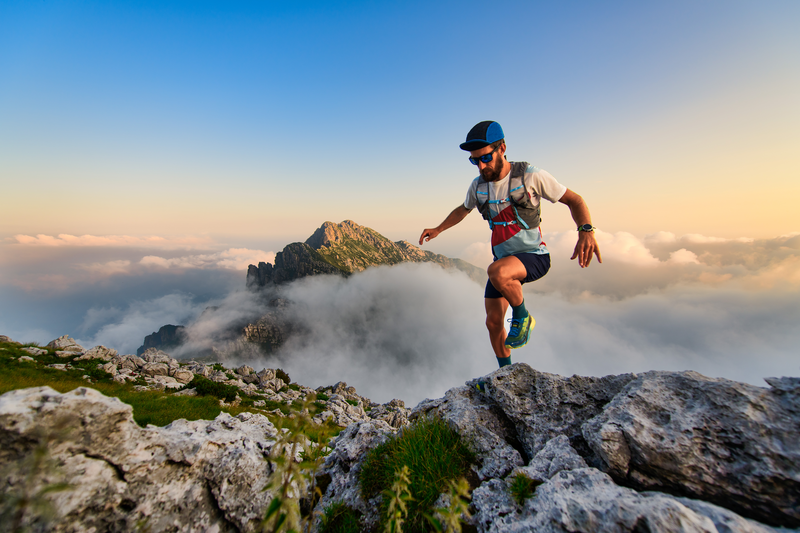
94% of researchers rate our articles as excellent or good
Learn more about the work of our research integrity team to safeguard the quality of each article we publish.
Find out more
EDITORIAL article
Front. Mar. Sci. , 16 March 2023
Sec. Coastal Ocean Processes
Volume 10 - 2023 | https://doi.org/10.3389/fmars.2023.1168296
This article is part of the Research Topic Human Impacts on River Catchments and Coastal Ecosystems: A Meta-Ecosystem Perspective. View all 5 articles
Editorial on the Research Topic
Human impacts on river catchments and coastal ecosystems: A meta-ecosystem perspective
Meta-ecosystems can be defined as a set of ecosystems connected by spatial flows of energy, matter and organisms (Loreau et al., 2003; Cid et al., 2022). A paradigmatic meta-ecosystem is the one formed by the interaction of river catchments and coastal ecosystems. For example, rivers transport enormous amounts of nutrients to coastal waters (Schlünz and Schneider, 2000; Li et al., 2020), determining primary and secondary productivity (Gibson et al., 2002; Garnier et al., 2010). Thus, estuaries are highly productive areas (Levin et al., 2001), with a higher value of ecosystem services per hectare than any other ecosystem (Costanza et al., 1997; Newton et al., 2018). At the same time, river basins are subjected to multiple human disturbances (e.g. hydrological disturbances, pollution, habitat degradation) that accumulate downstream until they reach the sea (Newton et al., 2012; Flo et al., 2019). This makes estuaries one of the most degraded ecosystems on Earth (Kaiser et al., 2011).
This meta-ecosystem perspective is extremely important to guide effective management efforts, since the environmental challenges that human societies face are not restricted to ecosystem boundaries. In the case of the river-coastal meta-ecosystem, this can be clearly illustrated with eutrophication (Smith, 2003; Smith et al., 2006). River catchments receive large phosphorus and nitrogen inputs from urban and industrial wastewater discharges and agricultural runoff (Meybeck and Helmer, 1989; Strokal et al., 2016). These nutrients accumulate in the estuaries and coastal zones, which receive more nutrients per unit area than any other ecosystem on Earth (Smith, 2003). Nutrient accumulation leads to changes in planktonic communities (Romero et al., 2013) that might trigger cascading effects, changing the ecosystem state (Scheffer et al., 2001). For example, eutrophication often stimulates toxic algal blooms such as some cyanobacteria and dinoflagellates (Wurtsbaugh et al., 2019) that could have cascading effects on local fisheries (Anderson et al., 2008). These blooms are influenced not only by the total concentration of nutrients but also by their ratio (C:Si:N:P), which determines the metabolism of phytoplankton (Redfield, 1958; Justić et al., 1995). Thus, the alteration of the nutrient ratio can favor different phytoplankton species depending on their metabolism (Glibert et al., 2018). In addition to toxic blooms, phytoplankton proliferation can cause the degradation of submerged vegetation through competition for light and nutrients (Munkes, 2005). Also, the excess of primary and secondary production is deposited in the sediments leading to hypoxic situations due to the great demand for oxygen by decomposing microorganisms (Díaz and Rosenberg, 2008). So, overall, when nutrients are added along the river catchment the coastal ecosystem might shift from a clear water state dominated by submerged vegetation into a turbid water state dominated by phytoplankton (including toxic species), leading to a general decline in aquatic biodiversity (Duarte, 2009; Rabalais et al., 2009; Rodríguez-Gallego et al., 2017).
