- 1School of Biological and Marine Sciences, University of Plymouth, Plymouth, United Kingdom
- 2Marine Programme, South African National Biodiversity Institute, Cape Town, South Africa
Degradation of the natural world and associated ecosystem services is attributed to a historical failure to include its ‘value’ in decision-making. Uncertainty in the quantification of the relationship between natural capital ‘assets’ that give rise to critical societal benefits and people is one reason for the omission of these values from natural resource management. As this uncertainty increases in marine systems and further still with distance from the coast, the connection between society and natural capital assets is less likely to be included adequately in decision-making. Natural capital assets of Areas Beyond National Jurisdiction (ABNJ), including those of the deep sea, are distant but are known to generate many benefits for society, from the diffuse and broad-scale benefits of climate regulation to the provision of wild fish for food. While our understanding of the precise relationships (the status of asset stocks, ecosystem functions and processes) that control the availability of ecosystem services and the flows of benefits is limited, this does not preclude opening a discourse on how these natural capital assets could best be managed to continue to benefit society. Here we apply a natural capital approach to the South East Atlantic ABNJ, one of the least scientifically understood regions of the planet, and develop a framework for risk assessment. We do this by describing the benefit flows from the natural capital assets of the region, appraising how activities are creating pressures on these flows and whether the controls for these pressures protect them. Our risk register highlights how governance currently favours the protection of direct (extractive) benefit flows from natural capital assets of the region, which are primarily targeted for financial benefit. Without a systems-based framework that can account for the cumulative pressures on natural capital assets their status, associated ecosystem services and benefits are at risk. Such an approach is essential to capture and protect the foundational and often diffuse connections between marine natural capital and global society.
1 Introduction
Decision-making in natural resource management typically involves cost-benefit analysis of an activity considering the balance of environmental, social and economic impacts. Cost-benefit analysis techniques tend to require that these impacts are quantified to understand the significance and acceptability of an activity (Dasgupta, 2021). The current state of knowledge for Areas Beyond National Jurisdiction (ABNJ), and deep-sea environments, complicates this calculation where information of exactly which ecological receptors are likely to be impacted by an activity and their respective function is commonly unavailable (Hooper et al., 2019; Howell et al., 2020; La Bianca et al., 2023). This lack of knowledge and the consequent uncertainty associated with environmental and social impact assessment often leads to assessments of significance being considered ‘insignificant’ owing to the relatively small unit of resource (e.g. seabed area) at risk compared to that comprising the ABNJ as a whole (Smith et al., 2020). This can lead to the approvals of unmonitored incremental ecological damage that may threaten the flow of ecosystem services critical to society. Historically, the ‘value’ of ecological damage has been ignored or weakly integrated into cost-benefit analysis and decision-making, and this is attributed to broadscale trends of ecological decline (Dasgupta, 2021).
1.1 Natural capital approaches
Natural capital approaches extend the focus on value common to ecosystem service frameworks to provide contextual information on the state of the system (Hooper et al., 2019). Such an approach attempts to describe the full ecological system, including humans and the complex relationships between abiotic and biotic elements that enable natural capital itself to persist (Mace, 2019), using all available knowledge regardless of information type (e.g. quantitative vs. qualitative). There is no single accepted methodology for the application of natural capital approaches but the accepted aim is to build understanding and where possible measure the extent, status, and value of natural capital assets and associated ecosystem services and benefits (Natural Capital Coalition, 2016; Natural Capital Committee, 2017; Hooper et al., 2019). In describing the system, information can be presented to inform on the extent and status of natural capital assets, the biotic (species, habitats) and abiotic (water, substrate) stocks or ‘building blocks’ that interact to deliver flows (ecosystem services) that in turn give rise to human benefit (Figure 1). This system view that includes humans and the relationships between humans and natural capital assets aligns with policy demands for an ecosystem approach to governance (Borja et al., 2016) and can support the application of the precautionary approach within decision-making. These qualities are enhanced by the flexibility of such approaches to integrate disparate information and describe ecological relationships where understanding may be incomplete, uncertain or unknown (Hooper et al., 2019). Information can then be included or excluded according to the required purpose of the approach taken to meet demands of accuracy or certainty supported by quantified data or the rich contextual description afforded by qualitative data.
The assimilation and incorporation of all available knowledge into a natural capital approach can assist decision-making in data poor scenarios such as in ABNJ through the provision of context and the identification of potential connections in the absence of detailed understanding of the precise ecological relationships that deliver benefits to people (Rees et al., 2012; Rees et al., 2022). This then enables risk assessment (i.e. the identification and evaluation of risk), either through tracking the extent and status of natural capital assets or through analysis of the sensitivity of assets to pressures (e.g. physical damage to the seabed from fishing activity), that may change the status (quantity and quality) of the natural capital asset. The asset status indicates its potential to perform the functions and processes that lead to the availability of ecosystem services and hence the availability of human benefit to society (Figure 1) (Mace et al., 2015; Rees et al., 2022). Risk assessment aims to compile available evidence, make the full (known and unknown) consequences of an action visible without the burden of mandatory tests of statistical significance such as that required within traditional environmental accounts such as the UN’s System of Environmental-Economic Accounting – Ecosystem Accounting (SEEA-EA) and provide a synthesis of the complexity of marine management to support decision-making (Hooper et al., 2019; Rees et al., 2022). Such an approach is particularly useful where available information is sparse or highly uncertain, as is the case in ABNJ and many marine environments.
1.2 The marine natural capital system
The flows between marine natural capital assets and people are among the most poorly understood ecological relationships and this is increasingly true as distance increases from the coast (Hooper et al., 2019). Areas Beyond National Jurisdiction (ABNJ) lie beyond the 200 nautical mile (nm) limit of state’s exclusive economic zones (EEZs) and comprise the seabed (the Area) and, water column (the High Seas) (UN, 1982). While ABNJ are often described as ‘unknown’ or an as yet uncrossed frontier (Ramirez-Llodra et al., 2011; Gjerde et al., 2016; Havice and Zalik, 2018) the natural capital assets of these regions are known to provide essential ecosystem services that give rise to human benefit (Thurber et al., 2014; La Bianca et al., 2023). Scientific consensus agrees that while ABNJ are inextricably linked to the provision of key ecosystem services the precise ecological and oceanographic relationships that deliver these remain largely unknown (Rees et al., 2012; Jobstvogt et al., 2014; Le et al., 2017).
A key feature of the marine system is its global interconnection, which enables planetary-scale processes and the diffuse flow of ecosystem services important for all humanity (e.g. climate regulation, many cultural services). Such ecosystem services arise from countless relationships between assets, operating cumulatively and synchronously across all spatial and temporal scales (Thurber et al., 2014; Drazen et al., 2020; Tilot et al., 2021; Melbourne-Thomas et al., 2023). Accordingly, key attributes of ABNJ include its position as the ‘connector’ of coastal environments and water masses to enable global ocean processes (e.g. large scale heat transport via currents) and its vast expanse that encompasses innumerable assets contributing to ecosystem services (FAO, 2020). The lack of understanding of the relative contribution of assets to ecosystem services challenges the prioritization and assessment of significance within decision-making. Combined with inabilities to meaningfully quantify globally accrued benefits arising from diffuse ecosystem services means that these critical flows are often omitted from assessments or risk or cost-benefit appraisal. A lack of oversight at scales relevant for such ecosystem services enables the degradation of individual assets which individually are viewed as likely insignificant but cumulatively could place these critical flows at risk.
2 Risk assessment framework
Currently available ecological baselines (including asset maps) for ABNJ are constrained to coarse resolution habitat models (Howell, 2010; McQuaid et al., 2020; Howell et al., 2022; McQuaid et al., 2023) or the limited areas of seafloor that have been acoustically mapped (Howell et al., 2022). While these baselines will likely improve over time with data collection, the timescales of this improvement are unlikely to match the pace at which exploitation activity is progressing (Jouffray et al., 2020). In the absence of habitat maps, knowledge of ecological relationships, and data to inform assessments of asset and ecosystem service status, a ‘matrix approach’ using literature review and expert opinion can link assets to ecosystem functions and processes, ecosystem services and benefits (Potts et al., 2014). Using this matrix approach, it is possible to focus on the elements of the system for which we have information and for which proxies can be identified to begin to compile a risk register (Figure 2) (Rees et al., 2022).

Figure 2 Schematic of the natural capital framework considered. Those components in black are the areas of interest for this study.
For ABNJ, readily available information to inform a risk register includes that relating to the broadscale benefits arising from the natural capital of ABNJ (e.g. a healthy climate, food, and spiritual wellbeing), the type of pressure exerted on assets, and the controls (governance) applied to manage these pressures (Figure 2). While this information is readily available, for some variables this is constrained to a more general or global understanding of benefit or pressure which limits spatially specific analysis. Despite limitations, compiling a risk register enables us to examine the interactions between pressures, natural capital assets, and benefits, and appraisal of pressure controls allows the assessment of risk to these benefits (Figure 3). Firstly, the interactions between the benefits are considered. This is informed by an analysis of which natural capital assets are known or likely to contribute to benefits and how pressures are known to affect these assets. This allows us to see the connections between pressures and benefits and understand how pressures from varying activities may combine to influence benefit availability. It also makes explicit those pressures that do not interact with benefit availability. Once relationships between pressure and benefit have been established, risk assessment is then possible. Risk assessment asks three questions of the policies controlling the pressures: 1) Are aims to protect benefits explicitly detailed within policies? 2) Do policies explicitly detail aims to protect natural capital assets? 3) How effective are the controls in achieving their stated aims? Examination of these questions highlights the gaps in governance that allow ongoing pressure and degradation of natural capital assets that may lead to diminished availability of benefits.
The benefits considered in this study include the 'large scale' benefits characterized in literature engaging with ecosystem services (e.g. Saunders et al., 2015; Rees et al., 2022). These include a healthy climate, food, marine genetic resources (MGR), marine mineral resources, cultural and/or spiritual wellbeing, opportunities for tourism and/or recreation and knowledge about the environment. Pressures are classified using the pressure definitions developed by The Marine Life Information Network (MARLIN) – the MareESA framework for pressures and benchmarks (Tyler-Walters et al., 2018; Garrad and Tyler-Walters, 2020; MARLIN, 2022; Tyler-Walters et al., 2022). Most pressures are exerted via multiple activities, those considered here include the major sectors active in ABNJ – carbon emissions, fishing, shipping cable laying, and scientific research (Durussel et al., 2018). We also consider the emerging pressures of MGR extraction and deep seabed mining (DSM). The policies governing these activities are considered to be the controls of the pressures on natural capital assets and benefit flows. The outcomes of the risk assessment depicted in Figure 3 is combined within a matrix (Table 1) to produce a final risk rating. Final risk ratings are determined by the highest level of risk indicated for a pressure to avoid masking (for detailed results by sector and policy see Supplementary Information).
2.1 Which natural capital assets of ABNJ contribute to human benefit?
2.1.1 A healthy climate
A healthy climate is maintained via global ocean processes that transport heat on a planetary scale (Garzoli and Matano, 2011; Lozier, 2012) the release and storage of water vapour (Stocker, 2013), and draw down atmospheric carbon dioxide (CO2) for long term storage. The water column, an abiotic asset, supports the drawdown of atmospheric CO2 via dissolution across the surface and vertical mixing into dense bottom waters. Biotic assets, such as those species involved in the ‘biological pump’ – an ecosystem service that transfers atmospheric carbon CO2 to the deep water and sediments (also referred to as blue carbon habitats, assets in and of themselves) – include the full food chain from apex predators to plankton and microbes (Ducklow et al., 2001; Herndl and Reinthaler, 2013). Deep-sea environments also play an important role in climate regulation including through significant contributions to nutrient cycling driven by microbes, viruses, protists and prokaryotes, and sponges (La Bianca et al., 2023), and carbon storage (Maier et al., 2021), cycling (Sweetman et al., 2019) and sequestration (Dunham et al., 2018).
2.1.2 Food
Fish, when extracted from the ocean, provide food and other nutritional products such as fishmeal. Fish stocks of commercial interest (biotic assets) are also reliant on other natural capital assets. These other assets include benthic habitat that acts as nursery (Costello et al., 2005; D’Onghia et al., 2012; Pham et al., 2015) and spawning grounds (Freese et al., 2003; Costello et al., 2005; Koenig et al., 2005; Marliave et al., 2009; D’Onghia et al., 2010; Pham et al., 2015). The bathymetry of the seafloor can drive upwelling which, similar to eddies and fronts between water bodies, creates shear and deliver nutrients to the surface (Snyder et al., 2017). Increased nutrient availability drives increased primary productivity (via biological assets such as phyto- and zooplankton concentrations) and make these areas important feeding grounds for target species (Sergi et al., 2020; Wright et al., 2021). Accordingly, the physical and chemical attributes of the water column (abiotic assets) can influence the availability of fish for food, such as the depth of the upper mixed layer, temperature and oxygen concentrations (Bernal et al., 2010; Arrizabalaga et al., 2015).
2.1.3 Marine genetic resources
Marine genetic resources (MGRs) refer to primary and secondary metabolites, with the former relating to genes, proteins and enzymes and the latter, biologically active molecules such as marine natural products (Harden-Davies, 2017). MGRs of the deep sea offer the potential for a variety of applications including the development of pharmaceutical products (Van Dover, 2014; Harden-Davies, 2017; Hesketh Best et al., 2019) and products for industries as diverse as agriculture and cosmetics (Leary et al., 2009; Martins et al., 2014). While many marine organisms (biotic assets) may host MGR of commercial or societal interest, deep-sea sponges (Porifera) are the most sampled marine phyla for biologically active molecules (Koch et al., 2021). Adaptations to deep-sea conditions of extreme pressure, no light and low oxygen concentrations can give rise to biochemical and physiological traits/characteristics unique to such environments (Skropeta and Wei, 2014). With some species of sponge filtering seawater at ratios of sponge to seawater of 1:50,000, they are in contact with vast quantities of debris, nutrients and planktonic bacteria making them valuable hosts of potential MGRs and valuable biotic assets (Weisz et al., 2008; Koch et al., 2021). The distribution of these targeted organisms is associated with silicate concentration, temperature, depth and availability of particulate organic carbon and accordingly is often limited to depths greater than 200m (Howell et al., 2016).
2.1.4 Mineral substance used for material purposes
The deep seabed is of increasing commercial interest for its deposits of manganese polymetallic nodules, seafloor massive sulphides and cobalt-rich crusts (Miller et al., 2018; Månberger and Johansson, 2019; Lèbre et al., 2020; Lee et al., 2020). The deposits of interest (abiotic assets) have formed over millennia and are associated with specific geomorphological features (Hein et al., 2013). Nodules form slowly by the adsorption of manganese from seawater or sediments, likely driven by bacterial oxidization (Blöthe et al., 2015), and comprise important habitat in the deep sea where they are often the only local hard substrate (Vanreusel et al., 2016; Miller et al., 2018). Seafloor massive sulphides are found at the sites of hydrothermal vents along ocean ridges and so their distribution is highly restricted (Miller et al., 2018).
2.1.5 Cultural and spiritual wellbeing
The ocean is important to many people, if not all of society. It provides wellbeing through relationships and rituals that shape identities, cultures and ways of life. The benefit of spiritual wellbeing accrues both directly to specific groups that can evidence their connection to the ocean, such as surfers or fishers, and also indirectly through intangible pathways that are not readily available for measurement (Boswell, 2022). For many Indigenous groups, nature, people and spirits are profoundly connected and exist in varying cosmologies and traditions (Childs, 2020). The health of the environment, including marine environments (e.g. Childs, 2020; DOSI, 2021), and its natural capital assets are therefore central to the cultural and spiritual wellbeing of these groups (e.g. Harmsworth and Awatere, 2013). The transmission and ‘life’ of this knowledge (frequently referred to as traditional or Indigenous knowledge/science) that perpetuate these traditions is often described as ‘place-based’ (Pascua et al., 2017) reflecting the specific and deep relationships between people and nature. With the natural capital assets of ABNJ contributing to many diffuse and globally important human benefits, their health is bound to that of coastal waters and integral to the climate experienced at a local level (Popova et al., 2019). Accordingly, the health and condition of ABNJ natural capital assets supports the cultural and spiritual wellbeing of groups located both at the coast and inland. A tangible illustration of this dependence is provided by the cultural significance of marine species that use these regions for part of their lifecycle such as sharks, marine mammals and tuna (Tilot et al., 2021).
