- 1Laboratório Marítimo da Guia, Marine and Environmental Sciences Centre (MARE) and Aquatic Research Network (ARNET), Faculdade de Ciências, Universidade de Lisboa, Cascais, Portugal
- 2Division of Biosphere Sciences and Engineering, Church Laboratory, California Institute of Technology, Carnegie Institution for Science, Pasadena, CA, United States
- 3Department of Life Sciences, Marine and Environmental Sciences Centre (MARE) and Aquatic Research Network (ARNET), University of Coimbra, Coimbra, Portugal
- 4AUT Lab for Cephalopod Ecology and Systematics (ALCES), School of Science, Auckland University of Technology, Auckland, New Zealand
- 5British Antarctic Survey (BAS), Natural Environment Research Council (NERC), Cambridge, United Kingdom
- 6Departamento de Biologia Animal, Faculdade de Ciências, Universidade de Lisboa, Lisboa, Portugal
Numerous terrestrial and marine organisms, including cephalopods, are capable of light emission. In addition to communication, bioluminescence is used for attraction and defense mechanisms. The present review aims to: (i) present updated information on the taxonomic diversity of luminous cephalopods and morphological features, (ii) describe large-scale biogeographic patterns, and (iii) show the research trends over the last 50 years on cephalopod bioluminescence. According to our database (834 species), 32% of all known cephalopod species can emit light, including oegopsid and myopsid squids, sepiolids, octopuses, and representatives of several other smaller orders (bathyteuthids, and the monotypic vampire “squid”, Vampyroteuthis infernalis and ram’s horn “squid”, Spirula spirula). Most species have a combination of photophores present in different locations, of which light organs on the head region are dominant, followed by photophores associated with the arms and tentacles and internal photophores. Regarding the biogeographic patterns of cephalopod species with light organs, the most diverse ocean is the Pacific Ocean, followed by the Atlantic and Indian Oceans. The least diverse are the Southern and the Arctic Oceans. Regarding publication trends, our systematic review revealed that, between 1971 and 2020, 277 peer-reviewed studies were published on bioluminescent cephalopods. Most research has been done on a single species, the Hawaiian bobtail squid Euprymna scolopes. The interest in this species is mostly due to its species-specific symbiotic relationship with the bacterium Vibrio fischeri, which is used as a model for the study of Eukaryote–Prokaryote symbiosis. Because there are many knowledge gaps about the biology and biogeography of light-producing cephalopods, new state-of-the-art techniques (e.g., eDNA for diversity research and monitoring) can help achieve a finer resolution on species’ distributions. Moreover, knowledge on the effects of climate change stressors on the bioluminescent processes is nonexistent. Future studies are needed to assess such impacts at different levels of biological organization, to describe the potential broad-scale biogeographic changes, and understand the implications for food web dynamics.
1 Introduction
Bioluminescence is the production of visible light by a living organism (Wilson & Hastings, 1998; Haddock et al., 2010). This phenomenon results from a chemical reaction that involves the release of energy—in this case, light (Hastings, 1995; Wilson & Hastings, 1998). It usually involves a light-producing molecule called luciferin and an enzyme to control the reaction, such as a luciferase or a photoprotein (Shimomura, 2006; Haddock et al., 2010). Light production generally occurs in an organ usually referred to as a “photophore” or “light organ” (Haddock et al., 2010); such organs can be autogenic, where the organism itself produces light, or bacteriogenic, where an organism is in symbiosis with bioluminescent bacteria and benefits from their light production.
Bioluminescence has evolved independently in several lineages including bacteria and fungi, and has arisen multiple times in animals (particularly within marine groups), such as arthropods, cnidarians, fishes, and cephalopods. Its functions are diverse since it can be used for attraction purposes (predation or reproduction), as a defense mechanism, or for communication (Widder, 1999; Haddock et al., 2010). Very few freshwater organisms are capable of light emission, and only a few representatives live on land, making the marine realm the most diverse environment in terms of bioluminescent organisms. Given that the oceans are vast and encompass a wide variety of environments—from the cold waters of the poles to the warm waters of the tropics and from the warm well-lit surface waters to the cold and dark deep sea—the diversity of bioluminescent biota in the ocean realm is probably underestimated. In fact, for most marine organisms, bioluminescence is the sole source of light in their environment, with a tendency to increase in occurrence below the euphotic zone (Widder, 1999; Haddock et al., 2010).
Cephalopods are one of the marine invertebrate groups that contain light-producing species. They include both autogenic (Takahashi & Isobe, 1994; Tsuji, 2002) and bacteriogenic organisms (Ruby & McFall-Ngai, 1992; Guerrero-Ferreira & Nishiguchi, 2009; Anderson et al., 2014) and the bioluminescence can be expressed in different locations throughout their body (Herring et al., 1981; Robison & Young, 1981; Herring et al., 2002; Robison et al., 2003; Kubodera et al., 2007). For example, the photophores can be internal and associated with the ink sac (e.g., Euprymna scolopes, Figure 1A-A’), around the mouth of the animal (e.g., Japetella diaphana, Figure 1B-B’), on the integument (e.g., Histioteuthis heteropsis, Figure 1C-C’) or even ocular (e.g., Abralia veranyi, Figure 1D-D’). In some cephalopods, photophores have developed from modified suckers (e.g., Stauroteuthis syrtensis, Figure 1E-E’); in others, they may be embedded within the tissue of the arms and/or tentacles (e.g., Chiroteuthis calyx, Figure 1F-F’) or at the tip of their arms (e.g., Taningia danae, Figure 1G-G’). Cephalopods use bioluminescence as a camouflage strategy or as an anti-predation method (Dilly & Herring, 1974; Herring et al., 2002; Robison et al., 2003; Jones & Nishiguchi, 2004; Bush et al., 2009). Some also use light as a communication or attraction mechanism (Robison & Young, 1981; Kubodera et al., 2007; Bush et al., 2009; Burford & Robison, 2020).
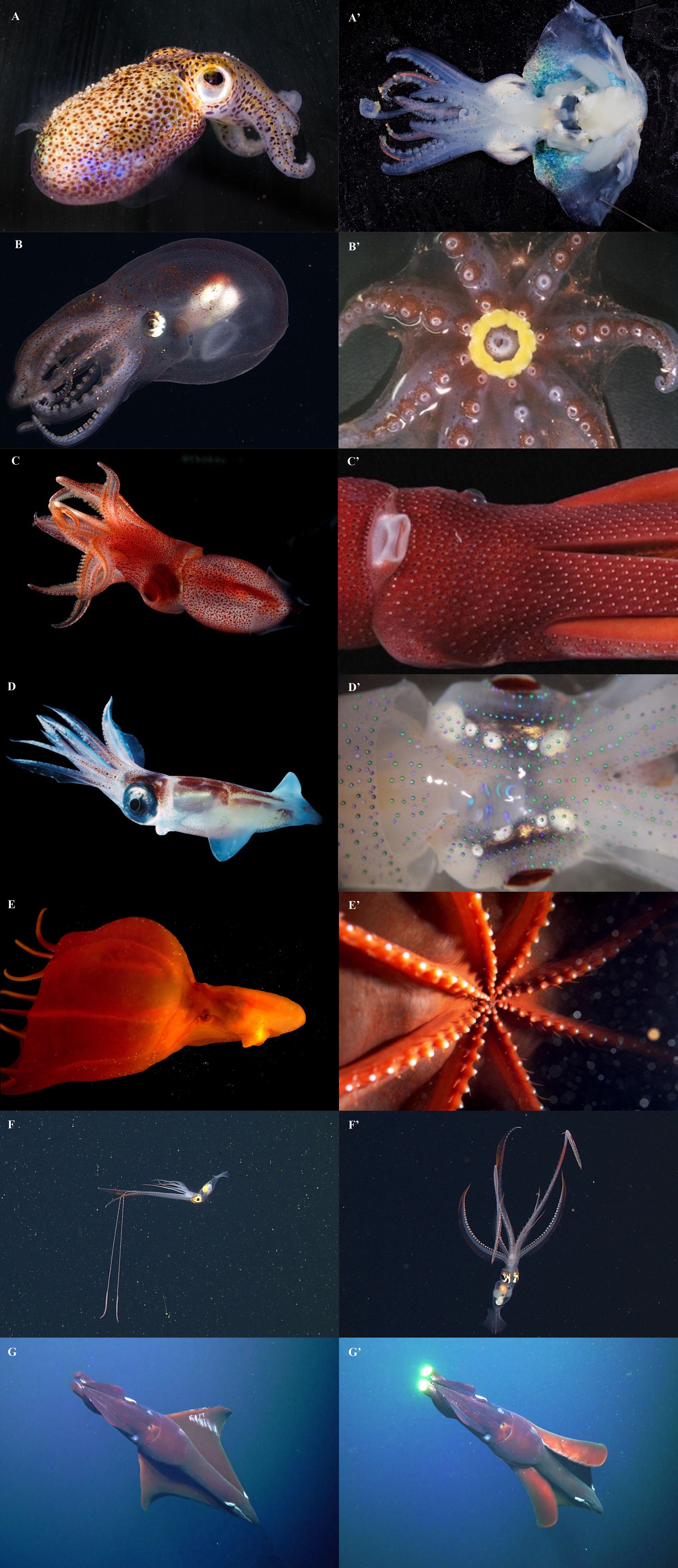
Figure 1 Bioluminescent cephalopods and the position of their respective light organs. (A-A’) The Hawaiian bobtail squid, Euprymna scolopes, with an internal light organ. (B-B’) The diaphanous pelagic octopod, Japetella diaphana, with a circumoral photophore. (C-C’) The strawberry squid, Histioteuthis heteropsis, with integumental photophores. (D-D’) the eye-flash squid, Abralia veranyi, with ocular photophores. (E-E’) the glowing sucker octopus, Stauroteuthis syrtensis, with modified light-emitting suckers. (F-F’) the swordtail squid, Chiroteuthis calyx, with photophores on the arms and tentacles. (G-G’) the Dana octopus squid, Taningia danae, with photophores on the arm tips. Photo credits: Margaret McFall-Ngai and Edward Ruby (A, A’), MBARI (B, F, F’), Michael Vecchione (B’, D, D’), Katie Thomas (C), Henk-Jan Hoving (C’), Edith Widder (E, E’), and the Schmidt Ocean Institute (G-G’).
In squids, autogenic photophores probably arose in a pelagic common ancestor and eventually diversified considerably in both morphology and position. In octopods, on the other hand, it appears that luminescence evolved separately in three different lines (Lindgren et al., 2012). The bacteriogenic photophores present in Loliginidae and Sepiolidae also appear to have evolved separately (Lindgren et al., 2012).
While many studies have been made on bioluminescence in cephalopods, a hundred years have passed since knowledge on this subject was first reviewed. Berry (1920a; 1920b) gathered information from 32 families to provide a guide on light production in this group. Out of these 32 families, he described 14 light-producing families (43.7%), including 128 bioluminescent species, out of a total of the 595 cephalopod species known at the time (21.3%) (Berry, 1920a; Berry, 1920b). Furthermore, Berry surveyed the positions and structures of photophores, showing that photophores could be found at several places in cephalopods, with the surface of the eyeball being the most common (Berry, 1920a; Berry, 1920b). Afterward, Herring published two reviews focused on cephalopods and other light-producing marine taxa (Herring, 1977b; Herring, 1988). More specifically, in 1977, Herring described luminescence found in several cephalopods (e.g. Teuthoidea comprising oegopsid and myopsid squids, Sepioidea and Vampyromorpha), with comparisons to luminescent fishes. He described: i) whether the luminescence was autogenic or bacteriogenic, ii) the position of the light organs as well as their structure, iii) how the light is produced and its functions, and iv) some information on the vertical distribution of some species (Herring, 1977b). Later, Herring combined knowledge about the luminous organs in other mollusks as well, reviewing bioluminescence in gastropods, bivalves, and cephalopods. He also gave more insights into the spectral emission found in luminous cephalopods (Herring, 1988).
Since then, bioluminescence has been described in many more cephalopod species, including new species (Collins & Henriques, 2000; Norman & Lu, 2000; Lipinski, 2001; Jereb & Roper, 2005a; Jereb & Roper, 2005b; Jereb et al., 2005; Lu, 2005; Young & Vecchione, 2005; Bolstad, 2007; Kubodera et al., 2007; Bolstad, 2010; Lindgren et al., 2012; Braid & Bolstad, 2015; Braid, 2016; Bolstad et al., 2018; Judkins et al., 2020; Reid, 2021; Lu & Okutani, 2022). Within this context, here we aim to: (i) present updated information on the taxonomic diversity of luminous cephalopods and morphological features; (ii) describe the global distribution of the bioluminescent cephalopods across different ocean basins (including coastal, epipelagic and mesopelagic realms), and last (iii) show the research trends (from 1971 to 2020) on bioluminescence in cephalopods (using a systematic PRISMA approach).
2 Diversity and morphology of luminous cephalopods
2.1 Diversity
Currently, the phylogeny of the Class Cephalopoda is still debated (Lindgren et al., 2004; Lindgren et al., 2012; Allcock et al., 2015; Allcock, 2017; Uribe & Zardoya, 2017; Sanchez et al., 2018; Anderson & Lindgren, 2021; Lindgren et al., 2023). In this review, we examined 10 extant orders, containing 50 families, with approximately 834 known species. Among them, 32% are presently understood to be bioluminescent (265 species, Figure 2, Supplementary Table 1 and Supplementary Material 1). At the order level, two are represented by a single species that is bioluminescent: the two monotypic orders Vampyromorpha (vampire “squid”, Vampyroteuthis infernalis) and Spirulida (ram’s horn “squid”, Spirula spirula). Idiosepida, Nautilida, and Sepiida appear to be the only orders that do not contain bioluminescent species. The most diverse group of cephalopods, in terms of families and genera, are the oegopsid squids (24 families, 82 genera). At the species level, there are 249 oegopsid species, of which 71% are bioluminescent. The order Bathyteuthida (with 2 families and 9 species) contains 67% light-producing species. The Myopsida (2 families, 48 species) and Sepiolida (2 families, 89 species) include 33% and 63% bioluminescent species, respectively. In contrast, only 3% of octopod species (15 families, 308 species) produce light (Figure 2).