As the human pressure on ecosystems increases (Díaz et al., 2019), an integrated management of river catchments and the coastal areas associated with them is becoming increasingly needed (Álvarez-Romero et al., 2011; Cantasano et al., 2020; Lewis et al., 2021). However, such approach can only be effective if it is based on a deep understanding on the links between freshwater and marine ecosystems. Within this context, this special issue provides insights into the interaction of river and coastal ecosystems, laying out the foundations of future research.
Pargaonkar and Vinayachandran show the presence of Irrawaddy River jets for the first time in the Andaman Sea during summer monsoon. The Irrawaddy is the largest river discharging into the Andaman Sea. River plumes are considered important in the shelf seas all across the world’s oceans (Kang et al., 2013; Milliman and Farnsworth, 2013; Horner-Devine et al., 2015). When the river meets the ocean at the coast the discharge from the river forms a region of freshwater with a distinct dynamical process. River discharge to the coastal ocean modifies the local circulation and associated biogeochemistry. This study presents the first comprehensive evidence through satellite and model simulation to show that during the summer monsoon the discharge from the Irrawaddy spreads as a freshwater jet oriented towards southeast and accumulates over the shelf at the eastern coast of the Andaman Sea.
The meta-ecosystem perspective of river and coastal ecosystems is very relevant from the point of view of ecosystems services, which often rely on the interaction between river and coastal dynamics. One good example is maritime transportation, which strongly contributes to local and regional economic development via global supply chains. In this Research Topic, Muñoz et al. show how sea-level rise and river flows interact to determine wetland inundation and vessel navigability. Their study demonstrates that an integrated management of river catchments and coastal areas is needed to find environmental-friendly solutions for increasing cargo transportation.
Deltas are areas of wide interest as they are usually widely populated and they harbour unique ecosystems characterized by an active mixing of seawater and freshwater, connecting the land and the ocean (Nicholls et al., 2020). As a result, delta systems such as the massive Ganga and Brahmaputra delta in India and Bangladesh (Allison, 1998), the Nile delta in Egypt (Negm, 2017), the Pearl delta in China (Chan et al., 2021), the Frazer delta in Canada (Hill and Lintern, 2021), and many others across the globe (Syvitski, 2008), are widely studied. In this Research Topic, Gallo-Vélez et al., provide a socioeconomic assessment of growing pollution in the Magdalena delta in Colombia. The authors looked into nitrogen, phosphorous, and biological oxygen demand, as well as fecal coliform and their impact on socio-economic development. The study found fecal coliforms were more than 4 orders of magnitude higher in untreated wastewater, while agriculture and livestock are theoretically the largest contributors of nitrogen (i.e., 14.84 t d-1 and 48.99 t d-1) and phosphorus (i.e., 5.90 t d-1 and 19.46 t d-1) in the basin. The study shows that waste generated within the study area has an adverse impact on the mangrove ecosystems, severely affecting the coastal population. The study highlights the state of contamination and pollution in the Magdalena delta, and its impact on increased cleaning maintenance on beaches, loss of tourism revenue, increased living cost, and loss of cultural values. Overall, the paper provides a comprehensive study of the socio-ecological system by identifying the drivers of contamination, pressure, and state of pollution, and its impact on livelihood. In the same vein, Magel and Francis evaluate the multi-benefit outcomes of potential management interventions to address population growth and development, which still remains a major management challenge for most coastal systems. Integrative decision support tools that consider socio-economic, cultural and ecological objectives remain overlooked. In this study, which focuses on the Puget Sound fjord-type estuary (USA), a conceptual model to represent the connections between human stressors and ecosystem components was developed. This allowed exploring alternative scenarios for accommodating human population growth. The study reported that moderate levels of coordinated interventions on both urban and rural lands had favorable outcomes for more ecosystem objectives, showcasing the value of qualitative tools for cross-habitat evaluations. Together, these studies showcase the vulnerability of deltas and estuaries in the Anthropocene and the need for developing integrated management actions that incorporate the complexity of socio ecological systems.