2.1.6 Opportunities for tourism and recreation
Tourism and recreation are described as spiritual acts that promote wellbeing (Jarratt and Sharpley, 2017) and are important components of many sustainable development programmes (Patil and Diez, 2016; Roberts and Ali, 2016; Rustomjee, 2017). The global ocean, including ABNJ is central to the control of climatic conditions that may make a destination desirable or a recreation activity attractive or possible (e.g. predictable winds for water sports). Furthermore, ABNJ plays an important, if largely unknown, role in the life histories of migratory mega-fauna that frequent coastal areas and are of great interest to tourists. For example, whale shark tourism has been shown to generate substantial income from seasonal aggregations (Cagua et al., 2014; Huveneers et al., 2017; Drew et al., 2021; Ziegler et al., 2021) with benefits extending beyond those actors immediately involved in boat-based activities (Huveneers et al., 2017; Norman et al., 2017). Beyond coastal tourism, small numbers of people will encounter marine species and benefit from the seascapes of the open ocean via cruise liner and yachts and also deep-sea submersibles (Ottaviani, 2020). Despite the low number of tourists involved in these activities, these industries all have the potential to generate economic benefit flowing to the areas where these individuals embark and disembark and the locations in which associated businesses are based.
2.1.7 Knowledge
ABNJ is one of the least studied areas of the planet (Howell et al., 2020) and whilst remote it offers a vast opportunity for new (secular) knowledge. The region can also be considered to be understood by non-secular sciences such as those held by Indigenous groups (Chao and Enari, 2021). This non-secular knowledge is often intangible and tightly woven to the cultures and spiritual wellbeing of Indigenous groups (see section 3.5.). The benefits of ‘new’ secular knowledge, which should also be developed to incorporate Indigenous sciences, could accrue to society as a whole where ground-breaking discoveries such as options for climate change mitigation or medical applications (e.g. vaccines) are developed. However, the benefit of knowledge does not necessarily depend on a healthy environment. A degraded environment can still offer the potential for knowledge accrual, however the option (bequest value) to understand natural capital assets in good status may have been lost. A more direct flow of knowledge benefit relates to education, formal scientific training and research or the existence value of knowing about remote marine systems or species. The latter may be developed through mediums targeting non-specialist audiences such as documentaries (Ankamah-yeboah et al., 2020). While the benefit of formal knowledge commonly accrues directly to those that have chosen to study the field, both in terms of intellectual fulfilment and remuneration (Ottaviani, 2020), the knowledge base developed through funding and research programmes by these individuals affords a degree of power. This power enables the knowledge-holders to make decisions that enable exploitation and decisions that may disadvantage other groups (Morgera, 2021). At present the benefit of knowledge is focussed on specific aspects of natural capital assets such as MGRs (section 3.3) and minerals (section 3.4.) where benefits have the potential to accrue to society as a whole, but currently tend towards small numbers of actors that have the power, agency and capital to exploit them (Blasiak et al., 2020).
2.2 Pressures on the natural capital assets of ABNJ
2.2.1 Climate change
Climate change is forced primarily by anthropogenic carbon emissions which are driving changes to the biological, chemical and physical composition of biotic and abiotic marine natural capital assets (Helm et al., 2011; Sweetman et al., 2017). A warming atmosphere is increasing the stratification of the upper ocean which inhibits mixing between the surface and deep waters (Keeling et al., 2010; Breitburg et al., 2018; Li et al., 2020). This has critical implications for the maintenance of the biological pump and global heat transport (Smith et al., 2008; Keeling et al., 2010; Buckley and Marshall, 2016; Sweetman et al., 2017) which potentially restrict the ability of the ocean to buffer against ongoing carbon emissions and could result in dramatic changes to climate globally (McGowan et al., 1998; Smith et al., 2008; Bindoff et al., 2019). Critical impacts influencing biotic assets include the expansion of hypoxic areas (oxygen minimum zones - OMZs) (Stramma et al., 2010; Schmidtko et al., 2017; Levin, 2018) which, through the constraint of energy acquisition, can restrict growth, reproduction, resilience against disease, and survival of many organisms (Breitburg et al., 2018; Limburg et al., 2020). The tolerance and response to reductions in oxygen vary widely and with oxygen availability governing the vertical, horizontal and temporal distribution of organisms, oxygen loss alters the structure of food webs as sessile organisms unable to adapt perish and mobile organisms avoid areas of low oxygen (Vaquer-Sunyer and Duarte, 2008). Habitat compression of mobile species can increase the energy required for feeding in new areas and lead to aggregation at shallow depths or at the margins of OMZs (Koslow et al., 2011; Stramma et al., 2012; Gilly et al., 2013) and for demersal species can lead to increased competition for prey (Casini et al., 2016). While some species will be able to adapt and take advantage of expanded OMZs (Breitburg et al., 2018), deoxygenation of the ocean is predicted to have a profound impact on benefits arising from biotic natural capital assets of ABNJ such as food and cultural wellbeing.
Similar to the effects of deoxygenation, ecological responses to acidification vary among species yet scientific consensus suggests a trend towards increasing biomass of primary producers and decreasing taxonomic diversity (Hall-Spencer et al., 2008; Sutton et al., 2018; Doney et al., 2020). Planktonic community structure is expected to drastically alter with acidification with consequences to the productivity of the entire food web (Bach et al., 2017; Taucher et al., 2017; Doney et al., 2020). Acidification corresponds with a lowering of the calcium carbonate saturation state, the threshold at which calcium carbonate is more likely to form or dissolve and likely impair the calcification of certain organisms including some zooplankton, corals, crustaceans and echinoderms (Mollica et al., 2018; Doney et al., 2020). Other consequences relate to increased susceptibility to bioerosion, increased prevalence of harmful algal blooms, lower survival rates, slower growth and development of organisms, particularly when acidification is experienced alongside warming temperatures (Kroeker et al., 2013; Doney et al., 2020). Similar to effects of deoxygenation, acidification looks set to drastically alter species composition and organization, and could drive irreversible changes in important environments such as deep-sea systems that depend on endemic species with little functional redundancy within species groups (Barry and Widdicombe, 2011). The shift towards reduced ecological complexity (and biodiversity) of pelagic systems (and biotic assets) as driven by acidification, deoxygenation and warming, and particularly where these threats combine (Gruber et al., 2021), is suggested to reduce biodiversity, productivity and the resilience that this imparts (Danovaro et al., 2008; Gascuel and Cheung, 2019).
2.2.2 Physical loss & damage of natural capital assets
Of the activities considered, fishing, DSM and cable laying are those most likely to implicate physical loss and damage of natural capital assets (habitat) in ABNJ (Carter et al., 2009; ICPC, 2016; Durussel et al., 2018; Miller et al., 2018; Bell et al., 2019). The permanent loss of assets precludes any future utility or benefit arising from these assets in addition to voiding all existence and bequest values imparted to all society and future generations.
2.2.2.1 Fishing and deep seabed mining
Demersal fisheries, particularly trawl-based fisheries can remove or damage benthic habitat affecting both biotic and abiotic assets, with seamounts particularly at risk (Bell et al., 2019). With recovery predicted to take decades to centuries after the cessation of fishing, associated impacts are often considered to be permanent (Koslow et al., 2001; Clark et al., 2016). DSM involves the direct removal of benthic assets as the target resource for the industry and damage to associated and proximal biotic assets (Jones et al., 2017). The abiotic aspects of these assets can include potential nursery grounds (Salinas-De-León et al., 2018) which in turn support biotic assets of fish populations, and seamounts which are important abiotic assets and host significant biotic assets such as fish and sponge aggregations. Associated and nearby faunal and microbial communities which contribute to nutrient regeneration and biogeochemical cycling through respiration and bioturbation could also be affected (Sweetman et al., 2019) For many assets, there is high uncertainty associated with recovery, which if possible is predicted to occur over extremely long time periods (Miljutin et al., 2011; Jones et al., 2017; Vonnahme et al., 2020).
Dewatering and vehicle-generated plumes from DSM operations are also anticipated to impact benthic and midwater biotic assets via potentially clogging respiratory and olfactory surfaces, reducing the availability of food materials, reducing plankton buoyancy, and increasing turbidity (Ellis, 2001; Boschen et al., 2013; Jones et al., 2017; Drazen et al., 2020). Consequences of these effects may include altered behaviour, disruptions to connectivity, a loss of reproductive capacity and modified trophic interactions (Washburn et al., 2019). Organisms of the deep sea are thought to be particularly sensitive to even relatively small increases in turbidity owing to extremely low baseline concentrations of suspended sediment among other factors (van der Grient and Drazen, 2022). The frequency and temporal persistence of plumes is estimated to last over 10 years and vary depending on the depth at which the plume occurs (Boschen et al., 2013; Washburn et al., 2019; van der Grient and Drazen, 2022). Despite a lack of understanding of the exact impacts associated with plumes, experts agree that they pose one of the highest risks for deep-sea environments and are expected to alter the structure of food webs and physically effect biotic and abiotic aspects of natural capital assets and thus the provision of associated benefits (Danovaro et al., 2008; Washburn et al., 2019).
2.2.2.2 Submarine cables
Submarine communication cables are the most common form of submarine infrastructure in ABNJ, with approximately 314,350km predicted to have been laid up to 2018 (Burnett and Carter, 2018). Impacts to benthic environments (and therefore natural capital assets) are managed via careful routing that minimizes any need for armouring or burial, and the use of inert marine grade sheaths. These management measures, coupled with a relatively low associated footprint support consensus that associated environmental impacts are low (Carter et al., 2009; ICPC, 2016; ISA, 2019d). Repair of cables is associated with a larger impact on benthic habitats, however the “fault” rate (requiring repair) is considered to be low with less than four repairs per year in ABNJ which represents two percent of global repairs with the remaining 98 percent of repairs required within EEZs (ISA, 2019d).
2.2.2.3 Research and marine genetic resources
Research and the extraction of MGR which is commonly undertaken as a secondary activity of other operations, such as the sampling programme of a research cruise in ABNJ (Humphries et al., 2020) also involve physical loss. However, the losses associated with research and sampling for MGR are highly targeted and thought to present a relatively small pressure on marine ecosystems (Hubert, 2011; Van Dover, 2014; Bernal and Simcock, 2016). Pressure pathways include the physical removal of target biotic assets via trawls, grabs and cores, or the use of human and remotely operated vehicles which also may cause ecological disturbance from noise and light (Hubert, 2011; Van Dover, 2014).
2.2.3 Physical pressure (other)
Other pressures include those relating to litter discharged by all seagoing activities. This includes litter from fishing activity, such as purposeful or accidental disposal of gear (Vieira et al., 2015), shipping (Ryan et al., 2019) and land-based sources (Ryan, 2014). Litter has been found at all depths, including the deep sea (Chiba et al., 2018) and pressures arising include entanglement of and ingestion by large animals (Kühn et al., 2015; Chiba et al., 2018), and those relating to chemical toxicity both through leaching into the receiving environment and, as micro-plastics are ingested and accumulated up the food web (Cole et al., 2013; Setälä et al., 2014; Chiba et al., 2018). Other sources of litter include ballast weights from remotely operated vehicles (commonly comprising solid steel plates or pellets), research instrument moorings and in some cases the instruments themselves which can smother benthic habitat (Ramirez-Llodra et al., 2011; Bernal and Simcock, 2016).
Further physical pressures relate to the introduction of noise and light by shipping and ship-based activity (Walker et al., 2019). Similarly, DSM operations, submarine cables and deep-sea research are also associated with the introduction of light, noise, and electromagnetic charges. Whilst these pressures are considered to be low risk in ABNJ, there is high uncertainty associated with assessments and understanding (Van Dover, 2014; Washburn et al., 2019). Potential impacts that have been observed in shallow water relate to larval recruitment and behavioural responses of animals (Gill, 2005; Lillis et al., 2013; Washburn et al., 2019). The presence of vessels and their activity has been shown to effect species distribution and movement, such as the attraction of seabirds and top predators to fishing vessels (Anderson et al., 2011; Hamer et al., 2012; Seco Pon et al., 2015) and the potential creation of barriers (Pirotta et al., 2019). With these pressures effecting biotic assets such as species and the habitats on which species are dependent, the benefits at risk include that of food and health and those related to populations of large marine animals such as tourism.
2.2.4 Pollution and other chemical changes
All ship-based activity presents the potential for pollution. Emissions from the global shipping industry accounted for approximately three percent of all anthropogenic CO2 emissions in 2018 (IMO, 2020) and discharges also include food waste, raw sewage, wastewater and other treated discharges (e.g. bilge water, ash from incinerated waste) (Butt, 2007; Zuin et al., 2009; Walker et al., 2019). For many accidental oil spills that may occur offshore ‘natural remediation’ is considered the most appropriate form of treatment (Walker et al., 2019).
Dewatering plumes, and to a lesser degree those arising from vehicle movement, from DSM operations present a risk of toxic heavy metal contamination (Miller et al., 2018). These metals can bioaccumulate and enter the food chain (Drazen et al., 2020) and can affect the functioning of microbial communities implicated in nutrient cycling (Orcutt et al., 2020). Plumes can also lead to nutrient and organic enrichment which can result in hypoxia and alter the structure of food webs.
For those pressures arising from DSM, please see Section 4.2.2.
2.2.5 Biological pressures
Many target species of commercial interest in ABNJ are vulnerable to overfishing. Many of these target species sit high in the food chains and their targeted removal is described as ‘fishing down’ marine food webs (Pauly et al., 2005) and is attributed to a range of impacts, reducing fish biomass and can lead to ecosystem regime shifts (Daskalov et al., 2007). Importantly, while the fishing of stocks in ABNJ does not commonly lead to benefits critical to human health and nutrition (Schiller et al., 2018), through reducing the availability of fish it can influence the availability of these benefits to smaller-scale fishers within jurisdictional waters (Block et al., 2011; Sumaila et al., 2015) for whom they may be essential.
Other pressures from fishing in ABNJ relate to bycatch of non-target species including seabirds, top predators and fishes. The removal of non-target species threatens associated direct benefits such as food and nutrition, spiritual wellbeing (Tilot et al., 2021) and opportunities for tourism and indirect benefits such as a healthy climate (Davison et al., 2013; Rhodes-Reese et al., 2021). As the complexity of ecosystems is reduced, through the removal of top predators, this corresponds with a reduced resilience of an ecosystem to adapt to other pressures such as changing ocean chemistry and temperature which exacerbates the risk of ecosystem service loss (Pauly et al., 1998; Myers and Worm, 2003; Davison et al., 2013; Daw et al., 2015; Cheung et al., 2018). Large marine migratory species are also at risk of ship strike (Walker et al., 2019), a risk which is unquantified for ABNJ owing to difficulties in detection and monitoring by the shipping industry (Walker et al., 2019; IWC, 2022). However, given the populations of some species are very small, the removal of even low numbers could influence ecosystem services (Cook et al., 2020; IWC, 2022).
Human activity in ABNJ poses a risk of the translocation and introduction of invasive species. Potential vectors for such species include the ballast of ships implicated in all activity in these regions (Walker et al., 2019) and that used to control the buoyancy of sampling equipment on human and remotely operated vehicles (Thaler et al., 2015). This risk is particularly relevant for the transfer of species between deep-sea habitats which can be extremely isolated and unique in their ecology (Van Dover, 2014).
3 Case study: risk assessment of South East Atlantic ABNJ
3.1 Region overview
Common to many regions of the southern hemisphere and remote ocean, there has been significantly less ecological and oceanographic study of the South East Atlantic (S.E. Atlantic) as compared to northern regions (Howell et al., 2020). The S.E. Atlantic ABNJ encompasses two major basins, the Angola basin to the north and Cape basin to the south, separated by the Walvis Ridge (Pérez-Díaz and Eagles, 2017) and bounded to the west by the Mid-Atlantic Ridge (MAR). Running north-east to south-west the Walvis Ridge has an associated chain of seamounts and terminates at the archipelago of Tristan da Cunha. The sub-tropical archipelagos of Ascension Island and Saint Helena are located to the north of the region. Another prominent, yet less studied, feature is the Agulhas Ridge, similarly host to seamounts (Le Roex et al., 2010). Seamounts are also found outside of these ridge systems and have been subject to varied degrees of sampling (Bridges et al., 2023). Almost all seamounts have been observed to host cold-water coral communities and large dead reefs in areas of the region (Durán-Muñoz et al., 2014; Bergstad et al., 2019; FAO, 2022). These varied habitats are associated with high benthic diversity (German et al., 2011) and coupled with important oceanographic features in the region such as areas of upwelling, and globally important currents are highly significant ecologically both in terms of productivity and for the lifecycles of threatened and highly migratory species (Durussel et al., 2018). Other important habitats in the S.E. Atlantic ABNJ include hydrothermal vents on the MAR (Beaulieu and Szafrański, 2020) and potential polymetallic nodule fields at abyssal depths in the Cape Basin (Hein et al., 2013). The limited knowledge of S.E. Atlantic ABNJ perhaps reflects the delay in commercial interest in the region as compared to other oceanic regions (Bridges et al., 2023). Whilst knowledge of the area is increasing, there are large swathes of the region including seamounts that remain to be studied, and ecological processes and functions are largely unknown (Clark et al., 2020).
