- 1Centre Oceanogràfic de les Balears (COB-IEO), CSIC, Palma, Spain
- 2Centro Oceanográfico de Cádiz (IEO), CSIC, Cádiz, Spain
- 3Institut de Ciències del Mar (ICM), CSIC, Ecopath International Initiative (EII), Barcelona, Spain
- 4AZTI, Marine Research Division, Basque Research and Technology Alliance (BRTA), Sukarrieta, Spain
- 5Marine Research Division, Agrupació Son Navata, Felanitx, Illes Balears, Spain
Within the context of the ecosystem approach to fisheries management, an ecosystem model was developed for the Balearic Islands in the early 2000s, covering from 0 to 800 m of depth. The aim of the study was to describe the structure and functioning of the ecosystem together with the fishing impacts. The results show that the biomass of the primary producers (PP), mainly dominated by Posidonia oceanica meadows and red algae beds, represented a high percentage of the biomass (39.20%) in the ecosystem. Most of the trophic flows occurred between PP (mostly benthic) and trophic level II. The mixed trophic impact analysis also highlighted a positive impact of the functional groups (FGs) at the base of the food web. Besides, there were important trophic flows between several demersal FGs, and many groups of the pelagic compartment, confirming the importance of the benthopelagic coupling previously reported. The mean trophic level of the catch (mTLc=2.76) was lower than those reported in adjacent areas due to the discard of benthic producers. By excluding benthic PP, the Balearic Islands showed the highest mTLc (3.44) among all the Mediterranean areas modelled, probably due to differences in the trophic structure, lower fisheries exploitation levels and lower development of the purse seine fleet targeting small pelagic fishes. The mean transfer efficiency (21.80%) was double than the global average (10%), which highlights the oligotrophy of the system, the importance of demersal production which has lower rates of transfer through the food web, and a more efficient use of nutrients in the ecosystem. Sponges, one of the most important groups of benthic invertebrates in the Balearic Archipelago, are known to recycle dissolved organic matter, which is reintroduced into the food web similar to the microbial loop, but the magnitude of this and other processes remains still unknown in this area. The present study is a first step to develop spatio-temporal simulations under different exploitation scenarios and to calculate ecological indicators to assess the state of the marine environment in this region.
1 Introduction
The need to apply an ecosystem approach to fisheries management (EAFM) is globally accepted. This holistic approach allows fisheries assessment and management to take into account the physical, biological and socio-economic complexities of living resources (Pikitch et al., 2004). The complementarity of EAFM to single-species assessment and management takes into account the maximisation and sustainability of the target species yields. However, EAFM approaches are still largely lacking in fisheries assessment and management (Patrick and Link, 2015). This situation is evident in the Mediterranean, where fisheries assessment and management is largely based on the single-species approach of few assessed stocks (GFCM, 2022; STECF, 2022). The current application of the European Multiannual Plan for fisheries exploiting demersal stocks in the Western Mediterranean (MAP; Regulation (EU) 2019/1022 of the Parliament and of the Council) is a clear example of the continued dominance of the single-species approach in Mediterranean fisheries, which uses the Maximum Sustainable Yield of the main target species as the main reference point for the implementation of management measures. This approach, which is perhaps the simplest but not the most effective given the diversity and complexity of the Mediterranean fisheries (Caddy, 1993; Farrugio et al., 1993; Lleonart and Maynou, 2003), may be one of the reasons for the long-term history of generalised overfishing in the area (Colloca et al., 2013).
Key tools for the implementation of the EAFM are marine ecosystem models. In this sense, trophodynamic models have become key tools for the multidimensional assessments required by the EAFM (Longo et al., 2015), as they allow the integration of available information on trophic relationships, interactions with environmental factors and anthropogenic pressures such as fishing. Among them, the Ecopath with Ecosim (EwE) approach (Christensen and Walters, 2004) is one of the most used ecological models worldwide and in the Mediterranean (Coll and Libralato, 2012; Colléter et al., 2015; Keramidas et al., 2023), where ecological models may be particularly important (Caddy, 1993; Lleonart and Maynou, 2003).
In the Western Mediterranean Sea, several Ecopath models have been implemented for the ecosystems of the mainland shore, including coastal, shelfs and deep sea areas (Coll et al., 2006; Coll et al., 2008; Banaru et al., 2013; Tecchio et al., 2013; Corrales et al., 2015; Coll et al., 2021). However, the functioning of the insular ecosystems in their eastern part (i.e. the Balearic Islands) is still unknown, which contrasts with the abundant information on their trophic webs (e.g. Cartes et al., 2008a; Madurell et al., 2008; Fanelli et al., 2009; Valls et al., 2014; Valls et al., 2017).
Due to their specificities, the Balearic Islands are considered as an individualised area for assessment and management purposes in the Western Mediterranean (Quetglas et al., 2012): the Geographical Subarea 5 (GSA 5) of the General Fisheries Commission for the Mediterranean (GFCM). The most important aspects for this differentiation are: (1) Geomorphology: The Balearic Islands are one of the most distant insular areas in the Mediterranean, separated from the mainland by 200 km and by bottom depths up to about 2000 m, except in the Ibiza Channel, the area closest to the mainland, where the maximum depth is about 800 m. The Archipelago is divided into two physiographic provinces, Eivissa-Formentera and Mallorca-Menorca, which are separated by the Mallorca Channel, with maximum depths of more than 600 m (Acosta et al., 2002); (2) Oligotrophy: the waters around the Archipelago, where there is no supply of nutrients from river runoff, are more oligotrophic than the adjacent waters off the Iberian coast and the Gulf of Lions (Estrada, 1996; Bosc et al., 2004); (3) Sediment types: the sediments on the Balearic shelf are mainly biogenic sands and gravels (Acosta et al., 2002), in contrast to the predominance of terrigenous mud on the mainland; (4) The presence of habitats: due to the clear waters in the Archipelago, the algae beds predominate down to a depth of 90 m, overlapping with the shallow shelf bottom trawl fishery, including essential and sensitive habitats such as the Peyssonnelia and rhodoliths beds, respectively (Ballesteros, 1992; Ballesteros, 1994; Ordines et al., 2009; Barberá et al., 2012; Ordines, 2015; Ordines et al., 2017); (5) The exploitation state of resources and ecosystems, which is better than in the adjacent mainland area due to a historically relatively less developed fishing fleet, in which the artisanal vessels represent a higher percentage than on the mainland (80% vs 60%, respectively) (Quetglas et al., 2012); and (6) The general lack of interaction with mainland fishing fleets, particularly in Mallorca and Menorca, where the fleets targeting demersal and small pelagic species are entirely local (Quetglas et al., 2012).
This study represents a first step in the characterisation of the Balearic Islands with an ecosystem view, including the Mallorca and Menorca subunit, using an ecosystem model. Our main objectives are: (1) describe the structure and functioning of the ecosystem using the Ecopath food-web model in early 2000s, (2) assess the impact of fishing activity using ecological indicators, and (3) describe the main specificities and differences with other modeled ecosystems in the Western Mediterranean Sea and adjacent waters.
2 Materials and methods
2.1 Study area and period
The Ecopath model developed in this study considered the Mallorca and Menorca subunit of the Balearic Promontory, and represents an average annual situation of the early 2000s (2001–2003; Figure 1). The Balearic Islands model includes the continental shelf and the middle slope (between 0 and 800 m), covering a total area of 15,197 km2. The islands of Ibiza and Formentera were not included in the model because of their different ecological and oceanographic conditions, but also due to data limitations. The Ibiza and Formentera sub-unit is still a poorly known area, where the international bottom trawl survey in the Mediterranean (MEDITS) started in 2021.
The Balearic Islands are the most distant insular area in the Western Mediterranean, separated from the Iberian Peninsula by a minimum distance of 95 nautical miles and with depths ranging from 800 to almost 2000 m (Acosta et al., 2002). The Archipelago delimits the Balearic sub-basin in the north from the Algerian sub-basin in the south. These sub-basins are characterised by different oceanographic conditions (Lehucher et al., 1995) and are connected by a series of channels that play an important role in the regional circulation, as passages for the exchange of water masses between them. Mesoscale frontal events between Mediterranean and Atlantic waters (Pinot et al., 1995) and the input of cold northern waters into the channels (Fernández de Puelles et al., 2004), may act as external fertilisation mechanisms that increase productivity off the Balearic Islands. The Archipelago has no rivers, and consequently the sediments of its shelf are mainly biogenic sands and gravels, with a high percentage of carbonates (Canals and Ballesteros, 1997). The lack of supply of nutrients from land runoff makes the oligotrophy around the Balearic Islands more pronounced than in the adjacent waters off the Iberian coast and the Gulf of Lions (Estrada, 1996; Bosc et al., 2004).
The main fisheries in the area are those exploited by the bottom trawl fleet and the artisanal fleet (Quetglas et al., 2016b). The bottom trawl fishery is highly multispecific and operates between 50 and 800 m depth, particularly in the continental shelf, targeting more than 60 species of fish, cephalopods and crustaceans (Palmer et al., 2009; Ordines et al., 2014). The main target species in the continental shelf and shelf break are the striped red mullet Mullus surmuletus and the European hake Merluccius merluccius, in the upper slope are the deep-rose shrimp Parapenaeus longirostris and the Norway lobster Nephrops norvegicus, and the red shrimp Aristeus antennatus in the middle slope. The artisanal fleet operates mainly on the continental shelf, using a variety of gears including trammel nets and gillnets (mainly targeting M. surmuletus, the spiny lobster Palinurus elephas, and the cuttlefish Sepia officinalis), handlines (targeting the squid Loligo vulgaris), long lines (mainly targeting Dentex dentex, sparid fishes and the scorpionfish Scorpaena scrofa), and specific purse seines targeting the dolphinfish Coryphaena hippurus and the transparent goby Aphia minuta (Quetglas et al., 2016b). The total landings of the commercial fleet were around 4000 t per year, but in addition to these catches there is a very important recreational fishery with catches ranging from 1200 to 2700 t per year (Morales-Nin et al., 2005; Morales-Nin et al., 2008).
2.2 Food-web model description: equations and basic parameters
The food-web model of the Balearic Islands was constructed using the Ecopath with Ecosim (EwE) software version 6.6.8 (Christensen and Walters, 2004; Christensen et al., 2008). We used the static component Ecopath that allowed us to provide a snapshot representation of the food web in terms of biomass and energy flows during the studied period.
Ecopath is based on the mass-balance assumption over the time period and it is parameterized by two master equations, describing the biological production (Eq. 1) and consumption (Eq. 2) of each functional group (thereafter FG).
Within each FG, three of the four basic parameters are required: biomass of group i (Bi), production/biomass ratio (P/Bi), consumption/biomass ratio (Q/Bi) and the ecotrophic efficiency (EEi) defined as the fraction of the production that is used in the system (Christensen et al., 2008). The fourth parameter is estimated through the mass-balance routine. Moreover, the catch by fleet and functional group (Yj) and the diet (DCij) of all groups are also needed. Further details on the algorithms and equations, and the main advantages and limitations of the approach are described in Christensen and Walters (2004); Christensen et al. (2008) and Heymans et al. (2016).
2.3 Input data and sources
2.3.1 Functional groups
In this model, the ecosystem is defined according to functional groups (FGs) trophically linked to each other. FGs can be constituted by a group of species with similar ecological features (i.e. habitats, feeding habits), a single species, or representing different ontogenetic phases of a species (i.e. juveniles and adults) (Christensen et al., 2008; Heymans et al., 2016). The definition of FGs in the Balearic Islands model was based on the commercial importance of the species, data availability and ecological traits. The main species targeted by the fishery (dolphinfish, hake, anglerfish, mullets, red shrimp, deep-rose shrimp and Norway lobster) were modeled separately. One multi-stanza FG was created for European hake (Merluccius merluccius) based on juvenile hake population, i.e. < 25 cm of total length and adult hake, i.e. ≥ 25 cm, with an age of transition of 18 months according to the National Programme of collection, management and use of data in the fisheries sector (Mellon-Duval et al., 2010) (Figure 1A). Overall, up to 994 species were grouped into 45 FGs, including 1 group of dolphins, 1 group of seabirds, 20 groups of fishes, 17 groups of invertebrates, 3 groups of primary producers (benthic primary producers, seagrass and phytoplankton) and 2 groups of detritus (discards and detritus) (Table 1).
2.3.2 Input data
Input parameters (biomass, production, consumption, diet composition and fisheries data) were principally obtained from published literature and unpublished information, mostly taken from local field studies of the Spanish Institute of Oceanography (IEO) (Table 2; Table A.1).
Biomasses were obtained from different sources and methodologies: (1) for demersal and benthopelagic species, distributed between 50 and 800 m depth, biomasses were estimated from information obtained from the BALAR bottom trawl research surveys conducted during spring in 2001, 2002 and 2003, which followed the MEDITS sampling protocol (Bertrand et al., 2002; Spedicato et al., 2019), including the stratified sampling strategy (Massutí and Renones, 2005); (2) for small benthic species (e.g. crabs, shrimps, echinoderms) and algae (e.g. benthic primary producers), biomasses were obtained from beam trawl research surveys in the continental shelf (CANAL, INDEMARES and DRAGONSAL projects; Barberá et al., 2012; Domínguez et al., 2013) and the slope (IDEADOS project: Massutí et al., 2014); (3) for coastal fish species (0-50 m) (Coll et al., 2003; Morey et al., 2003; Mallol and Goñi, 2004; Coll et al., 2007), marine mammals (Forcada et al., 2004), seabirds (Arcos et al., 2009) and sea turtles (Cardona et al., 2005) biomasses were taken from visual census; (4) phytoplankton biomass was calculated through images of chlorophyll-a data via satellite (MODIS). Biomass of microzooplankton & mesozooplankton was obtained from data estimated in the study area (Fernandez de Puelles et al., 2003; Fernandez de Puelles et al., 2007); (5) detritus biomass was calculated by the empirical equation of Christensen and Pauly (1993); and (6) for those FGs without biomass data in the area (FGs: medium pelagics, dolphinfish, mesopelagic fishes, suprabenthos and macrozooplankton) biomasses were estimated by mass balancing using the EE default value of 0.95. Annual biomass estimates were weighed to the total area modelled taking into account the area from each stratum and then averaged for the period 2001-2003.
