all were positive for Fusarium spp. The one additional swab from the asymptomatic neonate (i.e., negative control) had no fungal growth after 21 days.
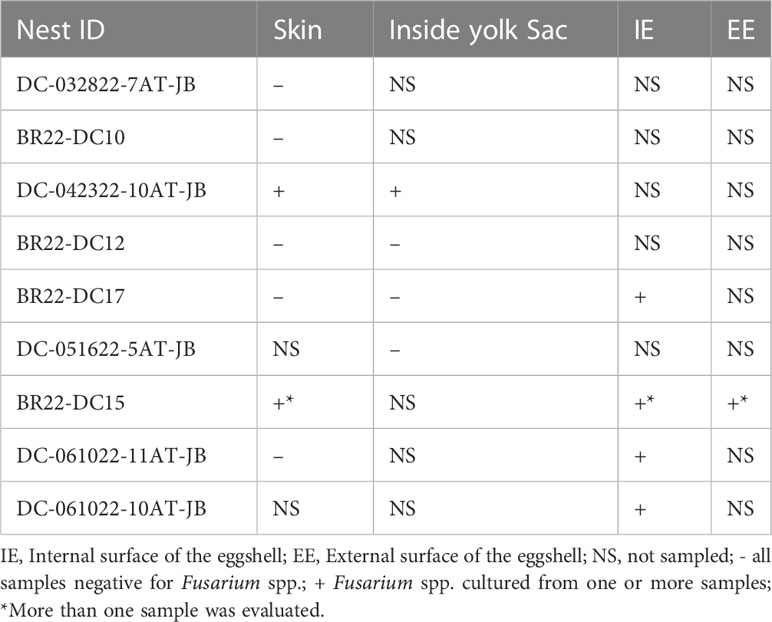
Table 3 Fungal culture results from dead-in-nest hatchling and dead-in-nest egg samples across 9 nests in 2022.
All Fusarium spp. colonies isolated on culture grew rapidly and had white to cream-colored aerial mycelium (Figure 7A). On microscopic examination macroconidia were curved, fusiform, and cylindrical (Figure 7B). Most macroconidia had 3 septae (Figure 7B). Abundant microconidia formed from long lateral phialides, were one or two-celled, and cylindrical or oval (Figure 7C). All findings were suggestive of Fusarium spp. within the FSSC.
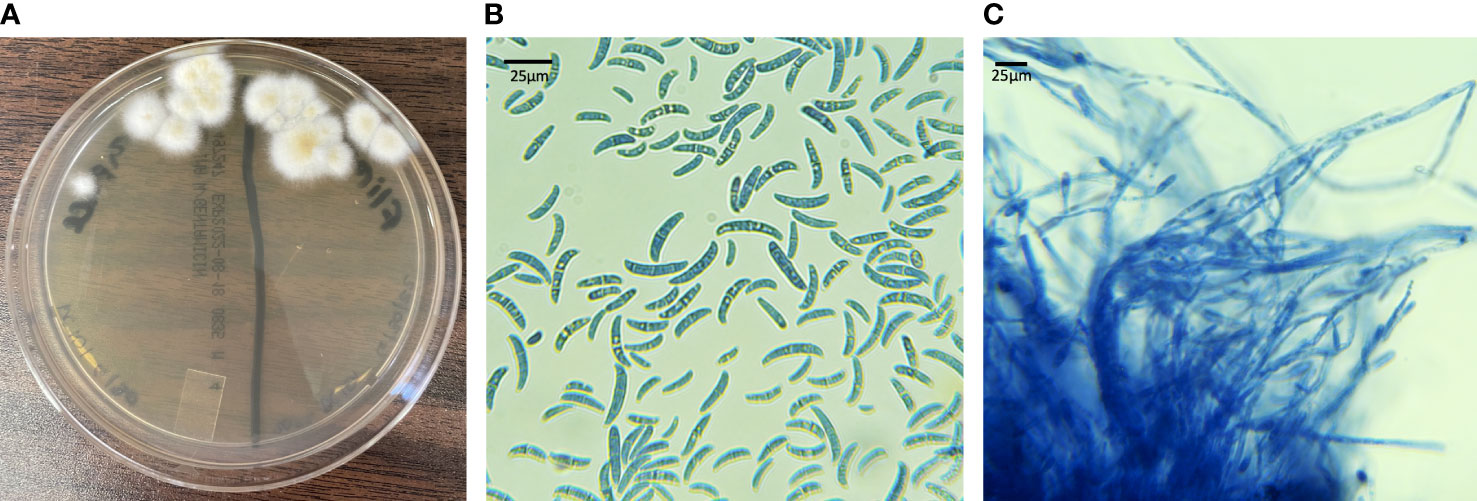
Figure 7 Fungal culture results from live captive leatherback neonates with fungal morphology characteristic of the FSSC. (A) IMA plate with white to cream colored aerial mycelium (B) macro and microconidia (lactophenol aniline blue dyed, 100X) (C) long lateral phialides forming one-celled microconidia (lactophenol aniline blue dyed, 100X).
Hatching success
Hatching success for all nests used in 2022 are included in Table 4. There was no significant difference in hatching success between nests that had Fusarium spp. identified or mycotic dermatitis in one or more live captive neonate, dead captive neonate, dead-in-nest hatchling or dead-in-nest egg samples and those that did not. However, comparing the 9 nests in which dead-in-nest hatchling and egg samples were obtained revealed that nests with dead-in-nest samples that tested positive for Fusarium spp. had significantly lower hatching success than those in which all dead-in-nest samples tested negative (p = 0.02; W = 20).