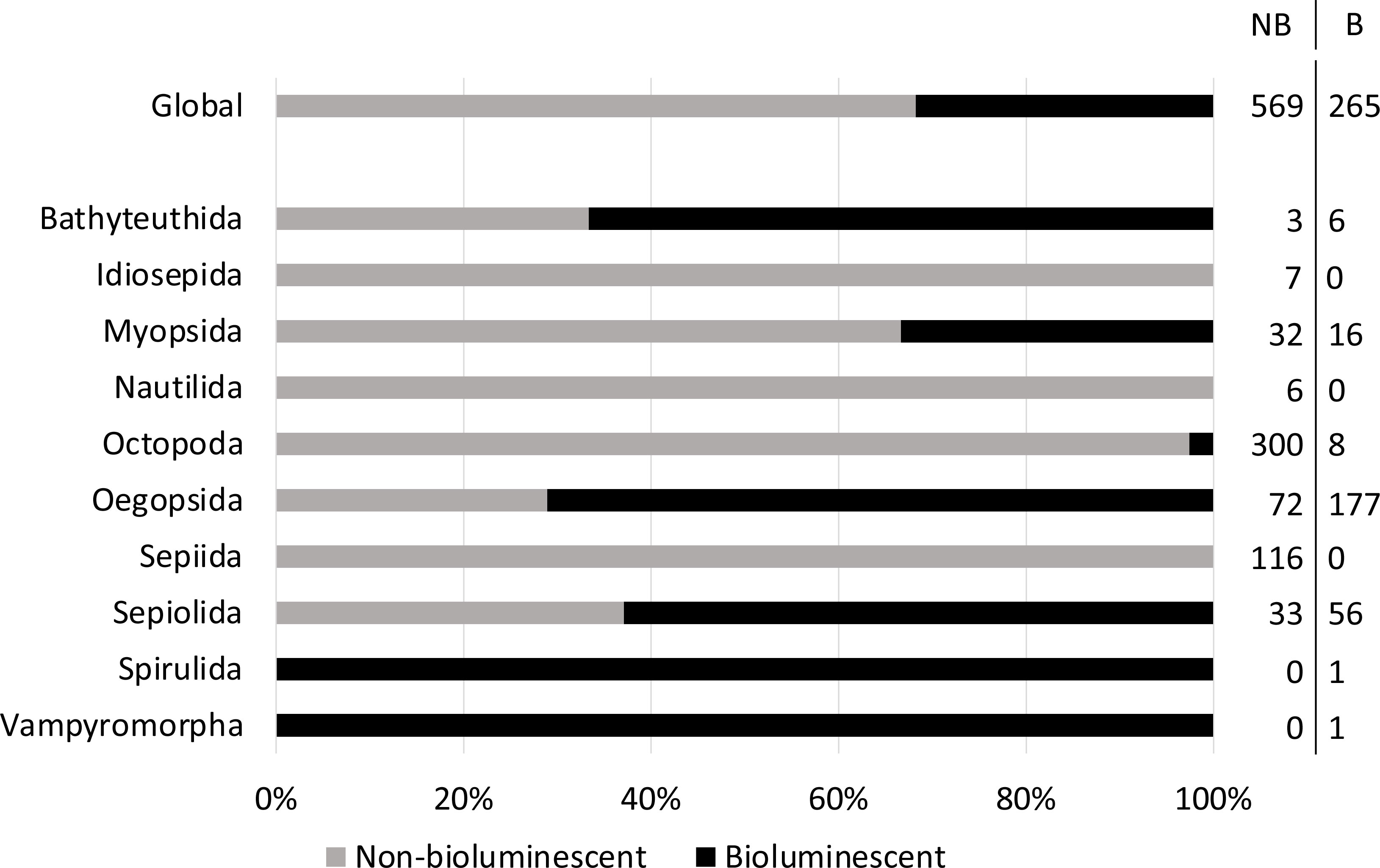
Figure 2 Percentage of known non-bioluminescent (grey) and bioluminescent (black) cephalopod species, globally and according to the cephalopod order. The number of non-bioluminescent (NB) and bioluminescent (B) species is detailed on the right of the figure.
Important differences arise at the family level (Figure 3). For example, amongst the Sepiolida, bioluminescence is only present in “bobtail squids” (Sepiolidae, 71%). Among the myopsid squids, 32% of Loliginidae and the sole species of Australiteuthidae (Australiteuthis aldrichi) are bioluminescent. In the Oegopsida, several families are 100% bioluminescent, such as Brachioteuthidae, Cranchiidae, Cycloteuthidae, Enoploteuthidae, Histioteuthidae, Lycoteuthidae, Octopoteuthidae, Pyroteuthidae, and the following species, each the sole representative of its family: Ancistrocheirus lesueurii (Ancistrocheiridae), Batoteuthis skolops (Batoteuthidae), Psychroteuthis glacialis (Psychroteuthidae) and Thysanoteuthis rhombus (Thysanoteuthidae). A few other oegopsid families are partially luminescent: Chiroteuthidae (65%), Mastigoteuthidae (81%), Ommastrephidae (44%), Onychoteuthidae (38%), and one species of the Gonatidae (Gonatus pyros, 5%). Finally, Stauroteuthis syrtensis (Stauroteuthidae), Japetella spp. (3 species) and Bolitaena pygmaea (Amphitretidae, 50%), as well as Cirroteuthis muelleri and Cirrothauma sp. (2 species, Cirroteuthidae, 75%) are the only known bioluminescent species of the order Octopoda.
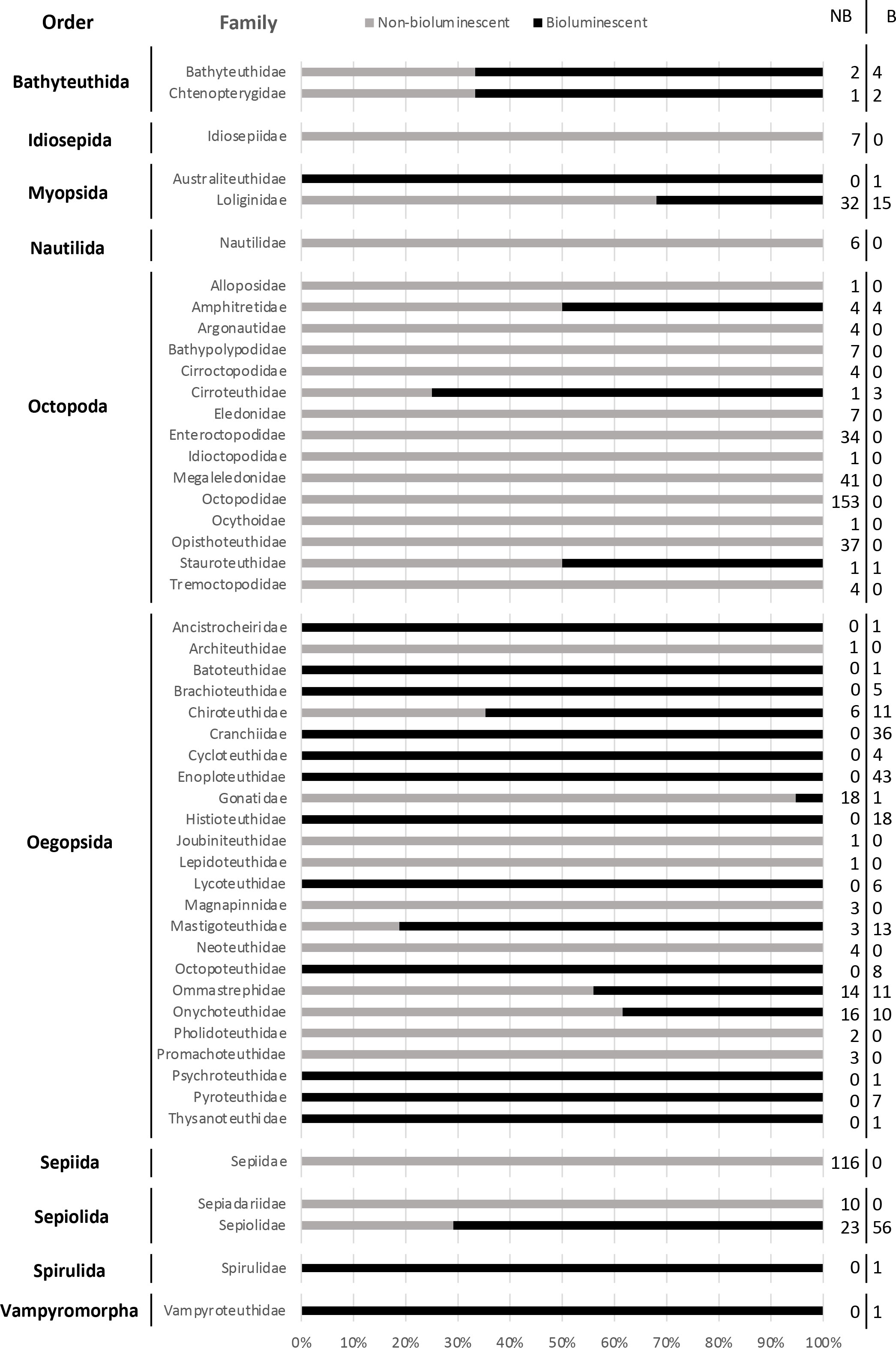
Figure 3 Percentage of known non-bioluminescent (grey) and bioluminescent (black) cephalopod species, according to each cephalopod family. The exact number of non-bioluminescent (NB) and bioluminescent (B) species is detailed on the right of the figure.
2.2 Photophores and spectra
To compare the diversity of bioluminescence among cephalopods, we assign photogenic tissue and secretions into six simplified categories: i) release of bioluminescent material to the surrounding environment; ii) strictly internal photophores that are associated with the ink sac or viscera; iii) circumoral photophores (around the mouth); iv) photophores on the arms and tentacles (comprising photophores on the arms and tentacles themselves, on the arm tips and tentacular stalks, at the base of the suckers and photophores with a sucker-like structure); v) photophores on the head region (combining the photophores on the head integument up to the base of the arms and internal photophores associated with the eyes and eyelids) and vi) photophores on the mantle region, comprising photophores both on the surface and embedded in the mantle, between and on the fins, as a strip along the ventral midline and/or on the funnel (Figure 4). In this comparison, we do not distinguish between bacteriogenic and autogenic photophores. We note that our grouping of photophores on the head integument with internal photophores associated with the eyes differs from previous reviews (Berry, 1920b; Herring, 1988), because they are thought to have mostly a similar role in counterillumination (Herring, 1988).
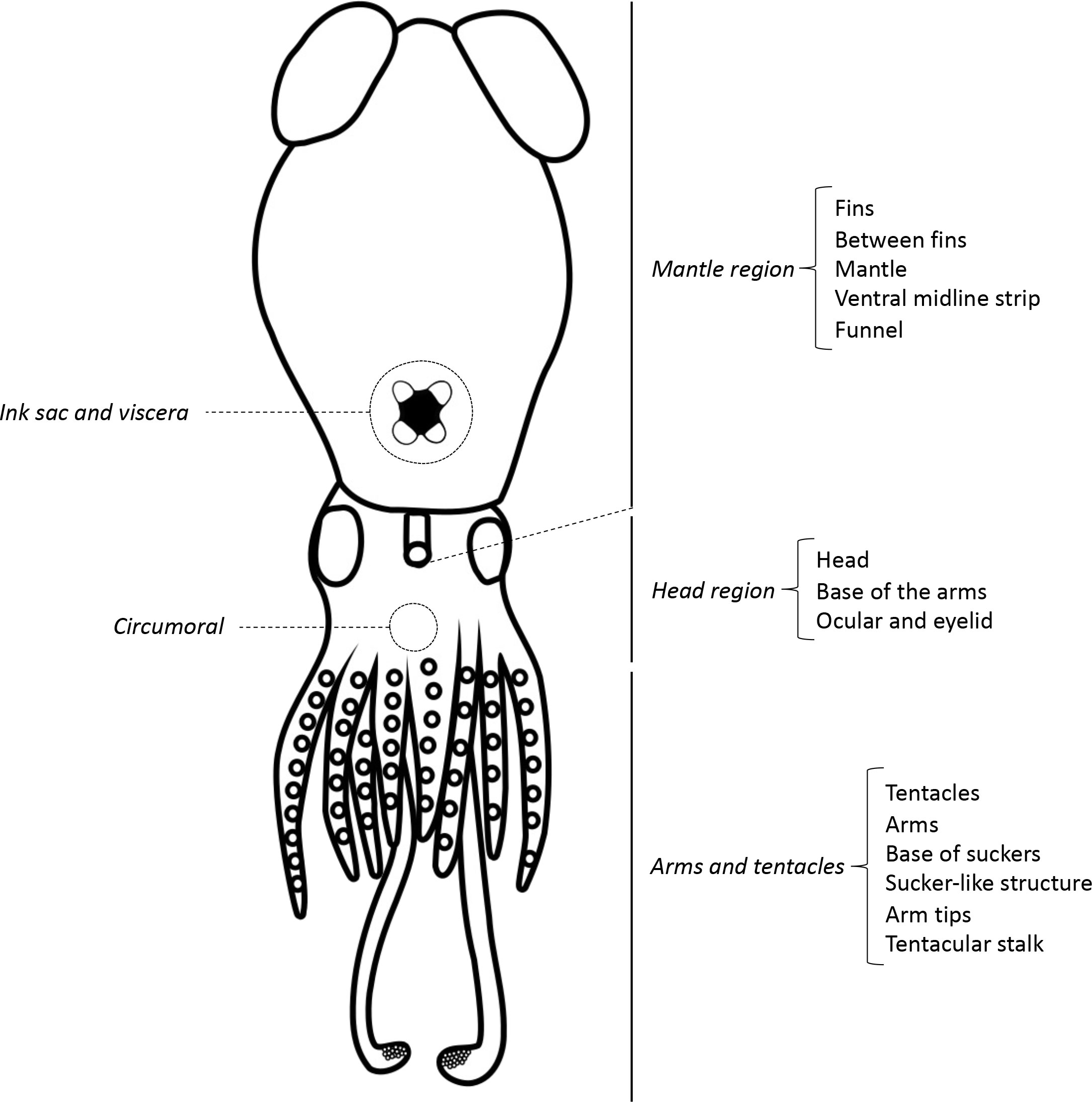
Figure 4 Schematic of all photophore positions. This schematic represents a fictitious cephalopod that combines all known photophore positions except for the release of bioluminescent material.