For the majority of FGs, production (P/B) and consumption (Q/B) rates were calculated using empirical equations. P/B parameters were obtained: (1) for target species (adult hake, mullets, red shrimp, white shrimp and Norway lobster) from fish stock assessments GSA 5; (2) for fishes, from the empirical equation of Pauly (1980) or Gascuel et al. (2011); (3) for most invertebrate species (i.e. octopus, cuttlefishes, other shrimps, crabs, echinoderms) was calculated following Brey’s model (Brey, 2001); (4) for FGs with no available data (i.e. dolphins, seabirds, loggerhead turtle, suprabenthos, gelatinous plankton, macrozooplankton, microzooplankton & mesozooplankton and benthic primary producers), we used P/B values from other models and corrected for the difference of mean temperature according to Opitz (1996).
Q/B was calculated: (1) for almost all the FGs using the empirical equation of Pauly et al. (1990); (2) for seabirds, consumption was calculated using the empirical equation proposed by Nilsson and Nilsson (1976); (3) in the case of dolphins, consumption was estimated using the empirical equation of Innes et al., 1987 and Trites et al., 1997; (4) for mesopelagic fishes FG, as no information was available, the consumption was calculated assuming their consumption is about 6 times higher than their production from the equation proposed by Christensen et al. (2008); (5) for the juvenile hake, consumption was estimated by the model (as a multistanza group); and (6) for other FGs (i.e. loggerhead turtle, octopus, cuttlefishes, squids, bivalves & gastropods, crabs (reptantia), echinodermata, other benthic invertebrates, annelids, suprabenthos, gelatinous plankton, macrozooplankton and micro-mesozooplankton), as information was not available for this area, the values were adapted from other models, which involved similar species.
The diet composition matrix used in the parameterisation of the model was constructed using local stomach content data of 71 species, including fish, cephalopods and crustaceans, obtained during the MEDITS and IDEADOS research surveys, together with published diet datasets (41 species) from the Balearic Islands (see references in Table A.2 and Table A.1). For those groups for which no trophic information available in the study area, data were collected from the literature. Migratory species (seabirds, marine turtles, pelagic sharks, medium and large pelagic fish, dolphinfish) were taken into account by modelling part of their diet composition as imports into the ecosystem, following Coll et al. (2006).
Bottom trawlers, artisanal boats, purse seiners, surface longliners and recreational boats were included in the model. Official landings were obtained from the daily sales records between 2001 and 2003, provided by OPMALLORCAMAR, the fisheries producers of Mallorca, which commercialises the catches of all the fishing fleets around the Island, and by the Regional Government of the Balearic Islands for the fishing fleets of Menorca. IUU and black market data were compiled from Carreras et al. (2015) and Estimated from Sea Around Us (seaaroundus.org) program datasets. Discards were calculated based on the data collected from sampling developed by scientific observers on board the local fishing fleet between 2001 and 2003. Finally, recreational catches were obtained from studies carried out in the Balearic Islands (Morales-Nin et al., 2005; Cabanellas-Reboredo et al., 2017), also supplemented with data from the Sea Around Us project (Carreras et al., 2015).
2.4 Pre-balancing and balancing analyses
An Ecopath model is considered mass-balanced if the following requirements are accomplished (Christensen and Walters, 2004; Christensen et al., 2008): (1) EE values are less than 1; (2) Gross efficiency of food conversion or P/Q values are between 0.1 - 0.35 with the possible exception of fast growing organisms, such as zooplankton, with larger values, and lower values for top predators; (3) Respiration/biomass ratio (R/B) values are between 1 – 10 year-1 for fishes and between 50 – 100 year-1 for planktonic organisms; (4) Respiration/assimilation ratio (R/A) values are lower than 1 (respiration should not exceed food assimilation); (5) Production/respiration ratio (P/R) values should not be higher than 1; and (6) the net efficiency (NE) of food conversion values are lower than 1; moreover, the net efficiency should be higher than the P/Q.
To achieve the balancing of the Balearic Islands model, a manual procedure was applied, following the best practices principles of the approach (Heymans et al., 2016) and a top-down strategy. The main deviations from the initial values were related to the biomass of juvenile hake, octopus, cuttlefishes, squids, bivalves, shrimps (red shrimp, white shrimp and other shrimps), crabs and annelids groups. Therefore, biomass values were readjusted as a condition for achieving a mass balance of the model for these groups with larger EE values. We corrected the estimations of biomass for epifauna (i.e. other shrimps) as beam-trawl sampling could underestimate it by a factor of up to ten (Reiss et al., 2006).
The Prebal diagnostics introduced by Link (2010) are used to ensure that the input data follow general ecological and fisheries principles. These diagnostics guide the balancing procedure, and are applied before the model is balanced and the dynamic application is run (Scotti et al., 2022). The aim is to increase the robustness and the reliability of the results generated. For this model, the Prebal diagnosis (Figure 2A) allowed us to check and visualise B, P/B, Q/B and P/Q per all FGs.
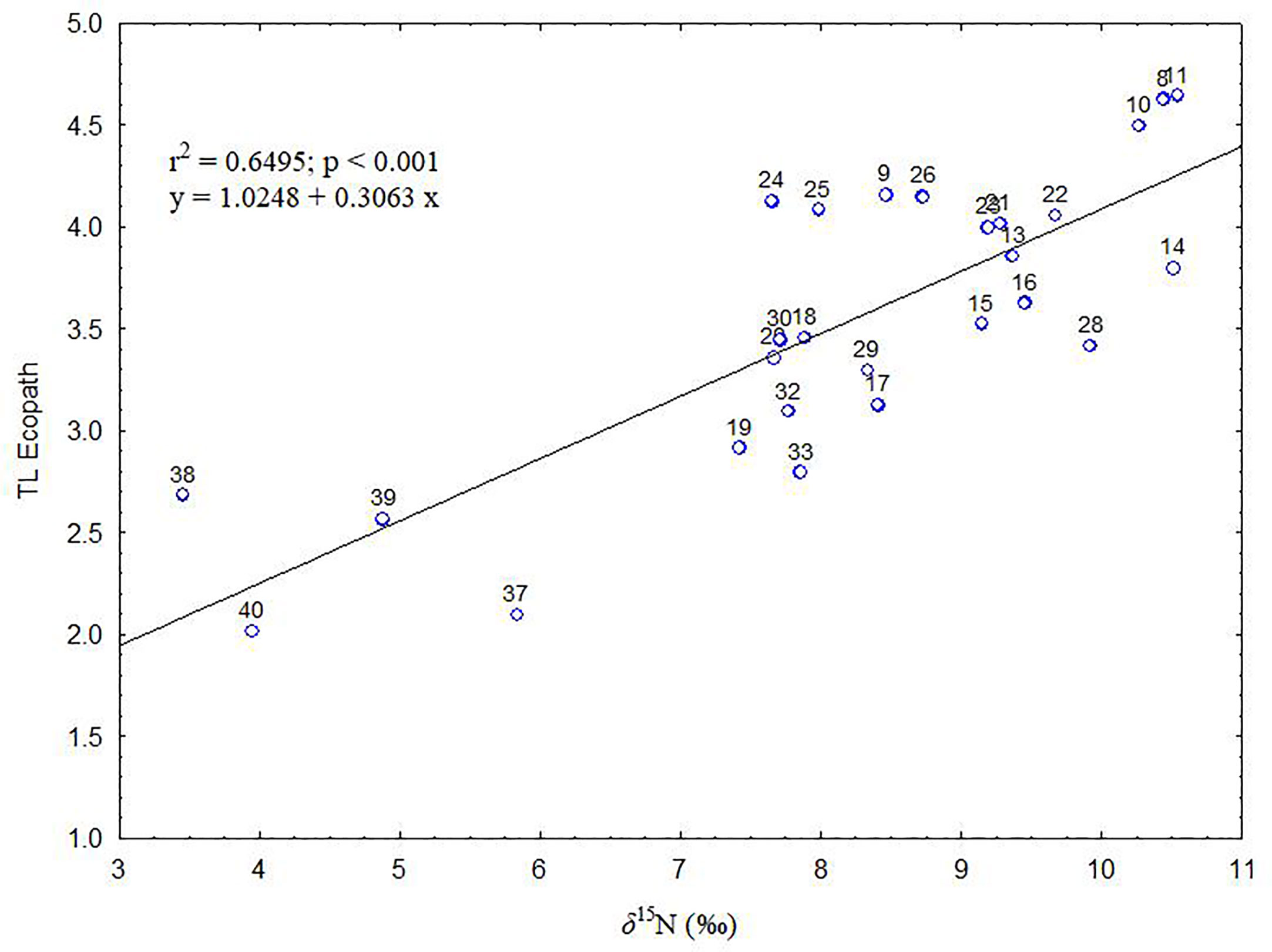
Figure 2 Relationship between the trophic levels (TLs) estimated by Ecopath and δ15N values (mean) calculated from stable isotopes analysis in the Balearic Islands. The solid line represents the regression line and the numbers correspond to the functional groups (see Table 1).
2.5 Model analysis and ecosystem indicators
Ecological indicators were used to analyze fishing impacts based on trophic flow analysis, thermodynamic concepts, information theory and trophodynamic indicators (Christensen and Walters, 2004).
2.5.1 Pedigree index and quality of the model
The Pedigree index (Christensen and Walters, 2004) was used to quantify the uncertainty associated with the input data and to validate the choices made during the balancing process. This index provides an uncertainty value or a confidence interval (CI) for each FG, which is calculated for the overall model. This index varies from 0 (low-quality model, i.e. values estimated from other models) to 1 (high-quality model, i.e. well-sampled and high-precision local input data).
The quality of the model was evaluated by comparing model outputs to independent data. Specifically, trophic levels (TLs) estimated by the model were compared to the δ15N values estimated by stable isotopes values (δ15N) of 130 species belonging to 27 FGs (Figure 2). For those FGs from the model with several δ15N values, they were weighted using biomass proportions of these species in each FG. TLs estimated were plotted against the FGs δ15N values, and a linear regression was performed to fit a model between the two variables and test their correlation. The comparison of TLs estimated by EwE with stable isotopes has been done previously as they are highly correlated (Navarro et al., 2011; Corrales et al., 2017).
2.5.2 Ecosystem properties and trophic flows
The trophic structure of the ecosystem was represented using the Lindeman spine (Lindeman, 1942), proposed by Ulanowicz (1995), in which the system is represented in a linear food chain form where biomasses and trophic flows are represented for each TL, whereas the detritus is separated from the primary producers to show the energy amount that flows through it.
In order to evaluate the status of the ecosystem and its stage of development and maturity, ecological indicators (Table 3) described by the theory of development of Odum (1969) were used: (1) the total system throughput (TST, t·km-2·year-1), which estimates the total flows of the ecosystem (Ulanowicz, 1986; Christensen and Pauly, 1993; Christensen and Walters, 2004), as the sum of consumption, flows to detritus, exports and respiration; (2) the sum of all production (TP, t·km-2·year-1); (3) the total primary production (TPP, t·km-2·year-1), which represents the total primary production of all producers; (4) the ratio of total primary production to total respiration (TPP/TR), which represents the stage of maturity of the ecosystem, where production tends to exceed respiration in immature systems, resulting in a ratio greater than 1 (Odum, 1971); (5) the net system production (TPP-TR, t·km-2·year-1), which represents the difference between total primary production and total respiration, with lower estimates indicating a more mature ecosystem (Christensen and Walters, 2004); (6) the ratio of total primary production to total biomass (TPP/TB), which represents maturity of the ecosystem under study, as in immature ecosystems the production exceeds the biomass; (7) the ratio of total biomass to total system throughput (TB/TST), which represents the degree of maturity, as it increases with the maturity of the ecosystem under study (Odum, 1971); (8) the total biomass (TB, t·km-2), excluding detritus; (9) the transfer efficiency (TE, %), that estimates the fraction of the overall flows at each TL which are either exported out of the ecosystem (e.g by the fishing activity) or transferred to higher trophic TLs as consumption; (10) the Connectance Index (CI), which is the ratio of the number of actual links to the number of possible links in a given food web, roughly proportional to the number of FGs in the system (Nee, 1990); and (11) the System Omnivory Index (SOI), indicates how feeding interactions are distributed across TLs and is defined as the average omnivory index of all consumers weighted by the logarithm of each consumer’s food intake (Pauly et al., 1993).
