- 1Centro Oceanográfico de Canarias, Instituto Español de Oceanografía - Consejo Superior de Investigaciones Científicas (IEO-CSIC), Santa Cruz de Tenerife, Canary Islands, Spain
- 2Unidad Océano y Clima, Instituto de Oceanografía y Cambio Global, IOCAG, Universidad de Las Palmas de Gran Canaria, ULPGC, Unidad Asociada ULPGC-CSIC, Las Palmas de Gran Canaria, Spain
- 3Global Fishing Watch (GFW), Research and Innovation Department, Washington, DC, United States
- 4Ifremer, University of Brest, CNRS, IRD, Laboratoire d’Océanographie Physique et Spatiale (LOPS), Plouzané, France
- 5National Institute of Oceanography and Applied Geophysics (OGS), Trieste, Italy
- 6Ifremer, Département Infrastructure Marines et Numériques, Brest, France
The Argo observation network is made up of approximately 4,000 drifting floats, which provide valuable information about the ocean and its role in the climate system. Each one of these floats work in continuous cycles, until their batteries run out. Due to its importance in operational forecasting and climate research, the Argo community continually assesses the status of the sensors mounted on each of the floats. Recovering floats would offer a great opportunity to gain insight into sensor performance and stability, although the economic and environmental costs of dedicating a ship exclusively to recover Argo floats make it unsustainable. In this work, the potential of world shipping traffic as float retrievers has been evaluated through an analysis of encounters based on the Automatic Identification System (AIS) of ships and the location of Argo floats in the years 2019 and 2020. About 18,500 and 28,500 encounters happened for both years, respectively. The Mediterranean Sea hosted the most encounters, and fishing ships were the most suitable type of ship aimed for potential recoveries. A total of 298 and 373 floats interacted with the world shipping traffic in favorable weather conditions in 2019 and 2020, respectively, a figure equivalent to 25% of the annual replacement rate of the Argo network. The same approach was applied to 677 floats affected by abrupt salinity drift (ASD), an issue that has recently come to the attention of the Argo community. It turned out that 59 and 103 ASD-affected floats interacted with ships of opportunity in both years.
1 Introduction
The interest in understanding the role that the ocean plays in the global climate system has led to the creation of the Global Ocean Observing System (GOOS), a multidisciplinary observation system that aims to monitor the essential physical and biogeochemical variables of the oceans before the turn of the 21st century (Moltmann and Coauthors, 2019). One of the main in-situ components of GOOS is the Argo International Program. Argo is a global observation network that collects, through its Core mission, data on pressure, salinity, temperature in the top 2000 meters of the ocean. This network is made up of approximately 4,000 autonomous floats in the global ocean, high latitudes, ice-covered areas and marginal seas. More than two million vertical profiles have been collected by the Argo network during the past two decades (Wong and Coauthors, 2020).
The challenges the Argo network has faced since its inception have been numerous, the continuity of the Core Argo mission being the biggest of them. By deploying several hundred floats per year, the global coverage of the oceans is sustained over time. According to the Joint Centre for Oceanography and Marine Meteorology in situ Observations Programmes Support (OceanOPS), this figure currently stands at an average of 830 floats per year. Another challenge is to assure the target accuracy, by addressing drifts in the pressure (Barker et al., 2011) and conductivity sensors. A notice in early 2021 from the leading manufacturer of the Argo CTDs (Sea-Bird Electronics), stated that an increased number of conductivity cells had salinity drifts due to a manufacturing change at the end of 2018 (Argo Program Office, 2021a). This is an example of sensor performance and stability that has been closely monitored by the Argo community to initiate rapid systematic problem detection procedures (Roemmich and Coauthors, 2009). These issues will likely increase as the network expands with biogeochemical sensors. Additionally, the Argo community has acknowledged the need for consistent post-mission CTD calibrations (Roemmich and Coauthors, 2009; D’Ortenzio and Coauthors, 2020) to facilitate an affordable way to discover and evaluate faults in batches of instruments deployed. To achieve this, it is necessary to recover floats.
The objective of this paper is to assess the viability and potential of the global shipping traffic monitored by the AIS as a key player in a global float recovery scenario, thus offering a means to evaluate the long-term performance of Argo float sensors and batteries. The remainder of this paper is organized as follows: in section 2. Methods, we describe the methodology used to estimate the number of encounters between the global fleet and the Argo floats. In section 3. Data, we describe the data sets used to develop the encounter analysis. In section 4. Results, the results of the encounter analysis are presented. In section 5. Discussion, the results are discussed and the conclusions are presented in a final section.
1.1 Technological advances that encourage float recovery
1.1.1 Bidirectional satellite communications and AIS
The NAOS (Novel Argo ocean Observing System) project focused on preparing and consolidating a Mediterranean Argo pilot study, using the recoveries as a resource to provide evaluation and characterization of the calibration and observational errors. Recovery ratios of 45% were achieved, which made it possible to refurbish and redeploy floats (D’Ortenzio and Coauthors, 2020). Even though part of the success was due to quick access to research ships for recoveries, it was also thanks to the two-way satellite communication and the Automatic Identification System AIS.
To date, there are a variety of transmission systems used by the Argo program, such as BEIDOU, ORBCOMM, ARGOS3 or IRIDIUM. The ARGOS (Advanced Research and Global Observation Satellite) transmission system (mostly pre-ARGOS 3 system) has been the most widely used along the history of the Argo program, however nowadays more than 90% (OceanOPS) of the Argo fleet uses the IRIDIUM transmission system (Argo Program Office, 2021b). Its constellation of satellites are positioned in Low Earth Orbit (LEO) and provides a pole-to-pole coverage. This allows requests to be transmitted from one satellite to another until they reach the satellite above the float, thus transmitting the data to Earth. At present, all transmission systems mentioned above offer this service. Before this two-way communication system got introduced, floats were pre-programmed before being deployed and because its settings could not be modified from a distance, it would keep doing its cycles until the battery died. The new system makes it possible to modify the float settings in real-time. In this way, it is possible for the floats to be placed on the surface when required (recovery mode), waiting for a possible recovery.
Satellite communications providers have a wide range of services, some of which meet the requirements of the Argo network itself. Among the services offered by IRIDIUM, the Short-Burst data (SBD) and the Router-based Unrestricted Digital Interworking Connectivity Solution (RUDICS) are the solutions that best fit the requirements of the Argo network, as both guarantee two-way communication for low data volume. For both solutions, two types of connections are generated: Mobile Originated (MO), meaning a message originated in a mobile phone, and Mobile Terminated (MT), meaning a message ended in a mobile phone. SBD allows messages to be transmitted to (MT) and from (MO) an Argo float, so the message sending protocol can be simply initiated through an email from the deployers’ system. RUDICS works in a similar way, since it facilitates two-way communication but it is based on an optimized circuit-switched data channel.
AIS is an automatic tracking system developed in the 1990s that operates in the VHF (Very High Frequency, 30-300 MHz) band assigned to shipping mobile services (156.025 MHz-162.025 MHz) through the use of transceivers and receivers to transmit real-time information of shipping traffic. AIS transceivers/receivers transmit/receive ship information at regular intervals. This information is broadcast, tracked and recorded (Wright, 2019); most of the applications dedicated to global ship tracking in real time do not make their data freely available. The IMO (International Maritime Organization) adopted a new regulation that required AIS to be fitted aboard all ships of 300 gross tonnage engaged on international voyages, cargo ships of 500 gross tonnage and upwards and all passenger ships, irrespective of size (IMO 2002). For the rest of the ships there is no obligation to use the AIS, but many countries and intergovernmental agencies are creating AIS requirements within their waters, so an even greater use of AIS is expected in the coming years (Global Fishing Watch, 2021a).
Shipboard AIS transceivers have a horizontal range of 40 nautical miles (74 km), as do transceivers that work in coastal domains, called terrestrial AIS (T-AIS). It largely depends on the height of the antenna, as VHF largely works on line of sight. Its vertical range reaches up to 400 km, a fundamental reason why in recent decades AIS stations have been incorporated into satellites, called Satellite-Based AIS (S-AIS). This has contributed to improved ship tracking, navigation and shipping safety with global coverage. In a latest review by (Emmens et al., 2021) a total of seven possible drawbacks of using AIS data were listed, of which we highlight: (1) AIS data contains noise, which means that the information can be erroneously communicated; (2) the quality of the data may be affected by the AIS equipment itself, which may not work correctly (Ou and Zhu, 2008; Kazimierski and Stateczny, 2015) (5) AIS does not work well in dense traffic areas due to possible collision of messages; (6) AIS data is vulnerable to weather/atmospheric conditions (Tsou, 2010; Last et al., 2015; Pelich et al., 2015); (7a) falsification and attacks through crafting a valid non-existing ship, known as spoofing; (7b) lack of coverage in certain areas and deliberate AIS switch off. Despite these potential drawbacks, the wide variety of applications for AIS data makes it worth exploiting its potential (Emmens et al., 2021).