3.2 Pressure policy controls in S.E. Atlantic ABNJ
Many of the pressures exerted on natural capital assets of the S.E. Atlantic ABNJ are globally pervasive and cannot be disassociated to consider only those spatially relevant to the case study. For those pressures where spatially specific information is available, that associated at a global level is considered. Results from the assessment of whether policy controls consider benefits are summarised in Tables 2 and 3 presents the risk register as it relates to the benefits arising from natural capital assets of the region.
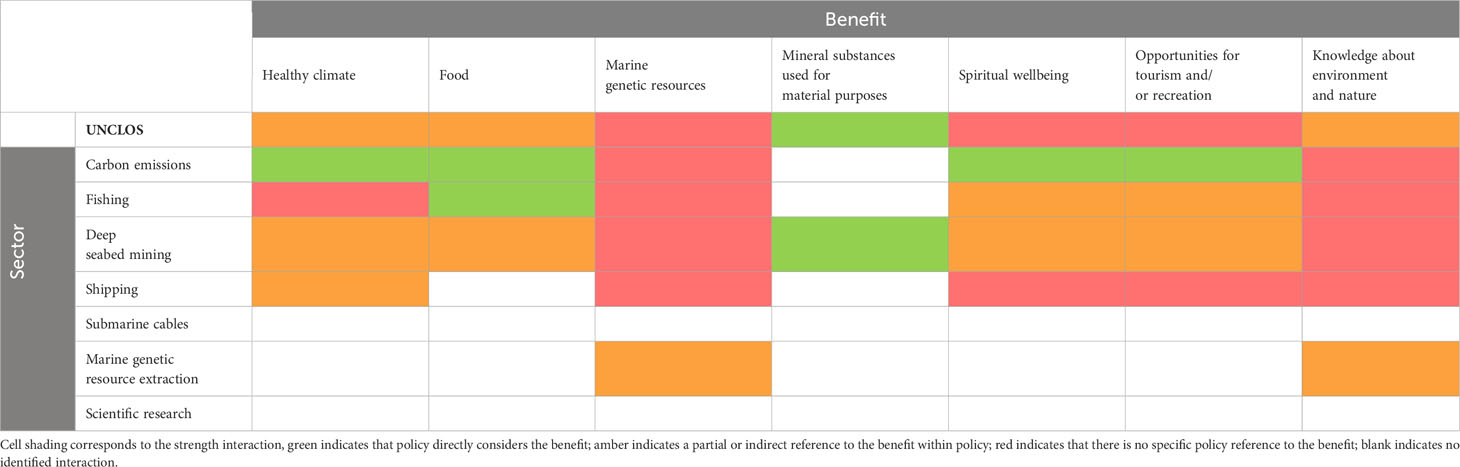
Table 2 Indicates whether sectoral policies specifically consider the benefits arising from the natural capital assets of the S.E. Atlantic ABNJ.
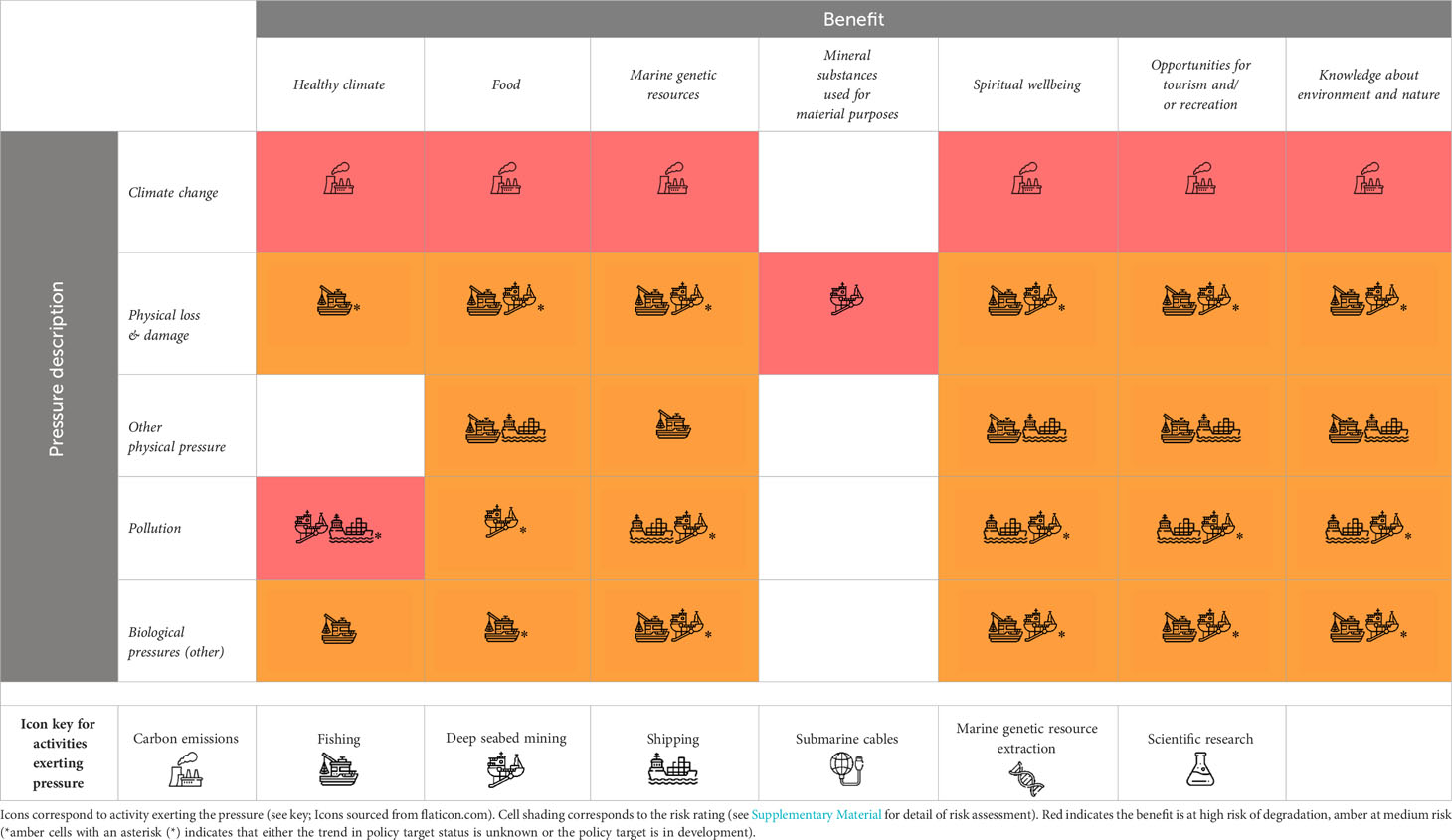
Table 3 A natural capital risk register. Cell shading indicates the risk of degradation of benefits arising from natural capital assets of the S.E. Atlantic ABNJ via pressure type.
3.2.1 Climate change
Climate governance centres around the reduction of carbon emissions and the agreed aim of the United Nations Framework Convention on Climate Change (UNFCCC) of preventing “dangerous anthropogenic interference with the climate system” (UNFCCC, 2015; UNFCCC, 2021b) where the system is considered to include the ocean (Morgera et al., 2023a). Despite explicit reference in commitments outlined in the UNFCCC the international climate regime is limited in its consideration of the ocean (Elsler et al., 2022; Morgera et al., 2023a). Ocean environments are formally recognised in the Preamble to both the Paris Agreement (UNFCCC, 2015) and the Glasgow Climate Pact (Lennan and Morgera, 2022) the latter of which casts marine ecosystems (among other natural systems) as sinks of greenhouse gases that require protection, conservation and restoration (UNFCCC, 2021a). The Glasgow Climate Pact includes a call to integrate and strengthen ocean-based action with work plans, and to hold an annual dialogue in support of this. This broadly sets the stage for climate action and a reduction of carbon emissions and touches on the need to ensure the ‘system’ (including ABNJ and the S.E. Atlantic) can continue to function and regulate global climate. Accordingly, the benefits afforded to humanity are considered at a high level, yet detail relating to the pressure pathways by which climate change may influence these benefits is absent.
Nations present their climate action strategies that respond to the aims set by the Paris Agreement (UNFCCC, 2015) through Nationally Determined Contributions (NDCs) (Bodansky, 2016; Bäckstrand et al., 2017). NDCs outline the operationalisation of the Paris Agreement through detailing commitments to climate action. In a 2016 review of NDCs, Gallo et al. (2018) found that the majority included marine issues but were notably absent for some countries with large EEZs. The authors also found, reflecting the concerns of others (see Harrould-Kolieb, 2020), that there is limited political attention on the issues effecting biogeochemical processes (Gallo et al., 2018) and therefore the pressure pathways that consider the benefits arising from natural capital assets of ABNJ. While reductions in CO2 emissions will reduce pressure on the natural capital of ABNJ, the region is outside the jurisdiction of the UNFCCC and so is not specifically considered within NDCs (Gallo et al., 2018). Assessment of end-of-century median warming suggests that we are currently on track to miss established targets (Climate action tracker, 2022), accordingly associated pressure from climate change on the S.E. Atlantic ABNJ is likely to continue.
3.2.2 Physical loss & damage of natural capital assets
The Biodiversity Beyond National Jurisdiction Agreement (BBNJ Agreement), agreed in 2023, seeks to ensure the conservation and sustainable use of marine biodiversity in ABNJ through addressing gaps in the preexisting UNCLOS regime that have allowed biodiversity (and natural capital asset) degradation (Gjerde et al., 2008). While the BBNJ Agreement does not control specific pressures, it sets out the need for the precautionary principle, the ecosystem approach and an approach that builds ecosystem resilience through an integrated governance approach using the best available science and traditional knowledge. Through the aspirations set within the text it aims to improve the status of natural capital assets within global ABNJ including the case study area of the S.E. Atlantic. It focusses on the sharing of the benefits of MGRs (Humphries et al., 2020), the development of standards and guidelines for EIAs (De Santo et al., 2020; Humphries and Harden-Davies, 2020) and area-based tools for management. While the BBNJ Agreement sets out the requirement to not undermine the effectiveness of pre-existing governance, it promotes the application of Strategic Environmental Assessment (SEA) of plans and programmes to understand cumulative effects and place EIA within context (UN, 2023). Whilst fishing is largely not subject to EIA, it’s effects should be incorporated into future SEA processes.
3.2.2.1 Fishing
Regional Fisheries Management Organisations (RFMOs) are required under the 1995 Fish Stock Agreement (UNFSA) to conserve high seas living resources (UNGA, 1995). This endeavour builds on the requirement of the Convention on Biological Diversity (CBD) taken forward in the Kunming-Montreal Global Biodiversity Framework adopted in 2022 (CBD, 2022) to sustainably manage marine living resources through the application of an ecosystem approach, the elimination of illegal unreported and unregulated fishing (IUU), the minimisation of detrimental impacts of fishing (CBD, 2010) among other international agreements including Sustainable Development Goal (SDG) 14.4 seeking to control destructive fishing practices (UN, 2015). In the S.E. Atlantic there are four regional fisheries bodies (RFBs), the International Commission for the Conservation of Atlantic Tunas (ICCAT), the Commission for the Conservation of Southern Bluefin Tuna (CCSBT), the South East Atlantic Fisheries Organisation (SEAFO) and the Fishery Committee for the Eastern Central Atlantic (CECAF), the latter of which is not a RFMO and as such only has an advisory mandate (Durussel et al., 2018). Both ICCAT and SEAFO have adopted an ecosystems-based fisheries management (EBFM) approach in line with the FAO voluntary code of conduct for responsible fisheries that seeks to “take into account all their relevant biological, technological, economic, social, environmental and commercial aspects” (FAO, 2015). In theory these aims imply that all associated benefits and pressures are included within decision-making surrounding fishing and fishing activities.
The areas of fishing interest in the region, particularly those targeting the top and slopes of seamounts in the S.E. Atlantic ABNJ are all known as biodiversity hotspots (Thompson et al., 2016; Bergstad et al., 2019; Bridges et al., 2023) and overlap with many areas classified as vulnerable marine ecosystems (VMEs; as called for by UNGA Resolution 61/105). To protect VMEs from significant adverse impacts arising from demersal fishing SEAFO has closed 12 areas to fishing (SEAFO, 2016) and applies exploratory fishing rules where fishing in new areas is subject to impact assessment and a ‘move on’ rule whereby fishing is halted in that area if VME indicators are encountered (SEAFO, 2016). Through the identification of VMEs and the setting out of protocols that mitigate damage to VMEs, ecologically important areas of benthic habitat (assets) are protected including areas that are important for lifecycles of species (Pham et al., 2015; Bell et al., 2019). However, associated reports have been found to be of varied quality, and commonly limited in their integration of assessment of wider ecosystem effects (e.g. migratory species) beyond direct impacts on VME habitats (Kaikkonen et al., 2022). Further, with bottom interaction (i.e. abrasion/penetration) still occurring outside of VMEs by trawling, bottom long-lining and IUU activity, ecosystem services associated with benthic natural capital assets, such as the provision of genetic material and the protection of lifecycles, habitat and gene pools among others will likely still be affected. Policies exist seeking to control IUU and alleviate associated pressures including the Port State Measures Agreement that restricts vessels in accessing land-based infrastructure to realise the value of their catch (FAO, 2016) and the FAO Compliance Agreement that holds flag States accountable for the compliance of their vessels with international conservation and management measures (FAO, 1995). In the S.E. Atlantic region SEAFO implements an authorized vessel list and contributed to the IUU vessel lists compiled by other Atlantic and Southern Ocean RFMOs (Bridges et al., 2023). However, while these policies seek to alleviate pressures on benthic natural capital assets their effectiveness is unknown owing to a lack of monitoring, low levels of compliance, cooperation and transparency (Bell et al., 2019; Guggisberg, 2022). While little information is available to appraise the pressure of fishing on the natural capital assets of the S.E. Atlantic ABNJ seamounts have been found to have severe damage by sea-urchin hyperabundance pointing to degradation from overfishing (Buhl-Mortensen et al., 2022; Bridges et al., 2023).
3.2.2.2 Deep seabed mining
While there are currently no exploration contracts for DSM in the case study region, potential targets for the future extraction of cobalt-rich ferromanganese crusts and polymetallic nodules have been identified (Hein et al., 2013).
The International Seabed Authority (ISA) established under UNCLOS (UN, 1982) and the 1994 Agreement (DOALOS, 2016) is the organisation through which State Parties to UNCLOS manage mineral resources in ABNJ for the “benefit of mankind as a whole” (UN, 1982). Through the ISA, State Parties to UNCLOS organise and control activities and resources in the Area (ISA, 2020). The ISA is in the process of developing a ‘Mining Code’ which is set to provide a comprehensive set of rules, regulations, and procedures to regulate all aspects of DSM activity in ABNJ from prospecting and exploration, to exploitation (ISA, 2019e). Regional Environment Management Plans (REMPs) are also being developed for ‘priority regions’ to provide the ISA with spatial information to ensure the protection of the marine environment, and regulations for exploitation, setting out the procedure for environmental impact assessment (EIA) in the Area are at a draft stage (ISA, 2022).
REMPs are proposed as a way to provide contractors and sponsoring States with strategic information to support “informed decision-making that balances resource development with the protection of marine environment at regional scale” (ISA, 2019c). A core function of REMPs is to identify Areas of Particular Environmental Interest (APEIs), a network of ecologically representative areas that if protected coherently “preserve [the] ecological balance of the marine environment” in ABNJ. To date a REMP has been developed and adopted for the Clarion Clipperton Zone (CCZ) in the Pacific and efforts are underway to detail a REMP for the Mid-Atlantic Ridge region. Data applied to inform the selection of APEIs for the CCZ was found to be constrained with respect to quality, validation and completeness when answering questions relating to the assessment of the representation and ecological coherence of the proposed APEI network (Wedding et al., 2015; ISA, 2019a). Although now partially addressed via subsequent expansion (Blanchard and Gollner, 2022), the network design recommended by scientific experts advising the ISA was compromised by modification to accommodate the exploration contracts (and areas of DSM interest) that had been previously granted (Wedding et al., 2015).
In addition to REMPs and APEIs, the impacts of DSM projects are set to be considered through an EIA process. This process will appraise the “biophysical, social and other relevant effects” of the project (ISA, 2019b) and will follow steps of screening and scoping to focus the Environmental Impact Statement on the most significant environmental issues and risks. Impact and risk analysis will demonstrate how projected effects will be managed “within acceptable levels” following an environmental management and monitoring plan. Assessment of impacts is set to follow the mitigation hierarchy of avoid, minimize, restore/rehabilitate and finally, offset of impacts.