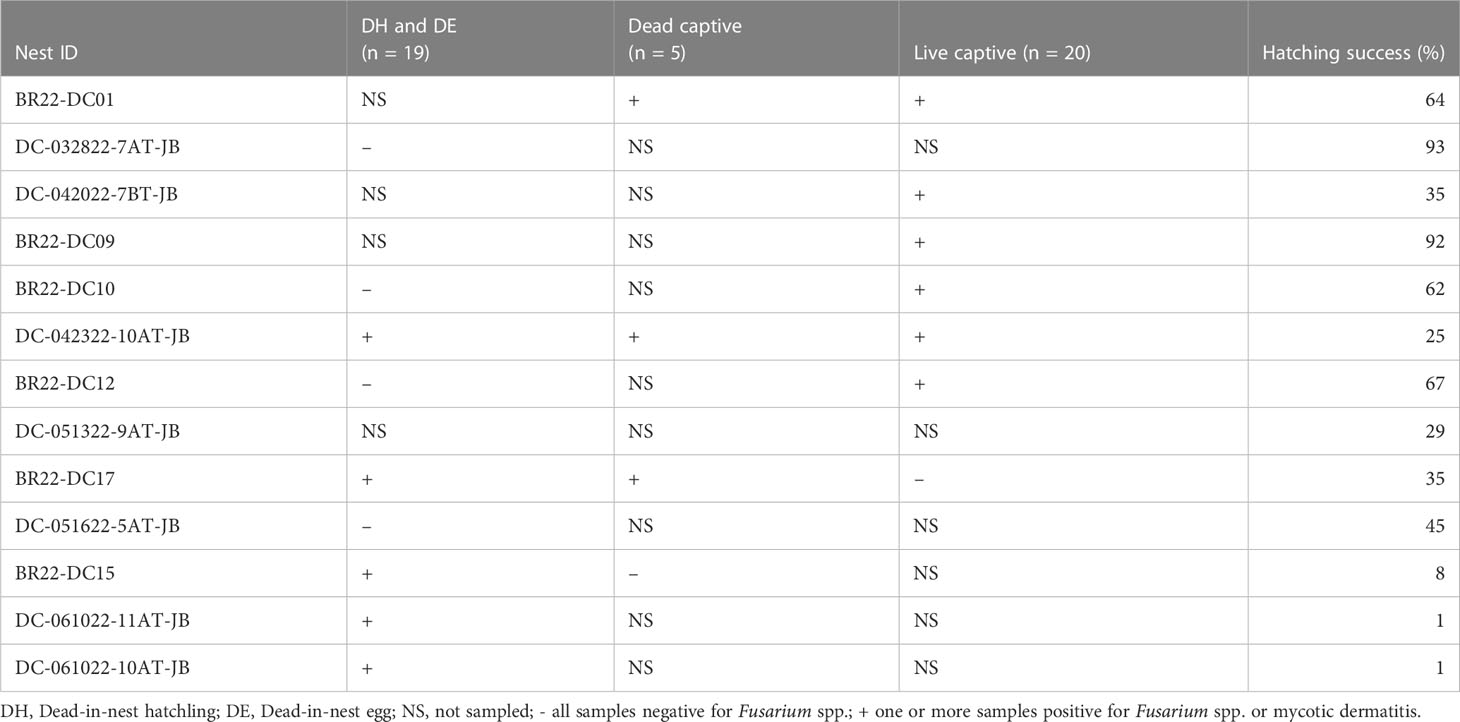
Table 4 Summary of all samples (i.e., swabs for fungal culture, histopathology) used to detect the presence of Fusarium spp. or mycotic dermatitis in 2022 and the hatching success of each individual nest.
Blood analysis
Packed cell volume was significantly lower at emergence in live captive neonates that developed mycotic dermatitis (p = 0.002, W = 435; Figure 8). There was no significant difference in estimated TP at emergence between neonates that developed mycotic dermatitis and those that did not. Gamma globulins were significantly higher at emergence in neonates that developed mycotic dermatitis (p = 0.005, W = 150; Figure 9). No other blood values were significantly different between groups.