1.1.2 The Argo network: a float’s recovery perspective
The growth of the Argo network has been successful and continuous, since the original program plan called for an array with global coverage of about 3,000 floats, achieved in 2007 (Riser and Coauthors, 2016) by deploying floats at a mean rate of a 1000 every year. At the time this paper is written, the Argo network is made up of a total of 3,869 floats according to OceanOPS, distributed throughout the oceans after more than two decades in operation. Each float is assigned a unique WMO identifier (WMO ID) and launched from a wide variety of platforms, like oceanographic ships, sailboats and commercial ships.
After more than two decades collecting, managing, and freely sharing in situ oceanographic measurements on a global scale, the current Argo network will expand to OneArgo (Owens et al., 2022). A new global design that envisions full spatial coverage to include polar sea ice zones and marginal seas, while increasing regional resolution in key areas such as western boundary currents and equatorial regions. In addition, it is planned to extend the network to ocean full-depth and add biogeochemical sensors to better understand the cycles of carbon, nutrients, and ocean ecosystems. These last two challenges are already a reality and are raised as the Deep-Argo and BioGeoChemical (BGC-Argo) missions, complementing the current Core mission. Deep Argo is currently made up of 188 floats with the capacity to sample up to 4,000 - 6,000 m depth, while BGC Argo is made up of 400 floats with the capacity to measure up to 6 biogeochemical parameters up to 2,000 m depth. Despite being consolidated missions, OneArgo intends to achieve greater accuracy through the development of new sensors in collaboration with commercial vendors. This is where post-mission analysis of sensor performance would strengthen all three missions, especially Deep and BGG Argo. Most floats operate in remote ocean regions with scarce ship traffic and with a distance between them of ~ 300 km (Argo Program Office, 2020). Some of these oceanic regions have very low density of floats. The Southern Ocean is a clear example of oceanic areas with a low density of floats according to OceanOPS, despite initiatives such as the SOCCOM (Southern Ocean Carbon and Climate Observations and Modeling) project, where more than 225 floats have been launched since 2014. Marginal seas are situated on the other side of the coin in terms of potential recoveries, since some of them are areas of considerable shipping traffic, with a high density of floats and with a short distance between ports. Although recoveries are not a common practice, there are some locations such as the Baltic Sea or the Mediterranean Sea where floats are frequently recovered (Cancouët et al., 2021) mostly by oceanographic research ships. No official statistics have been reported on the total number of floats that have been recovered globally by the Argo community. However, an audit carried out within the framework of the Euro-Argo RISE project revealed that more than 84 floats have been recovered at European level at the time this paper is written, highlighting the need to carry out the same work at an international level (Cancouët et al., 2021).
Thanks to the AIS system it is possible to obtain direct information on shipping routes such as departure and arrival ports, speed and course, estimated time of arrival and even GPS position in real time. This is relevant information that can be used in favor of potential recoveries of Argo floats without any limitation, being accessible worldwide.
1.1.3 The Virtual Fleet-recovery: an Argo float recovery helper
In practice, a simple way is needed to facilitate the encounter of an Argo float with a ship of opportunity. If the float is set to “recovery” mode, i.e., freely drifting at the surface and transmitting its position in real time, it can be assumed that a recovery ship is already nearby looking for the float. Recoveries should happen in a matter of hours or days. However, the earlier period when the float is in its last cycles and operators are considering switching it to “recovery” mode is also critical. In this case, the float can operate for one or a few more cycles, and nearby ships still navigate along predefined routes. Therefore, operators have to determine float/ship encounter probabilities with minimal re-routing of nearby vessels.
The goal of the Virtual Fleet-recovery software (Maze and Balem, 2023) is to make Argo floats trajectory predictions easily. The software automatically downloads 3D currents from ocean forecasting models and Argo float mission parameters, and produces a float position probability patch (of the next cycle surfacing position) that can be used to determine if nearby vessels can reasonably be re-routed toward the next float position for recovery. By varying the virtual Argo float mission parameters (such as the parking depth or cycle period), it is possible to explore several scenarios and to select mission parameters that will minimize re-routing of opportunity vessels.
2 Methods
Our analysis revolves around obtaining the number of encounters that have occurred between ships of opportunity and Argo floats that were in their last 10 cycles before losing connection due to dead batteries. The choice of the last 10 cycles is taken as a proxy of the float’s lifespan. In this way, avoiding set time ranges and battery levels, similar life stages of the floats are included in the analysis, regardless of the type of float and its configuration (configurations of 5 and 10-day cycles being most common).
While these assumptions may overestimate the outcomes of potential encounters in a real scenario, a 10-day window of opportunity was selected as a reasonable time frame for staging potential recoveries, at least on floats operating near shore. The encounters between shipping traffic and Argo floats were determined in space, by a circumference of 20 nautical miles radius around the float’s surfacing (Figure 1). When a ship enters in the area within two hours of the surfacing event, it is counted as an encounter. Overlap between two consecutive areas may occur as the surfacing of the floats themselves may not be equidistant from each other. On the other hand, it is possible that encounters occur with the same ship in different surfacings, depending on the trajectories of the ships and the floats. Depending on the ship’s route planning and time availability, it is accounted that a small change in its journey can be part of the improvisation margin of any route, equivalent to a change of course due to meteorological or unforeseen causes. However, this voluntary decision would depend entirely on the skipper of the ship in a real scenario. Under these assumptions, a float could be retrieved when the ship is within a 20-mile radius of action. By increasing the float’s GPS position frequency to intervals of minutes or seconds, the searching becomes more accurate, although GPS errors or unfavorable weather conditions can severely affect search times.
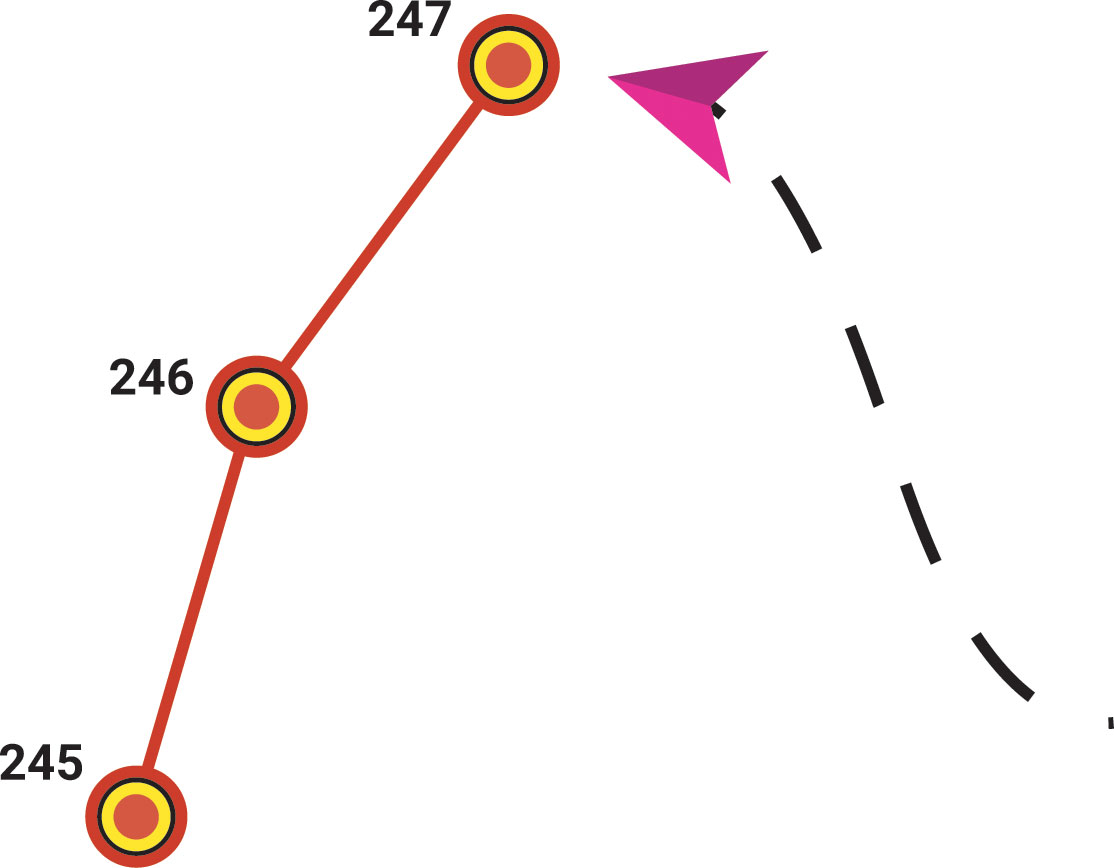
Figure 1 Simulation of an encounter. The waypoints on the left represent the Argo float surfacing. The path on the right represents the heading of a ship of opportunity.