Currently guidance is weak in its support of ecological baseline definition and in procedures for data collection, storage and management for both EIA and cumulative impact assessment. Given the shortcomings of previous EIA for seabed mining projects in effectively characterizing and assessing all potential impacts, agreed standards and thresholds for the industry are felt to be essential (Clark et al., 2019). While socioeconomic and sociocultural assessment are also required to be considered within impact assessment (ISA, 2019b), developing guidance of the ISA does not currently advise how these assessments should be undertaken.
In their current form, the developing controls for the emerging DSM industry are unlikely to safeguard natural capital assets of the S.E. Atlantic. Perhaps most significantly SEA of the region is not required (McQuaid et al., 2022). The absence of SEA undermines assessment of significance and acceptability of potential impacts. While providing a strategic attempt to protect important biodiversity, REMPs are unlikely to protect ecosystem services given that to date their design has focused on access to resource rather than the benefits arising from the natural capital assets they encompass (Wedding et al., 2015). Finally, including biodiversity offsetting within the deep-sea environments implicated by DSM is thought to be unfeasible owing to challenges in restoration and establishing equivalence of biodiversity (Van Dover et al., 2017; Niner et al., 2018). Accordingly, the inclusion of offsets within the processes of impact assessment will likely facilitate degradation of natural capital assets in these regions.
3.2.2.3 Submarine cables
Currently the activity of laying of submarine cables are not required to conduct an EIA under UNCLOS because they are not considered to “cause substantial pollution of or significant harmful changes to the marine environment” (UN, 1982). Current consensus is that submarine cables in ABNJ regions are unlikely to pose a threat to natural capital assets.
3.2.2.4 Research & Marine genetic resources
Current consensus is that the potential impacts arising from Research activity is negligible, however associated activity is commonly guided by internal risk assessment and the development of, and adherence to guidance (e.g. SEAFO, 2014) and sectoral best practice (e.g. Thaler et al., 2015).
3.2.3 Physical pressure (other)
SEAFO prohibit the intentional abandonment of fishing gear except under extreme circumstances, all vessels active under the Convention are also required to mark gear and to commit to retrieve lost or abandoned gear (SEAFO, 2019). However, the International Maritime Organisation (IMO) sets the regulatory frameworks for the shipping industry globally, including in ABNJ (IMO, 2019). Its role is to create a level playing field to ensure the safety, security and environmental performance of the sector. The International Convention for the Prevention of Pollution from Ships (MARPOL) is the main instrument of the IMO that addresses the environmental impacts of the sector. Particularly Sensitive Sea Areas (PSSAs) are identified under MARPOL where an area is recognised for its significant ecological, socio-economic or scientific attributes that may be vulnerable to damage by international shipping activities including the physical presence of vessels (IMO, 2005). Associated Protective Measures (APMs) including routing, speed and equipment restrictions in addition to discharge controls are applied within PSSAs. To date no proposals have been made for PSSAs in ABNJ (UNEP-WCMC, 2017). The levels of implementation and compliance with these policy targets seeking to control the discharge of litter and other forms of pollution are currently unknown. The Cape of Good Hope is of high importance for the global trade of goods and commodities, particularly that flowing between South and Central America, and Asia but also up to North America and Europe (Notteboom et al., 2022). With the African maritime transport sector identified as an opportunity for economic development (Okafor-Yarwood et al., 2020) these routes may increase in importance, thus increasing associated pressure in the S.E. Atlantic ABNJ through which they pass.
3.2.4 Pollution and other chemical changes
MARPOL regulates pollution arising from shipping including chemicals, sewage and emissions, and sets standards for vessel design and discharges and can define Special Areas where environmental sensitivities are felt to preclude ‘safe’ discharge. While the adoption of MARPOL has led to a decline in unregulated discharge of substances harmful to the marine environment, such as oil (Szepes, 2013) full compliance has not been achieved. Compliance has been hampered by the challenges and costs associated with monitoring and taking action against a violation in ABNJ. The link between pollution originating from shipping in ABNJ is not reported as a significant threat to natural capital assets of these regions. Accordingly for this case study, we consider the threat to be negligible whilst noting that the pressure is not effectively governed.
For those pressures arising from DSM, please see Section 4.2.2.
3.2.5 Biological pressures
Legally binding obligations to holistically protect the marine ecosystem from fishing are outlined in both UNCLOS (UN, 1982), UNFSA (UNGA, 1995) and the CBD which explicitly promotes the application of an ecosystem approach to management (CBD, 2010). These aims are supported by various SDGs seeking to decouple economic activity from environmental degradation (8.4) and specifically to end destructive fishing practices (14.4) (UN, 2015). RFMOs are set as the vehicle for fisheries management in ABNJ. While the development, application and understanding of the ecosystem approach varies between RFMO within the S.E. Atlantic ABNJ (FAO, 2015; ICCAT, 2015; Durussel et al., 2018), all RFMOs stipulate a precautionary approach to management (Durussel et al., 2018) and stipulate gear restrictions with ICCAT and SEAFO also closing areas according to temporal and/or spatial criteria (e.g. SEAFO, 2015; ICCAT, 2022). Quota limiting the amount of fish extracted are set according to research undertaken by RFMOs most commonly focussed on target stocks (Crespo et al., 2019), these are set to accord with the respective aim of the organisation (e.g. ICCAT set quota according to an aim of maximum sustainable catch and, SEAFO and CCSBT assign quotas according to a total allowable catch). Alongside pelagic species including tuna and shark, the only bottom fisheries active in 2019 in the region were that for red crab and Patagonian toothfish (Bridges et al., 2023).
In line with the objective of the voluntary FAO code of conduct for responsible fisheries (2015) to “promote [the] protection of living aquatic resources and their environments” and obligations under previously described instruments (e.g. UNCLOS) RFMOS are required to consider impacts to the wider marine ecosystem from their activities including cumulative contributions (see UNFSA). Despite a stipulation for an ecosystem approach to RFMO governance many species in the S.E. Atlantic ABNJ region are considered overfished (Cullis-Suzuki and Pauly, 2010; Gjerde et al., 2013; Spiteri et al., 2021), there are known impacts on non-target fish species (Crespo and Dunn, 2017), and bycatch management remains an issue often not extending beyond requirements for reporting (Gilman et al., 2014; Mucientes et al., 2022). A key issue for RFMO governance is a narrow focus on species of commercial importance that misses implications for the wider system influencing the 95 percent of fish species not targeted by fisheries (Wright et al., 2015; Juan-Jordá et al., 2018; Crespo et al., 2019).
Overfishing is recognised as a serious issue within ABNJ and is largely attributed to IUU fishing. The 1993 FAO Compliance Agreement (FAO, 1995) and 2009 Port State Measures Agreement (PSMA) (FAO, 2016) and the WTO Agreement on fisheries subsidies (WTO, 2022) seek to prevent this (Durussel et al., 2018). The FAO Compliance Agreement dictates the responsibilities of Flag States with respect to vessels bearing their flag, including compliance with international conservation and management measures, and to adequately report information relating to operations, catch and landings and cooperation on information sharing to detect IUU fishing activity. It also stipulates requirements for enforcement and imparts responsibility to issue sanctions that can lead to the refusal or withdrawal of permission to fish. The PSMA imparts the power to States to close ports to vessels suspected of IUU fishing, in the ABNJ identification of these vessels is undertaken in close cooperation with RFMOs. To date 75 countries have ratified the PSMA, implementation is ongoing and monitoring of its effectiveness is yet to be made available by the FAO (Guggisberg, 2022). However, political momentum to address IUU has been signalled by the recently agreed WTO Agreement on fisheries subsidies that prohibits subsidies that contribute to overfishing and IUU fishing.
Species-specific governance seeking to reduce pressure on endangered populations include The Convention on International Trade in Endangered Species of Wild Fauna and Flora (CITES) which stipulates measures that prevent or restrict the international import and export of listed species (CITES, 1973). While the effectiveness of CITES in reducing pressures on marine migratory species is difficult to quantify and restricted in its application to listed species (Lascelles et al., 2014; Vincent et al., 2014) it is thought to complement and strengthen existing fisheries management (Friedman et al., 2018; Friedman et al., 2020). The Convention on the Conservation of Migratory Species of Wild Animals (CMS) requires the conservation and restoration of the habitats of listed endangered migratory species, apply the mitigation hierarchy (avoid, minimize, compensate) in the management of impacts to these species and the prohibition of their ‘taking’ (CMS, 1983). The CMS is a framework agreement under which other instruments relate to a range of species including the Agreement on the Conservation of Albatrosses and Petrels 2004 (ACAP), (see Durussel et al. (2018) for a comprehensive list of these agreements) and has been criticized for its limited effectiveness (Sellheim and Schumacher, 2022).
In addition to MARPOL the IMO adopted the International Convention for the Control and Management of Ships’ Ballast Water and Sediments (Ballast Water Management Convention) in 2004 (IMO, 2004). It requires all ships to agree and implement a ballast water management plan, to carry a record book and to carry out management procedures to an agreed standard. The translocation of species by research, such as through remotely operative vehicles is self-governed, although community-developed best practice is available (e.g. Thaler et al., 2015). Other factors known to present a risk for the spread of invasive species include the introduction of hard substrate in regions of soft substrate where the introduction of new or different habitat can provide a ‘staging point’ from which invasive species may spread (Ramirez-Llodra et al., 2011). While this is a known risk, the minimisation of cable armouring, the activity most likely to introduce such hard material in the region, is thought to mitigate this risk to very low levels (Carter et al., 2009; ICPC, 2016).
4 Discussion
Remote oceanic regions are often portrayed as isolated and unknown, attributes that can ‘devalue’ the natural capital of ABNJ and facilitate their exploitation through a minimisation of societal oversight or interest (Jamieson et al., 2020; Smith et al., 2020; Kaikkonen and van Putten, 2021; Morais et al., 2022). The framework presented provides an easily interpretable visual demonstration of the relationships via which natural capital of ABNJ supports human wellbeing and the pressure pathways that set these benefits at risk and ultimately challenge misconceptions related to their (lack of) importance (Smith et al., 2020). Through the application of a case study of the S.E. Atlantic ABNJ arguably one of, if not the most, ‘ecologically unknown’ regions of the planet we highlight how even with high scientific uncertainty, assessment at a broad scale can inform impact assessment against global benefit availability, and tests of impact significance and acceptability at all scales.
In examining the policy goals protecting the natural capital assets of the S.E. Atlantic ABNJ we show that despite a plethora of policies in place that seek to protect natural capital assets of the region, these measures are often either unmonitored or ineffective. Risk is appraised as ‘medium’ for most pressures because of a lack of data collection or enforcement mandate, and thus an absence of oversight or knowledge over whether a control is effective or not. This is particularly the case where policies look to protect the wider ecosystem such as through an ecosystem approach, or assets that are not those of direct interest to actors engaged with an activity. An example of this would be commercial fishing where monitoring of asset health is required for stocks of commercial interest but requirements for monitoring of non-target species or bycatch (i.e. the wider system or biodiversity) are either absent or weak (Crespo et al., 2019). Similarly, the emerging governance system for the nascent DSM industry is focused on the development of processes that administratively protect commercial benefits through enabling rapid extraction rather than instilling precaution as a priority to understand the potential risks to the natural capital system. Overall, benefits, again outside of those of commercial interest, and particularly those that experienced diffusely by society, are not robustly considered within the policy controls seeking to protect natural capital assets.
The culturally focused benefits including spiritual wellbeing, opportunities for tourism and/or recreation, and knowledge about environment and nature, alongside that of MGRs are most weakly considered within policy. Their consideration is often restricted to an acknowledgement of the socio-economic dependence on a resource and the cultural importance of artisanal fishing or where a specific pressure (e.g. bycatch) relates to a specific connection (cultural importance of migratory species) (Vierros et al., 2020). The complex and inextricable connection to nature and the environment is a clear challenge for governance which commonly falls short of fully incorporating such connections in a meaningful or appropriate way. For many policy controls their reference to societal benefits is general such as that of the emerging DSM governance regime that states the “physicochemical, biological, socioeconomic and other relevant effects” will be considered (ISA, 2021). The benefit of MGRs is considered via an identified need to ensure equity in access as opposed to protection. Linked to the potential of MGRs for medical applications, the benefit of knowledge is weakly considered beyond the need to consider the needs of future generations as stipulated by UNCLOS (UN, 1982). While these targets call for broadscale integration of these values in impact assessment and consequent decision-making, it falls short of setting ambition to protect benefits or human wellbeing.
Furthermore, examining the breadth of policy in place to protect the natural capital assets of the S.E. Atlantic ABNJ, it is clear that despite intention within many policies for an ecosystem approach, the protection of ecosystem resilience is poorly operationalised (Mace et al., 2014). As highlighted by research elsewhere the climate regime poorly encapsulates the role of the ocean in global climate regulation and there is a lack of integration between climate and marine biodiversity governance (Harrould-Kolieb, 2021; Morgera et al., 2023b). The lack of integration is a key signal that the ecosystems approach required by various international and sectoral policies that sets a need to consider the connections among and between natural assets and humans is not being appropriately fulfilled (Borja et al., 2016).
With all benefits, excluding that of abiotic asset extraction (via DSM), affected by multiple pressure pathways the case for an integrated strategic approach that considers connections between the system is strengthened (Elsler et al., 2022). A crucial element of this strategic approach is robust cumulative assessment including comprehensive and appropriate stakeholder engagement. This engagement is particularly important given the poor representation of culturally focused or future-orientated benefits within existing governance regimes. Considering pressures in relation to their influence on benefits forces a cumulative view of impacts and a reflection on the form of consultation or participation required to appropriately consider these risks in decision-making. For ABNJ, where uncertainty is high and those affected globally dispersed, strategic assessment that sits above a project level is likely best placed to consider how the necessary participation is operationalized and to identify options to support the future-resilience of these systems (e.g. Craik and Gu, 2021).
Addressing the risk of cumulative pressures is recognised as a key area of weakness in ocean governance and calls for integration across the institutions governing the ocean are widespread (e.g. Global Biodiversity Framework, UNFCCC, BBNJ, SDGs). Cumulative impact assessment is complex and despite commonly required by policies it operationalisation often lacks a defined approach or procedure (Judd et al., 2015; Cormier et al., 2018; Stelzenmüller et al., 2020). It is challenged by inconsistencies in the definition of scope (i.e. which activities and ecosystem components should be included), available ecological data to support temporal and spatial analysis, and also by available science to support understanding of the relationship between effects which may be additive, synergistic or antagonistic (Stelzenmüller et al., 2018). While the application of the natural capital risk assessment framework to the S.E. Atlantic ABNJ does not assist in a detailed calculation of spatially and temporally linked impacts, it raises awareness of the scale and nature of risks to benefits arising from the combined pressure of activities in the region. The value of the framework is its systems view that enables the potential interactions between natural capital assets and people to be made visible even in the absence of information to quantify these relationships. It provides an easily interpretable visual demonstration of the multiple pressure pathways and indicates gaps in sectoral and regional governance, both in terms of target enforcement or compliance and where targets are being missed, and thus potential opportunities to understand or mitigate pressures. These attributes support the integration of governance across sectors and jurisdiction to enable strategic assessment and planning as demanded by calls for transformed governance of ABNJ (Christiansen et al., 2022).
There are several opportunities available to actively address some of the gaps highlighted in this study. Most obvious are those presented by the development of EIA frameworks under BBNJ and DSM but also extend to the potential investment in marine spatial planning and the development of emerging sectors of geoengineering and energy in such regions. Ensuring that these frameworks have adequate provisions for the integration of social-cultural values and are undertaken in the context of the cumulative picture of natural capital asset status will be essential. Further opportunities include those presented by the decisions made at the UNFCCC COP26 and 27. Such as the Glasgow Breakthrough Agenda to create an enabling environment for ‘clean’ industry (UKCOP26, 2021). This agenda has implications for activities in the S.E. Atlantic ABNJ, both through the control of vessel emissions but could also promote ocean science to inform supply chain impact assessment where products from these regions are used such as deep-sea minerals for clean technologies or MGR for medical, chemical or other applications. In addition, the agreement at COP27 to address slow onset events (Stuart-Watt, 2022) draws attention to the importance of the natural capital assets of the global ocean to society, reinforcing the need for diffuse benefit flows to humankind to be protected despite the challenges of quantification.
5 Conclusion
We show here, using the case study of the S.E. Atlantic ABNJ, that sectoral governance that does not take a systems view of natural capital presents risk to global society. Under current governance frameworks direct benefits, which for ABNJ largely comprise those that are benefitting economically from natural capital via extraction (e.g. fishing and the developing DSM industry), are favoured by current governance arrangements that are oriented towards the protection of resource access rather than system resilience. If remote ocean natural capital is to be governed for the benefit of society and future generations as required by international legislation, then a full systems approach to managing natural capital assets explicitly for the ecosystem services and benefits accrued to society must be considered. Strategic management processes such as SEA may be useful starting points for agreeing shared rights and responsibility to manage and protect the multiple benefits from natural capital assets. However, given the distance of ABNJ (physically and psychologically) from society, explicit consideration is needed on equitable decision making with regard to who benefits and how sectoral activities and pressures impact beneficiaries at multiple scales. The framework presented provides a basis from which to problematize the questions asked of SEA and identify the appropriate form of stakeholder consultation.