Most bioluminescent species have photophores at several different locations, with the head region being the most common position, followed by photophores associated with the arms and tentacles and internal photophores (Figure 5A). In the case of bathyteuthids, all luminous species have photophores on the head, and two of them (Chtenopteryx sepioloides and C. sicula) have an additional internal light organ (Figure 5B). On the other hand, the myopsid squids only possess internal photophores (Figure 5C). Octopoda is the only group with circumoral photophores, and some also possess light organs on the arms (Figure 5D). Oegopsid squids mainly present photophores on the head region and the arms and tentacles. However, many of these squids have additional light organs on the mantle region and internally (Figure 5E). Like the myopsids, sepiolid species possess internal photophores except for Nectoteuthis pourtalesi, the only sepiolid species to have photophores on the head region, and Stoloteuthis japonica, on the mantle region. Species of the genera Heteroteuthis (n=7) and Sepiolina nipponensis can also release bioluminescent material to their surroundings (Figure 5F). In the monotypic orders, Spirula spirula only presents photophores on the mantle area (Figure 5G), and Vampyroteuthis infernalis possesses photophores on the mantle region and the arms. The vampire squid can also release bioluminescent particles (Figure 5H).
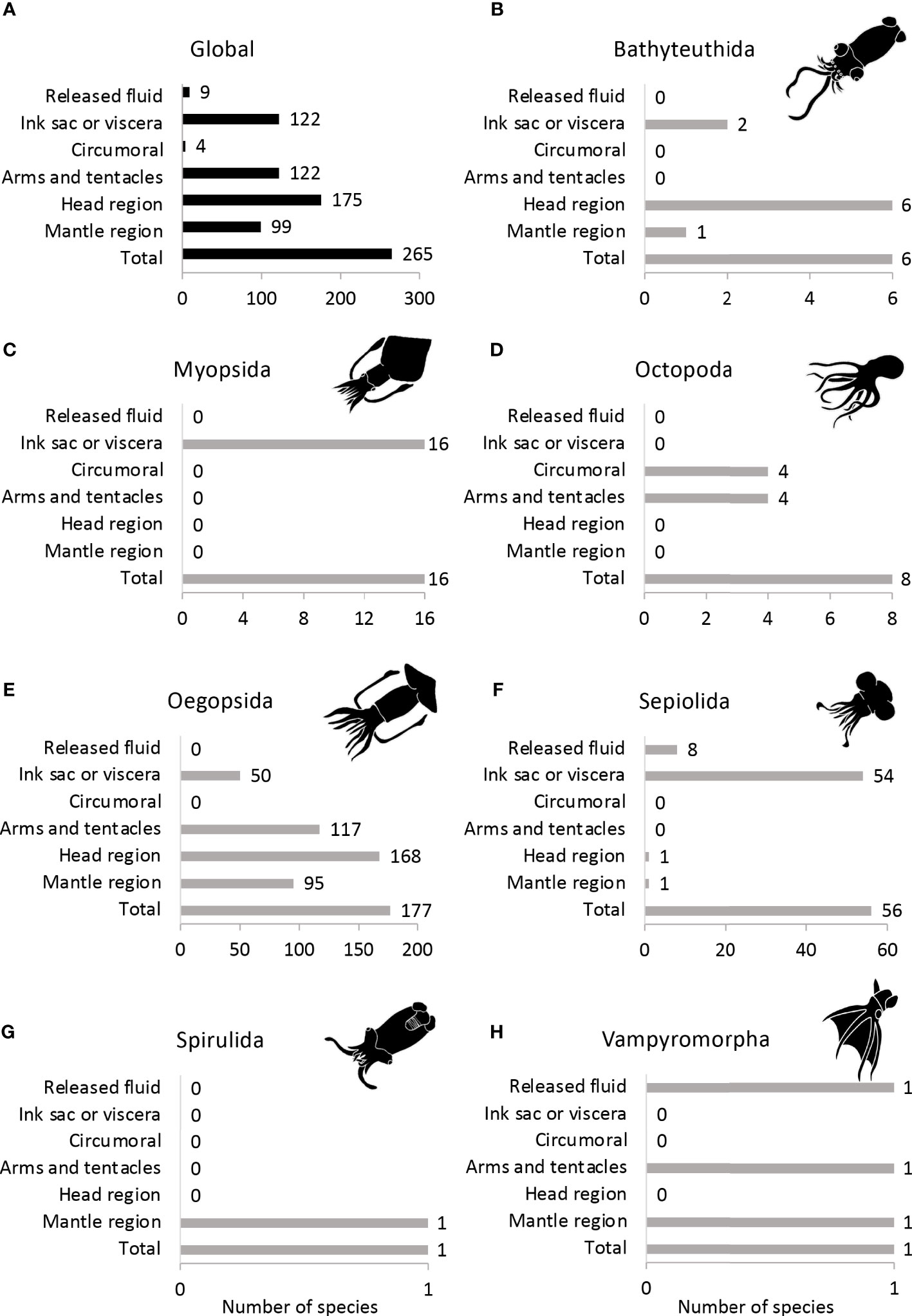
Figure 5 Morphological distribution of photophores in (A) all cephalopods, (B) Bathyteuthida, (C) Myopsida, (D) Octopoda, (E) Oegopsida, (F) Sepiolida, (G) Spirulida and (H) Vampyromorpha.
The bioluminescent material released by some of the above-mentioned species is ejected when the animal is disturbed. Therefore, these luminous clouds are likely used as a defense mechanism to startle or distract predators and allow the animal to escape (Robison et al., 2003). In the case of the sepiolids (Heteroteuthis sp.), this fluid contains a high concentration of bioluminescent bacteria that are released to the animal’s surroundings through the siphon, in combination with ink and mucus (Herring, 1977a; Herring, 1988; Herring, 2002). Vampyroteuthis releases bioluminescent material by a different process, ejecting glowing particles from the arm-tip photophores, in a stickier and more viscous matrix. This material can adhere to other surfaces, including potential predators, placing them at risk of secondary predation (Robison et al., 2003).
The function of the circumoral photophores remains unclear; the luminescence is only visible to the surrounding organisms and not to the emitting animal itself due to its position. Moreover, it is unlikely to be detectable by potential prey. Finally, as it is only present in female individuals, the most likely role of circumoral bioluminescence would be for sexual-recognition purposes (Herring et al., 1987). In terms of communication, the case of the oegopsid Dosidicus gigas (“Humboldt” or “jumbo” squid) is worth highlighting. In most cases, luminous cephalopods modify the light intensity for a single photophore, which usually glows in an outward direction. However, due to the tissue-embedded photophores of the Humboldt squid, in this case, light radiates through the tissues, causing the animal to luminesce entirely (Burford and Robison, 2020). This squid can reveal and conceal specific lighted body regions in any combination, using the overlying chromatophores. Such complex communication ability is probably crucial for group coordination and cohesion, which are usually only observed in well-lit environments (Burford and Robison, 2020).
Photophore position appears strongly correlated to cephalopods’ habitats. Here, coastal species were considered to be associated with, but not necessarily restricted to, continental shelves (bottom depths <200 m) at late ontogenetic stages (as in Rosa et al., 2008a; Rosa et al., 2019). Pelagic species living outside the continental shelf were considered oceanic (as in Rosa et al., 2008b). In the present database, 97% of the coastal bioluminescent cephalopods possess internal photophores, and only a very few have external light organs. Only 9% of the coastal luminous species release bioluminescent material (Figure 6). In oceanic light-producing species, on the other hand, photophores are mostly present externally, on the head region (88%), on the arms and tentacles (62%), or on the mantle region (50%). Light organs associated with the ink sac and viscera are less common in this group (only 30% of the oceanic luminous species) compared to coastal bioluminescent organisms (Figure 6).
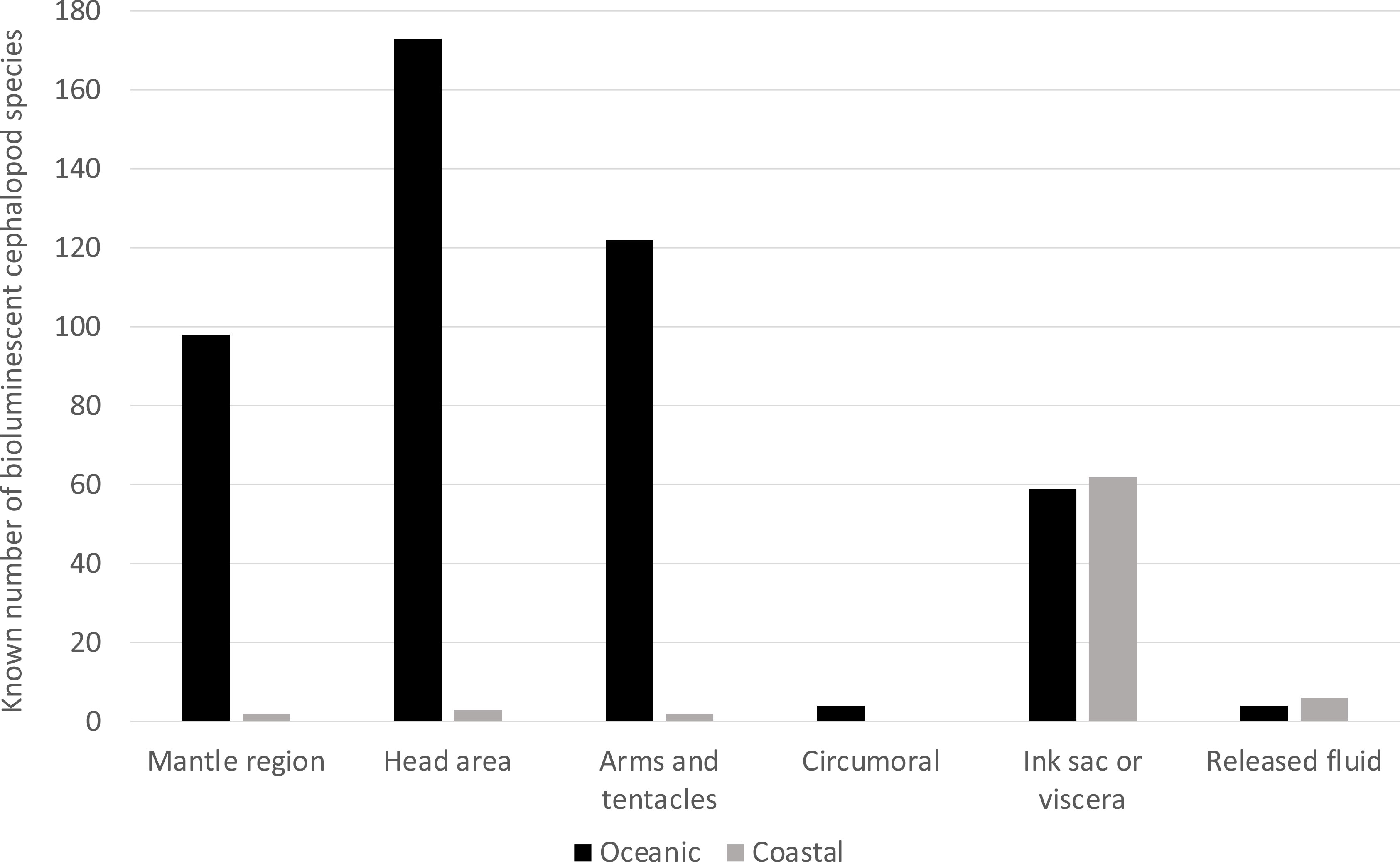
Figure 6 Diversity in photophore location according to the lifestyle of the cephalopods. Oceanic species are in black, and coastal ones are in grey.
Interestingly, these patterns are maintained when we divide the groups according to light origin (autogenic vs. bacteriogenic photophores). Coastal light-producing cephalopods mainly comprise myopsid and bobtail squids, namely the Loliginidae and Sepiolidae (see also section 4), which have bacteriogenic photophores (Nishiguchi et al., 2004; Guerrero-Ferreira & Nishiguchi, 2009; Lindgren et al., 2012). By contrast, oceanic luminous species usually have autogenic light organs (Lindgren et al., 2012). Similar trends have been observed in fishes, where a higher proportion of bacteriogenic light organs is found in species that live closer to the ocean floor and the coastal areas, compared to oceanic fishes (Morin, 1983; McFall-Ngai and Toller, 1991; Haygood, 1993; Paitio et al., 2016).
Finally, the characteristics of the light produced should be considered. Cephalopods whose emission spectra have been measured produce light mostly within blue-green wavelengths (450-490mm; Herring, 1983; Haddock et al., 2010). However, more extreme values are possible in some species (Widder et al., 1983), ranging from 420 nm (Chtenopteryx; Herring, 1983; Herring, 1988) to 535 nm (Abralia; Herring, 1988). Some species appear to emit light outside even these values, either directly such as the bright yellow arm-tip photophores of Taningia (Figure 1G-G’) or through colored filters (e.g., Abralia; Young and Tsuchiya, 2014). Some species of Abraliopsis and Abralia have also been observed to actively modify the emitted wavelength with temperature, which would correspond to temperature (and likely light intensity) variations encountered during vertical migration (Young and Mencher, 1980).
2.3 Bioluminescence disparities amongst cephalopod groups
In this section, we emphasize some ontogenetic, and inter- and intra-specific differences observed in cephalopod bioluminescence.
2.3.1 Bathyteuthida
Four species in the family Bathyteuthidae were considered bioluminescent, possessing photophores at the base of the arms. In 2020, the new species Bathyteuthis inopinatum and B. devoleii were described by Judkins et al. as lacking these basal arm photophores. In their third new species, B. numerosus, sexual dimorphism was observed, with photophores only present in males.
Within the Chtenopterygidae family, only Chtenopteryx sepioloides and C. sicula have been confirmed as bioluminescent, for which the large eyeball photophores can assist in identifying these species (Escánez et al., 2012; Escánez et al., 2018; Luna et al., 2021). The potential presence of photophores in C. canariensis remains debated (Escánez et al., 2012; Escánez et al., 2018; Luna et al., 2021).