A series of processing levels have been applied that aim to draw as much as possible the real conditions in which potential encounters would occur. The level (L1) is made up of a global raw data matrix that houses all kinds of alphanumeric data from the Argo and AIS datasets. Level (L2) filters encounters by ship size (Figure 2). Passenger, cargo and tanker (PCT) ships have been discarded due to their low maneuverability and tight schedules. All those ships greater than 60 meters have also been ruled out regardless of type, except NGO and oceanographic research ships. In level (L3) we included only the encounters that occurred in locations with favorable weather conditions for potential recoveries. The wave spectrum can be decomposed into wind-sea waves, which are directly affected by local winds, and swells, waves that were generated by wind at a different place and time. The ‘significant height of combined wind waves and swell’ parameter considers both effects. It represents the average height of the highest third of surface ocean/sea waves generated by wind and swell and it is four times the square root of the integral over all directions and all frequencies of the two-dimensional wave spectrum (Hersbach and Coauthors, 2020). Therefore, locations where encounters happened with wind values greater than 20 knots and significant height of combined wind waves and swell values greater than 2.5 m have been ruled out.
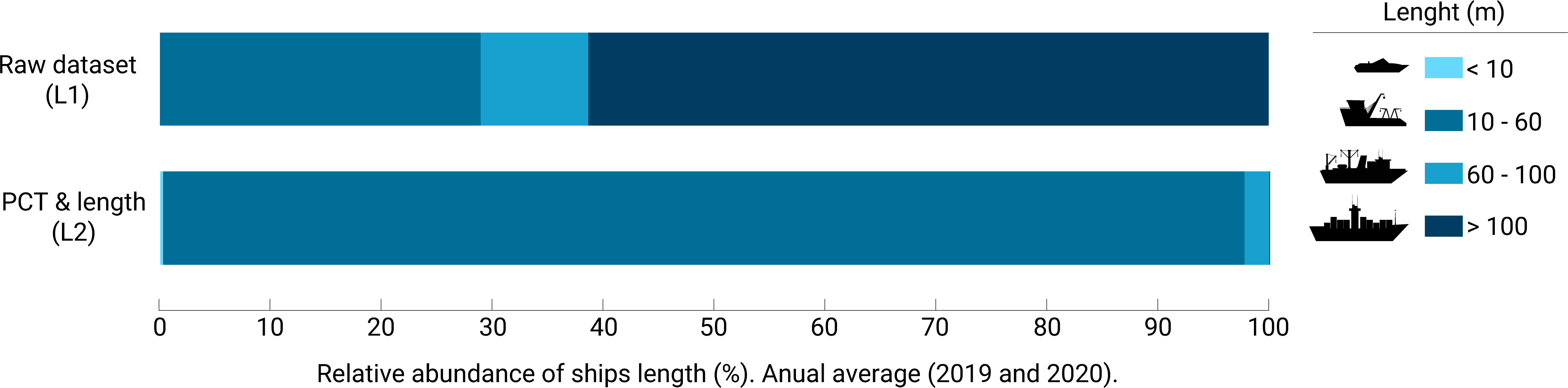
Figure 2 This classification corresponds to the size of the global fleet on average that interacted with Argo floats (encounters) in 2019 and 2020. The first stacked bar corresponds to the raw data from GFW (L1). The second stacked bar is obtained after applying the L2 processing level, discarding the PCT ships and all those ships > 60 m in length, except NGO and oceanographic ships.
The processing level (L4) filters those encounters that only occurred during daylight hours. We have assumed a time frame between half an hour after sunrise to half an hour before sunset, considering the different time zones in which the encounters took place. This assumption is based on the limited visibility during night navigation, in addition to the fact that any type of Argo floats do not have a flashing light to aid recovery in the dark.
3 Data
3.1 Argo and AIS data
The global Argo dataset (Argo, 2022) for years 2019 and 2020 used in this study was obtained on November 11th, 2021 through the Euro-Argo data selection tool (Euro-Argo 2021). No distinction was made between Core, Deep or BGC floats. Two-way communications was the only requirement to fulfill. These inputs were set on the Euro-Argo Data Selection Tool main menu and the resulting dataset was exported in Argo Original NetCDF format. The last 10 surfacing of all floats with Iridium transmission were selected for the analysis. On the other hand, all the surfacing of the floats (>10) contained in the Argo greylist (Argo Data Management Team, 2022) were also included in the dataset for the same time range. The recovery of these floats is desirable regardless of how much longer the battery may last, since their measurements are suspect or unreliable, mainly due to different technical issues related to the sensors.
The collection and processing of the AIS data was carried out by Global Fishing Watch (GFW). The raw AIS data used by GFW was provided by Spire Global. All ship types were included in the analysis (Figure 2), but with a focus on ship types that seem most suited for actually recovering floats, including all types of relatively small vessels (fishing boats, tugboats, yachts, sailboats, racing sailboats, pleasure crafts, coast guard and others), NGO (non-governmental organizations) ships and oceanographic research ships. According to GFW, the world’s fleet of ships on AIS was estimated at a total of 362,189 in 2019 and 365,412 ships in 2020. This fleet derived from AIS data includes ships active for at least one week a year and for which vessel characteristics (i.e. class, size) could be determined following the methods of Kroodsma et al. (2018). It was biased toward 1) large vessels; 2) upper-income and middle-income countries/territories; and 3) distant water fleets (Taconet et al., 2019). Although the majority of fishing vessels larger than 24 m use AIS (between 52% and 85%), relatively few fishing vessels between 12 and 24 m in length broadcast AIS (14% to 19%), and only a tiny fraction of vessels under 12 m do so (<0.4%). Broadcasting is predominantly from upper-income and upper middle-income countries because 1) the majority of vessels over 24 m are from these countries/territories and 2) these countries/territories generally have higher use of AIS, largely due to stronger regulations (Taconet et al., 2019). Last, AIS use is relatively high in distant water fleets (fleets of vessels fishing in the Exclusive Economic Zones (EEZ) of foreign nations or in the high seas).
3.2 Meteorological data
To evaluate the possible risks related to recovery operations due to weather conditions in locations where encounters occurred, a meteorological data analysis was carried out every hour for years 2019 and 2020. The ERA5 (Hersbach and Coauthors, 2020) product generated by the Copernicus Climate Change Service, which is part of the European Union Earth monitoring program and implemented by the ECMWF (European Centre for Medium-Range Weather Forecasts), was selected to develop the meteorological analysis. The ERA5 dataset is a new analysis of hourly weather conditions back to 1979, although for our study only the years 2019 and 2020 were selected. It combines a weather model with observational data from satellites and ground-based sensors to create a consistent long-term record of our climate. The global gridded datasets were obtained through the Copernicus Climate Data Store (Copernicus, 2021) and their coverage is global, with a resolution of 0.25° x 0.25° for wind data and 0.5° x 0.5° for ocean waves. The parameters selected for this analysis included the u and v components of the wind at a height of ten meters above the surface (m/s) and the significant height of combined wind waves and swell (m). The analysis simply crosses the Argo surfacing data with both meteorological sets in time and space, in such a way that we obtain the closest wind and wave values for each surfacing.
4 Results
4.1 Encounters analysis
A total of 18,615 encounters between ships and Argo floats occurred in 2019 and 28,488 in 2020. These figures decreased by 75% when levels of data processing were applied, meaning a total of 4,719 and 6,951 encounters respectively for both years (Figures 3A–D). The float 6902968 comprised 52% of all encounters globally for the year 2019. A similar scenario occurred in 2020 with floats 6903014 and 6902954; together they comprised 54% of the total encounters. Greylisted floats represented approximately 61% and 74% of the encounters (Table 1). The geographical areas that concentrated the most global encounters in both years were the Mediterranean Sea, the Indian Ocean, the Pacific Ocean and the Atlantic Ocean (Figures 3A–D). In 2019 and 2020, 66% and 53% of the total encounters happened with sailing-pleasure ships, while 25% (2019) and 35% (2020) happened with fishing ships (Figure 4).