Author contributions
HN, KH, SR contributed to the conception and design of the analysis. HN undertook the analysis, wrote the first draft and led on revisions. All authors contributed to the manuscript revision, read, and approved the submitted version.
Funding
All authors funded by UKRI GCRF One Ocean Hub (Grant Ref: NE/S008950/1).
Acknowledgments
The authors thank Prof. Elisa Morgera, University of Strathclyde, for detailed feedback in the development of this article.
Conflict of interest
The authors declare that the research was conducted in the absence of any commercial or financial relationships that could be construed as a potential conflict of interest.
Publisher’s note
All claims expressed in this article are solely those of the authors and do not necessarily represent those of their affiliated organizations, or those of the publisher, the editors and the reviewers. Any product that may be evaluated in this article, or claim that may be made by its manufacturer, is not guaranteed or endorsed by the publisher.
Supplementary material
The Supplementary Material for this article can be found online at: https://www.frontiersin.org/articles/10.3389/fmars.2023.1168686/full#supplementary-material
Glossary
References
Anderson O. R. J., Small C. J., Croxall J. P., Dunn E. K., Sullivan B. J., Yates O., et al. (2011). Global seabird bycatch in longline fisheries. Endanger. Species Res. 14, 91–106. doi: 10.3354/esr00347
Ankamah-yeboah I., Xuan B. B., Hynes S., Armstrong C. W., Armstrong C. W. (2020). Public perceptions of deep-sea environment: evidence from scotland and Norway. Frontiers in Marine Science 7. doi: 10.3389/fmars.2020.00137
Arrizabalaga H., Dufour F., Kell L., Merino G., Ibaibarriaga L., Chust G., et al. (2015). Global habitat preferences of commercially valuable tuna. Deep. Res. Part II. Top. Stud. Oceanogr. 113, 102–112. doi: 10.1016/j.dsr2.2014.07.001
Bach L. T., Alvarez-Fernandez S., Hornick T., Stuhr A., Riebesell U. (2017). Simulated ocean acidification reveals winners and losers in coastal phytoplankton. PloS One 12, e0188198. doi: 10.1371/journal.pone.0188198
Bäckstrand K., Kuyper J. W., Linnér B. O., Lövbrand E. (2017). Non-state actors in global climate governance: from Copenhagen to Paris and beyond. Env. Polit. 26, 561–579. doi: 10.1080/09644016.2017.1327485
Barry J. P., Widdicombe S. (2011). Effects of ocean acidification on marine biodiversity and ecosystem function. Ocean. Acidif. doi: 10.1093/oso/9780199591091.003.0015
Beaulieu S. E., Szafrański K. M. (2020). InterRidge global database of active submarine hydrothermal vent fields. doi: 10.1594/PANGAEA.917894
Bell J. B., Guijarro-Garcia E., Kenny A. (2019). Demersal fishing in areas beyond national jurisdiction: A comparative analysis of regional fisheries management organisations. Front. Mar. Sci. 6. doi: 10.3389/fmars.2019.00596
Bergstad O. A., Gil M., Høines Å.S., Sarralde R., Maletzky E., Mostarda E., et al. (2019). Megabenthos and benthopelagic fishes on Southeast Atlantic seamounts. Afr. J. Mar. Sci. 41, 29–50. doi: 10.2989/1814232X.2019.1571439
Bernal D., Sepulveda C., Musyl M., Brill R. (2010). “The eco-physiology of swimming and movement patterns of tunas, billfishes, and large pelagic sharks,” in Fish locomotion: an etho-ecological approach. Eds. Domenici P., Kapoor B. G. (Enfield: Science), 436–483.
Bernal P., Simcock A. (2016). “Marine Scientific Research,” in The First Global Integrated Marine Assessment. World Ocean Assessment I., eds. Inniss L., Simcock A. (New York), 1–18.
Bindoff N. L., Cheung W. W. L., Kairo J. G., Aristegui J., Guinder V. A., Hallberg R., et al. (2019). “Changing ocean, marine ecosystems, and dependent communities,” in IPCC special report on the ocean and cryosphere in a changing climate eds. Pörtner H.-O., Roberts D.C., Masson-Delmotte V., Zhai P., Tignor M., Poloczanska E., et al, 447–588. Available at: https://www.ipcc.ch/srocc/download-report/.
Blanchard C., Gollner S. (2022). Area-based management tools to protect unique hydrothermal vents from harmful effects from deep-sea mining: A review of ongoing developments. Front. Polit. Sci 4. doi: 10.3389/fpos.2022.1033251
Blasiak R., Wynberg R., Grorud-Colvert K., Thambisetty S., Bandarra N. M., Canário A. V. M., et al. (2020). The ocean genome and future prospects for conservation and equity. Nat. Sustain. 3, 588–596. doi: 10.1038/s41893-020-0522-9
Block B. A., Jonsen I. D., Jorgensen S. J., Winship A. J., Shaffer S. A., Bograd S. J., et al. (2011). Tracking apex marine predator movements in a dynamic ocean. Nature 475, 86–90. doi: 10.1038/nature10082
Blöthe M., Wegorzewski A., Müller C., Simon F., Kuhn T., Schippers A. (2015). Manganese-cycling microbial communities inside deep-sea manganese nodules. Environ. Sci. Technol. 49, 7692–7700. doi: 10.1021/es504930v
Bodansky D. (2016). The Paris Climate Change Agreement: A new hope? Am. J. Int. Law 110, 288–319. doi: 10.5305/amerjintelaw.110.2.0288
Borja A., Elliott M., Andersen J. H., Berg T., Carstensen J., Halpern B. S., et al. (2016). Overview of integrative assessment of marine systems: The ecosystem approach in practice. Front. Mar. Sci. 3. doi: 10.3389/fmars.2016.00020
Boschen R. E., Rowden A. A., Clark M. R., Gardner J. P. A. (2013). Mining of deep-sea seafloor massive sulfides: A review of the deposits, their benthic communities, impacts from mining, regulatory frameworks and management strategies. Ocean. Coast. Manage. 84, 54–67. doi: 10.1016/j.ocecoaman.2013.07.005
Boswell R. (2022). Salted identities: biocultural heritage for a rehumanised ocean management in South Africa. Anthropol. Humanism 47 (2), 363–380. doi: 10.1111/anhu.12402
Breitburg D., Levin L. A., Oschlies A., Grégoire M., Chavez F. P., Conley D. J., et al. (2018). Declining oxygen in the global ocean and coastal waters. Sci. (80-. ). 359, eaam7240. doi: 10.1126/science.aam7240
Bridges A. E. H., Howell K. L., Amaro T., Atkinson L., Barnes D. K. A., Bax N., et al. (2023). Review of the central and south atlantic shelf and deep-sea benthos: science, policy, and management. Oceanogr. Mar. Biol. 61, 127–218. doi: 10.1201/9781003363873-5
Buckley M. W., Marshall J. (2016). Observations, inferences, and mechanisms of the Atlantic Meridional Overturning Circulation: A review. Rev. Geophys. 54, 5–63. doi: 10.1002/2015RG000493
Buhl-Mortensen P., Braga-Henriques A., and Stevenson A. (2022). Polyp loss and mass occurrence of sea urchins on bamboo corals in the deep sea: an indirect effect of fishing impact? Ecology 103, 1–5. doi: 10.1002/ecy.3564
Burnett D. R., Carter L. (2018). International submarine cables and biodiversity of Areas Beyond National Jurisdiction: The Cloud Beneath the Sea. (Leiden: Brill). doi: 10.1163/24519359-12340002
Butt N. (2007). The impact of cruise ship generated waste on home ports and ports of call: A study of Southampton. Mar. Policy 31, 591–598. doi: 10.1016/j.marpol.2007.03.002
Cagua E. F., Collins N., Hancock J., Rees R. (2014). Whale shark economics: A valuation of wildlife tourism in South Ari Atoll, Maldives. PeerJ 2014, e515. doi: 10.7717/peerj.515
Carter L., Burnett D., Drew S., Marle G., Hagdorn L., Bartlett-McNeil D., et al. (2009). Submarine cables and the oceans: connecting the world. 66.
Casini M., Käll F., Hansson M., Plikshs M., Baranova T., Karlsson O., et al. (2016). Hypoxic areas, density-dependence and food limitation drive the body condition of a heavily exploited marine fish predator. R. Soc Open Sci. 3, 160416. doi: 10.1098/rsos.160416
CBD (2010) Decision adopted by the conference of the parties to the Convention on Biological Diversity at its tenth meeting. Available at: https://www.cbd.int/doc/decisions/cop-10/cop-10-dec-29-en.pdf (Accessed January 10, 2023).
CBD (2022) Kunming-Montreal Global biodiversity framework. Available at: https://www.cbd.int/conferences/2021-2022/insession (Accessed February 8, 2023).
Chao S., Enari D. (2021). Decolonising climate change: A call for beyond-human imaginaries and knowledge generation. eTropic 20, 32–54. doi: 10.25120/etropic.20.2.2021.3796
Cheung W. W. L., Jones M. C., Reygondeau G., Frölicher T. L. (2018). Opportunities for climate-risk reduction through effective fisheries management. Glob. Change Biol. 24, 5149–5163. doi: 10.1111/gcb.14390
Chiba S., Saito H., Fletcher R., Yogi T., Kayo M., Miyagi S., et al. (2018). Human footprint in the abyss: 30 year records of deep-sea plastic debris. Mar. Policy 96, 204–212. doi: 10.1016/j.marpol.2018.03.022
Childs J. (2020). Performing ‘blue degrowth’: critiquing seabed mining in Papua New Guinea through creative practice. Sustain. Sci. 15, 117–129. doi: 10.1007/s11625-019-00752-2
Christiansen S., Durussel C., Guilhon M., Singh P., Unger S. (2022). Towards an ecosystem approach to management in areas beyond national jurisdiction: REMPs for deep seabed mining and the proposed BBNJ instrument. Front. Mar. Sci. 9. doi: 10.3389/fmars.2022.720146
Clark M. R., Althaus F., Schlacher T. A., Williams A., Bowden D. A., Rowden A. A. (2016). The impacts of deep-sea fisheries on benthic communities: A review. ICES. J. Mar. Sci. 73, i51–i69. doi: 10.1093/icesjms/fsv123
Clark M. R., Durden J. M., Christiansen S. (2019). Environmental Impact Assessments for deep-sea mining: Can we improve their future effectiveness? Mar. Policy 1–9, 1–9. doi: 10.1016/j.marpol.2018.11.026
Clark M. R., Bernardino A. F., Roberts J. M., Narayanaswamy B. E., Snelgrove P., Tuhumwire J. T. (2020). Seamounts and pinnacles. In World Ocean Assessment II, Vol I New York. Available at: https://www.un.org/regularprocess/sites/www.un.org.regularprocess/files/2011859-e-woa-ii-vol-i.pdf.
Climate action tracker (2022) Temperatures. Available at: https://climateactiontracker.org/global/temperatures/ (Accessed November 14, 2022).
Cole M., Lindeque P., Fileman E., Halsband C., Goodhead R., Moger J., et al. (2013). Microplastic ingestion by zooplankton. Environ. Sci. Technol. 47, 6646–6655. doi: 10.1021/es400663f
Cook D., Malinauskaite L., Davíðsdóttir B., Ögmundardóttir H., Roman J. (2020). Reflections on the ecosystem services of whales and valuing their contribution to human well-being. Ocean. Coast. Manage. 186, 105100. doi: 10.1016/j.ocecoaman.2020.105100
Cormier R., Stelzenmüller V., Creed I. F., Igras J., Rambo H., Callies U., et al. (2018). The science-policy interface of risk-based freshwater and marine management systems: From concepts to practical tools. J. Environ. Manage. 226, 340–346. doi: 10.1016/j.jenvman.2018.08.053
Costello M. J., McCrea M., Freiwald A., Lundälv T., Jonsson L., Bett B. J., et al. (2005). “Role of cold-water Lophelia pertusa coral reefs as fi sh habitat in the NE Atlantic,” in Cold-water corals and ecosystems. Eds. Freiwald A., Roberts J. M. (Berlin, Heidelberg: Springer-Verlag), 771–805. Available at: www.ecoserve.ie/projects/aces/.
Craik N., Gu K. (2021) Implementing environmental impact asessment for deep sea mining: Lessons to be drawn from International and Domestic EIA processes. Available at: https://www.pewtrusts.org/-/media/assets/2021/06/craik–gu–implementing-environmental-impact-assessment-for-deep-sea-mining.pdf.
Crespo G. O., Dunn D. C. (2017). A review of the impacts of fisheries on open-ocean ecosystems. ICES. J. Mar. Sci. 74, 2283–2297. doi: 10.1093/icesjms/fsx084
Crespo G. O., Dunn D. C., Gianni M., Gjerde K., Wright G., Halpin P. N. (2019). High-seas fish biodiversity is slipping through the governance net. Nat. Ecol. Evol. 3, 1273–1276. doi: 10.1038/s41559-019-0981-4
Cullis-Suzuki S., Pauly D. (2010). Failing the high seas: A global evaluation of regional fisheries management organizations. Mar. Policy 34, 1036–1042. doi: 10.1016/j.marpol.2010.03.002
Danovaro R., Gambi C., Dell’Anno A., Corinaldesi C., Fraschetti S., Vanreusel A., et al. (2008). Exponential decline of deep-sea ecosystem functioning linked to benthic biodiversity loss. Curr. Biol. 18, 1–8. doi: 10.1016/j.cub.2007.11.056
Dasgupta P. (2021) The Economics of Biodiversity: The Dasgupta review. Available at: https://www.gov.uk/government/publications/final-report-the-economics-of-biodiversity-the-dasgupta-review.
Daskalov G. M., Grishin A. N., Rodionov S., Mihneva V. (2007). Trophic cascades triggered by overfishing reveal possible mechanisms of ecosystem regime shifts. Proc. Natl. Acad. Sci. U. S. A. 104, 10518–10523. doi: 10.1073/pnas.0701100104
Davison P. C., Checkley D. M., Koslow J. A., Barlow J. (2013). Carbon export mediated by mesopelagic fishes in the northeast Pacific Ocean. Prog. Oceanogr. 116, 14–30. doi: 10.1016/j.pocean.2013.05.013
Daw T. M., Coulthard S., Cheung W. W. L., Brown K., Abunge C., Galafassi D. (2015). Evaluating taboo trade-offs in ecosystems services and human well-being. Proceedings of the National Academy of Sciences 112 (22), 6949–6954. doi: 10.1073/pnas.1414900112
De Santo E. M., Mendenhall E., Nyman E., Tiller R. (2020). Stuck in the middle with you (and not much time left): The third intergovernmental conference on biodiversity beyond national jurisdiction. Mar. Policy 117, 103957. doi: 10.1016/j.marpol.2020.103957
DOALOS (2016) Agreement relating to the implementation of Part XI of the United Nations Convention on the Law of the Sea of 10 December 1982. Available at: https://www.un.org/Depts/los/convention_agreements/convention_overview_part_xi.htm (Accessed May 25, 2021).
Doney S. C., Busch D. S., Cooley S. R., Kroeker K. J. (2020). The impacts of ocean acidification on marine ecosystems and reliant human communities. Annu. Rev. Environ. Resour. 45, 83–112. doi: 10.1146/annurev-environ-012320-083019
D’Onghia G., Maiorano P., Carlucci R., Capezzuto F., Carluccio A., Tursi A., et al. (2012). Comparing deep-sea fish fauna between coral and non-coral “Megahabitats” in the santa maria di leuca cold-water coral province (Mediterranean sea). PloS One 7, e44509. doi: 10.1371/journal.pone.0044509
D’Onghia G., Maiorano P., Sion L., Giove A., Capezzuto F., Carlucci R., et al. (2010). Effects of deep-water coral banks on the abundance and size structure of the megafauna in the Mediterranean Sea. Deep. Res. Part II. Top. Stud. Oceanogr. 57, 397–411. doi: 10.1016/j.dsr2.2009.08.022
DOSI (2021) The necessity of traditional knowledge for management of deep-seabed mining. Available at: https://biblio.ugent.be/publication/8717205.