2.3.2 Myopsida
Only 16 species (33%) of myopsid squids are bioluminescent; of these, all species except for one are from the genus Uroteuthis (Loliginidae). As in most neritic cephalopods, loliginids have bacteriogenic bioluminescence (Guerrero-Ferreira and Nishiguchi, 2009). In terms of phylogeny, Uroteuthis are considered as closely related to Loliolus (non-bioluminescent species). In fact, it is suggested that these loliginids had a common ancestor that possessed bacteriogenic light organs, which were maintained in Uroteuthis but lost in Loliolus (Anderson et al., 2014).
Furthermore, it is also interesting to highlight that several different species of bioluminescent bacteria have been reported within a single squid species, Sepioteuthis lessoniana, which appears to be non-luminous (Zari et al., 2020). In this case, swabs were taken from the surface to the gut and ink sac of the non-bioluminescent “big-fin” reef squid (S. lessoniana). Although 5 luminous bacterial species were found, they do not appear to confer the capacity of light production to the squid (Zari et al., 2020).
2.3.3 Octopoda
Within the Octopoda, photophores are found in two locations, each unique to one evolutionary lineage and all potentially restricted to females. Circumoral photophores appear specific to the incirrate species (family Amphitretidae), while the cirrate octopods (Cirroteuthidae and Stauroteuthidae families) only have photophores on the arms (Jereb et al., 2005).
Stauroteuthis syrtensis is the only species of its genus confirmed to have bioluminescence, which is produced by modified suckers along the length of all arms. Only female S. syrtensis have been confirmed to luminesce (Collins and Henriques, 2000); if males prove non-luminescent, this species provides another example of sex-specific light production in cephalopods. Bioluminescence in Stauroteuthis gilchristi has not been reported to date, although its suckers appear similarly modified to those of S. syrtensis (Johnsen et al., 1999; Collins and Henriques, 2000).
The circumoral photophore found in Japetella diaphana and Bolitaena pygmaea (also previously known as Eledonella pygmaea), and potentially all derived species of the same genus, is also confirmed to be present only in mature females (Robison and Young, 1981; Herring et al., 1987). This strengthens the probability that sex-specific signaling is a primary function of octopod photophores.
2.3.4 Oegopsida
Within the cephalopods, oegopsid squids display the greatest variety of bioluminescent structures and positions, in some cases also varying with ontogeny. The diamondback squid, Thysanoteuthis rhombus (Thysanoteuthidae), is the only known bioluminescent cephalopod species where the photophore is present solely in immature squids and is lost by adulthood (Fernández-Álvarez et al., 2021). In this case, the light organ may play a role in pairing behavior, where immature male and female individuals pair up as young individuals and remain together through maturity (Guerra and Rocha, 1997; Jereb and Roper, 2005b).
For some oegopsids, bioluminescence is suspected but remains unconfirmed. For example, Grimalditeuthis bonplandii and Psychroteuthis glacialis were included as bioluminescent species in our analysis, due to the suspected photogenic nature of structures that develop at the arm tips of mature females, but further investigation into the nature of this tissue is needed (Jereb and Roper, 2005b; Hoving et al., 2013; Young and Roper, 2016). The onychoteuthid species Ancistroteuthis lichtensteinii has also been suggested (but not confirmed) to possess photogenic tissue on the ventral eye surface (Bolstad, 2010).
Another oegopsid worth mentioning is Gonatus pyros, the only luminescent member of the family Gonatidae (~20 species). This species possesses a large light organ on the ventral surface of each eye (Young, 1972; Bublitz, 1981). This raises questions about the evolution of its light organ, given that other members of Gonatidae (several sympatric with G. pyros) do not possess a photophore. Lindgren et al. (2005) reported G. pyros as being most closely related to several sequenced individuals attributed to G. kamtschaticus based on three mitochondrial loci, but the position of this clade within the family was not resolved and should be further investigated.
2.3.5 Sepiolida
Bioluminescence in Sepiolida is only present in the family Sepiolidae, which are hypothesized to have radiated from a common ancestor that possessed a bilobed light organ associated with bioluminescent bacteria. This structure was eventually lost for the genera Inioteuthis and Sepietta (Sanchez et al., 2021). In Heteroteuthis, however, where pelagic adults depart from the typical benthic sepiolid lifestyle, the autogenic origin of its bioluminescence suggests a convergent evolution with other pelagic bioluminescent cephalopods (Dilly and Herring, 1978; Lindgren et al., 2012; Sanchez et al., 2021). Heteroteuthis is also the only sepiolid known to secrete luminous fluid.
Interestingly, as in Sepioteuthis lessoniana, bioluminescent bacteria have been identified in a sepiolid species that is considered non-luminescent. Neorossia caroli lacks a light organ (Sanchez et al., 2021; Calogero et al., 2022); yet bioluminescent bacteria (Photobacterium kishitanii and P. leiognathi) were found in samples taken from the siphon and the mantle (Calogero et al., 2022). The relationships between the non-luminous cephalopods S. lessoniana and N. caroli and the respective bioluminescent bacteria found, in terms of light production, would then be facultative rather than obligate (Zari et al., 2020; Calogero et al., 2022). However, no studies have yet investigated such associations for purposes other than bioluminescence.
3 Biogeography
3.1 Database limitations and assumptions
The biogeographic patterns of bioluminescent cephalopod richness were derived using a conservative approach, using only species with well-known distributions (238 species, Supplementary Material 1) and excluding new or rare species [as described by Rosa et al. (2019)]. Moreover, it is important to note that many coastal and oceanic regions are still under-sampled, and the present patterns may also be driven by an underestimated cryptic diversity (Rosa et al., 2019). Here, we only included the coastal, epipelagic (<200m), and mesopelagic (between 200m and 1000m) realms, since they are the ones with a relatively solid global biogeographic classification (Spalding et al., 2007; Spalding et al., 2012 and Sutton et al., 2017, respectively). Yet, it is worth mentioning that at least half of the bioluminescent cephalopod species present in the mesopelagic realm can also be found in waters below 1000m depth.
3.2 Richness patterns per ocean and habitat
Our results show that the most diverse ocean, in terms of light-producing cephalopod species, is the Pacific Ocean (162 species), followed by the Atlantic (117 species) and Indian (112 species) Oceans. The least diverse oceans are the Southern and the Arctic Oceans (Figure 7A). These patterns are also consistent within the orders Bathyteuthida, Oegopsida, Spirulida, and Vampyromorpha (the two latter are not present in the Arctic or Southern Oceans (Figures 7B, E, G, H). However, in the case of myopsid squids, the highest number of luminescent species (14 species) are observed in the Indian Ocean, followed by the Pacific Ocean (10 species); they are absent from the three other Oceans (Figure 7C). On the other hand, bioluminescent octopods can be observed in all oceans, but mainly in the Atlantic and the Pacific Ocean (6 species, Figure 7D). Similarly, luminescent members of Sepiolida are mainly observed in the Atlantic Ocean (21 species), followed by the Pacific and the Indian Oceans (Figure 7F). Furthermore, one species (Sepiola atlantica) can be found as far north as Iceland, already part of the Arctic Ocean (Jereb & Roper, 2005a).
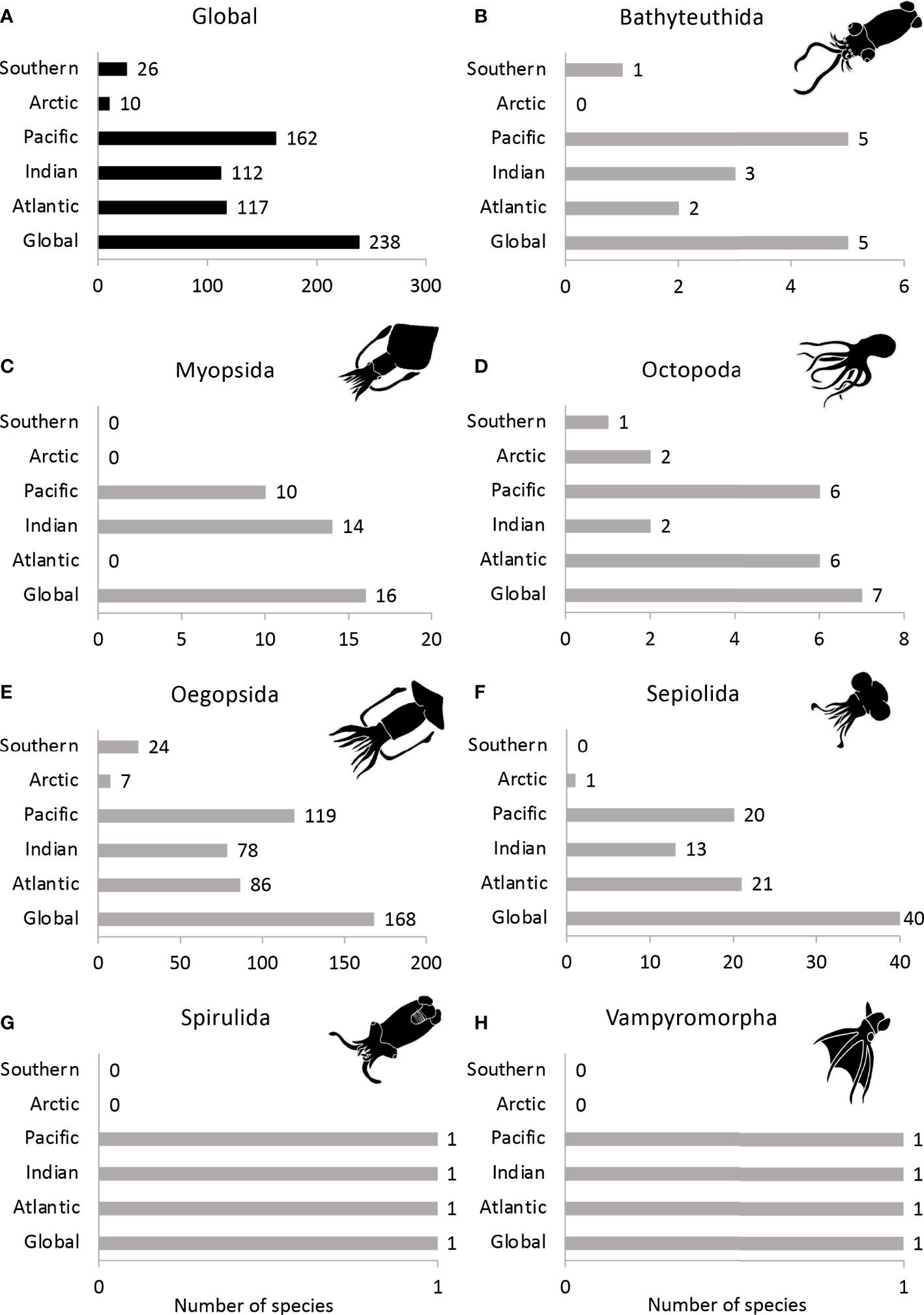
Figure 7 Total known number of bioluminescent cephalopod species for each ocean basin in (A) all cephalopods, (B) Bathyteuthida, (C) Myopsida, (D) Octopoda, (E) Oegopsida, (F) Sepiolida, (G) Spirulida and (H) Vampyromorpha.
Using 231 luminous cephalopod species with a well-characterized habitat preference, we identified that most are found in the oceanic zone, while only 23% occur in coastal waters (Figure 8). Yet, it is important to note that most oceanic species undergo both diel and ontogenetic vertical migrations and are reported to be vertically distributed across pelagic zones (Judkins and Vecchione, 2020). In fact, 53% of the bioluminescent cephalopod species are found both in the epipelagic and mesopelagic zones of the oceans, such as Abralia spp., Histioteuthis spp., and Leachia spp., amongst others.
Regarding coastal areas, and according to ecoregions (Spalding et al., 2007), the highest species richness of luminescent cephalopods is found in the Eastern Philippines ecoregion, with 13 species, followed by the Sulawesi Sea/Makassar Strait (11 species) and the ecoregion of Palawan/North Borneo (10 species, Figure 9A). These ecoregions are present in the delimitations of the Coral Triangle (Veron et al., 2009), a region of the ocean that is well known for being the most significant hotspot in terms of marine biodiversity (Bowen et al., 2013; Cowman et al., 2017), including cephalopods (Rosa et al., 2019). Following these three ecoregions, bioluminescent cephalopods are also highly present in the Aegean and Adriatic Sea, Ionian Sea, Alboran Sea, and Western Mediterranean ecoregions, with 8 or 9 species observed (Figure 9A). Indeed, bobtail squids (Sepiolidae) have the maximum species richness values in the Mediterranean Sea, constituting a biogeographic hotspot for this group (Bello, 2019; Rosa et al., 2019). Their high presence in the Mediterranean Sea can be explained by the separation 5.5 Mya of the Mediterranean Sea from the Atlantic as part of the “Messinian salinity crisis”, which induced the extinction of stenohaline species and started an increase in endemism in the area (Rosa et al., 2008a; Rosa et al., 2019). Bioluminescent coastal species are represented mainly by the Sepiolidae and Loliginidae (Uroteuthis sp.), in addition to the oegopsid squids Watasenia scintillans and Pyroteuthis margaritifera. Overall, the general biogeographic trends described here for luminescent cephalopods follow those described by Rosa et al. (2019) for all coastal cephalopods worldwide. Therefore, the distribution of cephalopod luminescent fauna seems to be mainly driven by well-known historical (geological) processes and species-area–energy relationships (Rosa et al., 2008a), rather than adaptations to specific coastal habitats or unique geographic regions.