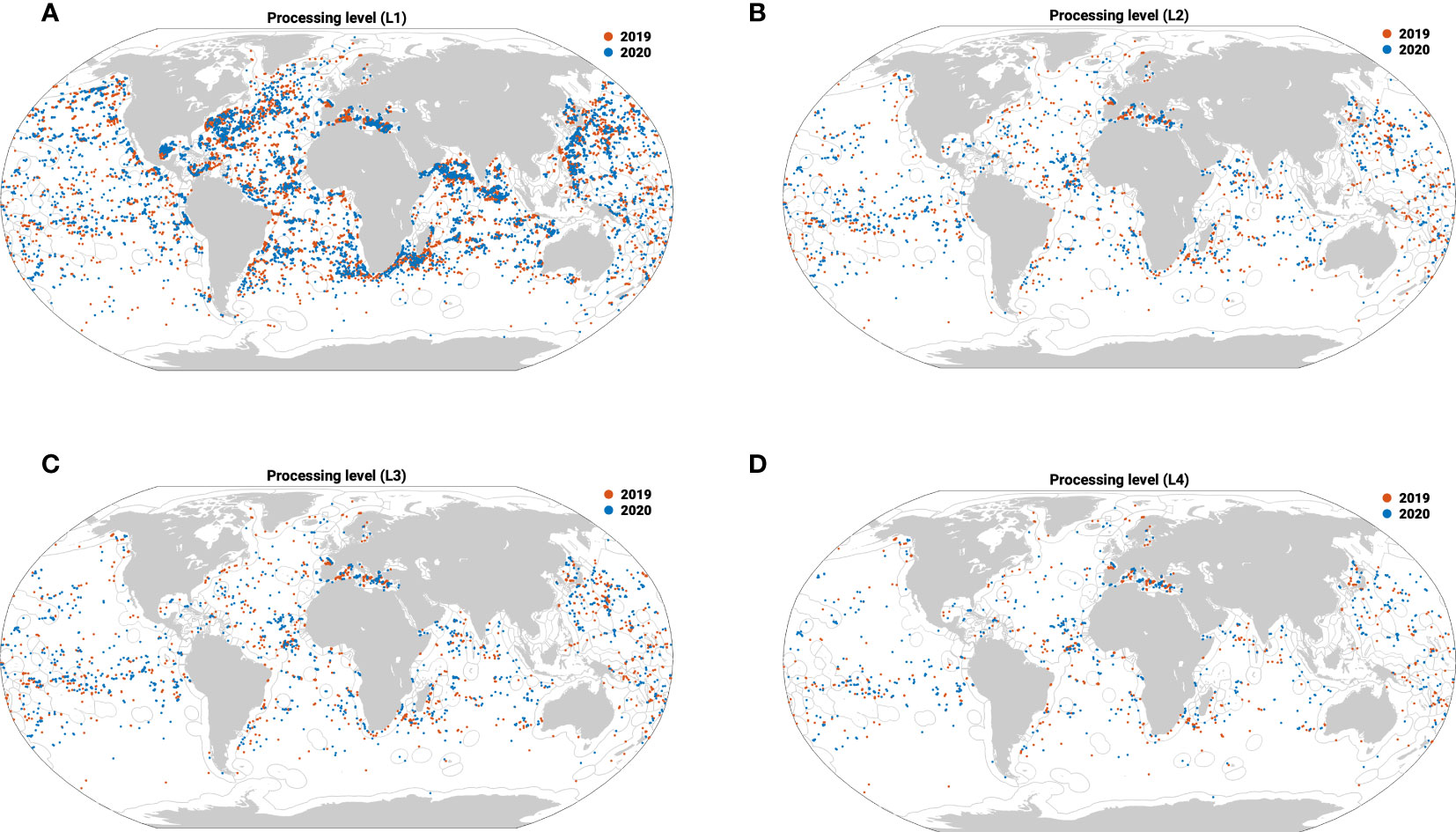
Figure 3 Encounters worldwide in 2019 (blue) and 2020 (red) by levels of processing: L1 (A), L2 (B), L3 (C) and L4 (D).
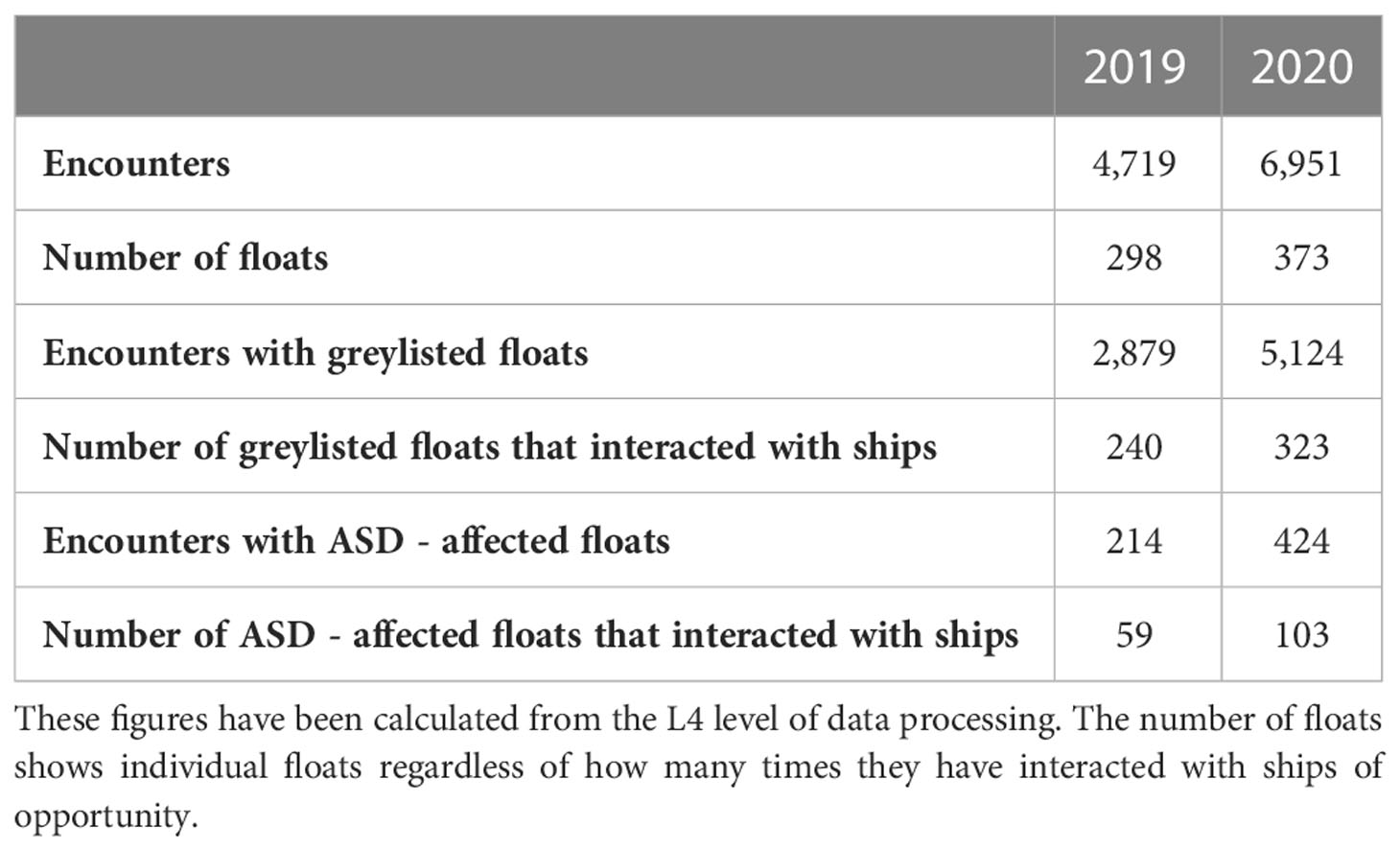
Table 1 Results of greylisted and ASD-affected floats interacted by ships worldwide in 2019 and 2020.