The study of river and coastal ecosystems as a continuum is still quite rare. Marine ecology and limnology have been developed as two separate fields of research that don’t communicate as often as they should, with estuarine environments (i.e. transitional waters sensu Water Framework Directive) being comparatively less studied than coasts and rivers. Thus, there are still many research needs to be covered, such as:
A good example of this is the northern Indian ocean, which has a complex circulation system that undergoes seasonal reversal and determines shelf biogeochemistry (Naqvi et al., 2006; Zhang et al., 2010). India has 7000 km coastline and several glacial rivers flowing through major cities that have the potential to change the coastal nutrient budget and ecosystem dynamics (Samanta et al., 2015). Further, freshwater inputs also have the potential to enhance ocean stratification thereby influencing the salinity budget (Seo et al., 2009). From a regional point of view, the response of the phytoplankton population to freshwater input and its influence in carbon dynamics can be important areas for future research. For example, how the nutrient stoichiometry changes in the freshwater system over time and its physical controls can be one interesting area of research.
Salinity is a key parameter determining the distribution of the species along the riverine-estuarine-coastal continuum (Remane, 1934; Whitfield et al., 2012). For example, magrove forests, which provide important ecosystem services such as carbon sequestration (Adame et al., 2021), are highly dependent on salinity dynamics (Bunt et al., 1982; Krauss et al., 2008). The water salinity along the river-coastal metaecosystem is heavily modified by humans through three different mechanisms: 1) sea-level rise associated with climate change (Pörtner et al., 2022); 2) groundwater overexploitation (Alfarrah and Walraevens, 2018); and 3) reductions in river discharge due to the proliferation of dams (Grill et al., 2019; Bellafiore et al., 2021). Understanding the complex hydrological dynamics resulting from the interaction of these three factors is urgently needed to preserve biodiversity and food and water security in the face of global change.
Many rivers around the world are getting saltier due to different human drivers such as agriculture, resource extraction and transportation (Cañedo-Argüelles et al., 2013; Thorslund et al., 2021). This might promote the invasion of brackishwater species from coastal and estuarine environments (Kefford et al., 2016). For example, the crustacean Gammarus tigrinus was able to colonize large sections of the River Werra in Germany due to the salinization caused by the potash mining industry (Koop and Grieshaber, 2000; Bäthe and Coring, 2011; Szöcs et al., 2014). Although the spread of invasive species in aquatic ecosystems has been well documented around the world (Williams and Grosholz, 2008; Gallardo et al., 2016), there is still little information on how the modification of meta-ecosystem dynamics by humans might affect the distribution of aquatic invasive species along the coast-river continuum (Lago et al., 2019).
Overall, it is clear that rivers and coasts should not be managed as separate ecosystems given the strong socioecological connections that they have (Lago et al., 2019; Gladstone-Gallagher et al., 2022). Therefore, we call freshwater and marine scientists to collaborate to produce an integrated perspective of aquatic ecosystems, which is urgently needed to halt global biodiversity loss and ecosystem degradation.
All authors contributed to conceptualizing the paper. MC-A led the writing process and AB, IS and RR also contributed to writing and providing critical comments. All authors contributed to the article and approved the submitted version.
MC-A was supported by a Ramón y Cajal contract funded by the Spanish Ministry of Science and Innovation (RYC2020-029829-I). AB was funded by the Fundação para a Ciência e a Tecnologia (FCT), through the Scientific Employment Stimulus Programme (CEECIND/0095/2017). This work also received further support from Fundação para a Ciência e a Tecnologia, through the strategic projects (UIDB/04292/2020, LA/P/0069/2020) granted to MARE and ARNET.
The authors declare that the research was conducted in the absence of any commercial or financial relationships that could be construed as a potential conflict of interest.
All claims expressed in this article are solely those of the authors and do not necessarily represent those of their affiliated organizations, or those of the publisher, the editors and the reviewers. Any product that may be evaluated in this article, or claim that may be made by its manufacturer, is not guaranteed or endorsed by the publisher.