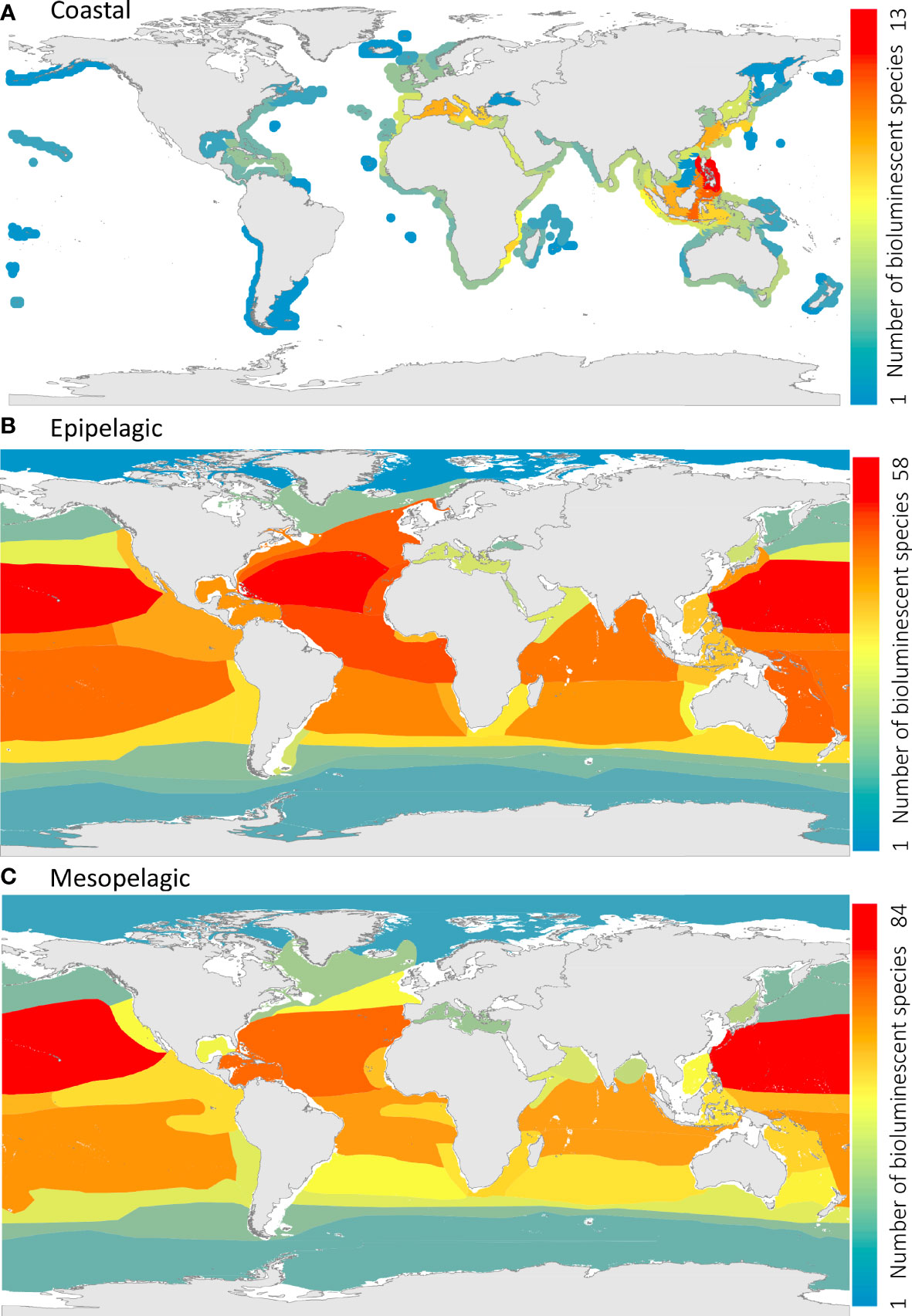
Figure 9 Species richness (known number of bioluminescent cephalopod species per ecoregion) of (A) coastal, (B) epipelagic, and (C) mesopelagic bioluminescent cephalopods. Ecoregions and provinces shown in the coastal panel, the epipelagic panel, and mesopelagic are based on Spalding et al. (2007); Spalding et al. (2012), and Sutton et al. (2017), respectively.
Regarding the epipelagic zone, and based on the biogeographic provinces of Spalding et al. (2012), most species are found in the Indo-Pacific Warmwater Realm (IPWR) and the Atlantic Warmwater Realm (AWR, Figure 9B). More specifically, the North Central Pacific (IPWR) and the North Central Atlantic (AWR) are the provinces with the highest concentration of bioluminescent cephalopods, with 58 species and 57 species, respectively. The Equatorial Atlantic and the Canary Current also present a high species richness, with 51 and 50 species, respectively, followed by the North Atlantic Current and the Southwest Pacific (49 species), the South-Central Pacific (48 species) and Northern Indian Ocean (46 species), the Equatorial Pacific and the Gulf Stream (45 species). The lowest species richness of bioluminescent cephalopods is found in the Arctic (Northern Coldwater Realm), with only two bioluminescent species observed (Cirroteuthis muelleri and Brachioteuthis riisei), and the Antarctic and Antarctic Polar Fronts (Southern Coldwater Realm), with 8 and 12 species observed, respectively. The epipelagic bioluminescent cephalopods are represented mainly by the oegopsid squids. The bioluminescent octopods Japetella spp. and Bolitaena pygmaea, in addition to the ram’s horn squid Spirula spirula, are also present in the epipelagic zone.
Finally, within the mesopelagic realm, the main luminescent cephalopods are oegopsid squids and pelagic octopods, in addition to the vampire squid Vampyroteuthis infernalis. According to the provinces outlined by Sutton et al. (2017), the Northern Central Pacific ecoregion is the hotspot for mesopelagic light-producing cephalopod richness, with 84 species, followed by the Central North Atlantic, with 69 species (Figure 9C). Following these ecoregions, 50 to 62 bioluminescent species are present in the Coral Sea, the Northern Indian Ocean, the Mid-Indian Ocean, the Tropical and West Equatorial Atlantic, and the Southern Central Pacific ecoregions. The Arctic and the Antarctic/Southern Ocean have the lowest species richness of luminescent cephalopods, with 8 and 14 species observed, respectively.
Several large-scale biogeographic studies have been made on pelagic cephalopods, but following different approaches, namely by: i) displaying the exact location of verified species records (e.g., Nesis, 1982; Nesis, 1985; Voss et al., 1998; Nesis, 2003; Collins and Rodhouse, 2006); ii) using presence–absence matrices condensed into latitudinal bands (Ibáñez et al., 2019), or iii) choosing different regional spatial units (provinces) (Rosa et al., 2008b). Although none of these approaches is directly comparable with the present findings, Rosa et al. (2008b) pointed out, for the Atlantic Ocean, that the highest levels of cephalopod pelagic diversity were found in Benguela Current System and the Southern convergence, and described a diversity impoverishment in some western oceanic regions, namely the South Sargasso Sea and South Atlantic Subtropical. As a result, these authors found a significant positive relationship between ocean net primary productivity and oceanic richness at regional scales and maximum oceanic richness at intermediate sea surface temperatures. Here, by contrast, bioluminescent cephalopods show: i) a decreased longitudinal richness, at subtropical latitudes, from the western to the eastern margins of both Atlantic and Pacific Oceans, namely towards the most important eastern boundary upwelling systems (EBUS) and ii) a decreased richness at higher latitudes (Figure 9). These patterns seem to corroborate the findings of Ibáñez et al. (2019) in the Eastern Pacific, since pelagic richness was higher across the tropics and decreased steadily towards both poles, apparently not showing any correlation with ocean productivity. Angel (1993) also pointed out that high species richness in the pelagic ecosystems may be associated with zones of low productivity lacking marked seasonality. Oxygen availability may also be a key limiting factor for the distribution of bioluminescent cephalopods since oxygen is one of the primary components in the chemical reaction that induces light production (Tsuji, 2002; Haddock et al., 2010). Oceanic oxygen minimum zones (OMZs), where oxygen concentrations are limited, are usually formed by high productivity at the surface of the oceans due to upwelling, which degrades and falls to the bottom while depleting the oxygen present in the water column (Levin, 2003). These zones can create major boundaries for species since the lack of oxygen prevents mobile organisms from traversing or living within these ocean zones unless they are strongly adapted (Levin, 2003; Rosa and Seibel, 2008; Rosa & Seibel, 2010). In fact, the west coasts of Central America, Peru (Humboldt Current), southwest Africa (Benguela Upwelling), the Arabian Sea and Bay of Bengal are well known for their seasonal or permanent OMZs (Levin, 2003; Stramma et al., 2008; Sutton et al., 2017). Although mid-water oxygen clines could explain the general longitudinal decrease in the diversity of light-producing cephalopods towards both EBUS (although not so clear along the northern epipelagic area of the California Current system) and the monsoon-influenced Arabian Sea (Figures 9B, C), it is worth noting that some bioluminescent cephalopods thrive within OMZs, including the vampire squid (Vampyroteuthis infernalis). This may indicate that productivity, and not oxygen per se, is the key environmental driver for the large-scale longitudinal trends in cephalopod oceanic diversity.
4 Research trends
Research on cephalopods and, more specifically, work focusing on light-producing species has increased substantially during the last 50 years (see below). Here, we aim to understand which research areas have dominated and identify the main studied species. To do so, we performed a systematic analysis to investigate the studies on bioluminescence in cephalopods, using the PRISMA (Preferred Reporting Items for Systematic Reviews and Meta-Analyses) approach (Moher et al., 2010). The literature considered in the present analysis was restricted to the Scopus and Web of Science scientific databases (detailed methods are explained in Supplemental Material 2, 3 and Figure S1).
Between 1971 and 2020, 277 peer-reviewed studies were published on bioluminescent cephalopods. Since the 1980s, there has been a steady increase in publications on this topic, with 126 studies (45% of the publications) published in the last decade (2011–2020; Figure 10A).
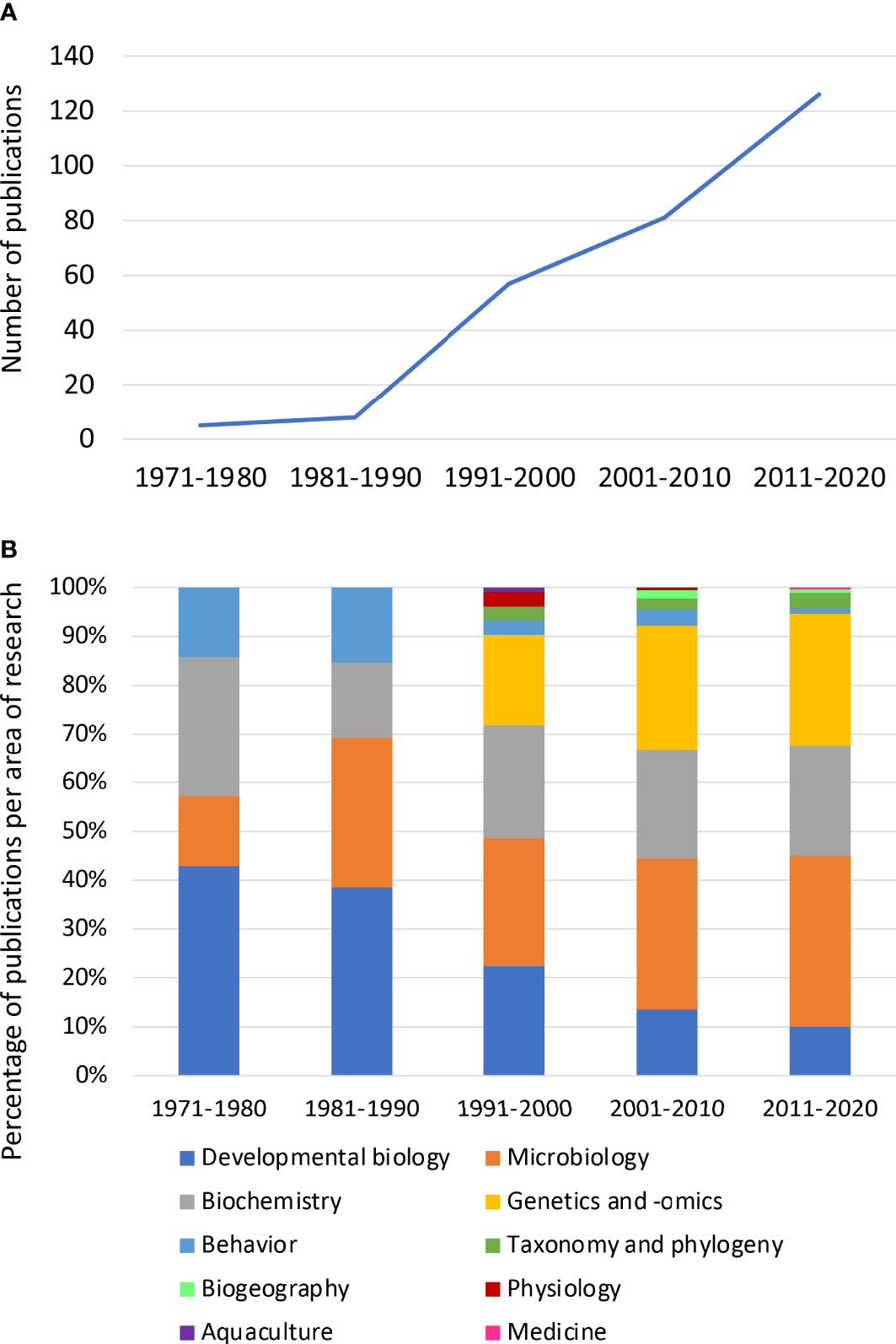
Figure 10 (A) Decadal publication trends (number of publications) on bioluminescence in cephalopods between 1971 and 2020. (B) Percentage of publications on bioluminescence in cephalopod research, between 1971 and 2020, according to the research area.
Studies on the fields of “Behavior” and “Developmental biology” within bioluminescent cephalopods have been decreasing over the years, being replaced by studies on genetics and -omics (e.g., genomic, transcriptomic, proteomic). In fact, the latter have been increasing since the 1990s (Figure 10B). The growing interest in this type of study comes along with the great improvements made in the field, e.g., the creation of GenBank® in 1982, and the notable reduction in cost for sequencing (Gužvić, 2013; Kulski, 2016). Together these factors have supported a vast increase in DNA and RNA sequences produced and uploaded to GenBank® since 1992 (Kulski, 2016).
Regarding the areas of “Microbiology” and “Biochemistry” of luminous cephalopods, the number of studies has largely held stable across the decades, except between 1971 and 1980, when the number of publications in “Microbiology” was lower compared to other areas. On the other hand, less than 10% of the studies included the fields “Aquaculture”, “Taxonomy and Phylogeny”, “Biogeography”, and “Physiology” (Figure 10B). It is worth noting that one recent study opened the doors of light-producing cephalopods to the field of “Medicine”, investigating the cytotoxicity effects of symbiotic bacteria against cancer cells (Luyon et al., 2017).