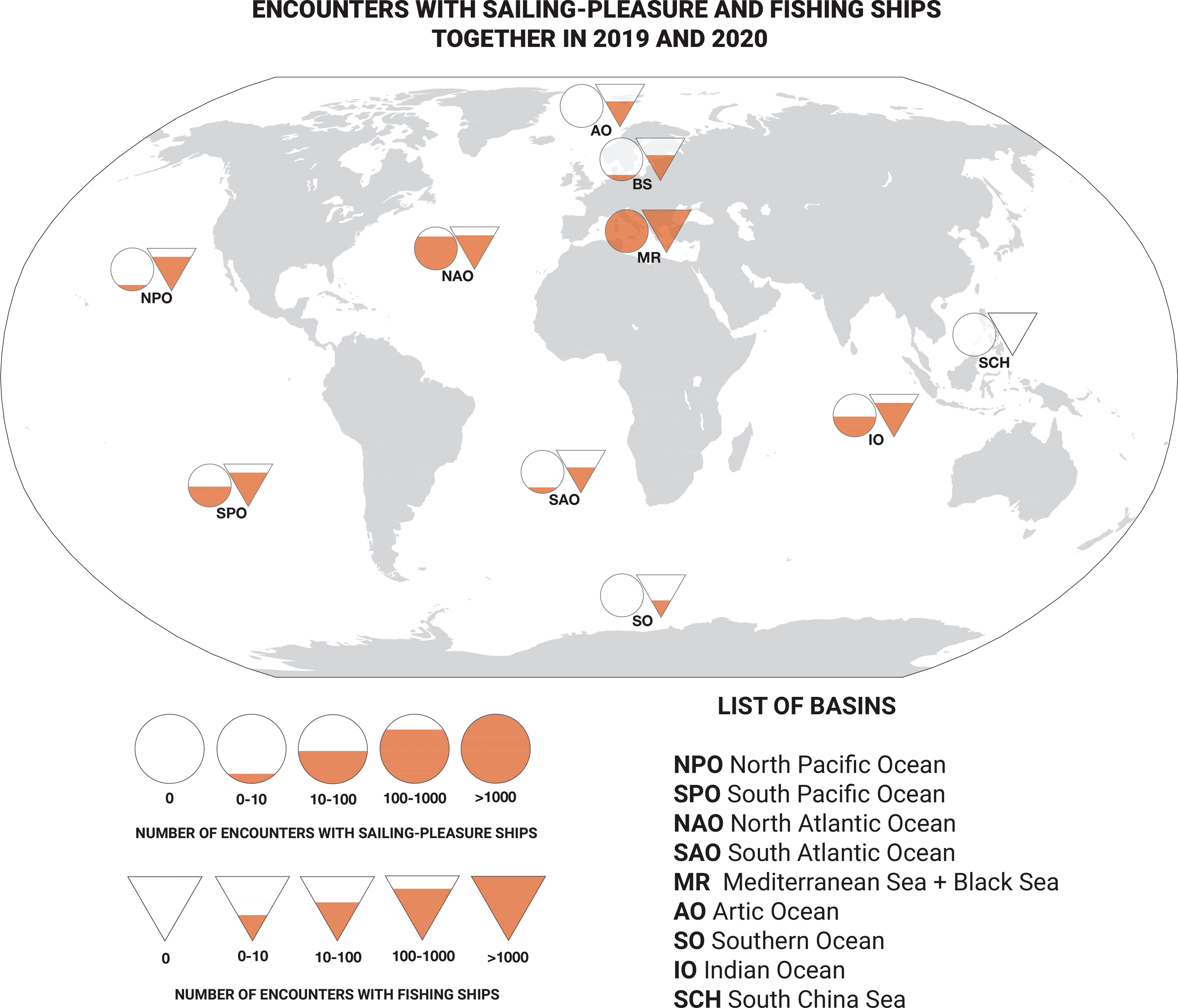
Figure 4 Encounters between Argo floats and sailing-pleasure ships (circles) and fishing ships (triangles) worldwide together in 2019 and 2020. The L4 processing level dataset has been used to generate this panel. Despite the fact that fishing vessels have a better global distribution (3612 encounters), the highest numbers of encounters occurred between Argo floats and sailing-pleasure ships (6814 encounters), especially in the Mediterranean Sea.
The distances given by the difference between both the floats and the ships’ GPS positions, were divided into 4 groups (Figure 5), from 0 nm to 20 nm. Roughly 4% of the encounters happened in distances of 0-5 nm and around 35% in distances of 10-15 nm in average for both years. A total of 19 and 26 encounters, respectively in 2019 and 2020, happened in distances shorter than 1nm. The highest figure of encounters (39.24%) happened for distances between 15-20 miles. The global shipping fleet was also filtered by ship timestamp, within the range of -2 and +2 hours around the Argo float’s surfacing (Figure 5). Although it is also a way to quantify the number of ships in a specific time frame, it shouldn’t be interpreted as navigation time to the float, since it depends on the ship’s speed and the drift of the float. In the shortest time frame (0-30 min), encounters rose to 21% in average for both years. In the following ranges (30-60 min and 60-90 min) no significant increase of encounters were found. Instead, when the time frame increases to 90-120 min., the initial encounter figures increased to 34%. The ‘number of floats’ parameter (Tables 1, 2) provides the number of floats that at least had one encounter; each float is counted individually regardless of how many times it has interacted with ships of opportunity. Based on the latter, a total of 298 and 373 floats interacted with ships in both years, respectively. These numbers represented roughly 25% of the current annual replacement rate of 830 floats required to maintain the Argo network in 2019 and 2020. On the other hand, 91% of these floats corresponded to the Core Argo mission, while the remaining 8.5% and 0.6% corresponded to the BGC and Deep Argo missions in 2019 and 2020.
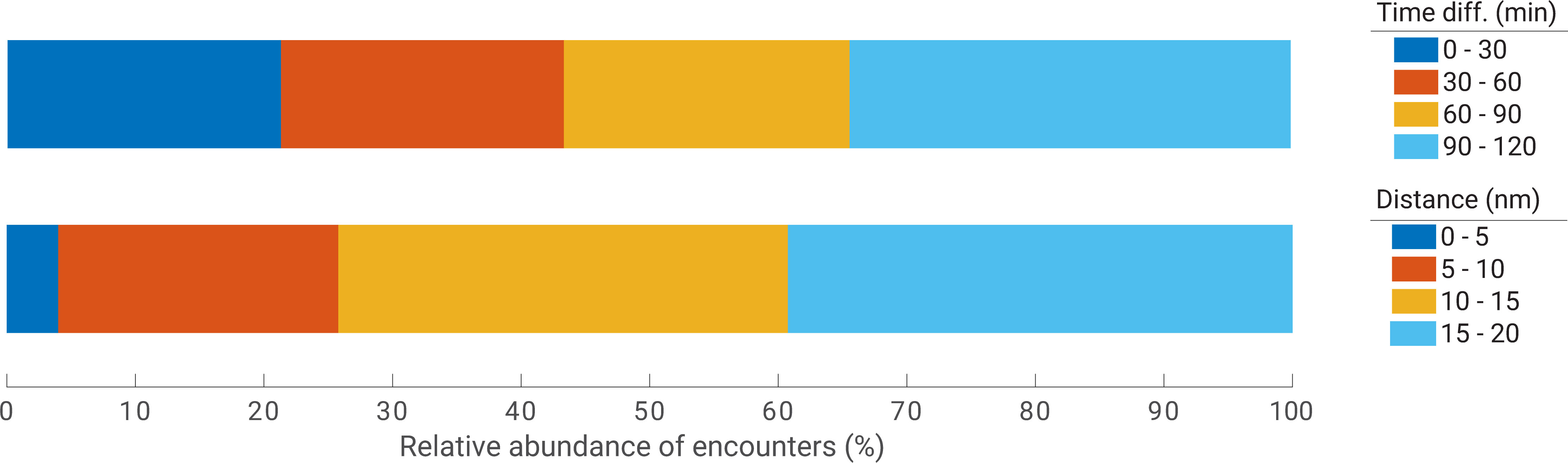
Figure 5 Distance and time difference between ships and floats for each encounter in 2019 and 2020 (averaged). The L4 processing level dataset has been used to generate this plot.
The Mediterranean region formed by the Mediterranean Sea and the Black Sea, was the basin that hosted the highest number of encounters for both years, 76.5% and 81.7% respectively (Figure 3D). Regarding the rest of the non-marginal areas, the North Pacific, North Atlantic and Indian oceans hosted the highest number of encounters between ships and Argo floats in both years (Figure 3D). Around 90% of the encounters took place in the exclusive economic zones (EEZ) both in 2019 and 2020 (Figure 3D).
4.2 Weather conditions
Weather conditions can determine the success of a recovery maneuver. Considering an operating range limit of a force wind of 5 on the Beaufort scale (~20 knots) and 2.5 meters of height for waves, a meteorological analysis in every location where an encounter took place was carried out. The averaged results for both years showed that about 96% of the observations occurred in areas with winds less than 20 knots for both years. Approximately 97% of the observations occurred in areas with values below the maneuverability limit, stipulated at 2.5 m of significant height of combined wind waves and swell, in average for both years (Figure 6).