Adame M. F., Connolly R. M., Turschwell M. P., Lovelock C. E., Fatoyinbo T., Lagomasino D., et al. (2021). Future carbon emissions from global mangrove forest loss. Global Change Biol. 27 (12), 2856–2866. doi: 10.1111/gcb.15571
Alfarrah N., Walraevens K. (2018). Groundwater overexploitation and seawater intrusion in coastal areas of arid and semi-arid regions. Water 10 (2), 143. doi: 10.3390/w10020143
Allison M. A. (1998). Geologic framework and environmental status of the Ganges-Brahmaputra delta. J. Coast. Res. 14 (3), 827–836.
Álvarez-Romero J. G., Pressey R. L., Ban N. C., Vance-Borland K., Willer C., Klein C. J., et al. (2011). Integrated land-sea conservation planning: the missing links. Annu. Rev. Ecol. Evol. Syst. 42, 381–409. doi: 10.1146/annurev-ecolsys-102209-144702
Anderson D. M., Burkholder J. M., Cochlan W. P., Glibert P. M., Gobler C. J., Heil C. A., et al. (2008). Harmful algal blooms and eutrophication: Examining linkages from selected coastal regions of the united states. Harmful Algae 8 (1), 39–53. doi: 10.1016/j.hal.2008.08.017
Bäthe J., Coring E. (2011). Biological effects of anthropogenic salt-load on the aquatic fauna: A synthesis of 17 years of biological survey on the rivers werra and weser. Limnologica 41 (2), 125–133. doi: 10.1016/j.limno.2010.07.005
Bellafiore D., Ferrarin C., Maicu F., Manfè G., Lorenzetti G., Umgiesser G., et al. (2021). Saltwater intrusion in a Mediterranean delta under a changing climate. J. Geophys. Res.: Oceans 126 (2), e2020JC016437. doi: 10.1029/2020JC016437
Bunt J. S., Williams W. T., Clay H. J. (1982). River water salinity and the distribution of mangrove species along several rivers in north Queensland. Aust. J. Bot. 30 (4), 401–412. doi: 10.1071/BT9820401
Cañedo-Argüelles M., Kefford B. J., Piscart C., Prat N., Schäfer R. B., Schulz C. J. (2013). Salinisation of rivers: an urgent ecological issue. Environ. Pollut. 173, 157–167. doi: 10.1016/j.envpol.2012.10.011
Cantasano N., Pellicone G., Ietto F. (2020). The coastal sustainability standard method: A case study in calabria (southern Italy). Ocean Coast. Manage. 183, 104962. doi: 10.1016/j.ocecoaman.2019.104962
Chan F. K. S., Yang L. E., Scheffran J., Mitchell G., Adekola O., Griffiths J., et al. (2021). Urban flood risks and emerging challenges in a Chinese delta: The case of the pearl river delta. Environ. Sci. Policy 122, 101–115. doi: 10.1016/j.envsci.2021.04.009
Cid N., Erős T., Heino J., Singer G., Jähnig S. C., Cañedo-Argüelles M., et al. (2022). From meta-system theory to the sustainable management of rivers in the anthropocene. Front. Ecol. Environ. 20 (1), 49–57. doi: 10.1002/fee.2417
Costanza R., d'Arge R., De Groot R., Farber S., Grasso M., Hannon B., et al. (1997). The value of the world's ecosystem services and natural capital. Nature 387 (6630), 253–260. doi: 10.1038/387253a0
Díaz R. J., Rosenberg R. (2008). Spreading dead zones and consequences for marine ecosystems. Science 321 (5891), 926–929. doi: 10.1126/science.1156401
Díaz S. M., Settele J., Brondízio E., Ngo H., Guèze M., Agard J., et al. (2019). The global assessment report on biodiversity and ecosystem services: Summary for policy makers. Available at: https://ipbes.net/system/tdf/inline/files/ipbes_global_assessment_report_summary_for_policymakers.pdf?file=1&type=node&id=36213.