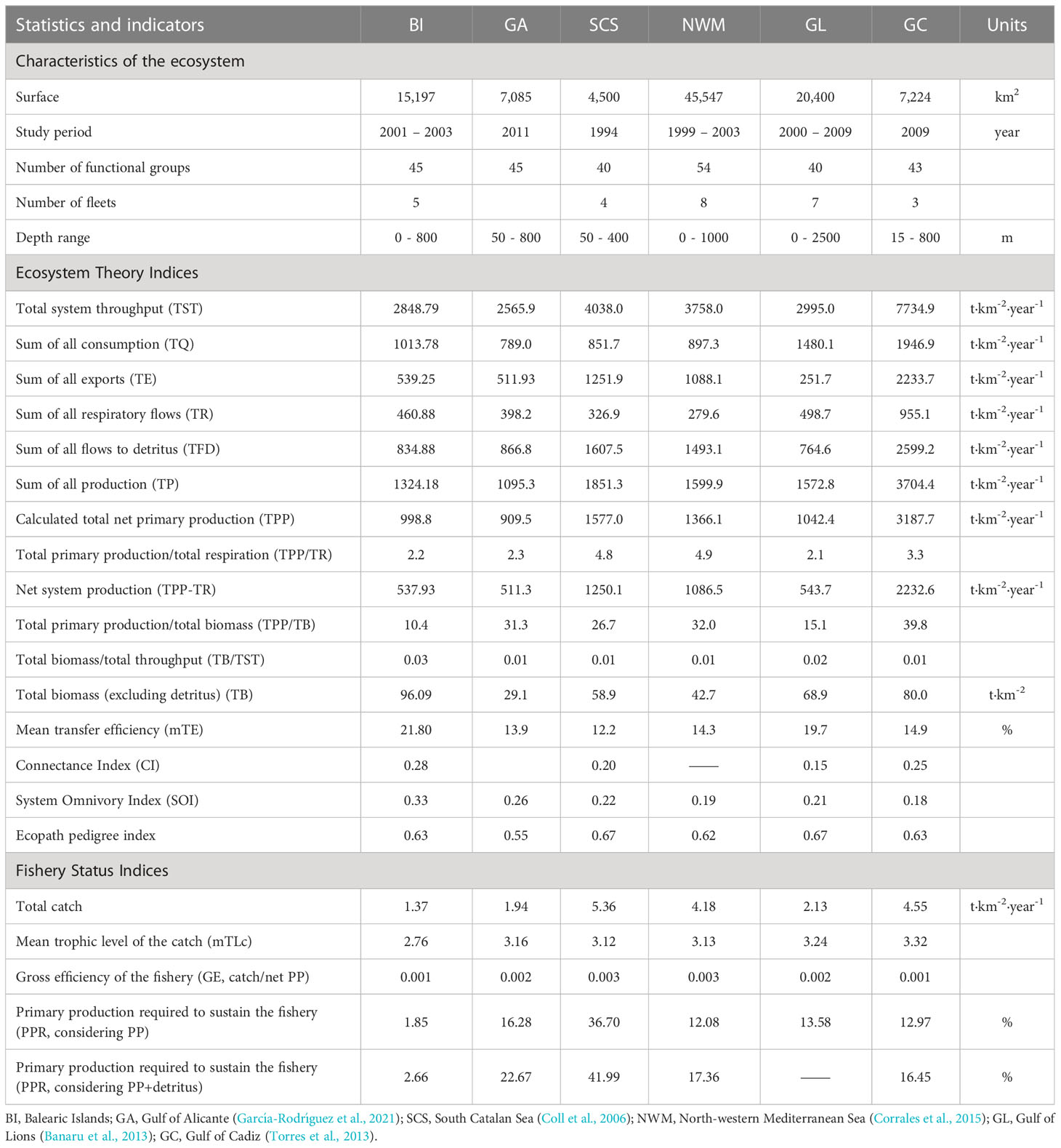
Table 3 Characteristics and ecosystem theory indices for the Balearic Islands and other neighboured models.
2.5.3 The fishery: exploitation status
The Mixed Trophic Impact (MTI) routine quantifies direct and indirect trophic impacts among all FGs in the ecosystem (Ulanowicz and Puccia, 1990), including fishing fleets. The MTI was also used to quantify the impact of fishing fleets on the FGs. In addition, to evaluate the exploitation status of the fisheries, the following indicators were analysed: (1) total catch (t·km-2·y-1); (2) mean trophic level of the catch (mTLc), which represents the strategy of a fishery in terms of selected food-web components and is calculated as the weighted average of the TL of harvested species (Pauly et al., 1998); (3) the exploitation rate (F/Z, fishing mortality/total mortality); (4) the gross efficiency of the fishery (GE), estimated as the ratio between total catch and total primary production; and (5) the primary production required to sustain the fishery (PPR, %), which represents the amount of primary productivity required to sustain commercial fisheries (Christensen and Pauly, 1995) and it is calculated as:
where Yi is the catch of a given group (i), TE is the mean transfer efficiency, TLi is the trophic level of group (i) and factor 1/9 is taken as the average conversion coefficient from wet weight to g C.
2.5.4 Comparison with other food-web models in the Western Mediterranean Sea and adjacent waters
Several Ecopath models have been previously constructed in the Mediterranean Sea close to the Balearic Islands, for example: the Catalan Sea (CS) (Coll et al., 2006), the Northwestern Mediterranean Sea (NWM) (Corrales et al., 2015), the Gulf of Lions (GL) (Banaru et al., 2013), and the Gulf of Alicante (GA) (García-Rodríguez et al., 2021); including the Gulf of Cadiz (GC) (Torres et al., 2013) in the Atlantic Ocean but closely connected to the Mediterranean (Table 3). Although most of these models were developed following similar criteria, comparisons among ecosystem models are difficult due to important differences in the characteristics of the models that can affect results (time period, fishing fleets, depth range and number and species composition of FGs (Heymans et al., 2014). Therefore, we only compared robust indicators following previous studies (Heymans et al., 2014; Corrales et al., 2015; Corrales et al., 2017). The indicators that allowed us to compare neighbouring models were: (1) total system throughput (TST, t·km-2·y-1), which is the sum of all consumption (TQ), sum of all exports (TE), sum of all respiratory flows (TR) and sum of all flows to detritus (TFD); (2) total net primary production (TPP, t·km-2·y-1); (3) total primary production/total respiration (TPP/TR); (4) net system production (TPP-TR, t·km-2·y-1); (5) total primary production/total biomass (TPP/TB); (6) total biomass/total system throughput (TB/TST) and (7) mean transfer efficiency (mTE, %).
3 Results
3.1 Quality and uncertainty of the model
The pedigree index had a value of 0.629 (Table 3), which indicates that the input data used in this model has an adequate quality. Most of the biomass and diet composition data, as well as landings and discards data were collected in the study area. The index values and confidence intervals used in this study are presented in Table A.2. Furthermore, the trophic levels estimated by the Ecopath model were highly and positively correlated with the δ15N values (R2 = 0.6495, p<0.001; Figure 2). Such correlation is related to the accuracy of the diet information used in the model for 27 out of 40 FGs among consumers.
3.2 Output parameters
The total biomass supported by the ecosystem (excluding detritus and discards) was 96.09 t·km-2 (Table 2). This biomass of producers is mainly composed of two FGs: benthic primary producers (36.52%) and seagrass (20.63%). The rest of representative percentages of biomass supported by the ecosystem are structured as follows: phytoplankton (5.69%), microzooplankton & mesozooplankton (5.20%), bivalves & gastropods (3.61%), other shrimps (3.46%) and mesopelagic fishes (3.28%), with other groups having smaller proportions.
Most FGs showed high Ecotrophic Efficiency values (EE > 0.90; Table 2), indicating that the production of each group was widely used within the ecosystem in terms of predation and/or exploitation by fisheries. For fishes, EE were high, except for anglerfish. In contrast, low values of EE (< 0.30) were observed for vulnerable species (dolphins and seabirds), indicating that a small fraction of the production of these groups is being used in the modeled ecosystem (by predation or by fishing). In addition, primary producers (benthic primary producers, seagrass and phytoplankton) also showed low EE values (0.14, 0.002 and 0.54), indicating that a large proportion of their production flows to the detritus compartment. Phytoplankton had the highest flow to detritus (422.5 t·km-2·year-1) (Table A.3) followed by microzooplankton & mesozooplankton (221.4 t·km-2·year-1), and both were much higher compared to seagrass (39.56 t·km-2·year-1) and benthic primary producers (33.77 t·km-2·year-1).
The values of the respiration – assimilation (R/A) (Table A.3) rate ranged from 0.50 for macrozooplankton to 0.99 for dolphins. Most of the highest values were associated with organisms with high TLs, whereas this rate was lower in organisms of lower TLs. The R/B ranged from 0.96 for echinodermata to 57.22 for microzooplankton & mesozooplankton. Most FGs had a ratio lower than 10, except suprabenthos (18.61), macrozooplankton (20.72), gelatinous plankton (27.00), seabirds (48.52) and micro & mesozooplankton. for which P/R values ranged from 0.01 (dolphins) to 1.00 (macrozooplankton). Finally, net efficiency (NE) ranged from 0.006 (dolphins) to 0.50 (macrozooplankton) and was higher than their production/consumption (P/Q) value for all FGs. The values of NE and P/Q were within the expected range (Christensen and Walters, 2004). Most of these high values were associated with fast growing groups, while they were low for dolphins, seabirds or loggerhead turtles.
3.3 Trophic levels and trophic flows
The FGs were included in four trophic levels, ranging from TL=1 for primary producers (benthic primary producers, seagrass and phytoplankton), to TL=4.9 for pelagic sharks. Cephalopods, including octopus, squids and cuttlefishes, together with demersal elasmobranch (rays, skates and sharks), displayed TL>4. For osteichthyes, the TL ranged between 2.9 for sardine and anchovy to TL≥4.5 for anglerfish (4.8), adult hake (4.7) and macrocarnivorous fishes (4.5). Decapod crustaceans encompassed less than one TL (from crabs TL=2.8 to Norway lobster TL=3.5). Plankton (gelatinous plankton, macrozooplankton and microzooplankton & mesozooplankton) and other invertebrates (echinoderms, annelids, suprabenthos and other benthic invertebrates) ranged from TL=2.1 to 2.2.
The trophic flows among the FGs observed from the Ecopath flow diagram (Figure 3) showed several links between some demersal FGs, such as juvenile and adult hake, anglerfish and demersal fishes, and many groups of the pelagic compartment, i.e. mesopelagic fishes, sardine & anchovy, gelatinous plankton, macrozooplankton and microzooplankton & mesozooplankton. The detritus appeared to be an important compartment of the ecosystem and closely linked to the demersal habitat in terms of biomass and production, based on the links of the diagram flow and on the biomass and flow values of this FG, represented in the Lindeman spine.
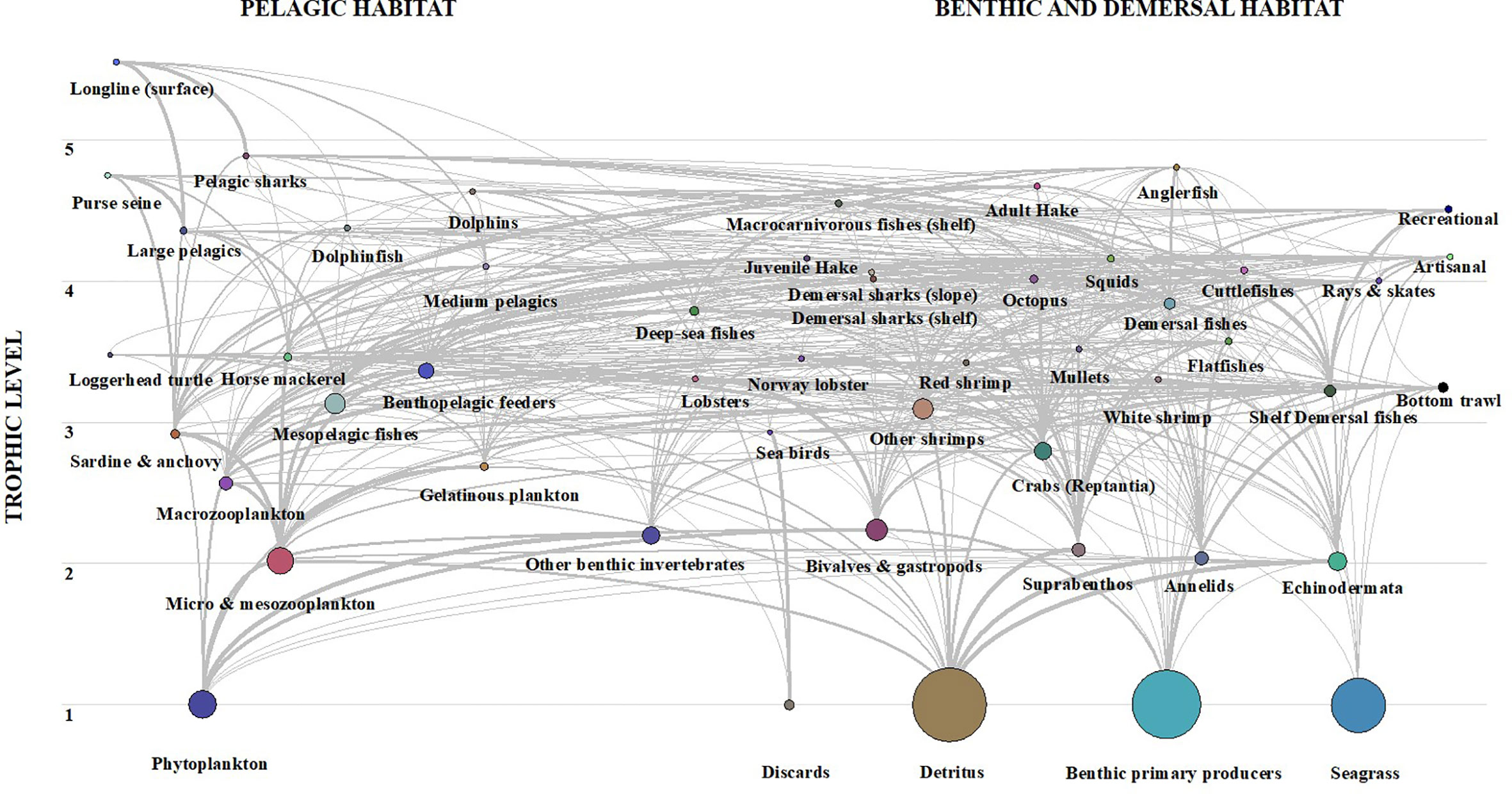
Figure 3 Flow diagram of Balearic Islands model separated by pelagic habitat (left) and benthic and demersal habitats (right). The size of each circle is proportional to the biomass of the FG.