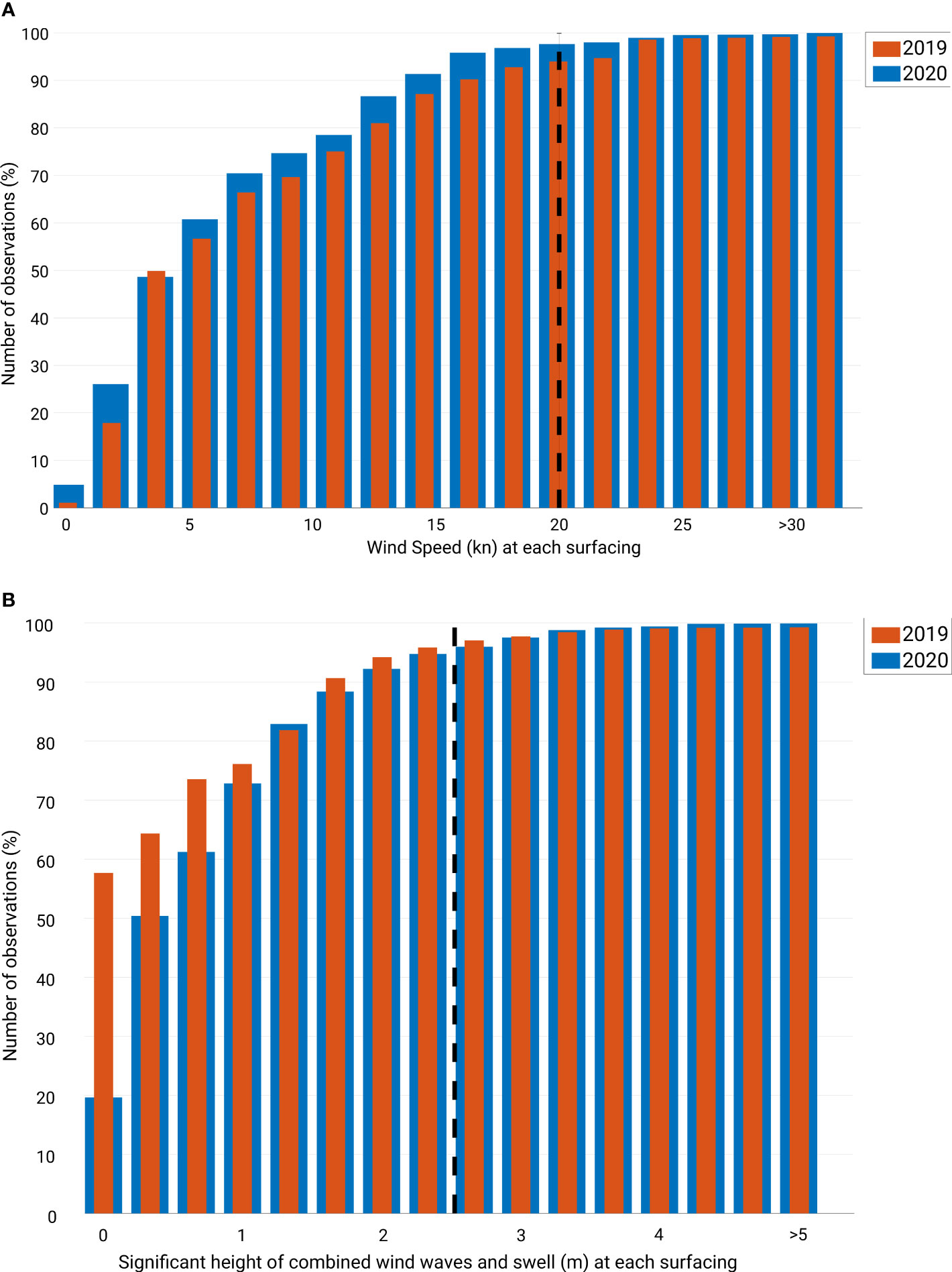
Figure 6 Cumulative diagrams of wind speed (A) and significant height of combined wind waves and swell (B) for both years. Both plots correspond to the L3 processing level dataset.
4.3 Abrupt salinity drift affected floats from an encounters analysis perspective
Through an extensive audit of the global network conducted by Euro-Argo, it was found that at least 677 of Sea-Bird Electronics CTDs sensors mounted on Argo floats caused abrupt salinity drift (ASD) due to a problem in their conductivity cell. At the time this study is carried out, there are still 463 affected floats currently transmitting data according to Euro-Argo. ASD appears prematurely in some cases and cannot be detected by the real-time quality controls. The issue is extended upstream when data is automatically transmitted to the General Telecommunications System (GTS) or to the Global Data Acquisition Centers (GDACS). Only after one year of data collection, solid corrections are guaranteed through exhaustive delayed mode quality control carried out by experts from the Argo community. The encounter analysis showed that global shipping traffic interacted with at least 59 and 103 floats suffering from ASD in 2019 and 2020 respectively (Table 1; Figure 7). Approximately 6% of these encounters occurred at a distance of less than 5 nautical miles in both years.

Figure 7 Relative averaged abundance of encounters between ASD-affected floats and world shipping traffic by distance. The L4 processing level dataset has been used to generate this plot.
5 Discussion
5.1 Battery life expectancy
It is still difficult to estimate with certainty when a float is approaching its end-of-life (EOL). Most floats are powered by lithium batteries. These types of batteries suffer a slight degradation in the long term (Gordon, 2017), which allows them to offer stable performance over 3 - 5 years in general. Making battery depletion predictions is critical to determine when a float should be recovered. Energy budget information provided by manufacturers is necessary to better understand energy consumption and determine the life expectancy of the float (Arduini and Coauthors, 2021). Hence, accurate predictions become complex as its depletion progresses to a point where the voltage drops dramatically, causing the float to go into an idle state. Battery consumption depends on many factors based on multi-parameter relationships (Arduini and Coauthors, 2021), such as battery type, number of sensors, type of float, transmission system type, mission configuration, bathymetry, measured variables, ocean basin, etc. Initiatives such as the one developed by Euro-Argo’s Fleet Monitoring Tool, constitute a step forward in EOL prediction. An alert trigger based on technical parameters collected by the float has been designed when the battery voltage drops below a certain threshold (Arduini and Coauthors, 2021), obtaining a window of opportunity to identify potential float’s last cycles. This information would be extremely valuable, as it could work as the primary source of data input to run an accurate encounters analysis. However, the objective of this analysis was not to determine the Argo floats life expectancy, but these considerations should be taken in account in the assessment of potential for float recovery.
5.2 Encounter analysis as a tool to facilitate potential recoveries
The determination of the encounters depends on (a) permanent factors at a global level, determined by activities that can affect the frequency of encounters, such as the number of ports or marinas on the coastline, existence of oil & gas extraction areas or large fishing grounds; (b) unpredictable factors at a global scale, such as the COVID-19 pandemic, which affected both shipping traffic activities (March et al., 2021) and the planning of the Argo float deployments; and (c) some other factors, such as the variability of ocean currents that cause the Argo float to drift. It exceptionally also depends on (d) the variety of operating configurations of the Argo floats, generally associated with scientific purposes of their owners.
In our study, PCT ship types and ships in general greater than 60 m length, showed an exceptional traffic density around the world (March et al., 2021) but are not eligible for recoveries given their size, maneuverability and tight schedules. However, we have demonstrated the validity of the proposed analysis to identify ships of opportunity that act as potential Argo floats retrievers. Once the aforementioned processing levels were applied, it was shown that sailing-pleasure (66.5% and 53%) and fishing ships (25% and 35%) were the types of ships with the greatest prominence in the encounters for the years 2019 and 2020 respectively (Figure 8). Despite the high number of encounters between sailing-pleasure ships and Argo floats, its global distribution is not homogeneous and concentrated especially in the marginal seas. Considering their fleet density and remarkably global distribution (even in remote areas of the ocean where the Argo floats operate), our study suggests that fishing ships may be the most feasible option for retrieving Argo floats, since they can perform float recovery maneuvers in better conditions than large or gross tonnage ships. A total of 1,182 and 2,430 encounters (Figure 4) for fishing ships may seem much lower compared to the initial figures (around 10,500 and 16,000 encounters in 2019 and 2020) before applying the processing levels. Also, AIS data includes only a small fraction (approximately 70,000 ships) of the world’s estimated 2.8 million fishing ships (Global Fishing Watch, 2021b). The lack of data (from many fishing boats i.e.), the non-interpolation of the boats’ tracks and the Argo floats that do not stay on the surface suggest that many more encounters occurred in a real scenario than those found in the analysis, but they are difficult to trace. Other factors such as the distance between the ship’s deck and the waterline (freeboard) for direct-recoveries or accounting with an auxiliary boat, are key in terms of fishing ship’s maneuverability. On the other hand, tug-type ships were mainly constrained to coastal areas, being an interesting option for floats located in boundary regions.