Drazen J. C., Smith C. R., Gjerde K. M., Haddock S. H. D., Carter G. S., Anela Choy C., et al. (2020). Midwater ecosystems must be considered when evaluating environmental risks of deep-sea mining. Proc. Natl. Acad. Sci. U. S. A. 117, 17455–17460. doi: 10.1073/pnas.2011914117
Drew J., Andrews K., Smith N. (2021). The potential for expansion of whale shark (Rhincodon typus) tourism in St. Helena: stakeholder engagement and willingness to pay. J. Ecotourism 21 (4), 354–365. doi: 10.1080/14724049.2021.1971684
Ducklow H. W., Steinberg D. K., Buesseler K. O. (2001). Upper ocean carbon export and the biological pump. Oceanography 14, 50–58. doi: 10.5670/oceanog.2001.06
Dunham A., Archer S. K., Davies S. C., Burke L. A., Mossman J., Pegg J. R., et al. (2018). Assessing condition and ecological role of deep-water biogenic habitats: Glass sponge reefs in the Salish Sea. Mar. Environ. Res. 141, 88–99. doi: 10.1016/j.marenvres.2018.08.002
Durán-Muñoz P., Sacau M., Del Río J. L., López-Abellán L. J., Sarralde R. (2014). “Poster: Seabed mapping and Vulnerable Marine Ecosystems protection in the highseas fisheries: Four case studies on progress in the Atlantic Ocean,” in ICES ASC 2014. Theme Session B, The Science and Tools for the Management of Networks of Marine Protected Areas, A Coruña, Spain.
Durussel C., Wright G., Wienrich N., Boteler B., Unger S., Rochette J. (2018). Strengthening regional ocean governance for the high seas: opportunities and challenges to improve the legal and institutional framework of the southeast atlantic and southeast pacific. pp 92. doi: 10.2312/iass.2018.025
Ellis D. V. (2001). A review of some environmental issues affecting marine mining. Mar. Georesour. Geotechnol. 19, 51–63. doi: 10.1080/10641190109353804
Elsler L. G., Oostdijk M., Levin L. A., Satterthwaite E. V., Pinsky M. L., Crespo G. O., et al. (2022). Protecting ocean carbon through biodiversity and climate governance. Front. Mar. Sci. 9. doi: 10.3389/fmars.2022.880424
FAO (1995). Agreement to promote compliance with international conservation and management measures by fishing vessels on the high seas.
FAO (2016). Agreement on Port State Measures to Prevent, Deter and Eliminate Illegal, Unreported and Unregulated Fishing / Accord relatif aux mesures du ressort de l’Etat du port visant à prévenir, contrecarrer et éliminer la pêche illicite, non déclarée et non régle (Rome).
FAO (2020). Terminal evaluation of the areas beyond national jurisdiction (ABNJ) Deep-Sea project, part of the “Sustainable fisheries management and biodiversity conservation of deep-sea living marine resources and ecosystems in ABNJ (Rome). Available at: https://www.fao.org/evaluation/evaluation-digest/evaluations-detail/en/c/1312175/#:~:text=. The marine areas beyond national,95 percent of its volume.
FAO (2022) Research vessel Dr Fridtjof Nansen sails beyond national borders to study corals and sponges in the depths of the southeast Atlantic Ocean. Available at: www.fao.org/in-action/eaf-nansen/news-events/detail-events/en/c/1538899/ (Accessed September 4, 2023).
Freese J., Lincoln, Wing B. L. (2003) Juvenile Red Rockfish, Sebastes sp., Associations with Sponges in the Gulf of Alaska Item Type article. Available at: http://hdl.handle.net/1834/26347.
Friedman K., Braccini M., Bjerregaard-Walsh M., Bonfil R., Bradshaw C. J. A., Brouwer S., et al. (2020). Informing CITES Parties: Strengthening science-based decision-making when listing marine species. Fish. Fish. 21, 13–31. doi: 10.1111/faf.12411
Friedman K., Gabriel S., Abe O., Adnan Nuruddin A., Ali A., Bidin Raja Hassan R., et al. (2018). Examining the impact of CITES listing of sharks and rays in Southeast Asian fisheries. Fish. Fish. 19, 662–676. doi: 10.1111/faf.12281
Gallo N. D., Victor D. G., Levin L. A. (2018). Ocean commitments under the Paris Agreement. Nat. Clim. Change 7 (11), 833–838. doi: 10.1038/nclimate3422
Garrad S. L., Tyler-Walters H. (2020). Habitat (biotope) sensitivity assessments for climate change pressures. Report from the Marine Life Information Network, to Dept. for Environment, Food and Rural Affairs (Defra) & Joint Nature Conservation Committee(JNCC) (Plymouth: Marine Biological Association of the United Kingdom).
Garzoli S. L., Matano R. (2011). The south atlantic and the atlantic meridional overturning circulation. Deep. Res. Part II. Top. Stud. Oceanogr. 58, 1837–1847. doi: 10.1016/j.dsr2.2010.10.063
Gascuel D., Cheung W. W. L. (2019). “Marine biodiversity and ecosystem services: the large gloomy shadow of climate change,” in Predicting future oceans. Eds. Cisneros-Montemayor A. M., Cheung W. W. L., Ota Y. (Amsterdam: Elsevier), 79–85. doi: 10.1037/a0023412
German C. R., Ramirez-Llodra E., Baker M. C., Tyler P. A., Baco-Taylor A., Boetius A., et al. (2011). Deep-water chemosynthetic ecosystem research during the census of marine life decade and beyond: A proposed deep-ocean road map. PloS One 6, e23259. doi: 10.1371/journal.pone.0023259
Gill A. B. (2005). Offshore renewable energy: Ecological implications of generating electricity in the coastal zone. J. Appl. Ecol. 42, 605–615. doi: 10.1111/j.1365-2664.2005.01060.x
Gilly W. F., Michael Beman J., Litvin S. Y., Robison B. H. (2013). Oceanographic and biological effects of shoaling of the oxygen minimum zone. Ann. Rev. Mar. Sci. 5, 393–420. doi: 10.1146/annurev-marine-120710-100849
Gilman E., Passfield K., Nakamura K. (2014). Performance of regional fisheries management organizations: Ecosystem-based governance of bycatch and discards. Fish. Fish. 15, 327–351. doi: 10.1111/faf.12021
Gjerde K. M., Currie D., Wowk K., Sack K. (2013). Ocean in peril: Reforming the management of global ocean living resources in areas beyond national jurisdiction. Mar. pollut. Bull. 74, 540–551. doi: 10.1016/j.marpolbul.2013.07.037
Gjerde K. M., Dotinga H., Hart S., Molenaar E. J., Rayfuse R., Warner R. (2008). Regulatory and governance gaps in the international regime for the conservation and sustainable use of marine biodiversity in areas beyond national jurisdiction. 70pp.
Gjerde K. M., Reeve L. L. N., Harden-Davies H., Ardron J., Dolan R., Durussel C., et al. (2016). Protecting Earth’s last conservation frontier: scientific, management and legal priorities for MPAs beyond national boundaries. Aquat. Conserv. Mar. Freshw. Ecosyst. 26, 45–60. doi: 10.1002/aqc.2646
Gruber N., Boyd P. W., Frölicher T. L., Vogt M. (2021). Biogeochemical extremes and compound events in the ocean. Nature 600, 395–407. doi: 10.1038/s41586-021-03981-7
Guggisberg S. (2022). Transparency in the activities of the Food and Agriculture Organization for sustainable fisheries. Mar. Policy 136, 1–10. doi: 10.1016/j.marpol.2021.104498
Hall-Spencer J. M., Rodolfo-Metalpa R., Martin S., Ransome E., Fine M., Turner S. M., et al. (2008). Volcanic carbon dioxide vents show ecosystem effects of ocean acidification. Nature 454, 96–99. doi: 10.1038/nature07051
Hamer D. J., Childerhouse S. J., Gales N. J. (2012). Odontocete bycatch and depredation in longline fisheries: A review of available literature and of potential solutions. Mar. Mammal. Sci. 28, e345-e374. doi: 10.1111/j.1748-7692.2011.00544.x
Harden-Davies H. (2017). Deep-sea genetic resources: New frontiers for science and stewardship in areas beyond national jurisdiction. Deep. Res. Part II. Top. Stud. Oceanogr. 137, 504–513. doi: 10.1016/j.dsr2.2016.05.005
Harmsworth G. R., Awatere S. (2013). Indigenous māori knowledge and perspectives of ecosystems. Ecosyst. Serv. New Zeal. – Cond. Trends, 274–286.
Harrould-Kolieb E. R. (2020). Framing ocean acidification to mobilise action under multilateral environmental agreements. Environ. Sci. Policy 104, 129–135. doi: 10.1016/j.envsci.2019.10.019
Harrould-Kolieb E. (2021). Enhancing synergies between action on ocean acidification and the post-2020 global biodiversity framework. Conserv. Biol. 35, 548–558. doi: 10.1111/cobi.13598
Havice E., Zalik A. (2018). Ocean frontiers: epistemologies, jurisdictions, commodifications. Int. Soc Sci. J. 68, 219–235. doi: 10.1111/issj.12198
Hein J. R., Mizell K., Koschinsky A., Conrad T. A. (2013). Deep-ocean mineral deposits as a source of critical metals for high- and green-technology applications: Comparison with land-based resources. Ore. Geol. Rev. 51, 1–14. doi: 10.1016/j.oregeorev.2012.12.001
Helm K. P., Bindoff N. L., Church J. A. (2011). Observed decreases in oxygen content of the global ocean. Geophys. Res. Lett. 38, 1–6. doi: 10.1029/2011GL049513
Herndl G. J., Reinthaler T. (2013). Microbial control of the dark end of the biological pump. Nat. Geosci. 6, 718–724. doi: 10.1038/ngeo1921
Hesketh Best P., Howell K., Upton M., Bishop A., Warburton P., Foster N., et al. (2019). Assessing and optimising culturing methods for the associated-bacteria of two species of deep-sea sponges (class Hexactinellida) for antimicrobial bioprospecting. Access Microbiol. 1. doi: 10.1099/acmi.ac2019.po0230
Hooper T., Börger T., Langmead O., Marcone O., Rees S. E., Rendon O., et al. (2019). Applying the natural capital approach to decision making for the marine environment. Ecosyst. Serv. 38, 100947. doi: 10.1016/j.ecoser.2019.100947
Howell K. L. (2010). A benthic classification system to aid in the implementation of marine protected area networks in the deep/high seas of the NE Atlantic. Biol. Conserv. 143, 1041–1056. doi: 10.1016/j.biocon.2010.02.001
Howell K., Bridges A., Graves K., Allcock L., la Bianca G., Ventura-Costa C., et al. (2022). Performance of deep-sea habitat suitability models assessed using independent data, and implications for use in area-based management. Mar. Ecol. Prog. Ser. 695, 33–51. doi: 10.3354/meps14098
Howell K. L., Hilário A., Allcock A. L., Bailey D. M., Baker M., Clark M. R., et al. (2020). A blueprint for an inclusive, global deep-sea ocean decade field program. Front. Mar. Sci. 7. doi: 10.3389/fmars.2020.584861
Howell K. L., Piechaud N., Downie A. L., Kenny A. (2016). The distribution of deep-sea sponge aggregations in the North Atlantic and implications for their effective spatial management. Deep. Res. Part I. Oceanogr. Res. Pap. 115, 309–320. doi: 10.1016/j.dsr.2016.07.005
Hubert A. M. (2011). The new paradox in marine scientific research: Regulating the potential environmental impacts of conducting ocean science. Ocean. Dev. Int. Law 42, 329–355. doi: 10.1080/00908320.2011.619368
Humphries F., Gottlieb H. M., Laird S., Wynberg R., Lawson C., Rourke M., et al. (2020). A tiered approach to the marine genetic resource governance framework under the proposed UNCLOS agreement for biodiversity beyond national jurisdiction (BBNJ). Mar. Policy 122. doi: 10.1016/j.marpol.2020.103910
Humphries F., Harden-Davies H. (2020). Practical policy solutions for the final stage of BBNJ treaty negotiations. Mar. Policy 122, 104214. doi: 10.1016/j.marpol.2020.104214
Huveneers C., Meekan M. G., Apps K., Ferreira L. C., Pannell D., Vianna G. M. S. (2017). The economic value of shark-diving tourism in Australia. Rev. Fish. Biol. Fish. 27, 665–680. doi: 10.1007/s11160-017-9486-x
ICCAT (2015) 2015-2020 SCRS science strategic plan. Available at: http://uso.edu/strategicplan/handbook/uso/k12.php.
ICCAT (2022) Compendium management recommendations and resolutions adopted by ICCAT for the conservation of Atlantic Tunas and Tuna-like species. Available at: https://www.iccat.int/en.RecRes.asp (Accessed November 1, 2022).
ICPC (2016) Submarine cables and BBNJ. Available at: http://www.un.org/depts/los/biodiversity/prepcom_files/ICC_Submarine_Cables_&_BBNJ_August_2016.pdf.
IMO (2004) International convention for the control and management of ships’ Ballast water and sediments. Available at: www.gov.uk/official-documents.
IMO (2005) Revised guidelines for the identification and designation of particularly sensitive sea areas. Available at: https://wwwcdn.imo.org/localresources/en/OurWork/Environment/Documents/A24-Res.982.pdf.
IMO (2019) Introduction to IMO. Available at: https://www.imo.org/en/About/Pages/Default.aspx (Accessed May 28, 2021).
IMO (2020) Fourth greenhouse gas study 2020. Available at: https://www.imo.org/en/OurWork/Environment/Pages/Fourth-IMO-Greenhouse-Gas-Study-2020.aspx (Accessed April 21, 2022).
ISA (2019a) Deep CCZ biodiversity synthesis workshop. Available at: https://www.isa.org.jm/files/files/documents/DeepCCZBiodiversitySynthesisWorkshopReport-Final-forposting-clean-1.pdf.
ISA (2019b) Draft regulations on exploitation of mineral resources in the Area. Available at: https://www.isa.org.jm/instruments-juridiques/ongoing-development-regulations-exploitation-mineral-resources-area.
ISA (2019c). Guidance to facilitate the development of Regional Environmental Management Plans (REMPs) (Kingston). Available at: https://www.isa.org.jm/files/files/documents/remp_guidance_.pdf.
ISA (2019d). ISA Technical Study: No. 24. Deep seabed mining and marine cables: developing practical options for the implementation of ‘due regard’ and ‘reasonable regard’ obligations under the United Nations Convention on the Law of the Sea: report of the second work (Kingston). Available at: https://www.isa.org.jm/node/19339.
ISA (2019e) The mining code. Available at: https://www.isa.org.jm/mining-code (Accessed January 5, 2020).
ISA (2020) About the international seabed authority. Available at: https://www.isa.org.jm/about-isa (Accessed May 17, 2022).
ISA (2022) Exploration contracts. Available at: https://www.isa.org.jm/exploration-contracts (Accessed May 17, 2022).
IWC (2022). Strategic plan to mitigate the impacts of ship strikes on cetacean populations: 2022-2032.
Jamieson A. J., Singleman G., Linley T. D., Casey S. (2020). Fear and loathing of the deep ocean: why don’t people care about the deep sea? ICES. J. Mar. Sci 78 (3), 797–809. doi: 10.1093/icesjms/fsaa234
Jarratt D., Sharpley R. (2017). Tourists at the seaside: Exploring the spiritual dimension. Tour. Stud. 17, 349–368. doi: 10.1177/1468797616687560
Jobstvogt N., Townsend M., Witte U., Hanley N. (2014). How can we identify and communicate the ecological value of deep-sea ecosystem services? PloS One 9, e100646. doi: 10.1371/journal.pone.0100646
Jones D. O. B., Kaiser S., Sweetman A. K., Smith C. R., Menot L., Vink A., et al. (2017). Biological responses to disturbance from simulated deep-sea polymetallic nodule mining. PloS One 12, e0171750. doi: 10.1371/journal.pone.0171750
Jouffray J., Blasiak R., Norstro A. V. (2020). Perspective the blue acceleration: the trajectory of human expansion into the ocean. One Earth 43, 43–54. doi: 10.1016/j.oneear.2019.12.016
Juan-Jordá M. J., Murua H., Arrizabalaga H., Dulvy N. K., Restrepo V. (2018). Report card on ecosystem-based fisheries management in tuna regional fisheries management organizations. Fish. Fish. 19, 321–339. doi: 10.1111/faf.12256
Judd A. D., Backhaus T., Goodsir F. (2015). An effective set of principles for practical implementation of marine cumulative effects assessment. Environ. Sci. Policy 54, 254–262. doi: 10.1016/j.envsci.2015.07.008
Kaikkonen L., van Putten I. (2021). We may not know much about the deep sea, but do we care about mining it? People Nat. 3, 843–860. doi: 10.1002/pan3.10224
Kaikkonen L., Victorero L., Gianni M., Amaro T., Aranha S. G. (2022) A review of impact assessments for deep-sea fisheries on the A review of impact assessments for deep-sea fisheries on the high seas high seas part of the marine biology commons, and the oceanography and atmospheric sciences and meteorology commons. Available at: https://nsuworks.nova.edu/occ_facreports.