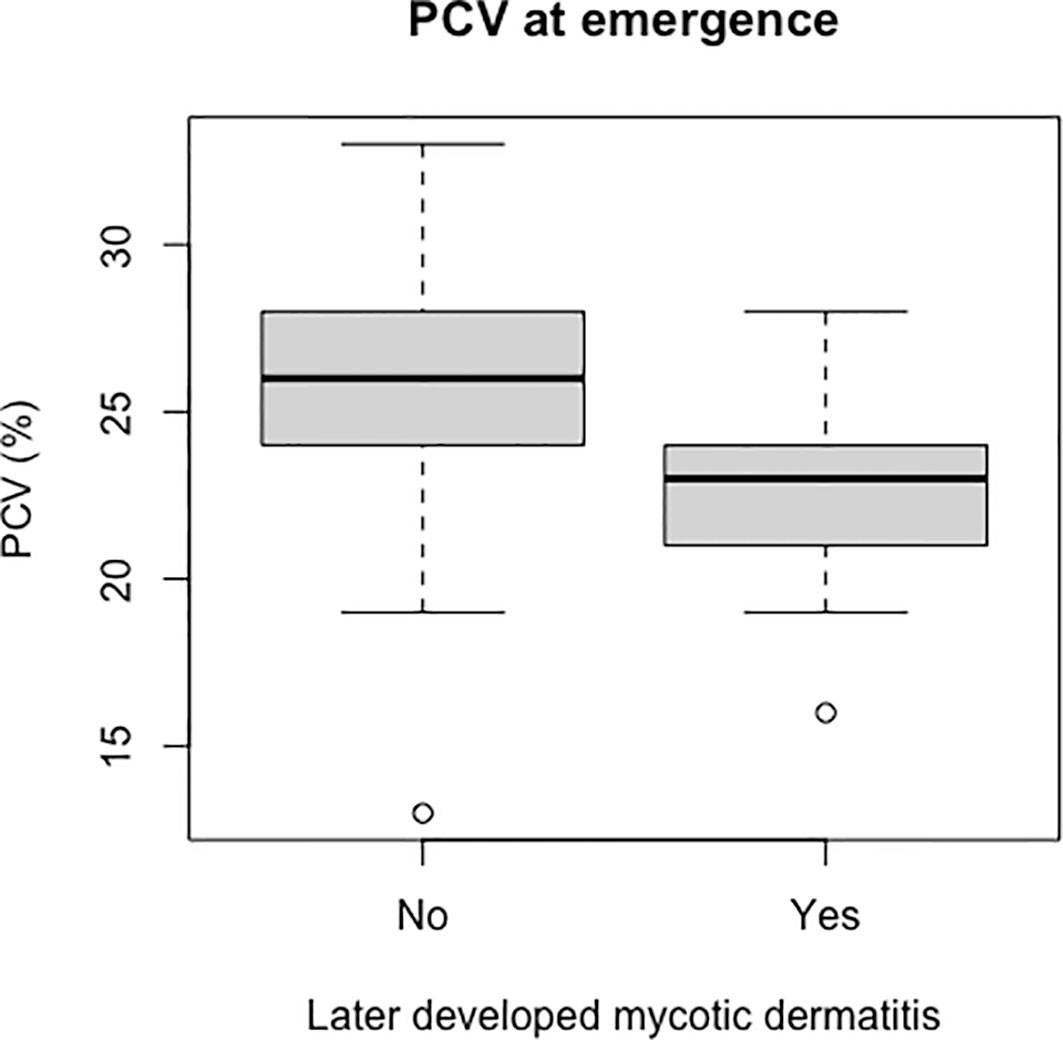
Figure 8 Boxplot of the PCV values from live captive leatherback neonates at emergence comparing values between those that later developed mycotic dermatitis and those that did not. PCV was significantly lower in neonates that developed mycotic dermatitis (p = 0.002, W = 435). Gray boxes are the first and third quartiles; thick black lines designate the median value; dashed lines are the minimum and maximum values; open circles are outliers. Outliers may have lower PCV due to undetected hemodilution from lymph contamination during venipuncture but were not removed because the Wilcoxon Rank Sum Test is resistant to outliers.
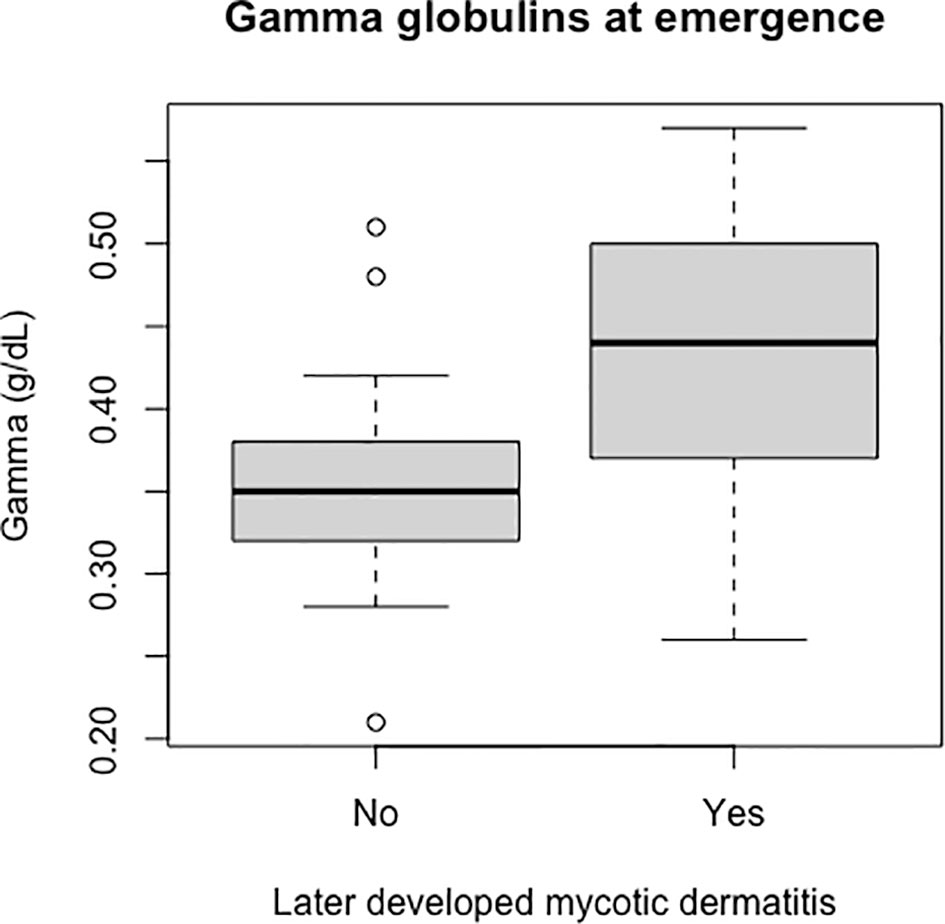
Figure 9 Boxplot of the gamma globulins values of live captive leatherback neonates at emergence comparing values between those that later developed mycotic dermatitis and those that did not. Gamma globulins were significantly higher in neonates that developed mycotic dermatitis (p = 0.005, W = 150). Boxplot format as in Figure 8. Outliers were not removed because the Wilcoxon Rank Sum Test is resistant to outliers.