The Lindeman spine representation (Figure 4) showed that most of the biomasses and trophic flows were between TL I and II. The primary producers had the highest biomass (60.38 t·km-2), followed by detritus (43.98 t·km-2) and TL II (17.78 t·km-2). The biomass located on the primary producers’ compartment (TL I) was 43.08%, whereas this percentage was lower in the adjacent modeled areas: 22.7% in North-western Mediterranean, 32.2% in Gulf of Lion and 25.2% in Gulf of Cadiz. Flows obtained from primary producers to detritus (502.5 t·km-2·year-1) were higher than the total flows of upper TLs to detritus (336.8 t·km-2year-1), with the higher contribution of TL II (279.8 t·km-2·year-1). Flow values from primary producers were almost equally distributed between detritus (495.9 t·km-2·year-1) and TL II (502.5 t·km-2·year-1). Respiration was higher for TL II, while the highest Transfer Efficiency (TE), export and catch flows were mainly concentrated between TL III and IV.
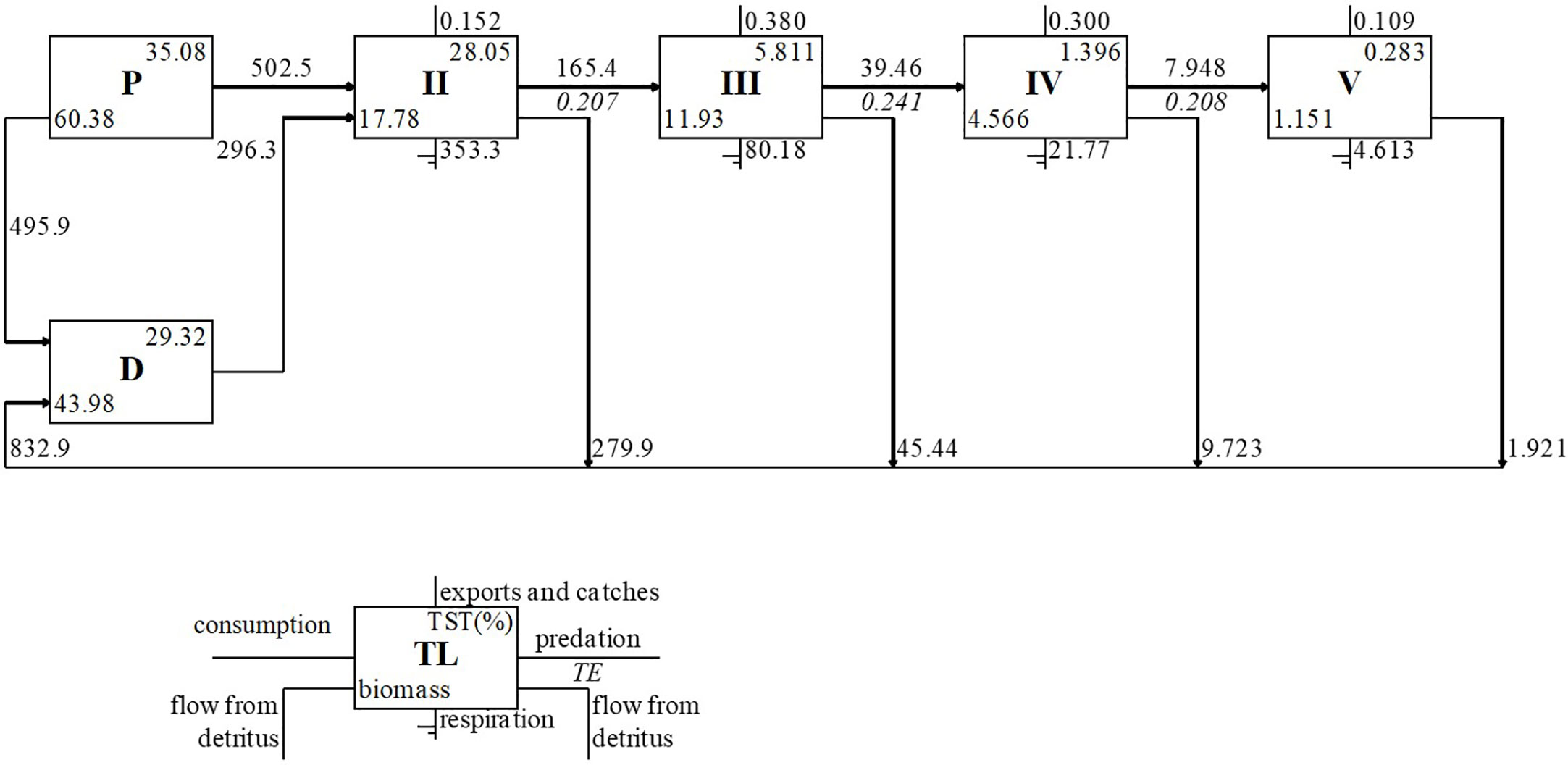
Figure 4 Lindeman spine representation of trophic flows from the Balearic Islands model. The flows are represented in t·km-2·year-1.
3.4 Ecosystem properties
Statistics and ecological indicators obtained are shown in Table 3. The total system throughput (TST) was 2848.79 t·km-2·year-1. In terms of flows, the total consumption (35.59%) and the flow to detritus (29.31%) were higher than exports (18.93%) and respiration (16.18%), meaning that a significant quantity of biomass ends up as detritus.
The sum of all production (TP) was 1324.18 t·km-2·year-1 and the total primary production (TPP) was 998.80 t·km-2·year-1. The total primary production/total respiration ratio (three times higher for the rest of ecosystems compared except for the GC, which was twice, TPP/TR), close to unity in mature ecosystems, was 2.2 t·km-2·year-1 in the Balearic Islands, revealed that the energy produced was two times higher than the total respiration needed to maintain the balance cost. The net system production (TPP-TR) was 537.92 t·km-2·year-1. The total primary production/total biomass (TPP/TB) ratio in the Balearic Islands showed that the productivity was ten and a half times higher than the biomass accumulated within the ecosystem (10.4). The total biomass/total system throughputs (TB/TST) ratio shows the energy needed by the model to sustain the biomass in the Balearic Islands ecosystem and it was 0.03. The Connectance Index (CI) was 0.28 and the System Omnivory Index (SOI) was 0.33. The mean transfer efficiency (mTE) of the system was high (21.80%) and TE values had their maximum between TL III and IV, according to the Lindeman spine (Figure 4).
3.5 Fisheries impacts on the ecosystem
The MTI showed that most FGs had a negative impact on themselves and on their main preys, due to competition for food resources and predation, respectively (Figure 5). For example, adult hake had a negative impact on itself and on juvenile hake. Demersal fishes had a negative impact on horse mackerel and mullets. Octopus had a negative impact on lobsters. FGs at the base of the food web (microzooplankton & mesozooplankton, phytoplankton and detritus) concentrated most of the positive impacts on other FGs. MTI also showed that the bottom trawl and the artisanal fleets had the most widespread impacts on the ecosystem and most of the highest negative impacts on several groups, mainly from the demersal compartments (Figure 5). Bottom trawls had negative impacts on anglerfish, demersal sharks (shelf), demersal sharks (slope), rays & skates and red shrimp. The artisanal fleet had negative impacts on vulnerable groups and/or groups located at the upper part of the TLs, such as dolphins, seabirds, loggerhead turtle, dolphinfish, anglerfish, mullets, rays & skates, octopuses, cuttlefishes and red shrimp. Purse seiners (including tuna purse seiners) and the recreational fishery had negative impacts on some of their target species, large pelagics and dolphinfish, respectively. Longline had a negative impact on pelagic sharks.
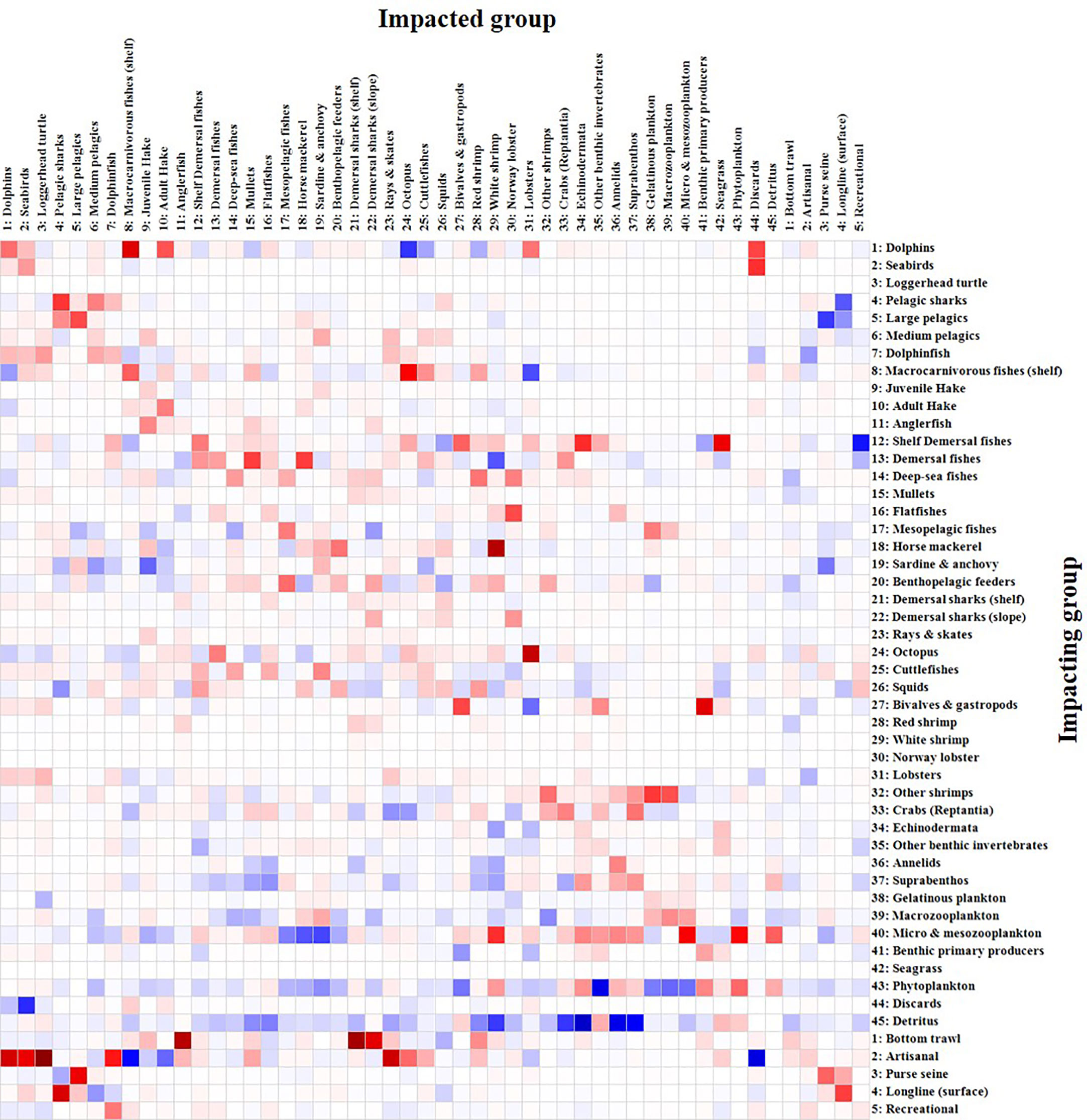
Figure 5 Mixed Trophic Impact (MTI) analysis of the Balearic Islands model. The size of the rectangles represents the size of the functional group trophic impact, while blue indicates positive impact and red negative impact. The five fishing fleets are also included.
The fishing mortality values (F) were higher (F>0.2) for anglerfish, mullets, pelagic sharks, red shrimp, demersal sharks (shelf), dolphinfish, juvenile hake, large pelagic fishes, demersal sharks (slope) and rays & skates, compared to the rest of FGs of the Balearic Islands (Table 2). The majority of FGs showed high predation mortality (M2) and it was the first cause of mortality for most groups of invertebrates (octopus, cuttlefishes, squids, bivalves & gastropods, red shrimp, white shrimp, Norway lobster, lobster, other shrimps, crabs and annelids) and fishes (macrocarnivorous fishes, medium pelagic fishes, mullets, juvenile hake, flatfishes, horse mackerel, adult hake, benthopelagic feeders, mesopelagic fishes, deep-sea fishes, demersal fishes, shelf demersal fishes and sardine & anchovy). Pelagic sharks, large pelagic fishes, dolphinfish, and demersal elasmobranchs (sharks, rays and skates) were the groups with higher fishing mortality than predation mortality (Table 2). The exploitation rates (F/Z) also showed high values >0.5 (i.e. more than 50% of mortality is produced by fishing) for loggerhead turtle, rays & skates, demersal sharks (slope), dolphinfish, pelagic sharks and large pelagic fishes. Some groups showed F/Z values ranging from 0.51 to 0.2: dolphins, demersal sharks (shelf), mullets, anglerfish, medium pelagic fishes, red shrimp and juvenile hake. Other FGs showed lower exploitation rates (Table 2).