Keeling R. F., Körtzinger A., Gruber N. (2010). Ocean deoxygenation in a warming world. Ann. Rev. Mar. Sci. 2, 199–229. doi: 10.1146/annurev.marine.010908.163855
Koch M. J., Hesketh-Best P. J., Smerdon G., Warburton P. J., Howell K., Upton M. (2021). Impact of growth media and pressure on the diversity and antimicrobial activity of isolates from two species of hexactinellid sponge. Microbiol. (United. Kingdom). 167, 001123. doi: 10.1099/MIC.0.001123
Koenig C. C., Shepard A. N., Reed J. K., Coleman F. C., Brooke S. D., Brusher J., et al. (2005). Habitat and fish populations in the deep-sea oculina coral ecosystem of the western atlantic. Am. Fish. Soc. Symp. 41, 795–805.
Koslow J. A., Goericke R., Lara-Lopez A., Watson W. (2011). Impact of declining intermediate-water oxygen on deepwater fishes in the California Current. Mar. Ecol. Prog. Ser. 436, 207–218. doi: 10.3354/meps09270
Koslow J. A., Gowlett-Holmes K., Lowry J. K., O’Hara T., Poore G. C. B., Williams A. (2001). Seamount benthic macrofauna off southern Tasmania: Community structure and impacts of trawling. Mar. Ecol. Prog. Ser 213, 111–125. doi: 10.3354/meps213111
Kroeker K. J., Kordas R. L., Crim R., Hendriks I. E., Ramajo L., Singh G. S., et al. (2013). Impacts of ocean acidification on marine organisms: Quantifying sensitivities and interaction with warming. Glob. Change Biol. 19, 1884–1896. doi: 10.1111/gcb.12179
Kühn S., Bravo Rebolledo E. L., Van Franeker J. A. (2015). “Deleterious effects of litter on marine life,” in Marine anthropogenic litter (Berlin: Springer International Publishing), 75–116. doi: 10.1007/978-3-319-16510-3_4
La Bianca G., Rees S., Attrill M. J., Lombard A. T., McQuaid K. A., Niner H. J., et al. (2023). A standardised ecosystem services framework for the deep sea. Front. Mar. Sci. 10. doi: 10.3389/fmars.2023.1176230
Lascelles B., Notarbartolo Di Sciara G., Agardy T., Cuttelod A., Eckert S., Glowka L., et al. (2014). Migratory marine species: Their status, threats and conservation management needs. Aquat. Conserv. Mar. Freshw. Ecosyst. 24, 111–127. doi: 10.1002/aqc.2512
Le J. T., Levin L. A., Carson R. T. (2017). Incorporating ecosystem services into environmental management of deep-seabed mining. Deep. Sea. Res. Part II. Top. Stud. Oceanogr. 137, 486–503. doi: 10.1016/j.dsr2.2016.08.007
Leary D., Vierros M., Hamon G., Arico S., Monagle C. (2009). Marine genetic resources: A review of scientific and commercial interest. Mar. Policy 33, 183–194. doi: 10.1016/j.marpol.2008.05.010
Lèbre É., Stringer M., Svobodova K., Owen J. R., Kemp D., Côte C., et al. (2020). The social and environmental complexities of extracting energy transition metals. Nat. Commun. 11, 4823. doi: 10.1038/s41467-020-18661-9
Lee J., Bazilian M., Sovacool B., Hund K., Jowitt S. M., Nguyen T. P., et al. (2020). Reviewing the material and metal security of low-carbon energy transitions. Renew. Sustain. Energy Rev. 124, 109789. doi: 10.1016/j.rser.2020.109789
Lennan M., Morgera E. (2022). The glasgow climate conference (COP26). Int. J. Mar. Coast. Law 37, 137–151. doi: 10.1163/15718085-bja10083
Le Roex A., Class C., O’Connor J., Jokat W. (2010). Shona and discovery aseismic ridge systems, South Atlantic: Traace element evidence for enriched mantle sources. J. Petrol. 51, 2089–2120. doi: 10.1093/petrology/egq050
Levin L. A. (2018). Manifestation, drivers, and emergence of open ocean deoxygenation. Ann. Rev. Mar. Sci. 10, 229–260. doi: 10.1146/annurev-marine-121916-063359
Li G., Cheng L., Zhu J., Trenberth K. E., Mann M. E., Abraham J. P. (2020). Increasing ocean stratification over the past half-century. Nat. Clim. Change 10, 1116–1123. doi: 10.1038/s41558-020-00918-2
Lillis A., Eggleston D. B., Bohnenstiehl D. R. (2013). Oyster larvae settle in response to habitat-associated underwater sounds. PloS One 8, e79337. doi: 10.1371/journal.pone.0079337
Limburg K. E., Breitburg D., Swaney D. P., Jacinto G. (2020). Ocean deoxygenation: A primer. One Earth 2, 24–29. doi: 10.1016/j.oneear.2020.01.001
Lozier M. S. (2012). Overturning in the north atlantic. Ann. Rev. Mar. Sci. 4, 291–315. doi: 10.1146/annurev-marine-120710-100740
Mace G. M., Hails R. S., Cryle P., Harlow J., Clarke S. J. (2015). Towards a risk register for natural capital. J. Appl. Ecol. 52, 641–653. doi: 10.1111/1365-2664.12431
Mace G. M., Reyers B., Alkemade R., Biggs R., Chapin F. S., Cornell S. E., et al. (2014). Approaches to defining a planetary boundary for biodiversity. Glob. Environ. Change 28, 289–297. doi: 10.1016/j.gloenvcha.2014.07.009
Mace G. M. (2019). Towards a risk register for natural capital. Oxford Rev. Econ. Policy 35, 54–67. doi: 10.1093/oxrep/gry023
Maier S. R., Mienis F., de Froe E., Soetaert K., Lavaleye M., Duineveld G., et al. (2021). Reef communities associated with ‘dead’ cold-water coral framework drive resource retention and recycling in the deep sea. Deep. Res. Part I. Oceanogr. Res. Pap. 31 (18), 175. doi: 10.1016/j.dsr.2021.103574
Månberger A., Johansson B. (2019). The geopolitics of metals and metalloids used for the renewable energy transition. Energy Strateg. Rev. 26, 100394. doi: 10.1016/j.esr.2019.100394
Marliave J. B., Conway K. W., Gibbs D. M., Lamb A., Gibbs C. (2009). Biodiversity and rockfish recruitment in sponge gardens and bioherms of southern British Columbia, Canada. Mar. Biol. 156, 2247–2254. doi: 10.1007/s00227-009-1252-8
MARLIN (2022) MarESA pressures and benchmarks. Available at: https://www.marlin.ac.uk/sensitivity/SNCB-benchmarks (Accessed September 8, 2022).
Martins A., Vieira H., Gaspar H., Santos S. (2014). Marketed marine natural products in the pharmaceutical and cosmeceutical industries: Tips for success. Mar. Drugs 12, 1066–1101. doi: 10.3390/md12021066
McGowan J. A., Cayan D. R., Dornman L. M. (1998). Climate-ocean variability and ecosystem response in the northeast pacific. Sci. (80-. ). 281, 210–217. doi: 10.1126/science.281.5374.210
McQuaid K. A., Attrill M. J., Clark M. R., Cobley A., Glover A. G., Smith C. R., et al. (2020). Using habitat classification to assess representativity of a protected area network in a large, data-poor area targeted for deep-sea mining. Front. Mar. Sci. 7. doi: 10.3389/fmars.2020.558860
McQuaid K. A., Bridges A. E. H., Howell K. L., Gandra T. B. R., de Souza V., Currie J. C., et al. (2023). Broad-scale benthic habitat classification of the South Atlantic. Progress in Oceanography 214, 103016. doi: 10.1016/j.pocean.2023.103016
McQuaid K. A., Howell K. L., Rees S. E., Niner H. J., La Bianca G., Gjerde K., et al. (2022) Policy brief: The need for Strategic Environmental Assessments and Regional Environmental Assessment in ABNJ for ecologically meaningful management. Plymouth. Available at: https://oneoceanhub.org/publications/policy-brief-the-need-for-strategic-environmental-assestements-and-regional-environmental-assessment-in-abnj-for-ecologically-meaningful-management/ (Accessed April 20, 2023).
Melbourne-Thomas J., Tommasi D., Gehlen M., Murphy E. J., Beckensteiner J., Bravo F., et al. (2023). Integrating human dimensions in decadal-scale prediction for marine social–ecological systems: lighting the grey zone. ICES. J. Mar. Sci. 80, 16–30. doi: 10.1093/icesjms/fsac228
Miljutin D. M., Miljutina M. A., Martı P. (2011). Deep-Sea Research I Deep-sea nematode assemblage has not recovered 26 years after experimental mining of polymetallic nodules. (Clarion-Clipperton Fracture. Zone. Trop. Eastern. Pacific). . 58, 885–897. doi: 10.1016/j.dsr.2011.06.003
Miller K. A., Thompson K. F., Johnston P., Santillo D. (2018). An overview of seabed mining including the current state of development, environmental impacts, and knowledge gaps. Front. Mar. Sci. 4. doi: 10.3389/fmars.2017.00418
Mollica N. R., Guo W., Cohen A. L., Huang K. F., Foster G. L., Donald H. K., et al. (2018). Ocean acidification affects coral growth by reducing skeletal density. Proc. Natl. Acad. Sci. U. S. A. 115, 1754–1759. doi: 10.1073/pnas.1712806115
Morais C., Moreira L., Teixeira A. S., Aguiar T. (2022). No waves from surface knowledge: diving into the social representations of the deep sea. Int. J. Sci. Educ. Part B. Commun. Public Engagem. 12, 22–41. doi: 10.1080/21548455.2021.2017507
Morgera E. (2021). “The relevance of the human right to science for the conservation and sustainable use of marine biodiversity of areas beyond national jurisdiction: a new legally binding instrument to support co-production of ocean knowledge across scales Introduction Thi,” in International law and marine areas beyond national jurisdiction: current status and future trends. Eds. Lucia V., Nguyen L., Elferink A. G. O. (Leiden & Boston, Brill), 1–28. doi: 10.2139/ssrn.3870399
Morgera E., Lennan M., Kulovesi K., La Bianca G., Niner H. J., Harrould-Kolieb E., et al. (2023a). Ocean-based climate action and human rights implications under the international climate change regime. Int. J. Mar. Coast. Law 38 (3), 1–36. doi: 10.1163/15718085-bja10142
Morgera E., McQuaid K., La Bianca G., Niner H., Shannon L., Strand M., et al. (2023b). Addressing the ocean-climate nexus in the BBNJ agreement: strategic environmental assessments, human rights and equity in ocean science. Int. J. Mar. Coast. Law 38 (3), 1–33. doi: 10.1163/15718085-bja10139
Mucientes G., Vedor M., Sims D. W., Queiroz N. (2022). Unreported discards of internationally protected pelagic sharks in a global fishing hotspot are potentially large. Biol. Conserv. 269, 109534. doi: 10.1016/j.biocon.2022.109534
Myers R. A., Worm B. (2003). Rapid worldwide depletion of predatory fish communities. Nature 423, 280–283. doi: 10.1038/nature01610
Niner H. J., Ardron J. A., Escobar E. G., Gianni M., Jaeckel A., Jones D. O. B., et al. (2018). Deep-sea mining with no net loss of biodiversity—An impossible aim. Front. Mar. Sci. 5. doi: 10.3389/fmars.2018.00053
Norman B. M., Holmberg J. A., Arzoumanian Z., Reynolds S. D., Wilson R. P., Rob D., et al. (2017). Undersea constellations: The global biology of an endangered marine megavertebrate further informed through citizen science. Bioscience 67, 1029–1043. doi: 10.1093/biosci/bix127
Notteboom T., Pallis A., Rodrigue J.-P. (2022). Port economics, management and policy (New York: Routledge).
Okafor-Yarwood I., Kadagi N. I., Miranda N. A. F., Uku J., Elegbede I. O., Adewumi I. J. (2020). The blue economy–cultural livelihood–ecosystem conservation triangle: the african experience. Front. Mar. Sci. 7. doi: 10.3389/fmars.2020.00586
Orcutt B. N., Bradley J. A., Brazelton W. J., Estes E. R., Goordial J. M., Huber J. A., et al. (2020). Impacts of deep-sea mining on microbial ecosystem services. Limnol. Oceanogr. 65, 1489–1510. doi: 10.1002/lno.11403
Ottaviani D. (2020). Economic value of ecosystem services form the deep seas and the areas beyond national jurisdiction (Rome: FAO). doi: 10.4060/ca8340en
Pascua P., McMillen H., Ticktin T., Vaughan M., Winter K. B. (2017). Beyond services: A process and framework to incorporate cultural, genealogical, place-based, and indigenous relationships in ecosystem service assessments. Ecosyst. Serv. 26, 465–475. doi: 10.1016/j.ecoser.2017.03.012
Patil P., Diez S. M. (2016) Grenada: blue growth coastal master plan (english). Available at: http://documents.worldbank.org/curated/en/358651480931239134/Grenada-Blue-growth-coastal-master-plan.
Pauly D., Christensen V., Dalsgaard J., Froese R., Torres F. (1998). Fishing down marine food webs. Sci. (80-. ). 279, 860–863. doi: 10.1126/science.279.5352.860
Pauly D., Watson R., Alder J. (2005)Global trends in world fisheries: impacts on marine ecosystems and food security on JSTOR (Accessed November 26, 2015).
Pérez-Díaz L., Eagles G. (2017). South Atlantic paleobathymetry since early Cretaceous. Sci. Rep. 7, 11819. doi: 10.1038/s41598-017-11959-7
Pham C. K., Vandeperre F., Menezes G., Porteiro F., Isidro E., Morato T. (2015). The importance of deep-sea vulnerable marine ecosystems for demersal fish in the Azores. Deep. Res. Part I. Oceanogr. Res. Pap. 96, 80–88. doi: 10.1016/j.dsr.2014.11.004
Pirotta V., Grech A., Jonsen I. D., Laurance W. F., harcourt R. G. (2019). Consequences of global shipping traffic for marine giants. Front. Ecol. Environ. 17, 39–47. doi: 10.1002/fee.1987
Popova E., Vousden D., Sauer W. H. H., Mohammed E. Y., Allain V., Downey-Breedt N., et al. (2019). Ecological connectivity between the areas beyond national jurisdiction and coastal waters: Safeguarding interests of coastal communities in developing countries. Mar. Policy 104, 90–102. doi: 10.1016/j.marpol.2019.02.050
Potts T., Burdon D., Jackson E., Atkins J., Saunders J., Hastings E., et al. (2014). Do marine protected areas deliver flows of ecosystem services to support human welfare? Mar. Policy 44, 139–148. doi: 10.1016/j.marpol.2013.08.011
Ramirez-Llodra E., Tyler P. A., Baker M. C., Bergstad O. A., Clark M. R., Escobar E., et al. (2011). Man and the last great wilderness: human impact on the deep sea. PloS One 6, e22588. doi: 10.1371/journal.pone.0022588
Rees S. E., Ashley M., Cameron A., Mullier T., Ingle C., Oates J., et al. (2022). A marine natural capital asset and risk register—Towards securing the benefits from marine systems and linked ecosystem services. J. Appl. Ecol. 59 (4), 1–12. doi: 10.1111/1365-2664.14121
Rees S., Austen M. C., Attrill M. J., Rodwell L. D. (2012). Incorporating indirect ecosystem services into marine protected area planning and management. Int. J. Biodivers. Sci. Ecosyst. Serv. Manage. 8, 273–285. doi: 10.1080/21513732.2012.680500
Rhodes-Reese M., Clay D., Cunningham C., Moriles-Miller J., Reese C., Roman J., et al. (2021). Examining the role of marine mammals and seabirds in southeast alaska’s marine ecosystem dynamics. Front. Mar. Sci. 8. doi: 10.3389/fmars.2021.720277
Roberts J. P., Ali A. (2016). The blue economy and small states (London: Commonwealth Secretariat). Available at: http://www.jgme.org/. doi: 10.4300/JGME-D-15-00414.1
Rustomjee C. (2017). Operationalizing the blue economy in small states: lessons from the early movers. Policy Br., 1–8.