Discussion
In this set of studies, we demonstrate that Fusarium spp. are likely present in leatherback sea turtle nests in Boca Raton and Juno Beach, Florida. Additionally, based on the occurrence of mycotic dermatitis in captive neonates (live and dead) it is likely that the presence of Fusarium spp. in nests is affecting leatherback neonates even after emergence. This result is consistent with the findings of Miller et al. (2009), as they interpreted finding microorganisms on histopathology of dead captive and dead-in-nest hatchlings as likely originating in the environment. Furthermore, the isolation of Fusarium spp. from the internal surface of intact eggshells suggests that STEF is infecting leatherback sea turtle eggs on the beaches in Boca Raton and Juno Beach, Florida. Sea turtle egg fusariosis can cause mass mortality events and has been implicated in decreased hatching success in all 7 species of sea turtles (Sarmiento-Ramírez et al., 2010; Sarmiento- Ramírez et al., 2014; Gleason et al., 2020). However, of the nests sampled during this study, there was no significant difference in hatching success between nests that had any Fusarium-positive sample and those that did not. This is likely due to the low number of nests without any Fusarium spp. detected across all sample types (i.e., dead-in-nest hatchlings and eggs, live captive neonates, and dead captive neonates). Of the 13 nests included in this study, only 2 had all samples negative for Fusarium spp. Notably, when we focused on the dead-in-nest samples alone, we found a significant decrease in hatching success in Fusarium-positive nests compared to those with no Fusarium spp. detected. This result leads us to infer that the relatively low emergence success of leatherback nests may reflect the negative impact of microbial stressors on hatchlings, which is consistent with findings from previous work (Sarmiento-Ramírez et al., 2014; Gleason et al., 2020). However, the unsterile nature of the samples used for dead-in-nest samples make it difficult to determine a definitive relationship between Fusarium spp. and hatching success. Future work should aim to further explore how Fusarium spp. impact hatching success.
Not only are Fusarium spp. a problem for eggs but they also appear to be a growing issue for leatherback neonates after emergence. After using fungal cultures for diagnosis during the 2022 hatching season, it is likely that Fusarium spp. are the causative agent of mycotic dermatitis in this population. Additional diagnostics (i.e., cytology and histopathology) in live captive neonates should be used to confirm this in the future. Furthermore, based on the consistent pathologic findings and gross descriptions across years, it is also likely that this same pathogen is the cause of most of the mycotic dermatitis and characteristic skin lesions seen in dead captive neonates since 2010. Unfortunately, the percent of occurrence of skin lesions and mycotic dermatitis in dead captive neonates is increasing. Mycotic dermatitis appears to be a significant threat to leatherback neonates. While the neonates in this study were in a captive setting and came from a single facility, this is currently the only large, representative population available for neonatal leatherback sea turtle research. Access to leatherback neonates in the wild is very limited due to their oceanic pelagic niche. Moreover, the profound absence of neonatal leatherback sightings at sea makes a wild assessment for mycotic dermatitis impossible.
Although the source of the Fusarium spp. responsible for mycotic dermatitis in captive leatherbacks is not fully understood, the detection of Fusarium spp. from dead-in-nest hatchlings and eggs suggests that exposure in the nest is likely. This theory is further supported by the alterations in blood values at emergence seen in live captive neonates that later developed mycotic dermatitis. In live captive neonates that developed mycotic dermatitis PCV was significantly lower while gamma globulins were significantly higher. A decreased PCV can be caused by chronic disease, systemic infections, inflammatory disease of the liver, kidney, or lungs, and gastrointestinal disease (Stacy et al., 2011). Generally, a decreased PCV is accompanied by a decrease in TP, but we found no significant differences in estimated TP between groups. However, this may be related to the method used to measure estimated TP (i.e., refractometry). Estimated TP values measured via refractometer can be skewed by blood glucose, urea, and triglycerides (Hunsaker et al., 2016; Legendre et al., 2017). The biuret method is the preferred method for measuring TP and should be considered in future work. Additionally, other biochemical tests and white blood cell counts should also be considered moving forward if blood volumes allow. These blood values could help determine if the hatchlings are suffering from other physiologic challenges at emergence.
Protein electrophoresis in non-traditional species is generally used to detect inflammation (Cray, 2021). Alterations in the different protein fractions are then used to characterize the inflammation (Cray, 2021). For example, increased gamma globulins are associated with humoral immunity and more specifically immunoglobulins (Eckersall, 2008; Cray, 2021). Reptile and avian species have immunoglobulin Y (IgY) as a major part of their humoral response against antigens (Pettinello and Dooley, 2014; Rodgers and Rice, 2018). In chickens there is a strong correlation between the IgY levels of the hen, the egg yolk, and the offspring serum (Sun et al., 2013). Based on this, it is possible that elevated gamma globulin values seen in the leatherbacks may be a consequence of high maternal transfer of IgY via the egg yolk. While high maternal transfer of antibodies is generally considered an advantage, in leatherbacks high maternal gamma globulins have been negatively associated with both hatching success and emergence success (Boulinier and Staszewski, 2008; Perrault et al., 2012). Maternal health status likely plays an important role in the long-term survival of hatchlings. While the increased gamma globulins may not be the primary problem in either the mother or the hatchlings, the increased maternal gamma globulin values may indicate poor maternal health (Perrault et al., 2012). A recent infection or physiologic strain in the adult female may have diverted physiologic energy and resources away from reproductive processes. Consequently, while a hatchling may have higher IgY, they may also be deficient in other key resources that were not available for reproductive use due to the mother’s physiologic strain.