The total catch in the model was 1.37 t·km-2·year-1 (Table 2) and it was dominated mainly by benthic primary producers (28.18%) and shelf demersal fishes (9.70%). Other FGs such as rays & skates, deep-sea fishes, sardine & anchovy, demersal fishes, large pelagic fishes, benthopelagic feeders and echinoderms showed high landing values too.
The mean trophic level of the catch (mTLc) was 2.76. Bottom trawl fleet represented the highest catch in the area (59.85%), but with the lowest trophic level (mTLc = 2.24) (Table 4). On the contrary, the longline (surface) fleet had the highest mTLc (4.55) but the lowest catch. It is important to highlight the weight of the recreational fleet, representing the second highest catch in the area (14.6%) and exploiting species situated at higher trophic level than the artisanal fleet (3.51 vs 3.17). When benthic primary producers were excluded from the catches, the mTLc of the bottom trawl fleet increased to 3.31, resulting in a global mTLc=3.44.
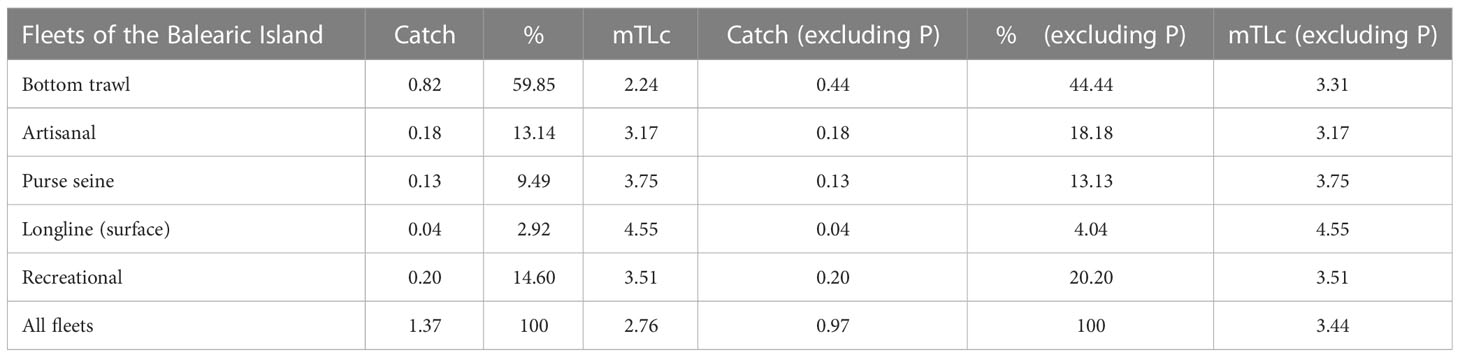
Table 4 Catch (t·km-2·year-1), their representative percentage (%) and mean trophic level of the catch (mTLc) for the fleets, with all FGs and excluding primary producers (P): benthic primary producers and seagrass.
Regarding the gross efficiency of the fishery (GE), the model exhibited a low value (0.001) (Table 3). The primary production required to sustain the fishery (PPR, %) was estimated at 1.85% during the period between 2001 and 2003. When this value takes into account the primary production together with the detritus, the value was 2.66% (Table 3).
4 Discussion
The different components of the marine ecosystem of the Balearic Islands have been widely studied, from the shallow continental shelf to deep waters, considering different approaches and including life history and population dynamics of the main target species of fisheries, benthic habitats, vulnerable species and trophic dynamics (e.g. Barberá et al., 2012; Massutí et al., 2014; Valls et al., 2014; Ordines et al., 2015; Quetglas et al., 2016a; Ramírez-Amaro et al., 2020). However, the present study describes, for the first time, the structure, functioning and fishing impacts of the Mallorca and Menorca’s marine ecosystem, using a food-web model.
The pedigree index obtained for the Balearic Islands model indicates that the input data used in this model has an adequate quality. In fact, it is located in the upper part of the rank of the models assessed worldwide by Colléter et al. (2015). Further, our results revealed a clear correlation between the TLs estimated by the Ecopath model and the measured stable isotope values of nitrogen (δ15N), validating the assessment of Ecopath output of TL, and highlighting that the diet information used in the model for 27 out of 40 FGs among consumers was accurate (Navarro et al., 2011; Corrales et al., 2017).
Biomasses were mostly estimated from local studies, using a variety of approaches such as underwater visual census in littoral areas and different sampling gears such as bottom trawl, beam trawl, plankton nets at deeper and offshore waters. However, and despite the large amount of scientific knowledge on the marine species and their environment around the Balearic Islands, there are still gaps for some important FGs and a generalized lack of information in terms of biological parameters. For that reason, the biomass of important groups, mainly fish inhabiting the pelagic domain and species at the base of the food web (i.e., medium pelagic fishes, dolphinfish, mesopelagic fishes, suprabenthos and macrozooplankton) had to be estimated by the model. Also, many input parameters such as P/B and Q/B were estimated by the model, calculated with empirical equations or taken from other models.
The mixed trophic impact (MTI) analysis highlighted the positive impact of the FGs found at the base of the food web (detritus, phytoplankton, microzooplankton & mesozooplankton and macrozooplankton). In oligotrophic areas, such as the Balearic Islands, with little supply of terrigenous nutrients and without advective inputs of organic matter via submarine canyons, trophic webs are supported to a greater extent by planktonic biomass, rather than by benthic biomass (Madurell et al., 2004; Madurell and Cartes, 2005). In the coastal areas of the Archipelago, stable carbon isotopes provided evidence of the contribution of Posidonia oceanica as carbon source for species dwelling seagrass meadows (<35 m depth) (Cardona et al., 2007). However, in deep waters one single carbon source of pelagic origin supports the food webs of the lower shelf and the upper and mid slope (Polunin et al., 2001; Valls et al., 2014). Such energy transfer highlighted the close coupling between surface and benthic production in deep waters. In terms of trophic interactions, the nycthemeral migrating community constitutes an important food source for many abundant demersal species in the Balearic Islands (Cartes et al., 2008b; Cartes et al., 2009; Valls et al., 2011; Valls et al., 2015; Valls et al., 2017), which represents an active transport of carbon and other nutrients to the benthic habitats in the modeled area. Mesopelagic fish and suprabenthos biomass estimates showed a large difference (one order of magnitude higher) compared to other ecosystems modeled using Ecopath in the Mediterranean, which reflects the importance of such groups in the study area. In fact, both FGs play an important role in these nycthemeral migrations and in the formation of the deep scattering layer and the benthic boundary layer in our study area (Olivar et al., 2012; Peña et al., 2014). However, biomass values have to be considered with caution as they were estimated by the model.
4.1 Ecosystem structure and function
The TST (2848.79 t·km-2·year-1) was only compared to the Gulf of Alicante considering the number of FGs (i.e. 45 FGs). Both models reflected similar values in the aggregation of trophic flows. In terms of TST (sum of all consumption, exports, respiratory flows and flows into detritus), the sum of all consumption (35.59%) and flow to detritus (29.31%) were the most important flows in the Balearic Islands model, highlighting the amount of biomass that finishes into the detritus compartment. Trophic flows from primary producers to detritus and to TL II were 50%. The sum of TP (1324.18 t·km-2·year-1) and TPP (998.8 t·km-2·year-1) were similar to the Gulf of Alicante.
In terms of system maturity indices, the TPP/TR ratio and TPP-TR were similar to that found in the Gulf of Lions (Banaru et al., 2013) and the Gulf of Alicante (García-Rodríguez et al., 2021). The TPP/TR ratio (2.2) represents the stage of maturity of the ecosystem (Odum, 1971), and showed that the energy produced was approximately two times greater than the maintenance costs. Lower estimates of TPP/TR and TPP-TR (537.93 t·km-2·year-1) indicated that the Balearic Island ecosystem is slightly closer to maturity (Christensen and Walters, 2004) compared to the neighbouring models. In the case of TPP/TB (10.4), the value was the lowest among published models, reflecting a higher level of biomass accumulation in the system compared to its productivity. TB/TST value (0.03) was 3 times higher when compared to these other ecosystems.
Regarding food-web complexity indices, the Connectance Index (0.28) was similar to that obtained for the Gulf of Cadiz (Torres et al., 2013) and suggested moderate-low inner linkages within the food web. Furthermore, the System Omnivory Index (0.33) was similar to the Gulf of Alicante (García-Rodríguez et al., 2021) and relatively higher than the values obtained in other models. The System Omnivory Index indicated a more complex predator-prey relationships than other neighbouring models, but lower than global averages (Christensen and Pauly, 1995). The Balearic Island ecosystem is in a medium-low stage of development. However, according to several indices values, it could be considered as a slightly more mature ecosystem than the rest of the Mediterranean neighbouring models in comparative terms (Table 3).
The biomass located on the primary producers’ compartment (TL I) was higher than in the adjacent modeled areas (North-western Mediterranean, Gulf of Lion and Gulf of Cadiz). The highest Transfer Efficiency (TE) in the present model occurred between the TL III and IV, and both compartments supported the highest and rather similar fishery exploitation. Such export flows contrast with those observed in Mediterranean and Atlantic areas where catches are mainly concentrated in TL II or III.
The mean transfer efficiency (mTE) in the Balearic Islands was 21.80%, which is higher than the average value of 10% reported worldwide (Christensen and Pauly, 1995). Such value can be explained by the oligotrophic character of the Mediterranean, more pronounced in the Balearic Islands (Bosc et al., 2004), compared to the adjacent waters off the Iberian Peninsula and the Gulf of Lions (Estrada, 1996; Bosc et al., 2004). In oligotrophic areas, food webs are known to recycle and use nutrients more efficiently through strong microbial loop (Pomeroy et al., 2007; Armengol et al., 2019).
4.2 The impact of fishing activities in the Balearic Islands
The present model showed lower values of mean trophic level of the catch (mTLc) and catches (t∙km-2∙year-1), compared to the models developed from adjacent areas. Whereas the mTLc for these models is between 3.12-3.32, in the Balearic Islands model the mTLc is 2.76. Such result was highly influenced by the low TLc of the bottom trawl fleet (2.24), which represents 59.85% of the total catch. The high biomass of benthic primary producers in the shelf bottoms, which constitute 45.9% of the catch, may explain the low TLc of the bottom trawl fleet calculated by the model. By excluding benthic primary producers and seagrass from mTLc calculation, the Balearic Islands showed the highest mTLc (3.44) among all the Mediterranean areas modelled. This may be the result of both differences in the trophic structure and a relatively better status of the ecosystem in the Balearic Islands due to a lower level of development of the fishing fleet, but also due to the scarce representation of purse seiners in this area. Purse-seiners fleet is characterized by large catches of small pelagic fish like sardine and anchovy and consequently has the lowest mTLc in the North-western Mediterranean model (Corrales et al., 2015). In adjacent areas, purse-seiners landings can represent up to 50% of the total landings in contrast to the Balearic Islands where purse-seiners landings do not reach 15% (Quetglas et al., 2012). Moreover, whereas mTLc of purse seiners in the North-western Mediterranean model shows the lowest values among the fleets operating there (2.99; Corrales et al., 2015), in the Balearic Islands its value (3.75) ranks second after longliners. In fact, even excluding algae and seagrass and from the catches, the mTLc of the bottom trawl fleet has a lower value (3.31) than in the adjacent area of the North-western Mediterranean (3.40; Corrales et al., 2015), probably due to the high importance of the middle slope fishery targeting red shrimp in the Balearic Islands, whose landings represented more than 20% of the total biomass landed by this fleet, composed mainly of crustaceans (Quetglas et al., 2012). The longline fleet, which targets large pelagic fish, represented the smallest proportion of the total catch but with the highest mTLc, similar to other longline fleets from the Western Mediterranean (Coll et al., 2006; Corrales et al., 2015). It is noteworthy the higher catches (14.60%) and mTLc (3.51) of the recreational fleet compared to the artisanal fleet in the Balearic Islands. Despite the important catches of the recreational fishery and its increasing trend (Carreras et al., 2015), there is a lack of information and robust data regarding the species targeted by this fleet. Such information is needed to properly account for its potential impact on the exploitation state on some target stocks (Quetglas et al., 2016b).
The Gross Efficiency of the Fishery (GE) for the Balearic Islands (0.001) was higher than the worldwide value (0.0002) calculated by Christensen et al. (2008) from different marine ecosystems, but similar to the values found in neighbouring areas of the Western Mediterranean and the Gulf of Cadiz (Table 3). This value points out the high impact of the fishing activity (Corrales et al., 2015) and the fact that fishing catches are less effective in converting primary production (Keramidas et al., 2022).
The primary production required to sustain the fisheries (PPR, considering PP) for the Balearic Islands ecosystem was 1.85%. This was the lowest value compared to nearby models (12-37%) (Coll et al., 2006; Banaru et al., 2013; Torres et al., 2013; Corrales et al., 2015; García-Rodríguez et al., 2021) and even compared to the usual values (24-35%) of other coastal systems throughout the world (Christensen and Pauly, 1995). This could be due to the high phytoplankton production relative to the low total catch (1.37 t·km-2·year-1). However, our values were similar to models from other oligotrophic areas such as those from the North Aegean Sea (3.45%; Tsagarakis et al., 2010) and the Gulf of Gabes (3.77%; Hattab et al., 2013) (Table 5).