4.1 Focus species in studies on cephalopod bioluminescence
Overall, amongst the 277 studies listed in our analysis, the Hawaiian bobtail squid Euprymna scolopes is the most studied species by far, with 214 publications (77%). Other studied bioluminescent cephalopods include E. tasmanica, Watasenia scintillans, Sthenoteuthis oualanienesis and Sepiola robusta, but each only with 6 to 10 studies (Figure 11A). The category “others” comprises 49 species or groups of species.
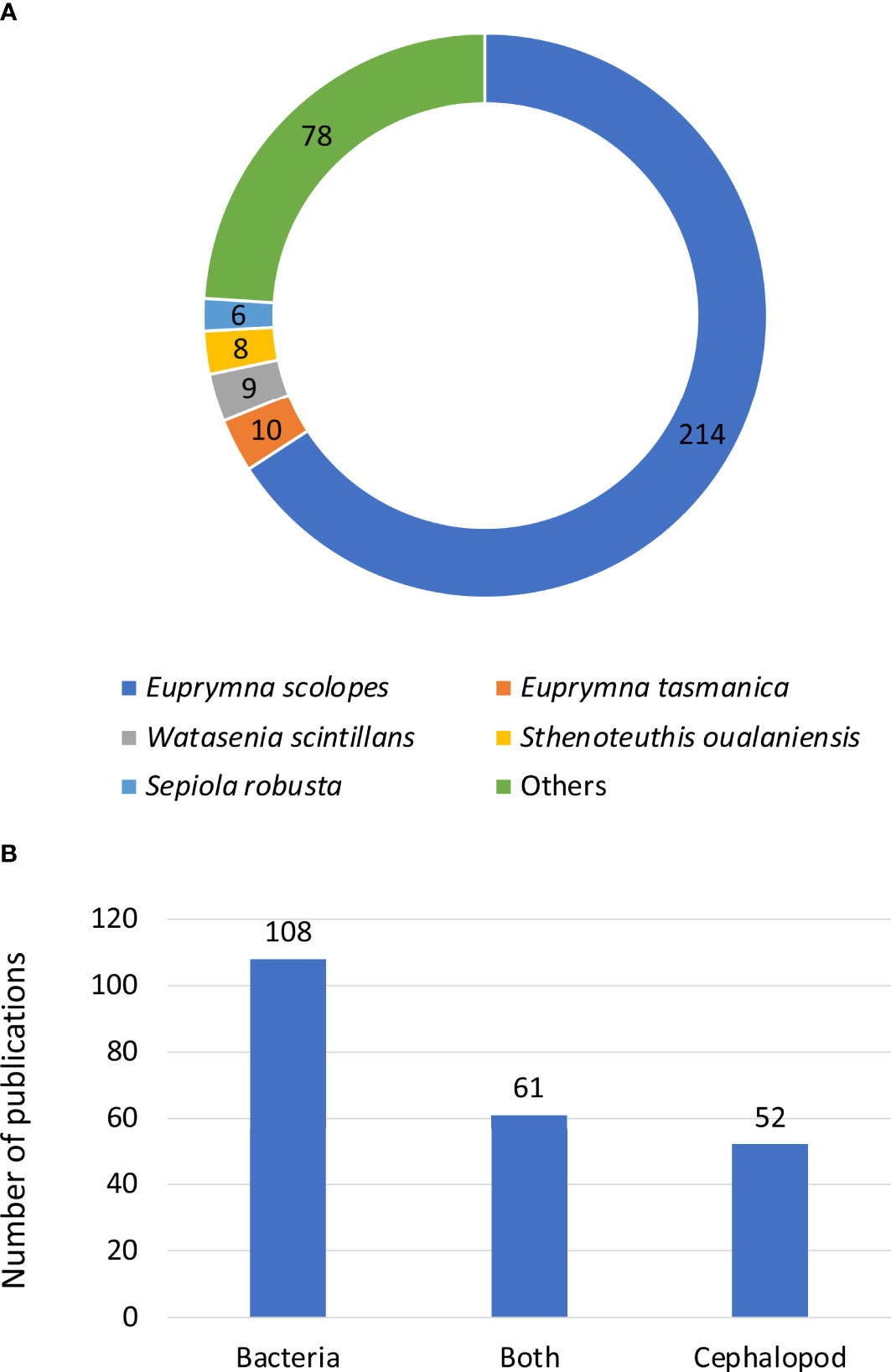
Figure 11 Number of publications on bioluminescent cephalopods: (A) according to the species of interest and (B) related to bacteriogenic bioluminescence, and according to the partner of interest, the symbiotic bacteria, the cephalopod or both partners.
Furthermore, it is interesting to highlight that nearly half of the studies about bacteriogenic bioluminescent species (221 studies) focused on the bacterial side of the symbiosis (49%, Figure 11B). In other words, these studies investigated the requirements of the bacterium, in order to successfully establish the symbiosis with the cephalopod. 24% of the studies focused on the cephalopod itself, and 28% focused on both symbiotic partners and their interaction with each other (Figure 11B).
4.2 Euprymna scolopes: the model organism
As most of the recent research on bioluminescent cephalopods focuses on Euprymna scolopes, we summarize in this section the state of knowledge for this species. Euprymna scolopes gains its bioluminescence through the single species-specific symbiosis with the bacterium Vibrio fischeri (McFall-Ngai and Ruby, 1998; Nyholm and McFall-Ngai, 2021). This sepiolid species is one of the best-studied cephalopods and both symbiotic partners have been the subject of research in the past decades (Nyholm and McFall-Ngai, 2021; Visick et al., 2021). In 2009, Lee et al. (2009) suggested considering this species a model organism as it offers many advantages for studies, including its overall small size, short lifespan, fast growth, and year-round availability. The interest in this species is mostly due to its species-specific symbiotic relationship with the bacterium V. fischeri, from which an easy comparison can be made for the study of eukaryote–prokaryote symbiosis (Nyholm and McFall-Ngai, 2004; Nyholm and McFall-Ngai, 2021). The symbiosis between these two partners is established at each generation, in the first hours after the bobtail squid hatches, and lasts for the entire life of the animal. When hatching, E. scolopes only possesses a rudimentary light organ associated with the ink sac, and the first encounter with the symbiont induces several steps, including organ morphogenesis, leading to a fully developed light organ and functional symbiosis (Nyholm & McFall-Ngai, 2004; Nyholm & McFall-Ngai, 2021). The first meeting between the two partners happens when the surrounding seawater, with free-living V. fischeri, flows through the mantle cavity of E. scolopes hatchlings (Visick et al., 2021). After aggregating at the surface of the light organ’s pores, bacteria are brought into the light organ’s crypts by the host’s ciliary beats. This recruitment of bacteria subsequently induces a series of morphogenesis events of the light organ in the next four days post-hatching (Nyholm & McFall-Ngai, 2004; Nyholm & McFall-Ngai, 2021). Over the following four weeks, the host light organ becomes fully mature, and the bobtail squid’s behavior changes from arrhythmic to nocturnally active (Nyholm & McFall-Ngai, 2004; Nyholm & McFall-Ngai, 2021). After hatching, the sepiolid quickly develops a diel behavioral pattern linked with the variation in bacterial density in the light organ, and the symbiont undergoes a metabolic change. The symbiont starts to shift its nutrient source to chitin at nighttime, causing the pH in the crypts of the light organ to decrease and, in turn, the luminescence to increase (Nyholm & McFall-Ngai, 2021). At dawn, bobtail squids expel between 90 and 95% of the symbiotic bacteria into the surrounding water, a process referred to as “venting”. The animal then remains buried until dusk, by which time the 5–10% remaining bacteria have multiplied to fully re-occupy the light organ. Finally, bobtail squids rise out of the sand at night to hunt, with a fully restored bacterial population and using the bacterial bioluminescence as camouflage (Jones and Nishiguchi, 2004; Nyholm and McFall-Ngai, 2004; Nyholm and McFall-Ngai, 2021).
The regularity of this behavior makes E. scolopes a prime subject for understanding morphological, physiological, biochemical, molecular, and genetic pathways associated with such host– microorganism interactions. Moreover, the ease of rearing both partners, as well as the possibility of raising individuals in aposymbiotic conditions (without the bacterial symbiont), allow a deep understanding of such a relationship (Nyholm and McFall-Ngai, 2004; Lee et al., 2009; Nyholm and McFall-Ngai, 2021). Euprymna scolopes’ genome availability is a reference in the field and set the interest in other species of this genus (Belcaid et al., 2019; Jolly et al., 2022). In fact, thanks to E. scolopes, there are now additional emerging models such as E. berryi and E. morsei (Jolly et al., 2022).
Finally, Vibrio fischeri colonizes the epithelia of Euprymna scolopes extracellularly, along the apical surface of this epithelium. This process is similar to how the microbiome cells associate with gut epithelia, including in humans (Nyholm and McFall-Ngai, 2021). Therefore, the squid–Vibrio system can also be used to gain insight into the mechanisms underlying the recruitment of gram-negative bacteria by the epithelia and the mechanisms by which they persist over the life of the host.
5 Conclusions and future directions
This study reviews the biodiversity of light-producing cephalopods and provides insights into their geographic distribution, following earlier reviews by Berry (1920a; 1920b) and Herring (1977b; 1988). We show that out of the 834 currently known cephalopod species, 32% are luminous with light organs most often occurring on the head region. Both coastal and oceanic light-producing cephalopods show clear latitudinal gradients of species richness, with higher diversity at lower latitudes. To understand the future geographical distribution of luminous cephalopods, new state-of-the-art techniques such as environmental DNA (eDNA, DNA obtained from environmental samples rather than organisms directly and that is released and accumulated in the environment by these organisms; Thomsen and Willerslev, 2015) could help obtain more complete temporal and biogeographical data. This eDNA could also be used for biodiversity research and monitoring (Thomsen and Willerslev, 2015). Across the past few decades, research on bioluminescent cephalopods has mostly focused on three main research areas (genetics and -omics, biochemistry, and microbiology) and mainly on the bobtail squid Euprymna scolopes. We have little ability to predict how bioluminescent cephalopods may respond to future anthropogenic pressures. At present, only two studies involving climate change-related stressors have been conducted to investigate the adaptation capabilities of Vibrio fischeri to temperature and pH stress, and how these affect the initiation of the symbiosis with Euprymna scolopes (Cohen et al., 2019; Cohen et al., 2020). Moreover, cephalopods, including bioluminescent species, are present in the diets of numerous top predators (Clarke, 1996; Klages, 1996; Smale, 1996). However, with climate change pressures, the geographical distribution of marine species such as cephalopods will change (Golikov et al., 2013), which would then affect food webs by impacting the top predators (Xavier et al., 2018). Thus, studying and understanding the marine food web dynamics in relation to future environmental climate is highly relevant. Many species-specific questions also need to be answered, such as: i) Which evolutionary processes led to a single species within a diverse family, such as Gonatus pyros, developing or retaining photophores? ii) which additional roles might luminous bacteria play within hosts that do not utilize their luminescence (e.g., Sepioteuthis lessoniana and Neorossia caroli)? iii) What additional bioluminescent cephalopods exist beyond those currently reported?
Finally, -omics research is becoming more and more dominant, bringing the possibility of a wide range of additional studies. The genome sequencing of cephalopods with bacteriogenic bioluminescence, such as E. scolopes, shows that these light organs can have a specific genomic signature, highlighting genes associated with host immunity and light mediation (Belcaid et al., 2019). However, as bacteriogenic species are limited to sepiolids and loliginids (Belcaid et al., 2019), more studies are required to analyze the genomic markers in cephalopods with autogenic bioluminescence. Moreover, such techniques have enabled, for example, a genus-level phylogenetic analysis of cephalopods, including bioluminescent species, using molecular markers (Sanchez et al., 2018). On the other hand, one striking feature of cephalopods is their ability to edit their messenger RNA, mostly converting adenosines to inosines (Alon et al., 2015; Albertin et al., 2022). While such research has mainly focused on non-luminous species, it would be interesting to focus future studies on species that have the capacity for light production as a comparison.
Author contributions
EO and RR contributed to the design of the study. EO organized the databases. All authors contributed to populating the databases. EO performed the analysis of the database and produced the figures, and VP produced the biogeographical distribution maps. EO and RR wrote the original draft and all authors commented on previous versions of the manuscript. All authors contributed to the article and approved the submitted version.
Funding
Portuguese national funds funded this study through FCT–Fundação para a Ciência e Tecnologia, I.P, within the PhD scholarships awarded to EO (UI/BD/151019/2021) and VP (2020.05435.BD), and the MARE strategic fund UIDB/04292/2020, project LA/P/0069/2020 (granted to the Associate Laboratory ARNET). The NIH funded MM-N and Edward Ruby through grants R37 AI50661, as well as OD11024 and GM135254.
Conflict of interest
The authors declare that the research was conducted in the absence of any commercial or financial relationships that could be construed as a potential conflict of interest.
Publisher’s note
All claims expressed in this article are solely those of the authors and do not necessarily represent those of their affiliated organizations, or those of the publisher, the editors and the reviewers. Any product that may be evaluated in this article, or claim that may be made by its manufacturer, is not guaranteed or endorsed by the publisher.