Moreover, the gamma globulin region can also be affected by the presence of acute phase proteins. Acute phase proteins are blood proteins that increase in response to infection, inflammation, trauma, or stress (Murata et al., 2004). One of the most recognized stressors on sea turtle eggs and hatchlings over the last few decades has been rising nest temperatures (Fuentes et al., 2009; Fleming et al., 2020). There is evidence that high nest temperatures increase physiologic stress on developing turtles and research in chickens has shown that increased thermal stress does increase acute phase proteins (Fleming et al., 2020; Hanafi et al., 2022; Perrault et al., 2022). Thermal stress could be increasing gamma globulin values and making developing turtles more susceptible to pathogens such as Fusarium spp. both in the nest and after emergence. In the future, tests to assess specific acute phase proteins used in conjunction with nest temperature data may be useful. Additionally, increased nest temperatures are likely altering the nest microbial profile (Bérard et al., 2011). As a result, Fusarium spp. may be outcompeting other microorganisms and overgrowing in nests. Other environmental factors (moisture, sand pH) and various anthropogenic effects may contribute to the potential for pathogenesis and should be investigated. Regardless, an important point is, given that Fusarium spp. were isolated from dead-in-nest hatchlings and eggs, it is possible that wild leatherback neonates emerging from nests affected by Fusarium spp. may be suffering from the same disease process found in the live captive and dead captive neonates.
Currently, the etiology and epidemiology of STEF is not fully understood but some hypothesize an anthropogenic source (Smyth et al., 2019). While one causative agent of STEF, Ff, is routinely found in soil, the other, Fk, had only previously been isolated from non-soil sources such as plumbing systems (Smyth et al., 2019). Given the potential for Fusarium spp. to affect nonhuman animals, plants, the environment, and humans, Fusarium spp. are truly a One Health problem (Sáenz et al., 2020; Thornton, 2020). Fusarium spp. in sand have the potential to not only affect eggs and pre-emergent hatchlings but this study has now shown Fusarium spp. also have the ability to negatively impact seemingly healthy leatherback neonates after emergence. Furthermore, reports of fungal infections in humans are rising, likely due to the implementation of new medical therapies such as antibiotics and immunosuppressive therapies (One Health, 2019; Batista et al., 2020; Thornton, 2020). It is possible that if beach Fusarium spp. burdens increase, the sand could potentially serve as a nidus of infection for other animals or humans using the beaches.
Moving forward, it is essential to further examine the presence and impact of STEF on the important sea turtle nesting beaches in Boca Raton and Juno Beach, Florida which, together host about1/3 of all leatherback nesting in the continental U.S.A. (Florida Statewide Nesting Beach Survey, 2021). Determining the prevalence of Fusarium spp. and STEF in the nests of all nesting species on these densely nested beaches is important for determining the full impact STEF may have on current conservation efforts. Performing additional robust studies using both fungal culture and next generation sequencing on samples from eggs and nests will aid in the understanding of the microbial community present in the nests and may provide a means to combat Fusarium spp. Nest temperature, moisture, and sand pH should also be investigated to identify any relationships with STEF. Additional studies on neonates after emergence following exposure to Fusarium spp. in the nest should also be undertaken to determine the long-term health effect on individual leatherback neonates (captive and wild) and its potential to impact the leatherback population. Furthermore, a more robust blood analysis after emergence could also help illuminate the pathogenesis of this disease in leatherback neonates and provide insight into mitigation measures for the development of mycotic dermatitis.
Data availability statement
The original contributions presented in the study are included in the article/supplementary material. Further inquiries can be directed to the corresponding author.
Ethics statement
This work was authorized under permit MTP-073 and the Florida Atlantic University protocol A21-31.
Author contributions
SK, JW, and DM initiated the paper by developing the hypothesis that Fusarium spp. was impacting leatherbacks in the study area. ET conducted nest inventories, provided all dead-in-nest specimens, and summarized/provided hatching success data. MK performed all dead-in-nest fungal cultures. SK performed all live captive examinations and fungal cultures. CC aided with protein electrophoresis interpretation. DM performed all histologic examinations. SK performed all data analysis and wrote all drafts. JW, DM, and CC edited final drafts. All authors approved the finalized text.
Funding
This study was funded in part by a grant awarded from the Florida Sea Turtle Grants Program (22-031R), the National Save the Sea Turtle Foundation, and Upwell Turtles. This study also received funding from Royal Caribbean Cruises, Ltd. The funder was not involved in the study design, collection, analysis, interpretation of data, the writing of this article or the decision to submit it for publication. All authors declare no other competing interests. Partial funding for open access to this research was provided by University of Tennessee's Open Publishing Support Fund.
Acknowledgments
The authors thank the Avian & Wildlife Laboratory (University of Miami) for assistance with the protein electrophoresis (CC), Loggerhead Marinelife Center’s research department and the Gumbo Limbo Nature Center Marine Turtle Specialists for essential assistance on the nesting beaches. The authors are grateful for support of the University of Tennessee Comparative and Experimental Medicine program and the University of Tennessee College of Veterinary Medicine’s Center of Excellence student summer research program (DM, SK). Essential funding to (JW) was provided by the Florida Sea Turtle Grants Program, National Save the Sea Turtle Foundation, Royal Caribbean Cruises, Ltd., and Upwell Turtles, whose mission is to protect imperiled sea turtles by reducing threats. This work was authorized under permit MTP-073 and the FAU protocol A21-31.