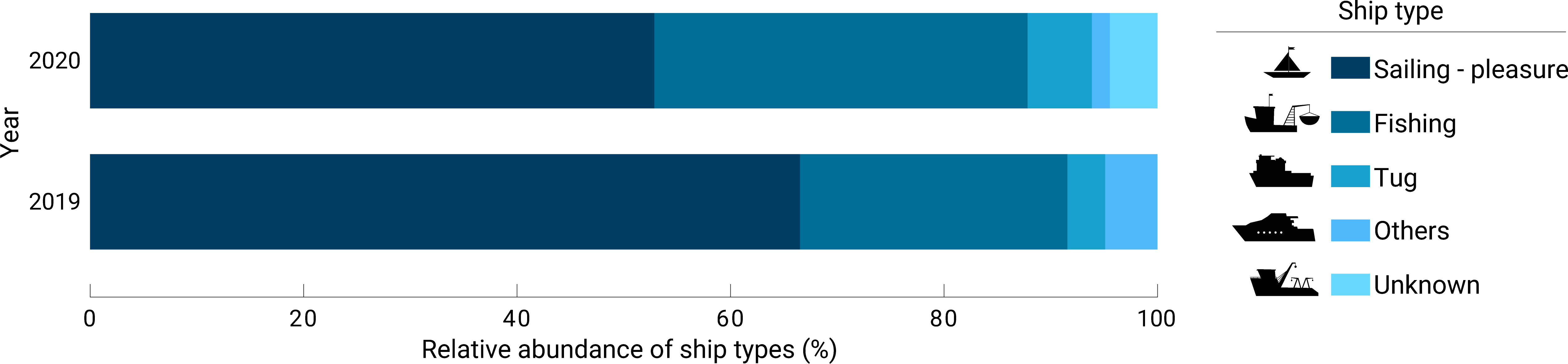
Figure 8 Relative abundance of types of ships of opportunity that interacted with Argo floats in both years. The L4 processing level dataset has been used to generate this plot.
In a potential recovery scenario, the weather forecast is an essential tool to guarantee the safety of the maneuver and crew’s integrity. Factors such as the number of crew members, type of ship or having an auxiliary boat, when necessary, may help dealing with weather conditions and therefore, determine if a recovery is feasible. Safety recommendations, warnings and basic procedures could be included in an open manual of good practices on recoveries produced by the Argo community, contributing significantly to appropriate decision-making. When encounters are sorted by geographic areas, the basins most likely to host encounters were the Mediterranean region, North Pacific, North Atlantic, and Indian Oceans, while the least likely was the Southern Ocean and the South China Sea. The main reason for not recovering many Argo floats nowadays is because of the economic and environmental costs of dedicating a ship exclusively for this purpose. The distance sailed by ships and the estimated time needed for a retrieval are fundamental factors that offer key information for correct decision-making. Our findings suggest that encounters between global shipping traffic and Argo floats can be taken as an indicator of the number of floats that could have been recovered. It is also worth noting that the Virtual Fleet-recovery software could be used by operators to optimize Argo float mission parameters to further increase the probability of float/ship encounters and to minimize rerouting. This last point may decrease the recovery environmental impact of extra carbon emission caused by rerouting ships not powered by wind.
This work also reveals that around 90% of the encounters took place in EEZ in both years. These areas, that span 200 miles from the coast, might not be considered remote regions when we take into account the extent of the global ocean. This finding suggests that the Argo community could count on national contributors to build a suitable very-first recovery pilot program as proof of concept using national resources as volunteer ships, such as coast guards, naval or oceanographic research ships.
The floats contained in the GreyList and those affected by ASD (Table 1) represent case studies that could encourage the creation of a global recovery program for Argo floats. Affected floats in both years numbered in the hundreds, reaching significant figures such as the 323 greylisted floats and the 103 ASD-affected floats that interacted with ships both in 2020. Some of these encounters occurred at distances less than 5 nm (Figure 7). In both case studies it would not be necessary to wait for the float batteries to enter EOL mode for a possible recovery. Since these floats show anomalous performance, their recovery is desirable at any time.
Assuming that the figures shown in this work represent a hypothetical scenario in recent years, and that the real scenarios probably involve more complexity due to the factors mentioned above, it serves to shed some light on initiatives that do not consider Argo floats as expendable goods. Some partners in the Argo community have already demonstrated on a small scale that a basic float recovery and refurbishment (exchanging batteries and critical components, basic cleaning and recalibration of sensors) would represent a significant saving compared to the overall cost of a new float. A study in the Mediterranean Sea presented a case scenario in which the cost per profile is reduced by around 25% for a standard float and almost 30% for a BGC float if both were recovered at 90% of its life expectancy. This represented savings of 64% and 72% respectively compared to the acquisition of new floats, including cruise costs (Poffa and Amice, 2018). Floats recovery and their respective redeployment may then provide short term alternatives to maintain the Argo array, bearing in mind some of the concerns raised in the Argo community: continued rising costs for floats’ procurement, areas of the global ocean insufficiently covered and the weakening of the Core Argo network for the benefit of the Deep-Argo and BGC Argo networks.
5.3 Encounters analysis considerations
The assumptions made at each level of data processing have been taken to obtain a figure that is closest to reality. Although the final number of encounters resulted in 1/4 of the original dataset, it is intended not to lose sight of the fact that the encounters are events that take place with ships of opportunity on a daily basis in oceanic and coastal areas around the planet. Due to their high frequency and wide spatial spectrum, we have shown that it could be a valuable resource in the recovery of Argo floats.
Despite its global scope, the encounter analysis has shown some sensitivity in certain geographical areas such as the Mediterranean Sea. The results showed by far that it was the most represented ocean basin by number of encounters globally. It could be due to being one of the busiest traffic lanes in the world (United Nations Environment Programme/Mediterranean Action Plan and Plan Bleu, 2020), but it is mainly due to two factors. These figures have a direct relationship with Argo float configurations based on certain specific scientific requirements. Especially those floats that worked with a period of cycles lower than the standard (5 or 10 days), causing an increase in encounter frequency. The greater the number of surfacings, the greater the chances of encountering a ship. The float number 6902968 was a clear example, which represented 52% of the encounters worldwide in 2019, despite having been operating on the high seas for less than a month and having performed 30 daily cycles. The float 6902954 showed to be a similar case, which accounted for 20.6% of the encounters globally in 2020, having cycled every 3 days for approximately 9 months. It was a float linked to the NAOS pilot project. On the other hand, the encounters took place especially in areas of intense shipping traffic related to ports and marinas. This last factor is fundamental, since a higher frequency of cycles can increase the chances of encounters, but it entirely depends on the existence of shipping traffic. Despite this peculiarity in the Mediterranean Sea, the analysis also recorded a multitude of encounters in the rest of the ocean basins, demonstrating its global reach (Figures 3A–D).
This study shows the analysis of encounters as one more tool for the Argo community to address the challenge of recovering floats with better guarantees. It could be valuable for the Argo community, in addition to other actors such as OceanOPS, another study focused on transcendental issues such as the fit of recoveries in the custom’s legal framework of each country, the commitment of the fishing community and their feedback, possible compensations in case of successful recovery, float’s batteries optimization prior to recovery, or the economic profitability estimation of a recovered float. Taking consolidated initiatives such as The Ship of Opportunity Program (SOOP) as a reference (Goni et al., 2010), the possible complexities of the Argo float recoveries could be answered through the creation of a pilot program.
6 Conclusions
While the technology of the Core mission is well established, recovery of floats via ships of opportunity constitutes one way to facilitate post-mission CTD calibrations and gain insight into sensor performance and stability, especially in the Deep Argo and BGC Argo missions, according to the challenges raised in OneArgo. Through an analysis of encounters based on the AIS of ships and the location of Argo floats in the years 2019 and 2020, this study reveals that a total of 298 and 373 floats interacted with the world shipping traffic in favorable weather conditions, a figure equivalent to 25% of the annual replacement rate of the Argo network. It was also found that encounters with 32% and 62% of ASD-affected floats occurred in 2019 and 2020 respectively.
After evaluating the global shipping fleet, sailing-pleasure and fishing vessels were found to be the best possible types of vessels to recover Argo floats, the latter being the most convenient given its fleet density and global distribution.
The Argo community has all the necessary elements to improve the accuracy of the network through a pilot recovery program, even though the remote regions of the global ocean in which the Argo floats are located continue to be the main drawback. Our analysis also showed that more than 150 floats interacted with ships of opportunity in the first 200 nautical miles from shore both in 2019 and 2020. If EEZs are considered as non-remote regions, a considerable number of floats close to its EOL can be reached. The possible complexities of the Argo float recoveries could be evaluated through a pilot program in specific regions with a high number of encounters and intense shipping traffic.
Data availability statement
The datasets presented in this study can be found in online repositories. The names of the repository/repositories and accession number(s) can be found in the article/supplementary material.
Author contributions
AG-S and MO contributed to conception and design of the study, from the analysis setup to data processing. TC worked on obtaining the AIS data and developing the encounter analysis. GM contributed to the development of section 1.1 of the paper. All authors contributed to the article and approved the submitted version.
Funding
Euro-Argo RISE project. Funded by the European Union’s Horizon 2020 research and innovation programme under grant agreement No 824131. Call reference/H2020-INFRADEV-2018-2020.
Acknowledgments
We would like to thank the European Commission and Euro-Argo ERIC for the implementation of the EA-RISE (Euro-Argo Research Infrastructure Sustainability and Enhancement) project. Special thanks to Rubén Marrero Gómez for his fruitful feedback whenever it was required and Clàudia Pérez Barrancos for her recommendations. Finally, we would like to thank the Global Fishing Watch organization, for working in partnership with us and without whom this work could not have gone ahead.