Duarte C. M. (2009). Coastal eutrophication research: A new awareness. Hydrobiologia 629 (1), 263–269. doi: 10.1007/s10750-009-9795-8
Flo E., Garcés E., Camp J. (2019). Land uses simplified index (LUSI): Determining land pressures and their link with coastal eutrophication. Front. Mar. Sci. 6, 18. doi: 10.3389/fmars.2019.00018
Gallardo B., Clavero M., Sánchez M. I., Vilà M. (2016). Global ecological impacts of invasive species in aquatic ecosystems. Global Change Biol. 22 (1), 151–163. doi: 10.1111/gcb.13004
Garnier J., Beusen A., Thieu V., Billen G., Bouwman L. (2010). N: P: Si nutrient export ratios and ecological consequences in coastal seas evaluated by the ICEP approach. Global Biogeochem. Cycles 24 (4), GB0A05. doi: 10.1029/2009GB003583
Gibson R. N., Barnes M., Atkinson R. J. A. (2002). Impact of changes in flow of freshwater on estuarine and open coastal habitats and the associated organisms. Oceanogr. Mar. Biol. an Annu. Rev. 40, 233.
Gladstone-Gallagher R. V., Tylianakis J. M., Yletyinen J., Dakos V., Douglas E. J., Greenhalgh S., et al. (2022). Social–ecological connections across land, water, and sea demand a reprioritization of environmental management. Elementa Sci. Anthropocene 10 (1), 00075. doi: 10.1525/elementa.2021.00075
Glibert P. M., Berdalet E., Burford M. A., Pitcher G. C., Zhou M. (2018). Global ecology and oceanography of harmful algal blooms Vol. 232 (Cham: Springer).
Grill G., Lehner B., Thieme M., Geenen B., Tickner D., Antonelli F., et al. (2019). Mapping the world’s free-flowing rivers. Nature 569 (7755), 215–221.
Hill P. R., Lintern D. G. (2021). Sedimentary processes at the mouth of a tidally-influenced delta: New insights from submarine observatory measurements, Fraser delta, Canada. Sedimentology 68 (6), 2649–2670. doi: 10.1111/sed.12868
Horner-Devine A. R., Hetland R. D., MacDonald D. G. (2015). Mixing and transport in coastal river plumes. Annu. Rev. Fluid Mechanics 47, 569–594. doi: 10.1146/annurev-fluid-010313-141408
Justić D., Rabalais N. N., Turner R. E., Dortch Q. (1995). Changes in nutrient structure of river-dominated coastal waters: Stoichiometric nutrient balance and its consequences. Estuar. Coast. Shelf Sci. 40 (3), 339–356. doi: 10.1016/S0272-7714(05)80014-9
Kaiser M. J., Jennings S., Thomas D. N., Barnes D. K. (2011). Marine ecology: Processes, systems, and impacts (Oxford, UK: Oxford University Press).
Kang Y., Pan D., Bai Y., He X., Chen X., Chen C. T. A., et al. (2013). Areas of the global major river plumes. Acta Oceanol. Sin. 32, 79–88. doi: 10.1007/s13131-013-0269-5
Kefford B. J., Buchwalter D., Cañedo-Argüelles M., Davis J., Duncan R. P., Hoffmann A., et al. (2016). Salinized rivers: Degraded systems or new habitats for salt-tolerant faunas? Biol. Lett. 12 (3), 20151072. doi: 10.1098/rsbl.2015.1072
Koop J. H. E., Grieshaber M. K. (2000). The role of ion regulation in the control of the distribution of gammarus tigrinus (Sexton) in salt-polluted rivers. J. Comp. Physiol. B 170, 75–83. doi: 10.1007/s003600050010
Krauss K. W., Lovelock C. E., McKee K. L., López-Hoffman L., Ewe S. M., Sousa W. P. (2008). Environmental drivers in mangrove establishment and early development: A review. Aquat. Bot. 89 (2), 105–127. doi: 10.1016/j.aquabot.2007.12.014
Lago M., Boteler B., Rouillard J., Abhold K., Jähnig S. C., Iglesias-Campos A., et al. (2019). Introducing the H2020 AQUACROSS project: Knowledge, assessment, and management for AQUAtic biodiversity and ecosystem services aCROSS EU policies. Sci. Total Environ. 652, 320–329. doi: 10.1016/j.scitotenv.2018.10.076
Levin L. A., Boesch D. F., Covich A., Dahm C., Erséus C., Ewel K. C., et al. (2001). The function of marine critical transition zones and the importance of sediment biodiversity. Ecosystems 4 (5), 430–451. doi: 10.1007/s10021-001-0021-4
Lewis S. E., Bartley R., Wilkinson S. N., Bainbridge Z. T., Henderson A. E., James C. S., et al. (2021). Land use change in the river basins of the great barrier reef 1860 to 2019: A foundation for understanding environmental history across the catchment to reef continuum. Mar. Pollut. Bull. 166, 112193. doi: 10.1016/j.marpolbul.2021.112193
Li L., Ni J., Chang F., Yue Y., Frolova N., Magritsky D., et al. (2020). Global trends in water and sediment fluxes of the world’s large rivers. Sci. Bull. 65 (1), 62–69. doi: 10.1016/j.scib.2019.09.012
Loreau M., Mouquet N., Holt R. D. (2003). Meta-ecosystems: A theoretical framework for a spatial ecosystem ecology. Ecol. Lett. 6 (8), 673–679. doi: 10.1046/j.1461-0248.2003.00483.x
Meybeck M., Helmer R. (1989). The quality of rivers: From pristine stage to global pollution. Palaeogeogr. Palaeoclimatol. Palaeoecol. 75 (4), 283–309. doi: 10.1016/0031-0182(89)90191-0
Milliman J. D., Farnsworth K. L. (2013). River discharge to the coastal ocean: A global synthesis (Cambridge, UK: Cambridge University Press).