Supplementary material
The Supplementary Material for this article can be found online at: https://www.frontiersin.org/articles/10.3389/fmars.2023.1161049/full#supplementary-material
References
Albertin C. B., Medina-Ruiz S., Mitros T., Schmidbaur H., Sanchez G., Wang Z. Y., et al. (2022). Genome and transcriptome mechanisms driving cephalopod evolution. Nat. Commun. 13 (1), 2427. doi: 10.1038/s41467-022-29748-w
Allcock A. L. (2017). “Systematics of cephalopods,” in Evolution of venomous animals and their toxins. Ed. Malhotra A. (Dordrecht: Springer Netherlands), 415–434. doi: 10.1007/978-94-007-6458-3_8
Allcock A. L., Lindgren A., Strugnell J. M. (2015). The contribution of molecular data to our understanding of cephalopod evolution and systematics: A review. J. Natural History 49 (21–24), 1373–1421. doi: 10.1080/00222933.2013.825342
Alon S., Garrett S. C., Levanon E. Y., Olson S., Graveley B. R., Rosenthal J. J. C., et al. (2015). The majority of transcripts in the squid nervous system are extensively recoded by A-to-I RNA editing. ELife 4, e05198. doi: 10.7554/eLife.05198
Anderson F. E., Bergman A., Cheng S. H., Pankey M. S., Valinassab T. (2014). Lights out: The evolution of bacterial bioluminescence in Loliginidae. Hydrobiologia 725 (1), 189–203. doi: 10.1007/s10750-013-1599-1
Anderson F. E., Lindgren A. R. (2021). Phylogenomic analyses recover a clade of large-bodied decapodiform cephalopods. Mol. Phylogenet. Evol. 156, 107038. doi: 10.1016/j.ympev.2020.107038
Angel M. V. (1993). Biodiversity of the Pelagic Ocean. Conserv. Biol. 7 (4), 760–772. doi: 10.1046/j.1523-1739.1993.740760.x
Belcaid M., Casaburi G., McAnulty S. J., Schmidbaur H., Suria A. M., Moriano-Gutierrez S., et al. (2019). Symbiotic organs shaped by distinct modes of genome evolution in cephalopods. Proc. Natl. Acad. Sci. 116 (8), 3030–3035. doi: 10.1073/pnas.1817322116
Bello G. (2019). The Mediterranean Sepiolidae (Mollusca Cephalopoda) diversity. Biodivers. J. 10 (4), 389–404. doi: 10.31396/Biodiv.Jour.2019.10.4.389.404
Berry S. (1920a). Light production in cephalopods, i. An introductory survey. Biol. Bull. 38 (3), 141–169. doi: 10.2307/1536213
Berry S. (1920b). Light production in cephalopods, II. An introductory survey. Biol. Bull. 38 (4), 171–195. doi: 10.2307/1536323
Bolstad K. S. R. (2007). Systematics and distribution of the New Zealand onychoteuthid fauna (Cephalopoda: Oegopsida), including a new species, Notonykia nesisi sp. nov. Rev. Fish Biol. Fish. 17 (2–3), 305–335. doi: 10.1007/s11160-007-9041-2
Bolstad K. S. R. (2010). Systematics of the Onychoteuthidae Gray 1847 (Cephalopoda: Oegopsida). Zootaxa 2696 (1), 1. doi: 10.11646/zootaxa.2696.1.1
Bolstad K. S. R., Braid H. E., Strugnell J. M., Lindgren A. R., Lischka A., Kubodera T., et al. (2018). A mitochondrial phylogeny of the family Onychoteuthidae (Cephalopoda: Oegopsida). Mol. Phylogenet. Evol. 128, 88–97. doi: 10.1016/j.ympev.2018.05.032
Bowen B. W., Rocha L. A., Toonen R. J., Karl S. A. (2013). The origins of tropical marine biodiversity. Trends Ecol. Evol. 28 (6), 359–366. doi: 10.1016/j.tree.2013.01.018
Braid H. E. (2016). Resolving the taxonomic status of asperoteuthis lui Salcedo-Vargas 1999 (Cephalopoda, Chiroteuthidae) using integrative taxonomy. Mar. Biodivers. 47 (3), 621–635. doi: 10.1007/s12526-016-0547-5
Braid H. E., Bolstad K. S. R. (2015). Systematics of the Mastigoteuthidae Verrill 1881 (Cephalopoda: Oegopsida) from New Zealand waters. New Z. J. Zool. 42 (3), 187–256. doi: 10.1080/03014223.2015.1063516
Bublitz C. G. (1981). Systematics of the Cephalopod family Gonatidae from the southeastern Bering Sea (University of Alaska).
Burford B. P., Robison B. H. (2020). Bioluminescent backlighting illuminates the complex visual signals of a social squid in the deep sea. Proc. Natl. Acad. Sci. 117 (15), 8524–8531. doi: 10.1073/pnas.1920875117
Bush S. L., Robison B. H., Caldwell R. L. (2009). Behaving in the dark: Locomotor, chromatic, postural, and bioluminescent behaviors of the deep-Sea squid Octopoteuthis deletron Young 1972. Biol. Bull. 216, 7–22. doi: 10.1086/BBLv216n1p7
Calogero R., Rizzo C., Arcadi E., Stipa M. G., Consoli P., Romeo T., et al. (2022). Isolation and identification of luminescent bacteria in deep sea marine organisms from Sicilian waters (Mediterranean Sea). J. Mar. Sci. Eng. 10 (8), 1113. doi: 10.3390/jmse10081113
Clarke M. R. (1996). Cephalopods as prey. III. Cetaceans. Philos. Trans. R. Soc. London Ser. B: Biol. Sci. 351 (1343), 1053–1065. doi: 10.1038/1981244a0
Cohen M. L., Mashanova E. V., Jagannathan S. V., Soto W. (2020). Adaptation to pH stress by Vibrio fischeri can affect its symbiosis with the Hawaiian bobtail squid (Euprymna scolopes). Microbiology 166 (3), 262–277. doi: 10.1099/mic.0.000884
Cohen M. L., Mashanova E. V., Rosen N. M., Soto W. (2019). Adaptation to temperature stress by Vibrio fischeri facilitates this microbe’s symbiosis with the Hawaiian bobtail squid (Euprymna scolopes). Evolution 73 (9), 1885–1897. doi: 10.1111/evo.13819
Collins M. A., Henriques C. (2000). A revision of the family Stauroteuthidae (Octopoda: Cirrata) with redescriptions of Stauroteuthis syrtensis and S. gilchristi. J. Mar. Biol. Assoc. United Kingdom 80 (4), 685–697. doi: 10.1017/S0025315400002514
Collins M. A., Rodhouse P. G. K. (2006). Southern Ocean Cephalopods. Adv. Mar. Biol. 50 (5), 191–265. doi: 10.1016/S0065-2881(05)50003-8
Cowman P. F., Parravicini V., Kulbicki M., Floeter S. R. (2017). The biogeography of tropical reef fishes: Endemism and provinciality through time. Biol. Rev. 92 (4), 2112–2130. doi: 10.1111/brv.12323
Dilly P. N., Herring P. J. (1974). The ocular light organ of Bathothauma lyromma (Mollusca: Cephalopoda). J. Zool. 172 (1), 81–100. doi: 10.1111/j.1469-7998.1974.tb04095.x
Dilly P. N., Herring P. J. (1978). The light organ and ink sac of Heteroteuthis dispar (Mollusca: Cephalopoda). J. Zool. 186 (1), 47–59. doi: 10.1111/j.1469-7998.1978.tb03356.x
Escánez A., González Á. F., Guerra Á. (2012). Discovering biodiversity: Is the Canary Islands the habitat of a new Chtenopteryx species? (Florianópolis: Cephalopod International Advisory Council Symposium (CIAC).
Escánez A., Roura Á., Riera R., González Á. F., Guerra Á. (2018). New data on the systematics of comb-fin squids Chtenopteryx spp. (Cephalopoda: Chtenopterygidae) from the Canary Islands. Zool. Stud. 57, 1–11. doi: 10.6620/ZS.2018.57-40
Fernández-Álvarez F. Á., Colmenero A. I., Barría C. (2021). First record of the elusive oceanic squid Thysanoteuthis rhombus Troschel 1857 (Cephalopoda: Thysanoteuthidae) in the Catalan coast. Graellsia 77 (1), e122. doi: 10.3989/graellsia.2021.v77.284
Golikov A. V., Sabirov R. M., Lubin P. A., Jørgensen L. L. (2013). Changes in distribution and range structure of Arctic cephalopods due to climatic changes of the last decades. Biodiversity 14 (1), 28–35. doi: 10.1080/14888386.2012.702301
Guerra A., Rocha F. (1997). On a floating egg mass of the diamond shaped squid Thysanoteuthis rhombus (Cephalopoda: Thysanoteuthidae) in the western Mediterranean. Iberus 15 (1), 125–130.
Guerrero-Ferreira R. C., Nishiguchi M. K. (2009). Ultrastructure of light organs of loliginid squids and their bacterial symbionts: A novel model system for the study of marine symbioses Vie Milieu 8. Life and Environment 59 (3/4), 307–313.
Gužvić M. (2013). The history of DNA sequencing. J. Med. Biochem. 32 (4), 301–312. doi: 10.2478/jomb-2014-0004
Haddock S. H. D., Moline M. A., Case J. F. (2010). Bioluminescence in the sea. Annu. Rev. Mar. Sci. 2 (1), 443–493. doi: 10.1146/annurev-marine-120308-081028
Hastings J. (1995). Bioluminescence. Cell Physiol. Source Book, 665–681. doi: 10.1016/b978-0-12-656970-4.50054-3
Haygood M. G. (1993). Light organ symbioses in fishes. Crit. Rev. Microbiol. 19 (4), 191–216. doi: 10.3109/10408419309113529
Herring P. J. (1977a). Bioluminescence of marine organisms. Nature 267, 788–793. doi: 10.1038/267788a0
Herring P. J. (1983). The spectral characteristics of luminous marine organisms. Proc. R. Soc. London Ser. B. Biol. Sci. 220 (1219), 183–217. doi: 10.1098/rspb.1983.0095
Herring P. J. (1988). “Luminescent organs,” in The Mollusca: form and function, vol. 11. (Elsevier), 449–489). doi: 10.1016/B978-0-12-751411-6.50021-7
Herring P. J., Clarke M. R., von Boletzky S., Ryan K. P. (1981). The light organs of Sepiola atlantica and Spirula spirula (Mollusca: Cephalopoda): Bacterial and intrinsic systems in the order Sepioidea. J. Mar. Biol. Assoc. United Kingdom 61 (4), 901–916. doi: 10.1017/S0025315400023043
Herring P. J., Dilly P. N., Cope C. (1987). The morphology of the bioluminescent tissue of the cephalopod Japetella diaphana (Octopoda Bolitaenidae). J. Zool. 212, 245–254. doi: 10.1111/j.1469-7998.1987.tb05987.x
Herring P. J., Dilly P. N., Cope C. (2002). The photophores of the squid family Cranchiidae (Cephalopoda: Oegopsida). J. Zool. 258 (1), 73–90. doi: 10.1017/S095283690200122X
Hoving H. J. T., Zeidberg L. D., Benfield M. C., Bush S. L., Robison B. H., Vecchione M. (2013). First in situ observations of the deep-sea squid Grimalditeuthis bonplandi reveal unique use of tentacles. Proc. R. Soc. B: Biol. Sci. 280 (1769), 20131463. doi: 10.1098/rspb.2013.1463
Ibáñez C. M., Braid H. E., Carrasco S. A., López‐Córdova D. A., Torretti G., Camus P. A., et al (2019). Zoogeographic patterns of Pelagic Oceanic cephalopods along the eastern Pacific Ocean. J Biogeogr 46 (6), 1260–1273. doi: 10.1111/jbi.13588
Jereb P., Roper C. F. E. (2005a). “Cephalopods of the world: An annotated and illustrated catalogue of cephalopod species known to date: vol. 1,” in Chambered nautiluses and sepioids (Rome: Food and Agriculture Organization of the United Nations).
Jereb P., Roper C. F. E. (2005b). “Cephalopods of the world: An annotated and illustrated catalogue of cephalopod species known to date: vol. 2,” in Myopsid and oegopsid squids (Rome: Food and Agriculture Organization of the United Nations).
Jereb P., Roper C. F. E., Norman M. D., Finn J. K., FAO (2005). “Cephalopods of the world: An annotated and illustrated catalogue of cephalopod species known to date: vol. 3,” in Octopods and vampire squids (Rome: Food and Agriculture Organization of the United Nations).
Johnsen S., Balser E. J., Fisher E. C., Widder E. A. (1999). Bioluminescence in the deep-sea cirrate octopod Stauroteuthis syrtensis Verrill (Mollusca: Cephalopoda). Biol. Bull. 197, 26–39. doi: 10.2307/1542994
Jolly J., Hasegawa Y., Sugimoto C., Zhang L., Kawaura R., Sanchez G., et al. (2022). Lifecycle, culture, and maintenance of the emerging cephalopod models Euprymna berryi and Euprymna morsei. Front. Mar. Sci. 9. doi: 10.3389/fmars.2022.1039775
Jones B. W., Nishiguchi M. K. (2004). Counterillumination in the Hawaiian bobtail squid, Euprymna scolopes Berry (Mollusca: Cephalopoda). Mar. Biol. 144 (6), 1151–1155. doi: 10.1007/s00227-003-1285-3
Judkins H., Lindgren A., Villanueva R., Clark K., Vecchione M. (2020). A description of three new bathyteuthid squid species from the North Atlantic and Gulf of Mexico. Bull. Mar. Sci. 96 (2), 281–296. doi: 10.5343/bms.2019.0051
Judkins H., Vecchione M. (2020). Vertical distribution patterns of cephalopods in the Northern Gulf of Mexico. Front. Mar. Sci. 7. doi: 10.3389/fmars.2020.00047
Klages N. T. W. (1996). Cephalopods as prey. II. Seals. Philos. Trans. R. Soc. London Ser. B: Biol. Sci. 351 (1343), 1045–1052. doi: 10.1098/rstb.1996.0093
Kubodera T., Koyama Y., Mori K. (2007). Observations of wild hunting behaviour and bioluminescence of a large deep-sea, eight-armed squid, Taningia danae. Proc. R. Soc. B: Biol. Sci. 274 (1613), 1029–1034. doi: 10.1098/rspb.2006.0236
Kulski J. K. (2016). “Next-generation sequencing–an overview of the history, tools, and “Omic” applications,” in Next generation sequencing–advances, applications and challenges. Ed. Kulski J. K. (InTech). doi: 10.5772/61964
Lee P. N., McFall-Ngai M. J., Callaerts P., de Couet H. G. (2009). The Hawaiian bobtail squid (Euprymna scolopes): A model to study the molecular basis of Eukaryote-Prokaryote mutualism and the development and evolution of morphological novelties in Cephalopods. Cold Spring Harbor Protoc. 4 (11), 1–9. doi: 10.1101/pdb.emo135
Levin L. A. (2003). Oxygen minimum zone benthos: Adaptation and community response to hypoxia. In Gibson R. N., Atkinson R. J. A. Eds. Oceanography and Marine Biology, An Annual Review, 1st ed, vol. 41, pp. 1–45. (London: CRC Press). doi: 10.1201/9780203180570-3
Lindgren A. R., Giribet G., Nishiguchi M. K. (2004). A combined approach to the phylogeny of Cephalopoda (Mollusca). Cladistics 20 (5), 454–486. doi: 10.1111/j.1096-0031.2004.00032.x
Lindgren A. R., Katugin O. N., Amezquita E., Nishiguchi M. K. (2005). Evolutionary relationships among squids of the family Gonatidae (Mollusca: Cephalopoda) inferred from three mitochondrial loci. Mol. Phylogenet. Evol. 36 (1), 101–111. doi: 10.1016/j.ympev.2004.12.009
Lindgren A. R., Pankey M. S., Hochberg F. G., Oakley T. H. (2012). A multi-gene phylogeny of Cephalopoda supports convergent morphological evolution in association with multiple habitat shifts in the marine environment. BMC Evol. Biol. 12 (1), 129. doi: 10.1186/1471-2148-12-129
Lindgren A. R., Pratt A., Vecchione M., Anderson F. E. (2023). Finding a home for the ram’s horn squid: Phylogenomic analyses support Spirula spirula (Cephalopoda: Decapodiformes) as a close relative of oegopsida. Organisms Diversity Evol. 23 (1), 91–101. doi: 10.1007/s13127-022-00583-7
Lipinski M. R. (2001). Preliminary description of two new species of Cephalopods (Cephalopoda: Brachioteuthidae) from South Atlantic and Antarctic waters. Bull. Sea Fish. Institute 1 (152).