Conflict of interest
The authors declare that the research was conducted in the absence of any commercial or financial relationships that could be construed as a potential conflict of interest.
Publisher’s note
All claims expressed in this article are solely those of the authors and do not necessarily represent those of their affiliated organizations, or those of the publisher, the editors and the reviewers. Any product that may be evaluated in this article, or claim that may be made by its manufacturer, is not guaranteed or endorsed by the publisher.
References
Anderson P. K., Cunningham A. A., Patel N. G., Morales F. J., Epstein P. R., Daszak P. (2004). Emerging infectious diseases of plants: pathogen pollution, climate change and agrotechnology drivers. Trends Ecol. Evol. 19 (10), 535–544. doi: 10.1016/j.tree.2004.07.021
Batista B. G., de Chaves M. A., Reginatto P., Saraiva O. J., Fuentefria A. M. (2020). Human fusariosis: an emerging infection that is difficult to treat. Rev. Da Sociedade Bras. Medicina Trop. 53, e20200013. doi: 10.1590/0037-8682-0013-2020
Bérard A., Bouchet T., Sévenier G., Pablo A. L., Gros R. (2011). Resilience of soil microbial communities impacted by severe drought and high temperature in the context of Mediterranean heat waves. Eur. J. Soil Biol. 47 (6), 333–342. doi: 10.1016/j.ejsobi.2011.08.004
Berger L., Speare R., Daszak P., Green D. E., Cunningham A. A., Goggin C. L., et al. (1998). Chytridiomycosis causes amphibian mortality associated with population declines in the rain forests of Australia and central America. Proc. Natl. Acad. Sci. 95 (15), 9031–9036. doi: 10.1073/pnas.95.15.9031
Boulinier T., Staszewski V. (2008). Maternal transfer of antibodies: raising immuno-ecology issues. Trends Ecol. Evol. 23 (5), 282–288. doi: 10.1016/j.tree.2007.12.006
Cafarchia C., Paradies R., Figueredo L. A., Iatta R., Desantis S., Di Bello A. V. F., et al. (2020). Fusarium spp. in loggerhead sea turtles (Caretta caretta): from colonization to infection. Veterinary Pathol. 57 (1), 139–146. doi: 10.1177/0300985819880347
Candan A. Y., Katılmış Y., Ergin Ç. (2021). First report of fusarium species occurrence in loggerhead sea turtle (Caretta caretta) nests and hatchling success in iztuzu beach, Turkey. Biologia 76 (2), 565–573. doi: 10.2478/s11756-020-00553-4
Casadevall A. (2018). Fungal diseases in the 21st century: the near and far horizons. Pathogens & immunity. 3, 183–196. doi: 10.20411/pai.v3i2.249
Cray C. (2021). Protein electrophoresis of non-traditional species: a review. Veterinary Clin. Pathol. 50 (4), 478–494. doi: 10.1111/vcp.13067
Desoubeaux G., Debourgogne A., Wiederhold N. P., Zaffino M., Sutton D., Burns R. E., et al. (2018). Multi-locus sequence typing provides epidemiological insights for diseased sharks infected with fungi belonging to the Fusarium solani species complex. Med. Mycology 56 (5), 591–601. doi: 10.1093/mmy/myx089
Eckersall P. D. (2008). “Proteins, proteomics, and the dysproteinemias,” in Clinical biochemistry of domestic animals, 6th ed. Eds. Kaneko J. J., Harvey J. W., Bruss M. L. (Academic Press), 117–155.
Fisher M. C., Daniel. A., Briggs C. J., Brownstein J. S., Madoff L. C., McCraw S. L., et al. (2012). Emerging fungal threats to animal, plant and ecosystem health. Nature 484 (7393). doi: 10.1038/nature10947
Fisher M. C., Gurr S. J., Cuomo C. A., Blehert D. S., Jin H., Stukenbrock E. H., et al. (2020). Threats posed by the fungal kingdom to humans, wildlife, and agriculture. MBio 11 (3), e00449–e00420. doi: 10.1128/mBio.00449-20
Fleming K. A., Perrault J. R., Stacy N. I., Coppenrath C. M., Gainsbury A. M. (2020). Heat, health and hatchlings: associations of in situ nest temperatures with morphological and physiological characteristics of loggerhead sea turtle hatchlings from Florida. Conserv. Physiol. 8 (1), coaa046. doi: 10.1093/conphys/coaa046
Florida Statewide Nesting Beach Survey (2021). Available at: https://myfwc.com/research/wildlife/sea-turtles/nesting/statewide/.
Fuentes M., Maynard J., Guinea M., Bell I., Werdell J., Hamann M. (2009). Proxy indicators of sand temperature help project impacts of global warming on sea turtles in northern Australia. Endangered Species Res. 9, 33–40. doi: 10.3354/esr00224
Garcia-Solache M. A., Casadevall A. (2010). Global warming will bring new fungal diseases for mammals. MBio 1 (1), e00061–e00010. doi: 10.1128/mBio.00061-10
Gauthier G. M., Keller N. P. (2013). Crossover fungal pathogens: the biology and pathogenesis of fungi capable of crossing kingdoms to infect plants and humans. Fungal Genet. Biol. 61, 146–157. doi: 10.1016/j.fgb.2013.08.016
Gleason F. H., Allerstorfer M., Lilje O. (2020). Newly emerging diseases of marine turtles, especially sea turtle egg fusariosis (SEFT), caused by species in the Fusarium solani complex (FSSC). Mycology 11 (3), 184–194. doi: 10.1080/21501203.2019.1710303
Hanafi S. A., Zulkifli I., Ramiah S. K., Chung E. L. T., Awad E. A., Sazili A. Q. (2022). Prenatal thermal stress affects acute-phase proteins, heat shock protein 70, and circulating corticosterone in developing broiler embryos and neonates. J. Thermal Biol. 109, 103328. doi: 10.1016/j.jtherbio.2022.103328
Higgins R. (2000). Bacteria and fungi of marine mammals: a review. Can. Veterinary J. 41 (2), 105–116.