Munkes B. (2005). Eutrophication, phase shift, the delay and the potential return in the greifswalder bodden, Baltic Sea. Aquat. Sci. 67 (3), 372–381. doi: 10.1007/s00027-005-0761-x
Naqvi S. W. A., Naik H., Pratihary A., D'souza W., Narvekar P. V., Jayakumar D. A., et al. (2006). Coastal versus open-ocean denitrification in the Arabian Sea. Biogeosciences 3 (4), 621–633. doi: 10.5194/bg-3-621-2006
Newton A., Brito A. C., Icely J. D., Derolez V., Clara I., Angus S., et al. (2018). Assessing, quantifying and valuing the ecosystem services of coastal lagoons. J. Nat. Conserv. 44, 50–65. doi: 10.1016/j.jnc.2018.02.009
Newton A., Carruthers T. J., Icely J. (2012). The coastal syndromes and hotspots on the coast. Estuar. Coast. Shelf Sci. 96, 39–47. doi: 10.1016/j.ecss.2011.07.012
Nicholls R. J., Adger W. N., Hutton C. W., Hanson S. E. (2020). Deltas Anthropocene, 282. doi: 10.1007/978-3-030-23517-8 Available at: https://library.oapen.org/handle/20.500.12657/22862.
Pörtner H. O., Roberts D. C., Poloczanska E. S., Mintenbeck K., Tignor M., Alegría A., et al. (2022). IPCC 2022: Summary for policymakers. (Cambridge, UK: Cambridge University Press)
Rabalais N. N., Turner R. E., Díaz R. J., Justić D. (2009). Global change and eutrophication of coastal waters. ICES J. Mar. Sci. 66 (7), 1528–1537. doi: 10.1093/icesjms/fsp047
Redfield A. C. (1958). The biological control of chemical factors in the environment. Am. Scientist 46 (3), 230A–2221.
Remane A. (1934). Die brackwasserfauna. Verhandlungen Der Deutschen Zoologischen Gesellschaft 36, 34–74.
Rodríguez-Gallego L., Achkar M., Defeo O., Vidal L., Meerhoff E., Conde D. (2017). Effects of land use changes on eutrophication indicators in five coastal lagoons of the southwestern Atlantic ocean. Estuar. Coast. Shelf Sci. 188, 116–126. doi: 10.1016/j.ecss.2017.02.010
Romero E., Garnier J., Lassaletta L., Billen G., Le Gendre R., Riou P., et al. (2013). Large-Scale patterns of river inputs in southwestern Europe: Seasonal and interannual variations and potential eutrophication effects at the coastal zone. Biogeochemistry 113 (1), 481–505. doi: 10.1007/s10533-012-9778-0
Samanta S., Dalai T. K., Pattanaik J. K., Rai S. K., Mazumdar A. (2015). Dissolved inorganic carbon (DIC) and its δ 13C in the Ganga (Hooghly) River estuary, India: Evidence of DIC generation via organic carbon degradation and carbonate dissolution. Geochimica et cosmochimica acta 165, 226–248.