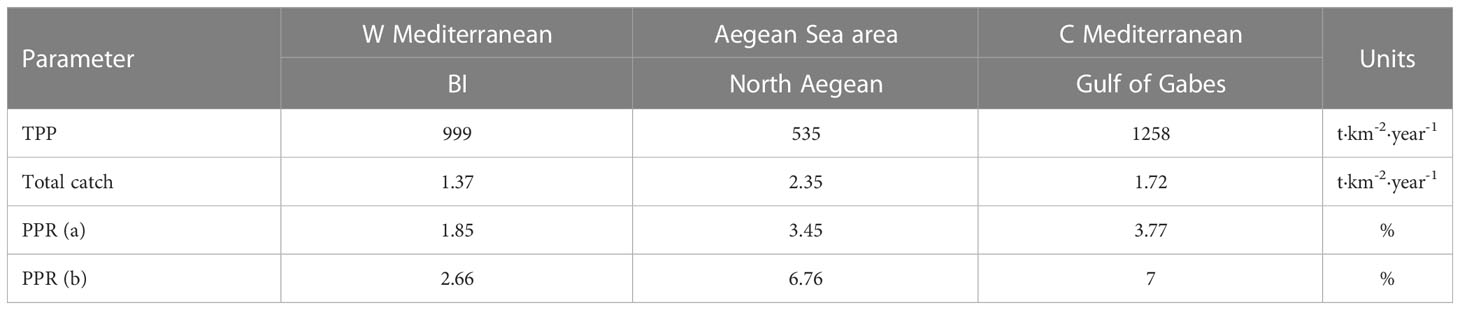
Table 5 Total net primary production (TPP), total catch, Primary production required to sustain the fishery (PPR) from primary producers (a) and from primary producers and detritus (b) of the Balearic Island model (BI) compared to other models of the Aegean Sea area: North Aegean (Tsagarakis et al., 2010), and Central Mediterranean: Gulf of Gabes (Hattab et al., 2013).
4.3 Limitations of Balearic Islands model
There are still gaps of knowledge on basic biological parameters for many species (target and non-target species) included in this model. In terms of biomass, whereas the data of many FGs was obtained from the MEDITS bottom trawl surveys, developed between 50 and 800 m depth, data from infralittoral bottoms shallower than 50 m depth is scarce and scattered. Biomass of demersal and benthic organisms are also better estimated (studied and sampled) than the biomass for pelagic groups located in the water column. The model had to calculate the biomass for some FGs, such as medium pelagic fishes, dolphinfish, mesopelagic fishes, suprabenthos and macrozooplankton, due to the lack of information. Finally, the biomass of phytoplankton and zooplankton, should be obtained from in situ sampling surveys in order to have more accurate data. This lack of information identifies potential targets of future research in the study area.
We gathered local information on the diet of many organisms in the Balearic Islands, obtained from the stomach content analyses carried out during the last decades from samples collected in the MEDITS surveys and previously published data in the area. For some FGs such as dolphins, turtles, pelagic sharks, large pelagic fishes, medium pelagic fishes, sardine & anchovy, bivalves & gastropods, annelids, suprabenthos, gelatinous plankton and zooplankton, the diet composition had to be completed with information from other areas of the Western Mediterranean. In spite of this, some studies developed in this area have revealed that the feeding behaviour of important species such as Merluccius merluccius (Rueda et al., 2019) or Galeus melastomus (Ordines et al., 2021) show differences even at medium spatial scales. Such results highlight that diet data should be improved with studies developed in the Balearic Islands in future research.
For the majority of FGs, production (P/B) and consumption (Q/B) parameters were calculated using empirical equations. For target species of fisheries developed in the Balearic Islands (adult hake, mullets, red shrimp, white shrimp and Norway lobster), Q/B parameters were obtained from the empirical equation of Pauly et al. (1990), whereas P/B parameters were obtained from the stock assessments models applied to these fisheries. This information should be updated in the future as more empirical data on FGs parameters and assessments are available.
The “other benthic invertebrates” FG considered in the present model is constituted by numerous species with many differences in ecological and biological traits. The role of some of the taxonomic groups inside this FG needs to be better determined and properly addressed in the future. For example, sponges are one of the most important groups of benthic invertebrates in the Balearic Archipelago (Ordines et al., 2017; Massutí et al., 2022). Considering the importance of the sponge loop in recycling dissolved organic carbon making it available for other organisms of the ecosystem (De Goeij et al., 2013), the magnitude of this and other processes such as the microbial loop, currently unknown in the area, should be also properly considered in the model.
Another limitation of the model is to explain how the benthic primary production flows from the infralittoral and circalittoral bottoms, which are mainly dominated by the highly diverse and productive Posidonia oceanica meadows and red algae beds, respectively (Ordines and Massutí, 2009; Álvarez et al., 2015), into the whole food web. In fact, both the diet matrix and the MTI analysis showed few possible ways in which the energy and matter of benthic primary producers could influence other FGs. In the model, benthic primary producers and seagrass have high biomass and low EE. According to Heymans et al. (2016), values of EE near to 0 are expected for functional groups, which suffer no predation and are not exploited by the fishery. In the Balearic Islands, benthic primary producers and seagrass are directly consumed by very few species. These peculiarities are supported by isotopic analysis and stomach contents. Further, benthic primary producers EE is higher than seagrass EE because the former can be found at depths up to 90-100 m and are harvested by bottom trawls. Much of the biomass of these FGs goes to detritus. In this sense, the magnitude of the microbial and sponges’ loops (De Goeij et al., 2013) which is currently unknown in the area, may be related to the functioning of biomass flows from seagrass and benthic PP to higher trophic levels. On the other hand, benthic primary producers have positive effects in structuring benthic communities of sedimentary bottoms. They act as “Essential Fish Habitats” for the demersal resources and increase their productivity in the Balearic Islands, particularly for the species inhabiting in red algae beds (Ordines and Massutí, 2009; Ordines et al., 2009; Ordines, 2015). These indirect roles and non-trophic interactions can be represented and captured in dynamic versions of the model (Horn et al., 2021).
In fact, the Ecopath model of the Balearic Islands presented in this study will be a first step to develop temporal and spatio-temporal dynamic simulations, with the aim of evaluating the impact of different fisheries management measures, particularly those related to the implementation of the ongoing multiannual plan for the fisheries exploiting demersal stocks in the Western Mediterranean Sea (Regulation (EU) 2019/1022). It can also be useful within the context of the Marine Strategy Framework Directive, which aims to more effectively protect the European marine environment and to achieve a good environmental status, enabling a sustainable use of marine goods and services (Piroddi et al., 2015). For that, it is required the application of an ecosystem-based approach to better link ecosystem components, anthropogenic pressures and impacts on the marine environment as it has been proposed in this study.
Data availability statement
The original contributions presented in the study are included in the article/Supplementary Material, further inquiries can be directed to the corresponding author/s.
Author contributions
PZ, MV, BG and FO conceived this work. MD-V, MV, MZ built the reference model and PZ, MV and FO updated the current model, while XC, EA, MT and MC provided support for the development. MV analyzed the stomach contents and provided the diet data. PZ, MV, MZ, BG and FO wrote the manuscript with the contribution of all the authors. All authors contributed to the article and approved the submitted version.
Funding
BALAR surveys were funded by the Instituto Español de Oceanografı́a (IEO) and later-on integrated in the MEDITS programme. These data, together with the information of landings and data from observers on board was collected within the National Programme of collection, management and use of data in the fisheries sector and support for scientific advice regarding the Common Fisheries Policy, co-funded by the IEO and the EU through the European Maritime and Fisheries Fund (EMFF). This research was partly funded by the European Union’s Horizon 2020 Research and Innovation Program (H2020-BG-10-2020-2), grant number No. 101000302 – EcoScope (Ecocentric management for sustainable fisheries and healthy marine ecosystems). CANAL project was co-funded by Fundación Biodiversidad and the EC (LIFE+ INDEMARES project). DRAGONSAL project was funded by the Balearic Islands Government (EU Fisheries Fund). IDEADOS project was funded by the Spanish Government (CTM2007-65844-C02-01-02-03). The project is a contribution to the project “Mejora del conocimiento cientı́fico-técnico para la sostenibilidad de las pesquerı́as demersales del Mediterráneo occidental”, funded by Next Generation European funds (Plan de Recuperación, Tranformación y Resiliencia), with an agreement between the Spanish Ministry of Agriculture, Fisheries and Food and CSIC by means of the IEO. MC would like to acknowledge the institutional support of the ‘Severo Ochoa Centre of Excellence’ accreditation (CEX2019-000928-S) and the European Union’s Horizon 2020 research and innovation programme under grant agreement No 869300 (FutureMares). The funders had no role in study design, data collection and analysis, decision to publish, or preparation of the manuscript.
Acknowledgments
The authors would like to acknowledge all the people who worked on the scientific surveys and the financial support by the following projects: European Union’s Horizon 2020 Research and Innovation Program, EcoScope, CANAL, DRAGONSAL, DISCARDLESS and IDEADOS. X.C. was supported by the Spanish National Program Juan de la Cierva-Formación (MICINN/AEI/10.13039/501100011033 FJC2020-044367-I) funded by the Government of Spain (Ministry of Science and Innovation (MICINN) and the State Research Agency (AEI)) and the European Union (NextGenerationEU/PRTR).
Conflict of interest
The authors declare that the research was conducted in the absence of any commercial or financial relationships that could be construed as a potential conflict of interest.
Publisher’s note
All claims expressed in this article are solely those of the authors and do not necessarily represent those of their affiliated organizations, or those of the publisher, the editors and the reviewers. Any product that may be evaluated in this article, or claim that may be made by its manufacturer, is not guaranteed or endorsed by the publisher.
Supplementary material
The Supplementary Material for this article can be found online at: https://www.frontiersin.org/articles/10.3389/fmars.2023.1166674/full#supplementary-material
References
Acosta J., Canals M., López-Martı́nez J., Muñoz A., Herranz P., Urgeles R., et al. (2002). The balearic promontory geomorphology (Western Mediterranean): morphostructure and active processes. Geomorphology 49, 177–204. doi: 10.1016/S0169-555X(02)00168-X
Álvarez E., Grau A. M., Marbà N., Carreras D. (2015) Praderas de angiospermas marinas de baleares. Available at: http://hdl.handle.net/10508/10464
Arcos J. M., Bécares J., Rodríguez B., Ruíz A. (2009). Áreas importantes para la conservación de las Aves marinas en españa (Madrid, Spain: Sociedad Española de Ornitologia (SEO/BirdLife).
Armengol L., Calbet A., Franchy G., Rodríguez-Santos A., Hernández-León S. (2019). Planktonic food web structure and trophic transfer efficiency along a productivity gradient in the tropical and subtropical Atlantic ocean. Sci. Rep. 9, 1–19. doi: 10.1038/s41598-019-38507-9
Ballesteros E. (1992). Els fons rocosos profunds amb osmundaria volubilis (Linné) r. e. Norris a les balears. Bolletí la Soc d’Història Nat. Balear. 35, 33–50. Available at: https://www.raco.cat/index.php/BolletiSHNBalears/article/view/168869.
Ballesteros E. (1994). The deep-water peyssonnelia beds from the Balearic islands (Western Mediterranean). Mar. Ecol. 15, 233–253. doi: 10.1111/j.1439-0485.1994.tb00055.x
Banaru D., Mellon-Duval C., Roos D., Bigot J. L., Souplet A., Jadaud A., et al. (2013). Trophic structure in the gulf of lions marine ecosystem (North-Western Mediterranean Sea) and fishing impacts. J. Mar. Syst. 111–112, 45–68. doi: 10.1016/j.jmarsys.2012.09.010
Barberá C., Moranta J., Ordines F., Ramón M., de Mesa A., Díaz-Valdés M., et al. (2012). Biodiversity and habitat mapping of menorca channel (Western Mediterranean): implications for conservation. Biodivers. Conserv. 21, 701–728. doi: 10.1007/s10531-011-0210-1
Bertrand J. A., Sola L. G. I. L. D. E., Papaconstantinou C., Relini G., Souplet A. (2002). The general specifications of the MEDITS surveys. Sci. Mar. 66, 9–17. doi: 10.3989/scimar.2002.66s29
Bosc E., Bricaud A., Antoine D. (2004). Seasonal and interannual variability in algal biomass and primary production in the Mediterranean Sea, as derived from 4 years of SeaWiFS observations. Global Biogeochem. Cycles 18, 1–17. doi: 10.1029/2003GB002034
Brey T. (2001) Population dynamics in benthic invertebrates. a virtual handbook. Available at: http://www.awi-bremerhaven.de/Benthic/Ecosystem/FoodWeb/Handbook/main.html.
Cabanellas-Reboredo M., Palmer M., Alós J., Morales-nin B. (2017). Estimating harvest and its uncertainty in heterogeneous recreational fisheries. Fish. Res. 188, 100–111. doi: 10.1016/j.fishres.2016.12.008
Caddy J. F. (1993). Some future perspectives for assessment and management of Mediterranean fisheries. Sci. Mar. 57 (2-3), 121–130
Canals M., Ballesteros E. (1997). Production of carbonate particles by phytobenthic communities on the mallorca-menorca shelf, Northwestern Mediterranean Sea. Deep Sea Res. Part II Top. Stud. Oceanogr. 44, 611–629. doi: 10.1016/S0967-0645(96)00095-1
Cardona L., Revelles M., Carreras C., San Félix M., Gazo M., Aguilar A. (2005). Western Mediterranean Immature loggerhead turtles: habitat use in spring and summer assessed through satellite tracking and aerial surveys. Mar. Biol. 147, 583–591. doi: 10.1007/s00227-005-1578-9
Cardona L., Revelles M., Sales M., Aguilar A., Borrell A. (2007). Meadows of the seagrass posidonia oceanica are a significant source of organic matter for adjoining ecosystems. Mar. Ecol. Prog. Ser. 335, 123–131. doi: 10.3354/meps335123
Carreras M., Coll M., Quetglas A., Massutí E., Oliver P., Goñi R., et al. (2015). Estimates of total fisheries removal for the Balearic Islands, (1950–2010). Fish. Cent. Res. Rep. 19, 1–45.