Hoh D., Lin Y., Liu W.-A., Mohamed Sidique S. N., Tsai I. (2020). Nest microbiota and pathogen abundance in sea turtle hatcheries. Fungal Ecol. 47, 100964. doi: 10.1016/j.funeco.2020.100964
Hoyt J. R., Kilpatrick A. M., Langwig K. E. (2021). Ecology and impacts of white-nose syndrome on bats. Nat. Rev. Microbiol. 19 (3). doi: 10.1038/s41579-020-00493-5
Hunsaker J. J. H., Wyness S. P., Snow T. M., Genzen J. R. (2016). Clinical performance evaluation of total protein measurement by digital refractometry and characterization of non-protein solute interferences. Pract. Lab. Med. 6, 14–24. doi: 10.1016/j.plabm.2016.08.001
Jain P., Gupta V., Misra A. K., Gaur R., Bajpai V., Issar S. (2011). Current status of Fusarium infection in human and animal. Asian J. Anim. Veterinary Adv. 6, 201–227. doi: 10.3923/ajava.2011.201.227
Kwon S., Park J., Choi W.-J., Koo K.-S., Lee J.-G., Park D. (2017). First case of ranavirus-associated mass mortality in a natural population of the huanren frog (Rana huanrenensis) tadpoles in south Korea. Anim. Cells Syst. 21 (5), 358–364. doi: 10.1080/19768354.2017.1376706
Legendre K. P., Leissinger M., Le Donne V., Grasperge B. J., Gaunt S. D. (2017). The effect of urea on refractometric total protein measurement in dogs and cats with azotemia. Veterinary Clin. Pathol. 46 (1), 138–142. doi: 10.1111/vcp.12464
Leslie J. F., Summerell B. A., Bullock S. (2006). The fusarium laboratory manual (John Wiley & Sons, Incorporated). Available at: http://ebookcentral.proquest.com/lib/utk/detail.action?docID=362021.
Lötters S., Kielgast J., Bielby J., Schmidtlein S., Bosch J., Veith M., et al. (2009). The link between rapid enigmatic amphibian decline and the globally emerging chytrid fungus. EcoHealth 6, 358–372. doi: 10.1007/s10393-010-0281-6
Miller D. L., Wyneken J., Rajeev S., Perrault J., Mader D. R., Weege J., et al. (2009). Pathologic findings in hatchling and post hatchling leatherback sea turltes (Dermochelys coriacea) from Florida. J. Wildlife Dis. 45 (4), 962–971. doi: 10.7589/0090-3558-45.4.962
Murata H., Shimada N., Yoshioka M. (2004). Current research on acute phase proteins in veterinary diagnosis: an overview. Veterinary J. 168 (1), 28–40. doi: 10.1016/S1090-0233(03)00119-9
Nucci M., Anaissie E. (2002). Cutaneous infection by fusarium species in healthy and immunocompromised hosts: implications for diagnosis and management. Clin. Infect. Dis. 35 (8), 909–920. doi: 10.1086/342328
O’Donnell K. (2000). Molecular phylogeny of the Nectria haematococca-Fusarium solani species complex. Mycologia 92 (5), 919–938. doi: 10.1080/00275514.2000.12061237
One health: Fungal pathogens of humans, animals, and plants: Report on an American Academy of Microbiology Colloquium (2019). American Society for microbiology (Washington (DC). Available at: http://www.ncbi.nlm.nih.gov/books/NBK549988/.
Palm Beach County (2023) Palm beach county interesting facts and figures. Available at: https://discover.pbcgov.org/pages/pbc_facts.aspx.
Perrault J. R., Miller D. L., Eads E., Johnson C., Merrill A., Thompson L. J., et al. (2012). Maternal health status correlates with nest success of leatherback sea turtles (Dermochelys coriacea) from Florida. PloS One 7 (2), e31841. doi: 10.1371/journal.pone.0031841
Perrault J. R., Page-Karjian A., Morgan A. N., Burns L. K., Stacy N. I. (2022). Morphometrics and blood analytes of leatherback sea turtle hatchlings (Dermochelys coriacea) from Florida: reference intervals, temporal trends with clutch deposition date, and body size correlations. J. Comp. Physiol. B 192 (2), 313–324. doi: 10.1007/s00360-021-01422-5
Pettinello R., Dooley H. (2014). The immunoglobulins of cold-blooded vertebrates. Biomolecules 4 (4), 1045. doi: 10.3390/biom4041045
Phillott A. D., Parmenter C. J. (2014). Fungal colonization of green sea turtle (Chelonia mydas) nests is unlikely to affect hatchling condition. Herpetological Conserv. Biol.
Phillott A. D., Parmenter C. J., Limpus C. J. (2001). Mycoflora identified from failed green (Chelonia mydas) and loggerhead (Caretta caretta) sea turtle eggs at heron island, Australia. Chelonian Conserv. Biol. 4, 170–172.