Scheffer M., Carpenter S., Foley J. A., Folke C., Walker B. (2001). Catastrophic shifts in ecosystems. Nature 413 (6856), 591–596. doi: 10.1038/35098000
Schlünz B. S. R. R., Schneider R. R. (2000). Transport of terrestrial organic carbon to the oceans by rivers: re-estimating flux-and burial rates. Int. J. Earth Sci. 88 (4), 599–606. doi: 10.1007/s005310050290
Seo H., Xie S. P., Murtugudde R., Jochum M., Miller A. J. (2009). Seasonal effects of Indian ocean freshwater forcing in a regional coupled model. J. Climate 22 (24), 6577–6596. doi: 10.1175/2009JCLI2990.1
Smith V. H. (2003). Eutrophication of freshwater and coastal marine ecosystems a global problem. Environ. Sci. Pollut. Res. 10 (2), 126–139. doi: 10.1065/espr2002.12.142
Smith V. H., Joye S. B., Howarth R. W. (2006). Eutrophication of freshwater and marine ecosystems. Limnol. Oceanogr. 51 (1part2), 351–355. doi: 10.4319/lo.2006.51.1_part_2.0351
Strokal M., Kroeze C., Wang M., Bai Z., Ma L. (2016). The MARINA model (Model to assess river inputs of nutrients to seAs): Model description and results for China. Sci. Total Environ. 562, 869–888. doi: 10.1016/j.scitotenv.2016.04.071
Szöcs E., Coring E., Bäthe J., Schäfer R. B. (2014). Effects of anthropogenic salinization on biological traits and community composition of stream macroinvertebrates. Sci. Total Environ. 468, 943–949. doi: 10.1016/j.scitotenv.2013.08.058
Thorslund J., Bierkens M. F., Oude Essink G. H., Sutanudjaja E. H., van Vliet M. T. (2021). Common irrigation drivers of freshwater salinisation in river basins worldwide. Nat. Commun. 12 (1), 4232. doi: 10.1038/s41467-021-24281-8
Whitfield A. K., Elliott M., Basset A., Blaber S. J. M., West R. J. (2012). Paradigms in estuarine ecology–a review of the remane diagram with a suggested revised model for estuaries. Estuarine Coast. Shelf Sci. 97, 78–90. doi: 10.1016/j.ecss.2011.11.026
Williams S. L., Grosholz E. D. (2008). The invasive species challenge in estuarine and coastal environments: marrying management and science. Estuaries Coasts 31, 3–20. doi: 10.1007/s12237-007-9031-6
Wurtsbaugh W. A., Paerl H. W., Dodds W. K. (2019). Nutrients, eutrophication and harmful algal blooms along the freshwater to marine continuum. Wiley Interdiscip. Reviews: Water 6 (5), e1373. doi: 10.1002/wat2.1373
Keywords: integrated catchment management (ICM), metaecosystem, estuaries, rivers, coastal, river plumes
Citation: Cañedo-Argüelles M, Brito AC, Sen I and Roy R (2023) Editorial: Human impacts on river catchments and coastal ecosystems: A meta-ecosystem perspective. Front. Mar. Sci. 10:1168296. doi: 10.3389/fmars.2023.1168296
Received: 17 February 2023; Accepted: 03 March 2023;
Published: 16 March 2023.
Edited and Reviewed by:
Marta Marcos, University of the Balearic Islands, SpainCopyright © 2023 Cañedo-Argüelles, Brito, Sen and Roy. This is an open-access article distributed under the terms of the Creative Commons Attribution License (CC BY). The use, distribution or reproduction in other forums is permitted, provided the original author(s) and the copyright owner(s) are credited and that the original publication in this journal is cited, in accordance with accepted academic practice. No use, distribution or reproduction is permitted which does not comply with these terms.
*Correspondence: Miguel Cañedo-Argüelles, bWlndWVsLmNhbmVkb0BpZGFlYS5jc2ljLmVz
Disclaimer: All claims expressed in this article are solely those of the authors and do not necessarily represent those of their affiliated organizations, or those of the publisher, the editors and the reviewers. Any product that may be evaluated in this article or claim that may be made by its manufacturer is not guaranteed or endorsed by the publisher.
Research integrity at Frontiers
Learn more about the work of our research integrity team to safeguard the quality of each article we publish.