Cartes J. E., Madurell T., Fanelli E., López-Jurado J. L. (2008a). Dynamics of suprabenthos-zooplankton communities around the Balearic Islands (Western Mediterranean): influence of environmental variables and effects on the biological cycle of aristeus antennatus. J. Mar. Syst. 71, 316–335. doi: 10.1016/j.jmarsys.2006.11.013
Cartes J. E., Maynou F., Fanelli E., Romano C., Mamouridis V., Papiol V. (2009). The distribution of megabenthic, invertebrate epifauna in the Balearic Basin (Western Mediterranean) between 400 and 2300 m: environmental gradients influencing assemblages composition and biomass trends. J. Sea Res. 61, 244–257. doi: 10.1016/j.seares.2009.01.005
Cartes J. E., Papiol V., Guijarro B. (2008b). The feeding and diet of the deep-sea shrimp aristeus antennatus off the Balearic Islands (Western Mediterranean): influence of environmental factors and relationship with the biological cycle. Prog. Oceanogr. 79, 37–54. doi: 10.1016/j.pocean.2008.07.003
Christensen V., Pauly D. (1993). Trophic models of aquatic ecosystems (International Center for Living Aquatic Resources Management (ICLARM), the International Council for the Exploration of the Sea, and the Danish International Development Agency: WorldFish).
Christensen V., Pauly D. (1995). Primary production required to sustain global fisheries. Nature 374, 255–257. doi: 10.1038/374255a0
Christensen V., Walters C. J. (2004). Ecopath with ecosim: methods, capabilities and limitations. Ecol. Modell. 172, 109–139. doi: 10.1016/j.ecolmodel.2003.09.003
Christensen V., Walters C., Pauley D., Forrest R. (2008). Ecopath with ecosim version 6: user guide. Lenfest Ocean Futur. Proj. 1–235.
Coll J., García-Rubies A., Hereu Fina B. (2003). Estat actual de les poblacions de peixos vulnerables a la pesca en els fons rocosos de l’àrea d’influència marina del parc natural de sa dragonera (SO de mallorca). Cent. d’Estudis Avançats Blanes. 1–46. Available at: http://hdl.handle.net/2445/22608.
Coll M., Libralato S. (2012). Contributions of food web modelling to the ecosystem approach to marine resource management in the Mediterranean Sea. Fish Fish. 13, 60–88. doi: 10.1111/j.1467-2979.2011.00420.x
Coll M., Ortega-Cerdà M., Mascarell-Rocher Y. (2021). Ecological and economic effects of COVID-19 in marine fisheries from the Northwestern Mediterranean Sea. Biol. Conserv. 255, 108997. doi: 10.1016/j.biocon.2021.108997
Coll M., Palomera I., Tudela S., Dowd M. (2008). Food-web dynamics in the South Catalan Sea ecosystem (NW Mediterranean) for 1978-2003. Ecol. Modell. 217, 95–116. doi: 10.1016/j.ecolmodel.2008.06.013
Coll M., Palomera I., Tudela S., Sardà F. (2006). Trophic flows, ecosystem structure and fishing impacts in the South Catalan Sea, Northwestern Mediterranean. J. Mar. Syst. 59, 63–96. doi: 10.1016/j.jmarsys.2005.09.001
Coll J., Rubies A. G., Morey G., Navarro O., Martino S., Munuera F. R., et al. (2007). “La reserva Marina del nord de menorca com a eina per a la gestió sostenible dels recursos marins: el cas de l’anfós epinephelus marginatus (Lowe 1834),” in V Jornades del medi ambient de les illes balears: ponències i resums (Societat d’Història natural de les balears), 272–273.
Colléter M., Valls A., Guitton J., Gascuel D., Pauly D., Christensen V. (2015). Global overview of the applications of the ecopath with ecosim modeling approach using the EcoBase models repository. Ecol. Modell. 302, 42–53. doi: 10.1016/j.ecolmodel.2015.01.025
Colloca F., Cardinale M., Maynou F., Giannoulaki M., Scarcella G., Jenko K., et al. (2013). Rebuilding Mediterranean fisheries: a new paradigm for ecological sustainability. Fish Fish. 14, 89–109. doi: 10.1111/j.1467-2979.2011.00453.x
Corrales X., Coll M., Tecchio S., Bellido J. M., Fernández Á.M., Palomera I. (2015). Ecosystem structure and fishing impacts in the northwestern Mediterranean Sea using a food web model within a comparative approach. J. Mar. Syst. 148, 183–199. doi: 10.1016/j.jmarsys.2015.03.006
Corrales X., Ofir E., Coll M., Goren M., Edelist D., Heymans J. J., et al. (2017). Modeling the role and impact of alien species and fisheries on the Israeli marine continental shelf ecosystem. J. Mar. Syst. 170, 88–102. doi: 10.1016/j.jmarsys.2017.02.004
De Goeij J. M., Van Oevelen D., Vermeij M. J. A., Osinga R., Middelburg J. J., De Goeij A. F. P. M., et al. (2013). Surviving in a marine desert: the sponge loop retains resources within coral reefs. Science 342, 108–110. doi: 10.1126/science.1241981
Domínguez M., Fontán A., Rivera J., Ramón M. (2013). Caracterización del ecosistema bentónico de la plataforma costera del área comprendida entre sa dragonera, cabrera y el cap de ses salines (Mallorca). Inst. Espanyol d’Oceanografia.
Fanelli E., Cartes J. E., Rumolo P., Sprovieri M. (2009). Food-web structure and trophodynamics of mesopelagic–suprabenthic bathyal macrofauna of the Algerian basin based on stable isotopes of carbon and nitrogen. Deep Sea Res. Part I Oceanogr. Res. Pap. 56, 1504–1520. doi: 10.1016/j.dsr.2009.04.004
Farrugio H., Oliver P., Biagi F. (1993). An overview of the history, knowledge, recent and future research trends in Mediterranean fisheries *. Sci. Mar. Trends Res. Mediterr. Fish. 57, 105–119.
Fernandez de Puelles M., Grás D., Hernández-León S. (2003). Annual cycle of zooplankton biomass, abundance and species composition in the neritic area of the Balearic Sea, Western Mediterranean. Mar. Ecol. 24, 123–139. doi: 10.1046/j.1439-0485.2003.03816.x
Fernandez de Puelles M. L., Morillas A., Font E. A., Vicente L. (2007). The spatial zooplankton distribution in the north balear front (Western mediterranean): early spring 2005. Rapp. Commun. Int. Mer Medit. 38, 473.
Fernández de Puelles M. L., Valencia J., Jansá J., Morillas A. (2004). Hydrographical characteristics and zooplankton distribution in the mallorca channel (Western mediterranean): spring 2001. ICES J. Mar. Sci. 61, 654–666. doi: 10.1016/j.icesjms.2004.03.031
Forcada J., Gazo M., Aguilar A., Gonzalvo J., Fernández-Contreras M. (2004). Bottlenose dolphin abundance in the NW Mediterranean: addressing heterogeneity in distribution. Mar. Ecol. Prog. Ser. 275, 275–287. doi: 10.3354/meps275275
García-Rodríguez E., Coll M., Vivas M., Bellido J. M., Esteban A., Torres M.Á. (2021). A food-web comparative modeling approach highlights ecosystem singularities of the gulf of alicante (Western Mediterranean Sea). J. Sea Res. 174, 102073. doi: 10.1016/j.seares.2021.102073
Gascuel D., Guénette S., Pauly D. (2011). The trophic-level-based ecosystem modelling approach: theoretical overview and practical uses. ICES J. Mar. Sci. 68, 1403–1416. doi: 10.1093/icesjms/fsr062
GFCM (2022). “General fisheries commission for the Mediterranean,” in Report of the twenty-third session of the scientific advisory committee on fisheries (Rome, Italy: FAO headquartes), 21–24. doi: 10.4060/cc3109en
Hattab T., Ben F., Lasram R., Albouy C., Salah M., Jarboui O., et al. (2013). An ecosystem model of an exploited southern Mediterranean shelf region ( gulf of gabes , Tunisia ) and a comparison with other Mediterranean ecosystem model properties. J. Mar. Syst. 128, 159–174. doi: 10.1016/j.jmarsys.2013.04.017
Heymans J. J., Coll M., Libralato S., Morissette L., Christensen V. (2014). Global patterns in ecological indicators of marine food webs: a modelling approach. PloS One 9, 1–16. doi: 10.1371/journal.pone.0095845
Heymans J. J., Coll M., Link J. S., Mackinson S., Steenbeek J., Walters C., et al. (2016). Best practice in ecopath with ecosim food-web models for ecosystem-based management. Ecol. Modell. 331, 173–184. doi: 10.1016/j.ecolmodel.2015.12.007
Horn S., Coll M., Asmus H., Dolch T. (2021). Food web models reveal potential ecosystem effects of seagrass recovery in the Northern Wadden Sea. Restor. Ecol. 29, 1–13. doi: 10.1111/rec.13328
Innes S., Lavigne D. M., Earle W. M., Kovacs K. M. (1987). Feeding rates of seals and whales. J. Anim. Ecol. 56 (1), 115–130. doi: 10.2307/4803
Keramidas I., Dimarchopoulou D., Eyal O., Scotti M., Tsikliras A. C., Gal G. (2023). Ecotrophic perspective in fi sheries management : a review of ecopath with ecosim models in European marine ecosystems. Front. Mar. Sci 10, 1–22. doi: 10.3389/fmars.2023.1182921
Keramidas I., Dimarchopoulou D., Tsikliras A. C. (2022). Modelling and assessing the ecosystem of the Aegean Sea, a major hub of the Eastern Mediterranean at the intersection of Europe and Asia. Reg. Stud. Mar. Sci. 56, 102704. doi: 10.1016/j.rsma.2022.102704
Lehucher P. M., Beautier L., Chartier M., Martel F., Mortier L., Brehmer P., et al. (1995). Progress from 1989 to 1992 in understanding the circulation of the Western Mediterranean Sea. Oceanol. Acta 18, 255–271. Available at: https://archimer.ifremer.fr/doc/00097/20776/.
Lindeman R. L. (1942). The trophic-dynamic aspect of ecology author ( s ): Raymond l . lindeman published by : ecological society of America stable URL : http://www.jstor.org/stable/1930126. Ecology 23, 399–417. doi: 10.2307/1930126
Link J. S. (2010). Adding rigor to ecological network models by evaluating a set of pre-balance diagnostics: a plea for PREBAL. Ecol. Modell. 221, 1580–1591. doi: 10.1016/j.ecolmodel.2010.03.012
Lleonart J., Maynou F. (2003). Fish stock assessments in the Mediterranean: state of the art. Sci. Mar. 67, 37–49. doi: 10.3989/scimar.2003.67s137
Longo C., Hornborg S., Bartolino V., Tomczak M. T., Ciannelli L., Libralato S., et al. (2015). Role of trophic models and indicators in current marine fisheries management. Mar. Ecol. Prog. Ser. 538, 257–272. doi: 10.3354/meps11502
Madurell T., Cartes J. E. (2005). Trophodynamics of a deep-sea demersal fish assemblage from the bathyal eastern Ionian Sea (Mediterranean Sea). Deep Sea Res. Part I Oceanogr. Res. Pap. 52, 2049–2064. doi: 10.1016/j.dsr.2005.06.013
Madurell T., Cartes J. E., Labropoulou M. (2004). Changes in the structure of fish assemblages in a bathyal site of the Ionian Sea (eastern Mediterranean). Fish. Res. 66, 245–260. doi: 10.1016/S0165-7836(03)00205-4
Madurell T., Fanelli E., Cartes J. E. (2008). Isotopic composition of carbon and nitrogen of suprabenthic fauna in the NW Balearic Islands (western Mediterranean). Mar. Syst. 71, 336–345. doi: 10.1016/j.jmarsys.2007.03.006
Mallol S., Goñi R. (2004). Seguimiento de las pesquerías (Punto Cero) en el ámbito de la futura Reserva Marina de Cala Rajada (Mallorca). Informe Técnico IEO-COB y SGPM. PESCALA I/04-1. Secreta.
Massutí E., Olivar M. P., Monserrat S., Rueda L., Oliver P. (2014). Towards understanding the influence of environmental conditions on demersal resources and ecosystems in the Western Mediterranean: motivations, aims and methods of the IDEADOS project. J. Mar. Syst. 138, 3–19. doi: 10.1016/j.jmarsys.2014.01.013
Massutí E., Renones O. (2005). Demersal resource assemblages in the trawl fishing grounds off the Balearic Islands (Western Mediterranean). Sci. Mar. 69, 167–181. doi: 10.3989/scimar.2005.69n1167
Massutí E., Sánchez-Guillamón O., Farriols M. T., Palomino D., Frank A., Bárcenas P., et al. (2022). Improving scientific knowledge of mallorca channel seamounts (Western Mediterranean) within the framework of natura 2000 network. Diversity 14, 1–71. doi: 10.3390/d14010004
Mellon-Duval C., De Pontual H., Métral L., Quemener L. (2010). Growth of European hake (Merluccius merluccius) in the gulf of lions based on conventional tagging. ICES J. Mar. Sci. 67, 62–70. doi: 10.1093/icesjms/fsp215
Morales-Nin B., Moranta J., Garcia C., Tugores P., Grau A. M. (2008). “Evaluation of the importance of recreational fisheries on a Mediterranean Island,” in Reconciling fisheries with conservation: Proceedings of the Fourth World Fisheries Congress Nielsen J., et al. American Fisheries Society Symposium (AMERICAN FISHERIES SOCIETY). 49, 1946.