Pirarat N., Sahatrakul K., Lacharoje S., Lombardini E., Chansue N., Techangamsuwan S. (2016). Molecular and pathological characterization of Fusarium solani species complex infection in the head and lateral line system of Sphyrna lewini. Dis. Aquat. Organisms 120 (3), 195–204. doi: 10.3354/dao03028
Rodgers M. L., Rice C. D. (2018). Monoclonal antibodies against loggerhead sea turtle, Caretta caretta, IgY isoforms reveal differential contributions to antibody titers and relatedness among other sea turtles. Dev. Comp. Immunol. 87, 12–15. doi: 10.1016/j.dci.2018.05.015
Sáenz V., Alvarez-Moreno C., Pape P. L., Restrepo S., Guarro J., Ramírez A. M. C. (2020). A one health perspective to recognize Fusarium as important in clinical practice. J. Fungi 6. doi: 10.3390/jof6040235
Salter C. E., Sutton D. A., Marancik D. P., Knowles S., Clauss T. M., Berliner A. L., et al. (2012). Dermatitis and systemic mycosis in lined seahorses Hippocampus erectus associated with a marine-adapted Fusarium solani species complex pathogen. Dis. Aquat. Organisms 101 (1), 23–31. doi: 10.3354/dao02506
Sarmiento-Ramírez J. M., Abella E., Martín M. P., Tellería M. T., López-Jurado L. F., Marco A., et al. (2010). Fusarium solani is responsible for mass mortalities in nests of loggerhead sea turtle, Caretta caretta, in boavista, cape Verde. FEMS Microbiol. Lett. 312 (2), 192–200. doi: 10.1111/j.1574-6968.2010.02116.x
Sarmiento-Ramírez J. M., Abella-Pérez E., Phillott A. D., Sim J., West P., Martín M. P., et al. (2014). Global distribution of two fungal pathogens threatening endangered sea turtles. PloS One 9 (1), e85853. doi: 10.1371/journal.pone.0085853
Sarmiento-Ramirez J. M., Sim J., West P. V., Dieguez-Uribeondo J. (2017). Isolation of fungal pathogens from eggs of the endangered sea turtle species chelonia mydas in ascension island. J. Mar. Biol. Assoc. United Kingdom 97 (4), 661–667. doi: 10.1017/S0025315416001478
Scognamiglio T., Zinchuk R., Gumpeni P., Larone D. H. (2010). Comparison of inhibitory mold agar to sabouraud dextrose agar as a primary medium for isolation of fungi. J. Clin. Microbiol. 48 (5), 1924–1925. doi: 10.1128/JCM.01814-09
Sidique S. N. M., Azuddin N., Joseph J. (2017). First report of fusarium species at nesting sites of endangered sea turtles in terengganu and melaka, Malaysia. Malaysian Appl. Biol. 46, 195–205.
Smyth C. W., Sarmiento-Ramírez J. M., Short D. P. G., Diéguez-Uribeondo J., O’Donnell K., Geiser D. M. (2019). Unraveling the ecology and epidemiology of an emerging fungal disease, sea turtle egg fusariosis (STEF). PloS Pathog. 15 (5), e1007682. doi: 10.1371/journal.ppat.1007682
Stacy N. I., Alleman A. R., Sayler K. A. (2011). Diagnostic hematology of reptiles. Clinics Lab. Med. 31 (1), 87–108. doi: 10.1016/j.cll.2010.10.006
Sun H., Chen S., Cai X., Xu G., Qu L. (2013). Correlation analysis of the total IgY level in hen serum, egg yolk and offspring serum. J. Anim. Sci. Biotechnol. 4 (1), 10. doi: 10.1186/2049-1891-4-10
Tanaka M., Izawa T., Kuwamura M., Nakao T., Maezono Y., Ito S., et al. (2012). Deep granulomatous dermatitis of the fin caused by Fusarium solani in a false killer whale (Pseudorca crassidens). J. Veterinary Med. Sci. 74 (6), 779–782. doi: 10.1292/jvms.11-0421
Thornton C. R. (2020). Chapter one - detection of the ‘Big five’ mold killers of humans: Aspergillus, fusarium, lomentospora, scedosporium and Mucormycetes. Adv. Appl. Microbiol. 110, 1–61. doi: 10.1016/bs.aambs.2019.10.003
Yadav S., Gettu N., Swain B., Kumari K., Ojha N., Gunthe S. S. (2020). Bioaerosol impact on crop health over India due to emerging fungal diseases (EFDs): an important missing link. Environ. Sci. pollut. Res. 27 (11), 12802–12829. doi: 10.1007/s11356-020-08059-x
Keywords: fusarium, sea turtle egg fusariosis (STEF), sea turtle, leatherback, mycotic dermatitis, emerging disease
Citation: Kuschke SG, Wyneken J, Cray C, Turla E, Kinsella M and Miller DL (2023) Fusarium spp. an emerging fungal threat to leatherback (Dermochelys coriacea) eggs and neonates. Front. Mar. Sci. 10:1170376. doi: 10.3389/fmars.2023.1170376
Received: 20 February 2023; Accepted: 03 July 2023;
Published: 14 August 2023.
Edited by:
Andrea D. Phillott, Flame University, IndiaReviewed by:
Daphne Goldberg, Instituto de Pesquisas Ambientais Littoralis, BrazilEsra Deniz Candan, Giresun University, Türkiye
Copyright © 2023 Kuschke, Wyneken, Cray, Turla, Kinsella and Miller. This is an open-access article distributed under the terms of the Creative Commons Attribution License (CC BY). The use, distribution or reproduction in other forums is permitted, provided the original author(s) and the copyright owner(s) are credited and that the original publication in this journal is cited, in accordance with accepted academic practice. No use, distribution or reproduction is permitted which does not comply with these terms.
*Correspondence: Samantha G. Kuschke, skuschke@vols.utk.edu