Ryan P. G. (2014). Litter survey detects the South Atlantic “garbage patch”. Mar. Pollut. Bull. 79, 220–224. doi: 10.1016/j.marpolbul.2013.12.010
Ryan P. G., Dilley B. J., Ronconi R. A., Connan M. (2019). Rapid increase in Asian bottles in the South Atlantic Ocean indicates major debris inputs from ships. Proc. Natl. Acad. Sci. U. S. A. 116, 20892–20897. doi: 10.1073/pnas.1909816116
Salinas-De-León P., Phillips B., Ebert D., Shivji M., Cerutti-Pereyra F., Ruck C., et al. (2018). Deep-sea hydrothermal vents as natural egg-case incubators at the Galapagos Rift. Sci. Rep. 8, 1–7. doi: 10.1038/s41598-018-20046-4
Saunders J., Potts T., Jackson E., Burdon D., Atkins J. P., Hastings E., et al. (2015). Linking ecosystem services of marine protected areas to benefits in human wellbeing? 167–190. doi: 10.1007/978-3-319-17214-9_9
Schiller L., Bailey M., Jacquet J., Sala E. (2018). High seas fisheries play a negligible role in addressing global food security. Sci. Adv. 4, 1–8. doi: 10.1126/sciadv.aat8351
Schmidtko S., Stramma L., Visbeck M. (2017). Decline in global oceanic oxygen content during the past five decades. Nature 542, 335–339. doi: 10.1038/nature21399
SEAFO (2014). Guidelines for fisheries research and basic marine science activity in the SEAFO Convention Area.
SEAFO (2015). Conservation measure 30/15 on bottom fishing activities and vulnerable marine ecosystems in the SEAFO convention area.
SEAFO (2016). Conservation measure 30/15 on bottom fishing activities and vulnerable marine ecosystems in the SEAFO convention area.
SEAFO (2019) System of observation, inspection, compliance and enforcement. Available at: https://www.fao.org/faolex/results/details/en/c/LEX-FAOC163878/ (Accessed September 8, 2022).
Seco Pon J. P., Copello S., Tamini L., Mariano-Jelicich R., Paz J., Blanco G., et al. (2015). “Seabird conservation in fisheries: Current state of knowledge and conservation needs for Argentine high-seas fleets,” in Seabirds and songbirds: Habitat preferences, conservation and migratory behavior, ed. Mahala G. (New York: NOVA Science Publishers Inc), 45–88.
Sellheim N., Schumacher J. (2022). Increasing the effectiveness of the bonn convention on the conservation of migratory species. J. Int. Wildl. Law Policy 25 (4), 367–383. doi: 10.1080/13880292.2022.2153461
Sergi S., Baudena A., Cotté C., Ardyna M., Blain S., d’Ovidio F. (2020). Interaction of the antarctic circumpolar current with seamounts fuels moderate blooms but vast foraging grounds for multiple marine predators. Front. Mar. Sci. 7. doi: 10.3389/fmars.2020.00416
Setälä O., Fleming-Lehtinen V., Lehtiniemi M. (2014). Ingestion and transfer of microplastics in the planktonic food web. Environ. pollut. 185, 77–83. doi: 10.1016/j.envpol.2013.10.013
Skropeta D., Wei L. (2014). Recent advances in deep-sea natural products. Nat. Prod. Rep. 31, 999–1025. doi: 10.1039/c3np70118b
Smith C. R., Levin L. A., Koslow A., Tyler P. A., Glover A. G., Division I. O., et al. (2008). “The near future of the deep seafloor ecosystems,” in Aquatic ecosystems trends and global prospects, vol. 334–349 . Ed. Polunin N. (Cambridge: Cambridge University Press).
Smith C. R., Tunnicliffe V., Colaço A., Drazen J. C., Gollner S., Levin L. A., et al. (2020). Deep-sea misconceptions cause underestimation of seabed-mining impacts. Trends Ecol. Evol. 35 (10), 1–5. doi: 10.1016/j.tree.2020.07.002
Snyder S., Franks P. J. S., Talley L. D., Xu Y., Kohin S. (2017). Crossing the line: Tunas actively exploit submesoscale fronts to enhance foraging success. Limnol. Oceanogr. Lett. 2, 187–194. doi: 10.1002/lol2.10049
Spiteri C., Senechal T., Hazin C., Hampton S., Greyling L., Boteler B. (2021). Study on the socio-economic importance of areas beyond national jurisdiction in the southeast atlantic region. doi: 10.48481/iass.2021.010
Stelzenmüller V., Coll M., Cormier R., Mazaris A. D., Pascual M., Loiseau C., et al. (2020). Operationalizing risk-based cumulative effect assessments in the marine environment. Sci. Total. Environ. 724, 138118. doi: 10.1016/j.scitotenv.2020.138118
Stelzenmüller V., Coll M., Mazaris A. D., Giakoumi S., Katsanevakis S., Portman M. E., et al. (2018). A risk-based approach to cumulative effect assessments for marine management. Sci. Total. Environ. 612, 1132–1140. doi: 10.1016/j.scitotenv.2017.08.289
Stocker T. F. (2013). “The ocean as a component of the climate system,” in Ocean circulation & Climate: A 21st century perspective. Eds. Siedler G., Griffies S. M., Gould J., Church J. A. (Oxford: Academic Press), 3–30.
Stramma L., Prince E. D., Schmidtko S., Luo J., Hoolihan J. P., Visbeck M., et al. (2012). Expansion of oxygen minimum zones may reduce available habitat for tropical pelagic fishes. Nat. Clim. Change 2, 33–37. doi: 10.1038/nclimate1304
Stramma L., Schmidtko S., Levin L. A., Johnson G. C. (2010). Ocean oxygen minima expansions and their biological impacts. Deep. Res. Part I. Oceanogr. Res. Pap. 57, 587–595. doi: 10.1016/j.dsr.2010.01.005
Stuart-Watt A. (2022) Why COP27 will be remembered as the Loss and Damage COP and what to expect next. LSE. Available at: https://www.lse.ac.uk/granthaminstitute/news/why-cop27-will-be-remembered-as-the-loss-and-damage-cop-and-what-to-expect-next/#:~:text= (Accessed January 9, 2023). While many thought it was,for “Loss and Damage”.
Sumaila U. R., Lam V. W. Y., Miller D. D., Teh L., Watson R. A., Zeller D., et al. (2015). Winners and losers in a world where the high seas is closed to fishing. Sci. Rep. 5, 8481. doi: 10.1038/srep08481
Sutton A. J., Feely R. A., Maenner-Jones S., Musielwicz S., Osborne J., Dietrich C., et al. (2018). Autonomous seawater pCO2 and pH time series from 40 surface buoys and the emergence of anthropogenic trends. Earth Syst. Sci. doi: 10.5194/essd-2018-77
Sweetman A. K., Smith C. R., Shulse C. N., Maillot B., Lindh M., Church M. J., et al. (2019). Key role of bacteria in the short-term cycling of carbon at the abyssal seafloor in a low particulate organic carbon flux region of the eastern Pacific Ocean. Limnol. Oceanogr. 64, 694–713. doi: 10.1002/lno.11069
Sweetman A. K., Thurber A. R., Smith C. R., Levin L. A., Mora C., Wei C. L., et al. (2017). Major impacts of climate change on deep-sea benthic ecosystems. Elementa 5. doi: 10.1525/elementa.203
Szepes M. (2013). MARPOL 73/78: The Challenges of Regulating Vessel-Source Oil Pollution. Manchester Student Law Rev. 2, 73–109.
Taucher J., Haunost M., Boxhammer T., Bach L. T., Algueró-Muñiz M., Riebesell U. (2017). Influence of ocean acidification on plankton community structure during a winter-tosummer succession: An imaging approach indicates that copepods can benefit from elevated CO2 via indirect food web effects. PloS One 12, e0169737. doi: 10.1371/journal.pone.0169737
Thaler A. D., Freitag A., Bergman E., Fretz D., Saleu W. (2015). Robots as vectors for marine invasions: best practices for minimizing transmission of invasive species via observation-class ROVs. Trop. Conserv. Sci. 8, 711–717. doi: 10.1177/194008291500800308
Thompson A., Sanders J., Tandstad M., Carocci F., Fuller J. (2016) Vulnerable marine ecosystems: Processes and practices in the high seas (Rome). Available at: https://www.fao.org/3/i5952e/i5952e.pdf (Accessed September 4, 2023).
Thurber A. R., Sweetman A. K., Narayanaswamy B. E., Jones D. O. B., Ingels J., Hansman R. L. (2014). Ecosystem function and services provided by the deep sea. Biogeosciences 11, 3941–3963. doi: 10.5194/bg-11-3941-2014
Tilot V., Willaert K., Guilloux B., Chen W., Mulalap C. Y., Gaulme F., et al. (2021). Traditional dimensions of seabed resource management in the context of deep sea mining in the pacific: learning from the socio-ecological interconnectivity between island communities and the ocean realm. Front. Mar. Sci. 8. doi: 10.3389/fmars.2021.637938
Tyler-Walters H., Tillin H. M., D’avack E. A. S., Perry F., Stamp T. (2018). Marine evidence-based sensitivity assessment (MarESA) – A guide (Plymouth: Marine Biological Association of the United Kingdom). Available at: https://www.marlin.ac.uk/publications.
Tyler-Walters H., Williams E., Mardle M. J., Lloyd K. A. (2022). Sensitivity assessment of contaminant pressures - approach development, application, and evidence reviews (Plymouth: Marine Biological Association of the United Kingdom). Available at: https://www.marlin.ac.uk/publications.
UKCOP26 (2021) Breakthrough Agenda - Launching an annual global checkpoint process in 2022. Available at: https://ukcop26.org/breakthrough-agenda-launching-an-annual-global-checkpoint-process-in-2022/ (Accessed January 10, 2023).
UN (1982) UNCLOS. Available at: http://www.ingentaselect.com/rpsv/cgi-bin/cgi?ini=xref&body=linker&req.
UN (2015) Sustainable development goals. Available at: http://www.un.org/sustainabledevelopment/sustainable-development-goals/ (Accessed October 31, 2017).
UN (2023). Agreement under the united nations convention on law of the sea on the conservation and sustainable use of marine biological diversity of areas beyond national jurisdiction.
UNEP-WCMC (2017). Governance of areas beyond national jurisdiction for biodiversity conservation and sustainable use: Institutional arrangements and cross-sectoral cooperation in the Western Indian Ocean and South East Pacific (Cambridge).
UNFCCC (2015) Paris agreement. Available at: https://unfccc.int/process-and-meetings/the-paris-agreement/the-paris-agreement.
UNFCCC (2021a) COP 26 glasgow climate pact. Available at: https://unfccc.int/sites/default/files/resource/cop26_auv_2f_cover_decision.pdf.
UNFCCC (2021b) What is the united nations framework convention on climate change? Available at: https://unfccc.int/process-and-meetings/the-convention/what-is-the-united-nations-framework-convention-on-climate-change (Accessed June 2, 2021).
UNGA (1995). Agreement for the implementation of the provisions of the united nations convention on the law of the sea of 10 december 1982 relating to the conservation and management of straddling fish stocks and highly migratory fish stocks (New York: United Nations General Assembly). Available at: https://documents-dds-ny.un.org/doc/UNDOC/GEN/N95/274/67/PDF/N9527467.pdf?OpenElement.
van der Grient J. M. A., Drazen J. C. (2022). Evaluating deep-sea communities’ susceptibility to mining plumes using shallow-water data. Sci. Total. Environ. 852, 158162. doi: 10.1016/j.scitotenv.2022.158162
Van Dover C. L. (2014). Impacts of anthropogenic disturbances at deep-sea hydrothermal vent ecosystems: A review. Mar. Environ. Res. 102, 59–72. doi: 10.1016/j.marenvres.2014.03.008
Van Dover C. L., Ardron J. A., Escobar E., Gianni M., Gjerde K. M., Jaeckel A., et al. (2017). Biodiversity loss from deep-sea mining. Nat. Geosci. 10, 464–465. doi: 10.1038/ngeo2983
Vanreusel A., Hilario A., Ribeiro P. A., Menot L., Arbizu P. M. (2016). Threatened by mining, polymetallic nodules are required to preserve abyssal epifauna. Sci. Rep. 6, 26808. doi: 10.1038/srep26808
Vaquer-Sunyer R., Duarte C. M. (2008). Thresholds of hypoxia for marine biodiversity. Proc. Natl. Acad. Sci. U. S. A. 105, 15452–15457. doi: 10.1073/pnas.0803833105
Vieira R. P., Raposo I. P., Sobral P., Gonçalves J. M. S., Bell K. L. C., Cunha M. R. (2015). Lost fishing gear and litter at Gorringe Bank (NE Atlantic). J. Sea. Res. 100, 91–98. doi: 10.1016/j.seares.2014.10.005
Vierros M. K., Harrison A. L., Sloat M. R., Crespo G. O., Moore J. W., Dunn D. C., et al. (2020). Considering Indigenous Peoples and local communities in governance of the global ocean commons. Mar. Policy 119, 104039. doi: 10.1016/j.marpol.2020.104039
Vincent A. C. J., Sadovy de Mitcheson Y. J., Fowler S. L., Lieberman S. (2014). The role of CITES in the conservation of marine fishes subject to international trade. Fish. Fish. 15, 563–592. doi: 10.1111/faf.12035
Vonnahme T. R., Molari M., Janssen F., Wenzhöfer F., Haeckel M., Titschack J., et al. (2020). Effects of a deep-sea mining experiment on seafloor microbial communities and functions after 26 years. Sci. Adv. 6, eaaz5922. doi: 10.1126/sciadv.aaz5922
Walker T. R., Adebambo O., Del Aguila Feijoo M. C., Elhaimer E., Hossain T., Edwards S. J., et al. (2019). Environmental effects of marine transportation. World Seas: An Environmental Evaluation eds. Sheppard C. (London: Academic Press), 505–530. doi: 10.1016/B978-0-12-805052-1.00030-9
Washburn T. W., Turner P. J., Durden J. M., Jones D. O. B., Weaver P., Van Dover C. L. (2019). Ecological risk assessment for deep-sea mining. Ocean. Coast. Manage. 176, 24–39. doi: 10.1016/j.ocecoaman.2019.04.014
Wedding L. M., Reiter S. M., Smith C. R., Gjerde K. M., Kittinger J. N., Friedlander A. M., et al. (2015). Managing mining of the deep seabed. Sci. (80-. ). 349, 144–145. doi: 10.1126/science.aac6647
Weisz J. B., Lindquist N., Martens C. S. (2008). Do associated microbial abundances impact marine demosponge pumping rates and tissue densities? Oecologia 155, 367–376. doi: 10.1007/s00442-007-0910-0
Wright G., Ardron J., Gjerde K., Currie D., Rochette J. (2015). Advancing marine biodiversity protection through regional fisheries management: A review of bottom fisheries closures in areas beyond national jurisdiction. Mar. Policy 61, 134–148. doi: 10.1016/j.marpol.2015.06.030
Wright S. R., Righton D., Naulaerts J., Schallert R. J., Bendall V., Griffiths C., et al. (2021). Fidelity of yellowfin tuna to seamount and island foraging grounds in the central South Atlantic Ocean. Deep. Res. Part I. Oceanogr. Res. Pap. 172, 103513. doi: 10.1016/j.dsr.2021.103513
WTO (2022) Agreement on fisheries subsidies. World trade organisation: (Geneva). Available at: https://www.wto.org/english/tratop_e/rulesneg_e/fish_e/fish_e.htm (Accessed January 10, 2023).
Ziegler J. A., Diamant S., Pierce S. J., Bennett R., Kis/Ka J. J. (2021). ECONOMIC VALUE AND PUBLIC PERCEPTIONS OF WHALE SHARK TOURISM IN NOSY BE, MADAGASCAR. Tour. Mar. Environ. 16, 167–182. doi: 10.3727/154427321X16223819324721
Keywords: natural capital, risk register, ecosystem services, ABNJ, ecosystem service governance, deep-sea ecosystem services, societal benefits, ecosystem approach
Citation: Niner HJ, Rees SE, La Bianca G, McQuaid KA and Howell KL (2024) A risk assessment for the remote ocean: the case of the South East Atlantic. Front. Mar. Sci. 10:1168686. doi: 10.3389/fmars.2023.1168686
Received: 17 February 2023; Accepted: 24 November 2023;
Published: 09 January 2024.
Edited by:
Miriam Von Thenen, Leibniz Institute for Baltic Sea Research (LG), GermanyReviewed by:
Maria E. Bravo, Environmental and Applied Geosciences (IGEBA), ArgentinaNezha Mejjad, National Centre for Nuclear Energy, Science and Technology, Morocco
Copyright © 2024 Niner, Rees, La Bianca, McQuaid and Howell. This is an open-access article distributed under the terms of the Creative Commons Attribution License (CC BY). The use, distribution or reproduction in other forums is permitted, provided the original author(s) and the copyright owner(s) are credited and that the original publication in this journal is cited, in accordance with accepted academic practice. No use, distribution or reproduction is permitted which does not comply with these terms.
*Correspondence: Holly J. Niner, holly.niner@plymouth.ac.uk