Morales-Nin B., Moranta J., García C., Tugores M. P., Grau A. M., Riera F., et al. (2005). The recreational fishery off Majorca island (western mediterranean): some implications for coastal resource management. ICES J. Mar. Sci. 62, 727–739. doi: 10.1016/j.icesjms.2005.01.022
Morey G., García-Rubies A., Hereu Fina B., Coll J. (2003). Estat actual de les poblacions de peixos vulnerables a la pesca en els fons rocosos de la futura Reserva Marina de Llevant (NE de Mallorca).
Navarro J., Coll M., Louzao M., Palomera I., Delgado A., Forero M. G. (2011). Comparison of ecosystem modelling and isotopic approach as ecological tools to investigate food webs in the NW Mediterranean Sea. J. Exp. Mar. Bio. Ecol. 401, 97–104. doi: 10.1016/j.jembe.2011.02.040
Nee S. (1990). Community construction. Trends Ecol. Evol. 5, 337–340. doi: 10.1016/0169-5347(90)90182-D
Nilsson S. G., Nilsson I. N. (1976). Numbers, food consumption, and fish predation by birds in lake möckeln, Southern Sweden. Ornis Scand. 7, 61–70. doi: 10.2307/3676175
Odum E. P. (1969). The strategy of ecosystem development: an understanding of ecological succession provides a basis for resolving man’s conflict with nature. Sci. (80-.). 164, 262–270. doi: 10.1126/science.164.3877.262
Odum E. P. (1971). Fundamentals of ecology (Saunders Philadelphia: International Council for the Exploration of the Sea (ICES)).
Olivar M. P., Bernal A., Molí B., Peña M., Balbín R., Castellón A., et al. (2012). Vertical distribution, diversity and assemblages of mesopelagic fishes in the western Mediterranean. Deep Sea Res. Part I Oceanogr. Res. Pap. 62, 53–69. doi: 10.1016/j.dsr.2011.12.014
Opitz S. (1996). Trophic interactions in Caribbean coral reefs (ICLARM, Manila, Philippines: WorldFish).
Ordines F. (2015) Habitats and nekto-benthic communities of the bottom-trawl fishery developed on the continental shelf of the Balearic Islands. Available at: http://hdl.handle.net/10508/10097.
Ordines F., Bauzá M., Sbert M., Roca P., Gianotti M., Massutí E. (2015). Red algal beds increase the condition of nekto-benthic fish. J. Sea Res. 95, 115–123. doi: 10.1016/j.seares.2014.08.002
Ordines F., Farriols M. T., Lleonart J., Guijarro B., Quetglas A., Massutí E. (2014). Biology and population dynamics of by-catch fish species of the bottom trawl fishery in the Western Mediterranean. Mediterr. Mar. Sci. 15 (3), 613–625. doi: 10.12681/mms.812
Ordines F., Massutí E. (2009). Relationships between macro-epibenthic communities and fish on the shelf grounds of the Western Mediterranean. Aquat. Conserv. Mar. Freshw. Ecosyst. 383, 370–383. doi: 10.1002/aqc
Ordines F., Quetglas A., Massutí E., Moranta J. (2009). Habitat preferences and life history of the red scorpion fish, scorpaena notata, in the Mediterranean. Estuar. Coast. Shelf Sci. 85, 537–546. doi: 10.1016/j.ecss.2009.09.020
Ordines F., Ramón M., Rivera J., Rodríguez-Prieto C., Farriols M. T., Guijarro B., et al. (2017). Why long term trawled red algae beds off Balearic Islands (Western Mediterranean) still persist? Reg. Stud. Mar. Sci. 15, 39–49. doi: 10.1016/j.rsma.2017.07.005
Ordines F., Valls M., Meléndez M. J., Ramírez-Amaro S., López E., Lloret J., et al. (2021). Potential factors influencing the condition of demersal sharks in the Mediterranean deep sea ecosystems. Deep. Res. Part I Oceanogr. Res. Pap. 176, 103603. doi: 10.1016/j.dsr.2021.103603
Palmer M., Quetglas A., Guijarro B., Moranta J., Ordines F., Massutí E. (2009). Performance of artificial neural networks and discriminant analysis in predicting fishing tactics from multispecific fisheries. Can. J. Fish. Aquat. Sci. 66, 224–237. doi: 10.1139/F08-208
Patrick W. S., Link J. S. (2015). Myths that continue to impede progress in ecosystem-based fisheries management. Fisheries 40, 155–160. doi: 10.1080/03632415.2015.1024308
Pauly D. (1980). On the interrelationships between natural mortality, growth parameters, and mean environmental temperature in 175 fish stocks. ICES J. Mar. Sci. 39, 175–192. doi: 10.1093/icesjms/39.2.175
Pauly D., Christensen V., Dalsgaard J., Froese R., Jr F. T. (1998). Fish. Down Mar. Food Webs 279, 860–863. doi: 10.1126/science.279.5352.860
Pauly D., Christensen V., Sambilay J. V. (1990). Some features of fish food consumption estimates used by ecosystem modelers (International Council for the Exploration of the Sea (ICES).
Pauly D., Soriano-Bratz M. L., Palomares M. L. D. (1993). “Improved construction, parameterization and interpretation of steady-state ecosystem models,” in Trophic models of aquatic ecosystems. Eds. Christensen V., Pauly D. (WorldFish), 1–13.
Peña M., Olivar M. P., Balbín R., López-Jurado J. L., Iglesias M., Miquel J. (2014). Acoustic detection of mesopelagic fishes in scattering layers of the Balearic Sea (Western Mediterranean). Can. J. Fish. Aquat. Sci. 71, 1186–1197. doi: 10.1139/cjfas-2013-0331
Pikitch E. K., Santora C., Babcock E. A., Bakun A., Bonfil R., Conover D. O., et al. (2004). Ecosystem-based fishery management. Sci. (80-. ). 305, 346–347. doi: 10.1126/science.1098222
Pinot J.-M., Tintoré J., Gomis D. (1995). Multivariate analysis of the surface circulation in the Balearic Sea. Prog. Oceanogr. 36, 343–376. doi: 10.1016/0079-6611(96)00003-1
Piroddi C., Teixeira H., Lynam C. P., Smith C., Alvarez M. C., Mazik K., et al. (2015). Using ecological models to assess ecosystem status in support of the European marine strategy framework directive. Ecol. Indic. 58, 175–191. doi: 10.1016/j.ecolind.2015.05.037
Polunin N. V. C., Morales-Nin B., Pawsey W. E., Cartes J. E., Pinnegar J. K., Moranta J. (2001). Feeding relationships in Mediterranean bathyal assemblages elucidated by stable nitrogen and carbon isotope data. Mar. Ecol. Prog. Ser. 220, 13–23. doi: 10.3354/meps220013
Pomeroy L. R., le Williams P. J. B., Azam F., Hobbie J. E. (2007). The microbial loop. Oceanography 20, 28–33. doi: 10.5670/oceanog.2007.45
Quetglas A., Guijarro B., Ordines F., Massutí E. (2012). Stock boundaries for fisheries assessment and management in the Mediterranean: the Balearic Islands as a case study. Sci. Mar. 76, 17–28. doi: 10.3989/scimar.2012.76n1017
Quetglas A., Merino G., González J., Ordines F., Garau A., Grau A. M., et al. (2016a). Regional implementation plan for demersal fisheries from the Balearic islands (Centro Oceanográfico de Baleares: Western Mediterranean).
Quetglas A., Merino G., Ordines F., Guijarro B., Garau A., Grau A. M., et al. (2016b). Assessment and management of western Mediterranean small-scale fisheries. Ocean Coast. Manage. 133, 95–104. doi: 10.1016/j.ocecoaman.2016.09.013
Ramírez-Amaro S., Ordines F., Esteban A., García C., Guijarro B., Salmerón F., et al. (2020). The diversity of recent trends for chondrichthyans in the Mediterranean reflects fishing exploitation and a potential evolutionary pressure towards early maturation. Sci. Rep. 10, 1–18. doi: 10.1038/s41598-019-56818-9
Reiss H., Kröncke I., Ehrich S. (2006). Estimating the catching efficiency of a 2-m beam trawl for sampling epifauna by removal experiments. ICES J. Mar. Sci. 63, 1453–1464. doi: 10.1016/j.icesjms.2006.06.001
Rueda L., Valls M., Hidalgo M., Guijarro B., Esteban A., Massutí E. (2019). From trophic ecology to fish condition: contrasting pathways for European hake in the western Mediterranean. Mar. Ecol. Prog. Ser. 623, 131–143. doi: 10.3354/meps13025
Scotti M., Opitz S., MacNeil L., Kreutle A., Pusch C., Froese R. (2022). Ecosystem-based fisheries management increases catch and carbon sequestration through recovery of exploited stocks: the western Baltic Sea case study. Front. Mar. Sci. 9. doi: 10.3389/fmars.2022.879998
Sea Around Us. Available at: https://www.seaaroundus.org/.
Spedicato M. T., Massutí E., Mérigot B., Tserpes G., Relini G., Tecnologia C. (2019). The MEDITS trawl survey specifications in an ecosystem approach to fishery management. Sci. Mar. 83, 9–20.
STECF (2022). “Scientific, technical and economic committee for fisheries (STECF)- stock assessments: demersal stocks in the western Mediterranean Sea (STECF-20-09),” in Publ. off. eur. union, luxemb. Available at: https://stecf.jrc.ec.europa.eu/documents/43805/1446742/STECF+16-14+-+Methods+for+MED+stock+assessments_JRC102680.pdf.
Tecchio S., Coll M., Christensen V., Company J. B., Ramírez-Llodra E., Sardà F. (2013). Food web structure and vulnerability of a deep-sea ecosystem in the NW Mediterranean Sea. Deep. Res. Part I Oceanogr. Res. Pap. 75, 1–15. doi: 10.1016/j.dsr.2013.01.003
Torres M.Á., Coll M., Heymans J. J., Christensen V., Sobrino I. (2013). Food-web structure of and fishing impacts on the gulf of cadiz ecosystem (South-Western Spain). Ecol. Modell. 265, 26–44. doi: 10.1016/J.ECOLMODEL.2013.05.019
Trites A. W., Christensen V., Pauly D. (1997). Competition between fisheries and marine mammals for prey and primary production in the Pacific Ocean. J. Northwest Atl. Fish. Sci. 22, 173–187. doi: 10.2960/J.v22.a14
Tsagarakis K., Coll M., Giannoulaki M., Somarakis S., Papaconstantinou C., Machias A. (2010). Food-web traits of the north Aegean Sea ecosystem (Eastern Mediterranean) and comparison with other Mediterranean ecosystems. Estuar. Coast. Shelf Sci. 88, 233–248. doi: 10.1016/j.ecss.2010.04.007
Ulanowicz R. E. (1986). Growth and development: ecosystem phenomenology. Springer Verlag 203. doi:10.1007/978-1-4612-4916-0
Ulanowicz R. E. (1995). The part-whole relation in ecosystems. Complex Ecol. Prentice-Hall New Jersey, 549–560.
Ulanowicz R. E., Puccia C. J. (1990). Mixed trophic impacts in ecosystems. Coenoses 5, 7–16. Available at: http://www.jstor.org/stable/43461017
Valls A., Coll M., Christensen V., Ellison A. M. (2015). Keystone species: toward an operational concept for marine biodiversity conservation. Ecol. Monogr. 85, 29–47. doi: 10.1890/14-0306.1
Valls M., Quetglas A., Ordines F., Moranta J. (2011). Feeding ecology of demersal elasmobranchs from the shelf and slope off the Balearic Sea (western Mediterranean). Sci. Mar. 75, 633–639. doi: 10.3989/scimar.2011.75n4633
Valls M., Rueda L., Quetglas A. (2017). Feeding strategies and resource partitioning among elasmobranchs and cephalopods in Mediterranean deep-sea ecosystems. Deep. Res. Part I Oceanogr. Res. Pap. 128, 28–41. doi: 10.1016/j.dsr.2017.09.002
Keywords: Balearic Islands, ecopath model, food web, trophic flows, fishing impacts, comparative model approach
Citation: Sánchez-Zulueta P, Valls M, Guijarro B, Ángeles Torres M, Ángeles Zapata M, Coll M, Corrales X, Andonegi E, Díaz-Valdés M, Massutí E and Ordines F (2023) Trophic structure and fishing impacts on an oligotrophic ecosystem in the Western Mediterranean: the Balearic Islands. Front. Mar. Sci. 10:1166674. doi: 10.3389/fmars.2023.1166674
Received: 15 February 2023; Accepted: 21 June 2023;
Published: 20 July 2023.
Edited by:
Georgios Sylaios, Democritus University of Thrace, GreeceReviewed by:
Bernardo Patti, National Research Council (CNR), ItalyGideon Gal, Israel Oceanographic and Limnological Research, Israel
Copyright © 2023 Sánchez-Zulueta, Valls, Guijarro, Ángeles Torres, Ángeles Zapata, Coll, Corrales, Andonegi, Díaz-Valdés, Massutí and Ordines. This is an open-access article distributed under the terms of the Creative Commons Attribution License (CC BY). The use, distribution or reproduction in other forums is permitted, provided the original author(s) and the copyright owner(s) are credited and that the original publication in this journal is cited, in accordance with accepted academic practice. No use, distribution or reproduction is permitted which does not comply with these terms.
*Correspondence: Paula Sánchez-Zulueta, cGF1bGEuc2FuY2hlei56dWx1ZXRhQGllby5jc2ljLmVz; María Valls, bWFyaWEudmFsbHNAaWVvLmNzaWMuZXM=