Lu C.-C. (2005). A new family of myopsid squid from Autralasian waters (Cephalopoda: Teuthida). Phuket Mar. Biol. Center Res. Bull. 66, 71–82.
Lu C.-C., Okutani T. (2022). Two new genera and species of sepioline squids (Cephalopoda: Sepiolidae) from Australia. Memoirs Museum Victoria 81, 1–23. doi: 10.24199/j.mmv.2022.81.01
Luna A., Rocha F., Perales-Raya C. (2021). A review of cephalopods (Phylum: Mollusca) of the canary current Large marine ecosystem (Central-East Atlantic, African coast). J. Mar. Biol. Assoc. United Kingdom 101 (1), 1–25. doi: 10.1017/S0025315420001356
Luyon J. P., Oyong G. G., Cabrera E. C. (2017). Selective cytotoxicity of bioluminescent bacteria isolated from fresh ink of Philippine squid on human colon cancer and normal cell lines. Malaysian J. Microbiol. 13 (3), 244–252. doi: 10.21161/mjm.93716
McFall-Ngai M. J., Ruby E. G. (1998). Sepiolids and Vibrios: When they first meet. BioScience 48 (4), 257–265. doi: 10.2307/1313352
McFall-Ngai M. J., Toller W. (1991). “Frontiers in the study of the biochemistry and molecular biology of vision and luminescence in fishes,” in Biochemistry and molecular biology of fishes, vol. 1. (Amsterdam: Elsevier), 77–107). doi: 10.1016/B978-0-444-89124-2.50008-7
Moher D., Liberati A., Tetzlaff J., Altman D. G. (2010). Preferred reporting items for systematic reviews and meta-analyses: The PRISMA statement. Int. J. Surg. 8 (5), 336–341. doi: 10.1016/j.ijsu.2010.02.007
Morin J. G. (1983). Coastal bioluminescence: Patterns and functions. Bull. Mar. Sci. 33 (4), 787–817.
Nesis K. N. (1982). “Zoogeography of the world ocean: Comparison of the zonation of the pelagic realm and regional subdivision of the shelf (concerning cephalopods),” in Marine biogeography: Subject, methods, principles of regionalization. Ed. Kussakin O. G. (Moskow: Nauka Press), 114–134.
Nesis K. N. (2003). Distribution of recent Cephalopoda and implications for Plio-Pleistocene events. Berliner Paläobiol. Abh. 3, 199–224.
Nishiguchi M. K., Lopez J. E., Boletzky S. V. (2004). Enlightenment of old ideas from new investigations: More questions regarding the evolution of bacteriogenic light organs in squids. Evol. Dev. 6 (1), 41–49. doi: 10.1111/j.1525-142X.2004.04009.x
Norman M. D., Lu C. C. (2000). Preliminary checklist of the cephalopods of the South China Sea. Raffles Bull. Zool. 8, 539–567.
Nyholm S. V., McFall-Ngai M. J. (2004). The winnowing: Establishing the squid–Vibrios symbiosis. Nat. Rev. Microbiol. 2 (8), 632–642. doi: 10.1038/nrmicro957
Nyholm S. V., McFall-Ngai M. J. (2021). A lasting symbiosis: How the Hawaiian bobtail squid finds and keeps its bioluminescent bacterial partner. Nat. Rev. Microbiol. 19, 666–679. doi: 10.1038/s41579-021-00567-y
Paitio J., Oba Y., Meyer-Rochow V. B. (2016). “Bioluminescent fishes and their eyes,” in Luminescence–an outlook on the phenomena and their applications. Ed. Thirumalai J. (InTech). doi: 10.5772/65385
Reid A. L. (2021). Two new species of Iridoteuthis (Cephalopoda: Sepiolidae: Heteroteuthinae) from the southwest pacific, with a redescription of Stoloteuthis maoria (Dell 1959). Zootaxa 5005 (4), 503–537. doi: 10.11646/zootaxa.5005.4.3
Robison B. H., Reisenbichler K. R., Hunt J. C., Steven H. D., Robison B. H., Reisenbichler K. I. M. R., et al. (2003). Light production by the arm tips of the deep-sea cephalopod Vampyroteuthis infernalis. Biol. Bull. 205 (2), 102–109. doi: 10.2307/1543231
Robison B. H., Young R. E. (1981). Bioluminescence in Pelagic Octopods. Pacific Sci. 35 (1), 39–44. Available at: http://hdl.handle.net/10125/537.
Rosa R., Dierssen H. M., Gonzalez L., Seibel B. A. (2008a). Ecological biogeography of cephalopod molluscs in the Atlantic Ocean: Historical and contemporary causes of coastal diversity patterns. Global Ecol. Biogeogr. 17 (5), 600–610. doi: 10.1111/j.1466-8238.2008.00397.x
Rosa R., Dierssen H. M., Gonzalez L., Seibel B. A. (2008b). Large-Scale diversity patterns of cephalopods in the Atlantic open ocean and deep sea. Ecology 89 (12), 3449–3461. doi: 10.1890/08-0638.1
Rosa R., Pissarra V., Borges F. O., Xavier J., Gleadall I., Golikov A., et al. (2019). Global patterns of species richness in coastal cephalopods. Front. Mar. Sci. 6 (469). doi: 10.3389/fmars.2019.00469
Rosa R., Seibel B. A. (2008). Synergistic effects of climate-related variables suggest future physiological impairment in a top oceanic predator. Proc. Natl. Acad. Sci. United States America 105 (52), 20776–20780. doi: 10.1073/pnas.0806886105
Rosa R., Seibel B. A. (2010). Metabolic physiology of the Humboldt squid, Dosidicus gigas: Implications for vertical migration in a pronounced oxygen minimum zone. Prog. Oceanogr. 86 (1–2), 72–80. doi: 10.1016/j.pocean.2010.04.004
Ruby E. G., McFall-Ngai M. J. (1992). A squid that glows in the night: Development of an animal-bacterial mutualism. J. Bacteriol. 174 (15), 4865–4870. doi: 10.1128/jb.174.15.4865-4870.1992
Sanchez G., Fernández-Álvarez F. Á., Taite M., Sugimoto C., Jolly J., Simakov O., et al. (2021). Phylogenomics illuminates the evolution of bobtail and bottletail squid (order Sepiolida). Commun. Biol. 4 (1), 819. doi: 10.1038/s42003-021-02348-y
Sanchez G., Setiamarga D. H. E., Tuanapaya S., Tongtherm K., Winkelmann I. E., Schmidbaur H., et al. (2018). Genus-level phylogeny of cephalopods using molecular markers: Current status and problematic areas. PeerJ 6, e4331. doi: 10.7717/peerj.4331
Shimomura O. (2006). Bioluminescence: Chemical principles and methods (World Scientific Publishing Co Pte Ltd). doi: 10.1142/6102
Smale M. J. (1996). Cephalopods as prey. IV. Fishes. Philos. Trans. R. Soc. London Ser. B: Biol. Sci. 351 (1343), 1067–1081. doi: 10.1098/rstb.1996.0094
Spalding M. D., Agostini V. N., Rice J., Grant S. M. (2012). Pelagic provinces of the world: A biogeographic classification of the world’s surface pelagic waters. Ocean Coast. Manage. 60, 19–30. doi: 10.1016/j.ocecoaman.2011.12.016
Spalding M. D., Fox H. E., Allen G. R., Davidson N., Ferdaña Z. A., Finlayson M., et al. (2007). Marine ecoregions of the world: A bioregionalization of coastal and shelf areas. BioScience 57 (7), 573–583. doi: 10.1641/B570707
Stramma L., Johnson G. C., Sprintall J., Mohrholz V. (2008). Expanding oxygen-minimum zones in the tropical oceans. Science 320 (5876), 655–658. doi: 10.1126/science.1153847
Sutton T. T., Clark M. R., Dunn D. C., Halpin P. N., Rogers A. D., Guinotte J., et al. (2017). A global biogeographic classification of the mesopelagic zone. Deep Sea Res. Part I: Oceanogr. Res. Papers 126, 85–102. doi: 10.1016/j.dsr.2017.05.006
Takahashi H., Isobe M. (1994). Photoprotein of luminous squid, Symplectoteuthis oualaniensis and reconstruction of the luminous system. Chem. Lett. 23 (5), 843–846. doi: 10.1246/cl.1994.843
Thomsen P. F., Willerslev E. (2015). Environmental DNA – an emerging tool in conservation for monitoring past and present biodiversity. Biol. Conserv. 183, 4–18. doi: 10.1016/j.biocon.2014.11.019
Tsuji F. I. (2002). Bioluminescence reaction catalyzed by membrane-bound luciferase in the “firefly squid,” Watasenia scintillans. Biochim. Biophys. Acta 1564 (1), 189–197. doi: 10.1016/S0005-2736(02)00447-9
Uribe J. E., Zardoya R. (2017). Revisiting the phylogeny of Cephalopoda using complete mitochondrial genomes. J. Molluscan Stud. 83 (2), 133–144. doi: 10.1093/mollus/eyw052
Veron J. E. N., Devantier L. M., Turak E., Green A. L., Kininmonth S., Stafford-Smith M., et al. (2009). Delineating the coral triangle. Galaxea J. Coral Reef Stud. 11 (2), 91–100. doi: 10.3755/galaxea.11.91
Visick K. L., Stabb E. V., Ruby E. G. (2021). A lasting symbiosis: How Vibrio fischeri finds a squid partner and persists within its natural host. Nat. Rev. Microbiol. 19, 654–665. doi: 10.1038/s41579-021-00557-0
Voss N. A., Vecchione M., Toll R. B., Sweeney M. J. (1998). Systematics and biogeography of cephalopods. volume II. Smithsonian Contributions to Zool. 586, 277–599. doi: 10.5479/si.00810282.586.277
Widder E. A. (1999). “Bioluminescence,” in Adaptive mechanisms in the ecology of vision. Eds. Archer S. N., Djamgoz M. B. A., Loew E. R., Partridge J. C., V. S. (Dordrecht: Springer), 555–581.
Widder E. A., Latz M. I., Case J. F. (1983). Marine bioluminescence spectra measured with an optical multichannel detection system. Biol. Bull. 165 (3), 791–810. doi: 10.2307/1541479
Wilson T., Hastings J. (1998). Bioluminescence. Annu. Rev. Cell Dev. Biol. 14 (1), 197–230. doi: 10.1146/annurev.cellbio.14.1.197
Xavier J. C., Cherel Y., Allcock L., Rosa R., Sabirov R. M., Blicher M. E., et al. (2018). A review on the biodiversity, distribution and trophic role of cephalopods in the Arctic and Antarctic marine ecosystems under a changing ocean. Mar. Biol. 165 (5), 1–26. doi: 10.1007/s00227-018-3352-9
Young R. E. (1972). The systematics and areal distribution of pelagic cephalopods from the seas off Sounthern California. Smithsonian contributions to zoology. Smithsonian Contributions to Zoology 97, 159. doi: 10.5479/si.00810282.97
Young R. E., Mencher F. M. (1980). Bioluminescence in mesopelagic squid: Diel color change during counterillumination. Science 208 (4449), 1286–1288. doi: 10.1126/science.208.4449.1286
Young R. E., Roper C. F. E. (2016). Grimalditeuthis joubin 1898. grimalditeuthis bonplandi (Verany 1839) (The Tree of Life Web Project). Available at: http://tolweb.org/Grimalditeuthis_bonplandi/19463/2016.02.27.
Young R. E., Tsuchiya K. (2014) Abraliopsis joubin 1896. The tree of life web project. Available at: http://tolweb.org/Abraliopsis/19644/2014.01.21.
Young R. E., Vecchione M. (2005). Narrowteuthis nesisi, a new genus and new species of the squid family Neoteuthidae (Mollusca: Cephalopoda). Proc. Biol. Soc. Washington 118 (3), 566–569. doi: 10.2988/0006-324X(2005)118[566:NNANGA]2.0.CO;2
Keywords: bioluminescence, mollusks, Cephalopoda, diversity, distribution, systematic review
Citation: Otjacques E, Pissarra V, Bolstad K, Xavier JC, McFall-Ngai M and Rosa R (2023) Bioluminescence in cephalopods: biodiversity, biogeography and research trends. Front. Mar. Sci. 10:1161049. doi: 10.3389/fmars.2023.1161049
Received: 07 February 2023; Accepted: 30 May 2023;
Published: 27 June 2023.
Edited by:
Michele Kiyoko Nishiguchi, University of California, Merced, United StatesReviewed by:
Edith Widder, Ocean Research & Conservation Association, United StatesMichael Vecchione, National Oceanic and Atmospheric Administration (NOAA), United States
Copyright © 2023 Otjacques, Pissarra, Bolstad, Xavier, McFall-Ngai and Rosa. This is an open-access article distributed under the terms of the Creative Commons Attribution License (CC BY). The use, distribution or reproduction in other forums is permitted, provided the original author(s) and the copyright owner(s) are credited and that the original publication in this journal is cited, in accordance with accepted academic practice. No use, distribution or reproduction is permitted which does not comply with these terms.
*Correspondence: Eve Otjacques, ZWJvdGphY3F1ZXNAY2llbmNpYXMudWxpc2JvYS5wdA==