Conflict of interest
The authors declare that the research was conducted in the absence of any commercial or financial relationships that could be construed as a potential conflict of interest.
Publisher’s note
All claims expressed in this article are solely those of the authors and do not necessarily represent those of their affiliated organizations, or those of the publisher, the editors and the reviewers. Any product that may be evaluated in this article, or claim that may be made by its manufacturer, is not guaranteed or endorsed by the publisher.
References
Arduini L., Coauthors (2021). Recommendations to increase the overall life expectancy of argo floats, based on at sea monitoring fleet behavior monitoring, assessment and report (including a review of metadata that impact life expectancy: specific floats configurations, batteries, etc. Zenodo. doi: 10.5281/zenodo.7101551
Argo. (2022). Argo float data and metadata from global data assembly centre (Argo GDAC). SEANOE. doi: 10.17882/42182
Argo Data Management Team. (2022). Argo user’s manual. Available at: https://archimer.ifremer.fr/doc/00187/29825/.
Argo Program Office. (2020). Argo’s environmental impact. Argo and the environment. Argo’s environmental impact statement. Available at: https://argo.ucsd.edu/about/argos-environmental-impact/ (Accessed Nov 2020).
Argo Program Office. (2021a) Telecommunications systems. Data transmission systems. Available at: https://argo.ucsd.edu/how-do-floats-work/telecommunications-systems/ (Accessed January 2020).
Argo Program Office. (2021b). Argo “Salty drift” salinity data issue notice 2021. Available at: https://argo.ucsd.edu/argo-salty-drift-salinity-data-issue-notice-2021/ (Accessed July 2021).
Barker P. M., Dunn J. R., Domingues C. M., Wijffels S. E. (2011). Pressure sensor drifts in argo and their impacts. J. Atmos Oceanic Technol. 28, 1036–1049. doi: 10.1175/2011JTECHO831.1
Cancouët R, Arduini Plaisant L., Garcia Juan A., Belbéoch M., Siiriä S.-M. (2021). Enrichments of monitoring tools to track and compare float configurations and estimate life expectancies. Zenodo. doi:https://zenodo.org/record/7101519.
D’Ortenzio F., Coauthors (2020). Biogeochemical argo: the test case of the NAOS Mediterranean array. Front. Mar. Sci. 7. doi: 10.3389/fmars.2020.00120
Emmens T., Amrit C., Abdi A., Ghosh M. (2021). The promises and perils of automatic identification system data. Expert Syst. Appl. 178, 114975. doi: 10.1016/j.eswa.2021.114975
Global Fishing Watch. (2021a). What vessels are required to use AIS? What are global regulations and requirements for vessels to carry AIS? Available at: https://globalfishingwatch.org/faqs/what-vessels-are-required-to-use-aiswhat-are-global-regulations-and-requirements-for-vessels-to-carry-ais/. (Accessed July 2021).
Global Fishing Watch. (2021). Datasets and code: apparent fishing effort. Available at: https://globalfishingwatch.org/dataset-and-code-fishingeffort/.
Goni G., Roemmich D., Molinari R., Meyers G., Sun C., Boyer T., et al. (2010). “The ship of opportunity program,” in Proceedings of OceanObs’09: sustained ocean observations and information for society, OceanObs’09: sustained ocean observations and information for society (European Space Agency), 366–383. Available at: https://www.researchgate.net/publication/281783976_THE_SHIP_OF_OPPORTUNITY_PROGRAM.
Gordon L. (2017). Recommendations for Reports About Float Batteries. 528. Available at: https://argo.ucsd.edu/wp-content/uploads/sites/361/2020/04/Argo-battery530report.pdf. (Accesed March 2021).
Hersbach H., Coauthors (2020). The ERA5 global reanalysis. Q.J.R Meteorol. Soc. 146, 1999–2049. doi: 10.1002/qj.3803
IMO. (2002). Available at: https://wwwcdn.imo.org/localresources/en/KnowledgeCentre/IndexofIMOResolutions/AssemblyDocuments/A.917(22).pdf.
Kazimierski W., Stateczny A. (2015). Radar and automatic identification system track fusion in an electronic chart display and information system. J. Navigation 68, 1141–1154. doi: 10.1017/S0373463315000405
Kroodsma D. A., Mayorga J., Hochberg T., Miller N. A., Boerder K., Ferretti F., et al (2018). Tracking the global footprint of fisheries. Science 359, 904–908. doi: 10.1126/science.aao5646
Last P., Hering-Bertram M., Linsen L. (2015). How automatic identification system (AIS) antenna setup affects AIS signal quality. Ocean Eng. 100, 83–89. doi: 10.1016/j.oceaneng.2015.03.017
March D., Metcalfe K., Tintoré J., Godley B. J. (2021). Tracking the global reduction of marine traffic during the COVID-19 pandemic. Nat. Commun. 12, 2415. doi: 10.1038/s41467-021-22423-6
Moltmann T., Coauthors (2019). A global ocean observing system (GOOS), delivered through enhanced collaboration across regions, communities, and new technologies. Front. Mar. Sci. 6. doi: 10.3389/fmars.2019.00291
Ou Z., Zhu J. (2008). AIS database powered by GIS technology for maritime safety and security. J. Navigation 61, 655–665. doi: 10.1017/S0373463308004888
Owens W. B., Zilberman N., Johnson K. S., Claustre H., Scanderbeg M., Wijffels S., et al. (2022). OneArgo: a new paradigm for observing the global ocean. Mar. Technol. Soc. J. 56, 84–90. doi: 10.4031/MTSJ.56.3.8
Pelich R., Longepe N., Mercier G., Hajduch G., Garello R. (2015). AIS-based evaluation of target detectors and SAR sensors characteristics for maritime surveillance. IEEE J. Sel Top. Appl. Earth Observations Remote Sens. 8, 3892–3901. doi: 10.1109/JSTARS.2014.2319195
Poffa N., Amice M. (2018). Personal communication. Available at: https://github.com/euroargodev/recovery/files/4386566/Recovering.Argo.Floats.-.N.Poffa.-.M.Amice.docx or https://github.com/euroargodev/recovery/issues/3.
Riser S. C., Coauthors (2016). Fifteen years of ocean observations with the global argo array. Nat. Clim Change 6, 145–153. doi: 10.1038/nclimate2872
Roemmich D., Coauthors (2009). The argo program: observing the global oceans with profiling floats. Oceanog 22, 34–43. doi: 10.5670/oceanog.2009.36
Taconet M., Kroodsma D., Fernandes J. A., Food and Agriculture Organization of the United Nations, Global Fishing Watch, AZTI-Tecnalia, et al. (2019). Global atlas of AIS-based fishing activity: challenges and opportunities. Available at: https://globalfishingwatch.org/fisheries/fao-atlas/.
Tsou M.-C. (2010). Discovering knowledge from AIS database for application in VTS. J. Navigation 63, 449–469. doi: 10.1017/S0373463310000135
United Nations Environment Programme/Mediterranean Action Plan and Plan Bleu. (2020). Available at: https://planbleu.org/wp-content/uploads/2021/04/SoED_fullreport.pdf.
Wong A. P. S., Coauthors (2020). Argo data 1999–2019: two million temperature-salinity profiles and subsurface velocity observations from a global array of profiling floats. Front. Mar. Sci. 7. doi: 10.3389/fmars.2020.00700
Keywords: encounters, ships of opportunity, recovery, sensor accuracy, AIS, Argo floats
Citation: González-Santana A, Oosterbaan M, Clavelle T, Maze G, Notarstefano G, Poffa N and Vélez-Belchí P (2023) Analysis of the global shipping traffic for the feasibility of a structural recovery program of Argo floats. Front. Mar. Sci. 10:1161580. doi: 10.3389/fmars.2023.1161580
Received: 08 February 2023; Accepted: 19 June 2023;
Published: 13 July 2023.
Edited by:
Gilles Reverdin, Center for the National Scientific Research (CNRS), FranceReviewed by:
Peter Strutton, University of Tasmania, AustraliaBlair John William Greenan, Fisheries and Oceans Canada (DFO), Canada
Paul Chamberlain, University of California, San Diego, United States
Copyright © 2023 González-Santana, Oosterbaan, Clavelle, Maze, Notarstefano, Poffa and Vélez-Belchí. This is an open-access article distributed under the terms of the Creative Commons Attribution License (CC BY). The use, distribution or reproduction in other forums is permitted, provided the original author(s) and the copyright owner(s) are credited and that the original publication in this journal is cited, in accordance with accepted academic practice. No use, distribution or reproduction is permitted which does not comply with these terms.
*Correspondence: Alberto González-Santana, alberto.gonzalez@ieo.csic.es